- 1Marine Biology Lab, Groningen Institute of Evolutionary Life Sciences, Rijksuniversiteit Groningen, Groningen, Netherlands
- 2Acoustic Ecology Lab, Scripps Institution of Oceanography, University of California, San Diego, La Jolla, CA, United States
- 3Faculteit der Natuurwetenschappen, Wiskunde en Informatica, University of Amsterdam, Amsterdam, Netherlands
- 4Juanes Lab, School of Biology, University of Victoria, Victoria, BC, Canada
- 5Suzuki Lab, Faculty of Science, Graduate School of Science, Kyoto University, Kyoto, Japan
- 6Canadian Wildlife Service, Environment and Climate Change Canada, Sidney, BC, Canada
- 7School of Biodiversity, One Health and Veterinary Medicine, University of Glasgow, Glasgow, United Kingdom
- 8School of Animal, Rural and Environmental Sciences, Nottingham Trent University, Southwell, United Kingdom
- 9School of Science Technology and Engineering, University of the Sunshine Coast, Petrie, QLD, Australia
- 10School of Biological Sciences, University of Bristol, Bristol, United Kingdom
- 11Centre for Environment, Fisheries and Aquaculture Science, Weymouth Laboratory, Weymouth, United Kingdom
- 12Fisheries Department, Faculty of Natural Resources, University of Guilan, Someh Sara, Iran
- 13School of Natural Sciences, Bangor University, Bangor, United Kingdom
- 14Behavioural Ecology Research Group, School of Life Sciences, Anglia Ruskin University, Cambridge, United Kingdom
Anthropogenic noise is a major pollutant in terrestrial and aquatic ecosystems. Since the industrial revolution, human activities have become increasingly noisy, leading to both acute and chronic disturbance of a wide variety of animals. Chronic noise exposure can affect animals over their lifespan, leading to changes in species interactions and likely altering communities. However, the community-level impacts of chronic noise are not well-understood, which impairs our ability for effective mitigation. In this review, we address the effects of chronic noise exposure on communities and explore possible mechanisms underlying these effects. The limited studies on this topic suggest that noise can affect communities by changing the behavior and/or physiology of species in a community, which results in direct or knock-on consequences for other species in the ecosystem. Major knowledge gaps remain due to the logistically complex and financially expensive nature of the long-term studies needed to address these questions. By identifying these gaps and suggesting approaches to answer them, we provide a road map toward mitigating the effects of a noisy world.
1. Chronic noise can change communities
1.1. Introduction
Anthropogenic noise is recognized as a major global pollutant that has considerable implications for human health (Matheson et al., 2003; Hammer et al., 2014; Mohamed et al., 2021) and the behavior, physiology and fitness of wildlife (Barber et al., 2010). Indeed, a substantial body of research has been published over the past two decades that has explored the effects of noise pollution on animals across terrestrial and aquatic ecosystems (Shannon et al., 2016b; Sordello et al., 2020; Duarte et al., 2021; Jerem and Mathews, 2021). Anthropogenic noise that significantly alters the acoustic environment has increased markedly since the Industrial Revolution both in terms of the level of sound exposure and geographical extent. The rise in noise pollution has been driven by population growth, mechanization, infrastructure development and increasing demand for resources (Barber et al., 2010). For example, in the US continent-wide changes in the soundscape extend well beyond the boundaries of urban environments (Mennitt and Fristrup, 2016), with 63% of protected areas experiencing sound exposure double that of the ambient level (Buxton et al., 2017). As such, noise has the potential to be a major selective force that can restructure wildlife communities (Swaddle et al., 2015). At the same time, noise does not persist long-term in the environment like many other pollutants—as was evidenced during the COVID-19 pandemic when lockdown measures led to sustained global quieting (Lecocq et al., 2020)—which can facilitate mitigation measures and the reestablishment of natural soundscapes. The latency of recovery following noise removal will depend on the specific behavioral and/or physiological mechanisms that restructure wildlife communities, as well as the flexibility of species to adapt to the return of a more natural acoustic environment.
Field-based studies and laboratory experiments have provided considerable evidence that exposure to noise can cause a wide range of ecological impacts to wildlife (Shannon et al., 2016b; Kunc and Schmidt, 2019; Jerem and Mathews, 2021). These include changing spatial distribution and deterring wildlife from important feeding and breeding areas, or interfering with crucial biological functions such as foraging performance (more food handling errors and discrimination errors), predator avoidance, prey detection and conspecific communication. Furthermore, there are direct physiological costs associated with exposure to noise from reduced sleep (Grunst et al., 2021) to increasing stress hormone levels (Troïanowski et al., 2017). These varied impacts may lead to negative consequences for individual fitness, population levels and community structure (Slabbekoorn et al., 2010; Francis and Barber, 2013). Similarly, human communities experience physiological and behavioral effects from noise exposure that include sleep deprivation, cognitive impairment, elevated stress and annoyance, and a higher incidence of cardiovascular disease (Stansfeld and Matheson, 2003; Szalma and Hancock, 2011; Hammer et al., 2014).
The behavioral effects of noise exposure are driven by four key mechanisms that are not mutually exclusive: (1) Noise masks critical sounds including communication of conspecifics and other relevant cues such as approaching danger; (2) noise distracts animals from attending to pertinent information in the environment; (3) noise is perceived as a direct threat, thereby altering behavioral responses of the animal; and/or (4) noise initiates chronic stress, leading to long-term behavioral and physiological changes in the animal. It is important to note that each of these mechanisms is capable of driving a change in animal distribution and habitat use, which can alter the composition and interaction of species at the community-level.
1.2. Chronic noise exposure
Although there have been considerable advances in our understanding of the biological responses associated with anthropogenic noise exposure over the past two decades, most are based on comparatively short-term experiments and observations (Shannon et al., 2016b; Jerem and Mathews, 2021). In addition, these studies have predominantly focused on a single species. However, transport networks, industry and urban environments are major sources of chronic anthropogenic noise that permeate natural and human transformed environments over the long-term. These noise sources are also characterized by acoustic energy mainly being concentrated in the low-frequency spectrum (<2 kHz), which travel further than high-frequency sounds and therefore potentially impact a wide range of species simultaneously.
Sound in nature is a complex and challenging physical phenomenon to measure, as it varies in terms of duration, frequency and amplitude. The acoustic characteristics of a given noise source and the perceptual capabilities (e.g., hearing sensitivity) of the species exposed to it will be instrumental in determining whether it is likely to significantly impact animal behavior and physiology (Francis and Barber, 2013). Indeed, the duration, spectral and temporal characteristics of chronic noise may ultimately be as important as the amplitude when it comes to mediating long-term effects on animal communities. It is therefore crucial that scientists and wildlife practitioners accurately measure and report the specific sound characteristics of the noise source, as this information is critical for comparing research findings and understanding the specific levels of exposure that can drive a biological response (McKenna et al., 2016). Currently, many of the noise metrics used in research and monitoring are focused on human perceptual abilities, rather than considering the varied hearing thresholds of animal communities.
To fully understand the impacts of chronic anthropogenic noise exposure on animal communities it is necessary to conduct long-term studies (Jerem and Mathews, 2021). These need to consider the effects of chronic exposure and lasting impacts after exposure has ended. However, a definition of chronic exposure is rarely given (Jerem and Mathews, 2021) and short-term and chronic exposure are on a continuum, making it difficult to classify exposure events in the field. For example, repeated short bursts of exposure (e.g., sonar) over longer periods (a few times a year for years) differ from medium-term exposure (continuous for weeks, e.g., construction site) in their effects. Additionally, what is considered chronic will also depend on the lifespan of the organism, which ranges from weeks to decades (Austad, 2010). Construction of a gas pipeline that takes a year would thus be multi-generation exposure for some animals, whilst only a brief exposure for others. Finally, the effects of chronic exposure are expected to impact multiple species in the community, and should therefore be long enough to affect species with varying lifespans. Chronic noise exposure can thus have a low or high duty cycle, as long as it occurs regularly or long enough to impact species with varying lifespans. Therefore, we suggest a rather broad, but practical chronic exposure definition: “Exposure throughout a significant part of the lifespan of an animal, at regular enough intervals to have the potential of lasting impacts from the individual- to community-scale.”
