- 1UMR 8187, CNRS, Laboratoire d’Océanologie et de Géosciences, Station Marine de Wimereux, University of Lille, University of Littoral Côte d’Opale, Lille, France
- 2UMR 1158 BioEcoAgro, TERRA Viollette, USC Anses, INRAE, Yncréa, University of Lille, University of Littoral Côte d’Opale, University of Artois, University of Picardie Jules Verne, University of Liège, Boulogne-sur-Mer, France
- 3Department of Biological Sciences, College of Science, Sungkyunkwan University, Suwon, South Korea
Copepods represent an interesting alternative or a complement live food to brine shrimps and rotifers commonly used in aquaculture. They constitute the natural prey of many fish species and therefore do not require a potential nutritional enrichment. But an optimization of the microalgal diets used to feed copepods is essential to improve their mass culture. This study examined the effects of seven microalgal diets, namely single-species diets of Rhodomonas salina (R), Tisochrysis lutea (T), and Pavlova lutheri (=Diacronema lutheri) (P), two-species diets (R + T, T + P, and R + P), and a three-species diet (R + T + P), on the fatty acid and monosaccharide composition of the cyclopoid copepod Paracyclopina nana as well as its reproductive investment. Experiments were run during 15 days in 10-L beakers; starting with nauplii collected from a large 300-L batch culture. Copepods fatty acid contents were studied, particularly the relative amounts of docosahexaenoic acid (DHA) and eicosa-pentaenoic acid (EPA). The R + T, R, and T diets induced the highest total fatty acid amount in copepods. R + T and R also generated the lowest DHA/EPA ratios in copepods due to high EPA contents. The highest value of total monosaccharides was found in copepods fed with R + T + P. Diets R + T and R induced the greatest prosome volumes and clutch volumes in ovigerous females. Both prosome volume and clutch volume in P. nana ovigerous females were correlated to the individual EPA amount. The results demonstrated that all diets including R. salina enhanced the productivity of P. nana in mass culture, particularly when combined with T. lutea. R. salina, and T. lutea induced complementary fatty acid and monosaccharide profiles, confirming that R + T represents the best microalgae combination for productive culture of P. nana. Conversely, P. lutheri did not enhance the nutritional profile nor the fecundity of P. nana in the culture. This study is the first to demonstrate that R. salina is a suitable microalga for productive mass culture of P. nana for use as live food in aquaculture.
Introduction
Copepods are the primary component of zooplankton and have a key role as the trophic link between phytoplankton and secondary consumers in marine ecosystems (Breteler et al., 1990; Mauchline, 1998; Støttrup, 2000). They serve as an important food source for numerous fish and crustacean larvae (Sun and Fleeger, 1995; Turner, 2004). Commercial fish species are bred in aquaculture on diets comprising brine shrimps and rotifers, as these organisms are easy to grow in large volumes at relatively low cost (Støttrup and McEvoy, 2008; Dhont et al., 2013). However, brine shrimp and rotifers are not the natural prey species of many of the fish species grown in aquaculture, and do not always induce optimal fish larval growth because of their inadequate size or fatty acid content, therefore nutritional enrichment is sometimes required (Fernández-Reiriz et al., 1993; Thinh et al., 1999; Sorgeloos et al., 2001; Lubzens et al., 2003). Mass culture of several marine copepod species for use as live feed for marine fish cultures has been explored by several research groups (Ohno et al., 1990; Støttrup and Norsker, 1997; Payne and Rippingale, 2000; Van der Meeren et al., 2014; Blanda et al., 2017). Copepods have been found to have superior nutritional value to that of commonly used live prey such as brine shrimp and rotifers, and to enhance the growth and development of many fish species of commercial or ornamental interest such as the Atlantic halibut, the Pink snapper or the Yellowtail clownfish (McEvoy et al., 1998; Shields et al., 1999; Payne and Rippingale, 2000; Payne et al., 2001; Olivotto et al., 2008). More recent studies confirmed that the use of small nauplii of copepods such as the calanoid Parvocalanus crassirostris in breeding of new ornamental fishes with very small mouth is required (Anzeer et al., 2019; Burgess et al., 2020).
Copepods have a high content of highly unsaturated fatty acids (HUFAs) such as docosahexaenoic acid (DHA) and eicosa-pentaenoic acid (EPA), which are particularly important nutrients for the development of fish larvae (Sargent et al., 1997; McEvoy et al., 1998; Pan et al., 2014; Mejri et al., 2021) thus, copepod species with a high lipid concentration and high fecundity are desirable from an aquaculture perspective. Copepod productivity can be measured by female fecundity and fatty acid content (Souissi et al., 2016; Lee et al., 2017), which is related to the amount of carbon that the copepods ingest. Monosaccharides, are the only type of carbohydrates that are rarely stored by crustaceans, but carbohydrates are also no less important than lipids because they are rapidly metabolized after ingestion for many vital functions and thus are key contributors to biological performance (Cuzon et al., 2000; Hohnke and Scheer, 2012). It is therefore essential to supply copepod mass cultures with optimal microalgal diets to induce maximal fecundity, larval development, and nutritional storage (Pan et al., 2014). Optimization of diets for copepod cultures has been investigated previously, but many questions remain to be explored. The appropriate microalgal diet has been investigated for copepods in the orders Harpacticoida (Pinto et al., 2001; Arndt and Sommer, 2014), Calanoida (Milione et al., 2007; Camus et al., 2009; Ohs et al., 2010; Jeyaraj and Santhanam, 2012; Pan et al., 2014; Siqwepu et al., 2017), and Cyclopoida (Lee et al., 2006; Rasdi and Qin, 2016; Pan et al., 2018). Results have shown that mixed algal diets are more effective than monoalgal diets. However, these previous studies did not use the same species combinations of microalga and cannot be generalized to all copepod species. Several studies have noted the beneficial effects of red microalgae from the genus Rhodomonas on female fecundity (Ohs et al., 2010; Siqwepu et al., 2017). The biochemical composition of Rhodomonas sp. is well characterized, especially the fatty acid profiles of species in this genus (Latsos et al., 2020). Renaud et al. (1999) found that lipids accounted for 18.7% of the dry weight of Rhodomonas sp. and that most of this lipid content comprised polyunsaturated fatty acids (65.8%). However, the carbohydrate profile of Rhodomonas has not been well characterized. Another micro-algae genus widely used in aquaculture is Isochrysis. The lipid content of Isochrysis sp. can reach 23.4% of dry weight (Renaud et al., 1999). The carbohydrate content of Tisochrysis lutea, a species in the genus Isochrysis, accounts for 23% of this organism’s dry weight and has been reported to be a complex mixture of polysaccharides such as glucose, galactose, mannose, xylose, arabinose, xylose, and rhamnose in various proportions, all of which are essential for copepod metabolism (Chu et al., 1982; Gnouma et al., 2017). The marine phytoflagellate Pavlova sp. (=Diacronema sp.) is also of great interest as it is known to be able to synthesize DHA and EPA in large amounts (Rehberg-Haas, 2014). Patil et al. (2007) stated that Pavlova sp. comprises around 13 mg/g of DHA and 18 mg/g of EPA and that these values can even be enhanced by accordingly adjusting its culture conditions.
The brackish water cyclopoid copepod Paracyclopina nana Smirnov 1935 has strong potential for use in the aquaculture industry (Lee et al., 2006, 2013; Ki et al., 2009). Native to the bays and estuaries of Eastern Asia (Japan, Taiwan, South Korea), it has a small size at adult stage (600 μm on average), high tolerance of salinity (from 5 to 30 ppt), endures culture at high densities (up to 20 ind./mL), and grows under a wide range of temperatures (from 15 to 30°C) (Lee et al., 2012b, 2017). Its small first naupliar stage (<80 μm) is also important when breeding fish species that have tiny first feeding larval stages. Recent studies conducted on P. nana attempted to establish the optimal diet for its growth in aquaculture (Min et al., 2006; Lee et al., 2012a). However, these studies focused on algal monoculture, while studies carried out on other copepod species demonstrated that microalgae quality and diversity can enhance copepods productivity (Pan et al., 2014). It is therefore essential to optimize copepod culture conditions by selecting the right combination of microalgae.
The aim of this study was therefore to examine which of a variety of different microalgal diets had optimal nutritive quality and maximized reproductive investment of P. nana populations grown in mass culture.
Materials and Methods
The section “Materials and Methods” used in this study are the same as those described in Dayras et al. (2020), as the results presented here were derived from the same set of experiments. Methods and materials are therefore summarized below and the reader is invited to refer to Dayras et al. (2020) for further details. On the contrary the volume measurement of copepod females as well as the nutritional profile of microalgae and copepods are only presented in this paper and therefore their methods were detailed.
Microalgae Species Selection
Three species of microalgae were individually grown in the laboratory using standardized methods: Rhodomonas salina (RCC20), T. lutea (RCC1349), and Pavlova lutheri (=Diacronema lutheri) (RCC1537), which were obtained from the Roscoff Culture Collection of Marine Microalgae1. R. salina and T. lutea are currently widely used in zooplankton cultures for their nutritional value and P. lutheri has a desirable fatty acid profile (DHA, EPA) (Coutteau, 1996; Brown, 2002). These three species belong to separate genera and families of microalgae. As a result, their physiology, and therefore their physico-chemical composition and nutritional quality, are assumed to be different (Brown, 2002).
To establish which species and combinations of microalgae species are the most beneficial to P. nana maintained in culture in terms of population growth and individual nutritional value, it is essential to construct microalgal diets where the species are combined in equivalent proportions relative to their chemical composition (Pan et al., 2014). Within the different defined diets, each microalgal species should provide equivalent amounts of carbon to test only the effect of the species.
We used carbon as a proxy to establish equivalent ratios among the three microalgae species (Pan et al., 2014). CHN analysis was performed using an elemental analyzer (FLASH 2000 Series CHNS/O Analyzer, Thermo Fisher Scientific, Waltham, MA, United States) to assess the basic chemical profiles of each of these three microalgae cultures and deduce their respective cellular carbon concentrations. All different possible microalgal diets of the combined species were then constructed so that the total feeding volume remained the same. Diets were provided for a period of 15 days to copepods starting from early naupliar stages.
Establishment of Microalgae Diets
CHN Analysis, Microalgal Cell Density, and Carbon Cellular Amount
Prior to experiments, CHN analysis was performed on samples of each of the three microalgal cultures at their exponential phase to determine the carbon content of each species. Microalgae culture conditions in the laboratory were standardized to growth in natural seawater salinity, a constant temperature of 18°C, and a 12-h light:12-h dark photoperiod. These culture conditions were used before CHN analysis and were maintained throughout the experiment. Microalgal cultures in exponential phase were used to feed copepods. To estimate the carbon content of single algal cells, the number of cells present in a 1-mL culture of each microalgal species was counted in five replicates. The average amount of carbon in each species in this volume was then divided by the total number of cells present in this volume. Further details can be found in Dayras et al. (2020).
Differences in carbon concentrations in each microalgal species compared with those reported in the literature are likely due to culture conditions (temperature, light, and salinity), which can affect carbon content (Renaud et al., 1995; Hu, 2004; Pal et al., 2011). For this reason, we used the results from our CHN analyses as reference values in this study.
Microalgal Diet Combinations and Relative Proportions of Microalgae
Given three species of microalgae [R. salina (R), T. lutea (T), and P. lutheri (P)], seven different diets combinations are possible:
– Three single-species diets: R, T, and P.
– Three two-species diets: R + T, T + P, and R + P.
– One three-species diet: R + T + P.
