- 1School of Basic Medical Sciences, Xianning Medical Colloge, Hubei University of Science and Technology, Xianning, China
- 2Dongying Municipal Bureau of Marine Development and Fisheries, Dongying, China
- 3Hubei Key Laboratory of Environmental and Health Effects of Persistent Toxic Substances, School of Environment and Health, Jianghan University, Wuhan, China
Persistent organic pollutants (POPs) and heavy metals in marine systems have been given more and more attention due to their high toxicity and bioaccumulation capacity. After discharging into the marine systems, large proportions of POPs and heavy metals are likely to remain in coastal seas, causing serious contamination and high ecological risks. However, the lack of systematic studies restricts our understanding of POP and heavy metal cycling in coastal regions. Herein, the coastal regions along the Chinese Bohai Sea (an important fishing and typically polluted area) were chosen to establish a study model for investigating long-term spatial–temporal variations, risk assessments, and controlling factors of hexachlorocyclohexanes (HCHs), dichlorodiphenyltrichloroethanes (DDTs), polycyclic aromatic hydrocarbons (PAHs), and heavy metals in coastal regions. The results showed that concentrations of HCHs, DDTs, and PAHs in sediment, and contents of heavy metals in seawater and sediment were higher than those in other coastal regions during 2015–2018 and that concentrations of As and Cu in approximately 95% and 20% of sampled organisms exceeded maximum permissible levels recommended by China and the WHO, respectively. The significantly temporal variations in HCH and PAH concentrations were indicative of increasing POP pollution, and no significantly temporal variations in most heavy metal concentrations were found. Meanwhile, the higher concentrations of POPs and heavy metals were mainly located in estuaries and other nearshore areas. The geo-accumulation index (Igeo), potential ecological risk index (PERI), and provisional tolerable daily intake (PTDI) showed that As, Cd and Hg should be listed as the primary heavy metal pollutants in sediment; higher dietary risk was posed by As through seafood consumption; the dietary risks posed by HCHs, DDTs, and PAHs were lower. Finally, the results of multiple regression, Pearson’s, and cluster analyses indicated that anthropogenic activities (e.g., industrial and agricultural discharges, and riverine input) played important roles in controlling HCH, DDT, PAH, and heavy metal distributions in coastal regions along the Chinese Bohai Sea. The outcomes of this study are expected to shed new light on an improved understanding of POP and heavy metal cycling and provide a scientific basis for contamination control in coastal systems.
1 Introduction
Persistent toxic substances (PTS), including persistent organic pollutants (POPs) and heavy metals, have been given more and more attention due to their high toxicity and bioaccumulation capacity, especially through the aquatic food chain (Ali et al., 2019; Sreedevi and Harikumar, 2023). The POPs, for instance, organochlorine pesticides (OCPs) including hexachlorocyclohexanes (HCHs) and dichlorodiphenyltrichloroethanes (DDTs), and polycyclic aromatic hydrocarbons (PAHs) [listed in the Stockholm Convention (UNEP, 2019)], in aquatic environments originate from anthropogenic sources (e.g., domestic sewage discharge and industrial and agricultural activities) (Schwarzenbach et al., 2010). Heavy metals in aquatic environments originate from both natural (e.g., geological activities) and anthropogenic sources (e.g., mining, industrial and agricultural activities, and atmospheric deposition) (Kennsih, 1997). Compared with natural sources, the increase in anthropogenic discharge is deemed to be the major cause of intensified PTS pollution in aquatic environments in the past several decades, posing a serious threat to human health, especially through the consumption of aquatic products (Kennsih, 1997; Schwarzenbach et al., 2010; Pan and Wang, 2012). The POPs and heavy metals released from these anthropogenic and natural sources then enter the aquatic environments via a variety of pathways, e.g., river input, atmospheric deposition, wastewater discharge, and coastal erosion.
The oceanic ecosystem, as the largest aquatic environment, plays important roles in controlling the biogeochemical cycling of PTS. After discharging into the marine systems, large proportions of POPs and heavy metals are likely to remain in coastal seas through settling to sediment bounding with suspended particulate matter and then release through resuspending/diffusing from sediment into the water column, resulting in long-term environmental risks (Palm et al., 2004; Zeng et al., 2019). The subsequent bioaccumulation by marine organisms in these areas makes coastal systems the “hot spots” for ecosystem risks of POPs and heavy metals (Harmesa et al., 2022). The toxicity and fate of PTS in coastal regions depend not only on the gross concentrations but also on its cycling processes and bioavailability. The POPs and heavy metals in marine systems are controlled by a variety of environmental factors, such as temperature, salinity, pH, suspended particulate matter (SPM), nutrients, organic matter, and redox condition (Hoffmann et al., 2012; Sunda, 2012; Yao et al., 2016; Zhang et al., 2018; Fernandes et al., 2019; Wu et al., 2020; Harmesa et al., 2022; Lu et al., 2022; Wang et al., 2024). These important factors have significant effects on the major biogeochemical processes, chemical speciation, and bioavailability of POP and heavy metal cycling, e.g., adsorption/desorption, diffusion at the sediment–water interface, sedimentation/resuspension, and exchange with nearby oceans (Aparicio-González et al., 2012). Elucidating the controlling factors and processes is crucial for understanding the cycling of POPs and heavy metals in coastal environments.
The Chinese Bohai Sea, a semi-closed coastal sea and a part of the Northwest Pacific Ocean, is an important fishing area in China with approximately 106 tons of fishery catch yield per year (Bureau of Fisheries and Administration, Ministry of Agriculture and Rural Affairs of the People’s Republic of China, 2019). The Chinese Bohai Sea abuts the northeastern terrestrial areas of China, which are among the three most populated and industrialized areas in China (Bohai Sea Economic Rim) (Figure 1) (Chen et al., 2022). With the economic development, pollutants including POPs and heavy metals are discharging into the Chinese Bohai Sea, especially the coastal areas of Bohai Bay and Laizhou Bay with several rivers including the Yellow River (the second largest river in China) discharging, causing serious contamination and subsequently posing potential risks to the ecosystem and human health (Gao et al., 2014; Zhang et al., 2016; Chen et al., 2022). Great efforts were exerted to investigate concentrations, distributions, and risk assessments of POPs and heavy metals in seawater, sediment, and organisms of the Chinese Bohai Sea (Wang et al., 2005; Wang and Wang, 2007; Gao and Chen, 2012; Hu et al., 2017; Li et al., 2017; Liu et al., 2018; Wang et al., 2020; Gong et al., 2021; Chen et al., 2022). However, a systematic study on POPs and heavy metals in seawater, sediment, and organisms in coastal regions along the Chinese Bohai Sea is lacking, and the magnitude of PTS contamination in this ecosystem remains unknown. Additionally, the long-term monitoring of POPs and heavy metals and relevant parameters in various matrixes present in coastal regions along the Chinese Bohai Sea is scarce. The lack of such important information restricts our understanding of long-term spatial–temporal variations, proper risk assessments, and controlling factors of POPs and heavy metals in coastal regions along the Chinese Bohai Sea.
The aims of this study were to 1) analyze long-term spatial–temporal variations of POPs (HCHs, DDTs, and PAHs) and heavy metals (As, Cd, Cu, Hg, Pb, and Zn) in seawater, sediment, and organisms in coastal regions along the Chinese Bohai Sea; 2) assess ecological risks posed by HCHs, DDTs, PAHs, and heavy metals; and 3) identify major factors controlling HCHs, DDTs, PAHs, and heavy metals in these regions. The outcomes of this study are expected to shed new light on an improved understanding of POP and heavy metal cycling and provide a scientific basis for PTS contamination control in coastal systems.
2 Materials and methods
2.1 Study area and sample collection
The Chinese Bohai Sea consists of three bays, namely, Bohai Bay, Laizhou Bay, and Liaodong Bay. Western Laizhou Bay and southern Bohai Bay are located in the southern region of the Chinese Bohai Sea, abutting the northeastern terrestrial areas, which are among the most industrialized areas in China. More than 10 rivers, including the Yellow Sea, which is the second largest river in China, carry pollutants including POPs and heavy metals, causing serious contamination in this coastal system. To evaluate spatial–temporal variations and environmental risk of HCHs, DDTs, PAHs, and heavy metals, a total of 538 seawater, 76 sediment, and 120 organism (Oratosquilla oratoria, Meretrix meretrix L., Scapharca subcrenata, Mactra veneriformis, Sinonovacula constricta, Sphyraenus, and Ruditapes philippinarum) samples were collected from the coastal regions along the Chinese Bohai Sea in spring, summer, autumn, and winter of 2015–2018 (Figure 1). Seawaters were collected using 4-L Niskin samplers at surface layers. Seawater with a volume of 500 mL was transported in a high-density polyethylene (HDPE) bottle, and HNO3 (trace metal grade, Fisher Inc., Waltham, MA, USA) was added to adjust to pH ~ 2. The acidized seawater sample was stored at 4°C for heavy metal analysis. Surface sediment (0–5 cm) was collected using a grab sampler, one aliquot of sample was transported into a plastic bag and stored at −20°C for heavy metal analysis, and another aliquot of sample was wrapped with aluminum foil and stored at −20°C for analyses of HCHs, DDTs, and PAHs. Fish muscle, mollusk, or arthropod soft tissue was sampled using a ceramic knife, washed with deionized water (DIW), and stored at −20°C for analyses of POPs and heavy metals.
2.2 Determinations of HCHs, DDTs, PAHs, and heavy metals in seawater, sediment, and organisms
The surface sediment was treated according to the United States Environmental Protection Agency (USEPA) Methods 3540C and 3630C (USEPA, 1996a, 1996b) for analyses of HCHs, DDTs, and PAHs. Briefly, 10 g of freeze-dried and sieved (180-μm) surface sediment and 10 g of Na2SO4 (Merck, Darmstadt, Germany) were mixed, followed by Soxhlet extraction in 300 mL of hexane (Merck, Germany) and acetone (Merck, Germany) solution (1:1 v/v) for 24 h at 6 cycles/h. The extract was cooled and concentrated using hexane by a rotary evaporator and then further concentrated to approximately 2 mL using N2. The solid-phase extraction tube including 1 g of SiO2 sorbent was pre-eluted with 4 mL hexane. After adding the extract, 10 mL of n-hexane (Merck, Germany) and diethyl ether (Merck, Germany) (1:1, v/v) were used for eluting the column. These two fractions were mixed and concentrated to approximately 1 mL using N2. The organism sample (fish muscle, mollusk, or arthropod soft tissue) was treated according to previous studies for analyses of HCHs, DDTs, and PAHs (Carro et al., 2002; Vives and Grimalt, 2002). Briefly, approximately 2 g of freeze-dried and homogenized soft tissue was extracted using 35 mL of n-hexane and acetone solution (4:1, v/v) and 5 mL KOH (1%). The samples were spiked with a mix of internal standards, including tetrabromobenzene (TBB) (Aldrich-Chemie, Germany) for the OCPs, and perylene-d12 and pyrene-d10 (Sigma-Aldrich, St. Louis, MO, USA) and anthracene-d10 and naphthalene-d8 (Acros Organics, Geel, Belgium) for PAHs. The extract was fractionated by adsorption chromatography with glass columns containing 5 g of Al2O3 (Sigma-Aldrich, USA) deactivated with DIW and 1 g of Na2SO4 (Merck, Germany). Two fractions were collected: F1, non-polar OCPs, eluting 8 mL of n-hexane-dichloromethane (19:1, v/v) and 2.5 mL of n-hexane-dichloromethane (1:2, v/v); and F2, polar OCPs and PAHs, adding 10 mL of n-hexane-CH2Cl2 (1:2, v/v). The OCPs and PAHs in samples were measured using gas chromatography–mass spectrometry (Agilent 7890A GC coupled with Agilent 5975C MS, Agilent, Santa Clara, CA, USA).
