- 1Department of Agricultural Economics, University Sistan and Baluchestan, Zahedan, Iran
- 2Department of Agricultural Economics, Faculty of Agricultural Engineering and Rural Development, Agricultural Sciences and Natural Resources University of Khuzestan, Mollasani, Iran
Introduction: Due to the expansion of population, economic progress, urbanization, increasing food demands, and diversification of food systems, resources are being excessively exploited and degraded. This is compounded by the challenges posed by climate change and limited resources, as well as inadequate management practices. The concept of water-energy-food (WEF) nexus management recognizes the interdependencies among various resources, such as water, food, and energy, in order to promote sustainable resource management. By establishing a harmonious balance among different objectives, this approach aims to safeguard the well-being of both human societies and the environment, ensuring the fulfillment of needs and the preservation of benefits for both parties.
Methods: In this study, the water-energy-food (WEF) nexus approach is applied to the Sistan plain, located in the southeastern region of Iran, to effectively redistribute production resources within the agricultural sector. The methodology employed is multi-objective programming, which incorporates various goals. These objectives encompass maximizing farmer revenue and energy derived from food production (measured in calories), while simultaneously minimizing greenhouse gas (GHG) emissions, irrigation water consumption, and overall energy consumption throughout the 2018–2019 crop year.
Results: The findings of this study demonstrate that implementing the water-energy-food (WEF) nexus approach in the Sistan plain yields positive outcomes. Despite a reduction in the cultivation area, there is a notable shift towards growing more nutritious crops. This shift not only contributes to food security but also increases crop calorie production from 457.16 million to 565.19 million. Consequently, there is a decrease in irrigation water consumption from 261.62 million to 260.48 million cubic meters, energy consumption from 1400.13 million to 1396.81 million MJ per hectare, and greenhouse gas (GHG) emissions from 0.014 million to 0.0139 million tons per hectare.
Discussion: Analyzing the physical and economic productivity reveals that GHG emissions had the highest productivity in terms of both physical and economic measures in Zahak County. As the WEF nexus approach aims to preserve and prevent environmental degradation, it is recommended to implement development and bio-balance policies utilizing this approach to ensure environmental conservation.
1 Introduction
Water, energy, and food are inextricably linked and essential for human existence and sustenance. The production of food requires both water and energy. Water plays a crucial role in ensuring food security for the growing human population and supporting economic activities (Sabouhi and Mardani, 2013). It is vital to acknowledge the interdependence of water security, energy security, and food security. Water serves as the foundation of this cycle, which ultimately culminates in food production through the utilization of energy.
Energy is also required for various processes related to water, including collection, delivery, pumping, distribution, and treatment. Conversely, water holds crucial significance for power generation, fossil fuel generation and processing, and waste treatment (Li et al., 2016; Zhang et al., 2018). However, the rapid expansion of urban and residential areas, coupled with the growth of transportation, commerce, industry, and agriculture, has significantly escalated the demand for water, making water security and energy consumption critical concerns (Artioli et al., 2017). Consequently, it becomes imperative to prioritize the efficient and sustainable management of water and energy resources. In an agricultural system, water and energy resources are indispensable for food production (Daccache et al., 2014). Water is essential for irrigation and food processing, while energy is utilized for tasks such as collecting, transferring, pumping, and discharging irrigation water. Additionally, energy is used for fertilization, tillage, and crop harvesting. Thus, water and energy are consumed in the production of food, which may also serve as raw materials for biofuel. Therefore, it is crucial to optimize the utilization of limited water, energy, and land resources in an agricultural system, particularly in developing countries that heavily rely on agriculture (Singh Vijay et al., 2014; Yang et al., 2016; Tian et al., 2018).
Indeed, agriculture plays a critical role in ensuring food security, but it is also the largest consumer of freshwater resources, which has implications for energy security (Tian et al., 2018). Globally, food production accounts for approximately 90 percent of freshwater consumption, while about 30 percent of energy consumption is related to food production and supply chain activities (Zhang & Vesselinov, 2016). However, with the projected global population growth, economic development, and the increasing demand for 50 percent more food, 40 percent more energy, and 30 percent more water by 2030, numerous challenges are expected to arise, including water and energy shortages (Yang et al., 2016).
In Iran, as in many other countries, the agricultural sector is not only a consumer of energy but also a significant energy supplier. As the main food producer in Iran, the agricultural sector faces the dual challenge of limited production resources on one hand and the responsibility to ensure food security for a growing population on the other. Therefore, the sustainable utilization of production resources is crucial for achieving sustainable development development (Hoolohan et al., 2018; Chai et al., 2020; Fabiani et al., 2020). Given these circumstances, the integrated management of limited water and energy resources becomes a critical concern for meeting the increasing food demand in the agricultural sector (Flammini, 2014). It is essential to develop strategies and practices that optimize the use of water and energy resources in agriculture while ensuring sustainable production and addressing environmental concerns. This can include adopting efficient irrigation techniques, promoting renewable energy use in farming operations, implementing precision agriculture practices, and integrating resource optimization approaches such as multi-objective programming (MOP) to find the best allocation of resources (Tian et al., 2018). By addressing the challenges of water and energy scarcity in the agricultural sector through sustainable resource management, it is possible to enhance food security, reduce environmental impacts, and contribute to overall sustainable development goals.
The primary driver behind global and national resource challenges lies in the inefficient and fragmented nature of the energy, water, and environmental sectors. As a result, sustainable development policies and plans now emphasize the integration of these three sectors. This has given rise to a new discipline called the energy-water-environment nexus, which seeks to provide comprehensive solutions for major global challenges. Experts argue that independent policymaking in any of these sectors is flawed and can have significant consequences for human wellbeing and national stability. They stress that true sustainability can only be achieved by adopting an integrated framework that considers the interconnectedness of energy, water, and climate (Li et al., 2016).
By adopting an integrated approach, policymakers can gain a deeper understanding of the intricate relationships between energy, water, and the environment. This understanding enables the development of more effective and holistic solutions that address multiple challenges simultaneously. It also helps identify synergies and trade-offs between different sectors, allowing for more efficient allocation of resources and improved decision-making. The energy-water-environment nexus approach underscores the importance of collaboration and cooperation among stakeholders from various sectors to achieve sustainable development goals. It recognizes that the challenges we face are interrelated, and therefore, integrated solutions are indispensable in ensuring a sustainable and resilient future for both human societies and the environment. By incorporating energy, water, and climate considerations into policymaking, significant progress can be made in overcoming resource challenges and promoting sustainable development. In the coming decades, the world will experience population growth and shifts in the food regime, leading to an increased demand for food, water, and energy in food production. These three elements are fundamental to human survival and are intricately interconnected. Water production relies on both water and energy resources, while energy generation requires water. Additionally, access to water necessitates energy, and food can also be used as an energy source. Given these interdependencies, it is crucial to consider all three systems together when making decisions (Sadeghi et al., 2020).
As the global population continues to grow, ensuring an adequate supply of water, food, and energy will be one of the most significant challenges we face. Agriculture, as the primary food producer, not only consumes substantial amounts of water and energy but also plays a crucial role in energy production. The introduction of the Water-Energy-Food (WEF) nexus approach at the Bonn Conference in 2011 has brought global attention to the need for integrated and sustainable development (Hoff, 2011; Kurian, 2017).
The WEF nexus approach recognizes the comprehensive and integrated relationships between water, energy, and food systems. It emphasizes the importance of considering these interdependencies in planning and managing resources. By adopting a holistic approach and understanding the synergies and trade-offs between these systems, policymakers can develop effective strategies to ensure the sustainable provision of water, food, and energy for future generations. The WEF nexus approach has become a valuable framework for guiding sustainable development policies and practices. It encourages collaboration among stakeholders from different sectors to achieve integrated and balanced outcomes. By taking into account the complex relationships between water, energy, and food, we can address future challenges and work towards a more sustainable and resilient future.
In a broader sense, systemic thinking aims to identify the interconnections among service flows associated with various sectors related to the environment, water, food, land, and energy. It is worth noting that Iran has the highest energy consumption intensity among all countries. This high energy consumption is accompanied by a significant energy waste estimate of over 50 percent, particularly in non-productive sectors. This waste can be attributed to the relatively low prices of energy carriers in Iran and the country’s lag in adopting updated technology compared to the rest of the world.
Considering the current rate of energy consumption, it is conceivable that Iran could potentially supply an economic volume four times greater than its current economy. However, it is important to recognize that this higher intensity of energy consumption exacerbates environmental emissions. Therefore, addressing energy efficiency and adopting cleaner technologies are crucial steps towards reducing environmental impact and promoting sustainable development in Iran. By improving energy efficiency and embracing updated technology, Iran can work towards achieving a more sustainable and environmentally conscious approach to energy consumption and economic growth. "Based on a comprehensive analysis of global data undertaken by the International Energy Agency, it becomes evident that the fuel distribution system in Iran suffers from inefficiency. This lack of efficiency has consequently resulted in reduced energy efficiency throughout the country. Remarkably, the intensity of energy consumption in Iran surpasses the global average energy intensity by a factor of three (Anonymous, 2018)".
