- 1College of Marine Science and Technology, Zhejiang Ocean University, Zhoushan, China
- 2Key Laboratory of Engineering Oceanography, Second Institute of Oceanography, Ministry of Natural Resource of the People’s Republic of China, Hangzhou, China
Heavy metals play an important role in marine ecosystems, but excessive heavy metals can harm aquatic life and human health. The Yangshan Port is located near an important agricultural and fishing area in China, with rich fishery resources. However, there is a lack of research on the impact of heavy metal pollution on local fishery and environment. This study assessed the concentrations of seven metals (Cu, Zn, Pb, Cr, Cd, As, Hg) in the water, sediments and two organisms (fish and benthic organisms) of Yangshan Port, aiming to get a comprehensive information about the heavy metal pollution in Yangshan Port and its adjacent sea areas. The results showed that the concentration of heavy metals in the sediments was higher than that observed in the water. The concentrations of heavy metals in water and sediments were influenced by human activities. The main reason for this regional distribution difference was human factors such as industrial wastewater discharges, household pollution, and agricultural and fishery discharges, followed by various natural factors, such as organic content and sulfate, as well as hydrodynamic self-disturbance. It is worth noting that As is affected not only by emissions from land-based sources, but also by riverine inputs and human marine engineering activities. The ecological risk indicated that two of seven metals (As and Hg) posed a risk to the marine environment in this region. In addition, the content and accumulation level of heavy metals in benthic organisms was significantly higher than that in fish. Human health risk assessments showed that the heavy metal posed a potential carcinogenic risk to human health, particularly to children. Overall, the health risks from seafood intake by humans were low, with As having the greatest impact on human health, and metal concentrations being the most sensitive factor affecting metal health risk assessment, with As being the most sensitive element. This study provided new insight into the heterogeneity of heavy metal pollution accumulation in the Yangshan Port and its adjacent sea areas and offered a scientific basis and favorable support for port ecological environment protection and prevention of heavy metal pollution-related ecological risks.
1 Introduction
Heavy metals are considered the primary source of pollution in the marine environment due to their high biological toxicity, wide distribution, and persistent accumulation characteristics. With the acceleration of urbanization and the development rapidly industry in coastal areas, heavy metals circulated continuously in the marine environment through river runoff and atmospheric deposition (Torres et al., 2008). Heavy metals entering marine environment were affected by a variety of factors, including domestic sewage, garbage discharge, automobile exhaust and agricultural and fishery wastewater, fertilizer residues, metal mining and smelting, industrial wastewater, etc (Islam et al., 2015), as well as antifouling coatings and fuel leaks from ships (Zhang et al., 2011; Li et al., 2014, Li et al., 2023). Excessive heavy metal contamination not only impacted the marine environment negatively, but also presented a threat to marine organisms and human health (Chow et al., 2005; Hope, 2006). Heavy metals circulated between seawater, sediment, and various organisms, and eventually preserved in sediment. Under specific hydrodynamic conditions, sediments may release heavy metals, creating a state of dynamic equilibrium (Shah, 2021). In marine environments, sediments provide habitats and food sources for benthic organisms. The transfer of heavy metals throughout food chains occurs via bioaccumulation and bio-magnification, resulting in indirect toxicity to aquatic organisms. This phenomenon has an impact on primary consumers, which in turn affect top predators in the food chain (Nour, 2020). Heavy metal contamination can lead to fatal effects or even mass mortality of fish in aquaculture areas (McGeer et al., 2000; Almeida et al., 2002; Tian et al., 2020), whose persistence, toxicity, and bio-accumulation can also damage various organs in humans and other living organisms (Zhang et al., 2014). Consequently, an examination of the distribution of heavy metals in the marine environment surrounding the source of seafood products can elucidate the impact of human activities on the ecosystem, while also facilitating an assessment of the risks associated with waste discharge (de Mora et al., 2004; Zheng et al., 2008; Li et al., 2020).
Several researches conducted on heavy metals in the major ports in China in recent years (Lu et al., 2015; Sun et al., 2015; Mao et al., 2017; Su et al., 2023). As these ports are key gateways for rapid economic development, human activities, and large-scale constructions inevitably have potential negative impacts on the surrounding environment (Pursiainen et al., 2021). Yangshan Port, situated in the eastern portion of Hangzhou Bay, serves as a spawning, feeding, and traditional fishing ground for a multitude of marine organisms. It is endowed with a plethora of natural resources and fishery resources, in addition to a strategic location, which collectively confer upon it a distinctive competitive advantage (Yu et al., 2022). However, with the advancement of the Yangshan Port Phase II project, the expansion of the port scale exacerbated the transportation pressure and also caused concerns about the ecological environment pollution of the surrounding waters (Li et al., 2014). However, the resulting risks are not yet known, especially since heavy metal contamination due to port expansion has not been reported yet. At the same time, the change in sea conditions will also hurt the port operation, especially since the research on the heavy metal pollution of Zhoushan and its adjacent sea areas in the second phase of the Yangshan Port construction is not sufficient. Therefore, the survey was conducted in November 2017, heavy metals including Hg, Cd, As, Pb, Cu, Cr, and Zn in seawater, sediments, and biological samples from the Yangshan Port and its adjacent sea areas were studied, and the sources of heavy metal pollution in the sea area were determined through ecological risk assessment, correlation analysis, and principal component analysis, while also assessing the ecotoxicological risks of heavy metals and potential threats to human health. Overall, these results provide new insights into heavy metal pollution from a risk-hazard perspective and provide valuable insights into the scientific use and management of port and fishing waters in complex pollution environments for marine ecological prevention and control and the green development of marine fisheries.
2 Article types
Advanced Monitoring, Modelling, and Analysis of Coastal Environments and Ecosystems
3 Materials and methods
3.1 Sample collection
The survey was conducted in November 2017 in the waters near Xiaoyangshan Island, Zhejiang. A total of 20 seawater quality monitoring stations, 10 marine sediment monitoring stations, and 12 marine biological sample collection stations were established (Figure 1). All samples were collected, stored, transported, and analyzed in strict accordance with the relevant guidelines, including the “Marine Survey Specifications” (GB/T 12763-2007) and the “Marine Monitoring Specifications” (GB 17378-2007). Seawater samples were collected using a Niskin sampler (General Oceanics, USA) at depths of 0.5 meters below the surface and 1 meter above the seabed. The collected seawater was immediately filtered through a vacuum filter with a 0.45 μm membrane, acidified to a pH 2 with nitric acid, and prepared for analysis. Sediment samples at each site were collected using a grab sampler (Lanke Ocean, China). The sediment samples were dried in a freeze-dryer (LC, China) at -75°C and 5 Pa, then ground into a fine powder using a hand-held agate mortar (LC, China), sieved through a 106 μm nylon mesh, and stored until digestion. At the 12 survey stations, representative samples of key local economic species from the local marine fauna were collected. A total of 39 samples of five common local economic fish and crustacean species were collected, with individuals of similar sizes selected for metal analysis. The collected biological samples were categorized, weighed, measured for length, and dissected. Muscle tissues were dried, ground, sieved, and stored in the same manner as the sediment samples until digestion. The ecological characteristics and morphological records of the collected organisms are shown in Supplementary Table S1.
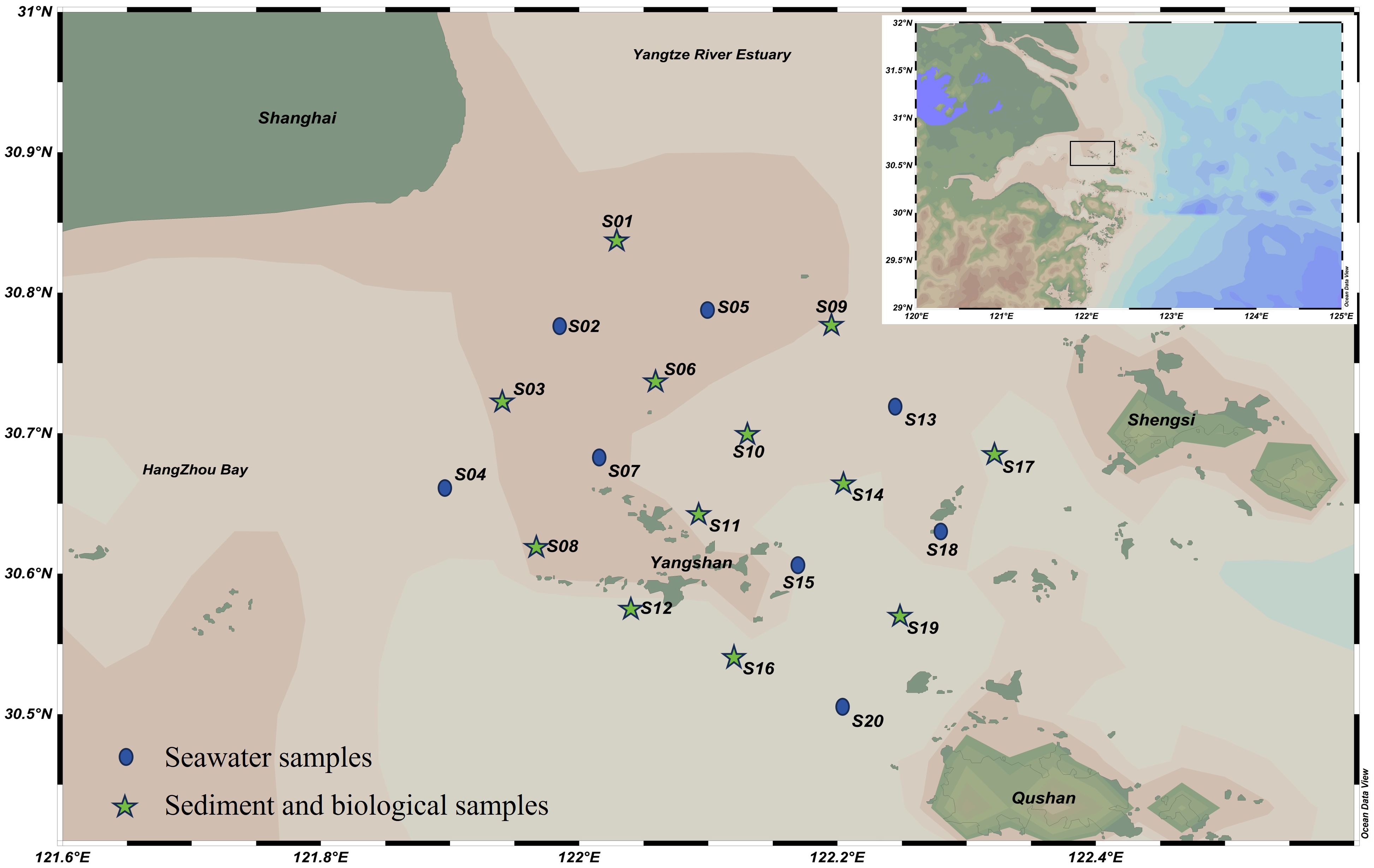
Figure 1. Sampling sites in Yangshan Port and its adjacent sea areas. The green five-pointed star indicates the location where sediment and biological samples were collected. Seawater samples were taken at all stations.
