- 1College of Fisheries, Guangdong Ocean University, Zhanjiang, China
- 2Faculty of Marine Science and Fisheries, Hasanuddin University, Makassar, Indonesia
This study examined the population dynamics and seasonal variation (winter-summer) in the biological characteristics of black sea bream (Acanthopagrus schlegelii) in Zhanjiang coastal waters, China. Key growth parameters were estimated using the von Bertalanffy growth model, revealing an asymptotic length (L∞) of 505.05 mm and a growth coefficient (K) of 0.26 year-¹, with an estimated longevity of 11.24 years. Mortality analysis showed a total mortality rate (Z) of 0.35 year-¹, primarily driven by natural causes (M = 0.33 year-¹) with minimal fishing impact (F = 0.02 year-¹), resulting in a low exploitation rate (E) of 0.05. Length-weight relationship (LWR) analysis indicated positive allometric growth in females and nearly isometric growth in males, with significant seasonal variations in condition factors (K). Yield Per Recruit (YPR) analysis identified a maximum sustainable exploitation rate (Emax) of 0.421, with a conservative management approach suggested at E0.1 = 0.355. Seasonal variations in reproductive indices, with higher Gonadosomatic Index (GSI) and Hepatosomatic Index (HSI) in winter, highlight the species’ reproductive cycle. The findings suggest that Acanthopagrus schlegelii is stable, with low fishing pressure. Seasonal GSI and HSI variations suggest fisheries management should time fishing to reduce impacts on reproductive success. Maintaining exploitation rates below Emax is essential for sustainable management, contributing valuable insights for fisheries conservation in coastal China.
1 Introduction
China’s coastal waters host diverse and economically vital fish species, making them essential to fisheries and marine ecosystems (Shan et al., 2020). However, they face significant sustainability challenges, including overfishing, habitat degradation, pollution, and climate change (Xu and Zhang, 2022). Overfishing has particularly impacted key fish stocks, notably the black sea bream (Acanthopagrus schlegelii), which once supported a thriving fishery (Jiatong et al., 2021). Since the late 20th century, the decline of black sea bream has had far-reaching consequences, disrupting not only the ecological balance by weakening invertebrate control and benthic health but also undermining local economies that once depended on the species for a stable fishery (Nip et al., 2003). The continued pressure on this species has exacerbated these challenges, making its recovery critical for marine ecosystem health and economic sustainability.
Globally, fisheries management strategies have been developed to address overexploitation and habitat degradation, focusing on setting fishing limits, improving gear selectivity, and restoring critical habitats. These approaches are essential for sustaining fish populations and supporting marine ecosystems. In China, similar strategies have been applied to various species, using stock assessment tools to monitor and manage populations (Wang et al., 2022). These tools help implement effective measures like habitat protection and sustainable fishing practices, aiming to restore overexploited species and maintain the health of coastal waters. Hatchery release programs have also been increasingly used, particularly for black sea bream, alongside research and conservation efforts (Wang et al., 2021). However, more efforts are needed to address the complex interplay of factors threatening the health and productivity of China’s coastal waters. Collaboration among scientists, policymakers, industry stakeholders, and local communities will be crucial in finding innovative solutions to ensure the long-term sustainability of China’s fisheries and marine resources. Given the species’ importance to local ecosystems and fisheries, updating its population status is crucial. Continuous assessments and refining management strategies are necessary to ensure the long-term sustainability of black sea bream and its role in marine ecosystem stability and economic viability.
Length and weight analysis are fundamental tools in fisheries science, providing critical insights into fish populations’ growth, condition, and health. Assessing the black sea bream population through these metrics may lay the groundwork for effective management. These assessments offer valuable data on growth rates, body condition, and overall population health. The weight of internal organs, especially the liver and gonads, is key for understanding the species’ reproductive status and its approach to energy allocation, especially in response to environmental conditions and fishing pressure (Akter et al., 2019; Ladisa et al., 2021; Zhang K. et al., 2022). These relationships allow for accurate modelling of population dynamics. Moreover, they help to detect shifts in population structure that may result from fishing pressure or environmental variations. Such insights are vital for designing strategies to sustain species and optimize stock enhancement efforts.
Building on the significance of length and weight analysis, this study aims to update the population status of black sea bream (Acanthopagrus schlegelii) by investigating key population dynamics parameters, including growth rates, mortality, capture probability, Virtual Population Analysis (VPA), and Yield Per Recruit (YPR). It also seeks to evaluate biological characteristics such as length-weight relationships, condition factors, and organ weights, including the Gonadosomatic Index (GSI) and Hepatosomatic Index (HSI). A particular focus is placed on the seasonal variations in these biological characteristics, comparing winter and summer seasons. By examining how these traits fluctuate across seasons, the study aims to provide a more comprehensive understanding of the factors affecting population sustainability and to inform more effective management strategies.
The uniqueness of this study lies in its integration of traditional growth and mortality metrics, vital for understanding population dynamics, with organ weight analysis (GSI, HSI), providing a more holistic view of the population’s reproductive and physiological health. This comprehensive approach is critical for refining stock management strategies and enabling informed decision-making. By combining these methodologies, the study evaluates how the analyzed factors affect the sustainability of black sea bream stocks. The findings from this research will offer valuable insights for setting appropriate fishing limits and improving gear selectivity to protect juvenile black sea bream better, thereby contributing to the long-term sustainability of the population. Moreover, these insights will strengthen stock management strategies by providing a thorough assessment of population health and dynamics, supporting effective species management and contributing to the overall stability of the marine ecosystem.
2 Materials and methods
2.1 Study area and data collection
Individuals of Acanthopagrus schlegelii were collected from fishing ports in Zhanjiang, China, between 2019 and 2021 (Figure 1). The sample represents the population as fish were sourced from multiple regional fishing ports, ensuring spatial variability. The three-year sampling period provided temporal coverage, capturing potential changes in the population over time. After collection, the fish were immediately placed on ice and transported to the laboratory to preserve their biological integrity. The specimens were measured in the laboratory for key biological and growth parameters. Total lengths were recorded to the nearest 0.01 mm using a digital caliper. Body weight, liver weight, and gonad weight were recorded to the nearest 0.01 g using an electronic balance.
2.2 Methods and analysis
2.2.1 ELEFAN method and empirical formulas
The ELEFAN (Electronic Length Frequency Analysis) method (FiSAT II software, version 1.2.2) (Gayanilo et al., 2005) was employed to estimate key growth parameters, Mortality and Exploitation rates, capture probabilities, Virtual population, and Yield Per Recruit parameters. Length frequencies of 1492 individuals were used for this analysis.
2.2.1.1 Growth parameters
The growth parameters, the asymptotic length (L∞), and the growth coefficient (K) were estimated using the von Bertalanffy Growth Function (VBGF) (Beverton and Holt, 1957), which is expressed as:
Where Lt is the length at age t, and t0 is the theoretical age at zero length.
The analyses for estimation of t0, longevity (tmax), and the Growth Performance Index (Φ) were conducted using formulas (2) (Pauly, 1983), (3) (Pauly, 1980), and 4 (Munro and Pauly, 1983), respectively. L∞ and K were used as inputs.
2.2.1.2 Mortality and exploitation analysis
Total Mortality (Z) was calculated using a length-converted catch curve in the FiSAT II method. Natural Mortality (M) was estimated using Pauly’s formula (Pauly, 1980), which considers L∞, K, and the study area’s annual average sea surface temperature (T), which was 27°C.
