- 1Center for Catastrophic Risk Management, Social Science Matrix, University of California, Berkeley, Berkeley, CA, United States
- 2Department Community and Ecosystem Ecology, Leibniz Institute of Freshwater Ecology and Inland Fisheries (IGB), Berlin, Germany
- 3Department of Geography, Humboldt-Universität zu Berlin, Berlin, Germany
- 4School of Life Sciences, Technical University of Munich, Freising, Germany
- 5Department of Landscape Architecture and Environmental Planning, College of Environmental Design, University of California, Berkeley, Berkeley, CA, United States
- 6Department of Wetland Ecology, Institute of Geography and Geoecology, Karlsruhe Institute of Technology, Rastatt, Germany
- 7Department of Conservation Biology and Social-Ecological Systems, Helmholtz Centre for Environmental Research (UFZ), Leipzig, Germany
- 8Department of Civil and Environmental Engineering, University of California Davis, Davis, CA, United States
- 9Center for Watershed Sciences, University of California Davis, Davis, CA, United States
- 10World Wildlife Fund, Washington, DC, United States
- 11California Department of Water Resources, Sacramento, CA, United States
- 12American Rivers, Washington, DC, United States
- 13River Partners, Sacramento, CA, United States
- 14Department of Environmental Science, Policy and Management, University of California, Berkeley, Berkeley, CA, United States
- 15Environmental Science Associates, Sacramento, CA, United States
- 16The Woods Institute for the Environment, Stanford University, Stanford, CA, United States
Conventional flood control has emphasized structural measures such as levees, reservoirs, and engineered channels—measures that typically simplify river channels and cut them off from their floodplain, both with adverse environmental consequences. Structural measures tend to be rigid and not easily adapted to increased flooding regimes resulting from environmental change. Such actions also limit the natural hydrologic benefits of floodplains such as storing floodwaters, improving water quality, providing habitat for invertebrates and fish during periods of inundation, and supporting a multitude of cultural services. As these benefits are more widely recognized, policies are being adopted to encourage projects that reduce flood risks and restore floodplain ecosystems, while acknowledging the social-ecological context. The number of such projects, however, remains small. We assessed four multi-benefit floodplain projects (two in California, United States, and two in Germany) and characterized their drivers, history, and measures implemented. In both United States cases, the dominant driver behind the project was flood risk reduction, and ecosystem restoration followed, in one case inadvertently, in the other as a requirement to receive a subsidy for a flood risk reduction project. One German case was motivated by ecosystem restoration, but it was more widely accepted because it also offered flood management benefits. The fourth case was conceived in terms of balanced goals of flood risk reduction, ecosystem restoration, and recreation. We conclude that projects that both reduce flood risk and restore ecosystems are clearly possible and often cost-effective, and that they could be more widely implemented. The principal barriers are often institutional and regulatory, rather than technical.
1 Introduction
Conventional flood risk management leans heavily on structural measures to decrease flood hazard, rather than integrative approaches such as giving more room to rivers and preventing development on flood-prone lands (Galloway and Lewis, 2012). In fact, structural measures such as levees (dikes), dams, and engineered channels tend to encourage urban and agricultural development on the floodplain because the public perceives that flood risk is eliminated (Auerswald et al., 2019). The result is more development on the floodplain, where houses are at risk from floods that exceed the capacity of the structural measures to control flooding, a coupled human-natural interaction termed the “levee effect” (White et al., 2001). As a result of the structural approaches, floodplains have been extensively altered by urban and agricultural development (Auerswald et al., 2019). Moreover, by preventing floodwaters from spreading out over floodplains, levees can concentrate flow and result in higher peak floods downstream (Lulloff, 2013) and upstream (Heine and Pinter, 2012), exacerbating flooding problems. Likewise, by cutting floodplains off from their river channels, levees and engineered channels can render environmental flows largely irrelevant, because these flows cannot reach and interact with floodplains, to the detriment of both aquatic and riparian ecosystems (Thoms, 2003).
