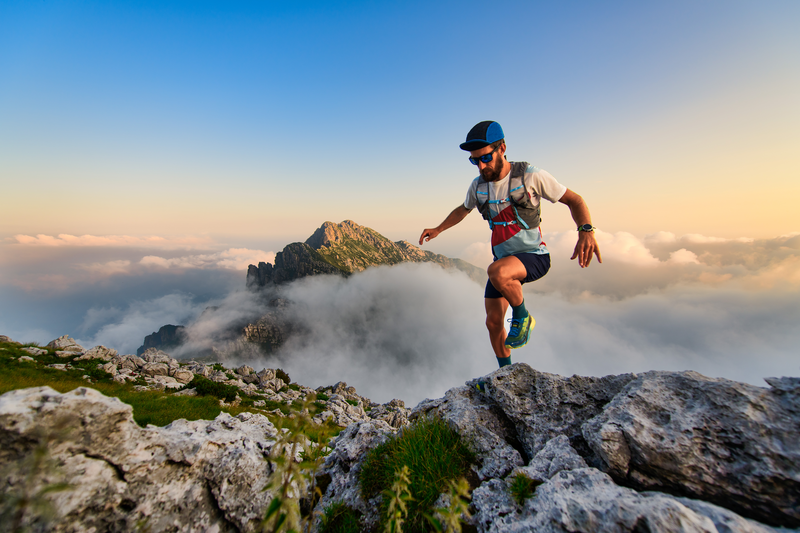
94% of researchers rate our articles as excellent or good
Learn more about the work of our research integrity team to safeguard the quality of each article we publish.
Find out more
ORIGINAL RESEARCH article
Front. Mar. Sci. , 26 May 2021
Sec. Marine Fisheries, Aquaculture and Living Resources
Volume 8 - 2021 | https://doi.org/10.3389/fmars.2021.688058
This article is part of the Research Topic Live Feed for Early Ontogenetic Development in Marine Fish Larvae View all 18 articles
Seahorses (Hippocampus spp.) are exceptional marine species considering their reproductive patterns and other features. Due to the iconic characteristics of these fishes, aquarium trade, and research efforts have increased in the last years. Consequently, novel rearing techniques have been developed; however, there is a need for improvements on a series of issues, namely reproduction success enhancement. The tropical species Hippocampus reidi is the most traded seahorse but many aspects of breeding and its impact on the quality of neonates are still poorly understood. In the present study, we assessed the effects of two pre-breeding diets on newborn quality and viability considering biochemical characteristics, energetic status, and ultrastructural aspects of muscular tissue. During the whole pre-breeding season (5 months), the breeders were fed on one of the following diets: M0 (adult non-enriched Artemia) and M5 (adult non-enriched Artemia + mysidaceans). From the onset of the reproduction period, all breeders were fed for 6 months on diet M5. Breeding success and energetic status (ATP, total adenylic nucleotides, AEC, and NAD) of newborns resulted considerably enhanced in treatment M5. However, initial differences in neonates quality did not affect further newborn performance (survival and growth until day 7 after male’s pouch release) while gaining access to high-quality preys (copepods). Besides, morphological alterations in muscle tissue were not observed. The reproduction in the species followed a capital–income continuum pattern characterized by an initial mixed capital-income period (until 70–100 days since the onset of the breeding season) followed by an income breeding period with progressive exhaustion of body reserves, especially in M0-newborns. Interestingly, the effects of pre-breeding diets were also noticed in the second half of the breeding period. Our results seemed to indicate that the requirements in essential fatty acids in H. reidi are lower than in other seahorse species (e.g., Hippocampus guttulatus). Globally, the results achieved revealed that high-quality pre-breeding diets enhanced reproduction success and would likely result advantageous to improve newborn endurance in conditions of moderate starvation or sub-optimal feeding.
Seahorses (Hippocampus spp.) are flagship fishes submitted to over-exploitation in nature due to their use in several human activities, habitat loss, and the aquarium and curio trade, compromising their conservation (Vincent, 1996; Foster and Vincent, 2004; Martin-Smith et al., 2004; Vincent et al., 2011). In recent years, concern over how to reduce the exploitation of wild populations has been increasing in parallel to rising efforts to provide reliable tools for their rearing in ex situ facilities (Koldewey and Martin-Smith, 2010; Olivotto et al., 2011b; Cohen et al., 2016). Breeding in captivity can partially replace the capture of wild seahorses and reduce the pressure over natural populations.
There are more than thirty seahorse species but only a few are currently reared with certain success in captivity. The tropical fish Hippocampus reidi Ginsburg, 1933 is one of the seahorse species most in demand for the marine aquarium trade, and one of the most promising for industrial cultivation. The geographic distribution of the species ranges from Florida to Rio de Janeiro (Brazil) and Gulf of Mexico (Kuiter, 2013). Adults measure up to 26 cm in TL and inhabit shallow inshore to moderately deep water (15–55 m) (Rosa et al., 2002).
A singular characteristic of seahorses is reproduction, in which females provide most nutrients to eggs whereas males take care and also supply nutrients to embryos developing from eggs transferred by females to their brood pouches (Carcupino et al., 2002; Skalkos et al., 2020; Wittington and Friesen, 2020). Mating and parturition are highly synchronized with egg maturation so that the release of eggs and newborn occur almost simultaneously and at similar time intervals, which vary depending on species, temperature, and breeder’s diet (Lin et al., 2006, 2007; Planas et al., 2010; Otero-Ferrer et al., 2020). In seahorses, the assessment of nutritional and energetic requirements in seahorse breeders is lacking. Only a few studies have focused on the impact of breeder’s diet on newborn quality and further viability. Most of them were carried out in H. guttulatus, H. hippocampus, and H. reidi by comparing diets differing in biochemical profiles [namely fatty acids (FAs)] and nutritional quality (e.g., Artemia, mysidaceans, and amphipods as prey) (Woods and Valentino, 2003; Murugan et al., 2009; Otero-Ferrer et al., 2012, 2016, 2020; Palma et al., 2012; Saavedra et al., 2014; Saavedra et al., 2015; Planas et al., 2020b).
The effect of breeder’s diet in fish have been usually focused on the impact of FAs and the resulting quality of fry (Bromage, 1995; Brooks et al., 1997; Izquierdo et al., 2001; Fernández-Palacios et al., 2011; Migaud et al., 2013). In seahorses, most studies have focused on the effects of diets provided during the breeding season (Woods and Valentino, 2003; Binh and Serrano, 2012; Otero-Ferrer et al., 2012, 2020; Palma et al., 2012, 2017; Planas et al., 2020b) or shortly before reproduction (Otero-Ferrer et al., 2016). In a previous study on H. guttulatus, we assessed isotopically the assimilation by breeders of three diets furnished during the whole pre-breeding period and their incorporation into newborns (Planas, 2021). We demonstrated that the species follows a capital-income breeding strategy ((Williams et al., 2017). The optimization of seahorse breeding also should consider how newborn development is affected by pre-breeding dietary regimes. For that, newborn characteristics and initial viability must be properly addressed. Condition indices, energy allocation, and metabolic scope in early ontogeny aim to evaluate the physiological status of fish (Cunha et al., 2003, 2007; Di Pane et al., 2019). Adenylic energy charge (AEC) was proposed as an indicator of the energy potentially available for cellular metabolism (Atkinson, 1977; Dickson and Franz, 1980; Harms, 1992). To our knowledge, the unique study available focused on adenylic nucleotides and energetic status in fish larvae was carried out in clownfish (Olivotto et al., 2011a).
In the present study, we assessed the effects of two dietary treatments on the reproduction of the seahorse H. reidi by analyzing (1) breeding success and general newborn characteristics (FA profiles, endogenous nucleosides, and AEC), and (2) determining the breeding pattern of the species. To our knowledge, this is the first multiapproach study on the effect of long-term pre-breeding diets on reproductive performance and newborn energetic status in seahorses.
Animal handling and sampling were conducted in compliance with all bioethics standards on animal experimentation of the Spanish Government (Real Decreto 1201/2005, 10th October) and the Regional Government Xunta de Galicia (REGA ES360570202001/15/FUN/BIOL.AN/MPO01).
Adult seahorses H. reidi Ginsburg, 1933 were kept in captivity at the facilities of Instituto de Investigaciones Marinas (IIM-CSIC) (Vigo, Spain) for at least two generations, or donated by l’Oceanogràfic aquarium (Valencia, Spain). The fish were maintained in 320 L aquaria (Planas et al., 2008) in a semi-closed system with a 10–14% daily renewal of seawater. Seawater temperature was maintained constant at 26 ± 0.5°C, and a natural-like photoperiod regime was applied (16L:8D). Water quality was checked periodically for NO2, NO3, and NH4/NH3 content (0 mg L–1). Salinity and pH levels were 37 ± 2 and 8.0 ± 0.2, respectively. Wastes and uneaten food were removed daily by siphoning the bottom of aquaria.
The fish were routinely fed twice daily on diets comprising enriched adult Artemia (Iberfrost, Spain; 40–70 Artemia seahorse–1 dose–1) and frozen mysidaceans Neomysis sp. (Ocean Nutrition, Spain). Adult Artemia was produced in 100 L units at 26–28°C with gentle aeration and constant light on a mixture consisting of live microalgae Phaeodactylum tricornutum and Isochrysis galbana, dried Spirulina, and subsequently long-time enrichment for at least 3 days (from day 16 onward) on the commercial product Red Pepper (Bernaqua, Belgium) (Planas et al., 2017).
Two groups of seahorses (groups M0 and M5; eight females and six males per group) were randomly selected from the seahorse broodstock and kept separate for 5 months (from November 1, 2017 to March 31, 2018) in two 320 L and maintained under the same chemical-physical conditions reported above. For each group, males and females were kept physically separate during the whole period.
