- Key Laboratory of Aquatic Nutrition and Feed Science of Jiangsu Province, College of Animal Science and Technology, Nanjing Agricultural University, Nanjing, China
This study was conducted to evaluate the effect of cholesterol (CHO) on ovarian development of Eriocheir sinensis through a feeding trial. Crabs (average weight 43.35 ± 0.05 g) were randomly fed with one of three diets: 0% CHO, 0.4% CHO and 1.6% CHO for 16 weeks. Results indicated that the hepatosomatic index (HSI) and gonad index (GSI) of crabs fed with 0.4% CHO diet were significantly higher than those in the control group. (P < 0.05). Meanwhile, 0.4% CHO can significantly increase the gene expression of vtg and vgr in ovary (P < 0.05), thus promoting the accumulation of Vtg. The protein expression of MEK1/2, ERK, p-ERK1/2, Cyclin B and CDC2 were significantly increased in the 0.4% CHO group, therefore dietary CHO might promote oocyte maturation by activating MAPK signal pathway and cooperating with maturation promoting factor (MPF). Further exploration results showed that 0.4% CHO was able to significantly up-regulate the protein expression of STAR and SR-B1 to promote the transport of CHO to ovary, thereby providing sufficient substrates for estradiol (E2) synthesis. In addition, the results in vivo and in vitro shown that CHO could regulate the activities of enzymes such as CYP11A1 and CYP19A1 through the cAMP-PKA-CREB/SF-1 signal pathway and then affects the level of E2 in the organism. In summary, supplementing the appropriate amount of CHO in the diet can improve the E2 level of the organism, thus increasing the accumulation of nutrients in the ovary, promoting the completion of meiosis, and finally achieving the purpose of promoting ovarian maturation.
Introduction
As one of the most important economic crustaceans, the Chinese mitten crab (Eriocheir sinensis) has a vast consumer market in China, and its output and economic benefits have been increasing continuously in the past few years (Wang et al., 2016; Chinese Fishery Statistical Yearbook, 2021). As the most popular edible part, the maturing status of ovary directly affects the economic value of Eriocheir sinensis (Long et al., 2020). In China, the most popular time for Eriocheir sinensis consumption is mainly around the Mid-Autumn Festival. But, during this period, compared with crabs fed with formula feed, the crabs fed with trash fish have higher gonad maturity, which significantly affected farmers’ income and thus hindered the promotion of formula feed (Djunaidah et al., 2003; Zheng et al., 2020; Fang et al., 2021). However, feeding trash fish for a long time will lead to environmental pollution and ecological damage (Wu et al., 1994). Therefore, looking for a nutrient that can promote the ovarian development, and then preparing a more comprehensive formula feed is of great significance to the sustainable development of the crab industry.
In fact, the ovarian maturation of Eriocheir sinensis is actually a process of nutrient accumulation, of which the most important substance is yolk protein (Wu et al., 2007). Therefore, the accumulation of yolk protein directly affects the maturity and economic value of Eriocheir sinensis (Wouters et al., 2001). The main component of yolk protein is vitellin (Vn), which can be processed by vitellogenin (Vtg), lipids and proteins (Okuno et al., 2002; Wu et al., 2017). Presently, there is still some controversy about the sites of Vtg synthesis in crustaceans, but for most shrimp and crabs, hepatopancreas and ovary are still the main sites of Vtg synthesis (Subramoniam, 2011). According to the different sites of Vtg synthesis, vitellogenesis can be divided into two stages: endogenous vitellogenesis and exogenous vitellogenesis (Wu et al., 2017). In the endogenous vitellogenesis stage, Vtg is mainly synthesized in the ovary, and in the exogenous vitellogenesis stage, the hepatopancreas is the main site of Vtg synthesis (Rani and Subramoniam, 1997; Lu et al., 2018). The Vtg synthesized in the hepatopancreas will be transported to the ovary through hemolymph after combining with steroid hormones, vitamins and lipids, and binds to vitellogenin receptor (VgR) on the surface of oocytes, then stored in oocytes through endocytosis (Soroka et al., 2000).
Besides the accumulation of nutrients, meiosis is also a critical event in the process of ovarian maturation (Song et al., 2014). However, during gametogenesis, immature oocytes will be blocked in the germinal vesicle (GV) stage, and the recovery of meiosis is a process of cell cycle transition induced by complex signal pathways (Cau et al., 1988; Lanot and Cledon, 1989). As the main promoter of cell cycle transformation, maturation promoting factor (MPF) is a heterodimeric protein kinase composed of the regulatory subunit Cyclin B and the catalytic subunit CDC2, which can catalyze the phosphorylation of various proteins, and then promote the transformation of oocytes from G2 phase to M phase (Wang et al., 2013; Song et al., 2014). Mitogen-activated protein kinase (MAPK) signaling pathway is a highly conservative signal transduction pathway, which widely exists in all kinds of eukaryotic cells. Many studies have shown that MAPK cascade signaling pathway through synergy with MPF and activate each other by positive feedback mechanism to jointly regulate meiotic cell cycle transition (Bodart et al., 2002; Ohashi et al., 2003).
The ovarian development of Eriocheir sinensis is regulated by many factors, among which hormone regulation plays a vital role (Malati et al., 2013). Estradiol (E2), as one of the most active reproductive hormones, has been identified in many decapod crustaceans, and the promoting effect on the ovarian maturation of crustaceans has also been reported (Martins et al., 2007; Huang et al., 2009). Previously, there was some controversy about whether crustaceans have the ability to synthesize E2. However, with the discovery of related enzymes in the process of E2 synthesis in crustaceans such as Macrobrachium rosenbergii, Procambarus clarkii and Eriocheir sinensis, it is widely believed that crustaceans can synthesize E2 with CHO as raw material under the action of a series of enzymes. (Teshima and Kanazawa, 1971; Warrier et al., 2001; Summavielle et al., 2003). Therefore, it may be an excellent method to solve the problem of delayed ovarian maturation of Eriocheir sinensis by supplementing exogenous nutrients to regulate the secretion of endogenous hormones.
CHO, as a necessary nutrient for aquatic animals, and the effects of growth performance has been widely studied (Suprayudi et al., 2012; Tian et al., 2020; Guo et al., 2022; Su et al., 2022). Meanwhile, as a substrate for the synthesis of E2, CHO could be of great significance for ovarian development (Teshima et al., 1983; Kumar et al., 2018). However, there are few reports about the effect of CHO on the ovarian development of Eriocheir sinensis yet. The synthesis of E2 occurs in the mitochondrial intima of the ovary (Jefcoate et al., 1992). In crustaceans, high-density lipoprotein (HDL) and scavenger receptor class B type 1 (SR-B1) are responsible for the transport of CHO into oocytes, and CHO in oocytes needs to be further transported into the inner mitochondrial membrane under the action of steroidogenic acute regulatory protein (STAR). (Becker et al., 1993; Miller, 2007). Subsequently, CHO produces pregnenolone under the action of CHO side-chain cleavage enzyme (CYP11A), which goes through a series of reactions to testosterone, and then to E2 under the further action of aromatase (CYP19A1). Therefore, STAR, CYP11A1 and CYP19A1 are three key substances catalyzed E2 synthesis (Miller, 2007; Zheng et al., 2020). cAMP/PKA is an important signal pathway for regulating E2 synthesis in the organism, SF-1 and CREB are the response factors of the cAMP/PKA signal pathway, which is responsible for regulating the activity of various enzymes in the process of E2 synthesis (Carlone and Richards, 1997; Stocco, 2001; Qu et al., 2008). In some aquatic animals, it has been reported that by controlling the substrate to regulate secretion of estrogen to influence the ovarian development, but this has been not reported in crustaceans (Jefcoate et al., 1992). Therefore, this experiment aims to evaluate whether the exogenous CHO can regulate the secretion of E2 through the cAMP-PKA-CREB/SF-1 signal pathway and then affect the ovarian maturation. Thereby solving the problems of delayed ovarian maturation of Eriocheir sinensis.
Materials and methods
The experimental design and all experimental operations of this study was approved by the Animal Care and Use Committee of Nanjing Agricultural University (Nanjing, China) (permit number: SYXK (Su) 2011–0036).
Experimental diets
Our previous study results showed that the CHO deposition in the hepatopancreas of Eriocheir sinensis was about 0.4%, so we designed a group with 0.4% CHO (Guo et al., 2022). In addition, in order to explore whether excessive CHO will affect ovarian development, a 1.6% CHO supplement group was set up. Therefore, three isonitrogenous and isolipid diets were formulated to contain CHO 0%, 0.4% and 1.6% (actually 0.06%, 0.44%, 1.64%) at the cost of soybean oil. The preparation method of experimental diets according to the previous study of our laboratory (Guo et al., 2022). Firstly, all raw materials were smashed and passed through a 60-mesh percolator, then mixed step by step. Put the weighed CHO and oil of each group into a beaker and mix them thoroughly with a glass rod. Subsequently, about 30% deionized water was added to the mixture to make a dough. At last, the feed pellets (2.5-mm diameter) were squeezed out through a single-screw meat grinder extruder. After being air-dried (27°C) for 24 hours, four diets were packed in vacuum plastic bags and stored at -20°C. The ingredient formulation and proximate composition of the diet was shown in Table 1.
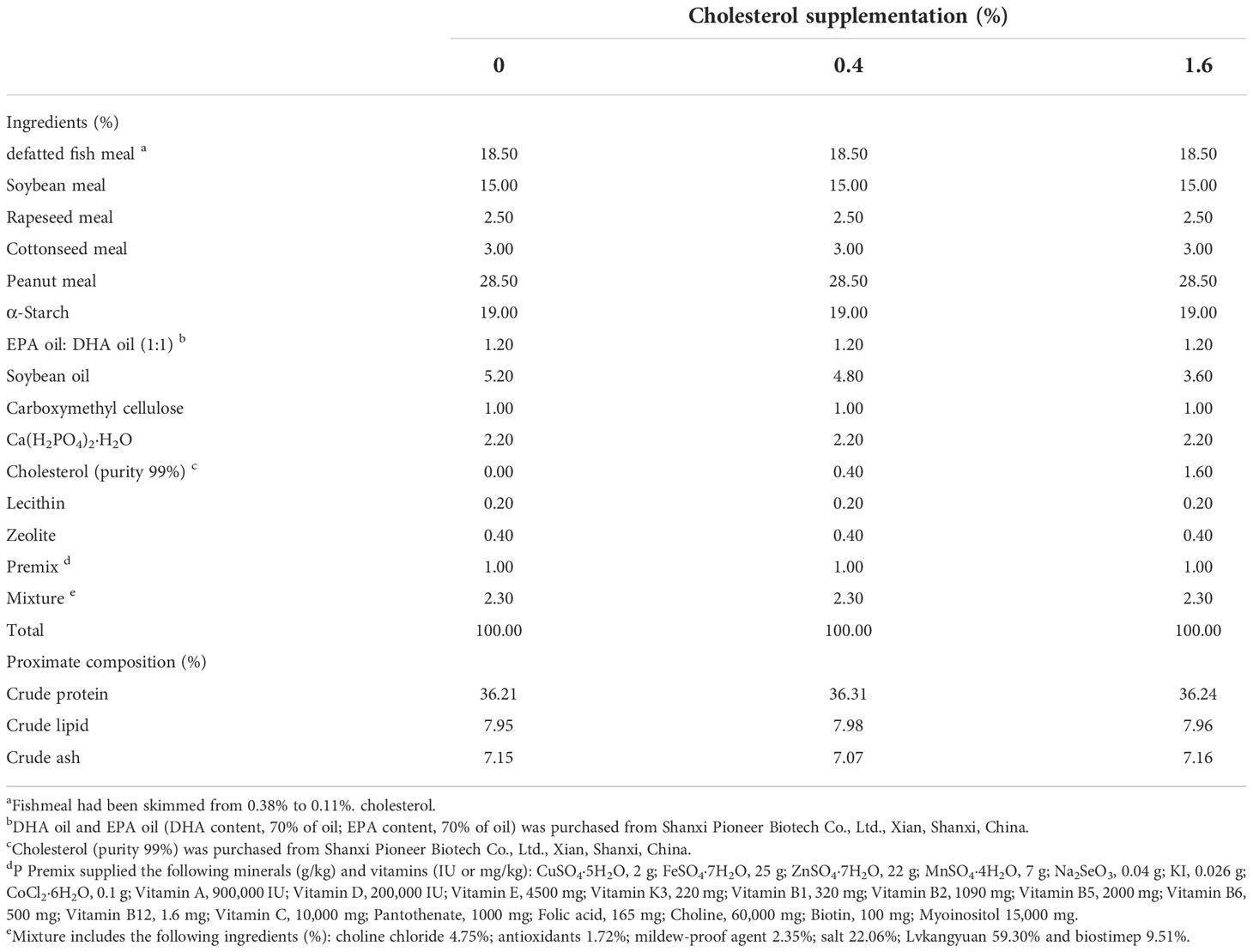
Table 1 Ingredient formulation and proximate composition (% dry matter) of the diet in this feeding trial.
