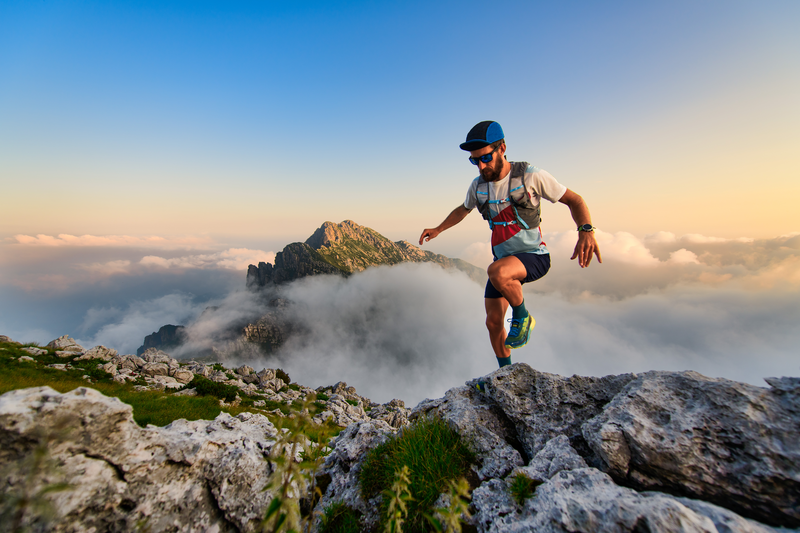
94% of researchers rate our articles as excellent or good
Learn more about the work of our research integrity team to safeguard the quality of each article we publish.
Find out more
ORIGINAL RESEARCH article
Front. Mar. Sci. , 16 March 2023
Sec. Marine Fisheries, Aquaculture and Living Resources
Volume 10 - 2023 | https://doi.org/10.3389/fmars.2023.1104870
This article is part of the Research Topic Beneficial Effects of Functional Ingredients in Feed on Immunity Improvement and Growth Promotion of Aquaculture Animals View all 11 articles
This study evaluated the growth performance and in vivo antioxidant capacity of the white shrimp, Litopenaeus vannamei, fed diets containing the grape extract Nor-grape 80. A control diet containing 1000 ppm vitamin E without added grape extract and four other diets containing 250, 500, 750 and 1000 ppm grape extract were formulated. Shrimp, twenty 0.02 g of individuals in each aquarium, were randomly assigned to one of the above five treatment diets in triplicate and fed three times daily to satiation for six weeks. The final weight, weight gain percentage and specific growth rate of the shrimp larvae fed diets containing 250 and 500 ppm Nor-grape 80 were significantly higher than those fed the other treatment diets. The levels of superoxide dismutase activity of the shrimp fed diets containing 250 and 500 ppm Nor-grape 80 were significantly higher than those of shrimp fed the control diet. On the other hand, the thiobarbituric acid-reactive substance levels of the shrimp fed diets containing 250 and 500 ppm of Nor-grape 80 were significantly lower than those fed the other diets. Furthermore, the highest levels of dietary Nor-grape 80, namely 750 and 1000 ppm, gave rise to reduced growth performance, as well as lower levels of in vivo antioxidant activity. Therefore, for the best growth performance and highest level of in vivo antioxidant activity, it is suggested that the optimal level of Nor-grape 80 as part of a white shrimp diet falls between 250 and 500 ppm.
The Pacific white shrimp Litopenaeus vannamei, a native species that is distributed along the East Pacific coasts of Mexico, Central America and Peru, has now become the most popular farmed penaeid shrimp species throughout the world (Dugassa and Gaetan, 2018; Lucas et al., 2019). In 2020, the global aquaculture production of white shrimp was more than 5,400,000 tonnes and this represents approximately 80% of the total aquaculture production of crustaceans (FAO, 2022). This shrimp shows rapid growth, good survival under high density culture, and a high tolerance to a broad range of salinities and temperatures, and therefore it is highly suitable for intensive culture systems. The shrimp has widely been employed commercially in Southeast Asia, including in India, Thailand, Vietnam, the People’s Republic of China and Taiwan (Briggs et al., 2004). Generally, the white shrimp require five to seven months of cultivation to reach a marketable size of 15 to 30 grams (Tacon et al., 2013). As part of feeding management practices, shrimp aquafeed includes costly fish meal that provides an excellent protein source within the formula; therefore, the cost of feeding these shrimp can reach as much as 40% to 60% of the total production costs when under semi-intensive and intensive systems (Hardy and Tacon, 2002).
In order to reduce dietary costs, several plant and animal protein sources have been successfully used as fish meal alternatives without compromising shrimp production (Davis and Arnold, 2000; Cummins et al., 2017). For white shrimp, it is known that the optimal dietary protein content ranges from 32% to 36% across the various growth stages (Lee and Lee, 2018). However, the effect of shrimp diets supplemented with phytochemicals, which are the bioactive components derived from plants and plant-based beverages, such as fruit, vegetables, grains, nuts, seeds, tea and wine (Chakraborty et al., 2013; Encarnação, 2016), has not yet been totally explored. Many of these phytogenics, which are classified as alkaloids, flavonoids, terpenoids, polyphenols, essential oils and pigments, have been exploited as feed additives in order to improve the growth performance of fish (Chakraborty et al., 2013; Encarnação, 2016; Artés-Hernández et al., 2021).