The analyses of six heavy metals (As, Cd, Cu, Hg, Pb, and Zn) in seawater and sediment were conducted according to the Specification for marine monitoring—Part 4: Seawater analysis (GB 17378.4–2007) (General Administration of Quality Supervision, Inspection, and Quarantine and Standardization Administration of the People’s Republic of China, 2008a) and Part 5: Sediment analysis (GB 17378.5–2007) (General Administration of Quality Supervision, Inspection, and Quarantine and Standardization Administration of the People’s Republic of China, 2008b). Briefly, the concentrations of Cd, Cu, Pb, and Zn in seawater were determined by anodic stripping voltammetry (797 VA Computrace, Wantong Inc., Switzerland); the concentrations of Hg and As were analyzed by atomic fluorescence spectrometry (AFS; AFS-820, Beijing Jitian Instrument Inc., Beijing, China). Approximately 0.2 g of freeze-dried and sieved (180-μm) surface sediment was weighed in a polytetrafluoroethylene (PTFE) tube followed by the addition of 2 mL HNO3 (trace metal grade) and 6 mL HCl (trace metal grade). The sample was digested by microwave (Master, Sineo, Shanghai, China) at 105°C for 10 min and 180°C for 25 min. The digestion was evaporated to 1 mL and then diluted to 50 mL with DIW for analysis of heavy metals. The concentrations of Cd, Cu, Pb, and Zn were determined by atomic absorption spectrometry (ZEENIT® 700P, Jena, Germany), and the concentrations of Hg and As were determined by AFS. Approximately 0.2 g of freeze-dried and homogenized soft tissue and 6 mL of 65% HNO3 (v/v) were added into a 50-mL PTFE tube for pre-digestion overnight. Subsequently, 1 mL of 30% H2O2 (v/v) was added to the tube, followed by microwave digestion for 1.5 h. After cooling, the digestion was diluted with DIW, and 5 mL of the solution was transferred to a centrifuge tube. Finally, concentrations of As, Cd, Cu, Pb, and Zn were measured using inductively coupled plasma–mass spectrometry (ICP-MS; iCAP RQ, Thermo Fisher, Waltham, MA, USA) (Chen et al., 2022). The concentration of Hg in organisms was digested by 10 mL HNO3 and 1 mL HClO4, then reduced using KHB4, and measured via AFS (GB 17378.6–2007) (General Administration of Quality Supervision, Inspection, and Quarantine and Standardization Administration of the People’s Republic of China, 2008c).
2.3 Measurements of other ancillary parameters
Temperature (T), salinity (S), pH, and redox potential (Eh) were in situ determined using a portable multi-parameter analytical instrument (SG98-FK2, Mettler-Toledo, Columbia, MD, USA). The dissolved oxygen (DO) was measured using the Winkler titration method (Zhang et al., 2015). The concentrations of total phosphorus (TP), total nitrogen (TN), nitrate (NO3−), nitrite (NO2−), ammonia nitrogen (NH4+–N), and silicate (SiO43−) were determined following the Specification for marine monitoring—Part 4: Seawater analysis (GB 17378.4–2007). Chl-a was extracted with 90% acetone (v/v) in the dark for 24 h and measured according to a previous study (Parsons et al., 1984). Water with a volume of 500 mL was vigorously blended and then filtered through a pre-weighed 0.45-μm glass fiber filter to measure the SPM. Total organic carbon (TOC) in the sediment was measured using a TOC analyzer (SSM-5000A, Shimadzu, Kyoto, Japan) (Chen et al., 2021). The sulfide in the sediment was analyzed using the methylene blue spectrophotometric method according to Specification for marine monitoring—Part 5: Sediment analysis (GB 17378.5–2007).
2.4 QA/QC
Quality assurance and control procedures were conducted during the analyses of HCHs, DDTs, PAHs, and heavy metals. For each of the 20 samples, three method blanks, three certified reference materials or three matrix spikes in water samples, and triplicates of one randomly chosen sample were included. The recoveries of PAHs and OCPs in the spiked samples ranged from 87% to 103% and 78% to 101%, respectively. Relative standard deviations (RSDs) of triplicates were less than 16% and 13% for the PAHs and OCPs, respectively. The limits of detections (LODs) of OCPs and PAHs were 0.01 μg/kg to 1.46 μg/kg and 0.04 μg/kg to 0.40 μg/kg, respectively. The recoveries of heavy metals in seawater (87%–110%), sediment (93%–107%), and organisms (88%–112%) were within the acceptable ranges. The RSD of triplicates ranged from 3.6% to 13.2%, which were also within the acceptable RSD. The LODs were 0.5 μg/L for As, 0.01 μg/L for Cd, 0.6 μg/L for Cu, 0.007 μg/L Hg, 0.1 μg/L for Pb, and 1.2 μg/L for Zn in seawater. The LODs were 0.06 mg/kg for As, 0.04 mg/kg for Cd, 2.0 mg/kg for Cu, 0.002 mg/kg Hg, 1.0 mg/kg for Pb, and 6.0 mg/kg for Zn in sediment. The LODs were 0.27 mg/kg for As, 0.01 mg/kg for Cd, 0.08 mg/kg for Cu, 0.002 mg/kg Hg, 0.01 mg/kg for Pb, and 1.60 mg/kg for Zn in organisms. The DIW was used throughout the sample collection and analysis. All HDPE bottles were soaked in 30% HNO3 for at least 96 h and then cleaned and filled with DIW until sampling heavy metals. Field and method blanks using the DIW were conducted during the sampling and analysis procedure. The concentrations of HCHs, DDTs, PAHs, and heavy metals in field and method blanks were comparable or lower than those of the LODs.
2.5 Statistical analysis
Distribution patterns of HCHs, DDTs, PAHs, and heavy metals in seawater and sediment were drawn using Ocean Data View (AWI, Bremerhaven, Germany). The temporal and spatial variations in concentrations of HCHs, DDTs, PAHs, and heavy metals were evaluated using the Mann–Whitney U-test by SPSS 19.0 (SPSS Inc., Armonk, NY, USA). A p-value of <0.05 indicates a significant difference. The cluster analysis and Pearson’s analysis were conducted to discover the relationships among HCHs, DDTs, PAHs, and heavy metals in seawater and sediment. The relationships between HCHs, DDTs, PAHs, heavy metals, and other ancillary parameters were detected by conducting Spearman’s analysis and multiple regression analyses to identify the controlling factors. The multicollinearity test was conducted before the multiple regression analyses, and the variance inflation factor (VIF) and condition index were calculated to detect the possible existence of collinearity among the input parameters (VIF < 10 and condition index <30). All of the above statistical analyses were performed using SPSS 19.0. The environmental risks posed by heavy metals in sediment and provisional tolerable daily intake of HCHs, DDTs, PAHs, and heavy metals through seafood consumption were calculated by the following methods.
2.5.1 Igeo
The geo-accumulation index (Igeo) was calculated to assess the pollution status of heavy metals in sediment of the coastal regions along the Chinese Bohai Sea (Equation 1).
where Ci is the concentration of each heavy metal in the sediment of the coastal regions along the Chinese Bohai Sea (mg/kg). Bi is the background value of each heavy metal in sediment (mg/kg). The values of 6.26 mg/kg, 0.032 mg/kg, 15.34 mg/kg, 0.019 mg/kg, 13.43 mg/kg, and 69.12 mg/kg were adopted for background concentrations of As, Cd, Cu, Hg, Pb, and Zn, respectively (Xiao et al., 2017).
2.5.2 PERI
The potential ecological risk index (PERI) was also calculated to assess the potential ecological risk posed by heavy metals following Equations 2 and 3 (Yu et al., 2023):
where PERI is the potential ecological risk index posed by all heavy metals; is the potential risk of each heavy metal; is the toxic response factor for each heavy metal (As = 10, Cd = 30, Cu = Pb = 5, Hg = 40, and Zn = 1) (Hilton et al., 1985); is the contamination factor (the ratio between present and background concentrations in sediment). The background values of 6.26 mg/kg, 0.032 mg/kg, 15.34 mg/kg, 0.019 mg/kg, 13.43 mg/kg, and 69.12 mg/kg were adopted for As, Cd, Cu, Hg, Pb, and Zn, respectively (Xiao et al., 2017).
2.5.3 PTDI
Provisional tolerable daily intake (PTDI) values of HCHs, DDTs, PAHs, and heavy metals by residents were calculated by Equation 4.
where PTDI is the provisional tolerable daily intake of HCHs, DDTs, PAHs, and heavy metals (μg kg−1 bw d−1); Ci is the concentrations of HCHs, DDTs, PAHs, and heavy metals in aquatic product (mg/kg); mv is the average consumption amount of aquatic products by adults in Shandong Province (32.6 g/d) (National Bureau of Statistics of China, 2022); bw is the average body weight of adults in Shandong Province (61.4 kg) (Health Commission of Shandong Province, 2015).
3 Results
3.1 Heavy metals in seawater
The concentrations of As, Cd, Cu, Hg, Pb, and Zn were 2.72 ± 1.01 μg/L, 0.25 ± 0.11 μg/L, 3.32 ± 0.80 μg/L, 0.06 ± 0.02 μg/L, 1.09 ± 0.83 μg/L, and 19.41 ± 10.78 μg/L in all seawater samples collected during 2015–2018, respectively. In comparison to those of other coastal systems, the concentrations of heavy metals in seawater of the coastal regions along the Chinese Bohai Sea were approximately 2–10 times higher than the background values of natural surface seawater (Wu and Zeng, 1983) and higher than those in the river estuaries, coastal bays, and open oceans in China and other countries (Supplementary Table 1). In general, the concentrations of heavy metals in seawater of the coastal regions along the Chinese Bohai Sea were at the highest rank among the reported coastal regions (at the higher 10th percentile) (Supplementary Table 1), suggesting the serious pollution status of heavy metals in this region.
