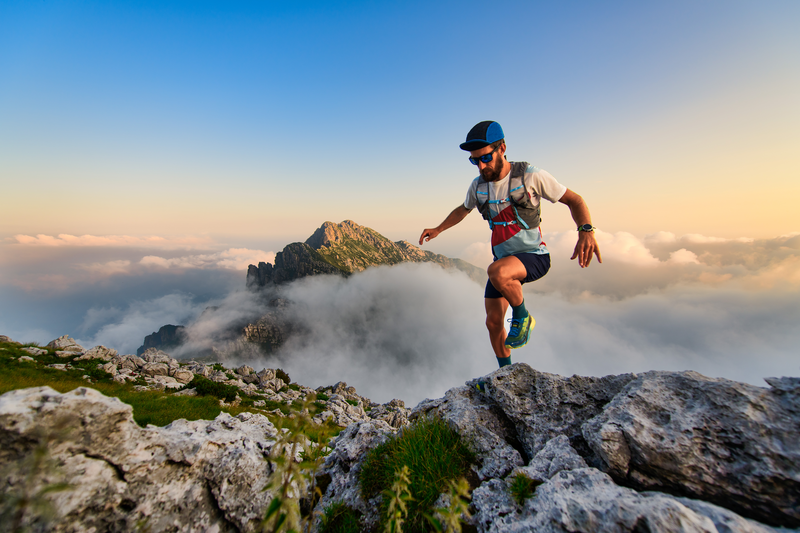
94% of researchers rate our articles as excellent or good
Learn more about the work of our research integrity team to safeguard the quality of each article we publish.
Find out more
SYSTEMATIC REVIEW article
Front. Sustain. Food Syst. , 12 January 2023
Sec. Waste Management in Agroecosystems
Volume 6 - 2022 | https://doi.org/10.3389/fsufs.2022.1012436
This article is part of the Research Topic Circular Economy and Sustainability in the Agro-Food System View all 12 articles
A correction has been applied to this article in:
Corrigendum: Industrial symbiosis and agri-food system: Themes, links, and relationships
Industrial symbiosis is an eco-innovative system concept that is based on a circular economy and industrial ecology ideas. This process comprises the movement of materials, energy, and skills across enterprises located within eco-industrial parks, and strives to provide economic, environmental, and social competitive advantages for all the involved parties. Considering that the agri-food system creates a vast quantity of by-products along the supply chain, it is a sector that has huge potential within material and energy recovery systems and fits well into eco-industrial parks. The current study is a literature review that aims to evaluate the interest exhibited so far by scientific research in the topic of industrial symbiosis in the agri-food sector and to highlight the primary analytical techniques that have been used for this topic. Using the paradigm of multiple correspondence analysis, a content analysis was conducted from which the major themes of the researched phenomena emerged. The results indicate that the topic areas are unrelated and somewhat distant from each other. The analyzed case studies have revealed that the authors had neglected the communicative and collaborative elements among stakeholders, and instead focused on the potential use of some tools and approaches. Furthermore, it has been hypothesized that the hiding of information within a supply chain prevents industrial symbiosis procedures from being implemented. This research suggests the necessity of creating communication and cooperation platforms among stakeholders, which would promote the introduction of new techniques and tools for the development of circular production systems.
The influence of the existing global agri-food system poses a threat to local ecosystems (Willett et al., 2019). The current agri-food production practices in developed nations are marked by industrialized and intensive agriculture, market-oriented production, and high water and energy usage (Senauer and Venturini, 2005).
As a result of the ongoing changes in land use, in CO2 emissions, energy and water consumption, and in chemical pollution, the continuous growth in the amount and quality of output required to sustain an appropriate level of nourishment has had detrimental effects (Di Vita et al., 2017; Willett et al., 2019).
As food-production areas have reached their maximum capacity (Dubois, 2011), innovative cultivation, production, and consumption methods that include a radical transformation of the system are required (Herrero et al., 2020).
A shift in food production and processing practices has the potential of being a powerful driver of the local and global transition to sustainable development (Willett et al., 2019).
In this regard, governments, businesses, research institutes, and non-governmental organizations (NGOs) are exploring new ways of reusing products, their components, and waste material through the adoption of closed-loop systems that are aimed at improving economic and environmental sustainability (Toop et al., 2017; Duque-Acevedo et al., 2020).
The notion of circular economy (CE) is seen as the guiding principle of eco-innovation (Hamam et al., 2021, 2022), which is aimed at achieving a zero-waste society and economy, whose raw materials are utilized to create new goods and uses.
However, CE is viewed as an “umbrella concept” (Blomsma and Brennan, 2017) which encompasses a variety of phenomena that promote closed-loop systems (Prieto-Sandoval et al., 2018), such as industrial ecology (IE), industrial ecosystems and industrial symbiosis (IS), natural capitalism, cradle-to-cradle, blue economy, biomimicry, and regenerative design (Prieto-Sandoval et al., 2018; Sehnem et al., 2019; Unay-Gailhard and Bojnec, 2019).
Although the circular economy is considered as a restorative system, the associated ideas are viewed as preventative systems that require efforts to reduce energy and material losses and/or optimize them inside the system (Borrello et al., 2020; Cembalo et al., 2020; Al-Thani and Al-Ansari, 2021; Atanasovska et al., 2022).
The development of CE would not be conceivable without IE ideas and techniques (Saavedra et al., 2018), and in particular the use of industrial symbiosis processes (Herczeg et al., 2018).
In fact, a circular economy encourages the development of the concept of industrial symbiosis (IS), which is an eco-innovative system approach that involves the transfer of such resources as matter, energy, water, by-products, skills, and competencies between traditionally separate industries to generate competitive advantages for all the involved territorial actors (Chertow, 2000; Graedel and Allenby, 2003; Haller et al., 2022).
The symbiosis process develops through systems of cooperation and synergies between different industrial enterprises, as well as through various resource exchange mechanisms at a local scale to support the transition to a circular economy (Imbert, 2017; Kalmykova et al., 2018; Kerdlap et al., 2019), so that the waste and/or by-products of one enterprise can become the input of another (Mulrow et al., 2017).
The distance between the waste producer and the potential consumer is in fact one of the most important economic factors that should be considered when assessing the viability of symbiosis (Marchi et al., 2017; Aschemann-Witzel and Stangherlin, 2021). However, if the cost of transit remains the same and is more than the cost of purchasing raw materials, a circular system cannot function.
IS, despite its complexity, has been applied in agriculture, aquaculture, and animal husbandry (Dumont et al., 2013; Alfaro and Miller, 2014).
The application of an industrial symbiosis process to agri-food supply chains represents a significant opportunity for systemic changes in various components of the food system, such as technologies, infrastructure, skills, and knowledge (Abson et al., 2017; Parker and Svantemark, 2019; Herrero et al., 2020; Poponi et al., 2022; Stillitano et al., 2022), as well as for the creation of interactions between economic agents within a district (Nowak et al., 2015; Unay-Gailhard and Bojnec, 2016).
In line with this, industrial symbiosis facilitation tools that use information and communication technology, as well as network optimisation techniques, have been created to uncover synergistic connections between industrial processes and businesses (Grant et al., 2010; Boix et al., 2015; Kastner et al., 2015; Fraccascia et al., 2016; Van Capelleveen et al., 2018; Yeo et al., 2019; Raimbault et al., 2020).
This is one of the first studies to have conducted a literature review on the issues and major research subjects linked to the idea of industrial symbiosis in the agri-food field. The aim of this review has been to help provide a baseline for the development of strategies that will lead to the creation of more environmentally, financially and socially sustainable industrial technologies and processes.
This work addresses the following research question:
(RQ1) What are the main themes and the main interrelations that are emerging from the link between industrial symbiosis and agribusiness system?
(RQ2) Does a true relationship exist between these systems?
A content analysis was undertaken using the text mining WordStat programme and the “bibliometrix” R package to facilitate the synthesis of qualitative data. This computer-assisted synthesis of the literature made it possible to identify the major research topics of the articles, as well as their interrelationships, and to obtain a holistic interpretation of the reference framework pertaining to the examined phenomena.
On the basis of the data analysis, the authors then developed a set of research-based suggestions that could be further explored in future studies. They thus proposed set of recommendations for researchers and policymakers to promote symbiotic exchange, and identified the tools currently available in industrial symbiosis cases so that all stakeholders can recognize the tangible benefits of this business model and engage economically in promoting its implementation.
The remainder of the paper is structured as follows: Section Agro-Ecological symbiosis strategies offers an assessment of the literature on agro-ecological symbiosis strategies. The methodology is described and the results are analyzed in Sections Methodology and Results. The implications of the results are discussed in Section Discussion and recommendations are provided for further research. The concluding remarks are provided in the last section.
Industrial ecology (IE) is a study and practice discipline that arose in the 1990s, which focuses on the establishment and management of a closed-loop industrial environment (Saavedra et al., 2018; Baldassarre et al., 2019; Al-Thani and Al-Ansari, 2021).
It examines the relationships that exist between industrial systems and the natural environment (Garner and Keoleian, 1995) to achieve economic, social, and environmental harmony (Trokanas et al., 2014), and proposes approaches and applied solutions for a more efficient management of material and energy flows in companies (Simboli et al., 2015).
Industrial ecosystems were described by Frosch and Gallopolus (1989), as a system in which “the consumption of energy and materials is optimized, and the effluents of one process serve as raw material for another process.” In fact, they hypothesized that an industrial system can learn about efficiency by watching the material and energy movements of natural ecosystems (Frosch and Gallopolus, 1989).
A few years later, in 1995, Graedel and Allenby (1995) were the first to describe EI as a mechanism that could be used to achieve an economic, cultural, and technical expansion, while preserving the carrying capacity of the environment.
Its goals include not only the optimisation of energy and material consumption, but also the reduction of waste and of the resulting contamination of the natural environment, via the transformation of waste and industrial by-products into inputs for other processes (Trokanas et al., 2014; Beaulieu, 2015).
Specifically, the concept of IE encompasses five elements: dematerialisation; long-term policy alignment; the creation of industrial ecosystems; industrial metabolism, which is defined as the transformation of a linear economic system into an integrated industrial ecosystem (Frosch and Gallopolus, 1989; Prendeville et al., 2018), i.e., an analysis of the flow of materials and energy that spans the entire life cycle of a particular product; and balancing inputs and outputs.
The primary applications of IE-based solutions in the agro-food sector include animal and vegetable waste, and by-products (Mirabella et al., 2014; Simboli et al., 2015).
IE concepts and tools may function at several levels, including the farm, local, regional, and supra-regional levels (Figure 1), with the goal of decreasing environmental effects at each step of production and consumption. Many of these techniques concentrate on “closing the loop” of material flows and rely on recovery and recycling (Despeisse et al., 2012); others include product and process design and technology, organizational and management strategies, and government activities (Frosch and Gallopolus, 1989; Allenby, 1996; Ayres and Ayres, 1996; Erkman, 1997).
