- 1Anthropology Department, University of California Santa Cruz, Santa Cruz, CA, United States
- 2Department of Anthropology, University College London, London, United Kingdom
- 3Department of Human Origins, Max Planck Institute for Evolutionary Anthropology, Leipzig, Germany
- 4School of Biological and Environmental Sciences, Liverpool John Moores University, Liverpool, United Kingdom
Introduction: Investigation into chimpanzee termite-fishing behavior has provided invaluable insights into the evolution of animal tool use and culture. However, research to date often overlooks how the ecology of termites may shape the nature of this predator–prey interaction. Though anecdotal links between meteorological factors, termite dispersal flights and the ability of chimpanzees to termite-fish have been made, these relationships were never empirically tested. In this study, we examined if and how the reproductive ecology of mound building termites (Macrotermes subhyalinus) affects the availability of this resource to termite-fishing chimpanzees in the Issa Valley, Tanzania.
Methods: We utilized in-person termite-fishing experiments, remote camera trap footage, as well as meteorological data to model both the availability of termite prey across seasons for chimpanzees as well as the timing of dispersal flights of the termite reproductive caste (alates). Additionally, we describe the predator diversity that characterizes alate swarming events during their seasonal dispersal flights.
Results: We identify strong relationships between meteorological factors, Macrotermes alate dispersal flights and vulnerability of the colony to predation. As rainfall accumulates, Macrotermes become more abundant in their flight holes and are thus more vulnerable to researcher termite-fishing experiments. This increased accessibility to Macrotermes continues until alate dispersal flights which we also find are linked to cumulative rainfall as well as negatively correlated to wind speed.
Discussion: This type of baseline data on Macrotermes ecology is fundamental when forming hypotheses and designing studies into topics such as chimpanzee culture, tool-use, and foraging cognition.
Introduction
In 1960, Jane Goodall observed chimpanzees (Pan troglodytes) at Gombe stream in western Tanzania construct tools out of vegetation to use them to “fish” for termites (van Lawick-Goodall, 1968). As termite-fishing was the first evidence of non-human animals making and using tools in the wild, much research has since been devoted towards studying this behavior, particularly cultural variations in technique and learning, across chimpanzee populations (Boesch et al., 2020). Researchers assign much significance to the study of chimpanzee termite-fishing with its implications for primate cognition, culture, tool-use, and even hominin evolution. Additionally, paleoanthropologists have used chimpanzee models of termite-fishing (Lesnik, 2014) along with evidence from stable-isotope analysis (Sponheimer et al., 2005) and experimental tool use-wear analysis (Backwell and d’Errico, 2001; Gardner, 2019) to hypothesize that termite-fishing was a foraging strategy employed by hominins (Paranthropus robustus). However, the influence of termite prey behavior and ecology within this predator–prey interaction is often overlooked.
In fact, the reproductive life cycle and ecology of termites may represent a significant obstacle for chimpanzees to utilize this nutritious food resource. Despite the diversity of termite genera present in chimpanzee habitats, chimpanzees primarily feed on the large-bodied genus of Macrotermes (Lesnik, 2014). Macrotermes (Macrotermitinae) is a genus of fungus-cultivating termites distributed across Africa and South-East Asia. Macrotermes are considered ecosystem engineers in part for their ability to concentrate nutrient-rich soils during the construction of their large, epigeal mound structures that can rise several meters out of the earth (Dangerfield et al., 1998; Jouquet et al., 2006; Abe et al., 2009). In mature colonies, the winged-reproductive caste, known as alates, annually disperse en mass from the epigeal surfaces of these mounds to establish new colonies. Macrotermes colonies spend much of their time deep underground to avoid desiccation and predation. In preparation for seasonal dispersal flights, however, the worker castes construct passageways between the nest interior and the surface of the mound and open flight holes for the alate caste to disperse (Darlington, 1986; Mitchell, 2007). These passageways are sealed off by freshly worked soil patches constructed by the worker caste in order to minimize invasions into the colony before and after flights.
In her pioneering work with chimpanzees at Gombe, van Lawick-Goodall (1968) wrote about the connection between Macrotermes seasonal dispersal flights at the onset of the rainy season and the ability of chimpanzees to effectively termite-fish. However, this initial field observation was never empirically tested. McGrew and colleagues (McGrew et al., 1979) cited these early observations and found a correlation between monthly rainfall patterns and the quantity of chimpanzee tools found on termite mounds at Mt. Assirik in Senegal and Gombe (McGrew et al., 1979). The relationship between the onset of the rainy season and the onset of the presence of termite-fishing tools suggested a relationship either with termite accessibility or Macrotermes annual reproductive cycle, or both. However, some chimpanzee populations continue to termite-fish for Macrotermes despite seasonal variations in rainfall patterns (Sanz and Morgan, 2013 for review). To date, though, we lack data on termite presence and accessibility throughout the annual cycle (McBeath and McGrew, 1982; Collins and McGrew, 1985; McGrew and Collins, 1985; Collins and McGrew, 1987; Koops et al., 2013). Termite dispersal flights are still occasionally referenced as a potential contributing factor to seasonal patterns of termite-fishing (Sanz et al., 2004; Sommer et al., 2017). Yet the relationship between the seasonal reproductive cycle of Macrotermes and Macrotermes-fishing by chimpanzees remains poorly understood. Such data are useful for primatologists seeking to understand the availability of insect food resources similar to how phenology data are collected on important fruiting trees to provide metrics of fruit availability (Marshall and Wich, 2013). Macrotermes represent key sources of protein, fat, and minerals to chimpanzees that feed on them (Deblauwe and Janssens, 2008; O’Malley and Power, 2014). Thus, tracking fluctuations in the availability of this resource across seasons is a key and yet overlooked measure of resource availability in chimpanzee habitats. Additionally, identifying the environmental conditions that lead to alate dispersal flights and the corresponding influx of Macrotermes soldiers near mound surfaces may clarify mechanisms that contribute to observed seasonality of chimpanzee termite-fishing in some communities (Koops et al., 2013; Sanz and Morgan, 2013).
By documenting changes in availability of Macrotermes to chimpanzee exploitation, we can more accurately test hypotheses on tool-use variation between chimpanzee communities. The particular driving factors of the emergence, maintenance, and intraspecific variation of tool use behaviors between great ape populations are uncertain (Fox et al., 1999; Sanz and Morgan, 2013). Fox and colleagues (Fox et al., 1999) have previously described three competing, but non-mutually exclusive hypotheses: the “necessity” hypothesis, the “opportunity” hypothesis, and the “limited-invention” hypothesis. That termite-fishing is practiced year-round by Fongoli chimpanzees is inferred to be an adaption to a lack of alternative protein sources (Bogart and Pruetz, 2011). This interpretation of the seasonality and intensity of this tool-use behavior within the Fongoli population supports the “necessity” hypothesis (Sanz and Morgan, 2013). However, to test which hypotheses best explain seasonality in termite-fishing in any chimpanzee community, we must first assess the extent to which, if any, Macrotermes is an ephemeral resource for a particular community of termite-fishing chimpanzees.
Termite alate dispersal flights are significant ecosystem events not only due to the alates themselves serving as a critical nutrient pulse for many animal species (Silva et al., 2021), but also due to the increased activity of the other castes close to the epigeal mound surface before and after dispersal flights, which leave them vulnerable to predation from termite-fishing chimpanzees. The ability to reliably track and observe alate dispersal patterns can therefore reveal valuable insight not only to entomologists but to ecologists, and primatologists in particular, who are interested in the availability of this food resource to higher trophic levels. Identifying which, if any, meteorological factors predict alate dispersal flights as well as the associated opening of flight holes atop mounds, is one avenue for understanding the availability of this resource. A few studies have examined the meteorological factors influencing Macrotermes spp. dispersal flights. Mitchell (2008) observed M. natalensis dispersal flights from 11 swarming seasons in Pretoria, South Africa. Rainfall was emphasized as the most important factor as a minimum 5mm threshold had to be met after a dry season for initial flights to begin. Additionally, flights occurred between 17–19°C, relative humidity range between 39%– 90%, and also occurred primarily during windless conditions (Mitchell, 2008). Neoh and Lee (2009) later reported that meteorological conditions also affected the dispersal flight timing of two sympatric Macrotermes species (M. gilvus and M. carbonarius) in Penang Island, Malaysia. Although both species dispersed during windless conditions, the authors emphasized interspecific differences in the timing of dispersal flights. For instance, M. gilvus flights generally occurred during the warmer and more humid months (March–September), before dawn, and lasted for hours. By contrast, M. carbonarius flights tended to occur between November–January, more often at dusk, and lasting for only a few minutes. The authors suggest these temporal niches reduce competition between these sympatric species as foraging intensity is highest during the period of alate production (Schuurman, 2006; Neoh and Lee, 2009). These studies of Macrotermes dispersal flights suggest the ecological shifts that trigger flights vary by region and species, and may commonly require windless conditions.
Dispersal flights are remarkable ecological phenomenon for entomologists, as well for those interested in the broader implications to the wider food web. This influx of nutrients may contribute to the increased relative abundance of some mammal species around Macrotermes mounds (Fleming and Loveridge, 2003; D’Ammando et al., 2022). Predator abundance may also play a role in the timing of alate dispersal flights (Nutting, 1969; Neoh and Lee, 2009). Thus, accounting for the diversity and abundance of predators during alate dispersal flights may positively contribute to an understanding of how Macrotermes contribute to the food web as well as the biotic pressures that may affect flight timing.
Reports on the diversity of predators at Macrotermes dispersal flights have been constrained by the difficulties associated with reliably observing these ephemeral events as well as possible bias introduced by human observer presence. Observations in person can take several months, and the synchrony of dispersal flights within a location makes it difficult to observe multiple dispersal flights in a season without a large team of researchers. Whilst trapping alates may be ideal for studies focused solely on recording the density and intensity of alate dispersion (Silva et al., 2021), insect trapping methods are limited in their ability to precisely chronicle dispersal flights by hour of day or observe surrounding ecological phenomena such as predation events. In the most detailed published report on predation events observed in person during Macrotermes flights, researchers recorded in person M. subhyalinus dispersal flights across two years in Kenya. The authors were able to identify multiple species including 26 bird, 3 reptiles, and 1 non-human mammal as predators during these events (Dial and Vaughan, 1987). The overwhelming bias of avian predators and relative paucity of terrestrial ones may be an artifact of researcher presence during these events, as larger bodied, terrestrial species may be more wary of approaching a termite mound with humans in the area (Lasky and Bombaci, 2023). Additionally, daytime observations of dispersal flights are overwhelmingly biased towards noting diurnal predators whereas nocturnal predators may also feed on these swarming insects. New approaches are required to observe the diversity of vertebrate predators that exploit alate dispersal flights. Remote observations made via camera-trap footage offer a unique opportunity to overcome the many limitations of human observers.
