- 1Centre for Marine Science and Technology, Curtin University, Bentley, WA, Australia
- 2Port Stephens Fisheries Institute, NSW Department of Primary Industries, Queanbeyan, NSW, Australia
- 3Northeast Fisheries Science Center, Woods Hole, MA, United States
- 4Australian Institute for Marine Science, Perth, WA, Australia
- 5Parks Australia, Canberra, ACT, Australia
Biological sources are significant contributors to aquatic soundscapes. Soniferous fish can dominate the soundscape in certain locations, at specific times and frequencies, particularly during the production of choruses. Passive acoustic monitoring of fish choruses can provide important ecological information about soniferous fish populations. This study presents the Australian Fish Chorus Catalogue, an inventory of fish choruses detected from 83 locations in Australian estuarine and marine waters. The Australian Fish Chorus Catalogue contains data on fish chorus occurrence and the spectral and temporal measurements, spectrographic images, and audio examples of 301 fish choruses. This catalogue has been developed to establish the foundations of an ongoing effort to document, quantify, compare, and track Australian fish choruses. We hope this open-access data depository will be used as a reference for future research and will facilitate an increase in understanding of fish choruses, which can then be applied to the monitoring and management of fish populations and their respective ecosystems.
1 Introduction
Fishes are prolific sound producers. Over 1,000 fish species have been documented to produce sound passively or actively (Slabbekoorn et al., 2010; Looby et al., 2022). Active fish sounds are typically produced through the oscillation of the swim bladder by specialised sonic muscles or through the stridulation of bony body parts such as fin rays or pharyngeal teeth (Kaatz, 2002; Kasumyan, 2008; Fine and Parmentier, 2015). Fishes produce sound in support of key life functions, including: feeding, courtship, breeding, aggregation, and territorial aggression (Moulton, 1958; Fish and Mowbray, 1970; Ladich, 2015). Many species produce sound en masse during these behavioural functions (Mok and Gilmore, 1983; Connaughton and Taylor, 1995; McCauley, 2012; Borie et al., 2019), which is referred to as a fish chorus.
A standardised definition of a fish chorus has yet to be established. The definition of a biological chorus outlined by Cato (1978) (“when noise from many individuals is continuously above background for an extended period using an equipment averaging time of 1 s”) is widely applied in fish chorus literature and, therefore, will be utilised for this study. However, it is important to note that there are variations across the literature regarding the exact parameters (and their quantities) that constitute a fish chorus. This variation is mainly in regards to the number of individuals producing the sound, the duration of the chorus, and the increase in sound levels within the fish chorus characteristic frequency band. Fish choruses can be species-specific (Mok and Gilmore, 1983; Luczkovich et al., 1999; Parsons et al., 2009) and are typically categorised into types based on spectral and temporal characteristics.
A variety of spectral parameters have previously been used to delineate fish chorus types. These include: the characteristic frequency band, peak frequency, and centre frequency of the chorus, as well as the call duration, pulse repetition frequency, and pulse number of the calls of which the chorus was comprised (Parsons et al., 2016; Parsons et al., 2017; McWilliam et al., 2018; Di Iorio et al., 2018). The spectral parameters of a fish chorus can be species-specific (Sprague et al., 1998; Luczkovich et al., 1999; Parsons et al., 2013c) as they can be related, in large part, to the sound-producing mechanisms of the individual fish (Brawn, 1961; Fine et al., 1997; Kaatz, 2002; Lagardère and Mariani, 2006; Parsons et al., 2013a; McCauley and Cato, 2016). For example, the swim bladder and its associated musculature have long been associated with sound production across many fish species (Brawn, 1961; Fine et al., 2001; Fine, 2012; Fine and Parmentier, 2015; Mok et al., 2020). Fish chorus types are also characterised by patterns in the chorusing activity.
Diel, lunar, seasonal, and annual patterns in fish chorus presence and activity have been used to define the temporal characteristics of fish choruses (Wall et al., 2013; Mahanty et al., 2016; Siddagangaiah et al., 2021a). Fish choruses commonly demonstrate characteristic and, in some circumstances, species-specific patterns in the presence or activity across these temporal extents (McCauley, 2012; Parsons et al., 2016; McWilliam et al., 2017; Siddagangaiah et al., 2021a). The nature of these temporal patterns often provides insights into the biological function of the respective chorus. For example, it has been hypothesised that choruses that are present every evening, year-round may be associated with feeding behaviours (McCauley, 2012; McCauley, 2001; McCauley and Cato, 2016; Panicker and Stafford, 2023); however, this has yet to be ground-truthed in the field. Fish choruses that demonstrate seasonality in their presence are more likely to be associated with courtship or breeding behaviours (McCauley, 2001; Van Hoeck et al., 2023; Montie et al., 2015; Connaughton and Taylor, 1995). The temporal distribution of these choruses and the biological functions they are associated with are typically driven by environmental rhythms.
Fish chorus activity has previously been associated with particular environmental cues. Siddagangaiah et al. (2021a), McWilliam et al. (2017), Parsons et al. (2016), McCauley (2012), Locascio and Mann (2011), and Parsons (2009) (just to name a few) have reported significant relationships between fish chorus activity and temperature, lunar phase, and tidal regime. Parsons (2009) identified salinity as one of the drivers of deviations observed in the mean sound pressure levels of a mulloway (Argyrosomus japonicus) chorus recorded in the Swan River, Western Australia, and Mann and Grothues (2009) reported a correlation between advection events in the mid-Atlantic Ocean and a significant reduction in fish chorusing. Luczkovich et al. (2024) determined that chorus activity was impacted by hypoxia events, and McWilliam et al. (2017) highlighted a significant negative relationship between wind speed and calling intensity of fish choruses recorded off Lizard Island, Queensland. Variations in fish chorus activity have also been associated with anthropogenic noise (Ceraulo et al., 2021; Siddagangaiah et al., 2021b) and significant natural events such as cyclones (Mahanty et al., 2019; Locascio and Mann, 2005). Identification of the patterns of fish chorus behaviour facilitates the tracking of the response of the chorus source species to these different types of environmental stimuli. Tracking these patterns over time will enable researchers and managers to understand how natural and anthropogenic changes may currently affect these fish populations and predict future impacts. For this to be achieved, the distribution, diversity, drivers, and characteristics of fish choruses need to be better understood.
Over 50 suspected fish chorus types have been identified in Australian waters since 1978 (Cato, 1978; Kelly et al., 1985; McCauley, 2001; McCauley and Cato, 2000; McCauley and Cato, 2016; Parsons et al., 2013b; Parsons et al., 2016; Parsons et al., 2017; McWilliam et al., 2018; Ward et al., 2019; Parsons, 2009; Hawkins et al., 2023). These fish choruses were recorded in aquatic environments ranging from riverine, estuarine, and coastal to coral reefs; islands; the continental shelf; deep offshore waters; and submarine canyons. Some Australian recording sites have demonstrated a high diversity of fish chorus types (Parsons et al., 2017; Parsons et al., 2016; Hawkins et al., 2023), varying across times of the day or during different seasons (Parsons et al., 2017; Parsons et al., 2016; McWilliam et al., 2017; Parsons et al., 2013b). Given the variety, abundance, and dominance of fish choruses in Australian soundscapes, documenting those of known and unknown origins provides valuable information for monitoring and management purposes (Di Iorio et al., 2021; Parsons et al., 2022). Documenting fish choruses can assist in delineating fish distribution (Pagniello et al., 2019; Rountree and Juanes, 2017) and habitat use (Walters et al., 2009; Ricci et al., 2017; Luczkovich et al., 2008), monitoring spawning aggregations (Monczak et al., 2017; Picciulin et al., 2020; Ricci et al., 2017), indicating biodiversity (Hawkins et al., 2023), and in certain circumstances, quantifying the abundance of fish populations (Parsons, 2009; Rowell et al., 2017; Sprague and Luczkovich, 2011). Baselines of fish chorus distribution and spatiotemporal patterns can be utilised for comparisons with future records to assess the impacts of natural and/or anthropogenic change. Future studies may also use fish chorus parameters and descriptions as part of a weight-of-evidence approach to identify chorus source species (McCauley and Cato, 2016). Large-scale mapping of fish choruses is also essential to enhance the scientific understanding of how acoustic communities interact and how they are related. To achieve this, fish choruses need to be identified and baselines of long-term distribution and chorusing patterns documented across significant spatial and temporal extents.
Historically, passive acoustic monitoring (PAM) data have been collected across limited spatial and temporal extents (Ross et al., 2023). However, there is now a movement to prioritise mapping, analysis, and comparison of soundscapes and particular soniferous species at a regional to global scale (Looby et al., 2022; Parsons et al., 2022; Looby et al., 2023a; Darras et al., 2024). The viability of collecting large acoustic datasets has traditionally been restrained by storage and data processing requirements, in addition to the time and effort required to manually analyse all of the data (Napier et al., 2024). Recent technological advances are starting to tackle these challenges, making PAM more accessible (Gibb et al., 2019). Cloud storage is evolving, facilitating the storage of larger volumes of data and allowing for much faster upload and download speeds (Napier et al., 2024). Additionally, machine learning and signal processing techniques are evolving rapidly, significantly shortening data analysis times, which is allowing large amounts of raw data to be analysed (Napier et al., 2024). Raw acoustic data are increasingly being shared with greater accessibility. For instance, federally funded acoustic data in the U.S. have been made available online through NOAA’s National Centres for Environmental Information (NCEI) (Wall et al., 2021), and long-term PAM data collected at several locations in Australian waters have been made available on the Australian Ocean Data Network (AODN), collected from a series of passive acoustic observatories as part of Australia’s Integrated Marine Observing System (IMOS, 2023). These are just a few examples; for a more comprehensive list of open-access underwater acoustic datasets, please refer to The UK Acoustics Network (2024). Despite this increase in data availability, PAM research is still hindered by the lack of standardisation and transparency in acoustic data collection, processing, and analysis protocols, as well as the limited availability of open-access reference sound libraries (Gibb et al., 2019).
