- 1The State Key Laboratory of Herbage Improvement and Grassland Agro-ecosystems, National Field Scientific Observation and Research Station of Grassland Agro-Ecosystems in Gansu Qingyang, College of Pastoral Agriculture Science and Technology, Lanzhou, China
- 2The UWA Institute of Agriculture, The University of Western Australia, Perth, WA, Australia
Introduction: Lucerne (Medicago sativa L.) is a crucial component in agricultural rotation systems due to its ability to enhance soil carbon (C) and nitrogen (N) contents. In China, conventional tillage (CT) is commonly used for lucerne termination, leading to soil structure degradation and C and N losses. While herbicide application has been suggested for lucerne termination in the United States and EU, its impact on lucerne growth suppression and soil C and N contents in China remains uncertain.
Methods: In this study, we examined the effects of herbicide type [glyphosate and 2,4-D (G + 2), glyphosate and dicamba (G + D), 2,4-D and dicamba (2 + D), with water (W) and CT as controls] and concentration (100, 200, 300%) on various lucerne and soil properties.
Results and Discussion: Our findings revealed that G + 2 and 2 + D treatments significantly reduced the regreening rate, and the recommended herbicide concentration (100%) was sufficient for lucerne termination. CT and W treatments resulted in a higher soil pH compared to herbicide treatments. Moreover, herbicide treatments exhibited higher soil organic C (SOC) and total N (TN) levels than the CT treatment. The 2 + D treatment demonstrated a higher SOC content compared to the G + 2 treatment, while the 200 and 300% herbicide concentrations reduced SOC and TN levels. The G + 2 treatment had the highest soil ammonium nitrogen content (7.94 ± 1.45 mg kg−1), while the CT treatment showed the lowest (6.46 ± 1.54 mg kg−1). In conclusion, our study suggests that applying the recommended herbicide dosage (100%) effectively terminates lucerne grassland on the Loess Plateau of China without negatively impacting soil C and N storage. Finally, it is important to acknowledge that one-year trials at a single site have inherent limitations, and the findings should be considered cautiously when informing policy decisions.
1. Introduction
Soil is the largest terrestrial carbon (C) pool (Scharlemann et al., 2014), with soil organic carbon (SOC) widely recognized as the core of soil quality, particularly in agricultural ecosystems due to its essential role in sustaining crop production and environmental resistance (Smith et al., 2016; Rumpel et al., 2018). Consequently, increasing SOC stocks could secure sustainable agricultural systems and food production and mitigate rising atmospheric carbon dioxide levels (Basile-Doelsch et al., 2020). Hence, optimizing agricultural management practices has been promoted to facilitate the SOC sequestration since agricultural management practices largely affect the turnover of SOC and relevant soil processes (Basile-Doelsch et al., 2020; Li et al., 2021).
Combined with minimum tillage, crop rotation increases agricultural SOC storage (Holland, 2004; Li et al., 2020). Studies have shown that legume-based rotations increase agricultural crop production (Zhao et al., 2022) and SOC content in global croplands (Liu et al., 2022). Incorporating legumes into cropping systems can improve system productivity under low fertilizer nitrogen (N) inputs and enhance soil C stabilization, particularly in N-limited deep subsoil (Peixoto et al., 2022). Deep-rooted perennial legumes can also improve subsoil C inputs and stabilization (Yuan et al., 2016).
Lucerne (Medicago sativa L.) is the most widely cultivated and used perennial leguminous forage worldwide (Wang et al., 2021). Lucerne’s abundant underground biomass and root exudates promote soil aggregate formation and improve microbial C utilization efficiency, reducing soil heterotrophic respiration and system carbon dioxide emissions (Drinkwater, 1999). In addition, crop rotations with lucerne can improve soil quality, increase SOC and total nitrogen (TN) contents, and enhance agricultural SOC sequestration (Bell et al., 2012; Eerd et al., 2014), with significance for ecological security (Yuan et al., 2016).
Lucerne takes up plenty of water and has a high transpiration coefficient (Fourie, 2017). Therefore, lucerne growth over time inevitably leads to soil water deficit and even deep soil desiccation in water-limited areas (Shen et al., 2009; Ali et al., 2021). Thus, lucerne/annual crop rotations can maximize the benefits of lucerne in rotation systems and avoid the formation of dry soil layers so as not to impede subsequent crop growth (Davies and Peoples, 2003; Bell et al., 2012). However, lucerne termination in the rotation system frustrates producers. Conventional techniques, such as moldboard plowing, subsoiling, and disking, are commonly used to terminate lucerne; however, they increase soil erosion (Mohr et al., 1999), break down soil aggregates, and eventually decrease SOC (Entz et al., 1995), offsetting the sequestrated SOC from lucerne in the rotation system.
As an alternative to conventional techniques, herbicide application could terminate lucerne growth in the rotation system (Randall et al., 2001), with increasing interest in this technique among producers in the Canadian Prairies and northern US Great Plains (Entz et al., 1995). Herbicides that inhibit lucerne growth include glyphosate [N-(phosphonomethyl) glycine] (Davis et al., 1978; Clayton, 1982), dicamba (3,6-dichloro-2-methoxybenzoic acid) (Button, 1994), 2,4-D [(2,4-dichloro phenoxy) acetic acid] (Moomaw and Martin, 1976), and clopyralid (3,6-dichloropicolinic acid) (Clayton, 1982; Button, 1994). However, the effect of herbicides on suppressing lucerne growth and its impact on soil C and N contents in China remains unclear.
Lucerne is an important crop in the dryland farming systems of the Loess Plateau, China, occupying more than 70% of the farmland in north-western China (Han et al., 2010). Therefore, assessing herbicide efficiency in inhibiting lucerne growth would be meaningful for optimizing lucerne in crop rotation systems. Thus, our hypothesis was that different combinations of herbicides applied at various doses (100, 200, 300%) might result in different effects on lucerne termination and on C and N cycles.
2. Materials and methods
2.1. Site characterization
This study was performed at the Loess Plateau Research Station of Lanzhou University, Gansu, China (35°40′ N, 107°51′ E, elevation 1,297 m), a typical rainfed agricultural area with a semiarid continental monsoon climate and more than 60% of the precipitation occurring from July to September. The mean annual temperature and precipitation (1970–2021) are 9°C and 561 mm, respectively (Li et al., 2022). The soil is classified as silty loam soil based on the FAO/UNESCO soil classification (Table 1). The soil pH is 8.4 (0–20 cm).
Lucerne grassland (Medicago sativa L. cv. Gannong No.3) was established in April 2016 at a seeding rate of 1.5 kg ha−1, sowing depth of 3 cm, and row spacing of 30 cm (Pan et al., 2016). The experimental field received 140 kg ha−1 N and 70 kg ha−1 P, as urea and calcium superphosphate at sowing stage, and no irrigation. Lucerne was harvested twice a year.
2.2. Experimental design and setup
We conducted a preliminary study based on previous research to determine the types and combinations of herbicide (Davies and Peoples, 2003; Clark et al., 2021), from which three herbicides were selected: 41% glyphosate isopropylamine salt solution (Shandong Shengpeng Technology Co., Ltd., China, referred to herein as glyphosate), 90% 2,4-D isooctyl emulsifiable concentrate (Tianjin Boke Baisheng Technology Co., Ltd., China, 2,4-D), and 48% dicamba water agent (Zhejiang Baike Biotechnology Co., Ltd., China, dicamba).
The factorial experiment comprised two factors: (1) three herbicide type combinations [glyphosate and 2,4-D (G + 2), glyphosate and dicamba (G + D), 2,4-D and dicamba (2 + D)], with water (W) and conventional tillage (CT) used as controls; (2) three herbicide concentrations at 100, 200, and 300% of the recommended dose. The recommended doses for glyphosate, 2,4-D, and dicamba are 0.55 mL m−2, 0.07 mL m−2, and 0.09 mL m−2, respectively. The experimental plots were arranged randomly with four replications of each treatment and 1 m walkways between each plot.
The treatments were applied on September 16, 2021, when the average height of lucerne was about 15 cm. The CT treatment was spaded to a depth of 40 cm to remove lucerne roots. Herbicide treatments involved spraying a tank mixture of the herbicide combination on the lucerne. Soil samples were collected in December 2021, January 2022, and March 2022 to assess dynamic changes in SOC and TN contents.
2.3. NDVI, SPAD, plant height, and regreening rate
The normalized difference vegetation index (NDVI) was measured on September 20, September 30, and October 24, 2021, using GreenSeeker (RT100B, N-Tech, United States). The relative leaf chlorophyll content (characterized by SPAD) was measured with a portable SPAD chlorophyll meter (SPAD-502, Konica Minolta, Japan) on September 30, 2021. Five leaves in each plot were randomly selected to determine SPAD values, which were averaged.
The number of lucerne plants in a 1 m2 quadrat was counted before the treatments were applied (September 2021) and after the regreening stage (April 2022) to calculate the regreening rate (Li, 2020):
Plant height was measured in May 2022 on four lucerne stems randomly selected in each plot, with the natural extension height from the root to the leaf tip measured with a ruler and the average value taken as plant height.
