- 1IGEPP, INRAE, Institut Agro, University Rennes, Rennes, France
- 2Innolea, Mondonville, France
Introduction: Animal nutritional strategies have been extensively studied in vertebrates, where generalism at the individual scale is the rule. In insect herbivores, the determinants of the nutritional strategy of individual-scale generalists remain poorly studied, and the focus has been placed mainly on the influence of plant defense. Moreover, the integration of a physiological dimension in such studies remains rare. Here, we investigated the determinants of the nutritional strategy of pre-diapausing pollen beetles, Brassicogethes aeneus, with a focus on the influence of macronutrients. Before their diapause, pollen beetles are known to feed from plants belonging to many different families. This raises three questions: (i) Is the generalism of pollen beetles a populational consequence of individuals specialized on different plant families? (ii) Do individuals feed at random on flowers available or do they have a particular nutritional strategy? and (iii) In case of non-random feeding choices, do pollen macronutrients explain this nutritional strategy?
Methods: To answer these questions, we used a series of laboratory experiments including feeding choice tests on flowers and artificial substrates, quantification of pollen nutrient content, quantification of the insect energetic budget, and performance experiments.
Results: We show that pollen beetles are generalist at the individual scale, and that clear and stable food preferences are established over a few hours in a multi-choice context. Pollen beetles prefer to feed on flowers with a carbohydrate-rich pollen, and this preference is adaptive since performance correlates positively with the plant carbohydrate content. This better performance may be explained by the fact that individuals feeding on carbohydrate-rich resources accumulate more glycogen and total energetic reserves.
Discussion: This study represents one of the few evidences of generalism at the individual scale in an herbivorous insect. It provides a better understanding of the nutritional strategy of a non-bee pollen feeder and shows the importance of carbohydrates in this strategy. It highlights the need to combine assessments of the plant macronutrient content and insect energetic budget in an adaptive framework to better understand the nutritional strategies of herbivores.
Introduction
Animals have evolved different strategies to exploit their food sources, ranging from strict specialism (i.e., feeding on a narrow range of resources) to generalism (i.e., feeding on a broad range of resources) (Futuyma and Moreno, 1988). Generalist species logically benefit from higher opportunities to find food sources compared to specialists (Bernays and Minkenberg, 1997; Richter and Cumming, 2006). The mixing of a variety of food items may be a strategy to dilute the intake of toxins (Freeland and Janzen, 1974) and to counterbalance nutritional deficiencies with complementary food sources (Pulliam, 1975; Westoby, 1978). However, Fox and Morrow (1981) and Bernays and Minkenberg (1997) drew attention to the fact that generalist species can be composed of specialized populations or individuals, and that a distinction should be made between generalist populations composed of specialist individuals and generalist populations composed of generalist individuals. Since the benefits of generalism are obviously not relevant for generalist populations composed of specialist individuals, this distinction appears crucial when deciphering nutritional strategies of generalist species.
These strategies have been extensively studied in vertebrates, where generalism at the individual scale is the rule (Bernays and Minkenberg, 1997). Indeed, examples of such generalist feeding strategies at the individual scale can be found in mammals (Wiggins et al., 2006; Cui et al., 2018; Takahashi et al., 2021; Windley et al., 2022), birds (Fargallo et al., 2020; Cady et al., 2021; Oortwijn et al., 2022), reptiles (Figueras et al., 2021), and fishes (Yeager et al., 2014; Laske et al., 2018). In herbivorous insects, however, where most species are specialists (Bernays and Graham, 1988), feeding strategies of generalist species have received much less attention. Among generalist herbivorous insects, most of which are pollinators (Fontaine et al., 2009), only nutritional strategies of bees have been thoroughly studied (Bonoan et al., 2020; Ghosh et al., 2020; Vaudo et al., 2020) while it is known that non-bee hymenopterans, as well as many dipterans, lepidopterans and coleopterans, are important pollen feeders too (Rader et al., 2020). Therefore, we lack studies aiming at deciphering nutritional strategies of the vast diversity of non-bee generalist pollen feeders.
Since generalist herbivorous insects have long been considered less able than specialists to deal with plant defenses, most studies on the nutritional strategies of generalists have focused on the influence of these defenses on the host selection process (Schoonhoven et al., 2005; Lankau, 2007; Kaplan et al., 2014; Brochu et al., 2020). By contrast, much less is known about the contribution of macronutrients to this process. However, a great interspecific heterogeneity exists in the nutrient content of plants (Ojima and Isawa, 1968; Ryder et al., 2021; Paź-Dyderska et al., 2022; Venjakob et al., 2022) and insects’ feeding preferences and performance can vary in response to such variation (Raubenheimer and Simpson, 1997; Schoonhoven et al., 1998; Wetzel et al., 2016; Vaudo et al., 2020). It has even been suggested that a low macronutrient content may act as a defense against insects, by reducing survival or fecundity and by increasing exposure to natural enemies as a result of a prolonged development (Berenbaum, 1995). Thus, the same coevolutionary process as observed with plant defenses might occur with plant macronutrients (Moran and Hamilton, 1980; Lundberg and Astrom, 1990; Augner, 1995; Berenbaum, 1995) and considering their content in plants is critical to understand host plant selection processes by generalist herbivorous insects.
The relationship between the plant macronutrient content and the insect fitness is a matter of physiology, covering digestion, storage, metabolism and usage of these nutrients and their by-products (Behmer, 2009). Central to this physiology and its study is the energetic budget of the insect. An insect energetic budget can be divided into four major categories of stored molecules, namely free sugars, glycogen, triglycerides, and proteins (Rivero and Casas, 1999). Free sugars can serve as direct energy (Beenakkers et al., 1984; Weber, 2011), glycogen and triglycerides as long-term energy that can be used in case of food deprivation (Arrese and Soulages, 2010), and proteins as energy available for a diversity of physiological processes (Gilbert, 1972; Telfer and Kunkel, 1991; Hahn and Denlinger, 2007). The geometric framework developed by Simpson and Raubenheimer (1993), which allows modeling nutritional strategies in a geometric space where food items are placed mostly depending on their nutrient content, has proven to be an effective tool to discover behavioral adaptations of multiple species. However, it does not integrate the physiological dimension of these strategies while it could give a greater realism and lead to a more complete picture of nutritional strategies in animals.
In this study, we decipher the determinants of the nutritional strategy of a generalist non-bee pollen feeder, the pollen beetle (Brassicogethes aeneus F. syn. Meligethes aeneus, Coleoptera: Nitidulidae). Before their summer diapause, adult pollen beetles feed on the pollen from plants belonging to many different families (Free and Williams, 1978; Marques and Draper, 2012; Ouvrard et al., 2016). A previous study has shown that the total macronutrient content of the food source is a key driver of pollen beetle feeding decisions at the inflorescence scale (Bellec et al., 2022). However, it is currently unknown whether macronutrients also influence the nutritional strategy of this species on a larger scale, i.e., when having the choice between plants of different species and families. The dominant flower associations recorded for pollen beetles belong to three main families: Brassicaceae, Ranunculaceae and Asteraceae (Free and Williams, 1978; Ouvrard et al., 2016; Herrera and Otero, 2021). We therefore chose different species among these families on which pollen beetles are commonly found just before entering diapause (Ouvrard et al., 2016 and Bellec, personal observation), and addressed the three following questions: (i) Is the generalism of pollen beetles a populational consequence of individuals specialized on different plant families? (ii) Do individuals feed at random on flowers available or do they follow a nutritional strategy? (iii) In case of non-random feeding choices, do pollen macronutrients explain this nutritional strategy? To answer these questions, we combine a set of manipulative laboratory experiments including behavioral experiments on flowers and artificial substrates, quantification of pollen nutrient content, quantification of the insect energetic budget and performance experiments.
Materials and methods
Plants and insects
Six species from three different families on which pollen beetles are found just before diapause were selected: Crepis capillaris and Senecio vulgaris (Asteraceae), Sisymbrium officinale and Sinapis arvensis (Brassicaceae), and Ranunculus acris and Ranunculus repens (Ranunculaceae). We only chose species with yellow flowers since color influences flower selection in this insect species and yellow is the preferred color (Döring et al., 2012). Plants were studied at the flowering stage, i.e., the period during which pollen beetles visit them. Since the cultivation of these plants is difficult to synchronize under controlled conditions, flowers were field-collected every day in the same local area in Rennes (Brittany, France), just before the experiments started.
