- 1Department of Marine, Earth and Atmospheric Sciences, North Carolina State University, Raleigh, NC, United States
- 2Nicholas School of the Environment, Duke University School of Law, Durham, NC, United States
- 3United States Department of Agriculture, Agricultural Research Service, Florence, SC, United States
- 4Department of Natural Resources and Environmental Design, North Carolina A&T State University, Greensboro, NC, United States
Concentrated animal feeding operations (CAFOs) have led to environmental challenges, specifically waste management. Swine CAFOs generate large amounts of waste, requiring proper treatment to avoid air and water pollution. Conventional waste management technologies, such as lagoon and spray field systems, do not prevent air and water pollution impacts. Research for the past few decades led to recommendations for waste treatment technologies superior to lagoons and spray fields. Private environmental sustainability initiatives focused on reducing greenhouse gas emissions in the food supply chain have implemented biogas digester projects for capturing methane in covered swine lagoons to reduce greenhouse gas emissions. However, research indicates that methane capture alone does not solve the broader pollution issues associated with lagoon and spray field systems still in use at these CAFOs to dispose of digested effluents. The Environmentally Superior Technologies (EST) initiative in North Carolina set public standards to eliminate waste discharge, reduce atmospheric emissions, and control odors and pathogens. Research has confirmed that technologies coupling solids separation with water treatments to remove volatile organic carbon, pathogens, and reactive forms of nitrogen can meet EST standards. A designated EST—the Super Soil System—substantially reduced odor by 99.9%; pathogens by 99.99%, nutrients (phosphorus and nitrogen) by >90%, and heavy metals (cooper and zinc) by 99%. The ammonia emissions were reduced by 94.4% for the warm and 99.0% for the cool season with respect to a conventional lagoon system. Corresponding greenhouse gas emission reductions were 96.7%. Components of designated EST can be applied to retrofit covered lagoons and anaerobic digestion systems with significant environmental benefits. Recommendations are proposed, based on the collective experience with EST and current trends in animal production concentration, for environmentally safe technologies to handle excess manure produced in the USA.
Introduction
Raising farm animals for meat and by-products has become an integral part of agriculture in the USA. To increase economic efficiency, small-scale farm animal production systems have given way to larger operations under corporate production management systems. This transformation, most prominent in poultry and swine production, has resulted in environmental and air quality challenges (Domingo et al., 2021). In the USA, large poultry farms are spread throughout the southeastern states. In contrast, large swine farms are located in a few states, such as Iowa, North Carolina, Missouri, and Minnesota. These poultry and swine operations are housed in high-density confined spaces called concentrated animal feeding operations (CAFOs).
A CAFO is defined to include waste management systems where animals are confined and fed for 45 days or more per year. The US Environmental Protection Agency defines swine CAFOs as an operation having over 2,500 pigs weighing more than 25 kg each, or an operation having over 10,000 pigs weighing less than 25 kg each (Copeland, 2010; USEPA, 2004). Swine CAFO facilities are not permitted to discharge waste/wastewater directly into the water supply or allow the wastewater to come into contact with the water bodies. Swine CAFO facilities produce enormous amounts of animal waste- a mixture of feces, urine, water, and spilled feed. Swine barns at CAFO facilities commonly have a slotted floor that permits the passage of excreted waste into a pit. In each case, a large volume of swine waste is created which must be properly managed and treated to protect human health and the environment.
Swine CAFO facilities typically treat liquid waste using a deep pit or lagoon system. In the deep pit system, swine waste is stored and treated under the swine housing itself primarily through anaerobic digestion. In the lagoon systems, a shallow holding area under the slatted floor holds waste which is then flushed into lagoons. Swine lagoons have aerobic layers when exposed to oxygen and anaerobic layers at deeper levels where oxygen is unavailable. The primary treatment processes in the lagoon are a combination of anaerobic and aerobic decomposition of waste by microbes. In turn this decomposition creates gases including methane and volatile organic compounds. In lagoon and sprayfield systems, liquid waste is disposed of by land application on cropland for crops such as Bermuda grass or corn. Lagoon and sprayfield systems generate noxious air pollutants, particulates, suspended dust, pathogens, and odorous compounds (Aneja et al., 2008d; Aneja et al., 2009; USEPA, 2014). Land application of waste coupled with extreme weather, such as too much rain during hurricanes, has been found to degrade surface and groundwater quality (Mallin, 2000; Hooda et al., 2000). Additional waste treatment technologies are required to supplement lagoons and sprayfields to prevent air and water pollution from the lagoon and sprayfield systems.
The objectives of this work are to review the negative environmental impacts of the conventional lagoon-sprayfield system used to manage manure in swine CAFOs in southeastern USA, versus the environmental benefits of: (1) using a covered lagoon for biogas capture and greenhouse gas (GHG) reduction, (2) a designated Environmentally Superior Technology system without a lagoon, and (3) covered lagoon and biogas digesters coupled with waste treatment technologies that are environmentally superior to lagoons and sprayfields to protect human health and the environment. We also propose recommendations and policy changes to address these findings.
The negative environmental impacts of the anaerobic lagoon-spray field system documented in this review for the southeastern USA are also of interest to other intensive swine production regions in the world that adopted the lagoon-spray field system, which was initially developed in North Carolina. The environmental benefits of Environmentally Superior Technologies, and their components or modules, developed in North Carolina 30 years later and documented in this review, should be of interest to all intensive swine production regions throughout the world that seek to reduce air and water pollution from manure management, especially concentrated areas that rely only on anaerobic digesters in their manure management.
Swine waste anaerobic lagoons create wastewater, sludge, and air pollutants
The United States Department of Agriculture’s Natural Resources Conservation Service has developed its Agricultural Waste Management Field Handbook (AWMFH) to provide guidelines for handling agricultural wastes, including animal wastes. The AWMFH-Animal Waste Management guidelines provide tools to estimate manure production, animal waste storage needs, and design standards for various types of animal waste treatment facilities, including waste lagoons (USDA, 2018a). Eastern North Carolina’s swine farms are dominated by lagoon and sprayfield systems, where lagoons are built for the biological treatment of wastewater and consist of an excavated pit with an earthen embankment or levee (Miller and Longest, 2020; USDA, 2019). The liquid manure is transferred from the barn to the lagoon to reduce water pollution potential for nitrogen, phosphorus, and biological oxygen demand (USDA, 2019). Relying on settled manure to provide sealing of lagoons is ineffective in soils with low clay content. Therefore, as part of the design, state regulations require clay or synthetic liners to avoid polluting surface and groundwater with lagoon seepage (USDA, 2008). Anaerobic lagoons depend on physical, chemical, and biological processes, but biological processes play the most significant role in the anaerobic decomposition of liquid manure (Hamilton et al., 2006). Anaerobic lagoon systems store and treat liquid manure wastes with high loads of organic matter in the absence of oxygen. These processes decompose organic matter but less efficiently than aerobic ones per-unit volume. The extent of organic matter degradation depends on influent characteristics and microbial communities in the anaerobic lagoon (Hamilton et al., 2006). They transform large organic compounds into smaller, volatile organic compounds and manure proteins and urea into ammonia gas. Ammonia (NH3), reduced sulfur (e.g., H2S), and volatile organic compounds (VOCs) contribute to emissions of air pollution and odor impacts from the lagoons (Aneja et al., 2000; Blunden et al., 2008; Blunden and Aneja, 2008; Aneja et al., 2008d; Rumsey et al., 2014; Rumsey and Aneja, 2014).
While lagoon waste liquid volume is drawn down by land-applying effluents during the crop season, lagoons must never become fully emptied—a minimum operating liquid level keeps biological degradation processes active in anaerobic lagoons (USDA, 2019). Specific lagoon design standards provide lagoon sizing with sufficient volume for sludge accumulation and waste treatment, plus volume for liquid manure storage and rainfall storage produced between drawdown events (ASABE, 2011). Lagoon systems were designed to have several advantages over the land disposal of raw animal waste. Compared to handling raw manure and urine, lagoon systems reduce labor and operating costs for removing manure from barns by allowing regular flushing and emptying of animal waste from the barns without removing the animals.
To maintain lagoon treatment performance, anaerobic lagoon design standards require the removal of sludge solids before sludge fills up the treatment volume. This is done through agitation of the sludge or dredging plus dewatering (Owusu-Twum and Sharara, 2020). However, the cost of removing sludge can become an economic challenge for the producers, especially when lagoon designs and wet weather create sludge management problems. Sludge management options in eastern North Carolina are limited since cropland areas near swine CAFO facilities have nutrient surpluses and the hauling costs associated with transporting the material to distant fields are large (Owusu-Twum and Sharara, 2020). The combination of sludge management challenges threatens the long-term use of lagoons and sprayfields as both contribute to the sludge management problem. Abundant nutrients from ongoing waste spraying reduces demand for sludge nutrients in nearby cropland, however, the use of lagoons increases sludge buildup over time.
Swine lagoon wastewater applied to sprayfields creates a pathway for water pollution
From the mid-1960s through the 1970s, researchers with backgrounds in agronomy and livestock management researched the impact of swine waste management on water quality (Humenik, 1972; Humenik et al., 1974). They identified several pathways for water pollution that needed to be addressed. When animal manure is land-applied to crops through sprayfields, there are physical and biological processes that are sensitive to miscalculation, operator error, or equipment failure, leading to over-application of waste beyond the crops and soils’ ability to take up the waste. When over-application occurs, nutrients accumulate and then run off into the stream network, causing eutrophication. Therefore, if nutrient applications are not precisely aligned with crop needs, degraded water quality results. If manure is applied aligned with crop nitrogen (N) requirements, phosphorus (P) may be in excess (Mallin and McIver, 2018). Because of the imbalance of N and P in animal manure (N:P < 4:1) relative to crop needs (N:P = 8:1), land application of lagoon effluents at optimal N rates for crop growth results in the accumulation of P in soils and insufficient use of plant nutrients by crops (Szogi et al., 2015). Phosphorus enrichment contributes to eutrophication in P-sensitive ecosystems and has been linked to Pfiesteria piscida outbreaks—a toxic microorganism causing mass fish kills—in North Carolina waters (Burkholder et al., 2007).
Nitrates can also escape crop uptake on sprayfields leading to surface or groundwater nitrate pollution. Elevated nitrate in drinking waters from wells has been known to cause methemoglobinemia or blue-baby syndrome (Johnson and Bonrud, 1988). USEPA found that 29 states have identified AFOs (Animal Feeding Operations) as contributing to water quality impairment (Copeland, 2010). In 1995, 22 million gallons of liquid swine waste entered North Carolina’s Neuse River and its estuary due to a waste lagoon rupture (Aneja et al., 2001a,b). Similarly, major CAFO accidents have occurred in Iowa, Maryland, and Missouri (Thu and Durrenberger, 1998; Mallin, 2000). Miralha et al. (2022) have monitored CAFOs in 16 states across the United States for N and P levels relating to water quality. When manure was land applied, high levels of N and P were found in watersheds where clusters of CAFOs were present. The United States Geological Survey (USGS) has conducted studies on animal manure’s impact on water quality in southern US states, and their reports indicated significant levels of nutrients, pathogens, and pharmaceutical chemicals contaminating streams and rivers. Also, pollutants can enter the groundwater through leakage from poorly constructed manure-holding lagoons. These open lagoons are prone to overflow during unexpected high rainfall or hurricane events.
Veterinary pharmaceuticals (Boxall et al., 2004) and hormones (Kjaer et al., 2007; Shappell et al., 2007) have also been found in animal manure (Abrol et al., 2017). Once manure is applied to agricultural fields, these compounds can persist in soils for days to years. Shappell et al. (2007) found that estradiol (hormone) equivalents in a manure pit (washed manure from a farrowing swine facility) ranged from 843 to 858 pM. Still, estradiol in lagoon wastewater levels ranged from 6.4 to 11.5 pM (37pM = 10 ng). Kjaer et al. (2007) found that 17 beta-estradiol (E2) and its degradation product estrone (E1) were 68.1 2.5 ng/L, respectively, were found in leachates from the root zone to tile drainage system after 3 months of field application of swine manure.
At least 17 classes of antimicrobials are approved for use in animals raised in the United States to control diseases (Anderson et al., 2003). The use of antimicrobials in animal production is a significant concern because of the potential for developing antimicrobial resistance. Ibekwe et al. (2016) reported that E. coli bacteria in swine wastewater were highly resistant to tetracycline, erythromycin, ampicillin, and streptomycin.
