- 1Department Biology & Biotechnology, Faculty of Mathematics and Natural Sciences, Halu Oleo University, Kendari, Indonesia
- 2Postgraduate Program, Halu Oleo University, Kendari, Indonesia
- 3Department Oceanography, Faculty of Fisheries and Marine Sciences, Diponegoro University, Semarang, Indonesia
- 4Department Marine Science, Faculty of Fisheries and Marine Sciences, Diponegoro University, Semarang, Indonesia
- 5Department Aquatic Resource Management, Faculty of Fisheries and Marine Sciences, Bogor Agriculture University, Bogor, Indonesia
- 6Department Information Technology, Faculty of Engineering, Halu Oleo University, Kendari, Indonesia
- 7Department Aquaculture, Faculty of Fisheries and Marine Sciences, Halu Oleo University, Kendari, Indonesia
- 8Ministry of Fisheries and Marine, Republic of Indonesia, Jakarta, Indonesia
- 9Transdisciplinary Science and Engineering, School of Environment and Society, Tokyo Institute of Technology, Tokyo, Japan
Mangroves play a vital role in the environment and contribute signific aptly to the well-being of coastal communities by providing goods and services. Unfortunately, the degradation and deforestation of mangroves has frequently occurred. Therefore, it is important to understand the vulnerability of mangroves and its impact on blue carbon storage for effective mangrove management and coastal planning. This study aims to assess the vulnerability of mangroves and its effect on blue carbon storage in the coral triangle region of Southeast Sulawesi, Indonesia. The vulnerability assessment included factors such as exposure, sensitivity, and adaptive capacity. Blue carbon storage was evaluated based on carbon stock in sediment, biomass, and total carbon stock in mangrove ecosystems in both protected and unprotected areas. The mangrove ecosystem in the protected area of Rawa Aopa Watumohai National (RAWN) Park showed lower vulnerability due to lower exposure, lower sensitivity, and higher adaptive capacity. On the other hand, mangrove ecosystems in unprotected areas such as Tinanggea (TNG), Kendari Bay (KDI), and Staring Bay (STR) exhibited moderate to high vulnerability due to higher exposure, sensitivity, and lower adaptive capacity. Mangroves in RAWN Park, which were less vulnerable, had higher blue carbon storage in sediment (381.64 tons C/ha), biomass (312.65 tons C/ha), and the entire ecosystem (706.76 tons C/ha). These values were significantly higher (p<0.05) compared to those in TNG (306.66 tons, 144.15 tons, and 448.37 tons C/ha, respectively), KDI (262.08 tons, 227.73 tons, and 470.76 tons C/ha, respectively), and STR (169.44 tons, 66.66 tons, and 253.27 tons C/ha, respectively). The high vulnerability of mangrove ecosystems resulted in reduced carbon storage in the coastal regions of Southeast Sulawesi. Therefore, efforts such as rehabilitation and restoration, legislation, and conservation should be prioritized to enhance blue carbon storage, and other ecosystem services provided by mangroves in the coral triangle region of Southeast Sulawesi.
1 Introduction
The Coral Triangle regions are renowned for hosting diverse coastal ecosystems containing mangroves, seagrasses, corals, and more, all of which offer invaluable ecosystem services to both society and the environment (Bandh et al., 2023; Gao et al., 2016). As a crucial blue carbon ecosystem, mangroves hold significant importance in climate change mitigation and adaptation efforts, given their remarkable ability to sequester substantial amounts of carbon in comparison to other forest ecosystems (Choudhary et al., 2024; Chowdhury et al., 2023, 2024; Azman et al., 2023; Bandh et al., 2023). Mangroves have been acknowledged as pivotal ecosystems for implementing nature-based solutions aimed at combating climate change (Sunkur et al., 2023; Stefanakis et al., 2021; O’Higgins et al., 2020; Seddon et al., 2020).
The mangrove ecosystem is highly dynamic, with multifaceted interacting processes involving terrestrial, atmospheric, coastal, and oceanic elements. This complexity results in significant biological activities and substantial inputs of carbon and nutrients. Playing a crucial role in controlling biogeochemical cycles, the mangrove ecosystem enhances the functioning of adjacent ecosystems through habitat connectivity (Kristensen et al., 2008; Nagelkerken et al., 2008). Numerous studies have demonstrated that mangrove ecosystems store and contribute significant amounts of blue carbon to coastal areas (Sharma et al. 2020; Kauffman et al. 2020; Azman et al., 2023; Analuddin et al., 2020; Bouillon, 2011; Breithaupt et al., 2012; Maher et al., 2013a). Globally, the deposition of organic matter in coastal sediments is a well-known process driven by the mineralization of organic carbon (Borges et al., 2003; Bouillon et al., 2007a).
Mangrove ecosystems in Southeast Asia are renowned for their significant carbon storage capacity, such as the mangroves of Malaysia (Lee et al., 2024), Vietnam (Mackenzie et al., 2016; Nam et al., 2016) and Ubi Island in Singapore (Phang et al., 2015). Several studies on mangrove blue carbon have been conducted in Indonesia, covering regions such as Kalimantan, Papua, Java, and Sumatera Islands (Kusumaningtyas et al., 2019; Murdiyarso et al., 2015), as well as Sulawesi Island (Analuddin et al., 2016, 2020, 2023a). However, data regarding the relationship between mangrove vulnerability and blue carbon storage remain limited.
Given the various goods and services provided by mangroves, a large population resides in coastal zones, heavily relying on coastal resources in unsustainable ways. Consequently, coastal ecosystems have experienced significant declines, leading to the deterioration of coastal and oceanic health over the course of several decades. Despite the numerous critical roles played by mangroves – such as acting as coastal buffers, fish spawning and nursery sites, pollutant filters, and carbon sequesters (Analuddin et al., 2017, 2023b; Bouillon, 2011; Spalding et al., 2010; Mumby et al., 2004), the rapid degradation of mangrove ecosystems and unsustainable practices have severely impacted the remaining habitats in recent times (Analuddin et al., 2023b; Giri et al., 2011a; Spalding et al., 2010). Both climate change and anthropogenic activities have exacerbated the effects of direct human pressures (Wong et al., 2014), contributing to the degradation and loss of essential coastal buffering systems. This loss diminishes the coastal protection they offer during extreme events, increasing vulnerability and significantly impacting coastal environments, economies, and socio-ecological systems.
Previous studies have primarily focused on assessing global ecosystem vulnerability in the context of climate change alone (Johnson and Marshall, 2007; Nitschke and Innes, 2008; Glick and Stein, 2010, etc.), while recent studies on mangrove ecosystem vulnerability have been investigated not only in relation to climate risk (Cinco-Castro and Herrera-Silveira, 2020;, Retnowati et al., 2019), mangrove vulnerability has investigated in relation to coastal reclamation and carbon loss (Slamet et al., 2020), mangrove land conversion to aquaculture (Munana et al., 2023), coastal vulnerability (Mondal et al., 2024) as well as intensive anthropogenic threats (Fan et al., 2024). However, investigations into the vulnerability of mangrove ecosystems concerning both climate change and human stressors, and their effects on mangrove carbon storage capacity in the Coral Triangle, remain limited (Johnson et al., 2023; RARE Indonesia, 2020). Therefore, evaluating the vulnerability of mangroves and its relation to the blue carbon storage is important for understanding vulnerability processes and their consequences for refining coastal ecosystem development and climate change adaptation strategies. Drawing from vulnerability interpretations (Remling and Persson, 2014), strategies, including reducing exposure through climate change mitigation and implementing technical and sector-specific adaptations, are developed to minimize adverse outcomes. Ecological resilience approaches are prominently featured in policies advocating for nature-based solutions (Chambers et al., 2019). The conservation of coastal ecosystems including mangroves can be considered as an ecological resilience approach, enabling the natural coastal environment to withstand and adjust to sea-level rise and coastal flooding (Garmestani et al., 2019).
The coastal zone of Southeast Sulawesi is a significant area within the Coral Triangle, hosting a wealth of coastal biodiversity that includes mangroves, seagrasses, and coral reefs. Mangroves within Southeast Sulawesi are situated in various locations, such as Rawa Aopa Watumohai National (RAWN) Park, Tinanggea, Kendari Bay, Staring Bay, among others. Despite the extensive scientific research conducted on the mangroves of Southeast Sulawesi (Analuddin et al., 2013, 2016, 2017, 2018, 2020, 2023a, Analuddin et al., 2023b; Rahim et al., 2024), there is a noticeable lack of studies focusing on mangrove vulnerability and its impact on blue carbon storage in the region.
Currently, mangrove ecosystems in Southeast Sulawesi, Indonesia, encompassing areas like Kendari Bay, Tinanggea, Staring Bay, among others, have faced significant negative impacts from human activities in Kendari city and Konawe Selatan Regency (CBSSS, 2021; Analuddin et al., 2023b). Over the past two decades, there has been a notable decline in these ecosystems, with the original mangrove area shrinking from 350 hectares to 146 hectares in Kendari Bay and from 4,433 hectares to 667 hectares in Tinanggea (Analuddin et al., 2023b). This decline is predominantly attributed to various human activities, such as the establishment of hotels, resorts, factories, industries, marine ports, as well as agricultural, aquaculture and mining operations, all of which contribute to the increased vulnerability of the mangrove ecosystems.
Given the significance of mangroves in supporting the livelihoods of numerous coastal communities through the provision of goods and services, it is important to understand the vulnerability status of mangroves and their impact on blue carbon storage in this region. This understanding is crucial for effective mangrove management and coastal planning, especially in the areas of climate change mitigation, adaptation, and coastal development. Hence, the insights from this study are important for the sustainable management of the coastal area in Southeast Sulawesi. The primary objectives of this study are as follows: (1) to ascertain the vulnerability status (exposure, sensitivity, and adaptive capacity components) of mangrove ecosystems in the coastal areas of Southeast Sulawesi, (2) to determine carbon storage within mangrove ecosystems, and (3) to elucidate the relationship between mangrove vulnerability and blue carbon storage within the Coral Triangle areas of Southeast Sulawesi, Indonesia.
2 Methods
2.1 Study site
The study was carried out across four distinct mangrove conditions in Southeast Sulawesi, specifically in the Rawa Aopa Watumohai National (RAWN) Park, Tinanggea (TNG), Kendari (KDI) Bay, and Staring (STR) Bay (Figure 1). The mangroves in RAWN Park were natural, exhibiting natural and well-defined zonation. These mangroves were intersected by the Lanowulu and Roraya rivers, which transported significant amounts of terrestrial sediment. On the other hand, mangroves in Tinanggea were severely impacted by land conversion for aquaculture purposes and faced pressures from various anthropogenic and climate change factors. The Tinanggea and Langkoawa rivers carried substantial sediment through this area. In Kendari Bay, the condition of the mangroves had significantly deteriorated due to land conversion for aquaculture, urban expansion, and the construction of settlements. The Wanggu River flowed through these mangroves, depositing significant sediment into the bay. Local governmental and non-governmental organizations had initiated the planting of Rhizophora spp. seedlings in certain areas of Kendari Bay. In contrast to the previous locations, the mangroves in Staring Bay lacked major rivers flowing through them, relying on water from small channels during the rainy seasons. Rhizophora spp. and Sonneratia spp. were prevalent in Staring Bay. Notably, new facilities, including a shipyard and a coal-fired power plant, had been established near the mangrove area. Settlements were closely situated to these mangroves, and some fishing ports were located within the mangrove ecosystem.
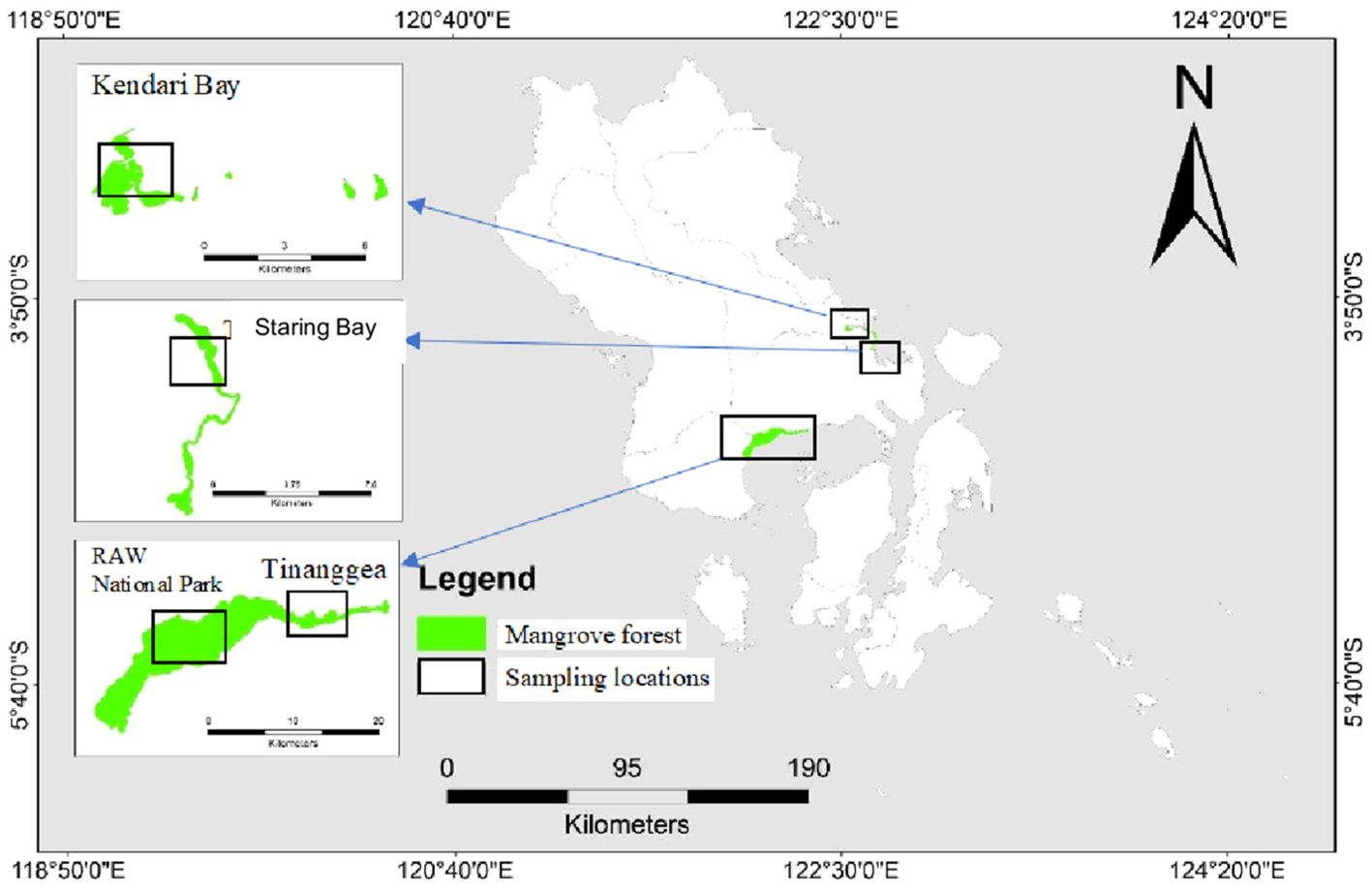
Figure 1. Map of study site in mangrove ecosystems at Rawa Aopa Watumohai National (RAWN) Park, Tinanggea (TNG), Kendari (KDI) Bay and Staring (STR) Bay, Southeast Sulawesi, Indonesia.
