- 1Department of Fishery Resources Conservation and Management, Khulna Agricultural University, Khulna, Bangladesh
- 2Department of Plant and Environmental Sciences, University of Copenhagen, Frederiksberg, Denmark
- 3Centre for Environment, Fisheries and Aquaculture Science, Weymouth, United Kingdom
- 4Biosciences, University of Exeter, Exeter, Uinted Kingdom
- 5Department of Food Resource and Economics, University of Copenhagen, Frederiksberg, Denmark
- 6Department of Fisheries and Marine Bioscience, Bangabandhu Sheikh Mujibur Rahman Science and Technology University, Gopalganj, Bangladesh
- 7Department of Aquaculture, Bangladesh Agricultural University, Mymensingh, Bangladesh
- 8Department of Fisheries Technology, Bangladesh Agricultural University, Mymensingh, Bangladesh
Farmed freshwater prawn (Macrobrachium rosenbergii) and black tiger shrimp (Penaeus monodon) comprise a significant portion of Bangladesh’s seafood exports, raising concerns about their environmental impacts. Freshwater prawn farms, which require a relatively high amount of feed supply, release 1.0 MT CO2-equivalents/year, equating to 18.8 kg CO2e/MT prawn, contributing significantly to global warming and climate change risks. Integrated Multi-Trophic Aquaculture (IMTA) offers an alternative farming method to conventional prawn farming systems, as it minimizes greenhouse gas (GHG) emissions and climate change impacts. Systematically reviewing 112 scientific articles on IMTA, this article offers recommendations for adopting IMTA to promote sustainable freshwater prawn farming in Bangladesh. IMTA is undergoing extensive experimentation and practice in many parts of the world, offering economic benefits, social acceptability, and environmental sustainability. In addition to native prawn species, various indigenous organic extractive freshwater mollusks, and inorganic extractive plants are available which can seamlessly be used to tailor the IMTA system. Extractive organisms, including aquatic mollusks and plants within prawn farms, can capture blue carbon effectively lowering GHG emissions and helping mitigate climate change impacts. Aquatic mollusks offer feed for fish and livestock, while aquatic plants serve as a dual food source and contribute to compost manure production for crop fields. Research on IMTA in Bangladesh was primarily experimented on finfish in freshwater ponds, with the absence of studies on IMTA in prawn farms. This necessitates conducting research at the prawn farmer level to understand the production of extractive aquatic mollusk and plants alongside prawn in the prawn-producing regions of southwestern Bangladesh.
1 Introduction
Globally, aquaculture has steadily boosted the food production sector over the past decades, considered the “blue revolution” (FAO, 2022; Engelhard et al., 2022; Khanjani et al., 2022; Thornber et al., 2022). The aquaculture production reached a record high of 122.6 million metric tons (MT) in 2020, contributing significantly to world’s nutrition and food security (FAO, 2022; Garlock et al., 2022). A total of 622 aquatic species are farmed across a variety of habitats and farming methods (Ahmed and Turchini, 2021). According to Jiang et al. (2022), since the late 1980s, there has been a 5-fold expansion in the global aquaculture production, and it has contributed significantly to the improvement of livelihood conditions, economic growth, job creation, and also the achievement of the United Nations Sustainable Development Goals (SDGs). Aquaculture production is projected to reach 140 million MT in 2050, to help meeting the world’s rapidly growing population and stagnation of capture fisheries (Ahmed and Turchini, 2021). Additionally, aquaculture is considered to have an enormous potential to make the world’s food systems more resilient in the face of climate change, rising demand for animal protein, and international trade (Troell et al., 2014). Despite its significance and rapid expansion, the aquaculture sector has globally raised serious environmental concerns (Haque et al., 2016; Yang H. et al., 2021). The negative impacts of aquaculture production include, among others, excessive water and energy use, habitat destruction (e.g., wetlands, agricultural fields, and mangroves), water pollution, eutrophication, disease and parasite transmission, biotic depletion, and greenhouse gas (GHGs) emissions (MacLeod et al., 2020; Ahmed and Turchini, 2021; Alam et al., 2022). These negative impacts are expected to grow continuously as aquaculture becomes more prevalent and resource-intensive over time, and it will continue to meet difficulties in terms of long-term environmental sustainability (Khanjani et al., 2022). Due to these expected adverse effects, the aquaculture industry has also been criticized in international media and by many non-governmental organizations (Alexander et al., 2016).
As the aquaculture industry intensifies the amounts of dissolved and solid effluents, such as fish feed waste, feces and other debris, increase (Irisarri et al., 2015; Kibria and Haque, 2018; Correia et al., 2020). These are high in nutrients, in particular phosphorus and nitrogen which once released into the environment can lead to the eutrophication of waterbodies (Troell et al., 2003; Zhou et al., 2006; Rosa et al., 2019; Tom et al., 2021). According to Avnimelech and Ritvo (2003), on average, only 13% of the carbon, 29% of the nitrogen, and 16% of the phosphorus in feeds utilized in aquaculture ponds are retained by the farmed animals; the rest ends up in the water, and sediment as waste. For example, Wang et al. (2020) reported that in China, the aquaculture waste discharged 1.6 MT/year of nitrogen and 0.2 MT/year of phosphorus into local freshwater and coastal regions between 2006 and 2017. The high biological density in intensive aquaculture systems leads to increased rates of respiration by crustaceans or fish in the ponds, but bacterial metabolism (respiration and methanogenesis by degradation of organic matter), also causes increased amounts of GHGs, such as CO2 (carbon dioxide), N2O (nitrous oxide) and CH4 (methane) emissions, resulting in adverse effects on climate change (Doney et al., 2012; Yang P. et al., 2021; Zhang et al., 2022; Xu et al., 2022).
Bangladesh is ranked as the fifth largest aquaculture producer worldwide, accounting for approximately 57% of the country’s total fish production, and contributing significantly to food and nutrition security in a national context (Jahan et al., 2015; Alam et al., 2022; AftabUddin et al., 2021; Haque et al., 2021; Bell et al., 2023). In Bangladesh, like other aquaculture producing countries, aquaculture is considered the fastest-growing animal protein generating industry, providing employment for about 18 million people (Alam et al., 2014; Haque et al., 2015; DoF, 2022). In total aquaculture production, finfish are sold domestically and consumed within the country, while shellfish, particularly freshwater prawn and black tiger shrimp, are primarily exported abroad (Ahmed et al., 2018). While the volume and production of freshwater prawn farming are relatively less than that of black tiger shrimp, the practice for farming freshwater prawns is relatively intensive. It involves cultivating freshwater prawn with other species of finfish, providing substantial amounts of feed, requiring significant manpower, and using various other inputs. According to the latest statistics by the Department of Fisheries (DoF), freshwater prawn and black tiger shrimp collectively account for 42 and 74% by volume and foreign currency earnings, respectively, of the total seafood export from Bangladesh (DoF, 2022). According to annual reports from DoF in Bangladesh, freshwater prawn and black tiger shrimp farming expanded sharply over the years due to their high nutritional value, good meat quality, high growth rate, and substantial international market demand. The farming area and yield of freshwater prawn and black tiger shrimp were 141,353 ha and 97,605 MT, respectively, in 2001–2002 (DoF, 2002), which have increased to 257,888 ha and 270,114 MT in 2019–2020 (DoF, 2020), respectively. This indicates farming area and production of these species have increased by 1.82 and 2.77 times, respectively over the last two decades.
In Bangladesh, farmers tend to grow prawn in polyculture systems, to optimize feeding efficiency and total pond biomass (Marques et al., 2016). Prawns consume supplied commercial feed, but they also consume fish faeces and unused fish feed, while filter-feeding fish (i.e., carps, tilapia) can reduce the amount of phytoplankton and zooplankton and hereby help minimizing the risk of low dissolved oxygen levels at night (Santos and Valenti, 2002; Ibrahim et al., 2015). Despite implementation of polyculture, there has been growing concern about the long-term environmental sustainability of prawn farming. It has been estimated that prawn farms release about 1.0 MT CO2-equivalents/year (Islam et al., 2021), corresponding to 18.8 kg CO2e/MT prawn. In similar prawn production systems in Vietnam, only 9.6 kg CO2e/MT prawn is produced (Jonell and Henriksson, 2015) and in China, shrimp farmers only produce 3.1 kg CO2e/MT shrimp (Cao et al., 2011). Prawn farming in Bangladesh has a high GHG footprint due to the traditional farming systems, contributing to increased global warming and climate change risks (Al-Amin and Alam, 2016).
The Integrated Multitrophic Aquaculture (IMTA) could represent an alternative farming approach to common polyculture systems in Bangladesh, since this technique is known to produce lower GHGs emissions. The IMTA concept is based on co-culturing aquaculture species from different trophic levels and with complementary ecosystem functions. In this way, uneaten feed, waste, and by-products of one species are utilized as fertilizers, feed, and energy for the other crops, and can take advantage of synergistic interactions among the species (Chopin et al., 2001; Troell et al., 2003; Neori et al., 2004; Chopin et al., 2008). A growing literature acknowledges that IMTA is an environmentally friendly and climate resilient technology compared to other forms of conventional aquaculture (Buck et al., 2018; Biswas et al., 2020). For example, in South Africa, the integration of seaweed into an abalone farm resulted in a reduction of GHG emissions from 350 to 290 MT CO2e/year (Nobre et al., 2010). This review explores the global evolution and principles of IMTA, its bioremediation capacity, and its role in sustainable aquaculture, focusing on how it reduces GHG emissions, enhances climate resilience, and addresses challenges to promote IMTA with prawn, with the goal of determining its applicability for improving prawn farming sustainability in Bangladesh.
2 Methodology: procedure of systematic review
2.1 Literature search and filtering
A systematic and extensive literature review was undertaken as recommended by Xiao and Watson (2019). The Preferred Reporting Items for Systematic Reviews and Meta-Analyses (PRISMA) framework protocol was used to assure a transparent and scientific quality, systematic review. Two databases—Web of Science and Scopus—were searched, as recommended by Green et al. (2006) and carried out, e.g., by Gambelli et al. (2019), and Anastasiou et al. (2023).
Only journal articles published in English-language after 2000 meeting the eligibility requirements, were allowed with an emphasis on this review articles. The criteria for eligibility and exclusion in the study selection process are listed in Table 1. We used relevant keywords and Boolean operators to search for IMTA fields, followed by Viana et al. (2022). The term “integrated multitrophic aquaculture” was searched in conjunction with the phrases “prawn farming,” “food safety,” “food security,” “productivity,” “profitability,” “sustainability,” “techniques,” “bioremediation,” “potentials,” “challenges,” “environmental consequences,” “GHG emissions,” and “climate change adaptation” for the literature selection in this review. Table 2 shows the search terms that were entered into each search engine. Accordingly, a total of 1,058 articles were retrieved and identified from the two databases through this process. A total of 883 studies were excluded due to duplication, language problems, and irrelevance to the research objectives. After the meticulous screening, 175 studies were identified and nominated for verification of appropriateness, among which another 61 articles were eliminated due to lack of full document access. Finally, 112 empirical studies were included in the comprehensive analysis for this research.
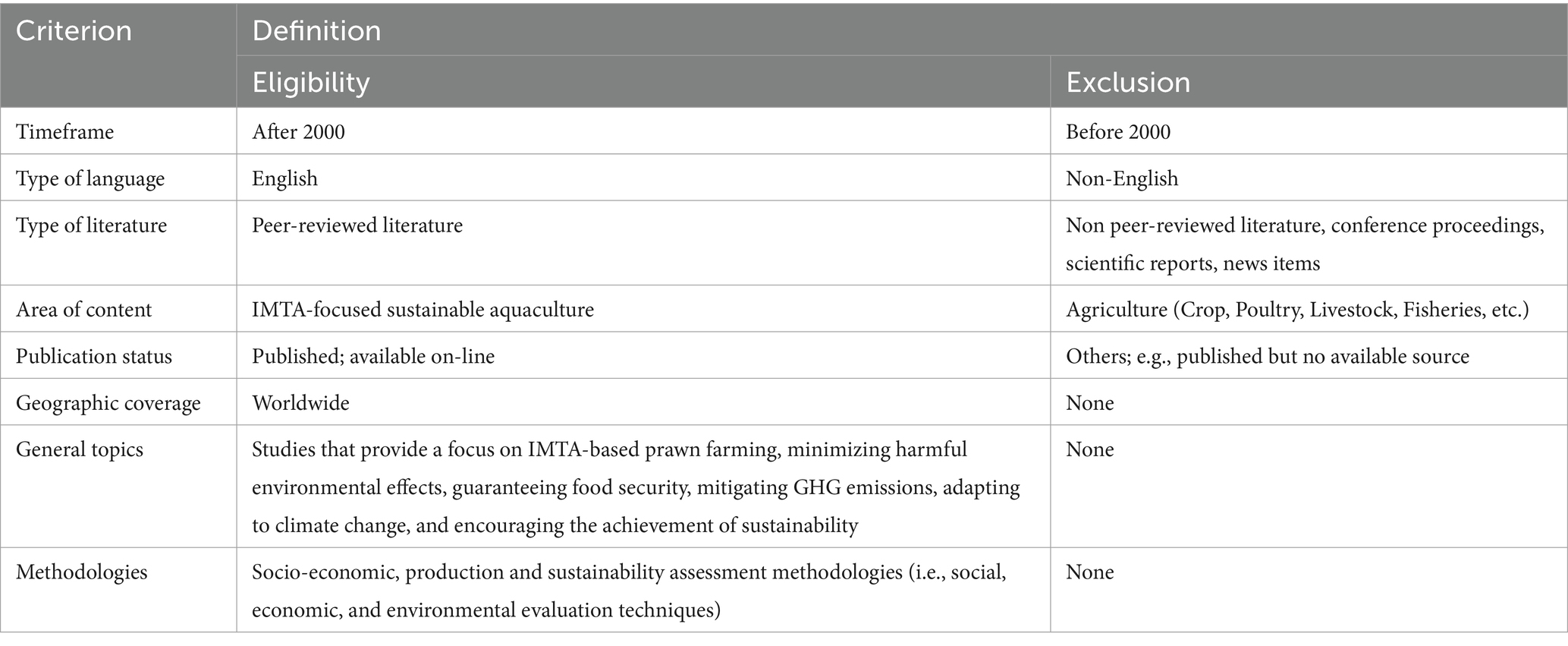
Table 1. The study’s eligibility and exclusion criteria (followed by Gambelli et al., 2019).
