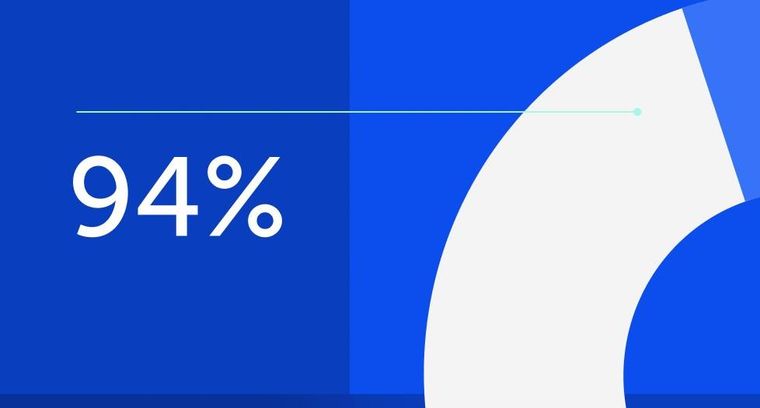
94% of researchers rate our articles as excellent or good
Learn more about the work of our research integrity team to safeguard the quality of each article we publish.
Find out more
ORIGINAL RESEARCH article
Front. Mar. Sci., 07 December 2022
Sec. Aquatic Physiology
Volume 9 - 2022 | https://doi.org/10.3389/fmars.2022.1038394
This article is part of the Research TopicThe Relationship Between Nutrition and Stress on Intestinal Health and Reproduction in Aquatic AnimalsView all 8 articles
The study aimed to investigate the effects of oregano oil (OO) supplement in the diet on the growth performance, disease resistance, immunity, and intestinal health of hybrid grouper. Six isonitrogenous and isolipidic diets were fed to the grouper with an initial weight of 19.10 ± 0.12 g for 8 weeks, of which the dietary OO levels were 0 (Control), 0.1 (OO-0.1), 0.3 (OO-0.3), 0.6 (OO-0.6), 0.9 (OO-0.9), or 1.2 (OO-1.2) g kg-1. The results showed that there were no significant differences in growth performance among the six groups, while the OO supplement could improve the disease resistance ability of grouper. Our findings have demonstrated that the OO supplement, especially 0.3 g kg-1, could improve the intestinal morphology via increasing the villus number and muscle thickness and elevate digestive enzyme activities such as those of amylase (AMS) and lipase. The 0.3-g kg-1 OO supplement also can activate the antioxidant defense system, reduce nuclear factor erythroid 2-related factor 2-like 2 (Nrf2)/Kelch-like-ECH-associated protein 1 (Keap1) expression, and then enhance the immune ability via acid phosphatase (ACP), lysozyme (LYS), complement C3 (C3), and tight junction-associated signaling pathways in hybrid grouper. Meanwhile, the relative abundance of Firmicutes and Bacteroidetes in the OO-0.3 group was significantly higher than that of Control group (P< 0.05), while the relative abundance of Proteobacteria showed a basically opposite trend (P< 0.05). These findings suggested that dietary OO supplementation could significantly improve intestinal health and induce the antioxidant defense system and immunity, which means that OO could be used as a functional feed additive to protect the intestine, and the appropriate addition level of OO should be 0.3 g kg-1.
Oregano oil (OO) is an aromatic volatile oil extracted from oregano and has many biological activities, such as anti-inflammatory, antibacterial, and antioxidative activities (Goulas and Kontominas, 2007; Scramlin et al., 2010; Rodriguez-Garcia et al., 2016). Therefore, it has attracted a lot of attention regarding its application in both humans (Avola et al., 2020) and animals, such as cows (Benchaar, 2020), mice (Gilling et al., 2014), and poultry (Al-Hijazeen et al., 2018; Migliorini et al., 2019; Zhou et al., 2019). Previous research has shown that the feed efficiency of turbot is improved when feeding OO by stimulating beneficial intestinal microorganisms, while it could inhibit the growth of pathogenic microorganisms, i.e., Escherichia coli and Staphylococcus aureus (Preuss et al., 2005). Furthermore, carvacrol and thymol, the principal compounds in OO, have preeminent antioxidant properties and are effective in retarding the process of lipid peroxidation in fatty food and scavenging free radicals (Quiroga et al., 2013; Rodriguez-Garcia et al., 2016; Asensio et al., 2017). However, despite the fact that previous studies have shown that OO also has growth-promoting effects in fish and the dose is a very important factor (Ferreira et al., 2014), research on OO in fish is still relatively limited and incomplete.
The intestine represents the largest compartment of the immune system, an extremely complex living system involved in protecting the host by providing a strong defense against stresses from the external environment (Bourlioux et al., 2003). The intestine always is a port of entry for many clinically important pathogens (Mowat and Agace, 2014), and intestinal homeostasis is therefore extremely cardinal for animals to maintain their organismal health, which is a process that requires a prudent balance between the host’s response to a beneficial intestinal microbial community and potentially pathogenic stimuli (Moreira Lopes et al., 2020). However, stimulation by numerous extracellular factors, such as inflammatory cytokines and reactive oxygen species (ROS), can disrupt the intestinal mucosal barrier and thus disrupt this balance, leading to a further inflammatory response and numerous diseases of the intestine (Rombout et al., 2011; Suzuki and Hara, 2011). OO has been shown to be effective in repairing the human intestinal mucosal barrier and maintaining homeostasis in the intestine (Llana-Ruiz-Cabello et al., 2015; Allaoua et al., 2018; Gaur et al., 2018; Kaschubek et al., 2018). Furthermore, the function of the intestinal mucosal barrier is tightly associated with the interaction of several barrier components, for example, the mucous layer and the tight junction (TJ) between adjacent epithelial cells (Turner, 2009; Ou et al., 2019). In turn, relevant studies have shown that damage to the TJ shield is a major determinant of intestinal barrier function and is associated with various diseases (Suzuki and Hara, 2011; Camilleri et al., 2012; Dokladny et al., 2016). Numerous studies have reported that TJ protein expression and binding to the actin cytoskeleton, which determines TJ permeability, were dynamically regulated by various intracellular signaling molecules and signaling pathways, such as protein kinases, protein phosphatases, and nuclear factor-κB (NF-κB) signaling pathway (Sheth et al., 2003; Seth et al., 2007; Suzuki et al., 2009; Zou et al., 2016). In addition, OO can strengthen the intestinal TJ functions and enhance the barrier integrity in pigs (Zou et al., 2016). However, deeper molecular mechanisms of dietary OO on TJ-associated signaling pathways in the fish intestinal have not been reported.
The hybrid grouper is a new species produced by the cross between giant grouper (Epinephelus lanceolatus ♂) and brown marbled grouper (Epinephelus fuscoguttatus ♀), which has been widely farmed in the southeast coast of China with the advantages of fast growth, strong disease resistance, excellent taste, and high economic value (Sun et al., 2016; Tan et al., 2018; Tan et al., 2020). In the present study, we hypothesized that dietary OO supplementation promotes the intestinal barrier integrity by regulating intestinal bacteria and inflammation. We thus use the hybrid grouper to test this hypothesis and study the morphology and permeability of the intestine, the composition of the intestinal microbiota, innate immunity, and disease resistance after OO supplementation.
