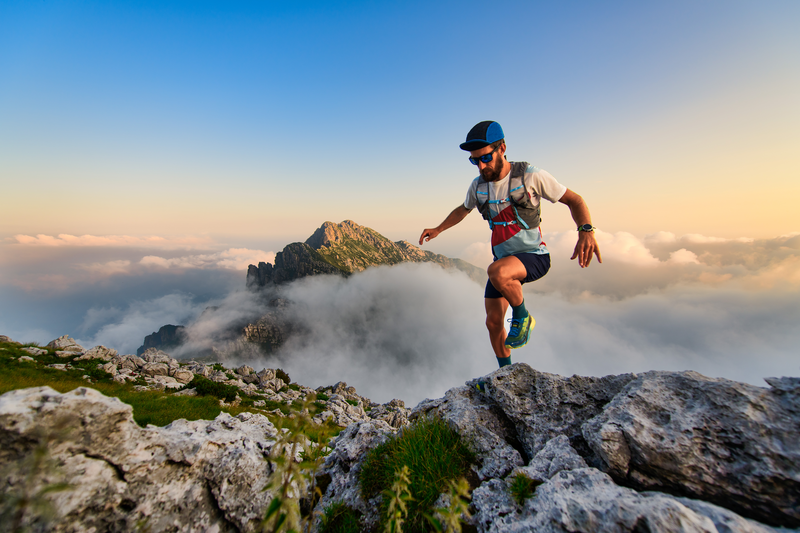
95% of researchers rate our articles as excellent or good
Learn more about the work of our research integrity team to safeguard the quality of each article we publish.
Find out more
ORIGINAL RESEARCH article
Front. Sustain. Food Syst. , 26 March 2025
Sec. Sustainable Food Processing
Volume 9 - 2025 | https://doi.org/10.3389/fsufs.2025.1545310
Introduction: Enset (Ensete ventricosum) is, a perennial crop native to Ethiopia, yields a variety of starch-based foods. It serves as a staple food for approximately 24 million people, which is roughly 20% of the Ethiopian population. This study aimed to investigate the impacts of different parts of Ensete ventricosum and pressing cycles on the proximate composition, functional properties, and mineral content of bulla extracted from the pseudostem and corm, across multiple processing stages.
Methods: The proximate composition including moisture content, ash, fat, fiber, and Crude protein were determined using the official AOAC method 920.87. Carbohydrates were measured according to the method outlined by FAO and World Health Organization, while gross energy content was calculated based on the Atwater conversion factors. The functional properties of bulla extracted from Enset (Ensete ventricosum) such as bulk density, water absorption capacity, oil absorption capacity Swelling power and solubility were also analyzed using different standard analytical methods. Mineral contents were analyzed using Scanning Electron Microscopy (SEM) coupled with Energy-Dispersive X-Ray Spectroscopy (EDS).
Results: The yield of Bulla from the first, second, and third pressings of the pseudostem was 89.1 grams (25.86%), 20 grams (5.80%), and 14 grams (4.06%), respectively. The proximate composition revealed moisture content ranging from 15.35 to 16.10%, ash content from 0.205 to 0.335%, crude fiber from 0.405 to 0.300%, protein from 0.595 to 0.760%, and carbohydrate content from 0.570 to 0.760%. The functional properties of Bulla, including bulk density (BD), water absorption (WA), solubility index (SI), oil absorption (OA), and swelling power (SWP), ranged from 0.645 ± 0.006 to 0.813 ± 0.009 (g/mL), 1.803 ± 0.077 to 4.413 ± 0.286 (%), 3.100 ± 0.424 to 6.391 ± 0.136 (%), 1.752 ± 0.011 to 2.043 ± 0.003 (mL/g), and 4.945 ± 1.133 to 7.430 ± 0.940, respectively. All values represent the mean ± SD of replicate analyses. The elemental composition of Bulla showed ranges for oxygen from 53.05 to 91.1, sodium from −2.45 to 2.33, magnesium from −0.99 to −0.02, potassium from 0.47 to 4.91, copper from −3.73 to 11.94, zinc from −1.87 to 3.96, selenium from −1.24 to 0.95, calcium from 0.02 to 0.43, and iron from −0.55 to 4.06. All mineral content results are presented in weight% and atomic %.
Discussion: The study revealed significant impacts of enset pressing cycles and fractions on the physicochemical, functional, and mineral composition of bulla derived from the pseudostem and corm of the yeshraqinqey enset plant. The carbohydrates dominate the composition, with moderate levels of fiber, protein, and fat contributing to its functionality as a food stabilizer and thickener. The corm was identified as a rich source of calcium and iron, enhancing its gelling and stabilizing properties, while the pseudostem bulla exhibited high potassium, supporting its viscosity-modifying potential. The pseudostem bulla showed a particular affinity for viscosity enhancement, while the corm-derived bulla excelled in gelling and stabilizing functions. The study contributes to a deeper understanding of the effects of different processing methods on the properties of bulla, paving the way for enhanced utilization of enset Bulla in various food applications.
Indigenous Ethiopian tuber crops, such as enset, represent unexploited sources of starch with promising applications in the starch-based foods (Dereje, 2021). Enset (Ensete ventricosum), perennial crop native to Ethiopia, yields a variety of starch-based foods, including kocho, bulla, and amicho. Enset is a rich source of starch, and the main foodstuffs obtained from it are known as kocho (fermented starch product of psedeo-stem and corm), bulla (dehydrated Enset starch juice product), and amicho (boiled starch product of corm) in the local community (Wondimu et al., 2014). These food products are rich in carbohydrates, minerals, and fiber and derived from the pseudostem and underground corms of the enset plant (Awol et al., 2020). However, its yield and nutritional composition of enset vary significantly depending on varieties, age, cultivation practices, and environmental factors (Daba and Shigeta, 2016).
Enset (Ensete ventricosum), resilient and versatile plant commonly known as the false banana, holds great significance in the agricultural, cultural, and economic landscape of certain regions, particularly Ethiopia. Its cultivation for food is primarily confined to the south and south-western highlands of Ethiopia, where it serves as a staple food for approximately 24 million people (roughly 20% of the Ethiopian population). This reliance on enset as a food source is further underscored by the fact that nearly one-fifth of Ethiopians currently depend upon it for sustenance (Jacobsen et al., 2018). According to the study of Yemata (2020) the unprocessed/raw pseudostem and corm of enset are rich in soluble carbohydrates (80%) and starch (65%), which significantly influence the physicochemical, rheological and textural properties of derived food products. Enset starch accounts for more than 90% of bulla (on dry weight basis) and composed of moisture (14.0%), ash (0.16%), fat (0.25%), protein (0.35%), and amylose (29.0%).
Enset starch has broad application in the pharmaceutical industries, where it’s used to bind tablets, help them dissolve, and create gels for drug delivery (Nigussu et al., 2013, Tesfay et al., 2020, Yemata, 2020). It can also service as a gelling agent, substituting agar (Ayenew et al., 2012), biodegradable films (Awol et al., 2022; Feyissa and Gudayu, 2023; Geleta et al., 2020; Majamo and Amibo, 2024) and its flour and starch substituting in different food products development. The global food industry is increasingly seeking novel and sustainable sources of functional ingredients. Ensete ventricosum, an indigenous plant primarily cultivated in Ethiopia, offers a unique source of starch (Bulla) that could help address the overexploitation of conventional starch sources and the health concerns associated with synthetic alternatives.
Various methods of bulla processing are employed across regions in Ethiopia. For instance, in the Hadiya area, bulla is prepared by squeezing liquid starch from scraped pseudostems and pulverized corms (Dilebo et al., 2022), while in Gedio, a starchy liquid is obtained from scraping leaf sheaths and grating corms (Brihanu and Zerihun, 2015). Other regions, such as Kembata-Tembaro and Cheha Woreda, employed, after the completion of decorticating and grating, the leaf sheath pulp is spread on fresh enset leaves covering the ground, followed by the grated corm. Subsequently, the mixture is thoroughly combined and placed into a pit lined with fresh enset leaves. After 7 to 15 days, the mixture is removed, the pulp is squeezed, and the liquid starch is collected (Ayenew et al., 2016). Moreover, in Gurage Zone, Southern Ethiopia, the extraction process involves adding the desired amount of water to the freshly decorticated pseudostem mass, pressing it by hand, allowing it to settle, decanting the water, and then filtering using hand and sieves (Urga et al., 1997; Tedla and Abebe, 1994). While, in Sebat-Bet Guraghe, Southwest Ethiopia, bulla is squeezed by hand from the decorticated and chopped enset, with water added to facilitate the extraction process (Wondimu and Kebede, 2022).
