- 1Department of Agriculture, Austin Peay State University, Clarksville, TN, United States
- 2Department of Plant and Soil Sciences, Oklahoma State University, Stillwater, OK, United States
Soil heavy metals (HM) contamination threatens soil and water quality, which significantly affects humans and animals. This study focuses on the competitive immobilization of zinc (Zn), lead (Pb), and cadmium (Cd) in soils from a contaminated site using ryegrass (Lolium perenne) in a potting experiment amended with biochars. Increased rates of switchgrass- (SGB) and poultry litter-derived biochars (PLB) were applied before ryegrass cultivation. Soil HM phytoavailability and HM concentrations in plant shoots were determined. Multivariate regression models were used to evaluate the influence of several soil chemical attributes on the HM phytoavailability. The increased rates of both biochars reduced the Zn, Pb, and Cd availability (p < 0.001). Langmuir models showed that the maximum HM immobilization (IMAX) was 169.2 ± 29.5 mg kg–1 for Zn with SGB, and 20 ± 7.4 (Pb) and 1.08 mg kg–1 (Cd) with PLB. The extended Langmuir model (EL) showed competitive HM immobilization since there was a decrease in the IMAX of Zn (∼90 ± 28 mg kg–1 from SGB) and Cd (∼0.70 ± 0.21 mg kg–1). Negative values of Pb and Cd immobilization at low rates of SGB indicated an increase in those HM availabilities and preferential immobilization for Zn. The reduced Zn and Pb uptake in ryegrass shoots ranged from 70% to 98% and were optimum at rates of 0.50% ± 0.00%–0.60% ± 0.06% for both biochars, and 1.6% ± 0.4% of SGB for Cd. The stepwise multiple linear regression (SMLR) and partial least squares (PLS) revealed that pH and organic matter (OM) were the most responsible factors for reducing Zn bioavailability while OM was more impactful in decreasing Pb and Cd levels. This suggests that the preferential immobilization for Zn relies on its higher sensitivity to the pH increase. Also, the ubiquitous positive relationship among the metals studied shows that competitive immobilization is ceased at high rates of biochars application. Path analysis (PA) showed that pH and OM were the common contributors from both biochars to simultaneously affect Zn, Pb, and Cd availability regardless of the contrasting physicochemical properties of the two bioproducts. This work proved the potential of applying low rates of two contrasting feedstock-derived biochars to remediate the contaminants and safely grow ryegrass.
1 Introduction
Excessive deposition of HM in the soil caused by human activities is highly hazardous to both the environment and living organisms. Gradual increase in both the level and type of HM has been observed in recent years (da Silva et al., 2022; Su, 2014). Lack of proper chemical remediation interventions as well as phytoremediation could lead to accumulation of HM in the farmlands (Ali et al., 2013; Antonangelo and Zhang, 2019; Yang et al., 2021). Soil quality and its ability to support crops and pasture for animals become compromised at certain higher concentration levels of HM. At these levels, they may even enter the food chain and endanger human health (Abbas et al., 2017; Yang et al., 2021). For instance, Pb is considered one of the most important toxic heavy metal because of its direct effect on humans through water pollution. Drinking Pb contaminated water can cause respiratory, digestive, nervous, blood, urinary and immune system symptoms of acute or chronic poisoning, and may even lead to death (Järup, 2003).
Current advancements in physical, chemical, and biological remediation technologies have greatly enhanced HM’s immobilization efforts. Implementation of simple remediation strategies such as using lime, clay, phosphates, silicates, organic compost, etc. have been found to greatly help immobilize heavy metals (Yang et al., 2021). In addition to helping lower HM’s level in the soil, implementation of these strategies has benefited soil in many other ways. Soil fertility, pH, cation exchange, etc. have been improved following the application of these soil remediation strategies (Ahmad et al., 2014; Sun et al., 2020). This study will focus on biochar (switchgrass and poultry litter) which are low-cost yet have high efficiency with regards to their effects on soil conditioning (Yang et al., 2021). Biochar is a carbonaceous material derived from different biowastes such as crop straw, wood chips, swine manure, etc. (Ahmad et al., 2014; Fan et al., 2018; Meng et al., 2018; Sun et al., 2020). Unique physicochemical properties of most biochars such as availability, high porosity, low cost, environmentally sustainable and high adsorption performance (Wang et al., 2017; He et al., 2019; Shaheen et al., 2019) makes them highly desirable.
Biochar application increases soil pH (Qiao et al., 2017; Sun et al., 2020; Yang et al., 2021), it can also adsorb various contaminants through ion exchange, precipitation and/or surface complexation (Bradl, 2004; Inyang et al., 2015; Wang et al., 2017). Most recent studies are pointing out the use of advanced technologies to modify biochar and improve its characteristics of soil contaminant immobilization (Liu et al., 2022a; Liu et al., 2022b; Liu et al., 2023) in addition to evaluating the recovery of such bioproducts and their potential reuse, which promotes, even more, the sustainable approach offered by these charcoal products. Of particular interest is its ability to reduce HM bioavailability in the soil (Park et al., 2011; Ahmad et al., 2012; Meng et al., 2018). Biochar has therefore been extensively used in remediation of HM contaminated soils (Zhang et al., 2017; Sun et al., 2020). The effectiveness of biochar in metal immobilization, however, depends on soil physicochemical properties, biochar properties, and metal species (Xie et al., 2015; Zeng et al., 2015; Weng et al., 2017; Meng et al., 2018). The bioavailability of HM has been found to be highly correlated with physicochemical characteristics of soil, including pH, organic matter, redox conditions, etc. (Weng et al., 2017).
It is important to understand the role that soil properties play on the behavior of HM. Fine-textured soils have been found to facilitate rapid metal mobility than coarse-textured soils (Fashola et al., 2020). High organic matter content can enhance metal adsorption thus reducing mobility (He et al., 2019). Acidic conditions can decrease soil cation exchange by increasing metal solubility and thus HM’s mobility (Fashola et al., 2020). The solubility of Zn, for example, increases 100-fold for each unit decrease in soil pH (Rengel, 2015). Changes in soil pH have greatly disrupted soil microbial activities by slowing pH-dependent enzymes, altering key nutrients availability, and/or HM’s mobility (Kapoor et al., 2015; Fashola et al., 2020).
In this study, the biochars generated from switchgrass (SGB) and poultry litter (PLB) were used to determine their effects on metal phytoavailability in the soil and their concentrations in plant (ryegrass) shoots. Qiao et al. (2017) found that when a biochar composite derived from Chinese herb medicine residue was added to soil, it not only reduced the bioaccumulation of Cd by the cabbage mustard plants but also promoted the growth and development of the plants. The influence of several soil chemical attributes such as pH, organic matter content, etc. on metals’ phytoavailability in the soil is also evaluated. This research becomes unique since multimetal contaminated sites must be remediated using a single practice able to immobilize all contaminants simultaneously. Hence, biochars produced from contrasting feedstocks were applied at reasonable rates in a potting experiment so that any potential results might be feasibly tested on a field scale. In the previous work of Antonangelo and Zhang (2021), it was proven low rates of biochar were sufficient to grow ryegrass at the same time Cd concentration was considered safe in ryegrass shoots for cattle grazing. This current work evaluates the competitive immobilization of additional metals to elucidate the viability of applying low rates of either SGB or PLB as multimetal remediation practices.
2 Materials and methods
2.1 Biochar production and properties
The biochars were produced from switchgrass (SGB; Panicum virgatum) and poultry litter (PLB) by using high-temperature slow pyrolysis (700°C). The feedstocks obtainment and their conversion into biochar are in Antonangelo and Zhang (2019). The biochar coarse materials were gently ground with a mortar and pastel before being sieved through 1- and 0.25-mm filter/strain for physicochemical analyses and potting experiment, respectively (Antonangelo et al., 2019). The SGB and PLB characterization including several physicochemical properties such as moisture, ash content, particle-size distribution, elemental composition, surface functional groups, chemical attributes, specific surface area (SSA), cation exchange capacity (CEC), and morphology can be found in Antonangelo et al. (2019).
2.2 Soil sampling and analysis
Heavy metal contaminated soil samples were collected from a depth of 0–15 cm in a residential yard near chat piles located in Picher, Ottawa County, Oklahoma using a shovel. Soil sample preparation is fully described in Antonangelo and Zhang (2019). Before and after the pot experiment, soil samples were analyzed for DTPA–extractable Cd, Pb, and Zn. The DTPA-extractable heavy metals have been considered the most efficient method to predict their phytoavailability in perennial ryegrass (Antonangelo and Zhang, 2019) and also a reliable extractant for potentially toxic metals in soils (da Silva et al., 2022). Before the potting experiment, the total Cd, Pb, and Zn contents were determined by an inductively coupled plasma-atomic emission spectroscopy, ICP-AES (SPECTRO Analytical Instruments GmbH, Boschstr. 10, 47533 Kleve, Germany) after digestion by concentrated HNO3 and H2O2 using EPA method 3050B (Church et al., 2017). The DTPA-extractable Cd, Pb, and Zn before the potting experiment were respectively 1.84 ± 0.03, 49.9 ± 1.7, and 220.6 ± 9.3 mg kg−1. The total Cd, Pb, and Zn were respectively 9.0 ± 0.6, 233.7 ± 10.8, and 1765 ± 221 mg kg−1. The total Cd content was about tenfold the maximum found in normal Oklahoma soils (Richards et al., 2012; Antonangelo and Zhang, 2021). The threshold of DTPA-extractable Cd was 0.03–0.16 mg kg−1 (Wu et al., 2021), which is over tenfold lower than the content found in the Tar Creek soil of this study. The total Zn and Pb contents were about twelvefold and sevenfold, respectively, the maximum found in normal Oklahoma soils (Richards et al., 2012). These reemphasize the immediate need for remediating those metals in the Tar Creek area.