3.2 HCHs, DDTs, PAHs, and heavy metals in sediment
The average concentrations of ∑HCHs, ∑DDTs, and ∑PAHs were 0.023 ± 0.032 mg/kg, 0.003 ± 0.002 mg/kg, and 0.26 ± 0.10 mg/kg in surface sediment collected from coastal regions of the Chinese Bohai Sea during 2015–2018, respectively. As shown in Figure 2, the compositions of HCHs showed a decreasing order of δ-HCH (41.8%) > α-HCH (32.4%) > γ-HCH (16.1%) > β-HCH (9.7%); compositions of DDTs were in order of OP′-DDT (44.5%) > PP′-DDE (28.3%) > PP′-DDT (14.9%) > PP′-DDD (12.3%); the dominant compositions of PAHs were FLU (25.3%), PHE (19.1%), NAP (11.1%), FLT (9.1%), ANT (7.8%), PYR (5.8%), and ANA (5.5%). Concentrations of As, Cd, Cu, Hg, Pb, and Zn were 10.38 ± 4.41 mg/kg, 0.20 ± 0.08 mg/kg, 14.35 ± 4.37 mg/kg, 0.02 ± 0.02 mg/kg, 16.70 ± 6.20 mg/kg, and 39.93 ± 11.51 mg/kg in all surface sediment samples collected during 2015–2018, respectively. Compared with those of other coastal regions, the concentrations of ∑HCHs, ∑DDTs, and ∑PAHs in surface sediment of the coastal regions along the Chinese Bohai Sea were at the highest rank; notably, the ∑HCHs and ∑DDTs levels were 3–20 times higher (Supplementary Table 2). The concentrations of As (at the higher 10th percentile) and Cd (at the higher 40th percentile) in surface sediment of the coastal regions along the Chinese Bohai Sea were higher, and comparable or lower concentrations of other measured heavy metals were observed (Supplementary Table 3). These results indicated that the sediment of the coastal regions along the Chinese Bohai Sea was seriously polluted by HCHs, DDTs, PAHs, As, and Cd.
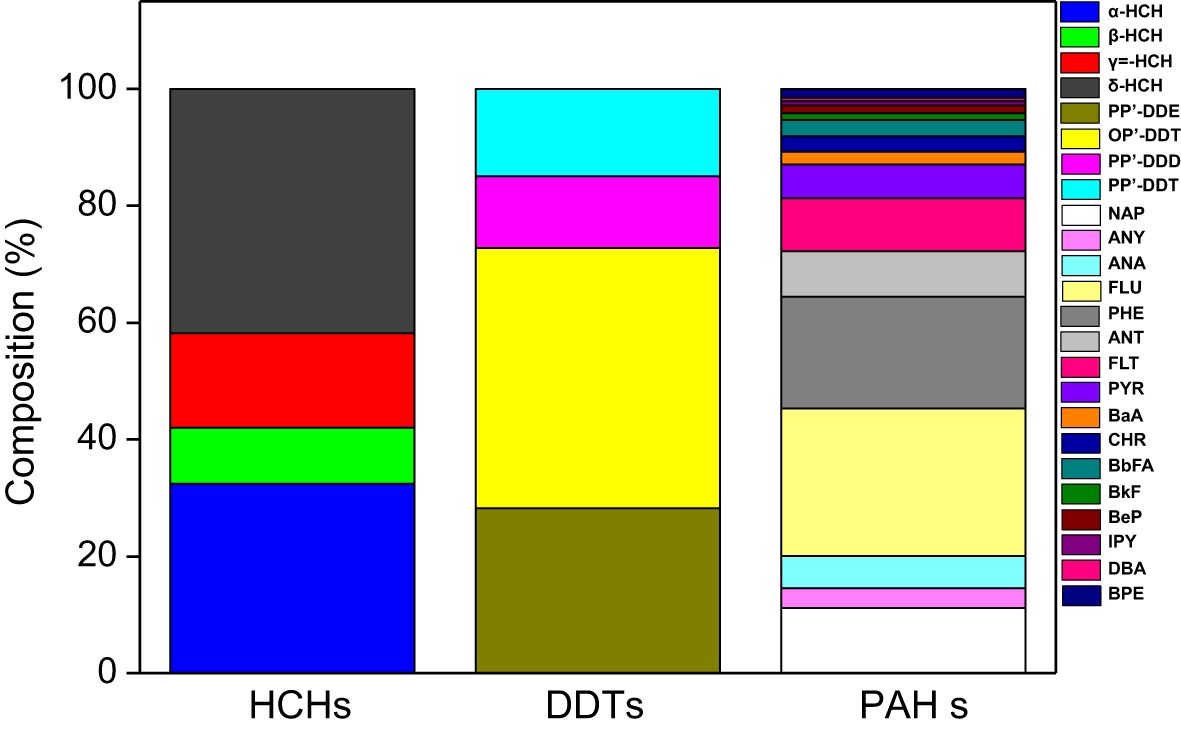
Figure 2 Compositions of HCHs, DDTs, and PAHs (%) in surface sediment of the coastal regions along the Chinese Bohai Sea. HCHs, hexachlorocyclohexanes; DDTs, dichlorodiphenyltrichloroethanes; PAHs, polycyclic aromatic hydrocarbons.
3.3 HCHs, DDTs, PAHs, and heavy metals in organisms
The average concentrations of HCHs, DDTs, and PAHs were respectively 0.62 ± 0.23 μg/kg, 6.81 ± 2.90 μg/kg, and 6.14 ± 1.60 μg/kg in arthropods and 1.98 ± 0.57 μg/kg, 2.17 ± 0.39 μg/kg, and 11.30 ± 9.91 μg/kg in mollusks. The average concentrations of As, Cd, Cu, Hg, Pb, and Zn were respectively 2.69 ± 0.62 mg/kg, 0.15 ± 0.03 mg/kg, 10.16 ± 1.41 mg/kg, 0.01 ± 0.002 mg/kg, 0.30 ± 0.04 mg/kg, and 18.58 ± 0.77 mg/kg in arthropods; 1.82 ± 0.61 mg/kg, 0.19 ± 0.16 mg/kg, 4.61 ± 3.61 mg/kg, 0.01 ± 0.006 mg/kg, 0.33 ± 0.09 mg/kg, and 12.36 ± 3.20 mg/kg in mollusks; and 1.52 ± 1.51 mg/kg, 0.07 ± 0.003 mg/kg, 0.31 ± 0.20 mg/kg, 0.02 ± 0.005 mg/kg, 0.20 ± 0.03 mg/kg, and 4.01 ± 1.99 mg/kg in fish. In comparison to those of other coastal regions, the concentrations of HCHs, DDTs, and PAHs were comparable or lower; however, the heavy metal levels in organisms collected from the coastal regions along the Chinese Bohai Sea were higher (Supplementary Table 4). Meanwhile, the levels of HCHs, DDTs, and PAHs in organisms collected from the coastal regions along the Chinese Bohai Sea were lower than the maximum permissible levels (MPLs) recommended in China (0.1 mg/kg for HCHs, 0.5 mg/kg for DDTs, and 5 μg/kg for PAHs) (National Health Commission, Ministry of Agriculture and Rural Affairs, State Administration of Market Regulation of the People's Republic of China, 2021; National Health Commission, State Administration of Market Regulation of the People's Republic of China, 2022). The concentrations of As in approximately 95% of the sampled organisms exceeded MPLs of 0.5 mg/kg recommended in China (National Health and Family Planning Commission, National Food and Drug Administration of the People’s Republic of China, 2017). Meanwhile, the concentrations of Cu in 20% of the sampled organisms exceeded MPLs of 10 mg/kg according to the guideline of the World Health Organization (Joint FAO/WHO Expert Committee on Food Additives, 1982), suggesting that organisms in the coastal regions along the Chinese Bohai Sea were contaminated with different levels of As and Cu.
4 Discussion
4.1 Spatial–temporal distributions and variations of HCHs, DDTs, PAHs, and heavy metals in coastal regions along the Chinese Bohai Sea
4.1.1 Spatial distributions of HCHs, DDTs, PAHs, and heavy metals in seawater and sediment
As shown in Figure 3, the concentrations of As, Pb, and Zn in seawater sampled from western Laizhou Bay were higher than those from southern Bohai Bay (Mann–Whitney U-test, p < 0.05), suggesting that pollution of these heavy metals in the former system was more serious than in the latter, while opposite variations were found in Cd and Cu concentrations (Mann–Whitney U-test, p < 0.05). No significant trend of Hg concentrations was found in these two regions. Generally, the concentrations of heavy metals displayed decreasing trends from nearshore to offshore. Several “hot spots” were located at the river estuaries, e.g., Yellow River and Xiaoqiang River (Figure 3), indicative of the effect of riverine input on heavy metal distributions. Moreover, some higher concentrations of heavy metals also were observed in offshore areas due to oceanic current transport, resuspension and diffusion from sediment, atmospheric deposition, and other processes.
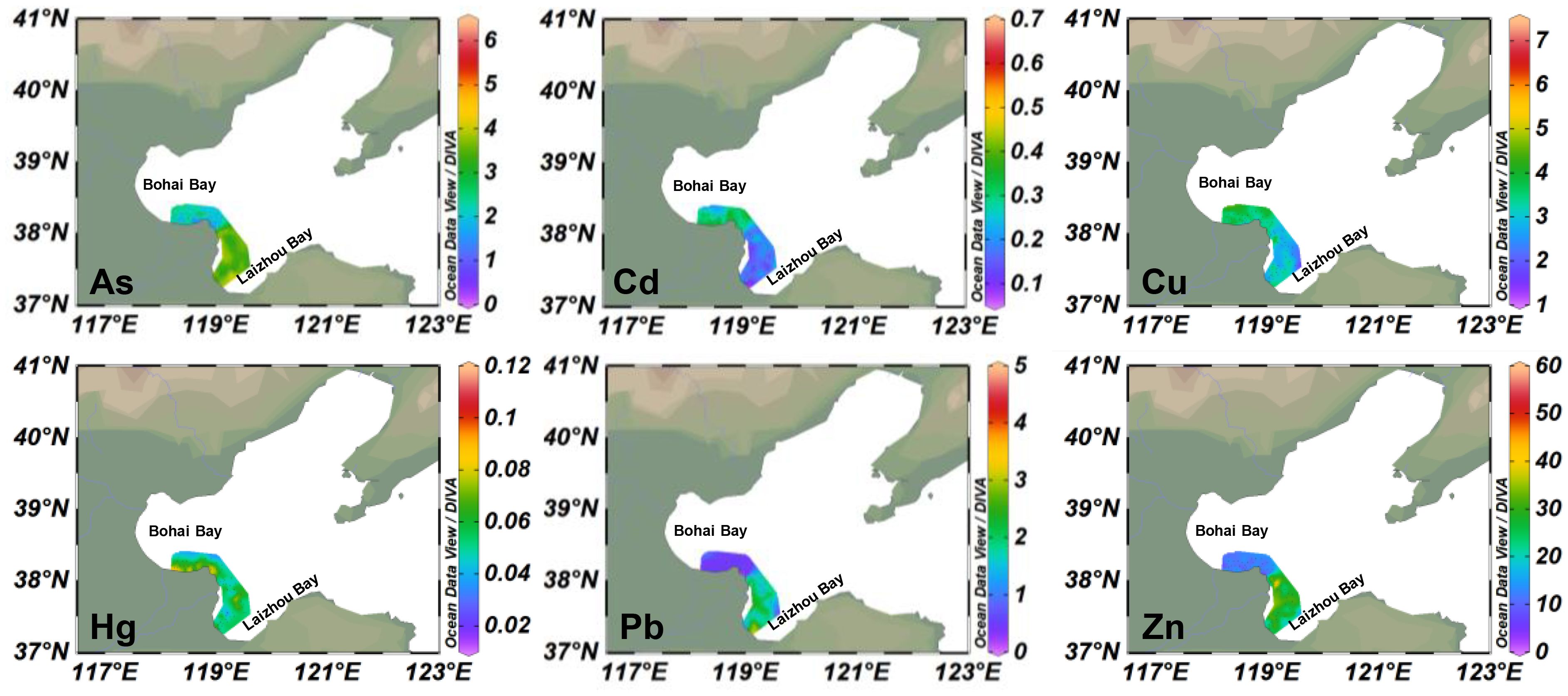
Figure 3 Spatial distributions of heavy metals in seawater (μg/L) of the coastal regions along the Chinese Bohai Sea.