Grapes, rich in polyphenols, are characterized as having antioxidant, antimicrobial, anti-inflammatory and anti-carcinogenic properties (Shi et al., 2003; Xia et al., 2010; Doshi et al., 2013). The most abundant phenolic components of grapes are present in their seeds, which contain 74% to 78% proanthocyanidin oligomers and 6% free flavanols (Shi et al., 2003; Burdock, 2005), followed by grape skins and grape pulp (Shi et al., 2003). It is worth noting that grape polyphenols have been recognized as being able to improve nutrient absorption and biological growth of terrestrial animals (Viveros et al., 2011; Aditya et al., 2018). Broiler chickens fed diets supplemented with grape seeds, grape pomace and pomace extract containing polyphenols have been shown to have enhanced growth performance. These findings have been attributed to improved gut morphology and a better intestinal microbiota (Viveros et al., 2011; Abu Hafsa and Ibrahim, 2018; Aditya et al., 2018). Grape seed proanthocyanidin extract has also been reported to elevate intestinal release of both glucagon-like peptide-1 and peptide YY, two hormones that regulate appetite and glucose homeostasis (Casanova-Marti et al., 2020). With aquatic animals, grape seeds and pomace extracts have served as dietary additives to improve the growth of tilapia (Oreochromis niloticus) and of Pacific white shrimp (Zhai et al., 2014; Niyamosatha et al., 2015).
Grape seeds and pomace would seem to act as powerful antioxidants that scavenge the free radicals causing oxidative stress in cells, both in vitro and in vivo (Bagchi et al., 1998; Bouhamidi et al., 1998; Bagchi et al., 2000; Jayaprakasha et al., 2003; Shi et al., 2003). The antioxidant potential of grape-seed procyanidins, in particular, is twenty fold more than that of vitamin E (Uchida, 1980; Shi et al., 2003). In several terrestrial animals, diets including either grape seeds or grape pomace extract have been found to result in improved antioxidant capacity, namely an increase in superoxide dismutase (SOD) activity and a decrease in the level of thiobarbituric acid-reactive substances (TBARS) or malodialdehyde (MDA) (Garcia et al., 2002; Park et al., 2014; Guerra-Rivas et al., 2016; Zhao et al., 2017; Aditya et al., 2018). In addition to enhanced SOD activity, reduced levels of TBARS or MDA, reactive compounds, which are the result of lipid peroxidation of polyunsaturated fatty acids, has been observed in broilers fed diets containing increasing amounts of grape seeds and grape pomace (Abu Hafsa and Ibrahim, 2018; Aditya et al., 2018). Surprisingly, penned rams fed diets containing wine grape extract have even shown significantly higher levels of SOD activity, as well as a lower level of MDA, in their testes when compared to those fed diets without wine grape extract (Zhao et al., 2017).
For most animals, although dietary grape extract has been known to improve their growth performance and in vivo antioxidant capacity (Viveros et al., 2011; Chamorro et al., 2013; Park et al., 2014; Zhai et al., 2014; Niyamosatha et al., 2015; Zhao et al., 2017; Abu Hafsa and Ibrahim, 2018; Aditya et al., 2018), these also seems to be adverse effects on the growth performance, related to protein and amino acid digestibility, if the dietary grape products are provided at a level higher than their optimal requirements (Chamorro et al., 2013; Zhai et al., 2014; Abu Hafsa and Ibrahim, 2018). Broilers fed the highest dietary levels of grape seeds or seed extracts (Chamorro et al., 2013; Abu Hafsa and Ibrahim, 2018), as well as tilapia (O. niloticus) fed a diet containing the highest level of grape extract (Zhai et al., 2014), showed reduced growth performance. Previously, white shrimp fed diets containing 150 and 200 ppm Nor-grape 80, a commercial grape extract that consists of 80% polyphenols, were found to have a significantly higher weight gain percentage than those fed diets containing less than 150 ppm of Nor-grape 80 (Cheng et al., 2017). As the amount of Nor-grape 80 in the shrimp diet was increased to the highest level of 200 ppm, an increase in SOD and a decrease in TBARS levels were correspondingly detected (Cheng et al., 2017). However, the optimal dietary concentration of Nor-grape 80 for white shrimp, in terms of the growth performance and antioxidant capacity, has not yet been determined. The aim of the present study was to examine a suitable level of this grape extract that will give the best growth performance for white shrimp. In vivo antioxidant activity, specifically SOD activity and TBARS levels, of the white shrimp were also evaluated when the shrimp were fed diets supplemented with Nor-grape 80 at a level greater than 200 ppm.
Five isonitrogenous (35% crude protein), isolipidic (9% crude lipid) and isoenergetic (300 Kcal/100 g) diets were formulated (Table 1). The control diet contained 1000 ppm vitamin E, while the four other diets contained 250 (N250), 500 (N500), 750 (N750) and 1000 ppm (N1000) of Nor-grape 80, an extract of whole Vitis vinefera grapes that was provided by Kaye Bio-tech Co., Taiwan. Nor-grape 80 has been shown to comprise 80% polyphenols of which 60% are proanthocyanidins and 0.75% are anthocyanins. Fish meal was used as the protein source. A mixture of 2:1 fish oil and corn oil (w/w) was used as the source of lipid. Wheat flour and corn starch were used as sources of carbohydrate and also acted as binders. Cellulose was also included to balance out the composition of the diet. On preparing the experimental diets, all dietary ingredients were first ground into small particles using a hammer mill and then passed through a 250 μm mesh sieve. The dry ingredients were then thoroughly mechanically mixed to insure homogeneity before the addition of the oil. The mixture, after an addition of distilled water, was blended until a consistent dough was formed. Each of the diets was extruded through a chopper (3.0 mm die diameter) to produce pellets. The pellets were then ground into small particles (<500 μm) and dried in an air oven at 60 0C for 12 hours and finally the five experimental diets were stored separately at 4 0C in a refrigerator until use.
