- Institute of Agricultural Resources and Environment, Sichuan Academy of Agricultural Sciences, Chengdu, China
Polycyclic aromatic hydrocarbon (PAH) accumulation in topsoil is getting particular concern with the rapid development of urbanization and industrialization, while the overall pollution status and related risk posed by PAHs received limited attentions at the national scale. This study conducted an overview of published data on 16 priority control PAHs by USEPA on the related peer-reviewed 207 research papers between 2000 and 2020 in 30 provinces of China. Based on that, the pollution levels, composition status, spatial distribution pattern, ecological risk, and human health risk posed by soil PAHs were evaluated. Monte Carlo simulation was adopted to model the probabilistic health risk and identify the contributor of such risks. Results demonstrated the concentrations of ∑16PAHs in soil varied from “undetected” to 261 μg g−1 with a mean value of 0.63 μg g−1, indicating the obvious accumulation of PAHs in topsoil of most provinces in China compared with the guideline value (0.2 μg g−1). The concentrations of ∑16PAHs in surface soil of China has obvious regional characteristics. Higher concentrations of soil PAHs are mainly distributed in north, northwest, and eastern regions, especially in Xinjiang, Shandong, Jiangsu, Sichuan, and Guangxi Province. Risk assessment indicates potential ecological and human health risk were posed by soil PAHs, therefore, reducing soil PAHs concentration and exposure frequency are the most effective pathways to protect human health. Despite the fact that risks posed by soil PAHs are generally low, concentrations of PAHs in some sites are relatively high. It is necessary to take effective measures to remediate soil PAHs pollution in certain areas to reduce concentration and associated risks.
1 Introduction
Soil contamination is one of the most concerned issues around the world currently and has attracted particular attentions (Lu et al., 2015; Daso et al., 2016). Pollutants in soil not only have direct harmful effects on ecology, but also pose potential hazard risks to mankind and animals (Salazar et al., 2012; Niu et al., 2013; Lestan, 2017). As reported by the first national soil survey report, the percentage of organic pollutants which exceeded its responding guideline values, ranked second followed by heavy metals (Ministry of Environment Protection and Ministry of Land Resources of the People’s Republic of China, 2014). The polycyclic aromatic hydrocarbon (PAHs) belongs to a group of organic compounds that are composed of more than two condensed aromatic rings, which are carcinogenic, mutagenic, teratogenic, and toxic to living beings (Franco et al., 2008; Ambade and Sethi, 2021). PAHs are widespread in the water, atmosphere, sediments, and soil, and are both from natural sources such as volcanoes and anthropogenic activities such as incomplete combustion of biomass fuels, fossil, and oil spills (Wolska et al., 2012; Yang et al., 2020; Ambade et al., 2022). PAHs tend to retain and accumulate in soil due to their high hydrophobicity. Soil PAHs contamination is getting increasing attention nowadays with a large number reports about high concentrations of PAHs from continuous emission of oil combustion and traffic (Leung et al., 2015; Alves et al., 2016; Chen et al., 2017). To address the overall contamination status, assessment of potential sources, and risks of soil PAHs are vital and essential for soil PAHs pollution remediation.
Risk assessment is the process of estimating the probability of harmful effects posed by contaminants (USEPA, 2016). According to the different end-point receptors, it can be classified into ecological risk and human health risk. Previous studies were mostly focused on the occurrence, source apportionment, and risk assessment of regional soil PAHs (Huang et al., 2014; Yang et al., 2014; Tong et al., 2018). These studies reported the occurrence of soil PAHs regionally, mainly due to human activities such as industrial emission, transportation vehicle, and/or e-waste dismantling. However, existed studies on soil PAHs were largely focused on small regions or areas, limited number of soil samples, or single soil type (Wang et al., 2014; Cai et al., 2017; Zheng et al., 2019). Besides, deterministic (the most likely) values are usually adopted in health risk assessment model of soil PAHs. However, variable of exposure parameters due to the individual differences and PAHs concentration differences from soil spatial heterogeneity would underestimate or overestimate the human health risk from traditional deterministic methods (Yang et al., 2014). Therefore, a comprehensive study taking into account concentration status, pollution level, and induced probabilistic risk modeling of soil PAHs at the national scale was urgent to conduct.
In the present study, a dataset of USEPA 16 priority PAHs in Chinese topsoil was compiled based on peer-reviewed literature. On the ground of the dataset compiled, the objectives of the study were to 1) reveal the overall accumulation and contamination status of PAHs in topsoil of China, 2) present the spatial distribution of soil PAHs concentration, and 3) evaluate the associated ecological and human health risk posed by soil PAHs and model probabilistic health risk by considering variability of exposure parameters. The results would provide basic reference for remediation and risk control of soil PAHs pollution.
2 Methods and materials
2.1 Data collection and development of soil PAHs concentrations dataset
The dataset of PAHs concentrations in topsoil (0–20 cm) was developed based on 14,161 soil samples in 207 research papers published between 2000 and 2020, covering 30 provinces across China (except Hainan, HongKong, Macao, and Taiwan). The keywords “soil PAHs” and “polycyclic aromatic hydrocarbons (PAHs) in soil” were searched on Web of Science, Science Direct, the China Wan Fang database, China Wei Pu Literature, and China National Knowledge Infrastructure Database to find related publications. The papers had to meet the criteria that sampling, analytical methods, quality control were strictly conducted to guarantee the accuracy of the soil PAHs concentrations. The information extracted from each paper included 1) sampling location (province, city, longitude, and latitude), sampling time and depth, number of soil samples; 2) mean value, range of ∑16PAHs and mean concentration of the sixteen PAHs congeners. The 16 priority PAHs identified by USEPA were acenaphthylene (ACY), naphthalene (NAP), acenaphthene (ACE), phenanthrene (PHE), fluorene (FL), anthracene (ANT), pyrene (PYR), fluoranthene (FLU), benzo[a] anthracene (BaA), benzo[b] fluoranthene (BbF), chrysene (CHR), benzo[k] fluoranthene (BkF), benzo[a]pyrene (BaP), indeno(1,2, 3-cd)pyrene (IND), dibenzo(a,h) anthracene (DBA), and benzo(g,h,i)perylene (BghiP). Detailed information for each publication are presented in Supplementary Table S1.