Experimental crabs and feed trial
The experimental crabs were obtained from a local farm in Pukou, Nanjing, China. This experiment was implemented in the Aquatic Teaching Base of Nanjing Agricultural University (Nanjing, Jiangsu, China). A total of 160 healthy female crabs (average weight, 43.35 ± 0.05 g) were randomly assigned to 16 cement pools (10 crabs in each cement pool, each treatment contains four cement pools). 12 pipes (20 cm long and 10 cm diameter) were put into each cement pool as shelters for crabs to hide. Prior to the 16-week breeding experiment, the crabs were fed in the cement pool (1.0×1.0×0.8m, L:W:H) for a week to adapt to the new environment. After a week of acclimation, crabs of each treatment were fed with their respective diets once daily (18:00) with 3%-5% of total body weight till obvious satiation. During the experiment period, the residual feed of the previous day was removed with a dirt absorber, and 1/3 volume of water should be changed in cement pool. The water quality was detected daily and maintain the water temperature at 24-28°C, dissolved oxygen at 5.0-7.0 mg/L, pH at 7.3-8.4, and ammonia nitrogen under 0.05 mg/L.
Sample collection
At the end of 16-week feeding trial, all crabs were starved for 24 hours. Subsequently, four individuals were randomly selected from each cement pool to collect hemolymph with a 1mL syringe according to the method in our laboratory (Cheng et al., 2020). Thereafter, the crabs were anesthetized on ice, then the ovaries and hepatopancreas were quickly collected and weighed to calculate hepatosomatic index (HSI) and gonad index (GSI). After weighing, part of the ovaries was fixed with 4% paraformaldehyde solution for histological analysis. The remaining samples were quickly frozen with liquid nitrogen and stored at -80°C for determining biochemical indicators and enzymes activities and protein expression. The calculation formulas of related parameters were as follows:
Proximate composition analysis
The content of crude protein, ash and moisture in the diet were determined according to the standard method (AOAC, 1995). The details detection of crude protein, ash and moisture were the same as Guo et al. (2022). The content of CHO in the feed was detected through the high-performance liquid chromatography (HPLC) method (Agilent ZORBAX Eclipse Plus, column C18 5 μm 4:6 × 150 mm). 100% methanol was used as a mobile phase pumped with a flow rate at 1 mL/min. The specific measurement method refers to Guo et al. (2022).
Gene expression analysis
The total RNA of the ovary and hepatopancreas were extracted using RNAiso Plus (TakaRa, Dalian, China), and treated with gDNA wiper Mix and HisScript III Qrt SuperMix (Cat. No. R323–01, Vazyme Biotech Co, China) to eliminate genomic DNA. Then, the purity of total RNA was estimated by spectrophotometry on the basis of OD 260/280 using a Nano Drop 2000 spectrophotometer (NanoDrop Technologies, Wilmington, DE). Subsequently, 1 µg RNA was reverse transcribed into first-standard cDNA immediately using HisScript III Qrt SuperMix (Vazyme Biotech Co, China) according to Wang et al. (2020). After reverse transcription, the target cDNA was amplified by the ChamQ Universal SYBR qPCR Master Mix Kit (Vazyme Biotech Co, China). The specific sequences of primers are given in Table 2. The reaction and protocol were set according to Guo et al. (2022). β-actin was the reference to do relative quantification of the target gene transcripts and the target gene expression of crabs in the group with 0% CHO as the correct factor. The transcript levels of target genes were analyzed by the 2−ΔΔCT method (Livak and Schmittgen, 2001).
Ovarian histology analysis
The ovaries were fixed in 4% paraformaldehyde for 24 hours, then placed in 70%, 75%, 80%, 90%, 95% and absolute ethanol for gradient dehydration. After that, transparently treated with xylene and embedded in paraffin. The embedded ovarian tissue was sliced with a microtome (LeicaRM2016, Berlin, Germany) according to the thickness of 6 μm. Then the slices were routinely dewaxed and rehydrated in absolute ethanol, 95% ethanol and 80% ethanol. After rehydration, soak in ultrapure water for 5 minutes and then hematoxylin-eosin (H&E) staining was performed according to Zheng et al. (2020). Finally, the film was sealed with neutral gum, and four individuals were observed under the optical microscope (Nikon Eclipse 80i, Tokyo, Japan), captured by a digital camera (Nikon DS-U2, Tokyo, Japan). Only oocytes with obvious nuclei were measured by Image-Pro Plus 6.0 (media cybernetics, USA). The parameters include the short diameter of an oocyte (SO), the long diameter of an oocyte (LO), the short diameter of nuclei (SN), the long diameter of nuclei (LN). The volume of an oocyte (VO), the volume of a nuclei (VN), and the nucleo-cytoplasmic ratio (NCR) were calculated according to the formula as follows (Wu et al., 2017; Zheng et al., 2020):
Where Wo is the maximum width of oocyte, Lo is the maximum length of oocyte, Wn is the maximum width of nuclei, Ln is the maximum length of nuclei.
Fluorescence assay of cholesterol
The ovarian was embedded in the optimal cutting temperature compound (OCT, Bioss, C2076), and then fixed on the sample holder. After the temperature of the microtome stabilized at -20°C, the tissue was cut into 6 μm thin slices, dipped in the slices with glass slides, and then washed away the excess OCT with PBS. The FILIPIN dye was dropped on the slices and incubated at room temperature for 30 minutes. In the end, washing away the excess staining solution with PBS, the slides were sealed and observed under confocal laser scanning microscope (ZEISSLMS900, Berlin, Germany).
Biochemical analysis of ovary
The ovarian samples were made into homogenate, centrifuged at 4°C and 6000 rpm for 5 minutes, and the supernatant was taken to detect the following indexes: total CHO (TC) (TC, Kit, NO. ml094937), estradiol (E2) (mouse E2 ELISA Kit, NO. ml001962), vitellogenin (Vtg) (crab Vtg ELISA Kit, NO. ml003467), Cytochrome P450 Family 11 Subfamily A Member 1 (CYP11A1) (insect CYP11A1 ELISA Kit, NO.ml122176), Cytochrome P450 Family 19 Subfamily A Member 1 (CYP19A1) (insect CYP19A1 ELISA Kit, NO. ml036941). The commercial kits (Shanghai Enzyme Bioengineering Co., Shanghai, China) were used to detect the above indicators, specific operation steps are strictly implemented in accordance with the instructions.
Western blot analysis
The process of western blot refers to Cao et al. (2019). Total protein of ovary was extract with a glass tissue grinder (Kimble Chase) on ice and lysed with RIPA lysis buffer (#ab156034, Abcam, United Kingdom). The protein concentration was detected by BCA protein assay kit (Beyotime Biotechnology, China). Heat denatured protein lysate (2 µg/µL) of 10 µL was loaded into each well, separated on 4-20% gradient SDS-PAGE electrophoresis gels for 30-35 minutes at 150 V using a Mini-Protean System (Bio-Rad, United States). The protein was transferred to PVDF membrane by electrophoresis at 105 V for 65 minutes. Each PVDF was blocked with 5% BSA in TBST (0.1% Tween 20, 500 mM NaCl, 20 mM pH=7.4 Tris-HCl) at room temperature for 2 hours, then incubated overnight at 4°C, with primary antibodies against STAR (#AC026, ABclonal), SR-B1 (#DF6479, Affinity), cAMP (#ab76238, Abcam), PKA (#ab75991, Abcam), SF-1 (#AF7895, Affinity), p-CREB (#AF3189, Affinity), CYP19A1 (#AF5229, Affinity), CYP11A1 (#DF4697, Affinity), MEK1/2 (#AF6385, Affinity), ERK1/2 (#AF0155 Affinity), p-ERK1/2 (#AF1015, Affinity), Cyclin B (#DF6786, Affinity), CDC2 (#DF6024, Affinity), VTG (#abs119855, Absin), α-Tubulin (#AF7010, Affinity). The next day, PVDF membranes were incubated with secondary antibodies (Goat Anti-Mouse IgG H&L: #ab6728, Abcam; Goat Anti-Rabbit IgG H&L: #ab6721, Abcam) for 2 hours at room temperature. The bands of the protein were detected by a ECL reagent (Beyotime Biotechnology, China), and captured with a luminescent image analyzer (Fujifilm LAS-3000, Japan). The intensity of target bands was analyzed by image J software (U.S. National Institutes of Health, Bethesda, MD, USA).
Immunofluorescence
Refer to the above steps of making paraffin sections, and treat the ovaries to the step of dewaxing and rehydrating. Then the slices were immersed in 0.01 M citrate solution and heated at 95°C for 5 minutes for antigen repair. After cooling to room temperature, rinse with PBS three times for 5 minutes each time. Then 5% goat serum was dripped and sealed at room temperature for 2 hours, and the excess sealing solution was removed. The first antibody was dripped and incubated in a wet box at 4°C for 12 hours. After returning to room temperature, rinsed with PBS three times for 5 minutes each time. Drop the fluorescent secondary antibody to cover the sample completely, incubate at 37°C for 1 h. Subsequently, DAPI was added and dye for 10 minutes at room temperature in the dark, then wash the excess dye off with PBS, seal the film and observed under confocal laser scanning microscope (ZEISSLMS900, Berlin, Germany).
In vitro tissue culture
The crabs were obtained from the Aquaculture Base of Nanjing Agriculture University. Before dissection, the female crabs were anesthetized on ice and disinfected with 75% ethanol. Ovaries were quickly taken out in a sterile environment and rinsed 8 times with PBS solution containing penicillin (100 IU/mL) and streptomycin (100 ug/mL), meanwhile, hemolymph and connective tissue were removed. Cut the ovaries into small pieces of ~1 mm3 and place into a 24-well cell culture plate with 0.5 mL L-15 medium. After 30 minutes of tissue attachment, the previous culture medium was sucked off, and 400 µL of L-15 medium containing double antibody was added again. The ovarian tissue was cultured in a constant temperature incubator at 26°C. The CHO was resuspended in ethanol absolute (EA) and mixed thoroughly with L-15 medium. Based on the pre-experiment, the final concentrations of CHO were set to 0 mg/L, 50 mg/L and 200 mg/L, the media and the EA were control group. Each treatment contains four replicates, after culturing at 26°C for 24 hours, removing the culture medium, then rinse the ovaries with PBS for three times, and quickly extract the total protein for western blot analysis.
Statistical analysis
The statistical analysis of the data in this study were analyzed using SPSS 23.0 (Chicago, IL USA). All date were presented as mean ± S.E.M. (standard error of the mean). Before analysis, the normality and homogeneity of data were evaluated by Kolmogorov-Smirnov test and Levene’s tests. Subsequently, a one-way analysis of variance was performed, and then, Duncan’s multiple comparison test to estimate the significant differences (P < 0.05).
Results
Growth performance
The parameters of growth performance were shown in Figure 1. Compared to the control group, crabs fed the diet with 0.4% CHO resulted in a significantly higher HSI and GSI, while no significant difference was observed in 1.6% CHO treatment group (P < 0.05).
