- 1Faculty of Science and Engineering, Southern Cross University, Lismore, NSW, Australia
- 2National Marine Science Centre, Southern Cross University, Coffs Harbour, NSW, Australia
- 3Southern Cross Plant Science, Southern Cross University, Lismore, NSW, Australia
First Nations people of Australia sustained complex grassland grain production systems prior to colonisation. The revival of these foodways could aid in mitigating the interlinked issues of land degradation, reduced landscape resilience and declining food security. For the Gamilaraay people, original custodians of the grasslands of north-west New South Wales and south-west Queensland, efforts are underway to bring their ancient food system into a modern context with authenticity and integrity. The aim of this transdisciplinary study was to investigate the nutritional quality of Australian native grains to identify functional properties that may help promote this nascent industry; complimented by using autoethnography to understand how the original custodians, like the Gamilaraay people, might equitably benefit. Ethnographic findings highlight that Gamilaraay people aspire to improve their health and wellbeing through economic development and consumption of native grains, particularly Elders who disproportionately suffer from non-communicable disease. However, many First Nations people have lived experiences of being systemically exploited and excluded, particularly in the food and agriculture space. To prioritise the interests of the Traditional Custodians, the species used in the biochemical assays were de-identified. Wholegrains from seven culturally significant species, with domesticated brown rice as control, were threshed, milled, and analysed in triplicate for proximate, elemental, non-starch fatty acids, and total free phenolic content. Compared to brown rice, protein was significantly higher in all native species (9.4–32.6 g/100 g); whilst carbohydrates were significantly lower (36.5–53.7 g/100 g). One of the native species had exceptionally high total phenolics (569 mg GAE/100 g) compared to brown rice (60 mg GAE/100 g). All native species had generally higher elemental content, with significantly higher levels of Ca, Fe, Zn, Cu, Mg, P, and K in two native species. All samples were dominated by unsaturated fats with significantly higher polyunsaturated fats in two native species. The generally low carbohydrates, high protein, good fats, high mineral content and high phenolic content suggests that native grains may be beneficial to human health by improving nutrient intake and protecting against non-communicable disease, thus marketable as functional foods. To ensure a flourishing industry where all of Australia benefits from these healthful grains, future research and industry development must be First Nations led.
1. Introduction
The global population is expected to approach 10 billion people by the year 2050 (United Nations, 2019), but ensuring equitable access to nutritious food remains an ongoing concern (Godfray et al., 2010; FAO, 2011; FAO et al., 2020). Slowing economies, rising costs of living, and limitations in accessing nutritious food is driving the combined prevalence of malnutrition and obesity in both developed and developing nations (FAO et al., 2019). Furthermore, after three decades of progress, world hunger is once again rising, driven by climate instability in the form of more frequent and intense drought and flood events (FAO et al., 2018). Resilience to climatic extremes in agricultural lands has been substantially reduced due to land clearing, land degradation, and declining diversity, in terms of both agricultural diversity and natural biodiversity (FAO, 2019); which has coincided with the globalisation of food and the displacement and dispossession of peasant farmers around the globe. This has resulted in the permanent loss of many unique cereal cultivars (Shiva, 2016). Combined with the need for more food, the loss of arable land, and the intrinsically linked acceleration of climate change, humanity is now approaching a precarious point in global food security.
Cereal crops have long been important in feeding the global population; with wheat, rice, and maize traditionally providing approximately 60% of the carbohydrate and protein in the average human diet (FAO, 1993). However, many domesticated cereals have been bred for higher yields, at the cost of reduced bioactive compounds and essential micronutrients (FAO et al., 2019). Unlike domesticated cereals, which have seen an estimated 75% decline in genetic diversity since 1900, wild cereals retain their genetic diversity and healthful nutritional qualities (FAO, 1993, 2019). The development of underutilised, ancient, and wild cereal crops could play a significant role in mitigating the interlinked issues of land degradation, reduced landscape resilience, and declining food security (Paustian et al., 2016; Hirsch et al., 2017; Jungers et al., 2019). Perennial grain crops, as an alternate to annuals which form the majority of cultivated cereal crops, have the potential to increase landscape resilience and improve essential ecosystem services, such as: supporting biodiversity, cycling nutrients, reducing erosion risk, increasing the water holding capacity of soils, and reducing risk of salinity (Glover et al., 2010; Six, 2013; Paustian et al., 2016). Furthermore, they require no pesticides, and no irrigation; they increase soil biodiversity, and biogeochemical cycling; require less labour input once established; can provide emergency stock feed during drought; reduce the need to purchase seed; reduce expenditure on fuel; and in Australia, current perennial crops have been grown without fertiliser (Cox, 2014; Personal Communication, 2021). While there is great promise with perennial crops, there is still much more research to be done to optimise these low input systems for food production.
Opportunity presents in native Australian grasses (Family: Poaceae), an underutilised resource that could be developed for cereal cultivation on semi-arid, marginal and degraded lands and could be important for future food security (Shapter et al., 2008, 2010; Cox et al., 2018; Henry, 2019). Many native Australian grasses were once widely utilised by the First Nations people of Australia (Allen, 1974; Tindale, 1974). Grindstones and seed fragments found in layer upon layer of stratum suggest native cereals have sustained First Nations people for at least 60,000 years, even in some of the most marginal and arid regions of Australia, and through times of great climatic change (Balme et al., 2001; Clarkson et al., 2017; Dilkes-Hall et al., 2019). For the Gamilaraay, Yuwaalaraay and Yuwaaliyaay people of north-west New South Wales and south-west Queensland, the scale and importance of these food systems is evidenced in the writings of early European explorers and settlers. The European explorer, Thomas Mitchell, remarked at the heaped piles of guli (Panicum spp.), that extended in all directions for miles, that had been expressly pulled for seed gathering along the grassed edges of Dharriwaa (the Narran Lakes, North-West NSW – an important gathering place for the Yuwaaliyaay-Yuwaalaraay-Gamilaraay people) and the Narran River that extends north (Mitchell, 1848). Slightly north, again on Yuwaaliyaay-Yuwaalaraay-Gamilaraay Country, the celebration of the ganalay (Astrebla spp.) harvest was reminiscent of traditional harvest celebrations seen around the world (Parker, 1905). Despite the long and significant importance of native grains in First Nations people’s diets and culture, and emerging contemporary interest in developing native crops (Cole and Johnston, 2006; Chivers et al., 2015; Drake et al., 2021), a native grains industry that empowers the Traditional Custodians is yet to materialise.
Whilst there will be requirements to conduct agronomic research to develop the native grain industry for human consumption, focusing purely on agronomy may be exclusionary of First Nations values and knowledge systems. However, success may be found in approaching research and development from a culturally appropriate framework. Furthermore, native grains may attract investment and support if they contain functional nutritional properties which make them more beneficial to health (Tan et al., 2010). Evidence supporting functional food properties of native grains may help improve quality of life by applying the knowledge generated to develop healthy food guidelines, and by promoting them as part of a healthy diet (Brand-Miller et al., 1993). Native grains, which grow in remote and marginal lands, may provide greater food security, and improve diets for First Nations people in Australia who experience disproportionate levels of diet related non-communicable disease and, in remote and regional communities, experience food insecurity due to lack of access and affordability. The lack of formalised industry gives First Nations people a window of opportunity to establish leadership and set a precedent in this industry. However, First Nations communities may face difficulty due to financial limitations (Metcalfe and Bui, 2016). Furthermore, while First Nations people have legal interests in approximately 57% of the Australian landmass, the majority of this land is amongst the most marginal and remote in Australia or cannot be used for economic activity due to the type of land tenure and government regulations (Jacobsen et al., 2020).
Information about the nutritional quality of native Australian grains is limited, focusing primarily on the proximate composition of species from arid and desert regions, and finding values that are comparable to domesticated cereals (Elphinstone, 1971; Cane, 1987; Brand-Miller et al., 1993; Smith and Smith, 2003; Wurm et al., 2012). However, there is a vast diversity of grains that have been traditionally used, from a broad range of bioregions, and some of these overlooked grains may have significantly better nutritional profiles compared to commonly cultivated domesticated cereals, like rice for example (Thorburn et al., 1987; Konczak et al., 2009). This study aims to provide a more comprehensive nutritional profile of seven species of native Australian grains, while reflecting on the challenges and aspirations of First Nations people looking to engage in this industry. Specifically, this project will quantify the total moisture, carbohydrates, protein, elemental composition, fatty acid composition, and total free phenolic content; and will compliment this quantitative data with qualitative autoethnography to reflect on the learning and engagement journey from a First Nations-researcher perspective. Significantly, to our knowledge, this project will provide the most comprehensive investigation into Australian native grain nutrition to date, whilst also optimistically attempting to showcase a higher standard of integrity, based on First Nations values, to help guide future research in this space.
2. Materials and methods
2.1. Respectful engagement
When commencing this research, it was understood that working with a food crop that has intangible cultural significance to Australia’s First Nations people could not be conducted purely in the lab and in the field, it also requires working with the relevant First Nations communities to ensure that cultural protocols associated with the use and research of native grains is understood and worked within. Therefore, using yarning as an established methodology (Barlo et al., 2021), the lead author, a Gamilaraay man, sought to understand from a Gamilaraay lens how this nascent industry might benefit the First Nations community by sitting and listening to First Nations Elders who were interested in speaking about native grains (HREC approval number: 2020/104). However, while the process of preparing for the yarning research provided an excellent foundation for understanding the ethical considerations and implications of conducting research for and about First Nations people, and provided excellent opportunity to build relationships with participants, the lead author chose to suspend the formal process of sociological data collection, to allow a more natural process to emerge.
Prior to colonisation, the Gamilaraay people relied significantly on native grains. The Gamilaraay ancestral homelands are the meandering rivers and grassy plains of north-west New South Wales and south-west Queensland. During this study, the lead author took field notes; engaged in cultural practice, including artefact creation; exchanged correspondence with thought leaders and industry experts; engaged in rich yarning and sharing with many First Nations and non-First Nations stakeholders; and spent time with Elders following the yarning process. Extracurricular learning was also undertaken, such as taking language classes and participating in international conferences. Furthermore, the author was immersed in the culture of his ancestors, spending time on Country (Gamilaraay homelands) identifying native grains, harvesting them, processing them, eating them, and sharing them with community. Throughout this process, the lead author became an active participant through the research, and his own personal journey of cultural immersion and transformation provided many opportunities for reflection and analysis (Frazer and Yunkaporta, 2019). With this in mind, an autoethnographic approach augmented the research journey (Houston, 2007), recognising the transformational capacity of research for empowering previously silenced voices (Wright, 2011).
While only the results of this process that are relevant to achieving nutritional outcomes for First Nations people will be discussed in this paper, the authors wish to highlight the importance of working to ensure that native grain research will benefit those who have been caring for these grains for millennia. It is intended that the full process of cultural engagement and results, including reasons for suspending the yarning research, will be reported separately as a standalone, yet complimentary, article.