2.2 Risk assessment models
2.2.1 Ecological risk assessment
Toxic equivalent concentrations (TEQBaP) was adopted to characterize ecological risk posed by soil PAHs. TEQBaP represents the total concentration of transformed individual PAHs congener by the toxic equivalency factor (TEF) (μg kg−1) of BaP (Lin et al., 2013). The TEQBaP was calculated by the Eq. 1:
where Ci means the concentration of PAHs congener i (μg kg−1) and
2.2.2 Human health risk assessment
Human health can be threatened by soil PAHs via exposure pathways including oral intake, inhalation, and dermal contact. The USEPA exposure model was adopted to assess the incremental lifetime cancer risk (ILCR) for adults and children exposed by soil PAHs (USEPA, 1989; USEPA, 2009a; USEPA, 2009b). The ILCR through each of the exposure pathways were calculated by the Eqs 2–4:
Where ILCRderm, ILCRoral, and ILCRinh are the exposure risks of soil PAHs via dermal contact, oral intake, and inhalation pathways, respectively (unitless), and ILCRs is the total human health risk exposed from all the three pathways (unitless). CEs is TEQBaP (ug kg−1), BW is the body weight (kg), AT is the average life span (d), IRinh as the soil inhalation rate (m3 d−1), IRing represents the soil particle intake rate (mg d−1), ED means the exposure duration (years), SA represents the surface area of dermal exposure (cm2), EF denotes the exposure frequency (d year−1), AF stands for the adherence factor (mg cm−2 h−1), ABS denotes the dermal adsorption fraction (unitless), PEF represents the particle emission factor (m3 kg−1), and CSFIng, CSFInh, and CSFDerm are the carcinogenic slope factor for ingestion, inhalation, and dermal contact respectively (mg kg−1 d−1)−1.
Determined values of each parameter were used in deterministic risk assessment. CEs is the mean values of TEQBaP in all sampled soil sites, and the value of other parameters used in Eqs 2–4 are listed in Supplementary Table S3.
For probabilistic risk modelling, Monte Carlo (MC) simulation was employed to handle variability in concentrations of PAHs and uncertainty of parameters with 10,000 iterations. Values of CEs were chosen from the compiled dataset of sampling sites for each iteration, and extreme values of CEs were removed to suit a lognormal distribution. The parameters (ED, EF, BW, SA, IRIng, and IRInh) were probabilistically treated by the defined distribution list in Supplementary Table S4. Other exposure parameters with less variability were still single-point values, as listed in Supplementary Table S3. During the MC simulation, sensitivity analyses was conducted to estimate the contribution of each input parameter to the total variance of probabilistic health risks.
2.3 Statistical analysis tools
Microsoft Excel 2016 and SPSS 16.0 were employed to perform basic statistical analysis. Figures were made by Origin 2019 Pro (Origin Lab, Northampton, MA). MC simulations and sensitivity analyses were conducted by Oracle Crystal Ball software (11.1.2.4). The spatial distribution maps of soil PAHs concentrations and risks were conducted by ArcGIS 10.7.
3 Results
3.1 Concentration and spatial distribution of PAHs in topsoil of China
The research areas of collected 207 papers distributed in 30 provinces of China (Supplementary Figure S1), which can reflect the overall soil PAHs pollution status nationally. The sampling sites were mainly located in the eastern and northern areas, especially Pearl River Delta, Yangtze River Delta, and the Beijing-Tianjin-Hebei region (Table 1). The concentration of ∑16PAHs in each collected literature is listed in Supplementary Table S1. Concentrations of ∑16PAHs in the topsoil of China ranged from “undetected” to 261 μg g−1 with a mean value of 0.63 μg g−1. The median value of ∑16PAHs was 17.94 μg g−1, which is much higher than the 0.05 μg g−1 of the Dutch government standard of soil PAHs (Agarwal, 2009), indicating PAH pollution in the topsoil was mainly affected by human activities. Figure 1 shows the content distribution of 16 PAHs congers. The 25%–75% interval distribution of 16 PAHs congers concentration ranged from 1.39 to 108.5 μg kg−1. The median concentrations of FL and PYR were 39.7 μg kg−1 and 33.21 μg kg−1, respectively, which were highest among the 16 PAHs congeners. Alternatively, the median concentrations of ACE and ACY were 4.85 μg kg−1 and 6.13 μg kg−1, which were the lowest.
At present, the threshold of soil PAHs pollution is not modulated in China. The pollution classification from Maliszewska-Kordybach (1996) on the base of the total concentrations of PAHs was adopted in this study. Four categories were classified by Maliszewska-Kordybach (1996): heavily contaminated (>1,000 μg kg−1), moderately contaminated (600–1,000 μg kg−1), weakly contaminated (200–600 μg kg−1), and non-contaminated (<200 μg kg−1). Based on the classification, 24.15% of the topsoil in China were categorized as non-contaminated, 39.13% of the soil as weakly contaminated, 14.98% of the soil as moderately contaminated and 21.74% as heavily contaminated. The heavily contaminated soil samples were mainly located in the eastern coastal and southern regions, especially in Yangtze River Delta area, while non-contaminated sites were scattered in across multiples areas of China (Figure 2A).
