- 1Integrated Statistics Contractor for NOAA’s Northeast Fisheries Science Center, Orono, ME, United States
- 2NOAA’s Restoration Center, Gloucester, MA, United States
- 3NOAA’s Northeast Fisheries Science Center, Orono, ME, United States
- 4NOAA’s Greater Atlantic Regional Fisheries Office, Protected Resources Division, Orono, ME, United States
- 5NOAA’s Northeast Fisheries Science Center, Woods Hole, MA, United States
- 6Smithsonian Environmental Research Center, Edgewater, MD, United States
Diadromous fishes play important ecological roles by delivering ecosystem services and making crucial connections along the watersheds-ocean continuum. However, it is difficult to fully understand the community-level impacts and cumulative benefits of diadromous fish migrations, as these species are most often considered individually or in small groups. Their interactions at a community level (e.g., interdependencies such as predation, co-migration, and habitat conditioning) and the connections between their ecosystem roles and functions (e.g., cumulative marine-derived nutrient contributions, impacts on stream geomorphology) are yet to be fully understood. Similarly, freshwater, estuarine, and marine ecosystems are often considered as independent parts, limiting understanding of the importance of connections across systems. We argue that not considering the ecosystem interdependence and importance of diadromous fish as a community currently hinders the implementation of the large-scale management required to increase ecosystem resilience and fish productivity across the full range of these species. We developed a conceptual model, the Diadromous Watersheds-Ocean Continuum (DWOC), that uses ecosystem services to promote a more holistic approach to the management of the diadromous community and encourages an integrated understanding of the ecosystem connections made by these species. DWOC provides a framework for discussions that can help identify research and management needs, discuss the trade-offs of different management options, and analyze what pressing questions impede the implementation of large-scale management solutions toward a more ecosystem-based management approach.
Introduction
Today, diadromous fishes abundance levels across the majority of their range are at near all-time lows (Limburg and Waldman, 2009; Nieland et al., 2015; Waldman and Quinn, 2022). Most of our understanding of the ecological roles of these species comes from the literature on Pacific salmon spp. (Oncorhynchus spp.), partly due to their widespread cultural importance and continued existence of significant commercial and recreational fisheries (Flecker et al., 2010; Pess et al., 2014; Walsh et al., 2020). However, there is no synthesis of the ecosystem services provided by diadromous fishes, which are defined as ecosystem contributions to human wellbeing (The Common International Classification of Ecosystem Services [CICES], 2022). As the abundance of these species diminished, people lost sight of their significance, accepting today’s low abundance (Waldman, 2010; Liebich et al., 2018).
Reasons for these continuous declines are linked to large-scale environmental changes and human activities (e.g., industrial fisheries, deforestation, urbanization, agriculture, pollution, and invasive species), causing profound habitat modifications and the loss of connectivity along the watersheds-ocean continuum (Hutchings and Reynolds, 2004; Hall et al., 2011; Hare et al., 2016; Crozier et al., 2019; Tickner et al., 2020). While indigenous communities modified landscapes, the land-use changes accelerated with colonization and became more extensive during the industrial revolution (Foster et al., 2003). Like in many places, the Northeast U.S. watersheds and waterways were transformed dramatically, with consequences like increased runoff conditions and sediment erosion and deposition rates (Happ et al., 1940; Hooke, 2000; Wilkinson, 2005; Kemp et al., 2020). Over the same period, dam construction became widespread (Walter and Merritts, 2008; Johnson et al., 2019). While these transformations were omnipresent, habitat responses were localized due to differences in glaciation history, topography, hydrography, and surface geology. Intensive reforestation since the late 1800s has dramatically changed land cover again, and many areas of the region are now heavily forested (Steyaert and Knox, 2008; Ramankutty et al., 2010; Thompson et al., 2013). Changes in land cover continue to impact runoff conditions with implications for channel forms and processes (Magilligan et al., 2008; Armstrong et al., 2014). Additional dam construction has modified hydrology and sediment transport (Magilligan et al., 2008; Zhang et al., 2016), as has urbanization and industrial agriculture (Leopold et al., 2005; Steyaert and Knox, 2008). Finally, changing climate has altered hydrology and sediment transport conditions, especially since the latter decades of the twentieth century (McCabe and Wolock, 2002; Hodgkins and Dudley, 2006; Rice and Hirsch, 2012; Cook et al., 2015). The magnitude and frequency of floods have increased- especially channel-forming flows impacting both river channels and diadromous fish habitats (Collins, 2009; Armstrong et al., 2012, 2014). Changes in river flood seasonality have also influenced migratory and resident fish populations (Collins, 2019).
Changes in marine and estuarine environments also impact diadromous species (Mattocks et al., 2017; Thorstad et al., 2021; Verhelst et al., 2021). Scientific understanding of these historical changes has improved in the last decade, but management actions to implement mitigation strategies remain elusive. Sea surface temperatures are warming mainly due to climate change, impacting ocean salinity and acidity (McCormick and Regish, 2018; Chen et al., 2020). Climate indices such as the North Atlantic Oscillation and the Atlantic Multidecadal Oscillation have shifted in amplitude or cyclicity (Greene et al., 2013; Drinkwater et al., 2014; Alexander et al., 2020). These changes impacted ocean productivity (Capotondi et al., 2012; Alexander et al., 2018), resulting in ecosystem shifts of predator and prey species of diadromous fishes and food webs in general (Lynch et al., 2015; Tommasi et al., 2015). The literature is scarcer for estuaries, especially in the context of diadromous fishes, as estuaries were primarily perceived as migratory corridors. However, estuaries are dynamic transition zones between freshwater and marine ecosystems where changing upstream conditions (flow, water temperature, and sediment) interact with changing downstream conditions (salinity, acidity, temperature, and current; Greene et al., 2005; Ray, 2005). Studies now indicate that diadromous fishes might use estuaries more than initially hypothesized (Stich et al., 2015; Stevens et al., 2021). These observations reinforce the urgency of improving our understanding of changing estuarine ecosystems, fish use, and their potential as emerging migration barriers (e.g., oxygen depletion zone, low-flow; Tétard et al., 2016; Malone and Newton, 2020).
Typically, diadromous species are considered individually or in small groups (e.g., river herrings). Their interactions at a community level (e.g., interdependencies such as predation, co-migration, habitat conditioning), connections between their ecosystem roles and functions (e.g., cumulative marine-derived nutrients contributions, impacts on stream geomorphology), and the overall spatio-temporal variability of these interactions are often ignored and understudied (McDowall et al., 2009; Levin et al., 2018). The bulk of evidence suggests that at historic abundance levels, the diadromous species community functioned in a complementary way that impacted the structure, function, and productivity of coastal watersheds (rivers and lakes), estuarine, marine, terrestrial, and aerial ecosystems (Saunders et al., 2006; Call, 2015; Mattocks et al., 2017; Zydlewski et al., 2021; Lopez et al., 2022). Many temperate watersheds host multiple diadromous species, sometimes over a dozen, with complex and varied life histories both within and between species. These complex and varied dynamics are intrinsically linked to the seasonal abundance of diadromous fishes, their migration pattern and timing, and the mosaic of habitats they used along what we describe as the diadromous watersheds-ocean continuum. The single species assessment and research approach to diadromous fishes explains why our understanding of these species’ ecosystem connections and services is poor. Moreover, the impacts of various human activities are derived from isolated studies and often do not reflect the scale needed to comprehend how these species interact along the watersheds-ocean continuum. This lack of comprehensive assessment limits our understanding of their role in connecting the various ecosystems that they inhabit, thereby preventing the management of the diadromous community as a whole. The fact that the community is not assessed as a whole, nor over the different ecosystems they use, is an obstacle that prevents implementing large-scale solutions as part of an ecosystem-based management approach (EBM). Managing diadromous fishes as a community is necessary to account for species interactions (e.g., habitat conditioning, co-migration, and predatory interactions) that may affect overall species productivity.
Ecosystems are often considered as independent units. While this setting can work for species inhabiting only one ecosystem, it is problematic from a migratory fish perspective and leads to underestimating the importance of the interactions between each ecosystem via the inherent connections that diadromous fishes make. While we recognize that accounting for the ecosystem connections can be a complex task, not focusing on the entire life cycle of these species and ignoring the interdependencies of the freshwater, estuarine, marine, terrestrial, and aerial ecosystems not only limit our understanding of these connections but also limits management actions (Mathewson et al., 2003; Tonra et al., 2016; Verhelst et al., 2021). This separation prevents the integrated assessment of the threats and implementation of solutions at scales larger than what is typically acted upon (i.e., across the distribution range of these migratory species). Management actions are, therefore, primarily targeted at freshwater systems and often limited to fisheries management within marine and estuarine environments (e.g., fishing regulation and bycatch limits in offshore fisheries). In freshwater, dam removal is often portrayed as the primary solution as it restores connectivity. However, a whole host of other management actions, such as the restoration of spawning and nursery habitat, pollution abatement, remediation, and fish passage improvement from the perspective of a single or suite of species are also employed (Hall et al., 2012; Lake et al., 2012; Hershey, 2021; Verhelst et al., 2021; Waldman and Quinn, 2022). A more integrated assessment of these actions and how they can be implemented in parallel (e.g., upstream pollution abatement while waiting on dam removal) can avoid implementing actions that directly impact single species and a small sub-section of the life cycle. The lack of this more integrated and holistic assessment and management actions for the diadromous community is perhaps due to the perception that EBM is complicated (e.g., Link and Browman, 2014), especially since we do not have a complete understanding of the interconnectedness of the productivity of these species or the connections with the ecosystems they inhabit.
Given this holistic disconnect, governance often remains ecosystem- and species-specific, resulting in (1) a poor assessment of the critical research gaps regarding diadromous life stages outside freshwater systems (Mcdowall, 1999; Greene K.E. et al., 2009; McDowall et al., 2009), (2) potential misalignment of research and management needs (Miles et al., 2014; Bisbal, 2022), and (3) an overall lack of coordination between different management actions and entities (Munsch et al., 2020). Thus, coordinated approaches designed to achieve results at a large-scale (i.e., over entire rivers, regions, or species distribution ranges) are very rare and often fail to adequately increase productivity for a suite of species, prompting calls to transition from ad hoc conservation and restoration efforts to a strategic approach to achieve far larger-scale results (De Eyto et al., 2016; Tickner et al., 2020). Finally, the need to show rapid results (e.g., within funding allocation and elected office timeframe) can also steer management away from ecosystem-scale projects, as it generally takes more time to observe changes at that scale. All these reasons explain why scientists and managers still struggle to some extent to implement large-scale management approaches such as EBM (DeFries and Nagendra, 2017; Link and Browman, 2017; Levin et al., 2018). This highlights the need for a tool that can guide such integrative approaches across species and ecosystems, while recognizing the ramifications of different management actions to avoid partitioned information and action plans.
To truly safeguard the future of the diadromous species and protect the ecosystems they inhabit, we propose a more explicit, dedicated, large-scale, and holistic management tool that can be used at different steps of elaborating integrated ecosystem management that paired with proven effective action, while also considering complex drivers of change (Kritzer et al., 2022). We present a conceptual model, the Diadromous Watersheds-Ocean Continuum (DWOC), to help management to implement incremental steps toward an EBM approach. Our model focuses on the spatio-temporal variability and diversity of ecosystem services that the diadromous community provides and its role in connecting and influencing various ecosystems. The watersheds-ocean continuum reflects that these migratory species are not bound to a single ecosystem and use a mosaic of habitats along this continuum. Therefore, coordinated research and management actions across their distribution range are needed. DWOC is a qualitative model that examines species and ecosystem connections through the lens of ecosystem services to encourage a more integrated understanding of the benefits that can be realized from a fully functioning diadromous community. Viewing these connections and dynamics through the lens of the ecosystem services provided also helps think about ecosystems more holistically by focusing on their functions and what, where, and when ecosystem services are necessary to support these functions. Before introducing our conceptual model, we provide a synthesis of the literature on the diadromous community and the ecological services they provide for the Northwest Atlantic region. We then discuss our conceptual model and provide an inventory of the overarching research and management needs as highlighted while synthesizing the information supporting DWOC. We finally provide a roadmap to move toward EBM and recognize the need to account for the roles of diadromous fishes when managing for ecosystem resilience. We explore DWOC within the Northwest Atlantic, where diadromous community productivity within a region where abundance and connections are lost; however, the pathways described toward increasing ecosystem resilience and diadromous community productivity can be applied broadly to other regions where diadromous fishes occur.
Diadromous community ecosystem services
This section first synthesizes the ecosystem services provided by the diadromous community as this is key to our conceptual model and provides a basis for identifying what research gaps may impede current management efforts. Diadromous fishes shaped many economic and socio-cultural activities by delivering a broad spectrum of ecosystem services (Table 1) across various ecosystems at specific times of the year. Indigenous communities and early colonizers mention diadromous runs of millions of fishes, enough to make rivers look silver or give the impression that one could cross rivers walking on fish (reviewed in Waldman, 2013). While the scale is poorly understood, there was widespread pre-colonial use of diadromous fishes (Goode, 1880; Denny and Fanning, 2016). These fish remain cultural keystones for many indigenous communities for at least 12,000 years (Atlas et al., 2021; Carothers et al., 2021). Atlantic salmon (Salmo salar), American shad (Alosa sapidissima), and river herrings were used by indigenous communities of the Atlantic coast (food, goods, fertilizer; Goode, 1880; Bassett, 2015; Denny and Fanning, 2016). While less documented by western science, American eels (Anguilla rostrata), sturgeons (Atlantic, Acipenser oxyrinchus oxyrinchus, and shortnose, Acipenser brevirostrum), and sea lamprey (Petromyzon marinus) likely played important societal and cultural roles (Beaty, 2014; Gribbin, 2015). While current abundance is low, these species remain culturally important and part of a long heritage for indigenous and non-indigenous peoples (Bassett, 2015; Drouineau et al., 2018a; Liebich et al., 2018).
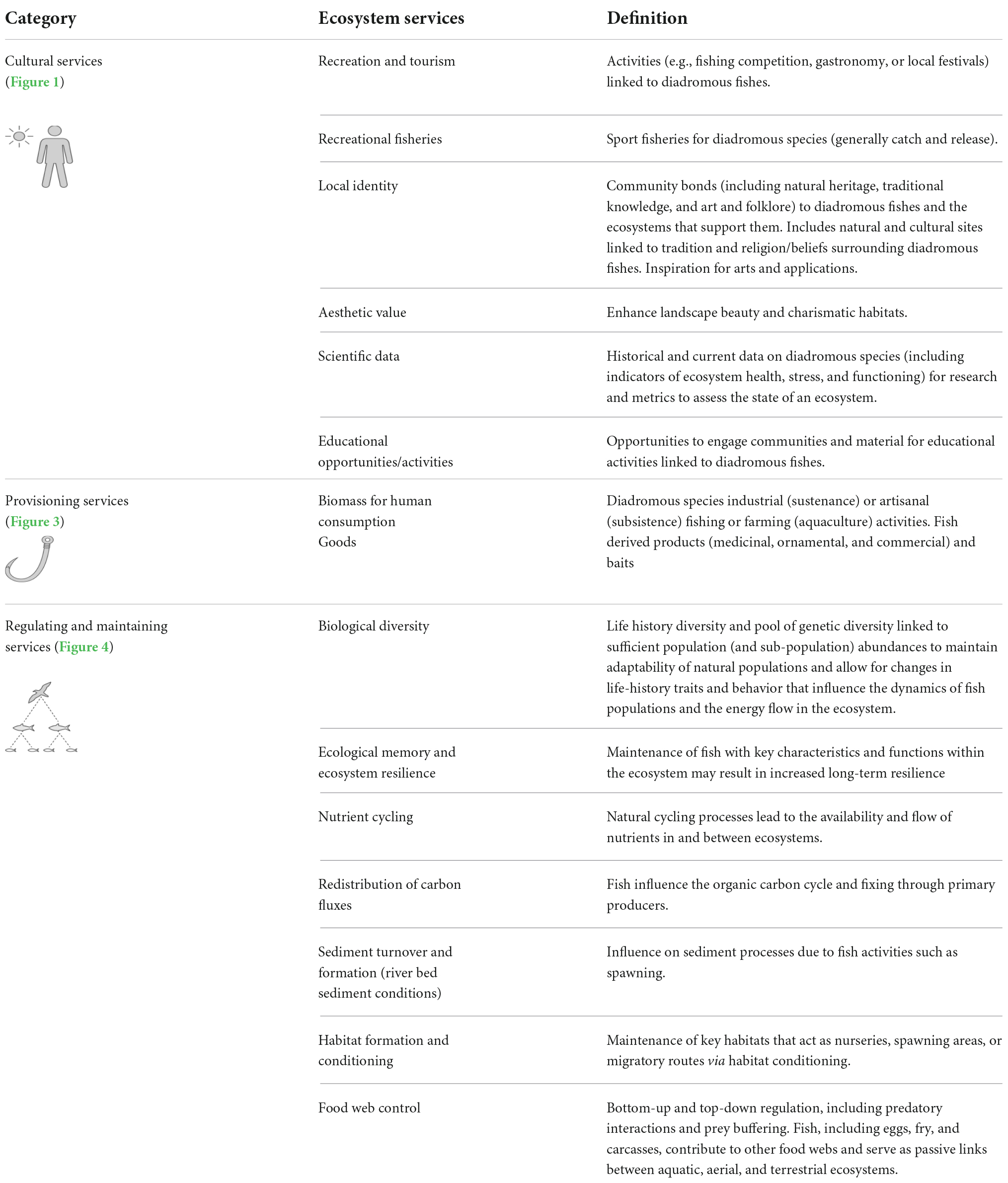
Table 1. Categorized list of ecosystem services linked to diadromous fishes and their definitions. Note that some for some ecosystem services, these are shortened versions of the CICES names.
Aside from a few ecosystem services (e.g., consumption and marine-derived nutrients), we do not have a good inventory or understanding of the full suite of ecosystem services the diadromous species provides, and more knowledge is needed. Given the low abundance of the diadromous community (Limburg and Waldman, 2009), it is difficult to decouple shifting baselines and knowledge gaps to evaluate the true ecological value of each service. Still, using ecosystem services to evaluate the diadromous community’s importance will help clarify the trade-offs among various human activities, which is central to an EBM approach (Dolan et al., 2016; Levin et al., 2018; Hare et al., 2021).
Diadromous watersheds-ocean continuum aids in visualizing the spatiotemporal nature of specific ecosystem services, which benefits can be tangible, e.g., increased fish biomass, or intangible, e.g., redistribution of carbon fluxes provided by fish species or groups of species (Table 1). They can be direct, e.g., predation on one species by another, or indirect, e.g., activities that increase stream primary production and ultimately affect species productivity (Table 1). Ecosystem services help synthesize available information, considering trade-offs between different management options and assessing the ecological and economic value of services provided by fish under present and future climates (DiadES, 2019; Hare et al., 2021). We use the Common International Classification for Ecosystem Services (CICES) to describe the three main categories: cultural, provisioning, and regulating and maintenance (Table 1). For this effort, we integrate the CICES list of aquatic ecosystem services to view them as direct humans and broader ecosystem benefits to support ecosystem resilience. Considering these services along the watersheds-ocean continuum provides a solid foundation for developing EBM. Ecosystem services provide a common language to help summarize the impacts of multiple species. They improve communication and provide a transparent method for assessing trade-offs associated with management alternatives and priorities (Granek et al., 2009). They also provide a systematic way to incorporate biogeophysical and socioeconomic information that is key to EBM (Hare et al., 2019; Langhans et al., 2019). Using an ecosystem services approach instead of a fish species focus allows for a more integrative evaluation of functions that need to be restored to increase fish species productivity and ecosystems’ resilience under changing conditions. The following subsections provide details on each service listed in Table 1.
Cultural services
Cultural services (Figure 1 and Table 1) are defined as the non-material benefits that people obtain from ecosystems and are challenging to integrate into management frameworks as they are often perceived as intangible and incorporate some level of subjectivity (Daniel et al., 2012). However, cultural services are a strong incentive for people to support environmental conservation, and there are more efforts to better integrate them into management frameworks (Phillips, 1998; Schaich et al., 2010; Hendee and Flint, 2014).