Fishing Mortality (F) was calculated by subtracting M from Z. Exploitation ratio (E) was calculated by the ratio of fishing mortality to total mortality (F/Z) (Gulland, 1971).
2.2.1.3 Gear-related analyses and YPR
The capture probability for Acanthopagrus schlegelii was estimated using FiSAT II, focusing on gear selectivity at key lengths. The software determined the lengths at which 25%, 50%, and 75% of the population were likely to be captured, known as L25, L50, and L75, respectively. Additionally, the maturity size L50 was estimated using the method described by (Hoggarth et al., 2006), while L95 was calculated following the approach outlined by (Prince et al., 2015), with the formulas provided below:
A Virtual Population Analysis (VPA) was conducted to evaluate the impact of fishing mortality on the population structure across various length groups. By combining VPA with capture probability and maturity assessments, insights were gained into how fishing pressures and gear selectivity influence different size classes. Additionally, a Yield Per Recruit (YPR) analysis, based on the Beverton and Holt model within the FiSAT II software, was performed to estimate the expected yield from a recruit. This analysis assessed various fishing mortality rates to identify the optimal level that maximizes YPR while ensuring the sustainability of the fish population.
2.2.2 Seasonal health and growth assessments
Seasonal comparisons (winter and summer) were made to understand fluctuations in the species’ health by performing LWR, Condition factor, Gonadosomatic and Hepatosomatic Indices Analysis.
2.2.2.1 LWR and condition factors
The length-weight relationship was analyzed in R software (version 4.3.3) to assess the growth patterns in Acanthopagrus schlegelii using the power function (Ricker, 1973):
Where W is the body weight (g), L is the total length (mm), and a and b are constants determined by the specific population.
The parameters a and b were estimated using a log-log regression model. The analysis involved identifying and removing outliers based on Cook’s Distance to ensure the robustness of the model. The model’s fit was assessed using the coefficient of determination (R²) and ANOVA. Confidence intervals for the parameters a and b were estimated using bootstrapping, providing a measure of the precision of these estimates. A t-test was conducted to evaluate the significance of slope b, including a comparison against an expected value of 3 to assess isometric growth. The p-value associated with the slope b was calculated to determine the statistical significance of this parameter.
Fulton’s Condition Factor (K) (Froese, 2006) was calculated in R software (version 4.3.3) to evaluate the health and condition of the fish using the formula:
Where W is the body weight of the fish (g) and L is the total length of the fish (cm). The condition factor (K) provided insights into the fish’s nutritional status and overall health, with values greater than 1.0 indicating better condition.
2.2.2.2 GSI and HSI
Descriptive statistics of paired data on body weight, gonad weight, and liver weight (winter: n = 75; summer: n = 79) were conducted using R software (version 4.3.3). These analyses supported the calculation of the Gonadosomatic Index (GSI) and Hepatosomatic Index (HSI) to evaluate reproductive and nutritional status across seasons (King, 2007).
Independent t-tests were conducted to determine whether there were significant differences in GSI and HSI between the two seasons. Welch’s t-test was used to account for unequal variances between groups. Additionally, non-parametric Wilcoxon rank-sum tests were performed to confirm the t-test results, as these tests do not assume normality and are robust to outliers. A one-way ANOVA was conducted further to assess the effect of season on GSI and HSI. Multiple linear regression models were applied to explore the relationships between GSI, HSI, and predictor variables (body weight, gonad weight, liver weight, and season). At the same time, Pearson correlation coefficients provided insights into the interrelationships among these variables.
3 Results
3.1 Growth parameters, mortality, and exploitation
The von Bertalanffy growth function analysis using FiSAT II (Figure 2) estimated an asymptotic length (L∞) of 505.05 mm, indicating the maximum potential size the species can reach. The growth coefficient (K) of 0.26 year-1 suggests a moderate growth rate, while the theoretical age at zero length (t0) was calculated to be -0.296 years. The species’ estimated longevity is approximately 11.24 years, highlighting its potential lifespan under natural conditions. The growth performance index (Φ) was determined to be 4.82.
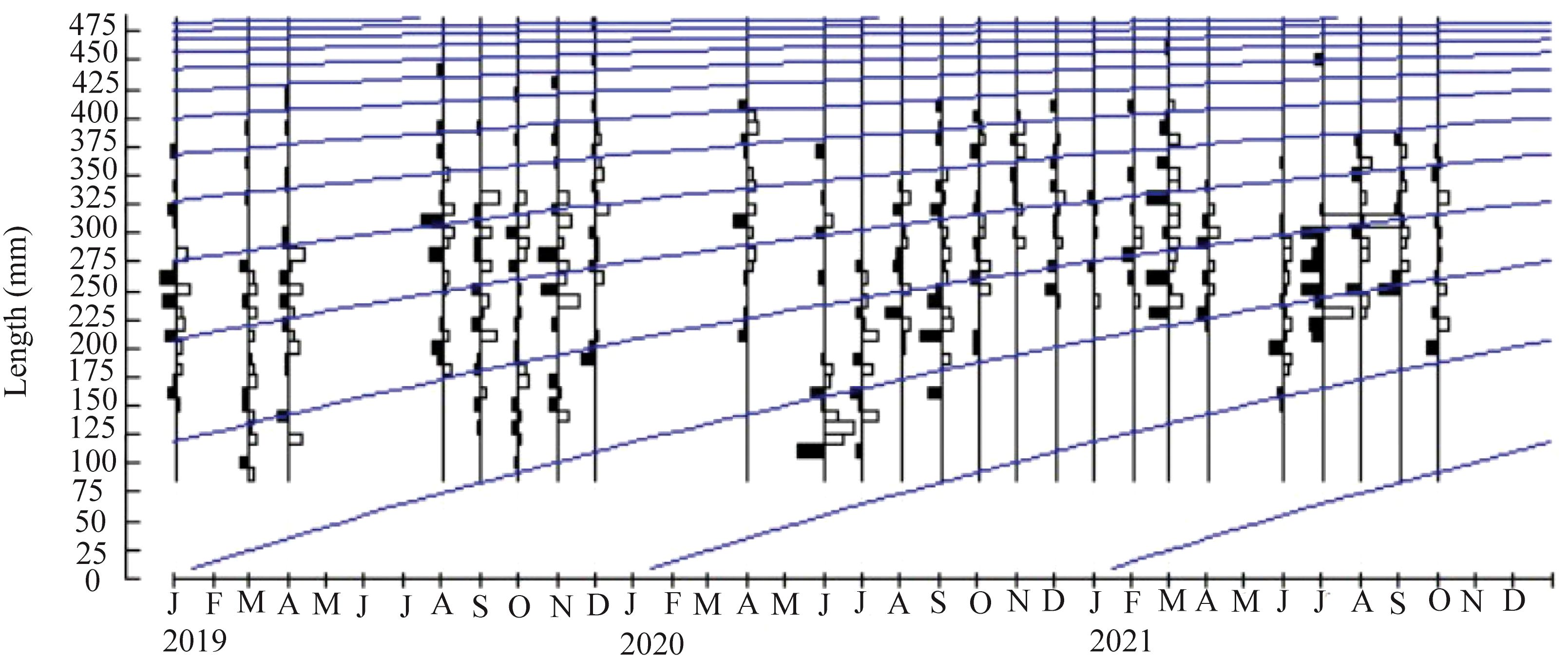
Figure 2. Monthly length-frequency distribution of Acanthopagrus schlegelii collected from 2019 to 2021. The horizontal main axis represents the months and years of data collection, while the vertical main axis shows fish length in mm. Blue growth curves have been fitted to the data, representing the progression of cohorts over time. Data points correspond to individual fish lengths recorded during each sampling month, and the distribution suggests multiple cohort recruitment events across the study period.