The breeders were fed (ad libitum) twice daily according to the following feeding scheme (Figure 1):
1. Group M0: adult non-enriched Artemia (Iberfrost, Spain).
2. Group M5: adult non-enriched Artemia and frozen mysidaceans (Neomysis sp.; Ocean Nutrition, United States) (1:1).
Figure 1. General scheme of the experimental framework (Hippocampus reidi breeders fed on diets M0 and M5) and sampling (dorsal fin and newborn). Seahorse illustration by Sergio Picazo Ferro (https://sergiopicazo.com/).
Prey lengths were 5–7 mm for Artemia and about 1–1.5 cm for mysidaceans.
Adult non-enriched Artemia was produced as reported above but not enriched with Red Pepper.
The mixed diet used in group M5 was selected considering that it provides the best trophic enrichment factors in syngnathids (Planas et al., 2020a). The lipid content and FAs profile of prey are provided in Table 1.
Table 1. Total lipids (% dry weight) and fatty acids (relative percentage) in prey (n = 2) for seahorse breeders.
Mean weight (DW) and standard length (SL) of breeders at the onset of Period 1 did not differ significantly across groups (K-W test, n = 32, p = 0.951 for DW and 0.878 for SL). Mean DW and SL were 6.17 ± 2.07 g and 14.15 ± 1.53 cm in M0, and 6.76 ± 3.09 g and 14.43 ± 2.22 cm in M5, respectively. At the end of the pre-breeding period, mean DW (9.18 ± 2.69 g in M0; 10.99 ± 2.96 g in M5), SL (16.03 ± 1.42 cm in M0; 17.01 ± 1.60 cm in M5) and G (daily weight-specific growth rates) (0.355 ± 0.196% day–1 in M0; 0.434 ± 0.221% day–1 in M0) were similar across groups (K-W test, n = 32; p = 0.242 for DW, 0.140 for SL, and 0.124 for G).
Males and females from the same experimental treatment were regrouped on April 1, 2018 and maintained until September 30, 2018 under the same conditions reported above for Period 1, except for the diet. During this period, both experimental groups were fed twice daily on a mixture of non-enriched adult Artemia and frozen mysidaceans Neomysis sp. (1:1).
Seahorses were monitored several times a day to check for newborn releases from male’s pouch. Samples of freshly released newborn were immediately collected for further analyses (electronic microscopy, total lipids, FAs, endogenous nucleotides, and energy charge calculation) and weight/size determination. Sampled juveniles were euthanized with Tricaine MS-222 (0.1 mg L–1, Sigma-Aldrich). For length and weight measurements, the juveniles were transferred to Petri dishes, photographed, and weighed individually on a Sartorius microbalance MC210P (±0.01 mg). Curved SL were measured (SL = head + trunk + curved tail) from digital photographs using image processing software (NIS, Nikon).
After sampling, the remaining released juveniles were carefully collected, counted, and transferred (2–5 juveniles L–1) to 30 L pseudo-Kreisel aquaria connected to a semi-opened recirculation system (Blanco et al., 2014). The newborns were reared until day 7 (7 DAR) after male’s pouch release (DAR) at 26°C under a constant 14L:10D photoperiod. Further details on the rearing system were reported by Planas et al. (2012).
From 0 to 5 DAR (days after male’s pouch release), newborn seahorses were fed on two daily doses of Acartia tonsa (0.67 copepods mL–1 dose–1), whereas one daily dose of A. tonsa (0.67 copepods mL–1 dose–1) and one daily dose of Artemia nauplii (1 Artemia mL–1 dose–1) were supplied on 6–7 DAR.
Dead seahorses were daily removed (8:00 a.m. and 3:00 p.m.) and counted. Final survivals of juveniles were recorded at 7 DAR, corresponding to complete mortality in unfed seahorses (personal observation).
Microalgae and live prey (copepods and Artemia nauplii) were cultured as reported by Planas et al. (2020b).
Total lipids from live prey and 0 DAR juveniles (10–20 mg dry weight per sample; n = 30–60) were extracted according to Bligh and Dyer (1959). Aliquots of total lipid extracts with known lipid content were centrifuged, resuspended with 0.5 M ammonium formate solution, freeze-dried and stored at −80°C. Total lipid content was quantified gravimetrically (Herbes and Allen, 1983). FA composition in total lipids was analyzed by gas-chromatography (GC) according to Christie (1982). Lipids were transmethylated (Lepage and Roy, 1986) and FA analyzed by GC (Perkin Elmer, GC-FID Clarus 500 gas chromatograph) (Planas et al., 2010).
Elemental composition (total C and N) were analyzed in dried newborn seahorses (n > 5; pooled individuals). The samples were measured by continuous flow isotope ratio mass spectrometry using a FlashEA1112 elemental analyzer (Thermo Finnigan, Italy) coupled to a Delta Plus mass spectrometer (FinniganMat, Bremen, Germany) through a Conflo II interface. Further details are provided by Planas (2021).
Whole seahorses, in five replicates each, were anesthetized and rapidly collected, frozen, and grounded in liquid nitrogen, weighed in aliquots, and stored at −80°C and processed according to Olivotto et al. (2011a).
All data in this work are referred to as grams of N2-powder (five fresh weight tissues). Tissue N2-powders (20–200 mg aliquots) were added with 6 mL g–1 of 0.4 M HClO4 containing 50 mM cAMP (300 nmol g–1) as internal standard and thawed by vortexing. The perchloric extracts were sonicated (30 s at 50 watts with 0.5-s pulses) and centrifuged (16.000g, 10 min at 4°C). The supernatants were neutralized by adding 0.29 vol of 1 M K2CO3 and centrifuged again to remove the precipitated KClO4. The clear supernatant, referred as to neutralized extract, was used for subsequent analyses of metabolites.
The endogenous nucleotides and the internal standard cAMP were quantified by UV C18-HPLC analysis, both under reverse-phase and ion-pairing conditions. For details see Mori et al. (2014). The column temperature was 8°C and the eluate absorbance was monitored by a diode-array detector (Shimadzu SPD-M10A). For quantitation, the integrated peak areas, expressed by the Shimadzu LC solution v1.24 software used as mAU s–1, were first converted into mAU × ml by appropriate factoring, then into nanomoles dividing by the appropriate extinction coefficient at 260 nm (18.6 mM–1 cm–1 for NAD; 15.4 mM–1 cm–1 for ATP, AMP, and cAMP, respectively). The extraction yield calculated from cAMP recovery was 95% in all samples.
For the AEC evaluation, the following equation was used (Atkinson and Walton, 1967):
Abdominal portions of newborns (three newborns per dietary treatment) were processed for Durcupan ACM (Fluka) resin embedding as follows: pieces fixed in 2.5% glutaraldehyde in 0.2 M phosphate-buffered saline (pH 7.4), for 2 h at 4°C, were washed repeatedly in the same buffer and postfixed in 1% osmium tetroxide in 0.2 M phosphate-buffered saline for 1 h at 4°C. Then, the tissues were dehydrated with increasing alcohol concentrations. The dehydrated pieces were embedded in Durcupan ACM (Squadrito et al., 2017). Finally, the sections were obtained with a Reichert Jung Ultracut E. Semi-thin sections (1 μm) were stained with toluidine blue and examined with a light microscope. Ultrathin sections (740 Å) were obtained from selected areas of the semi-thin sections, stained with uranyl acetate and lead citrate, and examined and photographed with a transmission electron microscope (JEOL JEM 1400 Flash) (Viña et al., 2014).
Statistical analyses were conducted in R v.3.6.3 (R Core Team, 2020a). Variability across treatments was examined by non-parametric Kruskal–Wallis test for independent samples (Kassambara, 2020a). When significant, the means were compared by Wilcoxon range test (Pgirmess v1.6.9 package of R) (Giraudoux et al., 2018) or Dunnet’s test (Kassambara, 2020a). Correlations (Pearson’s correlation coefficients) and hierarchical clustering linkage (complete method) of variables and samples for newborn batches were performed with factoextra v1.0.7 (Kassambara, 2020b) for computational analysis and igraph v1.2.6 (Csardi and Nepusz, 2006) for plotting. Additionally, principal component analyses (PCA) were performed to summarize and visualize the information newborn datasets. For that, we used factoMineR v2.3 (Husson et al., 2020), factoextra v1.0.7 (Kassambara, 2020b), and corrplot v0.8.4 (Wei et al., 2017) packages in R. The data values were standardized (mean = 0; SD = 1) for clustering and PCA. Data normality was checked with the Shapiro–Wilk normality test (stats v3.6.2 R package) (R Core Team, 2020b). Significance levels were set at p < 0.05 and results are presented as mean ± standard deviation. Graphics were constructed using ggplot2 v3.3.0 (Wickham et al., 2020) and lattice v0.20-41 (Sarkar et al., 2020) packages in R.
The present study was carried out on an endangered/threatened species. Bioethics referred to these organisms imply the use of a number of specimens as much reduced as possible (strictly the necessary). Besides this, the availability of endangered species (particularly breeders) and large aquaria is certainly limited. For that reason, only one aquarium was used for each dietary treatment. Consequently, the breeders were considered pseudoreplicates and the aquaria were the basic experimental units. There is literature available that justify the use of pseudoreplicates in some circumstances and recommends that pseudoreplication not be invoked when reviewing research manuscripts and research proposals (see Planas, 2021).
The total number of newborn batches produced in treatments M0 and M5 were 11 and 22, respectively, whereas total newborn production was 4,397 in M0 and 7,298 in M5 (Figure 2). The average batch size in treatments M0 (400 ± 182 newborns) and M5 (332 ± 155 newborns) were similar (K-W test, n = 36, p = 0.321). Survivals of juveniles at 7 DAR did not differ similarly across dietary treatments (K-W test, n = 33, p < 0.219), ranging from 86 ± 7% in group M5 to 88 ± 10% in group M0 (Figure 2).
Figure 2. Progressive changes (smoothed trends with 95% confidence intervals) in batch size (newborn), survival at 7 DAR (%), dry weight (DW; g), and standard length (SL; mm) along the breeding period in newborn released by seahorses from groups M0 and M5. Gray shadowed bars show inflection periods indicating a remarkable change (knee) on the smoothed trend.