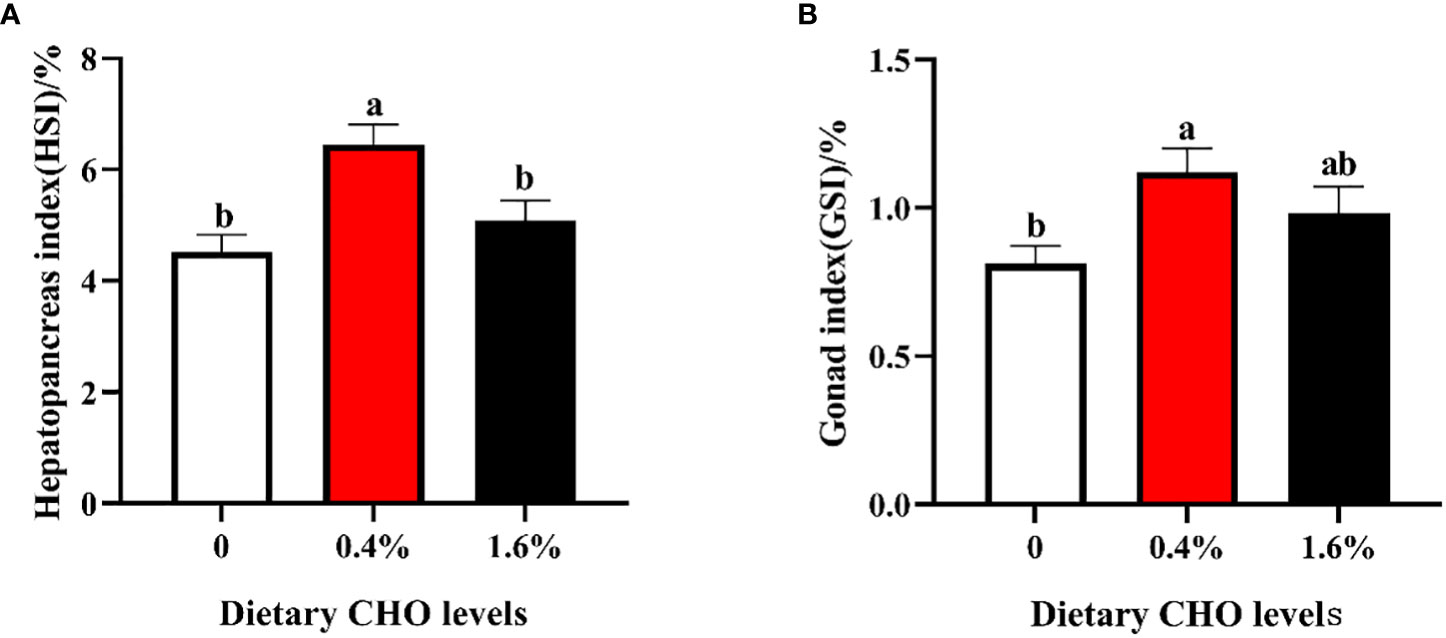
Figure 1 Effects of dietary CHO on (A) hepatopancreas index (HSI); (B) gonad index (GSI). The values are the Means ± SEM (n = 4). Different letters indicate significant differences (P < 0.05).
Histological analysis
The effect of dietary CHO on the ovarian histology of crabs was shown in Figure 2. Compared with the group without CHO, the volume of oocytes in the group supplemented with CHO was larger, and the oocytes were filled with yolk granules. Further observation showed that when the dietary CHO was 0.4%, the oocytes were squeezed and deformed, and the volume of oocytes and yolk granules was obviously larger.
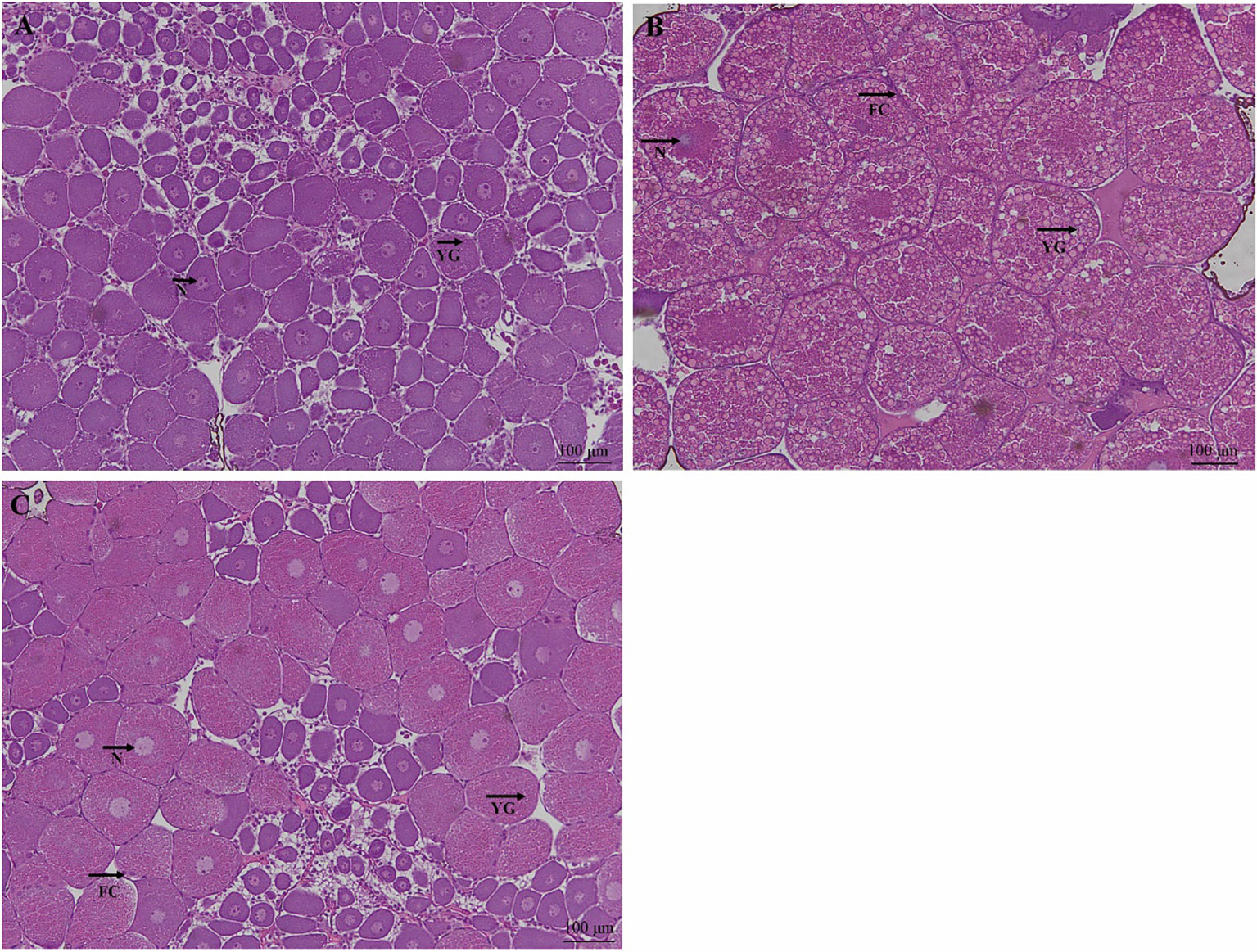
Figure 2 H&E staining of ovarian tissue from E. sinensis subjected to different levels of dietary CHO (means ± SEM, n = 4). Photomicrographs (10×) and scale bar (100 μm). (A): crabs fed with 0% CHO diets; (B): crabs fed with 0.4% CHO diets; (C) crabs fed with 1.6% CHO diets. N: nucleus; YG: yolk granule; FC: follicle cell.
The related parameters of oocytes were shown in Table 3. Compared with the control group, the LO, SO and VO in CHO treatment group were significantly increased (P < 0.05), and the maximum value appeared in the group with 0.4% CHO. For the nucleus, adding CHO has no effect on LN, but when the amount of CHO is 0.4%, SN and VN are significantly higher than the control group (P < 0.05). In contrast, after adding CHO, NCR was significantly lower than that of the control group (P < 0.05).
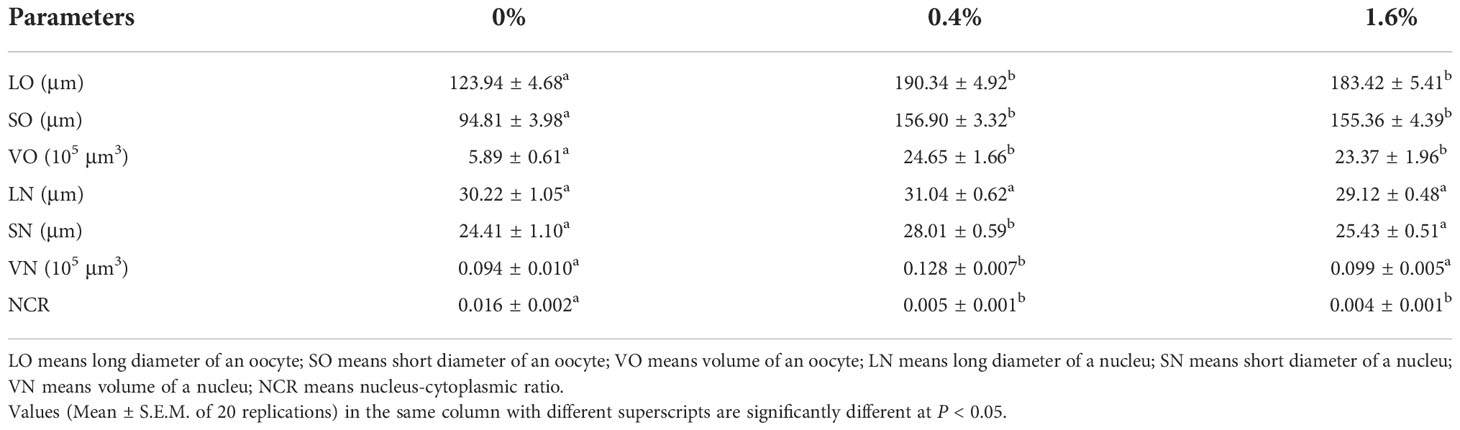
Table 3 Sizes of oocytes and nuclei in Eriocheir sinensis subjected to different levels of dietary CHO.
The expression and transport of VTG in the hepatopancreas and ovary
Dietary CHO had no significant effect on the content of VTG in the hepatopancreas (Figure 3B). While for the ovary, when the amount of CHO is 0.4%, the content of VTG was significantly increased (P < 0.05), and when crabs were fed the diet with 1.6% CHO, the content of VTG was significantly lower than the control group (P < 0.05) (Figure 3A). Compared with the control group, 0.4% CHO could significantly increase the mRNA expression level of vtg in ovary, but had no significant effect on the expression of vtg in hepatopancreas (P < 0.05) (Figures 3C, D). Furthermore, in 0.4% CHO group, the gene expression of vgr significantly increased in the ovary. In comparison, the expression of vgr shows a downward trend when the content of CHO reached 1.6% (P < 0.05) (Figure 3E). The results of protein expression showed that the protein expression level of VTG was significantly increased by adding CHO, and the protein expression level of VTG was the highest in the group with 0.4% CHO (Figures 3F, G).
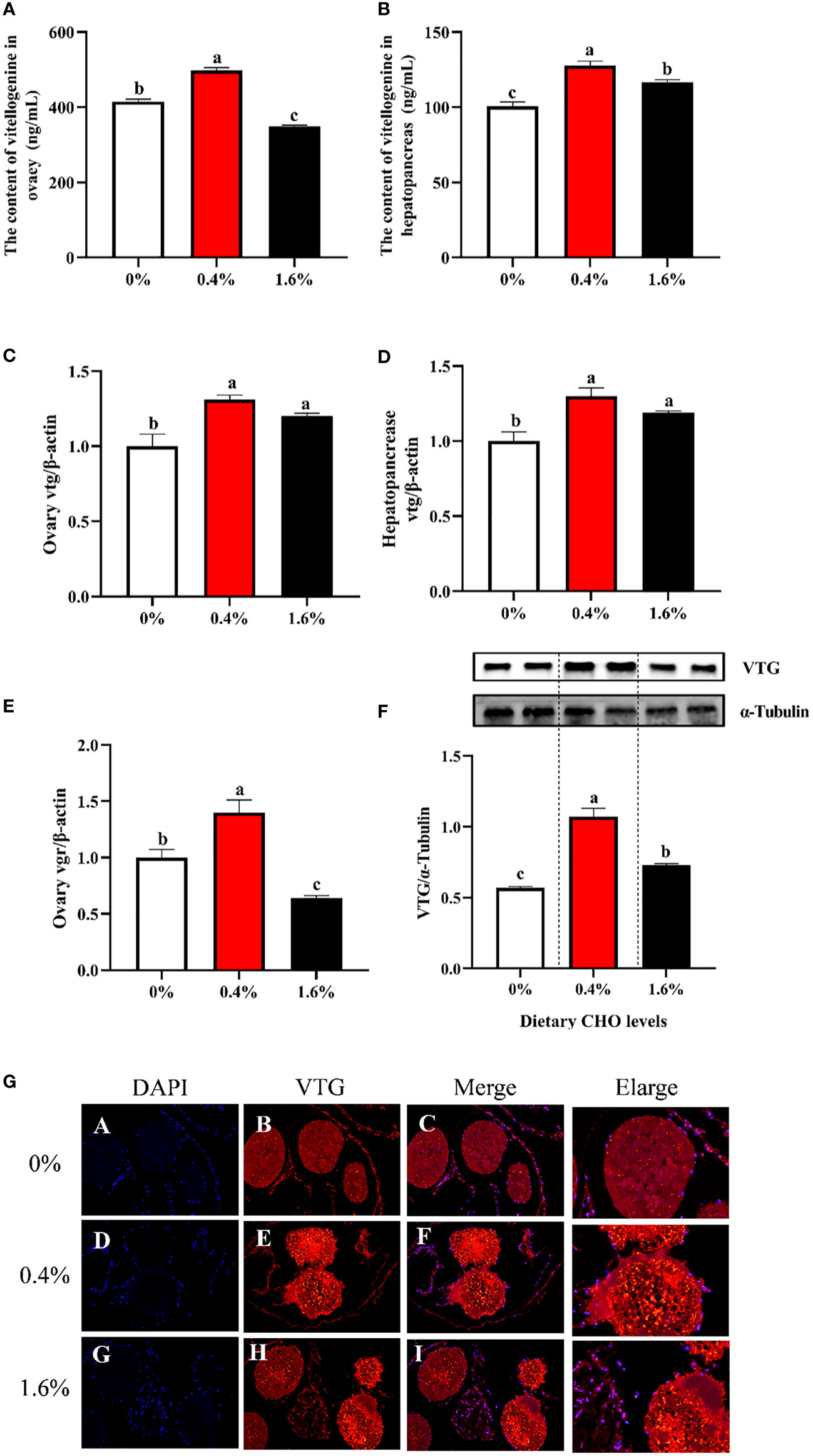
Figure 3 Effects of dietary CHO on (A) the content of Vtg in ovary; (B) the content of Vtg in hepatopancreas; (C) the mRNA expression of vtg in ovary; (D) the mRNA expression of vtg in hepatopancreas; (E) the mRNA expression of vgr in ovary; (F) the protein expression of VTG in ovary. (G) the immunofluorescence of VTG in ovarian tissue of E.sinensis after fed with diets supplemented different CHO levels (blue: nucleus; red: VTG). The values are the Means ± SEM (n = 4). Different letters indicate significant differences (P < 0.05).
