- 1Institute of Oceanographic Instrumentation, Qilu University of Technology (Shandong Academy of Sciences), Qingdao, China
- 2Laoshan Laboratory, Qingdao, China
- 3Department of Materials Science and Engineering, Ocean University of China, Qingdao, China
- 4Key Laboratory of Global Change and Marine Atmospheric Chemistry, Ministry of Natural Resources, Xiamen, China
- 5Third Institute of Oceanography, Ministry of Natural Resources, Xiamen, China
In recent years, microplastics, especially marine microplastic pollution, have received global attention as a new type of environmental problem. The establishment of accurate and efficient methods for the detection of microplastics is the basis for in-depth research on the transport, transformation, fate, and ecotoxicological effects of microplastics in the environment. Microplastics in seawater frequently mix with biological tissues, resulting in challenges when identifying samples. However, commonly used pretreatment protocols for microplastics often suffer from long digestion times, inadequate digestion, and the risk of potentially damaging microplastics. This study compared the digestion efficiencies of five digestion reagents and provided further insights into two advanced oxidation methods involving Fenton’s reagent and an innovative alkaline K2S2O8 protocol based on sulfate and hydroxyl radicals. Using Raman spectroscopy, scanning electron microscopy-energy dispersive spectroscopy (SEM−EDS), and carbonyl index (CI) analyses, the status of microplastics after pretreatment was evaluated. The results revealed that the alkaline K2S2O8 method could enhance the reaction efficiency while reducing the potential for functional group damage during microplastic pretreatment. Moreover, the proposed K2S2O8 method was applied to the pretreatment of field seawater samples, and field microplastics were effectively separated from biologically rich samples. Thus, a digestion protocol based on alkaline K2S2O8 provides an effective way to isolate seawater microplastics from biologically rich samples. This study contributes to the development of efficiently microplastic monitoring and provides valuable insights into access to reliable data for fate and inventory of oceanic microplastics.
1 Introduction
Microplastics, commonly defined as plastic particles, filaments, films, and fragments smaller than 5 mm, have garnered significant research attention in marine environmental monitoring studies (Auta et al., 2017; Prabhu et al., 2022; Medina et al., 2024). Polystyrene (PS) pellets were initially reported in British coastal waters during the 1970s (Carpenter et al., 1972). Numerous studies (Andrady, 2011; Enders et al., 2015; Zhou et al., 2022) have demonstrated the widespread presence of microplastics measuring less than 5 mm in the ocean. Due to their small particle size, microplastics can be ingested by marine organisms, including zooplankton, shellfish, fish, seabirds, and mammals, and subsequently, they can traverse the food chain, posing a potential health hazard to humans (Cole et al., 2015; Zurub et al., 2024). Marine microplastic pollution not only threatens marine biological ecosystems, the safety of the food chain and human health but also involves the impact of climate change (Li et al., 2024), transboundary cross-border pollution (Ramesh et al., 2024), industrial restructuring (Guerranti et al., 2019) and international governance (Thacharodi et al., 2024). Accurate and efficient monitoring and analysis of microplastics in the marine environment is an important basis for assessing the ecological risk of microplastics, evaluating the state of marine health, and regulating and managing microplastic pollution (Belioka and Achilias, 2023).
Microplastics in the marine environment have diverse compositions, complex chemical structures, and different appearances and morphologies, and under the constraints of different research objectives and experimental environments, there is no uniform analytical and identification standard at present. Spectroscopic methods have been developed as the most convenient and effective approaches for detecting microplastics in the environment (Lenz et al., 2015). However, microplastics in the environment often coexist with a large number of impurities that are far in excess of their own levels. The samples collected in the field are contaminated with biological impurities; hence, they cannot be directly analyzed by spectrum instruments. The quality of sample pretreatment significantly influences subsequent detection by the instrument (Zheng et al., 2024). Furthermore, there is a pressing need for the purification of microplastics, especially for methods of qualitative analysis performed on surfaces, such as Fourier transform infrared (FTIR) spectroscopy and Raman spectrometry (Adhikari et al., 2022). However, there is a particular lack of methods for purifying microplastics to prevent the interference of biological impurities during determination.
To achieve efficient digestion for microplastic purification, prior studies (Miller et al., 2017; Tagg et al., 2017; Prata et al., 2019) have examined factors facilitating the thorough treatment of large-volume microplastic samples in a short timeframe. Currently, the primary purification methods include physical separation and chemical digestion, which include stirring and rinsing with fresh water (Mcdermid and Mcmullen, 2004), acid digestion (Andrady, 2011; Liebezeit and Dubaish, 2012), alkaline digestion (Claessens et al., 2013), oxidative agents (Tagg et al., 2017; Klaus et al., 2024), enzyme digestion (Cole et al., 2014), ultrasonic cleaning (Cooper and Corcoran, 2010), and the pressurized fluid extraction technique (Fuller and Gautam, 2016). Among these methods, chemical digestion has proven to be the most effective, with Fenton’s reagent (H2O2 mixed with FeSO4) emerging as the superior choice (Tagg et al., 2017). This method can effectively remove biological impurities from a sample (chitin, lignin, etc.), especially from amphipod carapaces, which may be difficult to digest (Shim et al., 2016; Erni-Cassola et al., 2017). Moreover, damage to polymers caused by acid/alkaline-based methods can also be avoided (Cole et al., 2014; Tagg et al., 2015). However, the highly reactive Fenton’s reagent method easily causes bumping and danger, especially in the semi-closed system of an automatic digestion device. Therefore, the quest for a digestion reagent with a mild chemical reaction and a high reaction rate remains ongoing.
The aim of this work was to select an effective method that (1) efficiently digests biological impurities while minimizing damage to as many polymer types as possible and (2) has a simple operating procedure that is less prone to staining, microplastic loss, and operational hazards. The efficiency and potential risks of multiple digestion methods were tested by setting different digestion conditions and characterized by Raman spectroscopy, Fourier transform infrared spectroscopy (FTIR) and scanning electron microscopy-energy dispersive spectroscopy (SEM−EDS). The final selected method was evaluated in field seawater samples.
2 Materials and methods
2.1 General conditions
The method validation protocols were tested on chitin (C8H13O5N)n, which is considered a significant insoluble polysaccharide-based biopolymer material present in the samples (Cole et al., 2014). The digestion efficiency of five solutions, namely, 10% KOH, alkaline K2S2O8 (original solution, 0.2 mol L–1 K2S2O8 with 0.4 mol L–1 NaOH), 30% H2O2, Fenton’s reagent, and 2 mol L–1 NaOH, was assessed using a known weight of chitin fragments. All solutions were prepared in distilled water and used immediately after preparation. The potential degradation risks of the method were examined for a series of polymers derived from commonly used chemical materials in daily human life: polycarbonate (PC) (≤100 µm), polyamide-6/6 (PA6/6) (≤250 µm), polybutylene terephthalate (PBT) (≤150 µm), PS (≤250 µm), polyvinyl chloride (PVC) (≤250 µm), polypropylene (PP) (≤500 µm), polyethylene (PE) (≤1 mm), and ethylene-vinyl acetate copolymer (EVA) (≤5 mm). All experiments carried out in this study were conducted using glass flasks within a meticulously maintained laboratory environment. Synthetic-free clothing and powder-free gloves were worn to prevent contamination from clothing or the surrounding air.
2.2 Digestion efficiency of chitin
The initial test aimed to assess the digestion efficiency of the chosen protocols. For comparison, 0.1 g of chitin (Aladdin, Shanghai, China) was precisely weighed and deposited into 250 mL conical flasks, which were covered with watch glass to prevent contamination. For digestion, 40 mL of digestion reagent was added to each sample, which was heated at 65°C for 6 h (except for Fenton’s reagent). Fenton’s reagent was used in accordance with the protocol of Tagg et al. (2017). Following digestion, the mixed solution was promptly filtered. Two well-performing methods were chosen for further comparison, involving an increase in the volume of the digestion solution, with 60 mL of these solutions added to 0.1 g of chitin and heated as previously described. After assessing these methods, 0.1 g of chitin was subjected to testing with various volumes of alkaline K2S2O8 for screening purposes. Additionally, different doses of alkaline K2S2O8, determined by their proportions and concentrations, were evaluated by reducing the concentration of alkaline K2S2O8 by 0.75 times.