Second generation, pre-diapausing pollen beetles, were sampled in late July on various plant species in Rennes (Brittany, France) and maintained under controlled conditions as described in Bellec et al. (2022). Insects were starved in individual Petri dishes (∅ = 35 mm) containing a moistened filter paper until 50% of the individuals died (i.e., 5 days), ensuring that energy levels of individuals still alive were low, but not null, when experiments started (Supplementary Figure 1). No ethical approval was required for the use of this species.
Preference experiments
Feeding choice-tests on detached flowers—To assess food preferences of pollen beetles, choice tests were performed. One individual was placed in a Petri dish (∅ = 135 mm) with one flower from each of the six plant species. Since flowers of S. officinale were much smaller than the others, here and in the following experiments, six flowers (i.e., a total anther volume equivalent to that of a single anther of S. arvensis) were used instead of one, to avoid visual bias and to provide the same amount of resources as with the other species. Flowers of the six species were disposed randomly and at equal distances from each other on a circle where they were maintained in slightly moistened floral foam covering the bottom of the Petri dish. The experiment lasted 7 h per day and was performed over three consecutive days. To avoid flower degradation and to provide an unlimited amount of resources flowers were replaced every day with freshly collected flowers. The resource on which the individual was found was recorded every hour and just before flower replacement. Forty-five replicates were performed.
Feeding choice-tests on artificial substrates—To validate the involvement of plant macronutrients in the feeding preference of pollen beetles, choice tests with artificial substrates were performed. Artificial substrates consisted of 3% agar disks (∅ = 5 mm, thickness = 2 mm) supplemented with macronutrients (casein:whey 80:20 as protein source and sucrose as carbohydrate source). One individual was placed in a Petri dish (∅ = 55 mm) with six agar disks supplemented with macronutrients in the same concentration as found in anthers from the six plant species (see section “Results”). Since the preference experiment on detached flowers showed that the feeding strategy of the pollen beetles was established on the first day, the experiment on artificial substrates was conducted on a single day. The experiment lasted 5 h to avoid desiccation of the agar disks. The resource on which the individual was found was recorded every 30 min. Thirty replicates were performed.
Performance experiment
To assess the influence of the resource on the pollen beetle performance, a starved individual was placed in a Petri dish (∅ = 55 mm) with no food or a flower of one of the six species, and a moistened filter paper. The experiment lasted 3 days. To avoid flower degradation and to provide an unlimited amount of resources, flowers were replaced every day with freshly collected flowers. After the feeding period, flowers were removed and the performance was quantified as the survival time. The filter paper was moistened every day and the survival time was recorded through daily observations. Thirteen to fifteen replicates were performed per treatment.
Quantification of plant macronutrients
Sample preparation—Anthers from freshly, field-collected flowers were dissected and immediately frozen into liquid nitrogen. For each species, two to three samples of 100 mg FW of anthers coming from a large number of plants were used. The number of samples was limited by the difficulty to collect sufficient amounts of plant material. Samples were then lyophilized and ground into fine powder for 20 s at 3,500 rpm (BeadBug© homogenizer and 2.8 mm stainless beads). Macronutrients were quantified as described in Masuko et al. (2005) and Deans et al. (2018), with modifications as detailed below.
Soluble proteins—To 5 mg of dried powder, 250 μl of 0.1 M NaOH were added. Samples were then sonicated for 30 min and incubated for 15 min in a static water bath at 90°C, then centrifuged for 10 min at 13,000 rpm and the supernatant collected. The pellet was extracted a second time as above, and supernatants collected. The pH was neutralized by adding 5.5 μl of 5.8 M HCl. To precipitate proteins, 45 μl of 100% trichloroacetic acid was added and samples were cooled in ice for 30 min. Samples were centrifuged tubes for 10 min at 13,000 rpm, the supernatant discarded and the pellet washed with 50 μl of −20°C acetone that was quickly removed within 5 s. Samples were then dried under a fume hood for 30 min to ensure complete evaporation of the acetone. The dry residue was resuspended in 500 μl of 0.1 M NaOH and heated in a hot water bath, vortexed, and sonicated until the protein pellet was completely dissolved. Samples were then 20-fold diluted in distilled water. Quantification was performed using the Bradford method (Bradford, 1976) and standard solutions of bovine serum albumin as references, with 75 μl of sample mixed with 225 μl of Coomassie reagent and absorbance red at 595 nm.
Digestible carbohydrates—To 5 mg of dried powder, 500 μl of 0.1 M H2SO4 were added. Samples were incubated for 1 h in a static water bath at 90°C, cooled into tepid water and centrifuged for 10 min at 13,000 rpm. Samples were 10-fold diluted in distilled water. Quantification was performed using the phenol-sulfuric acid method (Masuko et al., 2005) and standard solutions of glucose as references, with 50 μl of sample mixed with 150 μl of H2SO4 μl and 30 μl of 5% phenol. Reading plates was incubated for 5 min in a static water bath at 90°C, cooled for 5 min at room temperature and absorbance red at 490 nm.
Quantification of the insect energetic budget
Sample preparation—To assess the influence of the resource on the pollen beetle energetic budget, the same experimental design as described in the performance experiment was followed, except that individuals were immediately and individually frozen at −20°C after the feeding period. Ten replicates were performed per plant species. Proteins, sugars, glycogen, and triglycerides were quantified in each individual using the following protocol adapted from Foray et al. (2012) and Hidalgo et al. (2016).
Proteins—Insects were crushed for 30 s at 3,500 rpm into 300 μl of an aqueous lysis buffer (100 mM KH2PO4, 1 mM DTT, and 1 mM EDTA in pure water), using a homogenizer and 2.8 mm stainless beads (BeadBug©). Samples were then briefly centrifuged at 180 g and 4°C. Protein quantification was performed using the Bradford method and standard solutions of bovine serum albumin as references, with 150 μl of sample mixed with 150 μl of Coomassie reagent and absorbance red at 595 nm.
Sugars and glycogen—To dissolve carbohydrates, 20 μl of 20% sodium sulfate solution (Na2SO4, Sigma) was added to the remaining homogenate, as well as 10 μl of the extraction buffer. This solution was then mixed with 1,200 μl of methanol:chloroform (1:2) to solubilize total lipids as well as sugars. Samples were then vortexed and centrifuged for 15 min at 180 g and 4°C. From the biphasic supernatant, 450 μl of the upper phase was collected for the quantification of sugars and 850 μl of the lower phase for the quantification of triglycerides, while the pellet was kept for the subsequent quantification of glycogen. Upper phases were evaporated under a fume hood for 24–48 h. The pellet was washed twice by adding 400 μl of 80% methanol, vortexing and centrifugating for 5 min at 13,000 rpm and 4°C. Sugars and glycogen were quantified using the anthrone reagent method (Van Handel, 1965) and standard solutions of glucose as references. For that purpose, 1 ml of anthrone reagent was added to each sample, then let incubating for 15 min in a water bath at 90°C and cooled in ice for 10 min. Quantification was realized on 250 μl of sample and absorbance red at 625 nm.
Triglycerides—The lower phase of the previous extraction protocol was evaporated under a fume hood for 24–48 h, then resuspended in 100 μl of BSA-Triton X100-water solution (0.3:0.02:1). Quantification of triglycerides was performed using a dedicated enzymatic kit (Cayman chemicals©) and standard solutions of triolein as references, with 100 μl of sample mixed with 100 μl of the kit, incubated for 30 min at 37°C for 30 min and absorbance red at 525 nm.
Energy—Total energy was calculated using the following conversion factors: proteins 0.0043 cal μg–1, sugars 0.0041 cal μg–1, glycogen 0.0041 cal μg–1, and triglycerides 0.0079 cal μg–1 (Beninger, 1984).
