- 1Laboratory of Aquatic Nutrition and Feed, College of Fisheries, Guangdong Ocean University, Zhanjiang, China
- 2Aquatic Animals Precision Nutrition and High-Efficiency Feed Engineering Research Centre of Guangdong Province, Zhanjiang, China
- 3Key Laboratory of Aquatic, Livestock and Poultry Feed Science and Technology in South China, Ministry of Agriculture, Zhanjiang, China
In this experiment, we aimed to evaluate the relationship between the addition of Schizochytrium limacinum to low fish meal diets on growth performance, apoptosis, autophagy, lipid metabolism, and intestinal health of Lipenaeus vanamei. The diet containing 25% fish meal was used as a positive control (FM) and the other three diets contained 15% fish meal and were supplemented with 0, 0.3, and 0.6% S. Limacinum (LF, LFLD, LFHD). The shrimp (0.22 ± 0.00 g) were divided into four replicates of 40 shrimp per tank and fed four times daily to apparent satiation for 8 weeks. Results showed that the final weight (FBW) and weight gain rate (WGR) of shrimp fed FM and LFHD diets were significantly increased compared to those fed the LFLD diet (P<0.05), and there was no significant difference in survival rate (SR) and feed conversion rate (FCR) among the groups (P>0.05). Supplementation of S. Limacinum in low fish meal diets had no effects on shrimp body composition (P<0.05). There were significant differences (P<0.05) in low-density lipoprotein (LDL-C) glucose (GLU), triglycerides (TG), and total cholesterol (TC) in the hemolymph of shrimp fed the LF diet compared to those fed the LFLD and LFHD diets. HE staining and transmission electron microscopy (TEM) results showed that the microvilli height, mucosal folds height, mucosal folds width and muscle layer thickness in the intestine of shrimp fed the LF diet were significantly reduced compared to those fed the other three diets (P<0.05). Swelling of the endoplasmic reticulum and irregular mitochondria in the gut of shrimp fed the LF diet was also observed by TEM, and the endoplasmic reticulum and mitochondria of shrimp fed the LFHD diet returned to a healthy state. Hepatopancreas genes expression results were showed that the gene expression of 5′ -AMP-activated protein kinase (ampk), stearoyl-CoA desaturase (scd1), acetyl-CoA carboxylase 1 (acc1), and malonyl-CoA decarboxylase (mcd) of shrimp fed the LF diet was significantly increased compared to those fed the FM diet (P<0.05). The gene expression of sterol regulatory element-binding protein (srbep) and carnitine palmitoyl transferase 1 (cpt-1) of shrimp fed the LFLD diet was significantly increased compared to those fed the LF diet (P<0.05). The gene expression of acc1, mcd and scd1 of shrimp fed the LFHD diet was significantly reduced compared to those fed the LF diet (P<0.05). Results of genes expression associated with apoptosis in the hepatopancreas showed that the gene expression of B lymphocytoma-2 (bcl-2), BCL2 associated X apoptosis regulator (bax) and cysteinyl aspartate specific proteinase 8 (caspase 8) of shrimp fed the LF diet was significantly reduced compared to those fed the FM diet (P<0.05). The gene expression of bcl-2 of shrimp fed the LFHD diet was significantly reduced compared to those fed the LF diet (P<0.05). Genes related to autophagy in the hepatopancreas showed that the expression of autophagy-related protein 12 (atg 12), autophagy-related protein 13 (atg 13) and beclin1 of shrimp fed LF the diet was significantly reduced compared to those fed the FM diet (P<0.05). The gene expression of atg 12 and atg 13 of shrimp fed the LFHD diet was significantly increased compared to those fed the LF diet (P<0.05). In summary, reducing fish meal is detrimental to the growth performance and intestinal health of shrimp, and 0.6% S. Limacinum supplementation can improve the growth performance, promotes hepatopancreas lipid metabolism, reduces apoptosis, promotes autophagy and improve intestinal health of Litopenaeus vannamei.
Introduction
Litopenaeus vannamei has the characteristics of fast growth, strong disease resistance, and delicious taste, which has important economic value, and the annual production has up to 5.8 million tons in 2020 (FAO, 2022; Xu et al., 2022a). Fish meal is rich in amino acids, vitamins, and minerals which are necessary for the growth of fish, shrimp, and crab, and has a special flavor that makes it good palatability (An et al., 2018). Nonetheless, the high cost of fish meal has increased the cost of feed Previous studies found that fish meal in aquatic animal feed can be substituted by different protein sources, such as soy protein peptide (Lin et al., 2022), soy protein concentrate (Zhu et al., 2020), fermented soy pulp (Kari et al., 2022), hydrolyzed fish protein powder (Hlordzi et al., 2022), bacterial protein meal (Chen et al., 2021b), concentrated dephenolization cottonseed protein (Zhao et al., 2021), blood meal (Kirimi et al., 2016), black soldier fly (Huang et al., 2022), meat and bone meal (Ai et al., 2006), and hydrolyzed feather meal (Campos et al., 2017). Plant proteins are widely available and inexpensive, which were favored by researchers for partial replacement of fish meal in aquatic animal feed (Liao et al., 2022). However, plant protein generally has the disadvantages of amino acid imbalance and high content of anti-nutritional factors (Jannathulla et al., 2019), and adding excessive plant protein can also affect the growth performance, intestinal microecology, and nutrition metabolism of aquatic animals (Ray et al., 2020). In our previous study, we found that the essential nutrient balance in low fish meal diets improved growth properties and intestinal fitness of shrimp (Xie et al., 2016; Xie et al., 2020c).
Fish meal contains high levels of fish oil, which is rich in n-3 long-chain polyunsaturated fatty acids, especially for docosahexaenoic acid (DHA) and eicosapentaenoic acid (EPA) (Cho and Kim, 2011). Consequently, the content of high-unsaturated fatty acids decreases accordingly in the low fish meal diets. Polyunsaturated fatty acids are essential fatty acids for fish and crustaceans, which cannot be synthesized in vivo and must be obtained from food (Dyall et al., 2022). DHA is a polyunsaturated fatty acid, which has various functional roles in regulating the physiological health of the body and has important regulatory influences on growth, inflammatory response, development, immune regulation, and antioxidation in aquatic animals (Ruyter et al., 2022). DHA is an essential fatty acid for many fish, shrimp, and shellfish larvae, previous study proved that dietary supplementation of DHA in the low fish meal diet could improve the immune system response and intestinal fitness of Penaeus monodon (Xie et al., 2020b).
Schizochytrium Limacinum (S. Limacinum), a type of marine algae, is rich in the highly unsaturated fatty acid DHA (Li et al., 2018). In contrast to other algae, S. Limacinum is produced by employing heterotrophic propagation and was regarded as microalgae with the potential to produce DHA in an industrial chain (Sarker et al., 2016; Osmond et al., 2021). Numerous studies have been conducted to demonstrate the improvement of the growth performance of aquatic animals by feeding diets containing S. Limacinum, such as Litopenaeus vannamei (Wang et al., 2017), Salmo Salar (Sprague et al., 2015), Ictalurus punctatus (Li et al., 2009), Danio rerio (Byreddy et al., 2019), Trachinotus Ovatus (Xie et al., 2019), Epinephelus Lanceolatus (García-Ortega et al., 2016). In addition, there have been several studies showing that dietary supplementation of S. Limacinum be significantly enhanced the non-specific immunity of Trachinotus Ovatus (Xie et al., 2019) and improve the intestinal health of Oreochromis niloticus (Souza et al., 2020) and Oncorhynchus mykiss (Lyons et al., 2016). The results of another study suggest that S. Limacinum can be a promising low-level substitute for fish meals, which could improve the fillet texture of largemouth bass (Liao et al., 2022).
Few studies have been reported on the effect of S. Limacinum addition in the low fish meal diet of Litopenaeus vannamei. Thus, the intention of this experiment was to assess the effects of low fish meal diet supplementation with S. Limacinum on growth performance, hemolymph biochemistry, intestinal health, lipid metabolism, apoptosis, and autophagy of Litopenaeus vannamei.
Materials and methods
Diet preparation
Two diets with different levels of the fish meal were formulated as the positive control (FM) and negative control (LF), and two levels of S. Limacinum were added to the LF, which were labeled as LFLD and LFHD. The nutritional composition of the four diets was shown in Table 1. Ingredients were crushed and passed through an 60 mesh sieve, weighed accurately, and blended well (M-256, South China University of Technology, Guangzhou), next were stirred well with pre-weighed distilled water, fish oil, soybean oil, soy lecithin, and pre-configured coated crystal amino acids as described by Xie et al. (Xie et al., 2020c). The 1.0 mm and 1.5 mm feeds were extruded by a twin-screw extruder (F-26, South China University of Technology, Guangzhou), followed by being heated in an oven at 90°C for 60 min and air-drying at room temperature, the diets were storage at -20°C until use (Li W. et al., 2022).
Experimental shrimp and management conditions
Juvenile Litopenaeus Vannamei were purchased from Zhanjiang Yuehai Seed Co. The shrimp was transferred to a pre-disinfected specimen pond for one month and acclimatized for one week to the experimental conditions before the start of the experiment. Then 640 healthy shrimp with similar body sizes (0.22 ± 0.00g) were randomly assigned to 16 fiberglass tanks (500 L) with 40 shrimp per tank. The shrimp were periodically fed four times a day (7:00, 11:00, 16:00, 21:00) for 8 weeks. During the time of trial, water temperature and salinity were to be measured daily, with the water temperature at 28.0-30.0°C, salinity at 26-30‰, pH at 7.6-8.1, and ammonia nitrogen level below 0.05 mg/L (He et al., 2017). In addition, each tank was aerated to ensure enough oxygen, and the water was changed by approximately 60% at 2h after the first feeding every day.
Sample collection and analysis
Growth indexes
At the end of 8 weeks of feeding, shrimp were starved and treated for 24 h before being anesthetized using MS-222 (Zhou et al., 2019; Xu et al., 2021). Samples were then collected, and shrimp in each tank was counted and weighed to determine the initial weight (IBW), final weight (FBW), survival rate (SR), feed conversion rate (FCR), and weight gain rate (WGR). Immediately after weighing, 10 shrimp were randomly selected from each tank, and blood was taken using a 1 mL sterile syringe, and placed in a 1.5 mL sterile centrifuge tube. The hemolymph was gathered by centrifugation at 1500 x g for 10 min at 4°C through a benchtop high-speed frozen centrifuge (JIDI-20R, Guangzhou JIDI Instruments Ltd. formula). Six shrimps were randomly detected from each tank, which were stored at -20°C for the shrimp body composition analysis using standard methods (Feldsine et al., 2002). Moisture was determined in a constant-weight oven at 105°C. The content of crude protein and Crude lipid were determined using the Kjeldahl method (Kjeltec™8400, Sweden) and the Soxhlet extraction method (extractant petroleum ether), respectively, according to the description of (Zhang et al., 2018; Liu et al., 2021).