1.3. State of the knowledge about chronic noise effects on communities
We conducted a search of peer-reviewed journal articles that have been published since 1970 and have addressed the effects of noise on animal communities. Our article search used the datasets provided in four previous systematic review papers (Shannon et al., 2016b; Sordello et al., 2020; Duarte et al., 2021; Jerem and Mathews, 2021), as well as using the Web of Science and Google Scholar search engines to identify any further papers that were omitted from the reviews or that have been published in the last 2 years. The search terms we used were “anthropogenic,” “noise,” “wildlife,” “animal,” and “community.” We specifically focused on studies that explored whether noise exposure affected community-level composition (e.g., changes in abundance and diversity of multiple species) over time. The dataset comprised 48 papers published between 1995 and 2021 (Table 1 and Supplementary Material 1), of which 16 were not included in the previous review papers. Overall, the most common method to explore the community effects of noise was through direct observation (n = 29) followed by playback (n = 10) and natural experiment (n = 9). Seventy-nine percent of the studies (n = 38) reported negative effects of noise exposure in one taxonomic group (decreased abundance/species richness, decreased nesting, and decreased offspring survival/hatching success) while only one study documented a positive effect (increased abundance/species richness). The remainder revealed either no effect (n = 7) or mixed effects within the same taxonomic group (n = 7), with the direction depending upon the species. Birds featured in 38 (79%) of the studies; mammals, amphibians, reptiles and invertebrates were the least represented, featuring in one study each. Seventy-nine percent of the studies were conducted in either North America or Europe (n = 38). Seventy-one percent of the studies (n = 34) explored whether noise influenced abundance and/or species diversity.

Table 1. Number of studies in the literature review (S2) that investigated the effects of noise on communities separated by taxonomic group.
The early research on community-level effects of noise was conducted by observing the abundance and diversity of bird species as a function of distance from a chronic noise source, such as a busy roadway. This proved an effective method for understanding the effects of noise with clear evidence that species composition, density and abundance were more negatively impacted the higher the noise levels (Reijnen and Foppen, 1995; Reijnen et al., 1995, 1996). Later studies highlighted how species with low frequency calls that overlapped considerably with traffic noise were likely to be impacted to a much greater extent than species with higher frequency calls [(e.g., lower occupancy (Goodwin and Shriver, 2011)]. However, there were challenges associated with this observational approach such as accounting for confounding variables, including habitat fragmentation, chemical pollution, elevated human activity, and increased mortality (e.g., vehicle strike), that also occur to a greater extent in close proximity to roads (Summers et al., 2011).
The expansion of gas extraction across North America led to the development of natural experiments where areas with noise-generating compressor stations could be compared to areas structurally equivalent to compressor stations but with quiet well pads. These natural experiments largely controlled for the confounding variables associated with other forms of disturbance — such as habitat transformation and human activity — and explicitly investigated the effects of noise on the abundance and diversity of bird species in an otherwise natural environment (Bayne et al., 2008). These studies demonstrated that occupancy, diversity and abundance of avian species were negatively impacted by anthropogenic noise (Bayne et al., 2008), while also revealing that species exhibited varying levels of sensitivity to noise exposure depending on the extent of vocal masking that they experienced (Francis et al., 2009, 2011a). Larger bodied birds with lower frequency calls were found to use noisy areas considerably less than smaller bodied species with higher frequency vocalizations, which presents a strong selective force shaping avian community structure and species interactions such as predator—prey relationships (Francis et al., 2009, 2011a). However, researchers working in grassland prairies documented an effect of the gas extraction infrastructure, rather than the noise that was associated with it (Nenninger and Koper, 2018), showing the complexity of the mechanisms underlying this disturbance. The physiological costs of noise have also been documented using this natural study system demonstrating impacts to glucocorticoid-signaling and reduced fitness across bird species (Kleist et al., 2018), while truly long-term cascading impacts of noise exposure were recently found in a study that revealed chronic noise exposure (15 years) impacted seedling recruitment and woody plant community structure (Phillips et al., 2021). These effects were still in evidence 2–4 years after the removal of noise.
Scientists have also employed playbacks across the landscape to assess experimentally the effects of introduced noise on wildlife communities. The advantages of the playback approach include the ability to control the specific location and duration of noise exposure, as well as the noise source sound level. Furthermore, playbacks allow for the effects of noise to be investigated in isolation of confounding variables that are generally associated with noise disturbance. The first of these landscape-level studies broadcast traffic noise along a 500 m “phantom road” in habitat favored by migratory birds, with the researchers documenting a 25% reduction in bird abundance during playback periods (McClure et al., 2013). Further work revealed that 31% of the bird community avoided the phantom road, while those individuals that remained experienced a reduction in body condition that was associated with an altered trade-off in foraging and vigilance (Ware et al., 2015). There were also age effects with younger birds being impacted by noise to a greater extent than adults (McClure et al., 2017). Subsequent research has demonstrated similar effects of introduced traffic noise on invertebrates, particularly species that rely on acoustic signals in the environment (Senzaki et al., 2020), while breeding birds in North American grassland prairies declined in abundance when exposed to playbacks of noise associated with energy extraction at the landscape scale (Cinto Mejia et al., 2019; Rosa and Koper, 2022). There appear to be complex interactions between infrastructure, noise and species-specific physiological and behavioral responses that can potentially mediate negative effects, and in some cases noise effects may be dwarfed by the effects of physical structures in natural areas [i.e., oil pumps: (Nenninger and Koper, 2018)]. However, we believe these complexities further highlight gaps in noise research on community ecology where interactions between different ecosystem components, noise, and the health of the community network still exist (Curry et al., 2018).