In this review, two authors individually conducted the literature selection process based on the result of the search outcomes. To begin with, the outcomes of the search strategies were filtered to produce a list of relevant studies. The final selection of papers was made after careful reading and analysis of the titles and abstracts. Each article was categorized at every stage based on its applicability and eligibility for the study. Two other authors have looked at further ambiguous classifications of the studies to reach a consensus. The framework applied for study selection is illustrated in Figure 1.
2.2 Data synthesis and analysis
After filtering, a descriptive synthesis was carried out by reading the titles, abstracts, and full texts to condense information from the included reviews. The data on the number of studies were entered into a Microsoft Excel spreadsheet, analyzed using descriptive statistics, and presented in graphical form. The study’s findings were recorded, synthesized, and scrutinized to ensure correct interpretation. The analyzed outcomes are presented using tables and line diagrams. The first author extracted the data; the other authors then verified all of the data. Any discrepancies identified in this study were clarified by discussion among the entire research team.
3 Results and discussion
3.1 Brief history of IMTA development
IMTA is not a novel idea; its roots are originated in Asia (Chopin, 2013; Alexander and Hughes, 2017) which can be traced back to ancient civilizations thousands of years ago in China (Park et al., 2018; Nederlof et al., 2022). The integration of fish with aquatic plants and vegetable production is detailed in several Chinese and Egyptian documents from 2200 to 1070 BC (Chopin, 2013). Moreover, Park et al. (2018) noted that Fan Li probably carried out IMTA-like approaches in China as earlier as 470 BC (Table 3). Several published papers documented that IMTA has long been practiced in Asia, especially in China, Japan, and South Korea, with the integrated cultivation of fish from various trophic levels and shellfish and seaweed1 (Kleitou et al., 2018). Lately, IMTA has been successfully practiced in Shangou Bay, China, since 1980 (Fang et al., 2016). Over time, it has become the world’s most extensive aquaculture practice (Zhou et al., 2006), and has gained widespread acceptance. The number of publications focusing on IMTA has risen consistently since 2006 (Figure 2).
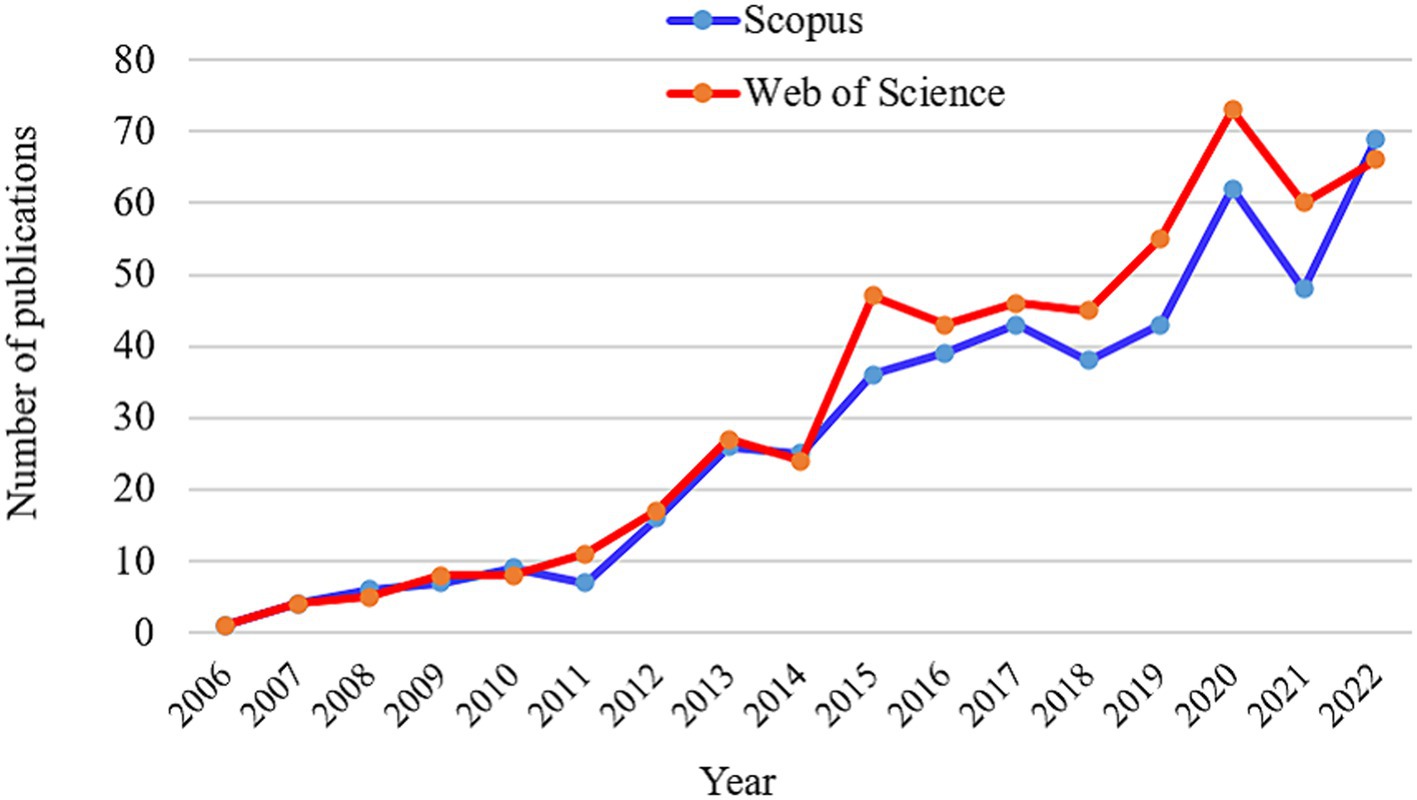
Figure 2. The number of publications within the scientific literature per year of IMTA publication since 2006.
Outside, Asia, the IMTA concept has also later been experimented in western countries, including Europe (Chopin et al., 2012; Kleitou et al., 2018). In the 1970s, John Ryther and his team at the Woods Hole Oceanographic Institution in the USA started research on land-based polyculture systems (Park et al., 2018; Strand et al., 2019), initially calling it “Integrated Waste-Recycling Marine Polyculture Systems”; hence, he is regarded as the grandfather of modern IMTA (Chopin, 2013). Since then, interest in IMTA has grown in the Western world and has slowly being implemented from pilot studies to commercial scale systems (Nederlof et al., 2022). In 2006, IMTA was acknowledged as a study priority for the advancement of aquaculture practices at the joint European Aquaculture Society and World Aquaculture Society Conference in Florence, Italy (Barrington et al., 2009).
Globally, IMTA practice has experienced several modifications from 1970s in the last century to open water systems on a broader scale in the 21st century (Fang et al., 2016; Strand et al., 2019). Ahmed and Glaser (2016) noted that IMTA is operated in over 40 countries on an experimental and commercial basis, including China, Japan, Canada, the USA, Chile, and many European nations.
In Israel, the IMTA approach is being adapted to intensive multi-species aquaculture in desert climates, focusing on water conservation and nutrient regulation (Strand et al., 2019). In Chile, land-based IMTA began in the late 1980s, integrating rainbow trout (Oncorhynchus mykiss), oyster (Crassostrea gigas), and seaweed (Gracilaria chilensis) (Buschmann et al., 2014). In Canada, an IMTA pilot experiment was carried out in the Bay of Fundy in 2001 using Atlantic salmon (Salmo salar), blue mussels (Mytilus edulis), and kelp (Saccharina latissima), and has since transitioned from research and development scale to a commercial scale of production (McVey et al., 2002; Buschmann et al., 2008; MacDonald et al., 2011; Liutkus et al., 2012). In South Korea, open-water IMTA practice started in 2012 and was conducted in small land-based systems (Park et al., 2018).
According to published literature, only two shrimp species, such as P. monodon and Litopenaeus vannamei, have been grown in IMTA with various extractive plant species at a pilot scale in India, Philippines, Taiwan, and Mexico (Yeh et al., 2017; Xiao and Watson, 2019; Strand et al., 2019; Biswas et al., 2019; Arriesgado et al., 2022). The outcomes of these investigations have been proven productive and economically viable, and also have shown a positive environmental bio-remediation effect. In Bangladesh, IMTA has only recently been taken into consideration for research and development to diversify production, using combinations of carps (Catla catla, Hypophthalmichthys molitrix, Labeo rohita, and Cirrhinus cirrhosus), stinging catfish (Heteropneustes fossilis), snails (Viviparus bengalensis), and water spinach (I. aquatica). However, no documented reports of the IMTA systems with freshwater prawn farming exist, though it is becoming an increasingly important targeted species in Bangladesh and elsewhere, and there is in enormous demand for this prawn in the export market.
3.2 Basic principles of IMTA
The principles of IMTA are based on nutrient recycling, whereby various complementary species at different trophic levels are grown in proximity, allowing the waste from one species to become the feed for another (Ellis and Tiller, 2019). Thus, IMTA involves raising organisms to enable one species’ uneaten feed, faeces and metabolic excretions, nutrients, and by-products to be recaptured and transformed into feed, fertilizer, and fertilizer energy for the development of the other species. FAO (2014) defines IMTA as “a practice in which by-products from one species are recycled to become inputs for another.” In a typical IMTA systems, finfish, shrimps or prawns are cultivated by allocation of feed, while extractive inorganic, such as aquatic vegetation or seaweeds, use the inorganic waste, and animals, e.g., mussels and oysters, utilize the organic waste materials (Ridler et al., 2007; Chopin et al., 2012; Sri-uam et al., 2016; Biswas et al., 2020), as illustrated in Figure 3.
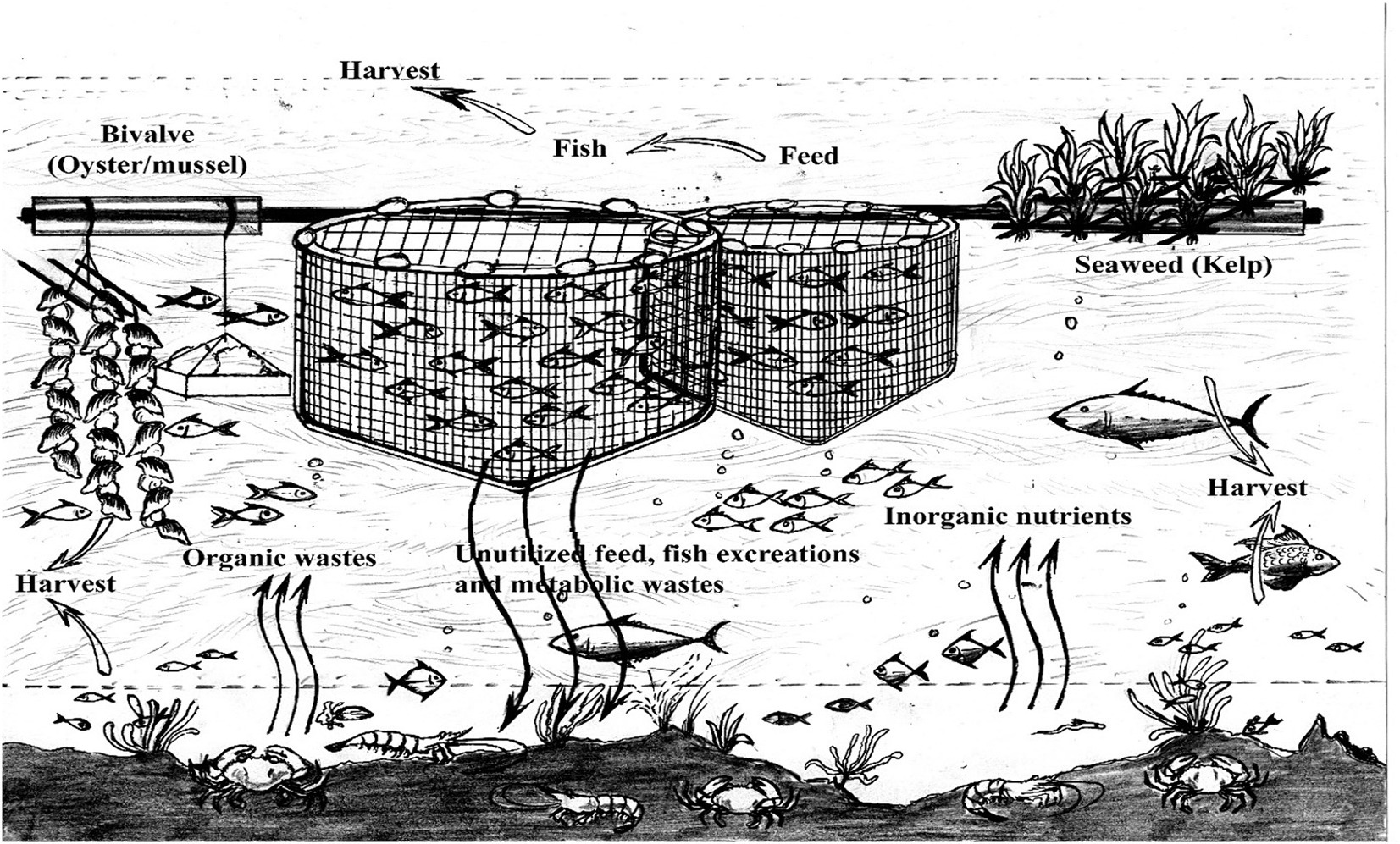
Figure 3. Sketch of integrated multitrophic aquaculture technique with a combination of trophic levels [adapted with permission from Hossain et al. (2017) © The authors of Hossain et al. (2017)].
Globally, various organic and inorganic extractive species are co-cultured with different species of fish in IMTA systems, as shown in Table 4. Organic and inorganic extractive species drastically reduce waste materials emitted by feed material in IMTA. Earlier studies demonstrated that mussels and oysters had filter-feeding capacity to significantly reduce the organic effluents that were released from fish farming (Irisarri et al., 2015). Mussels and oysters are cosmopolitan species and general suspension feeders cultured in dense aggregations. Similar studies have reported that mussels and oysters grew faster when absorbing the organic waste when co-cultured with finfish or shrimps (MacDonald et al., 2011; Sarà et al., 2012). Cranford et al. (2013) noted that the large biofiltration capacity of suspended mussels provided a rationale for their use in IMTA systems. Dissolved nutrients are not taken up by mussels, but can be reduced by absorption in aquatic plants to fuel growth and biomass production in the IMTA system. In addition to the uptake of nutrients, aquatic plants may also provide shelter and shade for other organisms and release allelopathic chemicals to resist toxic algal blooms. Photosynthetic bacteria can concurrently decrease the amount of nitrogen and phosphorus in the system and provide food for suspension feeders (Samocha et al., 2015; Yeh et al., 2017). Consequently, IMTA increases the production of both seaweeds or other vegetation and provides a better environment for cultured aquatic animals (Wu et al., 2015).