2.3 Degradation effects on common polymers
In the second stage, eight virgin microplastics were subjected to the selected solution to evaluate the effects on polymer integrity. The integrity of the microplastics was assessed by examining weight loss and surface alterations and conducting qualitative tests on polymers after exposure to alkaline K2S2O8 (the original solution) at 65°C for 6 h. Each sample underwent weighing after overnight drying and imaging at 4000x magnification using an SEM-EDS system (Thermo Fisher Scientific Apreo 2, USA). The microplastics’ spectra were obtained using a Raman spectrometer (Horiba HR Evolution, Japan) and an FTIR spectrometer (Thermo Fisher Scientific Nicolet iS5, USA). The carbonyl index (CI) was used to characterize the degree of aging of the microplastics. The CI is obtained using the specified area under band method (Almond et al., 2020) via Equation 1:
The integrated band absorbance area in the carbonyl (C=O) region (1650–1850 cm-1) was normalized by using the integrated band absorbance area at wavenumbers from 1420 to 1500 cm-1, which is the bending-extension vibration of methylene (CH2) (Almond et al., 2020). Hence, it can be used as a reference since it remains constant throughout the aging process. The area under the band was calculated by Omnic 9 software (Thermo Fisher Scientific Inc., USA) using the peak analysis tool.
2.4 Evaluation with field samples
To explore the potential application of the novel digestion method, the digestion method was assessed using field seawater samples collected at the Marine Experimental Station of the Institute of Oceanographic Instrumentation in Qingdao, China (120.30°E, 36.05°N). The site is situated on the east coast of Qingdao, near the tourist resort area, making it susceptible to microplastic contamination. Seawater samples (1 ton) were collected using a sampling pump and filtered through metal filters (40 mm in diameter, 50 µm). The samples on the metal filters were washed with distilled water three to four times before being subjected to digestion. These samples were immediately subjected to digestion with alkaline K2S2O8. The treatment of the field samples was the same as that for the integrity test performed in section 2.3. The compositions of the suspected microplastics were identified and photographed using a Raman imaging spectrometer (Thermo Scientific DXRxi, USA). Ultrapure water served as a negative control, while metal filters without samples were used as the blank control.
2.5 Statistical analysis
Each treatment was executed on duplicate samples. Origin 2019b software (OriginLab, USA) was used for statistical calculations. The standard deviation of the mean was used to determine the precision of the mean.
3 Results and discussion
3.1 Digestion efficiency of chitin exposed to the method
In the exploratory test conducted to assess the digestion efficiency of chitin, the removal rate for each solution is shown in Figure 1A, with Fenton’s reagent and the alkaline K2S2O8 method presenting higher digestion efficiencies than the other methods. Although the 10% KOH, 30% H2O2 and 1–10 mol L-1 NaOH methods have been widely employed in various surveys involving seawater, sediments, and biota (Cole et al., 2014; Bellas et al., 2016; Prata et al., 2019; Ghayebzadeh et al., 2021), the digestion efficiencies exhibited a low rate with insignificant changes throughout the entire incubation duration in this experiment. In comparison to previous studies, the relatively short incubation period (6 h) in this experiment may explain the lower digestion efficiency, as previous research utilized incubation periods of at least 24 h and, in some cases, up to a week (Dehaut et al., 2016). The ability of Fenton’s reagent to rapidly degrade organic compounds through oxidation (Tagg et al., 2017) has made it effective for isolating microplastics from biological tissue, sediments, and wastewater. Similarly, the alkaline K2S2O8 method operates based on the advanced scavenging effect of free radicals generated by chemical reactions (Wang and Wang, 2018). Persulfate (PS) can be activated by thermal activation (> 50°C) and alkaline activation. For heat activation, the energy input due to the rising temperature can cause the fission of O-O bonds to form sulfate radicals, but the degradation efficiency might decrease when the temperature is greater than 60°C (Wang and Wang, 2018). During alkaline activation, PS can decompose into sulfate radicals, and sulfate radicals can further transform into hydroxyl radicals (Liang and Su, 2009). The sulfate radical has a higher oxidation reduction potential (2.5–3.1 eV) than the hydroxyl radical produced by Fenton’s reagent, which may account for the higher efficiency of digestion in the alkaline K2S2O8 system (Qin et al., 2016). The production of free radicals by PS activation is highly important for removing biological impurities from microplastics.
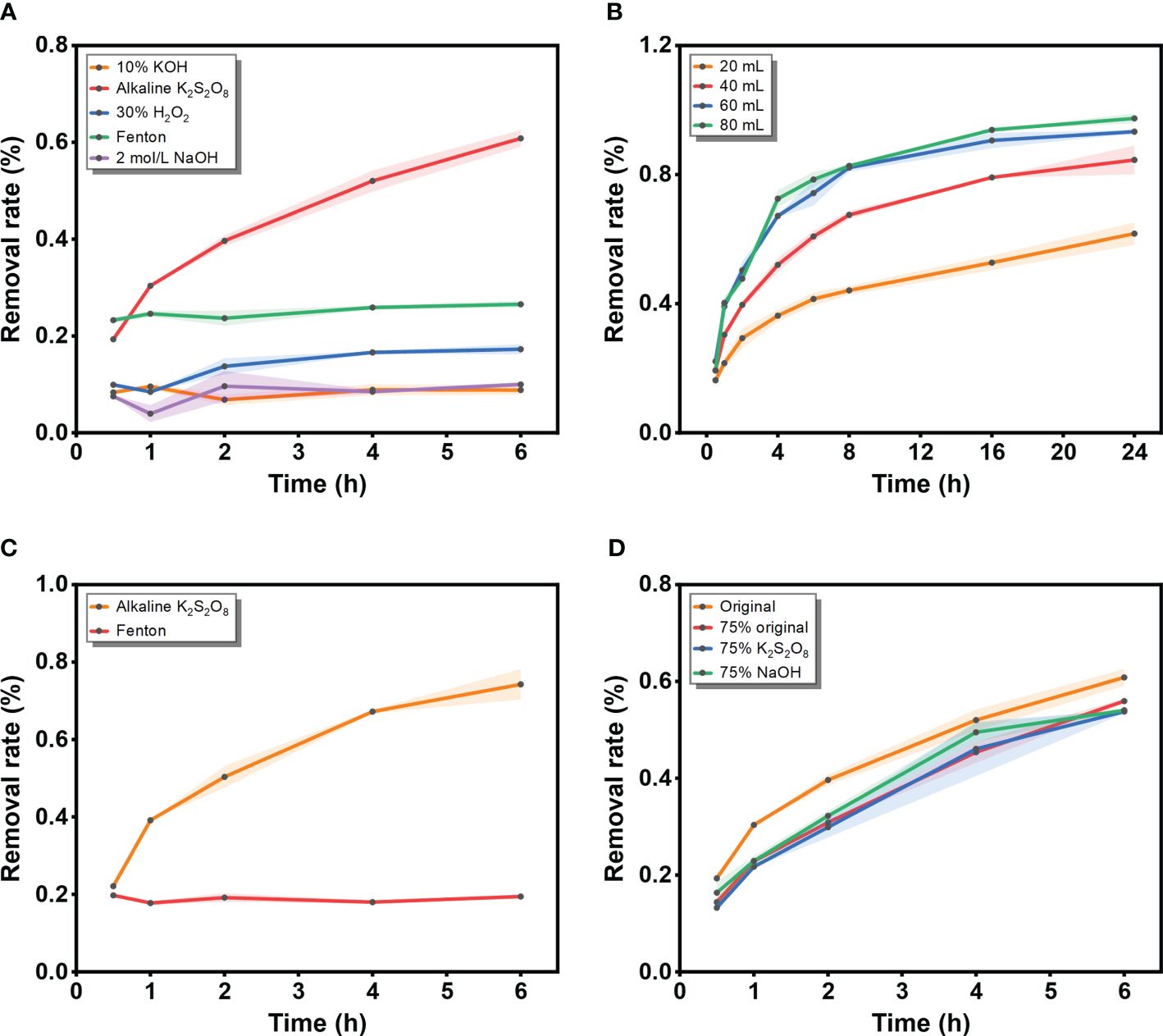
Figure 1 Digestion efficiency (%) of chitin exposed to different conditions. (A) Five solutions (10% KOH, alkaline K2S2O8 (original solution, 0.2 mol L-1 K2S2O8 with 0.4 mol L-1 NaOH), 30% H2O2, the Fenton’s reagent and 2 mol L-1 NaOH); (B) Different volumes of alkaline K2S2O8 (20 mL, 40 mL, 60 mL and 80 mL); (C) Two solutions (alkaline K2S2O8 and the Fenton’s reagent) at 60 mL; (D) Diluted groups of alkaline K2S2O8 (original solution, 75% original, 75% K2S2O8 and 75% NaOH).