Recreation, tourism, and recreational fisheries
Numerous recreational activities are oriented around diadromous fishes in the Northwest Atlantic (Figures 1A,B). Striped bass (Morone saxatilis) is one of the most important recreational fisheries from Florida to Maine, with harvest averaging 3.2 million fish per year (Atlantic States Marine Fisheries Commission [ASMFC], 2017a). American shad harvest occurs over most of its range and can be significant, with landings since 2016 being less than a quarter of a million pounds (Atlantic States Marine Fisheries Commission [ASMFC], 2020). A small recreational harvest of alewife (Alosa pseudoharengus) and blueback herring (Alosa aestivalis) is currently occurring in Maine, New York, and North Carolina (Atlantic States Marine Fisheries Commission [ASMFC], 2017c), as well as Rainbow smelt (Osmerus mordax) in New Hampshire and Maine (Enterline et al., 2012). Aside from fisheries, many diadromous species remain a living part of recreational and tourist activities. Many species hold special status within communities throughout their range to the extent that different festivals and species days are organized to honor these connections (e.g., Shadfest in Pennsylvania—Figure 1A, Gifton Shad Festival Gifton, Connecticut; Alewife Festival Damariscotta, Maine; Rainbow smelt festival Columbia Falls, Maine). Although it is difficult to evaluate the direct and indirect benefits of some of these activities, their prevalence throughout the range demonstrates the importance of these species to the cultural life of the Northeast Atlantic Coast.
Local identity and aesthetic value
Local identity is driven by an attachment to a place and results in people defining themselves in terms related to that specific place. Local identity may have deep cultural roots, but a place might represent different identities for different people. A wide array of literature suggests that physical attributes and social and psychological experiences help individuals develop a local identity (Stedman, 2002; Proshansky et al., 1983; Liebich et al., 2018).
Perhaps the most obvious example of local identity is the deep cultural connections among native, indigenous peoples and diadromous fishes. Relationships among many indigenous human communities, their food, and their homelands are deeply linked culturally, physically, emotionally, psychologically, and spiritually (Lynn et al., 2013). In a recent case study, Daigle et al. (2019) describe how Wabanaki stories involving the creation of the Penobscot River and people’s connection to the river and all its inhabitants may inform modern tribal members’ ethics and values. The authors noted: “These teachings reinforce the responsibility to foster quality of life for the next seven generations of humans and for all relations and are grounded in intergenerational experiences and observations of the environment.” These strong cultural links can also be seen in the names of landscape features. For instance, in Maine, many stream names are in Wabanaki, such as the Mattamiscontis stream, which translates as “alewife stream,” and the Kenduskeag river, which means “eel weir place” (Rutherford, 1970).
Despite decreased abundance of diadromous fishes across their range, many cities and towns still use diadromous fish as branding for their communities, a cultural heritage, to promote tourism, education, and awareness-raising (Figures 1C,E1,E2). Diadromous fishes may also provide added aesthetic value to rivers. For instance, fish community composition can alter specific rivers’ aesthetic value and importance (Leopold, 1969). Aesthetic values of various fishes can also influence the attention given to a particular species (Tribot et al., 2018), as species that are perceived as beautiful (e.g., Atlantic salmon, Striped bass) receive more attention than less attractive species (e.g., sea lamprey).
These social connections might seem trivial, but they may be key in overcoming the shifting baseline syndromes. Pauly (1995) described the danger of gradual accommodation of the creeping disappearance of resource species and reliance on inappropriate reference points for evaluating economic losses. Diadromous fish can connect the local to the global and engage the public to support management, restoration, and conservation efforts. Although some of these social connections are fading, it is possible to reawaken the social connections between fish and people through educational activities and fostering shared responsibility among constituencies to advocate for diadromous fish ecosystems (Waldman, 2010). Additionally, approaches such as Two-eyed Seeing that align indigenous, traditional, and western knowledge would maintain and grow social connections by providing a pathway to knowledge co-production for fish stewardship and management (Atlas et al., 2021; Reid et al., 2021). Such approaches are envisioned to be applied within local communities to reinforce people’s sense of responsibility toward natural resources and prevent ecosocial anomie (i.e., at low abundance, species lose both relevance to society and people, further hastening their decline; Limburg and Waldman, 2009; Vierros et al., 2020).
Scientific data and educational opportunities/activities
Between 2010 and 2020, 55,277 studies were published on diadromous fishes worldwide, with nearly 50% being on salmonidae spp., and 32% of the published literature (17,629) came from the U.S. (for keywords, see Supplementary Table 1 Web of Science search, period 2010–2020; Figure 2). Of the 4,461 publications addressing diadromous fishes topics on the east coast of the U.S., approximately half of them focused on Atlantic salmon in Maine, showing the effort given to this species alone. The search confirms the interest and scientific focus on the diadromous community (Figure 1D) and the strong focus on specific regions and species. However, the species and spatial disparities highlight the potential benefits of addressing diadromous fishes as a community so that all species across their entire range are considered.
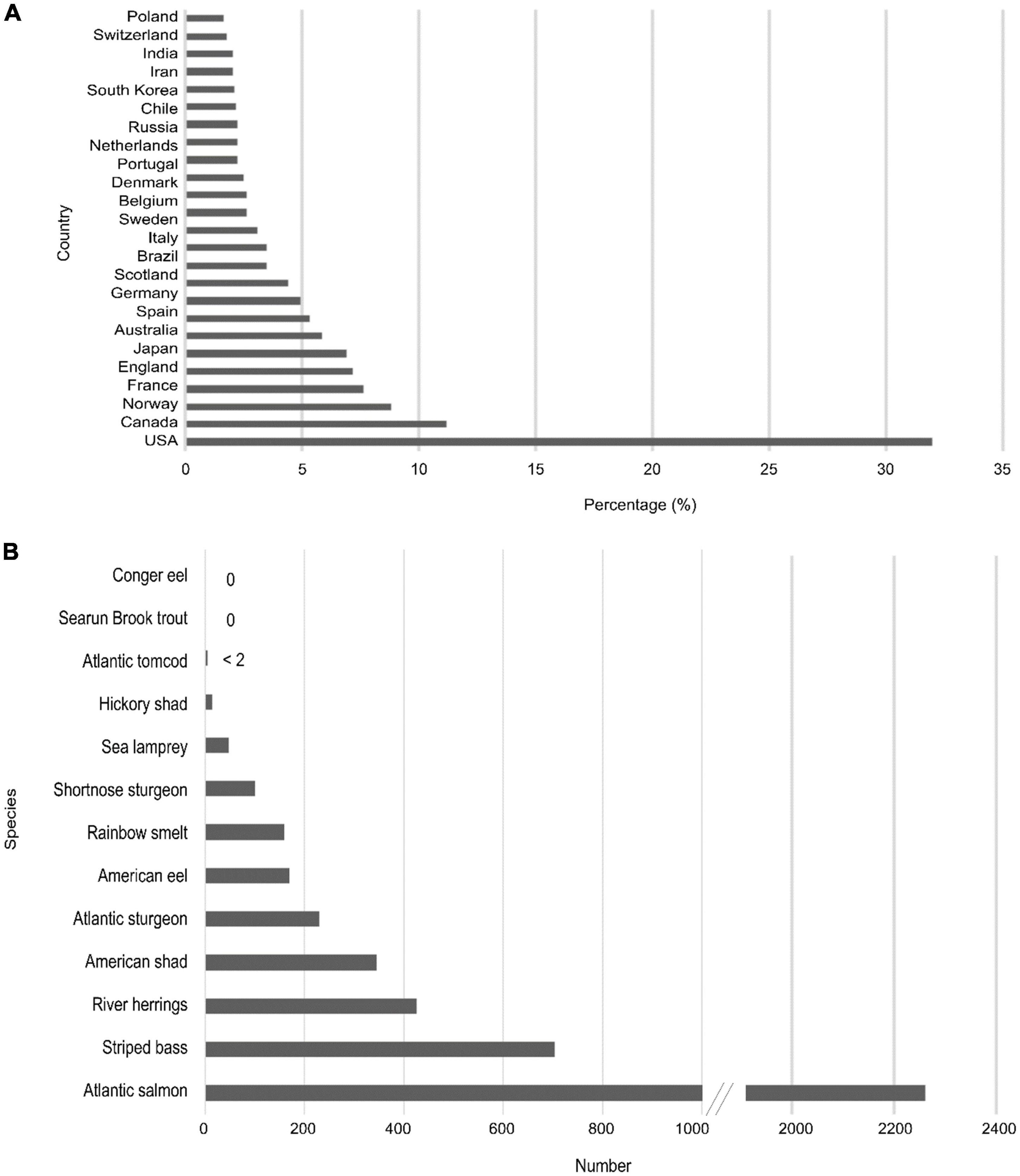
Figure 2. Web of Science data for publications involving diadromous species between 2010 and 2020 showing (A) percentage of published literature per country and (B) the number of publications by species for the East Coast U.S.
The suite of diadromous species across the east coast of the U.S. inhabit vast areas and varying habitats and undergo inspirational migrations that take them from freshwater to coastal to high seas environments. These amazing and fascinating life histories provide opportunities for enhanced educational value (Figures 1E1,E2) by facilitating the discussion of conservation and restoration in a broader spatial context by showing how distant ecosystems are connected. Examples of scientific communication efforts include children’s education from kindergarten through high school with programs such as Salmon and Shad in-Schools and Fish Friends (e.g., ASF, 2021; AWS, 2021; The Connecticut River Salmon Association [CRSA], 2021). These programs allow children to raise fish in class and learn about their life cycle, habitat restoration, and conservation while following a pre-developed curriculum. For the general public, products like the alewife trail map produced by Maine Rivers identifies locations where people can observe river herrings during their spring run and provides information about fish migration and restoration activities (Maine Rivers [MR], 2021). Thousands of people each year venture to local waterways to observe these migrations. In many cases, additional educational opportunities developed around these events to further educate the public about the current status and dynamics of these fishes. Another powerful education tool is the Stream Smart Table used throughout New-England (U.S.), which is a hands-on activity that mimics the dynamics of water flow within a model watershed where participants modify road crossings and other structures and see firsthand the changes to the stream channel and impact to fish migration (Figure 1E2). These are but a few examples of the available educational opportunities associated with diadromous fishes.
Provisioning services
Provisioning services are benefits that humans typically extract from nature (The Common International Classification of Ecosystem Services [CICES], 2022). These benefits are typically related to the food and good that these species can provide (Tables 1, 2 and Figure 3).
Provision of biomass for human consumption
Prior to the widespread collapse of multiple populations between the mid to late 1800s (Limburg and Waldman, 2009; Hall et al., 2012), diadromous fishes were a significant resource for human consumption and use (Figure 3 and Table 2). Table 2 shows that commercial diadromous fisheries in the U.S. began with annual landings in the late 1800s and early 1900s, yielding millions of pounds collectively for species like river herrings (Atlantic States Marine Fisheries Commission [ASMFC], 2017c), American shad (Atlantic States Marine Fisheries Commission [ASMFC], 2020), American eel (Atlantic States Marine Fisheries Commission [ASMFC], 2017a), Atlantic salmon (Saunders et al., 2006), Atlantic and shortnose sturgeons (Atlantic States Marine Fisheries Commission [ASMFC], 2017b; National Oceanic and Atmospheric Administration [NOAA], 2021b), and Rainbow smelt (Enterline et al., 2012). Among the species still being harvested for human consumption, Striped bass has the highest coastwide commercial landings, with an average of 4.7 million pounds since 2015 (Atlantic States Marine Fisheries Commission [ASMFC], 2019). Alewife (average of 1,354,463 pounds from the mid-1990s–2015; Figure 3B), blueback herring (average of 1,354,463 pounds from the mid-1990s to 2015; Figure 3B; Atlantic States Marine Fisheries Commission [ASMFC], 2017c; National Marine Fisheries Service [NMFS], 2019), and American shad (average of 493,835 pounds of bycatch harvested between 2007 and 2018; Atlantic States Marine Fisheries Commission [ASMFC], 2019) are also still harvested (Table 2). A modest commercial harvest of Rainbow smelt continues in Downeast Maine, primarily centered on the Pleasant River in Columbia Falls, where gill and bag nets are used in late winter (Enterline et al., 2012). American eels also continue to be harvested in some areas, although the significant downward trends in some survey areas have been noted as a cause for concern (Atlantic States Marine Fisheries Commission [ASMFC], 2017a). Given these species’ reduced harvest and abundance, many are being managed to restore stock abundance (Atlantic States Marine Fisheries Commission [ASMFC], 2017a,b,c, 2019; Shepherd et al., 2018). Many indigenous communities can engage in traditional hunting and fishing activities whether or not they choose to exert these rights. Today, most species cannot support commercial fisheries, with annual landings being only a small fraction of historical levels (e.g., Striped bass, Atlantic States Marine Fisheries Commission [ASMFC], 2019; National Oceanic and Atmospheric Administration [NOAA], 2021a) or have federal designations as threatened or endangered (e.g., Atlantic salmon, Atlantic sturgeon, shortnose sturgeon) under the Endangered Species Act (National Oceanic and Atmospheric Administration [NOAA], 2022). For most species, the decline in abundance occurred in parallel with a shift in their distribution (either shifting north or range reduction), especially for species like Atlantic salmon, shortnose sturgeon, and Rainbow smelt. For anadromous Brook trout (Salvelinus fontinalis), Atlantic tomcod (Microgadus tomcod), and Hickory shad (Alosa mediocris), data is insufficient (regional distribution and quality) to compare current and historical abundance (Fried and Schultz, 2006; Dauwalter et al., 2014; Rulifson and Batsavage, 2014; Table 2). For American conger eel (Conger oceanicus) and sea lamprey, even less data is available to attempt abundance and distribution work (Kynard and Horgan, 2019).
Goods
Fishes are used in an extensive range of manufacturing (e.g., fish glue and food packaging), industrial (e.g., absorbents and lubricants), technological (e.g., nanogenerators and pollutant treatment), and health care (e.g., toothpaste and burn wound treatment) related goods (Olden et al., 2020). Salmonids such as Atlantic salmon were widely used to produce clothing and associated goods historically produced from wild-caught specimens (Hatt and Taylor, 1969). Nowadays, they are mainly locally produced and originate from salmon reared within the commercial aquaculture industry (Grey et al., 2006; Olden et al., 2020). Historically, fish leather products were a significant import in the U.S., with an average of 725,000 fish-leather products worth over US$ 6 million imported annually in the early 2000s. In addition, sea lamprey is valuable for biological and medical research on neurological and spinal cord regeneration, locomotion, eyes, kidneys, blood research, and hormones (Kircheis, 2004). Harvests of diadromous fish for bait continue to be an important use. Adult alewives are preferred bait for Striped bass and lobster fisheries (National Marine Fisheries Service [NMFS], 2019), although there is no commercial distinction between alewife and blueback herring (Atlantic States Marine Fisheries Commission [ASMFC], 2017c). Estimated numbers of fish used as bait are not available, although reports suggest this is a significant part of the river herring harvests (Atlantic States Marine Fisheries Commission [ASMFC], 2017c).
Regulating and maintaining services
Regulating and maintaining services support the ecosystem’s functioning and productivity. They describe how diadromous fishes mediate or moderate their habitat (The Common International Classification of Ecosystem Services [CICES], 2022; Table 1 and Figure 4) and are linked to ecosystem resilience.
Biological diversity, ecological memory, and ecosystem resilience
Some studies highlighted diadromous fishes’ influence on biological diversity (Figure 4A). Atlantic salmon and sea lamprey have been shown to influence macroinvertebrate diversity and biomass via marine-derived nutrients (MDN) imports (see section “Nutrient cycling”; Guyette et al., 2014; Weaver et al., 2017, Weaver et al., 2018a). Diadromous species have also been shown to influence marine, estuarine, terrestrial, and areal ecosystems biodiversity, as they serve as prey for many marine and terrestrial mammals, marine and freshwater fishes, and birds (see Food web control section; Blackwell and Krohn, 1997; Dias et al., 2019). The literature on these services on East Coast populations is relatively recent and possibly limited due to the low abundance. Further quantification is needed to better understand the influence of the diadromous community on biodiversity across these ecosystems to accurately inform management strategies and decisions.
Diadromous fishes populations have a rich genetic diversity and display a wide range of life-history traits (Rieman and Dunham, 2000; Morris et al., 2003; Lage and Kornfield, 2006; Turner and Limburg, 2016; Stevens et al., 2021). This diversity contributes to biodiversity and ecosystem resilience through within and across species portfolio effects (Petitgas et al., 2010; Schindler et al., 2015; Secor et al., 2020). Genetic diversity can be important in allowing populations to face changing environmental conditions and adapt to climate changes (Crozier et al., 2008; Pess et al., 2014; Kocik et al., 2022). Ecological memory (i.e., the network of species and their dynamics that makes reorganization after disturbance possible) is a crucial component of ecological resilience and, coupled with the rich genetic diversity, is key to the adaptive capacity of the ecosystems they inhabit in the future.
Nutrient cycling
The impacts of MDN importation (Figure 4B) by diadromous species have primarily been characterized for Pacific salmon (Wipfli, 1997; Helfield and Naiman, 2003; Chaloner et al., 2004; Darimont et al., 2008; Lessard et al., 2009; Taylor et al., 2010; Schindler et al., 2013; Mattocks, 2016). The disparity between the Pacific and Atlantic knowledge base may be partially explained by the lower contemporary biomass estimates and/or the higher iteroparity of East coast diadromous populations. Northwestern Atlantic freshwater ecosystems are generally nutrient-poor, and there is relatively little information describing MDN dynamics for these systems. However, recent field studies have demonstrated that when rivers are enriched with MDN from diadromous fishes, the impacts include: changes in biofilm standing stock and productivity (Walters et al., 2009; Samways et al., 2015; Weaver et al., 2017), increased macroinvertebrates composition and abundance (Samways and Cunjak, 2015; Samways et al., 2017; Weaver et al., 2018a), and increased juvenile fish growth (Guyette et al., 2013; Weaver et al., 2016, 2018b; Samways et al., 2017). MDN have also been shown to directly (consumption of diadromous fishes) and indirectly (consumption of prey that consumed diadromous fishes) benefit various avian species (Dalton et al., 2009; Call, 2015). Similarly, MDN transfers have also been observed in fish species that prey on diadromous fishes (Macavoy et al., 2009) and marine mammals (Tonra et al., 2016; Dias et al., 2019). MDN can also be transferred to terrestrial ecosystems, impacting vegetation and insects (Mathewson et al., 2003; Lessard et al., 2009; Lopez et al., 2022). Recent modeling exercises have highlighted the significant MDN contributions that species such as American shad and river herrings could have if they were at historical abundance (Dias et al., 2019; Zydlewski et al., 2021). These nutrients transferred through the food web are hypothesized to translate into fish and birds increased reproductive success (Zhang et al., 2003; Tonra et al., 2015, 2016).
It is essential to better understand the importance of MDN for the ecosystems’ productivity and functioning for different species at comparable and varying biomasses. To do so, we need to know what we are missing regarding MDN (e.g., Zydlewski et al., 2021) and what impacts these services have on the systems’ productivity. For instance, models show that Alewives once supplied the majority of nitrogen and phosphorus to coastal lakes through their carcasses and excretion but now supply only 6–7% where runs remain (Twining et al., 2013). It has also been estimated that American Shad would contribute approximately 98,000 metric tons of MDN annually to U.S. East Coast rivers if these rivers were undammed, an input reduced by more than 33% given passage barriers (Zydlewski et al., 2021). Few studies have highlighted the role that fish migration may play in exporting nutrients from estuaries to coastal marine ecosystems (Deegan, 1993; Dame and Allen, 1996), and the implications have not been quantified (Petticrew et al., 2011). It has been hypothesized that smolts could export more nutrients than their parents import to freshwater ecosystems at low spawning densities (e.g., Moore and Schindler, 2004). These research gaps limit our understanding of the overall benefit of nutrient transfers across ecosystems.