Remote camera-trapping (CT) is an important methodology for non-invasively and cost-effectively collecting ecological data. In a 2015 review of CT studies, most studies focused on mammals (94.7%), and on carnivores (64.7%) in particular (Burton et al., 2015). Recently, however, researchers have demonstrated the use of CTs to observe insect–plant interactions (Steen, 2017; McElveen and Meyer, 2020; Pegoraro et al., 2020; Naqvi et al., 2022). Naqvi and colleagues showed that both automatic and scheduled recordings significantly outperformed human observers in collecting data on insect pollinator interactions (Naqvi et al., 2022). Additionally, CT in agricultural contexts may help the monitoring of pests (Lima et al., 2020; Preti et al., 2021). Despite these examples, CT is mostly constrained in its ability to monitor insect ecology due to the size of study organisms unable to trigger camera recordings. However, CT might still prove useful to ecologists interested in observing and chronicling termite alate dispersal flights due to the large abundance of swarming insects as well as the presence of vertebrate predators able to trigger the cameras. CT could offer a chronologically precise and resource-efficient alternative to human observers for monitoring dispersal flights events simultaneously across multiple termite mounds. Additionally, CT may be able to record predator interactions during alate dispersal flights that would otherwise remain unobserved.
In this study, we utilized multi-year CT footage, meteorological data, and termite-fishing experiments at 13 M. subhyalinus mounds in a miombo woodland mosaic ecosystem in western Tanzania. First, we employed observational and termite-fishing experiment data (emulating chimpanzee termite-fishing behavior) on termite activity at flight holes to monitor the seasonal availability of this food resource for the termite-fishing chimpanzee population in the area (Stewart and Piel, 2014; Almeida-Warren et al., 2017). Second, we sought to identify the meteorological predictors of M. subhyalinus alate dispersal flights by comparing the onset of dispersal flights to meteorological data recorded on site. Finally, we documented the diversity of fauna that predate on this highly-seasonal but abundant food resource. In order to understand the role of alate dispersal events on chimpanzee termite-fishing behavior, we first need to understand the relationship between ecological conditions and both the ability for researchers to extract termites and alate dispersal events. More specifically, we sought to test the following hypotheses and predictions:
- The abundance of Macrotermes occupying flight holes and available to termite-fishing chimpanzees is highly seasonal given chimpanzee termite-fishing is highly seasonal at the Issa Valley. We predicted that termites within flight holes would increase during the onset of heavy rains after the dry season and decrease during the late wet season after which alates no longer disperse.
- The occurrence of Macrotermes dispersal flights in the Issa Valley are significantly correlated to meteorological conditions. We predicted that dispersal flights would occur more after the first 100mm of rainfall and during both windless and rainless conditions and that flights would precipitously decrease as rain continues to accumulate into the late wet season.
- Multiple species of Issa vertebrates will feed on and compete for Macrotermes alates during their annual dispersal flights, as had been described in previous studies of Macrotermes alate dispersal events.
Methods
Study site
The Issa Valley is a ca. 85 km2 mosaic landscape located in the Tongwe West Forest Reserve in western Tanzania. The landscape consists of steep valleys and plateaus ranging from 900–1,800 meters above sea level. The mosaic of habitat types in the Issa Valley include miombo woodlands, swamps, grasslands, rocky outcrops and patches of riverine forests (Drummond-Clarke et al., 2022). The site is continuously monitored by a team of researchers. Issa Valley has marked seasonality with a distinct six month dry season (~May–October) in which rainfall is less than 100 mm/month and a wet season (~November–April) that accounts for most of the annual rainfall averaging 1,245 mm per year (Piel et al., 2017). Macrotermes mounds are abundant in both miombo and riverine forests throughout the site. Thirteen of the fourteen colonies of the species M. subhyalinus in this study were taxonomically identified by SP with support by Rudolf Scheffrahn at Fort Lauderdale Research & Education Center (Phillips et al., 2021).
Experimental termite-fishing
We collected observational data of Macrotermes activity at the surface of all study mounds in order to monitor termite surface activity across multiple seasons and years (2018–2022). Fourteen termite mounds were visited twice a month between October 2018 and January 2022 to collect data on the flight hole activity (FHA) of M. subhyalinus colonies. We define FHA by our ability to collect termites from alate flight holes at the epigeal surface of a mound by copying chimpanzee termite-fishing techniques in a controlled experimental setting. The term FHA is distinct to the term surface activity, which has been described in the literature as any Macrotermes activity (e.g., foraging) by the colony above the surface but not necessarily at the epigeal mound. For our systematic termite-fishing experiments, we constructed vegetative tools in the style of Issa chimpanzees (Stewart and Piel, 2014; Almeida-Warren et al., 2017) and then attempted to emulate Issa chimpanzee termite-fishing technique and insert these tools at 30 cm deep for exactly 10 seconds into 5 passageways atop of the mound for 5 trials during each mound visit. This often required first gently removing freshly laid soil patches to expose flight holes. We then noted if termites bit into the tool and were retrieved. FHA was defined as the ability of experimenters to retrieve termite prey in a trial. As a previous study suggested savanna termite activity generally increases with the rainy season (Davies et al., 2015), we increased our FHA sampling effort during the periods of October 2018–January 2019 as well as between August 2018 and January 2022 during which we visited each mound twice a week to conduct our termite-fishing experiment. For subsequent statistical analyses we included FHA as the overall proportion of successful experimental fishing trials, binned by week, as a response variable.
Camera traps monitoring swarming and predation events
Between 2016 and 2019, we deployed motion-triggered cameras (Bushnell Trophy Cam HD Aggressor, Bushnell CORE) in front of 13 Macrotermes mounds in an area of ca. 5 km2 in the Issa Valley. Seven of these cameras were in place and recorded continuously since 2016. In October 2018, six more cameras were placed in front of additional termite mounds based on direct observations of recent Macrotermes activity near the mound surface. All cameras were placed ca. 1m high on nearby trees and set to record in 60s intervals 24 hours a day with 1-s time intervals between videos every time motion is detected. SD cards and batteries were replaced twice monthly. All resulting camera trap videos (n = 42,384) were assessed by the authors and/or volunteer undergraduate students in the PEMA lab at University of California Santa Cruz (see Acknowledgements). We recorded all instances of alate dispersal flights detectable by numerous large (up to 45 mm) (Scheffrahn, 2008) winged insects departing in a vertical trajectory from the mound surface. In parallel to flight departure, we could often observe numerous alates moving around on the mound surface itself. These dispersal flight detections were often, but not always, associated with the presence of vertebrate predators who triggered the cameras. Any video in which one or more alate could be observed emerging from a flight hole a top the mound was considered as an alate dispersal flight for analysis (see Supplementary Video 1). We also identified the predator type investigating and feeding on dispersing termites (see Supplementary Video 2) and differentiated diurnal (7:00h to 18:00h) from nocturnal (18:00h to 7:00h) predation events. For subsequent statistical analyses we included the presence or absence of the start of an alate swarm at a given hour as a binomial response variable.
Meteorological data
We collected continuous weather data to observe whether meteorological factors significantly predicted the onset of Macrotermes dispersal flights. An environmental weather logger (HOBO RX3000 Remote Monitoring Station) was deployed at the central field camp at Issa Valley to continuously collect meteorological data. The station tracked daily precipitation, barometric pressure, temperature, wind speed, gust speed, and relative humidity in five-minute intervals year-round since 2017. For the year 2016, only precipitation data were gathered via the environmental weather logger.
Data analysis
We performed all statistical data analyses and data handling in R, version 3.4.4 (R Core Team, 2018). We first explored how precipitation predicts the presence of termites at flight holes (FHA) in our sample of Macrotermes mounds. We considered the variable of precipitation in two ways. First, we calculated the rolling weekly average of daily total precipitation (hereafter weekly rainfall) to consider how our response FHA is influenced by ongoing precipitation. Second, we calculated the total accumulated precipitation since the onset of the previous seasonal dry spell (hereafter cumulative rainfall) to address whether a rainfall threshold triggers termite activity. We included a quadratic term for cumulative rainfall in our model because we predicted that flight hole activity would increase as rainfall accumulates initially but then decline as rain continues to accumulate into the second half of the wet season as most mounds disperse their annual brood of alates. Annual seasonal dry spells were identified as the first period of 30 or more days without daily rainfall ≥ 0.3 mm. We tested for the Pearson’s correlation coefficient to observe to what degree these two rainfall variables correlated and whether one had to be dismissed for this analysis.
To model the influence of precipitation predictors on FHA, we then built a generalized linear model (GLM) with binomial distribution and logit link function by using the glmer function in R (lme4 package, Bates et al., 2015). The response variable FHA consisted of a two-column matrix with weekly counts of successes and failures during fishing-experiments attempting to collect termites from flight holes. As we did not observe a significant correlation between our predictors of weekly rainfall and cumulative rainfall, both terms were included as covariates into the model. The covariates were log transformed to reduce the skewness in their distributions. All data used in this model can be found in Supplementary Table S1. We tested for collinearity in our model by finding the variance inflation factors (VIF) in our model and we found values around one.
