- 1Department of Silviculture, Faculty of Forestry, Universitas Gadjah Mada, Yogyakarta, Indonesia
- 2Department of Animal Products Technology, Faculty of Animal Science, Universitas Gadjah Mada, Yogyakarta, Indonesia
- 3Center for Watershed Management and Protected Forests Ake Malamo, Ministry of Environment and Forestry, Ternate, Indonesia
- 4Department of Forest Management, Faculty of Forestry, Universitas Gadjah Mada, Yogyakarta, Indonesia
A new massive dieback case of Avicennia marina was observed in the area of the Sunda Strait, which started in 2020, and the cause is still unknown. This research was conducted to report the rate of mangrove degradation, measure biomass loss, and predict the causes of the dieback. A sequential imagery analysis (2019–2022) was conducted to interpret the area changes using a research area of 200 ha from the total 528.69-ha mangrove, using the plant senescence reflectance index (PSRI) and normalized difference vegetation index (NDVI). Field data were collected before and during the dieback event (2020 and 2021) at seven research stations including, live mangroves, partial dieback, and full dieback sites. Biomass loss was measured as standing stock volume (SSV) and total biomass carbon (TBC). A literature study was conducted to predict the cause of the dieback. Based on the results, the mangrove dieback caused a rapid and significant loss of healthy stands (results of the PSRI) and very-high-density stands (results of the NDVI). The rate of healthy stand loss was 13.43 ha month−1 (during July–October 2020) and that of very-high-density stand loss was 14.99 ha month−1 (October 2020–April 2021), which affected 126.62 ha or 24% of the total area (last measurement in January 2022). The SSV before dieback was 118.70 (±46.1) m3 ha−1, but within 14 months, it decreased to 20.8 (±6.0), 79.80 (±52.3), and only 1.0 (±1.0) m3 ha−1 for live, partial dieback, and full dieback stands, respectively. The TBC of live mangroves (before dieback) was 51.6 (±24.4) Mg C ha−1, but within the same period, it changed to 30.70 (±3.80), 69.50 (±14.6), and 51.60 (±9.4) Mg C ha−1 for live, partial dieback, and full dieback stands, respectively. Based on the literature study and field observation, the dieback was probably related to less flushing and long-term inundation by the decrease in rainfall intensity, in addition to nitrogen (NO3−N and NO2−N) enrichment, an over-supply of N from fishpond wastes. Sustainable silvofishery may be introduced to ameliorate water and soil quality.
1. Introduction
The mangrove is a special ecosystem in the tropics on the boundaries of coastal and terrestrial ecosystems and therefore plays an important role in preserving ecosystem stability on both sides (Suratman, 2008; Mohd et al., 2012; Suman, 2019). As Indonesia is an island country with vast mangrove areas (Kusmana, 2014), the role of mangrove ecosystems in Indonesia is essential in order to protect the land from abrasion (Onrizal and Kusmana, 2008; Fatimatuzzahroh et al., 2018) and tsunamis (Kathiresan and Rajendran, 2005; Yanagisawa et al., 2010), to conserve biodiversity (Mulyana et al., 2021; Damastuti et al., 2022) and carbon storage (Patil et al., 2014; Hossain et al., 2015; Budiadi, 2020), and to provide a source of livelihood for coastal communities (Budiadi et al., 2016; Damastuti et al., 2022). Regarding their important functions for people's livelihoods from the local to global scales, the mangrove ecosystems must be conserved and protected.
In addition to the important functions, mangroves are also prone to disturbances from natural and human or anthropological causes (Jayanthi et al., 2018; Chowdhury et al., 2019; Quevedo et al., 2020). Based on the eco-geomorphology study, mangrove species naturally grow in a cycle from pioneer species domination, mudflat alteration, mangrove dieback, and then new mangrove culmination (Fagherazzi et al., 2017; Nardin et al., 2021), although this case is still lesser known in academic records. The death of mangroves is a normal occasion, and they are replaced with a new generation of the same or different species as a part of ecosystem succession (see Proffitt and Devlin, 2005; Berger et al., 2006; Nardin et al., 2021). However, some reports have focused on the massive and rapid death of mangroves in different locations (Duke et al., 2017; Asbridge et al., 2019), highlighting the alarming loss of their essential functions. A sudden and massive dieback of mangroves is noticeable, but chronic and gradual degradation is sometimes more dangerous, unexpected, and widespread; these issues have become challenges for global scientists (Lewis et al., 2016; Krauss et al., 2018). Mangrove dieback causes the loss of ecosystem functions and services, including the loss of function for carbon sequestration, the nutrient cycle, coastal protection, and habitat for biodiversity (Lovelock et al., 2017; Sippo et al., 2020).
Mangrove dieback or die-off is defined as the loss of mangrove canopy for at least one growing season, which probably could pose its mortality (Sippo et al., 2018; McCarthy et al., 2020), whereas mangrove mortality itself is the condition of the dead forest as a result of environmental shifts or other disturbances, which also can be used to describe its severity status (Sippo et al., 2018; Svejkovsky et al., 2020; Gomes et al., 2021). Other scientists use the term “ghost forest” that means stressed mangrove forest in a long-term period which then forms an extensive mortality area (Krauss et al., 2018; Nardin et al., 2021). Some reports of massive mangrove dieback explain the possibility of general or specific causes of the dieback, mostly concerning the effect of climate change (Duke et al., 2017; Lovelock et al., 2017; Gomes et al., 2021). Despite the massive dieback reported in Australia (Duke et al., 2005, 2017; Lovelock et al., 2017; Asbridge et al., 2019), Brazil (Gomes et al., 2021; Melo et al., 2022), the Caribbean (Trujillo et al., 2021), the United States (Krauss et al., 2018), and Thailand (Vaiphasa et al., 2007), our field observation in the coastal area of Lampung Timur of Sumatra found a case of rapid and vast mangrove (especially Avicennia marina) dieback during the period of 2020 to early 2022 (Budiadi et al., 2021, unpublished data).
Events of mangrove dieback are frequently observed and reported in many locations and require greater attention from foresters and more effort to find proper restoration strategies. This research aimed to observe the massive mangrove dieback in East Lampung (which represents a mangrove ecosystem in the Sunda Strait), using spatial and terrestrial analyses, to examine area changes and biomass losses, and to discuss the possible causes of the dieback. This report also intended to provide site-specific information on the mangrove dieback with its vulnerability, which has to be considered in the future restoration program.
2. Materials and methods
2.1. Study area
This research was conducted in a mangrove area in Pasir Sakti Sub-District, East Lampung, Sumatra (5°31′56.98″ S, 105°49′8.59″ E), Indonesia, representing mangrove on the East Coast of Sumatra Island. The location was used as a successful mangrove restoration area in 2010–2020 (Marpaung, 2021), but then the dieback probably started in the middle of 2020. The total mangrove area was 528.69 ha, of which 200 ha or 37.8% of the area was set as the research site, representing dieback location (Figure 1). The maximum temperature of the site in the last 10 years was 34.20± 1.27°C; the minimum temperature was 22.23 ± 0.36°C; and the average rainfall was 2189.06 ± 381.13 mm year−1. The weather data were extracted from power.larc.nasa.gov on 29 January 2022. The dominant species in the area was Avicennia marina, with some Rhizophora stylosa and Rhizophora mucronata, and also a small number of Excoecaria agallocha (Marpaung et al., 2022). In addition, there were two emerging activities by the people in the location that may have impacted the mangrove ecosystem, that is, maintaining the fishponds and ecotourism.
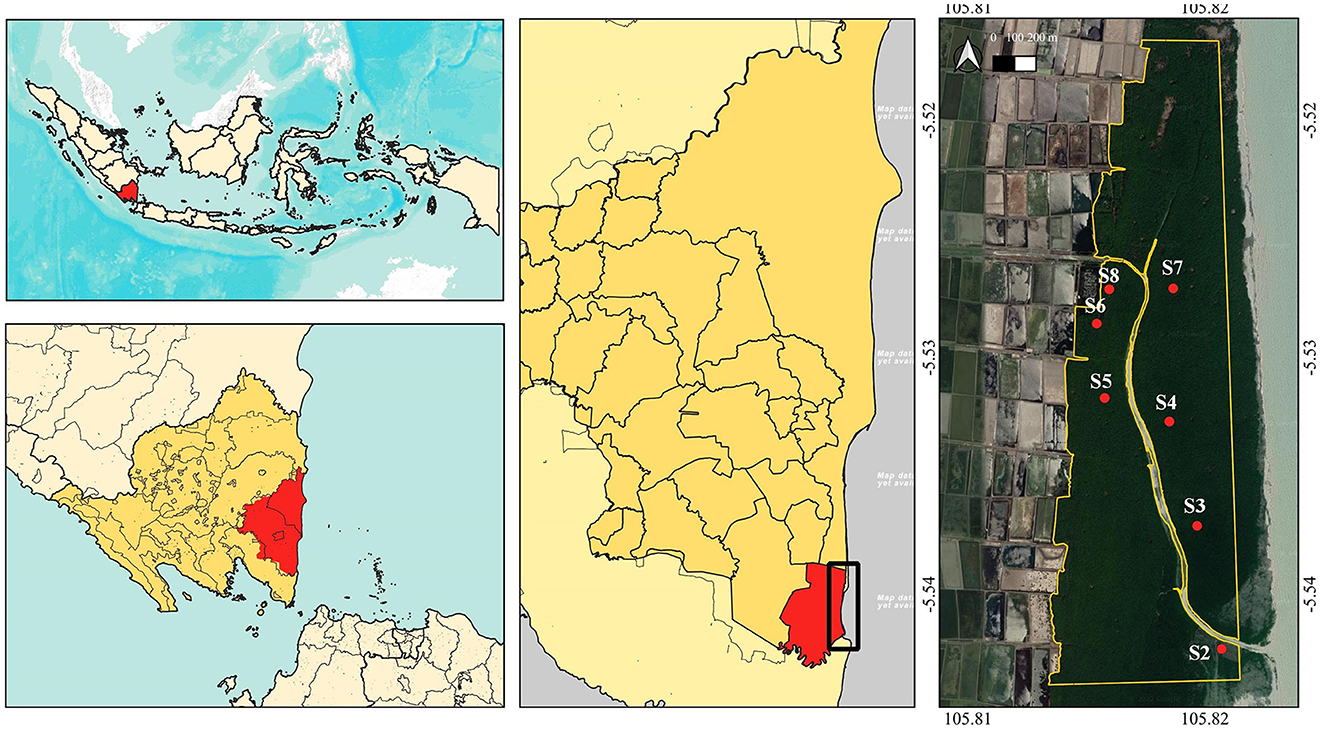
Figure 1. Map of the research location in East Lampung, Sumatra, Indonesia, research area, and research stations coordinate referred to Marpaung (2021) (map base: ESRI Terrain, Google Satellite on QGIS 3.24, accessed on 25 December 2022).