2.3 Potting experiment
Plastic pots received 1,200 g of 2-mm sieved soils and were added 0.0 (control), 0.5, 1.0, 2.0, and 4.0% (w/w) of 0.25-mm sieved SGB and PLB. The mixture was then incubated for 30 days at 75% of field capacity before ryegrass sowing in each pot at the seeding rate of 30 kg ha−1. Ryegrass was grown for 75 days in biochar-amended soils in an environmentally controlled growth chamber. The pots were arranged in a complete randomized block design (CRBD) with 3 replications (n = 3). Plots were weekly rotated to eliminate spatial variability in the growth chamber. A detailed description of all procedures conducted during the potting experiment can be found in Antonangelo and Zhang (2019).
2.4 Metal contents in ryegrass
Ryegrass shoots were separated after harvesting, washed with deionized (D.I.) water, and oven-dried at 105°C until constant weight. Dried plant materials were ground using a mechanical grinder then analyzed for Cd, Pb, and Zn using nitric acid digestion (as discussed earlier). To determine metal concentrations in plant tissues, 0.5 g of ground plant materials were predigested for 1 h with 10 mL of trace metal grade HNO3 in the HotBlock™ Environmental Express block digester (Environmental Express, 2345A Charleston Regional Parkway, Charleston, South Carolina 29492, SC, United States), and the digests were then heated to 115°C for 2 h and diluted with D.I. water to 50 mL (Jones and Case, 1990). Finally, the digested samples were filtered and determined for Cd, Pb, and Zn by an ICP-AES.
2.5 Data analysis
2.5.1 Descriptive statistics and ANOVA
Boxplot was plotted after normalizing the whole dataset of measurements (n = 15 for each biochar) to show soil chemical attributes (Antonangelo et al., 2022) affected by switchgrass and poultry litter-derived biochar application rates. The effects of Biochar, Rate, and the interaction Biochar × Rate were tested and a 2-way ANOVA was performed to compare treatment means using the Tukey test (p < 0.05).
2.5.2 Langmuir models
Single and extended Langmuir models were used to predict the maximum immobilization of heavy metals as a function of biochar application rates. The heterogeneous extended Langmuir model (EL) was verified because it predicts the adsorption from mixtures and applies the theory of multi-region or multisite adsorption, which is coherent with our study since SGB and PLB have shown several surface functional groups (Antonangelo et al., 2019). Langmuir models were then developed for SGB and PLB separately.
2.5.3 Non-linear regressions
Maximum responsive rates of biochar application to reduced metals uptake were determined by fitting the segmented polynomials linear–plateau (LP) and quadratic−plateau (QP) response models using the NLIN (non-linear) procedure of SAS version 9.4. The models were accepted only when the NLIN convergence criterion method successfully converged and the model was significant (p < 0.05). The standard error (SE) of joint points was used to verify if biochars had different maximum responses to increased application rates.
2.5.4 Stepwise multiple linear regression (SMLR)
For Stepwise Multiple Linear Regression (SMLR), all soil chemical attributes were used as independent variables to predict the available metal contents. The SMLR was generated with the whole dataset of measurements in JMP Pro 15 using the backward method. Initially, it incorporates all variables into the model followed by removal of the least important ones (Pelegrino et al., 2021). The remaining variables compose the final SMLR model. In our study, the stopping rule was tested for the p-value threshold, AICc (Akaike Information Criterion), and BIC (Bayesian Information Criterion), and the AICc was chosen because it is more appropriate in finding the best model for predicting future observations.
2.5.5 Partial least squares (PLS)
Partial Least Squares Regression (PLSR) was performed using the whole dataset of measurements in JMP Pro 15 to analyze the importance of factors affecting metal availability. PLSR is a multivariate statistical analysis method that integrates the advantages of principal component analysis (PCA), canonical correlation analysis, and multiple linear regression analysis (Yang et al., 2021). It has been widely used to conduct regression analyses with small sample sizes, multiple variables, and multicollinearity among variables (Wold et al., 2001; Fang et al., 2015; Tong et al., 2020). It also uses cross-validation results to determine the number of components and avoids overfitting. The importance of an independent variable is measured by the variable importance in projection (VIP) value (Shi et al., 2013). When VIP > 1 the independent variable is important for predicting the dependent variable; whereas it is less important when V < 0.5 (Shi et al., 2014). The +/− symbols for each of the regression coefficients (RC) in the model indicate the action direction of each independent variable (Onderka et al., 2012). According to Yang et al. (2021), PLSR presents multiple advantages over other methods because it has a stronger interpretative ability for independent variables and its results are more stable and reliable.
For most PLS models, different independent variables (soil chemical attributes) affecting heavy metals availability as well as their direction of effect (positive or negative) were used, as shown in Supplementary Tables S1, S2.
2.5.6 Path analysis
A path analysis (PA) model similar to that performed by Richards et al. (2012) was used to evaluate the relationships between the combined contents of available metals and soil chemical attributes. The selected independent variables of the models were Fe, K, OM, P, and soil pH for SGB; and soil pH and OM for PLB. Direct and indirect effects were obtained with the whole dataset of measurements by using multiple regression of soil chemical attributes on metal contents and between soil chemical attributes, respectively, using the CALIS procedure of SAS version 9.4.
Graphs were created in Microsoft Excel 2019, PowerPoint, and OriginPro 9.1.
2.5.7 Useful equations
1.
2.
3.
4.
Where: a, b, and c are the coefficients of the equation.
3 Results
3.1 Soil chemical attributes and heavy metals
The data distribution for all analyzed variables shows that, regardless of the biochar applied, soil pH and soil organic matter (SOM) increased as biochar application rates increased, and so did some macronutrients such as phosphorus (P) and potassium (K) (Figure 1). Meanwhile, a decrease in the availability of heavy metals (Zn, Pb, and Cd) was observed (Figure 1). As for the other soil chemical attributes the trends were non-uniform thus it was necessary to fully investigate which variables were affecting the metals’ availability and how increased biochar rates are affecting metals’ immobilization.
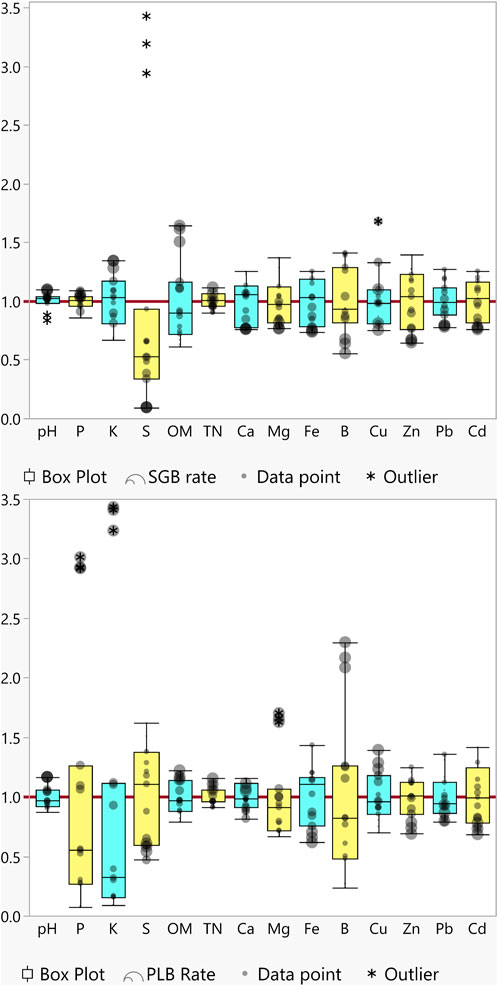
FIGURE 1. Boxplot of soil chemical attributes after switchgrass- and poultry litter-derived biochar application rates, SGB (top) and PLB (bottom). Data were normalized by dividing every measurement by the average of the whole dataset of measurements (y-axis). The Red line denotes the average. Boxes span the 25th to 75th data percentile, whiskers represent 1.5 × the interquartile range, and horizontal lines denote the median.
3.2 Metal immobilization
The simple Langmuir model was successfully fitted for Zn when SGB was applied and showed a maximum immobilization (IMAX) of 169.2 ± 29.5 mg kg−1 (Figure 2). This means that 89.5% of the Zn available can be immobilized when compared to the available content in the control (189.1 ± 10.0 mg kg−1 at 0% SGB). Such immobilization potential is estimated to occur when SGB beyond 4% is applied since the ANOVA returned an IMAX of 93.9 mg kg−1 for the highest rate of SGB application (4%) (Table 1), which is 49.7% immobilization in comparison to the available Zn content in the control. There was no fit when PLB was applied although an increment in the Zn immobilization is noticed (Figure 2). This is confirmed by ANOVA since the 4% PLB application rate provided the highest immobilization of 66.5 mg Zn kg−1, in comparison to other application rates, which is only 35.2% immobilization as compared to the available Zn content in the control (Table 1). Moreover, from a 1%–4% rate increment, the SGB immobilizes more Zn than PLB (Table 1). This shows that SGB has a higher potential to immobilize Zn although Zn availability decreases as both SGB and PLB application rates increase.