Animal care and treatment procedures in the current study were approved by the Animal Care and Use Committee of Guangdong Ocean University, China.
Six isonitrogenous and isolipidic experimental diets were formulated to contain 0 (Control), 0.1 (OO-0.1), 0.3 (OO-0.3), 0.6 (OO-0.6), 0.9 (OO-0.9), or 1.2 (OO-1.2) g kg-1 OO (Table 1). All dietary ingredients were ground into fine powder through a 320-μm mesh, and then they were thoroughly mixed with fish oil. After adding water, the stiff dough was produced, and it was pelleted with an experimental single-screw feed extruder. Finally, the feeds were dried for about 12 h in a ventilated oven at 45°C and then stored at −20°C.
Juvenile hybrid grouper (initial body weight 19.10 ± 0.12 g) were bought from a commercial farm in Zhanjiang, China. After being fed with the commercial diet for 2 weeks to acclimate to the experimental conditions, a total of 540 fish were fasted for 24 h and weighed, then they were randomly distributed to 18 cylindrical fiberglass tanks (300 L) with continuous aeration and circulating water. Each experimental feed was randomly assigned to triplicate tanks. Fish were slowly hand-fed to apparent satiation twice daily (7:00 a.m. and 7:00 p.m.). During the feeding period (8 weeks), seawater was disinfected with chlorine dioxide. The water quality factors were measured during the experiment period, and the water temperature was 25°C–27°C, salinity was 30–33‰, dissolved oxygen was more than 7 mg/L, and ammonia and nitrate remained below 0.03 mg/L.
After the 8-week feeding trial, the fish in each tank were fasted for 24 h and anesthetized using 100 mg/L tricaine methanesulfonate (MS-222, Sigma, USA). Then, the fish were weighed and counted to calculate the growth performance indexes such as weight gain rate (WG) and specific growth rate (SGR). Hepatosomatic index (HSI), viscerosomatic index (VSI) and condition factor (CF), gut-somatic index (GSI), and gut-length index (GLI) were determined from three individual fish by obtaining tissues (viscera and liver) and expression ratios as a percentage of body weight. The intestines of three fish were selected for histological analysis. The intestines of three fish in each tank were pulled into 1.5-ml frozen tubes randomly and then stored at −80°C for enzyme activity assay and gene expression analysis and microbial community analysis.
Before determining, the intestine was homogenized with normal saline and centrifuged at 6,000× g for 10 min at 4°C, and the supernatant was taken for the determination of biochemical parameters. A total protein quantitative assay kit was used by the Bicinchoninic Acid Assay (BCA) method to determine the total protein content of the intestine homogenate supernatant as a prerequisite for the calculation of biochemical parameters of the intestine. Amylase (AMS), lipase, trypsin, alkaline phosphatase (AKP), acid phosphatase (ACP), and lysozyme (LYS) were determined by assay kits (Nanjing Jiancheng Bioengineering Institute, China) and detected through a UV spectrophotometer.
The concentrations of complement 3 (C3) and complement 4 (C4) in the intestine were determined through enzyme-linked immunosorbent assay (ELISA) kits (Shanghai Jianglai Industry Co., Ltd., Shanghai, China) following the manufacturer’s instructions.
Intestine samples were fixed in Bouin’s solution for 24 h and then transferred to 70% ethanol. The samples were dehydrated in ethanol of different grades, followed by embedding in paraffin. Sections were stained with hematoxylin and eosin and viewed under a microscope (Nikon Ni-U, Japan). Subsequently, three parameters of intestine histology were measured using ImageJ software, including the villus length, muscle thickness, and goblet cells per villus, as well as the villus number. The average value of six parallels per parameter in each sample was measured randomly to determine significant differences among the test groups.
Total RNA was extracted from the intestine of fish with TRIzol (Takara Biotech, Kyoto, Japan), its quality and quantity were determined by a NanoDrop 2000 spectrophotometer (Thermo, MA, USA), and its integrity was assessed by 1% agarose gel electrophoresis (Wang et al., 2015). The cDNA was synthesized with PrimeScript reverse transcriptase (Takara Biotech, Kyoto, Japan) in a 20-μl reaction volume containing 1 μg total RNA. The reaction mixture was stored at −20°C for future use. Quantitative real-time RT-PCR (qRT-PCR) experiments were performed in a CFX96 Multicolor Real-Time PCR Detection System (Bio-Rad Laboratories, Inc., CA, USA) using SYBR Mixture (Takara Biotech, Kyoto, Japan). In this study, β-actin was used as an internal reference gene. Each sample was paralleled three times. PCRs were performed on a total reaction volume of 20 μl containing 4 μl of cDNA, 0.5 μl of primer, 10 μl of SYBR Mixture, and 5 μl of ddH2O. The thermal cycling program was as follows: activation at 95°C for 10 min, followed by 35 cycles of 95°C for 10 s, several annealing temperatures for 30 s, and 72°C for 32 s; melting curve detection was performed from 60°C to 95°C (increments of 0.5°C) for 5 s. Quantitative analyses were performed using the 2−ΔΔCt method. The PCR cycling protocol was 95°C for 60 s, 40 cycles of 94°C for 5 s and 60°C for 30 s, followed by 95.0°C for 5 s; melting curve analysis was performed from 65.0°C to 95.0°C (increments of 0.5°C) for 5 s. Quantitative analyses were performed using the 2−ΔΔCt method. The primer sequences are described in Table 2.
Intestine microbial DNA from three fish per tank in the Control and OO-0.3 groups was extracted with EZNA Fecal DNA Kit (Omega Bio-Tek, Inc., USA). The V3-V4 variable region of the 16S rRNA gene was amplified by PCR. Amplicons were separated on a 2% agarose gel, followed by purifying using the AxyPrep DNA Gel Extraction Kit (Axygen Biosciences, Union City, CA, USA) and quantifying using an ABI StepOnePlus real-time PCR instrument (Life Technologies, Carlsbad, USA). Then, purified amplicons were mixed in equimolar amounts, and paired-end sequencing (2 × 250) was conducted on an Illumina platform (HiSeq 2,500, Illumina, San Diego, CA, USA). After filtering for noisy sequences and checking for the presence of chimeras, data were assigned to the same operational taxonomic units (OTUs). Intergroup Venn analysis was conducted to identify endemic and shared OTUs using the microbial indicative analysis function of the Omicshare platform (https://www.omicshare.com/). Moreover, the overall microbial composition was conducted with beta analysis through partial least squares discriminant analysis in the Omicshare platform. The RDP classifier software was used for microbial species annotation (version 2.2, http://sourceforge.net/projects/rdpclassifier/).
Ten fish from each tank were randomly sampled after the 8-week feeding experiment for the bacterial challenge. Frozen stock culture of Vibrio harveyi was obtained from the Key Laboratory of Control for Disease of Aquatic Economic Animals of Guangdong Higher Education Institutes (Zhanjiang, China). According to our previous study (An et al., 2020), each fish was intraperitoneally injected with a dose of 200 μl phosphate-buffered saline (PBS) containing 6.7 × 107 CFU V. harveyi. The cumulative mortality was recorded until 72 h, and the relative percentage survival was calculated. The formula used was Cumulative mortality (%) = (100 × total mortality per treatment/total number of fish challenged), whereas that of the Percentage survival (%) = 100 × (1 – [percent mortality in treatment groups/percent mortality in Control group]).
