- 1Department of Microbiology, Cornell University, Ithaca, NY, United States
- 2Department of Microbiology and Immunology, College of Veterinary Medicine, Cornell University, Ithaca, NY, United States
- 3Independent Researcher, Freeland, WA, United States
Sea Star Wasting Disease (SSWD) describes a suite of disease signs that affected >20 species of asteroid since 2013 along a broad geographic range from the Alaska Peninsula to Baja California. Previous work identified the Sea Star associated Densovirus (SSaDV) as the best candidate pathogen for SSWD in three species of common asteroid (Pycnopodia helianthoides, Pisaster ochraceus, and Evasterias troscheli), and virus-sized material (<0.22 μm) elicited SSWD signs in P. helianthoides. However, the ability of virus-sized material to elicit SSWD in other species of asteroids was not known. Discordance between detection of SSaDV by qPCR and by viral metagenomics inspired the redesign of qPCR primers to encompass SSaDV and two densoviral genotypes detected in wasting asteroids. Analysis of asteroid samples collected during SSWD emergence in 2013–2014 showed an association between wasting asteroid-associated densoviruses (WAaDs) and SSWD in only one species (P. helianthoides). WAaDs were found in association with asymptomatic asteroids in contemporary (2016 and later) populations, suggesting that they may form subclinical infections at the times they were sampled. WAaDs were found in SSWD-affected P. helianthoides after being absent in asymptomatic individuals a year earlier at one location (Kodiak). Direct challenge of P. ochraceus, Pisaster brevispinus, and E. troscheli with virus-sized material from SSWD-affected individuals did not elicit SSWD in any trial. RNA viral genomes discovered in viral metagenomes and host transcriptomes had viral loads and metagenome fragment recruitment patterns that were inconsistent with SSWD. Analysis of water temperature and precipitation patterns on a regional scale suggests that SSWD occurred following dry conditions at several locations, but mostly was inconsistently associated with either parameter. Semi-continuous monitoring of SSWD subtidally at two sites in the Salish Sea from 2013 to 2017 indicated that SSWD in E. troscheli and P. ochraceus was associated with elevated water temperatures, but wasting in P. helianthoides occurred irrespective of environmental conditions. Our data therefore do not support that widespread SSWD is associated with potential viral pathogens in species other than P. helianthoides. Rather, we speculate that SSWD may represent a syndrome of heterogeneous etiologies between geographic locations, between species, or even within a species between locations.
Introduction
Sea star wasting disease (SSWD) describes a suite of common atypical gross morphological signs (loss of turgor, appearance of lesions, limb autotomy, and loss of body wall integrity) affecting members of the class Asteroidea, which in some cases result in animal mortality (Eckert et al., 1999; Staehli et al., 2008; Bates et al., 2009). Diseases have been reported more widely in Echinoidea (Jangoux, 1987), including during the catastrophic losses of the tropical urchin Diadema antillarum in the early 1980s (Lessios et al., 1984; Lessios, 1988, 1995, 2005). SSWD has been reported in northeast Pacific populations since at least 2008 (Bates et al., 2009; Gravem and Morgan, 2017), and in southern California since the late 1970s (Dungan et al., 1982). Beginning in mid- to late-2013, SSWD was observed in the northeast Pacific across a wide taxonomic diversity (>20 species) of asteroids. Mass mortality associated with the disease was first reported in the ochre star (Pisaster ochraceus) along the Olympic Peninsula, followed within 1–2 months by reports of mass mortality of the sunflower star Pycnopodia helianthoides and several other species in the Salish Sea and central California (Hewson et al., 2014). By mid-2016, the geographic range of the disease extended from south-central Alaska to Baja California (Multi-Agency Rocky Intertidal Network; http://www.seastarwasting.org). SSWD resulted in catastrophic declines in population density of some species, notably P. helianthoides in subtidal waters (Montecino-Latorre et al., 2016; Schultz et al., 2016). In intertidal communities, localized mass mortality events of Leptasterias hexactis and P. ochraceus were reported from 2010 and 2013 in Horseshoe Cove, CA (Gravem and Morgan, 2017). Mass mortality of P. ochraceus occurred from June to August 2014 and reduced asteroid densities 50–94% amongst sites in Oregon (Menge et al., 2016) and in the San Juan Islands to 25% of their pre-SSWD abundances (Eisenlord et al., 2016). The loss of subtidal and intertidal asteroids was correlated with an increase in the abundance of snails (Gravem and Morgan, 2017) and urchins (Schultz et al., 2016), normally prey of asteroids, suggesting that the losses of asteroids after mortality events due to SSWD caused a trophic cascade. Strong recruitment of P. ochraceus juveniles on intertidal coastlines indicate that such cascades may be short-lived (Menge et al., 2016). Hence, understanding the mechanisms by which SSWD occur is important for predicting its impact on coastal ecology.
The causative agent of SSWD has not been fully elucidated. The disease was associated, on a metacommunity level, with the Sea Star associated Densovirus (SSaDV) in P. helianthoides, P. ochraceus, and E. troscheli along its geographic range (Salish Sea to Southern California) when surveyed in 2013–2014 (Hewson et al., 2014). In addition, virus-sized material inoculated into one species (P. helianthoides) repeatedly elicited SSWD-like signs (Hewson et al., 2014) and were accompanied by enhanced expression of immune-related and tissue remodeling genes (Fuess et al., 2015; Gudenkauf and Hewson, 2015) and autocatalyzed cell death processes (Gudenkauf and Hewson, 2015). An apparent conundrum of SSWD is the presence (and in at least one asteroid species the active replication) of SSaDV in a large proportion of asymptomatic asteroids surveyed in 2013–2014, and detection of SSaDV in museum specimens since at least 1942, well before SSWD was first observed (Hewson et al., 2014). The rate of SSWD disease sign onset has been linked in experiments and some locations to elevated temperature (Bates et al., 2009; Eisenlord et al., 2016; Kohl et al., 2016), however elsewhere SSWD prevalence negatively correlated with water temperature (Hewson et al., 2014; Menge et al., 2016). SSWD is also associated with the frequency of heterozygous EF1α in P. ochraceus (Wares and Schiebelhut, 2016) and transcriptomic analyses of EF1α mutants suggest that these individuals have a greater cellular response to temperature stress (Chandler and Wares, 2017). Despite associations of SSaDV and temperature variation with SSWD, firm pathology of any etiological agent, environmental or pathogenic, has remained elusive. While there is evidence for the association between SSaDV and SSWD in P. helianthoides, the association between any pathogen and SSWD in other asteroid species is more tenuous and may be multifactorial.
The aims of the present study were to: (1) examine the association between SSaDV and SSWD in species not targeted in prior work (Hewson et al., 2014); (2) explore the association of SSWD with other viral agents, notably RNA viruses; and (3) to examine patterns of SSWD occurrence in relation to environmental conditions on a regional scale and at semi-continuously monitored sites. We approached these aims by re-analyzing patterns of SSaDV (and other wasting-associated densoviruses) occurrence and by performing challenge experiments similar to those performed by Hewson et al. (2014) on species other than P. helianthoides. We also examined the diversity of RNA viruses and probed their association with SSWD by direct quantification in tissue samples, and by fragment recruitment to viral metagenomes and host transcriptomes. These data do not provide a clear association between SSWD and viruses in species other than P. helianthoides as described in previous work (Hewson et al., 2014). Rather, SSWD in other species may be caused by heterogeneous etiologies which are related to unmeasured environmental variables or unstudied pathogenic agents.
Results and Discussion
Hewson et al. (2014) explored the relationship between potential viral pathogens and SSWD by three approaches: (1) examination of potential pathogens in asymptomatic and SSWD-affected individuals; (2) direct challenge of asymptomatic individuals with potential pathogens; and (3) studies of pathogen transmission and persistence in environmental reservoirs. We sought to further understand the relationship between viruses and SSWD through detailed study of viral occurrence and genome variation in tissues of SSWD-affected and asymptomatic individuals (see Supplemental Material). Based on these observations we refined our detection approach to target wasting asteroid-associated densoviruses (WAaDs) (including SSaDV) and to exclude related densoviruses associated with asymptomatic individuals. We then re-assessed epidemiological association between densoviruses targeted by our assay and SSWD through analysis of museum specimens, populations of asteroids affected during SSWD onset in 2013–2014, and contemporary (post 2015) populations. We also examined the persistence of asteroid densoviruses in environmental reservoirs to determine the likelihood of their transmission between geographically disparate asteroid populations. Finally, we examined the relationship between RNA viruses and environmental conditions (temperature, precipitation) and SSWD. Our data provide evidence that SSWD may only be associated with densoviruses at some locations and in certain species, but is unlikely to be related across the entire geographic range or all species. Furthermore, we found no compelling evidence for any association between RNA viruses and SSWD. While environmental conditions may be related to wasting mass mortality at some sites and in some species, there were no consistent patterns of association between instantaneous and historical water temperature or precipitation across the entire geographic range of SSWD.