Redistribution of carbon fluxes
Carbon fixation increases with high nutrient inputs and high primary production. Increased carbon fixation influences net carbon diffusion from the water to the atmosphere (Wetzel, 2001), and may influence the global carbon budget and help influence the climate change trajectory. Schindler et al. (1997) showed that the structure of fish communities could regulate the carbon-fixing capacity of nutrient-rich lakes, indirectly mediating the carbon flux between lakes and the atmosphere. By importing MDN in freshwater ecosystems (e.g., large biomass imports into lakes and ponds from spawning river herring populations), diadromous fishes could impact the net carbon flux at the interface of water and the atmosphere. This relationship has not been studied, but evaluating diadromous fishes’ specific contributions will help quantify their influence on carbon cycling (Li et al., 2017).
Sediments turnover and formation
A rich literature focuses on how Pacific salmon’s spawning activities influence sediment transport rates and channel stability (Figures 4C1,C2; Montgomery et al., 1996; Buffington et al., 2004; Gottesfeld et al., 2004; Buxton, 2018). Their spawning activity alters their and other aquatic species habitat (Gottesfeld et al., 2004; Fremier et al., 2018). Recent studies have shown that Pacific salmon spawning activity profoundly influenced the stream bed longitudinal profiles, channel erosion processes, and likely the evolution of entire watersheds (Buxton, 2018; Fremier et al., 2018). Similar observations have been made for Atlantic salmon and sea lamprey, which also displace and resuspend sediments during spawning (Figures 4C1,C2; Guyette et al., 2014; Samways and Cunjak, 2015), resulting in altered physical stream habitat, sediment transport, and increasing streambed complexity (Hogg et al., 2014; Weaver et al., 2018a). However, the potential for physical habitat impacts of other diadromous fishes has been neglected. Considering that these dynamics may exert fundamental influences on other organism habitats (see section “Habitat formation and conditioning”), it is essential to improve our understanding to help understand how sediment turnover by different species may influence evolution of various riverine habitat processes.
Habitat formation and conditioning
Fish-mediated habitat alterations have been shown to have associated biological consequences, and Atlantic salmon, and sea lamprey spawning activities have altered habitat, which has been correlated with higher biomass and more diverse macroinvertebrates as the loosened substrate provides improved microhabitat for many aquatic species (Figures 4D1,D2, Kircheis, 2004; Hogg et al., 2014). Substrate compactness may be reduced by mobilizing impacted silts and fine sediments (Saunders et al., 2006), which results in an improved flow of water and oxygen within the nest and increased microhabitats that can be used by salmonid fry and parr (Kircheis, 2004). Nesting areas become attractive for local species such as common shiners (Luxilus cornutus), fallfish (Semotilus corporalis), Atlantic salmon, and Brook trout, which have been shown to re-use these sea lamprey nests for their own spawning (Kircheis, 2004). Kircheis (2004) hypothesized that in rivers severely impacted with silts and fine sediments, lamprey could help recover native fish populations.
Migratory fishes can also serve a critical role for other species in completing their life cycle. The freshwater mussels (Margaritifera spp.) are widely distributed in Europe and the Northwestern Atlantic and are at risk of global extinction due to pollution and the lack of viable fish hosts (Galbraith et al., 2018; Kreeger et al., 2018). The larvae encysted on the gills of their host fish (e.g., American eel), relying on their host’s movement for dispersion while they grow into free-living juvenile mussels (Galbraith et al., 2018). While the role of freshwater mussels and the interactions with their hosts is well studied in Europe, this relationship and the ecosystem benefits (filtration and pollutant uptake in freshwater ecosystems and fish habitat conditioning) are understudied (Kreeger et al., 2018) in North America. Freshwater mussel restoration programs seek to rebuild the interactions with hosts, such as American eel. They are working to quantify the ecosystem services provided by freshwater mussels, such as filtration and habitat conditions to identify research gaps regarding the connections between freshwater mussels, their hosts, and the overall ecosystem benefits (Kreeger et al., 2018).
Food web control
Diadromous fishes directly impact food webs through various predator-prey interactions (Figures 4E1,E2, Montevecchi, 2007; Wippelhauser, 2021). The diadromous community supports food webs in all ecosystems via predatory interactions where diadromous fishes are prey to marine and terrestrial mammals (e.g., whales, seals, and bears), marine and freshwater fishes (e.g., cod, bluefin tuna, pickerel, and yellow perch) including other diadromous species (e.g., Striped bass and Atlantic salmon) and birds (e.g., osprey, bald eagle, and double-crested cormorant) (Juanes et al., 1993; Grout, 2006; Blackwell et al., 2018; Boulêtreau et al., 2020).
The most documented interactions between diadromous species are Striped bass foraging on Atlantic salmon smolts (Heimbuch, 2008; Davis et al., 2009), on post-spawning Atlantic salmon adults, and Rainbow smelts (Cunjak et al., 1998). It has been hypothesized that the broad declines in Rainbow smelt populations throughout Maine could partially explain the declining occurrence of Atlantic salmon repeat spawners (Saunders et al., 2006). American eels feed on other fish, including diadromous species such as sea lamprey ammocoetes (e.g., Denoncourt and Stauffer, 1993), but little is known about their freshwater diet.
During migrations, diadromous species can also display spatio-temporal overlaps offering protection from predators for some species and potentially acting as prey buffers (Saunders et al., 2006; Leach et al., 2022), especially when the abundance of one species of prey is significantly larger than the abundance of another. Upstream migratory adult river herring may divert predation pressure from native predators such as double-crested cormorants, river otters, and ospreys (Mather, 1998; US Atlantic Salmon Assessment Committee [USASAC], 2004) away from downstream migration salmon smolts. Similarly, upstream migration American shad and river herring may provide similar prey buffering for Atlantic salmon adults from river otters, harbor seals, and perhaps odontocete cetaceans (Odontoceti spp.) (Saunders et al., 2006). A recent study reported that seal-induced injuries on returning adult Atlantic salmon declined in the Penobscot River from 2012 to 2019 and was correlated with increasing river herring returns (Leach et al., 2022). The prey buffering hypothesis remains valid, but much more work is needed to quantify the dynamic influence across diadromous fish species at variable levels of relative abundance.
A diadromous watersheds-ocean continuum approach
As highlighted in the previous section, the diadromous community has impactful roles and connects the different ecosystems (marine, estuarine, freshwater, terrestrial, and aerial) they inhabit through migration and varying seasonal abundance across this mosaic of habitats. Our understanding of the diadromous community roles remains incomplete and will remain incomplete for the foreseeable future if the scientific community does not take a more holistic view of the diadromous community and the ecosystems they inhabit.
To help develop a more holistic view of the diadromous community and the ecosystems they inhabit, we present a conceptual model: the Diadromous Watersheds-Ocean Continuum concept (DWOC, Figure 5). DWOC highlights how the diadromous community connects the different ecosystems (marine, estuaries, freshwater, terrestrial, and aerial) they inhabit through migration and seasonal abundance, and the various ecosystem services they provide across this mosaic of habitats located from headwater streams to low river habitats and coastal streams, as well as lake, estuaries, nearshore, coastal shelf and high sea habitats. We propose that diadromous fishes have impactful roles in these ecosystems that vary over seasons, life stages, and locations, such that the community functions as a continuum varying across both time and space. Our model relies on modern portfolio theory, which can help unify our collective efforts to conserve the suite of diadromous species, the ecological services they deliver, and these species’ portfolios. As Schindler et al. (2015) describe, modern portfolio theory suggests that the dynamics of many biological systems are often more stable than the individual components they are comprised of. Therefore, identifying and managing biological processes that generate complexity (e.g., increasing connections between ecosystems and creating heterogeneity) would enhance management opportunities for individual species and their ecosystem functions (Schindler et al., 2015). Such integrated assessment includes understanding how to distribute the risks associated with the inevitable uncertainties that are inherent to managing complex species portfolios (i.e., genetic diversity and life history traits; Schindler et al., 2015; Levin et al., 2018), and evaluating management strategies viable for given system states and resilience stages (Folke et al., 2010; Camp et al., 2020). DWOC is a management tool consistent with modern portfolio theory and ecosystem resilience concepts. We suggest that its application to support EBM approaches would substantially increase the level of ecosystem services delivered by the diadromous community. Currently, some ecosystem services still exist, meaning they are delivered but are at a low level (i.e., low intensity) due to low abundance (Weiskopf et al., 2020). We postulate that increasing the diversity and abundance of the diadromous community will increase the intensity of ecosystem services delivered through the community’s distribution range and increase the community’s and ecosystems’ resilience to future perturbation.
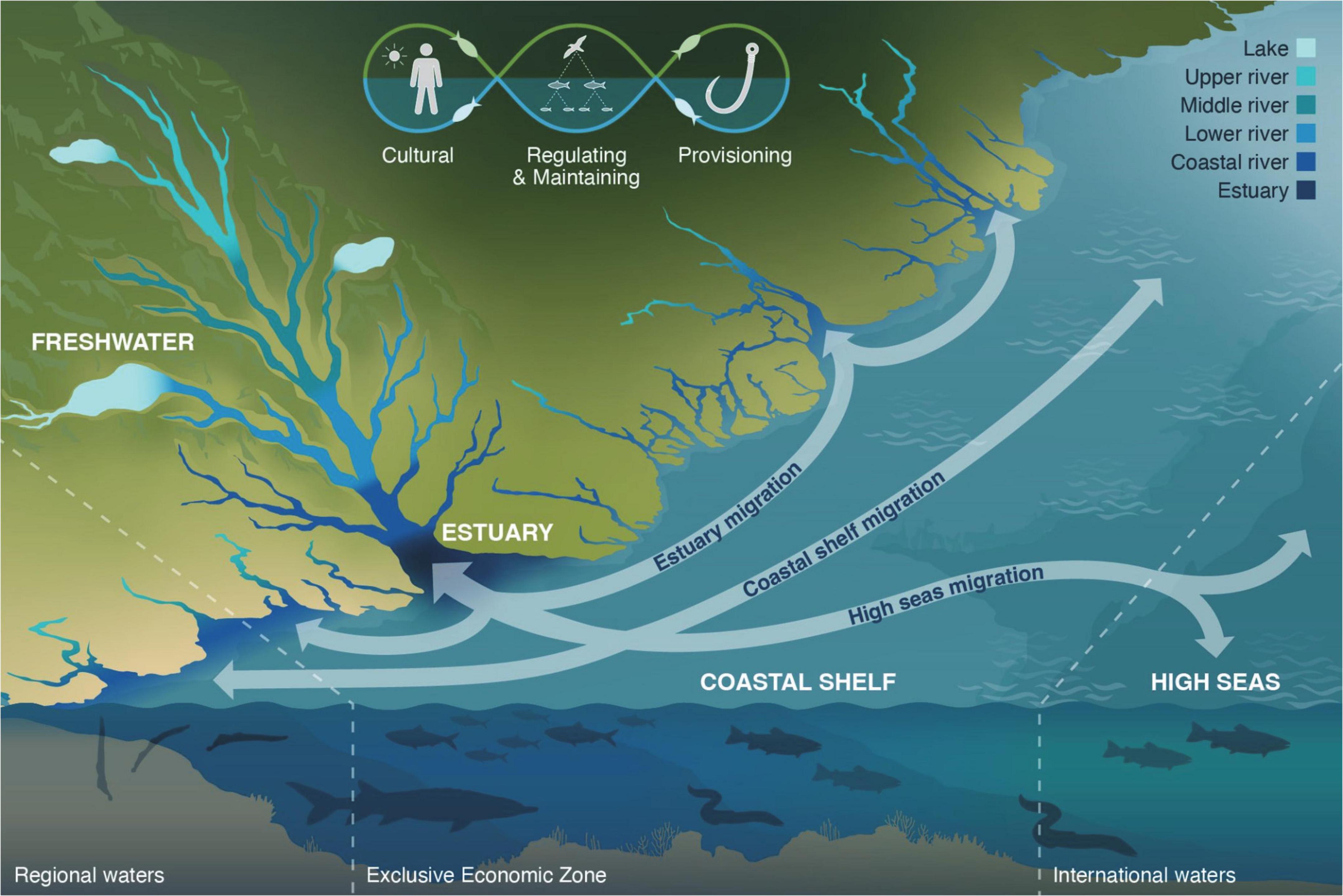
Figure 5. Diadromous watershed-ocean continuum (DWOC) highlighting the various ecosystems that diadromous fishes connect through the delivery of ecosystem services (cultural, regulation and maintaining, and provisioning), from lakes and upper river areas to coastal and high sea areas, across regional and international borders. The ecosystem services logo highlights how these services are connected through the diadromous community and provide essential connections between ecosystems, which vary spatio-temporally via the migrations paths and habitats occupied by diadromous fishes along the watershed-ocean continuum.
Diadromous watersheds-ocean continuum is an inexpensive and easily applicable tool that recognizes the diadromous community ecosystem services landscape and, by doing so, helps users to take a more holistic approach to diadromous community and ecosystem management. DOWC can be used as a qualitative model to identify research and management needs, define objectives and management targets while identifying competing objectives (e.g., need for hydropower vs. fish passage), and promote transparent discussion to reconcile these different objectives. Applying the DWOC can help visualize the relationships and spatial overlap of the various species/ecosystems and how obstacles such as dams may prevent the delivery of ecosystem services in different watershed areas. By promoting a large-scale view of the diadromous community, DWOC supports the development of EBM approaches for this diverse suite of fishes and the associated ecosystem services they provide. By using DWOC to do the integrated assessment of the watersheds-ocean continuum, it is possible to discuss the best way forward to improving ecosystem services delivery while also assessing the trade-offs (e.g., keeping a dam while improving fish passage for multiple species, etc.). Although we use specific examples and species of the Northwest Atlantic region to describe the ecosystem services, the DWOC approach can be applied globally to watersheds and regions hosting diadromous species as the list of services and connections can easily be adapted based on the regional species diversity and dynamics.
Migration patterns and abundance levels vary in space and time and thus strongly influence the intensity of ecosystem services delivered. For instance, MDN deposits in the headwater areas may come from Atlantic salmon, sea lamprey, and American eel, whereas deposits in the mid-river and lakes will come primarily from river herrings, American and Hickory shads, and Striped bass (Samways et al., 2015; Rosset et al., 2017; Twining et al., 2017). Some species, such as Atlantic tomcod, and conger eel, mainly deliver services in estuaries, while Atlantic and shortnose sturgeons make connections between estuaries (Smith et al., 2016). Some species (river herrings, Striped bass) embark on coastal migrations in the marine ecosystem, while others migrate throughout the high seas (Atlantic salmon and American eel; Greene E. et al., 2009; Verhelst et al., 2021). Species can also have multiple spatial impacts via different services; Atlantic salmon deposit MDN in upstream areas, may serve as prey for freshwater, avian, and marine predators, and may be harvested in marine fisheries (Guyette et al., 2014; Barber-O’Malley et al., 2022; Leach et al., 2022). The role of species such as Rainbow smelt may seem less significant at current low abundance, but if abundance were restored to high levels, they could play an important role for coastal streams (Samways et al., 2015). Thus, the ecosystem services delivered by different diadromous species depend on their abundance and distribution across these different ecosystems. Their spatiotemporal distribution is also highly relevant for species management, as species may cross borders and reach international waters, adding complexity to management strategies by involving multiple jurisdictions (Munsch et al., 2020; Kritzer et al., 2022).
In Figure 6, we present a conceptual model to explore how spatial, social, and biological scales increase concurrently with the expected quantity (i.e., how many services) and intensity (i.e., the “strength” of each service) of the ecosystem services delivered by the diadromous community. The portfolio effect is embedded within the DWOC as the varying scales (e.g., spatial, temporal, and taxonomic) affect the dynamics of genes, phenotypes, populations, and ecosystems and are strongly linked to ecosystem resilience (MacArthur, 1955; Schindler et al., 2015). We propose that diadromous species management issues at any scale, from the individual phenotype to an ecosystem, can be viewed through DWOC. Management actions are incredibly variable and can range from no defined management plan to a more integrated plan accounting for selected species. However, it is hard for managing entities to collaborate if there are no defined management plans or if the objectives are not clearly defined. DWOC is a tool that helps understand the species as a community and the ecosystem connections to enable the development of a holistic management approach that accounts for the ecosystem services provided by these species along the watersheds-ocean continuum. DWOC can help management move to a more holistic consideration of diadromous species and ecosystems and facilitate discussion and collaboration between managing entities. Figure 6 outlines the various scales where management actions may be warranted and how large-scale actions can support higher ecosystem resilience. This conceptual figure highlights that large-scale management may be required to improve diadromous community productivity. As management actions are enacted at varying scales, the resulting ecosystem services are assumed to intensify (i.e., become more numerous and variable) and provide higher and longer-term resilience when actions are undertaken at higher scales. However, this does not indicate that small-scale action may not have intense social relevance on a small scale. Ecosystem level changes may take more time as they involve higher organizational levels and more complex dynamics; however, they may correspond to increased benefits (e.g., ecosystem service intensity and ecosystem resilience). Changes at the community level and ecosystem scale generally operate on a longer timescale, which can be hard to ‘reconcile’ with our will to respond to threats in a timely manner or the realities of budget and political constraints. Using this portfolio-level approach also helps visualize how the social and cultural services are linked to scale. Managing at the sub-population or river level is expected to provide benefits for that specific river or the local communities, whereas working at a larger scale is expected to bring benefits to a broader regional area and the communities within.
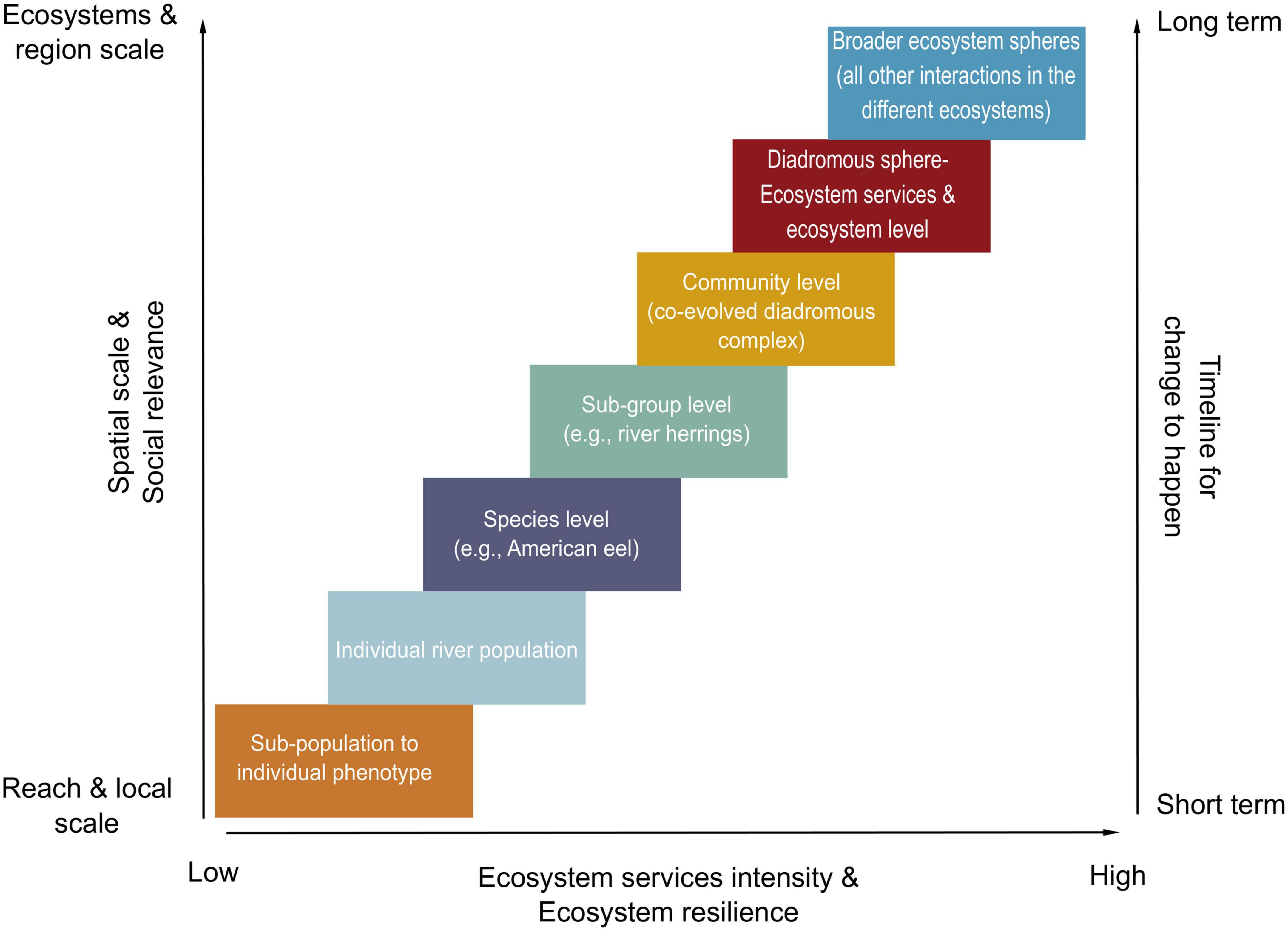
Figure 6. Conceptual model using the portfolio effect concept applied to the management of the diadromous species in relation to the scale of actions, social relevance, ecosystems’ resilience, ecosystem services intensity, and timeline for change. The figure is conceptual in nature, meaning that the various axes should not be quantitatively interpreted. The joint topics on the axes do not mean that they are explicitly linked, rather than operating on their own relative spatio-temporal scales. The diadromous sphere box refers to the diadromous community and essential freshwater habitat coupled with ALL the species they interact with (i.e., primary producers to predators), while the broader ecosystem sphere refers to all the other interactions, e.g., freshwater and marine harvest, marine predators, terrestrial insects, avian predators, etc.
