- 1Department of Entomology, Texas A&M University, College Station, TX, United States
- 2Department of Entomology, Michigan State University, East Lansing, MI, United States
- 3Ecology and Evolutionary Biology Program, Texas A&M University, College Station, TX, United States
- 4USDA, ARS, Southern Plains Agricultural Research Center, College Station, TX, United States
Plants attacked by insects may induce defenses locally in attacked plant tissues and/or systemically in non-attacked tissues, such as aboveground herbivory affecting belowground roots or belowground herbivory modifying aboveground tissues (i.e., cross-compartment systemic defense). Through induced systemic plant defenses, above-and belowground insect herbivores indirectly interact when feeding on a shared host plant. However, determining the systemic effects of herbivory on cross-compartment plant tissues and cascading consequences for herbivore communities remains underexplored. The goal of this study was to determine how belowground striped cucumber beetle (Acalymma vittatum) larval herbivory alters aboveground zucchini squash (Cucurbita pepo subsp. pepo) defenses and interactions with herbivores, including adult cucumber beetles and squash bugs (Anasa tristis). To explore this question, field and laboratory experiments were conducted to compare responses of aboveground herbivores to belowground larvae-damaged plants and non-damaged control plants. We also characterized changes in defensive chemicals and nutritional content of aboveground plant structures following belowground herbivory. We discovered belowground herbivory enhanced aboveground plant resistance and deterred aboveground foraging herbivores. We also found that larvae-damaged plants emitted higher amounts of a key volatile compound, (E)-β-ocimene, compared to non-damaged controls. Further investigation suggests that other mechanisms, such as plant nutrient content, may additionally contribute to aboveground herbivore foraging decisions. Collectively, our findings underscore connections between above-and belowground herbivore communities as mediated through induced systemic defenses of a shared host plant. Specifically, these findings indicate that belowground larval herbivory systemically enhances plant defenses and deters a suite of aboveground herbivores, suggesting larvae may manipulate aboveground plant defenses for their own benefit, while plants may benefit from enhanced systemic defenses against multi-herbivore attack.
Introduction
Following insect herbivory, plants induce defenses tailored to specific pests (War et al., 2012; Karban, 2020). Defense induction typically begins with changes in molecular signaling pathways of directly attacked plant tissues, modifying gene expression and activating downstream defenses (Erb and Reymond, 2019). Herbivore-induced defenses are not limited to plant tissues directly fed on by herbivores but can also systemically extend to non-attacked plant tissues (Heil and Ton, 2008; Wu and Baldwin, 2009; Kloth and Dicke, 2022). Systemic defenses can occur in the same types of plant tissues, such as foliar herbivory enhancing defenses in non-damaged leaves (Vos et al., 2013), as well as across different tissue types, such as aboveground herbivory affecting belowground roots or belowground herbivory modifying aboveground tissues (i.e., cross-compartment systemic responses; Biere and Goverse, 2016; Papadopoulou and van Dam, 2017). Cross-compartment systemic responses to herbivory can modify plant growth and defense, resulting in indirect interactions between above-and belowground herbivores feeding on a shared host plant (Barber, 2018). Above-and belowground herbivores can negatively impact or positively facilitate each other through indirect plant-mediated interactions (Erb et al., 2009a; Huang et al., 2013, 2014), although the outcomes of such herbivore-herbivore interactions and underlying cross-compartment mechanisms remain underexplored. Here, we examine the consequences of cross-compartment herbivory on plant and herbivore communities by characterizing systemic plant defense induction and herbivore behavior and performance.
Mounting evidence shows that systemic, non-attacked plant tissues play key roles in defense against herbivory (Erb et al., 2009b, 2015; Wang et al., 2019; Karssemeijer et al., 2020). These cross-compartment tissues, which are generally inaccessible to attacking herbivores, often act as metabolite sinks or sources that facilitate the defense process (Bezemer and van Dam, 2005; van Dam, 2009; Zhou et al., 2015). Plants can translocate nutrients (e.g., amino acids or carbohydrates) to cross-compartment tissues and away from attacking herbivores (Schultz et al., 2013), a phenomenon referred to as “herbivore-induced resource sequestration” (Orians et al., 2011; Steinbrenner et al., 2011). Plants can also withdrawal nutrients from cross-compartment plant tissues during defense activation, as seen in the depletion of root carbohydrates in Nicotiana attenuata following aboveground leaf herbivory (Machado et al., 2013, 2017). This movement of nutrients in systemic tissues can impact cross-compartment herbivores. For example, aboveground herbivory facilitates belowground plant parasitic nematode development through modified root carbohydrates in Nicotiana spp. (Kaplan et al., 2008a; Machado et al., 2018). Herbivory can also alter plant defense metabolites (e.g., toxic or repellent compounds) in cross-compartment systemic plant tissues. For instance, tobacco plants synthesize nicotine, a toxic secondary metabolite, in root tissues, but plant parasitic nematodes can interfere with nicotine biosynthesis thereby facilitating aboveground herbivory (Kaplan et al., 2008a,b). In addition to endogenous secondary metabolites, plants also emit volatile compounds following herbivory, and herbivore-induced changes in plant volatile emissions can attract or repel subsequent herbivores (De Moraes et al., 2001; Robert et al., 2012a; Grunseich et al., 2020). Intriguingly, a recent study determined that belowground herbivory enhances aboveground volatile emissions from Chinese tallow (Triadica sebifera), attracting aboveground conspecific herbivores, which enhance host-plant quality for belowground offspring (Sun et al., 2019). Beyond a handful of study systems, cross-compartment systemic plant responses to herbivory are relatively uncharacterized and the subsequent effects on herbivore communities feeding on different compartments remain poorly understood.
Plants in the gourd family (Cucurbitaceae) represent an excellent study system for cross-compartment interactions between above-and belowground herbivores. Cultivated cucurbits, such as zucchini squash, are vulnerable to a number of herbivorous pests that feed on different plant tissue types (Mondal et al., 2020). Cucumber beetles, in the genera Diabrotica and Acalymma, are important cucurbit herbivores, as larvae feed belowground on roots and adults feed aboveground on leaves, pollen, flowers, and fruits (Shapiro and Mauck, 2018). Cucumber beetles range from generalists that attack a wide variety of host plants, such as banded cucumber beetles (Diabrotica balteata LeConte), to specialists like the striped cucumber beetle (Acalymma vittatum F.) that exclusively feeds on cucurbits (Eben, 2022). For most cultivated cucurbits, crop breeding over evolutionary time resulted in an inability to produce defensive secondary metabolites called “cucurbitacins” (Theis et al., 2014; Brzozowski et al., 2019, 2020b), raising intriguing questions about how cucurbitacin loss alters interactions with herbivores. Recent evidence points towards plant volatile emissions as the major determinant of adult striped cucumber beetle host plant preference (Brzozowski et al., 2020a). Squash bugs (Anasa tristis DeGeer) are another major pest of cucurbits that feed solely on aboveground plant tissues as both juveniles and adults (Doughty et al., 2016). As a piercing-sucking herbivore, squash bugs inflict markedly different damage on host plants than chewing cucumber beetles (Neal, 1993; Mauck et al., 2015; Marmolejo et al., 2021). Both squash bugs and cucumber beetles are native to North America, sharing a long coexistence history with zucchini squash (Shapiro and Mauck, 2018). Zucchini squash likely evolved to anticipate and defend against simultaneous attack from multiple herbivores. Indeed, recent findings demonstrated that squash bugs are attracted to striped cucumber beetle aggregation pheromone (Brzozowski et al., 2022), highlighting how both herbivore species aggregate on shared hosts and suggesting multi-herbivore attack is a common challenge for cucurbit plants. Although a handful of studies have investigated cucurbit-mediated interactions between above-and belowground feeding cucumber beetles (Hladun and Adler, 2009; Barber et al., 2012, 2015; Milano et al., 2015), none to date have integrated squash bugs or investigated a range of possible mechanisms underlying cross-compartment, plant-mediated herbivore-herbivore interactions in zucchini squash.
The goal of this study was to determine how belowground striped cucumber beetle (Acalymma vittatum) larval herbivory alters cross-compartment zucchini squash (Cucurbita pepo subsp. pepo) defenses and interactions with aboveground herbivores, including adult cucumber beetles and squash bugs (Anasa tristis). Based on previous work indicating that belowground beetle larvae suppress defenses in cucumber roots for their own benefit (Grunseich et al., 2020), we predicted that larvae also systemically manipulate aboveground plant defense. Thus, we hypothesized larval herbivory would enhance cross-compartment plant defenses to deter aboveground herbivores, providing a competitive advantage to larvae when accessing shared plant resources. To explore this question, we determined how belowground larval herbivory affects the naturally occurring herbivore community in a squash agroecosystem. We further discerned how belowground herbivory influenced aboveground herbivore performance and host preference. We also explored belowground herbivore-induced systemic changes in aboveground plant volatile emissions, phytohormones, and nutrients. To understand how aboveground herbivory influences belowground larvae, we assessed belowground larval performance. Our study underscores connections between above-and belowground herbivore communities as mediated through induced systemic defenses of a shared host plant, shedding light on how herbivores utilize plant defenses for an advantage over competitors and how plants systemically respond to multi-herbivore attack.
Materials and methods
Plants and insect herbivores
Zucchini squash plants (Cucurbita pepo ssp. pepo cv. Raven) were grown from seed (Johnny’s Selected Seeds, Fairfield, ME, United States) in individual 10-cm diameter pots with topsoil mix (Hyponex Corporation, Marysville, United States) and 3 g Osmocote® fertilizer (Scotts, Marysville, United States). Plants were kept in an insect-free, climate-controlled growth room with supplemental lighting (16 h light: 8 h dark; 2.33 μmol/J; 29°C (light phase): 22°C (dark phase); 56% RH, Fluence, Austin, TX, United States). After 3–4 weeks of growth, plants were used in experiments. Striped cucumber beetles (Acalymma vittatum) and squash bugs (Anasa tristis) were maintained in separate laboratory colonies on cultivated cucumber (Cucumis sativus cv. Max Pack) and squash (C. pepo cv. Raven). Beetles were reared by separating adults and larvae—adults laid eggs in the soil surrounding cucurbit plants and once per week, soil containing eggs was removed from the adult colony to isolate emerging larvae. Larvae hatched, developed, and pupated in a separate colony until emergence as adult beetles. Adult beetles emerging from the soil were continually added to the adult beetle colony. Squash bug juveniles and adults were reared together in one colony by continually adding fresh cucurbit plants. Beetles were originally obtained from State College, PA, United States, and squash bugs were procured in College Station, TX, United States. Both colonies were intermittently supplemented with individuals collected near College Station, TX, United States and kept at 25°C on a 16 h light: 8 h dark schedule.
