- School of Grassland Science, Beijing Forestry University, Beijing, China
Objective: The effects of low-temperature-resistant lactic acid bacteria (LAB) and chemical additives on the quality and biogenic amine production of oat (Avena sativa L.) silage stored at low temperature were investigated.
Methods: The Lactobacillus plantarum strain Y28, isolated from oat silage, demonstrated robust growth at low temperature. Fresh and wilted oat forages were treated with no additives (Con), L. plantarum inoculant (Y28), propionic acid (PA), formic acid (FA) and sodium benzoate (SB). Silages were opened after 30 or 60 days of storage, and their quality and biogenic amine production were evaluated.
Results: After fermentation, putrescine, cadaverine and tyramine were present at the highest levels in oat silage stored at low temperature, constituting approximately about 90% of the total biogenic amines measured. Five other amines, tryptamine, phenethylamine, histamine, spermidine and spermine were mostly detected at concentrations below 30 mg/kg. The concentrations of tryptamine, phenethylamine, putrescine, cadaverine, histamine, tyramine, spermidine and total biogenic amines, but not spermine, were higher in fresh oat silages compared to wilted oat silages after 30 or 60 days of fermentation. The Y28 inoculant improved the fermentation quality of oat silage at low temperature by lowering the pH and ammonia nitrogen content while increasing lactate content. Oat silage treated with Y28, PA, FA and SB showed lower concentrations of putrescine, cadaverine, tyramine and total biogenic amines than the control in both fresh and wilted oat silage after 30 or 60 days of fermentation.
Conclusion: Among these treatments, FA was the most effective at suppressing the formation of tyramine, cadaverine and putrescine in oat silage stored at low temperature.
Introduction
Oat (Avena sativa L.) is a crucial annual crop with extensive application as forage crop across the world. In China, oats are primarily cultivated in regions with cool climates. Ensiling is a method of preserving forage crops through fermentation, which produces acids that help in maintaining the forage. However, low temperatures during the harvest stage can reduce the microbial activity, hinder the fermentation process (Zhou et al., 2016; Li et al., 2021), leading to inadequate acid production in silage (Cao et al., 2011; Zhang et al., 2021). This results in poor preservation of the oat forage, which may negatively affect the nutritional quality and safety of the silage. Despite the importance of ensuring high-quality silage, current research has largely overlooked the challenges associated with fermentation in cold environments, especially in regions with naturally low temperatures. Although bacterial inoculants are commonly used to enhance fermentation, Bernardes et al. (2018) pointed out that such bacterial inoculants may be less effective at low temperatures, as most silage inoculants are developed from bacterial strains that thrive in warmer conditions (Weinberg and Muck, 1996). This raises a critical issue in silage production in colder climates—existing additives and inoculants are often not suited for low-temperature conditions. Thus, it is essential to explore new methods and develop preparation techniques that can enhance fermentation quality at colder environment. Chemical additives such as formic acid (FA), propionic acid (PA) and sodium benzoate (SB) are effective in controlling spoilage and pathogenic microorganisms. While the majority of studies have focused on the effects of these chemical additives on silage fermentation at moderate temperatures, research on silage under low-temperature conditions remains limited, particularly with respect to biogenic amine formation. However, investigating their impact at low temperature is equally important, particularly for regions with colder climates or for situations where oat silage might be stored in less-than-ideal conditions.
Biogenic amines are low molecular weight nitrogenous organic compounds formed in silage through the decarboxylation of amino acids (Liang et al., 2024). This process is primarily facilitated by microorganisms during the fermentation of silage. Some biogenic amines such as putrescine, cadaverine, tyramine, histamine, and spermidine are commonly found in silage and can significantly impact its quality and safety (Scherer et al., 2015). The presence and concentration of these amines varies depending on factors such as the forage types, ensiling conditions, and the microbial populations present during fermentation process. An appropriate level of biogenic amines is essential for normal cell growth and differentiation, excessive amounts can adversely affect feed intake and livestock health (Driehuis et al., 2018). High levels of putrescine can decrease nitrogen degradability in the rumen of steers, which can impair protein utilization (Dawson and Mayne, 1997). Tyramine can raise the pH and increase the proportion of isovalerate in rumen fluid, which can affect rumen fermentation dynamics and animal performance (Dawson and Mayne, 1996). Significant levels of biogenic amines have been observed in silages made from perennial ryegrass, maize, cocksfoot, alfalfa and clovers (Van Os et al., 1996; Nishino et al., 2007; Skladanka et al., 2017). Temperature plays a crucial role in the formation of biogenic amines in fermented food (Ormanci and Colakoglu, 2017), with Peñas et al. (2010) specifically noted that low temperature can lead to the accumulation of biogenic amines due to slower acidification and altered microbial activity. Few studies have explored the role of low-temperature-resistant lactic acid bacteria (LAB) and the effectiveness of chemical additives in mitigating biogenic amine formation in low-temperature environment.
In the present study, we investigate the potential of low-temperature-resistant LAB and chemical additives (PA, FA, and SB) to improve silage quality during fermentation in cold conditions. Moreover, the possibility of decreasing the concentrations of biogenic amines during low-temperature fermentation with LAB and chemical additives was tested.
Materials and methods
Lactic acid bacteria strain
Strain Y28 was specifically isolated from oat silage and screened based on its growth performance in MRS medium and its distinct acid production ability at 10°C. The strain was identified as Lactobacillus plantarum through 16S ribosomal RNA sequencing. Strain Y28 is selected for its acid production ability at low temperatures, where most other strains show reduced activity. Strain Y28 might be suitable for improving silage quality in colder climates, where fermentation is typically slower and less effective. The morphological, physiological and biochemical tests conducted for strain Y28, following the methods described by Kozaki et al. (1992). In addition, the ability of Y28 to produce different biogenic amines was determined using the method described by Bover-Cid and Holzapfel (1999). A 0.1 mL aliquot of a pre-culture grown in MRS medium was inoculated in 10 mL of biogenic amine production medium. This medium consisted per litre of: meat extract 8 g, tryptone 5 g, yeast extract 4 g, glucose 1.5 g, fructose 1 g, Tween 80 0.5 g, MgSO4 0.2 g, FeSO4 0.04 g, MnSO4 0.05 g, CaCO3 0.1 g, tryptophane 2 g, phenylalanine 2 g, histidine 2 g, tyrosine 2 g, ornithine 2 g, lysine 2 g, pyridoxal phosphate 0.25 g, adjusted to pH 5.5, and incubated at 37°C for 48 h. The fermentation broth was centrifuged at 18,000 × g for 10 min, and the supernatant was stored at −20°C.
Ensiling
Oat (A. sativa L.) was grown in June 2017, in Shaogen town, Ar Horqin Banner, Chifeng city, Inner Mongolia Autonomous Region. The whole-crop oat was harvested in the early morning at the boot stage. The 50% of the grass was chopped to a theoretical length of 1–2 cm for fresh silage preparation. Another 50% of the grass was wilted on a polyethylene sheet for approximately 24 h and then chopped to a theoretical length of 1–2 cm for wilted silage preparation. Two types of ensiled materials, fresh and wilted oat grass, were treated with five additives: untreated (Con), L. plantarum inoculant (Y28), propionic acid (PA), formic acid (FA), sodium benzoate (SB). Y28 was inoculated at 1.0 × 106 cfu/g of fresh matter (FM). PA, FA and SB were applied at 4.0 g/kg of fresh matter. Three hundred grams of whole-crop oat forage was thoroughly mixed with the additives, packed into polyethylene film bags (30 × 40 cm; 0.19-mm thickness), and sealed using a vacuum sealer (FW3150; Fresh World Electric Co., Ltd., Guangzhou, China). All silos were stored in a 10°C incubator (SPX-250, Beijing Luxi Tech. Co. Ltd., Beijing, China). Each treatment was conducted in triplicate. After 30 and 60 days of ensiling, three bags per treatment were opened to evaluate fermentation end products, microbial and chemical compositions, and biogenic amine concentrations.
Fermentation quality analysis
We mixed the silage sample (20 g) with 180 mL of distilled water and homogenized in a juicer for 2 min. The mixture was then filtered through four layers of cheesecloth and one layer of qualitative filter paper. The filtrate was used to measure pH value and levels of organic acid and ammonia nitrogen. The pH was measured using a pH meter (PHS-3C, INESA Scientific Instrument Co., Ltd., Shanghai, China). Organic acids (lactate, acetate, propionate, and butyrate) were analyzed by high-performance liquid chromatography (HPLC) using a Shodex RS Pak KC-811 column (Showa Denko K.K., Kawasaki, Japan) with detection at 210 nm using a diode array detector (DAD, SPD-20A, Shimadzu Co., Ltd., Kyoto, Japan). The eluent used was 3 mmol/L HClO4, at a flow rate of 1.0 mL/min, and the column temperature was maintained at 50°C. Ammonia nitrogen (NH3-N) concentration was determined using the phenol and sodium hypochlorite method as described by Broderick and Kang (1980).