Because of variability in cellular carbon concentration and cell density in microalgae cultures, we used the volume of microalgal solution as a proxy for carbon concentration in our study. The total required volume of each diet for daily feeding was deduced from the standard volume of microalgae used to feed permanent copepod cultures maintained in the laboratory, and corresponded to a volume of 9.1 mL of microalgal culture per day for each large beaker of 10 L. This volume of algal cells corresponds to a carbon amount that is not limiting for P. nana (Lee et al., 2012a). To create the combination diets, volume ratios of each of the microalgal species that contained the same proportional amount of carbon in the same total volume of 9.1 mL were deduced based on the results of CHN analysis so that species parameter is the only parameter being tested.
Microalgal Cultures and Initial Sampling
Microalgae were grown in batch cultures in six different autoclaved 2 L flasks following the protocol of Sadovskaya et al. (2014). Specific culture conditions used are described in detail in Dayras et al. (2020). Three additional 2 L flasks were set up with the exact same protocol, one for each species, and were used exclusively for fatty acid and carbohydrate analyses. When exponential phase growth was reached by each of the three microalgal species, 1 mL samples were obtained and fixed with Lugol’s solution to count cells. The entire remaining volume of culture of each species was then centrifuged and lyophilized for further fatty acid and carbohydrate analyses.
Copepod Culture and Initial Sampling
Paracyclopina nana used in current experiments was originally obtained from J-SL from Sungkyunkwan University of South Korea in 2015. Copepods were grown in the laboratory in a 300-L cylindrical acrylic tank. Specific culture conditions and the collecting protocol are described in Dayras et al. (2020). Adult stages were removed and half the amount of nauplii present in the tank were kept for experiments.
Seven 10-L beakers containing 8 L of culture medium were set up, one for each of the diet combination being evaluated. The use of a single replicate of these large beakers was justified by our preliminary observations of low variability between replicate P. nana cultures. Each beaker was filled with 8 L of 15 ppt water and placed at a constant temperature of 18°C with a 12-h light:12-h dark photoperiod. The 1.2 L aliquot of concentrated nauplii was divided in 12× 100-mL aliquots. Seven of these aliquots were diluted in one of the seven beakers used in the experiments; two were individually filtered to remove any water, concentrated in cryotubes, and stored at −80°C for both fatty acid and carbohydrate analyses; and the remaining three aliquots of 100 mL were used for other analyses.
Experiment Monitoring
Daily Feeding With Microalgal Diets
Each beaker of copepods was fed daily with a total volume of 9.1 mL of each combination of microalgal diet containing relative proportions of the different microalgal species as described in Dayras et al. (2020).
Daily and Final Sampling
Each day, 1 mL of each microalgal culture was sampled to determine the exact daily amount of carbon provided after cellular counting. Corresponding data can be found in Dayras et al. (2020).
At the end of the experiment (15 days), two volumes of 500 mL were obtained from each of the seven copepod beakers. These 500-mL samples were individually filtered to remove water, and copepods were collected and concentrated in cryotubes and stored at −80°C for both fatty acid and carbohydrate analyses. This sampling was supplemented by sorting 20 ovigerous females for morphological measurements (prosome length and width) and fertility assessment (number and size of eggs per ovigerous female).
Ovigerous Female Morphological Measurements
The 20 fixed ovigerous females obtained from each diet condition were individually photographed and studied under an inverted microscope (model Olympus IX71, Tokyo, Japan) with 10× magnification coupled to a ToupCam camera (model UCMOS05100KPA, Zhejiang, China) connected to a computer using ToupView software from ToupTek Photonics (version 3.7). For each single ovigerous female, a photo of the whole body was taken for measurements. Egg sacs were then manually detached and the eggs were photographed.
Morphological measurements were assessed from photographs using ImageJ software (version 1.48v) as described in Souissi et al. (2016). Prosome length and width and egg diameter were manually measured for each ovigerous female. Prosome volume was then calculated using the formula used to calculate the volume of an ellipsoid, namely V = ab2 where a corresponds to half the body length and b corresponds to half the body width.
Fatty Acid Analysis
To investigate variations in internal fatty acid quantity induced by the different tested diets, fatty acid methyl esters (FAMEs) of copepods and microalgae samples stored at −80°C were prepared by direct transesterification reactions. We used the method detailed in Pan et al. (2018). Five milligrams of lyophilized copepods and microalgal pellets (n = 1 and n = 3, respectively) were evaluated. Extracted FAMEs were stored at −20°C until gas chromatography (GC) analysis, which was performed using a Trace GC ULTRA system (Thermo Fisher Scientific, Waltham, MA, United States) equipped with a capillary column NMTR-5MS (30 m × 0.25 mm) and FID detector using a temperature gradient of 170°C (3 min) → 250°C at a heating rate of 5°C/min. Peaks were identified with appropriate standards and samples previously analyzed by GC-MS (Pan et al., 2018). Copepod values were converted and quantified using the internal standard C17:0 quantity introduced in each sample (10 μg) and directly expressed in μg/mg DW (μg per mg of dry weight). The fatty acid amount was then estimated relative to the fatty acid quantity measured in a control sample (T0) at the beginning of the experiment. For microalgae, the number of cells in 1 mL triplicates was counted and converted to the initial tested volume of 1700 mL for each species, then extrapolated for 5 mg and a mean value was calculated. Results were then also expressed in μg/mg DW.
Carbohydrate Analysis
Internal carbohydrate quantities of the different copepod and microalgae samples were also measured. Monosaccharide analysis of cells was performed as described previously (Sadovskaya et al., 2014). After lyophilization of all samples, whole copepod samples and 5 mg of microalgal pellets in triplicate were used (n = 1 and n = 3, respectively), after recording sample weights. Samples were defatted by extraction with CHCl–MeOH, 2:1 and 1:2 (v/v), and then air-dried. Residue was hydrolyzed with 4 M TFA (110°C, 3 h) in the presence of a known amount of myo-inositol, the internal standard. Fifty microliters of myo-inositol concentrated to 180 μg/100 μL (corresponding to 90 μg) was used for microalgae samples and 50 μL of myo-inositol concentrated to 9 μg/100 μL (corresponding to 4.5 μg) was used for copepod samples. The acid was evaporated with nitrogen, and released monosaccharides were reduced with NaBH4 overnight. Excess NaBH4 was removed with 10% AcOH in MeOH and the solution was evaporated. The procedure was repeated twice more with 10% AcOH in MeOH and twice more with MeOH to remove borates. Alditols were then acetylated with 0.4 mL of Ac2O–0.4 mL pyridine mixture for 1 h at 100°C. Reagents were evaporated with toluene, residue was dissolved in chloroform, washed twice with water, filtered through a cotton filter, and concentrated prior to GC analysis. GC was performed using a Trace GC ULTRA system (Thermo Fisher Scientific, Waltham, MA, United States) equipped with a capillary column NMTR-5MS (30 m × 0.25 mm) and FID detector using a temperature gradient of 170°C (3 min) → 250°C at a heating rate of 5°C/min. Results were converted to μg/mg DW from the initial standard myo-inositol quantity introduced into each sample and mean values were then calculated.
Statistical Analysis
To test the effect of diet and potential correlations between the three parameters of prosome volume, clutch volume, and EPA amount in ovigerous females, Pearson’s chi-squared test was used and applied to each combination of two parameters. All statistical tests were conducted at the 95% confidence level. To test the effect of all treatments on female volume and clutch volume, we performed an ANOVA and MULTICOMPARE tests. These analyses were performed using the Matlab Software (Mathworks Inc., Version, 7.5).
Results
Effects of Microalgal Diet on Fatty Acid Composition of P. nana
A typical chromatogram illustrating the fatty acid profiles obtained by gas chromatography (GC) in copepod samples can be seen in Figure 1, corresponding to the copepods fed with the R + T diet. Predominant fatty acids in P. nana were the C14:0, C16:1, C16:0, C18:0, C20:5 (EPA), and C22:6 (DHA), and their detailed amounts in all microalgae and copepods samples are presented in Table 1. Tisochrysis lutea (T) had the highest total fatty acid content of the three species evaluated (85.38 ± 4.36 μg/mg DW), whereas, R. salina (R) had the lowest total fatty acid content (66.38 ± 7.22 μg/mg DW) and also the lowest amount of DHA (5.04 ± 0.62 μg/mg DW). Both of these microalgae contained particularly high amounts of C18:0 (22.35 ± 2.54 μg/mg DW for R and 23.62 ± 1.25 μg/mg DW for T), the double what P contained (11.22 ± 0.66 μg/mg DW of C18:0). T. lutea contained the highest DHA amount (8.31 ± 0.56 μg/mg DW) but the lowest EPA amount (0.69 ± 0.24 μg/mg DW) among the three tested microalgae species, resulting in the highest DHA/EPA ratio (12.1). P. lutheri (P) presented high levels for each individual fatty acid except C18:0 compared to the other two species and particularly concerning C16:1 and C16:0 (12.68 ± 2.02 μg/mg DW and 12.52 ± 1.90 μg/mg DW, respectively). P. lutheri especially contained a much higher EPA amount (25.43 ± 1.27 μg/mg DW) and a relatively high DHA amount (7.68 ± 0.84 μg/mg DW), resulting in the lowest DHA/EPA ratio among the three species (0.30).
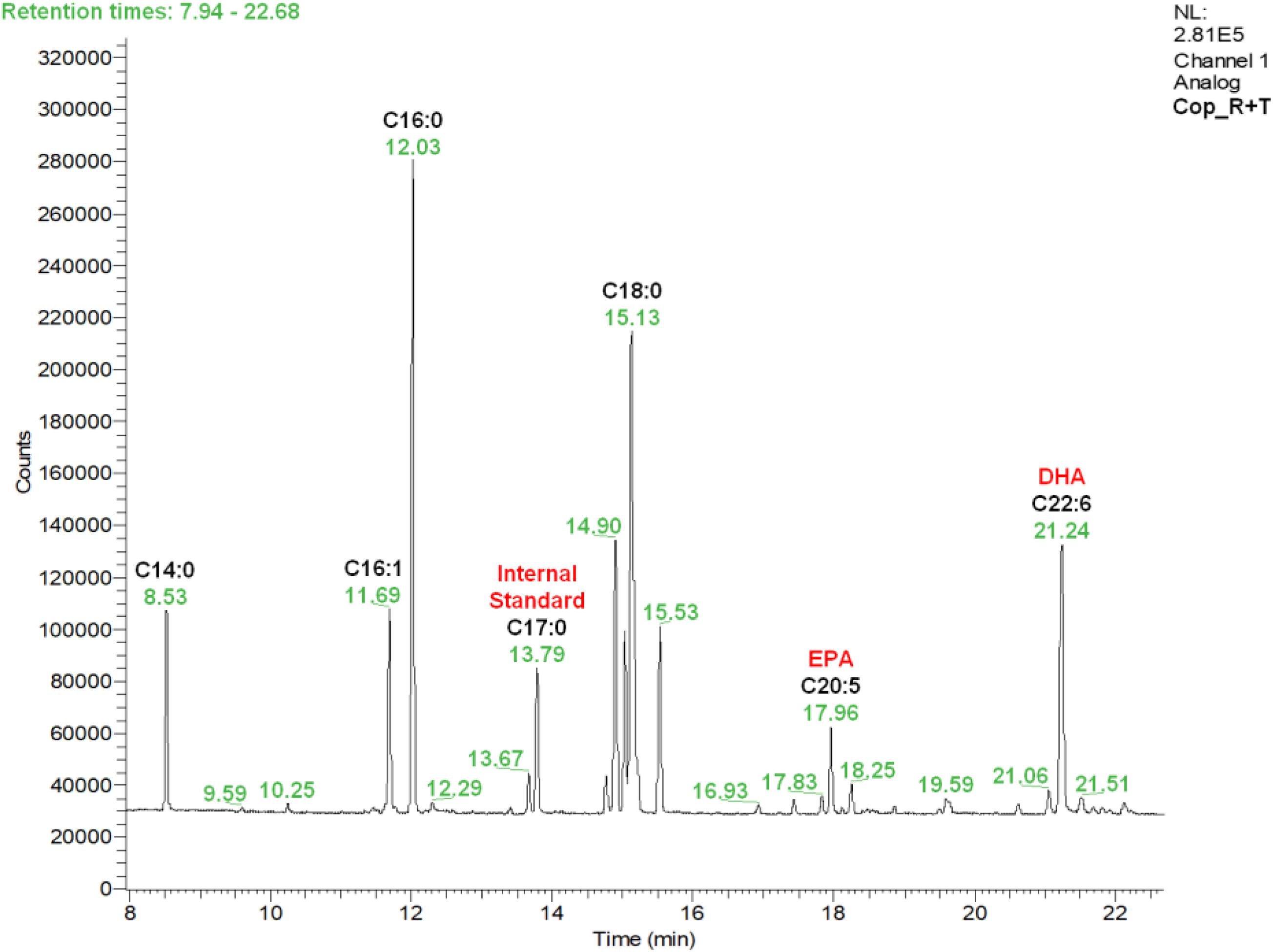
Figure 1. Fatty acid profile obtained by gas chromatography (GC) in Paracyclopina nana copepods fed with the microalgal diet R + T. Respective rentention times for each peak appear in green. Peaks corresponding to the identified fatty acids and the internal standard are annotated in bold black. R, Rhodomonas salina; T, Tisochrysis lutea.