2. Why do we see differences in community composition due to noise?
Noise can have a profound effect on community composition through a variety of mechanisms (Figure 1 and Supplementary Figure 1). It can directly impact local abundance of different species due to avoidance, increased mortality and decreased recruitment, while indirectly impacting predator—prey and parasite—host interactions, competitor interactions, and species-driven ecosystem structure. Noise can have such a broad impact because species within a community respond differently (e.g., declines, altered predator–prey relationships, etc.). This combination of direct and indirect effects and species variability in response alter composition and structure of the community
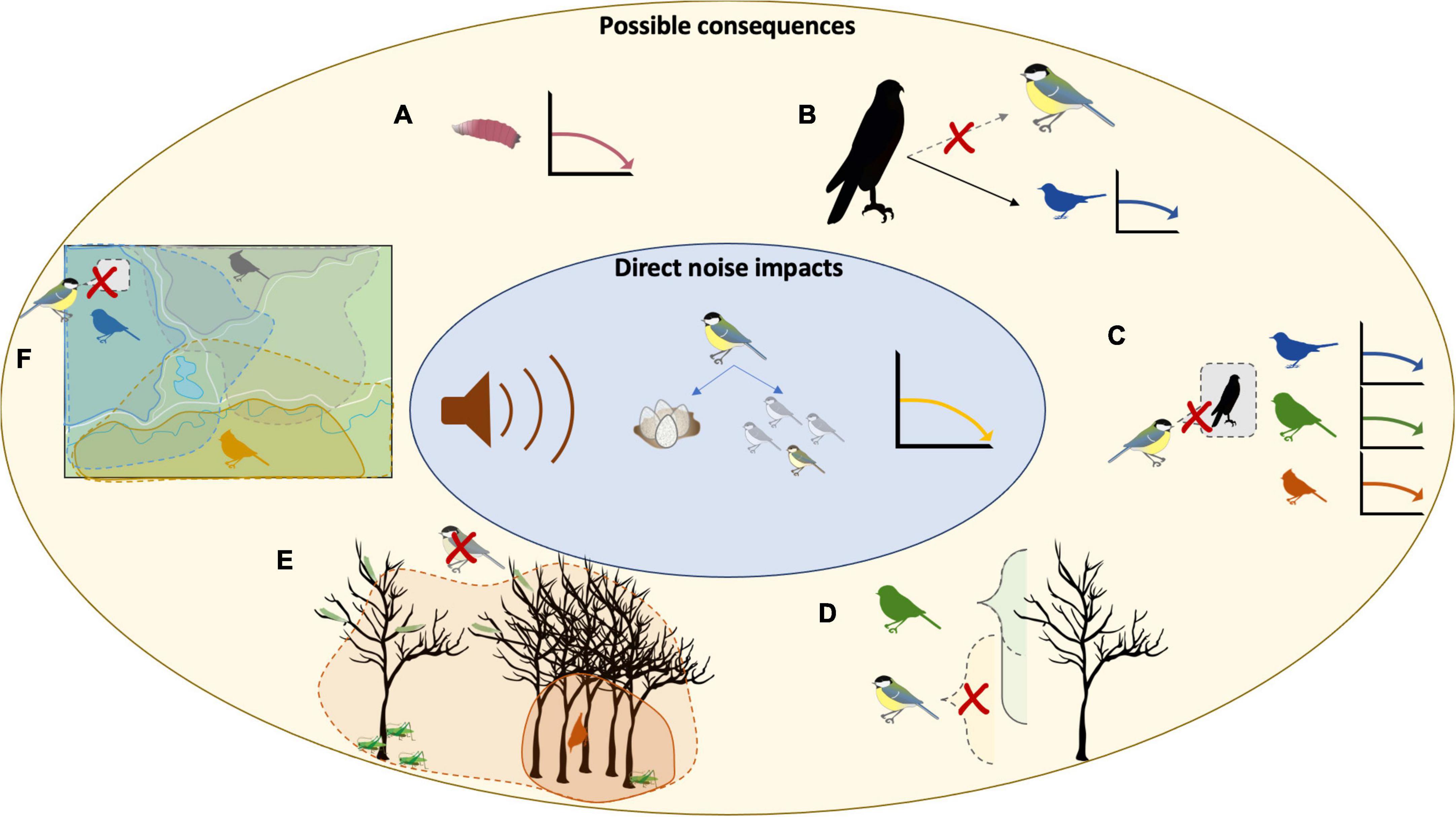
Figure 1. Theoretical knock-on consequences from direct noise impacts on the population of one species. This example is based on decline in recruitment as a result of lower fecundity in female great tits and lower nestling survival due to noise. The resulting decrease in the local population could affect a number of other species though a variety of mechanisms: (A) Removing hosts for parasites (e.g., blowfly larvae), (B) reducing prey availability resulting in prey switching, causing declines in sympatric species due to increased predation pressure, (C) removing anti-predator information resulting in higher mortality due to predation, or altered spatial use within a mixed-species community by (D) opening a niche previously used by the declining species (Cimprich and Grubb Jnr, 1994), (E) removing information/presence that allowed species to broaden their own microhabitat use when the declining species were present (Dolby and Grubb, 1998), or (F) removing information/perceived safety that allowed species to cross barriers such as open spaces (e.g., heterospecific using chick-a-dee calls to initiate movement across roads, man-made fields, etc.) (Sieving et al., 2004).
2.1. Direct effects
One of the direct influences of noise is avoidance behavior; many species will temporarily (Slotte et al., 2004; McClure et al., 2013; Ware et al., 2015; Bunkley et al., 2017; Carral-Murrieta et al., 2020) or permanently (Nicholson et al., 1992; Pearson et al., 1992; Morton and Symonds, 2002; Francis et al., 2009; Thompson et al., 2010; Herrera-Montes and Aide, 2011; Ciach and Fröhlich, 2017) avoid noisy areas. A variety of migrating bird species, for example, avoided stopping over in noisy areas, even though they used the same areas under quieter conditions (McClure et al., 2013; Ware et al., 2015), while many insects demonstrate reduced abundance in noisy areas (Bunkley et al., 2017). Species respond differently to noise (Shafiei Sabet et al., 2016; Voellmy et al., 2016; Bunkley et al., 2017). While some species may disappear entirely from a noisy area (Voellmy et al., 2016), others may not exhibit such a drastic decline, or, in some cases, may even increase (Francis et al., 2009; Voellmy et al., 2016; Bunkley et al., 2017), altering the composition and interactions of the species remaining.
While avoidance is a common strategy employed by many species, chronic noise can also alter species abundance through increased mortality. Noise can directly lead to individual mortality through noise-induced permanent injury (excluding hearing loss) or reduction of predator detection. To the best of our knowledge, no examples of noise-induced injury have been demonstrated in terrestrial systems; however, they are unfortunately common in marine and other aquatic systems (Duarte et al., 2021). Noise can kill by causing injuries such as swim bladder and kidney rupture in fish (National Academies of Sciences, Engineering and Medicine, 2011; Halvorsen et al., 2012; Jenkins et al., 2022), or through alterations in behavior that can lead to death [e.g., diving behavior (Fernández et al., 2005), strandings (Bernaldo de Quirós et al., 2019)]. This is likely in part due to the physical properties of the two environments. Sound pressure in water is relatively much higher than in air, due to the density difference between the two media (Popper and Hawkins, 2019). Additionally, sound travels further and faster underwater than in air, increasing the potential area of effect of noise. These factors have been exploited by many marine and freshwater species that have evolved sensitive specialized anatomical structures for hearing and sensing pressure (Popper and Hawkins, 2019). However, noisy activities such as pile driving, sonar, dredging and seismic airguns for oil and gas exploration are common in underwater environments and can overwhelm these highly adapted systems.
Though not as immediately fatal, animals living in noisy conditions often show altered levels of stress hormones, which are linked to increased stress-responsiveness (Leshyk et al., 2013). These can lead to poorer body condition (Anderson et al., 2011) and reduced reproductive success, such as lower hatching success (Kleist et al., 2018) and poorer growth and development in those offspring that survive (Injaian et al., 2018). Poorer body condition can be a result of increased glucocorticoid concentrations, decreased foraging success, and changes in social relationships and behavior. Noise can result in activation of the hypothalamic–pituitary–adrenocortical (HPA) axis. If the animal is unable to escape the source of stress, the HPA system remains activated (Pravosudov et al., 2001), and the short-term physiological or behavioral changes that ameliorate stress and promote survival can become deleterious. These effects have the potential to be far-reaching: for example, in birds, short-term corticosterone manipulation has been shown to reduce survivorship 2 years after the manipulation (Goutte et al., 2010). Extended bouts of stress do not only deplete energy reserves, but also impair growth and immune function, accelerate aging, negatively impact cognitive ability and increase atrophy of nerve cells in the brain (Wingfield et al., 1998; Sims and Holberton, 2000; Pravosudov et al., 2001; Wingfield and Kitaysky, 2002; Ellenberg et al., 2007). However, it is important to note that measuring stress response is inherently complex because stress is experienced on multiple levels—social, psychological, and physiological—and varies with life stage and life history, circadian rhythm, hormone interactions, and the extent and longevity of environmental disturbance [e.g., (Angelier et al., 2009; Dickens and Romero, 2013; Leshyk et al., 2013; Schultner et al., 2013)].
Aside from causing avoidance and increased mortality, noise can alter local populations by impacting reproductive success. This can occur through several mechanisms including decreased breeding rates and decreased juvenile recruitment, as well as altered resource allocation. For example, noise can reduce breeding rates through disrupting detection of potential mates [e.g., greater sage grouse (Blickley et al., 2012), ovenbirds, Seiurus aurocapilla (Habib et al., 2007)] and alter or reduce mating behavior [e.g., decreasing display, de Jong et al. (2018b)]. In a similar fashion to causing direct mortality and poor condition, noise can result in lower clutch size (Halfwerk et al., 2011), hatching success (Kleist et al., 2018), juvenile growth/condition (Lagardère, 1982; Kight et al., 2012), and juvenile survival (De Soto et al., 2013; Nedelec et al., 2017; de Jong et al., 2018a). Many of these effects are thought to be either a result of changes in parental behavior (e.g., increased aggression and decreased attention to young, Nedelec et al. (2017), increases in larval/juvenile mutation (Lagardère, 1982), and/or via direct or indirect interactions with elevated stress hormones resulting in higher mortality or poorer condition e.g., Silverin (1986); Injaian et al. (2018). In combination, this is especially damaging as populations under chronic stress are less likely to recover, due to increased mortality and decreased fitness of remaining adults. Fitness costs can fundamentally influence population dynamics; for instance, changes in breeding success can drastically alter population size and range by decreasing the numbers of new individuals coming into the population, and in dispersing species, potentially alter population range as a result of fewer individuals to disperse or lower survival whilst dispersing.