The expression of oocyte division-related proteins
As shown in Figure 4, with the increase of dietary CHO level, the expression levels of MEK1/2, ERK1/2, p-ERK1/2, Cyclin B and CDC2 showed a tendency to increase first and then decrease. And when crabs were fed the diet with 0.4% CHO, the expression of the above proteins in the ovary was significantly higher than that in the control group (P < 0.05).
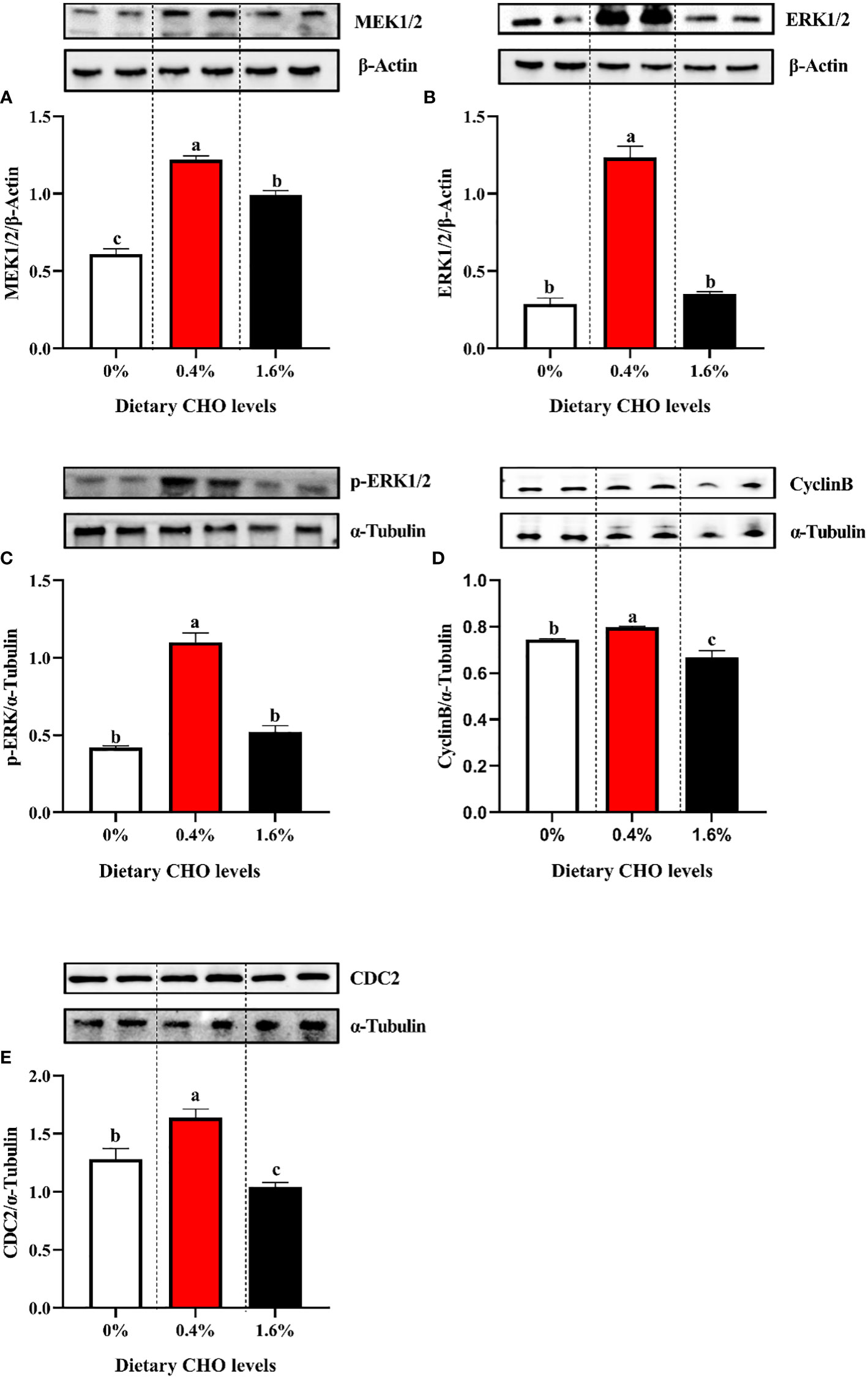
Figure 4 Western blot analysis of (A) the protein expression of MEK1/2 in ovary; (B) the protein expression of ERK1/2 in ovary; (C) the protein expression of p-ERK1/2 in ovary; (D) the protein expression of Cyclin B in ovary; (E) the protein expression of CDC2 in ovary. The values are the Means ± SEM (n = 4). Different letters indicate significant differences (P < 0.05).
The analysis of cholesterol transport
The results of western blot and immune fluorescence showed that CHO can significantly affect the protein expression of SR-B1 and STAR (P < 0.05) (Figures 5A–D). Compared with the control group, 0.4% CHO can significantly promote the protein expression of SR-B1 and STAR (P < 0.05), while 1.6% CHO will significantly suppress the expression of SR-B1 (P < 0.05). Through the staining and detection of CHO in the ovaries, it was found that after feeding a diet with 0.4% CHO, the amount of CHO deposition in the ovaries was significantly higher than that of other groups (P < 0.05).However, when the diet containing 1.6% CHO was fed to crabs, the content of CHO in the ovaries was significantly lower than that in the control group (P < 0.05) (Figures 5E, F).
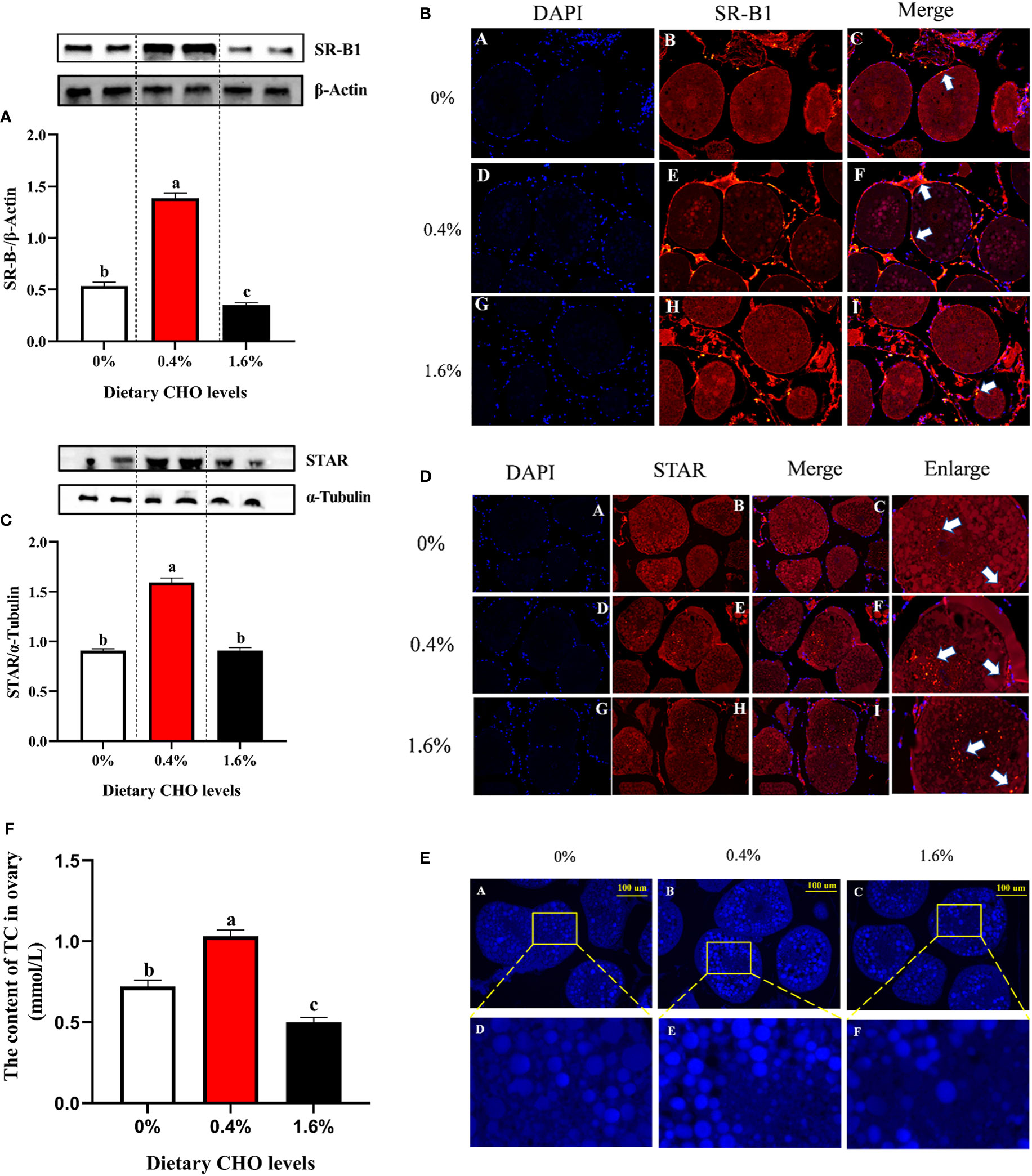
Figure 5 Effects of dietary CHO on (A) the protein expression of SR-B1 in ovary; (B) the immunofluorescence of SR-B1 in ovary (blue: nucleus; red: SR-B1); (C) the protein expression of STAR in ovary; (D) the immunofluorescence of STAR in ovary (blue: nucleus; red: STAR). The content of ovary CHO after crabs was fed diets with different CHO levels. (E) Representative confocal microscopic image of ovarian CHO stained by FILIPIN (blue), photomicrographs (200×) and scale bar (100 μm); (F) The content of TC in ovary. The values are the Means ± SEM (n = 4). Different letters indicate significant differences (P < 0.05).
The analysis of estradiol synthesis
The detection of E2 synthesis-related proteins found that with the increase of CHO content from 0% to 1.6% in the diet, the expression levels of cAMP, PKA, p-CREB, SF-1, CYP11A1 and CYP19A1 showed a tendency to increase first and then decrease (P < 0.05) (Figures 6A–F). In addition, 0.4% CHO can significantly increase the activity of enzymes CYP11A1 and CYP19A1 in the ovary compared to the control group (P < 0.05) (Figures 6G, H). The detection of E2 in the ovary showed that the content of E2 in CHO treated groups was significantly higher than that in the control group (P < 0.05), and the maximum value appeared in the group with 0.4% CHO (Figure 6I).
