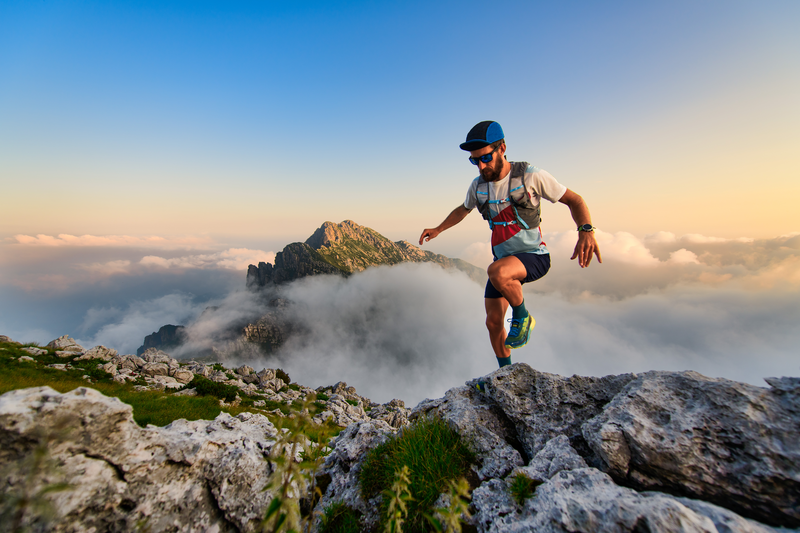
94% of researchers rate our articles as excellent or good
Learn more about the work of our research integrity team to safeguard the quality of each article we publish.
Find out more
BRIEF RESEARCH REPORT article
Front. Mar. Sci. , 04 June 2024
Sec. Marine Pollution
Volume 11 - 2024 | https://doi.org/10.3389/fmars.2024.1371196
This article is part of the Research Topic Environmental Behavior Processes and Mechanisms of Organic Pollutants in Global Coastal Waters View all 4 articles
The coastal area of Qinhuangdao, particularly the Changli Gold Coast Nature Reserve, is experiencing ecological degradation and frequent Harmful Algal Blooms (HABs). This study focuses on the changing phytoplankton communities in these coastal waters, examining them from both a macroscopic and microscopic perspective. Utilizing microscopy, molecular techniques, and pigment analysis, seasonal shifts were observed, with diatoms predominating in June and July, and dinoflagellates in August. Our morphological examination enabled the classification of 89 species into four distinct groups. The species Paralia sulcata and Pseudo-nitzschia pungens were most abundant in early summer, while Tripos furca, a dinoflagellate, dominated in August. This indicates a shift in phytoplankton communities due to environmental factors such as phosphate deficiency and high nitrogen/phosphorus ratios. Additionally, the study notes the impact of reduced river runoff and reintroduction of scallop farming contributing to nitrogen-rich eutrophication in August. Molecular analysis revealed a disparity between microscopic observations and the prevalence of Teleaulax blooms during early summer. Elevated concentrations of TN and DOC, coupled with limited water exchange, emerged as primary factors contributing to their occurrence. Sediment analysis revealed a high diversity but low abundance of dinoflagellates in August, with a significant presence of harmful species. The study highlights the shift from diatoms to harmful dinoflagellate populations, exacerbated by eutrophication and pollution, leading to HABs. These findings provide a theoretical basis for understanding toxic algal blooms and are crucial for environmental agencies in developing strategies to protect and sustainably develop offshore environments.
Qinhuangdao city, located in the northeast of Hebei province, China, is an important harbor city within the Bohai Rim economic zone. This port city is situated near the Bohai Sea, a semi-enclosed sea characterized by poor circulation (Tao, 2006; Qiao et al., 2017). In recent years, human activities have significantly affected the coastal waters of Qinhuangdao, making it one of the most polluted sea areas (Cao et al., 2016). Consequently, the occurrence of harmful algal blooms (HABs) in these coastal waters has become increasingly severe (Zhang et al., 2012; Cao et al., 2016; Ou et al., 2018; Wang et al., 2021).
HABs in the coastal waters of the Qinhuangdao region typically occur from May to August, mainly in summer (Oceanic Administration of Hebei Province, 2005–2021). However, the dominant species have varied considerably. Before 2000, the dominant species were primarily microphytoplankton, such as Skeletonema costatum and Pseudo-nitzschia delicatissima (Su and Zhou, 2001; Yu et al., 2020). Since 2000, the causative species of HABs have tended to become more diversified, harmful, and miniaturized, including Alexandrium catenella and Aureococcus anophagefferens (Kong et al., 2012; Zhang et al., 2012; Yu et al., 2019; Wang et al., 2021). Earlier studies predominantly focused on diatoms (usually > 20μm), identified and quantified primarily through light and electron microscopy. However, recent research has increasingly focused on harmful dinoflagellates and other nanophytoplankton (2μm - 20μm), which are not only being gathered from water samples but also from sediment samples (Xu et al., 2017; Yu et al., 2020; Wang et al., 2021). The presence of harmful dinoflagellates within communities can lead to harmful algal blooms (HABs), making it crucial to study the cysts deposited in sediment as a preventive measure. Obtaining samples of dinoflagellate cysts from sediment is thus imperative for this purpose. Additionally, novel methodologies, such as the pigment method (Kong et al., 2012) and molecular techniques (Metfies et al., 2010; Yu et al., 2020), have been developed to explore various aspects of HABs. However, there remains a lack of studies comparing the outcomes derived from these distinct methodologies.
The sampling site was located near the Changli coast of Qinhuangdao, positioned between the heavily polluted Yang River Estuary and Dai River Estuary. These regions are typical areas influenced by riverine discharge into the marine environment (Li, 2012). Additionally, this site is proximal to tourist and aquaculture areas, significantly impacted by human activities. It is also located in the Changli ecology-monitoring area, between the Gold Coast National Nature Reserve and the Mariculture zone, where HABs have recently occurred frequently, posing threats to the ecological environment and resources such as amphioxus and lagoons (Li, 2012). In our study, we combined traditional classification techniques with the pigment method and molecular techniques to investigate the abundance and diversity of phytoplankton. Our main objectives were: (1) to explore their response to environmental dynamics, and (2) to evaluate the marine ecological system through the utilization of indicator species and the analysis of alterations in community structure. Such investigations can provide valuable insights to relevant authorities for the formulation of effective conservation policies.