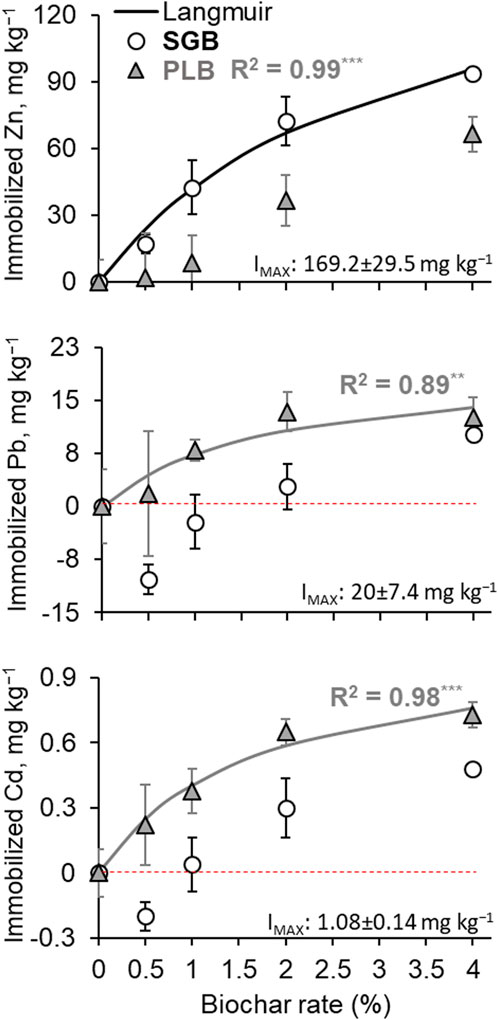
FIGURE 2. Langmuir models used to predict the maximum immobilization of heavy metals. No line means a non-significant fit of the model. Bars represent the standard deviation of the mean (n = 3). Points below the red dashed line (negative values) mean that heavy metal becomes more available than the control treatment (0% biochar application). IMAX = maximum immobilization for the fitted model. Values followed by ± are the standard deviation. **: p < 0.01; ***: p < 0.001.
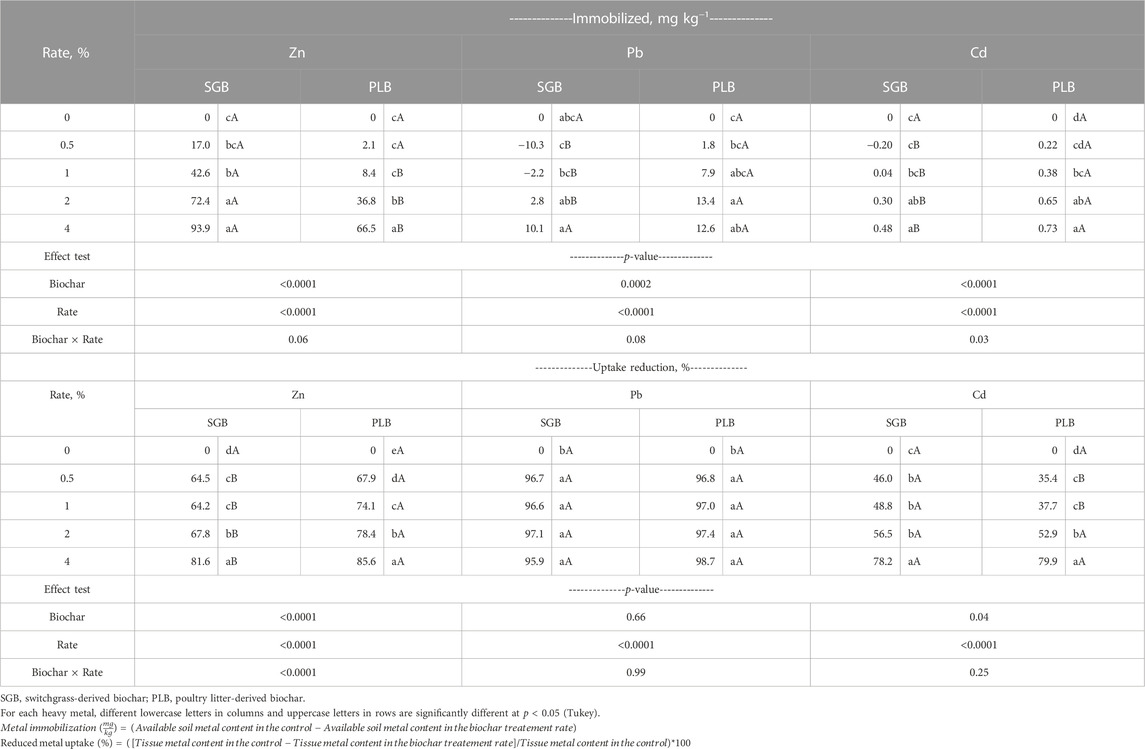
TABLE 1. ANOVA of soil heavy metal immobilization and uptake reduction by ryegrass shoots as a function of increased rates of two feedstock-derived biochars applied separately.
In the case of Pb and Cd, a simple Langmuir model was fitted only with PLB application rates (Figure 2). This was the first indication that SGB has preferable adsorption/immobilization for Zn. Further, negative values of Pb and Cd immobilization at low rates of SGB application indicate that Pb and Cd are becoming more available, then immobilization takes over when increased rates of SGB are applied (Figure 2; Table 1). This reinforces the idea that depending on the feedstock used to produce biochar there might be a preference for some metals adsorption in comparison to others when rates of biochar application are low. Such competition is suppressed when more functional groups are available to adsorb metals at higher rates of application.
The IMAX achieved for Pb with PLB application was 20 ± 7.4 mg kg−1 (Figure 2), which corresponds to 41.9% immobilization of the Pb available in the control. Such estimation overcome the PLB application rates used in this study since immobilized PLB ranged from 1.8 to 13.4 mg kg−1 (Table 1). For Cd, similar results were observed. The IMAX with PLB application was 1.08 ± 0.14 mg kg−1 (Figure 2), which corresponds to 65.3% immobilization of the Cd available in the control. This was greater than the ANOVA results showing 0.73 mg kg−1 of immobilized Cd (corresponding to 44.2% immobilization) (Table 1). The relationship between observed and predicted metal concentrations from the simple Langmuir models is shown in Supplementary Figure S1.
The extended Langmuir model (EL) successfully fitted for immobilized Zn as a function of increased rates of SGB application and Cd only with PLB addition (Figure 3). The results reinforced the existing competitive immobilization between metals since there was a decrease in the IMAX of Zn (∼90 ± 28 mg kg−1 from SGB) and Cd (∼0.70 ± 0.21 mg kg−1 from PLB). Moreover, with the EL, a fit was observed for Zn immobilization with PLB application rates as well (Figure 3). The IMAX obtained was 63.9 ± 22.7 mg kg−1 which corresponds to 33.8% immobilization as compared to the amount available in the control. Still, there is greater efficiency of SGB when comparing the IMAX of the EL for Zn immobilization, however, the PLB fit with this model demonstrates the existence of multisite adsorption for Zn since the fit was not successful with the simple Langmuir model. No further conclusion could be drawn for Pb since the EL did not converge for this metal for any of the biochars applied.
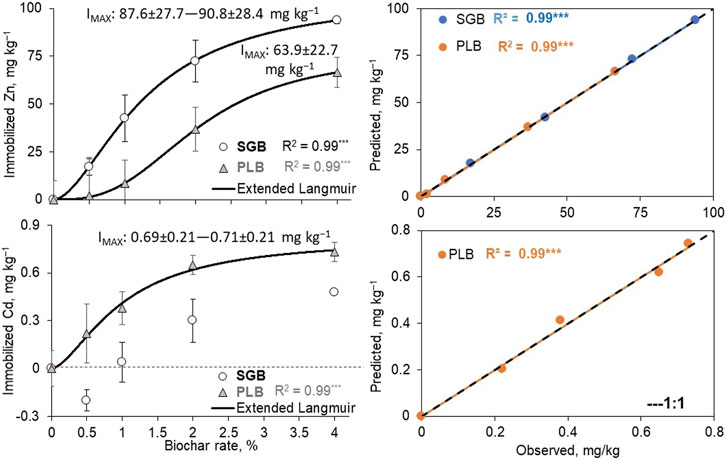
FIGURE 3. Extended Langmuir models used to predict the maximum immobilization of heavy metals as a function of biochar application rates (left), and plot of observed × predicted values from the fitted models (right). No line means a non-significant fit of the model. Bars represent the standard deviation of the mean (n = 3). Points below the red dashed line (negative values) mean that heavy metal becomes more available than the control treatment (0% biochar application). IMAX = maximum immobilization for the fitted model. Values followed by ± are the standard deviation. ***: p < 0.001.
3.3 Metal uptake
The linear–with–plateau model successfully converged for Zn reduced uptake and the optimum application rates were 0.55% ± 0.09% and 0.58% ± 0.06%, respectively for SGB and PLB (Figure 4). Those rates correspond to 71.2% ± 1.99% (SGB) and 79.4% ± 5.27% (PLB) of reduced Zn uptake (Figure 4). These results were emphasized with ANOVA, where no reduced uptake increment was observed after 1% of SGB application and 0.5% of PLB application (Table 1). The PLB was better than SGB in terms of reduced uptake for each application rate (Table 1). However, low rates can reduce Zn uptake at its optimum although the metal immobilization keeps increasing as rates of biochar application increase (Table 1; Figures 2, 3). This is also true for Pb because, at 0.5% of both SGB and PLB, the reduced uptake by ryegrass shoots was 96.6% ± 0.08%–97.5% ± 0.33% even though the immobilization was only 3.8% for PLB and Pb was more available than the control for SGB at the same application rate (Table 1).