2.2. Sample collection and preparation
Seven species of native Australian grasses were collected (Figure 1) with priority to sample from the field where possible, and within the limits of sampling permits (D2019/1181383; DM # 8979758), COVID-19 travel restrictions, and drought conditions at the time of sampling (December 2019 – March 2020). Kangaroo grass (Themeda triandra) was hand collected from locations within the Sunshine Coast and Toowoomba regions, Queensland, and the Narrabri region, New South Wales (n = 5), along with one population of native millet (Panicum decompositum) being sampled by hand, also from the Toowoomba region. Native rice (Oryza meridionalis) originated from the Cook region of Far North Queensland and was acquired through a material transfer agreement with the Queensland Alliance for Agriculture and Food Innovation. One population of weeping grass (Microlaena stipoides) came from the Gippsland region, Victoria, and was donated in good faith by a farm manager. Additional samples of T. triandra, native sorghum (Sorghum leiocladum) and M. stipoides were donated by Diversity Native Seeds, Coonabarabran, New South Wales. Further samples of barley Mitchell grass (Astrebla pectinata), curly Mitchell grass (A. lappacea), and M. stipoides were purchased from commercial seed supplier, Austrahort; and A. pectinata and M. stipoides from commercial supplier, Nindethana. Commercially cultivated domesticated wholegrain rice (O. sativa) was purchased at a major food retailer and used as the control.
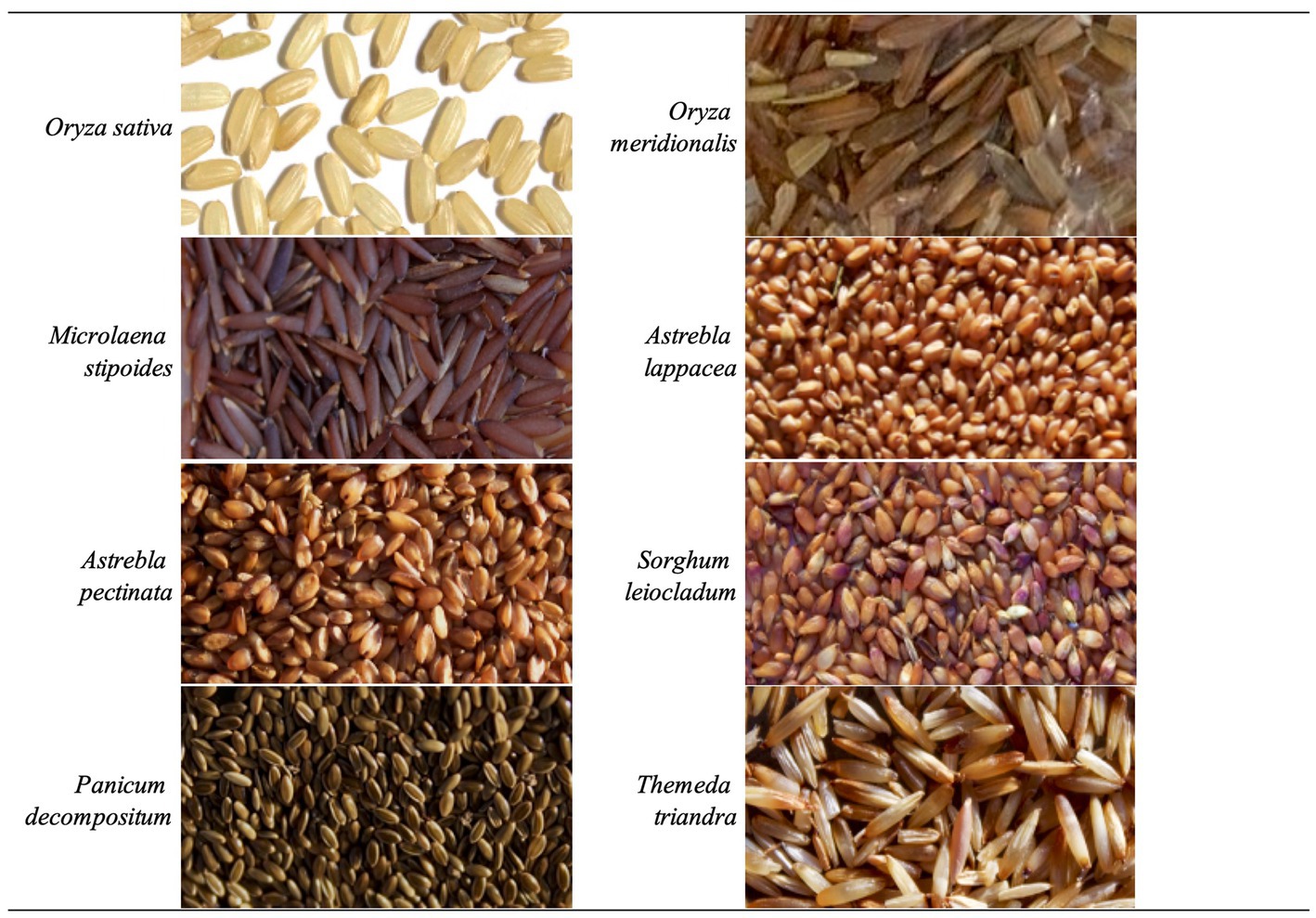
Figure 1. Images of grain samples from seven native Australian grass species, and Oryza sativa, ready for milling and analysis. Images have been captured using different lenses and magnification. Therefore, sizes are not relative or to scale.
Seed was stored at 4°C before being threshed, manually by hand, to separate the grain from the chaff. Panicum decompositum seed was left with the hull intact as per traditional methods of preparation (Latz, 1995). Each population was then milled and analysed in triplicate, except T. triandra which was compiled into a composite sample due to low seed quantity. To ensure standardisation for statistical analyses, replicates were randomly selected from across populations to represent each species (n = 3). The wholegrain samples were ground into flour using a laboratory ball mill (Retsch MM301) set at 30 r/s for 30 s. M. stipoides samples were milled for an extra 15 s due to their coarseness after 30 s. Aliquots of milled sample were sealed in airtight tubes and stored at 4°C for a maximum of 3 weeks before being used in the following assays. Samples were analysed fresh, still containing their inherent moisture content.
2.3. Elemental analysis and moisture
Macro- and micronutrient analysis, and moisture content, was completed by the Environmental Analysis Laboratory (EAL, Southern Cross University, Lismore, NSW, Australia, NATA accreditation no. 14960). Each sample of milled wholegrain was weighed (0.5 ± 0.05 g) into aliquots and provided as triplicate samples. Moisture was determined by change in weight after being oven dried at 105°C for a minimum of 12 h until no further weight loss. Total nitrogen, total carbon and total sulphur were analysed via the Dumas method using a LECO Trumac CNS2000 Analyser, following an EAL in-house method. All other elemental nutrients were determined by microwave digestion with nitric acid and analysed using an inductively coupled plasma mass spectrometer (ICP-MS) following an in-house method. Crude protein was determined by multiplying the total nitrogen (TN %) by the conservative nitrogen to protein conversion factor for cereals of 5.4 [Crude Protein (%) = TN (%) x 5.4] according to Mariotti et al. (2008).
2.4. Available carbohydrates
For available carbohydrate analysis, each sample of milled flour was accurately weighed out (0.1 ± 0.002 g) into flat-bottom glass vials in triplicate. An ‘Available Carbohydrates and Dietary Fiber’ (K-ACHDF) assay kit (Megazyme Ltd) was used to analyse total available carbohydrates. The assay protocol, which is based on AOAC methods developed by Lee et al. (1992) and Prosky et al. (1988), was followed with some minor modifications. Briefly, the enzymatic digestion assay was scaled down to 1:40 to adjust for the small sample size and the available carbohydrates were enzymatically hydrolysed into sugar. Once the carbohydrates had been hydrolysed, the solution was removed and diluted at a 1:200 ratio to allow for the absorbance to be measured at 340 nm using a microplate reader, where the reading was then converted to carbohydrate concentration by comparison to a standard curve of D-glucose and D-fructose.
2.5. Total free phenolics
For total free phenolics, each sample of milled flour was accurately weighed (0.05 ± 0.001 g) out into 5 mL Eppendorff tubes in triplicate prior to analysis of free phenolics following the modified methods of Ti et al. (2014) using the Folin-Ciolteau phenol reagent (Merck, Darmstadt, Germany). The endpoint absorbance was then measured at 760 nm in a Clariostar microplate reader. A linear curve was created from nine standard dilutions of gallic acid (Sigma-Aldrich, St. Louis, MO, United States) ranging from 2 mg/mL – 0.0078125 mg/mL.
2.6. Non-starch fatty acids by FAMEs
For the determination of non-starch fatty acids, each sample of milled flour was weighed (0.1 ± 0.002 g) into flat-bottom glass vials in triplicate. The fatty acids were determined following the modified methods of Liu et al. (2014) and Ahmad et al. (2019). Briefly, fats were first extracted using chloroform/methanol (2:1, v/v; 1.5 mL) for 30 min. After 30 min the vials were centrifuged at 3000 rpm (~1300 RCF) for 5 min, then the supernatant (~1 mL) was transferred into a clean flat-bottom HPLC vial and dried under nitrogen gas. This process of extraction (using 1 mL of chloroform/methanol) and drying was repeated two more times (a total of three extracts). Samples were then stored at −20°C overnight before analysis.
An internal standard of 31.3 μL of 7.3 mg/mL C17:0 (heptadecanoic acid) in hexane was added to each sample and dried under nitrogen prior to esterification to convert the complex fats into volatile methyl esters using 14% boron trifluoride in methanol (200 μL). Fatty acid methyl esters (FAMEs) were then detected by gas chromatography using a Hewlett-Packard 7683 Series Injector mounted onto a Hewlett-Packard HP 6890 Series GC System with Flame Ionisation Detection (GC-FID). FAMEs in the analysed samples were identified by benchmarking with the internal standard and comparing order and peak retention time to a FAMEs reference standard mix (Supelco 37-Component FAME Mix CRM47885). A linear curve was created from known concentrations of heptadecanoic acid.
2.7. Statistical analysis
The data generated in the laboratory analyses were tested for statistical differences using PRIMERe v7+ software. A one-factor design was used to test significant differences between species. Any significant differences detected were further tested using a pair-wise post-hoc test to determine significant differences between pairs of species. When <100 unique permutations were possible, the Monte-Carlo test was used. Euclidian distance similarity matrices were generated for univariate data sets (protein, carbohydrates, phenolics and moisture), and for multivariate data sets (morphometrics, fatty acid composition and macro−/micronutrient composition) after the data was transformed using a fourth-root in order to increase the weighting of minor constituents. Each variable in the multivariate data sets was also tested individually as a univariate data set. Principle coordinate ordination (PCO) plots, with vector overlays, were used to visually represent the trends in the fatty acid composition and macro−/micronutrient composition data.
