- 1School of Agriculture and Environment, College of Sciences, Massey University, Palmerston North, New Zealand
- 2The New Zealand Institute for Plant and Food Research Limited, Christchurch, New Zealand
Exotic invasive plants are present in almost all terrestrial ecosystems. Their spread and ability to colonize new habitats are predicted to increase with human travel, global trade, and climate change. These plants alter the environments they invade in multiple ways, affecting surrounding species. Chemically, invasive plants can modify their environment by releasing secondary metabolites such as root exudates (liquid) or airborne (gaseous) volatile organic compounds (VOCs). The allelopathic effect of invasive species' root exudates is well studied and acknowledged as a trait contributing to invasiveness. However, less is known about the effects of invasive species' VOCs, which are likely to play important ecological roles. Therefore, we conducted a systematic review of the literature during the last decade (2012–2022) to explore what is known about the ecological aspects of VOCs emitted by invasive plants, focusing on the factors affecting their emission (genetic, biotic, and abiotic), and their role on plant-plant and plant-insect interactions. We found 29 studies matching our search criteria. These studies suggest that invasive species are more “chemically diverse” than their native counterparts and have different chemical behavior in native compared to invaded ranges. The studies further highlight that chemical traits are heritable and contribute to invasiveness. Multiple biotic and abiotic factors affecting invasive plants' VOC emission have been explored (e.g., herbivory, soil microorganisms, warming, and CO2). The studies indicate that invasive plants may experience less variation in their VOC emissions in response to environmental change than natives, with trade-offs between growth, reproduction and defense influencing VOC emissions. Regarding the impact on native species and their interactions, the allelopathic role of invasive plants' VOCs on native plants is well documented, consistently showing phytotoxic effects. There is also evidence of their involvement in neighbor detection. While volatile-mediated interactions between invasive plants and native insects remain poorly studied, the existing evidence shows that invasive plant VOCs have the potential to disrupt communication between native plants and insects. But insects also use multiple cues to make foraging/oviposition decisions, compensating for the disruptive effect of invasive plant VOCs. To conclude, we identify knowledge gaps and propose avenues for future research.
1. Introduction
The introduction and establishment of exotic species into new habitats have skyrocketed during the last decades, with 37% of the first records reported since 1970 (Seebens et al., 2017). Increased human travel and trade favor deliberate and accidental dispersal of species outside their native ranges, with e-commerce contributing substantially to the propagation and sale of alien species in recent years (Mack et al., 2000; Mainka and Howard, 2010; Humair et al., 2015; Turbelin et al., 2017). Habitat destruction and climate change also affect species distribution, reproduction, and behavior, often creating suitable environments for exotic species, some of which will become invasive (see Box 1). While biosecurity (i.e., preventing the introduction and spread of harmful organisms) is a national priority in some countries such as the US, Australia, South Africa, and New Zealand, in other countries (mainly developing nations) biological invasions receive little attention due to limited resources and competing priorities (Pyšek et al., 2020).
Box 1. Defining biological invasion.
There are many definitions of “biological invasion,” and some disagreements persist, but two main criteria currently in use are based on either species distribution (geographic criterion) or their impact (Valéry et al., 2008), both of which have limitations. For instance, the movement of species into new ranges is not new and has been facilitated by humans for centuries. Most cultivated species grow and thrive in environments where they did not evolve. Yet, these are not considered invasive (in fact, this has contributed to the development of human society). The “impact” argument somewhat addresses this issue, but it tends to have an anthropocentric view, i.e., impact on humans, and the definition of impact is often vague. For regulatory purposes, there has been an effort to reconcile these views, e.g., the Convention of Biological Diversity (CBD) defines invasive alien species as those “whose introduction and/or spread outside their natural past or present distribution threatens biological diversity,” the International Union for Conservation of Nature (IUCN) as “animals, plants or other organisms that are introduced into places outside their natural range, negatively impacting native biodiversity, ecosystem services or human well-being” and the Center for Agriculture and Bioscience International (CABI) as “species whose establishment and spread threaten ecosystems, habitats or species with economic or environmental harm.” These definitions typically emphasize the negative impacts of invasive species. However, some authors (Pearce, 2016) highlight the remarkable traits of invasive species that allow them to persist above others in highly anthropogenically disturbed environments and the important role they may play in maintaining ecosystems in the future. Others promote a neutral view of invasive species not as inherently ‘bad' or ‘good' but rather as an ecological phenomenon that requires scientific study to further our understanding of species interactions and responses to environmental change (Brown and Sax, 2004). In this paper, we take a broad approach, including papers where the authors have identified plant species as being invasive, independently of the definition they follow. We also included the terms “exotic”, “alien” and “weed” as part of our search criteria, as these terms are often associated with invasive species.
Exotic plants constitute an important percentage of invasive species worldwide, almost outnumbering native flora in islands such as Hawaii and New Zealand (Simberloff, 2010; Pyšek et al., 2020), and in many cases, invasion is widespread, and thus eradication no longer feasible (Souza-Alonso et al., 2017). While the negative ecological impacts of invasive plants are well documented, interactions with invasive plants do not always have adverse outcomes for native species. Evidence of facilitation (e.g., through nutrient enrichment, food source diversification, increased pollination, competitive release, or predator release) is increasing (Rodriguez, 2006; Oduor et al., 2018; Effah et al., 2020b; Galappaththi et al., 2022). Also, the impacts of invasive species can change over time, and ecological and evolutionary processes may increase or attenuate the effects of an invader (Strayer et al., 2006; Dostál et al., 2013). Regardless, native plants are under selective pressure to adapt to competition with invasives (Callaway et al., 2005; Leger, 2008; Oduor, 2022). Therefore, a broader perspective, including multiple co-occurring species and different time-frames, is needed when exploring the ecological impacts of invasive species (Barney et al., 2015).
Exotic invasive plants (henceforth invasive plants) can, directly and indirectly, alter the environments they colonize in multiple ways. For example, they can affect geomorphology, fire regimes, hydrology, microclimate, nutrient cycling and productivity (Dukes and Mooney, 2004; Weidenhamer and Callaway, 2010) and engage in multiple interactions with native and other introduced species (Bezemer et al., 2014; Bajwa et al., 2016; Tallamy et al., 2021). Chemically, invasive plants can alter their invaded habitats by releasing secondary metabolites, mainly through root exudates and airborne emissions, which act as semiochemicals mediating intra- and interspecies communication (Kost, 2008; Heil, 2014; Reinecke and Hilker, 2014). The allelopathic potential (i.e., the production of chemicals by a plant species that can affect the growth, survival, development, reproduction, or behavior of neighboring organisms) of root exudates has been extensively studied and acknowledged as a key trait contributing to invasive plants' ecological success (Chengxu et al., 2011; Chen et al., 2017; Kalisz et al., 2021). However, less is known about volatile (airborne) allelochemicals, which are also likely to play crucial ecological roles (Xie et al., 2021; de Souza et al., 2022).
Plants are prolific producers and emitters of volatile organic compounds (VOCs) from reproductive and vegetative parts, constituting the plant's scent. These are typically lipophilic products with low molecular mass (<300) and can be assigned to different classes according to their biosynthetic origin (e.g., terpenoids, fatty acid derivatives, phenylpropanoids/benzenoids, C-5 branched compounds, and nitrogen and sulfur-containing compounds and others classes) (Dudareva et al., 2004, 2013). Plant VOC production and release are phenotypically plastic, being species-specific but also highly responsive to environmental change (Agrawal, 2001; Clavijo McCormick, 2016; Campbell et al., 2019). VOC production is metabolically costly, so trade-offs between VOC emission, other forms of defense, reproduction and growth are known to occur (Ballhorn et al., 2008; Schiestl et al., 2014; Zhang et al., 2020).
Plant VOCs play a crucial role in plant-insect interactions by mediating host location and acceptance by pollinators, herbivores, and their natural enemies (Pichersky and Gershenzon, 2002; Bruce et al., 2005). VOCs also mediate plant-plant interactions, including kin-recognition, priming, and competition (Baldwin et al., 2006; Heil and Kost, 2006; Kegge and Pierik, 2010; Kigathi et al., 2013; Effah et al., 2019). Given the importance of plant VOCs, we explored the literature published on ecological aspects of invasive plants' VOCs during the last decade (2012–2022), focusing on factors affecting their emission and their ecological impact on plant-plant and plant-insect interactions.
2. Search criteria
To gather information for this review, we initially consulted the Google Scholar search engine using the criteria “invasive plant” OR “exotic plant” OR “alien plant” OR “weed” AND “VOCs” OR “volatile” OR “scent.” We then paired those terms with each one of the following terms of interest, i.e., “biotic,” “abiotic,” “allelopathy,” “warming,” “temperature,” “UV-radiation,” “ultraviolet,” “CO2,” “carbon dioxide,” “ozone,” “O3,” “pollution,” “moisture,” “drought,” “soil nutrients,” “gene,” “genetics,” “herbivore,” “herbivory,” “predator,” “parasitoid,” “natural enemy,” “pollinator,” “pollination,” “microorganism,” “microbe,” “microbial,” “pathogen.” The terms of interest were selected based on previous literature reporting the impacts of environmental and genetic factors on plant VOC emissions and their known ecological roles (Holopainen, 2004; Figueiredo et al., 2008; Holopainen and Gershenzon, 2010; Blande et al., 2014; Clavijo McCormick, 2016; Effah et al., 2019; Ninkovic et al., 2021). As a limitation, we are aware that other studies relevant to the topic may not have been found using the chosen terms and that further studies might exist in languages other than English.
The search range included publications from 2012 to 2022. While the initial search was conducted in October 2022, a revised search was further conducted in January 2023 to incorporate data from the whole year. We decided to focus on work from the last decade but recognize that studies conducted before 2012 may yield further light on the findings of this review. The search was limited to full documents (i.e., conference abstracts were excluded) in English, reporting observational or experimental studies under laboratory or field conditions and published in reputable journals, having undergone peer-review (pre-prints were not included). We excluded reviews and work solely addressed to developing control methods for weeds (e.g., introduced biological control agents or use of plant extracts as bioherbicides) as this is out of scope for our review. However, we recognize that introduced biocontrol agents and neighboring plants can modify the VOC emissions of invasive plants. We also excluded studies focused on plant extracts (from above or below-ground tissue) because some solvents do not favor plant VOC extraction. Others are not selective, i.e., include volatile and non-volatile compounds, making it difficult to assert if VOCs alone are responsible for the observed activity. While we acknowledge and provide some examples of work on essential oils, we did not conduct an exhaustive revision on this topic since the elevated concentration of VOCs in essential oils makes it challenging to assess their ecological roles under natural conditions.
We found 29 studies matching our search criteria (Supplementary material). The following sections summarize and critically discuss our findings, identifying knowledge gaps and avenues for further research.
3. Factors affecting the VOC emissions of invasive plants
Invasive species are known to have high phenotypic plasticity (i.e., the ability to produce different phenotypes from a single genotype when exposed to varying environmental conditions), contributing to their ecological success and competitive advantage (Yu-Peng et al., 2004; Richards et al., 2006; Davidson et al., 2011; Gratani, 2014; Liao et al., 2016). Plant-VOC synthesis and emission embody this phenotypical plasticity, having a genetic component but being highly responsive to multiple biotic and abiotic factors such as herbivory, temperature, UV-radiation, drought, and their complex interactions (Maja et al., 2016; Campbell et al., 2019; De Lange et al., 2019; Rieksta et al., 2021). These factors may play an even more critical role in invasion scenarios where habitats are likely to differ from those in the invasive plants' native ranges. In this section, we reviewed studies exploring the impacts of genetics, abiotic and biotic factors (summarized in Figure 1) on the emission of VOCs by invasive plants.