While DWOC is presented as a conceptual model, it can evolve to be applied quantitatively. This quantification could be achieved by juxtaposing GIS layers of fish abundance, movement, habitats, and quantitative measures of relevant ecosystem services (e.g., abundance level required to provide an adequate prey buffer, dam passage performance standards to facilitate abundance levels that may support fisheries and predator needs, biomass imports required to provide MDN inputs necessary to increase diadromous species productivity through increased primary production) to quantitatively define management targets and support management actions that take into account all of these interdependencies. If the required input data were available, the quantification of DWOC would allow for a data-based definition of management objectives and a prioritization of research and management needs. As with the qualitative application, the quantification of DWOC would be region-specific and dependent on the ecosystem services and species present.
Aligning research and management needs
Synthesizing the available information about ecosystem services provided by diadromous fishes through the lens of DWOC, helps identify research gaps that may hinder the development of large-scale management actions. We identified three main areas of research needed to better inform management and understand diadromous fishes community productivity (Figure 7). The general research groups target the knowledge gaps on ecosystem services (inventory and quantification), species interactions (individual level and cumulative impacts), and changing habitat conditions (in relation to past and global changes). This list is not exhaustive, as the intent is to highlight some of the overarching gaps as a starting point. A more in-depth application of DWOC would help define more region-specific gaps and needs. Using Figures 5, 6 as a guide, research needs can be developed to align with the management needs at multiple scales, thereby accommodating the need to work at the reach, river, and region-wide scales. The DWOC process can facilitate discussions between scientists and managers on what are the most pressing issues and how to address them. When assessing the ecosystem service of MDN delivery at the river scale, a clear research need that may be identified would be the quantification of species-specific MDN contributions. Addressing this service at a larger may result in the investigation of the cumulative impact of MDN on the productivity of multiple species. Identifying research needs is particularly important for some of the ecosystem services that have been less studied (e.g., aesthetic value, ecosystem resilience, and carbon fluxes) or for species we have little knowledge of.
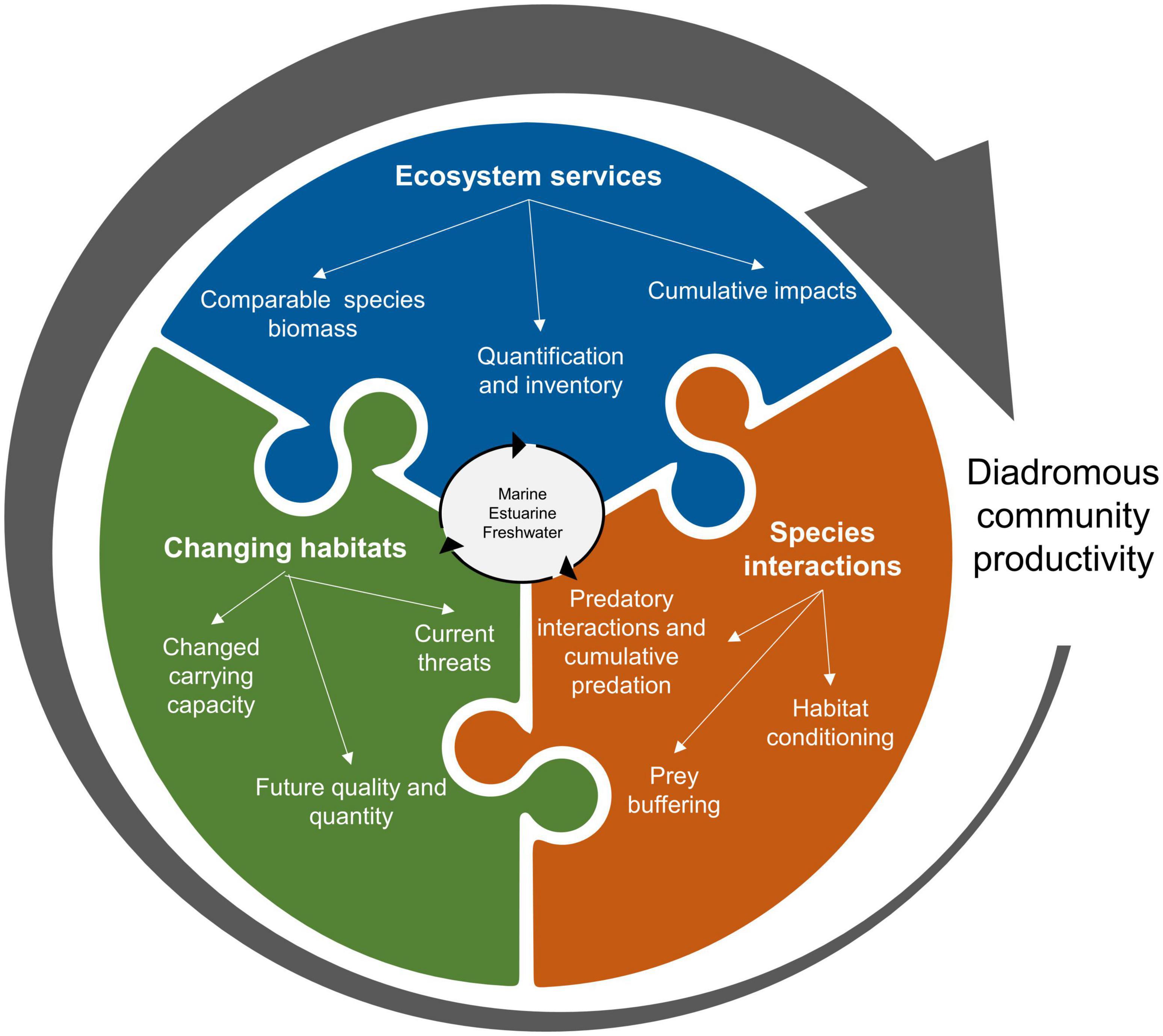
Figure 7. Some research gap groups must be addressed to inform large-scale management of the diadromous community sphere (Figure 6).
As ecosystem services are a primary focus within DWOC, the first group of research needs focuses on the need to quantify ecosystem services (Table 1) for the different species across all the habitats they occupy. A full inventory of ecosystem services would be required to ensure that all important services are identified (especially for understudied species). To better understand the role of the diadromous fishes as a community, we need an improved knowledge of the role of species we do not know much about (e.g., Rainbow smelt, Atlantic tomcod, American eel, sturgeons, and shads), the relative importance of the services they provide and their cumulative impacts. In some instances, we need a better understanding of the basic biology of the species (e.g., conger eel), while in other instances, we need a better understanding of the services that may be provided in specific ecosystems (e.g., estuarine services provided by tomcod or sturgeons). While in other situations, we need a better understanding of the bi-directional transfers of services (e.g., MDN is focused on marine imports with little known about estuary inputs or marine exports). We have also identified ecosystem services that we know little about (e.g., carbon flux impacts on climate changes and habitat conditioning). Further, garnering a better understanding of the impact of various cultural services may be of benefit as these services can facilitate greater public support for diadromous species management and ecosystem restoration efforts. Accounting for cultural services requires a more social science-based approach to stop conceiving these services as immaterial and unquantifiable and to use one of the many existing frameworks that clarify their practical assessment across diverse contexts (e.g., Fish et al., 2016). Applying DWOC shows that these knowledge gaps impede our understanding of the connections between these species and the ecosystems they inhabit, therefore hindering the development of management actions that can achieve the goal of restoring the diadromous community as a whole and the full suite of ecosystem services they provide.
The second group of research needs is related to species interactions. Critical knowledge of the estuarine and marine habitats used and how species interact in these habitats is currently lacking, preventing a more holistic understanding of these connections. A better understanding of these connections would also inform progress toward EBM implementation. Indeed, species interactions are crucial in establishing how species can influence each other’s productivity (Petitgas et al., 2010). As an example, some studies have begun to investigate the link between forage fish such as river herrings and the productivity of marine groundfish species (Lotze and Milewski, 2004; Petitgas et al., 2010; Mcdermott et al., 2015; Smith et al., 2016), although more information is needed (Daniels et al., 2019). In addition, a greater understanding of the impacts of cumulative predation pressure (including from non-native species; Baumann and Kwak, 2011; Schmitt et al., 2017) is needed. Indeed, one predation source might seem trivial, but all sources may represent a more significant cause of mortality than initially estimated, and this misunderstanding could render management actions targeting mortality ineffective. The potential for species to offer protection from predation via prey buffering, and the impacts of habitat conditioning by species occupying similar habitats deserve further research. Sea lamprey is hypothesized to condition habitats, making them more attractive and productive for salmon spawning (Saunders et al., 2006), although we do not have the empirical data to demonstrate this. Studies have shown that increased MDN benefits juvenile salmon growth (Guyette et al., 2013; Samways et al., 2017); however, we do not know whether these benefits result in increased survival and overall productivity. We also need to understand how diadromous fishes interact with other species, such as freshwater mussels, which are also at risk of extinction across most of their range and can improve fish habitat quality by biofiltration (Galbraith et al., 2018; Kreeger et al., 2018). Synthesizing the available information through DWOC highlighted a limited quantification of the ecosystem services in these ecosystems and their spatiotemporal delivery, as it is barely known where and what habitat fish use. This critical research gap prevents an understanding of how modifications in coastal watersheds can affect coastal habitats for species such as sturgeons, sea-run Brook trout, and river herrings. A further complicating factor to our understanding of these research gaps is the idea of ecological thresholds (e.g., how much MDN inputs are needed before seeing a productivity increase? What recovery threshold is required to see a shift in predation interactions?). Increasing our knowledge base in relation to these various species interactions could influence management priorities by increasing the awareness of how species need to be considered as a community rather than singularly.
The third group of research needs we identified is related to issues associated with habitat use, which influences where fish will deliver ecosystem services. Changing habitats, driven by current and future climate change, as well as a whole suite of disturbances (e.g., forestry, barrier construction, forestation, water withdrawal, and invasive species), is perhaps one of the most critical challenges as changing conditions can reverberate throughout the entire ecosystem through complex interactions. Habitats have changed tremendously over the last centuries through human modifications of the land surface, direct manipulations of river channels, introduced contaminants, and changing climates (Thompson et al., 2021). The adverse effects on diadromous species populations are generally known for some human impacts (e.g., dam construction; Limburg and Waldman, 2009; Waldman and Quinn, 2022), but many impacts are not sufficiently understood to inform management at the species or community levels. First, it is necessary to understand how ecosystems have changed (section “Diadromous community ecosystem services”) and influenced habitat quality and ecosystem carrying capacity. When making such assessments, we need to recognize that habitat responses to the same stressor may vary considerably across a region because of changes in regional or local conditions (relief, surficial geology, land cover, seasonal oceanic circulation patterns, etc.). Understanding these local constraints and their impact can help define local management objectives in broader EBM planning. This knowledge can also help define the current habitat trajectories and develop models to inform on changing conditions and potential responses to management actions. Understanding the complex ramifications of changing habitat conditions is no small task. For instance, changing thermal conditions can directly impact fish by causing thermal stress or indirectly by affecting primary production or the risk of infectious diseases. Current challenges also include understanding the cumulative impacts of changing conditions impacting diadromous fishes across the range of habitats they utilize, while characterizing the impact of emerging threats such as new pollutants (e.g., 6PPD-quinone and other chemicals found in urban watersheds and microplastics (Hiki et al., 2021; Johannessen et al., 2021; Parker et al., 2021). This needs to be assessed jointly with ocean acidification, warming, and changes in salinity (Huntington, 2010; Lynch et al., 2015; Le Bris et al., 2018; Wright-Fairbanks et al., 2020). Coastal habitats are often highly vulnerable to degradation (e.g., eutrophication and warming) due to increased watershed urbanization and agricultural use, and toxic episodes have already been observed in estuaries like the Chesapeake Bay (Brush et al., 2020; Malone and Newton, 2020). Runoff from agricultural and urban areas is one of the main reasons for these oxygen depletion events (Somers et al., 2013; Tétard et al., 2016; Brush et al., 2020; Timm et al., 2020, 2021). Toxic episodes in estuaries can reduce fish migration (Tétard et al., 2016), but the extent of the impact is still yet to be sufficiently understood. Additionally, threats such as increasing demands for water (including groundwater) (e.g., Van Vliet et al., 2013), changes in precipitation patterns, and drought and flood dynamics (Lytle and Poff, 2004; Cassardo and Jones, 2011) that directly impact habitats need to be integrated altogether so we can truly assess if habitat restoration projects can mitigate some of the anthropogenic changes and if these gains be sustained for future climate conditions.
Roadmap to ecosystem-based management approach
The challenges of restoring and conserving the community of diadromous fishes in a changing ecosystem are daunting. As such, a more holistic understanding of the (1) role of the diadromous community in connecting ecosystems and the ecosystem services they provide, (2) range of threats affecting the diadromous community, and (3) global, local, and cumulative impacts of these stressors is essential. As scientists increase our understanding of these aspects, opportunities to strengthen EBM implementation are emerging (Hare et al., 2021; Link, 2021; Watz et al., 2021). The complexity of working at the ecosystem scale can be overwhelming (DeFries and Nagendra, 2017), but we propose a four steps DWOC roadmap (Figure 8) to explore the broken links within the watersheds-ocean continuum. This roadmap can be adapted to specific regions to inform ecosystem-scale project integration and help coordinate complex questions spanning multiple disciplines to enhance our understanding of the causality of diadromous fish productivity in a changing environment. Implementing these steps would represent tangible progress toward EBM and should increase diadromous species productivity and ecosystems’ resilience by restoring the delivery of a diverse array of ecosystem services.
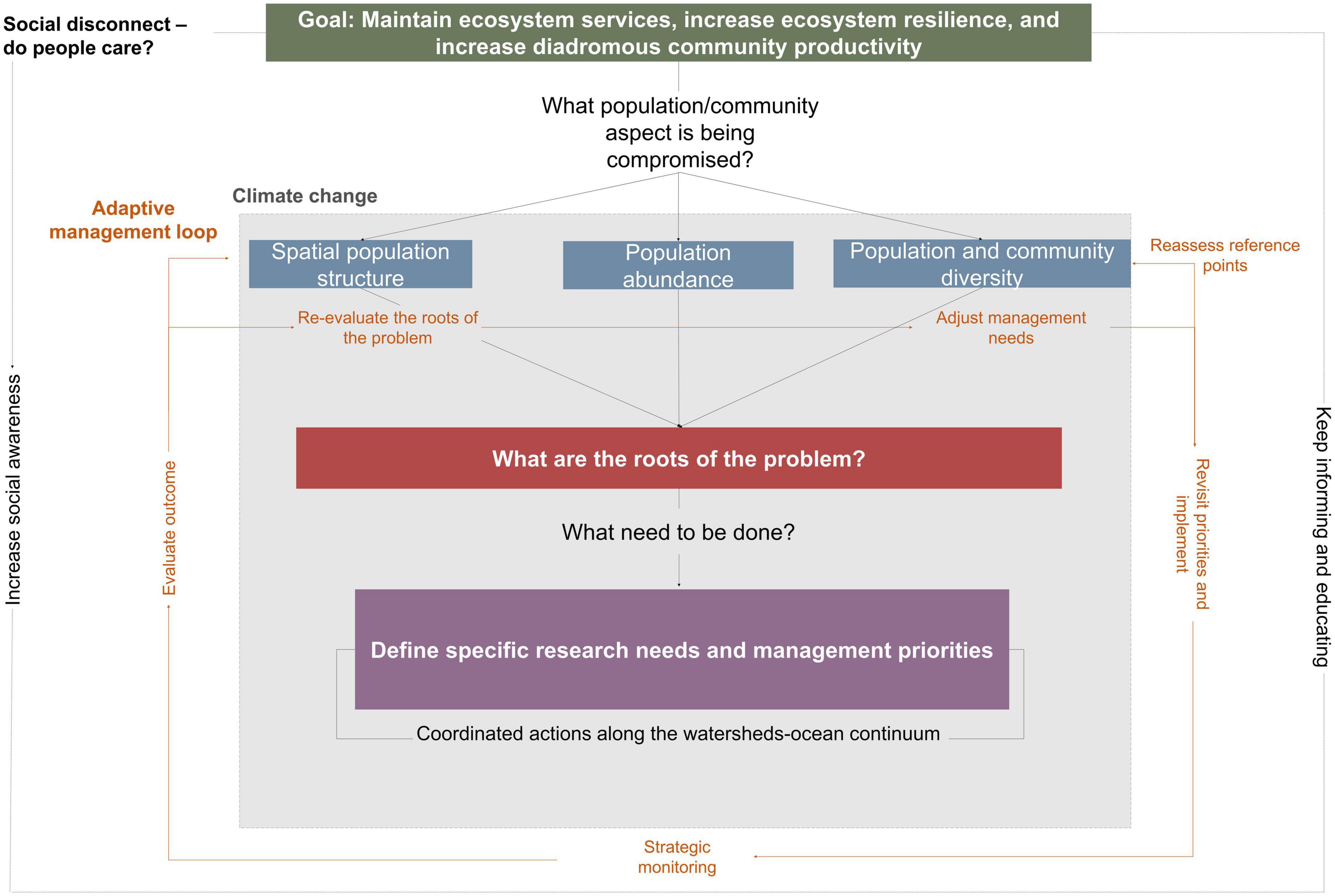
Figure 8. Diadromous Watersheds-Ocean Continuum (DWOC) roadmap for ecosystem-based management implementation to foster increased diadromous community productivity, resilience, and improved ecosystem services delivery. Climate change affects all aspects of the diadromous community across all spatial scales. As the management process (e.g., evaluation of the roots of the problem, management actions implementation) progresses, there is a need to adopt an adaptive management approach where the situation is reassessed, and management approaches are modified accordingly. The question of if people care is an ongoing issue that may be directly related to willingness to support ongoing management intervention.
The overarching goal of the DWOC roadmap is to improve ecosystem service delivery and resilience and increase diadromous community productivity. These three aspects are intrinsically linked together, as a productive community is required to ensure a higher intensity of ecosystem services that can support ecosystem resilience, while higher resilience can also be linked to higher productivity (Worm et al., 2006; Kerr et al., 2010; Petitgas et al., 2010; Waldman et al., 2016). Therefore, the first step toward this goal is to assess the spatial structure of the community across all life stages, population abundances, and population and community diversity (including life-history traits and genetic diversity), to define what aspects of the community are compromised. We recognize that this is an ambitious and complex task, but progress can be made by breaking it down into pieces (e.g., what part of the life cycle and spatiotemporal specific aspects are the most impacted) and prioritizing the more crucial gaps for future research.
