- 1Key Lab of Urban Environment and Health, Institute of Urban Environment, Chinese Academy of Sciences, Xiamen, China
- 2Zhongke Dingshi Environmental Engineering Co., Ltd, Beijing, China
- 3School of Environmental Sciences, University of East Anglia, Norwich, United Kingdom
- 4School of Water, Energy and Environment, Cranfield University, Cranfield, United Kingdom
Introduction: Cadmium (Cd) has been recognized as a significant contributor to the pollution of farmland soils in China, and biochars have been reported to be effective in mitigating soil Cd pollution. However, most studies have been conducted in laboratory or greenhouse settings, not at a field scale, and the biochars used have been applied at unrealistically high amounts (>10 t/ha).
Methods: In this research, three biochars: rice straw biochar (RSB), pig manure biochar (PMB) and rice husk biochar (RHB) were produced from readily available farm residues. Then the effects at low-level application (1.8 and 3.6 t/ha) on Cd were investigated in a field experiment cropped with rape (Brassica campestris L.).
Results: Batch adsorption experiments indicated Cd adsorption capacity of three biochars followed the order of RSB (43.5 mg/g) > PMB (33.3 mg/g) > RHB (24.4 mg/g). Field experiment indicated biochar amendments could slightly change soil pH and cation exchange capacity (CEC); yet led to considerable and significant decreases in extractable Cd concentrations [reductions of: 43%–51% (PMB), 29%–35% (RSB) and 17%–19% (RHB)]. Reduced extractable Cd correlated with lower Cd concentrations in rape plants. PMB and RSB were more effective in decreasing Cd phytoaccumulation into edible parts of rape (>68% reduction) than RHB.
Discussion: Low-level application of PMB or RSB could efficiently decrease the phytoaccumulation of Cd from soils into crops. These results demonstrate the reality of biochar-based remediation solutions to contribute to the mitigation of diffuse Cd contamination in farmland. The results also highlight the need to trail biochars in the presence of the soil to be targeted for remediation.
Highlights
• Low application rates, 1.8 t/ha of biochar reduced Cd bioavailability in field soils
• Doubling field application rates of biochar increased Cd immobilization by 10%
• PMB and RSB amendment resulted in lower Cd concentrations in rape than RHB
1 Introduction
Due to industrialization and urbanization, soil cadmium (Cd) pollution is a global issue. Often this pollution is localized in areas of industrial activity (Liao et al., 2015). However, long range transport from industrial sources and the use of tainted water resources for irrigation have resulted in farmland accumulating high levels of contaminants (Khan et al., 2021). In China the latest official soil surveys conclude that 19.4% of national farmland soils (based on the sampling points) has been contaminated (MEP, 2014). Cd, along with mercury (Hg), arsenic (As), copper (Cu), lead (Pb), chromium (Cr), zinc (Zn) and nickel (Ni), are recognized to be priority risk drivers. Cd can migrate from contaminated soils to food crops, which can significantly increase human health risk associated with Cd poisoning (Xiao et al., 2018). There is therefore an urgent need to develop efficient and scalable techniques for the remediation of farmland diffusely polluted with potentially toxic elements (PTEs).
Biochar is the product of the pyrolysis of organic solid wastes under anoxic or limited oxygen conditions (Gao et al., 2022). Several types of biochars have been reported to be efficient in immobilizing heavy metals through mechanisms such as adsorption, surface precipitation and electrostatic interaction (Alam et al., 2018). Biochar influence on Cd availability has been extensively studied over the last decade. For example, Rehman et al. (2018), Li et al. (2019) and Bashir et al., 2018 all reported decreases in Cd bioavailability and reduced Cd accumulation into plants following biochar application to soil. In addition, biochars have been reported to improve soil properties, enhance soil fertility and increase crop yields (Rehman et al., 2018). Thus, biochar amendment is considered as a cost-effective strategy for soil improvement and pollution abatement.
However, most of these studies were conducted in laboratory or greenhouse settings (Rees et al., 2014; Mohamed et al., 2017; Bashir et al., 2018). There is to date little information and guidance on field-scale in situ applications of biochar. Moreover, where field trial results have been reported they have shown inconsistent outcomes (O’Connor et al., 2018); these different outcomes being attributed to variable field conditions and contrasting biochar properties. The properties of biochars can be varied widely according to feedstock type used and the pyrolysis conditions applied (Tomczyk et al., 2020; Zong et al., 2021). In addition, after being applied to soils, different biochar types give rise to contrasting changes to the soil properties such as pH and cation exchange capacity (CEC) (Yuan et al., 2021). These soil factors further influence metal ion behaviour. Xiao et al. (2019) produced wheat straw biochar (WS500) and rice straw biochar (RS500) at 500°C, and found the pH, ash content and CEC of two biochars were differed. After applied into two alkaline soils, the decrease of concentration of DTPA extracted Cd in WS500 treatments were kept higher than those in RS500 treatments, and the Cd accumulation into ryegrass grew in WS500 amend soils were 1.04–1.17 times as those in RS500 amended soils.