The growth parameters and morphological indices were calculated as follows:
All analyses were carried out using the SPSS software (version 26.0). The data were assessed for homogeneity of variances, then were analyzed by one-way analysis of variance (ANOVA) followed by Tukey's test. For statistically significant differences, P< 0.05 was required. All data were expressed as means ± SEM.
As shown in Table 3, there were no significant differences in WG, SGR, FCR, VSI, HSI, GLI, FI, and SR among the six groups (P > 0.05). With the increasing dietary OO levels, the GSI increased clearly and the OO group was significantly higher than that in the Control group except the OO-0.3 group (P< 0.05). CF showed a similar pattern and reached the maximum value in the OO-0.6 group.
Table 3 Effect of dietary OO on growth performance and body indices of juvenile hybrid grouper fed the experimental diets for 8 weeks.
The results of the V. harveyi challenge test were shown in Figure 1. At 72 h after injection, the cumulative mortality rate of fish fed the OO-supplemented diet was significantly lower than that of fish fed the control diet, and the OO-0.1 and OO-0.3 groups showed higher SR compared with the other groups.
Figure 1 Effect of dietary oregano oil (OO) on cumulative mortality of juvenile hybrid grouper fed the experimental diets for 8 weeks after the Vibrio harveyi challenge.
The effects of dietary OO on intestinal morphology in juvenile hybrid grouper were presented in Table 4 and Figure 2. Compared to the Control group, the villus length distinctly increased in the OO-0.1 group (P< 0.05) and slightly rose in the OO-0.3 and OO-0.6 groups while consequently declined in the OO-0.9 and OO-1.2 groups with no significant difference (P > 0.05). The muscle thickness of the intestine of the OO groups except the OO-0.6 group was significantly higher than that in the Control group, and there was no significant difference among the OO-0.6 and Control groups. The number of goblet cells increased first and then decreased with the dietary OO increasing, and the number of goblet cells in the OO groups except the OO-1.2 group was significantly higher than those in the Control group (P< 0.05). Additionally, our study found that the villus number in the OO-0.3 group increased significantly, whereas it diminished remarkably in the OO-0.9 and OO-1.2 groups (P< 0.05), which showed no significant changes among the Control, OO-0.1, and OO-0.6 groups (P > 0.05).
Figure 2 Effect of dietary oregano oil (OO) on the intestinal morphology of juvenile hybrid grouper fed the experimental diets for 8 weeks. Black arrow: goblet cell; red arrow: epithelioid cell. Scale size: 200 μm.
Digestive enzyme activities of the intestine were shown in Figure 3A. The AMS activity of the OO-0.1, OO-0.3, and OO-0.6 groups was significantly higher in comparison with that of the Control group (P< 0.05), while the maximum value was obtained in the OO-0.3 group, and there was no significant difference between the other groups and the Control group. As the dietary OO level increased to more than 0.3 g kg-1, the activity of AMS was gradually decreased, but there was no apparent difference in the OO-0.6, OO-0.9, and OO-1.2 groups (P > 0.05). However, the activity of lipase was obviously increased in the OO-0.3, OO-0.6, and OO-1.2 groups (P< 0.05) and reached the highest level in the OO-0.3 group by approximately 2-fold relative to that of the Control group, whereas no significant difference was observed among the Control, OO-0.1, and OO-0.9 groups regarding the activity of lipase (P > 0.05). The addition of OO in the diet significantly increased the activity of trypsin in the intestine. From Figure 3A, we can see that the trypsin activity of all OO-treated groups was higher than that of the Control group (P< 0.05). Consistent with the results of AMS and lipase, the activity of trypsin also exhibited the highest value in the OO-0.3 group.
Figure 3 Effect of dietary oregano oil (OO) on the digestive enzyme activities and immunity capacity in the intestine of juvenile hybrid grouper fed the experimental diets for 8 weeks. (A) The content of amylase (AMS), lipase and trypsin. (B) The content of alkaline phosphatase (AKP), acid phosphatase (ACP),lysozyme (LYS), complement 3 (C3) and complement 4 (C4). Different lowercase letters show significant differences among treatments by Tukey’s test (P < 0.05).
Results of immunological parameters of the intestine were shown in Figure 3B. It is worth noting that the AKP activity was apparently higher (P< 0.05) in the OO-0.6 group compared to the OO-0.1, OO-0.9, and Control groups, while the activity of AKP marginally decreased in the OO-0.1 group compared to that of the Control group, and there was no significant difference in AKP activity in the OO-0.3, OO-0.6, and OO-1.2 groups. Our study demonstrated that the activity of ACP in the groups adding OO was significantly higher than that in the Control group (P< 0.05), and the level of ACP reached a maximum in the OO-0.3 group, which was nearly 1.5 times than that in the Control group. In addition, the activity of LYS obviously increased in the OO-0.3 and OO-0.6 groups (P< 0.05), while there was no significant difference among the Control, OO-0.1, OO-0.9, and OO-1.2 groups (P > 0.05). The concentrations of C3 and C4 in the intestine were consistently significantly higher in all OO-treated groups than that in the Control group (P< 0.05). The concentration of C3 in the intestine increased first and then decreased, and the highest value was obtained in the OO-0.3 group, and then decreased distinctly in the OO-0.9 and OO-1.2 groups (P< 0.05). Furthermore, the concentration of C4 increased significantly from group OO-0.1 to group OO-0.3 (P< 0.05) and reached its highest concentration in group OO-0.3, which was consistent with the result of C3. Afterward, the concentration of C4 decreased significantly in the OO-0.6 and OO-0.9 groups (P< 0.05) and finally increased in the OO-1.2 group, but there was no significant difference in the intestinal C4 concentration among the last three groups.
As shown in Figure 4, the mRNA expressions of glutathione peroxidase (GPx), catalase (CAT), and glutathione reductase (GR) were outstandingly upregulated in the OO-treated groups compared to those in the Control group (P< 0.05). It is worth noting that GPx and CAT mRNA expression levels were highest in the OO-0.3 group, and the mRNA expression of GPx and CAT showed no difference among the OO-0.1, OO-0.6, OO-0.9, and OO-1.2 groups. Interestingly, the expression of GR in all OO-treated groups showed no change (P< 0.05).
Figure 4 Effect of dietary oregano oil (OO) on the relative expression of antioxidant genes in the intestine of hybrid grouper. Different lowercase letters show significant differences among treatments by Tukey’s test (P < 0.05).
The nuclear factor erythroid 2-related factor 2-like 2 (Nrf2) mRNA expression levels reached the minimum in the OO-0.1 and OO-0.3 groups, which was significantly lower than that in the Control, OO-0.6, OO-0.9, and OO-1.2 groups (P< 0.05). At the same time, Kelch-like-ECH-associated protein 1 (Keap1) showed a similar pattern compared with Nrf2. The Keap1 mRNA expression level of the intestine was suppressed by 0.1 and 0.3 g kg-1 OO in the diet and significantly lower than that in the OO-0.9, OO-1.2, and Control groups (P< 0.05).