As the importance of the hydrologic and ecological functions of floodplains becomes better understood, there are increasing calls to restore connectivity of floodplains, so that they actively flood. This not only supports native riparian and aquatic species, but it also accomodates floodwaters, thereby reducing flood peaks downstream (Anderson et al., 1996; Tockner and Stanford, 2002; Opperman et al., 2009; van Rees et al., 2021). In addition, increasing recreational use of floodplains is contributing to greater awareness of the values and services these ecosystems support (Geist, 2011). New policies are also being adopted that recognize the ecological benefits of floodplain inundation and prioritize projects that achieve both flood risk reduction and ecological restoration (USEOP (United States, Executive Office of the President), 1977; EC (European Commission), 2000; USEOP (United States, Executive Office of the President and Barbour), 2015; DWR (California Department of Water Resources), 2017; CRS (Congressional Research Service), 2020; FEMA (Federal Emergency Management Agency), 2021). Despite the rapidly increasing number of ecosystem restoration projects worldwide, few are planned in a way to provide multiple benefits, such as combining flood risk reduction, ecosystem restoration, and adaptability to climate change. Learning from successful examples can thus greatly improve future approaches of floodplain management. Consequently, this paper addresses the issue of how to restore floodplains as multi-benefit, coupled Social-Ecological Systems. We propose a framework to better understand the role of multi-benefit projects for flood risk reduction in the context of social-ecological systems (Section 2); examine the guiding policies that have encouraged, enabled, and sometimes inhibited such projects (Section 3); analyze four well documented cases, two in California and two in Germany, two regions that have been at the forefront of multi-benefit floodplain management (Section 4); and identify enablers and barriers for these particular projects (Section 5). These case studies have been selected because they are well documented sites that provide flood risk reduction while preserving or restoring ecosystem functions. For each case study, we trace the original motivation for the project, the implementing agency, methods used, funding sources, and limitations. Each case study has unique elements, but collectively they highlight the challenges and opportunities to reduce flood risks and restore ecosystems through multi-benefit floodplain projects.
2 The Role of Multi-Benefit Projects in Social-Ecological Systems
2.1 Defining Floodplains
The term “floodplain” has different connotations to different disciplines, and to the public in different places. Not surprisingly given the diversity of meanings ascribed to the term “floodplain”, there are often misunderstandings in communication among different experts and with the public. Hydrologists may refer to different “floodplains” defined by the return period of their inundation (e.g., 100 years, 200 years, 500 years). Geomorphologists often identify floodplains based on presence of alluvium (sediment deposited by fluvial transport) and frequent inundation, while ecologists may identify floodplains based on ecological processes and presence of indicator species. Some countries use different terms to distinguish the “natural” floodplain from the “administrative” boundaries of the regulatory flood prone area (Figure 1). For example, the regulatory flood prone area is referred to as “flood zone” (zona inundable/zone inondable) in Spain and Quebec (Canada), as “reference flood” (crue de reference) in France, or as “flood hazard areas” (Hochwassergefahrenflächen) in Germany. In contrast, in the United States the word floodplain is widely used to refer to the regulatory flood hazard area, which corresponds to jurisdictional boundaries related to flood insurance and land use. As defined by the Federal Emergency Management Agency (FEMA), this corresponds to the area inundated by the flood with a return period of 100 years, i.e., the “100-year floodplain” (Klein 2019) or the “FEMA floodplain”. Properties that lie outside these designated flood hazard areas are often said to be “out of the floodplain,” even though they may be at considerable risk of flooding. Using the word “floodplain” in this context—even in academia-has created misunderstandings of flood risk and what a floodplain actually is.
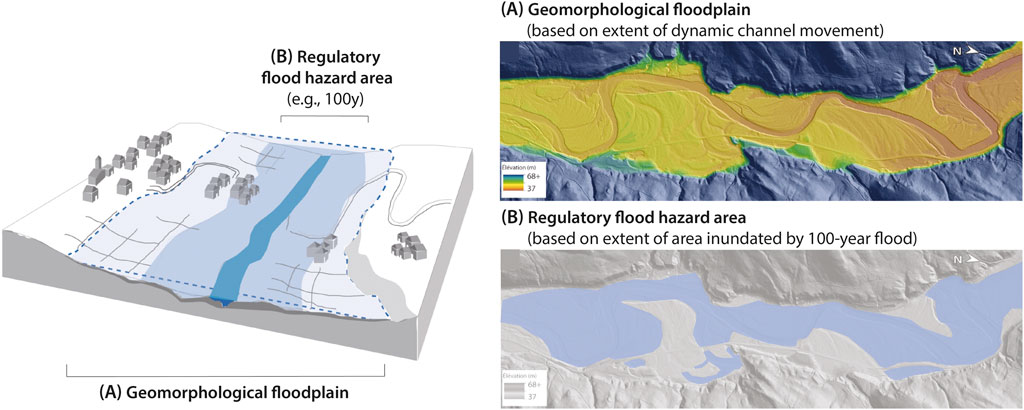
FIGURE 1. Regulatory “floodplain” (flood hazard area) as distinct from the geomorphological (natural) floodplain. (A) Extent of dynamic channel movement over time (geomorphological floodplain) vs. (B) extent of area inundated by the 100-year flood as defined with hydrologic and hydraulic models (modified from Serra-Llobet et al., 2022).