There are also issues pertaining to the realism of biochar application rates applied into field soils in many of the published reports (Rajkovich et al., 2012; Cui et al., 2016). Many authors have used biochar at very large application amounts, for example, 10–40 t ha−1 (Cui et al., 2016; O’Connor et al., 2018). In a few studies, application rates of biochar have been even higher (equivalent to more than 90 t/ha; Rajkovich et al., 2012; Rondon et al., 2007). Given the limit availability of biochar resource and the associated cost of biochar application, such large rates of biochar application at a field scale would likely be unfeasible. Importantly, it has been reported that decreases in metal availability, following biochar application, are not proportional to the amount of biochar applied (Bian et al., 2014); and, in Jeffery’s meta-analysis (1,483 studies), a poor relationship between biochar application rate and crop yield was reported (r2 = .1; Jeffery et al., 2011). It might therefore be concluded that high application rates of biochar may not be necessary to achieve desired outcomes. Thus, there is a need to evaluate biochar efficacy to mitigate Cd phytoaccumulation using low application rates of biochar under field relevant conditions.
In this study, three biochars including rice straw biochar (RSB), rice husk biochar (RHB) and pig manure biochar (PMB) were prepared and evaluated for their Cd adsorption capacities in the laboratory. The biochars were then used, in a field experiment at two low application rates (1.8 and 3.6 t/ha), under a crop of rape (Brassica campestris L.), to: 1) evaluate their influence upon the accumulation of Cd from field soils into the rape roots and edible parts; 2) explore the mechanisms influencing Cd phytoaccumulation, and; 3) provide evidence to assist the selection of biochar feedstock and biochar application rate for utilization to abate risks associated with Cd pollution in farmland soils.
2 Materials and methods
2.1 Biochar preparation
Rice straw biochar (RSB), rice husk biochar (RHB) and pig manure biochar (PMB) were produced using pilot-scale biochar pyrolysis equipment (L 4.5 m × W .8 m × H 2 m). The pyrolysis equipment comprised a pyrolysis chamber, a heating chamber, a feedstock supply system and a biochar output system. The equipment had an overall production capacity of 20 ton per year. Feedstocks were pyrolyzed in the pyrolysis chamber at 500°C for 1 h under limited oxygen conditions. Thereafter, the biochars were ground through a 2 mm sieve. The physico-chemical properties of the three biochars were assessed using methods described in previous work (Zhang and Luo, 2014). Cd adsorption capacities of the biochars were characterized using batch equilibration adsorption experiments (Zhang and Luo, 2014; Zhang et al., 2019) (full details are provided in the supporting information). Briefly, biochar (50 mg) was shaken with Cd(NO3)2 solution (5 mL) with Cd2+ concentration of 25 mol/L–500 mol/L at 25°C at 150 rpm for 48 h. Thereafter the suspensions were collected, and the concentration of Cd measured by inductively coupled plasma optical emission spectrometer (ICP-OES) (Optima 7000DV; PerkinElmer Inc.).
2.2 Field study
The field experiment was conducted on an upland farm located in Zhouzai village (24°23′26.08″N, 117°43′26.25″E), Zhangzhou city, Fujian province in southern China. The concentration of Cd in local soil was .38 mg/kg. This Cd concentration of soils exceeded the regulatory limit for agricultural soils (.30 mg/kg; MEP, 2018). Thus, the Cd concentration was less than twice of the value of regulatory limit, which suggests the experimental soil was not heavily polluted. In China, such marginal exceedance is far more common, than heavy pollution in polluted farmland soils (Teng et al., 2014).
In November 2016, soil (0–20 cm) was ploughed. Biochars were then spread on the soil surface, and thoroughly mixed with the soils using a tillage machine (1GQN-120; Weifang shengxuan machines corporation). The applications of biochar were 0, 1.8 and 3.6 t/ha. Each treatment was produced in triplicate. The area of each plot was 4 m2. Two weeks after biochar application, the land was rolled, and rape (Brassica campestris L.) seeds were sown. Irrigation and fertilizer management were performed according to the conventional practices of the local farmers and were identical on all plots, in which the metal concentrations of fertilizers used in the experiment fitted the requirement of limit value of Chinese standard of ecological index of arsenic, cadmium, lead, chromium and mercury for fertilizers (AQSIQ, 2009).
2.3 Sampling and analysis
In January 2017, the rape plants were harvested. Three composite rape samples, each consisting of 10 plants randomly selected from each plot, were collected (resulting in nine composite rape samples for each treatment regime). Following their transfer to the laboratory, the rape samples were washed with deionized water. Thereafter, the rape samples were cut into two parts (the edible part and the root), and dried in the oven at 90°C. For the analysis of Cd concentrations in the plants, subsamples of the edible part, or root, were crushed, ground, and passed through a .2 mm sieve. Samples of the edible part or root were then digested with HNO3 (65%, Merck, EMSURE™) using a protocol modified from Zhu et al. (2008). For quality control, plant reference material (GBW 10015; purchased from the National Research Center for Standards in China) was digested and measured alongside the experimental samples, as were reagent blanks. The concentration of Cd in the digestates were analysed using an inductively coupled plasma mass spectrometry (ICP-MS 7500cx; Agilent Inc.). Concentrations of Cd were reported on a dry weight basis. The recovery of Cd from the reference material was 108%–113%. Details of quality control of ICP-MS measurement were described in the Supplementary materials.