The post larvae shrimp of 20 days after hatch were obtained from a local aquafarm (Pintung, Taiwan), where the water temperature and salinity ranged from 28°C to 30°C and 30‰ to 33‰, respectively, and then acclimatized in a 2000 L fiberglass tank at National Taiwan Ocean University for one week. During the acclimatization phase, the shrimp were cultured under 26-28 °C and fed the control diet. At the beginning of the feeding trial, the shrimp, which had initial weights of 0.02 g, were starved for 24 h before they were randomly distributed into the 15 glass aquaria (57 x 35 x 30 cm), each of which finally contained twenty shrimp. Three replicate groups of shrimp were fed one of the five treatment diets three times a day. They were fed 30% of their body weight daily until satiation for six weeks. The experiment aquaria were closed systems with continuous aeration. Half of the water in each tank was renewed daily to maintain water quality. The water temperature, salinity, dissolved oxygen and pH ranged between 25 and 28°C, 31‰ and 33‰, 5.6 mg/L and 7.0 mg/L and 8.1 and 8.5, respectively. The concentration of total ammonia-nitrogen was maintained at less than 0.05 ppm during the entire feeding trial period. Uneaten feed and feces were siphoned off from each tank every day. At the end of the feeding trial, feeding was stopped for 24 h prior to weighing. Each shrimp was individually weighed, sacrificed by placing it in ice water and then carefully dissected. The muscle tissue of individual shrimp that had been cultivated in the same aquarium was pooled, dried and homogenized (n=3) in order to carry out the proximate analysis twice. In parallel, the hepatopancreas of the shrimp from each aquarium was isolated and pooled to measure SOD activity and the level of TBARS (n=3).
The growth parameters of the shrimp were calculated according to the following equations:
, and , where W0 is the initial mean body weight (g), Wt is the final mean body weight (g).
The compositions of the experimental diets and the shrimp muscle samples were analyzed according to the method of AOAC (1984). Crude protein was determined using the Kjeldahl system (Kjeldahl system 1002, Tecator, Sweden) after acid digestion. Crude lipid was measured by the chloroform and methanol (2:1, v/v) extraction method (Folch et al., 1957). Crude fiber was determined by acid and alkaline digestion using the Fibertec M 1020 system (Foss Tecator, Sweden). Moisture and ash were determined by conventional methods using an oven at 105 0C and a muffle furnace at 540 0C, respectively. Nitrogen-free extract (NFE) was calculated as follows: NFE= [100 – (crude protein + crude lipid + crude fiber + ash)] %. A proximate analysis of the experimental diets is presented in Table 2. The crude protein, crude lipid, ash, crude fiber and NFE of the treatment diets ranged from 37.85% to 38.03%, 8.86% to 9.03%, 14.11% to 14.23%, 11.90% to 12.29% and 26.59% to 27.77%, respectively.
The SOD activity of each pooled hepatopancreas sample was measured using SOD assay kits (19160 SOD determination kit, Sigma, USA). In brief, a total of 0.5 g of hepatopancreas tissue was homogenized in 0.1 M phosphate buffer using a T25 homogenizer (IKA, Germany). The homogenate was then centrifuged at 3000 rpm for 10 min and the supernatant was collected. From this point onwards, the procedure described in the instruction manual of the SOD assay kit was followed. The working solution in the kit, namely, WST-1 (2- (4-iodopheny) - 3- (4-nitophenyl) -5- (2, 4-disulfophenyl) -2 H tetrazolium), reacts with the superoxide radicals generated in the presence of oxygen and the enzyme, xanthine oxidase. The superoxide radicals would be reduced and this results in the formation of a yellowish water-soluble formazan dye. However, the superoxide anions are also reduced by SOD and such reaction will decrease the amount of formazan formed. Thus, after the optical density has been measured at 450 nm using a spectrophotometer (Synergy HT, Biotek, USA), the SOD activity is able to be expressed as a percentage inhibition rate.
TBARS was measured using the method of Kornbrust and Mavis (1980). A total of 1 g hepatopancreas tissue was homogenized in a buffer solution (0.15M KCL and 5 mM Tris-maleate, pH 7.4) using a T25 homogenizer (IKA, Germany). Next, 1 ml of ascorbic acid (2 mM) was added and the mixture was incubated at 37 0C for 30 min. After the addition of 5 ml HCL (0.7 M) and 5 ml thiobarbituric acid (0.05 M), the samples were boiled for 25 min and then placed on ice. Next, 5 ml of trichloroacetic acid (200 g/L) was added and then the samples were centrifuged at 495 g for 5 min. The supernatant was collected to measure the amount of TBARS present using a U-1800 spectrophotometer (Hitachi, Japan) at 530 nm. The concentration of TBARS was expressed as micrograms of malondialdehyde equivalent per milligram of tissues of the hepatopancreas. Standard MDA solutions (Sigma T-1642) were used to create a calibration curve in order to measure the concentration of MDA in each pooled shrimp hepatopancreas sample.