Opperman et al. (2010) defined floodplains as “a landscape feature that is periodically inundated by water from an adjacent river,” and further emphasized floodplains as “geomorphic features—formed and influenced by river flows and sediment—upon which ecosystems develop and operate.” Thus, floodplain environments can be defined by their dynamic conditions, where the hydrodynamics of floodwaters moving across a complex floodplain landscape create shifting inundation, scour, and deposition patterns as flows rise and fall in the river. As a result, naturally functioning floodplains are productive habitats that support some of the highest biodiversity in freshwater systems (Ward et al., 1999) and provide abundant food resources to invertebrates, fish, riparian birds, and other animals that utilize the habitat (Sommer et al., 2001; Grosholz and Gallo, 2006; Jeffres et al., 2008; Limm and Marchetti, 2009). For example, the experimental reconnection of the unregulated Cosumnes River with its floodplain (California) demonstrates how riparian and aquatic ecosystems can respond to increased floodplain connectivity. Following the partial removal and setback of levees that disconnected the Cosumnes River from its historical floodplain, renewed flooding enhanced flow diversity across the floodplain, increasing geomorphic response and evolution (Florsheim and Mount, 2002; Nichols and Viers, 2017) (i.e., diversity of flow depths and velocities), which in turn promoted riparian vegetation establishment (Trowbridge, 2007). Collectively, these dynamic physical processes supported positive responses from the native fish and aquatic ecosystem (Moyle et al., 2003; Opperman et al., 2010). In particular, the floodplain supported increased growth rates of juvenile fish relative to the main river channel (Jeffres et al., 2008) and increased primary productivity (i.e., food for fish) as a function of flood frequency and period of inundation (Ahearn et al., 2006; Grosholz and Gallo, 2006).
In addition to the ecological benefits of reconnecting a river with its floodplain (Pander et al., 2018), there can be hydrological benefits beyond flood storage and conveyance. Where infiltration of surface water is possible over large areas, such as where deposits of sand and gravel occur at the surface, extended river flows and floodplain inundation provide increased recharge to the underlying aquifer (Maples et al., 2019). Active and connected floodplains also promote carbon storage in the soil, as heterogeneous fluvial deposition over previous floodplain deposits traps and stabilizes organic carbon (D’Elia et al., 2017; Steger et al., 2019), and restore soil microbial metabolic pathways, such as denitrification (Hoagland et al., 2019). Restoring the hydrologic connectivity and function is a prerequisite to restoring ecological functions, but not sufficient alone. The heterogeneity of the floodplain surface is likewise important (Scown et al., 2015; Pander et al., 2018), and biotic interactions such as proximity to sources of colonizing organisms exert a strong control on the potential for recovery of floodplain ecosystems (Lake et al., 2007). When floodplains are fully functional, they provide multiple benefits (termed ¨co-benefits¨ following the terminology of the European Commission), which refer to 1) benefits to ecosystems, and 2) a diversity of ecosystem services that benefit society (Figure 2). The latter can include flood risk reduction, but also other social benefits such as improved water and air quality, recreation, aesthetics, or economic opportunities (e.g., agriculture) (Scholz et al., 2012a; Auerswald et al., 2019; Pugliese et al., 2020; Perosa et al., 2021).