In Bangladesh, freshwater prawn is naturally farmed together with an abundance of various species of mollusks and aquatic weeds. Various mollusks species including apple snails, freshwater snails are available in prawn farms, which are commercially important with good food sources for fish and livestock. Aquatic weeds, being emergent, floating anchored, free-floating or submerged, are also present in most prawn gher (modified rice fields to enable the culture of prawn, finfish together with rice), providing a food source, habitat, carbon dioxide removal and oxygen production through photosynthesis. Many of these organic and inorganic extractive species may be acceptable candidates for IMTA practices in coastal prawn farms in Bangladesh. However, their potential advantageous effects in integrated practices in research fields have remained mostly untouched and unexplored. To adequately ensure additional food production and multiple uses of the same land, extensive research is needed to select suitable candidate species of mollusk and aquatic plants for IMTA practices in coastal prawn farms.
3.3 IMTA for sustainable aquaculture
Sustainable aquaculture is a dynamic concept integrating three key pillars: i.e., economically profitable, environmentally friendly, and socially equitable (FAO, 2010; FAO, 2017). The concept of IMTA could meet most of these criteria including sustainable production as it focuses on a circular economy approach, minimization of energy losses and environmental deterioration (Pereira and Yarish, 2008). Recent literature reported that sustainable aquaculture also contributes to the achievement of the UN SDGs2 (Jiang et al., 2022). In particular, IMTA robustly supports the achievement of SDG 1 (no poverty), SDG 2 (zero hunger) and SDGs14 (life below water) by helping to reduce poverty, provide food for people, and conserve sustainable use of the oceans, but IMTA also indirectly achieves SDG 3 (good health and well-being) and SDG 13 (climate action) by providing rich proteins diets and maintaining a healthy weather (Naylor et al., 2021). IMTA contributes to environmental, economic, and social advantages through product diversification, possible price premiums, increased economic resilience, and nutrient cycling (Kumar et al., 2000; Chopin et al., 2012; Hughes and Black, 2016; Correia et al., 2020; Hossain et al., 2022). Buck et al. (2018) emphasize that extractive species absorb significant amounts of waste nutrients, control eutrophication, improve aquatic ecosystems’ health and stability, and promote an ecologically sound aquaculture practice and resource management through a balanced coastal ecosystem approach.
IMTA appears as an economically viable technique that reduces risks through crop diversification and production in different seasons (Chopin et al., 2012). Literature reveals positive prospects of cost-effectiveness at the farm level and economic viability of IMTA systems through product diversification, faster production cycles, and higher product prices (Hossain et al., 2022). IMTA has been demonstrated to boost individual revenue in favorable market conditions and foster economic resilience in challenging times (Whitmarsh et al., 2006). Barrington et al. (2009) mentioned that Canadian consumers prefer IMTA products and are willing to pay an additional 10% for IMTA-labeled products for better quality and food safety. Carras et al. (2020) observed that the yield of salmon in monoculture and with IMTA techniques with a combination of mussels and kelps was unchanged in Canada in an experimental setting; however, the extra production of the mussel and kelp increased the total biomass in IMTA systems, which aided to boost revenues by selling the mussel and kelp providing a higher net present value than salmon monoculture. Fraga-Corral et al. (2022) noted that the IMTA approach is best adapted to foster a circular economy based on the economic viability of integrated aquaculture species. Knowler et al. (2020) reported that the sale of seaweed generated an additional US$ 34,000 in annual revenue, corresponding to about US$ 0.28 per kg of fish, in salmon IMTA farms. They also demonstrated the economic viability of IMTA, since the NPV (net present value) for the IMTA system was 24% higher than the NPV of the monoculture operation, when assuming a 10-year period and a 5% discount rate. Fonseca et al. (2017) assessed IMTA farming with different combinations of species, including shrimp, oysters, and seahorses, and reported that it is economically feasible. In this system, the internal rate of return was 131.1% and payback period for the investment was <2.0 years.
IMTA techniques have gained social acceptance and satisfied the key aquaculture stakeholders in many countries (MacLeod et al., 2020). Extensive literature has recognized that IMTA is a societally acceptable farming technique due to better management practices, improved regulatory governance, and appreciation of differentiated and safe products (Ridler et al., 2007; Troell et al., 2009; Chopin et al., 2012; Correia et al., 2020). In North America, the IMTA method has achieved social acceptance over conventional fish monoculture as seafood produced using this system was regarded as better for the environment and animal welfare and, to a lesser extent, safer and healthier (Kibria and Haque, 2018).
Prawn farming (monoculture and polyculture) has several impacts on employment generation, food supply, export earnings, strengthened livelihoods and ultimate consequences for the economy of Bangladesh. The prawn cultivation has become a multimillion-dollar business in coastal Bangladesh and is called “white gold” due to its international market export value (Islam, 2008; Alexander et al., 2016; Sukhdhane et al., 2018). However, this growing industry faces various bottlenecks, such as dependency on the imported feeds ingredients, a lack of quality feeds and adulteration-free ingredients, hatchery-produced quality seed, water quality problems and high GHG emissions from the farms. If the IMTA approach is implemented into the prawn farming, this farming system can be a sustainable strategy the existing problems in the coastal aquaculture of Bangladesh.
3.4 Extraction/bioremediation capacity of species used in IMTA systems
This literature review demonstrated that many organisms may serve as extractive species, but some organisms are more efficient than others. In addition to extraction and bioremediation capacity, the most suitable organisms should ideally also serve as producers of valuable food in IMTA systems (Park et al., 2021; Hossain et al., 2022; Hargrave et al., 2022). Efficient extractive species for organic and inorganic compounds are shown in Table 5. The most efficient extractive species for organic material in IMTA are mussels, oysters, and clams (Chopin, 2011; Diana et al., 2013). Sicuro et al. (2020) made a similar statement, describing bivalves to play a pivotal role in aquatic ecosystems due to their high filtration capacity and ability to recycle nutrients and mix sediments. Earlier studies reported that blue mussels (M. edulis) and other filter feeding bivalves (Diplodon chilensis, M. galloprovincialis) can uptake nitrogen, and reduce levels of chlorophyll a, phosphate, and ammonia (Hossain et al., 2022; Hargrave et al., 2022). Bivalves may consume dissolved compounds (branchial and urinary losses), reducing the concentration of nutrients and preventing the overgrowth of phytoplankton (Papageorgiou et al., 2023). Hargrave et al. (2021) observed that bivalves M. edulis, and Magallana gigas significantly reduced kelp3 biofouling by as much as 50% in IMTA systems. The freshwater mussel, Sinanodonta woodiana is highly efficient for bioremediation since it has been shown to reduce seston (suspended particulate organic matter) loads and regulate eutrophication in waterbodies (Douda and Čadková, 2018). Moreover, in IMTA cultures, the two oyster species, C. gigas and C. rhizophorae efficiently utilized organic matter (fish feed waste and fish feces) and eliminated total suspended solids and chlorophyll-a contents from the waterbody (Ramos et al., 2009; Jiang et al., 2013).
Seaweeds are widely used in IMTA systems due to their high affinity for nutrient absorption, reducing eutrophication and contributing to bioremediation (Wu et al., 2015; Kang et al., 2021; Hargrave et al., 2022). Macchiavello and Bulboa (2014) discovered that the seaweeds, G. chilensis and U. lactuca, in an IMTA system absorbed almost 100% of ammonia (NH3), nitrate (NO3), and phosphate (PO43−) that was generated by red abalone (Haliotis rufescens) in the IMTA. Similarly, Marinho-Soriano et al. (2009) showed that the red seaweed G. birdiae had high biofiltration capacity and substantially reduced concentrations of PO43−, NH4+, and NO3− in IMTA cultivation. Mao et al. (2009) demonstrated that the red alga G. lemaneiformis had a high nutrient assimilation and bioremediation efficiency and assimilative capacity and served efficiently in an IMTA techniques by reducing ammonium and phosphorus loading. When the seaweed S. latissima was co-cultivated with the bivalves Chlamys farreri, the concentration of nitrate and ammonium was significantly reduced (Hargrave et al., 2021). A similar effect was observed for the macroalgae, Agardhiella subulata which in different IMTA systems significantly decreased the concentration of dissolved inorganic nutrients in the water, such as NH3, NO3−, NO2−, and PO43− (Lohroff et al., 2021). According to Biswas et al. (2019), water spinach, I. aquatica, may have an important role in bioremediation and removal of inorganic nitrogenous (NO2-N, NO3-N, and TAN) and phosphate-phosphorus (PO4-P) from organically polluted waters. Supporting this, water spinach has also been used to treat aquaculture wastewater successfully. For removal of mainly ammonium from wastewater, the application of Ulva spp. in the IMTA systems is also widely practiced and may be combined by recirculation of valuable elements to save pumping costs (Neveux et al., 2018). Yang et al. (2005) highlighted that G. lemaneiformis had multiple effects on pond IMTA systems; it reduced turbidity and phytoplankton biomass, and it provided a substantial reduction of nutrients by assimilation of NO2−-N, PO4−3-P.
Mollusks may also be important in IMTA in Bangladesh. Potential mollusk species in prawn farms and coastal waterbodies in Bangladesh include various mussels (M. edulis, C. gigas, Perna viridis), clams (Pila globosa, Viviparous bengalensis, Meretrix meretrix), and the oysters C. madrasensis (Shahabuddin et al., 2010; Ahmed and Glaser, 2016). These mollusks are considered ecosystem engineers with the potential to create, modify or maintain habitats and ecosystem processes (Hossain et al., 2013). For extraction of inorganic nutrients, various freshwater and brackish water aquatic weeds grow in prawn ghers. Ahmed and Taparhudee (2005) identified seven potential seaweeds in coastal areas of Bangladesh suitable for IMTA practices (genera: Caulerpa, Enteromorpha, Gelidiella, Gelidium, Halymenia, Hydroclathrus, Hypnea, and Sargassum). The mollusk and aquatic weed industries in Bangladesh are still at the infancy stage. However, these species in IMTA practices are highly promising in coastal Bangladesh due to suitable habitats, their high capacity to remove nutrients, improve water quality, and provide better economic return during co-culture with fish and other aquatic animals.
3.5 Contribution to food safety and security
Global food security is a dynamic concept evolving over decades with its definition and policy implementation (Sultana et al., 2023). IMTA systems contribute significantly to food and nutrition security through a diversified production of fish and crustaceans, and besides they deliver valuable products at different trophic levels, including oysters, mussels, clams, and seaweed. These extractive species, being bivalves and seaweeds, have high food, nutritional and economic value. Among important organic extractive species are mussels due to their high content of proteins and essential polyunsaturated fatty acids, such as eicosapentaenoic acid and docosahexaenoic acid that appear beneficial for healthy human development and prevention of diseases (Orban et al., 2002; Carboni et al., 2019). Mussels also contain other vitamins and minerals than most other meat-based protein sources, such as vitamins B and trace minerals (Venugopal and Gopakumar, 2017). Subasinghe et al. (2019) noted that the oyster C. madrasensis is an ideal source of omega-3 fatty acids and is rich in protein and low in calories and fat. The freshwater mussels Anodonta pseudodopsis and Unio tigridis may also have healthy properties due to their content of mono- and polyunsaturated fatty acids (Şereflişan and Ersoy-Altun, 2018). Moniruzzaman et al. (2021) determined that the apple snail P. globosa contain 50% protein and 3% lipid, which means that it represents a good source of protein for human consumption.
For seaweeds, Barbier et al. (2020) reported that they are viewed as promising, sustainable and healthy food sources and can contribute to achieving future policy goals related to blue growth and food security. Seaweeds contain essential nutrients, including proteins, lipids, vitamins, and minerals for human growth and development (Mahadevan, 2015). Yang H. et al. (2021) reported that humans consume seaweeds worldwide because of their nutritional value and abundance of proteins, vitamins, minerals, and other organic compounds. According to Shannon and Abu-Ghannam (2019), the red seaweed Porphyra tenera contains high-protein contents, accounting for approximately 47% protein in dry weight. The available literature reported numerous essential fatty acids in seaweed; approximately 50% are polyunsaturated fatty acids (Dembitsky et al., 2003). Eicosapentaenoic and arachidonic acids are abundant in Rhodophyta and Phaeophyta, whereas oleic, hexadecatetraenoic, and palmitic acids are prevalent in Chlorophyta, such as Ulva pertusa (Ortiz et al., 2006). Mišurcová et al. (2011) reported that seaweeds contained abundant water-soluble and fat-soluble vitamins, including thiamine, riboflavin, cobalamin, ascorbic acid, folic acid, and its derivatives, tocopherols, and carotenoids. Furthermore, seaweeds have low lipid and carbohydrate content, contain essential amino acids and are rich in carotenes, vitamin C and vitamin B12 (Rajapakse and Kim, 2011; Slegers et al., 2021).
IMTA has arisen as an efficient method for safe production of high-quality food production through biological elimination of waste, improvement of water quality, maintenance of ecological services, diversification of the production, and maximization of resource uses (Kim et al., 2022). Hargrave et al. (2022) demonstrated the advantage of integrating seaweeds alongside blue mussels in IMTA systems rather than in monoculture to benefit both yields and quality (Irisarri et al., 2015; Moniruzzaman et al., 2021). Therefore, in Bangladesh comprehensive research on integration and combination of species and their nutritional value can accelerate the production and economic significance of IMTA systems. In prawn farms, IMTA practices may increase the total pond biomass through a diversified production of prawn, fish, mollusks and aquatic plants. Although some tribal people consume bivalves, most farmers extensively use bivalve meat, mainly snails, for feed in freshwater prawn farming in the southwest of Bangladesh (Baby et al., 2010). Currently, fish feed in Bangladesh mainly depends on imports because most feed ingredients come from international feed markets. If mollusk farming can be practiced sustainably in an IMTA approach, the import pressure of fish feed on prawn farming can be reduced to a greater extent (Mamun-Ur-Rashid et al., 2013).