Building upon the findings of previous experiments, the two well-performing methods were further assessed by increasing the solution volume to 60 mL (Figure 1B). During digestion, an increasing trend in digestion efficiency was observed for the alkaline K2S2O8 solution. The digestion efficiency of the alkaline K2S2O8 treatment significantly improved with increasing amounts of reagent (40 mL: 0.5 h: 19.32%, 6 h: 60.83%; 60 mL: 0.5 h: 22.12%, 6 h: 74.26%). With respect to the increase in volume, Fenton’s reagent maintained a constant digestion efficiency (approximately 20%) during the incubation, with the digestion endpoint occurring immediately at the beginning of the reaction. Considering digestion efficiency, weight loss, and spectral variations, Prata et al. (2019) regarded Fenton’s reagent as an effective and suitable protocol for removing organic matter from microplastic samples, successfully achieving 100% removal of algae. Notably, the completeness of digestibility varied depending on the organic matter composition. Similar to the PS activation mechanism, Wang et al. (2024) investigated an oxidation system using Co2+ to activate peroxymonosulfate. The time required to isolate micro/nanoplastics from organic pollutants was only 30 min, much shorter than in this study. However, the effectiveness of the peroxymonosulfate system in eliminating chitin remains unknown. Kallenbach et al. (2021) also evaluated four protocols for dissolving organisms with chitin exoskeletons. The combination of H2O2 and chitinase enzyme was highly effective, but required an incubation period exceeding 24 h. As demonstrated in the present study, alkaline K2S2O8 exhibited greater digestion efficiency than Fenton’s reagent throughout the entire incubation period, particularly for the most insoluble organic matter, chitin. The volume test revealed that the digestion efficiency increased with increasing volume. Moderate resolution efficiency was achieved with 40 mL of digestion solution, while nearly the same high digestion efficiency was maintained when the volume was increased to 60 mL and 80 mL (Figure 1C). The optimal concentration of the digestion solution was found to be 0.2 mol L–1 saturated K2S2O8 at room temperature (20°C) with 0.4 mol L–1 NaOH, which resulted in higher digestion efficiencies than those of the diluted groups (Figure 1D). Therefore, the ratio of K2S2O8 to NaOH was controlled at 1:2, with the goal of achieving better efficiency.
3.2 Assessment of microplastic weight loss
Prior to conducting quantitative or qualitative detection of microplastic samples, it is essential to eliminate the interference of biological impurities while ensuring that the sample itself remains undamaged. During digestion, samples are transferred, filtered, and exposed to air or the environment, resulting in sample loss. On the other hand, digestion reagents should be able to remove biological matter without damaging microplastics. Thus, after evaluating the digestion efficiency of several protocols, we proceeded to evaluate the effect of the selected digestion method on the weight loss of the microplastics. The effect of the preferred digestion reagents on the mass loss of microplastics was evaluated. The weight loss experiment, as depicted in Figure 2, revealed changes in the weight of various microplastics and the average recoveries. The microplastics exhibited a recovery rate of more than 95%. This observation can be attributed to two factors. First, it underscores the mild nature of the digestion agent, which does not substantially dissolve microplastics. Second, the findings were made on standard microplastic samples that had not undergone weathering or corrosion in the environment. Consequently, the functional groups on the surface of the microplastics remained relatively stable without alteration. Notably, the PC sample exhibited the lowest recovery rate. This could be attributed to the following factor: the particle size of PC is the smallest, making it more susceptible to significant sample contamination loss during the experiment compared to other microplastics. Kühn et al. (2017) reported that a mass loss of 7% occurred in PE after 48 h of treatment in KOH, whereas the loss was smaller in the research of Prata et al. (2019) and may be related to the limited exposure time. Prata et al. (2019) considered that some of the loss of microplastics may be related to surface heterogeneity of weathered microplastics. This result agrees with the present study. Nevertheless, Zheng et al. (2024) reported that the mass of PE decreased by approximately 4% after 30% H2O2 digestion and approximately 8% after 10% KOH digestion. This mass loss of PE was related only to the digestion reagent but not to the particle size.
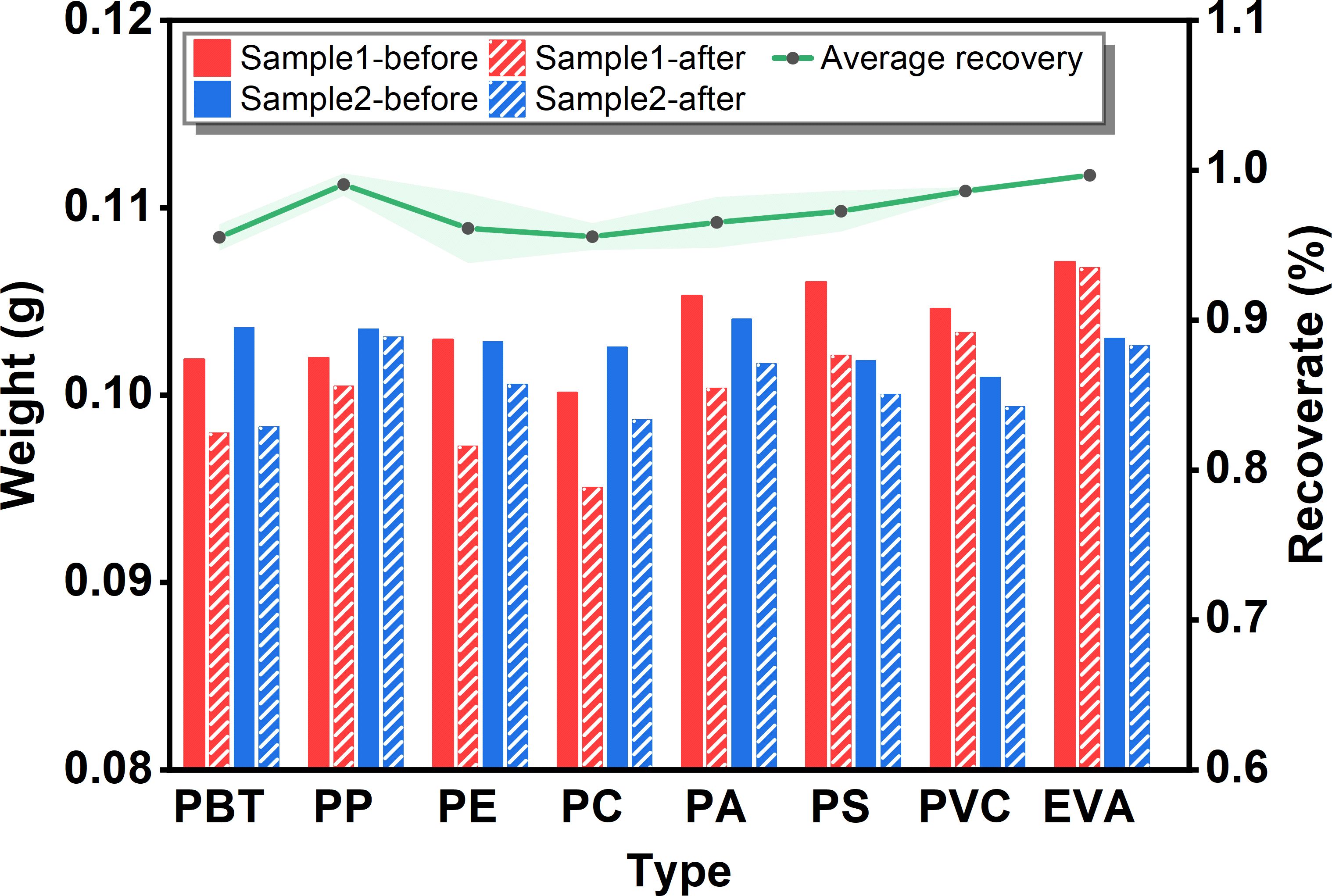
Figure 2 Weight loss of common microplastic types (PBT, PP, PE, PC, PA, PS, PVC and EVA) before and after alkaline K2S2O8 treatment.