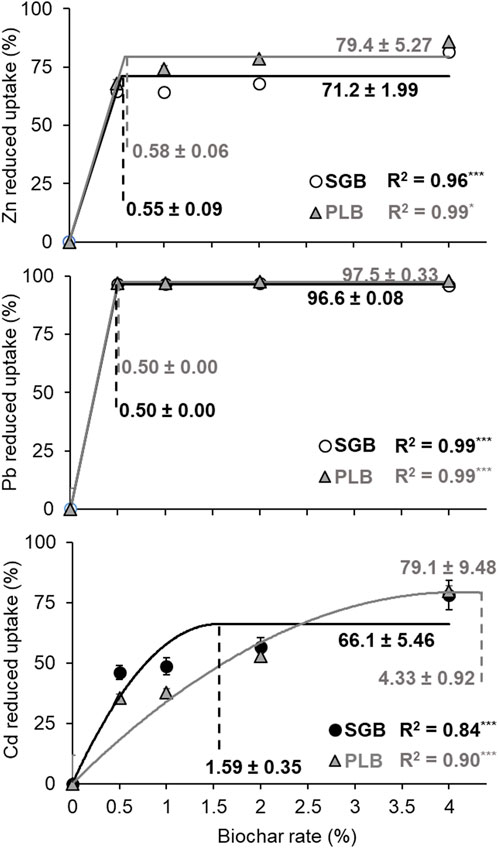
FIGURE 4. The trends of heavy metal uptake reduction by biochar application rates increase. Significant fits to the linear- and quadratic-with-upper-plateau statistical model were obtained. Bars represent the standard deviation of the mean (n = 3). *: p < 0.05; ***: p < 0.001. Values followed by ± are the standard deviation.
In the case of Cd, different results were observed. First, the quadratic–with–plateau model was successfully fitted instead of the linear–with–plateau one (Figure 4). Secondly, the optimum application rate for SGB was 1.59% ± 0.35% reducing Cd uptake by 66.1% ± 5.46%; and 4.33% ± 0.08% for PLB, thus overcoming the maximum application rate and reducing Cd uptake by 79.1% ± 9.48% (Figure 4). The reduced Cd uptake by 66.1% ± 5.46% with SGB application falls within the range found by Liang et al. (2022), who observed a reduced Cd concentration in brown rice by 61.2%–65.4% after the application of biochar derived from crop residues.
In both cases (SGB and PLB), the biochar application rates necessary to reduce Cd uptake at its maximum were higher than the rates necessary to reduce the Zn and Pb uptake at their maximum. This may demonstrate that ryegrass has a preferable tolerance for Cd uptake than the other two metals. This is further evidenced by Antonangelo and Zhang (2021), where the transfer of Cd from ryegrass roots to ryegrass shoots was higher than other metals, which was also proved in the work of Ji et al. (2020). All equations from the linear–and quadratic−with–upper–plateau statistical models to predict metal uptake reduction are presented in Table 2.
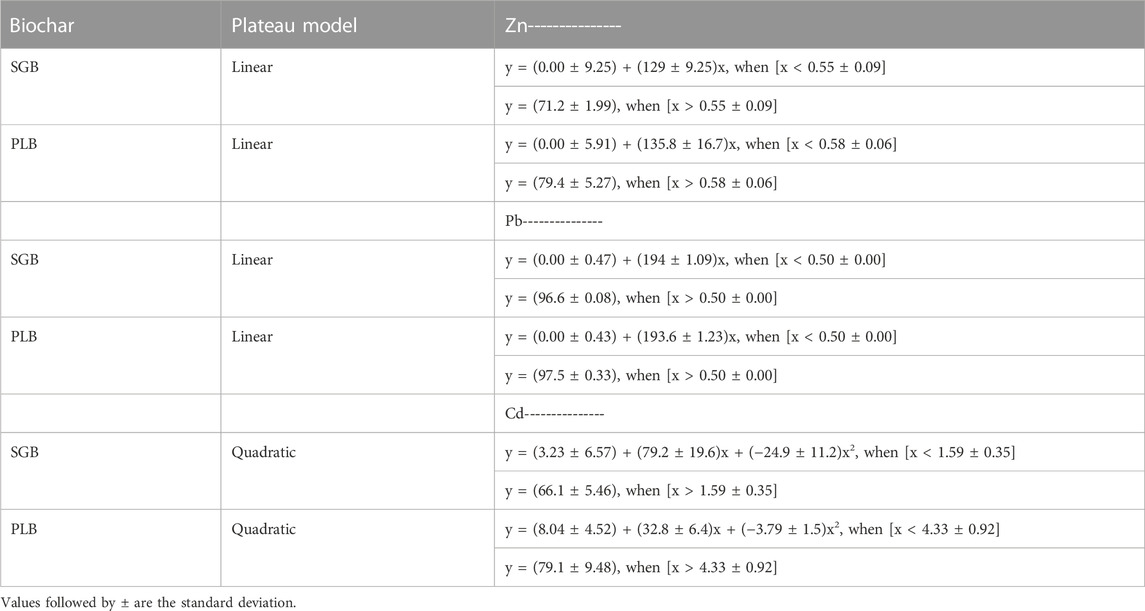
TABLE 2. Equations from the linear- and quadratic-with-upper-plateau statistical models to predict uptake reduction (%) of heavy metals by ryegrass shoots.
3.4 Soil chemical attributes affecting metals availability
3.4.1 Stepwise multiple linear regression
From the results of stepwise multiple linear regression (SMLR), it is unquestionable that the soil chemical attributes interfering with the availability of each metal are different when comparing the two biochars given their contrasting characteristics, except for Cd, which gives a positive relationship for Zn and Pb availability regardless of the biochar (Table 3). Considering that results comprise the whole dataset of measurements, which means all the increased rates of biochar application are included, it seems that any possible competition ceases when higher rates of biochar are applied. In that case, it is safe to assume that metals are simultaneously immobilized at 2%+ of SGB and PLB application rates. Besides, Cd availability is explained by a positive relationship with available Zn and Pb (SGB) and only Zn (PLB) (Table 3). The negative relationship between Pb availability and available Zn with SGB application partially explains the preferable immobilization for Zn from SGB, and the considerable negative values of Pb immobilization with low rates of SGB reinforce this assumption (Table 1). This indicates competition between those two metals for immobilization into the same adsorption sites. The relationship between observed and predicted metal concentrations from the SLMR models is shown in Supplementary Figure S2.
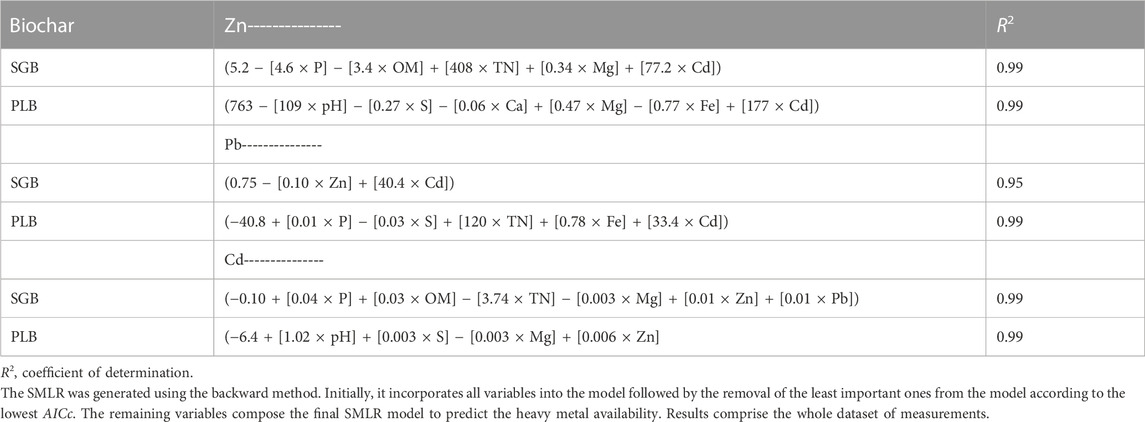
TABLE 3. Equations from stepwise multiple linear regression models (SMLR) based on the lowest adjusted Akaike information criterion (AICc).
Surprisingly, no significant effect of soil organic matter (OM) and soil pH was observed on Pb availability with either the biochars (Table 3). On the other hand, soil OM and pH negatively impacted Zn availability, respectively for SGB and PLB, and positively for Cd (Table 3). The positive relationship of those two soil chemical attributes for Cd availability suggests that, with the preferred immobilization for Zn as a consequence of OM and pH increase, Cd becomes more available since OM and pH are acting directly in reducing Zn availability. However, knowing that OM and pH were substantially responsible for decreasing those metals’ availability (Antonangelo and Zhang, 2019), further statistical analyses were performed to elucidate Zn, Cd, and Pb immobilization dynamics.
3.4.2 Partial least squares
The partial least squares (PLS) regression showed that soil OM was a major factor in the immobilization of Zn, Pb, and Cd for both biochars, which is reflected by the negative regression coefficients and the VIP >1.0 (Figures 5, 6). Soil pH did not perform as well as OM for Pb and Cd for any of the biochars application but was effective in immobilizing Zn (Figures 5, 6). In that scenario, one might assume that the preferential immobilization for Zn might rely on its higher sensitivity to the pH increase. The PLS confirmed a positive and significant relationship among the metals studied leading to the conclusion that competition stops when increased rates of biochar are applied. The same is also observed for the relationship between those metals (Zn, Pb, and Cd) and available iron (Fe)—although Fe is not a contaminant in the studied soil (Figures 5, 6).