2.2 Exposure component analysis
The parameters and criteria ranking for exposure component analysis were adopted from Ellison (2015) and Subur et al. (2011). Parameters for the exposure component were identified, encompassing elevation, water depth/tidal level, substrate type, sedimentation, and the distance of mangroves from settlements. The elevation of the mangrove habitat was assessed at each mangrove stand in every study location by measuring the angle at a distance of 10–20 meters using a semicircular ruler, and subsequently calculating the slope or elevation. The results of this assessment were then classified into different categories: very low elevation (<20 cm, score 5), low elevation (21–40 cm, score 4), medium elevation (41–60 cm, score 3), high elevation (61–80 cm, score 2), and very high elevation (>80 cm, score 1).
The tidal level was determined during high tide using a pole meter for each mangrove stand at the study site. The measured results were classified as either very high (>3 m, score 1), high (2–3 m, score 2), medium (1.5–2 m, score 3), low (1–1.5 m, score 4), or very low (<1 m, score 5). Substrate type was directly assessed on-site and categorized as follows: soft mud (very suitable, score 1), sandy mud (suitable, score 2), muddy sand (moderate, score 3), sand (less suitable, score 4), and sandy with corals (unsuitable, score 5). Sedimentation status was visually inspected on-site at the river mouth in each location and categorized as very high (score 1), high (score 2), medium (score 3), low (score 4), or very low (score 5). The distance of the mangrove ecosystem from settlements was estimated using the Google Earth application and classified as very close (<0.5 km, score 5), close (0.5–2 km, score 4), medium (2–5 km, score 3), far (5–7.5 km, score 2), or very far (>7.5 km, score 5).
2.3 Sensitivity data
The parameters and criteria ranking for sensitivity component analysis were adopted from Ellison (2015) and Subur et al. (2011). Mangrove sensitivity data consisted of mangrove condition, and mangrove composition and structure.
a. Mangrove condition
The parameters and criteria ranking for mangrove condition including mangrove reduction area, and mortality were adopted from Ellison (2015), while the parameters and criteria ranking other mangrove condition parameters including vegetation thickness and species richness were adopted from Subur et al. (2011). The parameters of mangrove condition, encompassing aspects like mangrove reduction area, mortality, vegetation thickness, and species richness, was assessed in RAWN Park, TNG, KDI Bay, and STR Bay. Data on mangrove reduction area, which included information on land converted to aquaculture and urban development, was collected through direct field observations from January to July 2022. Land conversion was classified as follows: no conversion (score 1), slight conversion (score 2), moderate conversion (score 3), significant conversion (score 4), and extensive conversion (score 5). Mangrove mortality was also noted and categorized as follows: no mortality (score 1), low mortality (score 2), moderate mortality (score 3), high mortality (score 4), and very high mortality (score 5). Mangrove vegetation thickness was assessed using the Google Earth application and categorized into very thick (>2000 m, score 1), thick (1000–2000 m, score 2), medium (500–1000 m, score 3), thin (100–500 m, score 4), and very thin (<100 m, score 5). Lastly, species richness was observed and classified as very rich (>15 species, score 1), rich (11–15 species, score 2), moderate (7–10 species, score 3), low (4–6 species, score 4), and very low (<4 species, score 5).
b. Mangrove composition and structure
The parameters and criteria ranking for sensitivity component analysis of mangrove composition and structure were adopted from Ellison (2015) including tree density, tree size and basal area, while the parameters and criteria ranking for mangrove species dominant and mangrove recruitment were adopted from Subur et al. (2011). Mangrove composition and structure in each study location were assessed through tree censuses using a transect method. These censuses were conducted between January and July 2022 in RAWN Park, TNG, KDI Bay, and STR Bay mangrove forests. Tree density was calculated and classified as very high (>2000 individuals/ha, score 1), high (1500–2000 individuals/ha, score 2), medium (1000–1500 individuals/ha, score 3), low (500–1000 individuals/ha, score 4), and very low (<500 individuals/ha, score 5). Basal area was determined from the diameter at breast height (DBH) of trees in each study plot, averaged, and categorized as very high (>25 m²/ha, score 1), high (15–25 m²/ha, score 2), medium (10–15 m²/ha, score 3), low (5–10 m²/ha, score 4), and very low (<5 m²/ha, score 5). The dominant species were identified as follows: Avicennia sp. (score 1), Sonneratia sp. (score 2), Rhizophora spp. (score 3), Bruguiera sp. (score 4), and Pallem (score 5). Finally, mangrove recruitment was determined based on seedlings in each study plot and classified as very high (score 1), high (score 2), medium (score 3), low (score 4), and very low (score 5).
2.4 Adaptive capacity component
The parameters and criteria ranking for adaptive capacity component analysis were adopted from Ellison (2015) and Subur et al. (2011). The data regarding the adaptive capacity components includes elevation above the mangroves, available space behind the mangroves, community management capacity, stakeholder involvement, and mangrove protection and legislation. The area designated for mangrove migration, based on elevation above the mangroves, was determined and classified as fully available for migration (score 1), mostly available for migration (score 2), moderately available for migration (score 3), minimally available for migration (score 4), and not available for migration (score 5). Community management activities, specifically the engagement of local communities in mangrove conservation and management, were evaluated and categorized as very good (score 1), good (score 2), moderate (score 3), poor (score 4), and very poor or non-existent (score 5). Stakeholder involvement, which focused on the engagement of NGOs and other groups in mangrove conservation efforts, was also assessed and categorized as very good (score 1), good (score 2), moderate (score 3), poor (score 4), and very poor or non-existent (score 5). Lastly, the status of mangrove protection and legislation was scrutinized and classified as very good (score 1), good (score 2), moderate (score 3), poor (score 4), and very poor or non-existent (score 5).
2.5 Vulnerability status
A mangrove vulnerability status was determined by averaging ranking values from exposure (E), sensitivity (S), and adaptive capacity (A) components following the methods of Ellison (2015) and Subur et al. (2011). Here, a score of 1 signifies very low vulnerability, a score of 2 indicates low vulnerability, a score of 3 reflects moderate vulnerability, a score of 4 signifies high vulnerability, and a score of 5 indicates very high vulnerability.
2.6 Blue carbon sequestration analysis
a. Sediment carbon stock sampling
Sediment sampling for estimating carbon stock was conducted in the unprotected mangrove areas of Tinanggea, Kendari Bay, and Staring Bay. However, data on sediment carbon stock in the protected area of RAWN Park was obtained from Analuddin et al. (2023a). Thirteen sediment cores were extracted from mangroves in the unprotected areas: five cores from Tinanggea, five from Kendari Bay, and three from Staring Bay. Sediment cores were collected up to a depth of 100 cm using a 5.5 cm diameter open-face peat gouge auger during low tide. These cores were segmented into depth intervals of 0–20, 20–40, 40–60, 60–80, and 80–100 cm. The total depth of the sediment cores was noted to represent the endpoint of each interval, and a one-centimeter subsection of sediment was collected from each of the five depth intervals. The sediment samples were placed in labeled plastic bags and then transported to the Biology Laboratory at the University of Halu Oleo, Kendari.
b. Preparation of sediment samples
The sediment samples were prepared for analyzing sediment bulk density (SBD) and concentrations of soil organic carbon (SOC) content at the Laboratory of Halu Oleo University. Initially, the sediment samples were dried in an oven at 60°C until a constant weight was reached to determine the SBD in grams per cubic centimeter (g cm-3). The SBD for each sample was calculated by dividing the dried weight by the volume. Approximately 20 grams of sediment from each sample was then ground using a mortar and pestle, passed through a 0.5-mm sieve to eliminate large roots and inorganic debris, and readied for the analysis of SOC concentrations.
c. Determination of SOC concentration
Determination of SOC concentration was done by following the method of Suleman and Eviati (2009) and Analuddin et al. (2021) as follows:
2.6.1 Sediment dry process
A 5.0 g sediment sample was collected and placed in an aluminum dish with the weight noted. It was then dried in an oven at 105°C for 3 hours. Subsequently, the dried samples were moved to a desiccator to cool, weighed, and the loss in sample weight was identified as the mass of water.
2.6.2 Analysis of SOC concentration
The soil organic carbon (SOC) content was determined based on the reduction of Cr6+ (orange color) to Cr3+ (green) in an acidic environment following the method described by Suleman and Eviati (2009), which was adopted from Walkley and Black (1934). A 100 mL volumetric flask was filled with 0.5 g of fine sediment sample (particle size< 0.5 mm), to which 5 mL of 1 N K2Cr2O7 was added, shaken, followed by the addition of 7.5 mL of concentrated H2SO4. This mixture was shaken and left to stand for 30 minutes. Subsequently, the flask was topped up with ion-free water to reach a volume of 100 mL and left to cool overnight at room temperature or for approximately 12 hours until a clear solution was formed. The solution was then analyzed using a spectrophotometer at a wavelength of 561 nm and compared with standard solutions of 0 and 250 ppm. To prepare the standard solutions, 0 and 5 mL of a 5,000 ppm standard solution were diluted to 100 mL using a measuring flask, following the same procedure as the sample assessment but without the sample. The organic carbon content (%) was calculated as described below:
Where: ppm curve = sample levels obtained from the curve of the relationship between the grades of series standard with its reading after corrected blank.
d. Quantification of sediment carbon stock
Sediment C stock (Mg C ha-1) was determined by multiplying SOC (%) by SBD (g cm-3) in each interval sampled following the method of Analuddin et al. (2023a) as follows:
e. Estimation of biomass carbon stock
The carbon stock in mangrove biomass was determined by considering the above and below-ground biomass of each mangrove species present at the study site. Various allometric equations were utilized for estimating the biomass of each mangrove species. These included allometric models for estimating the biomass of Rhizophora apiculata, R. mucronata, and Ceriops tagal (source: Analuddin et al., 2018), R. stylosa (source: Analuddin et al., 2020), Sonneratia sp (source: Kusmana et al., 2018), and Bruguiera sp (source: Perera et al., 2013). Additionally, the above-ground carbon (AGC) stock was calculated using the methodology outlined by the Eggleton et al. (2006) as follows:
Where; AGC is above-ground carbon stock, B is above-ground biomass and the 0.47 is constant value of C in organic matter (IPCC 2006).
The below-ground carbon (BGC) stock was estimated following the method of Cairns et al. (1997) by using the following equation:
Where BGC is below-ground C, AGC is above-ground carbon stock, and the constant of 0.216 is the ratio of BGC to AGC (Cairns et al., 1997).
Biomass carbon stock (BCS) was estimated using the following equation:
Furthermore, Total carbon stock (TCS) in mangrove ecosystem was estimated as follows:
2.7 Statistical analysis
Statistical descriptive analysis was done to estimate the mean ± standard error of exposure, sensitivity, adaptive capacity, vulnerability, SOC, SCS, BCS, and TCS. One-way ANOVA and a post-hoc LSD test were performed for comparing all of these parameters among sites. All analyses were done using statistical software packages of KaleidaGraph 4.0 (Synergy Software, USA).