3.3 Assessment of the degradation effects of microplastics
In addition to quality loss, microplastic degradation is generally recognized as the most unfavorable effect following digestion, resulting from violent reactions caused by highly oxidizing agents. For example, sulfate radicals produced from the decomposition of K2S2O8 under heating and alkaline conditions might cause the isolation of microplastics. Hence, it is important to test whether alkaline K2S2O8 will cause damage to the surface functional groups of microplastics. Further microscopic analysis was conducted to assess potential damage to the microplastics caused by the reagents. Changes in characteristics indicated that the tested reagents did not cause significant damage to the microplastics. Under magnification at 4000×, images captured before and after digestion demonstrated that the sample surface retained its smooth appearance without displaying noticeable corrosion, cracks, depressions, aggregation effects, and so on (Figure 3).
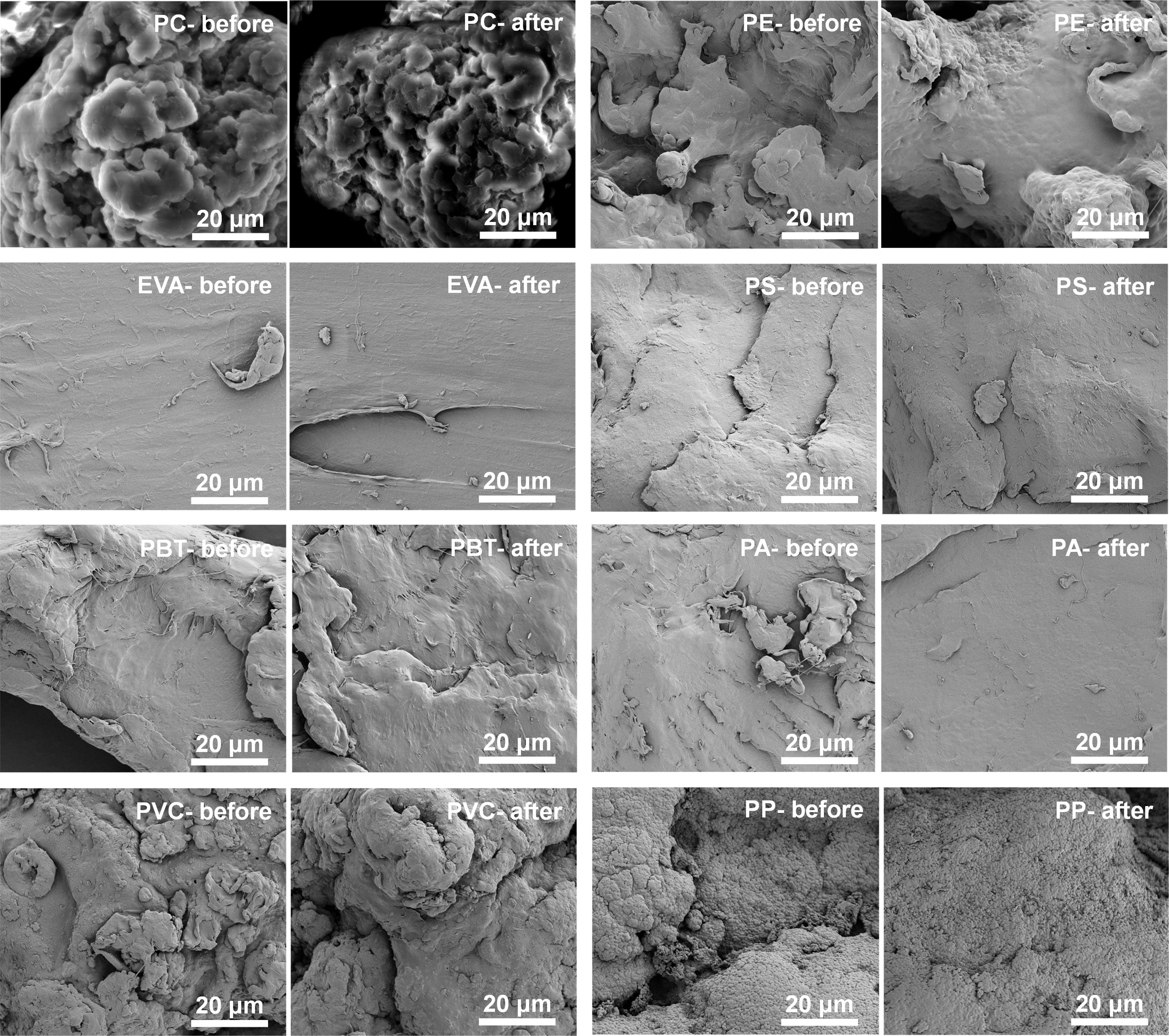
Figure 3 Surface images of microplastics (PC, PE, EVA, PS, PBT, PA, PVC and PP) before and after alkaline K2S2O8 treatment.
Nevertheless, surface examination alone cannot conclusively establish the harmlessness of the digestion agent to microplastics. Qualitative testing is essential to confirm whether chemical treatment impacts the integrity of key absorbance bands in the spectrum. Both virgin and digested microplastics were analyzed via Raman spectroscopy and FTIR. As depicted in the Raman identification results (Figure 4), no new characteristic peaks were observed, and the changes in the spectrum before and after the alkaline K2S2O8 treatment were not obvious. Furthermore, the presence and positions of key absorbance bands in the functional groups of the microplastics showed no significant misalignment. Any slight intensity changes observed in the spectrum can primarily be attributed to the displacement of the focused laser point, as alterations in sample surface morphology and location can lead to variations in intensity (Lenz et al., 2015). Zheng et al. (2024) also reported varying degrees of enhancement and attenuation of the characteristic peak intensity after digestion by HNO3, KOH, and H2O2 solutions. The Raman spectroscopy results of PA and PVC after H2O2 and KOH digestion reported by Karami et al. (2017) might be attributed to the longer exposure time.
