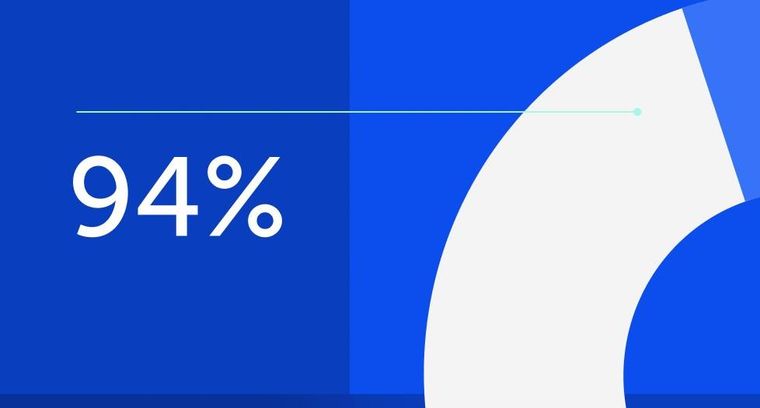
94% of researchers rate our articles as excellent or good
Learn more about the work of our research integrity team to safeguard the quality of each article we publish.
Find out more
ORIGINAL RESEARCH article
Front. Ecol. Evol., 03 March 2022
Sec. Paleoecology
Volume 10 - 2022 | https://doi.org/10.3389/fevo.2022.788101
The Oldowan represents the earliest recurrent evidence of human material culture and one of the longest-lasting forms of technology. Its appearance across the African continent amid the Plio-Pleistocene profound ecological transformations, and posterior dispersal throughout the Old World is at the foundation of hominin technological dependence. However, uncertainties exist concerning the degree to which the Oldowan constitutes an environment-driven behavioral adaptation. Moreover, it is necessary to understand how Oldowan technology varied through time in response to hominin ecological demands. In this study, we present the stone tool assemblage from Ewass Oldupa, a recently discovered archeological site that signals the earliest hominin occupation of Oldupai Gorge (formerly Olduvai) ∼2.03 Ma. At Ewass Oldupa, hominins underwent marked environmental shifts over the course of a ∼200 kyr period. In this article, we deployed an analysis that combines technological and typological descriptions with an innovative quantitative approach, the Volumetric Reconstruction Method. Our results indicate that hominins overcame major ecological challenges while relying on technological strategies that remained essentially unchanged. This highlights the Oldowan efficiency, as its basic set of technological traits was able to sustain hominins throughout multiple environments.
The long-lasting Oldowan tradition signals the onset of technological dependence among hominins. Understanding the Oldowan’s origins and development is key for examining the factors that differentiated hominins from non-human primates, allowing them to thrive through an efficient exploitation of Plio-Pleistocene environments despite strong ecological competition. The earliest known Oldowan tool kits occur at ca. 2.6 Ma, as identified at the Ethiopian sites of Bokol Dora (Braun et al., 2019) and Gona (Semaw et al., 1997). With eastern Africa as their presumed cradle, Oldowan-bearing cultures seem geographically widespread by ∼2.0 Ma, following dispersal into the temperate zone in places like Algeria (Sahnouni et al., 2018) and South Africa (Kuman and Clarke, 2000; Figure 1A), galvanizing in contexts occupied by diverse hominin genera such as Australopithecus, Paranthropus, and Homo (Leakey et al., 1964; Leakey, 1971; Wood, 1997; Blumenschine et al., 2003). Importantly, we still do not know which species is responsible for the development of this technology, or if it appeared simultaneously in different territories and among different hominin groups (Wood, 1997; Tennie et al., 2017). Stone tool technologies comparable to the African Oldowan are found across Eurasia (Baena et al., 2010; Ferring et al., 2011; Mgeladze et al., 2011; Moyano et al., 2011; de Lombera-Hermida et al., 2015; Mosquera et al., 2018; Zhu et al., 2018) from ∼2.0 Ma onward, thus pointing to the Oldowan as the first widespread technological system in the Old World.
Figure 1. (A) Map of Early Oldowan African sites: 1, Lomekwi; 2, Bokol Dora; 3, Gona; 4, Hadar (AL666); 5, Lokalalei; 6, Omo; 7, Ain Boucherit; 8, Kanjera South; 9, Koobi Fora; 10, Oldupai Gorge; 11, Fejej; 12, Melka Kunture; 13, Sterkfontein; 14, Ain Hanech; 15, El Kherba. (B) Detail map on Oldupai Gorge and the location of Ewass Oldupa in relation with DK, FLK, Loc. 68, Naibor Soit and Nasiusiu. (C) Stratigraphic section from Ewass Oldupa, subdivided into two segments: Lower Bed I (i) and Upper Bed I (ii). See Mercader et al. (2021) for detailed descriptions. Maps modified from Tinted Hillshade using ESRI ArcGIS Desktop 10.5.1 (https://desktop.arcgis.com) and Images ServicesWorldElevation/Terrain Server Tinted Hillside Airbus, USGS, NGA, NASA, CGIAR, NCEAS, NLS, OS, NMA, Geodatastyrelsen, GSA, GSI and the GIS user community (CC BY-SA 4.0; https://creativecommons.org/licenses/by/4.0/).
The Oldowan hominins evolved amid climatic and ecological variability across the Plio-Pleistocene, with cooling and drying leading to open habitats, species turnover, and dispersal (Potts, 1991, 1998; Plummer, 2004). Roughly paralleling these climatic and environmental changes, hominins began to rely on stone tool technologies based on cobbles, cores, and flakes (Potts, 1991). Variation in Oldowan tool kits has been documented (de la Torre and Mora, 2008; Stout et al., 2010), yet there is uncertainty as to what type of lithic diversity emanates from environmental pressure, cognition, and/or behavioral complexity (Stout et al., 2010). Some of the underlying causes for Oldowan technological variability remain unknown owing to two reasons: (1) the scarcity of high-resolution, multi-episode localities amenable to diachronic study, firmly dated, and in ecological context and (2) the inferential challenges derived from conflicting classification and nomenclature systems in the study of stone technology, a probable consequence of the overuse of Leakey’s (1971) typological criteria.
To partly address these problems, we conducted new archeological research in the region where the Oldowan was first identified, Oldupai Gorge (formerly Olduvai), Tanzania. The Oldupai site complex is arguably one of the richest and best-dated geological, paleontological, and archeological archives from the last ∼2.4 million years (Hay, 1976; Deino, 2012; McHenry, 2012; Deino et al., 2020; McHenry et al., 2020; Stanistreet et al., 2020). Most of the information about hominin activity at Oldupai comes from the eastern side of the basin, where the Oldowan record is younger (Leakey, 1971) than the early Oldowan from Kenya and Ethiopia. Recent work (Mercader et al., 2021; Stollhofen et al., 2021), however, has shown that the earliest Oldowan yet known at Oldupai occurs in outcrops from the western side of the gorge (Figure 1B), sometimes comprising multi-stratified, high-resolution sites.
The newly reported site of Ewass Oldupa (Mercader et al., 2021), occupied as early as 2.03 Ma, represents the earliest hominin occupation so far identified at Oldupai Gorge. Archeological assemblages were recovered from five trenches, each representing discrete sedimentary environments over a geological package >40 m thick and encompassing five occupation phases (I–V) (Figure 1C).
Phase I corresponds with the earliest geological deposits, in a fluvial context of mafic sands over the extruded Naabi ignimbrite, which gives the assemblage an age of ∼ 2.038 ± 0.005 Ma (Deino, 2012). During this phase, the sedimentary environment is characterized by high-energy volcanics (McHenry et al., 2008); then it stabilized, supporting fluvial systems through the floodplain. Phytolith analysis (Mercader et al., 2021) indicates a pioneering floral context of ferns, facilitating subsequent colonization by other plant groups. No faunal remains were recovered. Phase II is documented within an excavation area of 91 m2. Stratigraphically, it represents the uppermost part of the Coarse Feldspar Crystal Tuff compositional zone (CFCTcz) dated to 2.015 ± 0.006 Ma (Deino, 2012), in a sedimentary environment that precedes the deposition of lowermost Bed I and the earliest paleolake in outcrop, 4.6 m below Tuff IA. Plant waxes, stable isotopes from dental enamel, and phytolith analysis (Mercader et al., 2021) suggest an environment consisting of short woodland mosaics. Phase III is in a sedimentary environment of waxy clays from the earliest expansion of paleolake Oldupai, excavated over 31 m2. This occupation lies 4 m below Tuff IA, dated to ∼ 2 Ma (Deino, 2012). Phytoliths indicate a mixed landscape of woodlands and palms (Mercader et al., 2021). Animal bones represent diverse habitats occupied by Antilopini, Bovini, Hippotragini, and Tragelaphini. In addition, Chelonia and Crocodylus demonstrate the proximity of water bodies and a typical Upper Bed I, lake-margin context.