3 Results
3.1 Vulnerability components
3.1.1 Exposure
The exposure parameters (environmental factors) related to mangroves in this study are presented in Table 1. The land elevations of the mangrove habitats in RAWN Park, TNG, KDI Bay, and STR Bay exhibited variation. For instance, at RAWN Park, the mangrove habitat elevations ranged from 17 cm to 96 cm, with the highest elevation observed at the Rhizophora mucronata (Rm) mangrove stand and the lowest at the Ceriops tagal (Ct) mangrove stand. Similarly, the elevations of mangrove habitats in TNG, KDI Bay, and STR Bay ranged from 43 cm to 67 cm, 23 cm to 52 cm, and 0.18 to 0.30 m, respectively. Moreover, seawater depths during high tide varied from 0.1 to 1.22 m in RAWN Park, 0.73 to 1.13 m in TNG, 0.49 to 0.81 m in KDI Bay, and 0.54 to 0.75 m in STR Bay. Significant differences (p< 0.05) were noted in land elevations and seawater depths among mangrove stands at each research site.
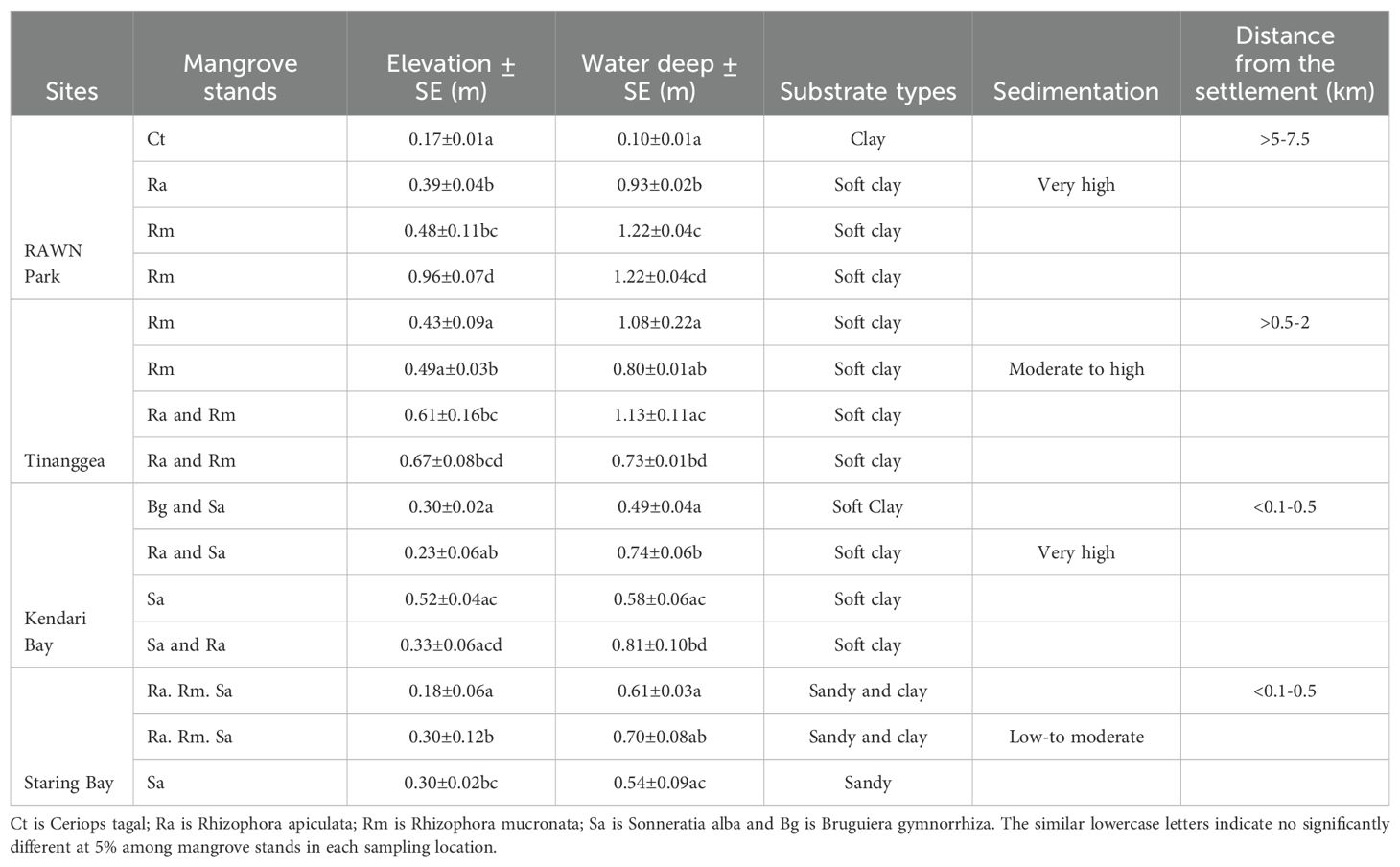
Table 1. Trends of the exposure component (elevation. water deep, substrate types, sedimentation, and distance from the settlement within the mangrove ecosystems) of the Rawa aopa Watumohai National (RAWN) Park, Tinanggea, Kendari Bay and Staring Bay, Southeast Sulawesi, Coral Triangle areas, Indonesia.
The substrate in the mangrove habitats at RAWN Park, TNG, and KDI Bay predominantly consisted of soft clay. In contrast, at STR Bay, the substrate was a mix of sand and clay, with sand being the dominant component in the mangrove areas populated by Sonneratia spp. Sedimentation levels were notably high in RAWN Park and KDI Bay, while sedimentation in TNG experienced moderate. In contrast, sedimentation at STR Bay was rated as low to very low.
The distance from human settlements varied significantly for each mangrove habitat. The mangrove forests at RAWN Park and TNG ranged 5–7.5 km away from settlements, while the distance from of mangroves in TNG ranged 0.5–2 km a little bit far from settlements, In contrast, the mangrove habitats in KDI and STR Bays were notably close to settlements, ranging from 0.1 to 0.5 km.
All the measured exposure parameters exhibited varying rank values (see Figure 2A). The majority of exposure parameters in the mangrove ecosystem of RAWN Park had rank values below 3, with the exception of tidal levels. Conversely, most exposure parameters in the STR mangrove ecosystem received high scores (4 and 5), except for elevation, which fell into the medium category (score 3). Tidal level and distance from settlements were notably ranked high for the mangrove ecosystems in TNG, KDI Bay, and STR Bay. When considering the average rank values of the measured exposure parameters (see Figure 2B), RAWN Park had a significantly lower value (1.4) compared to the other sites (p< 0.05), suggesting lower environmental pressure in RAWN Park. There were no significant differences in the average rank values of exposure parameters (p > 0.05) among the mangrove ecosystems in TNG (3.4), KDI Bay (2.8), and STR Bay (4.2).
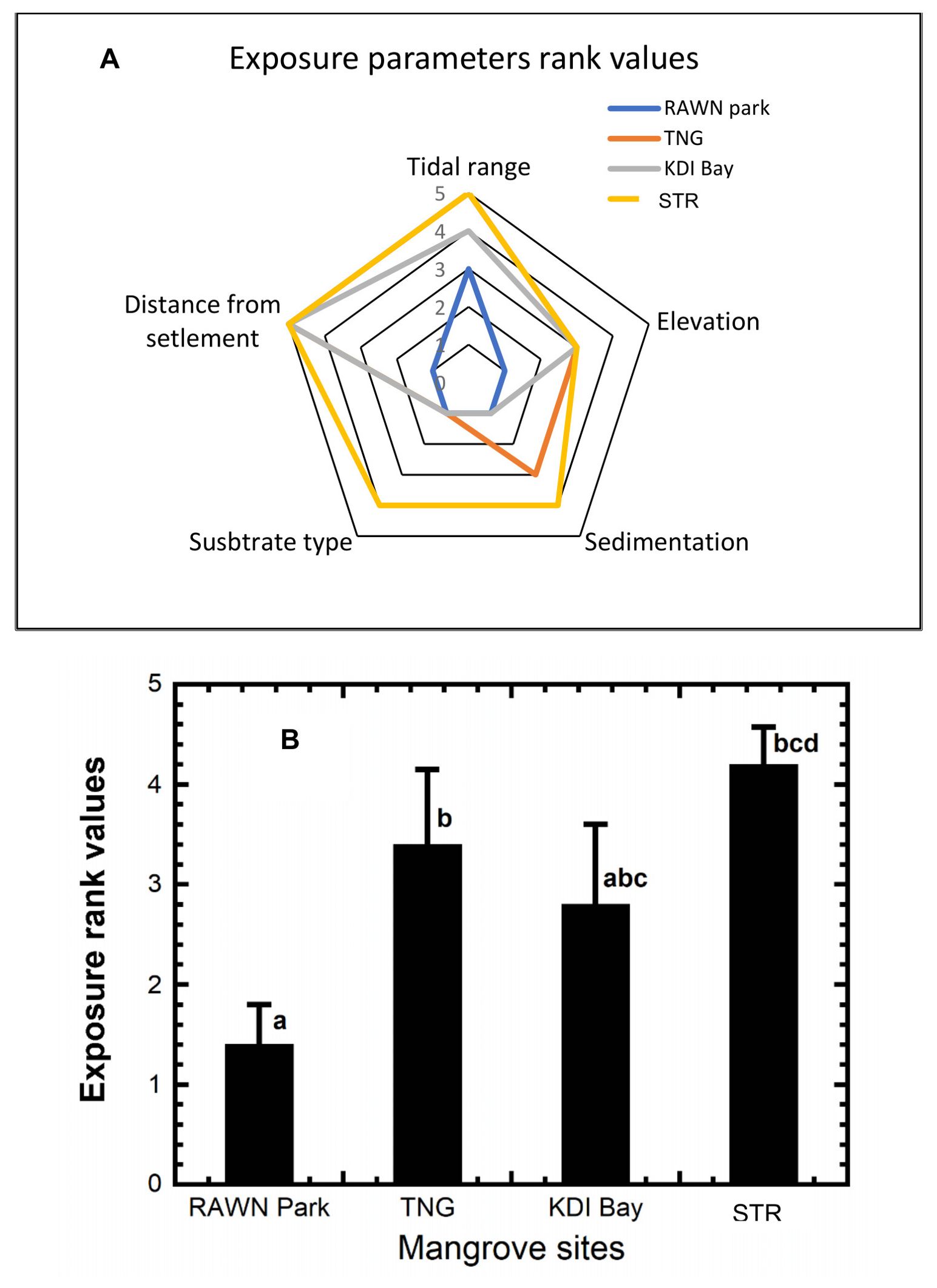
Figure 2. Diagram of the exposure parameters rank values (A) and average exposure rank values (B) at RAWN Park, TNG, KDI Bay and STR Bay in Southeast Sulawesi, Indonesia. The different lowercase letters indicate significantly different at 5% among mangrove sites.
3.1.2 Sensitivity
Mangrove sensitivity data, including (a) mangrove condition, and (b) mangrove composition and structure, are presented in Table 2 and Table 3, respectively. Table 2 outlines the reduction area, thickness, species richness, and mortality rates of mangroves at the study sites. There was no reduction in mangrove area at RAWN Park, and the mortality rate was low. In contrast, significant reductions in area and high mangrove mortality rates were observed in Tinanggea and Kendari Bay. At STR Bay, the mangrove reduction area was small, and the mangrove mortality rate was moderate. Regarding mangrove vegetation thickness, the thickest vegetation was in RAWN Park (nearly 5 km wide), while much thinner mangrove vegetation was recorded in Tinanggea and STR Bay (< 100 m). The highest species richness (18 species) of mangroves was observed in RAWN Park, with the other research sites displaying medium species richness (10 species).

Table 2. Sensitivity component of mangrove condition (reduction area, thickness, species richness, and mortality) in Rawa Aopa Watumohai National (RAWN) Park, Tinanggea, Kendari Bay and Staring in Southeast Sulawesi, Coral Triangle areas. Indonesia.
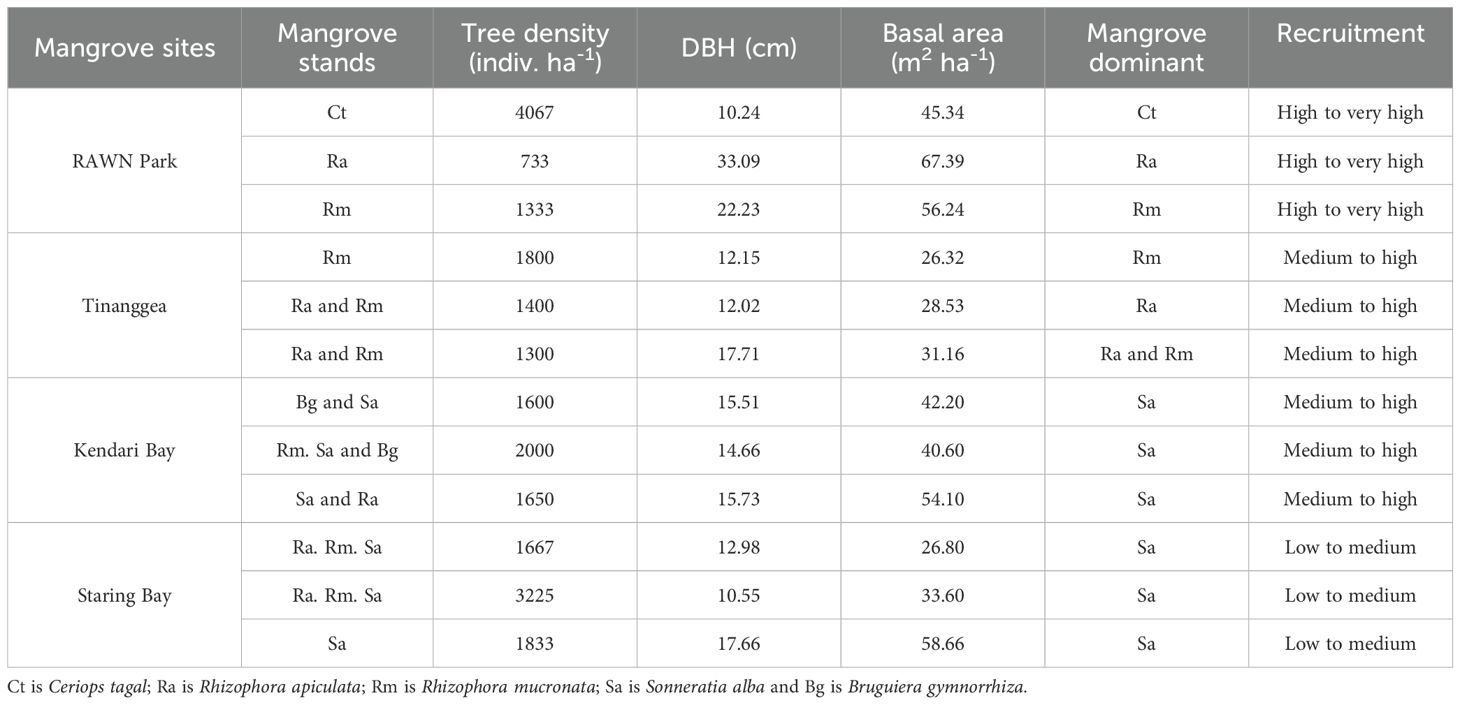
Table 3. The sensitivity component of mangrove structure in Rawa Aopa Watumohai National (RAWN) Park, Tinanggea, Kendari Bay and Starings in Southeast Sulawesi, Coral Triangle areas, Indonesia.