The second step is to evaluate the roots of the problem impacting the spatial structure, abundance, and diversity of the diadromous community. Although impacts and appropriate responses may be species and region-specific, they should still be viewed at the larger scale across the species distribution range. DWOC provides a discussion framework to facilitate the development of a holistic understanding of the roots of the problem as well as a multi-solution approach applied at the community level (Figure 6), while exploring the ramifications of the different solutions along the ecosystem continuum.
With this comprehensive understanding (steps 1 and 2), clear objectives can be defined (e.g., improving population genetic diversity) and evaluate what reference points (baseline) should be used (step 3). Each objective should include a set of quantitative targets of diadromous fish abundance, diversity, and distribution to support ecosystem services delivery. These targets can be used to align and coordinate monitoring programs to measure whether objectives are reached or what prevents achieving them. Clear and transparent communication between all parties involved is key to defining objectives (Cook et al., 2013; Link and Browman, 2017). In a project where multiple entities are represented, everyone must state their specific objectives clearly and acknowledge their jurisdiction to help identify a more focused set of governance conditions to facilitate the operationalization of an EBM plan (Link and Browman, 2017). Such transparency can also allow for an open discussion about the trade-offs among multiple project directions and management objectives (Harvey et al., 2017), improving the project’s chances of success. This also facilitates the discussion of conflicting interests, which will likely increase in the future due to climate change, water-use demands, invasive species, and other anthropogenic impacts that can directly or indirectly affect the diadromous community. We acknowledge that natural and social context can complicate this process, but the abundant literature on participatory frameworks can help users decide on how to best proceed forward (e.g., identifying the leadership needs, how to maintain participation, and deal with conflicts (Brosius and Russell, 2003; Jentoft, 2007; Berghöfer et al., 2008; Gray and Hatchard, 2008; Orach and Schlüter, 2021).
Concurrent with these previous steps, assessing the connections between people and the diadromous fish community in the affected area is required to estimate the expected level of social support for any future management actions. Gauging and then fostering increased public support for diadromous fishes can be leveraged to increase political support and funding (e.g., Ostergren et al., 2008). According to Soga and Gaston (2018), shifting baselines could be one of the fundamental reasons behind society’s tolerance to the continuing destruction and degradation of the natural environment, leading to society not fully appreciating ecosystem services or intrinsic values and thereby not supporting the need for conservation and restoration efforts to protect these ecosystems. This loss of connection and awareness can directly affect the perspective of the importance of the ecosystem services delivered by the diadromous community (Waldman, 2010; Liebich et al., 2018). Therefore, throughout the effort, there should be a focus on evaluating if there is social support to address the issues and, if not, to evaluate the socio-economic reasons for the disconnect (Figure 8, dotted line loop). Cultural services are critical in this assessment as they measure the connection between these fish species and people, with low levels of cultural services often being associated with a more substantial social disconnection (Schaich et al., 2010; Daniel et al., 2012). Societal views and expectations may also influence the definition of a baseline. Taking advantage of the cultural services that the diadromous community provides to participate in cultural events, promote citizen engagement through arts, fishing events, education, and outreach activities, are ways to increase social awareness and maintain connections between citizens and the diadromous community.
There is an abundance of literature establishing that connectivity, overfishing, habitat quality, and decreased marine survival are among the primary causes of reduced diadromous species abundances (Mattocks et al., 2017; Link, 2021; Tillotson et al., 2021; Verhelst et al., 2021; Waldman and Quinn, 2022). Of these, reduced watersheds-ocean connectivity is a particularly critical factor impeding ecosystem services delivery and species abundance (Dias et al., 2019; Merg et al., 2020; Zydlewski et al., 2021). With more than 90,000 dams in the U.S. alone, and almost 10,000 in the North Atlantic region (USACE, 2020), dams remain a significant threat and will remain so for the foreseeable future (Waldman and Quinn, 2022). Therefore, using approaches such as DWOC will assist with more holistic considerations of the connections between the different roots of the problems and the strategy to address them. DWOC can help visualize the ramification of the problems (e.g., dams can also result in increased predation at the dam location, decreased stream flow, etc.), as well as the ramification of restoration to maximize the positive outcomes of the management actions. DWOC also helps show that connectivity loss is not just a dam issue, let alone a longitudinal issue; it is also lateral in nature (Cluer and Thorne, 2014). Complex interactions with agricultural, urban, and other altered landscapes include channelization, diversions, and other factors that impede natural lateral connectivity in watersheds. Lateral barriers (e.g., dykes) also affect fish movement between the river and its floodplain (e.g., Roy and Le Pichon, 2017). Additionally, thermal barriers linked with land use (e.g., absence of riparian vegetation to provide shading and urban areas; Baruch et al., 2018; Ouellet et al., 2020) and oxygen depletion affecting estuaries; Tétard et al., 2016; Malone and Newton, 2020) are challenging to identify. Nonetheless, they affect connectivity within freshwater, estuary, and marine ecosystems as well as upland habitats. The cumulative impacts of all migration barriers must be assessed and mitigated along the entire watersheds-ocean continuum.
Climate change is an overarching threat that may directly impact diadromous species productivity in many regions but also synergistically interact with all the root problems simultaneously (Figure 8; Drouineau et al., 2018b; Lennox et al., 2019; Gilligan-Lunda et al., 2021). Most systems have been changed due to human activities or are at least impacted by climate change. The human dimension is vital in evaluating the roots of the problem. Such an approach will allow for large-scale connectivity improvements while addressing the other roots of the problem or mitigation that occurs to conserve species in the interim. Discussion on the roots of the problems and the trade-offs regarding solution implementations can be structured using a “resist-adapt-direct” (RAD) framework approach that can help deliberate and make strategic decisions while addressing profound ecosystem changes linked to climate change (Jackson, 2021; Lynch et al., 2021; Thompson et al., 2021).
Future conditions can be predicted using models addressing species movement and interactions at different scales and for different habitat conditions to understand how changing conditions and management actions may affect populations. Such integrated multispecies and ecosystem modeling can support management at the local and ecosystem levels by forecasting changing conditions. Quantitative models can explicitly account for the ecosystem connections and dynamics and provide quantitative management targets (e.g., x number of fish to get y tones of MDN), also helping with prioritization. Knowledge of status, trends, and risks are essential information in assessing urgency (Levin et al., 2018), and both measured and modeled data can help assess this. However, quality data are needed to feed these models, and there is a strong need to identify the most important model inputs and develop uniform protocols for data collection and monitoring so data sets can be compared across all spatial scales.
The fourth step is to define management actions coordinated across species, life cycle stages, and ecosystems, encompassing specific governance boundaries to increase the productivity of all diadromous fishes. The prioritization of these actions can be done by considering criteria such as feasibility, logistics, governance, public and institutional support, reversibility of the roots of the problem, costs of implementation, management, and lack of recovery (Wilson et al., 2006; Levin et al., 2018). Some objectives can be small-scale and reach-specific (e.g., addressing point source pollution), but still should be viewed within a larger ecosystem-scale perspective to achieve progress toward EBM (e.g., improving overall watershed habitat quality). The DWOC approach reinforces the need to manage all diadromous spaces across their entire life cycle, ecosystems, jurisdictions, and borders. Such implementation requires trans-disciplinary, regional, and international collaborations (biologists, climatologists, engineers, etc.), across all stakeholders (managers, scientists, stakeholders, and policymakers) while using Two-Eyed Seeing to incorporate indigenous research, knowledge, and governance as appropriate (Andrachuk et al., 2021; Cooke et al., 2021; Reid et al., 2021). Achieving such collaborations requires effective communication, bi-directional knowledge transfer (Cook et al., 2013; Andrachuk et al., 2021), and knowledge co-production (Cooke et al., 2021).
To fully implement strategies such as these and to fully embrace large-scale management, some consideration of governance structures is clearly warranted. Transparent discussion and coordination across larger regions encompassing partners’ jurisdictional boundaries can be facilitated by “boundary organizations” (environmental organizations that span the boundary between science and management; Cook et al., 2013). Boundary organizations can simply facilitate exchanges and improve management strategies by facilitating domestic and international cooperation and helping the community to have the latest scientific updates (e.g., North Atlantic Salmon Conservation Organization; NASCO, 2022) or operate in both scientific and practical spheres but retain distinct lines of accountability to both groups (Guston, 2001). Unfortunately, we are lacking a boundary organization for all diadromous species across their distribution range at present times, but this should not impede progress in the short term. Over the long term, developing boundary organization(s) targeting the suite of diadromous species and covering the diversity of ecosystem services they provide would facilitate progress toward EBM.
Finally, adaptive management is critical (Figure 8) and needs to be planned as part of an integrated monitoring design that accounts for effective monitoring of entire systems and promotes efficient use of funds that support high-impact works (Bisbal, 2003; Arciszewski et al., 2021). Focusing on applying resilient concepts supports adaptive management and favors management structures more capable of sustaining ecosystem services provided by the diadromous community under changing conditions (Camp et al., 2020). As progress is being made and data and knowledge are gained, there is a need to re-evaluate the root problems and adjust objectives, targets, management priorities, and actions, which will guide future implementation and monitoring. An adaptive management approach may be essential to maintain continuous progress and should also include reporting on levels of target achievement routinely (e.g., every year) to maintain participation and engagement. Long-term monitoring and study design covering before, during, and after implementation of management actions over a long time period (>10 years) is an essential ingredient for effective adaptive management. More specifically, Arciszewski et al. (2021) propose a framework for integrated monitoring that includes defining attributes such as program flexibility, strength of inference, sensitivity to errors, and administrative burden that can be used to define the integrated monitoring plan while looking at the timing (study cycle) and the type of data required. Although funding can be restrictive, when there is a base of social support for these efforts, local resources in the form of interested individuals or watershed groups can often be mobilized to bolster available resources to achieve this (e.g., Wippelhauser, 2021).
Working toward the DWOC overarching goal across large regions is a daunting task, akin to reaching for the golden ring. However, our approach can be adapted to work on smaller scales and local issues by starting on a smaller suite of ecosystem services for instance, while embedding these steps into that large-scale holistic understanding of the diadromous communities and ecosystem connections. Building from each other’s work and looking at how different entities can address different objectives within that large-scale framework are also key aspects of progress. When it comes down to making step-by-step progress toward DWOC large-scale goal, size matters not.
Conclusion
Diadromous fish conservation remains incredibly challenging. We provide a conceptual model, DWOC, to advance a more holistic management of diadromous species across the watersheds-ocean continuum to further aid our collective efforts to increase the productivity of this suite of species to restore and enhance the ecosystem services they provide. More holistic approaches (i.e., looking at diadromous species as a community and including their interactions with other species across ecosystems) may support the development of management actions that increase ecosystems resilience and maximize ecosystem service delivery across the continuum. While we review DWOC through the lens of the Northwestern Atlantic region, DWOC can be applied more globally to support EBM of the diadromous community.
The DWOC approach and roadmap provide a pathway to a more holistic way of thinking about the pressing questions impeding the diadromous community productivity. It promotes a more integrated understanding of ecosystem functions, while considering the ecosystem services needed to support these functions. This holistic view of the diadromous community provides a base to assess the pros and cons of different management actions, thereby facilitating informed decision-making. Engaging a broader coalition of communities, sectors, and discipline is an essential element of the DWOC application, as highlighted in step 3, as involving all stakeholders and expertise is the only way to properly assess the diadromous community and the ecosystem services they can provide across the entire watersheds-ocean continuum. The DWOC tool can help support the development and application of EBM in the face of continued and increasing threats from global change, with the portfolio effect firmly embedded within as intervention at different scales can build toward a broader ecosystem resilience.
There is an increased recognition that small-scale and single species management actions are woefully inadequate at addressing the ongoing and future challenges associated with global climate change and the multitudinous anthropogenic and natural resource conflicts. Through DWOC, we directly tackle this challenge by developing a tool to facilitate a more holistic way of thinking about the challenges that diadromous fishes face and how different management actions impact ecosystem resilience to improve our decision-making and collaborative work. DWOC helps visualize how different ecosystem dynamics fit together, providing the information needed to support the development of coordinated management interventions at large-scales; moving toward fostering greater ecosystem resilience. The challenges to evolving our science and management structure toward a DWOC approach are significant and will take significant resources and real dedication to change. However, many of the pieces needed to more holistically assess and manage the ecosystem services diadromous species provide are currently available. Initiating a DWOC approach with the available data and information today would result in all stakeholders garnering a more holistic view of the diadromous community and could support the evolution of our single species small-scale management toward a more integrated approach across species and ecosystems. As our diadromous species knowledge base increases, the continual evolution of the DWOC application will foster a concurrent evolution of our EBM management approach toward our desired goal of increased diadromous species productivity, which will support increased ecosystem resilience and result in the maintenance of the full suite of ecosystem services that this community provides.
Author contributions
VO created the conceptual model, figures, and project design, organized the database, and wrote the first draft of the manuscript. TS and RS performed the language and structure review. All authors contributed to the project’s conception, contributed to the manuscript revision, read, and approved the submitted version.
Funding
This work was funded by NOAA Office of Habitat Conservation, Northeast Fisheries Science Center, and Greater Atlantic Regional Fisheries Office.
Acknowledgments
Between juggling work, family obligations, and more, time is a precious commodity. We would like to thank everyone for taking the time to meet with VO, and exchange ideas and information; the diadromous community is lucky to have such passionate people involved.
Conflict of interest
The authors declare that the research was conducted in the absence of any commercial or financial relationships that could be construed as a potential conflict of interest.
Publisher’s note
All claims expressed in this article are solely those of the authors and do not necessarily represent those of their affiliated organizations, or those of the publisher, the editors and the reviewers. Any product that may be evaluated in this article, or claim that may be made by its manufacturer, is not guaranteed or endorsed by the publisher.
Supplementary material
The Supplementary Material for this article can be found online at: https://www.frontiersin.org/articles/10.3389/fevo.2022.1007599/full#supplementary-material
References
Alexander, M. A., Scott, J. D., Friedland, K. D., Mills, K. E., Nye, J. A., Pershing, A. J., et al. (2018). Projected sea surface temperatures over the 21 st century : Changes in the mean, variability and extremes for large marine ecosystem regions of Northern Oceans. Elem. Sci. Anth. 6:9.
Alexander, M. A., Shin, S. I., Scott, J. D., Curchitser, E., and Stock, C. (2020). The response of the Northwest Atlantic Ocean to climate change. J. Clim. 33, 405–428. doi: 10.1175/JCLI-D-19-0117.1
Andrachuk, M., Kadykalo, A. N., Cooke, S. J., Young, N., and Nguyen, V. M. (2021). Fisheries knowledge exchange and mobilization through a network of policy and practice actors. Environ. Sci. Policy 125, 157–166.
Arciszewski, T. J., Roberts, D. R., Munkittrick, K. R., and Scrimgeour, G. J. (2021). Challenges and benefits of approaches used to integrate regional monitoring programs. Front. Environ. Sci. 9:666698. doi: 10.3389/fenvs.2021.666698
Armstrong, W. H., Collins, M. J., and Snyder, N. P. (2012). Increased frequency of low-magnitude floods in New England. J. Am. Water Resour. Assoc. 48, 306–320.
Armstrong, W. H., Collins, M. J., and Snyder, N. P. (2014). Hydroclimatic flood trends in the Northeastern United States and linkages with large-scale atmospheric circulation patterns. Hydrol. Sci. J. 59, 1636–1655. doi: 10.1080/02626667.2013.862339
ASF (2021). Fish Friends. Maine Counc. - Atl. Salmon Fed. Available online at: https://fishfriends.me/ (accessed November 20, 2021).
Atlantic States Marine Fisheries Commission [ASMFC] (1985). Management Plan for American Shad and River Herrings. Arlington, VA: Atlantic States Marine Fisheries Commission.
Atlantic States Marine Fisheries Commission [ASMFC] (2007). American Shad Stock Assessment Report for Peer Review, Vol. 1. Arlington, VA: Atlantic States Marine Fisheries Commission.
Atlantic States Marine Fisheries Commission [ASMFC] (2010). Amendment 3 to the Interstate Fishery Management Plan for Shad and River Herring (American Shad Management). Arlington, VA: Atlantic States Marine Fisheries Commission.
Atlantic States Marine Fisheries Commission [ASMFC] (2017a). 2017 American Eel Stock Assessment Update. Arlington, VA: Atlantic States Marine Fisheries Commission, 110.
Atlantic States Marine Fisheries Commission [ASMFC] (2017b). 2017 Atlantic Sturgeon Benchmark Stock Assessment and Peer Review Report. Arlington, VA: Atlantic States Marine Fisheries Commission.
Atlantic States Marine Fisheries Commission [ASMFC] (2017c). River Herring Stock Assessment Update, Volume I: Coastwide Summary. Arlington, VA: Atlantic States Marine Fisheries Commission.
Atlantic States Marine Fisheries Commission [ASMFC] (2019). Summary of the 2019 Benchmark Stock Assessment for Atlantic Striped Bass. Arlington, VA: Atlantic States Marine Fisheries Commission.
Atlantic States Marine Fisheries Commission [ASMFC] (2020). 2020 American Shad Benchmark Stock Assessment and Peer Review Report. Arlington, VA: Atlantic States Marine Fisheries Commission.
Atlas, W. I., Ban, N. C., Moore, J. W., Tuohy, A. M., Greening, S., Reid, A. J., et al. (2021). Indigenous systems of management for culturally and ecologically resilient Pacific Salmon (Oncorhynchus spp.) Fisheries. Bioscience 71, 186–204. doi: 10.1093/biosci/biaa144
AWS (2021). Schools in Schools- Shad Restoration. Anacostia Watershed Soc. Available online at: https://www.anacostiaws.org/what-we-do/education/school-based-programs/schools-in-schools-shad-restoration.html (accessed November 3, 2021).
Barber-O’Malley, B., Lassalle, G., Lambert, P., and Quinton, E. (2022). Dataset on European diadromous species distributions from 1750 to present time in Europe, North Africa and the Middle East. Data Brief 40:107821. doi: 10.1016/j.dib.2022.107821
Baruch, E. M., Voss, K. A., Blaszczak, J. R., Delesantro, J., Urban, D. L., and Bernhardt, E. S. (2018). Not all pavements lead to streams: Variation in impervious surface connectivity affects urban stream ecosystems. Freshw. Sci. 37, 673–684. doi: 10.1086/699014
Bassett, E. (2015). Sipayik environmental department: Cultural importance of river herring to the passamaquoddy people. Perry, ME: Pleasant point reservation.
Baumann, J. R., and Kwak, T. J. (2011). Trophic relations of introduced flathead catfish in an Atlantic river. Trans. Am. Fish. Soc. 140, 1120–1134. doi: 10.1080/00028487.2011.607046
Beaty, J. (2014). Fisheries {Then}: {American} {Eel}. Maine Sea Grant Publications. 73. Downeast Fish. Trail website. Orono, ME: The University of Maine.
Berghöfer, A., Wittmer, H., and Rauschmayer, F. (2008). Stakeholder participation in ecosystem-based approaches to fisheries management: A synthesis from European research projects. Mar. Policy 32, 243–253. doi: 10.1016/j.marpol.2007.09.014
Bisbal, G. A. (2003). The best available science for the management of anadromous salmonids in the Columbia River Basin. Can. J. Fish. Aquat. Sci. 59, 1952–1959. doi: 10.1139/F02-157
Bisbal, G. A. (2022). The researcher’s lament: Why do they ignore my science? Ecosphere 13, 1–8. doi: 10.1002/ecs2.4044
Blackwell, B. F., and Krohn, W. B. (1997). Spring foraging distribution and habitat selection by Double-crested Cormorants on the Penobscot River, Maine USA. Waterbirds 20, 66–76. doi: 10.2307/1521765
Blackwell, B. F., Krohn, W. B., and Allen, R. B. (2018). Foods of nestling double-crested cormorants in Penobscot Bay, Maine, USA : Temporal and spatial comparisons. Colon. Waterbirds 18, 199–208.