Chemical composition analysis
The oat material and silage samples were oven-dried for 48 h at 65°C to determine the dry matter (DM) content. After drying, samples were milled using a vertical pulverizer and passed through a 1.0-mm screen. Then they were analyzed for water-soluble carbohydrate (WSC), crude protein (CP), neutral detergent fiber (NDF), acid detergent fiber (ADF), and hemicellulose (HC). WSC levels were measured using the anthrone method (Murphy, 1958). CP content was determined following method 976.05 of AOAC International (2012). NDF and ADF levels were measured according to Van Soest et al. (1991), with heat-stable α-amylase and sodium sulfite added during NDF quantification. HC content was estimated by subtracting the ADF value from the NDF value.
Biogenic amine analysis
Lyophilized oat silage samples were utilized to measure the concentrations of eight biogenic amines: tryptamine, phenylethylamine, putrescine, cadaverine, histamine, tyramine, spermidine and spermine. Initially, 2.5 g of lyophilized powder were mixed with 10 mL of 50 g/L trichloroacetic acid and shaken for 30 min in a table concentrator. The mixture was then centrifuged for 10 min at 1800 × g, and the supernatant was filtered through filter paper. This process was repeated by adding another 10 mL of trichloroacetic acid to the residue, shaking it again and centrifuging at the same speed. The supernatant from this second centrifugation was also filtered. The combined filtrate volume was adjusted to 25 mL with 50 g/L trichloroacetic acid. Next, one millilitre of the extract was transferred to a 5-mL volumetric flask. Then, 200 μL of 2 N sodium hydroxide, 300 μL of saturated sodium bicarbonate, and 1 mL of 10 mg/mL dansyl chloride solution were added. The mixture was incubated in the dark at 40°C for 45 min. To neutralize the residual dansyl chloride, 100 μL of 25% ammonium hydroxide was added, and the solution was left at ambient temperature for 30 min. The final volume of the reaction mixture was adjusted to 5 mL with acetonitrile. This mixture was then centrifuged for 5 min at 5,000 × g. The supernatant was filtered through a 0.22 μm syringe filter and analyzed using high performance liquid chromatography (HPLC).
The separation was conducted on a C18 column (Reprosil-Pur Basic, 5 μm, 250 mm × 4.6 mm, Dr. Maisch GmbH) equipped with a diode-array detecter (DAD). Gradient elution was utilized with acetonitrile (solvent A) and 0.1 mol/L ammonium acetate (solvent B). The gradient program was as follows: starting at 50% A and 50% B at 0.01 min, transitioning to 90% A and 10% B at 25 min, maintaining 90% A and 10% B until 35 min, and returning to 50% A and 50% B at 45 min. The flow rate was set at 0.8 mL/min, with the column maintained at 30°C, and detection was performed at a wavelength of 254 nm. The injection volume for each sample was 20 μL. For the analysis of biogenic amines in the fermentation broth, 1 mL of the broth was mixed with an equal volume of 100 g/L trichloroacetic acid. The mixture was then processed using the same procedure as previously described.
Statistical analysis
Data on fermentation quality, chemical and microbial composition, and biogenic amines were analyzed using ANOVA with the general linear model-univariate procedure in SPSS19.0 software (SPSS Inc., Chicago, IL, United States). The analyses considered grass types (W) and additives (A) as the two main effects, including their interaction. Duncan’s multiple range test was employed to compare mean values, with significance defined as p < 0.05. The Pearson correlation coefficient was utilized to assess the relationship between biogenic amines and ammonia nitrogen in oat silage.
Results
Characteristics of Y28 used in experiments
The morphological, physiological and biochemical characteristics of Y28 are presented in Table 1. The phylogenetic tree based on partial 16S rRNA sequences of the isolated strain Y28 is displayed in Figure 1. The L. plantarum strain Y28, isolated from oat silage, demonstrated robust growth at 10°C. Additionally, strain Y28 was found to produce putrescine in synthetic screening media containing precursor amino acids.
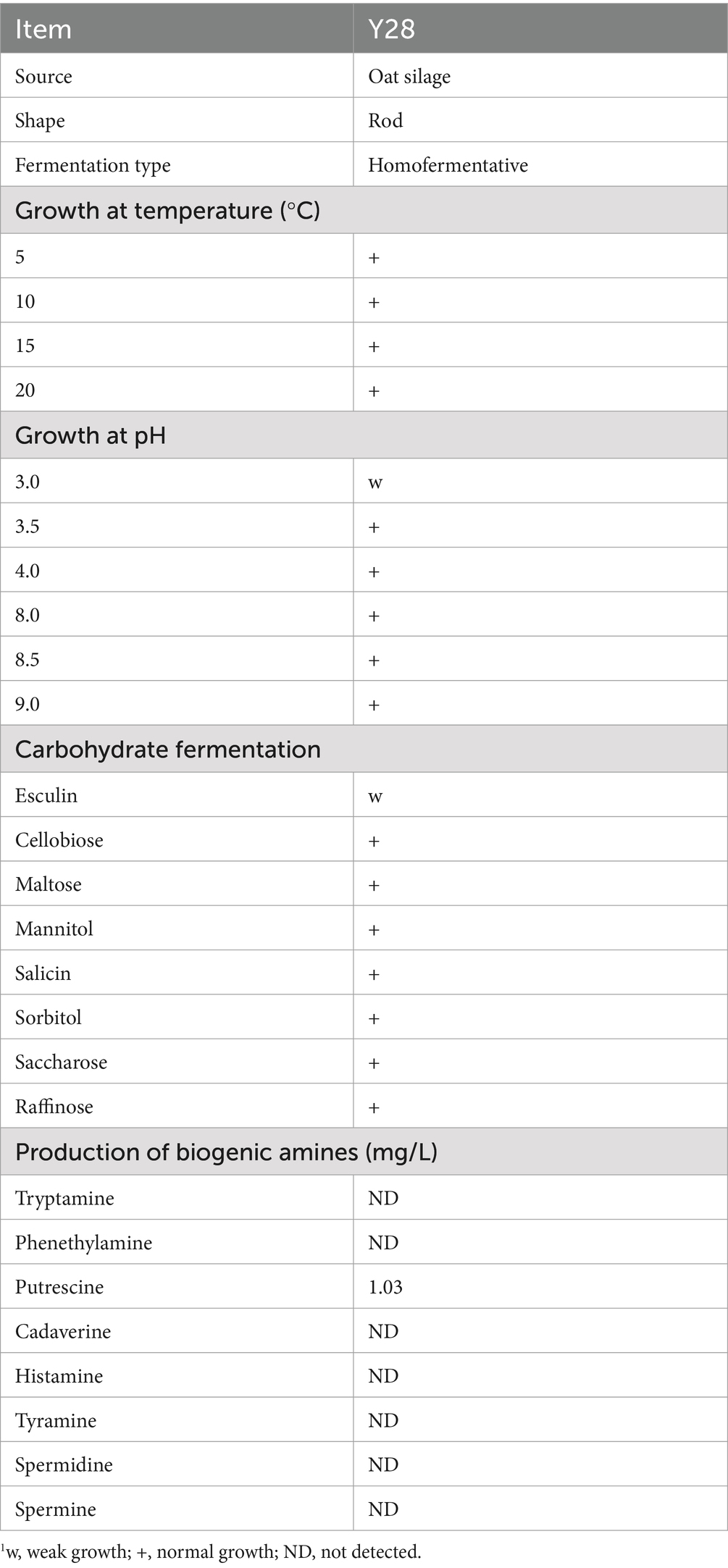
Table 1. Characteristics of the Y28 strains used in experiments.1
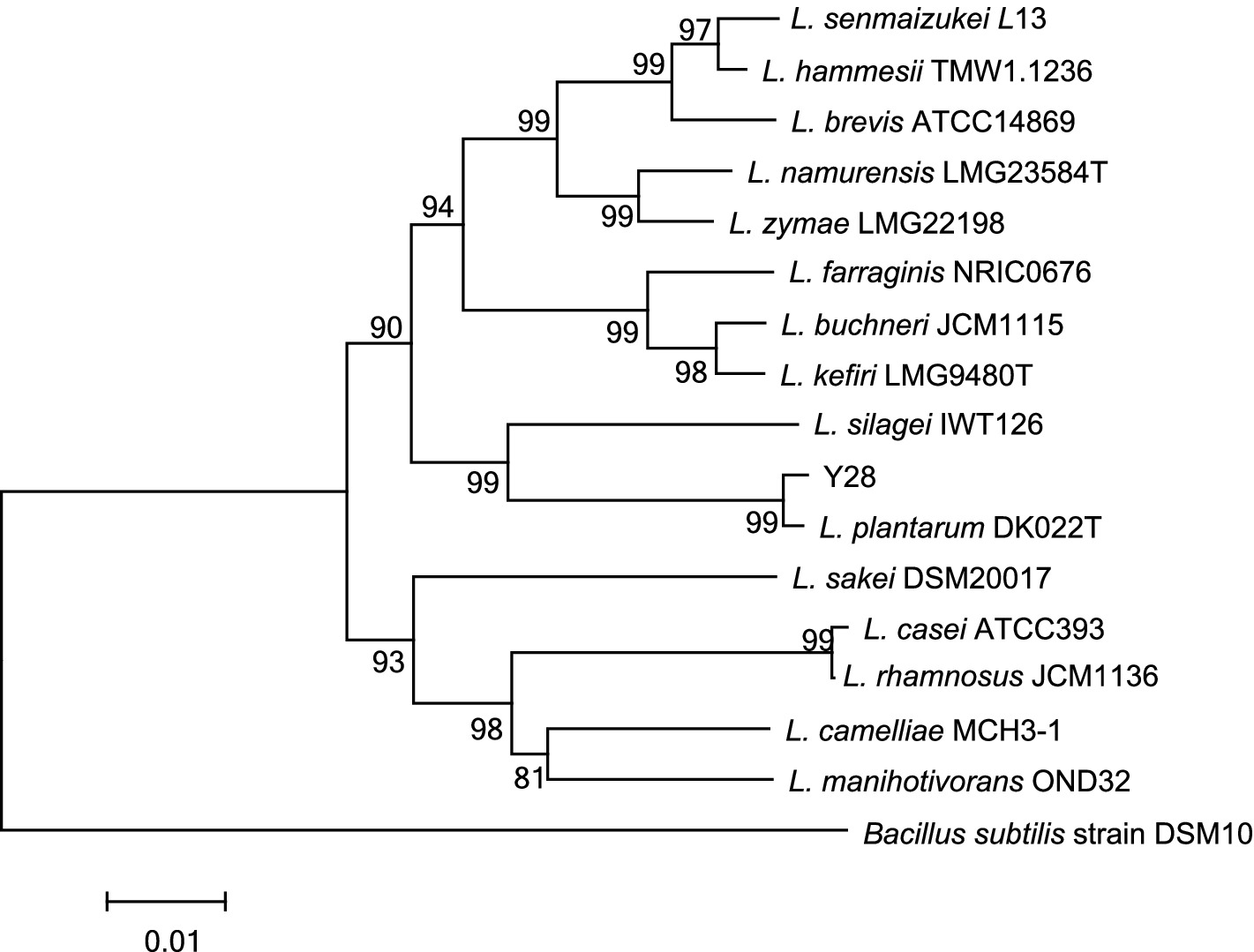
Figure 1. Phylogenetic tree of partial 16 S rDNA sequences of the isolated strain Y28 and sequences of identified bacteria in the GenBank nucleotide database. The bar indicates 1% sequence divergence.