Nine soil samples (0–20 cm) were collected from each treatment (3 samples drawn from each plot replicate). Soil samples were air-dried in the laboratory at room temperature, and then ground and passed through a 2 mm sieve. Subsamples of soils were ground and passed through a .15 mm sieve. The pH of soil samples was measured using a pH meter at the ratio of 1 g soil: 2.5 mL deionized water. The CEC of soil was measured using the NH4OAc-NaOAc method (U.S. Environmental Protection Agency 1986). The concentrations of available Cd in soils were determined using a .01 mol/L CaCl2 extraction method (Houba et al., 1996). Briefly, soil samples were extracted with .01 mol/L CaCl2 solution at the ratio of 1 g soil : CaCl2 solution (10 mL). After 2 h shaking, the suspensions were centrifuged, and the concentrations of Cd in the supernatant were measured using inductively coupled plasma mass spectrometry (ICP-MS 7500cx; Agilent Inc.).
2.4 Data management and statistic analysis
The data were analysed using Microsoft Excel (Microsoft Corp., United States), OriginLab OriginPro 2018 software (OriginLab Corp., United States) and IBM SPSS Statistics 22.0 software (IBM Corp., United States). The fitting of batch adsorption data was performed with OriginLab 2018 software. Statistical analysis of the data was performed with IBM SPSS Statistics 22.0 software. Significant differences among treatments were analysed using one-way analysis of variance (ANOVA) (at p < .05 level).
3 Results
3.1 Biochar characterisation
Properties varied across the three biochar types (Table 1). pH was always alkaline and ranged between 9.87 and 10.7; RSB had the highest pH (10.7). Carbon content in RSB or RHB was much higher than the content in PMB, while N and O contents in PMB were the highest amongst the three biochars (1.51% and 20%, respectively). The surface topography and pore structure of the biochars also varied (Table 1; Supplementary Figures S1, S2). The surface area of biochars followed the order of RSB > PMB >> RHB, and the average pore volume of biochars followed the order of PMB = RSB >>> RHB. Based on these chemical and physical properties, it was hypothesised that the RSB (with high pH, high carbon content and high surface area) would offer the best performance for Cd immobilisation (Chen et al., 2011; Shen et al., 2017; O’Connor et al., 2018).
Results of the batch equilibrium experiment indicated that Cd adsorption capacity of the three biochars followed the order: RSB > PMB > RHB (Figure 1; Supplementary Table S1). Cd adsorption onto both PMB and RHB were better fitted by the Freundlich isotherm model (rather than Langmuir isotherm model), while the RSB was fitted well with both the Langmuir and Freundlich isotherm models (Supplementary Table S1). As fitted by the Langmuir isotherm model, the saturation adsorption capacity of RSB, PMB and RHB were 43.5 mg/g, 33.3 mg/g and 24.4 mg/g, respectively. In addition, based on the fitting results, it was concluded that Cd adsorption onto the three biochars was likely attributable to chemical adsorption on heterogeneous surfaces (Zhang and Luo 2014). The value of 1/n in the Freundlich isotherm model followed the order of RSB < PMB < RHB. Since 1/n represented the adsorption affinity of metal ions onto adsorbent, this result confirmed that the adsorption of Cd2+ ion onto RSB was the greatest, while RHB showed lowest affinity for Cd ions.
3.2 Effects of biochar amendment on the properties of field soils
The pH in biochar amended soils were increased (Figure 2A). However, no significant difference (p > .05) in soil pH were observed among three biochar treatments.
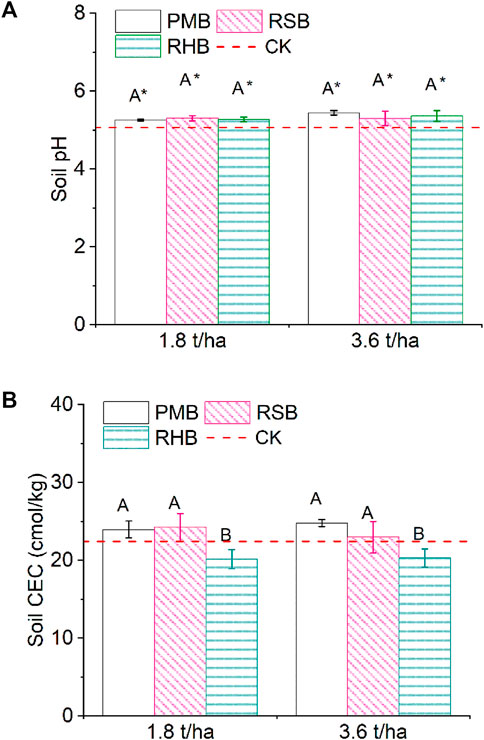
FIGURE 2. Changes in the properties of soil and soil amended with biochars (A) soil pH and (B) CEC. “*” indicates a value to be significantly different to the control (CK) value (p < .05).
The CEC in the PMB and RSB amended soils were slightly higher, but not significantly different (p > .05), to that in the control (Figure 2B). In contrast, soil amended with RHB had a lower CEC (20.0 cmol/kg–20.3 cmol/kg), and it was not significantly different (p > .05) from that in the control.