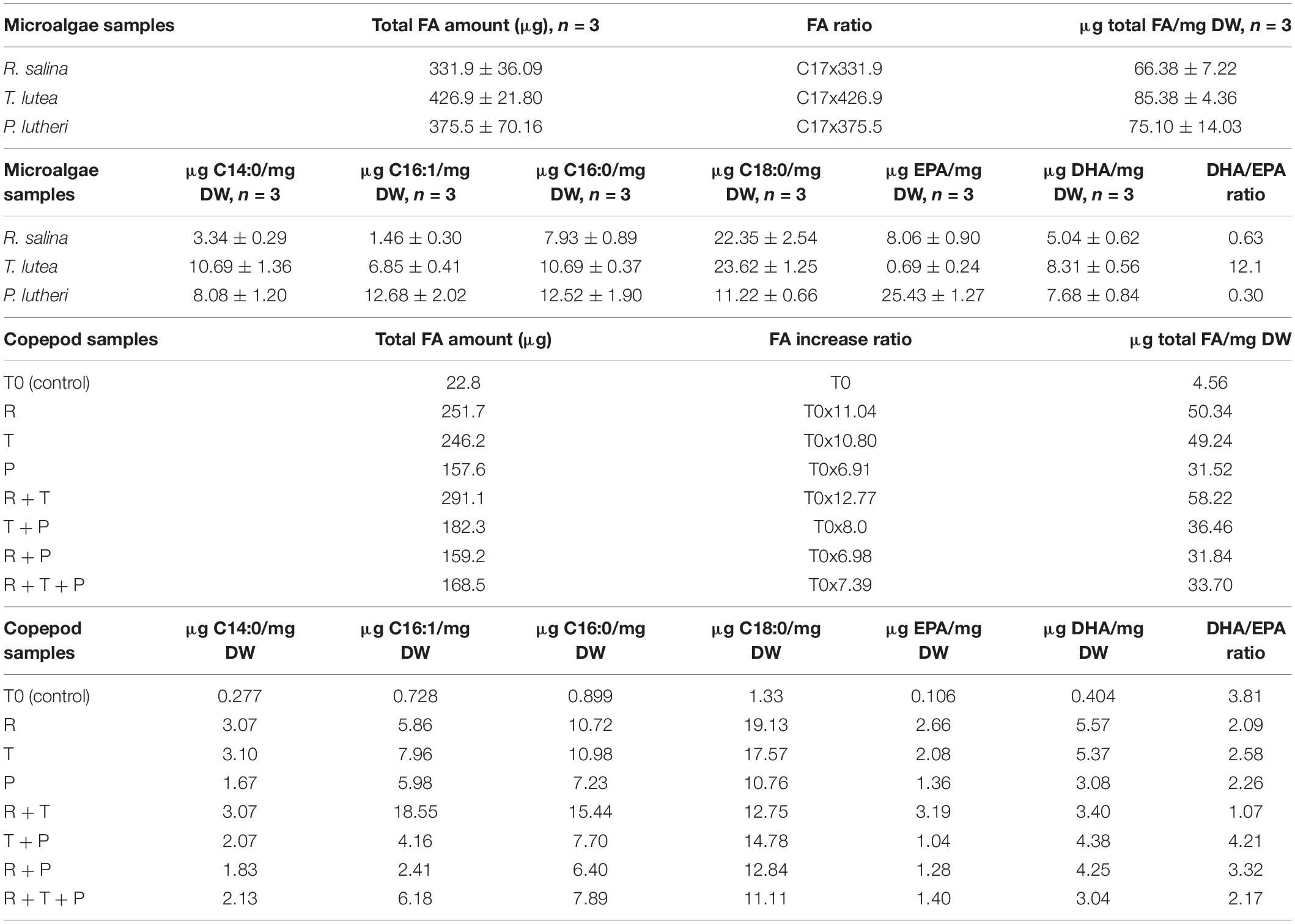
Table 1. Total fatty acid amounts and respective EPA and DHA contents in each copepod and microalgae lyophilized sample.
Copepods fed on diets R + T (291.1 μg/mg DW), R (251.7 μg/mg DW), and T (246.2 μg/mg DW) for 15 days had the highest amounts of fatty acids; these values correspond to increase ratios of 12.77, 11.04, and 10.80, respectively. Diets R and T both engendered high levels of C16:0 (10.72 μg/mg DW and 10.98 μg/mg DW, respectively) and the highest C18:0 levels (19.13 μg/mg DW and 17.57 μg/mg DW, respectively) in copepods. The highest amounts of C16:1 (18.55 μg/mg DW) and C16:0 (15.44 μg/mg DW) in copepods have been induced by the R + T diet. Diets that induced the smallest fatty acid increases in copepods were R + T + P (168.5 μg/mg DW), R + P (159.2 μg/mg DW), and P (157.6 μg/mg DW), corresponding to increase ratios of 7.39, 6.98 and 6.91, respectively. Overall, the R + P and R + T + P diets engendered lower levels of each fatty acid than the other diets, and particularly the P diet which led to copepods containing some of the lowest levels of each individual studied fatty acid. R + T also induced the highest EPA amount of 3.19 μg in copepods among all seven tested diets, and the lowest DHA/EPA ratio (1.07). The highest DHA amounts were detected in copepods fed the R and T diets at 5.57 μg/mg DW and 5.37 μg/mg DW, respectively. A high level of C18:0 (14.78 μg/mg DW) and also the highest DHA/EPA ratio were found in copepods fed the T + P diet due to the low amount of EPA this diet induced (1.04 μg/mg DW).
Effects of Microalgal Diet on the Monosaccharide Composition of P. nana
Detailed amounts of seven specific monosaccharides in microalgae and copepods samples are shown in Table 2. Rhodomonas salina (R) had the highest total monosaccharide content of the three species (46.60 ± 4.71 μg/mg DW), whereas, T. lutea (T) had the lowest total monosaccharide content (28.28 ± 9.20 μg/mg DW). R had a higher amount of glucose than the other two species (36.46 ± 3.31 μg/mg DW) and was also the only species in which fucose was detected (1.23 ± 0.17 μg/mg DW). T contained the highest amounts of mannose (3.35 ± 1.03 μg/mg DW), galactose (7.55 ± 2.82 μg/mg DW), arabinose (9.55 ± 2.53 μg/mg DW), and xylose (2.66 ± 1.22 μg/mg DW). P contained the smallest amounts of each of the different monosaccharides evaluated with the exception of ribose (3.96 ± 1.50 μg/mg DW).
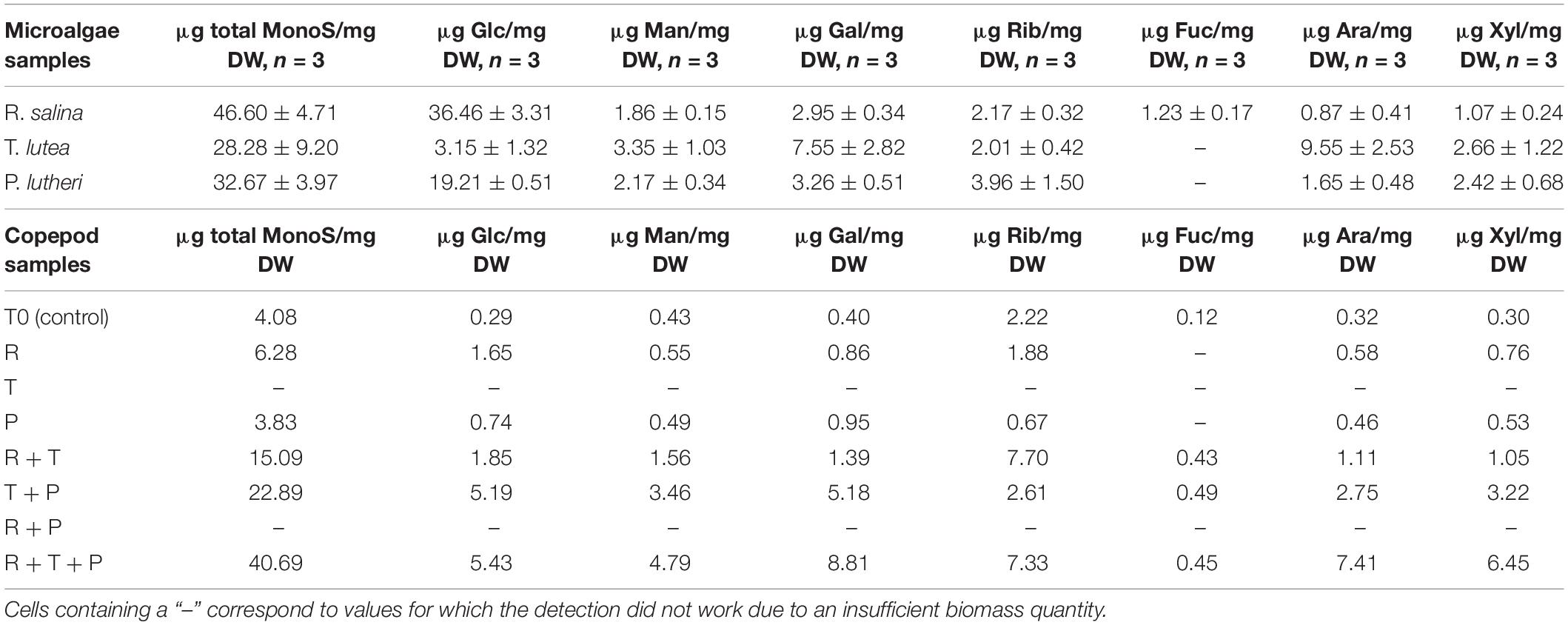
Table 2. Total monosaccharide amounts and respective amounts of seven specific monosaccharides for each copepod and microalgae lyophilized sample.
The R + T + P diet induced the highest total monosaccharide amount in copepods after 15 days of feeding among the seven tested diets at 40.69 μg/mg DW. T + P (22.89 μg/mg DW) and R + T (15.09 μg/mg DW) diets also resulted in a high total monosaccharide content in copepods. Fucose was only detected in copepods fed these three diets : R + T + P, T + P, R + T). R + T + P and T + P diets both led to relatively high amounts of each of the studied monosaccharides in comparison with the five other diets, with the monosaccharide quantities generally highest for the R + T + P diet with the exception of ribose, which was present in the highest quantity in copepods fed the R + T diet (7.70 μg/mg DW). Diet P was associated with the lowest total monosaccharide amount in copepods (3.83 μg/mg DW) in comparison with all treatments, which was even less than the initial amount present in the T0 copepod sample (4.08 μg/mg DW). Furthermore, diet P was associated with the lowest amount of monosaccharides among the seven tested diets except for galactose (0.95 μg/mg DW).