2.2. Indirect effects
Changes in one species’ local presence can have indirect consequences across trophic and taxonomic lines, especially if those relocating are predators or parasites. For example, while bird abundance and diversity decreased near chronic playback of traffic noise, grasshoppers and odonates decreased in areas far from the traffic noise, likely because their predators relocated to those places (Senzaki et al., 2020). Changes in the predator assemblage can alter both the communities they move to (increased/different predation pressure) and those they move from (lower/different predation pressure). They may alter the predator–prey relationships with third parties as predators may be forced to switch to uncommonly eaten prey or start eating prey they have never hunted before. Indirect effects of noise can also fundamentally alter the habitat entire species communities live in, for example, if important species like seed dispersers are impacted. By altering the presence of both pollinators and seed predators/dispersers, noise has been shown to alter both the tree and flower communities that are at the foundation of most terrestrial ecosystems (Francis et al., 2012; Phillips et al., 2021).
Noise can also change the interaction with other species in the community. It can alter predator—prey interactions, both through the increase of mortality by predation and through changes in foraging success. Similarly, it can change parasite–host interactions by affecting parasite species’ ability to find their hosts (Berkhout et al., 2023). By masking important cues (Siemers and Schaub, 2011; Mason et al., 2016; Senzaki et al., 2016; Jung et al., 2020) or reducing hearing abilities e.g., Wysocki and Ladich (2005); Ladich (2013); Kastelein et al. (2016), noise can reduce the distance before which a predator or prey is perceived, and likely impedes localization of a host (McMahon et al., 2017; Phillips et al., 2019). Noise can distract from responses to predator or prey, e.g., by increasing food handling and food discrimination errors (Purser and Radford, 2011; Shafiei Sabet et al., 2015), or by distracting from alarm signals in another sensory modality (Hasan et al., 2018).
Noise has both been shown to reduce responses to predation in some species (Simpson et al., 2015, 2016) but also to increase anti-predator behavior in others (Neo et al., 2014; Shannon et al., 2014, 2016a; Voellmy et al., 2014; van der Knaap et al., 2022). These changes in the effectiveness in which predators hunt their prey can have multiple outcomes. If predators in a noisy area preferentially switch to new or less commonly hunted prey as they become easier prey, this might exacerbate that prey’s local decline, while less drastically affected species may then be temporarily released from predation pressure. Therefore, the outcome of the changes in predator—prey interactions can only be predicted on a case-by-case basis. Furthermore, many of the studies that showed these effects only investigated short-term noise exposure, and might therefore have studied behavioral changes that fade over time.
3. Future directions
There is growing evidence that the impact of chronic noise on animals affects the composition of communities. Direct effects of chronic noise exposure on one species, such as population declines and long-term alterations in behavior, can lead to knock-on consequences for other species in the community. By differentially affecting species, chronic noise has the ability to drastically change community structure and function. Although the growing body of work on this topic is commendable, given the expensive and logistically complex nature of long-term studies, some key knowledge gaps remain. These gaps need to be addressed to ensure effective mitigation of chronic noise exposure. Below, we discuss the main gaps that we see, and suggest possible approaches to fill these.
There is a discrepancy in our understanding of how chronic noise affects different types of communities. The majority of the literature that we reviewed (90%, Table 1) investigated terrestrial ecosystems and diurnal species. While studying freshwater and marine ecosystems is more challenging than studying terrestrial systems, the effects of sound are likely to be different for aquatic communities. Sound propagates further in water than on land, so the same noise source will affect a larger area in the water. Furthermore, terrestrial species can often compensate for acoustic information loss by visual signals and perception. This option is less readily available for aquatic species, as light attenuates rapidly in water. Similarly, nocturnal communities might suffer larger impacts from chronic noise disturbance because of their dependence on acoustic signals.
To understand community impacts of noise properly, we must gain a better understanding of the mechanisms underlying species differences in response to the same noise source (Gunn et al., 2022). While in some instances there is a clear link between species traits and the impact of noise, in other cases this link has not yet been established. For instance, while the effect of chronic compressor noise on birds could be linked to the overlap between their vocalizations and the noise (Francis et al., 2009, 2011a), it is unknown why changes in fish anti-predator behavior in response to noise exposure vary from diminished responses to faster responses (Voellmy et al., 2014; Simpson et al., 2015, 2016; McCormick et al., 2018; Kok et al., 2021).
Besides species differences in response, changing species interactions are likely to be an important influence on community change. Parasites that cannot locate their hosts (McMahon et al., 2017; Phillips et al., 2019), predators that leave an area (Fröhlich and Ciach, 2019), seed dispersers that decline in abundance (Francis et al., 2012), all will directly impact the species that they are interacting with, whether or not that species is sensitive to noise itself. Because these interactions lead to indirect effects of noise on the community, the outcome may be difficult to predict. When a predator leaves, does that always lead to a release of predation pressure for the prey, or do other predators fill up the gap? Conversely, when the prey leaves, does the predator switch prey type, or does it follow the prey to the new area?
While a lot of the effects of chronic noise on communities have been uncovered with observational studies, understanding the mechanisms behind these effects will require long-term experimental studies. Current experimental studies focus mostly on short-term changes due to noise exposure, while long-term changes in species interactions are likely to have a more profound effect on the community. These long-term changes may be quite different from the changes observed on a short time scale (Kok et al., 2021). A decrease in foraging behavior, for example, might not persist over time, but might be compensated for when the noise stops. While long-term experimental exposure studies are expensive and logistically challenging, the recent developments in citizen science and technology have opened up possibilities that were previously unheard of, such as continental-scale analysis of acoustic niche partitioning in frogs (Allen-Ankins and Schwarzkopf, 2022) or biodiversity monitoring of insect communities for which many species are undescribed (van Klink et al., 2022). Additionally, existing differences in chronic noise exposure in natural settings can be exploited to study community effects, as has been done for the gas compressor stations in North America (Francis et al., 2009, 2011a,2011b,2012; Bunkley et al., 2017; Phillips et al., 2021).
Finally, to mitigate effects of chronic noise pollution effectively, we need to know what happens when the noise stops. In contrast to other forms of pollution, noise pollution does not leave long-term traces in the environment once removed. However, does the community immediately change back to pre-noise conditions once noise is removed, or is the changes that occurred due to the noise exposure permanent? The few studies that investigated this have shown mixed results. While white-crowned sparrows (Zonotrichia leucophrys) reverted back to low frequency songs (Derryberry et al., 2020) and spiny chromis (Acanthochromis polyacanthus) larvae had increased survival rates (Nedelec et al., 2022) in quieted conditions, juniper and pinyon seedling recruitment did not recover following the removal of noise (Phillips et al., 2021). The persistence of effects might depend on the time it takes for the effect to reverse, as well as the behavioral plasticity of the affected species. While the COVID-19 pandemic inadvertently created a natural experiment to test the effects of global quieting (Montgomery et al., 2021), we should also start experimentally removing noise from communities, to see if the effects of noise can truly be removed by noise mitigation. Those data will be vital in developing effective conservation strategies for the future.
Data availability statement
The original contributions presented in this study are included in the article/Supplementary material, further inquiries can be directed to the corresponding author.
Author contributions
AK, GS, MS, SS, NK, NC, and CW wrote the manuscript. GS, MS, and SS performed the literature research. NC made the figures. AK led the collaboration and guided the writing process. All authors critically reviewed the manuscript, conceived the idea behind the manuscript, approved the submitted version, and revised the manuscript.