Lagoon and sprayfield systems create a pathway for air pollution
In the mid-1980s, livestock management experts reported that storage of livestock feces and urine under swine confinement housing created toxic gasses and hazardous particulates and recommended ventilating these harmful air pollutants to the ambient environment to protect the swine and humans working in the confinement housing (Barker et al., 1986). Air pollutants are released in each of the swine waste management steps at swine CAFO facilities using a lagoon and sprayfield treatment system. Swine CAFOs generate noxious air pollutants such as ammonia (NH3), hydrogen sulfide (H2S), volatile organic compounds (VOCs), organic acids, particulate matter, and other gases (Aneja et al., 2000; Aneja et al., 2008c; Aneja et al., 2009; Heinzen, 2015). Swine waste also contains hormones, antibiotics, heavy metals, nutrients, solids, pathogens, and particulate dust (Scanes, 2018). The air around CAFOs can be contaminated with high concentrations of particulates or suspended dust, of which one-third is respirable (PM2.5 and PM10). Casey et al. (2006) described in detail the measurements of air emission methods and emission rate calculations from different animal facilities in different states.
Recent studies have used a mass balance approach to estimate NH3 emission rates from liquid swine manure and have found that swine houses represent a more significant source than previously thought (Doorn et al., 2002). Based on a review of published data, the loss of NH3 from swine houses was estimated to be around 15% of the total N excreted (Westerman et al., 2000). This study (Westerman et al., 2000) also used a mass balance approach to estimate NH3-N emissions from different components of advanced manure treatment and lagoon spray technologies, as well as N excretion rates, based on swine population and feed data. Normalizing emissions by N excretion rate, percentage reductions in NH3-N emissions are determined for water-holding structures, barns, and the whole farm for each Environmentally Superior Technologies (EST) facility from their estimated values for the appropriate Lagoon and Sprayfield Technology (LST) farm (Aneja et al., 2008a,b,c). Aneja et al. (2000) measured ammonia fluxes seasonally from a 2.5 hectares (ha) hog waste storage lagoon in NC. They found higher ammonia fluxes in summer (mean NH3 flux 4017 ± 987 μg N m−2 m−1) followed by a decrease in spring, then fall followed by winter as 1706 ± 552 μg N m−2 m−1, 844 ± 401 μg N m−2 m−1, and 305 ± 154 μg N m−2 m−1, respectively. Griffing et al. (2004) used the mass balance method to estimate that approximately 80% of NH3 loss was due to volatilization from liquid waste storage systems. Szögi and Vanotti (2007) found that 80% of annual nitrogen loss was as NH3 volatilization after anaerobic decomposition in lagoons.
The NH3 released from swine waste has a mean life in its gaseous state of about 14–36 h, depending on weather conditions. It reacts rapidly with acidic agents such as sulfuric acid, nitric acid, and hydrochloric acid to form fine-particulate ammonium (NH4) salts (i.e., PM2.5) (Renard et al., 2004; Wiegand et al., 2022). These NH4 salts are the main components of smog aerosols, causing visibility problems. These particulates linger in the atmosphere for days and can be transported hundreds of miles. Also, these fine particulates will lead to respiratory problems in humans. Ammonia is deposited by dry (wind drift) or wet (precipitation) mechanisms. When ammonia is deposited on soils, consequences include soil acidification, nitrification, NH4 adsorption to the clay particles, and subsequent runoff along with soil particles. Nitrate from nitrification is soluble and contaminates ground water through leaching processes. Ammonia is also an important contributor to surface water eutrophication (Whitall et al., 2003).
In addition to NH3, other gases produced by manure decomposition create odor impacts on humans and other animals. Odor generation is a complex process that depends on feed types, manure storage method and length of storage, manure temperature, pH, and bacterial species. Bacterial species and communities act on manure and produce an extensive range of volatile organic compounds which are responsible for odors emitting from storage pits or lagoons (Aneja et al., 2000; Blunden et al., 2008; Rumsey and Aneja, 2014).
Hydrogen sulfide (H2S) is an air pollutant of great concern. It is also a toxic gas with a characteristic odor. It is produced in all anaerobic manure storage systems such as underground storage tanks and outside earthen manure-holding lagoons. H2S results from sulfur-containing proteins present in manure. Liu et al. (2014) measured H2S emitting from deep pits, recharge pits, and lagoons receiving wastewater from farrowing, gestation, and finishing houses. They found that farrowing houses showed higher emission rates of H2S (2.5 kg yr.−1pig−1) than other houses. Rumsey and Aneja (2014) measured H2S seasonally from swine lagoon at CAFOs and found that H2S fluxes were higher in summer (3.81 ± 3.24 ug m−2 m−1) and lowest in winter (0.08 ± ug m−2 m−1). After exposing subjects to either control or to diluted swine waste odorous compounds, Schiffman et al. (2005) found that test subjects were 4.1 (p = 0.001) times more likely to report headaches, 6.1 (p = 0.004) times more likely to report eye irritation, and 7.8 (p = 0.014) times more likely to report nausea in the swine air (experimental) condition than in the control condition (Schiffman et al., 2005).
Lagoon and sprayfield systems create greenhouse gases from swine waste
Methane (CH4) is a potent greenhouse gas and the second most important GHG contributor to climate change following carbon dioxide (CO2). On a 100-year timescale, methane has 28–34 times greater global warming potential than carbon dioxide and is 84–86 times more potent on a 20-year timescale (UNECE, 2024). Methane is oxidized to CO2 in the presence of water vapor. The emissions of CH4 occur when liquid manure is collected in underground pits in animal confinement operations in an anaerobic digester. During this period CH4 and CO2 emissions occur due to the organic matter decomposition. The rate of CH4 emissions depends on the type of manure (percent of volatile solids) and retention time in storage pits, tanks, or lagoons. Estimating CH4 emission rates in field conditions is difficult due to expensive equipment, environmental variables (wind speed, wind direction, temperature, pH), and seasonality. Therefore, highly variable rates may be observed between farms. Methane emission rates from deep swine pits have been measured at 5.5 + 1.1 kg CH4 per finished pig (Liu et al., 2014), 0.24 to 63 mg m−2 min−1 (Park et al., 2006), and 15 mg m−2 min−1 by Zhan et al. (2001). An inventory of 15 different digestates showed an average residual gas formation of CH4 of 5 m3 ton−1 (range 1-10 m3 CH4 ton−1 digestate) for central manure systems (Van Lier et al., 2008). Methane emissions vary over the year, because methane production is exponentially related to temperature (Harper et al., 2014). Methane fluxes from an anaerobic swine lagoon ranged from 1 to 500 kg ha−1 d−1 and the average flux for the year was 52.3 kg of CH4 ha−1 d−1 (Sharpe and Harper, 1999). Biogas digester systems are touted for their ability to reduce CH4 emissions (Miller and Longest, 2020).
Nitrous oxide (N2O) is a significant greenhouse gas with 298 times more global warming potential than CO2 over 100 years (Ren et al., 2017). N-containing substances in the manure are converted to ammonium (NH4) during the manure decomposition process. In aerobic conditions, NH4 is oxidized to nitrate (NO3), which can then undergo reduction to NO− N2O and N2 if anaerobic conditions exist. Nitrous oxide is generated during anaerobic digestion and manure application and in partially aerobic soil that has received swine manure slurry (Onema et al., 2005). Eighty-five selected publications and their meta-analytical results showed that animal manure amendments to agricultural soils have significantly increased N2O emissions from agricultural soils by 17.7% (Shakoor and Mehmood, 2021), while Vac et al. (2013) concluded ~37% of global N2O emissions are from land application and storage of animal manure. Nitrous oxide (N2O) is a potent greenhouse gas and essentially an inert gas in the atmosphere. It has no significant sinks at the Earth’s surface and may be transported to the stratosphere (Muller, 2021).
Anaerobic digestion for biogas production and GHG emission reduction
Anaerobic digestion (AD) is a method for converting biomass into methane biogas, which can produce energy or bioenergy. Livestock manure is a commonly used biomass material to produce bioenergy. Many livestock (hog and cattle) manure treatment systems rely on open lagoons where the CH4, CO2, NH3, and other gases, such as reduced sulfur compounds, and volatile organic compounds (VOCs), are emitted into the atmosphere. When these open systems are covered, gaseous emissions are reduced, which results in the effluent leaving the anaerobic digester, known as digestate, with a modified chemical content [e.g., total solids, carbon, ammonia, ammonium (NH4+), and pH], relative to waste from a conventional open lagoon system. The digestate contains more NH4+ due to a reduction in ammonia emissions from the anaerobic digester (i.e., covered lagoon) to the atmosphere and has less degradable biomass carbon than the substrate in an open lagoon resulting in changes in GHG and NH3 emissions (Clemens et al., 2006).
In 2017, the large retail superstore Wal-Mart launched Project Gigaton, an initiative of its corporate environmental sustainability commitments (Miller and Longest, 2020). Under this initiative, Wal-Mart has recruited its suppliers to reduce their greenhouse gas emissions in order to help Wal-Mart reach a Gigaton reduction from 2017 levels by 2030 (Miller and Longest, 2020). One of Wal-Mart’s suppliers, Smithfield Foods, has implemented a number of swine waste biogas digester projects in North Carolina, seeking credit for greenhouse gas reductions by capturing methane that would otherwise escape from its swine waste lagoons (Miller and Longest, 2020). Since first announcing its commitment to Project Gigaton, Smithfield joined with the large energy utility Dominion in a commitment to invest more than $500 million to build biogas infrastructure and projects. Notably absent from these commitments were promises to reduce the impact of swine waste from noxious air pollutants, particulates, suspended dust, pathogens, odorous compounds, water pollutants, or groundwater contamination. Biogas digesters do not solve the pollution problems characteristic of the lagoon and sprayfield systems, as research conducted under the Smithfield Agreement demonstrated (Miller and Longest, 2020). Research has demonstrated that lagoons and sprayfields are inadequate to protect human health, especially the health of people who live nearby, and to protect the environment (Miller and Longest, 2020). Implementing biogas digesters should be coupled with waste treatment technologies that are environmentally superior to lagoons and sprayfields to protect human health and the environment.
Producer agreements funded research to replace lagoon systems with designated ESTs
New swine CAFO facilities using lagoons and sprayfields as their waste management systems were widely deployed across North Carolina. Between 1989 and 1995, vertically integrated corporations and their contract growers built 700 CAFO facilities in Eastern North Carolina while 7,000 smaller hog farmers went out of business (Miller and Longest, 2020). The number of swine being raised per farm increased dramatically while the total number of farms decreased just as dramatically. Subsequent policy-making attention was directed to environmental, social, and political issues related to this growth in size of operations and concentration geographically. Research agendas were developed to study the impacts of swine waste management systems on the total environment as well as systems and treatment technologies superior to the lagoon and sprayfield system (Longest, 2005).
Concerns were raised by North Carolina-based News and Observer in a series of articles captioned “Boss Hog” in 1995 (Longest, 2005). These articles showcased how North Carolina’s environmental managers had allowed excess growth in the industry coupled with lax regulation. Following the publication of these articles, several lagoons and sprayfield systems experienced disastrous physical failures, including the Oceanview Farm spill estimated at more than 25 million gallons of swine waste spilled into a local waterway (Longest, 2005). Regulators inspected swine farms and legislators enacted restrictions on new farms, adding construction setback distance requirements to lagoon and spray field sites. Massive fish kills linked to nutrient overloads in coastal water basins prompted more stringent actions. In 1997, the North Carolina General Assembly enacted a moratorium on swine waste system construction while it studied the impacts of swine waste management.
On July 25, 2000, the Smithfield Agreement was signed between the Attorney General of North Carolina and Smithfield Foods as well as its subsidiary companies operating in North Carolina. The Smithfield Agreement provided resources from Smithfield for the implementation of innovative swine waste management systems and evaluation of “Environmentally Superior Technologies” (“EST”) through a framework led by a research team from North Carolina State University and other research universities (Agreement, 2000). A subsequent agreement was signed with Premium Standard Farms, the second largest producer, as well as an organization representing swine farmers (Longest, 2005). The “Designee” under the Smithfield Agreement, Dr. Mike Williams, led the effort to identify EST including research partnerships and stakeholder engagements (Williams, 2009). EST was defined by the Agreement as any technology or combination of technologies that is permittable by the appropriate governmental agencies, is determined to be technically, operationally, and economically feasible, and meets the following environmental performance standards taken from the North Carolina General Assembly’s standards under the moratorium:
1. Eliminate the discharge of animal waste to surface waters and groundwater through direct discharge, seepage, or runoff.
2. Substantially eliminate atmospheric emissions of ammonia.
3. Substantially eliminate the emission of odor that is detectable beyond the boundaries of the parcel or tract of land on which the swine farm is located.