3.6 Reduction of GHG emissions
While aquaculture contributes to resolving food crises, it does also generate GHGs emissions (Alam et al., 2022; Chen et al., 2023), and this has attracted negative attention from scientists, researchers, organizations and the public worldwide (Ziegler et al., 2013). Carbon dioxide, methane, and nitrous oxide are three major GHGs, which are increasingly generated from the intensified aquaculture (Xu et al., 2022). It is not uncommon that aquaculture farmers apply a large volume of industrial feed during intensive farming, resulting in addition of unutilized feed, semi-digested feed, excretions of metabolites, and mucous into the water. This organic matter stimulates microbial degradation that potentially trigger methane and nitrous oxide production that eventually generate GHGs (Yang et al., 2019; Zhao et al., 2021; Pu et al., 2022). Earlier studies confirm that aquaculture intensification may lead to eutrophication, causing depletion of the oxygen in the water and correlating positively with methane and carbon dioxide emissions (Li et al., 2021; Malyan et al., 2022). Thus, the global and rapid expansion of aquaculture has become a critical driver of global warming and climate change (Ahmed and Turchini, 2021; Xu et al., 2022).
IMTA has been documented as a potential solution to adapt and mitigate GHG emissions through nutrient absorption and blue carbon4 sequestration (Abisha et al., 2022). Combining shellfish, i.e., mussels and oysters, and seaweeds in IMTA techniques significantly enhances aquatic ecosystems functions by sequestering CO2 and removing it by fixing carbon in solid form and hereby minimizing GHG emissions (Ahmed et al., 2017a; Macreadie et al., 2017; Fodrie et al., 2017; Ye et al., 2022). Shellfish serve as an important carbon sink and can help sequester blue carbon (SARF, 2017). Ahmed et al. (2017b) reported that the estimated global mollusk production was 16.1 million MT in 2014, potentially sequestering 0.97–1.93 million MT of blue carbon each year. Seaweed farming is a global mitigation approach to combat carbon emissions and promote blue growth initiatives (Froehlich et al., 2019). In the latest scientific literature, seaweeds are highly regarded elements in a potential blue carbon adaptation strategy, because they aid in controlling ocean acidification and deoxygenation and contributes to carbon sequestration, coastal safety, and serve as a carbon sink (Turan and Neori, 2010; Chung et al., 2013; Jagtap and Meena, 2022; Farghali et al., 2023).
In freshwater prawn farms, farmers typically apply artificial feeds that retain a large quantity of organic matter from residual feed to feces into the pond water, generating a higher GHG footprint than traditional aquaculture systems. This enlarged GHG footprint is not only a challenge to Bangladesh, it also affects export options to global markets that demand not only high animal welfare, ecolabelling and food safety, but also a sustainable production of farmed seafood, including prawns (Ahmed et al., 2018). Therefore, adaptation strategies must be developed to cope with the challenges. The IMTA approach is a potential solution to resolve environmental and climate change problems by inclusion of different extractive species together with prawn. Priorities should focus on a comprehensive research investigation to determine the amount of carbon that can be sequestered by various mollusks, bivalves and aquatic plants in farms to make the farming of prawns sustainable and ensure a reduced emission of GHG.
3.7 IMTA on climate change resilience
Climate change is a global challenge and has substantially impacted aquaculture and mariculture production, including hatchery operations, through temperature fluctuation, erratic rainfall, sea-level rise, salinity intrusion, hypoxia, and ocean acidification (Fraga-Corral et al., 2022; Siddique et al., 2022a; Siddique et al., 2022b; Mahalder et al., 2023). In recent years, the impacts of climate change on sustainable aquaculture have drawn attention since they threaten global food security, nutrition supplement, and livelihood status (Maulu et al., 2021). IMTA provides potential solutions and adaptation options for sustainable aquaculture in the context of climate change (Ahmed et al., 2017a; Tan and Zheng, 2020). Thus, as mentioned above, IMTA can improve the marine environments by diminishing ocean acidification, deoxygenation, and reduction of carbon emissions (Ye et al., 2022). In IMTA, bivalves like oysters can act as “ecosystem engineers,” by reducing negative environmental impacts directly or indirectly. They do this through carbon sequestration, enhancing nutrient removal from eutrophic areas, and contributing to habitat formation for other species (Mcleod et al., 2011; Fuentes-Santos et al., 2021). Oyster shells act as carbon sink, playing vital role in mitigating the effects of climate change, particularly by reducing ocean acidification (Papageorgiou et al., 2023). Oysters are crucial to global ocean ecosystems, providing shelter and habitat for various estuarine species, filtering and purifying water, preventing bank erosion, and serving as buffers against extreme climatic events (Grabowski and Peterson, 2007). Aquatic plants in IMTA reduce carbon dioxide levels and mitigate the effects of global warming and climate change over the long term (Farghali et al., 2023). Sultana et al. (2023) reviewed how seaweed can reduce the concentration of pCO2 (partial pressure of carbon dioxide) in seawater by converting dissolved inorganic carbon through photosynthesis. Carbon sequestration via seaweed culture can potentially contribute significantly to global warming and climate change mitigation (Duarte et al., 2017). Farghali et al. (2023) noted that one MT of dry seaweed biomass can absorb approximately 960 kg of carbon dioxide during culture period. Seaweed can also fix phosphorus, potassium, and nitrogen in IMTA approaches, minimizing ocean acidification and increasing oxygen levels to revitalize and restore water habitats (Yong et al., 2022). Duan et al. (2019) reported that seaweed farming of G. lemaneiformis removed approximately 1,192 MT, 15.89 MT, and 128.10 MT of carbon, phosphorus, and nitrogen, respectively, from Yantian Bay, China.
In conclusion mollusks and aquatic plants are sustainable, climate-friendly elements in aquaculture production and they serve as nutrient-rich protein for human consumption (Jones et al., 2022). In addition, neither mollusks nor plants depend on feeds, like fish or prawn do, meaning that they reduce land-based emissions from agricultural products, such as fish feeds. It should be emphasized that the inclusion of extractive species together with fed species (e.g., prawn or fish) do not exacerbate climate change, including floods, sea level rise, salinity intrusion, and mangrove deforestation, rather they represent proactive climate-friendly practices that will help create sustainable environmental, social and economic solutions. Therefore, available mollusk and aquatic plants in prawn farms used in the IMTA approach can provide strong resilience to climate change in coastal Bangladesh by sequestering blue carbon and reducing CO2 emissions.
3.8 IMTA for prawn farming in Bangladesh
IMTA is an environmentally friendly aquaculture approach as by sequestering blue carbon it tackles climate change mitigation. The main advantage of IMTA is its flexible and versatile nature as it can be practiced in land-based freshwater, coastal and marine water adopting several species combinations (Chopin and Sawhney, 2009). Seaweeds and bivalves comprise about half of all aquaculture production globally, and their production have high market and non-market economic value (van der Schatte Olivier et al., 2020). Open ocean IMTA has also been recently practiced where seaweed was integrated together with fed species. This technique might attract an increased economic interest once high-value seaweed species can be cultivated and serve as novel human food products. The IMTA approach aligns with EU (European Union) directions for blue growth and the blue economy, and it works well within the global ambition of circularity in food production (Papageorgiou et al., 2023). Expansion of the IMTA approach with prawn farming in coastal areas could boost the blue economy, which is the top priority sector of Bangladesh government. Prawn farming with IMTA technique could ensure a safer and more sustainable production accelerating the export potential of freshwater prawns from Bangladesh to the global market. Locally available freshwater mollusks (e.g., P. globosa, V. bengalensis, Bellamya dissimilis, etc.) can be farmed in IMTA system due to their potential role to sustainable diversification of food production and extensive ecosystem benefits, including nutrient remediation, and carbon sequestration (Seitz et al., 2013; van der Schatte Olivier et al., 2020). These mollusk species can inhabit various niches in prawn farms, including the floating water column, substrates, and the bottom of the waterbodies. Similarly, locally available aquatic plants (e.g., I. aquatica, Oxalis corniculate, Azolla pinnata, Lemna minor, etc.) have the potential to be cultured in IMTA alongside freshwater prawn. These plants can then be used as feed for livestock, replacing expensive protein sources and helping to reduce the overall cost of meat and milk production (Froehlich et al., 2019).
3.9 Challenges of IMTA approach within prawn farms in Bangladesh
Despite the more sustainable side compared to monoculture and climate change adaptability, some drawbacks are becoming evident as IMTA is experimented more widely in the world (Troell et al., 2009; Khanjani et al., 2022). Scaling up the IMTA technique in coastal prawn farms is likely to face some technological and environmental challenges. Adopting IMTA in prawn farms in coastal areas could result in various social problems, such as theft, robbery, and vandalism due to valuable produces in a small area (Ahmed and Glaser, 2016). The number of appropriate species used for the IMTA system may be minimal in some coastal waterbodies (Rosa et al., 2020). The selection of the most appropriate species to co-culture with freshwater prawn is a great challenge; therefore, one of the priorities to implement IMTA is the need for extensive research to identify the most appropriate aquatic plant and mollusk species to evaluate the densities and circumstances for optimum revenues (Granada et al., 2016). Achieving consumer acceptance of aquatic weeds and mollusk will be a big challenge because these are entirely new food items for the local people. Another challenge is making aquatic plants and mollusk produced in IMTA affordable and available to the domestic and export markets (Biswas et al., 2020; Sultana et al., 2023). An important limitation to IMTA notably in Bangladesh is the lack of farmer level research, technical knowledge, experience, and training in operational practices to prawn farmers. Facilities, including action research opportunities, extension services, technical aid, credit support, marketing promotion of the produces, are required for the farmers to adopt IMTA practices. The government and non-government organizations, particularly the Bangladesh Fisheries Research Institute (BFRI) and WorldFish, other research local and international research institutes, and the Department of Fisheries and relevant extension agencies together, can assist by implementing action research at the farmers level and providing extension service and technical support to promote IMTA technique in the prawn farming region.
4 Conclusion
Freshwater prawn farming is the export oriented farming in coastal areas of Bangladesh by virtue of extensive polyculture and integration with rice/vegetables. Freshwater prawn has established a strong global trade, particularly in the European Union and the United States of America. Despite having broader economic advantages, traditional prawn farming raises concerns about long-term environmental sustainability due to GHG emissions. Prawn farming with IMTA represents an eco-friendly method under a circular economy approach, minimizing energy losses, environmental degradation, GHG emissions, and can alleviate negative effects of climate change. Mollusks and aquatic plants in IMTA can sequester blue carbon, reduce global warming, and mitigate climate change impacts. Additionally, these extractive organisms grown in prawn farms can decrease emission of waste materials, such as unutilized feed, excretions, and metabolic wastes, by feeding aquaculture material to improve water quality and the aquatic ecosystem functions. Farming extractive species in IMTA increase food and nutritional value; therefore, they can be used instead of other expensive protein sources to optimize the cost of meat, milk, and other animal products. The IMTA approach in prawn farms can fit well with the goal of circular food production and has great potential to contribute to solve the food crisis, blue growth, and the blue economy. Despite the current technological and environmental limitations of IMTA approaches, adapting this technique to prawn farming can enhance export potential and be socially, economically, and ecologically sustainable. To fully optimize the potential of prawn farming in Bangladesh, it is essential to identity and combine the most suitable extractive species (e.g., aquatic mollusks and plants) within the IMTA strategy. This requires comprehensive research and development initiatives, which should be a future priority in the national policy. The National Fisheries Policy, developed by the DoF (1998), requires a significant reformation, as it did not adequately address aquaculture research and development issues (DoF, 1998). Updating this policy with a clear aquaculture policy guideline that seriously considers advanced techniques like IMTA is essential. In line with this, government and non-governmental research and extension organizations (i.e., BFRI, WorldFish, DoF) can collaborate to revise national policy, and implement action research to develop IMTA model in prawn farms, and provide training and technical support to the farmers to foster the adoption of this sustainable technique in coastal regions of Bangladesh.
Author contributions
MMA: Writing – review & editing, Writing – original draft, Methodology, Formal analysis, Data curation, Conceptualization. NOGJ: Writing – review & editing, Writing – original draft, Methodology, Conceptualization. DB: Writing – review & editing, Writing – original draft, Methodology, Conceptualization. MS: Writing – review & editing, Writing – original draft, Methodology. MN: Writing – review & editing, Writing – original draft, Conceptualization. MAR: Writing – review & editing, Writing – original draft, Data curation. NAH: Writing – review & editing, Writing – original draft, Data curation. ALB: Writing – review & editing, Writing – original draft. AB: Writing – review & editing, Writing – original draft. MIH: Writing – review & editing, Writing – original draft. LHH: Writing – review & editing, Writing – original draft. MMH: Writing – review & editing, Writing – original draft, Supervision, Methodology, Funding acquisition, Conceptualization.
Funding
The author(s) declare that financial support was received for the research, authorship, and/or publication of this article. This study was conducted under a collaborative project “Climate-Friendly and Climate-Resilient Prawn Farming in Bangladesh (ECOPRAWN)” funded by the Danish International Development Agency (DANIDA), Denmark, Grant No. DFC File No. 21-01-KU (Project No. 2022/3/DANIDA). The work was also supported by the Ocean Country Partnership Programme (OCPP) under the project “Shrimp Health in Coastal Aquaculture of Bangladesh (Project No, 2022/21/Other),” funded through Official Development Assistance (ODA) as part of the UK’s Blue Planet Fund.
Conflict of interest
The authors declare that the research was conducted in the absence of any commercial or financial relationships that could be construed as a potential conflict of interest.
Publisher’s note
All claims expressed in this article are solely those of the authors and do not necessarily represent those of their affiliated organizations, or those of the publisher, the editors and the reviewers. Any product that may be evaluated in this article, or claim that may be made by its manufacturer, is not guaranteed or endorsed by the publisher.
Footnotes
1. ^Seaweeds are autotrophic organisms of simple structure with little or no cellular differentiation and complex tissues, grow in the ocean as well as in rivers, lakes, and other water bodies, and being used in human consumption, hydrocolloids extraction, fertilizers, extracts for cosmetics and pharmaceuticals, biofuels and wastewater treatment (Müssig, 2009; Peñalver et al., 2020).
2. ^SDGs (Sustainable Development Goals), adopted by all UN Member States in 2015, serve as a blueprint for a sustainable future, targeting poverty eradication, inequality reduction, climate crisis mitigation, peace and justice promotion, and environmental protection, with all 17 interlinked goals to be achieved by 2030 to ensure that no one is left behind.
3. ^Kelp is referred to as large cold-water brown algae of the family Laminariaceae, used as food, medicines, and various manufacturing processes (Fraser, 2012).
4. ^Blue carbon is an integral part of the global carbon cycle. It refers to organic carbon absorbed and deposited by the oceans and coastal ecosystems, especially vegetated coastal ecosystems, such as seagrass meadows, tidal marshes, and mangrove forests (Ahmed et al., 2017a; Macreadie et al., 2019).