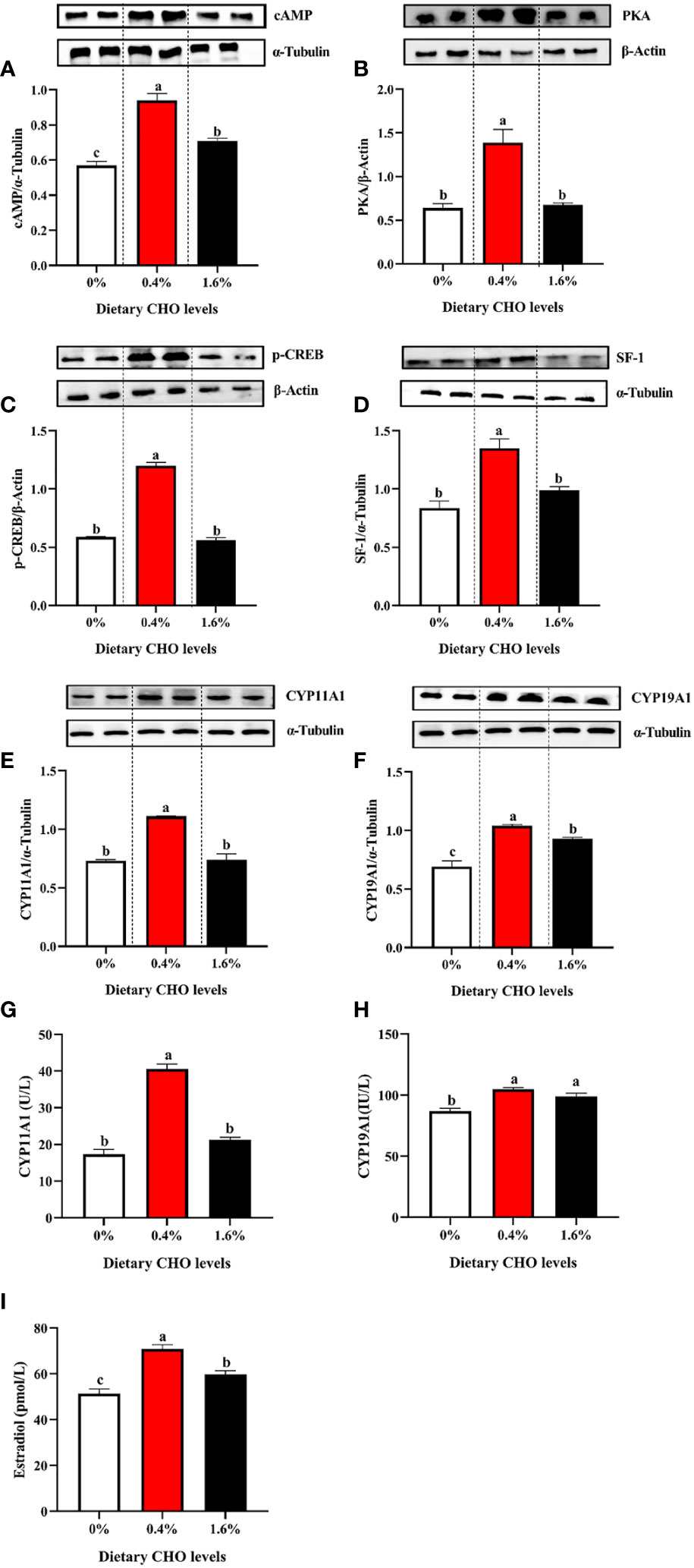
Figure 6 The protein expression of (A) cAMP; (B) PKA, (C) p-CREB, (D) SF-1, (E) CYP11A1 and (F) CYP19A1 in the ovary of Eriocheir sinensis fed with the experimental diets. (G) The activity of CYP11A1 in the ovary; (H) the activity of CYP19A1 in ovary; (I) the content of E2 in ovary. The values are the Means ± SEM (n = 4). Different letters indicate significant differences (P < 0.05).
Protein expression in vitro
The proteins expression of cAMP, PKA, p-CREB, SF-1, CYP11A1 and CYP19A1 in the ovary exposed to 0, 50 and 200 mg/L CHO were shown in Figure 7. Compared with the control group, the expression levels of cAMP and PKA in the CHO treated groups were significantly increased (Figures 7A, B) (P < 0.05). Moreover, 50 mg/L CHO could significantly up-regulate the expression levels of p-CREB, SF-1, CYP11A1 and CYP19A1 (P < 0.05), while when the concentration of CHO reached 200 mg/L, the expression of p-CREB, SF-1, CYP11A1 and CYP19A1 decreased significantly (P < 0.05) (Figures 7C–F).
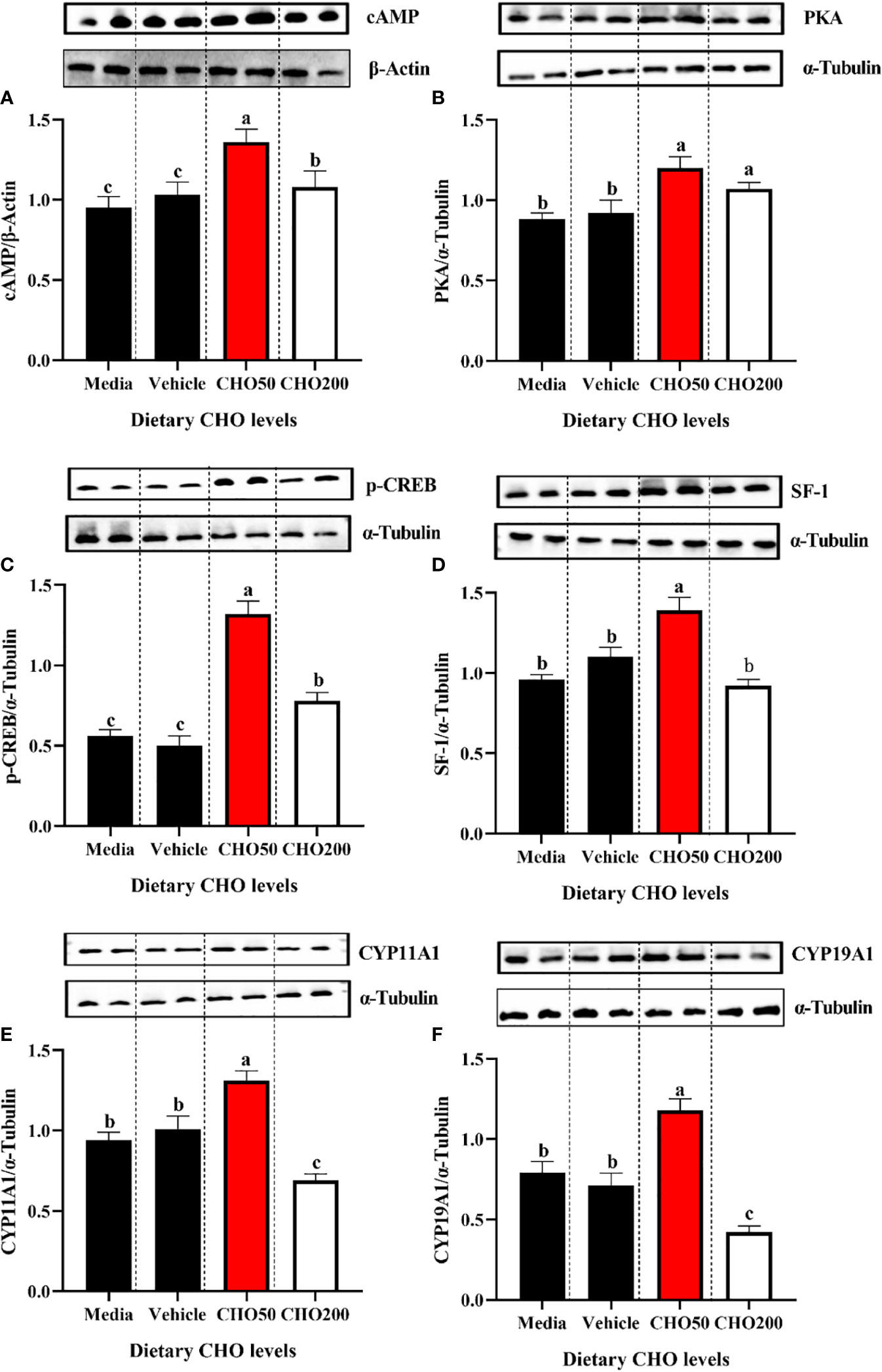
Figure 7 The protein expression of (A) cAMP; (B) PKA, (C) p-CREB, (D) SF-1, (E) CYP11A1 and (F) CYP19A1 in the ovary exposed to 0, 50 and 200 mg/L CHO. The values are the Means ± SEM (n = 4). Different letters indicate significant differences (P < 0.05).
Discussion
CHO, as an essential nutrient for aquatic animals, widely exists in various animal tissues. Especially for crustaceans, which have limited ability to synthesize CHO on their own and can only obtain it from food to meet their own needs (Kumar et al., 2018). Therefore, most previous studies on CHO in crustaceans have focused on survival, growth, and lipid metabolism (Sheen et al., 1994; Teshima et al., 1997; Niu et al., 2012; Tian et al., 2020). Our previous study showed that 0.27% CHO could significantly improve the weight gain and survival ratio of Eriocheir sinensis. However, 0.27% CHO may be able to meet the growth requirement of Eriocheir sinensis, but may be insufficient for the demand of ovarian development (Tao et al., 2014; Guo et al., 2022). For Eriocheir sinensis, the HSI increases gradually before the gonad enters the rapid development stage. However, when the gonad enters the rapid development period, the GSI will gradually increase, while the HSI will decrease accordingly. Therefore, it is generally believed that the nutrients in hepatopancreas are the energy pool to support the gonad development of crab (Wu et al., 2017; Jiang et al., 2022). In this study, the GSI and HSI in the group that fed diet containing 0.4% CHO were significantly higher than those in the control group, while this difference was no longer significantly at high doses of CHO. This result may be because dietary CHO affects the E2 synthesis, leading to the difference in ovarian development (Fairs et al., 1990; Janer and Porte, 2007; Pan et al., 2018).
The ovarian maturation of Eriocheir sinensis is often accompanied by increased oocyte volume (Chen et al., 2004; Zheng et al., 2021). In this study, under the same magnification of light microscope, the number of oocytes observed in 0.4% CHO group was much less than that in the other two groups, and the yolk granules were fuller. Further measurement results also showed that the LO, SO and VO of oocytes in 0.4% CHO group were significantly higher than those in the other two groups. This further proves that an appropriate amount of CHO plays an important role in promoting ovarian maturation. In crustaceans, it has been reported that the increase of oocyte size is closely related to vitellogenesis (Wu et al., 2017). For example, substantial quantities of vitellin accumulates in the oocyte resulted in the active growth of oocytes in Macrobrachium rosenbergii (Du et al., 1995; Lee and Chang, 1997). In this experiment, 0.4% CHO significantly increased the content VTG in the ovary. According to this, this study speculated that the reason why adding an appropriate amount of CHO makes oocytes grow actively by promoting the deposition of VTG in ovaries (Xue et al., 1987; Zheng et al., 2020).
In terms of nutrition, the development of ovary in crustaceans is actually a process of nutrient accumulation, of which the main nutrient is Vtg (Yano and Hoshino, 2006; Matozzo et al., 2008). In the process of vitellogenesis, Vtg can provide nutrients such as lipids, protein, vitamins and mineral salts for oocytes to meet the needs for ovarian development. Therefore, the content of Vtg is usually considered as an important indicator for judging the ovarian development of crustacean (Meusy, 1980; Subramoniam, 2011). The results of the present study showed that the addition of 0.4% CHO could significantly promote the transcription level of vtg and vgr and thus increase the content of Vtg in the ovary, while when the amount of CHO reached 1.6%, it no longer had promoting effect, which was consistent with the results of protein expression, and further confirmed that the appropriate amount of CHO could promote the ovarian development of Eriocheir sinensis by promoting the accumulation of VTG. We speculated that there might be two reasons for this result. On the one hand, CHO, as an important component of Vtg, is crucial to the formation of VTG (Okuno et al., 2002). Previous studies have reported that CHO in hemolymph decreases rapidly during vitellogenesis in female ghost crab and Litopenaeus Vannamei, at the same time the content of CHO in the ovary increases significantly, which may be due to the organism transporting CHO to the ovaries to compensate for CHO depletion (Teshima et al., 1986; Vinagre et al., 2007). On the other hand, as a substrate for synthetic E2, CHO may promote Vtg synthesis and transport by affecting the synthesis of endogenous hormones (Kumar et al., 2018). Although there is no report on CHO promoting ovarian development of Eriocheir sinensis at present, the promoting effect of estrogen on vitellogenesis of crustaceans has been demonstrated. Warrier et al. (2001) found a positive correlation between the level of E2 in hemolymph of crustacean and the stage of vitellogenesis. Yano and Hoshino (2006) reported that exogenous E2 could promote ovarian development in Litopenaeus vannamei and Portunus trituberculatus by in vivo injection. Therefore, we believe that the appropriate amount of CHO can promote the synthesis of VTG, then promote the transport of various nutrients to the ovary, and finally achieve the goal of promoting ovarian maturation.