4. Substantially eliminate the release of disease-transmitting vectors and airborne pathogens.
5. Substantially eliminate nutrient and heavy metal contamination of soil and groundwater.
In addition to the environmental standards, economic and operational feasibility criteria were considered in a process overseen by the Designee (Agreement, 2000; Williams, 2004; Williams, 2009). The Designee oversaw advisory board meetings, project operation, technology evaluation, and economic data review. Following this process, the Designee issued three technology determination reports with only one on-farm liquid waste treatment technology designated as meeting the EST process for new swine farms in North Carolina: the Super Soils Technology (Williams, 2009). Several technologies for processing separated solids were also approved. No biogas digester technologies met EST determination on their own, but some anaerobic digester technologies were identified as needing additional treatment systems to meet the standards (Williams, 2009).
Following the issuance of the Phase Three Report from the Designee, Dr. Williams, some farms continued to develop improvements on their technologies. In 2007, the North Carolina General Assembly enacted Senate Bill 1465 (N.C. Sess. Law § 2007-523) (General Assembly of North Carolina Session, 2007). Senate Bill 1465 assisted farmers financially in installing EST systems while also making permanent the requirements that new swine farms and swine waste management systems meet the standards of the EST process (NC Legislature, 2007). It also established a swine farm methane-capture pilot program. The outcome of the EST research initiative was recognized worldwide for its impact on engineering, air quality, animal science, food safety, and climate change (Vanotti M. et al., 2009). But existing farms were allowed to keep operating indefinitely under the law without converting to technologies which met the EST.
Super Soils technology background
This was the only on-farm technology of 18 evaluated that met all the EST standards under the Smithfield Agreement. The Super Soils on-farm system treated the liquid waste stream from the swine using a solid–liquid separation and nitrogen and phosphorus removal processes that replaced traditional anaerobic lagoons with a system that produces a clean, deodorized, and disinfected effluent (Figure 1). Treated water was reused in the barns and as irrigation water. Solid–liquid separation up-front in a treatment train allows recovery of the organic compounds that can be used for the manufacture of compost materials, peat substitutes, biochars, etc.
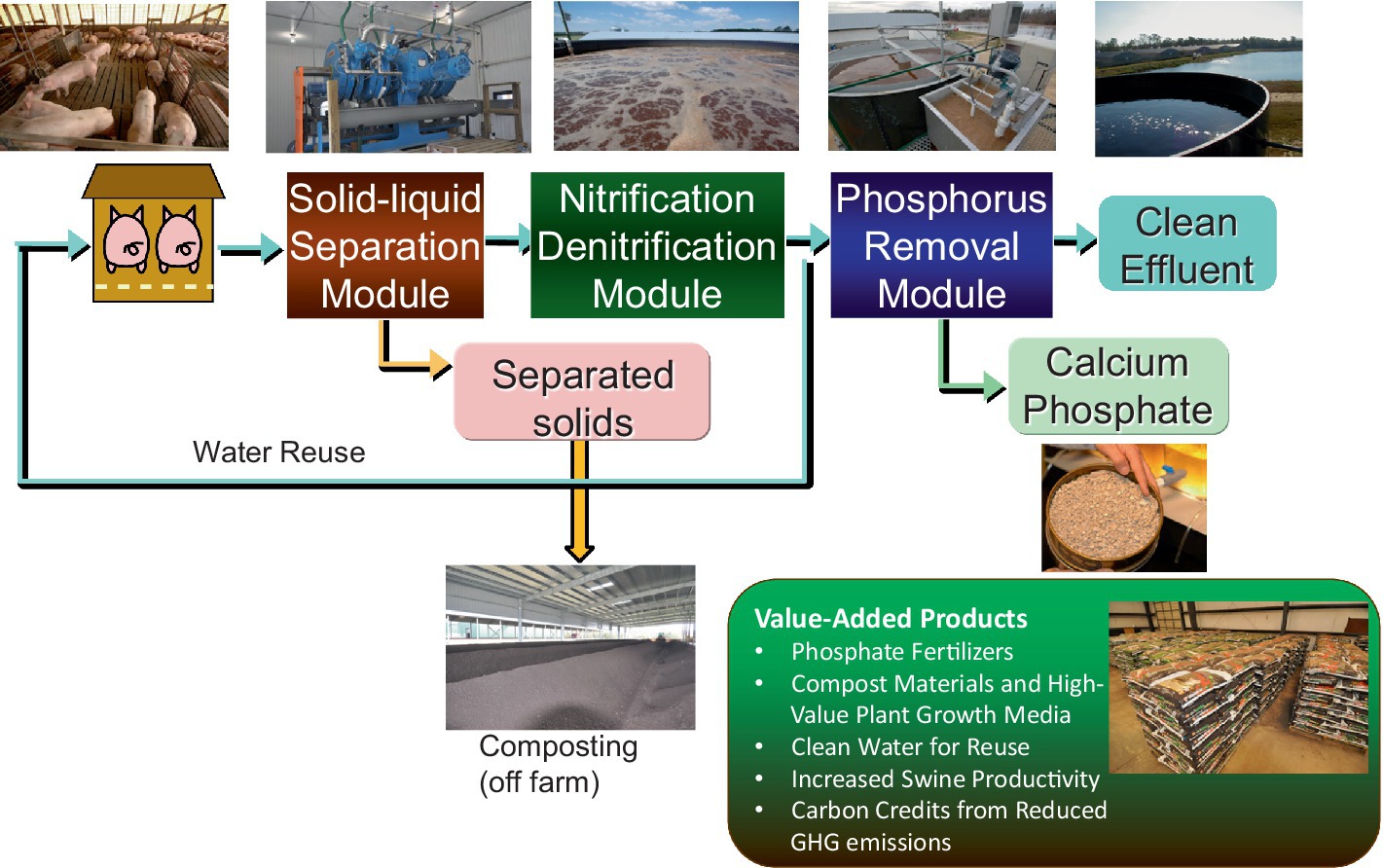
Figure 1. Schematic of the Super Soils system (Vanotti et al., 2005; Vanotti et al., 2010) applied to systems without lagoons and value-added products. The on-farm system used three modules: the first to separate organic solids from the liquid manure, the second to remove the ammonia and odors, and the third to separate phosphorus and disinfect the effluent. A closed loop recycled treated water was used to recharge the barn pits. The organic solids were further processed off-farm in a centralized composting facility.
The liquid treatment begins with separating the solid and liquid portions of the waste stream. Solids separation is accomplished using polyacrylamide, a flocculating agent. The liquid portion of the waste stream goes to a nitrogen module where the liquid flows between tanks in a circulating loop undergoing denitrification due to anaerobic activity in one tank, and nitrification through the use of concentrated nitrifying bacteria in the second tank under aerobic conditions. Nitrogen is removed from the waste stream during this stage of the process. The liquid then flows to a settling tank, where phosphorus is removed through the addition of calcium hydroxide and a dewatering bag system. Calcium phosphate, which has value as a fertilizer, precipitates during this process, providing a value-added product. During phosphorus removal, the pH of the liquid is raised to 9.5–10.5 using lime, which precipitates the soluble P and disinfects the effluent. Roughly 80% of the liquid is recycled through the hog houses, while 20% is used to irrigate crop fields.
The Super Soils project was completed with composting of the separated manure solids, which was done in a centralized facility in Sampson County, NC (Aneja et al., 2008b) that processed dewatered solids from the three full-scale projects. The composting process used a mixture of manure and cotton gin residue and produced class-A composts and value-added organic products for use in horticultural markets (Vanotti et al., 2006). The Super Soils compost system also met the environmental standards of an EST. Three other technologies that processed separated swine solids in centralized facilities also met EST requirements under the Smithfield Agreement: they used high-solids anaerobic digestion or gasification processes (Williams, 2009).
First-generation Super Soils system was demonstrated to meet EST standards
Super Soils was the first on-farm system certified by the Designee as an EST due to its efficacy in reducing ammonia emissions, excess nitrogen and phosphorus, pathogens, odors, and heavy metals (Williams, 2009). It was invented by USDA and is covered by US Patents 6,893,567 and 7,674,379 (Vanotti et al., 2005; Vanotti et al., 2010) that are now available for public use. The first-generation version of the Super Soils technology was first pilot-tested for 2 years at the North Carolina State University’s Lake Wheeler Rd. Swine Unit (Vanotti et al., 2001). Subsequently, the same system was scaled up (125:1) for performance verification of EST. It was installed and demonstrated at full-scale for 2 years on Goshen Ridge farm, a 4,360-head finishing swine operation with 6 barns in Duplin Co., North Carolina (Vanotti et al., 2007; Vanotti and Szogi, 2008). The manure was collected under the barns using slatted floors and a pit-recharge system. With the new treatment system, the flow of raw wastewater into the lagoons was discontinued; instead, all the raw wastewater was sent to the treatment plant (Figure 2).
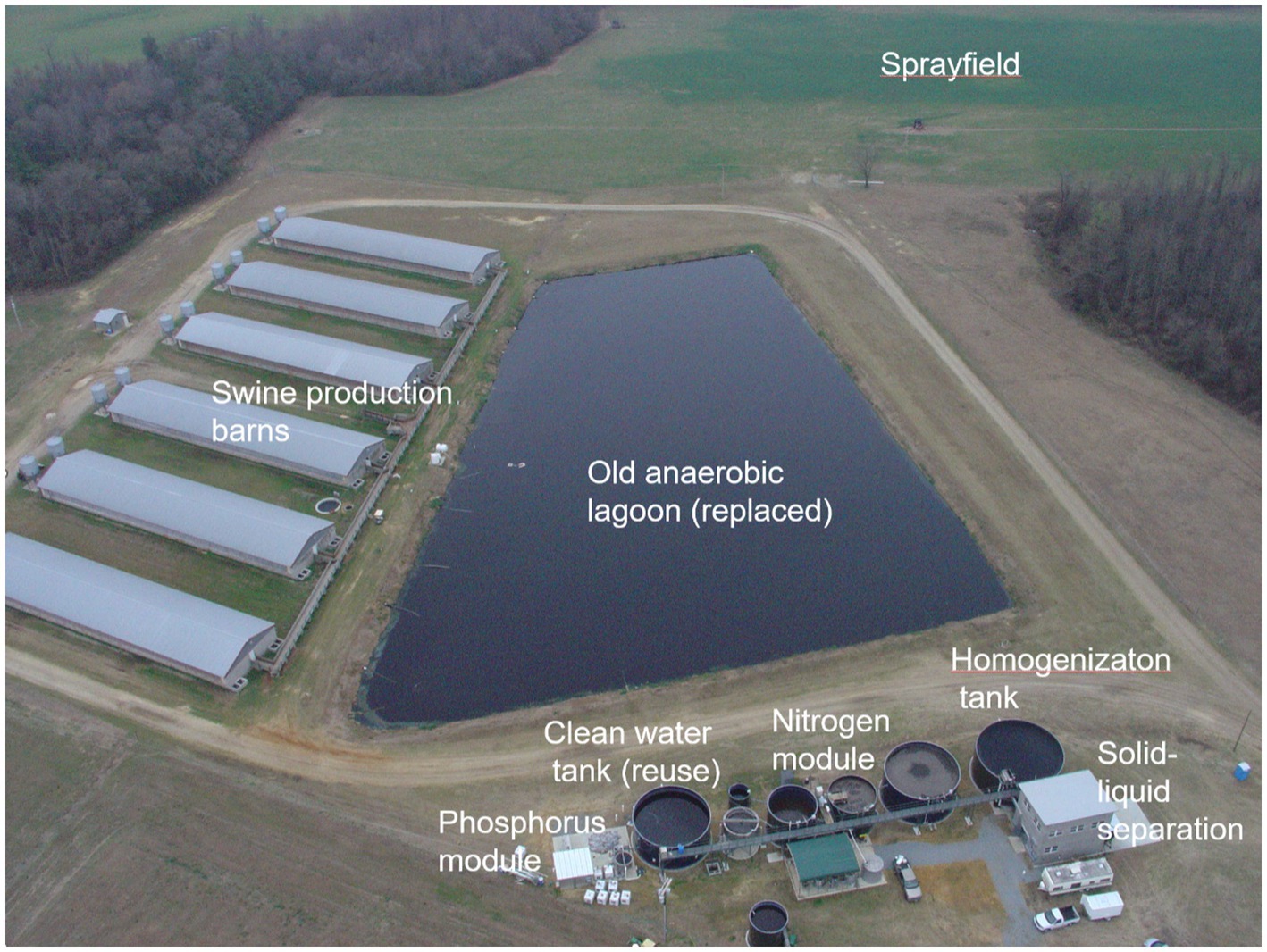
Figure 2. First-generation EST on-farm system using solid–liquid separation, nitrogen, and phosphorus modules treating all the animal waste from a 4,360-head swine finishing farm in Duplin County, NC.