Aboveground systemic Cucurbita pepo resistance in an agroecosystem
To evaluate how belowground herbivory affects aboveground plant resistance under natural conditions, a field study was completed in 2020 at the USDA-ARS Southern Plains Agricultural Research Center in College Station, TX, United States. Zucchini squash plants (C. pepo cv. Raven) were grown from seed in a greenhouse for 4 weeks prior to transplantation in the field on 24-April-2020. Plants (n = 72) were transplanted within 8 rows with 9 plants per row. Rows were spaced approximately 2.04 m apart and plants within a row were spaced approximately 2 m apart (Supplementary Figure S1). Plants were watered individually and daily with a hose for 2 weeks. Thereafter, plants were watered by flood irrigation on 5-May-2020 and 10-May-2020. On 11-May-2020, after 3 weeks of plant growth in the field, second-instar striped cucumber beetle (A. vittatum) larvae were removed from the laboratory colony and 6 larvae were placed at the soil/plant interface of each plant in 4 rows (n = 36 plants). Plants were treated with 6 larvae as this density was within the range previously shown to induce plant defense and herbivore response in a related herbivore species (Robert et al., 2012b). Plants in the other 4 rows were not treated with larvae and served as controls (n = 36 plants). After 48 h of belowground larval herbivory, 160 adult spotted cucumber beetles (Diabrotica undecimpunctata howardi Barber) were released from the center of the field on 13-May-2020 (Supplementary Figure S1). Spotted cucumber beetles are a generalist pest of cucurbits that feed on aboveground plant tissues as adults. Adult beetles were collected 5 days prior from a nearby alfalfa (Medicago sativa) field, maintained on squash in a laboratory, and starved for 24 h prior to release in the field. Numbers of adult cucumber beetles on control or belowground larvae-damaged plants were monitored, but very few individuals of those released or other beetle species were recovered (Supplementary Figure S2). Due to the low numbers of cucumber beetles foraging in the field and limited recovery of spotted cucumber beetles that were released, aboveground banded cucumber beetles (Diabrotica balteata) were caged on leaves of control (n = 12) or larvae-damaged (n = 12) plants on 14-May-2020 (i.e., after 72 h of belowground larval herbivory). Banded cucumber beetles were collected at the same time as the spotted cucumber beetles and maintained in a laboratory until field deployment. For each row, three plants were randomly selected and one leaf of similar size from each plant was baged with one adult banded cucumber beetle (Supplementary Figure S1). After 3 days of aboveground beetle herbivory, bags and leaves were removed and the area of feeding damage was quantified using ImageJ (National Institute of Health, Bethesda, United States) as previously described in detail by Marmolejo et al. (2021).
Aboveground insect herbivore preference and performance assays
To examine whether belowground herbivory alters aboveground herbivore foraging, dual-choice (larvae-damaged or non-damaged control plants) behavioral bioassays were conducted in the laboratory. To establish larvae-damaged plants, 8 second-instar striped cucumber beetle (A. vittatum) larvae were added to each plant and allowed to feed for 48 h, while control plants received no larvae. After 48 h of belowground herbivory, 1 non-damaged control and 1 larvae-damaged plant were placed in opposite corners of a mesh cage. For adult striped cucumber beetles (A. vittatum), small mesh cages (30.5 × 30.5 × 30.5 cm) were used and 6 adult female beetles, which were starved for 24 h prior, were introduced to each cage (n = 14). Adult female beetles were allowed to forage and feed for 48 h, then the location of each beetle in each cage was recorded to determine plant preference. Damaged leaves from all plants were removed and the area of feeding damage was determined using methods described above.
Adult squash bugs (A. tristis) were presented with the same choices (non-damaged controls or belowground larvae-damaged plants) as adult beetles. For squash bug bioassays, we placed 2 male and 2 female adult squash bugs into each large mesh cage (60 × 40 × 40 cm; n = 6) and allowed the bugs to mate, feed, and lay eggs. After 5 days, larvae-damaged plants in all cages were supplemented with another group of 8 larvae to sustain herbivory as the first cohort of larvae were likely close to pupation. Thirteen days after squash bugs were introduced to cages, the location of each squash bug was recorded within each cage. Considering squash bugs lay visible eggs on the underside of leaf tissue, numbers of eggs laid on each plant were also recorded to determine squash bug plant preference.
To evaluate aboveground herbivore performance on belowground larvae-damaged plants, an assay with nymphal squash bugs was carried out in the laboratory. Individual plants were each placed in a mesh cage (30.5 × 30.5 × 30.5 cm), and plants were left as non-damaged controls (n = 5) or treated with 8 second-instar belowground larvae (n = 5). After 48 h of belowground larval herbivory, 5 pre-weighed first-instar squash bug nymphs were introduced to each cage because they are gregarious feeders and cannot survive alone on host plants (Mauck et al., 2015). Each group of 5 nymphs was weighed together and allowed to feed for 5 days, at which time they were removed from plants and reweighed as a group.
Aboveground systemic Cucurbita pepo defense induction
To determine how belowground herbivory systemically modifies aboveground defense and host plant quality, we quantified belowground herbivore-induced changes in aboveground zucchini squash volatile emissions, phytohormones, and nutrients in the laboratory. For aboveground volatile collections, plants were left as non-damaged controls (n = 4) or treated with 6 striped cucumber beetle (A. vittatum) larvae (n = 4). Belowground larvae fed for 24 h, then individual plants were each introduced to separate 4-L glass domes (Rogers Custom Glass, Warriors Mark, United States). A dynamic headspace sampling method was used to collect plant volatiles every 8 h across multiple days as Grunseich et al. (2020) previously established 8 h as a duration for volatile collection in this system. Volatiles were first collected from control and larvae-damaged plants after 24 h of herbivory during photophase (14:00–22:00). The next collection period was during scotophase (22:00–6:00), and then another collection took place the following day during photophase (6:00–14:00). Collections continued every 8 h throughout the 80-h period of belowground larval herbivory. During collections, filtered air was pushed into each dome at 1.8 l/min and air was simultaneously pulled out of each dome through a volatile filter trap containing 45 mg of HayeSep® Q (Hayes Separations Inc., Bandera, United States) at 1.0 l/min. After the final collection, aboveground plant tissues were harvested and dried at 35°C to quantify volatiles per gram of plant tissue.
Volatiles collected on the filter traps were eluted with 150 μl dichloromethane and 5 μl of nonyl acetate (80 ng/μL) was added to each sample as an internal standard. Samples were analyzed on a GC–MS using previously described methods (Grunseich et al., 2020; Marmolejo et al., 2021). Target compounds were tentatively identified by comparison with mass spectral libraries (NIST17 and Adams2 [Allured Publishing Corporation]), and structure assignments were confirmed where possible by comparison of mass spectra and retention times with authentic standards (Helms et al., 2019). Compounds were quantified relative to standard concentrations and converted to ng/g dried aboveground plant tissue.
To further describe aboveground systemic defense following belowground larval herbivory, phytohormone concentrations in the leaves of zucchini squash plants were quantified, focusing specifically on the antiherbivore defense-related phytohormones: jasmonic acid (JA) and salicylic acid (SA; Helms et al., 2017; Harth et al., 2018). Plants were left as non-damaged controls (n = 5) or challenged by 5–8 second-instar striped cucumber beetle (A. vittatum) larvae (n = 5). Following 24 or 72 h of larval herbivory, ~100 mg of aboveground leaf tissue was harvested from each plant and flash-frozen in liquid nitrogen. Leaf samples were ground using a tissue homogenizer (Bullet Blender Storm 24, Next Advance, NY, United States) and stored in a-80°C freezer until analysis. To extract and quantify JA and SA from leaf tissues, we followed procedures previously described in Schmelz et al. (2003, 2004). Phytohormones were extracted and derivatized to methyl esters, and methyl esters were isolated using vapor-phase extraction. Phytohormone concentrations were analyzed with GC/CI-MS (Agilent Technologies, Santa Clara, CA, United States) using isobutane and selected ion monitoring (SIM). Phytohormones were confirmed by comparing the retention times and spectra with standards, and quantified by adding 100 ng of internal standard for JA (i.e., dihydro-JA) and SA (i.e., 2-Hydroxybenzoic Acid-d6) to each sample.
To determine how belowground herbivory modifies aboveground host-plant quality, we quantified protein and non-structural carbohydrate content in leaves of non-damaged controls (n = 20) and plants treated with 8 second-instar striped cucumber beetle (A. vittatum) larvae (n = 20). Larvae fed on roots for 48 h, then leaf tissue was collected from each plant, flash-frozen in liquid nitrogen, and stored at −80°C until analysis. Tissues were lyophilized and ground using a mortar and pestle. Following the methods of Deans et al. (2018), a modified Bradford assay was used to quantify protein content and the phenol-sulfuric acid method was used to assess non-structural carbohydrate content.
Aboveground insect herbivore β-ocimene preference assays
To isolate the effect of the dominant volatile from the herbivore-induced volatile blend on foraging adult beetles and squash bugs, dual-choice preference bioassays were conducted in the laboratory using β-ocimene dispensers formulated with biologically relevant β-ocimene levels (i.e., levels emitted by belowground larvae-damaged squash plants). Volatile dispensers were created by preparing a 1 mg/ml solution of β-ocimene (purity ≥99.5%; Sigma-Aldrich), which contained a mixture of (Z)- and (E)-β-ocimene, in dichloromethane and adding 150 μl to individual 2-mL glass vials wrapped with aluminum foil to prevent UV light degradation. Control vials contained 150 μl dichloromethane. The rubber septum of each vial cap was pierced with a 5-μL glass capillary tube (12.8 mm) to dispense volatiles.
For adult beetle assays, two plants (1 control and 1 plant supplemented with a β-ocimene dispenser) were placed in opposite corners of each small mesh cage (30.5 × 30.5 × 30.5 cm; n = 8). Female beetle settling location and feeding damage were recorded following methods described above.