References
Abisha, R., Krishnani, K. K., Sukhdhane, K., Verma, A. K., Brahmane, M., and Chadha, N. K. (2022). Sustainable development of climate-resilient aquaculture and culture-based fisheries through adaptation of abiotic stresses: a review. J. Water Clim. Chang. 13, 2671–2689. doi: 10.2166/wcc.2022.045
AftabUddin, S., Hussain, M. G., Abdullah, A. M., Failler, P., and Drakeford, B. M. (2021). On the potential and constraints of mariculture development in Bangladesh. Aquac. Int. 29, 575–593. doi: 10.1007/s10499-020-00643-9
Ahmed, N., Bunting, S. W., Glaser, M., Flaherty, M. S., and Diana, J. S. (2017a). Can greening of aquaculture sequester blue carbon? Ambio 46, 468–477. doi: 10.1007/s13280-016-0849-7
Ahmed, N., Cheung, W. W. L., Thompson, S., and Glaser, M. (2017b). Solutions to blue carbon emissions: shrimp cultivation, mangrove deforestation and climate change in coastal Bangladesh. Mar. Policy 82, 68–75. doi: 10.1016/j.marpol.2017.05.007
Ahmed, N., and Glaser, M. (2016). Can “integrated multi-trophic aquaculture (IMTA)” adapt to climate change in coastal Bangladesh? Ocean Coast. Manag. 132, 120–131. doi: 10.1016/j.ocecoaman.2016.08.017
Ahmed, N., and Taparhudee, W. (2005). Seaweed cultivation in Bangladesh: problems and potentials. Fish. Res. Bull. Kasetsart Univ. 28, 13–21.
Ahmed, N., Thompson, S., and Glaser, M. (2018). Transforming organic prawn farming in Bangladesh: potentials and challenges. J. Clean. Prod. 172, 3806–3816. doi: 10.1016/j.jclepro.2017.06.110
Ahmed, N., and Turchini, G. M. (2021). Recirculating aquaculture systems (RAS): environmental solution and climate change adaptation. J. Clean. Prod. 297:126604. doi: 10.1016/j.jclepro.2021.126604
Alam, M. M., and Haque, M. M. (2021). Presence of antibacterial substances, nitrofuran metabolites and other chemicals in farmed pangasius and tilapia in Bangladesh: probabilistic health risk assessment. Toxicol. Rep. 8, 248–257. doi: 10.1016/j.toxrep.2021.01.007
Alam, M., Haque, M., and Shikha, F. (2014). Studies on public health and hygiene condition of retailers at fish markets in south-central Bangladesh. J. Bangladesh Agric. Univ. 12, 411–418. doi: 10.3329/jbau.v12i2.28702
Alam, M. M., Tikadar, K. K., Hasan, N. A., Hasan, N. A., Akter, R., Bashar, A., et al. (2022). Economic viability and seasonal impacts of integrated Rice-prawn-vegetable farming on agricultural households in Southwest Bangladesh. Water 14:2756. doi: 10.3390/w14172756
Al-Amin, A., and Alam, G. (2016). Sustainability challenges to Giant freshwater prawn (GFP) firming sector in Bangladesh: lesson to ensure sustainable economic growth. Asian J. Agric. Res. 10, 132–143. doi: 10.3923/ajar.2016.132.143
Alexander, K. A., Angel, D., Freeman, S., Israel, D., Johansen, J., Kletou, D., et al. (2016). Improving sustainability of aquaculture in Europe: stakeholder dialogues on integrated multi-trophic aquaculture (IMTA). Environ. Sci. Pol. 55, 96–106. doi: 10.1016/j.envsci.2015.09.006
Alexander, K. A., and Hughes, A. D. (2017). A problem shared: technology transfer and development in European integrated multi-trophic aquaculture (IMTA). Aquaculture 473, 13–19. doi: 10.1016/j.aquaculture.2017.01.029
Anastasiou, E., Balafoutis, A. T., and Fountas, S. (2023). Applications of extended reality (XR) in agriculture, livestock farming, and aquaculture: a review. Smart Agric. Techn. 3:100105. doi: 10.1016/j.atech.2022.100105
Arriesgado, E. M., dela Peña, M., Pinaso, R., Tering, J., Navarro, V., Eballe, R., et al. (2022). Identifying the suitable integrated multi-trophic aquaculture (IMTA) species combination for Penaeus monodon (Fabricius, 1798) for a sustainable pond aquaculture. Philipp. J. Sci. 151, 2497–2507. doi: 10.56899/151.6B.14
Avnimelech, Y., and Ritvo, G. (2003). Shrimp and fish pond soils: processes and management. Aquaculture 220, 549–567. doi: 10.1016/S0044-8486(02)00641-5
Baby, R., Hasan, I., Kabir, K., and Naser, M. (2010). Nutrient analysis of some commercially important molluscs of Bangladesh. J. Sci. Res. 2, 390–396. doi: 10.3329/jsr.v2i2.3362
Barbier, M., Araújo, R., Rebours, C., Jacquemin, B., Holdt, S. L., and Charrier, B. (2020). Development and objectives of the Phycomorph European guidelines for the sustainable aquaculture of seaweeds (Pegasus). Bot. Mar. 63, 5–16. doi: 10.1515/bot-2019-0051
Barrington, K., Chopin, T., and Robinson, S., (2009). “Integrated multi-trophic aquaculture (IMTA) in marine temperate waters.” in Integrated mariculture: a global review FAO Fisheries and Aquaculture Technical Paper. 529, 7–46.
Bell, A. G., Thornber, K., Chaput, D. L., Hasan, N. A., Alam, M. M., Haque, M. M., et al. (2023). Metagenomic assessment of the diversity and ubiquity of antimicrobial resistance genes in Bangladeshi aquaculture ponds. Aquac. Rep. 29:101462. doi: 10.1016/j.aqrep.2023.101462
Biswas, G., Kumar, P., Ghoshal, T. K., Kailasam, M., De, D., Bera, A., et al. (2020). Integrated multi-trophic aquaculture (IMTA) outperforms conventional polyculture with respect to environmental remediation, productivity and economic return in brackishwater ponds. Aquaculture 516:734626. doi: 10.1016/j.aquaculture.2019.734626
Biswas, G., Kumar, P., Kailasam, M., Ghoshal, T. K., Bera, A., and Vijayan, K. K. (2019). Application of integrated multi trophic aquaculture (IMTA) concept in Brackishwater ecosystem: the first exploratory trial in the Sundarban, India. J. Coast. Res. 86, 49–55. doi: 10.2112/SI86-007.1
Buck, B. H., Troell, M. F., Krause, G., Angel, D. L., Grote, B., and Chopin, T. (2018). State of the art and challenges for offshore integrated multi-trophic aquaculture (IMTA). Front. Mar. Sci. 5:165. doi: 10.3389/fmars.2018.00165
Buschmann, A. H., Prescott, S., Potin, P., Faugeron, S., Vásquez, J. A., Camus, C., et al. (2014). “Chapter six-the status of kelp exploitation and marine agronomy, with emphasis on Macrocystis pyrifera, in Chile” in Advances in botanical research. ed. N. Bourgougnon (Vannes, France: Academic Press), 161–188.
Buschmann, A. H., Varela, D. A., Hernández-González, M. C., and Huovinen, P. (2008). Opportunities and challenges for the development of an integrated seaweed-based aquaculture activity in Chile: determining the physiological capabilities of Macrocystis and Gracilaria as biofilters. J. Appl. Phycol. 20, 571–577. doi: 10.1007/s10811-007-9297-x
Cao, L., Diana, J. S., Keoleian, G. A., and Lai, Q. (2011). Life cycle assessment of Chinese shrimp farming systems targeted for export and domestic sales. Environ. Sci. Technol. 45, 6531–6538. doi: 10.1021/es104058z
Carboni, S., Kaur, G., Pryce, A., Mckee, K., Desbois, A. P., Dick, J. R., et al. (2019). Mussel consumption as a “food first” approach to improve Omega-3 status. Nutrients 11:1381. doi: 10.3390/nu11061381
Carras, M. A., Knowler, D., Pearce, C. M., Hamer, A., Chopin, T., and Weaire, T. (2020). A discounted cash-flow analysis of salmon monoculture and integrated multi-trophic aquaculture in eastern Canada. Aquac. Econ. Manag. 24, 43–63. doi: 10.1080/13657305.2019.1641572
Chatzivasileiou, D., Dimitriou, P. D., Theodorou, J., Kalantzi, I., Magiopoulos, I., Papageorgiou, N., et al. (2022). An IMTA in Greece: co-culture of fish, bivalves, and holothurians. J. Mar. Sci. Eng. 10:776. doi: 10.3390/jmse10060776
Chen, G., Bai, J., Bi, C., Wang, Y., and Cui, B. (2023). Global greenhouse gas emissions from aquaculture: a bibliometric analysis. Agric. Ecosyst. Environ. 348:108405. doi: 10.1016/j.agee.2023.108405
Cheshuk, B. W., Purser, G. J., and Quintana, R. (2003). Integrated open-water mussel (Mytilus planulatus) and Atlantic salmon (Salmo salar) culture in Tasmania, Australia. Aquaculture 218, 357–378. doi: 10.1016/S0044-8486(02)00640-3
Chopin, T. (2011). Progression of the integrated multi-trophic aquaculture (IMTA) concept and upscaling of IMTA systems towards commercialization. Aquac. Europe 36, 5–12.
Chopin, T. (2013). Integrated multi-trophic aquaculture–ancient, adaptable concept focuses on ecological integration. Glob. Aquacult. Adv. 16, 16–19.
Chopin, T., Buschmann, A. H., Halling, C., Troell, M., Kautsky, N., Neori, A., et al. (2001). Integrating seaweeds into marine aquaculture systems: a key toward sustainability. J. Phycol. 37, 975–986. doi: 10.1046/j.1529-8817.2001.01137.x
Chopin, T., Cooper, J. A., Reid, G., Cross, S., and Moore, C. (2012). Open-water integrated multi-trophic aquaculture: environmental biomitigation and economic diversification of fed aquaculture by extractive aquaculture. Rev. Aquac. 4, 209–220. doi: 10.1111/j.1753-5131.2012.01074.x
Chopin, T., and Robinson, S. M. (2004). Defining the appropriate regulatory and policy framework for the development of integrated multi-trophic aquaculture practices: introduction to the workshop and positioning of the issues. Bull. Aquac. Assoc. Can. 104:4.
Chopin, T., Robinson, S. M. C., Troell, M., Neori, A., Buschmann, A. H., and Fang, J. (2008). “Multitrophic integration for sustainable marine aquaculture” in Encyclopedia of ecology. eds. S. E. Jørgensen and B. D. Fath (Elsevier, Oxford: Academic Press), 2463–2475.
Chopin, T., and Sawhney, M. (2009). “Seaweeds and their Mariculture” in Encyclopedia of ocean sciences (second edition). ed. J. H. Steele (Elsevier, Oxford: Academic Press), 317–326.
Chung, I. K., Oak, J. H., Lee, J. A., Shin, J. A., Kim, J. G., and Park, K. S. (2013). Installing kelp forests/seaweed beds for mitigation and adaptation against global warming: Korean project overview. ICES J. Mar. Sci. 70, 1038–1044. doi: 10.1093/icesjms/fss206
Correia, M., Azevedo, I. C., Peres, H., Magalhães, R., Oliva-Teles, A., Almeida, C. M. R., et al. (2020). Integrated multi-trophic aquaculture: a laboratory and hands-on experimental activity to promote environmental sustainability awareness and value of aquaculture products. Front. Mar. Sci. 7:156. doi: 10.3389/fmars.2020.00156
Costa, L. C. O., Poersch, L. H. S., and Abreu, P. C. (2021). Biofloc removal by the oyster Crassostrea gasar as a candidate species to an integrated multi-trophic aquaculture (IMTA) system with the marine shrimp Litopenaeus vannamei. Aquaculture 540:736731. doi: 10.1016/j.aquaculture.2021.736731
Cranford, P. J., Reid, G. K., and Robinson, S. M. C. (2013). Open water integrated multi-trophic aquaculture: constraints on the effectiveness of mussels as an organic extractive component. Aquac. Environ. Interact. 4, 163–173. doi: 10.3354/aei00081
Cunha, M. E., Quental-Ferreira, H., Parejo, A., Ribeiro, L., Moreira, M., Monteiro, I., et al. (2019). Understanding the individual role of fish, oyster, phytoplankton and macroalgae in the ecology of integrated production in earthen ponds. Aquaculture 512:734297. doi: 10.1016/j.aquaculture.2019.734297
Dembitsky, V. M., Řezanková, H., Řezanka, T., and Hanuš, L. O. (2003). Variability of the fatty acids of the marine green algae belonging to the genus Codium. Biochem. Syst. Ecol. 31, 1125–1145. doi: 10.1016/S0305-1978(03)00043-7
Diana, J. S., Egna, H. S., Chopin, T., Peterson, M. S., Cao, L., Pomeroy, R., et al. (2013). Responsible aquaculture in 2050: valuing local conditions and human innovations will be key to success. Bioscience 63, 255–262. doi: 10.1525/bio.2013.63.4.5
DoF (1998). National Fisheries Policy. Bangladesh: Department of Fisheries, Ministry of Fisheries and Livestock, Dhaka, 18.
DoF (2002). “Yearbook of fisheries statistics of Bangladesh, 2001-02” in Fisheries resources survey system (FRSS), Department of Fisheries, vol. 19 (Bangladesh: Ministry of Fisheries and Livestock), 41.
DoF (2020). “Yearbook of fisheries statistics of Bangladesh, 2019-20” in Fisheries resources survey system (FRSS), Department of Fisheries, vol. 37 (Bangladesh: Ministry of Fisheries and Livestock), 141.
DoF (2022). “Yearbook of fisheries statistics of Bangladesh, 2020-21” in Fisheries resources survey system (FRSS), Department of Fisheries, vol. 38 (Bangladesh: Ministry of Fisheries and Livestock), 138.