Chemical compositions of oat materials
The chemical compositions of the oat forages before ensiling are shown in Table 2. The dry matter (DM) of fresh oat forage was 16.4%, which increased by 10.0% after wilting. For fresh oat forage, the levels of water-soluble carbohydrates (WSC), crude protein (CP), neutral detergent fiber (NDF), acid detergent fiber (ADF), and hemicellulose (HC) were 8.51, 12.2, 53.9, 32.6, and 21.4% of the DM, respectively. For wilted oat forage, these levels were 9.31, 12.5, 53.2, 31.8, and 21.4% of the DM, respectively.

Table 2. Chemical and microbial compositions of oat materials prior to ensiling.1
Fermentation quality of oat silages
The fermentation products and pH values of oat silages ensiled for 30 and 60 days are shown in Tables 3, 4. After both 30 or 60 days of fermentation, silages treated with Y28 exhibited significantly lower (p < 0.05) pH values and ammonia nitrogen content and significantly higher (p < 0.05) lactate content compared to the control in both fresh and wilted oat forage. Silages treated with FA also had significantly lower (p < 0.05) pH values, lactate and ammonia nitrogen content compared to the control. Except for the PA treatment, propionate levels in all other treatments were below the detection limit. Silages treated with SB had significantly lower (p < 0.05) ammonia nitrogen concentration than the control and PA treatments. Butyrate was not detected in any of the treatments.
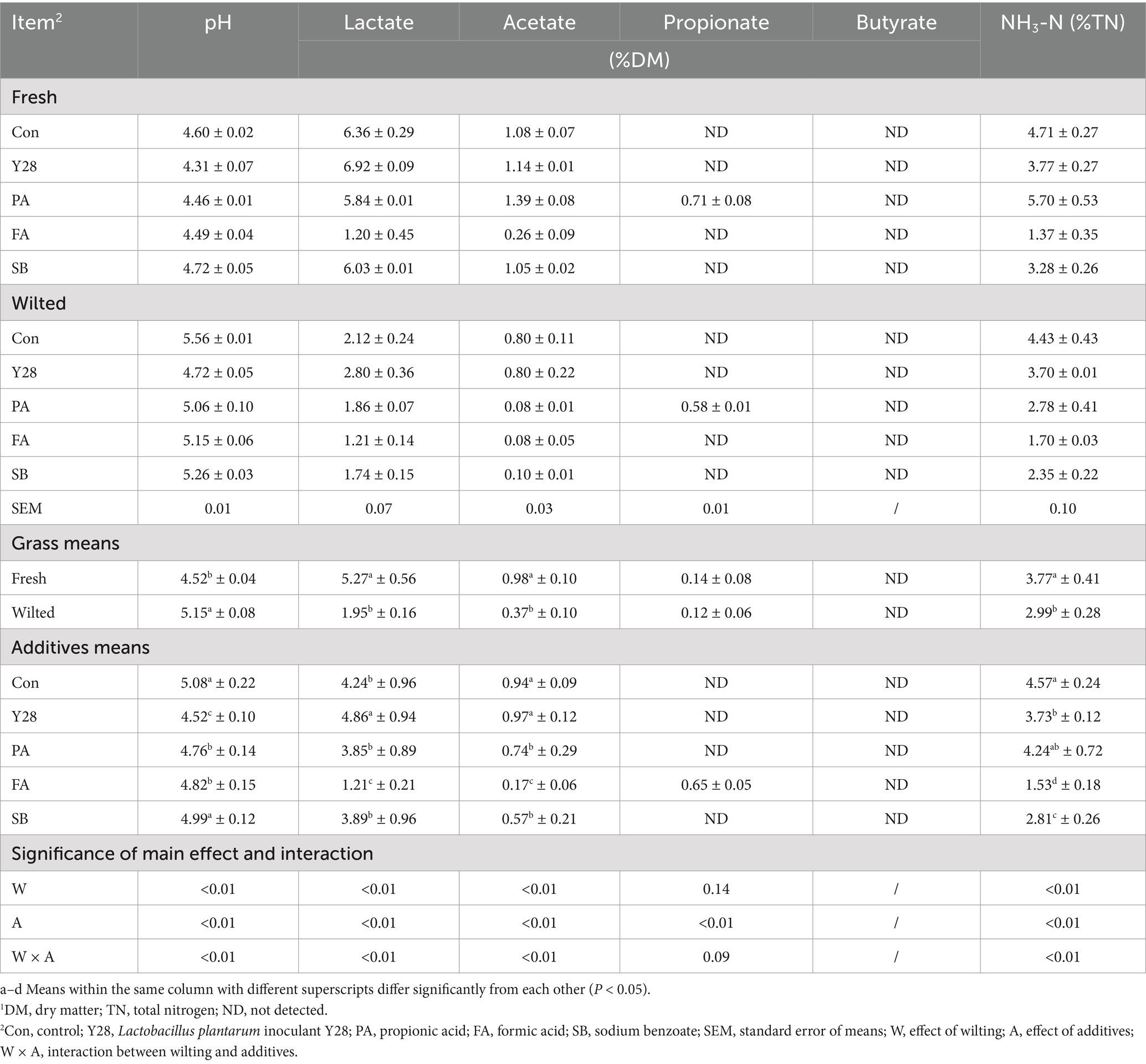
Table 3. Fermentation quality of oat silages at low temperature after ensiling for 30 days.1
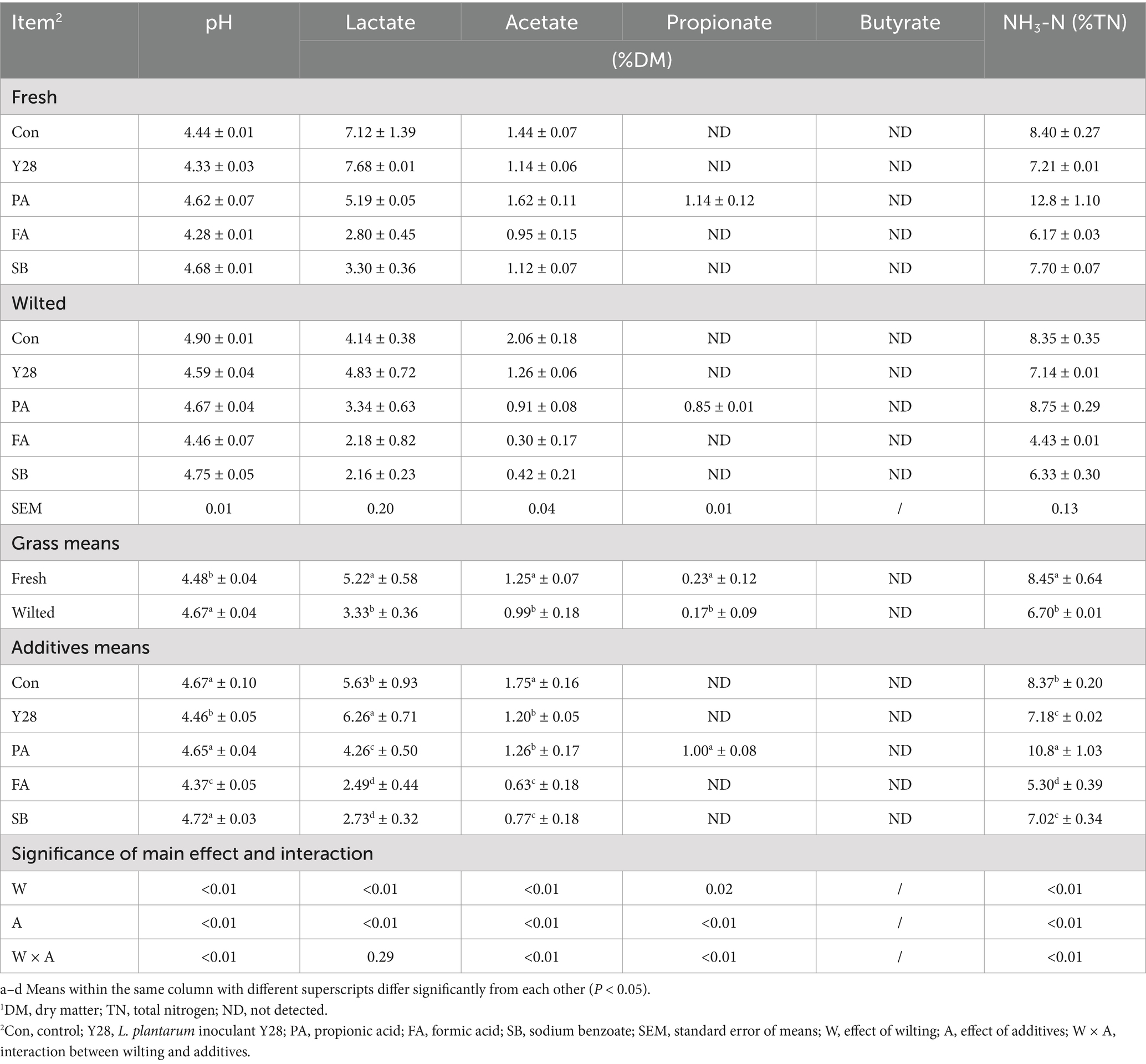
Table 4. Fermentation quality of oat silages at low temperature after ensiling for 60 days.1
Chemical compositions of oat silages
The chemical compositions of oat silages ensiled for 30 and 60 days are shown in Tables 5, 6. Following 30 or 60 days of fermentation, the levels of DM, CP, NDF, ADF, and HC in both fresh and wilted oat silage showed no major changes compared to the unfermented materials. However, the WSC content decreased significantly after low-temperature fermentation compared to the unfermented material. Among all treatments, the FA treatment had the highest WSC content. There were no significant differences observed among the five treatments regarding their effects on the DM, NDF and ADF content in fresh and wilted oat forage.