Effects of Microalgal Diet on the Reproductive Investment of P. nana Ovigerous Females
Ovigerous Female Prosome Volume and Clutch Volume
The distribution of ovigerous females prosome volumes after 15 days of receiving one of the seven diets is shown in Figure 2A. R + T and R diets induced the greatest prosome volumes in ovigerous females (R + T: 8.40 × 10–3 ± 0.66 × 10–3 mm3, R: 7.82 × 10–3± 0.82 × 10–3 mm3), whereas, the R + P and T diets were associated with the smallest prosome volumes (R + P: 7.37 × 10–3 ± 1.16 × 10–3 mm3, T: 6.82 × 10–3± 0.93 × 10–3 mm3). The average clutch volume of these same ovigerous females (Figure 2B) was also the greatest for copepods fed the R + T, R, and R + T + P diets (R + T: 2.59 × 10–3 ± 0.55 × 10–3 mm3, R: 2.36 × 10–3 ± 0.37 × 10–3 mm3, R + T + P: 2.27 × 10–3 ± 0.47 × 10–3 mm3), whereas, the P diet resulted in the smallest clutch volume (1.09 × 10–3 ± 0.31 × 10–3 mm3).
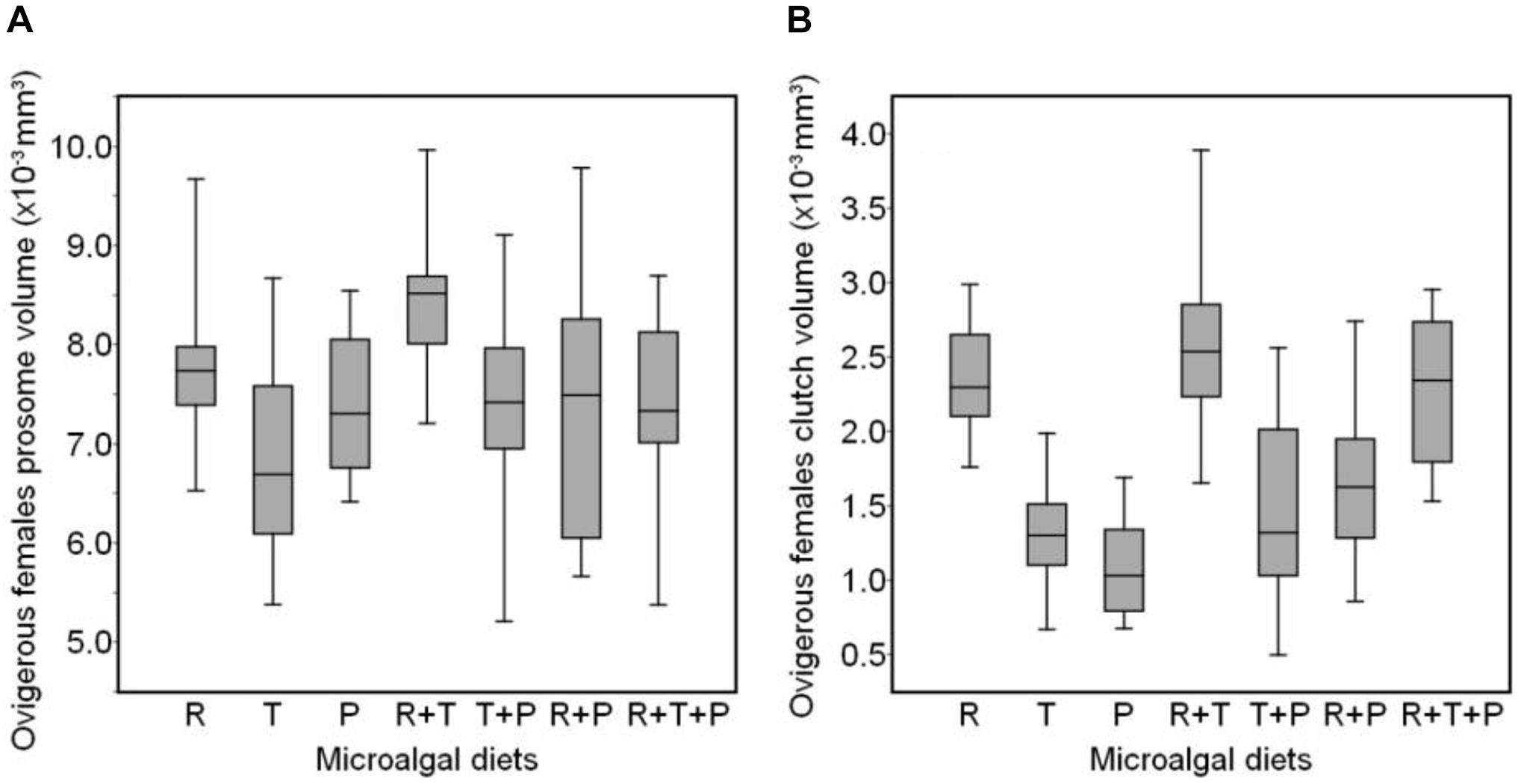
Figure 2. Boxplot showing the distribution of prosome volume (A) and clutch volume (B) of Paracyclopina nana ovigerous females fed on different microalgal diets. Shaded boxes represent the first and third quartiles with the middle bar indicating the median. The end of the whiskers extends from the hinge to the lowest and highest value within a 1.5 inter-quartile range. R, Rhodomonas salina; T, Tisochrysis lutea; P, Pavlova lutheri.
A plot of clutch volume versus prosome volume for each microalgal diet confirmed a linear relationship between female clutch volume and prosome volume (Figure 3). In particular, the R + T diet resulted in the highest values for both parameters and a linear relationship between ovigerous females clutch volume and prosome volume (y = 0.9163x - 5.069) with a significant correlation coefficient (R2 = 0.5841, p < 0.01). Both of these morphological parameters were also evaluated with regard to the EPA data; clutch volume and prosome volume were each plotted against EPA amount for each microalgal diet (Figures 4, 5, respectively). As can be seen from these plots, R + T and R diets yielded the highest values. A linear relationship was found between ovigerous female clutch volume and EPA amount (y = 0.4781x + 0.9374), with a significant correlation coefficient (R2 = 0.446, p < 0.01), as well as between ovigerous female prosome volume and EPA amount (y = 0.387x + 6.8058), with a significant correlation coefficient (R2 = 0.4202, p < 0.01). The other tested relationships, in particular DHA amount or total fatty acid amount, did not show any significant correlations with clutch volume or prosome volume.
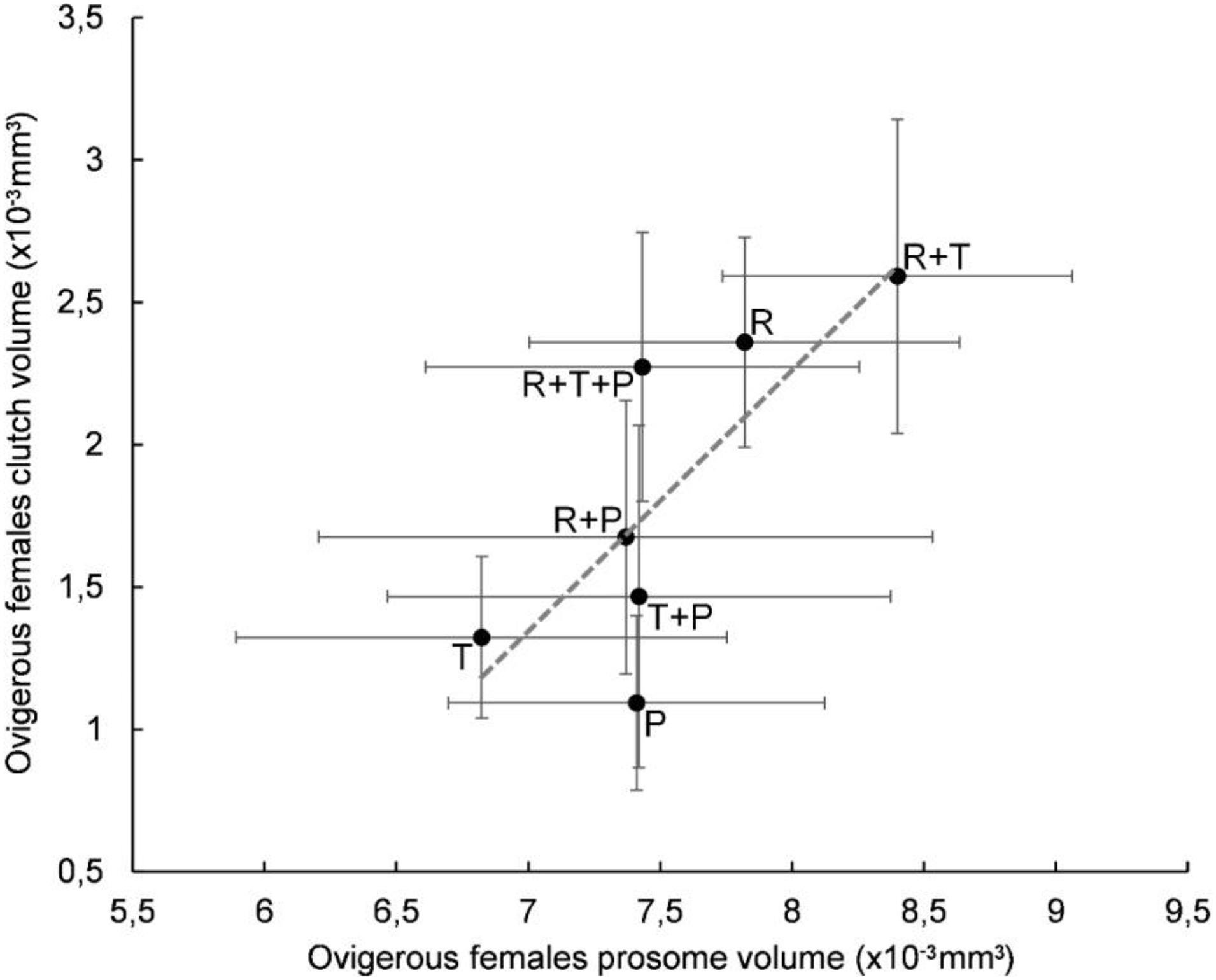
Figure 3. Relationship between average prosome volume and clutch volume in Paracyclopina nana ovigerous females for each microalgal diet. Error bars show the standard deviations obtained from n = 20 females. Dashed line corresponds to the linear regression (EV = 0.9163 X PV – 5.069, R2 = 0.5841, p < 0.01). Pearson’s test was performed to determine the significance of the coefficient of determination R2. R: Rhodomonas salina, T: Tisochrysis lutea, P: Pavlova lutheri.