Doney, S. C., Ruckelshaus, M., Duffy, J. E., Barry, J. P., Chan, F., English, C. A., et al. (2012). Climate change impacts on marine ecosystems. Annu. Rev. Mar. Sci. 4, 11–37. doi: 10.1146/annurev-marine-041911-111611
Douda, K., and Čadková, Z. (2018). Water clearance efficiency indicates potential filter-feeding interactions between invasive Sinanodonta woodiana and native freshwater mussels. Biol. Invasions 20, 1093–1098. doi: 10.1007/s10530-017-1615-x
Duan, Y., Yang, N., Hu, M., Wei, Z., Bi, H., Huo, Y., et al. (2019). Growth and nutrient uptake of Gracilaria lemaneiformis under different nutrient conditions with implications for ecosystem services: a case study in the laboratory and in an enclosed mariculture area in the East China Sea. Aquat. Bot. 153, 73–80. doi: 10.1016/j.aquabot.2018.11.012
Duarte, C. M., Wu, J., Xiao, X., Bruhn, A., and Krause-Jensen, D. (2017). Can seaweed farming play a role in climate change mitigation and adaptation? Front. Mar. Sci. 4:100. doi: 10.3389/fmars.2017.00100
Ellis, J., and Tiller, R. (2019). Conceptualizing future scenarios of integrated multi-trophic aquaculture (IMTA) in the Norwegian salmon industry. Mar. Policy 104, 198–209. doi: 10.1016/j.marpol.2019.02.049
Engelhard, G. H., Howes, E. L., Pinnegar, J. K., and Le Quesne, W. J. F. (2022). Assessing the risk of climate change to aquaculture: a national-scale case study for the Sultanate of Oman. Clim. Risk Manag. 35:100416. doi: 10.1016/j.crm.2022.100416
Fang, J., Zhang, J., Xiao, T., Huang, D., and Liu, S. (2016). Integrated multi-trophic aquaculture (IMTA) in Sanggou Bay, China. Aquac. Environ. Interact. 8, 201–205. doi: 10.3354/aei00179
FAO (2010). The state of the world fisheries and aquaculture. Rome, Italy: Food and Agricultural Organization of the United Nations, 197.
FAO (2014). The state of world fisheries and aquaculture 2014. Rome: Opportunities and challenges, 223.
FAO (2017). Aquaculture development. 7. Aquaculture governance and sector development. FAO Technical Guidelines for Responsible Fisheries N. 5 Suppl. 7. Rome, Italy. 2017:50.
FAO (2022). The state of world fisheries and aquaculture 2022. Rome, FAO: Towards Blue Transformation, 266.
Farghali, M., Mohamed, I. M. A., Osman, A. I., and Rooney, D. W. (2023). Seaweed for climate mitigation, wastewater treatment, bioenergy, bioplastic, biochar, food, pharmaceuticals, and cosmetics: a review. Environ. Chem. Lett. 21, 97–152. doi: 10.1007/s10311-022-01520-y
Fodrie, F. J., Rodriguez, A. B., Gittman, R. K., Grabowski, J. H., Lindquist, N. L., Peterson, C. H., et al. (2017). Oyster reefs as carbon sources and sinks. Proc. R. Soc. 284:20170891. doi: 10.1098/rspb.2017.0891
Fonseca, T., David, F. S., Ribeiro, F. A. S., Wainberg, A. A., and Valenti, W. C. (2017). Technical and economic feasibility of integrating seahorse culture in shrimp/oyster farms. Aquac. Res. 48, 655–664. doi: 10.1111/are.12912
Fossberg, J., Forbord, S., Broch, O. J., Malzahn, A. M., Jansen, H., Handå, A., et al. (2018). The potential for upscaling kelp (Saccharina latissima) cultivation in Salmon-driven integrated multi-trophic aquaculture (IMTA). Front. Mar. Sci. 5:418. doi: 10.3389/fmars.2018.00418
Fraga-Corral, M., Ronza, P., Garcia-Oliveira, P., Pereira, A. G., Losada, A. P., Prieto, M. A., et al. (2022). Aquaculture as a circular bio-economy model with Galicia as a study case: how to transform waste into revalorized by-products. Trends Food Sci. Technol. 119, 23–35. doi: 10.1016/j.tifs.2021.11.026
Fraser, C. I. (2012). Is bull-kelp kelp? The role of common names in science. N. Z. J. Mar. Freshw. Res. 46, 279–284. doi: 10.1080/00288330.2011.621130
Freitas, J. R. C., Morrondo, J. M. S., and Ugarte, J. C. (2016). Saccharina latissima (Laminariales, Ochrophyta) farming in an industrial IMTA system in Galicia (Spain). J. Appl. Phycol. 28, 377–385. doi: 10.1007/s10811-015-0526-4
Froehlich, H. E., Afflerbach, J. C., Frazier, M., and Halpern, B. S. (2019). Blue growth potential to mitigate climate change through seaweed offsetting. Curr. Biol. 29, 3087–3093.e3. doi: 10.1016/j.cub.2019.07.041
Fuentes-Santos, I., Labarta, U., Fernández-Reiriz, M. J., Kay, S., Hjøllo, S. S., and Alvarez-Salgado, X. A. (2021). Modeling the impact of climate change on mussel aquaculture in a coastal upwelling system: a critical assessment. Sci. Total Environ. 775:145020. doi: 10.1016/j.scitotenv.2021.145020
Gambelli, D., Vairo, D., Solfanelli, F., and Zanoli, R. (2019). Economic performance of organic aquaculture: a systematic review. Mar. Policy 108:103542. doi: 10.1016/j.marpol.2019.103542
Garlock, T., Asche, F., Anderson, J., Ceballos-Concha, A., Love, D. C., Osmundsen, T. C., et al. (2022). Aquaculture: the missing contributor in the food security agenda. Glob. Food Sec. 32:100620. doi: 10.1016/j.gfs.2022.100620
Grabowski, J. H., and Peterson, C. H. (2007). “15 - restoring oyster reefs to recover ecosystem services” in Theoretical ecology series. eds. K. Cuddington, J. E. Byers, W. G. Wilson, and A. Hastings (Elsevier, United Kingdom: Academic Press), 281–298.
Granada, L., Sousa, N., Lopes, S., and Lemos, M. F. L. (2016). Is integrated multitrophic aquaculture the solution to the sectors’ major challenges? – a review. Rev. Aquac. 8, 283–300. doi: 10.1111/raq.12093
Green, B. N., Johnson, C. D., and Adams, A. (2006). Writing narrative literature reviews for peer-reviewed journals: secrets of the trade. J. Chiropr. Med. 5, 101–117. doi: 10.1016/S0899-3467(07)60142-6
Guerrero, S., and Cremades, J., (2012). Integrated multi-trophic aquaculture (IMTA): a sustainable, pioneering alternative for marine cultures in Galicia. Regional Government of Galicia (Spain); pp. 111.
Haque, M. M., Alam, M. R., Alam, M. M., Basak, B., Sumi, K. R., Belton, B., et al. (2015). Integrated floating cage aquageoponics system (IFCAS): an innovation in fish and vegetable production for shaded ponds in Bangladesh. Aquac. Rep. 2, 1–9. doi: 10.1016/j.aqrep.2015.04.002
Haque, M. M., Alam, M. M., Hoque, M. S., Hasan, N. A., Nielsen, M., Hossain, M. I., et al. (2021). Can Bangladeshi pangasius farmers comply with the requirements of aquaculture certification? Aquac. Rep. 21:100811. doi: 10.1016/j.aqrep.2021.100811
Haque, M. M., Belton, B., Alam, M. M., Ahmed, A. G., and Alam, M. R. (2016). Reuse of fish pond sediments as fertilizer for fodder grass production in Bangladesh: potential for sustainable intensification and improved nutrition. Agric. Ecosyst. Environ. 216, 226–236. doi: 10.1016/j.agee.2015.10.004
Hargrave, M. S., Ekelund, A., Nylund, G. M., and Pavia, H. (2021). Filtration and fertilisation effects of the bivalves Mytilus edulis and Magallana gigas on the kelp Saccharina latissima in tank culture. J. Appl. Phycol. 33, 3927–3938. doi: 10.1007/s10811-021-02553-6
Hargrave, M. S., Nylund, G. M., Enge, S., and Pavia, H. (2022). Co-cultivation with blue mussels increases yield and biomass quality of kelp. Aquaculture 550:737832. doi: 10.1016/j.aquaculture.2021.737832
Hossain, M.S., Chowdhury, S.R., and Sharifuzzaman, S.M., (2017). Blue economic development in Bangladesh: A policy guide for marine fisheries and aquaculture. Institute of Marine Sciences and Fisheries, University of Chittagong, Bangladesh, 32 pp.
Hossain, M. S., Rothuis, A., Chowdhury, S. R., Smaal, A., Ysebaert, T., Sharifuzzaman, S. M., et al. (2013). Oyster aquaculture for coastal defense with food production in Bangladesh. Aquacult. Asia 18, 15–24.
Hossain, A., Senff, P., and Glaser, M. (2022). Lessons for coastal applications of IMTA as a way towards sustainable development: a review. Appl. Sci. 12:11920. doi: 10.3390/app122311920
Hughes, A. D., and Black, K. D. (2016). Going beyond the search for solutions: understanding trade-offs in European integrated multi-trophic aquaculture development. Aquac. Environ. Interact. 8, 191–199. doi: 10.3354/aei00174
Ibrahim, A.N.A.F., Noll, M.S.M.C., and Valenti, W.C., (2015). Zooplankton capturing by Nile Tilapia, Oreochromis niloticus (Teleostei: Cichlidae) throughout post-larval development. Zoologia (Curitiba). 32.
Irisarri, J., Fernández-Reiriz, M. J., Labarta, U., Cranford, P. J., and Robinson, S. M. C. (2015). Availability and utilization of waste fish feed by mussels Mytilus edulis in a commercial integrated multi-trophic aquaculture (IMTA) system: a multi-indicator assessment approach. Ecol. Indic. 48, 673–686. doi: 10.1016/j.ecolind.2014.09.030
Irisarri, J., Fernández-Reiriz, M. J., Robinson, S. M. C., Cranford, P. J., and Labarta, U. (2013). Absorption efficiency of mussels Mytilus edulis and Mytilus galloprovincialis cultured under integrated multi-trophic aquaculture conditions in the bay of Fundy (Canada) and Ría Ares-Betanzos (Spain). Aquaculture 388-391, 182–192. doi: 10.1016/j.aquaculture.2013.01.034
Islam, M. S. (2008). In search of “white gold”: environmental and agrarian changes in rural Bangladesh. Soc. Nat. Resour. 22, 66–78. doi: 10.1080/08941920801942255
Islam, I., Nielsen, M., Badiuzzaman, B., and Schulze-Ehlers, B. (2021). Knowledge transfer from experienced to emerging aquaculture industries in developing countries: the case of shrimp and pangasius in Bangladesh. J. Appl. Aquac. 33, 73–95. doi: 10.1080/10454438.2020.1716914
Jagtap, A. S., and Meena, S. N. (2022). “Chapter 23 - seaweed farming: a perspective of sustainable agriculture and socio-economic development” in Natural resources conservation and advances for sustainability. eds. M. K. Jhariya, R. S. Meena, A. Banerjee, and S. N. Meena (Amsterdam, Netherlands: Elsevier), 493–501.
Jahan, K., Belton, B., Ali, H., Dhar, G.C., and Ara, I., (2015). Aquaculture technologies in Bangladesh: An assessment of technical and economic performance and producer behavior. Penang, Malaysia: WorldFish. Program Report: 2015-52.
Jiang, Q., Bhattarai, N., Pahlow, M., and Xu, Z. (2022). Environmental sustainability and footprints of global aquaculture. Resour. Conserv. Recycl. 180:106183. doi: 10.1016/j.resconrec.2022.106183
Jiang, Z., Wang, G., Fang, J., and Mao, Y. (2013). Growth and food sources of Pacific oyster Crassostrea gigas integrated culture with Sea bass Lateolabrax japonicus in Ailian Bay, China. Aquac. Int. 21, 45–52. doi: 10.1007/s10499-012-9531-7
Jonell, M., and Henriksson, P. J. G. (2015). Mangrove–shrimp farms in Vietnam—comparing organic and conventional systems using life cycle assessment. Aquaculture 447, 66–75. doi: 10.1016/j.aquaculture.2014.11.001
Jones, A. R., Alleway, H. K., McAfee, D., Reis-Santos, P., Theuerkauf, S. J., and Jones, R. C. (2022). Climate-friendly seafood: the potential for emissions reduction and carbon capture in marine aquaculture. Bioscience 72, 123–143. doi: 10.1093/biosci/biab126
Kang, Y. H., Kim, S., Choi, S. K., Lee, H. J., Chung, I. K., and Park, S. R. (2021). A comparison of the bioremediation potential of five seaweed species in an integrated fish-seaweed aquaculture system: implication for a multi-species seaweed culture. Rev. Aquac. 13, 353–364. doi: 10.1111/raq.12478
Khanjani, M. H., Zahedi, S., and Mohammadi, A. (2022). Integrated multitrophic aquaculture (IMTA) as an environmentally friendly system for sustainable aquaculture: functionality, species, and application of biofloc technology (BFT). Environ. Sci. Pollut. Res. 29, 67513–67531. doi: 10.1007/s11356-022-22371-8
Kibria, A. S. M., and Haque, M. M. (2018). Potentials of integrated multi-trophic aquaculture (IMTA) in freshwater ponds in Bangladesh. Aquac. Rep. 11, 8–16. doi: 10.1016/j.aqrep.2018.05.004
Kim, J. K., Kraemer, G. P., and Yarish, C. (2014). Field scale evaluation of seaweed aquaculture as a nutrient bioextraction strategy in Long Island sound and the Bronx River estuary. Aquaculture 433, 148–156. doi: 10.1016/j.aquaculture.2014.05.034
Kim, D.-Y., Shinde, S. K., Kadam, A. A., Saratale, R. G., Saratale, G. D., Kumar, M., et al. (2022). Advantage of species diversification to facilitate sustainable development of aquaculture sector. Biology 11:368. doi: 10.3390/biology11030368
Kleitou, P., Kletou, D., and David, J. (2018). Is Europe ready for integrated multi-trophic aquaculture? A survey on the perspectives of European farmers and scientists with IMTA experience. Aquaculture 490, 136–148. doi: 10.1016/j.aquaculture.2018.02.035
Knowler, D., Chopin, T., Martínez-Espiñeira, R., Neori, A., Nobre, A., Noce, A., et al. (2020). The economics of integrated multi-trophic aquaculture: where are we now and where do we need to go? Rev. Aquac. 12, 1579–1594. doi: 10.1111/raq.12399
Kumar, M., Clarke, S., and Sierp, M., (2000). Linkage between wastewater treatment and aquaculture: Initiatives by the South Australian Research and Development Institute (SARDI). 153–159.