Funding
This manuscript was conceived during an interdisciplinary workshop entitled “Allying Conservation & Welfare Research on the Impacts of Human-Generated Sounds on Wild & Captive Animals” funded by the Association for the Study of Animal Behaviour. The funding for the publication of this manuscript was provided by the Canadian Wildlife Service, Environment and Climate Change Canada. During the writing of this manuscript NC was supported by two grants: Japan Society for the Promotion of Science no. PE21015 and Mitacs Accelerate IT29508.
Acknowledgments
We thank the organizers of the workshop for bringing us together and providing a platform for new ideas. We also thank Patrick O’Hara and the Canadian Wildlife Service, Environment and Climate Change Canada for the funds to publish this manuscript.
Conflict of interest
The authors declare that the research was conducted in the absence of any commercial or financial relationships that could be construed as a potential conflict of interest.
Publisher’s note
All claims expressed in this article are solely those of the authors and do not necessarily represent those of their affiliated organizations, or those of the publisher, the editors and the reviewers. Any product that may be evaluated in this article, or claim that may be made by its manufacturer, is not guaranteed or endorsed by the publisher.
Supplementary material
The Supplementary Material for this article can be found online at: https://www.frontiersin.org/articles/10.3389/fevo.2023.1130075/full#supplementary-material
References
Allen-Ankins, S., and Schwarzkopf, L. (2022). Using citizen science to test for acoustic niche partitioning in frogs. Sci. Rep. 12, 1–7. doi: 10.1038/s41598-022-06396-0
Anderson, P. A., Berzins, I. K., Fogarty, F., Hamlin, H. J., and Guillette, L. J. (2011). Sound, stress, and seahorses: The consequences of a noisy environment to animal health. Aquaculture 311, 129–138. doi: 10.1016/j.aquaculture.2010.11.013
Angelier, F., Clément-Chastel, C., Welcker, J., Gabrielsen, G. W., and Chastel, O. (2009). How does corticosterone affect parental behaviour and reproductive success? A study of prolactin in black-legged kittiwakes. Funct. Ecol. 23, 784–793. doi: 10.1111/j.1365-2435.2009.01545.x
Austad, S. N. (2010). Cats, “Rats,” and Bats: The comparative biology of aging in the 21st century. Integr. Comp. Biol. 50, 783–792. doi: 10.1093/icb/icq131
Barber, J. R., Crooks, K. R., and Fristrup, K. M. (2010). The costs of chronic noise exposure for terrestrial organisms. Trends Ecol. Evol. 25, 180–189. doi: 10.1016/j.tree.2009.08.002
Bayne, E. M., Habib, L., and Boutin, S. (2008). Impacts of chronic anthropogenic noise from energy-sector activity on abundance of songbirds in the boreal forest. Conserv. Biol. 22, 1186–1193. doi: 10.1111/j.1523-1739.2008.00973.x
Berkhout, B. W., Budria, A., Thieltges, D. W., and Slabbekoorn, H. (2023). Anthropogenic noise pollution and wildlife diseases. Trends Parasitol. 39, 181–190. doi: 10.1016/j.pt.2022.12.002
Bernaldo de Quirós, Y., Fernandez, A., Baird, R. W., Brownell, R. L., Aguilar de Soto, N., et al. (2019). Advances in research on the impacts of anti-submarine sonar on beaked whales. Proc. Biol. Sci. 286:20182533. doi: 10.1098/rspb.2018.2533
Blickley, J. L., Blackwood, D., and Patricelli, G. L. (2012). Experimental evidence for the effects of chronic anthropogenic noise on abundance of greater sage-grouse at leks. Conserv. Biol. 26, 461–471. doi: 10.1111/j.1523-1739.2012.01840.x
Bunkley, J. P., McClure, C. J. W., Kawahara, A. Y., Francis, C. D., and Barber, J. R. (2017). Anthropogenic noise changes arthropod abundances. Ecol. Evol. 7, 2977–2985. doi: 10.1002/ece3.2698
Buxton, R. T., McKenna, M. F., Mennitt, D., Fristrup, K., Crooks, K., Angeloni, L., et al. (2017). Noise pollution is pervasive in U.S. protected areas. Science 356, 531–533. doi: 10.1126/science.aah4783
Carral-Murrieta, C. O., García-Arroyo, M., Marín-Gómez, O. H., Sosa-López, J. R., and Macgregor-Fors, I. (2020). Noisy environments: Untangling the role of anthropogenic noise on bird species richness in a Neotropical City. Avian Res. 11:32. doi: 10.1186/s40657-020-00218-5
Ciach, M., and Fröhlich, A. (2017). Habitat type, food resources, noise and light pollution explain the species composition, abundance and stability of a winter bird assemblage in an urban environment. Urban Ecosyst. 20, 547–559. doi: 10.1007/s11252-016-0613-6
Cimprich, D. A., and Grubb Jnr, T. C. (1994). Consequences for Carolina chickadees of foraging with tufted titmice in winter. Ecology 75, 1615–1625. doi: 10.2307/1939622
Cinto Mejia, E., McClure, C. J. W., and Barber, J. R. (2019). Large-scale manipulation of the acoustic environment can alter the abundance of breeding birds: Evidence from a phantom natural gas field. J. Appl. Ecol. 56, 2091–2101. doi: 10.1111/1365-2664.13449
Curry, C. M., Des Brisay, P. G., Rosa, P., and Koper, N. (2018). Noise source and individual physiology mediate effectiveness of bird songs adjusted to anthropogenic noise. Sci. Rep. 8:3942. doi: 10.1038/s41598-018-22253-5
de Jong, K., Amorim, M. C. P., Fonseca, P. J., and Heubel, K. U. (2018b). Noise affects multimodal communication during courtship in a marine fish. Front. Ecol. Evol. 6:113. doi: 10.3389/fevo.2018.00113
de Jong, K., Amorim, M. C. P., Fonseca, P. J., Fox, C. J., and Heubel, K. U. (2018a). Noise can affect acoustic communication and subsequent spawning success in fish. Environ. Pollut. 237, 814–823. doi: 10.1016/j.envpol.2017.11.003
De Soto, N. A., Delorme, N., Atkins, J., Howard, S., Williams, J., and Johnson, M. (2013). Anthropogenic noise causes body malformations and delays development in marine larvae. Sci. Rep. 3:2831. doi: 10.1038/srep02831
Derryberry, E. P., Phillips, J. N., Derryberry, G. E., Blum, M. J., and Luther, D. (2020). Singing in a silent spring: Birds respond to a half-century soundscape reversion during the COVID-19 shutdown. Science 370, 575–579. doi: 10.1126/science.abd5777
Dickens, M. J., and Romero, L. M. (2013). A consensus endocrine profile for chronically stressed wild animals does not exist. Gen. Comp. Endocrinol. 191, 177–189. doi: 10.1016/j.ygcen.2013.06.014
Dolby, A. S., and Grubb, T. C. (1998). Benefits to satellite members in mixed-species foraging groups: An experimental analysis. Anim. Behav. 56, 501–509. doi: 10.1006/anbe.1998.0808
Duarte, C. M., Chapuis, L., Collin, S. P., Costa, D. P., Devassy, R. P., Eguiluz, V. M., et al. (2021). The soundscape of the Anthropocene ocean. Science 371:eaba4658. doi: 10.1126/science.aba4658
Ellenberg, U., Setiawan, A. N., Cree, A., Houston, D. M., and Seddon, P. J. (2007). Elevated hormonal stress response and reduced reproductive output in yellow-eyed penguins exposed to unregulated tourism. Gen. Comp. Endocrinol. 152, 54–63. doi: 10.1016/j.ygcen.2007.02.022
Fernández, A., Edwards, J. F., Rodríguez, F., Espinosa de los Monteros, A., Herráez, P., Castro, P., et al. (2005). Gas and fat embolic syndrome”’ involving a mass stranding of beaked whales (family ziphiidae) exposed to anthropogenic sonar signals. Vet. Pathol. 42, 446–457. doi: 10.1354/vp.42-4-446