3.1. Genetics
The ability of plants to produce certain VOCs is determined by their biosynthetic machinery, which is regulated by genetic traits from the species' unique evolutionary history. Hence, plant metabolites can be reliable indicators of plant taxonomy. However, multiple factors influence gene expression leading to considerable variation in VOC production and emission within species (Figueiredo et al., 2008). Therefore, genetics alone may not explain an invasive plant's unique and dynamic chemical behavior. Metabolomics studies reveal that invasive plant species have more unique metabolomic profiles than native congeners and higher chemical diversity than conspecifics growing in the native range (Macel et al., 2014; Skubel et al., 2020). While not targeting VOCs exclusively, these studies suggest that a genetic predisposition to chemical uniqueness may favor invasive behavior. Exposure to new environments may also lead to a rapid evolution of chemical traits in invasive plants.
A recent study conducted in Europe (Lin et al., 2021) aimed to understand the effect of genetic and evolutionary aspects on an invasive species' VOC emission. The authors explored different populations of ragwort Jacobaea vulgaris from its native range in Europe and invaded ranges in Australia, New Zealand, and Western North America. Seeds were sourced from different origins and grown under controlled conditions, where their constitutive and herbivore-induced VOC emissions were explored. The findings show that, under the same experimental conditions, plants from invaded ranges released more constitutive than herbivore-induced VOCs, making them more attractive to a specialist herbivore but less so its parasitoid. Constitutive VOCs can act as deterrents against generalist insects but can be used as host cues by specialist herbivores. In contrast, herbivore-induced VOCs are linked to the attraction of natural enemies (McCormick et al., 2012; Kessler, 2015). The authors posit that in the absence of specialist herbivores and their natural enemies, selection for induced VOC emission may be relaxed when invasive plants invade new areas, favoring the emission of more constitutive VOCs.
Within-population genetic variation in VOC emission was investigated for the invasive Mayweed chamomile (Anthemis cotula), which is a significant problem in the US Pacific Northwest due to its high abundance and lack of control options (Adhikari et al., 2021). The authors investigated trait variation and heritability, including seedling emergence, biomass, date and duration of flowering and number of flower heads and floral scent on 300 plants from six half-sib families (i.e., coming from seeds of the same mother plant) from 10 different populations (infested farms or sites). The results showed significant differences in the flowering period, which could differ by a month between some half-sib families, and floral VOCs. Trait heritability was high for most phenotypic traits, including VOCs, suggesting that individual plants differ in scent traits with potential impacts on reproductive success.
Altogether, these studies suggest that (a) invasive plants are likely to have higher VOC diversity than native counterparts, which may contribute to their invasiveness, (b) plants of the same species show different chemical behavior in invaded and native areas, and (c) chemical diversity within species is heritable, and if it presents a competitive advantage, individuals who reproduce more successfully will pass on these traits to their offspring.
3.2. Abiotic factors
Multiple studies have explored the role of abiotic factors on plant VOC emission in natural and productive systems. Temperature (warming), carbon dioxide (CO2), ozone (O3), drought and UV radiation are all known to influence VOCs (Holopainen, 2004; Figueiredo et al., 2008; Holopainen and Gershenzon, 2010; Blande et al., 2014; Clavijo McCormick, 2016; Effah et al., 2019; Ninkovic et al., 2021). Within our search criteria, we found studies exploring the impact of warming, CO2, and UV radiation on invasive plants' VOC emissions.
Warming is one of the most studied factors influencing VOCs, typically causing a consistent and significant increase in volatile emissions (Peñuelas and Staudt, 2010; Bao et al., 2022). Exploring the effects of warming is essential to understand species' responses, robustness, and resilience to climate change. The effect of elevated temperature on VOC emission of an invasive plant, Alternanthera philoxeroides (alligator weed) and its native congener, A. sessilis was investigated in the Henan Province of China (Liu et al., 2021). Simulated warming was conducted under field conditions using 2000 W infrared heaters suspended 2 m above the plants, which increased air temperature by 0.86°C (close to the predicted temperature increase for this region due to climate change). In contrast to other reports, the authors found that warming suppressed VOC emissions for both species. Still, this effect was less pronounced for the invasive species, where only undecane, (E)-3-hexen-1-ol and acetate azulene were suppressed, while multiple compounds were suppressed under the same treatment for the native plant. The results suggest that invasive plants may be more stable in their VOC emissions under warming conditions than their native counterparts.
Carbon dioxide (CO2) from burning fossil fuels and other human-related activities accumulates in the atmosphere, contributing to greenhouse effects and thus being a climate change contributor. Elevated CO2 is also a well-known factor influencing VOCs, but available evidence so far indicates that its impacts vary greatly across different studied systems (e.g., Peñuelas and Staudt, 2010; Bao et al., 2022). For instance, Oster et al. (2015) investigated the effect of elevated CO2 (an addition of 300 ppm for a total of 700 ppm—nearly twice the ambient level), warming (temperature raised an ~3°C with infrared heaters) and their combination on damage-induced VOC emissions of Centaurea solstitialis (yellow star thistle), a major invasive weed in western North America. They found that, under the measured conditions, CO2, warming, or their combination, did not have a significant impact on the levels of individual or total VOCs, contrasting other studies (Yuan et al., 2009; Peñuelas and Staudt, 2010; Copolovici et al., 2021; Bao et al., 2022). Again, this study supports the notion that invasive species VOCs may be more stable (i.e., fluctuate less) in response to environmental variation. Whether this is a species-specific trait, or a common feature of invasive species remains to be further investigated.
UV radiation is also known to influence invasive plants' VOC emissions. In moderate quantities, it can be a potent elicitor of secondary metabolites (including VOCs), but high levels can damage the biosynthetic machinery of the plant (Fini et al., 2011; Llusia et al., 2012; Liu et al., 2015; Maja et al., 2016). A New Zealand study (Effah et al., 2020d) experimentally manipulated UV radiation to explore its impacts on VOC emissions of the invasive weed Calluna vulgaris (heather). UV radiation varies starkly between the native range of this plant (UK), where the UV index rarely exceeds 7, and the invaded range (New Zealand), where summer daytime indexes often reach 12 and can exceed 13 in the far North. Using tunnel houses clad with UV-selective filters, VOCs produced by field-collected heather were measured under New Zealand ambient, 20% attenuated and 95% attenuated solar UV treatments. Plants with lower UV exposure (95% attenuated) produced significantly more sesquiterpenes than those under ambient UV and 20% attenuation, showing that high levels of UV radiation can compromise VOC emissions of invasive plants. This study shows that UV radiation may be a critical factor influencing the behavior of invasive plants coming from regions with different UV intensities.
3.3. Biotic factors
Herbivory is well known to have significant effects on plant VOC profiles, leading to the production and release of herbivore-induced plant volatiles (HIPVs). HIPVs play an essential role in indirect plant defense by attracting natural enemies of the herbivores and providing precise information about the location, identity, abundance, and developmental stage of their insect host or prey (McCormick et al., 2012; Clavijo McCormick et al., 2014a,b). Under invasion scenarios, introduced plants will interact with native herbivores that they did not co-evolve with, and their natural enemies may be unattracted to or unable to interpret the herbivore-induced volatile signals (Bezemer et al., 2014). Therefore, it is assumed that invasive plants will invest fewer resources in producing HIPVs and produce more constitutive volatiles (direct defenses) instead. The study by Lin et al. (2021), discussed earlier, supports this assumption. However, a study conducted in Italy (Cozzolino et al., 2015) shows that the production of herbivore-induced VOCs may not always negatively impact invasive plant fitness since invasive plants relying on arthropods for reproduction can use herbivore-induced signals to their advantage. The authors investigated the impact of herbivory by a native generalist insect Spodoptera littoralis on the floral signaling of an invasive weed Silene latifolia (white campion) in Southern Italy. They found that herbivore-infested plants emitted higher amounts of (Z)-3-hexenyl acetate and β-ocimene, which increased nocturnal pollination, leading to higher fruit production.
Another possible scenario is the interaction between alien invasive plants and insects, which is predicted to increase due to global trade and climate change (Johnson et al., 2009). A series of studies (Tun et al., 2020, 2021, 2022; Jones et al., 2021) investigated the resistance, growth, reproduction, and VOC emission of 15 introduced willow clones in New Zealand in response to the attack of the giant willow aphid Tuberolachnus salignus (of Asian origin). These willow clones were mainly from Europe and North America, with some having invasive traits. The studies found that only two clones were naturally resistant to this attacker. Aphid attack negatively impacted growth for the remaining clones but caused compensatory reproduction, evidenced by a higher number of catkins and longer flowering times. Interestingly, there was no sign of induced VOC emission, and VOC reduction was even observed in some clones. Possible explanations for this phenomenon include (1) inability of the plant to recognize and respond to the attacker, (2) active suppression of plant defenses by the introduced herbivore as documented for other aphid species (Pareja et al., 2012; Najar-Rodriguez et al., 2015), and (3) a trade-off between induced defenses, growth, and reproduction.
The presence of introduced biological control agents, typically specialists that co-evolved with the plants in their native range, is also likely to influence the energy budget allocation of invasive plants and their herbivore-induced VOC emissions. A New Zealand study (Effah et al., 2020d) showed that herbivory by the biocontrol agent Lochmaea suturalis on the invasive weed C. vulgaris affected different compound groups throughout the seasons, reflecting changes in the phenology of the plant and developmental stages of the herbivore. In general, herbivory caused a moderate but significant increase in terpenoid production, but during the peak of the flowering season, a strong and significant reduction in VOC emissions was observed, suggesting potential trade-offs between herbivore-induced VOC emission and reproduction. Additional evidence supporting the cost of induced defenses for invasive plants comes from invasive Cynoglossum officinale L. (hounds tongue plants) in North America, where exogenous application of methyl jasmonate (MeJa) increased the emission of plant VOCs and doubled the production of trichomes (mechanical defense structures), but resulted in fewer and smaller leaves, and lighter nutlets (seeds) (Runyon and Birdsall, 2016). These studies suggest that, like other species, invasive plants experience trade-offs between different forms of defense (indirect vs. direct or chemical vs. mechanical) and between defense, growth, and reproduction.
Another fundamental aspect of invasion biology is the impact of soil microorganisms. Mutualist and antagonist bacteria can facilitate or hinder the success of invasive plants by modifying the availability of soil nutrients, influencing plant metabolism, and shaping plant defense traits, among other mechanisms (Reinhart and Callaway, 2006). A recent study (Kalske et al., 2022) investigated the impact of soil microbiota on herbivore resistance in native (US) and invasive (Finnish) populations of Lupinus polyphyllus, including the emission of VOCs. The authors assessed VOC emissions on plants from both populations grown on intact or autoclaved soil from the invaded range. They found that soil inoculum treatment significantly affected the composition of VOC emissions for both populations, mainly by increasing the emission rates of green leaf volatiles, which are known to mediate direct and indirect plant defense. However, plants from the invaded range showed higher resistance to herbivory (snails) than native ones, suggesting that invasive plants can associate with and benefit from soil microbiota from distant locations, thus influencing VOC emissions and plant defense.
The impact of neighboring plants on invasive plants' VOCs is discussed in Section 4.2.