Significant differences among treatments were analyzed by analysis of variance (ANOVA) after confirming the homogeneity of variance using the Levene’s test (Snedecor and Cochran, 1980). A one-way analysis of variance was performed to examine differences in weight gain percentage, SGR, and survival in the five treatments. When a significant difference was observed, Tukey’s range test was used to compare differences among treatments. Weight gain percentage data were log-transformed while others were arcsine-transformed before statistical analysis. Polynomial contrast procedures were applied to detect linear and quadratic trends between the growth parameters of the shrimp and the dietary levels of Nor-grape 80. The significant level was set at p< 0.05 and all statistical analyses were conducted using a SAS software program for Windows (V.9.3., SAS Institute, Cary, NC, U.S.A.).
The growth performance and survival of the white shrimp fed the experimental diets for six weeks are shown in Table 3. The survival of white shrimp fed the treatment diets was in all cases more than 90%. The shrimp fed diets containing different levels of Nor-grape 80 showed better growth performance than those fed the control diet that contained 1000 ppm vitamin E without Nor-grape 80. The final weight, weight gain percentage and SGR of the white shrimp fed diets containing 250 and 500 ppm Nor-grape 80 were significantly higher than those of shrimp fed the other diets. However, no significant differences were found in the final weight, weight gain percentage and SGR of white shrimp fed diets containing 250 and 500 ppm Nor-grape 80. Only the quadratic trends analyzed using polynomial contrast procedures between the growth parameters and Nor-grape 80 levels showed a significant difference.
Table 3 Initial weight, final weight, weight gain percentage, SGR and survival of white shrimp fed dietary treatments for 6 weeks.
The proximate analysis of the muscle of white shrimp fed the treatment diets for six weeks is shown in Table 4. The crude protein content of the muscle tissue from shrimp fed with the treatment diets was all above 80%. The crude lipid of muscle from shrimp fed the treatment diets ranged from 6.81 to 7.38%, The ash of the muscle tissue from shrimp fed the treatment diets ranged from 6.61 to 6.91%.
The levels of SOD activity and the amounts of TBARSs present in the hepatopancreas samples from white shrimp fed the experimental diets for six weeks are shown in Table 5. The highest SOD activity and the lowest TBARS level were found in the hepatopancreas of shrimp fed the diet containing 250 ppm of Nor-grape 80. Both the SOD value and the TBARS level in the hepatopancreas of shrimp fed the diet containing 250 ppm Nor-grape 80 are significantly different from those of shrimp fed the control diet containing 1000 ppm vitamin E. However, the hepatopancreatic SOD values of shrimp fed the diets containing 250 and 500 ppm of Nor-grape 80 were not significantly different. By way of contrast, the hepatopancreatic TBARS level of shrimp fed the diet containing 250 ppm of Nor-grape 80 was significantly lower than that of shrimp fed the other diets. A decrease in SOD activity and an increase in TBARS level in the hepatopancreas of shrimp fed the treatment diets, without the control diet being compared, was found to be proportional to the increase in the amount of Nor-grape 80 in the shrimp diets.
Table 5 SOD activity and TBARS levels in the hepatopancreas of white shrimp fed dietary treatments for 6 weeks.
Positive and negative effects of grape extract were observed in relation to the growth performance of the white shrimp. In this study, white shrimp fed the diets supplemented with 250 and 500 ppm Nor-grape 80 showed an equivalent best growth performance, and a decrease in growth performance was observed among the white shrimp fed diets containing 750 and 1000 ppm Nor-grape 80. Both tilapia and shrimp fed diets supplemented with grape by-products, depending on the dosage used, showed either an improved or a reduced growth performance (Zhai et al., 2014; Rosas et al., 2022). In tilapia, O. niloticus, the weight gain increased as the diets contained increasing amounts (0, 200 and 400 ppm) of grape seed proanthocyanidins and this was followed by a decrease as the dietary grape seed proanthocyanidins were increased to 600 and 800 ppm (Zhai et al., 2014). Similarly, white shrimp fed a diet containing 2.5 x 104 ppm grape bagasse had the highest growth parameters, while shrimp fed a diet containing the highest 1.5 x 105 ppm grape bagasse showed the lowest growth performance (Rosas et al., 2022). Although grape polyphenols, when included in diets at a lower dose, have previously been described as benefiting the growth performance of a wide range of animals (Dolara et al., 2005; Rhodes et al., 2006; Kao et al., 2010; Tucsek et al., 2011; Viveros et al., 2011; Fiesel et al., 2014; Zhai et al., 2014; Shimizu, 2017; Abu Hafsa and Ibrahim, 2018; Ao and Kim, 2020), it is also obvious that there are detrimental effects on the growth performance of animals fed diets containing high levels of grape by-products. This could be due to an increase in the antinutritional factors present in grapes, such as alkaloids, saponins and tannins (Shi et al., 2003; Iriti and Faoro, 2006), as well as intensified grape polyphenol-protein interactions that are likely to reduce the nutritional value of the protein present in food matrix (Shi et al., 2003). As a result, we suggested that the dietary level of Nor-grape 80 fed to 0.02 g white shrimp should not be greater than 500 ppm in order to prevent the above adverse effects on the shrimp growth performance.