Figure 1. Industrial ecology structure. Source: Chertow (2000).
At the inter-firm level, industrial symbiosis is the process of promoting the evolution of synergy networks between different organizations (Chertow, 2000) and the efficiency of the physical exchange of materials and resources, including energy, water, and by-products, in the local and regional industrial ecosystem (Chertow, 2007; Li, 2018).
Industrial symbiosis (IS) is a progression of the industrial ecosystem idea, which Frosch and Gallopoulos initially introduced in 1989. It adheres to the concepts of industrial ecology, that is, to promote developing sustainable methods to boost production cycle efficiency and reduce the usage of non-renewable resources (Pagotto and Halog, 2016).
Consequently, it identifies business opportunities (Mantese and Amaral, 2018) that leverage on the synergistic exchange of such resources as water, energy, material flows, residues, waste, and under-utilized by-products (Chertow, 2000; Lombardi and Laybourn, 2012) between actors in co-located companies (Chertow et al., 2004; Wolf et al., 2007; Simboli et al., 2015; De Angelis et al., 2018; Mantese and Amaral, 2018; Yenipazarli, 2019).
The execution of an IS project can have several positive effects, not only from an environmental point of view, but also socially and economically (Neves et al., 2020). The environmental benefits are primarily related to the reduction of the impacts associated with waste disposal processes and methods, as well as the extraction and importing of virgin raw materials, which result in greenhouse gas emissions, the depletion of natural resources, and waste that would otherwise end up in landfills and incinerators.
These effects, which are verifiable at several levels, demonstrate that developing synergy is not only an exchange or pooling of resources, but also a new value generation process for all the involved parties. Consequently, the global value generated through such a synergy will be larger than the value provided by organizations working separately (Lowe, 1997).
The social advantages are a result of the development of new employment through the processing of leftovers and by-products, and the valorisation of labor resources through decreased raw material prices.
In addition to social and environmental advantages, an IS project also results in economic gains, due to a decreased cost of the raw materials and waste treatment.
Resource sharing may occur in three ways: by-product exchange, service/infrastructure sharing, or shared service purchase (Ehrenfeld and Gertler, 1997; Mirata and Emtairah, 2005; Chertow, 2007).
By-product reuse is simply an interchange of industrial material flows between two or more businesses, in which any surplus material from one operation is utilized to substitute raw materials or commercial goods in the other (Walmsley et al., 2019). Service/infrastructure sharing refers to the joint management and use of essential resources, including water, electricity, and wastewater. Similarly, the collaborative supply of services includes coordination, such as shared transit arrangements, food provision, and other requirements shared by neighboring firms and industries (Chertow, 2007).
However, this should entail the participation and interaction of historically different sectors, if the aim is to develop an integrated and collective strategy to interchange resource flows, create competitive advantage, and maximize resource efficiency (Chertow, 2000).
Chertow (2007), in order to differentiate industrial symbiosis from other types of exchange, considered a “3–2 heuristic,” which recognizes complex relationships in which at least three distinct entities should be involved in the exchange of at least two distinct resources, none of which should be engaged in recycling.
In addition, one of the characteristics of industrial symbiosis is the capacity to consider different types of industries, including not only farms, but also their upstream and downstream partners (Fernandez-Mena et al., 2016), and to make their specific input requirements and supply capacities explicit. An additional benefit of these systems is their ability to handle a variety of convertible materials, chemicals, and energy fluxes. It is possible to design locally effective recycling cycles by analyzing how and to what extent these various resources are handled, created, and changed within agent clusters (Fernandez-Mena et al., 2016).
Companies may employ internal IS strategies, whereby waste from one production process is used to replace virgin raw material inputs in other production processes inside the firm, or external IS strategies, whereby waste is sent to other companies that will then utilize it in their manufacturing processes (Fraccascia et al., 2016).
Yu et al. (2014) provided a summary of the recent advancements in industrial symbiosis procedures. From 1997 to 2005, scientific research on industrial symbiosis (IS) comprised just a small portion of the Industrial Ecology (IE) literature and focused on the concept of IS, the evaluation of eco-industrial park (EIP) projects (Garner and Keoleian, 1995), and the development of waste treatment and recycling networks.
Cases of industrial symbiosis may be split into three distinct categories: (1) area symbiosis, that is, the experiences of industrial symbiosis districts (bottom-up), such as those of Kalundborg, Denmark, or Eco-industrial Parks, which are characterized by the implementation, in more or less extensive territorial areas, of several subjects which, over time, carry out specific interventions for the closure and optimisation of cycles; (2) networked symbiosis, i.e., cases of industrial symbiosis based on cognitive/relational tools intended to facilitate the meeting between the demand and supply of resources (matter, energy, water, by-products, capacity, skills) between interlocutors whose economic and social activities would not otherwise have the chance to take place; (3) resource diagnostics, i.e., programmes that attempt to map business resources and find internal efficiency improvement solutions, as well as to enable the identification of potential input and output synergies with external interlocutors. A business that follows this route should conduct an integrated study of its resource management system in order to achieve internal and external efficiency benefits.
Organic substances have rarely been the subject of investigation in classic cases of industrial symbiosis. However, the concept may also be extended to agri-food chains and energy systems (Koppelmäki et al., 2019; Onu and Mbohwa, 2021).
The term “agroecological symbiosis” (AES) was first used for the redesign of a production system in the Finnish town of Palopuro (Koppelmäki et al., 2016). It is derived from the concept of industrial symbiosis applied to the food production and processing chain that operates with renewable energy, derived from its own raw materials, and in which farms, food processors, and energy producers operate in an integrated manner (Helenius et al., 2020), thereby strengthening local socio-economic ties.
When applying the concept of industrial symbiosis in the food processing sector, the first step is the identification, quantification, and characterization of residues, which are typically by-products or waste from biorefineries, agro-industries, or bio-oriented chemical industries (Ehrenfeld and Gertler, 1997; Van Beers et al., 2007), and then the determining of the recovery steps and technologies that can be applied for their processing (Galanakis, 2012).
There are instances in the literature (Zabaniotou et al., 2015) of systems that were built on a circular economy and industrial symbiosis framework in firms, such as the study of Pagotto and Halog (2016), who used input-output methodologies to analyse the Australian agri-food sector.
Industrial symbiosis is a crucial instrument for industrial activities in geographic regions where several enterprises may collaborate synergistically to produce more sustainably.
The transfer of resources from one firm to another provide economic benefits: the transferring company obtains a reduction in yearly waste management expenses and a profit upon sale, while the receiving company experiences a decrease in production costs.
The objective of the literature review was to select relevant research from the academic literature and synthesize the important findings on industrial symbiosis in the agricultural field Figure 2 shows a Preferred Reporting Items for Systematic Review and Meta-Analysis (PRISMA) flowchart to illustrate how the selection criteria were created using a systematic and repeatable process to identify articles that explored the topic (Maesano et al., 2022).
The literature review was undertaken by searching the Scopus and Web of Science (WoS) databases for pertinent papers.
The following keywords were used to extract articles published between 2007 and 2022: “industrial symbiosis” AND “agri-food” OR “agrifood” OR “agro-food” OR “agrofood” OR “food.” The search was run in May 2022 on titles, abstracts, and keywords, without any regard for the year. A total of 87 Scopus articles and 96 WoS papers, for a total of 183, were found and then submitted to a selection process.
The exclusion criteria included books, chapters, proceedings, editorials, and studies (31). In addition, any duplicates (93) and non-English language items (4) were removed. In all, 55 studies were ultimately considered.
A thorough search revealed that 55 publications satisfied the inclusion criteria, i.e., case studies and reviews, for the purpose of this study's literature review.
The authors first proposed a representation of the evolution of the publications over time, the most frequent keywords over the years, and a classification of the scientific papers.
Subsequently, to find the primary issues, a multiple correspondence analysis (MCA) was used to identify the reoccurring themes of the articles.
The overall conceptual framework of industrial symbiosis research in the agri-food industry was investigated using k-means clustering and correspondence analysis (Mitsuhiro and Yadohisa, 2015). Multiple correspondence analysis (MCA) is a multidimensional statistical approach that dates back to Hirschfeld (1935) and it is commonly utilized in marketing, and in particular for multidimensional mapping (Raimondo et al., 2022).
Through a graphical representation of a data matrix of qualitative variables, it is presented as a paradigm for exploratory bibliometric analysis to assess the dependency between keywords and discover clusters (Batagelj and Cerinšek, 2013).
The research was conducted using the “bibliometrix” R package (Aria and Cuccurullo, 2017), which enabled us to input text files and generate a data matrix, a conceptual structure map, and a dendrogram. “Keywords Plus” was used as input for the study as it includes fewer content-specific descriptors of the articles and, therefore, conveys wider meanings, thus making it acceptable for conceptual structure analysis. A k-means clustering technique was used for the data to determine the clusters in the MCA-obtained conceptual framework.
Co-occurrence analyses were conducted for the keywords and clusters to determine the strength of the relationships between the keywords and clusters that had emerged.
Co-occurrence analysis is an well-known technique that is used for mapping scientific knowledge (Radhakrishnan et al., 2017; Spina et al., 2021; Vindigni et al., 2021; Bellia et al., 2022), in which words or categories that tend to be repeated together are combined through a clustering process.
Each keyword is represented by a node, and a link between two nodes indicates their co-occurrence.
The frequency with which two keywords are used together is therefore illustrated by the weight of an edge. By applying network clustering algorithms, any mutual associations between them can be identified and illustrated.
The rise in the number of publications on industrial symbiosis in the agri-food industry from 2002 to 2022 is shown in Figure 3.
Research on the phenomena was rarely conducted prior to 2019. However, the quantity of published papers has increased significantly since 2019. The graph demonstrates the correlation between the rise in publications on industrial symbiosis and the interest in closed-loop production systems and sustainability.
In fact, the European Commission launched the European Green Deal in that very year with the intention of turning the climate crisis into a chance for a new model of development. The aim was to become the first carbon-neutral continent by 2050 by means of a socially and just ecological transition and through an industrial revolution that could guarantee sustainable productions (European Commission, 2020). It is realistic to imagine that there will be more papers on these themes in the future, given the increased interest in these topics among scientists.
Figure 4 depicts a one-year log scale to illustrate the trends in the development and sustainability study themes over the previous decade.
Figure 4. Trend keywords in the last 10 years. Source: our elaboration using “bibliometrix” R package.
The use of the terms “industrial symbiosis” and “industrial ecology” increased in 2015 and 2016, respectively. In 2019, the most frequent term was “waste management,” followed by “food waste” in 2020 and “eco-industrial park” in 2021.
The analysis of the publications revealed that 29 publications were case studies, 17 were reviews, five were articles, two were full-length, one referred to hypothesis and theory, and one to decision assistance, as shown in Figure 5.