3.4. Multiple co-occurring factors
Under natural conditions, invasive plants are simultaneously exposed to multiple biotic and abiotic factors. Therefore, more field studies exploring the effects of co-occurring factors on VOC emissions are essential to advance our understanding of the chemical behavior of invasive plants in their “new” environment. A field study on the North Island Central Plateau, New Zealand (Effah et al., 2020a) explored the relationship between VOC emissions of the invasive plant C. vulgaris and some biotic and abiotic factors (herbivory, temperature, soil nutrients and soil moisture). The study found that soil nutrients were the most significant factor associated with C. vulgaris VOC emissions in this region, having a strong positive correlation with the emission of most compounds tested. Temperature was the second most important factor positively correlated with the emission of some green leaf volatiles and the homoterpene (E)-4,8-dimethyl-1,3,7-nonatriene (DMNT). Herbivory was low and only correlated with the emission of hexyl acetate. No correlation was found between soil water content and VOC emissions. A similar study conducted in Brazil (Sampaio and Costa, 2018) also explored the correlation between multiple abiotic environmental factors and VOC emissions by the Mexican sunflower, Tithonia diversifolia, with outcomes supporting the role of soil nutrients and temperature in modulating VOC emissions. While these studies did not investigate direct causation, they suggest that soil nutrients are essential drivers of VOC emissions by invasive plants, and further support other studies showing a relationship between soil nutrients or soil type and VOC emissions (Ormeño and Fernandez, 2012). Invasive plants can colonize and have high performance and nutrient-use efficiency in depleted soils, and over time, can modify soil chemistry and biota (Weidenhamer and Callaway, 2010; Matzek, 2011; Funk, 2013). Plant-soil feedback is known to change during the course of invasion, influencing the invasive plants' competitive ability against native plant communities (Oduor et al., 2022). Therefore, more studies are needed to understand how changes in soil chemistry and biota influence invasive plants' VOC emissions over time.
Other factors including drought, ozone (O3) and increasingly polluted atmospheres could also influence VOC emissions of invasive plants (Pinto et al., 2010; Blande et al., 2014; Rissanen et al., 2022). However, more studies are required to understand how invasive plants respond chemically to these factors.
4. Invasive plants' VOCs in plant-plant interactions
Invasive plants must adapt to new environmental factors but also compete for resources with other plant species (both native and introduced), with which they may not have co-evolved. Invasive plants share multiple traits that facilitate their ecological success, such as rapid growth and prolific reproduction, and it is increasingly acknowledged that their allelopathic potential is one of such attributes (Chengxu et al., 2011; Chen et al., 2017; Kalisz et al., 2021). In competition scenarios, VOCs allow plants to obtain information about neighbors' identities (e.g., kin vs. non-kin) and physiological and health status (e.g., healthy vs. herbivore-damaged) and to respond accordingly (Effah et al., 2019). However, this phenomenon has been poorly explored under biological invasion scenarios. This section aims to document and discuss the current state of knowledge of invasive plants' VOCs in plant-plant interactions, concentrating on allelopathy and neighbor detection.
4.1. VOC-mediated allelopathy by invasive plants
Invasive plants often outcompete natives partly due to the possession of performance-related traits, including rapid growth and reproduction and physiological tolerance to a wide range of environmental factors (Van Kleunen et al., 2010). To enhance their competitiveness, invasive plants also release novel biochemicals into their surroundings that inhibit native plants' germination and growth, with the magnitude of the effect being regulated by co-evolution (Callaway and Ridenour, 2004; Macias et al., 2007; Thorpe et al., 2009). A significant number of studies have explored the allelopathic properties of invasive plants' root exudates or extracts, which may include volatile and non-volatile compounds (e.g., Callaway and Ridenour, 2004; Thorpe et al., 2009; Kim and Lee, 2011; Darji et al., 2021; Kato-Noguchi and Kurniadie, 2021, 2022). Another prolific area exploring allelopathic properties of invasive plants is the study of their essential oils (i.e., highly concentrated volatile compounds). Research on essential oils often shows inhibition of germination and growth of other plants (e.g., Shao et al., 2013; Costa et al., 2019; Abd-ElGawad et al., 2020; Han et al., 2021). These studies suggest that VOCs (mainly terpenoids) released by invasive plants can have phytotoxic effects on natives in a dose-dependent manner. However, essential oils are highly concentrated, making comparisons with natural emissions difficult.
Interestingly, growing evidence shows that VOCs at natural concentrations also exhibit allelopathic properties. Owing to their physicochemical properties, movements (i.e., diffusion) of VOCs in the atmosphere are less restricted and could affect several nearby plants compared to non-volatile compounds, producing cost-effective outcomes for emitters.
Table 1 summarizes studies during the last decade reporting VOC-mediated allelopathic effects of invasive plants. These studies suggest that invasive species have the potential to severely affect the chemical environments of native plants by releasing volatile allelochemicals into the surrounding that reduce or inhibit the germination and growth of native species. This can be achieved by allelopathic compounds reducing the photosynthetic efficiency of receiver plants or affecting cellular division processes (mitosis) (Romagni et al., 2000; Aşkin Çelik and Aslantürk, 2010; Araniti et al., 2017; Han et al., 2021). The underlying mechanism seems to be associated with allelochemicals triggering the release of reactive oxygen species in the receiver, which initiate signaling cascades, ultimately leading to genome-wide changes in gene expression (Bais et al., 2003). More studies are needed to elucidate the mode(s) of action of volatile allelochemicals.
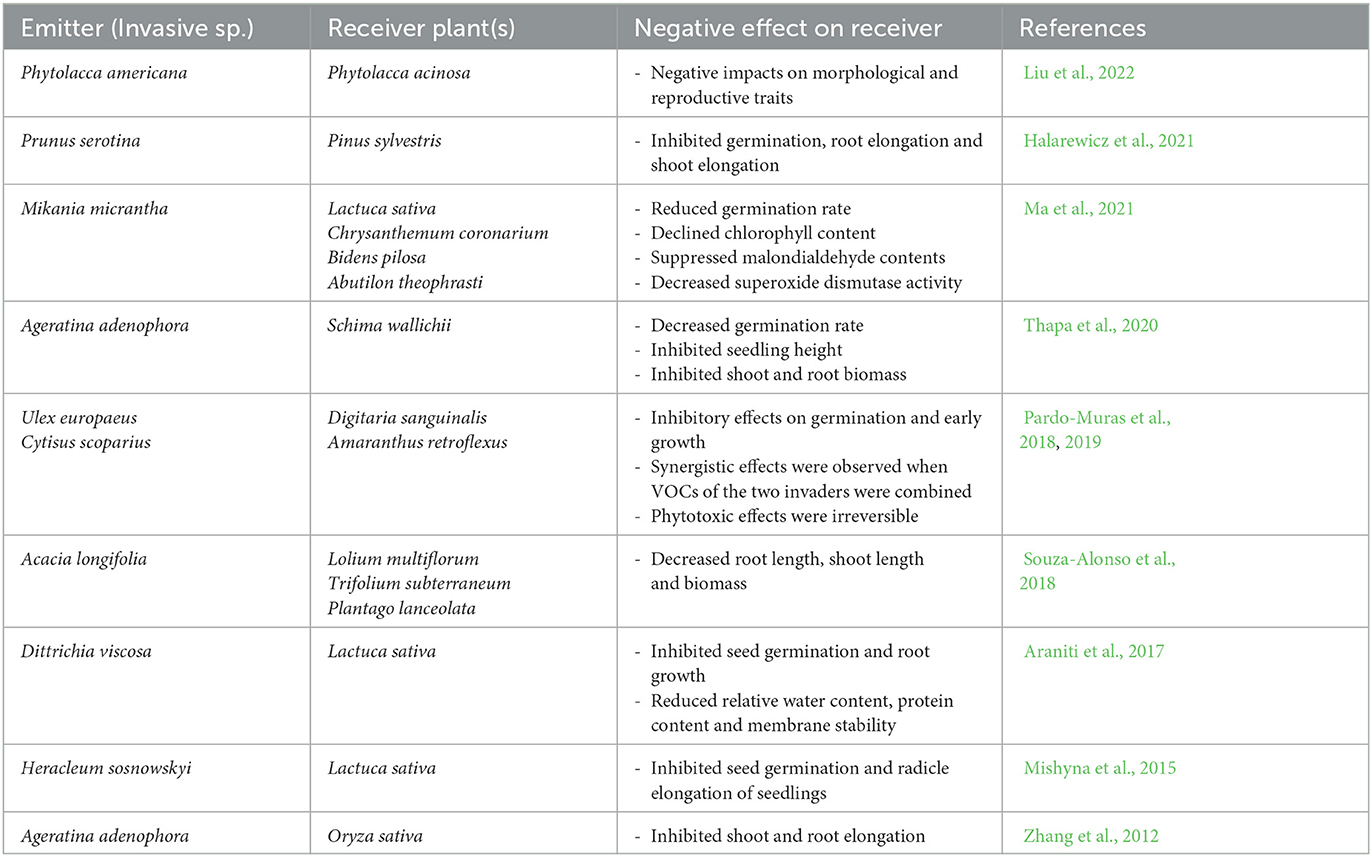
Table 1. Studies exploring the effect of invasive plants aboveground VOCs on recipient plants during the last decade (see search criteria).
Invasive species volatile allelochemicals can also be deposited in the rhizosphere through litter. Litter volatile compounds are known to be persistent and can still be detected after a long time following litter deposition (Kainulainen and Holopainen, 2002). A pioneer study outside the scope of this review (Inderjit et al., 2011) suggests that volatile compounds present in the litter of an invasive species play a crucial role in its invasiveness. Therefore, we encourage further studies to investigate the allelopathic potential of invasive plants' litter VOCs on native species and elucidate how long residues remain bioactive after invasive plants have been removed.
4.2. Invasive plants' VOCs and neighbor detection
The ability of plants to detect the presence and identity of neighbors is critical for resource acquisition and competitive decisions. Plants sense their neighbors through mechanosensing and by assessing light quality, and nutrient availability (Volkov and Ranatunga, 2006; Pierik et al., 2013; Pierik and de Wit, 2014). Plant root exudates also contribute to plant-plant signaling and neighbor recognition (Badri and Vivanco, 2009; Pierik et al., 2013; Semchenko et al., 2014; Wang et al., 2021). However, there is increasing evidence that plants also use VOCs in neighbor recognition (Li, 2016).
Airborne emissions (VOCs) can provide vital information about the emitter to other plants. Nearby plants can therefore decode this information to establish the emitter's identity (kin or not kin), phenology, and even health status and adjust their behavior accordingly (Heil and Kost, 2006; Kigathi et al., 2013; Karban et al., 2016; Moreira and Abdala-Roberts, 2019). This is well-established in plant defense against herbivores, whereby VOCs emitted by damaged plants trigger defense-related responses in neighbors in a phenomenon known as priming (Heil and Kost, 2006). Unfortunately, such information is scarce in plant invasion contexts.
The existing evidence suggests that invasive plants can detect neighbors' identities and regulate their own chemical profiles accordingly. A classic study tested the chemical defense strategy of the invasive weed, Centaurea maculosa, in response to different neighbors and found that C. maculosa accumulated higher levels of defense-related compounds when growing with conspecifics relative to heterospecifics under both controlled and field conditions (Broz et al., 2010). However, VOCs were not explicitly addressed in this study. A field study conducted in New Zealand found that the invasive C. vulgaris modulated its VOC emission when growing in conspecific versus heterospecific stands. C. vulgaris lowered its emission when growing with heterospecifics, particularly another invasive species, Cytisus scoparius (Scotch broom) (Effah et al., 2020a). This example highlights that invasive plants can adjust the quality and quantity of their VOCs in response to their neighbors' cues.
Since VOCs act as both signaling and bioactive molecules, receiver native plants are also expected to alter their chemical profiles in response to VOCs emanating from invasive plants. However, this remains largely obscure, and to the best of our knowledge, only two studies have investigated native plants VOC emissions in response to invasive plants. Under natural conditions, Effah and co-workers (Effah et al., 2020c) found that the New Zealand native plant Leptospermun scoparium (mānuka) emitted lower amounts of VOCs at sites where invaders (C. vulgaris or C. scoparius) were present but not when neighboring conspecifics or another native plant (Dracophyllum subulatum). In a follow-up semi-field experiment, where environmental factors were controlled and above or below-ground contact between plants was prevented, L. scoparium displayed the same behavior, reducing its VOC emissions when the neighbors were invasive species, but not when surrounded by conspecifics (Effah et al., 2022). These studies demonstrate that VOCs from invasive plants can influence the VOC emissions of native plants. Still, the underlying mechanisms behind the observed reduction in VOC emissions and the impacts of these changes on native plants' growth, reproduction, and interactions with other organisms remain to be investigated.