In a second model, we investigated whether some of the environmental predictors described by Mitchell (2008) were associated with alate dispersal flights (ADF). In particular we considered wind speed, atmospheric pressure, temperature, relative humidity, and precipitation on the outcome variable ADF similar to Mitchell (2008). Additionally, we included cumulative rainfall since the onset of latest dry spell (see above) as another predictor variable. Again, we included a quadratic term for cumulative rainfall to account for the predicted quadratic nature of our model response. Each of these meteorological variables were included as a mean value of the hourly data preceding our variable ADF. For our analysis of alate dispersal flights, we constructed a data frame including all instances when a dispersal flight was documented and the corresponding meteorological data from the preceding hour of the start time of each event. Additionally, we randomly selected one hour from each day in which no dispersal event was observed along with the corresponding meteorological variables for that hour. Since alate dispersal flights are rare events and thus can lead to non-converging models, we ran a modified GLM with a binomial distribution and logit link function using the logistf function in the logistf package (“logistf: Firth’s Bias-Reduced Logistic Regression”) with the argument “flic=TRUE”. This function and argument reduce bias in logistic regression of rare events by a post-hoc adjustment of the intercept (Puhr et al., 2017). We systematically checked for correlations between all predictor variables. We found that relative humidity correlated with temperature and precipitation. We chose to exclude relative humidity from our model as we judged that Macrotermes colonies are more likely to respond to changes in temperature or direct rainfall rather than changes in relative humidity alone. The final covariates in this model were all zero-transformed and included the following: wind speed, temperature, precipitation, barometric pressure, cumulative rainfall, as well as a quadratic term for the cumulative rainfall. Our response variable was a binomial (0 = no, 1 = yes) whether a dispersal flight began at the hour in our data frame. All data used in this model can be found in Supplementary Table S2. We tested the VIF in our model and found all values around one.
Results
Experimental termite-fishing
We conducted 1,924 termite-fishing trials from 2018–2022 to determine when Macrotermes occupied termite mound flight holes. Of these experiments, 363 were successful in extracting termites occupying flight holes. There was only one mound in which experimenters were never able to extract termites during the study period. The results of our logistic regression analysis on FHA suggested that both weekly rainfall and cumulative rainfall had significant effects on the weekly proportion of FHA (Table 1). FHA significantly increased as weekly rainfall increased (Log-odds = 0.12, p = .006) (see Figure 1). FHA was also significantly affected by cumulative rainfall (Log-odds = −0.85, p < .001). In this case, the proportion of successful experimental trials increased as rainfall accumulated until 200 mm, but decreased sharply thereafter (see Figure 2).
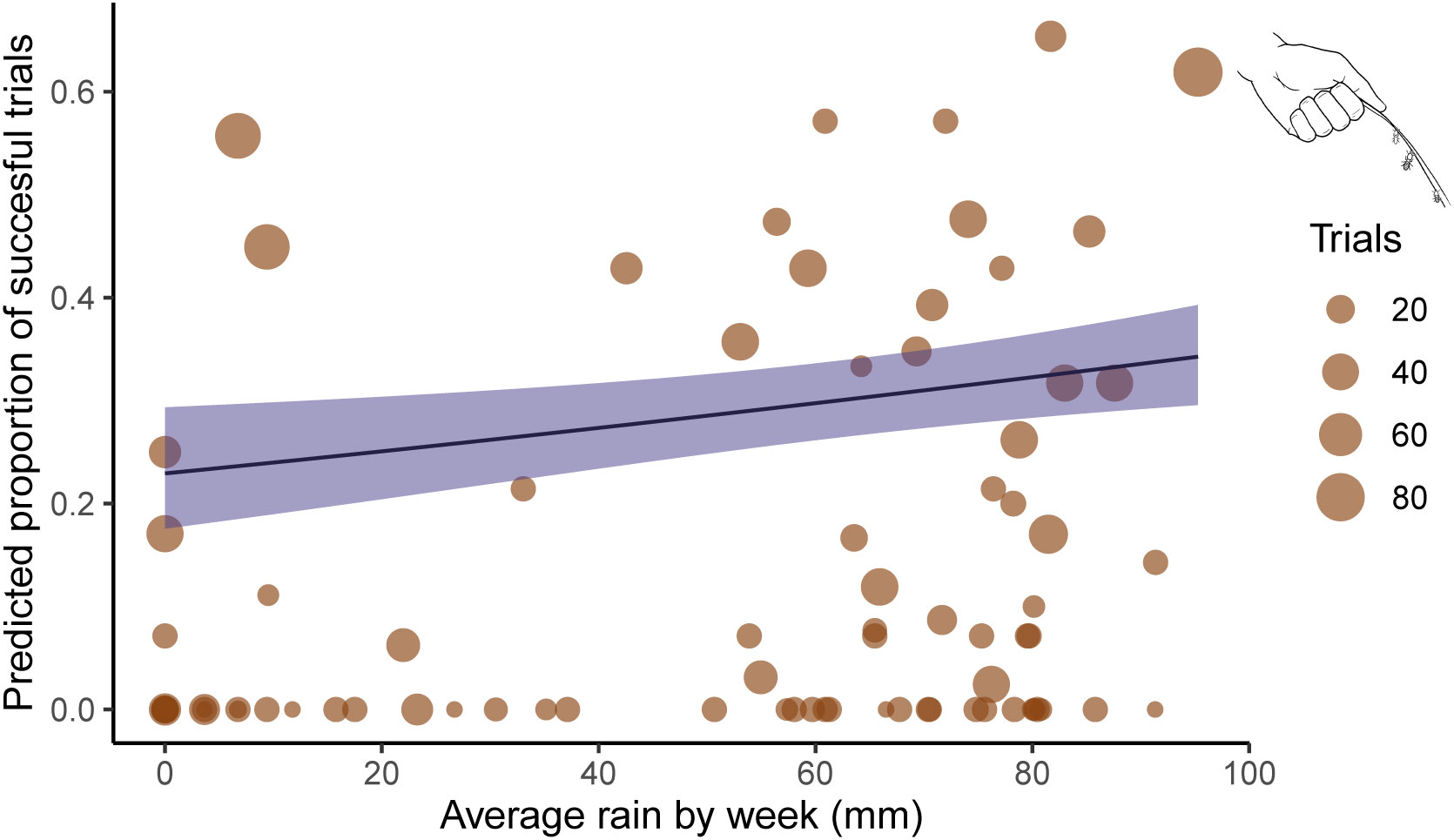
Figure 1 Simulation of mean weekly precipitation against proportion of termite-fishing success at flight holes. Each brown dot represents the termite fishing trials binned per week and their size represents the number of trials. The black line represents the predicted proportion of success based on 1,000 randomly simulated data points when the other covariate is held at 0. Blue ribbon represents the 5 and 95 confidence intervals.
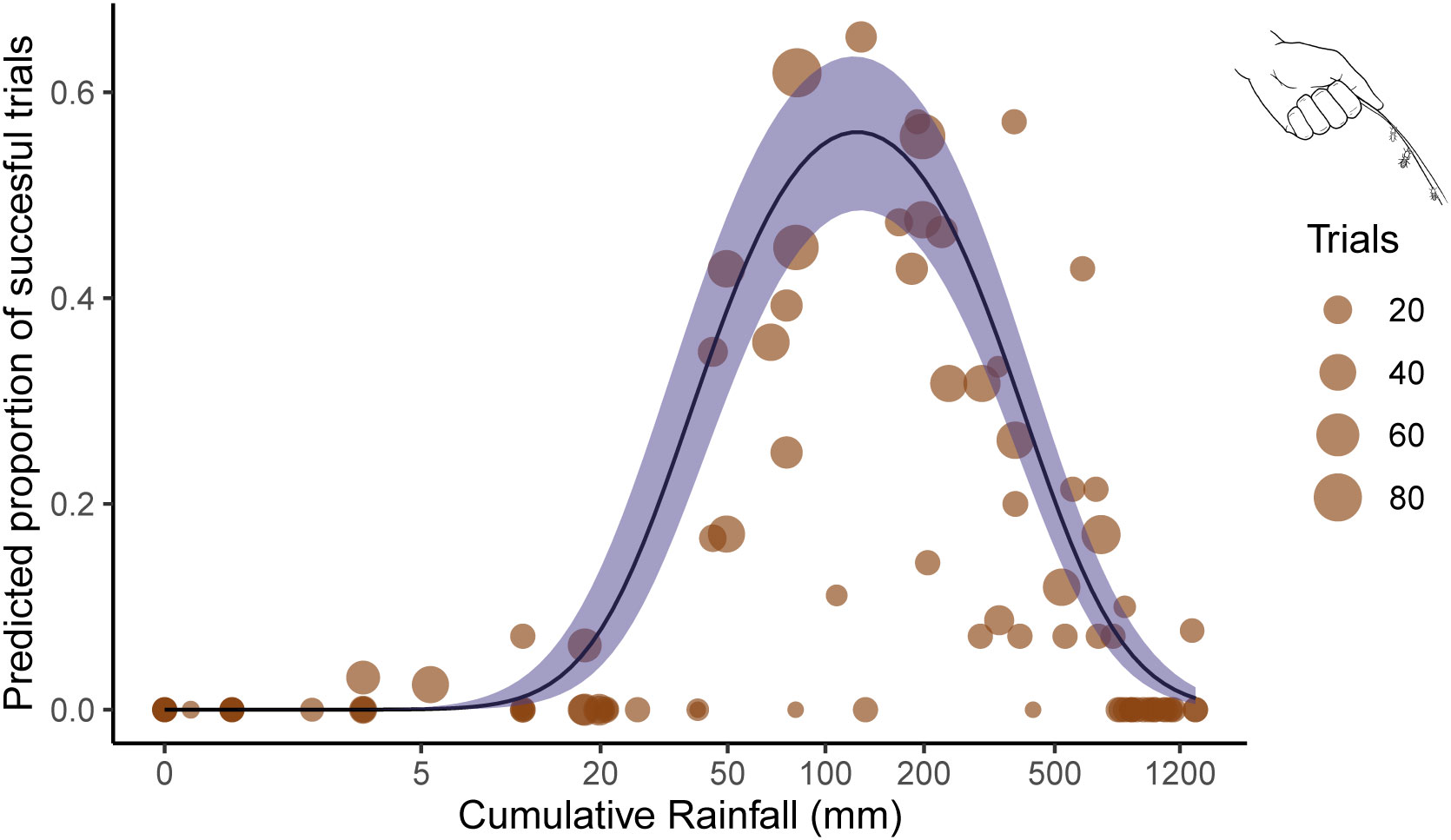
Figure 2 Simulation of total rain accumulation since the latest onset of a dry season against the proportion of termite-fishing success at flight holes. Each brown dot represents the termite fishing trials binned per week and their size represents the number of trials. The black line represents predicted proportion of success based on 1,000 randomly simulated data when the other covariate is held at 0. Blue ribbon represents the 5 and 95 confidence intervals. Cumulative rainfall is presented on the logarithmic scale in order to retain detail of trial success in early rain accumulation while also visualizing the entire breadth of cumulative rainfall on site.