Local pollutants, such as NOx, SOx, CO2, and suspended particles, have significantly contaminated the atmosphere of urban areas. Furthermore, the emissions of greenhouse gases (GHGs) are disproportionately high in relation to Iran’s population and economy (Sadeghi et al., 2020; Kellner, 2023). However, research on the Water-Energy-Food (WEF) network within a comprehensive framework is still in its early stages. The majority of studies have primarily focused on uncovering correlations and mechanisms related to water, energy, and food systems (Howells et al., 2013; Ringler et al., 2016; Singh, 2017; Cai et al., 2018).
1.1 Review of literature
Numerous studies have been conducted to examine the Water-Energy-Food (WEF) nexus, utilizing various indicators for this purpose. Below are some examples of studies conducted in the field of WEF network: Various approaches have been employed to optimize Water-Energy-Food Nexus (WEFN) systems. These methods include the use of random optimization in research studies Namany et al. (2018) and the application of multi-objective optimization techniques (Li et al., 2019b) Multi-objective optimization is a subset of multi-criteria decision-making and is utilized to address problems with conflicting objectives. These approaches aim to find an optimal solution for a set of objective functions simultaneously (Campana et al., 2018; Nie et al., 2019) In their study, Radmehr et al. (2021) utilized multi-objective optimization techniques to maximize the benefits of the agricultural, urban, and industrial sectors while minimizing the utilization of groundwater resources. They carefully considered the constraints imposed by water and energy resources. Through the application of this approach, they derived a set of solutions that were Pareto optimal. Additionally, they employed multi-criteria decision-making methods and relevant indicators related to the Water-Energy-Food Nexus (WEFN) to select the most optimal solution and propose an appropriate cropping pattern".
In their study on designing an integrated local production system and investigating the Water-Energy-Food (WEF) nexus, Leung Pah Hang et al. (2016) employed the partial equilibrium approach to explore the development of engineering system tools in conjunction with the concept of energy-using resources for the purpose of designing a local production system. The proposed method, which encompasses the fundamental principles for designing a local production system, presents a case study on the integrated design of the WEF nexus within the ecosystem of a city in the UK.
In their study Artioli et al. (2017) used the partial equilibrium approach to investigate the WEF nexus to provide an integrated plan and implications for urban governance and reported that the political tests and its results and challenges can be used beyond the water, energy, and food sectors to distribute expertise and coordinated capacity. Similarly, Rasul and Sharma (2016) explored the nexus approach to WEF security as a potential adaptation option for addressing climate change. They revealed that the nexus approach has the potential to facilitate the development of national plans and decisions for sustainable adaptation support. By promoting cooperation, minimizing resource trade-offs, and increasing political coherence among the three sectors, the nexus approach can contribute to maximizing the effectiveness of adaptation efforts.
In their study, Wicaksono and Kang (2019) investigated the Water-Energy-Food (WEF) nexus through simulation in South Korea and Indonesia. The research aimed to assess the reliability of resources and evaluate the study outcomes in these two countries. The findings demonstrated that simulation models can effectively analyze the security of water, energy, and food resources under future conditions with a high level of reliability. These models can be valuable tools for decision-makers, providing them with important insights to inform their decision-making processes.
The studies conducted by Esteve et al. (2015) have indeed contributed to our understanding of the WEF nexus, particularly in the context of the Guadiana basin in Portugal and Spain. Their use of nonlinear mathematical programming models provided insights into the complex interdependencies between water, energy, and environmental systems in that region.
The concept of the WEF nexus has gained significant traction in both developed and developing countries, as it recognizes the interconnectedness of these systems and promotes integrated planning and management. Rather than treating water, energy, and environmental control as separate entities, the nexus approach emphasizes the need for holistic and coordinated strategies to address the challenges and opportunities that arise from their interconnections.
Iran, given its geographical conditions and the impacts of industrial and economic development, faces significant water, energy, and climatic challenges. The country’s unique position at the center of these challenges underscores the importance of adopting a comprehensive approach like the WEF nexus. By considering the interdependencies and synergies between water, energy, and climate systems, Iran can enhance its resilience, improve resource efficiency, and mitigate the negative impacts of development.
The application of the WEF nexus in Iran can help identify innovative solutions to address water scarcity, energy security, and climate change. By integrating planning and management strategies across these sectors, Iran can work towards a more sustainable and balanced approach to development, ensuring the efficient use of resources while minimizing environmental degradation.
The interactions between these sectors are complex, and making decisions for each sector in isolation without considering their interrelationships and interactions can have critical consequences. (Bizikova et al., 2014). Considering the arid to semi-arid climate in Middle Eastern countries, including Iran, there are substantial challenges in terms of water supply and distribution, especially in the agricultural sector. Therefore, it is crucial to develop strategies that ensure the efficient use of water resources.
Therefore, this study aims to investigate the water-energy-food nexus in the Sistan plain, located in the southeast of Iran, with specific goals and limitations different from previous studies. This study contributes to the existing literature in two main aspects. Firstly, the water-energy-food nexus approach has not been previously applied to the agricultural sector in this basin. Secondly, a novel method for calculating environmental pollutant variables has been introduced, allowing for a better understanding of the interaction between environmental and economic variables. This calculation method is particularly useful considering the limited data available on greenhouse gas emissions from agricultural activities. Nexus thinking is a crucial approach to achieve a sustainable balance in resource production and consumption, offering advantages such as improved resource efficiency and mitigation of adverse impacts resulting from single-sector development policies. The model employed in this study addresses the following hypotheses:
• Hypothesis 1: Implementing WEF nexus management will lead to a reduction in greenhouse gas (CO2) emissions in the Sistan plain.
• Hypothesis 2: The proposed model will result in changes to the cultivation areas of crops compared to the current situation.
• Hypothesis 3: The application of the WEF nexus approach will decrease irrigation water consumption.
• Hypothesis 4: The reallocation of agricultural production resources will increase farmers' gross profits in the Sistan plain.
• Hypothesis 5: WEF nexus management will improve food security.
• Hypothesis 6: The utilization of the proposed model will reduce energy consumption in crop production.
The aim is to provide valuable information that can contribute to the development of appropriate strategies for mitigating environmental impacts and increasing farmers' income in the study area.
1.2 Case study
The Sistan plain, which is the focus of this study (Figure 1), encompasses over 135,000 ha of arable land. However, only 50–70 percent of this land is currently cultivated with agricultural and horticultural crops, depending on the allocated water resources. The region is bordered by Afghanistan to the east and north, Nosratabad County in Sistan and Baluchistan province to the south, and Nehbandan County in South Khorasan province and Dasht-e Lut to the west. Approximately 150,000 ha of the plain’s land holds agricultural potential and can be utilized for food production. The Sistan plain experiences a hot and arid climate according to various climatic classifications. The Sistan plain has a mean annual temperature of 21°C, a mean relative humidity of 38 percent, and receives an average precipitation of about 58 mm. This amount accounts for approximately 25% of the mean precipitation in Iran and only 7% of the mean global precipitation. Consequently, the limited precipitation has little significant impact on improving agriculture in this region. Moreover, the annual evapotranspiration in the Sistan plain is estimated to be 80 times higher than its precipitation, reaching levels of 4500–5,000 mm. One of the most notable meteorological phenomena in the area is the 120-day winds, which typically blow from northwest to southeast, starting in early June and lasting until late September. These winds, with speeds exceeding 100 kg/h, contribute to sand transfer, worsen erosion, and increase evaporation, among other effects (Anonymous, 2018).
The river’s drainage basin is situated in Afghanistan. It enters Sistan from Afghanistan along the eastern border of Iran. Originating from the southern foothills of the Hindu Kush Range near Kabul, the river travels approximately 1000 km within Afghanistan with an average slope of 1 m/3 km. It enters Iranian territory around 1 km upstream of Doroudi. To meet a portion of Sistan’s water demand, the Chahnimeh reservoirs are supplied by the Hirmand River. Chahnimeh refers to natural pits that serve as reservoirs for floodwater after undergoing minor construction modifications. Currently, there are three Chahnimeh reservoirs in the region, covering an area of 4700 ha and having a combined approximate capacity of 600 million cubic meters. These reservoirs have been in use since 1982. The decline in crop cultivation in the Sistan region can be attributed to the lack of reliable water resources. Over the years, water resources in the region have been overexploited due to a decrease in rainfall caused by the climatic conditions of the area. This has led to the drying up of surface and seasonal streams, including the Hirmand River, as well as a decline in the water level of the International Hamun Wetland and Chahnimeh reservoirs. Additionally, the depletion of freshwater springs has contributed to the scarcity of water resources. On the one hand, Sistani farmers have engaged in agricultural activities such as wheat, barley, watermelon, and melon cultivation to maximize their economic profits. These activities heavily rely on the limited water resources available in the region. On the other hand, the inefficient use of water resources by farmers, who predominantly use traditional irrigation methods like flood and furrow irrigation, further exacerbates the issue. Smallholder farmers, who form the majority in the region, often lack the financial means to adopt modern irrigation systems like drip and sprinkler systems. Moreover, suboptimal cropping patterns at the farm and regional levels pose a challenge, as they not only threaten water resources but also contribute to soil degradation and erosion. The excessive use of chemical fertilizers and pesticides to increase crop yields, driven by economic goals, worsens water and soil pollution, degrades plant and animal ecosystems, and has adverse environmental impacts. The research site, Sistan, is located between longitudes 60°15′and 61°50′E and latitudes 30°05′and 31°28′N in the northeast of Sistan and Baluchistan province. It covers an area of 15,197 km2, accounting for 8.1% of the total area of the province (Anonymous, 2018).