Reference libraries are an essential tool for the application of PAM in ecological research. An audio reference library is defined as “a collection of (annotated) audio recordings with known species identities, used as type-specimen references for identifying species in new recordings” (Ross et al., 2023). These annotated collections provide essential baselines for the comparison of known and unknown sounds (Browning et al., 2017; Parsons et al., 2022). Several open-access reference libraries feature recordings of fish calls (Fish and Mowbray, 1970; Vigness-Raposa et al., 2012; Kaschner, 2012; Scholes, 2015; Looby et al., 2023b, Ocean Networks Canada, 2024; Sonothèque, 2024, The British Library, 2024; Tierstimmenarchiv, 2024; FonoZoo, 2024); however, to the authors’ knowledge, limited effort has been made to create a library of fish choruses beyond a local-scale extent. In this data paper, we present the Australian Fish Chorus Catalogue (2005–2023) (AFCC, Hawkins et al. (2024)). This catalogue is the first benchmark reference library of 301 described fish choruses, produced by predominantly unknown fish species and recorded at 83 locations in marine and estuarine waters around Australia. Each fish chorus entry includes audio and spectrographic records, seasonal presence data, and measurements of chorus spectral parameters extracted using reproducible methodologies. The catalogue is an open-access data repository available on the AODN. Development of the AFCC is ongoing, and future work will focus on expanding the coverage of Australian soundscapes, undertaking classification of fish chorus types, and identifying source species.
2 Methods
The AFCC has been developed to document previously unreported Australian fish choruses and collate them alongside previously reported Australian fish choruses in a format that allows for comparisons with existing and future fish chorus recordings. The catalogue was created in four stages, and these are conceptualised in Figure 1.
2.1 Stage 1: data collection
The acoustic recordings analysed in this study were collected from 169 underwater acoustic recorder deployments undertaken at 83 locations in Australian marine and estuarine waters from 2005 to 2023 (Supplementary Table S1; Figure 2). Data collection was undertaken by the Centre for Marine Science and Technology (CMST), the Australian Institute of Marine Science (AIMS), the New South Wales Department of Primary Industries (NSW DPI), Parks Australia, and the National Oceanic and Atmospheric Administration (NOAA). Recording lengths ranged from 8 days to over a year in duration, sampling frequencies ranged from 3 to 96 kHz, and sampling schedules varied between locations (Supplementary Table S1). Twenty deployments were undertaken as part of the Australian Integrated Marine Observing System (IMOS (2022)). The IMOS is a nationally coordinated program that consists of a network of oceanographic observing equipment (historically including passive acoustic observatories at selected sites) deployed throughout Australia’s coastal areas and open oceans (IMOS, 2022; Lynch et al., 2010). Recordings from the IMOS deployments are freely accessible under a Creative Commons Attribution 4.0 International License (IMOS, 2023). Seven types of acoustic recorders were used to collect the acoustic recordings. The details of these recorder types are outlined in Table 1. These include the Centre for Marine Science and Technology and Defence Science and Technology Organisation underwater sound recorders (CMST-DSTO USRs, McCauley et al. (2017)) and Ocean Instruments SoundTraps. CMST-DSTO USRs were most commonly deployed recorder type (Supplementary Table S1); these included all the IMOS deployments. The remaining acoustic recordings were acquired using SoundTrap ST500, ST300, or ST202s. Data pre-processing and analysis were tailored to the respective recorder type.
2.2 Stage 2: data pre-processing
The acoustic recordings were pre-processed using a MATLAB (The MathWorks Inc., Natick, MA, United States) toolbox, the CHaracterisation Of Recorded Underwater Sound (CHORUS) (Gavrilov and Parsons, 2014). CHORUS and its respective User Guide are available free for download at http://cmst.curtin.edu.au/products/chorussoftware/ under a single-user license agreement (Gavrilov and Parsons, 2014). This toolbox was designed and built at CMST. Recorder-specific settings were used to pre-process the data. The timing data of each recording were extracted, the calibrated power spectral density (PSD) of each recording file was calculated, and the PSDs were corrected for the frequency response of the respective hydrophone and system. The CHORUS toolbox pre-processing functions that were used in this process included the following: 1) CMST_LF_data_preprocessing, 2) CMST_HF_data_preprocessing, and 3) ST_data_preprocessing. The CHORUS_GUI function was then used to manually identify fish choruses.
2.3 Stage 3: data analysis
2.3.1 Manual fish chorus identification
For each dataset, fish choruses were identified via manual scrutiny of 1) long-term spectral averages (LTSA, 7 days in length at a time), 2) figures of high-resolution relative acoustic amplitude (referred to as oscillograms), and 3) high-resolution spectrograms created using the CHORUS_GUI function (Figure 3). LTSAs were visualised in CHORUS with a constant time window of 1,000 s. Spectrograms were plotted on a logarithmic scale ranging from 5 to 48,000 Hz, with a colour scale from 40 to 120 dB re 1 μPa2/Hz. Fish calling events identified in the CHORUS_GUI were classified as a fish chorus if they met all of the following criteria:
1. It consisted of overlapping fish calls from many individuals, to the extent that, at times, individual calls within the chorus could not be distinguished.
2. It increased background sound levels within a characteristic frequency band (when using an averaging time of 1 s) to an observable degree in a 7-day LTSA (Hamming window = sampling frequency of respective recording and no overlap).
3. It displayed a prolonged duration (approximately 1 hour or longer).
4. It displayed temporal variation on a diel, lunar, seasonal, or annual scale.
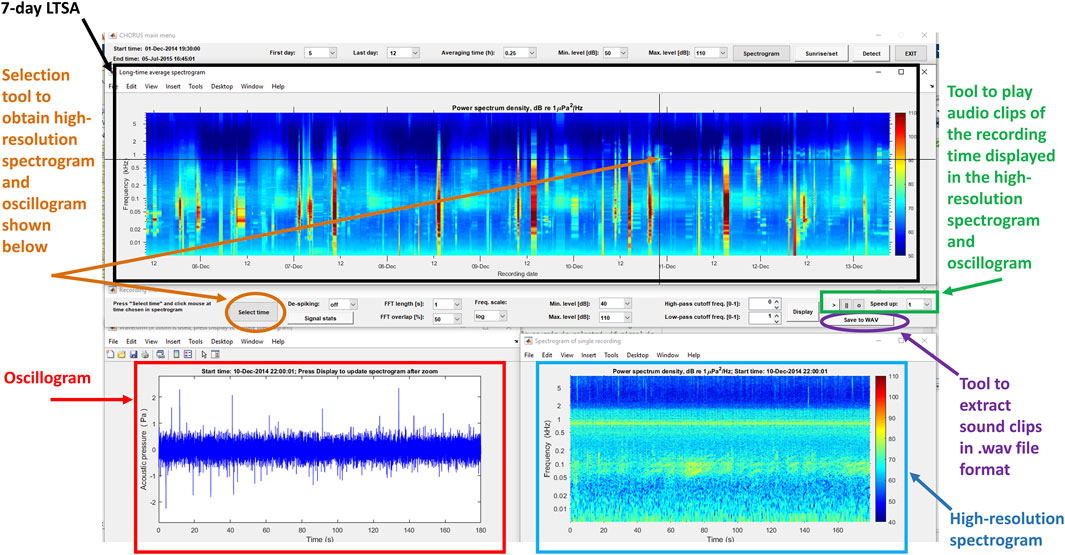
Figure 3. Annotated screenshot of the CHORUS_GUI function interface. It displays the tools available in the interface that were used to identify and extract the fish chorus spectrographic and audio records from the AFCC datasets.
The criteria used for this study were based on a review of fish chorus definitions in the literature; examples of some of these definitions are outlined in Table 2. Fish choruses were distinguished from other biological choruses using characteristic features of fish calls. Fish calls are typically low frequency (<5 kHz), contain repetitive sound elements of short duration, and can often demonstrate multiple frequency harmonics (Amorim et al., 2006; Fish and Mowbray, 1970).
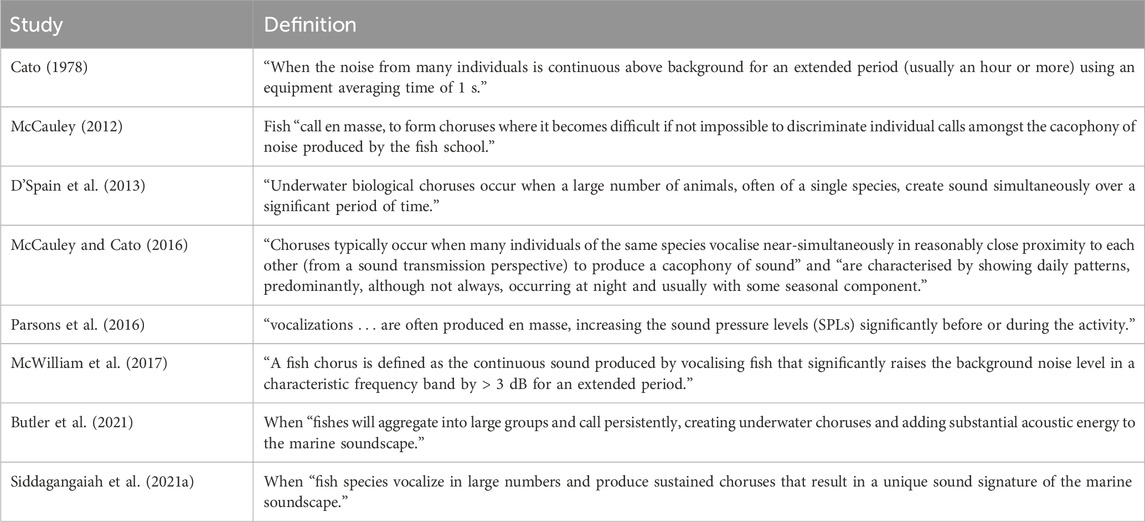
Table 2. Examples of common fish chorus definitions and descriptions reported in scientific literature.
All datasets were reviewed twice. The first round of fish chorus identifications was undertaken by five operators (Authors, L.H., M.J.P., J.M., D.W K., and R.W). M.J.P analysed the Port Hedland and Darwin Harbour datasets; J.M. analysed the Lizard Island sets (except for Lizard Island North Point 2); D.W K analysed the Bonaparte Gulf 2, North West Shelf 1, North West Shelf 3, North West 1, Timor Sea, and Thevenard 1 datasets; R.W analysed the Fowlers Bay 1 and 2 datasets; and L.H analysed all other datasets. The manual chorus identification process outlined above was followed by all operators. To address observer bias and ensure all fish choruses had been identified, all datasets were reviewed a second time, and the above process was repeated for all 169 datasets by L.H.
2.3.2 Fish chorus naming convention
Each fish chorus was assigned a unique identifier. The identifier was associated with the recording location of the specific fish chorus and followed the naming convention “SiteLabel_FishChorusNumber.” For example, the first fish chorus identified at the Bremer Bay recording site was named “BB_1.” Australian fish choruses described in eight previous studies were also included in the catalogue. These choruses and their corresponding catalogue identifiers are outlined in Table 3. The catalogue also provides descriptions of the fish choruses identified by Hawkins et al. (2023). The recording site, label, and number of each fish chorus reported by Hawkins et al. (2023) correspond to the unique identifier of the respective chorus in the AFCC. Please note that only previous studies where raw data were available could be included; thus, several previously recorded choruses are not included here. These include (but are not limited to) fish choruses reported by Cato (1978), Cato (1980), Kelly et al. (1985), McCauley and Cato (2000), and McCauley (2001).