Available Cd concentration in the control soil was 21.5 ± 1.9 μg/kg. Following biochar amendment, the available Cd concentrations were significantly lowered in all treatments (p < .05). However, greatest reductions in bioavailable Cd in soil were observed with PMB amendment (43% and 51% reduction at 1.8 and 3.6 t/ha) and RSB amendment (29% and 35% reduction at 1.8 and 3.6 t/ha) (Figure 3).
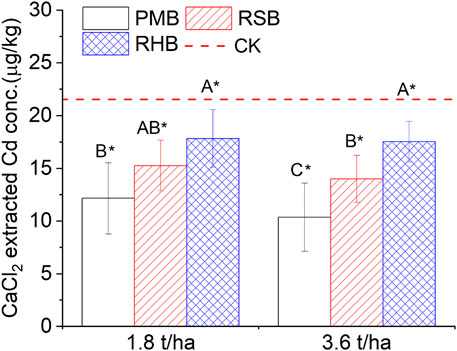
FIGURE 3. Available Cd concentrations in soil and soil amended with biochars. “*” indicates a value to be significantly different to the control (CK) value (p < .05).
3.3 Effects of biochar amendment on the growths of rape plants in filed
Biochar amendment influenced the growth of rape plants. Increases in dry weight of edible parts (Supplementary Figure S3A) and dry weight of roots (Supplementary Figure S3B) were observed in all instance following biochar amendment. However, these increases in biomass were marginal, and none were significantly different (p > .05) when compared to the control (except for the dry weight of root, in the treatment with 1.8 t/ha amendment of PMB).
3.4 Effects of biochar amendment on Cd accumulation into plants
In all instances, biochar amendment resulted in a decrease in the concentration of Cd in rape plants (Figure 4). For the edible part of rape (Figure 4A), the concentrations of Cd in the treatments with PMB and RSB were significantly (p < .05) decreased; these levels being 68%–70% of the control value. In the RHB treatments, the Cd concentrations were approximately 89% of the control value, which were higher than the concentrations in treatment with PMB or RSB amendment.
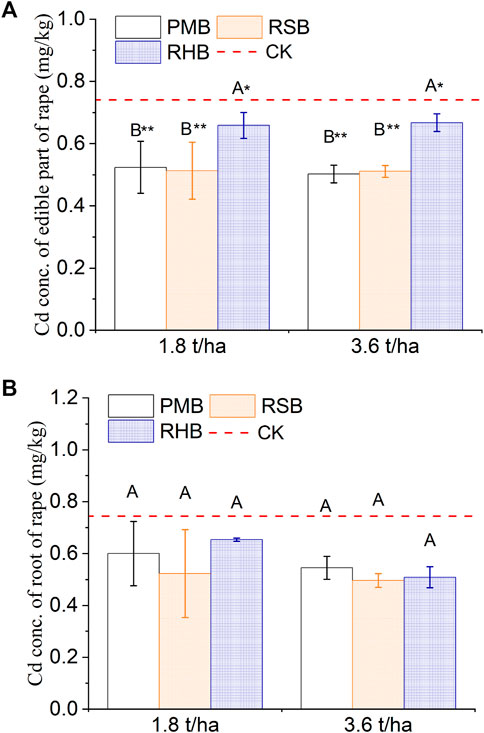
FIGURE 4. Cd concentrations in edible parts (A) and roots (B) of rape plants grown in soil and soil amended with biochars. “*” indicates a value to be significantly different to the control (CK) value (p < .05).
Regarding Cd concentrations in roots (Figure 4B), the Cd concentrations in the treatments with PMB, RSB and RHB were all observed to decrease. Where application rate was equivalent, no significant differences (p > .05) were observed across the three biochar types.
4 Discussion
The physico-chemical properties of three biochars were different. PMB contained lower C and higher N than RSB and RHB prepared from plant residues, which was in agreement with previous reports (Zornoza et al., 2016; Bashir et al., 2018). Such differences have previously been attributed to differences in the element composition of feedstocks (Zhao et al., 2013). In addition, the three biochars had different surface topography and pore structure, where PMB and RHB offer higher pore structures than RHB. This finding is in good agreement with previous literature highlighting that contrasting feedstock sources produced biochars with different physical and chemical properties (Tomczyk et al., 2020).
The properties of biochar including surface area, distribution of surface functional groups, ash content and pH, play important roles during metal adsorption (Jiang et al., 2016). As a consequence of differing physical and chemical properties, the adsorption capacities of the three biochars varied (Figure 1). Complexation of metals, through ion exchange interactions, with ionized surfaces and oxygen-containing functional groups [i.e., carboxyl (–COOH), hydroxyl (–OH), phenol (R–OH) groups] has been suggested as an important mechanism for metal adsorption by biochar (Kołodyńska et al., 2017). In this study, RSB had highest surface area and highest content of surface functional groups, available to prompt interactions with metal ions. Similarly, since the BET surface area and the content of surface functional groups of RHB were lowest among three biochars, the adsorption of Cd2+ ion onto RHB was correspondingly lower. In addition, the pH of three biochars also influenced the equilibrium pH and thus the adsorption of Cd2+ ions (Supplementary Figure S4). The adsorption of more Cd2+ ion onto biochar with increasing pH is consistent with previous reports (Jiang et al., 2016). In the batch experiments, the addition of RSB with highest pH lead to highest equilibrium pH among three biochars (Supplementary Table S4B); this, likely, underpinned the greatest adsorption of Cd2+ ions onto RSB. Overall the equilibrium pH in RHB system was lower than RSB or PMB, and its Cd2+ ion adsorption was also lower.