3. Results
3.1. Yarning and autoethnography
The yarning with Elders and ethnographic research made it obvious that health and holistic outcomes are at the front of mind for the Gamilaraay community. However, it became apparent during autoethnographic reflection that the Gamilaraay people lack the ability to participate within this emerging industry and to effectively capitalise and benefit from the sharing of native grain knowledge that seeks to accelerate the growth of the industry. A direct question was asked of the lead author about how the knowledge of nutrition would benefit the Gamilaraay people and who would really benefit from making the results of this study available (Gamilaraay Elder, February 1, 2023). Therefore, considering the implications of sharing knowledge that can be directly linked to a specific species of grass, particularly those that could be considered functional foods, the lead author chose to deidentify the native species. For the subsequent reporting of results, all native grain species have been deidentified. Native species will be referred to as ‘Native_1’, ‘Native_2’, … ‘Native_7’. The reasoning behind this decision will be elucidated further in the discussion.
3.2. Proximate composition and phenolics
Moisture content varied significantly between the species (Pseudo-F = 44.965; p = <0.001); with O. sativa recording the highest moisture content (17.2 ± 0.1%), whilst all native species recorded significantly lower moisture content, ranging from 6.9 ± 0.0–12.3 ± 0.2 (Table 1).
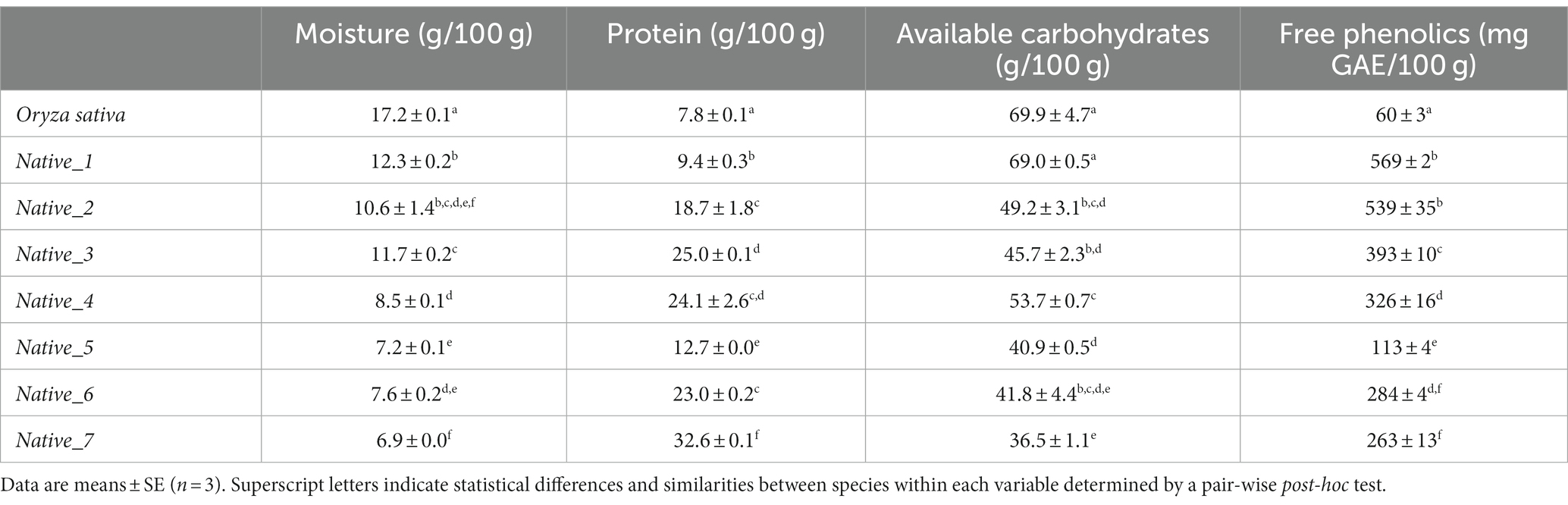
Table 1. Results for univariate analyses (moisture, protein, carbohydrates, and phenolics) for seven species of native Australian grains, including Oryza sativa as control.
Crude protein, as an expression of total nitrogen (TN x 5.4) was significantly different between species (Pseudo-F = 59.033; p = <0.001); and ranged broadly between the native species, from 9.4 ± 0.3 g/100 g (Native_1) to 32.6 ± 0.1 g/100 g (Native_7). Native_2 and Native_4 showed a greater amount of intraspecific variability compared to other species. Post-hoc pair-wise comparisons revealed all native species to have significantly higher protein than O. sativa (Table 1).
Analysis of available carbohydrates (ACH) revealed a significant dissimilarity between species (Pseudo-F = 21.564; p = <0.001), and a large amount of intraspecific variability for the majority of species (Table 1). O. sativa has the highest ACH content at 69.9 ± 4.7% and shows statistical similarity with Native_1. All other native species have significantly lower carbohydrates compared to O. sativa, with Native_7 recording the lowest ACH at 36.5 ± 1.1% (Table 1).
The total free phenolic content varied significantly between species (Pseudo-F = 149.52; p = <0.001), and ranged very broadly, from 60 ± 3 mg GAE/100 g (O. sativa) to 569 ± 2 mg GAE/100 g (Native_1) (Table 1). The post-hoc pair-wise comparisons revealed Native_1 and Native_2 had significantly higher phenolic content compared to all other species. All native species had significantly higher phenolics than O. sativa (Table 1).
3.3. Macro- and micronutrients
Analysis of the elemental composition revealed a significant amount of variability between species for macronutrient composition (Pseudo-F = 70.402; p = <0.001) and micronutrient composition (Pseudo-F = 89.593; p = <0.001), including higher intraspecific variation in Native_2 and Native_4 compared to other species (Table 2). This is illustrated in the PCO plots which show a generally clear clustering of samples within species with very little overlap between species, whereas samples from Native_2 and Native_4 are more widely dispersed (Figures 2A,B).
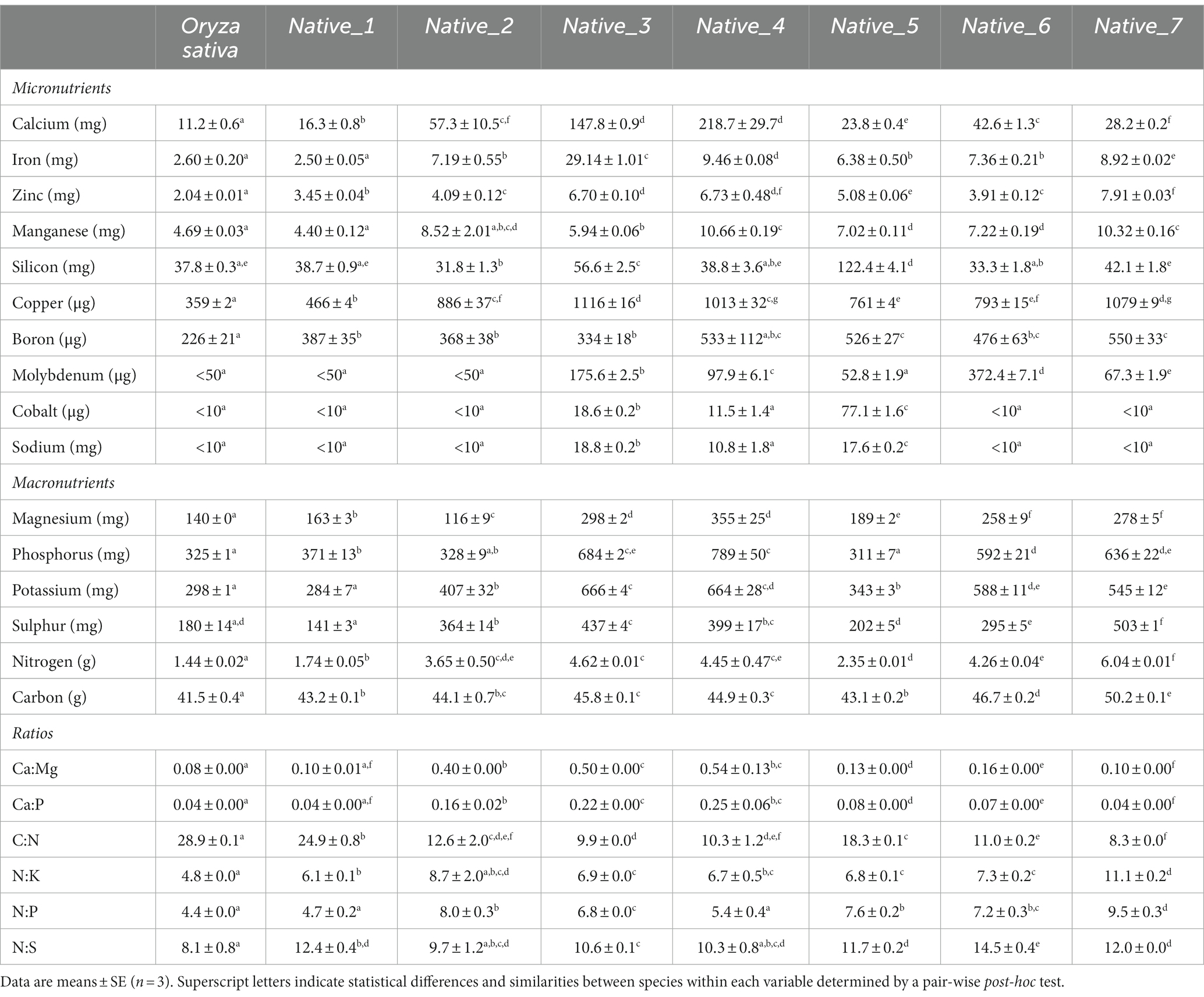
Table 2. Elemental composition per 100 g of milled wholegrain for seven species of native Australian grains, including Oryza sativa as control.