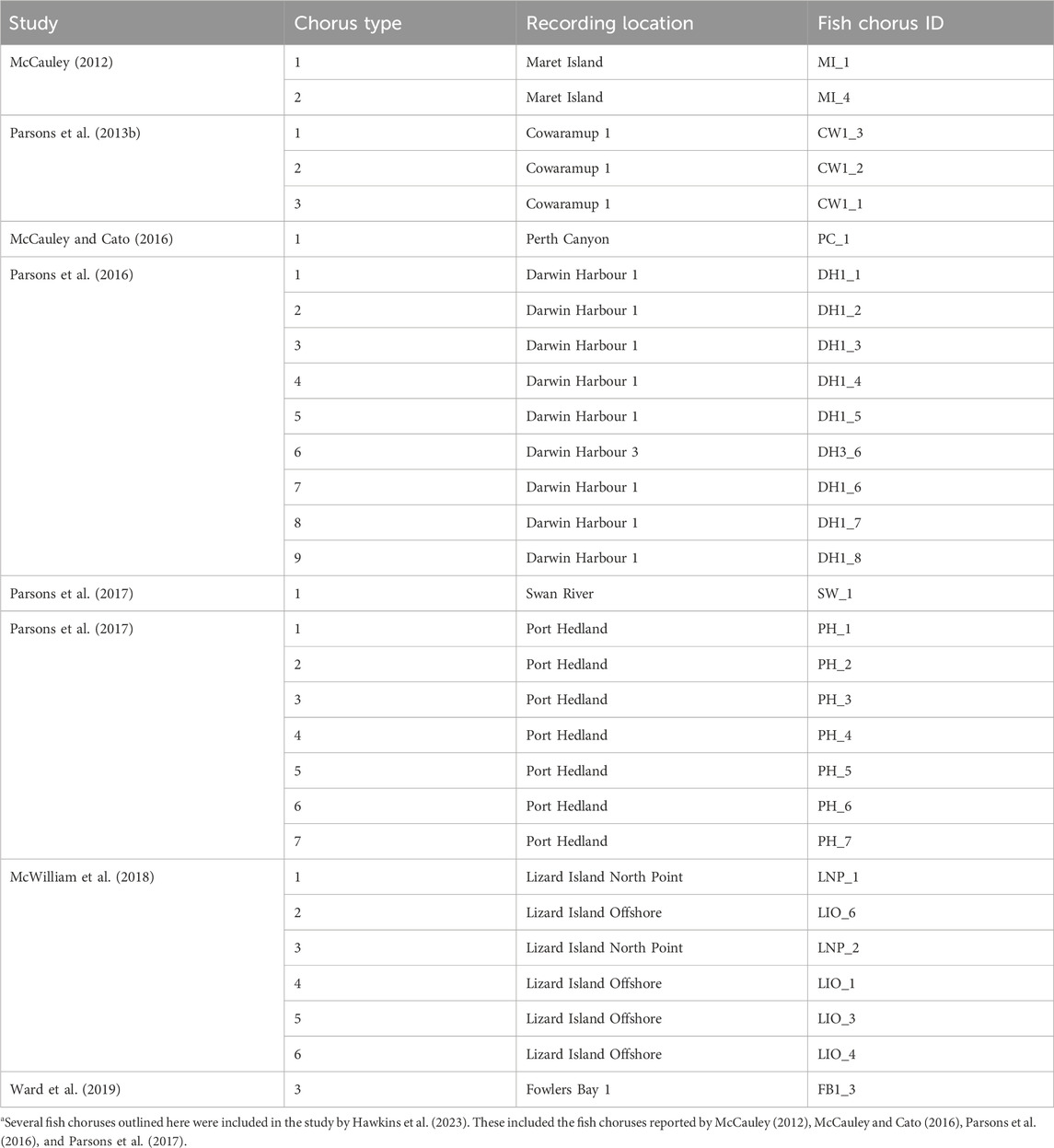
Table 3. Australian fish choruses described in previous studies in relation to the fish choruses reported in the AFCC. Chorus type refers to the type number assigned to the chorus in its respective publicationa.
2.3.3 Recording of presence/absence
Daily fish chorus presence/absence was recorded in Microsoft Excel spreadsheets after the manual inspection of LTSAs of each dataset (one 7-day spectrogram at a time). Each analysed day in every recording was designated one of four classifications: 0 = fish chorus absent, 1 = fish chorus present, 2 = unable to discern presence due to masking from ambient noise, and 3 = unable to discern presence due to lack of available recordings. These daily categorisations provided a record of the seasonal presence/absence for each fish chorus, at every location, and were subsequently collated to provide a monthly presence/absence measure. If the respective fish chorus was detected on any day of a particular month, it was marked as present (1), and if the fish chorus was not detected across any days of the respective month, it was marked as absent (0). Fish chorus monthly presence/absence has been made available as part of the catalogue.
2.3.4 Extraction of spectrographic and audio records
Each fish chorus entry in the AFCC includes spectrographic and audio records. The spectrographic records for each chorus include the following: 1) a soundscape spectrogram, 2) a high-resolution chorus spectrogram, 3) a composition of a spectrogram and oscillogram of the individual call/s making up the specific chorus, and 4) a mean power spectrum plot. The spectrograms and oscillograms were extracted manually from LTSAs created in the CHORUS_GUI (Figure 3) during the fish chorus identification process. The mean power spectrum plots were created using a custom-written MATLAB function to illustrate the frequency content of the respective fish chorus. Two audio records for each fish chorus were included in the catalogue. The first was an audio recording featuring the chorus, and the second was a shorter recording, featuring the individual call/s of the respective fish chorus. Both audio record types were also extracted manually using the CHORUS_GUI function (Figure 3) and saved as .wav files.
2.4 Stage 4: spectral measurements
2.4.1 Spectral characteristic extraction
A series of parameters were measured for each fish chorus to describe the spectral characteristics of the chorus. These parameters included the following: minimum frequency, maximum frequency, peak frequency, 3 dB bandwidth, 3 dB bandwidth low frequency, 3 dB bandwidth high frequency, 10 dB bandwidth, 10 dB bandwidth low frequency, 10 dB bandwidth high frequency, 20 dB bandwidth, 20 dB bandwidth low frequency, 20 dB bandwidth high frequency, centre frequency, root-mean-square (RMS) bandwidth, RMS bandwidth low frequency, RMS bandwidth high frequency, 90% energy bandwidth, 90% energy signal duration, peak chorus band level, ambient level, and chorus band level increase over ambient level (SNR). Descriptions and definitions of each spectral parameter are outlined in Supplementary Table S2. The start time, end time, minimum frequency, and maximum frequency of each fish chorus were manually selected from spectrograms in a conservative manner (i.e., by over-predicting the boundary box and thus over-predicting duration and bandwidth) by operator L.H. The more robust and exact measurements (such as 90% energy duration and 90% energy bandwidth) were automatically computed within the boundary boxes using custom software in MATLAB (this software can be accessed in the AFCC data repository, titled “AFCC_Mcode.m” (Hawkins et al., 2024)). Centre frequency was calculated using the equation provided by Au (1993) (page 217, Equation 10.3). RMS bandwidth low and high frequencies, 90% energy duration, and 90% energy bandwidth were calculated using their respective equations taken from Erbe et al. (2022). It is important to note that if the fish chorus signal-to-noise ratio (SNR) was low (i.e., below 3, 10, or 20 dB), the spectral variables 3 dB bandwidth, 3 dB bandwidth low frequency, 3 dB bandwidth high frequency, 10 dB bandwidth, 10 dB bandwidth low frequency, 10 dB bandwidth high frequency, 20 dB bandwidth, 20 dB bandwidth low frequency, and 20 dB bandwidth high frequency could not be measured; this occurrence is noted in the spectral measurement spreadsheet as NA.
Measurements of each parameter were taken over the course of one chorusing event per fish chorus (generally within a 24-h period) and, therefore, are not representative of the fish chorus over the entire recording period of the specific recorder deployment. The extent of these calling events is defined in the spectral measurement spreadsheet (see Section 3.3). The chorusing events used for these measurements typically occurred during a period of high chorusing activity with minimal masking from ambient noise sources. On occasion, full spectral analysis of fish choruses was impossible due to masking from ambient noise or if the fish chorus exceeded the Nyquist frequency of the sampling regime. In the case of the former, fish choruses were partially analysed during periods of minimal or no masking. Where ambient noise masked the chorus to an extent that spectral characteristics could not be accurately determined at any point in the recording or when a fish chorus exceeded the frequency cut-off, the maximum and minimum frequency of the chorus were estimated from the scrutiny of the LTSAs, and all other spectral characteristics were omitted as the entire chorus energy could not be sampled.
2.5 Technical validation
The analyses undertaken in this study followed previously reported protocols that have been adapted in this study where appropriate. The technical quality of the catalogue data is assured by the use of these protocols, which have been validated through the peer-review process. Such protocols include the pre-processing techniques outlined by McCauley and Cato (2016) and McCauley et al. (2017) for data recorded by the CMST-DSTO USRs, McWilliam et al. (2018) and Marley et al. (2017) for data recorded by SoundTraps (ST500 and ST300 STD), and Gavrilov and Parsons (2014) for the use of the CHORUS toolbox in the data pre-processing and fish chorus identification stages of the analysis. The fish chorus identification process followed protocols established by Parsons et al. (2016), Parsons et al. (2017), and McWilliam et al. (2018). During the identification process, each acoustic dataset was reviewed twice to ensure all fish choruses present in the soundscape were identified. The spectral measurements of each fish chorus were validated manually. Validation was undertaken through the scrutiny of a series of spectrograms, and PSD and mean spectrum figures were created during the spectral measurement process.
3 Data records
The AFCC has been deposited at the AODN repository (https://doi.org/10.26198/qfj2-jj93, Hawkins et al. (2024)). This is a full copy of all data at the date of submission of this publication. The catalogue holds an inventory of fish chorus records and online spreadsheets, including a file for each fish chorus, which contains the respective spectrographic records and audio files. Three online spreadsheets have been made available; these provide deployment information and the seasonal presence, duration, and spectral measurements of each fish chorus.