The barn pits were flushed once a week as before, but the flushed manure (barns 1–6) was diverted into a homogenization tank that mixed the manure before the solid–liquid separation step. The separated liquid moved into the nitrogen module that contained polymer-immobilized nitrifiers. The nitrified wastewater was continually recycled into the DN (denitrification) tank using a Modified Ludzack-Ettinger (MLE) configuration. In the DN tank, suspended denitrifying bacteria used abundant soluble manure carbon and volatile odorous compounds in the separated manure to transform oxidized nitrogen into N2 gas. In the third step of the system—the phosphorus treatment/disinfection module—the soluble P was recovered as a calcium phosphate solid, and pathogens were substantially reduced by the alkaline environment (pH 10.5). A portion of the water after ammonia treatment was used to recharge the barn pits for flushing. The first-generation system removed from the wastewater 97.6% of the suspended solids, 99.7% of BOD, 98.5% of total Kjeldahl nitrogen (TKN), 98.7% of ammonia (NH4+-N), 95.0% of total P, 98.7% of copper, 99.0% of zinc, 97.9% of malodorous aromatic compounds, and produced a sanitized effluent with a reduction in the number of pathogenic bacteria to non-detectable levels (Vanotti et al., 2007). According to Aneja et al. (2008b), the NH3 emissions for this first-generation wastewater treatment plant were reduced by 94.4% for the warm season and 99.0% for the cool season with respect to a conventional lagoon system. In addition, the system transformed the old lagoon into an aerobic reservoir within a year and significantly reduced lagoon odor (Loughrin et al., 2006). Recommendations were also made to evaluate an improved, redesigned second-generation version of the wastewater treatment system. Therefore, a lower-cost, second-generation treatment system was designed and demonstrated full-scale on B&B Tyndall farm, a 5,145-head finishing swine operation in Sampson County, North Carolina.
Second and third-generation Super Soils system was demonstrated to meet EST standards with reduced costs
The second-generation system (Figure 3 and Table 1) provided improvements while meeting EST standards (Vanotti M. B. et al., 2009). Data in Table 1 shows the key contributions of each component of the technology toward the total efficiency of the system. Solid–liquid separation with polymers was effective in separating suspended solids, oxygen-demanding organic compounds, and organic nutrients and heavy metals by capturing the suspended particles. This efficient removal of suspended solids early in the treatment train is a significant departure from treatment typically used in municipal wastewater systems because it recovers most of the organic carbon and organic nutrient compounds contained in the liquid manure, therefore enabling the conservation and generation of value-added products. The system recovered most (97 and 95%, respectively) of the organic phosphorus and nitrogen contained in the flushed manure, which left the farm and left the swine production farm and were incorporated into compost products. ICF International (2013) provided the total cost of the technology for a 6,000-head finishing farm in NC as well the various processes in the system: Total capital costs: (1) Waste evacuation: $20,000, (2) Solid removal module: $300,000, (3) Soluble N removal module: $180,000, and Soluble P removal module: $56,000, resulting in a total capital cost of $556,000. The yearly operations and maintenance costs were $ 58,000 total, separated as follows: (1) electricity: $12,000, Maintenance: $10,000, and all other costs: $36,000.
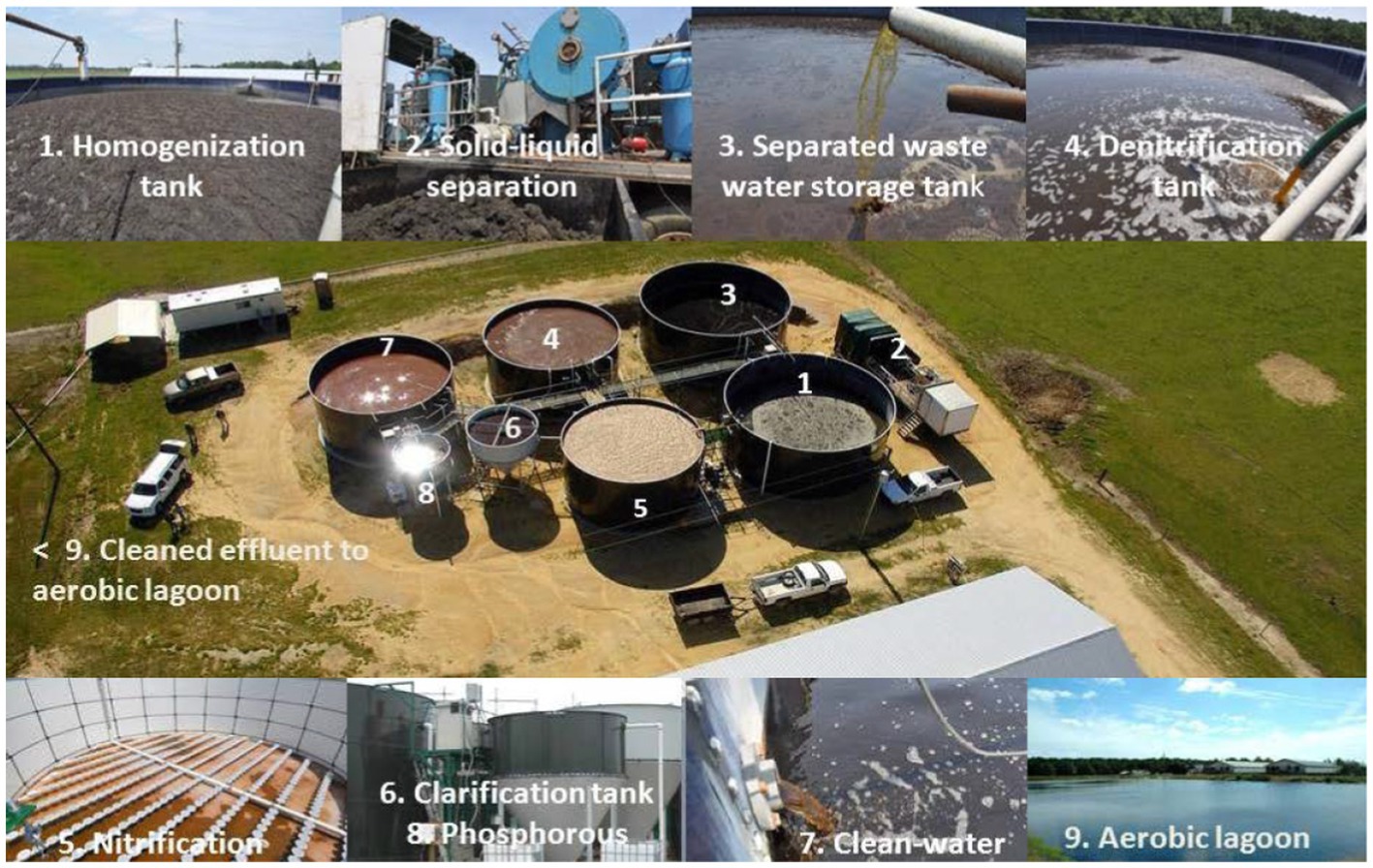
Figure 3. Aerial picture of the second-generation EST on-farm system using solid–liquid separation, nitrogen, and phosphorus modules treating all the animal waste from a 5,145-head swine finishing farm in Sampson County, NC.
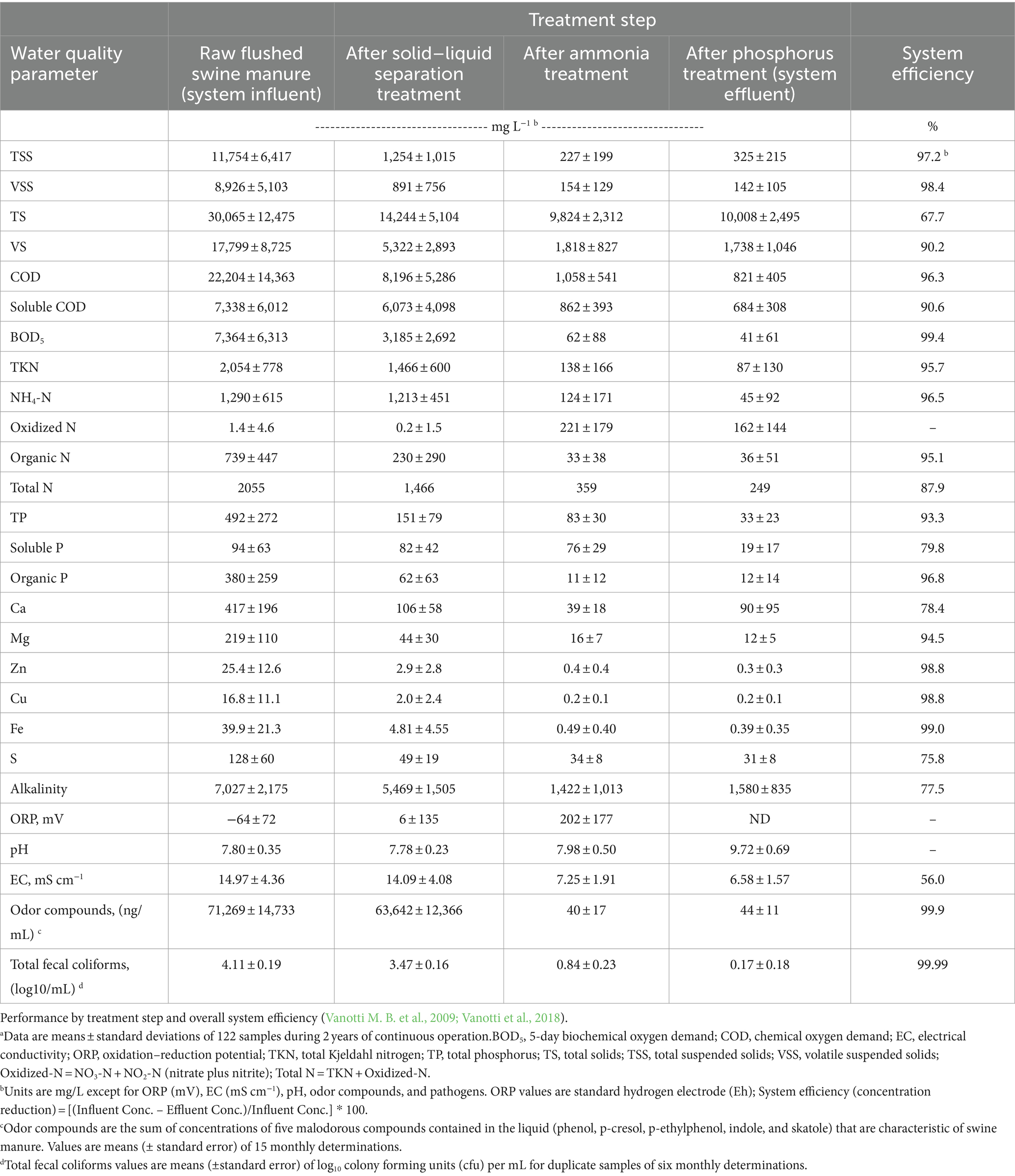
Table 1. Second-generation USDA technology using solid–liquid separation, nitrogen, and phosphorus modules for the treatment of swine manure (Super Soils system).a
The third generation of Super Soils was designed to further reduce the cost of manure treatment through economies of scale by installing it on a larger farm and to test the adaptation of the system to farms using a flushing system (Barker, 1996a) that used flush tanks to evacuate manure from the barn several times per day, producing much-diluted manure, compared to pit-recharge systems (Barker, 1996b) that evacuate manure from the barn once per week producing higher strength manure. The adaptation in the third-generation project consisted of pre-concentration of the diluted manure using a decanting tank before polymer application (Vanotti M. et al., 2013). The project sponsor was North Carolina’s Clean Water Management Trust Fund.
The third-generation technology was demonstrated full-scale on a 1,168,200 kg steady-state live weight (SSLW) Farrow-to-Finish farm that produced 30,450 hogs per year in Wayne County, North Carolina. The treatment system was operated by the farmer. The treatment system was contained in tanks and replaced two anaerobic lagoons (Figure 4). The system treated the waste stream from two operations: (1) a 1,200-sow Farrow-to-Feeder operation (Sow farm) that used a flushing system and generated 141 m3 of manure per day (TSS = 1.3 g/L), and (2) a 12,960 Feeder-to-Finish operation (Finishing farm) that used a pit recharge system and generated 157 m3 of manure per day (TSS = 10.1 g/L). A decanting tank was added in this project to the flushing system waste stream (Sow farm) to pre-concentrate the manure before solid–liquid separation with flocculants. Highly efficient treatment performance was obtained in the 3rd generation Super Soils with both high hydraulic loads typical of flushing systems and high-strength wastewater typical of the pit-recharge systems (Vanotti M. et al., 2013). With regard to the solid–liquid separation process, the decanting tank was effective in concentrating the diluted manure in the flushing waste stream. It reduced the total manure volume processed by the solid separator press by 25,860 gal/day, and it increased polymer use efficiency 5.4 times (from 52 to 279 g/g). This is one of the major advances of this project: It increased solid separator press capacity and lowered operating expenses. With regard to water quality performance, the system removed on a mass basis 98.6% of the total suspended solids (TSS), 98.1% of the COD, 99.3% of the TKN, 96.7% of the total N, 100% of ammonia, 91.9% of total phosphorus, 95.4% of copper, and 97.0% of zinc. With regard to killing pathogens, due to the high pH in the phosphorus module, the system was effective: it met the new Swine Waste Management System Performance Standards (15A NCAC 02T, 2010) for pathogens (Fecal coliforms <7,000 MPN/100 mL) (<3.84 log10). With regards to odor standards, the treatment system removed 100% of odorous compounds in the liquid including skatole, phenol, total cresol, indole, and volatile fatty acids (acetate, propionate, isobutyric).