The sampling cruise (119.418°E; 39.743°N) was conducted in the vicinity of the Changli coast of the Qinhuangdao (Figure 1). During the summer months of 2017 (June, July, and August), a total of nine samples were collected, consisting of six water samples and three sediment samples. Detailed information can be found in Figure 1.
Figure 1 Map of study area and sampling station in Bohai Sea showing the Hebei Changli Gold Coast National Nature Reserve and scallop culture area.
A CTD profiler (Seabird SBE 911 CTD) installed onto a rosette sampler was used to measure the in-situ temperature (T), pH, salinity, dissolved oxygen (DO) and total nitrogen (TN). Samples for measurement of inorganic nutrients and size-fractionated chlorophyll a (Chl-a) concentrations were collected from the two layers (0 m and 6 m) in the water column and passed through a 200 µm mesh filter to remove large zooplankton and debris. For nutrient analysis, water samples were collected into 100 mL HCl-rinsed plastic bottles and stored at 4°C. For Chl-a measurements, 500 mL of seawater from each layer was filtered through GF/F filter paper and preserved in liquid nitrogen until analysis. Under negative pressure of < 100mm Hg, 5 l of seawater was filtered through a 25 mm GF/F filter membrane. The filter membrane obtained was then wrapped in tin foil and stored in a -80°C refrigerator (or in liquid nitrogen). In the laboratory, the filter membrane was thawed and extracted with 100% methanol and analyzed by High-Performance Liquid Chromatography (HPLC) (Mackey et al., 1996; Schlüter et al., 2011). To collect DNA, 800 mL of seawater was filtered through 0.22 µm GTTP filters (Millipore, Eschbonn, Germany) under a low-pressure vacuum. Sediment samples for further DNA analysis were collected using a bottom sampler, placed into 2 mL microtubes, and immediately frozen in liquid nitrogen. For microphytoplankton estimation, water samples were placed into 250 mL polyethylene (PE) bottles, fixed with a 1% final concentration formaldehyde solution, and stored in the dark until further identification in the laboratory. Surface sediments were collected using a grab sampler (June sediment (JUNsedi); July sediment (JULsedi); August sediment (AUGsedi)), following the methods of Gu et al. (2010). Dinoflagellate cyst abundance was measured as the number of cysts per gram of wet weight sediment.
In the laboratory, inorganic nutrient concentrations including ammonium (NH4-N), nitrate (NO3-N), nitrite (NO2-N), silicate (SiO3-Si), and phosphate (PO4-P) were determined using a Technicon AA3 Auto-Analyzer (Bran+Luebbe, Norderstedt, Germany) with standard procedures (Guo et al., 2016). Dissolved inorganic carbon (DIC) and dissolved organic carbon (DOC) concentrations were measured using high-temperature combustion in a Shimadzu TOC-V analyzer equipped with an inline chemiluminescence nitrogen detector (Davis and Benner, 2005). For the determination of Chl-a concentrations, 500 mL water samples were filtered sequentially through 20 μm, 2 μm and 0.72 μm Waterman GF/F filter (each 25 mm in diameter). This process facilitated the classification of Chl-a into micro-, nano-, and pico-sized fractions. For total Chl-a determination, another sample was filtered through a 20μm Waterman GF/F filter, followed by 2 μm GF/F filter, specifically targeting the nano-sized Chl-a fraction. These filters were then placed into aluminum-foil bags and kept in the dark at -20°C. For pigment extraction, filters were submerged in 20 mL vials containing 90% acetone for 24 h at 4°C. Subsequently, the concentration of Chl-a was determined using a Trilogy (CHL NA, Model #046) fluorometer following the method described by Parsons et al. (1984).
The water-column samples of phytoplankton were placed in Utermöhl counting chambers (100 mL), allowed to settle for 24 h, and then examined and enumerated using an inverted microscope (Motic BA300) at ×200 or ×400 magnification. Sample volume was optimized to ensure that settled cells did not overlap. Phytoplankton morphotypes were identified following the standard identification keys (Sun et al., 2002).
For dinocyst community analysis, surface sediment samples were processed using the previously published protocol (Gu et al., 2010) with modifications. Approximately 3 g of wet sediment was mixed with filtered seawater (20 mL) and sonicated for 2 min to dislodge detritus particles. The obtained slurry was then passed through two-tier (100 and 20 µm) sieves. The fraction accumulated on the 20 µm sieve was washed, suspended in 1 mL filtered seawater, and used for quantification of dinocysts under an inverted microscope (Motic BA300) at ×200 magnification. Dinocyst morphotypes were identified using published literature (Accoroni et al., 2016). Dinocyst abundance was measured as the number of cysts per gram of wet weight sediment (cysts g-1 wet weight).
Total genomic DNA samples were extracted using a DNA Extraction Kit, following the established protocols (Bio-Rad, Hercules, CA, USA). The purity and concentration of DNA were assessed with NanoDrop (ThermoFisher, Waltham, MA, USA) and agarose gel electrophoresis. These validated DNA samples were diluted to 1 ng/μl and stored at -20°C until they were used as templates for PCR amplification of eukaryotic 18S rRNA genes using barcoded primers and HiFi Hot Start Ready Mix (KAPA Biosystems, Roche, Basel, Switzerland). To analyze eukaryote diversity, variable regions of the 18S rRNA genes were amplified using universal primers for the V9 region, namely 817F (5’- TTAGCATGGAATAATRRAATAGGA-3’) and 1196R (5’- TCTGGACCTGGTGAAGTTTCC-3’) (Rousk et al., 2010). Amplicon quality was visualized via gel electrophoresis. The PCR products were then purified using AMPure XP beads (Agencourt, Beckman Coulter, Brea, CA) and subjected to another round of PCR amplification. The final amplicon concentration was quantified using the Qubit dsDNA assay kit (ThermoFisher). Equal amounts of the purified amplicons were pooled for subsequent sequencing.