Table 3 shows the structure of mangroves (tree density, diameter at breast height (DBH), basal area, dominant species, and recruitment status) across the study sites, namely RAWN Park, TNG, KDI Bay, and STR Bay in Southeast Sulawesi. The mangrove densities ranged from 733 to 4067 individuals per hectare in RAWN Park, 1300 to 1800 individuals per hectare in TNG, 1600 to 2000 individuals per hectare in KDI Bay, and 1667 to 3225 individuals per hectare in STR Bay. The average DBH of mangroves varied from 10.24 to 33.29 cm in RAWN Park, 12.02 to 17.71 cm in TNG, 14.66 to 15.73 cm in KDI Bay, and 10.55 to 17.66 cm in STR Bay. Basal areas of mangroves spanned from 45.34 to 67.39 m2 per hectare in RAWN Park, 26.31 to 31.16 m2 per hectare in TNG, 40.60 to 54.10 m2 per hectare in KDI Bay, and 26.80 to 58.66 m2 per hectare in STR Bay. The dominant mangrove species were Rhizophora sp in RAWN Park and TNG, while Sonneratia sp was dominant mangrove species in KDI Bay and STR Bay. Mangrove recruitment statuses varied with high to very high in RAWN Park, medium to high in TNG and KDI Bay, and low to medium in STR Bay.
Figure 3A presents the rank values of sensitivity parameters for mangrove ecosystems in RAWN Park, TNG, KDI Bay, and STR Bay in Southeast Sulawesi. Most sensitivity parameters assessed in RAWN Park were ranked very low, indicating lower mangrove sensitivity at this location. In contrast, sensitivity parameters in KDI Bay, such as mortality, spatial reduction in mangrove area, and adjacency to coral and seagrass ecosystems, received high rankings (score 5), signifying very high mangrove sensitivity. For sensitivity parameters in TNG, such as mangrove thickness, spatial reduction in mangrove area, and mortality were ranked high. Meanwhile, in STR Bay, only mangrove thickness received a high ranking among all assessed sensitivity parameters, with the remaining parameters falling within the medium and low rank values. However, spatial reduction in the mangrove area and mangrove recruitment were rated high (score 4). The overall sensitivity (as shown in Figure 3B) of mangrove ecosystems exhibited significant differences among the sites (p< 0.05), with RAWN Park demonstrating significantly lower sensitivity (1.67) compared to TNG (3.1) and KDI Bay (3.2). Nonetheless, there were no differences (p > 0.05) in sensitivity among mangroves in TNG, KDI Bay, and STR Bay.
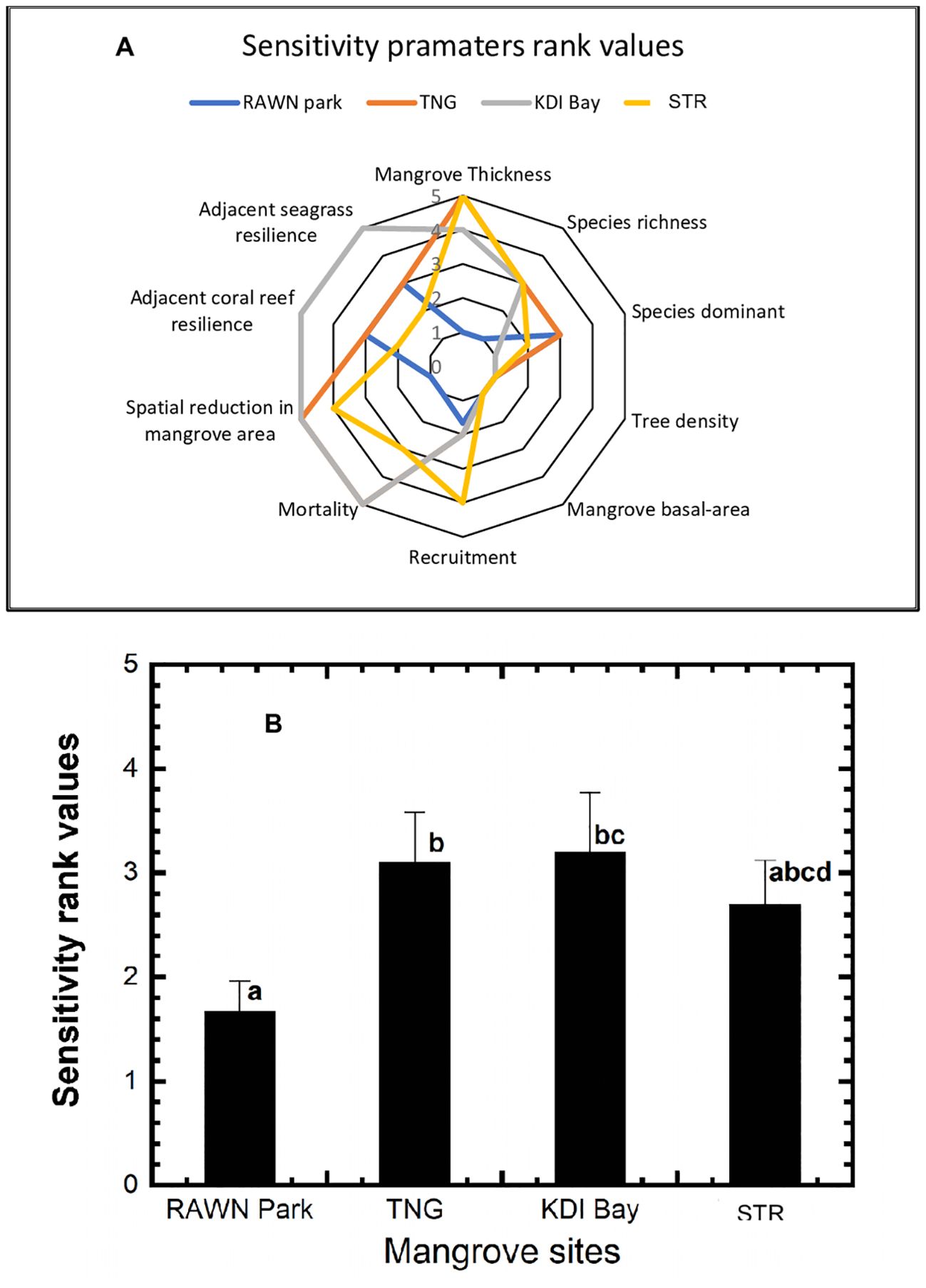
Figure 3. Diagram of the sensitivity parameters rank values (A) and average sensitivity rank values (B) at RAWN Park, TNG, KDI Bay and STR Bay in Southeast Sulawesi, Indonesia. The different lowercase letters indicate significantly different at 5% among mangrove sites.
3.1.3 Adaptive capacity
Table 4 shows the adaptive capacity status of mangrove ecosystems as outlined in this study. The availability of migration areas above the mangroves was high in RAWN Park but not present in TNG, KDI Bay, and STR Bay. In terms of community management capacity, RAWN Park demonstrated a strong capacity, whereas TNG and STR Bay exhibited poor capacities. Community management capacity in mangroves of KDI Bay displayed a moderate level. Stakeholder involvement and mangrove protection legislation were robust in RAWN Park but lacking in TNG and STR Bay. However, stakeholder involvement was relatively strong while mangrove protection legislation was at a moderate level in KDI Bay.

Table 4. The adaptive capacity component of mangrove ecosystems in the present study of Rawa Aopa Watumohai National (RAWN) Park, Tinanggea, Kendari Bay, and Staring Bay, Southeast Sulawesi, Indonesia.
Figure 4A shows the rank values of adaptive capacity parameters for mangrove ecosystems in RAWN Park, TNG, KDI Bay, and STR Bay, Southeast Sulawesi. All adaptive capacity parameters for the mangrove ecosystem in RAWN Park were rated below 2, indicating a high adaptive capacity in this location. In contrast, all measured adaptive parameters for the mangrove ecosystem in STR Bay scored very high (scores 4 and 5), signifying a very low adaptive capacity for mangroves in that area. Tinanggea’s adaptive capacity parameters were mostly high, except for elevation above the mangroves, which was rated very high, indicating a low adaptive capacity for mangroves in that region. KDI Bay displayed medium-ranked adaptive capacity parameters, except for elevation above the mangroves, which was ranked very high. The average rank values of adaptive capacity (as shown in Figure 4B) for the mangrove ecosystem in RAWN Park (1.17) were significantly lower (p< 0.05) than in TNG (4.25), KDI Bay (3.25), and STR Bay (STR) (4.75). Nonetheless, there were no differences (p > 0.05) in adaptive capacity among the mangrove ecosystems of TNG, KDI Bay, and STR Bay.
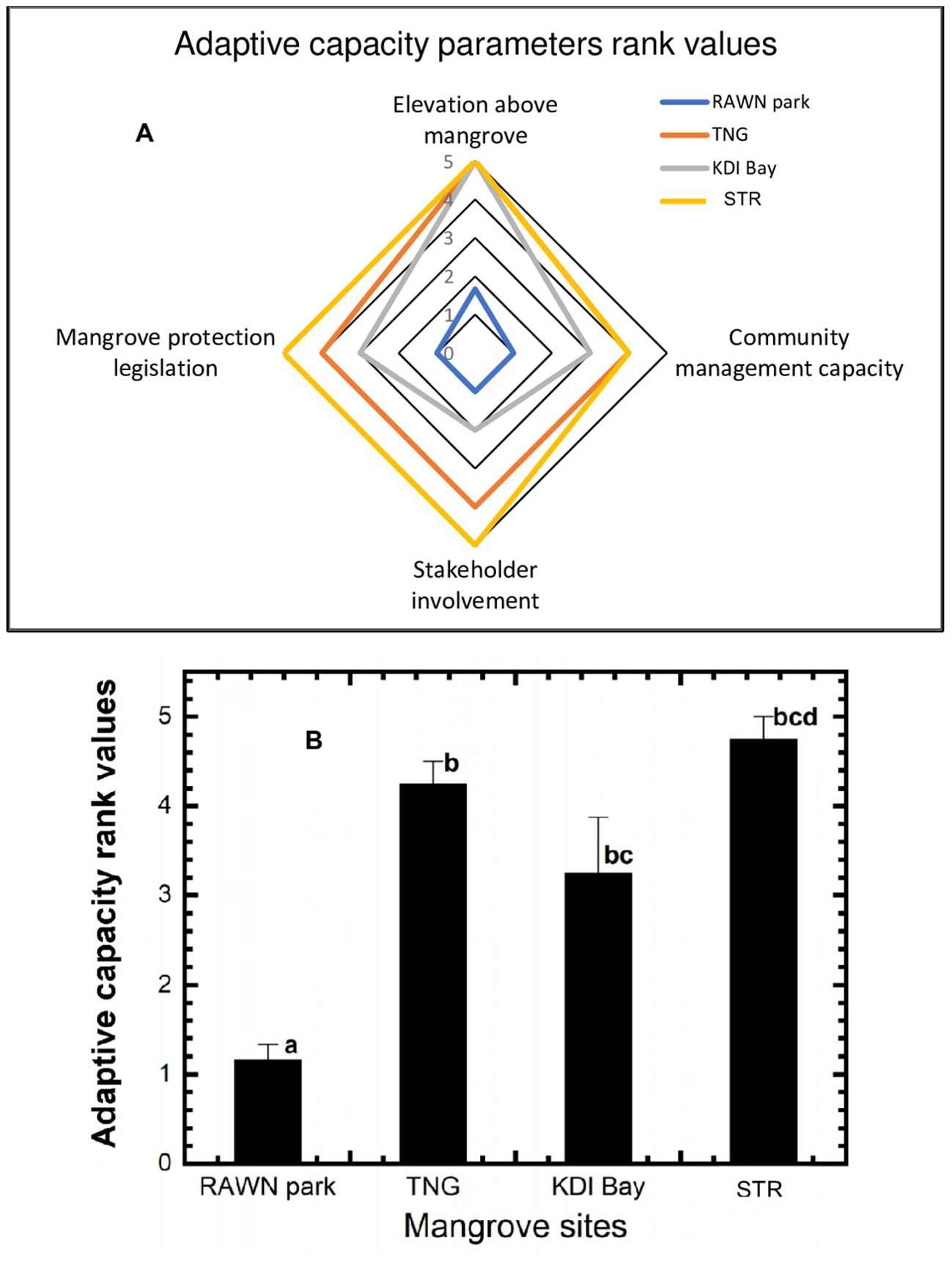
Figure 4. Diagram of the adaptive capacity parameters rank values (A) and average adaptive capacity rank values (B) at RAWN Park, TNG, KDI Bay and STR Bay in Southeast Sulawesi, Indonesia. The different lowercase letters indicate significantly different at 5% among mangrove sites.