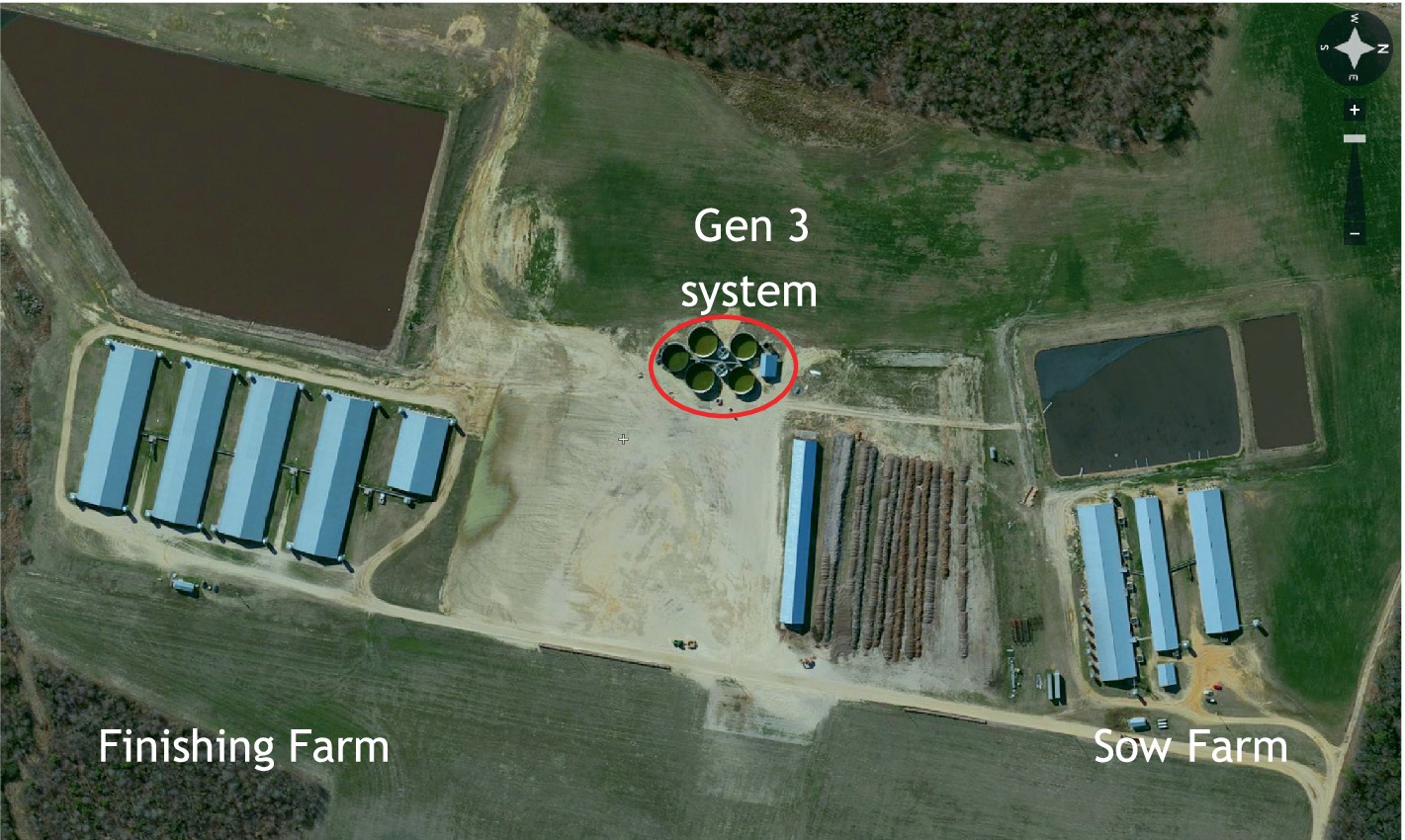
Figure 4. Third generation EST swine waste treatment system (red circle) that replaced the lagoon system (existing lagoons shown above the barns) using solid–liquid separation, nitrogen, and phosphorus modules. The new system provided treatment to all the manure from two operations: a 1,200-sow farrow-to-feeder farm that used flushing (right), and a 12,960-head feeder-to-finish farm that used pit-recharge (left).
Animal health improvements documented with Super Soils system
Reusing cleaner, sanitized water (after ammonia treatment, Table 2) to refill barn pits reduced ammonia concentration in the air of the barns and improved the growing environment (Vanotti et al., 2019). The recycled water in the barns had low ammonia, reduced total fecal coliforms (0.84 ± 0.23 log10 mL−1), and substantial oxidized N (211 ± 179 mg L−1) (Table 2, column “after ammonia treatment”). It replaced the dirtier lagoon liquid charged with ammonia used for flushing the barns under traditional lagoon management. The enhanced animal productivity was demonstrated in the second-generation project by comparing five production cycles using traditional lagoon management (before conversion to the new system) and five production cycles after conversion to the new technology. Since the recycled wastewater was oxidized liquid low in ammonia, ambient ammonia levels in the barns dropped an average of 75 percent. As a result, animal health and productivity were enhanced (Vanotti et al., 2019). Daily weight gain increased by 6.1 percent, and feed conversion improved by 5.1 percent. Animal mortality decreased by 47 percent, and cull weight was reduced by 80 percent. The farmer sold an average of 5,265 pigs per growing cycle, which resulted in a 516,300-kg net gain per cycle. Using the second-generation system instead of the lagoon system, the farmer sold 28,100 kg more hogs—a 5.8 percent increase—per growing cycle. Additional economic benefits from improvements in animal productivity and health were calculated to $91,920 per year [$120.15/454 kg (1,000 lbs.) live animal weight/year] (Vanotti and Szogi, 2007).
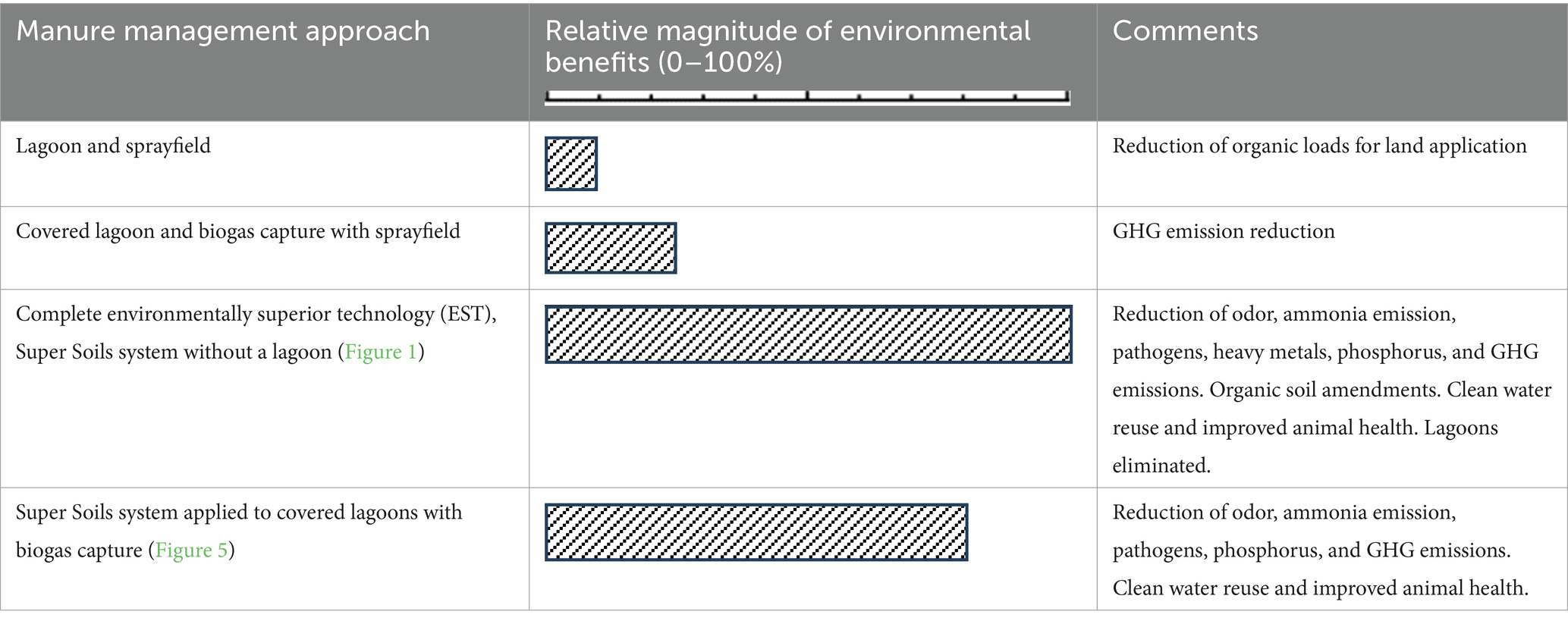
Table 2. Assessment of the relative magnitude of environmental benefits from alternative manure management technologies for NC swine farms.
Greenhouse gas elimination demonstrated with Super Soils system
Although the greenhouse gas emissions of manure processing technologies are very important now, it was not an environmental standard under the EST designation process, which addressed only the elimination of pathogens, ammonia emissions, odor, heavy metals, phosphorus, and discharge to surface and groundwater. However, Super Soils System was demonstrated to be very effective in reducing methane emissions. Using protocols adopted through the United Nations Framework Convention on Climate Change (UNFCCC), Vanotti et al. (2008) estimated a 96.9% reduction in GHG emissions (CH4 and N2O) by replacement of the traditional lagoon-spray field technology with the cleaner, aerobic Super Soils technology (on-farm liquid treatment plus composting). The GHG emissions reductions due to the installation of this technology in a 6,000-head swine farm in NC were 6,732 tonnes of CO2-eq per year, valued at $26,930 per year from carbon offsets markets at the time of evaluation (Vanotti et al., 2008). The solid–liquid separation alone and subsequent diversion of the separated solids away from the lagoon to other aerobic processes can reduce CH4 emissions from swine manure by 65.5% (Sohoulande et al., 2024).
Waste treatment methods to treat effluents from anaerobic digesters for biogas
A wide variety of waste treatment methods have been designed to improve swine waste management which can be used in conjunction with anaerobic digesters (AD) to reduce air and water pollution concerns.
The gas-permeable membrane process (Vanotti and Szogi, 2015) has been used for removing and recovering nitrogen from AD manure effluents. Gas-permeable membranes have effectively recovered more than 97% of NH4+ from cover lagoon swine wastewater (Dube et al., 2016). By coupling the ammonia recovery technology with anaerobic digestion, the methane yield increased by up to 28% (González-García et al., 2021).
Removal of N by nitrification/denitrification in aerobic/anaerobic conditions can effectively reduce N concentrations. However, removing N efficiently at high rates requires controlling pH, O2, temperature, nitrifying bacteria, and organic carbon at favorable levels. Cold temperatures slow nitrification, and to circumvent this problem, a high-performance nitrifying sludge (HPNS) has been introduced for treating swine wastewater (Vanotti M. B. et al., 2013). Using this system enabled the removal of >95% NH3 from swine wastewater containing 1,000–2,700 mg NH3 L−1 (Vanotti M. B. et al., 2009). Nitrification–denitrification systems using continuous flow intermittent aeration (Hu et al., 2003) and sequencing batch reactor (Zhang et al., 2006) removed N by 80 and 97.5%, respectively. A Solepur process has been developed to provide low-cost biological N treatment of swine effluents using the soil as a living reactor (Martinez, 1997). Partial nitrification coupled with anammox (deammonification process) can reduce aeration costs 58% providing effective biological ammonia treatment with no carbon requirement (Magri et al., 2012) thus it is especially suited for anaerobic digestion effluents with low carbon remaining.