2.4. Soil sample collection and analysis
Soil samples at 0–10, 10–20, 20–30, 30–60, and 60–100 cm were collected with a soil drill (5 cm diameter). Part of the sample was air-dried and ground to pass through a 0.2 mm sieve to determine SOC and TN contents. Soil for ammonium-N (NH4+-N) and nitrate-N (NO3−-N) analysis was stored at 4°C.
SOC content was determined using the potassium dichromate external heating method (K2Cr2O7-H2SO4 oxidation method) (Chan et al., 2001) and subsequently titrated with o-phenanthroline indicator and 0.2 mol L−1 ferrous sulfate (FeSO4) solution (Mccarty et al., 2010). TN content was determined using the Kjeldahl method (Kjeldahl, 1883). NO3−-N and NH4+-N contents were determined with an automatic high flux intermittent chemical analyzer (smart 450 auto analyst, AMS, Italy) with 50 mL of 2 mol L−1 KCl extraction (Li et al., 2012). Soil pH was measured with an intelligent portable redox potentiometer (LD-QX6530, Laide-en, China).
2.5. Data analysis
NDVI, SPAD, and plant height at the regreening stage, regreening rate, and soil pH were analyzed by two-way ANOVA for herbicide type and concentration. SOC, TN, and NO3−-N and NH4+-N contents were analyzed by three-way ANOVA for herbicide type, herbicide concentration, and sampling date. The data were tested for normality and homogeneity before performing the ANOVA. Significant results were compared using Tukey’s HSD method with a significance level of 0.05. All data analyses were undertaken in the Agricola package (De Mendiburu, 2014) and plotted with the ‘ggplot2’ package (Wickham, 2016) in R (v4.1.3).
3. Results
3.1. Effect of herbicide type and concentration on lucerne growth
Herbicide type, herbicide concentration, and their interaction significantly affected the NDVI of lucerne grassland (Figure 1), which decreased over time (p < 0.05). The G + 2 treatment had a lower NDVI than the 2 + D treatment (p < 0.05). Regardless of herbicide type and concentration, the herbicide treatments had lower NDVI values than the W control (NDVI = 0.918 ± 0.010, p < 0.05).
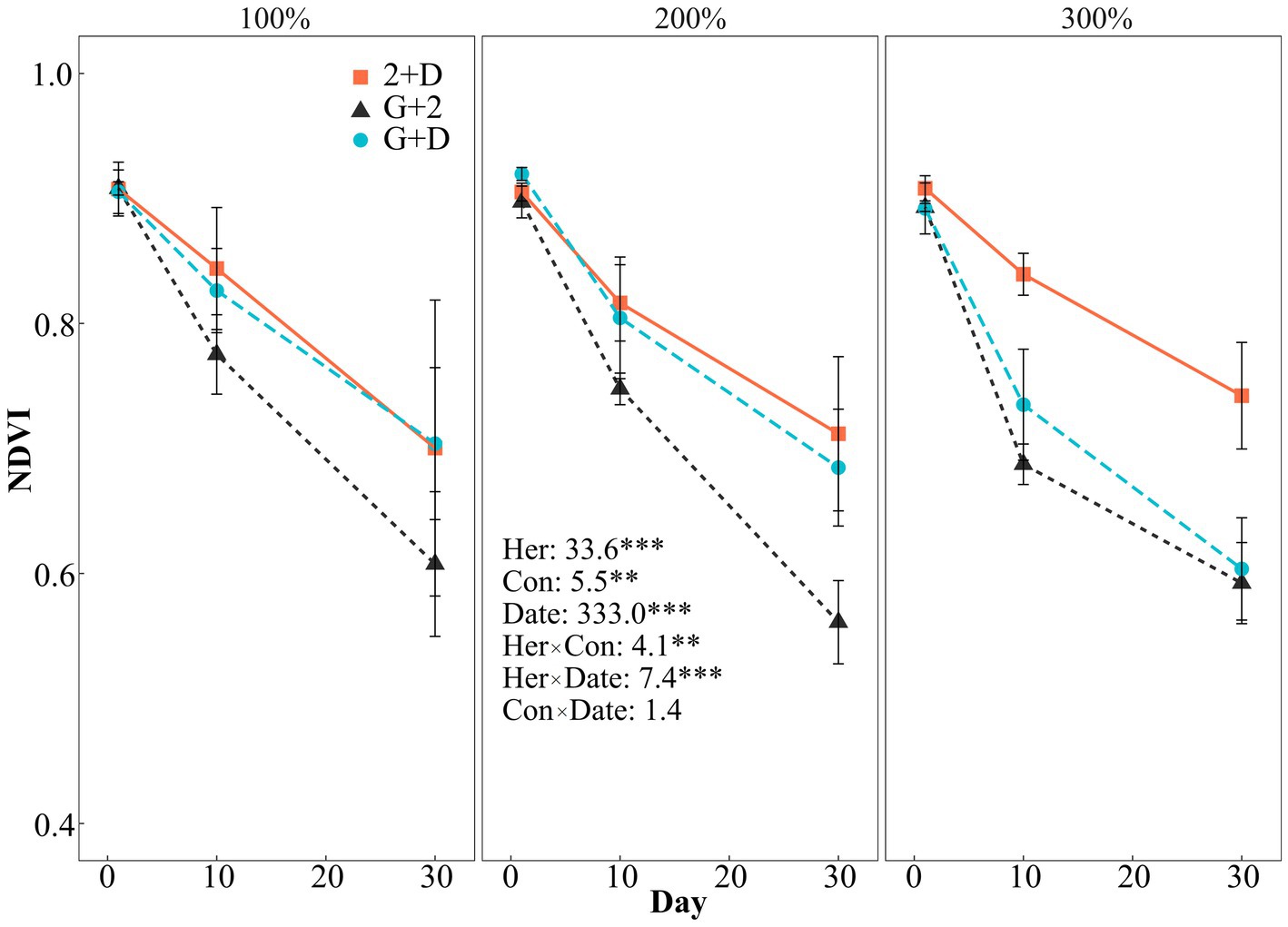
Figure 1. Effect of herbicide (2 + D, G + D, G + 2) and concentration (100%, 200%, 300%) on the NDVI of lucerne for 30 days following herbicide application. Dots are means (bars represent standard deviation, n = 4). F value is marked in the figure. *, **, and *** indicate significance levels at p < 0.05, 0.01, and 0.001. Note the y-intercept is not 0. The three herbicide types were combined glyphosate and 2,4-D (G + 2), glyphosate and dicamba (G + D), and 2,4-D and dicamba (2 + D). Water (W) and conventional tillage (CT) was used as the control. The three herbicide concentrations were 100, 200, and 300% of the recommended dose.
Herbicide type, herbicide concentration, and their interaction significantly affected the SPAD value of lucerne (Figure 2). Except for the G + 2 (100%) treatment, the herbicide treatments had significantly lower SPAD values than the W control (49.91 ± 8.68). The G + 2 (200%) and G + 2 (300%) treatments had lower SPAD values than the G + D (200%) treatments (p < 0.05).
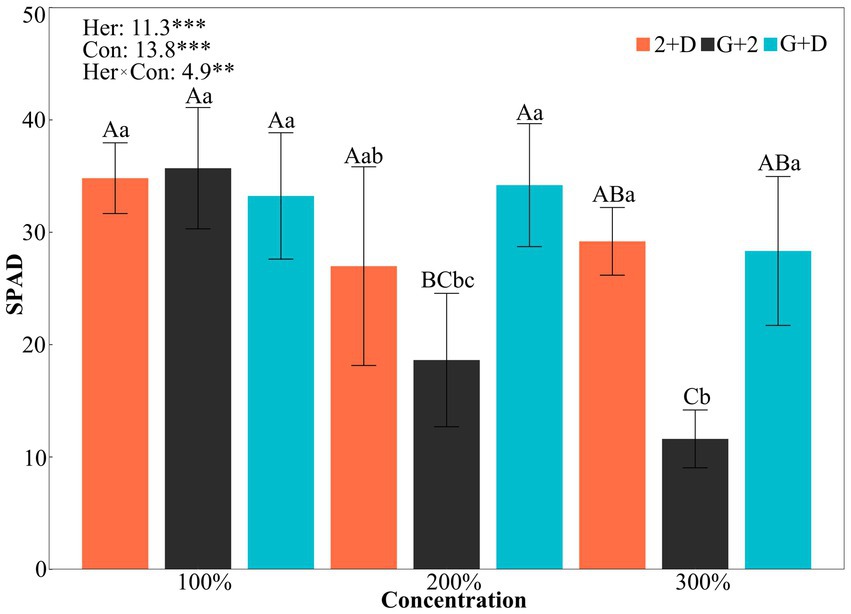
Figure 2. Effect of herbicide (2 + D, G + D, G + 2) and concentration (100, 200, 300%) on SPAD value of lucerne 14 days after treatment application. Values are means of four replicates (± standard deviation). F value is marked in the figure. *, **, and *** indicate significance levels at p < 0.05, 0.01, and 0.001. Different upper case letters indicate significant differences in SPAD values of lucerne under different concentrations of the same herbicide, and different lower case letters indicate significant differences in SPAD values of lucerne under different herbicide types with the same concentration (p < 0.05). For a description of the abbreviations, refer to the Figure 1 caption.