Hemolymph biochemical indexes and enzyme activity analysis
Hemolymph biochemical indicators were measured using kits developed by Nanjing Jiancheng Institute of Biology (China), the content of high-density lipoprotein (HDL-C), low-density lipoprotein (LDL-C), glucose (GLU), triglyceride (TG), total protein (TP), and total cholesterol (TC), and the activities of aspartate aminotransferase (AST), alanine aminotransferase (ALT) were measured using a full-wavelength enzyme marker (Thermo, Multiskan GO 1510). The commercial kit IDs were as follows: A112-1-1, A113-1-1, A154-1-1, A110-1-1, A045-2, A111-1, C009-2-1, and C009-2-1, respectively. The methods of the kit were tested strictly according to the description of (Gui et al., 2019; Wu et al., 2021).
Quantitative real time PCR analysis
Total RNA was obtained from the hepatopancreas and intestine of Litopenaeus vannamei using the TransZol Up Plus RNA kit (Transgen, China), and the extracted RNA was assayed by NanoDrop2000 (Thermo USA), and the massification of RNA was determined by the A260/280 ratio (Zhu et al., 2021; Zhang et al., 2022a). Reverse transcription was performed using the PrimeScriptTM RT kit (Takara, Japan) according to the manufacturer’s instructions. Oligo dt (18T) primer random 6 mers, PrimeScriptTM RT enzyme mixture I, 5× PrimeScriptTM buffer, and RNase-free water were used to reverse transcribe 1000 ng of RNA at 37°C for 15 min, followed by inactivation at 85°C for 5 s (Li Y. et al., 2022; Xu et al., 2022c).
The polymerase chain reaction was performed using SYBR® Green Premix Pro Taq HS qPCR Kit II (Accurate Biotechnology (Hunan) Co., Ltd.) and the system was quantified fluorescently on a LightCycler 480 (Roche Applied Science) according to the set procedure for 10 The fluorescence quantification of the samples was performed on a LightCycler 480 (Roche Applied Science) according to a set procedure. The 10 μl system consisted of 0.5 μM of forward and reverse specific primers, 5 μl of 2× SYBR® Green Pro Taq HS Premix II, 10 ng of cDNA template and RNase-free water (Chi et al., 2017). The denaturation step lasted for the 30s at 95°C, followed by 40 amplification cycles, denaturation at 95°C for 5s, and annealing at 60°C for 30s before analysis of the solubility curves. The relative gene expression was calculated by the 2-ΔΔCt method using ef-1α as the internal reference gene (Chen et al., 2021a; Wang et al., 2022). (The information of the primers used in this study was shown in Table 2).
Intestinal tissue analysis
Intestine of four shrimp was randomly selected from each tank for hematoxylin-eosin (H&E) stain and transmission electron microscopy (TEM) analysis. The intestine was stored in Bouin’s solution for 24 h and then dehydrated in 75% ethanol. Then the tissue was dehydrated and washed with xylene, The samples were cleaned in toluene followed by embedding in paraffin to make solid wax blocks. A rotary microtome was used to cut the solid wax blocks into transverse section blocks into 5 μm sections followed by mounting on the slide and staining with hematoxylin-eosin (H&E). The slides were observed under a microscope (Olympus, BX51, Tokyo, Japan) and the built-in software was used to measure the thickness of the muscle layer, the height of the mucosal fold, and the width of the mucosal fold. TEM was performed as the method described before (Xie et al., 2018): intestines were fixed with 2.5% glutaraldehyde for 2h, washed and fixed with osmium acid for 3h, dehydrated and embedded with resin, and ultrathin sections were made of resin blocks, followed by staining with saturated uranyl acetic acid solution for 30 min and distilled water washing followed by lead citrate for 30 min. Finally, the cytoarchitecture was observed by TEM (Hitachi HT7700 TEM, Japan) and the length of microvilli was measured with Image-Pro Plus 6.3 software (Media Cybernetics, Inc., Rockville, USA).
Calculations and statistical analysis
These statistics are obtained as follows:
The results are expressed as mean ± SEM. There was a one-way ANOVA for all data and a Duncan’s multiple tests was performed using SPSS 21.0 to identify significant between treatment differences. The probability value of P<0.05 is statistically significant and indicates a significant difference in the results.
Result
Growth performance
The results in Table 3 show that the FBW and WGR of shrimp fed the LFLD diet were significantly lower than those fed the FM diet (P < 0.05), dietary supplementation of 0.6% S. Limacinum significantly increased the growth of shrimp (P<0.05). The SR and FCR of shrimp were similar among the four groups.
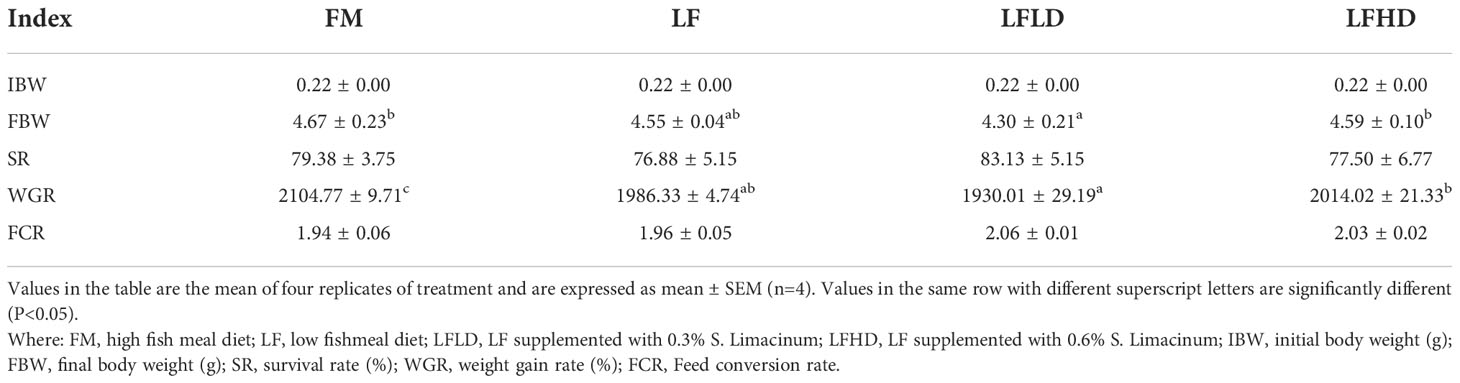
Table 3 Effect of low fish meal diet supplemented with Schizochytrium limacinum on the growth performance of Litopenaeus vannamei.
Analysis of whole shrimp body composition
The results in Table 4 show the whole shrimp body composition.

Table 4 Effect of low fish meal diet supplemented with Schizochytrium limacinum on the body composition of Litopenaeus vannamei.
Hemolymph biochemical indexes
As shown in Table 5, the HDL-C content of shrimp fed the LF diet was significantly increased compared to those fed the FM and LFHD diets (P < 0.05). The LDL-C content of shrimp fed the LF and FM diets were significantly increased compared to those fed the LFLD and LFHD diets (P < 0.05). The GLU content of shrimp fed the LFLD and LFHD diets were significantly lower than those fed the FM and LF diets (P < 0.05). The TG content in the hemolymph of shrimp fed the LF diet was significantly lower than those fed the LFDH diet (P < 0.05), and the TG content of shrimp fed the LFLD diet was significantly lower than those fed the other diets (P < 0.05). AST and ALT activity in the hemolymph of shrimp fed the LF diet were significantly increased compared to those fed the other diets (P < 0.05). TC levels in the hemolymph of shrimp fed the FM diet were significantly increased compared to those fed the other diets (P < 0.05).
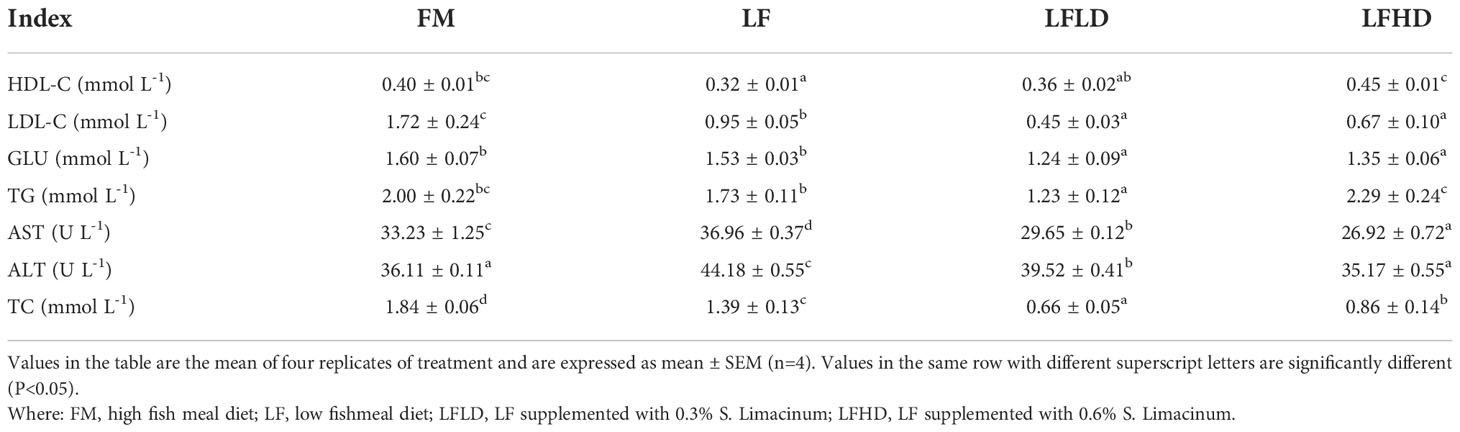
Table 5 Effect of low fish meal diet supplemented with Schizochytrium limacinum on hemolymph biochemical parameters of Litopenaeus vannamei.
The expression levels of the lipid metabolism, apoptosis, and autophagy-related genes
Lipid metabolism-related gene expression levels were shown in Figure 1. The gene expression of ampk of shrimp fed the LFLD diet was significantly increased compared to those fed the FM and LFHD diets (P<0.05). The gene expression of srbep was significantly higher in shrimp fed the LFLD and LFHD diets than those fed the FM and LF diets, which is decreased with the dietary S. Limacinum supplementation (P<0.05). The gene expression of acc1 and scd1 of shrimp fed the LFLD and LFHD diets were significantly increased compared to those fed the FM diet, which is decreased with the dietary S. Limacinum supplementation (P<0.05). The gene expression of mcd and cpt-1 of shrimp fed the LFLD diet was significantly increased compared to those fed the FM and LFHD diets (P<0.05). the gene expression of fas of shrimp fed the LFLD and LFHD diets were significantly reduced compared to those fed the FM and LF diets (P<0.05).