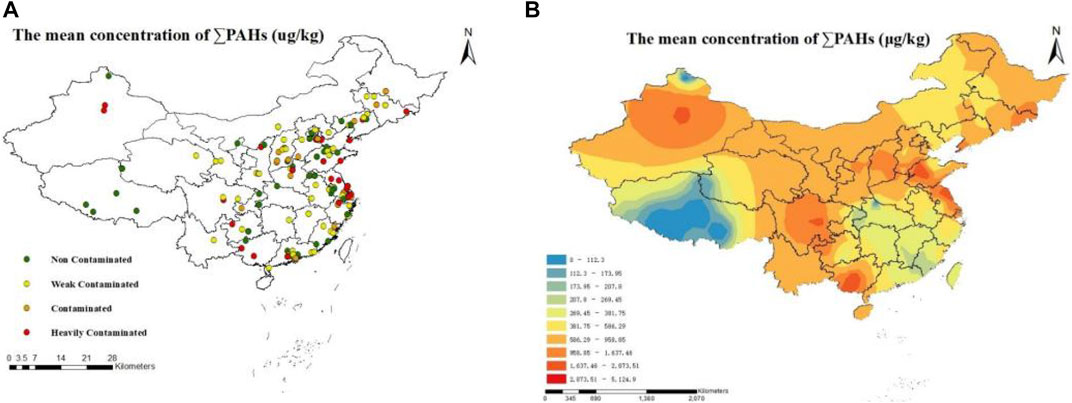
FIGURE 2. The spatial distribution of ∑16PAHs concentrations in China from collected data [(A): sampling sites (B): interpolation].
The concentrations of soil PAHs in China varied greatly among different regions. The median values of ∑16PAHs concentration in different regions ranked as: North China (Tianjin, Beijing, Hebei, Shaanxi, Inner Mongolia) (690.3 μg kg−1) > Northeast China (Heilongjiang, Jilin, Liaoning) (497.5 μg kg−1) > East China (Shandong, Jiangsu, Fujian, Zhejiang, Shanghai, Anhui, Jiangxi) (356.47 μg kg−1) >Northwest China (Shanxi, Gansu, Xinjiang, Ningxia, Qinghai) (318.4 μg kg−1) > South China (Guangxi, Guangdong) (318.2 μg kg−1) > Southwest China (Guizhou, Yunnan, Tibet, Sichuan, Chongqing) (217.79 μg kg−1) > Central China (Henan, Hunan, Hubei) (129.5 μg kg−1). The spatial distribution of ∑16PAHs in Chinese topsoil was mapped by the Inverse Distance Weight (IDW) interpolation method (Figure 2B). The higher concentrations of ∑16PAHs were mainly distributed in north, northwest and east regions, especially Xinjiang, Shandong, Jiangsu, Sichuan, and Guangxi Province, while lower concentrations prevailed in the western areas, such as Tibet, where industrial activities had lesser effects.
3.2 Risk assessment of soil PAHs
3.2.1 Potential ecological risk assessment
The potential ecological risk posed by soil PAHs was assessed by Toxic equivalent concentrations of BaP (TEQBaP). Results showed the TEQBaP of soil PAHs in China ranged from 0.19 to 1,268.01 μg kg−1 with a mean value of 93.65 μg kg−1. Overall, 52.98% of the soil samples exceeded the ecological risk guideline value (33 μg kg−1), indicating moderately ecological risks in topsoil of China were posed by PAHs. The spatial distribution map of TEQBaP of soil PAHs shared similar pattern with concentration (Figure 3). Higher concentrations of TEQBaP were mainly located in north, southwest, and eastern areas. TEQBaP of soil PAHs in most regions of China were greater than 33 μg kg−1, especially in Shaanxi, Sichuan, and Jiangsu Provinces.
3.2.2 Human health risk assessment
ILCR model was adopted to assess the human health risk posed by soil PAHs for children and adults. ILCRs ranged from 2.56E-09 to 1.67E-05, with a mean value of 1.24E-06, and from 1.67E-09 to 1.09E-05, with a mean value of 8.05E-07 for adults and children, respectively (Table 2). ILCRs higher than 10–4 is considered as high risk, ILCRs lower than 10–6 is regarded as no risk, and ILCRs range from 10–6 to 10–4 is considered as potential but acceptable risk posed (USEPA, 2016). The health risk assessment results showed that 35.10% and 20.53% of sampling sites had ILCRs exceeding 10–6 for children and adults, respectively, with no site having ILCRs more than 10–4 both for children and adults, which indicate potential human health risks were posed by soil PAHs, but risks were acceptable. Among the three exposure pathways, the ILCRs exposed from ingestion and dermal contact were 4–5 orders of magnitude higher than the inhalation pathway. Therefore, ingestion and dermal contact are the dominant pathways of ILCRs, while inhalation is negligible. The spatial distribution maps of ILCRs of adults and children shared the similar distribution pattern with higher values in north, southwest, central and eastern regions, especially in Shaanxi, Sichuan, and Jiangsu provinces, while lower risks prevailed in west and southeast areas (Supplementary Figure S2).
3.2.3 MC simulation and sensitivity analyses for probabilistic health risk
The probabilistic health risks of adults and children exposed to soil PAHs were simulated by MC and results are showed in Figure 4. The probabilistic ILCRs ranged from 2.34 E-11 to 5.61E-05 with a mean value of 6.51E-08for adults, and from 1.35E-12to 3.27E-07 with a mean value of 4.31E-09 for children exposed from soil PAHs through oral intake, dermal contact, and inhalation pathways. Overall, 0.53% of ILCRs exceeded 10−6 for adults, and no ILCRs exceeded 10−6 for children. The results were common with deterministic risk assessment, where potential health risks were posed by surface soil PAHs but risks were acceptable.
The sensitivity of variables to ILCRs for adults and children was analyzed together with MC (Figure 5). Among the parameters, EF (exposure frequency) contributed the most to the total variance of ILCRs, which accounted for 60.0% and 57.1% to ILCRs for adults and children, respectively. CEs contributed the second highest to total variance of ILCRs, followed by IRing, BW, IRinh, and SA. The total contributions of EF and CEs to ILCRs accounted for 98.5% and 94.6% of the sum of variance for adults and children, respectively. IRinh and SA contributed least to the total variance of ILCRs, confirming that inhalation is a negligible pathway for ILCRs exposure.