3.1 Spectrographic records
Each fish chorus file presented in the catalogue includes four spectrographic records. These records provide a range of graphical representations of each fish chorus. The first spectrographic record is a 7-day LTSA, and it displays the PSD (dB re 1 μPa2/Hz) of the fish chorus across time in days (x-axis) and frequency in kHz (y-axis). These records are created using a Hamming fast-Fourier transform (FFT) window equal to the sampling frequency of the respective recording (so that the frequency resolution equaled 1 Hz) with no overlap (Gavrilov and Parsons, 2014). The recording-specific sampling frequency can be found in Supplementary Table S1. This record demonstrates the diel pattern of a respective fish chorus over 1 week, at local time. If there were multiple sound sources visible in the 7-day LTSA, the respective fish chorus was highlighted using a black or white box as demonstrated in the example record, shown in Figure 4. Please note that the time scale of the LTSA may be slightly shorter for some fish choruses due to limitations of the respective recording schedules.
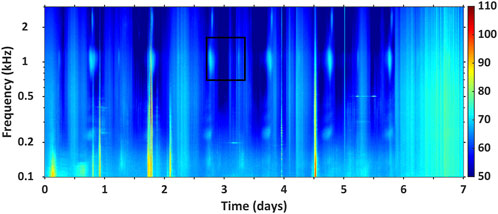
Figure 4. Example of the 7-day LTSA record provided for each fish chorus in the AFCC. This figure displays the PSD (dB re 1 μPa2/Hz) and diel presence of fish chorus BB_1 (highlighted in the black box) over 7 days at local time. This spectrogram was produced with an averaging time of 0.25 h and a FFT window length of 300 s. The acoustic data at this location were collected at a 6 kHz sampling frequency.
The second spectrographic record features a high-resolution spectrogram of a respective fish chorus, displaying the PSD (dB re 1 μPa2/Hz) of the fish chorus across frequency (kHz) and time over a time scale ranging 100–600 s. These spectrograms are produced with an FFT window length of 0.5 s and an overlap of 50 or 60%. An example of this record is shown in Figure 5. The third record is a composition of a high-resolution spectrogram again depicting PSD (dB re 1 μPa2/Hz) across time and frequency (with time scales ranging from 1 to 100 s) and a corresponding oscillogram to display the energy and relative amplitude of the individual call/s making up the respective fish chorus (if possible). The spectrograms of these figures were produced with an FFT length of 0.05 or 0.1 s and an overlap of 80 or 90%. The FFT window length and overlap combination were dependent on the peak frequency of the fish chorus calls. An example of this composition figure is demonstrated in Figure 6. It is important to note that it is often difficult to distinguish single calls within a chorus. Therefore, for some fish chorus records, the call composition figures have yet to be included in the catalogue.
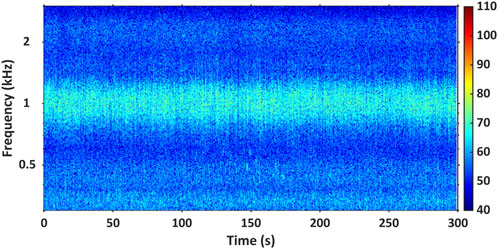
Figure 5. Example of the high-resolution spectrogram record provided for each fish chorus in the AFCC. It displays the PSD (dB re 1 μPa2/Hz) of fish chorus BB_1 over a 300 s resolution. This spectrogram was produced with an FFT window length of 0.5 s and 60% overlap. The acoustic data shown here were collected at a 6 kHz sampling frequency.
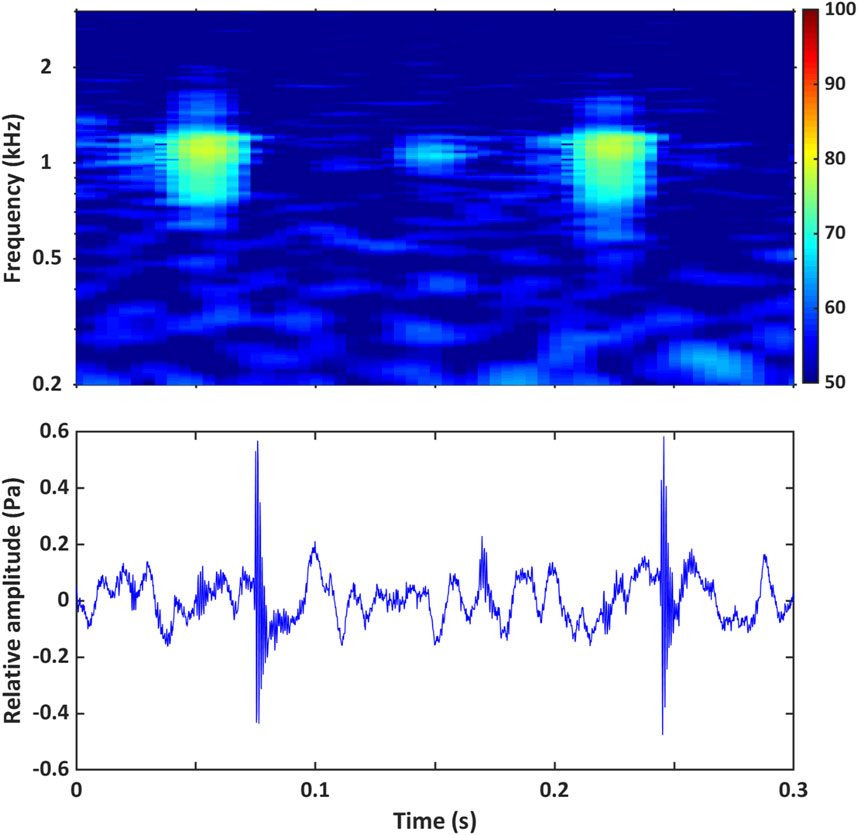
Figure 6. Example of the call composition record provided for each fish chorus in the AFCC. It comprises a high-resolution spectrogram and oscillogram, displaying the PSD (dB re 1 μPa2/Hz) of the fish chorus calls (collected over 0.3 s) and the relative amplitude of the fish chorus calls of chorus BB_1, respectively. The spectrogram component of the figure was produced with an FFT window length of 0.01 s and 90% overlap. The acoustic data shown here were collected at a 6 kHz sampling frequency.
The final spectrographic record is a mean power spectrum plot of a respective fish chorus. It displays the mean (i.e., averaged over the duration of the chorus) frequency content of the fish chorus over one chorusing event per fish chorus (generally within a 24-h period). The two black lines on each mean power spectrum plot show the maximum and minimum frequencies of the respective fish chorus (Figure 7). The timing and extent of each chorusing event were chosen to exclude (as much as possible) the contribution of ambient sound to the chorus frequency band. On the occasion that a fish chorus was significantly masked by ambient sounds, a mean spectrum plot was not produced for that particular chorus. This was also the case for fish choruses with a frequency that exceeded the Nyquist frequency of the respective USR. As the mean power spectrum plot was produced from one chorusing event only, it is important to note that it is not representative of the frequency content of the respective fish chorus over the entire recording period. The extent of each calling event is defined in the spectral measurement spreadsheet (see Section 3.3).
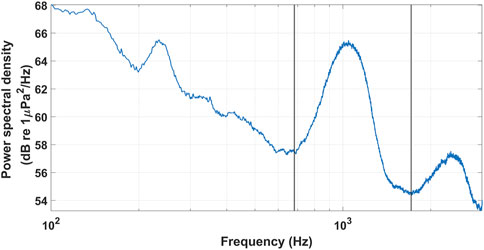
Figure 7. Example of the mean power spectrum record that is provided for each fish chorus in the AFCC. It displays the spectral content of the BB_1 fish chorus. The black lines denote the minimum and maximum frequency of the fish chorus, respectively. The acoustic data shown here were collected at a 6 kHz sampling frequency.
Each spectrographic record is available in the .tif file format. The records are named using the following conventions:
1. ChorusIdentifier_soundscape_spectrogram.tif.
2. ChorusIdentifier_chorus_spectrogram.tif.
3. ChorusIdentifier_call_composition.tif.
4. ChorusIdentifier_meanspectrum.tif.
3.2 Audio records
A 100–600 s .wav audio file of each fish chorus is featured in the AFCC. Each chorus audio record is named using the convention “ChorusIdentifier_chorus_audiofile.wav.” This audio file aligns with the sounds shown in the respective chorus spectrogram figure. If possible, an additional, shorter .wav audio file of the individual calls making up the chorus is also provided. These audio records were named using the convention “ChorusIdentifier_call_audiofile.wav.” The shorter audio file aligns with the sounds shown in the respective call composition figure.
3.3 Online spreadsheets
Three online spreadsheets are featured in the AFCC. They include 1) deployment information, 2) fish chorus seasonal presence/absence, and 3) fish chorus duration and spectral measurements. The online deployment information table contains the latitude, longitude, start time, and end time of each deployment. This table has been made available in the .pdf file format. The file is named “AFCC_deployment_information.pdf.” The seasonal presence/absence spreadsheet has been made available as a Microsoft Excel spreadsheet in the .csv file format. The columns of the spreadsheet are as follows:
• “Loc”: location (the name of the location where the recording of the fish chorus was taken).
• “Label”: a shortened version of the location name to help with the fish chorus naming conventions.
• “ID”: chorus identifier (the unique name for each fish chorus).
• “Seasonal presence”: the seasonal presence or absence of each fish chorus at the recording location. Each letter corresponds to the months of the year in order from January to December, 0 indicates fish chorus absence, 1 indicates fish chorus presence, 2 indicates that the presence of the fish chorus could not be discerned due to masking by ambient sound sources, and 3 indicates that the presence of the fish chorus could not be discerned due to the lack of acoustic recordings available for that respective period.
The spreadsheet file is named “AFCC_seasonal_presence.csv.” A snapshot of this spreadsheet is displayed in Table 4.

Table 4. Snapshot of the seasonal presence spreadsheet made available in the AFCC. This snapshot displays the seasonal presence of the fish choruses identified at the Bremer Bay recording location from January (J) to December (D). 0 = fish chorus absent and 1 = fish chorus present.
The fish chorus spectral measurement spreadsheet has been made available in a Microsoft Excel spreadsheet in the .csv file format. The file is named “AFCC_measurement_spreadsheet.csv.” The definitions of each measurement variable are outlined in Supplementary Table S2. In addition to the variables shown in Supplementary Table S2, the following columns are also included in the online spreadsheet:
• “SD”: extraction start date and time (start date and time of fish chorus recording used for spectral measurements in Universal Coordinated Time (UTC)).
• “ED”: extraction end date and time (end date and time of fish chorus recording used for spectral measurements in UTC).