In order to meet the strict environmental performance standards of ammonia, phosphorus, odors, and pathogen elimination of an EST (Williams, 2009), the crucial modules of the Super Soils system are the nitrification and phosphorus modules (Vanotti et al., 2005; Vanotti, 2010). Szogi and Vanotti (2009) used a nitrification module followed by a phosphorus module to efficiently remove ammonia and phosphorus and disinfect the effluent from anaerobic lagoon effluents in 10 diverse swine farms in North Carolina. These modules can also be applied to retrofit covered lagoons and anaerobic digestion systems (Figure 5) with significant environmental benefits (Table 2) to jointly remove odor, ammonia emission, pathogens, phosphorus, and GHG emissions, generating clean water for reuse, biogas, and phosphorus fertilizer. A management issue is the removal of the sludge solids from existing lagoons that have been covered. However, in Brazil, Cândido et al. (2022) developed a full-scale system comprised of an efficient first module for anaerobic digestion with biogas production using a continuous stirred tank reactor (CSTR) and covered lagoon biodigesters (CLBs), and the critical Super Soils modules: a nitrogen module using nitrification and denitrification (MLS configuration), and a phosphorus module with calcium phosphate recovery. It provided treatment to a farrow-to-wean swine farm with 6,655 sows and generated 1880 kWh d−1 of energy, removed 98.6% of nitrogen and 89.7% of phosphorus present in the manure, and produced a clean, disinfected effluent for reuse in the barns.
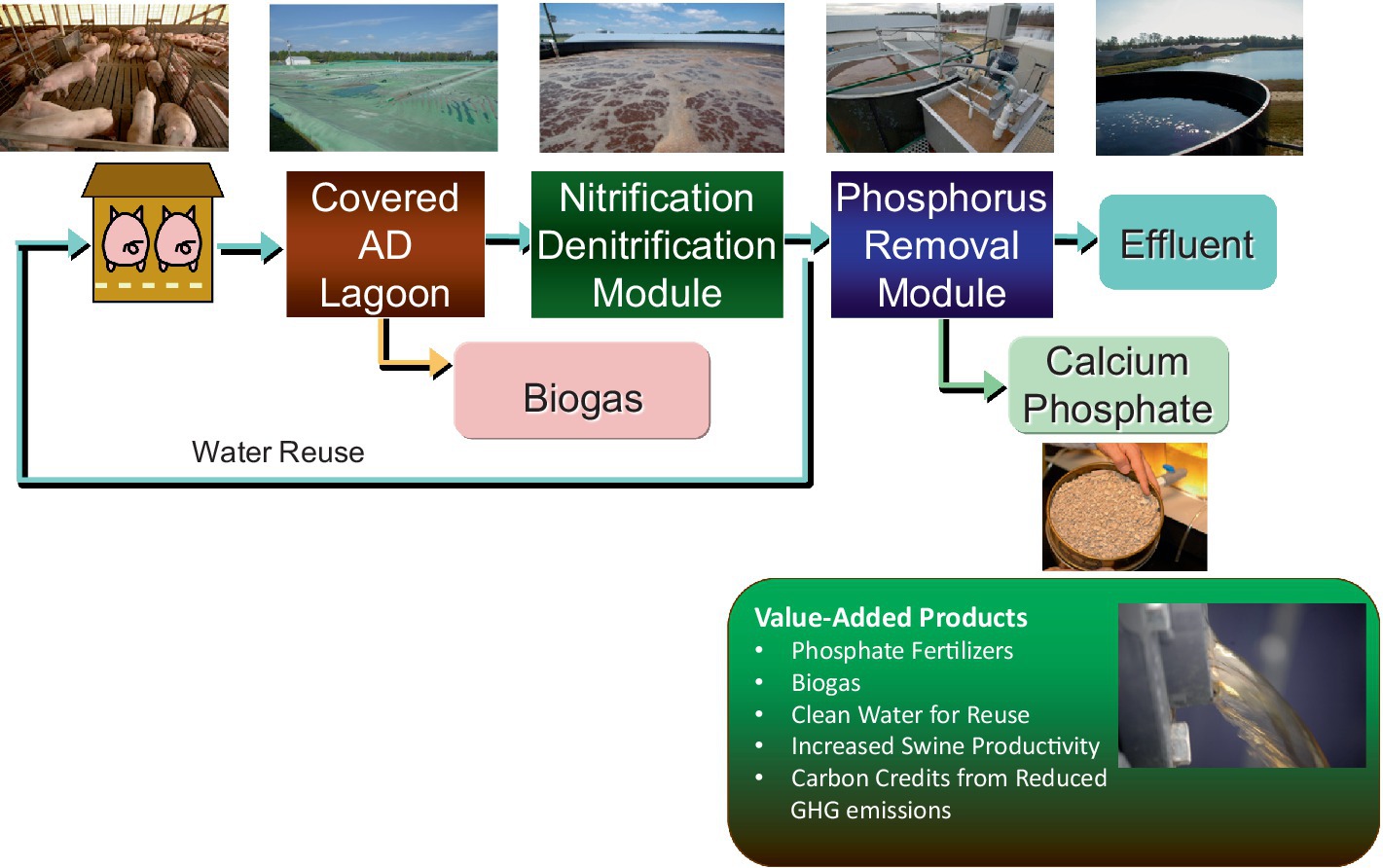
Figure 5. Schematic of the Super Soils system (Vanotti et al., 2005; Vanotti et al., 2010) applied to systems with covered lagoons and value-added products. The on-farm system uses three modules: the first to separate organic solids from the liquid manure using anaerobic digestion (AD), the second to remove the ammonia and odors with biological nitrification/denitrification, and the third to separate phosphorus and disinfect the effluent. A closed loop recycles treated water to recharge the barn pits.
Recommendations and policy
Significant concerns about the impact of land-applied manure nutrients on soil and air resources in North Carolina arose during the late 1980s to mid-1990s due to the swine industry’s rapid growth within relatively small geographic areas (Williams, 2009). These concerns led to stricter pollution controls on swine and new performance criteria demanding that new farms use technologies that substantially eliminated ammonia, odors, pathogens, nutrient pollution, and heavy metals. The first generation of Super Soils farm-tested technology met these standards and subsequent generations improved performance while reducing costs.
Along with the industry’s expansion in the USA, Federal and State governments’ environmental policies under the Clean Water Act supported required compliance with land application of treated wastewater or manure solids based on realistic crop yield expectations and nutrient loading rates through comprehensive nutrient management plans (CNMPs). However, nutrient assessment and geographic distribution of animal manure revealed that several counties had enough animal manure to exceed by over a hundred percent the nitrogen and phosphorus requirements of all non-legume agronomic crops and forages (Zublena and Barker, 1992). Information derived from this assessment served extension practitioners to educate producers on strategies to reduce environmental pollution due to land application of manure nutrients above cropland needs. These strategies included dispersing livestock operations to avoid localized nutrient saturation in soils, integrating manure into existing commercial fertilizer operations, or transporting manure long distances to solve distribution problems of manure nutrients. None of these were attractive to pork producers and industry because of the high production and transportation costs for exporting nutrients in raw manure. No government policy in the United States has incentivized transporting nutrients from areas of surplus production such as eastern North Carolina to places with nutrient deficits, hundreds of miles distant. Policy directives from private firms, including Wal-Mart, are incentivizing more production of swine wastes processed through biogas digesters.
With the urgent need to address alternatives to lagoon treatment and land application of swine manure in North Carolina (the second largest swine-producing State in the USA), the North Carolina Attorney General started the “Environmentally Superior Technology” (EST) research initiative in 2000. The EST initiative propelled a more comprehensive policy as a strategy to reduce CAFOs’ environmental impacts. The new animal waste management methods developed under the EST protect the environment and allow manure management to switch to a current recycling view of manure handling within the food chain’s circular economy.
In the USA, cost-share programs are available through State and Federal agencies to help producers install conservation practices, including waste management in CAFOs. The Farm Bill provides financial and technical assistance to America’s agricultural producers addressing natural resource concerns and delivering environmental benefits, such as improved water and air quality through the Environmental Quality Incentives Program (EQIP) and Conservation Innovation Grants (CIG) (USDA, 2018b). These cost-share and matching requirement programs award competitive grants to develop and implement new tools, technologies, and strategies in soil, water, and air conservation efforts. These programs could help implement proven ESTs or their components, contributing to greenhouse gas reductions and improved water quality.
The following recommendations are proposed, based on the collective experience with the EST in North Carolina and current trends in animal production concentration, for environmentally safe technologies to handle excess manure produced in CAFOs:
1. Seek public policies and government programs to incentivize building new systems, like the Super Soil EST, to create manufacturing jobs for wastewater treatment equipment, construction, installation, and service maintenance, providing economic opportunities in rural communities (Gereffi et al., 2008).
2. Establish a follow-up to the Smithfield Agreement so that technologies that have made engineering improvements may be evaluated.
3. Biogas capture and sale offers opportunities for an unprecedented market of a by-product of the lagoon components—greenhouse gas emissions. Revenues from this market should be sufficient to pay for technologies added to the lagoon and sprayfield systems to substantially eliminate ammonia, odorous compounds, pathogens, nutrient pollution, and heavy metal contamination.
4. New funding sources, such as the Farm Bill and Inflation Reduction Act in the USA, must take care to ensure that the biogas projects they promote do not ignore the problems posed by lagoon and sprayfield systems to human health and the environment. Instead, these biogas projects should be required to meet EST standards.
Author contributions
VA: Writing – original draft, Writing – review & editing. RL: Writing – original draft, Writing – review & editing. MV: Writing – original draft, Writing – review & editing. AS: Writing – original draft, Writing – review & editing. GR: Writing – original draft, Writing – review & editing.
Funding
The author(s) declare no financial support was received for the research, authorship, and/or publication of this article.
Acknowledgments
We thank Dr. C. Mike Williams for all his management and research efforts to help us understand animal waste management technologies. The authors are also grateful to Dr. C. Ray Campbell for his help with the evaluation of the four Super Soils full-scale projects. We acknowledge Chapter 10, Superior Technologies, in Industrial Farm Animal Production, the Environment and Public Health, Forthcoming Johns Hopkins University Press. Mention of trade names or commercial products in this article is solely for the purpose of providing specific information and does not imply recommendation or endorsement by the U.S. Department of Agriculture.
Conflict of interest
The authors declare that the research was conducted in the absence of any commercial or financial relationships that could be construed as a potential conflict of interest.
The author(s) declared that they were an editorial board member of Frontiers, at the time of submission. This had no impact on the peer review process and the final decision.
Publisher’s note
All claims expressed in this article are solely those of the authors and do not necessarily represent those of their affiliated organizations, or those of the publisher, the editors and the reviewers. Any product that may be evaluated in this article, or claim that may be made by its manufacturer, is not guaranteed or endorsed by the publisher.
References
Abrol, Y. P., Adhya, T. K., Aneja, V. P., Raghuram, N., Pathak, H., Kulshrestha, U., et al. (2017). The Indian nitrogen assessment: Sources of reactive nitrogen, environmental and climate effects, management options, and policies. 1st Edn. ScienceDirect, Elsevier. Available at: https://www.sciencedirect.com/book/9780128118368/the-indian-nitrogen-assessment
Agreement, (2000). Agreement between the Attorney General of North Carolina and Smithfield Foods and its subsidiary companies to develop and implement Environmentally Superior Technologies for the management of swine waste in North Carolina. Available at:https://www.northcarolinahealthnews.org/wp-content/uploads/2022/12/3.-Smithfield-Agreement.pdf (Accessed August 31, 2024).
Anderson, A. D., Nelson, J. M., Rossiter, S., and Angulo, F. J. (2003). Public health consequences of use of antimicrobial agents in food animals in the United States. Microbial Drug Resist. 9, 373–379. doi: 10.1089/107662903322762815
Aneja, V. P., Arya, S. P., Kim, D. S., Rumsey, I. C., Arkinson, H. L., Semunegus, H., et al. (2008a). Characterizing Ammonia emissions from swine farms in eastern North Carolina: part 1—conventional lagoon and spray Technology for Waste Treatment. J. Air Waste Mgmt. Ass. 58, 1130–1144. doi: 10.3155/1047-3289.58.9.1130
Aneja, V. P., Arya, S. P., Rumsey, I. C., Kim, D. S., Arkinson, H. L., Semunegus, H., et al. (2008b). Characterizing Ammonia emissions from swine farms in eastern North Carolina: part 2—potential environmentally superior Technologies for Waste Treatment. J. Air Waste Manage. Assoc. 58, 1145–1157. doi: 10.3155/1047-3289.58.9.1145
Aneja, V. P., Arya, S. P., Rumsey, I. C., Kim, D. S., Bajwa, K. S., and Williams, C. M. (2008c). Characterizing ammonia emissions from swine farms in eastern North Carolina: reduction of emissions from water-holding structures at two candidate superior technologies for waste treatment. Atmos. Environ. 42, 3291–3300. doi: 10.1016/j.atmosenv.2007.08.037
Aneja, V. P., Bunton, B. J., Walker, J. T., and Malik, B. P. (2001a). Measurements and analysis of atmospheric ammonia emissions from anaerobic lagoons. Atmos. Environ. 35, 1949–1958. doi: 10.1016/S1352-2310(00)00547-1
Aneja, V. P., Chauhan, J. P., and Walker, J. T. (2000). Characterization of atmospheric ammonia emissions from swine waste storage and treatment lagoons. J. Geophy. Res. 105, 11535–11545. doi: 10.1029/2000JD900066
Aneja, V. P., Roelle, P. A., Murray, G. C., Southerland, J., Erisman, J. W., Fowler, D., et al. (2001b). Atmospheric nitrogen compounds II: emissions, transport, transformation, deposition and assessment. Atmospheric Environ. 35, 1903–1911. doi: 10.1016/S1352-2310(00)00543-4
Aneja, V. P., Schlesinger, W. H., and Erisman, J. W. (2008d). Farming pollution. Nat. Geosci. 1, 409–411. doi: 10.1038/ngeo236
Aneja, V. P., Schlesinger, W. H., and Erisman, J. W. (2009). Effects of agriculture upon the air quality and climate: research, policy, and regulations. Environ. Sci. Technol. 43, 4234–4240. doi: 10.1021/es8024403
ASABE (2011). Design of anaerobic lagoons for animal waste management : American National Standard; ANSI/ASAE EP403.4 FEB2011 (R2020). St. Joseph, MI, USA: American Society of Agricultural and Biological Engineers.