When biochar were amended to soil, the increases of soil pH by the addition of three biochars were not significantly different (p > .05), and soil CEC were limited changed after biochar application. These outcomes are likely due to the low amendment levels (1.8 and 3.6 t/ha). Nonetheless, all three biochars significantly (p < .05) reduced extractable Cd concentrations. It is suggested, therefore, that changes in Cd availability were most likely linked to Cd ion interaction with biochar (rather than changes to the soil chemical environment). Reduced concentrations of available Cd were translated into observed correlated reductions in Cd content in rape plants (Supplementary Table S2). Importantly, the three types of biochar led to different outcomes for Cd-plant interactions. Treatment with RHB was relatively ineffective, while amendment with PMB or RSB resulted in much more effective abatement of soil to plant transfer of Cd. With a doubling in application rates, the decrease of soil available Cd concentrations were increased (on average by approximately extra 10%). This observation suggesting, even at the lowest application rate (1.8 t/ha), that PMB and RSB were effective ameliorants. The literature, in many cases, has reported metal-biochar-soil-plant interactions could result in large decreases in phytoaccumulation of metals into numerous crop types (Mohamed et al., 2017; Zeeshan et al., 2020); while other cases this ameliorative influence has been reported to be minimal (Rees et al., 2014; Zhang et al., 2017; Wang et al., 2019). However, in the present study, RSB and PMB (applied at low-levels: 1.8 and 3.6 t/ha) were established to be effective for the control of Cd phytoaccumulation, while RHB was observed to have only a limited effect.
The performance of these biochars in the batch adsorption experiments and in real soil systems were not consistent, where there was no significant difference between the decreased magnitude of Cd concentrations in rape plants of RSB and PMB treatments. This outcome is consistent with Uchimiya et al. (2010) who reported biochars produced from broiler litter manure at 350°C (350BL) removed more Ni2+ and Cd2+ ions than biochars produced at 700°C (700BL); but when applied (at 5%–10% (w/w)) to soil, the 350BL treatment contained higher soluble metal concentrations than the 700BL treated soils. Although the 350BL had a higher adsorption capacity than the 700BL, its lower ability to increase soil pH underpinned the less effective immobilisation of metals in soil by 350BL. Similarly, the pH increase, rather than primary Cd-biochar interaction, has also been proposed by other researchers (Rees et al., 2014). Thus, it is recommended that, before field scale deployment, biochar adsorption capacity should be established in the presence of the soil it is intended to remediate. In addition, given that soil chemical properties (pH and CEC) were largely unchanged, it is suggested that the primary interactions between Cd and the biochars were likely responsible for the outcomes observed.
It is highlighted that in the present research, the soil was not heavily contaminated, which is typical soil pollution condition in farmlands of China. The soil Cd concentration (.38 mg/kg) only just exceeding the regulatory limit of .30 mg/kg (MEP 2018). It is therefore emphasized that the low application rates of biochar were directed at a small excess of Cd in the soil system (this likely underpinned the successful outcomes observed) and that the lower application rate was sufficient to accommodate the excess of Cd. It follows that should prevailing soil Cd concentrations be much higher, such an outcome might not transpire and larger applications of biochar could be needed to accommodate a greater excess of Cd in the soil system. This said, Nie et al. (2018) reported the low level (1.5–3.0 t/ha) application of sugarcane bagasse biochar decreased the concentrations of Cd, Pb and Cu in pak choi by 62%–76%, 17.3%–49.1% and 15%–38%, respectively. In contrast to the present study, the concentrations of Cd, Cu and Pb in this experimental soil were 1.4 mg/kg, 278 mg/kg and 348 mg/kg; Cd being more than 4 times the regulatory limit. Overall, the results of the current research support low-level application (i.e., 1.8 t/ha) of biochar to mitigate the transfer of Cd from soil to rape plants.
It is also highlighted that many of the results reported in the literature relate to biochars that are produced in small quantities in the laboratory (Bashir et al., 2018; Meng et al., 2022). In contrast, the present study considered biochars produced using a larger pyrolysis system having an output capacity of 20 ton per year. Thus, assuming an application rate of 2 t/ha, such a unit could service 10 ha p.a. So, it is emphasised that this scale of production, and low application rates, represents a realistic approach to support the production of biochar in quantities that would allow for meaningful field scale application.
5 Conclusion
Biochars derived from different feedstocks, under identical pyrolysis conditions, showed different adsorption capacities for Cd2+ ions. Following amendment to field soil, even at low application rates (1.8 t/ha), all biochars were observed to reduce the bioavailable concentrations of Cd in soil. Biochars derived from pig manure (PMB) or rice straw (RSB) led to much lower Cd concentrations in rape plants when compared to outcomes for rice husk biochar (RHB). This research has validated an approach, wherewith low-level biochar application could efficiently remediate Cd-tainted farmland. These results also highlight the need to trail biochars, in the presence of the soil to be targeted for remediation, before full scale deployment is undertaken. Further field verifications with different biochars at low application rate shall be conducted in different climates with contrasting soil properties and metal concentrations.