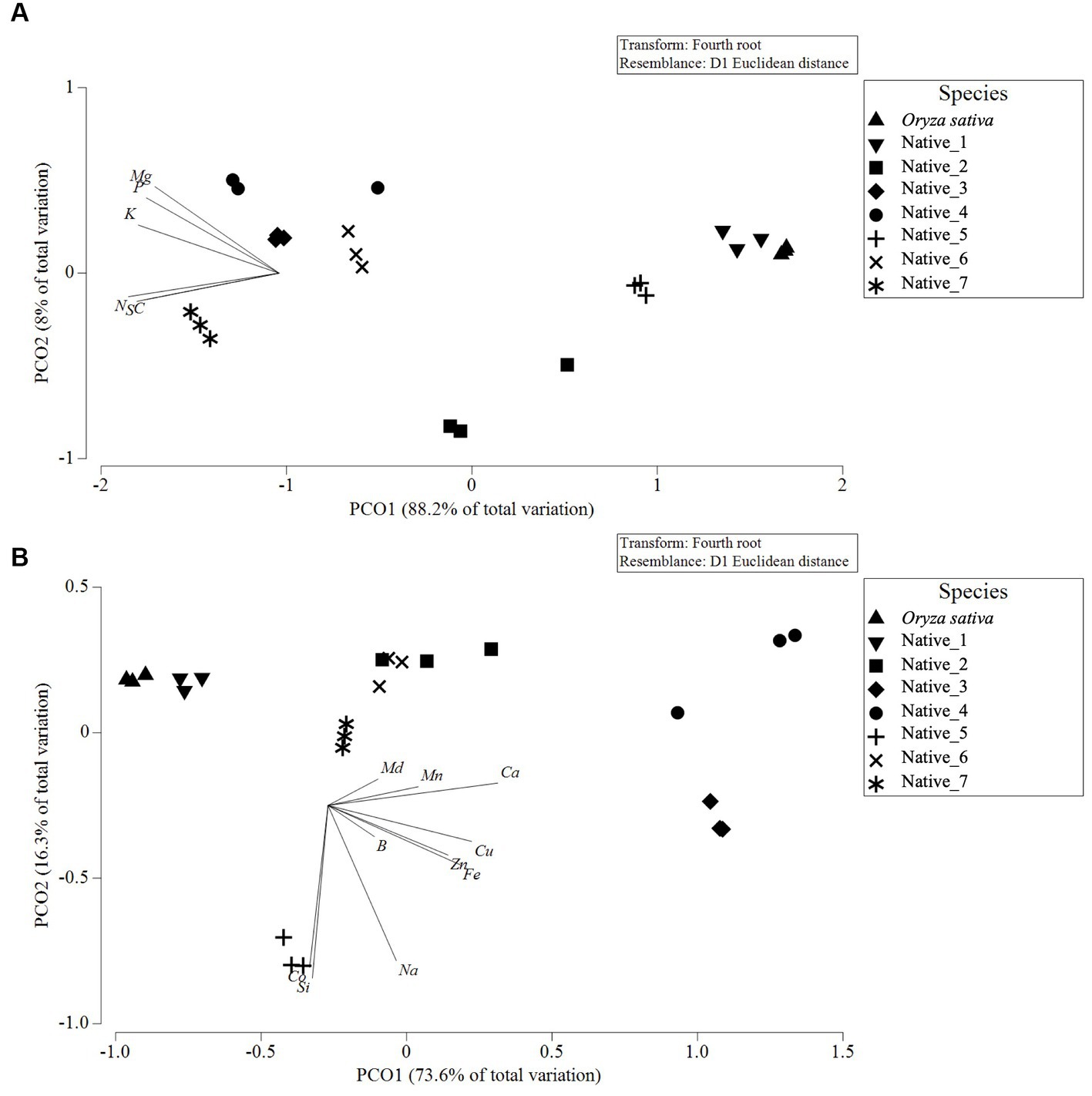
Figure 2. Principle coordinate ordination (PCO) plot for macronutrient composition (A) and micronutrient composition (B) of milled wholegrains from seven species of Australian native grains, with Oryza sativa as control. Vector overlay, based on Pearson’s correlation (r > 0.8), indicates a greater concentration of macronutrients in the samples to the left of the (A) PCO plot, with greater Mg and P towards the top left vs. greater S, N and C towards the bottom left; and greater concentration of micronutrients in the samples to the right and bottom of the (B) PCO plot, with greater Md, Mn and Ca in the top right vs. greater B, Zn, Fe and Cu in the bottom right vs. greater Co, Si and Na towards the bottom.
All native species had higher values than O. sativa for calcium (Ca), copper (Cu), zinc (Zn), boron (B), nitrogen (N) and carbon (C) (Table 2). For the remaining elements, the majority of native species exceeded values recorded in O. sativa, except Native_1, which showed the greatest similarity to O. sativa and was the only native species to record lower values for iron (Fe), manganese (Mn), potassium (K), and sulphur (S) (Table 2). In the native species, calcium (Ca) ranged from 16.3 ± 0.8 mg/100 g in Native_1 up to 218.7 ± 29.7 mg/100 g in Native_4 (Table 2); iron (Fe) ranged from 2.50 ± 0.05 mg/100 g in Native_1 up to 29.14 ± 1.01 mg/100 g in Native_3 (Table 2); and zinc (Zn) ranged from 3.45 + 0.04 mg/100 g in Native_1 up to 7.91 ± 0.03 mg/100 g in Native_7 (Table 3). Native_3 and Native_4 standout as the most nutrient dense of the species tested, with each variable generally averaging at least two times greater concentration than O. sativa. The differences in Native_3 and Native_4 are driven by generally higher macronutrients Mg, P, and to a lesser extent K (Figure 2A); and higher micronutrients Ca, Cu and to a lesser extent Zn, B, Mn and Fe (Figure 2B). Native_5 also showed unique qualities, driven primarily by high values for silicon (Si) and cobalt (Co) (Figure 2B).
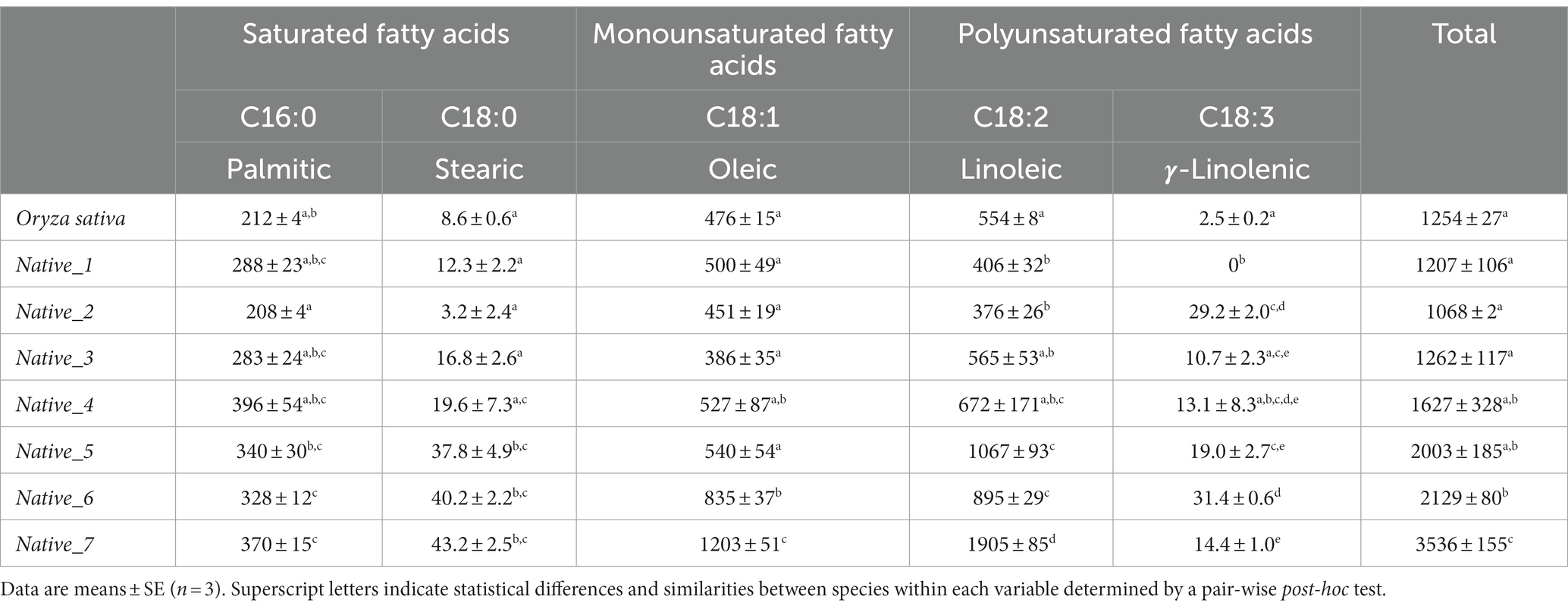
Table 3. Identified fatty acids (mg) per 100 g of milled wholegrain for seven species of native Australian grains, including Oryza sativa as control.
Analysis of the nutrient ratios found a great deal of variability between species, and a great deal of intraspecific variability within Native_2 and Native_4 (Table 2). All native species had higher ratios of Ca:Mg, N:P, N:K, and N:S compared to O. sativa. The Ca:Mg ratio revealed all native species had values closer to 1:1 than O. sativa; with values ranging from 0.10 ± 0.01 (Native_1) to 0.54 ± 0.13 (Native_4) (Table 2). The Ca:P ratios for the seven native cereal species revealed similar trends seen in the Ca:Mg ratio.
3.4. Fatty acid composition
Analysis of FAMEs revealed that all samples eluted peaks at identical retention times which, when correlated with the standard mix, corresponded to the known fatty acids; C16:0, C18:0, C18:1, C18:2, & C18:3; with C17:0 present as the internal control (Supplementary material - Appendix 1). However, peak heights and ratios of fatty acids vary between species. Several minor peaks were present but were not quantifiable. The composition of individual fatty acids varies significantly between samples (Table 3; Pseudo-F = 22.441; p = <0.001). This is illustrated in the PCO plot which shows, despite a large amount of intraspecific variation and overlapping in species, Native_1, Native_2 and Native_7 are dispersed away from the central cluster (Figure 3). Whilst many species were statistically similar (Table 3), Native_7 is significantly different to all species which is driven by higher proportions of C16:0, C18:0, C18:1, and C18:2 (Figure 3).