Francis, C. D., and Barber, J. R. (2013). A framework for understanding noise impacts on wildlife: An urgent conservation priority. Front. Ecol. Environ. 11, 305–313. doi: 10.1890/120183
Francis, C. D., Kleist, N. J., Ortega, C. P., and Cruz, A. (2012). Noise pollution alters ecological services: Enhanced pollination and disrupted seed dispersal. Proc. Biol. Sci. 279, 2727–2735. doi: 10.1098/rspb.2012.0230
Francis, C. D., Ortega, C. P., and Cruz, A. (2009). Noise pollution changes avian communities and species interactions. Curr. Biol. 19, 1415–1419. doi: 10.1016/j.cub.2009.06.052
Francis, C. D., Ortega, C. P., and Cruz, A. (2011a). Noise pollution filters bird communities based on vocal frequency. PLoS One 6:e27052. doi: 10.1371/journal.pone.0027052
Francis, C. D., Paritsis, J., Ortega, C. P., and Cruz, A. (2011b). Landscape patterns of avian habitat use and nest success are affected by chronic gas well compressor noise. Landsc. Ecol. 26, 1269–1280. doi: 10.1007/s10980-011-9609-z
Fröhlich, A., and Ciach, M. (2019). Nocturnal noise and habitat homogeneity limit species richness of owls in an urban environment. Environ. Sci. Pollut. Res. 26, 17284–17291. doi: 10.1007/s11356-019-05063-8
Goodwin, S. E., and Shriver, W. G. (2011). Effects of traffic noise on occupancy patterns of forest birds. Conserv. Biol. 25, 406–411. doi: 10.1111/j.1523-1739.2010.01602.x
Goutte, A., Angelier, F., Welcker, J., Moe, B., Clément-Chastel, C., Gabrielsen, G. W., et al. (2010). Long-term survival effect of corticosterone manipulation in black-legged kittiwakes. Gen. Comp. Endocrinol. 167, 246–251. doi: 10.1016/j.ygcen.2010.03.018
Grunst, M. L., Grunst, A. S., Pinxten, R., and Eens, M. (2021). Variable and consistent traffic noise negatively affect the sleep behavior of a free-living songbird. Sci. Total Environ. 778:146338. doi: 10.1016/j.scitotenv.2021.146338
Gunn, R. L., Hartley, I. R., Algar, A. C., Niemelä, P. T., and Keith, S. A. (2022). Understanding behavioural responses to human-induced rapid environmental change: A meta-analysis. Oikos 2022:4. doi: 10.1111/oik.08366
Habib, L., Bayne, E. M., and Boutin, S. (2007). Chronic industrial noise affects pairing success and age structure of ovenbirds Seiurus aurocapilla. J. Appl. Ecol. 44, 176–184. doi: 10.1111/j.1365-2664.2006.01234.x
Halfwerk, W., Holleman, L. J. M., Lessells, C. M., and Slabbekoorn, H. (2011). Negative impact of traffic noise on avian reproductive success. J. Appl. Ecol. 48, 210–219. doi: 10.1111/j.1365-2664.2010.01914.x
Halvorsen, M. B., Casper, B. M., Matthews, F., Carlson, T. J., and Popper, A. N. (2012). Effects of exposure to pile-driving sounds on the lake sturgeon, Nile tilapia and hogchoker. Proc. Biol. Sci. 279, 4705–4714. doi: 10.1098/rspb.2012.1544
Hammer, M. S., Swinburn, T. K., and Neitzel, R. L. (2014). Environmental noise pollution in the United States: Developing an effective public health response. Environ. Health Perspect. 122, 115–119. doi: 10.1289/ehp.1307272
Hasan, M. R., Crane, A. L., Ferrari, M. C. O., and Chivers, D. P. (2018). A cross-modal effect of noise: The disappearance of the alarm reaction of a freshwater fish. Anim. Cogn. 21, 419–424. doi: 10.1007/s10071-018-1179-x
Herrera-Montes, M. I., and Aide, T. M. (2011). Impacts of traffic noise on anuran and bird communities. Urban Ecosyst. 14, 415–427. doi: 10.1007/s11252-011-0158-7
Injaian, A. S., Taff, C. C., Pearson, K. L., Gin, M. M. Y., Patricelli, G. L., and Vitousek, M. N. (2018). Effects of experimental chronic traffic noise exposure on adult and nestling corticosterone levels, and nestling body condition in a free-living bird. Horm. Behav. 106, 19–27. doi: 10.1016/j.yhbeh.2018.07.012
Jenkins, A. K., Dahl, P. H., Kotecki, S. E., Bowman, V., Casper, B., Boerger, C., et al. (2022). Physical effects of sound exposure from underwater explosions on Pacific mackerel (Scomber japonicus): Effects on non-auditory tissues. J. Acoust. Soc. Am. 151, 3947–3956. doi: 10.1121/10.0011587
Jerem, P., and Mathews, F. (2021). Trends and knowledge gaps in field research investigating effects of anthropogenic noise. Conserv. Biol. 35, 115–129. doi: 10.1111/cobi.13510
Jung, H., Sherrod, A., LeBreux, S., Price, J. M., and Freeberg, T. M. (2020). Traffic noise and responses to a simulated approaching avian predator in mixed-species flocks of chickadees, titmice, and nuthatches. Ethology 126, 620–629. doi: 10.1111/eth.13013
Kastelein, R. A., Hoek, L., and Gransier, R. (2016). “The cumulative effects of exposure to continuous and intermittent sounds on temporary hearing threshold shifts induced in a harbor porpoise (Phocoena phocoena),” in The effects of noise on aquatic life II Advances in experimental medicine and biology, eds A. N. Popper and A. Hawkins (Hungary: Springer Science + Business Media), 523–528. 93 doi: 10.1007/978-1-4939-2981-8
Kight, C. R., Saha, M. S., and Swaddle, J. P. (2012). Anthropogenic noise is associated with reductions in the productivity of breeding Eastern Bluebirds (Sialia sialis). Ecol. Appl. 22, 1989–1996. doi: 10.1890/12-0133.1
Kleist, N. J., Guralnick, R. P., Cruz, A., Lowry, C. A., and Francis, C. D. (2018). Chronic anthropogenic noise disrupts glucocorticoid signaling and has multiple effects on fitness in an avian community. Proc. Natl. Acad. Sci. U.S.A. 115, E648–E657. doi: 10.1073/pnas.1709200115
Kok, A. C. M., van Hulten, D., Timmerman, K. H., Lankhorst, J., Visser, F., and Slabbekoorn, H. (2021). Interacting effects of short-term and long-term noise exposure on antipredator behaviour in sand gobies. Anim. Behav. 172, 93–102. doi: 10.1016/j.anbehav.2020.12.001
Kunc, H. P., and Schmidt, R. (2019). The effects of anthropogenic noise on animals: A meta-analysis. Biol. Lett. 15:20190649. doi: 10.1098/rsbl.2019.0649
Ladich, F. (2013). “Effects of noise on sound detection and acoustic communication in fishes,” in Animal communication and noise, ed. K. Brumm (Heidelberg: Springer), 65–90.
Lagardère, J. P. (1982). Effects of noise on growth and reproduction of Crangon crangon in rearing tanks. Mar. Biol. 71, 177–185.
Lecocq, T., Hicks, S. P., Van Noten, K., van Wijk, K., Koelemeijer, P., M De Plaen, R. S., et al. (2020). Global quieting of high-frequency seismic noise due to COVID-19 pandemic lockdown measures. Science 369, 1338–1343. doi: 10.1126/science.abd2438
Leshyk, R., Nol, E., Chin, E. H., and Burness, G. (2013). Adult Ovenbirds (Seiurus aurocapilla) show increased stress-responsiveness in logged forests. Gen. Comp. Endocrinol. 194, 295–299. doi: 10.1016/j.ygcen.2013.10.001
Mason, J. T., McClure, C. J. W., and Barber, J. R. (2016). Anthropogenic noise impairs owl hunting behavior. Biol. Conserv. 199, 29–32. doi: 10.1016/j.biocon.2016.04.009
Matheson, M. P., Stansfeld, S. A., and Haines, M. M. (2003). The effects of chronic aircraft noise exposure on children’s cognition and health: 3 field studies. Noise Health 5, 31–40.