Barker, J. C. (1996a). Swine production facility manure management: underfloor flush - lagoon treatment. Raleigh, NC: North Carolina Cooperative Extension Service. Pub. No. EBAE 129.88. Available at: http://www.bae.ncsu.edu/programs/extension/publicat/wqwm/ebae129_88.html
Barker, J. C. (1996b). Swine production facility manure management: pit recharge- lagoon treatment. Raleigh, NC: North Carolina Cooperative Extension Service. Pub. No. EBAE 128.88. Available at: http://www.bae.ncsu.edu/programs/extension/publicat/wqwm/ebae128_88.html
Barker, J. C., Curtis, S., Hogsett, O., and Humenik, F. (1986). Safety in swine production systems. Cooperative extension service extension bulletin E-1948, Michigan State University. Available at: https://archive.lib.msu.edu/DMC/Ag.%20Ext.%202007-Chelsie/PDF/e1948-1986.pdf (Accessed August 31, 2024).
Blunden, J., and Aneja, V. P. (2008). Characterizing ammonia and hydrogen sulfide emissions from a swine waste treatment lagoon in North Carolina. Atmos. Environ. 42, 3277–3290. doi: 10.1016/j.atmosenv.2007.02.026
Blunden, J., Aneja, V. P., and Overton, J. H. (2008). Modeling hydrogen sulfide emissions across the gas–liquid interface of an anaerobic swine waste treatment storage system. Atmos. Environ. 42, 5602–5611. doi: 10.1016/j.atmosenv.2008.03.016
Boxall, A. B. A., Fogg, L. A., Blackwell, P. A., Kay, P., Pemberton, E. J., and Croxford, A. (2004). Veterinary medicines in the environment. Rev. Environ. Contam. Toxicol. 180, 1–91. doi: 10.1007/0-387-21729-0_1
Burkholder, J., Libra, B., Weyer, P., Heatheote, S., Koplin, D., Thorne, P., et al. (2007). Impacts of waste from concentrated animal feeding operations on water quality. Environ. Health Perspect. 115, 308–312. doi: 10.1289/ehp.8839
Cândido, D., Bolsan, A. C., Hollas, C. E., Venturin, B., Tápparo, D. C., Bonassa, G., et al. (2022). Integration of swine manure anaerobic digestion and digestate nutrients removal/recovery under a circular economy concept. J. Environ. Manag. 301:113825. doi: 10.1016/j.jenvman.2021.113825
Casey, K. D., Bicudi, J. R., Schmidt, D. R., Singh, A., Gay, S. W., Gates, R. S., et al. (2006). “Air quality and emissions from livestock and poultry production/ waste management systems” in Animal agriculture and the environment. eds. J. M. Rice, D. F. Caldwell, and F. J. Humenick (St. Joseph, MI: National Center for Manure and Animal Waste Management White Papers, ASABE), 1–40.
Clemens, J., Trimborn, M., Weiland, P., and Amon, B. (2006). Mitigation of greenhouse gas emissions by anaerobic digestion of cattle slurry. Agric. Ecosyst. Environ. 112, 171–177. doi: 10.1016/j.agee.2005.08.016
Copeland, C. (2010, 2010). Animal waste and water quality: EPA regulation of concentrated animal feeding operations (CAFOs). Congressional research service report for congress. Order Code RL :31851.
Domingo, N. G. G., Balasubramanian, S., Thakrar, S. K., Clark, M. A., Adams, P. J., Marshall, J. D., et al. (2021). Air quality–related health damages of food. PNAS 118:e2013637118. doi: 10.1073/pnas.2013637118
Doorn, M. R. J., Natschke, D. F., Thorneloe, S. A., and Southerland, J. (2002). Development of an emission factor for Ammonia emissions from U.S. swine farms based on field tests and application of a mass balance method. Atmos. Environ. 36, 5619–5625. doi: 10.1016/S1352-2310(02)00689-1
Dube, P. J., Vanotti, M. B., Szogi, A. A., and García-González, M. C. (2016). Enhancing recovery of ammonia from swine manure anaerobic digester effluent using gas-permeable membrane technology. Waste Manag. 49, 372–377. doi: 10.1016/j.wasman.2015.12.011
General Assembly of North Carolina Session (2007). Session Law 2007–523. Available at: https://www.ncleg.net/EnactedLegislation/SessionLaws/HTML/2007-2008/SL2007-523.html (Accessed August 31, 2024).
Gereffi, G., Dubay, K., and Lowe, M. (2008). Manufacturing climate solutions. Carbon-reducing technologies and US jobs : Duke University, Center on Globalization, Governance, and Competitiveness. Durham,NC, USA: Duke University.
González-García, I., Riaño, B., Molinuevo-Salces, B., Vanotti, M. B., and García-González, M. C. (2021). Improved anaerobic digestion of swine manure by simultaneous ammonia recovery using gas-permeable membranes. Water Res. 190:116789. doi: 10.1016/j.watres.2020.116789
Griffing, E., Overcash, M., and Kim, S. (2004). Environmental analysis of swine waste processing technologies using the life-cycle method, report no. 350. Raleigh, NC: Water Resources Research Institute of the University of North Carolina, 44–50.
Hamilton, D.W., Fathepure, B., Fulhage, C.D., Clarkson, W., and Lalman, J. (2006). Treatment lagoons for animal agriculture. ASABE Pub. Number 913C0306. pp. 547–574. Available at: http://osuwastemanage.bae.okstate.edu/articles-1/lagoonwp.pdf (Accessed August 31, 2024).
Harper, L. A., Weaver, K. H., and De Visscher, A. (2014, 2014). Dinitrogen and methane gas production 487 during the anaerobic/anoxic decomposition of animal manure. Nutr. Cycl. Agroecosyst. 100, 53–64. doi: 10.1007/s10705-014-9626-9
Heinzen, T. (2015). Quantification and regulation of air emissions from animal feeding operations. Curr. Environ. Health Rep. 2, 25–32. doi: 10.1007/s40572-014-0038-4
Hooda, P. S., Edwards, A. C., Anderson, H. A., and Miller, A. A. (2000). Review of water quality concerns in livestock farming areas. Sci. Total Environ. 250, 143–167. doi: 10.1016/S0048-9697(00)00373-9
Hu, Z., Mota, C.R., and de los Reyes, L III., Cheng, J (2003). Optimization of nitrogen removal from anaerobically pretreated swine wastewater in intermittent aeration tanks. Proceedings of the 9th international symposium on animal, agricultural, and food processing wastes (ISAAFPW 2003), research Triangle Park, NC. American Society of Agricultural Engineers: St. Joseph, MI, USA. pp. 590–595.
Humenik, F. J. (1972). Animal waste characterization and evaluation of swine waste treatment alternatives : Report No. 61, Chapel Hill, NC, USA: Water Resources Research Institute of the University of North Carolina.
Humenik, F., Overcash, M. R., Triggers, L. B., and Kriz, G. J. (1974). Cleaning the animal farm environment. Environ. Sci. Technol. 8, 984–989. doi: 10.1021/es60097a610
Ibekwe, A. M., Murinda, S. E., DeRoy, C., and Reddy, G. B. (2016). Potential pathogens, antimicrobial patterns, and genotypic diversity of Escherichia coli isolated in constructed wetlands treating swine wastewater. FEMS Microbiol Ecol. 92:fiw006. doi: 10.1093/femsec/fiw006
ICF International (2013). Greenhouse gas mitigation options and costs for agricultural land and animal production within the United States. Report prepared for US Department of Agriculture by ICF International, Washington, DC. Available at: https://www.climatehubs.usda.gov/sites/default/files/GHG_Mitigation_Options.pdf (Accessed August 31, 2024).
Johnson, C. J., and Bonrud, P. (1988). Methemoglobinemia: is it coming back to haunt us? Health Environ. Digest. 1, 3–4.
Kjaer, J., Olsen, P., Bach, K., and Bariebo, H. C. (2007). Leaching of estrogenic hormones from manure-treated structured soils. Environ. Sci. Technol. 41, 3911–3917. doi: 10.1021/es0627747
Liu, J., Power, W., Murphy, J., and Maghirang, R. (2014). Ammonia and hydrogen sulfide emissions from swine production facilities in Northern America: a meta-analysis. J. Anim. Sci. 92, 1656–1665. doi: 10.2527/jas.2013-7160
Longest, Ryke . (2005). Legal Developments Regarding North Carolina’s Swine Farms. National Environmental Enforcement Journal NAAG (2005).
Loughrin, J. H., Szogi, A. A., and Vanotti, M. B. (2006). Reduction of malodorous compounds from a treated swine anaerobic lagoon. J. Environ. Qual. 35, 194–199. doi: 10.2134/jeq2005.0035
Magri, A., Vanotti, M. B., Szogi, A. A., and Cantrell, K. B. (2012). Partial nitritation of swine wastewater in view of its coupling with the anammox process. J. Environ. Qual. 41, 1989–2000. doi: 10.2134/jeq2012.0092
Mallin, M. A. (2000). Impacts of industrial scale swine and poultry production on rivers and estuaries. Am. Sci 88, 26–37. doi: 10.1511/2000.1.26
Mallin, M. A., and McIver, M. R. (2018). Season matters when sampling streams for swine CAFO waste pollution impacts. J. Water Health 16:1.
Martinez, J. (1997). Solepur: a soil treatment process for pig slurry with subsequent denitrification of drainage water. J. Agric. Eng. Res. 66, 51–62. doi: 10.1006/jaer.1996.0116
Miller, D., and Longest, R. (2020). Reconciling environmental justice with climate change mitigation: case study of NC swine CAFOS. Vermont J. Environ. Law 21, 523–543.
Miralha, L., Siddique, S., and Muenich, R. L. (2022). The spatial organization of CAFOs and its relationship to water quality in the United States. J. Hydrol. 613:128301. doi: 10.1016/j.jhydrol.2022.128301
Muller, R. (2021). Impact of the rise in atmosphere nitrous oxide on stratospheric ozone. Ambio 50, 35–39. doi: 10.1007/s13280-020-01428-3
NC Legislature (2007). Performance standards for animal waste management systems that serve swine farms; lagoon and sprayfield systems prohibited. NC Legislature, Enacted Legislation 143–215. Available at: https://www.ncleg.net/EnactedLegislation/Statutes/pdf/BySection/Chapter_143/GS_143-215.10I.pdf (Accessed August 31, 2024).