Statistical analyses
All statistical analyses were performed using the R software 4.2.1 (R Core Team, 2022). Plot state sequence was used to visualize the behavioral feeding sequence of pollen beetles at the individual scale (R package “TraMineR,” Gabadinho et al., 2011). A Shannon entropy index was used as a measure of inter-individual heterogeneity in food choices, and the relationship between this index and time was tested using a Pearson’s correlation test (R package “TraMineR”). The proportions of individuals feeding on the different resources (flowers or artificial diets depending on the experiment) were compared using a likelihood ratio test (LRTest) applied on a Generalized Linear Model (family: binomial, link: logit) including the resource, time and their interaction as independent variables. This model allowed performing pairwise comparisons of Estimated Marginal Means (EMMeans) and testing whether trends observed time differed significantly from zero (R package “emmeans”; Lenth, 2019). p-Values were adjusted using the false discovery rate (FDR) correction (Benjamini and Hochberg, 1995) when performing pairwise comparisons. The macronutrient content of anthers was compared between plant species using ANOVAs performed on the protein content, carbohydrate content, total content, and protein:carbohydrate ratio. All responses but the protein:carbohydrate ratio were square-root transformed to ensure a better model fit. Pearson’s correlation tests were performed between all combinations of a food preference index, performance, components of the plant macronutrient content (i.e., digestible carbohydrates and soluble proteins) and components of the insect energetic budget (i.e., free sugars, glycogen, proteins, triglycerides, and total energy). In order to focus on the establishment of the nutritional strategy, the preference index was computed as the proportion of individuals feeding on each resource at the last record of the first day of the multi-choice experiment (i.e., once the nutritional strategy was established) minus the proportion of individuals feeding on the these resources at the first record (i.e., before the feeding strategy was established).
Results
Pollen beetles are generalists at the individual scale
In feeding choice tests with flowers of different species and families, 96% of individuals fed on more than one plant species and 82% of individuals fed on more than one plant family (Figure 1). Therefore, pollen beetles shifted from the resource they exploited over time at the individual scale (Supplementary Figure 2).
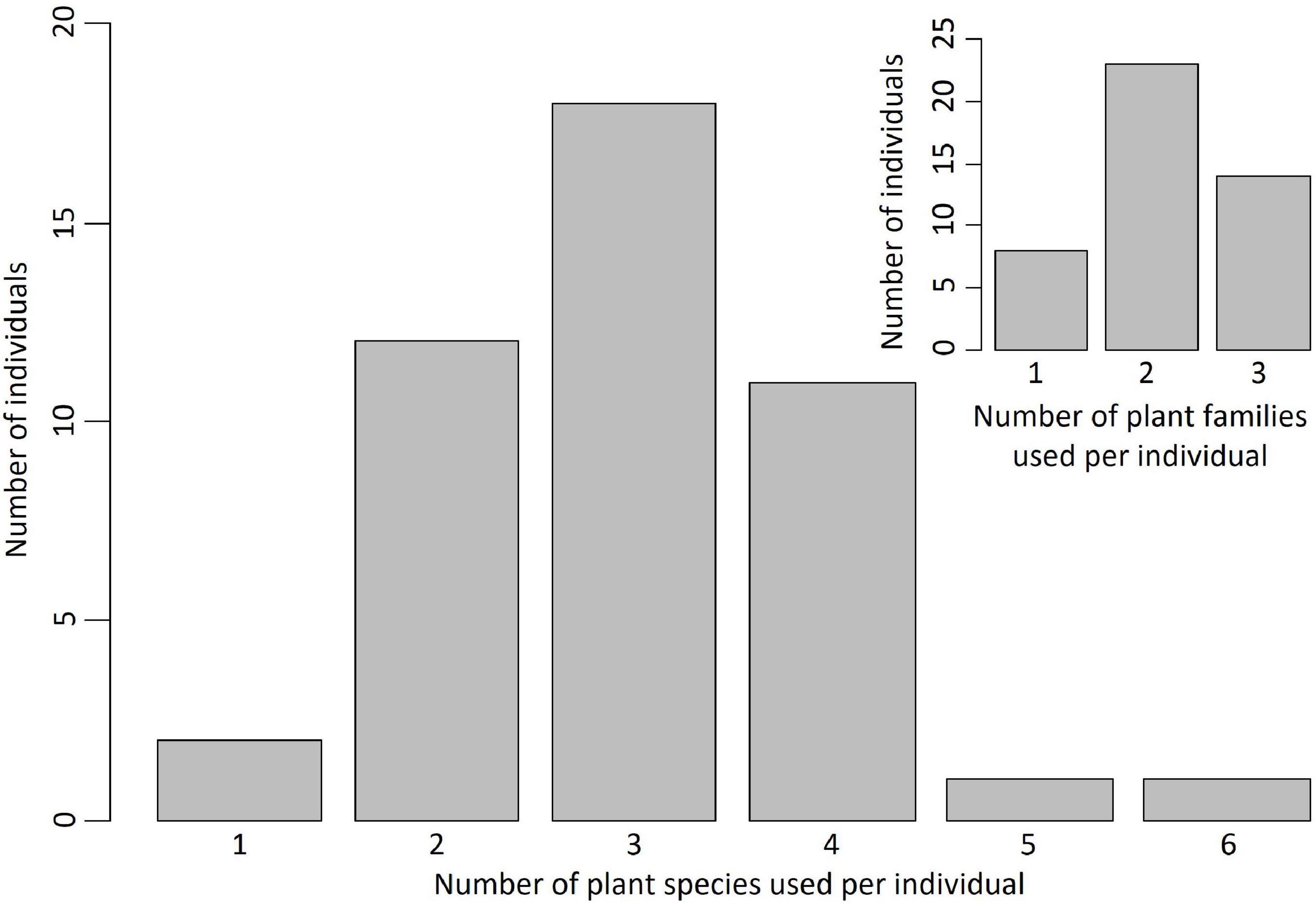
Figure 1. Pollen beetles feed on plants from different species and families at the individual scale. Distribution of the number of species/families used per individual. n = 45.
Pollen beetles adopt a common nutritional strategy with a preferential feeding on Ranunculaceae
The heterogeneity in food choices between individuals decreased significantly over time (r = −0.73, df = 22, p < 0.001), meaning that individuals progressively adopted a more uniform feeding strategy (Supplementary Figure 3). Indeed, although individuals fed equally on all resources at the first record, a significant preference for Ranunculaceae was established by the end of the first day and this remained constant throughout the rest of the experiment (Figure 2 and Supplementary Figure 4).
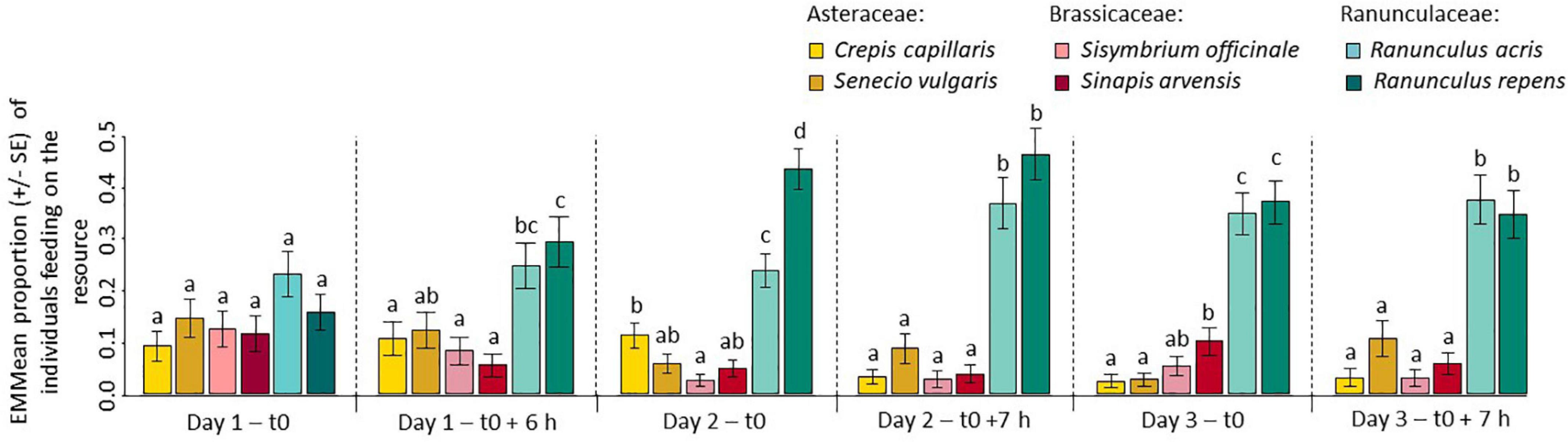
Figure 2. The proportion of pollen beetles feeding on Ranunculaceae increases quickly over time and remains constant. Estimated Marginal Mean (EMMean) proportion (±SE) of individuals feeding on each resource at the first and last record of each day of the experiment. Different letters indicate significantly different EMMeans. n = 45.