2.2. Satellite imagery interpretation
Spatial analysis was conducted using Sentinel-2 images, and imagery data were accessed via https://earthexplorer.usgs.gov/, with 10 m accuracy (Le et al., 2020). Cloud cover affected the spatial frequency of the cloud-free images and caused spatial inconsistency (Carrasco et al., 2019) during the period of 2019–2022, which resulted in the selection of seven Sentinel data (Table 1). A pre-processing image was used for atmospheric correction using the Semi-Automatic Classification Plugin (SCP) of the QGIS software (Yulianto et al., 2016; Obodai et al., 2019) version 3.06.10.
Sentinel data were analyzed using the plant senescence reflectance index (PSRI) and the normalized difference vegetation index (NDVI). The PSRI was used to predict leaf aging (Zhen et al., 2021) in order to measure the stress level of the mangroves. The formula for the PSRI was derived from Sims and Gamon (2002) and Sorboni et al. (2019), and then, plant health was classified into three categories: 0.1–0.075 (healthy); 0.075–0.175 (unhealthy); and >0.175 (critically unhealthy) based on the PRSI range in Asbridge et al. (2019). The NDVI was calculated by measuring the visible red (R) and near-infrared (NIR) (Guo et al., 2021) color bands that are sensitive to green and dead objects (Asbridge et al., 2019), and it was analyzed using the formula from Le et al. (2020) and Zaitunah et al. (2021), with the categories derived from Zaitunah et al. (2021). A value of <0.0 indicates sand or bare land (Tovar, 2011; Gandhi et al., 2015), corresponding to an extremely low density in this research, with a very high density corresponding to a value of 1.0. Imagery analyses were conducted using ArcGIS 10.8 software.
2.3. Ground check and field data collection
The pre-dieback condition of the mangrove ecosystem could be seen in Marpaung et al. (2022). Field data collection was conducted over a 2-year period in August 2020 and November 2021, including vegetation measurement (species identification, tree diameter, and height measurement) and microsite data measurement using 10 x 10 m measurement plots with three replications for each research station, at Station 2 (S2) to Station 8 (S8) (see Marpaung et al., 2022). Station 1 is a mangrove recruitment area or seedling plantation area, which was then excluded from this research. A total of 21 measurement plots were employed. Direct aerial photographs were taken in November 2021 using an unmanned aerial vehicle (UAV), the DJI Phantom 4 Pro. Due to the massive dieback of the mangrove in the location, based on field data and aerial photograph observation, the measurement plots of the year 2021 were classified into three categories, that is, live (three plots), partial dieback (10 plots), and full dieback (eight plots) (Figure 2).
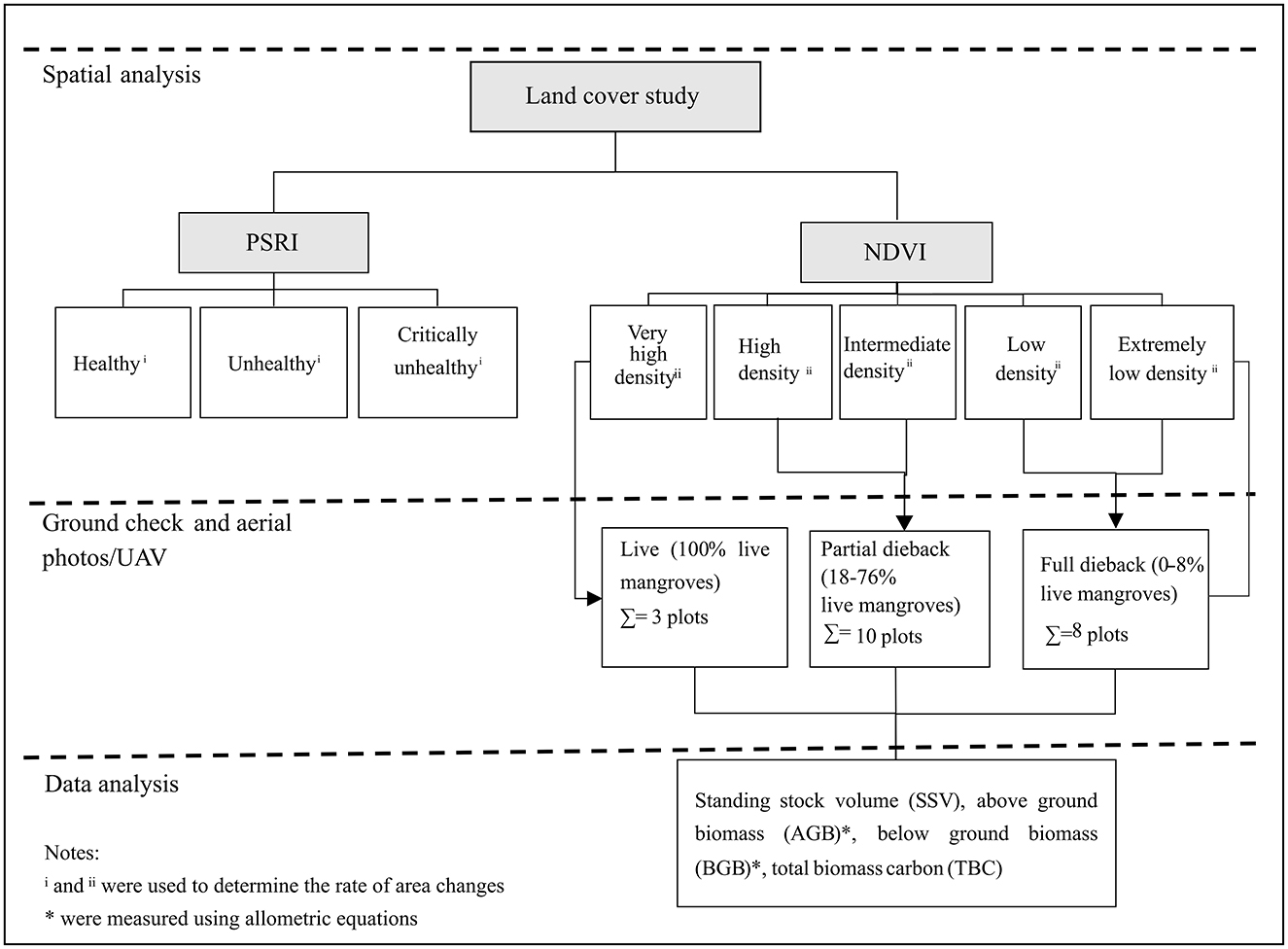
Figure 2. Scheme for the data collection and analyses of the mangrove dieback in East Lampung, Sumatra.
2.4. Data analysis
Based on the PSRI and NDVI analyses, the rate of area change (ha month−1) was calculated using the ratio of changed mangrove area to number of months.
In each plot, data analysis was conducted for the standing stock volume (SSV), aboveground biomass (AGB), belowground biomass (BGB), and total biomass carbon (TBC). The SSV of the remaining trees was measured using the formula from Hardjana (2015), with the mangrove species form factors derived from Njana (2017), with a note that Rhizophora spp. were estimated using the form factor of R. mucronata. Carbon storage estimation was conducted using a non-destructive method (Budiadi, 2020) by employing AGB and BGB allometric equations derived from Komiyama et al. (2008), as follows:
AGB (kg) allometric equations:
A. marina = 0.308 DBH 2.11
Rhizophora spp. = 0.128 DBH 2.06
BGB (kg) allometric equations:
A. marina = 1.28 DBH 1.17
Rhizophora spp. = 0.00974 (D2H)1.05
Notes: DBH (diameter at breast height, cm); H (height, m)
For dead trees, AGB was estimated based on decay status 1 derived from Kauffman and Donato (2012) and BGB was estimated using similar allometric equations for living trees (see Senger et al., 2021). Regarding AGB and BGB conversion to aboveground carbon (AGC) and belowground carbon (BGC), the procedure in Kauffman and Donato (2012) was followed. Total biomass carbon (TBC) was calculated by summing AGC and BGC.
The differences between the SSV, AGB, BGB, and TBC among the categories were analyzed using one-way ANOVA in R software version 4.1.2. The dynamics of the SSV and TBC were visualized using a diagram, by comparing the value of each mangrove category in the observed months to the live stands before dying (the year 2020). Finally, the prediction of the cause of the mangrove dieback in the location was discussed using literature studies. The most frequently used academic search engine, Google Scholar was accessed to retrieve publications (Gusenbauer, 2019) by employing several keywords, such as “Avicennia marina dieback,” “Avicennia marina mortality,” “mangrove dieback,” “mangrove mortality,” and “mangrove succession,” only publications with closely related topics and written in English were included. A total of 33 publications were used to provide adequate information to predict the dieback cause.