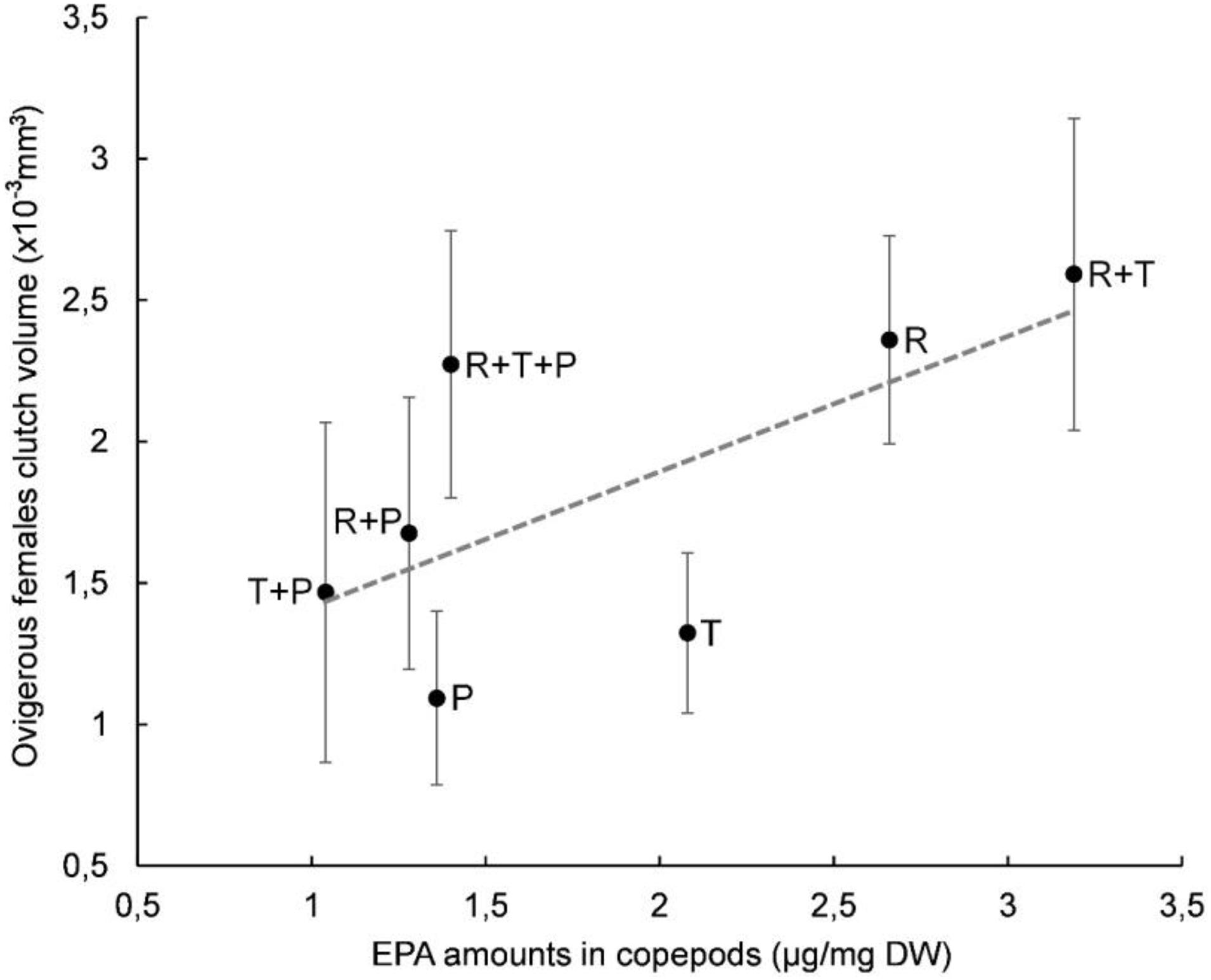
Figure 4. Relationship between average clutch volume and EPA amount in Paracyclopina nana ovigerous females for each microalgal diet. Error bars show the standard deviations obtained from n = 20 females. Dashed line corresponds to the linear regression (EV = 0.4781 X PV + 0.9374, R2 = 0.446, p < 0.01). Pearson’s test was performed to determine the significance of the coefficient of determination R2. R, Rhodomonas salina; T, Tisochrysis lutea; P, Pavlova lutheri.
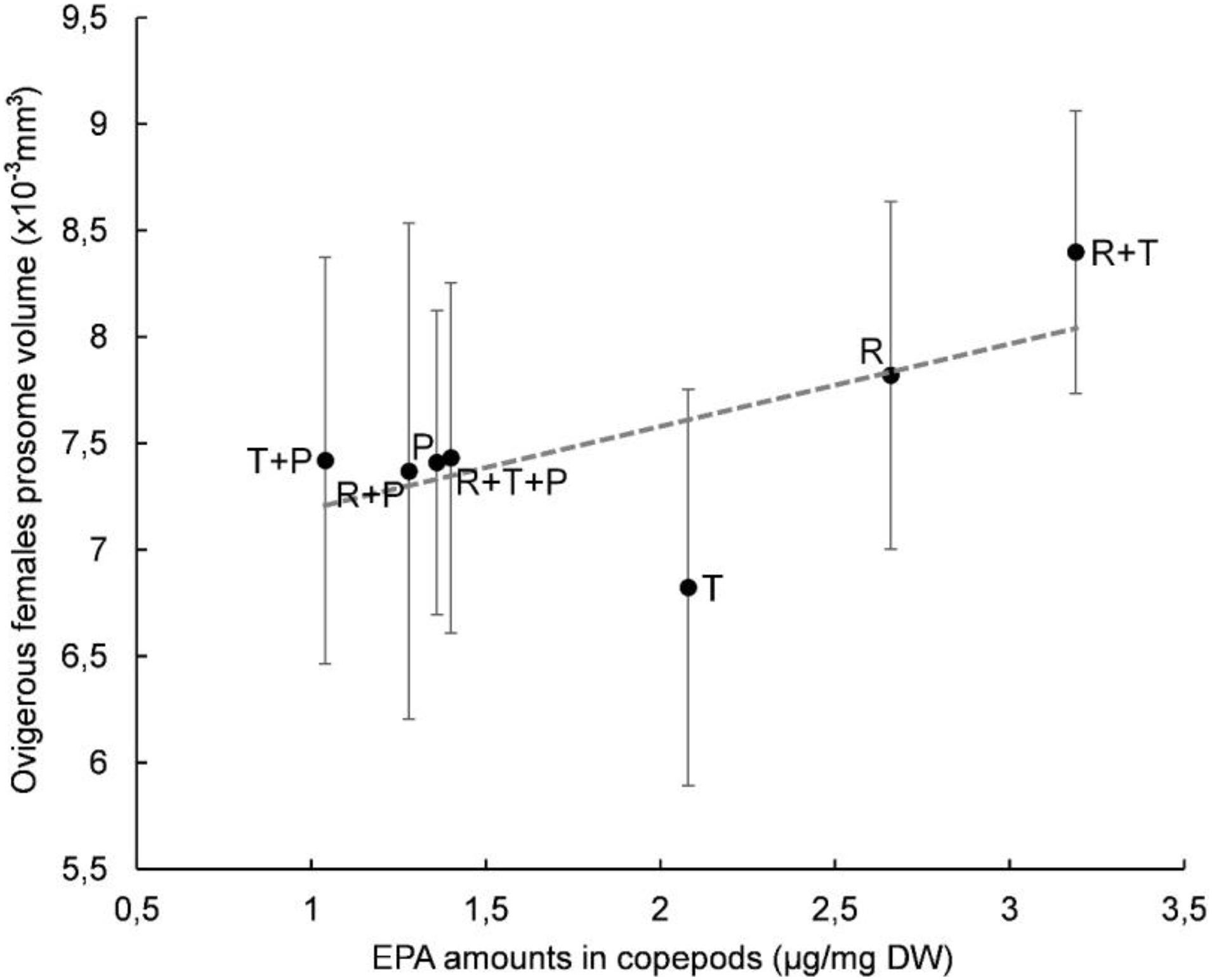
Figure 5. Relationship between average prosome volume and EPA amount in Paracyclopina nana ovigerous females for each microalgal diet. Error bars show the standard deviations obtained from n = 20 females. Dashed line corresponds to the linear regression (EV = 0.387 X PV + 6.8058, R2 = 0.4202, p < 0.01). Pearson’s test was performed to determine the significance of the coefficient of determination R2. R, Rhodomonas salina; T, Tisochrysis lutea; P, Pavlova lutheri.
Representative photographs of the morphology of the different P. nana ovigerous females fed each diet are presented in Figure 6. R-fed and R + T-fed ovigerous females visually looked the biggest and had the biggest egg sacs. Females fed the R + T + P diet and the R + P diet had the next largest egg sacs and body sizes. The smallest ovigerous females with the smallest egg sacs were those that received the T + P diet, the T diet and finally, the P diet.
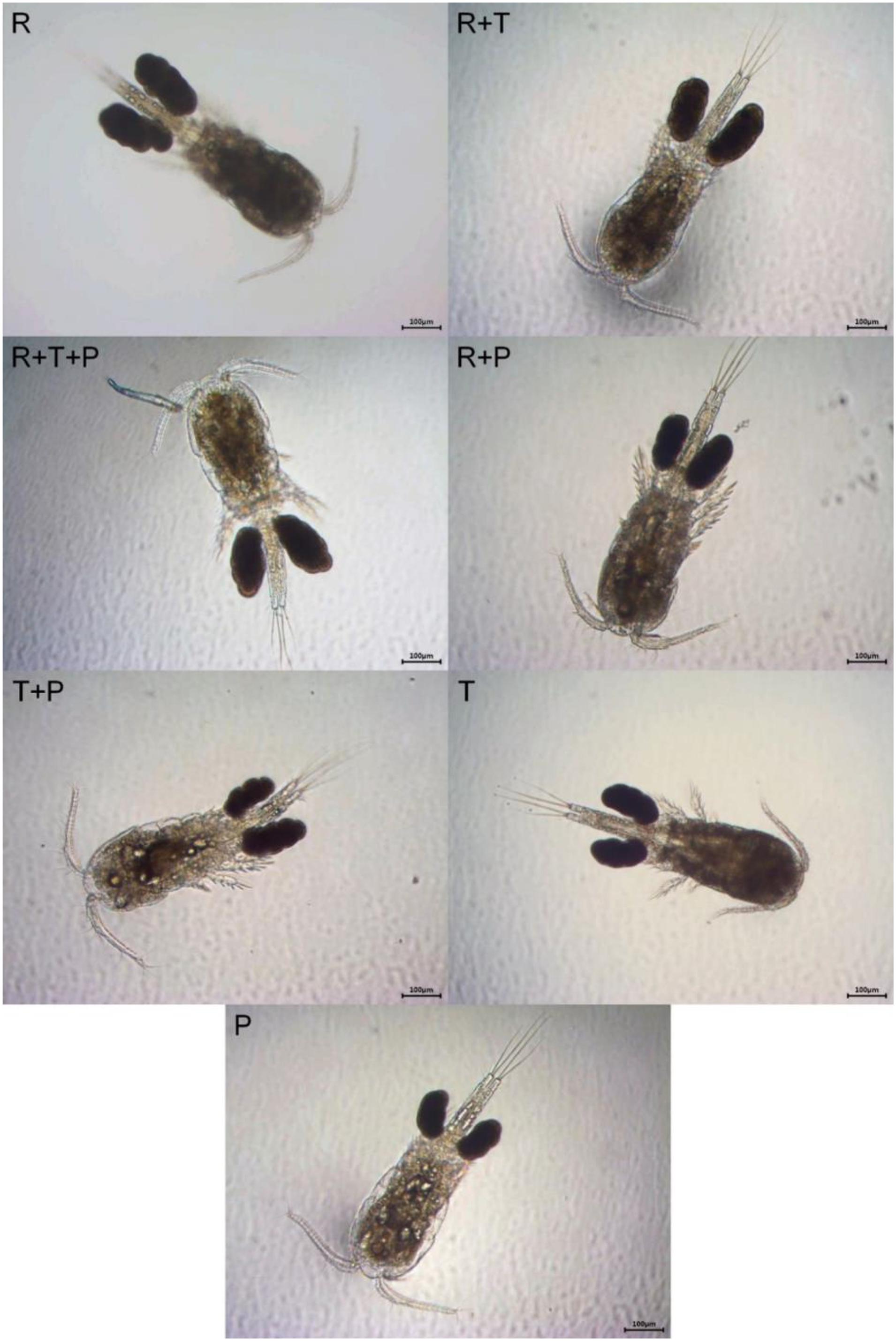
Figure 6. Visual comparison of the body size and the size of the egg sacs of Paracyclopina nana ovigerous females fed on different microalgal diets. Additional supporting data on ovigerous females respective prosome length and clutch size can be found in Dayras et al. (2020). R, Rhodomonas salina; T, Tisochrysis lutea; P, Pavlova lutheri.