The nutritional strategy of pollen beetles is adaptive and driven by the plant carbohydrate content
The macronutrient content differs between anthers from the six plant species
The quantification of the macronutrient content of anthers from flowers of the six species revealed no difference in soluble protein (P) concentration [F(1,10) = 2.10, p = 0.148] but a significant difference in digestible carbohydrate (C) concentration [F(1,10) = 10.13, p < 0.01], with a 2.8–8.1 times higher concentration in anthers from R. repens than in other species (Figure 3). Consequently, organs also differed in their P:C ratio [F(5,10) = 5.55, p < 0.05], and total P + C amount [F(5,10) = 9.74, p < 0.01; Supplementary Figure 5].
The plant carbohydrate content correlates positively with insect preference, performance, and energetic budget
The preference of pollen beetles in the multi-choice experiment correlated positively with the performance of individuals fed with single species (Figure 4, raw values in Supplementary Table 1), showing that their feeding strategy is adaptive. In addition, both the preference and performance correlated positively with the carbohydrate content of anthers (Figure 4). Lastly, individuals feeding on resources containing more carbohydrates accumulated significantly more glycogen and total energetic reserves (Figure 4). The array of positive correlations obtained here suggests that the preference of pollen beetles is driven by the plant carbohydrate content, which increases the performance of individuals through an increase of energy available.
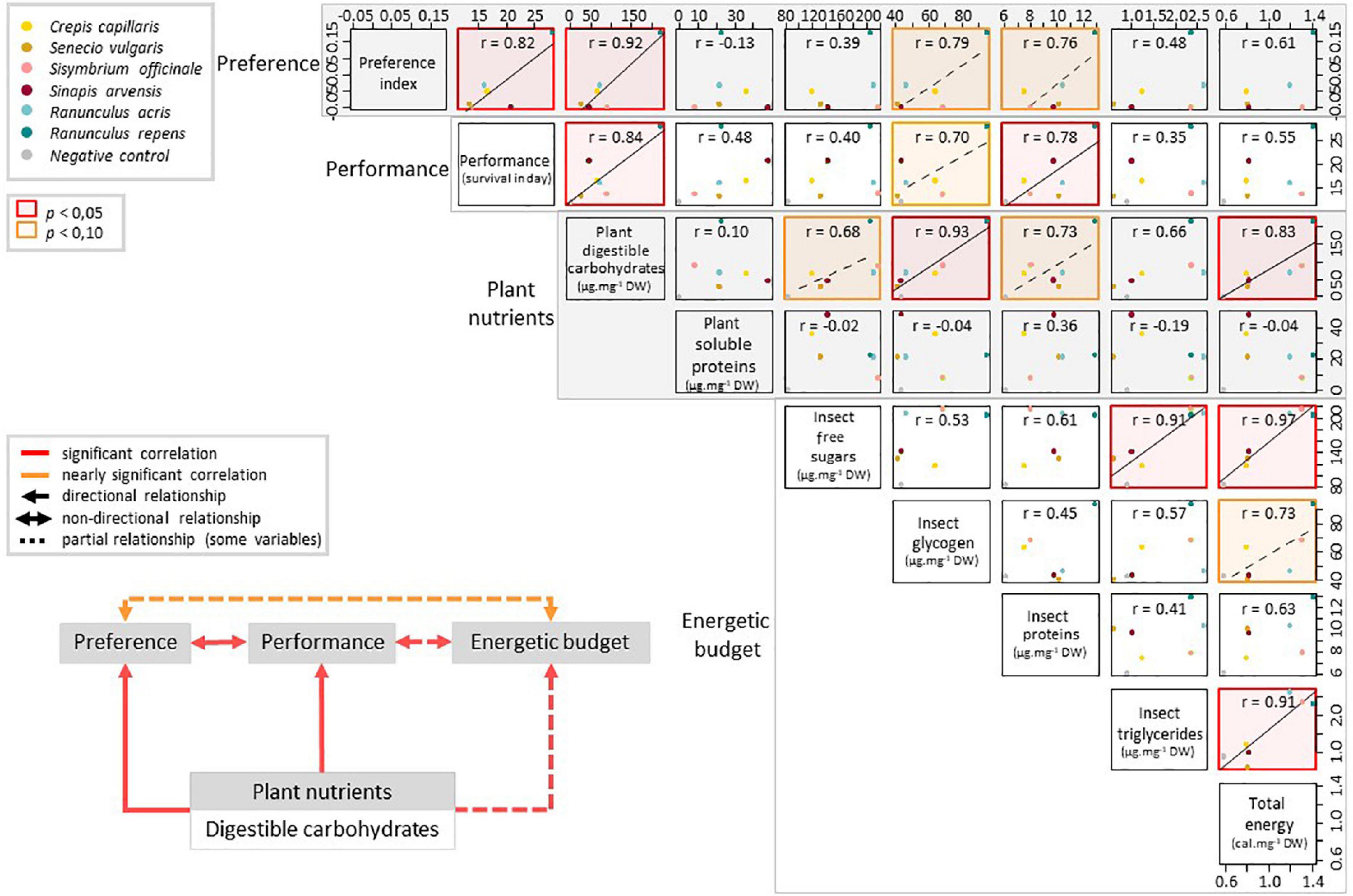
Figure 4. Relationships between the plant digestible carbohydrate content, the insect preference observed in the multi-choice experiment, and the performance and energetic budget of individuals fed with single species. Pearson’s correlation coefficients (r) indicate the strength and direction of the relationship. Axis values are the values of the measured variables. Results of the correlation tests are summarized in the chart at the bottom left, with putative directional relationships.
Pollen beetles prefer the macronutrient content of anthers from their preferred plant species
To validate the involvement of the plant macronutrient content in the feeding preference of pollen beetles, a choice test offering artificial substrates containing macronutrients in the same concentration and ratios as in anthers from the six plant species was performed. The proportion of individuals feeding on each food source varied significantly with time (χ2 = 24, df = 5, p < 0.001). Pollen beetles fed significantly more on the diet containing macronutrients in the same concentration and ratios as in anthers from R. repens at the first record, and this preference increased over time (Figure 5 and Supplementary Figure 6).
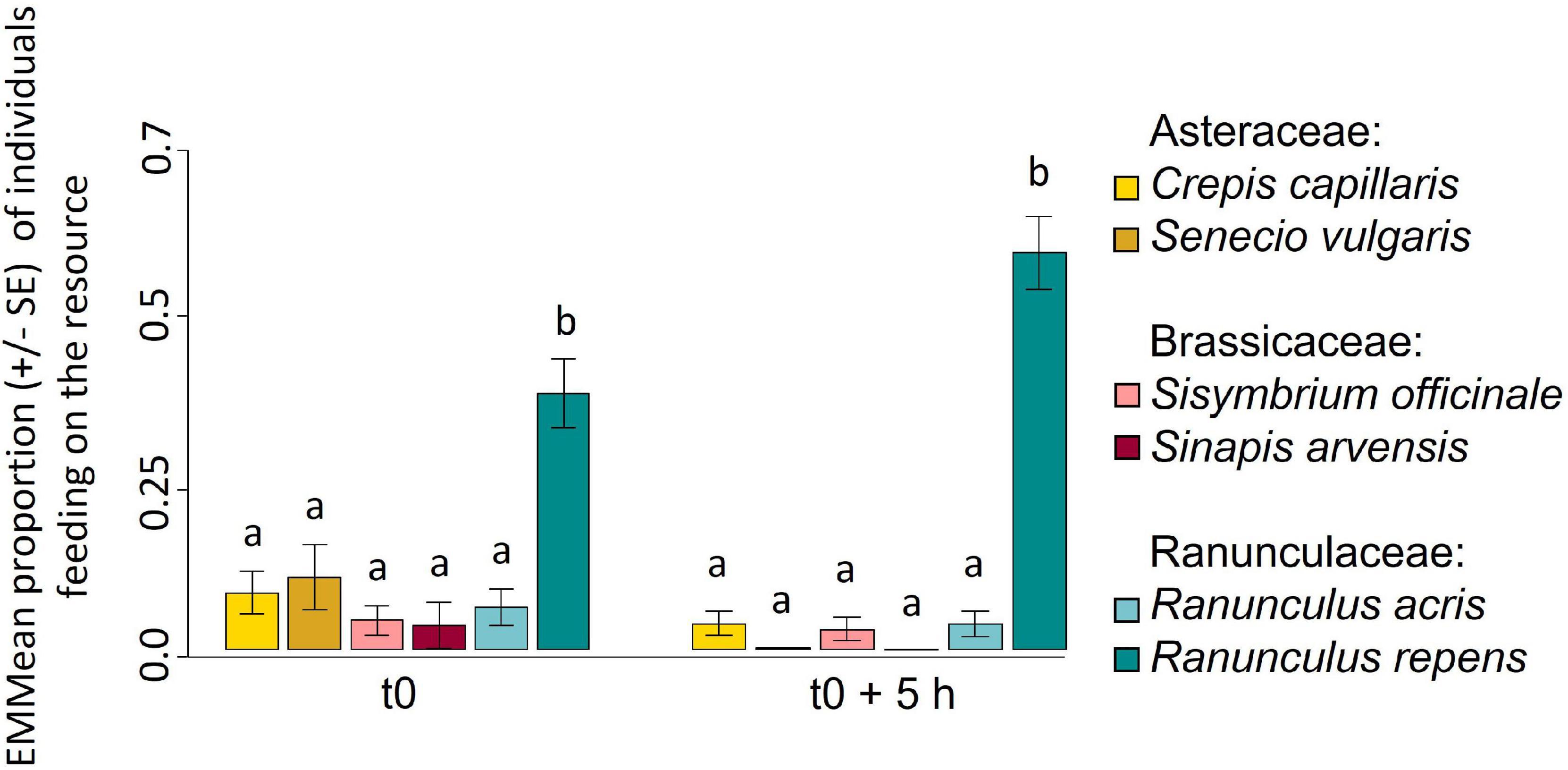
Figure 5. The proportion of pollen beetles feeding on artificial diets containing macronutrients in the same concentrations as found in anthers from Ranunculus repens increases over time. Estimated Marginal Mean (EMMean) proportion (±SE) of individuals feeding on each resource at the first and last record of the experiment. Different letters indicate significantly different EMMeans. n = 30.