Despite these traditional methods, significant research gaps remain regarding the optimization of bulla production processes. There is a lack of standardized information on key parameters necessary for producing quality bulla, such as the optimal water amount, pressing force, and number of pressing cycles. Additionally, there is no established guideline on which specific parts of the enset plant such as the pseudostem or corm are most effective for producing high-quality bulla. This study aims to systematically investigate these factors to enhance traditional practices and improve the overall efficiency of bulla production for food applications.
Samples of fresh Enset corms and pseudostems were collected from the Central Ethiopia region, Gurage Zone, Wolkite City, Gubre Sub-City/Woreda. Gubre is located in the Central Ethiopia Regional State and has an elevation of 1,918 meters. The distance to the regional capital, Hosa’ina, is approximately 72.5 km (45.0 mi). The distance from Gubre to the capital of Ethiopia, Addis Ababa, is approximately 138.8 km (86.2 mi). Both the pseudostem and corms were manually cleaned using a stainless-steel knife thoroughly to remove any dirt, debris, and outer layers/skins according to Seid et al. (2022). The selections of these parts are based on starch storing Enset parts (Hirose et al., 2010).
The selection of the Yeshiraqinqe corm and pseudostem was guided by multiple significant factors. Firstly, their prevalence and frequent consumption within local communities render them pivotal in unravelling the cultural and dietary importance of enset. Then, focusing on landraces favoured by communities due to their disease resistance provides valuable insights into preferences and utilization practices. Moreover, the widespread distribution of these landraces indicates their adaptability and potential for broader cultivation. Examining these extensively dispersed landraces allows for an assessment of their suitability across various agro-ecological zones, potentially aiding in the expansion of enset production. Lastly, the consistent vernacular names for the Yeshiraqinqe corm and pseudostem across different districts of the Gurage Zone and neighboring regions underscore their cultural and linguistic significance. This shared recognition positions them as prime candidates for further research and conservation endeavors.
The enset corms, which are the underground part of the plant, were divided into outer and inner parts. Both parts were sliced into thin sections, 2–3 mm in thickness using stainless steel knives according to Seid et al. (2022) modified. These sections were then soaked in sodium meta bisulphite solution1% (w/v) for duration of 5–10 min. The solution contains 1 g of sodium meta bisulphite dissolved in 1 L of distilled water. It serves as both an antioxidant and a preservative, particularly for the internal corm part, which immediately changes color when exposed to air. However, this solution helps maintain the color of the corm by inhibiting this change and aimed to preserve the quality of the slices during subsequent drying. The after, both the outer and inner corm slices were subjected to sun drying to remove moisture. Sun drying was chosen as an energy-efficient method to facilitate the drying process while minimizing energy consumption. The dried corm powder from both parts was then milled to produce enset bulla flour, which was sieved through a 0.3 mm mesh size. In general, by dividing the enset corm into outer and inner parts, both components undergo the same processing steps of immersion, drying, grinding, and sieving to obtain enset bulla flour.
First, the enset pseudostem, which is the above-ground part of the plant, underwent a series of processing steps to obtain bulla flour. The pseudostem was thoroughly cleaned to remove any dirt or impurities. Next, the cleaned pseudostems were scribed to create small incisions. The scribed pseudostems were then pressed multiple times using a ratio of 2 liters of water per 1.15 kg of enset pseudostem bulk, conducted over three extraction cycles. Throughout all three cycles, the pressure applied during the pressing was maintained at a constant 450 kPa. To achieve this, a PCM 304 compressor pressure model instrument was used, resulting in the extraction of liquid bulla. After that, liquid bulla was placed in a sun drying system. Sun drying was chosen as an energy-efficient method to facilitate the drying process while minimizing energy consumption. Following sun drying, bulla from pseudostem samples were carefully laid out on an aluminum foil tray and subjected to oven-drying at a temperature of 120°C for duration of 40 min. Then, the flour was grinded and was subsequently sieved with a 0.3 mm sieve. Finally, the powdered samples were then put in zipped polyethylene bags and was delivered to the Addis Ababa science and technology University Center for chemical engineering laboratories and kept at room temperature until it were needed for physicochemical, functional properties, and minerals contents characterization as food applications.
The Moisture content of samples was determined in accordance with the AOAC official method 925.09 (AOAC, 2000). Using the cleaned crucibles were dried in an oven at 105°C for 1 h and cooled in a desiccator. The weight of dried crucibles (W1) was recorded. Approximately 5 grams of each sample were weighed in the dried crucibles (W2) and subsequently crucible with the sample was dried in an oven at 105°C for 1 h. Finally, samples were transferred in to desiccators and weighed (W3). The moisture content was calculated using the equation:
Where: Mc = moisture content (%), W1 = wheight of crucibles (g), W2 = weight of fresh samples and crucibles (g), and W3 = weight of dry samples and crucibles (g).
Total Ash content was determined using the AOAC official method 923.03 (AOAC, 2000). Using the Clean porcelain crucibles were dried at 120°C in an oven, ignited at 550°C in a muffle furnace for 3 h, cooled in desiccators and weighed (M1). Then about 2.0 grams of the samples were weighed into previously dried and weighed porcelain crucibles (M2). The samples were dried in an oven at 120°C for 1 h and carbonized until the contents turn black. The crucibles containing the samples were placed in a Muffle furnace (Gallenkamp, ModelFSL340-0100, U.K.) set to 550°C for 1 h until complete ashing. After ashing period, the crucible were removed from the furnace and cooled in desiccators. The cooled crucibles with the residues were weighed (M3). The total ash content was expressed as a percentage of the initial sample weight on a dry matter basis as follows:
Where: (M2-M1) is sample mass in gram on dry base.
And (M3-M1) mass of ash in gram.
To determine the crude fat content of the samples, AOAC method (2000) was followed. Empty extraction flasks were cleaned, dried at 92°C for 1 h and then kept in the desiccator for 30 min to cool. The weight of cooled flasks was recorded (W1). Approximately 5 grams of the sample were weighed (W2) and placed into each thimbles lined with fat-free cotton at both upper and bottom ends. The thimbles containing the samples were placed in the Soxhlet extraction chamber. A 40 mL of ether were added to each flask, and extraction process was doing for approximately 4 h. Then the flasks with their contents were removed from the Soxhlet apparatus and placed in drying oven at 92°C for 1 h. The flasks were then placed in desiccators for 30 min. The masses of each flask together with its fat contents were weighed immediately after it was taken out of the desiccator (W3).
The crude fat content was calculated using following the equation:
The crude fat content was calculated from the equation:
Where: W1 = Weight of the extraction flask (g),
W2 = Weight of the extraction flask plus the dried crude fat (g),
and, W3 = Weight of the initial sample (g).
To quantify the crude fiber content of the samples, the AOAC method (AOAC, 2000) was employed. Approximately, 2.0 grams of defatted sample were weighed into 600 mL beakers. A 200 mL of 1.25% sulfuric acid solution were added to each beaker and boiled on hot plate for 30 min by rotating and stirring periodically. During boiling, the solution level was maintained by addition of hot distilled water. After 30 min, 20 mL of 28% potassium hydroxide solution was added in to each beaker, and the boiling process was continued for another 30 min. The solution in each beaker was then filtered through crucibles by placing each of them on Buchner funnel fitted with rubber stoppers. During filtration the sample was washed with hot distilled water. The final residue was sequentially washed with 1% sulphuric acid solution, hot distilled water, 1% sodium hydroxide solution and finally with acetone. The crucibles with their contents were dried at 130°C for 2 h., allowed to cooled in desiccators and weighed (W1). The crucibles were subsequently ashed in furnace at 550 ° C for 30 min., cooled in desiccators, and reweighed (W2). Crude fiber content was calculated using the following equation:
Where: (M2-M1) is sample mass in gram on dry base.
and (M3-M1) mass of ash in gram.