4 Discussion
The PAHs concentrations in soil varied greatly across China. The standard deviation (SD) of soil PAHs concentration was 77,455.85 μg kg−1 and coefficient of variation (CV) was 122.98, which reflects strong variety of soil PAHs concentration and huge anthropogenic activities influence (Zhang et al., 2007). According to a former review involving nearly 100 publications, the total concentrations of PAHs in China ranged from 65 to 23,603 μg kg−1 with a mean value of 2,802 μg kg−1 for urban soil, and 47% of soil were heavily contaminated (Yu et al., 2019). The mean concentration and heavily pollution rate reported by Yu et al. (2019) were both greater than the corresponding values in the present study, mainly because urban soil was influenced by intense anthropogenic activities such as industrial emission and heavy traffic. Sun et al. (2017) reviewed the concentration status of organic contaminants in agricultural soil of China, and their results revealed the concentrations of 16 PAHs ranged from “undetected” to 27,580 ng g−1, with a mean value of 772 ng g−1, which is slightly higher than the present study. Compared to other countries, the mean value of soil PAHs in China in the present study (629.83 μg kg−1) was higher than such locations as of urban soil in Viseu, Portugal (169 μg kg−1) (Cachada et al., 2012) and Belgrade, Serbia (375 μg kg−1) (Crnkovic et al., 2007) but was much lower than the mean concentration of PAHs in the UK (11,930 μg kg−1) (Morillo et al., 2007), urban traffic soils in Delhi, India (4,694 μg kg−1) (Agarwal, 2009), and an e-waste dismantling area in Accra, Ghana (5,627 μg kg−1) (Daso et al., 2016). Pollution levels of soil PAHs all around the world vary greatly, higher concentrations mostly being influenced by anthropogenic activities. Higher soil PAHs concentrations were generally detected in industrial areas and roadsides, while lower levels were found in residential areas (Zhang and Chen, 2017). The PAHs concentrations were also different from various types of sampling sites (Zhang and Chen, 2017). The PAHs concentrations in surface soil in Kunming showed higher values in industrial area and roadside, while lower in green space and education area (Lin et al., 2013). Peng et al. (2016) investigate the distribution of soil PAHs in Beijing with different land uses, results showed soil PAHs concentrations decreased by roadsides, green areas and agricultural areas.
Different migration and enrichment behaviors of PAHs were recorded with different ring numbers (Wild and Jones, 1995). Among the 16 PAHs congeners, FL and PYR had the highest concentrations, while ACE and ACY had the lowest. The finding is consistent with previous studies reported by Zhang and Chen. (2017). Li et al. (2016) analyzed the composition of PAHs in the Yangtze River Delta area, and reported that soil PAHs were dominated by four to five ring PAHs. They mainly related to low saturated vapor pressure, solubility and high Kow coefficient of HMW PAHs, and thus easily absorbed and enriched to soil particles, while LMW PAHs have lower molecular weight, and are easy to volatilize and be degraded by microorganisms (Liu et al., 2011; Dumanoglu et al., 2017). Moreover, PAHs compositions also differed between urban and rural areas. HMW PAHs in urban and suburban areas were usually higher than rural regions mainly due to the different energy types consumed (Wang et al., 2012).
The spatial distribution of soil PAHs in China is heterogenous, where concentrations of soil PAHs in north area was greater than the south. The result was in line with previous studies by Zhang et al. (2019), who reported higher concentrations of soil PAHs in north and northeast region, while lower concentrations in southwest and central areas. There are many factors influencing the concentration and distribution of soil PAHs, such as local economic development, land use, population density, soil organic matter, and traffic conditions (Gao et al., 2012; Wang et al., 2015). Higher concentrations of soil PAHs usually occurred in industry developed areas, and lower in tourist spots (Zhang et al., 2019). In addition to industry and economy development, climate also affect the concentrations of soil PAHs. The relatively low temperature, humidity, and weak ultraviolet radiation of north China decreased microbial and photolysis degradation of soil PAHs (Zhang and Chen, 2017). Identifying potential influencing factors for soil PAHs is essential to control and minimize adverse effects. Generally, the ratios of various PAHs congeners concentration, called diagnostic ratios, are often used to identify between petroleum and combustion sources (Yunker et al., 2002). Diagnostic ratios are used to differentiate origins, by composition and concentration of pollutants produced by different pollution sources (He et al., 2019). In the present study, the ratios of IND/(IND + BghiP) are ranged between 0.028 and 0.992, with a mean value of 0.472. Furthermore, ratios of FLU/(FLU + PYR) are ranged from 0.010 to 0.920 with the mean value of 0.333. As shown in Supplementary Figure S3, most of the soil samples were with the ratios of IND/(IND + BghiP) > 0.2, the ratios of FLU/(FLU + PYR) were scattered distributed between 0.2 and 1, suggesting that soil PAHs in China were mainly resulted from the mix combustion of biomass, petroleum, and coal (Cai et al., 2017). Identical results were also reported by Yu et al. (2019), both indicating coal, biomass, and oil combustion contributed more to soil PAHs distribution. Therefore, the huge amount of coal usage in north China contributes to higher soil PAHs concentrations possibly. The interpolation map of soil PAHs concentration showed the higher values in southwest and northwest area, especially Sichuan and Xinjiang Province, in contrast to the order of median values in different regions. It was possibly due to the limited number of soil samples collected in these areas and the high concentrations of soil PAHs in these samples. Besides, higher concentrations also occurred in Shaanxi and Shandong Province, which might be caused by massive consumption of coal and heavy traffic emissions (Ambade et al., 2021). The mean concentration of soil PAHs in Tibet is relatively low, and the sampling soils were all non-contaminated. As reported by Tao et al. (2011), PAHs in the Tibetan Plateau mostly come from long-range atmospheric transport.