Kunzmann, A., Beltran-Gutierrez, M., Fabiani, G., Namukose, M., and Msuya, F. E. (2018). Integrated seaweed–sea cucumber farming in Tanzania. West. Indian Ocean J. Mar. Sci. 17, 35–50. doi: 10.4314/wiojms.v17i2.4
Largo, D. B., Diola, A. G., and Marababol, M. S. (2016). Development of an integrated multi-trophic aquaculture (IMTA) system for tropical marine species in southern Cebu, Central Philippines. Aquac. Rep. 3, 67–76. doi: 10.1016/j.aqrep.2015.12.006
Li, Y., Shang, J., Zhang, C., Zhang, W., Niu, L., Wang, L., et al. (2021). The role of freshwater eutrophication in greenhouse gas emissions: a review. Sci. Total Environ. 768:144582. doi: 10.1016/j.scitotenv.2020.144582
Liutkus, M., Robinson, S., MacDonald, B., and Reid, G. (2012). Quantifying the effects of diet and mussel size on the biophysical properties of the blue mussel, Mytilus spp., feces egested under simulated IMTA conditions. J. Shellfish Res. 31, 69–77. doi: 10.2983/035.031.0109
Lohroff, T. J., Gillette, P. R., Close, H. G., Benetti, D. D., and Stieglitz, J. D. (2021). Evaluating the potential bioextractive capacity of South Florida native macroalgae Agardhiella subulata for use in integrated multi-trophic aquaculture (IMTA). Aquaculture 544:737091. doi: 10.1016/j.aquaculture.2021.737091
Macchiavello, J., and Bulboa, C. (2014). Nutrient uptake efficiency of Gracilaria chilensis and Ulva lactuca in an IMTA system with the red abalone Haliotis rufescens. Lat. Am. J. Aquat. Res. 42, 523–533. doi: 10.3856/vol42-issue3-fulltext-12
MacDonald, B. A., Robinson, S. M. C., and Barrington, K. A. (2011). Feeding activity of mussels (Mytilus edulis) held in the field at an integrated multi-trophic aquaculture (IMTA) site (Salmo salar) and exposed to fish food in the laboratory. Aquaculture 314, 244–251. doi: 10.1016/j.aquaculture.2011.01.045
MacLeod, M. J., Hasan, M. R., Robb, D. H. F., and Mamun-Ur-Rashid, M. (2020). Quantifying greenhouse gas emissions from global aquaculture. Sci. Rep. 10:11679. doi: 10.1038/s41598-020-68231-8
Macreadie, P. I., Anton, A., Raven, J. A., Beaumont, N., Connolly, R. M., Friess, D. A., et al. (2019). The future of blue carbon science. Nat. Commun. 10:3998. doi: 10.1038/s41467-019-11693-w
Macreadie, P. I., Serrano, O., Maher, D. T., Duarte, C. M., and Beardall, J. (2017). Addressing calcium carbonate cycling in blue carbon accounting. Limnol. Oceanogr. 2, 195–201. doi: 10.1002/lol2.10052
Mahadevan, K. (2015). “Chapter 13 - seaweeds: a sustainable food source” in Seaweed sustainability. eds. B. K. Tiwari and D. J. Troy (Amsterdam, Netherlands: Academic Press), 347–364.
Mahalder, B., Haque, M. M., Siddique, M. A. B., Hasan, N. A., Alam, M. M., Talukdar, M. M. N., et al. (2023). Embryonic and larval development of stinging catfish, Heteropneustes fossilis, in relation to climatic and water quality parameters. Life 13:583. doi: 10.3390/life13020583
Mahmood, T., Fang, J., Jiang, Z., and Zhang, J. (2016). Carbon and nitrogen flow, and trophic relationships, among the cultured species in an integrated multi-trophic aquaculture (IMTA) bay. Aquac. Environ. Interact. 8, 207–219. doi: 10.3354/aei00152
Malyan, S. K., Singh, O., Kumar, A., Anand, G., Singh, R., Singh, S., et al. (2022). Greenhouse gases trade-off from ponds: an overview of emission process and their driving factors. Water 14:970. doi: 10.3390/w14060970
Mamun-Ur-Rashid, M., Belton, B., Phillips, M., and Rosentrater, K.A., (2013). Improving aquaculture feed in Bangladesh: From feed ingredients to farmer profit to safe consumption. WorldFish.
Mao, Y., Yang, H., Zhou, Y., Ye, N., and Fang, J. (2009). Potential of the seaweed Gracilaria lemaneiformis for integrated multi-trophic aquaculture with scallop Chlamys farreri in North China. J. Appl. Phycol. 21, 649–656. doi: 10.1007/s10811-008-9398-1
Marinho, G. S., Holdt, S. L., Birkeland, M. J., and Angelidaki, I. (2015). Commercial cultivation and bioremediation potential of sugar kelp, Saccharina latissima, in Danish waters. J. Appl. Phycol. 27, 1963–1973. doi: 10.1007/s10811-014-0519-8
Marinho-Soriano, E., Nunes, S. O., Carneiro, M. A. A., and Pereira, D. C. (2009). Nutrients' removal from aquaculture wastewater using the macroalgae Gracilaria birdiae. Biomass Bioenergy 33, 327–331. doi: 10.1016/j.biombioe.2008.07.002
Marques, H. L. A., New, M. B., Boock, M. V., Barros, H. P., Mallasen, M., and Valenti, W. C. (2016). Integrated freshwater prawn farming: state-of-the-art and future potential. Rev. Fish. Sci. Aquac. 24, 264–293. doi: 10.1080/23308249.2016.1169245
Maulu, S., Hasimuna, O. J., Haambiya, L. H., Monde, C., Musuka, C. G., Makorwa, T. H., et al. (2021). Climate change effects on aquaculture production: sustainability implications, mitigation, and adaptations. Front. Sustain. 5:609097. doi: 10.3389/fsufs.2021.609097
Mcleod, E., Chmura, G. L., Bouillon, S., Salm, R., Björk, M., Duarte, C. M., et al. (2011). A blueprint for blue carbon: toward an improved understanding of the role of vegetated coastal habitats in sequestering CO2. Front. Ecol. Environ. 9, 552–560. doi: 10.1890/110004
McVey, J., Stickney, R., Yarish, C., and Chopin, T. (2002). Aquatic polyculture and balanced ecosystem management: New paradigms for seafood production. Elsevier, Amsterdam: CABI International, 91–104.
Mišurcová, L., Machů, L., and Orsavová, J. (2011). “Chapter 29 - seaweed minerals as nutraceuticals” in Advances in food and nutrition research. ed. S.-K. Kim (Waltham, USA: Academic Press), 371–390.
Moniruzzaman, M., Sku, S., Chowdhury, P., Tonu, M. B., Yeasmine, S., Hossen, M. N., et al. (2021). Nutritional evaluation of some economically important marine and freshwater mollusc species of Bangladesh. Heliyon 7:e07088. doi: 10.1016/j.heliyon.2021.e07088
Müssig, K. (2009). “Chapter 93 - iodine-induced toxic effects due to seaweed consumption” in Comprehensive handbook of iodine. eds. V. R. Preedy, G. N. Burrow, and R. Watson (Boston, USA: Academic Press), 897–908.
Naylor, R. L., Hardy, R. W., Buschmann, A. H., Bush, S. R., Cao, L., Klinger, D. H., et al. (2021). A 20-year retrospective review of global aquaculture. Nature 591, 551–563. doi: 10.1038/s41586-021-03308-6
Nederlof, M. A. J., Verdegem, M. C. J., Smaal, A. C., and Jansen, H. M. (2022). Nutrient retention efficiencies in integrated multi-trophic aquaculture. Rev. Aquac. 14, 1194–1212. doi: 10.1111/raq.12645
Neori, A., Chopin, T., Troell, M., Buschmann, A. H., Kraemer, G. P., Halling, C., et al. (2004). Integrated aquaculture: rationale, evolution and state of the art emphasizing seaweed biofiltration in modern mariculture. Aquaculture 231, 361–391. doi: 10.1016/j.aquaculture.2003.11.015
Neori, A., Guttman, L., Israel, A., and Shpigel, M. (2019). Israeli-developed models of marine integrated multi trophic aquaculture (IMTA). J. Coast. Res. 86, 11–20. doi: 10.2112/SI86-003.1
Neori, A., Shpige, M., Guttman, L., and Israel, A. (2017). Development of polyculture and integrated multi-trophic aquaculture (IMTA) in Israel: a review. Isr. J. Aquacult. Bamidgeh 69, 1–19. doi: 10.46989/001c.20874
Neveux, N., Bolton, J.J., Bruhn, A., Roberts, D.A., and Ras, M., (2018). The bioremediation potential of seaweeds: recycling nitrogen, phosphorus, and other waste products. Blue Biotechnology, 217–239.
Nobre, A. M., Robertson-Andersson, D., Neori, A., and Sankar, K. (2010). Ecological–economic assessment of aquaculture options: comparison between abalone monoculture and integrated multi-trophic aquaculture of abalone and seaweeds. Aquaculture 306, 116–126. doi: 10.1016/j.aquaculture.2010.06.002
Orban, E., Di Lena, G., Nevigato, T., Casini, I., Marzetti, A., and Caproni, R. (2002). Seasonal changes in meat content, condition index and chemical composition of mussels (Mytilus galloprovincialis) cultured in two different Italian sites. Food Chem. 77, 57–65. doi: 10.1016/S0308-8146(01)00322-3
Ortiz, J., Romero, N., Robert, P., Araya, J., Lopez-Hernández, J., Bozzo, C., et al. (2006). Dietary fiber, amino acid, fatty acid and tocopherol contents of the edible seaweeds Ulva lactuca and Durvillaea Antarctica. Food Chem. 99, 98–104. doi: 10.1016/j.foodchem.2005.07.027
Papageorgiou, N., Dimitriou, P. D., Chatzivasileiou, D., Tsapakis, M., and Karakassis, I. (2023). Can IMTA provide added ecosystem value services in the fish farms of Greece? Front. Mar. Sci. 9:1083099. doi: 10.3389/fmars.2022.1083099
Park, H. J., Han, E., Lee, W. C., Kwak, J. H., Kim, H. C., Park, M. S., et al. (2015). Trophic structure in a pilot system for the integrated multi-trophic aquaculture off the east coast of Korean peninsula as determined by stable isotopes. Mar. Pollut. Bull. 95, 207–214. doi: 10.1016/j.marpolbul.2015.04.019
Park, M., Shin, S. K., Do, Y. H., Yarish, C., and Kim, J. K. (2018). Application of open water integrated multi-trophic aquaculture to intensive monoculture: a review of the current status and challenges in Korea. Aquaculture 497, 174–183. doi: 10.1016/j.aquaculture.2018.07.051
Park, J.-S., Shin, S. K., Wu, H., Yarish, C., Yoo, H. I., and Kim, J. K. (2021). Evaluation of nutrient bioextraction by seaweed and shellfish aquaculture in Korea. J. World Aquacult. Soc. 52, 1118–1134. doi: 10.1111/jwas.12786
Peñalver, R., Lorenzo, J. M., Ros, G., Amarowicz, R., Pateiro, M., and Nieto, G. (2020). Seaweeds as a functional ingredient for a healthy diet. Mar. Drugs 18:301. doi: 10.3390/md18060301
Pereira, R., and Yarish, C. (2008). “Mass production of marine macroalgae” in Encyclopedia of ecology. eds. S. E. Jørgensen and B. D. Fath (Oxford: Elsevier), 2236–2247.
Pu, Y., Zhang, M., Jia, L., Zhang, Z., Ziao, W., Liu, S., et al. (2022). Methane emission of a lake aquaculture farm and its response to ecological restoration. Agric. Ecosyst. Environ. 330:107883. doi: 10.1016/j.agee.2022.107883
Putro, S. P., Widowati, W., Suhartana, S., and Muhammad, F. (2015). The application of integrated multi trophic aquaculture (IMTA) using stratified double net rounded cage (SDFNC) for aquaculture sustainability. Int. J. Sci. Eng. 9, 85–89. doi: 10.12777/ijse.9.2.85-89
Rajapakse, N., and Kim, S. K. (2011). “Chapter 2 - nutritional and digestive health benefits of seaweed” in Advances in food and nutrition research. ed. S.-K. Kim (Waltham, USA: Academic Press), 17–28.
Ramos, R., Vinatea, L., Seiffert, W., Beltrame, E., Silva, J. S., and Costa, R. H. R. (2009). Treatment of shrimp effluent by sedimentation and oyster filtration using Crassostrea gigas and C. rhizophorae. Braz. Arch. Biol. Technol. 52, 775–783. doi: 10.1590/S1516-89132009000300030
Ratcliff, J. J., Wan, A. H. L., Edwards, M. D., Soler-Vila, A., Johnson, M. P., Abreu, M. H., et al. (2016). Metal content of kelp (Laminaria digitata) co-cultivated with Atlantic salmon in an integrated multi-trophic aquaculture system. Aquaculture 450, 234–243. doi: 10.1016/j.aquaculture.2015.07.032
Ren, J. S., Stenton-Dozey, J., Plew, D. R., Fang, J., and Gall, M. (2012). An ecosystem model for optimising production in integrated multitrophic aquaculture systems. Ecol. Model. 246, 34–46. doi: 10.1016/j.ecolmodel.2012.07.020
Ridler, N., Wowchuk, M., Robinson, B., Barrington, K., Chopin, T., Robinson, S., et al. (2007). Integrated multi − trophic aquaculture (IMTA): a potential strategic choice for farmers. Aquac. Econ. Manag. 11, 99–110. doi: 10.1080/13657300701202767
Robertson-Andersson, D. V., Potgieter, M., Hansen, J., Bolton, J. J., Troell, M., Anderson, R. J., et al. (2008). Integrated seaweed cultivation on an abalone farm in South Africa. J. Appl. Phycol. 20, 579–595. doi: 10.1007/s10811-007-9239-7
Rosa, J., Leston, S., Crespo, D., Freitas, A., Pouca, A. S. V., Barbosa, J., et al. (2020). Uptake of enrofloxacin from seawater to the macroalgae Ulva and its use in IMTA systems. Aquaculture 516:734609. doi: 10.1016/j.aquaculture.2019.734609
Rosa, J., Leston, S., Freitas, A., Pouca, A. S. V., Barbosa, J., Lemos, M. F. L., et al. (2019). Oxytetracycline accumulation in the macroalgae Ulva: potential risks for IMTA systems. Chemosphere 226, 60–66. doi: 10.1016/j.chemosphere.2019.03.112
Samocha, T. M., Fricker, J., Ali, A. M., Shpigel, M., and Neori, A. (2015). Growth and nutrient uptake of the macroalga Gracilaria tikvahiae cultured with the shrimp Litopenaeus vannamei in an integrated multi-trophic aquaculture (IMTA) system. Aquaculture 446, 263–271. doi: 10.1016/j.aquaculture.2015.05.008
Santos, M. J. M., and Valenti, W. C. (2002). Production of Nile Tilapia Oreochromis niloticus and freshwater prawn Macrobrachium rosenbergii stocked at different densities in polyculture Systems in Brazil. J. World Aquacult. Soc. 33, 369–376. doi: 10.1111/j.1749-7345.2002.tb00513.x
Sarà, G., Reid, G. K., Rinaldi, A., Palmeri, V., Troell, M., and Kooijman, S. A. L. M. (2012). Growth and reproductive simulation of candidate shellfish species at fish cages in the southern Mediterranean: dynamic energy budget (DEB) modelling for integrated multi-trophic aquaculture. Aquaculture 324-325, 259–266. doi: 10.1016/j.aquaculture.2011.10.042
SARF (2017). Carbon footprint of Scottish suspended mussels and intertidal oysters, Scottish aquaculture research Forum (SARF):30.