McClure, C. J. W., Ware, H. E., Carlisle, J. D., and Barber, J. R. (2017). Noise from a phantom road experiment alters the age structure of a community of migrating birds. Anim. Conserv. 20, 164–172. doi: 10.1111/acv.12302
McClure, C. J. W., Ware, H. E., Carlisle, J., Kaltenecker, G., and Barber, J. R. (2013). An experimental investigation into the effects of traffic noise on distributions of birds: Avoiding the phantom road. Proc. Biol. Sci. 280:20132290. doi: 10.1098/rspb.2013.2290
McCormick, M. I., Allan, B. J. M., Harding, H. R., and Simpson, S. D. (2018). Boat noise impacts risk assessment in a coral reef fish but effects depend on engine type. Sci. Rep. 8:3847. doi: 10.1038/s41598-018-22104-3
McKenna, M. F., Shannon, G., and Fristrup, K. (2016). Characterizing anthropogenic noise to improve understanding and management of impacts to wildlife. Endanger. Species Res. 31, 279–291. doi: 10.3354/esr00760
McMahon, T. A., Rohr, J. R., and Bernal, X. E. (2017). Light and noise pollution interact to disrupt interspecific interactions. Ecology 98, 1290–1299. doi: 10.1002/ecy.1770
Mennitt, D. J., and Fristrup, K. M. (2016). Influential factors and spatiotemporal patterns of environmental sound levels. Noise Control Eng. J. 64, 342–353.
Mohamed, A.-M. O., Paleologos, E. K., and Howari, F. M. (2021). “Noise pollution and its impact on human health and the environment,” in Pollution assessment for sustainable practices in applied sciences and engineering, eds A. O. Mohamed, E. K. Paleologos, and F. M. Howari (Amsterdam: Elsevier), 975–1026. doi: 10.1016/B978-0-12-809582-9.00019-0
Montgomery, R. A., Raupp, J., and Parkhurst, M. (2021). Animal behavioral responses to the COVID-19 quietus. Trends Ecol. Evol. 36, 184–186. doi: 10.1016/j.tree.2020.12.008
Morton, A. B., and Symonds, H. K. (2002). Displacement of Orcinus orca (L.) by high amplitude sound in British Columbia, Canada. ICES J. Mar. Sci. 59, 71–80. doi: 10.1006/jmsc.2001.1136
National Academies of Sciences, Engineering and Medicine (2011). Hydroacoustic impacts on fish from pile installation. Washington, DC: National Academies Press. doi: 10.17226/14596
Nedelec, S. L., Radford, A. N., Gatenby, P., Davidson, I. K., Velasquez Jimenez, L., Travis, M., et al. (2022). Limiting motorboat noise on coral reefs boosts fish reproductive success. Nat. Commun. 13:2822. doi: 10.1038/s41467-022-30332-5
Nedelec, S. L., Radford, A. N., Pearl, L., Nedelec, B., McCormick, M. I., Meekan, M. G., et al. (2017). Motorboat noise impacts parental behaviour and offspring survival in a reef fish. Proc. Biol. Sci. 284:20170143. doi: 10.1098/rspb.2017.0143
Nenninger, H. R., and Koper, N. (2018). Effects of conventional oil wells on grassland songbird abundance are caused by presence of infrastructure, not noise. Biol. Conserv. 218, 124–133. doi: 10.1016/j.biocon.2017.11.014
Neo, Y. Y., Seitz, J., Kastelein, R. A., Winter, H. V., ten Cate, C., and Slabbekoorn, H. (2014). Temporal structure of sound affects behavioural recovery from noise impact in European seabass. Biol. Conserv. 178, 65–73. doi: 10.1016/j.biocon.2014.07.012
Nicholson, M., Rackham, B., and Miston, R. (1992). “Measuring the effect of underwater radiated noise on trawl catches,” in Proceedings of the ICES FTFB and FAST joint working group meeting (Bergen: ICES).
Pearson, W. H., Skalski, J. R., and Malme, C. I. (1992). Effects of sounds from a geophysical survey device on behavior of captive rockfish (Sebastes spp.). Can. J. Fish. Aquat. Sci. 49, 1343–1356. doi: 10.1139/f92-150
Phillips, J. N., Ruef, S. K., Garvin, C. M., Le, M. L. T., and Francis, C. D. (2019). Background noise disrupts host–parasitoid interactions. R. Soc. Open Sci. 6:190867. doi: 10.1098/rsos.190867
Phillips, J. N., Termondt, S. E., and Francis, C. D. (2021). Long-term noise pollution affects seedling recruitment and community composition, with negative effects persisting after removal. Proc. Biol. Sci. 288:20202906. doi: 10.1098/rspb.2020.2906
Popper, A. N., and Hawkins, A. D. (2019). An overview of fish bioacoustics and the impacts of anthropogenic sounds on fishes. J. Fish. Biol. 94, 692–713. doi: 10.1111/jfb.13948
Pravosudov, V. V., Kitaysky, A. S., Wingfield, J. C., and Clayton, N. S. (2001). Long-term unpredictable foraging conditions and physiological stress response in mountain chickadees (Poecile gambeli). Gen. Comp. Endocrinol. 123, 324–331. doi: 10.1006/gcen.2001.7684
Purser, J., and Radford, A. N. (2011). Acoustic noise induces attention shifts and reduces foraging performance in three-spined sticklebacks (gasterosteus aculeatus). PLoS One 6:e17478. doi: 10.1371/journal.pone.0017478
Reijnen, R., and Foppen, R. (1995). The effects of car traffic on breeding bird populations in Woodland. IV. Influence of population size on the reduction of density close to a highway. J. Appl. Ecol. 32, 481–491.
Reijnen, R., Foppen, R., and Meeuwsen, H. (1996). The effects of traffic on the density of breeding birds in Dutch agricultural grasslands. Biol. Conserv. 75, 255–260.
Reijnen, R., Foppen, R., and Thissen, J. (1995). The effects of car traffic on breeding bird populations in Woodland. III. Reduction of density in relation to the proximity of main roads. J. Appl. Ecol. 32, 187–202.
Rosa, P., and Koper, N. (2022). Impacts of oil well drilling and operating noise on abundance and productivity of grassland songbirds. J. Appl. Ecol. 59, 574–584. doi: 10.1111/1365-2664.14075
Schultner, J., Kitaysky, A. S., Gabrielsen, G. W., Hatch, S. A., and Bech, C. (2013). Differential reproductive responses to stress reveal the role of life-history strategies within a species. Proc. Biol. Sci. 280:20132090. doi: 10.1098/rspb.2013.2090
Senzaki, M., Kadoya, T., and Francis, C. D. (2020). Direct and indirect effects of noise pollution alter biological communities in and near noise-exposed environments. Proc. Biol. Sci. 287:20200176. doi: 10.1098/rspb.2020.0176
Senzaki, M., Yamaura, Y., Francis, C. D., and Nakamura, F. (2016). Traffic noise reduces foraging efficiency in wild owls. Sci. Rep. 6:30602. doi: 10.1038/srep30602
Shafiei Sabet, S., Neo, Y. Y., and Slabbekoorn, H. (2015). The effect of temporal variation in sound exposure on swimming and foraging behaviour of captive zebrafish. Anim. Behav. 107, 49–60. doi: 10.1016/j.anbehav.2015.05.022
Shafiei Sabet, S., Van Dooren, D., and Slabbekoorn, H. (2016). Son et lumière: Sound and light effects on spatial distribution and swimming behavior in captive zebrafish. Environ. Pollut. 212, 480–488. doi: 10.1016/j.envpol.2016.02.046
Shannon, G., Angeloni, L. M., Wittemyer, G., Fristrup, K. M., and Crooks, K. R. (2014). Road traffic noise modifies behaviour of a keystone species. Anim. Behav. 94, 135–141. doi: 10.1016/j.anbehav.2014.06.004
Shannon, G., McKenna, M. F., Angeloni, L. M., Crooks, K. R., Fristrup, K. M., Brown, E., et al. (2016b). A synthesis of two decades of research documenting the effects of noise on wildlife. Biol. Rev. Camb. Philos. Soc. 91, 982–1005. doi: 10.1111/brv.12207
Shannon, G., Crooks, K. R., Wittemyer, G., Fristrup, K. M., and Angeloni, L. M. (2016a). Road noise causes earlier predator detection and flight response in a free-ranging mammal. Behav. Ecol. 27, 1370–1375. doi: 10.1093/beheco/arw058
Siemers, B. M., and Schaub, A. (2011). Hunting at the highway: Traffic noise reduces foraging efficiency in acoustic predators. Proc. Biol. Sci. 278, 1646–1652. doi: 10.1098/rspb.2010.2262
Sieving, K. E., Contreras, T. A., and Maute, K. L. (2004). Heterospecific facilitation of forest-boundary crossing by mobbing understory birds in North-Central Florida. Auk 121, 738–751.