Crude protein was conducted in accordance with the official AOAC method 920.87 (AOAC, 2000). Initially, One gram of each sample was precisely weighed into a digestion flask (a round-bottom flask with a long neck, similar to a volumetric flask but without a calibration line). The sample was digested by addition of 5 mL of concentrated H2SO4 (an oxidizing agent which digests the organic material in the sample), anhydrous Na2SO4 or K2SO4 (to raising the boiling point of H2SO4) and a catalyst (CuSO4, selenium, titanium or mercury) to speed up the reaction. Often 1 g of catalyst mixture made of K2SO4 with anhydrous CuSO4 in the ratio of 10:1 was used.
2.0gm of the samples were weighed into a digestion flask and 0.5 g of catalyst mixture (K2SO4 and selenium) was added. 25 mL of concentrated H2SO4 were added and the flasks were shaken to mix the contents uniformly. The flasks were then placed on a digestion burner for 8 h and heated to 370°C allowing digestion until the solution turned green and clear. The digested samples were transferred to a 100 mL volumetric flask and filled to the mark with distilled water. 25 mL of 2% boric acid were pipetted into a 250 mL conical flask, followed by 2 drops of mixed indicator solution (20 mL of bromocresol green and 4 mL of methyl red) and 15 mL of 40% NaOH solution was added into the decomposition chamber of the distillation apparatus. 10 mL of the digested sample solution were then placed into a Kjeldahl flask and the condenser tip was dipped into the boric acid solution contained in the conical flask. The ammonia in the sample solution was then distilled into the boric acid until it completely changed to bluish green. Finally, the distillate was titrated with standardized 0.1 N sulphuric acid (H2SO4) to a reddish color. The percentage of total nitrogen and crude protein were calculated using the equation:
Where;
V1 = Volume of the standard sulphuric acid solution used in the titration of the blank.
B = Volume of sulfuric acid consumed blank.
N = N is the normality of HC1 used often is about 0.1 N and 14.00 is the molecular weight of nitrogen Wo = Weight of the sample, g.
Where: the conversion factor in most cases is 6.25.
Protein content (%, w/w) = % Nitrogen*Factor specific for different products.
Total utilizable carbohydrates determined by the method of FAO and World Health Organization (1998). The utilizable carbohydrate content was calculated by differences using the formula:
.
The gross energy content was calculated based on the Atwater conversion factors for the major macronutrients: crude protein, crude fat, and digestible carbohydrates. The formula used was:
.
To determine the bulk density of the samples, the method outlined by Adebowale et al. (2005) and Giami and Bekebain (1992) was employed. An empty calibrated centrifuge tube was filled with the sample to 5 mL through constant tapping, and the weight of the sample alone was calculated by difference. This process was repeated three times to ensure accuracy, and average bulk density for each samples were recorded. The volume of the sample (V) was recorded, and the filled tube was weighed (W2). The Bulk density was calculated from the values obtained as follows:
Water absorption capacity of bulla starch was determined according to (Umaru et al., 2010). Twenty-five millilitres of distilled water was added to a 3-g bulla powder (W1) in a pre-weighed centrifuge tube (W2) and stirred six times for 1 min at 10-min intervals. The mixture was centrifuged at 3000 rpm for 25 min and the clear supernatant were decanted and discarded. The sediments were dried at 50°C for 25 min and the adhered drop of water was removed and reweighed (W3). The weight gain was recorded as The amount of water absorbed by the sample and expressed as weight of water bounded by 100-g of dried flour. The weight difference between the initial and final weights represented the weight of absorbed water (Koroskenyi and McCarthy, 2001).
The oil absorption capacity was determined following the method outlined by Ocloo et al. (2010). Ten millilitres of refined oil, with a density of 0.92 g/cm3, were added to 1.0 g of the sample in a 25-mL centrifuge tube. The suspension was stirred using a magnetic stirrer (Model MS-12B/17B/22B) for 5 min. The suspension obtained was then centrifuged at 3555 rpm for 30 min and the supernatant was measured in a 10–mL graduated cylinder. Oil absorption was calculated as the difference between the initial volume of oil added to the sample and the volume of the supernatant.
Swelling power and solubility determination were evaluated within the temperature range of 60–90°C following the method of Leach et al. (1959). About 1 gram of bulla flour sample was accurately weighed and transferred into a clean, dried test tube and weighed (w1). 10 mL of distilled water was added and the mixture gently stirred at low speed for 5 min. The slurry was then heated in a thermostatically controlled water bath (YCW-0125) at 80°C for 30 min, gentle stirring to prevent lump formation. Then, the cooled test tube (20°C) was centrifuged at 2200 rpm for 15 min. The supernatant was decanted immediately after centrifuging into a pre-weighed evaporating dish and evaporated at 100°C until a constant weight was achieved, approximately for 4 h. The weight of the sediment was recorded as (W2) representing the swollen mass. The solubility was calculated by the following equation (Li et al., 2022): Solubility% = m*2.5/w*100%. Where m is the weight of soluble component in 10 g upper solution, W is the total weight of bulla.
Energy-Dispersive X-ray Spectroscopy (EDS) was an analytical technique used in conjunction with scanning electron microscopy (SEM) to analyse the elemental composition of a sample. Elemental Distribution: EDS analysis can provide information about the distribution of elements within a sample. The samples for mineral analysis first grinded, polished, and coated with a conductive material (such as carbon or) to prevent charging during imaging. The minerals were analysed using SEM-EDS to investigate their elemental compositions. The SEM-EDS instrument utilized for this study was the JSM 6500F. The operating conditions were set with an acceleration voltage of 15.0 kV and a magnification of 10,000 xs. The signal mode utilized was Secondary Electron Imaging (SEI). The working distance (WD) was 12.2 mm, and the micron bar thickness and marker size were 107 μm and 1 μm, respectively. The font size and display mode were set to 24 and 48, respectively. By employing the all elements analyzed (Normalized)” processing option with 2 iterations, a comprehensive understanding of the mineral composition can be achieved. The standards utilized included O: Oxygen; Compound or mineral: SiO2 (Silicon dioxide or silica), Na: Sodium; Compound or mineral: Albite (a type of sodium feldspar), Mg: Magnesium; Compound or mineral: MgO (Magnesium oxide), K: Potassium; Compound or mineral: MAD-10 Feldspar (a specific type of potassium feldspar), Ca: Calcium, Compound or mineral: Wollastonite (a calcium silicate mineral), Fe: Iron; Compound or mineral; Zn: Zinc; Compound or mineral: Zn (Zinc), Se: Selenium; Compound or mineral: Se (Selenium),
Experimental data obtained from the experiments were statistically analyzed and expressed as mean ± standard deviation. One-way analysis of variance (ANOVA) was used to compare differences between the parameters of bulla starch. Fisher’s least significant difference (LSD) was employed to identify significant difference between means with 95% confidence intervals. The statistical analysis was performed using the Minitab 20.3 software (Minitab, LLC, and State College, PA, United States). All tests were performed in duplicates.
The mass of the bulk of enset, on a dry basis, was determined to be 344.6 grams. During the first pressing, which involved extracting soluble components from the enset bulk using water, the amount of bulla obtained was 89.1 grams. In the second pressing, the yield of bulla decreased to 20 grams, and in the third pressing, it further reduced to 14 grams. The remaining bulk consisted of fiber, with a total mass of 221.5 grams.
To express these yields as percentages, the calculation for the first pressing was as follows: (89.1 g / 344.6 g) * 100 = 25.86%. Similarly, the percentage of bulla obtained from the second pressing was (20 g / 344.6 g) * 100 = 5.80%, and for the third pressing, it was (14 g / 344.6 g) * 100 = 4.06%. These calculations provide insight into the distribution of bulla during the pressing process.
The decrease in the amount of bulla during successive pressings is primarily due to the leaching of water-soluble components during the first pressing (Yemataw et al., 2014). As the pressed enset materials are repeatedly squeezed, the concentration of these soluble components diminishes, resulting in lower yields in subsequent cycles. Consequently, the efficiency of the pressing process declines with each cycle, as the pseudostem becomes less effective at yielding bulla after the initial press. This underscores the importance of optimizing the pressing technique to maximize extraction during the first cycle (Ndiritu et al., 2017).