Risk assessment is a valuable tool to characterize the adverse effects of soil pollutants, and quantify exposure risk, both for ecology and human health from soil PAHs, thus providing reference for remediation and management of soil PAHs pollution (Yang et al., 2019; Liu et al., 2021; Li et al., 2022). TEQBaP of soil PAHs assessment in this study shows that about half of the soil posed ecological risks, which was in accordance with a previous study that about 50% of the TEQBaP of sampling soils in Shanghai were above the safe level (Wang et al., 2013). Therefore, pollution of soil PAHs should not be ignored in China, remediation measures should be adopted in certain areas to guarantee ecological safety. Soil PAHs not only have direct harmful effects on the environment, but also pose potential risk to human health via various exposure pathways. The exposure of humans in contaminated soil usually occurs via three pathways, including oral intake, (e.g., incidental hand to mouth intake of soil particle when working or playing on the ground), inhalation and dermal contact with soil particles. As a result, quantifying the risk contribution and identifying the crucial exposure parameters can help provide effective risk control measures towards soil PAHs pollution (Peng et al., 2019). Both deterministic human health risk, where single point value of parameters were adopted, and probabilistic human health risk, where parameters were probability treated, were conducted to quantify the human health risk of soil PAHs in the present study. The results revealed that potential risks were posed to adults and children, but risks were acceptable. The spatial distribution of soil PAHs concentrations showed higher values in Urumqi, where the health risks were relatively low. This was mainly related to the limited number of soil samples collected in this area and the concentration difference of PAHs congeners that resulted in the low value of TEQBaP. When considering the variability of exposure parameters (EF, ED, BW, SA, IRInh, and IRIng), the percentages of ILCRs surpassing 10–6 for adults and children were both on the decline, confirming that human health risk would be overestimated when single values of parameters were adopted. Similar result was found by Jia et al. (2013), who assessed the risk of naphthalene and benzo(a) pyrene, and found the deterministic risk was four to six folds higher than probabilistic risk. Contrary to that, the deterministic risk assessment of PAHs using the most likely values of exposure parameters and PAHs concentrations were lower than that of the probabilistic risk obtained by MC method (Tong et al., 2018). Probabilistically treated key parameters can provide probability health risk, which is more reliable especially in vast regions, where PAHs concentrations and exposure parameters vary greatly. The sensitivity analysis demonstrated that EF and CEs were the dominant parameters contributing to the ILCRs. Therefore, reducing contact frequency and concentration of soil PAHs are the main and effective method to lower exposure risks.
5 Conclusion
The concentration, composition, spatial distribution, and risks of soil PAHs were systematically analyzed at the national scale based on a robust dataset compiled from literature review. The concentrations of ∑16PAHs ranged from “undetected” to 261 μg g−1, with a mean value of 0.63 μg g−1 and a median value of 17.94 μg g−1. According to the contamination classification criteria, about 21.74% of the soils were classified as heavily contaminated, 14.98% of the soils were moderately contaminated, 39.13% of the soils were weakly contaminated, and 24.15% of the soils were uncontaminated. Higher concentrations of soil PAHs were located in north and northeast China, and mainly derived from the mix combustion of coal and oil. The TEQBaP assessment indicated moderate ecological risks were posed by soil PAHs, where higher TEQBaP were mainly located in Sichuan and Jiangsu provinces. Human health risk assessment results indicated that potential health risks of adults and children were posed by soil PAHs, but risks were acceptable. Sensitivity analysis showed that exposure frequency and concentration of soil PAHs contributed most to the variability of the total risks. Therefore, reducing exposure frequency PAHs concentration from soil by taking remediation actions are effective pathways to protect environmental quality and human health.
6 Limitations and perspectives
We conducted a systematic overview of soil PAHs pollution status and assessed related potential ecological and human health risks at national scale. The data were extracted from previous published literature, and therefore, uncertainties exist inevitably to some extent. Firstly, the sampling time, sampling method, and laboratory analysis of soil PAHs in each publication varies greatly. Nevertheless, the concentrations of soil PAHs in each publication were compared at current time scale. Additionally, the mean concentrations of soil PAHs in each study were used to estimate and interpolate to depict a spatial distribution map. As a result, the actual concentration and induced risks of topsoil PAHs in China could have been underestimated to some extent. Besides, the total concentrations of soil PAHs were used to assess human health risk, while, bioaccessibility and bioavailability of soil PAHs are considered reliable in assessing real exposure risk as reported by Munir Hussain et al. (2011) and Dong et al. (2016). Such scenario could cause an overestimation of human health risks of soil PAHs. Results from this study could enhance the knowledge of soil PAHs contamination status, potential ecological and human health risks across China. However, further work is needed to overcome the existing limitations in this study. Consequently, it would be better to collect soil samples across China at the same time and analyze soil PAHs concentration by same procedure and method to obtain the actual concentration status of soil PAHs in the future. Besides, establishing a pollution classification criterion based on the actual soil properties of China is vital for further contamination assessment and remediation. In addition, assessing human health risk by using bioaccessibility and bioavailability of soil PAHs instead of the total concentrations can help obtain more reliable results. Overall, by combining soil samples, bioaccessibility and bioavailability experiments, probabilistic risk simulation will exert reliable results of soil PAHs contamination status and risk level.
Data availability statement
The raw data supporting the conclusion of this article will be made available by the authors, without undue reservation.
Author contributions
MH: Conceptualization, methodology, manuscript writing. YS: Software, data curation. ZZ: Data curation. SG: Software. HY: Data collection. KC: Data collection. XZ: Review and editing. YQ: Conceptualization and supervision.
Funding
This work was financially supported by the National Natural Science Foundation of China (42107021).
Conflict of interest
The authors declare that the research was conducted in the absence of any commercial or financial relationships that could be construed as a potential conflict of interest.
Publisher’s note
All claims expressed in this article are solely those of the authors and do not necessarily represent those of their affiliated organizations, or those of the publisher, the editors and the reviewers. Any product that may be evaluated in this article, or claim that may be made by its manufacturer, is not guaranteed or endorsed by the publisher.