For assays with squash bugs, pairs of plants (1 control and 1 plant supplemented with a β-ocimene dispenser) were placed in opposite corners of mesh cages (60 × 40 × 40 cm; n = 16), and four adult squash bugs (2 males and 2 females) were introduced to the center of each cage and allowed to feed, mate, and lay eggs for 13 days. All dispensers in each cage were replaced with fresh dispensers every 2 days to ensure more consistent volatile emissions throughout the experiment. Squash bug locations were recorded after 48 h and 13 days, and the number of eggs laid on control and β-ocimene-supplemented plants were counted on the last assessment day.
Belowground insect herbivore performance assays
To investigate the influence of aboveground herbivory on belowground root-feeding striped cucumber beetle (A. vittatum) larvae, larval performance assays were conducted in the laboratory on control or aboveground herbivore-damaged plants. For adult striped cucumber beetles (A. vittatum), control plants (n = 6) were left non-damaged, while damaged plants had 5 adult female beetles, which were starved for 24 h prior to experimentation, caged on separate leaves (n = 6). Aboveground adult beetles fed for 72 h, then roots of control and adult beetle-damaged plants were collected, gently washed with water to remove soil, and patted dry with paper towels. Approximately 2.5 g root tissue was weighed out for each plant, moistened with filtered water, and placed in separate petri dishes. One pre-weighed second-instar larva was placed in each petri dish. Dishes were closed, sealed with parafilm, and covered with foil to exclude light cues and induce natural belowground larval feeding behavior. After 24 h of feeding, both larvae and root tissues were reweighed for larval mass gain and root mass consumption.
For larval performance assays with squash bugs, each squash bug-damaged plant (n = 14) received 12 third-instar squash bug nymphs, while control plants (n = 14) received no nymphs. Each control and squash bug-damaged plant was held separately in small mesh cages. Bugs fed for 11 days, at which point they were removed from plants. To assess how bugs alter host-plant growth, we recorded numbers of leaves, male flowers, and female flowers on control and squash bug-damaged plants. Roots of control and squash bug-damaged plants were harvested and carefully washed with water to remove surrounding soil. Roots from each plant were patted dry with paper towels and ~ 1 g of root tissues were weighed out for each plant and placed in separate petri dishes for larval feeding assays. Remaining root tissues were flash frozen and set aside for plant nutrient analyses as described above. We followed the same procedure to evaluate larvae mass gain and root mass consumption as described above. Dead larvae or those weighing less than their starting mass were removed from the dataset (5 replicates were removed for controls and 2 replicates were removed for squash bug-damaged plants).
Statistical analyses
All statistical analyses were conducted in the software program R (R Version 4.0.3, R Core Team, 2021). To meet assumptions of normality, data were log- or square-root transformed as necessary. For adult beetle feeding damage in a field setting, we used a two-way ANOVA with treatment and row as fixed and random factors, respectively. For settling behavior of adult beetles and squash bugs in choice-tests, we used generalized linear models with a quasi-likelihood function to compensate for over-dispersion (Robert et al., 2012a). For adult beetle feeding damage in choice-tests, we used paired t-tests. For squash bug oviposition preference in choice-tests, we used Chi-square goodness-of-fit tests. One-way ANOVAs were used to analyze the volatile, phytohormone, nutrient, larval mass, and root consumption data. For leaf and flower count data, generalized linear models with a Poisson distribution were used to compare control and squash bug-damaged plants.
Results
Belowground insect herbivory reduces aboveground insect herbivore feeding in a squash agroecosystem
Larvae-damaged plants sustained less aboveground herbivore feeding damage compared to non-damaged controls in a field setting (Figure 1; ANOVA, F = 5.69, p = 0.03).
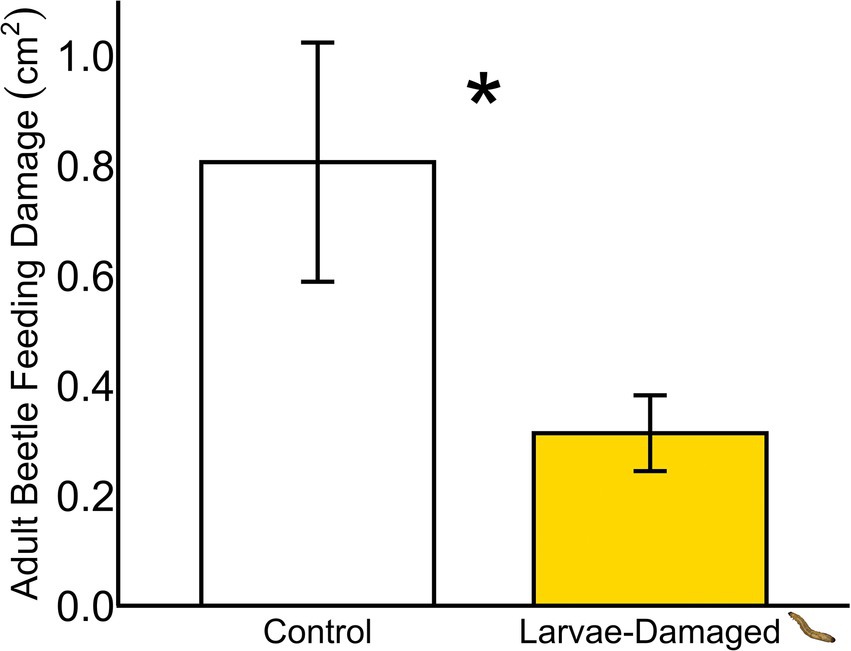
Figure 1. Belowground larval herbivory in the field reduces aboveground beetle feeding (*p ≤ 0.05). Means ± SE are presented.
Belowground insect herbivory repels aboveground insect herbivores
Significantly more aboveground female adult beetles were recovered on non-damaged controls than larvae-damaged plants (Figure 2A; GLM t = −3.15, p = 0.008), and there was a trend toward greater adult beetle feeding on controls relative to larvae-damaged plants (Figure 2B; paired t-test t = 1.87, p = 0.08). At the conclusion of the experiment, more adult squash bugs were recovered on non-damaged control plants than larvae-damaged plants (Figure 2C; GLM t = −2.41, p = 0.008). Female squash bugs also avoided ovipositing on larvae-damaged plants and laid more eggs on controls (Figure 2D; Chi-square goodness-of-fit X2 = 20.19, p < 0.001). However, squash bug nymph mass gain did not differ between control and larvae-damaged plants (Supplementary Figure S3; F = 0.02, p = 0.89).
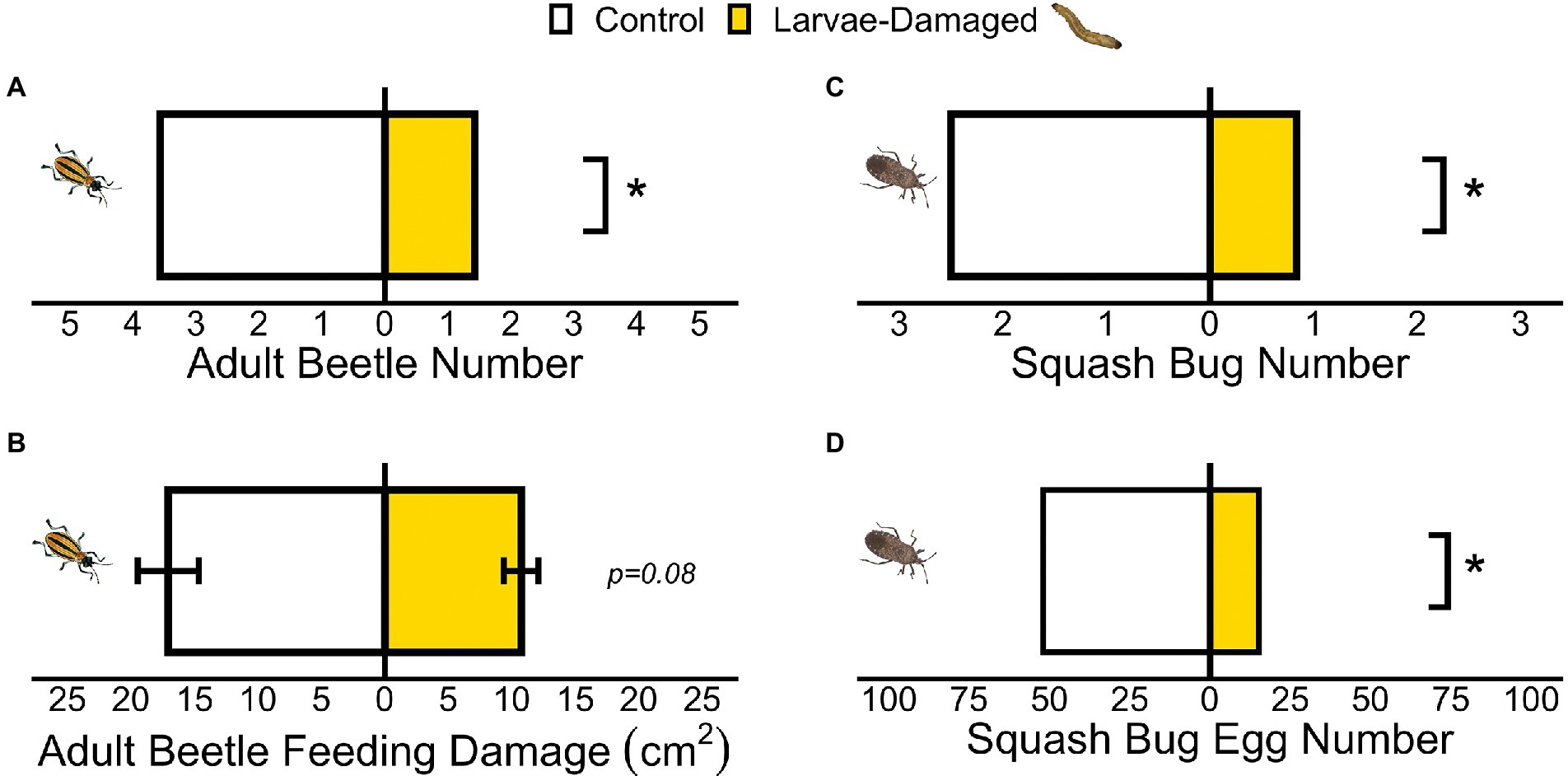
Figure 2. (A) Belowground larval herbivory deterred aboveground foraging adult beetles. (B) Adult beetles fed similarly when presented the choice between control and larvae-damaged plants. Squash bugs avoided (C) settling on and (D) laying eggs on belowground larvae-damaged plants. (*p ≤ 0.05) Means ± SE are presented.