Considering the critical water conditions in the Sistan region, this research endeavors to develop a Water-Energy-Food (WEF) nexus framework specifically tailored for this basin. To date, there has been a notable absence of research addressing this particular topic. Therefore, the primary objective of this research is to propose a multi-objective mathematical programming model based on the WEF nexus approach. The model aims to optimize various factors, including maximizing profit and calorie production, as well as minimizing CO2 emissions, energy consumption, and irrigation water usage in crop production. These objectives are crucial given the limitations imposed by water and land availability, ensuring an optimal allocation of resources to different crops.
2 Materials and methods
The water-energy-food (WEF) nexus optimization model utilized in this research is a multi-stage socioeconomic model that relies on linear mathematical programming, as highlighted by Zhang and Vesselinov (2016). This model enables the prediction of WEF production costs over multiple time periods, taking into account resource availability, as well as social, economic, and environmental demands. The WEF forecasts are derived through a straightforward algorithm aimed at achieving optimal solutions, as explained by Bizikova et al. (2014). In the scientific literature, optimization methods for the WEF nexus are classified into various categories, including multi-criteria, multi-objective, competing objectives, and multi-attribute approaches. These methods share the common characteristic that reaching a unanimous agreement on a specific goal is not easily attainable.
"The coefficients used in the proposed research model, aiming to minimize CO2 greenhouse gas emissions, are obtained from the study conducted by Li et al. (2019b) To calculate the energy consumption of inputs in the agricultural sector, the energy equivalents of various factors involved in production are taken into account. These factors include labor, fuel, water, seeds, chemical fertilizers, pesticides, and agricultural machinery. Therefore, multiple sources, including studies by Morankar et al. (2013), Yilmaz et al. (2005) and Gündoğmuş (2006), have been used to estimate the energy consumption of inputs in agricultural crop production in the Sistan basin."
2.1 The conceptual framework of the nexus model
Figure 2 illustrates the WEF nexus, which highlights the interconnectedness of water, energy, and food systems. According to this nexus, water plays a crucial role in irrigating crops, processing food, and generating energy. Similarly, energy is utilized in various agricultural activities such as collecting, transferring, pumping, and discharging irrigation water, as well as fertilization, tillage, and crop harvesting.
The nexus triangle in Figure 2 serves as the basis for this research, aiming to propose a multi-objective mathematical programming model using the WEF nexus approach in the Sistan plain study site. This study site includes Zabol, Zahak, Hirmand, and Hamun and faces limitations in water and land resources for optimal distribution to crops. The model aims to maximize profit, maximize calorie production, minimize CO2 emissions, reduce energy consumption, and minimize irrigation water usage for crop production.
The key outputs of the WEF nexus model are the variables related to cultivation area, energy allocation, surface water allocation, system profit, and the rates of P, N, and K fertilization for each region. Figure 2 emphasizes the importance of optimal utilization of limited water, energy, and land resources in agricultural systems, particularly in developing countries that heavily rely on agriculture.
Figure 2 provides a conceptual framework for the nexus model in this research. The primary objective is to propose a mathematical programming model using the multi-objective mathematical programming (MOP) technique for the WEF nexus, which can facilitate managerial decision-making. The model not only addresses economic considerations but also focuses on controlling greenhouse gas (GHG) emissions.
The counties of Zabol, Zahak, Hirmand, and Hamun are of particular importance as they are major crop-producing regions within the study site. To gather data for the research, various sources were utilized, including the Agriculture Organization, journals, reports, agricultural statistical annals for 2018–2019, as well as interviews with experts from each county and consultation companies, ensuring comprehensive coverage.
The main goals of the proposed model are to minimize water consumption, energy consumption, and CO2 emissions while simultaneously enhancing the economic profitability of the system and increasing crop calorie production in the Sistan plain. This multi-objective approach aims to optimize various aspects of the agricultural system, promoting sustainability and efficiency.
“The research was conducted in three main phases, following the research algorithm. In the initial phase, data was collected from various organizations. The subsequent phase focused on examining the relationships and interactions among the water, energy, and food systems, forming the WEF nexus triangle. Finally, the model’s outcomes were determined based on the objectives and limitations within the selected watershed. The study targeted the implementation of the proposed model in the cities of Zabol, Zahak, Hirmand, and Hamun. Due to the recent separation of the Nimiruz district and the decentralized presence of relevant agricultural offices, data from both Nimiruz and Zabol were merged for this research. Furthermore, the study specifically emphasized agricultural products, particularly common crops such as wheat, barley, alfalfa, onions, melons, and watermelons, which are extensively grown in the region.”
2.1.1 Multi-objective programming (MOP) model
The proposed model encompasses the cultivated lands of the Sistan plain. In this model, the water needed for crop irrigation is sourced from surface water resources. Electricity is utilized to extract and pump the required water for food production. Additionally, surface water resources and electricity are essential for food production and processing. Greenhouse gas emissions, particularly CO2, arise from activities like power generation, food production, irrigation, fertilization, and pesticide application. The subsequent section introduces the decision variables, objective functions, and constraints of the mathematical programming model. The collected data and statistical information from relevant organizations and agencies in the study site were organized using the MS Excel software package. Afterward, the data underwent analysis using the proposed mathematical programming model in the GAMS (ver. 24.1) software package. This software package is highly proficient in solving various types of problems, including linear programming, nonlinear programming, mixed integer programming, mixed integer nonlinear programming, and linear complementary problems.
2.1.2 Objective functions
“The objective functions and constraints of the proposed model are derived from studies conducted by (Li and Guo, 2014; Li, Fu, Singh, Liu, et al., 2019b). These studies provide the foundation for determining the goals and limitations incorporated into the model. By referring to the findings and methodologies presented in (Li et al., 2019b) research, the proposed model is designed to align with established scientific knowledge and best practices in the field”. In the WEF nexus approach, the modeling conducted in this research takes into account the economic, social, and environmental dimensions. It aims to address various objectives related to the water, food, and energy systems, considering their different aspects. The model’s flexibility enables the pursuit of diverse objectives from different dimensions, even in situations where decision-makers may have differing viewpoints. This research focuses on five objectives, which are to maximize gross profit and calorie production from foods, and to minimize CO2 emissions, irrigation water usage, and energy consumption. Before presenting the algebraic form of the mathematical programming model, the symbols used in it are described (Table 1). The algebraic forms representing these objectives are presented in Eqs 1–5. These equations provide a mathematical representation of the goals the model aims to achieve.
The first objective of the model is to maximize the gross profit of the system. This objective takes into account the total revenue generated from crop production while deducting the total costs involved. These costs include the expenses associated with water usage for food production, energy usage for food production, and water usage for crop production. By maximizing the gross profit, the model aims to optimize the financial outcomes of the system and ensure efficient resource allocation.
The second objective of the model is to increase crop calories. Considering the regional conditions, ensuring food security and environmental preservation necessitates the substitution of crops that are strategically and nutritionally valuable, while also having lower water and energy requirements. By focusing on increasing crop calories, the model aims to enhance the nutritional value of food production while simultaneously optimizing resource efficiency to support sustainable agricultural practices.
The third objective of the model is to alleviate greenhouse gas (GHG) emissions. This objective is driven by the need for environmental conservation and pollution reduction in the agricultural sector. The model aims to reduce GHGs, including carbon dioxide (CO2), methane (CH4), and other relevant gases. By focusing on minimizing GHG emissions, the model contributes to efforts aimed at mitigating climate change and promoting sustainable agricultural practices that have a lower environmental impact.
The fourth objective of the model is to reduce the consumption of surface water for crop production in the Sistan plain. This objective is motivated by the regional conditions, including recent droughts and the lack of groundwater tables in the area. Recognizing the importance of water conservation, the model aims to minimize the use of surface water resources. By focusing on reducing surface water consumption, the model contributes to sustainable water management practices in the region, addressing the challenges posed by water scarcity and promoting long-term water security.
The fifth objective of the model is to reduce total energy consumption. Crop production requires energy for various purposes, such as labor, machinery, diesel, fertilizers, pesticides, and more. Taking into account the energy equivalents of these inputs, this objective aims to minimize the overall energy usage in the system.
Considering these multiple objectives, the model is developed within the framework of Multi-Objective Programming (MOP). By incorporating and balancing these objectives, the model aims to find optimal solutions that simultaneously address the goals of maximizing profit, increasing crop calories, alleviating GHG emissions, reducing surface water consumption, and minimizing energy consumption. This approach allows for a comprehensive analysis of the system, considering the trade-offs and synergies between these objectives to support decision-making processes.