Epidemiological Assessment of the Association between SSWD and Wasting Asteroid Associated Densoviruses (WAaDs) During Initial Asteroid Mass Mortality (2013–2014)
Discordance between detection of SSaDV by qPCR and by viral metagenomics in asymptomatic asteroids inspired the redesign of qPCR primers to encompass SSaDV and two densoviral genotypes detected in wasting asteroids and exclude apparently asymptomatic genotypes (see Supplemental Material). To compare densoviral abundances detected using the Wasting Asteroid associated Densoviruses (WAaDs) primer/probe set with abundances measured with previous NS1 and VP4 primer/probe sets, we assayed tissue extracts of asymptomatic (n = 20 for each species) and SSWD-affected (n = 20 for each species) P. ochraceus, P. helianthoides, and E. troscheli from 2013 to 2014 that were analyzed in previous work (Hewson et al., 2014). We included in this analysis WAaDs abundance in both body wall tissues and in the pyloric caeca of individuals, since initial qPCR results using NS1 and VP4 primer sets against a range of samples indicated the presence of SSaDV in these tissue types. Loads of asteroid densoviruses measured using the WAaDs primer/probe pair were mostly lower than measured by VP4, but there was no clear relationship between WAaDs loads and loads measured by NS1 (Supplemental Figure 1). In no sample that was VP4 or NS1 negative were WAaDs detected, although several samples that were VP4 or NS1 positive were not detected by the WAaDs primer/probe pair. Thus, it is likely that VP4 or NS1 either amplified densoviruses that were homologous to SSaDV, or spuriously amplified DNA that was not associated with SSaDV.
The mean loads of WAaDs were significantly higher in both SSWD-affected body wall and pyloric caeca tissues of P. helianthoides than asymptomatic tissues (Student's t-test, p < 0.05, df = 20), however there were no significant differences in WAaDs loads between SSWD-affected and asymptomatic individuals of P. ochraceus or E. troscheli (Figure 1). Prevalence (% of samples tested bearing any detectable load of WAaDs) was higher in SSWD-affected pyloric caeca of P. ochraceus and body wall of E. troscheli, but not different in body wall tissues of P. ochraceus and lower in both pyloric caeca and body wall of P. helianthoides. This data contrasts with previous work showing significantly higher loads and prevalence of SSaDV (based on VP4 primers) across all species. On a metapopulation level, our data support an association between asteroid densovirus load (as measured by the WAaDs primer/probe set) and SSWD only in P. helianthoides, but not in either P. ochraceus nor E. troscheli.
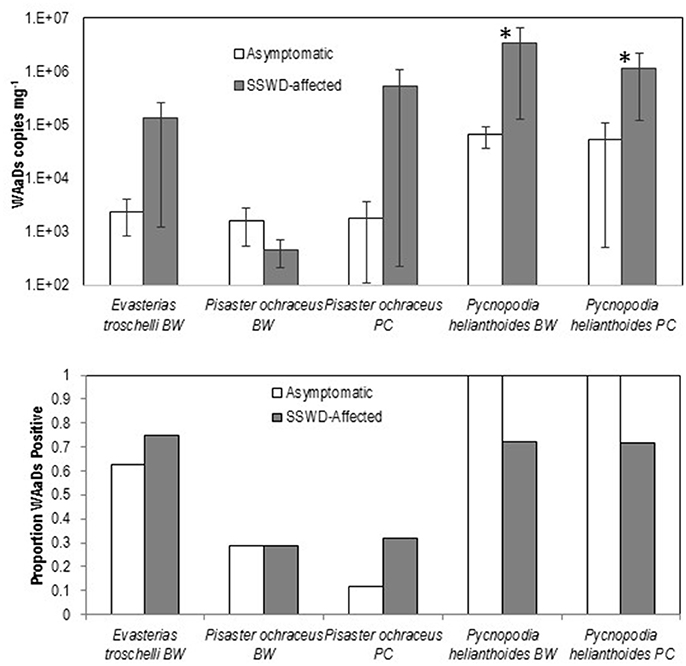
Figure 1. Comparison of WAaDs load (Top) and prevalence (Bottom) between asymptomatic and SSWD-affected individuals in body wall (BW) and pyloric caeca (PC) tissues. Error Bars = SE. *p < 0.05 (Student's t-test, df = 20).
To reassess the spatial and temporal distribution of asteroid densoviruses, we applied the WAaDs qPCR approach to contemporary samples collected over a wide geographic range (Supplemental Table 1) and museum specimens (Supplemental Table 2) in which VP4 and NS1 were both detected. With the exception of Asterias amurensis from Shandong, China, and Asterias forbesii from the Gulf of Maine (used to design the WAaDs primer/probe set), WAaDs were not detected in any geographic sample. Furthermore, WAaDs were not detected in any museum specimen tested from 1905 to 2010. Importantly, WAaDs were also not detected in samples of P. helianthoides, Dermasterias imbricata, Pisaster brevispinus, Pisaster giganteus, P. ochraceus, or Patiria miniata collected from Pigeon Point, CA (37.1822°N, 122.3961°W) in June 2012. Asteroids at Pigeon Point were affected by SSWD in August-September 2013. These data indicate that previous reports of SSaDV (detected by concurrent VP4 and NS1 qPCR) as long ago as 1942 (Hewson et al., 2014) represented non-specific detection.
Our results confirm that SSWD is associated with WAaDs on a metapopulation level only in contemporary (2013–present) P. helianthoides. We have no evidence that WAaDs are associated with SSWD on a population level in P. ochraceus or E. troscheli. In previous efforts the relationship between SSWD in P. ochraceus and SSaDV was the weakest on both population level, and transcription of the SSaDV capsid (VP4) gene was ~5 times higher in asymptomatic individuals than symptomatic individuals (Hewson et al., 2014). Despite a significantly higher load and prevalence of WAaDs in SSWD-affected asteroids when compared with asymptomatic individuals, a very large proportion of asymptomatic individuals also bore WAaDs (almost 80% of asymptomatic E. troscheli, and 100% of asymptomatic P. helianthoides) suggesting that not all infections may lead to SSWD, or that the outcome of WAaDs infections may be influenced by unmeasured parameters or occur sometime after exposure. The early finding of SSaDV in nearly every population of asteroid sampled and in a wide number of asymptomatic individuals (Hewson et al., 2014) suggested subclinical exposure to the virus, since asymptomatic individuals were collected either from regions that bore SSWD-affected individuals, or were amongst populations that wasted a short time later or after collection (Eisenlord et al., 2016; Kohl et al., 2016). Hence, we sought to determine the distribution of WAaDs in populations where SSWD was absent.
Mapping the Contemporary (Post-2015) Distribution of WAaDs in Northeast Pacific
We examined asteroids to determine the geographic distribution of SSWD and WAaDs in contemporary (2015–2017) asteroid populations that were also affected by SSWD during the 2013–2015 mass mortality event. We performed a survey of asteroids at 13 stations in deep (30–45 m) subtidal waters of the Salish Sea in January 2016. A total of 108 individuals were collected across all stations, where most individuals were the non-SSWD affected Henricia spp. (n = 61), but also several taxa (P. helianthoides, E. troscheli, Mediaster aequalis, and Orthasterias kohleri) that experienced significant population declines by mid-2015 (Kohl et al., 2016; Montecino-Latorre et al., 2016) (Supplemental Table 3). SSWD was not observed in any individual at any site. However, WAaDs were detected in several specimens, with greatest load in P. brevispinus and P. helianthoides, two species heavily affected by SSWD in terms of population density (Montecino-Latorre et al., 2016) (Figure 2). WAaDs were detected at sites heavily affected by SSWD between 2013–2015, including the central Puget Sound and the San Juan Islands (Figure 3). The observation of WAaDs in asteroids that lacked SSWD (which were collected during winter), and also in several species that were not known to be affected by SSWD in the 2013–2015 event (e.g., Crossaster papposus, Pteraster tesselatus, and Henricia spp.) suggests that WAaDs may have a wider host range than stars affected by SSWD. If associated with SSWD in these species, WAaDs may cause subclinical infections during winter months. We also examined the presence of WAaDs in sediments and net plankton collected at the same sites and times as asteroid samples, but WAaDs were not detected in any sample suggesting WAaDs do not form environmental reservoirs in these compartments. In addition to asymptomatic populations in the Salish Sea, we also examined samples of asymptomatic (n = 57) and SSWD-affected (n = 68) P. ochraceus collected from coastal Oregon, and asymptomatic (n = 6) and SSWD-affected (n = 6) Leptasterias spp. from northern California (Supplemental Table 4), and did not detect WAaDs in any sample. Finally, we sought to determine whether asteroids from deeper habitats adjacent to affected populations bore WAaDs. Several species of asteroid (including Rathbunaster californicus, a sister species to P. helianthoides) were obtained by ROV in waters from 150–690 m on the Southern California Borderland in July 2016 (Supplemental Table 3). However, no tissue samples from these deep waters bore WAaDs.