Research also indicates that pressing impacts the texture, moisture content, and nutritional value of certain food products (Olasunkanmi et al., 2017; Ndiritu et al., 2017). Additionally, the study by Sarangapani et al. (2016) shows that the pressure applied during squeezing or pressing can affect changes in the chemical composition and physicochemical properties of food. Therefore, optimizing squeezing or pressing conditions is crucial to ensure the desired quality and nutritional value of the final food product.
The proximate composition of bulla from Ensete ventricosum, including ash, moisture, protein, fat, fiber, carbohydrate, and energy content, was evaluated to understand its nutritional potential and application in food systems. The analysis revealed notable variations across the different plant parts (corm and pseudostem) and pressing stages, highlighting their diverse properties.
The mean moisture content values obtained from Table 1 reveals significant variations among different parts of the enset plant (corm and pseudostem), ranging from 15.35 to 16.10% on a dry basis. The highest moisture content was found in raw pseudostem first-pressed bulla, while the lowest moisture content was recorded in samples prepared from the internal corm bulla and second-pressed pseudostem bulla (Table 1).
Table 1. Proximate compositions (%) and gross energy (Kcal/100 g) of the native Bulla from different parts of enset (corm and pseudostem).
Notably, the moisture content reported in this study is higher than that documented by Hirose et al. (2010), who reported 14.3% moisture w/w in their analysis of enset starch proximate compositions. The moisture content potato tubers which exhibited a moisture content of 11.2% (Assefa et al., 2016), while starch extracted from anchote contained 9.06% moisture (Abera et al., 2019), and starch from dioscorea abyssinica had a moisture content of 12.5% (Gebre-Mariam and Schmidt, 1998). However, it is comparable with study on modified starches with moisture content of 15% serve as effective gelling agents in confectionery products such as jellybeans and Turkish delight. These starches can be used as a substitute for gelatin in gelatin-based jelly beans, where minimal hardness, good elasticity, and transparency are desired and are promising thickening agents for jelly confectioneries (Kibar et al., 2024). In the context of stabilizing, thickening, and gelling agents, moisture content is a critical factor as it can influence the texture, consistency, and overall quality of the final product. Ingredients with optimal moisture content levels can better perform these functions by interacting with other components in the food matrix.
The mean values of total ash content from the prepared corm and pseudostem samples exhibited variations ranging between 0.205 and 0.335% (Table 1). The highest total ash content was observed in bulla derived from the internal corm (0.335%), followed by the bulla sample from the second-pressed pseudostem (0.3%). This agrees with starch extracted from anchote showed an ash content of 0.30% (Abera et al., 2019). Conversely, the lowest total ash value, amounting to 0.205%, was recorded in the corm sample prepared from the first-pressed pseudostem (see Table 1). This finding aligns with the results of Hirose et al. (2010), which report a typical ash content of 0.2% w/w this suggesting that ingredient likely exhibits superior properties for stabilizing, thickening, and potentially gelling in food applications. while bulla from externan corm is 0.255 ± 0.035bc followed to first pressed bulla ash content. High ash content may indicate the presence of minerals and other impurities that can affect the performance and stability of the starch in various applications. Starch sourced from the tubers of Ethiopian potato exhibited an ash content of 0.14% (Assefa et al., 2016), while Godare starch contained 0.45% ash (Adane et al., 2006). Plectranthus edulis displayed an ash content of 0.14% (Assefa et al., 2016), and enset starch was reported to have 0.16% ash (Gebre-Mariam et al., 1996). These variations in ash content among different starch sources underline the diverse characteristics and potential applications of these ingredients in food processing and product development.
The mean values of crude fat content obtained from the prepared corm and pseudostem samples displayed variations ranging from 0.405 to 0.300% (refer to Table 1). The highest fat content was recorded in the bulla derived from the internal corm and the first-pressed bulla from the pseudostem, both registering 0.405%. Following that, the bulla sample from the outer part of the corm, which showed a fat content of 0.34%. In contrast, the lowest crude fat content, at 0.300%, was documented in the sample prepared from the second-pressed pseudostem (as shown in Table 1).
When comparing these findings with those of other researchers, it was noted that Godare recorded a fat content of 0.22% (Adane et al., 2006), while Plectranthus edulis displayed a fat content of 0.12% (Assefa et al., 2016). Additionally, enset showed a fat content of 0.25% (Gebre-Mariam et al., 1996), and starch extracted from anchote contained 0.20% fat (Abera et al., 2019). However, all results from this research are lower than the fat content of 1% reported by Gebre-Mariam and Schmidt (1998) and According to Dilebo et al. (2023) who stated the crude fat content ranges from 0.61 to 0.89%.
The fat content results shed light on these samples and their potential implications for their utility as food stabilizers, thickeners, and gelling agents. Higher fat content may contribute to richness and creaminess in foods and may offer some stabilizing properties in emulsions. Based on this, the crude fat contents of these samples suggest their appropriateness for food applications. The variations in fat content underscore the importance of understanding the composition of these components for nutritional evaluations and product development purposes.
The mean crude fiber content in the raw corm and pseudo stem samples of enset parts was shown (Table 1). The crude fiber value of samples revealed a remarkable variation, ranging from 0.595 to 0.760%. The bulla sample made from internal part of the corm had the highest crude fiber content, followed by bulla made from second pressed pseudo stem (0.665%). Whereas bulla from corm outer part contains 0.605%, while the lowest crude fiber contents were observed bulla sample from the first pressed pseudo stem 0.595%. This agrees with the work of Tsegaye et al. (2015), who reported a lower crude fiber values range from 0.8 to 1.1% but disagrees with the study of Parmar et al. (2017). Fiber content can contribute to the texture, viscosity, and stability of food formulations, making it an important component in various applications. Therefore, samples of corm external and first pressed bulla are likely to be more effective in enhancing the stabilizing, thickening, and potentially gelling properties. Generally, all samples show significant fiber content that can contribute positively to the functionality in various food applications.
The mean crude protein content of bulla samples obtained from different parts of the pseudostem and corm of enset is summarized in Table 1. The protein concentrations range from 0.76 to 0.570%, providing insights into the protein content of these samples and their potential applications as food stabilizers, thickeners, and gelling agents. According to study by Dilebo et al. (2023), the crude protein content ranges from 2.43 to 11.90% and all results found fall in this range. In contrast, starch extracted from the tubers of Ethiopian potatoes contains 0.43% (Assefa et al., 2016), while anchote has 0.34% (Abera et al., 2019), Dioscorea abyssinica contains 0.5% (Gebre-Mariam and Schmidt, 1998), enset has 0.35% (Gebre-Mariam et al., 1996), Plectranthus edulis contains 0.43% (Assefa et al., 2016), and Godare has 0.48% protein (Adane et al., 2006). In the context of food stabilizers, thickeners, and gelling agents, protein content is a significant factor that interacts with other ingredients to form networks, contributing to the desired texture and stability in food products. Generally, the protein contents of these samples indicate they are greater than those found in many tubers and starchy foods. This suggests that these products are suitable for various food application purposes.
The mean carbohydrates content of bulla samples obtained from different parts of the pseudostem and corm of enset is summarized in Table 1. The carbohydrates values of samples revealed minimum variation, ranging from 81.935 to 82.60. The bulla sample made from second pressed Bulla from pseudostem (82.6%) and the bulla sample of outer part of the corm had the highest carbohydrates content, followed by bulla made from internal part of bulla (82.395%). In contrast, the lowest carbohydrates contents were observed in the bulla sample from the first pressed pseudo stem (81.935%).
The results indicate that total carbohydrates represent the largest proximate composition present in the outer parts corms of enset in this study. This provides insights into the carbohydrate content of these samples and their potential applications as food stabilizers, thickeners, and gelling agents. These results are lower than the 99.2% starch content reported by Assefa et al. (2016) for tubers of Ethiopian potatoes, but greater than the 80.89–89.92% carbohydrate content revealed in the study by Dilebo et al. (2023).