Breeders from group M5 produced larger newborn both in SL (K-W test, n = 36, p = 0.002) and DW (K-W test, n = 36, p < 0.001) (Figure 2). Mean weights of newborns were 0.22 ± 0.03 mg in M0 and 0.28 ± 0.04 mg in M5, whereas mean SL were 8.0 ± 0.3 mm in M0 and 8.6 ± 0.6 mm in M5.
Overall, total lipids content in newborns ranged from 14.6 ± 1.7 to 14.4 ± 1.6% dry weight and did not differ among treatments (K-W test, n = 33, p = 0.942) but decreased continuously in group M5, especially during the first half of the breeding season (Figure 3).
Figure 3. Progressive changes (smoothed trends with 95% confidence intervals) in total lipids (dry weight percentage), fatty acid series (percentage of total FA) and fatty acid ratios along the breeding period in newborn released by seahorses from groups M0 and M5. Gray shadowed bars show inflection periods indicating a remarkable change (knee) on the smoothed trend. AA, EPA, DPA, and DHA: 20:4n-6, 20:5n-3, 22:5n-3, and 22:6n-3, respectively.
Regarding FA groups across dietary treatments, the relative content (percentage of total FA) in saturates (37.4–37.7%), monoenes (20.6–23.7%) and polyenes (38.9–41.7%) was similar (K-W test, n = 33; p = 0.643, 0.417 and 0.153, respectively) (Figure 3). Total n-3 HUFA accounted for 28.4–29.6% (K-W test, n = 33, p = 0.942).
DHA/EPA (range: 3.33–3.36), DHA/AA (4.31–4.70) and DHA/DPA (4.73–4.91) values gradually increased during the breeding season but average ratios for the whole period did not differ among treatments (K-W test, n = 33, p = 0.817, 0.113 and 0.589, respectively) (Figure 3).
Statistical mean comparisons for individual FAs (area percentage) for the whole Period 1 only revealed significant differences in 16:0 (K-W test, n = 33, p = 0.021), 18:0 (p = 0.025), 18:3n-3 (p = 0.045), 20:2n-6 (p = 0.049), and 22:4n-6 (p < 0.001) (Figure 4).
Figure 4. Progressive changes (smoothed trends with 95% confidence intervals) for fatty acids (percentage of total FA) along the breeding period in newborn released by seahorses from groups M0 and M5. Gray shadowed bars show inflection periods indicating a remarkable change on the smoothed trend.
Figure 5 reports the progress of endogenous nucleotide (AMP, ADP, ATP, and NAD) content and AEC in newborn seahorses along the breeding season. With regards to both AMP and ADP content, no significant differences were detected between M0 (5.49 ± 3.42 and 8.33 ± 3.52 pmol/μg of proteins for AMP and ADP, respectively) and M5 (5.60 ± 3.08 and 8.63 ± 2.75 pmol/μg of proteins for AMP and ADP, respectively) groups. Differently, M0-group showed significantly (n = 19; p < 0.05) lower values of ATP (13.26 ± 5.51 pmol/μg of proteins) and NAD (2.33 ± 0.81 pmol/μg of proteins) content as compared to M5-group (30.16 ± 10.41 and 3.35 ± 0.82 pmol/μg of proteins for ATP and NAD, respectively). Finally, M0-group (0.62 ± 0.14) evidenced a significantly (p = 0.001) lower AEC compared to M5-group (0.78 ± 0.05).
Figure 5. Progressive changes (smoothed trends with 95% confidence intervals) in AMP, ADP, ATP, total adenylic nucleotides, and NAD content (pmol/μg of proteins), and in adenylic energy charge (AEC) along the breeding period in newborn released by seahorses from groups M0 and M5.
ATP/ADP ratios in M5 and M0 treatments (3.69 ± 1.20 and 1.82 ± 0.83, respectively) were significantly different (n = 17; p = 0.005).
Pooled adenylic nucleotides were significantly correlated with total C (df = 15, r = 0.587, p = 0.01), ADP (r = 0.641, p = 0.006), and especially ATP (r = 0.925, p < 0.0001) and NAD (r = 0.630, p < 0.007). Furthermore, NAD was also positively correlated with total C (r = 0.544, p = 0.024), AMP (r = 0.541, p = 0.025), ADP (r = 0.693, p < 0.002) and ATP (r = 0.630, p = 0.007). Also, AEC was positively correlated with ATP (r = 0.640, p = 0.006) and ATP/ADP ratio (r = 0.722, p = 0.001), and negatively correlated with AMP (r = −0.556, p = 0.020).
Average values for the whole breeding period in the variables assessed in newborns did not differ significantly in most cases. However, the smoothed trends showed in Figures 2–6 revealed that the diverse characteristics of the batches released differed along the breeding season. Accordingly, the characteristics of batches released in the first part of the season (Period 1) differed in most cases from those released later (Period 2). Hence, a comparison between both periods was more informative than the analyses performed over the entire breeding period.
An intermediate phase, tentatively ranging from day 70 to day 100 (see gray bars in Figures 2–4), would indicate a transitional change between stages 1 and 3. The comparative analyses revealed that DW and SL in newborn released on stage 3 were lower in M0 than in M5 batches (K-W test, n = 33, p < 0.01). However, survivals at 7 DAR did not differ with treatments nor stages (K-W test, n = 33, p = 0.370). Concerning total lipids and FAs, the most significant differences occurred between stages 1–2 and 3, whereas MO and M5 batches released in stage 1 showed similar profiles and wide ranges for most FA. Interestingly, DHA (22:6n-3), AA (20:4n-6), and 11-eicosenoic acid (20:1n-9) contents in M0 and M5 batches from stage 1 were significantly lower than those in stage 3 (n = 20, p = 0.002).
About AMP, ADP, ATP, NAD, and AEC across groups M0 and M5, no statistically significant differences were revealed by the comparisons carried out on newborn batches produced on the first and second periods of the breeding season.
Additional data and results on statistical tests performed on newborn characteristics, lipid and FAs content, endogenous nucleotides, AEC and NAD are provided in Supplementary Figures 1–4. Data on the content in total C, total N and protein are shown in Supplementary Figure 5.
Ultrathin sections of abdominal portions of newborn seahorses were studied by transmission electron microscopy (TEM) to analyze the ultrastructural aspects of muscular tissue. Longitudinal sections from groups M0 and M5 showed the sarcoplasm filled with myofibrils oriented parallel to the long axis of the cell. The sarcomeres displayed the characteristic Z lines, light isotrope bands I, dark anisotrope bands A, and central H-bands bisected by M lines. That sarcoplasmatic reticule appeared regularly spaced. The sarcotubules formed a continuous system around and between individual myofibrils. Sarcoplasmic reticulum cisternae in contact with invaginations of the sarcolemma, T tubules, where they formed the sarcoplasmatic triad have, also, been observed. These were located at the Z line level of sarcomeres (Figures 6a,b, Upper).
Figure 6. Transmission electron micrographs of skeletal muscle in newborn seahorses from groups (a) M5 and (b) M0. Upper: Longitudinal sections. Sarcomeres with characteristic Z lines, I bands, A bands, and central H-bands bisected by M lines. Triad (T) located at the Z line level. Sarcoplasmic reticulum (Sr). Glycogen granules (arrowheads). Scale bars: 400 nm. Middle: Oblique sections at various levels through the sarcomeres. Light isotrope band (I); dark anisotrope band (A); myonucleus (N); mitochondria (asterisks); muscle satellite cell (S). Scale bars: 1 μm. Lower: Transverse sections. Myofibrils (Mf); mitochondria (asterisks). Sarcoplasmic reticule (Sr). Glycogen granules (arrowheads). Scale bars: 500 nm.
The oblique (Figures 6a,b, Middle) and transverse sections (Figures 6a,b, Lower) from groups M0 and M5 of a striated muscle through the A and I bands showed that the myofibrils sectioned at various levels through the sarcomeres revealed the regular different arrays of filaments. The myofibrils were represented by closely packed irregular polygonal profiles and this hexagonal organization determined by the arrangement of the thick and thin of the myofilaments was clearly visible. These are separated by a small amount of sarcoplasm containing rows of mitochondria regularly organized among the sarcomeres. The nuclei of fiber muscles, myonucleus, were located in the peripheral layer of the fiber beneath the sarcolemma. Numerous satellite cells, identified by their location inside the basal lamina and outside the sarcolemma, have been seen. They were characterized by a very little cytoplasm around the nucleus, devoid of myofilaments and few small mitochondria. Glycogen granules were also observed (Figures 6a,b, Upper and Lower).
The PCA performed on datasets (see variables included in Figure 7) revealed that factors 1 and 2 explained 61.4% of total data variability. Newborn batches released on the first half of the breeding season were displayed on the left side of the plot, whereas samples released on the second half were closer to each other and located on the right side. Factor 1 was positively associated with time (days elapsed since the onset of the breeding period), 17:0, 20:1n-9, 20:4 n-6, 22:6n-3, DHA/EPA, and DHA/DPA, and negatively related with 16:1n-7 and 22:5n-3. The plot also showed a higher similarity between samples collected on the second half of the breeding period (batches M0-2 and M5-2) compared to those on the first half of that period (M0-1 and M5-1). Factors 3 and 4 accounted for 18.2% of total variability, which was mostly explained by the content in 18:0 (M0 < M5).