3.2 Sample extraction and analysis
The analytical methods used in this study strictly followed the “Marine Monitoring Specifications” (GB 17378-2007). In this research, the concentrations of heavy metals in seawater (μg/L), sediments (mg/kg, dry weight), fish, and crustaceans (mg/kg, wet weight) were measured. The heavy metal content of acidified water samples was analyzed using an Agilent ICP-MS 7700x inductively coupled plasma mass spectrometer (ICP-MS). For sediment samples, 0.10 g of sediment was digested with a mixed acid solution (HNO3: HClO4: HF = 7:1:4). After freeze-drying, grinding, and sieving through a 100-mesh screen, the resulting mixture was further enriched for heavy metals and analyzed using ICP-MS. For biological samples, metal analysis followed the procedure outlined below. Approximately 0.4 g of dry biological sample was mixed with 9 mL of concentrated nitric acid and 2 mL of 30% hydrogen peroxide in a polytetrafluoroethylene (PTFE) digestion vessel and allowed to pre-digest for 0.5~1 hour. The digestion vessel was then transferred to a microwave digestion system (Anton Paar, Austria) for digestion. After digestion, the vessel was heated on a hotplate to reduce the remaining acid volume to approximately 1 mL. The remaining solution was transferred to a polypropylene tube and diluted to 25 mL with Milli-Q water. The resulting digested samples were then analyzed for heavy metal concentrations using ICP-MS. Each sample was analyzed in triplicate, ensuring consistency in the reagents used for each analysis batch, including blank measurements.
3.3 QA/QC
All laboratory tools were soaked in diluted nitric acid solution for 72 hours and rinsed three times with Milli-Q water before use. During all analyses, reagent blanks, method blanks, and parallel samples were used. Each test batch was evaluated using internal quality control methods, and verification was performed to ensure compliance with the defined internal quality control standards. Reference materials used in this study included seawater samples, where Cu, Pb, Zn, Cd, Cr (GBW (E) 080040), Hg standard solution (GBW08617), and As (GBW (E) 080117) were used as calibration standards (China BZWZ). Chinese standard reference materials, including GBW10050 (GSB-28) shrimp, GBW10024 (GSB-15) scallops, and the China Marine Sediment Standard Sample (GBW07314), were analyzed to verify the accuracy of sediment and biological sample measurements. All results were consistent with certified values, with the recovery rate for reference samples ranging from 80.4% to 119.2%, and the relative standard deviation (RSD) within ±5%.
3.4 Data analysis
3.4.1 Distribution coefficient and bioaccumulation
The distribution coefficient of sediment/water (Kd) was employed to assess the capacity of heavy metals to be adsorbed (Yavar Ashayeri and Keshavarzi, 2019).
where Cwater represents the concentration of heavy metals in water, in μg/L; and Csediment indicates the concentration of heavy metals in the sediment, in mg/kg. A high Kd indicates that the metal is preferentially retained by the sediment, while a low Kd suggests that most of the metal is retained in the water.
The accumulation of heavy metals by marine organisms in sediments and seawater was evaluated, and biota-sediment accumulation factor (BSAF) and bioaccumulation factors (BAF) were calculated. A BSAF value of lower than 1 and a BAF value of lower than 100 indicate that the organism’s ability to accumulate heavy metals is weak (Thomann et al., 1992; Zhang et al., 2015) (Supplementary Table S2).
where Cbiomass is the concentration of heavy metals in organisms, mg/kg. Cwater is the concentration of heavy metals in water. Csediment is heavy metal concentration in sediment.
3.4.2 Risk assessment of heavy metal in seawater
The Species Sensitivity Distribution (SSD) is a tool that can be used to assess the ecological risk of heavy metals in seawater. This allows for the evaluation of the extent of ecological harm caused by pollutants to the marine ecosystem as a whole. In this study, the Heavy Metal Risk Quotient (RQ) was employed to evaluate the ecological risk associated with the presence of heavy metals in seawater (Hou et al., 2024).
In the formula, HC5 (5%SSD value) is the maximum heavy metal concentration when 95% of the species in the ecosystem are not affected, Usually, only Single Factor and Nemerow indexing methods are available. The simplest of these calculations was used in this study for validation, and HC5 for the data is shown in Supplementary Table S3 (Li et al., 2019a). SF (assessment factor) is the uncertainty factor with a value of 5 (Li et al., 2019b; Liu et al., 2020). RQ > 1 indicated a potential ecological risk, but RQ < 1 indicated an acceptable level of ecological risk (Supplementary Table S2).
3.4.3 Ecological risk assessment
Given that different metals display varying degrees of toxicity, this study employed the Potential Ecological Risk Index (PERI), as proposed by Hakanson, to evaluate the ecological risk associated with each heavy metal and its mixture deposited in surface sediments (Hakanson, 1980). The extent of the harm is illustrated in Supplementary Table S4. The potential ecological harm index (Ei) of a single heavy metal is calculated according to the following equation:
The potential ecological hazard index RI of multiple heavy metals is calculated as shown in the equation:
where Ei represents the potential ecological hazard index of a single heavy metal; Ci represents the average concentration of heavy metals (mg/kg); C0 is the reference value, in this paper, C0 is selected as the reference value of pre-industrial heavy metal concentration; Ti is the toxicity coefficient of heavy metals.
In 1979, the German scientist Muller proposed the Geological Accumulation Index (Igeo) as a means of evaluating the status of heavy metal pollution in surface sediments (Xu et al., 2016; Zhang et al., 2016), which can enable a quantitative evaluation of the degree of heavy metal pollution in sediments. The degree of hazard is shown in Supplementary Table S4.
where Ci represents the average concentration of heavy metals (mg/kg); Bi represents the average geochemical background value of the measured element. In this paper, Bi is the background values of sediments in the coastal waters of Zhejiang Province were selected (Zhang et al., 2005; Jiang et al., 2021) (Table 1). k is the coefficient of background fluctuation caused by rock formation movement, and it is 1.5 to reduce the influence of background value variation (Anbuselvan et al., 2018).
3.4.4 Health risk assessment
Human health risk assessments can show the health risks that organisms pose when exposed to heavy metals (Ali et al., 2021; Peng et al., 2022). In this study, the assessment of the harm of heavy metals to humans was conducted utilizing four key indices: hazard quotient (HQ), hazard index (HI), and carcinogenic risk (CR). This study was conducted using the model recommended by the USEPA’s Water Environment Health Risk Assessment, which includes both carcinogenic and non-carcinogenic risks. In addition, the population was divided into two groups (children and adults) due to physiological and behavioral differences. The assessment model is located in the seafood zone and therefore focuses only on non-carcinogenic and carcinogenic risks through oral intake. Non-carcinogenic risk assessment with HQ and HI. The formula for calculating HQ and HI is:
where Ci represents the concentration of heavy metals in the organism (mg/kg); IR represents the average daily food intake (mg/day) of an organism; EF represents the frequency of exposure (days); ED is the total exposure time (years); BW represents the average body weight (kg); AT represents the average age life expectancy (days). where RfD is the standard reference dose of metal (mg/kg/day). HI ≥ 1 indicated that heavy metals will cause harm to human health; HI < 1 indicated that they will not cause harm to human health (Rinklebe et al., 2019). The individual parameters are detailed in the attached Supplementary Table S5.
Carcinogenic risk (CR) can assess the carcinogenic hazards of exposure to heavy metals in different populations over their life expectancy.
where Cbiomass is the concentration of heavy metals in the organisms (mg/kg). Supplementary Table S5 presents the factor values used in the risk assessment equation. SF is a carcinogenic slope factor (Cd is 6.3, Pb is 0.0085, Cr is 05, As is 1.5) (Liu et al., 2020; Guan et al., 2024).
Monte Carlo simulation has advantages in reducing event uncertainty and is widely used in Health risk assessment (HRA) (Ali et al., 2022; Lei et al., 2022; Guan et al., 2024). Random independent variables for each group were selected through a random number generator and the function values were determined based on the relationship between the dependent and independent variables. The probability distribution of the dependent variable of the function was obtained by repeated independent sampling. Based on the distribution function of each variable, non-carcinogenic and carcinogenic risks were calculated by taking random values after 10,000 model iterations (Özkaynak et al., 2022). Parameters of the influencing factors in the health risk assessment model refer to Supplementary Table S6.
3.5 Statistical analysis
The raw data were processed and computed using Microsoft Excel 2021 software while the data were statistically analyzed using IBM SPSS Statistics 23 software. Data were visualized using ODV and Origin 2021 through Principal Component Analysis (PCA) and Pearson’s correlation analysis using R. In this study, Oracle Crystal Ball 11.0 was used to process Monte Carlo simulations for probabilistic human health risk assessment.
4 Results and discussion
4.1 Distribution of heavy metals in seawater, sediments, and organisms
The concentration of Cu, Pb, Zn, Cd, Cr, Hg, and As in seawater ranged from 0.8 to 1.7, 0.09 to 0.99, 8.4 to 19.3, 0.02 to 0.27, ND to 1.1, 0.005 to 0.035, and 0.7 to 3.2 μg/L, respectively. The mean concentration of the elements in seawater was found to be Zn > Cu > As > Cr > Pb > Cd > Hg. From a distribution of heavy metal pollution in seawater (Figure 2), the high concentrations of heavy metals were found in the northern part of the Yangshan Port because the input of the Yangtze River and areas of high concentrations in anthropogenic areas to the east and south near Yangshan Port. It is worth noting that the Hg all have high concentrations areas in this area. The increased concentration of Cu and Zn observed in the vicinity of the Yangtze River estuary and upstream of the port can be attributed to the accumulation of these pollutants, facilitated by runoff into the bay in the estuary region (Hou et al., 2022). In contrast, Pb and Cd were observed in the southern region of Yangshan Port in the vicinity of Zhoushan Island, as well as in the eastern section in the proximity of Shengsi. This is due to the confluence of diluted water from the Qiantang and Yangtze rivers, which increases heavy metal concentration (Feng et al., 2021; Liu et al., 2024). The concentrations of heavy metals in seawater within the region were significantly impacted by the catchment of rivers at the mouths of the Yangtze River and Hangzhou Bay. Comparison with the average concentrations of heavy metals in seawater from other ports shows that the concentrations of the seven heavy metals in seawater from Yangshan Port and its nearby waters are generally low (Liu et al., 2015; Lao et al., 2019). Therefore, its seawater has fewer heavy metals than in other ports.