The evidence gathered in this section shows that (a) VOC-mediated allelopathy is common in invasive plants, (b) invasive plants can detect neighbors and adjust their VOC emissions accordingly, and (c) native plants can perceive and respond to invasive plants' VOCs by modulating their own emissions. Further studies using different plant species combinations (both invasive and natives) and additional trophic levels are also required to improve our knowledge of the ecological impact of invasive plants airborne chemical cues on native plants and their associated fauna. We acknowledge that plant roots are prolific emitters of VOCs that can affect neighboring plants' germination, growth, and resource allocation (Ens et al., 2009; Insam and Seewald, 2010; Gfeller et al., 2019). But we limited the evidence in this section to VOCs from aboveground organs due to the difficulties in disentangling the effects of VOCs and root exudates in below-ground interactions. Thus, further work on this topic is encouraged.
5. Invasive plants' VOCs in plant-insect interactions
Insects rely on a very sophisticated olfactory perception mechanism and olfactory information processing to detect and elucidate VOC-coded information (Schmidt and Benton, 2020). This complex olfactory system allows insects to identify and choose suitable host plants against a heterogeneous chemical background of compounds that are constantly being emitted by non-host plants (Bruce et al., 2005; De Bruyne and Baker, 2008; Bruce and Pickett, 2011). Natural enemies also use their sensitive sense of smell to obtain information about their prey or insect host coded into plant VOCs (McCormick et al., 2012; Clavijo McCormick et al., 2014a,b). The signal (plant VOCs) and receptor (olfactory system) have been shaped by co-evolution leading to highly-specific interactions and also remarkable variation in plant odors and insects' olfactory systems (Hansson and Stensmyr, 2011; Schiestl and Johnson, 2013; Jones et al., 2022).
In their invaded range, invasive plants will interact with insect species (both beneficial and antagonistic) they did not co-evolve with (unless these species are naturally present or have also been introduced) (Bezemer et al., 2014; Litt et al., 2014; Sunny et al., 2015). Thus, it is of interest to explore how invasive plants' VOCs could affect the behavior of native insects. Harvey and Fortuna formulated some hypotheses on how structural and chemical characteristics of invasive plants may affect the plant-finding abilities of native insect herbivores and their natural enemies (Harvey and Fortuna, 2012). The chemical complexity in a given habitat is closely related to the vegetation structure and plant diversity within that habitat (Randlkofer et al., 2010). Therefore, the authors used two different plant-community ecology scenarios, (a) diverse plant community containing native and invasive plants and (b) a community dominated by invaders, to suggest possible responses of native herbivores (and higher trophic levels associated with them) to the VOCs of invasive plants. We summarize and expand on these scenarios (a–e) and propose new ones (f and g) below:
(a) In a diverse plant community, VOCs emitted by the invasive plant could act as repellents for the native herbivores (and even their natural enemies), even if the plant is nutritionally suitable. If VOCs are repellent to native herbivores, the invasive plant will reduce herbivore pressure (as there may still be accidental landings or chance encounters).
(b) In the same scenario, if the invasive plant colonizes habitats containing closely related native species that share similarities in terms of nutritional suitability and VOC composition (qualitative and quantitative), the scent of the invasive could attract native herbivores (and the natural enemies of these herbivores) that could use them as alternative food/oviposition sources leading to potential “host range expansion.”
(c) Alternatively, in a diverse plant community, invasive plants unrelated to native ones may have overlapping or similar scents that are attractive to native herbivores (or their natural enemies) but are not suitable for their development, negatively impacting fitness and leading to an “evolutionary trap.” Such “evolutionary traps” could exert selection against the use of invasive plants by native insects (Bezemer et al., 2014).
(d) In highly disturbed habitats, invasive plants could spread rapidly and become dominant if they are fast-growing and early successional species. Suppose their scent is attractive in the absence of native hosts. In that case, this could promote herbivory by local species (most likely generalists) and “host shifts” leading to new adaptations and responses to the invasive plant's VOCs over time.
(e) If the invasive plant is dominant and its scent is repellent (and the plant nutritionally unsuitable), native insect species unable to adapt will likely face stark population reductions or even extinction, effectively creating an “enemy-free space.”
(f) If an invasive plant is introduced into a native plant community, where there are few or no related plant species present, the plant may be indifferent to the native insect, and it may not be attracted nor repelled by it. In the case of natural enemies, they may be unable to decode the information contained in the herbivore-induced VOC blend of an invasive plant.
(g) Another possible situation, especially if the invasive species is dominant or a prolific VOC emitter, is that VOC blends emitted by the invasive plant “confuse” or “overwhelm” native insects by causing sensory fatigue, altering background odors, masking the scent of the native plants or another mechanism. This might negatively affect the associations between the native plants and their co-adapted insects.
In addition to plant chemistry and habitat complexity, the degree of host specialization of an insect is expected to influence the outcome. This is illustrated in a study conducted in Europe (Fortuna et al., 2013), showing that when offered a choice, the specialist lepidopteran herbivore Pieris brassicae preferred the native crucifer Sinapis arvensis to the taxonomically related invasive Bunias orientalis. Female butterflies preferred to oviposit on the native plant, and their offspring survival and performance were higher on the native compared to the exotic plant. Chemical analyses revealed qualitative and quantitative differences in the VOC blends of both plant species. Yet, despite this dissimilarity, the generalist parasitoid Cotesia glomerata could not discriminate between the herbivore-induced VOC blends released by the two plants in flight-tent bioassays. However, under semi-field conditions, the parasitism rate of P. brassicae larvae by C. glomerata was higher when feeding on the native plant (about 40%) compared to when feeding on the invasive plant (about 30%), suggesting that other cues or sources of information influence insect's decisions. This study showed that C. glomerata could potentially parasitize its insect host when feeding on the invasive plant, but follow-up studies are needed to test the long-term success of C. glomerata when developing on insects fed on the invasive plant to assess whether this situation could represent an “evolutionary trap.”
Another study conducted in the US (Oster et al., 2014) investigated the responses of two generalist predatory beetles feeding on slugs, one native to US (Scaphinotus interruptus) and the other from Europe (Pterostichus melanarius), toward the VOCs of the invasive weed C. solstitialis (native to Europe) in response to damage by the garden slug Deroceras reticulatum (also introduced in Europe). The results show that the introduced beetle showed a significant preference for the odor of damaged C. solstitialis relative to the odor of intact plants, while the native predator did not. This study supports the notion that some native natural enemies may not be able to discriminate the information coded in the herbivore-induced VOCs of invasive plants due to the lack of shared evolutionary history.
A New Zealand Study (Effah et al., 2022) explored the influence of VOCs of the native mānuka and that of an unrelated invasive plant of European origin (C. vulgaris) on herbivores, under controlled conditions. Researchers offered host-plant and non-host VOCs versus clean air, and their combination in Y-tube olfactometer tests to two chrysomelid beetles, the generalist native Pyronota festiva (mānuka beetle), and the specialist introduced biocontrol agent Lochmaea suturalis (heather beetle). They also performed preference/feeding tests in Petri dishes with fresh plant material. Results indicated that the native beetle could not discriminate between host and non-host plants based on plant volatile cues only, although these plants are not closely related. The invasive plant alone was unattractive, suggesting some form of “confusion” in line with hypothesis (g). However, they performed relatively well when offered other cues (i.e., visual, gustatory, or tactile) in the Petri dish tests. This was in contrast to the introduced beetle, which showed high host-specificity in both Y-tube and Petri dish assays. This study showed that invasive plants' VOCs can disrupt communication between native insects and their host plants, but the use of other cues may compensate for this disruptive effect. The mechanism behind the inability of the native beetle to recognize its host plant based on olfactory cues only remains to be elucidated. Further studies should test whether the disruption of plant-plant communication and host-searching behavior of native insects by invasive VOCs reported by these authors is common in other systems.
In the context of climate change, it would be interesting to explore how invasive plants' VOC emission changes affect native insect behavior and its interaction with native plants. A previously cited study from China (Liu et al., 2021) investigated the effect of warming on the VOC emission of invasive A. philoxeroides and its native congener A. sessilis. The authors observed that, unlike invasive plants, native plants were strongly impacted by warming, decreasing their VOC emissions. In China (the native range of A. sessilis), native herbivores such as the generalist moth Spodoptera litura and oligophagous beetle Cassida piperata are known to use both plant species for feeding and oviposition. Therefore, authors recorded the behavior of these native herbivores toward the scent of both plants under warming and control conditions in Y-tube olfactometer bioassays. Both insects preferred the scent of the native plant to the invader, under control and warming treatments, suggesting that warming does not disrupt the ability of insects to find their preferred host. However, in oviposition assays, increased temperature caused a shift in S. litura oviposition behavior, resulting in significantly higher egg loads on the native plant, showing that warming could disproportionally increase herbivore pressure on natives.
These studies suggest that (a) plant chemistry, habitat complexity, and the degree of host specialization of an insect could shape native insect responses to invasive plants' VOCs, (b) invasive plants' VOCs can disrupt communication between native insects and their host-plants, but the use of multiple information sources by insects in making foraging/oviposition decisions may compensate for this disruption, (c) while climate change may not impair the insects' ability to find their preferred host(s) using VOCs in invasion scenarios, it may still affect insects' interactions with their host plants.
Despite the vital role of VOCs in mediating the relationship between plants and their pollinators (Pichersky and Gershenzon, 2002; Bouwmeester et al., 2019; Kantsa et al., 2019), and the knowledge that many invasive plants co-opt native pollinators and increase the number of pollinators over time (Pyšek et al., 2011), we found only one study exploring how native pollinators interact with invasive plant VOCs. The study (Cozzolino et al., 2015), discussed earlier, shows that native pollinators are attracted to herbivore-induced VOCs of an invasive plant, leading to higher fruit production and contributing to the potential spread of the invasive plant. A recent review (Stout and Tiedeken, 2017) raises awareness about the importance of exploring the interactions between native pollinators and invasive plants and the role of native pollinators in facilitating invasion. The authors propose trait-based approaches to guide future studies, and we suggest including VOC profiles as part of those traits.
6. Conclusions and future directions
This work presents a snapshot of the work on ecological aspects of invasive plant VOCs during the last decade. In the following paragraphs, we summarize key findings, highlight knowledge gaps, and suggest avenues for future research.
Evidence suggests invasive plants have more “chemical uniqueness” or “diversity” than their native counterparts. Still, it remains to be elucidated if this “chemical uniqueness” is a pre-condition to their invasive success or if exposure to a new environment favors this unique chemical behavior. Some abiotic factors affecting the VOC emission of invasive plants have been investigated (warming, CO2, UV radiation). The published studies suggest that invasive plants may be more resilient than natives to climate change and that soil nutrients play a key role in influencing invasive plants' VOC emissions. UV radiation can be important if the plant is invading areas with substantially different levels of UV exposure and responses to herbivory vary between systems. A better understanding of how invasive plant biochemistry, including VOC emission changes in response to “new” environments, could help improve the safety and effectiveness of biological control efforts, e.g., allowing to predict if an invasive plant will remain attractive and nutritionally suitable to an introduced biocontrol agent after experiencing chemical changes (Barrett et al., 2021).