Discussion
This study assessed the influence of plant macronutrients on the foraging behavior, energetic budget and resulting fitness of a generalist pollen feeder. Pollen beetles are known to be generalist pollen feeders at the species level, especially in late summer when new-generation individuals feed before seeking diapause sites (Free and Williams, 1978). However, it was previously unknown whether individuals are generalist themselves or whether they are specialized on different plant species or families. We found that pollen beetles feed, at the individual scale, on several plant species and even families during the course of the day, meaning that they are true generalists. Considering the level at which generalism occurs (i.e., species, populations, or individuals) is critical to understand nutritional strategies in animals (Fox and Morrow, 1981; Bernays and Minkenberg, 1997). Indeed, the benefits of being generalist (e.g., nutrient balancing, toxin dilution) are mostly relevant at the individual scale, and do not apply in generalist species composed of individuals specialized on different food sources. However, although individual-scale generalism has been widely described in grasshoppers and caterpillars (Barbosa and Krischik, 1987; Bernays and Minkenberg, 1997; Singer, 2001; Bernays and Singer, 2002; Singer et al., 2004), we currently lack studies that investigate this aspect in other insect taxa.
Overall, individual generalism does not mean that individuals feed randomly or that they accept all plants belonging to their host spectrum indifferently and marked feeding preferences have been documented (Scriber, 1979; Barbosa and Krischik, 1987; Karowe, 1989). In the present study, we found that individuals progressively move toward a common nutritional strategy consisting in a preferential feeding on the two species of Ranunculaceae we tested, and that this strategy is established over a few hours. These results are in line with field observations performed by Free and Williams (1978), Ouvrard et al. (2016), and Herrera and Otero (2021) on pollen beetles. However, the reasons behind the clear feeding preference for Ranunculaceae remained unexplained so far.
Bellec et al. (2022) demonstrated that the plant macronutrient content is a key driver of pollen beetles feeding strategy at the inflorescence scale. In the present study, the quantification of the macronutrient content of anthers from the six species tested revealed significant differences in the amount of digestible carbohydrates, which result in differences both in the total content and the ratio of macronutrients. In other pollen feeders, mainly bees, an influence of the pollen macronutrient content on the insect feeding behavior has been reported (Cook et al., 2004; Vaudo et al., 2016; Ghosh et al., 2020). Here, we found a positive relationship between food preferences of pollen beetles on flowers and anther carbohydrate content. Feeding tests on artificial substrates mimicking the macronutrient content of the six species corroborate observations on flowers, especially the clear preference for R. repens. Although these results can be explained by both the carbohydrate content and the proteins to carbohydrates ratio, these being highly correlated since the protein content were quite similar between all species, correlations shown here as well as conclusions of Bellec et al. (2022) clear point toward an influence of the carbohydrate content itself. While testing the influence of nutrients on insect preferences and fitness using artificial substrates in the laboratory is common and has proven successful (Simpson and Raubenheimer, 2012), our study brings further ecological realism by reproducing real plants’ content. Our results contrast with studies on other pollen feeders, mainly bees and bumblebees, where a substantial influence of protein quantity and quality on food preferences has been reported (Hanley et al., 2008; Kitaoka and Nieh, 2009; Leonhardt and Blüthgen, 2012; Ghosh et al., 2020). The usual emphasis on proteins to explain feeding behavior of pollinators is mainly because pollen is primarily considered a source of proteins, while nectar is a source of carbohydrates (Nicolson, 2011; Donkersley et al., 2017). However, as demonstrated here, host-plant selection by pollen feeders can also be determined by pollen carbohydrates. Overall, this work supports Le Gall et al. (2021, 2022) who highlighted the need to bridge field observations of host-plant selection with characterizations of the ambient nutrient landscape to better understand nutritional strategies in herbivores.
Performance experiments further showed that the preference of pollen beetles for carbohydrates-rich flowers is adaptive, as it positively correlates with survival. Although linking food preferences, performance and plant content is the common approach to decipher nutritional strategies of herbivorous insects, it is not integrative as it lacks a physiological intermediary that would give sense to the relationships observed. In particular, fitness is not only dependent on the uptake of food macronutrients but also on their allocation to different life-history traits through their conversion to energy-providing molecules (Heino and Kaitala, 1999; Tomlinson et al., 2014). However, the incorporation of energetic budget of insects in nutritional ecology studies remains rare [but see Body et al. (2019)]. Here, we found a positive relationship between the plant carbohydrate content and the total energy available in pollen beetles after feeding, with a particular correlation with the glycogen reserves. Such a view on the insect energetic budget allows increasing the ecological relevance of hypothetical nutritional strategies. Indeed, we found that pollen beetles feed preferentially on carbohydrate-rich plants, and it is known that glycogen reserves are mainly derived from dietary carbohydrates (Arrese and Soulages, 2010). Additionally, it has been widely documented that prior to diapause, such as here, many insects accumulate important glycogen reserves that will serve for fueling catabolism and eventual cryoprotection during diapause (Hahn and Denlinger, 2007, 2011). Since maximization of nutrient intake, including carbohydrates, has also been found in individuals feeding on oilseed rape inflorescences in spring, i.e., the reproductive period (Bellec et al., 2022), it seems that this strategy is not only a mean to accumulate energy for egg maturation or survival in spring, or to survive diapause in late summer. Indeed, it can be hypothesized that it is the overall nutritional strategy of the pollen beetle throughout its whole life cycle. Further investigations on the nutritional strategy of pollen beetles at other moments of their life cycle would be necessary to confirm this hypothesis.
Taken together, our results strongly suggest that food preferences of pollen beetles at the individual scale and when given the choice between phylogenetically distant plant species, is mainly driven by the plant carbohydrate content. According to the optimal foraging theory, individuals should forage to maximize their fitness (Pyke, 2019). Pollen beetles should then feed only on the optimal resource, i.e., R. repens here. However, we found that individuals still feed polyphagously, with a particular interest for R. acris, although it is not optimal from a carbohydrate maximization perspective. As shown by Lefcheck et al. (2013) in a large-scale meta-analysis, in more than half of the cases the mixing of several food sources does not result in any fitness gain compared to the feeding on the single optimal species. The maintenance of generalism in the pollen beetle, as well as in other species, might be due to associative learning (Desouhant et al., 2010; Simcock et al., 2014; Hollis and Guillette, 2015), individual personalities (Tremmel and Müller, 2013), ecological reasons such as spatial and temporal variations in resource availability and presence of natural enemies (Rusch et al., 2011; Skellern and Cook, 2018) or the presence of visual (shape, color, and resistance) and chemical (volatiles, surface, and internal compounds) cues (Fraenkel, 1959; Freeland and Janzen, 1974; Prokopy and Owens, 1983). Further studies are needed to characterize and assess the influence of other factors such as these to decrypt the nutritional strategy of pollen beetles in more depth.