The concentrations of Cu, Pb, Zn, Cd, Cr, Hg, and As in the sediment ranged from 23.0 to 32.4, 22.9 to 31.7, 73.5 to 94.8, 0.09 to 0.14, 38.7 to 47.3, 0.038 to 0.091, and 7.7 to 17.7 mg/kg, respectively. The mean concentrations were found to be Zn > Cr > Pb > Cu > As > Cd > Hg. According to the spatial distribution of heavy metal concentration in sediments (Figure 3), the spatial distribution of seven heavy metals in the sediments was similar, showing higher locations near the center of the port as well as to the north and south of the port, and lower locations in the east near Hangzhou Bay. However, Hg and Cd concentrations were generally elevated in the northern and southern parts of the Yangshan port, with a significant high value near Shanghai and the mouth of the Yangtze River in the northern of the port. Compared with the adjacent island of Zhoushan (Fang et al., 2019), the Shengsi Sea area, Daishan Sea area (Zhuo et al., 2019), the concentration of As was higher in the study area and demonstrated a gradual decline from Hangzhou Bay to the port, from west to east. This finding is consistent with the results of other studies, which have also demonstrated elevated levels of As in the Yangtze River estuary and Hangzhou Bay (Fang et al., 2016; Jiang et al., 2021). The reason for this situation may be trace metal pollution produced by the ports, industrial emissions, sewage outlets, shipyards, and oil depots (Feng et al., 2021). The heavy metal levels in the sediments were higher than the background levels of the soils in the coastal waters of Zhejiang, indicating possible pollution problems in the Yangshan Port area.
According to Figure 4A, the concentration of heavy metals in benthic organisms was higher than that in fish, because benthic organisms live on top of or inside the sediment and can easily take up metals from the sediment (Zhao et al., 2018). Both Cu and Zn in fish and benthic organisms are high in comparison to other heavy metals since these two metals are essential elements and micronutrients in living organisms (Zhao et al., 2017). In this study, the As concentrations were found in organisms 0.96 ± 0.11 mg/kg for benthic and 0.24 ± 0.01 mg/kg for fish, respectively. This was higher than the national food safety standard limit values for contaminants in food (0.5 mg/kg for aquatic animals and 0.1 mg/kg for fish) (Vu et al., 2017). Therefore, it is recommended that these organisms be consumed with caution.
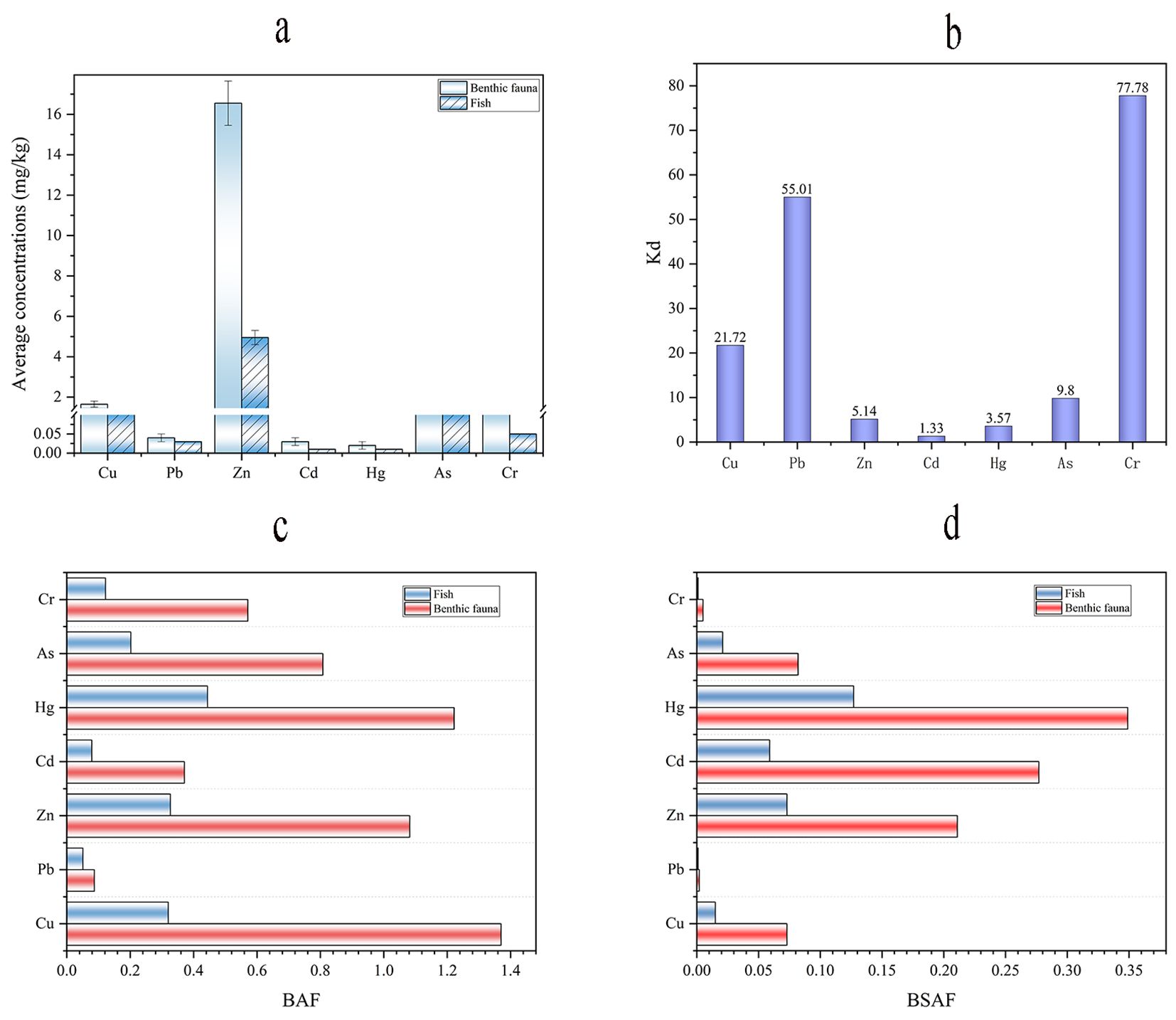
Figure 4. Mean heavy metal concentrations in different organisms (A), seawater and sediment distribution coefficients (B), and bioaccumulation factors (C, D).
4.2 Accumulation, transfer, and evaluation of heavy metals
As indicated in Figure 4B, the Kd of heavy metals between sediments and seawater is as follows: Cr (77.78) > Pb (55.01) > Cu (21.72) > As (9.80) > Zn (5.14) > Hg (3.57) > Cd (1.33), the results show that heavy metals Cr, Pb, and Cu have a stronger affinity for sediments than for seawater. Such differences can be attributed to several factors, including differences in solubility, adsorption effects of particulate matter, and biological processes, such as sand and clay, which also have a significant effect on the biological nature of Pb, Cr, and Cu (Enk and Mathis, 1977; Barak and Mason, 1989; Peng et al., 2022). It has also been shown that heavy metals tend to accumulate in sediments, and these accumulated heavy metals re-enter the ecological cycle through the absorption of animals and plants (Yi et al., 2011). Heavy metals can be taken up and stored by aquatic organisms, which can have a significant impact on the bioavailability of these elements. Dead deposition of aquatic organisms is an important component of the heavy metal uptake process (Luoma and Bryan, 1979; Liu et al., 2017; Sahu et al., 2023). In addition, according to the distribution of water bodies and sediments mentioned above, the heavy metal concentrations in the sediments of the bay area near the port and the anthropogenic area are higher than those in other study areas, and this area is affected by the sediment transport from the Yangtze River and the Qiantang River (Feng et al., 2021), as well as by other factors, such as agricultural activities and aquaculture (Alloway, 2013), which corroborate that the heavy metals in this area would be more accumulated in the sediments.
The results of BAF and BSAF indicated that Cu, Zn, Hg, and As were accumulated in organisms, and most of them came from water (Figures 4C, D). The Hg is mainly enriched in crustaceans, while As is mainly bioaccumulated in crustaceans and fishes. We will discuss the dangers of these two heavy metals later. This study also found that the concentration of heavy metals in benthic animals was greater than that in fish, which was consistent with previous studies that the biological accumulation of heavy metals in fish is closer to the concentration of heavy metals in seawater, while benthic animals were more inclined to accumulate and enrich heavy metal deposits (Chen and Folt, 2000; Zhao et al., 2018). In addition, differences in metabolic and detoxification mechanisms resulted in lower assimilation rates in fish than in benthic animals (Wang and Pan, 2004). Fish will show a stronger dilution effect on heavy metals during rapid growth (Zhang et al., 2015).
4.3 Traceability analysis of heavy metals
Significant correlations between heavy metals can also indicate similar levels of contamination or the same source. Pearson correlation analyses can thus be employed to identify potential sources of heavy metals in water and sediment (Johansson et al., 2001). According to the data in Figures 5A, seawater was analyzed by Mantel’s test and the results showed that Pb had a significant positive correlation with Cd and a negative correlation with Cu and Zn among heavy metals. In addition, environmental factors (temperature, salinity, and DIN) in seawater were significantly correlated with Cu and Pb, and the RSP was correlated with Pb, and the oils were significantly correlated with Cu. Since the adsorption of heavy metals is an exothermic process and the release of heavy metals is an absorptive process, an increase in temperature can facilitate the release of heavy metals into the water column (Fu et al., 2022). Since the ionic strength increases with the increase of salinity, the competition between heavy metal ions and the competition for adsorption sites between heavy metal ions and other metal cations increases. The heavy metals will be detached from the adsorption into the overlying water, and the high ionic strength will also cause other substances to be ionized and disintegrated (Yi, 2017). In addition, the correlation between DIN and RSP shows that eutrophication phenomena can clarify the impact of exogenous emissions from human activities in the sea area (Ali et al., 2019). According to the data in Figures 5B, the sediment Mantel test showed a significant positive correlation between heavy metals Cd, Hg, Cr, Pb, Zn, and Cu, and a significant negative correlation between Pb and As. Whereas salinity showed a positive and significant correlation with Pb, pH with Pb and As, sulphates showed a significant positive correlation with Cu, Pb, Zn, Hg, and Cr. With the increase of pH, the adsorption capacity of heavy metals in sediments increases, and the dissolved heavy metals will be adsorbed and settled with the increase of pH and other physicochemical effects, which leads to the increase of heavy metal content in sediments (Lu and Yan, 2010). In addition, the correlation between heavy metals in sediments and sulphates contributes to a deeper understanding of the influence of intrinsic factors affecting sediments on heavy metals (Fang et al., 2019). From the above correlation analysis between environmental factors and heavy metals, it was shown that the main source of heavy metals in the sediments of the study area is exogenous input.