The development and maturity of oocytes require the coordinated regulation of various signal pathways in the cells, in which the activation of MPF is the central link to initiate oocyte maturation (Gavet and Pines, 2010). As a key substance to promote oocyte maturation, MPF mainly exists in mitotic cells and is highly conservative among different species. The dephosphorylation of CDC2 and the recruitment of Cyclin B are two important factors to maintain the activity of MPF (Bodart et al., 2002). CDC2 contains three phosphorylation sites: Thr-14, Tyr-15 and Thr-161. The dephosphorylation of Thr-14 and Thr-15 is the prerequisite for activating MPF. In the cell cycle, CDC2 through phosphorylation and dephosphorylation, combined with Cyclin B to regulate the activity of MPF (Pirino et al., 2009; Feng et al., 2020). In this study, 0.4% CHO treatment group has the highest ovarian maturity,and the protein expression levels of Cyclin B and CDC2 are significantly higher than those in the control group. Previous study on Penaeus monodon has found that estrogen can increase the expression of Cyclin B, promote the dephosphorylation of Thr-14 and Thr-15, and then induce the combination of cyclin B and dephosphorylated CDC2 to transform oocytes from G2 phase to M phase (Loukaci et al., 2001; Pirino et al., 2009). In this experiment, after 0.4% CHO treatment, the level of E2 in the organism was significantly increased. Therefore, we speculate that CHO may promote ovarian maturity by promoting E2 synthesis. Besides MPF, MAPK also plays an irreplaceable role in the regulation of oocyte cycle. MAPK belongs to serine/threonine protein kinase family, ERK1/2 is one of the subtribes that is mainly responsible for the recovery of meiosis (Fan et al., 2009). Previous studies have pointed out that the maturation promoting effect of MAPK is to enhance the activity of MPF and then promote the germinal vesicle breakdown (GVBD), because the activation of MAPK is highly consistent with the synthesis of Cyclin B in time (Frank-Vaillant et al., 2001; Dupré et al., 2011). After MAPK is activated, the translation of Cyclin B increases immediately, MAPK can positively regulate the translation and synthesis of Cyclin B, and stabilize the activity of MPF by inhibiting the degradation of Cyclin B (Frank-Vaillant et al., 2001; Maller et al., 2001). The results of this study show that compared with the other two groups, 0.4% CHO can significantly increase the protein expression of MEK1/2. which is a specific protein kinase. MEK1/2 is a specific protein kinase, which uses MAPK as the only substrate and catalyzes the phosphorylation of threonine and tyrosine, thus ensuring the activation of MAPK. Activated MAPK can enter the nucleus and regulate the expression of cell cycle-related proteins such as Cyclin B and CDC2 (Gotoh and Nishida, 1995; Kishimoto, 2018). Zheng et al. (2020) reported that estrogen could promote the activation of MAPK signal pathway in the ovary of Eriocheir sinensis. Therefore, an appropriate amount of CHO may promote E2 synthesis, then induce the activation of the MAPK signal pathway, and cooperate with MPF to start ovarian maturation (Ohashi et al., 2003; Nagahama and Yamashita, 2008).
As a substrate for the synthesis of E2, CHO must be transported from the storage site to the ovary through hemolymph circulation in the organism (Rodenburg and Van der Horst, 2005). In crustaceans, lipid transport through the hemolymph is accomplished by HDL (Komatsu and Ando, 1998). HDL combines with CHO in peripheral tissues to form high-density lipoprotein CHO (HDL-C), which is transported to the ovary through hemolymph. Previous studies have reported that exogenous chemicals can reduce the content of HDL in hemolymph, then affect the content of CHO transported to the ovary, thus interfere with the synthesis of E2 (Sharpe et al., 2007; Reading et al., 2014). SR-B1 is the first HDL receptor to be identified, and it is an important substance to maintain the homeostasis of CHO metabolism in the organism. When SR-B1 gene is knocked out in mouse, it will lead to the disorder of CHO metabolism, and the female will be infertile (Kolmakova et al., 2010). In this experiment, 0.4% CHO can significantly increase the content of total CHO in the ovary, and when the amount of CHO reached 1.6%, the content of CHO in the ovary shows a downward trend, which may be due to the inhibition of SR-B1 expression by high doses CHO, thereby reducing the endocytosis of CHO (Ji et al., 1997). Due to the synthesis of E2 on the inner mitochondrial membrane, CHO in oocytes needs to be transported to the inner mitochondrial membrane by STAR to start the E2 synthesis pathway, this process which is a speed limit step for E2 synthesis (Jefcoate et al., 1992). STAR is a transporter of CHO, which is mainly involved in the absorption and metabolism of CHO. The damage of STAR will lead to E2 synthesis paths being blocked, thus the level of E2 in the organism will drop sharply (Stocco, 2000). In addition, the expression level of STAR is easily affected, for example, exposing goldfish to 200 µg/g β-sitosterol will significantly reduce the transcription level of STAR, thus reducing the content of CHO in the mitochondrial inner membrane (Sharpe et al., 2007). The results of this experiment also found that an appropriate amount of CHO could promote the transcription of STAR, while high doses of CHO would block the transcription of STAR. In short, an appropriate amount of CHO can promote the expression of proteins such as SR-B1 and STAR to provide sufficient raw materials for the synthesis of E2.
The conversion of CHO to E2 requires the catalysis of a series of enzymes, among which CYP11A1 and CYP19A1, two members of CYP450 family, are key enzymes (Ankley et al., 2012). CYP11A1 through cleaving the side chain of CHO, catalytic generated a precursor of steroid hormones. CYP19A1 is another rate-limiting enzyme in E2 synthesis, which is responsible for the conversion of testosterone to E2 (Hu et al., 2010). Ankley et al. (2012) found that the addition of non-specific inhibitors of CYP450 can significantly reduce the activity of different CYPs in the E2 synthesis pathway, of which CYP11A1 and CYP19A1 are the primary targets. In this experiment, 0.4% CHO can significantly increase the activity of CYP11A1 and CYP19A1, thus increasing the level of estradiol in the organism. The activity regulation of CYP11A1 and CYP19A1 depends on cAMP/PKA signal transduction pathway. When the cAMP/PKA signal pathway is activated, it can regulate the expression of steroidogenic enzymes related to transcription factors such as SF-1 and CREB (Lapointe and Boerboom, 2011; Manna and Stocco, 2011). It has been confirmed that CREB can regulate the activity of CYP19A1 through phosphorylation and dephosphorylation of serine at position 133. SF-1 can activate the expression of all CYPs involved in steroid hormone production, and SF-1 is also involved in regulating CHO transport in steroid-producing cells. Therefore, SF-1 is the key regulatory factor of CYP11A1, CYP19A1, STAR and other substances (Huang et al., 2022). Zheng et al. (2020) reported that the addition of icariin can regulate the expression level of p-CREB and SF-1, thereby affecting estradiol synthesis in Eriocheir sinensis. In this study, the results of in vivo and in vitro experiments showed that after appropriate CHO treatment, the cAMP/PKA signaling pathway was activated, and the protein expression levels of p-CREB and SF-1 were significantly increased. Therefore, we speculate that appropriate CHO can regulate the activity of key enzymes in the process of steroid hormone production through cAMP-PKA-CREB/SF-1 signaling pathway, and then affect the secretion of E2 in the organism.
Conclusion
In conclusion, the results of this study show that 0.4% CHO in the diet can promote the gene and protein expression of VTG in the ovary and hepatopancreas, thus promoting the accumulation of nutrients. On the other hand, an appropriate amount of CHO can induce the activation of the MAPK signal pathway, improve the activity of MPF, promote the completion of meiosis of oocytes, and then promote the ovarian maturation of Eriocheir sinensis. Further studies have found that an appropriate amount of CHO can increase the activity of E2 synthesis-related enzymes such as CYP11A1 and CYP19A1 in vivo and in vitro by increasing the expression of cAMP/PKA pathway related-proteins to increase the level of E2 in the organism, which may be the main reason for the improvement of ovarian maturity of Eriocheir sinensis (Figure 8).
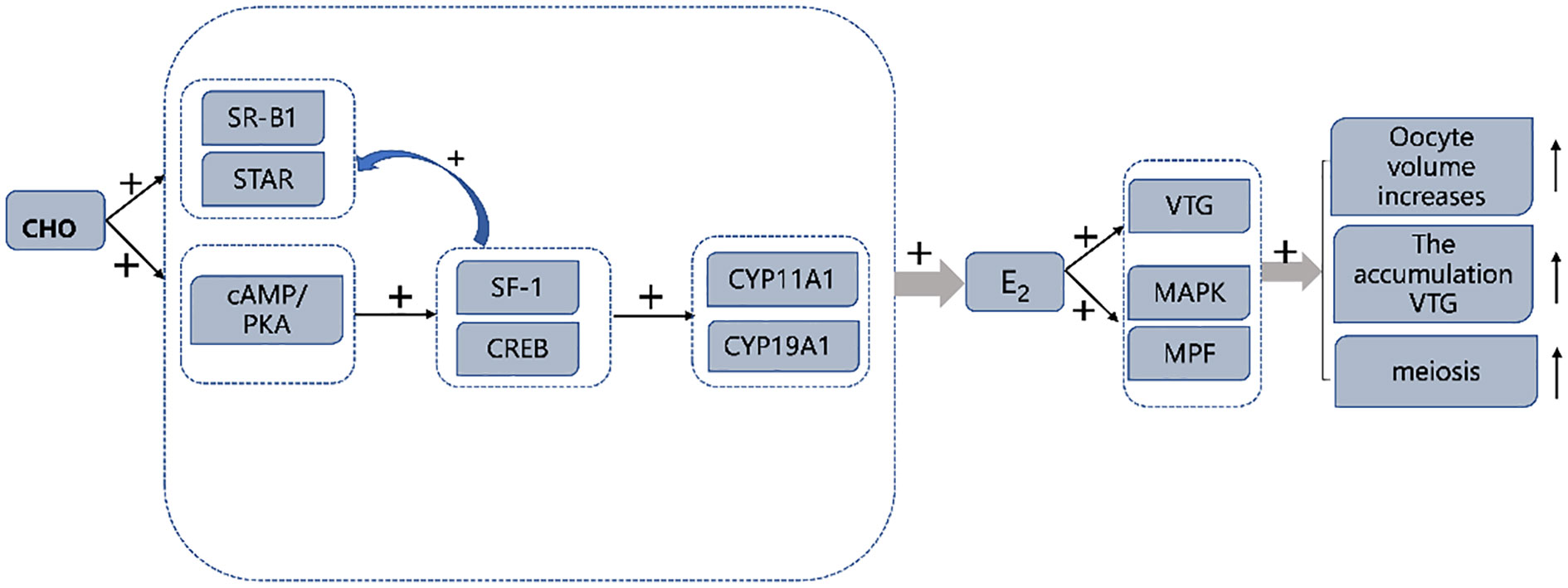
Figure 8 Graphical summary of CHO promotes ovarian maturation in Eriocheir sinensis. “+” symbol: expression was up-regulated by CHO.
Data availability statement
The datasets presented in this study can be found in online repositories. The names of the repository/repositories and accession number(s) can be found below: https://www.ncbi.nlm.nih.gov/, KM244725.1.
Ethics statement
The animal study was reviewed and approved by the Animal Care and Use Committee of Nanjing Agricultural University (Nanjing, China) (permit number: SYXK (Su) 2011–0036).
Author contributions
HG, GJ and WL designed the experiment. HG and MW completed the breeding experiment. XW and KX assisted in data analysis. HG drafted the manuscript. KA, WX, HH and YH polished the manuscript. All authors contributed to the article and approved the submitted version.
Funding
This research was supported by Agriculture Technical System of Jiangsu, China (JATS [2021]474), the Development Plan Project in Key Areas of Guangdong Province (Project No: 2020B0202010001) and Jiangsu Haipurui Feed Co., Ltd.
Conflict of interest
The authors declare that the research was conducted in the absence of any commercial or financial relationships that could be construed as a potential conflict of interest.
Publisher’s note
All claims expressed in this article are solely those of the authors and do not necessarily represent those of their affiliated organizations, or those of the publisher, the editors and the reviewers. Any product that may be evaluated in this article, or claim that may be made by its manufacturer, is not guaranteed or endorsed by the publisher.
References
Ankley G. T., Cavallin J. E., Durhan E. J., Jensen K. M., Kahl M. D., Makynen E. A., et al. (2012). A time-course analysis of effects of the steroidogenesis inhibitor ketoconazole on components of the hypothalamic-pituitary-gonadal axis of fathead minnows. Aquat. Toxicol. 114, 88–95. doi: 10.1016/j.aquatox.2012.02.012
AOAC (1995). Official methods of analysis of the association of official analytical chemists. 16th ed (Arlington, VA, USA: ACOC).
