Erratum: Screening of volatile organic compounds emitted from different packaging materials: case study on fresh-cut artichokes
- Department of Agriculture, Food, Natural Resources and Engineering (DAFNE), University of Foggia, Foggia, Italy
In the present work, the emission of volatile organic compounds (VOCs) from plastic packaging materials and their migration to the packaged product was investigated. Fresh-cut artichokes were chosen as a case study owing to their nutritional profile, including phenolic compounds, antioxidants, inulin and fiber, and the high market demand. Artichokes were cut into quarters, packaged in active modified atmosphere (5% O2 and 10% CO2) in three different micro-perforated (MP) packages (polypropylene (PP), polypropylene/polyamide (PP/PA) and polylactic acid (PLA)) and stored for 10 days at 5° C. For the detection of VOCs on the packaging materials and in the packaged product, three approaches were considered. First, VOCs emitted from a piece of package were extracted by solid phase micro extraction (SPME), at 30°C, and analyzed by gas-chromatography coupled to mass spectrometry (GC–MS). Second, VOCs from the headspace of the empty closed bag were extracted by SPME, at 5°C, to understand if any packaging volatile compound was transferred into the package atmosphere in this temperature condition. Finally, packaged artichokes were also analyzed by SPME/GC–MS to detect any presence of packaging volatiles compared to the fresh product. Emitted VOCs were different according to the material; in particular PP/PA emitted the greatest number of VOCs, most of them belonging to the class of branched alkanes and alkenes, such as 4-methyl-heptane, 2,4-dimethyl-heptane, 4-methyl-octane and 2,4 dimethyl heptene; PP emitted octane, aldehydes, and 1-metoxy-2-propanol; PLA emitted aldehydes and propanoic acid. Some VOCs were found into the atmosphere of the empty bags as well as on packaged artichokes, while they were not found in the fresh samples before packaging, suggesting that a study on the emission of VOCs from different plastic materials in contact with food and on the effect of different storage conditions is very critical for a better understanding of this issue.
1. Introduction
The demand of food products with a high quantity of bioactive components is rising in the food market. In this perspective, globe artichoke (Cynara cardunculus L. var. scolymus), whose cultivation was previously confined to the Mediterranean region, is now becoming incredibly common and demanded by customers worldwide for its taste and its functional content (Lombardo et al., 2018). Modern society’s lifestyle choices have boosted consumer desire for minimally processed goods, that are perceived as value-added products in terms of quality, simplicity, nutrient content, and easy preparation convenience (Giménez-Berenguer et al., 2022). The main edible fraction of artichoke plant is the inner part of the flower heads, representing only the 15–20% of total fresh head weight and the remaining part is considered as waste. Therefore, artichoke processing as a fresh-cut product is highly appreciated due to the complex and time consuming trimming and cutting operations. Conversely, the cutting promotes the increase of respiration, and the enzymatic browning, resulting in a shelf-life decrease (Cabezas-Serrano et al., 2009).
As a result, various options for limiting postharvest quality deterioration of artichokes have been developed, including washing, and dipping in antioxidant and antimicrobial solutions. The application of modified atmosphere packaging (MAP) in conjunction with low temperature storage, low pH, and the use of antimicrobials and anti-browning chemicals can significantly increase the storage life of this particular product (Sánchez-Guijarro et al., 2019). Recently, La Zazzera et al. (2015) designed and optimized MAP solutions for fresh-cut artichokes with the help of micro perforation, which permitted to create and maintain the desired gas conditions in micro perforated (MP) polypropylene (PP), MP-polypropylene/polyamide (PP/PA) and MP-polylactic acid (PLA), regardless of the used material. MAP allowed to store cut artichokes in absence of any stabilizing treatment for 9 days.
The most common material used for packaging, particularly for MAP, is plastic containing various substances that can migrate from package to food (Nerin et al., 2013). In the European Union (EU), various additives listed in Regulation 10/2011/EU can be intentionally used for the manufacturing of food contact materials (FCMs), in order to enhance their durability and physical characteristics. This list, which includes 885 intentionally added substances (IAS) and is maintained on a regular basis, also allocates specific migration limits (SML) to ingredients that have the ability to transfer into food at concentration levels that could pose a health risk or cause an undesirable change in food composition or sensory quality (European regulation 10/2011/EU Commission, 2011). Although these regulatory steps, numerous studies have been published on the potential transmission of some of these additives and ingredients to food and food simulants (Onghena et al., 2014; Carrero-Carralero et al., 2019; Xu et al., 2020; Tsochatzis et al., 2021).
Food packaging can also contain non-intentionally added substances (NIAS) as a result of the interactions between different compounds in the packaging materials, and between food and FCM substances (for example, additives). NIAS originate from different sources and include breakdown products of FCMs, impurities of starting materials, unwanted side-products and various contaminants from recycling processes (Nerin et al., 2013; Wrona and Nerín, 2020). Although several techniques have been used to get a comprehensive picture of the material’s safety, there is still the need for specific focuses for the detection of NIAS. Particular attention should be paid to low molecular weight substances, as it is generally accepted that substances with molecular weight over 1,000 Da are not absorbed by the gastro-intestinal tract (EFSA Panel on Food Contact Materials et al., 2008).
Furthermore, many literature studies focused on the identification of potential migrants in plastic packaging or their migration into food simulants rather than food. In these studies, organic solvents, such as acetonitrile, are generally used (Ibarra et al., 2019) together with high temperatures (more than 40°C) of extraction, which do not correspond to the normal packaging usage conditions.
Therefore, in this work, the emission of volatile organic compounds (VOCs) from plastic packages in contact with artichoke and their eventual presence in the artichoke was investigated by means of Headspace Solid-Phase Micro-Extraction (HS-SPME) in combination with gas chromatography–mass spectrometry (GC–MS), under real storage conditions.
2. Materials and methods
2.1. Materials and sample preparation
A total of 16 commercial analytical standards were used for confirmation purposes. 2,3-Dimethyl- heptane (≥98%) 2,4-dimethyl-heptane (≥98%), 2-ethyl-1-hexanol (≥99%) butylated hydroxytoluene (BHT) (≥99%), acetic acid (≥99.8%), heptanal (≥95%), and 2-methly-1-pentene, 4-methyl-heptane, nonadecane, octane, 3,5 dimethyl-octane, 1-methoxy-2-propanol, decanal, octanal, nonyl aldehyde, 1-octanol, all of analytical standard, were purchased from Sigma-Aldrich (Milan, Italy).