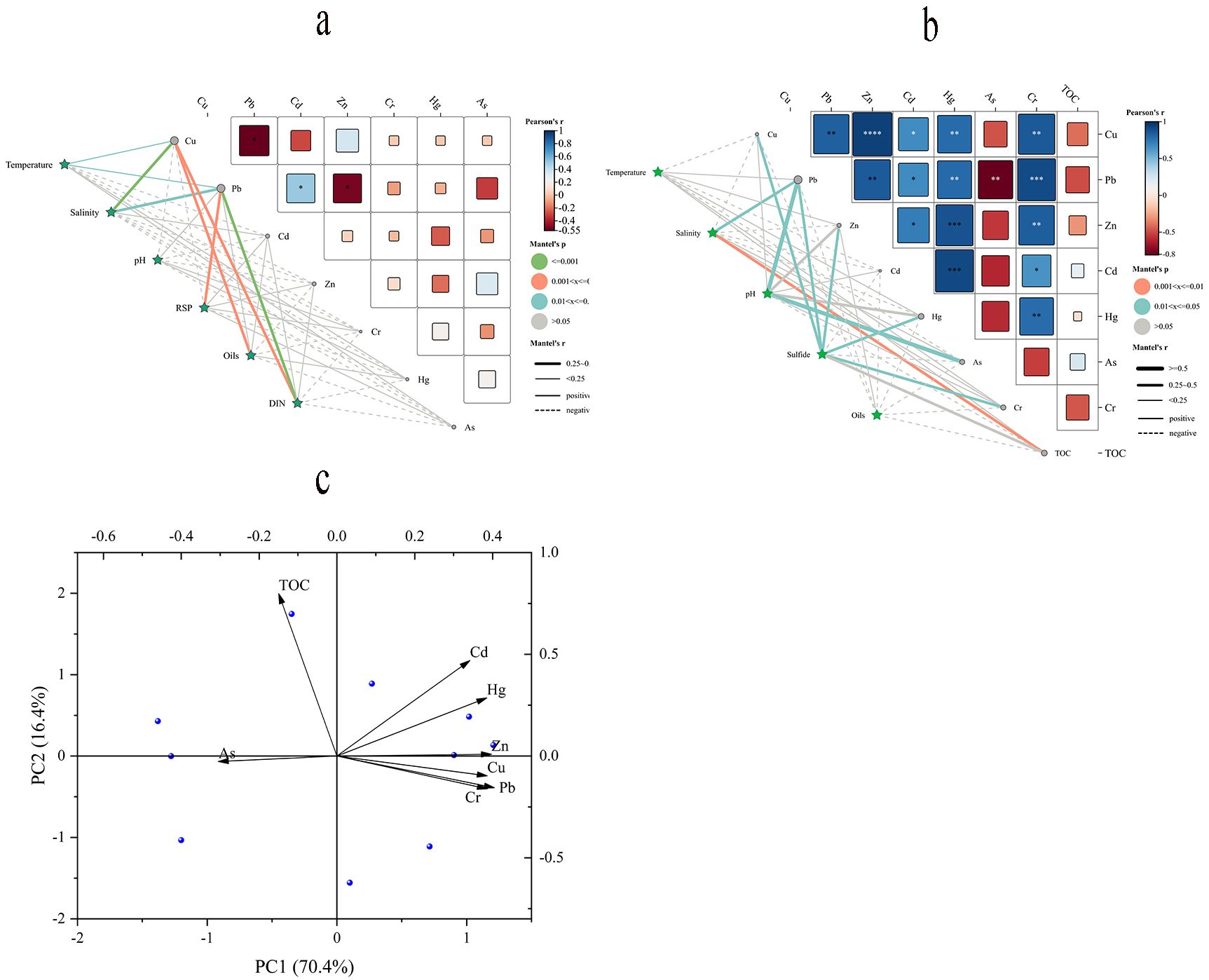
Figure 5. The relationship between heavy metals and other indicators in water (A) and sediment (B) was analyzed by Mantel test and PCA of variables for sediment heavy metals (C).
The PCA can further explain the main controlling factors of heavy source and distribution of heavy metals in this sea area (Figure 5C). According to 0.612 > 0.5 and Bartlett test result of 0.000, it is suitable for principal component analysis. The variation of TOC content and composition can determine the homology and distribution of heavy metals in sediments (Borg and Jonsson, 1996). The PCA was performed on the heavy metals in the sediments (Supplementary Table S7), and it showed that the contribution of the first principal component was 70.36%, which was significantly characterized by high positive loadings in the factor variables of Cu, Pb, Zn, Cd, Hg and Cr, while the above correlations showed that Cu, Pb, Zn, Cd, Hg and Cr were significantly positively correlated. The results of correlation analyses with the environment were consistent with human activities. However, the higher loadings for arsenic and the negative correlation with the associated lead suggest that arsenic is derived from the influence of other factors.
Previous studies have shown that Pb, Cd, and Zn are affected by industrial pollution and combustion of marine fuels, emissions from ship shipping, and degradation of organic matter (Zhong et al., 2006; Tian et al., 2017), and Cu and Zn are present in metal facilities used for aquaculture (Evans, 1994). The Cr enters the water column through agriculture, aquaculture, and fertilizers (Anik et al., 2022), while Cd is mainly influenced by anthropogenic factors such as agriculture, municipal waste and sewage sludge, industrial pollution, and natural factors such as inputs from terrestrial runoff and biological activities (Yan and Li, 2023). The Hg is mainly influenced by anthropogenic pollution, status quo, particle size composition, organic matter content, and sulphates (Zhang, 2015), but has also been found to be present in the loading and unloading of goods at terminals and from construction Cu and Hg are also present in residues (Ye et al., 2014). Thus, the contribution of the first principal component essentially reflects the impact of pollution of marine sediments by industrial and agricultural activities as well as by domestic sewage. It is noteworthy that the 2017 Marine Environment Bulletin of Zhejiang Province reported elevated levels of arsenic from the Yong and Qiantang rivers. Furthermore, it was determined that the concentration of As in the marine environment of the Yangtze River estuary is identical to that observed in this region (Zhang, 2014). Additional findings suggest that the As levels in this area are significantly elevated due to the construction of marine engineering and other human-made structures (Cai et al., 2024). Therefore, it can be inferred that the concentration of As in marine environments is influenced not only by the discharge of terrestrial resources but also by riverine inputs and human-induced marine engineering activities.
The variance contribution of the second principal component was 16.42%, in which TOC was the high loading and Hg was the medium loading, which indicated that organic matter was an important binding agent for metal ions, and secondly, it also indicated that Hg also had another source, combined with the results of the correlation analysis, It also confirms that the source of contamination may be different from other metals and may be associated with sulphate and organic matter (Fang et al., 2019). In the marine, heavy metals influence the formation of metal-organic complexes with TOC and sulphates through surface adsorption, cation-exchange reactions, and chelation (Miranda et al., 2021). The researchers concluded that slight changes in TOC during regional hydrodynamic transport have a depositional effect on heavy metals, causing particulate matter to be deposited on the seafloor. This process leads to resuspension of sediments from the ocean surface (Talling et al., 2023). And also in the sparsely distributed area of islands, tidal kinetic energy affects the deposition process of heavy metals (Cai et al., 2024). Thus, the second component is influenced by a variety of natural factors, such as organic content and sulphate, as well as hydrodynamic self-disturbance. From the study, it was found that heavy metals in the region are strongly influenced by anthropogenic factors such as land-based resource discharges and human marine engineering activities, and to a lesser extent by riverine inputs and a wide range of other natural factors, so the potential impacts of industrial effluents, domestic wastewater, energy consumption, and pesticide use, as well as the impacts of sediment inputs from estuarine areas, should be taken into account in the identification of sources of heavy metals in the future.
4.4 Ecological risk assessment of heavy metals
The ecological risk posed by these heavy metals to seawater was assessed by calculating RQ (Figure 6A), the RQ values in this study were lower than 1. It showed that the ecological risk posed by heavy metals in the seawater did not pose a significant ecological risk and was within acceptable levels.
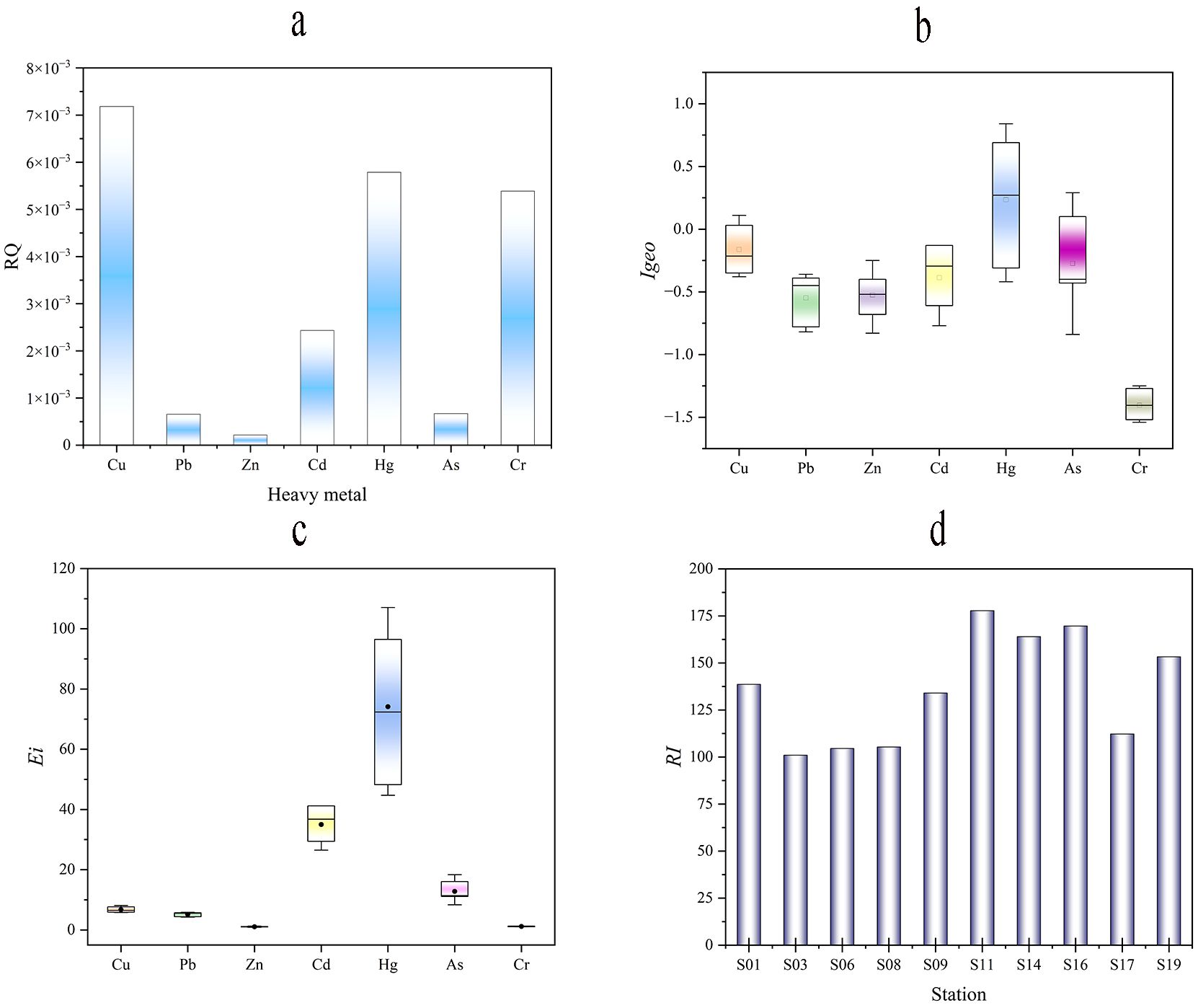
Figure 6. Sediment heavy metal pollution index and ecological risk red line. Heavy metal risk quotient values (A). The geoaccumulation index (Igeo) values (B). Ecological risk coefficient (Eir) value of each factor (C). Potential ecological risk index value (RI)(D).