As shown in Figure 4, the concentrations of PAHs in sediment sampled from the southern of Bohai Bay were higher than those in western Laizhou Bay (Mann–Whitney U-test, p < 0.05), indicative of more serious PAH pollution. The concentrations of As and Zn in sediment collected from western Laizhou Bay were higher than those in southern Bohai Bay (Mann–Whitney U-test, p < 0.05), also indicative of serious pollution in Laizhou Bay than in Bohai Bay. There were no significant spatial variations in concentrations of HCHs, DDTs, and other heavy metals between these two bays. The highest concentrations of HCHs, DDTs, PAHs, and heavy metals in sediment were mainly measured at river estuaries around the coastal regions along the Chinese Bohai Sea (Figure 4). The riverine water carries pollutants including POP and heavy metal discharges into the marine systems, and large proportions of pollutants remain in coastal regions with the settlement of suspended particulate matter.
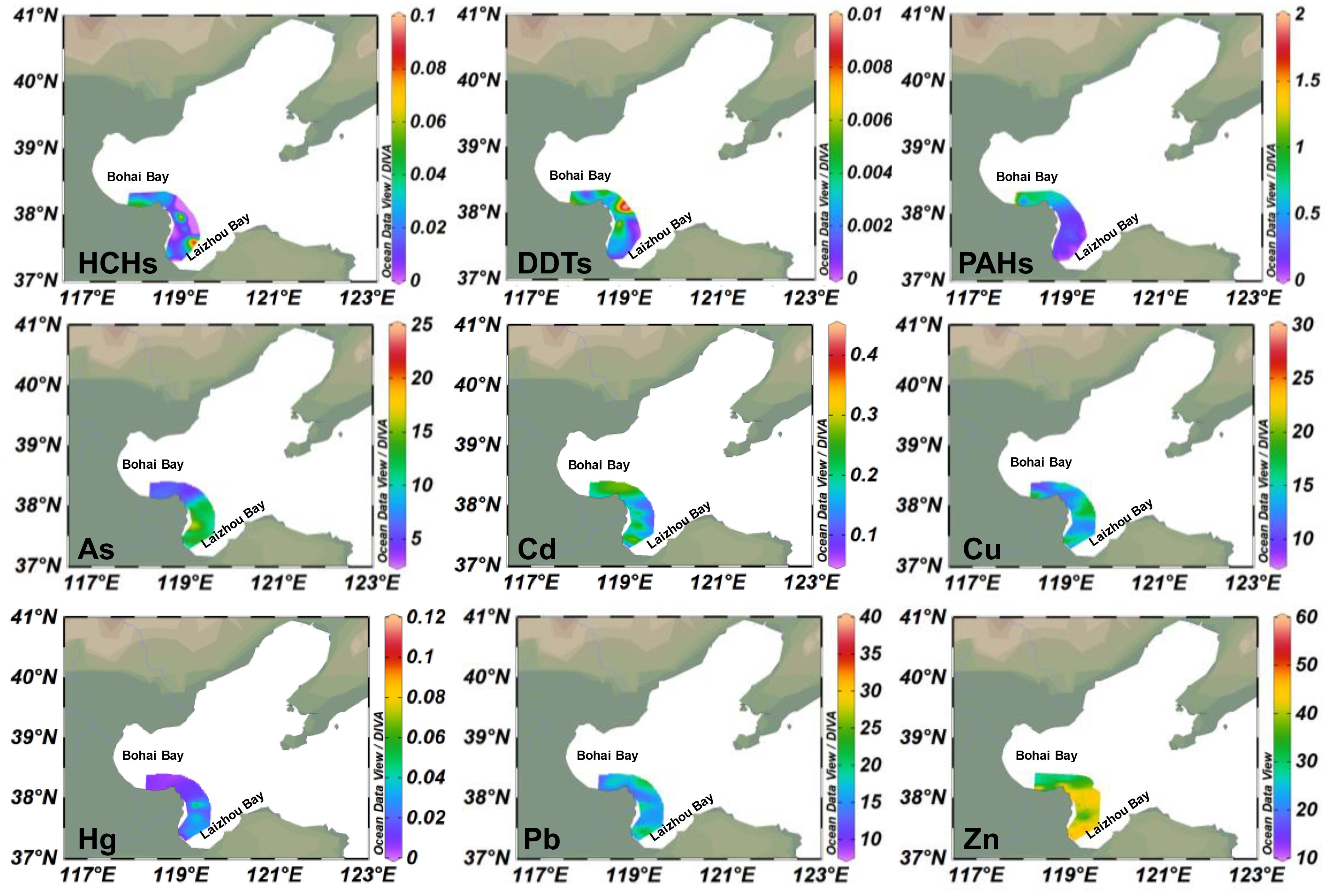
Figure 4 Spatial distributions of HCHs, DDTs, PAHs, and heavy metals in sediment (mg/kg) of the coastal regions along the Chinese Bohai Sea. HCHs, hexachlorocyclohexanes; DDTs, dichlorodiphenyltrichloroethanes; PAHs, polycyclic aromatic hydrocarbons.
4.1.2 Temporal variations of HCHs, DDTs, PAHs, and heavy metals in seawater and sediment
As shown in Figure 5A, the concentrations of HCHs were 0.004 ± 0.003 mg/kg, 0.011 ± 0.006 mg/kg, 0.027 ± 0.026 mg/kg, and 0.042 ± 0.047 mg/kg in surface sediment collected in 2015, 2016, 2017, and 2018, respectively (Supplementary Table 5), which showed significantly increasing trends (Mann–Whitney U-test, p < 0.01). The concentrations of PAHs in surface sediment increased from 0.15 ± 0.08 mg/kg in 2015 to 0.30 ± 0.07 mg/kg (Mann–Whitney U-test, p < 0.05), and no significantly temporal variations in DDT concentrations were found during the sampling period (Mann–Whitney U-test, p > 0.05) (Supplementary Table 5). These results suggested that the POP pollution, especially the HCHs and PAH, is becoming more and more serious in the coastal regions of the Chinese Bohai Sea. The temporal variations in heavy metal concentrations in seawater and surface sediment (Figures 5B, C) and results of the Mann–Whitney U-test showed that the concentrations of Zn in seawater in 2018 (14.53 ± 2.74 μg/L) were lower than those during the other years (p < 0.05) (Supplementary Table 5). The highest average concentrations of As, Cd, Cu, Hg, and Pb in seawater were 2.89 ± 0.39 μg/L, 0.32 ± 0.10 μg/L, 3.40 ± 0.79 μg/L, 0.06 ± 0.02 μg/L, and 1.23 ± 0.85 μg/L in 2015, 2018, 2016, 2015, and 2018, respectively. Except for slight fluctuations, no significantly temporal variations were found in the concentrations of these metals in seawater (Mann–Whitney U-test, p > 0.05) (Supplementary Table 5), indicating a relatively stable status of heavy metals in seawater of the coastal regions along the Chinese Bohai Sea during the sampling periods (Figure 5B). The highest average concentration of As in surface sediment was 16.78 ± 4.57 mg/kg in 2015 and showed a decreasing trend to 8.26 ± 1.42 mg/kg during 2018 (Mann–Whitney U-test, p < 0.05) (Figure 5C and Supplementary Table 5). The highest average concentration of Hg in surface sediment was 0.03 ± 0.03 mg/kg measured in 2017, and the highest average concentrations of other heavy metals (Cd, 0.22 ± 0.10 mg/kg; Cu, 17.26 ± 5.65 mg/kg; Pb, 21.34 ± 5.76 mg/kg; Zn, 44.84 ± 3.71 mg/kg) were measured during 2015, and no significantly temporal variations were found (Mann–Whitney U-test, p > 0.05) (Figure 5C and Supplementary Table 5).
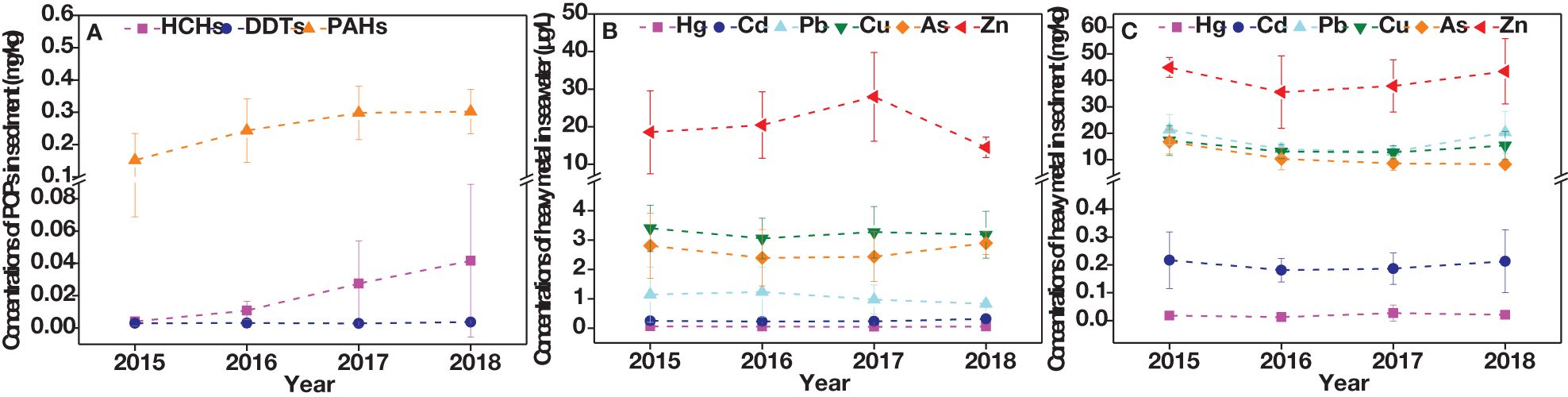
Figure 5 Temporal variations of HCHs, DDTs, and PAHs in sediment (A), heavy metals in seawater (B), and sediment (C) of the coastal regions along the Chinese Bohai Sea. HCHs, hexachlorocyclohexanes; DDTs, dichlorodiphenyltrichloroethanes; PAHs, polycyclic aromatic hydrocarbons.