Phase IV associates with a meandering stream, part of a multistory channel belt stacked 3 m below Tuff IA (∼2 Ma) and excavated over 21 m2. Phytoliths derive from woodlands and grasslands, while fauna comprises bovids, equids, suids, and primates (cf. Theropithecus oswaldi) (Mercader et al., 2021). Chelonia, Crocodylus, and Hippopotamus denote semipermanent water bodies. Lastly, Phase V comprises the youngest Oldowan assemblage at the site, recovered from an excavated area of 42 m2. This phase is sandwiched between Tuffs IB and IC, the latter dating to 1.832 ± 0.003 Ma (Deino, 2012). Environmentally speaking, this occupation corresponds with a transitional phase between woodland mosaics and the steppe habitats above it. No fauna was recovered.
This remarkably diverse paleoenvironmental context, spanning ∼200,000 years, makes Ewass Oldupa an ideal locality to examine questions of technological variability. Therefore, in this study, we present a qualitative and quantitative analysis of these early Oldowan lithic assemblages, offering a diachronic perspective on Oldowan technological change and revealing new aspects of the adaptive behavior of Early Pleistocene hominins.
Ewass Oldupa’s lithic assemblage includes 565 artifacts excavated in 2018 (n = 28) and 2019 (n = 537). Stone tools were measured following both their maximum morphological dimensions and technological dimensions (based on a minimal rectangle with the platform at the bottom and the dorsal surface upward). We used technological measurements for our analysis. No size cutoff point was applied when analyzing the assemblage. The weights of every artifact were recorded. Raw material was analyzed macroscopically to determine morphology, Munsell color, grain size, texture, gloss, transparency, mineral composition, impurities, postdepositional alterations, and foliation. Archeological assemblage raw material was compared with the reference collection created and curated by the Stone Tools, Diet, and Sociality project in the Tropical Archeology Lab (TAL; Earth Sciences 811, University of Calgary). Descriptive techno-typological analysis of artifacts was conducted in a database built on the Logical Analytical System (SLA) (Carbonell et al., 1983) and then modified comprehensively, adapting to the particularities of Oldowan assemblages (Mosquera et al., 2018). The nomenclature is standard in lithic analysis and broad enough to allow for external comparisons if needed.
We used nine techno-typological categories: (1) Complete flakes: knapping products that present dorsal and ventral surfaces and platform of percussion, no fractures and >20 mm of technological length; (2) Debris: knapping products <20 mm of technological length; (3) Fragmented flakes: knapping products that, while presenting one or more fractures, still preserve dorsal and ventral surfaces and a percussion platform; (4) Flake fragments: knapping products that, while preserving dorsal and ventral surfaces, do not present a platform of percussion due to previous fracture; (5) Cores: volumes managed by a specific knapping method with the purpose of removing flakes, presenting removals on their exploitation surfaces, and impact marks on their percussion surfaces; (6) Core fragments: volumes managed by a specific knapping method with the purpose of removing flakes, presenting removals on their exploitation surfaces, impact marks on their percussion surfaces, and one or more fractures not attributable to the dynamics of the knapping method; (7) Tools: volumes modified by a specific shaping pattern not solely directed toward obtaining flakes. This category refers to tool making from unmodified original blanks; (8) Retouched pieces: knapping products that have been modified postextraction following specific shaping patterns; and (9) Percussive material: blanks presenting an abundance of use wear on their surfaces, indicating pounding activities.
The classifications of fragmented flakes/retouched flakes follow these criteria: (1) Platform cortex: non-cortical, cortical; (2) Platform type: plain, lineal, point-shaped, facetted; (3) Number of platform facets, if present; (4) Platform measurements: length and width; (5) Platform angle: angle between the platform and the ventral surface; (6) Bulb type: pronounced or diffuse; (7) Ventral surface delineation: straight, concave, convex, sinuous; (8) Termination: step, hinge, feather, overtaken; (9) Dorsal cortex: non-cortical, non-cortical dominant over cortical, cortical dominant over non-cortical, complete cortex; (10) Cortex location; (11) Dorsal surface number of scars; (12) Arrangement of scars: unipolar, bipolar, centripetal, transversal, etc., and (13) Edge length in mm (full sum).
The retouched flakes were analyzed adapting the criteria of Laplace’s (1972) analytic typology: (1) Perimeter configuration (%); (2) Retouch orientation regarding the platform; (3) Retouch mode: simple, abrupt, plain; (4) Retouch amplitude regarding the edge; (5) Retouch depth regarding the surface; (6) Retouch direction: direct, inverse, alternate, alternating; (7) Retouch continuity: continuous, discontinuous, notch, denticulate; (8) Edge delineation: straight, concave, convex, sinuous; and (9) Retouched length in mm (complete sum). Each retouched piece was identified with a Primary Type code and then translated to broader public nomenclature.
For cores, we studied several aspects: (1) Faciality: unifacial, bifacial, multifacial; (2) Cortex: non-cortical, non-cortical dominant, cortical dominant, cortical; (3) Qualitative reduction stage: tested, initial, medium, exhausted; (4) Hierarchical surface organization; (5) Product predetermination; (6) Rotation (when possible): bifacial alternating, bifacial alternate, bifacial continuous, bifacial hierarchical; (7) Extraction negatives: unipolar, bipolar, multipolar, centripetal; (8) Angular relationship between percussion platform and removal surface; and (9) Perimeter (%).
We identified seven knapping methods in core exploitation: (1) Multipolar multifacial: core whose volumetric management involves a minimum of four surfaces exploited in at least four different directions; (2) Unipolar longitudinal: a single surface was exploited according to unidirectional schemes; (3) Bifacial orthogonal: knapping of two opposed surfaces unidirectionally; (4) Bifacial centripetal: exploitation of two surfaces separated by a horizontal plane, each with a minimum of three removals following a centripetal scheme; (5) Unifacial centripetal: exploitation of one surface with a minimum of three removals following a directional scheme toward the center of the surface; (6) Bipolar-on-anvil: Technique in which the positioning of the blank is on anvil; and (7) Unifacial bipolar: one single surface was exploited following an opposed bidirectional scheme.
The artifact weathering was recorded on a scale from 0 to 3: 0 = Fresh; 1 = Less than half is eroded; 2 = More than half is eroded; and 3 = Full weathering. Knapping accidents were located following the artifacts technological measurements. Patina was classified from 0 to 3: 0 = No patina; 1 = Less than half presents patina; 2 = More than half presents patina; and 3 = Full patina. Concretion: 0–3, where 0 = No concretion; 1 = Less than half presents concretion; 2 = More than half presents concretion; and 3 = Full concretion.
The software employed for techno-typological descriptive statistics were Excel and PAST (Hammer et al., 2006). The technical schemes on cores were made using Inkscape Open Source Vector Software.