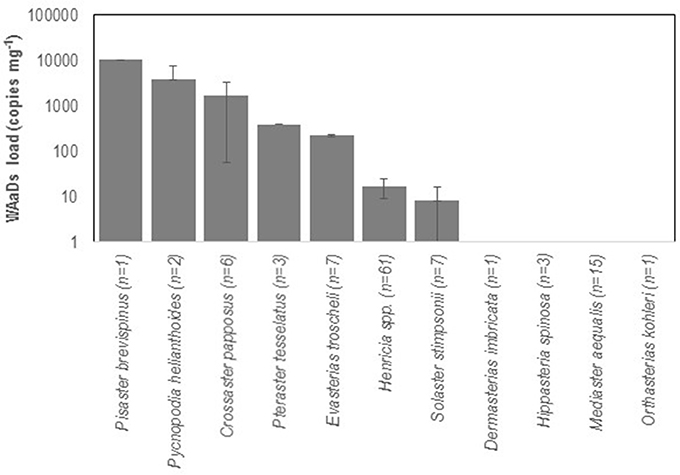
Figure 2. WAaDs abundance in sea star tissue samples collected from the Salish Sea in January 2016. The number of individuals tested is indicated for each species. Error bars = SE of all individuals tested.
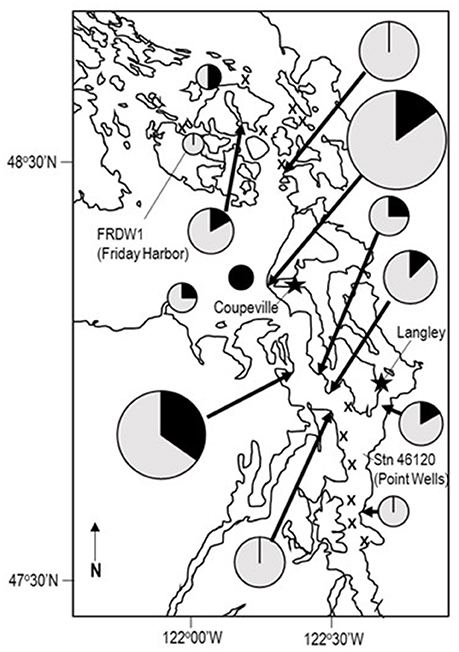
Figure 3. Distribution of WAaDs amongst asteroids collected in the Salish Sea in January 2016. The size of the pie chart is proportional to the number of individuals sampled across all species. X marks stations where asteroids were not recovered. The proportion of individuals bearing WAaDs are indicated in dark gray, while WAaDs-negative individuals are indicated in light gray. The location of oceanographic buoys FRDW1 and Station 46120, as well as Langley and Coupeville are also indicated.
Because WAaDs were not intimately associated with SSWD in the field beyond P. helianthoides, potentially due to subclinical infection at certain times of the year, or perhaps in exposed by asymptomatic individuals within populations, we sought to find unexposed populations of SSaDV that could be tracked as the disease appeared and populations of asteroids that bore SSaDV/WAaDs which lacked SSWD altogether. Asteroids (n = 89) were collected from Dutch Harbor and Kodiak Island in March 2015 (Supplemental Table 3); these represented the furthermost accessible populations from the current SSWD event affecting stars during the 2013–2015 event. qPCR using both SSaDV and WAaDs primer sets did not detect these in any individual. However, in August 2016 asteroids around Kodiak Island, including those maintained in a flow-through aquarium at the National Fisheries Service office on Near Island (sampled in March 2015) experienced widespread wasting. Four individuals tested (2 P. helianthoides, 1 E. troscheli, and 1 Henricia sp.) were positive for WAaDs and had tissue loads of 3.3–6.5 × 103 copies mg−1, which are amongst the highest loads measured in any tissue sample in this study. SSWD has not been observed (as of October 2017) in Dutch Harbor, nor further west on the Aleutian Island chain. Samples of asymptomatic P. helianthoides, E. troscheli, and S. stimpsoni from Adak, Attu, and Yunaska Island collected from June 2017 (Supplemental Table 3) yielded no significant WAaDs hits in any tissues. Additional samples of asymptomatic asteroids collected from Ketchikan, AK, in March 2017 (a site which experienced SSWD mortality during the period from 2014–present) were positive for WAaDs only rarely; Only 1 of 8 P. ochraceus, 1 of 10 D. imbricata, 2 of 5 P. helianthoides, and 2 of 12 E. troscheli had a detectable WAaDs level (≥10 copies mg−1) in any tissue sampled.
The presence of WAaDs in asymptomatic asteroids in southeast Alaska and the Salish Sea may suggest that WAaDs do not elicit disease in those species in which they are detected (i.e., asymptomatic infections), or that these represent exposed asteroids which do not develop SSWD until sometime later. The appearance of WAaDs during the arrival of SSWD on Kodiak Island in summer 2016 (which were absent in a survey performed a year earlier) is consistent with an association between the virus and the disease. However, lack of detection of WAaDs in SSWD-affected P. ochraceus and Leptasterias spp. in coastal California and Oregon suggest that WAaDs may not be associated with SSWD in these species, or any association is specific to certain locations.
Viral Challenge of Asymptomatic Asteriids
Previous experimental challenge of P. helianthoides with virus-sized (<0.2 μm) homogenate (VSH) from symptomatic tissues of the same species yielded disease signs within 7–10 d, and further challenge on asymptomatic individuals with VSH from experimentally-induced SSWD-affected individuals on other asymptomatic individuals yielded similar results (Hewson et al., 2014). Challenge experiments on the other two species of focus (P. ochraceus and E. troscheli) were not performed as part of the previous study.
To confirm the absent correlation between WAaDs and SSWD exhibited by qPCR detection, we performed direct challenge of asymptomatic E. troscheli, P. ochraceus, P. brevispinus, and the Atlantic species A. forbesii with VSH from SSWD-affected asteroids (Supplemental Table 5). A key hurdle in interpreting the response of challenged asteroids to VSH was the lack of SSaDV-naïve individuals for experimental challenge. Based on surveys of SSaDV prevalence (see above) we collected E. troscheli from Dutch Harbor in September 2015 (Supplemental Table 3). These E. troscheli, along with P. ochraceus, P. brevispinus collected from Whidbey Island, Puget Sound, and A. forbesii collected from the Gulf of Maine, when challenged with VSH, did not develop SSWD signs in most experiments. Wet weights, used as a proxy for animal deflation, were not significantly different between control individuals and challenged individuals in any experiment (Supplemental Figures 2, 3). Righting time, which was used to estimate animal activity (Watts and Lawrence, 1990; Gutowsky et al., 2016), was not different between control and VSH inoculated A. forbesii (Supplemental Figure 4). While solitary lesions (i.e., one per individual tested) and arm curling were induced in P. ochraceus challenged with tissues from a severely diseased individual of the same species in one experiment, the lack of progression to a higher SSWD category or even appearance of additional lesions after 7 d of monitoring suggest that the signs observed were not consistent with SSWD in field populations (Menge et al., 2016).
Data from repeated challenge experiments provide no evidence that VSH elicit SSWD in any northeastern Pacific asteroid examined in this study. In particular, the lack of inducible SSWD with viral challenge in E. troscheli and P. ochraceus is consistent with epidemiology-level study showing no association between WAaDs and SSWD in field samples. These data contrast with previous work on P. helianthoides in which affected animals experienced arm curling, deflation, and the appearance of multiple lesions (and in some cases autotomy and death) (Hewson et al., 2014) and suggest non-uniform etiologies for SSWD across different asteroid species.
Investigation of Densoviral Stability and Potential for Viral Transmission
The spread of WAaDs between populations (e.g., appearing in Kodiak in August 2017 after being previously absent, and being present mainly in samples in the central Salish Sea) raise interesting questions about how WAaDs may be transmitted between individuals in the wild. The rapid onset of SSWD and apparent wide geographic distribution of SSaDV in 2013–2014 amongst SSWD-affected populations suggested that the virus was highly transmissible between individuals, or that subclinical infections were widespread before the onset of mass mortality (Hewson et al., 2014). Viruses may spread between individuals through direct contact, as free particles through the water column, vertically to offspring, and through secondary hosts, fomites or other vectors. Initial movement of SSWD along coasts in Southern California and the Salish Sea in 2013, and observations of SSWD in aquariums suggested the presence of a potentially waterborne agent. The sand filters through which incoming waters into public aquaria were passed contained detectable SSaDV, and effective pore size of such filters indicated that the agent was likely submicron in size (Hewson et al., 2014). Furthermore, aquarium systems that contained incoming waters that were UV irradiated reportedly did not experience SSWD, while asteroids in aquaria receiving non-UV treated waters wasted at the same facility (Hewson et al., 2014). These observations suggested that transmission of the pathogenic agent occurred through the water column as free submicron sized particles. Our lack of detection of WAaDs in sediments or plankton of the Salish Sea at 9 sites where WAaDs were detected in animal tissues, and at an additional 4 sites were WAaDs were not detected, suggests that pelagic vectors (especially zooplankton) are unlikely to move WAaDs geographically or that the viruses are maintained on benthic surfaces.
To understand the persistence of SSaDV as free particles in the water column, we examined the decay of SSaDV in seawater incubations that were unfiltered and those which had been filtered to remove particles >0.22 μm. Previous studies attribute the decay of marine viruses to labile organic matter and UV light exposure (Heldal and Bratbak, 1991; Noble and Fuhrman, 1997). We found that SSaDV decayed very rapidly, with only 5–10% of initial SSaDV abundances remaining after 6 h incubation. Calculated decay rates were greatest for control incubations (3.3% h−1) and least for incubations with particles removed (2.8% h−1) (Supplemental Figure 5). The range of both incubations is in line with estimates of viral decay in coastal waters (Weinbauer, 2004), but is faster than other viruses that form resistant capsids that promote transmission when host densities are low (e.g., nucleopolyhedroviruses Peng et al., 1999; Holmes et al., 2008). However, the contact rates between free viruses suspended in seawater and benthic asteroids is low, so it is likely that almost all viruses would decay after release and before contacting new hosts.