4.2 Risk assessments of HCHs, DDTs, PAHs, and heavy metals in coastal regions along the Chinese Bohai Sea
4.2.1 Pollution status and ecological risks of heavy metals in sediment
The geo-accumulation index (Igeo) was calculated by Equation 1 and can be divided into seven grades: 1) unpolluted, Igeo ≤ 0; 2) unpolluted to moderately polluted, 0 < Igeo < 1; 3) moderately polluted, 1 < Igeo < 2; 4) moderately to strongly polluted, 2 < Igeo < 3; 5) strongly polluted, 3 < Igeo < 4; 6) strongly to extremely polluted, 4 < Igeo < 5); and 7) extremely polluted, Igeo > 5 (Wang et al., 2018). The average Igeo values of heavy metals in sediment of the coastal regions along the Chinese Bohai Sea were in order of As (2.65 ± 11.73) > Cd (0.61 ± 0.35) > Hg (−0.24 ± 2.82) > Zn (−0.76 ± 0.23) > Pb (−2.64 ± 9.08) > Cu (−3.97 ± 18.81) (Supplementary Table 6). These results suggested that the sediment in coastal regions along the Chinese Bohai Sea was moderately to strongly polluted by Cd and As, which was consistent with previous studies (Xiao et al., 2017; Liu et al., 2023).
The ecological risk of heavy metals in sediment based on the PERI values can be divided into five grades: 1) low risk, PERI < 150; 2) moderate risk, 150 < PERI < 300; 3) high risk, 300 < PERI < 600; and 4) significantly high risk, PERI > 600 (Ferreira et al., 2022). The average PERI value calculated by Equation 2 was 254.96 ± 89.92 (ranging from 106.04 to 494.94), suggesting that the ecological risk posed by heavy metals was moderate. As shown in Figure 6A, the higher PERI values were mainly located at the Yellow River estuary and western nearshore areas of Laizhou Bay, indicative of the effects of anthropogenic discharge on heavy metal risks in the sediment. According to Equation 3, the ecological risk () of As, Cd, Cu, Hg, Pb, and Zn were 16.58 ± 7.04, 185.12 ± 76.17, 4.68 ± 1.42, 41.79 ± 40.53, 6.22 ± 2.31, and 0.58 ± 0.17, respectively (Supplementary Table 6), indicating that the ecological risks posed by Cd and Hg were high (160≤ <320) and moderate (40≤<80) in sediment of the coastal regions along the Chinese Bohai Sea. Meanwhile, the indicated that the contribution of each heavy metal on the total PERI was in the order of Cd (71.92%) > Hg (16.09%) > As (7.18%) > Pb (2.56%) ≈ Cu (1.99%) > Zn (0.26%) (Figure 6B and Supplementary Table 6). To summarize, based on the PERI and Igeo methods, As, Cd, and Hg should be listed as the primary heavy metal pollutants in the sediment of the coastal regions along the Chinese Bohai Sea, while the ecological risks posed by Cd and Hg are worthy of attention. Additionally, the concentrations of ∑HCHs, ∑DDTs, and ∑PAHs in surface sediment of this study were at the highest rank in comparison to those of other coastal systems (Supplementary Table 2), suggesting that POP pollution in coastal regions along the Chinese Bohai Sea is serious, especially HCHs and DDTs.
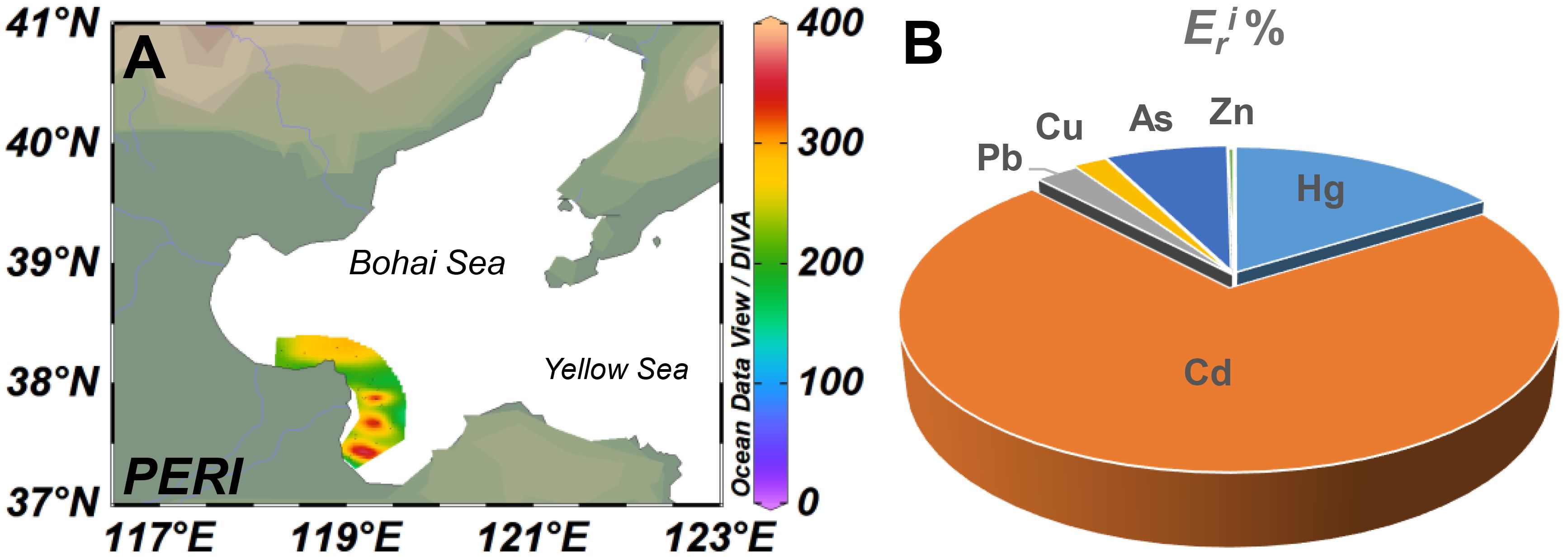
Figure 6 The distribution of potential ecological risk index (PERI) of all heavy metals (A) and contribution of each heavy metal (B).
4.2.2 Dietary risks of HCHs, DDTs, PAHs, and heavy metals in organisms
As shown in Table 1, the average PTDI values of HCHs, DDTs, and PAHs in arthropods sampled from the coastal regions along the Chinese Bohai Sea were respectively (3.3 ± 1.2) × 10−4 μg kg−1 bw d−1, (3.6 ± 1.5) × 10−3 μg kg−1 bw d−1, and (3.0 ± 0.8) × 10−3 μg kg−1 bw d−1. Higher PTDI values of (1.0 ± 0.3) × 10−3 μg kg−1 bw d−1, (5.1 ± 0.2) × 10−3 μg kg−1 bw d−1, and 0.12 ± 0.05 μg kg−1 bw d−1 by HCHs, DDTs, and PAHs, respectively, in mollusks were estimated. The calculated PTDI values were lower than the RfD recommended by the USEPA (Table 1), suggesting lower dietary risk posed by HCHs, DDTs, and PAHs in aquatic products sampled from the coastal regions along the Chinese Bohai Sea. The average PTDI values of As, Cd, Cu, Hg, Pb, and Zn in arthropods were 1.43 ± 0.33 μg kg−1 bw d−1, 0.08 ± 0.02 μg kg−1 bw d−1, 5.39 ± 0.75 μg kg−1 bw d−1, 0.007 ± 0.001 μg kg−1 bw d−1, 0.16 ± 0.02 μg kg−1 bw d−1, and 9.86 ± 0.41 μg kg−1 bw d−1; 0.97 ± 0.33 μg kg−1 bw d−1, 0.10 ± 0.09 μg kg−1 bw d−1, 2.45 ± 1.92 μg kg−1 bw d−1, 0.005 ± 0.003 μg kg−1 bw d−1, 0.17 ± 0.05 μg kg−1 bw d−1, and 6.56 ± 1.70 μg kg−1 bw d−1 in mollusks; and 0.81 ± 0.80 μg kg−1 bw d−1, 0.04 ± 0.002 μg kg−1 bw d−1, 0.16 ± 0.11 μg kg−1 bw d−1, 0.01 ± 0.02 μg kg−1 bw d−1, 0.10 ± 0.02 μg kg−1 bw d−1, and 2.13 ± 1.06 μg kg−1 bw d−1 in fish, respectively. Generally, the dietary risks posed by heavy metals in organisms displayed an order of anthropod > mollusk > fish for most heavy metals except for Hg and Pb (Table 1). As shown in Table 1, the PTDI by As in arthropods, mollusks, and fish exceeded the RfD, and others were below the recommended values by USEPA, indicative of high dietary risks posed by As in organisms from the coastal regions along the Chinese Bohai Sea. Although As has no biological functions in living organisms (Siegel, 2003), both inorganic and organic As are absorbed by living organisms in the gastrointestinal tract. The concentrations of As in tissues of aquatic organisms vary widely, with particularly high concentrations in lipids, liver, and muscles, posing high health risks to humans through the consumption of aquatic products (Sloth and Julshamn, 2008).
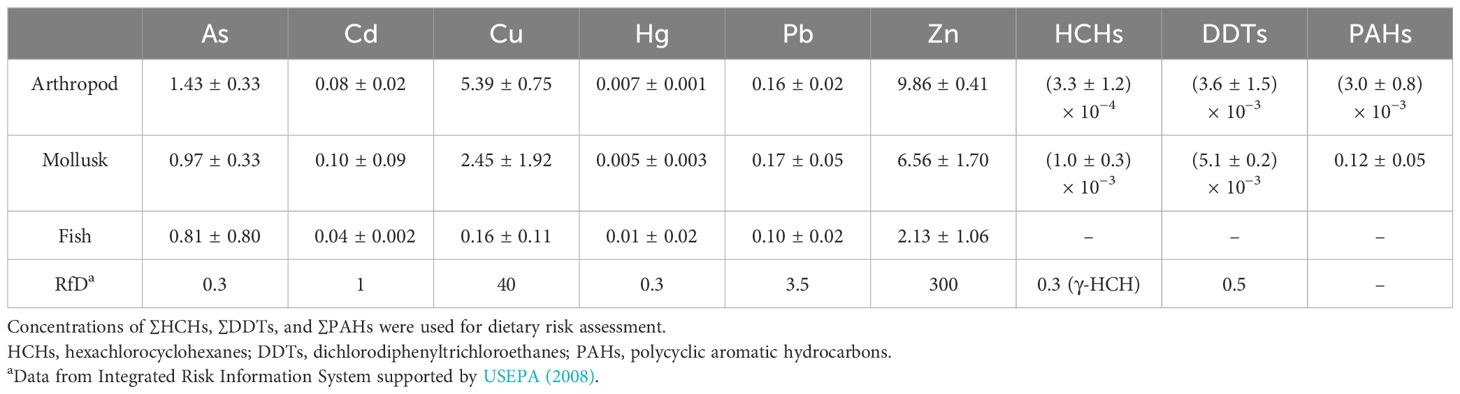
Table 1 Dietary risks posed by HCHs, DDTs, PAHs, and heavy metals in organisms collected from the coastal regions along the Chinese Bohai Sea.