Figure 6 represents the outcome of the multiple correspondence analysis as a map of the conceptual structure, which consists of five clusters: food supply chain, eco-industrial parks, life cycle assessment, greenhouse gas emissions, and anaerobic digestion, in relationship to the x and y axes.
MCA has two dimensions, one horizontal (x), and one vertical (y), which represent orthogonal latent dimensions.
The size of the map reflects the typical poles of the topical orientation within the industrial symbiosis concept, while the center of the map indicates the average location of all the keywords and, hence, the center of the search field. For instance, the terms “food waste,” “waste management,” and “circular economy” are located near to the center, since a significant number of publications on industrial symbiosis in the agri-food industry addressed these concerns.
Both of the latent dimensions are described by the fifty keywords that emerged from the research.
The total inertia, or total explained variability, is equal to the addition of the inertia of the two dimensions. The first dimension (x-axis) provides for most of the inertia (39.08%), while the second dimension (y-axis) contributes by 18.9%.
The first horizontal dimension divides the terms that emphasize production and emission processes (left) from those related to the management and recovery of by-products (right).
The second vertical dimension differentiates the keywords that emphasize heat processes due to emissions and waste recovery (at the top) from those that emphasize waste assessment and recovery systems (at the bottom).
The closeness between the keywords is proportional to the percentage of them that are mentioned together in the articles, while the greater the distance between them, the lower the proportion of keywords that are discussed together.
The figure enables linkages and disassociations to be determined between terms by analyzing their closeness.
The centroid concept guides the interpretation of the keyword points, according to which the keyword coordinates are the weighted average of the surrounding coordinates.
The dendrogram in Figure 7 illustrates the hierarchical link between clusters and variables. It is often generated for hierarchical clustering, and its primary use is to determine the optimal approach to allocate variables to clusters. The arrangement of keywords is based on how similar or distinct they are to one another and to other clusters.
Figure 7. Dendrogram of hierarchical link between clusters. Source: our elaboration using “bibliometrix” R package.
As evaluated by Pearson's correlation coefficient, clusters that are nearly the same height are comparable, while clusters with differing heights are distinct.
As shown in the dendrogram, the clusters of anaerobic digestion, greenhouse gas emissions, the food supply chain, life cycle assessments, and eco-industrial parks are distinct, due to their differing branch lengths.
Figure 8 illustrates the keyword co-occurrence network. Industrial symbiosis and industrial ecology are the primary nodes, and they are followed by food waste, waste management, sustainable development, symbiosis, and environmental effects.
Figure 8. Co-occurrence network for keywords. Source: our elaboration using “bibliometrix” R package.
The co-occurrence network connecting the groups found by means of the multiple correspondence analysis is shown in Figure 9. The anaerobic digestion and food supply chain clusters often co-occur (3,451), as does the food supply chain with greenhouse gas emission cluster (4,253).
The previously presented research questions may be addressed by considering the results of the outcome analysis. It was considered feasible to pinpoint the primary emergent themes and their potential relationships using multiple correspondence analysis. As a result, the interpretation of the findings demonstrates that there are still extremely weak, if any, correlations between the methodologies used in the literature, and that the actual implementation of industrial symbiosis processes in the agri-food system is still a long way off.
A reading of the discovered clusters is provided hereafter.
The CE Action Plan supports the deployment of biotechnologies and practices to transform a range of value bio-based goods (Maina et al., 2017; Zabaniotou and Kamaterou, 2019).
In fact, the loss and waste of food along the agri-food chain is a critical economic, environmental, and social aspect that requires immediate legislative action (Teigiserova et al., 2020).
Adopting the idea of circular economy, which encompasses several closed-loop solutions, such as industrial symbiosis, is one of the measures that can be taken to avoid and control food loss and waste (Raimondo et al., 2018).
Examples of resource recovery within agri-food supply chains in the CE have emerged from among the analyzed studies (Do et al., 2021), with special reference to the valorisation of bioresidues from the brewing, dairy, slaughter, and forestry sectors (Gregg et al., 2020).
IS is suggested as a suitable technique for recovering by-products from catering and retail services, even in situations other than food production systems.
For instance, the research by Filimonau and Ermolaev (2022) suggested a model for food waste recovery in food services to investigate the potential of the industrial symbiosis of reducing food waste in food services in Russia. A favorable attitude was established, through interviews with food service providers and farmers, toward the industrial symbiosis model as a food waste recovery method and as a chance to build the social and network capital of food service providers and farmers.
In fact, food service providers acknowledged the ability of the model to optimize their operational expenses by decreasing the cost of solid waste collection and by supplying fresher and more affordable farm goods. The possibility of a cost reduction and the development of new food supply and processing chains also clearly and favorably affected the farmers' perception of the concept.
Food waste also occurs in the retail sector, due to the unpredictability of the consumers' demand.
In this respect, Lee and Tongarlak (2017) investigated how a symbiosis process could minimize food waste in a retail environment and how it interacts with other waste reduction measures, including the waste disposal cost and the food donation tax credit.
StunŽenas and Kliopova (2021) proposed an integrated food waste management model, based on the IE principle, which demonstrates that numerous prevention and technological solutions, such as dematerialisation and industrial symbiosis models, can be implemented to reduce environmental impacts, thereby enabling a management approach that is close to the natural one.
Alfaro and Miller (2014) applied IS principles to small farms in a region of West Africa, using optimisation techniques to maximize agricultural production and minimize waste, such as integrated farming, which views the farm as a system of new technologies that increases agricultural production and makes use of established IS tools to create alternative pathways, based on symbiotic relationships, to increase production.
System integration of individual unit processes demonstrates increased productivity and decreased waste, thus indicating that there are still unrealised opportunities for IS in developing countries and that the integration of IS techniques into smallholder farming operations has the potential to influence sustainable development.
The cluster analysis revealed that the research is centered on a few specific cases and the agri-food sector as a whole. Moreover, there is a lack of research on certain industries, such the manufacturing of soft drinks or seafood, as opposed to wine or cereal.
Logistical concerns are other factors that have been overlooked in the research. For instance, there are few case studies that have dealt with the quantitative measurement of food waste generated by businesses. Another obstacle to the adoption of industrial symbiosis processes is the issue of geographical closeness to other businesses, which has not received enough attention in the literature.
Life cycle assessment (LCA) has been used extensively to measure the environmental benefits and costs of industrial symbiosis networks. Seven of the mentioned case studies evaluated the potential advantages of establishing industrial symbiosis processes using LCA as an analytical method.
For example, Kerdlap et al. (2020) introduced a methodology for modeling and analyzing the life-cycle environmental impacts of ISNs (Industrial Symbiosis Networks). This methodology enables models to be constructed that can be used to conduct multilevel assessments of the environmental performance of an individual or a set of waste-resource exchanges. An LCA was used to evaluate a prospective ISN for food waste-to-energy conversion in Singapore. The case study demonstrated that the technique is able to evaluate the environmental performance of a complete ISN.
Through a life cycle evaluation and a life cycle cost assessment, Diaz et al. (2021) investigated three major possible measures: energy recovery from waste via anaerobic digestion, the incorporation of renewable energy sources in warehouses, and the replacement of auxiliary equipment at a store. They discovered that the recovery of energy from food waste by means of anaerobic digestion and cogeneration offers the greatest advantages to the supply chain. Using a traditional life-cycle cost analysis, they determined that energy generation, through the utilization of waste for anaerobic digestion, was the most economically viable alternative.
Bhambhani et al. (2022) analyzed the advantages and disadvantages of the LCSA (Life Cycle Sustainability Assessment) methodology when used to evaluate the sustainability of water sector resource recovery systems. They identified three aspects of the LCSA that could be modified to better serve resource recovery solutions: its damage-based framework, its treatment of economic and natural capital as interchangeable, and its lack of environmental thresholds and historical emissions in its environmental assessment methodology.
Strazza et al. (2015) investigated a possible new turbo-drying technique for the recovery of cruise ship food waste for use as aquaculture feeds. They investigated the potential advantages of substituting standard salmon feed formulas with food waste, produced and processed on board a ship, by means of a comparative life-cycle evaluation.
Simboli et al. (2015) examined the potential growth of EI-based techniques in an Italian agri-food industry. The empirical data they used demonstrated that it was feasible to execute effective solutions via material substitution, repair, and recycling, as well as through the use of collaborative tactics between agriculture and industrial firms in the region.
According to the cluster analysis, LCA is now the most popular technique used for evaluating the environmental performance of industrial symbiosis processes that turn food waste into energy. This technique, together with other tools that encourage symbiotic interaction, might aid a variety of stakeholders in implementing eco-efficient systems in businesses and in obtaining the evidence-based information required to measure success, which will in turn assist in shaping laws and regulations.
Industrial ecosystems, eco-industrial networks and eco-industrial parks have been identified as the physical expressions of industrial symbiosis (Horn and Proksch, 2022).
Eco-industrial parks, which are based on a “top-down” approach, since they are developed from regulatory initiatives, represent communities of manufacturing and service firms in close locational proximity that coordinate to exchange material and informational resources in order to reduce waste, optimize the use of raw materials and energy, and promote multidimensional sustainable relationships between firms and key actors (Winans et al., 2017). The same concept is applied to industrial symbiosis and eco-industrial networks, where it is extended to a larger geographical area, even of the size of a state/province or nation (Winans et al., 2017).
Through cooperation, the business community seeks a greater collective advantage than the sum of the individual gains each company would obtain if it optimized its performance alone (Erkman, 1997; Lowe, 1997).
Denmark's Kalundborg eco-park is one of the most well-known examples of industrial symbiosis (Garner and Keoleian, 1995). The first network of exchanges, the first of water resources and then also of commodities and energy, arose in this region in the 1960s with twelve enterprises who considered IS at the core of their operations. This industrial ecosystem was created without the use of any specialized planning tools, but rather through bilateral agreements among several local businesses.
Significant environmental and economic advantages emerged from this IS situation. In fact, 14 million euros, 635 thousand tons of carbon dioxide, 3.6 cubic meters of water, 100 gigawatt hours of electricity, and 87,000 tons of materials were saved (Ehrenfeld and Gertler, 1997). Since then, other occurrences of eco-industrial parks have been documented (Mirata, 2004; Roberts, 2004; Zhu et al., 2007; Park et al., 2008).
Most of the case studies analyzed in this article suggest and have evaluated industrial symbiosis models for the development of eco-industrial parks to assess their technical, environmental, social, and economic viability (Frone and Frone, 2017; Hu et al., 2020).
For instance, Genc et al. (2020) suggested a novel design strategy for eco-industrial parks that takes into consideration the possibility of waste exchanges among co-located companies to mitigate the detrimental effects of market and company dynamism.