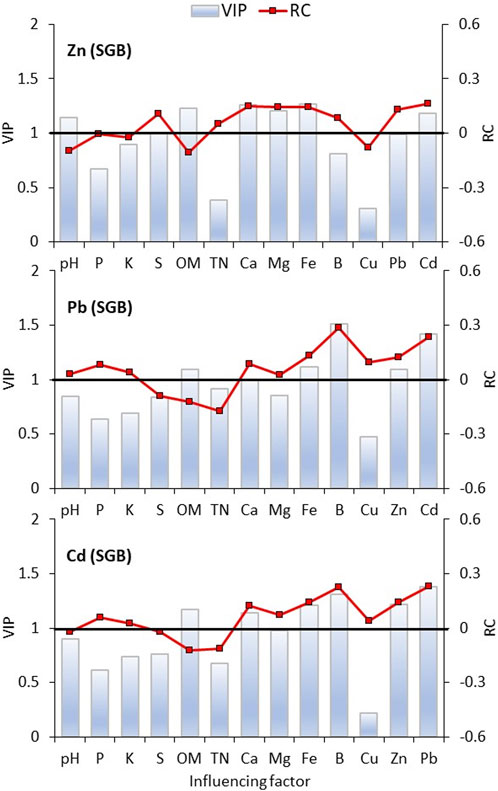
FIGURE 5. Partial Least Square Regression model (PLSR) of factors influencing the content of heavy metals in a soil amended with switchgrass-derived biochar (SGB). VIP is Variable Importance in Projection. When VIP >1, it means that the independent variable is important for predicting the dependent variable; whereas it is less important at VIP <0.5. The regression coefficients (RC) indicate the action direction of each independent variable. Results comprise the whole dataset of measurements.
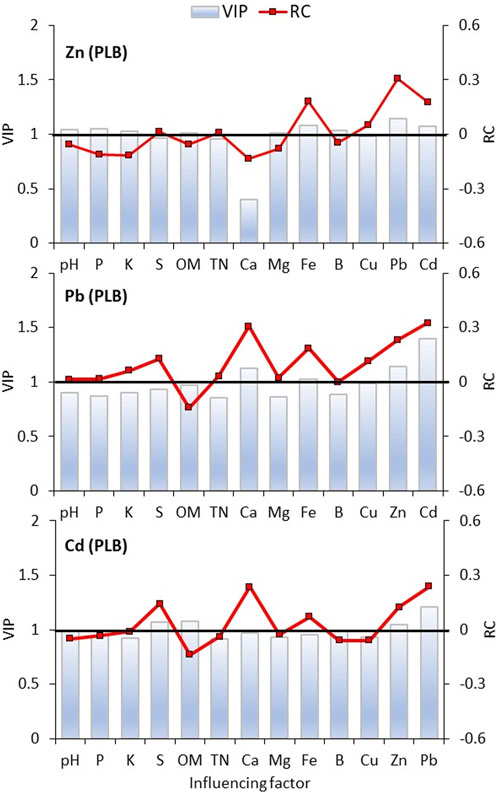
FIGURE 6. Partial Least Square Regression model (PLSR) of factors influencing the content of heavy metals in a soil amended with poultry litter-derived biochar (PLB). VIP is Variable Importance in Projection. When VIP >1, it means that the independent variable is important for predicting the dependent variable; whereas it is less important at VIP <0.5. The regression coefficients (RC) indicate the action direction of each independent variable. Results comprise the whole dataset of measurements.
Even though P and K considerably increased as both SGB and PLB application rates increased (Figure 1), non-effect was observed for those macronutrients over the metals’ availability, except for the punctual finding of available Zn with PLB application (Figure 6). This can be partially explained by the substantially greater amount of P and K in the PLB composition in comparison to SGB (Antonangelo et al., 2019), which might negatively affect the Zn levels in the soil to a higher extent than it affects Pb and Cd.
The relationship between observed and predicted metal concentrations from the PLS models is shown in Supplementary Figure S3.
3.4.3 Path analysis
The path analysis (PA) was conducted to verify which soil chemical attributes are interfering simultaneously with the availability of Zn, Pb, and Cd in the contaminated soil. Soil pH and OM are universally impacting the immobilization of those metals disregarding the biochar type (Figure 7). Only those two variables (Soil pH and OM) are affecting the combined contents of Zn, Cd, and Pb when PLB is applied. The significant and negative path coefficient for soil pH and Zn with both SGB and PLB shows a higher sensitivity of Zn to soil pH. This is further illustrated by the non-relationship of soil pH and Cd for any of the biochars and the absent relationship of OM and Zn with the application of PLB (Figure 7). Finally, soil pH did not show any relationship with Pb after PLB application and was positively related to the same metal when SGB was applied (Figure 7).
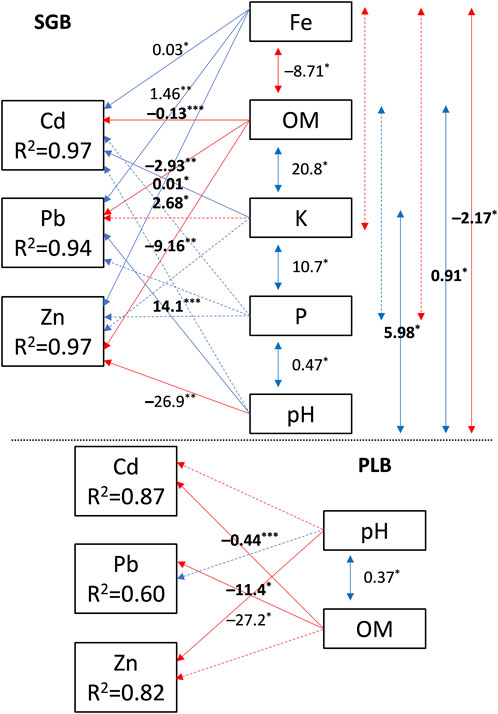
FIGURE 7. Path diagram for the relationship between soil attributes and metals availability. R2 denotes the coefficient of determination for Squared Multiple Correlations. The direct effects of soil attributes on metals availability are represented by single-headed arrows while the indirect effects of soil attributes are shown by double-headed arrows. Numbers near the arrows are the path coefficients (unstandardized solution). The solid-line path indicates that the effect is significant, and a dashed-line path indicates that the effect has no significance. Blue and red represent positive and negative correlations, respectively. SGB and PLB are switchgrass- and poultry litter-derived biochar, respectively. *: p < 0.05; **: p < 0.01; ***: p < 0.001.
4 Discussion
4.1 Metals immobilization
The IMAX was possible to determine only for Zn when SGB was applied (Figure 1). A maximum immobilization of 89.5% at a higher rate than the upper end in the range of applied rates (0.5%–4%) shows that SGB has a preference to immobilize Zn. This preferred immobilization for Zn is also reinforced when observing the 0 and 0.5% rates of SGB application because, at such low rates, Pb and Cd have their availability increased given the negative immobilization values (Figure 2; Table 1). In that scenario, Zn is more immobilized, consequently, Pb and Cd become more available. Since the levels of DPTA-extractable Zn were showing a drastic higher content in comparison to normal values found in Oklahoma soils, about twelvefold in contrast to tenfold and sevenfold for Cd and Pb respectively (Richards et al., 2012), it is expected that Zn–OM complexes are formed to a greater extent than Pb/Cd–OM complexes. This preferentially immobilizes Zn when low rates of SGB are applied. It was also observed by Geleto et al. (2022) that the competition among toxic heavy metals for adsorption sites was more intense at higher concentrations of Zn when contrasting biochars were applied. At higher rates of biochar application, the amounts of immobilization are not limited and Cd and Pb availability are reduced when 2%–4% of SGB is applied (Figure 2; Table 1). Indeed, there is competition among Zn, Pb, and Cd when biochar is applied, which is attributed to differences in feedstock properties, nutrient concentration, active functional groups, and pH (Wu et al., 2020).
Zinc is more sensitive to being immobilized by SGB, evidenced by the fact that SGB promoted Zn immobilization to a greater extent than PLB (Figures 2, 3; Table 1). On the other hand, PLB showed a preference for Cd and Pb immobilization although it did not increase Zn availability at low rates of application since no negative values were observed. In that case, we suggest that the mechanisms that PLB offer to immobilize metals go beyond the affinity to adsorption sites.
The extended Langmuir models (EL) also showed that Zn immobilization is more favored with SGB than PLB since the IMAX achieved was ∼90 ± 28 mg kg−1 for the former and 63.9 ± 28 mg kg−1 for the latter (Figure 3). The reason that the EL fitted for Zn as a function of PLB application but the simple Langmuir model did not, rely on the fact that a multi-component adsorption behavior is present in PLB or, in the case of our study, multiple mechanisms for Zn immobilization. In another study, the lower Zn availability was significantly affected by soil pH, EC, P, K, and Mg when PLB was applied, indicating significant adsorption by electrostatic interaction and ion exchange with the biochar surface and/or soil surface exchange sites (Tsai and Chang, 2022).
Considering that the IMAX of Cd after PLB application was lower with the EL (0.70 ± 0.20 mg kg−1) in comparison to the simple Langmuir model (1.08 ± 0.14 mg kg−1) it can be assured that competition between Zn and Cd takes place when the animal manure-derived biochar is applied (Figures 2, 3), mainly because Zn has shown an IMAX of 63.9 ± 28 mg kg−1 with PLB application and Pb did not present any relationship with the EL (Figure 3). One reason behind the competition among these metals might be the existence of multi-mechanisms to immobilize Zn and Cd and not Pb when PLB is applied. In that sense, when looking at the simple Langmuir model fitted with PLB, the reduced availability of Cd in comparison to the control was higher (65.3%) while with Pb it was lower (41.9%). The work of Wei et al. (2022) also showed that levels of available DTPA-extractable Cd decreased to a higher extent than DTPA-extractable Pb when biochar was applied. For Zn×Pb, the maximum adsorption capacity and strength of adsorption of Pb decreased in the presence of Zn when testing the aging effect of several biochar applications in a sandy calcareous soil (Raeisi et al., 2020).