Belowground insect herbivory modifies aboveground plant defense
Few volatile compounds were detected from non-damaged control and belowground larvae-damaged plants (Supplementary Table S1). Of the volatile compounds detected, larvae-damaged plants emitted greater amounts of (E)-β-ocimene from aboveground tissues compared to non-damaged controls (Figure 3A; ANOVA F = 15.12, p = 0.008) following 48 h of larval herbivory (Supplementary Figure S4). In contrast, no differences between larvae-damaged and non-damaged plants were observed for α-pinene—the other volatile found during collections (Supplementary Figure S5). Belowground herbivory did not modify aboveground concentrations of jasmonic acid (JA) or salicylic acid (SA; Supplementary Figure S6). However, a trend was observed toward belowground herbivory reducing aboveground leaf carbohydrate content (Figure 3B; ANOVA F = 2.93, p = 0.10). Belowground herbivory elevated aboveground leaf protein content (Figure 3C; ANOVA F = 9.08, p = 0.005) and increased the aboveground ratio of protein: carbohydrates in leaves (Figure 3D; ANOVA F = 7.85, p = 0.008)—a ratio which can be an important driver of herbivore host-plant selection and consumption (Behmer, 2009).
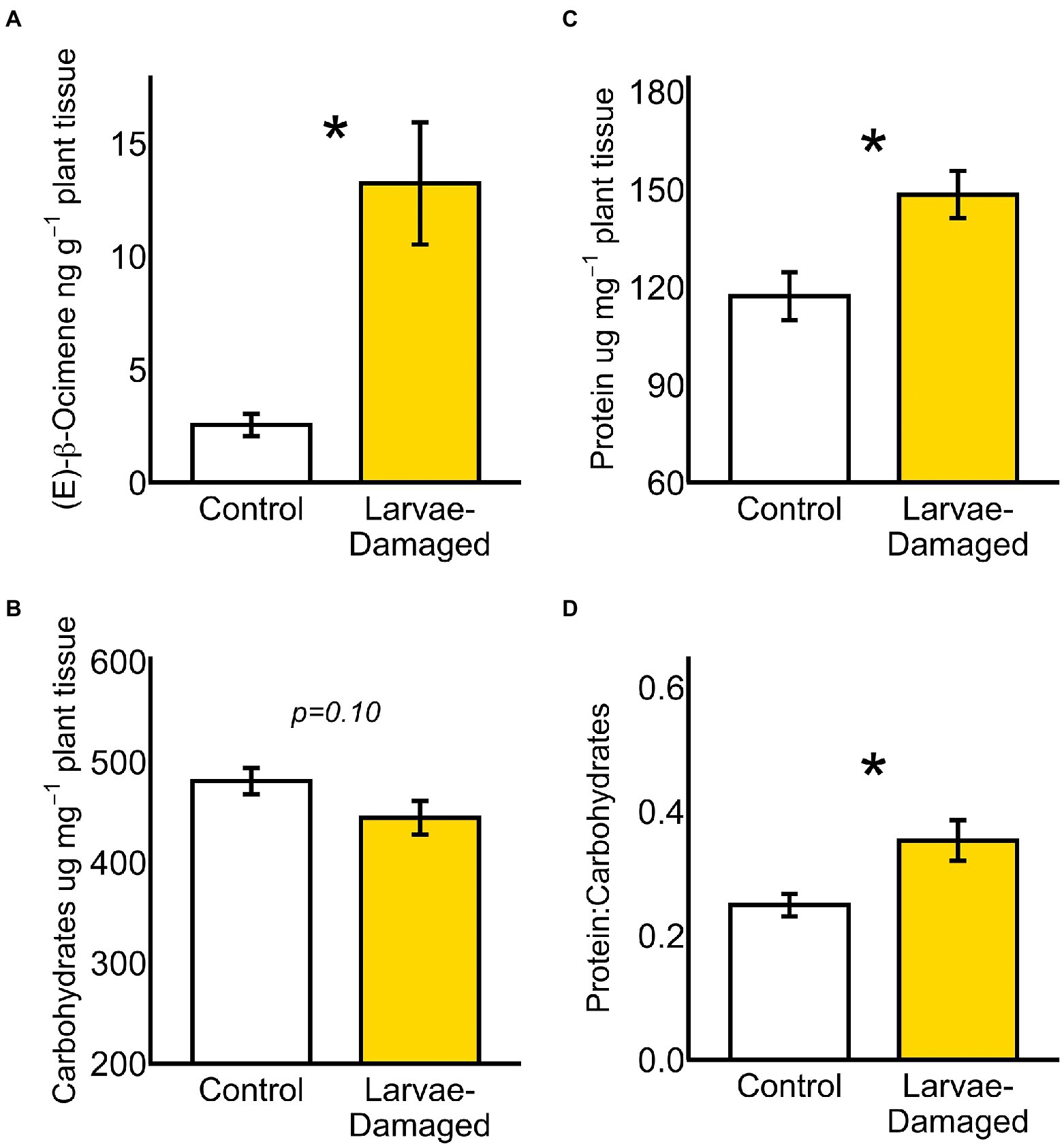
Figure 3. (A) Belowground larval herbivory induced systemic increases in aboveground (E)-β-ocimene emission, but did not significantly modify aboveground (B) carbohydrates. Belowground larval herbivory enhanced aboveground leaf (C) protein content and (D) the ratio of protein: carbohydrates. (*p ≤ 0.05) Means ± SE are presented.
Aboveground adult beetles are not deterred by β-ocimene alone while foraging squash bugs initially avoid higher β-ocimene emissions
Similar numbers of aboveground adult beetles were recovered on controls and plants supplemented with β-ocimene-dispensers (Figure 4A; GLM t = −0.62, p = 0.54), and aboveground adult beetle feeding damage did not differ between the two groups of plants (Figure 4B; paired t-test t = 0.57, p = 0.58). However, squash bugs avoided plants with β-ocimene-dispensers and preferred to settle on controls after 48 h of exposure (Figure 4C; GLM t = 2.080 p = 0.05), but after 13 days, more squash bugs were recovered on plants with β-ocimene-dispensers (Figure 4D; GLM t = −2.519 p = 0.01). Significantly more squash bug eggs were also found on β-ocimene-dispenser treated plants after 13 days of exposure (Figure 4E; Chi-square goodness-of-fit X2 = 8.19, p = 0.004).
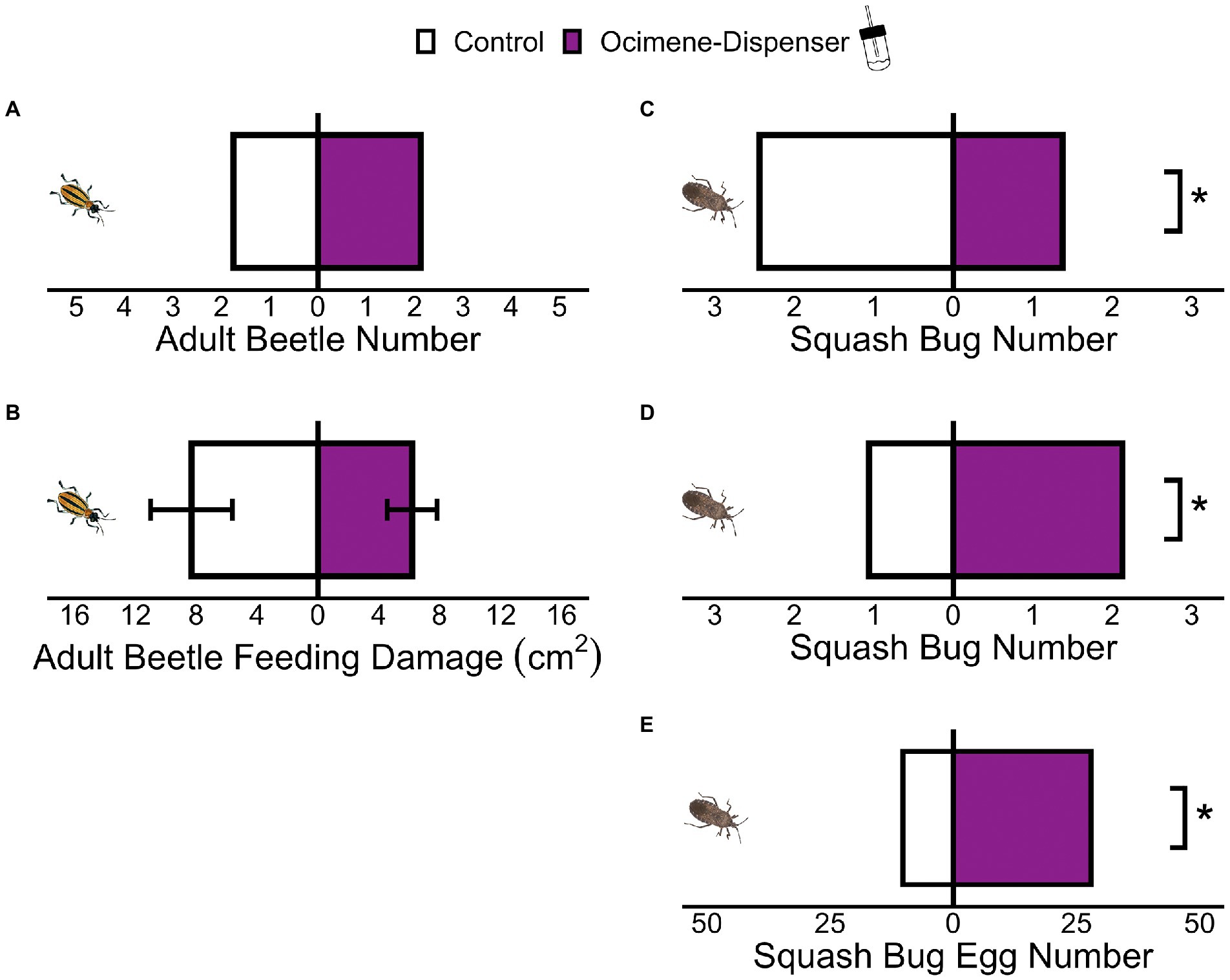
Figure 4. Adult beetles (A) settled and (B) fed equally on plants with and without supplemental β-ocimene. (C) Squash bugs avoided plants supplemented with β-ocimene after 48 h of exposure. After 13 days, squash bugs (D) preferred and (E) laid more eggs on plants supplemented with β-ocimene. (*p ≤ 0.05) Means ± SE are presented.