In this study, it is hypothesized that dietary grape extract enhances the growth performance of animals and that this might be due to the phenolic components present in the supplement, which appear to promote intestinal health. Several phenolic components in grape extract are known to possess antibacterial activity that can alter the intestinal microbiota (Dolara et al., 2005; Rhodes et al., 2006; Kao et al., 2010; Viveros et al., 2011; Zhai et al., 2014; Abu Hafsa and Ibrahim, 2018; Ao and Kim, 2020), as well as being able to attenuate intestinal inflammation (Kao et al., 2010; Tucsek et al., 2011; Fiesel et al., 2014; Shimizu, 2017). Anthocyanins and their metabolites when interacting with the microbiota have been reported to enhance the growth of Bifidobacteria spp. and Lactobacillus-Enterococcus spp. (Hidalgo et al., 2012). In rats, broilers and Pekin ducks, ingestion of red wine polyphenols and grape seeds has been shown, not only to increase the ileal and colonic numbers of probiotic bacteria such as Lactobacilli and Bifidobacteria spp., but also to inhibit the intestinal growth of detrimental bacteria such as Escherichia coli, Clostridia and Streptococcus (Dolara et al., 2005; Abu Hafsa and Ibrahim, 2018; Ao and Kim, 2020). Additionally, grape phenolic compounds have been found to be capable of modulating the intestinal morphology in poultry (Viveros et al., 2011; Ao and Kim, 2020). In post larvae stage of the freshwater prawn, Macrobrachium rosenbergii, the activity of digestive enzymes has been observed to increase whengrape waste is included in the diets (Bhavan et al., 2013). The dominant intestinal microbiota of white shrimp fed diets containing tannins, which are part of the polyphenols present in grapes, have recently been characterized and found to include Proteobacteria and Bacteroidota (Bolívar-Ramírez et al., 2022); these are groups of bacteria that are normally present in the intestinal tracts of heathy shrimps (Xiong et al., 2017; Ayiku et al., 2020; Schleder et al., 2020). Taking these findings together, we suggest that grape polyphenols improve nutrient absorption by directly affecting the digestive tracts of animals and thus enhance the growth performance of these animals, including the white shrimp in our case.
Previously, white shrimp fed the diets containing 50 to 200 ppm of Nor-grape 80 have been shown to exhibit an increase in SOD activity and a decrease in TBARS levels (Cheng et al., 2017). In the present study, the in vivo antioxidant capacity of the hepatopancreas of white shrimp fed diets containing 250 to 1000 ppm of Nor-grape 80 also showed trends involving a decrease in SOD activity and an increase in TBARS levels as the level of Nor-grape 80 increased. To date, the adverse effects of dietary grape by-products on in vivo antioxidant activity have not been investigated in terrestrial animals (Abu Hafsa and Ibrahim, 2018; Aditya et al., 2018; Ao and Kim, 2020). Pekin ducks fed diets containing 0, 0.01 and 0.02% grape seed extract showed an increase in growth parameters and serum SOD levels, and a decrease in serum TBARS values (Ao and Kim, 2020). Similar increases in SOD activity and the reduction in TBARS levels were also found in the serum and the meat of the broilers fed diets supplemented with increasing amounts of grape pomace and grape seeds (Abu Hafsa and Ibrahim, 2018; Aditya et al., 2018). On the other hand, Rosas et al. (2022) have shown a decrease in the hepatopancreatic TBARS level of white shrimp fed the diets containing 0, 2.5 and 5% grape bagasse; this was accompanied by a slight increase in the hepatopancreatic TBARS level of the white shrimp, as the dietary grape bagasse was increased from 5% to 15%. Therefore, it is possible that animals fed diets containing grape extract have an optimal level of such supplementation and going above this optimal level will result in both a reduced growth performance and a detrimental effect on in vivo antioxidant capacity.
Feed manufacturers have used vitamin E as an antioxidant in aquafeeds for many decades. In terms of highest weight gain, optimal SOD activity and lowest TBARS levels, the findings of the present study indicated that 250 ppm of Nor-grape 80 is more suitable than 1000 ppm of vitamin E when producing an aquafeed for white shrimp. In the present study, the weight gain of the shrimp fed a diet supplemented with 250 ppm Nor-grape 80 was almost 2.5 times higher than that of shrimp fed a diet containing 1000 ppm vitamin E. As a powerful antioxidant, the grape extract Nor-grape 80, at an optimal level, is not only able to promote the growth performance and in vivo antioxidant capacity of white shrimp, but using Nor-grape 80 will also lower the dietary cost of feeding white shrimp in an aquaculture system. In 2022, the prices for Nor-grape 80 and vitamin E in the Taiwan market were US $ 10/kg and US $ 30/kg, respectively. If 1000 ppm of vitamin E is completely replaced with a minimum of 250 ppm of Nor-grape 80, one that allows the white shrimp to reach their highest yield, the dietary cost of the aquafeed would be reduced by US $ 27.5 per tonne. Notwithstanding the above, the optimal dietary level of Nor-grape 80 for white shrimp that will give the maximum growth performance appears to be in the range between 250 and 500 ppm. This is a wide range and further large feeding trials of the white shrimp are needed to pinpoint exactly the optimal dietary level of Nor-grape 80 that gives the best weight gain. This would need to include a cost-benefit analysis and the optimal diet might change depending on the market price of Nor-grape 80, the total feed needed to reach a marketable size for white shrimp and the price that can be obtained for such white shrimp in the market place.
The raw data supporting the conclusions of this article will be made available by the authors, without undue reservation, upon request by any qualified researcher.