4.2 Metals uptake
The reduced Zn and Pb uptake in ryegrass shoots ranged from ∼70%–98% and were optimum at rates of 0.50% ± 0.00%–0.60% ± 0.06% for both biochars, and 1.6% ± 0.4% of SGB for Cd (Figure 4). Interestingly, from previous results, the HM immobilization achieves the IMAX when higher rates were applied. It means that, even though the HM immobilization increases as rates of biochar increase up to 4%+, the ryegrass uptake ceases at 0.5%–1.5% rates, except for Cd when PLB is applied (Figure 4). Such behavior might be explained by the rhizosphere effect. According to Wang et al. (2022), the rhizosphere effect of wheat accelerated the aging process of biochar, even in the short term, and increased the number of surface functional groups (C=O and O=C–OH) on the aged biochar, in turn, promoting the adsorption capacity of rice husk- and sludge-derived biochar for Cd and Pb. In our study, though, the reduced uptake of Pb was greater than Cd regardless of the biochar (Figure 4). This may be related to a more pronounced rhizosphere effect over Pb in comparison to Cd. Zhu et al. (2022) showed higher microbial Pb fixation and Pb immobilization when a bio-composite produced from wheat straw biochar (pyrolyzed at 700°C) was applied, which sequentially transformed labile Pb into a more stable fraction.
In another study, the 3% application rate of green waste-derived biochars can effectively reduce the uptake of Cd and Pb by Brassica chinensis (Houssou et al., 2022). This is within the range found in our study since the reduced uptake by ryegrass reached the plateau when SGB, a green waste-derived biochar, was applied at 0.50 ± 0.00 (Pb) and 1.59 ± 0.35 (Cd) (Figure 4). This higher rate of SGB needed for optimum reduced Cd uptake was the first evidence that ryegrass has more tolerance for Cd absorption than Pb and Zn. Later, with the application of animal manure-derived biochar (PLB), the plateau is reached only at 4.33% ± 0.92% of PLB application, while it was 0.58 ± 0.06 for Zn and 0.50 ± 0.00 for Pb with the same amendment (Figure 4). Thus, ryegrass demonstrates a preferable tolerance for Cd uptake than Zn and Pb.
It is worth to note that nitrogen (N) has a direct relationship with Cd accumulation in ryegrass shoots. First, the higher affinity to Cd2+ by the membrane transporter of Italian ryegrass was observed after urea application (increased the Cd uptake) which contributed to the effective Cd accumulation in plant shoots (Ji et al., 2020). Second, ryegrass N and Cd concentrations were strongly related after PLB application at rates of 0.5% and 1%, and the lowest levels of Cd accumulation were observed only at 4% PLB (Antonangelo and Zhang, 2021). It is also noticeable that the total nitrogen (TN) content from PLB is greater than SGB (Antonangelo et al., 2019). Therefore, it is not surprising that the reduced Cd uptake by ryegrass terminates only at the rate of 4.33% ± 0.92% with PLB application (Figure 4).
4.3 Metals availability affected by soil chemical attributes
4.3.1 PLS and SMLR
From the PLS regression, the soil OM was inherently the most important soil attribute that negatively affected the soil HM availability, which was true for both biochars used in this study (Figures 5, 6). The soil OM increased with biochar application rates (Figure 1) partially explains this behavior. There are several direct and indirect effects of soil OM on the bioavailability of metals. To highlight some, dissolved OM is directly involved in the complexation of HM and their removal from the bioavailable fraction. In addition, soil biodiversity and/or microbial communities are increased following soil OM enhancement—thus the effects of microbes on the availability of HMs and their immobilization (Bravin et al., 2012; Seshadri et al., 2015; Sun et al., 2019). In this study, although we were not able to test the microbial community and activity the soil OM increment from the control to the highest rates of SGB and PLB application, strongly suggests that soil biota benefited from biochar application.
Soil pH increment has contributed to decreasing Zn levels no matter which biochar was applied, to reduce available Cd only with PLB application, and had no effect on Pb (Figures 5, 6). This is not in agreement with the previous work of Antonangelo and Zhang (2019), where soil pH increment was one of the most important factors affecting the availability of all those metals, including Pb, regardless of the biochar applied. One reason behind this is the fact that no other soil chemical attributes were considered in the model of Antonangelo and Zhang (2021). Therefore, other variables must be responsible for affecting the dynamics of HM in contaminated soils.
For Zn, the soil pH increase affecting its availability after the application of any biochar shows a higher sensitivity of this metal for soil pH in comparison to other metals. Although both biochars presented a considerable soil pH increment as a function of increased application rates, the buffering capacity of SGB is much lower than PLB (Antonangelo et al., 2019), still it can immobilize Zn. It somehow explains why Zn is more susceptible to soil pH and thus is immobilized by SGB (Figures 2, 3). In the case of Cd, the soil pH effect is only pronounced with PLB application, even considering that pH is the major factor influencing Cd bioavailability (Liang et al., 2022). However, the influence of the increased soil pH by biochar on the immobilization of Cd is dependent on the physicochemical properties of biochar (Wei et al., 2023). In our study, the negative relationship between pH and Cd for PLB is then explained by the greater buffering capacity offered by this biochar in comparison to SGB.
Another trend that was similar for all HM was the positive relationship among them, and between them and available Fe (Figures 5, 6). Since PLS regression comprises the whole dataset of measurements, and thus the increased rates of each biochar application, then it is confirmed that any competition among metals, when immobilization resources are limited (low rates of soil amendment application), is disappeared at high application rates because there will be many immobilization mechanisms to reduce the bioavailability of HM. Positive relationships among DTPA-extractable metals in the soil, after biochar application, were also found elsewhere in the literature (da Silva et al., 2022; Wei et al., 2022; Liu et al., 2022c). Finally, such trends were similarly observed with the SLMR in our study as well (Table 3). Other soil chemical attributes did not exhibit a general trend to explain better the HM availability, so it was necessary to perform path analysis (PA) using the combined availability of all three HMs to determine the overall chemical attributes impacting the simultaneous availability of Zn, Pb, and Cd.
4.3.2 Path analysis
It was confirmed that soil OM is universally impacting the immobilization of those metals disregarding the biochar type (Figure 7). Additionally, when using the combined response variables (Zn + Pb + Cd), soil pH was also impacting metals’ availability although trends were different depending on the metals and were not always negative as it was with OM (Figure 7).
The significant inverse path coefficient (−) for soil pH and Zn with both SGB and PLB suggests a higher sensitivity of Zn to soil pH in comparison to other metals, also there is no relationship between soil pH and Cd for any of the biochars and no relationship between soil pH and Pb with PLB application (Figure 7). This means that the pH path moves negatively toward Zn, affecting preferentially its availability. Moreover, the non-relationship of OM and Zn with the application of PLB means higher Zn sensitivity for pH than for OM (Figure 7). Finally, soil pH was positively related to Pb when SGB was applied (Figure 7). This can be justified by the fact that pH increment is immobilizing Zn more than other metals thus Pb is becoming more available, until SOM dictates the Pb immobilization.
It is understandable that OM mainly contributes to the immobilization of all metals when SGB is applied (Figure 7) given its greater total carbon (TC) and lower ash content in comparison to PLB (Antonangelo et al., 2019). Further, the omnipresent positive relationship between Fe and Zn, Pb, and Cd (Figure 7) makes it safe to assume that, since the contents of those heavy metals fall into the toxic levels, OM acts in their complexation rather than non-toxic metals such as Fe, presenting a lower degree of availability. Indeed, in the path OM→Fe→HM, there is a negative relationship (p < 0.05) between OM and Fe and a positive relationship between Fe and HM (0.05 > p < 0.01) (Figure 7).
Surprisingly, no soil chemical attributes other than pH and OM affected the availability of HM simultaneously with PLB application (Figure 7). This was expected since PLB presented a higher ash content and drastic increase of soil available nutrients, mainly P and K, in comparison to SGB (Figure 7). We assume that lower levels of those nutrients in the soil are enough to cause some threat to HM availability and their slight increment will impact a single metal instead of all of them concomitantly. Both reasons are presented in this study. First, negative effects of P and K were observed only on Zn availability with PLB application when using PLS regression; and non-consistent effects of P, S, and Mg over Zn, Pb, and Cd, depending on the biochar, were shown when performing SLMR. Also, the lower available soil P and K with SGB in comparison to PLB demonstrated a significant effect on the immobilization of all metals when conducting PA (Figure 7). Further research is required to determine key variables in the soil affecting multi-metal immobilization on a global scale after soil organic amendments are applied.
5 Conclusion
In this study, we evaluated the application of biochar from switchgrass and poultry litter in the soil and their effectiveness on metal immobilization and HM concentrations in the plant (ryegrass) shoots. The competitive immobilization of Zn, Pb, and Cd from the Tar Creek superfund site was verified using several multivariate techniques. The simple Langmuir models showed that the maximum HM immobilization (IMAX) was 169.2 ± 29.5 mg kg−1 for Zn with SGB application, and 20 ± 7.4 and 1.08 mg kg−1 for Pb and Cd, respectively, with PLB application. On the other hand, the extended Langmuir model (EL) showed competitive immobilization between metals since there was a decrease in the IMAX of Zn, from 169.2 ± 29.5 to 90 ± 28 mg kg−1 with SGB application, and Cd from 1.08 to 0.70 ± 0.21 mg kg−1 with PLB application. The potential of applying low rates such as 0.5%–1% w/w of two contrasting feedstock-derived biochars to remediate the contaminants and safely grow perennial ryegrass was elucidated since Zn, Pb, and Cd uptakes are respectively reduced by ∼71.2–79.4%, ∼96.6 to 97.5, and ∼37.5–50% with that range of both biochars application (Figure 4). Although it is incontestable that OM and soil pH play a significant role in the combined HM immobilization, their direct/indirect mechanisms to do so remain unclear because many other soil chemical attributes may also affect HM dynamics. This study may encourage and serve as a basis for future research performing the similar techniques in more representative soil samples, containing contrasting textures and using both soils naturally contaminated due to anthropogenic activities and soils spiked with known concentrations of several HM. Also, the combined application of HM to soil samples could be compared to those samples receiving only a single metal. In such cases, more accurate results can be achieved when evaluating the many soil chemical attributes affecting HM availability.