Polypropylene (PP) with two lines of micro-perforations (MP), polypropylene/polyamide with two lines of micro-perforations (MP-PP/PA) and polylactic acid (PLA) with one line of micro-perforation (MP) were selected as packaging materials, optimizing product-weight/packaging dimensions, according to La Zazzera et al. (2015). Characteristics of packaging materials are shown in Table 1.
Artichokes (Cynara scolymus L. var. Opal) were grown in Foggia and harvested at the commercial maturity. All bench surfaces, utensils and plastic containers were washed with sodium hypochlorite before use. Artichokes were hand trimmed using sharp stainless-steel knives in order to remove external bracts, leaves and stalks; heads were then washed to eliminate residues of soil and insects. After washing, head trimming was completed by further removing external greener and tougher bracts, and the upper half part (inedible part) to keep just the innermost tender bracts. Artichoke hearts were then cut into quarters and 150 g of product was packed in MP-PP/PA and in MP-PP, whereas 75 g of product was packed in MP-PLA as optimized in La Zazzera et al. (2015), on the basis of product respiration rate and package permeability. The product was packed by means of a Tecnovac packaging machine (Mod. T520, Grassobbio, BG; Italy), in active MAP with concentrations of 5% O2 and 10% CO2, and stored for 6 days at 5°.
2.2. Volatile organic compounds (VOCs) extraction
For the detection of VOC emitted by the plastic material and the interaction with the product, three approaches were considered, including the study of the material itself, the headspace and the product (Figure 1). First, for the material, a 2.5×2.5 cm package squared piece was cut, inserted into a 20 mL capped solid-phase microextraction vial and conditioned at 30°C for 10 min, in the autosampler thermostat. VOCs emitted from the packaging were then extracted by a divinilbenzene/carboxen/polidimetilsiloxane (DVB/CAR/PDMS) solid phase micro extraction (SPME) fiber for 20 min, at 30°C, and analyzed by gas-chromatography coupled to mass spectrometry (GC–MS). An empty vial was used as control.
Second, a DVB/CAR/PDMS SPME fiber was inserted into the whole empty bag headspace through an adhesive rubber septum to extract VOCs, for 30 min, at 5°C, to understand if any packaging volatile compound was transferred into the package atmosphere in this temperature condition.
Finally, VOCs from the packed artichokes were analyzed by SPME/GC–MS to detect any presence of volatiles belonging to the plastic material. The fresh-cut artichokes were stored for 6 days at 5°C, then a representative 4 g-sample was introduced into a 20 mL SPME vial and incubated for 10 min at 30°C. Afterwards, a PDMS/CAR/DVB fiber was exposed for 20 min, at 30°C, to the vial headspace. Before storage in the packaging, fresh artichokes VOCs were analyzed for the comparison. All volatile samples were prepared in triplicate.
The precision of the whole method was evaluated in terms of repeatability (intra-day precision) and reproducibility (inter-day precision) expressed as percent relative standard deviation (%RSD). Intra-day precision was assessed by the application of the SPME/GC–MS to the same sample (MP-PP/PA), on the same day and by the same analyst (n = 8). Inter-day precision was evaluated with a similar procedure, by analyzing the same sample on 8 days (n = 8). Blank runs were carried out after three runs to check for eventual contamination of the fiber using an empty vial.
2.3. Gas chromatography–mass spectrometry (GC–MS) conditions
All SPME samples were introduced into the GC injector port for the desorption at 250°C for 4 min in the splitless injection mode. An Agilent 6890 Series gas chromatograph coupled to an Agilent 5975C network mass selective detector (Agilent Technologies, Palo Alto, CA, United States) was used to analyze the samples. Analytes were separated on a INNOWAX capillary column (60 m x 250 μm x 0.25 μm; J&W Scientific Inc., Folsom, CA, United States) by applying the following temperature program: 40°C for 4 min, then up to 140°C at the rate of 3°C/min and hold for 10 min. The transfer line temperature was 280°C. Mass detector conditions were as follows: electronic impact mode at 70 eV, source temperature at 230°C, scanning rate 2.88 scan/s and a mass scanning range of m/z 30–400. The carrier gas, helium, was used at a rate of 1.0 mL/min. Data were collected with HP Chem-station Software (Agilent Technologies). Volatile compounds were identified by comparing their retention times and mass spectra with those of pure compounds analyzed under the same conditions. Otherwise, comparison of MS fragmentation patterns with those included in the National Institute for Standards and Technology database (NIST 02, p > 80) were utilized to achieve tentative identification (Table 2). The semi-quantitative analysis of individual compounds was performed using the integrated peak area data from the trace GC–MS.