• “HRS”: the number of hours ahead (+) or behind (−) the UTC of the recording location of the respective fish chorus.
• “ANLS”: analysis type was designated with one of four classifications: 1) full analysis, 2) partial analysis due to ambient noise masking, 3) no analysis undertaken due to the chorus frequency exceeding the Nyquist frequency of the sampling regime, where the minimum frequency was estimated from the scrutiny of spectrograms, and 4) no analysis undertaken due to ambient noise masking, where the minimum and maximum frequency were estimated from the scrutiny of spectrograms.
4 Discussion and future work
The AFCC is the first open-access data repository of fish chorus data at a national scale. It holds spectral, spatial, and temporal distribution records of 301 fish choruses recorded across 83 locations in Australian estuarine and marine waters. The majority of these 83 locations are spread across marine waters all around the continent; however, few or no acoustic recordings could be sourced for several key marine areas. These include the Gulf of Carpentaria, the Great Barrier Reef, waters around Tasmania, and waters off the western side of the Great Australian Bight. Studies have shown that Australian recording sites with similar environments exhibit high fish chorus diversity (Parsons et al., 2017; McWilliam et al., 2018; Ward et al., 2019; Hawkins et al., 2023). This suggests a significant potential for identifying new types of fish choruses in these areas. The same can be said for Australian estuarine and freshwater environments. The AFCC contains very few fish choruses recorded in estuarine environments and none from freshwater environments. This is also due to a lack of available acoustic recordings. However, fish choruses have been extensively documented in estuarine environments globally (Lagardère and Mariani, 2006; Luczkovich et al., 2008; Montie et al., 2015; Zhi-Tao et al., 2017), and acoustic recordings in an Australian estuary have demonstrated a particularly high fish chorus diversity (Parsons et al., 2016). Little is known regarding fish chorus contributions to freshwater soundscapes. Only a few freshwater fish choruses have been described (Borie et al., 2014; Linke et al., 2018; Borie et al., 2019), including a fish chorus recorded in the Einasleigh River in Northern Australia (Linke et al., 2018). Many more fish choruses likely exist in these yet-to-be-recorded marine, estuarine, and freshwater environments. Consequently, the authors recommend prioritising future acoustic data collection in these under-represented areas. Fish choruses will continue to be added to the AFCC using a standardised methodology as more acoustic recordings become available.
A standardised methodology for the extraction of these records has been outlined here to ensure reproducibility to facilitate analysis of new recordings and comparisons with new fish chorus data. The spectral and temporal measurements included in the repository are indicative characterisations of the respective fish choruses; however, it is important to note that while these measurements represent the spectral characteristics of these fish choruses in acoustic conditions as close to ideal as possible, the authors acknowledge that the spectral measurements of these fish choruses may vary with time. This may be associated with alterations in the behaviour of the source species or may be due to changes in the acoustic habitat at the respective recording location. As such, this potential for variation needs to be taken into account when using the AFCC records for comparison. The spectral and temporal measurements have been used to differentiate fish chorus types per recording location within the AFCC, but they can also be used in combination with the spectrographic and audible records for fish chorus identification. The AFCC records can be compared with ground-truthed fish vocalisations in established sound libraries, such as the Audio Gallery (Discovery of Sound in the Sea) (Vigness-Raposa et al., 2012), Fish Sounds (Fish and Mowbray, 1970), The SOUND Table (Kaschner, 2012), the Macaulay Library (Scholes, 2015), Ocean Networks Canada (Ocean Networks Canada, 2024), Sonothèque (Sonothèque, 2024), the British Library Sound Archive (The British Library, 2024), FishSounds (Looby et al., 2023b), the Animal Sound Archive (Tierstimmenarchiv, 2024), and FonoZoo (FonoZoo, 2024), to assist with the identification of fish chorus source species and delineation of their distribution. Identification of fish chorus source species enhances the effectiveness of using ecological information from the AFCC records to monitor and manage these species.
The AFCC has contributed to the development of PAM of fish choruses to be applied to the monitoring and management of fish populations in Australian waters. The presence, distribution, and diversity of Australian fish choruses have been mapped and made accessible for managers and researchers, not only as a reference library but also as a source of ecological data. If the source species and biological function of the AFCC choruses are discerned, the AFCC can provide information on the distribution, behaviour, habitat use, and spawning dynamics of specific fish species. The value of PAM of ground-truthed fish choruses has been demonstrated in several monitoring applications, including the delineation of spawning seasons (Connaughton and Taylor, 1995; Barrios, 2004; Tellechea et al., 2011; Ricci et al., 2017; Monczak et al., 2017), identification of important spawning habitats (Saucier and Baltz, 1993; Luczkovich et al., 1999; Luczkovich et al., 2008; Ricci et al., 2017), and the tracking of invasive species (Rountree and Juanes, 2017). This would be particularly valuable for important commercial fish species (Stratoudakis et al., 2024) or for endangered or cryptic species (Mann and Grothues, 2009; Parsons et al., 2017; Rowell et al., 2015). Even if the AFCC fish choruses are not ground-truthed to species, the records of these choruses still provide important ecological information.
The value of identifying and documenting unknown underwater biological sounds has recently been brought to attention (Parsons et al., 2022). Unidentified sounds not only assist in the process of source species elucidation but can also be used to measure biodiversity and indicate habitat conditions (Rountree et al., 2012; Krause and Farina, 2016; Di Iorio et al., 2018; Lin et al., 2019; Parsons et al., 2022). The AFCC provides valuable baselines of the distribution, diversity, seasonal presence, and spectral and temporal characteristics of Australian fish choruses, which can be applied to a variety of research or monitoring efforts. The potential application of the AFCC is demonstrated in the study by Hawkins et al. (2023). The study counted the number of fish choruses recorded at 29 locations that are also included within the AFCC. This measure of fish chorus diversity was used to highlight potentially important foraging and spawning habitats for fish aggregations and to identify spatial drivers of fish chorus diversity (Hawkins et al., 2023). The AFCC records can also be used as a baseline to monitor fish chorus contributions to a specific location over time. Fish can be used as effective indicators of ecosystem conditions (Harris, 1995; Schiemer, 2000; Whitfield and Elliott, 2002; Dulvy et al., 2008). Long-term monitoring of fish choruses has the potential to reveal impacts of environmental and anthropogenic change on soniferous fish populations and their respective habitats (Locascio and Mann, 2005; Indeck et al., 2015; Luczkovich et al., 2024) and be used to predict the response of vulnerable species to climate change (Monczak et al., 2019; Vieira et al., 2022). The AFCC aims to not only support future research, monitoring, and management efforts in Australian waters but also to inspire similar studies on fish choruses and their contributions to underwater soundscapes worldwide, even when the source species are yet to be confirmed.
Moving forward, the creation and development of fish chorus reference libraries will need to address several issues. Standardisation in acoustic data collection, processing, and analysis methods needs to be prioritised, facilitating comparisons and ensuring consistency and reliability across various studies (Browning et al., 2017; Gibb et al., 2019; Parsons et al., 2022). Enhanced collaboration among researchers and institutions needs to be encouraged to pool resources and share data and knowledge, thereby expanding the availability, diversity, and volume of raw acoustic data and of ground-truthed fish sounds. For example, the spatial scope of the AFCC would not have been possible without raw data contributions from CMST, AIMS, NSW DPI, Parks Australia, and NOAA. Advanced technologies, such as machine learning, signal processing, and artificial intelligence, need to be integrated into reference library development and maintenance. This will facilitate automated analysis and classification of sounds, making it easier and less time-consuming to identify and catalogue fish species (Napier et al., 2024). A strong emphasis needs to be placed on metadata quality to enhance the usability of the libraries for comparative research purposes and for monitoring efforts (Bradbury et al., 1999; Parsons et al., 2022). Additionally, data continuity needs to be safeguarded. Long-term financial security is needed to ensure the maintenance of these libraries (Parsons et al., 2022), such as the support provided for the NOAA NCEI passive acoustic data archive (Wall et al., 2021). Finally, the promotion of open-access needs to be prioritised to facilitate acoustic data availability to a wider audience, fostering greater innovation, continued development of data analysis techniques, and knowledge sharing in this field (Parsons et al., 2022).
Data availability statement
The datasets presented in this study can be found in online repositories. The names of the repository/repositories and accession number(s) can be found in the article/Supplementary Material.
Ethics statement
The animal study was approved by the Curtin University Animal Ethics Committee, the New South Wales Department of Primary Industries, Parks Australia, and the Great Barrier Reef Marine Park Authority. The study was conducted in accordance with the local legislation and institutional requirements.
Author contributions
LH: conceptualization, data curation, formal analysis, investigation, methodology, project administration, software, validation, visualization, writing–original draft, and writing–review and editing. CE: conceptualization, methodology, software, supervision, writing–review and editing, and resources. AB: resources and writing–review and editing. CB: resources and writing–review and editing. JeM: writing–review and editing and resources. JaM: writing–review and editing and formal analysis. IP: writing–review and editing and supervision. MP: resources, writing–review and editing, conceptualization, data curation, and formal analysis. NR: resources and writing–review and editing. RW: resources and writing–review and editing. DW-K: formal analysis and writing–review and editing. RM: methodology, resources, supervision, and writing–review and editing.
Funding
The author(s) declare financial support was received for the research, authorship, and/or publication of this article. Publication was supported by CMST.