Data availability statement
The raw data supporting the conclusion of this article will be made available by the authors, without undue reservation.
Author contributions
JA and HZ contributed to the conception and design of the study. JA organized the database. JA performed the statistical analysis. JA, HZ, and IS wrote the first draft of the manuscript. JA and IS wrote sections of the manuscript. JA contributed to the methodology. JA contributed to visualization. JA contributed to the data validation and data curation. HZ contributed to the resources. JA, HZ, and IS contributed to writing the first draft, reviewing, and editing. HZ contributed to supervision, project administration, and funding acquisition. All authors contributed to the manuscript revision, and read, and approved the submitted version.
Funding
This work was supported by the Oklahoma Agricultural Experiment Station.
Conflict of interest
The authors declare that the research was conducted in the absence of any commercial or financial relationships that could be construed as a potential conflict of interest.
Publisher’s note
All claims expressed in this article are solely those of the authors and do not necessarily represent those of their affiliated organizations, or those of the publisher, the editors and the reviewers. Any product that may be evaluated in this article, or claim that may be made by its manufacturer, is not guaranteed or endorsed by the publisher.
Supplementary material
The Supplementary Material for this article can be found online at: https://www.frontiersin.org/articles/10.3389/fenvs.2023.1170427/full#supplementary-material
References
Abbas, T., Rizwan, M., Ali, S., Zia-ur-Rehman, M., Farooq Qayyum, M., Abbas, F., et al. (2017). Effect of biochar on cadmium bioavailability and uptake in wheat (triticum aestivum L.) grown in a soil with aged contamination. Ecotoxicol. Environ. Saf. 140, 37–47. doi:10.1016/j.ecoenv.2017.02.028
Ahmad, M., Rajapaksha, A. U., Lim, J. E., Zhang, M., Bolan, N., Mohan, D., et al. (2014). Biochar as a sorbent for contaminant management in soil and water: A review. Chemosphere 99, 19–33. doi:10.1016/j.chemosphere.2013.10.071
Ahmad, M., Soo Lee, S., Yang, J. E., Ro, H.-M., Han Lee, Y., and Sik Ok, Y. (2012). Effects of soil dilution and amendments (Mussel Shell, Cow Bone, and biochar) on PB availability and phytotoxicity in military shooting range soil. Ecotoxicol. Environ. Saf. 79, 225–231. doi:10.1016/j.ecoenv.2012.01.003
Ali, H., Khan, E., and Sajad, M. A. (2013). Phytoremediation of heavy metals—Concepts and applications. Chemosphere 91 (7), 869–881. doi:10.1016/j.chemosphere.2013.01.075
Antonangelo, J. A., Souza, J. L., Whitaker, A., Arnall, B., and Zhang, H. (2022). Evaluation of mehlich-3 as a multi-element extractant of micronutrients and sulfur in a soil–ryegrass system amended with varying biochar rates from two feedstocks. Land 11, 1979. doi:10.3390/land11111979
Antonangelo, J. A., and Zhang, H. (2019). Heavy Metal phytoavailability in a contaminated soil of northeastern Oklahoma as affected by Biochar Amendment. Environ. Sci. Pollut. Res. 26, 33582–33593. doi:10.1007/s11356-019-06497-w
Antonangelo, J. A., Zhang, H., Sun, X., and Kumar, A. (2019). Physicochemical properties and morphology of biochars as affected by feedstock sources and pyrolysis temperatures. Biochar 1, 325–336. doi:10.1007/s42773-019-00028-z
Antonangelo, J., and Zhang, H. (2021). Influence of biochar derived nitrogen on cadmium removal by ryegrass in a contaminated soil. Environments 8, 11. doi:10.3390/environments8020011
Bradl, H. B. (2004). Adsorption of heavy metal ions on soils and soils constituents. J. Colloid Interface Sci. 277, 1–18. doi:10.1016/j.jcis.2004.04.005
Bravin, M. N., Garnier, C., Lenoble, V., Gérard, F., Dudal, Y., and Hinsinger, P. (2012). Root-induced changes in ph and dissolved organic matter binding capacity affect copper dynamic speciation in the rhizosphere. Geochimica Cosmochimica Acta 84, 256–268. doi:10.1016/j.gca.2012.01.031
Church, C., Spargo, J., and Fishel, S. (2017). Strong acid extraction methods for “total phosphorus” in soils: EPA method 3050B and EPA method 3051. Agric. Environ. Lett. 2, 160037. doi:10.2134/ael2016.09.0037
da Silva, A. P., Silva, A. O., Lima, F. R., Benedet, L., Franco, A., Souza, J. K., et al. (2022). Potentially toxic elements in iron mine tailings: Effects of reducing soil ph on available concentrations of toxic elements. Environ. Res. 215, 114321. doi:10.1016/j.envres.2022.114321
Fan, Q., Sun, J., Chu, L., Cui, L., Quan, G., Yan, J., et al. (2018). Effects of chemical oxidation on surface oxygen-containing functional groups and adsorption behavior of biochar. Chemosphere 207, 33–40. doi:10.1016/j.chemosphere.2018.05.044
Fang, N., Shi, Z., Chen, F., and Wang, Y. (2015). Partial least squares regression for determining the control factors for runoff and suspended sediment yield during rainfall events. Water 7, 3925–3942. doi:10.3390/w7073925
Fashola, M. O., Ngole-Jeme, V. M., and Babalola, O. O. (2020). Physicochemical properties, heavy metals, and metal-tolerant bacteria profiles of abandoned gold mine tailings in Krugersdorp, South Africa. Can. J. Soil Sci. 100 (3), 217–233. doi:10.1139/cjss-2018-0161
Geleto, M. A., Forján, R., Arco-Lázaro, E., Covelo, E. F., Marcet, P., and Cerqueira, B. (2022). Influence of pyrolysis temperature and feedstock biomass on cu2+, PB2+, and zn2+ sorption capacity of biochar. Int. J. Environ. Sci. Technol. 19, 11857–11866. doi:10.1007/s13762-022-03989-0
He, L., Zhong, H., Liu, G., Dai, Z., Brookes, P. C., and Xu, J. (2019). Remediation of heavy metal contaminated soils by biochar: Mechanisms, potential risks and applications in China. Environ. Pollut. 252, 846–855. doi:10.1016/j.envpol.2019.05.151
Houssou, A. A., Jeyakumar, P., Niazi, N. K., Van Zwieten, L., Li, X., Huang, L., et al. (2022). Biochar and soil properties limit the phytoavailability of lead and cadmium by brassica chinensis l. in contaminated soils. Biochar 4, 5. doi:10.1007/s42773-021-00126-x
Inyang, M. I., Gao, B., Yao, Y., Xue, Y., Zimmerman, A., Mosa, A., et al. (2015). A review of biochar as a low-cost adsorbent for aqueous heavy metal removal. Crit. Rev. Environ. Sci. Technol. 46, 406–433. doi:10.1080/10643389.2015.1096880
Järup, L. (2003). Hazards of heavy metal contamination. Br. Med. Bull. 68, 167–182. doi:10.1093/bmb/ldg032
Ji, S., Gao, L., Chen, W., Su, J., and Shen, Y. (2020). Urea application enhances cadmium uptake and accumulation in Italian ryegrass. Environ. Sci. Pollut. Res. 27, 34421–34433. doi:10.1007/s11356-020-09691-3
Jones, J. B., and Case, V. W. (1990). Sampling, handling, and analyzing plant tissue samples. SSSA Book Ser. 3, 389–427. doi:10.2136/sssabookser3.3ed.c15
Kapoor, V., Li, X., Elk, M., Chandran, K., Impellitteri, C. A., and Santo Domingo, J. W. (2015). Impact of heavy metals on transcriptional and physiological activity of nitrifying bacteria. Environ. Sci. Technol. 49 (22), 13454–13462. doi:10.1021/acs.est.5b02748
Liang, T., Zhou, G., Chang, D., Wang, Y., Gao, S., Nie, J., et al. (2022). Co-incorporation of Chinese milk vetch (astragalus sinicus L.), rice straw, and biochar strengthens the mitigation of CD uptake by rice (Oryza sativa L.) Sci. Total Environ. 850, 158060. doi:10.1016/j.scitotenv.2022.158060
Liu, L., Li, X., Wang, X., Wang, Y., Shao, Z., Liu, X., et al. (2022a). Metolachlor adsorption using walnut shell biochar modified by soil minerals. Environ. Pollut. 308, 119610. doi:10.1016/j.envpol.2022.119610
Liu, L., Wang, X., Fang, W., Li, X., Shan, D., and Dai, Y. (2022b). Adsorption of metolachlor by a novel magnetic illite–biochar and recovery from soil. Environ. Res. 204, 111919. doi:10.1016/j.envres.2021.111919
Liu, Q., Huang, L., Chen, Z., Wen, Z., Ma, L., Xu, S., et al. (2022c). Biochar and its combination with inorganic or Organic Amendment on growth, uptake and accumulation of cadmium on lettuce. J. Clean. Prod. 370, 133610. doi:10.1016/j.jclepro.2022.133610
Liu, X., Shao, Z., Wang, Y., Liu, Y., Wang, S., Gao, F., et al. (2023). New use for lentinus edodes bran biochar for tetracycline removal. Environ. Res. 216, 114651. doi:10.1016/j.envres.2022.114651