In conclusion, this work represents the first evidence of generalism at the individual scale in the pollen beetle, a strategy described for herbivorous insects in a few orders only. It provides information on the nutritional strategy of generalist herbivores more specifically of pollen feeders other than bees, and highlights the importance of macronutrients, particularly carbohydrates, in this strategy that is uncommon in pollinivores. By originally combining the assessment of the plant nutrient content and the insect energetic budget in an adaptive framework, it also shows that nutritional ecology studies can gain in ecological and physiological realism.
Data availability statement
The original contributions presented in this study are included in the article/Supplementary material, further inquiries can be directed to the corresponding author.
Author contributions
MH, A-MC, SF, and LB conceived the ideas and designed the methodology. LB and TG collected the data. MH and LB analyzed the data. LB wrote the first draft of the manuscript. All authors contributed to revisions and gave final approval for publication.
Acknowledgments
We are very grateful to Marie Trabalon for her help in the quantification of plant nutrients and insect energetic budget.
Conflict of interest
LB and SF were employed by the company Innolea.
The remaining authors declare that the research was conducted in the absence of any commercial or financial relationships that could be construed as a potential conflict of interest.
Publisher’s note
All claims expressed in this article are solely those of the authors and do not necessarily represent those of their affiliated organizations, or those of the publisher, the editors and the reviewers. Any product that may be evaluated in this article, or claim that may be made by its manufacturer, is not guaranteed or endorsed by the publisher.
Supplementary material
The Supplementary Material for this article can be found online at: https://www.frontiersin.org/articles/10.3389/fevo.2022.1050321/full#supplementary-material
References
Arrese, E. L., and Soulages, J. L. (2010). Insect fat body: Energy, metabolism, and regulation. Annu. Rev. Entomol. 55, 207–225. doi: 10.1146/annurev-ento-112408-085356
Augner, M. (1995). Low nutritive quality as a plant defence: effects of herbivore-mediated interactions. Evol. Ecol. 9, 605–616. doi: 10.1007/BF01237658
Barbosa, P., and Krischik, V. A. (1987). Influence of alkaloids on feeding preference of eastern deciduous forest trees by the gypsy moth Lymantria dispar. Am. Nat. 130, 53–69. doi: 10.1086/284697
Beenakkers, A. M. T., Van der Horst, D. J., and Van Marrewijk, W. J. A. (1984). Insect flight muscle metabolism. Insect Biochem. 14, 243–260. doi: 10.1016/0020-1790(84)90057-X
Behmer, S. T. (2009). Insect herbivore nutrient regulation. Annu. Rev. Entomol. 54, 165–187. doi: 10.1146/annurev.ento.54.110807.090537
Bellec, L., Seimandi-Corda, G., Menacer, K., Trabalon, M., Ollivier, J., Lunel, C., et al. (2022). Factors driving the within-plant patterns of resource exploitation in a herbivore. Funct. Ecol. 36, 1700–1712. doi: 10.1111/1365-2435.14058
Beninger, P. G. (1984). Seasonal variations of the major lipid classes in relation to the reproductive activity of two species of clams raised in a common habitat: Tapes decussatus L. (Jeffreys, 1863) and T. philippinarum (Adams & Reeve, 1850). J. Exp. Mar. Biol. Ecol. 79, 79–90. doi: 10.1016/0022-0981(84)90031-5
Benjamini, Y., and Hochberg, Y. (1995). Controlling the false discovery rate: a practical and powerful approach to multiple testing. J. R. Stat. Soc. Series B Stat. Methodol. 57, 289–300. doi: 10.1111/j.2517-6161.1995.tb02031.x
Berenbaum, M. R. (1995). Turnabout is fair play: Secondary roles for primary compounds. J. Chem. Ecol. 21, 925–940. doi: 10.1007/BF02033799
Bernays, E. A., and Minkenberg, P. J. M. (1997). Insect herbivores: different reasons for being a generalist. Ecology 78, 1157–1169. doi: 10.1890/0012-96581997078[1157:IHDRFB]2.0.CO;2
Bernays, E., and Graham, M. (1988). On the evolution of host specificity in phytophagous arthropods. Ecol. 69, 886–892. doi: 10.2307/1941237
Bernays, E., and Singer, M. (2002). Contrasted foraging in two species of polyphagous caterpillars. Acta Zool. Hung. 48, 117–135.
Body, M. J. A., Behmer, S. T., Pelisson, P.-F., Casas, J., and Giron, D. (2019). Field application of the geometric framework reveals a multistep strategy of nutrient regulation in a leaf-miner. bioRxiv [Preprint]. doi: 10.1101/777367
Bonoan, R. E., Gonzalez, J., and Starks, P. T. (2020). The perils of forcing a generalist to be a specialist: lack of dietary essential amino acids impacts honey bee pollen foraging and colony growth. J. Apic. Res. 59, 95–103. doi: 10.1080/00218839.2019.1656702
Bradford, M. M. (1976). A rapid and sensitive method for the quantitation of microgram quantities of protein utilizing the principle of protein-dye binding. Anal. Biochem. 72, 248–254. doi: 10.1016/0003-2697(76)90527-3
Brochu, K. K., van Dyke, M. T., Milano, N. J., Petersen, J. D., McArt, S. H., Nault, B. A., et al. (2020). Pollen defenses negatively impact foraging and fitness in a generalist bee (Bombus impatiens: Apidae). Sci. Rep. 10:3112. doi: 10.1038/s41598-020-58274-2
Cady, S. M., Davis, C. A., Fuhlendorf, S. D., Scholtz, R., Uden, D. R., and Twidwell, D. (2021). Generalist bird exhibits site-dependent resource selection. Ecol. Evol. 11, 12714–12727. doi: 10.1002/ece3.8016
Cook, S. M., Murray, D. A., and Williams, I. H. (2004). Do pollen beetles need pollen? The effect of pollen on oviposition, survival, and development of a flower-feeding herbivore. Ecol. Entomol. 29, 164–173. doi: 10.1111/j.0307-6946.2004.00589.x
Cui, Z.-W., Wang, Z.-L., Shao, Q., Raubenheimer, D., and Lu, J.-Q. (2018). Macronutrient signature of dietary generalism in an ecologically diverse primate in the wild. Behav. Ecol. 29, 804–813. doi: 10.1093/beheco/ary003
Deans, C. A., Sword, G. A., Lenhart, P. A., Burkness, E., Hutchison, W. D., and Behmer, S. T. (2018). Quantifying plant soluble protein and digestible carbohydrate content, using corn (Zea mays) as an exemplar. J. Vis. Exp. 58164. doi: 10.3791/58164
Desouhant, E., Navel, S., Foubert, E., Fischbein, D., Théry, M., and Bernstein, C. (2010). What matters in the associative learning of visual cues in foraging parasitoid wasps: colour or brightness? Anim. Cogn. 13, 535–543. doi: 10.1007/s10071-009-0304-2
Donkersley, P., Rhodes, G., Pickup, R. W., Jones, K. C., Power, E. F., Wright, G. A., et al. (2017). Nutritional composition of honey bee food stores vary with floral composition. Oecologia 185, 749–761. doi: 10.1007/s00442-017-3968-3
Döring, T. F., Skellern, M., Watts, N., and Cook, S. M. (2012). Colour choice behaviour in the pollen beetle Meligethes aeneus (Coleoptera: Nitidulidae). Physiol. Entomol. 37, 360–378. doi: 10.1111/j.1365-3032.2012.00850.x
Fargallo, J. A., Navarro-López, J., Palma-Granados, P., and Nieto, R. M. (2020). Foraging strategy of a carnivorous-insectivorous raptor species based on prey size, capturability and nutritional components. Sci. Rep. 10:7583. doi: 10.1038/s41598-020-64504-4
Figueras, M. P., Green, T. M., and Burke, R. L. (2021). Consumption patterns of a generalist omnivore: eastern box turtle diets in the long island Pine Barrens. Divers 13:345. doi: 10.3390/d13080345
Fontaine, C., Thébault, E., and Dajoz, I. (2009). Are insect pollinators more generalist than insect herbivores? Proc. R. Soc. B. 276, 3027–3033. doi: 10.1098/rspb.2009.0635