Acknowledgments
The authors would like to acknowledge the traditional owners of land and sea country across Australia. The authors pay their respects to elders past, present, and emerging, and they extend this respect to all Aboriginal and Torres Strait Islander peoples. The authors of this study would like to acknowledge data collection by Dr. Claire Charlton and Dr. Angela Recalde-Salas, data pre-processing undertaken by Rachel Courts and Dr. Marcos Rossi dos Santos, and the contribution made by Hannah Pemberton in the commission of the Figure 1 illustration. They also acknowledge Dr. Jason Gedamke, formerly of the Australian Antarctic Division, for the organisation of the deployment of a sea noise logger on a CSIRO mooring south of Tasmania. While no financial support was received for the research and authorship of this article, the authors would like to acknowledge the contributions from several organisations that supported the collection of the historical PAM datasets utilised in this study. The authors thank the SeaWorld & Busch Gardens Conservation Fund for funding the acoustic recordings in Bremer Bay. They thank BHP for funding the research in Port Hedland through their Minerals Australia Social Investment Program – Environment Portfolio and for data collected at Ningaloo and Keppel Islands through the Australian Coral Reef Resilience Initiative (ACRRI), which incorporates the Reef Song Project and Woppaburra Coral Project. Acoustic data collected as part of projects “2010-004: Passive acoustic techniques to monitor aggregations of sound producing fish species” (PI Miles Parsons, Curtin University) and “2004-051: Management and monitoring of fish spawning aggregations within the West Coast Bio-region of Western Australia” (PI Michael Mackie, Curtin University) were funded by the Fisheries Research and Development Corporation on behalf of the Australian Government. Acoustic data collected at the Point Samson West, Point Samson East, and Broome North recording sites were collected as part of AIMS’ North West Shoals to Shore Research Program and were supported by Santos as part of the company’s commitment to better understand Western Australia’s marine environment. Acoustic recordings collected within the Cod Grounds, Murat, Solitary Island, and Two Rocks Australian Marine Parks were funded by the Director of National Parks, Australia. The authors would like to thank the New South Wales Department of Primary Industries, the South Australian Department of Primary Industries and Regions, and the Western Australian Department of Primary Industries and Regional Development for their assistance in the deployment and retrieval of acoustic recorders across these Australian Marine Parks. Acoustic data were also sourced from Australia’s Integrated Marine Observing System (IMOS), which is enabled by the National Collaborative Research Infrastructure Strategy (NCRIS) (IMOS, 2022). The IMOS is operated by a consortium of institutions as an unincorporated joint venture, with the University of Tasmania as the Lead Agent. The authors would also like to thank the Australian Institute of Marine Science, the Centre for Marine Science and Technology, Chevron Australia, Fugro, Inpex (Parsons et al., 2016; Comrie-Greig and Abdo, 2014), Woodside, the Western Australian Department of Biodiversity, Conservation, and Attractions, the New South Wales Department of Primary Industries, the NSW Recreational Fishing Trust, the Holsworth Wildlife Research Endowment–Equity Trustees Charitable Foundation and the Ecological Society of Australia (Grant number HWRE 2016R2085CONT- PI, Jamie McWilliam), the Lizard Island Reef Research Foundation (PI, Jamie McWilliam), the Australian Acoustical Society (PI, Jamie McWilliam), the Australian Acoustical Society Western Australian Division (PI, Rhianne Ward), and Chandra Salgado Kent for supporting data collection.
Conflict of interest
The authors declare that the research was conducted in the absence of any commercial or financial relationships that could be construed as a potential conflict of interest.
Publisher’s note
All claims expressed in this article are solely those of the authors and do not necessarily represent those of their affiliated organizations, or those of the publisher, the editors, and the reviewers. Any product that may be evaluated in this article, or claim that may be made by its manufacturer, is not guaranteed or endorsed by the publisher.
Supplementary material
The Supplementary Material for this article can be found online at: https://www.frontiersin.org/articles/10.3389/frsen.2024.1473168/full#supplementary-material
References
Barrios, A. T. (2004). “Use of passive acoustic monitoring to resolve spatial and temporal patterns of spawning activity for red drum, Sciaenops ocellatus, in the Neuse River Estuary, North Carolina,” in Degree of master of science. Raleigh, NA: North Carolina State University.
Borie, A., Hungria, D. B., Ali, H., Doria, C. R., Fine, M. L., and Travassos, P. E. (2019). Disturbance calls of five migratory Characiformes species and advertisement choruses in Amazon spawning sites. J. Fish Biol. 95, 820–832. doi:10.1111/jfb.14078
Borie, A., Mok, H.-K., Chao, N. L., and Fine, M. L. (2014). Spatiotemporal variability and sound characterization in silver croaker Plagioscion squamosissimus (Sciaenidae) in the Central Amazon. PLOS One 9, e99326. doi:10.1371/journal.pone.0099326
Bradbury, J., Budney, G. F., Stemple, D. W., and Kroodsma, D. E. (1999). Organizing and archiving private collections of tape recordings. Anim. Behav. 57, 1343–1344. doi:10.1006/anbe.1999.1113
Brawn, V. M. (1961). Sound production by the Cod (Gadus callarias L.). Behaviour 18, 239–255. doi:10.1163/156853961x00150
Browning, E., Gibb, R., Glover-Kapfer, P., and Jones, K. E. 2017. Passive acoustic monitoring in ecology and conservation.
Butler, J., Pagniello, C. M. L. S., Jaffe, J. S., Parnell, P. E., and Širović, A. (2021). Diel and seasonal variability in kelp forest soundscapes off the southern California coast. Front. Mar. Sci. 8. doi:10.3389/fmars.2021.629643
Cato, D. H. (1978). Marine biological choruses observed in tropical waters near Australia. J. Acoust. Soc. Am. 64, 736–743. doi:10.1121/1.382038
Cato, D. H. (1980). Some unusual sounds of apparent biological origin responsible for sustained background noise in the Timor Sea. J. Acoust. Soc. Am. 68, 1056–1060. doi:10.1121/1.384989
CENTRE FOR MARINE SCIENCE AND TECHNOLOGY (2021). CHORUS software. Available at: http://cmst.curtin.edu.au/products/chorus-software/(Accessed February 8, 2021).
Ceraulo, M., Moyano, M. P. S., Hidalgo, F. J., Bazterrica, M. C., Mazzola, S. M., Gavio, M. A., et al. (2021). Boat noise and black drum vocalizations in Mar Chiquita coastal Lagoon (Argentina). J. Mar. Sci. Eng. 9, 44. doi:10.3390/jmse9010044
Comrie-Greig, J., and Abdo, L. (2014). Ecological studies of the Bonaparte Archipelago and Browse Basin, Perth, WA: INPEX Operations Australia Pty Ltd.
Connaughton, M. A., and Taylor, M. H. (1995). Seasonal and daily cycles in sound production associated with spawning in the weakfish, Cynoscion regalis. Environ. Biol. Fishes 42, 233–240. doi:10.1007/bf00004916
Darras, K. F. A., Rountree, R., Wilgenburg, S. V., Dong, L., Gasc, A., Pitz, F., et al. (2024). Worldwide soundscape ecology patterns across realms. bioRxiv 10, 588860. . doi:10.1101/2024.04.10.588860
Di Iorio, L., Audax, M., Deter, J., Holon, F., Lossent, J., Gervaise, C., et al. (2021). Biogeography of acoustic biodiversity of NW Mediterranean coralligenous reefs. Sci. Rep. 11, 16991. doi:10.1038/s41598-021-96378-5
Di Iorio, L., Raick, X., Parmentier, E., Boissery, P., Valentini-Poirier, C.-A., and Gervaise, C. (2018). Posidonia meadows calling’: a ubiquitous fish sound with monitoring potential. Remote Sens. Ecol. Conservation 4, 248–263. doi:10.1002/rse2.72
D'Spain, G., Batchelor, H., Helble, T. A., and McCarty, P. (2013). “New observations and modeling of an unusual spatiotemporal pattern of fish chorusing off the southern California coast,” in Proceedings of Meetings on Acoustics, June 2–7, 2013. Montreal, Canada: Acoustical Society of America.
Dulvy, N. K., Rogers, S. I., Jennings, S., Stelzenmüller, V., Dye, S. R., and Skjoldal, H. R. (2008). Climate change and deepening of the North Sea fish assemblage: a biotic indicator of warming seas. J. Appl. Ecol. 45, 1029–1039. doi:10.1111/j.1365-2664.2008.01488.x
Erbe, C., Duncan, A., Hawkins, L., Terhune, J. M., and Thomas, J. A. (2022). “Introduction to acoustic terminology and signal processing,” in Exploring animal behavior through sound. Methods. Editors C. ERBE, and J. A. THOMAS (Cham, Switzerland: Springer International Publishing), 1, 111–152. doi:10.1007/978-3-030-97540-1_4
Fine, M. L. (2012). Swimbladder sound production: the forced response versus the resonant bubble. Bioacoustics 21, 5–7. doi:10.1080/09524622.2011.647453
Fine, M. L., Friel, J. P., Mcelroy, D., King, C. B., Loesser, K. E., and Newton, S. (1997). Pectoral spine locking and sound production in the channel catfish Ictalurus punctatus. Copeia 1997, 777–790. doi:10.2307/1447295
Fine, M. L., Malloy, K. L., King, C., Mitchell, S. L., and Cameron, T. M. (2001). Movement and sound generation by the toadfish swimbladder. J. Comp. Physiology A 187, 371–379. doi:10.1007/s003590100209
Fine, M. L., and Parmentier, E. (2015). “Mechanisms of fish sound production,” in Sound communication in fishes. Editor F. LADICH (Vienna: Springer).
Fish, M. P., and Mowbray, W. H. (1970). “Sounds of Western North Atlantic Fishes,” in A reference file of biological underwater sounds. Baltimore, MA: Rhode Island University Kingston Narragansett Marine Lab.
FONOZOO (2024). Fonoteca zoológica. Mus. Nac. Ciencias Nat. Madr. (Spain). Available at: http://www.fonozoo.com/index.php.
Gavrilov, A. (2021). A MATLAB toolbox for characterisation of recorded underwater sound (CHORUS) User's Guide. Centre Mar. Sci. Technol. Available at: https://cmst.curtin.edu.au/wp-content/uploads/sites/4/2021/06/2021-04-CHORUS-V8-Users-Guide.pdf.
Gavrilov, A., and Parsons, M. (2014). A MATLAB tool for the characterisation of recorded underwater sound (CHORUS). Acoust. Aust. 42, 190–196.
Gibb, R., Browning, E., Glover-Kapfer, P., and Jones, K. E. (2019). Emerging opportunities and challenges for passive acoustics in ecological assessment and monitoring. Methods Ecol. Evol. 10, 169–185. doi:10.1111/2041-210x.13101
Harris, J. H. (1995). The use of fish in ecological assessments. Aust. J. Ecol. 20, 65–80. doi:10.1111/j.1442-9993.1995.tb00523.x
Hawkins, L. A., Erbe, C., Becker, A., Browne, C. E., McCordic, J., McWilliam, J., et al. (2024). The Australian Fish Chorus Catalogue (2005 - 2023). Aust. Ocean Data Netw. doi:10.26198/qfj2-jj93
Hawkins, L. A., Saunders, B. J., Landero Figueroa, M. M., McCauley, R. D., Parnum, I. M., Parsons, M. J., et al. (2023). Habitat type drives the spatial distribution of Australian fish chorus diversity. J. Acoust. Soc. Am. 154, 2305–2320. doi:10.1121/10.0021330
IMOS (2022). Integrated Marine Observing System. Available at: https://imos.org.au/(Accessed August 20, 2022).