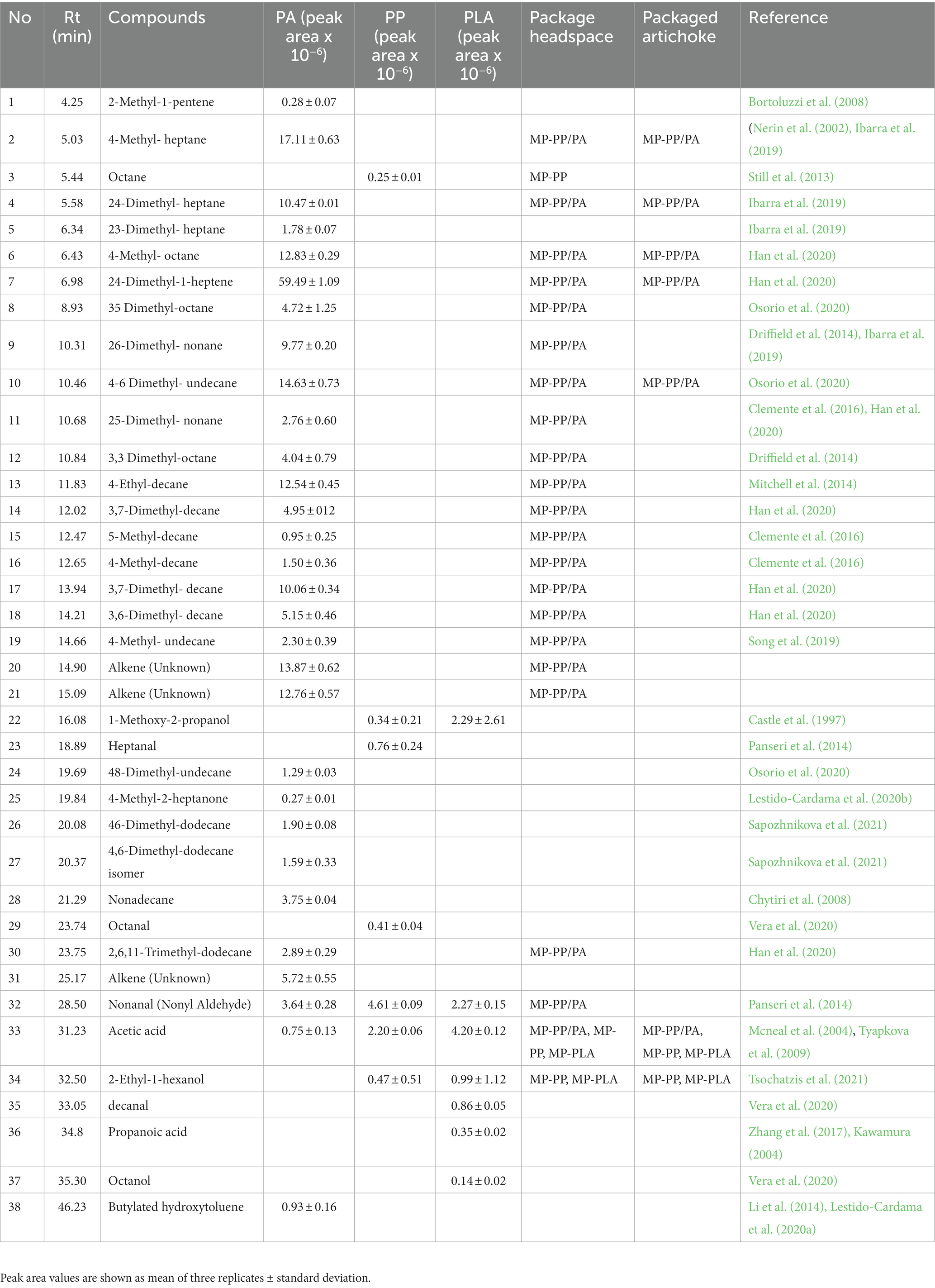
Table 2. Number, retention times, and name of identified compounds in MP-PA/PP, MP-PP and MP-PLA, peak area, presence in package headspace and in packed artichoke, and the references relevant to each compound.
2.4. FCCmigex database
FCCmigex Database (Geueke et al., 2022) was helpful to find the literature relevant to each identified compound. FCCmigex Database contains, up to the present investigation, 3,000 food contact chemicals, and was developed by studying 1,210 scientific studies, 731 regarding plastic (2,210 chemicals from plastic). The search was carried out against each investigated material and against each tentatively identified compound.
3. Results and discussion
VOCs emission from all packaging materials was investigated by means of an untargeted SPME-GC/MS analysis. Initially, package sample was maintained intentionally at 30°C in SPME vial to detect the compounds having potential to migrate from package to headspace and eventually to the food. Whereas migration tests are generally carried out at hard conditions to guarantee an adequate safety to the products, here the aim was to have insights on the released compounds in real storage conditions. Then, volatile compounds were sampled in the packaging empty space by using SPME at 5°C in order to detect compounds that most easily migrated into the package atmosphere, in real storage conditions of fresh-cut products. Finally, the volatile profile of packed artichoke was analyzed in order to track VOCs migration on the product (Figure 1). Table 2 summarizes all data showing the 38 identified compounds, their retention times, peak area, and the references relevant to each compound. Sixteen compounds were identified using standard injections, 22 compounds were tentatively identified using the NIST mass spectra library, including alkanes, alkenes, aldehydes, alcohols and acids. Intra-day precision ranged from 5 to 25%, inter-day precision ranged from 10 to 30%.
In the Figure 2, VOCs profiles of PDMS/CAR/DVB fiber (trace A), MP-PP/PA (trace B), MP-PP (trace C) and PP-PLA (trace D) and the labels of the identified compounds are reported. Package materials showed different VOCs profiles; the package MP-PP/PA emitted the highest number of compounds, crowding the chromatogram in the range 4–25 min of elution time, followed by MP-PP (trace C) and PP-PLA.