Figure 5 exhibited the effect of OO on the mRNA expression of junction-associated protein genes in the intestine of hybrid grouper. The mRNA expression of ZO-1 was significantly upregulated in all OO-treated groups compared to that of the Control group (P< 0.05), peaking at the OO-0.6 group, and the ZO-1 mRNA expression was significantly higher than that of the other OO-treated groups. Compared with the OO-1.2 and Control groups, dietary supplementation with 0.1, 0.3, 0.6, and 0.9 g kg-1 OO significantly upregulated the mRNA expression of ZO-2 and ZO-3 (P< 0.05). Meanwhile, the expression of ZO-2 reached its highest level in the OO-0.3 group and consistent with the trend of ZO-1 expression in the OO-0.6 group, and there was no significant difference in the ZO-3 mRNA expression among the OO-0.1, OO-0.3, OO-0.6, and OO-0.9 groups. Furthermore, the occludin mRNA expression level in the fish fed with the diet supplemented with 0.1, 0.3, and 0.6 g kg-1 OO was remarkably higher than that in the OO-0.9, OO-1.2, and Control groups (P< 0.05) and reached its highest level in the OO-0.3 group. Additionally, the mRNA expression of Claudin-3a obviously increased in the OO-0.1, OO-0.3, OO-0.6, and OO-1.2 groups compared to that of the OO-0.9 and Control groups (P< 0.05), reaching the peak at the OO-0.3 group.
Figure 5 Effect of dietary oregano oil (OO) on the relative expression of tight junction-associated protein genes in the intestine of hybrid grouper. Different lowercase letters show significant differences among treatments by Tukey’s test (P < 0.05).
As shown in Figure 6A, the unique operational taxonomic units (OUTs) for the Control and OO-0.3 groups were 347 and 491, respectively. Figures 6B, C showed the relative abundance of intestinal microbiota at the phylum and genus levels induced by different diets. At the phylum level, Firmicutes, Proteobacteria, Bacteroidetes, Actinobacteria, and Fusobacteria were the top 5 ranked phyla in both groups. The relative abundance of Firmicutes and Bacteroidetes in fish fed the OO-0.3 diet was significantly higher than that fed the control diet (P< 0.05), while the relative abundance of Proteobacteria in fish fed the control diet was significantly higher than that fed the OO-0.3 diet (P< 0.05). At the genus level, Vibrio, Muribaculaceae, Streptococcus, Lachnospiraceae_NK4A136_group, and Clostridium_sensu_stricto_1 were the top 5 ranked genera in the two groups. The relative abundance of Vibrio was significantly higher in fish fed the control diet than that fed the OO-0.3 diet (P< 0.05).
Figure 6 Effect of dietary oregano oil (OO) on the composition of intestinal microbiota of juvenile hybrid grouper fed the control and OO-0.3 diets for 8 weeks. Venn diagrams analysis at the (A) OUT level, (B) phylum level, and (C) genus level.
The LDA Effect Size (LEfSe) analysis results of the Control and OO-0.3 groups are shown in Figure 7A. Overall, 5 and >60 biomarkers were detected in the Control and OO-0.3 groups, respectively. Additional order-, family-, and genus-level biomarkers of the Control and OO-0.3 groups are shown in Figure 7B.
Figure 7 Effect of dietary oregano oil (OO) on the differences in the intestinal microbiota of juvenile hybrid grouper fed the control and OO-0.3 diets for 8 weeks. (A) The linear discriminant analysis score of the abundance of taxa. (B) Cladogram showing differences in the abundance of taxa (phylum to genus) in the intestinal microbiota.
In this study, OO supplementation did not affect the growth performance of fish. The current study is consistent with a previous study that showed that feeding with OO supplementation also did not significantly affect the growth performance of Black Sea salmon, Salmo labrax (Ozel et al., 2022). However, some research (Magouz et al., 2022) reported that 0.5 and 1 g OO/kg diet could not only relieve the impacts of heat stress but also markedly enhance the final weight, weight gain, and SGR of Nile tilapia. Additionally, OO was proven to enhance the growth performance, immunity, and antioxidative status of Oreochromis niloticus reared under intensive systems (Shourbela et al., 2021). This difference might be due to the different species and different environments in the study.
Bacterial infection and climatic changes are the most important factors impacting the aquaculture industry and resulting in low productivity and high economic loss (Wang et al., 2012; Xin et al., 2022). The cumulative mortality rate after the challenge by pathogenic bacteria is commonly used as an indicator of disease resistance in animals (Deng et al., 2013). In this study, the V. harveyi challenge was used to assess the disease resistance of fish according to previous research (An et al., 2020). Fish fed the OO diet had a lower cumulative mortality rate than those fed the control diet, indicating that the disease resistance to V. harveyi was significantly correlated with the dietary OO supplementation. Similar to our results, other previous studies had also proven that OO had a pronounced influence on the innate immunity in Tilapia zillii and increased the fish resistance to Vibrio anguillarum (Mabrok and Wahdan, 2018). Moreover, OO also could reduce Vibrio virulence and mediate antivirulence therapy against Vibrio in Penaeus vannamei (Dominguez-Borbor et al., 2020). These data gave clear insight into the potential use of OO in prophylactic strategies against threatening pathogens.
The intestine is one of the most important organs for aquatic organisms to absorb nutrients and the primary “frontline” physical barrier for protection, which played an essential role with intestinal microflora in fish health by stimulating the immune system and handling in nutrient absorption (Ye et al., 2019; Shi et al., 2022). Previous studies have shown that the increase in villus height and number contributed to facilitating the intestinal absorption capacity, which had a positive impact on nutrient utilization (Xu et al., 2020). This was consistent with the fact that the OO-0.3 diet remarkably increased the intestinal villus height of juvenile hybrid grouper compared with the control diet in this study. As the physiological and immunologic barrier to a variety of microorganisms and foreign objects, the intestinal mucosa’s overlying mucus-gel layer is the first line of defense formed by the secretion of goblet cells (Xia et al., 2022). Our study found that the diet supplemented with 0.3 and 0.6 g kg-1 OO significantly increased the number of goblet cells. Most noteworthy, the diet added 0.6 g kg-1 OO also apparently boosts the villus number, which is similar to the results of turbot (Scophthalmus maximus) after supplementation with OO (Guangxin et al., 2022). Consequently, we believe that 0.3 g kg-1 and 0.6 g kg-1 of OO were the suitable dietary supplements for improving the intestinal metabolism through elevating the intestinal development of hybrid grouper.
Intestinal digestive enzymes are often used as an indicator of the digestive capacity and nutritional status of fish. In some cases, fish can regulate the activity of digestive enzymes in response to food sources and dietary nutrient concentrations (Abolfathi et al., 2012). Previous studies have shown that adding OO to the fish’s diet improved the growth performance (Shourbela et al., 2021; Guangxin et al., 2022); therefore, we thought that one of the possible reasons was the activation of digestive enzymes. Furthermore, OO supplementation in the diet made a contribution to the secretion of digestive enzymes in poultry (Jang et al., 2007). In our study, the OO supplementation in the diet increased the digestive enzyme activity to some extent in the hybrid grouper. It shall be noted that adding 0.3 g kg-1 of OO into the diet of hybrid grouper significantly increased the activities of AMS, lipase, trypsin, ACP, and LYS, while the dietary 0.6 g kg-1 OO obviously increased the activities of AKP, which is consistent with the results of the koi carp (Cyprinus carpio) with the diet supplemented with OO (Zhang et al., 2020). Interestingly, the activities of AMS, AKP, and LYS of the hybrid grouper fed with a high concentration of OO (0.9 and 1.2 g kg-1) showed no significant difference from those of the Control group, thereby, we suggested that the hybrid grouper was less sensitive to high concentrations of OO (0.9 and 1.2 g kg-1).