Alate dispersal flights
From the analysis of the camera trap footage, we observed 59 dispersal events across 44 different days. All dispersal flights occurred during the wet season. Two of the mounds had no evidence of dispersal flights during the entire study period. When a flight was captured for a mound during a given season, 76% of the time we recorded at least one subsequent flight (range 1–5) at that mound over the season. We found that wind speed and cumulative rainfall had significant effects on the timing of dispersal flights (Table 2). The likelihood of a dispersal flight occurring decreased significantly as wind speed increased (Log-odds = −0.88, p < .001). The likelihood of a dispersal flight occurring significantly increased initially as cumulative rainfall increased but then began to decrease after approximately 400 mm of rainfall (Log-odds = −1.06, p < .001).
Predator diversity
We observed a total of 104 predation events with at least 18 predator species across nine orders. All observations of predator abundance and diversity are listed in Table 3 and specific examples are shown in Figure 3.
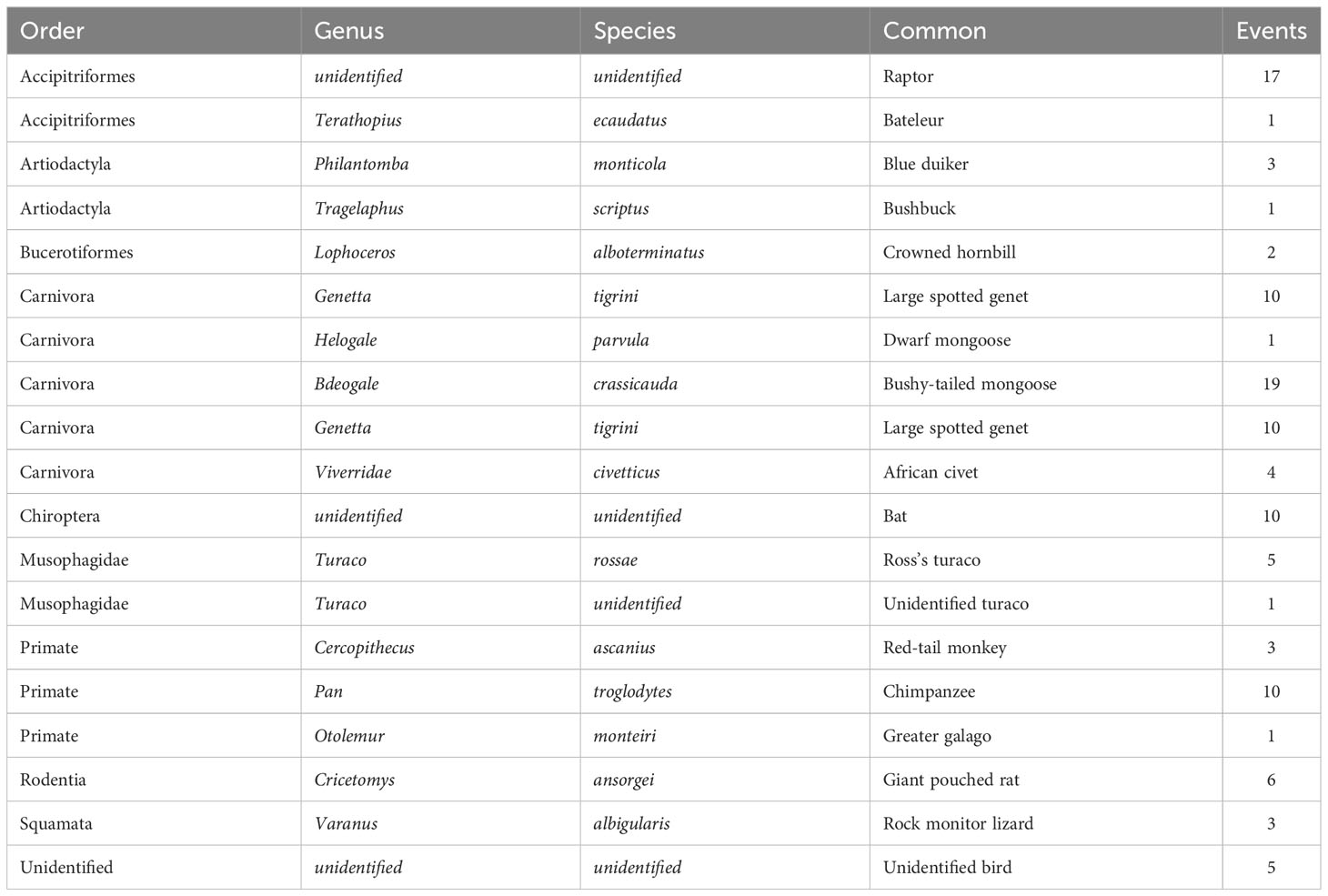
Table 3 Taxonomy of alate predators and number of predation events observed for Macrotermes dispersal flights between 2016 and 2019.
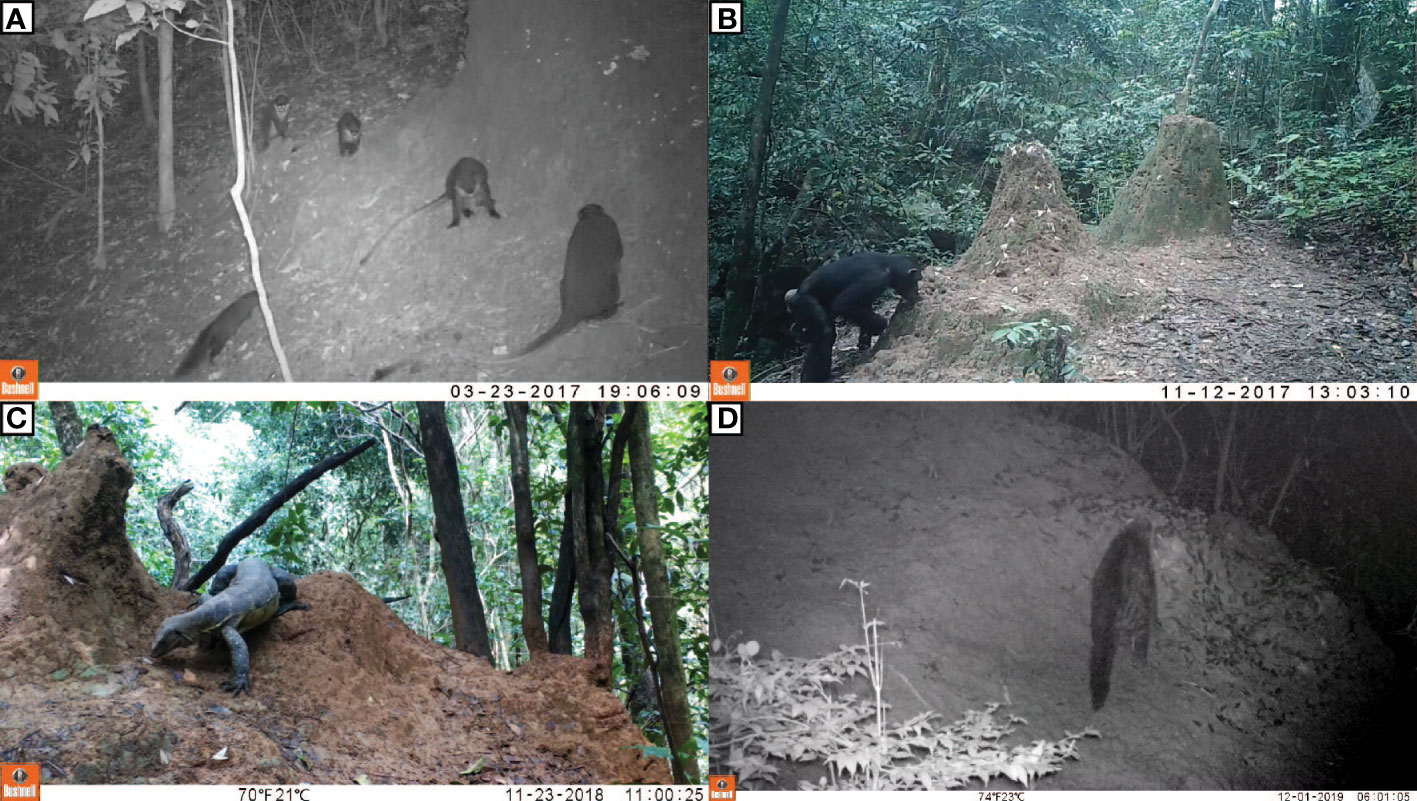
Figure 3 Predation events at Macrotermes mounds observed via camera trap footage. (A) Red tailed monkeys alongside Bushy tailed mongoose, (B) chimpanzee eating an alate with termite-fishing tool in hand, (C) rock monitor lizard, (D) African civet.
Discussion
Our findings suggest that there is a strong link between meteorological factors, particularly cumulative rainfall, and Macrotermes reproductive ecology. The termites’ preparation for annual dispersal flights during the early onset of the wet season appears to offer Issa chimpanzees an opportunity to exploit Macrotermes colonies using their specialized fishing tools. We show that the dispersal flights themselves offer additional opportunities for chimpanzees, as well as a wide variety of vertebrates, to opportunistically exploit this nutrient dense resource. This study presents an empirical analysis linking seasonal Macrotermes ecology with the ability to extract termites in the style of one chimpanzee tool-use tradition. Similar studies at other termite-fishing chimpanzee sites are warranted to further clarify reported differences in the seasonality of this tool-use behavior.