4.3 Controlling factors and potential sources of HCHs, DDTs, PAHs, and heavy metals in coastal regions along the Chinese Bohai Sea
4.3.1 Controlling factors of heavy metals in seawater
To investigate the factors controlling the distributions of heavy metals in seawater of the coastal regions along the Chinese Bohai Sea, multiple regression analyses of heavy metal concentrations in seawater against physicochemical parameters were performed. Spearman’s correlation analysis was first conducted to choose the parameters significantly correlated to heavy metal concentrations (Supplementary Table 7), and these parameters were adopted in subsequent multiple regression analyses. As shown in Table 2, the results of multiple regression analyses showed that SPM, TN, chemical oxygen demand (COD), and TP were parameters controlling distributions of Cd, Cu, Pb, and Zn in seawater of the coastal regions along the Chinese Bohai Sea, respectively; T, COD, TP, SiO43−, T, and SPM dominated distributions of As and Hg. Nutrients, phosphorus, and silicate in coastal systems are mainly discharged into riverine water, industrial and sewage wastewaters, and groundwater (Slomp and Van Cappellen, 2004; Archana et al., 2018); moreover, the chemical oxygen demand generally reveals the content of organic pollutants (Kamarudin et al., 2020). The significantly positive relationships between these parameters and heavy metals in seawater observed in this study suggested that anthropogenic discharge plays important roles in controlling the distributions of heavy metals in coastal regions along the Chinese Bohai Sea. The significantly positive correlations between As, Hg, and T may be due to more riverine input and wet deposition of pollutants in the warm season (Peng, 2015), also indicative of the important effects of anthropogenic discharge on heavy metal distributions. The high affinity to be absorbed and transported with the suspended particulate matter may result in positive correlations of Hg and Cd with SPM, which was consistent with previous studies (Zhang et al., 2018; Lao et al., 2019).
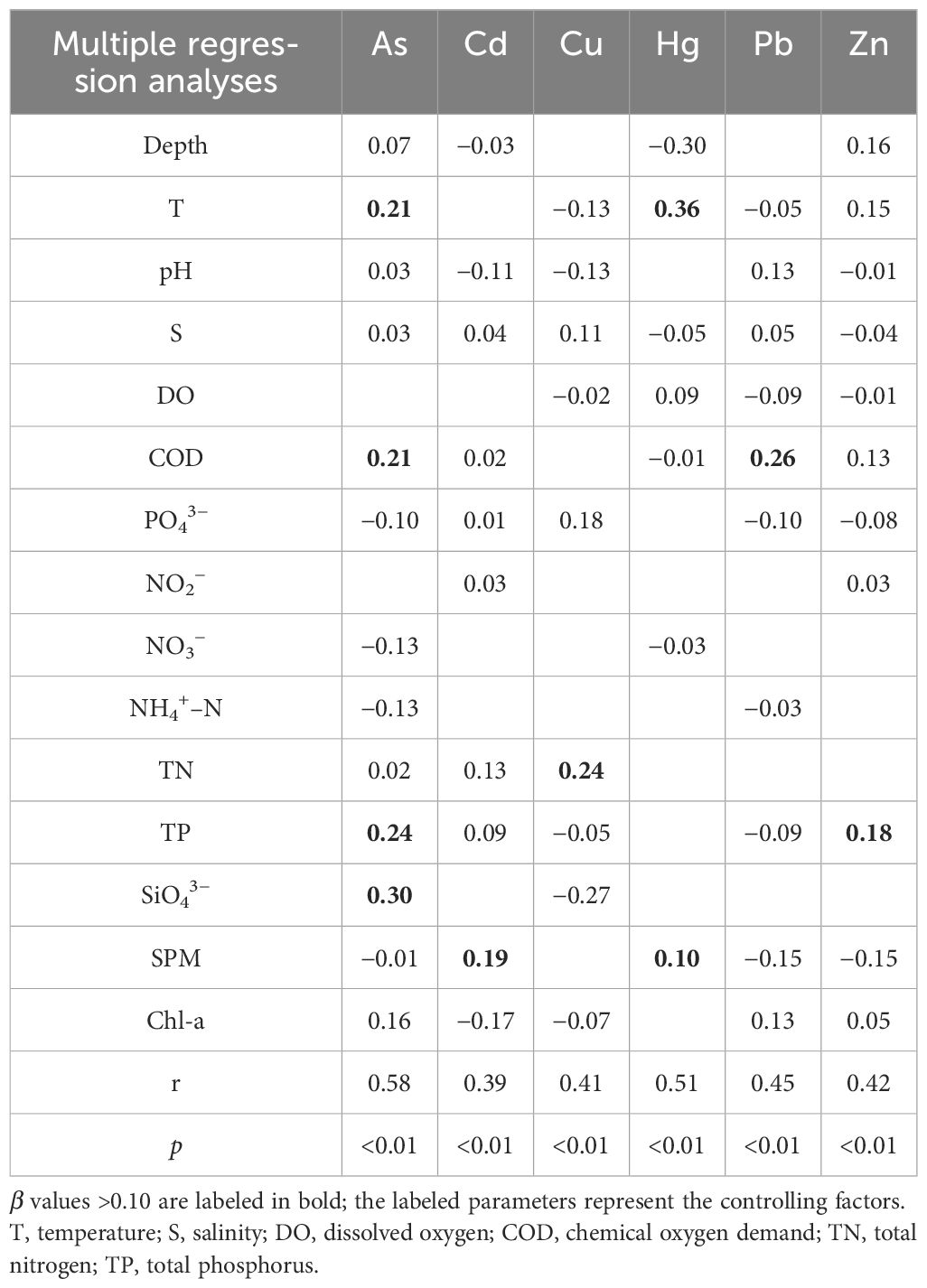
Table 2 Factors controlling heavy metals in seawater of the coastal regions along the Chinese Bohai Sea.
4.3.2 Potential sources of HCHs, DDTs, PAHs, and heavy metals in sediment
As shown in Supplementary Table 8, the results of Pearson’s analysis showed that HCHs, DDTs, PAHs, and heavy metals were significantly positive with organic matter (p < 0.01). Previous studies reported that the terrestrial organic matter and pollutants were transported by the rivers, discharged, and retained in the sediment of the coastal regions (Gao and Chen, 2012; Gao et al., 2012). These results also indicated that anthropogenic discharge plays important roles in controlling the distributions of POPs and heavy metals in coastal regions along the Chinese Bohai Sea. Moreover, the cluster analysis and Pearson’s analysis can be used to identify similar sources or environmental behavior of pollutants (Li et al., 2013). The cluster analysis on the concentrations of HCHs, DDTs, PAHs, and heavy metals in surface sediment of the coastal regions along the Chinese Bohai Sea is presented in Figure 7. Three clusters were identified from HCHs, DDTs, PAHs, and heavy metal enrichment dendrograms. The first cluster contains HCHs, DDTs, PAHs, Hg, and Cd; Pb, Cu, and As were identified in the second cluster; Zn was identified in the third cluster. The results of Pearson’s correlation analysis (Supplementary Table 8) showed significantly positive relationships (p < 0.01) among Hg-DDTs (0.41), DDTs-HCHs (0.49), HCHs-Cd (0.39), and Cd-PAHs (0.37) in the sediment of the coastal regions along the Chinese Bohai Sea (Supplementary Table 8). Agricultural fertilizers and pesticides, domestic and industrial sewage, and river water carrying pollutants may be the most important sources of POPs and heavy metals in coastal regions along the Chinese Bohai Sea. Except for the point source, the atmospheric deposition also contributed input of Hg and Cd into these regions. Moreover, the same cluster of Cd and Hg in sediment and the significant correlations between them and SPM in seawater (Table 2) indicated that anthropogenic discharge dominated Cd and Hg and that SPM plays important roles in their biogeochemical cycling in coastal regions along the Chinese Bohai Sea.
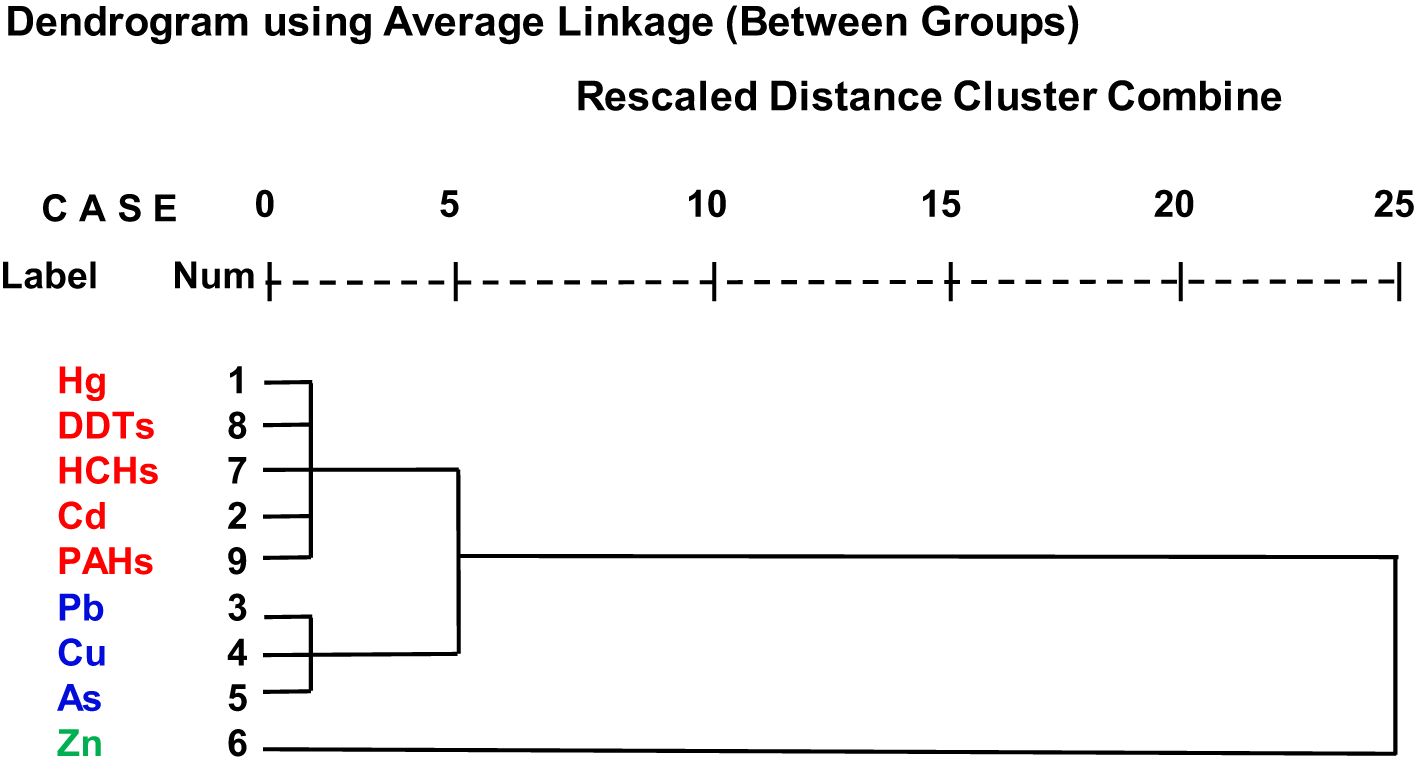
Figure 7 Cluster analyses of HCHs, DDTs, PAHs, and heavy metal concentrations in surface sediment of the coastal regions along the Chinese Bohai Sea. HCHs, hexachlorocyclohexanes; DDTs, dichlorodiphenyltrichloroethanes; PAHs, polycyclic aromatic hydrocarbons.