To further analyze its ecological risk, this paper presents a multi-parameter analysis of the ecological risk of sediments. According to the Igeo results (Figure 6B), the risk levels of the heavy metal were Hg > Cu > As > Cd > Cr > Zn > Pb. The sediments of the study area exhibited mild contamination with heavy metals, including As, Hg, and Cu. However, the area was not contaminated with Cr. The Eir of heavy metals in sediments were Hg>Cr>As>Cd>Pb>Cu>Zn (Figure 6C). The potential ecological risk indices of Cr, As, Cd, Pb, Cu, and Zn at all stations were lower than 40, which showed low risk, and Hg was in the medium-high risk status. The Igeo focuses on the comparison of background values. The risk assessment of the Igeo showed that the overall heavy metal in the marine area was relatively low risk. In particular, sediments had no effect on Pb, Cd, Cr, and Zn. Cu, As, and Hg showed mild pollution. The study demonstrated that Cd and Hg had a greater risk and As had a higher ecological risk for seawater, especially Hg, despite its relatively low levels, it was still considered to pose a moderate potential ecological risk due to its high level of toxicity (Du et al., 2013; Sun et al., 2015). The RI value ranged between 100.95 to 177.75, with an average of 136.02, which is at a low potential ecological risk level as a whole (Figure 6D). However, some stations show moderately low risk and require our attention. Such as stations S11, S14, S16, and S19 in the sea area near Yangshan Port and Qushan Island.
The comprehensive ecological risk level of heavy metals in sediments was low, and Hg was the main contributing element. This matched the results of previous studies on marine heavy metals (Wang et al., 2021; Cai et al., 2024). At the same time, the same results were also found in the waters of Shantou Bay (Zhao et al., 2020) and Beibu Gulf (Teng et al., 2020). Combined with the results of multi-parameter analyses of seawater and sediments, which negated the possibility of transient heavy metal pollution (Kwok et al., 2014; Peng et al., 2022). Since the toxicity coefficients of Hg differ significantly from those of other elements, and Hg is the most important ecological hazard factor, while the risk of other heavy metals is relatively low, Hg should be used as the main object of monitoring and ecological research in the port area as a neighboring aquaculture area, especially in the vicinity of the Port close to the aquaculture area.
4.5 Probabilistic health risk assessment
Consumption of contaminated aquatic products can lead to trace metal poisoning, which can be harmful to human health, so it is necessary to assess the hazards caused by trace metals. In this paper, important seafood products such as fish widely consumed by local coastal residents collected from the sea area near Yangshan Port were selected. Based on the traditional deterministic approach, the study assessed the non-carcinogenic and carcinogenic risks of heavy metals in seafood consumed by adults and children using Monte Carlo simulation, and the probability distribution curves of HQ, HI, and CR were calculated (Figure 7).
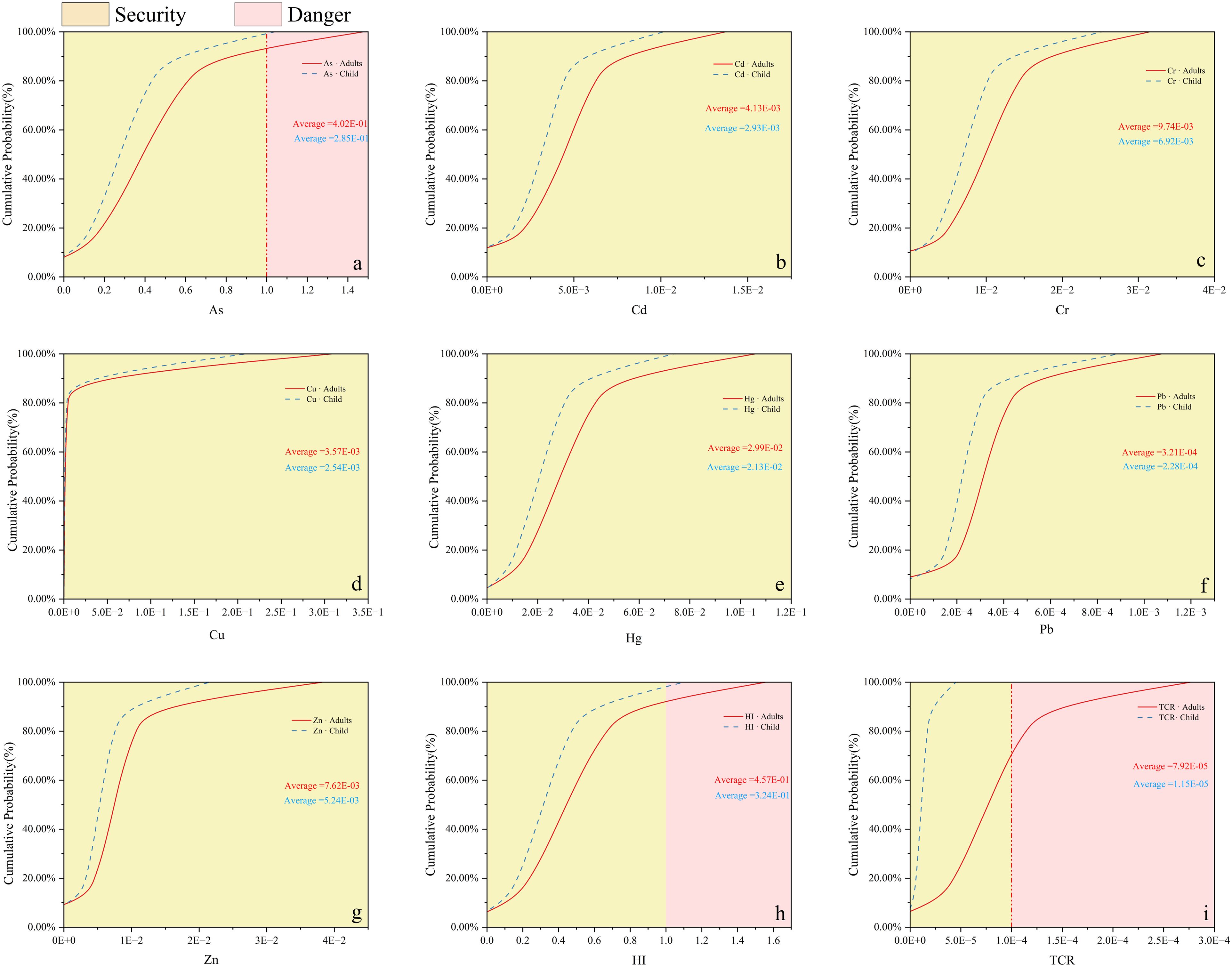
Figure 7. Probability distribution of heavy metal (A): As; (B): Cd; (C): Cr; (D): Cu; (E): Hg; (F): Pb; (G): Zn; (H): HI; (I): TCR) contamination levels in adults and children. Yellow and carmine represent their hazardous levels.
The mean HI values for adults and children were less than the USEPA guideline value of 1, indicating a negligible non-carcinogenic risk for these heavy metal stains. However, of all 10,000 Monte Carlo-simulated random output risk values, 2.25% and 0.05% of the HI values for adults and children, respectively, were above the acceptable thresholds, suggesting that the cumulative noncarcinogenic risk for adults is higher than that for children in the extreme cases and that the heavy metals may pose a potential noncarcinogenic risk for adults. Individual heavy metal studies showed that there was a 1.41% probability of exceedance for adults by the oral route of intake for As, and for the other six heavy metals, the cumulative frequency was already 100% when HQ did not reach threshold 1, indicating that there is no non-carcinogenic risk in different populations. Thus, the heavy metal As poses a potential non-carcinogenic risk to adults and may.
Studies have found that chronic exposure to As also poses serious health risks as it affects multiple systems in the body, including the respiratory system, skin, liver, nervous system, and cardiovascular system (Balali-Mood et al., 2021). In addition, recent studies have found that As levels below national safety limits also increase the risk of disease, and further research is needed to investigate the relationship between As in the environment and health risks in exposed populations (Saint-Jacques et al., 2018).
The results of CR cumulative frequency curves show that adults and children have negligible CR (mean value less than threshold 1E-04) for Cr, Cd, and Pb in the region; however, 19.89% of the output risk values for As have CR values for adults above the acceptable threshold (1E-04). In addition, as indicated by the TCR data, nearly 31.55% of the heavy metals in adults were above 1E-04, surfacing that adults in the area are exposed to higher concentrations of heavy metals and that the area is more affected by As, which is at low risk. This is consistent with previous studies that exposure yields high levels of carcinogenic effects (Lei et al., 2022).
It is worth noting that the value of Pb is small and can be ignored. The mean adult CR for Cd is 9.30E-06 and for Cr is 5.22E-06 and 8.26E-06 for both adults and children, with values above the minimum threshold of 1E-06. Studies have shown that Cd disrupts the endocrine system of the human body and induces oxidative stress, cell death, inflammation, and other cytotoxic responses, and chronic exposure to Cd significantly increases the risk of renal dysfunction and osteochondrosis (McElroy et al., 2017). Cr is considered an essential element for living organisms, including humans, and its deficiency or excess will greatly affect their metabolism (Rincon, 2015), but Cr is also a very soluble and highly toxic substance causing even more damaging and destructive effects on animals and humans (Nakkeeran et al., 2018), and its contribution to heavy metal-related health risks in adults and children cannot be ignored.