Experimental termite-fishing
The first objective of this study was to identify if there is a relationship between precipitation patterns and the availability of Macrotermes prey for termite-fishing chimpanzees in the Issa Valley. We found that precipitation was associated with flight hole activity of Macrotermes both as a weekly average and as a cumulative value (Table 1). Given the large dispersion of data observable in Figure 1, it does not appear that heavy precipitation during one week is necessarily a better predictor for termite extraction compared to a week with less, but still some, precipitation. Rather, that there is at least some recent precipitation is the more reliable predictor for termite extraction (i.e., after the onset of the wet season). This indicates that FHA is much higher once the wet season has started compared to dry season experiments, which aligns with reported seasonality of termite-fishing from many (Sanz and Morgan, 2013), but not all (Bogart and Pruetz, 2011; Sanz et al., 2014) chimpanzee communities.
While the dry versus wet season distinction on FHA is important to demonstrate empirically, we gain even higher resolution on FHA seasonality by analyzing cumulative rainfall as a predictor of FHA. When comparing Figures 1, 2, it is clear that cumulative rainfall is a better predictor of FHA than weekly average rainfall. We did observe that some colonies open their flight holes and occupy them with soldiers after the initial onset of rains aligning with previous reports of Macrotermes pre-flight behavior (Mitchell, 2008). However, the proportion of mounds with active flight holes was highest between roughly 50–200 mm of cumulative rainfall (Figure 2). During this period, colony members are closer to the surface in anticipation of dispersal flights and are especially vulnerable to intrusion from predators, including termite-fishing chimpanzees. FHA then decreases during the late wet season after most alates have dispersed so as to reduce risk to the colony. By gathering data on the termite availability, we can begin to address the dynamic role termites play as part of a seasonal multi-species interaction rather than as a consistent resource for exploitation by chimpanzees.
Our results add to the discussion on observed chimpanzee tool use variation specifically by examining how ecological factors play a role in seasonality in one community. Researchers have posited that Fongoli chimpanzees will termite-fish in response to lack of other available protein sources (Bogart and Pruetz, 2011) and this explanation aligns with the “necessity” hypothesis of tool-use variation between great ape sites (Sanz and Morgan, 2013). However, in the Issa Valley, Giuliano (2022) found that both general food availability and hunting of mammalian prey by chimpanzees was highest during the transitionary period between the late dry season and early wet season or August–December (Giuliano, 2022), coinciding with peak FHA reported in this study. These results do not support a “necessity” hypothesis that termite-fishing by chimpanzees in the Issa Valley is a response to nutritional scarcity. Instead, we find that the ability for researchers to experimentally extract termites is strongly linked to Macrotermes behavior, itself driven by meteorological variables during early wet season. Therefore “opportunity” rather than “necessity” appears to best explain the seasonality of termite-fishing by chimpanzees in the Issa Valley. Macrotermes are a rewarding source of nutrition that are sought by Issa chimpanzees when they are available even in times of relative food abundance.
However, some chimpanzee communities do exploit Macrotermes year-round, which indicates that the temporally limiting opportunity to reliably termite-fish described in this study is not a uniform phenomenon across chimpanzee communities. Even chimpanzees at Gombe Stream, which is ~ 140 km away from the Issa Valley, are documented to successfully termite-fish year-round. Still, termite-fishing at Gombe Stream peaks in the early wet season suggesting the intensity of this behavior is also influenced by the flight preparation behavior of Macrotermes colonies (Goodall, 1986; McGrew, 1992). Additionally, there are communities of central chimpanzees (P. t. troglodytes) that utilize termite-fishing “tool-sets”, which are more sophisticated than the termite-fishing tools reported in eastern chimpanzees (P. t. schweinfurthii) (Sanz et al., 2014). Sanz and Morgan (2013) concluded that termite-fishing in these communities does not increase in response to preferred food scarcity. Instead, termites are considered a high-quality resource that would be energetically rewarding year-round and consistent access is suggested to be feasible due to the particular tools used by Goualougo chimpanzees in the Republic of Congo (Sanz and Morgan, 2013). As discussed above, western chimpanzees (P. t. verus) from Fongoli, Senegal are also known to forage for termites throughout the year but their tool-sets are not as sophisticated and consist mainly of fishing probes and rarely perforation sticks (Bogart, 2005). Our results suggest that Issa chimpanzees are unable to reliably extract Macrotermes year-round with their termite-fishing tools which consist only of the fishing probe (Almeida-Warren et al., 2017). Whether a more complex tool-set with perforating and/or puncturing sticks would enable year-round access is currently unclear, although preliminary experimental attempts to use robust puncturing and perforating sticks at the study mounds at Issa suggest that they would not reliably help to increase access to Macrotermes due to the hard ground (S. Phillips, unpublished). A “limited invention” hypothesis of tool use variation posits that tool use is simply rare and unlikely to be invented and maintained (Sanz and Morgan, 2013). Our interpretation from preliminary experiments, however, is that soil is likely to be much tougher and less penetrable in the miombo woodland ecosystem, even during the wet season, than what is found in the rainforests of the Republic of Congo. Future avenues of research could include comparative termite-fishing experiments with various tool-set traditions at sites where chimpanzees are known to predate on Macrotermes year-round perhaps by following a protocol inspired by Lesnik and Thackery’s experiments with bone tools at Trinevitermes mounds (Lesnik and Thackeray, 2007). Such experiments could further clarify documented distinctions in Macrotermes seasonality and the extent of its effect on chimpanzee cultural variability.
Macrotermes in the Issa Valley are only available for a brief period, but chimpanzees may improve their access with effective foraging skills. Though evidence of termite consumption at the Issa Valley is a seasonal behavior (Stewart and Piel, 2014; Giuliano, 2022), whether or not attempts to termite-fish by this community are similarly biased towards the onset of the wet season has yet to be documented. Dr. Karline Janmaat argues for the importance of measuring a primate’s inspection events as well as their nonevents at a resource as one way to measure a primate’s expectations for resource availability and cognitive abilities (Janmaat, 2019). Chimpanzees may use weather cues, particularly rainfall, similarly to effectively forage for termites in the Issa Valley. For instance, we can hypothesize that Issa chimpanzee termite-fishers taking cues from environmental conditions would attempt to fish after the initial onset of rains following a long dry spell and generally not during the late wet season or dry season. This use of indirect weather cues would save time and energy constructing tools, visiting mounds, and attempting to extract termites from inaccessible termite colonies. Data collection efforts to test this hypothesis in the Issa Valley are underway.
Alate dispersal flights
Our second objective was to test whether meteorological patterns predict alate dispersal flights. By doing so, we further clarify how seasonal meteorological patterns influence Macrotermes ecology and, in turn, chimpanzee exploitation of these colonies. We tested the effects of multiple meteorological predictors on Macrotermes ADF. We found that flights most often occurred during the first few hundred millimeters of seasonal rainfall and noticeably decline in frequency after ~ 400 mm (Figure 4). There are several, non-mutually exclusive, explanatory mechanisms by which cumulative rainfall predicts the dispersal of Macrotermes alates. The saturation of soils likely aids the ability for alates to establish subterranean colonies. Moisture hastens the development of alates in some species, and the timing of flights based on rain accumulation may synchronize with the most productive time for workers in young colonies to forage for food (Sands, 1965; Nutting, 1969). Perhaps ~200 mm of rainfall in the Issa Valley is the best time for nuptial pairing of alates to connect, reproduce, and build colonies in time for workers to forage when vegetative resources that Macrotermes prefer at Issa are most abundant (Phillips et al., 2021). That dispersal flights peak in a similar range of cumulative rainfall to the ability of researchers to successfully extract termites from flight holes further links chimpanzee termite-fishing ability to Macrotermes reproductive ecology. Researchers could consider tracking the alate dispersal flights of Macrotermes at other chimpanzee termite-fishing sites to test whether their reproductive cycle is correlated to peak termite-fishing intensity.
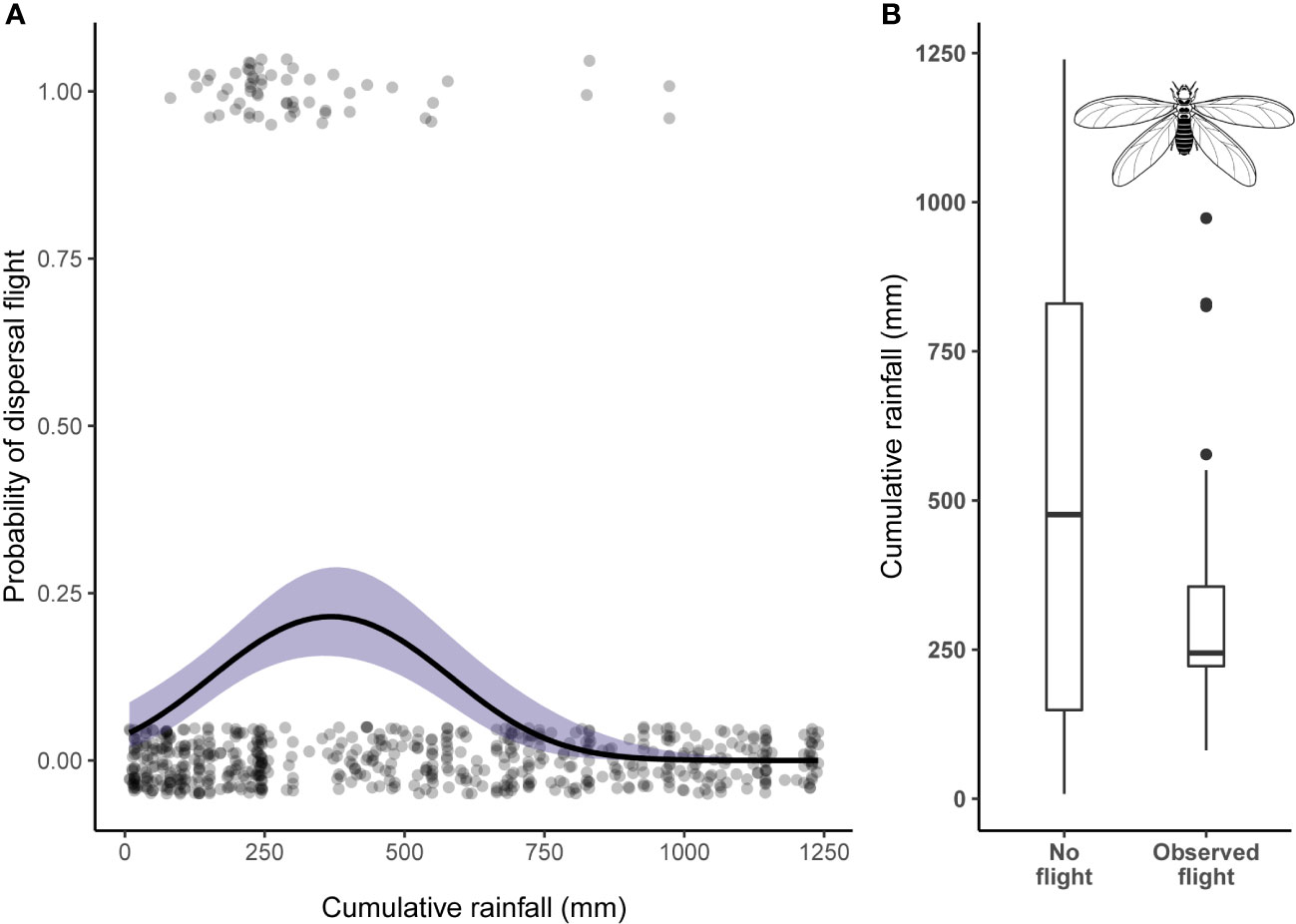
Figure 4 (A) Scatterplot of dispersal flight binomial data with superimposed logistic regression fit and confidence intervals for the effect of cumulative rainfall. Each dot scattered around the value 0 represents no observation and each dot scattered around value 1 represents an observation of an onset of a dispersal flight. Points are jittered to visualize the number of observations for this binary dataset. (B) Box and whisker diagram for cumulative rainfall values. Horizontal lines represent lower 25% quartile, median, and upper 25% quartile.