MiSeq sequencing (Illumina, San Diego, CA, USA) was used to generate raw reads. to the initial step in analyzing microbial diversity sequencing involved stitching the original double-end sequencing data and removing irrelevant materials. Subsequently, low-quality and fuzzy base sequences were excised, and chimera sequences were identified and removed using RStudio. After the preliminary filtration, the resulting high-quality sequences were considered valid tags and used for further analysis. The remaining effective tags were clustered using VSEARCH (v2.4.2) (Torbjørn et al., 2016), with sequences exhibiting ≥97% similarity deemed operational taxonomic units (OTUs) (Edgar, 2010). Subsequently, the OTU classification table were generated through comparison with the Silva database (v. 123). Species comparison annotations were performed using the RDP classifier software, retaining annotation results with confidence intervals greater than 0.7.
Raw sequences obtained from Miseq were deposited in theNCBI Sequence Read Archive under accession number PRJNA503792. For each sample, the relative abundance of phytoplankton OTUs was calculated by dividing the number of sequences from the same taxa by the total number of phytoplankton sequences. Richness was calculated as the number of OTUs from the same taxa over total number of algal OTUs.
Redundancy analysis (RDA) is a constrained principal component analysis, which combines correspondence analysis with multiple regression analysis. In this study, nutrient paraments were used as environmental factors, and various indicators were used to elucidate the relationship between phytoplankton abundance. The RDA analysis was performed using CANOCO 5.0 software. Significant differences between phytoplankton abundance and environmental factors were determined using SPSS one-way ANOVA. The Shannon-Wiener Biodiversity (H’) and Pielou Evenness (J) indices were calculated using the “DIVERSITY” function of the “vegan” package in R v3.6.1 software (R Development Core Team, 2008). The results of HPLC pigment analysis were evaluated using the CHEMTAX program developed by Mackey et al. (1996). CHEMTAX calculated the contributions from different phytoplankton groups to Chl-a based on ratios between accessory pigments and Chl-a, which were input into the program together with the field measurements of pigment concentrations.
A total of 89 phytoplankton species belonging to 43 taxa were identified from the water samples through conventional microscopic analysis. Diatoms (Bacillariophyceae) were predominant, comprising 56 species (65.2% of the total phytoplankton), while dinoflagellates, with 30 species, contributed 33.7%. Additionally, a small number of Dictyochophyceae were observed (Figure 2A). Within the diatoms, the genus Chaetoceros was notably abundant, with 9 species representing 15.5% of the total diatom assemblage. In contrast, the genus Protoperidinium predominated among the dinoflagellates, constituting 33.3% of its total. During June and July, Paralia sulcata and Pseudo-nitzschia pungens were the most abundant phytoplankton species, comprising approximately 40% of the total phytoplankton. Conversely, in August, Tripos furca (a dinoflagellates) emerged as the dominant species, constituting up to 70% of the total. The number of Tripos furca reached to 4.68×104 cells/L in AUG0m layer, accounting for over 80% of the total phytoplankton population. Phytoplankton diversity index, measured by Shannon-Wiener diversity index, varied from 1.433 to 3.899 (average 2.647), with the highest diversity observed in June and the lowest in August. The Pielou evenness index ranged from 0.039 to 0.186, with an average of 0.089.
Figure 2 Phytoplankton structure based on (A) Morphological examination; (B) 18S rRNA; (C) Pigment analysis.
Regarding trophic modes, mixotrophic and heterotrophic species predominantly characterized the dinoflagellates assemblage in the region. Nineteen dinocyst species from 8 genera were identified in the surface sediments. Notably, Alexandrium species (mainly A. minutum) dominated the cyst assemblage in June, with a concentration of 75 cysts g-1 wet weight, but their prevalence decreased over time (Figure 3). These 19 dinocyst species were categorized as autotrophs (8 species), heterotrophs (9 species), and mixotrophs (2 species). The abundance of heterotrophs and mixotrophs decreased monthly towards August. The dinocyst diversity index (Shannon-Wiener diversity index) ranged from 2.654 to 3.039 (average 2.810), with the highest diversity observed in August and the lowest in June. The Pielou evenness index ranged from 0.649 to 0.798, with an average of 0.757.
In total, 305,018 effective tags were included in our study after quality control. The sequencing coverages (C) exceeded 99.9% for all, suggesting adequate depth for 18S rRNA gene diversity analysis. Clustering the unique sequences at a 97% similarity threshold resulted in 292 OTUs, with individual sample OTUs ranging from 94 to 186. To accentuate the abundance and richness of eukaryotic phytoplankton, non-algal and unclassified OTUs were excluded, yielding a total of 118,325 sequences with ≥97% similarity to the eukaryotic algae reference sequence from 9 samples. These accounted for 39% of the total sequences across 53 OTUs.
Phylogenetic analysis indicated that these 53 OTUs spanned five major types of marine algae: Cryptophyceae, Bacillariophyceae, Dinophyceae, Prymnesiophyceae and Dictyochophyceae (Figure 2B). Notably, diatoms and dinoflagellates each had 15 species, representing 57% of the total OTUs. A minor disparity in OTU richness between samples suggested a relative stable composition of the eukaryotic phytoplankton community at higher taxonomic levels. However, the relative abundance of different eukaryotic phytoplankton groups varied considerably between samples (Figure 2B). Most sequences in the water samples were attributed to Cryptophyceae, which accounted for 71.65–94.40% of phytoplankton community, except in August. The genus Teleaulax was the dominant species within Cryptophyceae. Notably, the abundance of dinoflagellates in water samples increased rapidly from 0.89% (JUL0m) to 68.09% (AUG0m), with the genera Amoebophrya and Ceratium being the most prevalence. In the sediments, dinoflagellates were the most abundant, though their prevalence significantly diminished in August, similar to the diatoms. The sediment diversity index (Shannon-Wiener diversity index) ranged from 2.23 to 3.53 (average 2.98), with the highest diversity observed in August and the lowest in July. Dinoflagellates dominated in July, accounting for approximately 74.50% of the total sequences, but their dominance rapidly decreased from July (74.50%) to August (46.15%).