The high decay rates of SSaDV, and absence of WAaDs in environmental reservoirs (plankton and sediments) of at least 9 sites where WAaDs were detected in asteroid tissues suggest that asteroid densoviruses are unlikely to be transmitted directly through the water column. Hence, transmission of asteroid densoviruses is likely due to direct contact between infected and uninfected individuals. Therefore, our findings are not consistent with the apparent geographic pattern of SSWD occurrence in 2013–2014 (Salish Sea and Central California, etc.), since it is unlikely that WAaDs / SSaDV were able to transmit across such broad geographic distances in the timeframe of SSWD occurrence (days to weeks). We cannot, however, discount the possibility that WAaDs/SSaDV may also have a secondary host that moved the viruses between geographically disparate locations.
Investigation of RNA Viruses Associated with SSWD-Affected and Asymptomatic Asteroids
Early efforts to understand the viral consortia associated with asymptomatic and SSWD-affected asteroids focused on DNA viruses (Hewson et al., 2014). Hewson et al. (2014) also examined RNA virus composition in two asymptomatic and SSWD-affected P. helianthoides from the Seattle Aquarium in October 2013. They found that most RNA viruses were Picornaviruses and Dicistroviruses, but that no RNA viral family was significantly different (in terms of representation in libraries) between health states. We further examined these libraries to identify specific viral genomes which may have contributed to the disease. We found 11 contigs resembling Picornaviruses and chose two (PhaDiV1 and PhaDiV3; both most similar to Dicistroviruses) based on their coverage within metaviromes, completion of genomes, and presence in host transcriptomes prepared from material from the Seattle Aquarium (Gudenkauf and Hewson, 2015) and Friday Harbor (Fuess et al., 2015). We then performed qRT-PCR across a broad range of SSWD-affected and asymptomatic samples from 2013 used to determine pattern of SSaDV association with SSWD (Hewson et al., 2014). There was no significant relationship between PhaDiV1 and PhaDiV3 abundance and the occurrence of SSWD in P. ochraceus and P. helianthoides, however PhaDiV3 was only detected in SSWD-affected E. troscheli (Supplemental Figure 6). Similarly, recruitment of RNA viral metatranscriptomes prepared from P. helianthoides (Hewson et al., 2014) did not show a consistent pattern in association with disease (Supplemental Table 5). Both PhaDiV1 and PhaDiV3 had significant (e < 1e-10) alignments to P. helianthoides control incubations prepared in the San Juan Islands, however they were not observed in VSH-challenged libraries (Gudenkauf and Hewson, 2015). Hence, the association between either PhaDiV1 or PhaDiV3 and SSWD across all species is unclear.
We performed additional RNA viral metagenomic surveys to identify RNA viruses in P. ochraceus. Metagenomes were prepared from 2 asymptomatic and 4 SSWD-affected P. ochraceus collected from the Olympic National Park and Santa Cruz in October 2013 (Supplemental Table 6). Metaviromes bore a total of 72 contigs which were similar to known RNA viruses based on BLASTx against RNA-dependent RNA polymerases (RdRps) of known picornaviruses, and envelope proteins of coronaviruses, flaviviruses, and nodaviruses available from NCBI. Of these, over half (38) were found in all 6 libraries based on read recruitment. All viral contigs present in SSWD-affected libraries were also present in asymptomatic libraries. We speculated that if the geographically widespread SSWD in 2013 and beyond was the result of infection by an RNA virus, then that putative pathogen would be found in both P. ochraceus and P. helianthoides. Hence, we examined the presence of contig sequences that were the same between species, regardless of their health status. Sixteen contigs were homologous in metagenomes prepared from both species. Re-assembly of these contiguous sequences resulted in 4 RNA viral genomes (ARV1, ARV2, ARV3, and ARV4; Supplemental Table 7). Recruitment of the 6 P. ochraceus RNA metaviromes prepared in this study and 4 P. helianthoides libraries (Hewson et al., 2014) revealed no differences in recruitment pattern between asymptomatic and SSWD-affected P. ochraceus for ARV3 and ARV4, with only a handful of recruits from P. helianthoides (Supplemental Table 8). ARV2 was primarily present in asymptomatic P. ochraceus and in P. helianthoides, while ARV1 demonstrated no clear pattern in recruitment. Recruitment against transcriptomes prepared from P. helianthoides likewise revealed no clear pattern with disease occurrence or treatment, with all 4 viruses present mostly in control or asymptomatic libraries. Hence, it is unlikely that any of these associated RNA viruses is associated with SSWD in P. ochraceus or P. helianthoides.
The lack of clearly associated RNA viral genotypes with SSWD in P. helianthoides and P. ochraceus suggests that agents other than viral pathogens may have been associated with SSWD occurrence in 2013–2014. Cellular inclusions in lesions were not observed by histopathology (Hewson et al., 2014), and kinetoplastids were not detected in SSWD-affected individuals, suggesting eukaryotic protozoa were not involved in SSWD. Furthermore, cell-size fraction (>0.2 μm) metagenomes available in GenBank (BioProjects SAMN08012634-SAMN08012636) do not bear consistent nor distinctive eukaryotic protozoan signals that might indicate eukaryotic associations with SSWD. Genera of bacteria inhabiting asteroids which include pathogenic representatives (notably Mollicutes, Spirochaetes and Vibrio spp.-like Gammaproteobacteria) do not correspond with SSWD occurrence, and did not elicit SSWD by challenge (see Supplemental Materials). However, SSWD may also be influenced by, or be the direct result of environmental conditions.
How Did Mass Mortality Correspond with Environmental Conditions?
Concern that warming oceans may elicit increased disease and mass mortality by altering the relationship between pathogens and hosts (Burge et al., 2014) fueled concerns that SSWD was a result of higher water temperatures. Two studies have examined the impacts of elevated temperature on SSWD appearance in aquarium studies of asteroids (Eisenlord et al., 2016; Kohl et al., 2016). While both studies noted mortality of individuals after collection, elevated temperature (Eisenlord et al., 2016) and decreased temperature (Kohl et al., 2016) significantly increased and decreased, respectively, the rate at which SSWD signs appeared. Furthermore, field observations of SSWD in natural populations of the San Juan Islands demonstrated correspondence with late summer and hence elevated water and air temperature and SSWD appearance (Eisenlord et al., 2016). In contrast, Menge et al. (2016) found that SSWD in P. ochraceus was associated with colder water temperature in Oregon, and SSWD intensified in winter months along the pacific coast of the Olympic peninsula (Hewson et al., 2014). These studies suggest complex interactions of environmental variables may exacerbate or even elicit SSWD.
To expand on previous studies to determine if environmental variables influenced disease progression, we investigated correspondence between two abiotic parameters (water temperature and precipitation) and SSWD occurrence at both regional- and local-scales. On the regional scale, we consulted National Oceanographic Data Center Buoy data (NOAA, NWS, USDOC, 1971) from six locations in the northeastern Pacific (see section Methods) from 2011 to 2016, encompassing the onset of mass mortality in 2013–2014. Across all 6 locations, the first report of disease (the absolute onset of SSWD is unknown) was preceded by >2 months of below 5-year average water temperatures (Figure 4). In only two of six locations was the water temperature at or about disease onset higher than the six year (2011–2017) mean; in Friday Harbor water temperature was also higher than mean temperature 2 months prior the disease event but cooler than mean temperature the month before disease onset. Neither absolute water temperature nor monthly rate of temperature change corresponded with the disease onset across all locations. Logistic regression of approximate disease occurrence (exact onset is uncertain at most locations) against temperature was not significant at any location (p > 0.05, Wald test, df = 11), and the same analyses performed with +1 month and −1 month offsets of temperature were again not significant at any location (p > 0.05, Wald test, df = 11). Temperature in the 4 months preceding disease onset was not significantly different (p > 0.05, Student's t-test, df = 23) than temperature in the 4 months after disease onset across all sites. Because of uncertainties in the date of mass mortality onset, we qualitatively observed the same subtidal asteroid populations at two locations on Whidbey Island (Coupeville Wharf and Langley Harbor, WA) semi-continuously from November 2013 to October 2017. SSWD appeared in Langley Harbor at a low incidence (<10% of individuals) before the survey began in late 2013, however intensified (>10% of individuals affected) in February 2014 in P. helianthoides and in June 2014 for E. troscheli and P. ochraceus (Figure 5). Wasting at Coupeville Wharf in both E. troscheli and P. ochraceus was observed at a similar time. The occurrence of high incidence SSWD in E. troscheli and P. ochraceus corresponded with elevated water temperature in late summer and into fall, however, high incidence P. helianthoides mortality occurred in Spring and into Summer and did not correspond with overall water temperature. After mass mortality of adult P. helianthoides in Spring 2014, the abundance of juvenile P. helianthoides increased over time, however the site experienced considerable population decrease between July and October 2015 which corresponded with elevated water temperature. However, logistic regression of disease in all species at both Langley and Coupeville against absolute temperature were not significant (p > 0.05, Wald test, df = 56 for Langley and df = 35 for Coupeville). These analyses both suggest that while some proportion of wasting may be influenced by warm and cool water temperature anomalies, and that wasting in some species occurs consistently with season (notably P. ochraceus and E. troscheli), our analysis could not identify a single or combination of parameters of environmental conditions that universally correspond with disease across multiple species or an entire geographic range.