Becker M., Staab D., Von Bergmann K. (1993). Treatment of severe familial hypercholesterolemia in childhood with sitosterol and sitostanol. J. Pediatr. 122, 292–296. doi: 10.1016/S0022-3476(06)80136-8
Bodart J.-F. L., Gutierrez D. V., Nebreda A. R., Buckner B. D., Resau J. R., Duesbery N. S. (2002). Characterization of MPF and MAPK activities during meiotic maturation of xenopus tropicalis oocytes. Dev. Biol. 245, 348–361. doi: 10.1006/dbio.2002.0647
Cao X. F., Dai Y. J., Liu M. Y., Yuan X. Y., Wang C. C., Huang Y. Y., et al. (2019). High-fat diet induces aberrant hepatic lipid secretion in blunt snout bream byactivating endoplasmic reticulum stress-associated IRE1/XBP1 pathway. Biochim. Biophys. Acta (BBA) - Mol. Cell Biol. Lipids 1864, 213–222. doi: 10.1016/j.bbalip.2018.12.005
Carlone D. L., Richards J. S. (1997). Evidence that functional interactions of CREB and SF-1 mediate hormone regulated expression of the aromatase gene in granulosa cells and constitutive expression in R2C cells. J. Steroid Biochem. Mol. Miology 61, 223–231. doi: 10.1016/S0960-0760(97)80016-7
Cau A., Davini M. A., Deiana A. M., Salvadori S. (1988). Gonadal structure and gametogenesis in Scyllarus ardus (L.)(Crustacea, decapoda). Ital. J. Zoology 55, 299–306. doi: 10.1080/11250008809386627
Cheng H. H., Liu W. B., Yuan X. Y., Jia E. T., Zhang D. D., Jiang G. Z. (2020). Effects of dietary cottonseed meal protein hydrolysate on growth, antioxidants and immunity of Chinese mitten crab Eriocheir sinensis. J. Oceanol. Limnol. 38, 869–882. doi: 10.1007/s00343-019-9152-3
Chen L., Jiang H., Zhou Z., Li K., Li K., Deng G. Y., et al. (2004). Purification of vitellin from the ovary of Chinese mitten-handed crab (Eriocheir sinensis) and development of an antivitellin ELISA. Comp. Biochem. Physiol. Part B: Biochem. Mol. Biol. 138, 305–311. doi: 10.1016/j.cbpc.2004.04.012
Chinese Fishery Statistical Yearbook (2021). Fisheries agency of China agriculture ministry Vol. 24 (Beijing: China Agriculture Press).
Djunaidah I. S., Wille M., Kontara E., Sorgeloos P. (2003). Reproductive performance and offspring quality in mud crab (Scylla paramamosain) broodstock fed different diets. Aquacult. Int. 11, 3–15. doi: 10.1023/A:1024188507215
Du N., Lai W., Nan C. (1995). The morphology and ultrastructure of the mature egg of Eriocheir sinensis (Crustacea, decapoda). Acta Zool. Sin. 41 (26), 229–234. https://europepmc.org/article/CBA/282067
Dupré A., Haccard O., Jessus C. (2011). Mos in the oocyte: how to use MAPK independently of growth factors and transcription to control meiotic divisions. J. Signal transduction 2011, 350412. doi: 10.1155/2011/350412
Fairs N., Quinlan P., Goad L. (1990). Changes in ovarian unconjugated and conjugated steroid titers during vitellogenesis in Penaeus monodon. Aquaculture 89, 83–99. doi: 10.1016/0044-8486(90)90235-F
Fang L., Huang Z., Fan L., Hu G., Qiu L., Song C., et al. (2021). Health risks associated with sulfonamide and quinolone residues in cultured Chinese mitten crab (Eriocheir sinensis) in China. Mar. pollut. Bull. 165, 112184. doi: 10.1016/j.marpolbul.2021.112184
Fan H. Y., Liu Z., Shimada M., Sterneck E., Johnson P. F., Hedrick S. M., et al. (2009). MAPK3/1 (ERK1/2) in ovarian granulosa cells are essential for female fertility. Science 324, 938–941. doi: 10.1126/science.1171396
Feng H., Dong Y. T., Liu X., Qiu G. F. (2020). Cyclin b protein undergoes increased expression and nuclear relocation during oocyte meiotic maturation of the freshwater prawn Macrobrachium rosenbergii and the Chinese mitten crab Eriocheir sinensis. Gene 758, 144955. doi: 10.1016/j.gene.2020.144955
Frank-Vaillant M., Haccard O., Ozon R., Jessus C. (2001). Interplay between Cdc2 kinase and the c-Mos/MAPK pathway between metaphase I and metaphase II in xenopus oocytes. Dev. Biol. 231, 279–288. doi: 10.1006/dbio.2000.0142
Gavet O., Pines J. (2010). Progressive activation of CyclinB1-Cdk1 coordinates entry to mitosis. Dev. Cell 18 (4), 533–543. doi: 10.1016/j.devcel.2010.02.013
Gotoh Y., Nishida E. (1995). Activation mechanism and function of the MAP kinase cascade. Mol. Reprod. Dev. 42, 486–492. doi: 10.1002/mrd.1080420417
Guo H. X., Jiang G. Z., Dai Y. J., Huang Y. Y., Wang X., Xiao K., et al. (2022). Effect of dietary cholesterol on growth performance, cholesterol deposition, and lipid metabolism in adult Chinese mitten crab (Eriocheir sinensis). Aquacult. Nutr. 2022, 2012958. doi: 10.1155/2022/2012958
Huang H., Ye H., Han S., Wang G. (2009). Profiles of gonadotropins and steroid hormone-like substances in the hemolymph of mud crab Scylla paramamosain during the reproduction cycle. Mar. Freshw. Behav. Physiol. 42, 297–305. doi: 10.1080/10236240903174792
Huang Y., Zhang W., Cui N., Xiao Z., Zhao W., Wang R., et al. (2022). Fluorene-9-bisphenol regulates steroidogenic hormone synthesis in H295R cells through the AC/cAMP/PKA signaling pathway. Ecotoxicol. Environ. Saf. 243, 113982. doi: 10.1016/j.ecoenv.2022.113982
Hu J., Zhang Z., Shen W.-J., Azhar S. (2010). Cellular cholesterol delivery, intracellular processing and utilization for biosynthesis of steroid hormones. Nutr. Metab. 7, 1–25. doi: 10.1186/1743-7075-7-47
Janer G., Porte C. (2007). Sex steroids and potential mechanisms of non-genomic endocrine disruption in invertebrates. Ecotoxicology 16, 145–160. doi: 10.1007/s10646-006-0110-4
Jefcoate C. R., McNamara B. C., Artemenko I., Yamazaki T. (1992). Regulation of cholesterol movement to mitochondrial cytochrome P450scc in steroid hormone synthesis. J. Steroid Biochem. Mol. Biol. 43, 751–767. doi: 10.1016/0960-0760(92)90305-3
Jiang X., Pan K., Yang Y., Shu-Chien A. C., Wu X. (2022). Dietary DHA oil supplementation promotes ovarian development and astaxanthin deposition during the ovarian maturation of Chinese mitten crab Eriocheir sinensis. Aquacult. Nutr. 2022, 9997317. doi: 10.1155/2022/9997317
Ji Y., Jian B., Wang N., Sun Y., de la Llera Moya M., Phillips M. C., et al. (1997). Scavenger receptor BI promotes high density lipoprotein-mediated cellular cholesterol efflux. J. Biol. Chem. 272, 20982–20985. doi: 10.1074/jbc.272.34.20982
Kishimoto T. (2018). MPF-based meiotic cell cycle control: Half a century of lessons from starfish oocytes. Proc. Japan Academy Ser. B 94, 180–203. doi: 10.2183/pjab.94.013
Kolmakova A., Wang J., Brogan R., Chaffin C., Rodriguez A. (2010). Deficiency of scavenger receptor class b type I negatively affects progesterone secretion in human granulosa cells. Endocrinology 151, 5519–5527. doi: 10.1210/en.2010-0347
Komatsu M., Ando S. (1998). A very-high-density lipoprotein with clotting ability from hemolymph of sand crayfish, Ibacus ciliatus. Biosci. Biotech. Biochem. 62, 459–463. doi: 10.1271/bbb.62.459
Kumar V., Sinha A. K., Romano N., Allen K. M., Bowman B. A., Thompson K. R., et al. (2018). Metabolism and nutritive role of cholesterol in the growth, gonadal development, and reproduction of crustaceans. Rev. Fish. Sci. Aquacult. 26, 254–273. doi: 10.1080/23308249.2018.1429384
Lanot R., Cledon P. (1989). Ecdysteroids and meiotic reinitiation in Palaemon serratus (Crustacea decapoda natantia) and in Locusta migratoria (Insecta orthoptera). A comparative study. Invertebrate Reprod. Dev. 16, 169–175. doi: 10.1080/07924259.1989.9672074
Lapointe E., Boerboom D. (2011). WNT signaling and the regulation of ovarian steroidogenesis. Front. Bioscience-Scholar 3, 276–285.