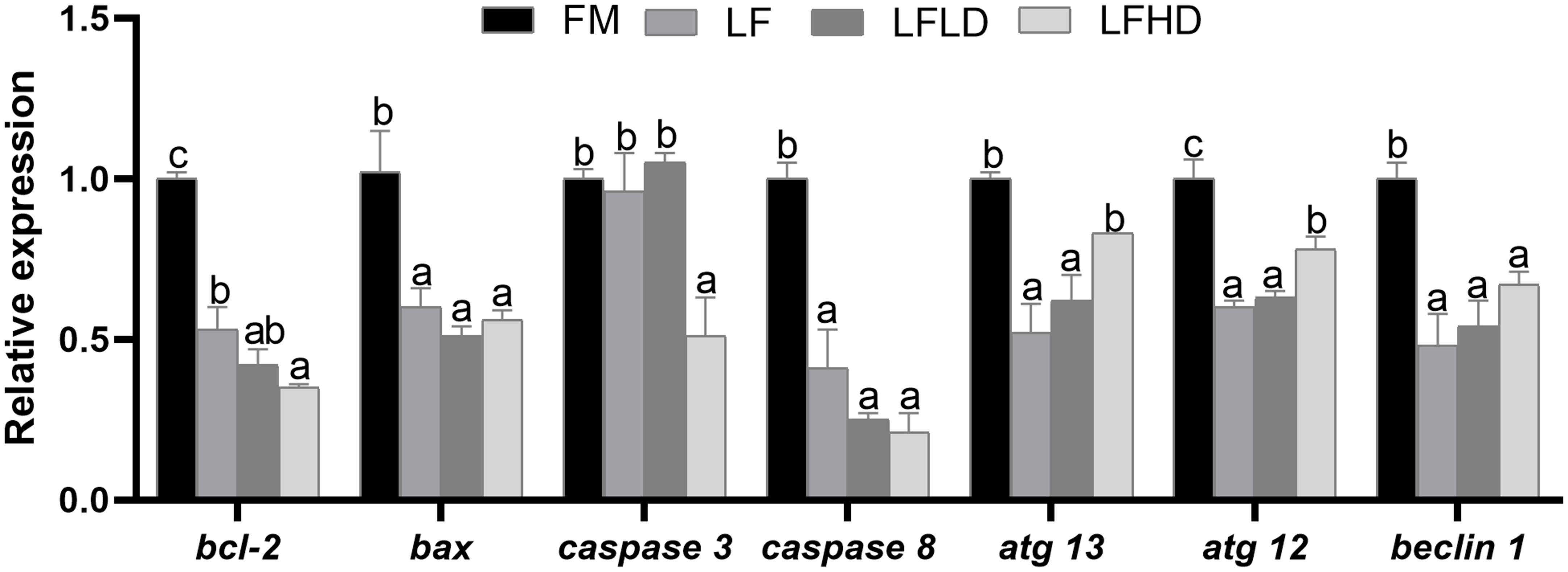
Figure 1 Effect of low fish meal diet supplemented with Schizochytrium Limacinum on the expression levels of hepatopancreas lipid metabolism-related genes in Litopenaeus vannamei. Vertical bars represent the mean ± SEM (n = 5). Data marked with letters differ significantly (P < 0.05) among groups. Where: FM, high fish meal diet; LF, low fishmeal diet; LFLD, LF supplemented with 0.3% S. Limacinum; LFHD, LF supplemented with 0.6% S. Limacinum.
Apoptosis and autophagy-related gene expression levels were shown in Figure 2. The gene expression of bax and caspase 8 of shrimp fed the other three diets were significantly reduced compared to those fed the FM diet (P<0.05). The gene expression of caspase 3 of shrimp fed the LF diet was significantly reduced compared to those fed the other three diets (P<0.05). The gene expression of bcl-2 of shrimp fed the LF and LFHD diets were significantly reduced compared to those fed the FM diet (P<0.05). The gene expression of beclin1 of shrimp fed the other three diets were significantly reduced compared to those fed the FM diet (P<0.05). The gene expression of atg 12 of shrimp fed the LFLD and LFHD diets were significantly reduced compared to those fed the FM diet, which is decreased with the dietary S. Limacinum supplementation (P<0.05). The gene expression of atg 13 of shrimp fed the LF and LFLD diets was significantly reduced compared to those fed the FM and LFHD diets (P<0.05).
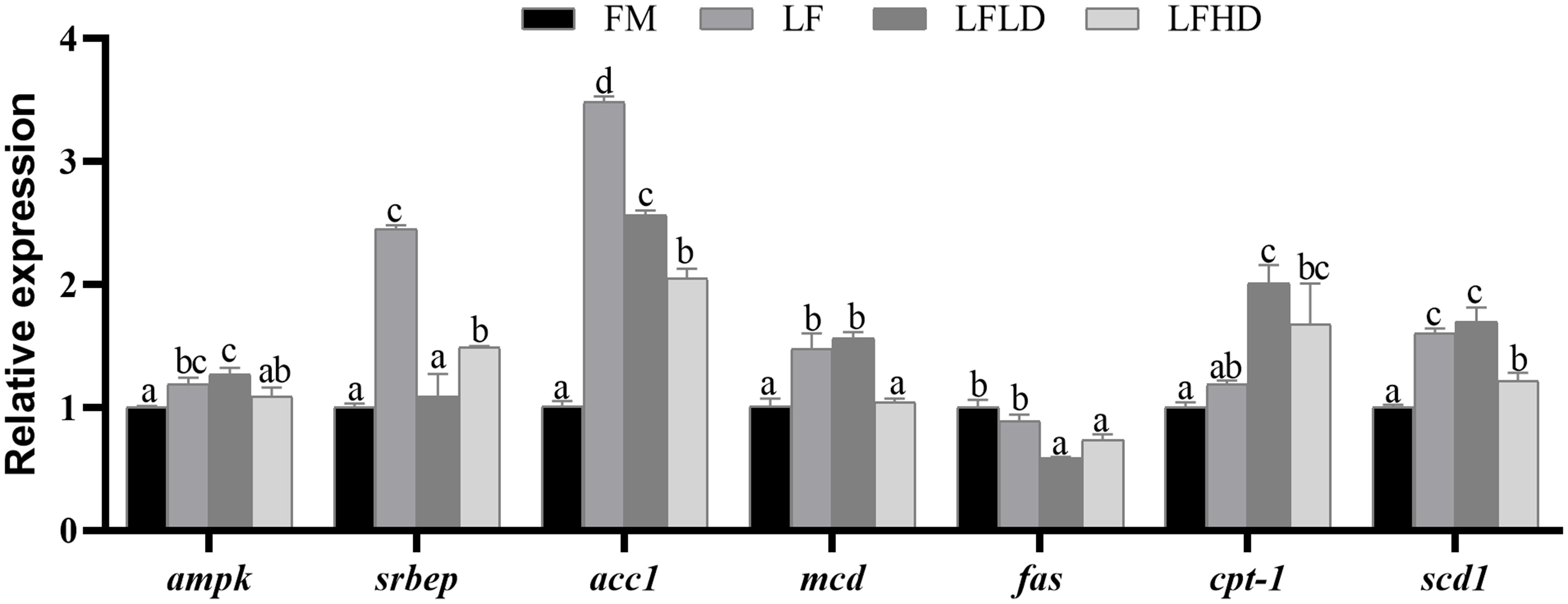
Figure 2 Effect of low fish meal diet supplemented with Schizochytrium Limacinum on the expression levels of hepatopancreas apoptosis and autophagy-related genes in Litopenaeus vannamei. Vertical bars represent the mean ± SEM (n = 5). Data marked with letters differ significantly (P < 0.05) among groups. Where: FM, high fish meal diet; LF, low fishmeal diet; LFLD, LF supplemented with 0.3% S. Limacinum; LFHD, LF supplemented with 0.6% S. Limacinum.
Intestinal histology
The statistical results of the intestinal histology are shown in Table 6. The microvilli height, mucosal fold height, mucosal fold width, and muscle layer thickness of shrimp fed the LF diet were significantly reduced compared to those fed the other diets (P<0.05). The mucosal fold height, mucosal fold width, and muscle layer thickness of shrimp fed the LFLD diet were higher than those fed the other diets. As shown in Figure 3, TEM results showed that with the decrease in dietary fish meal levels, the endoplasmic reticulum and mitochondria of shrimp fed the LF diet were found to be swollen, in which the mitochondrial matrix became irregularly arranged and tended to dissolve. After the supplementation of 0.3% S. Limacinum in the low fish meal diet, the endoplasmic reticulum recovered to its original state, but the mitochondria were irregular and the mitochondrial matrix was marginalized. After supplementation with 0.6% S. Limacinum, the mitochondria recovered to the level of fish meal.
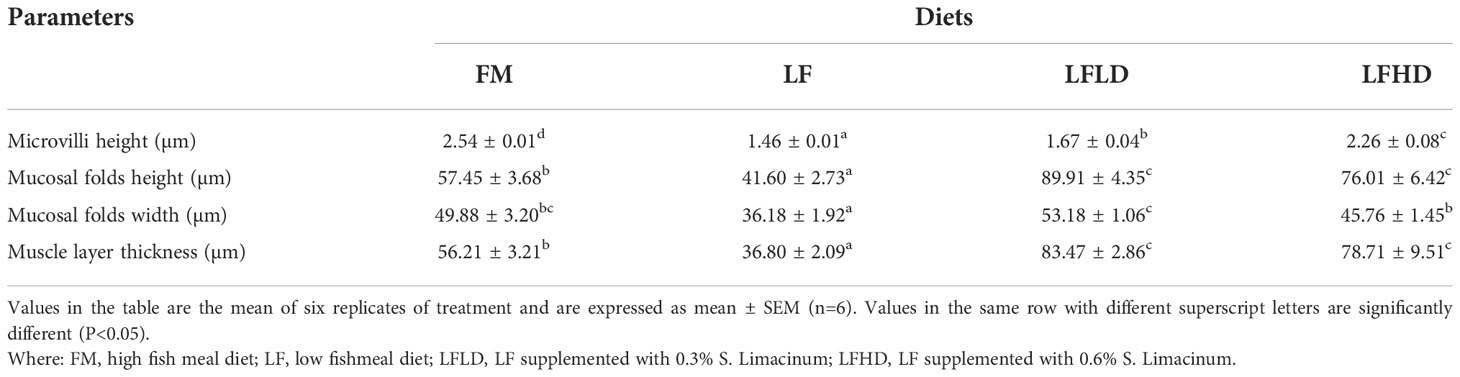
Table 6 Effect of low fish meal diet supplemented with Schizochytrium limacinum on the intestinal tissues of Litopenaeus vannamei.