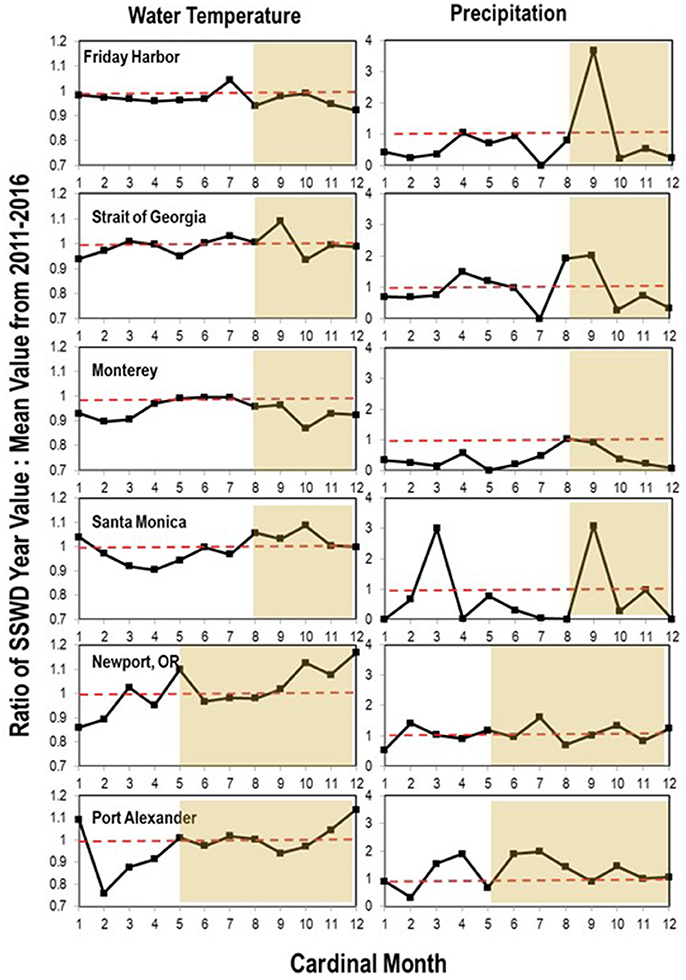
Figure 4. Ratio of water temperature and precipitation relative to 6-year average during SSWD onset (gray shaded region) at 6 locations. The month of onset has been re-ordered to include 5–8 months of pre-SSWD data. Data was obtained from the National Climatic Data Center and National Weather Service (https://www.ncdc.noaa.gov/), and Environment Canada (https://weather.gc.ca/).
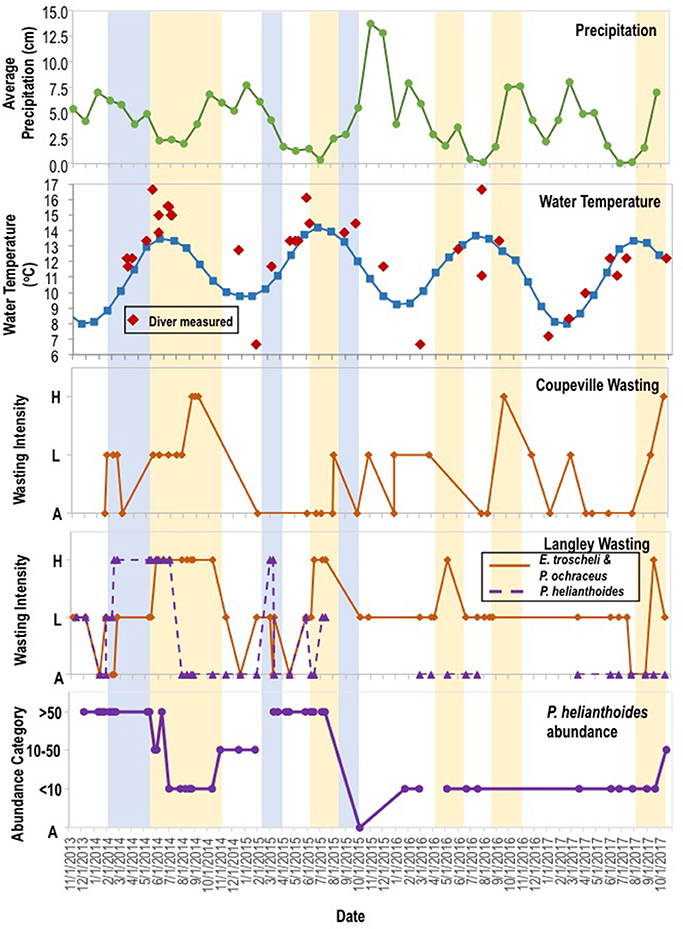
Figure 5. Variation in precipitation, water temperature, incidence of asteroid wasting, and P. helianthoides abundance from Nov 2013–2014. Temperature data was derived from Station 46120 (Pt Wells, WA), and also measured by divers. Wasting intensity was categorized as: (H)igh when >10% of individuals exhibited SSWD signs; (L)ow when <10% of individuals exhibited SSWD signs; and (A)bsent when not observed. The abundance (individuals observed per dive) was categorized into >50, 10–50, <10 and (A)bsent. The blue shaded region indicates the periods of high mortality in P. helianthoides, while the orange shaded regions indicate periods of high mortality in E. troscheli and P. ochraceus.
Environmental variation has been hypothesized to alter the outcome of pathogens and their hosts. An obvious question regarding SSWD is whether the effects of densoviruses on hosts (if they are pathogens) may be exacerbated by temperature anomalies. To address this, we performed two experiments in which temperature was elevated in aquaria containing asteroids that were exposed to asteroid densoviruses (and were positive via qPCR to WAaDs). However, elevated temperature or controls kept at ambient temperature resulted in no SSWD signs in E. troscheli and P. ochraceus (Supplemental Figures 8, 9) after 14 d incubation. Our data is therefore inconsistent with previous reports of temperature eliciting SSWD, suggesting that temperature is not the sole cause of SSWD in the field, or that the impact of WAaDs on SSWD progression is exacerbated by elevated temperature.
It is worthwhile noting that SSWD mass mortality onset occurred concomitant with or immediately following drought conditions in both the Salish Sea region and in central California. Likewise, onset of SSWD mass mortality in Oregon (which occurred after both the former regions) occurred during a period of drought. To investigate the relationship between rainfall and SSWD occurrence, we examined meteorological records from each of the six stations using data from the National Climatic Data Center (https://www.ncdc.noaa.gov/) and Environment Canada (https://weather.gc.ca/). In all 6 locations, SSWD onset corresponded with or was followed by elevated rainfall, and was preceded by lower than 5-year (2011–2016) average rainfall for 1–2 months in 3 of 6 locations. In the Salish Sea (Friday Harbor and Strait of Georgia) and Southern California, rainfall was over 2 times greater than the 5-year average after mass mortality onset. Logistic regression of SSWD occurrence against precipitation was not significant (p > 0.05, Wald test, df = 11) at any location, nor was it significant with offsets of +1 or −1 month (to account for uncertainty in disease onset). At Whidbey Island, precipitation demonstrated little pattern with the occurrence of SSWD mass mortality, and logistic regression of disease state against precipitation was not significant at Langley or Coupeville. Despite this, precipitation in the 4 months prior to disease onset was significantly lower (p = 0.030, Student's t-test, df = 23) than the 4 months after disease onset across all locations, but this relationship was not significant at any individual location when considered separately. Rainfall events at the end of drought conditions potentially bring large amounts of contaminants from terrestrial and freshwater habitats into contact with coastal marine habitats. For example, rainfall from urban habitats brings large concentrations of human pathogenic bacteria and viruses into coastal waters (Noble et al., 2006). Recently, freshwater microcystins have been associated with mortality in sea otter populations in central California (Miller et al., 2010), and incubation of several invertebrates in aquaria containing microcystin resulted in biological accumulation of microcystins (Preece et al., 2017). We sought to examine concentrations of microcystins in asteroid tissues experiencing SSWD from October 2013 in the Puget Sound. Microcystin concentrations were highest in tissues of P. helianthoides and lower in P. ochraceus, however there was no significant relationship in mean microcystin concentration between asymptomatic and SSWD-affected asteroids (Supplemental Figure 7). Direct challenge of P. ochraceus with microcystin-LF (12.5 μg individual−1) yielded no SSWD after 14 d incubation. Therefore, while microcystin is unlikely to cause SSWD, our observations demonstrate that freshwater-derived toxins were present in asteroids during the wasting event and may represent an additional source of organism stress.