Discussion
Effects of Microalgal Diet on the Fatty Acid Composition of P. nana
The fatty acid profile of copepods is directly linked to their diet and its fatty acid composition, as these nutrients are stored in the copepod body as lipid droplets (Lee et al., 2017). It is therefore essential to investigate both copepod and diet fatty acid composition to determine the optimal diet for copepod aquaculture (Van der Meeren et al., 2008) and to gain insight into the trophic ecology of copepods in marine ecosystems (El-Sabaawi et al., 2009).
In the present study, copepods fed the R + T, R, and T diets had the highest final fatty acid amounts. Diets R and T both induced particularly high C18:0 amounts in copepods and this is consistent with the C18:0 amounts in both microalga R. salina and T. lutea that were originally really high. Diets associated with the lowest fatty acids amounts in copepods were the R + T + P, R + P, and P diets, confirming that P. lutheri is not the best microalgal prey species for P. nana. However, P. lutheri contained a large amount of fatty acids while R. salina contained the lowest amount of fatty acids among the three microalgal species evaluated. This implies that the fatty acid profile of copepods is not determined by the relative quantity of fatty acids in their diet, but rather the nature and quality of dietary fatty acids. This is supported by the DHA/EPA ratio results we obtained. The highest DHA/EPA ratio was found in T. lutea due to the very low amount of EPA present in this species, but the T diet yielded some of the highest DHA and EPA amounts in P. nana individuals. This implies that copepods have the ability to bio-convert EPA to DHA. Desvilettes et al. (1997) previously reported that P. nana could convert high amounts of α-linolenic acid (ALA) from Tetraselmis suecica to EPA and DHA when fed on it. In our study, another conversion effect is observed in P. nana as the individuals fed R + T present the highest amounts of C16:1 and C16:0, whereas, our results showed that both R. salina and T. lutea contain lower amounts of these two fatty acids than P. lutheri. Lee et al. (2020) also showed that the fatty acid contents of P. nana individuals were affected by the fatty acid composition of their microalgal diet. Results from food selectivity experiments of different microalgae species demonstrated that P. nana fed Nannochloropsis oculata contained long-chain saturated fatty acids (C20:0 and C22:0), although this microalgae did not contain any C20:0 and C22:0 fatty acids, indicating that P. nana can biosynthesize saturated fatty acids. Thus, cyclopoid copepods can incorporate shorter chain fatty acids from microalgae into EPA and DHA. Nielsen et al. (2019) investigated the potential ability of the cyclopoid copepod Apocyclops royi to biosynthesize polyunsaturated fatty acids (PUFA) through feeding experiments using PUFA-poor Dunaliella tertiolecta and PUFA-rich Isochrysis galbana. Results indicated that the copepods always contained high contents of DHA, and no significant differences in absolute DHA content were detected between treatments, even when copepods were starved of DHA for two generations. Gene expression analysis revealed significantly higher expression of two desaturases in copepods fed PUFA-poor microalgae compared to copepods fed PUFA-rich microalgae. This suggested active PUFA biosynthesis and DHA production capability in A. royi fed low-PUFA diets, confirming the idea that cyclopoid copepods have the ability to bio-convert dietary essential fatty acids into complex storage fatty acids such as EPA and DHA.
In our study, the lowest DHA/EPA ratio was found in P. lutheri because of its high EPA amount, but copepods fed the P diet did not have elevated amounts of EPA and in fact had some of the lowest DHA and EPA amounts among all tested diets. Although P. lutheri contains potential precursor fatty acids for DHA synthesis, these precursors may not be efficiently absorbed by copepods. A similar phenomenon was observed for the other fatty acids studied since the copepods fed with the P diet presented some of the lowest levels of each individual fatty acid while P. lutheri is the microalga containing the highest amounts of C16: 1 and C16: 0 among the three microalgae studied. This may be related to the fact that P. lutheri is the smallest microalgal species among the three tested here (4–6 μm) (Kamiyama and Arima, 2001; Rehberg-Haas, 2014). It is more than twice as small as R. salina, which measures 12.9 × 7.7 μm (average coefficient of variation 19.4%) (Renaud et al., 1999; Schipp et al., 1999). This likely makes P. lutheri the most expensive of the three microalgal species to graze on for P. nana, or P. nana may not have the adaptions required to digest this microalgal species. The genus Pavlova is also known to be a very fragile one. Most common strains of Pavlova are known to be sensitive against shear force and high temperature (>28°C). They appear to be very sensitive to downstreaming processes and have a very short shelf-life, and for this reason it has not been possible to produce Pavlova sp. in an industrial scale so far (Rehberg-Haas, 2014). This fragility may also justify why P. lutheri was not the most performant microalga of our experiment.
In our work, R. salina had average DHA and EPA amounts, but its diet combination with T. lutea (R + T) led to the lowest DHA/EPA ratio in copepods, with a record EPA amount, and its combination with P. lutheri (R + P) led to the highest DHA/EPA ratio in copepods, with a high DHA amount. The R diet alone resulted in a record DHA amount and one of the highest EPA amounts in P. nana. Many studies have pointed out the crucial roles of dietary DHA and EPA in fish development (Rainuzzo et al., 1997; Sargent et al., 1999; Izquierdo et al., 2000; Mejri et al., 2021). These particular fatty acids help maintain the function of visual and neural cells as well as cell membrane structure. Therefore, a diet deficient in DHA and EPA could lead to delayed growth and increased mortality in fish larvae (Mejri et al., 2021). DHA is of greater importance than EPA in marine fish as the former is present in high concentrations in fish larval tissue and promotes development to a greater extent than EPA. The relative proportion of DHA to EPA in larval diets is an important component to consider, and the optimal ratio of DHA to EPA has been established to be 2:1 (Sargent et al., 1997). In the present study, the ratio of DHA to EPA in P. nana was always higher than 2:1 among the different tested microalgal diets, except for R + T (1:1). Particularly high DHA-to-EPA ratios were observed in copepods fed the T + P and R + P diets, probably because of the contributions of P. lutheri, which had by far the highest amount of EPA that was then converted to DHA.
Comparison of microalgae and copepods fatty acid profiles confirmed that P. nana has fatty acid conversion capability. Based on our results, R + T and R diets appear optimal for culture and enrichment of P. nana as these diets induced record amounts of DHA and EPA in this copepod species and globally increased its fatty acid content. Diets T + P and R + P are preferable diets to feed P. nana cultured for use in aquaculture if the aim is to obtain copepods with the highest DHA/EPA ratio.
Effects of Microalgal Diet on the Monosaccharide Composition of P. nana
Carbohydrates in aquatic animals have been mostly studied in fish species to investigate the ability of fish to use dietary carbohydrates; few studies have been performed on crustaceans and even fewer on copepods. Most research has focused on the carbohydrate metabolism of shrimp and crab species because of their use as high quality protein sources in human diets (Wang et al., 2016). Carbohydrates in copepods are poorly characterized and previous investigations provided conflicting results, likely because carbohydrate content in copepods varies widely according to study conditions and various intrinsic factors (Kleppel, 1993). Carbohydrates are rarely stored in crustaceans, but are rather rapidly metabolized for several vital functions (Cuzon et al., 2000).
The three tested microalgal species had different monosaccharide profiles. R. salina had the highest total monosaccharide content per milligram, with almost one third more than the two others species. However, the individual amounts of monosaccharides were always the lowest in this species except for glucose, which was present at a high concentration in R. salina, which positively correlated with the high total monosaccharide content of this microalgal species. R. salina therefore seems to be a glucose-specialized microalga. Conversely, T. lutea had the lowest total monosaccharide content, with 10-fold less glucose per milligram than R. salina, but generally had the highest individual amounts of each of the individual monosaccharides evaluated. P. lutheri had an intermediate monosaccharide profile characterized by a moderately glucose content and lower concentrations of the individual monosaccharides than T. lutea.
When monosaccharide content in copepods was evaluated, copepods fed the R + T + P diet combination had the highest total monosaccharide content and also, in general, the highest amounts of each individual monosaccharide. These results suggest an additive effect of each microalgal species on monosaccharide richness; the higher the amounts of various monosaccharides in the diet, the greater the concentration of monosaccharides in P. nana. Both T + P and R + T diets induced high monosaccharide concentrations in P. nana. Diets that yielded the lowest total monosaccharide contents in copepods were the single-species diets, often resulting in individual monosaccharide quantities less than 1 μg/mg. Diet P was the least efficient diet in term of monosaccharide content in P. nana, which is consistent with the very low concentrations of monosaccharides found in this microalgal species.
Comparing both fatty acids and monosaccharides profiles in microalgae (Appendix A), R. salina had the highest monosaccharide content but lowest fatty acid content. Inversely, T. lutea had the lowest monosaccharides content but the highest fatty acid content. The same phenomenon was observed in copepods fed these diets. R + T + P induced the highest monosaccharide concentration in copepods but a low fatty acid content, whereas, R + T and R diets resulted in the highest fatty acid concentration in copepods but not monosaccharide content. Our results therefore suggest that, for fish larvae rearing purposes, it is preferable to use a varied diet comprising several species of microalgae; we recommend the R+T diet as the best compromise diet for productive culture of P. nana.
Effects of Microalgal Diet on Reproductive Investment by P. nana Ovigerous Females
The three diets of R + T, R, and R + T + P gave the best results in terms of clutch volume and ovigerous female prosome volume in P. nana. We also demonstrated that these three diets were associated with the highest egg numbers per ovigerous female. As shown in Figure 3, diets R + T, R, and R + T + P induced both large females with bulky prosomes and a large clutch size. The photographs shown in Figure 6 also confirm that it is the ovigerous females fed with the diets R, R + T, and R + T + P which ended to look the biggest and obtained the largest egg sacs. These observations are also consistent with the conclusions from the prosome length and clutch size analyses which can be found in Dayras et al. (2020). In our present study, these three diets were associated with the lowest DHA/EPA ratios in copepods. A low DHA/EPA ratio and high EPA content therefore appear to be linked to increased fecundity in P. nana copepod females, both in terms of number of eggs and morphological quality of eggs and females. Copepods fed the R + T and R diets had the highest EPA amounts and also the highest total fatty acid amounts. Ovigerous females rich in fatty acids and particularly in EPA appeared to be the largest and most fertile females.
The P diet was associated with the lowest clutch volumes and lowest egg numbers in ovigerous females. P-fed females were also the smallest and carrying the smallest egg sacs. All the diets in which P. lutheri was involved were the lowest ones in terms of EPA content in copepods, confirming again its poor performances in the culture of P. nana.
Ovigerous females prosome volume and clutch volume seem to be not only appear to be influenced in the same way by EPA richness and to be correlated with each other. We demonstrated a significant linear relationship between prosome volume and clutch volume in P. nana ovigerous females and between each one of these two parameters and EPA amount. Thus, EPA affects the fecundity of P. nana. Arendt et al. (2005) found a positive relationship between the EPA amount ingested by the copepod Temora longicornis and its egg production rate. It appears that the higher the EPA content of the copepod, the higher its egg production. Previous studies have shown that copepod fecundity may be influenced by the DHA and EPA content of their diets (Støttrup and Jensen, 1990; Kleppel et al., 2005). Similar to the findings of Pan et al. (2018) for Apocyclops royi, our results indicate that dietary DHA levels did not have a large effect on P. nana fecundity, and may rather play an indirect role. Consistent with Lee et al. (2006) findings, P. nana appears to be able to synthesize DHA from short-chain fatty acids. This ability would allow this copepod species to remain reproductively active even in the absence of DHA in its diet.