3. Result
3.1. Field condition and spatial analysis
Based on the UAV photos, several conditions of mangrove stand in the East Lampung were found, for example, healthy conditions, partially dead, and completely dead. No indications of A. marina dieback at research station 2 (4- to 6-year-old stands). Whereas mangrove dieback affected 24–82% of 8- to 10-year-old A. marina at plots of research stations 3, 4, and 5. The most severe area was found at research stations 6, 7, and 8 (11- and 14-year-old stands) with the percentage of dead A. marina accounted for 92–100% in each plot. Mature mangroves (at the northern part of the plantation) were the earliest in indicating canopy loss, which then spread out to the nearby stands, eventually expanding almost to the entire research area. In other words, the dieback patterns in this location mainly occurred in mature mangroves (8- to 14-year-old stands) and were generally located non-adjacent to the water flows, while fringe mangroves were less affected (Figure 3).
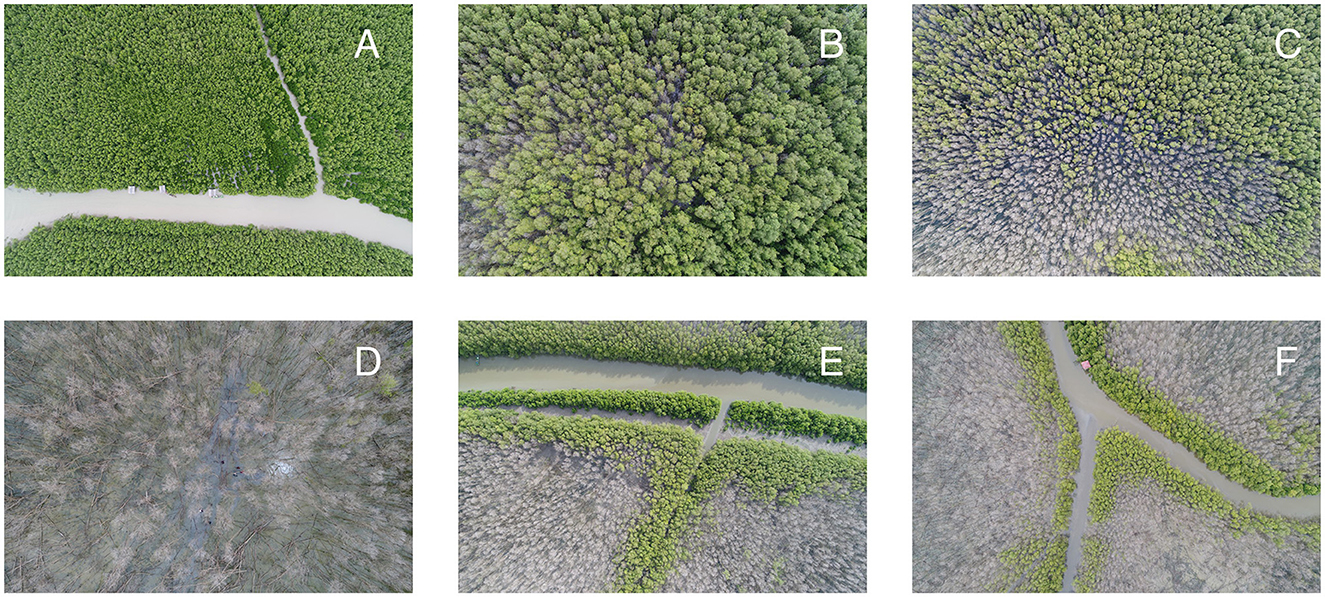
Figure 3. Findings of field conditions of the mangrove ecosystem in East Lampung, Sumatra (aerial photographs were taken using a UAV on 11–13 November 2021), no dieback cases were found in younger stands and mangrove along the riverbank, whereas dieback occurred in the site of 8- to 14-year-old A. marina as: (A) Station 2, live mangrove; (B) Station 3, partial dieback; (C) Station 4, partial dieback and full dieback; (D) Station 7, dead mangrove zoomed in; (E, F) fringe mangrove.
During the observation period of August 2019–January 2022, mangrove degradation was evaluated based on the declining stand health and stand density. The stand health was determined based on the PSRI changes (Figure 4), and in correlation with the declining stand density in the NDVI (Figure 5) at the research site (200 ha). In April 2020, mangrove stress did not affect the death of the trees, as there was no relationship with the declining density in the NDVI analysis of the same month. The optimum condition of the healthy site was observed in a 195.7 ha area in July 2020 (PSRI), and that for the very-high-density stands was observed in a 194.52 area in April 2020 (NDVI). A decreasing health condition of the mangroves was observed in July 2020, estimated at 4.02 ha of the unhealthy stands, which spread out, further extending until January 2022 to an area of 84.8 ha. In the early stress period (July 2020), a very-high-density stand of 188.60 ha was observed, which decreased to 177.36 ha in October 2020 and further to only 87.40 ha in the next 6 months (April 2021), remaining at 72.34 ha in January 2022. Areas of extremely low density and low density totaled 42.53 and 42.22 ha in January 2022.
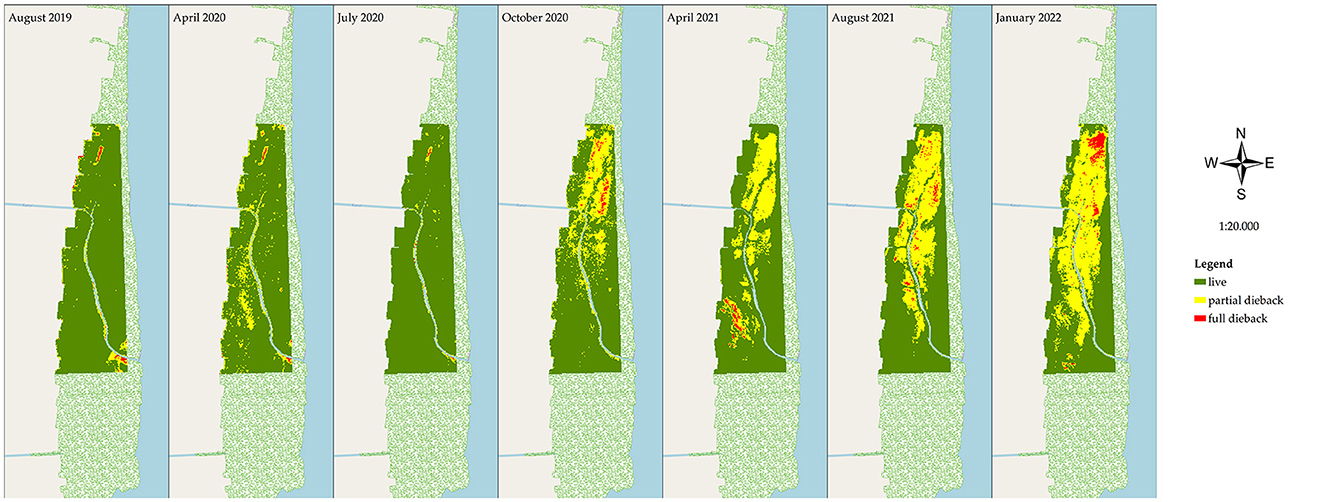
Figure 4. Results from PSRI analysis of the mangrove condition in East Lampung, Sumatra, in different months in August 2019–January 2022 (map base: ESRI OpenStreetMap by ArcMap 10.8, accessed on March 12, 2022).
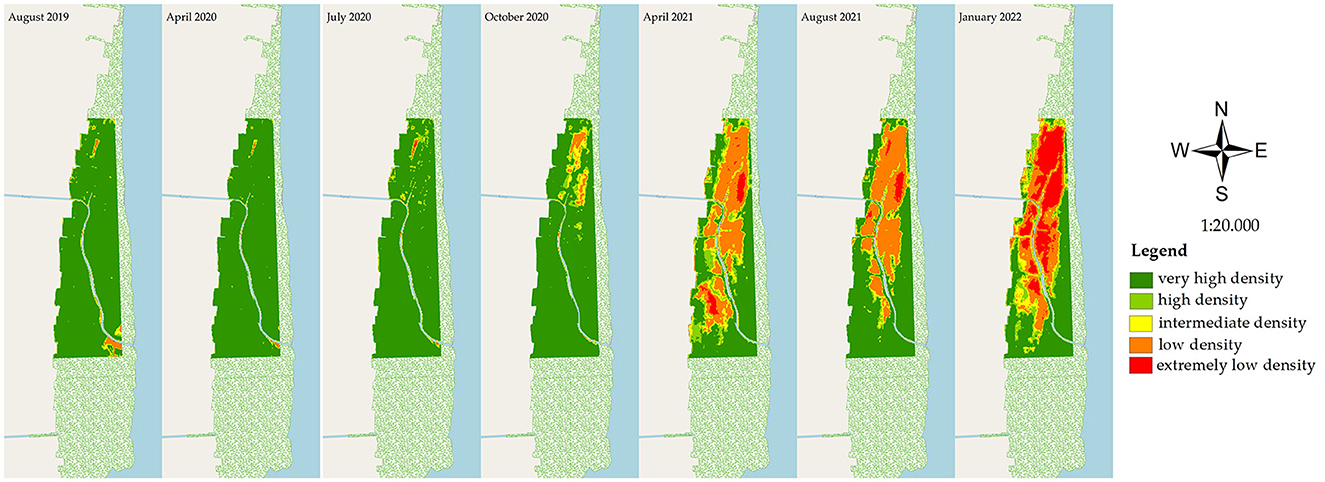
Figure 5. Results from NDVI analysis of the mangrove condition in East Lampung, Sumatra, in different months in August 2019–January 2022 (map base: ESRI OpenStreetMap by ArcMap 10.8, accessed on March 12, 2022).
3.2. Changes and accumulative area of mangrove dieback
Based on the PSRI and NDVI analyses, the rate of land cover changes in the mangrove area varied during the period of August 2019–January 2022 (Table 2). Rapid changes in stand health were observed from July 2020 to October 2020, where the healthy stand area decreased by 13.43 ha month−1, and the area of unhealthy and critically unhealthy stands increased by 12.88 and 0.58 ha month−1, respectively. In the same period, the very-high-density stands decreased by 3.75 ha month−1. More rapid changes in the stand condition were observed in the next period (October 2020–April 2021), with the very-high-density stands decreasing, on average, at a rate of 14.99 ha month−1, with increases in the areas of high-density, intermediate-density, low-density, and extremely-low-density stands in this period. The most rapid increase in extremely-low-density stands was observed in the period of August 2021–January 2022, with a value of 7.66 ha month−1.