IMOS (2023). IMOS - ANMN passive acoustic observatories sub-facility. Available at: https://catalogue-imos.aodn.org.au/geonetwork/srv/api/records/e850651b-d65d-495b-8182-5dde35919616 (Accessed January, 2023).
Indeck, K. L., Simard, P., Gowans, S., Lowerre-Barbieri, S., and Mann, D. A. (2015). A severe red tide (Tampa Bay, 2005) causes an anomalous decrease in biological sound. R. Soc. Open Sci. 2, 150337. doi:10.1098/rsos.150337
Kaatz, I. M. (2002). Multiple sound-producing mechanisms in teleost fishes and hypotheses regarding their behavioural significance. Bioacoustics 12, 230–233. doi:10.1080/09524622.2002.9753705
Kaschner, K. (2012). The SOUNDS table in FishBase. Editors R. Froese, and D. Pauly (QLD, Australia: World Wide Web Electronic Publication).
Kasumyan, A. O. (2008). Sounds and sound production in fishes. J. Ichthyology 48, 981–1030. doi:10.1134/s0032945208110039
Kelly, L. J., Kewley, D. J., and Burgess, A. S. (1985). A biological chorus in deep water northwest of Australia. J. Acoust. Soc. Am. 77, 508–511. doi:10.1121/1.392363
Krause, B., and Farina, A. (2016). Using ecoacoustic methods to survey the impacts of climate change on biodiversity. Biol. Conserv. 195, 245–254. doi:10.1016/j.biocon.2016.01.013
Lagardère, J. P., and Mariani, A. (2006). Spawning sounds in meagre Argyrosomus regius recorded in the Gironde estuary, France. J. Fish Biol. 69, 1697–1708. doi:10.1111/j.1095-8649.2006.01237.x
Lin, T.-H., Chen, C., Watanabe, H. K., Kawagucci, S., Yamamoto, H., and Akamatsu, T. (2019). Using soundscapes to assess deep-sea benthic ecosystems. Trends Ecol. & Evol. 34, 1066–1069. doi:10.1016/j.tree.2019.09.006
Linke, S., Decker, E., Gifford, T., and Desjonquères, C. (2018). Diurnal variation in freshwater ecoacoustics: implications for site-level sampling design. Freshw. Biol. 65, 86–95. doi:10.1111/fwb.13227
Locascio, J. V., and Mann, D. A. (2005). Effects of hurricane Charley on fish chorusing. Biol. Lett. 1, 362–365. doi:10.1098/rsbl.2005.0309
Locascio, J. V., and Mann, D. A. (2011). Diel and seasonal timing of sound production by black drum (Pogonias cromis). Fish. Bull. 109, 327–338.
Looby, A., Cox, K., Bravo, S., Rountree, R., Juanes, F., Reynolds, L., et al. (2022). A quantitative inventory of global soniferous fish diversity. Rev. Fish Biol. Fish. 32, 581–595. doi:10.1007/s11160-022-09702-1
Looby, A., Erbe, C., Bravo, S., Cox, K., Davies, H. L., Di Iorio, L., et al. (2023a). Global inventory of species categorized by known underwater sonifery. Sci. Data 10, 892. doi:10.1038/s41597-023-02745-4
Looby, A., Riera, A., Vela, S., Cox, K., Bravo, S., Rountree, R., et al. (2023b). FishSounds. Available at: http://www.fishsounds.net (Accessed March 27, 2023).
Luczkovich, J. J., Pullinger, C. R., Johnson, S. E., and Sprague, M. W. (2008). Identifying sciaenid critical spawning habitats by the use of passive acoustics. Trans. Am. Fish. Soc. 137, 576–605. doi:10.1577/t05-290.1
Luczkovich, J. J., Sprague, M. W., Johnson, S. E., and Pullinger, R. C. (1999). Delimiting spawning areas of weakfish Cynoscion regalis (Family Sciaenidae) in Pamlico Sound, North Carolina using passive hydroacoustic surveys. Bioacoustics 10, 143–160. doi:10.1080/09524622.1999.9753427
Luczkovich, J. J., Sprague, M. W., and Paerl, H. W. (2024). Bottom water hypoxia suppresses fish chorusing in estuaries. J. Acoust. Soc. Am. 155, 2014–2024. doi:10.1121/10.0025289
Lynch, T., Mclaughlin, D., Hughes, D., Darby, I., de Boer, P., and Marouchos, A. (2010). Overview of CSIRO oceanographic moorings. IEEE: OCEANS 2010 MTS/IEEE SEATTLE, 1–9.
Mahanty, M. M., Latha, G., and Edwards, D. P. (2019). Impact of tropical cyclone Vardah on the fish chorus in shallow waters of Southwest Bay of Bengal. Ecol. Indic. 96, 288–292. doi:10.1016/j.ecolind.2018.09.012
Mahanty, M., Latha, G., and Durai, E. 2016. Soundscapes in the shallow waters off Southwest coast of India during pre-monsoon season the Journal of Acoustical Society of India, 43, 1–9.
Mann, D. A., and Grothues, T. M. (2009). Short-term upwelling events modulate fish sound production at a mid-Atlantic ocean observatory. Mar. Ecol. Prog. Ser. 375, 65–71. doi:10.3354/meps07720
Marley, S. A., Erbe, C., Salgado Kent, C. P., Parsons, M. J. G., and Parnum, I. M. (2017). Spatial and temporal variation in the acoustic habitat of bottlenose dolphins (Tursiops aduncus) within a highly urbanized estuary. Front. Mar. Sci. 4. doi:10.3389/fmars.2017.00197
McCauley, R. D. (2001). Biological sea noise in northern Australia: patterns of fish calling. Perth, WA: James Cook University. Ph.D.
McCauley, R. D., and Cato, D. H. (2000). Patterns of fish calling in a nearshore environment in the Great Barrier Reef. Philosophical Trans. R. Soc. Lond. Ser. B Biol. Sci. 355, 1289–1293. doi:10.1098/rstb.2000.0686
McCauley, R. D., and Cato, D. H. (2016). Evening choruses in the Perth Canyon and their potential link with Myctophidae fishes. J. Acoust. Soc. Am. 140, 2384–2398. doi:10.1121/1.4964108
McCauley, R. (2012). “Fish choruses from the Kimberley, seasonal and lunar links as determined by long term sea noise monitoring,” in Proceedings of Acoustics 2012. Fremantle. Western Australia: Australian Acoustical Society.
McCauley, R., Thomas, F., Parsons, M., Erbe, C., Cato, D., Duncan, A., et al. (2017). Developing an underwater sound recorder: the long and short (time) of it. Acoust. Aust. 45, 301–311. doi:10.1007/s40857-017-0113-8
McWilliam, J. N., McCauley, R. D., Erbe, C., and Parsons, M. J. G. (2018). Soundscape diversity in the Great Barrier Reef: Lizard Island, a case study. Bioacoustics 27, 295–311. doi:10.1080/09524622.2017.1344930
McWilliam, J., McCauley, R., Erbe, C., and Parsons, M. (2017). Patterns of biophonic periodicity on coral reefs in the Great Barrier Reef. Sci. Rep. 7, 1–13. doi:10.1038/s41598-017-15838-z
Mok, H. K., and Gilmore, R. G. (1983). Analysis of sound production in estuarine aggregations of Pogonias cromis, Bairdiella chrysoura, and Cynoscion nebulosus (Sciaenidae). Bull. Inst. Zoology, Acad. Sinica 22, 157–186.
Mok, H.-K., Wu, S.-C., Sirisuary, S., and Fine, M. L. (2020). A sciaenid swim bladder with long skinny fingers produces sound with an unusual frequency spectrum. Sci. Rep. 10, 18619. doi:10.1038/s41598-020-75663-9
Monczak, A., Berry, A., Kehrer, C., and Montie, E. W. (2017). Long-term acoustic monitoring of fish calling provides baseline estimates of reproductive timelines in the May River estuary, southeastern USA. Mar. Ecol. Prog. Ser. 581, 1–19. doi:10.3354/meps12322
Monczak, A., Ji, Y., Soueidan, J., and Montie, E. W. (2019). Automatic detection, classification, and quantification of sciaenid fish calls in an estuarine soundscape in the Southeast United States. PLOS One 14, e0209914. doi:10.1371/journal.pone.0209914
Montie, E. W., Vega, S., and Powell, M. (2015). Seasonal and spatial patterns of fish sound production in the May River, South Carolina. Trans. Am. Fish. Soc. 144, 705–716. doi:10.1080/00028487.2015.1037014
Moulton, J. M. (1958). The acoustical behavior of some fishes in the Bimini area. Biol. Bull. 114, 357–374. doi:10.2307/1538991
Napier, T., Ahn, E., Allen-Ankins, S., Schwarzkopf, L., and Lee, I. (2024). Advancements in preprocessing, detection and classification techniques for ecoacoustic data: a comprehensive review for large-scale Passive Acoustic Monitoring. Expert Syst. Appl. 252, 124220. doi:10.1016/j.eswa.2024.124220
Ocean Instruments NZ (2021a). SoundTrap ST300 and ST4300 series User Guide. Available at: http://www.oceaninstruments.co.nz/wp-content/uploads/2015/04/ST-User-Guide.pdf.
Ocean Instruments NZ (2021b). SoundTrap ST500 User Guide. Available at: http://www.oceaninstruments.co.nz/wp-content/uploads/2018/03/ST500-User-Guide.pdf.
Ocean Networks Canada (2024). Ocean. Netw. Can. SoundCloud. Available at: https://soundscloud.com/oceannetworkscanada.
Pagniello, C. M. L. S., Cimino, M. A., and Terrill, E. (2019). Mapping fish chorus distributions in southern California using an autonomous wave glider. Front. Mar. Sci. 6. doi:10.3389/fmars.2019.00526
Panicker, D., and Stafford, K. M. (2023). Temporal variability of a soundscape near a mid-oceanic atoll in the northern Indian ocean. Prog. Oceanogr. 214, 103033. doi:10.1016/j.pocean.2023.103033
Parsons, M. J., Lin, T.-H., Mooney, T. A., Erbe, C., Juanes, F., Lammers, M., et al. (2022). Sounding the call for a global library of underwater biological sounds. Front. Ecol. Evol. 10, 810156. doi:10.3389/fevo.2022.810156
Parsons, M. J., McCauley, R. D., and Mackie, M. C. (2013c). Characterisation of mulloway Argyrosomus japonicus advertisement sounds. Acoust. Aust. 41.