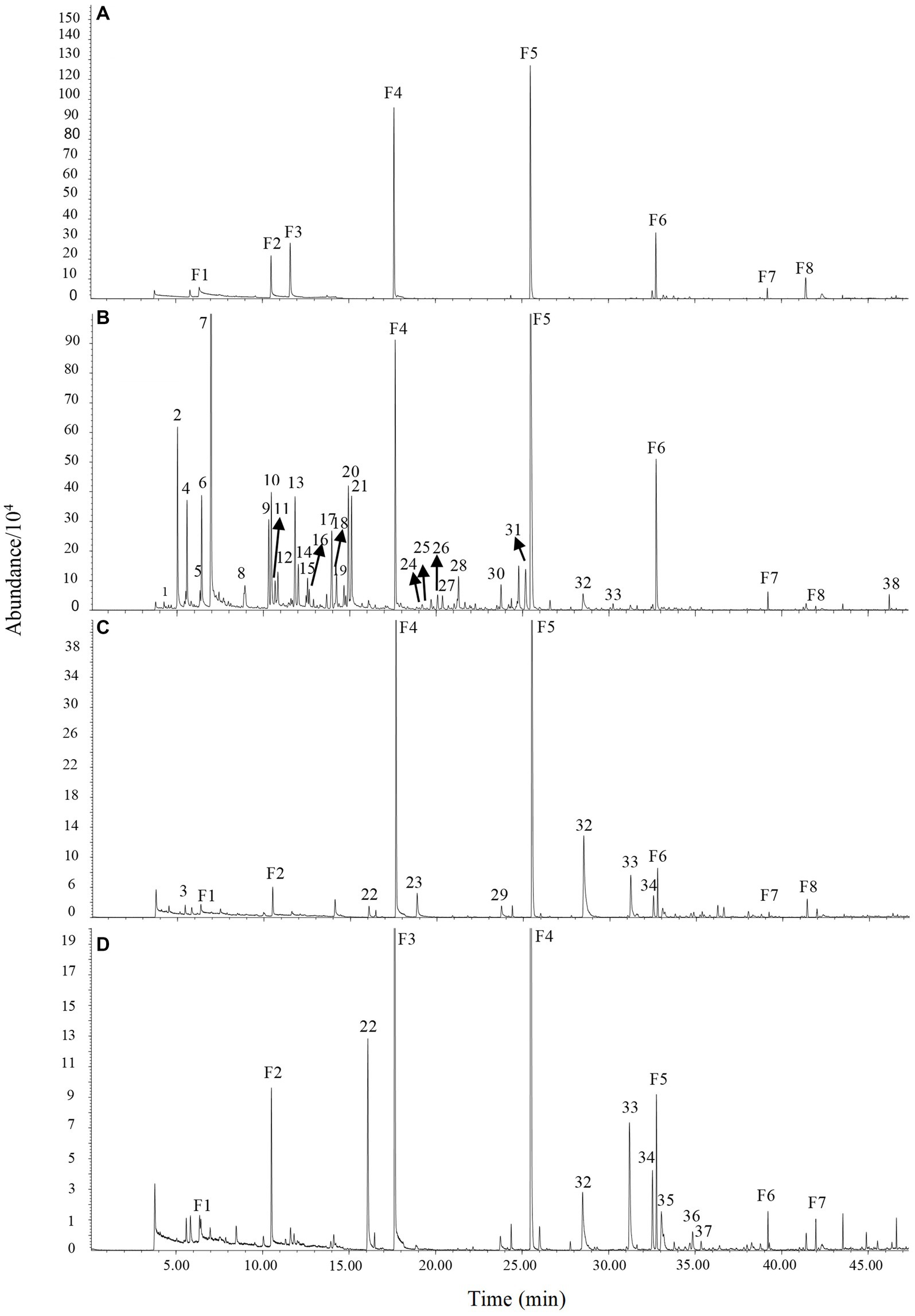
Figure 2. SPME-GC/MS chromatograms relevant to PDMS/CAR/DVB fiber (A), PP/PA material (B), PP material (C), PLA material (D). Labels F1-F8 on the chromatograms refer to fibre compounds; the other labels correspond to the identified compounds reported in table 2.
3.1. MP-PP/PA volatiles
Most of MP-PP/PA volatiles belong to the class of aliphatic branched alkanes (Table 2). These compounds are reported to be due to the PP polymerization and pelleting processing in industry (Bortoluzzi et al., 2008) and likely derive from broken polymeric chains or are compounds commonly used as raw materials in the polymer production. Other compounds may derive from the oxidation which can take place during the material manufacture, due to the interactions between the polymeric material and the oxygen (Ibarra et al., 2019). Within the list of the compounds reported in this work (Table 2), compounds 3,3 dimethyl octane and 2,6-dimethyl-nonane were previously found by Driffield et al. (2014) in PP samples in contact with food submitted to gamma irradiation. 4,6-dimethyl-dodecane was identified as NIAS in migration experiments on PP trays for microwave (Sapozhnikova et al., 2021) and previously found in hydrocarbon liquids from polypropylene by microwave pyrolysis (Jing et al., 2017). The compound 3,6 dimethyldecane was demonstrated to be released from polyolefin resin used in plastic food packaging and in the additives to produce resin pellets by Han et al. (2020). 2,4-dimethyl-heptane, 3,5-dimethyl octane, 4,6-dimethyl undecane, nonadecane, 2,6,11-trimethyl-dodecane were found in melamine with bamboo filler by Osorio et al. (2020), whereas Song et al. (2019) found nonadecane and 4 methyl-undecane in polysterene samples.
MP-PP/PA also emitted some branched alkenes including 2-methyl-1-pentene and 2,4-dimethyl-1-heptene, as previously reported by Bortoluzzi et al. (2008). The latter compound 2,4-dimethyl-1-heptene corresponded to the most abundant peak, assuming a response factor equal to 1 for all the compounds. Alkanes and alkenes were, then, reported in several materials and different conditions; however, under our soft extraction conditions, they constituted the volatile fingerprint only of MP-PP/PA (Figure 2, trace B).
A reliable recognition of all alkanes and alkenes was quite difficult due to the significant similarity in the mass spectral information of the isomers, therefore the complete identification of some compounds (peaks 20, 21 and 31) was not possible with the tools used in this work. Nevertheless, some information about their structure could be obtained. Peaks 20 and 21, eluting, respectively, at 14.9 and 15.1 min, showed both a mass spectrum (data not shown) characterized by main ions at m/z 125, 111, 97, 83, 69 and 55, diagnostic of a hydrocarbon chain containing a double bond, whereas the ion at m/z 168 was indicative of a branched alkene. The unknown peak at 25.17 min showed the same ions as the peaks 20 and 21 with a different proportion; moreover, the ion at m/z 154 suggested the occurrence of a branching site different from the previous one. Finally, butylated hydroxytoluene was identified in MP-PP/PA in our conditions, which is a frequently used in both package and food as antioxidant (Kang et al., 2018; Tortosa et al., 2020).
According to Cramer rules, alkanes and alkenes belong to class I of toxicity, corresponding to a low level, however, a maximum daily intake of 1800 (μg/day) or 30 (μg/kg bw/day) for this class is recommended (Munro et al., 1996). In general, acute toxic signs like nausea, headaches, and eye and nose irritation are linked to VOC exposure. The potential of mutagenicity and carcinogenicity is also reported to rise with prolonged exposure to VOCs. Furthermore, a lot of VOCs are also undesirable smelly compounds (Hirota et al., 2004). The possible reason of the highest emission of VOCs from PP/PA could be the thermal treatment applied for the lamination of bilayers causing a major degradation.