Aboveground insect herbivory does not affect belowground larval performance
Aboveground adult beetle herbivory on plant leaves did not affect belowground larval mass gain (Supplementary Figure S7; ANOVA F = 0.06, p = 0.82) or alter larval root consumption (Supplementary Figure S7; ANOVA F = 0.02, p = 0.91). Squash bug herbivory did not alter plant leaf number (Figure 5A; GLM z = −0.69, p = 0.49), male flower number (Figure 5B; GLM z = −1.51, p = 0.13), female flower number (Figure 5C; GLM z = −1.51, p = 0.13), or root protein content (Figure 5D; ANOVA F = 0.06, p = 0.80). Aboveground squash bug herbivory did not modify belowground larval mass gain (Figure 5E; ANOVA F = 0.50, p = 0.49), but there was a trend toward larvae consuming more root tissue on aboveground squash bug-damaged plants relative to non-damaged controls (Figure 5F; ANOVA F = 3.49, p = 0.06).
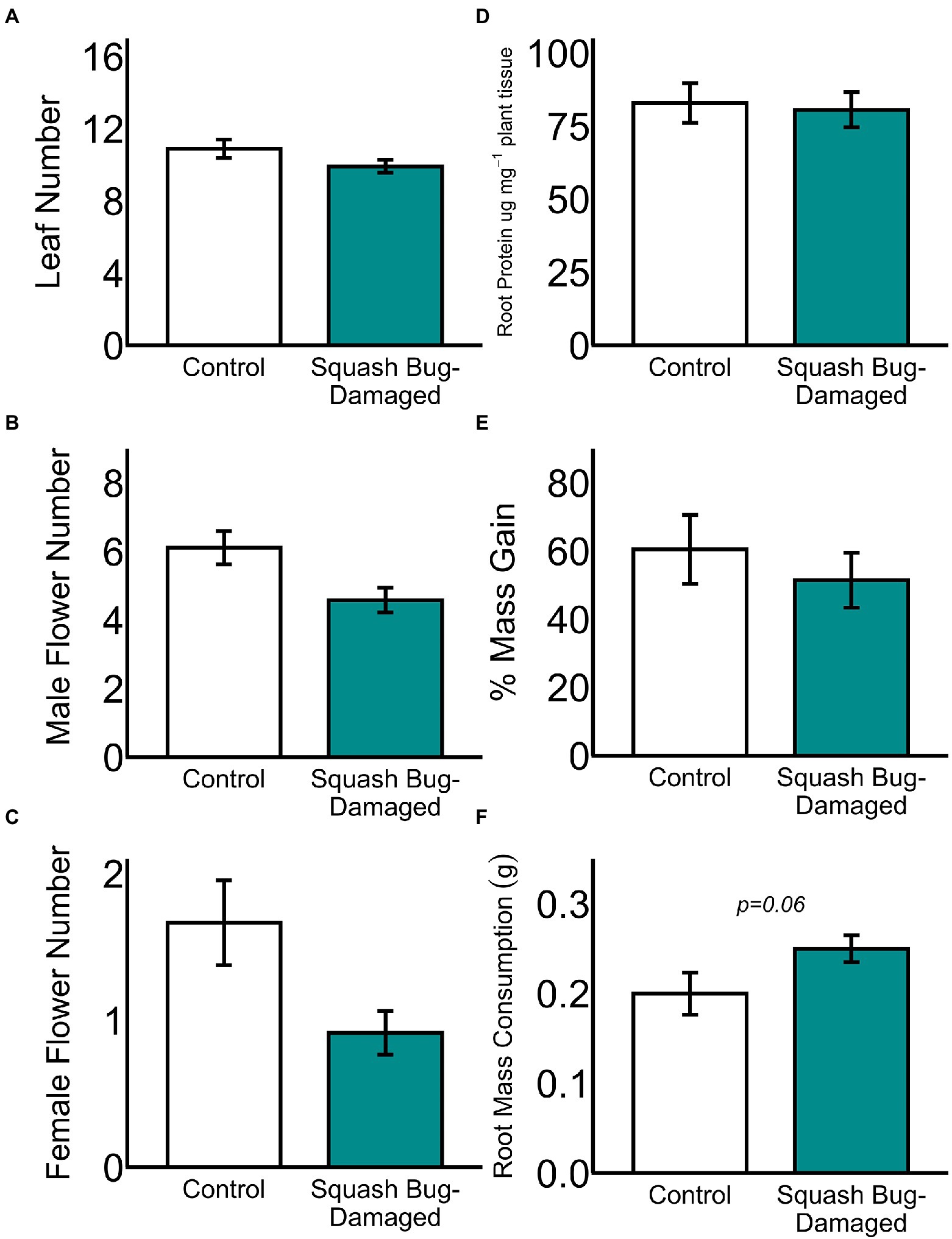
Figure 5. Aboveground squash bug herbivory does not modify (A) leaf number, (B) male flower number, (C) female flower number, (D) root protein content, (E) belowground larval mass gain, or (F) belowground larval root mass consumption. (*p ≤ 0.05) Means ± SE are presented.
Discussion
Plants induce defenses against insect herbivory, which can affect subsequent later-arriving herbivores (Erb et al., 2011; Huang et al., 2017). Although multi-herbivore attack is common, surprisingly little is known about the outcomes of such interactions across different plant-herbivore systems (Fernández de Bobadilla et al., 2022). For example, plants must frequently navigate tradeoffs between enhancing resistance against one herbivore while possibly increasing susceptibility to the following herbivore (Stam et al., 2014). Plant defense induction impacts both local and systemic plant tissues (Biere and Goverse, 2016), and potential tradeoffs in defense induction are further influenced by attack from above-and belowground herbivores (Karssemeijer et al., 2022). Our study examined how belowground herbivory shapes cross-compartment zucchini squash defenses and interactions with aboveground herbivores. Belowground striped cucumber beetle (A. vittatum) larval herbivory enhanced aboveground plant resistance (Figure 1) and deterred aboveground foraging herbivores (Figures 2A,C,D). We detected changes in aboveground volatile emissions and nutrients from larvae-damaged plants relative to non-damaged controls (Figures 3A,C,D), with mixed evidence for the role of plant volatiles in aboveground herbivore host-plant selection (Figures 4A–E). Although aboveground adult beetle herbivory did not impact belowground larval performance (Supplementary Figures S7A,B), we found a trend toward aboveground squash bug herbivory increasing larval root consumption (Figure 5F). Collectively, our findings demonstrate that belowground insect herbivory enhances cross-compartment systemic plant defense in zucchini squash and suggest plants and/or cucumber beetle larvae optimize defense induction to thwart subsequent aboveground herbivores.
The outcomes of multi-herbivore attack are predicted to correspond to traits of the attacking herbivores, such as feeding guild (Kaplan and Denno, 2007). Chewing and piercing-sucking herbivores often activate contrasting plant defenses, which are negatively correlated through phytohormone crosstalk, and often result in chewing herbivores enhancing plant susceptibility to piercing-sucking herbivores and vice versa (Soler et al., 2012a). However, phytohormone crosstalk is not always observed and cannot fully explain how plants deal with multi-herbivore attack (Ali and Agrawal, 2014; Davidson-Lowe et al., 2019), particularly when considering plant-mediated interactions between above-and belowground herbivores (Kafle et al., 2017; Mbaluto et al., 2021). Intriguingly, recent evidence points toward the evolutionary history between plants and their associated herbivore community as playing a crucial role in plant defense against multiple herbivores, as plants prioritize defenses against herbivores that are common in their environments (Mertens et al., 2021). All herbivores used in our study are common pests of zucchini squash in North America, sharing a long co-existence history with the plant and suggesting that squash plants are likely adapted to face predictable bouts of multi-herbivore attack both above-and belowground. In the current study, regardless of herbivore host-plant range (e.g., generalist or specialist) or feeding guild (e.g., chewing or piercing-sucking), belowground herbivory enhanced aboveground plant resistance (Figure 1) and deterred aboveground herbivores (Figures 2A,C,D). These findings could indicate that belowground herbivory from striped cucumber beetle larvae provides a predictable cue to zucchini squash that aboveground attack is eminent, particularly from adult beetles which emerge from the soil after pupation and begin feeding on aboveground plant tissues. Previous research documented consistent reductions in numbers of flowers, pollinator visits, and fruit set in cucurbit plants attacked by belowground striped cucumber beetle larvae (Barber et al., 2012, 2015), demonstrating a major fitness cost to larvae-damaged plants. Due to the fitness costs induced by belowground herbivory, a defense strategy of broad-spectrum resistance in aboveground tissues may be favorable to zucchini squash to mitigate additional damage and fitness costs.
Belowground herbivory can modify numerous aspects of aboveground plant defense (van Dam, 2009; Soler et al., 2012b). In some cases, the distribution pattern of belowground herbivores on roots can further modify systemic aboveground defenses (Tsunoda et al., 2018). Although we did not characterize the larval distribution pattern in our study, we did find belowground larval feeding increased aboveground plant volatile (E)-β-ocimene emissions (Figure 3A), elevated leaf protein content (Figure 3C), and altered the protein: carbohydrate ratio of aboveground leaves (Figure 3D). These findings indicate that changes in volatile (E)-β-ocimene emissions may serve as a signal of reduced host plant nutritional quality to aboveground herbivores. For example, many aphid species rely on plant volatiles to discriminate between host plants of differing nutritional quality (Bruce and Pickett, 2011). Alternatively, (E)-β-ocimene alone could serve as a direct defense and repel aboveground foraging herbivores. Recent evidence from tea plants demonstrated β-ocimene emissions enhance plant resistance to foraging herbivores (Jing et al., 2021). For our study, to test the hypothesis that (E)-β-ocimene directly repels herbivores, we conducted dual-choice preference bioassays with β-ocimene-dispensers and found mixed support for (E)-β-ocimene serving as a direct defense. On one hand, adult striped cucumber beetles showed no preference for plants with or without β-ocimene-dispensers (Figures 4A,B), revealing adult beetles are not directly repelled by (E)-β-ocimene emissions alone. On the other hand, squash bugs initially avoided plants with β-ocimene-dispensers (Figure 4C), but following additional days of exposure during the bioassay, we recovered more squash bug adults and eggs on plants with supplemental ocimene than plants without (Figures 4D,E). The initial avoidance behavior of adult squash bugs provides some evidence that (E)-β-ocimene functions as a direct defense, but we suspect the bugs likely habituated to the supplemental ocimene over time. Considering squash bugs habituated to the volatile emissions, we speculate that (E)-β-ocimene does not serve as a direct defense. Instead, (E)-β-ocimene may provide some information to aboveground herbivores about host-plant quality, but it is possible that changes in plant nutritional quality alone drive herbivore avoidance of larvae-damaged plants. Future research should investigate the nutritional preferences of adult striped cucumber beetles and squash bugs to determine their foraging responses to changes in plant nutrients.