Silverin, B. (1986). Corticosterone-Binding proteins and behavioral effects of hi plasma levels of corticosterone during the breeding period in t pied flycatcher. Gen. Comp. Endocrinol. 64, 67–74. doi: 10.1016/0016-6480(86)90029-8
Simpson, S. D., Purser, J., and Radford, A. N. (2015). Anthropogenic noise compromises antipredator behaviour in European eels. Glob. Chang. Biol. 21, 586–593. doi: 10.1111/gcb.12685
Simpson, S. D., Radford, A. N., Nedelec, S. L., Ferrari, M. C. O., Chivers, D. P., McCormick, M. I., et al. (2016). Anthropogenic noise increases fish mortality by predation. Nat. Commun. 7:10544. doi: 10.1038/ncomms10544
Sims, C. G., and Holberton, R. L. (2000). Development of the corticosterone stress response in young Northern Mockingbirds (Mimus polyglottos). Gen. Comp. Endocrinol. 119, 193–201. doi: 10.1006/gcen.2000.7506
Slabbekoorn, H., Bouton, N., van Opzeeland, I., Coers, A., ten Cate, C., and Popper, A. N. (2010). A noisy spring: The impact of globally rising underwater sound levels on fish. Trends Ecol. Evol. 25, 419–427. doi: 10.1016/j.tree.2010.04.005
Slotte, A., Hansen, K., Dalen, J., and Ona, E. (2004). Acoustic mapping of pelagic fish distribution and abundance in relation to a seismic shooting area off the Norwegian west coast. Fish Res. 67, 143–150. doi: 10.1016/j.fishres.2003.09.046
Sordello, R., Ratel, O., de Lachapelle, F. F., Leger, C., Dambry, A., and Vanpeene, S. (2020). Evidence of the impact of noise pollution on biodiversity: A systematic map. Environ. Evid. 9, 1–27. doi: 10.1186/s13750-020-00202-y
Stansfeld, S. A., and Matheson, M. P. (2003). Noise pollution: Non-auditory effects on health. Br. Med. Bull. 68, 243–257. doi: 10.1093/bmb/ldg033
Summers, P. D., Cunnington, G. M., and Fahrig, L. (2011). Are the negative effects of roads on breeding birds caused by traffic noise? J. Appl. Ecol. 48, 1527–1534. doi: 10.1111/j.1365-2664.2011.02041.x
Swaddle, J. P., Francis, C. D., Barber, J. R., Cooper, C. B., Kyba, C. C. M., Dominoni, D. M., et al. (2015). A framework to assess evolutionary responses to anthropogenic light and sound. Trends Ecol. Evol. 30, 550–560. doi: 10.1016/j.tree.2015.06.009
Szalma, J. L., and Hancock, P. A. (2011). Noise effects on human performance: A meta-analytic synthesis. Psychol. Bull. 137, 682–707. doi: 10.1037/a0023987
Thompson, P. M., Lusseau, D., Barton, T. R., Simmons, D., Rusin, J., and Bailey, H. (2010). Assessing the responses of coastal cetaceans to the construction of offshore wind turbines. Mar. Pollut. Bull. 60, 1200–1208. doi: 10.1016/j.marpolbul.2010.03.030
Troïanowski, M., Mondy, N., Dumet, A., Arcanjo, C., and Lengagne, T. (2017). Effects of traffic noise on tree frog stress levels, immunity, and color signaling. Conserv. Biol. 31, 1132–1140. doi: 10.1111/cobi.12893
van der Knaap, I., Ashe, E., Hannay, D., Bergman, A. G., Nielsen, K. A., Lo, C. F., et al. (2022). Behavioural responses of wild Pacific salmon and herring to boat noise. Mar Pollut Bull 174:113257. doi: 10.1016/j.marpolbul.2021.113257
van Klink, R., August, T., Bas, Y., Bodesheim, P., Bonn, A., Fossøy, F., et al. (2022). Emerging technologies revolutionise insect ecology and monitoring. Trends Ecol. Evol. 37, 872–885. doi: 10.1016/j.tree.2022.06.001
Voellmy, I. K., Purser, J., Simpson, S. D., and Radford, A. N. (2014). Increased noise levels have different impacts on the anti-predator behaviour of two sympatric fish species. PLoS One 9:e102946. doi: 10.1371/journal.pone.0102946
Voellmy, I. K., Purser, J., Simpson, S. D., and Radford, A. N. (2016). “Effects of previous acoustic experience on behavioral responses to experimental sound stimuli and implications for research,” in The effects of noise on aquatic life II, Advances in experimental medicine and biology, eds A. N. Popper and A. Hawkins (New York, NY: Springer), 1191–1196. doi: 10.1007/978-1-4939-2981-8_149
Ware, H. E., McClure, C. J. W., Carlisle, J. D., Barber, J. R., and Daily, G. C. (2015). A phantom road experiment reveals traffic noise is an invisible source of habitat degradation. Proc. Natl. Acad. Sci. U.S.A. 112, 12105–12109. doi: 10.1073/pnas.1504710112
Wingfield, J. C., and Kitaysky, A. S. (2002). Endocrine responses to unpredictable environmental events: Stress or anti-stress hormones? Integ. Comp. Biol. 42, 600–609.
Wingfield, J. C., Maney, D. L., Breuner, C. W., Jacobs, J. D., Lynn, S., Ramenofsky, M., et al. (1998). Ecological bases of hormone-behavior interactions: The “emergency life history stage” 1. Amer. Zool. 38, 191–206.
Keywords: anthropogenic noise, chronic, community, behavior, ecology
Citation: Kok ACM, Berkhout BW, Carlson NV, Evans NP, Khan N, Potvin DA, Radford AN, Sebire M, Shafiei Sabet S, Shannon G and Wascher CAF (2023) How chronic anthropogenic noise can affect wildlife communities. Front. Ecol. Evol. 11:1130075. doi: 10.3389/fevo.2023.1130075
Received: 22 December 2022; Accepted: 15 March 2023;
Published: 05 April 2023.
Edited by:
Jarle Tryti Nordeide, Nord University, NorwayReviewed by:
Maria Clara P. Amorim, University of Lisbon, PortugalAnn Bowles, Hubbs-SeaWorld Research Institute, United States
Copyright © 2023 Kok, Berkhout, Carlson, Evans, Khan, Potvin, Radford, Sebire, Shafiei Sabet, Shannon and Wascher. This is an open-access article distributed under the terms of the Creative Commons Attribution License (CC BY). The use, distribution or reproduction in other forums is permitted, provided the original author(s) and the copyright owner(s) are credited and that the original publication in this journal is cited, in accordance with accepted academic practice. No use, distribution or reproduction is permitted which does not comply with these terms.
*Correspondence: Annebelle C. M. Kok, YS5jLm0ua29rQHJ1Zy5ubA==