All herbicide types and concentrations significantly decreased the regreening rate of lucerne compared to the W control (regreening rate > 90%) (Table 2). There was no significant difference between herbicide types and concentration levels, and regreening rates under CT and herbicide treatments irrespective of types and concentrations were lower than 8%.
All herbicide types and concentrations significantly decreased lucerne height at the regreening stage compared to the W control (45.25 ± 1.13 cm) (Table 3). There was no significant difference between herbicide types and concentrations, and lucerne height under CT and herbicide treatments irrespective of types and concentrations were in the range of 10–25 cm, which was significantly lower than the W control.
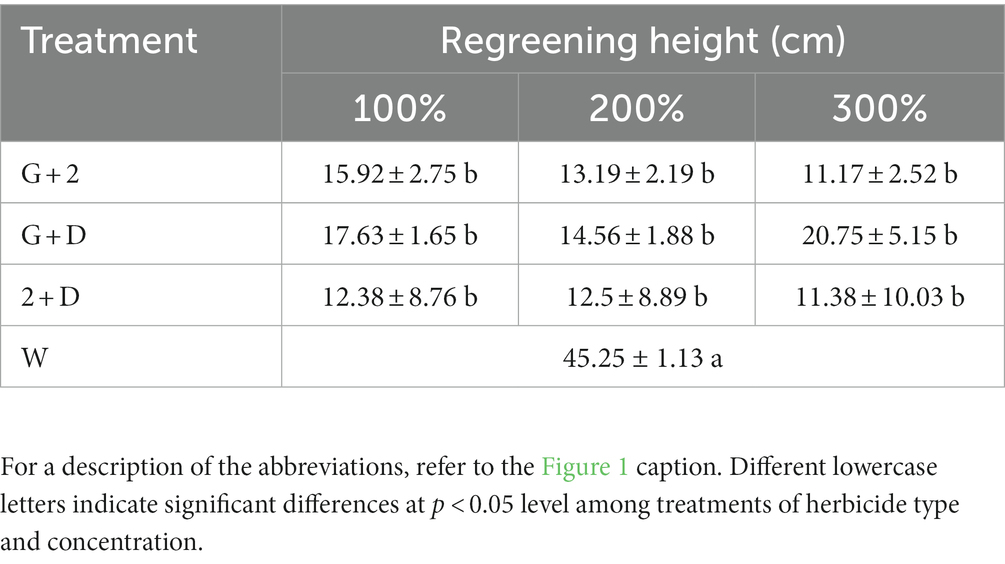
Table 3. Effect of herbicide type and concentration on lucerne height at regreening stage (mean ± SD, n = 4).
3.2. Effect of herbicide type and concentration on soil pH and soil C and N contents
Herbicide type, herbicide concentration, and their interaction significantly affected soil pH from 0–100 cm (Figure 3). The CT and W controls had significantly higher soil pH values than the herbicide treatments. The G + D treatment had the lowest soil pH (p < 0.05). The herbicide treatments at 100% concentration had significantly higher soil pH values than the 200 and 300% concentrations, with no significant difference between the 200 and 300% concentrations. The 2 + D treatment (100, 200 and 300%) had higher soil pH values than the G + D treatment (100 and 300%, p < 0.05).
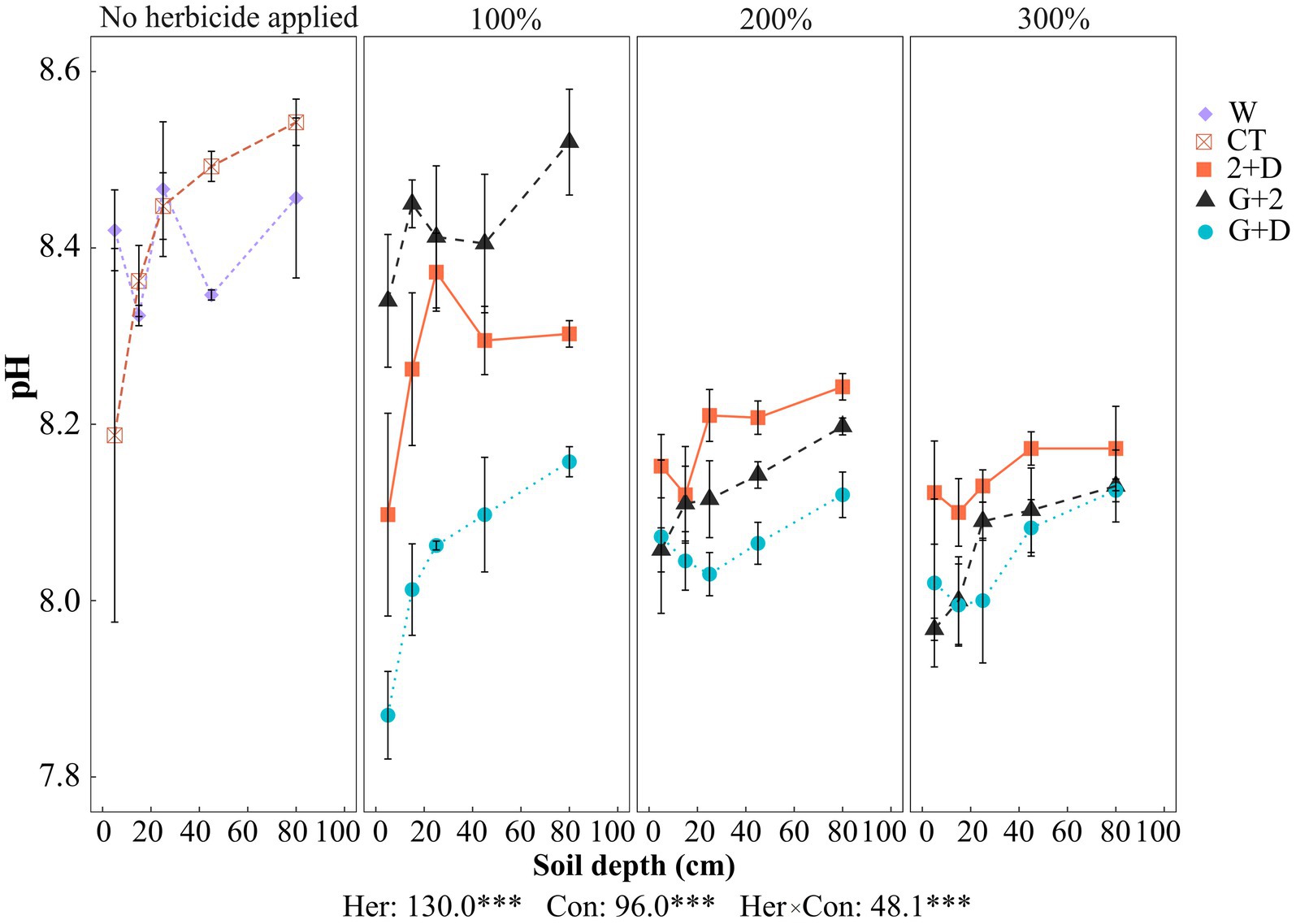
Figure 3. Soil pH at different depths (0–10, 10–20, 20–30, 30–60, 60–100 cm) under different herbicides (2 + D, G + D, G + 2) and concentrations (100, 200, 300%) treatments at the end of this study in 2022. Values are means of four replicates (± standard deviation). *** indicate significance levels at p < 0.001. Note the y-intercept is not 0. For a description of the abbreviations, refer to the Figure 1 caption.
Herbicide type, sampling date, and their interaction significantly affected SOC content (Figure 4). The 2 + D treatment had higher SOC content than the G + 2 treatment and W control (p < 0.05). The 2 + D treatment had the highest SOC content from 30–100 cm (p < 0.05). December had higher SOC contents from 0–10 cm than January and March, while March had higher SOC contents from 20–100 cm than January and December (p < 0.05). The 100% herbicide concentration had the highest SOC contents from 0–10 cm (10.65 ± 1.50 g kg−1), even higher than the CT and W controls (p < 0.05).