In the context of food stabilizers, thickeners, and gelling agents, carbohydrate content is crucial as it can influence the texture, viscosity, and overall functionality of the product. Higher carbohydrate content generally indicates better thickening and stabilizing properties in food products.
The energy content in bulla samples obtained from different enset parts (corm and pseudo stem) was not significantly different (p < 0.05). The letters following the mean values indicate the no statistical significance among the samples. Energy content is an important factor in food products as it provides calories and contributes to the overall nutritional value of the product. However, all samples show significant energy values that can play a role in the functionality and nutritional aspects in various food applications.
Functional properties, such as water-holding, oil-holding, and swelling capacities, are critical for food quality (Tosif et al., 2022). Smaller particle sizes can increase the surface area, leading to enhanced water absorption and gelation (Romero and Zhang, 2019). In contrast, larger particle sizes can result in reduced texture and increased toughness. Overall, both particle size and chopping technique play a crucial role in determining the quality and nutritional value of food (Romero and Zhang, 2019; Raigar and Mishra, 2015). The proportions of different components in a food formulation can also affect its nutritional content (Li et al., 2022). Furthermore, how ingredients are mixed including the order in which they are added and the mixing speed can influence the final product’s quality (Jeddou et al., 2017).
As indicated in Table 2, the outer part of the corm exhibits a mean bulk density of 0.645, which reflects a lower density compared to outer part of corm bulla. In contrast, the inner part of the corm bulla reveals a slightly higher mean bulk density of 0.710, suggesting that it is denser. Notably, while there is no significant difference in bulk density between the first and the second pressed bulla, the bulk density relative to corms shows an increase, reaching at 0.813. This indicants, a significant variation in material composition when compared to with earlier samples. Furthermore, in both cases bulk density of samples is greater than that of Tarap seed flour which contains bulk density of 0.57 g/cm3 (Noorfarahzilah et al., 2017). Ingredients with higher bulk density contribute to gels with greater strength and firmness due to more compact structures and closer particle packing. As a result, this leads to firmer and more resilient gels in food products. Overall, these results provide a comprehensive understanding of variability in bulk density among the tested samples. This information is valuable for quality control, product development, and material characterization. By understanding and controlling bulk density, food manufacturers can optimize these properties to achieve the desired texture, stability, and sensory attributes in the final product.
The water absorption capacity of bulla flours are varies as demonstrated in Table 2. Water absorption indicates the ability of an ingredient to absorb water. The water absorption capacities of bulla flour for pseudo stem first and second pressed and bulla from internal and external corm were 1.803, 1.854, 4.413, and 3.529%, respectively. The water absorption of each variety has a significant difference (p < 0.05), except between bulla pseudo stem first and second pressed. Ingredients with higher water absorption can retain moisture and contribute to the texture and hydration of food products. The outer part of corm bulla, with a mean absorption of 3.529, demonstrates a moderate level of water absorption, while the inner part of corm bulla, with a mean absorption of 4.413, exhibits the highest water absorption capacity. A result indicates less values than the water absorption capacities of Tarap seed flour were 2.61 (Noorfarahzilah et al., 2017). In comparison, the first and second pressed bulla (1.803 and 1.854), have lower water absorption. The result obtained by this study, was much lower than reported by Suresh Chandra and Samsher (2013). Understanding the water absorption characteristics of ingredients is crucial for formulators in optimizing gelling, stabilizing, and thickening properties in various food applications. By selecting ingredients with appropriate water absorption capacities, food manufacturers can tailor the texture, structure, and overall quality of their products to meet consumer preferences and industry standards effectively.
As illustrated in Table 2, the oil absorption values range 2.043 to 1.752, indicating that there is no statistically significant difference among the groups. This result lower than the findings of Irena et al. (2020), who reported that blending kocho with flaxseed varieties resulted in oil absorption capacities of 2.48, 2.58, 2.40, and 2.65 mL/g, respectively. Conversely, the results of this research are greater than the oil absorption capacities of yanbule, gewada, zereta, and messena, which were 0.85, 0.9, 0.6, and 0.7 g/g, respectively (Bekele, 2015). Furthermore, the values observed in this study exceed the findings of Abebe et al. (2007), where the highest oil absorption capacities recorded for Gewada and Zereta ranged from 0.6 to 0.9 mL/g. In contrast, cassava flour has oil absorption capacities ranging from 0.65 to 1.9 mL/g (Abebe et al., 2007).
These variations highlight the importance of balancing oil absorption with other functional attributes to meet diverse food production requirements. Ingredients with higher oil absorption capacities can effectively bind or incorporate fats and oils, thereby enhancing the texture, flavor, and mouthfeel of food products. Consequently, food manufacturers must carefully optimize oil absorption to create culinary products that not only meet but also exceed consumer expectations for taste, consistency, and sensory appeal.
The outer part of the corm and the second-pressed bulla from the pseudo stem, have no significance difference in a solubility index. However, exhibit the highest solubility index incorporation to others. This slightly higher solubility, when compared to the other samples, suggests a versatile behavior that could influence the texture and structure of food products. The tendency of these phospholipids to form complexes with amylose and amylopectin leads to low solubility of starch (Han et al., 2025; Ogunsona et al., 2018).
In contrast, the first-pressed bulla stands out with a solubility index of 3.100, indicating lower solubility. According to Irena et al. (2020), their investigation showed that the functional properties of kocho blended with flaxseed varieties Belay 96, Jeldu, and Kulumusa exhibited solubility values of 3.90, 4.20, and 4.06%, respectively. This lower solubility may impact its gelling, stabilizing, and thickening abilities, potentially leading to unique textural outcomes compared to samples with higher solubility indexes.
As depicted in Table 2, the swelling power results ranges from 4.945–7.430, suggesting that there are no statistically significant differences in swelling power among the samples tested in this analysis. However, these values are greater than the swelling power of bulla flour, which is fermented for 30 days. Among the varieties, the swelling power values were 1.65, 1.62, 1.62, and 1.6% for yanbule, gewada, zereta and messena, respectively (Bekele, 2015).
In comparison, a study by Irena et al. (2020) demonstrated that the functional properties of blends of kocho with flaxseed varieties exhibited swelling power values of 5.03, 5.23, and 6.31%, respectively. Similarly, the study conducted by Gebre-Mariam et al. (1996) revealed that both enset and maize starches showed lower solubility values compared to potato starch. Swelling in starch is closely related to the presence of amylopectin, as amylose inhibits swelling (Ogunsona et al., 2018). Furthermore, the small fractional lipid content in starch granules significantly reduces swelling, as reported by Morrison and Azudin (1987).
According to Suresh Chandra and Samsher (2013), the swelling power observed in this study is lower than the value reported for potato starch. While swelling power is not directly synonymous with gelling, stabilizing, and thickening properties, it plays a crucial role in these functions. Ingredients with higher swelling power tend to absorb more water, which can be advantageous for creating gels, stabilizing, and thickening food products. Moreover, starch with amylose-lipid complexation exhibits considerable swelling ability but with low dissolution capacity (Schirmer et al., 2015).
Several factors influence the degree of swelling power, including the amylose-to-amylopectin ratio, chain length, molecular weight distribution, degree and length of branching, and overall conformation (Gao et al., 2023). Based on the provided data on the functional properties of the samples, the swelling power indicates their potential applications in various food applications.
The following Figures illustrate the elemental distribution map obtained through EDS analysis, showcasing the mineral composition within the samples A and B: Bulla from the supermarket; C and D: Pseudostem bulla; E and F: Bulla extracted from the corm of enset. The analysis highlights the interplay between structural features and mineral compositions in determining the functional properties of bulla, suggesting that the choice of enset part can be tailored to specific food applications.
The microstructural and compositional properties of bulla derived from various parts of Ensete ventricosum specifically from the pseudostem, corm, and supermarket sources were analyzed using Scanning Electron Microscopy (SEM) coupled with Energy-Dispersive X-Ray Spectroscopy (EDS). SEM provided detailed insights into the surface morphology of bulla, while EDS facilitated the identification and quantification of elemental compositions.