Complement plays an important part in the regulation and enhancement of humoral immunity (Kuroda et al., 2000). However, C3 and C4 had a vital effect in the activation of the complement system, which were non-catalytic but essential components of the C3 convertases as alternative and classical pathways, respectively (Nakao and Yano, 1998). Previous studies have shown that OO plays a momentous role in enhancing nonspecific immune responses (Mabrok and Wahdan, 2018), suggesting that dietary supplementation with OO was important in the innate immunity of fish. We found that the concentration of C3 and C4 significantly increased in the intestine of hybrid grouper with the diet supplemented with OO. Interestingly, 0.3 g kg-1 of OO distinctly increased the concentrations of C3 and C4 in the intestine, which was consistent with the finding in the koi carp (Zhang et al., 2020). Consequently, these data implied that optimum dietary OO supplementation (0.3 g kg-1) could enhance the immune response in fish.
Oxidative stress is the result of the imbalance of oxidants and antioxidants in the internal environment, which can cause irreversible damage to macromolecules and cells and cause serious damage to the body (Gonsette, 2008). Nevertheless, fish have the ability of scavenging free radicals and dealing with oxidative stress by enzyme (such as GPx, CAT, and GR) and non-enzyme antioxidant defense such as Nrf2/Keap1 system (Sinha et al., 2014). In the present study, OO-treated diets increased the levels of GPx, CAT, and GR expression in the intestine of hybrid grouper. It should be noted that the highest expression of GPx and CAT was observed in the grouper hybrids fed the 0.3 g kg-1 OO diet, remarkably higher than those in the other groups. As a basic leucine zipper redox-sensitive transcriptional factor, Nrf2 always plays an important central role in antioxidant response elements that mediated the induction of antioxidant enzymes. These data suggested that the diet supplemented with OO enhanced the Nrf2-mediated antioxidant status of hybrid grouper, which was consistent with the findings in Nile tilapia (O. niloticus) (Shourbela et al., 2021) and koi carp (Zhang et al., 2020).
Intestinal epithelial cells and TJ-associated protein are the main components of the mechanical epithelial barrier, which plays a tremendous part in preventing the entry of harmful microorganisms, antigens, and related toxins (Groschwitz and Hogan, 2009; Lee, 2015). A previous study has shown that dietary benzoic acid, Bacillus coagulans, and OO supplementation is beneficial to improve the immune status and intestinal barrier integrity and microflora of piglets (Pu et al., 2020). In this study, we observed crucial alterations in the gene expression of TJ-associated proteins. Our study found that OO supplementation contributed to upregulating the expression level of ZO-1 in the intestine of hybrid grouper. It is worth noting that the highest expression levels of ZO-2, Occludin, and Claudin-3a were found in fish fed 0.3 g kg-1 dietary OO and remarkably higher than those of the other groups. These results implied that optimum dietary OO supplementation could reduce the intestinal permeability and enhance the intestinal barrier function, thus strengthening the intestinal tract resistance to the pathogenic bacterial infection.
Another biological barrier of the intestine is the microbiota, which means that the stable intestinal microbiota and intact intestine structure contribute to a healthy intestine, and it thus always affects physiology, immune functions, nutrition, and metabolism (Tran et al., 2018). Firmicutes and Proteobacteria are commonly the most stable bacteria located in the intestine regardless of diet changes in tilapia and barramundi (Lates calcarifer) (Siddik et al., 2020; Li et al., 2022), which was consistent with our study. We also found that the relative abundance of Firmicutes in fish fed the OO-0.3 diet was significantly higher than that fed the control diet. A previous study showed that Firmicutes can oxidize sugar through lactic acid fermentation to fight the invasion of external pathogens, which is beneficial to the body’s growth and intestinal health (Huang et al., 2018). There are also some evidence showing that the increase of Firmicutes of hybrid snakehead fed dietary arginine may be regarded to effectively improve the intestinal microbiota composition and maintain the intestinal microbiota balance (Li et al., 2022). Additionally, in this research, the relative abundance of Vibrio was significantly higher in fish fed the control diet than that fed the OO-0.3 diet. Lots of previous studies showed that Vibrio is known as an opportunistic bacterial pathogen in invertebrate species and marine fish, which was consistent with this study (Austin and Austin, 1999; He et al., 2022). Therefore, this study suggested that the OO-0.3 diet can increase the abundance of beneficial bacteria and decrease that of harmful bacteria in the intestinal microbiota of fish, which means reducing the Vibrio bacteria and affecting the balance of the intestinal microbiota.
In conclusion, our findings have demonstrated that the OO-supplemented diet has no significant effect on the growth performance, while OO could improve the disease resistance ability of grouper. In this study, 0.3 g kg-1 OO could improve the intestinal morphology via increasing the villus number and muscle thickness and elevate the digestive enzyme activities such as AMS and lipase. The 0.3-g kg-1 OO supplement also can activate the antioxidant defense system, reduce the Nrf2/Keap1 expression, and then enhance the immune ability via ACP, LYS, C3, and TJ-associated signaling pathways in hybrid grouper. The relative abundance of Firmicutes and Bacteroidetes in fish fed the OO-0.3 diet was significantly higher than that fed the control diet (P< 0.05), while the relative abundance of Proteobacteria in fish fed the control diet was significantly higher than that fed the OO-0.3 diet (P< 0.05). All of these findings suggested that OO supplementation could significantly improve intestinal health and induce the antioxidant defense system and immunity, which means that OO could be used as a functional feed additive to protect the intestine, and the optimal addition level of OO should be 0.3 g kg-1.
The original contributions presented in the study are included in the article/Supplementary Material. Further inquiries can be directed to the corresponding author.
Animal care and treatment procedures in the current study were approved by the Animal Care and Use Committee of Guangdong Ocean University, China. Written informed consent was obtained from the owners for the participation of their animals in this study.
YX and XD: conceptualization. YX, HL, XY, and WH: methodology. YX, MZ, BL, and YY: formal analysis. YX: writing. XD: review, supervision and funding. BT: review and funding. All the authors read and approved the submitted version.
This research was supported by the Research and Demonstration of Precision Functional Compound Feed Technology of Major Cultured Fishes and Shrimps in South China (2021B0202050002), the General Program of Natural Science Foundation of Guangdong Province (2021A1515011165), the Department of Education of Guangdong Province (2021ZDZX4005), the Nation Natural Science Fund (31972808), Science and Technology Bureau of Zhanjiang (2020A03010 & 2020A05003) and the China Agriculture Research System (CARS-47).
The authors declare that the research was conducted in the absence of any commercial or financial relationships that could be construed as a potential conflict of interest.
All claims expressed in this article are solely those of the authors and do not necessarily represent those of their affiliated organizations, or those of the publisher, the editors and the reviewers. Any product that may be evaluated in this article, or claim that may be made by its manufacturer, is not guaranteed or endorsed by the publisher.