The Oldowan stone technology has been surmised as an expedient or least-effort reduction system (Toth, 1985; Isaac, 1997; Schick and Toth, 2006). Yet, until recently, few studies aimed to quantify lifespan and curation history behind core reduction to prove this assumption. Previous studies relied on technological indices from variables like the number of removals on a core, cortex remaining, or average platform angles, among other proxies of reduction intensity (Clarkson, 2013; Douglass et al., 2018). We opted for the Volumetric Reconstruction Method (VRM) (Lombao et al., 2020, 2021), grounded in geometrical formulae specific to quartzite-based assemblages and the three-dimensional shapes under scrutiny. The VRM is specifically designed to examine Oldowan technology, addressing common problems such as the overlapping effect of stone reduction processes (Lombao et al., 2019), while allowing for comparisons among multiple knapping methods. Moreover, the VRM is a holistic method that requires the integration of both core and flake analysis to maximize resolution. The VRM performed quantitative analysis of cores in terms of reduction intensity, according to their knapping method, and their distribution by occupation phase (Phases I–IV, as no cores were recovered in Phase V). We did not try intra-phase comparisons, due to the small sample size for Phases I and IV. We restricted our VRM study to quartzite cores on tabular clasts, which constitutes the largest group (94.9%). Statistical analyses of VRM data were processed with R (R Core Team, 2013). For full details on the VRM method, we refer the reader to its original source (Lombao et al., 2020), summarizing here the application procedure:
• Record mean and median of thickness and platform thickness. Shapiro–Wilk normality tests were conducted to evaluate distribution (Supplementary Tables 1–3).
• Measure core morphological dimensions by its minimal rectangle.
• Identify the number of generations of flake removals that modified the maximum dimension of each product length and width axes. The number of generations involved is called a “correction unit.”
• Apply correction units to length and width. Multiply the number of identified correction units by mean/median of the flake’s platform thickness. To correct the thickness axis, multiply the number of identified flake generations by the mean/median of the flake’s thickness.
• Add these values to core dimensions (Then use these corrected dimensions to apply the ellipsoid volume formula).
• Multiply the original estimated volume by the density of each core. This converted the volume in mass values, resulting in an estimate of original mass.
• Divide the remaining core mass, which signals mass upon discard, by the estimated original mass of the blank prior to knapping; then, multiply the results by 100 to obtain percentages.
• To estimate the percentage of extracted mass, subtract the percentage of remaining mass from 100%.
A comprehensive description of lithics is shown in Table 1, with classification criteria detailed under Methods. Quartzite is the dominant raw material (97.7%), with minor input from ignimbrite (1.6%), gneiss (0.5%), and basalt (0.2%). The quartzite assemblage exhibits different grain sizes along with tabular to subangular morphologies with no evidence of rounded surfaces/cortex, thus indicating primary procurement. Sourcing was determined by geochemistry (Mercader et al., 2021). Quartzite was derived from regional and local inselbergs (Naibor Soit, ∼12 km/Naisiusiu, 0.4 km). Knapping sequences encompass nine techno-typological categories. Cores (n = 59, 10.4%) represent various reduction methods, such as bifacial centripetal (n = 3), unifacial centripetal (n = 4), multipolar multifacial (n = 19), bipolar on anvil (n = 3), unipolar longitudinal (n = 16), unifacial bipolar (n = 1), and bifacial orthogonal (n = 14). Heavy-duty tools consist of 10 quartzite spheroids (1.8%) and two ignimbrite chopper/chopping-tools (0.35%). Retouched pieces were totally six (1.1%); all of them on quartzite: notches (n = 3), side scrapers (n = 2), and a denticulate (n = 1). Complete flakes (>20 mm technological length) (n = 234, 41.4%) are abundant, the majority of which are on quartzite, with only two examples in ignimbrite and gneiss. Debris (<20 mm technological length) (n = 57, 10.1%) is only present in the three phases with the largest accumulation of stone artifacts. Percussive materials are scarce (n = 15, 2.65%), but display the highest raw material diversity.
Table 1. Ewass Oldupa lithic distribution by artifact classification, occupation phase, and raw material.
At Phase I, the excavation (82 m2) exposed 10 artifacts clustered in 7 m2 (Figure 2). All artifacts were made on quartzite, and the material shows little to minimal rounding. Two flakes have metric values of 47.2 × 39.3 × 17.2 mm and 63.8 × 58.1 × 25.5 mm. Their platforms are single facetted with non-cortical dorsal surfaces. The six cores document four knapping methods: unipolar longitudinal, bifacial orthogonal, unifacial centripetal, and multipolar multifacial. The original blanks were tabular clasts. The core metrics and the number of removals per knapping method are given in Supplementary Table 4.
Figure 2. Selection of stone tools from Phase I. (A) Quartzite multipolar multifacial core with technical schemes. (B) Quartzite bifacial orthogonal core with technical schemes. (C) Quartzite unifacial centripetal core with technical schemes. (D,E) Quartzite flakes. Red arrows indicate the directions of flake’s removals.
Phase II is documented within an excavation area of 91 m2. The artifacts (n = 201) (Figure 3) were scattered over 40 m2 and constitute the largest lithic accumulation at the site. The artifacts present little to minimal rounding, with medium to high rounding in 16 pieces (7.9%). The stone tools from this horizon encompass the highest raw material variability with ignimbrite, basalt, and gneiss, albeit quartzite is still prevalent (95.5%). Complete flakes are the most common product. Most are in quartzite, with only one example of a flake made of gneiss. The average dimensions of flakes and SDs are 34 ± 13.5 × 32 ± 14 × 14 ± 6.5 mm. The platform average dimensions and SDs are 21.1 ± 11.4 × 11.6 ± 6.2 mm. The platforms are mainly single facetted (85.8%), with some bi-facetted (9.5%) and cortical examples (4.7%). The flakes present mainly non-cortical dorsal surfaces (78.5%), with other categories being rare and including slight cortex (6.3%), cortical dominance (7.6%), and fully cortical (7.6%). Dorsal surfaces support 0 - 6 scars (X = 2.4), derived from unipolar, bipolar, and centripetal extractions. Debris is exclusively on quartzite and relatively scarce. Fragmented flakes present almost exclusively lateral fractures, with evidence of two pieces with longitudinal fractures. Retouched flakes are scarce: three quartzite pieces (1.5%) supporting a notch, a side scraper, and a denticulate, measuring 63.5 × 61.4 × 23.7 mm, 47.0 × 40.4 × 26.2 mm, and 31.3 × 55.8 × 15.8 mm, respectively. Perimeter configuration is scarce; retouch is not extensive in any piece. It covers 20 mm in the notch, 34 mm on the side scraper, and 38 mm in the denticulate. The three pieces present retouch coming from the ventral surface toward the dorsal one, with ∼ 90-degree angles. With respect to cores, quartzite is the preferred knapping material, with only one core in ignimbrite. The original blanks were tabular clasts, other than two cores on flakes. Most cores are non-cortical (82.2%), while a low proportion of cores have slight cortical cover (10.7%) or are dominated by cortex (7.1%). Five knapping methods were identified: (1) multipolar multifacial, unipolar longitudinal, bifacial orthogonal, bipolar on anvil, and bifacial centripetal. The core metrics and number of removals per knapping method are in given Supplementary Table 5. Phase II is the richest in classic Oldowan tools, including eight quartzite spheroids and heavy-duty tools represented by two ignimbrite chopper/chopping tools. Technologically, these two artifacts represent unifacial and bifacial reduction strategies. However, the convexity of their edges and the angular relationship between their surfaces are more acute in comparison to cores sharing the same type of reduction schemes. These observations, along with the very low number of ignimbrite flakes in the assemblage, suggest that these artifacts result from tool configuration, not just core exploitation. Phase II has the highest number of percussive materials. This includes eight hammerstones and four pounding fragments, mostly in quartzite along with two ignimbrite implements, one in gneiss, plus the only basalt artifact we retrieved. Hammerstones average dimensions and SDs are 94.7 ± 31.3 mm × 75.9 ± 31.9 mm × 58.7 ± 26.6 mm. Percussion marks are systematically present on the rounded ends of the hammerstones. Three of the pounding fragments present lateral fractures, with the remaining presenting multiple fractures.
Figure 3. Selection of stone tools from Phase II. (A) Ignimbrite chopping tool with technical schemes. (B) Ignimbrite chopper with technical schemes. (C) Quartzite unipolar longitudinal core with technical schemes. (D) Quartzite multipolar multifacial core with technical schemes. (E) Quartzite unifacial centripetal core with technical schemes. (F,G) Quartzite flakes. (H) Basalt percussive material. Detail shows impact marks on the surface. Red arrows indicate the directions of flake’s removals.