3.2. MP-PP and MP-PLA volatiles
The emission of MP-PP and MP-PLA packages was characterized by the same compounds, with some exception. Both packages released 1-metoxy-2-propanol, nonanal, acetic acid and 2-ethyl-1-hexanol. Acetic acid was reported (Philippart et al., 1995; Kang et al., 2020) as a product of PP photooxidation and as a product of PLA decomposition (Kopinke et al., 1996). In the present work, heptanal and octanal were found only in MP-PP, whereas decanal, propanoic acid and octanol were found only in MP-PLA. Octanal and nonanal with grassy, green, citrus-like and fatty odour were previously found by Tyapkova et al. (2009) in PP; octanal, heptanal and nonanal were also found as odor-active constituents in cardboard (Czerny and Buettner, 2009) as well as a result of thermal decomposition in polyethylene (Wiedmer et al., 2017). The compound 1-metoxy-2-propanol was previously found in kitchen paper towels (Castle et al., 1997). Acetic acid, propanoic acid as well as the aldehyde compounds (octanal, decanal, nonanal) were reported by Vera et al. (2020) as representative odour compounds detected by sniffers in polyethylene and polypropylene materials, whereas octanol with moss and mushroom notes was found only in PP. 2-Ethyl-1-hexanol, corresponding to green odour was found in PP and in paper, and is used as precursor for production of plasticizers. Although all these compounds are safe for food contact materials, they have been showed to be perceived by the sniffers, therefore, they could change the organoleptic characteristics of packaged food (Vera et al., 2020).
As regards to PLA, the quantification of VOCs produced during extrusion and after thermoforming was carried out by Salazar et al. (2017) showing the presence of eight compounds all different from the ones found in this work except for the acetic acid. Ubeda et al. (2019) identified the main volatile and semi-volatile compounds of a PLA/polyester blend intended for food packaging, analysing them after total sample dissolution/precipitation and by migration tests with food simulants. Also in this case, the compounds here reported were all different with the exception of nonanal, but can be explained by the different method applied and the presence of polyester.
3.3. VOCs migration from package to headspace and fresh-cut artichokes
The last part of the study regarded the analysis of the migration from packaging to the headspace and to the product. To this aim, volatile compounds in the headspace of empty packages were extracted by SPME sampling at 5°C, in order to detect compounds that most easily migrated into the package atmosphere, in real storage conditions. Ratio between package surface and headspace volume was different according to the type of material since packaging condition was optimized for each material to reach at the equilibrium the desired target atmosphere; therefore semi-quantitative comparison among the packages can not be performed. Volatiles detected for MP-PP/PA were the compounds from 2 to 21 except compounds number 5, and also compounds 24, 25, 26, 27, 28 and 38 (Table 2). As for MP-PP, the volatiles were octane, nonanal, acetic acid and 2-ethyl-hexanol, whereas for MP-PLA, only acetic acid and 2-ethyl-hexanol, which were present in trace amounts.
In order to track VOCs migration on the packed artichoke, the volatile profile of packed artichoke was analyzed, by sampling the empty space of a vial containing cut artichokes with SPME technique. Figure 3 reported, as an example, the SPME-GC/MS profiles of fresh artichoke (Figure 3A), which was not in contact with any package material, of MP-PP/PA packed artichokes (Figure 3B) after 10 days of storage at 5°C, and of MP-PP/PA (Figure 3C), which was not in contact with any food. Compounds octane (peak 3), 2,4-dimethyl-heptane (peak 4), 4-methyl-octane (peak 6), 2,4-dimethyl-heptene (peak 7) and 4,6-dimethyl undecane (peak 10) found in MP-PP/PA profile were also detected in MP-PP/PA packed artichokes, whereas they were absent in fresh artichoke. As for MP-PP and MP-PLA packed artichokes, acetic acid and 2-ethyl-hexanol not found in the fresh samples before packaging, were found; however, these compounds could derive also from vegetables (Saucier et al., 2014).
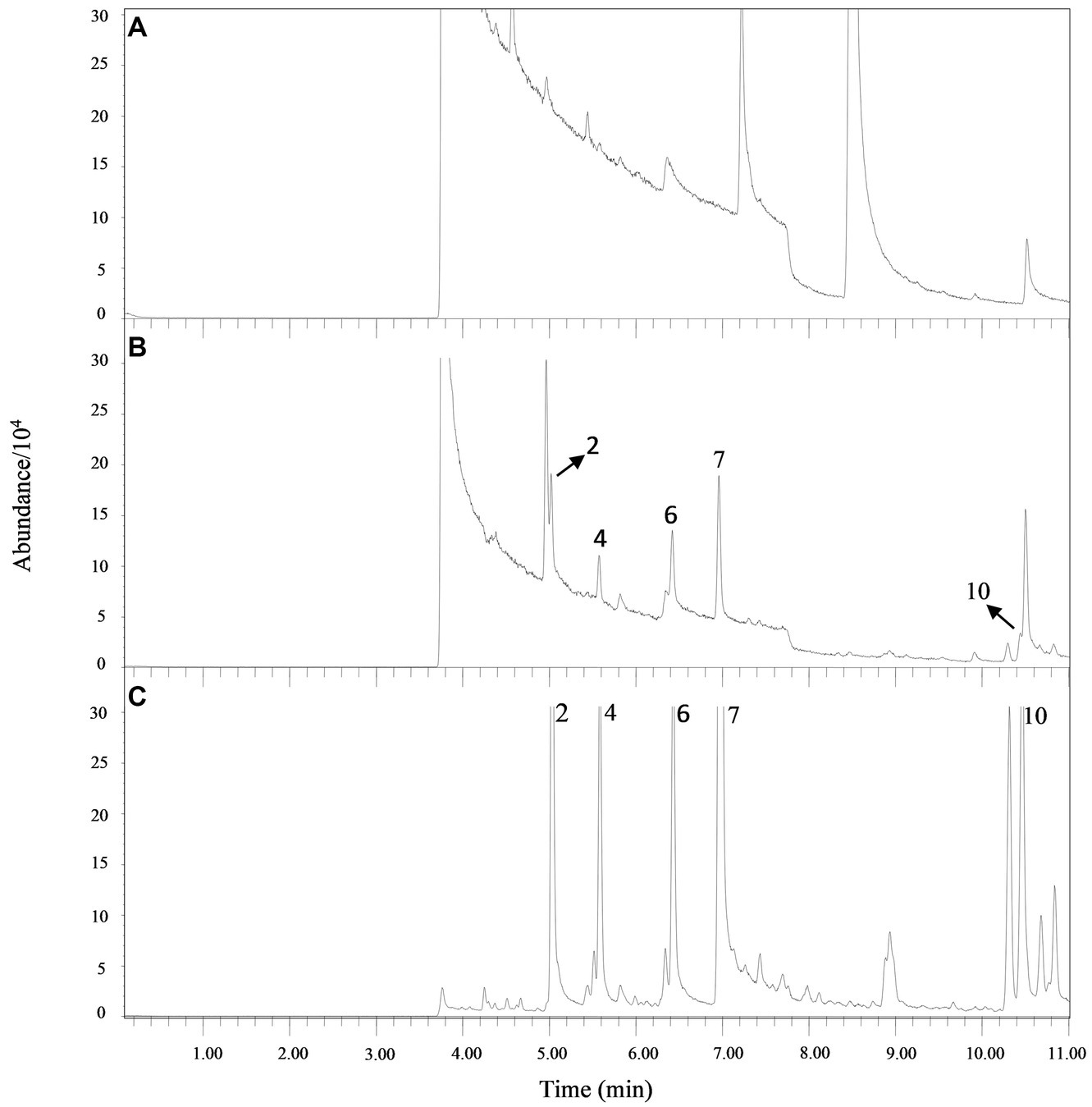
Figure 3. SPME-GC/MS chromatograms relevant to fresh artichoke (A), MP-PP/PA packed artichoke (B), MP-PP/PA material (C). Labels on the chromatograms refer to the following identified compounds: 2; 4-Methyl- heptane, 4; 2,4 Dimethyl- heptane, 6; 4-Methyl- octane, 7; 2,4 Dimethyl-1-heptene, 10; 4–6 Dimethyl- undecane.