5 Conclusions
HCHs, DDTs, PAHs, and heavy metals in seawater, surface sediment, and organisms in coastal regions along the Chinese Bohai Sea were measured during 2015–2018. The results showed that the concentrations of HCHs, DDTs, and PAHs in surface sediment and concentrations of heavy metals in seawater and surface sediment were higher than the background values and the other coastal regions and that concentrations of As and Cu in approximately 95% and 20% of the sampled organisms exceeded MPLs recommended in China and the WHO, respectively, indicative of serious heavy metal pollution in coastal regions along the Chinese Bohai Sea. Significantly temporal variations in HCH and PAH concentrations and no variations in most heavy metal concentrations were found, and the higher concentrations were mainly located at estuaries and other nearshore areas, suggesting the important effects of anthropogenic discharges on POPs and heavy metal distributions in this coastal system. As, Cd and Hg were identified to be the primary heavy metal pollutants in sediment using Igeo and PERI methods, and the dietary risk posed by As in organisms is worthy of attention according to higher PTDI values. Finally, anthropogenic activities (e.g., industrial and agricultural discharges, and riverine and sewage inputs) played important roles in controlling HCHs, DDTs, PAHs, and heavy metal distributions in coastal regions along the Chinese Bohai Sea by multiple regression, Pearson’s, and cluster analyses.
Data availability statement
The original contributions presented in the study are included in the article/Supplementary Material. Further inquiries can be directed to the corresponding authors.
Ethics statement
The manuscript presents research on animals that do not require ethical approval for their study.
Author contributions
YS: Writing – original draft, Writing – review & editing, Formal analysis, Data curation, Methodology. WW: Writing – original draft, Investigation, Formal analysis. YYS: Writing – original draft, Investigation. XW: Writing – original draft, Investigation. HZ: Writing – original draft, Investigation. ZL: Writing – review & editing, Writing – original draft, Visualization, Supervision, Resources, Conceptualization. YY: Writing – review & editing, Visualization, Supervision, Resources, Project administration, Methodology, Conceptualization.
Funding
The author(s) declare financial support was received for the research, authorship, and/or publication of this article. This work was supported by the Hubei University of Science and Technology Program (BK202416) and the National Natural Science Foundation of China (32000906, 42307332).
Acknowledgments
We thank the editor and reviewer for their valuable comments and suggestions. We are also thankful to our lab fellow and all those who assisted during this study.
Conflict of interest
The authors declare that the research was conducted in the absence of any commercial or financial relationships that could be construed as a potential conflict of interest.
Publisher’s note
All claims expressed in this article are solely those of the authors and do not necessarily represent those of their affiliated organizations, or those of the publisher, the editors and the reviewers. Any product that may be evaluated in this article, or claim that may be made by its manufacturer, is not guaranteed or endorsed by the publisher.
Supplementary material
The Supplementary Material for this article can be found online at: https://www.frontiersin.org/articles/10.3389/fmars.2024.1450208/full#supplementary-material
References
Ali H., Khan E., Ilahi I. (2019). Environmental chemistry and ecotoxicology of hazardous heavy metals: environmental persistence, toxicity, and bioaccumulation. J. Chem. 2019, 1–14. doi: 10.1155/2019/6730305
Aparicio-González A., Duarte C. M., Tovar-Sánchez A. (2012). Trace metals in deep ocean waters: a review. J. Mar. Syst. 100-101, 26–33. doi: 10.1016/j.jmarsys.2012.03.008
Archana A., Thibodeau B., Geeraert N., Xu M. N., Kao S. J., Baker D. M. (2018). Nitrogen sources and cycling revealed by dual isotopes of nitrate in a complex urbanized environment. Water Res. 142, 459–470. doi: 10.1016/j.watres.2018.06.004
Bureau of Fisheries and Administration, Ministry of Agriculture and Rural Affairs of the People's Republic of China (2019). China Fishery Statistical Yearbook (Beijing, China: China Agriculture Press).
Carro N., Garcia I., Ignacio M. C., Llompart M., Yebra M. C., Mouteira A. (2002). Microwave-assisted extraction and mild saponification for determination of organochlorine pesticides in oyster samples. Anal. Bioanal. Chem. 374, 547–553. doi: 10.1007/s00216-002-1437-1
Chen L. F., Cai X. Y., Cao M. X., Liu H. W., Liang Y., Hu L. G., et al. (2022). Long-term investigation of heavy metal variations in mollusks along the Chinese Bohai Sea. Ecotoxicol. Environ. Saf. 236, 113443. doi: 10.1016/j.ecoenv.2022.113443
Chen L. F., Zhang X., Cao M. X., Pan Y., Xiao C. L., Wang P., et al. (2021). Release of legacy mercury and effect of aquaculture on mercury biogeochemical cycling in highly polluted Ya-Er Lake, China. Chemosphere 275, 130011. doi: 10.1016/j.chemosphere.2021.130011
Fernandes L. L., Kessarkar P. M., Rao V. P., Suja S., Parthiban G., Kurian S. (2019). Seasonal distribution of trace metals in suspended particulate and bottom sediments of four microtidal river estuaries, west coast of India. Hydrolog. Sci. J. 64, 1519–1534. doi: 10.1080/02626667.2019.1655147
Ferreira S. L. C., da Silva J. B., dos Santos I. F., de Oliveira O. M. C., Cerda V., Queiroz A. F. S. (2022). Use of pollution indices and ecological risk in the assessment of contamination from chemical elements in soils and sediments-practical aspects. Trends Environ. Anal. 35, e00169. doi: 10.1016/j.teac.2022.e00169
Gao X., Chen C. T. (2012). Heavy metal pollution status in surface sediments of the coastal Bohai Bay. Water Res. 46, 1901–1911. doi: 10.1016/j.watres.2012.01.007
Gao X. L., Yang Y. W., Wang C. Y. (2012). Geochemistry of organic carbon and nitrogen in surface sediments of coastal Bohai Bay inferred from their ratios and stable isotopic signatures. Mar. pollut. Bull. 64, 1148–1155. doi: 10.1016/j.marpolbul.2012.03.028
Gao X. L., Zhou F. X., Chen C. T. (2014). Pollution status of the Bohai Sea: an overview of the environmental quality assessment related trace metals. Environ. Int. 62, 12–30. doi: 10.1016/j.envint.2013.09.019
General Administration of Quality Supervision, Inspection, and Quarantine and Standardization Administration of the People’s Republic of China (2008a). The specification for marine monitoring-Part 4: Seawater analysis (GB 17378.4-2007) (in Chinese) Beijing: Standards Press of China.
General Administration of Quality Supervision, Inspection, and Quarantine and Standardization Administration of the People’s Republic of China (2008b). The specification for marine monitoring-Part 5: Sediment analysis (GB 17378.5-2007) (in Chinese) Beijing: Standards Press of China.
General Administration of Quality Supervision, Inspection, and Quarantine and Standardization Administration of the People’s Republic of China (2008c). The specification for marine monitoring-Part 6: Organism analysis (GB 17378.6-2007) (in Chinese) Beijing: Standards Press of China.
Gong W., Wang J., Cui W., Zhu L. (2021). Distribution characteristics and risk assessment of TBBPA in seawater and zooplankton in northern sea areas, China. Environ. Geochem. Health 43, 4759–4769. doi: 10.1007/s10653-021-00948-5
Harmesa W., Lestari A. J., Taufiqurrahman E. (2022). Variability of trace metals in coastal and estuary: distribution, profile, and drivers. Mar. pollut. Bull. 174, 113173. doi: 10.1016/j.marpolbul.2021.113173
Health Commission of Shandong Province (2015). Available online at: http://wsjkw.shandong.gov.cn/zwgk/zdmsgysy/.
Hilton J., Davison W., Ochsenbein U. (1985). A mathematical model for analysis of sediment core data: implications for enrichment factor calculations and trace-metal transport mechanisms. Chem. Geol. 48, 281–291. doi: 10.1016/0009-2541(85)90053-1
Hoffmann L. J., Breitbarth E., Boyd P. W., Hunter K. A. (2012). Influence of ocean warming and acidification on trace metal biogeochemistry. Mar. Ecol. Prog. Ser. 470, 191–205. doi: 10.3354/meps10082
Hu N. J., Huang P., Zhang H., Wang X. J., Zhu A. M., Liu J. H., et al. (2017). Geochemical source, deposition, and environmental risk assessment of cadmium in surface and core sediments from the Bohai Sea, China. Environ. Sci. pollut. Res. Int. 24, 827–843. doi: 10.1007/s11356-016-7800-0
Joint FAO/WHO Expert Committee on Food Additives (1982). Toxicological Evaluation of Certain Food Additives and Contaminants. WHO Food Additives Series No 17 (Cambridge, UK: Cambridge University Press), 28–35.
Kamarudin M. K. A., Abd Wahab N., Bati S. N. A. M., Toriman M. E., Saudi A. S. M., Umar R., et al. (2020). Seasonal variation on dissolved oxygen, biochemical oxygen demand and chemical oxygen demand in Terengganu River basin, Malaysia. J. Environ. Sci. Manag. 23, 1–7. doi: 10.47125/jesam/
Kennsih M. J. (1997). Heavy metals: practical handbook of estuarine and marine pollution. 253–298 London: CRC Press.