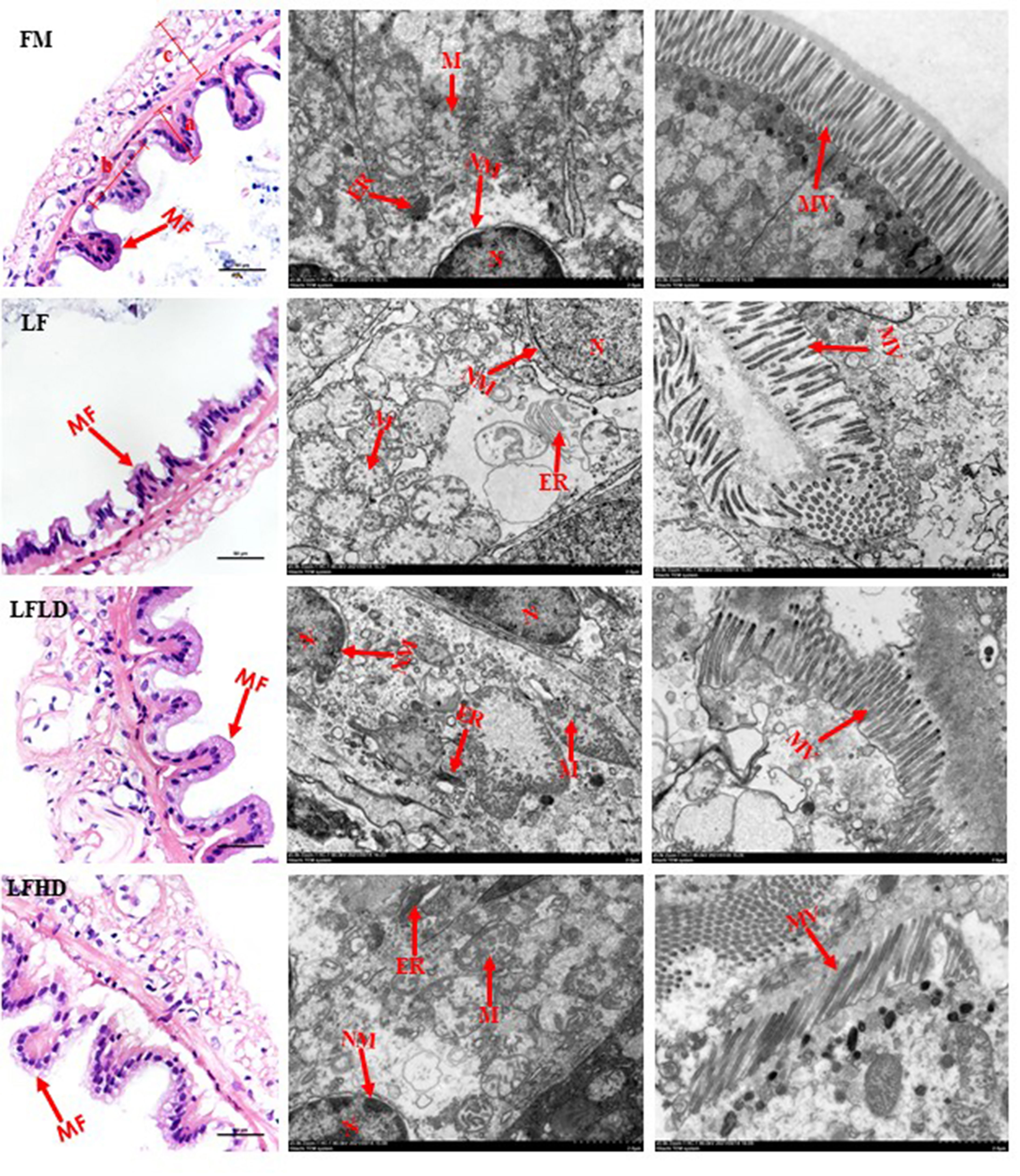
Figure 3 Effect of low fish meal diet supplemented with Schizochytrium limacinum on the histology of the midgut of Litopenaeus vannamei. Where: FM, high fish meal diet; LF, low fishmeal diet; LFLD, LF supplemented with 0.3% S. Limacinum; LFHD, LF supplemented with 0.6% S. Limacinum. MF, mucosal folds; a, width of mucosal folds; b, height of mucosal folds; c, thickness of the annular sarcolemma; MV, microvilli; ER, endoplasmic reticulum; N, nucleus; NM, nuclear membrane; M, mitochondria.
Discussion
SPC has been proven as a high-quality protein source to replace fish meal protein in the feed of a wide range of aquatic animal species (Paripatananont et al., 2001; Chen et al., 2019; Zhang et al., 2022b). Several researches have pointed out that satisfactory growth and feed utilization were obtained in juvenile cobia (El-Saidy and Gaber, 2003) and juvenile starry flounder (Li et al., 2015) when dietary SPC inclusion was below 60%, and even further increased SPC content in the diet can cause lower dietary efficiency and higher death rates in fish. However, Zhao et al. showed that the SR and SGR of Nile tilapia were not affected even if the fish meal was totally replaced with SPC (Salze et al., 2010; Zhao et al., 2010). Earlier studies reported that it was possible to reduce the dietary fish meal content from 20% to 5% with SPC without negatively affecting the growth of Litopenaeus vannamei (Ray et al., 2020). The results of Paripatananont et al. showed that 50% substitution of fish meal with SPC in the diet of Penaeus monodon could support the normal growth of shrimp (Paripatananont et al., 2001), which was similar to the results of the current research. Results of this trial showed that the FBW of Litopenaeus vannamei was not influenced when the dietary fish meal content was reduced from 25% to 15%, after amino acids, micronutrients, and fish oil was supplemented in the low fish meal diet to balance the nutritional profile. Several similar studies have also been done on Epinephelus lanceolatus, Salmon, and Seriola rivoliana (Ai et al., 2006; Perez-Velazquez et al., 2018; Katerina et al., 2020). Some studies reported that dietary supplementation with 4% S. Limacinum improved the growth performance of Litopenaeus vannamei (Wang et al., 2016), 3% S. Limacinum improved the growth performance of Trachinotus ovatus (Xie et al., 2019). Interestingly, (Xie et al., 2020b) found that 0.75% S. Limacinum supplementation in a low fish meal diet improved the growth performance of Penaeus monodon. High levels of nutrients in S. Limacinum such as DHA, docosapentaenoic acid (EPA), and carotenoids may contribute to the growth of animals (Xie et al., 2019). However, some studies have also found that supplementation with high levels of S. Limacinum is detrimental to the growth performance of Litopenaeus vannamei and blunt snout bream (Wang et al., 2016; Wang C. et al., 2020). Our results showed that the supplementation of 0.6% S. Limacinum had a positive effect on the FBW and WGR of shrimp.
Hemolymph is important for fat absorption and transport, and TG is transported as an energy substance between adipose tissue and the liver via hemolymph (Gyan et al., 2021). The fitness of aquatic animals which can be assessed using blood parameters (Lemaire et al., 1991). Several studies have shown that SPC substitution for fish meal affects blood indicators (Zhang et al., 2019; Wang J. et al., 2020; Zhang Q. et al., 2021). TG and TC are important indexes of lipid sedimentation in animals. LDL-C is responsible for transporting liver cholesterol to tissue cells throughout the body, and HDL-C is responsible for transporting excess cholesterol from blood or tissues to the liver pancreas (Yepiz-Plascencia et al., 2000; Hamilton-Reeves et al., 2007). In the present trial, the levels of TC and TG in the hemolymph of Litopenaeus vannamei fed the LFLD diet were significantly reduced, and when the addition level of S. Limacinum increased to 0.6%, the levels of TC and TG increased significantly. Studies have shown that unsaturated fatty acids are effective in lowering hemolymph cholesterol levels (Pentieva et al., 2003) and high levels of dietary unsaturated fatty acids prevent triglycerides from penetrating the lipoprotein particles of the liver, resulting in lower levels of TG secreted by hepatocytes into the hemolymph (Yu et al., 2012). According to (Zhang X. et al., 2021), diets supplemented with 0.5%-2.0% S. Limacinum significantly increased the hemolymph TG content of Litopenaeus vannamei and had no significant effect on the TC content, which was similar to the present study supplemented with 0.6% S. Limacinum but the TC content first decreased significantly and then increased. Hemolymph GLU is the most important energy substance in the hemolymph and is the direct source of energy required for all types of activities in aquatic animals (Boonanuntanasarn et al., 2016). Supplementation of S. Limacinum under the present experimental conditions decreased the hemolymph GLU content of shrimp, a result that is inconsistent with the results of studies on Pelodiscus sinensis (Zhang X. et al., 2021), possibly because of the species and possibly because of the enhanced energy consumption of the substance metabolism. HDL-C and LDL-C are able to be transported in shrimp for lipids (Yepiz-Plascencia et al., 2000). Whereas HDL-C removes TC from the blood and sedimentation in the liver, which is then excreted from the animal, LDL-C plays an important part in the immune system of shrimp (Yue et al., 2012; Chen et al., 2018). The current research showed a decrease in HDL-C and LDL-C levels and a significant decrease in HDL-C in the hemolymph of Litopenaeeus vannamei fed the LF diet, which is different from the results hemolymph in Micropterus salmoides fed low-level fish meal diet supplemented with 4% S. Limacinum (Liao et al., 2022). Reasons for this may be the differences in diet composition, species, and amount of S. Limacinum supplementation. AST and ALT activity are important health parameters of liver function in invertebrates (Song et al., 2018). AST and ALT are the two most important transaminases in the body and are generally present in the liver (Zhou et al., 2013; Liu et al., 2019). When the liver is damaged, the AST and ALT stored in the liver will be transferred to the blood (Barcellos et al., 2004). In the current research, the AST and ALT activities in the hemolymph of Litopenaeus vannamei fed the LFLD and LFHD diets were significantly reduced, a result consistent with the fact that dietary supplementation with 0.8%-1.2% S. Limacinum reduced the blood AST/ALT ratio of Cyprinus carpio var. Jian (Liu et al., 2016) and dietary supplementation with 1% S. Limacinum reduced the AST and ALT activities in the blood of Labidochromis caeruleus (Cui et al., 2018). These results indicated that the supplementation of S. Limacinum in the diet can decrease liver injury in aquatic animals.
Due to the altered hemolymph biochemical parameters in shrimp, we further investigated the effect of low fish meal diet supplementation with S. Limacinum on the expression of genes related to apoptosis, lipid metabolism, and autophagy in Litopenaeus vannamei. Lipid metabolism refers to the process of fat synthesis and catabolism, the digestion of fat, which is subject to the action of a variety of enzymes and bile (Serrano et al., 2021; Su et al., 2022). Lipid metabolism is mainly in the liver, and research has found that DHA can regulate the molecular mechanism of lipid metabolism and promote hepatocytes to stimulate the synthesis of lipoprotein lipase to further promote lipid metabolism (Morabito et al., 2019; Deragon et al., 2021). Ampk is a modulator of energy metabolism in cells. Under low energy regulation, ampk inhibits TG synthesis and activates the β-oxidation process of fatty acids to produce more ATP (Gaidhu et al., 2010; Wang et al., 2018; Xu et al., 2022b). The findings of this research revealed that the gene expression of ampk and cpt-1 in shrimp fed the LF diet showed an increasing trend compared to those fed the FM diet but the gene expression of ampk and cpt-1 increased and then decreased after supplementation with S. Limacinum, which indicated an increase in energy production from lipolysis. The gene expression of fas in shrimp fed the LFLD and LFHD diets was significantly lower than those fed the FM diet, and the gene expression of acc1 and cpt-1 in shrimp fed the LFLD and LFHD diets was significantly higher and then significantly lower than those fed the FM diet, suggesting a decrease in lipid synthesis after supplementation with S. Limacinum. The combined effect of lipid synthesis genes and lipolysis genes resulted in reduced lipid deposition. Srebp is a major regulator of cholesterol and fatty acids and a critical gene in lipid synthesis. (Eberle et al., 2004; Kamisuki et al., 2009). From the experimental results, shrimp fed the high SPC increased the expression of genes related to lipid synthesis, probably due to the ability of soy protein amphiphilic globulin to adsorb lipids (Lusas and Riaz, 1995). It may also be due to DHA’s inherent property of lowering lipids (Horrocks and Yeo, 1999). Studies in human cardiovascular disease have found that DHA reduces total blood cholesterol and triglyceride levels (Mozaffarian and Wu, 2011), and in mice, DHA has been found to reduce the size of fat cells and lower body fat levels (Lu et al., 2015). Dietary supplementation with S. Limacinum decreased the expression of genes related to lipid synthesis, suggesting that S. Limacinum can reduce lipid synthesis. This is similar to the results of Zhu et al. (Zhu et al., 2013) which indicated that supplementation of S. Limacinum in the diet promoted lipid metabolism and inhibited fat deposition.