Regarding the impact of biotic factors, invasive plants will likely interact with insects they have not co-evolved with, and local natural enemies may not be attracted to or able to interpret the plant's herbivore-induced VOCs. This may trigger changes in the invasive plant's defense strategy favoring direct (constitutive VOCs) over indirect defense (herbivore-induced VOCs). However, induced-VOCs may be co-opted as signals to attract native pollinators in some systems, reflecting trade-offs between different defense modes. Likewise, trade-offs between defense, growth, and reproduction may influence invasive plants' VOC emissions. The complexity added by multiple attackers, including introduced insects, is open for exploration. Invasive plants will also interact with and modify microbial communities (predominantly in the soil). How invasive plants behave chemically over time in response to these changes is also an exciting area for future study.
Moving forward, we strongly encourage investigating the impact of co-occurring abiotic factors across trophic levels. While exploring this complexity may be challenging from an experimental perspective, the number of studies incorporating multiple factors and trophic levels in other areas is increasing, and we predict that this trend will extend to include invasive plant VOCs. Also, validating lab results in the field will become increasingly important for this information to be useful for management and decision-making.
Allelopathic effects of invasive plant-VOCs on native species are well documented, showing consistently that terpene-rich emissions have a phytotoxic activity on the seed germination and growth of other plants. Allelopathy is a common trait of many invasive plants contributing to their ecological success. Exploring the allelopathic properties of leaf litter VOCs is an interesting and promising avenue for future research. Evidence is building and suggests that invasive plant VOCs also play a role in neighbor detection and preparedness for competition, but the mechanisms behind this phenomenon remain to be elucidated.
While the ecological role of VOCs in plant-insect interactions is widely acknowledged, only a few studies have explored the responses of native insects to invasive plants' VOCs. Existing studies show that invasive plants' VOCs can disrupt communication between native plants and insects. However, native insects use multiple cues to inform their foraging/oviposition decisions, potentially compensating for the negative impacts of invasive plants' VOCs. Theoretical studies have developed multiple hypotheses on the impact of invasive plants on plant-insect communication, yet these remain largely untested. We suggest empirical studies exploring the different scenarios proposed here.
More studies are needed to explore how native insects respond to invasive plant VOCs (for example, if they are innately attracted or repelled by them, whether their presence could disrupt the host-finding process, impacting their fitness or if they can rapidly learn and avoid the scents of invasive plants that are unsuitable). Another topic of interest is how insects respond to changes in the VOC profiles of their hosts due to the direct or indirect influence of invasive plants. The impact of invasive plants' VOCs on herbivore species with different dietary breadth (specialists vs. generalists), feeding modes (phloem feeders vs. chewers), taxonomic affiliation, and geographical distribution (restricted distribution/endemic vs. cosmopolitan) is another avenue for future exploration. Multitrophic and food web approaches will allow a better understanding of the direct and indirect effects of invasive plants on native ecosystems to inform conservation and management efforts (e.g., to understand the non-target impacts of weed management, predict disruption of ecosystem processes by invasives, prioritize management of certain species to preserve organisms at risk, etc.).
The majority of the studies in this review have been conducted in Europe, North America (US or Canada), Oceania, and East Asia. This may reflect a bias toward journals published in English. However, it seems more likely that this obeys socio-economic factors limiting access to information, technology, research funding and the need to prioritize other research topics (e.g., food security) over biological invasions in developing nations from Africa, Western Asia, and Latin America. While acknowledging limitations, we would like to encourage international research collaborations to expand our knowledge of invasive species' chemical ecology in different latitudes. Many invasive species colonize a wide variety of habitats across the globe, and simultaneous studies with comparable research methods would deepen our understanding of the chemical behavior of invasive plants and the factors affecting it. Species included in the list of the 100 world's worst invasive species, which have multiple economic, environmental, and social impacts, would be a good starting point (Lowe et al., 2000).
As methods continue to develop, we hope to see more studies using innovative approaches to measure invasive species VOC and assess their effects. Transdisciplinary approaches, such as the integration of omics (genomics, proteomics, metabolomics, metagenomics, phenomics and transcriptomics) in future research may help elucidate the complex mechanisms behind the observed ecological phenomena (e.g., van Dam and van der Meijden, 2011; Macel et al., 2014; Jud et al., 2018; Mounger et al., 2021). Understanding these mechanisms in the face of rapid environmental changes like global warming will be one of the most significant challenges in the following decades. Advanced techniques, such as genomics, transcriptomics, and metabolomics, could be associated with behavioral data using novel tools, such as network analysis and machine learning, which will undoubtedly advance our knowledge and understanding of the ecological roles of VOCs in biological invasions.
Data availability statement
The original contributions presented in the study are included in the article/Supplementary material, further inquiries can be directed to the corresponding author.
Author contributions
All authors listed have made a substantial, direct, and intellectual contribution to the work and approved it for publication.
Funding
This work was funded by a Fast-Start Marsden grant from the Royal Society Te Apārangi awarded to AC (MAU2004).
Acknowledgments
The authors wish to thank Fern Kumeroa for her assistance with the literature search and the editors of this Special Issue for showcasing the contributions of women to the field of chemical ecology.
Conflict of interest
The authors declare that the research was conducted in the absence of any commercial or financial relationships that could be construed as a potential conflict of interest.
Publisher's note
All claims expressed in this article are solely those of the authors and do not necessarily represent those of their affiliated organizations, or those of the publisher, the editors and the reviewers. Any product that may be evaluated in this article, or claim that may be made by its manufacturer, is not guaranteed or endorsed by the publisher.
Supplementary material
The Supplementary Material for this article can be found online at: https://www.frontiersin.org/articles/10.3389/fevo.2023.1059125/full#supplementary-material
References
Abd-ElGawad, A. M., El Gendy, A. E.-N. G., Assaeed, A. M., Al-Rowaily, S. L., Omer, E. A., Dar, B. A., et al. (2020). Essential oil enriched with oxygenated constituents from invasive plant Argemone ochroleuca exhibited potent phytotoxic effects. Plants 9, 998. doi: 10.3390/plants9080998
Adhikari, S., Burke, I. C., Revolinski, S. R., Piaskowski, J., and Eigenbrode, S. D. (2021). Within-population trait variation in a globally invasive plant species Mayweed chamomile (Anthemis cotula): implications for future invasion and management. Front. Agronom. 3, 640208. doi: 10.3389/fagro.2021.640208
Agrawal, A. A. (2001). Phenotypic plasticity in the interactions and evolution of species. Science 294, 321–326. doi: 10.1126/science.1060701
Araniti, F., Lupini, A., Sunseri, F., and Abenavoli, M. R. (2017). Allelopatic potential of Dittrichia viscosa (L.) W. Greuter mediated by VOCs: a physiological and metabolomic approach. PLoS One 12, e0170161. doi: 10.1371/journal.pone.0170161
Aşkin Çelik, T., and Aslantürk, Ö. S. (2010). Evaluation of cytotoxicity and genotoxicity of Inula viscosa leaf extracts with Allium test. J. Biomed. Biotechnol. 2010, 252. doi: 10.1155/2010/189252
Badri, D. V., and Vivanco, J. M. (2009). Regulation and function of root exudates. Plant Cell Environ. 32, 666–681. doi: 10.1111/j.1365-3040.2009.01926.x
Bais, H. P., Vepachedu, R., Gilroy, S., Callaway, R. M., and Vivanco, J. M. (2003). Allelopathy and exotic plant invasion: from molecules and genes to species interactions. Science 301, 1377–1380. doi: 10.1126/science.1083245
Bajwa, A. A., Chauhan, B. S., Farooq, M., Shabbir, A., and Adkins, S. W. (2016). What do we really know about alien plant invasion? a review of the invasion mechanism of one of the world's worst weeds. Planta 244, 39–57. doi: 10.1007/s00425-016-2510-x
Baldwin, I. T., Halitschke, R., Paschold, A., Von Dahl, C. C., and Preston, C. A. (2006). Volatile signaling in plant-plant interactions:” talking trees” in the genomics era. science 311, 812–815. doi: 10.1126/science.1118446
Ballhorn, D. J., Kautz, S., Lion, U., and Heil, M. (2008). Trade-offs between direct and indirect defences of lima bean (Phaseolus lunatus). J. Ecol. 96, 971–980. doi: 10.1111/j.1365-2745.2008.01404.x
Bao, X., Zhou, W., Xu, L., and Zheng, Z. (2022). A meta-analysis on plant volatile organic compound emissions of different plant species and responses to environmental stress. Environ. Pollut. 22, 120886. doi: 10.1016/j.envpol.2022.120886
Barney, J. N., Tekiela, D. R., Barrios-Garcia, M. N., Dimarco, R. D., Hufbauer, R. A., Leipzig-Scott, P., et al. (2015). Global Invader Impact Network (GIIN): toward standardized evaluation of the ecological impacts of invasive plants. Ecol. Evol. 5, 2878–2889. doi: 10.1002/ece3.1551
Barrett, D. P., Fowler, S. V., Subbaraj, A. K., Groenteman, R., and Clavijo-McCormick, A. (2021). Metabolomic analysis of host plant biochemistry could improve the effectiveness and safety of classical weed biocontrol. Biologic. Control 160, 104663. doi: 10.1016/j.biocontrol.2021.104663
Bezemer, T. M., Harvey, J. A., and Cronin, J. T. (2014). Response of native insect communities to invasive plants. Ann. Rev. Entomol. 59, 119. doi: 10.1146/annurev-ento-011613-162104
Blande, J. D., Holopainen, J. K., and Niinemets, Ü. (2014). Plant volatiles in polluted atmospheres: stress responses and signal degradation. Plant Cell Environ. 37, 1892–1904. doi: 10.1111/pce.12352
Bouwmeester, H., Schuurink, R. C., Bleeker, P. M., and Schiestl, F. (2019). The role of volatiles in plant communication. Plant J. 100, 892–907. doi: 10.1111/tpj.14496
Brown, J. H., and Sax, D. F. (2004). An essay on some topics concerning invasive species. Austr. Ecol. 29, 530–536. doi: 10.1111/j.1442-9993.2004.01340.x
Broz, A. K., Broeckling, C. D., De-la-Peña, C., Lewis, M. R., Greene, E., Callaway, R. M., et al. (2010). Plant neighbor identity influences plant biochemistry and physiology related to defense. BMC Plant Biol. 10, 1–14. doi: 10.1186/1471-2229-10-115
Bruce, T. J., and Pickett, J. A. (2011). Perception of plant volatile blends by herbivorous insects–finding the right mix. Phytochemistry 72, 1605–1611. doi: 10.1016/j.phytochem.2011.04.011
Bruce, T. J., Wadhams, L. J., and Woodcock, C. M. (2005). Insect host location: a volatile situation. Trends Plant Sci. 10, 269–274. doi: 10.1016/j.tplants.2005.04.003
Callaway, R. M., and Ridenour, W. M. (2004). Novel weapons: invasive success and the evolution of increased competitive ability. Front. Ecol. Environ. 2, 436–443. doi: 10.1890/1540-9295(2004)0020436:NWISAT2.0.CO
Callaway, R. M., Ridenour, W. M., Laboski, T., Weir, T., and Vivanco, J. M. (2005). Natural selection for resistance to the allelopathic effects of invasive plants. J. Ecol. 93, 576–583. doi: 10.1111/j.1365-2745.2005.00994.x
Campbell, D. R., Sosenski, P., and Raguso, R. A. (2019). Phenotypic plasticity of floral volatiles in response to increasing drought stress. Annals Bot. 123, 601–610. doi: 10.1093/aob/mcy193
Chen, B.-M., Liao, H.-X., Chen, W.-B., Wei, H.-J., and Peng, S.-L. (2017). Role of allelopathy in plant invasion and control of invasive plants. Allelopathy J. 41, 155–166. doi: 10.26651/2017-41-2-1092
Chengxu, W., Mingxing, Z., Xuhui, C., and Bo, Q. (2011). Review on allelopathy of exotic invasive plants. Procedia Eng. 18, 240–246. doi: 10.1016/j.proeng.2011.11.038
Clavijo McCormick, A. (2016). Can plant–natural enemy communication withstand disruption by biotic and abiotic factors? Ecol. Evolut. 6, 8569–8582. doi: 10.1002/ece3.2567
Clavijo McCormick, A., Boeckler, G. A., Köllner, T. G., Gershenzon, J., and Unsicker, S. B. (2014a). The timing of herbivore-induced volatile emission in black poplar (Populus nigra) and the influence of herbivore age and identity affect the value of individual volatiles as cues for herbivore enemies. BMC Plant Biol. 14, 1–14. doi: 10.1186/s12870-014-0304-5
Clavijo McCormick, A., Irmisch, S., Reinecke, A., Boeckler, G. A., Veit, D., Reichelt, M., et al. (2014b). Herbivore-induced volatile emission in black poplar: Regulation and role in attracting herbivore enemies. Plant Cell Environ. 37, 1909–1923. doi: 10.1111/pce.12287
Copolovici, L., Popitanu, A. C., and Copolovici, D.-M. (2021). Volatile organic compound emission and residual substances from plants in light of the globally increasing CO2 level. Curr. Opin. Environ. Sci. Health 19, 100216. doi: 10.1016/j.coesh.2020.10.004
Costa, R. O., Jose, C. M., Grombone-Guaratini, M. T., and Matos, D. M. S. (2019). Chemical characterization and phytotoxicity of the essential oil from the invasive Hedychium coronarium on seeds of Brazilian riparian trees. Flora 257, 151411. doi: 10.1016/j.flora.2019.05.010
Cozzolino, S., Fineschi, S., Litto, M., Scopece, G., Trunschke, J., and Schiestl, F. P. (2015). Herbivory increases fruit set in Silene latifolia: a consequence of induced pollinator-attracting floral volatiles? J. Chem. Ecol. 41, 622–630. doi: 10.1007/s10886-015-0597-3
Darji, T. B., Adhikari, B., Pathak, S., Neupane, S., Thapa, L. B., Bhatt, T. D., et al. (2021). Phytotoxic effects of invasive Ageratina adenophora on two native subtropical shrubs in Nepal. Scientific Rep. 11, 1–9. doi: 10.1038/s41598-021-92791-y
Davidson, A. M., Jennions, M., and Nicotra, A. B. (2011). Do invasive species show higher phenotypic plasticity than native species and, if so, is it adaptive? a meta-analysis. Ecol. Lett. 14, 419–431. doi: 10.1111/j.1461-0248.2011.01596.x
De Bruyne, M., and Baker, T. C. (2008). Odor detection in insects: volatile codes. J. Chemic. Ecol. 34, 882–897. doi: 10.1007/s10886-008-9485-4
De Lange, E. S., Salamanca, J., Polashock, J., and Rodriguez-Saona, C. (2019). Genotypic variation and phenotypic plasticity in gene expression and emissions of herbivore-induced volatiles, and their potential tritrophic implications, in cranberries. J. Chemic. Ecol. 45, 298–312. doi: 10.1007/s10886-018-1043-0
de Souza, A. L. C., de Matos, C. H. C., and Campos e Silva, R. (2022). “Volatile Allelochemicals,” in Essential Oils (New York, NY: Springer), 247–261.