Conclusion
Overall, our experimental results indicate that R. salina is the diet that induces the highest productivity of P. nana in culture, especially when combined with T. lutea. A mixed diet composed of R. salina and T. lutea induced the highest total fatty acid content in P. nana, a good monosaccharide profile, and the greatest prosome and clutch volumes in ovigerous females. Conversely, P. lutheri does not appear to be an effective microalgal prey species for P. nana raised for aquaculture purposes. This study is the first to explore the effects of different diets on the fatty acid and monosaccharide profile of P. nana, and to demonstrate that R. salina is a suitable microalgal species for productive and effective mass culture of this copepod species for use as a live prey in aquaculture.
Data Availability Statement
The raw data supporting the conclusions of this article will be made available by the authors, without undue reservation.
Author Contributions
SS and J-SL conceived the experiment, helped in drafting the manuscript and approved the current version. PD conducted the expertiments and drafted the manuscript. CB contributed to all experiments and commented on the manuscript. IS realized fatty acid and monosaccharides analyses and commented on the manuscript. M-CL contributed to writting of the manuscript and commented on the manuscript in earlier version. All authors contributed to the article and approved the submitted version.
Funding
This study is a part of the Ph.D. thesis of PD from the University of Lille, funded in equal parts by the University of Lille and the region Hauts-de-France.
Conflict of Interest
The authors declare that the research was conducted in the absence of any commercial or financial relationships that could be construed as a potential conflict of interest.
Publisher’s Note
All claims expressed in this article are solely those of the authors and do not necessarily represent those of their affiliated organizations, or those of the publisher, the editors and the reviewers. Any product that may be evaluated in this article, or claim that may be made by its manufacturer, is not guaranteed or endorsed by the publisher.
Acknowledgments
We are grateful to Gwendoline Duong and Dominique Menu from the LOG laboratory for their respective help with CHN analysis and the installation and maintenance of the experimental space. This work is a contribution to the COPEFISH project (an Interdisciplinary and partnership-based STIMULE Project of the Hauts-de-France Regional Council and a long-term partnership between Aquarium Nausicaa and University of Lille) and also to the CPER 2014-2020 MARCO and the CPER 2021-2027 IDEAL projects funded by Europe FEDER, French government the region Hauts-de-France and IFREMER. We thank the Agglomeration Community of Boulogne-sur-Mer for providing experimental facilities in the business incubator Haliocap.
Footnotes
References
Anzeer, F. M., Aneesh, K. S., Abraham, M. V., Darsana, S., Santhosh, B., Anil, M. K., et al. (2019). Breeding, early development and larval rearing of cloudy damsel, Dascyllus carneus Fischer, 1885. Aquaculture 505, 374–385.
Arendt, K. E., Jónasdóttir, S. H., Hansen, P. J., and Gärtner, S. (2005). Effects of dietary fatty acids on the reproductive success of the calanoid copepod Temora longicornis. Mar. Biol. 146, 513–530. doi: 10.1007/s00227-004-1457-9
Arndt, C., and Sommer, U. (2014). Effect of algal species and concentration on development and fatty acid composition of two harpacticoid copepods, Tisbe sp. and Tachidius discipes, and a discussion about their suitability for marine fish larvae. Aquac. Nutr. 20, 44–59. doi: 10.1111/anu.12051
Blanda, E., Drillet, G., Huang, C. C., Hwang, J.-S., Højgaard, J. K., Jakobsen, H. H., et al. (2017). An analysis of how to improve production of copepods as live feed from tropical Taiwanese outdoor aquaculture ponds. Aquaculture 479, 432–441. doi: 10.1016/j.aquaculture.2017.06.018
Breteler, W. K., Schogt, N., and Gonzalez, S. R. (1990). On the role of food quality in grazing and development of life stages, and genetic change of body size during cultivation of pelagic copepods. J. Exp. Mar. Biol. Ecol. 135, 177–189. doi: 10.1016/0022-0981(90)90117-U
Brown, M. R. (2002). Nutritional value and use of microalgae in aquaculture. Avances en Nutrición Acuícola VI. Memorias del VI Simposium Int. Nutr. Acuícola 3, 281–292.
Burgess, A. I., Callan, C. K., Touse, R., and Delos Santos, M. (2020). Increasing survival and growth in larval leopard coral grouper (Plectropomus leopardus) using intensively cultured Parvocalanus crassirostris nauplii. J. World Aquac. Soc. 51, 171–182. doi: 10.1111/jwas.12635
Camus, T., Zeng, C., and McKinnon, A. D. (2009). Egg production, egg hatching success and population increase of the tropical paracalanid copepod, Bestiolina similis (Calanoida: Paracalanidae) fed different microalgal diets. Aquaculture 297, 169–175. doi: 10.1016/j.aquaculture.2009.09.018
Chu, F. L. E., Dupuy, J. L., and Webb, K. L. (1982). Polysaccharide composition of five algal species used as food for larvae of the American oyster, Crassostrea virginica. Aquaculture 29, 241–252.
Coutteau, P. (1996). Micro-Algae. Manual on the Production and use of Live Food for Aquaculture. FAO Fisheries Technical Paper, 361. Rome: FAO, 7–48.
Cuzon, G., Rosas, C., Gaxiola, G., Taboada, G., and Van Wormhoudt, A. (2000). “Utilization of carbohydrates by shrimp. Avances en nutrición acuícola V,” in Memorias del V Simposium Internacional de Nutrición Acuícola, (Mérida), 19–22.
Dayras, P., Bialais, C., Lee, J. S., and Souissi, S. (2020). Effects of microalgal diet on the population growth and fecundity of the cyclopoid copepod Paracyclopina nana. J. World Aquac. Soc. 51, 1386–1401. doi: 10.1111/jwas.12685
Desvilettes, C., Bourdier, G., and Breton, J. C. (1997). On the occurrence of a possible bioconversion of linolenic acid into docosahexaenoic acid by the copepod Eucyclops serrulatus fed on microalgae. J. Plankton Res. 19, 273–278.
Dhont, J., Dierckens, K., Støttrup, J., Van Stappen, G., Wille, M., and Sorgeloos, P. (2013). Rotifers, Artemia and copepods as live feeds for fish larvae in aquaculture. Adv. Aquac. Hatchery Technol. 2013, 157–202. doi: 10.1533/9780857097460.1.157
El-Sabaawi, R., Dower, J. F., Kainz, M., and Mazumder, A. (2009). Characterizing dietary variability and trophic positions of coastal calanoid copepods: insight from stable isotopes and fatty acids. Mar. Biol. 156, 225–237. doi: 10.1007/s00227-008-1073-1
Fernández-Reiriz, M. J., Labarta, U., and Ferreiro, M. J. (1993). Effects of commercial enrichment diets on the nutritional value of the rotifer (Brachionus plicatilis). Aquaculture 112, 195–206. doi: 10.1016/0044-8486(93)90445-5
Gnouma, A., Sadovskaya, I., Souissi, A., Sebai, K., Medhioub, A., Grard, T., et al. (2017). Changes in fatty acids profile, monosaccharide profile and protein content during batch growth of Isochrysis galbana (T. iso). Aquac. Res. 48, 4982–4990. doi: 10.1111/are.13316
Hohnke, L., and Scheer, B. T. (2012). Carbohydrate metabolism in crustaceans. Chem. Zool. 5, 147–164.
Hu, Q. (2004). Environmental Effects On Cell Composition, Vol. 1. Oxford: Blackwell Science Ltd, 83–93.
Izquierdo, M. S., Socorro, J., Arantzamendi, L., and Hernández-Cruz, C. M. (2000). Recent advances in lipid nutrition in fish larvae. Fish Physiol. Biochem. 22, 97–107. doi: 10.1023/A:1007810506259
Jeyaraj, N., and Santhanam, P. (2012). Influence of algal diet on population density, egg production and hatching succession of the calanoid copepod, Paracalanus parvus (Claus, 1863). J. Algal Biomass Utln 4, 1–8.
Kamiyama, T., and Arima, S. (2001). Feeding characteristics of two tintinnid ciliate species on phytoplankton including harmful species: effects of prey size on ingestion rates and selectivity. J. Exp. Mar. Biol. Ecol. 257, 281–296. doi: 10.1016/S0022-0981(00)00341-5
Ki, J.-S., Park, H. G., and Lee, J.-S. (2009). The complete mitochondrial genome of the cyclopoid copepod Paracyclopina nana: a highly divergent genome with novel gene order and atypical gene numbers. Gene 435, 13–22. doi: 10.1016/j.gene.2009.01.005
Kleppel, G. S. (1993). On the diets of calanoid copepods. Mar. Ecol. Progr. Ser. 99, 183–183. doi: 10.3354/meps099183
Kleppel, G. S., Hazzard, S. E., and Burkart, C. A. (2005). “Maximizing the nutritional values of copepods in aquaculture: managed versus balanced nutrition,” in Copepods in Aquaculture, eds C. S. Lee, P. J. O’Bryen, and N. H. Marcus (Hoboken. NJ: Willy), 352. doi: 10.1002/9780470277522
Latsos, C., Van Houcke, J., and Timmermans, K. R. (2020). The effect of nitrogen starvation on Biomass Yield and Biochemical Constituents of Rhodomonas sp. Front. Mar. Sci. 7:900. doi: 10.3389/fmars.2020.563333
Lee, K.-W., Dahms, H.-U., Park, H. G., and Kang, J. H. (2013). Population growth and productivity of the cyclopoid copepods Paracyclopina nana, Apocyclops royi and the harpacticoid copepod Tigriopus japonicus in mono and polyculture conditions: alaboratory study. Aquac. Res. 44, 836–840. doi: 10.1111/j.1365-2109.2011.03071.x
Lee, K.-W., Kang, J. H., and Park, H. G. (2012a). Effect of food concentration on grazing, growth and fecundity of cyclopoid copepod Paracyclopina nana. J. Korea Acad. Ind. Coop. Soc. 13, 5206–5210. doi: 10.5762/KAIS.2012.13.11.5206
Lee, K.-W., Park, H. G., Lee, S. M., and Kang, H. K. (2006). Effects of diets on the growth of the brackish water cyclopoid copepod Paracyclopina nana smirnov. Aquaculture 256, 346–353. doi: 10.1016/j.aquaculture.2006.01.015
Lee, K.-W., Rhee, J.-S., Han, J., Park, H. G., and Lee, J.-S. (2012b). Effect of culture density and antioxidants on naupliar production and gene expression of the cyclopoid copepod, Paracyclopina nana. Comp. Biochem. Physiol. Part A Mol. Integr. Physiol. 161, 145–152. doi: 10.1016/j.cbpa.2011.10.019
Lee, M.-C., Choi, H., Park, J. C., Yoon, D. S., Lee, Y., Hagiwara, A., et al. (2020). A comparative study of food selectivity of the benthic copepod Tigriopus japonicus and the pelagic copepod Paracyclopina nana: a genome-wide identification of fatty acid conversion genes and nitrogen isotope investigation. Aquaculture 521, 734930. doi: 10.1016/j.aquaculture.2020.734930
Lee, S.-H., Lee, M.-C., Puthumana, J., Park, J. C., Kang, S., Hwang, D.-S., et al. (2017). Effects of salinity on growth, fatty acid synthesis, and expression of stress response genes in the cyclopoid copepod Paracyclopina nana. Aquaculture 470, 182–189. doi: 10.1016/j.aquaculture.2016.12.037
Lubzens, E., Zmora, O., Stottrup, J., and McEvoy, L. (2003). “Production and nutritional value of rotifers,” in Live feeds in marine aquaculture, eds J. Stottrup and L. McEvoy (Hoboken. NJ: Willy), 300–303.
Mauchline, J. (ed.) (1998). “The biology of calanoid copepods,” in. Advances in Marine Biology, Vol. 33, (London: Academic Press), 170.