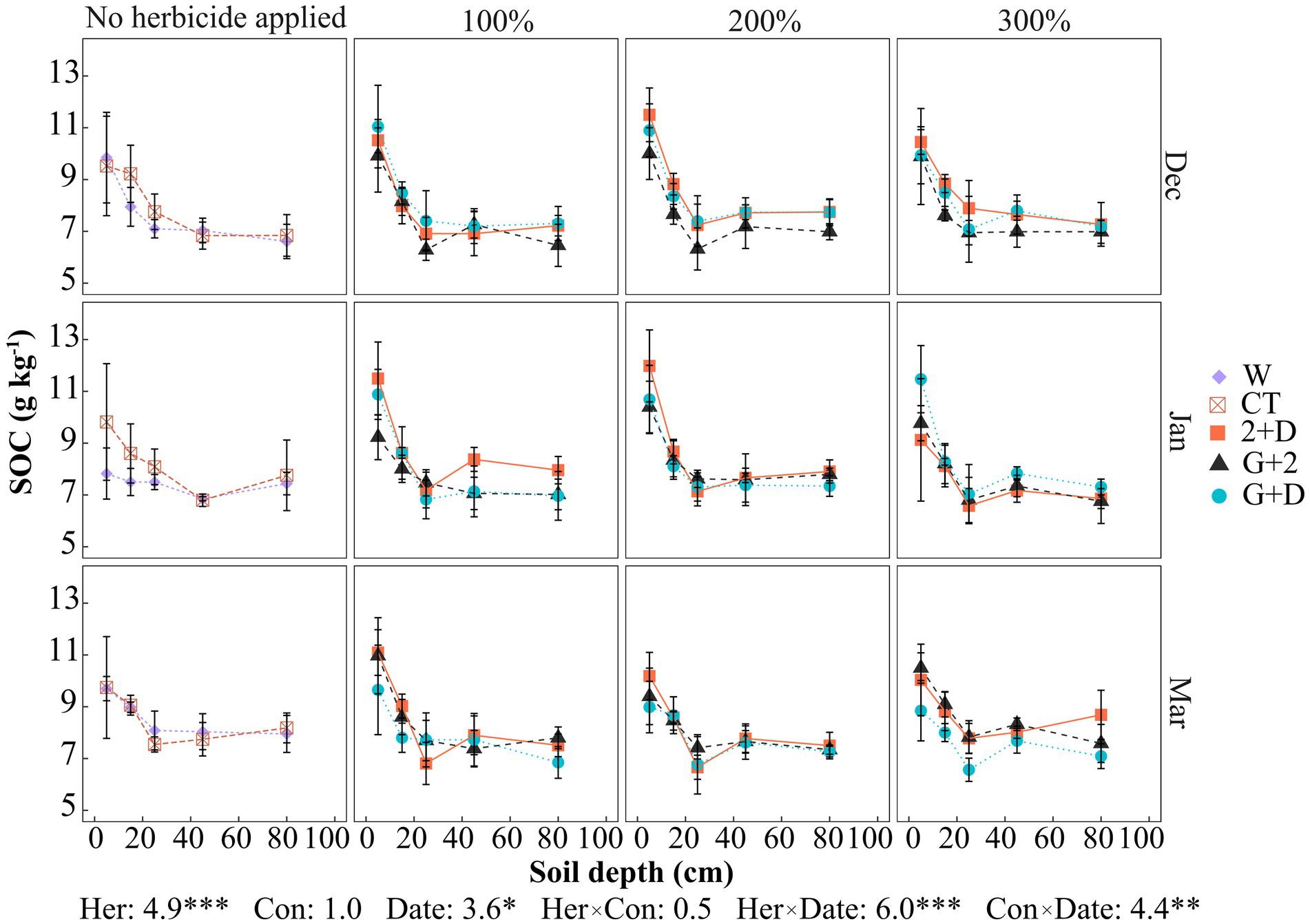
Figure 4. Effect of herbicide (2 + D, G + D, G + 2) and concentration (100%, 200%, 300%) on soil organic carbon (SOC) content at different depths (0–10, 10–20, 20–30, 30–60, 60–100 cm) in December 2021, January 2022, and March 2022. *, **, and *** indicate significance levels at p < 0.05, 0.01, and 0.001. Values are means of four replicates (± standard deviation). F value is marked in the figure. Note the y-intercept is not 0. For a description of the abbreviations, refer to the Figure 1 caption.
Herbicide type, sampling date, and their interaction significantly affected soil TN content (Figure 5). The CT control and 2 + D and G + D treatments had higher TN contents than the W control (p < 0.05). Soil TN contents did not significantly differ in December and January but were higher than in March (p < 0.05). The herbicide types had similar soil TN contents in December, higher than the W control (p < 0.05). In January, the CT control had significantly higher TN content than the G + 2 treatment and W control, while the W control had significantly lower TN content than the G + D and 2 + D treatments. In March, no significant differences in TN content occurred between herbicide types.
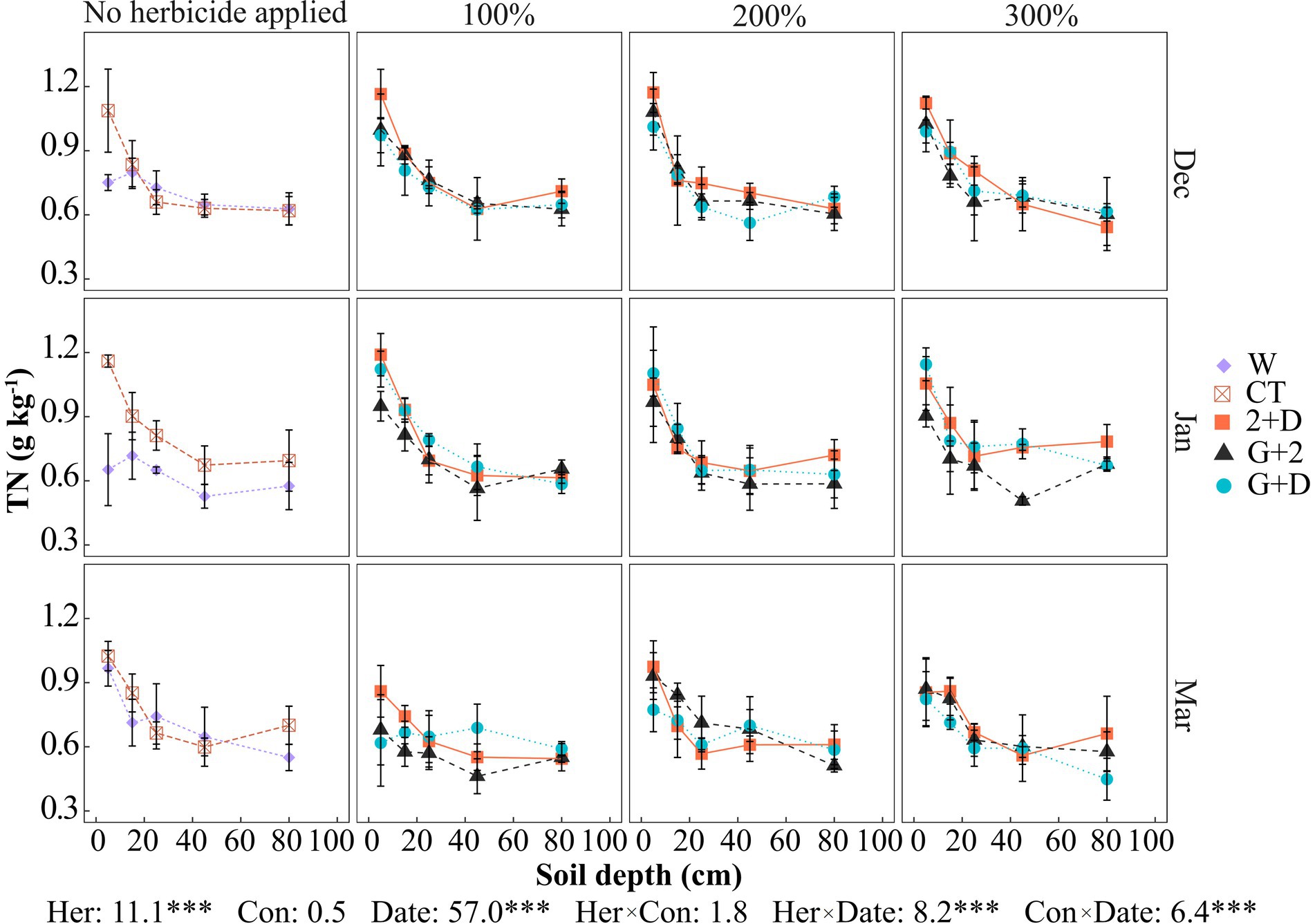
Figure 5. Effect of herbicide (2 + D, G + D, G + 2) and concentration (100%, 200%, 300%) on soil total N (TN) content at different depths (0–10, 10–20, 20–30, 30–60, 60–100 cm) in December 2021, January 2022, and March 2022. Values are means of four replicates (± standard deviation). F value is marked in the figure.*** indicate significance levels at p < 0.001. Note the y-intercept is not 0. For a description of the abbreviations, refer to the Figure 1 caption.
Herbicide type, herbicide concentration, sampling date, and their interaction significantly affected soil NO3−-N content (Figure 6). All herbicide treatments significantly increased soil NO3−-N content compared to the W control but did not significantly differ from the CT control (p > 0.05). The 100% herbicide concentration increased soil NO3−-N content more than the 200 and 300% concentrations (p < 0.05). January had higher soil NO3−-N contents than December (p < 0.05). Regardless of herbicide type, herbicide application produced higher soil NO3−-N contents than the W control in January and March (p < 0.05). The G + D (100%) and G + 2 (300%) treatments had higher soil NO3−-N contents than the W control (p < 0.05).