Figure 7. Factor score plots for the principal component analysis (PCA) on newborn seahorses released by breeders in groups M0 and M5, considering batches released on the first (M0-1 and M5-1; 0–100 DAR) and second (M0-2 and M5-2; >100 DAR) half of the breeding season. Only the most contributing variables (cos2 > 0.6) are shown. Ellipses correspond to 95% confidence intervals. Original variable names were automatically modified by R software. Variables in upper PCA plots: days elapsed since the onset of the breeding period (Time), SL, DW, survival at 7 DAR, total lipids, saturated FA (S), monoenes (M), polyunsaturated FA (P), n-3 HUFA, n-3 FA, n-6 FA, n-3/n-6 (n3.n6), DHA/EPA, DHA/AA, and individual FA. saturated FA (S), monoenes (M), polyenes (P), n-3 HUFA, DHA/EPA, DHA/AA, DHA/DPA, and individual FA. Variables in lower PCA plots: Time, SL, DW, survival at 7 DAR, AMP, ADP, ATP, NAD, and AEC (Adenylic energy charge).
%
With regards to PCA results on endogenous nucleotides and energy charge in newborn seahorses (Figure 7), Factor 1 (36.8%) was positively associated with NAD, ATP, and newborn weight, whereas factor 2 (21.7%) was positively related to time and survival at 7 DAR, and negatively associated with lipid content. The plot showed clear discrimination among treatments, with lower endogenous nucleotide levels in treatment M0, particularly in M0-2. The main finding in factors 3 (12.9%) and 4 (9%) biplot was the higher energy charge in newborn from treatment M5 during the whole breeding season.
The results achieved on the assessment of effects of dietary treatments on the pre-breeding season in the tropical seahorse H. reidi denoted significant improvements in size, weight, endogenous nucleotide content and AEC in newborn, as well as several changes in the biochemical composition (FA profiles). The use of Artemia as the unique prey (treatment M0) resulted in lower initial fitness in newborns as compared to those fed on the mixed diet including mysidaceans (treatment M5). Since some aspects of the influence of the diet on reproductive potential and success in H. reidi have been assessed previously (Planas, 2021), this discussion will be mostly focused on biochemical characteristics, energetic status and tissue ultrastructure in newborns.
The experimental diets were efficiently incorporated into the breeder’s tissues as assessed by stable isotopes (Planas, 2021). Hence, the results achieved would rely mainly on the biochemical characteristics of the diets provided during the pre-breeding season. The negative effect of low-quality diets (i.e., non-enriched adult Artemia) on fish breeding has been demonstrated in many fish species (Izquierdo et al., 2000, 2001; Piccinetti et al., 2012). Providing a mixed diet including a potential high-quality prey (i.e., mysidaceans, amphipods, or carideans) or supplementary enrichment (Murugan et al., 2009; Otero-Ferrer et al., 2012; Saavedra et al., 2014) would certainly enhance breeding success as reported in H. guttulatus (Palma et al., 2012, 2017; Planas et al., 2020b). Neither average lipid content nor initial survivals for the whole reproduction season resulted significantly affected by the type of pre-breeding diet offered to breeders. However, overall breeding performance (spawning events and total newborn produced), and neonates size and weight were markedly enhanced in breeders fed on the mixed diet (M5-group).
We did not observe premature egg clutch dropping nor premature newborn release in breeders fed exclusively on Artemia, which is in agreement with previous findings in H. guttulatus (Planas et al., 2020b) but not with those in H. hippocampus (Otero-Ferrer et al., 2012; Saavedra et al., 2015) or Hippocampus kuda (Saavedra et al., 2014). Therefore, the quality of breeder’s diet during the pre-reproduction period should not affect postnatal viability if offspring are subsequently fed on high-quality prey such as copepods. However, it is known that the quality of male’s diet offered from 1 month before conception may result in large neonates with low viability (Otero-Ferrer et al., 2016). An effect of diet quality in males has also been reported on other syngnathids (Sagebakken et al., 2017). Our findings might not be extrapolated to other syngnathid species as the early performance of newborns would likely depend upon specific characteristics such as the balance between brood size, newborn size, and survival (Planas et al., 2020b), and on parental size and age (Dzyuba et al., 2006; Faleiro et al., 2016). In that respect, our breeder’s groups were similar in average weight but there were within-group differences in ranges for both size and age. Since those characteristics seem to affect further juvenile performances, it would have been interesting to analyze both growth and survival until more advanced developmental stages and to identify parents involved in each bath produced as suggested by the results obtained by Dzyuba et al. (2006). Unfortunately, parental identities for each batch produced could not be identified in the present study.
The importance of essential FAs, namely n-3 HUFA, to marine and freshwater fish larvae has been studied extensively (Sargent et al., 1999; Izquierdo et al., 2000; Kanazawa, 2003; Olivotto et al., 2010; Tocher, 2010; Zarantoniello et al., 2018), but only a few studies have been published in eggs or newborn seahorses. FA profiles in seahorse eggs, neonates and also adults largely differ depending on species, temperature and breeder’s diet (Chang and Southgate, 2001; Lin et al., 2008; Faleiro and Narciso, 2010; Otero-Ferrer et al., 2010, 2012; Planas et al., 2010, 2020b; Saavedra et al., 2014, 2015; Buen-Ursue et al., 2015), hence, comparative analyses are difficult to assess. Despite the large differences in the proportion of dietary FA groups, average relative proportions in saturates, monoenes, polyenes, and n-3 HUFA in newborn seahorses were rather similar across our dietary treatments. That finding indicates that the production of eggs/neonates in H. reidi is accomplished following a conservative plan for global FA groups, essential FAs (arachidonic and docosahexaenoic acids) and FA ratios. Interestingly, the shapes of the patterns followed by those FAs and ratios in H. reidi resembled those in H. guttulatus if time lags and differences in values are not considered (Figure 8 and Supplementary Figure 6). Conversely to AA and DHA, EPA values in both species along the second phase of the breeding season followed different patterns. These three FA, especially DHA and AA, are essential biochemical compounds in marine fish larvae, being highly dominant within the total pool of FA (Rainuzzo, 1993; Rainuzzo et al., 1997; Sargent et al., 1999). DHA is the main FA in both vitellogenin and developing embryos of marine fishes (Sargent et al., 1999; Tocher, 2003). However, their stores in young seahorses may be partially used as fuel, especially EPA, which is consumed at a higher rate (Faleiro and Narciso, 2010). Differences in EPA catabolism compared to AA and DHA were likely reflected in differences in the patterns of change discussed above. When comparing H. reidi and H. guttulatus, time lags observed for certain FA and ratios (e.g., AA, DHA/EPA, and DHA/DPA) might be due to species-specific differences on inter-batch intervals and temperature thresholds. The ratio DHA/EPA and the content in AA seem to be higher in species from warmer water temperatures (Dey et al., 1993; Ogata et al., 2004; Ibáñez, 2018) but the validity of those assumptions would likely depend on a series of factors other than temperature (e.g., breeder’s diet).
Figure 8. Progressive changes (smoothed trends) in essential FA (AA, EPA, and DHA) and FA ratios (DHA/EPA, DHA/AA, and DHA/DPA) along the breeding period in newborn released by H. reidi (red line; present study) from group M0. For comparison purposes, the same data are provided for H. guttulatus breeders (blue line; Planas et al., 2020b) submitted to a similar dietary treatment. The black curve shows the loess fitting with the confidence interval (95%) in the gray shading for pooled M0-samples from both species.
The interest of analyzing by TEM the ultrastructure of muscular tissue in newborns was due to a previous study in which newborn of H. guttulatus released by breeders fed on Artemia during the reproduction season showed the presence of ultrastructural muscle alterations (Planas et al., 2020b), likely due to deficiencies in DHA (Cong-cong et al., 2019). The observations by TEM have not detected morphological alterations nor differences as for both analyzed groups M0 and M5, suggesting that both administered diets ensured the regular development of muscular tissue.
The energy status of an organism is fully charged when it consists of ATP alone (adenylate charge = 1.0). A charge value of 0.5 indicates that only ADP is present, whereas the energy charge (AEC) is zero when all adenine nucleotide pool is in form of AMP (system fully discharged) (De la Fuente et al., 2014). The AEC might be a relatively conservative property and changes in AEC are always accompanied if not preceded by changes in the quantity of adenylate nucleotides (Atkinson, 1968). Commonly, AEC values in unstressed organisms are above 0.80 (Ivanovici, 1980; Vetter and Hodson, 1982), so that AEC was proposed as an indicator of the energy potentially available for cellular metabolism (Atkinson, 1977; Dickson and Franz, 1980). Interestingly, ATP concentration, total adenylate concentration, and AEC might be useful indices of environmental impact (i.e., stress) (Vetter and Hodson, 1982; Dehn and Schirf, 1986; Methling et al., 2017) and condition factor in fishes (Giesy, 1988). However, AEC level did not always reflect the growth of organisms, as reported by Harms (1992) in sub-optimally fed larvae of the crab Hyas araneus, in which pooled adenylic nucleotides were found to be correlated with the content in total C and protein, reflecting differences in the experimental diets. In our study, pooled adenylic nucleotides were correlated with total C, ATP, and NAD but not with total N (protein content: 65.9 ± 1.4 and 65.1 ± 0.8% in M5 and M0 newborn, respectively). Hence, increases in ATP were reflected in increases in AEC values and total adenylate concentration rather than in declines in ADP or AMP (Vetter and Hodson, 1982). The pool of free amino acids from yolk proteolysis increases in demersal fish eggs during ripening (Craik and Harvey, 1984; Greeley Calder and Wallace et al., 1986). In many teleosts, the pool constitutes the main energy source at that stage, during embryogenesis, and in newly hatched larvae throughout the stage of endogenous nutrition (mostly in larvae lacking oil globules) (Rønnestad et al., 1999). However, lipids are an important initial fuel store in many early developing fish larvae, especially in lecithotrophic larvae with oil globules (Rainuzzo et al., 1997). Our results on nucleotides and AEC in newborn seahorses seem to confirm those findings.
Total adenylic nucleotides pools (mostly reflected in ATP content) and AEC values in batches from M5-treatment were quite stable along the breeding season and similar between batches, approaching 0.8 for AEC (0.78 ± 0.05). In treatment M0, the values were lower (0.62 ± 0.14 for AEC) and much more variable, suggesting a worse energetic fitness in newborn. The findings support the statement of Atkinson (1968), who pointed out that the AEC might be a relatively conservative property and that changes in AEC are always preceded or accompanied by changes in the level of adenylate nucleotides. The differences achieved between treatments for AEC and total adenylic nucleotides pools reflected the food conditions of parents during the pre-breeding period.