4. Conclusion
The results of this study confirm how important is the investigation on migration applied to the real food, such as fresh cut products, and not only using food simulants. The packaging used for fresh-cut artichokes emitted different VOCs according to the material. PP/PA emitted the greatest number of VOCs, most of them belonging to the class of branched alkanes and alkenes; PP emitted octane, aldehydes, and 1-metoxy-2-propanol; PLA emitted aldehydes and propanoic acid. Some VOCs were found into the headspace of the empty bags as well as on packaged artichokes, while they were not found in the fresh samples before packaging. Before designing a packaging solution, the study on the emission of VOCs from different plastic materials in contact with food and on the effect of different storage conditions is desirable to optimize the packaging considering both quality of stored food and VOCs release from packaging.
Data availability statement
The raw data supporting the conclusions of this article will be made available by the authors, without undue reservation.
Author contributions
JA managed the literature searches, carried out the laboratory work, elaborated and interpreted the results, and wrote the first draft of the manuscript. SP designed the study, assisted in the result elaboration and interpretation, and wrote and revised the manuscript. DF carried out the laboratory work. MA designed the study, revised the manuscript, and secured funds for the research. GC designed the study, revised the manuscript, and secured funds for the research. All authors contributed to the article and approved the submitted version.
Funding
This research was funded by Italian Ministry of University and Research (MUR), project “Conservabilità, qualità e sicurezza dei prodotti ortofrutticoli ad alto contenuto di servizio - ARS01_00640 – POFACS,” D.D. 1211/2020 and 1104/2021.
Acknowledgments
This contribution is based upon work from the COST Action CA19124, Rethinking Packaging for Circular and Sustainable Food Supply Chains of the Future (CIRCUL-A-BILITY) supported by COST (European Cooperation in Science and Technology).
Conflict of interest
The authors declare that the research was conducted in the absence of any commercial or financial relationships that could be construed as a potential conflict of interest.
Publisher’s note
All claims expressed in this article are solely those of the authors and do not necessarily represent those of their affiliated organizations, or those of the publisher, the editors and the reviewers. Any product that may be evaluated in this article, or claim that may be made by its manufacturer, is not guaranteed or endorsed by the publisher.
References
Bortoluzzi, J. H., Cristiano, R., Gallardo, H. A., Carasek, E., and Soldi, V. (2008). Use of the SPME-GC-MS technique to study the thermal degradation of isotactic polypropylene: effects of temperature and reaction time, and analysis of the reaction mechanism. E-Polymers 8:193. doi: 10.1515/epoly.2008.8.1.193
Cabezas-Serrano, A. B., Amodio, M. L., Cornacchia, R., Rinaldi, R., and Colelli, G. (2009). Screening quality and browning susceptibility of five artichoke cultivars for fresh-cut processing. J. Sci. Food Agric. 89, 2588–2594. doi: 10.1002/jsfa.3759
Carrero-Carralero, C., Escobar-Arnanz, J., Ros, M., Jiménez-Falcao, S., Sanz, M. L., and Ramos, L. (2019). An untargeted evaluation of the volatile and semi-volatile compounds migrating into food simulants from polypropylene food containers by comprehensive two-dimensional gas chromatography-time-of-flight mass spectrometry. Talanta 195, 800–806. doi: 10.1016/j.talanta.2018.12.011
Castle, L., Offen, C. P., Baxter, M. J., and Gilbert, J. (1997). Migration studies from paper and board food packaging materials. 1. Food Addit Contam 14, 35–44. doi: 10.1080/02652039709374495
Chytiri, S., Goulas, A. E., Badeka, A., Riganakos, K. A., Petridis, D., and Kontominas, M. G. (2008). Determination of radiolysis products in gamma-irradiated multilayer barrier food packaging films containing a middle layer of recycled LDPE. Radiat. Phys. Chem. 77, 1039–1045. doi: 10.1016/j.radphyschem.2008.04.007
Clemente, I., Aznar, M., Nerín, C., and Bosetti, O. (2016). Migration from printing inks in multilayer food packaging materials by GC-MS analysis and pattern recognition with chemometrics. Food Addit Contam Part A Chem Anal Control Expo Risk Assess. 33, 703–714. doi: 10.1080/19440049.2016.1155757
Czerny, M., and Buettner, A. (2009). Odor-active compounds in cardboard. J. Agric. Food Chem. 57, 9979–9984.
Driffield, M., Bradley, E., Leon, I., Lister, L., Speck, D., Castle, L., et al. (2014). Analytical screening studies on irradiated food packaging. Food Addit. Contam. Part A Chem. Anal. Control Expo Risk Assess 31, 556–565. doi: 10.1080/19440049.2013.865087
Efsa Panel On Food Contact MaterialsFlavourings Aids, P., Silano, V., Bolognesi, C., Castle, L., Cravedi, J. P., et al. (2008). Note for guidance for the preparation of an application for the safety assessment of a substance to be used in plastic food contact materials. EFSA J. 6:21r. doi: 10.2903/j.efsa.2008.21r
EU Commission (2011). Commission regulation (EU) no 10/2011 of 14 January 2011 on plastic materials and articles intended to come into contact with food. Off. J. Eur. Union 12, 1–89.