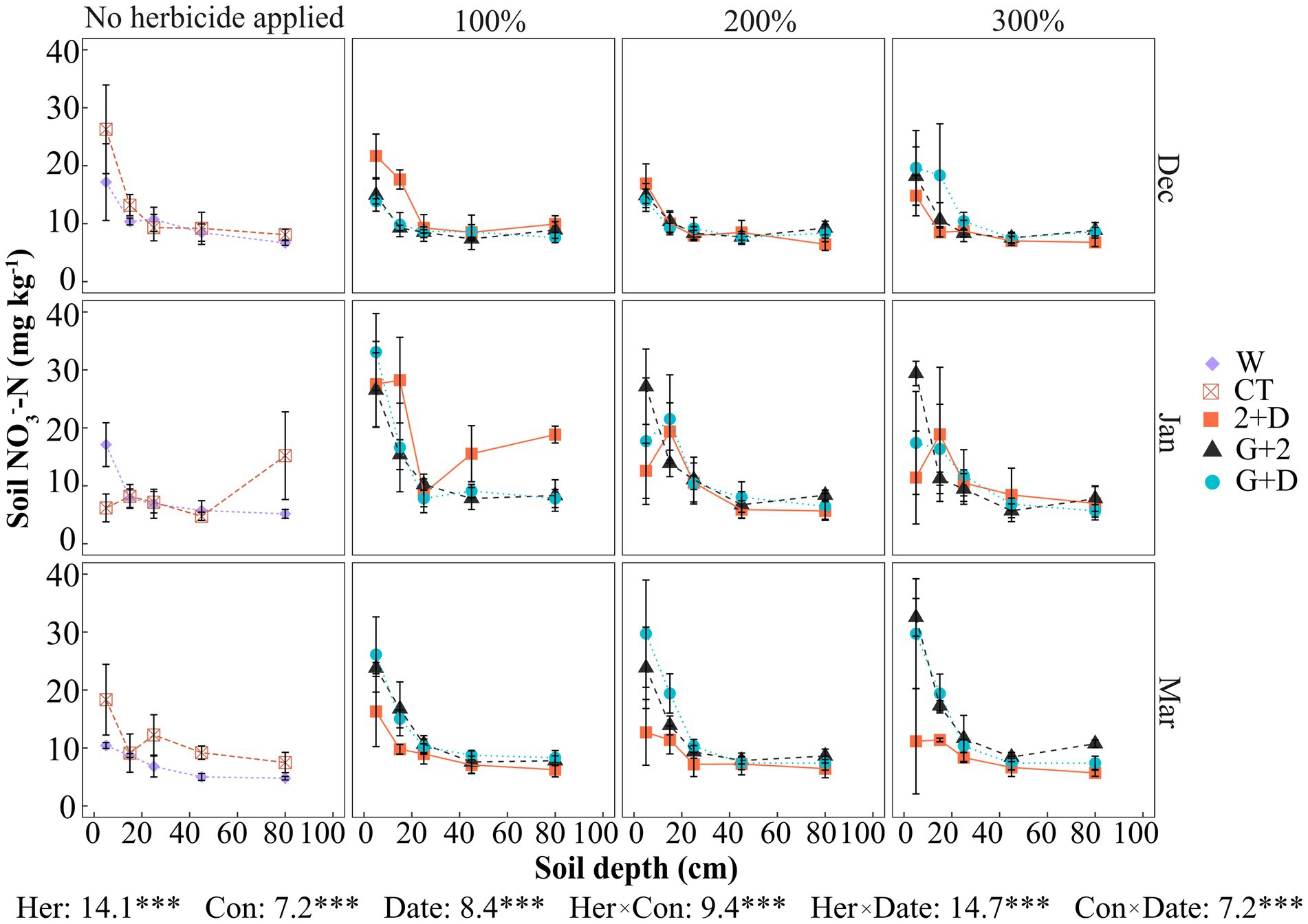
Figure 6. Effect of herbicide (2 + D, G + D, G + 2) and concentration (100%, 200%, 300%) on soil nitrate-N (NO3−-N) content at different depths (0–10, 10–20, 20–30, 30–60, 60–100 cm) in December 2021, January 2022, and March 2022. *** indicate significance levels at p < 0.001. Values are means of four replicates (± standard deviation). F value is marked in the figure. For a description of the abbreviations, refer to the Figure 1 caption.
Herbicide type, herbicide concentration, sampling date, and their interactions significantly affected soil NH4+-N content (Figure 7). The G + 2 treatment had the highest soil NH4+-N contents (7.94 ± 1.45 mg kg−1) (Figure 7, p < 0.05), while CT had the lowest (6.46 ± 1.54 mg kg−1). Regardless of herbicide concentration, the herbicide treatments had higher soil NH4+-N contents than the CT and W controls (p < 0.05). December and March had lower soil NH4+-N contents than January (p < 0.05).
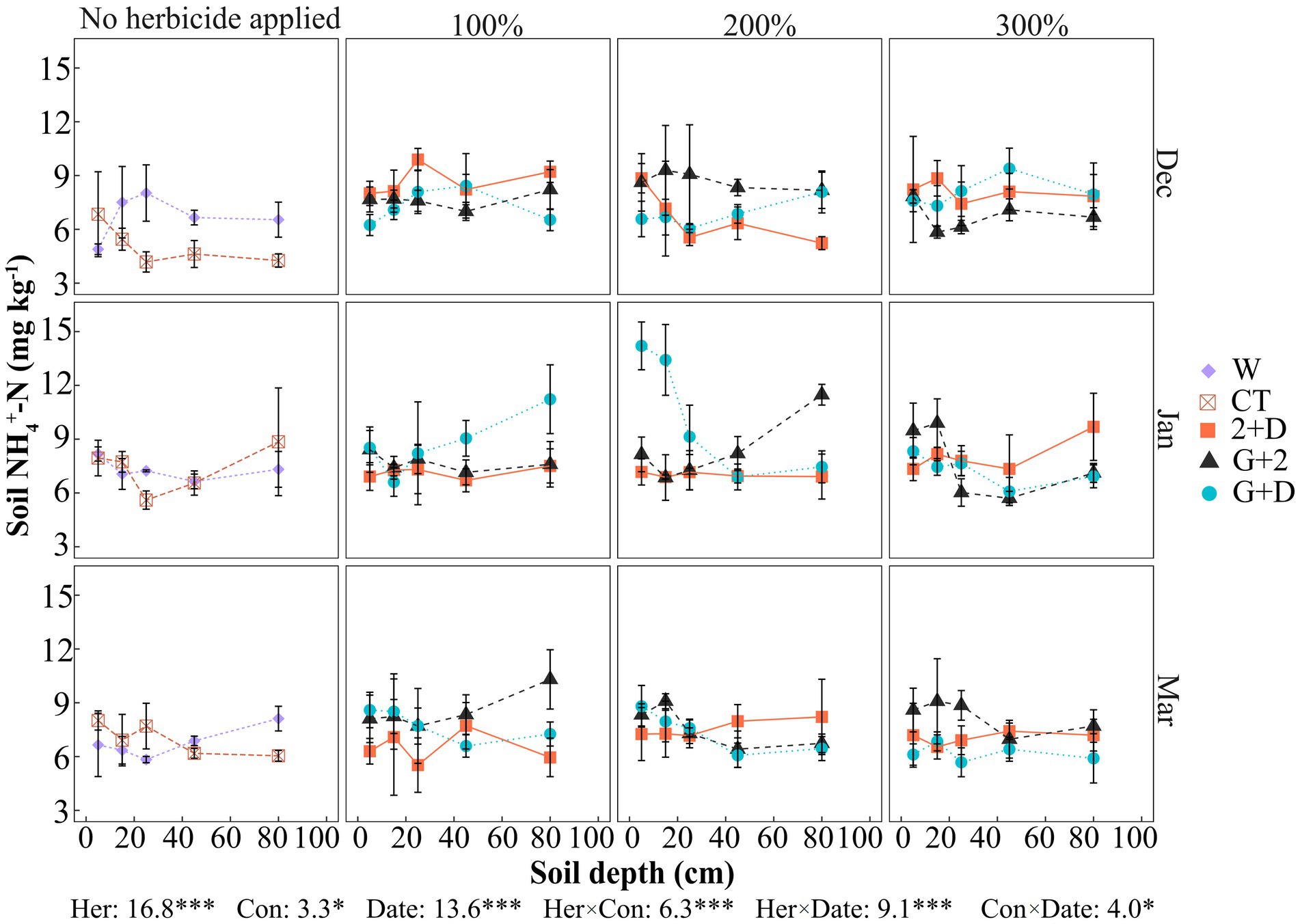
Figure 7. Effect of herbicide (2 + D, G + D, G + 2) and concentration (100%, 200%, 300%) on soil ammonium-N (NH4+-N) content at different depths (0–10, 10–20, 20–30, 30–60, 60–100 cm) in December 2021, January 2022, and March 2022. * and *** indicate significance levels at p < 0.05 and 0.001. Values are means of four replicates (± standard deviation). F value is marked in the figure. Note the y-intercept is not 0. For a description of the abbreviations, refer to the Figure 1 caption.