Seitz, R. D., Wennhage, H., Bergström, U., Lipcius, R. N., and Ysebaert, T. (2013). Ecological value of coastal habitats for commercially and ecologically important species. ICES J. Mar. Sci. 71, 648–665. doi: 10.1093/icesjms/fst152
Şereflişan, H., and Ersoy-Altun, B. (2018). Amino acid and fatty acid composition of freshwater mussels, Anodonta pseudodopsis and Unio tigridis. Pakistan J. Zool. 50, 2153–2158. doi: 10.17582/journal.pjz/2018.50.6.2153.2158
Shahabuddin, A., Wahab, M., Miah, M., and Salam, M. (2010). Abundance, distribution and culture potentials of three commercially important mollusks species along the coast of Bay of Bengal. J. Agric. Biol. Sci. 6, 754–762. doi: 10.5555/20113079807
Shannon, E., and Abu-Ghannam, N. (2019). Seaweeds as nutraceuticals for health and nutrition. Phycologia 58, 563–577. doi: 10.1080/00318884.2019.1640533
Shpigel, M., Ari, T. B., Shauli, L., Odintsov, V., and Ben-Ezra, D. (2016). Nutrient recovery and sludge management in seabream and grey mullet co-culture in integrated multi-trophic aquaculture (IMTA). Aquaculture 464, 316–322. doi: 10.1016/j.aquaculture.2016.07.007
Sicuro, B., Castelar, B., Mugetti, D., Pastorino, P., Chiarandon, A., Menconi, V., et al. (2020). Bioremediation with freshwater bivalves: a sustainable approach to reducing the environmental impact of inland trout farms. J. Environ. Manag. 276:111327. doi: 10.1016/j.jenvman.2020.111327
Siddique, M. A. B., Ahammad, A. K. S., Bashar, A., Hasan, N. A., Mahalder, B., Alam, M. M., et al. (2022a). Impacts of climate change on fish hatchery productivity in Bangladesh: a critical review. Heliyon 8:e11951. doi: 10.1016/j.heliyon.2022.e11951
Siddique, M. A. B., Ahammad, A. K. S., Mahalder, B., Alam, M. M., Hasan, N. A., Bashar, A., et al. (2022b). Perceptions of the impact of climate change on performance of fish hatcheries in Bangladesh: an empirical study. Fishes. 7:270. doi: 10.3390/fishes7050270
Slegers, P. M., Helmes, R. J. K., Draisma, M., Broekema, R., Vlottes, M., and van den Burg, S. W. K. (2021). Environmental impact and nutritional value of food products using the seaweed Saccharina latissima. J. Clean. Prod. 319:128689. doi: 10.1016/j.jclepro.2021.128689
Sri-uam, P., Donnuea, S., Powtongsook, S., and Pavasant, P. (2016). Integrated multi-trophic recirculating aquaculture system for Nile Tilapia (Oreochlomis niloticus). Sustain. For. 8:592. doi: 10.3390/su8070592
Stenton-Dozey, J. M. E., Heath, P., Ren, J. S., and Zamora, L. N. (2021). New Zealand aquaculture industry: research, opportunities and constraints for integrative multitrophic farming. N. Z. Mar. Freshw. Res. 55, 265–285. doi: 10.1080/00288330.2020.1752266
Strand, Ø., Jansen, H. M., Jiang, Z., and Robinson, S. M. C. (2019). “Perspectives on bivalves providing regulating Services in Integrated Multi-Trophic Aquaculture” in Goods and Services of Marine Bivalves. eds. A. C. Smaal, J. G. Ferreira, J. Grant, J. K. Petersen, and Ø. Strand (Cham, Switzerland: Springer International Publishing), 209–230.
Subasinghe, M. M., Jinadasa, B. K. K. K., Navarathne, A. N., and Jayakody, S. (2019). Seasonal variations in the total lipid content and fatty acid composition of cultured and wild Crassostrea madrasensis in Sri Lanka. Heliyon 5:e01238. doi: 10.1016/j.heliyon.2019.e01238
Sukhdhane, K., Kripa, V., Divu, D., Vase, V., and Mojjada, S. (2018). Integrated multi-trophic aquaculture systems: a solution for sustainability. Aquac. Asia Magazine 22, 26–29.
Sultana, F., Wahab, M. A., Nahiduzzaman, M., Mohiuddin, M., Iqbal, M. Z., Shakil, A., et al. (2023). Seaweed farming for food and nutritional security, climate change mitigation and adaptation, and women empowerment: a review. Aquac. Fish. 8, 463–480. doi: 10.1016/j.aaf.2022.09.001
Tan, K., and Zheng, H. (2020). Ocean acidification and adaptive bivalve farming. Sci. Total Environ. 701:134794. doi: 10.1016/j.scitotenv.2019.134794
Thornber, K., Bashar, A., Ahmed, M. S., Bell, A., Trew, J., Hasan, M., et al. (2022). Antimicrobial resistance in aquaculture environments: unravelling the complexity and connectivity of the underlying societal drivers. Environ. Sci. Technol. 56, 14891–14903. doi: 10.1021/acs.est.2c00799
Tom, A. P., Jayakumar, J. S., Biju, M., Somarajan, J., and Ibrahim, M. A. (2021). Aquaculture wastewater treatment technologies and their sustainability: a review. Energy Nexus 4:100022. doi: 10.1016/j.nexus.2021.100022
Troell, M., Halling, C., Neori, A., Chopin, T., Buschmann, A. H., Kautsky, N., et al. (2003). Integrated mariculture: asking the right questions. Aquaculture 226, 69–90. doi: 10.1016/S0044-8486(03)00469-1
Troell, M., Joyce, A., Chopin, T., Neori, A., Buschmann, A. H., and Fang, J. G. (2009). Ecological engineering in aquaculture — potential for integrated multi-trophic aquaculture (IMTA) in marine offshore systems. Aquaculture 297, 1–9. doi: 10.1016/j.aquaculture.2009.09.010
Troell, M., Naylor, R. L., Metian, M., Beveridge, M., Tyedmers, P. H., Folke, C., et al. (2014). Does aquaculture add resilience to the global food system? Proc. Natl. Acad. Sci. 111, 13257–13263. doi: 10.1073/pnas.1404067111
Turan, G., and Neori, A. (2010). “Intensive Sea weed aquaculture: a potent solution against global warming” in Seaweeds and their role in globally changing environments. eds. J. Seckbach, R. Einav, and A. Israel (Netherlands: Springer), 357–372.
van der Schatte Olivier, A., Jones, L., Vay, L. L., Christie, M., Wilson, J., and Malham, S. K. (2020). A global review of the ecosystem services provided by bivalve aquaculture. Rev. Aquac. 12, 3–25. doi: 10.1111/raq.12301
Venugopal, V., and Gopakumar, K. (2017). Shellfish: nutritive value, health benefits, and consumer safety. Compr. Rev. Food Sci. Food Saf. 16, 1219–1242. doi: 10.1111/1541-4337.12312
Viana, C. M., Freire, D., Abrantes, P., Rocha, J., and Pereira, P. (2022). Agricultural land systems importance for supporting food security and sustainable development goals: a systematic review. Sci. Total Environ. 806:150718. doi: 10.1016/j.scitotenv.2021.150718
Viji, C. S., Chadha, N. K., Kripa, V., Prema, D., Prakash, C., Sharma, R., et al. (2014). Can oysters control eutrophication in an integrated fish-oyster aquaculture system? J. Mar. Biol. Assoc. India 56, 67–73. doi: 10.6024/jmbai.2014.56.2.01811-10
Wang, J., Beusen, A. H. W., Liu, X., and Bouwman, A. F. (2020). Aquaculture production is a large, spatially concentrated source of nutrients in Chinese freshwater and coastal seas. Environ. Sci. Technol. 54, 1464–1474. doi: 10.1021/acs.est.9b03340
Whitmarsh, D. J., Cook, E. J., and Black, K. D. (2006). Searching for sustainability in aquaculture: an investigation into the economic prospects for an integrated salmon–mussel production system. Mar. Policy 30, 293–298. doi: 10.1016/j.marpol.2005.01.004
Wu, H., Huo, Y., Han, F., Liu, Y., and He, P. (2015). Bioremediation using Gracilaria chouae co-cultured with Sparus macrocephalus to manage the nitrogen and phosphorous balance in an IMTA system in Xiangshan Bay, China. Mar. Pollut. Bull. 91, 272–279. doi: 10.1016/j.marpolbul.2014.11.032
Xiao, Y., and Watson, M. (2019). Guidance on conducting a systematic literature review. J. Plan. Educ. Res. 39, 93–112. doi: 10.1177/0739456X17723971
Xu, C., Su, G., Zhao, K., Xu, X., Li, Z., Hu, Q., et al. (2022). Current status of greenhouse gas emissions from aquaculture in China. Water Biol. Secur. 1:100041. doi: 10.1016/j.watbs.2022.100041
Yang, H. S. (2000). Comparison of efficiencies of different culture systems in the shallow sea along Yantai. J. Fish. Sci. China : 24, 140–145.
Yang, H., Huo, Y., Yee, J. C., and Yarish, C. (2021). Germplasm cryopreservation of macroalgae for aquaculture breeding and natural resource conservation: a review. Aquaculture 544:737037. doi: 10.1016/j.aquaculture.2021.737037
Yang, P., Lai, D. Y. F., Yang, H., Tong, C., Lebel, L., Huang, J., et al. (2019). Methane dynamics of aquaculture shrimp ponds in two subtropical estuaries, Southeast China: dissolved concentration, net sediment release, and water oxidation. J. Geophys. Res. Biogeosci. 124, 1430–1445. doi: 10.1029/2018JG004794
Yang, P., Zhao, G., Tong, C., Tang, K. W., Lai, D. Y. F., Li, L., et al. (2021). Assessing nutrient budgets and environmental impacts of coastal land-based aquaculture system in southeastern China. Agric. Ecosyst. Environ. 322:107662. doi: 10.1016/j.agee.2021.107662
Yang, H., Zhou, Y., Mao, Y., Li, X., Liu, Y., and Zhang, F. (2005). Growth characters and photosynthetic capacity of Gracilaria lemaneiformis as a biofilter in a shellfish farming area in Sanggou Bay, China. J. Appl. Phycol. 17, 199–206. doi: 10.1007/s10811-005-6419-1
Ye, W., Sun, H., Li, Y., Zhang, J., Zhang, M., Gao, Z., et al. (2022). Greenhouse gas emissions from fed mollusk mariculture: a case study of a Sinonovacula constricta farming system. Agric. Ecosyst. Environ. 336:108029. doi: 10.1016/j.agee.2022.108029
Yeh, S. L., Dahms, H. U., Chiu, Y. J., Chang, S. J., and Wang, Y. K. (2017). Increased production and water remediation by land-based farm-scale sequentially integrated multi-trophic aquaculture systems—an example from southern Taiwan. Sustain. For. 9:2173. doi: 10.3390/su9122173
Yokoyama, H., and Ishihi, Y. (2010). Bioindicator and biofilter function of Ulva spp. (Chlorophyta) for dissolved inorganic nitrogen discharged from a coastal fish farm — potential role in integrated multi-trophic aquaculture. Aquaculture 310, 74–83. doi: 10.1016/j.aquaculture.2010.10.018
Yong, W. T. L., Thien, V. Y., Rupert, R., and Rodrigues, K. F. (2022). Seaweed: a potential climate change solution. Renew. Sust. Energ. Rev. 159:112222. doi: 10.1016/j.rser.2022.112222
Zhang, Y., Tang, K. W., Yang, P., Yang, H., Tong, C., Song, C., et al. (2022). Assessing carbon greenhouse gas emissions from aquaculture in China based on aquaculture system types, species, environmental conditions and management practices. Agric. Ecosyst. Environ. 338:108110. doi: 10.1016/j.agee.2022.108110
Zhao, J., Zhang, M., Xiao, W., Jia, L., Zhang, X., Wang, J., et al. (2021). Large methane emission from freshwater aquaculture ponds revealed by long-term eddy covariance observation. Agric. For. Meteorol. 308–309:108600. doi: 10.1016/j.agrformet.2021.108600
Zhou, Y., Yang, H., Hu, H., Liu, Y., Mao, Y., Zhou, H., et al. (2006). Bioremediation potential of the macroalga Gracilaria lemaneiformis (Rhodophyta) integrated into fed fish culture in coastal waters of North China. Aquaculture 252, 264–276. doi: 10.1016/j.aquaculture.2005.06.046
Keywords: integrated multitrophic aquaculture, prawn farming, GHG emission, climate change, sustainability, Bangladesh
Citation: Alam MM, Jørgensen NOG, Bass D, Santi M, Nielsen M, Rahman MA, Hasan NA, Bablee AL, Bashar A, Hossain MI, Hansen LH and Haque MM (2024) Potential of integrated multitrophic aquaculture to make prawn farming sustainable in Bangladesh. Front. Sustain. Food Syst. 8:1412919. doi: 10.3389/fsufs.2024.1412919
Edited by:
Pallab Sarker, University of California, Santa Cruz, United StatesReviewed by:
Luis Poersch, Federal University of Rio Grande, BrazilHazrat Ali, WorldFish (Bangladesh), Bangladesh
Copyright © 2024 Alam, Jørgensen, Bass, Santi, Nielsen, Rahman, Hasan, Bablee, Bashar, Hossain, Hansen and Haque. This is an open-access article distributed under the terms of the Creative Commons Attribution License (CC BY). The use, distribution or reproduction in other forums is permitted, provided the original author(s) and the copyright owner(s) are credited and that the original publication in this journal is cited, in accordance with accepted academic practice. No use, distribution or reproduction is permitted which does not comply with these terms.
*Correspondence: Mohammad Mahfujul Haque, bW1oYXF1ZS5hcUBiYXUuZWR1LmJk