Although (E)-β-ocimene emissions did not solely determine aboveground herbivore foraging decisions, it is worth noting that (E)-β-ocimene plays important roles in other ecological interactions involving cucurbit plants. When damaged by herbivores, plants emit characteristic blends of volatiles, which can attract insect predators and parasitoids (i.e., natural enemies) to kill herbivores and thereby serve as an indirect defense (Turlings and Erb, 2018; Thompson et al., 2022). Cucumber (Cucumis sativus) plants damaged by spider mites emit greater quantities of (E)-β-ocimene, as well as other volatile compounds (Kappers et al., 2010, 2011), which play important roles in the attraction of natural enemies for indirect defense (Agrawal et al., 2002). In our study, we cannot exclude the possibility that (E)-β-ocimene recruits aboveground natural enemies for indirect plant defense against adult cucumber beetles and/or squash bugs. Indeed, previous research on Brassica nigra revealed belowground herbivory alters aboveground plant volatile emissions, which influence foraging decisions of Cotesia glomerata, an aboveground parasitoid of the leaf herbivore Pieris brassicae (Soler et al., 2007). Future research should aim to characterize the behavioral response of aboveground natural enemies to (E)-β-ocimene, with particular emphasis on natural enemies specialized to attack adult cucumber beetles and squash bugs. Alternatively, volatiles of herbivore-damaged plants can also influence the defense response of neighboring plants (i.e., volatile-mediated interplant communication; Karban, 2021). The first study to investigate interplant communication in cucurbits recently determined that (E)-β-ocimene plays an important role in triggering defense responses in neighboring plants (Marmolejo et al., 2021), which could indicate that aboveground volatiles of larvae-damaged plants provide information on the risk of herbivory to neighboring plants. Exploring more possibilities for the role of (E)-β-ocimene in aboveground ecological interactions will provide additional insights on its ecological function.
One such possibility is that belowground striped cucumber beetle larvae manipulate aboveground plant defenses for their own benefit. Previous research in cucumbers demonstrated belowground striped cucumber beetle herbivory induces root volatile emissions, which attract belowground natural enemies for indirect defense, but sustained larval herbivory over an extended time period suppresses volatile emissions and eliminates natural enemy attraction (Grunseich et al., 2020). Suppressed volatile emissions are likely a form of herbivore manipulation of plant defense (Lin et al., 2021), allowing herbivores to evade their natural enemies. In conjunction with our findings, it is possible that striped cucumber beetle larvae manipulate plant defenses both below- and aboveground, reducing the attraction of natural enemies and competitors to host plants. Although we did not find any changes in larval performance on plants damaged by aboveground adult beetles (Supplementary Figures S7A,B), we did detect a trend towards altered larval feeding behavior on plants damaged by aboveground squash bugs (Figure 5F). Squash bug herbivory could modify belowground root tissues, which could explain the trend towards increased larval root consumption (Figure 5F). Future research should investigate changes in other aspects of larval performance as well, such as rate of development or growth over longer periods of time. Additionally, recent evidence from Chinese tallow showed aboveground herbivore effects on belowground root nutrient content, as well as herbivore performance, were dependent on the identity and density of aboveground herbivores (Wan et al., 2022). Future research should investigate different densities of aboveground striped cucumber beetles and squash bugs to discern if there are any density-dependent effects on belowground striped cucumber beetle larval performance. It is also plausible that although larval performance did not change on aboveground herbivore-damaged plants, aboveground herbivory could modify larval host-plant preference. Previous work on striped cucumber beetle larvae found that larvae avoided cucumber plants damaged by aboveground herbivores, including adult striped cucumber beetles (Milano et al., 2015). In maize, aboveground herbivory modifies belowground volatile emissions which repel belowground foraging herbivores (Robert et al., 2012a). It is also important to note that both adult striped cucumber beetles and squash bugs are vectors for lethal plant pathogens, bacterial wilt (Erwinia tracheiphila) and yellow vine decline (Serratia marcescens), respectively (Bruton et al., 2003; Rojas et al., 2015). Larval performance on pathogen-infected cucurbits remains unknown, but since these plant pathogens are particularly virulent and ultimately kill host plants, it is likely that pathogen infection reduces belowground larval performance. It is possible that larvae manipulate aboveground plant defenses to deter vectors of plant pathogens, rather than to decrease direct competition with aboveground herbivores.
Conclusion
Our study investigated how belowground herbivory influences aboveground zucchini squash defense and interactions with aboveground herbivores. Overall, we found that belowground larval herbivory modified aboveground plant defense and repelled foraging herbivores, although exact mechanisms regulating aboveground herbivore host-plant preference remain elusive. We conclude that zucchini squash likely evolved to optimize its defensive strategy against commonly occurring above-and belowground herbivores, allowing plants to overcome multi-herbivore attack. We also provide an alternative explanation for our findings, namely that belowground striped cucumber beetle larvae may actively manipulate aboveground plant defenses for their own benefit. Our study provides novel insights into how plants navigate multi-herbivore attack both above-and belowground, shedding light on whole-plant defense strategies and plant-mediated interactions between herbivores.
Data availability statement
The original contributions presented in the study are included in the article/Supplementary material, further inquiries can be directed to the corresponding author.
Author contributions
MT, CS, and AH conceived the ideas and designed the methodology. MT, JG, NA, LM, and PB collected the data. MT analyzed the data. All authors contributed to the article and approved the submitted version.
Funding
MT was funded in part by a Texas A&M University College of Agriculture & Life Sciences Merit Fellowship and a John A. Jackman Endowed Scholarship. This research was supported by funding from the Texas Ecological Laboratory Program, Texas A&M University, and HATCH project TEX0-1-9066 awarded to AH.
Acknowledgments
We thank the Helms lab members for assistance with maintaining plants and colonies for experiments. We thank Mike O’Neil and USDA-ARS staff for assistance with the field experiment. We also extend extreme gratitude to the Fluckey lab members, particularly Patrick Ryan, for assistance with nutrient assays.
Conflict of interest
The authors declare that the research was conducted in the absence of any commercial or financial relationships that could be construed as a potential conflict of interest.
Publisher’s note
All claims expressed in this article are solely those of the authors and do not necessarily represent those of their affiliated organizations, or those of the publisher, the editors and the reviewers. Any product that may be evaluated in this article, or claim that may be made by its manufacturer, is not guaranteed or endorsed by the publisher.
Supplementary material
The Supplementary material for this article can be found online at: https://www.frontiersin.org/articles/10.3389/fevo.2022.1033730/full#supplementary-material
References
Agrawal, A. A., Janssen, A., Bruin, J., Posthumus, M. A., and Sabelis, M. W. (2002). An ecological cost of plant defence: attractiveness of bitter cucumber plants to natural enemies of herbivores. Ecol. Lett. 5, 377–385. doi: 10.1046/j.1461-0248.2002.00325.x
Ali, J. G., and Agrawal, A. A. (2014). Asymmetry of plant-mediated interactions between specialist aphids and caterpillars on two milkweeds. Funct. Ecol. 28, 1404–1412. doi: 10.1111/1365-2435.12271
Barber, N. A. (2018). “Cross-compartment herbivory effects on antagonists and mutualists and their consequences for plant fitness,” in Aboveground–Belowground Community Ecology. eds. T. Ohgushi, S. Wurst, and S. N. Johnson (Switzerland: Springer International Publishing), 247–269.