The SEM analysis revealed distinct structural differences among bulla samples. Bulla extracted from the pseudostem as depicted at Figure 1 exhibited a porous structure with a higher potassium content, which is known to influence the ionic strength and solubility of polysaccharides in food matrices. This suggests enhanced functional properties for the pseudostem bulla, making it suitable for applications requiring viscosity modifiers.
Figure 1. Pseudostem-Bulla, (A) indicate the regions where the elemental composition analysis was performed using scanning electron microscope (SEM) image -EDS., (B) Elements detected in the analyzed regions, x-axis shows the energy in keV (kiloelectronvolts), while the y-axis shows the counts (intensity of detected X-rays).
In contrast, corm-derived bulla displayed on Figure 2 a more compact and dense structure with fewer surface irregularities, correlating with its higher calcium content. Calcium plays a crucial role in crosslinking polysaccharides and forming stable gel networks, thus enhancing the gelling and stabilizing properties of the corm bulla, making it promising for food products that require rigidity and structural integrity.
Figure 2. Bulla extracted from corm of enset. (A) Indicate the regions where the elemental composition analysis was performed using scanning electron microscope (SEM) image -EDS., (B) Elements detected in the analyzed regions, x-axis shows the energy in keV (kiloelectronvolts), while the y-axis shows the counts (intensity of detected X-rays).
Market-obtained bulla samples Figure 3 exhibited variability, likely due to differences in processing methods, leading to mixed morphological features. These samples displayed intermediate functional attributes, contributing to their ability to form viscous solutions and emulsify fats.
Figure 3. Bulla from super market, (A) indicate the regions where the elemental composition analysis was performed using scanning electron microscope (SEM) image -EDS., (B) Elements detected in the analyzed regions, x-axis shows the energy in keV (kiloelectronvolts), while the y-axis shows the counts (intensity of detected X-rays).
The mineral compositions of bulla from different parts of Ensete ventricosum (supermarket, pseudostem, and corm) are summarized in Table 3. These analyses indicate that bulla derived from these sources is a rich source of essential minerals, significantly contributing to human nutrition.
The supermarket bulla and pseudostem bulla was less concentrated in trace elements except higher in selenium (Se). These trace minerals are essential for numerous enzymatic and biochemical processes, including those associated with antioxidant defense and the stabilization of emulsions (Bekele et al., 2023). Although their concentrations are relatively lower compared to major minerals, their synergistic effects on protein and enzyme interactions may indirectly influence emulsification and stabilization processes in food matrices.
Notably, potassium (K) concentrations were highest in the pseudostem bulla, consistent with findings from Atlabachew and Chandravanshi (2008), who reported similar trends in other bulla foodstuffs. The high potassium content enhances the capacity for forming thicker solutions, an advantageous property in food applications requiring viscosity modifiers (Bekele et al., 2023). There is similarity with studied the concentration of K was highest minerals in Tarap flour was potassium (Noorfarahzilah et al., 2017). Consequently, the high potassium content observed in the pseudostem may contribute to its enhanced capacity for forming thicker solutions, which is a desirable attribute in food applications requiring viscosity modifiers (Bekele et al., 2023).
Furthermore, the corm bulla stands out for its elevated calcium and iron levels, making it a valuable dietary component for addressing micronutrient deficiencies. The calcium-rich environment promotes gel formation, enhancing emulsion stability that is critical for food systems requiring structural integrity. Corm bulla’s sodium ions are known to enhance the ionic strength of aqueous solutions, promoting the solubility of polysaccharides and improving viscosity (Zhao et al., 2023). Calcium, as a polyvalent cation, forms strong cross-linked networks with negatively charged polymers, such as pectin or alginate-like polysaccharides, enhancing emulsion stability and promoting gel formation. Furthermore, calcium ions enhance the rigidity of polysaccharide gels, particularly when interacting with compounds like alginates, underscoring the corm’s relevance in applications where structural integrity is critical. In addition to calcium, the corm bulla exhibits higher levels of trace elements such as copper (Cu), zinc (Zn), and selenium (Se).
The enset (Ensete ventricosum) is an important starch source. The proximate compositions, functional properties and mineral content of bulla extracted from corm and psedostems were investigated. The proximate composition analysis underscores the diversity of nutritional components, with carbohydrates being the largest contributor, followed by appreciable levels of protein and fiber. Based on the provided data on the functional properties of ingredients, including bulk density, water absorption, solubility index, oil absorption, and swelling power, indicates their potential properties in food application areas. Functional properties, including water absorption and swelling power, validate the application of bulla as a thickener and stabilizer in various food products. The pseudostem bulla exhibited higher concentrations of potassium, enhancing its viscosity-modifying capabilities, while the corm was rich in calcium and iron, supporting its superior gelling and stabilizing properties. This study underscores the importance of enset bulla as a versatile and sustainable food ingredient with significant nutritional and industrial applications potential.
For future research, it is recommended to investigate the optimization of extraction methods for bulla flour, which could enhance yield and quality. Additionally, exploring the use of flour in specific product formulations may reveal its potential as a stabilizing, thickening, and gelling agent in various food applications. Further studies should focus on the pasting properties, thermal properties, particle size distribution, and morphological characteristics of flour. These investigations will contribute to the development and characterization of Bulla, ultimately improving its application in the food industry.
The original contributions presented in the study are included in the article/supplementary material, further inquiries can be directed to the corresponding author.
GW: Investigation, Methodology, Writing – original draft, Writing – review & editing. ET: Conceptualization, Methodology, Supervision, Validation, Visualization, Writing – original draft. HA: Methodology, Supervision, Writing – review & editing. MA: Formal analysis, Methodology, Software, Writing – review & editing.
The author(s) declare that no financial support was received for the research and/or publication of this article.
The authors declare that the research was conducted in the absence of any commercial or financial relationships that could be construed as a potential conflict of interest.
The author(s) declare that no Gen AI was used in the creation of this manuscript.
All claims expressed in this article are solely those of the authors and do not necessarily represent those of their affiliated organizations, or those of the publisher, the editors and the reviewers. Any product that may be evaluated in this article, or claim that may be made by its manufacturer, is not guaranteed or endorsed by the publisher.
Abebe, Y., Bogale, A., Hambidge, K. M., Stoecker, B. J., Bailey, K., and Gibson, R. S. (2007). Phytate, zinc, iron and calcium content of selected raw and prepared foods consumed in rural Sidama, southern Ethiopia, and implications for bioavailability. J. Food Compos. Anal. 20, 161–168. doi: 10.1016/j.jfca.2006.09.003
Abera, G., Woldeyes, B., Demash, H. D., and Miyake, G. M. (2019). Comparison of physicochemical properties of indigenous Ethiopian tuber crop (Coccinia abyssinica) starch with commercially available potato and wheat starches. Int. J. Biol. Macromol. 140, 43–48. doi: 10.1016/j.ijbiomac.2019.08.118
Adane, M., Endale, A., Bultosa, G., Abdel-Mohsen, M. G., and Gebre-Mariam, T. (2006). Isolation and physicochemical characterization of Godare (Colocasia esculenta) starch from Ethiopia. Ethiopian Pharma. J. 24, 13–22. doi: 10.4314/epj.v24i1.35094
Adebowale, K. O., Afolabi, T. A., and Olu-Owolabi, B. I. (2005). Hydrothermal treatments of finger millet (Eleusine coracana) starch. Food Hydrocoll. 19, 974–983. doi: 10.1016/j.foodhyd.2004.12.007
AOAC (2000). Official methods of analysis. 17th Edn. Rome, Italy: Association of Official Analytical Chemists.