Abolfathi M., Hajimoradloo A., Ghorbani R., Zamani A. (2012). Effect of starvation and refeeding on digestive enzyme activities in juvenile roach, rutilus rutilus caspicus. Comp. Biochem. Physiol. A Mol. Integr. Physiol. 161 (2), 166–173. doi: 10.1016/j.cbpa.2011.10.020
Al-Hijazeen M., Mendonca A., Lee E. J., Ahn D. U. (2018). Effect of oregano oil and tannic acid combinations on the quality and sensory characteristics of cooked chicken meat. Poult Sci. 97 (2), 676–683. doi: 10.3382/ps/pex285
Allaoua M., Etienne P., Noirot V., Carayon J. L., Téné N., Bonnafé E., et al. (2018). Pharmacokinetic and antimicrobial activity of a new carvacrol-based product against a human pathogen, Campylobacter jejuni. J. Appl. Microbiol. 125 (4), 1162–1174. doi: 10.1111/jam.13915
An W. Q., Dong X. H., Tan B. P., Yang Q. H., Chi S. Y., Zhang S., et al. (2020). Effects of dietary n-3 highly unsaturated fatty acids on growth, non-specific immunity, expression of some immune-related genes and resistance to Vibrio harveyi in hybrid grouper (♀ Epinephelus fuscoguttatus × ♂Epinephelus lanceolatu). Fish Shellfish Immunol. 96, 86–96. doi: 10.1016/j.fsi.2019.11.072
Asensio C. M., Paredes A. J., Martin M. P., Allemandi D. A., Nepote V., Grosso N. R. (2017). Antioxidant stability study of oregano essential oil microcapsules prepared by spray-drying. J. Food Sci. 82 (12), 2864–2872. doi: 10.1111/1750-3841.13951
Austin B., Austin B. (1999). Bacterial fish pathogens: Disease of farmed and wild fish. (New York-Chichester: Springer-Praxis Publishing).
Avola R., Granata G., Geraci C., Napoli E., Graziano A. C. E., Cardile V. (2020). Oregano (Origanum vulgare l.) essential oil provides anti-inflammatory activity and facilitates wound healing in a human keratinocytes cell model. Food Chem. Toxicol. 144, 111586. doi: 10.1016/j.fct.2020.111586
Benchaar C. (2020). Feeding oregano oil and its main component carvacrol does not affect ruminal fermentation, nutrient utilization, methane emissions, milk production, or milk fatty acid composition of dairy cows. J. Dairy Sci. 103 (2), 1516–1527. doi: 10.3168/jds.2019-17230
Bourlioux P., Koletzko B., Guarner F., Braesco V. (2003). The intestine and its microflora are partners for the protection of the host: Report on the danone symposium "The intelligent intestine," held in Paris, June 14, 2002. Am. J. Clin. Nutr. 78 (4), 675–683. doi: 10.1093/ajcn/78.4.675
Camilleri M., Madsen K., Spiller R., Greenwood-Van Meerveld B., Verne G. N. (2012). Intestinal barrier function in health and gastrointestinal disease. Neurogastroenterol Motil. 24 (6), 503–512. doi: 10.1111/j.1365-2982.2012.01921.x
Deng J. M., Kang B., Tao L. L., Rong H., Zhang X. (2013). Effects of dietary cholesterol on antioxidant capacity, non-specific immune response, and resistance to aeromonas hydrophila in rainbow trout (Oncorhynchus mykiss) fed soybean meal-based diets. Fish Shellfish Immunol. 34 (1), 324–331. doi: 10.1016/j.fsi.2012.11.008
Dokladny K., Zuhl M. N., Moseley P. L. (2016). Intestinal epithelial barrier function and tight junction proteins with heat and exercise. J. Appl. Physiol. 120 (6), 692–701. doi: 10.1152/japplphysiol.00536.2015
Dominguez-Borbor C., Sanchez-Rodriguez A., Sonnenholzner S., Rodriguez J. (2020). Essential oils mediated antivirulence therapy against vibriosis in Penaeus vannamei. Aquaculture 529, 1–14. doi: 10.1016/j.aquaculture.2020.735639
Ferreira P. D. M. F., Nascimento L. D. S., Dias D. C., Moreira D. M. D. V., Zuanon J. A. S. (2014). Essential oregano oil as a growth promoter for the yellowtail tetra, Astyanax altiparanae. J. World Aquaculture Soc. 45 (1), 28–34. doi: 10.1111/jwas.12094
Gaur S., Lopez E. C., Ojha A., Andrade J. E. (2018). Functionalization of lipid-lased nutrient supplement with β-cyclodextrin inclusions of oregano essential oil. J. Food Sci. 83 (6), 1748–1756. doi: 10.1111/1750-3841.14178
Gilling D. H., Kitajima M., Torrey J. R., Bright K. R. (2014). Antiviral efficacy and mechanisms of action of oregano essential oil and its primary component carvacrol against murine norovirus. J. Appl. Microbiol. 116 (5), 1149–1163. doi: 10.1111/jam.12453
Gonsette R. E. (2008). Neurodegeneration in multiple sclerosis: the role of oxidative stress and excitotoxicity. J. Neurol. Sci. 274 (1-2), 48–53. doi: 10.1016/j.jns.2008.06.029
Goulas A. E., Kontominas M. G. (2007). Combined effect of light salting, modified atmosphere packaging and oregano essential oil on the shelf-life of sea bream (Sparus aurata): Biochemical and sensory attributes. Food Chem. 100 (1), 287–296. doi: 10.1016/j.foodchem.2005.09.045
Groschwitz K. R., Hogan S. P. (2009). Intestinal barrier function: Molecular regulation and disease pathogenesis. Allergy Clin. Immunol. 124, 123–120. doi: 10.1016/j.jaci.2009.06.003
Guangxin G., Li K., Zhu Q., Zhao C., Li C., He Z., et al. (2022). Improvements of immune genes and intestinal microbiota composition of turbot (Scophthalmus maximus) with dietary oregano oil and probiotics. Aquaculture 547, 737442. doi: 10.1016/j.aquaculture.2021.737442
He Y. F., Liang J. F., Dong X. H., Liu H. Y., Yang Q. H., Zhang S., et al. (2022). Soybean beta-conglycinin and glycinin reduced growth performance and the intestinal immune defense and altered microbiome in juvenile pearl gentian groupers Epinephelus fuscoguttatus female x Epinephelus lanceolatus male. Anim. Nutr. 9, 193–203. doi: 10.1016/j.aninu.2021.11.001
Huang Y. C., Shi X., Li Z. Y., Shen Y., Shi X. X., Wang L. Y., et al. (2018). Possible association of firmicutes in the gut microbiota of patients with major depressive disorder. Neuropsychiatr. Dis. Treat 14, 3329–3337. doi: 10.2147/Ndt.S188340
Jang I. S., Ko Y. H., Kang S. Y., Lee C. Y. (2007). Effect of a commercial essential oil on growth performance, digestive enzyme activity and intestinal microflora population in broiler chickens. Anim. Feed Sci. Technol. 134 (3-4), 304–315. doi: 10.1016/j.anifeedsci.2006.06.009
Kaschubek T., Mayer E., Rzesnik S., Grenier B., Bachinger D., Schieder C., et al. (2018). Effects of phytogenic feed additives on cellular oxidative stress and inflammatory reactions in intestinal porcine epithelial cells1. J. Anim. Sci. 96 (9), 3657–3669. doi: 10.1093/jas/sky263