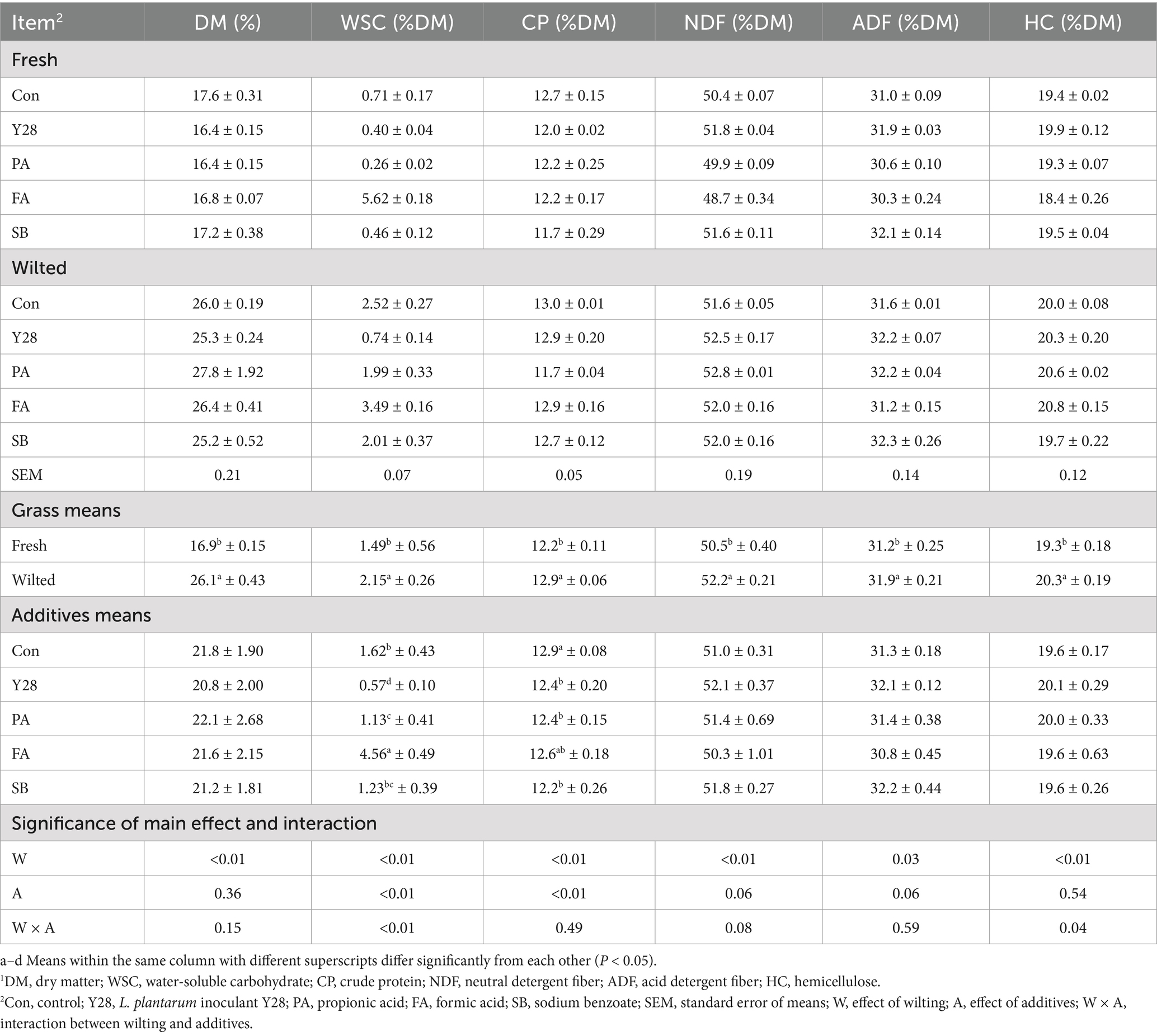
Table 5. Chemical composition of oat silages at low temperature after ensiling for 30 days.1
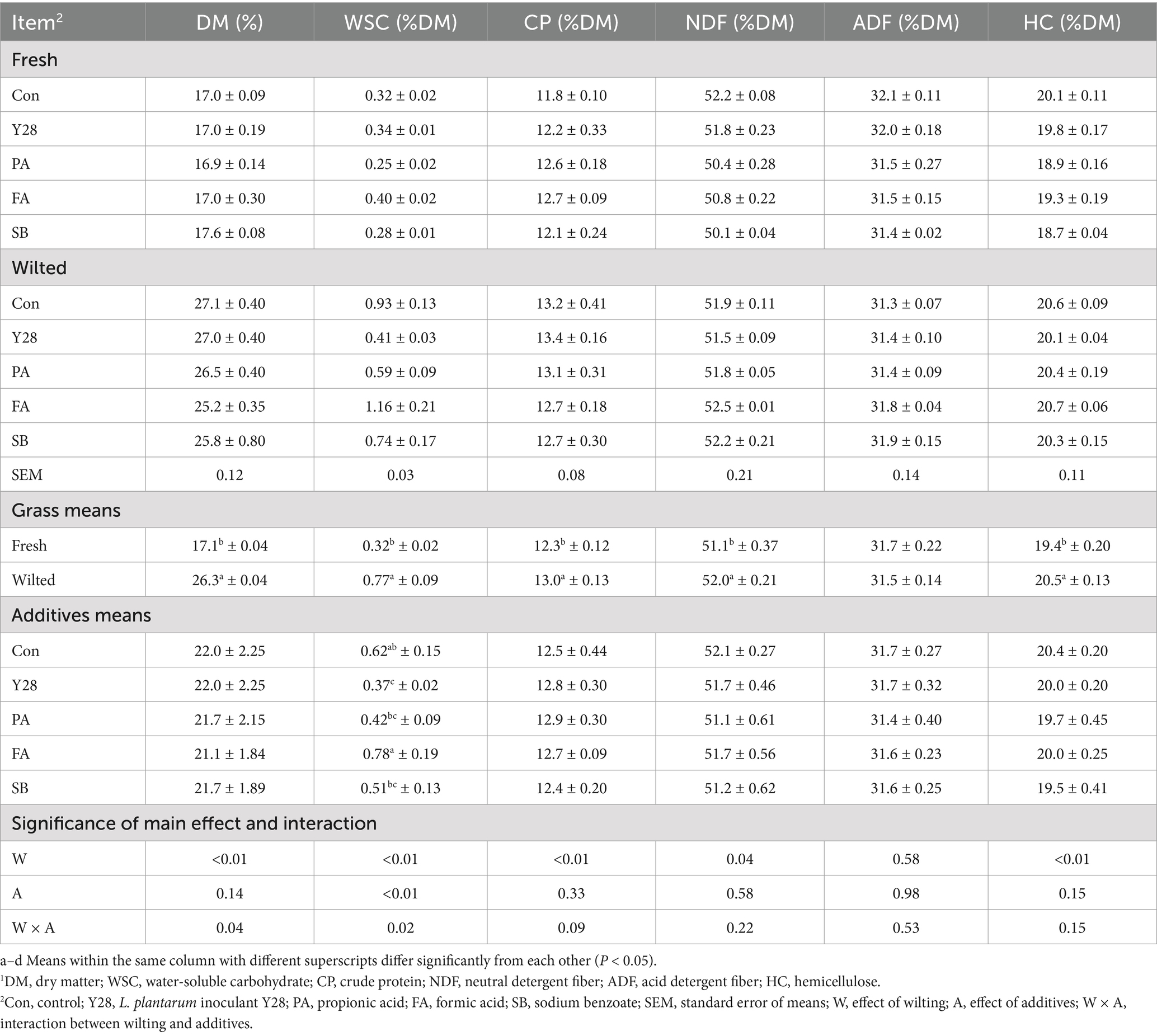
Table 6. Chemical composition of oat silages at low temperature after ensiling for 60 days.1
Biogenic amine concentrations of oat silage
The individual and total biogenic amine levels in oat silages ensiled for 30 and 60 days are presented in Tables 7, 8. After 30 or 60 days of fermentation, putrescine, cadaverine, and tyramine were consistently found in all oat silages fermented at low temperature, while tryptamine, phenethylamine, histamine, spermidine and spermine were detected at very low levels. At the end of fermentation, the highest levels of putrescine, cadaverine, tyramine and total biogenic amines were observed in the control samples of fresh oat silage, measuring 270.2, 637.9, 595.7, and 1539.4 mg/kg DM, respectively. In the same control samples, tryptamine, phenethylamine, histamine, spermidine and spermine were present at levels of 8.9, 10.3, 9.2, 6.0, and 1.3 mg/kg DM, respectively. Oat silages treated with Y28, PA, FA and SB showed significantly lower (p < 0.05) concentrations of putrescine, cadaverine, histamine, tyramine and total biogenic amines compared to the control after 30 or 60 days of fermentation. Among these treatments, FA treatments had the lowest levels of putrescine, cadaverine and total biogenic amine. The concentrations of tryptamine, phenethylamine, putrescine, cadaverine, tyramine, spermidine and total biogenic amines were significantly higher (p < 0.05) in fresh oat silages than in wilted oat silages after 30 or 60 days of fermentation, with the exception of spermine. The contribution of individual biogenic amines to the total levels in oat silage stored at low temperature is shown in Figure 2. Putrescine, cadaverine and tyramine were the predominant amines, accounting for approximately 90% of the total biogenic amines measured. The five amines—tryptamine, phenethylamine, histamine, spermidine and spermine—contributed less significantly to the total biogenic amines. Among the treatments, PA and FA were particularly effective at suppressing cadaverine content in oat silage.
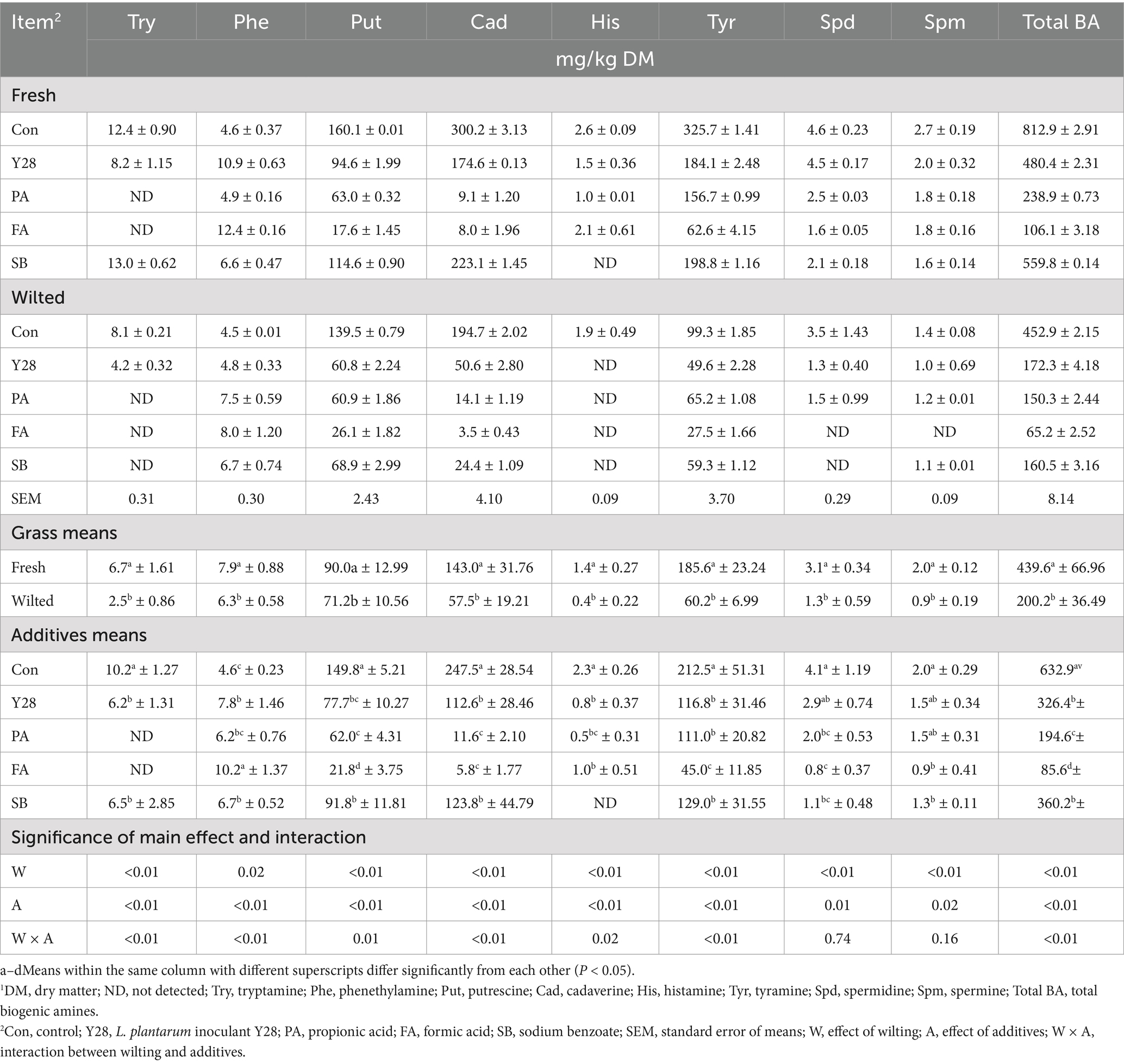