The HPLC analysis identified a total of 18 pigment components in water samples, with the content incrementally increasing monthly at the station. CHEMTAX analysis revealed seven phytoplankton groups present, including diatoms, Cyanophyceae, Dinophyceae, Cryptophyceae, Chrysophyceae, Prymnesiophyceae, and Chlorophyceae (Figure 2C). In June, pigment distribution at JUN0m was predominantly composed of diatoms (fucoxanthin 0.825 ug/L), Chlorophyceae (peridinin 0.820 ug/L), and Cryptophyceae (alloanthin 0.503 ug/L), with pigment/Chl-a ratios of 35%, 34% and 21% respectively. Conversely, at JUN6m, diatoms dominated, exhibiting a fucoxanthin/Chl-a ratio of 58.8%. In July and August, surface and bottom waters displayed similar trends. Specifically, in July, diatoms were prevalent in water samples with an average concentration of 2.637 ug/L and an average fucoxanthin/Chl-a ratio of 68%. In August, dinoflagellates predominated with an average concentration of 5.884 ug/L, and the peridinin/Chl-a ratio averaged 89%.
The RDA analysis of both conventional microscopic and molecular data identified SiO32-, TN (total nitrogen), PO43-, NO2- and NO3- as the most influential factors shaping the composition of phytoplankton assemblages, accounting for up to 97% of the total variation. In the conventional microscopic analysis, all these factors exhibited a significant positive correlation with diatoms. Conversely, dinoflagellates, demonstrated a positive correlation with SiO32-, TN and NO3-, but a negative correlation with PO43- and NO2-. Please refer to Table 1 for specific environmental parameters.
Table 1 Variations in environmental parameters at the sampling station in the coastal waters of Qinhuangdao.
During June and July, diatoms were the predominant species, whereas dinoflagellates became more prevalent in August. Specifically, P. sulcata and P. pungens were the most abundant phytoplankton species during the earlier months, constituting approximately 40% of the total. In contrast, by August, T. furca (a dinoflagellate) accounted for up to 70% of the total phytoplankton. This transition was validated through pigment and molecular analyses, indicating that the shift was largely attributed to phosphate deficiency. Nutrients serve as the foundational basis for phytoplankton growth, and terrestrial rivers inputs significantly influence the content and structure of marine nutrients (Strokal et al., 2017; Kubo et al., 2018). Notably, the average precipitation during the flood season in Hebei Province in 2017 decreased by 12% compared to the previous year, indicating a drier year (https://mip.ys137.com/zhishi/fanwen/15581844.html). Additionally, runoff monitoring at the Yang hydrological control station revealed that the annual runoff of the Yang River in 2017 was 0.2823 million cubic meters, a substantial 52% decrease from 2016 (China Water Resources Bulletin). This reduction in river runoff led to lower levels of DIN (Dissolved Inorganic Nitrogen, DIN) and DIP (Dissolved Inorganic Phosphorus), especially DIP, in 2017 compared to preceding years. In August, the area showed a stoichiometric limitation of phosphate. However, nutrient concentration analyses indicated no nitrogen limitation near the scallop farming waters during the sampling period. The high levels of nitrogen concentration might be attributed to the regeneration from scallop farming through direct excretion of ammonium (Prins and Smaal, 1994; Kong et al., 2022). Phosphorus, a fundamental component of nucleic acids, cell membranes, and high-energy compounds like adenosine triphosphate, plays a critical role in cellular functions. Notably, dinoflagellates exhibit a higher tolerance to low phosphate levels compared to diatoms (Egge, 1998; Liang et al., 2019). Our findings corroborate previous research indicating that the peak cell densities of Tripos consistently occur subsequent to a diatom bloom, as nutrients are provided through diatom decomposition (Holligan and Harbour, 1977). Another reason was that the higher N/P ratio significantly contributes to the displacement of diatoms by dinoflagellates (Wei et al., 2004; Xu et al., 2010; Kong et al., 2022). Particularly in aquaculture areas of the Bohai Sea, the elevated N/P ratio and phosphate deficiency are emerging concerns, adversely affecting phytoplankton growth and shellfish farming (Xu et al., 2010; Kong et al., 2022).
High-throughput sequencing revealed that the dominant species in the water samples belonged to the phylum Cryptophyta, with the highest quantity reaching 108 DNA copies. Pigment analysis corroborated this finding, showing that the concentration of alloxanthin, the characteristic pigment of cryptophytes, was 0.503 μg/L. This constituted a ratio of 21% to Chl-a in June, slightly lower than that of diatoms (35%) and dinoflagellates (34%). However, microscopic examination presented a contrasting result from the high-throughput sequencing. The discrepancy primarily arises because cryptophytes are nano-sized phytoplankton, challenging to be observed under a light microscope (Robinson et al., 1999; Novarino, 2005). Additionally, the cells of Teleaulax lack a cellulose-based cell wall and tare morphologically variable, complicating their qualitatively and quantitatively identification using conventional microscopy (Cerino and Zingone, 2007; Metfies et al., 2010).