Supplementary material
The Supplementary Material for this article can be found online at: https://www.frontiersin.org/articles/10.3389/fenvs.2023.1114027/full#supplementary-material
References
Agarwal, T. (2009). Concentration level, pattern and toxic potential of PAHs in traffic soil of Delhi, India. J. Hazard. Mat. 171, 894–900. doi:10.1016/j.jhazmat.2009.06.081
Alves, C. A., Vicente, A. M. P., Gomes, J., Nunes, T., Duarte, M., and Bandowe, B. A. M. (2016). Polycyclic aromatic hydrocarbons (PAHs) and their derivatives (oxygenated-PAHs, nitrated-PAHs and azaarenes) in size-fractionated particles emitted in an urban road tunnel. Atmos. Res. 180, 128–137. doi:10.1016/j.atmosres.2016.05.013
Ambade, B., Sethi, S. S., Giri, B., Biswas, J. K., and Bauddh, K. (2022). Characterization, behavior, and risk assessment of polycyclic aromatic hydrocarbons (pahs) in the estuarysediments. Bull. Environ. Contam. Toxicol. 108 (2), 243–252. doi:10.1007/s00128-021-03393-3
Ambade, B., and Sethi, S. S. (2021). Health risk assessment and characterization of polycyclic aromatic hydrocarbon from the hydrosphere. J. Hazard. Toxic. Radioact. Waste. 25 (2), 50200081–50200111. doi:10.1061/(asce)hz.2153-5515.0000586
Ambade, B., Sethi, S. S., Kurwadkar, S., Kumar, Amit., and Sankar, T. K. (2021). Toxicity and health risk assessment of polycyclic aromatic hydrocarbons in surface water, sediments and groundwater vulnerability in Damodar river basin. Groundw. Sustain. Dev. 13, 100553. doi:10.1016/j.gsd.2021.100553
Cachada, A., Pato, P., Rocha-Santos, T., daSilva, E. F., and Duarte, A. C. (2012). Levels, sources and potential human health risks of organic pollutants in urban soils. Sci. Total Environ. 430, 184–192. doi:10.1016/j.scitotenv.2012.04.075
Cai, C., Li, J. Y., Di, W., Wang, X. L., Tsang, D. C. W., Li, X. D., et al. (2017). Spatial distribution, emission source and health risk of parent PAHs and derivatives in surface soils from the Yangtze River Delta, eastern China. Chemosphere 178, 301–308. doi:10.1016/j.chemosphere.2017.03.057
Chen, Y., Zhang, J., Zhang, F., Li, F., and Zhou, M. (2017). Polycyclic aromatic hydrocarbons in farmland soils around main reservoirs of Jilin province, China: Occurrence, sources and potential human health risk. Environ. Geochem Hlth. 40, 791–802. doi:10.1007/s10653-017-0024-5
Crnkovic, D., Ristic, M., Jovanovic, A., and Antonovic, D. (2007). Levels of PAHs in the soils of Belgrade and its environs. Environ. Monit. Assess. 125, 75–83. doi:10.1007/s10661-006-9240-7
Daso, A. P., Akortia, E., and Okonkwo, J. O. (2016). Concentration profiles, source apportionment and risk assessment of polycyclic aromatic hydrocarbons (PAHs) in dumpsite soils from Agbogbloshie e-waste dismantling site, Accra, Ghana. Environ. Sci. Pollut. Res. 23 (11), 10883–10894. doi:10.1007/s11356-016-6311-3
Dong, Z., Yan, K., Liu, Y., Naidu, R., Duan, L., Wijayawardena, A., et al. (2016). A meta-analysis to correlate lead bioavailability and bioaccessibility and predict lead bioavailability. Environ. Int. 92-93, 139–145. doi:10.1016/j.envint.2016.04.009
Dumanoglu, Y., Gaga, E. O., Gungormus, E., Sofuoglu, S. C., and Odabasi, M. (2017). Spatial and seasonal variations, sources, air-soil exchange, and carcinogenic risk assessment for PAHs and PCBs in air and soil of Kutahya, Turkey, the province of thermal power plants. Sci. Total Environ. 580, 920–935. doi:10.1016/j.scitotenv.2016.12.040
Franco, S. S., Nardocci, A. C., and Günther., W. (2008). PAH biomarkers for human health risk assessment: A review of the state-of-the-art. Cad. Saúde Pública 24 (4), a569–s580. doi:10.1590/s0102-311x2008001600009
Gao, B., Guo, H., Wang, X. M., Zhao, X. Y., Ling, Z. H., Zhang, Z., et al. (2012). Polycyclic aromatic hydrocarbons in PM2.5 in guangzhou, southern China: Spatiotemporal patterns and emission sources. J. Hazard. Mat. 240, 78–87. doi:10.1016/j.jhazmat.2012.07.068
He, M. J., Yang, S. Y., Zhao, J., Collins, C., Xu, J. M., and Liu, X. M. (2019). Reduction in the exposure risk of farmer from e-waste recycling site following environmental policy adjustment: A regional scale view of PAHs in paddy fields. Environ. Int. 133, 105136. doi:10.1016/j.envint.2019.105136
Huang, D. Y., Liu, C. P., Li, F. B., Liu, T. X., Liu, C. S., Tao, L., et al. (2014). Profiles, sources, and transport of polycyclic aromatic hydrocarbons in soils affected by electronic waste recycling in Longtang, south China. Environ. Monit. Assess. 186 (6), 3351–3364. doi:10.1007/s10661-014-3622-z
Hussain, Z., Codling, E. E., Scheckel, K. G., and Chaney, R. L. (2011). In vitro and in vivo approaches for the measurement of oral bioavailability of lead (Pb) in contaminated soils: A review. Environ. Pollut. 159 (10), 2320–2327. doi:10.1016/j.envpol.2011.04.043
Jia, X., Xia, T., Zhong, M., and Liang, J. (2013). Comparing deterministic and probabilistic risk assessment at a coking plant site in China. Adv. Mat. Res. 610-613, 798–803. doi:10.4028/www.scientific.net/amr.610-613.798