The excavation at Phase III retrieved 188 artifacts (Figure 4), the second richest stone tool assemblage at the site. The assemblage is almost exclusively composed of quartzite artifacts (97.9%). Most materials are fresh (64.4%), with the rest presenting minimal rounding. Complete flakes are dominant. Most of them are made on quartzite, with one flake in ignimbrite. Flake average dimensions and SDs are 34.8 ± 12.8 mm × 31.7 ± 11.5 mm × 14.2 ± 5.8 mm. Platforms average dimensions and SDs are 19.1 ± 8.7 mm × 10.5 ± 5.2 mm. As in Phase II, single-facetted platforms are common (80.5%), along cortical (17.8%) and bi-facetted ones (1.7%). The flakes present mainly non-cortical dorsal surfaces (73.7%), with fewer examples of slight cortex cover (6.6%) and complete cortical surfaces (19.7%). Dorsal surfaces range from 0 to 6 scars (X = 1.7), involving unipolar, bipolar, and centripetal reduction. The number of knapping debris is the highest of all phases. Fragmented flakes exhibit lateral, distal, and longitudinal fractures in equal proportions. Retouched flakes are scarce, with only two examples having notches, measuring 48.1 × 29.2 × 14.2 mm and 59.8 × 51 × 24.5 mm. Perimeter configuration amounts to 22 and 29 mm. In both cases, retouch comes from the ventral surface toward the dorsal one, in ∼ 90-degree angles. The second largest core sample came from Phase III. Quartzite is the dominant raw material, with just two specimens in ignimbrite. Three quarters of the cores were on tabular clasts, while the remainder was on flakes. The core metrics and number of removals per knapping method are given in Supplementary Table 6. Most of the cores (65%) present non-cortical surfaces, with lower proportions of slight cortical presence (20%) and cortical dominance (15%). The knapping methods identified in Phase III are multipolar multifacial, unipolar longitudinal, bifacial orthogonal, bifacial centripetal, unifacial centripetal, and unifacial bipolar. Heavy-duty tools consist of two quartzite spheroids. These percussion implements are hammerstones in quartzite and ignimbrite, measuring 73.3 × 51.5 × 37.8 mm and 70.7 × 68.8 × 67.2 mm, respectively. Percussion marks concentrate on the rounded ends of both pieces. There is one instance of utilization of an artifact as both bifacial orthogonal core and percussive material.
Figure 4. Selection of stone tools from Phase III. (A) Quartzite bifacial orthogonal core with technical schemes. Detail shows impact marks on the surface. (B) Quartzite multipolar multifacial core with technical schemes. (C) Quartzite unifacial centripetal core with technical schemes. (D) Quartzite spheroid. (E–H) Quartzite flakes. Red arrows indicate the directions of flake’s removals.
At Phase IV, we recovered 151 stone tools (Figure 5). The assemblage is entirely made in quartzite. Most of the material presents no rounding (74.8%), with a minority being slightly rounded, and only two artifacts presenting heavy rounding. Complete flakes are the most common product. The flakes average dimensions and SDs are 30 ± 8.1 mm × 25.6 ± 7.2 mm × 12.1 ± 4.1 mm. Platforms average dimensions and SDs are 15.8 ± 6.9 mm × 9.2 ± 4.1 mm. Single-facetted platforms are dominant (78.5%), followed by bi-facetted (15.4%) and cortical platforms (6.1%). The flakes exhibit high proportions of non-cortical dorsal surfaces (83.1%), with lower proportions of slight cortex (12.3%) and complete cortical surfaces (4.6%). Scars on dorsal surfaces range from 0 to 4 (X = 2.1), involving unipolar, bipolar, and centripetal extractions. There are 23 debris fragments. As in previous phases, fragmented flakes present lateral (19%), distal (47.6%), and longitudinal (33.3%) fractures. No retouched flakes were recovered in Phase IV. The number of cores is low, and each core represents a different knapping method: multipolar multifacial, unipolar longitudinal, bifacial orthogonal, bifacial centripetal, and bipolar on anvil. The core metrics and number of removals per knapping method are given in Supplementary Table 7. All cores were on quartzite. Four of them occurred on tabular clasts, except for one (bifacial orthogonal) on flake. Percussive implements are limited to one quartzite hammerstone, measuring 87.8 × 57.3 × 47.9 mm.
Figure 5. Selection of stone tools from Phase IV. (A) Quartzite bifacial orthogonal core with technical schemes. (B) Quartzite multipolar multifacial core with technical schemes. (C–F) Quartzite flakes. (G) Quartzite unipolar longitudinal core with technical schemes. Red arrows indicate the directions of flake’s removals.
The stone tool assemblage of Phase V was scattered within 9 m2. The artifacts are limited to 15 quartzite lithics. The artifacts present no signs of erosion. Complete flakes are dominant, with average dimensions and SDs of 32 ± 9.5 mm × 29.5 ± 8 mm × 12.5 ± 3 mm. Platform average dimensions and SDs are 17.2 ± 9.7 mm × 8.8 ± 4.1 mm. The platforms are mostly single-facetted, with two examples of cortical platforms and one of bi-facetted platform. The flakes have non-cortical dorsal surfaces almost exclusively, except for two cortical flakes. Dorsal surfaces record scars ranging from 1 to 7 scars (X = 2.4), involving unipolar and centripetal reduction. No debris was recovered from this phase. Retouched flakes include one side scraper preserving dorsal cortex and measuring 50 × 39.8 × 14.2 mm. Perimeter configuration amounts to 64 mm. Retouch is inverse, from the dorsal surface toward the ventral one, and ∼ 90° angle.
At Ewass Oldupa, the VRM shows that both blank size and extracted mass are constant throughout the assemblage (Supplementary Tables 8–11). Most cores fall along a similar continuum: 200–1,400 g (Figure 6A). At face value, the mass removed from the cores (Figure 6B) seems widely variable, with cores in Phases II - III having ∼ 20% – ∼ 80% of their mass removed. Kolmogorov–Smirnov tests, however, show no significant differences in distribution [K-S (p) > 0.05] (Supplementary Tables 12, 13).
Figure 6. Quantitative results obtained through the Volumetric Reconstruction Method (VRM). (A) Boxplot and jitter plot of Estimated Original Mass (in grams) of cores according to Phase. (B) Histogram of estimated percentage of extracted mass (%) of cores according to occupation Phases. (C) Boxplot and jitter plot of estimated original mass (in grams) of cores according to knapping method. (D) Boxplot jitter plot of estimated percentage of extracted mass (%) of cores according to knapping method. In (A,C,D), the horizontal bars in the box represent the median. The box covers 50% of the central values, from the 1st (lower edge of the box) to the 3rd quartile (upper edge of the box). Upper whisker length represents data that are not 1.5 times greater than the third quartile. Lower whisker length represents data that are not less than 1.5 times the first quartile. Points represent values 1.5 greater or less than the 75th or 25th, respectively.
The larger blanks underwent multipolar multifacial reduction (n = 18; Median = 671.8 g). The smaller blanks used either bifacial orthogonal (n = 10; Median = 305.2 g) or bifacial centripetal (n = 2; Median = 459.8 g) methods, and especially, bipolar-on-anvil (n = 2; Median = 312.2 g) (Supplementary Table 14). Multipolar multifacial cores also show higher variability in original mass (Figure 6C), with statistically significant differences for bifacial orthogonal versus multipolar multifacial, as well as bifacial orthogonal versus unipolar longitudinal (Table 2).
Table 2. Upper triangle: Mann–Whitney pairwise tests (p) comparing the Estimated Original Mass (EOM) between knapping strategy; Lower triangle: Mann–Whitney pairwise tests (p) comparing the Estimated Percentage of Extracted Mass (EPEM) between knapping strategy.
Our estimates of extracted mass per knapping method (Figure 6D) show that unifacial longitudinal cores are the most highly variable at the time of discard: 20–80% reduction intensity (Supplementary Table 15). In contrast, multipolar multifacial cores (n = 18; Median = 72.6%) and bifacial centripetal cores (n = 2; Median = 67.8%) are both heavily reduced, but not exhausted. Mann-Whitney pairwise testing demonstrates that these differences are statistically significant on their central values (Table 2), with larger values for multifacial multipolar than for unifacial longitudinal, bifacial orthogonal, and bipolar-on-anvil.