The length-converted catch curve analysis revealed a total mortality rate (Z) of 0.35 year-¹ (Figure 3A). Natural mortality (M) was the predominant factor, contributing 0.33 year-¹, while the fishing mortality rate (F) was low at 0.02 year-¹. This resulted in an exploitation rate (E) of 0.05, indicating that 5% of total mortality was attributable to fishing activities. These mortality rates provide a quantitative assessment of the factors influencing the population of Acanthopagrus schlegelii under current conditions.
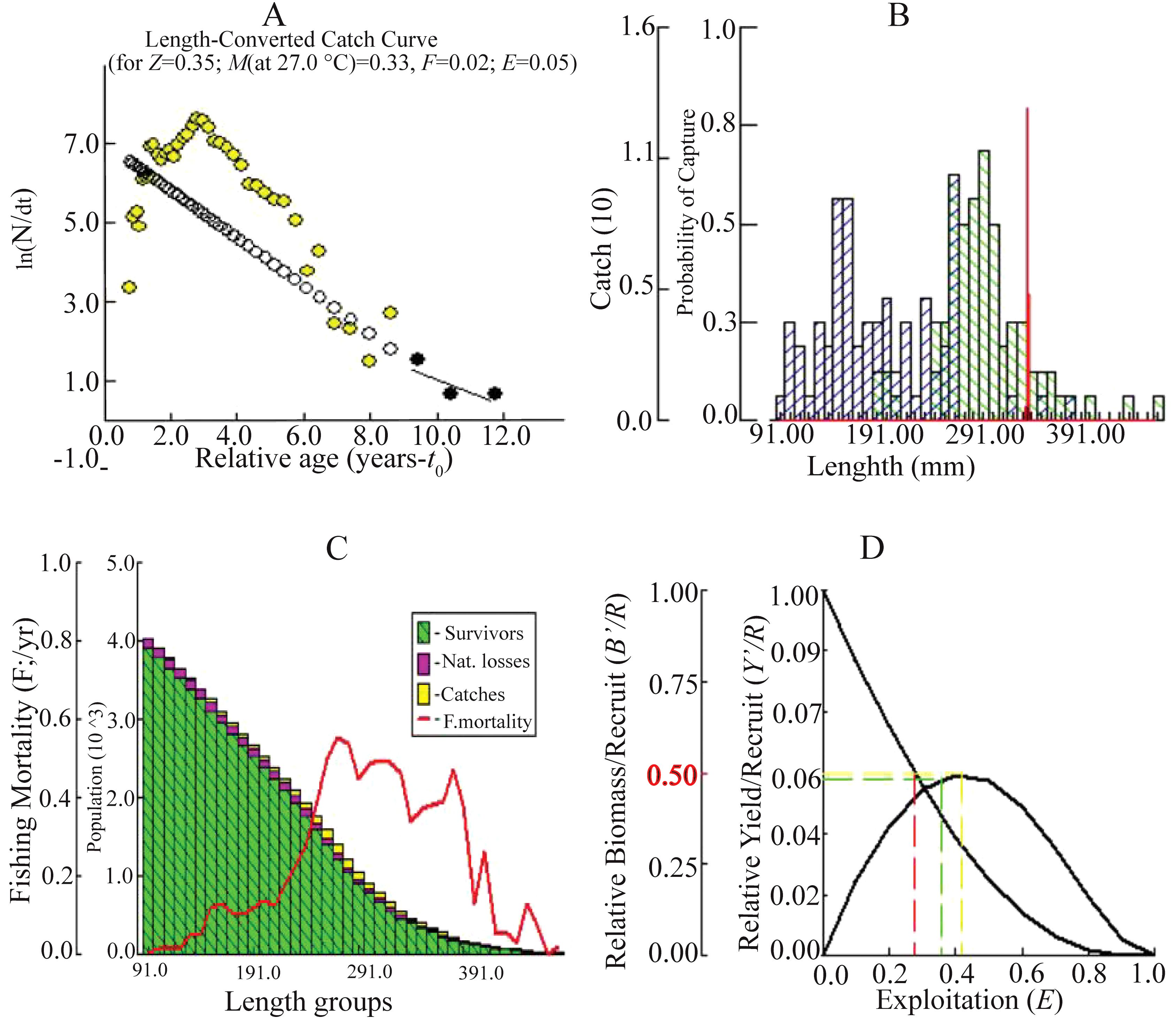
Figure 3. Outputs from the FiSAT II analysis of Acanthopagrus schlegelii. (A) Length-Converted Catch Curve Analysis with Estimated Mortality Rates (Z, M, F) and Exploitation Rate (E). (B) Length-frequency distribution and gear selectivity with capture probabilities (L25, L50, L75). (C) Population structure and fishing mortality across length groups (D) Relationship between Exploitation Ratio (E), Relative Yield per Recruit (Y’/R), and Relative Biomass per Recruit (B’/R).
3.2 Gear selectivity
Figure 3B illustrates the length-frequency distribution alongside the probability of capture, showing that most fish caught were within the 150 mm to 350 mm range, with a peak around 290 mm. The lengths at which 25%, 50%, and 75% of the fish population were likely to be captured were estimated as L25 at approximately 220 mm, L50 at 290 mm, and L75 at 340 mm, respectively. The capture probability reached its maximum (close to 1.0) around 370 mm, with the size at first maturity Lm estimated at 336.7 mm and 95% maturity (L95) at 370.37 mm. These findings suggest that the gear is highly selective for fish that are near or have reached maturity.
Figure 3C shows that the population decreases steadily as fish length increases, with the highest survival rate in the smallest length group (around 91.0 mm) and fewer survivors in larger groups. Natural losses are fairly consistent but more pronounced in larger-size classes. Catch rates peak around 290 mm before dropping sharply. Fishing mortality (F), indicated by the red line, increases with length, peaking at 320 mm, then fluctuates and decreases in the largest groups. These findings underscore selective fishing pressure on mid-sized fish, with smaller and larger fish facing lower fishing mortality, highlighting the impact of the gear on the population.
3.3 Seasonal health and growth assessment
3.3.1 LWR and conditional factors
The growth patterns of Acanthopagrus schlegelii, as indicated by length-weight relationships, differ by sex and season (Table 1, Figure 4). Females show slope (b) values above 3 in both winter (3.053) and summer (3.217), indicating positive allometric growth, with weight increasing at a greater rate than length, especially in summer. In contrast, males exhibit slope (b) values just below 3 in winter (2.883) and summer (2.912), indicating nearly isometric growth, where weight and length increase at almost proportional rates.