Geueke, B., Groh, K. J., Maffini, M. V., Martin, O. V., Boucher, J. M., Chiang, Y.-T., et al. (2022). Systematic evidence on migrating and extractable food contact chemicals: Most chemicals detected in food contact materials are not listed for use. Crit. Rev. Food Sci. Nutr. 18, 1–11. doi: 10.1080/10408398.2022.2067828
Giménez-Berenguer, M., García-Pastor, M. E., García-Martínez, S., Giménez, M. J., and Zapata, P. J. (2022). Evaluation of ‘Lorca’Cultivar aptitude for minimally processed artichoke. Agronomy 12:515. doi: 10.3390/agronomy12020515
Han, X., Wu, Y., Chen, G., Wang, X., Yuan, W., and Lv, Z. (2020). Optimization of a headspace solid-phase microextraction-gas chromatography–mass spectrometry procedure for odor compounds from polyolefin resin used in plastic food packaging. Packag. Technol. Sci. 33, 515–529. doi: 10.1002/pts.2532
Hirota, K., Sakai, H., Washio, M., and Kojima, T. (2004). Application of electron beams for the treatment of VOC streams. Ind. Eng. Chem. Res. 43, 1185–1191. doi: 10.1021/ie0340746
Ibarra, V. G., De Quirós, A. R. B., Losada, P. P., and Sendón, R. (2019). Non-target analysis of intentionally and non intentionally added substances from plastic packaging materials and their migration into food simulants. Food Packag. Shelf Life 21:100325. doi: 10.1016/j.fpsl.2019.100325
Jing, X., Zhao, Y., Wen, H., and Xu, Z. (2017). High olefin yield in pyrolysis of heavier hydrocarbon liquids using microwave as heat supplier. Energy Fuel 31, 2052–2062. doi: 10.1021/acs.energyfuels.6b02734
Kang, K., Chang, Y., Choi, J. C., Park, S. J., and Han, J. (2018). Migration study of butylated hydroxytoluene and Irganox 1010 from polypropylene treated with severe processing conditions. J. Food Sci. 83, 1005–1010. doi: 10.1111/1750-3841.14104
Kang, P., Wu, P., Jin, Y., Shi, S., Gao, D., Chen, G., et al. (2020). Formation and emissions of volatile organic compounds from Homo-PP and co-PP resins during manufacturing process and accelerated photoaging degradation. Molecules 25:2761. doi: 10.3390/molecules25122761
Kawamura, Y. (2004). Effects of gamma irradiation on polyethylene, polypropylene, and polystyrene. ACS Publications, Washington, D.C.
Kopinke, F.-D., Remmler, M., Mackenzie, K., Möder, M., and Wachsen, O. (1996). Thermal decomposition of biodegradable polyesters—II. Poly (lactic acid). polymer Degrad. Stability 53, 329–342. doi: 10.1016/0141-3910(96)00102-4
La Zazzera, M., Amodio, M. L., and Colelli, G. (2015). Designing a modified atmosphere packaging (MAP) for fresh-cut artichokes. Designing modif. atmosphere packag. (MAP) fresh-cut artichokes 29, 24–29. doi: 10.13128/ahs-21301
Lestido-Cardama, A., Bernaldo, R., de Quirós, A., Bustos, J., Lomo, M. L., Paseiro Losada, P., et al. (2020a). Estimation of dietary exposure to contaminants transferred from the packaging in fatty dry foods based on cereals. Foods 9:1038. doi: 10.3390/foods9081038
Lestido-Cardama, A., Sendón, R., Bustos, J., Lomo, M. L., Losada, P. P., and De Quirós, A. R. B. (2020b). Dietary exposure estimation to chemicals transferred from milk and dairy products packaging materials in Spanish child and adolescent population. Foods 9:1554. doi: 10.3390/foods9111554
Li, C., Li, Y., Chen, Z., Liang, F., Chen, X., Wu, S., et al. (2014). Simultaneous determination of antioxidants and ultraviolet absorbers by ultra-performance liquid chromatography in food simulants. Food Anal. Methods 7, 1755–1762. doi: 10.1007/s12161-014-9811-0
Lombardo, S., Pandino, G., and Mauromicale, G. (2018). The influence of pre-harvest factors on the quality of globe artichoke. Sci. Hortic. 233, 479–490. doi: 10.1016/j.scienta.2017.12.036
Mcneal, T., Komolprasert, V., Buchalla, R., and Begley, T. (2004). “Effects of ionizing radiation on food contact materials” in Chapter 14 on Irradiation of Food and Packaging ACS Symposium Series (Washington, DC: ACS Publications. Konolprasert and Morehouse), U84.