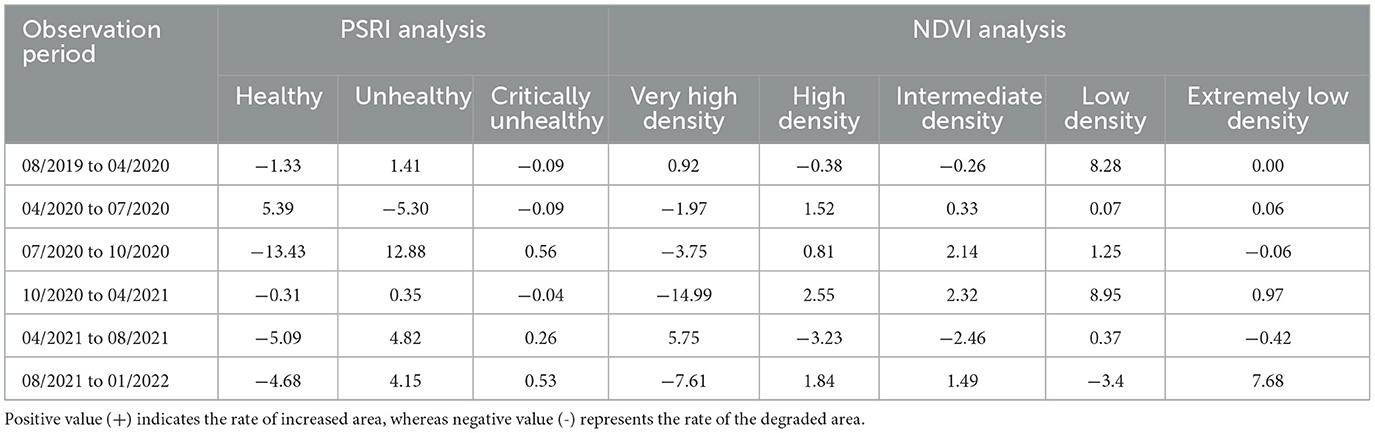
Table 2. Rate of land cover changes (ha month−1) in the mangrove area of East Lampung, Sumatra, based on PSRI and NDVI analyses in August 2019–January 2022.
In the PSRI analysis (Figure 6A), healthy stands decreased from 95.04% (in August 2019) to 54.90% (in January 2022), and unhealthy stands increased from 4.31% to 42.35% in the same period, although no significant changes were observed in critically unhealthy stands. In the NDVI analysis (Figure 6B), very-high-density stands decreased from 94.08% (in August 2019) to 36.35% (in January 2022). In addition, from October 2020 to January 2022, changes in the high-density, intermediate-density, low-density, and extremely-low-density stands were observed at 10.20, 10.35, 21.72, and 21.37%, as a result of the decrease in the very-high-density stands. Up to January 2022, an area of 126.62 ha (or 24% of the total mangrove area in East Lampung) was affected by the dieback event.
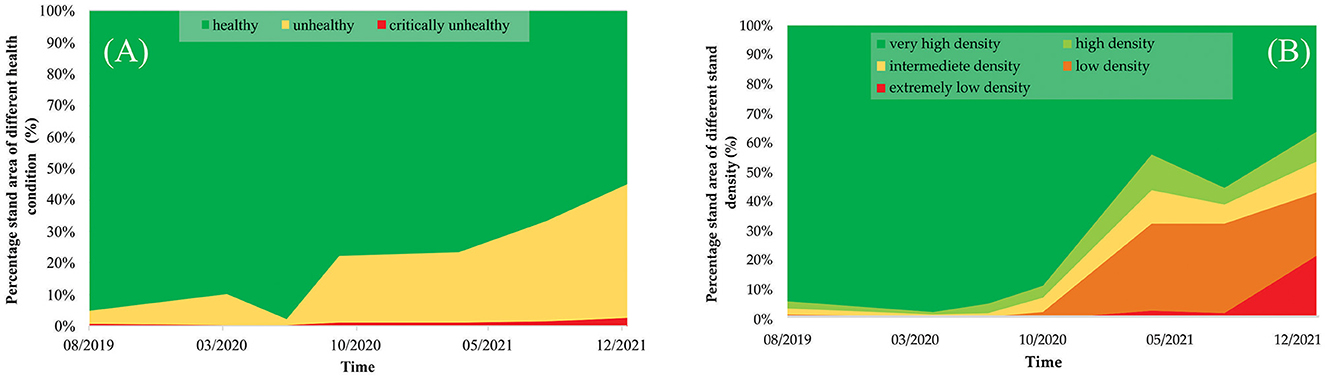
Figure 6. Accumulated areas of mangrove stand based on (A) the stand health condition from the PSRI analysis and (B) the stand density from the NDVI analysis of the mangrove ecosystem in East Lampung in August 2019–January 2022.
3.3. Biomass loss due to dieback
Mangrove dieback reduced the number of trees per ha (F = 54.69, p < 0.05) and decreased the standing stock volume (SSV) (F = 106.3, p < 0.05) (Table 3). Under the optimum condition prior to the dieback (in August 2020), the SSV was 118.7±46.1 m3 ha−1, which decreased to 49.9 ± 58.8 m3ha−1 (in November 2021). Based on the cumulative number of living and dead trees, the dieback reduced the number of trees per ha (F = 26.8, p < 0.05), aboveground biomass (AGB) (F = 24.8, p < 0.05), belowground biomass (BGB) (F = 2.5, p> 0.05), and total biomass carbon (TBC) (F = 15.2, p < 0.05). The TBC in the dieback event in 2021 was mostly stored in partial dieback stands at 69.5 ±1 4.6 Mg C ha−1.
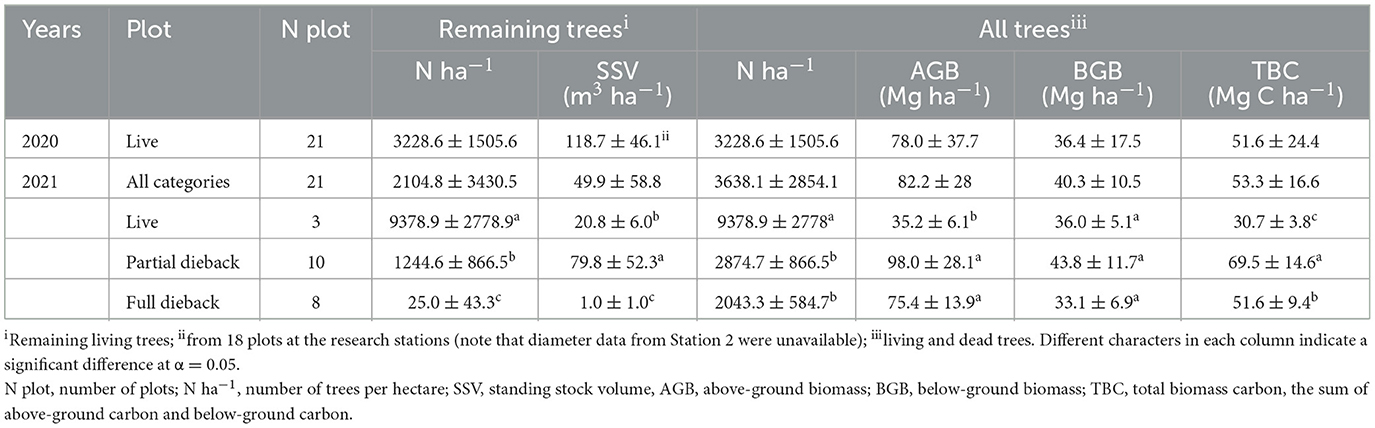
Table 3. Standing stock volume (SSV) and carbon stock estimation in mangrove stands in East Lampung during 2020–2021 (mean ± SD).
The mangrove dieback affected the reduction in the SSV of the live stands (Figure 7A), which started in October 2020 and remained at 8586.79 m3 in January 2022. The TBC of both living and dead trees accumulated in live, partial dieback, and full dieback stands (Figure 7B). The maximum TBC in the live stands was 10037.26 Mg C in April 2020, which then decreased gradually until October 2020 and drastically to 3732.76 Mg C in January 2022. Conversely, the TBC in the partial dieback stands started to increase in October 2020 to 2840.73 Mg C by January 2022. The TBC in the full dieback stands also steadily increased from 3330.96 Mg C in April 2021 to 3320.70 Mg C in August 2021, finally reaching 4424.56 Mg C in January 2022. In January 2022, the highest TBC was found in the full dieback stands (compared with the live and partial dieback stands), which was proportional to the increase in extremely-low-density and low-density stands (Figure 6B).