Genc et al. (2019) presented two approaches to analyse the robustness, redundancy, connectedness, and cyclicity of eco-parks in a Turkish industrial zone and to assess any variations in network topologies regarding prospective industrial symbiosis implementations. They also envisaged the possible future co-location of businesses in the industrial zone to facilitate the establishment of an industrial symbiotic network. The findings demonstrate that the approach may be used to evaluate the robustness of an industrial network.
Chatterjee et al. (2021) were the first to examine the advantages of using layered systems to achieve IS goals. They used a vast dataset, obtained from hypothetical and real industrial water networks, to demonstrate that highly layered designs cut resource use by a substantial amount. The findings indicate that the nesting concept may be an effective quantitative design principle for IS.
In 2015, Puente et al. (2015) showed the potential of small and medium-sized firms, concentrated in industrial districts or parks in northern Spain, for systemic eco-innovation via industrial symbiosis techniques.
Sanyé-Mengual et al. (2018) conducted a transnational comparative review, between Europe and South America, of eight case studies to obtain a more precise theoretical viewpoint on the prospective deployment of rooftop greenhouses in business parks. They used the life cycle and geographic information system assessment technique to estimate both the potential and anticipated advantages of building rooftop greenhouses. They discovered that business parks are better than industrial parks as urban locations for such undertakings.
Helenius et al. (2020) proposed agro-ecological symbiosis (AES) as a strategy to reconfigure primary food production in agriculture, food processing, and food community development in order to achieve system-level sustainability. Through sustained and robust collaboration and a co-creative process with trans-disciplinary actors, including food producers and processors as well as policy actors, they designed a food system model, based on networks of AES, that has the potential of facilitating the development of place-based food systems which advance the sustainability agenda.
The research of Brehm and Layton (2021) focused on the metric of nestedness, which is an ecological approach that resorts to the placement of linkages between nodes in a network to maximize network cyclicity for a given number of links. This measure provides numerous benefits for the design and study of EINs (Eco-Industrial networks), including maturity independence, size normalization, and strong statistical documentation of ecological systems with a high degree of mutualism.
The application of nestedness to EINs has revealed a lower occurrence of nested structures and a greater degree of unpredictability than is generally seen for food waste. Industrial networks also exhibit a link between high nesting and internal cycles, thus indicating that the reuse of materials and energy in EINs may be enhanced as a result of nesting structures more deeply.
Hardy and Graedel (2002) applied the food web theory to nineteen eco-industrial parks and biosystems, both real and fictional. They discovered a linear relationship by connecting the number of industrial participants and the number of linkages between them.
Wright et al. (2009) examined the possibilities for greater inter-disciplinary cooperation by determining whether the quantitative analytic approaches used in community ecology research were also applicable in an industrial environment in Nova Scotia. Their findings demonstrated that these methods are also applicable for industrial ecology.
Some research has focused on the growth of eco-industrial parks in eastern nations. Yu et al. (2015), for instance, used the Rizhao Economic and Technological Development Area as a case study to introduce the industrial symbiosis development process, research the evolution of industrial symbiosis and eco-industrial park construction, and summarize the factors and characteristics of industrial symbiosis development in China.
In addition, Shi et al. (2010) conducted a case study of the Tianjin Economic-Technological Development Area that summarized the characteristics of eco-industrial parks in a developing nation and assessed the environmental advantages of major symbiotic exchanges.
On the other hand, Ong et al. (2021) provided an overview of the existing management practices of industrial solid waste and the usual obstacles to the construction of an eco-industrial park in Malaysia.
Case studies related to eco-industrial parks make up the bulk of the cluster content analysis. Apart from exemplary instances, most of them serve as templates for possible eco-industrial parks that may be used in industrial symbiosis processes; empirical cases, on the other hand, are still very infrequent. The body of literature on this subject currently shows certain gaps.
Global fossil carbon emissions have grown dramatically since the turn of the century, and the European Union currently ranks third for global emissions (Boden et al., 2017).
In this perspective, these emissions comprise 24% of the global greenhouse gas emissions from the agriculture industry (IPCC, 2014). Recent IPCC findings indicate that maintaining global warming below 2°C may be achieved by reducing GHG emissions from several sectors, including the agricultural industry (IPCC, 2019).
Burg et al. (2021) conducted a regional investigation on the availability of manure as a feedstock for biogas plants and as a greenhouse heat source. In their research, they correlated the potential supply of waste heat from biogas derived from manure with the peak heat demand of the greenhouses.
In addition, they determined the area-based heating requirement of greenhouses for year-round tomato production and the possible heat supply from manure biogas.
Kikuchi et al. (2016) instead suggested an effective IS concept after conducting a thermodynamic study of energy fluxes in a sugar mill.
Martin et al. (2022), through a life cycle evaluation, quantified the environmental performance of synergies linked to energy integration and the circular use of materials in vertical farming systems of a fictitious urban farm situated in the basement of a residential building in Stockholm.
Sanyé-Mengual et al. (2018) discovered that business parks are better than industrial parks as urban locations for such undertakings. In addition, the deployment of insulated greenhouses on rooftops in Europe and South America led to high production values, CO2 reductions, and food independence.
According to the literature study, linear industrial processes are emerging as the primary issue that is causing greenhouse gas emissions. Assessments have been conducted to quantify the emissions from the agri-food sector via several case studies. As previously mentioned, LCA is one of the main tools in this sector. However, it seems that little progress has been made in creating strategic models that can be used in manufacturing to cut down these emissions.
Anaerobic digestion is an effective and eco-friendly waste treatment method (Capson-Tojo et al., 2016) that permits energy recovery and digestate recycling (Slorach et al., 2019; Zabaniotou and Kamaterou, 2019; Battista et al., 2020).
To address the special features of bio-based value chains, the establishment of new bio-based value chains would need collaboration across hitherto unconnected industries. Most of the traits are attributable to the primary production of biological resources in value chains. Such processes are often characterized by seasonality, decentralization, and underlying quality changes resulting from environmental variables (De Meyer et al., 2014; Ghosh, 2016). Because of the low density and tendency to decompose of biomass, its transportability is often hampered. The transformation of primary biomass has been anticipated to take place at a regional scale and to be characterized by several dissimilar characteristics (De Angelis et al., 2018).
However, primary biomass is excellent for industrial symbiosis, since the process can be done on a modest scale in any geographic region (Ingrao et al., 2018; Muradin et al., 2018).
Several research works have confirmed the advantages of setting up agro-industrial symbiosis networks (Santos and Magrini, 2018) and have offered decision support tools to find the most desirable inputs, processes, and outputs for biorefining (Tsakalova et al., 2015; Moncada and Aristizábal, 2016; Yu et al., 2017).
Teigiserova et al. (2019) suggested that the economics of scope, based on cascade production, are beneficial for small- and medium-sized and short-chain biorefineries whose productions depend on food waste. Moreover, large-scale biorefineries with significant transport distances and a lengthy value chain witness a decrease in the quality of raw materials and elevated transport emissions. Smaller facilities, on the other hand, have lower related transit costs and fewer infrastructure constraints for sorting, storage, and transport (Mak et al., 2020), while their output is accelerated to boost value addition (Banerjee et al., 2018; Barampouti et al., 2019).
Ometto et al. (2007) determined that the replacement of fossil fuels with bioalcohol in agricultural, animal, and food activities is advantageous in sugarcane agriculture to ensure economic returns, environmental quality, and higher social equality.
Sheppard et al. (2019) analyzed the challenges and potential associated with resource sharing between food sectors and biorefineries. The purpose of the case study was to determine and assess the resource efficiencies and economics of co-location between a coffee bean roasting enterprise and the biorefining of its downstream byproduct, i.e., discarded coffee grounds. The analysis demonstrated that there may be substantial advantages.
Zhang et al. (2021) investigated the potential advantages of adopting the industrial symbiosis strategy in agriculture and horticulture for a possible Eco-Industrial Park in Canada consisting of dairy farming, greenhouse vegetable cultivation, and mushroom cultivation. They considered the anaerobic digestion of dairy manure to create biogas and digestate.
An analysis of the literature has shown that anaerobic digestion processes now appear to be the most environmentally, socially, and economically beneficial means of converting waste into energy. Researchers, together with stakeholders, should make more efforts to develop new methods for the reuse of by-products.
The purpose of this article has been to identify the main themes that emerged from a review of the literature and to identify the main barriers to promoting industrial symbiosis in order to encourage innovative policies and ways to support its development.
The analysis of obstacles and drivers in this study provides valuable information for the research community and for business decision makers on the importance of implementing IS systems.
Despite the exemplary cases of industrial symbiosis, it remains to be understood why progress in IS implementation has been so hesitant and gradual.
The results of the study by Domenech et al. (2019) reveal, for example, that IS exchanges continue to face a number of challenges in Europe, some of which are related to risk and uncertainty, while others are related to poor IS project commercial margins and transaction costs.
Therefore, better policies that foster collaborations and reduce transaction costs, for example, through the use of indicators, and encourage ambitious goals, such as trust, geographic proximity, and knowledge and information exchange, are essential to promote IS deployment and foster the development of large-scale initiatives.
The latter appears to be a particularly limiting component of industrial symbiosis operations.
Supply chain management pays little attention to knowledge concealment (Fang, 2017; Butt and Ahmad, 2019; Connelly et al., 2019; Pérez-Salazar et al., 2019).
The concealment of information limits the transmission and interchange of information held by internal and external stakeholders about the utility, origin, and availability of food waste by-products (Butt and Ahmad, 2019; Singh, 2019; Mangla et al., 2021).
In encouraging industrial symbiosis, some authors (Raabe et al., 2017; Low et al., 2018) have emphasized the need for collaborative platforms that provide the necessary information to support the physical exchange of by-products between companies.
Moreover, information management should be closely linked to information exchange. In fact, if organizations hide information related to top-down supply chain operations, supply chains could become vulnerable and isolated (Butt and Ahmad, 2019; Singh, 2019).
Hence, information exchange is a crucial aspect of supply chain management to promote organizational responsiveness and creativity, as well as to improve the ability of organizations to cope with unforeseen challenges (Timpanaro et al., 2012; Di Vita et al., 2015; Pandey et al., 2020).
Instead, what emerges from the literature review presented in this paper is that the case studies have addressed the application of potentially applicable methods and tools to an industrial symbiosis system, but have avoided the collaborative aspect between actors, due to a lack of communication between them. We believe that collaboration and information exchange systems among stakeholders is an aspect that still requires studying, and needs more attention as it is one of the main barriers to the introduction of symbiotic systems.
For this reason, we suggest that an analysis that looks at the system as a whole, and which succeeds in identifying the links between different areas of study, and in proposing tools for collaboration between stakeholders through the design of innovative communication platforms, would lead to a better understanding of what the entry and exit points between different companies could be, and thus improve the collaborative efficiency between different production sectors to establish closer relationships and enable the sustainability and development of industrial symbiosis processes (Dora, 2019).