Apoptosis is divided into the endogenous mitochondrial pathway, the endoplasmic reticulum stress pathway, and the exogenous receptor apoptosis pathway (Sitarek et al., 2022). In the endogenous mitochondrial pathway, bcl-2 family proteins (anti-apoptotic protein bcl-2 and pro-apoptotic protein bax) control outer mitochondrial membrane permeability by regulating mitochondrial membrane potential (Green, 2022). Bax is normally found in the cytoplasm and when it receives an endogenous apoptotic signal, it relocates to the surface of mitochondria and constitutes a trans-mitochondrial membrane pore, which releases apoptotic factors. It has been suggested that the opening of the membrane pore causes a decrease in membrane potential and also leads to mitochondrial swelling and deformation (Chipuk et al., 2012). Caspase 3 is also one of the downstream effectors of the endogenous pathway (Samali et al., 1999). It has been shown that a decrease in mitochondrial membrane potential leads to an upregulation of the bax/bcl-2 ratio and promotes caspase 3 activation (Zorova et al., 2018). In addition, caspase 8 also activates caspase 3 directly or indirectly in exogenous signal-regulated pathways (Bridgham et al., 2003). The results of the current research showed that supplementation with 0.6% S. Limacinum appeared to reduce the expression of caspase 3 and thus inhibit the apoptosis in the hepatopancreas of shrimp; the expression of caspase 8 was also significantly reduced and thus inhibit apoptosis. Dietary supplementation with 0.6% S. Limacinum activated the expression of bcl-2 in the hepatopancreas of shrimp to further inhibit apoptosis. It has been suggested that autophagy eliminates damaged proteins and damaged organelles from the body (Tesseraud et al., 2021). Cellular autophagy is a highly conserved metabolic process that degrades its components through lysosomes to maintain cellular homeostasis and plays an important role in degrading damaged organelles, resisting pathogenic infections, and regulating inflammatory responses (Deretic, 2021). The process of cellular autophagy includes the formation of segmented membranes, the formation of autophagosomes, membrane fusion of autophagosomes with lysosomes, and the digestion of inclusions by autophagosomes (Cao et al., 2021). Atg 13 and beclin1 are participating in the startup of autophagic bubbles (Kabeya et al., 2005; Hosokawa et al., 2009), and atg 12 is responsible for the formation of autophagosomes (Radoshevich et al., 2010). In this experiment, dietary supplementation of 0.6% S. Limacinum significantly increased hepatopancreas atg 12 and atg 13 gene expression in shrimp, and it is hypothesized that S. Limacinum has an effect of promoting autophagy. Researches have shown that hepatic autophagy promotes glycolipid metabolism and protein turnover (Song et al., 2010). Several studies have shown that autophagy is involved in intracellular nonspecific immune responses, that an appropriate numbers of autophagy is a self-protective function driving cell survival (Shi and Kehrl, 2008), and S. Limacinum could activate autophagy-related gene expression to improve the immune response in shrimp.
Intestinal morphology and structure are important for nutritional intake and sustain normal intestinal function (Gao et al., 2013; Vizcaíno et al., 2014), and intestinal morphology is associated with shrimp health (Tang et al., 2009). The height of the mucosal fold and the width of the mucosal folds can be used as a measure of the functional characteristics of the intestinal walls. (Emami et al., 2012). Muscle layer thickness also plays an essential role in the metabolic digestion and absorptivity of the intestine, and increasing the thickness of the muscles can increase the absorptivity and metabolic digestion of nutrients in the intestine (Chen et al., 2021b). The results of this research showed that S. Limacinum supplementation in low fish meal diets improved the intestinal morphology and structure of Litopenaeus vannamei, with an increase in mucosal folds length, mucosal folds width, and muscle layer thickness. Meanwhile, the TEM results showed that the intestinal microvilli height of shrimp fed the LFLD and LFHD diets were significantly increased compared to those fed the LF diet, indicating that dietary supplementation of S. Limacinum improved the intestinal microvilli structures. However, this result is contrary to previous studies, which showed that dietary supplementation with 1.2% S. Limacinum did not affect intestinal microvilli structure in Nile tilapia, possibly due to the different amounts of S. Limacinum supplementation and interspecific differences (Souza et al., 2020). The TEM results also showed that shrimp fed a LF diet showed endoplasmic reticulum stress, irregular mitochondrial ridges, and significant swelling in the intestine, which was improved when the diet was supplemented with S. Limacinum, and the intestinal mitochondria and endoplasmic reticulum recovered well when supplemented with 0.6% S. Limacinum. Earlier researches have demonstrated that reducing fish meal levels can damage the intestinal epithelial structures (Xie et al., 2018) and upregulate the gene expression associated with endoplasmic reticulum stress, which leads to the severity of endoplasmic reticulum stress in the intestine (Xie et al., 2020a). Some studies have reported that dietary supplementation with 3% and 6% defatted S. Limacinum can increase intestinal villi height, but excessive levels can impede nutrient absorption (Xiao et al., 2021). Supplementation with 0.75% S. Limacinum was found to improve intestinal health and enhance immunity in Penaeus monodon (Xie et al., 2020b). The low fish meal diet supplemented with 0.6% S. Limacinum in this study not only promoted the early development of the intestinal tract of Litopenaeus vannamei but also improved the digestive capacity of the intestine, improved the endoplasmic reticulum and mitochondrial structure, further improving the intestinal health and contributing to the healthy growth of shrimp.
Conclusion
The present study revealed that supplementation with 0.6% S. Limacinum in the low fish meal diet levels improved the growth performance, reduced hepatopancreatic cell apoptosis, promoted autophagy, and improved intestinal health in shrimp.
Data availability statement
The original contributions presented in the study are included in the article/supplementary material. Further inquiries can be directed to the corresponding author.
Author contributions
XY and SX designed the experiments. XY carried out the experiments and drafted the manuscript. BT was accountable for some aspects (such as ingredients and sites) of the work in ensuring that experiments can be carried out properly. YiL, MS, LC, KQ and YuL were analyzed for data. SX reviewed and revised the manuscript. All authors contributed to the article and approved the submitted version.
Funding
This study was supported financially by the National Natural Science Foundation of China (32002402), the Characteristic innovation projects of ordinary universities in Guangdong (2022KTSCX049), Science and Technology Bureau of Zhanjiang (2020A05003) and program for scientific research start-up funds of Guangdong Ocean University.
Acknowledgments
We would like to thank all our lab mates for their great support and everything involved in the experiment. We are particularly grateful to the Key Laboratory of Aquatic, Livestock and Poultry Feed Science and Technology in South China, Ministry of Agriculture, for providing specialized technical assistance.
Conflict of interest
The authors declare that the research was conducted in the absence of any commercial or financial relationships that could be construed as a potential conflict of interest.
Publisher’s note
All claims expressed in this article are solely those of the authors and do not necessarily represent those of their affiliated organizations, or those of the publisher, the editors and the reviewers. Any product that may be evaluated in this article, or claim that may be made by its manufacturer, is not guaranteed or endorsed by the publisher.
References
Ai Q., Mai K., Tan B., Xu W., Duan Q., Ma H., et al. (2006). Replacement of fish meal by meat and bone meal in diets for large yellow croaker, Pseudosciaena crocea. Aquaculture 260, 255–263. doi: 10.1016/j.aquaculture.2006.06.043
An W. Q., Li W. W., Tan B. P., Yang Q. H., Dong X. H., Liu H. Y., et al. (2018). Optimum calcium and phosphorus supplemental levels in diets of large size Litopenaeus vannamei. J. Guangdong Ocean Univ. 38, 8–19. doi: 10.3969/j.issn.1673-9159.2018.04.002
Barcellos L. J. G., Kreutz L. C., de Souza C., Rodrigues L. B., Fioreze I., Quevedo R. M., et al. (2004). Hematological changes in jundiá (Rhamdia quelen quoy and gaimard pimelodidae) after acute and chronic stress caused by usual aquacultural management, with emphasis on immunosuppressive effects. Aquaculture 237, 229–236. doi: 10.1016/j.aquaculture.2004.03.026
Boonanuntanasarn S., Wongsasak U., Pitaksong T., Chaijamrus S. (2016). Effects of dietary supplementation with β-glucan and synbiotics on growth, haemolymph chemistry, and intestinal microbiota and morphology in the pacific white shrimp. Aquaculture Nutr. 22, 837–845. doi: 10.1111/anu.12302
Bridgham J. T., Wilder J. A., Hollocher H., Johnson A. L. (2003). All in the family: evolutionary and functional relationships among death receptors. Cell Death Differ. 10, 19–25. doi: 10.1038/sj.cdd.4401174
Byreddy A. R., Yoganantharjah P., Gupta A., Gibert Y., Puri M. (2019). Suitability of novel algal biomass as fish feed: accumulation and distribution of omega-3 long-chain polyunsaturated fatty acid in zebrafish. Appl. Biochem. Biotechnol. 188, 112–123. doi: 10.1007/s12010-018-2906-0
Campos I., Matos E., Marques A., Valente L. M. P. (2017). Hydrolyzed feather meal as a partial fishmeal replacement in diets for European seabass (Dicentrarchus labrax) juveniles. Aquaculture 476, 152–159. doi: 10.1016/j.aquaculture.2017.04.024