The proliferation of Teleaulax during this period can be ascribed to several factors. Firstly, nutrient conditions were sufficient to the growth of cryptophyte in early summer (Sommer, 1989). High concentrations of TN, PO4-P, and elevated temperature all supported growth in June (Šupraha et al., 2014). The proximity of the survey site to a scallop culture area led to an accumulation of excrement and food residue during the early summer growing season. On one hand, the direct excretion by shellfish and release of substantial amounts of NH4-N from bottom sediments significantly increased NH4-N concentrations. These high levels of NH4-N, often exceeding 4 μmol/L, inhibited the uptake and utilization of NO3-N, thus limiting the growth of micro-sized phytoplankton like dinoflagellates and promoting the proliferation of smaller primary producers (Dugdale et al., 2012). On the other hand, limited exchange and higher DOC were also conducive to the growth of bacteria, providing sufficient food for the proliferation of Teleaulax (Jeong et al., 2013; Yoo et al., 2017). The dominance of cryptophytes has been documented in various studies, including pigmentation measurements in the central North Sea and the Weddell-Scotia confluence area in the 1980s and 1990s, as well as in Masan Bay, Korea in 2004–2005 (Gieskes and Kraay, 1983; Buma et al., 1992; Jeong et al., 2013; Yoo et al., 2017). Cryptophytes have been identified as the primary cause of red tides in Masan Bay, with densities reaching 108 cells/L. Along the coast of British Columbia, marine photosynthetic cryptophytes were observed as the dominant species in summer surface water. Molecular technology has further confirmed that Teleaulax acuta and Plagioselmis prolonga, both belonging to the phylum Cryptophyta, are the causative species of algal blooms in various coastal waters, including those in Zhejiang Province, Xiamen Port, Hong Kong’s Tolo Harbour, and the Mediterranean Sea (Cerino and Zingone, 2007; Environmental Protection Department, Hong Kong, 2005–2006; Šupraha et al., 2014). Consequently, it is plausible that Teleaulax-dominated blooms occurred in our survey area during early summer.
In China, a latitudinal gradient was observed, with a decrease in the abundance of dinoflagellate cysts from north to south, as noted by Chen et al. (2011) and Shao et al. (2012). Molecular and microscopic analyses revealed that the number of dinoflagellate cysts in sediment diminished with rising temperatures, reaching its lowest point in August. This aligns with previous studies suggesting that elevated temperatures promote the germination of dinoflagellate cysts (Estrada and Berdalet, 1997; Smayda, 1997; Rose and Caron, 2007). Concurrently, TN reached its highest level in August, corroborating extensive research that confirms nitrogen as a primary factor influencing cyst germination (Qi et al., 1997; Wang et al., 2011; Figueroa et al., 2015). Significantly, the trophic mode of specific dinoflagellate critically influences the dynamics and impacts of resultant harmful algal blooms, which can inflict economic, ecological, and health repercussions (Park et al., 2013). Microscopic analysis indicated that heterotrophic dinoflagellate cysts were prevalent, constituting over 40% of the species during summer. Heterotrophic dinoflagellates can obtain adequate nutrients through feeding in nutrient-deficient waters, potentially leading to the formation of red tides under favorable environmental conditions (Jeong et al., 2010). Moreover, they have the capacity to foster the successive emergence of multiple dominant species within a single red tide through their feeding activities (Jeong et al., 2005). Furthermore, the proportion of toxic dinoflagellate cysts exceeded 85%, displaying a gradual decreasing trend from June to August. An analysis of species symbiosis revealed that parasitic marine dinoflagellate Syndiniales comprised half of the total OTUs of dinoflagellate algae in the water sample. These parasites terminate their hosts and release copious independent planktonic spores (Coats and Park, 2002; Guillou et al., 2010). In the sediment, Alexandrium cysts, prevalent in Bohai Bay, China, predominated. Notably, species like Gonyaulax spinifera, Lingulodinium polyedra, and Protoceratium reticulatum were present, known for producing Yessotoxins (YTXs). These toxins, not typical shellfish toxins, inflict damage on the heart, liver, nervous system, and other organs (Paz et al., 2008; Alvarez et al., 2016). The environmental concentration and form of phosphorus notably affect the growth and toxicity of dinoflagellates capable of producing paralytic shellfish poison. Low levels of DIP create favorable conditions for the accumulation of toxins (Touzet et al., 2007). Based on 18S rRNA and light microscope analysis, Polykrikos was identified as highly abundant among dinoflagellates in the sediment samples from August. Previous research also recognized Polygonum cysts, such as P. schwartzio and Polykrikos kofoidii, as common in Bohai Bay, China (Wang et al., 2004). In our study, P. schwartzio cysts were detected in all sediment samples, with the highest abundance occurring in August. Additionally, the prevalence of Polykrikos cysts can serve as indicators of eutrophication or high primary productivity (D’Silva et al., 2013; Narale and Anil, 2017). The presence, absence, and abundance of heterotrophic algae cysts also serve as markers for these conditions (D’Silva et al., 2013; Narale and Anil, 2017). The predominant species in the sediment samples of the survey area, all belonging to the same type, suggest a high level of eutrophication, significant pollution, and poor water quality in the survey area.
During the summer survey period, we observed a notable decline in phytoplankton diversity in the Qinhuangdao Changli coastal area. The dominant phytoplankton populations underwent a significant shift from diatom predominance to a dinoflagellate-dominated ecosystem, with cryptophytes emerging as a dominant species in early summer. They thrived in an environment rich in organic matter. The sediments were characterized by a wide variety of harmful dinoflagellate cysts exhibiting high richness. Specifically, Polykrikos, an indicator of water eutrophication, was notably abundant in August sediment samples. Additionally, various dinoflagellate cysts capable of producing YTXs were present in all sediment samples. These findings suggest that the marine ecosystem has suffered serious degradation. Scallop farming may be under threat, and the region faces an increased risk of harmful algal blooms in the future. Cryptophyte blooms are likely to occur in the most polluted stations. Furthermore, the area serves as a habitat for amphioxus, and changes in the marine environment could jeopardize this species, leading to a reduction in its density and biomass. This potential impact warrants attention in future research endeavors.
The datasets presented in this study can be found in online repositories. The names of the repository/repositories and accession number(s) can be found in the article/supplementary material.
XS: Writing – review & editing, Writing – original draft, Investigation, Formal Analysis, Data curation. SY: Data curation, Writing – review & editing. JS: Visualization, Supervision, Resources, Project administration, Methodology, Conceptualization, Writing – review & editing.
The author(s) declare that financial support was received for the research, authorship, and/or publication of this article.
This work was supported by the Natural Science Foundation of China (No. 42206087, 41676112) and the Changjiang Scholar Program of Chinese Ministry of Education (T2014253) to JS. We also thank our colleagues from Dr. Guicheng Zhang and Dr. Dai Jia at Tianjin University of Science and Technology who provided helps on water sampling.
The authors declare that the research was conducted in the absence of any commercial or financial relationships that could be construed as a potential conflict of interest.