This research contributes to the identification of the most widely used techniques for industrial symbiosis, and can thus help scholars and practitioners in the study and modeling of IS.
A content analysis was conducted to gather qualitative evidence from the literature. We ascertained that although interest seems to have increased in recent years, the number of publications is still rather modest. Emerging topics include food waste, life cycle assessment, eco-industrial parks, anaerobic digestion, and greenhouse gas emissions. Most research suggests industrial symbiosis models that can be used within eco-industrial parks.
However, to implement industrial symbiosis and create a circular economy, it is necessary to assess the connections between the production and consumption stages of the economic system. Since there is no link between emerging sectors and the capabilities associated with them, the results indicate that the hiding of information is a barrier that disconnects the system and slows down the spread of industrial symbiosis processes.
In this regard, we propose that the exchange of knowledge and information among stakeholders will help the development of industrial symbiosis processes and enable the most appropriate methods for converting a company's waste into secondary raw materials for other companies.
The application of this new business model can be a key industrial policy, as it creates significant economic and environmental benefits for the business system and the community as a whole through an increase in the overall competitiveness of local production systems and a reduction in pressure on ecosystem services.
In spite of the aim of providing a comprehensive review and synthesis of the literature, it was not possible to conceptualize several issues as thoroughly as we would have wanted to.
The main limitations of this search stem from the methods that we used to identify relevant papers. By focusing on only English-language publications, it is likely that several key articles on this topic were overlooked. Moreover, since only research articles and reviews were considered to ensure the quality of the reviewed publications, it is possible that there are conferences, books, and/or public papers on IS that have not been reported.
The raw data supporting the conclusions of this article will be made available by the authors, without undue reservation.
MH: conceptualization, data curation, methodology, software, writing—original draft preparation, and writing—review and editing. DS: methodology, software, writing—original draft preparation, and writing—review and editing. MR and RZ: writing—original draft preparation and writing—review and editing. GD, GC, and JT: writing—original draft preparation and writing—review, editing, and supervision. MD'A: writing—review and editing, supervision, project administration, and funding acquisition. All authors contributed to the article and approved the submitted version.
This research was supported by the project PRIN DRASTIC Driving The Italian AgriFood System Into A Circular Economy Model, PRIN-MIUR (2017 JYRZFF) and funded by the Italian Ministry of Education, University and Research (MIUR); GRINS Foundation, Growing Resilient, Inclusive and Sustainable; National Research, Development and Innovation Office, Hungary, Grant—K 143370 Agricultural Climate Change Adaptation Behavior and Circular Economy.
The authors declare that the research was conducted in the absence of any commercial or financial relationships that could be construed as a potential conflict of interest.
All claims expressed in this article are solely those of the authors and do not necessarily represent those of their affiliated organizations, or those of the publisher, the editors and the reviewers. Any product that may be evaluated in this article, or claim that may be made by its manufacturer, is not guaranteed or endorsed by the publisher.
Abson, D. J., Fischer, J., Leventon, J., Newig, J., Schomerus, T., Vilsmaier, U., et al. (2017). Leverage points for sustainability transformation. Ambio 46, 30–39. doi: 10.1007/s13280-016-0800-y
Alfaro, J., and Miller, S. (2014). Applying industrial symbiosis to smallholder farms: modeling a case study in Liberia, West Africa. J. Ind. Ecol. 18, 145–154. doi: 10.1111/jiec.12077
Allenby, B. R. (1996). A design for environment methodology for evaluating materials. Environ. Qual. Manag. 5, 69–84. doi: 10.1002/tqem.3310050409
Al-Thani, N. A., and Al-Ansari, T. (2021). Comparing the convergence and divergence within industrial ecology, circular economy, and the energy-water-food nexus based on resource management objectives. Sustain. Prod. Consum. 27, 1743–1761. doi: 10.1016/j.spc.2021.04.008
Aria, M., and Cuccurullo, C. (2017). bibliometrix: an R-tool for comprehensive science mapping analysis. J. Informetr. 11, 959–975. doi: 10.1016/j.joi.2017.08.007
Aschemann-Witzel, J., and Stangherlin, I. D. C. (2021). Upcycled by-product use in agri-food systems from a consumer perspective: a review of what we know, and what is missing. Technol. Forecast. Soc. Change 168, 120749. doi: 10.1016/j.techfore.2021.120749
Atanasovska, I., Choudhary, S., Koh, L., Ketikidis, P. H., and Solomon, A. (2022). Research gaps and future directions on social value stemming from circular economy practices in agri-food industrial parks: insights from a systematic literature review. J. Clean. Prod. 354, 131753. doi: 10.1016/j.jclepro.2022.131753
Baldassarre, B., Schepers, M., Bocken, N., Cuppen, E., Korevaar, G., and Calabretta, G. (2019). Industrial symbiosis: towards a design process for eco-industrial clusters by integrating circular economy and industrial ecology perspectives. J. Clean. Prod. 216, 446–460. doi: 10.1016/j.jclepro.2019.01.091
Banerjee, S., Ranganathan, V., Patti, A., and Arora, A. (2018). Valorisation of pineapple wastes for food and therapeutic applications. Trends Food Sci. Technol. 82, 60–70. doi: 10.1016/j.tifs.2018.09.024
Barampouti, E. M., Mai, S., Malamis, D., Moustakas, K., and Loizidou, M. (2019). Liquid biofuels from the organic fraction of municipal solid waste: a review. Renew. Sustain. Energy Rev. 110, 298–314. doi: 10.1016/j.rser.2019.04.005
Batagelj, V., and Cerinšek, M. (2013). On bibliographic networks. Scientometrics 96, 845–864. doi: 10.1007/s11192-012-0940-1
Battista, F., Frison, N., Pavan, P., Cavinato, C., Gottardo, M., Fatone, F., et al. (2020). Food wastes and sewage sludge as feedstock for an urban biorefinery producing biofuels and added-value bioproducts. J. Chem. Technol. Biotechnol. 95, 328–338. doi: 10.1002/jctb.6096
Beaulieu, L. (2015). Circular Economy: A Critical Literature Review of Concepts. Centre Interuniversitaire de Recherche sur le Cycle de vie des Produits, Procédés et Services. Montréal, QC.
Bellia, C., Bacarella, S., and Ingrassia, M. (2022). Interactions between street food and food safety topics in the scientific literature—a bibliometric analysis with science mapping. Foods 11, 789. doi: 10.3390/foods11060789
Bhambhani, A., van der Hoek, J. P., and Kapelan, Z. (2022). Life cycle sustainability assessment framework for water sector resource recovery solutions: strengths and weaknesses. Resour. Conserv. Recycl. 180, 106151. doi: 10.1016/j.resconrec.2021.106151
Blomsma, F., and Brennan, G. (2017). The emergence of circular economy: a new framing around prolonging resource productivity. J. Ind. Ecol. 21, 603–614. doi: 10.1111/jiec.12603
Boden, T. A., Marland, G., and Andres, R. J. (2017). National CO2 Emissions From Fossil-Fuel Burning, Cement Manufacture, and Gas Flaring: 1751-2014. Tennessee: Carbon Dioxide Information Analysis Center, Oak Ridge National Laboratory, US Department of Energy.
Boix, M., Montastruc, L., Azzaro-Pantel, C., and Domenech, S. (2015). Optimization methods applied to the design of eco-industrial parks: a literature review. J. Clean. Prod. 87, 303–317. doi: 10.1016/j.jclepro.2014.09.032
Borrello, M., Pascucci, S., and Cembalo, L. (2020). Three propositions to unify circular economy research: a review. Sustainability 12, 4069. doi: 10.3390/su12104069
Brehm, C., and Layton, A. (2021). Nestedness of eco-industrial networks: exploring linkage distribution to promote sustainable industrial growth. J. Ind. Ecol.25, 205–218. doi: 10.1111/jiec.13057
Burg, V., Golzar, F., Bowman, G., Hellweg, S., and Roshandel, R. (2021). Symbiosis opportunities between food and energy system: the potential of manure-based biogas as heating source for greenhouse production. J. Ind. Ecol. 25, 648–662. doi: 10.1111/jiec.13078
Butt, A. S., and Ahmad, A. B. (2019). Are there any antecedents of top-down knowledge hiding in firms? Evidence from the United Arab Emirates. J. Knowl. Manag. 23, 1605–1627. doi: 10.1108/JKM-04-2019-0204
Capson-Tojo, G., Rouez, M., Crest, M., Steyer, J. P., Delgenès, J. P., and Escudié, R. (2016). Food waste valorization via anaerobic processes: a review. Rev. Environ. Sci. Biotechnol. 15, 499–547. doi: 10.1007/s11157-016-9405-y
Cembalo, L., Borrello, M., De Luca, A. I., Giannoccaro, G., and D'Amico, M. (2020). Transitioning agri-food systems into circular economy trajectories. Aestimum 199–218. doi: 10.13128/aestim-8860
Chatterjee, A., Brehm, C., and Layton, A. (2021). Evaluating benefits of ecologically-inspired nested architectures for industrial symbiosis. Resour. Conserv. Recycl. 167, 105423. doi: 10.1016/j.resconrec.2021.105423
Chertow, M., Ashton, W., and Kuppalli, R. (2004). The Industrial Symbiosis Research Symposium at Yale: Advancing the Study of Industry and Environment. Yale School of the Environment Publications Series 23. New Haven, CT.