Lestan, D. (2017). Novel chelant-based washing method for soil contaminated with pb and other metals: A pilot-scale study. Land. Degrad. Dev. 28, 2585–2595. doi:10.1002/ldr.2818
Leung, A. O. W., Cheung, K. C., and Wong, M. H. (2015). Spatial distribution of polycyclic aromatic hydrocarbons in soil, sediment, and combusted residue at an e-waste processing site in southeast China. Environ. Sci. Pollut. Res. 22 (12), 8786–8801. doi:10.1007/s11356-013-1465-8
Li, J., Wu, D., Xu, Y., Li, X., Wang, X., Zeng, C., et al. (2016). Recent distribution and sources of polycyclic aromatic hydrocarbons in surface soils from Yangtze River Delta. Environ. Sci. 37, 253–261. doi:10.13227/j.hjkx.2016.01.033
Li, X. N., Yi, S. Y., Cundy, A. B., and Chen, W. P. (2022). Sustainable decision-making for contaminated site risk management: A decision tree model using machine learning algorithms. J. Clean. Prod. 371, 133612. doi:10.1016/j.jclepro.2022.133612
Lin, C., Liu, J., Wang, R., Wang, Y., Huang, B., and Pan, X. (2013). Polycyclic aromatic hydrocarbons in surface soils of kunming, China: Concentrations, distribution, sources, and potential risk. J. Soil Contam. 22, 753–766. doi:10.1080/15320383.2013.768201
Liu, G. Q., Yu, L. L., Li, J., Liu, X. A., and Zhang, G. (2011). PAHs in soils and estimated air soil exchange in the Pearl River Delta, South China. Environ. Monit. Assess. 173, 861–870. doi:10.1007/s10661-010-1429-0
Liu, J., Wang, Y., Liu, X., and Xu, J. (2021). Occurrence and health risks of heavy metals in plastic-shed soils and vegetables across China. Agr. Ecosyst. Environ. 321, 107632. doi:10.1016/j.agee.2021.107632
Lu, Y. L., Song, S., Wang, R. S., Liu, Z. Y., Meng, J., Sweetman, A. J., et al. (2015). Impacts of soil and water pollution on food safety and health risks in China. Environ. Int. 77 (1), 5–15. doi:10.1016/j.envint.2014.12.010
Maliszewska-Kordybach, B. (1996). Polycyclic aromatic hydrocarbons in agricultural soils in Poland: Preliminary proposals for criteria to evaluate the level of soil contamination. Appl. Geochem. 11, 121–127. doi:10.1016/0883-2927(95)00076-3
Ministry of Environment Protection and Ministry of Land Resources of the People's Republic of China (2014). Nationwide soil pollution survey report. Avaliable At: http://www.zhb.gov.cn/gkml/hbb/qt/201404/t20140417_270670.htm.
Morillo, E., Romero, A. S., Maqueda, C., Madrid, L., Ajmone-Marsan, F., Grcman, H., et al. (2007). Soil pollution by PAHs in urban soils: A comparison of three European cities. J. Environ. Monit. 9, 1001–1008. doi:10.1039/b705955h
Niu, L., Yang, F., Xu, C., Yang, H., and Liu, W. (2013). Status of metal accumulation in farmland soils across China: From distribution to risk assessment. Environ. Pollut. 176 (5), 55–62. doi:10.1016/j.envpol.2013.01.019
Peng, C., Wang, M., Zhao, Y., and Chen, W. (2016). Distribution and risks of polycyclic aromatic hydrocarbons in suburban and rural soils of beijing with various land uses. Environ. Monit. Assess. 188 (3), 1–12. doi:10.1007/s10661-016-5156-z
Peng, H., Chen, Y., Weng, L., Ma, J., Ma, Y., Li, Y., et al. (2019). Comparisons of heavy metal input inventory in agricultural soils in north and south China: A review. Sci. Total Environ. 660, 776–786. doi:10.1016/j.scitotenv.2019.01.066
Salazar, M. J., Rodriguez, J. H., Leonardo, N. G., and Pignata, M. L. (2012). Effects of heavy metal concentrations (Cd, Zn and Pb) in agricultural soils near different emission sources on quality, accumulation and food safety in soybean (glycine max (L.) merrill). J. Hazard. Mat. 10, 244–253. doi:10.1016/j.jhazmat.2012.07.026
Samburova, V., Zielinska, B., and Khlystov, A. (2017). Do 16 polycyclic aromatic hydrocarbons represent PAH air toxicity? Toxics 5 (3), 17. doi:10.3390/toxics5030017
Sun, J., Pan, L., Tsang, D. C. W., Zhan, Y., and Li, X. (2017). Organic contamination and remediation in the agricultural soils of China: A critical review. Sci. Total Environ. 615, 724–740. doi:10.1016/j.scitotenv.2017.09.271
Tao, S., Wang, W., Liu, W., Zuo, Q., Wang, X., Wang, R., et al. (2011). Polycyclic aromatic hydrocarbons and organochlorine pesticides in surface soils from the Qinghai-Tibetan plateau. J. Environ. Monit. 13, 175–181. doi:10.1039/c0em00298d
Tong, R., Yang, X., Su, H., Pan, Y., Zhang, Q., Wang, J., et al. (2018). Levels, sources and probabilistic health risks of polycyclic aromatic hydrocarbons in the agricultural soils from sites neighboring suburban industries in Shanghai. Sci. Total Environ. 616-617, 1365–1373. doi:10.1016/j.scitotenv.2017.10.179
USEPA (1989). “Risk assessment guidance for superfund,” in Human health evaluation manual (Part A) (Washington, DC: US Environmental Protection Agency, Offifice of Emergency and Remedial Response).
USEPA (2009a). Risk assessment guidance for superfund (RAGS), volume I: Human health evaluation manual (Part E, supplemental guidance for dermal risk assessment) interim. Washington, DC: EPA.