Table 7. Biogenic amine concentrations of oat silages at low temperature after ensiling for 30 days.1
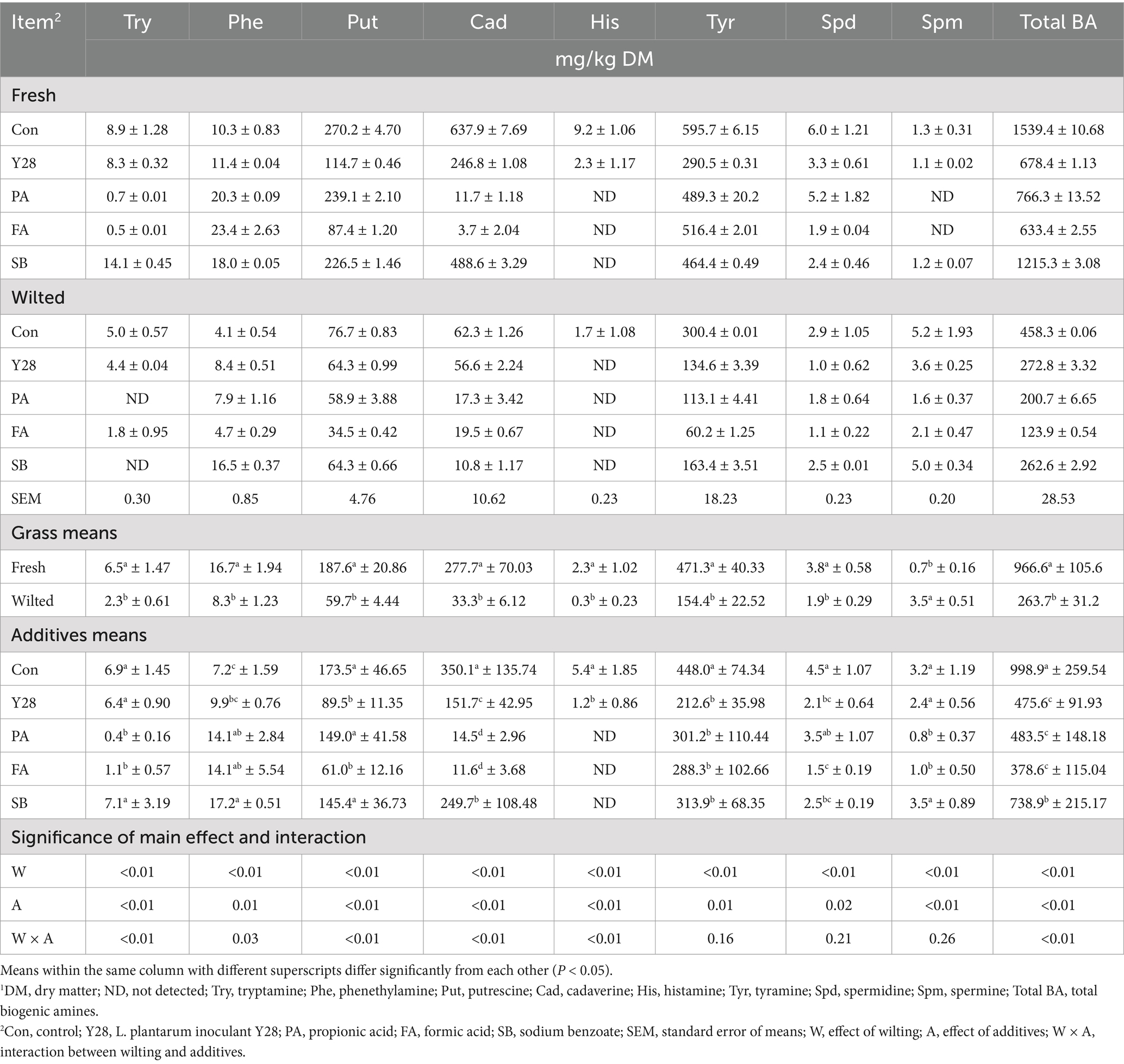
Table 8. Biogenic amine concentrations of oat silages at low temperature after ensiling for 60 days.1
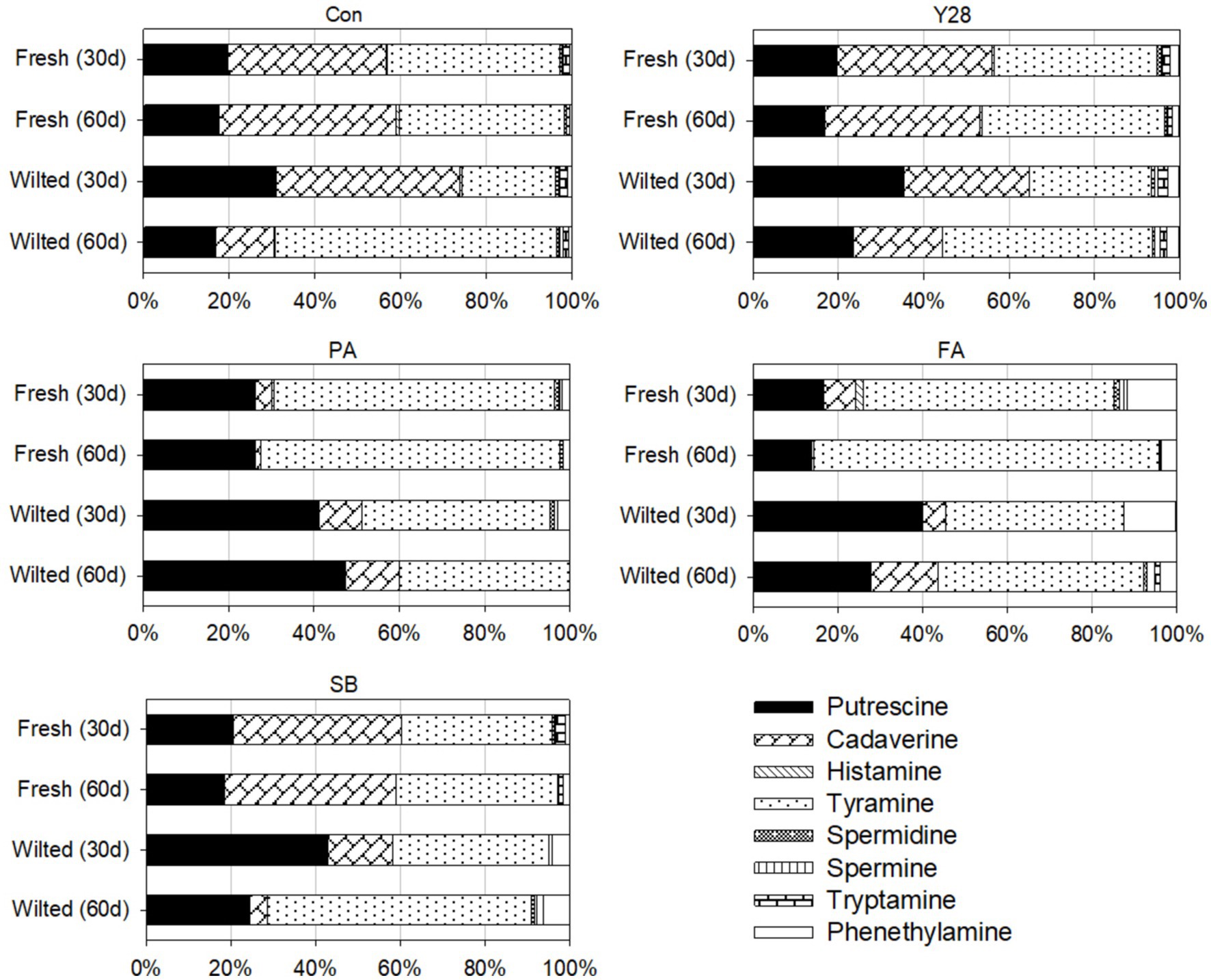
Figure 2. Contribution of individual biogenic amines to total levels in oat silages stored at low temperature. Con, control; Y28, L. plantarum inoculant Y28; PA, propionic acid; FA, formic acid; SB, sodium benzoate.
Relationships between ammonia nitrogen and biogenic amine
Significant positive correlations were observed between biogenic amines and ammonia nitrogen (Table 9). Specifically, individual amines such as phenethymine, putrescine, tyramine, and spermidine presented significant positive correlations with ammonia nitrogen, with correlation coefficients of R = 0.36 (p < 0.01), R = 0.56 (p < 0.01), R = 0.62 (p < 0.01), and R = 0.44 (p < 0.01), respectively. The total biogenic amine content also had a significant positive correlation with ammonia nitrogen, with a correlation coefficient of R = 0.49 (p < 0.01).
Discussion
Low temperature restricts silage fermentation (Li et al., 2021), while extensive studies have been conducted on silage at moderate and high temperatures, research on silage at low temperature is limited. This study explored methods to improve oat silage quality under low-temperature conditions. We isolated the low-temperature-resistant strain Y28 and applied it, along with chemical additives propionic acid (PA), formic acid (FA) and sodium benzoate (SB), to oat silage to enhance its quality at low-temperature. Additionally, the production of biogenic amines during low-temperature ensiling was investigated.
The isolated strain Y28 exhibited robust growth capabilities at low temperature (10°C) and low pH (3.5), demonstrating psychrotolerance and acid tolerance. Inoculating oat forage with strain Y28 during ensiling notably improved the fermentation quality of oat silage under low-temperature conditions. The application of Y28 significantly reduced the presence of putrescine, cadaverine, histamine, tyramine, spermidine and total biogenic amines in silage. Amino acid decarboxylase activity assays revealed that strain Y28 has the capability to produce putrescine in synthetic screening media containing precursor