Chertow, M. R. (2000). Industrial symbiosis: literature and taxonomy. Annu. Rev. Energy Environ. 25, 313–337. doi: 10.1146/annurev.energy.25.1.313
Chertow, M. R. (2007). Uncovering industrial symbiosis. J. Ind. Ecol. 11, 11–30. doi: 10.1162/jiec.2007.1110
Connelly, C. E., Cerne, M., Dysvik, A., and Škerlavaj, M. (2019). Understanding knowledge hiding in organizations. J. Organ. Behav. 40, 779–782. doi: 10.1002/job.2407
De Angelis, R., Howard, M., and Miemczyk, J. (2018). Supply chain management and the circular economy: towards the circular supply chain. Prod. Plan. Control. 29, 425–437. doi: 10.1080/09537287.2018.1449244
De Meyer, A., Cattrysse, D., Rasinmäki, J., and Van Orshoven, J. (2014). Methods to optimise the design and management of biomass-for-bioenergy supply chains: a review. Renew. Sust. Energ. Rev. 31, 657–670. doi: 10.1016/j.rser.2013.12.036
Despeisse, M., Ball, P. D., Evans, S., and Levers, A. (2012). Industrial ecology at factory level–a conceptual model. J. Clean. Prod. 31, 30–39. doi: 10.1016/j.jclepro.2012.02.027
Di Vita, G., Pilato, M., Pecorino, B., Brun, F., and D'Amico, M. (2017). A review of the role of vegetal ecosystems in CO2 capture. Sustainability 9, 1840. doi: 10.3390/su9101840
Di Vita, G. D., Allegra, V., and Zarbà, A. S. (2015). Building scenarios: a qualitative approach to forecasting market developments for ornamental plants. Int. J. Bus. Glob. 15, 130–151. doi: 10.1504/IJBG.2015.071152
Diaz, F., Vignati, J. A., Marchi, B., Paoli, R., Zanoni, S., and Romagnoli, F. (2021). Effects of energy efficiency measures in the beef cold chain: a life cycle-based study. Rigas Tehn. Univer. Zinatniskie Raksti 25, 343–355. doi: 10.2478/rtuect-2021-0025
Do, Q., Ramudhin, A., Colicchia, C., Creazza, A., and Li, D. (2021). A systematic review of research on food loss and waste prevention and management for the circular economy. Int. J. Prod. Econ. 239, 108209. doi: 10.1016/j.ijpe.2021.108209
Domenech, T., Bleischwitz, R., Doranova, A., Panayotopoulos, D., and Roman, L. (2019). Mapping industrial symbiosis development in Europe_ typologies of networks, characteristics, performance and contribution to the circular economy. Resour. Conserv. Recycl. 141, 76–98. doi: 10.1016/j.resconrec.2018.09.016
Dora, M. (2019). Collaboration in a circular economy: learning from the farmers to reduce food waste. J. Enterp. Inf. Manag. 33, 769–789. doi: 10.1108/JEIM-02-2019-0062
Dubois, O. (2011). The State of the World's Land and Water Resources for Food and Agriculture: Managing Systems at Risk. Rome; Eathscan.
Dumont, B., Fortun-Lamothe, L., Jouven, M., Thomas, M., and Tichit, M. (2013). Prospects from agroecology and industrial ecology for animal production in the 21st century. Animals 7, 1028–1043. doi: 10.1017/S1751731112002418
Duque-Acevedo, M., Belmonte-Ureña, L. J., Plaza-Úbeda, J. A., and Camacho-Ferre, F. (2020). The management of agricultural waste biomass in the framework of circular economy and bioeconomy: an opportunity for greenhouse agriculture in Southeast Spain. Agronomy 10, 489. doi: 10.3390/agronomy10040489
Ehrenfeld, J., and Gertler, N. (1997). Industrial ecology in practice: the evolution of interdependence at Kalundborg. J. Ind. Ecol. 1, 67–79. doi: 10.1162/jiec.1997.1.1.67
Erkman, S. (1997). Industrial ecology: an historical view. J. Clean. Prod 5, 1–10. doi: 10.1016/S0959-6526(97)00003-6
European Commission (2020). Un Nuovo Piano D'azione Per L'economia Circolare. Per un'Europa più pulita e più competitiva, COM/2020/98 final. Bruxelles: European Commission.
Fang, Y. H. (2017). Coping with fear and guilt using mobile social networking applications: knowledge hiding, loafing, and sharing. Telemat. Inform. 34, 779–797. doi: 10.1016/j.tele.2017.03.002
Fernandez-Mena, H., Nesme, T., and Pellerin, S. (2016). Towards an agro-industrial ecology: a review of nutrient flow modelling and assessment tools in agro-food systems at the local scale. Sci. Total Environ. 543, 467–479. doi: 10.1016/j.scitotenv.2015.11.032
Filimonau, V., and Ermolaev, V. A. (2022). Exploring the potential of industrial symbiosis to recover food waste from the foodservice sector in Russia. Sustain. Prod. Consum 29, 467–478. doi: 10.1016/j.spc.2021.10.028
Fraccascia, L., Magno, M., and Albino, V. (2016). Business models for industrial symbiosis: a guide for firms. Proc. Environ. Sci. Eng. Manag. 3, 83–93.
Frone, D. F., and Frone, S. (2017). “Circular economy in Romania: an industrial synergy in the agri-food sector,” in Scientific Papers Series Management, Economic Engineering in Agriculture and Rural Development. 17.
Frosch, R. A., and Gallopolus, N. (1989). Strategies for manufacturing. Sci. Am. 261, 94. doi: 10.1038/scientificamerican0989-144
Galanakis, C. M. (2012). Recovery of high added-value components from food wastes: conventional, emerging technologies and commercialized applications. Trends Food Sci. Technol. 26, 68–87. doi: 10.1016/j.tifs.2012.03.003
Garner, A., and Keoleian, G. A. (1995). Industrial Ecology: An Introduction, University of Michigan. Ann Arbor, MI: National Pollution Prevention Center for Higher Education.
Genc, O., Kurt, A., Yazan, D. M., and Erdis, E. (2020). Circular eco-industrial park design inspired by nature: an integrated non-linear optimization, location, and food web analysis. J. Environ. Manage. 270, 110866. doi: 10.1016/j.jenvman.2020.110866
Genc, O., van Capelleveen, G., Erdis, E., Yildiz, O., and Yazan, D. M. (2019). A socio-ecological approach to improve industrial zones towards eco-industrial parks. J. Environ. Manage. 250, 109507. doi: 10.1016/j.jenvman.2019.109507
Ghosh, S. K. (2016). Biomass & bio-waste supply chain sustainability for bio-energy and bio-fuel production. Proc. Environ. Sci. 31, 31–39. doi: 10.1016/j.proenv.2016.02.005
Graedel, T. E., and Allenby, B. R. (2003). Industrial Ecology. Upple Saddle River, NJ: Prentice Hall.
Grant, G. B., Seager, T. P., Massard, G., and Nies, L. (2010). Information and communication technology for industrial symbiosis. J. Ind. Ecol. 14, 740–753. doi: 10.1111/j.1530-9290.2010.00273.x
Gregg, J. S., Jürgens, J., Happel, M. K., Strøm-Andersen, N., Tanner, A. N., Bolwig, S., et al. (2020). Valorization of bio-residuals in the food and forestry sectors in support of a circular bioeconomy: a review. J. Clean. Prod 267, 122093. doi: 10.1016/j.jclepro.2020.122093
Haller, H., Fagerholm, A. S., Carlsson, P., Skoglund, W., van den Brink, P., Danielski, I., et al. (2022). Towards a resilient and resource-efficient local food system based on industrial symbiosis in härnösand: a Swedish case study. Sustainability 14, 2197. doi: 10.3390/su14042197
Hamam, M., Chinnici, G., Di Vita, G., Pappalardo, G., Pecorino, B., Maesano, G., et al. (2021). Circular economy models in agro-food systems: a review. Sustainability 13, 3453. doi: 10.3390/su13063453
Hamam, M., D'Amico, M., Zarbà, C., Chinnici, G., and Tóth, J. (2022). Eco-Innovations transition of agri-food enterprises into a circular economy. Front. Sustain. Food. Syst. 6, 845420. doi: 10.3389/fsufs.2022.845420
Hardy, C., and Graedel, T. E. (2002). Industrial ecosystems as food webs. J. Ind. Ecol. 6, 29–38. doi: 10.1162/108819802320971623
Helenius, J., Hagolani-Albov, S. E., and Koppelmäki, K. (2020). Co-creating agroecological symbioses (AES) for sustainable food system networks. Front. Sustain. Food Syst. 4, 588715. doi: 10.3389/fsufs.2020.588715
Herczeg, G., Akkerman, R., and Hauschild, M. Z. (2018). Supply chain collaboration in industrial symbiosis networks. J. Clean. Prod. 171, 1058–1067. doi: 10.1016/j.jclepro.2017.10.046
Herrero, M., Thornton, P. K., Mason-D'Croz, D., Palmer, J., Benton, T. G., Bodirsky, B. L., et al. (2020). Innovation can accelerate the transition towards a sustainable food system. Nat. Food 1, 266–272. doi: 10.1038/s43016-020-0074-1
Hirschfeld, H. O. (1935). A connection between correlation and contingency. Math. Proc. Camb. Philos. Soc. 31, 520–524. doi: 10.1017/S0305004100013517
Horn, E., and Proksch, G. (2022). Symbiotic and regenerative sustainability frameworks: moving towards circular city implementation. Front. Built Environ. 7, 780478. doi: 10.3389/fbuil.2021.780478
Hu, W., Tian, J., Li, X., and Chen, L. (2020). Wastewater treatment system optimization with an industrial symbiosis model: a case study of a Chinese eco-industrial park. J. Ind. Ecol. 24, 1338–1351. doi: 10.1111/jiec.13020
Imbert, E. (2017). Food waste valorization options: opportunities from the bioeconomy. Open Agric. 2, 195–204. doi: 10.1515/opag-2017-0020
Ingrao, C., Faccilongo, N., Di Gioia, L., and Messineo, A. (2018). Food waste recovery into energy in a circular economy perspective: a comprehensive review of aspects related to plant operation and environmental assessment. J. Clean. Prod. 184, 869–892. doi: 10.1016/j.jclepro.2018.02.267
Kalmykova, Y., Sadagopan, M., and Rosado, L. (2018). Circular economy–from review of theories and practices to development of implementation tools. Resour. Conserv. Recycl. 135, 190–201. doi: 10.1016/j.resconrec.2017.10.034
Kastner, C. A., Lau, R., and Kraft, M. (2015). Quantitative tools for cultivating symbiosis in industrial parks; a literature review. Appl. Energy 155, 599–612. doi: 10.1016/j.apenergy.2015.05.037
Kerdlap, P., Low, J. S. C., and Ramakrishna, S. (2019). Zero waste manufacturing: a framework and review of technology, research, and implementation barriers for enabling a circular economy transition in Singapore. Resour. Conserv. Recycl. 151, 104438. doi: 10.1016/j.resconrec.2019.104438
Kerdlap, P., Low, J. S. C., Tan, D. Z. L., Yeo, Z., and Ramakrishna, S. (2020). M3-IS-LCA: a methodology for multi-level life cycle environmental performance evaluation of industrial symbiosis networks. Resour. Conserv. Recycl. 161, 104963. doi: 10.1016/j.resconrec.2020.104963
Kikuchi, Y., Kanematsu, Y., Ugo, M., Hamada, Y., and Okubo, T. (2016). Industrial symbiosis centered on a regional cogeneration power plant utilizing available local resources: a case study of Tanegashima. J. Ind. Ecol. 20, 276–288. doi: 10.1111/jiec.12347
Koppelmäki, K., Eerola, M., Albov, S., Kivelä, J., Helenius, J., Winquist, E., et al. (2016). “Palopuro agroecological symbiosis: a pilot case study on local sustainable food and farming (Finland),” in Challenges for the New Rurality in a Changing World Proceedings from the 7th International Conference on Localized Agri-Food Systems 8-10 May 2016 (Stockholm: Södertörn University).