Assefa, A., Belete, A., and Gebre-Mariam, T. (2016). Physicochemical characterization of starch isolated from ethiopian potato (plectranthus edulis). Ethiopian J. Sci. 39, 11–20. Available at: https://www.ajol.info/index.php/sinet/article/view
Atlabachew, M., and Chandravanshi, B. S. (2008). Levels of major, minor and trace elements in commercially available enset (Ensete ventricosum (Welw.), Cheesman) food products (Kocho and bulla) in Ethiopia. J. Food Compos. Anal. 21, 545–552. doi: 10.1016/j.jfca.2008.05.001
Awol, A. M., Waghray, K., PG, P. R., and Math, R. G. (2022). Preparation and characterization of Enset starch (ES) based films: plasticized by glycerol and sorbitol. Iran. J. Chem. Chem. Eng. 41, 446–454. Available at: https://www.ijcce.ac.ir/article_245942
Awol, A. M., Waghray, K., Prabhakar, R. P., and Rudrayya, G. (2020). Characterizing physicochemical properties of Enset starch. J. Textiles Polym. 8, 43–52. Available at: https://www.itast.ir/article_101708.html
Ayenew, B., Mengesha, A., Tadesse, T., and GebreMariam, E. (2012). Ensete ventricosum (Welw.) Cheesman: a cheap and alternative gelling agent for pineapple (Ananas comosus var. smooth cayenne) in vitro propagation. J. Microbiol. Biotechnol. Food Sci. 2, 640–652.
Ayenew, A., Mulatu, A., Lemma, B., and Girma, D. (2016). An ethnobotanical study of enset (Ensete ventricosum (Welw) Cheesman) in angacha woreda, kembata-tembaro zone, south region, Ethiopia. Am. J. Life Sci. 4, 195–204. doi: 10.11648/j.ajls.20160406.18
Bekele, H. (2015). Effect of enset (ensete ventricosum (wele) cheesman) variety and fermentation on nutritional composition, anti-nutritional factors, physicochemical characteristics and functional property of bulla. Addis Ababa, Ethiopia: Addis Ababa University.
Bekele, T. H., Trijsburg, L., Brouwer, I. D., de Vries, J. H., Covic, N., Kennedy, G., et al. (2023). Dietary recommendations for ethiopians on the basis of priority diet-related diseases and causes of death in ethiopia: an umbrella review. Adv. Nutr. 14, 895–913.
Brihanu, Z. T., and Zerihun, B. G. (2015). Community indigenous knowledge on traditional fermented enset product preparation and utilization practice in Gedeo zone. JBES 5. Available at: https://www.researchgate.net/publication
Daba, T., and Shigeta, M. (2016). Enset (Ensete ventricosum) production in Ethiopia: its nutritional and socio-cultural values. Agric. Food Sci. Res. 3, 66–74. doi: 10.20448/journal.512/2016.3.2/512.2.66.74
Dereje, B. (2021). Composition, morphology and physicochemical properties of starches derived from indigenous Ethiopian tuber crops: a review. Int. J. Biol. Macromol. 187, 911–921. doi: 10.1016/j.ijbiomac.2021.07.188
Dilebo, T., Feyissa, T., Asfaw, Z., and Zewdu, A. (2022). “Indigenous knowledge of farmers on classification, utilization and on-farm management of Enset (Ensete ventricosum)” in Diversity in Hadiya (Southern Ethiopia: Research square).
Dilebo, T., Feyissa, T., Asfaw, Z., and Zewdu, A. (2023). Analysis of proximate composition, mineral contents, and anti-nutritional factors of enset (Ensete ventricosum) landraces commonly used for amicho preparation in Hadiya zone, southern Ethiopia: implications for food security and mineral bioavailability. J. Agric. Food Res. 14:100771. doi: 10.1016/j.jafr.2023.100771
FAO and World Health Organization (1998). “Carbohydrates in human nutrition: report of a joint FAO/WHO expert consultation, Rome, 14-18 April 1997” in Carbohydrates in human nutrition: Report of a joint FAO/WHO expert consultation, Rome, Italy: Food and Agriculture Organization (FAO) World Health Organization, (WHO). 14–18.
Feyissa, E. M., and Gudayu, A. D. (2023). Synthesis of starch-derived biopolymer reinforced Enset fiber green composite packaging films: processes and properties optimization. Compos. Adv. Mat. 32:26349833231200907. doi: 10.1177/26349833231200907
Gao, L., Van Bockstaele, F., Lewille, B., Haesaert, G., and Eeckhout, M. (2023). Characterization and comparative study on structural and physicochemical properties of buckwheat starch from 12 varieties. Food Hydrocoll. 137:108320. doi: 10.1016/j.foodhyd.2022.108320
Gebre-Mariam, T., Abeba, A., and Schmidt, P. (1996). Isolation and physico-chemical properties of enset starch. Starch-Stärke 48, 208–214. doi: 10.1002/star.19960480603
Gebre-Mariam, T., and Schmidt, P. (1998). Some physico-chemical properties of Dioscorea starch from Ethiopia. Starch-Stärke 50, 241–246. doi: 10.1002/(SICI)1521-379X(199806)50:6<241::AID-STAR241>3.0.CO;2-R
Geleta, T. T., Habtegebreil, S. A., and Tolesa, G. N. (2020). Physical, mechanical, and optical properties of enset starch from bulla films influenced by different glycerol concentrations and temperatures. J. Food Proc. Preserv. 44:e14586. doi: 10.1111/jfpp.14586
Giami, S. Y., and Bekebain, D. A. (1992). Proximate composition and functional properties of raw and processed full-fat fluted pumpkin (Telfairia occidentalis) seed flour. J. Sci. Food Agric. 59, 321–325. doi: 10.1002/jsfa.2740590308
Han, F., Wang, Y., Zhang, H., and Zhang, S. (2025). Physicochemical properties of Alisma starch. Front. Nutr. 12:1513814. doi: 10.3389/fnut.2025.1513814
Hirose, R., Tezuka, N., Kondo, T., Hirao, K., Hatta, T., Nemoto, K., et al. (2010). Characteristic physico-chemical properties and potential uses of Enset (Ensete ventricosum) starch: comparative studies with starches of potato, sago, and corn. J. Appl. Glycosci. 57, 185–192. doi: 10.5458/jag.57.185
Irena, G., Abera, S., and Weledesemayat, G. (2020). Effect of functional property and anti-nutritional factor of Kocho-flaxseed flat bread in Ethiopia. World J. Nutr. Food Sci. 1:1005. Available at: https://www.researchgate.net/publication/350994698
Jacobsen, K., Blomme, G., Tawle, K., Muzemil, S., and Yemataw, Z. (2018). Dietary diversity associated with different enset [Ensete ventricosum (Welw.) Cheesman]-based production systems in Ethiopia. Fruits 73, 356–364. doi: 10.17660/th2018/73.6.6
Jeddou, K. B., Bouaziz, F., Zouari-Ellouzi, S., Chaari, F., Ellouz-Chaabouni, S., Ellouz-Ghorbel, R., et al. (2017). Improvement of texture and sensory properties of cakes by addition of potato peel powder with high level of dietary fiber and protein. Food Chem. 217, 668–677. doi: 10.1016/j.foodchem.2016.08.081
Kibar, E. A. A., Aslan, Ö., Bakan, A., and Özer, H. (2024). Determination of physicochemical and functional properties of cross-linked, Hydroxypropylated, and dual modified corn starch and investigation of its use as gelling agent in soft confectionery. Starch-Stärke 76:2300036. doi: 10.1002/star.202300036
Koroskenyi, B., and McCarthy, S. P. (2001). Synthesis of acetylated konjac glucomannan and effect of degree of acetylation on water absorbency. Biomacromolecules 2, 824–826. doi: 10.1021/bm010014c
Leach, R. M., Dam, R., Zeigler, T. R., and Norris, L. C. (1959). The effect of protein and energy on the potassium requirement of the chick. J. Nutr. 68, 89–100. doi: 10.1093/jn/68.1.89
Li, L., Zhou, W., Wu, A., Qian, X., Xie, L., Zhou, X., et al. (2022). Effect of Ginkgo Biloba powder on the physicochemical properties and quality characteristics of wheat dough and fresh wet noodles. Food Secur. 11:698. doi: 10.3390/foods11050698
Majamo, S. L., and Amibo, T. A. (2024). Study on extraction and characterization of anchote (Coccinia abyssinica) starch and reinforced enset (Ensete ventricosum) fiber for the production of reinforced bioplastic film. Heliyon 10:e23098. doi: 10.1016/j.heliyon.2023.e23098