amino acids. However, this potential does not necessarily translate to putrescine production under the complex conditions of ensiling, as confirmed by our experiments. It suggests that the impact of LAB species like Y28 on biogenic amine production may not solely stem from their own enzymatic activities, but also from their broader effects on fermentation quality. Min et al. (2007) reported that the organic acid, such as lactic acid, can reduce biogenic amines in meat. In this study, Y28 significantly increased lactic acid production, which could potentially inhibit the growth of pathogenic microorganisms and thereby prevent excessive accumulation of biogenic amines in silage. There is limited literature on the effects of different additives on the concentrations of individual and total biogenic amine in silage at low temperature. In fresh oat silages, Y28, PA, FA and SB treatments resulted in reductions of total biogenic amines by 55.9, 50.2, 58.9, and 21.1%, respectively, by the end of fermentation compared to the control oat silage. For wilted oat silages, the reduction percentages of total biogenic amine were 40.5, 56.2, 73.0, and 42.7%, respectively, for Y28, PA, FA, and SB treatment by the end of fermentation compared to the control. Clearly, Y28, PA, FA, and SB effectively inhibited biogenic amine formation in oat silage, with the lowest biogenic amine concentrations observed in the FA treatment. Specifically, when FA was applied, only 61.0 mg/kg DM of putrescine and 11.6 mg/kg DM of cadaverine were produced, compared to 173.5 mg/kg DM of putrescine and 350.1 mg/kg DM of cadaverine in the untreated control. Many bacteria genera, such as Bacillus, Clostridium, Escherichia, Proteus, Citrobacter, Klebsiella, Pseudomonas, Salmonella, Shigella, Photobacterium, as well as lactic acid bacteria like Lactobacillus, Pediococcus, Streptococcus, along with certain types of yeast, are known for their ability to decarboxylation amino acid (Pereira et al., 2001; Scherer et al., 2015). FA, PA, and SB are well known for their antimicrobial properties and are commonly used as additives in feed and food preservation (Rooke and Hatfield, 2003). The antimicrobial properties of FA, PA and SB probably inhibited decarboxylase activity, thereby reducing biogenic amines in oat silage.