The stone tool assemblages from the western Pleistocene basin at Oldupai Gorge (Habermann et al., 2016; Mercader et al., 2021; Stollhofen et al., 2021) constitute the earliest record in the outcrop of hominin presence in the area. Ewass Oldupa is one of a few multi-stratified, high-resolution records of the Early Oldowan and the Early Pleistocene, underscoring ecological pioneering behavior, that is, the first occupation of a territory after a catastrophic event, and displaying a stable technological record amid changing environments. Hominins utilized recurrent technological strategies throughout the sequence, denoting consistent land-use patterns, raw material sourcing, and lithic manufacturing over the course of ∼200,000 years. This behavioral adaptation manifest itself in two ways.
First, in every phase, lithic technology was produced mostly on quartzite, with other materials being less frequent. Macroscopic and petrographic data, compared to published reference collections (Favreau et al., 2020; Soto et al., 2020a,b), determined that the most likely sources for the raw materials employed at Ewass Oldupa were the outcrops of Naisiusiu, Naibor Soit, Endonyo Osunyai, and/or Oittii. However, chemical characterization and Linear Discriminant Analysis of Oldowan materials showed that up to three-quarters of the quartzite came from Naibor Soit, while strictly local rock from Naisiusiu was less commonly employed (Mercader et al., 2021). This is an interesting behavioral feature, as Naibor Soit is located ∼ 12 km away from Ewass Oldupa, supporting the view that differential raw material transport and management was a key characteristic already present in the early Oldowan and sustained through time (Stout et al., 2005; Negash et al., 2006; Braun et al., 2008b; Harmand, 2009; Goldman-Neuman and Hovers, 2012). In contrast, younger Oldowan sites from the eastern basin, such as at Douglas Korongo (DK), reveal a focus on volcanic rocks (Leakey, 1971; Ludwig, 1999; Kimura, 2002; Mora and de la Torre, 2005; Sánchez-Yustos, 2021).
Second, the lithic production consistently relied on the same knapping methods, with three of them (multipolar multifacial, unipolar longitudinal, and bifacial orthogonal) recurrently appearing in four phases. Centripetal knapping strategies came about throughout the sequence. Bipolar-on-anvil knapping is present, but in marginal proportions. The platform preparation was minimal, with single-facetted platforms prevailing and demonstrating that hominins pursued the extraction of flakes as the main goal of core reduction. Retouched flakes are scarce. Classic heavy-duty/chopping tools, such as those from the eastern basin (Leakey, 1971), are uncommon in the west (Habermann et al., 2016; Stollhofen et al., 2021).
The lithic record from Ewass Oldupa shares many commonalities with other Oldowan assemblages (Leakey, 1971; Toth, 1985; Isaac, 1997; Semaw et al., 1997; Semaw, 2000; de la Torre, 2004; Delagnes and Roche, 2005; Schick and Toth, 2006; Barsky et al., 2011; Braun et al., 2019; Reeves et al., 2021; Stollhofen et al., 2021). Overall, there is a preference for unmodified flakes, along with unifacial, bifacial, and multifacial cores exploited unidirectionally, bidirectionally, and multidirectionally. Retouch is infrequent. This set of technological features is displayed throughout Oldowan assemblages since its very origin. Thus, our data support recent analyses suggesting that Oldowan stone technology exhibits limited temporal variation (Braun et al., 2019; Mercader et al., 2021). Assemblage differences among distinct archeological sites seem to be based on relative artifact frequency, not on the types themselves.
In this article, we deployed a quantitative approach to lithic analysis known as VRM (Lombao et al., 2020, 2021), which facilitates inter-assemblage comparability using geometric principles and is potentially applicable to any lithic assemblage regardless of context and techno-typological affiliation. Given the qualitative nature of conventional techno-typological analysis, we argue in this study for a quantitative approach to interpret lithic assemblages (Diez-Martín et al., 2010; de la Torre et al., 2021). This aims to overcome the lack of shared analysis nomenclature, compensating for the abovementioned conflicting classification criteria derived from the overreliance on typology that sometimes drives traditional approaches to the Oldowan, as pointed out by several authors (de la Torre, 2004; Delagnes and Roche, 2005; Braun et al., 2008a).
At Ewass Oldupa, raw material provenance and techno-typology indicate recurrent technological strategies during the early Oldowan, from knapping methods to artifact types. The VRM findings do point toward consistent blank size selection over time (i.e., larger blanks were systematically procured to support multipolar multifacial exploitation). This correlation between blank size and knapping method indicates that hominins were most likely adapting their tool kit to the size, shape, and quality constraints encountered in raw materials. The core reduction schemes were stable over time. Intensity of stone reduction was manifest. The VRM results demonstrate that the selected knapping methods were effective when intensively reducing a large variety of original morphologies. In addition, similar findings come from Kanjera South (Douglass et al., 2018; Reeves et al., 2021), where the lack of cores abandoned during initial reduction stage (0–20%) is notable. This might be due to transport distance: blank testing was performed in the primary capitation source with blanks arriving at the site already partially reduced.
At the same time, the Oldowan technological recurrence could also indicate some limitations in technical capability. The continuous adaptation of knapping methods to raw material characteristics might indicate incapacity to impose blank preparation, hierarchical reduction, and control of expected outcomes regardless of the natural constrains of the rocks, a feature later seen in Acheulean assemblages (Sánchez-Yustos, 2021). In spite of the shared operative (Cueva-Temprana et al., 2019) and artifactual (Gallotti and Mussi, 2015; Sánchez-Yustos et al., 2018) bases suggestive of a “gradual transition” (Duke et al., 2021; Sánchez-Yustos, 2021) from the Oldowan to the Acheulean, it has been said (Faisal et al., 2010; Stout et al., 2015) that distinct biological and neurological systems separate them (Isaac, 1969; Wynn, 1989; Sánchez-Yustos, 2021).
This becomes especially relevant when considering the authorship of the Oldowan technology, which remains a puzzling question. Based on the moment of its appearance and its geographical dispersal, it is difficult to relate the Oldowan with an individual species. At Oldupai Gorge, a minimum of two, Paranthropus boisei (Leakey, 1971) and Homo habilis (Leakey, 1971; Blumenschine et al., 2003; Stanistreet et al., 2018; de la Torre et al., 2021) inhabited the basin penecontemporaneously. In fact, some authors have even posited that the often-mentioned “stasis” in Oldowan toolmaking roughly parallels the evolutionary trajectory of one of these species: Paranthropus boisei (Semaw et al., 1997; Wood, 1997). However, this discussion is applicable not only to Oldupai Gorge, but also to the whole Oldowan world. Similar scenarios occur in earlier Oldowan sites, such as in the Hatayae Member of The Bouri Formation, where the alleged 2.5 Ma cut marks are contemporaneous with the presence of Australopithecus garhi (de Heinzelin et al., 1999; Sahle et al., 2017). Recently, it has been argued (Tennie et al., 2017) that the Oldowan might be an example of the so called “latent solutions,” that is, behavioral traits susceptible to appear and disperse without the influence of high-fidelity social learning. One implication of this could be that Oldowan technology possesses a relatively reduced technical complexity that renders possible independent, temporal and geographical, appearances. If this were the case, the Oldowan would be susceptible to have appeared multiple times and among several hominin species. Moreover, the fact that Oldowan technology remains virtually unchanged for more than a million years supports the “latent solution” idea, as it is unlikely to develop technological innovations while relying on limited forms of social transmission (Morgan et al., 2015; Snyder et al., 2021).
In summary, our work has shown that Oldowan hominins at Ewass Oldupa lived through drastic environmental change without a concomitant transformation of their stone technology at times of ecological upheaval, whether this could be postvolcanic landscapes 2.03 Ma, woodland/grassland mosaics during lake transgression 1.9 Ma, open woodlands 1.85 Ma, or steppe biomes (Mercader et al., 2021). This diachronic outlook, along with technological characteristics shared by multiple stone tool assemblages across the Old World, suggests that the Oldowan itself constitutes a versatile adaptive trait, requiring no more than minor technological adjustments to effectively satisfy hominin ecological demands throughout different types of environments.