4. Discussion
4.1. Herbicides inhibited lucerne growth
The NDVI and SPAD values indicated that the three herbicide combinations had a higher efficiency of terminating and inhibiting lucerne growth in a short time. While it is difficult to successfully terminate lucerne growth with a single herbicide, selecting an appropriate herbicide combination can inactivate lucerne roots (Buhler and Mercurio, 1988). Studies have reported a synergistic relationship between glyphosate and auxin herbicides, which may induce lucerne withering (Wehtje and Walker, 1997; Flint and Barrett, 1998).
All herbicide treatments significantly inhibited lucerne’s regreening rate and plant height at the regreening stage, with the lowest NDVI and SPAD values in the G + 2 treatment (Figure 2), particularly at higher concentrations (200, 300%). The selected herbicides in this experiment are relatively safe. For instance, glyphosate has a half-life in soil and water that is relatively short (averaging about 30 d in temperate climates), and it does not bioaccumulate in organisms due to its high water solubility (Duke, 2020). Commercial 2,4-D is easily soluble in water and other solvents result in rapid penetration through the leaves/roots, and runoff of 2,4-D can be proper irrigation management (Islam et al., 2018). Dicamba is a chlorobenzene acid herbicide, and the half-life of dicamba in soil would be <12 weeks under typical conditions (Caux et al., 1993; Ochsner et al., 2006). Nonetheless, the recommended dosage, 100%, already significantly decreased regreening rate (Table 2) and height at the regreening stage (Table 3). Thus, in light of environmental and economic costs, applying the recommended dosage of glyphosate and 2,4-D in autumn can mostly terminate lucerne growth on the Loess Plateau. However, the general outcome of this study may vary depending on the climate and the specific aspect being studied. Further research is warranted to validate the relevant findings across multiple sites and years. It is worth noting that one-year trials have limitations in terms of their ability to fully capture the long-term effects of treatments, and the results should be interpreted with caution when making policy decisions.
4.2. Effect of different treatments on soil pH and soil C and N contents
Soil C and N contents in the herbicide treatments significantly differed from the W and CT controls (Figures 4–7). For instance, the 2 + D treatment had significantly higher SOC contents from 30–100 cm than the CT and W controls (Figure 4), possibly because the tillage destroyed soil aggregate structure, releasing and mineralizing SOC. A study on lucerne showed that the herbicide treatment had higher SOC content than the tillage treatment (Malhi et al., 2010). In our study, the herbicide treatments had lower soil pH values than the CT and W controls, possibly due to the degradation of lucerne roots (killed by the herbicides) by microorganisms, subsequently releasing carbon dioxide from organic acids and reducing soil pH (Hinsinger et al., 2003). This suggested that lucerne roots are gradually apoptotic, releasing C and N substances in the herbicide treatments. However, soil C and N contents did not significantly differ between the different herbicide treatments (Figure 8). This may be because soil organic and labile compounds can persist not for weeks but years, with the initial stages of litter decomposition unable to explain the persistence of soil organic compounds in the long term (Schmidt et al., 2011). Therefore, treatment effects were not evident for organic C inputs or outputs during the seven-month experiment, possibly because significant changes in SOC take time. Additionally, this study did not monitor gaseous soil C and N losses that significantly contribute to soil C and N dynamics. Thus, further study is warranted to assess gaseous and labile soil C and N dynamics in response to lucerne termination with herbicides.
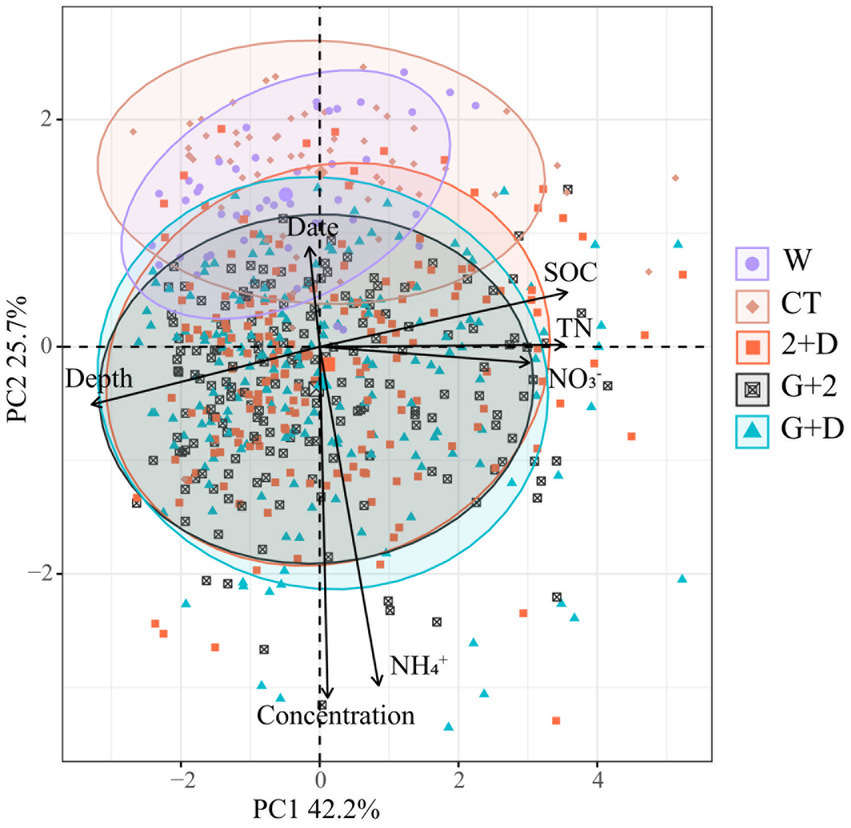
Figure 8. Principal component analysis of herbicide (2 + D, G + D, G + 2) and concentration (100%, 200%, 300%) effects on SOC, TN, NO3−-N, NH4+-N content at different depths (0–10, 10–20, 20–30, 30–60, 60–100 cm) in December 2021, January 2022, and March 2022.
The herbicide treatments and CT control increased soil TN and NO3−-N contents in lucerne grassland relative to the W control, with significantly higher soil NO3−-N content in the CT control than the herbicide treatments. This may be because CT adversely affects soil structure, disturbing aggregate formation (Six et al., 2002), increasing soil aeration, and releasing physically protected organic matter, thus promoting organic residue decomposition and mineralization (Jia, 2013). Nonetheless, lucerne termination with CT generally causes soil erosion, with large amounts of NO3−-N runoff due to leaching and denitrification (Malhi and Nyborg, 1986; Nyborg et al., 1997). Herbicide-based lucerne termination can improve soil water conservation capacity, reduce water and soil erosion, and reduce carbon dioxide and nitrous oxide emissions relative to CT (Toderi et al., 2022). Although the termination effect of CT treatment was acceptable in this experiment, spading at 40 cm is a high energy consumption tillage operation, that is difficult to achieve in high clay soils.
4.3. Sampling date significantly affected soil C and N contents
March had significantly higher SOC contents from 20–100 cm under lucerne grassland than January and December. From December to March, the soils on the Loess Plateau experience severe freezing–thawing cycles (Li and Fan, 2014), which can increase SOC content by destroying soil aggregate structure and releasing physically protected SOC (Wang et al., 2012; Ma et al., 2021). Soil freezing also facilitates root death (Henry, 2007; Kreyling et al., 2012), increasing SOC content. The effect of soil frost on root mortality or function can also affect C dynamics because root respiration accounts for 40–60% of soil C losses (Hogberg et al., 2001).
Similarly, January had higher soil NO3−-N and NH4+-N contents than December, with soil NH4+-N content decreasing in March relative to January, possibly due to the increasing temperature from January to March increasing nitrification (Hoyle et al., 2006; Wang et al., 2006), thus decreasing NH4+-N content (Nicolardot et al., 2001; Wang et al., 2006). Besides, the decrease in soil pH could convert NH4+ to NH3, reducing soil N content. Changes in soil TN content depend on N accumulation and consumption. In this study, the change in soil TN content lagged behind that of mineral N, reflecting a relatively stable soil TN content, but the change in soil N supply capacity is difficult to predict over a short period (Kaye et al., 2002).