Koppelmäki, K., Parviainen, T., Virkkunen, E., Winquist, E., Schulte, R. P., and Helenius, J. (2019). Ecological intensification by integrating biogas production into nutrient cycling: modeling the case of agroecological symbiosis. Agric. Syst. 170, 39–48. doi: 10.1016/j.agsy.2018.12.007
Lee, D., and Tongarlak, M. H. (2017). Converting retail food waste into by-product. Eur. J. Oper. Res. 257, 944–956. doi: 10.1016/j.ejor.2016.08.022
Li, X. (2018). “Industrial ecology and industrial symbiosis-definitions and development histories,” in Industrial Ecology and Industry Symbiosis for Environmental Sustainability (London: Cham). doi: 10.1007/978-3-319-67501-5_2
Lombardi, D. R., and Laybourn, P. (2012). Redefining industrial symbiosis: crossing academic–practitioner boundaries. J. Ind. Ecol. 16, 28–37. doi: 10.1111/j.1530-9290.2011.00444.x
Low, J. S. C., Tjandra, T. B., Yunus, F., Chung, S. Y., Tan, D. Z. L., Raabe, B., et al. (2018). A collaboration platform for enabling industrial symbiosis: application of the database engine for waste-to-resource matching. Proc. CIRP 69, 849–854. doi: 10.1016/j.procir.2017.11.075
Lowe, E. A. (1997). Creating by-product resource exchanges: strategies for eco-industrial parks J. Clean. Prod. 5, 57–65. doi: 10.1016/S0959-6526(97)00017-6
Maesano, G., Milani, M., Nicolosi, E., D'Amico, M., and Chinnici, G. (2022). A network analysis for environmental assessment in wine supply chain. Agronomy 12, 211. doi: 10.3390/agronomy12010211
Maina, S., Kachrimanidou, V., and Koutinas, A. (2017). From waste to bio-based products: a roadmap towards a circular and sustainable bioeconomy. Curr. Opin. Green Sustain. Chem. 8, 18–23. doi: 10.1016/j.cogsc.2017.07.007
Mak, T. M., Xiong, X., Tsang, D. C., Iris, K. M., and Poon, C. S. (2020). Sustainable food waste management towards circular bioeconomy: policy review, limitations and opportunities. Bioresour. Technol. 297, 122497. doi: 10.1016/j.biortech.2019.122497
Mangla, S. K., Börühan, G., Ersoy, P., Kazancoglu, Y., and Song, M. (2021). Impact of information hiding on circular food supply chains in business-to-business context. J. Bus. Res. 135, 1–18. doi: 10.1016/j.jbusres.2021.06.013
Mantese, G. C., and Amaral, D. C. (2018). Agent-based simulation to evaluate and categorize industrial symbiosis indicators. J. Clean. Prod. 186, 450–464. doi: 10.1016/j.jclepro.2018.03.142
Marchi, B., Zanoni, S., and Zavanella, L. E. (2017). Symbiosis between industrial systems, utilities and public service facilities for boosting energy and resource efficiency. Energy Proc. 128, 544–550. doi: 10.1016/j.egypro.2017.09.006
Martin, M., Weidner, T., and Gullstrom, C. (2022). Estimating the potential of building integration and regional synergies to improve the environmental performance of urban vertical farming. Front. Sustain. Food Syst. 6, 849304. doi: 10.3389/fsufs.2022.849304
Mirabella, N., Castellani, V., and Sala, S. (2014). Current options for the valorization of food manufacturing waste: a review J. Clean. Prod. 65, 28–41. doi: 10.1016/j.jclepro.2013.10.051
Mirata, M. (2004). Experiences from early stages of a national industrial symbiosis programme in the UK: determinants and coordination challenges. J. Clean. Prod. 12, 967–983. doi: 10.1016/j.jclepro.2004.02.031
Mirata, M., and Emtairah, T. (2005). Industrial symbiosis networks and the contribution to environmental innovation: the case of the landskrona industrial symbiosis programme. J. Clean. Prod. 13, 993–1002. doi: 10.1016/j.jclepro.2004.12.010
Mitsuhiro, M., and Yadohisa, H. (2015). Reduced k-means clustering with MCA in a low-dimensional space. Comput. Stat. 30, 463–475. doi: 10.1007/s00180-014-0544-8
Moncada, J., and Aristizábal, V. (2016). Design strategies for sustainable biorefineries. Biochem. Eng. J. 116, 122–134. doi: 10.1016/j.bej.2016.06.009
Mulrow, J. S., Derrible, S., Ashton, W. S., and Chopra, S. S. (2017). Industrial symbiosis at the facility scale. J. Ind. Ecol. 21, 559–571. doi: 10.1111/jiec.12592
Muradin, M., Joachimiak-Lechman, K., and Foltynowicz, Z. (2018). Evaluation of eco-efficiency of two alternative agricultural biogas plants. Appl. Sci. 8, 2083. doi: 10.3390/app8112083
Neves, A., Godina, R., Azevedo, S. G., and Matias, J. C. (2020). A comprehensive review of industrial symbiosis. J. Clean. Prod. 247, 119113. doi: 10.1016/j.jclepro.2019.119113
Nowak, B., Nesme, T., David, C., and Pellerin, S. (2015). Nutrient recycling in organic farming is related to diversity in farm types at the local level. Agric. Ecosyst. Environ. 204, 17–26. doi: 10.1016/j.agee.2015.02.010
Ometto, A. R., Ramos, P. A. R., and Lombardi, G. (2007). The benefits of a Brazilian agro-industrial symbiosis system and the strategies to make it happen. J. Clean. Prod. 15, 1253–1258. doi: 10.1016/j.jclepro.2006.07.021
Ong, J., Mahmood, N. Z., and Musa, S. N. (2021). Challenges to promoting eco-industry parks in Malaysia: a case study of rawang integrated industrial park. J. Mater. Cycles Waste Manag. 23, 1258–1269. doi: 10.1007/s10163-021-01199-3
Onu, P., and Mbohwa, C. (2021). Industry 4.0 opportunities in manufacturing SMEs: sustainability outlook. Mater. Today 44, 1925–1930. doi: 10.1016/j.matpr.2020.12.095
Pagotto, M., and Halog, A. (2016). Towards a circular economy in Australian agri-food industry: an application of input-output oriented approaches for analyzing resource efficiency and competitiveness potential. J. Ind. Ecol. 20, 1176–1186. doi: 10.1111/jiec.12373
Pandey, P., Valkenburg, G., Mamidipudi, A., and Bijker, W. (2020). Responsible research and innovation in the global south: agriculture, renewable energy and the pursuit of symmetry. Sci. Technol. Soc. 25, 215–222. doi: 10.1177/0971721820902961
Park, H. S., Rene, E. R., Choi, S. M., and Chiu, A. S. (2008). Strategies for sustainable development of industrial park in Ulsan, South Korea—from spontaneous evolution to systematic expansion of industrial symbiosis. J. Environ. Manage. 87, 1–13. doi: 10.1016/j.jenvman.2006.12.045
Parker, T., and Svantemark, M. (2019). Resilience by industrial symbiosis? A discussion on risk, opportunities and challenges for food production in the perspective of the food-energy-water nexus. Sustain. Earth 2, 1–16. doi: 10.1186/s42055-019-0016-7
Pérez-Salazar, M. D. R., Aguilar-Lasserre, A. A., Cedillo-Campos, M. G., Juárez-Martínez, U., and Posada-Gómez, R. (2019). Processes and measurement of knowledge management in supply chains: an integrative systematic literature review. Int. J. Prod. Res. 57, 2136–2159. doi: 10.1080/00207543.2018.1521530
Poponi, S., Arcese, G., Pacchera, F., and Martucci, O. (2022). Evaluating the transition to the circular economy in the agri-food sector: selection of indicators. Resour. Conserv. Recycl. 176, 105916. doi: 10.1016/j.resconrec.2021.105916
Prendeville, S., Cherim, E., and Bocken, N. (2018). Circular cities: mapping six cities in transition. Environ. Innov. Soc. Transit. 26, 171–194. doi: 10.1016/j.eist.2017.03.002
Prieto-Sandoval, V., Jaca, C., and Ormazabal, M. (2018). Towards a consensus on the circular economy. J. Clean. Prod. 179, 605–615. doi: 10.1016/j.jclepro.2017.12.224
Puente, M. R., Arozamena, E. R., and Evans, S. (2015). Industrial symbiosis opportunities for small and medium sized enterprises: preliminary study in the Besaya region (Cantabria, Northern Spain). J. Clean. Prod. 87, 357–374. doi: 10.1016/j.jclepro.2014.10.046
Raabe, B., Low, J. S. C., Juraschek, M., Herrmann, C., Tjandra, T. B., Ng, Y. T., et al. (2017). Collaboration platform for enabling industrial symbiosis: application of the by-product exchange network model. Proc. CIRP 61, 263–268. doi: 10.1016/j.procir.2016.11.225
Radhakrishnan, S., Erbis, S., Isaacs, J. A., and Kamarthi, S. (2017). Novel keyword co-occurrence network-based methods to foster systematic reviews of scientific literature. PLoS ONE 12, e0172778. doi: 10.1371/journal.pone.0172778
Raimbault, J., Broere, J., Somveille, M., Serna, J. M., Strombom, E., Moore, C., et al. (2020). A spatial agent based model for simulating and optimizing networked eco-industrial systems. Resour. Conserv. Recycl. 155, 104538. doi: 10.1016/j.resconrec.2019.104538
Raimondo, M., Caracciolo, F., Cembalo, L., Chinnici, G., Pecorino, B., and D'Amico, M. (2018). Making virtue out of necessity: managing the citrus waste supply chain for bioeconomy applications. Sustainability 10, 4821. doi: 10.3390/su10124821
Raimondo, M., Hamam, M., D'Amico, M., and Caracciolo, F. (2022). Plastic-free behavior of millennials: An application of the theory of planned behavior on drinking choices. Waste Manage. 138, 253–61. doi: 10.1016/j.wasman.2021.12.004
Roberts, B. H. (2004). The application of industrial ecology principles and planning guidelines for the development of eco-industrial parks: an Australian case study. J. Clean. Prod. 12, 997–1010. doi: 10.1016/j.jclepro.2004.02.037
Saavedra, Y. M., Iritani, D. R., Pavan, A. L., and Ometto, A. R. (2018). Theoretical contribution of industrial ecology to circular economy. J. Clean. Prod. 170, 1514–1522. doi: 10.1016/j.jclepro.2017.09.260
Santos, V. E. N., and Magrini, A. (2018). Biorefining and industrial symbiosis: a proposal for regional development in Brazil. J. Clean. Prod. 177, 19–33. doi: 10.1016/j.jclepro.2017.12.107
Sanyé-Mengual, E., Martinez-Blanco, J., Finkbeiner, M., Cerd,à, M., Camargo, M., Ometto, A. R., et al. (2018). Urban horticulture in retail parks: Environmental assessment of the potential implementation of rooftop greenhouses in European and South American cities. J. Clean. Prod 172, 3081–3091. doi: 10.1016/j.jclepro.2017.11.103