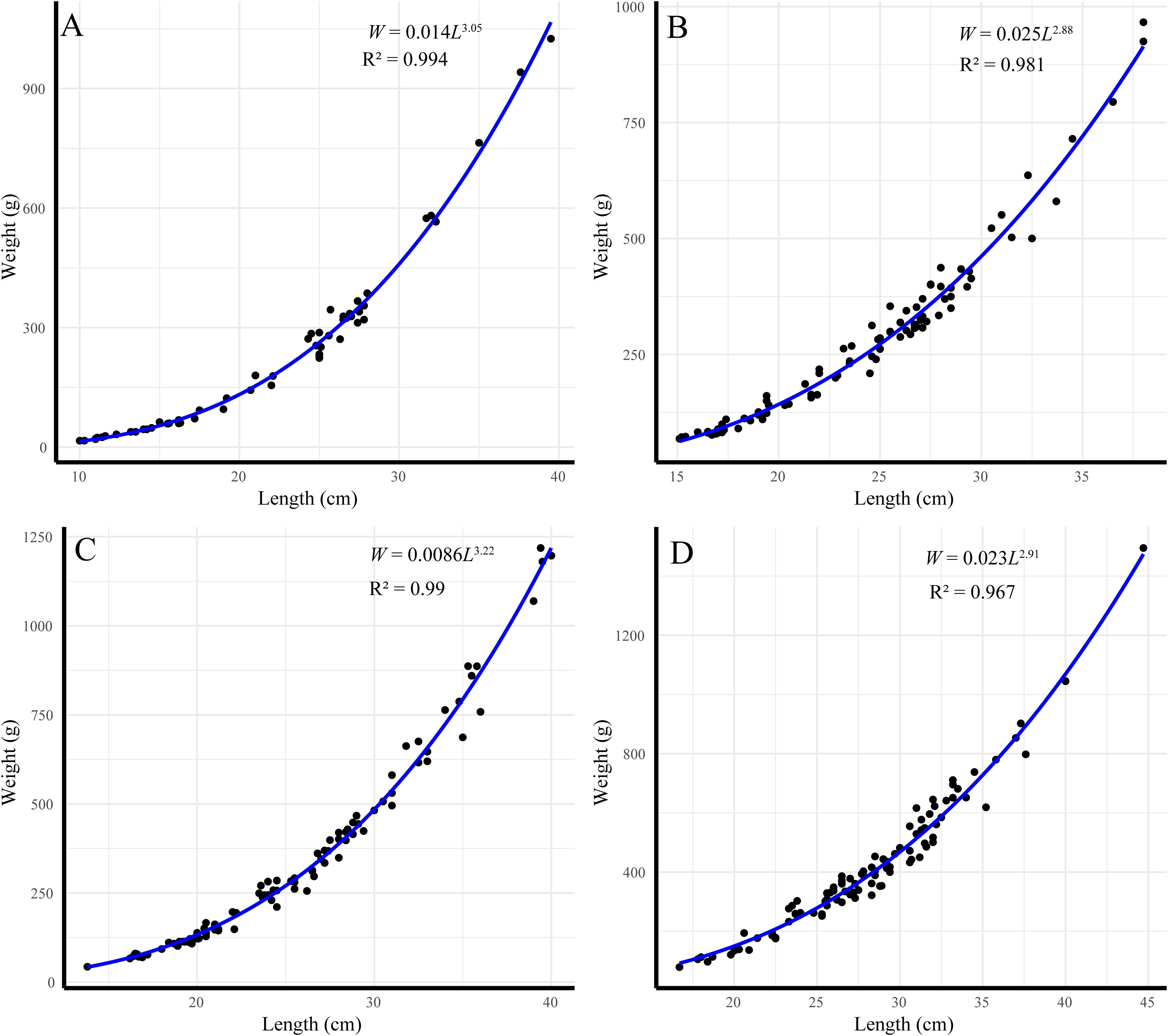
Figure 4. Length-weight relationship of Acanthopagrus schlegelii in the Zhanjiang coastal waters. (A) Female, winter season (B) Male, winter season (C) Female, summer season (D) Male, summer season.
The condition factor (K) of fish, used as a metric for their well-being, was shown to have differing values based on sex and season. For females, the mean (K) was higher in summer (1.726) than in winter (1.655), suggesting that females were in better condition during the summer. In contrast, males consistently had higher mean (K) values in both winter (1.757) and summer (1.754) compared to females, indicating that males were generally in better physical condition throughout the year.
ANOVA and t-tests confirmed that the differences in growth patterns and condition factors between sexes and seasons were statistically significant. ANOVA results revealed a significant difference in mean condition factors, showing that females were in better condition during summer, while males maintained a stable condition year-round. The t-tests validated the distinctions between sexes, highlighting the biological significance of these seasonal and sex-based variations.
3.3.2 GSI and HSI
In summer, the mean GSI was 0.226% (SD = 0.166%), and the mean HSI was 0.89% (SD = 0.387%). In winter, the mean GSI increased to 4.49% (SD = 2.21%), and the mean HSI increased to 1.34% (SD = 0.546%). Statistical analyses, as summarized in Table 2, revealed significant seasonal differences.
The t-test and Wilcoxon rank-sum test confirmed a significant difference in GSI and HSI between summer and winter. ANOVA also demonstrated a significant seasonal effect on both GSI and HSI. Multiple linear regression models accounted for 91.31% of the variance in GSI and 85.35% of the variance in HSI. Significant predictors for both GSI and HSI were body weight, gonad weight, liver weight, and season. The Pearson correlation coefficient between GSI and HSI was moderate (r = 0.42). The full correlation matrix can be found in Table 3.
4 Discussion
This study provides an updated assessment of the black sea bream (Acanthopagrus schlegelii) population in the Northern South China Sea, where the species holds considerable commercial and socio-economic significance. The analysis focused on the population dynamics and biological characteristics, comprehensively evaluating the species’ status. Growth rates, mortality, and capture probability were assessed alongside the Virtual Population Analysis (VPA) and Yield Per Recruit (YPR), providing a detailed picture of the population’s response to current fishing pressures. Key biological characteristics, such as length, weight, and organ weights (liver and gonads), were evaluated through the Hepatosomatic Index (HSI) and Gonadosomatic Index (GSI). The indices helped reveal the species’ energy allocation strategies and reproductive health, particularly the seasonal variation between winter and summer. The condition factors have added valuable context to the population’s overall health. The species’ decline in the 1980s prompted significant management interventions, including hatchery releases aimed at stock enhancement. This study provides updated insights into these efforts, helping to refine current management strategies and inform future decisions. By integrating population dynamics with key biological metrics, the study contributes essential information for sustaining black sea bream stocks and supporting the long-term viability of the fishery.
4.1 Growth and population structure analysis
The estimated growth parameters offer valuable perspectives on the population dynamics and overall health of Acanthopagrus schlegelii. The asymptotic length (L∞) of 505.05 mm indicates the species’ potential to reach a substantial size in optimal conditions. According to previous studies on this species in the Northern South China Sea (Table 4), large asymptotic lengths indicate species exhibiting traits associated with healthy and robust populations, such as fast growth rates and significant contributions to stock enhancement efforts. The moderate growth coefficient (K) of 0.26 year-¹ suggests that the species experiences a relatively slow but steady growth rate which is consistent with its estimated longevity of 11.24 years. Longevity indicates that Acanthopagrus schlegelii can sustain itself over extended periods, provided that environmental conditions remain favorable and that fishing pressure is managed appropriately. The growth performance index (Φ) of 4.82, a key indicator of the species’ growth efficiency, suggests that Acanthopagrus schlegelii is well-adapted to its environment. A high growth performance index (Φ) indicates rapid growth and the ability to reach large sizes, key factors for boosting stock productivity and faster biomass recovery. This study’s t0 value of -0.296 implies earlier growth to measurable sizes than in past studies (Table 4). Early growth, marked by rapid size increases, is vital for population sustainability. It helps reduce predation, enhances reproductive success, and boosts resilience to environmental changes (Beverton and Holt, 1957; Lorenzen, 2000). These factors are key in stock assessment models, allowing for more accurate predictions of biomass, recruitment, and stock health (Lorenzen, 2000). Early growth and high growth performance guide management strategies, particularly size limits and fishing pressure adjustments, ensuring individuals reach reproductive size before harvest. As observed in this study, a stable growth rate and long lifespan suggest stock resilience if fishing mortality remains within sustainable limits (Chang et al., 2022).