Ethical review and approval were not required for the animal study because the research utilized the invertebrate Litopenaeus vannamei.
AC conceptualized, wrote the manuscript and designed the study. SS secured funding and supervised the study. YC performed the experiments, data collection. RK wrote and revised sections of the manuscript. All authors contributed to the article and approved the submitted version.
This research was supported by funds from the Council of Agriculture, Executive Yuang, Taiwan, under COA grants 110AS-10.1.8-FA-F1.
We would like to thank Kaye Bio-tech Co., Taiwan and Miss Ivan Chiu for providing the Nor-grape 80.
The authors declare that the research was conducted in the absence of any commercial or financial relationships that could be construed as a potential conflict of interest.
All claims expressed in this article are solely those of the authors and do not necessarily represent those of their affiliated organizations, or those of the publisher, the editors and the reviewers. Any product that may be evaluated in this article, or claim that may be made by its manufacturer, is not guaranteed or endorsed by the publisher.
Abu Hafsa S. H., Ibrahim S. A. (2018). Effect of dietary polyphenol-rich grape seed on growth performance, antioxidant capacity and ileal microflora in broiler chicks. J. Anim. Physiol. Anim. Nutr. 102, 268–275. doi: 10.1111/jpn.12688
Aditya S., Ohh S. J., Ahammed M., Lohakare J. (2018). Supplementation of grape pomace (Vitis vinifera) in broiler diets and its effect on growth performance, apparent total tract digestibility of nutrients, blood profile, and meat quality. Anim. Nutr. 4, 210–214. doi: 10.1016/j.aninu.2018.01.004
Ao X., Kim I. H. (2020). Effects of grape seed extract on performance, immunity, antioxidant capacity, and meat quality in pekin ducks. Poultry Sci. 99, 2078–2086. doi: 10.1016/j.psj.2019.12.014
Artés-Hernández F., Castillejo N., Martínez-Zamora L., Martínez-Hernández G. B. (2021). Phytochemical fortification in fruit and vegetable beverages with green technologies. Foods 10, 2534. doi: 10.3390/foods10112534
Ayiku S., Shen J. F., Tan B. P., Dong X. H., Liu H. Y. (2020). Effects of dietary yeast culture on shrimp growth, immune response, intestinal health and disease resistance against Vibrio harveyi. Fish Shellfish Immunol. 102, 286–295. doi: 10.1016/j.fsi.2020.04.036
Bagchi D., Bagchi M., Stohs S. J., Das D. K., Ray D., Kuszynski C. A., et al. (2000). Free radicals and grape seed proanthocyanidin extract: importance in human health and disease prevention. Toxicology 148, 187–197. doi: 10.1016/S0300-483X(00)00210-9
Bagchi D., Garg A., Krohn R. L., Bagchi M., Bachi D. J., Balmoori J., et al. (1998). Protective effects of grape seed proanthocyanidins and selected antioxidants against TPA-induced hepatic and brain lipid peroxidation and DNA fragmentation, and peritoneal macrophage activation in mice. Gen. Pharmacol-Vasc. S. 30, 771–776. doi: 10.1016/S0306-3623(97)00332-7
Bernhart F. W., Tomarelli R. M. (1966). A salt mixture supplying the national research council estimates of mineral requirements of the rat. J. Nutr. 89, 495–500. doi: 10.1093/jn/89.4.495
Bhavan P. S., Devim N. N., Muralisankar T., Manickam N., Radhakrishnan S., Srinivasan V. (2013). Effects of Myristica fragrans, glycyrrhiza glabra and Quercus infectoria on growth promotion in the prawn Macrobrachium rosenbergii. Int. J. Life Sc. Bt. Pharm. Res. 2, 169–182.
Bolívar-Ramírez N. C., Mallmann A. S., Schleder D. D., Machado C., Seiffert W. Q., Vieira F. (2022). Tannins as a food additive in pacific white shrimp diet. Aquaculture 556, 738232. doi: 10.1016/j.aquaculture.2022.738232
Bouhamidi R., Prevost V., Nouvelot A. (1998). High protection by grape seed proanthocyanidins (GSPC) of polyunsaturated fatty acids against UV-c induced peroxidationHaute protection par les proanthocyanidines de pépins de raisin contre la peroxydation des acides gras polyinsaturés induite par les UV-c. CR Acad. Sci. III-Vie 321, 31–38. doi: 10.1016/s0764-4469(97)89623-0
Briggs M., Funge-Smith S., Subasinghe R., Rhillips M. (2004). Introductions and movenemnt of penaeus vannamei and penaeus stylirostis in Asia and the pacific (Bangkok, Thailand: FAO Regional office for Asia and the Pacific).
Burdock G. A. (2005). Fenaroli’s handbook of flavor ingredients. fifth Edn (Boca Raton, Florida: CRC Press).