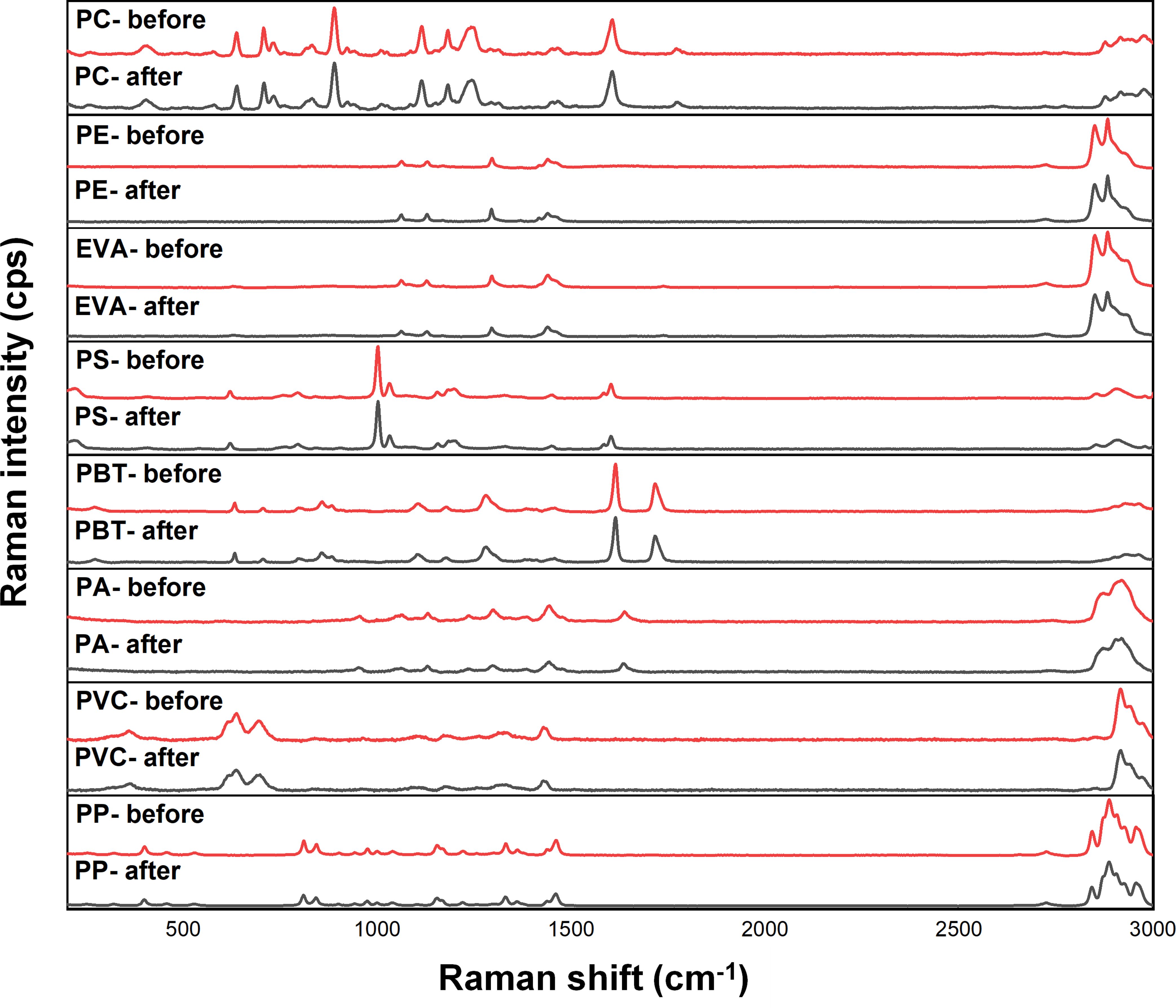
Figure 4 Raman spectra of microplastics (PC, PE, EVA, PS, PBT, PA, PVC and PP) before and after the digestion procedure.
According to the degradation mechanism of polymers, many different chemical groups are formed during their degradation, mainly producing different carbonyl and hydroxyl functional groups. Among them, the carbonyl group is the one that causes the most obvious changes in the infrared spectra and is ideal for tracking its degradation process because of its fixed position, high intensity and lack of interference. To determine the possible degradation of the microplastics under alkaline K2S2O8 treatment, the CI of individual samples was calculated (Figure 5). However, the calculation of the CI varied depending on the selection of the reference peak and the calculation of the peak integral area. Therefore, the C and O distributions on the surface of the microplastics obtained from EDS elemental analysis were combined to assess whether the microplastics underwent age-related degradation after alkaline K2S2O8 digestion (Figure 6). Most studies have shown that the CI of microplastics after prolonged advanced oxidation treatment generally increases significantly compared to that of virgin microplastics (Liu et al., 2019; Ren et al., 2021; Yang et al., 2024).
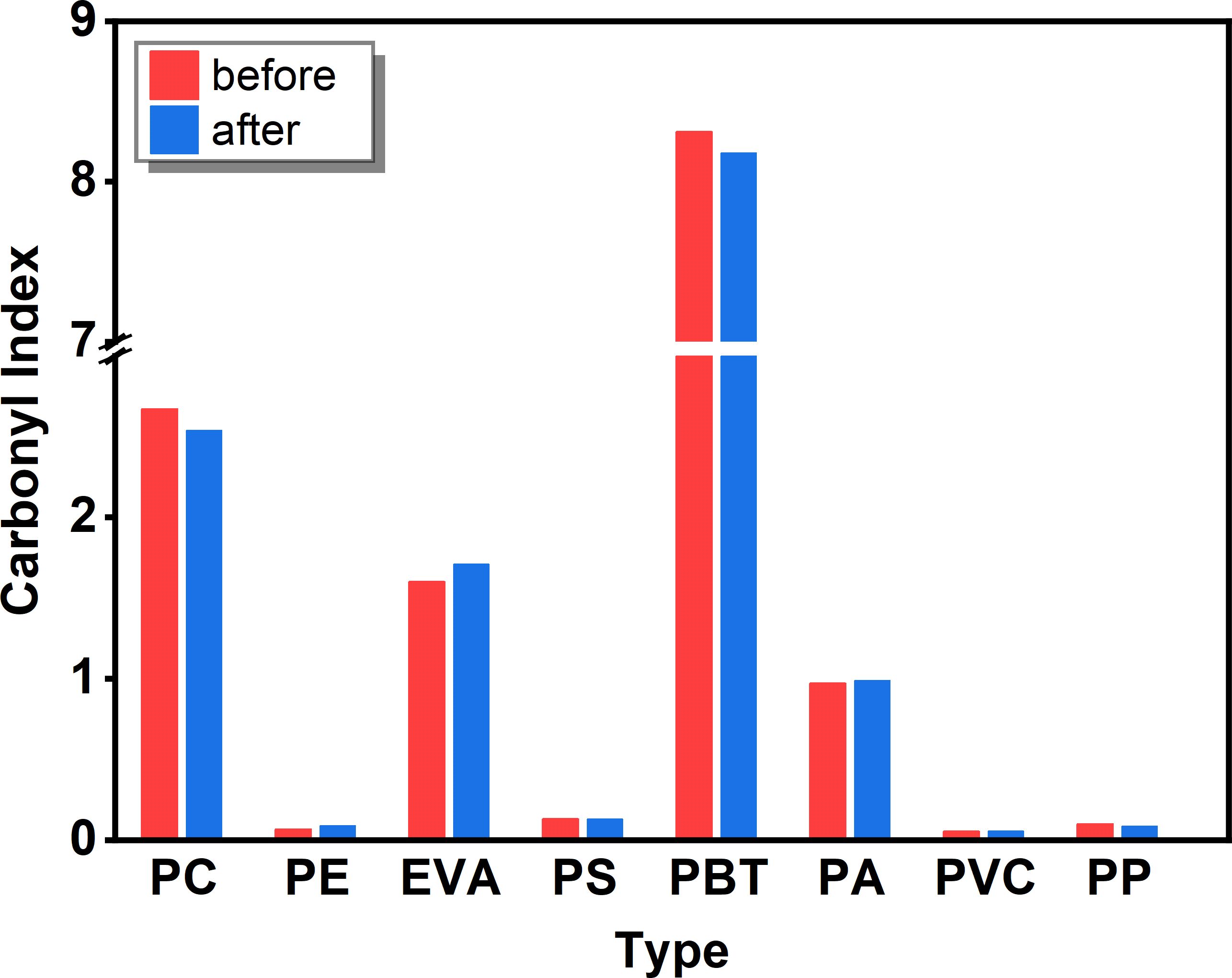
Figure 5 Changes in the CI of microplastics (PC, PE, EVA, PS, PBT, PA, PVC and PP) exposed to alkaline K2S2O8.