Barber, N. A., Adler, L. S., Theis, N., Hazzard, R. V., and Kiers, E. T. (2012). Herbivory reduces plant interactions with above-and belowground antagonists and mutualists. Ecology 93, 1560–1570. doi: 10.1890/11-1691.1
Barber, N. A., Milano, N. J., Kiers, E. T., Theis, N., Bartolo, V., Hazzard, R. V., et al. (2015). Root herbivory indirectly affects above-and below-ground community members and directly reduces plant performance. J. Ecol. 103, 1509–1518. doi: 10.1111/1365-2745.12464
Behmer, S. T. (2009). Insect herbivore nutrient regulation. Annu. Rev. Entomol. 54, 165–187. doi: 10.1146/annurev.ento.54.110807.090537
Bezemer, T. M., and van Dam, N. M. (2005). Linking aboveground and belowground interactions via induced plant defenses. Trends Ecol. Evol. 20, 617–624. doi: 10.1016/j.tree.2005.08.006
Biere, A., and Goverse, A. (2016). Plant-mediated systemic interactions between pathogens, parasitic nematodes, and herbivores above-and belowground. Annu. Rev. Phytopathol. 54, 499–527. doi: 10.1146/annurev-phyto-080615-100245
Bruce, T. J. A., and Pickett, J. A. (2011). Perception of plant volatile blends by herbivorous insects – finding the right mix. Phytochemistry 72, 1605–1611. doi: 10.1016/j.phytochem.2011.04.011
Bruton, B., Mitchell, F., Fletcher, J., Pair, S., Wayadande, A., Melcher, U., et al. (2003). Serratia marcescens, a phloem-colonizing, squash bug-transmitted bacterium: causal agent of cucurbit yellow vine disease. Plant Dis. 87, 937–944. doi: 10.1094/PDIS.2003.87.8.937
Brzozowski, L. J., Gardner, J., Hoffmann, M. P., Kessler, A., Agrawal, A. A., and Mazourek, M. (2020a). Attack and aggregation of a major squash pest: parsing the role of plant chemistry and beetle pheromones across spatial scales. J. Appl. Ecol. 57, 1442–1451. doi: 10.1111/1365-2664.13689
Brzozowski, L. J., Gore, M. A., Agrawal, A. A., and Mazourek, M. (2020b). Divergence of defensive cucurbitacins in independent CUCURBITA PEPO domestication events leads to differences in specialist herbivore preference. Plant Cell Environ. 43, 2812–2825. doi: 10.1111/pce.13844
Brzozowski, L. J., Mazourek, M., and Agrawal, A. A. (2019). Mechanisms of resistance to insect herbivores in isolated breeding lineages of Cucurbita pepo. J. Chem. Ecol. 45, 313–325. doi: 10.1007/s10886-019-01046-8
Brzozowski, L. J., Weber, D. C., Wallingford, A. K., Mazourek, M., and Agrawal, A. A. (2022). Trade-offs and synergies in management of two co-occurring specialist squash pests. J. Pest. Sci. 95, 327–338. doi: 10.1007/s10340-021-01379-y
Davidson-Lowe, E., Szendrei, Z., and Ali, J. G. (2019). Asymmetric effects of a leaf-chewing herbivore on aphid population growth: insect feeding guild interactions. Ecol. Entomol. 44, 81–92. doi: 10.1111/een.12681
De Moraes, C. M., Mescher, M. C., and Tumlinson, J. H. (2001). Caterpillar-induced nocturnal plant volatiles repel conspecific females. Nature 410, 577–580. doi: 10.1038/35069058
Deans, C. A., Sword, G. A., Lenhart, P. A., Burkness, E., Hutchison, W. D., and Behmer, S. T. (2018). Quantifying plant soluble protein and digestible carbohydrate content, using corn (Zea mays) as an exemplar. J. Vis. Exp. 138:58164. doi: 10.3791/58164
Doughty, H., Wilson, J. M., Schultz, P. B., and Kuhar, T. P. (2016). Squash bug (Hemiptera: Coreidae): biology and management in cucurbitaceous crops. J. Integr. Pest Manag. 7:1. doi: 10.1093/jipm/pmv024
Eben, A. (2022). Ecology and evolutionary history of Diabrotica beetles—overview and update. Insects 13:156. doi: 10.3390/insects13020156
Erb, M., Flors, V., Karlen, D., de Lange, E., Planchamp, C., D’Alessandro, M., et al. (2009a). Signal signature of aboveground-induced resistance upon belowground herbivory in maize. Plant J. 59, 292–302. doi: 10.1111/j.1365-313X.2009.03868.x
Erb, M., Lenk, C., Degenhardt, J., and Turlings, T. C. (2009b). The underestimated role of roots in defense against leaf attackers. Trends Plant Sci. 14, 653–659. doi: 10.1016/j.tplants.2009.08.006
Erb, M., and Reymond, P. (2019). Molecular interactions between plants and insect herbivores. Annu. Rev. Plant Biol. 70, 527–557. doi: 10.1146/annurev-arplant-050718-095910
Erb, M., Robert, C. A. M., Hibbard, B. E., and Turlings, T. C. J. (2011). Sequence of arrival determines plant-mediated interactions between herbivores: sequence-specific plant-insect interactions. J. Ecol. 99, 7–15. doi: 10.1111/j.1365-2745.2010.01757.x
Erb, M., Robert, C. A. M., Marti, G., Lu, J., Doyen, G. R., Villard, N., et al. (2015). A physiological and behavioral mechanism for leaf herbivore-induced systemic root resistance. Plant Physiol. 169, 2884–2894. doi: 10.1104/pp.15.00759
Fernández de Bobadilla, M., Vitiello, A., Erb, M., and Poelman, E. H. (2022). Plant defense strategies against attack by multiple herbivores. Trends Plant Sci. 27, 528–535. doi: 10.1016/j.tplants.2021.12.010
Grunseich, J. M., Thompson, M. N., Hay, A. A., Gorman, Z., Kolomiets, M. V., Eubanks, M. D., et al. (2020). Risky roots and careful herbivores: sustained herbivory by a root-feeding herbivore attenuates indirect plant defences. Funct. Ecol. 34, 1779–1789. doi: 10.1111/1365-2435.13627
Harth, J. E., Ferrari, M. J., Helms, A. M., Tooker, J. F., and Stephenson, A. G. (2018). Zucchini yellow mosaic virus infection limits establishment and severity of powdery mildew in wild populations of Cucurbita pepo. Front. Plant Sci. 9:792. doi: 10.3389/fpls.2018.00792
Heil, M., and Ton, J. (2008). Long-distance signalling in plant defence. Trends Plant Sci. 13, 264–272. doi: 10.1016/j.tplants.2008.03.005
Helms, A. M., De Moraes, C. M., Tröger, A., Alborn, H. T., Francke, W., Tooker, J. F., et al. (2017). Identification of an insect-produced olfactory cue that primes plant defenses. Nat. Commun. 8:337. doi: 10.1038/s41467-017-00335-8
Helms, A. M., Ray, S., Matulis, N. L., Kuzemchak, M. C., Grisales, W., Tooker, J. F., et al. (2019). Chemical cues linked to risk: cues from below-ground natural enemies enhance plant defences and influence herbivore behaviour and performance. Funct. Ecol. 33, 798–808. doi: 10.1111/1365-2435.13297
Hladun, K. R., and Adler, L. S. (2009). Influence of leaf herbivory, root herbivory, and pollination on plant performance in Cucurbita moschata. Ecol. Entomol. 34, 144–152. doi: 10.1111/j.1365-2311.2008.01060.x
Huang, W., Robert, C. A. M., Hervé, M. R., Hu, L., Bont, Z., and Erb, M. (2017). A mechanism for sequence specificity in plant-mediated interactions between herbivores. New Phytol. 214, 169–179. doi: 10.1111/nph.14328
Huang, W., Siemann, E., Xiao, L., Yang, X., and Ding, J. (2014). Species-specific defence responses facilitate conspecifics and inhibit heterospecifics in above–belowground herbivore interactions. Nat. Commun. 5:4851. doi: 10.1038/ncomms5851
Huang, W., Siemann, E., Yang, X., Wheeler, G. S., and Ding, J. (2013). Facilitation and inhibition: changes in plant nitrogen and secondary metabolites mediate interactions between above-ground and below-ground herbivores. Proc. R. Soc. B 280:20131318. doi: 10.1098/rspb.2013.1318
Jing, T., Qian, X., Du, W., Gao, T., Li, D., Guo, D., et al. (2021). Herbivore-induced volatiles influence moth preference by increasing the β-ocimene emission of neighbouring tea plants. Plant Cell Environ. 44, 3667–3680. doi: 10.1111/pce.14174
Kafle, D., Hänel, A., Lortzing, T., Steppuhn, A., and Wurst, S. (2017). Sequential above-and belowground herbivory modifies plant responses depending on herbivore identity. BMC Ecol. 17, 1–10. doi: 10.1186/s12898-017-0115-2
Kaplan, I., and Denno, R. F. (2007). Interspecific interactions in phytophagous insects revisited: a quantitative assessment of competition theory. Ecol. Lett. 10, 977–994. doi: 10.1111/j.1461-0248.2007.01093.x
Kaplan, I., Halitschke, R., Kessler, A., Rehill, B. J., Sardanelli, S., and Denno, R. F. (2008a). Physiological integration of roots and shoots in plant defense strategies links above-and belowground herbivory. Ecol. Lett. 11, 841–851. doi: 10.1111/j.1461-0248.2008.01200.x
Kaplan, I., Halitschke, R., Kessler, A., Sardanelli, S., and Denno, R. F. (2008b). Constitutive and induced defenses to herbivory in above-and belowground plant tissues. Ecology 89, 392–406. doi: 10.1890/07-0471.1
Kappers, I. F., Hoogerbrugge, H., Bouwmeester, H. J., and Dicke, M. (2011). Variation in herbivory-induced volatiles among cucumber (Cucumis sativus L.) varieties has consequences for the attraction of carnivorous natural enemies. J. Chem. Ecol. 37, 150–160. doi: 10.1007/s10886-011-9906-7
Kappers, I. F., Verstappen, F. W., Luckerhoff, L. L., Bouwmeester, H. J., and Dicke, M. (2010). Genetic variation in jasmonic acid-and spider mite-induced plant volatile emission of cucumber accessions and attraction of the predator Phytoseiulus persimilis. J. Chem. Ecol. 36, 500–512. doi: 10.1007/s10886-010-9782-6
Karban, R. (2020). The ecology and evolution of induced responses to herbivory and how plants perceive risk. Ecol. Entomol. 45, 1–9. doi: 10.1111/een.12771
Karban, R. (2021). Plant Communication. Annu. Rev. Ecol. Evol. Syst. 52, 1–24. doi: 10.1146/annurev-ecolsys-010421-020045
Karssemeijer, P. N., Reichelt, M., Gershenzon, J., Loon, J., and Dicke, M. (2020). Foliar herbivory by caterpillars and aphids differentially affects phytohormonal signalling in roots and plant defence to a root herbivore. Plant Cell Environ. 43, 775–786. doi: 10.1111/pce.13707
Karssemeijer, P. N., Winzen, L., van Loon, J. J. A., and Dicke, M. (2022). Leaf-chewing herbivores affect preference and performance of a specialist root herbivore. Oecologia 199, 243–255. doi: 10.1007/s00442-022-05132-9
Kloth, K. J., and Dicke, M. (2022). Rapid systemic responses to herbivory. Curr. Opin. Plant Biol. 68:102242. doi: 10.1016/j.pbi.2022.102242
Lin, P., Chen, Y., Chaverra-Rodriguez, D., Heu, C. C., Zainuddin, N. B., Sidhu, J. S., et al. (2021). Silencing the alarm: an insect salivary enzyme closes plant stomata and inhibits volatile release. New Phytol. 230, 793–803. doi: 10.1111/nph.17214
Machado, R. A. R., Arce, C. C. M., McClure, M. A., Baldwin, I. T., and Erb, M. (2018). Aboveground herbivory induced jasmonates disproportionately reduce plant reproductive potential by facilitating root nematode infestation: induced jasmonates benefit parasitic nematodes. Plant Cell Environ. 41, 797–808. doi: 10.1111/pce.13143
Machado, R. A. R., Ferrieri, A. P., Robert, C. A. M., Glauser, G., Kallenbach, M., Baldwin, I. T., et al. (2013). Leaf-herbivore attack reduces carbon reserves and regrowth from the roots via jasmonate and auxin signaling. New Phytol. 200, 1234–1246. doi: 10.1111/nph.12438
Machado, R. A. R., Zhou, W., Ferrieri, A. P., Arce, C. C. M., Baldwin, I. T., Xu, S., et al. (2017). Species-specific regulation of herbivory-induced defoliation tolerance is associated with jasmonate inducibility. Ecol. Evol. 7, 3703–3712. doi: 10.1002/ece3.2953
Marmolejo, L. O., Thompson, M. N., and Helms, A. M. (2021). Defense suppression through interplant communication depends on the attacking herbivore species. J. Chem. Ecol. 47, 1049–1061. doi: 10.1007/s10886-021-01314-6
Mauck, K. E., Smyers, E., De Moraes, C. M., and Mescher, M. C. (2015). Virus infection influences host plant interactions with non-vector herbivores and predators. Funct. Ecol. 29, 662–673. doi: 10.1111/1365-2435.12371
Mbaluto, C. M., Ahmad, E. M., Mädicke, A., Grosser, K., van Dam, N. M., and Martínez-Medina, A. (2021). Induced local and systemic defense responses in tomato underlying interactions between the root-knot nematode Meloidogyne incognita and the potato aphid Macrosiphum euphorbiae. Front. Plant Sci. 12:632212. doi: 10.3389/fpls.2021.632212
Mertens, D., Fernández de Bobadilla, M., Rusman, Q., Bloem, J., Douma, J. C., and Poelman, E. H. (2021). Plant defence to sequential attack is adapted to prevalent herbivores. Nat. Plants 7, 1347–1353. doi: 10.1038/s41477-021-00999-7
Milano, N. J., Barber, N. A., and Adler, L. S. (2015). Conspecific and heterospecific aboveground herbivory both reduce preference by a belowground herbivore. Environ. Entomol. 44, 317–324. doi: 10.1093/ee/nvv003
Mondal, B., Mondal, C. K., and Mondal, P. (eds.) (2020). “Insect pests and non-insect pests of cucurbits,” in Stresses of Cucurbits: Current Status and Management. (Singapore: Springer), 47–113.