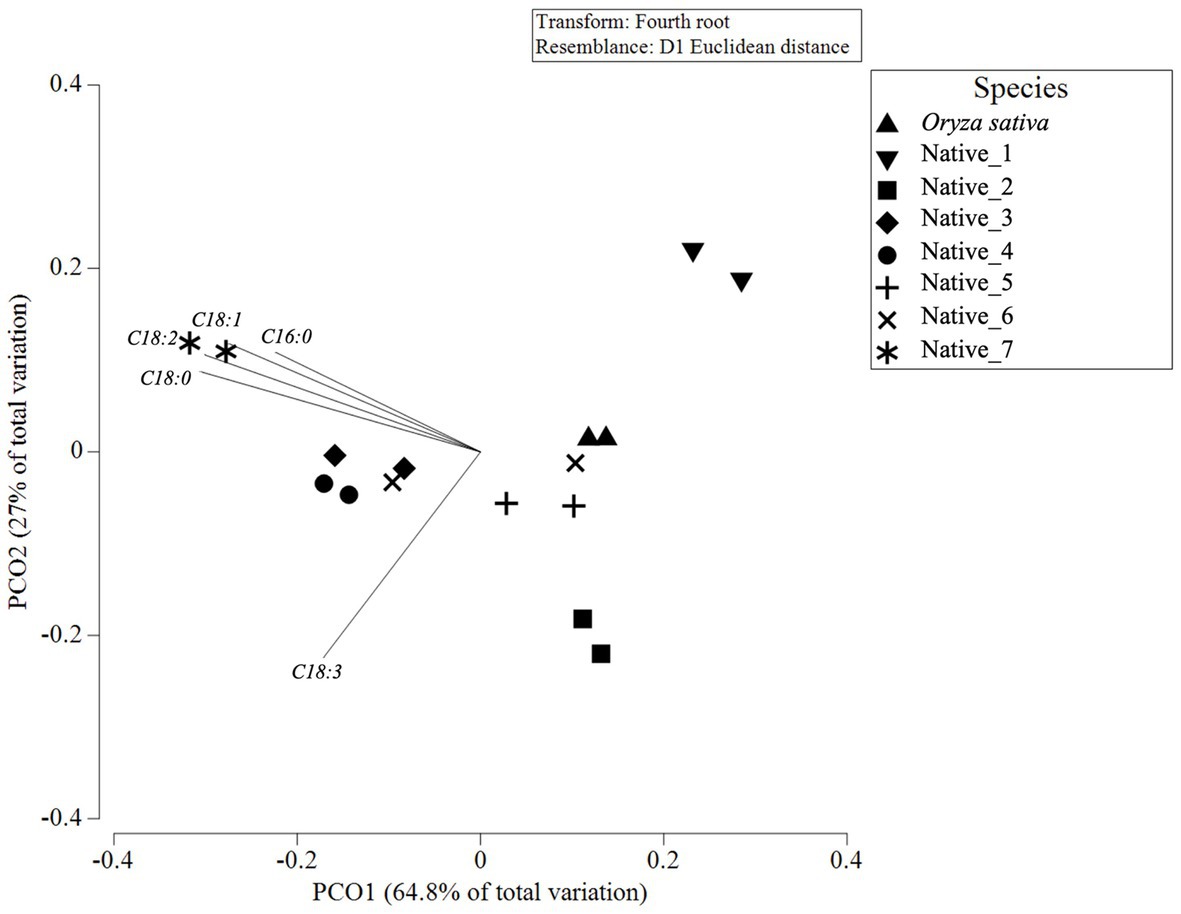
Figure 3. Principle coordinate ordination (PCO) plot of fatty acid composition in milled wholegrain samples for replicates of seven native grains, with Oryza sativa as control. Vector overlay, based on Pearson’s correlation (r > 0.8), indicates a greater concentration of fatty acids in the samples to the left of the PCO plot, and greater C16:0, C18:0, C18:1, and C18:2 towards the top left vs. greater C18:3 towards the bottom left.
Stearic acid (C16:0) and 𝜸-linolenic (C18:3) are only present in relatively low concentrations. Interestingly, all samples, except Native_1, recorded the omega-3 polyunsaturated fat (PUFA) C18:3; with Native_6 recording the highest C18:3 value (31.4 ± 0.6 mg/100 g). Oleic acid (C18:1) ranged from 386 ± 35 mg/100 g (Native_3) up to 1,203 ± 51 mg/100 g (Native_7) (Table 3). Linoleic acid (C18:2) ranged from 376 ± 26 mg/100 g (Native_2) up to 1905 ± 85 mg/100 g (Native_7) (Table 3). The total fatty acids in the native species ranges from 1.07 ± 0.00 g (Native_2) to 3.54 ± 0.16 g (Native_7) (Table 3).
Comparison of percentage composition of saturated fats (SFAs), mono-unsaturated fats (MUFAs), and PUFA’s presents some dissimilarity between species. There were higher proportions of total unsaturated fats compared to saturated fats observed in all species with ratios ranging from 2.85:1 (Native_4) up to 7.55:1 (Native_7). The majority of species, except Native_1 and Native_2, also had higher proportions of PUFAs than MUFAs. Notably, the species with the greatest proportion of PUFAs were Native_7 and Native_5, with PUFAs making up 54.3 and 54.2% of their total fatty acid composition, respectively.
4. Discussion
Native grains have significant potential to contribute to human health and nutrition. The biochemical analyses of the Australian native grains in this study have revealed that, in general, they provide a more nutritionally dense food when compared to Oryza sativa (brown rice). Compared to brown rice, the percentage of protein was up to four-fold higher in native species; the phenolic content was up to nine-fold higher; zinc (Zn) was up to three-fold higher; iron (Fe) was up to 11-fold higher; calcium (Ca) was up to 19-fold higher; moisture was at least 4% lower; and the fatty acid profiles were dominated by healthy unsaturated fats. It is becoming increasingly acknowledged that ancestral foods contain an important suite of essential nutrients and bioactive compounds, such as polyunsaturated fatty acids and phenolics, that act in synergy with our microbiome to protect against non-communicable illness like heart disease, diabetes, and cancer, whilst also contributing to overall health and mental wellbeing (Jew et al., 2009; Ahmed et al., 2022). Furthermore, it is also becoming apparent that the human metabolic profile, genetic coding, and the microbiome have co-evolved to be optimally adapted to ancestral foods (Logan et al., 2015; Parolo et al., 2017). While native grains offer a nutritious and novel food for mainstream markets, they could have profoundly important health implications for Australia’s First Nations people, who have co-evolved within grassland ecosystems, eating native grains.
Whilst this study highlights the potential benefits of native grain consumption, it also highlights a danger. Without research and industry development goals co-designed with First Nations communities, an emerging native grains industry may fail to provide any benefit back to First Nations people. This is because, in general, First Nations people lack the governance literacy and infrastructure; they lack access to land that can be used for economic activities; they find it difficult to access capital and lack intergenerational wealth; and they lack intergenerational business acumen and networks to be able to participate equitably within this industry (Gorman et al., 2020; Jarvis et al., 2022). Furthermore, First Nations aspirations to balance social, cultural, environmental, and economic outcomes is a divergent way of doing business compared to the dominant culture which prevents First Nations people from participating within the food and agriculture industry on their own terms (Gorman et al., 2023). Sharing scientific knowledge publicly about native grains stands to only benefit those who can adeptly operate within the dominant culture system and who have the existing capabilities and capacity to capitalise on the sharing of knowledge. For example, native grains currently grown on Gamilaraay country are not generating any revenue for the Gamilaraay community; instead the grains are transported 1000 kms from where they are grown to be processed into flour and sold for $350/kg, far beyond the access of Gamilaraay Elders (Personal Communication, April 26, 2023). The concern is that promoting native grains as functional foods will generate more mainstream interest and embolden unethical activities within the industry. For example, non-Indigenous entities seeking to capitalise on Kakadu plum (Terminalia ferdinandiana), which has garnered global interest for its functional properties, will persist until they find loopholes and backdoors to access product and claim cultural license (Robinson and Raven, 2017). Withholding species information in this paper gives the Gamilaraay people, and other First Nations people whose grains have been included in this study, time to set up their own infrastructure to self-determine this industry on their homelands.
This research also sought to understand what a native grain industry should look like from a Gamilaraay perspective and how the research outcomes could be clearly related back to First nations participants. One of the priorities of Indigenist research is to maintain relationships with the participants and ensure it is very clear how the research relates to them (Martin, 2003). The author found that the current research priorities for Gamilaraay Elders is understanding how research and development around ancestral foods and medicines might return tangible nutritional and health outcomes for their communities. Some relevant points relating to nutrition came out of the yarning with Gamilaraay Elders. For one Elder, she had seen the health benefits of eating native grains on gut health and aspired to take our ancestral grains back on to country to heal women (Gamilaraay Elder, June 27, 2020). Another Elder said, given the opportunity, he’d rather focus on traditional medicines (Gamilaraay Elder, personal communication, July 8, 2020). One consistent theme that could be generalised for the Gamilaraay people is that ancestral medicines are sought after and regarded for their healing properties. While it is important for First Nations peoples to maintain use of, and connection with, their ancestral medicines, this research has found there is an opportunity and need to promote ancestral foods as medicine to prevent the onset of non-communicable disease, rather than looking to treat the symptoms of illness.
The majority of the native grains tested in this study have remarkably high grain protein content, exceeding 20% protein in the wholegrains, with Native_7 recording over 32% crude protein. Modern cereals, such as wheat and barley, have been domesticated with preference for increasingly higher yields, but higher yields result in a higher ratio of digestible starch in the grain, at the expense of other important nutrients like protein (Hebelstrup, 2017). To our knowledge, protein exceeding 20% has not yet been reported in the literature for any native Australian grass species (Table 4). However, higher protein content is consistently observed in ancient and wild grains when compared to their modern counterparts; for example, average values of >20% protein have been observed amongst populations of wild emmer wheat (Triticum turgidum ssp. dicccoides) (Chatzav et al., 2010). For the majority of the native species, a high protein content correlated with a low level of available carbohydrates. Conversely, Native_1 and Native_5 had relatively low protein values (7.8–13.9%).