5. Conclusion
Our findings indicate that the application of combined herbicides is effective for terminating lucerne growth on the Loess Plateau. We recommend using the suggested dosage (100%) of glyphosate and 2,4-D in autumn, considering both environmental and economic factors. Both conventional tillage and herbicide treatments contribute to increased SOC, soil TN, and NO3−-N contents in lucerne grasslands. Herbicide treatments, compared to CT, further enhance SOC and soil NH4+-N content; however, higher herbicide concentrations (200 and 300%) may lead to decreased soil C and N levels. Future research should explore gaseous soil C and N losses, as well as the yield of subsequent crops such as maize (Zea mays) and spring wheat (Triticum aestivum), to assess the overall impact of herbicide application on lucerne termination. Additionally, it is worth noting that one-year trials have limitations in terms of their ability to fully capture the long-term effects of treatments, and the results should be interpreted with caution when making policy decisions. Therefore, further research, including multi-year trials, is needed to validate these findings and provide more robust evidence for policy stakeholders.
Data availability statement
The raw data supporting the conclusions of this article will be made available by the authors, without undue reservation.
Author contributions
YZ: conceptualization, methodology, investigation, writing–original draft, formal analysis, and visualization. JG: methodology, data curation, and visualization. SL: conceptualization, methodology, and writing–review. KS: writing–review & editing, validation, and visualization. YL and YS: conceptualization, writing–review & editing, supervision, project administration, and funding acquisition. All authors contributed to the article and approved the submitted version.
Funding
This work was supported by the Key Technology Integration and Demonstration for Improving Quality and Efficiency of High-Quality Forage Grass in Arid Regions of Huan County (2022YFD1602102), Natural Science Foundation of China (32101431, 31872416), ‘Innovation Star’ Project for Excellent Postgraduates in Gansu Province (2022CXZX-089), and Natural Science Foundation of Gansu Province (22JR5RA455).
Conflict of interest
The authors declare that the research was conducted in the absence of any commercial or financial relationships that could be construed as a potential conflict of interest.
Publisher’s note
All claims expressed in this article are solely those of the authors and do not necessarily represent those of their affiliated organizations, or those of the publisher, the editors and the reviewers. Any product that may be evaluated in this article, or claim that may be made by its manufacturer, is not guaranteed or endorsed by the publisher.
References
Ali, G., Wang, Z., Li, X., Jin, N., Chu, H., and Jing, L. (2021). Deep soil water deficit and recovery in alfalfa fields of the loess plateau of China. Field Crop Res. 260:107990. doi: 10.1016/j.fcr.2020.107990
Basile-Doelsch, I., Balesdent, J., and Pellerin, S. (2020). Reviews and syntheses: the mechanisms underlying carbon storage in soil. Biogeosciences 17, 5223–5242. doi: 10.5194/bg-17-5223-2020
Bell, L. W., Sparling, B., Tenuta, M., and Entz, M. H. (2012). Soil profile carbon and nutrient stocks under long-term conventional and organic crop and alfalfa-crop rotations and re-established grassland. Agric. Ecosyst. Environ. 158, 156–163. doi: 10.1016/j.agee.2012.06.006
Buhler, D. D., and Mercurio, J. C. (1988). Vegetation management and corn growth and yield in untilled mixed-species perennial sod. Agron. J. 80, 454–462. doi: 10.2134/agronj1988.00021962008000030013x
Caux, P. Y., Kent, R. A., Tache, M., Grande, C., Fan, G. T., and MacDonald, D. D. (1993). Environmental fate and effects of dicamba: a Canadian perspective. Rev. Environ. Contam. Toxicol. 133, 1–58. doi: 10.1007/978-1-4613-9529-4_1
Chan, K. Y., Bowman, A., and Oates, A. (2001). Oxidizible organic carbon fractions and soil quality changes in an Oxic Paleustalf under different pasture leys. Soil Sci. 166, 61–67. doi: 10.1097/00010694-200101000-00009
Clark, J. D., Yost, M. A., Cardon, G. E., Ransom, C. V., and Creech, J. E. (2021). Tillage method and glyphosate-resistant alfalfa termination timing affect soil properties and subsequent corn yield. Agron. J. 113, 321–334. doi: 10.1002/agj2.20478
Davies, S. L., and Peoples, M. B. (2003). Identifying potential approaches to improve the reliability of terminating a lucerne pasture before cropping: a review. Aust. J. Exp. Agric. 43, 429–447. doi: 10.1071/EA02042
Davis, H., Fawcett, R., and Harvey, R. (1978). Effect of fall frost on the activity of glyphosate on alfalfa (Medicago sativa) and quackgrass (Agropyron repens). Weed Sci. 26, 41–45. doi: 10.1017/S0043174500032665
De Mendiburu, F. (2014). Agricolae: statistical procedures for agricultural research. R package version 1, 1–4.
Drinkwater, L. (1999) in Using plant species composition to restore soil quality and ecosystem function. Designing and testing crop rotations for organic farming. eds. J. E. Olesen, R. Eltun, M. J. Gooding, E. S. Jensen, and U. Kopke, 37–46.
Duke, S. O. (2020). Glyphosate: environmental fate and impact. Weed Sci. 68, 201–207. doi: 10.1017/wsc.2019.28
Eerd, L. L. V., Congreves, K. A., Hayes, A., Verhallen, A., and Hooker, D. C. (2014). Long-term tillage and crop rotation effects on soil quality, organic carbon, and total nitrogen. Can. J. Soil Sci. 94, 303–315. doi: 10.4141/cjss2013-093
Entz, M., Bullied, W., and Katepa-Mupondwa, F. (1995). Rotational benefits of forage crops in Canadian prairie cropping systems. J. Prod. Agric. 8, 521–529. doi: 10.2134/jpa1995.0521
Flint, J., and Barrett, M. (1998). Effects of glyphosate combinations with 2, 4-D or dicamba on field bindweed. Weed Sci. 37, 12–18. doi: 10.1017/S0043174500055776
Fourie, K.L., (2017). Evaluation of salinity and irrigation guidelines for lucerne, University of the Free State. Bloemfontein
Han, L., Tsunekawa, A., and Tsubo, M. (2010). Monitoring near-surface soil freeze–thaw cycles in northern China and Mongolia from 1998 to 2007. Int. J. Appl. Earth Obs. Geoinf. 12, 375–384. doi: 10.1016/j.jag.2010.04.009
Henry, H. A. L. (2007). Soil freeze-thaw cycle experiments: trends, methodological weaknesses and suggested improvements. Soil Biol. Biochem. 39, 977–986. doi: 10.1016/j.soilbio.2006.11.017
Hinsinger, P., Plassard, C., Tang, C. X., and Jaillard, B. (2003). Origins of root-mediated pH changes in the rhizosphere and their responses to environmental constraints: a review. Plant Soil 248, 43–59. doi: 10.1023/A:1022371130939
Hogberg, P., Nordgren, A., Buchmann, N., Taylor, A. F. S., Ekblad, A., Hogberg, M. N., et al. (2001). Large-scale forest girdling shows that current photosynthesis drives soil respiration. Nature 411, 789–792. doi: 10.1038/35081058
Holland, J. M. (2004). The environmental consequences of adopting conservation tillage in Europe: reviewing the evidence. Agric. Ecosyst. Environ. 103, 1–25. doi: 10.1016/j.agee.2003.12.018
Hoyle, F. C., Murphy, D. V., and Fillery, I. R. P. (2006). Temperature and stubble management influence microbial CO2-C evolution and gross N transformation rates. Soil Biol. Biochem. 38, 71–80. doi: 10.1016/j.soilbio.2005.04.020
Islam, F., Wang, J., Farooq, M. A., Khan, M. S. S., Xu, L., Zhu, J., et al. (2018). Potential impact of the herbicide 2, 4-dichlorophenoxyacetic acid on human and ecosystems. Environ. Int. 111, 332–351. doi: 10.1016/j.envint.2017.10.020
Jia, S.W., (2013). Effects of conventional tillage and conservation tillage on soil organic carbon, 2nd international conference on energy and environmental protection (ICEEP 2013). Advanced Materials Research, Guilin, China, pp. 3832–3836.