USEPA (2009b). Risk assessment guidance for superfund volume I: Human health evaluation manual (Part F, supplemental guidance for inhalation risk assessment). Washington, DC: EPA.
USEPA (2016). Risk assessment guidance for superfund, Volume 1, Human health evaluation manual (Part B, Development of risk-based preliminary remediation goals). Washington, DC: EPA. Avaliable At: https://www.epa.gov/expobox_1034 (Accessed February 5, 2017).
Wang, C., Wang, X., Gong, P., and Yao, T. (2014). Polycyclic aromatic hydrocarbons in surface soil across the Tibetan plateau: Spatial distribution, source and air-soil exchange. Environ. Pollut. 184, 138–144. doi:10.1016/j.envpol.2013.08.029
Wang, L., Xu, X., and Lu, X. (2015). Composition, source and potential risk of polycyclic aromatic hydrocarbons (PAHs) in vegetable soil from the suburbs of xianyang city, northwest China: A case study. Environ. Earth Sci. 75, 56–13. doi:10.1007/s12665-015-4853-1
Wang, X. T., Miao, Y., Zhang, Y., Li, Y. C., Wu, M. H., and Yu, G. (2013). Polycyclic aromatic hydrocarbons (PAHs) in urban soils of the mega city Shanghai: Occurrence, source apportionment and potential human health risk. Sci. Total Environ. 447, 80–89. doi:10.1016/j.scitotenv.2012.12.086
Wang, Z., Yang, P., Wang, Y., and Ma, X. (2012). Urban fractionation of polycyclic aromatic hydrocarbons from Dalian soils. Environ. Chem. Lett. 10, 183–187. doi:10.1007/s10311-011-0341-3
Wild, S. R., and Jones, K. C. (1995). Polynuclear aromatic hydrocarbons in the United Kingdom environment: A preliminary source inventory and budget. Environ. Pollut. 88, 91–108. doi:10.1016/0269-7491(95)91052-M
Wolska, L., Mechlińska, A., Rogowska, J., and Namieśnik, J. (2012). Sources and fate of PAHs and PCBs in the marine environment. Crit. Rev. Environ. Sci. Technol. 42, 1172–1189. doi:10.1080/10643389.2011.556546
Yang, J., Sun, P., Zhang, X., Wei, X. Y., Huang, Y., Du, W. N., et al. (2020). Source apportionment of pahs in roadside agricultural soils of a megacity using positive matrix factorization receptor model and compound-specific carbon isotope analysis. J. Hazard Mate 403, 123592. doi:10.1016/j.jhazmat.2020.123592
Yang, S. Y., Zhao, J. A., Scott, X., Chris, C., Xu, J. M., and liu, X. M. (2019). Status assessment and probabilistic health risk modeling of metals accumulation in agriculture soils across China: A synthesis. Environ. Int. 128, 165–174. doi:10.1016/j.envint.2019.04.044
Yang, W., Lang, Y., and Li, G. (2014). Cancer risk of polycyclic aromatic hydrocarbons (PAHs) in the soils from Jiaozhou Bay Wetland. Chemosphere 112, 289–295. doi:10.1016/j.chemosphere.2014.04.074
Yu, H., Li, T., Liu, Y., and Ma, L. (2019). Spatial distribution of polycyclic aromatic hydrocarbon contamination in urban soil of China. Chemosphere 230, 498–509. doi:10.1016/j.chemosphere.2019.05.006
Yunker, M. B., Macdonald, R. W., Vingarzan, R., Mitchell, R. H., Goyette, D., and Sylvestre, S. (2002). PAHs in the fraser river basin: A critical appraisal of PAH ratios as indicators of PAH source and composition. Org. Geochem. 33, 489–515. doi:10.1016/S0146-6380(02)00002-5
Zhang, P., and Chen, Y. G. (2017). Polycyclic aromatic hydrocarbons contamination in surface soil of China: A review. Sci. Total Environ. 605-606, 1011–1020. doi:10.1016/j.scitotenv.2017.06.247
Zhang, X. Y., Sui, Y. Y., Zhang, X. D., Meng, K., and Herbert, S. J. (2007). Spatial variability of nutrient properties in black soil of northeast China. Pedosphere 17, 19–29. doi:10.1016/S1002-0160(07)60003-4
Zhang, Y., Peng, C., Guo, Z., Xiao, X., and Xiao, R. (2019). Polycyclic aromatic hydrocarbons in urban soils of China: Distribution, influencing factors, health risk and regression prediction. Environ. Pollut. 254, 112930. doi:10.1016/j.envpol.2019.07.098
Keywords: polycyclic aromatic hydrocarbons, risk assessment, spatial distribution, Monte Carlo, China
Citation: He M, Shangguan Y, Zhou Z, Guo S, Yu H, Chen K, Zeng X and Qin Y (2023) Status assessment and probabilistic health risk modeling of polycyclic aromatic hydrocarbons (PAHs) in surface soil across China. Front. Environ. Sci. 11:1114027. doi: 10.3389/fenvs.2023.1114027
Received: 13 December 2022; Accepted: 19 January 2023;
Published: 01 February 2023.
Edited by:
Nsikak U. Benson, Université Claude Bernard Lyon 1, FranceReviewed by:
Balram Ambade, National Institute of Technology, Jamshedpur, IndiaHui Wang, Shenyang University, China
Copyright © 2023 He, Shangguan, Zhou, Guo, Yu, Chen, Zeng and Qin. This is an open-access article distributed under the terms of the Creative Commons Attribution License (CC BY). The use, distribution or reproduction in other forums is permitted, provided the original author(s) and the copyright owner(s) are credited and that the original publication in this journal is cited, in accordance with accepted academic practice. No use, distribution or reproduction is permitted which does not comply with these terms.
*Correspondence: Yusheng Qin, c2hlbmd5dXFAMTI2LmNvbQ==