Casanova-Marti A., Gonzalez-Abuin N., Serrano J., Blay M. T., Terra X., Frost G., et al. (2020). Long term exposure to a grape seed proanthocyanidin extract enhances l-cell differentiation in intestinal organoids. Mol. Nutr. Food Res. 64, 2000303. doi: 10.1002/mnfr.202000303
Chakraborty S. B., Horn P., Hancz C. (2013). Application of phytochemicals as growth-promoters and endocrine modulators in fish culture. Rev. Aquac. 5, 1–19. doi: 10.1111/raq.12021
Chamorro S., Viveros A., Centeno C., Romero C., Arija I., Brenes A. (2013). Effects of dietary grape seed extract on growth performance, amino acid digestibility and plasma lipids and mineral content in broiler chicks. Anim. 7, 555–561. doi: 10.1017/S1751731112001851
Cheng Y. C., Sheen S. S., Chien A. (2017). The effects of dietary grape extract on growth performance and antioxidant activities of white shrimp. Litopenaeus vannamei. J. Fish. Soc Taiwan 44, 229–237. doi: 10.29822/JFST.201712_44(4).0002
Cummins V. C., Rawles S. D., Thompson K. R., Velasquez A., Kobayashi Y., Hager J., et al. (2017). Evaluation of black soldier fly (Hermetia illucens) larvae meal as partial or total replacement of marine fish meal in practical diets for pacific white shrimp (Litopenaeus vannamei). Aquaculture 473, 337–344. doi: 10.1016/j.aquaculture.2017.02.022
Davis D. A., Arnold C. R. (2000). Replacement of fish meal in practical diets for the pacific white shrimp, Litopenaeus vannamei. Aquaculture 185, 291–298. doi: 10.1016/S0044-8486(99)00354-3
Dolara P., Luceri. C., De Filippo C., Femia A. P., Giovannelli L., Caderni G., et al. (2005). Red wine polyphenols influence carcinogenesis, intestinal microflora, oxidative damage and gene expression profiles of colonic mucosa in F344 rats. Mutat. Res.-Fund Mol. M. 591, 237–246. doi: 10.1016/j.mrfmmm.2005.04.022
Doshi A., Adsule P., Banerjee K., Oulkar D. (2013). Phenolic compounds, antioxidant activity and insulinotropic effect of extracts prepared from grape (Vitis vinifera) by-products. J. Food Sci. Technol. 52, 181–190. doi: 10.1007/s13197-013-0991-1
Dugassa H., Gaetan D. G. (2018). Biology of white leg shrimp, Penaeus vannamei: Review. World J. Fish Mar. Sci. 10, 5–17. doi: 10.5829/idosi.wjfms.2018.05.17
Encarnação P. (2016). “Functional feed additives in aquaculture feeds,” in Aquafeed formulation. Ed. Nates S. F. (San Diego: Academic Press), 217–237.
Fiesel A., Gessner D. K., Most E., Eder K. (2014). Effects of dietary polyphenol-rich plant products from grape or hop on pro-inflammatory gene expression in the intestine, nutrient digestibility and faecal microbiota of weaned pigs. BMC Veter. Res. 10, 196. doi: 10.1186/s12917-014-0196-5
Folch J., Lees M., Stanley G. H. S. (1957). A simple method for the isolation and purification of total lipids from animal tissues. J. Biol. Chem. 266, 497–509. doi: 10.1016/S0021-9258(18)64849-5
Garcia J., Nicodemus N., Carabano R., De Blass J. C. (2002). Effects of inclusion of defatted grape seed meal in the diet on digestion and performance of growing rabbits. J. Anim. Sci. 80, 162–170. doi: 10.2527/2002.801162x
Guerra-Rivas C., Vieira C., Rubio B., Martinez B., Gallardo B., Mantecon A. R., et al. (2016). Effects of grape pomace in growing lamb diets compared with vitamin E and grape seed extract on meat shelf life. Meat Sci. 116, 221–229. doi: 10.1016/j.meatsci.2016.02.022
Hardy R. W., Tacon A. G. J. (2002). “Fish meal: historical uses, production trends and future outlook for sustainable supplies,” in Responsible marine aquaculture. Eds. Stickney R. R., McVey J. P. (New York: CABI publishing), 311–325.
Hidalgo M., Oruna-Concha M. J., Kolida S., Walton G. E., Kallithraka S., Spencer J. P. E., et al. (2012). Metabolism of anthocyanins by human gut microflora and their influence on gut bacterial growth. J. Agric. Food Chem. 60, 3882–3890. doi: 10.1021/jf3002153
Iriti M., Faoro F. (2006). Grape phytochemicals: A bouquet of old and new nutraceuticals for human health. Med. Hypotheses 67, 833–838. doi: 10.1016/j.mehy.2006.03.049
Jayaprakasha G. K., Selvi T., Sakariah K. K. (2003). Antibacterial and antioxidant activities of grape (Vitis vinifera) seed extracts. Food Res. Int. 36, 117–122. doi: 10.1016/S0963-9969(02)00116-3
Kao T. T., Tu H. C., Chang W. N., Chen B. H., Shi Y. Y., Chang T. C., et al. (2010). Grape seed extract inhibits the growth and pathogenicity of Staphylococcus aureus by interfering with dihydrofolate reductase activity and folate-mediated one-carbon metabolism. Int. J. Food Microbiol. 141, 17–27. doi: 10.1016/j.ijfoodmicro.2010.04.025
Kornbrust D. J., Mavis R. D. (1980). Relative susceptibility of microsomes from lung, heart, liver, kidney, brain and testes to lipid peroxidation: correlation with vitamin E content. Lipids 15, 315–322. doi: 10.1007/BF02533546
Lee C., Lee K. J. (2018). Dietary protein requirement of pacific white shrimp Litopenaeus vannamei in three different growth stages. Fisheries Aquat. Sci. 21, 30. doi: 10.1186/s41240-018-0105-0
Lucas J. S., Southgate P. C., Tucher C. S. (2019). Aquaculture: Farming aquaculture animals and plants. 3rd ed. (Hoboken, NJ: John Wiley & Sons Ltd).