This study’s growth parameters align with regional findings but show variations (Table 4) typical among sparids, often due to differences in habitat quality and fishing pressures (Law and Sadovy de Mitcheson, 2018). For example, populations in Hongkong report lower asymptotic lengths (403–437 mm) and growth coefficients (K = 0.22–0.28 year-¹), while a study in China observed a higher L∞ (543 mm) with a lower K (0.15 year-¹). Higher K values and greater longevity suggest reduced fishing pressure. Phylogenetic analyses reveal genetic diversity within the Sparidae family, providing insights into the evolutionary adaptations of the species (Wu et al., 2018). These findings underscore the importance of early growth traits and high growth performance indices in distinguishing Acanthopagrus schlegelii for its adaptive strategies and contributions to stock recovery. Furthermore, they emphasize the need for sustainable fishing practices and environmental conservation to ensure the resilience and long-term sustainability of Acanthopagrus schlegelii populations.
4.2 Mortality patterns and fishery exploitation
The mortality rates offer further insights into the current status of the Acanthopagrus schlegelii population (Figure 3A). The results highlight the dominance of natural mortality (M) over fishing mortality (F), suggesting that natural factors play a critical role in shaping population dynamics. With a minimal contribution of fishing to total mortality, the exploitation rate remains low, underscoring the relatively low impact of current fishing practices. These findings suggest that the population is stable and that the existing fishing pressure is sustainable. However, careful monitoring is required to ensure that any future increases in fishing activity do not compromise this stability. Sustainable management practices should continue to prioritize maintaining low fishing pressure to support the long-term health of the stock (Melnychuk et al., 2021).
The observed dominance of natural mortality in this population is likely driven by a combination of ecological factors, including habitat degradation, water quality fluctuations, predation, and disease. Environmental stressors, such as seasonal changes in temperature, low dissolved oxygen, and habitat degradation, may exacerbate stress on juveniles and sub-adults, leading to higher mortality rates. These drivers are likely to have a more significant impact on population dynamics than fishing mortality, particularly under conditions of low exploitation. For example, seasonal temperature fluctuations can affect metabolic rates, while hypoxic events may lead to localized die-offs, especially in nursery habitats. In addition, predation pressure, both from natural predators and the increased vulnerability of stressed individuals, can further elevate natural mortality rates. Furthermore, disease outbreaks can cause significant population losses, particularly when fish are already physiologically stressed by environmental factors. These ecological drivers likely regulate the population more effectively than fishing pressure alone. Continued research into these factors is essential for identifying key stressors and informing targeted management strategies that can mitigate their effects. By addressing these challenges such as habitat quality and predation pressures, alongside maintaining low fishing pressure, we can enhance the long-term resilience and sustainability of Acanthopagrus schlegelii populations.
4.3 Capture efficiency and yield optimization
The analysis of capture probability and selectivity, as illustrated in Figures 3B–D, reveals important insights into the interaction between the species and the fishing gear used. The results suggest that the gear is selective for individuals approaching maturity, which is encouraging for sustainable fisheries management (Froese, 2004). The estimated lengths at which fish were likely to be captured indicate that the fishing gear targets fish that have already reached a significant size, thereby reducing the chance of catching smaller, immature individuals. Moreover, the close match between L50 and the peak capture probability suggests that current fishing practices are sustainable. By targeting fish that are near or have already reached maturity, the gear helps ensure that individuals have the opportunity to reproduce before being caught (Froese, 2004). This is vital for preserving the population’s reproductive potential and ensuring long-term stock viability. Moreover, the findings suggest that any changes in gear selectivity, such as increasing mesh size, could further enhance the sustainability of the fishery by allowing more fish to reach maturity before being caught (Gatti et al., 2019).
The Yield Per Recruit (YPR) analysis findings call for careful control of fishing efforts in the Acanthopagrus schlegelii fishery. It shows that yield increases with exploitation, peaking at Emax = 0.421, after which it declines Figure 3D. Exceeding this rate risks overfishing, making it essential to keep exploitation below Emax for sustainability. Two important reference points have been identified in the analysis. The first, E0.5 = 0.278, indicates the level at which the biomass per recruit is reduced to 50% of its unfished level, reflecting the effects of moderate exploitation on the population. The second, E0.1 = 0.355, represents a more conservative threshold that aims to balance yield with sustainability, helping to minimize the risk of overexploitation. These thresholds provide vital guidance for maintaining healthy fish stocks (Beverton and Holt, 1957).
4.4 Length-weight correlation and condition assessment
The growth patterns of Acanthopagrus schlegelii, as assessed through the length-weight relationship (LWR), highlight notable differences between sexes and seasons. Females demonstrate positive allometric growth, which may indicate higher energy allocation towards growth and reproductive activities, particularly during the summer. In contrast, males exhibit nearly isometric growth, suggesting a more balanced energy allocation between growth and other physiological processes (Figure 4). This difference between sexes may reflect different energy allocation strategies, with females potentially investing more in reproductive tissues, while males maintain a more balanced growth pattern throughout the year (Ladisa et al., 2021; Rljnsdorp and Ibelings, 1989).
The condition factor (K) further highlights these seasonal and sex-based differences. Females were found to be in better condition during the summer than in the winter, likely due to the increased energy demands of reproduction. On the other hand, males consistently exhibited higher condition factors in both seasons, suggesting that males maintain a generally better physical condition year-round. The statistical significance of these differences, confirmed by ANOVA and t-tests, underscores the biological relevance of these findings. The seasonal variations in K-values may be related to environmental factors such as food availability and water temperature, which can influence the energy reserves and overall health of the fish (Morato et al., 2001).
The LWR and K analyses provide a crucial understanding of the growth and health of Acanthopagrus schlegelii, indicating potential seasonal energy allocation shifts between males and females. Investigating the mechanisms behind these shifts could offer deeper insight into their effects on this species’ population dynamics.
4.5 Reproductive and nutritional health indicators
The GSI and HSI values provide valuable insights into the reproductive and nutritional status of Acanthopagrus schlegelii across seasons (Table 2). The significant increase in GSI during winter (mean GSI = 4.49%) compared to summer (mean GSI = 0.226%) suggests that the winter season is a critical period for reproductive activity (Figure 5A) (Zhang H. et al., 2022). This seasonal variation in GSI indicates the species’ spawning cycle, where energy is allocated towards gonad development in preparation for spawning. Temperature seems to affect sexual activity and breeding in fish (Davies and Bromage, 2002; Kawai et al., 2022). The higher GSI in winter aligns with the observed increase in HSI during the same period (mean HSI = 1.34% in winter versus 0.89% in summer), suggesting that the liver, which stores energy reserves, plays a crucial role in supporting reproductive processes during the colder months (Figure 5B) (Abderrazik et al., 2016; Caballero-Huertas et al., 2022). The seasonal increase in GSI observed in Acanthopagrus schlegelii, peaking during winter, aligns with the reproductive cycle of Acanthopagrus latus, which also spawns during cooler seasons (Tang et al., 2023). This pattern suggests an adaptive energy allocation strategy, where both species prioritize gonad development during peak reproductive periods under optimal environmental conditions, likely to maximize offspring survival. However, a study on Acanthopagrus schlegelii from Japan reports a spawning period from April to June, indicating an extended spawning season as a response to varying ecological pressures (Yamashita et al., 2015). These findings highlight the importance of region-specific reproductive management strategies tailored to the unique spawning cycles of each species.