The initial ontogeny of fishes is characterized by low assimilation efficiencies, very high growth rates and high energy-consumption for hunting and swimming. The first complete energy allocation budget (discriminating costs of maintenance, growth, and activity) available for early larval stages of marine teleost fish species was carried out by Cunha et al. (2007) in turbot (Psetta maxima) larvae. Those authors reported a daily weight-specific growth rate of 29.6% day–1, and suggested a trade-off between fast growth and viability in the larvae. With growth rates of up to about 25% day–1, the energy demands for growth represent a large fraction of the energy available (Pedersen, 1997). The ATP/ADP ratio is a robust readout of the cellular energy status and its value should remain high in order to maximize the energy status and cell metabolism. Conversely, energy depletion with an accumulation of AMP would deplete the cell’s stores of ATP and ADP leading to cell death. Considering the higher AEC values in M5-newborn compared to M0-newborn (3.69 ± 1.20 and 1.82 ± 0.83, respectively), a greater amount of energy would be available in the former for growth and cell metabolism. The values of ATP/ADP ratio in newborn seahorses support that statement, showing that suboptimal feeding conditions in breeders were reflected in the nucleotide pool and energy charge of newborn. In this context, higher initial growth rates would be expected in M5-newborns. However, neither survivals nor specific growth rates until day 7 differed significantly (SGR: 17.7 ± 7.4 and 20.8 ± 4.5% day–1 in M5- and M0-juveniles, respectively) (data not provided). Those growth rates were high but markedly lower than in larvae of other fish species, likely due to low digestion and assimilation efficiencies in early developing seahorses (Novelli et al., 2015; Ofelio et al., 2018; Valladares and Planas, 2021). Seahorse newborn from treatment M0 enhanced their AEC values along the first stage of the breeding season, suggesting a progressive energetic improvement. Increasing AEC values in newborn might facilitate a rapid resumption of synthetic activity when accessing high-quality food (Harms, 1992) such as copepods (Olivotto et al., 2008; Palma et al., 2017; Randazzo et al., 2018).
NAD plays an important role as energy and signal transducer in cellular metabolism and can be synthesized from NMN (nicotinamide mononucleotide) and ATP. Overall, ATP, total adenylic nucleotides and endogenous NAD in seahorse newborns were highly correlated, and their levels in group M5 were about 2. 3-, 1. 7-, and 1.4-fold higher than in newborns from group M0. Studies on this subject in marine fish larvae are extremely scarce but our findings agree with those reported in developing clownfish (Amphiprion ocellaris) larvae by Olivotto et al. (2011a). In that study, the authors suggested that the enhancement in HUFA content in preys may improve larval development through the presence of better-structured mitochondria, and higher energy status and ATP synthesis compared to the control. Consequently, growth performance and survivals enhanced in clownfish larvae supplemented with HUFA and the larval phase was shortened, resulting in better larval condition to undertake metamorphosis.
In seahorse neonates, yolk reserves are lacking or almost exhausted by the time of male’s pouch release (Novelli et al., 2015; Ofelio et al., 2018). Hence, the quantity of lipid of energy reserves would be particularly important for the newborns to withstand starvation or low-quality prey supply, especially in species lacking yolk sac at hatching (e.g., seahorses). However, the presence of ontogenetic shifting in the energy allocation strategy (growth vs storage) demonstrated in other fish larvae (Cunha et al., 2007; Olivotto et al., 2011a; Di Pane et al., 2019) is unknown in seahorses, deserving further investigation.
The pattern of change throughout the breeding season was investigated to identify and assess the effects with time of pre-breeding feeding on the variables analyzed (see Figures 2–5 and Supplementary Figure 6) and the timing of energy allocation to reproduction. The detection of knee-phases and their onset on general patterns for each variable were used to determine the breeding strategy followed by H. reidi. In a previous study, we applied a similar assessment to the temperate seahorse H. guttulatus, whose breeders fed on different diets (but similar to those used in the present study) during the breeding season (Planas et al., 2020b). Our results in H. reidi were highly similar to those achieved in H. guttulatus. Both species displayed a mixed capital-income strategy, which implies a progressive decrease in newborn quality along the breeding season (McBride et al., 2015; Griffen, 2018). Due to species-specific characteristics concerning temperature and inter-brood intervals, the knee-phase in H. reidi occurred about 40–50 days earlier than in the temperature species. Inter-brood intervals are about 14–16 days at 25–27°C in H. reidi (Otero-Ferrer et al., 2016) and 27–35 days at 18–20°C in H. guttulatus (Planas et al., 2010).
In most cases, knee-periods identified in general trends for H. reidi were located within the period comprising days 70 and 100 since the onset of the breeding season, depending on the variable and treatment considered. Hence, samples of newborn released before day 100 were notably different to those subsequently released, as confirmed by the PCS analysis and the hierarchical cluster analysis provided in Figure 9. The similarity dendrograms also showed high differences among groups from both dietary treatments, particularly for those released before day 100. Accordingly, three stages can be recognized along the whole breeding period:
1. Period 1 (mixed capital-income sources): this stage would extend until days 70–100 since the onset of the breeding season. Most energy and nutritional resources allocated to eggs/newborn originated from the diet provided before the onset of the breeding period (capital breeding). However, the importance of income breeding (sources gained to the experimental diet) increases gradually.
2. Inflection period (income sources): this is a transitory period shown by a knee on smoothed curves. Considering the whole datasets, the duration of this stage would be about 1 month, indicating the end of the capital-income breeding period and the use of nutritional resources from the breeder’s diet.
3. Period 2 (income sources and exhaustion of body reserves): globally, the similarity between dietary treatments increased compared to samples released in Period 1. This statement is especially true for FAs profiles. Conversely, differences for total adenylic nucleotides and NAD were maximal by the end of this period. The drop observed in those products and in survivals from group M0 was noticeable. These findings together with the values for DHA and DHA/EPA in late batches suggest different levels of body resources exhaustion, particularly notorious in M0-newborn as the result of limitation in the availability of nutrients for egg/newborn formation. In this period, brood size and newborn size, and weight were considerably lower in treatment M0.
Figure 9. Hierarchical cluster plot (complete linkage, Pearson method) for samples (left) and variables (right) analyzed for fatty acids, nucleotides, and elemental composition (C and N) in newborn batches. Original variable names were automatically modified by R software. Sample identifiers Mx-y indicate experimental group (Mx) and days elapsed since the onset of the breeding period (y). Variables: days elapsed since the onset of the breeding period (Time), batch size (Batch), length (SL), dry weight (DW), survival at 7 DAR (SUR), AMP, ADP, ATP, Pooled (AMP + ADP + ATP), NAD, adenylate energy charge (EC), total C, total N, total lipids (LIP), fatty acids (>1% percentage), DHA/EPA, DHA/AA, and DHA/DPA.
Breeding patterns are a conditional reproductive strategy since patterns along the capital–income continuum may shift with ontogeny or environmental conditions (McBride et al., 2015; Williams et al., 2017). Shiftings on the acquisition of nutritional and energy supplies for newborns along the breeding season were reported in H. guttulatus (Planas et al., 2020b) and validated for H. reidi in the present study. Shifting patterns, timing and effective duration of feeding treatments are crucial factors to understand breeding success in synchronous species such as seahorses. The duration of the improvement in eggs or fry quality is a pivotal aspect that must be precisely determined. However, the data available are rather contradictory. Some characteristics in newborn resulted notably enhanced in most M5-batches along the whole reproductive period compared to those from treatment M0. This finding agrees with our previous study on H. guttulatus. However, the effect of enhanced diets provided to H. kuda females on egg size was limited to the first spawning (Saavedra et al., 2014). From a nutritional and energetical point of view, the roles played by seahorse males and females in the production of eggs and neonates remain unclear, even though the contribution of males (and the effect of their diets) seems to be important in nutritional supply (Skalkos et al., 2020) and a key determinant in newborn quality (Otero-Ferrer et al., 2020).
The results achieved in this study in H. reidi demonstrate the importance of feeding breeders on a high-quality diet (M5-treatment) during the pre-breeding period. The improved diet, including Artemia and mysidaceans during the whole breeding season, resulted in more reproductive events, and larger size and higher energetic status (ATP, total adenylic nucleotides, AEC, and NAD) in neonates. Initially, the effect of dietary treatments on n-3 and n-6 HUFA, especially on DHA, were lower than expected but noticed in FA ratios. This finding suggests (but not demonstrates) lower requirements in n-3 HUFA than other seahorse species. The species displayed a mixed capital-income breeding continuum, as previously demonstrated in H. guttulatus, in which the effects of the pre-reproductive diet were mainly noticed on the first 2–3 months of the breeding season. Even though the further newborn performance was not jeopardized when the juveniles gained access to high-quality prey, the effect of parental diets should deserve further investigation under low quality or availability of food to seahorse juveniles.
The datasets presented in this study can be found in online repositories (http://dx.doi.org/10.17632/n6zt5zw22w.1).
The animal study was reviewed and approved by the Xunta de Galicia (REGA ES360570202001/15/FUN/BIOL.AN/MPO01).
MP: funding acquisition, project administration, conceptualization, methodology, fatty acids and other analyses, data curation, formal analysis, visualization, writing—original draft preparation, and writing—review and editing. IO: formal analysis, data curation, visualization, and writing—review and editing. MG: methodology, fatty acids analysis, and data curation. RL: methodology, TEM analysis, formal analysis, visualization, and writing—review and editing. CA and AA: methodology, nucleotides analysis, data curation, and writing—review and editing. MZ: methodology, nucleotides analysis, data curation, formal analysis, and writing—review and editing. All authors contributed to the article and approved the submitted version.