2.1.3 Constraints
The objective functions in the model are subject to certain constraints, which are outlined below. One of the constraints relates to the allocation of surface water to crops in each region. It ensures that the total amount of surface water allocated to all crops does not exceed the available surface water resources from various water supply projects, such as the water transfer project, the water storage project, and the water exploitation project. This constraint is mathematically expressed in Eq. 6 of the model. By incorporating this constraint, the model ensures that the allocation of surface water remains within the sustainable limits of the available water supply projects.
Energy usage in the model primarily encompasses activities such as surface water abstraction, groundwater pumping, drainage, and irrigation. To ensure sustainable energy consumption, it is important to adhere to certain constraints. Specifically, the electricity consumed should not exceed the allowable quantity for each subarea and the total available energy resources.
Given the scarcity of underground water in the Sistan plain, the energy used in this region is solely dedicated to irrigation water. This constraint can be expressed as follows in the model formulation.
The maximum and minimum arable land for all crops are determined by Eq. 9 in the model. This equation sets the upper and lower bounds on the land area that can be allocated to each crop. By defining these limits, the model ensures that the allocation of land resources for crop production remains within the specified range. These bounds play a crucial role in optimizing the allocation of arable land and achieving the objectives of the system.
Eventually, the nonnegative set of the model variables is given in Eq. 10.
2.1.4 Nomenclature
Here is a list of symbols commonly used in the model for defining sets, variables, and parameters: These symbols are commonly used to formulate and solve optimization models for crop allocation, water allocation, energy consumption, and soil moisture management in agricultural systems.
2.2 Data processing
According to the report by the Agriculture Jahad Organization of Sistan and Baluchistan, the Sistan plain has a total of 135,000 ha (ha) of arable land. However, only 50–70 percent of this land is cultivated by agricultural and horticultural crops, depending on the amount of water allocated. The decline in cultivation area is primarily attributed to fluctuations in the flow rate of the Hirmand River and the lack of reliable water resources in the region (Anonymous, 2018).
Table 2 in the report provides information about the cultivation areas of agricultural and horticultural crops in the Sistan region, as well as the share of the plain in the total agricultural and horticultural production of Sistan and Baluchistan province.
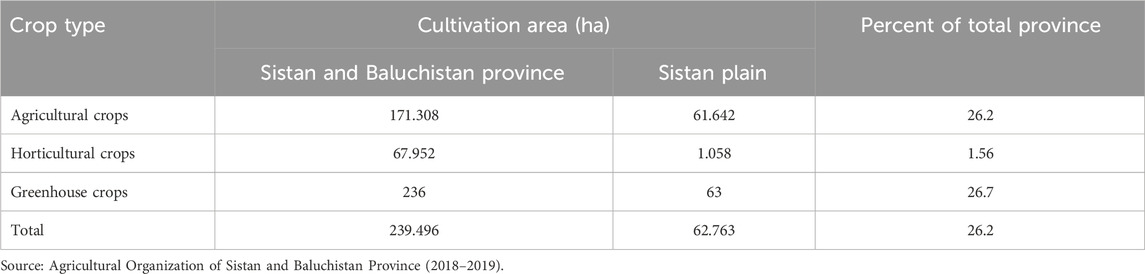
Table 2. The total cultivation area of agricultural and horticultural crops in the Sistan plain in the base year (2018–2019).
According to the Agricultural Organization of Sistan and Baluchistan Province’s report for the year 2018–2019, here are some key findings:
Crop Cultivation: The report provides information on the cultivation areas of various crops in the province. This includes both agricultural and horticultural crops.
Production Share: The report also highlights the share of the Sistan plain in the total agricultural and horticultural production of the province. This gives an understanding of the contribution of the plain to the overall agricultural output in the region.
Water Allocation: The report might discuss the allocation of water resources for agricultural purposes in the province. It may highlight the challenges faced due to fluctuating flow rates of the Hirmand River and the shortage of reliable water resources.
Agricultural Performance: The report might include data on the overall performance of the agricultural sector in terms of yields, production, and productivity. This information can provide insights into the state of agriculture in Sistan and Baluchistan Province.
Based on the results, the main crops investigated in this study were wheat, barley, alfalfa, onion, melon, and watermelon, as shown in Table 3. The data on crop yields revealed that melon and watermelon had the highest yields, with 19 tons per hectare (t/ha), while barley had the lowest yield of 2.6 t/ha. Among the studied crops, barley had the lowest rate of pesticide application, which includes herbicides, insecticides, and pesticides.
In terms of calorie production per hectare, barley had the highest value at 3,890 kg/ha, while watermelon had the lowest value at 200 kg/ha. Wheat and barley had the lowest input costs, which include fertilizers, pesticides, machinery, labor, and land.
Regarding water requirements, alfalfa had the highest water requirement at 12,100 cubic meters per hectare (m3/ha), while barley and wheat had lower water requirements at 3,240 m3/ha and 3,520 m3/ha, respectively.
These findings provide insights into the yields, pesticide application rates, calorie production, input costs, and water requirements of different crops studied in the region.
3 Results
The study in the Sistan plain proposed a nonlinear programming model based on the multiple objectives of the Water-Energy-Food (WEF) nexus approach for different crops. The data were calculated by comparing the total variations in the multi-objective cultivation area with the current conditions, as shown in Table 4.
According to the results, the multi-objective cultivation area showed a decrease in various regions compared to the current conditions. Specifically, Zabol, Zahak, Hirmand, and Hamun experienced decreases of 5.81%, 1.77%, 16.41%, and 11.43% respectively. It is important to note that the variations in the cultivation area were analyzed separately for each crop and region.
Interestingly, the study also revealed slight increases in the cultivation areas of wheat in Zabol and Zahak, as well as an increase in the cultivation area of barley across all regions.
These findings provide insights into the changes in the multi-objective cultivation areas for different crops in the Sistan plain, highlighting the potential impacts on water, energy, and food resources within the WEF nexus framework.
The observed increase in the cultivation areas of wheat and barley in Zabol and Zahak can be attributed to higher rates of water and input consumption, as well as reduced input costs for these crops. Additionally, in Zahak, the multi-objective cultivation areas of alfalfa and onion also experienced slight increases compared to the current cultivation pattern.
Similar slight increases were observed in the cultivation area of watermelon in Hirmand and melon in Hamun. These findings suggest potential shifts in crop choices and cultivation patterns based on the multi-objective approach of the study.
In Zabol, Zahak, Hirmand, and Hamun counties, the study identified the lowest total cultivation areas for objectives such as minimum water consumption, minimum CO2 emission, and minimum energy consumption. These were calculated as 2988.99 ha, 65.99 ha, 3963.405 ha, and 3599.94 ha, respectively.
On the other hand, the highest total cultivation areas were observed for the objectives of profit maximization and calorie maximization. These were determined as 9797.128 ha, 10544.67 ha, 17634 ha, and 14161.24 ha, respectively, in the mentioned counties.
These results provide insights into the varying cultivation areas based on different objectives, such as water consumption, CO2 emissions, energy consumption, profit maximization, and calorie maximization, for the different regions of Zabol, Zahak, Hirmand, and Hamun.
Based on the results presented in Table 4, it was observed that there were declines in the total cultivation areas for the studied crops in all four regions: Zabol, Zahak, Hirmand, and Hamun. These declines were consistent across the goals of minimum water consumption, CO2 emission, and energy consumption, indicating limitations for cultivating these crops in terms of economic, environmental, and other studied objectives.
However, it is important to note that contrasting trends were observed for the goals of maximum profit and maximum calories, where the cultivation areas showed increases. This suggests that from an economic and nutritional perspective, there may still be potential for cultivating certain crops in these target counties.
Overall, these findings highlight the challenges and limitations associated with cultivating the studied crops in terms of the economy, environment, and other objectives. Further analysis and consideration of alternative approaches may be necessary to address these limitations and optimize the cultivation patterns in these regions.
Crops play a crucial role in ensuring food security for societies, and in the future, arable lands are expected to be a more limiting factor for food production compared to other resources (Tichenor et al., 2017).
Therefore, it is essential to enhance the efficiency of their utilization. The optimization of the mathematical programming model, considering the Water-Energy-Food (WEF) nexus, leads to economic improvements on one hand, and the optimization of chemical fertilization, cost reduction, and GHG emission reduction on the other.
In light of this, allocating more cultivation areas to wheat and barley can increase profits and reduce environmental impacts in the study site. The proposed model in this study not only focuses on profit maximization but also takes into account the goals of reducing GHG emissions and energy consumption.
Table 5 presented important variables and their variations for the mathematical modeling of the Water-Energy-Food (WEF) nexus. The results indicated a decrease in the cultivation areas of the target crops in Zabol, Zahak, Hirmand, and Hamun. The reasons for this decline can be attributed to regional climate change and the reduction of input water from Chahnimehs. Additionally, according to Table 5, there was a descending trend in the difference in available energy in the multi-objective model across Zabol, Zahak, Hirmand, and Hamun. On the other hand, the rate of fertilizer consumption (N, P, and K) showed an ascending trend in the studied counties. Given the decline in cultivation areas, one of the main techniques employed to increase production and profit has been the excessive use of fertilizers and pesticides. This excessive use is aimed at boosting production and yield, which explains the increase in this parameter.