Biogenic amines and ammonia nitrogen are primarily end products resulting from the degradation of amino acids. Studies by Van Os et al. (1996) and Nishino et al. (2007) have highlighted a strong correlation between ammonia nitrogen content and total biogenic amine concentrations in silages. In this study, a significant correlation (R = 0.49, p < 0.01) was observed between ammonia nitrogen and total biogenic amine concentrations. Ammonia nitrogen is produced through deamination reactions, whereas biogenic amines are generated via decarboxylation reaction (Kung et al., 2003; Xia et al., 2018). In the present study, wilted oat silages exhibited lower levels of total biogenic amines and ammonia nitrogen compared to fresh oat silages. Similar findings of reduced biogenic amine levels in wilted silages have been reported by Steidlová and Kalač (2002). Wilting appears to reduce the concentrations of biogenic amines and ammonia nitrogen in silage, this effect may be attributed to the reduction in water activity of forage. The decrease in water activity may inhibit the activity of both plant-and microbially-derived proteolytic enzymes, which are crucial for the breakdown of proteins into amino acids. This suppression of protein degradation may limit the availability of precursor amino acids for biogenic amine formation during fermentation. Additionally, the lower moisture content may create an environment that is less favorable for the growth and activity of microorganisms responsible for biogenic amine production. High levels of ammonia nitrogen and biogenic amines have been noted in clostridial silages (Tveit et al., 1992). However, the findings from the current study indicate that butyric acid was not detected despite the significant formation of biogenic amines. This suggests that the production of biogenic amines does not necessarily coincide with butyric acid formation.
It has been documented that biogenic amines accumulate in sauerkraut stored at low temperatures (Peñas et al., 2010), and concentrations of cadaverine and putrescine increase in pork meat during low-temperature storage (Halász et al., 1994) However, relatively little attention has been given to biogenic amine formation in silage. Biogenic amine concentrations in silage can indicate undesirable changes in forage (Scherer et al., 2015). In this study, eight biogenic amines (tryptamine, phenethylamine, putrescine, cadaverine, histamine, tyramine, spermidine and spermine) were detected in oat silages fermented at low temperature. Putrescine, cadaverine, and tyramine were the predominant amines, collectively representing approximately 90% of the total biogenic amines measured. Specifically, putrescine, cadaverine and tyramine in oat silages at low temperature were found at concentrations of up to 270.2, 637.9, and 595.7 mg/kg DM, respectively. The levels of biogenic amines observed in the present study exceed those reported by Steidlová and Kalač (2004) for untreated false oat (Arrhenatherum elatius) silages, where putrescine, cadaverine and tyramine levels were 182, 218 and 120 mg/kg DM, respectively. The maximum phenethylamine content in the present study was approximately 23.4 mg/kg DM, similar to levels found in low dry matter perennial ryegrass silage (phenethylamine 17 mg/kg DM) as reported by Van Os et al. (1996). Significant amounts of spermidine (51.5 mg/kg DM) and spermine (24.8 mg/kg DM) were previously detected in untreated oat silage (Křížek, 1993); however, in the present study, only trace amounts of spermidine and spermine were detected in oat silage fermented at low temperature. In the present study, the maximum levels of tryptamine and histamine were approximately 14.1 and 9.2 mg/kg DM, respectively, which are similar to the findings of Scherer et al. (2019), who reported tryptamine and histamine levels of 14.4 and 3.0 mg/kg DM, respectively, in maize var. Tereza silage. Five amines—tryptamine, phenethylamine and histamine, spermidine and spermine—were mostly detected at concentrations below 30 mg/kg DM, with some samples having levels below detection limits. Consequently, the biological effects of these amines on cattle may be considered limited. The type and amount of biogenic amine formation are influenced by various factors, including fermentation temperature, raw material quality, dry matter concentration, the addition of silage additives, and the microorganisms present (Křížek, 1993; Steidlová and Kalač, 2002; Scherer et al., 2019). Currently, there are no regulations governing the biogenic amine content in silages, and there is a lack of information on the minimum concentrations of biogenic amines that may negatively affect animal health. Tveit et al. (1992) found that the dry matter intake (DMI) of early lactation cows decreased when 100 g/day putrescine was infused intraruminally. Scherer et al. (2019) reported no reduction in feed intake in goats within a total biogenic amine concentration range of 1.2 to 4.1 g/kg DM. Van Os et al. (1995) observed that supplementing grass silage was a mixture of biogenic amines at 2.8 g/kg DM tended to reduce the DMI in sheep. Phuntsok et al. (1998) found the ruminal DM digestibility, volatile fatty acids, and intake decreased in steers fed diets that included more alfalfa silage, which contained 2,953 mg/kg DM of putrescine, 3,987 mg/kg DM of cadaverine, 376 mg/kg DM of tryptamine, 2,803 mg/kg DM of phenylethylamine, 12 mg/kg DM of spermine, and 3,078 mg/kg DM of histamine. The maximum total biogenic amine content in our study was 1539.4 mg/kg DM, which is significantly lower than the levels known to reduce voluntary intake of a silage-based diet. However, young animals may be more sensitive to biogenic amines than adults. Fusi et al. (2004) found that supplementing a diet with 1.4 g of mixture biogenic amines per day reduced the DMI and growth rate of weaned Saanen kids. From 30 to 60 days, our study showed an increase in total biogenic amines in both fresh and wilted oat forage. Consequently, the concentrations of total biogenic amines may be higher during later stages of silage feeding than those reported in Tables 7, 8. Therefore, the risk of detrimental health effects on animals consuming oat silages fermented at low temperature cannot be excluded.
Conclusion
In conclusion, the application of Lactobacillus plantarum strain Y28 enhanced the fermentation quality of oat silage at low temperature by decreasing pH and ammonia nitrogen content, while increasing the lactate content. This study also demonstrated that significant amounts of biogenic amines are formed during the low-temperature fermentation of oat silage, with fresh oat silage exhibiting higher levels of biogenic amines than wilted oat silage. The application of Y28, PA, FA and SB effectively inhibited biogenic amine accumulation, thereby improving the safety and quality of oat silage. Among these treatments, FA was the most effective in suppressing the formation of tyramine, cadaverine and putrescine. This research contributes valuable insights into the mechanisms by which L. plantarum Y28 and various additives can modulate the fermentation process and reduce the risk of biogenic amine formation in silage. Furthermore, it opens up new avenues for future research focused on optimizing microbial inoculants and additives to enhance silage preservation, safety, and nutritional quality, particularly in low-temperature environments. Future studies could explore the underlying metabolic pathways involved in biogenic amine production, as well as investigate the long-term effects of such interventions on animal health and productivity.
Data availability statement
The original contributions presented in the study are included in the article/supplementary material, further inquiries can be directed to the corresponding author.
Author contributions
YH: Conceptualization, Supervision, Writing – review & editing. TJ: Conceptualization, Data curation, Formal analysis, Funding acquisition, Investigation, Methodology, Project administration, Resources, Software, Supervision, Validation, Visualization, Writing – original draft, Writing – review & editing.
Funding
The author(s) declare that financial support was received for the research, authorship, and/or publication of this article. This work was financially supported by the Grass Seed Industry Core Technology Research Project (SJCZFY2022-5), the Fundamental Research Funds for the Central Universities (BLX202267), and Innovation and Entrepreneurship Training Program for College Students (S202410022238).
Conflict of interest
The authors declare that the research was conducted in the absence of any commercial or financial relationships that could be construed as a potential conflict of interest.
Publisher’s note
All claims expressed in this article are solely those of the authors and do not necessarily represent those of their affiliated organizations, or those of the publisher, the editors and the reviewers. Any product that may be evaluated in this article, or claim that may be made by its manufacturer, is not guaranteed or endorsed by the publisher.
References
AOAC International (2012). Official methods of analysis. 19th Edn. Gaithersburg, MD: AOAC International.