Mitchell, G., Higgitt, C., and Gibson, L. T. (2014). Emissions from polymeric materials: characterised by thermal desorption-gas chromatography. Polym. Degrad. Stab. 107, 328–340. doi: 10.1016/j.polymdegradstab.2013.12.003
Munro, I. C., Ford, R. A., Kennepohl, E., and Sprenger, J. G. (1996). Correlation of structural class with no-observed-effect levels: a proposal for establishing a threshold of concern. Food Chem. Toxicol. 34, 829–867. doi: 10.1016/S0278-6915(96)00049-X
Nerin, C., Acosta, D., and Rubio, C. (2002). Potential migration release of volatile compounds from plastic containers destined for food use in microwave ovens. Food Addit. Contam. 19, 594–601. doi: 10.1080/02652030210123887
Nerin, C., Alfaro, P., Aznar, M., and Domeño, C. (2013). The challenge of identifying non-intentionally added substances from food packaging materials: a review. Anal. Chim. Acta 775, 14–24. doi: 10.1016/j.aca.2013.02.028
Onghena, M., Van Hoeck, E., Vervliet, P., Scippo, M. L., Simon, C., Van Loco, J., et al. (2014). Development and application of a non-targeted extraction method for the analysis of migrating compounds from plastic baby bottles by GC-MS. Food Addit. Contam. Part A Chem. Anal. Control Expo. Risk Assess 31, 2090–2102. doi: 10.1080/19440049.2014.979372
Osorio, J., Aznar, M., Nerín, C., Birse, N., Elliott, C., and Chevallier, O. (2020). Ambient mass spectrometry as a tool for a rapid and simultaneous determination of migrants coming from a bamboo-based biopolymer packaging. J. Hazard. Mater. 398:122891. doi: 10.1016/j.jhazmat.2020.122891
Panseri, S., Chiesa, L. M., Zecconi, A., Soncini, G., and De Noni, I. (2014). Determination of volatile organic compounds (VOCs) from wrapping films and wrapped PDO Italian cheeses by using HS-SPME and GC/MS. Molecules 19, 8707–8724. doi: 10.3390/molecules19078707
Philippart, J.-L., Posada, F., and Gardette, J.-L. (1995). Mass spectroscopy analysis of volatile photoproducts in photooxidation of polypropylene. Polym. Degrad. Stab. 49, 285–290. doi: 10.1016/0141-3910(95)87010-5
Salazar, R., Domenek, S., Plessis, C., and Ducruet, V. (2017). Quantitative determination of volatile organic compounds formed during Polylactide processing by MHS-SPME. Polym. Degrad. Stab. 136, 80–88. doi: 10.1016/j.polymdegradstab.2016.12.010
Sánchez-Guijarro, M., García-Gómez, P., Almela, L., Nuñez-Delicado, E., and Gabaldón, J. (2019). Improvement of the shelf life of minimally processed artichoke through antimicrobial and antioxidants agents. X Int. Symposium Artichoke, Cardoon their Wild Relatives 1284, 205–220. doi: 10.17660/ActaHortic.2020.1284.28
Sapozhnikova, Y., Nuñez, A., and Retired, J. J. (2021). Screening of chemicals migrating from plastic food contact materials for oven and microwave applications by liquid and gas chromatography-Orbitrap mass spectrometry. J. Chromatogr. A 1651:462261. doi: 10.1016/j.chroma.2021.462261
Saucier, C., Polidoro, A. S., Santos, A. L., Shneider, J. K., Caramão, E. B., and Jacques, R. A. (2014). Comprehensive two-dimensional gas chromatography with mass spectrometry applied to the analysis of volatiles in artichoke (Cynara scolymus L.) leaves. Ind. Crop. Prod. 62, 507–514. doi: 10.1016/j.indcrop.2014.09.023
Song, X.-C., Wrona, M., Nerin, C., Lin, Q.-B., and Zhong, H.-N. (2019). Volatile non-intentionally added substances (NIAS) identified in recycled expanded polystyrene containers and their migration into food simulants. Food Packag. Shelf Life 20:100318. doi: 10.1016/j.fpsl.2019.100318
Still, M., Schlummer, M., Gruber, L., Fiedler, D., and Wolz, G. (2013). Impact of industrial production and packaging processes on the concentration of per-and polyfluorinated compounds in milk and dairy products. J. Agric. Food Chem. 61, 9052–9062. doi: 10.1021/jf4020137
Tortosa, V., Pietropaolo, V., Brandi, V., Macari, G., Pasquadibisceglie, A., and Polticelli, F. (2020). Computational methods for the identification of molecular targets of toxic food additives. Butylated hydroxytoluene as a case study. Molecules 25:2229. doi: 10.3390/molecules25092229
Tsochatzis, E. D., Lopes, J. A., Gika, H., Dalsgaard, T. K., and Theodoridis, G. (2021). A fast SALLE GC–MS/MS multi-analyte method for the determination of 75 food packaging substances in food simulants. Food Chem. 361:129998. doi: 10.1016/j.foodchem.2021.129998
Tyapkova, O., Czerny, M., and Buettner, A. (2009). Characterisation of flavour compounds formed by γ-irradiation of polypropylene. Polym. Degrad. Stab. 94, 757–769. doi: 10.1016/j.polymdegradstab.2009.02.006
Ubeda, S., Aznar, M., Alfaro, P., and Nerín, C. (2019). Migration of oligomers from a food contact biopolymer based on polylactic acid (PLA) and polyester. Anal. Bioanal. Chem. 411, 3521–3532. doi: 10.1007/s00216-019-01831-0
Vera, P., Canellas, E., and Nerín, C. (2020). Compounds responsible for off-odors in several samples composed by polypropylene, polyethylene, paper and cardboard used as food packaging materials. Food Chem. 309:125792. doi: 10.1016/j.foodchem.2019.125792
Wiedmer, C., Velasco-Schön, C., and Buettner, A. (2017). Characterization of off-odours and potentially harmful substances in a fancy dress accessory handbag for children. Sci. Rep. 7, 1–11. doi: 10.1038/s41598-017-01720-5
Wrona, M., and Nerín, C. (2020). Analytical approaches for analysis of safety of modern food packaging: a review. Molecules 25:752. doi: 10.3390/molecules25030752
Xu, Z., Xiong, X., Zhao, Y., Xiang, W., and Wu, C. (2020). Pollutants delivered every day: phthalates in plastic express packaging bags and their leaching potential. J. Hazard. Mater. 384:121282. doi: 10.1016/j.jhazmat.2019.121282
Keywords: packaging, artichoke, volatile organic compounds, sustainability, gas-chromatography-mass spectrometry, branched alkanes, alkenes
Citation: Ashraf JZ, Pati S, Fatchurrahman D, Amodio ML and Colelli G (2023) Screening of volatile organic compounds emitted from different packaging materials: case study on fresh-cut artichokes. Front. Sustain. Food Syst. 7:1178104. doi: 10.3389/fsufs.2023.1178104
Edited by:
B. N. Dar, Islamic University of Science and Technology, IndiaReviewed by:
Lochan Singh, National Institute of Food Technology Entrepreneurship and Management, IndiaAnnalisa Apicella, University of Salerno, Italy
Copyright © 2023 Ashraf, Pati, Fatchurrahman, Amodio and Colelli. This is an open-access article distributed under the terms of the Creative Commons Attribution License (CC BY). The use, distribution or reproduction in other forums is permitted, provided the original author(s) and the copyright owner(s) are credited and that the original publication in this journal is cited, in accordance with accepted academic practice. No use, distribution or reproduction is permitted which does not comply with these terms.
*Correspondence: Pati Sandra, Sandra.pati@unifg.it