Foray, V., Pelisson, P.-F., Bel-Venner, M.-C., Desouhant, E., Venner, S., Menu, F., et al. (2012). A handbook for uncovering the complete energetic budget in insects: The van Handel’s method (1985) revisited. Physiol. Entomol. 37, 295–302. doi: 10.1111/j.1365-3032.2012.00831.x
Fox, L. R., and Morrow, P. A. (1981). Specialization: species property or local phenomenon? Science 211, 887–893. doi: 10.1126/science.211.4485.887
Fraenkel, G. S. (1959). The raison d’ětre of secondary plant substances; these odd chemicals arose as a means of protecting plants from insects and now guide insects to food. Science 129, 1466–1470. doi: 10.1126/science.129.3361.1466
Free, J. B., and Williams, I. H. (1978). The responses of the pollen beetle, Meligethes aeneus, and the seed weevil, Ceuthorhynchus assimilis, to oilseed rape, Brassica napus, and other Plants. J. Appl. Ecol. 15:761. doi: 10.2307/2402773
Freeland, W. J., and Janzen, D. H. (1974). Strategies in herbivory by mammals: the role of plant secondary compounds. Am. Nat. 108, 269–289. doi: 10.1086/282907
Futuyma, D. J., and Moreno, G. (1988). The evolution of ecological specialization. Annu. Rev. Ecol. Evol. Syst. 19, 207–233. doi: 10.1146/annurev.es.19.110188.001231
Gabadinho, A., Ritschard, G., Müller, N. S., and Studer, M. (2011). Analyzing and visualizing state sequences in R with TraMineR. JSS 40, 1–37. doi: 10.18637/jss.v040.i04
Ghosh, S., Jeon, H., and Jung, C. (2020). Foraging behaviour and preference of pollen sources by honey bee (Apis mellifera) relative to protein contents. J. Ecol. Environ. 44:4. doi: 10.1186/s41610-020-0149-9
Gilbert, L. E. (1972). Pollen feeding and reproductive biology of Heliconius butterflies. Proc. Natl. Acad. Sci. U.S.A. 69, 1403–1407. doi: 10.1073/pnas.69.6.1403
Hahn, D. A., and Denlinger, D. L. (2007). Meeting the energetic demands of insect diapause: Nutrient storage and utilization. J. Insect Physiol. 53, 760–773. doi: 10.1016/j.jinsphys.2007.03.018
Hahn, D. A., and Denlinger, D. L. (2011). Energetics of Insect Diapause. Annu. Rev. Entomol. 56, 103–121. doi: 10.1146/annurev-ento-112408-085436
Hanley, M. E., Franco, M., Pichon, S., Darvill, B., and Goulson, D. (2008). Breeding system, pollinator choice and variation in pollen auality in British herbaceous plants. Funct. Ecol. 22, 592–598.
Heino, M., and Kaitala, V. (1999). Evolution of resource allocation between growth and reproduction in animals with indeterminate growth. J. Evol. Biol. 12, 423–429. doi: 10.1046/j.1420-9101.1999.00044.x
Herrera, C. M., and Otero, C. (2021). Plant phylogeny as a major predictor of flower visitation by nitidulid beetles, a lineage of ancestral angiosperm pollinators. J. Poll. Ecol. 29, 179–188. doi: 10.26786/1920-76032021636
Hidalgo, K., Siaussat, D., Braman, V., Dabiré, K. R., Simard, F., Mouline, K., et al. (2016). Comparative physiological plasticity to desiccation in distinct populations of the malarial mosquito Anopheles coluzzii. Parasit. Vectors 9:565. doi: 10.1186/s13071-016-1854-1
Hollis, K. L., and Guillette, L. M. (2015). What associative learning in insects tells us about the evolution of learned and fixed behavior. Int. J. Comparat. Psychol. 28, 1–18. doi: 10.46867/ijcp.2015.28.01.07
Kaplan, I., McArt, S. H., and Thaler, J. S. (2014). Plant defenses and predation risk differentially shape patterns of consumption, growth, and digestive efficiency in a guild of leaf-chewing insects. PLoS One 9:e93714. doi: 10.1371/journal.pone.0093714
Karowe, D. N. (1989). Facultative monophagy as a consequence of prior feeding experience: Behavioral and physiological specialization in Colias philodice larvae. Oecologia 78, 106–111. doi: 10.1007/BF00377204
Kitaoka, T. K., and Nieh, J. C. (2009). Bumble bee pollen foraging regulation: Role of pollen quality, storage levels, and odor. Behav. Ecol. Sociobiol. 63, 625–625. doi: 10.1007/s00265-008-0707-0
Lankau, R. A. (2007). Specialist and generalist herbivores exert opposing selection on a chemical defense. New. Phytol. 175, 176–184. doi: 10.1111/j.1469-8137.2007.02090.x
Laske, S. M., Rosenberger, A. E., Wipfli, M. S., and Zimmerman, C. E. (2018). Generalist feeding strategies in Arctic freshwater fish: A mechanism for dealing with extreme environments. Ecol. Freshw. Fish. 27, 767–784. doi: 10.1111/eff.12391
Le Gall, M., Beye, A., Diallo, M., and Cease, A. J. (2022). Generational variation in nutrient regulation for an outbreaking herbivore. Oikos 2022:e09096. doi: 10.1111/oik.09096
Le Gall, M., Word, M. L., Beye, A., and Cease, A. J. (2021). Physiological status is a stronger predictor of nutrient selection than ambient plant nutrient content for a wild herbivore. CRIS 1:100004. doi: 10.1016/j.cris.2020.100004
Lefcheck, J. S., Whalen, M. A., Davenport, T. M., Stone, J. P., and Duffy, J. E. (2013). Physiological effects of diet mixing on consumer fitness: A meta-analysis. Ecology 94, 565–572. doi: 10.1890/12-0192.1
Leonhardt, S., and Blüthgen, N. (2012). The same, but different: Pollen foraging in honeybee and bumblebee colonies. Apidologie 43, 449–464. doi: 10.1007/s13592-011-0112-y
Lundberg, P., and Astrom, M. (1990). Low nutritive quality as a defense against optimally foraging herbivores. Am. Nat. 135, 547–562. doi: 10.1086/285061
Marques, I., and Draper, D. (2012). Pollination activity affects selection on floral longevity in the autumnal-flowering plant, Narcissus serotinus L. Botany 90, 283–291. doi: 10.1139/b11-110
Masuko, T., Minami, A., Iwasaki, N., Majima, T., Nishimura, S.-I., and Lee, Y. C. (2005). Carbohydrate analysis by a phenol–sulfuric acid method in microplate format. Anal. Biochem. 339, 69–72. doi: 10.1016/j.ab.2004.12.001
Moran, N., and Hamilton, W. D. (1980). Low nutritive quality as defense against herbivores. J. Theor. Biol. 86, 247–254. doi: 10.1016/0022-5193(80)90004-1
Nicolson, S. W. (2011). Bee food: the chemistry and nutritional value of nectar, pollen and mixtures of the two. Afr. Zool. 46, 197–204. doi: 10.1080/15627020.2011.11407495
Ojima, K., and Isawa, T. (1968). The variation of carbohydrates in various species of grasses and legumes. Can. J. Bot. 46, 1507–1511. doi: 10.1139/b68-208
Oortwijn, T., de Fouw, J., Petersen, J. M., and van Gils, J. A. (2022). Sulfur in lucinid bivalves inhibits intake rates of a molluscivore shorebird. Oecologia 199, 69–78. doi: 10.1007/s00442-022-05170-3
Ouvrard, P., Hicks, D. M., Mouland, M., Nicholls, J. A., Baldock, K. C. R., Goddard, M. A., et al. (2016). Molecular taxonomic analysis of the plant associations of adult pollen beetles (Nitidulidae: Meligethinae), and the population structure of Brassicogethes aeneus. Genome 59, 1101–1116. doi: 10.1139/gen-2016-0020
Paź-Dyderska, S., Żytkowiak, R., and Jagodziń;ski, A. M. (2022). Intra- and interspecific variability of non-structural carbohydrates and phenolic compounds in flowers of 70 temperate trees and shrubs. Forests 13:1256. doi: 10.3390/f13081256
Prokopy, R. J., and Owens, E. D. (1983). Visual detection of plants by herbivorous insects. Annu. Rev. Entomol. 28, 337–364. doi: 10.1146/annurev.en.28.010183.002005
Pulliam, H. R. (1975). Diet optimization with nutrient constraints. Am. Nat. 109, 765–768. doi: 10.1086/283041
Pyke, G. (2019). “Optimal foraging theory: an introduction,” in Encyclopedia of animal behavior, ed. J. C. Choe (Amsterdam: Elsevier Academic Press), 111–117. doi: 10.1016/B978-0-12-809633-8.01156-0
R Core Team (2022). R: A language and environment for statistical computing. Vienna: R Foundation for Statistical Computing.