Bernardes, T. F., Daniel, J. L. P., Adesogan, A. T., McAllister, T. A., Drouin, P., Nussio, L. G., et al. (2018). Silage review: Unique challenges of silages made in hot and cold regions. J. Dairy Sci. 101, 4001–4019. doi: 10.3168/jds.2017-13703
Bover-Cid, S., and Holzapfel, W. H. (1999). Improved screening procedure for biogenic amine production by lactic acid bacteria. Int. J. Food Microbiol. 53, 33–41. doi: 10.1016/s0168-1605(99)00152-x
Broderick, G. A., and Kang, J. H. (1980). Automated simultaneous determination of ammonia and total amino acids in ruminal fluid and in vitro media. J. Dairy Sci. 63, 64–75. doi: 10.3168/jds.S0022-0302(80)82888-8
Cao, Y., Cai, Y. M., Hirakubo, T., Fukui, H., and Matsuyama, H. (2011). Fermentation characteristics and microorganism composition of total mixed ration silage with local food by-products in different seasons. Anim. Sci. J. 82, 259–266. doi: 10.1111/j.1740-0929.2010.00840.x
Dawson, L. E. R., and Mayne, C. S. (1996). The effect of intraruminal infusions of amines and gamma amino butyric acid on rumen fermentation parameters and food intake of steers offered grass silage. Anim. Feed Sci. Technol. 63, 35–49. doi: 10.1016/S0377-8401(96)01039-5
Dawson, L. E. R., and Mayne, C. S. (1997). The effect of infusion of putrescine and gamma amino butyric acid on the intake of steers offered grass silage containing three levels of lactic acid. Anim. Feed Sci. Technol. 66, 15–29. doi: 10.1016/S0377-8401(96)01149-2
Driehuis, F., Wilkinson, J. M., Jiang, Y., Ogunade, I., and Adesogan, A. T. (2018). Silage review: animal and human health risks from silage. J. Dairy Sci. 101, 4093–4110. doi: 10.3168/jds.2017-13836
Fusi, E., Rossi, L., Rebucci, R., Cheli, F., Giancamillo, A. D., Domeneghini, C., et al. (2004). Administration of biogenic amines to Saanen kids: effects on growth performance, meat quality and gut histology. Small Rumin. Res. 53, 1–7. doi: 10.1016/j.smallrumres.2003.07.009
Halász, A., Baráth, Á., Simon-Sarkadi, L., and Holzapfel, W. (1994). Biogenic amines and their production by microorganisms in food. Trends Food Sci. Tech. 5, 42–49. doi: 10.1016/0924-2244(94)90070-1
Kozaki, M., Uchimura, T., and Okada, S. (1992). Experimental manual for lactic acid bacteria. Tokyo, Japan: Asakurasyoten.
Křížek, M. (1993). Biogenic amines in silage. 1. The occurrence of biogenic amines in silage. Arch. Anim. Nutr. 43, 169–177. doi: 10.1080/17450399309386032
Kung, L., Stokes, M. R., and Lin, C. J. (2003). “Silage additives” in Silage Science and Technology. eds. D. R. Buxton, R. E. Muck, and J. H. Harrison (Madison, WI: American Society of Agronomy,Inc), 305–360.
Li, X. M., Chen, F., Wang, X. K., Sun, L., Guo, L. N., Xiong, Y., et al. (2021). Impacts of low temperature and ensiling period on the bacterial community of oat silage by SMRT. Microorganisms. 28:274. doi: 10.3390/microorganisms9020274
Liang, Z. Q., Chu, H., Gao, L., Sun, X. T., Guo, S. N., Guo, W. K., et al. (2024). Effects of probiotics on biogenic amines content in fermented milk during fermentation and storage. J. Food Compos. 127:105985. doi: 10.1016/j.jfca.2024.105985
Min, J. S., Lee, S. O., Jang, A. C., and Lee, M. (2007). Control of microorganisms and reduction of biogenic amines in chicken breast and thigh by irradiation and organic acids. Poult. Sci. 86, 2034–2041. doi: 10.1093/ps/86.9.2034
Murphy, R. P. (1958). A method for the extraction of plant samples and the determination of total soluble carbohydrates. J. Sci. Food Agric. 9, 714–717. doi: 10.1002/jsfa.2740091104
Nishino, N., Hattori, H., Wada, H., and Touno, E. (2007). Biogenic amine production in grass, maize and total mixed ration silages inoculated with Lactobacillus casei or Lactobacillus buchneri. J. Appl. Microbiol. 103, 325–332. doi: 10.1111/j.1365-2672.2006.03244.x
Ormanci, H. B., and Colakoglu, F. A. (2017). Changes in biogenic amines levels of Lakerda (salted Atlantic bonito) during ripening at different temperatures. J. Food Process. Preserv. 41:e12736. doi: 10.1111/jfpp.12736
Peñas, E., Frias, J., Sidro, B., and Vidal-Valverde, C. (2010). Impact of fermentation conditions and refrigerated storage on microbial quality and biogenic amine content of sauerkraut. Food Chem. 123, 143–150. doi: 10.1016/j.foodchem.2010.04.021
Pereira, C. I., Crespo, M. T. B., and Romão, M. V. S. (2001). Evidence for proteolytic activity and biogenic amines production in Lactobacillus curvatus and L. homohiochii. Int. J. Food Microbiol. 68, 211–216. doi: 10.1016/S0168-1605(01)00534-7
Phuntsok, T., Froetschel, M. A., Amos, H. E., Zheng, M., and Huang, Y. W. (1998). Biogenic amines in silage, apparent postruminal passage, and the relationship between biogenic amines and digestive function and intake by steers. J. Dairy Sci. 81, 2193–2203. doi: 10.3168/jds.S0022-0302(98)75798-4
Rooke, J. A., and Hatfield, R. D. (2003). “Biochemistry of ensiling” in Silage science and technology. eds. S. Science, D. R. Technology, and R. E. Buxton (Madison, WI: ASA), 95–139.
Scherer, R., Gerlach, K., and Südekum, K. H. (2015). Biogenic amines and gamma-amino butyric acid in silages: formation, occurrence and influence on dry matter intake and ruminant production. Anim. Feed Sci. Tech. 210, 1–16. doi: 10.1016/j.anifeedsci.2015.10.001
Scherer, R., Gerlach, K., Taubert, J., Adolph, S., Weiß, K., and Südekum, K. (2019). Effect of forage species and ensiling conditions on silage composition and quality and the feed choice behaviour of goats. Grass Forage Sci. 74, 297–313. doi: 10.1111/gfs.12414
Skladanka, J., Adam, V., Zitka, O., Mlejnkova, V., Kalhotka, L., Horky, P., et al. (2017). Comparison of biogenic amines and mycotoxins in alfalfa and red clover fodder depending on additives. Int. J. Env. Res. Pub. He. 14:418. doi: 10.3390/ijerph14040418
Steidlová, Š., and Kalač, P. (2002). Levels of biogenic amines in maize silages. Anim. Feed Sci. Technol. 102, 197–205. doi: 10.1016/S0377-8401(02)00217-1
Steidlová, Š., and Kalač, P. (2004). The effects of lactic acid bacteria inoculants and formic acid on the formation of biogenic amines in grass silages. Arch. Anim. Nutr. 58, 245–254. doi: 10.1080/00039420410001701378
Tveit, B., Lingaas, F., Svendsen, M., and Sjaastad, Ø. V. (1992). Etiology of acetonemia in Norwegian cattle. 1. Effect of ketogenic silage, season, energy level, and genetic factors. J. Dairy Sci. 75, 2421–2432. doi: 10.3168/jds.S0022-0302(92)78003-5
Van Os, M., Dulphy, J. P., and Baumont, R. (1995). The effect of protein degradation products in grass silages on feed intake and intake behaviour in sheep. Br. J. Nutr. 73, 51–64. doi: 10.1079/BJN19950008
Van Os, M., Van Wikselaar, P. G., and Spoelstra, S. F. (1996). Formation of biogenic amines in well fermented grass silages. J. Agric. Sci. 127, 97–107. doi: 10.1017/S0021859600077418
Van Soest, P. J., Robertsom, J. B., and Lewis, B. A. (1991). Methods for dietary fiber, neutral detergent fiber and nonstarch polysaccharides in relation to animal nutrition. J. Dairy Sci. 74, 3583–3597. doi: 10.3168/jds.S0022-0302(91)78551-2
Weinberg, Z. G., and Muck, R. E. (1996). New trends and opportunities in the development and use of inoculants for silage. FEMS Microbiol. Rev. 19, 53–68. doi: 10.1016/0168-6445(96)00025-3
Xia, X. L., Luo, Y., Zhang, Q. W., Huang, Y., and Zhang, B. (2018). Mixed starter culture regulates biogenic amines formation via decarboxylation and transamination during Chinese rice wine fermentation. J. Agric. Food Chem. 66, 6348–6356. doi: 10.1021/acs.jafc.8b01134
Zhang, M., Wang, L., Wu, G. F., Wang, X., Lv, H. X., Chen, J., et al. (2021). Effects of Lactobacillus plantarum on the fermentation profile and microbiological composition of wheat fermented silage under the freezing and thawing low temperatures. Front. Microbiol. 12:671287. doi: 10.3389/fmicb.2021.671287
Keywords: oat silage, lactic acid bacterial, additives, biogenic amine, low temperature
Citation: Huang Y and Jia T (2025) Effects of lactic acid bacterial and chemical additives on the quality and biogenic amine production of oat silage at low temperature. Front. Sustain. Food Syst. 9:1499741. doi: 10.3389/fsufs.2025.1499741
Edited by:
Raul Avila-Sosa, Benemérita Universidad Autónoma de Puebla, MexicoReviewed by:
Qingli Dong, University of Shanghai for Science and Technology, ChinaMaria Simona Chis, University of Agricultural Sciences and Veterinary Medicine of Cluj-Napoca, Romania
Copyright © 2025 Huang and Jia. This is an open-access article distributed under the terms of the Creative Commons Attribution License (CC BY). The use, distribution or reproduction in other forums is permitted, provided the original author(s) and the copyright owner(s) are credited and that the original publication in this journal is cited, in accordance with accepted academic practice. No use, distribution or reproduction is permitted which does not comply with these terms.
*Correspondence: Tingting Jia, amlhdHRAYmpmdS5lZHUuY24=