Kuroda N., Naruse K., Shima A., Nonaka M., Sasaki M., Nonaka M. (2000). Molecular cloning and linkage analysis of complement C3 and C4genes of the Japanese medaka fish. Immunogenetics 51 (2), 117–128. doi: 10.1007/s002510050020
Lee S. H. (2015). Intestinal permeability regulation by tight junction: implication on inflammatory bowel diseases. Intest Res. 13 (1), 11–18. doi: 10.5217/ir.2015.13.1.11
Li S. L., Wang C., Wu Z. L. (2022). Dietary l-arginine supplementation of tilapia (Oreochromis niloticus) alters the microbial population and activates intestinal fatty acid oxidation. Amino Acids 54 (3), 339–351. doi: 10.1007/s00726-021-03018-3
Llana-Ruiz-Cabello M., Gutiérrez-Praena D., Puerto M., Pichardo S., Jos Á., Cameán A. M. (2015). In vitro pro-oxidant/antioxidant role of carvacrol, thymol and their mixture in the intestinal caco-2 cell line. Toxicol. In Vitro 29 (4), 647–656. doi: 10.1016/j.tiv.2015.02.006
Mabrok M. A., Wahdan A. (2018). The immune modulatory effect of oregano (Origanum vulgare l.) essential oil on tilapia zillii following intraperitoneal infection with vibrio anguillarum. Aquaculture Int. 26 (4), 1147–1160. doi: 10.1007/s10499-018-0274-y
Magouz F. I., Amer A. A., Faisal A., Sewilam H., Aboelenin S. M., Dawood M. A. O. (2022). The effects of dietary oregano essential oil on the growth performance, intestinal health, immune, and antioxidative responses of Nile tilapia under acute heat stress. Aquaculture 548(1-8), 73763210 doi: 10.1016/j.aquaculture.2021.737632
Migliorini M. J., Boiago M. M., Roza L. F., Barreta M., Arno A., Robazza W. S., et al. (2019). Oregano essential oil (Origanum vulgare) to feed laying hens and its effects on animal health. Acad. Bras. Cienc 91 (1), e20170901. doi: 10.1590/0001-3765201920170901
Moreira Lopes T. C., Mosser D. M., Goncalves R. (2020). Macrophage polarization in intestinal inflammation and gut homeostasis. Inflammation Res. 69 (12), 1163–1172. doi: 10.1007/s00011-020-01398-y
Mowat A. M., Agace W. W. (2014). Regional specialization within the intestinal immune system. Nat. Rev. Immunol. 14 (10), 667–685. doi: 10.1038/nri3738
Nakao M., Yano T. (1998). Structural and functional identification of complement components of the bony fish, carp (Cyprinus carpio). Immunol. Rev. 166 (1), 27–38. doi: 10.1111/j.1600-065x.1998.tb01250.x
Ou W. H., Hu H. B., Yang P., Dai J. H., Ai Q. H., Zhang W. B., et al. (2019). Dietary daidzein improved intestinal health of juvenile turbot in terms of intestinal mucosal barrier function and intestinal microbiota. Fish Shellfish Immunol. 94, 132–141. doi: 10.1016/j.fsi.2019.08.059
Ozel O. T., Cakmak E., Gurkan S. E., Coskun I., Ture M. (2022). Evaluation of oregano (Origanum vulgare) essential oil supplementation on growth performance, digestive enzymes, intestinal histomorphology and gut microbiota of black Sea salmon, Salmo labrax. Ann. Anim. Sci. 22 (2), 763–772. doi: 10.2478/aoas-2021-0068
Preuss H. G., Echard B., Enig M., Brook I., Elliott T. B. (2005). Minimum inhibitory concentrations of herbal essential oils and monolaurin for gram-positive and gram-negative bacteria. Mol. Cell Biochem. 272 (1-2), 29–34. doi: 10.1007/s11010-005-6604-1
Pu J. N., Chen D. W., Tian G., He J., Zheng P., Mao X. B., et al. (2020). Effects of benzoic acid, Bacillus coagulans and oregano oil combined supplementation on growth performance, immune status and intestinal barrier integrity of weaned piglets. Anim. Nutr. 6 (2), 152–159. doi: 10.1016/j.aninu.2020.02.004
Quiroga P. R., Grosso N. R., Nepote V. (2013). Antioxidant effect of poleo and oregano essential oil on roasted sunflower seeds. J. Food Sci. 78 (12), S1904–S1912. doi: 10.1111/1750-3841.12306
Rodriguez-Garcia I., Silva-Espinoza B. A., Ortega-Ramirez L. A., Leyva J. M., Siddiqui M. W., Cruz-Valenzuela M. R., et al. (2016). Oregano essential oil as an antimicrobial and antioxidant additive in food products. Crit. Rev. Food Sci. Nutr. 56 (10), 1717–1727. doi: 10.1080/10408398.2013.800832
Rombout J. H., Abelli L., Picchietti S., Scapigliati G., Kiron V. (2011). Teleost intestinal immunology. Fish Shellfish Immunol. 31 (5), 616–626. doi: 10.1016/j.fsi.2010.09.001
Scramlin S. M., Newman M. C., Cox R. B., Sepe H. A., Alderton A. L., O'Leary J., et al. (2010). Effects of oregano oil brine enhancement on quality attributes of beef longissimus dorsi and semimembranosus muscles from various age animals. J. Food Sci. 75 (2), S89–S94. doi: 10.1111/j.1750-3841.2009.01459.x
Seth A., Sheth P., Elias B. C., Rao R. (2007). Protein phosphatases 2A and 1 interact with occludin and negatively regulate the assembly of tight junctions in the CACO-2 cell monolayer. J. Biol. Chem. 282 (15), 11487–11498. doi: 10.1074/jbc.M610597200
Sheth P., Basuroy S., Li C., Naren A. P., Rao R. K. (2003). Role of phosphatidylinositol 3-kinase in oxidative stress-induced disruption of tight junctions. J. Biol. Chem. 278 (49), 49239–49245. doi: 10.1074/jbc.M305654200
Shi H. T., Zhao S. Z., Wang K. L., Fan M. X., Han Y. Q., Wang H. L. (2022). Effects of dietary astragalus membranaceus supplementation on growth performance, and intestinal morphology, microbiota and metabolism in common carp (Cyprinus carpio). Aquaculture Rep. 22, 100955. doi: 10.1016/j.aqrep.2021.100955
Shourbela R. M., El-Hawarry W. N., Elfadadny M. R., Dawood M. A. O. (2021). Oregano essential oil enhanced the growth performance, immunity, and antioxidative status of Nile tilapia (Oreochromis niloticus) reared under intensive systems. Aquaculture 542(1-8), 736868. doi: 10.1016/j.aquaculture.2021.736868
Siddik M. A. B., Chaklader M. R., Foysal M. J., Howieson J., Fotedar R., Gupta S. K. (2020). Influence of fish protein hydrolysate produced from industrial residues on antioxidant activity, cytokine expression and gut microbial communities in juvenile barramundi Lates calcarifer. Fish Shellfish Immunol. 97, 465–473. doi: 10.1016/j.fsi.2019.12.057
Sinha A. K., AbdElgawad H., Giblen T., Zinta G., De Rop M., Asard H., et al. (2014). Anti-oxidative defences are modulated differentially in three freshwater teleosts in response to ammonia-induced oxidative stress. PLoS One 9 (4), e95319. doi: 10.1371/journal.pone.0095319