These findings highlight the trade-offs and challenges associated with the WEF nexus in the study area. While excessive fertilizer and pesticide use may lead to short-term gains, it is important to consider the long-term environmental and sustainability implications. Balancing the use of inputs while optimizing production and profit is crucial for achieving sustainable and efficient agricultural practices in the region. It is important to highlight that in the multi-objective (MOP) model, GHG emissions in Zabol decreased by 0.00295 (0.18%) compared to the current model. This indicates that the optimal Water-Energy-Food (WEF) nexus model will effectively reduce GHG emissions in Zabol, Zahak, Hirmand, and Hamun.
Furthermore, Table 5 demonstrates that crop calories increase in the MOP model compared to the current conditions in Zabol, Zahak, Hirmand, and Hamun by 11.55%, 33.03%, 15.75%, and 26.84%, respectively. This suggests that the implementation of the mathematical programming pattern of the WEF nexus in the Sistan plain study site not only facilitates sustainable resource management but also promotes increased crop calorie production in the mentioned areas.
Overall, by considering the available resources and emphasizing environmental conservation, the implementation of the optimal WEF nexus model in the study site of the Sistan plain would contribute to the reduction of water, food, and energy consumption. This approach ensures a more sustainable and efficient use of resources while addressing the environmental conservation goals.
In the study, economic productivity was assessed by dividing the gross profit in each region by the corresponding target variable in the model. For instance, the economic productivity of water in the model was calculated by dividing the gross profit in each region by the amount of water consumption (as shown in Figure 3).
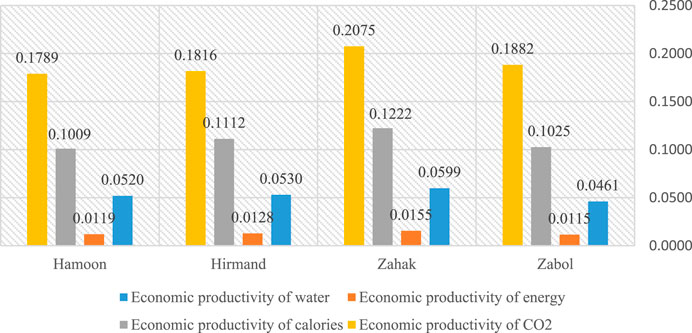
Figure 3. The economic productivity of some variables in optimal MOP conditions in different regions.
Based on the results, the CO2 variable exhibited the highest economic productivity in the optimal model. Specifically, the economic productivity of this variable was found to be higher in Zahak compared to the other regions. On the other hand, the variable of energy had the lowest economic productivity across all regions, with Zabol having the lowest economic productivity for this variable.
These findings provide insights into the economic efficiency and productivity of different variables within the WEF nexus framework. It indicates the potential for economic gains and highlights the variation in economic productivity across different regions and variables. Such information can be valuable for decision-making and resource allocation strategies to enhance economic performance while considering the water, energy, and food interlinkages.
Figure 3 displays the physical productivity of key variables within the proposed model’s approach. Physical productivity can be estimated using a straightforward method, such as performance per volume unit. For instance, the physical efficiency of water consumption can be determined by calculating the amount of product produced per unit volume of water consumed, typically expressed in kilograms per cubic meter.
According to Figure 4, Zahak city exhibits the highest physical productivity among all variables, with a value of 190,805.9 kg MJ per hectare related to the energy variable. On the other hand, Zabol city demonstrates the lowest physical productivity, with a value of 11,727 kg CO2 per hectare associated with the CO2 variable.
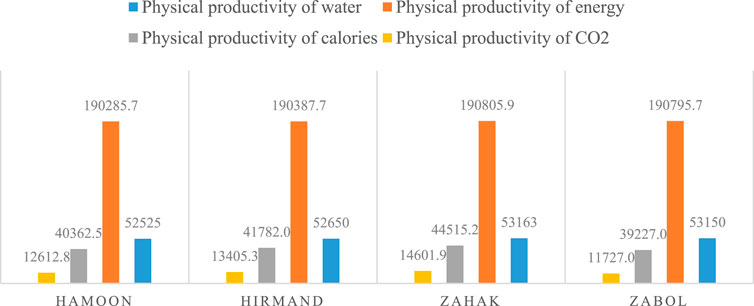
Figure 4. The physical productivity of some variables in optimal MOP conditions in different regions.
These physical productivity measurements provide insights into the efficiency and output achieved per unit of input within the WEF nexus framework. It indicates the varying levels of physical productivity across different variables and regions, shedding light on the potential gains and performance disparities. These findings can aid decision-making processes and resource management strategies to enhance physical productivity while considering the interdependencies of water, energy, and food systems.
The implementation of the WEF nexus approach resulted in a reduction of greenhouse gas (GHG) emissions from 0.01494 to 0.01301 million t/ha. Before the implementation of the nexus approach, GHG emissions were at a high level in almost all counties. However, after its implementation, GHG emissions decreased in all counties, as shown in Figure 5. It can be inferred that there was a 0.49 percent decrease in GHG emissions after implementing the nexus approach compared to the current conditions. This reduction indicates the positive impact of the WEF nexus approach on mitigating GHG emissions and addressing environmental pollution. The optimal WEF nexus model’s implementation in Zabol, Zahak, Hirmand, and Hamun played a significant role in reducing GHG emissions and mitigating environmental pollution. These findings highlight the potential of the nexus approach as a valuable tool for achieving sustainability goals and improving environmental performance in the water-energy-food sectors.
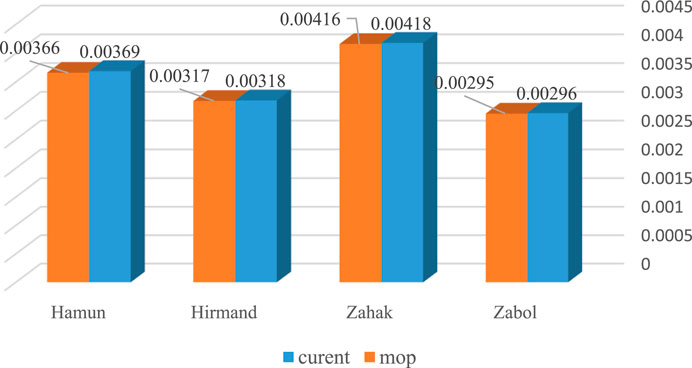
Figure 5. The comparison of greenhouse gas (GHG) emissions before and after implementing the Water-Energy-Food (WEF) nexus approach in the regions of Zabol, Zahak, Hirmand, and Hamun has been conducted.
"Today’s modern agriculture is centered around managing water resources and reducing CO2 greenhouse gas emissions and environmental pollutants. In order to accomplish this, it is crucial to minimize energy input consumption in the agricultural sector, as demonstrated by the findings of the current research through the proposed approach in the study area. The model’s results indicate that reducing energy input consumption leads to a decrease in CO2 greenhouse gas emissions in the basin. Consequently, reducing environmental pollutants in the study area can have positive long-term effects on the economic, environmental, and resource management sectors. The outcome of this model contributes to the attainment of sustainable agricultural development goals by optimizing the continuous and sustainable utilization of resources and production factors".
In a broader context, it can be argued that this approach ensures the optimal utilization of water, energy, and food resources while promoting sustainable and equitable access to these resources and mitigating environmental pollution. Consequently, this approach contributes to the attainment of sustainable development goals.
4 Discussion
This section discusses the research hypotheses and compare them with the results of other studies (Table 6).
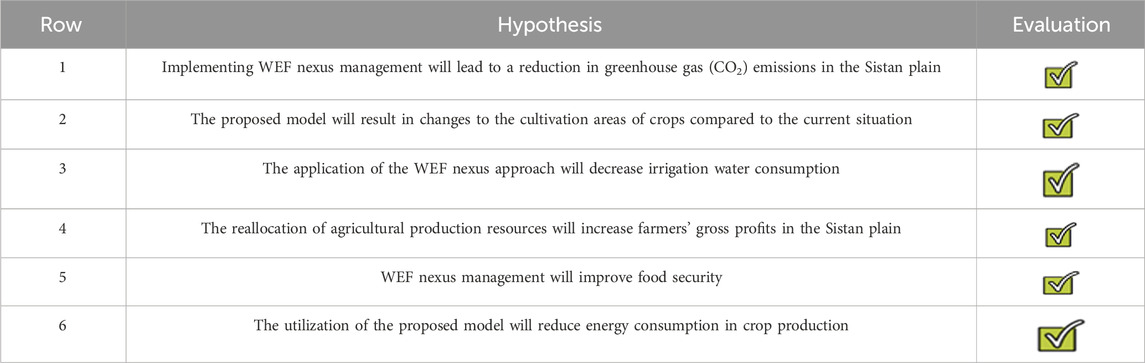
Table 6. Evaluation of hypotheses based on confirmation or disconfirmation of the results obtained from the proposed model.