Morrison, W. R., and Azudin, M. N. (1987). Variation in the amylose and lipid contents and some physical properties of rice starches. J. Cereal Sci. 5, 35–44. doi: 10.1016/S0733-5210(87)80007-3
Ndiritu, A. K., Kinyuru, J. N., Kenji, G. M., and Gichuhi, P. N. (2017). Extraction technique influences the physico-chemical characteristics and functional properties of edible crickets (Acheta domesticus) protein concentrate. J. Food Meas. Charact. 11, 2013–2021. doi: 10.1007/s11694-017-9584-4
Nigussu, E., Belete, A., and Gebre-Mariam, T. (2013). Acetylation and characterization of enset starch and evaluation of its direct compression and drug release sustaining properties. Int. J. Pharm. Sci. Res. 4:4397. Available at: https://www.researchgate.net/publication/289379993
Noorfarahzilah, M., Mansoor, A., and Hasmadi, M. (2017). Proximate composition, mineral content and functional properties of Tarap (Artocarpus odoratissimus) seed flour. Food Res. 1, 89–96. doi: 10.26656/fr.2017.3.025
Ocloo, F. C. K., Bansa, D., Boatin, R., Adom, T., and Agbemavor, W. S. (2010). Physico-chemical, functional and pasting characteristics of flour produced from jackfruits (Artocarpus heterophyllus) seeds. Agric. Biol. J. N. Am. 1, 903–908. doi: 10.5251/abjna.2010.1.5.903.908
Ogunsona, E., Ojogbo, E., and Mekonnen, T. (2018). Advanced material applications of starch and its derivatives. Eur. Polym. J. 108, 570–581. doi: 10.1016/j.eurpolymj.2018.09.039
Olasunkanmi, G. S., Omolayo, F. T., and Olusegun, O. T. (2017). Fatty acid profile, physico-chemical and functional properties of oil and protein isolate simultaneously extracted from sesame (Sesamum indicum) seed. Ann. Food Sci. Technol. 18, 1–10. Available at: https://www.researchgate.net/publication/316035947
Parmar, A., Sturm, B., and Hensel, O. (2017). Crops that feed the world: production and improvement of cassava for food, feed, and industrial uses. Food Secur. 9, 907–927. doi: 10.1007/s12571-017-0717-8
Raigar, R., and Mishra, H. (2015). Effect of moisture content and particle sizes on physical and thermal properties of roasted B engal gram flour. J. Food Proc. Preserv. 39, 1839–1844. doi: 10.1111/jfpp.12419
Romero, H. M., and Zhang, Y. (2019). Physicochemical properties and rheological behavior of flours and starches from four bean varieties for gluten-free pasta formulation. J. Agric. Food Res. 1:100001. doi: 10.1016/j.jafr.2019.100001
Sarangapani, C., Thirumdas, R., Devi, Y., Trimukhe, A., Deshmukh, R. R., and Annapure, U. S. (2016). Effect of low-pressure plasma on physico–chemical and functional properties of parboiled rice flour. LWT-Food Sci. Technol. 69, 482–489. doi: 10.1016/j.lwt.2016.02.003
Schirmer, M., Jekle, M., and Becker, T. (2015). Starch gelatinization and its complexity for analysis. Starch-Stärke 67, 30–41. doi: 10.1002/star.201400071
Seid, N., Griesheimer, P., and Neumann, A. (2022). Investigating the processing potential of ethiopian agricultural residue enset/Ensete ventricosum for biobutanol production. Bioengineering 9:133. doi: 10.3390/bioengineering9040133
Suresh Chandra, S. C., and Samsher, S. (2013). Assessment of functional properties of different flours. Food Safety and Standards: A Comprehensive Approach. New Delhi, India: Springer.
Tedla, M., and Abebe, Y. (1994). Study of Enset processing and development of Enset processing tools in the southern region of Ethiopia. Ethiopia: Food Safety and Quality Control, Ethiopian Journal of Health Development Addis Ababa.
Tesfay, D., Abrha, S., Yilma, Z., Woldu, G., and Molla, F. (2020). Preparation, optimization, and evaluation of Epichlorohydrin cross-linked Enset (Ensete ventricosum (Welw.) Cheeseman) starch as drug release sustaining excipient in microsphere formulation. Biomed. Res. Int. 2020:2147971. doi: 10.1155/2020/2147971
Tosif, M. M., Najda, A., Klepacka, J., Bains, A., Chawla, P., Kumar, A., et al. (2022). A concise review on taro mucilage: extraction techniques, chemical composition, characterization, applications, and health attributes. Polymers 14:1163. doi: 10.3390/polym14061163
Tsegaye, M., Assefa, F., and Zeleke, J. (2015). Symbiotic and phenotypic characterization of rhizobium isolates nodulating fenugreek (Trigonellafoenum-graecum L.) from north and east Shewa, Ethiopia. Int. J. Appl. Agric. Res. 7, 93–104.
Umaru, H. A., Iya, S. A., Obidah, W., and Dahiru, D. (2010). Taurocholate binding capacity and water holding capacity of some wild leafy vegetables of northern Nigeria. Pak. J. Nutr. 9, 987–989.
Urga, K., Fite, A., and Biratu, E. (1997). Natural fermentation of Enset (Ensete ventricosum) for the production of Kocho. Ethiop. J. Health Dev. 11. Available at: https://www.ajol.info/index.php/ejhd/article/view
Wondimu, H., and Kebede, A. (2022). Cultural, economic, and symbolic value of the enset crop in Sebat-bet Guraghe, Southwest Ethiopia: a qualitative study. Human. Soc. Sci. Commun. 9, 1–11. doi: 10.1057/s41599-022-01179-4
Wondimu, A., Molla, F., Dinda, S. C., Gebre-Samuel, N., and Tadese, E. T. (2014). Literature review on enset starch: Physico-chemical properties and pharmaceutical applications. J. Drug Deliv. Therap. 4, 1–6. doi: 10.22270/jddt.v4i3.822
Yemata, G. (2020). Ensete ventricosum: a multipurpose crop against hunger in Ethiopia. Sci. World J. 2020, 1–10. doi: 10.1155/2020/6431849
Yemataw, Z., Mohamed, H., Diro, M., Addis, T., and Blomme, G. (2014). Enset (Ensete ventricosum) clone selection by farmers and their cultural practices in southern Ethiopia. Genet. Resour. Crop. Evol. 61, 1091–1104. doi: 10.1007/s10722-014-0093-6
Keywords: enset, bulla, proximate composition, functional properties, food applications
Citation: Weyya G, Tadesse E, Admassu H and Adem M (2025) Physicochemical and functional properties of bulla from Ensete ventricosum for food applications. Front. Sustain. Food Syst. 9:1545310. doi: 10.3389/fsufs.2025.1545310
Received: 14 December 2024; Accepted: 27 February 2025;
Published: 26 March 2025.
Edited by:
C. K. Sunil, National Institute of Food Technology, Entrepreneurship and Management, Thanjavur (NIFTEM-T), IndiaReviewed by:
N. Baskaran, Indian Institute of Food Processing Technology, IndiaCopyright © 2025 Weyya, Tadesse, Admassu and Adem. This is an open-access article distributed under the terms of the Creative Commons Attribution License (CC BY). The use, distribution or reproduction in other forums is permitted, provided the original author(s) and the copyright owner(s) are credited and that the original publication in this journal is cited, in accordance with accepted academic practice. No use, distribution or reproduction is permitted which does not comply with these terms.
*Correspondence: Getu Weyya, Z2V0dXdleWFAZ21haWwuY29t; Eneyew Tadesse, ZW5leWV3LnRhZGVzc2VAYWFzdHUuZWR1LmV0
†ORCID: Getu Weyya, https://orcid.org/0009-0008-5964-0404
Eneyew Tadesse, https://orcid.org/0000-0002-6128-3759
Habtamu Admassu, https://orcid.org/0000-0001-9933-3988
Muhammed Adem, https://orcid.org/0000-0002-2263-822X
Disclaimer: All claims expressed in this article are solely those of the authors and do not necessarily represent those of their affiliated organizations, or those of the publisher, the editors and the reviewers. Any product that may be evaluated in this article or claim that may be made by its manufacturer is not guaranteed or endorsed by the publisher.
Research integrity at Frontiers
Learn more about the work of our research integrity team to safeguard the quality of each article we publish.