Parsons, M. J., McCauley, R. D., Mackie, M. C., Siwabessy, P., and Duncan, A. J. (2009). Localization of individual mulloway (Argyrosomus japonicus) within a spawning aggregation and their behaviour throughout a diel spawning period. ICES J. Mar. Sci. 66, 1007–1014. doi:10.1093/icesjms/fsp016
Parsons, M. J. G. (2009). An investigation into active and passive acoustic techniques to study aggregating fish species. Thesis (PhD). Curtin University.
Parsons, M. J. G., Salgado-Kent, C. P., Marley, S. A., Gavrilov, A. N., and Mccauley, R. D. (2016). Characterizing diversity and variation in fish choruses in Darwin Harbour. ICES J. Mar. Sci. 73, 2058–2074. doi:10.1093/icesjms/fsw037
Parsons, M. J. G., Salgado Kent, C. P., Recalde-Salas, A., and McCauley, R. D. (2017). Fish choruses off Port Hedland, Western Australia. Bioacoustics 26, 135–152. doi:10.1080/09524622.2016.1227940
Parsons, M., Anning, J., and Parnum, I. (2024). “Passive and active acoustic monitoring of mulloway in the Swan River,” in Proceeding of Acoustics 2017, November 19–22, 2017. Perth, Australia: Australian Acoustical Society.
Parsons, M., Longbottom, S., Lewis, P., McCauley, R., and Fairclough, D. (2013a). Sound production by the West Australian dhufish (Glaucosoma hebraicum). J. Acoust. Soc. Am. 134, 2701–2709. doi:10.1121/1.4818775
Parsons, M., McCauley, R., and Thomas, F. (2013b). The sounds of fish off Cape Naturaliste, Western Australia. Acoust. Aust. 41, 58–64.
Picciulin, M., Fiorin, R., Facca, C., and Malavasi, S. (2020). Sound features and vocal rhythms as a proxy for locating the spawning ground of Sciaena umbrain the wild. Aquatic Conservation Mar. Freshw. Ecosyst. 30, 1299–1312. doi:10.1002/aqc.3340
Ricci, S., Eggleston, D., and Bohnenstiehl, D. (2017). Use of passive acoustic monitoring to characterize fish spawning behavior and habitat use within a complex mosaic of estuarine habitats. Bull. Mar. Sci. 93, 439–453. doi:10.5343/bms.2016.1037
Ross, S. R. P. J., O'Connell, D. P., Deichmann, J. L., Desjonquères, C., Gasc, A., Phillips, J. N., et al. (2023). Passive acoustic monitoring provides a fresh perspective on fundamental ecological questions. Funct. Ecol. 37, 959–975. doi:10.1111/1365-2435.14275
Rountree, R. A., Juanes, F., Goudey, C. A., and Ekstrom, K. E. (2012). Is biological sound production important in the deep sea? Springer, 181–183.
Rountree, R., and Juanes, F. (2017). Potential of passive acoustic recording for monitoring invasive species: freshwater drum invasion of the Hudson River via the New York canal system. Biol. Invasions 19, 2075–2088. doi:10.1007/s10530-017-1419-z
Rowell, T. J., Demer, D. A., Aburto-Oropeza, O., Cota-Nieto, J. J., Hyde, J. R., and Erisman, B. E. (2017). Estimating fish abundance at spawning aggregations from courtship sound levels. Sci. Rep. 7, 3340. doi:10.1038/s41598-017-03383-8
Rowell, T. J., Nemeth, R. S., Schärer, M. T., and Appeldoorn, R. S. (2015). Fish sound production and acoustic telemetry reveal behaviors and spatial patterns associated with spawning aggregations of two Caribbean groupers. Mar. Ecol. Prog. Ser. 518, 239–254. doi:10.3354/meps11060
Saucier, M. H., and Baltz, D. M. (1993). Spawning site selection by spotted seatrout, Cynoscion nebulosus, and black drum, Pogonias cromis, in Louisiana. Environ. Biol. Fishes 36, 257–272. doi:10.1007/bf00001722
Schiemer, F. (2000). Fish as indicators for the assessment of the ecological integrity of large rivers. Hydrobiologia 422-423, 271–278. doi:10.1007/978-94-011-4164-2_22
Scholes, E. (2015). Macaulay library Audio and video collection [online]. Ithaca, NY: Cornell Lab of Ornithology. [Accessed via GBIF.org on 1st October 2024].
Siddagangaiah, S., Chen, C.-F., Hu, W.-C., Danovaro, R., and Pieretti, N. (2021a). Silent winters and rock-and-roll summers: the long-term effects of changing oceans on marine fish vocalization. Ecol. Indic. 125, 107456. doi:10.1016/j.ecolind.2021.107456
Siddagangaiah, S., Chen, C. F., Hu, W. C., and Pieretti, N. (2021b). Impact of pile-driving and offshore windfarm operational noise on fish chorusing. Remote Sens. Ecol. Conservation 8, 119–134. doi:10.1002/rse2.231
Slabbekoorn, H., Bouton, N., Van Opzeeland, I., Coers, A., Ten Cate, C., and Popper, A. N. (2010). A noisy spring: the impact of globally rising underwater sound levels on fish. Trends Ecol. & Evol. 25, 419–427. doi:10.1016/j.tree.2010.04.005
SONOTHÈQUE (2024). La Sonothèque du Muséum National d’Histoire Naturelle, Résultats des Espèces. Available at: https://sonotheque.mnhn.fr/?q=&tbs=qr:e.
Sprague, M. W., and Luczkovich, J. J. (2011) “Modeling fish aggregation sounds in very shallow water to estimate numbers of calling fish in aggregations,” in 161 st Meeting Acoustic Society of America, May 23–27, 2011. Seattle, Washington: Acoustical Society of America.
Sprague, M. W., Luczkovich, J. J., and Johnson, S. E. (1998). Using fish sounds to identify spawning activity of weakfish (Cynoscion regalis) and red drum (Sciaenops ocellatus) in nature. J. Acoust. Soc. Am. 103, 3001. doi:10.1121/1.421734
Stratoudakis, Y., Vieira, M., Marques, J. P., Amorim, M. C. P., Fonseca, P. J., and Quintella, B. R. (2024). Long-term passive acoustic monitoring to support adaptive management in a sciaenid fishery (Tagus Estuary, Portugal). Rev. Fish Biol. Fish. 34, 491–510. doi:10.1007/s11160-023-09825-z
Tellechea, J., Bouvier, D., and Norbis, W. (2011). Spawning sounds in whitemouth croaker (Sciaenidae): seasonal and daily cycles. Bioacoustics 20, 159–168. doi:10.1080/09524622.2011.9753641
The British Library (2024). Environment & nature sounds. Br. Libr. Available at: https://sounds.bl.uk/Environment.
The UK Acoustics Network (2024). Open access underwater acoustics data. Available at: https://acoustics.ac.uk/open-access-underwater-acoustics-data/.
Tierstimmenarchiv (2024). The Animal Sound Archive (Tierstimmenarchiv) at the Museum für Naturkunde in Berlin. Available at: https://www.tierstimmenarchiv.de/.
Van Hoeck, R. V., Rowell, T. J., Dean, M. J., Rice, A. N., and Van Parijs, S. M. (2023). Comparing Atlantic Cod temporal spawning dynamics across a biogeographic boundary: insights from passive acoustic monitoring. Mar. Coast. Fish. 15. doi:10.1002/mcf2.10226
Vieira, M., Amorim, M. C. P., Marques, T. A., and Fonseca, P. J. (2022). Temperature mediates chorusing behaviour associated with spawning in the sciaenid Argyrosomus regius. Mar. Ecol. Prog. Ser. 697, 109–124. doi:10.3354/meps14128
Vigness-Raposa, K. J., Scowcroft, G., Miller, J. H., and Ketten, D. (2012). “Discovery of sound in the Sea: an online resource,” in The effects of noise on aquatic life, 2012//2012. Editors A. N. POPPER, and A. HAWKINS (New York: Springer), 135–138.
Wall, C. C., Haver, S. M., Hatch, L. T., Miksis-Olds, J., Bochenek, R., Dziak, R. P., et al. (2021). The next wave of passive acoustic data management: how centralized access can enhance science. Front. Mar. Sci. 8, 703682. doi:10.3389/fmars.2021.703682
Wall, C. C., Simard, P., Lembke, C., and Mann, D. (2013). Large-scale passive acoustic monitoring of fish sound production on the West Florida Shelf. Mar. Ecol.-Prog. Ser. 484, 173–188. doi:10.3354/meps10268
Walters, S., Lowerre-Barbieri, S., Bickford, J., and Mann, D. (2009). Using a passive acoustic survey to identify spotted seatrout spawning sites and associated habitat in Tampa Bay, Florida. Trans. Am. Fish. Soc. 138, 88–98. doi:10.1577/t07-106.1
Ward, R., McCauley, R. D., Gavrilov, A. N., and Charlton, C. M. (2019). Underwater sound sources and ambient noise in Fowlers Bay, South Australia, during the austral winter. Acoust. Aust. 47, 21–32. doi:10.1007/s40857-019-00150-9
Whitfield, A. K., and Elliott, M. (2002). Fishes as indicators of environmental and ecological changes within estuaries: a review of progress and some suggestions for the future. J. Fish Biol. 61, 229–250. doi:10.1006/jfbi.2002.2079
Keywords: fish chorus, unknown fish sounds, passive acoustics, spatiotemporal distribution, soundscape, spectral analysis, data repository
Citation: Hawkins LA, Erbe C, Becker A, Browne CE, McCordic J, McWiliam J, Parnum IM, Parsons MJ, Rivero N, Ward R, White-Kiely D and McCauley RD (2024) The Australian fish chorus catalogue (2005–2023). Front. Remote Sens. 5:1473168. doi: 10.3389/frsen.2024.1473168
Received: 30 July 2024; Accepted: 25 October 2024;
Published: 12 December 2024.
Edited by:
Delwayne Roger Bohnenstiehl, North Carolina State University, United StatesReviewed by:
T. Aran Mooney, Woods Hole Oceanographic Institution, United StatesAude Pacini, University of Hawaii at Manoa, United States
Joseph John Luczkovich, East Carolina University, United States
Copyright © 2024 Hawkins, Erbe, Becker, Browne, McCordic, McWiliam, Parnum, Parsons, Rivero, Ward, White-Kiely and McCauley. This is an open-access article distributed under the terms of the Creative Commons Attribution License (CC BY). The use, distribution or reproduction in other forums is permitted, provided the original author(s) and the copyright owner(s) are credited and that the original publication in this journal is cited, in accordance with accepted academic practice. No use, distribution or reproduction is permitted which does not comply with these terms.
*Correspondence: Lauren Amy Hawkins, bGF1cmVuaGF3a2luczc5OUBnbWFpbC5jb20=