Wind speed is another factor clearly relevant to the dispersal of the alate caste. Previous studies noted that Macrotermes flights tend to occur during windless conditions (Darlington, 1986; Mitchell, 2008; Neoh and Lee, 2009). However, this is the first study to empirically report the influence of wind speed on Macrotermes dispersal flight timing. Decreased wind speeds are a factor influencing termite dispersal flight timing but the phenomenon shows variability. For example, Odontotermes formosanus in Southern China actually dispersed during high winds, travelling as far as 743 m with the aid of prevailing wind (Hu et al., 2007). Messenger and Mullings found that Copototermes formosanus in New Orleans (USA) traveled as far as a kilometer with wind (Messenger and Mullins, 2005). In these cases, wind apparently aids the dispersal of termite alates by increasing the distance they can travel. However, such species appear to be the exception rather than the rule for termites. It has been previously suggested less wind may increase the likelihood of post flight pairings whereas alates that disperse during high wind conditions would likely be too scattered to reliably connect (Nutting, 1969; Neoh and Lee, 2009). In the present study, we find that Macrotermes subhyalinus most commonly disperse at wind speeds less than 0.5 mph and always less than 1 mph (Figure 5). It strikes us as unlikely that chimpanzees, or other predators, respond to a decrease in wind speed by visiting Macrotermes mounds in hopes of capturing alate prey given the rarity of these events.
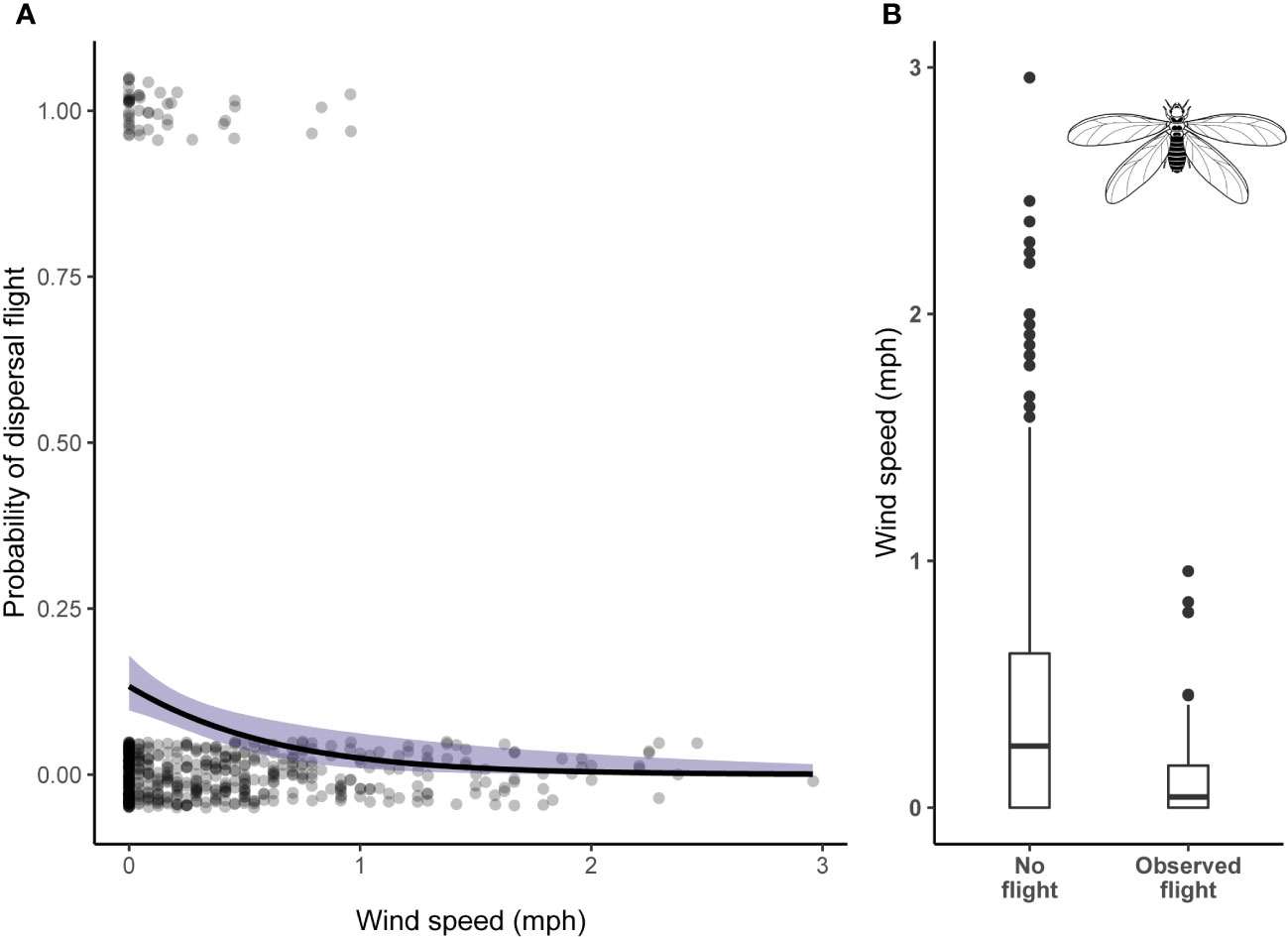
Figure 5 (A) Scatterplot of dispersal flight binomial data with superimposed logistic regression fit and confidence intervals for the effect of wind speed. Each dot on 0 represents failure to observe onset of a dispersal flight and each dot at 1 represents a success (total n = 59). Points are jittered around 0 and 1 to better visualize the number of observations for this binary dataset. (B) Box and whisker diagram for wind speed values. Horizontal lines represent lower 25% quartile, median, and upper 25% quartile.
While our results illuminate some of the mechanisms driving dispersal flights, they surely do not account for the full picture as flights are still quite rare even under ideal circumstances (Figures 5, 4). Notably, light and time of day is frequently considered a major factor for flight timing of many species (Nutting, 1969; Mitchell, 2008) though we did not consider those factors in this study. In addition to the abiotic factors that we considered, there are likely several biotic factors affecting ADF. Predation pressure, for instance, may influence termite flight timing (Nutting, 1969; Neoh and Lee, 2009). We can see that flights began in both nocturnal (n = 27) and diurnal (n = 32) conditions and thus were similarly vulnerable to both types of predators. However, we only recorded the presence of predators during these flights and did not analyze feeding duration or estimate number of alates consumed. Future studies may consider analyzing these factors from camera trap footage to gain insight on whether predator pressure may play an important role in alate dispersal flight timing.
Predator diversity
Our third objective in this study was to observe and document the diversity of predators of Macrotermes alates during dispersal flights. We observed a minimum of 19 distinct species predating on alates (Table 3). Among the most common predators to feed on dispersing alates was the chimpanzee (n = 10). By examining alate predation events, we discovered another way in which Macrotermes colonies positively contribute to the diet of chimpanzees. The alate caste of Macrotermes may be particularly valuable to chimpanzees due to their relatively high dietary fat content compared to other insect prey options (O’Malley and Power, 2014). Generally, we observed that chimpanzees would arrive to the mounds already with a tool, indicating their intention to termite-fish, and then opportunistically feed on alates. Additionally, many other miombo woodland mammals exploit Macrotermes mounds during dispersal flights (Table 3). The high abundance of an accessible and nutrient-rich prey likely influences the diversity and frequency of broad mammalian visits to termite mounds (Fleming and Loveridge, 2003; D’Ammando et al., 2022).
Camera trap evidence of Macrotermes dispersal flights offer ecologists a unique opportunity to observe rare inter-specific interactions (see Figure 3). We observed twenty-two incidences in which two or more species of predators occurred simultaneously at a mound during a dispersal flight. Half of these species-interaction events involved bats as well as a terrestrial predator (e.g., African civet, bushy tailed mongoose, large spotted genet) concurrently feeding. However, there were eleven dispersal events in which one predator supplanted another predator on the mound. Four of these events involved a bushy-tailed mongoose that displaced a giant pouched rat and two more when the same species of mongoose displaced a large spotted genet. In another case, a group of red-tailed monkeys displaced a bushy-tailed mongoose. We documented only one incident in which a chimpanzee directly overlapped with another predator and, in this case, it supplanted a red-tailed monkey. Our camera trap evidence indicates that alate dispersal flights are an ecological phenomenon that attract many vertebrates at once creating an ecological hotspot of activity.