4.1 Reduction of GHG emissions
The concept of the Water-Energy-Food (WEF) nexus has been explored in previous research at various levels, including the country (Wang et al., 2018; Jin et al., 2019; Xu et al., 2019), province, or basin (Chen et al., 2018; Li, Fu, Singh, Ji, et al., 2019a; Si et al., 2019). Given that the present study observes a reduction in energy consumption for food production, it is expected that this reduction would contribute to sustainable development and environmental protection in the agricultural sector, leading to a decrease in CO2 greenhouse gas emissions. According to the results, under optimal conditions, CO2 emissions in the Sistan plain have decreased by 0.49 percent compared to the current conditions. Furthermore, under the goal of CO2 minimization, this decrease amounts to 67.7 percent compared to the current status. This significant difference is attributed to the trade-off between objectives in the proposed multi-objective model, where weights are assigned to each objective. In other words, in the proposed model for the Sistan region, the reduction in cultivation areas, irrigation water consumption, and energy input consumption all contribute to the reduction in CO2 emissions. Some studies have reported even greater reductions in CO2 emissions (Xie et al., 2017; Yu et al., 2019). For instance, Li et al. (2019a) conducted a study with the objectives of maximizing profit and minimizing CO2 emissions, and they achieved a reduction of 20.5 percent in greenhouse gas (GHG) emissions. This reduction in GHG emissions contributes to a more sustainable and less polluted environment. Therefore, the hypothesis of a decrease in GHG emissions, specifically CO2 emissions in this research, is supported.
4.2 Variations in cultivation areas
One crucial factor in farmers' adaptation to various resource and climatic constraints is changing the cropping pattern, which can shed light on different aspects of the Water-Energy-Food (WEF) nexus. In all of the studied areas, the total cultivated area and irrigation water usage have decreased. This indicates that a reduction in available water can lead to a decrease in the cultivated area. Since one of the objectives of the model is to maximize gross profit, the model approach ensures that the cultivated area does not shift towards crops that require more water. Instead, the cropping pattern is shifted towards crops like wheat and barley, resulting in a decrease in the total cultivated area in the study area. Specifically, we observed an 8.4% decrease in the total cultivation area in the Sistan plain. Researchers such as (Leung Pah Hang et al., 2016; Xu et al., 2022) suggest that a decline in the cultivation area is a common outcome when agricultural production resources are optimally utilized using different approaches. However, this decline primarily pertains to the total cultivation area, and the analysis of individual crop cultivation areas does not necessarily reveal uniform decreases or increases. For instance, in our proposed model, the cultivation areas of wheat and barley have increased in almost all regions. This finding can be attributed to the increased water consumption, input usage, and reduced input costs for these two crops. Therefore, in the study area, expanding the cultivation areas of wheat and barley would lead to higher profits, reduced environmental impacts, and decreased GHG emissions. According to Xu et al. (2022), the most significant decrease in the cultivation area was observed in grain corn, which also resulted in the highest rate of GHG emissions. In their study, they replaced grain corn with vegetable crops as a strategy to reduce environmental pollutants. Therefore, our hypothesis regarding variations in cultivation areas in the proposed WEF nexus model is supported. These variations align with the objective of decreasing the overall cultivation area.
4.3 Reallocation of irrigation water resources
In the current scenario, policymakers are increasingly focusing on integrated water management and addressing the challenge of water scarcity. Given that the Sistan plain is situated in a hot and arid region, effective water resource management is crucial across all sectors including drinking water, agriculture, and industry. However, water resource management becomes even more critical in the agricultural sector, as it is the largest consumer of water and the region is facing groundwater and treated water shortages. Therefore, it is necessary to allocate resources to crops that are less water-intensive and more productive. After implementing the WEF nexus approach, water consumption by the agricultural sector in the Sistan plain decreased from 261.62 to 260.48 million m3. The results obtained from our proposed model indicate a 0.43% decrease in water allocation to crops in the Sistan plain. This decrease in water consumption following the management of irrigation water resources has been reported in several studies, including (Tian et al., 2018; Zhang et al., 2018; Yu et al., 2019; Yu et al., 2020). Although this decline may not be substantial, it has a positive impact on water allocation planning. This finding is consistent with the study by Yu et al. (2020), which highlighted that even a 1.49% decrease in irrigation water consumption is desirable. Therefore, the results partially support our hypothesis regarding the decrease in irrigation water consumption.
4.4 Variations in gross profit
Although the implementation of the proposed model in the Sistan plain has resulted in a decrease in the cultivation area and crop production, it has led to an increase in farmers' income from 2,640,466 to 2,912,731 million IRR, representing a 10.3% increase. Similar findings have been reported by several researchers who have explored the reallocation of agricultural production resources, such as Mardani (Mardani Najafabadi et al., 2019; Wang et al., 2023). For instance, Shirzadi Laskookalayeh et al. (2022) reported a 27% reduction in crop cultivation areas, a 4.90% decrease in irrigation water consumption, and a 35% increase in gross profit. In our study, the observed increase in farmers' income can be attributed to the shift in some cities within the basin towards cultivating seasonal water-intensive crops like watermelon and melon, which provide higher profitability. It is important to note that farmers prioritize increasing their profits. Given that the proposed model assigns significant weight to the amount of water consumption based on regional conditions, it is likely that these two crops were excluded from the cultivation pattern. However, to address this contradiction and ensure that farmers' income is not negatively impacted, a series of adjustments and facilitations are necessary. Therefore, the results support our hypothesis regarding the positive effect of the proposed model on increasing farmers' gross income.
4.5 Food security and energy consumption
With the increasing population, agricultural lands play a crucial role in food production, making them the largest ecosystem for sustaining food security and ensuring survival. Strategic crops, which are pivotal for the food security of communities, become essential in this context. In the Sistan plain, wheat and barley are considered strategic crops, and it is crucial to prioritize their improvement to maintain food security. The results of our study demonstrate that the proposed model has successfully increased food calories by 23.6% through the enhanced production of strategic crops like wheat and barley, thus contributing to food security. In the past, chemical fertilizers were extensively used in agriculture to increase crop yield. However, the overuse of chemical fertilizers is not an environmentally friendly choice as it depletes soil health and poses a global threat to agricultural production. Consequently, modern agriculture has shifted towards water resource management patterns, aiming to reduce CO2 emissions and environmental pollutants. In the agricultural sector, it is necessary to minimize the consumption of energy inputs, including labor, machinery, fertilizers, pesticides, and diesel, to achieve sustainability. In the Sistan plain, energy consumption in the agricultural sector has decreased by 0.25%. This aligns with the findings of, Cansino-Loeza et al. (2022), who reported a 26% increase in crop food security, and Karamian et al. (2023), who found a 12.71% reduction in energy consumption within the agricultural sector. Thus, our study confirms the last two hypotheses, indicating an improvement in food security and a decrease in energy consumption. Overall, the proposed model has successfully contributed to both food security and sustainable agricultural practices by focusing on strategic crop production and reducing energy inputs.
5 Conclusion
“The current research has focused on the analysis and examination of agricultural products. However, it is worth noting that the proposed model can also be applied to products that have the potential to be grown but have not been cultivated yet, such as lentils and mash. Before applying the model to these new products, it is essential to conduct expert and technical research specific to these crops to ensure its effectiveness. Moreover, the proposed model can be extended to other areas that share similarities with the study basin. Comparing the results of these areas with the present research can provide valuable insights. It is important to consider that the limitations and objective functions of the model should be calibrated based on the unique characteristics of the new area. Looking ahead, the conceptual framework established in the current research can serve as a basis for future studies involving horticultural, livestock, and even medicinal products within the basin. By considering the WEFN correlation, the model can be adapted to address the specific requirements and challenges associated with these different sectors. In summary, the proposed model has potential applications for unexplored agricultural products, other comparable areas, and diverse sectors within the basin, paving the way for future research and development”.
In this research, the evaluation of the Water-Energy-Food (WEF) nexus approach was conducted in the discharge basin of Sistan under two scenarios: nexus and non-nexus. The aim of this approach is to promote sustainable development by directing investments and resources towards the sustainability of ecosystem services based on the integrated management of vital environmental resources.
Based on the findings, it can be concluded that prior to the implementation of the WEF nexus approach, water and energy consumption in the basin were suboptimal. However, after the implementation of the approach, there was a reduction in the cultivated area, leading to decreased rates of water and energy consumption and their more efficient utilization. Moreover, the implementation of the approach resulted in a shift in the cropping pattern, where water-intensive crops were partially replaced with less water-intensive alternatives.
Additionally, the implementation of the WEF nexus approach has had a positive impact on environmental conservation. Before its implementation, greenhouse gas (GHG) emissions in the Sistan plain were increasing. However, after the approach was implemented, there was a decrease in emissions. This indicates that the nexus approach not only contributes to environmental pollution reduction but also plays a role in achieving sustainable development goals, while promoting food security and optimizing the use of water and energy resources.
In summary, the WEF nexus approach, as evaluated in the Sistan basin, has demonstrated its potential to reduce environmental pollution, contribute to sustainable development, and enhance food security by optimizing the use of water and energy resources.
Based on the results of mathematical modeling of the WEF nexus, it is evident that the implementation of this approach can lead to several positive outcomes, including a decrease in crop cultivation areas, energy consumption, GHG emissions, and water consumption in certain regions. In the Sistan region, specifically, the nexus approach successfully minimized GHG emissions.