Taken together, these data suggest that environmental conditions may influence SSWD, but that the relationship is not predictable based on any combination of factors examined in this study to explain the disease across its entire geographic range. The strongest relationship between environmental conditions and SSWD occurrence was in P. ochraceus and E. troscheli, but temperature manipulations did not result in SSWD signs under experimental settings. Environmental conditions were least clearly tied to SSWD in P. helianthoides. Hence, we speculate that mass mortality caused by SSWD at Langley and Coupeville, and indeed between other geographic regions, may have complex relationships to environmental settings, or may be influenced by environmental factors other than instantaneous or historical temperature.
Conclusions and Synthesis
Our analyses demonstrate that SSWD does not have a consistent etiology across asteroid species or locations, and may be related instead to a diversity of potential pathogens or environmental conditions. While WAaDs are associated on a metapopulation level with SSWD in P. helianthoides, SSWD is not epidemiologically related to WAaDs in other species. The high ambient decay rate of densoviruses suggests that they may only be transmitted when population densities are high. The apparent absence of other promising viral pathogens and failure to induce SSWD by viral challenge suggests that SSWD may not consistently be related to any viral candidate pathogen in species other than P. helianthoides. Furthermore, in areas experiencing mass mortality of P. ochraceus in intertidal zones, the cause of SSWD may be different to those causing mass mortality in predominately subtidal species, such as P. helianthoides. Perturbation experiments indicated that temperature did not elicit SSWD in E. troscheli or P. ochraceus. Similarly, excursions of water temperature from interannual means did not consistently correspond with SSWD occurrence at all locations. The presence of freshwater-derived toxins in subtidal and intertidal species demonstrates that both may be affected by precipitation, and SSWD occurred in regions experiencing drought, however this was not the case across its entire geographic range. It is therefore unlikely that SSWD results from a single etiology or is the same disease across its entire geographic range and across all species in which it is observed. Rather, “sea star wasting disease” describes a similar, though not identical, set of disease signs (i.e., “syndrome”) that afflict the same species between different geographic regions, or between species at the same location. We propose that SSWD be re-named “Asteroid Idiopathic Wasting Syndrome” (AIWS) to account for this possibility. The cause of AIWS is therefore likely a complex tango of diverse potential pathogens and environmental conditions.
Methods
Surveys of SSaDV and WAaDs Presence in Contemporary Samples
Asteroids were collected during several research expeditions and by collaborators at remote field sites (Supplemental Tables 3, 4). Samples were collected either by hand in the intertidal zone, by SCUBA diver, or by rock dredge. For the latter, a rock dredge was submerged to a target depth between 30 and 45 meters, and then towed behind the research vessel (R/V Clifford A. Barnes) for a period of 10 min at 2 kts, collecting approximately 600 m2 of material. Dredge materials were emptied on deck, cleaned using a saltwater hose and asteroids were recovered by hand. In all cases, retrieved asteroids were subsampled or frozen within 2 h of collection. For samples from Unalaska, biopsy punches (3 mm) were used to collect body wall tissues, which were immediately placed into 2 ml of 95% EtOH. For other samples, individual animals were frozen at −80°C or in liquid N2. Additional samples from deeper waters in the Southern California Borderland were retrieved using the ROV Argus and retrieved to the surface within 2 h of collection. There, animals were photographed and placed into −80°C freezer and transported on liquid N2 to Cornell. During the January 2016 Salish Sea expedition, a 5 min plankton tow using a 200 μm mesh net was performed, and 50 ml of concentrated plankton placed into sterile 50 ml tubes and immediately frozen on liquid N2. Additionally, a Van Veen Grab was used to obtain surface sediment samples from each station where asteroids were retrieved. A 2 ml syringe core of material was collected and frozen in liquid N2. DNA was extracted from tissue and plankton samples using the Zymo Tissue & Insect Kit and from sediments using the Zymo Soil DNA kit, then subjected to qPCR for WAaDs as described above.
Viral Challenge Experiments
Challenge experiments were performed to study the impacts of virus-sized homogenates (<0.2 μm; VSH) on asteroid health (Supplemental Table 9). Six experiments were performed using VSH of various tissues, including epidermis (defined as material scraped using a sterile razor blade from the surface of animals including papillae, pedicellaria, paxillae, and other easily dislodged tissues), ovaries, ventral papillae (collected with forceps) and body wall+pyloric caeca. In addition, one experiment was performed using whole tissue which was placed into mussels used to feed animals. The material used to challenge stars originated from SSWD-affected asteroids of three species (P. ochraceus, E. troscheli, and Solaster stimpsoni). Target animals included SSaDV-naïve E. troscheli collected from shallow (~0.2 m) waters of Dutch Harbor, Alaska in September 2015, P. brevispinus and P. ochraceus collected from Whidbey Island, WA in May 2016, and A. forbesii collected from Coos Bay, ME in March 2017. Homogenates of SSWD-affected individuals were prepared by placing tissues into 30 ml artificial seawater, which was then homogenized in a blender (NutriBullet). The homogenate was filtered over a 0.2 μm filter and inoculated into 4–6 asymptomatic individuals in separate 40 L aquaria. Asteroids were maintained at 14°C in darkness. Response variables depended on the experiment, as did length of observation. In all experiments, asteroid wet weight (after shaking off excess water) and SSWD category was measured ~daily. In one experiment (using A. forbesii), animal righting time (Watts and Lawrence, 1990) was measured by measuring the time taken for individual asteroids to move their central disc vertically after being placed completely upside-down. SSWD staging categories employed by the Multi-Agency Rocky Intertidal Network (http://www.seastarwasting.org) were used to determine animal health: Category 0—asymptomatic; Category 1—limb curling/twisting and loss of turgor; Category 2—small lesion on a single ray; Category 3—multiple lesions on multiple rays but no limb autotomy; Category 4—body wall erosions, internal organ protrusion, limb autotomy; and Category 5—animal mortality.
Viral Decay Experiment
Decay of SSaDV was measured using the approach of Heldal and Bratbak (1991). Tissue homogenate was prepared from a sample of P. helianthoides collected from Gower Point, BC, Canada on 17 October 2013 by placing 1 g of body wall and pyloric caeca in 10 ml of 0.02 μm filtered seawater. The homogenate was subsequently filtered over 0.2 μm Durapore filters to obtain the virus-sized fraction, and then frozen in liquid N2. Eight 2 L acid washed and seawater rinsed polycarbonate bottles were filled with seawater. Four incubations were immediately filtered over 0.2 μm Durapore filters to remove particles, while two bottles remained unfiltered. Bottle incubations (two filtered and two unfiltered) were each inoculated with 2 ml of virus-sized asteroid homogenate, while the remaining filtered and unfiltered incubations served as controls. Samples (200 ml) were withdrawn from incubations at 0, 6, 12, 24, and 48 h and filtered by positive pressure (peristaltic pump) sequentially through 0.2 μm Durapore and 0.02 μm Anotop filters. The filters were immediately frozen in liquid N2 before transport to the laboratory at Cornell University. Viral DNA was extracted from the Anotop filters using the Zymo Viral DNA kit, and SSaDV abundance measured by qPCR as described above.
Analysis of P. helianthoides RNA Metaviromes
For P. helianthoides-derived RNA viruses (Hewson et al., 2014), sequence reads (BioProject SAMN02867503-SAMN02867506) were assembled using the CLC Genomics Workbench 4.0 using parameters of 0.5 overlap and 0.8 identity. Resulting contiguous sequences were compared against an in-house database of RNA viral RNA-dependent RNA polymerases (RdRp), coronavirus and flavivirus envelope proteins, and coat proteins of nodaviruses collected from NCBI Genbank at 16 May 2016. Contigs matching these databases by BLASTx at e < 10−10 were further examined by BLASTn against the non-redundant database to exclude spurious hits. Resulting contigs (n = 11) were then vetted for completeness of the polycistronic ORF (resulting in 4 contigs). The four contigs were then compared against four transcriptomes prepared as part of a prior study (Fuess et al., 2015). From these four, two recruited reads only from a single transcriptome, while the remaining two (PhaDiV1 and PhaDiV3) produced alignments from all transcriptomes (Supplemental Table 5). PhaDiV1 and PhaDiV3 were selected for further quantitation by qRT-PCR. PhADiV1 and 3 were also compared against transcriptomes prepared in a separate study (Fuess et al., 2015) examining viral challenge and its impacts on host gene transcription.
qRT-PCR of PhaDiV1 and PhaDiV3
Quantitative reverse transcriptase PCR (qRT-PCR) TaqMan primers and probes were designed around the RdRp region of both PhaDiV1 and PhaDiV3 using Primer3 (Rozen and Skaletsky, 2000). RNA extracts used to determine transcription of SSaDV (Hewson et al., 2014) were used to determine the prevalence and load of these RNA viral phylotypes (Supplemental Table 10). Briefly, RNA was subject to reverse transcription by Superscript III following manufacturer's protocols. A no-RT blank was included for each sample to account for co-extracted DNA. cDNA was subject to qPCR using primers and probes for each viral phylotype (Supplemental Table 11), where each 25 μl reaction contained 1X TaqMan Mastermix (no UNG; Life Technologies), 100 pmol of each primer/probe, and 2 μl cDNA template. qRT-PCR was run in duplicate for each sample against duplicate oligonucleotide standards spanning 1010 to 101 copies per reaction. Viral copies (subtracting no-RT values) were accounted for total extraction volume and weight of tissue used for extraction.