Lao Q. B., Su Q. Z., Liu G. Q., Shen Y. L., Chen F. J., Lei X. T., et al. (2019). Spatial distribution of and historical changes in heavy metals in the surface seawater and sediments of the Beibu Gulf, China. Mar. pollut. Bull. 146, 427–434. doi: 10.1016/j.marpolbul.2019.06.080
Li G. G., Hu B. Q., Bi J. Q., Leng Q. N., Xiao C. Q., Yang Z. C. (2013). Heavy metals distribution and contamination in surface sediments of the coastal Shandong Peninsula (Yellow Sea). Mar. pollut. Bull. 76, 420–426. doi: 10.1016/j.marpolbul.2013.08.032
Li L., Wang X. J., Liu J. H., Shi X. F. (2017). Dissolved trace metal (Cu, Cd, Co, Ni, and Ag) distribution and Cu speciation in the southern Yellow Sea and Bohai Sea, China. J. Geophys. Res. Oceans 122, 1190–1205. doi: 10.1002/2016JC012500
Liu H. J., Ding C. L., Zhang G. C., Guo Y. Y., Song Y. Y., Thangaraj S., et al. (2023). Dissolved and particulate heavy metal pollution status in seawater and sedimentary heavy metals of the Bohai Bay. Mar. Environ. Res. 191, 106158. doi: 10.1016/j.marenvres.2023.106158
Liu L., Tang J., Zhong G., Zhen X., Pan X., Tian C. (2018). Spatial distribution and seasonal variation of four current-use pesticides (CUPs) in air and surface water of the Bohai Sea, China. Sci. Total Environ. 621, 516–523. doi: 10.1016/j.scitotenv.2017.11.282
Lu Y. X., Pan D. W., Yang T. T., Wang C. C. (2022). Distribution characteristics and controlling factors of typical heavy metals in Huanghe River estuary, China. J. Oceanol. Limnol. 41, 150–165. doi: 10.1007/s00343-021-1320-6
National Bureau of Statistics of China (2022). Available online at: https://www.stats.gov.cn/.
National Health and Family Planning Commission, National Food and Drug Administration of the People's Republic of China (2017). National standards for food safety: Limits of contaminants in food (GB 2762-2017) (in Chinese) Beijing: Standards Press of China.
National Health Commission, Ministry of Agriculture and Rural Affairs, State Administration of Market Regulation of the People's Republic of China (2021). National food safety standard-Maximum residue limits for pesticides in food (GB 2763-2021) (in Chinese) Beijing: Standards Press of China.
National Health Commission, State Administration of Market Regulation of the People's Republic of China (2022). National standards for food safety: Limits of contaminants in food (GB 2762-2022) (in Chinese) Beijing: Standards Press of China.
Palm A., Cousins I., Gustafsson O., Axelman J., Grunder K., Broman D., et al. (2004). Evaluation of sequentially-coupled POP fluxes estimated from simultaneous measurements in multiple compartments of an air-water-sediment system. Environ. pollut. 128, 85–97. doi: 10.1016/j.envpol.2003.08.023
Pan K., Wang W. X. (2012). Trace metal contamination in estuarine and coastal environments in China. Sci. Total Environ. 421-422, 3–16. doi: 10.1016/j.scitotenv.2011.03.013
Parsons T. R., Maita Y. R., Lalli C. M. (1984). A manual of chemical & biological methods for seawater analysis (Oxford: Pergamin Press), 173.
Peng S. T. (2015). The nutrient, total petroleum hydrocarbon and heavy metal contents in the seawater of Bohai Bay, China: temporal-spatial variations, sources, pollution statuses, and ecological risks. Mar. pollut. Bull. 95, 445–451. doi: 10.1016/j.marpolbul.2015.03.032
Schwarzenbach R. P., Egli T., Hofstetter T. B., von Gunten U., Wehrli B. (2010). Global water pollution and human health. Annu. Rev. Environ. Resour. 35, 109–136. doi: 10.1146/annurev-environ-100809-125342
Siegel F. R. (2003). Environmental geochemistry of potentially toxic metals (New York: Springer Verlag). doi: 10.1007/978-3-662-04739-2
Slomp C. P., Van Cappellen P. (2004). Nutrient inputs to the coastal ocean through submarine groundwater discharge: controls and potential impact. J. Hydrol. 295, 64–86. doi: 10.1016/j.jhydrol.2004.02.018
Sloth J. J., Julshamn K. (2008). Survey of total and inorganic arsenic content in blue mussels (Mytilus edulis L.) from Norwegian fiords: revelation of unusual high levels of inorganic arsenic. J. Agric. Food Chem. 56, 1269–1273. doi: 10.1021/jf073174+
Sreedevi M. A., Harikumar P. S. (2023). Occurrence, distribution, and ecological risk of heavy metals and persistent organic pollutants (OCPs, PCBs, and PAHs) in surface sediments of the Ashtamudi wetland, south-west coast of India. Reg. Stud. Mar. Sci. 64, 103044. doi: 10.1016/j.rsma.2023.103044
Sunda W. G. (2012). Feedback interactions between trace metal nutrients and phytoplankton in the ocean. Front. Microbiol. 3, 204. doi: 10.3389/fmicb.2012.00204
UNEP (2019). “Stockholm convention on persistent organic pollutants (POPS)-texts and annexes,” in Secr. Stock. Conv, vol. 79. Available at: http://chm.pops.int/.
USEPA (1996a). SW-846 Test Method 3540C: Soxhlet Extraction. Available online at: https://www.epa.gov/hw-sw846/sw-846-test-method-3540c-soxhlet-extraction.
USEPA (1996b). SW-846 Test Method 3630C: Silica Gel Cleanup. Available online at: https://www.epa.gov/hw-sw846/sw-846-test-method-3630c-silica-gel-cleanup.
USEPA (2008). Integrated Risk Information System. Available online at: https://cfpub.epa.gov/ncea/risk/recordisplay.cfm?deid=2776&wd=&eqid=edfe8f3f000319aa00000004647818c3.
Vives I., Grimalt J. O. (2002). Method for integrated analysis of polycyclic aromatic hydrocarbons and organochlorine compounds in fish liver. J. Chromatogr. B. 768, 247–254. doi: 10.1016/S1570-0232(01)00585-2
Wang X. J., Fu R. L., Li H. L., Zhang Y., Lu M. Q., Xiao K., et al. (2020). Heavy metal contamination in surface sediments: a comprehensive, large-scale evaluation for the Bohai Sea, China. Environ. pollut. 260, 113986. doi: 10.1016/j.envpol.2020.113986
Wang A. B., Guo X. Y., Ding X. K., Shi J., Tang J. H. (2024). Effect of hydrodynamic and ecosystem conditions on persistent organic pollutant temporal-spatial variations in the Yellow Sea. J. Hazard. Mater. 469, 134051. doi: 10.1016/j.jhazmat.2024.134051
Wang Y. W., Liang L. N., Shi J. B., Jiang G. B. (2005). Study on the contamination of heavy metals and their correlations in mollusks collected from coastal sites along the Chinese Bohai Sea. Environ. Int. 31, 1103–1113. doi: 10.1016/j.envint.2005.02.005
Wang C. C., Pan D. W., Han H. T., Hu X. P. (2018). Vertical profile, contamination assessment of mercury and arsenic in sediment cores from typical intertidal zones of China. Environ. Monit. Assess. 190, 366. doi: 10.1007/s10661-018-6732-1
Wang C. Y., Wang X. L. (2007). Spatial distribution of dissolved Pb, Hg, Cd, Cu and as in the Bohai sea. J. Environ. Sci. 19, 1061–1066. doi: 10.1016/S1001-0742(07)60173-9
Wu Z. L., Lin T., Guo T. F., Li Y. Y., Li Z. X., Guo Z. G. (2020). Occurrence, air-sea exchange, and gas-particle partitioning of atmospheric polybrominated diphenyl ethers from East Asia to the Northwest Pacific Ocean. Chemosphere 240, 124933. doi: 10.1016/j.chemosphere.2019.124933
Wu Y. R., Zeng J. Y. (1983). Heavy metal pollution and the background value on the estuaries, bays and coastal waters. Mar. Environ. Sci. 12, 60–67. doi: CNKI:SUN:HYHJ.0.1983-04-009
Xiao C. L., Jian H. M., Chen L. F., Liu C., Gao H. Y., Zhang C. S., et al. (2017). Toxic metal pollution in the Yellow Sea and Bohai Sea, China: distribution, controlling factors and potential risk. Mar. pollut. Bull. 119, 381–389. doi: 10.1016/j.marpolbul.2017.03.027
Yao Q. Z., Wang X. J., Jian H. M., Chen H. T., Yu Z. G. (2016). Behavior of suspended particles in the Changjiang Estuary: size distribution and trace metal contamination. Mar. pollut. Bull. 103, 159–167. doi: 10.1016/j.marpolbul.2015.12.026
Yu M. Y., Chen L. F., Tao H., Cao M. X., Zhang X., Liang Y., et al. (2023). Pollution status and risk assessment of heavy metals in the sediment of a historically contaminated lake treated by oxidation pond in China. Environ. Sci. pollut. Res. 30, 41794–41805. doi: 10.1007/s11356-023-25205-3
Zeng J., Han G. L., Wu Q. X., Tang Y. (2019). Heavy metals in suspended particulate matter of the Zhujiang River, Southwest China: contents, sources, and health risks. Int. J. Environ. Res. Public Health 16, 1843. doi: 10.3390/ijerph16101843
Zhang Y., Lu X. Q., Wang N. L., Xin M. N., Geng S. W., Jia J., et al. (2016). Heavy metals in aquatic organisms of different trophic levels and their potential human health risk in Bohai Bay, China. Environ. Sci. pollut. Res. Int. 23, 17801–17810. doi: 10.1007/s11356-016-6948-y
Zhang L., Shi Z., Zhang J. P., Jiang Z. J., Wang F., Huang X. P. (2015). Spatial and seasonal characteristics of dissolved heavy metals in the east and west Guangdong coastal waters, South China. Mar. pollut. Bull. 95, 419–426. doi: 10.1016/j.marpolbul.2015.03.035
Keywords: persistent organic pollutants, heavy metals, spatial-temporal variations, risk assessments, controlling factors, coastal regions along the Chinese Bohai Sea
Citation: Su Y, Wang W, Su Y, Wang X, Zhao H, Li Z and Yu Y (2024) Long-term investigation of spatial–temporal variations, risk assessments, and controlling factors of persistent organic pollutants (HCHs, DDTs, and PAHs) and heavy metals in coastal regions along the Chinese Bohai Sea. Front. Mar. Sci. 11:1450208. doi: 10.3389/fmars.2024.1450208
Received: 17 June 2024; Accepted: 01 July 2024;
Published: 26 July 2024.
Edited by:
Zeming Zhang, Ningbo University, ChinaReviewed by:
Guicheng Zhang, Tianjin University of Science and Technology, ChinaDongliang Lu, Beibu Gulf University, China
Copyright © 2024 Su, Wang, Su, Wang, Zhao, Li and Yu. This is an open-access article distributed under the terms of the Creative Commons Attribution License (CC BY). The use, distribution or reproduction in other forums is permitted, provided the original author(s) and the copyright owner(s) are credited and that the original publication in this journal is cited, in accordance with accepted academic practice. No use, distribution or reproduction is permitted which does not comply with these terms.
*Correspondence: You Yu, youyu12345@whu.edu.cn; Zhunjie Li, zjli@jhun.edu.cn
†These authors have contributed equally to this work and share first authorship