Cao W., Li J., Yang K., Cao D. (2021). An overview of autophagy: Mechanism, regulation and research progress. Bull. Cancer 108, 304–322. doi: 10.1016/j.bulcan.2020.11.004
Chen Y., Chi S., Zhang S., Dong X., Yang Q., Liu H., et al. (2021a). Effect of black soldier fly (Hermetia illucens) larvae meal on lipid and glucose metabolism of pacific white shrimp Litopenaeus vannamei. Br. J. Nutr. 128, 1–15. doi: 10.1017/S0007114521004670
Chen Y., Chi S., Zhang S., Dong X., Yang Q., Liu H., et al. (2021b). Replacement of fish meal with methanotroph (Methylococcus capsulatus, bath) bacteria meal in the diets of pacific white shrimp (Litopenaeus vannamei). Aquaculture 541, 736801. doi: 10.1016/j.aquaculture.2021.736801
Chen H., Liu S., Xu X. R., Diao Z. H., Sun K. F., Hao Q. W., et al. (2018). Tissue distribution, bioaccumulation characteristics and health risk of antibiotics in cultured fish from a typical aquaculture area. J. Hazard Mater 343, 140–148. doi: 10.1016/j.jhazmat.2017.09.017
Chen Y., Ma J., Huang H., Zhong H. (2019). Effects of the replacement of fishmeal by soy protein concentrate on growth performance, apparent digestibility, and retention of protein and amino acid in juvenile pearl gentian grouper. PloS One 14, e0222780. doi: 10.1371/journal.pone.0222780
Chipuk J. E., McStay G. P., Bharti A., Kuwana T., Clarke C. J., Siskind L. J., et al. (2012). Sphingolipid metabolism cooperates with BAK and BAX to promote the mitochondrial pathway of apoptosis. Cell 148, 988–1000. doi: 10.1016/j.cell.2012.01.038
Chi S., Zhong J., He Y., Li J., Luo Y. (2017). Cloning and tissue expression of amino acid transporter B0AT1 gene in Epinephelus coioides. J. Guangdong Ocean Univ. 37, 1–6. doi: 10.3969/j.issn.1673-9159.2017.06.001
Cho J. H., Kim I. H. (2011). Fish meal-nutritive value. J. Anim. Physiol. Anim. Nutr. (Berl) 95, 685–692. doi: 10.1111/j.1439-0396.2010.01109.x
Cui P., Sheng Y., Yang Y., Sun J. (2018). Effects of dietary algae Dunaliella salina, Schizochytrium limacinum and Chlorella vulgaris on growth performance, body color and partial biochemical indices of Labidochromis caeruleus. J. Dalian Ocean Univ. 33, 6. doi: 10.16535/j.cnki.dlhyxb.2018.06.006
Deragon E., Schuler M., Aiese Cigliano R., Dellero Y., Si Larbi G., Falconet D., et al. (2021). An oil hyper-accumulator mutant highlights peroxisomal ATP import as a regulatory step for fatty acid metabolism in aurantiochytrium limacinum. Cells 10, 2680. doi: 10.3390/cells10102680
Deretic V. (2021). Autophagy in inflammation, infection, and immunometabolism. Immunity 54, 437–453. doi: 10.1016/j.immuni.2021.01.018
Dyall S. C., Balas L., Bazan N. G., Brenna J. T., Chiang N., da Costa Souza F., et al. (2022). Polyunsaturated fatty acids and fatty acid-derived lipid mediators: Recent advances in the understanding of their biosynthesis, structures, and functions. Prog. Lipid Res. 86, 101165. doi: 10.1016/j.plipres.2022.101165
Eberle D., Hegarty B., Bossard P., Ferre P., Foufelle F. (2004). SREBP transcription factors: master regulators of lipid homeostasis. Biochimie 86, 839–848. doi: 10.1016/j.biochi.2004.09.018
El-Saidy D. M., Gaber M. M. (2003). Replacement of fish meal with a mixture of different plant protein sources in juvenile Nile tilapia, Oreochromis niloticus (L.) diets. Aquaculture Res. 34, 1119–1127. doi: 10.1046/j.1365-2109.2003.00914.x
Emami N. K., Samie A., Rahmani H., Ruiz-Feria C. (2012). The effect of peppermint essential oil and fructooligosaccharides, as alternatives to virginiamycin, on growth performance, digestibility, gut morphology and immune response of male broilers. Anim. feed Sci. Technol. 175, 57–64. doi: 10.1016/j.anifeedsci.2012.04.001
Feldsine P., Abeyta C., Andrews W. H. (2002). AOAC international methods committee guidelines for validation of qualitative and quantitative food microbiological official methods of analysis. J. AOAC Int. 85, 1187–1200. doi: 10.1093/jaoac/85.5.1187
Gaidhu M. P., Anthony N. M., Patel P., Hawke T. J., Ceddia R. B. (2010). Dysregulation of lipolysis and lipid metabolism in visceral and subcutaneous adipocytes by high-fat diet: role of ATGL, HSL, and AMPK. Am. J. Physiol. Cell Physiol. 298, C961–C971. doi: 10.1152/ajpcell.00547.2009
Gao Y., Han F., Huang X., Rong Y., Yi H., Wang Y. (2013). Changes in gut microbial populations, intestinal morphology, expression of tight junction proteins, and cytokine production between two pig breeds after challenge with escherichia coli K88: a comparative study. Journal of animal science 91, 5614–5625. doi: 10.2527/jas.2013-6528
García-Ortega A., Kissinger K. R., Trushenski J. T. (2016). Evaluation of fish meal and fish oil replacement by soybean protein and algal meal from Schizochytrium limacinum in diets for giant grouper Epinephelus lanceolatus. Aquaculture 452, 1–8. doi: 10.1016/j.aquaculture.2015.10.020
Green D. R. (2022). The mitochondrial pathway of apoptosis part II: The BCL-2 protein family. Cold Spring Harb. Perspect. Biol. 14, a041046. doi: 10.1101/cshperspect.a041046
Gui L. C., Mai H. B., Chi S. Y., Zhou W. H., Yong-Juan L. I., Tan B. P., et al. (2019). Effects of yeast culture on growth performance, hematological parameters, immunity and disease resistance of litopenaeus vannamei. J. Guangdong Ocean Univ. 39, 30–37. doi: 10.3969/j.issn.1673-9159.2019.03.005
Gyan W., Yang Q., Tan B., Xiaohui D., Chi S., Liu H., et al. (2021). Effects of replacing fishmeal with dietary dried distillers grains with solubles on growth, serum biochemical indices, antioxidative functions, and disease resistance for Litopenaeus vannamei juveniles. Aquaculture Rep. 21, 100821. doi: 10.1016/j.aqrep.2021.100821
Hamilton-Reeves J. M., Rebello S. A., Thomas W., Slaton J. W., Kurzer M. S. (2007). Isoflavone-rich soy protein isolate suppresses androgen receptor expression without altering estrogen receptor-β expression or serum hormonal profiles in men at high risk of prostate cancer. J. Nutr. 137, 1769–1775. doi: 10.1093/jn/137.7.1769
He Y. F., Chi S. Y., Tan B. P., Zhang H. L., Dong X. H., Yang Q. H., et al. (2017). Effect of yeast culture on intestinal microbiota of Litopenaeus vannamei. J. Guangdong Ocean Univ. 37, 21–27. doi: 10.3969/j.issn.1673-9159.2017.04.004
Hlordzi V., Wang J., Kuebutornye F. K. A., Yang X., Tan B., Li T., et al. (2022). Hydrolysed fish protein powder is better at the growth performance, hepatopancreas and intestinal development of pacific white shrimp (Litopenaeus vannamei). Aquaculture Rep. 23, 101025. doi: 10.1016/j.aqrep.2022.101025
Horrocks L. A., Yeo Y. K. (1999). Health benefits of docosahexaenoic acid (DHA). Pharmacol. Res. 40, 211–225. doi: 10.1006/phrs.1999.0495
Hosokawa N., Sasaki T., Iemura S., Natsume T., Hara T., Mizushima N. (2009). Atg101, a novel mammalian autophagy protein interacting with Atg13. Autophagy 5, 973–979. doi: 10.4161/auto.5.7.9296
Huang B., Zhang S., Dong X., Chi S., Yang Q., Liu H., et al. (2022). Effects of fishmeal replacement by black soldier fly on growth performance, digestive enzyme activity, intestine morphology, intestinal flora and immune response of pearl gentian grouper (Epinephelus fuscoguttatus ♀ x Epinephelus lanceolatus ♂). Fish Shellfish Immunol. 120, 497–506. doi: 10.1016/j.fsi.2021.12.027
Jannathulla R., Rajaram V., Kalanjiam R., Ambasankar K., Muralidhar M., Dayal J. S. (2019). Fishmeal availability in the scenarios of climate change: Inevitability of fishmeal replacement in aquafeeds and approaches for the utilization of plant protein sources. Aquaculture Res. 50, 3493–3506. doi: 10.1111/are.14324
Kabeya Y., Kamada Y., Baba M., Takikawa H., Sasaki M., Ohsumi Y. (2005). Atg17 functions in cooperation with Atg1 and Atg13 in yeast autophagy. Mol. Biol. Cell. 16, 2544–2553. doi: 10.1091/mbc.e04-08-0669
Kamisuki S., Mao Q., Abu-Elheiga L., Gu Z., Kugimiya A., Kwon Y., et al. (2009). A small molecule that blocks fat synthesis by inhibiting the activation of SREBP. Chem. Biol. 16, 882–892. doi: 10.1016/j.chembiol.2009.07.007
Kari Z. A., Kabir M. A., Dawood M. A. O., Razab M. K. A. A., Ariff N. S. N. A., Sarkar T., et al. (2022). Effect of fish meal substitution with fermented soy pulp on growth performance, digestive enzyme, amino acid profile, and immune-related gene expression of African catfish (Clarias gariepinus). Aquaculture 546, 737418. doi: 10.1016/j.aquaculture.2021.737418
Katerina K., Berge G. M., Turid M., Aleksei K., Grete B., Trine Y., et al. (2020). Microalgal Schizochytrium limacinum biomass improves growth and filet quality when used long-term as a replacement for fish oil, in modern salmon diets. frontiers in marine science. Frontiers in Marine Science 7, 57. doi: 10.3389/fmars.2020.00057
Kirimi J. G., Musalia L. M., Munguti J. M. (2016). Effect of replacing fish meal with blood meal on chemical composition of supplement for nile tilapia (Oreochromis niloticus). East Afr. Agric. Forestry J. 82, 1–9. doi: 10.1080/00128325.2016.1158898
Lemaire P., Drai P., Mathieu A., Lemaire S., Carriere S., Giudicelli J., et al. (1991). Changes with different diets in plasma enzymes (GOT, GPT, LDH, ALP) and plasma lipids (cholesterol, triglycerides) of sea-bass (Dicentrarchus labrax). Aquaculture 93, 63–75. doi: 10.1016/0044-8486(91)90205-L
Liao H., Liu P., Deng Y., Zhang W., Pan C., Jia Y., et al. (2022). Feeding effects of low-level fish meal replacement by algal meals of Schizochytrium limacinum and Nannochloropsis salina on largemouth bass (Micropterus salmoides). Aquaculture 557, 738311. doi: 10.1016/j.aquaculture.2022.738311
Li Y., Huang J., Chen Y., Wen Z., Ou G., Huang J., et al. (2022). Effects of low-temperature stress on serum biochemical, antioxidant enzymes activities and apoptosis-related gene expression in liver of juvenile cobia (Rachycentron canadum). J. Guangdong Ocean Univ. 42, 18–26. doi: 10.3969/j.issn.1673-9159.2022.05.003