Data availability statement
The original contributions presented in the study are included in the article/Supplementary Material; further inquiries can be directed to the corresponding author upon reasonable request.
Author contributions
Conceptualization, YZ and CC; Methodology, YZ and SLIN; Writing-original draft: YZ; Writing-review and editing, YZ, SLIU, BR, FC, and CC. All authors have read and agreed to the published version of the manuscript.
Funding
This work was financially supported by grants from National Key R&D Project (2016YFD0800706), the Science and Technology Project of Xiamen city (3502Z20182001), the STS Program of Fujian-Chinese Academy of Sciences (2021T3006) and the Science and Technology Project of Fujian province (2021N0031).
Acknowledgments
We thank J.J. Fan and Y.W. Hou for supporting in set-up of field trial. We also thank the editors and anonymous experts for their valuable comments.
Conflict of interest
Author SLIU was employed by Zhongke Dingshi Environmental Engineering Co., Ltd.
The remaining authors declare that the research was conducted in the absence of any commercial or financial relationships that could be construed as a potential conflict of interest.
Publisher’s note
All claims expressed in this article are solely those of the authors and do not necessarily represent those of their affiliated organizations, or those of the publisher, the editors and the reviewers. Any product that may be evaluated in this article, or claim that may be made by its manufacturer, is not guaranteed or endorsed by the publisher.
Supplementary material
The Supplementary Material for this article can be found online at: https://www.frontiersin.org/articles/10.3389/fenvs.2022.1114335/full#supplementary-material
References
Alam, M. S., Gorman-Lewis, D., Chen, N., Flynn, S. L., Ok, Y. S., Konhauser, K. O., et al. (2018). Thermodynamic analysis of Nickel(II) and Zinc(II) adsorption to biochar. Environ. Sci. Technol. 52 (11), 6246–6255. doi:10.1021/acs.est.7b06261
AQSIQ (General Administration of Quality Supervision, Inspection and Quarantine of the People’s Republic of China) (2009). Ecological index of arsenic, cadmium, lead, chromium and mercury for fertilizers. Beijing, China. (GB/T 23349–2009).
Bashir, S., Hussain, Q., Shaaban, M., and Hu, H. (2018). Efficiency and surface characterization of different plant derived biochar for cadmium (Cd) mobility, bioaccessibility and bioavailability to Chinese cabbage in highly contaminated soil. Chemosphere 211, 632–639. doi:10.1016/j.chemosphere.2018.07.168
Bian, R., Joseph, S., Cui, L., Pan, G., Li, L., Liu, X., et al. (2014). A three-year experiment confirms continuous immobilization of cadmium and lead in contaminated paddy field with biochar amendment. J. Hazard. Mater. 272, 121–128. doi:10.1016/j.jhazmat.2014.03.017
Chen, X. C., Chen, G. C., Chen, L. G., Chen, Y. X., Lehmann, J., McBride, M. B., et al. (2011). Adsorption of copper and zinc by biochars produced from pyrolysis of hardwood and corn straw in aqueous solution. Biores. Technol. 102, 8877–8884. doi:10.1016/j.biortech.2011.06.078
Cui, L., Pan, G., Li, L., Bian, R., Liu, X., Yan, J., et al. (2016). Continuous immobilization of cadmium and lead in biochar amended contaminated paddy soil: A five-year field experiment. Ecol. Eng. 93, 1–8. doi:10.1016/j.ecoleng.2016.05.007
Gao, Y., Wu, P., Jeyakumar, P., Bolan, N., Wang, H., Gao, B., et al. (2022). Biochar as a potential strategy for remediation of contaminated mining soils: Mechanisms, applications, and future perspectives. J. Environ. Manag. 313, 114973. doi:10.1016/j.jenvman.2022.114973
Houba, V. J. G., Lexmond, T. M., Novozamsky, I., and van der Lee, J. J. (1996). State of the art and future developments in soil analysis for bioavailability assessment. Sci. Total Environ. 178 (1-3), 21–28. doi:10.1016/0048-9697(95)04793-X
Jeffery, S., Verheijen, F. G. A., van der Velde, M., and Bastos, A. C. (2011). A quantitative review of the effects of biochar application to soils on crop productivity using meta-analysis. Agric. Ecosyst. Environ. 144 (1), 175–187. doi:10.1016/j.agee.2011.08.015
Jiang, S., Huang, L., Nguyen, T. A. H., Ok, Y. S., Rudolph, V., Yang, H., et al. (2016). Copper and zinc adsorption by softwood and hardwood biochars under elevated sulphate-induced salinity and acidic pH conditions. Chemosphere 142, 64–71. doi:10.1016/j.chemosphere.2015.06.079
Khan, S., Naushad, M., Lima, E. C., Zhang, S., Shaheen, S. M., and Rinklebe, J. (2021). Global soil pollution by toxic elements: Current status and future perspectives on the risk assessment and remediation strategies - a review. J. Hazard. Mater. 417, 126039. doi:10.1016/j.jhazmat.2021.126039
Kołodyńska, D., Krukowska, J., and Thomas, P. (2017). Comparison of sorption and desorption studies of heavy metal ions from biochar and commercial active carbon. Chem. Eng. J. 307, 353–363. doi:10.1016/j.cej.2016.08.088
Li, S., Wang, M., Zhao, Z., Li, X., and Chen, S. (2019). Use of soil amendments to reduce cadmium accumulation in rice by changing Cd distribution in soil aggregates. Environ. Sci. Pollut. Res. 26 (20), 20929–20938. doi:10.1007/s11356-019-05431-4
Liao, Q. L., Liu, C., Wu, H. Y., Jin, Y., Hua, M., Zhu, B. W., et al. (2015). Association of soil cadmium contamination with ceramic industry: A case study in a Chinese town. Sci. Total Environ. 514, 26–32. doi:10.1016/j.scitotenv.2015.01.084
Meng, Z., Huang, S., Lin, Z., Mu, W., Ge, H., and Huang, D. (2022). Cadmium long-term immobilization by biochar and potential risks in soils with different pH under combined aging. Sci. Total Environ. 825, 154018. doi:10.1016/j.scitotenv.2022.154018
MEP (Ministry of Environmental Protection of the People's Republic of China) (2018). Soil environmental quality: Risk control standard for contaminated agricultural soils (GB 15618-2018). Beijing, China.