Rader, R., Cunningham, S. A., Howlett, B. G., and Inouye, D. W. (2020). Non-bee insects as visitors and pollinators of crops: Biology, ecology, and management. Annu. Rev. Entomol. 65, 391–407. doi: 10.1146/annurev-ento-011019-025055
Raubenheimer, D., and Simpson, S. J. (1997). Integrative models of nutrient balancing: Application to insects and vertebrates. Nutr. Res. Rev. 10, 151–179. doi: 10.1079/NRR19970009
Richter, H. V., and Cumming, G. S. (2006). Food availability and annual migration of the straw-colored fruit bat (Eidolon helvum). J. Zool. 268, 35–44. doi: 10.1111/j.1469-7998.2005.00020.x
Rivero, A., and Casas, J. (1999). Incorporating physiology into parasitoid behavioral ecology: the allocation of nutritional resources. Popul. Ecol. 41, 39–45. doi: 10.1007/PL00011981
Rusch, A., Valantin-Morison, M., Sarthou, J.-P., and Roger-Estrade, J. (2011). Multi-scale effects of landscape complexity and crop management on pollen beetle parasitism rate. Landscape Ecol. 26, 473–486. doi: 10.1007/s10980-011-9573-7
Ryder, J. T., Cherrill, A., Thompson, H. M., and Walters, K. F. A. (2021). Lower pollen nutritional quality delays nest building and egg laying in Bombus terrestris audax micro-colonies leading to reduced biomass gain. Apidologie 52, 1033–1047. doi: 10.1007/s13592-021-00885-3
Schoonhoven, L. M., Jermy, T., and van Loon, J. J. A. (1998). Insect-plant biology: From physiology to evolution. London: Chapman and Hall.
Scriber, J. M. (1979). The effects of sequentially switching food plants upon biomass and nitrogen utilization by polyphagous and stenophagous papilio larvae. Entomol. Exp. Appl. 25, 203–215. doi: 10.1111/j.1570-7458.1979.tb02872.x
Simcock, N. K., Gray, H. E., and Wright, G. A. (2014). Single amino acids in sucrose rewards modulate feeding and associative learning in the honeybee. J. Insect Physiol. 69, 41–48. doi: 10.1016/j.jinsphys.2014.05.004
Simpson, S. J., and Raubenheimer, D. (1993). A multi-level analysis of feeding behaviour: the geometry of nutritional decisions. Philos. Trans. R. Soc. Lond. B 342, 381–402. doi: 10.1098/rstb.1993.0166
Simpson, S. J., and Raubenheimer, D. (2012). The nature of nutrition: a unifying framework from animal adaptation to human obesity. Princeton, NJ: Princeton University Press. doi: 10.1515/9781400842803
Singer, M. S. (2001). Determinants of polyphagy by a woolly bear caterpillar: A test of the physiological efficiency hypothesis. Oikos 93, 194–204. doi: 10.1034/j.1600-0706.2001.930203.x
Singer, M. S., Rodrigues, D., Stireman, J. O., and Carrière, Y. (2004). Roles of food quality and ennemy-free space in host use by a generalist insect herbivore. Ecology 85, 2747–2753. doi: 10.1890/03-0827
Skellern, M. P., and Cook, S. M. (2018). The potential of crop management practices to reduce pollen beetle damage in oilseed rape. Arthropod. Plant Interact. 12, 867–879. doi: 10.1007/s11829-017-9571-z
Takahashi, M. Q., Rothman, J. M., Raubenheimer, D., and Cords, M. (2021). Daily protein prioritization and long-term nutrient balancing in a dietary generalist, the blue monkey. Behav. Ecol. 32, 223–235. doi: 10.1093/beheco/araa120
Telfer, W. H., and Kunkel, J. G. (1991). The function and evolution of insect storage hexamers. Annu. Rev. Entomol. 36, 205–228. doi: 10.1146/annurev.en.36.010191.001225
Tomlinson, S., Arnall, S. G., Munn, A., Bradshaw, S. D., Maloney, S. K., Dixon, K. W., et al. (2014). Applications and implications of ecological energetics. Trends Ecol. Evol. 29, 280–290. doi: 10.1016/j.tree.2014.03.003
Tremmel, M., and Müller, C. (2013). Insect personality depends on environmental conditions. Behav. Ecol. 24, 386–392. doi: 10.1093/beheco/ars175
Van Handel, E. (1965). Microseparation of glycogen, sugars, and lipids. Anal. Biochem. 11, 266–271. doi: 10.1016/0003-2697(65)90014-X
Vaudo, A. D., Stabler, D., Patch, H. M., Tooker, J. F., Grozinger, C. M., and Wright, G. A. (2016). Bumble bees regulate their intake of essential protein and lipid pollen macronutrients. J. Exp. Biol. 219, 3962–3970. doi: 10.1242/jeb.140772
Vaudo, A. D., Tooker, J. F., Patch, H. M., Biddinger, D. J., Coccia, M., Crone, M. K., et al. (2020). Pollen protein: lipid macronutrient ratios may guide broad patterns of bee species floral preferences. Insects 11:132. doi: 10.3390/insects11020132
Venjakob, C., Ruedenauer, F. A., Klein, A. -M., and Leonhardt, S. D. (2022). Variation in nectar quality across 34 grassland plant species. Plant. Biol. J. 24, 134–144. doi: 10.1111/plb.13343
Weber, J.-M. (2011). Metabolic fuels: Regulating fluxes to select mix. J. Exp. Biol. 214, 286–294. doi: 10.1242/jeb.047050
Westoby, M. (1978). What are the biological bases of varied diets? Am. Nat. 112, 627–631. doi: 10.1086/283303
Wetzel, W. C., Kharouba, H. M., Robinson, M., Holyoak, M., and Karban, R. (2016). Variability in plant nutrients reduces insect herbivore performance. Nature 539, 425–427. doi: 10.1038/nature20140
Wiggins, N. L., McArthur, C., Davies, N. W., and McLean, S. (2006). Behavioral responses of a generalist mammalian folivore to the physiological constraints of a chemically defended diet. J. Chem. Ecol. 32, 1133–1147. doi: 10.1007/s10886-006-9076-1
Windley, H. R., Starrs, D., Stalenberg, E., Rothman, J. M., Ganzhorn, J. U., and Foley, W. J. (2022). Plant secondary metabolites and primate food choices: A meta-analysis and future directions. Am. J. Primatol. 84:e23397. doi: 10.1002/ajp.23397
Yeager, L. A., Layman, C. A., and Hammerschlag-Peyer, C. M. (2014). Diet variation of a generalist fish predator, grey snapper Lutjanus griseus, across an estuarine gradient: trade-offs of quantity for quality?: foraging trade-offs for a generalist predator. J. Fish. Biol. 85, 264–277. doi: 10.1111/jfb.12416
Keywords: herbivorous insect, plant macronutrients, feeding behavior, energetic budget, performance
Citation: Bellec L, Cortesero A-M, Giguère T, Faure S and Hervé MR (2022) Food preferences in a generalist pollen feeder: A nutritional strategy mainly driven by plant carbohydrates. Front. Ecol. Evol. 10:1050321. doi: 10.3389/fevo.2022.1050321
Received: 21 September 2022; Accepted: 10 November 2022;
Published: 24 November 2022.
Edited by:
Cesar Gemeno, Universitat de Lleida, SpainReviewed by:
Torsten Meiners, Julius Kühn-Institut, GermanyFranziska Beran, Max Planck Institute for Chemical Ecology, Germany
Copyright © 2022 Bellec, Cortesero, Giguère, Faure and Hervé. This is an open-access article distributed under the terms of the Creative Commons Attribution License (CC BY). The use, distribution or reproduction in other forums is permitted, provided the original author(s) and the copyright owner(s) are credited and that the original publication in this journal is cited, in accordance with accepted academic practice. No use, distribution or reproduction is permitted which does not comply with these terms.
*Correspondence: Laura Bellec, laura.bellec@innolea.fr