This study was financially supported by the Spanish Government with Project Hippoeco (Ref. CGL2015-68110-R, MINECO) and Fondos FEDER.
The authors declare that the research was conducted in the absence of any commercial or financial relationships that could be construed as a potential conflict of interest.
We acknowledge the support of the publication fee by the CSIC Open Access Publication Support Initiative through its Unit of Information Resources for Research (URICI). We are grateful to Alexandro Chamorro, David Costas, Isabel Medina, and the staff of the Aquaria Service at IIM-CSIC for assistance in the maintenance of seahorses in captivity and technical support. We are also grateful to the Regional Government Xunta de Galicia for providing permission for the capture of wild adult seahorses Hippocampus guttulatus. Finally, we are very grateful to Sergio Picazo (https://sergiopicazo.com/) for providing usage rights of seahorse illustration in Figure 1.
The Supplementary Material for this article can be found online at: https://www.frontiersin.org/articles/10.3389/fmars.2021.688058/full#supplementary-material
Atkinson, D. E. (1968). The energy charge of the adenylate pool as a regulatory parameter with feedback modifiers. Biochemistry 7, 4030–4034. doi: 10.1021/bi00851a033
Atkinson, D. E. (1977). Cellular Energy Metabolism and Its Regulation. New York, NY: Acadamic Press, 293.
Atkinson, D. E., and Walton, G. M. (1967). Adenosine triphosphate conservation in metabolism regulation. J. Biol. Chem. 242, 3239–3241. doi: 10.1016/S0021-9258(18)95956-9
Binh, M. N., and Serrano, A. E. Jr. (2012). Optimum level of fish liver oil as enrichment for Artemia fed to the tiger tail seahorse Hippocampus comes for reproduction and juvenile survival. AACL Bioflux 5, 249–258.
Blanco, A., Chamorro, A., and Planas, M. (2014). Implications of physical key factors in the early rearing of the long-snouted seahorse Hippocampus guttulatus. Aquaculture 433, 214–222. doi: 10.1016/j.aquaculture.2014.06.019
Bligh, E. G., and Dyer, W. J. (1959). A rapid method of total lipid extraction and purification. Can. J. Biochem. Physiol. 37, 911–917. doi: 10.1139/o59-099
Bromage, N. R. (1995). “Broodstock management and seed quality – general considerations,” in Broodstock Management and Egg and Larval Quality, eds N. R. Bromage and R. J. Roberts (London: Blackwell), 1–24.
Brooks, S., Tyler, C. R., and Sumpter, J. P. (1997). Egg quality in fish: what makes a good egg? Rev. Fish Biol. Fisher. 7, 387–416. doi: 10.1023/A:1018400130692
Buen-Ursue, S. M. A., Azuma, T., Arai, K., and Coloso, R. M. (2015). Improved reproductive performance of tiger tail seahorse, Hippocampus comes, by mysid shrimp fed singly or in combination with other natural food. Aquacult. Int. 23, 29–43. doi: 10.1007/s10499-014-9795-1
Carcupino, M., Baldacci, A., Mazzini, M., and Franzoi, P. (2002). Functional significance of the male brood pouch in the reproductive strategies of pipefishes and seahorses: a morphological and ultrastructural comparative study on three anatomically different pouches. J. Fish Biol. 61, 1465–1480. doi: 10.1111/j.1095-8649.2002.tb02490.x
Chang, M., and Southgate, P. C. (2001). Effects of varying dietary fatty acid composition on growth and survival of seahorse, Hippocampus sp., juveniles. Aquarium Sci. Conserv. 3, 205–214. doi: 10.1023/A:1011363807074
Christie, W. W. (1982). Lipid Analysis: Isolation, Separation, Identification, and Structural Analysis. Oxford: Pergamon Press, 207.
Cohen, F. P. A., Valenti, W. C., Planas, M., and Calado, R. (2016). Seahorse aquaculture, biology and conservation: Knowledge gaps and research opportunities. Rev. Fish. Sci. Aquac. 25, 1–12. doi: 10.1080/23308249.2016.1237469
Cong-cong, W., Wen-bin, L., Yang-yang, H., Xi, W., Xiang-fei, L., Ding-dong, Z., et al. (2019). Dietary DHA affects muscle fiber development by activating AMPK/Sirt1 pathway in blunt snout bream (Megalobrama amblycephala). Aquaculture 518:734835. doi: 10.1016/j.aquaculture.2019.734835
Craik, J. C. A., and Harvey, S. M. (1984). Biochemical changes occurring during final maturation of eggs of some marine and freshwater teleosts. J. Fish Biol. 2, 599–610. doi: 10.1111/j.1095-8649.1984.tb04830.x
Csardi, G., and Nepusz, T. (2006). The igraph software package for complex network research. Inter J. Complex Syst. 1695, 1–9.
Cunha, I., Conceição, L. E. C., and Planas, M. (2007). Energy allocation and metabolic scope in early turbot, Scophthalmus maximus, larvae. Mar. Biol. 151, 1397–1405. doi: 10.1007/s00227-006-0576-x
Cunha, I., Saborido-Rey, F., and Planas, M. (2003). Use of multivariate analysis to assess the nutritional condition of fish larvae from nucleic acids and protein content. Biol. Bull. 204, 339–349. doi: 10.2307/1543604
De la Fuente, I. M., Cortés, J. M., Valero, E., Desroches, M., Rodrigues, S., Malaina, I., et al. (2014). On the dynamics of the adenylate energy system: Homeorhesis vs Homeostasis. PLoS One 9:e108676. doi: 10.1371/journal.pone.0108676
Dehn, P. F., and Schirf, V. R. (1986). Energy metabolism in largemouth bass (Micropterus floridanus salmoides) from stressed and non-stressed environments: adaptations in the secondary stress response. Comp. Biochem. Physiol. A Comp. Physiol. 84, 523–528. doi: 10.1016/0300-9629(86)90360-9
Dey, I., Buda, C., Wiik, T., Harvel, J. E., and Farkas, T. (1993). Molecular and structural composition of phospholipid membranes in livers of marine and freshwater fish in relation to temperature. Proc. Natl. Acad. Sci. U.S.A. 90, 7498–7502. doi: 10.1073/pnas.90.16.7498
Di Pane, J., Joly, L., Koubbi, P., Giraldo, C., Monchy, S., Tavernier, E., et al. (2019). Ontogenetic shift in the energy allocation strategy and physiological condition of larval plaice (Pleuronectes platessa). PLoS One 14:e0222261. doi: 10.1371/journal.pone.0222261
Dickson, G. W., and Franz, R. (1980). Respiration rates, ATP turnover and adenylate energy charge in excised gills of surface and cave crayfish. Comp. Biochem. Physiol. 65A, 375–379. doi: 10.1016/0300-9629(80)90048-1
Dzyuba, B., Van Look, K. J. W., Cliffe, A., Koldewey, H. J., and Holt, W. V. (2006). Effect of parental age and associated size on fecundity, growth and survival in the yellow seahorse Hippocampus kuda. J. Exp. Biol. 209, 3055–3061. doi: 10.1242/jeb.02336
Faleiro, F., Almeida, A. J., Ré, P., and Narciso, L. (2016). Size does matter: an assessment of reproductive potential in seahorses. Anim. Reprod. Sci. 170, 61–67. doi: 10.1016/j.anireprosci.2016.04.003
Faleiro, F., and Narciso, L. (2010). Lipid dynamics during early development of Hippocampus guttulatus seahorses: Searching for clues on fatty acid requirements. Aquaculture 37, 56–64. doi: 10.1016/j.aquaculture.2010.07.005
Fernández-Palacios, H., Norberg, B. G., Izquierdo, M., and Hamre, K. (2011). “Effects of broodstock diets on eggs and larvae,” in Larval Fish Nutrition, ed. J. Holt (London: Wiley-Blackwell), 153–181. doi: 10.1002/9780470959862.ch5
Foster, S. J., and Vincent, A. C. J. (2004). Life history and ecology of seahorses: implications for conservation and management. J. Fish Biol. 65, 1–61. doi: 10.1111/j.1095-8649.2004.00429.x
Giesy, J. (1988). Phosphoadenylate concentrations and adenylate energy charge of largemouth bass (Micropterus salmoides): relationship with condition factor and blood cortisol. Comp. Biochem. Physiol. A Comp. Physiol. 90, 367–377. doi: 10.1016/0300-9629(88)90204-6
Giraudoux, P., Antonietti, J. P., Beale, C., Pleydell, D., and Treglia, M. (2018). Spatial Analysis and Data Mining for Field Ecologists v. 1.6.9. Available online at: https://cran.r-project.org/web/packages/pgirmess/index.html (accessed January 16, 2018).
Greeley, M. S. Jr., Calder, D. R., and Wallace, R. A. (1986). Changes in teleost yolk proteins during oocyte maturation: correlation of yolk proteolysis with oocyte hydration. Comp. Biochem. Physiol. B 84, 1–9. doi: 10.1016/0305-0491(86)90262-2
Griffen, B. D. (2018). The timing of energy allocation to reproduction in an important group of marine consumers. PLoS One 13:e0199043. doi: 10.1371/journal.pone.0199043
Harms, J. (1992). Effects of nutrition (herbivore vs carnivore) on energy charge and nucleotide composition in Hyas araneus larvae. Helgolander Meeresunters 46, 29–44. doi: 10.1007/BF02366210
Herbes, S. E., and Allen, C. P. (1983). Lipid quantification of freshwater invertebrates: method modification for microquantitation. Can. J. Fish. Aquat. Sci. 40, 1315–1317. doi: 10.1139/f83-150
Husson, F., Josse, J., Le, S., and Mazet, J. (2020). FactoMineR: Multivariate Exploratory Data Analysis and Data Mining. Available online at: https://CRAN.R-project.org/package=FactoMineR (accessed March 20, 2020).