4.6 Sensitivity analysis
The sensitivities of metal concentrations, life expectancy (ED), intake rate (IR), and body weight (BW) were taken into account in the calculation of CR and HI for adults and children (Figure 8A, B). It was found that IR showed a positive correlation with TCR and HI, indicating that the more intake of its hazardous hazards, and like IR, ED showed a positive correlation with TCR, indicating that the total carcinogenic risk of heavy metals increases with the duration of exposure. BW showed a negative correlation with annual CR in both adults and children, but the effect of these factors was small (Yang et al., 2022). In addition, sensitivity analyses of heavy metals with HI and CR found (Figure 8C) that health risks for adults and children showed similar sensitivities. For TCR, As and Cd were positively correlated with TCR and were the main contributors to carcinogenic risk, probably because of their large carcinogenic slope factors. Similarly, among the non-carcinogenic risks, As concentration had the highest contribution to HI. In summary, As concentration was the most sensitive factor in human health risk assessment. Therefore, the health risks caused by exposure to heavy metals in the Yangshan Port area are not negligible and should be considered for further control.
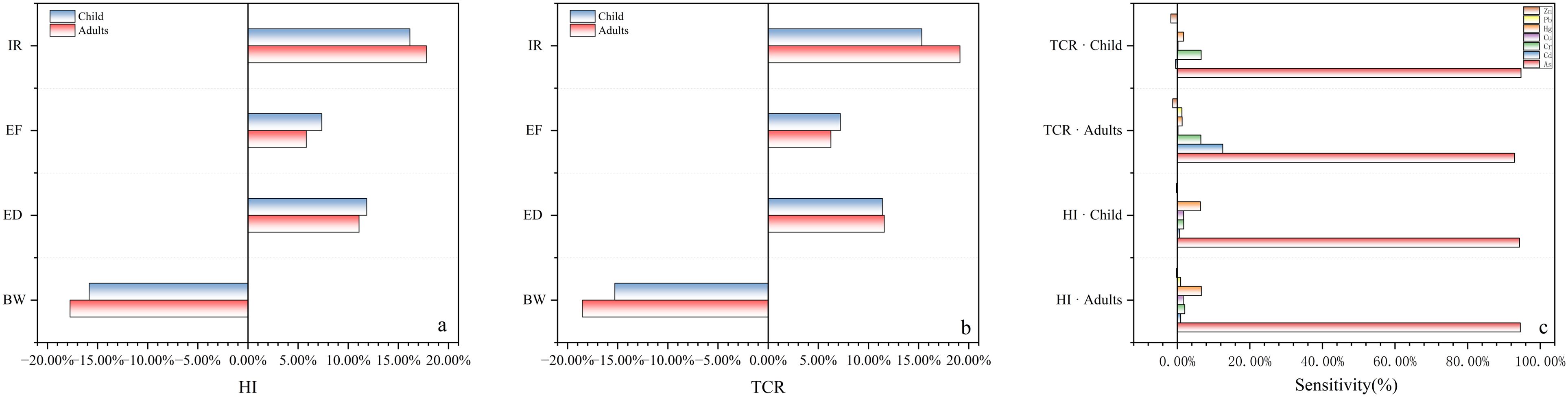
Figure 8. Sensitivity analysis of output variables to HI (A) and TCR (B) and heavy metal concentration to HI and TCR (C).
It is worth noting that non-oral carcinogenic metals such as Zn, Cu, and Hg were not considered in this study. However, widespread exposure to mercury in the environment can lead to various health problems, especially in infants and young children (Ferrante et al., 2018). Therefore, subsequent studies need to improve the accuracy of heavy metal determinations in carcinogenic risk assessment in addition to probability distributions of human life expectancy based on expanded sample sizes and reduced uncertainty in the results.
5 Conclusion
This study analyzed heavy metals in water, sediments, and marine organisms in the Yangshan Port and its adjacent sea areas. The results of the study showed that the heavy metal pollution in the Yangshan Port was characterized by significant spatial and temporal variations. The concentration of heavy metals is higher in estuaries, in proximity to ports, and in areas where human activity is prevalent. Heavy metal concentrations in sediments were generally higher than in water. Correlation and principal component analyses of heavy metals have revealed that the primary sources of pollution are anthropogenic, including industrial wastewater discharges, domestic pollution, and agricultural and fisheries discharges. Secondary sources of pollution are influenced by various natural factors, such as organic content and sulphate, and hydrodynamic self-disturbance. The distribution and accumulation of heavy metals differed between marine areas and aquatic organisms. Benthic animals have a high capacity to accumulate, while fish have a relatively low capacity to accumulate. These differences can be attributed to many different factors, such as metabolic pathways and diets of different organisms in different marine environments. Overall, the health risk of heavy metals from seafood consumption by coastal residents in the region is low. Metal concentration is the most sensitive factor for assessing the health risk of a single metal, and the As has the greatest impact on human health. This study provided new insight into the heterogeneity of heavy metal pollution accumulation in the Yangshan Port and its adjacent sea areas and offered a scientific basis and favorable support for port ecological environment protection and prevention of heavy metal pollution-related ecological risks.
Data availability statement
The original contributions presented in the study are included in the article/Supplementary Material. Further inquiries can be directed to the corresponding author.
Ethics statement
Ethical approval was not required for the study involving humans in accordance with the local legislation and institutional requirements. Written informed consent to participate in this study was not required from the participants or the participants’ legal guardians/next of kin in accordance with the national legislation and the institutional requirements. The manuscript presents research on animals that do not require ethical approval for their study.
Author contributions
WZ: Conceptualization, Data curation, Visualization, Writing – original draft, Writing – review & editing, Formal analysis, Supervision. JC: Data curation, Visualization, Writing – original draft, Writing – review & editing, Conceptualization, Methodology. WM: Formal analysis, Methodology, Visualization, Writing – review & editing. BL: Methodology, Software, Validation, Writing – review & editing. WF: Conceptualization, Data curation, Formal analysis, Investigation, Resources, Writing – review & editing, Supervision.
Funding
The author(s) declare financial support was received for the research, authorship, and/or publication of this article. This research was supported by the Bureau of Science and Technology of Zhoushan under Grant No.2023C41024, and the Zhejiang Provincial Natural Science Foundation of China under Grant No. LQ22D060003.
Acknowledgments
We would like to express our gratitude to the staff members of the Second Institute of Oceanography, Ministry of Natural Resources of the People’s Republic of China. We are also grateful to all the reviewers for their valuable comments and advice.
Conflict of interest
The authors declare that the research was conducted in the absence of any commercial or financial relationships that could be construed as a potential conflict of interest.
Generative AI statement
The author(s) declare that no Generative AI was used in the creation of this manuscript.
Publisher’s note
All claims expressed in this article are solely those of the authors and do not necessarily represent those of their affiliated organizations, or those of the publisher, the editors and the reviewers. Any product that may be evaluated in this article, or claim that may be made by its manufacturer, is not guaranteed or endorsed by the publisher.
Supplementary material
The Supplementary Material for this article can be found online at: https://www.frontiersin.org/articles/10.3389/fmars.2024.1512115/full#supplementary-material
References
Ali S., Ali H., Pakdel M., Ghale Askari S., Mohammadi A. A., Rezania S. (2022). Spatial analysis and probabilistic risk assessment of exposure to fluoride in drinking water using GIS and Monte Carlo simulation. Environ. Sci. pollut. Res. 29, 5881–5890. doi: 10.1007/s11356-021-16075-8
Ali A. Y. A., Idris A. M., Eltayeb M. A. H., El-Zahhar A. A., Ashraf I. M. (2021). Bioaccumulation and health risk assessment of toxic metals in red algae in Sudanese Red Sea coast. Toxin Rev. 40, 1327–1337. doi: 10.1080/15569543.2019.1697886
Ali H., Khan E., Ilahi I. (2019). Environmental chemistry and ecotoxicology of hazardous heavy metals: environmental persistence, toxicity, and bioaccumulation. J. Chem. 2019, 6730305. doi: 10.1155/2019/6730305
Alloway B. (2013). Heavy metals in soils: trace metals and metalloids in soils and their bioavailability (Switzerland: Springer International Publishing). doi: 10.1007/978-94-007-4470-7
Almeida J. A., Diniz Y. S., Marques S. F. G., Faine L. A., Ribas B. O., Burneiko R. C., et al. (2002). The use of the oxidative stress responses as biomarkers in Nile tilapia (Oreochromis niloticus) exposed to in vivo cadmium contamination. Environ. Int. 27, 673–679. doi: 10.1016/S0160-4120(01)00127-1
Anbuselvan N., Nathan D.S., Sridharan M. (2018). Heavy metal assessment in surface sediments off Coromandel Coast of India: Implication on marine pollution. Mar. pollut. Bull. 131, 712–726. doi: 10.1016/j.marpolbul.2018.04.074
Anik A. H., Khan R., Hossain S., Siddique M. A. B., Tamim U., Islam A. R. M. T., et al. (2022). Reconciling the geogenic and non-crustal origins of elements in an Indo-Bangla transboundary river, Atrai: Pollution status, sediment quality, and preliminary risk assessment. Environ. Res. 214, 114134. doi: 10.1016/j.envres.2022.114134
Balali-Mood M., Naseri K., Tahergorabi Z., Khazdair M. R., Sadeghi M. (2021). Toxic mechanisms of five heavy metals: mercury, lead, chromium, cadmium, and arsenic. Front. Pharmacol. 12. doi: 10.3389/fphar.2021.643972
Barak A. E., Mason C. F. (1989). Heavy metals in water, sediment and invertebrates from rivers in eastern England. Chemosphere 19, 1709–1714. doi: 10.1016/0045-6535(89)90514-6
Borg H., Jonsson P. (1996). Large-scale metal distribution in Baltic Sea sediments. Mar. pollut. Bull. 32, 8–21. doi: 10.1016/0025-326X(95)00103-T
Cai L., Jin J., Yu D., Jiang B. (2024). Distribution and ecological risk assessment of heavy mentals in surface sediments in Zhou shan coast area. People’s Yangtze River 55, 57–65. doi: 10.16232/j.cnki.1001-4179.2024.05.008
Chen C. Y., Folt C. L. (2000). Bioaccumulation and diminution of arsenic and lead in a freshwater food web. Environ. Sci. Technol. 34, 3878–3884. doi: 10.1021/es991070c
Chow T. E., Gaines K. F., Hodgson M. E., Wilson M. D. (2005). Habitat and exposure modelling for ecological risk assessment: A case study for the raccoon on the Savannah River Site. Ecol. Model. 189, 151–167. doi: 10.1016/j.ecolmodel.2005.04.001
de Mora S., Fowler S. W., Wyse E., Azemard S. (2004). Distribution of heavy metals in marine bivalves, fish and coastal sediments in the Gulf and Gulf of Oman. Mar. pollut. Bull. 49, 410–424. doi: 10.1016/j.marpolbul.2004.02.029