Boulêtreau, S., Carry, L., Meyer, E., Filloux, D., Menchi, O., Mataix, V., et al. (2020). High predation of native sea lamprey during spawning migration. Sci. Rep. 10:6122. doi: 10.1038/s41598-020-62916-w
Brosius, J. P., and Russell, D. (2003). Conservation from aboveimposing transboundary conservation. J. Sustain. For. 17, 39–65. doi: 10.1300/J091v17n01_04
Brush, M. J., Giani, M., Totti, C., Testa, J. M., Faganeli, J., Ogrinc, N., et al. (2020). “Eutrophication, harmful algae, oxygen depletion, and acidification,” in Coastal ecosystems in transition: A comparative analysis of the northern adriatic and chesapeake bay, eds T. C. Malone, A. Malej, and J. Faganeli (Washington, DC: American Geophysical Union). doi: 10.1002/9781119543626.ch5
Buffington, J. M., Montgomery, D. R., and Greenberg, H. M. (2004). Basin-scale availability of salmonid spawning gravel as influenced by channel type and hydraulic roughness in mountain catchments. Can. J. Fish. Aquat. Sci. 61, 2085–2096. doi: 10.1139/F04-141
Buxton, T. H. (2018). Flume simulations of salmon bioturbation effects on critical shear stress and bedload transport in rivers. River Res. Appl. 34, 357–371. doi: 10.1002/rra.3250
Call, E. (2015). River Birds as Indicator of Change in Riverine Ecosystems. Doctor of Philosophy (Ph D Thesis). Orono, ME: The University of Maine.
Camp, E. V., Kaemingk, M. A., Ahrens, R. N. M., Potts, W. M., Iii, W. E. P., Weyl, O. L. F., et al. (2020). Resilience management for conservation of inland recreational fisheries. Front. Ecol. Evol. 7:498. doi: 10.3389/fevo.2019.00498
Capotondi, A., Alexander, M. A., Bond, N. A., Curchitser, E. N., and Scott, J. D. (2012). Enhanced upper ocean stratification with climate change in the CMIP3 models. J. Geophys. Res. Ocean. 117:C04031. doi: 10.1029/2011JC007409
Carothers, C., Black, J., Langdon, S. J., Donkersloot, R., Ringer, D., Coleman, J., et al. (2021). Indigenous peoples and salmon stewardship: A critical relationship. Ecol. Soc. 26:16. doi: 10.5751/ES-11972-260116
Cassardo, C., and Jones, J. A. A. (2011). Managing water in a changing world. Water 3, 618–628. doi: 10.3390/w3020618
Chaloner, D. T., Lamberti, G. A., Merritt, R. W., Mitchell, N. L., Ostrom, P. H., and Wipfli, M. S. (2004). Variation in responses to spawning Pacific salmon among three south-eastern Alaska streams. Freshw. Biol. 49, 587–599. doi: 10.1111/j.1365-2427.2004.01213.x
Chen, Z., Kwon, Y. O., Chen, K., Fratantoni, P., and Gawarkiewicz, G. (2020). Long - Term SST variability on the Northwest Atlantic continental shelf and slope. Geophys. Res. Lett. 47:e2019GL085455. doi: 10.1029/2019GL085455
Cluer, B., and Thorne, C. (2014). A stream evolution model integrating habitat and ecosystem benefits. River Res. Appl. 30, 135–154. doi: 10.1002/rra.2631
Collins, M. J. (2009). Evidence for changing flood risk in New England since the late 20th century. J. Am. Water Resour. Assoc. 45, 279–290. doi: 10.1111/j.1752-1688.2008.00277.x
Collins, M. J. (2019). River flood seasonality in the Northeast United States: Characterization and trends. Hydrol. Process. 33, 687–698. doi: 10.1002/hyp.13355
Cook, C. N., Mascia, M. B., Schwartz, M. W., Possingham, H. P., and Fuller, R. A. (2013). Achieving conservation science that bridges the knowledge-action boundary. Conserv. Biol. 27, 669–678. doi: 10.1111/cobi.12050
Cook, T. L., Yellen, B. C., Woodruff, J. D., and Miller, D. (2015). Contrasting human versus climatic impacts on erosion. Geophys. Res. Lett. 42, 6680–6687. doi: 10.1002/2015GL064436
Cooke, S. J., Nguyen, V. M., Chapman, J. M., Reid, A. J., Landsman, S. J., Young, N., et al. (2021). Knowledge co-production: A pathway to effective fisheries management, conservation, and governance. Fisheries 46, 89–97. doi: 10.1002/fsh.10512
Crocker, J., Miller, M., Kircheirs, D., Harris, A., and Kocik, J. (2020). Atlantic salmon (Salmo salar) 5-Year Review: Summary and Evaluation 2020. Washington, DC: National Oceanic and Atmospheric Administration.
Crozier, L. G., Hendry, A. P., Lawson, P. W., Quinn, T. P., Mantua, N. J., Battin, J., et al. (2008). Potential responses to climate change in organisms with complex life histories: Evolution and plasticity in Pacific salmon. Evol. Appl. 1, 252–270. doi: 10.1111/j.1752-4571.2008.00033.x
Crozier, L. G., McClure, M. M., Beechie, T., Bograd, S. J., Boughton, D. A., Carr, M., et al. (2019). Climate vulnerability assessment for Pacific salmon and steelhead in the California Current Large Marine Ecosystem. PLoS One 14:e0217711. doi: 10.1371/journal.pone.0217711
Cunjak, R. A., Prowse, T. D., and Parrish, D. L. (1998). Atlantic salmon (Salmo salar) in winter: “The season of parr discontent”? Can. J. Fish. Aquat. Sci. 55, 161–180. doi: 10.1139/d98-008
Daigle, J. J., Michelle, N., Ranco, D. J., and Emery, M. R. (2019). Traditional lifeways and storytelling: Tools for adaptation and resilience to ecosystem change. Hum. Ecol. 47, 777–784. doi: 10.1007/s10745-019-00113-8
Dalton, C. M., Ellis, D., and Post, D. M. (2009). The impact of double-crested cormorant (Phalacrocorax auritus) predation on anadromous alewife (Alosa pseudoharengus) in south-central Connecticut, USA. Can. J. Fish. Aquat. Sci. 66, 177–186. doi: 10.1139/F08-198
Dame, R. F., and Allen, D. M. (1996). Between estuaries and the sea. J. Exp. Mar. Bio. Ecol. 200, 169–185. doi: 10.1016/S0022-0981(96)02642-1
Daniel, T. C., Muhar, A., Arnberger, A., Aznar, O., Boyd, J. W., Chan, K. M. A., et al. (2012). Contributions of cultural services to the ecosystem services agenda. Proc. Natl. Acad. Sci. U.S.A. 109, 8812–8819. doi: 10.1073/pnas.1114773109
Daniels, J., Sutton, S., Webber, D., and Carr, J. (2019). Extent of predation bias present in migration survival and timing of Atlantic salmon smolt (Salmo salar) as suggested by a novel acoustic tag. Anim. Biotelemetry 7:16. doi: 10.1186/s40317-019-0178-2
Darimont, C. T., Paquet, P. C., and Reimchen, T. E. (2008). Spawning salmon disrupt trophic coupling between wolves and ungulate prey in coastal British Columbia. BMC Ecol. 8:14. doi: 10.1186/1472-6785-8-14
Dauwalter, D. D., and McGurrin, J. (2013). Status Assessment of Coastal and Anadromous Brook Trout in the United States. Final report to National Fish and Wildlife Foundation. Arlington, VA: Trout Unlimited.
Dauwalter, D. D., McGurrin, J., Gallagher, M., and Hurley, S. (2014). “Status assessment of coastal and anadromous brook trout in the United States,” in Proceedings of the Wild Trout Symposium XI - Looking Back and Moving Forward (West Yellowstone, MT), 192–199.
Davis, J. P., Schultz, E. T., and Vokoun, J. (2009). Assessment of river herring and striped bass in the connecticut river: Abundance, population structure, and predator/prey interactions. EEB Articles. Paper 26. Available online at: http://digitalcommons.uconn.edu/eeb_articles/26 (accessed Oct 20, 2022).
De Eyto, E., Dalton, C., Dillane, M., Jennings, E., McGinnity, P., O’Dwyer, B., et al. (2016). The response of North Atlantic diadromous fish to multiple stressors, including land use change: A multidecadal study. Can. J. Fish. Aquat. Sci. 73, 1759–1769. doi: 10.1139/cjfas-2015-0450
Deegan, L. A. (1993). Nutrient and energy transport between estuaries and coastal marine ecosystems by fish migration. Can. J. Fish. Aquat. Sci. 50, 74–79.
DeFries, R., and Nagendra, H. (2017). Ecosystem management as a wicked problem. Science 356, 265–270. doi: 10.1126/science.aal1950
Denny, S. K., and Fanning, L. M. (2016). A Mi’kmaw perspective on advancing salmon governance in Nova Scotia, Canada: Setting the stage for collaborative co-existence. Int. Indig. Policy J. 7, 1–25. doi: 10.18584/iipj.2016.7.3.4
Denoncourt, C. E., and Stauffer, J. R. (1993). Feeding selectivity of the American Eel Anguilla rostrata (LeSueur) in the Upper Delaware River. Am. Midl. Nat. 129:301. doi: 10.2307/2426511
DiadES (2019). DiadES project - When fish and humans are looking for a better future. Available online at: https://diades.eu/ (accessed October 12, 2022).
Dias, B. S., Frisk, M. G., and Jordaan, A. (2019). Opening the tap: Increased riverine connectivity strengthens marine food web pathways. PLoS One 14:e0217008. doi: 10.1371/journal.pone.0217008
Dolan, T. E., Patrick, W. S., and Link, J. S. (2016). Delineating the continuum of marine ecosystem-based management : A US fisheries reference point perspective. ICES J. Mar. Sci. 73, 1042–1050.
Drinkwater, K. F., Miles, M., Medhaug, I., Helge, O., Kristiansen, T., Sundby, S., et al. (2014). The Atlantic Multidecadal Oscillation : Its manifestations and impacts with special emphasis on the Atlantic region north of 60 ° N. J. Mar. Syst. 133, 117–130. doi: 10.1016/j.jmarsys.2013.11.001
Drouineau, H., Carter, C., Rambonilaza, M., Beaufaron, G., Bouleau, G., Gassiat, A., et al. (2018a). River continuity restoration and diadromous fishes: Much more than an ecological issue. Environ. Manage. 61, 671–686. doi: 10.1007/s00267-017-0992-3
Drouineau, H., Durif, C., Castonguay, M., Mateo, M., Rochard, E., Verreault, G., et al. (2018b). Freshwater eels: A symbol of the effects of global change. Fish Fish. 19, 903–930. doi: 10.1111/faf.12300
Enterline, C. L., Chase, B. C., Street, P., Bedford, N., and Street, M. (2012). A Regional Conservation Plan for Anadromous Rainbow Smelt in the U. S. Gulf of Maine. Washington, DC: National Oceanic and Atmospheric Administration.
Fish, R., Church, A., and Winter, M. (2016). Conceptualising cultural ecosystem services: A novel framework for research and critical engagement. Ecosyst. Serv. 21, 208–217. doi: 10.1016/j.ecoser.2016.09.002
Flecker, A. S., McIntyre, P. B., Moore, J. W., Anderson, J. T., Taylor, B. W., and Hall, R. O. Jr. (2010). Migratory fishes as material and process subsidies in riverine ecosystems. Am. Fish. Soc. Symp. 73, 559–592.
Folke, C., Carpenter, S. R., Walker, B., Scheffer, M., Chapin, T., and Rockström, J. (2010). Resilience thinking : Integrating resilience, adaptability and transformability. Ecol. Soc. 15:20.
Foster, D., Swanson, F., Aber, J., Burke, I., Brokaw, N., Tilman, D., et al. (2003). The importance of land-use legacies to ecology and conservation. Bioscience 53, 77–88. doi: 10.1641/0006-35682003053[0077:TIOLUL]2.0.CO;2
Fremier, A. K., Yanites, B. J., and Yager, E. M. (2018). Sex that moves mountains: The influence of spawning fish on river profiles over geologic timescales. Geomorphology 305, 163–172. doi: 10.1016/j.geomorph.2017.09.033
Fried, H. A., and Schultz, E. T. (2006). Anadromous rainbow smelt and tomcod in connecticut: Assessment of populations, conservation status, and need for restoration plan. EEB Articles. 18. Available online at: https://opencommons.uconn.edu/eeb_articles/18 (accessed Oct 20, 2022).
Galbraith, H. S., Devers, J. L., Blakeslee, C. J., Cole, J. C., St. John White, B., Minkkinen, S., et al. (2018). Reestablishing a host–affiliate relationship: Migratory fish reintroduction increases native mussel recruitment. Ecol. Appl. 28, 1841–1852. doi: 10.1002/eap.1775
Gilligan-Lunda, E. K., Stich, D. S., Mills, K. E., Bailey, M. M., and Zydlewski, J. D. (2021). Climate change may cause shifts in growth and instantaneous natural mortality of American shad throughout their native range. Trans. Am. Fish. Soc. 150, 407–421. doi: 10.1002/tafs.10299
Goode, G. B. (1880). The use of agricultural fertilizers by the American Indians and the early English colonists. Am. Nat. 14, 473–479.
Gottesfeld, A. S., Hassan, M. A., Tunnicliffe, J. F., and Poirier, R. W. (2004). Sediment dispersion in salmon spawning streams: The influence of floods and salmon redd construction. J. Am. Water Resour. Assoc. 40, 1071–1086. doi: 10.1111/j.1752-1688.2004.tb01068.x
Granek, E. F., Polasky, S., Kappel, C. V., Reed, D. J., Stoms, D. M., Koch, E. W., et al. (2009). Ecosystem services as a common language for coastal ecosystem-based management. Conserv Biol. 24, 207–216. doi: 10.1111/j.1523-1739.2009.01355.x
Gray, T., and Hatchard, J. (2008). A complicated relationship: Stakeholder participation and the ecosystem-based approach to fisheries management. Mar. Policy 32, 158–168. doi: 10.1016/j.marpol.2007.09.002
Greene, C. H., Meyer-Gutbrod, E., Monger, B. C., McGarry, L. P., Pershing, A. J., Belkin, I. M., et al. (2013). Remote climate forcing of decadal-scale regime shifts in Northwest Atlantic shelf ecosystems. Limnol. Oceanogr. 58, 803–816. doi: 10.4319/lo.2013.58.3.0803
Greene, C. M., Jensen, D. W., Pess, G. R., Steel, E. A., and Beamer, E. (2005). Effects of environmental conditions during stream, estuary, and ocean residency on chinook salmon return rates in the Skagit River, Washington. Trans. Am. Fish. Soc. 134, 1562–1581. doi: 10.1577/t05-037.1
Greene, E., Zimmerman, J. L., Laney, R. W., and Thomas-Blate, J. C. (2009). Atlantic Coast Diadromous Fish Habitat: A Review of Utilization, Threats, Recommendations for Conservation, and Research Needs. Washington, DC: Atlantic States Marine Fisheries Commission.
Greene, K. E., Zimmerman, J. L., Laney, R. W., and Thomas-Blate, J. C.. 2009. Atlantic coast diadromous fish habitat: A review of utilization, threats, recommendations for conservation, and research needs. Atlantic states marine fisheries commission habitat management series No. 9. Atlantic States Marine Fisheries Commission Habitat Management: Washington, DC.
Grey, M., Blais, A. M., Hunt, B., and Vincent, A. C. J. (2006). The USA’s international trade in fish leather, from a conservation perspective. Environ. Conserv. 33, 100–108. doi: 10.1017/S0376892906003092
Gribbin, J. O. (2015). The glass eeling : Maine’s glass eel and elver regulations and their effects on maine’s native American tribes. Ocean Coast. Law J. 20:83.
Grout, D. E. (2006). Interactions between striped bass (Morone saxatilis) rebuilding programmes and the conservation of Atlantic salmon (Salmo salar) and other anadromous fish species in the USA. ICES J. Mar. Sci. 63, 1346–1352. doi: 10.1016/j.icesjms.2006.03.021
Guston, D. H. (2001). Boundary organizations in environmental policy and science: An introduction. Sci. Technol. Hum. Values 26, 399–408. doi: 10.1177/016224390102600401
Guyette, M. Q., Loftin, C. S., and Zydlewski, J. (2013). Carcass analog addition enhances juvenile Atlantic salmon (Salmo salar) growth and condition. Can. J. Fish. Aquat. Sci. 70, 860–870. doi: 10.1139/cjfas-2012-0496
Guyette, M. Q., Loftin, C. S., Zydlewski, J., and Cunjak, R. (2014). Carcass analogues provide marine subsidies for macroinvertebrates and juvenile Atlantic salmon in temperate oligotrophic streams. Freshw. Biol. 59, 392–406. doi: 10.1111/fwb.12272
Hall, C. J., Jordaan, A., and Frisk, M. G. (2011). The historic influence of dams on diadromous fish habitat with a focus on river herring and hydrologic longitudinal connectivity. Landsc. Ecol. 26, 95–107. doi: 10.1007/s10980-010-9539-1
Hall, C. J., Jordaan, A., and Frisk, M. G. (2012). Centuries of anadromous forage fish loss: Consequences for ecosystem connectivity and productivity. Bioscience 62, 723–731. doi: 10.1525/bio.2012.62.8.5
Happ, S. C., Dobson, G. C., and Rittenhouse, G. (1940). Some Principles of Accelerated Stream and Valley Sedimentation. U.S. Dept. Agr. Tech. Bull (No. 695). Washington, DC: U.S. Dept. of Agriculture.
Hare, J. A., Borggaard, D. L., Alexander, M. A., Bailey, M. M., Bowden, A. A., Damon-Randall, K., et al. (2021). A review of river herring science in support of species conservation and ecosystem restoration. Mar. Coast. Fish. 13, 627–664. doi: 10.1002/mcf2.10174
Hare, J. A., Kocik, J. F., and Link, J. S. (2019). Atlantic salmon recovery informing and informed by ecosystem-based fisheries management. Fisheries 44, 403–411. doi: 10.1002/fsh.10262
Hare, J. A., Morrison, W. E., Nelson, M. W., Stachura, M. M., Teeters, E. J., Griffis, R. B., et al. (2016). A vulnerability assessment of fish and invertebrates to climate change on the northeast u.s. continental shelf. PLoS One 11:e0146756. doi: 10.1371/journal.pone.0146756
Harvey, C. J., Kelble, C. R., and Schwing, F. B. (2017). Implementing “the IEA”: Using integrated ecosystem assessment frameworks, programs, and applications in support of operationalizing ecosystem-based management. ICES J. Mar. Sci. 74, 398–405. doi: 10.1093/icesjms/fsw201
Hatt, G., and Taylor, K. (1969). Arctic skin clothing in eurasia and america an ethnographic study. Artic Anthropol. 5, 3–132.
Heimbuch, D. G. (2008). Potential effects of striped bass predation on juvenile fish in the hudson river. Trans. Am. Fish. Soc. 137, 1591–1605. doi: 10.1577/t07-236.1
Helfield, J. M., and Naiman, R. J. (2003). Effects of salmon-derived nitrogen on riparian forest growth and implications for stream productivity. Ecology 84, 3396–3401. doi: 10.1890/02-3121
Hendee, J. T., and Flint, C. G. (2014). Incorporating cultural ecosystem services into forest management strategies for private landowners: An Illinois case study. For. Sci. 60, 1172–1179. doi: 10.5849/forsci.13-710
Hershey, H. (2021). Updating the consensus on fishway efficiency: A meta-analysis. Fish Fish. 22, 735–748. doi: 10.1111/faf.12547
Hiki, K., Asahina, K., Kato, K., Yamagishi, T., Omagari, R., Iwasaki, Y., et al. (2021). Acute toxicity of a tire rubber-derived chemical, 6PPD quinone, to freshwater fish and crustacean species. Environ. Sci. Technol. Lett. 8, 779–784. doi: 10.1021/acs.estlett.1c00453
Hildebrand, S. F. (1963). “Family clupeidae,” in Fishes of the Western North Atlantic, part 3, ed. Y. H. Olsen (New Haven, CT: Yale University), 257–454.