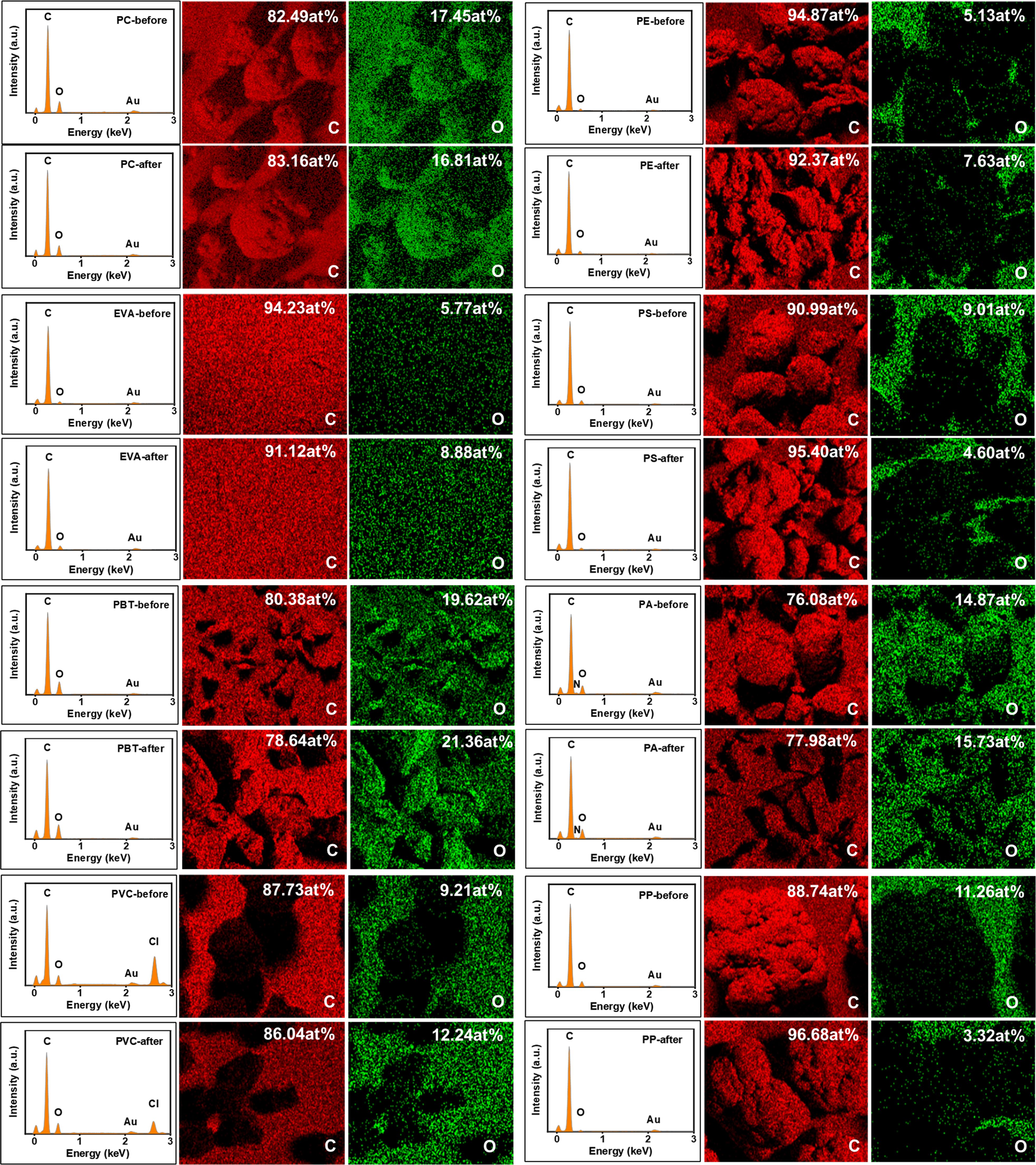
Figure 6 EDS of microplastic surfaces (PC, PE, EVA, PS, PBT, PA, PVC and PP) before and after alkaline K2S2O8 treatment.
The results of this experiment showed that the CI results of most of the microplastics were consistent with the results of EDS elemental analysis, and none of the changes were significant. The CI and O distribution of PE, EVA, PA, and PVC slightly increased, while a slight decrease was observed for PC. The O element distribution of PS decreased, and the opposite trend of CI value and O element distribution of PBT was observed with very little variation. The decrease in the O distribution of PP was more obvious, but the CI value decreased only slightly. Considering the error of individual samples, we inferred that the reagents did not significantly affect the chemical groups on the microplastic surfaces. However, as indicated by previous studies, certain digestion reagents, such as HNO3 (Claessens et al., 2013) and NaOH (Dehaut et al., 2016), have been shown to be detrimental to some microplastics. Roch and Brinker (2017) disintegrated the gastrointestinal tissue of fish using NaOH and HNO3 and found that PA is easily dissolved by HNO3. The reason may be that, first, the samples used in our test are granular and standard, the surface area is small, and the samples will not be cracked like environmental samples due to weathering. Cracks and grooves on microplastics will expose more adsorption sites on the surface, which is more likely to cause further etching. Prata et al. (2019) reported that the CI of fibrous PE but not that of ordinary PE increased after Fenton’s reagent treatment. Second, a shorter incubation time (6 h) may decrease the susceptibility of the microplastics to chemical alteration during pretreatment. Liu et al. (2019) reported an increase in the CI of PS and PE microplastics under heat-activated K2S2O8 treatment after 1 d of incubation, and the results indicated a significant alteration in the CI of the microplastics after 5 d of incubation. Overall, we concluded that the spectra of the microplastics treated with alkaline K2S2O8 did not affect the identification results, while the impurities in the samples were completely removed. Therefore, the method proposed in this experiment appears to be relatively effective and reliable.
3.4 Application of the method to field samples
To test the application of this method under real conditions, microplastics collected from seawater samples were processed using the alkaline K2S2O8 method. The digested samples showed no noticeable biological residue, and impurities were significantly reduced after treatment with alkaline K2S2O8. A total of 7 microplastics were detected on the metal filter, identified as PE, EVA, PS, and PA particles. The size of the collected microplastics ranged from 85–600 µm and included irregular particles and fragments. The detected microplastics exhibited translucent, white, and yellow colors (Figure 7). The present method enabled programmed treatment of biological impurities in microplastics, achieving convenient and efficient separation (within 6 h), with the isolated microplastics being easily identifiable. This study further demonstrates the suitability and effectiveness of alkaline K2S2O8 for seawater microplastic samples.
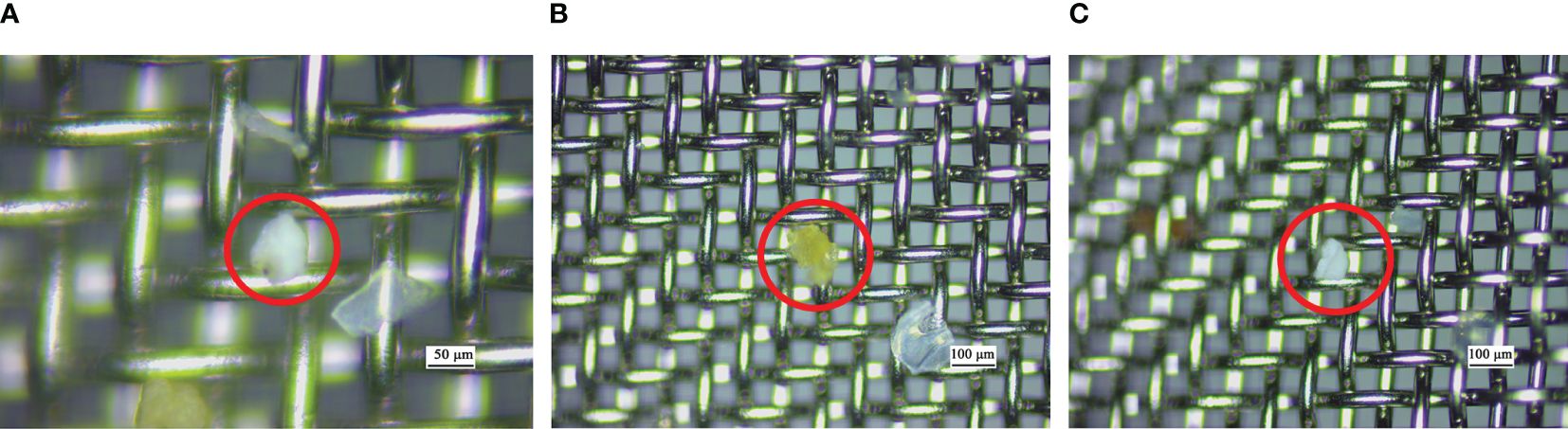
Figure 7 Several microplastics recovered from the seawater samples, using the described digestion method. (A) EVA, (B) PS and (C) PE.