Lee F. Y., Chang C. F. (1997). The concentrations of vitellogenin (vitellin) and protein in hemolymph, ovary and hepatopancreas in different ovarian stages of the freshwater prawn, Macrobrachium rosenbergii. Comp. Biochem. Physiol. Part A: Physiol. 117, 433–439. doi: 10.1016/S0300-9629(96)00260-5
Lin Z. D., Han F. L., Lu J. T., Guo J. L., Qi C. L., Wang C. L., et al. (2020). Influence of dietary phospholipidon growth performance, body composition, antioxidant capacity and lipid metabolism of Chinese mitten crab, Eriocheir sinensis. Aquaculture 516, 734653. doi: 10.1016/j.aquaculture.2019.734653
Livak K. J., Schmittgen T. D. (2001). Analysis of relative gene expression data using real-time quantitative PCR and the 2– ΔΔCT method. Methods 25, 402–408. doi: 10.1006/meth.2001.1262
Long X., Guo Q., Wang X., Francis D. S., Cheng Y., Wu X. (2020). Effects of fattening period on ovarian development and nutritional quality of adult female Chinese mitten crab Eriocheir sinensis. Aquaculture 519, 734748. doi: 10.1016/j.aquaculture.2019.734748
Loukaci A., Le Saout I., Samadi M., Leclerc S., Damiens E., Meijer L., et al. (2001). Coscinosulfate, a CDC25 phosphatase inhibitor from the sponge coscinoderma mathewsi. Bioorganic Medicinal Chem. 9, 3049–3054. doi: 10.1016/S0968-0896(01)00208-5
Lu Y., Liu M. M., Gong J., Cheng Y. X., Wu X. G. (2018). Effect of exogenous estrogen on the ovarian development and gene expression in the female swimming crab Portunus trituberculatus (Miers 1876) (Decapoda: Brachyura: Portunidae). J. Crustacean Biol. 38, 367–373. doi: 10.1093/jcbiol/ruy013
Malati E. F., Heidari B., Zamani M. (2013). The variations of vertebrate-type steroid hormones in the freshwater narrow-clawed crayfish Astacus leptodactylus (Eschscholtz 1823)(Decapoda, astacidae) during oocyte development. Crustaceana 86, 129–138. doi: 10.1163/15685403-00003141
Maller J. L., Gross S. D., Schwab M. S., Finkielstein C. V., Taieb F. E., Qian Y. W. (2001). Cell cycle transitions in early Xenopus development. Cell Cycle Development: Novartis Foundation Symposium 237, 58–78. doi: 10.1002/0470846666.ch6
Manna P. R., Stocco D. M. (2011). The role of specific mitogen-activated protein kinase signaling cascades in the regulation of steroidogenesis. J. Signal transduction 2011, 821615. doi: 10.1155/2011/821615
Martins J., Ribeiro K., Rangel-Figueiredo T., Coimbra J. (2007). Reproductive cycle, ovarian development, and vertebrate-type steroids profile in the freshwater prawn Macrobrachium rosenbergii. J. Crustacean Biol. 27, 220–228. doi: 10.1651/C-2597.1
Matozzo V., Gagné F., Marin M. G., Ricciardi F., Blaise C. (2008). Vitellogenin as a biomarker of exposure to estrogenic compounds in aquatic invertebrates: A review. Environ. Int. 34, 531–545. doi: 10.1016/j.envint.2007.09.008
Meusy J. (1980). Vitellogenin, the extraovarian precursor of the protein yolk in Crustacea: A review. Reprod. Nutr. Développement 20, 1–21. doi: 10.1051/rnd:19800101
Miller W. L. (2007). Steroidogenic acute regulatory protein (StAR), a novel mitochondrial cholesterol transporter. Biochim. Biophys. Acta (BBA)-Molecular Cell Biol. Lipids 1771, 663–676. doi: 10.1016/j.bbalip.2007.02.012
Nagahama Y., Yamashita M. (2008). Regulation of oocyte maturation in fish. Development Growth Differentiation 50, S195–S219. doi: 10.1111/j.1440-169X.2008.01019.x
Niu J., Chen P. F., Tian L. X., Liu Y. J., Lin H. Z., Yang H. J., et al. (2012). Excess dietary cholesterol may have an adverse effect on growth performance of early post-larval Litopenaeus vannamei. J. Anim. Sci. Biotechnol. 3, 1–5. doi: 10.1186/2049-1891-3-19
Ohashi S., Naito K., Sugiura K., Iwamori N., Goto S., Naruoka H., et al. (2003). Analyses of mitogen-activated protein kinase function in the maturation of porcine oocytes. Biol. Reprod. 68, 604–609. doi: 10.1095/biolreprod.102.008334
Okuno A., Yang W. J., Jayasankar V., Saido-Sakanaka H., Huong D. T. T., Jasmani S., et al. (2002). Deduced primary structure of vitellogenin in the giant freshwater prawn, Macrobrachium rosenbergii, and yolk processing during ovarian maturation. J. Exp. Zoology 292, 417–429. doi: 10.1002/jez.10083
Pan J., Liu M., Chen T., Cheng Y., Wu X. (2018). Immunolocalization and changes of 17beta-estradiol during ovarian development of Chinese mitten crab Eriocheir sinensis. Cell Tissue Res. 373, 509–520. doi: 10.1007/s00441-018-2834-x
Pirino G., Wescott M. P., Donovan P. J. (2009). Protein kinase a regulates resumption of meiosis by phosphorylation of Cdc25B in mammalian oocytes. Cell Cycle 8, 665–670. doi: 10.4161/cc.8.4.7846
Qu J. H., Hong X., Chen J. F., Wang Y. B., Sun H., Xu X. L., et al. (2008). Fenvalerate inhibits progesterone production through cAMP-dependent signal pathway. Toxicol. Lett. 176, 31–39. doi: 10.1016/j.toxlet.2007.09.004
Rani K., Subramoniam T. (1997). Vitellogenesis in the mud crab Scylla serrata–an in vivo isotope study. J. Crustacean Biol. 17, 659–665. doi: 10.2307/1549368
Reading B. J., Hiramatsu N., Schilling J., Molloy K. T., Glassbrook N., Mizuta H., et al. (2014). Lrp13 is a novel vertebrate lipoprotein receptor that binds vitellogenins in teleost fishes. J. Lipid Res. 55, 2287–2295. doi: 10.1194/jlr.M050286
Rodenburg K. W., Van der Horst D. J. (2005). Lipoprotein-mediated lipid transport in insects: analogy to the mammalian lipid carrier system and novel concepts for the functioning of LDL receptor family members. Biochim. Biophys. Acta (BBA)-Molecular Cell Biol. Lipids 1736, 10–29. doi: 10.1016/j.bbalip.2005.07.002
Sharpe R. L., Woodhouse A., Moon T. W., Trudeau V. L., MacLatchy D. L. (2007). β-sitosterol and 17β-estradiol alter gonadal steroidogenic acute regulatory protein (StAR) expression in goldfish, Carassius auratus. Gen. Comp. Endocrinol. 151, 34–41. doi: 10.1016/j.ygcen.2006.11.005
Sheen S.-S., Liu P.-C., Chen S.-N., Chen J.-C. (1994). Cholesterol requirement of juvenile tiger shrimp (Penaeus monodon). Aquaculture 125, 131–137. doi: 10.1016/0044-8486(94)90289-5
Song Y. N., Shi L. L., Liu Z. Q., Qiu G. F. (2014). Global analysis of the ovarian microRNA transcriptome: implication for miR-2 and miR-133 regulation of oocyte meiosis in the Chinese mitten crab, Eriocheir sinensis (Crustacea: Decapoda). BMC Genomics 15, 1–10. doi: 10.1186/1471-2164-15-547
Soroka Y., Milner Y., Sagi A. (2000). The hepatopancreas as a site of yolk protein synthesis in the prawn Macrobrachium rosenbergii. Invertebrate Reprod. Dev. 37, 61–68. doi: 10.1080/07924259.2000.9652400
Stocco D. (2000). The role of the StAR protein in steroidogenesis: challenges for the future. J. Endocrinol. 164, 247–253. doi: 10.1677/joe.0.1640247
Stocco D. M. (2001). StAR protein and the regulation of steroid hormone biosynthesis. Annu. Rev. Physiol. 63, 193–213. doi: 10.1146/annurev.physiol.63.1.193
Subramoniam T. (2011). Mechanisms and control of vitellogenesis in crustaceans. Fish. Sci. 77, 1–21. doi: 10.1007/s12562-010-0301-z
Su C., Li J., Lu Y., Wang Y., Ding Y., Pan L., et al. (2022). Interactive effects of dietary cholesterol and bile acids on the growth, lipid metabolism, immune response and intestinal microbiota of litopenaeus vannamei: Sparing effect of bile acids on cholesterol in shrimp diets. Aquaculture 547, 737412. doi: 10.1016/j.aquaculture.2021.737412
Summavielle T., Monteiro P. R. R., Reis-Henriques M. A., Coimbra J. (2003). In vitro metabolism of steroid hormones by ovary and hepatopancreas of the crustacean penaeid shrimp Marsupenaeus japonicus. Scientia Marina 67, 299–306. doi: 10.3989/scimar.2003.67n3299
Suprayudi M. A., Takeuchi T., Hamasaki K. (2012). Cholesterol effect on survival and development of larval mud crab Scylla serrata. Hayati J. Biosci. 19, 1–5. doi: 10.4308/hjb.19.1.1
Tao X., Wang C., Wei H., Ren Z., Ma X., Lu W. (2014). Effects of dietary cholesterol levels on moulting performance, lipid accumulation, ecdysteroid concentration and immune enzymes activities of juvenile Chinese mitten crab Eriocheir sinensis. Aquacult. Nutr. 20, 467–476. doi: 10.1111/anu.12097
Teshima S., Ishikawa M., Koshio S., Kanazawa A. (1997). Assessment of cholesterol requirements in the prawn, Penaeus japonicus. Aquacult. Nutr. 3, 247–253. doi: 10.1046/j.1365-2095.1997.00097.x
Teshima S.-I., Kanazawa A. (1971). Biosynthesis of sterols in the lobster, panulirus japonica, the prawn, Penaeus japonicus, and the crab, Portunus trituberculatus. Comp. Biochem. Physiol. Part B: Comp. Biochem. 38, 597–602. doi: 10.1016/0305-0491(71)90314-2
Teshima S. I., Kanazawa A., Kakuta Y. (1986). Effects of dietary phospholipids on growth and body composition of the juvenile prawn. bull. Japanese Soc. Fish. Sci. 52, 155–158. doi: 10.2331/suisan.52.155
Teshima S.-I., Kanazawa A., Sasada H. (1983). Nutritional value of dietary cholesterol and other sterols to larval prawn, Penaeus japonicus bate. Aquaculture 31, 159–167. doi: 10.1016/0044-8486(83)90310-1
Tian H., Yang C., Yu Y., Yang W., Lu N., Wang H., et al. (2020). Dietary cholesterol level affects growth, molting performance and ecdysteroid signal transduction in Procambarus clarkii. Aquaculture 523, 735198. doi: 10.1016/j.aquaculture.2020.735198
Vinagre A. S., do Amaral A. P. N., Ribarcki F. P., da Silveira E. F., Périco E. (2007). Seasonal variation of energy metabolism in ghost crab Ocypode quadrata at siriú beach (Brazil). Comp. Biochem. Physiol. Part A: Mol. Integr. Physiol. 146, 514–519. doi: 10.1016/j.cbpa.2006.02.004
Wang S., He Y., Wang Y., Tao N., Wu X., Wang X., et al. (2016). Comparison of flavour qualities of three sourced Eriocheir sinensis. Food Chem. 200, 24–31. doi: 10.1016/j.foodchem.2015.12.093
Wang C. C., Liu W. B., Huang Y. Y., Wang X., Li X. F., Zhang D. D., et al. (2020). Dietary DHA affects muscle fiber development by activating AMPK/Sirt1 pathway in blunt snout bream (Megalobrama amblycephala). Aquaculture 518, 734835. doi: 10.1016/j.aquaculture.2019.734835
Wang L. M., Zuo D., Lv W. W., Wang D. L., Liu A. J., Zhao Y. (2013). Characterization of Cdc2 kinase in the red claw crayfish (Cherax quadricarinatus): Evidence for its role in regulating oogenesis. Gene 515, 258–265. doi: 10.1016/j.gene.2012.11.082
Warrier S. R., Tirumalai R., Subramoniam T. (2001). Occurrence of vertebrate steroids, estradiol 17β and progesterone in the reproducing females of the mud crab Scylla serrata. Comp. Biochem. Physiol. Part A: Mol. Integr. Physiol. 130, 283–294. doi: 10.1016/S1095-6433(01)00385-3
Wouters R., Lavens P., Nieto J., Sorgeloos P. (2001). Penaeid shrimp broodstock nutrition: an updated review on research and development. Aquaculture 202, 1–21. doi: 10.1016/S0044-8486(01)00570-1
Wu R., Lam K., MacKay D., Lau T., Yam V. (1994). Impact of marine fish farming on water quality and bottom sediment: a case study in the sub-tropical environment. Mar. Environ. Res. 38, 115–145. doi: 10.1016/0141-1136(94)90004-3
Wu X., Liu M., Pan J., Chen H., Zeng C., Cheng Y. (2017). The ovarian development pattern of pond-reared Chinese mitten crab, Eriocheir sinensis h. Milne-Edward. Crustaceana 90, 449–470. doi: 10.1163/15685403-00003662
Wu X., Yao G., Yang X., Cheng Y., Wang C. (2007). A study on the ovarian development of Portunus trituberculatus in East China Sea during the first reproductive cycle. Acta Oceanologica Sin. 29, 120–127.
Xue L., Du N., Lai W. (1987). Histology of female reproductive system in Chinese mitten-handed crab, Eriocheir sinensis (Crustacea, decapoda). J. East China Univ. Sci. Technology(Natural Sci. Edition) 3, 88–97.
Yano I., Hoshino R. (2006). Effects of 17 β-estradiol on the vitellogenin synthesis and oocyte development in the ovary of kuruma prawn (Marsupenaeus japonicus). Comp. Biochem. Physiol. Part A: Mol. Integr. Physiol. 144, 18–23. doi: 10.1016/j.cbpa.2006.01.026
Zheng X., Liu W., Liu J., Zhang C., Zhang L., Gao F., et al. (2020). Dietary supplementation with icariin affects estrogen synthesis, vitellogenesis, and oocyte development in the Chinese mitten crab, Eriocheir sinensis. Front. Mar. Sci. 7, 161. doi: 10.3389/fmars.2020.00161
Zheng X., Zhang L., Jiang W., Abasubong K. P., Zhang C., Zhang D., et al. (2021). Effects of dietary icariin supplementation on the ovary development-related transcriptome of Chinese mitten crab (Eriocheir sinensis). Comp. Biochem. Physiol. Part D: Genomics Proteomics 37, 100756. doi: 10.1016/j.cbd.2020.100756
Keywords: Eriocheir sinensis, cholesterol, ovarian development, estradiol synthesis, vitellogenesis
Citation: Guo H, Wang M, Wang X, Xiao K, Huang Y, Hua H, Xiong W, Liu W, Abasubong KP, Qiang W, Chi C and Jiang G (2022) Effect of dietary cholesterol on ovarian development of Chinese mitten crabs (Eriocheir sinensis). Front. Mar. Sci. 9:1070829. doi: 10.3389/fmars.2022.1070829
Received: 15 October 2022; Accepted: 18 November 2022;
Published: 07 December 2022.
Edited by:
Zhendong Qin, Zhongkai University of Agriculture and Engineering, ChinaReviewed by:
Xinhua Chen, Fujian Agriculture and Forestry University, ChinaYao Zheng, Freshwater Fisheries Research Center (CAFS), China
Copyright © 2022 Guo, Wang, Wang, Xiao, Huang, Hua, Xiong, Liu, Abasubong, Qiang, Chi and Jiang. This is an open-access article distributed under the terms of the Creative Commons Attribution License (CC BY). The use, distribution or reproduction in other forums is permitted, provided the original author(s) and the copyright owner(s) are credited and that the original publication in this journal is cited, in accordance with accepted academic practice. No use, distribution or reproduction is permitted which does not comply with these terms.
*Correspondence: Guangzhen Jiang, jianggz@njau.edu.cn