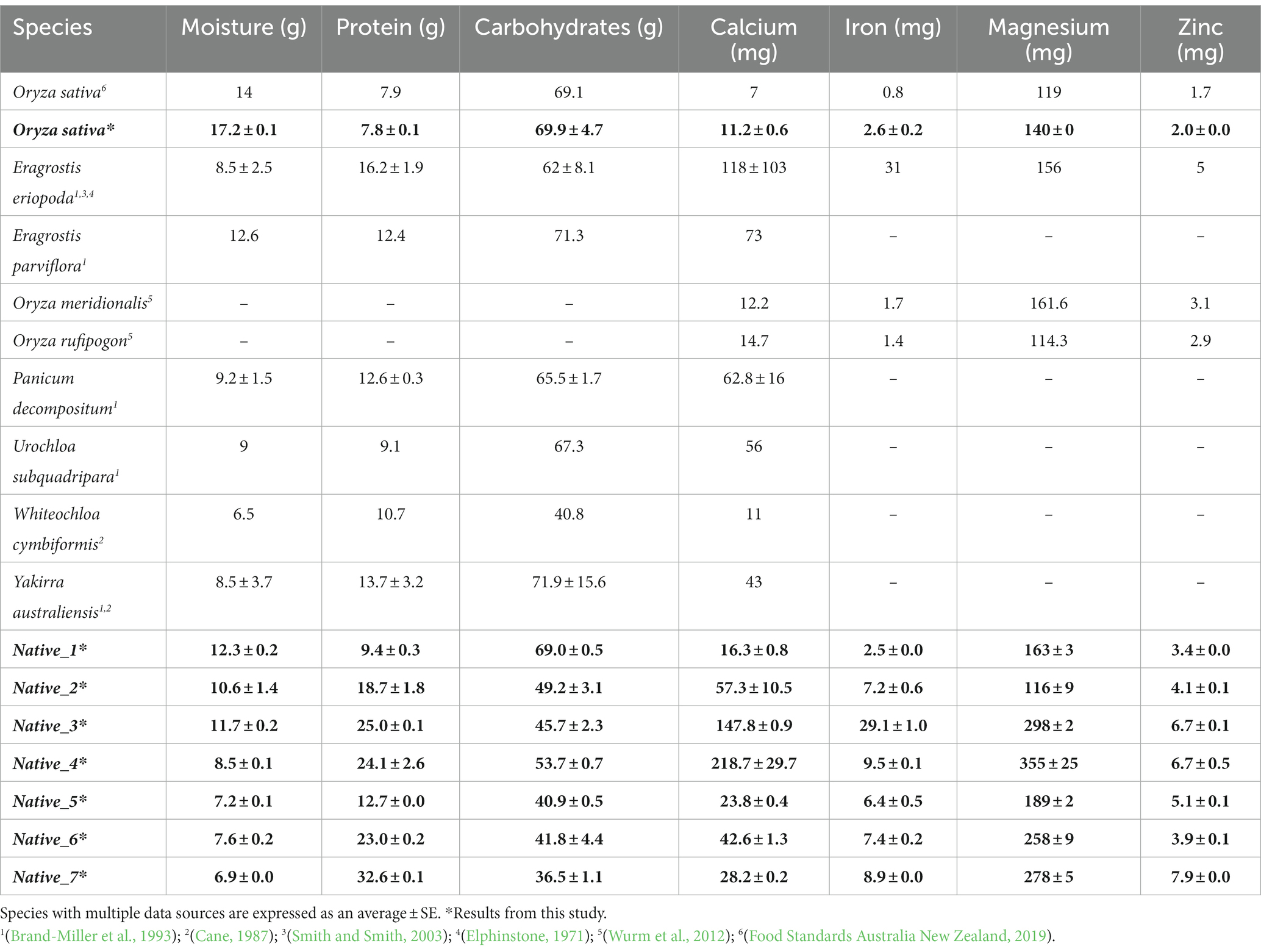
Table 4. Compilation of known and available nutritional data for native Australian grains (per 100 g), with Oryza sativa for comparison.
The highest phenolic content in this study, ranging from 539 to 569 mg GAE/100 g, came from Native_1 and Native_2. These results are comparible with wild rice (Zizania spp.) which have been found to have a high phenolic content, ranging from 407 to 588 mg GAE/100 g (Qiu et al., 2009, 2010). Zizania is an important genus of cereal that has been consumed by the First Peoples of North America for at least 10,000 years (Surendiran et al., 2014), and in China for at least 3,000 years (Yan et al., 2018). The Zizania spp. also have overall good nutritional profiles with protein values ranging from 10 to 18% (Zhai et al., 2001; Surendiran et al., 2014); calcium ranging from 11 to 25 mg/100 g; iron ranging from 1.2–5.1 mg/100 g; and zinc ranging from 1.2–12.0 mg/100 g (Surendiran et al., 2014; Yan et al., 2018). The consumption of Zizania spp. grain has been found to be effective in reducing insulin resistance and lipotoxicity, and in protecting against heart disease. These positive health benefits are thought to be directly related to the high phenolic content acting synergistically with the overall good nutritional profile (Yan et al., 2018; Yu et al., 2020). Subsequently, Zizania spp. grain is now promoted as a functional food with focus on its high phenolic content, and other related bioactive compounds (Surendiran et al., 2014; Yu et al., 2020). The phenolic results, complemented with the good overall nutritional profiles in Native_1 and Native_2, correlate well with existing nutritional data from Zizania spp. Therefore, based on the reported health benefits of Zizania spp., this provides an excellent foundation to popularise Native_1 and Native_2 as functional foods. Further research would be beneficial to explore the antioxidant activity and other bioactive compounds in native Australian grains.
All the native grains analysed in this study revealed relatively low total fatty acid content (<1.5% of the total proximate composition), with the exception of Native_7 which had approximately 3.5% lipids. Native_7 also had the lowest ratio of saturated fats to unsaturated fats. A diet consisting of higher proportions of unsaturated fats to saturated fats reduces the risk of heart disease, with polyunsaturated fatty acids (PUFAs) providing better health outcomes than monounsaturated fatty acids (MUFAs) (Clifton and Keogh, 2017). All native grains investigated in this study were found to have less than 30% saturated fatty acids, and a higher proportion of PUFAs to MUFAs. Whilst PUFAs like omega-6 and omega-3 are critical for good health, modern diets are high in omega-6 and there is some evidence suggesting high ratios (>10:1) of omega-6 to omega-3 can increase the risk of non-communicable disease (Simopoulos, 2016; Jandacek, 2017). Consumption of omega-6 and omega-3 in ratios of <4:1 (omega-6 to omega-3) are considered the most beneficial for reducing risk of non-communicable disease (Simopoulos, 2002, 2010). However, omega-6 is still an essential fatty acid and in a balanced diet can be protective against heart disease, type-2 diabetes and depression (Alabdulkarim et al., 2012). Omega-6, found in high quantities in olive oil, is considered a healthy alternative to saturated fats, particularly when consumed alongside carbohydrates, such as the Mediterranean diet where bread and pasta often goes hand in hand with olive oil (DiNicolantonio and O’Keefe, 2022). Therefore, it appears that the fatty acid content of native Australian grains can be considered as being generally healthy and complimentary components within their overall nutritional composition.
The native grains appear to be good sources of essential minerals and trace elements, with some species showing exceptional values that also exceed results found in previous studies into native grain nutrition (Table 4). For example, Native_3 and Native_4 had exceptional levels of calcium in a much more ideal calcium to magnesium ratio, and good levels of iron and zinc. It has been suggested that having a balanced ratio of calcium to magnesium (1:1), and calcium to phosphorus (1:1), is more beneficial for bone health compared to disproportionate ratios (Sax, 2001; Loughrill et al., 2017). Having access to a cereal that is high in calcium, iron and zinc content could be important for the growing movement of people taking up vegetarian and vegan diets, and those who are actively avoiding dairy products due to rising intolerances. It is also of particular importance for women who disproportionately experience lower levels of calcium, iron, and zinc. During the yarning and autoethnographic research, the author found many Elders from the Gamilaraay community have reported having chronic joint pain, rheumatoid arthritis, and osteoporosis to the point these conditions were debilitating and having major implications on their quality of life. Overall, Native _3 and Native_4 could be promoted as functional foods, particularly for bone and joint health, based on their good mineral ratios and high concentrations of calcium, magnesium, phosphorus, potassium, zinc and boron (Palacios, 2006). Again, this is highly relevant for the Gamilaraay people.
The rise of the paleo diet and gluten intolerances has resulted in cereals being considered unnecessary, and even incompatible, for optimum human health (Zopf et al., 2018); the paleo diet in particular considers grains to have only become an important part of the human diet some 10,000 years ago (Cordain, 1999). However, research out of Mozambique, Africa has shown starch grains from wild sorghum (Sorghum spp.) on stone tools dating back 105,000 years, strongly suggesting cereals have been an important part of modern human diets for a significant amount of time (Mercader, 2009). Furthermore, analysis of dental calculus from ancient human teeth found in East Asia has revealed modern humans were eating grass seed anywhere between 80,000 and 120,000 years ago (Wu et al., 2022). Meanwhile, in Australia, we already established that starch grains have been found on grindstones dating back at least 60,000 years. In fact, carbohydrate rich foods, such as wilds cereals, roots, tubers, culms, and nuts, may have been the driving factor in the evolution of primitive humans to modern humans (Hardy et al., 2015; Fellows Yates et al., 2021). Therefore, better understanding the nutritional profile of ancient grains, like those in this study, may help improve our understanding of optimal human nutrition and highlight the importance of returning to more ancient and wild varieties of cereals.
The results of this study show nutritional profiles can vary significantly within and between species. Intraspecies differences could be driven by biotic and/or abiotic conditions, such as differing soil types, historical uses of the surrounding landscape, nutrient availability, pest pressure, climatic conditions, landscape management techniques, the presence or absence of coinciding plants or plant communities, and moisture availability. Understanding the relative impact of genetic and environmental influences on the nutrient profile could include the use of models to analyse larger sample sizes from populations of known provenance, under varying conditions across the species range. This future research direction diverges from the typical research for commodification outcomes, and instead could be used to prioritises health outcomes for local communities.
This research has been undertaken to support the emerging native grain industry in Australia through promotion of native grains as functional foods. However, it is absolutely essential to acknowledge the interests of First Nations people who have a cultural legacy that stretches back 60,000 years. Promoting Australian native grains as a re-emerging industry to improve health outcomes, particularly for First Nations people, is both the challenge and the opportunity. Given the widening health disparity experienced by First Nations people, there is an opportunity to re-establish ancestral native grain food systems and use it to begin shifting the paradigm for First Nations people, whilst also improving biodiversity, regional economies, social cohesion, and cultural continuance. Several grasses investigated in this study are well adapted to Australia’s arid and variable climate, and if an industry is viable here, it could also be applicable to arid parts of Africa and Asia where many cosmopolitan species, such as T. triandra, are found (Dunning et al., 2017). However, achieving these goals will require the industry to be led by First Nations people, and investment that takes into consideration the necessary timelines to achieve long term and sustained success (Packer et al., 2019). Without consideration and leadership, this re-emerging industry may fail to achieve the highest benefits. Realising these benefits requires scientists, farmers, government, and industry to work alongside our First Nations communities authentically, guided by First Nations leadership and integrity. This research has led to careful consideration of our responsibilities as scientists, including how this research will be shared and used in the future. By deidentifying the results of this study, the author’s hope to bring attention to these important considerations and to inspire greater integrity within this emerging industry. Done the right way, there is every opportunity, and likely a necessity, to make these native grains relevant for the next 60,000 years of human history.
5. Conclusion
While many novel foods find success as functional foods based on one or two excellent nutritional components, this study has shown that Australian native grains provide excellent overall composition of bioactive compounds, trace elements, minerals, healthy fats, protein, and carbohydrates. This is extremely important as the world’s population is becoming increasingly overfed and undernourished; and highly relevant to Australia’s First Nations people who continue to disproportionately suffer from diet related non-communicable disease. The results of this study provide evidence to support an emerging native grain industry based on the nutritional superiority of grain that has been grown without inputs on marginal land. Many of the grains studied in this paper are hardy and may present an alternative food source to crops that are less resilient to reduced rainfall, soil degradation, and increased seasonal variability. Most importantly, those supporting the development of a native grains industry must set aside the structures that have historically excluded First Nations peoples and instead identify how this industry can be authentically led by Traditional Custodians, like the Gamilaraay, and with it, encourage the re-emergence of Australia’s first food systems.