Niyamosatha H., Chuchird N., Rairat T. (2015). Effect of dietary polyphenol-rich feed additive from grape pomace on growth, survival and tolerance to Vibrio infection in pacific white shrimp (Litopenaeus vannamei). Kasetsart Uni. Fish. Res. Bull. 39, 1–9.
Park J. C., Lee S. H., Hong J. K., Cho J. H., Kim I. H., Park S. K. (2014). Effect of dietary supplementation of procyanidin on growth performance and immune response in pigs. Asian-Australas J. Anim. Sci. 27, 131. doi: 10.5713/ajas.2013.13359
Rhodes P. L., Mitchell J. W., Wilson M. W., Melton L. D. (2006). Antilisterial activity of grape juice and grape extracts derived from Vitis vinifera variety. Ribier Int. J. Food Microbiol. 107, 281–286. doi: 10.1016/j.ijfoodmicro.2005.10.022
Rosas V. T., Mureb R. A., Monserrat J. M., Wasielesky W. Jr., Tesser M. B. (2022). Inclusion of grape bagasse (Vitis sp.) in the diet of white shrimp (Litopenaeus vannamei) and its effects on growth and antioxidant system. Aquac. Res. 53, 4805–4813. doi: 10.1111/are.15972
Schleder D. D., Blank M., Peruch L. G. B., Poli M. A., Goncalves P., Rosa K. V., et al. (2020). Impact of combinations of brown seaweeds on shrimp gut microbiota and response to thermal shock and white spot disease. Aquaculture 519, 734779. doi: 10.1016/j.aquaculture.2019.734779
Shi J., Yu J., Pohorly J. E., Kakuda Y. (2003). Polyphenolics in grape seeds-biochemistry and functionality. J. Med. Food 6, 291–299. doi: 10.1089/109662003772519831
Shimizu M. (2017). Multifunctions of dietary polyphenols in the regulation of intestinal inflammation. J. Food Drug Anal. 25, 93–99. doi: 10.1016/j.jfda.2016.12.003
Snedecor G. W., Cochran W. G. (1980). Statistical methods (Ames, Iowa: The Iowa University Press, Blackwell Publishing).
Tacon A. G. J., Jory D., Nunes A. (2013). “Shrimp feed management; issues and perspectives,” in On-farm feeding and feed management in aquaculture. Eds. Hasan M. R., News M. B. (Rome: FAO), 481–488.
Tucsek Z., Radnai B., Racz B., Debreceni B., Prib J. K., Dolowschiak T., et al. (2011). Suppressing LPS-induced early signaltransduction in macrophages by apolyphenol degradation product: a criticalrole of MKP-1. J. Leukocyte Biol. 89, 105–111. doi: 10.1189/jlb.0610355
Viveros A., Chamorro S., Pizarro M., Arija L., Centeno C., Brenes A. (2011). Effects of dietary polyphenol-rich grape products on intestinal microflora and gut morphology in broiler chicks. Poultry Sci. 90, 566–578. doi: 10.3382/ps.2010-00889
Xia E. Q., Deng G. F., Guo Y. J., Li H. B. (2010). Biological activities of polyphenols from grapes. Int. J. Mol. Sci. 11, 622–646. doi: 10.3390/ijms11020622
Xiong J., Dai W., Zhu J., Liu K., Dong C., Qiu Q. (2017). The underlying ecological processes of gut microbiota among cohabitating retarded, overgrown and normal shrimp. Microb. Ecol. 73, 988–999. doi: 10.1007/s00248-016-0910-x
Zhai S. W., Lu J. J., Chen X. H. (2014). Effects of dietary grape seed proanthocyanidins on growth performance, some serum biochemical parameters and body composition of tilapia (Oreochromis niloticus) fingerlings. Ital. J. Anim. Sci. 13, 536–540. doi: 10.4081/ijas.2014.3357
Keywords: SOD, TBARS, weight gain percentage, white shrimp, grape extract
Citation: Chien A, Cheng YC, Sheen SS and Kirby R (2023) Dietary grape extract can, at an appropriate level, improve the growth performance and antioxidant activity of the white shrimp Litopenaeus vannamei. Front. Mar. Sci. 10:1104870. doi: 10.3389/fmars.2023.1104870
Received: 22 November 2022; Accepted: 06 March 2023;
Published: 16 March 2023.
Edited by:
Jianchun Shao, Fujian Agriculture and Forestry University, ChinaReviewed by:
Amalia Pérez-Jiménez, University of Granada, SpainCopyright © 2023 Chien, Cheng, Sheen and Kirby. This is an open-access article distributed under the terms of the Creative Commons Attribution License (CC BY). The use, distribution or reproduction in other forums is permitted, provided the original author(s) and the copyright owner(s) are credited and that the original publication in this journal is cited, in accordance with accepted academic practice. No use, distribution or reproduction is permitted which does not comply with these terms.
*Correspondence: Angela Chien, YW5nZWxhY2hpZW4xMjIxQGdtYWlsLmNvbQ==
Disclaimer: All claims expressed in this article are solely those of the authors and do not necessarily represent those of their affiliated organizations, or those of the publisher, the editors and the reviewers. Any product that may be evaluated in this article or claim that may be made by its manufacturer is not guaranteed or endorsed by the publisher.
Research integrity at Frontiers
Learn more about the work of our research integrity team to safeguard the quality of each article we publish.