Our results are markedly different from those of Dial and Vaughn (1987), who reported on predator diversity during Macrotermes flights in Kenya. Most notably, they report little evidence of mammalian predators during flights observed in person while simultaneously reporting much higher bird diversity than what we found. We attribute most of this difference to the different methodologies utilized between these two studies. In-person observations would be heavily biased towards diurnal predators as well as biased against elusive species. Additionally, larger bodied wildlife are more likely to flee from humans (Lasky and Bombaci, 2023). However, our method introduces its own bias by limiting the scope of observation to animal occurrences within the view of the camera. Still, camera trap monitoring adds considerable value by providing an opportunity to assess temporal niche partitioning of both diurnal and nocturnal predators that are unlikely to occur simultaneously when human observers are present. Though our method may not be ideal for entomologists primarily concerned in the abundance of alates dispersed, ecologists benefit from broad environmental monitoring that CTs allows, and primatologists gain access to data that reveals how the seasonality of Macrotermes dispersal flights influence chimpanzee termite-fishing behavior.
Conclusion
In summary, the results of this study indicate that the seasonality of termite-fishing in the Issa Valley is likely driven in large part by the seasonal reproductive ecology of Macrotermes, which is in turn driven by meteorological factors, in particular cumulative rainfall. This explanation aligns with the “opportunity” hypothesis of cultural variation in chimpanzee tool use behavior (Fox et al., 1999; Sanz and Morgan, 2013) in this case on seasonality. Similar research on Macrotermes reproductive life cycle is warranted at other chimpanzee termite-fishing sites in order to clarify differences in seasonal exploitation of this resource. Researchers can then further examine the appropriateness of hypotheses on tool use variation between cultures by testing whether alternative tool sets work equally as well across chimpanzee habitats in which the seasonal availability of Macrotermes has been described. Additionally, establishment of this resource availability enables us to closely examine chimpanzee foraging cognition by analyzing how inspection rates and strategies change across seasons. We suggest a dynamical interplay between seasonal meteorological factors, Macrotermes reproductive ecology, chimpanzee tool-use, and opportunistic predation events.
Data availability statement
The original contributions presented in the study are included in the article/Supplementary Material. Further inquiries can be directed to the corresponding author.
Ethics statement
Ethical approval was not required for the study involving animals in accordance with the local legislation and institutional requirements because the methods used in this study involving vertebrates in the wild were strictly non-invasive (camera trap footage). Experimental procedures were only carried out on invertebrates (termites) which are not protected and subject to ethical approvals.
Author contributions
SP: Conceptualization, Methodology, Writing – original draft, Writing – review & editing. AP: Methodology, Writing – review & editing. FS: Writing – review & editing. VO: Conceptualization, Funding acquisition, Methodology, Writing – review & editing.
Funding
The author(s) declare financial support was received for the research, authorship, and/or publication of this article. Funding for this study was provided by the University of California Santa Cruz (UCSC) to VO. The Issa station is supported by the UCSD/Salk Institute Center for Academic Research and Training in Anthropogeny (CARTA) and the Department of Human Origins at the Max Plank Institute for Evolutionary Anthropology (Leipzig, Germany).
Acknowledgments
We thank all field researchers and visiting researchers affiliated with GMERC from 2018–2022 for their assistance, insight, and companionship during the 5 years of research for this study. Specifically, we thank Giacomo D’Ammando, Camille Giuliano, Patrick Hassan, Joffrey Lucas, Bethan Mason, Kidsoi Rarent, Allison Rogers, Payton Sime, Simon Sungara, Kyle Sweeney, and Ramadhani Tumaini for their assistance in data collection as well as their on-site expertise. We thank Gaëlle Bocksberger for generous assistance with data management and R. We thank Rudolf Scheffrahn for his expert assistance and training on Macrotermes spp. identification in addition to his encouragement and inspiration to report the diversity of predators that feed on dispersing alates at this site, and Joshua Zupan for his work on illustrations. SP is grateful to the late Sebastien Ramirez Amaya for his invaluable feedback on this project. We would also like to thank the UCSC Chimpanzee Video Coding Team for their effort in coding camera trap footage. Specifically, we’d like to thank Warren Ashburn, Maria Borges, Julie Elliot, Tony Estrella, Marjorie Kouma, Vanessa Morgan, and Payton Sime for their keen eyes in identifying dispersing alates. This research is dedicated to the lives and memories of Kidosi Rarent and Sebastian Ramirez Amaya, who both had a direct impact on the success of this project in addition to being truly wonderful and inspirational human beings.
Conflict of interest
The authors declare that the research was conducted in the absence of any commercial or financial relationships that could be construed as a potential conflict of interest.
The author(s) declared that they were an editorial board member of Frontiers, at the time of submission. This had no impact on the peer review process and the final decision.
Publisher’s note
All claims expressed in this article are solely those of the authors and do not necessarily represent those of their affiliated organizations, or those of the publisher, the editors and the reviewers. Any product that may be evaluated in this article, or claim that may be made by its manufacturer, is not guaranteed or endorsed by the publisher.
Supplementary material
The Supplementary Material for this article can be found online at: https://www.frontiersin.org/articles/10.3389/fevo.2023.1289433/full#supplementary-material
References
Abe S. S., Yamamoto S., Wakatsuki T. (2009). Soil-particle selection by the mound-building termite Macrotermes bellicosus on a sandy loam soil catena in a Nigerian tropical savanna. J. Trop. Ecol. 25, 449–452. doi: 10.1017/S0266467409006142
Almeida-Warren K., Sommer V., Piel A. K., Pascual-Garrido A. (2017). Raw material procurement for termite fishing tools by wild chimpanzees in the Issa Valley, Western Tanzania. Am. J. Phys. Anthropol. 164, 292–304. doi: 10.1002/ajpa.23269
Backwell L. R., d’Errico F. (2001). Evidence of termite foraging by Swartkrans early hominids. Proc. Natl. Acad. Sci. 98, 1358–1363. doi: 10.1073/pnas.98.4.1358
Bates D., Mächler M., Bolker B., Walker S. (2015). Fitting linear mixed-effects models using lme4. J Stat Softw 67 (1), 1-48.
Boesch C., Kalan A. K., Mundry R., Arandjelovic M., Pika S., Dieguez P., et al. (2020). Chimpanzee ethnography reveals unexpected cultural diversity. Nat. Hum. Behav. 4 (9), 1–7. doi: 10.1038/s41562-020-0890-1
Bogart S. L. (2005). Insectivory, termite diversity, and tool use of the Fongoli chimpanzees (Pan troglodytes verus) (West Africa: Senegal).
Bogart S. L., Pruetz J. D. (2011). Insectivory of savanna chimpanzees (Pan troglodytes verus) at Fongoli, Senegal. Am. J. Phys. Anthropol. 145, 11–20. doi: 10.1002/ajpa.21452
Burton A. C., Neilson E., Moreira D., Ladle A., Steenweg R., Fisher J. T., et al. (2015). Wildlife camera trapping: a review and recommendations for linking surveys to ecological processes. J. Appl. Ecol. 52, 675–685. doi: 10.1111/1365-2664.12432
Collins D., McGrew W. (1987). Termite fauna related to differences in tool-use between groups of chimpanzees (Pan troglodytes). Primates 28, 457–471. doi: 10.1007/BF02380861
Collins D. t, McGrew W. (1985). Chimpanzees’ (Pan troglodytes) choice of prey among termites (Macrotermitinae) in western Tanzania. Primates 26, 375–389. doi: 10.1007/BF02382454
D’Ammando G., Caro T., Oelze V. M., Phillips S., Sime P., Stewart F. A., et al. (2022). Ecological drivers of habitat use by meso mammals in a miombo ecosystem in the Issa Valley, Tanzania. Front. Ecol. Evol. 197. doi: 10.3389/fevo.2022.773568
Dangerfield J., McCarthy T., Ellery W. (1998). The mound-building termite Macrotermes michaelseni as an ecosystem engineer. J. Trop. Ecol. 14, 507–520. doi: 10.1017/S0266467498000364
Darlington J. (1986). Seasonality in mature nests of the termite Macrotermes michaelseni in Kenya. Insectes sociaux 33, 168–189. doi: 10.1007/BF02224596
Davies A. B., Eggleton P., van Rensburg B. J., Parr C. L. (2015). Seasonal activity patterns of African savanna termites vary across a rainfall gradient. Insectes Sociaux 62, 157–165. doi: 10.1007/s00040-014-0386-y
Deblauwe I., Janssens G. P. (2008). New insights in insect prey choice by chimpanzees and gorillas in southeast Cameroon: the role of nutritional value. Am. J. Phys. Anthropol. 135, 42–55. doi: 10.1002/ajpa.20703
Dial K., Vaughan T. (1987). Opportunistic predation on alate termites in Kenya. Biotropica 19, 185–187. doi: 10.2307/2388744
Drummond-Clarke R. C., Kivell T. L., Sarringhaus L., Stewart F. A., Humle T., Piel A. K. (2022). Wild chimpanzee behavior suggests that a savanna-mosaic habitat did not support the emergence of hominin terrestrial bipedalism. Sci. Adv. 8, eadd9752. doi: 10.1126/sciadv.add9752
Fleming P. A., Loveridge J. P. (2003). Miombo woodland termite mounds: resource islands for small vertebrates? J. Zool. 259, 161–168. doi: 10.1017/S0952836902003084
Fox E. A., Sitompul A. F., Van Schaik C. P. (1999). Intelligent tool use in wild Sumatran orangutans. mental. gorillas orangutans 480, 99–116. doi: 10.1017/CBO9780511542305.005
Gardner S. (2019). “Analysis of experimental bone tools from Swartkrans Cave, South Africa." Thesis, Georgia State University. doi: 10.57709/14345550
Giuliano C. (2022). Chimpanzee (Pan troglodytes schweinfurthii) behavioral responses to resource scarcity in the savanna-woodland environment of Issa Valley, Tanzania: study of feeding, ranging, and grouping patterns (United Kingdom: Liverpool John Moores University).
Hu J., Zhong J.-H., Guo M.-F., et al. (2007). Alate dispersal distances of the black-winged subterranean termite Odontotermes formosanus (Isoptera: Termitidae) in southern China. 50, 1-8.