MEP (Ministry of Environmental Protection of the People's Republic of China) (2014). Q&A for nationwide soil pollution survey report. Beijing, China. (In Chinese).
Mohamed, B. A., Ellis, N., Kim, C. S., and Bi, X. (2017). The role of tailored biochar in increasing plant growth, and reducing bioavailability, phytotoxicity, and uptake of heavy metals in contaminated soil. Environ. Pollut. 230, 329–338. doi:10.1016/j.envpol.2017.06.075
Nie, C., Yang, X., Niazi, N. K., Xu, X., Wen, Y., Rinklebe, J., et al. (2018). Impact of sugarcane bagasse-derived biochar on heavy metal availability and microbial activity: A field study. Chemosphere 200, 274–282. doi:10.1016/j.chemosphere.2018.02.134
O’Connor, D., Peng, T., Zhang, J., Tsang, D. C. W., Alessi, D. S., Shen, Z., et al. (2018). Biochar application for the remediation of heavy metal polluted land: A review of in situ field trials. Sci. Total Environ. 619-620, 815–826. doi:10.1016/j.scitotenv.2017.11.132
Rajkovich, S., Enders, A., Hanley, K., Hyland, C., Zimmerman, A. R., and Lehmann, J. (2012). Corn growth and nitrogen nutrition after additions of biochars with varying properties to a temperate soil. Biol. Fertil. Soils 48 (3), 271–284. doi:10.1007/s00374-011-0624-7
Rees, F., Simonnot, M. O., and Morel, J. L. (2014). Short-term effects of biochar on soil heavy metal mobility are controlled by intra-particle diffusion and soil pH increase: Heavy metal mobility in biochar-amended soils. Eur. J. Soil Sci. 65 (1), 149–161. doi:10.1111/ejss.12107
Rehman, M. Z., Rizwan, M., Hussain, A., Saqib, M., Ali, S., Sohail, M. I., et al. (2018). Alleviation of cadmium (Cd) toxicity and minimizing its uptake in wheat (Triticum aestivum) by using organic carbon sources in Cd-spiked soil. Environ. Pollut. 241, 557–565. doi:10.1016/j.envpol.2018.06.005
Rondon, M. A., Lehmann, J., Ramírez, J., and Hurtado, M. (2007). Biological nitrogen fixation by common beans (Phaseolus vulgaris L.) increases with bio-char additions. Biol. Fertil. Soils 43 (6), 699–708. doi:10.1007/s00374-006-0152-z
Shen, Z., Zhang, Y., McMillan, O., Jin, F., and Al-Tabbaa, A. (2017). Characteristics and mechanisms of nickel adsorption on biochars produced from wheat straw pellets and rice husk. Environ. Sci. Pollut. Res. 24 (14), 12809–12819. doi:10.1007/s11356-017-8847-2
Teng, Y., Jin, W., Lu, S., Wang, Y., Jiao, X., and Song, L. (2014). Soil and soil environmental quality monitoring in China: A review. Environ. Int. 69, 177–199. doi:10.1016/j.envint.2014.04.014
Tomczyk, A., Sokołowska, Z., and Boguta, P. (2020). Biochar physicochemical properties: Pyrolysis temperature and feedstock kind effects. Rev. Environ. Sci. Bio/Technology 19 (1), 191–215. doi:10.1007/s11157-020-09523-3
Uchimiya, M., Lima, I. M., Thomas, K., Chang, S., Wartelle, L. H., and Rodgers, J. E. (2010). Immobilization of heavy metal ions (CuII, CdII, NiII, and PbII) by broiler litter-derived biochars in water and soil. J. Agric. Food Chem. 58 (9), 5538–5544. doi:10.1021/jf9044217
U. S. Environmental Protection Agency (1986). Cation-exchange capacity of soils (sodium acetate) (SW846 Method 9081). Washington, DC, USA.