Figure 5A presents the triangular diagrammatic rank values of vulnerability components (exposure, sensitivity, and adaptive capacity) for mangrove ecosystems in the present study. The vulnerability component rank values for the mangrove ecosystem in RAWN Park were lower, indicating lower mangrove vulnerability in this area. Conversely, mangrove ecosystems in the unprotected areas of TNG, KDI Bay, and STR Bay sites exhibited high rank values, signaling raised vulnerability. These results suggest less vulnerability of the mangrove ecosystem in the protected area of RAWN Park, while the mangrove ecosystems in the unprotected regions of Southeast Sulawesi displayed moderate to high vulnerability statuses.
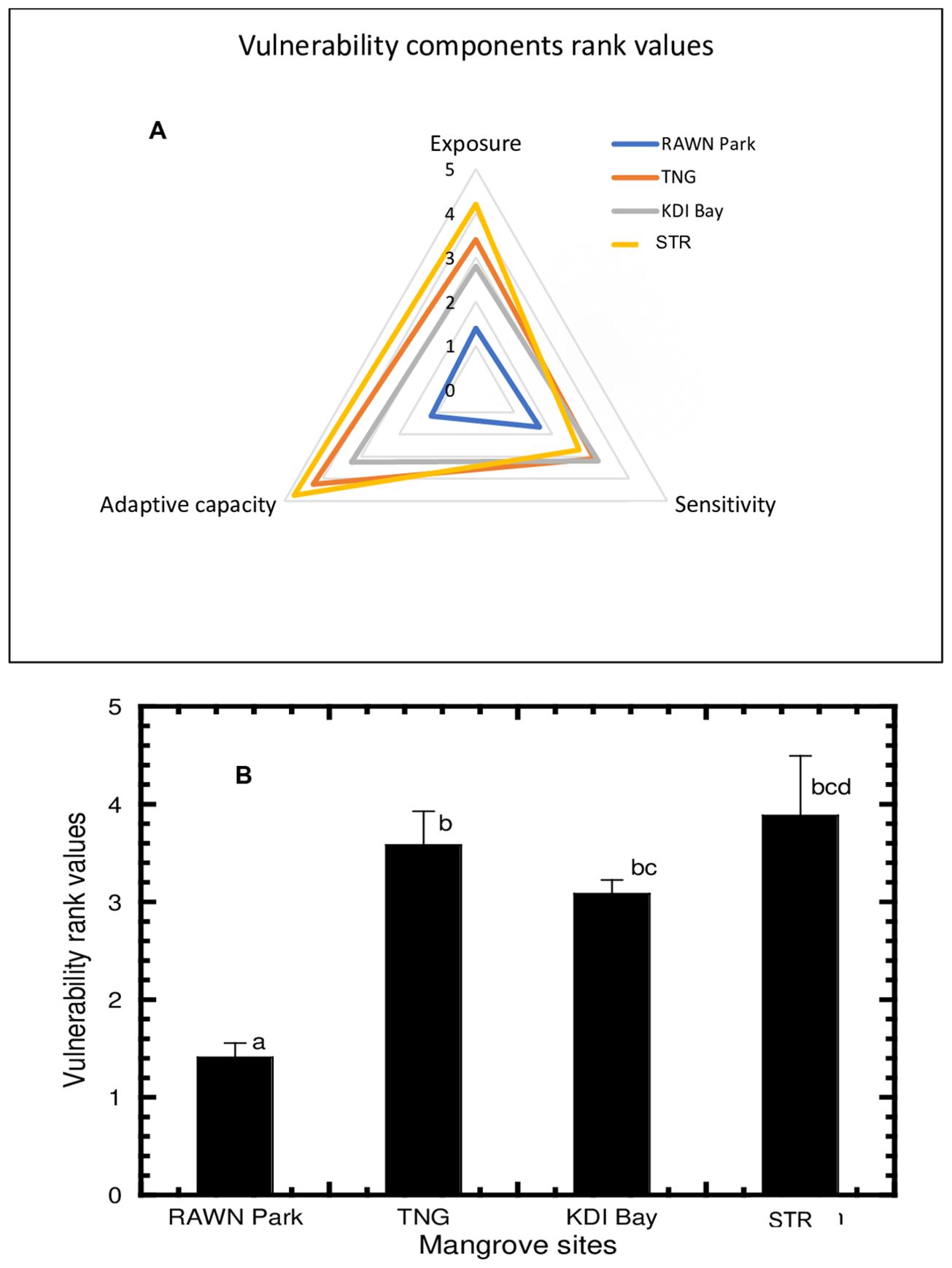
Figure 5. Triangular diagrammatic rank values of vulnerability components: exposure, sensitivity, and adaptive capacity (A) and average vulnerability rank values (B) at RAWN Park, TNG, KDI Bay and STR Bay in Southeast Sulawesi, Indonesia. The different lowercase letters indicate significantly different at 5% among mangrove sites.
3.2 Carbon storage
3.2.1 Carbon stock in sediment
The sediment carbon content varied from 1.33 to 8.65% (see Table 5). RAWN Park (8.65%) exhibited significantly higher values (p<0.05, refer to Figure 6A) compared to KDI Bay (3.77%) and STR Bay (1.33%), although there was no significant difference between RAWN and TNG (7.50%). Similarly, sediment carbon stock (SCS) ranged from 169.44 to 381.45 tons per hectare (refer to Table 5). The SCS in the mangrove area of RAWN Park (381.45 tons/ha) showed a significant difference (p<0.05, see Figure 6B) compared to KDI Bay (262.02 tons/ha) and STR Bay (169.44 tons/ha), although there was no significant difference (p>0.05) between RAWN Park and TNG (306.67 tons/ha).
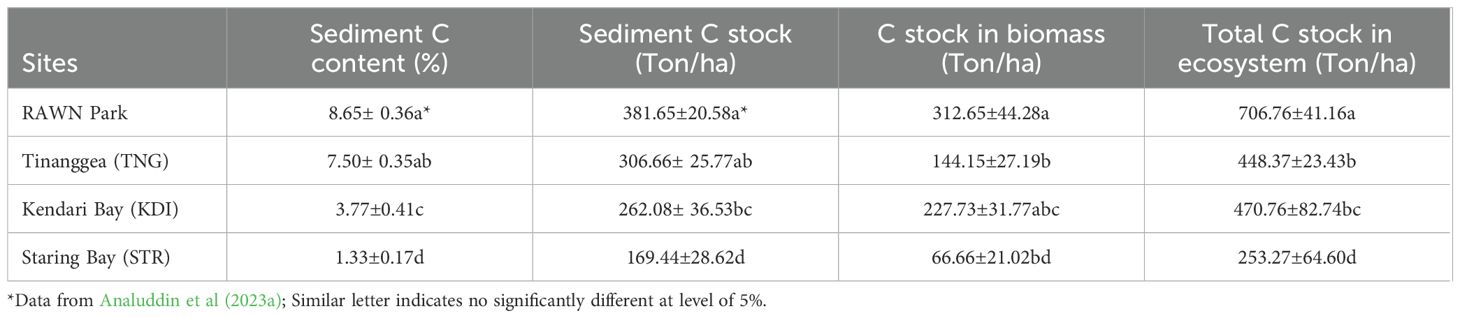
Table 5. Organic carbon storage in the mangrove ecosystems of Rawa Aopa Watumohai National (RAWN) Park, Tinanggea, Kendari Bay, and Staring Bay, Southeast Sulawesi, Indonesia.
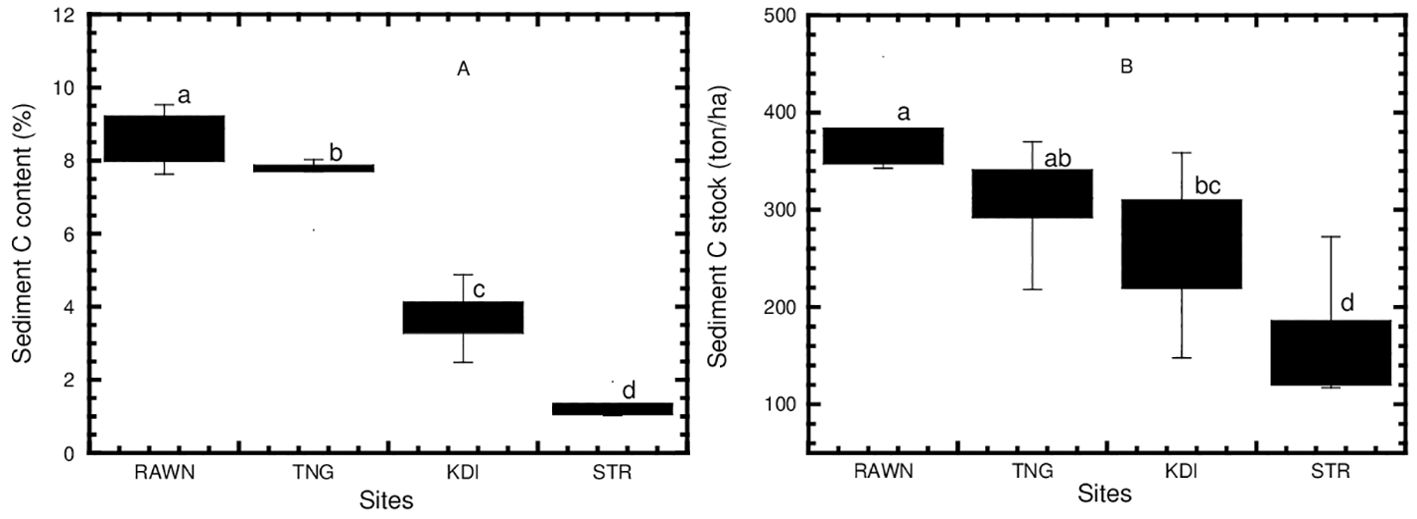
Figure 6. Concentration of organic carbon (A) and organic carbon stock (B) in sediments of mangrove ecosystems at RAWN Park, TNG, KDI Bay and STR Bay in Southeast Sulawesi, Indonesia. The different lowercase letters indicate significantly different at 5% among mangrove sites.
3.2.2 Carbon stock in biomass
The carbon stock in the biomass of mangroves in the current study ranged from 66.66 to 312.65 tons per hectare (see Table 5), showing significant differences (p<0.05, see Figure 7A) among the sites. The carbon stock in the biomass of mangroves in RAWN Park (312.65 tons/ha) was significantly higher (p<0.05) than in TNG (144.15 tons/ha) and STR Bay (66.66 tons/ha), although the carbon stock in biomass of mangroves did not differ significantly (p>0.05) between RAWN Park and KDI Bay (227.73 tons/ha). Additionally, the carbon stock in the biomass of mangroves in TNG did not differ significantly (p>0.05) from KDI Bay and STR Bay. However, the carbon stock in the biomass of mangroves in KDI Bay was significantly higher (p<0.05) than in STR Bay.
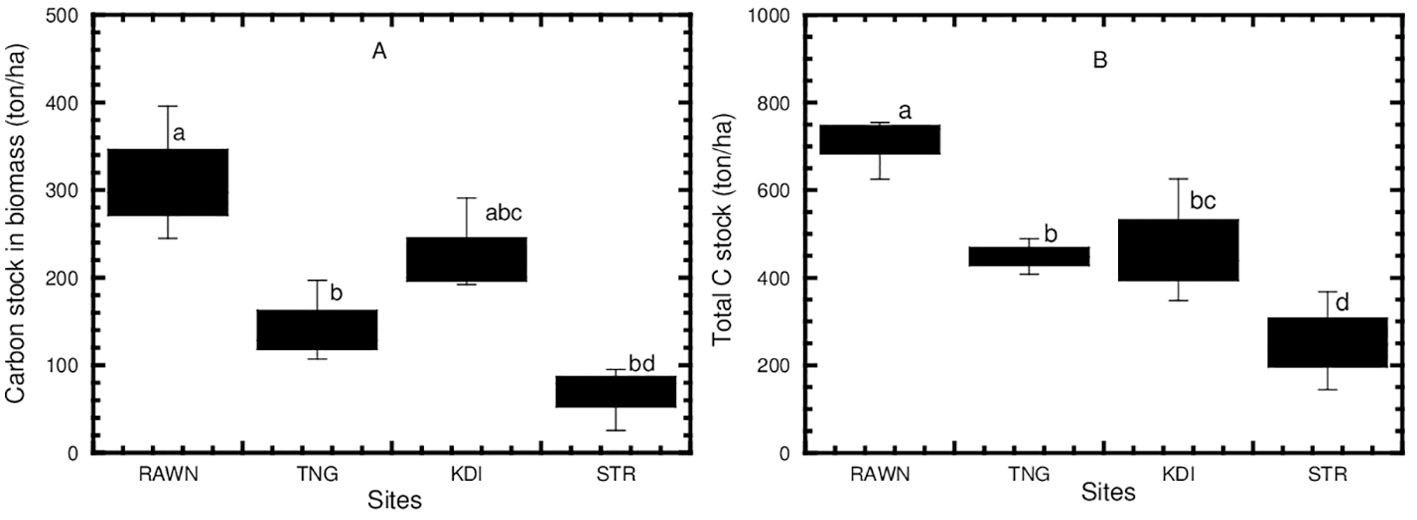
Figure 7. Organic carbon stock in mangrove biomass (A) and total organic carbon stock (B) in mangrove ecosystems at RAWN Park, TNG, KDI Bay and STR Bay in Southeast Sulawesi, Indonesia. The different lowercase letters indicate significantly different at 5% among mangrove sites.