Kaye, J., Barrett, J., and Burke, I. (2002). Stable nitrogen and carbon pools in grassland soils of variable texture and carbon content. Ecosystems 5, 461–471. doi: 10.1007/s10021-002-0142-4
Kjeldahl, J. (1883). Neue Methode zur Bestimmung des Stickstoffs in organischen Krpern. Z. Anal. Chem. 22, 366–382. doi: 10.1007/BF01338151
Kreyling, J., Persoh, D., Werner, S., Benzenberg, M., and Woellecke, J. (2012). Short-term impacts of soil freeze-thaw cycles on roots and root-associated fungi of Holcus lanatus and Calluna vulgaris. Plant Soil 353, 19–31. doi: 10.1007/s11104-011-0970-0
Li, M., (2020). Evaluation on alfalfa resistance to diseases in Guyuan, Ningxia. MA thesis Thesis, Lanzhou University. China
Li, G. Y., and Fan, H. M. (2014). Effect of freeze-thaw on water stability of aggregates in a black soil of Northeast China. Pedosphere 24, 285–290. doi: 10.1016/S1002-0160(14)60015-1
Li, Y., Li, Z., Chang, S. X., Cui, S., Jagadamma, S., Zhang, Q., et al. (2020). Residue retention promotes soil carbon accumulation in minimum tillage systems: implications for conservation agriculture. Sci. Total Environ. 740:140147. doi: 10.1016/j.scitotenv.2020.140147
Li, Y., Li, Z., Cui, S., Liang, G., and Zhang, Q. (2021). Microbial-derived carbon components are critical for enhancing soil organic carbon in no-tillage croplands: a global perspective. Soil Tillage Res. 205:104758. doi: 10.1016/j.still.2020.104758
Li, Y., Wang, G., Shurpali, N. J., and Shen, Y. (2022). Nitrogen addition affects nitrous oxide emissions of rainfed lucerne grassland. Int. J. Environ. Res. Public Health 19:7789. doi: 10.3390/ijerph19137789
Li, K. Y., Zhao, Y. Y., Yuan, X. L., Zhao, H. B., Wang, Z. H., Li, S. X., et al. (2012). Comparison of factors affecting soil nitrate nitrogen and ammonium nitrogen extraction. Commun. Soil Sci. Plant Anal. 43, 571–588. doi: 10.1080/00103624.2012.639108
Liu, X., Tan, S., Song, X., Wu, X., Zhao, G., Li, S., et al. (2022). Response of soil organic carbon content to crop rotation and its controls: a global synthesis. Agric. Ecosyst. Environ. 335:108017. doi: 10.1016/j.agee.2022.108017
Ma, R., Jiang, Y., Liu, B., and Fan, H. (2021). Effects of pore structure characterized by synchrotron-based micro-computed tomography on aggregate stability of black soil under freeze-thaw cycles. Soil Tillage Res. 207:104855. doi: 10.1016/j.still.2020.104855
Malhi, S. S., Lemke, R., and Schoenau, J. J. (2010). Influence of time and method of alfalfa stand termination on yield, seed quality, N uptake, soil properties and greenhouse gas emissions under different N fertility regimes. Nutr. Cycl. Agroecosyst. 86, 17–38. doi: 10.1007/s10705-009-9271-x
Malhi, S., and Nyborg, M. (1986). Increase in mineral N in soils during winter and loss of mineral N during early spring in north-Central Alberta. Can. J. Soil Sci. 66, 397–409. doi: 10.4141/cjss86-042
Mccarty, G.W., Iii, J., Yost, R., and Doraiswamy, P.C., (2010). Evaluation of methods for measuring soil organic carbon in West African soils. Afr. J. of Agric. Res. 5, 2169–2177.
Mohr, R. M., Entz, M. H., Janzen, H. H., and Bullied, W. J. (1999). Plant-available nitrogen supply as affected by method and timing of alfalfa termination. Agron. J. 91, 622–630. doi: 10.2134/agronj1999.914622x
Moomaw, R., and Martin, A. (1976). Herbicides for no-tillage corn in alfalfa sod. Weed Sci. 24, 449–453. doi: 10.1017/S0043174500066431
Nicolardot, B., Recous, S., and Mary, B. (2001). Simulation of C and N mineralisation during crop residue decomposition: a simple dynamic model based on the C: N ratio of the residues. Plant Soil 228, 83–103. doi: 10.1023/A:1004813801728
Nyborg, M., Laidlaw, J. W., Solberg, E. D., and Malhi, S. S. (1997). Denitrification and nitrous oxide emissions from a black chernozemic soil during spring thaw in Alberta. Can. J. Soil Sci. 77, 153–160. doi: 10.4141/S96-105
Ochsner, T. E., Stephens, B. M., Koskinen, W. C., and Kookana, R. S. (2006). Sorption of a hydrophilic pesticide: effects of soil water content. Soil Sci. Soc. Am. J. 70, 1991–1997. doi: 10.2136/sssaj2006.0091
Pan, J., Fan, Y., Li, R., Chen, L., and Hu, X. (2016). Screening of high efficient symbiotic rhizobium for Medicago sativa cv. Gannong no. 3 and M. sativa cv. Longdong. Pratacultural Sci. 33, 1536–1549. doi: 10.11829/j.issn.1001G0629.2016G0072
Peixoto, L., Olesen, J. E., Elsgaard, L., Enggrob, K. L., Banfield, C. C., Dippold, M. A., et al. (2022). Deep-rooted perennial crops differ in capacity to stabilize C inputs in deep soil layers. Sci. Rep. 12:10. doi: 10.1038/s41598-022-09737-1
Randall, G., Vetsch, J., and Murrell, T. (2001). Corn response to phosphorus placement under various tillage practices. Better Crops 85, 12–15.
Rumpel, C., Amiraslani, F., Koutika, L.-S., Smith, P., Whitehead, D., and Wollenberg, E., (2018). Put more carbon in soils to meet Paris climate pledges. Nature Publishing Group, 32–34, London.
Scharlemann, J. P. W., Tanner, E. V. J., Hiederer, R., and Kapos, V. (2014). Global soil carbon: understanding and managing the largest terrestrial carbon pool. Carbon Manag. 5, 81–91. doi: 10.4155/cmt.13.77
Schmidt, M. W. I., Torn, M. S., Abiven, S., Dittmar, T., Guggenberger, G., Janssens, I. A., et al. (2011). Persistence of soil organic matter as an ecosystem property. Nature 478, 49–56. doi: 10.1038/nature10386
Shen, Y., Li, L., Chen, W., Robertson, M., Unkovich, M., Bellotti, W., et al. (2009). Soil water, soil nitrogen and productivity of lucerne–wheat sequences on deep silt loams in a summer dominant rainfall environment. Field Crop Res. 111, 97–108. doi: 10.1016/j.fcr.2008.11.005
Six, J., Feller, C., Denef, K., Ogle, S. M., Sa, J. C. D., and Albrecht, A. (2002). Soil organic matter, biota and aggregation in temperate and tropical soils -effects of no-tillage. Agronomie 22, 755–775. doi: 10.1051/agro:2002043
Smith, P., House, J. I., Bustamante, M., Sobocká, J., Harper, R., Pan, G., et al. (2016). Global change pressures on soils from land use and management. Glob. Chang. Biol. 22, 1008–1028. doi: 10.1111/gcb.13068
Toderi, M., Dottavio, P., Francioni, M., Kishimoto-Mo, A. W., Santilocchi, R., and Trozzo, L. (2022). Short-term response of soil greenhouse gas fluxes to alfalfa termination methods in a Mediterranean cropping system. Soil Sci. Plant Nutr. 68, 124–132. doi: 10.1080/00380768.2021.1983869
Wang, E., Cruse, R. M., Chen, X., and Daigh, A. (2012). Effects of moisture condition and freeze/thaw cycles on surface soil aggregate size distribution and stability. Can. J. Soil Sci. 92, 529–536. doi: 10.4141/cjss2010-044
Wang, K., Liu, Y., Teng, F., Cen, H., Yan, J., Lin, S., et al. (2021). Heterogeneous expression of Osa-MIR156bc increases abiotic stress resistance and forage quality of alfalfa. Crop J. 9, 1135–1144. doi: 10.1016/j.cj.2020.11.009
Wang, C., Wan, S., Xing, X., Zhang, L., and Han, X. (2006). Temperature and soil moisture interactively affected soil net N mineralization in temperate grassland in northern China. Soil Biol. Biochem. 38, 1101–1110. doi: 10.1016/j.soilbio.2005.09.009
Wehtje, G., and Walker, R. H. (1997). Interaction of glyphosate and 2, 4-DB for the control of selected morningglory (Ipomoea spp.) species. Weed Technol. 11, 152–156. doi: 10.1017/S0890037X00041506
Yuan, Z.-Q., Yu, K.-L., Guan, X.-K., Fang, C., Li, M., Shi, X.-Y., et al. (2016). Medicago sativa improves soil carbon sequestration following revegetation of degraded arable land in a semi-arid environment on the loess plateau, China. Agric. Ecosyst. Environ. 232, 93–100. doi: 10.1016/j.agee.2016.07.024
Keywords: lucerne/alfalfa termination, herbicide, tillage, soil carbon, soil nitrogen
Citation: Zhao Y, Guo J, Luo S, Shen Y, Siddique KHM and Li Y (2023) Optimizing lucerne (Medicago sativa) termination on the Loess Plateau, China: a comparative analysis of conventional tillage and herbicide treatments. Front. Sustain. Food Syst. 7:1194724. doi: 10.3389/fsufs.2023.1194724
Edited by:
Xiukang Wang, Yan'an University, ChinaReviewed by:
Rui Dong, Guizhou University, ChinaCalogero Schillaci, Joint Research Centre (JRC), Netherlands
Copyright © 2023 Zhao, Guo, Luo, Shen, Siddique and Li. This is an open-access article distributed under the terms of the Creative Commons Attribution License (CC BY). The use, distribution or reproduction in other forums is permitted, provided the original author(s) and the copyright owner(s) are credited and that the original publication in this journal is cited, in accordance with accepted academic practice. No use, distribution or reproduction is permitted which does not comply with these terms.
*Correspondence: Yuan Li, yuanli@lzu.edu.cn