Dostál, P., Müllerov,á, J., Pyšek, P., Pergl, J., and Klinerová, T. (2013). The impact of an invasive plant changes over time. Ecol. Lett. 16, 1277–1284. doi: 10.1111/ele.12166
Dudareva, N., Klempien, A., Muhlemann, J. K., and Kaplan, I. (2013). Biosynthesis, function and metabolic engineering of plant volatile organic compounds. New Phytol. 198, 16–32. doi: 10.1111/nph.12145
Dudareva, N., Pichersky, E., and Gershenzon, J. (2004). Biochemistry of plant volatiles. Plant Physiol. 135, 1893–1902. doi: 10.1104/pp.104.049981
Dukes, J. S., and Mooney, H. A. (2004). Disruption of ecosystem processes in western North America by invasive species. Revista chilena de historia natural 77, 411–437. doi: 10.4067/S0716-078X2004000300003
Effah, E., Barrett, D. P., Peterson, P. G., Godfrey, A. J. R., Potter, M. A., Holopainen, J. K., et al. (2020a). Natural variation in volatile emissions of the invasive weed Calluna vulgaris in New Zealand. Plants 9, 283. doi: 10.3390/plants9020283
Effah, E., Barrett, D. P., Peterson, P. G., Potter, M. A., Holopainen, J. K., and Clavijo McCormick, A. (2020b). Effects of two invasive weeds on arthropod community structure on the central plateau of New Zealand. Plants 9, 919. doi: 10.3390/plants9070919
Effah, E., Barrett, D. P., Peterson, P. G., Potter, M. A., Holopainen, J. K., and Clavijo McCormick, A. (2020c). Seasonal and environmental variation in volatile emissions of the New Zealand native plant Leptospermum scoparium in weed-invaded and non-invaded sites. Scientific Rep. 10, 1–11. doi: 10.1038/s41598-020-68386-4
Effah, E., Barrett, D. P., Peterson, P. G., Wargent, J. J., Potter, M. A., Holopainen, J. K., et al. (2020d). Herbivory and attenuated UV radiation affect volatile emissions of the invasive weed Calluna vulgaris. Molecules 25, 3200. doi: 10.3390/molecules25143200
Effah, E., Holopainen, J. K., and McCormick, A. C. (2019). Potential roles of volatile organic compounds in plant competition. Perspect. Plant Ecol. Evol. Systemat. 38, 58–63. doi: 10.1016/j.ppees.2019.04.003
Effah, E., Svendsen, L., Barrett, D. P., and Clavijo McCormick, A. (2022). Exploring plant volatile-mediated interactions between native and introduced plants and insects. Scientific Rep. 12, 1–12. doi: 10.1038/s41598-022-18479-z
Ens, E.-J., Bremner, J. B., French, K., and Korth, J. (2009). Identification of volatile compounds released by roots of an invasive plant, bitou bush (Chrysanthemoides monilifera spp. rotundata), and their inhibition of native seedling growth. Biologic. Invas. 11, 275–287. doi: 10.1007/s10530-008-9232-3
Figueiredo, A. C., Barroso, J. G., Pedro, L. G., and Scheffer, J. J. (2008). Factors affecting secondary metabolite production in plants: volatile components and essential oils. Flav. Fragran. J. 23, 213–226. doi: 10.1002/ffj.1875
Fini, A., Brunetti, C., Di Ferdinando, M., Ferrini, F., and Tattini, M. (2011). Stress-induced flavonoid biosynthesis and the antioxidant machinery of plants. Plant Signal. Behav. 6, 709–711. doi: 10.4161/psb.6.5.15069
Fortuna, T. M., Woelke, J. B., Hordijk, C. A., Jansen, J. J., van Dam, N. M., Vet, L. E., et al. (2013). A tritrophic approach to the preference–performance hypothesis involving an exotic and a native plant. Biologic. Invas. 15, 2387–2401. doi: 10.1007/s10530-013-0459-2
Funk, J. L. (2013). The physiology of invasive plants in low-resource environments. Conserv. Physiol. 1, 26. doi: 10.1093/conphys/cot026
Galappaththi, H. S., de Silva, W., and Clavijo Mccormick, A. (2022). A mini-review on the impact of common gorse in its introduced ranges. Tropic. Ecol. 22, 1–25. doi: 10.1007/s42965-022-00239-9
Gfeller, V., Huber, M., Förster, C., Huang, W., Köllner, T. G., and Erb, M. (2019). Root volatiles in plant–plant interactions I: High root sesquiterpene release is associated with increased germination and growth of plant neighbours. Plant Cell Environ. 42, 1950–1963. doi: 10.1111/pce.13532
Gratani, L. (2014). Plant phenotypic plasticity in response to environmental factors. Adv. Bot. 2014, 747. doi: 10.1155/2014/208747
Halarewicz, A., Szumny, A., and Baczek, P. (2021). Effect of Prunus serotina Ehrh. volatile compounds on germination and seedling growth of Pinus sylvestris L. Forests 12, 846. doi: 10.3390/f12070846
Han, C., Shao, H., Zhou, S., Mei, Y., Cheng, Z., Huang, L., et al. (2021). Chemical composition and phytotoxicity of essential oil from invasive plant, Ambrosia artemisiifolia L. Ecotoxicol. Environ. Safety 211, 111879. doi: 10.1016/j.ecoenv.2020.111879
Hansson, B. S., and Stensmyr, M. C. (2011). Evolution of insect olfaction. Neuron 72, 698–711. doi: 10.1016/j.neuron.2011.11.003
Harvey, J. A., and Fortuna, T. M. (2012). Chemical and structural effects of invasive plants on herbivore–parasitoid/predator interactions in native communities. Entomologia Experimentalis et Applicata 144, 14–26. doi: 10.1111/j.1570-7458.2012.01252.x
Heil, M. (2014). Herbivore-induced plant volatiles: targets, perception and unanswered questions. New York, NY: Wiley Online Library.
Heil, M., and Kost, C. (2006). Priming of indirect defences. Ecol. Lett. 9, 813–817. doi: 10.1111/j.1461-0248.2006.00932.x
Holopainen, J. K. (2004). Multiple functions of inducible plant volatiles. Trends Plant Sci. 9, 529–533. doi: 10.1016/j.tplants.2004.09.006
Holopainen, J. K., and Gershenzon, J. (2010). Multiple stress factors and the emission of plant VOCs. Trends Plant Sci. 15, 176–184. doi: 10.1016/j.tplants.2010.01.006
Humair, F., Humair, L., Kuhn, F., and Kueffer, C. (2015). E-commerce trade in invasive plants. Conserv. Biol. 29, 1658–1665. doi: 10.1111/cobi.12579
Inderjit, E., vans, H., Crocoll, C., Bajpai, D., Kaur, R., Feng, Y.-L., et al. (2011). Volatile chemicals from leaf litter are associated with invasiveness of a neotropical weed in Asia. Ecology 92, 316–324. doi: 10.1890/10-0400.1
Insam, H., and Seewald, M. S. (2010). Volatile organic compounds (VOCs) in soils. Biol. Fertil. Soils 46, 199–213. doi: 10.1007/s00374-010-0442-3
Johnson, P. T., Olden, J. D., Solomon, C. T., and Vander Zanden, M. J. (2009). Interactions among invaders: community and ecosystem effects of multiple invasive species in an experimental aquatic system. Oecologia 159, 161–170. doi: 10.1007/s00442-008-1176-x
Jones, L. C., Rafter, M. A., and Walter, G. H. (2022). Host interaction mechanisms in herbivorous insects–life cycles, host specialization and speciation. Biologic. J. Linnean Soc. 137, 1–14. doi: 10.1093/biolinnean/blac070
Jones, T. G., Min Tun, K., Minor, M., and Clavijo McCormick, A. (2021). The giant willow aphid (Tuberolachnus salignus) and its effects on the survival and growth of willows. Agricult. For. Entomolo. 23, 420–428. doi: 10.1111/afe.12443
Jud, W., Winkler, J. B., Niederbacher, B., Niederbacher, S., and Schnitzler, J.-P. (2018). Volatilomics: a non-invasive technique for screening plant phenotypic traits. Plant Methods 14, 1–18. doi: 10.1186/s13007-018-0378-4
Kainulainen, P., and Holopainen, J. (2002). Concentrations of secondary compounds in Scots pine needles at different stages of decomposition. Soil Biol. Biochemistr. 34, 37–42. doi: 10.1016/S0038-0717(01)00147-X
Kalisz, S., Kivlin, S. N., and Bialic-Murphy, L. (2021). Allelopathy is pervasive in invasive plants. Biologic. Invas. 23, 367–371. doi: 10.1007/s10530-020-02383-6
Kalske, A., Blande, J. D., and Ramula, S. (2022). Soil microbiota explain differences in herbivore resistance between native and invasive populations of a perennial herb. J. Ecol. 110, 2649–2660. doi: 10.1111/1365-2745.13975
Kantsa, A., Raguso, R. A., Lekkas, T., Kalantzi, O. I., and Petanidou, T. (2019). Floral volatiles and visitors: a meta-network of associations in a natural community. J. Ecol. 107, 2574–2586. doi: 10.1111/1365-2745.13197
Karban, R., Wetzel, W. C., Shiojiri, K., Pezzola, E., and Blande, J. D. (2016). Geographic dialects in volatile communication between sagebrush individuals. Ecology 97, 2917–2924. doi: 10.1002/ecy.1573
Kato-Noguchi, H., and Kurniadie, D. (2021). Allelopathy of Lantana camara as an invasive plant. Plants 10, 1028. doi: 10.3390/plants10051028
Kato-Noguchi, H., and Kurniadie, D. (2022). Allelopathy and allelochemicals of Leucaena leucocephala as an invasive plant species. Plants 11, 1672. doi: 10.3390/plants11131672
Kegge, W., and Pierik, R. (2010). Biogenic volatile organic compounds and plant competition. Trends Plant Sci. 15, 126–132. doi: 10.1016/j.tplants.2009.11.007
Kessler, A. (2015). The information landscape of plant constitutive and induced secondary metabolite production. Curr. Opin. Insect Sci. 8, 47–53. doi: 10.1016/j.cois.2015.02.002
Kigathi, R. N., Weisser, W. W., Veit, D., Gershenzon, J., and Unsicker, S. B. (2013). Plants suppress their emission of volatiles when growing with conspecifics. J. Chemic. Ecol. 39, 537–545. doi: 10.1007/s10886-013-0275-2
Kim, Y. O., and Lee, E. J. (2011). Comparison of phenolic compounds and the effects of invasive and native species in East Asia: support for the novel weapons hypothesis. Ecologic. Res. 26, 87–94. doi: 10.1007/s11284-010-0762-7
Leger, E. A. (2008). The adaptive value of remnant native plants in invaded communities: an example from the Great Basin. Ecologic. Applicat. 18, 1226–1235. doi: 10.1890/07-1598.1
Li, T. (2016). “Neighbour recognition through volatile-mediated interactions,” in Deciphering Chemical Language of Plant Communication (New York, NY: Springer), 153–174.