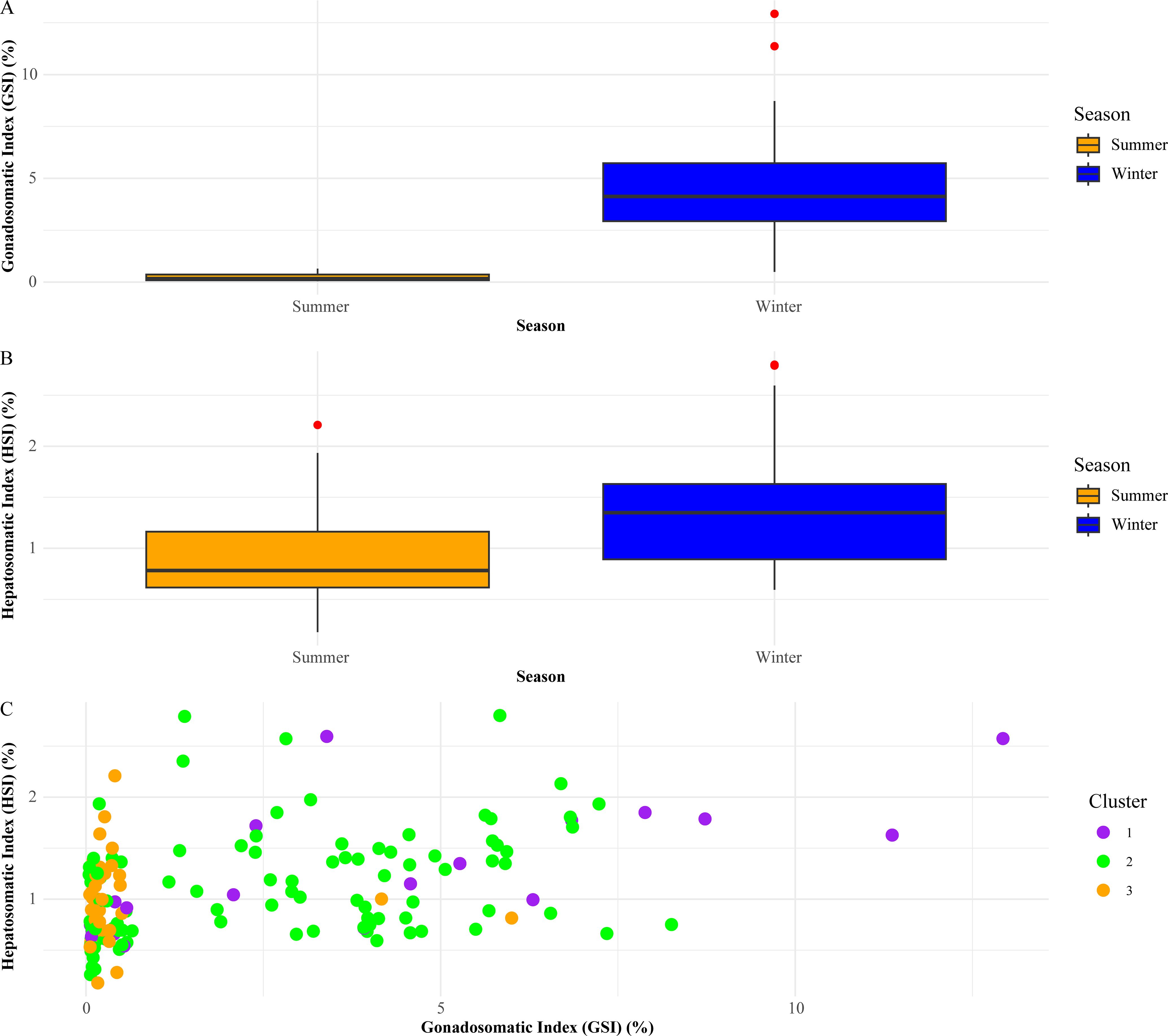
Figure 5. Seasonal variations in reproductive and nutritional status of fish. (A) Gonadosomatic Index (GSI) (B) Hepatosomatic Index (HSI) (C) Cluster analysis of GSI and HSI.
The significant seasonal differences in GSI and HSI, as confirmed by t-tests, Wilcoxon rank-sum tests, and ANOVA, highlight the adaptive strategies employed by Acanthopagrus schlegelii and other sparids to optimize reproductive success and energy utilization. The moderate Pearson correlation coefficient between GSI and HSI (Table 3) further reflects the energy trade-offs that Acanthopagrus schlegelii must balance between growth, reproduction, and survival, a challenge common across regional sparids. The cluster analysis presented in Figure 5C supports these findings by grouping the fish into distinct clusters based on their GSI and HSI values. These clusters illustrate the variability in reproductive and nutritional investments among individual fish, underscoring the species’ ability to adapt to varying environmental conditions, similar to its sparid relatives (Barrett et al., 2015; Sokołowska and Kulczykowska, 2006).
4.6 Implications for fisheries management and ecosystem health
The findings of this study carry important implications for managing Acanthopagrus schlegelii and its broader ecosystem.
i. The growth and mortality parameters, coupled with the observed reproductive and nutritional indicators, suggest that the current population of Acanthopagrus schlegelii is stable and shows resilience to current fishing pressure. However, maintaining this stability requires continuous monitoring to ensure that fishing activities and environmental changes do not negatively affect population dynamics.
ii. The low exploitation rate and the use of selective fishing gear indicate that the fishery is sustainable under current conditions. Nonetheless, changes in fishing efforts or environmental factors could disrupt the balance, influencing growth, reproduction, and survival. Management adjustments, such as refining gear regulations or implementing harvest restrictions, may become necessary if such shifts occur.
iii. The observed seasonal variations in GSI, HSI, condition factor (K), and length-weight relationships provide evidence of critical reproductive and energy allocation periods. Specifically, winter represents a peak reproductive season for Acanthopagrus schlegelii, as indicated by increased GSI and HSI values, with energy directed toward gonad development and storage. Seasonal fishing moratoriums during this period would be particularly beneficial, as they could protect spawning individuals, reduce fishing pressure during peak reproduction times, and enhance recruitment. By protecting fish during this critical reproductive window, we could ensure that a larger proportion of individuals reach maturity and contribute to future population replenishment.
Additionally, implementing size-based harvest restrictions, such as minimum size limits, would help prevent the capture of immature fish that have not yet reproduced. This would allow more individuals to contribute to the reproduction output of the population, thus supporting long-term population growth. Size-based restrictions are particularly important in ensuring that fish are allowed to grow to their full reproductive potential before being harvested.
Together, seasonal fishing moratoriums and size-based harvest restrictions would provide a dual strategy to sustain reproductive output while maintaining a balanced population structure. These measures are crucial for managing Acanthopagrus schlegelii populations and ensuring the sustainability of regional fisheries.
The growth and reproductive traits of Acanthopagrus schlegelii are crucial considerations for fisheries management. Variations in growth rates and reproductive timing, especially in comparison to other sparid species, underscore the need for adaptive management strategies. Flexible size limits and seasonal closures that reflect these differences are essential for ensuring the sustainability of regional fisheries and protecting reproductive stocks.