Ibáñez, N. (2018). Efecto de la Biogeografía en los Perfiles de Ácidos Grasos de caballitos de mar Recién Nacidos. Ph. D. Thesis. Vigo: University of Vigo, 43.
Ivanovici, A. M. (1980). Application of adenylate energy charge to problems of environmental impact assessment in aquatic organisms. Helgolander Meeresunters 33, 556–565. doi: 10.1007/BF02414779
Izquierdo, M., Socorro, J., Arantzamendi, L., and Hernández-Cruz, C. M. (2000). Recent advances in lipid nutrition in fish larvae. Fish Physiol. Biochem. 22, 97–107. doi: 10.1023/A:1007810506259
Izquierdo, M. S., Fernández-Palacios, H., and Tacon, A. G. J. (2001). Effect of broodstock nutrition on reproductive performance of fish. Aquaculture 197, 25–42. doi: 10.1016/S0044-8486(01)00581-6
Kanazawa, A. (2003). Nutrition of marine fish larvae. J. Appl. Aquacult. 13, 103–143. doi: 10.1300/J028v13n01_05
Kassambara, A. (2020a). rstatix: Pipe-Friendly Framework for Basic Statistical Tests. Available online at: https://cloud.r-project.org/web/packages/rstatix/index.html (accessed July 13, 2020).
Kassambara, A. (2020b). factoextra: Extract and Visualize the Results of Multivariate Data Analyses. Available online at: https://CRAN.R-project.org/package=factoextra (accessed February 23, 2020).
Koldewey, H. J., and Martin-Smith, K. M. (2010). A global review of seahorse aquaculture. Aquaculture 302, 131–152. doi: 10.1016/j.aquaculture.2009.11.010
Lepage, G., and Roy, C. C. (1986). Direct transesterification of all classes of lipids in a one-step reaction. J. Lipid Res. 27, 114–120. doi: 10.1016/S0022-2275(20)38861-1
Lin, Q., Gao, Y. L., Sheng, J. Q., Chen, Q. X., Zhang, B., and Lu, J. Y. (2007). The effect of food and the sum of effective temperature on the embryonic development of the seahorse, Hippocampus kuda Bleeker. Aquaculture 262, 481–492. doi: 10.1016/j.aquaculture.2006.11.011
Lin, Q., Lin, J., Lu, J., and Li, B. (2008). Biochemical composition of six seahorse species, Hippocampus sp., from the Chinese Coast. J. World Aquacult. Soc. 29, 225–234. doi: 10.1111/j.1749-7345.2008.00159.x
Lin, Q., Lu, J., Gao, Y., Shen, L., Cai, J., and Luo, J. (2006). The effect of temperature on gonad, embryonic development and survival rate of juvenile seahorses, Hippocampus kuda Bleeker. Aquaculture 254, 701–713. doi: 10.1016/j.aquaculture.2005.11.005
Martin-Smith, K. M., Samoilys, M. A., Meeuwig, J. J., and Vincent, A. C. J. (2004). Collabo-rative development of management options for an artisanal fishery for seahorses in thecentral Philippines. Ocean Coast. Manag. 47, 165–193. doi: 10.1016/J.OCECOAMAN.2004.02.002
McBride, R. S., Somarakis, S., Fitzhugh, G. R., Albert, A., Yaragina, N. A., Wuenschel, M. J., et al. (2015). Energy acquisition and allocation to egg production in relation to fish reproductive strategies. Fish Fish. 16, 23–57. doi: 10.1111/faf.12043
Methling, C., Skov, P. V., and Madsen, N. (2017). Reflex impairment, physiological stress, and discard mortality of European plaice Pleuronectes platessa in an otter trawl fishery. ICES J. Mar. Sci. 74, 1660–1671. doi: 10.1093/icesjms/fsx004
Migaud, H., Bell, G., Cabrita, E., McAndrew, B., Davie, A., Bobe, J., et al. (2013). Gamete quality and broodstock management in temperate fish. Rev. Aquacult. 5(Suppl. 1), S194–S223. doi: 10.1111/raq.12025
Mori, V., Amici, A., Mazzola, F., Di Stefano, M., Conforti, L., Magni, G., et al. (2014). Metabolic profiling of alternative NAD biosynthetic routes in mouse tissues. PLoS One 9:e113939. doi: 10.1371/journal.pone.0113939
Murugan, A., Dhanya, S., Sreepada, R. A., Rajagopal, S., and Balasubramanian, T. (2009). Breeding and mass scale rearing of three spotted seahorse, Hippocampus trimaculatus Leach under captive conditions. Aquaculture 290, 87–96. doi: 10.1016/j.aquaculture.2009.01.033
Novelli, B., Socorro, J. A., Caballero, M. J., Otero-Ferrer, F., Segade-Botella, A., and Molina Domínguez, L. (2015). Development of seahorse (Hippocampus reidi, Ginsburg 1933): histological and histochemical study. Fish Physiol. Biochem. 41, 1233–1251. doi: 10.1007/s10695-015-0082-5
Ofelio, C., Díaz, A. O., Radaelli, G., and Planas, M. (2018). Histological characterization of early developmental stages in the seahorse Hippocampus guttulatus. J. Fish Biol. 93, 72–87. doi: 10.1111/jfb.13668
Ogata, H. Y., Emata, A. C., Garibay, E. S., and Furuita, H. (2004). Fatty acid composition of five candidate aquaculture species in Central Philippines. Aquaculture 236, 361–375. doi: 10.1016/j.aquaculture.2003.10.015
Olivotto, I., Avella, M., Sampaolesi, G., Piccinetti, C. C., Ruiz, P., and Carnevali, O. (2008). Breeding and rearing the longsnout seahorse Hippocampus reidi: rearing and feeding studies. Aquaculture 283, 92–96. doi: 10.1016/j.aquaculture.2008.06.018
Olivotto, I., Di Stefano, M., Rosetti, S., Cossignani, L., Pugnaloni, A., Giantomassi, F., et al. (2011a). Live prey enrichment, with particular emphasis on HUFAs, as limiting factor in false percula clownfish (Amphiprion ocellaris, Pomacentridae) larval development and metamorphosis: molecular and biochemical implications. Comp. Biochem. Physiol. A Mol. Integr. Physiol. 159, 207–218. doi: 10.1016/j.cbpa.2011.02.004
Olivotto, I., Planas, M., Simões, N., Holt, G. J., Avella, M. A., and Calado, R. (2011b). Advances in breeding and rearing marine ornamentals. J. World Aquacult. Soc. 42, 135–166. doi: 10.1111/j.1749-7345.2011.00453.x
Olivotto, I., Tokle, N. E., Nozzi, V., Cossignani, L., and Carnevali, O. (2010). Preserved copepods as a new technology for the marine ornamental fish aquaculture: a feeding study. Aquaculture 308, 124–131. doi: 10.1016/j.aquaculture.2010.08.033
Otero-Ferrer, F., Izquierdo, M., Fazeli, A., Band, F., and Holt, W. V. (2016). Embryonic developmental plasticity in the long-snouted seahorse (Hippocampus reidi, Ginsburg 1933) in relation to parental preconception diet. Reprod. Fert. Dev. 28:1020. doi: 10.1071/rd14169
Otero-Ferrer, F., Lättekivi, F., Ord, J., Reimann, E., Kõks, S., Izquierdo, M., et al. (2020). Time-critical influences of gestational diet in a seahorse model of male pregnancy. J. Exp. Biol. 2020:jeb210302. doi: 10.1242/jeb.210302
Otero-Ferrer, F., Molina, L., Socorro, J., Fernández-Palacios, H., and Izquierdo, M. (2012). Effect of different live prey on spawning quality of short-snouted seahorse, Hippocampus hippocampus (Linnaeus, 1758). J. World Aquacult. Soc. 43, 174–186. doi: 10.1111/j.1749-7345.2012.00550.x
Otero-Ferrer, F., Molina, L., Socorro, J., Herrera, R., Fernández-Palacios, H., and Izquierdo, M. (2010). Live prey first feeding regimes for short-snouted seahorse Hippocampus hippocampus (Linnaeus, 1758) juveniles. Aquacult. Res. 41, e8–e19. doi: 10.1111/j.1365-2109.2010.02505.x
Palma, J., Andrade, J. P., and Bureau, D. P. (2012). Growth, reproductive performances, and brood quality of long snout seahorse, Hippocampus guttulatus, fed enriched shrimp diets. J. World Aquacult. Soc. 43, 802–813. doi: 10.1111/j.1749-7345.2012.00611.x
Palma, J., Andrade, J. P., and Bureau, D. P. (2017). The impact of dietary supplementation with astaxanthin on egg quality and growth of long snout seahorse (Hippocampus guttulatus) juveniles. Aquacult. Nutr. 23, 304–312. doi: 10.1111/anu.12394
Pedersen, B. H. (1997). The cost of growth in young fish larvae, a review of new hypotheses. Aquaculture 155, 259–269. doi: 10.1016/S0044-8486(97)00127-0
Piccinetti, C. C., Ricci, L. A., Tokle, N., Radaelli, G., Pascoli, F., Cossignani, L., et al. (2012). Malnutrition may affect common sole (Solea solea L.) growth, pigmentation and stress response: molecular, biochemical and histological implications. Comp. Biochem. Physiol. A Mol. Integr. Physiol. 161, 361–371. doi: 10.1016/j.cbpa.2011.12.009
Planas, M. (2021). Carry-over effects of pre-breeding diets on seahorse (Hippocampus reidi) reproductive success. Aquaculture 533:736148. doi: 10.1016/j.aquaculture.2020.736148
Planas, M., Blanco, A., Chamorro, A., Valladares, S., and Pintado, J. (2012). Temperature-induced changes of growth and survival in the early development of the seahorse Hippocampus guttulatus. J. Exp. Mar. Bio. Ecol. 438, 154–162. doi: 10.1016/j.jembe.2012.10.003
Planas, M., Chamorro, A., Paltrinieri, A., Campos, S., Jiménez, A., Nedelec, K., et al. (2020a). Effect of diet on breeders and inheritance in syngnathids: application of isotopic experimentally derived data to field studies. Mar. Ecol. Prog. Ser. 650, 107–123. doi: 10.3354/meps13315