The original contributions presented in the study are included in the article/Supplementary Material, further inquiries can be directed to the corresponding author/s.
JM and PB: project direction. JM, PB, NB, and MP: funding. JM, PB, MS, MI, AC-T, and MP: excavations. AC-T: lithic analysis. DL and AC-T: quantitative analysis. AC-T, DL, MS, MP, and JM: article conceptualization. AC-T, DL, and MS: visualization. All authors: writing and revision.
This work was supported by the Canadian Social Sciences and Humanities Research Council under its Partnership Grant Program no. 895-2016-1017. DL is beneficiary of Ph.D. research fellowship AGAUR/ESF “ESF, Investing in Your Future” (2020 FI_B2 00164), and was supported by AGAUR (project no. 2017 SGR-1040). IPHES-CERCA has received financial support from the Spanish Ministry of Science and Innovation through the “María de Maeztu” program for Units of Excellence (CEX2019-000945-M). MS research was funded by the 2019 UAM-Tomás y Valiente Program. NB, JM, MP, and AC-T are grateful to the Max Planck Society for funding.
The authors declare that the research was conducted in the absence of any commercial or financial relationships that could be construed as a potential conflict of interest.
All claims expressed in this article are solely those of the authors and do not necessarily represent those of their affiliated organizations, or those of the publisher, the editors and the reviewers. Any product that may be evaluated in this article, or claim that may be made by its manufacturer, is not guaranteed or endorsed by the publisher.
We acknowledge the essential contributions by the Maasai communities at Oldupai Gorge concerning the knowledge presented herein. The Tanzania Commission for Science and Technology authorized this work under permit no. 2018-112-NA-2018-36. The Tanzanian Ministry of Natural Resources and Tourism, through its Antiquities Division, granted us permission to carry out this work (14/2017/2018) and authorities at the Ngorongoro Conservation Area allowed us to enter the protected area (BE.504/620/01/53). The export license for the materials presented in this study were obtained from the Antiquities Division (EA.150/297/01: 5/2018/2019) and the Tanzanian Executive Secretary from the Mining Commission (00001258). We would like to thank the support provided by the University of Dar es Salaam. We would also like thank Mohammad Javad Shoaee and Julien Favreau for their assistance with photography, and reviewer’s comments and editor’s work during the revision process, which has undoubtedly improved this manuscript.
The Supplementary Material for this article can be found online at: https://www.frontiersin.org/articles/10.3389/fevo.2022.788101/full#supplementary-material
Baena, J., Lordkipanidze, D., Cuartero, F., Ferring, R., Zhvania, D., Martín, D., et al. (2010). Technical and technological complexity in the beginning: the study of Dmanisi lithic assemblage. Quat. Int. 223, 45–53. doi: 10.1016/j.quaint.2010.01.019
Barsky, D., Chapon-Sao, C., Bahain, J. J., Beyene, Y., Cauche, D., Celiberti, V., et al. (2011). The early oldowan stone-tool assemblage from Fejej FJ-1A, Ethiopia. J. Afr. Archaeol. 9, 207–224. doi: 10.3213/2191-5784-1019
Blumenschine, R. J., Peters, C. R., Masao, F. T., Clarke, R. J., Deino, A. L., Hay, R. L., et al. (2003). Late Pliocene Homo and hominid land use from western Olduvai Gorge, Tanzania. Science 299, 1217–1221. doi: 10.1126/science.1075374
Braun, D. R., Aldeias, V., Archer, W., Arrowsmith, J. R., Baraki, N., Campisano, C. J., et al. (2019). Earliest known Oldowan artifacts at >2.58 Ma from Ledi-Geraru, Ethiopia, highlight early technological diversity. Proc. Natl. Acad. Sci. U.S.A. 116, 11712–11717. doi: 10.1073/pnas.1820177116
Braun, D. R., Plummer, T., Ditchfield, P., Ferraro, J. V., Maina, D., Bishop, L. C., et al. (2008a). Oldowan behavior and raw material transport: perspectives from the Kanjera formation. J. Archaeol. Sci. 35, 2329–2345. doi: 10.1016/j.jas.2008.03.004
Braun, D. R., Tactikos, J. C., Ferraro, J. V., Arnow, S. L., and Harris, J. W. (2008b). Oldowan reduction sequences: methodological considerations. J. Archaeol. Sci. 35, 2153–2163. doi: 10.1016/j.jas.2008.01.015
Carbonell, E., Guilbaud, M., and Mora, R. (1983). Utilización de la lógica analítica para el estudio de tecno-complejos a cantos tallados. Cah. Noir 1, 1–63.
Clarkson, C. (2013). Measuring core reduction using 3D flake scar density: a test case of changing core reduction at Klasies River Mouth, South Africa. J. Archaeol. Sci. 40, 4348–4357. doi: 10.1016/j.jas.2013.06.007
Cueva-Temprana, A., Lombao, D., Morales, J. I., Geribàs, N., and Mosquera, M. (2019). Gestures during knapping: a two-perspective approach to Pleistocene technologies. Lithic Technol. 44, 74–89. doi: 10.1080/01977261.2019.1587255
de Heinzelin, J., Clark, J. D., White, T., Hart, W., Renne, P., Woldegabriel, G., et al. (1999). Environment and behavior of 2.5-million year-old Bouri hominids. Science 284, 625–629. doi: 10.1126/science.284.5414.625
de la Torre, I. (2004). Omo revisited. Evaluating the technical skills of Pliocene hominins. Curr. Anthropol. 45, 439–465. doi: 10.1086/422079
de la Torre, I., Benito-Calvo, A., Martín-Ramos, C., McHenry, L. J., Mora, R., Njau, J. K., et al. (2021). New excavations in the MNK Skull site, and the last appearance of the Oldowan and Homo habilis at Olduvai Gorge, Tanzania. J. Anthropol. Archaeol. 61:101255. doi: 10.1016/j.jaa.2020.101255
de la Torre, I., and Mora, R. (2008). “Remarks on the current theoretical and methodological approaches to the study of early technological strategies in Eastern Africa,” in Interdisciplinary Approaches to the Oldowan, eds E. Hovers and D. R. Braun (Berlin: Springer Science Business Media), doi: 10.1007/978-1-4020-9059-2
de Lombera-Hermida, A., Bargalló, A., Terradillos-Bernal, M., Huguet, R., Vallverdú, J., García-Antón, M. D., et al. (2015). The lithic industry of Sima del Elefante (Atapuerca, Burgos, Spain) in the context of Early and Middle Pleistocene technology in Europe. J. Hum. Evol. 82, 95–106. doi: 10.1016/j.jhevol.2015.03.002
Deino, A. L. (2012). 40Ar/39Ar dating of Bed I, Olduvai Gorge, Tanzania, and the chronology of early Pleistocene climate change. J. Hum. Evol. 63, 251–273. doi: 10.1016/j.jhevol.2012.05.004
Deino, A. L., Heil, C. Jr., King, J., McHenry, L. J., Stanistreet, I. G., Stollhofen, H., et al. (2020). Chronostratigraphy and age modeling of Pleistocene drill cores from the Olduvai Basin, Tanzania (Olduvai Gorge Coring Project). Palaeogeogr. Palaeoclimatol. Palaeoecol. 571:109990. doi: 10.1016/j.palaeo.2020.109990
Delagnes, A., and Roche, H. (2005). Late Pliocene hominid knapping skills: the case of Lokalalei 2C, West Turkana, Kenya. J. Hum. Evol. 48, 435–472. doi: 10.1016/j.jhevol.2004.12.005
Diez-Martín, F., Yustos, P. S., Domínguez-Rodrigo, M., Mabulla, A. Z. P., Bunn, H. T., Ashley, G. M., et al. (2010). New insights into hominin lithic activities at FLK North Bed I, Olduvai Gorge, Tanzania. Quat. Res. 74, 376–387. doi: 10.1016/j.yqres.2010.07.019
Douglass, M. J., Lin, S. C., Braun, D. R., and Plummer, T. W. (2018). Core use-life distributions in lithic assemblages as a means for reconstructing behavioral patterns. J. Archaeol. Method Theory 25, 254–288. doi: 10.1007/s10816-017-9334-2
Duke, H., Feibel, C., and Harmand, S. (2021). Before the Acheulean: the emergence of bifacial shaping at Kokiselei 6 (1.8 Ma), West Turkana, Kenya. J. Hum. Evol. 159:103061. doi: 10.1016/j.jhevol.2021.103061
Faisal, A., Stout, D., Apel, J., and Bradley, B. (2010). The manipulative complexity of Lower Paleolithic stone toolmaking. PLoS One 5:e13718. doi: 10.1371/journal.pone.0013718
Favreau, J., Soto, M., Nair, R., Bushozi, P. M., Clarke, S., DeBuhr, C. L., et al. (2020). Petrographic characterization of raw material sources at Oldupai Gorge, Tanzania. Front. Earth Sci. 8:158. doi: 10.3389/feart.2020.00158
Ferring, R., Oms, O., Agustí, J., Berna, F., Nioradze, M., Shelia, T., et al. (2011). Earliest human occupations at Dmanisi (Georgian Caucasus) dated to 1.85–1.78 Ma. Proc. Natl. Acad. Sci. U.S.A. 108, 10432–10436. doi: 10.1073/pnas.1106638108
Gallotti, R., and Mussi, M. (2015). The unknown Oldowan: ∼1.7-million-year-old standardized obsidian small tools from Garba IV, Melka Kunture, Ethiopia. PLoS One 10:e0145101. doi: 10.1371/journal.pone.0145101
Goldman-Neuman, T., and Hovers, E. (2012). Raw material selectivity in late Pliocene Oldowan sites in the Makaamitalu Basin, Hadar, Ethiopia. J. Hum. Evol. 62, 353–366. doi: 10.1016/j.jhevol.2011.05.006
Habermann, J. M., Stanistreet, I. G., Stollhofen, H., Albert, R. M., Bamford, M. K., Pante, M. C., et al. (2016). In situ ∼2.0 Ma trees discovered as fossil rooted stumps, lowermost Bed I, Olduvai Gorge, Tanzania. J. Hum. Evol. 90, 74–87. doi: 10.1016/j.jhevol.2015.09.011
Hammer, Ø., Harper, D. A., and Ryan, P. D. (2006). PAST—PAlaeontological STatistics, ver. 1.38. Software Documentation.