4.7 Stock enhancement and management in Zhanjiang coastal waters
Over the past few years, a noticeable quantity of black sea bream, especially juvenile fish of at least 5 cm in length, have been released into the Zhanjiang Sea region as part of a stock enhancement initiative. This is a key strategy for rebuilding the population, which had been under historical pressure. Despite the adoption of new fishing methods by both fishermen and anglers, the intensity of traditional fishing operations, such as trawling and gill netting, has remained stable. This is primarily due to the successful implementation of enhanced fisheries protection measures in Zhanjiang Port, which have kept overall fishing pressure relatively low.
The low exploitation rate and fishing mortality observed in our study support the conclusion that fishing pressure, in terms of fishing mortality, is not a primary driver of the fluctuations in the black sea bream population. Instead, natural mortality is the dominant factor influencing population dynamics. This finding underscores the need for ongoing stock enhancement efforts and continued habitat protection, rather than solely reducing fishing activity.
Moreover, the successful recovery of the black sea bream population in certain areas (Lauden et al., 2024) is likely a result of the combined effect of stock enhancement and controlled fishing. Measures such as seasonal closures and restrictions on fishing gear have proven effective in reducing fishing pressure during critical life stages, such as spawning seasons, and facilitating the population to regenerate. The enforcement of these management measures is essential for ensuring the long-term sustainability of the fishery. We emphasize that maintaining and strengthening these protections, especially during key reproductive periods is vital for the continued recovery and stability of the black sea bream population in Zhanjiang’s coastal waters.
5 Conclusion
This study provides a comprehensive assessment of the population dynamics, reproductive health, and growth patterns of Acanthopagrus schlegelii. The findings underscore the importance of sustainable fisheries management practices that consider not only the current population status but also the broader ecological context in which the species exists. By integrating growth, mortality, reproductive, and nutritional data, this study offers valuable insights that can inform future management decisions, ensuring that the species remains healthy and productive within its marine habitat.
Data availability statement
The raw data supporting the conclusions of this article will be made available by the authors, without undue reservation.
Ethics statement
The animal study was reviewed and approved by the Animal Experimental Ethics Committee of Guangdong Ocean University, China. The study was conducted in accordance with the local legislation and institutional requirements.
Author contributions
HL: Conceptualization, Software, Writing – original draft, Methodology. SL: Data curation, Formal analysis, Writing – original draft. AA: Data curation, Formal analysis, Software, Writing – original draft. HM: Data curation, Formal analysis, Writing – original draft. JA: Formal analysis, Writing – review & editing. NC: Writing – review & editing, Supervision. XW: Conceptualization, Funding acquisition, Supervision, Writing – review & editing.
Funding
The author(s) declare financial support was received for the research, authorship, and/or publication of this article. This work was financially supported by Sino-Indonesian technical cooperation in coastal marine ranching, Asian Cooperation Fund Program (12500101200021002), Asian Cooperation Fund Program on Modern Fishery Cooperation between China and Other Coastal States of the South China Sea (102125241620040000001), and the program for scientific research start-up funds of Guangdong Ocean University (060302022301; 060302022302).
Acknowledgments
We appreciate the valuable comments provided by the reviewers. We also gratefully acknowledge the Guangdong provincial government, China, for awarding HNL (PhD) and AA (Master’s) scholarships for the 2024-2025 academic year.
Conflict of interest
The authors declare that the research was conducted in the absence of any commercial or financial relationships that could be construed as a potential conflict of interest.
Generative AI statement
The author(s) declare that no Generative AI was used in the creation of this manuscript.
Publisher’s note
All claims expressed in this article are solely those of the authors and do not necessarily represent those of their affiliated organizations, or those of the publisher, the editors and the reviewers. Any product that may be evaluated in this article, or claim that may be made by its manufacturer, is not guaranteed or endorsed by the publisher.
References
Abderrazik W., Baali A., Schahrakane Y., Tazi O. (2016). Study of reproduction of sardine, Sardina Pilchardus in the north of Atlantic Moroccan area. AACL Bioflux 9, 507–517.
Akter Y., Hosen M. H. A., Idris Miah M., Ahmed Z. F., Chhanda M. S., Shahriar S. I. M. (2019). Impact of gonad weight on the length-weight relationships of river catfish (Clupisoma garua) in Bangladesh. Egypt J. Aquat. Res. 45, 375–379. doi: 10.1016/j.ejar.2019.10.003
Barrett T. J., Brasfield S. M., Carroll L. C., Doyle M. A., Heuvel M. R., Den V., et al. (2015). Reproductive strategies and seasonal changes in the somatic indices of seven small-bodied fishes in Atlantic Canada in relation to study design for environmental effects monitoring. Environ. Monit. Assess. 187, 305. doi: 10.1007/s10661-015-4496-4
Beverton R. J. H., Holt S. J. (1957). On the dynamics of exploited fish populations. Fish. Invest. Minist. Agric. Fish Food G. B. Ser. 219, 1–533.
Caballero-Huertas M., Frigola-Tepe X., Viñas J., Muñoz M. (2022). Somatic condition and reproductive potential as a tandem in european sardine: an analysis with an environmental perspective in the northern adriatic (Gulf of trieste). Fishes 7, 105. doi: 10.3390/fishes7030105
Chang S. K., Chou Y. T., Hoyle S. D. (2022). Length-weight relationships and otolith-based growth curves for brushtooth lizardfish off Taiwan with observations of region and aging–material effects on global growth estimates. Front. Mar. Sci. 9. doi: 10.3389/fmars.2022.921594
Davies B., Bromage N. (2002). The effects of fluctuating seasonal and constant water temperatures on the photoperiodic advancement of reproduction in female rainbow trout, Oncorhynchus mykiss. Aquaculture 205, 183–200. doi: 10.1016/S0044-8486(01)00665-2
Froese R. (2004). Keep it simple: Three indicators to deal with overfishing. Fish Fish. 5, 86–91. doi: 10.1111/j.1467-2979.2004.00144.x
Froese R. (2006). Cube law, condition factor and weight–length relationships: history, meta-analysis and recommendations. J. Fish Biol. 22, 241–253. doi: 10.1111/j.1439-0426.2006.00805.x
Froese R., Pauly D. (2024). FishBase (World Wide Web electronic publication). Available online at: www.fishbase.org (Accessed 20 Oct. 2024).
Gatti P., Sonia M., Morandeau F., Morfin M., Robert M. (2019). Reducing discards of demersal species using a 100 mm square mesh cylinder : Size selectivity and catch comparison analysis. Mar. Policy 116, 103777. doi: 10.1016/j.marpol.2019.103777
Gayanilo F. C. Jr., Sparre P., Pauly D. (2005). “FAO-ICLARM stock assessment tools II (FiSAT II); user’s guide,” in FAO computerized information series (Fisheries), no. 8, revised version (FAO, Rome, Italy), 168.
Hoggarth D., Abeyasekera S., Arthur R., Beddington J. R., Burn R. W., Halls A. S., et al. (2006). Stock assessment for fishery management. FAO Fish. Tech. Pap. 487, 261.
Jiatong G., Teng W., Defang C., Yong L., Qiumei Q., Jiujiang W., et al. (2021). Feeding habits of Acanthopagrus schlegeli in the Daya Bay. J. Fish. Sci. China 28, 1041–1050. doi: 10.12264/JFSC2020-0576
Kawai K., Fujita H., Deville D., Umino T. (2022). Spawning time of black sea bream Acanthopagrus schlegelii, related to underwater photoperiodism in oyster farms. J. Fish Biol. 101, 1142–1149. doi: 10.1111/jfb.15179
King M. (2007). Fisheries biology, assessment and management. 2nd ed (Oxford, UK: Blackwell Publishing).