Neal, J. J. (1993). Xylem transport interruption by Anasa tristis feeding causes Cucurbita pepo to wilt. Entomol. Exp. Appl. 69, 195–200. doi: 10.1111/j.1570-7458.1993.tb01741.x
Orians, C. M., Thorn, A., and Gómez, S. (2011). Herbivore-induced resource sequestration in plants: why bother? Oecologia 167, 1–9. doi: 10.1007/s00442-011-1968-2
Papadopoulou, G. V., and van Dam, N. M. (2017). Mechanisms and ecological implications of plant-mediated interactions between belowground and aboveground insect herbivores. Ecol. Res. 32, 13–26. doi: 10.1007/s11284-016-1410-7
R Core Team (2021). R: A language and environment for statistical computing. Vienna R. Foundation for Statistical Computing.
Robert, C. A. M., Erb, M., Duployer, M., Zwahlen, C., Doyen, G. R., and Turlings, T. C. J. (2012a). Herbivore-induced plant volatiles mediate host selection by a root herbivore. New Phytol. 194, 1061–1069. doi: 10.1111/j.1469-8137.2012.04127.x
Robert, C. A. M., Erb, M., Hibbard, B. E., French, B. W., Zwahlen, C., and Turlings, T. C. J. (2012b). A specialist root herbivore reduces plant resistance and uses an induced plant volatile to aggregate in a density-dependent manner. Funct. Ecol. 26, 1429–1440. doi: 10.1111/j.1365-2435.2012.02030.x
Rojas, E. S., Batzer, J. C., Beattie, G. A., Fleischer, S. J., Shapiro, L. R., Williams, M. A., et al. (2015). Bacterial wilt of cucurbits: resurrecting a classic pathosystem. Plant Dis. 99, 564–574. doi: 10.1094/PDIS-10-14-1068-FE
Schmelz, E. A., Engelberth, J., Alborn, H. T., O’Donnell, P., Sammons, M., Toshima,, et al. (2003). Simultaneous analysis of phytohormones, phytotoxins, and volatile organic compounds in plants. Proc. Natl. Acad. Sci. U. S. A. 100, 10552–10557. doi: 10.1073/pnas.1633615100
Schmelz, E. A., Engelberth, J., Tumlinson, J. H., Block, A., and Alborn, H. T. (2004). The use of vapor phase extraction in metabolic profiling of phytohormones and other metabolites. Plant J. 39, 790–808. doi: 10.1111/j.1365-313X.2004.02168.x
Schultz, J. C., Appel, H. M., Ferrieri, A. P., and Arnold, T. M. (2013). Flexible resource allocation during plant defense responses. Front. Plant Sci. 4:324. doi: 10.3389/fpls.2013.00324
Shapiro, L. R., and Mauck, K. E. (2018). “Chemically-mediated interactions among cucurbits, insects and microbes,” in Chemical Ecology of Insects. ed. J. Tabata (Boca Raton: CRC Press), 55–90.
Soler, R., Badenes-Pérez, F. R., Broekgaarden, C., Zheng, S.-J., David, A., Boland, W., et al. (2012a). Plant-mediated facilitation between a leaf-feeding and a phloem-feeding insect in a brassicaceous plant: from insect performance to gene transcription. Funct. Ecol. 26, 156–166. doi: 10.1111/j.1365-2435.2011.01902.x
Soler, R., Harvey, J. A., Kamp, A. F., Vet, L. E., van der Putten, W. H., van Dam, N. M., et al. (2007). Root herbivores influence the behaviour of an aboveground parasitoid through changes in plant-volatile signals. Oikos 116, 367–376. doi: 10.1111/j.0030-1299.2007.15501.x
Soler, R., van der Putten, W. H., Harvey, J. A., Vet, L. E., Dicke, M., and Bezemer, T. M. (2012b). Root herbivore effects on aboveground multitrophic interactions: patterns, processes and mechanisms. J. Chem. Ecol. 38, 755–767. doi: 10.1007/s10886-012-0104-z
Stam, J. M., Kroes, A., Li, Y., Gols, R., van Loon, J., Poelman, E. H., et al. (2014). Plant interactions with multiple insect herbivores: from community to genes. Annu. Rev. Plant Biol. 65, 689–713. doi: 10.1146/annurev-arplant-050213-035937
Steinbrenner, A. D., Gómez, S., Osorio, S., Fernie, A. R., and Orians, C. M. (2011). Herbivore-induced changes in tomato (Solanum lycopersicum) primary metabolism: a whole plant perspective. J. Chem. Ecol. 37, 1294–1303. doi: 10.1007/s10886-011-0042-1
Sun, X., Siemann, E., Liu, Z., Wang, Q., Wang, D., Huang, W., et al. (2019). Root-feeding larvae increase their performance by inducing leaf volatiles that attract above-ground conspecific adults. J. Ecol. 107, 2713–2723. doi: 10.1111/1365-2745.13196
Theis, N., Barber, N. A., Gillespie, S. D., Hazzard, R. V., and Adler, L. S. (2014). Attracting mutualists and antagonists: plant trait variation explains the distribution of specialist floral herbivores and pollinators on crops and wild gourds. Am. J. Bot. 101, 1314–1322. doi: 10.3732/ajb.1400171
Thompson, M. N., Medina, R. F., Helms, A. M., and Bernal, J. S. (2022). Improving natural enemy selection in biological control through greater attention to chemical ecology and host-associated differentiation of target arthropod pests. Insects 13:160. doi: 10.3390/insects13020160
Tsunoda, T., Grosser, K., and van Dam, N. M. (2018). Locally and systemically induced glucosinolates follow optimal defence allocation theory upon root herbivory. Funct. Ecol. 32, 2127–2137. doi: 10.1111/1365-2435.13147
Turlings, T. C. J., and Erb, M. (2018). Tritrophic interactions mediated by herbivore-induced plant volatiles: mechanisms, ecological relevance, and application potential. Annu. Rev. Entomol. 63, 433–452. doi: 10.1146/annurev-ento-020117-043507
van Dam, N. M. (2009). Belowground herbivory and plant defenses. Annu. Rev. Ecol. Evol. Syst. 40, 373–391. doi: 10.1146/annurev.ecolsys.110308.120314
Vos, I. A., Verhage, A., Schuurink, R. C., Watt, L. G., Pieterse, C. M., and Van Wees, S. C. (2013). Onset of herbivore-induced resistance in systemic tissue primed for jasmonate-dependent defenses is activated by abscisic acid. Front. Plant Sci. 4:539. doi: 10.3389/fpls.2013.00539
Wan, J., Yi, J., Tao, Z., Ren, Z., Otieno, E. O., Tian, B., et al. (2022). Species-specific plant-mediated effects between herbivores converge at high damage intensity. Ecology 103:e3647. doi: 10.1002/ecy.3647
Wang, G., Hu, C., Zhou, J., Liu, Y., Cai, J., Pan, C., et al. (2019). Systemic root-shoot signaling drives jasmonate-based root defense against nematodes. Curr. Biol. 29, 3430–3438.e4. doi: 10.1016/j.cub.2019.08.049
War, A. R., Paulraj, M. G., Ahmad, T., Buhroo, A. A., Hussain, B., Ignacimuthu, S., et al. (2012). Mechanisms of plant defense against insect herbivores. Plant Signal. Behav. 7, 1306–1320. doi: 10.4161/psb.21663
Wu, J., and Baldwin, I. T. (2009). Herbivory-induced signalling in plants: perception and action. Plant Cell Environ. 32, 1161–1174. doi: 10.1111/j.1365-3040.2009.01943.x
Keywords: above-belowground interactions, insect herbivory, herbivore-induced plant volatiles, phytohormones, systemic plant defense
Citation: Thompson MN, Grunseich JM, Marmolejo LO, Aguirre NM, Bradicich PA, Behmer ST, Suh CP-C and Helms AM (2022) Undercover operation: Belowground insect herbivory modifies systemic plant defense and repels aboveground foraging insect herbivores. Front. Ecol. Evol. 10:1033730. doi: 10.3389/fevo.2022.1033730
Edited by:
Maria L. Pappas, Democritus University of Thrace, GreeceReviewed by:
Junji Takabayashi, Kyoto University, JapanJulian Chen, Institute of Plant Protection (CAAS), China
Copyright © 2022 Thompson, Grunseich, Marmolejo, Aguirre, Bradicich, Behmer, Suh and Helms. This is an open-access article distributed under the terms of the Creative Commons Attribution License (CC BY). The use, distribution or reproduction in other forums is permitted, provided the original author(s) and the copyright owner(s) are credited and that the original publication in this journal is cited, in accordance with accepted academic practice. No use, distribution or reproduction is permitted which does not comply with these terms.
*Correspondence: Anjel M. Helms, amhelms@tamu.edu