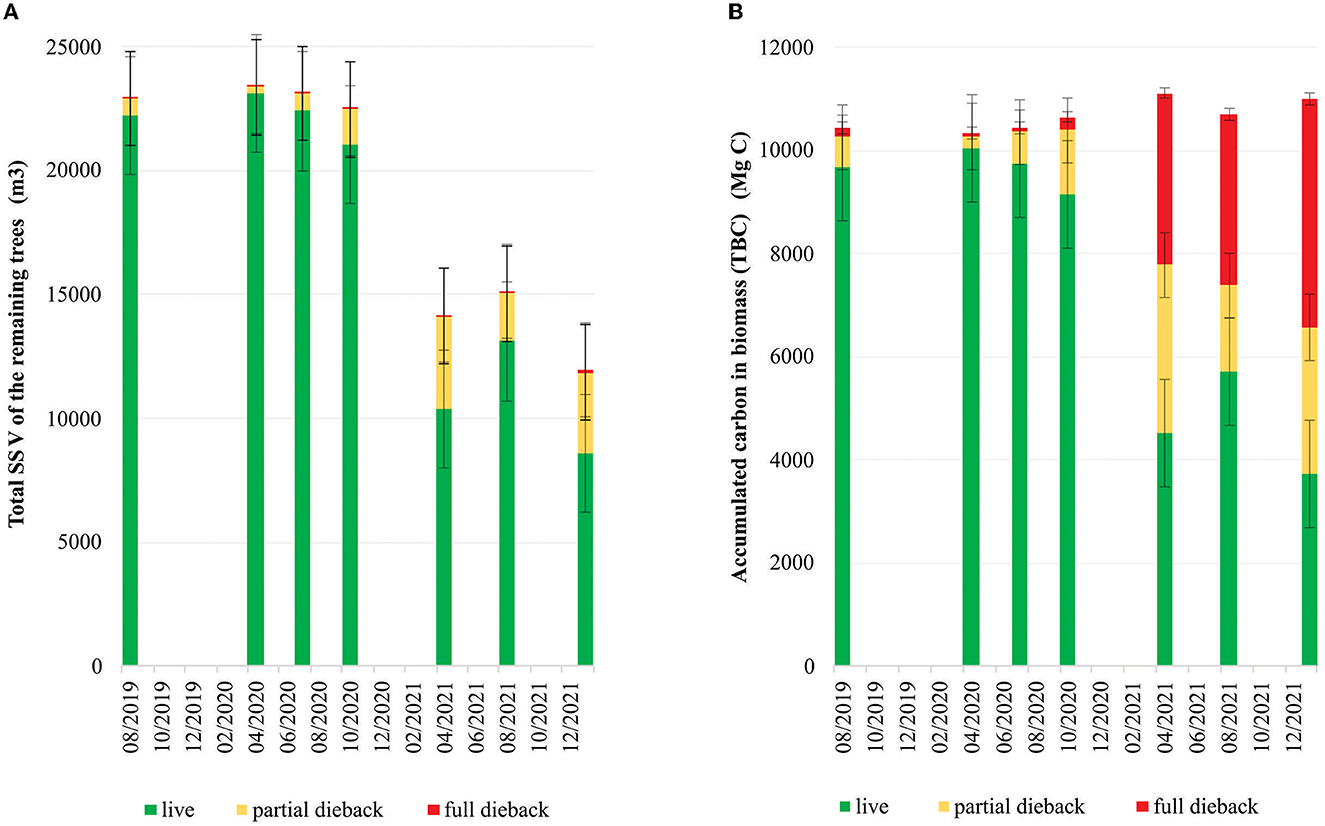
Figure 7. (A) Changes in the standing stock volume (SSV) of the remaining trees. (B) Accumulated total biomass carbon in the research area (200 ha) due to the dieback event in the mangrove ecosystem in East Lampung (August 2019–January 2022).
4. Discussions
4.1. Field condition and spatial analysis
Dieback events have also been reported in various locations, such as Mangrove Bay, Mackay, Carpentaria Bay, and Kakadu National Park in Australia (Duke et al., 2005, 2017; Lovelock et al., 2017; Asbridge et al., 2019), Piraquê-Açú-Mirim in Brazil (Gomes et al., 2021; Melo et al., 2022), Ciénaga Grande de Santa Marta in the Southern Caribbean (Trujillo et al., 2021), Macro Island in the USA (Krauss et al., 2018), and Pak Phanang in Thailand (Vaiphasa et al., 2007). Mangrove dieback is classified into two types, that is, multispecies dieback (e.g., Krauss et al., 2018; Duke et al., 2020) and species-specific dieback, for example, A. marina (Duke et al., 2005; Asbridge et al., 2019). The mangrove dieback in East Lampung mainly affected A. marina, although some R. stylosa trees were also found to be affected. In addition, mangrove dieback in East Lampung is similar to the case in Carpentaria Bay and Kakadu National Park, where dieback was found at the edge of the mainland, while the areas near river flows and the seashore were not affected (Duke et al., 2017; Asbridge et al., 2019), and only mangroves that were close to water flows were able to regenerate (Sippo et al., 2020). Dieback causes the spread of stressed stands and a decrease in stand density, as dieback affects the rapid decline in the NDVI value (Lovelock et al., 2017; Asbridge et al., 2019).
4.2. Massive dieback cases and their severity
The dieback rate varies among locations, and a pattern is yet to be found. For example, in 2002, mangrove dieback was reported in Pioneer River, Mackay, which affected 97% of A. marina (Duke et al., 2005). Dieback in Ciénaga Grande de Santa Marta in the Caribbean affected 8,700 ha of the mangrove area between 2015 and 2017 (Trujillo et al., 2021). A massive and sudden mangrove dieback was also reported in Carpentaria Bay from December 2015 to January 2016, which affected 6% or 7,500 ha of the area (Duke et al., 2017). In addition, mangrove dieback can occur rapidly and severely in many locations (Duke et al., 2005, 2017), for example, in Kakadu National Park, the dieback affected 14.7% or 183.4 km2 of the mangrove area (Asbridge et al., 2019). There were no specific dieback rates and patterns reported on the references, similar to an irregular pattern of the dieback events observed in East Lampung from October 2020 to January 2022.
4.3. Biomass loss due to mangrove dieback
We observed the SSV of the remaining trees at full dieback stands was only 1.0 m3 ha−1 with a TBC value of 51.6 Mg C ha−1 composed of dead and live stands. In the dead sites of mangroves, the number of remaining trees is usually very low (Krauss et al., 2018). In regular conditions, the biomass carbon of living trees of A. marina in the tropics is 49.8 Mg C ha−1 (TBC) in Baros, 79.8 Mg C ha−1 (AGC) in Karimunting Bay, 48.1 Mg C ha−1 (AGC) in Pengarengan, and 18,4 Mg C ha−1 (AGC) in Jor Bay (Budiadi, 2020; Dinilhuda et al., 2020; Mulyana et al., 2021; Zulhalifah et al., 2021), and dieback cases can result in as much loss of carbon as the amount of carbon sequestered and stored in healthy stands. Another study stated that dieback impacted the loss of total ABC by 49.4% and the loss of total BGC by 61.2% (Gomes et al., 2021). In Macro Island, mangrove dieback caused the loss of 233.4 Mg C ha−1 of carbon (standing stock and soil carbon), which, regarding the small amount of additional carbon stocks every year, proves the difficulties in conserving and restoring carbon in post-dieback mangrove stands (Krauss et al., 2018). In line with the other cases based on several references, the dieback event in East Lampung has experienced the loss of SSV and the decline of accumulated biomass, which led to carbon loss.
4.4. Prediction of dieback causes and follow-up
A. marina is a salt-tolerant woody plant, but some environmental conditions are detrimental to A. marina (Cheng et al., 2020; Li et al., 2020) and in conjunction with several nutrients that can inhibit its growth (Alongi, 2009, 2011, 2021; Reef et al., 2010; Chowdhury et al., 2019). Some researchers have predicted and discussed mangrove dieback causes (e.g., Sippo et al., 2018; Duke et al., 2021); the causes of mangrove dieback in some locations to predict dieback events in East Lampung are as follows:
a. Mangrove (dominated by A. germinans) dieback affected by hydrological shifts and climate change was reported in Ciénaga Grande de Santa Marta in the Southern Caribbean, which was linked with the development of a coastal road and embankment, and affected the increase in seawater supply and the decrease in the freshwater supply in the river (Monroy et al., 2011; Trujillo et al., 2021). The recent case of dieback (2015–2017) in Ciénaga Grande de Santa Marta was predicted to be due to a lack of water channel maintenance, the effect of El Nino 2015–2016 that increased sediment and water salinity, and other hydrological changes as reported in Trujillo et al. (2021). However, this hypothesis remains weak due to no indications of hypersaline and an increase in seawater level during the dieback event in East Lampung (Budiadi et al., 2021, unpublished data).
b. The increase in soil sedimentation and oil pollutants covering the antenna roots of Avicennia can lead to its mortality. About 10 cm of soil cover of A. marina roots can affect its death due to oxygen deficiency in the roots (Ellison, 1999; Nardin et al., 2021); in addition, large amounts of sediment decrease its growth and cause mangrove death (Sidik et al., 2016; Fagherazzi et al., 2017). However, some reports also discussed the capability of A. marina to survive in unfavorable conditions. For example, in terms of sedimentation, Sidik et al. (2016) and Okello et al. (2020) stated that A. marina is able to develop cable roots and its antenna roots can grow higher than the surface of the new sediment. Oil contamination can damage the xylem of the fine roots and decrease the root length of A. marina, but the species can adapt by developing new adventive roots (Ye and Tam, 2007; Naidoo et al., 2010; Naidoo and Naidoo, 2017). However, there were no indications of sudden soil sediment alteration in the East Lampung mangrove, or the presence of oil pollutants; thus, it may not be the cause of the dieback.
c. Dieback is probably a stage in mangrove succession; in this case, A. marina will be replaced by other secondary mangroves. A. marina is a pioneer in mangrove ecosystems (Naidoo and Naidoo, 2017; Cheng et al., 2020), and it can modify the environment to allow the development of secondary species (Fagherazzi et al., 2017). However, in some cases, its arduous to associate the case of dieback with natural succession, due to the recruitment of new seedlings, is very low during the dieback, which highlights the decrease in reproduction ability (Lovelock et al., 2017). Sippo et al. (2020) reported that plots of almost 100% mangrove dieback had low regeneration capability. Also, in dieback plots in Pioneer Estuary, there were no indications of vegetation recovery based on observation of unsuccessful recruitment (Duke et al., 2005). Therefore, the dieback case in East Lampung may not be related to ecosystem succession, because there is no natural indication of the introduction of other mangrove species and no intermediate stands to carry the succession process from A. marina to other new species.
d. A. marina dieback in East Lampung, Sumatra was probably affected by lower annual rainfall and anthropogenic activities in the upstream.