Planas, M., Chamorro, A., Quintas, P., and Vilar, A. (2008). Establishment and maintenance of threatened long-snouted seahorse, Hippocampus guttulatus, broodstock in captivity. Aquaculture 283, 19–28. doi: 10.1016/j.aquaculture.2008.06.023
Planas, M., Olivotto, I., González, M. J., Laurà, R., and Zarantoniello, M. (2020b). A multidisciplinary experimental study of the effects of breeders diet on newborn seahorses (Hippocampus guttulatus). Front. Mar. Sci. 7:638. doi: 10.3389/fmars.2020.00638
Planas, M., Quintas, P., Chamorro, A., and Silva, C. (2010). Female maturation, egg characteristics and fatty acids profile in the seahorse Hippocampus guttulatus. Anim. Reprod. Sci. 122, 66–73. doi: 10.1016/j.anireprosci.2010.07.008
Planas, M., Silva, C., Quintas, P., Chamorro, A., and Piñero, S. (2017). Ongrowing and enhancement of n-3 HUFA profile in adult Artemia: short- vs long-time enrichment. J. Appl. Phycol. 29, 1409–1420. doi: 10.1007/s10811-016-1016-z
R Core Team (2020a). The R Project for Statistical Computing Vienna, Austria. Available online at: http://www.R-project.org (accessed April 23, 2020)
R Core Team (2020b). Stats: The R stats package, Vienna, Austria. Available online at: https://www.rdocumentation.org/packages/stats/versions/3.6.2 (accessed February 23, 2018)
Rainuzzo, J. R. (1993). “Fatty acid and lipid composition of fish eggs and larvae,” in Fish Farming Techonology, eds H. Reinertsen, L. A. Dahle, J. Jørgensen, and K. Tvinnereim (Rotterdam: Balkema), 43–48. doi: 10.1201/9781003077770-8
Rainuzzo, J. R., Reitan, K. I., and Olsen, Y. (1997). The significance of lipids at early stages of marine fish?: a review. Aquaculture 155, 103–115. doi: 10.1016/S0044-8486(97)00121-X
Randazzo, B., Rolla, L., Ofelio, C., Planas, M., Gioacchini, G., Vargas-Abúndez, J. A., et al. (2018). The influence of diet on the early development of two seahorse species (H. guttulatus and H. reidi): Traditional and innovative approaches. Aquaculture 490, 75–90. doi: 10.1016/j.aquaculture.2018.02.029
Rønnestad, I., Thorsen, A., and Finn, R. N. (1999). Fish larval nutrition: a review of recent advances in the roles of amino acids. Aquaculture 177, 201–216. doi: 10.1016/s0044-8486(99)00082-4
Rosa, I. L., Dias, T. L., and Baum, J. K. (2002). Threatened fishes of the world: Hippocampus reidi Ginsburg, 1933 (Syngnathidae). Environ. Biol. Fish. 64, 378. doi: 10.1023/A:1016152528847
Saavedra, M., Batista, H., and Pousão-Ferreira, P. (2015). Dietary fatty acid enrichment during the spawning season increases egg viability and quality in Hippocampus hippocampus. Aquacult. Nutr. 22, 343–351. doi: 10.1111/anu.12252
Saavedra, M., Masdeu, M., Hale, P., Sibbons, C. M., and Holt, W. V. (2014). Dietary fatty acid enrichment increases egg size and quality of yellow seahorse Hippocampus kuda. Anim. Reprod. Sci. 145, 54–61. doi: 10.1016/j.anireprosci.2013.08.004
Sagebakken, G., Kvarnemo, C., and Ahnesjö, I. (2017). Nutritional state – a survival kit for brooding pipefish fathers. Biol. J. Linn. Soc. 121, 312–318. doi: 10.1093/biolinnean/blx002
Sargent, J., McEvoy, L., Estévez, A., Bell, G., Bell, M., Henderson, J., et al. (1999). Lipid nutrition of marine fish during early development: current status and future directions. Aquaculture 179, 217–229. doi: 10.1016/S0044-8486(99)00191-X
Sarkar, D., Andrews, F., Wright, K., Klepeis, N., and Murrell, P. (2020). lattice: Trellis graphics for R. Available online at: https://cran.r-project.org/web/packages/lattice/index.html (accessed September 17, 2019).
Skalkos, Z. M. G., Van Dyke, J. U., and Whittington, C. M. (2020). Paternal nutrient provisioning during male pregnancy in the seahorse Hippocampus abdominalis. J. Comp. Physiol. B 190, 547–556. doi: 10.1007/s00360-020-01289-y
Squadrito, F., Micali, A., Rinaldi, M., Irrera, N., Marini, H., Puzzolo, D., et al. (2017). Polydeoxyribonucleotide, an adenosine-A2 A receptor agonist, preserves blood testis barrier from cadmium-induced injury. Front. Pharmacol. 7:537. doi: 10.3389/fphar.2016.00537
Tocher, D. R. (2003). Metabolism and functions of lipids and fatty acids in Teleost. Rev. Fish. Sci. 11, 107–184. doi: 10.1080/713610925
Tocher, D. R. (2010). Fatty acid requirements in ontogeny of marine and freshwater fish. Aquac. Res. 41, 717–732. doi: 10.1111/j.1365-2109.2008.02150.x
Valladares, S., and Planas, M. (2021). Nutrient incorporation in first feeding seahorses evidenced by stable carbon isotopes. Animals 11:470. doi: 10.3390/ani11020470
Vetter, R. D., and Hodson, R. E. (1982). Use of adenylate concentrations and adenylate energy charge as indicators of hypoxic stress in estuarine fish. Can. J. Fish. aquat. Sci. 39, 535–541. doi: 10.1139/f82-076
Viña, E., Parisi, V., Abbate, F., Cabo, R., Guerrera, M. C., Laurà, R., et al. (2014). Acid-sensing ion channel 2 (ASIC2) is selectively localized in the cilia of the non-sensory olfactory epithelium of adult zebrafish. Histochem. Cell Biol. 143, 59–68. doi: 10.1007/s00418-014-1264-4
Vincent, A. C. J. (1996). The International Trade in Seahorses. Cambridge: Traffic International, 170.
Vincent, A. C. J., Foster, S. J., and Koldewey, H. J. (2011). Conservation and management of seahorses and other Syngnathidae. J. Fish Biol. 78, 1681–1724. doi: 10.1111/j.1095-8649.2011.03003.x
Wei, T., Simko, V., Levy, M., Xie, Y., Jin, Y., and Zemla, J. (2017). corrplot: Visualization of a Correlation Matrix. Available online at: https://CRAN.R-project.org/package=corrplot (accessed March 6, 2020).
Wickham, H., Chang, W., Henry, L., Pedersen, T. L., Takahashi, K., Wilke, C., et al. (2020). ggplot2: Create Elegant Data Visualisations Using the Grammar of Graphics. Available online at: https://CRAN.R-project.org/package=ggplot2 (accessed September 17, 2019).
Williams, C. T., Klaassen, M., Barnes, B. M., Buck, C. L., Arnold, W., Giroud, S., et al. (2017). Seasonal reproductive tactics: annual timing and the capital-to-income breeder continuum. Philos. Trans. R Soc. B 372:20160250. doi: 10.1098/rstb.2016.0250
Wittington, C. M., and Friesen, C. R. (2020). The evolution and physiology of male pregnancy in syngnathid fishes. Biol. Rev. 95, 1252–1272. doi: 10.1111/brv.12607
Woods, C. M. C., and Valentino, F. (2003). Frozen mysids as an alternative to live Artemia in culturing seahorses Hippocampus abdominalis. Aquacult. Res. 34, 757–763. doi: 10.1046/j.1365-2109.2003.00882.x
Zarantoniello, M., Bruni, L., Randazzo, B., Vargas, A., Gioacchini, G., Truzzi, C., et al. (2018). Partial dietary inclusion of Hermetia illucens (Black Soldier Fly) full-fat prepupae in zebrafish feed: biometric, histological, biochemical, and molecular implications. Zebrafish 15, 519–532. doi: 10.1089/zeb.2018.1596
Keywords: Syngnathidae, seahorses, Hippocampus reidi, breeder’s diet, newborn, fatty acids, energy charge, histology
Citation: Planas M, Olivotto I, González MJ, Laurà R, Angeletti C, Amici A and Zarantoniello M (2021) Pre-breeding Diets in the Seahorse Hippocampus reidi: How Do They Affect Fatty Acid Profiles, Energetic Status and Histological Features in Newborn? Front. Mar. Sci. 8:688058. doi: 10.3389/fmars.2021.688058
Received: 30 March 2021; Accepted: 04 May 2021;
Published: 26 May 2021.
Edited by:
Sami Souissi, Lille University of Science and Technology, FranceReviewed by:
Md. Abdul Wahab, WorldFish, BangladeshCopyright © 2021 Planas, Olivotto, González, Laurà, Angeletti, Amici and Zarantoniello. This is an open-access article distributed under the terms of the Creative Commons Attribution License (CC BY). The use, distribution or reproduction in other forums is permitted, provided the original author(s) and the copyright owner(s) are credited and that the original publication in this journal is cited, in accordance with accepted academic practice. No use, distribution or reproduction is permitted which does not comply with these terms.
*Correspondence: Miquel Planas, bXBsYW5hc0BpaW0uY3NpYy5lcw==
Disclaimer: All claims expressed in this article are solely those of the authors and do not necessarily represent those of their affiliated organizations, or those of the publisher, the editors and the reviewers. Any product that may be evaluated in this article or claim that may be made by its manufacturer is not guaranteed or endorsed by the publisher.
Research integrity at Frontiers
Learn more about the work of our research integrity team to safeguard the quality of each article we publish.