Du J., Zhao J., Chen B., Chen M., Yu W., Ma Z., et al. (2013). Assessing ecological risks of heavy metals to marine organisms by species sensitivity distributions[J]. Asian journal of ecotoxicology. Asian J. Ecotoxicology 8, 561–570. doi: 10.7524/AJE.1673-5897.20120525007
Enk M. D., Mathis B. J. (1977). Distribution of cadmium and lead in a stream ecosystem. Hydrobiologia 52, 153–158. doi: 10.1007/BF00036437
Evans R. D. (1994). Empirical evidence of the importance of sediment resuspension in lakes. Hydrobiologia 284, 5–12. doi: 10.1007/BF00005727
Fang L., Ge C., Jiang H., Ye G., Tang Y. J., Xie L. (2019). Spatial distribution, source and potential ecological risk assessment of heavy metals in surface sediments of Zhoushan sea area. Chin. J. Of Mar. Environ. Sci. 38, 769–775. doi: 10.12111/j.mes20190518
Fang H., Huang L., Wang J., He G., Reible D. (2016). Environmental assessment of heavy metal transport and transformation in the Hangzhou Bay, China. J. Hazardous Materials 302, 447–457. doi: 10.1016/j.jhazmat.2015.09.060
Feng W., Xu H., Zhu W., Zhang H., Zhang D., Wang Z. (2021). Trace metal contamination and species-specific bioaccumulation in the Zhoushan Fishery, northwestern East China Sea. Environ. Sci. pollut. Res. 28, 34642–34654. doi: 10.1007/s11356-021-12923-9
Ferrante M., Signorelli S., Ferlito S., Grasso A., Angela D., Copat C. (2018). Groundwater-based water wells characterization from Guinea Bissau (Western Africa): A risk evaluation for the local population. Sci. Total Environ. 619, 916–926. doi: 10.1016/j.scitotenv.2017.11.176
Fu T., Liang H., Niu L. (2022). Characteristics of heavy metals and their influential factors between sediments and water of the Zhujiang River Estuary in summer. Haiyang Xuebao 010), 044. doi: 10.12284/hyxb2022166
Guan C., Zhao X., Qu T., Sun B., Zhong Y., Hou C., et al. (2024). Quantitative and predictive evaluation of heavy metal pollution in bay ecosystems of northern China: characteristics, accumulation, and risk assessment. ACS ES&T Water 4, 57–67. doi: 10.1021/acsestwater.3c00334
Hakanson L. (1980). An ecological risk index for aquatic pollution control.a sedimentological approach. Water Res. 14, 975–1001. doi: 10.1016/0043-1354(80)90143-8
Hope B. K. (2006). An examination of ecological risk assessment and management practices. Environ. Int. 32, 983–995. doi: 10.1016/j.envint.2006.06.005
Hou W., Chen X., Wu J., Zhang C., Yu J., Bai J., et al. (2022). Sources and spatiotemporal variations of nitrogen and phosphorus in Liaodong Bay, China. Mar. pollut. Bull. 185, 114191. doi: 10.1016/j.marpolbul.2022.114191
Hou W., Wang Q., Xiang Z., Jia N., Hu J., Wu Z., et al. (2024). Comprehensive assessment of occurrence, temporal-spatial variations, and ecological risks of heavy metals in Jiaozhou Bay, China: A comprehensive study. Mar. pollut. Bull. 198, 115883. doi: 10.1016/j.marpolbul.2023.115883
Islam M. S., Ahmed M. K., Raknuzzaman M., Habibullah -Al- Mamun M., Islam M. K. (2015). Heavy metal pollution in surface water and sediment: A preliminary assessment of an urban river in a developing country. Ecol. Indic. 48, 282–291. doi: 10.1016/j.ecolind.2014.08.016
Jiang W., Liang B., Meng Q., Sun C. G. (2021). Concentration distribution and pollution assessment of heavy metals in surface sediments in Hangzhou bay. Chin. J. Of Mar. Environ. Sci. 40, 555–561. doi: 10.13634/j.cnki.mes.2021.04.011
Johansson H., Lindström M., Håkanson L. (2001). On the modelling of the particulate and dissolved fractions of substances in aquatic ecosystems — sedimentological and ecological interactions. Ecol. Model. 137, 225–240. doi: 10.1016/S0304-3800(00)00439-7
Kwok C. K., Liang Y., Wang H., Dong Y. H., Leung S. Y., Wong M. H. (2014). Bioaccumulation of heavy metals in fish and Ardeid at Pearl River Estuary, China. Ecotoxicology Environ. Saf. 106, 62–67. doi: 10.1016/j.ecoenv.2014.04.016
Lao Q., Su Q., Liu G., Shen Y., Chen F., Lei X., et al. (2019). Spatial distribution of and historical changes in heavy metals in the surface seawater and sediments of the Beibu Gulf, China. Mar. pollut. Bull. 146, 427–434. doi: 10.1016/j.marpolbul.2019.06.080
Lei M., Zhou J., Zhou Y., Sun Y., Ji Y., Zeng Y. (2022). Spatial distribution, source apportionment and health risk assessment of inorganic pollutants of surface water and groundwater in the southern margin of Junggar Basin, Xinjiang, China. J. Environ. Manage. 319, 115757. doi: 10.1016/j.jenvman.2022.115757
Li X., Chi W., Tian H., Zhang Y., Zhu Z. (2019a). Probabilistic ecological risk assessment of heavy metals in western Laizhou Bay, Shandong Province, China. PloS One 14, e0213011. doi: 10.1371/journal.pone.0213011
Li X., Chi W., Tian H., Zhang Y., Zhu Z. (2019b). Probabilistic ecological risk assessment of heavy metals in western Laizhou Bay, Shandong Province, China. PloS One 14, e0213011. doi: 10.1371/journal.pone.0213011
Li J., Cui Y., Fan Q., Li Z., Su L., Xue J. (2014). Annual monitoring and evaluation of heavy metal and petroleum hydrocarbon pollution in surface seawater of Yangshan Port. China J. Shanghai Ocean Univ. 23, 95–101.
Li Z., Liu P., Chen S., Liu X., Yu Y., Li T., et al. (2023). Bioinspired marine antifouling coatings: Antifouling mechanisms, design strategies and application feasibility studies. Eur. Polymer J. 190, 111997. doi: 10.1016/j.eurpolymj.2023.111997
Li Y., Zhang X., Meng J., Chen J., You X., Shi Q., et al. (2020). Molecular responses of an estuarine oyster to multiple metal contamination in Southern China revealed by RNA-seq. Sci. Total Environ. 701, 134648. doi: 10.1016/j.scitotenv.2019.134648
Liu J., Cao L., Dou S. (2017). Bioaccumulation of heavy metals and health risk assessment in three benthic bivalves along the coast of Laizhou Bay, China. Mar. pollut. Bull. 117, 98–110. doi: 10.1016/j.marpolbul.2017.01.062
Liu X., Ding C., Qin H., Zhang Y., Jiang Y., Li Z., et al. (2024). Pollution characteristics, distribution and risk level of heavy metals in sediments of the Yangtze River estuary. Heliyon 10, e28796. doi: 10.1016/j.heliyon.2024.e28796
Liu R., Jiang W., Li F., Pan Y., Wang C., Tian H. (2020). Occurrence, partition, and risk of seven heavy metals in sediments, seawater, and organisms from the eastern sea area of Shandong Peninsula, Yellow Sea, China. J. Environ. Manage. 279, 111771. doi: 10.1016/j.jenvman.2020.111771
Liu F., Yan W., Miao L., Xu W. (2015). Distribution and pollution assessment of heavy metals in seawater and surface sediments of Zhanjiang Port. China J. Mar. Technol. 34, 74–82.
Lu M., Hairy T., Lin X., Yang Y., Zhu Y. (2015). Pollution characteristics and ecological risk analysis of 6 heavy metals in surface sediments of Xiangshan Port. China J. Biol. 32, 30–34. doi: 10.3969/.issn.2095-1736.2015.04.030
Lu Y. Z., Yan B. X. (2010). Competitive adsorption of heavy metals on songhua river sediments and effect of pH. Res. Environ. Sci. 23, 20–25. doi: 10.13198/j.res.2010.01.22.luyzh.005
Luoma S. N., Bryan G. W. (1979). Trace metal bioavailability: Modeling chemical and biological interactions of sediment-bound zinc. Chem. modeling aqueous systems. 93, 577–609. doi: 10.1021/bk-1979-0093.ch026
Mao L., Ye H., Li F., Tao H., Shi L., Chen L., et al. (2017). Source-oriented variation in trace metal distribution and fractionation in sediments from developing aquaculture area—A case study in south Hangzhou bay, China. Mar. pollut. Bull. 125, 389–398. doi: 10.1016/j.marpolbul.2017.09.046
McElroy J. A., Kruse R. L., Guthrie J., Gangnon R. E., Robertson J. D. (2017). Cadmium exposure and endometrial cancer risk: A large midwestern U.S. population-based case-control study. PloS One 12, e0179360. doi: 10.1371/journal.pone.0179360
McGeer J. C., Szebedinszky C., McDonald D. G., Wood C. M. (2000). Effects of chronic sublethal exposure to waterborne Cu, Cd or Zn in rainbow trout. 1: Iono-regulatory disturbance and metabolic costs. Aquat. Toxicol. 50, 231–243. doi: 10.1016/S0166-445X(99)00105-8
Miranda L. S., Wijesiri B., Ayoko G. A., Egodawatta P., Goonetilleke A. (2021). Water-sediment interactions and mobility of heavy metals in aquatic environments. Water Res. 202, 117386. doi: 10.1016/j.watres.2021.117386
Nakkeeran E., Patra C., Shahnaz T., Rangabhashiyam S., Selvaraju N. (2018). Continuous biosorption assessment for the removal of hexavalent chromium from aqueous solutions using Strychnos nux vomica fruit shell. Bioresource Technol. Rep. 3, 256–260. doi: 10.1016/j.biteb.2018.09.001
Nour H. E. S. (2020). Distribution and accumulation ability of heavy metals in bivalve shells and associated sediment from Red Sea coast, Egypt. Environ. Monit. Assess. 192, 353. doi: 10.1007/s10661-020-08285-3
Özkaynak Ö.H., İçemer G. T., Merdun H. (2022). Determination of the risk on human health of heavy metals contained by ship source bilge and wastewater discharged to the sea on the mediterranean by monte carlo simulation. Sustainability. 14, 8408. doi: 10.3390/su14148408
Peng Z., Guo Z., Wang Z., Zhang R., Wu Q., Gao H., et al. (2022). Species-specific bioaccumulation and health risk assessment of heavy metal in seaweeds in tropic coasts of South China Sea. Sci. Total Environ. 832, 155031. doi: 10.1016/j.scitotenv.2022.155031
Pursiainen A., Veneranta L., Kuningas S., Saarinen A., Kallasvuo M. (2021). The more sheltered, the better – Coastal bays and lagoons are important reproduction habitats for pike in the northern Baltic Sea. Estuarine Coast. Shelf Sci. 259, 107477. doi: 10.1016/j.ecss.2021.107477
Rincon J. (2015). Toxicidad y bioacumulación de Cromo (Cr+6) en la almeja Polymesoda solida del sistema estuarino Lago de Maracaibo. Boletín del Centro Investigaciones Biologicas 49, 5–25.