Given that the fundamental objective of the nexus is environmental conservation and the prevention of degradation, it is recommended to implement development and bio-balance policies using the WEF nexus approach to further enhance environmental conservation efforts.
In the context of the Sistan region, the following additional recommendations can be made.
1. Promote new irrigation methods: To improve economic productivity and increase irrigation efficiency, it is suggested to adopt new irrigation methods suitable for the Sistan region. These methods should consider the specific needs and constraints of the area. Providing necessary arrangements and facilities for farmers to adopt these new irrigation methods is essential for their successful implementation.
2. Address the contradiction of irrigation efficiency (Mardani and Ashktorab, 2022): The study highlights a contradiction regarding irrigation efficiency. While increasing irrigation efficiency can lead to increased water storage, it may also result in an expansion of the cultivated area. To address this contradiction, it is recommended to provide bank facilities specifically tied to water consumption reduction when granting support for new irrigation methods. This approach ensures that the focus remains on optimizing water usage while avoiding the unintended expansion of cultivated areas.
3. Consider environmental considerations and resource optimization: The implementation of the WEF nexus approach allows farmers to consider environmental factors and optimize the use of resources, including water, food, and energy. By integrating environmental considerations into farming practices, it becomes possible to achieve sustainable and efficient resource utilization while preserving economic income.
4. Conduct expert and technical research for new crops: The mathematical modeling presented in this research can be used to identify potentially cultivable crops that have not yet been explored in the region. However, before introducing new crops, it is crucial to conduct a series of expert and technical research to assess their feasibility and suitability. This research will provide valuable insights into the viability and potential benefits of introducing new crops into the agricultural system.
In summary, it is recommended to implement development and bio-balance policies using the WEF nexus approach for environmental conservation in the Sistan region. Additionally, promoting new irrigation methods, addressing the contradiction of irrigation efficiency, considering environmental considerations, and conducting research for new crops are important steps towards achieving sustainable agricultural practices and optimal resource utilization in the region.
Data availability statement
The original contributions presented in the study are included in the article/supplementary material, further inquiries can be directed to the corresponding author.
Author contributions
HB: Data curation, Methodology, Software, Writing–original draft. SH: Conceptualization, Formal Analysis, Methodology, Supervision, Visualization, Writing–review and editing. MH: Data curation, Methodology, Writing–original draft. MN: Data curation, Methodology, Project administration, Supervision, Writing–review and editing.
Funding
The author(s) declare that no financial support was received for the research, authorship, and/or publication of this article.
Conflict of interest
The authors declare that the research was conducted in the absence of any commercial or financial relationships that could be construed as a potential conflict of interest.
Publisher’s note
All claims expressed in this article are solely those of the authors and do not necessarily represent those of their affiliated organizations, or those of the publisher, the editors and the reviewers. Any product that may be evaluated in this article, or claim that may be made by its manufacturer, is not guaranteed or endorsed by the publisher.
References
Anonymous (2018). Agriculture Jahad organization of sistan and baluchistan province, unpublished final results of annual statistics. (accessed 8.July.23), https://sbaj.ir/.
Artioli, F., Acuto, M., and McArthur, J. (2017). The water-energy-food nexus: an integration agenda and implications for urban governance. Polit. Geogr. 61, 215–223. doi:10.1016/j.polgeo.2017.08.009
Bizikova, L., Roy, D., Venema, H., and McCandles, M. (2014). The water-energy-food nexus and agricultural investment: a sustainable development guidebook. Int. Inst. Sustain. Dev 1, 1–46. doi:10.13140/2.1.2200.6566
Cai, X., Wallington, K., Shafiee-Jood, M., and Marston, L. (2018). Understanding and managing the food-energy-water nexus – opportunities for water resources research. Adv. Water Resour. 111, 259–273. doi:10.1016/j.advwatres.2017.11.014
Campana, P. E., Zhang, J., Yao, T., Andersson, S., Landelius, T., Melton, F., et al. (2018). Managing agricultural drought in Sweden using a novel spatially-explicit model from the perspective of water-food-energy nexus. J. Clean. Prod. 197, 1382–1393. doi:10.1016/j.jclepro.2018.06.096
Cansino-Loeza, B., Munguía-López, A. d. C., and Ponce-Ortega, J. M. (2022). A water-energy-food security nexus framework based on optimal resource allocation. Environ. Sci. Policy 133, 1–16. doi:10.1016/j.envsci.2022.03.006
Chai, J., Shi, H., Lu, Q., and Hu, Y. (2020). Quantifying and predicting the Water-Energy-Food-Economy-Society-Environment Nexus based on Bayesian networks - a case study of China. J. Clean. Prod. 256, 120266. doi:10.1016/j.jclepro.2020.120266
Chen, J., Yu, X., Qiu, L., Deng, M., and Dong, R. (2018). Study on vulnerability and coordination of water-energy-food system in northwest China. Sustainability 10 (10), 3712. doi:10.3390/su10103712
Daccache, A., Ciurana, J. S., Rodriguez Diaz, J. A., and Knox, J. W. (2014). Water and energy footprint of irrigated agriculture in the Mediterranean region. Environ. Res. Lett. 9 (12), 124014. doi:10.1088/1748-9326/9/12/124014
Esteve, P., Varela-Ortega, C., Blanco-Gutiérrez, I., and Downing, T. E. (2015). A hydro-economic model for the assessment of climate change impacts and adaptation in irrigated agriculture. Ecol. Econ. 120, 49–58. doi:10.1016/j.ecolecon.2015.09.017
Fabiani, S., Vanino, S., Napoli, R., and Nino, P. (2020). Water energy food nexus approach for sustainability assessment at farm level: an experience from an intensive agricultural area in central Italy. Environ. Sci. Policy 104, 1–12. doi:10.1016/j.envsci.2019.10.008
Flammini, A., Puri, M., Pluschke, L., and Dubois, O. (2014). Walking the nexus talk: assessing the water-energy-food nexus in the context of the sustainable energy for all initiative. FAO. Rome, Italy.
Gündoğmuş, E. (2006). Energy use on organic farming: a comparative analysis on organic versus conventional apricot production on small holdings in Turkey. Energy Convers. Manag. 47 (18), 3351–3359. doi:10.1016/j.enconman.2006.01.001
Hoff, H. (2011). Understanding the nexus. Stockholm, Sweden: Background paper for the Bonn 2011Nexus Environment Institute.
Hoolohan, C., Larkin, A., McLachlan, C., Falconer, R., Soutar, I., Suckling, J., et al. (2018). Engaging stakeholders in research to address water–energy–food (WEF) nexus challenges. Sustain. Sci. 13 (5), 1415–1426. doi:10.1007/s11625-018-0552-7
Howells, M., Hermann, S., Welsch, M., Bazilian, M., Segerström, R., Alfstad, T., et al. (2013). Integrated analysis of climate change, land-use, energy and water strategies. Nat. Clim. Change 3 (7), 621–626. doi:10.1038/nclimate1789
Jin, L., Chang, Y., Ju, X., and Xu, F. (2019). A study on the sustainable development of water, energy, and food in China. Int. J. Environ. Res. Public Health 16 (19), 3688. doi:10.3390/ijerph16193688
Karamian, F., Mirakzadeh, A. A., and Azari, A. (2023). Application of multi-objective genetic algorithm for optimal combination of resources to achieve sustainable agriculture based on the water-energy-food nexus framework. Sci. Total Environ. 860, 160419. doi:10.1016/j.scitotenv.2022.160419
Kellner, E. (2023). Identifying leverage points for shifting Water-Energy-Food nexus cases towards sustainability through the Networks of Action Situations approach combined with systems thinking. Sustain. Sci. 18 (1), 135–152. doi:10.1007/s11625-022-01170-7
Kurian, M. (2017). The water-energy-food nexus: trade-offs, thresholds and transdisciplinary approaches to sustainable development. Environ. Sci. Policy 68, 97–106. doi:10.1016/j.envsci.2016.11.006
Leung Pah Hang, M. Y., Martinez-Hernandez, E., Leach, M., and Yang, A. (2016). Designing integrated local production systems: a study on the food-energy-water nexus. J. Clean. Prod. 135, 1065–1084. doi:10.1016/j.jclepro.2016.06.194