Liao, H., D'Antonio, C. M., Chen, B., Huang, Q., and Peng, S. (2016). How much do phenotypic plasticity and local genetic variation contribute to phenotypic divergences along environmental gradients in widespread invasive plants? a meta-analysis. Oikos 125, 905–917. doi: 10.1111/oik.02372
Lin, T., Vrieling, K., Laplanche, D., Klinkhamer, P. G., Lou, Y., Bekooy, L., et al. (2021). Evolutionary changes in an invasive plant support the defensive role of plant volatiles. Curr. Biol. 31, 3450–6. doi: 10.1016/j.cub.2021.05.055
Litt, A. R., Cord, E. E., Fulbright, T. E., and Schuster, G. L. (2014). Effects of invasive plants on arthropods. Conserv. Biol. 28, 1532–1549. doi: 10.1111/cobi.12350
Liu, D., Chen, L., Chen, C., Zhou, Y., Xiao, F., Wang, Y., et al. (2022). Effect of plant VOCs and light intensity on growth and reproduction performance of an invasive and a native Phytolacca species in China. Ecol. Evol. 12, e8522. doi: 10.1002/ece3.8522
Liu, D., Gao, Y., Li, X.-X., Li, Z., and Pan, Q.-H. (2015). Attenuated UV radiation alters volatile profile in Cabernet Sauvignon grapes under field conditions. Molecules 20, 16946–16969. doi: 10.3390/molecules200916946
Liu, Z., Zhang, C., Ma, L., Zhou, X., Sun, X., and Ding, J. (2021). Elevated temperature decreases preferences of native herbivores to an invasive plant. Entomol. Gen. 41, 137–146. doi: 10.1127/entomologia/2020/1050
Llusia, J., Llorens, L., Bernal, M., Verdaguer, D., and Penuelas, J. (2012). Effects of UV radiation and water limitation on the volatile terpene emission rates, photosynthesis rates, and stomatal conductance in four Mediterranean species. Acta Physiologiae Plantarum 34, 757–769. doi: 10.1007/s11738-011-0876-8
Lowe, S., Browne, M., Boudjelas, S., and De Poorter, M. (2000). 100 of the world's worst invasive alien species: a selection from the global invasive species database. Invasive Species Specialist Group Auckland.
Ma, H., Chen, Y., Chen, J., Ji, J., and He, H. (2021). Identification and comparison of allelopathic effects from leaf and flower volatiles of the invasive plants Mikania micrantha. Chemoecology 31, 355–365. doi: 10.1007/s00049-021-00356-2
Macel, M., de Vos, R. C., Jansen, J. J., van der Putten, W. H., and van Dam, N. M. (2014). Novel chemistry of invasive plants: exotic species have more unique metabolomic profiles than native congeners. Ecol. Evol. 4, 2777–2786. doi: 10.1002/ece3.1132
Macias, F. A., Molinillo, J. M., Varela, R. M., and Galindo, J. C. (2007). Allelopathy—a natural alternative for weed control. Pest Manage. Sci. Form. Pesticide Sci. 63, 327–348. doi: 10.1002/ps.1342
Mack, R. N., Simberloff, D., Mark Lonsdale, W., Evans, H., Clout, M., and Bazzaz, F. A. (2000). Biotic invasions: causes, epidemiology, global consequences, and control. Ecologic. Applicat. 10, 689–710. doi: 10.1890/1051-0761(2000)0100689:BICEGC2.0.CO
Mainka, S. A., and Howard, G. W. (2010). Climate change and invasive species: double jeopardy. Integrat. Zool. 5, 102–111. doi: 10.1111/j.1749-4877.2010.00193.x
Maja, M. M., Kasurinen, A., Holopainen, T., Julkunen-Tiitto, R., and Holopainen, J. K. (2016). The effect of warming and enhanced ultraviolet radiation on gender-specific emissions of volatile organic compounds from European aspen. Sci. Total Environ. 547, 39–47. doi: 10.1016/j.scitotenv.2015.12.114
Matzek, V. (2011). Superior performance and nutrient-use efficiency of invasive plants over non-invasive congeners in a resource-limited environment. Biologic. Invas. 13, 3005–3014. doi: 10.1007/s10530-011-9985-y
McCormick, A. C., Unsicker, S. B., and Gershenzon, J. (2012). The specificity of herbivore-induced plant volatiles in attracting herbivore enemies. Trends Plant Sci. 17, 303–310. doi: 10.1016/j.tplants.2012.03.012
Mishyna, M., Laman, N., Prokhorov, V., Maninang, J. S., and Fujii, Y. (2015). Identification of octanal as plant growth inhibitory volatile compound released from Heracleum sosnowskyi fruit. Nat. Product Commun. 10, 1934578X1501000518. doi: 10.1177/1934578X1501000518
Moreira, X., and Abdala-Roberts, L. (2019). Specificity and context-dependency of plant–plant communication in response to insect herbivory. Curr. Opin. Insect Sci. 32, 15–21. doi: 10.1016/j.cois.2018.09.003
Mounger, J., Ainouche, M. L., Bossdorf, O., Cavé-Radet, A., Li, B., Parepa, M., et al. (2021). Epigenetics and the success of invasive plants. Philosophic. Transact. Royal Soc. B 376, 20200117. doi: 10.1098/rstb.2020.0117
Najar-Rodriguez, A. J., Friedli, M., Klaiber, J., and Dorn, S. (2015). Aphid-deprivation from Brassica plants results in increased isothiocyanate release and parasitoid attraction. Chemoecology 25, 303–311. doi: 10.1007/s00049-015-0199-0
Ninkovic, V., Markovic, D., and Rensing, M. (2021). Plant volatiles as cues and signals in plant communication. Plant Cell Environ. 44, 1030–1043. doi: 10.1111/pce.13910
Oduor, A. M. (2022). Native plant species show evolutionary responses to invasion by Parthenium hysterophorus in an African savanna. New Phytol. 233, 983–994. doi: 10.1111/nph.17574
Oduor, A. M., Adomako, M. O., Yuan, Y., and Li, J. M. (2022). Older populations of the invader Solidago canadensis exhibit stronger positive plant-soil feedbacks and competitive ability in China. Am. J. Bot. 109, 1230–1241. doi: 10.1002/ajb2.16034
Oduor, A. M., Long, H., Fandohan, A. B., Liu, J., and Yu, X. (2018). An invasive plant provides refuge to native plant species in an intensely grazed ecosystem. Biologic. Invas. 20, 2745–2751. doi: 10.1007/s10530-018-1757-5
Ormeño, E., and Fernandez, C. (2012). Effect of soil nutrient on production and diversity of volatile terpenoids from plants. Curr. Bioact. Compounds 8, 71–79. doi: 10.2174/157340712799828188
Oster, M., Beck, J. J., Furrow, R. E., Yeung, K., and Field, C. B. (2015). In-field yellow starthistle (Centaurea solstitialis) volatile composition under elevated temperature and CO2 and implications for future control. Chemoecology 25, 313–323. doi: 10.1007/s00049-015-0200-y
Oster, M., Smith, L., Beck, J. J., Howard, A., and Field, C. B. (2014). Orientation behavior of predaceous ground beetle species in response to volatile emissions identified from yellow starthistle damaged by an invasive slug. Arthropod-Plant Interact. 8, 429–437. doi: 10.1007/s11829-014-9322-3
Pardo-Muras, M. G, Puig, C., and Pedrol, N. (2019). Cytisus scoparius and Ulex europaeus produce volatile organic compounds with powerful synergistic herbicidal effects. Molecules 24, 4539. doi: 10.3390/molecules24244539
Pardo-Muras, M., Puig, C. G., López-Nogueira, A., Cavaleiro, C., and Pedrol, N. (2018). On the bioherbicide potential of Ulex europaeus and Cytisus scoparius: Profiles of volatile organic compounds and their phytotoxic effects. PLoS One 13, e0205997. doi: 10.1371/journal.pone.0205997
Pareja, M., Qvarfordt, E., Webster, B., Mayon, P., Pickett, J., Birkett, M., et al. (2012). Herbivory by a phloem-feeding insect inhibits floral volatile production. PLoS One 7, e31971. doi: 10.1371/journal.pone.0031971
Pearce, F. (2016). The New Wild: Why Invasive Species will be Nature's Salvation. Boston: Beacon press.