Janmaat K. R. (2019). What animals do not do or fail to find: A novel observational approach for studying cognition in the wild. Evolution. Anthropol.: Issues News Rev. 28, 303–320. doi: 10.1002/evan.21794
Jouquet P., Dauber J., Lagerlöf J., Lavelle P., Lepage M. (2006). Soil invertebrates as ecosystem engineers: intended and accidental effects on soil and feedback loops. Appl. Soil Ecol. 32, 153–164. doi: 10.1016/j.apsoil.2005.07.004
Koops K., McGrew W. C., Matsuzawa T. (2013). Ecology of culture: do environmental factors influence foraging tool use in wild chimpanzees, Pan troglodytes verus? Anim. Behav. 85, 175–185. doi: 10.1016/j.anbehav.2012.10.022
Lasky M., Bombaci S. (2023). Human-induced fear in wildlife: A review. J. Nat. Conserv. 74, 126448. doi: 10.1016/j.jnc.2023.126448
Lesnik J. J. (2014). Termites in the hominin diet: A meta-analysis of termite genera, species and castes as a dietary supplement for South African robust australopithecines. J. Hum. Evol. 71, 94–104. doi: 10.1016/j.jhevol.2013.07.015
Lesnik J., Thackeray J. (2007). The efficiency of stone and bone tools for opening termite mounds: implications for hominid tool use at Swartkrans. South Afr. J. Sci. 103, 354–356.
Lima M. C. F., de Almeida Leandro M. E. D., Valero C., Coronel L. C. P., Bazzo C. O. G. (2020). Automatic detection and monitoring of insect pests—a review. Agriculture 10, 161. doi: 10.3390/agriculture10050161
Marshall A. J., Wich S. (2013). Characterization of primate environments through assessment of plant phenology. Primate Ecol. conserv.: A Handb. tech., 103–127. doi: 10.1093/acprof:oso/9780199659449.003.0007
McBeath N. M., McGrew W. C. (1982). Tools used by wild chimpanzees to obtain termites at Mt Assirik, Senegal: the influence of habitat. J. Hum. Evol. 11, 65–72. doi: 10.1016/S0047-2484(82)80032-8
McElveen D., Meyer R. T. (2020). An effective and affordable camera trap for monitoring flower-visiting butterflies in sandhills: with implications for the frosted elfin (Callophrys irus). J. Pollin. Ecol. 26. doi: 10.26786/1920-7603(2020)573
McGrew W. C. (1992). Chimpanzee material culture: implications for human evolution (Cambridge: Cambridge University Press).
McGrew W. C., Collins D. A. (1985). Tool use by wild chimpanzees (Pan troglodytes) to obtain termites (Macrotermes herus) in the Mahale Mountains, Tanzania. Am. J. Primatol. 9, 47–62. doi: 10.1002/ajp.1350090106
McGrew W. C., Tutin C. E., Baldwin P. (1979). Chimpanzees, tools, and termites: cross-cultural comparisons of Senegal, Tanzania, and Rio Muni. Man 14 (2), 185–214. doi: 10.2307/2801563
Messenger M. T., Mullins A. J. (2005). New flight distance recorded for Coptotermes formosanus (Isoptera: Rhinotermitidae). Florida Entomol. 88, 99–100. doi: 10.1653/0015-4040(2005)088[0099:NFDRFC]2.0.CO;2
Mitchell J. (2007). Swarming and pairing in the fungus-growing termite, Macrotermes natalensis (Haviland) (Isoptera: Macrotermitinae). Afr. Entomol. 15, 153–160. doi: 10.4001/1021-3589-15.1.153
Mitchell J. (2008). Swarming flights of the fungus-growing termite, Macrotermes natalensis (Haviland) (Isoptera: Macrotermitinae), and the environmental factors affecting their timing and duration. Afr. Entomol. 16, 143–152. doi: 10.4001/1021-3589-16.2.143
Naqvi Q., Wolff P. J., Molano-Flores B., Sperry J. H. (2022). Camera traps are an effective tool for monitoring insect–plant interactions. Ecol. Evol. 12, e8962. doi: 10.1002/ece3.8962
Neoh K.-B., Lee C.-Y. (2009). Flight activity of two sympatric termite species, Macrotermes gilvus and Macrotermes carbonarius (Termitidae: Macrotermitinae). Environ. entomol. 38, 1697–1706. doi: 10.1603/022.038.0623
Nutting W. (1969). Flight and colony foundation. Biol. termites, 233–282. doi: 10.1016/B978-0-12-395529-6.50012-X
O’Malley R. C., Power M. L. (2014). The energetic and nutritional yields from insectivory for Kasekela chimpanzees. J. Hum. Evol. 71, 46–58. doi: 10.1016/j.jhevol.2013.09.014
Pegoraro L., Hidalgo O., Leitch I. J., Pellicer J., Barlow S. E. (2020). Automated video monitoring of insect pollinators in the field. Emerg. Topics Life Sci. 4, 87–97. doi: 10.1042/ETLS20190074
Phillips S., Scheffrahn R. H., Piel A., Stewart F., Agbor A., Brazzola G., et al. (2021). Limited evidence of C4 plant consumption in mound building Macrotermes termites from savanna woodland chimpanzee sites. PloS One 16, e0244685. doi: 10.1371/journal.pone.0244685
Piel A. K., Strampelli P., Greathead E., Hernandez-Aguilar R. A., Moore J., Stewart F. A. (2017). The diet of open-habitat chimpanzees (Pan troglodytes schweinfurthii) in the Issa Valley, western Tanzania. J. Hum. Evol. 112, 57–69. doi: 10.1016/j.jhevol.2017.08.016
Preti M., Verheggen F., Angeli S. (2021). Insect pest monitoring with camera-equipped traps: strengths and limitations. J. Pest Sci. 94, 203–217. doi: 10.1007/s10340-020-01309-4
Puhr R., Heinze G., Nold M., Lusa L., Geroldinger A. (2017). Firth’s logistic regression with rare events: accurate effect estimates and predictions? Stat Med. 36, 2302–2317. doi: 10.1002/sim.7273
R Core Team (2018). R: A language and environment for statistical computing. R Foundation for Statistical Computing, Vienna, Austria. Available at: https://www.R-project.org/.
Sands W. (1965). Alate development and colony foundation in five species of Trinervitermes (Isoptera, Nasutitermitinae) in Nigeria, West Africa. Insectes Sociaux 12, 117–130. doi: 10.1007/BF02223758
Sanz C. M., Deblauwe I., Tagg N., Morgan D. B. (2014). Insect prey characteristics affecting regional variation in chimpanzee tool use. J. Hum. Evol. 71, 28–37. doi: 10.1016/j.jhevol.2013.07.017
Sanz C. M., Morgan D. B. (2013). Ecological and social correlates of chimpanzee tool use. Philos. Trans. R. Soc. B: Biol. Sci. 368, 20120416. doi: 10.1098/rstb.2012.0416
Sanz C. M., Morgan D., Gulick S. (2004). New insights into chimpanzees, tools, and termites from the Congo Basin. Am. Nat. 164, 567–581. doi: 10.1086/424803
Scheffrahn R. H. (2008). “Encyclopedia of Entomology,” in Termites (Isoptera). Ed. Capinera J. L. (Dordrecht: Springer Netherlands), 3737–3747. doi: 10.1007/978-1-4020-6359-6_2400
Schuurman G. (2006). Foraging and distribution patterns in a termite assemblage dominated by fungus-growing species in semi-arid northern Botswana. J. Trop. Ecol. 22, 277–287. doi: 10.1017/S0266467405003044
Silva I. S., Lucena E. F., Moura F. M., Vasconcellos A. (2021). Termite flights seasonally promote nutrient pulses in the Caatinga dry forest in northeastern Brazil. Appl. Soil Ecol. 166, 104066. doi: 10.1016/j.apsoil.2021.104066
Sommer V., Buba U., Jesus G., Pascual-Garrido A. (2017). Sustained myrmecophagy in Nigerian chimpanzees: Preferred or fallback food? Am. J. Phys. anthropol. 162, 328–336. doi: 10.1002/ajpa.23122
Sponheimer M., Lee-Thorp J., de Ruiter D., Codron D., Codron J., Baugh A. T., et al. (2005). Hominins, sedges, and termites: new carbon isotope data from the Sterkfontein valley and Kruger National Park. J. Hum. Evol. 48, 301–312. doi: 10.1016/j.jhevol.2004.11.008
Steen R. (2017). Diel activity, frequency and visit duration of pollinators in focal plants: in situ automatic camera monitoring and data processing. Methods Ecol. Evol. 8, 203–213. doi: 10.1111/2041-210X.12654
Stewart F. A., Piel A. K. (2014). Termite fishing by wild chimpanzees: new data from Ugalla, western Tanzania. Primates 55, 35–40. doi: 10.1007/s10329-013-0362-6
Keywords: termite-fishing, tool-use, swarming behavior, opportunistic predation, experimental primatology, Pan troglodytes schweinfurthii
Citation: Phillips S, Piel AK, Stewart FA and Oelze VM (2023) A chimpanzee’s time to feast: seasonality of Macrotermes flight hole activity and alate dispersal flights detected by termite-fishing experiments and camera traps in the Issa Valley, Tanzania. Front. Ecol. Evol. 11:1289433. doi: 10.3389/fevo.2023.1289433
Received: 05 September 2023; Accepted: 09 October 2023;
Published: 17 November 2023.
Edited by:
Pedro Dias, Universidad Veracruzana, MexicoReviewed by:
Julie Lesnik, Wayne State University, United StatesRobert OMalley, Harvard Medical School, United States
Copyright © 2023 Phillips, Piel, Stewart and Oelze. This is an open-access article distributed under the terms of the Creative Commons Attribution License (CC BY). The use, distribution or reproduction in other forums is permitted, provided the original author(s) and the copyright owner(s) are credited and that the original publication in this journal is cited, in accordance with accepted academic practice. No use, distribution or reproduction is permitted which does not comply with these terms.
*Correspondence: Seth Phillips, c2VjcGhpbGxAdWNzYy5lZHU=