Rinklebe J., Antoniadis V., Shaheen S. M., Rosche O., Altermann M. (2019). Health risk assessment of potentially toxic elements in soils along the Central Elbe River, Germany. Environ. Int. 126, 76–88. doi: 10.1016/j.envint.2019.02.011
Sahu A. K., Dung M. S. D., Sahoo S. K., Mir S. A., Nayak B., Baitharu I. (2023). Ecological and human health risk associated with heavy metals in sediments and bioaccumulation in some commercially important fishes in Mahanadi River, Odisha, India. Environ. Chem. Ecotoxicology 5, 168–177. doi: 10.1016/j.enceco.2023.08.001
Saint-Jacques N., Brown P., Nauta L., Boxall J., Parker L., Dummer T. J. B. (2018). Estimating the risk of bladder and kidney cancer from exposure to low-levels of arsenic in drinking water, Nova Scotia, Canada. Environ. Int. 110, 95–104. doi: 10.1016/j.envint.2017.10.014
Shah S. (2021). Heavy metals in the marine environment—An overview. 1–26 (Switzerland: Springer International Publishing). doi: 10.1007/978-3-030-73613-2_1
Su Q., Lei X., Shin Y., Sun Y., Zhou P. (2023). Pollution characteristics of heavy metals in different marine functional areas of Tieshangang bay, Guangxi. Chin. J. Of Mar. Environ. Sci. 42, 876–885. doi: 10.13634/j.cnki.mes.2023.06.003
Sun N., Huang W., Yu H. (2015). Analysis and assessment of heavy metals accumulation feature of sediments and marine organisms from Zhanjiang Harbo. Chin. J. Mar. Environ. Sci. 34, 669–672. doi: 10.13634/j.cnki.mes.2015.05.005
Talling P. J., Cartigny M. J. B., Pope E., Baker M., Clare M. A., Heijnen M., et al. (2023). Detailed monitoring reveals the nature of submarine turbidity currents. Nat. Rev. Earth Environ. 4, 642–658. doi: 10.1038/s43017-023-00458-1
Teng D., Cui Z., Yuan X., Zhang L. (2020). Distribution patterns and potential ecological risk evaluation of heavy metals in the surface sediments of Beibu Gulf. Geological Survey China 7, 79–85. doi: 10.19388/j.zgdzdc.2020.06.10
Thomann R. V., Connolly J. P., Parkerton T. F. (1992). An equilibrium model of organic chemical accumulation in aquatic food webs with sediment interaction. Environ. Toxicol. Chem. 11, 615–629. doi: 10.1002/etc.5620110505
Tian Y., Chen J., Huang J., Lu S. (2017). Analysis and disposal technology of pollutants in ship antifouling paint. China J. Environ. Eng. 11, 1164–1170. doi: 10.12030/j.cjee.201601127
Tian K., Wu Q., Liu P., Hu W., Huang B., Shi B., et al. (2020). Ecological risk assessment of heavy metals in sediments and water from the coastal areas of the Bohai Sea and the Yellow Sea. Environ. Int. 136, 105512. doi: 10.1016/j.envint.2020.105512
Torres M. A., Barros M. P., Campos S. C. G., Pinto E., Rajamani S., Sayre R. T., et al. (2008). Biochemical biomarkers in algae and marine pollution: A review. Ecotoxicology Environ. Saf. 71, 1–15. doi: 10.1016/j.ecoenv.2008.05.009
Vu C. T., Lin C., Yeh G., Villanueva M. C. (2017). Bioaccumulation and potential sources of heavy metal contamination in fish species in Taiwan: assessment and possible human health implications. Environ. Sci. pollut. Res. 24, 19422–19434. doi: 10.1007/s11356-017-9590-4
Wang H., Li Z., Hao Q., Bao J. (2021). Analysis and evaluation of heavy metals in sediments in the northern waters of Zhoushan Port, Ningbo. Environ. Sci. Manage. China 46, 60–64. doi: 10.1007/s12665-020-09361-0
Wang W.-X., Pan J. (2004). The transfer of metals in marine food chains: A review. Acta Ecologica Sin. 24, 599.
Xu X., Cao Z., Zhang Z., Li R., Hu B. (2016). Spatial distribution and pollution assessment of heavy metals in the surface sediments of the Bohai and Yellow Seas. Mar. pollut. Bull. 110, 596–602. doi: 10.1016/j.marpolbul.2016.05.079
Yan J., Li Y. (2023). Research progress on the biogeochemical cycling of cadmium in the ocean. Environ. Chem. 42 (3), 720–732. doi: 10.7524/j.issn.0254-6108.2022041906
Yang Y., Zhang M., Chen H., Mai B., Xu J. (2022). Research progress in environmental exposure factors of chinese population. Res. Environ. Sci. 35, 2205–2215. doi: 10.13198/j.issn.1001-6929.2022.06.13
Yavar Ashayeri N., Keshavarzi B. (2019). Geochemical characteristics, partitioning, quantitative source apportionment, and ecological and health risk of heavy metals in sediments and water: A case study in Shadegan Wetland, Iran. Mar. pollut. Bull. 149, 110495. doi: 10.1016/j.marpolbul.2019.110495
Ye R., Ming-li Q., Yong-ping C., Hong-wei G., Xian-sen Y. (2014). Source analysis and ecological risk assessment for heavy metals in the sediments of the Yangshan Deepwater Harbor in autumn and winter. Mar. Sci. Bull. 16, 59–72. doi: 10.11840/j.issn.1001-6392.2015.01.011
Yi W. (2017). Evaluation of heavy metals in oyster (Crassostrea rivularis) from Qinzhou Harbor, Beibu Gulf. J. Fisheries China 41, 806–815. doi: 10.11964/jfc.20160810499
Yi Y., Yang Z., Zhang S. (2011). Ecological risk assessment of heavy metals in sediment and human health risk assessment of heavy metals in fishes in the middle and lower reaches of the Yangtze River basin. Environ. pollut. 159, 2575–2585. doi: 10.1016/j.envpol.2011.06.011
Yu W., Zhang D., Liao J., Ma L., Zhu X., Zhang W., et al. (2022). Linking ecosystem services to a coastal bay ecosystem health assessment: A comparative case study between Jiaozhou Bay and Daya Bay, China. Ecol. Indic. 135, 108530. doi: 10.1016/j.ecolind.2021.108530
Zhang X. (2014). Distribution characteristics and Controlling Factors of Heavy Metals in the Yangtze River Estuary the Yellow River Estuary and Adjacent seas. Ocean Univ. China (OUC).
Zhang H.J. (2015). Transformation and environmental effect of mercury and some heavy metalsin yangtze river estuary and its adjacent area (Qingdao, China: Ocean University of China (OUC). doi: 10.7666/d.D326582
Zhang Z., LI S., Dong Y., Wang Q., Xiao F., LU J. (2005). Sediment deposition rate and geochemistry in coastal waters of Zhejiang Province. Mar. Geology Quaternary Geology China 03), 15–24. doi: 10.16562/j.cnki.0256-1492.2005.03.003
Zhang L., Shi Z., Jiang Z., Zhang J., Wang F., Huang X. (2015). Distribution and bioaccumulation of heavy metals in marine organisms in east and west Guangdong coastal regions, South China. Mar. pollut. Bull. 101, 930–937. doi: 10.1016/j.marpolbul.2015.10.041
Zhang C., Sun S., Chen C. (2011). Heavy Metals in Seawater, Surface Sediment and Shellfishes inTideland ofZhanjiang Harbor. China J. Guangdong Ocean Univ. 31, 67–72.
Zhang D., Xu Z., Gao Q., Chen J. (2016). Effects of tide and water masses on the distribution of zooplankton in different parts of Hangzhou Bay. Acta Ecologica Sin. 36, 133–140. doi: 10.1016/j.chnaes.2015.12.007
Zhang C., Yu Z. G., Zeng G. M., Jiang M., Yang Z. Z., Cui F., et al. (2014). Effects of sediment geochemical properties on heavy metal bioavailability. Environ. Int. 73, 270–281. doi: 10.1016/j.envint.2014.08.010
Zhao C., Hu J., Li I., Liu L., Lu W., Fu J., et al. (2020). Distribution and risk assessment of heavy metal content in surface sediments of Shantou Bay, Guangdong Province. China J. Appl. Oceanography 39, 408–418. doi: 10.3969/J.ISSN.2095-4972.2020.03.012
Zhao B., Wang X., Jin H., Feng H., Shen G., Cao Y., et al. (2018). Spatiotemporal variation and potential risks of seven heavy metals in seawater, sediment, and seafood in Xiangshan Bay, Chin[amp]]ndash;2016). Chemosphere 212, 1163–1171. doi: 10.1016/j.chemosphere.2018.09.020
Zhao P., Zhang R., Qin X., Lan W., Chen B., Hu B., et al. (2017). Evaluation of heavy metals in oyster (Crassostrea rivularis) from Qinzhou Harbor, Beibu Gulf. J. Fisheries China 41, 806–815. doi: 10.11964/jfc.20160810499
Zheng N., Wang Q., Liang Z., Zheng D. (2008). Characterization of heavy metal concentrations in the sediments of three freshwater rivers in Huludao City, Northeast China. Environ. pollut. 154, 135–142. doi: 10.1016/j.envpol.2008.01.001
Zhong A. P., Guo S. H., Li F. M., Li G., Jiang K. X. (2006). Impact of anions on the heavy metals release from marine sediments. J. Environ. Sci. 18, 1216–1220. doi: 10.1016/S1001-0742(06)60065-X
Keywords: heavy metal, Yangshan port, pollution assessment, source apportionment, health risk
Citation: Zhu W, Cai J, Ma W, Li B and Feng W (2025) Distribution, sources and risk assessment of heavy metals in Yangshan port and its adjacent sea areas. Front. Mar. Sci. 11:1512115. doi: 10.3389/fmars.2024.1512115
Received: 16 October 2024; Accepted: 13 December 2024;
Published: 08 January 2025.
Edited by:
Qinsheng Wei, Ministry of Natural Resources, ChinaReviewed by:
Meng Chuan Ong, University of Malaysia Terengganu, MalaysiaYanbin Li, Ocean University of China, China
Copyright © 2025 Zhu, Cai, Ma, Li and Feng. This is an open-access article distributed under the terms of the Creative Commons Attribution License (CC BY). The use, distribution or reproduction in other forums is permitted, provided the original author(s) and the copyright owner(s) are credited and that the original publication in this journal is cited, in accordance with accepted academic practice. No use, distribution or reproduction is permitted which does not comply with these terms.
*Correspondence: WeiHua Feng, fengweihua1203@163.com