3.2.3 Total carbon stock in mangrove ecosystem
The total carbon stock (TCS) in mangrove ecosystems ranged from 253.27 to 706.76 tons per hectare and exhibited significant differences (p<0.05, see Figure 7B) among the sites. The TCS in the mangrove ecosystems of RAWN Park (706.76 tons/ha) was significantly higher (p<0.05) than in TNG (448.37 tons/ha), KDI Bay (470.76 tons/ha), and STR (253.27 tons/ha). Moreover, the TCS in the mangrove ecosystem of STR Bay was notably lower than that in TNG and KDI Bay. Nevertheless, the TCS in the mangrove ecosystems did not show significant differences (p>0.05) between TNG and KDI Bay.
4 Discussion
The vulnerability assessment of mangrove ecosystems in Southeast Sulawesi involved an analysis of exposure, sensitivity, and adaptive capacity. Variances in exposure parameters of the mangrove ecosystems (as depicted in Figure 2A) were observed. The proximity of mangrove ecosystems to settlements indicated very high pressures on the mangrove ecosystems in unprotected areas due to various human interference’s in relation to mangrove forests. High sediment loads were noted in RAWN Park, KDI Bay, and TNG, mainly attributed to significant rivers flowing within these mangrove ecosystems. In contrast, STR Bay exhibited low sediment supply as it was only fed by smaller rivers (refer to Table 1). Among the sites, STR Bay exhibited strong exposure to environmental factors, followed by TNG and KDI Bay, while RAWN Park showed lower exposure levels. The raised exposure pressures in mangrove ecosystem of STR Bay were linked to factors such as lower tidal depths, sandy and clay substrate types, decreased sediment input, and proximity to settlements. However, the moderate exposure status of the mangrove ecosystems in TNG and KDI bay might be associated by their close proximity to settlements, indicating potential impacts from various human activities. Amaral et al. (2023) highlighted that human factors intensified the vulnerability of mangroves in the Bahamian sub-region, with mangroves facing a risk of damage up to four times higher when situated closer to human infrastructure.
The sensitivity component of mangroves also exhibited significant variations across all study sites (refer to Figure 3). Sensitivity components such as mangrove condition, basal area change, and mortality received high rankings in TNG and KDI Bay. In TNG, land conversion for aquaculture and illegal extraction led to severe consequences like mangrove degradation and reduction, while in KDI Bay, extensive conversion of mangrove areas for human settlement, aquaculture, and road construction was observed, resulting in similar impacts. Reduction in mangrove areas and increased mortality were also noted in both TNG and KDI Bay study areas. Conversely, there was minimal to no impact on the mangrove ecosystem in RAWN Park due to its good mangrove condition, higher basal area, and natural mortality occurrences. Some sensitivity components of the mangroves in KDI Bay, such as mangrove mortality, mangrove area reduction, and the condition of neighboring ecosystems, were rated very high, indicating a heightened sensitivity status. In STR Bay, mangrove thickness alone received a very high ranking, while mangrove recruitment and spatial area reduction were also rated highly, signifying a high sensitivity status. However, the sensitivity status of mangroves was lowest in RAWN Park, followed by STR Bay and KDI Bay, while the highest sensitivity status observed in TNG. Thus, our study found that high sensitivity of mangroves was happen due to mostly anthropogenic pressure.
The rank values of adaptive capacity parameters, including elevation above the mangroves, community management capacity, stakeholder involvement, and mangrove protection legislation, were notably low in RAW National Park (refer to Figure 4). In the protected mangrove area of RAWN Park, there were lower-elevation zones behind or above the mangroves that could potentially facilitate the growth of new mangrove seedlings when sea levels rise to be happen. Furthermore, community management capacity, stakeholder involvement, and mangrove protection legislation were highly commendable in this area. Conversely, the adaptive capacity of mangrove ecosystems in TNG and STR Bay was ranked as high, indicating a lower adaptive capacity. Kendari Bay exhibited a moderate rank in terms of adaptive capacity. The sequence of adaptive capacity status in the study sites followed this order: RAWN Park > Kendari Bay > Tinanggea > Staring Bay. Unprotected areas lacked low-elevation land behind or above the mangrove zones, or if present, it was often in a degraded state. Additionally, STR Bay suffered from insufficient community management capacity, stakeholder involvement, and mangrove protection legislation. Tinanggea displayed a significantly low adaptive capacity, while Kendari Bay showed some involvement of stakeholders and presence of legislation to a certain degree.
Overall, the vulnerability status (refer to Figure 5) of mangroves was highest in STR Bay, followed by TNG and KDI Bay, with the lowest vulnerability observed in RAWN Park. The heightened vulnerability in STR Bay, Southeast Sulawesi, stemmed from significant exposure (Figure 2B) and limited adaptive capacity (Figure 4B). Instances of mangrove degradation were noted in the mangrove ecosystems of TNG, KDI Bay, and STR Bay, rendering these areas highly vulnerable. Conversely, the mangrove ecosystem within the protected confines of RAWN Park exhibited lower vulnerability (refer to Figure 5). Tinanggea and Staring Bay’s mangroves faced high vulnerability, primarily due to a dearth of legislation and stakeholder involvement. Additional factors contributing to the high vulnerability of the mangrove ecosystems in these study areas included urban development, marine ports incorporating power infrastructure, shipyards, among others. Previous research on mangrove vulnerability emphasized the impact of various environmental factors such as geomorphology, climate, forest structure, and human driving forces in determining mangrove vulnerability status and resilience (Amaral et al., 2023), which occurred also in Southeast Sulawesi representing a major challenge to mangrove sustainability in this region. The conversion of mangrove lands for aquaculture and urban development posed a significant threat to extensive mangrove areas in various countries like Thailand, Indonesia, Vietnam, and the Philippines (Primavera, 2000; Richards and Friess, 2016; Slamet et al., 2020; Munana et al., 2023). Other studies have highlighted the considerable risk that macro-tidal ranges pose to mangroves (Mumby et al., 2004; Wong et al., 2014; Bouillon, 2011; Giri et al., 2011a; Spalding et al., 2010; Kelly and Adger, 2000). The high vulnerability of mangroves stemming from habitat degradation and biodiversity loss presents a significant challenge to mangrove ecosystems (Arkema et al., 2015). In Tinanggea and Kendari Bay, over half of the original mangrove areas vanished due to conversion for aquaculture and urban expansion, potentially resulting in both biodiversity loss and reduced species diversity, ultimately leading to diminished functional diversity within mangrove ecosystems. Some studies mentioned that mangrove vulnerability have been associated with exposure aspect of tidal ranges, i.e. a greater tidal range is associated with less vulnerability, while a smaller range is associated with greater vulnerability (Mondal et al., 2024; Gorokhovich et al., 2014; Dwarakish et al., 2008). Bahuguna et al. (2008) found that high mortality of mangroves in the Andaman group of islands was happen due to prolonged inundation, while Prabakaran (2020) has observed that high vulnerability of mangroves in Car Nicobar Island was found due to sea level rise and land subsidence, Moreover, Veettil et al (2020) found that mangrove forests in the South and Middle Andaman Islands’ were susceptible to sea level rise due to low elevation. Therefore, vulnerability status of mangroves have been associated with multiple aspects of anthropogenic and natural pressures.
The concentration of soil organic carbon in mangrove ecosystems within RAWN Park and Tinanggea was notably higher (refer to Figure 6A) compared to other sites. This elevation could be linked to substantial organic carbon inputs from mangrove leaf litter (4.68 tons of carbon per hectare per year) in the protected environment of RAWN Park (Analuddin et al., 2021) and 12.34 tons of carbon per hectare per year in the unprotected area of Tinanggea (Syahrir, 2021). Additionally, the greater organic carbon stock in sediment within mangroves in RAWN Park and Tinanggea as compared to Kendari Bay and Staring Bay (refer to Table 5, Figure 6B), may be due to the presence of pristine mangrove areas in protected zones, as well as ample organic carbon inflows from terrestrial sources, which could contribute to increased organic carbon content in the sediments of these areas. Conversely, the lower organic carbon concentration in Kendari Bay and Staring Bay (as shown in Figures 6A, B) might be linked to diminished mangrove coverage (Analuddin et al., 2023b) and reduced organic carbon inputs from terrestrial sources and tidal periods. Furthermore, the higher organic carbon deposition in sediments within RAWN Park and Tinanggea might be tied to increased organic carbon inputs from sediment materials from nickel mining and agricultural activities on terrestrial lands in Southeast Sulawesi, Indonesia.
Numerous prior studies have indicated that sediment carbon storage in mangrove habitats correlates with the mineral and physical properties of the soil (Rasmussen et al., 2007), temperature and precipitation (McKenzie et al. 2021). Clay-rich sediments tend to exhibit higher carbon storage levels (Schimel et al., 1994), while Kirwan and Blum (2011) discovered a connection between the organic carbon content in coastal habitat sediments and the biomass of coastal plants and their productivity. However, the process of carbon sequestration in the sediment of mangrove habitats occurs over extended time-frames and influences carbon stock levels (Callaway et al., 2012; Hansen and Nestlerode, 2014). Other studies have indicated that carbon stock in sediments can be rapidly affected by both natural and human-induced processes (DeLaune and White, 2012; Macreadie et al., 2014). The sediment carbon stock in the mangrove ecosystems has been associated with mangrove productivity, sediment fertility, deposition of suspended materials, as well as edaphic conditions (Sanderman et al., 2018; Twilley et al., 2018). Moreover, Analuddin et al. (2023a) found that the high organic carbon storage in the sediments of mangroves ecosystems at RAWN Park, Southeast Sulawesi, Indonesia, has been associated to the high carbon input together with the suitable physical and chemical conditions of sediment in the coastal region. Thus, the sediment organic carbon stock in mangroves in the present study may be linked to various factors, including substrate type, hydrological conditions, mineral and physical properties, as well as natural and anthropogenic processes within the region. The carbon stock in both biomass (refer to Figure 7A) and the entire ecosystem (refer to Figure 7B) of mangroves in protected areas was notably higher than in unprotected areas, possibly due to factors such as larger tree sizes, reduced degradation, and improved soil conditions. According to the IPCC (2006), the concentration of carbon in vegetation is influenced by factors such as biomass, carbon absorption, soil fertility, plant diversity, and density. The organic carbon stock in mangrove ecosystem were correlated with the mangrove maturity and stand age (Tang et al., 2023; Chowdhury et al., 2023). Sharma et al. (2020) pointed out that deforestation reduced total ecosystem carbon stocks of intact mangroves by 60%, while suggested that mangrove plantation of mangroves after 20–40 years can restore carbon up to 75% (Bourgeois et al., 2024).
Our study revealed that the less vulnerable mangrove ecosystem in RAWN Park exhibited higher carbon storage in comparison to the more vulnerable mangrove ecosystems in Tinanggea and Staring Bay (refer to Figure 7A). It appears that the less vulnerable mangroves were able to sustain their growth and development due to lower exposure to environmental conditions and reduced mangrove sensitivity. However, high adaptive capacity, including effective protection and legislation, played a crucial role in enhancing blue carbon storage in mangrove ecosystems. Nonetheless, the degradation of mangrove ecosystems significantly reduced blue carbon storage, particularly in unprotected areas of Southeast Sulawesi. Slamet et al. (2020) found that low carbon stock in Serang, Jakarta Bay was occurred due to the sand mining. Therefore, our study provides essential insights into the importance of conserving and protecting mangrove ecosystems to mitigate mangrove vulnerability and maintain carbon storage in this region. The understanding of mangrove vulnerability and carbon storage could pave the way for a sensible ecological approach in mangrove ecosystem investigations, closely intertwined with studies of other coastal ecosystems. The findings of our study could be pertinent to the reforestation of degraded mangroves to reduce vulnerability status and enhance carbon storage, especially in the unprotected areas of Southeast Sulawesi. The environmental conditions in Tinanggea appear conducive for planting new mangrove seedlings (Rahim et al., 2024). However, efforts toward mangrove restoration need to be supported by an understanding of methodological, environmental, and social factors influencing mangrove survival (Christensen et al., 2003). Moreover, both natural and artificial regeneration methods should be implemented in suitable locations as strategies to restore degraded or lost mangroves, particularly in highly vulnerable mangrove areas with low carbon storage in the unprotected regions of the Coral Triangle in Southeast Sulawesi, Indonesia, recognized as a global biodiversity hot spot. Additionally, conducting further analyses focusing on social-ecological system aspects will be beneficial in gaining comprehensive insights into mangrove ecosystem services for more effective development strategies and identifying conservation priorities to reduce mangrove vulnerability and enhance blue carbon sequestration in this region.