McEvoy, L. A., Naess, T., Bell, J. G., and Lie, Ø (1998). Lipid and fatty acid composition of normal and malpigmented Atlantic halibut (Hippoglossus hippoglossus) fed enriched Artemia: A comparison with fry fed wild copepods. Aquaculture 163, 237–250. doi: 10.1016/S0044-8486(98)00237-3
Mejri, S., Tremblay, R., Audet, C., Wills, P. S., and Riche, M. (2021). Essential fatty acid requirements in tropical and cold-water marine fish larvae and juveniles. Front. Mar. Sci. 8:557. doi: 10.3389/fmars.2021.680003
Milione, M., and Zeng, C., Tropical Crustacean Aquaculture Research Group. (2007). The effects of algal diets on population growth and egg hatching success of the tropical calanoid copepod. Acartia sinjiensis. Aquaculture 273, 656–664. doi: 10.1016/j.aquaculture.2007.07.014
Min, B. H., Park, H. G., and Hur, S. B. (2006). Grazing of brackish water cyclopoid copepod Paracyclopina nana on different microalgae species and concentrations. J. Aquac. 19, 310–314.
Nielsen, B. L. H., Gøtterup, L., Jørgensen, T. S., Hansen, B. W., Hansen, L. H., Mortensen, J., et al. (2019). n-3 PUFA biosynthesis by the copepod Apocyclops royi documented using fatty acid profile analysis and gene expression analysis. Biol. Open 8:bio038331. doi: 10.1242/bio.038331
Ohno, A., Takahashi, T., and Taki, Y. (1990). Dynamics of exploited populations of the calanoid copepod. Acartia tsuensis. Aquaculture 84, 27–39. doi: 10.1016/0044-8486(90)90297-Z
Ohs, C. L., Chang, K. L., Grabe, S. W., DiMaggio, M. A., and Stenn, E. (2010). Evaluation of dietary microalgae for culture of the calanoid copepod Pseudodiaptomus pelagicus. Aquaculture 307, 225–232. doi: 10.1016/j.aquaculture.2010.07.016
Olivotto, I., Avella, M. A., Sampaolesi, G., Piccinetti, C. C., Ruiz, P. N., and Carnevali, O. (2008). Breeding and rearing the longsnout seahorse Hippocampus reidi: rearing and feeding studies. Aquaculture 283, 92–96. doi: 10.1016/j.aquaculture.2008.06.018
Pal, D., Khozin-Goldberg, I., Cohen, Z., and Boussiba, S. (2011). The effect of light, salinity, and nitrogen availability on lipid production by Nannochloropsis sp. Appl. Microbiol. Biotechnol. 90, 1429–1441. doi: 10.1007/s00253-011/3170-1
Pan, Y.-J., Sadovskaya, I., Hwang, J.-S., and Souissi, S. (2018). Assessment of the fecundity, population growth and fatty acid composition of Apocyclops royi (Cyclopoida, Copepoda) fed on different microalgal diets. Aquac. Nutr. 24, 970–978. doi: 10.1111/anu.12633
Pan, Y.-J., Souissi, S., Souissi, A., Wu, C. H., Cheng, S. H., and Hwang, J.-S. (2014). Dietary effects on egg production, egg-hatching rate and female life span of the tropical calanoid copepod Acartia bilobata. Aquac. Res. 45, 1659–1671. doi: 10.1111/are.12113
Patil, V., Källqvist, T., Olsen, E., Vogt, G., and Gislerød, H. R. (2007). Fatty acid composition of 12 microalgae for possible use in aquaculture feed. Aquac. Int. 15, 1–9. doi: 10.1007/s10499-006-9060-3
Payne, M. F., and Rippingale, R. J. (2000). Rearing West Australian seahorse, Hippocampus subelongatus, juveniles on copepod nauplii and enriched Artemia. Aquaculture 188, 353–361. doi: 10.1016/S0044-8486(00)00349-5
Payne, M. F., Rippingale, R. J., and Cleary, J. J. (2001). Cultured copepods as food for West Australian dhufish (Glaucosoma hebraicum) and pink snapper (Pagrus auratus) larvae. Aquaculture 194, 137–150. doi: 10.1016/S0044-8486(00)00513-5
Pinto, C. S., Souza-Santos, L. P., and Santos, P. J. P. (2001). Development and population dynamics of Tisbe biminiensis (Copepoda: Harpacticoida) reared on different diets. Aquaculture 198, 253–267. doi: 10.1016/S0044-8486(00)00582-2
Rainuzzo, J. R., Reitan, K. I., and Olsen, Y. (1997). The significance of lipids at early stages of marine fish: a review. Aquaculture 155, 103–115. doi: 10.1016/S0044-8486(97)00121-X
Rasdi, N. W., and Qin, J. G. (2016). Improvement of copepod nutritional quality as live food for aquaculture: a review. Aquac. Res. 47, 1–20. doi: 10.1111/are.12471
Rehberg-Haas, S. (2014). Utilization of the Microalga pavlova sp. In Marine fish Nutrition (Doctoral Dissertation). Kiel: Selbstverlag des Instituts für Tierzucht und Tierhaltung der Christian-Albrechts-Universität zu Kiel.
Renaud, S. M., Thinh, L. V., and Parry, D. L. (1999). The gross chemical composition and fatty acid composition of 18 species of tropical Australian microalgae for possible use in mariculture. Aquaculture 170, 147–159. doi: 10.1016/S0044-8486(98)00399-8
Renaud, S. M., Zhou, H. C., Parry, D. L., Thinh, L. V., and Woo, K. C. (1995). Effect of temperature on the growth, total lipid content and fatty acid composition of recently isolated tropical microalgae Isochrysis sp., Nitzschia closterium, Nitzschia paleacea, and commercial species Isochrysis sp. (clone T. ISO). J. Appl. Phycol. 7, 595–602. doi: 10.1007/BF00003948
Sadovskaya, I., Souissi, A., Souissi, S., Grard, T., Lencel, P., Greene, C. M., et al. (2014). Chemical structure and biological activity of a highly branched (1→ 3, 1→ 6)-β-d-glucan from Isochrysis galbana. Carbohydr. Polym. 111, 139–148. doi: 10.1016/j.carbpol.2014.04.077
Sargent, J., McEvoy, L., Estevez, A., Bell, G., Bell, M., Henderson, J., et al. (1999). Lipid nutrition of marine fish during early development: current status and future directions. Aquaculture 179, 217–229. doi: 10.1016/S0044-8486(99)00191-X
Sargent, J. R., McEvoy, L. A., and Bell, J. G. (1997). Requirements, presentation and sources of polyunsaturated fatty acids in marine fish larval feeds. Aquaculture 155, 117–127. doi: 10.1016/S0044-8486(97)00122-1
Schipp, G. R., Bosmans, J. M., and Marshall, A. J. (1999). A method for hatchery culture of tropical calanoid copepods. Acartia spp. Aquaculture 174, 81–88. doi: 10.1016/S0044-8486(98)00508-0
Shields, R. J., Bell, J. G., Luizi, F. S., Gara, B., Bromage, N. R., and Sargent, J. R. (1999). Natural copepods are superior to enriched Artemia nauplii as feed for halibut larvae (Hippoglossus hippoglossus) in terms of survival, pigmentation and retinal morphology: relation to dietary essential fatty acids. J. Nutr. 129, 1186–1194. doi: 10.1093/jn/129.6.1186
Siqwepu, O., Richoux, N. B., and Vine, N. G. (2017). The effect of different dietary microalgae on the fatty acid profile, fecundity and population development of the calanoid copepod Pseudodiaptomus hessei (Copepoda: Calanoida). Aquaculture 468, 162–168. doi: 10.1016/j.aquaculture.2016.10.008
Sorgeloos, P., Dhert, P., and Candreva, P. (2001). Use of the brine shrimp. Artemia spp., in marine fish larviculture. Aquaculture 200, 147–159. doi: 10.1016/S0044-8486(01)00698-6
Souissi, A., Souissi, S., and Hansen, B. W. (2016). Physiological improvement in the copepod Eurytemora affinis through thermal and multi-generational selection. Aquac. Res. 47, 2227–2242. doi: 10.1111/are.12675
Støttrup, J., and McEvoy, L. (Eds) (2008). Live Feeds in Marine Aquaculture. Hobken NJ: John Wiley and Sons.
Støttrup, J. G. (2000). The elusive copepods: their production and suitability in marine aquaculture. Aquac. Res. 31, 703–711. doi: 10.1046/j.1365-2109.2000.318488.x
Støttrup, J. G., and Jensen, J. (1990). Influence of algal diet on feeding and egg-production of the calanoid copepod acartia tonsa dana. J. Exp. Mar. Biol. Ecol. 141, 87–105. doi: 10.1016/0022-0981(90)90216-Y
Støttrup, J. G., and Norsker, N. H. (1997). Production and use of copepods in marine fish larviculture. Aquaculture 155, 231–247. doi: 10.1016/S0044-8486(97)00120-8
Sun, B., and Fleeger, J. W. (1995). Sustained mass culture of Amphiascoides atopus a marine harpacticoid copepod in a recirculating system. Aquaculture 136, 313–321. doi: 10.1016/0044-8486(95)01064-5
Thinh, L. V., Renaud, S. M., and Parry, D. L. (1999). Evaluation of recently isolated Australian tropical microalgae for the enrichment of the dietary value of brine shrimp, Artemia nauplii. Aquaculture 170, 161–173. doi: 10.1016/S0044-8486(98)00400-1
Turner, J. T. (2004). The importance of small planktonic copepods and their roles in pelagic marine food webs. Zool. Stud. 43, 255–266.
Van der Meeren, T., Karlsen, Ø, Liebig, P. L., and Mangor-Jensen, A. (2014). Copepod production in a saltwater pond system: a reliable method for achievement of natural prey in start-feeding of marine fish larvae. Aquac. Eng. 62, 17–27. doi: 10.1016/j.aquaeng.2014.07.003
Van der Meeren, T., Olsen, R. E., Hamre, K., and Fyhn, H. J. (2008). Biochemical composition of copepods for evaluation of feed quality in production of juvenile marine fish. Aquaculture 274, 375–397. doi: 10.1016/j.aquaculture.2007.11.041
Wang, X., Li, E., and Chen, L. (2016). A review of carbohydrate nutrition and metabolism in crustaceans. North Am. J. Aquac. 78, 178–187. doi: 10.1080/15222055.2016.1141129
Appendix
Keywords: copepod, fatty acid composition, live prey, microalgae, monosaccharide composition, Paracyclopina nana, reproductive investment
Citation: Dayras P, Bialais C, Sadovskaya I, Lee M-C, Lee J-S and Souissi S (2021) Microalgal Diet Influences the Nutritive Quality and Reproductive Investment of the Cyclopoid Copepod Paracyclopina nana. Front. Mar. Sci. 8:697561. doi: 10.3389/fmars.2021.697561
Received: 19 April 2021; Accepted: 26 July 2021;
Published: 13 August 2021.
Edited by:
Ana Maulvault, Portuguese Institute for Sea and Atmosphere (IPMA), PortugalReviewed by:
Vanessa M. Lopes Letria, Center for Marine and Environmental Sciences (MARE), PortugalHans Uwe Dahms, Kaohsiung Medical University, Taiwan
Copyright © 2021 Dayras, Bialais, Sadovskaya, Lee, Lee and Souissi. This is an open-access article distributed under the terms of the Creative Commons Attribution License (CC BY). The use, distribution or reproduction in other forums is permitted, provided the original author(s) and the copyright owner(s) are credited and that the original publication in this journal is cited, in accordance with accepted academic practice. No use, distribution or reproduction is permitted which does not comply with these terms.
*Correspondence: Sami Souissi, sami.souissi@univ-lille.fr; Jae-Seong Lee, jslee2@skku.edu