Ladisa C., Ma Y., Habibi H. R. (2021). Seasonally related metabolic changes and energy allocation associated with growth and reproductive phases in the liver of male goldfish (Carassius auratus). J. Proteomics 241, 104237. doi: 10.1016/j.jprot.2021.104237
Lauden H. N., Xu X., Lyu S., Alfatat A., Lin K., Zhang S., et al. (2024). Fish stock status and its clues on stocking : A case study of acanthopagrus schlegelii from zhanjiang coastal waters, China. Fishes-Basel 9, 381. doi: 10.3390/fishes9100381
Law C. S. W., Sadovy de Mitcheson Y. (2018). Age and growth of black seabream Acanthopagrus schlegelii (Sparidae) in Hong Kong and adjacent waters of the northern South China Sea. J. Fish Biol. 93, 382–390. doi: 10.1111/jfb.13774
Lorenzen K. (2000). Allometry of natural mortality as a basis for assessing optimal release size in fish-stocking programmes. Can. J. Fish. Aquat. Sci. 57, 2374–2381. doi: 10.1139/f00-215
Melnychuk M. C., Kurota H., Mace P. M., Pons M., Minto C., Osio G. C., et al. (2021). Identifying management actions that promote sustainable fisheries. Nat. Sustain. 4, 440–449. doi: 10.1038/s41893-020-00668-1
Morato T., Afonso P., Lourinho P., Barreiros J. P., Santos R. S., Nash R. D. M. (2001). Length-weight relationships for 21 coastal fish species of the Azores, north-eastern atlantic. Fish. Res. 50, 297–302. doi: 10.1016/S0165-7836(00)00215-0
Munro J. L., Pauly D. (1983). A simple method for comparing the growth of fishes and invertebrates. ICLARM Fishbyte 1, 5–6.
Nip T. H. M., Ho W., Wong C. K. (2003). Feeding ecology of larval and juvenile black seabream (Acanthopagrus. Environ. Biol. Fish. 66, 197–209. doi: 10.1023/A:1023611207492
Pauly D. (1980). On the interrelationships between natural mortality, growth parameters, and mean environmental temperature in 175 fish stocks. ICES J. Mar. Sci. 39, 175–192. doi: 10.1093/icesjms/39.2.175
Pauly D. (1983). Some simple methods for the assessment of tropical fish stocks. FAO Fish. Tech. Pap. (Rome) 234, 52.
Prince J., Victor S., KloulChad V., Hordyk A. (2015). Length based SPR assessment of eleven Indo-Pacific coral reef fish populations in Palau. Fish. Res. 171, 42–58. doi: 10.1016/j.fishres.2015.06.008
Ricker W. E. (1973). Linear regressions in fishery research. J. Fish. Res. Board Can. 30, 409–434. doi: 10.1139/f73-072
Rljnsdorp A. D., Ibelings B. (1989). Sexual dimorphism in the energetics of reproduction and growth of North Sea plaice, Pleuronectes platessa L. J. Fish Biol. 35, 401–415. doi: 10.1111/j.1095-8649.1989.tb02992.x
Shan B., Liu Y., Song N., Ji D., Yang C., Zhao Y., et al. (2020). Genetic diversity and population structure of black sea bream (Acanthopagrus schlegelii) based on mitochondrial control region sequences: the genetic effect of stock enhancement. Reg. Stud. Mar. Sci. 35, 101188. doi: 10.1016/j.rsma.2020.101188
Sokołowska E., Kulczykowska E. (2006). Annual reproductive cycle in two free living populations of three-spined stickleback (Gasterosteus aculeatus L.): patterns of ovarian and testicular development. Oceanologia 48, 103–124.
Tang G., He Z., Liu Y., Xiao Y., Bi S., Sun Y., et al. (2023). Acanthopagrus latus migration patterns and habitat use in Wanshan Islands, Pearl River Estuary, determined using otolith microchemical analysis. Front. Mar. Sci. 10. doi: 10.3389/fmars.2023.1104528
Wang X., Weng Z., Yang Y., Hua S., Zhang H., Meng Z. (2021). Genetic evaluation of black sea bream (Acanthopagrus schlegelii) stock enhancement in the south China sea based on microsatellite DNA markers. Fishes-Basel 6, 47. doi: 10.3390/fishes6040047
Wang Z., Tang H., Xu L., Zhang J. (2022). A review on fishing gear in China : Selectivity and application. Aquac. Fish. 7, 345–358. doi: 10.1016/j.aaf.2022.02.006
Wu R., Zhang H., Liu J., Niu S., Xiao Y., Chen Y. (2018). DNA barcoding of the family Sparidae along the coast of China and revelation of potential cryptic diversity in the Indo-West Pacific oceans based on COI and 16S rRNA genes. J. Oceanol. Limnol. 36, 1753–1770. doi: 10.1007/s00343-018-7214-6
Xu W., Zhang Z. (2022). Impact of coastal urbanization on marine pollution: evidence from China. Int. J. Env. Res. Pub. He. 19, 1–26. doi: 10.3390/ijerph191710718
Yamashita H., Katayama S., Komiya T. (2015). Age and growth of black sea bream Acanthopagrus schlegelii (Bleeker 1854) in Tokyo Bay. Asian Fisheries Sci. 28, 47–59. doi: 10.33997/j.afs.2015.28.2.001
Zhang K., Geng P., Li J., Xu Y., Kalhoro M. A., Sun M., et al. (2022). Influences of fisheries management measures on biological characteristics of threadfin bream (Nemipterus virgatus) in the Beibu Gulf, South China Sea. Acta Oceanol. Sin. 41, 24–33. doi: 10.1007/s13131-021-1925-9
Zhang S., Lin L., Wang X. (2021). Optimization of a marine fish release strategy: A case study of black sea bream acanthopagrus schlegelii in the zhanjiang estuary, northern south China sea. Front. Env. Sci-Switz. 9. doi: 10.3389/fenvs.2021.779544
Keywords: Acanthopagrus schlegelii, condition factors, exploitation rate, gonadosomatic index, hepatosomatic index, reproductive indices, Zhanjiang coastal waters
Citation: Lauden HN, Lyu S, Alfatat A, Mwemi HM, Alam JF, Chen N and Wang X (2025) Population dynamics and seasonal variation in biological characteristics of black sea bream (Acanthopagrus schlegelii) in Zhanjiang coastal waters, China. Front. Mar. Sci. 11:1515753. doi: 10.3389/fmars.2024.1515753
Received: 23 October 2024; Accepted: 16 December 2024;
Published: 13 January 2025.
Edited by:
Fabio Carneiro Sterzelecki, Federal Rural University of the Amazon, BrazilReviewed by:
José Pedro Andrade, University of Algarve, PortugalSávio Lucas De Matos Guerreiro, Federal Rural University of the Amazon, Brazil
Copyright © 2025 Lauden, Lyu, Alfatat, Mwemi, Alam, Chen and Wang. This is an open-access article distributed under the terms of the Creative Commons Attribution License (CC BY). The use, distribution or reproduction in other forums is permitted, provided the original author(s) and the copyright owner(s) are credited and that the original publication in this journal is cited, in accordance with accepted academic practice. No use, distribution or reproduction is permitted which does not comply with these terms.
*Correspondence: Xuefeng Wang, d2FuZ3h1ZWZAZ2RvdS5lZHUuY24=; Ning Chen, Y2hlbm5pbmdAZ2RvdS5lZHUuY24=