5 Conclusion
The current study has presented an important analysis of mangrove vulnerability status and blue carbon storage in the Coral Triangle areas of Southeast Sulawesi, Indonesia. Less vulnerable mangroves were identified in protected regions, whereas more vulnerable mangroves were observed in the unprotected areas of Southeast Sulawesi. The high vulnerability status of mangroves in unprotected areas has been linked to various anthropogenic disturbances such as land conversion for aquaculture and urban development, alongside hydrological considerations. Consequently, the high vulnerability of mangrove ecosystems notably reduces blue carbon storage and diminishes mangrove ecological services. Hence, it is imperative to develop adaptive strategies to protect mangroves from anthropogenic disturbances through effective legislation and mangrove conservation, aiming to reduce vulnerability status while enhancing blue carbon storage in the Coral Triangle area of Southeast Sulawesi, Indonesia. Efforts should focus on restoring mangroves in declining areas to mitigate future vulnerability risks and bolster their capacities for blue carbon storage, ultimately promoting the fulfillment of the Sustainable Development Goals (SDGs) in the Coral Triangle of Southeast Sulawesi, Indonesia.
Data availability statement
The raw data supporting conclusion of this article will not be made available by author.
Author contributions
KA: Conceptualization, Funding acquisition, Investigation, Methodology, Writing – original draft, Data curation, Writing – review & editing. MH: Conceptualization, Funding acquisition, Methodology, Writing – review & editing. RP: Conceptualization, Methodology, Writing – review & editing. LA: Conceptualization, Methodology, Supervision, Writing – review & editing. LG: Conceptualization, Investigation, Methodology, Writing – review & editing. WI: Formal analysis, Investigation, Methodology, Writing – original draft. NS: Conceptualization, Supervision, Writing – review & editing. AS: Formal analysis, Investigation, Methodology, Writing – original draft. KN: Conceptualization, Funding acquisition, Supervision, Writing – review & editing. TN: Conceptualization, Funding acquisition, Supervision, Writing – review & editing.
Funding
The author(s) declare that financial support was received for the research, authorship, and/or publication of this article. We declare that all sources of funding received for the research being submitted. This research was supported by grants from the Ministry of Research, Technology, and Higher Education of the Republic of Indonesia with grant no. 0054/E5/AK.04/2022, 0217/E5/PG.02.00/2023, and JST Japan for supporting the research collaboration by the E-Asia Joint Research Program.
Acknowledgments
We also thank the Rector and Research Center Director of Halu Oleo University.
Conflict of interest
The authors declare that the research was conducted in the absence of any commercial or financial relationships that could be construed as a potential conflict of interest.
Publisher’s note
All claims expressed in this article are solely those of the authors and do not necessarily represent those of their affiliated organizations, or those of the publisher, the editors and the reviewers. Any product that may be evaluated in this article, or claim that may be made by its manufacturer, is not guaranteed or endorsed by the publisher.
References
Amaral C., Poulter B., Lagomasino D., Fatoyinbo T., Taillie P., Lizcano G., et al. (2023). Drivers of mangrove vulnerability and resilience to tropical cyclones in the North Atlantic Basin Cibele. Sci. Tot. Environ. 898, 165413. doi: 10.1016/j.scitotenv.2023.165413
Analuddin K., Armid A., Ruslin R., Sharma S., Kadidae L. O., Yasir L. O. M., et al. (2023b). The carrying capacity of estuarine mangroves in maintaining the coastal urban environmental health of Southeast Sulawesi, Indonesia. Egyp. J. Aqua. Res. 49, 327–338. doi: 10.1016/j.ejar.2023.03.002
Analuddin K., Jamili, Septiana A., Raya R., Rahim S. (2013). The spatial trends in the structural characteristics of mangrove forests at the Rawa Aopa Watumohai National Park, Southeast Sulawesi, Indonesia. Inter. Res. J. Plant Sci. 4, 214–221.
Analuddin K., Jamili, Septiana A., Raya R., Sahidin I., Usman R., Rahim S., et al. (2016). Allometric model and aboveground biomass of mangrove Lumnitzera racemosa Willd. Forest at the Rawa Aopa Watumohai National Park, Southeast Sulawesi, Indonesia. For. Sci. Tech. 12, 43–50. doi: 10.1080/21580103.2015.1034191
Analuddin K., Jamili, Septiana A., Sahidin I., Usman R., Rahim S., Sharma S., et al. (2017). Heavy metals bioaccumulation in mangrove ecosystem at the Coral Triangle Ecoregion, Southeast Sulawesi, Indonesia. Mar. Poll. Bull. 125, 472–480. doi: 10.1016/j.marpolbul.2017.07.065
Analuddin K., Kadidae L. O., Yasir L. O. M., Septiana A., Sahidin I., Syahrir L., et al. (2020). Aboveground biomass, productivity and carbon sequestration in Rhizopora stylosa mangrove forest of Southeast Sulawesi, Indonesia. J. Biodiver. 21, 1316–1325. doi: 10.13057/biodiv/d210407
Analuddin K., Septiana A., Syahrir L., Rahim S., Pratama D., Hasidu L. O. A., et al. (2021). Blue carbon dynamics in mangroves and conservation of their services in the Coral Triangle Ecoregion, Southeast Sulawesi, Indonesia. J. Physics: Conf. Ser. 1899, 012016. doi: 10.1088/1742-6596/1899/1/012016
Analuddin K., Sharma S., Jamili, Septiana A., Raya R., Sahidin I., Rianse U., et al. (2018). Trends in allometric model and aboveground biomass of family Rhizophoraceae mangroves in Coral Triangle Ecoregion, Southeast Sulawesi, Indonesia. J. Sustain. Forest. 37, 681–711. doi: 10.1080/10549811.2018.1453843
Analuddin K., Sharma S., Kadidae L. O., Yasir L. O. M., Septiana S., Rahim S., et al. (2023a). Blue carbon stock in sediments of mangroves and seagrass ecosystems at Southeast Sulawesi, Indonesia. Ecol. Res. 38, 508–520. doi: 10.1111/1440-1703.12374
Arkema K. K., Verutes G. M., Wood S. A., Clarke-Samuels C., Rosado S., Canto M., et al. (2015). Embedding ecosystem services in coastal planning leads to better outcomes for people and nature. Proc. Natl. Acad. Sci. 112, 7390–7395. doi: 10.1073/pnas.1406483112
Azman M. S., Sharma S., Hamzah M. L., Zakaria R. M., Palaniveloo K., macKenzie R. A. (2023). Total ecosystem blue carbon stocks and sequestration potential along a naturally regenerated mangrove forest chronosequence. For. Ecol. Manage. 527, 120611. doi: 10.1016/j.foreco.2022.120611
Bahuguna A., Nayak S., Roy D. (2008). Impact of the tsunami and earthquake of 26th December 2004 on the vital coastal ecosystems of the Andaman and Nicobar Islands assessed using RESOURCESAT AWiFS data. Int. J. Appl. Earth Obs. Geoinf. 10, 229–237. doi: 10.1016/j.jag.2008.02.010
Bandh S. A., Malla F. A., Qayoom I., Mohi-Ud-Din H., Butt A. K., Altaf A., et al. (2023). Importance of blue carbon in mitigating climate change and plastic/microplastic pollution and promoting circular economy. Sustain. 15, 2682. doi: 10.3390/su15032682
Borges A. V., Djenidi S., Lacroix G., Théate J., Delille B., Frankignoulle M. (2003). Atmospheric CO2 flux from mangrove surrounding waters. Geo. Res. Let. 30, 1558. doi: 10.1029/2003GL017143
Bouillon S., Dehairs F., Velimirov B., Abril G., Borges A. V. (2007a). Dynamics of organic and inorganic carbon across contiguous mangrove and sea grass systems (Gazi Bay, Kenya). J. Geophys. Res. 112G02018, 1–14. doi: 10.1029/2006JG000325
Bourgeois C. F., MacKenzie R. A., Sharma S., Bhomia R. K., Johnson N. G., Rovai A. S., et al. (2024). Four decades of data indicate that planted mangroves stored up to 75% of the carbon stocks found in intact mature stands. Science Advances. 10 (27), eadk5430.
Breithaupt J. L., Smoak J. M., Smith T. J., Sanders C. J., Hoare A. (2012). Organic carbon burial rates in mangrove sediments: Strengthening the global budget. Glob. Biogeochem. Cyc. 26GB3011, 1–11. doi: 10.1029/2012GB004375
Cairns M. A., Brown S., Helmer E. H., Balimgardner G. A. (1997). Root biomass allocation in the world’s upland forests. Oecologia 111, 1–11. doi: 10.1007/s004420050201
Callaway J. C., Borgnis E. L., Turner R. E., Milan C. S. (2012). Carbon sequestration and sediment accretion in San Francisco Bay tidal wetlands. Estuar. Coast. 35, 1163–1181. doi: 10.1007/s12237-012-9508-9
CBSSS (2021). Southeast sulawesi in figures 2021 (Southeast Sulawesi, Indonesia: Central Bureau of Statistics).
Chambers J. C., Allen C. R., Cushman S. A. (2019). Operationalizing ecological resilience concepts for managing species and ecosystems at risk. Frontier Ecol. Evol. 7, 246. doi: 10.3389/fevo.2019.00241
Choudhary B., Dhar V., Pawase A. S. (2024). Blue carbon and the role of mangroves in carbon sequestration: Its mechanisms, estimation, human impacts and conservation strategies for economic incentives. doi: 10.1016/j.seares.2024.102504
Chowdhury A., Naz A., Maiti S. K. (2024). Community-based, cost-effective multispecies mangrove restoration innovation to maximize soil blue carbon pool and humic acid and fulvic acid concentrations at Indian Sundarbans. Environ. Sci. pollut. Res. Inpress.doi: 10.1007/s11356-024-33918-2
Chowdhury A., Naz A., Sharma S. B., Dasgupta R. (2023). Changes in salinity, mangrove community ecology, and organic blue carbon stock in response to cyclones at Indian sundarbans. Life 13, 1539. doi: 10.3390/life13071539
Christensen L., Ecklet N., Kasperson J. X., Luers A., Martello M. L., Polsky C., et al. (2003). A framework for vulnerability analysis in sustainability science. Proc. Nat. Acad. Sci. 100, 8074–8079. doi: 10.1073/pnas.1231335100
Cinco-Castro S., Herrera-Silveira J. (2020). Vulnerability of mangrove ecosystems to climate change effects: The case of the Yucatan Peninsula. Ocean Coast. Manage. 192, 105196. doi: 10.1016/j.ocecoaman.2020.105196
DeLaune R. D., White J. R. (2012). Will coastal wetlands continue to sequester carbon in response to an increase in global sea level? A case study of the rapidly subsiding Mississippi river deltaic plain. Climate Change 110, 297–314. doi: 10.1007/s10584-011-0089-6
Dwarakish G. S., Vinay S. A., Dinakar S. M., Pai J. B., Mahaganesh K., Natesan U. (2008). Integrated coastal zone management plan for Udupi coast using remote sensing, geographical information system and global position system. J. Appl. Remote Sens. 2, 023515. doi: 10.1117/1.2919101
Ellison J. C. (2015). Vulnerability assessment of mangroves to climate change and sea-level rise impacts. Wetland. Ecol. Manage. 23, 115–137. doi: 10.1007/s11273-014-9397-8
Eggleton S., Buendia L., Miwa K., Ngara T., Tanabe K. (2006). Intergovernmental Panel on Climate Change (IPCC): Guidelines for National Greenhouse Gas Inventories. Institute for Global Environmental Strategies, Kanagawa, Japan.
Fan B., Li Y., Zhang Z., Li Y. (2024). Exploring cumulative vulnerability of mangrove forests to intensive coastal anthropogenic threats. Ecos. Health Sustain. 10, 0153. doi: 10.34133/ehs.0153
Gao Y., Yu G., Yang T., Jia Y., He N., Zhuang J. (2016). New insight into global blue carbon estimation under human activity in land-sea interaction area: A case study of China. Earth-Sci. Rev. 159, 36–46. doi: 10.1016/j.earscirev.2016.05.003
Garmestani A., Robin K. Craig R. K., Gilissen H. K., McDonald J., Soininen N., van Doorn-Hoekveld W. J., et al. (2019). The role of social-ecological resilience in coastal zone management: A comparative law approach to three coastal nations. Frontier Ecol. Evol. 7, 410. doi: 10.3389/fevo.2019.00410
Giri C., Ochieng E., Tieszen L. L., Zhu Z., Singh A., Loveland T., et al. (2011a). Status and distribution of mangrove forests of the world using earth observation satellite data. Glob. Ecol. Biogeogr. 20, 154–159. doi: 10.1111/j.1466-8238.2010.00584.x
Glick P., Stein B. A. (2010). Scanning the conservation horizon: a guide to climate change vulnerability assessment (Washington DC: National Wildlife Federation).
Gorokhovich Y., Leiserowitz A., Dugan D. (2014). Integrating coastal vulnerability and community-based subsistence resource mapping in Northwest Alaska. J. Coast. Res. 30, 158–169. doi: 10.2112/JCOASTRES-D-13-00001.1
Hansen V. D., Nestlerode J. A. (2014). Carbon sequestration in wetland soils of the northern Gulf of Mexico coastal region. Wetland. Ecol. Manage. 22, 289–303. doi: 10.1007/s11273-013-9330-6
Johnson J. E., Marshall P. A. (2007). Climate change and the great barrier reef: a vulnerability assessment (Townsville: Great Barrier Reef Marine Park Authority and Australian Greenhouse Office).