Onema, O., Wrage, N., Velthof, G. L., Groenigen, J. W., Dolfing, J., and Kurman, P. J. (2005). Trends in global nitrous oxide emissions from animal production systems. Nutrient Cycling Agrosyst. 72, 51–65. doi: 10.1007/s10705-004-7354-2
Owusu-Twum, M. Y., and Sharara, M. A. (2020). Sludge management in anaerobic swine lagoons: a review. J. Environ. Manag. 271:110949. doi: 10.1016/j.jenvman.2020.110949
Park, K. H., Thompson, A. G., Marinier, M., Clark, K., and Wagner, R. C. (2006). Greenhouse gas emissions from stored liquid swine manure in cold climate. Atmos. Environ. 40, 618–627. doi: 10.1016/j.atmosenv.2005.09.075
Ren, F., Zhang, X., Liu, J., Sun, N., Wu, L., Li, Z., et al. (2017). A synthetic analysis of greenhouse gas emissions from manure amended agricultural soils in China. Sci. Rep. 7:8123. doi: 10.1038/s41598-017-07793-6
Renard, J. J., Calidonna, S. E., and Henley, M. (2004). Fate of ammonia in the atmosphere- a review for applicability to hazardous releases. J. Hazard. Mater. 108, 29–60. doi: 10.1016/j.jhazmat.2004.01.015
Rumsey, I., and Aneja, V. (2014). Measurement and modeling of hydrogen sulfide lagoon emissions from a concentrated swine animal feeding operation. Environ. Sci. Technol. 48, 1609–1617. doi: 10.1021/es403716w
Rumsey, I. C., Aneja, V. P., and Lonneman, W. A. (2014). Characterizing reduced sulfur compounds emissions from a swine concentrated animal feeding operation. Atmos. Environ. 94, 458–466. doi: 10.1016/j.atmosenv.2014.05.041
Scanes, C. G. (2018). Impact of agricultural animals on the environment, in Animals and Human Society. Elsevier Publication, Science Direct. 427–449. Available at: https://doi.org/10.1016/B978-0-12-805247-1.00025-3 (Accessed August 31, 2024).
Schiffman, S. S., Studwell, C. E., Landerman, L. R., Berman, K., and Sundy, J. S. (2005). Symptomatic effects of exposure to diluted air sampled from a swine confinement atmosphere on healthy human subjects. Environ. Health Perspect. 113, 567–576. doi: 10.1289/ehp.6814
Shakoor, A., and Mehmood, T. (2021). Nitrous oxide emission from agricultural soils: application of animal manure or Biochar A global meta-analysis. J. Env. Manag. 1:112170. doi: 10.1016/j.jenvman.2021.112170
Shappell, N. W., Billey, L. O., Forbes, D., Matheny, T. A., Poach, M. E., Reddy, G. B., et al. (2007). Estrogenic activity and steroid hormones in swine wastewater through a lagoon constructed wetland systems. Environ. Sci. Technol. 41, 444–450. doi: 10.1021/es061268e
Sharpe, R. R., and Harper, L. A. (1999). Methane emissions from an anaerobic swine lagoon. Atmos. Environ. 33, 3627–3633. doi: 10.1016/S1352-2310(99)00104-1
Sohoulande, C. D. D., Vanotti, M. B., and Szogi, A. A. (2024). Estimating methane emissions from swine waste treatment lagoons and the reduction through solid-liquid separation: a multiscale case evaluation. Cleaner Waste Syst. 7:100133. doi: 10.1016/j.clwas.2024.100133
Szögi, A. A., and Vanotti, M. B. (2007). Abatement of ammonia emissions from swine lagoons using polymer-enhanced solid-liquid separation. Appl. Eng. Agric. 23, 837–845. doi: 10.13031/2013.24053
Szogi, A. A., and Vanotti, M. B. (2009). Removal of phosphorus from livestock effluents. J. Environ. Qual. 38:576. doi: 10.2134/jeq2007.0641
Szogi, A. A., Vanotti, M. B., and Ro, K. S. (2015). Methods for treatment of animal manures to reduce nutrient pollution prior to soil application. Curr. Pollut. Rep. 1, 47–56. doi: 10.1007/s40726-015-0005-1
Thu, K. M., and Durrenberger, E. P. (1998). Pigs, profits, and rural communities : Albany State University of New York Press. New York, USA: SUNY Press. Available at: https://sunypress.edu/Books/P/Pigs-Profits-and-Rural-Communities2
UNECE (2024). Methane management. The United Nations Economic Commission for Europe (UNECE). Available at: https://unece.org/challenge (Accessed August 31, 2024).
USDA (2008). Chapter 10, Agricultural management system component design. Washington, DC: Agricultural Waste Management Field Handbook Part 651.
USDA (2018b). Farmers’ guide to 2018 USDA farm bill programs. Available at: https://www.fsa.usda.gov/Assets/USDA-FSA-Public/usdafiles/Farm-Bill/pdf/farmbill-2018-brochure.pdf (Accessed August 31, 2024).
USEPA (2004). Managing manure nutrients at concentrated animal feeding operations. US Environmental Protection Agency, Office of Water (4303T), Washington, DC. Available at: https://ndep.nv.gov/uploads/water-wpc-permitting-cafo-docs/epa-nutrient-mgmt-guidance-2004.pdf (Accessed August 31, 2024).
USEPA (2014). Animal feeding operations: compliance and enforcement. Washington, DC: U.S. Environmental Protection Agency.
Vac, S. C., Popita, G. E., Frunzeti, N., and Popovici, A. (2013). Evaluation of greenhouse gas emission from animal manure using the enclosed chamber method for gas fluxes. Nol. Bot. Horti Agrobot. 41, 576–581. doi: 10.15835/nbha4129259
Van Lier, J. B., Mahmoud, N., and Zeeman, G. (2008). Anaerobic wastewater treatment. Biological Wastewater Treatment: Principles, Modelling and Design (IWA Publishing). pp. 415–547.
Vanotti, MB . (2010). Producer benefits using Terra Blue treatment technology: increased pig productivity, expansion, and carbon credits. Proceedings of the National Poultry and Animal Waste Management Symposium. NCSU (CD-ROM). Greensboro, NC.
Vanotti, M., Hunt, P., Rice, M., Kunz, A., and Loughrin, J. (2013). Evaluation of generation 3 treatment technology for swine waste—A North Carolina’s Clean Water Management Trust Fund project. Final Environmental Performance Report for the Director, NCSU Animal and Poultry Waste Management Center. 50 pp. Available at: https://www.ars.usda.gov/ARSUserFiles/60820500/manuscripts/2013/man927.pdf (Accessed August 31, 2024).
Vanotti, M.B., Millner, P.D., Szogi, A.A., Campbell, C.R., and Fetterman, L.M. (2006). Aerobic composting of swine manure solids mixed with cotton gin trash. ASABE Paper #064061. Available at: https://www.ars.usda.gov/ARSUserFiles/60820000/Manuscripts/2006/Man729.pdf (Accessed August 31, 2024).
Vanotti, M.B., Rice, J.M., Hunt, P.G., Humenik, F.J., Ellison, A.Q., Baird, C.A., et al. (2001). Evaluation of polymer solids separation, nitrification-denitrification and soluble phosphorus removal system for treating swine manure. 4 Proc. Int’l. Symp. Addressing animal production and environmental issues, Raleigh, NC. (CD-ROM).
Vanotti, M. B., Ro, K. S., Szogi, A. A., Loughrin, J. H., and Millner, P. D. (2018). High-rate solid-liquid separation coupled with nitrogen and phosphorus treatment of swine manure: effect on water quality. Front. Sustain. Food Syst. 2:49. doi: 10.3389/fsufs.2018.00049
Vanotti, M., and Szogi, A.A. (2007). Evaluation of environmental superior technology contingent determination – second generation super soil technology. Final Report for NC Department of Justice – Office of the Attorney General Environmental Enhancement Fund Program. 48 pp. Available at: https://www.ars.usda.gov/ARSUserFiles/60820000/Manuscripts/2007/Man760.pdf (Accessed August 31, 2024).
Vanotti, M. B., and Szogi, A. A. (2008). Water quality improvements of CAFO wastewater after advanced treatment. J. Environ. Qual. 37, S86–S96. doi: 10.2134/jeq2007.0384
Vanotti, M.B., and Szogi, A.A. (2015). Systems and methods for reducing ammonia emissions from liquid effluents and for recovering ammonia. U.S. Patent No. 9,005,333.
Vanotti, M.B., Szogi, A.A., and Ducey, T.F. (2013). High performance nitrifying sludge for high ammonium concentration and low temperature wastewater treatment. U.S. Patent No. 8,445,253.
Vanotti, M.B., Szogi, A.A., and Fetterman, L., (2010). Wastewater treatment system with simultaneous separation of phosphorus sludge and manure solids. U.S. Patent No. 7,674,379.
Vanotti, M.B., Szogi, A.A., and Hunt, P.G. (2005). Wastewater treatment system. U.S. Patent No. 6,893,567.
Vanotti, M. B., Szogi, A. A., Hunt, P. G., Millner, P. D., and Humenik, F. J. (2007). Development of environmentally superior treatment system to replace anaerobic swine waste lagoons in the USA. Bioresour. Technol. 98, 3184–3194. doi: 10.1016/j.biortech.2006.07.009
Vanotti, M. B., Szogi, A. A., Millner, P. D., and Loughrin, J. H. (2009). Development of a second-generation environmentally superior technology for treatment of swine manure in the USA. Bioresour. Technol. 100, 5406–5416. doi: 10.1016/j.biortech.2009.02.019
Vanotti, M.B., Szogi, A.A., Millner, P.D., and Loughrin, J.H. (2019). Improved health and productivity of swine with treatment of ammonia from manure. Proc. XIXth International Congress of ISAH (International Society for Animal Hygiene). p. 145–148. Wroclaw University of Environmental and Life Sciences, Sept. 8-12, Wroclaw, Poland. Available at: https://www.ars.usda.gov/ARSUserFiles/60820500/Manuscripts/2019/ISAH_2019_Vanotti_paper.pdf (Accessed August 31, 2024).
Vanotti, M., Szogi, A., Pilar Bernal, M., and Martinez, J. (2009). Livestock waste treatment systems of the future: a challenge to environmental quality, food safety, and sustainability. OECD Workshop. Bioresource Technol. 100, 5371–5373. doi: 10.1016/j.biortech.2009.07.038
Vanotti, M. B., Szogi, A. A., and Vives, C. A. (2008). Greenhouse gas emission reduction and environmental quality improvement from implementation of aerobic waste treatment systems in swine farms. Waste Manag. 28, 759–766. doi: 10.1016/j.wasman.2007.09.034
Westerman, P. W., Bicudo, J. R., and Kantardjieff, A. (2000). Upflow biological aerated filters for the treatment of flushed swine manure. Bioresour. Technol. 74, 181–190. doi: 10.1016/S0960-8524(00)00028-6
Whitall, D., Hendrickson, B., and Paerl, H. (2003). Importance of atmospherically deposited nitrogen to the annual nitrogen budget of the Neuse River estuary, North Carolina. Environ. Int. 29, 393–399. doi: 10.1016/S0160-4120(02)00175-7
Wiegand, R., Battye, W., Bray, C., and Aneja, V. P. (2022). Ammonia and fine particulate matter (PM2.5) pollution in intensive agricultural regions of North Carolina: satellite analysis and integrated ground-based measurements. Atmos. 13:821. doi: 10.3390/atmos13050821
Williams, C.M. (2004). Development of environmentally superior technologies: phase 1 report for technology determinations per agreements between the attorney general of North Carolina and Smithfield Foods, Premium Standard Farms, and Frontline Farmers, July 26, 2004. Available at: https://citeseerx.ist.psu.edu/document?repid=rep1&type=pdf&doi=46a34c77b5c9c16d24921d9babd2f25c4eb4654c (Accessed August 31, 2024).
Williams, C. M. (2009). Development of environmentally superior technologies in the US and policy. Bioresour. Technol. 100, 5512–5518. doi: 10.1016/j.biortech.2009.01.067
Zhan, J. A., Dispirito, A. A., Do, Y. S., Brooks, B. E., Cooper, E. E., and Hatfield, J. L. (2001). Correlation of human olfactory responses to air born concentrations of malodorous volatile organic compounds emitted from swine effluent. J. Environ. Qual. 30, 624–634. doi: 10.2134/jeq2001.302624x
Zhang, Z., Zhu, J., and Li, W. (2006). A two-step fed SBR for treating swine manure. Process Biochem. 41, 892–900. doi: 10.1016/j.procbio.2005.11.005
Keywords: concentrated animal feeding operation, Environmentally Superior Technology, lagoon-sprayfield swine waste management, swine manure management in USA, swine manure treatment
Citation: Aneja VP, Longest R, Vanotti MB, Szogi AA and Reddy GB (2024) From pollution to resource: advancing swine waste treatment in the USA. Front. Sustain. Food Syst. 8:1450649. doi: 10.3389/fsufs.2024.1450649
Edited by:
Raul Moral, Miguel Hernández University of Elche, SpainReviewed by:
Alison Rollett, Agricultural Development Advisory Service, United KingdomLuciano Orden, Miguel Hernández University of Elche, Spain
Copyright © 2024 Aneja, Longest, Vanotti, Szogi and Reddy. This is an open-access article distributed under the terms of the Creative Commons Attribution License (CC BY). The use, distribution or reproduction in other forums is permitted, provided the original author(s) and the copyright owner(s) are credited and that the original publication in this journal is cited, in accordance with accepted academic practice. No use, distribution or reproduction is permitted which does not comply with these terms.
*Correspondence: Matias B. Vanotti, bWF0aWFzLnZhbm90dGlAdXNkYS5nb3Y=