In 2007–2009 recorded four to six dry months (with <100 mm/month) of the years, or the annual rainfall intensities below the decade average caused less water flushing inside the plantation. Based on the assessment of water quality on August 2020, in the dieback area, the concentration of total suspended solids (TSS) ranged from 175.33 to 652.00 mg/L and water turbidity ranged from 226.4 to 915.0 NTU (Marpaung et al., 2022). The concentration of TSS was greatly exceeding than the seawater quality standard in Indonesia (80 mg/L) and the concentration in other locations, such as mangroves in Malaysia, the Gulf of Jakarta, and the Gulf of Lampung (Marpaung, 2021), indicating the occurrence of water inundation assessed by the abundance of dissolved material in the water. A. marina is the most vulnerable species to waterlog stress compared with other mangrove species (Zhu et al., 2019), such as reported by Mbense et al. (2016); prolonged inundation and low rainfall intensity caused 90–95% of A. marina dieback in Kobonqaba Estuary.
In addition, the dieback location is adjacent to community's fishponds, while big amount of waste from the fishponds flows to the stream without a pre-treatment (Marpaung, 2021). The waste from extensive fishponds contains an accumulation of residual of inorganic and organic fertilizers, and feeds (Páez-Osuna, 2001; Hasan et al., 2007), including nitrogen (N) compound that excessively enters the mangrove ecosystem. Before the dieback event, the inorganic nitrogen in the locations is as follows: NO3−N content ranged from 0.7 to 1.1 (mg/L) or greater than a threshold of seawater quality standards in Indonesia (0.008 mg/L), while NO2−N content ranged from 0.4 to 0.7 (mg/L) (Marpaung, 2021; Marpaung et al., 2022), as an initial indicator of N enrichment.
Additional N to some extent can increase the growth; for instance, A. marina is optimum at the rate of N supply 10 mmol Nm−2d−1 in a tidal treatment with regular flushing (Naidoo, 2009; Alongi, 2011). However, supply of N above a threshold (known as N enrichment) has detrimental effects on changing N cycle, reduces ecosystem capability to fix N2, induces dead of roots, loss of foliage, and mangrove dieback (Lovelock et al., 2009; Reef et al., 2010; Romero et al., 2012; Reis et al., 2017; Miah and Moula, 2019). A. marina dieback resulting from urea herbicide toxicity has been reported in Mackay, Australia (Bell and Duke, 2005; Duke et al., 2005); another dieback case in Pak Phanang, Thailand, was caused by the deposition of shrimp ponds wastes (Vaiphasa et al., 2007). The waste is accumulated in mangrove sediment inside the plantation and potentially extends across the mangrove area and is exacerbated by the decrease in rainfall intensity (Vaiphasa et al., 2007; Lovelock et al., 2009). A. marina is known to be more susceptible to chemical contaminants than other mangrove species (Bell and Duke, 2005). However, fringe A. marina along the water streams was less affected, probably due to continuous water flows that washed away the pollutants among the stands (see Lovelock et al., 2009).
Among the predictive causes of the dieback, less water flushing and N enrichment from fishpond wastes are the most presumed reason for the dieback case. However, direct observation cannot be conducted yet, as the location is under investigation by the local authority to map the dynamic of the dieback and to search for the potential regrowth of the presumed dead trees. A long-term observation is needed to measure the effect of the wastes from fishponds and N toxicity, for example, 3- to 12-year period (Lovelock et al., 2009), in order to plan a restoration program of the damaged mangroves due to the dieback event. In the meantime, sustainable silvofishery systems can be introduced in the location, which may help to remove N and P residuals (De-León-Herrera et al., 2015), prohibit nutrient enrichment and ammonia accretion (Musa et al., 2020a), and ameliorate water and soil quality (Musa et al., 2020b).
Data availability statement
The original contributions presented in the study are included in the article/supplementary material, further inquiries can be directed to the corresponding author.
Author contributions
BB: conceptualization, methodology, formal analysis, writing—original draft, writing—review and editing, and visualization. AP: methodology, formal analysis, and writing—original draft. LL and AJ: data curation, formal analysis, and writing—original draft. BM: formal analysis and writing—original draft. SP: spatial data curation, formal analysis, and visualization. All authors have read and agreed to the published version of the manuscript.
Acknowledgments
The authors would like to thank the Ministry of Research, Technology, and Higher Education (RISTEKDIKTI) for supporting the research through the Grant Penelitian Terapan Unggulan Perguruan Tinggi 2021. The authors also thank the mangrove community group in Pasir Sakti, Lampung, for their support during the fieldwork.
Conflict of interest
The authors declare that the research was conducted in the absence of any commercial or financial relationships that could be construed as a potential conflict of interest.
Publisher's note
All claims expressed in this article are solely those of the authors and do not necessarily represent those of their affiliated organizations, or those of the publisher, the editors and the reviewers. Any product that may be evaluated in this article, or claim that may be made by its manufacturer, is not guaranteed or endorsed by the publisher.
References
Alongi, D. M. (2011). Early growth responses of mangroves to different rates of nitrogen and phosphorus supply. J. Exp. Marine Biol. Ecol. 397, 85–93. doi: 10.1016/j.jembe.2010.11.021
Alongi, D. M. (2021). Macro-and micronutrient cycling and crucial linkages to geochemical processes in mangrove ecosystems. J. Marine Sci. Engin. 5, 456. doi: 10.3390/jmse9050456
Asbridge, E. F., Bartolo, R., Finlayson, C. M., Lucas, R. M., Rogers, K., and Woodroffe, C. D. (2019). Assessing the distribution and drivers of mangrove dieback in Kakadu National Park, northern Australia. Estuarine Coast. Shelf Sci. 228, 106353. doi: 10.1016/j.ecss.2019.106353
Bell, A. M., and Duke, N. C. (2005). Effects of photosystem II inhibiting herbicides on mangroves—preliminary toxicology trials. Marine Pollut. Bullet. 51:1, 297–307. doi: 10.1016/j.marpolbul.2004.10.051
Berger, U., Adams, M., Grimm, V., and Hildenbrandt, H. (2006). Modelling secondary succession of neotropical mangroves: causes and consequences of growth reduction in pioneer species. Perspect. Plant Ecol. Evolut. Sys. 3, 1. doi: 10.1016/j.ppees.2005.08.001
Budiadi, B. (2020). Pendugaan Simpanan Karbon pada Kawasan Rehabilitasi Pesisir Selatan Pulau Jawa. J. Ilmu Kehutanan 1, 71–83. doi: 10.22146/jik.57473
Budiadi, B., Nurjanto, H. H., Hardiwinoto, S., and Primananda, E. (2016). Strategi pemilihan jenis tanaman untuk mendukung rehabilitasi pesisir berdasarkan karakteristik fisik makro di muara sungai progo (strategy of plant-species selection for coastal rehabilition based on macro-physical characteristics in progo estuary). J. Manusia Dan Lingkungan 3, 349–359. doi: 10.22146/jml.18809
Budiadi, L. L.D., Marpaung, B. A., and Jihad, A. N. (2021). Annual Report of the Changes of Water Salinity, Precipitation and Sea Water Level in Mangrove Areas in East Lampung. [Unpublished manuscript].
Carrasco, L., O'Neil, A. W., Morton, R. D., and Rowland, C. S. (2019). Evaluating combinations of temporally aggregated Sentinel-1, Sentinel-2 and Landsat 8 for land cover mapping with Google Earth Engine. Remote Sens. 11:3,288. doi: 10.3390/rs11030288
Cheng, H., Inyang, A., Li, C. D., Fei, J., Zhou, Y. W., and Wang, Y. S. (2020). Salt tolerance and exclusion in the mangrove plant Avicennia marina in relation to root apoplastic barriers. Ecotoxicology 6, 676–683. doi: 10.1007/s10646-020-02203-6
Chowdhury, R., Sutradhar, T., Begam, M., Mukherjee, C., Chatterjee, K., Basak, S. K., et al. (2019). Effects of nutrient limitation, salinity increase, and associated stressors on mangrove forest cover, structure, and zonation across Indian Sundarbans. Hydrobiologia 1, 191–217. doi: 10.1007/s10750-019-04036-9
Damastuti, E., de Groot, R., Debrot, A. O., and Silvius, M. J. (2022). Effectiveness of community-based mangrove management for biodiversity conservation: a case study from Central Java, Indonesia. Trees Forests People 7, 100202. doi: 10.1016/j.tfp.2022.100202
De-León-Herrera, R., Flores-Verdugo, F., Flores-de-Santiago, F., and González-Farías, F. (2015). Nutrient removal in a closed silvofishery system using three mangrove species (Avicennia germinans, Laguncularia racemosa, and Rhizophora mangle). Marine Pollution Bulletin 1, 243–248. doi: 10.1016/j.marpolbul.2014.11.040
Dinilhuda, A., Akbar, A. A., and Herawaty, H. (2020). Potentials of mangrove ecosystem as storage of carbon for global warming mitigation. Biodive. J. Biol. Div. 21, 11. doi: 10.13057/biodiv/d211141
Duke, Bell, A. M., Pederson, D. K., Roelfsema, C. M., and Nash, S. B. (2005). Herbicides implicated as the cause of severe mangrove dieback in the Mackay region, NE Australia: consequences for marine plant habitats of the GBR world heritage area. Marine Pollut. Bullet. 1–4, 308–324. doi: 10.1016/j.marpolbul.2004.10.040
Duke, Kovacs, J. M., Griffiths, A. D., Preece, L., Hill, D. J. E., van Oosterzee, P., Mackenzie, J., et al. (2017). Large-scale dieback of mangroves in Australia's Gulf of Carpentaria: a severe ecosystem response, coincidental with an unusually extreme weather event. Marine Freshwater Res. 10, 1816–1829. doi: 10.1071/MF16322
Duke, Mackenzie, J., Kovacs, J., Staben, G., Coles, R., Wood, A., and Castle, Y. (2020). Assessing the Gulf of Carpentaria mangrove dieback 2017–2019. Aerial Surv. 1, 56.