RNA Metaviromes
Purified viruses were prepared from six P. ochraceus (Supplemental Table 6) identically to DNA metaviromes (see Supplemental Methods). Viral RNA was extracted using the Zymo Viral RNA kit following manufacturer's protocols. Viral RNA was then amplified by the TransPlex whole transcriptome amplification kit (WTA) kit. Resulting DNA was sequenced on a Illumina MiSeq 2 × 150 bp following Nextera library preparation. Libraries were subject to assembly (0.5 overlap, 0.8 identity) using the CLC Genomics Workbench 4.0. Contigs spectra were then compared by BLASTx against the in-house RdRp and envelope protein databases as described above for PhaDiV1 and PhaDiV3. Sequence data is available at GenBank under BioProject accessions SAMN08012653-SAMN08012654.
Temperature Experiments
The impact of elevated temperature was examined in three experiments targeting two species that experienced SSWD (P. ochraceus and E. troscheli) that had been exposed to WAaDs (Pyloric caeca tissue loads of all 8 individuals used in the experiments were ~103 copies mg−1). Individual asteroids were placed into replicate 37 L aquaria containing InstantOcean, and in which TopFin Power 10 filter units operated continuously. For each species, two asteroids were subject to elevated water temperature using aquarium heaters, resulting in water temperatures of 17°C (c.f. 11°C for control incubations). The wet weight of individuals was measured daily, and asteroids were visually inspected for SSWD signs.
Analysis of Climate and Oceanographic Buoy Data
To investigate the relationship between SSWD and ambient environmental conditions, water temperature data was collected from 6 National Oceanographic Data Center buoys in Friday Harbor (FRDW1), Monterey (MYXC1), Santa Monica (ICAC1), Newport OR (NWP03), and Port Alexander AK (PLXA2) (NOAA, NWS, USDOC, 1971), in addition to Fisheries and Oceans Canada Buoy 46146 (Halibut Bank) (Environment Canada; https://weather.gc.ca/). Data was obtained for the period from January 2011 through August 2017. Five-year mean water temperatures were calculated for each month from Jan 2011 through Jan 2016, and the ratio of water temperature to mean temperature expressed for 5–6 months prior and after to SSWD onset (Figure 4). Meteorological data on precipitation were also obtained from Friday Harbor Airport, Monterey Peninsula Airport, Los Angeles International Airport, Newport Municipal Airport, Sitka Airport (National Climatic Data Center; https://www.ncdc.noaa.gov/), and Vancouver International Airport (Environment Canada; https://weather.gc.ca/) and treated the same way.
Analyses of SSWD Presence and Intensity at Coupeville Wharf and Langley Harbor
The appearance of SSWD in asteroid populations, along with an estimate of overall population density of each asteroid species, was made by recreational SCUBA divers at two sites on Whidbey Island (Salish Sea) periodically from November 2013 to October 2017. SCUBA divers visited the same locations within the dive area and dives lasted for approximately the same duration. Divers collected qualitative information about: (1) Number of asteroids observed (categories = <10, 10–50, or 50+ per dive); and (2) Disease intensity (>10% of individuals of a given species affected, <10% of individuals of a given species affected, or absence of disease); and (3) Whether most individuals were juveniles or adults. The qualitative (or semi-quantitative for abundance of P. helianthoides) data was then compared against water temperature derived from NODC buoy Station 46120 located off Point Wells, WA (NOAA, NWS, USDOC, 1971) and several diver-measured water temperatures in situ, along with precipitation data collected from the nearby Whidbey Island Naval Air Station (National Weather Service; https://www.ncdc.noaa.gov/).
Detection and Quantification of Microcystins in Asteroid Tissues
Samples of asymptomatic (n = 5) and SSWD-affected (n = 4) P. helianthoides from the Seattle Aquarium (collected 10/26/13), and asymptomatic (n = 5) and SSWD-affected (n = 5) P. ochraceus from the Olympic National Park (collected on 9/19/2013) were used to determine microcystin concentration using the Microcystins ELISA kit (PN520012A; Abraxis) following manufacturer's recommendations. In addition to measuring ambient microcystin concentrations, P. ochraceus was also challenged with microcystin in an aquarium experiment. Individual P. ochraceus were placed into separate 37 L aquaria containing InstantOcean, and in which TopFin Power 10 filter units operated continuously. Two individuals were inoculated with 12.5 μg microcystin-LF (Enzo Life Sciences), while two served as controls. Animal health was monitored daily for 14 d by visual inspection for SSWD signs.
Design of Wasting Asteroid Associated Densoviruses (WAaDs) and Bacterial Survey
Additional information about the design of WAaDs may be found in Supplementary Materials. Geographic samples (Supplemental Table 12) and densoviral genome fragments (Supplemental Table 13), were aligned (Supplemental Figure 10) and used to design the WAaDs qPCR primers. General PCR primers (Supplemental Table 14) for SSaDV were designed to test for the presence of SSaDV in historical and geographic sea star tissue samples. Bacteria (Supplemental Tables 15–16) were identified based on 16S rRNA, and by cultivation on sea star tissue extracts.
Author Contributions
IH, KB, EQ, JB, PF, JF, JK, and CL: Designed and executed research; IH, KB, EQ, JB, PF, JF, and CL: Analyzed and interpreted results. All authors contributed to the drafting of the work, approval of final manuscript, and agree to be accountable for data integrity.
Funding
This work was supported by a Hatch/Multistate supplemental grant awarded to PF, NSF grants OCE-1537111, OCE-1737127, and OCE-1401727 awarded to IH, and a grant from the Atkinson Center for Sustainable Futures awarded to IH.
Conflict of Interest Statement
The authors declare that the research was conducted in the absence of any commercial or financial relationships that could be construed as a potential conflict of interest.
Acknowledgments
The authors are grateful to: Elliot Jackson (Cornell) for comments on an early manuscript and for performing field and lab work; Victor Biguma, Emily Chei, Jamie Peterson, Arjun Pillai Hausner, Brooke Travis, Braulio Castillo, Phillip Fargo, Sierra Helmann, Mitchell Johnson, Jacob Sangren, Alex Vompe (Cornell University) for performing laboratory analysis in support of this project; Sarah Cohen (SFSU), Sarah Gravem and Bruce Menge (OSU) for providing samples of SSWD-affected and asymptomatic P. ochraceus and Leptasterias spp.; Christina Piotrowski and Richard Mooi (California Academy of Sciences) and Cathy Graves and Gordon Hendler (Museum of Natural History, Los Angeles) for providing museum specimens; Melissa Good and Joshua Good (Alaska SeaGrant) for assistance with sample collection in Dutch Harbor; Brenda Konar (UAF) and Rob Foy (NFS Kodiak) for sample collection in the Aleutian Islands and Kodiak, respectively; Lesanna Lahner for specimen collection on Whidbey Island, WA; Mike Donnellan (AK Department of Fish & Game) for collection in southeast Alaska; Yoanna Eissler (U Valparaiso), David Baker (U Hong Kong), Corinna Brussaard (NIOZ), Matthias Middelboe (U Copenhagen), Morgan Pratchett (James Cook University), Qiang Xu (U Hainan), and Charles Griffiths (U Cape Town) for providing samples for geographic analysis; Roxanne Beinart (U Rhode Island) and the Ocean Exploration Trust for providing deepwater asteroids; The crews of the R/V Clifford A. Barnes, R/V Atlantic Explorer and E/V Nautilus; and to Christopher Mah for assistance in identifying deepwater asteroids. Collection of specimens occurred under Alaska Department of Fish and Game Permits FRP CF-15-056, CF-15-127, and CF-17-034, California Fish and Wildlife Permit SC-13144 and Washington Fish and Game Permit 17-080.
Supplementary Material
The Supplementary Material for this article can be found online at: https://www.frontiersin.org/articles/10.3389/fmars.2018.00077/full#supplementary-material
References
Bates, A. E., Hilton, B. J., and Harley, C. D. (2009). Effects of temperature, season and locality on wasting disease in the keystone predatory sea star Pisaster ochraceus. Dis. Aquat. Organ. 86, 245–251. doi: 10.3354/dao02125
Burge, C. A., Mark Eakin, C., Friedman, C. S., Froelich, B., Hershberger, P. K., Hofmann, E. E., et al. (2014). “Climate change influences on marine infectious diseases: implications for management and society,” in Annual Review of Marine Science, Vol. 6, eds C. A. Carlson and S. J. Giovannoni (Palo Alto, CA: Annual Reviews), 249–277.
Chandler, V. K., and Wares, J. P. (2017). RNA expression and disease tolerance are associated with a “keystone mutation” the ochre sea star Pisaster ochraceus. PeerJ 5:e3696. doi: 10.7717/peerj.3696
Dungan, M. L., Miller, T. E., and Thomson, D. A. (1982). Catastrophic decline of a top carnivore in the Gulf of California rocky intertidal zone. Science 216, 989–991. doi: 10.1126/science.216.4549.989
Eckert, G., Engle, J. M., and Kushner, D. (1999). “Sea star disease and population declines at the Channel Islands,” in Proceedings of the 6th California Islands Symposium (Ventura, CA), 435–441.