Li W., Li L., Liu H., Tan B., Dong X., Yang Q., et al. (2022). Effects of clostridium butyricum on growth, antioxidant capacity and non-specific immunology of Litopenaeus vannamei fed with concentrated cottonseed protein replacement of fishmeal. J. Guangdong Ocean Univ. 42, 29–37. doi: 10.3969/j.issn.1673-9159.2022.02.004
Li Z., Meng T., Ling X., Li J., Zheng C., Shi Y., et al. (2018). Overexpression of malonyl-CoA: ACP transacylase in schizochytrium sp. to improve polyunsaturated fatty acid production. J. Agric. Food Chem. 66, 5382–5391. doi: 10.1021/acs.jafc.8b01026
Li M. H., Robinson E. H., Tucker C. S., Manning B. B., Khoo L. (2009). Effects of dried algae schizochytrium sp., a rich source of docosahexaenoic acid, on growth, fatty acid composition, and sensory quality of channel catfish Ictalurus punctatus. Aquaculture 292, 232–236. doi: 10.1016/j.aquaculture.2009.04.033
Lin H., Tan B., Ray G., Zeng M., Li M., Chi S., et al. (2022). A challenge to conventional fish meal: effects of soy protein peptides on growth, histomorphology, lipid metabolism and intestinal health for juvenile pompano Trachinotus ovatus. Front. Mar. Sci. 8. doi: 10.3389/fmars.2021.815323
Liu H., Cheng Z., Zhai S., Lin C., Wang J. E., Qiao X., et al. (2016). Effects of dietary Schizochytrium on the growth performance, serum nonspecific immunity indices, and disease resistance of Cyprinus carpio var. Jian. Prog. Fishery Sci. 37, 42–28. doi: 10.11758/yykxjz.20151227001
Liu H., Li L., Stephen A., Tang Z., Fan W., Tan B., et al. (2021). Effects of dietary yeast culture supplementation on growth, intestinal morphology, immunity, and disease resistance in Epinephelus fuscoguttatus♀× Epinephelus lanceolatu♂. J. Guangdong Ocean University. 41, 1–11. doi: 10.3969/j.issn.1673-9159.2021.03.001
Liu T., Zhang G., Feng Y., Kong C., Ayisi C. L., Huang X., et al. (2019). Dietary soybean antigen impairs growth and health through stress-induced non-specific immune responses in pacific white shrimp, Litopenaeus vannamei. Fish Shellfish Immunol. 84, 124–129. doi: 10.1016/j.fsi.2018.09.062
Li P. Y., Wang J. Y., Song Z. D., Zhang L. M., Zhang H., Li X. X., et al. (2015). Evaluation of soy protein concentrate as a substitute for fishmeal in diets for juvenile starry flounder (Platichthys stellatus). Aquaculture 448, 578–585. doi: 10.1016/j.aquaculture.2015.05.049
Lusas E. W., Riaz M. N. (1995). Soy protein products: processing and use. J. Nutr. 125, 573S–580S. doi: 10.1093/jn/125.suppl_3.573S
Lu T., Zheng Z., Zhang J., Xue M., Ge Y. (2015). The inhibitory effects DHA on immune inflammatory obesity acta academiae medicinae QingDao universitatis. Acta Academiae Medicinae QingDao Universitatis 51, 445–447. doi: 10.13361/j.qdyxy.201504023
Lyons P. P., Turnbull J. F., Dawson K. A., Crumlish M. (2016). Effects of low-level dietary microalgae supplementation on the distal intestinal microbiome of farmed rainbow trout Oncorhynchus mykiss (Walbaum). Aquaculture Res. 48, 2438–2452. doi: 10.1111/are.13080
Morabito C., Bournaud C., Maes C., Schuler M., Aiese Cigliano R., Dellero Y., et al. (2019). The lipid metabolism in thraustochytrids. Prog. Lipid Res. 76, 101007. doi: 10.1016/j.plipres.2019.101007
Mozaffarian D., Wu J. H. (2011). Omega-3 fatty acids and cardiovascular disease: effects on risk factors, molecular pathways, and clinical events. J. Am. Coll. Cardiol. 58, 2047–2067. doi: 10.1016/j.jacc.2011.06.063
Osmond A. T. Y., Arts M. T., Hall J. R., Rise M. L., Bazinet R. P., Armenta R. E., et al. (2021). Schizochytrium sp. (T18) oil as a fish oil replacement in diets for juvenile rainbow trout (Oncorhynchus mykiss): Effects on growth performance, tissue fatty acid content, and lipid-related transcript expression. Anim. (Basel). 11, 1185. doi: 10.3390/ani11041185
Paripatananont T., Boonyaratpalin M., Pengseng P., Chotipuntu P. (2001). Substitution of soy protein concentrate for fishmeal in diets of tiger shrimp Penaeus monodon. Aquaculture Res. 32, 369–374. doi: 10.1046/j.1355-557x.2001.00045.x
Pentieva K., McKillop D., Duffy N., de Deckere E., Jacobs R., van der Put N., et al. (2003). Acute absorption of folic acid from a fortified low-fat spread. Eur. J. Clin. Nutr. 57, 1235–1241. doi: 10.1038/sj.ejcn.1601674
Perez-Velazquez M., Gatlin D. M., González-Félix M. L., García-Ortega A. (2018). Partial replacement of fishmeal and fish oil by algal meals in diets of red drum sciaenops ocellatus. Aquaculture 487, 41–50. doi: 10.1016/j.aquaculture.2018.01.001
Radoshevich L., Murrow L., Chen N., Fernandez E., Roy S., Fung C., et al. (2010). ATG12 conjugation to ATG3 regulates mitochondrial homeostasis and cell death. Cell 142, 590–600. doi: 10.1016/j.cell.2010.07.018
Ray G., Liang D., Yang Q., Tan B., Dong X., Chi S., et al. (2020). Effects of replacing fishmeal with dietary soybean protein concentrate (SPC) on growth, serum biochemical indices, and antioxidative functions for juvenile shrimp Litopenaeus vannamei. Aquaculture 516, 734630. doi: 10.1016/j.aquaculture.2019.734630
Ruyter B., Bou M., Berge G. M., Mørkøre T., Sissener N. H., Sanden M., et al. (2022). A dose-response study with omega-3 rich canola oil as a novel source of docosahexaenoic acid (DHA) in feed for Atlantic salmon (Salmo salar) in seawater; effects on performance, tissue fatty acid composition, and fillet quality. Aquaculture 561, 738733. doi: 10.1016/j.aquaculture.2022.738733
Salze G., McLean E., Battle P. R., Schwarz M. H., Craig S. R. (2010). Use of soy protein concentrate and novel ingredients in the total elimination of fish meal and fish oil in diets for juvenile cobia, Rachycentron canadum. Aquaculture 298, 294–299. doi: 10.1016/j.aquaculture.2009.11.003
Samali A., Cai J., Zhivotovsky B., Jones D. P., Orrenius S. (1999). Presence of a pre-apoptotic complex of pro-caspase-3, Hsp60 and Hsp10 in the mitochondrial fraction of jurkat cells. EMBO J. 18, 2040–2048. doi: 10.1093/emboj/18.8.2040
Sarker P. K., Kapuscinski A. R., Lanois A. J., Livesey E. D., Bernhard K. P., Coley M. L. (2016). Towards sustainable aquafeeds: Complete substitution of fish oil with marine microalga schizochytrium sp. improves growth and fatty acid deposition in juvenile Nile tilapia (Oreochromis niloticus). PloS One 11, e0156684. doi: 10.1371/journal.pone.0156684
Serrano E., Simpfendorfer R., Medina A., Sandoval C., Martínez A., Morales R., et al. (2021). Partially replacing fish oil with microalgae (Schizochytrium limacinum and Nannochloropsis oceanica) in diets for rainbow trout (Oncorhynchus mykiss ) reared in saltwater with reference to growth performance, muscle fatty acid composition and liver ultrastructure. Aquaculture Res. 52, 4401–4413. doi: 10.1111/are.15279
Shi C., Kehrl J. H. (2008). MyD88 and trif target beclin 1 to trigger autophagy in macrophages. J. Biol. Chem. 283, 33175–33182. doi: 10.1074/jbc.M804478200
Sitarek P., Synowiec E., Kowalczyk T., Bangay G., Sliwinski T., Picot L., et al. (2022). Anticancer properties of Plectranthus ornatus-derived phytochemicals inducing apoptosis via mitochondrial pathway. Int. J. Mol. Sci. 23, 11653. doi: 10.3390/ijms231911653
Song L., Hu B., Wang A., Mao S., Zhang Y., Pan X. (2010). Effects of antibacterial peptide on growth and immunity of penaeus vannamei. J. Guangdong Ocean University. 30, 28–32.
Song Z., Li P., Wang J., Sun Y., Wang C. (2018). Dietary inclusion of hydrolyzed soybean and cottonseed meals influence digestion, metabolic enzymes, and growth-related hormones and growth of juvenile turbot (Scophthalmus maximus). Aquaculture Int. 26, 1017–1033. doi: 10.1007/s10499-018-0265-z
Souza F. P., Lima E. C. S., Urrea-Rojas A. M., Suphoronski S. A., Facimoto C. T., Bezerra Junior J. D. S., et al. (2020). Effects of dietary supplementation with a microalga (Schizochytrium sp.) on the hemato-immunological, and intestinal histological parameters and gut microbiota of Nile tilapia in net cages. PloS One 15, e0226977. doi: 10.1371/journal.pone.0226977
Sprague M., Walton J., Campbell P. J., Strachan F., Dick J. R., Bell J. G. (2015). Replacement of fish oil with a DHA-rich algal meal derived from schizochytrium sp. on the fatty acid and persistent organic pollutant levels in diets and flesh of Atlantic salmon (Salmo salar, l.) post-smolts. Food Chem. 185, 413–421. doi: 10.1016/j.foodchem.2015.03.150
Su C., Li J., Lu Y., Wang Y., Ding Y., Pan L., et al. (2022). Interactive effects of dietary cholesterol and bile acids on the growth, lipid metabolism, immune response and intestinal microbiota of Litopenaeus vannamei: Sparing effect of bile acids on cholesterol in shrimp diets. Aquaculture, 547, 737412. doi: 10.1016/j.aquaculture.2021.737412
Tang L., Wang G. X., Jiang J., Feng L., Yang L., Li S. H., et al. (2009). Effect of methionine on intestinal enzymes activities, microflora and humoral immune of juvenile jian carp (Cyprinus carpio var. jian). Aquaculture Nutr. 15, 477–483. doi: 10.1111/j.1365-2095.2008.00613.x
Tesseraud S., Avril P., Bonnet M., Bonnieu A., Cassar-Malek I., Chabi B., et al. (2021). Autophagy in farm animals: Current knowledge and future challenges. Autophagy 17, 1809–1827. doi: 10.1080/15548627.2020.1798064
Vizcaíno A., López G., Sáez M., Jiménez J., Barros A., Hidalgo L., et al. (2014). Effects of the microalga scenedesmus almeriensis as fishmeal alternative in diets for gilthead sea bream, Sparus aurata, juveniles. Aquaculture 431, 34–43. doi: 10.1016/j.aquaculture.2014.05.010
Wang J., Liang D., Yang Q., Tan B., Dong X., Chi S., et al. (2020). The effect of partial replacement of fish meal by soy protein concentrate on growth performance, immune responses, gut morphology and intestinal inflammation for juvenile hybrid grouper (Epinephelus fuscoguttatus ♀ x Epinephelus lanceolatus ♂). Fish Shellfish Immunol. 98, 619–631. doi: 10.1016/j.fsi.2019.10.025
Wang Y., Li M., Filer K., Xue Y., Ai Q., Mai K. (2016). Evaluation of Schizochytrium meal in microdiets of pacific white shrimp (Litopenaeus vannamei) larvae. Aquaculture Res. 48, 2328–2336. doi: 10.1111/are.13068