4 Conclusion
In summary, to identify an efficient method for digesting biological impurities in microplastic samples, several adapted methods as well as digestion conditions for impurities in microplastics were compared in this study. Our laboratory tests and experiments conducted under real conditions confirmed that the alkaline K2S2O8 method is an effective approach for purifying microplastics, even though H2O2 and Fenton’s reagents have long been regarded as highly effective methods for removing impurities from microplastics. This method is capable of meeting the demands of digesting biological impurities in microplastics and addresses the limitation of time consumption for achieving higher digestion efficiency than H2O2 (Prata et al., 2019). Moreover, compared with Fenton’s reagent, this method does not cause bumping or dissolution of microplastics (compared with the effects of HNO3, etc.) (Claessens et al., 2013), which may cause danger or change the properties of microplastics. This finding holds significant promise for further automatic purification in equipment development. Typically, 60 mL of digestion agent is adequate for removing impurities from microplastics. To ensure effective digestion of substantial impurities, it is advisable to extend the digestion time, but the risk of microplastic degradation should be considered.
While achieving perfect digestion results can be challenging, this method appears to offer a better compromise when considering interference factors and achievable objectives. However, it is important to acknowledge the limitations of this experiment, including the feasibility of detecting fibrous microplastics, which can be more challenging in the environment, and potential interferences with microplastics of various colors. Considering the time, cost, reagent dosage, and safety of the experimental operation, the alkaline K2S2O8 method is recommended.
Data availability statement
The original contributions presented in the study are included in the article/Supplementary Material. Further inquiries can be directed to the corresponding authors.
Author contributions
NG: Conceptualization, Data curation, Funding acquisition, Methodology, Writing – original draft. XK: Data curation, Formal analysis, Methodology, Writing – review & editing. YMZ: Methodology, Writing – review & editing. YG: Investigation, Writing – review & editing. YBZ: Supervision, Writing – review & editing. YL: Funding acquisition, Writing – review & editing.
Funding
The author(s) declare financial support was received for the research, authorship, and/or publication of this article. This work was supported by the National Natural Science Foundation of China (U2006209), the Project (GCMAC2303) supported by the Key Laboratory of Global Change and Marine Atmospheric Chemistry (GCMAC) of Ministry of Natural Resources (MNR), the Basic Research Project (2022PX069) and the Science Education Industry Integration Pilot Major Innovation Project (2023HTZX01) of Qilu University of Technology (Shandong Academy of Sciences), and the Natural Science Foundation of Shandong Province (No. ZR2022QD013).
Acknowledgments
We thank the Shiyanjia Lab (www.shiyanjia.com) for the language editing of this manuscript.
Conflict of interest
The authors declare that the research was conducted in the absence of any commercial or financial relationships that could be construed as a potential conflict of interest.
Publisher’s note
All claims expressed in this article are solely those of the authors and do not necessarily represent those of their affiliated organizations, or those of the publisher, the editors and the reviewers. Any product that may be evaluated in this article, or claim that may be made by its manufacturer, is not guaranteed or endorsed by the publisher.
Supplementary material
The Supplementary Material for this article can be found online at: https://www.frontiersin.org/articles/10.3389/fmars.2024.1450330/full#supplementary-material
References
Adhikari S., Kelkar V., Kumar R., Halden R. U. (2022). Methods and challenges in the detection of microplastics and nanoplastics: a mini-review. Polym Int. 71, 543–551. doi: 10.1002/pi.6348
Almond J., Sugumaar P., Wenzel M. N., Hill G., Wallis C. (2020). Determination of the carbonyl index of polyethylene and polypropylene using specified area under band methodology with ATR-FTIR spectroscopy. e-Polymers. 20, 369–381. doi: 10.1515/epoly-2020-0041
Andrady A. L. (2011). Microplastics in the marine environment. Mar. pollut. Bull. 62, 1596–1605. doi: 10.1016/j.marpolbul.2011.05.030
Auta H. S., Emenike C. U., Fauziah S. H. (2017). Distribution and importance of microplastics in the marine environment: a review of the sources, fate, effects, and potential solutions. Environ. Int. 102, 165–176. doi: 10.1016/j.envint.2017.02.013
Belioka M., Achilias D. S. (2023). Microplastic pollution and monitoring in seawater and harbor environments: A meta-analysis and review. Sustainability. 15, 9079. doi: 10.3390/su15119079
Bellas J., Martínez-Armental J., Martínez-Cámara A., Besada V., Martínez-Gómez C. (2016). Ingestion of microplastics by demersal fish from the Spanish Atlantic and Mediterranean coasts. Mar. pollut. Bull. 109, 55–60. doi: 10.1016/j.marpolbul.2016.06.026
Carpenter E. J., Anderson S. J., Harvey G. R., Miklas H. P., Peck B. (1972). Polystyrene spherules in coastal waters. Science. 17, 749–750. doi: 10.1126/science.178.4062.749
Claessens M., Van Cauwenberghe L., Vandegehuchte M. B., Janssen C. R. (2013). New techniques for the detection of microplastics in sediments and field collected organisms. Mar. pollut. Bull. 70, 227–233. doi: 10.1016/j.marpolbul.2013.03.009
Cole M., Lindeque P., Fileman E., Halsband C., Galloway T. S. (2015). The impact of polystyrene microplastics on feeding, function and fecundity in the marine copepod calanus helgolandicus. Environ. Sci. Technol. 49, 1130–1137. doi: 10.1021/es504525u
Cole M., Webb H., Lindeque P. K., Fileman E. S., Halsband C., Galloway T. S. (2014). Isolation of microplastics in biota-rich seawater samples and marine organisms. Sci. Rep. 4, 4528. doi: 10.1038/srep04528
Cooper D. A., Corcoran P. L. (2010). Effects of mechanical and chemical processes on the degradation of plastic beach debris on the island of Kauai, Hawaii. Mar. pollut. Bull. 60, 650–654. doi: 10.1016/j.marpolbul.2009.12.026
Dehaut A., Cassone A., Frère L., Hermabessiere L., Himber C., Rinnert E., et al. (2016). Microplastics in seafood: Benchmark protocol for their extraction and characterization. Environ. pollut. 215, 223–233. doi: 10.1016/j.envpol.2016.05.018
Enders K., Lenz R., Stedmon C. A., Nielsen T. G. (2015). Abundance, size and polymer composition of marine microplastics ≥10 μm in the Atlantic Ocean and their modelled vertical distribution. Mar. pollut. Bull. 100, 70–81. doi: 10.1016/j.marpolbul.2015.09.027
Erni-Cassola G., Gibson M. I., Thompson R. C., Christie-Oleza J. A. (2017). Lost, but found with Nile red; a novel method to detect and quantify small microplastics (20 µm–1 mm) in environmental samples. Environ. Sci. Technol. 51, 13641–13648. doi: 10.1021/acs.est.7b04512
Fuller S., Gautam A. (2016). A procedure for measuring microplastics using pressurized fluid extraction. Environ. Sci. Technol. 50, 5774. doi: 10.1021/acs.est.6b00816
Ghayebzadeh M., Taghipour H., Aslani H. (2021). Abundance and distribution of microplastics in the sediments of the estuary of seventeen rivers: Caspian southern coasts. Mar. pollut. Bull. 164, 112044. doi: 10.1016/j.marpolbul.2021.112044