Eisenlord, M. E., Groner, M. L., Yoshioka, R. M., Elliott, J., Maynard, J., Fradkin, S., et al. (2016). Ochre star mortality during the 2014 wasting disease epizootic: Role of population size structure and temperature. Philos. Trans. R. Soc. B Biol. Sci. 371:20150212. doi: 10.1098/rstb.2015.0212
Fuess, L. E., Eisenlord, M. E., Closek, C. J., Tracy, A. M., Mauntz, R., Gignoux-Wolfsohn, S., et al. (2015). Up in arms: Immune and nervous system response to sea star wasting disease. PLoS ONE 10:e0133053. doi: 10.1371/journal.pone.0133053
Gravem, S. A., and Morgan, S. G. (2017). Shifts in intertidal zonation and refuge use by prey after mass mortalities of two predators. Ecology 98, 1006–1015. doi: 10.1002/ecy.1672
Gudenkauf, B. M., and Hewson, I. (2015). Metatranscriptomic analysis of Pycnopodia helianthoides (Asteroidea) affected by sea star wasting disease. PLoS ONE 10:e0128150. doi: 10.1371/journal.pone.0128150
Gutowsky, L. F. G., Brownscombe, J. W., Wilson, A. D. M., Szekeres, P., and Cooke, S. J. (2016). Improved performance, within-individual consistency and between-individual differences in the righting behaviour of the Caribbean sea star, Oreaster reticulatus. Behaviour 153, 1763–1776. doi: 10.1163/1568539X-00003401
Heldal, M., and Bratbak, G. (1991). Production and decay of viruses in aquatic environments. Mar. Ecol. Prog. Ser. 72, 205–212. doi: 10.3354/meps072205
Hewson, I., Button, J. B., Gudenkauf, B. M., Miner, B., Newton, A. L., Gaydos, J. K., et al. (2014). Densovirus associated with sea-star wasting disease and mass mortality. Proc. Natl. Acad. Sci. U.S.A. 111, 17276–17283. doi: 10.1073/pnas.1416625111
Holmes, S. B., Fick, W. E., Kreutzweiser, D. P., Ebling, P. M., England, L. S., and Trevors, J. T. (2008). Persistence of naturally occurring and genetically modified Choristoneura fumiferana nucleopolyhedroviruses in outdoor aquatic microcosms. Pest Manag. Sci. 64, 1015–1023. doi: 10.1002/ps.1600
Jangoux, M. (1987). Diseases of echinodermata. 1. Agents microorganisms and protistans. Dis. Aquat. Org. 2, 147–162. doi: 10.3354/dao002147
Kohl, W. T., McClure, T. I., and Miner, B. G. (2016). Decreased temperature facilitates short-term sea star wasting disease survival in the keystone intertidal sea star Pisaster ochraceus. PLoS ONE 11:e0153670. doi: 10.1371/journal.pone.0153670
Lessios, H. A. (1988). Mass mortality of Diadema antillarum in the Caribbean-what have we learned. Annu. Rev. Ecol. Syst. 19, 371–393. doi: 10.1146/annurev.es.19.110188.002103
Lessios, H. A. (1995). Diadema antillarum 10 years after mass mortality-still rare, despite help from a competitor. Proc. R. Soc. B Biol. Sci. 259, 331–337. doi: 10.1098/rspb.1995.0049
Lessios, H. A. (2005). Diadema antillarum populations in Panama twenty years following mass mortality. Coral Reefs 24, 125–127. doi: 10.1007/s00338-004-0443-5
Lessios, H. A., Cubit, J. D., Robertson, D. R., Shulman, M. J., Parker, M. R., Garrity, S. D., et al. (1984). Mass mortality of Diadema antillarum on the Caribbean coast of Panama. Coral Reefs 3, 173–182. doi: 10.1007/BF00288252
Menge, B. A., Cerny-Chipman, E. B., Johnson, A., Sullivan, J., Gravem, S., and Chan, F. (2016). Sea star wasting disease in the keystone predator Pisaster ochraceus in Oregon: insights into differential population impacts, recovery, predation rate, and temperature effects from long-term research. PLoS ONE 11:e0153994. doi: 10.1371/journal.pone.0153994
Miller, M. A., Kudela, R. M., Mekebri, A., Crane, D., Oates, S. C., Tinker, M. T., et al. (2010). Evidence for a novel marine harmful algal bloom: Cyanotoxin (microcystin) transfer from land to sea otters. PLoS ONE 5:e12576. doi: 10.1371/journal.pone.0012576
Montecino-Latorre, D., Eisenlord, M. E., Turner, M., Yoshioka, R., Harvell, C. D., Pattengill-Semmens, C. V., et al. (2016). Devastating transboundary impacts of sea star wasting disease on subtidal asteroids. PLoS ONE 11:e0163190. doi: 10.1371/journal.pone.0163190
NOAA, NWS, USDOC. (1971). Meteorological and Oceanographic Data Collected from the National Data Buoy Center Coastal-Marine Automated Network (C-MAN) and Moored (Weather) Buoys. National Oceanographic Data Center.
Noble, R. T., and Fuhrman, J. A. (1997). Virus decay and its causes in coastal waters. Appl. Environ. Microbiol. 63, 77–83.
Noble, R. T., Griffith, J. F., Blackwood, A. D., Fuhrman, J. A., Gregory, J. B., Hernandez, X., et al. (2006). Multitiered approach using quantitative PCR to track sources of fecal pollution affecting Santa Monica Bay, California. Appl. Environ. Microbiol. 72, 1604–1612. doi: 10.1128/AEM.72.2.1604-1612.2006
Peng, F., Fuxa, J. R., Richter, A. R., and Johnson, S. J. (1999). Effects of heat-sensitive agents, soil type, moisture, and leaf surface on persistence of Anticarsia gemmatalis (Lepidoptera : Noctuidae) nucleopolyhedrovirus. Environ. Entomol. 28, 330–338. doi: 10.1093/ee/28.2.330
Preece, E. P., Hardy, F. J., Moore, B. C., and Bryan, M. (2017). A review of microcystin detections in estuarine and marine waters: environmental implications and human health risk. Harmful Algae 61, 31–45. doi: 10.1016/j.hal.2016.11.006
Rozen, S., and Skaletsky, H. (2000). “Primer3 on the WWW for general users and for biologist programmers,” in Bioinformatics Methods and Protocols, eds S. Krawertz and S. Misener (Totowa, NJ: Humana Press), 365–386.
Schultz, J. A., Cloutier, R. N., and Côté, I. M. (2016). Evidence for a trophic cascade on rocky reefs following sea star mass mortality in British Columbia. PeerJ 4:e1980. doi: 10.7717/peerj.1980
Staehli, A., Schaerer, R., Hoelzle, K., and Ribi, G. (2008). Temperature indiced disease in the starfish Astropecten jonstoni. Mar. Biodivers. Rec. 2:e78. doi: 10.1017/S1755267209000633
Wares, J. P., and Schiebelhut, L. M. (2016). What doesn't kill them makes them stronger: an association between elongation factor 1-alpha overdominance in the sea star Pisaster ochraceus and “sea star wasting disease”. PeerJ 4:e1876. doi: 10.7717/peerj.1876
Watts, S. A., and Lawrence, J. M. (1990). The effect of temperature and salinity interactions on righting, feeding and growth in the sea star Luidia clathrata (SAY). Mar. Behav. Physiol. 17, 159–165. doi: 10.1080/10236249009378765
Keywords: sea star wasting, Asteroidea, densovirus, RNA virus, pathogen
Citation: Hewson I, Bistolas KSI, Quijano Cardé EM, Button JB, Foster PJ, Flanzenbaum JM, Kocian J and Lewis CK (2018) Investigating the Complex Association Between Viral Ecology, Environment, and Northeast Pacific Sea Star Wasting. Front. Mar. Sci. 5:77. doi: 10.3389/fmars.2018.00077
Received: 29 November 2017; Accepted: 21 February 2018;
Published: 07 March 2018.
Edited by:
Cosimo Solidoro, National Institute of Oceanography and Experimental Geophysics, ItalyReviewed by:
Colette J. Feehan, Montclair State University, United StatesGorka Bidegain, University of Southern Mississippi, United States
Copyright © 2018 Hewson, Bistolas, Quijano Cardé, Button, Foster, Flanzenbaum, Kocian and Lewis. This is an open-access article distributed under the terms of the Creative Commons Attribution License (CC BY). The use, distribution or reproduction in other forums is permitted, provided the original author(s) and the copyright owner are credited and that the original publication in this journal is cited, in accordance with accepted academic practice. No use, distribution or reproduction is permitted which does not comply with these terms.
*Correspondence: Ian Hewson, hewson@cornell.edu
†Present Address: Jason B. Button, Illumina Corporation, San Diego, CA, United States
Parker J. Foster, Department of Pathology, Johns Hopkins School of Medicine, Baltimore, MD, United States
Chaunte K. Lewis, College of Veterinary Medicine, The Ohio State University, Columbus, OH, United States