Harmand, S. (2009). “Variability in raw material selectivity at the Late Pliocene sites of Lokalalei, West Turkana, Kenya,” in Interdisciplinary Approaches to the Oldowan, eds E. Hovers and D. R. Braun (Dordrecht: Springer), 85–97. doi: 10.1007/978-1-4020-9060-8_8
Isaac, G. L. (1969). Studies of early culture in East Africa. World Archaeol. 1, 1–28. doi: 10.1080/00438243.1969.9979423
Isaac, G. L. (1997). Koobi Fora Research Project, Plio-Pleistocene Archaeology, Vol. 5. Oxford: Clarendon Press.
Kimura, Y. (2002). Examining time trends in the Oldowan technology at Beds I and II, Olduvai Gorge. J. Hum. Evol. 43, 291–321. doi: 10.1006/jhev.2002.0576
Kuman, K., and Clarke, R. J. (2000). Stratigraphy, artefact industries and hominid associations for Sterkfontein, Member 5. J. Hum. Evol. 38, 827–847. doi: 10.1006/jhev.1999.0392
Laplace, G. (1972). La typologie analytique et structurale: base rationnelle d’étude des industries lithiques et osseuses. Banques Données Archéol. 932, 91–143.
Leakey, L. S., Tobias, P. V., and Napier, J. R. (1964). A new species of the genus Homo from Olduvai Gorge. Nature 202, 7–9. doi: 10.1038/202007a0
Leakey, M. D. (1971). Olduvai Gorge: Excavations in Beds I and II, 1960 - 1963, Vol. 3. Cambridge: Cambridge University Press.
Lombao, D., Cueva-Temprana, A., Mosquera, M., and Morales, J. I. (2020). A new approach to measure reduction intensity on cores and tools on cobbles: the volumetric reconstruction method. Archaeol. Anthropol. Sci. 12:122. doi: 10.1007/s12520-020-01154-7
Lombao, D., Cueva-Temprana, A., Rabuñal, J. R., Morales, J. I., and Mosquera, M. (2019). The effects of blank size and knapping strategy on the estimation of core’s reduction intensity. Archaeol. Anthropol. Sci. 11, 5445–5461. doi: 10.1007/s12520-019-00879-4
Lombao, D., Rabuñal, J. R., Morales, J. I., Ollé, A., Carbonell, E., and Mosquera, M. (2021). The technological behaviours of Homo antecessor: core management and reduction intensity at Gran Dolina – TD6.2 (Atapuerca, Spain). OSF [Preprints] doi: 10.31219/osf.io/tez2y
Ludwig, B. V. (1999). A Technological Reassessment of East African Plio-Pleistocene Lithic Artifact Assemblages. Ph.D. dissertation. Camden, NJ: Rutgers, The State University of New Jersey.
McHenry, L. J. (2012). A revised stratigraphic framework for Olduvai Gorge Bed I based on tuff geochemistry. J. Hum. Evol. 63, 284–299. doi: 10.1016/j.jhevol.2011.04.010
McHenry, L. J., Mollel, G. F., and Swisher, C. C. (2008). Compositional and textural correlations between Olduvai Gorge Bed I tephra and volcanic sources in the Ngorongoro Volcanic Highlands, Tanzania. Quat. Int. 178, 306–319. doi: 10.1016/j.quaint.2007.01.004
McHenry, L. J., Stanistreet, I. G., Stollhofen, H., Njau, J. K., Toth, N., and Schick, K. (2020). Tuff fingerprinting and correlations between OGCP cores and outcrops for Pre-Bed I and Beds I/II at Olduvai Gorge, Tanzania. Palaeogeogr. Palaeoclimatol. Palaeoecol. 548:109630. doi: 10.1016/j.palaeo.2020.109630
Mercader, J., Akuku, P., Boivin, N., Bugumba, R., Bushozi, P., Camacho, A., et al. (2021). Earliest Olduvai hominins exploited unstable environments ∼ 2 million years ago. Nat. Commun. 12:3. doi: 10.1038/s41467-020-20176-2
Mgeladze, A., Lordkipanidze, D., Moncel, M. H., Despriee, J., Chagelishvili, R., Nioradze, M., et al. (2011). Hominin occupations at the Dmanisi site, Georgia, Southern Caucasus: raw materials and technical behaviours of Europe’s first hominins. J. Hum. Evol. 60, 571–596. doi: 10.1016/j.jhevol.2010.10.008
Mora, R., and de la Torre, I. (2005). Percussion tools in Olduvai Beds I and II (Tanzania): implications for early human activities. J. Anthropol. Archaeol. 24, 179–192. doi: 10.1016/j.jaa.2004.12.001
Morgan, T. J., Uomini, N. T., Rendell, L. E., Chouinard-Thuly, L., Street, S. E., Lewis, H. M., et al. (2015). Experimental evidence for the co-evolution of hominin tool-making teaching and language. Nat. Commun. 6:6029. doi: 10.1038/ncomms7029
Mosquera, M., Ollé, A., Rodríguez-Álvarez, X. P., and Carbonell, E. (2018). Shedding light on the early Pleistocene of TD6 (Gran Dolina, Atapuerca, Spain): the technological sequence and occupational inferences. PLoS One 13:e0190889. doi: 10.1371/journal.pone.0190889
Moyano, I. T., Barsky, D., Cauche, D., Celiberti, V., Grégoire, S., Lebegue, F., et al. (2011). The archaic stone tool industry from Barranco León and Fuente Nueva 3 (Orce, Spain): evidence of the earliest hominin presence in southern Europe. Quat. Int. 243, 80–91. doi: 10.1016/j.quaint.2010.12.011
Negash, A., Steven Shackley, M., and Alene, M. (2006). Source provenance of obsidian artifacts from the Early Stone Age (ESA) site of Melka Konture, Ethiopia. J. Arch. Sci. 33, 1647–1650. doi: 10.1016/j.jas.2005.11.001
Plummer, T. (2004). Flaked stones and old bones: biological and cultural evolution at the dawn of technology. Yearb. Phys. Anthropol. 47, 118–164. doi: 10.1002/ajpa.20157
Potts, R. (1991). Why the Oldowan? Plio-Pleistocene toolmaking and the transport of resources. J. Anthropol. Res. 47, 153–176.