Duke, N. C., Hutley, L. B., Mackenzie, J. R., and Damien, Burrows. (2021). “Processes and factors driving change in mangrove forests: an evaluation based on the mass dieback event in Australia's Gulf of Carpentaria,” in Ecosystem Collapse and Climate Change (Issue July, p. 221). doi: 10.1007/978-3-030-71330-0_9
Ellison, J. C. (1999). Impacts of sediment burial on mangroves. Marine Pollut. Bullet. 12, 420–426. doi: 10.1016/S0025-326X(98)00122-2
Fagherazzi, S., Bryan, K. R., and Nardin, W. (2017). Buried alive or washed away: the challenging life of mangroves in the Mekong Delta. Oceanography. 30:3, 48–59. doi: 10.5670/oceanog.2017.313
Fatimatuzzahroh, F., Hadi, S. P., and Purnaweni, H. (2018). Mangrove cultivation for dealing with coastal abrasion case study of Karangsong. E3S Web of Conf. 31, 8028. doi: 10.1051/e3sconf/20183108028
Gandhi, G. M., Parthiban, S., Thummalu, N., and Christy, A. (2015). Ndvi: vegetation change detection using remote sensing and gis—A case study of vellore district. Procedia Comp. Sci. 57, 1199–1210. doi: 10.1016/j.procs.2015.07.415
Gomes, L. E., de, O., Sanders, C. J., Nobrega, G. N., Vescovi, L. C., Queiroz, H. M., et al. (2021). Ecosystem carbon losses following a climate-induced mangrove mortality in Brazil. J. Environ. Management, 297, 113381. doi: 10.1016/j.jenvman.2021.113381
Guo, X., Wang, M., Jia, M., and Wang, W. (2021). Estimating mangrove leaf area index based on red-edge vegetation indices: a comparison among UAV, WorldView-2 and Sentinel-2 imagery. Int. J. App. Earth Observ. Geoinform. 103, 102493. doi: 10.1016/j.jag.2021.102493
Gusenbauer, M. (2019). Google Scholar to overshadow them all? Comparing the sizes of 12 academic search engines and bibliographic databases. Scientometrics 1, 177–214. doi: 10.1007/s11192-018-2958-5
Hardjana, A. K. (2015). Kapasitas stok biomassa tegakan dipterokarpa dan non-dipterokarpa berdasarkan kondisi tutupan vegetasi hutan di khdtk labanan, kabupaten berau, kalimantan timur. Prosid. Sem. Nasi. Masyarakat Biodiv. Indon. 3, 590–596. doi: 10.13057/psnmbi/m010335
Hasan, M. R., Hecht, T., de Silva, S. S., and Tacon, A. G. J. (2007). Study and Analysis of Feeds and Fertilizers for Sustainable Aquaculture Development. Rome: Food and Agriculture Organization of the United Nations.
Hossain, M., Siddique, M. R. H., Saha, S., and Abdullah, S. M. R. (2015). Allometric models for biomass, nutrients and carbon stock in Excoecaria agallocha of the Sundarbans, Bangladesh. Wetlands Ecol. Manag. 4, 765–774. doi: 10.1007/s11273-015-9419-1
Jayanthi, M., Thirumurthy, S., Nagaraj, G., Muralidhar, M., and Ravichandran, P. (2018). Spatial and temporal changes in mangrove cover across the protected and unprotected forests of India. Estu. Coastal Shelf Sci. 213, 81–91. doi: 10.1016/j.ecss.2018.08.016
Kathiresan, K., and Rajendran, N. (2005). Coastal mangrove forests mitigated tsunami. Estu. Coastal and Shelf Sci. 3, 601–606. doi: 10.1016/j.ecss.2005.06.022
Kauffman, J. B., and Donato, D. C. (2012). Protocols for the Measurement, Monitoring and Reporting of Structure, Biomass, and Carbon Stocks in Mangrove Forests. Bogor: Center of International Forestry Research.
Komiyama, A., Ong, J. E., and Poungparn, S. (2008). Allometry, biomass, and productivity of mangrove forests: a review. Aquatic Bot. 2, 128–137. doi: 10.1016/j.aquabot.2007.12.006
Krauss, K. W., Demopoulos, A. W. J., Cormier, N., From, A. S., Mcclain-counts, J. P., and Iii, R. R. L. (2018). Ghost forests of Marco Island: Mangrove mortality driven by belowground soil structural shifts during tidal hydrologic alteration. Estu. Coast. Shelf Sci. 212, 51–62. doi: 10.1016/j.ecss.2018.06.026
Kusmana, C. (2014). Distribution and current status of mangrove forests in Indonesia. In Mangrove ecosystems of Asia (pp. 37–60). Springer. doi: 10.1007/978-1-4614-8582-7_3
Le, H. T., Tran, T. V, Gyeltshen, S., Nguyen, C., Tran, D. X., Luu, T. H., and Duong, M. B. (2020). Characterizing spatiotemporal patterns of mangrove forests in Can Gio biosphere reserve using Sentinel-2 imagery. Applied Sci. 12,4058. doi: 10.3390/app10124058
Lewis, III, R. R., Milbrandt, E. C., Brown, B., Krauss, K. W., Rovai, A. S., Beever, III, J. W., et al. (2016). Stress in mangrove forests: Early detection and preemptive rehabilitation are essential for future successful worldwide mangrove forest management. Mar. Pollut. Bull. 2, 764–771. doi: 10.1016/j.marpolbul.2016.03.006
Li, H., Li, Z., Shen, Z. J., Luo, M. R., Liu, Y. L., Wei, M. Y., et al. (2020). Physiological and proteomic responses of mangrove plant Avicennia marina seedlings to simulated periodical inundation. Plant Soil 1, 231–254. doi: 10.1007/s11104-020-04474-8
Lovelock, C. E., Ball, M. C., Martin, K. C., and Feller, I. (2009). Nutrient enrichment increases mortality of mangroves. PLoS ONE 5,e5600. doi: 10.1371/journal.pone.0005600
Lovelock, C. E., Feller, I. C., Reef, R., Hickey, S., and Ball, M. C. (2017). Mangrove dieback during fluctuating sea levels. Scient. Reports 1, 1–8. doi: 10.1038/s41598-017-01927-6
Marpaung, B. A. (2021). Preferensi Habitat Mangrove Berdasarkan Sifat Edafik Dan Kualitas Air (Studi Kasus: Mangrove Desa Purworejo Kecamatan Pasir Sakti Kabupaten Lampung Timur). Universitas Gadjah Mada.
Marpaung, B. A., Budiadi, B., Pratiwiningrum, A., Lestari, L. D., Nurjanto, H. H., and Widiyatno, W. (2022). Interspecific associations of mangrove species and their preferences for edaphic factors and water quality. Biodiv. J. Biol. Div. 23, 9. doi: 10.13057/biodiv/d230929
Mbense, S., Rajkaran, A., Bolosha, U., and Adams, J. (2016). Rapid colonization of degraded mangrove habitat by succulent salt marsh. South Af. J. Bot. 107, 129–136. doi: 10.1016/j.sajb.2016.06.011
McCarthy, M. J., Jessen, B., Barry, M. J., Figueroa, M., McIntosh, J., Murray, T., et al. (2020). Automated high-resolution time series mapping of mangrove forests damaged by hurricane Irma in Southwest Florida. Remote Sens. 11, 1740. doi: 10.3390/rs12111740
Melo, H., Tiago, Q., Ferreira, O., Asensio, V., Okuma, I., Ferraz, B., et al. (2022). Changes in soil iron biogeochemistry in response to mangrove dieback. Biogeochemistry 3, 357–372. doi: 10.1007/s10533-022-00903-1
Miah, M. A. Q., and Moula, M. G. (2019). Effect of NPK fertilizers on seedling growth of mangrove species. J. Biosci. Agricult. Res. 01, 1687–1693. doi: 10.18801/jbar.200119.205
Mohd, O., Suryanna, N., Sahibuddin, S. S., Abdollah, M. F., and Selamat, S. R. (2012). Thresholding and fuzzy rule-based classification approaches in handling mangrove forest mixed pixel problems associated with in QuickBird remote sensing image analysis. Int. J. Agric. For 2, 300–306. doi: 10.5923/j.ijaf.20120206.06
Monroy, R., Robert, R., Eguren, A., Moya, C., Monroy, C., Victoria, L., et al. (2011). Aventuras y desventuras en Macondo: rehabilitación de la Ciénaga Grande de Santa Marta, Colombia. Ecotropicos 2, 72–93
Mulyana, B., Purwanto, R. H., Sari, P. I., Hidayatullah, M. F., Marpaung, A. A., Putra, I. S. R., et al. (2021). The environmental services of Pangarengan mangrove forest in Cirebon, Indonesia: conserving biodiversity and storing carbon. Biodiv. J. Biol. Div. 22, 12. doi: 10.13057/biodiv/sd221246
Musa, M., Lusiana, E. D., Buwono, N. R., Arsad, S., and Mahmudi, M. (2020a). The effectiveness of silvofishery system in water treatment in intensive whiteleg shrimp (Litopenaeus vannamei) ponds, probolinggo district, East Java, Indonesia. Biodiv. J. Biol. Div. 21, 10. doi: 10.13057/biodiv/d211031
Musa, M., Mahmudi, M., Arsad, S., and Buwono, N. R. (2020b). Feasibility study and potential of pond as silvofishery in coastal area: Local case study in Situbondo Indonesia. Reg. Stud. Marine Sci. 33, 100971. doi: 10.1016/j.rsma.2019.100971
Naidoo, G. (2009). Differential effects of nitrogen and phosphorus enrichment on growth of dwarf Avicennia marina mangroves. Aquatic Botany 2, 184–190. doi: 10.1016/j.aquabot.2008.10.001
Naidoo, G., and Naidoo, K. (2017). Are pioneer mangroves more vulnerable to oil pollution than later successional species? Marine Pollut. Bullet. 1, 135–142. doi: 10.1016/j.marpolbul.2017.05.067
Naidoo, G., Naidoo, Y., and Achar, P. (2010). Responses of the mangroves Avicennia marina and Bruguiera gymnorrhiza to oil contamination. Flora Morphol. Distribut. Funct. Ecol. Plants, 5, 357–362. doi: 10.1016/j.flora.2009.12.033