The author(s) declared that they were an editorial board member of Frontiers, at the time of submission. This had no impact on the peer review process and the final decision.
All claims expressed in this article are solely those of the authors and do not necessarily represent those of their affiliated organizations, or those of the publisher, the editors and the reviewers. Any product that may be evaluated in this article, or claim that may be made by its manufacturer, is not guaranteed or endorsed by the publisher.
Accoroni S., Romagnoli T., Pichierri S., Totti C. (2016). Effects of the bloom of harmful benthic dinoflagellate Ostreopsis cf. ovata on the microphytobenthos community in the northern Adriatic Sea. Harmful Algae 55, 179–190. doi: 10.1016/j.hal.2016.03.003
Alvarez G., Uribe E., Regueiro J., Blanco J., Fraga S. (2016). Gonyaulax taylorii, a new yessotoxins-producer dinoflagellate species from Chilean waters. Harmful Algae 58, 8–15. doi: 10.1016/j.hal.2016.07.006
Buma A. G. J., Gieskes W. W. C., Thomsen H. A. (1992). Abundance of Cryptophyceae and chlorophyll b-containing organisms in the Weddell-Scotia confluence area in the spring 1988. Polar Biol. 12, 43–52. doi: 10.1007/BF00239964
Cao X., Yu Z., Song X., Qiu L. (2016). Controlling harmful algae blooms using aluminum-modified clay. Mar. pollut. Bull. 103, 211–219. doi: 10.1016/j.marpolbul.2015.12.017
Cerino F., Zingone A. (2007). “Decrypting cryptomonads: a challenge for molecular taxonomy,” in Unravelling the algae: the past, present, and future of algal systematics. Eds. Brodie J., Lewis J., 197–214.
Chen B. Z., Irwin A. J., Finkel Z. V. (2011). Biogeographic distribution of diversity and size-structure of organic-walled dinoflagellate cysts. Mar. Ecol. Prog. Ser. 425, 35–45. doi: 10.3354/meps08985
Coats D. W., Park M. G. (2002). Parasitism of photosynthetic dinoflagellates by three strains of Amoebophrya (Dinophyta): Parasite survival, infectivity, generation time, and host specificity. J. Phycol. 38, 520–528. doi: 10.1046/j.1529-8817.2002.01200.x
Davis J., Benner R. (2005). Seasonal trends in the abundance, composition and bioavailability of particulate and dissolved organic matter in the Chukchi/Beaufort Seas and western Canada Basin. Deep Sea Res. Part II Topical Stud. Oceanogr. 52, 3396–3410. doi: 10.1016/j.dsr2.2005.09.006
D’Silva M. S., Anil A. C., Sawant S. S. (2013). Dinoflagellate cyst assemblages in recent sediments of Visakhapatnam harbor, east coast of India: influence of environmental characteristics. Mar. pollut. Bull. 66, 59–72. doi: 10.1016/j.dsr2.2005.09.006
Dugdale R., Parker A. E., Marchi A., Wilkerson F., Taberski K. (2012). River flow and ammonium discharge determine spring phytoplankton blooms in an urbanized estuary. Estuarine Coast. Shelf Sci. 115, 187–199. doi: 10.1016/j.ecss.2012.08.025
Edgar R. C. (2010). Search and clustering orders of magnitude faster than BLAST. Bioinformatics 26, 2460–2461. doi: 10.1093/bioinformatics/btq461
Egge J. K. (1998). Are diatoms poor competitors at low phosphate concentrations? J. Mar. Syst. 16, 191–198. doi: 10.1016/S0924-7963(97)00113-9
Figueroa R. I., Vázquez J. A., Massanet A., Murado M. A., Bravo I. (2015). Interactive effects of salinity and temperature on planozygote and cyst formation of Alexandrium minutum (Dinophyceae) in culture. J. Phycol. 47, 13–24. doi: 10.1111/j.1529-8817.2010.00937.x
Gieskes W. W. C., Kraay G. W. (1983). Dominance of Cryptophytes the phytoplankton spring bloom in the central North Sea detected by HPLC analysis of pigments. Mar. Biol. 75, 179–185. doi: 10.1007/BF00406000
Gu H., Sun J., Kooistra W. H. C. F., Zeng R. (2010). Phylogenetic position and morphology of thecae and cysts of Scrippsiella (Dinophyceae) species in the East China Sea. J. Phycol. 44, 478–494. doi: 10.1111/j.1529-8817.2008.00478.x
Guillou L., Viprey M., Chambouvet A., Welsh R. M., Worden A. Z. (2010). Widespread occurrence and genetic diversity of marine parasitoids belonging to Syndiniales (Alveolata). Environ. Microbiol. 10, 3349–3365. doi: 10.1111/j.1462-2920.2008.01731.x
Guo S., Sun J., Zhao Q., Feng Y., Huang D., Liu S. (2016). Sinking rates of phytoplankton in the Changjiang (Yangtze River) estuary: A comparative study between Prorocentrum dentatum and Skeletonema dorhnii bloom. J. Mar. Syst. 154, 5–14. doi: 10.1016/j.jmarsys.2015.07.003
Holligan P. M., Harbour D. S. (1977). The vertical distribution and succession of phytoplankton in the western English Channel in 1975 and 1976. J. Mar. Biol. Assoc. United Kingdom 57, 1075–1093. doi: 10.1017/S002531540002614X
Jeong H. J., Yoo Y. D., Kim J. S., Seong K. A., Kang N. S., Kim T. H. (2010). Growth, feeding and ecological roles of the mixotrophic and heterotrophic dinoflagellates in marine planktonic food webs. Ocean Sci. J. 45, 65–91. doi: 10.1007/s12601-010-0007-2
Jeong H. J., Yoo Y. D., Lee K. H., Kim T. H., Seong K. A., Kang N. S., et al. (2013). Red tides in Masan Bay, Korea in 2004–2005: I. Daily variations in the abundance of red-tide organisms and environmental factors. Harmful Algae 30, S75–S88. doi: 10.1016/j.hal.2013.10.008