Hodgkins, G. A., and Dudley, R. W. (2006). Changes in the timing of winter-spring streamflows in eastern North America, 1913-2002. Geophys. Res. Lett. 33, 1–5. doi: 10.1029/2005GL025593
Hogg, R. S., Coghlan, S. M., Zydlewski, J., and Simon, K. S. (2014). Anadromous sea lampreys (Petromyzon marinus) are ecosystem engineers in a spawning tributary. Freshw. Biol. 59, 1294–1307. doi: 10.1111/fwb.12349
Hood, P. B., Able, K. W., and Grimes, C. B. (1988). Biology of the conger eel Conger oceanicus in the Mid-Atlantic Bight. Mar. Biol. 98, 587–596. doi: 10.1007/bf00391549
Hooke, R. L. (2000). On the history of human as geomorphic agents. Geology 28, 843–846. doi: 10.1130/0091-76132000028<0843:OTHOHA<2.3.CO;2
Hume, J. B., Almeida, P. R., Buckley, C. M., Criger, L. A., Madenjian, C. P., Robinson, K. F., et al. (2021). Managing native and non-native sea lamprey (Petromyzon marinus) through anthropogenic change: A prospective assessment of key threats and uncertainties. J. Great Lakes Res. 47, S704–S722. doi: 10.1016/j.jglr.2020.08.015
Huntington, T. G. (2010). Climate warming-induced intensification of the hydrologic cycle. An assessment of the published record and potential impacts on agriculture. Adv. Agron. 109, 1–53. doi: 10.1016/B978-0-12-385040-9.00001-3
Hutchings, J. A., and Reynolds, J. D. (2004). Marine fish population collapses: Consequences for recovery and extinction risk. Bioscience 54, 297–309. doi: 10.1641/0006-35682004054[0297:MFPCCF]2.0.CO;2
Jackson, S. T. (2021). Transformational ecology and climate change. Science 373, 1085–1086. doi: 10.1057/978-1-137-48377-5_10
Jentoft, S. (2007). Limits of governability: Institutional implications for fisheries and coastal governance. Mar. Policy 31, 360–370. doi: 10.1016/j.marpol.2006.11.003
Johannessen, C., Helm, P., Lashuk, B., Yargeau, V., and Metcalfe, C. D. (2021). The tire wear compounds 6PPD-Quinone and 1,3-Diphenylguanidine in an Urban Watershed. Arch. Environ. Contam. Toxicol. 82, 171–179. doi: 10.1007/s00244-021-00878-4
Johnson, K. M., Snyder, N. P., Castle, S., Hopkins, A. J., Waltner, M., Merritts, D. J., et al. (2019). Legacy sediment storage in New England river valleys: Anthropogenic processes in a postglacial landscape. Geomorphology 327, 417–437. doi: 10.1016/j.geomorph.2018.11.017
Juanes, F., Marks, R. E., McKown, K. A., and Conover, D. O. (1993). Predation by Age-0 Bluefish on Age-0 Anadromous Fishes in the Hudson River Estuary. Trans. Am. Fish. Soc. 122, 348–356. doi: 10.1577/1548-86591993122<0348:pbaboa<2.3.co;2
Kemp, D. B., Sadler, P. M., and Vanacker, V. (2020). The human impact on North American erosion, sediment transfer, and storage in a geologic context. Nat. Commun. 11:6012. doi: 10.1038/s41467-020-19744-3
Kerr, L. A., Cadrin, S. X., and Secor, D. H. (2010). The role of spatial dynamics in the stability, resilience, and productivity of an estuarine fish population. Ecol. Appl. 20, 497–507. doi: 10.1890/08-1382.1
Kircheis, F. W. (2004). Sea lamprey (Petromyzon marinus Linnaeus 1758). Carmel, ME: Fish and Wildlife.
Klauda, R. J., Moos, R. E., and Schmidt, R. E. (1988). “Life history of Atlantic tomcod, Microgadus tomcod, in the Hudson River Estuary, with emphasis on spatio-temporal distributions and movements,” in Fisheries Research in the Hudson River, ed. C. L. Smith (Albany, NY: State University of New York Press), 219–251.
Kocik, J. F., Hayes, S. A., Carlson, S. M., and Cluer, B. (2022). A Resist-Accept-Direct (RAD) future for Salmon in Maine and California: Salmon at the southern edge. Fish. Manag. Ecol. 29, 456–474. doi: 10.1111/fme.12575
Kreeger, D. A., Gatenby, C. M., and Bergstrom, P. W. (2018). Restoration potential of several native species of bivalve molluscs for water quality improvement in mid-atlantic watersheds. J. Shellfish Res. 37, 1121–1157. doi: 10.2983/035.037.0524
Kritzer, J. P., Hall, C. J., Hoppe, B., Ogden, C., and Cournane, J. M. (2022). Managing small fish at large scales: The emergence of regional policies for river herring in the Eastern United States. Fish. Magaz. 47, 435–445. doi: 10.1002/fsh.10802
Kynard, B., and Horgan, M. (2019). Long-term studies on restoration of Connecticut River anadromous sea lamprey, Petromyzon marinus Linnaeus 1758: Trend in annual adult runs, abundance cycle, and nesting. J. Appl. Ichthyol. 35, 1154–1163. doi: 10.1111/jai.13967
Lage, C., and Kornfield, I. (2006). Reduced genetic diversity and effective population size in an endangered Atlantic salmon (Salmo Salar) population from Maine, USA. Conserv. Genet. 7, 91–104. doi: 10.1007/s10592-005-8669-5
Lake, T. R. T., Ravana, K. R., and Saunders, R. (2012). Evaluating changes in diadromous species distributions and habitat accessibility following the penobscot river restoration project. Mar. Coast. Fish. 4, 284–293. doi: 10.1080/19425120.2012.675971
Langhans, S. D., Domisch, S., Balbi, S., Delacámara, G., Hermoso, V., Kuemmerlen, M., et al. (2019). Combining eight research areas to foster the uptake of ecosystem-based management in fresh waters. Aquat. Conserv. Mar. Freshw. Ecosyst. 29, 1161–1173. doi: 10.1002/aqc.3012
Le Bris, A., Mills, K. E., Wahle, R. A., Chen, Y., Alexander, M. A., Allyn, A. J., et al. (2018). Climate vulnerability and resilience in the most valuable North American fishery. Proc. Natl. Acad. Sci. U.S.A. 115, 1831–1836. doi: 10.1073/pnas.1711122115
Leach, L., Simpson, M., Stevens, J. R., and Cammen, K. (2022). Examining the impacts of pinnipeds on Atlantic salmon: The effects of river restoration on predator–prey interactions. Aquat. Conserv. Mar. Freshw. Ecosyst. 32, 645–657. doi: 10.1002/aqc.3783
Leim, A., and Scott, W. B. (1966). Fishes of the Atlantic coast of Canada. Fish. Resour. Can. Board Bull. No 155. Ottawa: Fisheries Research Board of Canada.
Lennox, R. J., Paukert, C., Aarestrup, K., Auger-Methe, M., Baumgartner, L., Birnie-Gauvin, K., et al. (2019). 100 Pressing Questions on the Future of Global Fish Migration Science, Conservation, and Policy. Front. Ecol. Evol. 7:286. doi: 10.3389/FEVO.2019.00286
Leopold, L. B. (1969). Quantitative Comparison of Some Aesthetic Factors Among Rivers. U. S. G. Survey, Circular 620. Washington, D.C: U.S. Geological Survey.
Leopold, L. B., Huppman, R., and Miller, A. (2005). Effects of urbanization in geomorphic forty-one years of observation. Proc. Am. Philos. Soc. 149, 349–371.
Lessard, J. A. L., Merritt, R. W., and Berg, M. B. (2009). Investigating the effect of marine-derived nutrients from spawning salmon on macroinvertebrate secondary production in southeast Alaskan streams. J. North Am. Benthol. Soc. 28, 683–693. doi: 10.1899/08-141.1
Levin, P. S., Essington, T. E., Marshall, K. N., Koehn, L. E., Anderson, L. G., Bundy, A., et al. (2018). Building effective fishery ecosystem plans. Mar. Policy 92, 48–57. doi: 10.1016/j.marpol.2018.01.019
Li, M., Peng, C., Wang, M., Xue, W., Zhang, K., Wang, K., et al. (2017). The carbon flux of global rivers: A re-evaluation of amount and spatial patterns. Ecol. Indic. 80, 40–51. doi: 10.1016/j.ecolind.2017.04.049
Liebich, K. B., Kocik, J. F., and Taylor, W. W. (2018). Reclaiming a space for diadromous fish in the public psyche and sense of place. Fisheries 43, 231–240. doi: 10.1002/fsh.10063
Limburg, K. E., and Waldman, J. R. (2009). Dramatic declines in north Atlantic diadromous fishes. Bioscience 59, 955–965. doi: 10.1525/bio.2009.59.11.7
Link, J. S. (2021). Evidence of ecosystem overfishing in U.S. large marine ecosystems. ICES J. Mar. Sci. 78, 3176–3201.
Link, J. S., and Browman, H. I. (2014). Integrating what? Levels of marine ecosystem-based assessment and management. ICES J. Mar. Sci. 71, 1170–1173. doi: 10.1093/icesjms/fsu026
Link, J. S., and Browman, H. I. (2017). Operationalizing and implementing ecosystem-based management. ICES J. Mar. Sci. 74, 379–381. doi: 10.1093/icesjms/fsw247
Lopez, J. W., Allen, D. C., and Vaughn, C. C. (2022). White-tailed deer consumption of emergent macrophytes mediates aquatic-to-terrestrial nutrient flows. Ecol. Evol. 12:e9257. doi: 10.1002/ece3.9257
Lotze, H. K., and Milewski, I. (2004). Two centuries of multiple human impacts and successive changes in a north Atlantic food web. Ecol. Appl. 14, 1428–1447. doi: 10.1890/03-5027
Lynch, A. J., Thompson, L. M., Beever, E. A., Cole, D. N., Engman, A. C., Hawkins Hoffman, C., et al. (2021). Managing for RADical ecosystem change: Applying the resist-accept-direct (RAD) framework. Front. Ecol. Environ. 19:461–469. doi: 10.1002/fee.2377
Lynch, P. D., Nye, J. A., Hare, J. A., Stock, C. A., Alexander, M. A., Scott, J. D., et al. (2015). Projected ocean warming creates a conservation challenge for river herring populations. ICES J. Mar. Sci. 72, 374–387. doi: 10.1093/icesjms/fsu134
Lynn, K., Daigle, J., Hoffman, J., Lake, F., Michelle, N., Ranco, D., et al. (2013). The impacts of climate change on tribal traditional foods. Clim. Change 120, 545–556. doi: 10.1007/s10584-013-0736-1
Lytle, D. A., and Poff, N. L. (2004). Adaptation to natural flow regimes. Trends Ecol. Evol. 19, 94–100.
MacArthur, R. (1955). Fluctuations of animal populations and a measure of community stability. Ecology 36, 533–536.
Macavoy, S. E., Garman, G. C., and Macko, S. A. (2009). Anadromous fish as marine nutrient vectors. Fish. Bull. 107, 165–174. doi: 10.1007/s004420050980
Magilligan, F. J., Nislow, K. H., Fisher, G. B., Wright, J., Mackey, G., and Laser, M. (2008). The geomorphic function and characteristics of large woody debris in low gradient rivers, coastal Maine, USA. Geomorphology 97, 467–482. doi: 10.1016/j.geomorph.2007.08.016
Maine Rivers [MR] (2021). Alewife Trail Map. Maine Rivers. Available online at: https://mainerivers.org/ (accessed November 20, 2021).
Malone, T. C., and Newton, A. (2020). The globalization of cultural eutrophication in the coastal ocean: Causes and consequences. Front. Mar. Sci. 7:670. doi: 10.3389/fmars.2020.00670
Mather, M. E. (1998). The role of context-specific predation in understanding patterns exhibited by anadromous salmon. Can. J. Fish. Aquat. Sci. 55, 232–246. doi: 10.1139/d98-002
Mathewson, D. D., Hocking, M. D., and Reimchen, T. E. (2003). Nitrogen uptake in riparian plant communities across a sharp ecological boundary of salmon density. BMC Ecol. 3:4. doi: 10.1186/1472-6785-3-4
Mattocks, S. R. (2016). Ecological Consequences of Lost Anadromous Forage Fish in Freshwater Ecosystems. Masters Theses. Amherst, MA: University of Massachusetts Amherst.
Mattocks, S., Hall, C. J., and Jordaan, A. (2017). Damming, lost connectivity, and the historical role of anadromous fish in freshwater ecosystem dynamics. Bioscience 67, 713–728. doi: 10.1093/biosci/bix069
McCabe, G. J., and Wolock, D. M. (2002). A step increase in streamflow in the conterminous United States. Geophys. Res. Lett. 29, 8–11. doi: 10.1029/2002GL015999
McCormick, S. D., and Regish, A. M. (2018). Effects of ocean acidification on salinity tolerance and seawater growth of Atlantic salmon Salmo salar smolts. J. Fish Biol. 93, 560–566. doi: 10.1111/jfb.13656
Mcdermott, S. P., Bransome, N. C., Sutton, S. E., Smith, B. E., Link, J. S., and Miller, T. J. (2015). Quantifying alosine prey in the diets of marine piscivores in the Gulf of Maine. J. Fish Biol. 86, 1811–1829. doi: 10.1111/jfb.12692
Mcdowall, R. M. (1999). Short communication Di ff erent kinds of diadromy : Different kinds of conservation problems. ICES J. Mar. Sci. 56, 410–413.
McDowall, R. M., Haro, A., Smith, K. L., Rulifson, R. A., Moffitt, C. M., Klauda, R. J., et al. (2009). “Making the best of two worlds: Diadromy in the evolution, ecology, and conservation of aquatic organisms,” in Proceedings of the International Symposium “Challenges for Diadromous Fishes in a Dynamic Global Environment”. American Fisheries Society Symposium 69, (Bethesda, MD: American Fisheries Society), 1–22.
Merg, M. L., Dézerald, O., Kreutzenberger, K., Demski, S., Reyjol, Y., Usseglio-Polatera, P., et al. (2020). Modeling diadromous fish loss from historical data: Identification of anthropogenic drivers and testing of mitigation scenarios. PLoS One 15:e0236575. doi: 10.1371/journal.pone.0236575
Miles, N. G., Walsh, C. T., Butler, G., Ueda, H., and West, R. J. (2014). Australian diadromous fishes - Challenges and solutions for understanding migrations in the 21st century. Mar. Freshw. Res. 65, 12–24. doi: 10.1071/MF12340
Montevecchi, W. A. (2007). Binary dietary responses of northern gannets Sula bassana indicate changing food web and oceanographic conditions. Mar. Ecol. Prog. Ser. 352, 213–220. doi: 10.3354/meps07075
Montgomery, D. R., Buffington, J. M., Peterson, N. P., Schuett-Hames, D., and Quinn, T. P. (1996). Stream-bed scour, egg burial depths, and the influence of salmonid spawning on bed surface mobility and embryo survival. Can. J. Fish. Aquat. Sci. 53, 1061–1070. doi: 10.1139/f96-028
Moore, J. W., and Schindler, D. E. (2004). Nutrients export from freshwater ecosystems by anadromous sockeye salmon (oncorhynchus nerka). Can. J. Aquat. Sci. 61, 1582–1589.
Morris, J. A., Rulifson, R. A., and Toburen, L. H. (2003). Life history strategies of striped bass, Morone saxatilis, populations inferred from otolith microchemistry. Fish. Res. 62, 53–63. doi: 10.1016/S0165-7836(02)00246-1
Munsch, S. H., Greene, C. M., Johnson, R. C., Satterthwaite, W. H., Imaki, H., Brandes, P. L., et al. (2020). Science for integrative management of a diadromous fish stock: Interdependencies of fisheries, flow, and habitat restoration. Can. J. Fish. Aquat. Sci. 77, 1487–1504. doi: 10.1139/cjfas-2020-0075
NASCO (2022). Conserving and Restoring Wild Atlantic Salmon. Edinburgh: North Atlantic Salmon Conservation Organization.
National Marine Fisheries Service [NMFS] (2019). Status Review Report: Alewife (Alosa pseudoharengus) and Blueback Herring (Alosa aestivalis). Final Report to the National Marine Fisheries Service, Office of Protected Resources. Silver Spring, MD: National Marine Fisheries Service, 160.
National Oceanic and Atmospheric Administration [NOAA] (2021a). Listing Species Under the Endangered Species Act. Washington, DC: National Oceanic and Atmospheric Administration.
National Oceanic and Atmospheric Administration [NOAA] (2021b). Shortnose sturgeong. NOAA - Species Directory, 4. Available online at: https://www.fisheries.noaa.gov/species/shortnose-sturgeon (accessed May 17, 2021).
National Oceanic and Atmospheric Administration [NOAA] (2022). Laws & Policies: Endangered Species Act. Available online at: https://www.fisheries.noaa.gov/topic/laws-policies#endangered-species-act (accessed October 12, 2022).
Nieland, J. L., Sheehan, T. F., and Saunders, R. (2015). Assessing demographic effects of dams on diadromous fish: A case study for Atlantic salmon in the Penobscot River, Maine. ICES J. Mar. Sci. 72, 2423–2437. doi: 10.4135/9781412953924.n678
Olden, J. D., Vitule, J. R. S., Cucherousset, J., and Kennard, M. J. (2020). There’s more to fish than just food: Exploring the diverse ways that fish contribute to human society. Fisheries 45, 453–464. doi: 10.1002/fsh.10443
Orach, K., and Schlüter, M. (2021). Understanding the dynamics of fish politics: The role of diverse actor interactions in transformations towards co-management. Environ. Sci. Policy 124, 195–205. doi: 10.1016/j.envsci.2021.06.010
Ostergren, D. M., Abrams, J. B., and Lowe, K. A. (2008). Fire in the forest: Public perceptions of ecological restoration in north-central arizona. Ecol. Restor. 26, 51–60.
Ouellet, V., St-hilaire, A., Dugdale, S. J., Hannah, D. M., Krause, S., Ouellet-P, S., et al. (2020). River temperature research and practice: Recent challenges and emerging opportunities for managing thermal habitat conditions in stream ecosystems. Sci. Total Environ. 736:139679. doi: 10.1016/j.scitotenv.2020.139679
Parker, B., Andreou, D., Green, I. D., and Britton, J. R. (2021). Microplastics in freshwater fishes: Occurrence, impacts and future perspectives. Fish Fish. 22, 467–488. doi: 10.1111/faf.12528
Pauly, D. (1995). Anecdotes and the shifting baseline syndrome of fisheries. Trends Ecol. Evol. 10:430. doi: 10.1016/s0169-5347(00)89171-5
Pess, G. R., Quinn, T. P., Gephard, S. R., and Saunders, R. (2014). Re-colonization of Atlantic and Pacific rivers by anadromous fishes: Linkages between life history and the benefits of barrier removal. Rev. Fish Biol. Fish. 24, 881–900. doi: 10.1007/s11160-013-9339-1
Petitgas, P., Secor, D. H., McQuinn, I., Huse, G., and Lo, N. (2010). Stock collapses and their recovery: Mechanisms that establish and maintain life-cycle closure in space and time. ICES J. Mar. Sci. 67, 1841–1848. doi: 10.1093/icesjms/fsq082
Petticrew, E. L., Rex, J. F., and Albers, S. J. (2011). Bidirectional delivery of organic matter between freshwater and marine systems: The role of flocculation in Pacific salmon streams. J. North Am. Benthol. Soc. 30, 779–786. doi: 10.1899/10-133.1
Phillips, A. (1998). The nature of cultural landscapes - nature conservation perspective. Landsc. Res. 23, 21–38. doi: 10.1080/01426399808706523
Proshansky, H. M., Fabian, A. K., and Kaminoff, R. (1983). Place-identity: Physical world socialization of the self. J. Environ. Psychol. 3, 57–83. doi: 10.4324/9781315816852
Ramankutty, N., Heller, E., and Rhemtulla, J. (2010). Prevailing myths about agricultural abandonment and forest regrowth in the United States. Ann. Assoc. Am. Geogr. 100, 502–512. doi: 10.1080/00045601003788876
Ray, G. C. (2005). Connectivities of estuarine fishes to the coastal realm. Estuar. Coast. Shelf Sci. 64, 18–32. doi: 10.1016/j.ecss.2005.02.003
Reid, A. J., Eckert, L. E., Lane, J. F., Young, N., Hinch, S. G., Darimont, C. T., et al. (2021). “Two-Eyed Seeing”: An indigenous framework to transform fisheries research and management. Fish Fish. 22, 243–261. doi: 10.1111/faf.12516
Rice, K. C., and Hirsch, R. M. (2012). Spatial and Temporal Trends in Runoff at Long-Term Streamgages within and Near the Chesapeake Bay Watershed. Reston, VA: U.S. Geological Survey, doi: 10.3133/sir20125151
Rieman, B. E., and Dunham, J. B. (2000). Metapopulations and salmonids: A synthesis of life history patterns and empirical observations. Ecol. Freshw. Fish 9, 51–64. doi: 10.1034/j.1600-0633.2000.90106.x
Rosset, J., Roy, A. H., Gahagan, B. I., Whiteley, A. R., Armstrong, M. P., Sheppard, J. J., et al. (2017). Temporal patterns of migration and spawning of river herring in coastal Massachusetts. Trans. Am. Fish. Soc. 146, 1101–1114. doi: 10.1080/00028487.2017.1341851
Roy, M. L., and Le Pichon, C. (2017). Modelling functional fish habitat connectivity in rivers: A case study for prioritizing restoration actions targeting brown trout. Aquat. Conserv. Mar. Freshw. Ecosyst. 27, 927–937. doi: 10.1002/aqc.2786
Rulifson, R. A. (1994). “Status of anadromous Alosa along the East Coastof North America,” in Anadromous Alosa Symposium, eds J. E. Cooper, R. T. Eades, R. J. Klauda, and J. G. Loesch (Bethesda, MD: American Fisheries Society), 134–158.