Wang, Y., Zhong, B., Shafi, M., Ma, J., Guo, J., Wu, J., et al. (2019). Effects of biochar on growth, and heavy metals accumulation of moso bamboo (Phyllostachy pubescens), soil physical properties, and heavy metals solubility in soil. Chemosphere 219, 510–516. doi:10.1016/j.chemosphere.2018.11.159
Xiao, R., Wang, P., Mi, S., Ali, A., Liu, X. Y., Li, Y. M., et al. (2019). Effects of crop straw and its derived biochar on the mobility and bioavailability in Cd and Zn in two smelter-contaminated alkaline soils. Ecotoxicol. Environ. Saf. 181, 155–163. doi:10.1016/j.ecoenv.2019.06.005
Xiao, W. D., Xuezhu, Y., Qi, Z., De, C., Jing, H., and Na, G. (2018). Evaluation of cadmium transfer from soil to leafy vegetables: Influencing factors, transfer models, and indication of soil threshold contents. Ecotoxicol. Environ. Saf. 164, 355–362. doi:10.1016/j.ecoenv.2018.08.041
Yuan, C., Gao, B., Peng, Y., Gao, X., Fan, B., and Chen, Q. (2021). A meta-analysis of heavy metal bioavailability response to biochar aging: Importance of soil and biochar properties. Sci. Total Environ. 756, 144058. doi:10.1016/j.scitotenv.2020.144058
Zeeshan, M., Ahmad, W., Hussain, F., Ahamd, W., Numan, M., Shah, M., et al. (2020). Phytostabalization of the heavy metals in the soil with biochar applications, the impact on chlorophyll, carotene, soil fertility and tomato crop yield. J. Clean. Prod. 255, 120318. doi:10.1016/j.jclepro.2020.120318
Zhang, R. H., Li, Z. G., Liu, X. D., Wang, B., Zhou, G. L., Huang, X. X., et al. (2017). Immobilization and bioavailability of heavy metals in greenhouse soils amended with rice straw-derived biochar. Ecol. Eng. 98, 183–188. doi:10.1016/j.ecoleng.2016.10.057
Zhang, Y. C., Fan, J. J., Fu, M. L., Ok, Y. S., Hou, Y. W., and Cai, C. (2019). Adsorption antagonism and synergy of arsenate (V) and cadmium (II) onto Fe-modified rice straw biochars. Environ. Geochem. Health 41 (4), 1755–1766. doi:10.1007/s10653-017-9984-8
Zhang, Y. C., and Luo, W. S. (2014). Adsorptive Removal of heavy metal from acidic wastewater with biochar produced from anaerobically digested residues: Kinetics and surface complexation modeling. BioResources 9 (2), 2484–2499. doi:10.15376/biores.9.2.2484-2499
Zhao, L., Cao, X., Mašek, O., and Zimmerman, A. (2013). Heterogeneity of biochar properties as a function of feedstock sources and production temperatures. J. Hazard. Mater. 256–257, 1–9. doi:10.1016/j.jhazmat.2013.04.015
Zhu, Y. G., Sun, G. X., Lei, M., Teng, M., Liu, Y. X., Chen, N. C., et al. (2008). High percentage inorganic arsenic content of mining impacted and non-impacted Chinese rice. Environ. Sci. Technol. 42 (13), 5008–5013. doi:10.1021/es8001103
Zong, Y., Xiao, Q., and Lu, S. (2021). Biochar derived from cadmium-contaminated rice straw at various pyrolysis temperatures: Cadmium immobilization mechanisms and environmental implication. Bioresour. Technol. 321, 124459. doi:10.1016/j.biortech.2020.124459
Zornoza, R., Moreno-Barriga, F., Acosta, J. A., Munoz, M. A., and Faz, A. (2016). Stability, nutrient availability and hydrophobicity of biochars derived from manure, crop residues, and municipal solid waste for their use as soil amendments. Chemosphere 144, 122–130. doi:10.1016/j.chemosphere.2015.08.046
Keywords: biochar, cadmium, field application, farmland, Brassica
Citation: Zhang Y, Liu S, Lin S, Reid BJ, Coulon F and Cai C (2023) Field verification of low-level biochar applications as effective ameliorants to mitigate cadmium accumulation into Brassica campestris L from polluted soils. Front. Environ. Sci. 10:1114335. doi: 10.3389/fenvs.2022.1114335
Received: 02 December 2022; Accepted: 29 December 2022;
Published: 16 January 2023.
Edited by:
Lizhi He, Zhejiang Agriculture and Forestry University, ChinaReviewed by:
Hanbo Chen, Shenyang Agricultural University, ChinaBoling Li, Suzhou University of Science and Technology, China
Copyright © 2023 Zhang, Liu, Lin, Reid, Coulon and Cai. This is an open-access article distributed under the terms of the Creative Commons Attribution License (CC BY). The use, distribution or reproduction in other forums is permitted, provided the original author(s) and the copyright owner(s) are credited and that the original publication in this journal is cited, in accordance with accepted academic practice. No use, distribution or reproduction is permitted which does not comply with these terms.
*Correspondence: Chao Cai, ccai@iue.ac.cn