Sehnem, S., Vazquez-Brust, D., Pereira, S. C. F., and Campos, L. M. (2019). Circular economy: benefits, impacts and overlapping. Supply Chain Manag. 24, 784–804. doi: 10.1108/SCM-06-2018-0213
Senauer, B., and Venturini, L. (2005). The globalization of food systems: a conceptual framework and empirical patterns. J. Food Agric. Environ. 389, 197. doi: 10.22004/ag.econ.14304
Sheppard, P., Garcia-Garcia, G., Angelis-Dimakis, A., Campbell, G. M., and Rahimifard, S. (2019). Synergies in the co-location of food manufacturing and biorefining. Food Bioprod. Process. 117, 340–359. doi: 10.1016/j.fbp.2019.08.001
Shi, H., Chertow, M., and Song, Y. (2010). Developing country experience with eco-industrial parks: a case study of the Tianjin economic-technological development area in China. J. Clean. Prod. 18, 191–199. doi: 10.1016/j.jclepro.2009.10.002
Simboli, A., Taddeo, R., and Morgante, A. (2015). The potential of industrial ecology in agri-food clusters (AFCs): a case study based on valorisation of auxiliary materials. Ecol. Econ. 111, 65–75. doi: 10.1016/j.ecolecon.2015.01.005
Singh, S. K. (2019). Territoriality, task performance, and workplace deviance: empirical evidence on role of knowledge hiding. J. Bus. Res. 97, 10–19. doi: 10.1016/j.jbusres.2018.12.034
Slorach, P. C., Jeswani, H. K., Cuéllar-Franca, R., and Azapagic, A. (2019). Environmental sustainability of anaerobic digestion of household food waste. J. Environ. Manage. 236, 798–814. doi: 10.1016/j.jenvman.2019.02.001
Spina, D., Vindigni, G., Pecorino, B., Pappalardo, G., D'Amico, M., and Chinnici, G. (2021). Identifying themes and patterns on management of horticultural innovations with an automated text analysis. Agronomy 11, 1103. doi: 10.3390/agronomy11061103
Stillitano, T., Falcone, G., Iofrida, N., Spada, E., Gulisano, G., and De Luca, A. I. (2022). A customized multi-cycle model for measuring the sustainability of circular pathways in agri-food supply chains. Sci. Total Environ. 844, 157229. doi: 10.1016/j.scitotenv.2022.157229
Strazza, C., Magrassi, F., Gallo, M., and Del Borghi, A. (2015). Life cycle assessment from food to food: a case study of circular economy from cruise ships to aquaculture. Sustain. Prod. Consum. 2, 40–51. doi: 10.1016/j.spc.2015.06.004
StunŽenas, E., and Kliopova, I. (2021). Industrial ecology for optimal food waste management in a region. Environ. Res. Eng. 77, 7–24. doi: 10.5755/j01.erem.77.1.27605
Teigiserova, D. A., Hamelin, L., and Thomsen, M. (2019). Review of high-value food waste and food residues biorefineries with focus on unavoidable wastes from processing. Resour. Conserv. Recycl. 149, 413–426. doi: 10.1016/j.resconrec.2019.05.003
Teigiserova, D. A., Hamelin, L., and Thomsen, M. (2020). Towards transparent valorization of food surplus, waste and loss: clarifying definitions, food waste hierarchy, and role in the circular economy. Sci. Total Environ. 706, 136033. doi: 10.1016/j.scitotenv.2019.136033
Timpanaro, G., Di Vita, G., Foti, V. T., and Branca, F. (2012). Landraces in Sicilian peri-urban horticulture: a participatory approach to Brassica production system. Acta Horticult. 1005, 213–220. doi: 10.17660/ActaHortic.2013.1005.22
Toop, T. A., Ward, S., Oldfield, T., Hull, M., Kirby, M. E., and Theodorou, M. K. (2017). AgroCycle–developing a circular economy in agriculture. Energy Proc. 123, 76–80. doi: 10.1016/j.egypro.2017.07.269
Trokanas, N., Cecelja, F., and Raafat, T. (2014). Semantic input/output matching for waste processing in industrial symbiosis. Comput. Chem. Eng. 66, 259–268. doi: 10.1016/j.compchemeng.2014.02.010
Tsakalova, M., Lin, T. C., Yang, A., and Kokossis, A. C. (2015). A decision support environment for the high-throughput model-based screening and integration of biomass processing paths. Ind. Crops Prod. 75, 103–113. doi: 10.1016/j.indcrop.2015.05.035
Unay-Gailhard, I., and Bojnec, Š. (2016). Sustainable participation behaviour in agri-environmental measures. J. Clean. Prod. 138, 47–58. doi: 10.1016/j.jclepro.2015.09.003
Unay-Gailhard, I., and Bojnec, Š. (2019). The impact of green economy measures on rural employment: green jobs in farms. J. Clean. Prod. 208, 541–551. doi: 10.1016/j.jclepro.2018.10.160
Van Beers, D., Bossilkov, A., Corder, G., and Van Berkel, R. (2007). Industrial symbiosis in the Australian minerals industry: the cases of Kwinana and Gladstone. J. Ind. Ecol. 11, 55–72. doi: 10.1162/jiec.2007.1161
Van Capelleveen, G., Amrit, C., and Yazan, D. M. (2018). “A literature survey of information systems facilitating the identification of industrial symbiosis,” in From Science to Society, eds B. Otjacques, P. Hitzelberger, S. Naumann, and V. Wohlgemuth (Cham: Springer), 155–169. doi: 10.1007/978-3-319-65687-8_14
Vindigni, G., Mosca, A., Bartoloni, T., and Spina, D. (2021). Shedding light on peri-urban ecosystem services using automated content analysis. Sustainability 13, 9182. doi: 10.3390/su13169182
Walmsley, T. G., Ong, B. H., Klemeš, J. J., Tan, R. R., and Varbanov, P. S. (2019). Circular integration of processes, industries, and economies. Renew. Sustain. Energy Rev. 107, 507–515. doi: 10.1016/j.rser.2019.03.039
Willett, W., Rockström, J., Loken, B., Springmann, M., Lang, T., Vermeulen, S., et al. (2019). Food in the anthropocene: the EAT–lancet commission on healthy diets from sustainable food systems. Lancet 393, 447–492. doi: 10.1016/S0140-6736(18)31788-4
Winans, K., Kendall, A., and Deng, H. (2017). The history and current applications of the circular economy concept. Renew. Sustain. Energy Rev. 68, 825–833. doi: 10.1016/j.rser.2016.09.123
Wolf, A., Eklund, M., and Söderström, M. (2007). Developing integration in a local industrial ecosystem–an explorative approach. Bus. Strat. Environ. 16, 442–455. doi: 10.1002/bse.485
Wright, R. A., Côté, R. P., Duffy, J., and Brazner, J. (2009). Diversity and connectance in an industrial context: the case of Burnside Industrial Park. J. Ind. Ecol. 13, 551–564. doi: 10.1111/j.1530-9290.2009.00141.x
Yenipazarli, A. (2019). Incentives for environmental research and development: consumer preferences, competitive pressure and emissions taxation. Eur. J. Oper. Res. 276, 757–769. doi: 10.1016/j.ejor.2019.01.037
Yeo, Z., Masi, D., Low, J. S. C., Ng, Y. T., Tan, P. S., and Barnes, S. (2019). Tools for promoting industrial symbiosis: a systematic review. J. Ind. Ecol. 23, 1087–1108. doi: 10.1111/jiec.12846
Yu, C., Davis, C., and Dijkema, G. P. (2014). Understanding the evolution of industrial symbiosis research: a bibliometric and network analysis (1997–2012). J. Ind. Ecol. 18, 280–293. doi: 10.1111/jiec.12073
Yu, F., Han, F., and Cui, Z. (2015). Evolution of industrial symbiosis in an eco-industrial park in China. J. Clean. Prod. 87, 339–347. doi: 10.1016/j.jclepro.2014.10.058
Yu, H., Román, E., and Solvang, W. D. (2017). “A value chain analysis for bioenergy production from biomass and biodegradable waste: a case study in northern Norway,” in Energy Systems and Environment, ed I. P. Tsvetkov (London: IntechOpen), 183–206. doi: 10.5772/intechopen.72346
Zabaniotou, A., and Kamaterou, P. (2019). Food waste valorization advocating circular bioeconomy-A critical review of potentialities and perspectives of spent coffee grounds biorefinery. J. Clean. Prod. 211, 1553–1566. doi: 10.1016/j.jclepro.2018.11.230
Zabaniotou, A., Rovas, D., Libutti, A., and Monteleone, M. (2015). Boosting circular economy and closing the loop in agriculture: case study of a small-scale pyrolysis–biochar based system integrated in an olive farm in symbiosis with an olive mill. Environ. Dev. Sustain. 14, 22–36. doi: 10.1016/j.envdev.2014.12.002
Zhang, S., Wang, H., Bi, X., and Clift, R. (2021). Synthesis and assessment of a biogas-centred agricultural eco-industrial park in British Columbia. J. Clean. Prod. 321, 128767. doi: 10.1016/j.jclepro.2021.128767
Keywords: industrial symbiosis, industrial ecology, circular economy, agri-food, content analysis, MCA
Citation: Hamam M, Spina D, Raimondo M, Di Vita G, Zanchini R, Chinnici G, Tóth J and D'Amico M (2023) Industrial symbiosis and agri-food system: Themes, links, and relationships. Front. Sustain. Food Syst. 6:1012436. doi: 10.3389/fsufs.2022.1012436
Received: 05 August 2022; Accepted: 10 October 2022;
Published: 12 January 2023.
Edited by:
Maurizio Cellura, University of Palermo, ItalyReviewed by:
Štefan Bojnec, University of Primorska, SloveniaCopyright © 2023 Hamam, Spina, Raimondo, Di Vita, Zanchini, Chinnici, Tóth and D'Amico. This is an open-access article distributed under the terms of the Creative Commons Attribution License (CC BY). The use, distribution or reproduction in other forums is permitted, provided the original author(s) and the copyright owner(s) are credited and that the original publication in this journal is cited, in accordance with accepted academic practice. No use, distribution or reproduction is permitted which does not comply with these terms.
*Correspondence: Daniela Spina, danispina@gmail.com
Disclaimer: All claims expressed in this article are solely those of the authors and do not necessarily represent those of their affiliated organizations, or those of the publisher, the editors and the reviewers. Any product that may be evaluated in this article or claim that may be made by its manufacturer is not guaranteed or endorsed by the publisher.
Research integrity at Frontiers
Learn more about the work of our research integrity team to safeguard the quality of each article we publish.