Nardin, W., Vona, I., and Fagherazzi, S. (2021). Sediment deposition affects mangrove forests in the Mekong delta, Vietnam. Contt. Shelf Res. 213, 104319. doi: 10.1016/j.csr.2020.104319
Njana, M. A. (2017). Indirect methods of tree biomass estimation and their uncertainties. Southern Forests: J. Forest Sci. 1, 41–49. doi: 10.2989/20702620.2016.1233753
Obodai, J., Adjei, K. A., Odai, S. N., and Lumor, M. (2019). Land use/land cover dynamics using landsat data in a gold mining basin-the Ankobra, Ghana. Remote Sens. Appl. Soc. Environ. 13, 247–256. doi: 10.1016/j.rsase.2018.10.007
Okello, J. A., Kairo, J. G., Dahdouh-Guebas, F., Beeckman, H., and Koedam, N. (2020). Mangrove trees survive partial sediment burial by developing new roots and adapting their root, branch and stem anatomy. Trees – Struct. Funct. 1, 37–49. doi: 10.1007/s00468-019-01895-6
Onrizal, O., and Kusmana, C. (2008). Ecological study on mangrove forest in East Coast of North Sumatra. Biodiv. J. Biol. Div. 9, 1. doi: 10.13057/biodiv/d090107
Páez-Osuna, F. (2001). The environmental impact of shrimp aquaculture: causes, effects, and mitigating alternatives. Environ. Manage. 1, 131–140. doi: 10.1007/s002670010212
Patil, V., Singh, A., Naik, N., and Unnikrishnan, S. (2014). Estimation of carbon stocks in Avicennia marina stand using allometry, CHN analysis, and GIS methods. Wetlands 2, 379–391. doi: 10.1007/s13157-013-0505-y
Proffitt, C. E., and Devlin, D. J. (2005). Long-term growth and succession in restored and natural mangrove forests in southwestern Florida. Wetlands Ecol. Manag. 5, 531–551. doi: 10.1007/s11273-004-2411-9
Quevedo, J. M. D., Uchiyama, Y., and Kohsaka, R. (2020). Perceptions of local communities on mangrove forests, their services and management: Implications for Eco-DRR and blue carbon management for Eastern Samar, Philippines. J. Forest Res. 1, 1–11. doi: 10.1080/13416979.2019.1696441
Reef, R., Feller, I. C., and Lovelock, C. E. (2010). Nutrition of mangroves. Tree Physiol. 9, 1148–1160. doi: 10.1093/treephys/tpq048
Reis, C. R. G., Nardoto, G. B., and Oliveira, R. S. (2017). Global overview on nitrogen dynamics in mangroves and consequences of increasing nitrogen availability for these systems. Plant Soil 1, 1–19. doi: 10.1007/s11104-016-3123-7
Romero, I. C., Jacobson, M., Fuhrman, J. A., Fogel, M., and Capone, D. G. (2012). Long-term nitrogen and phosphorus fertilization effects on N2 fixation rates and nifH gene community patterns in mangrove sediments. Marine Ecol. 1, 117–127. doi: 10.1111/j.1439-0485.2011.00465.x
Senger, D. F., Saavedra Hortua, D. A., Engel, S., Schnurawa, M., Moosdorf, N., and Gillis, L. G. (2021). Impacts of wetland dieback on carbon dynamics: a comparison between intact and degraded mangroves. Sci. Total Environ. 753, 141817. doi: 10.1016/j.scitotenv.2020.141817
Sidik, F., Neil, D., and Lovelock, C. E. (2016). Effect of high sedimentation rates on surface sediment dynamics and mangrove growth in the Porong River, Indonesia. Marine Pollut. Bullet. 1, 355–363. doi: 10.1016/j.marpolbul.2016.02.048
Sims, D. A., and Gamon, J. A. (2002). Relationships between leaf pigment content and spectral reflectance across a wide range of species, leaf structures and developmental stages. Remote Sens. Environ. 2, 337–354. doi: 10.1016/S0034-4257(02)00010-X
Sippo, J. Z., Lovelock, C. E., Santos, I. R., Sanders, C. J., and Maher, D. T. (2018). Estuarine, Coastal and Shelf Science Mangrove mortality in a changing climate. An Overview. 215, 241–249. doi: 10.1016/j.ecss.2018.10.011
Sippo, J. Z., Santos, I. R., Sanders, C. J., Gadd, P., Hua, Q., Lovelock, C., et al. (2020). Linking climatic-driven iron toxicity and water stress to a massive mangrove dieback. Biogeosci. Discu. 2020, 1–27. doi: 10.5194/bg-2019-478
Sorboni, N. G., Pahlavani, P., and Bigdeli, B. (2019). Vegetation mapping of sentinel-1 and 2 satellite images using convolutional neural network and random forest with the aid of dual-polarized and optical vegetation indexes. Int. Arch. Photogram. Remote Sens. Spatial Inform. Sci. 42, 435–440. doi: 10.5194/isprs-archives-XLII-4-W18-435-2019
Suman, D. O. (2019). Chapter 31 - Mangrove Management: Challenges and Guidelines (G. M. E. Perillo, E. Wolanski, D. R. Cahoon, and C. S. B. T.-C. W. eds Second E. Hopkinson, pp. 1055–1079). Elsevier.
Suratman, M. N. (2008). “Carbon sequestration potential of mangroves in Southeast Asia,” in Managing Forest Ecosystems: The Challenge of Climate Change (Springer) (pp. 297–315). doi: 10.1007/978-1-4020-8343-3_17
Svejkovsky, J., Ogurcak, D. E., Ross, M. S., and Arkowitz, A. (2020). Satellite image-based time series observations of vegetation response to Hurricane Irma in the lower Florida Keys. Estuaries Coasts 43:5, 1058–1069. doi: 10.1007/s12237-020-00701-8
Trujillo, L. V. P., Mancera-pineda, J. E., Medina-calderon, J. H., and Zimmer, M. (2021). Massive loss of aboveground biomass and its effect on sediment organic carbon concentration: less mangrove, more carbon? Estu. Coastal Shelf Sci. 2, 106888. doi: 10.1016/j.ecss.2020.106888
Vaiphasa, C., Boer, W. F., de, Skidmore, A. K., Panitchart, S., Vaiphasa, T., Bamrongrugsa, N., et al. (2007). Impact of solid shrimp pond waste materials on mangrove growth and mortality: a case study from Pak Phanang, Thailand. Ecotropicos, 47–57. doi: 10.1007/s10750-007-0783-6
Yanagisawa, H., Koshimura, S., Miyagi, T., and Imamura, F. (2010). Tsunami damage reduction performance of a mangrove forest in Banda Aceh, Indonesia inferred from field data and a numerical model. J. Geophys. Res. Oceans 115:C6. doi: 10.1029/2009JC005587
Ye, Y., and Tam, N. F. Y. (2007). Effects of used lubricating oil on two mangroves Aegiceras corniculatum and Avicennia marina. J. Environ. Sci. 11, 1355–1360. doi: 10.1016/S1001-0742(07)60221-6
Yulianto, F., Prasasti, I., Pasaribu, J. M., Fitriana, H. L., Zylshal, Haryani, N. S., and Sofan, P. (2016). The dynamics of land use/land cover change modeling and their implication for the flood damage assessment in the Tondano watershed, North Sulawesi, Indonesia. Modeling Earth Sys. Environ. 1, 47. doi: 10.1007/s40808-016-0100-3
Zaitunah, A., Meliani, S., Syahputra, O. K., Budiharta, S., Susilowati, A., Rambe, R., et al. (2021). Mapping of mangrove forest tree density using SENTINEL 2A satelit image in remained natural mangrove forest of Sumatra eastern coastal. IOP Conf. Ser. Earth Environ. Sci. 1,12001. doi: 10.1088/1755-1315/912/1/012001
Zhen, J., Jiang, X., Xu, Y., Miao, J., Zhao, D., Wang, J., et al. (2021). Mapping leaf chlorophyll content of mangrove forests with Sentinel-2 images of four periods. Int. J. Appl. Earth Observ. Geoinform. 102, 102387. doi: 10.1016/j.jag.2021.102387
Zhu, X., Hou, Y., Weng, Q., and Chen, L. (2019). Integrating UAV optical imagery and LiDAR data for assessing the spatial relationship between mangrove and inundation across a subtropical estuarine wetland. ISPRS J. Photogram. Remote Sens. 149, 146–156. doi: 10.1016/j.isprsjprs.2019.01.021
Keywords: mangrove dieback, spatial analysis, N enrichment, Avicennia marina, silvofishery
Citation: Budiadi B, Pertiwiningrum A, Lestari LD, Jihad AN, Marpaung BA and Prasetyo S (2023) Land cover changes, biomass loss, and predictive causes of massive dieback of a mangrove plantation in Lampung, Sumatra. Front. For. Glob. Change 6:1150949. doi: 10.3389/ffgc.2023.1150949
Received: 25 January 2023; Accepted: 31 March 2023;
Published: 27 April 2023.
Edited by:
Rambod Abiri, Putra Malaysia University, MalaysiaReviewed by:
Fernanda Adame, Griffith University, AustraliaAbu Hena Mustafa Kamal, University of Malaysia Terengganu, Malaysia
Henti Hendalastuti Rachmat, National Research and Innovation Agency (BRIN), Indonesia
Copyright © 2023 Budiadi, Pertiwiningrum, Lestari, Jihad, Marpaung and Prasetyo. This is an open-access article distributed under the terms of the Creative Commons Attribution License (CC BY). The use, distribution or reproduction in other forums is permitted, provided the original author(s) and the copyright owner(s) are credited and that the original publication in this journal is cited, in accordance with accepted academic practice. No use, distribution or reproduction is permitted which does not comply with these terms.
*Correspondence: Budiadi Budiadi, budiadi@ugm.ac.id