Sun Y., Guo C. Y., Wang D. D., Li X. F., Xiao L., Zhang X., et al. (2016). Transcriptome analysis reveals the molecular mechanisms underlying growth superiority in a novel grouper hybrid (Epinephelus fuscogutatus♀ × E. lanceolatus ♂). BMC Genet. 17, 24. doi: 10.1186/s12863-016-0328-y
Suzuki T., Elias B. C., Seth A., Shen L., Turner J. R., Giorgianni F., et al. (2009). PKC eta regulates occludin phosphorylation and epithelial tight junction integrity. Proc. Natl. Acad. Sci. U.S.A. 106 (1), 61–66. doi: 10.1073/pnas.0802741106
Suzuki T., Hara H. (2011). Role of flavonoids in intestinal tight junction regulation. J. Nutr. Biochem. 22 (5), 401–408. doi: 10.1016/j.jnutbio.2010.08.001
Tan X. H., Sun Z. Z., Liu Q. Y., Ye H. Q., Zou C. Y., Ye C. X., et al. (2018). Effects of dietary ginkgo biloba leaf extract on growth performance, plasma biochemical parameters, fish composition, immune responses, liver histology, and immune and apoptosis-related genes expression of hybrid grouper (Epinephelus lanceolatus ♂ ×Epinephelus fuscoguttatus ♀) fed high lipid diets. Fish Shellfish Immunol. 72, 399–409. doi: 10.1016/j.fsi.2017.10.022
Tan X. H., Sun Z. Z., Ye C. X. (2020). Dietary ginkgo biloba leaf extracts supplementation improved immunity and intestinal morphology, antioxidant ability and tight junction proteins mRNA expression of hybrid groupers (Epinephelus lanceolatus ♂ × Epinephelus fuscoguttatus ♀) fed high lipid diets. Fish Shellfish Immunol. 98, 611–618. doi: 10.1016/j.fsi.2019.09.034
Tran N. T., Xiong F., Hao Y. T., Zhang J., Wu S. G., Wang G. T. (2018). Starvation influences the microbiota assembly and expression of immunity-related genes in the intestine of grass carp (Ctenopharyngodon idellus). Aquaculture 489, 121–129. doi: 10.1016/j.aquaculture.2018.02.016
Turner J. R. (2009). Intestinal mucosal barrier function in health and disease. Nat. Rev. Immunol. 9 (11), 799–809. doi: 10.1038/nri2653
Wang L., Wang X. R., Liu J., Chen C. X., Liu Y., Wang W. N. (2015). Rab from the white shrimp Litopenaeus vannamei: Characterization and its regulation upon environmental stress. Ecotoxicology 24 (7-8), 1765–1774. doi: 10.1007/s10646-015-1481-1
Wang L., Wu J., Wang W. N., Cai D. X., Liu Y., Wang A. L. (2012). Glutathione peroxidase from the white shrimp Litopenaeus vannamei: characterization and its regulation upon pH and cd exposure. Ecotoxicology 21 (6), 1585–1592. doi: 10.1007/s10646-012-0942-z
Xia R., Hao Q., Xie Y. D., Zhang Q. S., Ran C., Yang Y. L., et al. (2022). Effects of dietary saccharomyces cerevisiae on growth, intestinal and liver health, intestinal microbiota and disease resistance of channel catfish (Ictalurus punctatus). Aquaculture Rep. 24, 101157. doi: 10.1016/j.aqrep.2022.101157
Xin Y., Yang Z. X., Zhu Y. K., Li Y. X., Yu J., Zhong W. Q., et al. (2022). Hypoxia induces oxidative injury and apoptosis via mediating the nrf-2/Hippo pathway in blood cells of largemouth bass (Micropterus salmoides). Front. Ecol. Evol. 10. doi: 10.3389/fevo.2022.841318
Xu C., Zhong X. Q., Li X. F., Shi H. J., Liu W. B. (2020). Regulation of growth, intestinal microflora composition and expression of immune-related genes by dietary supplementation of Streptococcus faecalis in blunt snout bream (Megalobrama amblycephala). Fish Shellfish Immunol. 105, 195–202. doi: 10.1016/j.fsi.2020.07.011
Ye Q., Feng Y. Y., Wang Z. L., Zhou A. G., Xie S. L., Fan L. F., et al. (2019). Effects of dietary gelsemium elegans alkaloids on intestinal morphology, antioxidant status, immune responses and microbiota of Megalobrama amblycephala. Fish Shellfish Immunol. 94, 464–478. doi: 10.1016/j.fsi.2019.09.048
Zhang R., Wang X. W., Liu L. L., Cao Y. C., Zhu H. (2020). Dietary oregano essential oil improved the immune response, activity of digestive enzymes, and intestinal microbiota of the koi carp, Cyprinus carpio. Aquaculture 518, 734781. doi: 10.1016/j.aquaculture.2019.734781
Zhou R., Wu J. P., Zhang L. P., Liu L. S., Casper D. P., Jiao T., et al. (2019). Effects of oregano essential oil on the ruminal pH and microbial population of sheep. PLoS One 14 (5), e0217054. doi: 10.1371/journal.pone.0217054
Zou Y., Xiang Q. H., Wang J., Peng J., Wei H. K. (2016). Oregano essential oil improves intestinal morphology and expression of tight junction proteins associated with modulation of selected intestinal bacteria and immune status in a pig model. BioMed. Res. Int. 2016, 5436738. doi: 10.1155/2016/5436738
Keywords: oregano essential oil, hybrid grouper, intestinal immunity, intestinal microbiota, tight junction-associated signaling pathways
Citation: Xin Y, Liu H, Yan X, Huang W, Pan S, Zhou M, Lu B, Tan B, Dong X and Yang Y (2022) Effect of dietary oregano oil on growth performance, disease resistance, intestinal morphology, immunity, and microbiota of hybrid grouper (Epinephelus fuscoguttatus ♀ × Epinephelus lanceolatus ♂). Front. Mar. Sci. 9:1038394. doi: 10.3389/fmars.2022.1038394
Received: 07 September 2022; Accepted: 15 November 2022;
Published: 07 December 2022.
Edited by:
Jun Qiang, Freshwater Fisheries Research Center (CAFS), ChinaReviewed by:
Jian-An Xian, Institute of Tropical Bioscience and Biotechnology, ChinaCopyright © 2022 Xin, Liu, Yan, Huang, Pan, Zhou, Lu, Tan, Dong and Yang. This is an open-access article distributed under the terms of the Creative Commons Attribution License (CC BY). The use, distribution or reproduction in other forums is permitted, provided the original author(s) and the copyright owner(s) are credited and that the original publication in this journal is cited, in accordance with accepted academic practice. No use, distribution or reproduction is permitted which does not comply with these terms.
*Correspondence: Xiaohui Dong, ZG9uZ3hpYW9odWkyMDAzQDE2My5jb20=
Disclaimer: All claims expressed in this article are solely those of the authors and do not necessarily represent those of their affiliated organizations, or those of the publisher, the editors and the reviewers. Any product that may be evaluated in this article or claim that may be made by its manufacturer is not guaranteed or endorsed by the publisher.
Research integrity at Frontiers
Learn more about the work of our research integrity team to safeguard the quality of each article we publish.