Guerranti C., Martellini T., Perra G., Scopetani C., Cincinelli A. (2019). Microplastics in cosmetics: Environmental issues and needs for global bans. Environ. Toxicol. Phar. 68, 75–79. doi: 10.1016/j.etap.2019.03.007
Kallenbach E. M. F., Hurley R. R., Lusher A., Friberg N. (2021). Chitinase digestion for the analysis of microplastics in chitinaceous organisms using the terrestrial isopod Oniscus asellus L. as a model organism. Sci. Total. Environ. 786, 147455. doi: 10.1016/j.scitotenv.2021.147455
Karami A., Golieskardi A., Choo C. K., Romano N., Ho Y. B., Salamatinia B. (2017). A highperformance protocol for extraction of microplastics in fish. Sci. Total Environ. 578, 485–494. doi: 10.1016/j.scitotenv.2016.10.213
Klaus J., Seeger M., Bigalke M., Weber C. J. (2024). Microplastics in vineyard soils: First insights from plastic-intensive viticulture systems. Sci. Total. Environ. 10, 174699. doi: 10.1016/j.scitotenv.2024.174699
Kühn S., van Werven B., van Oyen A., Meijboom A., Reblledo E. L. B., van Franeker J. A. (2017). The use of potassium hydroxide (KOH) solution as a suitable approach to isolate plastics ingested by marine organisms. Mar. pollut. Bull. 115, 86–90. doi: 10.1016/j.marpolbul.2016.11.034
Lenz R., Enders K., Stedmon C. A., Mackenzie D. M. A., Nielsen T. G. (2015). A critical assessment of visual identification of marine microplastic using Raman spectroscopy for analysis improvement. Mar. pollut. Bull. 100, 82–91. doi: 10.1016/j.marpolbul.2015.09.026
Li K., Du L., Qin C., Bolan N., Wang H., Wang H. (2024). Microplastic pollution as an environmental risk exacerbating the greenhouse effect and climate change: a review. Carbon. Res. 3, 9. doi: 10.1007/s44246-023-00097-7
Liang C. J., Su H. W. (2009). Identification of sulfate and hydroxyl radicals in thermallyactivated persulfate. Ind. Eng. Chem. Res. 48, 472–475. doi: 10.1021/ie9002848
Liebezeit G., Dubaish F. (2012). Microplastics in beaches of the East Frisian islands Spiekeroog and Kachelotplate. B. Environ. Contam. Tox. 89, 213–217. doi: 10.1007/s00128-012-0642-7
Liu P., Qian L., Wang H., Zhan X., Lu K., Gu C., et al. (2019). New insights into the aging behavior of microplastics accelerated by advanced oxidation processes. Environ. Sci. Technol. 53, 3579–3588. doi: 10.1021/acs.est.9b00493
Mcdermid K. J., Mcmullen T. L. (2004). Quantitative analysis of small-plastic debris on beaches in the Hawaiian Archipelago. Mar. pollut. Bull. 48, 790–794. doi: 10.1016/j.marpolbul.2003.10.017
Medina L., Zaliznyak T., Taylor G. (2024). From the Caribbean to the Arctic, the most abundant microplastic particles in the ocean have escaped detection. Mar. pollut. Bull. 202, 116338. doi: 10.1016/j.marpolbul.2024.116338
Miller M. E., Kroon F. J., Motti C. A. (2017). Recovering microplastics from marine samples: A review of current practices. Mar. pollut. Bull. 123, 6–18. doi: 10.1016/j.marpolbul.2017.08.058
Prabhu P., Pan K., Krishnan J. N. (2022). Microplastics: Global occurrence, impact, characteristics and sorting. Front. Mar. Sci. 9. doi: 10.3389/fmars.2022.893641
Prata J. C., da Costa J. P., Girão A. V., Lopes I., Duarte A. C., Rocha-Santos T. (2019). Identifying a quick and efficient method of removing organic matter without damaging microplastic samples. Sci. Total. Environ. 686, 131–139. doi: 10.1016/j.scitotenv.2019.05.456
Qin W., Fang G., Wang Y., Wu T., Zhu C., Zhou D. (2016). Efficient transformation of DDT by peroxymonosulfate activated with cobalt in aqueous systems: Kinetics, products, and reactive species identification. Chemosphere. 148, 68–76. doi: 10.1016/j.chemosphere.2016.01.020
Ramesh K., Chellam P. V., Sundaram B. (2024). Transport of layered and spherical microplastics in aqueous ecosystems: a review. Environ. Chem. Lett. 22, 1221–1255. doi: 10.1007/s10311-024-01730-6
Ren Z., Gui X., Wei Y., Chen X., Xu X., Zhao L., et al. (2021). Chemical and photo-initiated aging enhances transport risk of microplastics in saturated soils: key factors, mechanisms, and modeling. Water. Res. 202, 117407. doi: 10.1016/j.watres.2021.117407
Roch S., Brinker A. (2017). Rapid and efficient method for the detection of microplastic in the gastrointestinal tract of fishes. Environ. Sci. Technol. 51, 4522. doi: 10.1021/acs.est.7b00364
Shim W. J., Song Y. K., Hong S. H., Jang S. (2016). Identification and quantification of microplastics using Nile Red staining. Mar. pollut. Bull. 113, 469–476. doi: 10.1016/j.marpolbul.2016.10.049
Tagg A. S., Harrison J. P., Ju-Nam Y., Sapp M., Bradley E. L., Sinclair C. J., et al. (2017). Fenton’s reagent for the rapid and efficient isolation of microplastics from wastewater. Chem. Commun. 53, 372–375. doi: 10.1039/C6CC08798A
Tagg A. S., Sapp M., Harrison J. P., Ojeda J. J. (2015). Identification and quantification of microplastics in wastewater using focal plane array-based reflectance micro-FT-IR imaging. Anal. Chem. 87, 6032–6040. doi: 10.1021/acs.analchem.5b00495
Thacharodi A., Hassan S., Meenatchi R., Bhat M. A., Hussain N., Arockiaraj J., et al. (2024). Mitigating microplastic pollution: A critical review on the effects, remediation, and utilization strategies of microplastics. J. Environ. Manage. 351, 119988. doi: 10.1016/j.jenvman.2023.119988
Wang J., Wang S. (2018). Activation of persulfate (PS) and peroxymonosulfate (PMS) and application for the degradation of emerging contaminants. Chem. Eng. J. 334, 1502–1517. doi: 10.1016/j.cej.2017.11.059
Wang S., Tan X., Wu Y., Zhang J., Tian Z., Ma J. (2024). Isolating micro/nanoplastics from organic-rich wastewater: Co/PMS outweighs Fenton system. J. Hazard. Mater. 463, 132840. doi: 10.1016/j.jhazmat.2023.132840
Yang Z., Li Y., Zhang G. (2024). Degradation of microplastic in water by advanced oxidation processes. Chemosphere. 357, 141939. doi: 10.1016/j.chemosphere.2024.141939
Zheng S., Zhou B., Guo N., Li N., Wu J., Cheng Y., et al. (2024). Optimization and application of pretreatment method of microplastics detection in municipal solid waste landfills. Waste Manage. 183, 260–270. doi: 10.1016/j.wasman.2024.05.013
Zhou Z., Wan L., Cai W., Tang J., Wu Z., Zhang K. (2022). Species-specific microplastic enrichment characteristics of scleractinian corals from reef environment: Insights from an in-situ study at the Xisha Islands. Sci. Total Environ. 815, 152845. doi: 10.1016/j.scitotenv.2021.152845
Keywords: microplastic, alkaline K2S2O8, seawater, pretreatment, spectrometer analysis
Citation: Gao N, Kong X, Zhang Y, Gao Y, Zhang Y and Liu Y (2024) Isolation of seawater microplastics from biologically rich samples using an alkaline K2S2O8 method. Front. Mar. Sci. 11:1450330. doi: 10.3389/fmars.2024.1450330
Received: 17 June 2024; Accepted: 22 July 2024;
Published: 06 August 2024.
Edited by:
Zeming Zhang, Ningbo University, ChinaReviewed by:
Liuqingqing Liu, Ocean University of China, ChinaWenchao Yang, National Marine Environmental Monitoring Center, China
Copyright © 2024 Gao, Kong, Zhang, Gao, Zhang and Liu. This is an open-access article distributed under the terms of the Creative Commons Attribution License (CC BY). The use, distribution or reproduction in other forums is permitted, provided the original author(s) and the copyright owner(s) are credited and that the original publication in this journal is cited, in accordance with accepted academic practice. No use, distribution or reproduction is permitted which does not comply with these terms.
*Correspondence: Nan Gao, nangao@qlu.edu.cn; Yan Liu, sdqdliuyan@126.com