Data availability statement
The original contributions presented in the study are included in the article/Supplementary material, further inquiries can be directed to the corresponding author.
Ethics statement
The studies involving human participants were reviewed and approved by Southern Cross University Human Research Ethics Committee. Written informed consent for participation was not required for this study in accordance with the national legislation and the institutional requirements.
Author contributions
JB, KB, and LL contributed to the conception and design of the biochemical assays. JB and HL contributed to the conception and design of the yarning and autoethnography. LL acquired the Oryza meridionalis sample. JB acquired all other samples and conducted the biochemical assays, statistical analysis, yarning and autoethnography, and wrote the first draft of the manuscript. KB provided critical review. All authors contributed to sections of the manuscript, revision, and approved the submitted version.
Acknowledgments
The authors wish to acknowledge the donation of sample by Diversity Native Seeds and the donation of sample from Queensland Alliance for Agriculture and Food Innovation. The authors also acknowledge with deep gratitude the generosity of the First Nations people involved in this study.
Conflict of interest
The authors declare that the research was conducted in the absence of any commercial or financial relationships that could be construed as a potential conflict of interest.
Publisher’s note
All claims expressed in this article are solely those of the authors and do not necessarily represent those of their affiliated organizations, or those of the publisher, the editors and the reviewers. Any product that may be evaluated in this article, or claim that may be made by its manufacturer, is not guaranteed or endorsed by the publisher.
Supplementary material
The Supplementary material for this article can be found online at: https://www.frontiersin.org/articles/10.3389/fsufs.2023.1237862/full#supplementary-material
References
Ahmad, T. B., Rudd, D., Kotiw, M., Liu, L., and Benkendorff, K. (2019). Correlation between fatty acid profile and anti-inflammatory activity in common Australian seafood by-products. Mar. Drugs 17:155. doi: 10.3390/md17030155
Ahmed, N., Dable-Tupas, G., Rahmatullah, M., Ratta, K., Moses, M., and Ghosh, R. (2022). “Bioactive compounds in diabetes care and prevention” in Role of nutrigenomics in modern-day healthcare and drug discovery. eds. G. Dable-Tupas and C. Egbuna (USA: Elsevier), 387–438.
Alabdulkarim, B., Bakeet, Z. A. N., and Arzoo, S. (2012). Role of some functional lipids in preventing diseases and promoting health. J. King Saud Univ. Sci. 24, 319–329. doi: 10.1016/j.jksus.2012.03.001
Allen, H. (1974). The Bagundji of the darling basin: cereal gatherers in an uncertain environment. World Archaeol. 5, 309–322. doi: 10.1080/00438243.1974.9979576
Balme, J., Garbin, G., and Gould, R. A. (2001). Residue analysis and palaeodiet in arid Australia. Aust. Archaeol. 53, 1–6. doi: 10.1080/03122417.2001.11681714
Barlo, S., Boyd, W. E., Hughes, M., Wilson, S., and Pelizzon, A. (2021). Yarning as protected space: relational accountability in research. AlterNative Int. J. Indigenous Peoples 17, 40–48. doi: 10.1177/1177180120986151
Brand-Miller, J., James, K. W., and Maggiore, P. M. A. (1993). Tables of composition of Australian aboriginal foods. Canberra, ACT: Aboriginal Studies Press.
Cane, S. (1987). Australian aboriginal subsistence in the Western Desert. Hum. Ecol. 15, 391–434. doi: 10.1007/BF00887998
Chatzav, M., Peleg, Z., Ozturk, L., Yazici, A., Fahima, T., Cakmak, I., et al. (2010). Genetic diversity for grain nutrients in wild emmer wheat: potential for wheat improvement. Ann. Bot. 105, 1211–1220. doi: 10.1093/aob/mcq024
Chivers, I., Warrick, R., Bornman, J., and Evans, C. (2015). Native grasses make new products: a review of current and past uses and assessment of potential. (Barton, ACT: Rural Industries Research and Development Corporation).
Clarkson, C., Jacobs, Z., Marwick, B., Fullagar, R., Wallis, L., Smith, M., et al. (2017). Human occupation of northern Australia by 65,000 years ago. Nature 547, 306–310. doi: 10.1038/nature22968
Clifton, P. M., and Keogh, J. B. (2017). A systematic review of the effect of dietary saturated and polyunsaturated fat on heart disease. Nutr. Metab. Cardiovasc. Dis. 27, 1060–1080. doi: 10.1016/j.numecd.2017.10.010
Cole, I. A., and Johnston, W. H. (2006). Seed production of Australian native grass cultivars: an overview of current information and future research needs. Aust. J. Exp. Agric. 46:361. doi: 10.1071/EA04107
Cordain, L. (1999). Cereal grains: humanity’s double-edged sword. World Rev. Nutr. Diet. 84, 19–73. doi: 10.1159/000059677
Cox, S. (2014). “Perennial crops for food security” in Perennial crops for food security: proceedings of the FAO expert workshop. eds. C. Batello, L. Wade, S. Cox, N. Pogna, A. Bozzini, and J. Choptiany (Rome, Italy: FAO).
Cox, S., Nabukalu, P., Paterson, A. H., Kong, W., and Nakasagga, S. (2018). Development of perennial grain sorghum. Sustainability 10:172. doi: 10.3390/su10010172
Dilkes-Hall, I. E., O'Connor, S., and Balme, J. (2019). People-plant interaction and economic botany over 47,000 years of occupation at Carpenter’s Gap 1, south central Kimberley. Aust. Archaeol. 85, 30–47. doi: 10.1080/03122417.2019.1595907
DiNicolantonio, J. J., and O’Keefe, J. H. (2022). Monounsaturated fat vs saturated fat: effect on cardio-metabolic health and obesity. Mo. Med. 119, 69–73.
Drake, A., Keitel, C., and Pattison, A. (2021). The use of Australian native grains as a food: a review of research in a global grains context. Rangeland J. 43, 223–233. doi: 10.1071/RJ21030
Dunning, L. T., Liabot, A.-L., Olofsson, J. K., Smith, E. K., Vorontsova, M. S., Besnard, G., et al. (2017). The recent and rapid spread of Themeda triandra. Botany Lett. 164, 327–337. doi: 10.1080/23818107.2017.1391120
Elphinstone, J. J. (1971). The health of Australian aborigines with no previous associations with Europeans. Med. J. Aust. 2, 293–301. doi: 10.5694/j.1326-5377.1971.tb50563.x
FAO (1993). Harvesting nature’s diversity. Rome, Italy: Food and Agriculture Organization of the United Nations.
FAO (2011). The state of the world’s land and water resources for food and agriculture (SOLAW) – managing systems at risk. Rome & London: Food and Agriculutre Organization of the United Nations & Earthscan.
FAO, IFAD, UNICEF, WFP, and WHO (2018). The state of food security and nutrition in the world 2018: Building climate resilience for food security. Rome: FAO.
FAO, IFAD, UNICEF, WFP, and WHO (2019). The state of food security and nutrition in the world 2019: safeguarding against economic slowdowns and downturns. Rome: FAO.
FAO, IFAD, UNICEF, WFP, and WHO (2020). The state of food security and nutrition in the world 2020. Available at: http://www.fao.org/documents/card/en/c/ca9692en
Fellows Yates, J. A., Velsko, I. M., Aron, F., Posth, C., Hofman, C. A., Austin, R. M., et al. (2021). The evolution and changing ecology of the African hominid oral microbiome. Proc. Natl. Acad. Sci. 118:1. doi: 10.1073/pnas.2021655118
Food Standards Australia New Zealand (2019). Australian food composition database. Available at: https://www.foodstandards.gov.au/science/monitoringnutrients/afcd/Pages/fooddetails.aspx?PFKID=F007648 (Accessed December 06, 2019).
Frazer, B., and Yunkaporta, T. (2019). Wik pedagogies: adapting oral culture processes for print-based learning contexts. Australian J. Indigenous Educ. 50, 88–94. doi: 10.1017/jie.2018.24
Glover, J. D., Reganold, J. P., Bell, L. W., Borevitz, J., Brummer, E. C., Buckler, E. S., et al. (2010). Agriculture. Increased food and ecosystem security via perennial grains. Science 328, 1638–1639. doi: 10.1126/science.1188761
Godfray, H. C. J., Beddington, J. R., Crute, I. R., Haddad, L., Lawrence, D., Muir, J. F., et al. (2010). Food security: the challenge of feeding 9 billion people. Science 327, 812–818. doi: 10.1126/science.1185383
Gorman, J. T., Bentivoglio, M., Brady, C., Wurm, P., Vemuri, S., and Sultanbawa, Y. (2020). Complexities in developing Australian Aboriginal enterprises based on natural resources. The Rangeland J. 42, 113–128. doi: 10.1071/RJ20010
Gorman, J., Ennis, G., Wurm, P., Bentivoglio, M., and Brady, C. (2023). Aboriginal community views about a native plant-based enterprise development in Northern Australia. Land 12:1096. doi: 10.3390/land12051096
Hardy, K., Brand-Miller, J., Brown, K. D., Thomas, M. G., and Copeland, L. (2015). The importance of dietary carbohydrate in human evolution. Q. Rev. Biol. 90, 251–268. doi: 10.1086/682587
Hebelstrup, K. H. (2017). Differences in nutritional quality between wild and domesticated forms of barley and emmer wheat. Plant Sci. 256, 1–4. doi: 10.1016/j.plantsci.2016.12.006
Henry, R. J. (2019). Australian wild rice populations: a key resource for global food security. Front. Plant Sci. 10:1354. doi: 10.3389/fpls.2019.01354
Hirsch, P., Jhurreea, D., Williams, J., Murray, P., Scott, T., Misselbrook, T., et al. (2017). Soil resilience and recovery: rapid community responses to management changes. Plant Soil 412, 283–297. doi: 10.1007/s11104-016-3068-x
Houston, J. (2007). Indigenous autoethnography: formulating our knowledge, our way. Australian J. Indigenous Educ. 36, 45–50. doi: 10.1017/S1326011100004695
Jacobsen, R., Howell, C., and Read, S. (2020). Australia’s indigenous land and forest estate: separate reporting of indigenous ownership, management and other special rights. (ABARES Technical Report: Canberra, ACT).