Meng, J., Tao, M., Wang, L., Liu, X., and Xu, J. (2018). Changes in heavy metal bioavailability and speciation from a pb-zn mining soil amended with biochars from co-pyrolysis of rice straw and swine manure. Sci. Total Environ. 633, 300–307. doi:10.1016/j.scitotenv.2018.03.199
Onderka, M., Wrede, S., Rodný, M., Pfister, L., Hoffmann, L., and Krein, A. (2012). Hydrogeologic and landscape controls of dissolved inorganic nitrogen (DIN) and dissolved silica (DSI) fluxes in heterogeneous catchments. J. Hydrology 450-451, 36–47. doi:10.1016/j.jhydrol.2012.05.035
Park, J. H., Choppala, G. K., Bolan, N. S., Chung, J. W., and Chuasavathi, T. (2011). Biochar reduces the bioavailability and phytotoxicity of heavy metals. Plant Soil 348, 439–451. doi:10.1007/s11104-011-0948-y
Pelegrino, M. H., Silva, S. H., de Faria, Á. J., Mancini, M., Teixeira, A. F., Chakraborty, S., et al. (2021). Prediction of soil nutrient content via pXRF spectrometry and its spatial variation in a highly variable tropical area. Precis. Agric. 23, 18–34. doi:10.1007/s11119-021-09825-8
Qiao, Y., Wu, J., Xu, Y., Fang, Z., Zheng, L., Cheng, W., et al. (2017). Remediation of cadmium in soil by biochar-supported iron phosphate nanoparticles. Ecol. Eng. 106, 515–522. doi:10.1016/j.ecoleng.2017.06.023
Raeisi, S., Motaghian, H., and Hosseinpur, A. R. (2020). Effect of the soil biochar aging on the sorption and desorption of pb2+ under competition of zn2+ in a sandy calcareous soil. Environ. Earth Sci. 79, 148. doi:10.1007/s12665-020-8891-y
Rengel, Z. (2015). Availability of Mn, Zn and Fe in the rhizosphere. J. soil Sci. plant Nutr. 15 (2), 0–409. doi:10.4067/s0718-95162015005000036
Richards, J. R., Schroder, J. L., Zhang, H., Basta, N. T., Wang, Y., and Payton, M. E. (2012). Trace elements in benchmark soils of Oklahoma. Soil Sci. Soc. Am. J. 76, 2031–2040. doi:10.2136/sssaj2012.0100
Seshadri, B., Bolan, N. S., and Naidu, R. (2015). Rhizosphere-induced heavy metal(loid) transformation in relation to bioavailability and remediation. J. soil Sci. plant Nutr. 15, 0. doi:10.4067/s0718-95162015005000043
Shaheen, S. M., Niazi, N. K., Hassan, N. E. E., Bibi, I., Wang, H., Tsang, D. C. W., et al. (2019). Wood-based biochar for the removal of potentially toxic elements in water and wastewater: A critical review. Int. Mater. Rev. 64, 216–247. doi:10.1080/09506608.2018.1473096
Shi, Z. H., Ai, L., Li, X., Huang, X. D., Wu, G. L., and Liao, W. (2013). Partial least-squares regression for linking land-cover patterns to soil erosion and sediment yield in watersheds. J. Hydrology 498, 165–176. doi:10.1016/j.jhydrol.2013.06.031
Shi, Z. H., Huang, X. D., Ai, L., Fang, N. F., and Wu, G. L. (2014). Quantitative analysis of factors controlling sediment yield in mountainous watersheds. Geomorphology 226, 193–201. doi:10.1016/j.geomorph.2014.08.012
Su, C. (2014). A review on heavy metal contamination in the soil worldwide: Situation, impact and remediation techniques. Environ. Skeptics Critics 3 (2), 24. Available at: https://scholar.google.com/scholar_lookup?title=A%20review%20on%20heavy%20metal%20contamination%20in%20the%20soil%20worldwide%3A%20Situation%2C%20impact%20and%20remediation%20techniquesandjournal=Environ%20Skept%20Critandvolume=3andpages=24-38andpublication_year=2014andauthor=Su%2CCandauthor=Jiang%2CLQandauthor=Zhang%2CWJ
Sun, J., Fan, Q., Ma, J., Cui, L., Quan, G., Yan, J., et al. (2020). Effects of biochar on cadmium (CD) uptake in vegetables and its natural downward movement in saline-alkali soil. Environ. Pollut. Bioavailab. 32, 36–46. doi:10.1080/26395940.2020.1714487
Sun, X., Li, Z., Wu, L., Christie, P., Luo, Y., and Fornara, D. A. (2019). Root-induced soil acidification and cadmium mobilization in the rhizosphere of Sedum plumbizincicola: Evidence from a high-resolution imaging study. Plant Soil 436, 267–282. doi:10.1007/s11104-018-03930-w
Tong, L. S., Fang, N. F., Xiao, H. B., and Shi, Z. H. (2020). Sediment deposition changes the relationship between soil organic and inorganic carbon: Evidence from the Chinese loess plateau. Agric. Ecosyst. Environ. 302, 107076. doi:10.1016/j.agee.2020.107076
Tsai, C.-C., and Chang, Y.-F. (2022). Poultry Litter Biochar as a gentle soil amendment in multi-contaminated soil: Quality evaluation on nutrient preservation and contaminant immobilization. Agronomy 12, 405. doi:10.3390/agronomy12020405
Wang, B., Gao, B., and Fang, J. (2017). Recent advances in engineered biochar productions and applications. Crit. Rev. Environ. Sci. Technol. 47, 2158–2207. doi:10.1080/10643389.2017.1418580
Wang, J., Liu, J., Zhou, J., Chang, L., Shen, Z., Shi, L., et al. (2022). Rhizosphere effect on physicochemical properties and immobilization performance of biochar. Water, Air, and Soil Pollut. 233, 438. doi:10.1007/s11270-022-05902-4
Wei, B., Peng, Y., Jeyakumar, P., Lin, L., Zhang, D., Yang, M., et al. (2023). Soil ph restricts the ability of biochar to passivate cadmium: A meta-analysis. Environ. Res. 219, 115110. doi:10.1016/j.envres.2022.115110
Wei, T., Li, X., Li, H., Gao, H., Guo, J., Li, Y., et al. (2022). The potential effectiveness of mixed bacteria-loaded biochar/activated carbon to remediate CD, PB co-contaminated soil and improve the performance of Pakchoi Plants. J. Hazard. Mater. 435, 129006. doi:10.1016/j.jhazmat.2022.129006
Weng, Z., Van Zwieten, L., Singh, B. P., Tavakkoli, E., Joseph, S., Macdonald, L. M., et al. (2017). Biochar built soil carbon over a decade by stabilizing rhizodeposits. Nat. Clim. Change 7 (5), 371–376. doi:10.1038/nclimate3276
Wold, S., Sjöström, M., and Eriksson, L. (2001). PLS-regression: A basic tool of chemometrics. Chemom. Intelligent Laboratory Syst. 58, 109–130. doi:10.1016/s0169-7439(01)00155-1
Wu, J., Song, Q., Zhou, J., Wu, Y., Liu, X., Liu, J., et al. (2021). Cadmium threshold for acidic and multi-metal contaminated soil according to Oryza sativa L. cadmium accumulation: Influential factors and prediction model. Ecotoxicol. Environ. Saf. 208, 111420. doi:10.1016/j.ecoenv.2020.111420
Wu, P., Wang, Z., Wang, H., Bolan, N. S., Wang, Y., and Chen, W. (2020). Visualizing the emerging trends of biochar research and applications in 2019: A scientometric analysis and review. Biochar 2, 135–150. doi:10.1007/s42773-020-00055-1
Xie, T., Reddy, K. R., Wang, C., Yargicoglu, E., and Spokas, K. (2015). Characteristics and applications of biochar for environmental remediation: A review. Crit. Rev. Environ. Sci. Technol. 45, 939–969. doi:10.1080/10643389.2014.924180
Yang, P., Hu, Z., and Shu, Q. (2021). Factors affecting soil organic carbon content between natural and reclaimed sites in Rudong Coast, Jiangsu Province, China. J. Mar. Sci. Eng. 9, 1453. doi:10.3390/jmse9121453
Zeng, G., Wu, H., Liang, J., Guo, S., Huang, L., Xu, P., et al. (2015). Efficiency of biochar and compost (or composting) combined amendments for reducing CD, Cu, Zn and PB bioavailability, mobility and ecological risk in wetland soil. RSC Adv. 5, 34541–34548. doi:10.1039/c5ra04834f
Zhang, R.-H., Li, Z.-G., Liu, X.-D., Wang, B., Zhou, G.-L., Huang, X.-X., et al. (2017). Immobilization and bioavailability of heavy metals in greenhouse soils amended with rice straw-derived biochar. Ecol. Eng. 98, 183–188. doi:10.1016/j.ecoleng.2016.10.057
Keywords: metals immobilization, biochar (BC), switchgrass, poultry litter, multivariate techniques
Citation: Antonangelo JA, Zhang H and Sitienei I (2023) Biochar amendment of a metal contaminated soil partially immobilized Zn, Pb, and Cd and reduced ryegrass uptake. Front. Environ. Sci. 11:1170427. doi: 10.3389/fenvs.2023.1170427
Received: 20 February 2023; Accepted: 06 April 2023;
Published: 13 April 2023.
Edited by:
Xing Yang, Hainan University, ChinaReviewed by:
Yingjie Dai, Northeast Agricultural University, ChinaHongfen Zhu, Shanxi Agricultural University, China
Copyright © 2023 Antonangelo, Zhang and Sitienei. This is an open-access article distributed under the terms of the Creative Commons Attribution License (CC BY). The use, distribution or reproduction in other forums is permitted, provided the original author(s) and the copyright owner(s) are credited and that the original publication in this journal is cited, in accordance with accepted academic practice. No use, distribution or reproduction is permitted which does not comply with these terms.
*Correspondence: Joao Arthur Antonangelo, YW50b25hbmdlbG9qQGFwc3UuZWR1