Wang Y., Li M., Filer K., Xue Y., Ai Q., Mai K. (2017). Replacement of fish oil with a DHA-rich Schizochytrium meal on growth performance, activities of digestive enzyme and fatty acid profile of pacific white shrimp (Litopenaeus vannamei) larvae. Aquaculture Nutr. 23, 1113–1120. doi: 10.1111/anu.12479
Wang C., Liu W.-b., Huang Y., Wang X., Li X., Zhang D., et al. (2020). Dietary DHA affects muscle fiber development by activating AMPK/Sirt1 pathway in blunt snout bream (Megalobrama amblycephala). Aquaculture 518, 734835. doi: 10.1016/j.aquaculture.2019.734835
Wang Q., Liu S., Zhai A., Zhang B., Tian G. (2018). AMPK-mediated regulation of lipid metabolism by phosphorylation. Biol. Pharm. Bull. 41, 985–993. doi: 10.1248/bpb.b17-00724
Wang L., Zhou Q., Ma Q., Yang Y., Yang E., Chen G., et al. (2022). Effects of salinity acclimation on expression of MMP2 and MMP9 in regenerated scales of young rainbow trout (Oncorhynchus mykiss). J. Guangdong Ocean Univ. 42, 110–115. doi: 10.3969/j.issn.1673-9159.2022.05.014
Wu Y., Li R., Shen G., Huang F., Yang Q., Tan B., et al. (2021). Effects of dietary small peptides on growth, antioxidant capacity, nonspecific immunity and ingut microflora structure of litopenaeus vannamei. J. Guangdong Ocean Univ. 41, 1–9. doi: 10.3969/j.issn.1673-9159.2021.05.001
Xiao F., Xing J., Li H., Xu X., Hu Z., Ji H. (2021). Effects of the defatted schizochytrium sp. on growth performance, fatty acid composition, histomorphology and antioxidant status of juvenile mirror carp (Cyprinus carpio var. specularis). Aquaculture Res. 52, 3062–3076. doi: 10.1111/are.15150
Xie J., Fang H., Liao S., Guo T., Yin P., Liu Y., et al. (2019). Study on schizochytrium sp. improving the growth performance and non-specific immunity of golden pompano (Trachinotus ovatus) while not affecting the antioxidant capacity. Fish Shellfish Immunol. 95, 617–623. doi: 10.1016/j.fsi.2019.10.028
Xie S., Liu Y., Tian L., Niu J., Tan B. (2020a). Low dietary fish meal induced endoplasmic reticulum stress and impaired phospholipids metabolism in juvenile pacific white shrimp, Litopenaeus vannamei. Front. Physiol. 11. doi: 10.3389/fphys.2020.01024
Xie S., Liu Y., Zeng S., Niu J., Tian L. (2016). Partial replacement of fish-meal by soy protein concentrate and soybean meal based protein blend for juvenile pacific white shrimp, Litopenaeus vannamei.. Aquaculture 464, 296–302. doi: 10.1016/j.aquaculture.2016.07.002
Xie S., Wei D., Chen S., Zhuang Z., Yin P., Liu Y., et al. (2020c). Dietary fishmeal levels affect anti-oxidative ability and metabolomics profile of juvenile pacific white shrimp, Litopenaeus vannamei. Aquaculture Nutr. 26, 978–989. doi: 10.1111/anu.13055
Xie S., Wei D., Tan B., Liu Y., Tian L., Niu J. (2020b). Schizochytrium limacinum supplementation in a low fish-meal diet improved immune response and intestinal health of juvenile Penaeus monodon. Front. Physiol. 11. doi: 10.3389/fphys.2020.00613
Xie S., Zheng L., Wan M., Niu J., Liu Y., Tian L. (2018). Effect of deoxynivalenol on growth performance, histological morphology, anti-oxidative ability and immune response of juvenile pacific white shrimp, Litopenaeus vannamei. Fish Shellfish Immunol. 82, 442–452. doi: 10.1016/j.fsi.2018.08.053
Xu D., Wu J., Sun L., Qin X., Fan X. (2021). Comparison on anesthetic efficacy of eugenol and MS-222 on shrimp Litopenaeus vannamei. J. Guangdong Ocean Univ. 41, 44–52. doi: 10.3969/j.issn.1673-9159.2021.05.006
Xu D., Wu J., Sun L., Qin X., Fan X. (2022a). Physiological response and metabolic regulation of Litopenaeus vannamei exposed to combination stress of acute cold exposure and chronic waterless duration. J. Guangdong Ocean Univ. 42, 20–28. doi: 10.3969/j.issn.1673-9159.2022.01.004
Xu J., Xie S., Chi S., Zhang S., Cao J., Tan B. (2022b). Short-term dietary antibiotics altered the intestinal microbiota and improved the lipid metabolism in hybrid grouper fed medium and high-lipid diets. Aquaculture, 547, 737453. doi: 10.1016/j.aquaculture.2021.737453
Xu J., Yao X., Li X., Xie S., Chi S., Zhang S., et al. (2022c). Bile acids reduced the lipid deposition in fatty degenerated hepatocytes of pearl gentian grouper (Epinephelus fuscoguttatus♂ × Epinephelus lanceolatus♀) in vitro. Front. Mar. Science. 9. doi: 10.3389/fmars.2022.861117
Yepiz-Plascencia G., Vargas-Albores F., Higuera-Ciapara I. (2000). Penaeid shrimp hemolymph lipoproteins. Aquaculture 191, 177–189. doi: 10.1016/S0044-8486(00)00427-0
Yin X., Wei W., Zhuang X., Li Z., Liu C., Ou M., et al. (2021). Determining the function of LvSmad3 on Litopenaeus vannamei in response to acute low temperature stress. Dev. Comp. Immunol. 125, 104209. doi: 10.1016/j.dci.2021.104209
Yue Y., Liu Y., Tian L., Gan L., Yang H., Liang G. (2012). Effects of replacing fish meal with soybean meal and peanut meal on growth, feed utilization and haemolymph indexes for juvenile white shrimp Litopenaeus vannamei, Boone. Aquaculture Res. 43, 1687–1696. doi: 10.1111/j.1365-2109.2011.02976.x
Yu Y., Jiang S., Lin H., Wang Y., Wang A. (2012). Effects of dietary lipid sources on the morphology and biochemical index of carassius auratus gibelio. J. Hunan Agric. Univ. 38, 192–197. doi: 10.3724/SP.J.1238.2012.00192
Zhang W., Tan B., Deng J., Yang Q., Chi S., Pang A., et al. (2022b). Soybean protein concentrate causes enteritis in juvenile pearl gentian groupers (Epinephelus fuscoguttatus ♀ × Epinephelus lanceolatus ♂). Anim. Nutr. doi: 10.1016/j.aninu.2022.08.006
Zhang W., Tan B., Pang A., Deng J., Yang Q., Zhang H. (2022a). Screening of potential biomarkers for soybean meal induced enteritis in pearl gentian grouper (Epinephelus fuscoguttatus♀× Epinephelus lanceolatus♂). J. Guangdong Ocean Univ. 42, 1–12. doi: 10.3969/j.issn.1673-9159.2022.04.001
Zhang H. T., Xue-Song P. U., Yang Q. H., Tan B. P., Dong X. H., Chi S. Y., et al. (2018). Effects of replacing fish meal with high-protein cottonseed meal on growth performance, non-specific immune index and disease resistance for litopenaeus vannamei. J. Guangdong Ocean Univ. 38, 20–26. doi: 10.3969/j.issn.1673-9159.2018.04.003
Zhang Q., Zhang S., Cong G., Zhang Y., Madsen M. H., Tan B., et al. (2021). Effects of soy protein concentrate in starter phase diet on growth performance, blood biochemical indices, carcass traits, immune organ indices and meat quality of broilers. Anim. (Basel) 11, 281. doi: 10.3390/ani11020281
Zhang X., Zhao L., Hu X. (2021). Progress on nutritional value of Schizochytrium limacinum and its application in animal production. J. Henan Agric. Sci. 50, 1–10. doi: 10.15933/j.cnki.1004-3268.2021.03.001
Zhang J., Zhong L., Peng M., Chu W., Liu Z., Dai Z., et al. (2019). Replacement of fish meal with soy protein concentrate in diet of juvenile rice field eel Monopterus albus. Aquaculture Rep. 15, 100235. doi: 10.1016/j.aqrep.2019.100235
Zhao H., Jiang R., Xue M., Xie S., Wu X., Guo L. (2010). Fishmeal can be completely replaced by soy protein concentrate by increasing feeding frequency in Nile tilapia (Oreochromis niloticus GIFT strain) less than 2 g. Aquaculture Nutr. 16, 648–653. doi: 10.1111/j.1365-2095.2009.00708.x
Zhao W., Liu Z., Niu J. (2021). Growth performance, intestinal histomorphology, body composition, hematological and antioxidant parameters of Oncorhynchus mykiss were not detrimentally affected by replacement of fish meal with concentrated dephenolization cottonseed protein. Aquaculture Rep. 19, 100557. doi: 10.1016/j.aqrep.2020.100557
Zhou W., Rahimnejad S., Lu K., Wang L., Liu W. (2019). Effects of berberine on growth, liver histology, and expression of lipid-related genes in blunt snout bream (Megalobrama amblycephala) fed high-fat diets. Fish Physiol. Biochem. 45, 83–91. doi: 10.1007/s10695-018-0536-7
Zhou Q., Wang Y., Wang H., Tan B. (2013). Dietary threonine requirements of juvenile pacific white shrimp, Litopenaeus vannamei. Aquaculture, 392, 142–147. doi: 10.1016/j.aquaculture.2013.01.026
Zhu X., Deng Q., Guo H., Li G., Zhu C. (2021). Effect of hydrolyzable tannins on hemolymph and cellular immunological responses of Litopenaeus vannamei challenged by vibrio parahaemolyticus. J. Guangdong Ocean Univ. 41, 12–19. doi: 10.3969/j.issn.1673-9159.2021.03.002
Zhu C., Jiang B., Zhou Q. (2013). The effects of different levels of EPA and DHA on lipids metabolism, growth and reproductive performance of rice field eel (Monopterus albus). Acta Hydrobiologica Sin. 37, 648–655. doi: 10.7541/2013.76
Zhu R., Li L., Li M., Yu Z., Wang H., Wu L. (2020). The effects of substituting fish meal with soy protein concentrate on growth performance, antioxidant capacity and intestinal histology in juvenile golden crucian carp, Cyprinus carpio × Carassius auratus. Aquaculture Rep. 18, 100435. doi: 10.1016/j.aqrep.2020.100435
Keywords: Litopenaeus vannamei, soy protein concentrate, Schizochytrium limacinum, lipid metabolism, apoptosis, autophagy, intestinal health
Citation: Yao X, Lin Y, Shi M, Chen L, Qu K, Liu Y, Tan B and Xie S (2022) Effect of Schizochytrium limacinum supplementation to a low fish-meal diet on growth performance, lipid metabolism, apoptosis, autophagy and intestinal histology of Litopenaeus vannamei. Front. Mar. Sci. 9:1090235. doi: 10.3389/fmars.2022.1090235
Received: 08 December 2022; Accepted: 24 November 2022;
Published: 08 December 2022.
Edited by:
Jianchun Shao, Fujian Agriculture and Forestry University, ChinaReviewed by:
Min Jin, Ningbo University, ChinaH-Michael Habte-Tsion, University of Maine, United States
Copyright © 2022 Yao, Lin, Shi, Chen, Qu, Liu, Tan and Xie. This is an open-access article distributed under the terms of the Creative Commons Attribution License (CC BY). The use, distribution or reproduction in other forums is permitted, provided the original author(s) and the copyright owner(s) are credited and that the original publication in this journal is cited, in accordance with accepted academic practice. No use, distribution or reproduction is permitted which does not comply with these terms.
*Correspondence: Shiwei Xie, eHN3enNkeEAxNjMuY29t
†These authors have contributed equally to this work