Rulifson, R. A., and Batsavage, C. F. (2014). Population demographics of hickory shad (Alosa mediocris) during a period of population growth. Fish. Bull. 112, 221–236. doi: 10.7755/FB.112.2-3.8
Rutherford, P. R. (1970). The Dictionnary of Maine Place-Names. Freeport, ME: The Bond Wheelwright Company.
Samways, K. M., and Cunjak, R. A. (2015). Increases in benthic community production and metabolism in response to marine-derived nutrients from spawning Atlantic salmon (Salmo salar). Freshw. Biol. 60, 1647–1658. doi: 10.1111/fwb.12597
Samways, K. M., Blair, T. J., Charest, M. A., and Cunjak, R. A. (2017). Effects of spawning Atlantic salmon (Salmo salar) on total lipid content and fatty acid composition of river food webs. Ecosphere 8:e01818. doi: 10.1002/ecs2.1818
Samways, K. M., Quiñones-Rivera, Z. J., Leavitt, P. R., and Cunjak, R. A. (2015). Spatiotemporal responses of algal, fungal, and bacterial biofilm communities in Atlantic rivers receiving marine-derived nutrient inputs. Freshw. Sci. 34, 881–896. doi: 10.1086/681723
Saunders, R., Hachey, M. A., and Fay, C. W. (2006). Maine’s diadromous fish community: Past, present, and implications for Atlantic salmon recovery. Fisheries 31, 537–547. doi: 10.1577/1548-8446200631[537:MDFC]2.0.CO;2
Schaich, H., Bieling, C., and Plieninger, T. (2010). Linking ecosystem services with cultural landscape research. Ecol. Perspect. Sci. Soc. 19, 269–277. doi: 10.14512/gaia.19.4.9
Schindler, D. E., Armstrong, J. B., and Reed, T. E. (2015). The portfolio concept in ecology and evolution. Front. Ecol. Environ. 13:257–263. doi: 10.1890/140275
Schindler, D. E., Armstrong, J. B., Bentley, K. T., Jankowski, K., Lisi, P. J., and Payne, L. X. (2013). Riding the crimson tide: Mobile terrestrial consumers track phenological variation in spawning of an anadromous fish. Biol. Lett. 9:20130048. doi: 10.1098/rsbl.2013.0048
Schindler, D. E., Carpenter, S. R., Cole, J. J., Kitchell, J. F., and Pace, M. L. (1997). Influence of food web structure on carbon exchange between lakes and the atmosphere. Science 277, 248–251. doi: 10.1126/science.277.5323.248
Schmitt, J. D., Hallerman, E. M., Bunch, A., Moran, Z., Emmel, J. A., and Orth, D. J. (2017). Predation and prey selectivity by nonnative catfish on migrating alosines in an atlantic slope estuary. Mar. Coast. Fish. 9, 108–125. doi: 10.1080/19425120.2016.1271844
Secor, D. H., O’Brien, M. H. P., Gahagan, B. I., Carter Watterson, J., and Fox, D. A. (2020). Differential migration in Chesapeake Bay striped bass. PLoS One 15:e0233103. doi: 10.1371/journal.pone.0233103
Shepherd, G. R., Nelson, G. A., Rago, P. J., Richards, R. A., and Goodyear, C. P. (2018). NOAA Technical Memorandum NMFS-NE-246 A Chronicle of Striped Bass Population Restoration and Conservation in the Northwest Atlantic, 1979-2016. Washington, DC: National Oceanic and Atmospheric Administration.
Smith, K. M., Byron, C. J., and Sulikowski, J. A. (2016). Modeling predator-prey linkages of diadromous fishes in an estuarine food web. Mar. Coast. Fish. 8, 476–491. doi: 10.1080/19425120.2016.1194920
Soga, M., and Gaston, K. J. (2018). Shifting baseline syndrome: Causes, consequences, and implications. Front. Ecol. Environ. 16:222–230. doi: 10.1002/fee.1794
Somers, K. A., Bernhardt, E. S., Grace, J. B., Hassett, B. A., Sudduth, E. B., Wang, S., et al. (2013). Streams in the urban heat island: Spatial and temporal variability in temperature. Freshw. Sci. 32, 309–326. doi: 10.1899/12-046.1
Stedman, R. C. (2002). Toward a social psychology of place: Predicting behavior from place-based cognitions, attitude, and identity. Environ. Behav. 34, 561–581. doi: 10.1177/0013916502034005001
Stevens, J. R., Saunders, R., and Duffy, W. (2021). Evidence of life cycle diversity of river herring in the penobscot river estuary. Maine. Mar. Coast. Fish. 13, 292–305. doi: 10.1002/mcf2.10157
Steyaert, L. T., and Knox, R. G. (2008). Reconstructed historical land cover and biophysical parameters for studies of land-atmosphere interactions within the eastern United States. J. Geophys. Res. Atmos. 113:D02101. doi: 10.1029/2006JD008277
Stich, D. S., Zydlewski, G. B., Kocik, J. F., and Zydlewski, J. D. (2015). Linking behavior, physiology, and survival of atlantic salmon smolts during estuary migration. Mar. Coast. Fish. 7, 68–86. doi: 10.1080/19425120.2015.1007185
Taylor, P., Wipfli, M. S., and Baxter, C. V. (2010). Subsidies in salmonid watersheds linking ecosystems, food webs, and fish production : Subsidies in salmonid watersheds suministros alimenticios que controlan la productividad en peces : Modelación del flujo de presas en redes fluviales. Fisheries 35, 373–387. doi: 10.1577/1548-8446-35.8.373
Tétard, S., Feunteun, E., Bultel, E., Gadais, R., Bégout, M. L., Trancart, T., et al. (2016). Poor oxic conditions in a large estuary reduce connectivity from marine to freshwater habitats of a diadromous fish. Estuar. Coast. Shelf Sci. 169, 216–226. doi: 10.1016/j.ecss.2015.12.010
The Common International Classification of Ecosystem Services [CICES] (2022). Towards a Common Classification of Ecosystem Services. Common Int. Classif. Ecosyst. Serv. Eur. Environ. Agency. Available online at: https://cices.eu/ (accessed January 3, 2022).
The Connecticut River Salmon Association [CRSA] (2021). SALMON-IN-SCHOOLS. Available online at: https://www.ctriversalmon.org/salmon-in-schools/ (accessed November 3, 2021).
Thompson, J. R., Carpenter, D. N., Cogbill, C. V., and Foster, D. R. (2013). Four centuries of change in Northeastern United States forests. PLoS One 8:e72540. doi: 10.1371/journal.pone.0072540
Thompson, L. M., Lynch, A. J., Beever, E. A., Engman, A. C., Falke, J. A., Jackson, S. T., et al. (2021). Responding to ecosystem transformation: Resist, accept, or direct? Fisheries 46, 8–21. doi: 10.1002/fsh.10506
Thorstad, E. B., Bliss, D., Breau, C., Damon-Randall, K., Sundt-Hansen, L. E., Hatfield, E. M. C., et al. (2021). Atlantic salmon in a rapidly changing environment—Facing the challenges of reduced marine survival and climate change. Aquat. Conserv. Mar. Freshw. Ecosyst. 31, 2654–2665. doi: 10.1002/aqc.3624
Tickner, D., Opperman, J. J., Abell, R., Acreman, M., Arthington, A. H., Bunn, S. E., et al. (2020). Bending the curve of global freshwater biodiversity loss: An emergency recovery plan. Bioscience 70, 330–342. doi: 10.1093/biosci/biaa002
Tillotson, M. D., Sheehan, T. F., Ellingson, B., Haas-Castro, R. E., Olmos, M., and Mills, K. E. (2021). Non-stationary effects of growth on the survival of North American Atlantic salmon (Salmo salar). ICES J. Mar. Sci. 78, 2967–2982. doi: 10.1093/icesjms/fsab174
Timm, A., Ouellet, V., and Daniels, M. (2020). Swimming through the urban heat island: Can thermal mitigation practices reduce the stress? River Res. Appl. 36, 1973–1984. doi: 10.1002/rra.3732
Timm, A., Ouellet, V., and Daniels, M. D. (2021). Riparian land cover, water temperature variability and thermal stress for aquatic species in urban streams. Water 13:2731. doi: 10.3390/w13192732
Tommasi, D., Nye, J., Stock, C., Hare, J. A., Alexander, M., and Drew, K. (2015). Effect of environmental conditions on juvenile recruitment of alewife (Alosa pseudoharengus) and blueback herring (Alosa aestivalis) in fresh water: A coastwide perspective. Can. J. Fish. Aquat. Sci. 72, 1037–1047. doi: 10.1139/cjfas-2014-0259
Tonra, C. M., Sager-Fradkin, K., and Marra, P. P. (2016). Barriers to salmon migration impact body condition, offspring size, and life history variation in an avian consumer. Ecography 39, 1056–1065. doi: 10.1111/ecog.02014
Tonra, C. M., Sager-Fradkin, K., Morley, S. A., Duda, J. J., and Marra, P. P. (2015). The rapid return of marine-derived nutrients to a freshwater food web following dam removal. Biol. Conserv. 192, 130–134. doi: 10.1016/j.biocon.2015.09.009
Tribot, A. S., Carabeux, Q., Deter, J., Claverie, T., Villéger, S., and Mouquet, N. (2018). Confronting species aesthetics with ecological functions in coral reef fish. Sci. Rep. 8:11733. doi: 10.1038/s41598-018-29637-7
Turner, S. M., and Limburg, K. E. (2016). Juvenile river herring habitat use and marine emigration trends: Comparing populations. Oecologia 180, 77–89. doi: 10.1007/s00442-015-3443-y
Twining, C. W., Palkovacs, E. P., Friedman, M. A., Hasselman, D. J., and Post, D. M. (2017). Nutrient loading by anadromous fishes: Species-specific contributions and the effects of diversity. Can. J. Fish. Aquat. Sci. 74, 609–619. doi: 10.1139/cjfas-2016-0136
Twining, C. W., West, D. C., and Post, D. M. (2013). Historical changes in nutrient inputs from humans and anadromous fishes in New England’s coastal watersheds. Limnol. Oceanogr. 58, 1286–1300. doi: 10.4319/lo.2013.58.4.1286
US Atlantic Salmon Assessment Committee [USASAC] (2004). Annual report of the U.S. Atlantic Salmon Assessment Committee Report 16—2003 Activities. Woods Hole, MA: U.S. Atlantic Salmon Assessment Committee.
US Atlantic Salmon Assessment Committee [USASAC] (2020). Annual Report of the U.S. Atlantic Salmon Assessment Committee No 32: 2019 Activities. Portland, ME: US Atlantic Salmon Assessment Committee.
USACE (2020). National inventory of dams. U.S. Army corp Eng. Available online at: https://nid.sec.usace.army.mil/#/ (accessed January 3, 2022).
Van Vliet, M. T. H., Franssen, W. H. P., Yearsley, J. R., Ludwig, F., Haddeland, I., Lettenmaier, D. P., et al. (2013). Global river discharge and water temperature under climate change. Glob. Environ. Chang. 23, 450–464. doi: 10.1016/j.gloenvcha.2012.11.002
Verhelst, P., Reubens, J., Buysse, D., Goethals, P., Van Wichelen, J., and Moens, T. (2021). Toward a roadmap for diadromous fish conservation: The Big Five considerations. Front. Ecol. Environ. 19:396–403. doi: 10.1002/fee.2361
Vierros, M. K., Harrison, A. L., Sloat, M. R., Crespo, G. O., Moore, J. W., Dunn, D. C., et al. (2020). Considering indigenous peoples and local communities in governance of the global ocean commons. Mar. Policy 119:104039. doi: 10.1016/j.marpol.2020.104039
Waldman, J. (2010). The Natural World Vanishes: How Species Cease To Matter. New Haven, CT: Yale Environment 360.
Waldman, J. R. (2013). Running Silver: Restoring Atlantic Rivers And Their Great Fish Migrations. Guilford, CT: Lyons Press.
Waldman, J. R., and Quinn, T. P. (2022). North American diadromous fishes : Drivers of decline and potential for recovery in the Anthropocene. Sci Adv. 8:eabl5486. doi: 10.1126/sciadv.abl5486
Waldman, J., Wilson, K. A., Mather, M., and Snyder, N. P. (2016). A resilience approach can improve anadromous fish restoration. Fisheries 41, 116–126. doi: 10.1080/03632415.2015.1134501
Walsh, J. C., Pendray, J. E., Godwin, S. C., Artelle, K. A., Kindsvater, H. K., Field, R. D., et al. (2020). Relationships between Pacific salmon and aquatic and terrestrial ecosystems: Implications for ecosystem-based management. Ecology 101:e03060. doi: 10.1002/ecy.3060
Walter, R. C., and Merritts, D. J. (2008). Natural streams and the legacy of water-powered mills. Science 319, 299–304. doi: 10.1126/science.1151716
Walters, A. W., Barnes, R. T., and Post, D. M. (2009). Anadromous alewives (Alosa pseudoharengus) contribute marine-derived nutrients to coastal stream food webs. Can. J. Fish. Aquat. Sci. 66, 439–448. doi: 10.1139/F09-008
Watz, J., Aldvén, D., Andreasson, P., Aziz, K., Blixt, M., Calles, O., et al. (2021). Atlantic salmon in regulated rivers: Understanding river management through the ecosystem services lens. Fish Fish. 1–14. doi: 10.1111/faf.12628
Weaver, D. M., Coghlan, S. M., and Zydlewski, J. (2016). Sea lamprey carcasses exertlocal and variable food web effects in a nutrient-limited Atlantic coastal stream. Can. J. Fish. Aquat. Sci. 73, 1616–1625. doi: 10.1139/cjfas-2015-0506
Weaver, D. M., Coghlan, S. M., and Zydlewski, J. (2018a). Effects of sea lamprey substrate modification and carcass nutrients on macroinvertebrate assemblages in a small atlantic coastal stream. J. Freshw. Ecol. 33, 19–30. doi: 10.1080/02705060.2017.1417168
Weaver, D. M., Coghlan, S. M., and Zydlewski, J. (2018b). The influence of nutrients from carcasses of sea lamprey (Petromyzon marinus) on larval growth and spawner abundance. Fish. Bull. 116, 142–152. doi: 10.7755/FB.116.2.3
Weaver, D., Coghlan, S. M., and Zydlewski, J. (2017). Sea lamprey carcasses exert local and variable food web effects in a nutrient-limited 7 Atlantic Coastal Stream. J. Chem. Inf. Model. 53, 21–25.
Weiskopf, S. R., Rubenstein, M. A., Crozier, L. G., Gaichas, S., Grif, R., Halofsky, J. E., et al. (2020). Science of the total environment climate change effects on biodiversity, ecosystems, ecosystem services, and natural resource management in the United States. Sci Total Environ. 733:137782. doi: 10.1016/j.scitotenv.2020.137782
Wilkinson, B. H. (2005). Humans as geologic agents: A deep-time perspective. Geology 33, 161–164. doi: 10.1130/G21108.1
Wilson, K. A., McBride, M. F., Bode, M., and Possingham, H. P. (2006). Prioritizing global conservation efforts. Nature 440, 337–340. doi: 10.1038/nature04366
Wipfli, M. S. (1997). Terrestrial invertebrates as salmonid prey and nitrogen sources in streams: Contrasting old-growth and young-growth riparian forests in southeastern Alaska, U.S.A. Can. J. Fish. Aquat. Sci. 54, 1259–1269. doi: 10.1139/f97-034
Wippelhauser, G. (2021). Recovery of diadromous fishes: A Kennebec River Case Study. Trans. Am. Fish. Soc. 150, 277–290. doi: 10.1002/tafs.10292
Worm, B., Barbier, E. B., Beaumont, N., Duffy, J. E., Folke, C., Halpern, B. S., et al. (2006). Impacts of biodiversity loss on ocean ecosystem services. Science 314, 787–790. doi: 10.1126/science.1132294
Wright-Fairbanks, E. K., Miles, T. N., Cai, W. J., Chen, B., and Saba, G. K. (2020). Autonomous observation of seasonal carbonate chemistry dynamics in the mid-atlantic bight. J. Geophys. Res. Ocean 125:e2020JC016505. doi: 10.1029/2020JC016505
Zhang, Q., Hirsch, R. M., and Ball, W. P. (2016). Long-term changes in sediment and nutrient delivery from conowingo dam to chesapeake bay: Effects of reservoir sedimentation. Environ. Sci. Technol. 50, 1877–1886. doi: 10.1021/acs.est.5b04073
Zhang, Y., Negishi, J. N., Richardson, J. S., and Kolodziejczyk, R. (2003). Impacts of marine-derived nutrients on stream ecosystem functioning. Proc. R. Soc. B Biol. Sci. 270, 2117–2123. doi: 10.1098/rspb.2003.2478
Keywords: conceptual model, sea-run fish population, fish community, ecosystem services, ecosystem connections, ecosystem-based management, portfolio effect
Citation: Ouellet V, Collins MJ, Kocik JF, Saunders R, Sheehan TF, Ogburn MB and Trinko Lake T (2022) The diadromous watersheds-ocean continuum: Managing diadromous fish as a community for ecosystem resilience. Front. Ecol. Evol. 10:1007599. doi: 10.3389/fevo.2022.1007599
Received: 30 July 2022; Accepted: 10 October 2022;
Published: 08 November 2022.
Edited by:
Diana Hamilton, Mount Allison University, CanadaReviewed by:
Sukran Yalcin Ozdilek, Çanakkale Onsekiz Mart University, TurkeyTim Arciszewski, Alberta Environment and Parks, Canada
Copyright © 2022 Ouellet, Collins, Kocik, Saunders, Sheehan, Ogburn and Trinko Lake. This is an open-access article distributed under the terms of the Creative Commons Attribution License (CC BY). The use, distribution or reproduction in other forums is permitted, provided the original author(s) and the copyright owner(s) are credited and that the original publication in this journal is cited, in accordance with accepted academic practice. No use, distribution or reproduction is permitted which does not comply with these terms.
*Correspondence: Valerie Ouellet, dmFsZXJpZS5vdWVsbGV0QG5vYWEuZ292