Li, M., Fu, Q., Singh, V. P., Ji, Y., Liu, D., Zhang, C., et al. (2019a). An optimal modelling approach for managing agricultural water-energy-food nexus under uncertainty. Sci. Total Environ. 651, 1416–1434. doi:10.1016/j.scitotenv.2018.09.291
Li, M., Fu, Q., Singh, V. P., Liu, D., and Li, T. (2019b). Stochastic multi-objective modeling for optimization of water-food-energy nexus of irrigated agriculture. Adv. Water Resour. 127, 209–224. doi:10.1016/j.advwatres.2019.03.015
Li, M., and Guo, P. (2014). A multi-objective optimal allocation model for irrigation water resources under multiple uncertainties. Appl. Math. Model. 38 (19), 4897–4911. doi:10.1016/j.apm.2014.03.043
Li, M., Guo, P., Singh, V. P., and Zhao, J. (2016). Irrigation water allocation using an inexact two-stage quadratic programming with fuzzy input under climate change. JAWRA J. Am. Water Resour. Assoc. 52 (3), 667–684. doi:10.1111/1752-1688.12415
Mardani, M., and Ashktorab, N. (2022). Mathematical programming approaches for modeling a sustainable cropping pattern under uncertainty: a case study in Southern Iran. Environ. Dev. Sustain. 25, 9731–9755. doi:10.1007/s10668-022-02458-5
Mardani Najafabadi, M., Ziaee, S., Nikouei, A., and Ahmadpour Borazjani, M. (2019). Mathematical programming model (MMP) for optimization of regional cropping patterns decisions: a case study. Agric. Syst. 173, 218–232. doi:10.1016/j.agsy.2019.02.006
Morankar, D., Komaragiri, S. R., and Kumar, D. N. (2013). Integrated sustainable irrigation planning with multiobjective fuzzy optimization approach. Water Resour. Manag. 27, 3981–4004. doi:10.1007/s11269-013-0391-3
Namany, S., Al-Ansari, T., and Govindan, R. (2018). “Integrated techno-economic optimization for the design and operations of energy, water and food nexus systems constrained as non-cooperative games,” in Computer aided chemical engineering. Editors M. R. Eden, M. G. Ierapetritou, and G. P. Towler (Elsevier, Amsterdam, Netherlands), 44, 1003–1008. doi:10.1016/B978-0-444-64241-7.50162-2
Nie, Y., Avraamidou, S., Xiao, X., Pistikopoulos, E. N., Li, J., Zeng, Y., et al. (2019). A Food-Energy-Water Nexus approach for land use optimization. Sci. Total Environ. 659, 7–19. doi:10.1016/j.scitotenv.2018.12.242
Radmehr, R., Ghorbani, M., and Ziaei, A. N. (2021). Quantifying and managing the water-energy-food nexus in dry regions food insecurity: new methods and evidence. Agric. Water Manag. 245, 106588. doi:10.1016/j.agwat.2020.106588
Rasul, G., and Sharma, B. (2016). The nexus approach to water–energy–food security: an option for adaptation to climate change. Clim. Policy 16 (6), 682–702. doi:10.1080/14693062.2015.1029865
Ringler, C., Willenbockel, D., Perez, N., Rosegrant, M., Zhu, T., and Matthews, N. (2016). Global linkages among energy, food and water: an economic assessment. J. Environ. Stud. Sci. 6 (1), 161–171. doi:10.1007/s13412-016-0386-5
Sabouhi, M., and Mardani, M. (2013). Application of robust optimization approach for agricultural water resource management under uncertainty. J. Irrigation Drainage Eng. 139, 571–581. doi:10.1061/(ASCE)IR.1943-4774.0000578
Sadeghi, S. H., Sharifi Moghadam, E., Delavar, M., and Zarghami, M. (2020). Application of water-energy-food nexus approach for designating optimal agricultural management pattern at a watershed scale. Agric. Water Manag. 233, 106071. doi:10.1016/j.agwat.2020.106071
Shirzadi Laskookalayeh, S., Mardani Najafabadi, M., and Shahnazari, A. (2022). Investigating the effects of management of irrigation water distribution on farmers' gross profit under uncertainty: a new positive mathematical programming model. J. Clean. Prod. 351, 131277. doi:10.1016/j.jclepro.2022.131277
Si, Y., Li, X., Yin, D., Li, T., Cai, X., Wei, J., et al. (2019). Revealing the water-energy-food nexus in the Upper Yellow River Basin through multi-objective optimization for reservoir system. Sci. Total Environ. 682, 1–18. doi:10.1016/j.scitotenv.2019.04.427
Singh, V. P. (2017). Challenges in meeting water security and resilience. Water Int. 42 (4), 349–359. doi:10.1080/02508060.2017.1327234
Singh Vijay, P., Khedun Chundun, P., and Mishra Ashok, K. (2014). Water, environment, energy, and population growth: implications for water sustainability under climate change. J. Hydrologic Eng. 19 (4), 667–673. doi:10.1061/(ASCE)HE.1943-5584.0000866
Tian, H., Lu, C., Pan, S., Yang, J., Miao, R., Ren, W., et al. (2018). Optimizing resource use efficiencies in the food–energy–water nexus for sustainable agriculture: from conceptual model to decision support system. Curr. Opin. Environ. Sustain. 33, 104–113. doi:10.1016/j.cosust.2018.04.003
Tichenor, N. E., van Zanten, H. H. E., de Boer, I. J. M., Peters, C. J., McCarthy, A. C., and Griffin, T. S. (2017). Land use efficiency of beef systems in the Northeastern USA from a food supply perspective. Agric. Syst. 156, 34–42. doi:10.1016/j.agsy.2017.05.011
Wang, Q., Li, S., He, G., Li, R., and Wang, X. (2018). Evaluating sustainability of water-energy-food (WEF) nexus using an improved matter-element extension model: a case study of China. J. Clean. Prod. 202, 1097–1106. doi:10.1016/j.jclepro.2018.08.213
Wang, Y., Lu, Y., Xu, Y., Zheng, L., and Fan, Y. (2023). A factorial inexact copula stochastic programming (FICSP) approach for water-energy- food nexus system management. Agric. Water Manag. 277, 108069. doi:10.1016/j.agwat.2022.108069
Wicaksono, A., and Kang, D. (2019). Nationwide simulation of water, energy, and food nexus: case study in South Korea and Indonesia. J. Hydro-environment Res. 22, 70–87. doi:10.1016/j.jher.2018.10.003
Xie, Y., Xia, D., Ji, L., and Huang, G. H. (2017). An inexact stochastic-fuzzy optimization model for agricultural water allocation and land resources utilization management under considering effective rainfall. Ecol. Indic. 92, 301–311. doi:10.1016/j.ecolind.2017.09.026
Xu, S., He, W., Shen, J., Degefu, D. M., Yuan, L., and Kong, Y. (2019). Coupling and coordination degrees of the core water–energy–food nexus in China. Int. J. Environ. Res. Public Health 16 (9), 1648. https://www.mdpi.com/1660-4601/16/9/1648. doi:10.3390/ijerph16091648
Xu, Y., Tan, J., Wang, X., Li, W., He, X., Hu, X., et al. (2022). Synergetic management of water-energy-food nexus system and GHG emissions under multiple uncertainties: an inexact fractional fuzzy chance constraint programming method. Agric. Water Manag. 262, 107323. doi:10.1016/j.agwat.2021.107323
Yang, Y. C. E., Ringler, C., Brown, C., and MondalAlam, M. H. (2016). Modeling the agricultural water–energy–food nexus in the indus river basin, Pakistan. J. Water Resour. Plan. Manag. 142 (12), 04016062. doi:10.1061/(ASCE)WR.1943-5452.0000710
Yilmaz, I., Akcaoz, H., and Ozkan, B. (2005). An analysis of energy use and input costs for cotton production in Turkey. Renew. Energy 30 (2), 145–155. doi:10.1016/j.renene.2004.06.001
Yu, L., Li, Y. P., and Huang, G. H. (2019). Planning municipal-scale mixed energy system for stimulating renewable energy under multiple uncertainties - the City of Qingdao in Shandong Province, China. Energy 166, 1120–1133. doi:10.1016/j.energy.2018.10.157
Yu, L., Xiao, Y., Zeng, X. T., Li, Y. P., and Fan, Y. R. (2020). Planning water-energy-food nexus system management under multi-level and uncertainty. J. Clean. Prod. 251, 119658. doi:10.1016/j.jclepro.2019.119658
Zhang, J., Campana, P. E., Yao, T., Zhang, Y., Lundblad, A., Melton, F., et al. (2018). The water-food-energy nexus optimization approach to combat agricultural drought: a case study in the United States. Appl. Energy 227, 449–464. doi:10.1016/j.apenergy.2017.07.036
Keywords: cropping pattern, multi-objective programming, production resources optimization, Sistan plain cropping pattern, Sistan plain
Citation: Badih Barzin H, Hoseini SM, Hashemitabar M and Mardani Najafabadi M (2024) The allocation of crop production resources in the southeast of Iran: the application of the water-energy-food nexus approach. Front. Environ. Sci. 12:1349095. doi: 10.3389/fenvs.2024.1349095
Received: 04 December 2023; Accepted: 09 February 2024;
Published: 22 February 2024.
Edited by:
Olusola Oluwayemisi Ololade, University of the Free State, South AfricaReviewed by:
Jerry Dlamini, University of the Free State, South AfricaYing Zhu, Xi’an University of Architecture and Technology, China
Copyright © 2024 Badih Barzin, Hoseini, Hashemitabar and Mardani Najafabadi. This is an open-access article distributed under the terms of the Creative Commons Attribution License (CC BY). The use, distribution or reproduction in other forums is permitted, provided the original author(s) and the copyright owner(s) are credited and that the original publication in this journal is cited, in accordance with accepted academic practice. No use, distribution or reproduction is permitted which does not comply with these terms.
*Correspondence: S. M. Hoseini, aG9zc2VpbmlAZWNvLnVzYi5hYy5pcg==