Jandacek, R. (2017). Linoleic acid: a nutritional quandary. Healthcare 5:25. doi: 10.3390/healthcare5020025
Jarvis, D., Maclean, K., and Woodward, E. (2022). The Australian indigenous-led bush products sector: insights from the literature and recommendations for the future. Ambio 51, 226–240. doi: 10.1007/s13280-021-01542-w
Jew, S., AbuMweis, S. S., and Jones, P. J. H. (2009). Evolution of the human diet: linking our ancestral diet to modern functional foods as a means of chronic disease prevention. J. Med. Food 12, 925–934. doi: 10.1089/jmf.2008.0268
Jungers, J. M., Dehaan, L. H., Mulla, D. J., Sheaffer, C. C., and Wyse, D. L. (2019). Reduced nitrate leaching in a perennial grain crop compared to maize in the Upper Midwest, USA. Agric. Ecosyst. Environ. 272, 63–73. doi: 10.1016/j.agee.2018.11.007
Konczak, I., Zabaras, D., Dunstan, M., Aguas, P., Roulfe, P., and Pavan, A. (2009). Health benefits of Australian native foods: an evaluation of health-enhancing compounds. Australia: Rural Industries Research and Development Corporation.
Latz, P. K. (1995). Bushfires and bushtucker: aboriginal plant use in Central Australia. Alice Springs, NT: IAD Press.
Lee, S. C., Prosky, L., and DeVries, J. W. (1992). Determination of total, soluble, and insoluble dietary fiber in foods - enzymatic-gravimetric method, MES-TRIS buffer: collaborative study. J. Assoc. Offic. Anal. Chem. 75, 395–416. doi: 10.1093/jaoac/75.3.395
Liu, L., Tong, C., Bao, J., Waters, D. L. E., Rose, T. J., and King, G. J. (2014). Determination of starch lysophospholipids in rice using liquid chromatography-mass spectrometry (LC-MS). J. Agric. Food Chem. 62, 6600–6607. doi: 10.1021/jf500585j
Logan, A. C., Katzman, M. A., and Balanzá-Martínez, V. (2015). Natural environments, ancestral diets, and microbial ecology: is there a modern "paleo-deficit disorder"? Part II. J. Physiol. Anthropol. 34:9. doi: 10.1186/s40101-014-0040-4
Loughrill, E., Wray, D., Christides, T., and Zand, N. (2017). Calcium to phosphorus ratio, essential elements and vitamin D content of infant foods in the UK: possible implications for bone health. Matern. Child Nutr. 13:e12368. doi: 10.1111/mcn.12368
Mariotti, F., Tomé, D., and Mirand, P. P. (2008). Converting nitrogen into protein - beyond 6.25 and Jones’ factors. Crit. Rev. Food Sci. Nutr. 48, 177–184. doi: 10.1080/10408390701279749
Martin, K. (2003). Ways of knowing, being and doing: a theoretical framework and methods for indigenous and indigenist re-search. J. Aust. Stud. Voicing Dissent 27, 203–214. doi: 10.1080/14443050309387838
Mercader, J. (2009). Mozambican grass seed consumption during the middle stone age. Science 326, 1680–1683. doi: 10.1126/science.1173966
Metcalfe, D. J., and Bui, E. N. (2016). Australia state of the environment 2016: land, independent report to the Australian Government Minister for the Environment and Energy. Australian Government Department of the Environment and Energy: Canberra, Australia.
Mitchell, T. L. (1848). Journal of an expedition into the interior of tropical Australia: in search of a route from Sydney to the Gulf of Carpentaria. London, UK: Longman, Brown, Green and Longmans.
Packer, J., Turpin, G., Ens, E., Venkataya, B., Mbabaram Community, Yirralka Rangers, et al. (2019). Building partnerships for linking biomedical science with traditional knowledge of customary medicines: a case study with two Australian indigenous communities. J. Ethnobiol. Ethnomed. 15:69. doi: 10.1186/s13002-019-0348-6
Palacios, C. (2006). The role of nutrients in bone health, from A to Z. Crit. Rev. Food Sci. Nutr. 46, 621–628. doi: 10.1080/10408390500466174
Parker, K. L. (1905). The Euahlayi tribe a study of Aboriginal life in Australia. London: Archibald Constable and Company, Ltd.
Parolo, S., Lacroix, S., Kaput, J., and Scott-Boyer, M.-P. (2017). Ancestors’ dietary patterns and environments could drive positive selection in genes involved in micronutrient metabolism-the case of cofactor transporters. Genes Nutr. 12:28. doi: 10.1186/s12263-017-0579-x
Paustian, K., Lehmann, J., Ogle, S., Reay, D., Robertson, G. P., and Smith, P. (2016). Climate-smart soils. Nature 532, 49–57. doi: 10.1038/nature17174
Prosky, L., Asp, N.-G., Schweizer, T. F., DeVries, J. W., and Furda, I. (1988). Determination of insoluble, soluble, and total dietary fiber in foods and food products: interlaboratory study. J. Assoc. Offic. Anal. Chem. 71, 1017–1023. doi: 10.1093/jaoac/71.5.1017
Qiu, Y., Liu, Q., and Beta, T. (2009). Antioxidant activity of commercial wild rice and identification of flavonoid compounds in active fractions. J. Agric. Food Chem. 57, 7543–7551. doi: 10.1021/jf901074b
Qiu, Y., Liu, Q., and Beta, T. (2010). Antioxidant properties of commercial wild rice and analysis of soluble and insoluble phenolic acids. Food Chem. 121, 140–147. doi: 10.1016/j.foodchem.2009.12.021
Robinson, D., and Raven, M. (2017). Identifying and preventing biopiracy in Australia: patent landscapes and legal geographies for plants with indigenous Australian uses. Australian Geogr. 48, 311–331. doi: 10.1080/00049182.2016.1229240
Sax, L. (2001). The Institute of Medicine’s “dietary reference intake” for phosphorus: a critical perspective. J. Am. Coll. Nutr. 20, 271–278. doi: 10.1080/07315724.2001.10719047
Shapter, F. M., Chivers, I. H., and Henry, R. J. (2010). A new niche cereal may offer on-farm diversification that mitigates risks associated with climate variability In From passion to profit: new rural industries Australia conference. Gold Coast, QLD.
Shapter, F. M., Malory, S., Chivers, I., and Henry, R. J. (2008). Accelerated domestication of Australian grasses as new sustainable food and fodder crops. In: Proceedings of the 58th Australian cereal chemistry conference. Gold Coast, QLD.
Shiva, V. (2016). Stolen harvest: the hijacking of the global food supply. Lexington, Kentucky: The University Press of Kentucky.
Simopoulos, A. P. (2002). The importance of the ratio of omega-6/omega-3 essential fatty acids. Biomed. Pharmacother. 56, 365–379. doi: 10.1016/S0753-3322(02)00253-6
Simopoulos, A. P. (2010). The omega-6/omega-3 fatty acid ratio: health implications. Oléagineux Corps Gras Lipides 17, 267–275. doi: 10.1051/ocl.2010.0325
Simopoulos, A. P. (2016). An increase in the omega-6/omega-3 fatty acid ratio increases the risk for obesity. Nutrients 8:128. doi: 10.3390/nu8030128
Smith, R., and Smith, P. A. (2003). An assessment of the composition and nutrient content of an Australian aboriginal Hunter-gatherer diet. Aust. Aborig. Stud. 2, 39–52.
Surendiran, G., Alsaif, M., Kapourchali, F. R., and Moghadasian, M. H. (2014). Nutritional constituents and health benefits of wild rice (Zizania spp.). Nutr. Rev. 72, 227–236. doi: 10.1111/nure.12101
Tan, A., Konczak, I., Sze, D., and Ramzan, I. (2010). Towards the discovery of novel phytochemicals for disease prevention from native Australian plants: an ethnobotanical approach. Asia Pac. J. Clin. Nutr. 19, 330–334. doi: 10.6133/apjcn.2010.19.3.06
Thorburn, A. W., Brand, J. C., and Truswell, A. S. (1987). Slowly digested and absorbed carbohydrate in traditional bushfoods: a protective factor against diabetes? Am. J. Clin. Nutr. 45, 98–106. doi: 10.1093/ajcn/45.1.98
Ti, H., Li, Q., Zhang, R., Zhang, M., Deng, Y., Wei, Z., et al. (2014). Free and bound phenolic profiles and antioxidant activity of milled fractions of different indica rice varieties cultivated in southern China. Food Chem. 159, 166–174. doi: 10.1016/j.foodchem.2014.03.029
Tindale, N. B. (1974). Aboriginal tribes of Australia: their terrain, environmental controls, distribution, limits, and proper names. Canberra, ACT: Australian National University Press.
Wright, M. (2011). Research as intervention: engaging silenced voices. Action Learn. Action Res. J. 17:25. doi: 10.3316/informit.463130742827571
Wu, Y., Tao, D., Wu, X., Liu, W., and Cai, Y. (2022). Diet of the earliest modern humans in East Asia. Front. Plant Sci. 13:989308. doi: 10.3389/fpls.2022.989308
Wurm, P. A., Campbell, L. C., Batten, G. D., and Bellairs, S. M. (2012). Australian native rice: a new sustainable wild food enterprise. Barton, ACT: Australian Government.
Yan, N., du, Y., Liu, X., Chu, C., Shi, J., Zhang, H., et al. (2018). Morphological characteristics, nutrients, and bioactive compounds of Zizania latifolia, and health benefits of its seeds. Molecules 23:1561. doi: 10.3390/molecules23071561
Yu, X., Chu, M., Chu, C., du, Y., Shi, J., Liu, X., et al. (2020). Wild rice (Zizania spp.): a review of its nutritional constituents, phytochemicals, antioxidant activities, and health-promoting effects. Food Chem. 331:127293. doi: 10.1016/j.foodchem.2020.127293
Zhai, C. K., Lu, C. M., Zhang, X. Q., Sun, G. J., and Lorenz, K. J. (2001). Comparative study on nutritional value of Chinese and North American wild rice. J. Food Compos. Anal. 14, 371–382. doi: 10.1006/jfca.2000.0979
Keywords: Australian native grains, ancient grain nutrition, First Nations foodways, bushfood industry, indigenous health outcomes, perennial cereal crops, functional foods
Citation: Birch J, Benkendorff K, Liu L and Luke H (2023) The nutritional composition of Australian native grains used by First Nations people and their re-emergence for human health and sustainable food systems. Front. Sustain. Food Syst. 7:1237862. doi: 10.3389/fsufs.2023.1237862
Edited by:
Martin Kussmann, Bayerische Landesanstalt für Landwirtschaft (LfL), GermanyReviewed by:
Lori Hoagland, Purdue University, United StatesHarriet Kuhnlein, Guangzhou University of Traditional Chinese Medicine, China
Copyright © 2023 Birch, Benkendorff, Liu and Luke. This is an open-access article distributed under the terms of the Creative Commons Attribution License (CC BY). The use, distribution or reproduction in other forums is permitted, provided the original author(s) and the copyright owner(s) are credited and that the original publication in this journal is cited, in accordance with accepted academic practice. No use, distribution or reproduction is permitted which does not comply with these terms.
*Correspondence: Jacob Birch, jacob.birch@scu.edu.au