Jeong H. J., Yoo Y. D., Park J. Y., Song J. Y., Kim S. T., Lee S. H., et al. (2005). Feeding by the phototrophic red tide dinoflagellates: five species newly revealed and six species previously known to be mixotrophic. Aquat. Microbial Ecol. 40, 133–155. doi: 10.3354/ame040133
Kong N., Liu Z., Yu Z., Fu Q., Li H., Zhang Y., et al. (2022). Dynamics of phytoplankton community in scallop farming waters of the Bohai Sea and north yellow sea in China. BMC Ecol. Evol. 22, 48. doi: 10.1186/s12862-022-02002-z
Kong F. Z., Yu R. C., Zhang Q. C., Yan T., Zhou M. J. (2012). Pigment characterization for the 2011 bloom in Qinhuangdao implicated “brown tide” events in China. Chin. J. Oceanol. Limnol. 30, 361–370. doi: 10.1007/s00343-012-1239-z
Kubo A., Hashihama F., Kanda J., Horimoto-Miyazaki N., Ishimaru T. (2018). Long-term variability of nutrient and dissolved organic matter concentrations in Tokyo Bay between 1989 and 2015. Limnol. Oceanogr. 64, 209–222. doi: 10.1002/lno.10796
Li Z. W. (2012). Contaminative conditions of main rivers flowing into the sea and their effect on seashore of Qinhuangdao. Ecol. Environ. Sci. 21, 1285–1288. doi: 10.16258/j.cnki.1674-5906(2012)07-1285-04
Liang Y., Zhang G., Wan A., Zhao Z., Wang S., Liu Q. (2019). Nutrient-limitation induced diatom dinoflagellate shift of spring phytoplankton community in an offshore shellfish farming area. Mar. pollut. Bull. 141, 1–8. doi: 10.1016/j.marpolbul.2019.02.009
Mackey M. D., Mackey D. J., Higgins H. W., Wright S. W. (1996). CHEMTAX - A program for estimating class abundances from chemical markers: Application to HPLC measurements of phytoplankton. Mar. Ecol. Prog. Ser. 144, 265–283. doi: 10.3354/meps144265
Metfies K., Gescher C., Frickenhaus S., Niestroy R., Wichels A., Gerdts G., et al. (2010). Contribution of the class Cryptophyceae to phytoplankton structure in the German bight. J. Phycol. 46, 1152–1160. doi: 10.1111/j.1529-8817.2010.00902.x
Narale D. D., Anil A. C. (2017). Spatial distribution of dinoflagellates from the tropical coastal waters of the south Andaman, India: Implications for coastal pollution monitoring. Mar. pollut. Bull. 115, 498–506. doi: 10.1016/j.marpolbul.2016.11.035
Novarino G. (2005). Nanoplankton protists from the western Mediterranean Sea. II. Cryptomonads (Cryptophyceae = Cryptomonadea). Scientia Marina 69, 47–74. doi: 10.3989/scimar.2005.69n1
Ou L., Liu X., Li J., Qin X., Cui L., Lu S. (2018). Significant activities of extracellular enzymes from a brown tide in the coastal waters of Qinhuangdao, China. Harmful Algae 74, 1–9. doi: 10.1016/j.hal.2018.03.005
Park J., Jeong H. J., Yoo Y. D., Yoon E. Y. (2013). Mixotrophic dinoflagellate red tides in Korean waters: distribution and ecophysiology. Harmful Algae 30, S28–S40. doi: 10.1016/j.hal.2013.10.004
Parsons T. R., Takahashi M., Hargrave B. (1984). ERRATUM - Biological Oceanographic Processes, 3rd ed. 331.
Paz B., Daranas A. H., Norte M., Riobó P., Franco J. M., Fernández J. J. (2008). Yessotoxins, a group of marine polyether toxins: an overview. Mar. Drugs 6, 73–102. doi: 10.3390/md6020073
Prins T., Smaal A. (1994). The role of the blue mussel Mytilus edulis in the cycling of nutrients in the Oosterschelde estuary (The Netherlands). Hydrobiologia 282, 413–429. doi: 10.1007/BF00024645
Qi Y. Z., Zheng L., Wang R. (1997). The life cycle of Scrippsiella trochoidea and its physiol-ecological control. Oceanologia Limnologia Sin. 28, 588–593.
Qiao Y., Feng J., Cui S., Zhu L. (2017). Long-term changes in nutrients, chlorophyll a and their relationships in a semi-enclosed eutrophic ecosystem, Bohai Bay, China. Mar. pollut. Bull. 117, 222. doi: 10.1016/j.marpolbul.2017.02.002
R Development Core Team (2008). R: A language and environment for statistical computing (Vienna, Austria: R Foundation for Statistical Computing). Available at: http://www.R-project.org.
Robinson C., Archer S., Williams P. (1999). Microbial dynamics in coastal waters of east Antarctica: plankton production and respiration. Mar. Ecol. Prog. Ser. 180, 23–36. doi: 10.3354/meps180023
Rose J. M., Caron D. A. (2007). Does low temperature constrain the growth rates of heterotrophic protists? Evidence and implications for algal blooms in cold waters. Limnol. Oceanogr. 52, 886–895. doi: 10.4319/lo.2007.52.2.0886
Rousk J., BååTh E., Brookes P. C., Lauber C. L., Lozupone C., Caporaso J. G., et al. (2010). Soil bacterial and fungal communities across a pH gradient in an arable soil. Int. Soc. Microbial Ecol. J. 4, 1340–1351. doi: 10.1038/ismej.2010.58
Schlüter L., Henriksen P., Nielsen T. G., Jakobsen H. H. (2011). Phytoplankton composition and biomass across the southern Indian Ocean. Deep Sea Res. Part I: Oceanographic Res. Papers 58, 546–556.
Shao H. B., Shi Y. J., Liu D. Y. (2012). Study on species diversity of dinoflagellate cysts in the central of north Yellow Sea and Sishili Bay. Mar. Sci. Bull. 31, 557–565.