Potts, R. (1998). Environmental hypotheses of hominin evolution. Yearb. Phys. Anthropol. 41, 93–136. doi: 10.1002/(sici)1096-8644(1998)107:27+<93::aid-ajpa5>3.0.co;2-x
R Core Team (2013). R: a Language and Environment for Statistical Computing. Vienna: R Foundation for Statistical Computing.
Reeves, J. S., Braun, D. R., Finestone, E. M., and Plummer, T. W. (2021). Ecological perspectives on technological diversity at Kanjera South. J. Hum. Evol. 158:103029. doi: 10.1016/j.jhevol.2021.103029
Sahle, Y., El Zaatari, S., and White, T. D. (2017). Hominid butchers and biting crocodiles in the African Plio–Pleistocene. Proc. Natl. Acad. Sci. U.S.A. 114, 13164–13169. doi: 10.1073/pnas.1716317114
Sahnouni, M., Parés, J. M., Duval, M., Cáceres, I., Harichane, Z., Van der Made, J., et al. (2018). 1.9-million- and 2.4-million-year-old artefacts and stone tool-cutmarked bones from Ain Boucherit, Algeria. Science 362, 1297–1301. doi: 10.1126/science.aau0008
Sánchez-Yustos, P. (2021). Knocking on Acheulean’s door. DK revisited (Bed I, Olduvai, Tanzania). J. Archaeol. Sci. Rep. 35:102763. doi: 10.1016/j.jasrep.2020.102763
Sánchez-Yustos, P., Diez-Martín, F., Domínguez-Rodrigo, M., Fraile, C., Duque, J., Díaz, I., et al. (2018). Acheulean without handaxes? Assemblage variability at FLK West (Lowermost Bed II, Olduvai, Tanzania). J. Anthropol. Sci. 18, 1–22. doi: 10.4436/JASS.96007
Schick, K., and Toth, N. (eds). (2006). The Oldowan: Case Studies into the Earliest Stone Age. Gosport, IN: Stone Age Institute Press.
Semaw, S. (2000). The world’s oldest stone artefacts from Gona, Ethiopia: their implications for understanding stone technology and patterns of human evolution between 2.6–1.5 million years ago. J. Archaeol. Sci. 27, 1197–1214. doi: 10.1006/jasc.1999.0592
Semaw, S., Renne, P., Harris, J. W., Feibel, C. S., Bernor, R. L., Fesseha, N., et al. (1997). 2.5-million-year-old stone tools from Gona, Ethiopia. Nature 385, 333–336. doi: 10.1038/385333a0
Snyder, W. D., Reeves, J. S., and Tennie, C. (2021). Early knapping techniques do not necessitate cultural transmission. OSF [Preprint]. doi: 10.31219/osf.io/ph6gw
Soto, M., Favreau, J., Campeau, K., Carter, T., Abtosway, M., Bushozi, P. M., et al. (2020a). Fingerprinting of quartzitic outcrops at Oldupai Gorge, Tanzania. J. Archaeol. Sci. Rep. 29:102010. doi: 10.1016/J.JASREP.2019.102010
Soto, M., Favreau, J., Campeau, K., Carter, T., Durkin, P. R., Hubbard, S. M., et al. (2020b). Systematic sampling of quartzites in sourcing analysis: intra-outcrop variability at Naibor Soit, Tanzania (part I). Archaeol. Anthropol. Sci. 12, 1–14. doi: 10.1007/s12520-020-01054-w
Stanistreet, I. G., Boyle, J. F., Stollhofen, H., Deocampo, D. M., Deino, A., McHenry, L. J., et al. (2020). Palaeosalinity and palaeoclimatic geochemical proxies (elements Ti, Mg, Al) vary with Milankovitch cyclicality (1.3 to 2.0 Ma), OGCP cores, Palaeolake Olduvai, Tanzania. Palaeogeogr. Palaeoclimatol. Palaeoecol. 546:109656. doi: 10.1016/j.palaeo.2020.109656
Stanistreet, I. G., Stollhofen, H., Njau, J. K., Farrugia, P., Pante, M. C., Masao, F. T., et al. (2018). Lahar inundated, modified, and preserved 1.88 Ma early hominin (OH24 and OH56) Olduvai DK site. J. Hum. Evol. 116, 27–42. doi: 10.1016/j.jhevol.2017.11.011
Stollhofen, H., Stanistreet, I. G., Toth, N., Schick, K. D., Rodríguez-Cintas, A., Albert, R. M., et al. (2021). Olduvai’s oldest Oldowan. J. Hum. Evol. 150:102910. doi: 10.1016/j.jhevol.2020.102910
Stout, D., Hecht, E., Khreisheh, N., Bradley, B., and Chaminade, T. (2015). Cognitive demands of Lower Paleolithic toolmaking. PLoS One 10:e0121804. doi: 10.1371/journal.pone.0121804
Stout, D., Quade, J., Semaw, S., Rogers, M. J., and Levin, N. E. (2005). Raw material selectivity of the earliest stone toolmakers at Gona, Afar, Ethiopia. J. Hum. Evol. 48, 365–380. doi: 10.1016/j.jhevol.2004.10.006
Stout, D., Semaw, S., Rogers, M. J., and Cauche, D. (2010). Technological variation in the earliest Oldowan from Gona, Afar, Ethiopia. J. Hum. Evol. 58, 474–491. doi: 10.1016/j.jhevol.2010.02.005
Tennie, C., Premo, L. S., Braun, D. R., and McPherron, S. P. (2017). Early stone tools and cultural transmission: resetting the null hypothesis. Curr. Anthropol. 58, 652–672. doi: 10.1086/693846
Toth, N. (1985). The Oldowan reassessed A close look at early stone artifacts. J. Archaeol. Sci. 12, 101–120. doi: 10.1016/0305-4403(85)90056-1
Wynn, T. (1989). The Evolution of Spatial Competence, Vol. 14. Urbana: University of Illinois Press.
Keywords: Oldowan, Oldupai Gorge, Early Pleistocene, stone tool technology, environmental shifts
Citation: Cueva-Temprana A, Lombao D, Soto M, Itambu M, Bushozi P, Boivin N, Petraglia M and Mercader J (2022) Oldowan Technology Amid Shifting Environments ∼2.03–1.83 Million Years Ago. Front. Ecol. Evol. 10:788101. doi: 10.3389/fevo.2022.788101
Received: 01 October 2021; Accepted: 31 January 2022;
Published: 03 March 2022.
Edited by:
Pasquale Raia, University of Naples Federico II, ItalyReviewed by:
Antoine Souron, UMR 5199 De la Préhistoire à l’Actuel: Culture, Environnement et Anthropologie (PACEA), FranceCopyright © 2022 Cueva-Temprana, Lombao, Soto, Itambu, Bushozi, Boivin, Petraglia and Mercader. This is an open-access article distributed under the terms of the Creative Commons Attribution License (CC BY). The use, distribution or reproduction in other forums is permitted, provided the original author(s) and the copyright owner(s) are credited and that the original publication in this journal is cited, in accordance with accepted academic practice. No use, distribution or reproduction is permitted which does not comply with these terms.
*Correspondence: Arturo Cueva-Temprana, cuevatemprana@shh.mpg.de; Michael Petraglia, petraglia@shh.mpg.de; Julio Mercader, mercader@ucalgary.ca
Disclaimer: All claims expressed in this article are solely those of the authors and do not necessarily represent those of their affiliated organizations, or those of the publisher, the editors and the reviewers. Any product that may be evaluated in this article or claim that may be made by its manufacturer is not guaranteed or endorsed by the publisher.
Research integrity at Frontiers
Learn more about the work of our research integrity team to safeguard the quality of each article we publish.