Peñuelas, J., and Staudt, M. (2010). BVOCs and global change. Trends Plant Sci. 15, 133–144. doi: 10.1016/j.tplants.2009.12.005
Pichersky, E., and Gershenzon, J. (2002). The formation and function of plant volatiles: perfumes for pollinator attraction and defense. Curr. Opin. Plant Biol. 5, 237–243. doi: 10.1016/S1369-5266(02)00251-0
Pierik, R., and de Wit, M. (2014). Shade avoidance: phytochrome signalling and other aboveground neighbour detection cues. J. Experiment. Bot. 65, 2815–2824. doi: 10.1093/jxb/ert389
Pierik, R., Mommer, L., and Voesenek, L. A. (2013). Molecular mechanisms of plant competition: neighbour detection and response strategies. Funct. Ecol. 27, 841–853. doi: 10.1111/1365-2435.12010
Pinto, D. M., Blande, J. D., Souza, S. R., Nerg, A.-M., and Holopainen, J. K. (2010). Plant volatile organic compounds (VOCs) in ozone (O3) polluted atmospheres: the ecological effects. J. Chem. Ecol. 36, 22–34. doi: 10.1007/s10886-009-9732-3
Pyšek, P., Hulme, P. E., Simberloff, D., Bacher, S., Blackburn, T. M., Carlton, J. T., et al. (2020). Scientists' warning on invasive alien species. Biologic. Rev. 95, 1511–1534. doi: 10.1111/brv.12627
Pyšek, P., Jarošík, V. A., Chytr,ý, M., Danihelka, J.í., Kühn, I., Pergl, J., et al. (2011). Successful invaders co-opt pollinators of native flora and accumulate insect pollinators with increasing residence time. Ecologic. Monograph. 81, 277–293. doi: 10.1890/10-0630.1
Randlkofer, B., Obermaier, E., Hilker, M., and Meiners, T. (2010). Vegetation complexity—the influence of plant species diversity and plant structures on plant chemical complexity and arthropods. Basic Appl. Ecol. 11, 383–395. doi: 10.1016/j.baae.2010.03.003
Reinecke, A., and Hilker, M. (2014). Plant semiochemicals–perception and behavioural responses by insects. Ann. Plant Rev. Insect-Plant Interact. 47, 115–153. doi: 10.1002/9781118829783.ch4
Reinhart, K. O., and Callaway, R. M. (2006). Soil biota and invasive plants. New phytol. 170, 445–457. doi: 10.1111/j.1469-8137.2006.01715.x
Richards, C. L., Bossdorf, O., Muth, N. Z., Gurevitch, J., and Pigliucci, M. (2006). Jack of all trades, master of some? on the role of phenotypic plasticity in plant invasions. Ecol. Lett. 9, 981–993. doi: 10.1111/j.1461-0248.2006.00950.x
Rieksta, J., Li, T., Michelsen, A., and Rinnan, R. (2021). Synergistic effects of insect herbivory and changing climate on plant volatile emissions in the subarctic tundra. Glob. Change Biol. 27, 5030–5042. doi: 10.1111/gcb.15773
Rissanen, K., Aalto, J., Gessler, A., Hölttä, T., Rigling, A., Schaub, M., et al. (2022). Drought effects on volatile organic compound emissions from Scots pine stems. Plant Cell Environ. 45, 23–40. doi: 10.1111/pce.14219
Rodriguez, L. F. (2006). Can invasive species facilitate native species? Evidence of how, when, and why these impacts occur. Biol. Invasions 8, 927–939. doi: 10.1007/s10530-005-5103-3
Romagni, J. G., Allen, S. N., and Dayan, F. E. (2000). Allelopathic effects of volatile cineoles on two weedy plant species. J. Chemic. Ecol. 26, 303–313. doi: 10.1023/A:1005414216848
Runyon, J. B., and Birdsall, J. L. (2016). Costs of induced defenses for the invasive plant houndstongue (Cynoglossum officinale L.) and the potential importance for weed biocontrol. Arthropod-Plant Interact. 10, 383–391. doi: 10.1007/s11829-016-9449-5
Sampaio, B. L., and Costa, F. B. D. (2018). Influence of abiotic environmental factors on the main constituents of the volatile oils of Tithonia diversifolia. Revista Brasileira de Farmacognosia 28, 135–144. doi: 10.1016/j.bjp.2018.02.005
Schiestl, F. P., and Johnson, S. D. (2013). Pollinator-mediated evolution of floral signals. Trends Ecol. Evol. 28, 307–315. doi: 10.1016/j.tree.2013.01.019
Schiestl, F. P., Kirk, H., Bigler, L., Cozzolino, S., and Desurmont, G. A. (2014). Herbivory and floral signaling: phenotypic plasticity and tradeoffs between reproduction and indirect defense. New Phytol. 203, 257–266. doi: 10.1111/nph.12783
Schmidt, H. R., and Benton, R. (2020). Molecular mechanisms of olfactory detection in insects: beyond receptors. Open Biol. 10, 200252. doi: 10.1098/rsob.200252
Seebens, H., Blackburn, T. M., Dyer, E. E., Genovesi, P., Hulme, P. E., Jeschke, J. M., et al. (2017). No saturation in the accumulation of alien species worldwide. Nat. Commun. 8, 1–9. doi: 10.1038/ncomms14435
Semchenko, M., Saar, S., and Lepik, A. (2014). Plant root exudates mediate neighbour recognition and trigger complex behavioural changes. New Phytol. 204, 631–637. doi: 10.1111/nph.12930
Shao, H., Zhang, Y., Nan, P., Huang, X., and Zhang, C. (2013). Chemical composition and phytotoxic activity of the volatile oil of invasive Xanthium italicum Moretti from Xinjiang, China. J. Arid Land 5, 324–330. doi: 10.1007/s40333-013-0170-2
Simberloff, D. (2010). Invasive species. Conserv. Biol. 10, 131–152. doi: 10.1093/acprof:oso/9780199554232.003.0008
Skubel, S. A., Su, X., Poulev, A., Foxcroft, L. C., Dushenkov, V., and Raskin, I. (2020). Metabolomic differences between invasive alien plants from native and invaded habitats. Scientific Rep. 10, 1–9. doi: 10.1038/s41598-020-66477-w
Souza-Alonso, P., González, L., López-Nogueira, A., Cavaleiro, C., and Pedrol, N. (2018). Volatile organic compounds of Acacia longifolia and their effects on germination and early growth of species from invaded habitats. Chemistr. Ecol. 34, 126–145. doi: 10.1080/02757540.2017.1404584
Souza-Alonso, P., Rodríguez, J., González, L., and Lorenzo, P. (2017). Here to stay. Recent advances and perspectives about Acacia invasion in Mediterranean areas. Annal. For. Sci. 74, 1–20. doi: 10.1007/s13595-017-0651-0
Stout, J. C., and Tiedeken, E. J. (2017). Direct interactions between invasive plants and native pollinators: evidence, impacts and approaches. Functional Ecology 31, 38–46. doi: 10.1111/1365-2435.12751
Strayer, D. L., Eviner, V. T., Jeschke, J. M., and Pace, M. L. (2006). Understanding the long-term effects of species invasions. Trends Ecol. Evol. 21, 645–651. doi: 10.1016/j.tree.2006.07.007
Sunny, A., Diwakar, S., and Sharma, G. P. (2015). Native insects and invasive plants encounters. Arthropod-Plant Interact. 9, 323–331. doi: 10.1007/s11829-015-9384-x
Tallamy, D. W., Narango, D. L., and Mitchell, A. B. (2021). Do non-native plants contribute to insect declines? Ecologic. Entomol. 46, 729–742. doi: 10.1111/een.12973
Thapa, L. B., Kaewchumnong, K., Sinkkonen, A., and Sridith, K. (2020). Airborne and belowground phytotoxicity of invasive Ageratina adenophora on native species in Nepal. Plant Ecol. 221, 883–892. doi: 10.1007/s11258-020-01048-7
Thorpe, A. S., Thelen, G. C., Diaconu, A., and Callaway, R. M. (2009). Root exudate is allelopathic in invaded community but not in native community: field evidence for the novel weapons hypothesis. J. Ecol. 9, 641–645. doi: 10.1111/j.1365-2745.2009.01520.x
Tun, K. M., Clavijo McCormick, A., Jones, T., and Minor, M. (2021). Seasonal abundance of Tuberolachnus salignus and its effect on flowering of host willows of varying susceptibility. J. Appl. Entomol. 145, 543–552. doi: 10.1111/jen.12866
Tun, K. M., Minor, M., Jones, T., and Clavijo McCormick, A. (2022). Direct and Indirect Impacts of the Tuberolachnus salignus (Hemiptera: Aphididae) Invasion in New Zealand and Management Alternatives. J. Integrat. Pest Manage. 13, 22. doi: 10.1093/jipm/pmac018
Tun, K. M., Minor, M., Jones, T., and McCormick, A. C. (2020). Volatile profiling of fifteen willow species and hybrids and their responses to giant willow aphid infestation. Agronomy 10, 1404. doi: 10.3390/agronomy10091404
Turbelin, A. J., Malamud, B. D., and Francis, R. A. (2017). Mapping the global state of invasive alien species: patterns of invasion and policy responses. Global Ecol. Biogeograph. 26, 78–92. doi: 10.1111/geb.12517
Valéry, L., Fritz, H., Lefeuvre, J.-C., and Simberloff, D. (2008). In search of a real definition of the biological invasion phenomenon itself. Biological Invas. 10, 1345–1351. doi: 10.1007/s10530-007-9209-7
van Dam, N. M., and van der Meijden, E. (2011). A role for metabolomics in plant ecology. Ann. Plant Rev.: Biol. Plant Metabolom. 43, 87–107. doi: 10.1002/9781444339956.ch4
Van Kleunen, M., Weber, E., and Fischer, M. (2010). A meta-analysis of trait differences between invasive and non-invasive plant species. Ecology Lett. 13, 235–245. doi: 10.1111/j.1461-0248.2009.01418.x
Volkov, A. G., and Ranatunga, D. R. A. (2006). Plants as environmental biosensors. Plant Signal. Behav. 1, 105–115. doi: 10.4161/psb.1.3.3000
Wang, N. Q., Kong, C. H., Wang, P., and Meiners, S. J. (2021). Root exudate signals in plant–plant interactions. Plant Cell Environ. 44, 1044–1058. doi: 10.1111/pce.13892
Weidenhamer, J. D., and Callaway, R. M. (2010). Direct and indirect effects of invasive plants on soil chemistry and ecosystem function. J. Chem. Ecol. 36, 59–69. doi: 10.1007/s10886-009-9735-0
Xie, Y., Tian, L., Han, X., and Yang, Y. (2021). Research advances in allelopathy of volatile organic compounds (VOCs) of plants. Horticulturae 7, 278. doi: 10.3390/horticulturae7090278
Yuan, J. S., Himanen, S. J., Holopainen, J. K., Chen, F., and Stewart Jr, C. N. (2009). Smelling global climate change: mitigation of function for plant volatile organic compounds. Trends Ecol. Evol. 24, 323–331. doi: 10.1016/j.tree.2009.01.012
Yu-Peng, G., Wen-Ju, Z., Bo, L., and Jia-Kuan, C. (2004). Phenotypic plasticity and invasiveness of alien plants. Biodiv. Sci. 12, 447. doi: 10.17520/biods.2004055
Zhang, F., Chen, F., Liu, W., Guo, J., and Wan, F. (2012). ρ-Cymene inhibits growth and induces oxidative stress in rice seedling plants. Weed Sci. 60, 564–570. doi: 10.1614/WS-D-12-00029.1
Keywords: alien plants, environmental change, plant-plant interactions, plant-insect interactions, semiochemicals
Citation: Clavijo McCormick A, Effah E and Najar-Rodriguez A (2023) Ecological aspects of volatile organic compounds emitted by exotic invasive plants. Front. Ecol. Evol. 11:1059125. doi: 10.3389/fevo.2023.1059125
Received: 30 September 2022; Accepted: 27 February 2023;
Published: 23 March 2023.
Edited by:
Dani Lucas-Barbosa, Research Institute of Organic Agriculture (FiBL), SwitzerlandReviewed by:
Viviane Cordovez, Netherlands Institute of Ecology (NIOO-KNAW), NetherlandsAyub Oduor, Technical University of Kenya, Kenya
Copyright © 2023 Clavijo McCormick, Effah and Najar-Rodriguez. This is an open-access article distributed under the terms of the Creative Commons Attribution License (CC BY). The use, distribution or reproduction in other forums is permitted, provided the original author(s) and the copyright owner(s) are credited and that the original publication in this journal is cited, in accordance with accepted academic practice. No use, distribution or reproduction is permitted which does not comply with these terms.
*Correspondence: Andrea Clavijo McCormick, a.c.mccormick@massey.ac.nz