Johnson J. E., Welch D. J., van Hooidonk R., Tracey D., Chandrasa G., Molinari B., et al. (2023). Climate change implications for the Arafura and Timor Seas region: assessing vulnerability of marine systems to inform management and conservation. Climatic Change 176, 88. doi: 10.1007/s10584-023-03554-9
Kauffman J. B., Adame M. F., Arifanti V. B., Schile‐Beers L. M., Bernardino A. F., Bhomia R. K., et al. (2020). Total ecosystem carbon stocks of mangroves across broad global environmental and physical gradients. Ecological Monographs 90 (2), e01405.
Kelly P. M., Adger W. N. (2000). Theory and practice in assessing vulnerability to climate change and facilitating adaptation. Climate Change 47, 325–352. doi: 10.1023/A:1005627828199
Kirwan M. L., Blum L. K. (2011). Enhanced decomposition offsets enhanced productivity and soil carbon accumulation in coastal wetlands responding to climate change. Biogeosci. 8, 987–993. doi: 10.5194/bg-8-987-2011
Kristensen E., Bouillon S., Dittmar T., Marchand C. (2008). Organic carbon dynamics in mangrove ecosystems: a review. Aquat. Bot. 89, 201–219. doi: 10.1016/j.aquabot.2007.12.005
Kusmana C., Hidayat T., Tiryana T., Omo Rusdiana O., Istomo (2018). Allometric models for above- and below-ground biomass of Sonneratia spp. Glob. Ecol. Conser. 15, e00417. doi: 10.1016/j.gecco.2018.e00417
Kusumaningtyas M. A., Hutahaean A. A., Fischer H. W., Pérez-Mayo M., Ransby D., jennerjah T. C. (2019). Variability in the organic carbon stocks, sources, and accumulation rates of Indonesian mangrove ecosystems. Estuar. Coast. Shelf Sci. 218, 310–323. doi: 10.1016/j.ecss.2018.12.007
Lee S. L., Chee S. Y., Huxham M., Jamilah M., Choo J., Kaur C. R., et al. (2024). Blue Carbon Ecosystems in Malaysia–Status, Threats, and the Way Forward for Research and Policy. The Journal of Environment & Development. 10704965241284366.
Mackenzie R. A., Foulk P. B., Klump J. V., Weckerly K., Purbospito J., Murdiyarso D., et al. (2016). Sedimentation and belowground carbon accumulation rates in mangrove forests that differ in diversity and land use: A tale of two mangroves. Wetland. Ecol. Manage. 24, 245–261. doi: 10.1007/s11273-016-9481-3
Macreadie P. I., York P. H., Sherman C. D. H., Keough M. J., Ross D. J., Ricart A. M., et al. (2014). No detectable impact of small-scale disturbances on “blue carbon. within sea grass beds. Mar. Biol. 161, 2939–2944.
Maher D., Santos I., Golsby-Smith L., Gleeson J., Eyre B. (2013a). Groundwater- derived dissolved inorganic and organic carbon exports from a mangrove tidal creek: The missing mangrove carbon sink? Limnol. Oceanog. 58, 475–488. doi: 10.4319/lo.2013.58.2.0475
Mondal B., Bhomia R. K., Saha A. K., MacKenzie R. A. (2024). Assessment of coastal and mangrove vulnerability in the Andaman Island, Indian Ocean. Geosci. Front. 15, 101820. doi: 10.1016/j.gsf.2024.101820
Mumby P. J., Edwards A. J., Arias-Gonzalez J. E., Lindeman K. C., Blackwell P. G., Gall A., et al. (2004). Mangroves enhance the biomass of coral reef fish communities in the Caribbean. Nature 427, 533–536. doi: 10.1038/nature02286
Murdiyarso D., Purbopuspito J., Kauffman J. B., Warren M. W., Sasmito S. D., Donato D. C, et al. (2015). Supplementary information. The potential of Indonesian mangrove forests for global climate change mitigation. Nature Climate Change 5, 1089–1092.
Munana N., Pribadhi R., Suryanto C. A. (2023). Vulnerability assessment of mangroves using the coastal vulnerability index in timbulsloko village, sayung, demak. J. Kelautan Tropis 26, 565–570. doi: 10.14710/jkt.v26i3.20156
Nagelkerken I., Blaber S. J. M., Bouillon S., Green P., Haywood M., Kirton L. G., et al. (2008). The habitat function of mangroves for terrestrial and marine fauna: A review. Aquat. Bot. 89, 155–185. doi: 10.1016/j.aquabot.2007.12.007
Nam V. N., Sasmito S. D., Murdiyarso D., Purbopuspito J., MacKenzie R. A. (2016). Carbon stocks in artificially and naturally regenerated mangrove ecosystems in the Mekong Delta. Wetland. Ecol. Manage. 24, 231–244. doi: 10.1007/s11273-015-9479-2
Nitschke C. R., Innes J. L. (2008). Integrating climate change into forest management in South-Central British Columbia: an assessment of landscape vulnerability and development of a climate-smart framework. For. Ecol. Manage. 256, 313–327. doi: 10.1016/j.foreco.2008.04.026
O’Higgins T. G., Lago M., DeWitt T. H. (2020). Ecosystem-based management, ecosystem services and aquatic biodiversity: theory, tools and applications. Springer Nat., 580. doi: 10.1007/978-3-030-45843-0
Phang V. X. H., Chou L. M., Friess D. A. (2015). Ecosystem carbon stocks across a tropical intertidal habitat mosaic of mangrove forest, seagrass meadow, mudflat and sandbar. Earth Surf. Proc. Landf. 40, 1387–1400. doi: 10.1002/esp.v40.10
Prabakaran N. (2020). Mangrove community response to subsidence inflicted sea level change in Car Nicobar Island, India. Bot. Mar. 63, 419–427. doi: 10.1515/bot-2019-0088
Primavera J. H. (2000). Development and conservation of Philippine mangroves: Institutional issues. Ecol. Econ. 35, 91–106. doi: 10.1016/S0921-8009(00)00170-1
Rahim S., Iswandi M. R., Widayati W., Helmi M., Analuddin K. (2024). Environmental quality and carrying capacity for restoration of estuarine mangrove ecosystem in the coral triangle ecoregion, Southeast Sulawesi, Indonesia. Inter. J. Environ. Studies. 81 (2): 587–606.
RARE Indonesia (2020). Climate change vulnerability assessment: Southeast Sulawesi (Indonesia: Kendari).
Rasmussen C., Matsuyama N., Dahlgren R. A., Southard R. J., Brauer. N. (2007). Soil genesis and mineral transformation across an environmental gradient on andesitic lahar. Soil Sci. Soc Amer. J. 71, 225–237. doi: 10.2136/sssaj2006.0100
Remling E., Persson A. (2014). Who is an adaptation for? Vulnerability and adaptation benefits in proposals approved by the UNFCCC Adaptation Fund. Clim Dev. 7 (1), 16–34. doi: 10.1080/17565529.2014.886992
Retnowati E., Yusri. S., Idris M. P. S., Fakhrurrozi (2019). Vulnerability analysis to climate change in Lembeh Island, North Sulawesi. IOP Conf. Ser. Earth Env. Sci. 363, 12011. doi: 10.1088/1755-1315/363/1/012011
Richards D. R., Friess D. A. (2016). Rates and drivers of mangrove deforestation in Southeast Asia 2000-2012. Proc. Nat. Acad. Sci. 113, 344–349. doi: 10.1073/pnas.1510272113
Sanderman J., Hengl T., Fiske G., Solvik K., Adame M., Benson L., et al. (2018). A global map of mangrove forest sediment carbon at 30 m spatial resolution. Environ. Res. Let. 13, 055002. doi: 10.1088/1748-9326/aabe1c
Schimel D. S., Braswell B. H., Holland E. A., McKeown R., Ojima D. S., Painter T. H., et al. (1994). Climatic, edaphic, and biotic controls over storage and turnover of carbon in soils. Global Biogeochem. 8, 279–293. doi: 10.1029/94GB00993
Seddon N., Chausson A., Berry P., Girardin C. A., Smith A., Turner B. (2020). Understanding the value and limits of nature-based solutions to climate change and other global challenges. Philos. Trans. R. Soc B 375. doi: 10.1098/rstb.2019.0120
Slamet N. S., Dargusch P., Wadley D. (2020). Mangrove vulnerability and potential carbon stock loss from land reclamation in Jakarta Bay, Indonesia. Ocean Coast. Manage. 195, 105283. doi: 10.1016/j.ocecoaman.2020.105283
Sharma S., MacKenzie R. A., Tieng T., Soben K, Tulyasuwan N, Resanond A, et al. (2020). The impacts of degradation, deforestation and restoration on mangrove ecosystem carbon stocks across Cambodia. Science of the Total Environment. 706, 135416.
Stefanakis A. I., Calheiros C. S., Nikolaou I. (2021). Nature-based solutions as a tool in the new circular economic model for climate change adaptation. Circ. Econ. Sustain. 1, 303–318. doi: 10.1007/s43615-021-00022-3
Subur R., Fredinan Y., Achmad F., Susilo S. B. (2011). Adaptive capacity of the seagrass ecosystem in the guraici island cluster, south halmahera regency (In bahasa). J. Agrisains 12, 207–215.
Suleman, Eviati (2009). Analisis kimia tanah, tanaman, air dan pupuk. (Balai Penelitian Tanah. Bogor: Petunjuk Teknis. Edisi 2).
Sunkur R., Kantamaneni K., Bokhoree C., Shirish Ravan S. (2023). Mangroves’ role in supporting ecosystem-based techniques to reduce disaster risk and adapt to climate change: A review. J. Sea Res. 196, 102449. doi: 10.1016/j.seares.2023.102449
Syahrir L. (2021). Carbon and nitrogen dynamics in the mangroves of Tinanggea, South Konawe Regency, Southeast Sulawesi. Master Thesis. Postgraduate Program (Halu Oleo University, Kendari, Southeast Sulawesi, Indonesia).
Tang D., Liu X., Xia Z., Hou J., Yang X., Li P., et al. (2023). Sources of organic matter and carbon stocks in two mangrove sediment cores and surface sediment samples from Qinglan Bay, China. Sci. Tot. Environ. 893, 164897. doi: 10.1016/j.scitotenv.2023.164897
Twilley R. R., Rovai A. S., Riul P. (2018). Coastal morphology explains global blue carbon distributions. Front. Ecol. Environ. 16, 1–6. doi: 10.1002/fee.2018.16.issue-9
Veettil B. K., Van D. D., Quang N. X., Hoai P. N. (2020). Spatiotemporal dynamics of mangrove forests in the Andaman and Nicobar Islands (India). Reg. Stud. Mar. Sci. 39, 101455. doi: 10.1016/j.rsma.2020.101455
Walkley A., Black I. A. (1934). An examination of the Degtjareff Method for Determining Soil Organic Matter, and a proposed Modification of the Chromic Acid Titration Method. Soil Sci. 37, 29–38. doi: 10.1097/00010694-193401000-00003
Wong P. P., Losada I. J., Gattuso J.-P., Hinkel J., Khattabi A., McInnes K. L., et al. (2014). “Coastal systems and low-lying areas. In: Climate Change 2014: Impacts, Adaptation, and Vulnerability,” in Part A: global and sectoral aspects. Contribution of working group II to the fifth assessment report of the intergovernmental panel on climate change. Eds. Field C. B., Barros V. R., Dokken D. J., Mach K. J., Mastrandrea M. D., Bilir T. E., Chatterjee M., Ebi K. L., Estrada Y. O., Genova R. C., Girma B., Kissel E. S., Levy A. N., MacCracken S., Mastrandrea P. R., White L. L. (Cambridge University Press, Cambridge, United Kingdom and New York, NY, USA), 361–409.
Keywords: mangrove ecosystem, vulnerability status, carbon storage, mangrove conservation, coral triangle area, Southeast Sulawesi, Indonesia
Citation: Analuddin K, Helmi M, Pribadi R, Adrianto L, Jaya LMG, Iba W, Susetyo Adi N, Septiana A, Nadaoka K and Nakamura T (2024) Mangrove vulnerability and blue carbon storage in the Coral Triangle Areas, Southeast Sulawesi, Indonesia. Front. Ecol. Evol. 12:1420827. doi: 10.3389/fevo.2024.1420827
Received: 21 April 2024; Accepted: 10 October 2024;
Published: 07 November 2024.
Edited by:
Sahadev Sharma, University of Malaya, MalaysiaReviewed by:
Abhiroop Chowdhury, O. P. Jindal Global University, IndiaM. Belal Hossain, Noakhali Science and Technology University, Bangladesh
Copyright © 2024 Analuddin, Helmi, Pribadi, Adrianto, Jaya, Iba, Susetyo Adi, Septiana, Nadaoka and Nakamura. This is an open-access article distributed under the terms of the Creative Commons Attribution License (CC BY). The use, distribution or reproduction in other forums is permitted, provided the original author(s) and the copyright owner(s) are credited and that the original publication in this journal is cited, in accordance with accepted academic practice. No use, distribution or reproduction is permitted which does not comply with these terms.
*Correspondence: Kangkuso Analuddin, analuddin_biotek@uho.ac.id
†ORCID: Kangkuso Analuddin, orcid.org/0000-0003-0258-9376
L.M. Golok Jaya, orcid.org/0000-0003-0752-1207
Andi Septiana, orcid.org/0009-0003-4294-9855