Smayda T. J. (1997). Harmful algal blooms: their ecophysiology and general relevance to phytoplankton blooms in the sea. Limnol. Oceanogr. 42, 1137–1153. doi: 10.4319/lo.1997.42.5_part_2.1137
Sommer U. (1989). “The role of competition for resources in phytoplankton succession,” in Plankton ecology: succession in plankton communities. Ed. Sommer U. (Springer Berlin Heidelberg, Berlin), 57–106.
Strokal M., Kroeze C., Wang M., Lin M. (2017). Reducing future river export of nutrients to coastal waters of China in optimistic scenarios. Sci. Total Environ. 579, 517–528. doi: 10.1016/j.scitotenv.2016.11.065
Su J. L., Zhou M. J. (2001). Laboratory of ocean dynamic and processes. Harmful algal bloom research in China. Bull. Chin. Acad. Sci. 15, 222–224.
Sun J., Liu D. Y., Qian S. B. (2002). A quantitative research and analysis method for marine phytoplankton: an introduction to utermhl method and its modification. J. Oceanogr. Huanghai Bohai Seas 20, 105–112.
Šupraha L., Bosak S., Ljubešic Z., Mihanović H., Olujić G., Mikac I., et al. (2014). Cryptophyte bloom in a Mediterranean estuary: high abundance of Plagioselmis cf. prolonga in the Krka River estuary (eastern Adriatic Sea). Scientia Marina 78, 329–338. doi: 10.3989/scimar.03998.28C
Tao J. H. (2006). Numerical simulation of aquatic Eco-environment of Bohai bay. J. Hydrodynamics 18, 34–42. doi: 10.1016/S1001-6058(06)60027-9
Torbjørn R., Tomáš F., Nichols B., Quince C., Mahé F. (2016). VSEARCH: A versatile open source tool for metagenomics. PeerJ 4, e2584. doi: 10.7717/PEERJ.2584
Touzet N., Franco J. M., Raine R. (2007). Influence of inorganic nutrition on growth and PSP toxin production of Alexandrium minutum (Dinophyceae) from Cork Harbour, Ireland. Toxicon 50, 106–119. doi: 10.1016/j.toxicon.2007.03.001
Wang Z. H., Cao Y., Zhang Y. J. (2011). The fluxes of dinoflagellate cyst from sediment traps deployed in Dapeng-Ao area of Daya Bay from 2005 to 2006. J. Trop. Oceanogr. 30, 113–118. doi: 10.11978/j.issn.1009-5470.2011.01.113
Wang Z. H., Matsuoka K., Qi Y. Z., Chen J. (2004). Dinoflagellate cysts in recent sediments from Chinese Coastal Waters. Mar. Ecol. 25, 289–311. doi: 10.1111/j.1439-0485.2004.00035.x
Wang D., Zhang S., Zhang H., Lin S. (2021). Omics study of harmful algal blooms in China: Current status, challenges, and future perspectives. Harmful Algae 1), 102079. doi: 10.1016/j.hal.2021.102079
Wei H., Sun J., Moll A., Zhao L. (2004). Phytoplankton dynamics in the Bohai Sea-observations and modelling. J. Mar. Syst. 44, 233–251. doi: 10.1016/j.jmarsys.2003.09.012
Xu S., Song J., Li X., Yuan H., Li N., Duan L., et al. (2010). Changes in nitrogen and phosphorus and their effects on phytoplankton in the Bohai Sea. Chin. J. Oceanol. Limnol. 28, 945–952. doi: 10.1007/s00343-010-0005-3
Xu X., Yu Z. M., He L. Y., Cheng F. J., Cao X. H., Song X. X. (2017). Nano- and microphytoplankton community characteristics in brown tide bloom-prone waters of the Qinhuangdao coast, Bohai Sea, China. Sci. China Earth Sci. 60, 1189–1200. doi: 10.1007/s11430-017-9036-0
Yoo Y. D., Seong K. A., Jeong H. J., Yih W., Rho J., Nam S. W., et al. (2017). Mixotrophy in the marine red-tide cryptophyte Teleaulax amphioxeia, and ingestion and grazing impact of cryptophytes on natural populations of bacteria in Korean coastal waters. Harmful Algae 68, 105–117. doi: 10.1016/j.hal.2017.07.012
Yu R. C., Li S. H., Qi Y. Z., Zhou M. J. (2020). Progress and perspectives of harmful algal bloom studies in China. Oceanologia Limnologia Sin. 51, 768–788. doi: 10.11693/hyhz20200400127
Yu L., Zhang Y., Li M., Wang C., Lin S. (2019). Comparative metatranscriptomic profiling and microRNA sequencing to reveal active metabolic pathways associated with a dinoflagellate bloom. Sci. Total Environ. 699, 134323. doi: 10.1016/j.scitotenv.2019.134323
Keywords: scallop farming, phytoplankton community, cryptophytes, dinoflagellate cysts, 18S rRNA
Citation: Shang X, Yang S and Sun J (2024) Succession of phytoplankton communities from macro-scale to micro-scale in coastal waters of Qinhuangdao, China. Front. Mar. Sci. 11:1371196. doi: 10.3389/fmars.2024.1371196
Received: 16 January 2024; Accepted: 22 May 2024;
Published: 04 June 2024.
Edited by:
Hong-Hai Zhang, Ocean University of China, ChinaReviewed by:
Aijun Ma, Chinese Academy of Fishery Sciences (CAFS), ChinaCopyright © 2024 Shang, Yang and Sun. This is an open-access article distributed under the terms of the Creative Commons Attribution License (CC BY). The use, distribution or reproduction in other forums is permitted, provided the original author(s) and the copyright owner(s) are credited and that the original publication in this journal is cited, in accordance with accepted academic practice. No use, distribution or reproduction is permitted which does not comply with these terms.
*Correspondence: Jun Sun, cGh5dG9wbGFua3RvbkAxNjMuY29t
Disclaimer: All claims expressed in this article are solely those of the authors and do not necessarily represent those of their affiliated organizations, or those of the publisher, the editors and the reviewers. Any product that may be evaluated in this article or claim that may be made by its manufacturer is not guaranteed or endorsed by the publisher.
Research integrity at Frontiers
Learn more about the work of our research integrity team to safeguard the quality of each article we publish.