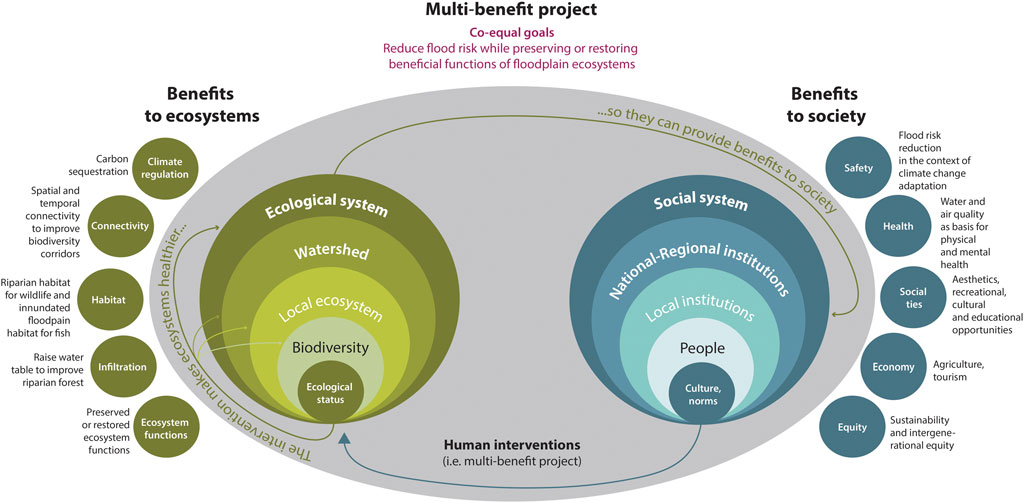
FIGURE 2. Conceptual diagram of the role of multi-benefit projects in the context of social-ecological systems (Source: modified from EEA (European Environment Agency), 2010).
With so many floodplains cut off from their river channels, restoration of floodplain connectivity is an increasingly important river restoration objective to enhance the functionality of environmental flows (Yarnell et al., 2015). In addition to the hydrologic connection effected by overbank flows, allowing channel margins to erode, fresh gravel and sand bars to deposit, and flood flows to rework floodplain surfaces can yield rapid ecological benefits (Thoms et al., 2017). These measures increase the width of the active river corridor, increasing the “process space” available to the river (Ciotti et al., 2021), and support dynamic river-wetland corridors that contribute to the functionality, biodiversity, and resilience of river systems broadly (Wohl et al., 2021). Thus, approaches to land use design and conservation planning are essential for the effectiveness of multi-benefit projects (Erős and Bányai 2020). These ideas can be conceptualized as shown in Figure 2.
2.2 Multi-Benefit Projects in Social-Ecological Systems
Figure 2 illustrates the characteristics of multi-benefit floodplain projects in the context of social-ecological systems. It conveys the idea that the human intervention—the multi-benefit project—restores riverine processes, making the ecosystem “healthier” by protecting or restoring natural functions that are critical to the well-functioning of the ecosystem. Such measures are for example: increasing infiltration of flood waters, which raise the water table, improving riparian forest health; creating riparian habitat for wildlife, including birds, and inundated habitat for invertebrates and fish; improving spatial (lateral, longitudinal and vertical) and temporal connectivity to improve abiotic and biotic functioning (e.g., improving groundwater recharge or creating biodiversity corridors); improving climate regulation functions (e.g., carbon sequestration); and providing biogeochemical functions associated with floodplains (e.g., water purification, nutrient cycling). The project thus seeks to make ecosystems healthier, and the ecosystems then provide the benefits to society. These social benefits can be related to: safety (e.g., increasing the area of flow conveyance to reduce peak flows); health (improvement of water and air quality contributing to better physical and mental health); social ties (providing aesthetics, recreational, cultural and educational opportunities); economy (creating opportunities for agriculture, tourism, groundwater recharge); or equity (promoting sustainability and intergenerational equity).
Multi-benefit projects build on actions implemented for one purpose to create other benefits. For example, a levee setback by itself is not a multi-benefit project but it creates an ideal situation for a river restoration project. Setting the levee back restores periodic inundation of the floodplain surface, which when coupled with dynamic flows and riparian restoration will produce inundated floodplain habitat. Even flooded farmland can be managed to yield high quality habitat for migratory birds and fish. Thus, the increased lateral connectivity brings multiple benefits: to the ecosystems (by preserving or restoring ecosystem functions) and to society from the restored ecosystem (e.g., flood risk reduction and other social benefits mentioned before). A levee setback without restoring or preserving ecosystem function can increase the area of conveyance reducing flood risk and provide a benefit to society, but it may not create the additional ecological benefits available from a “healthy” ecosystem—although some ecological benefits may be inadvertently created by flooding farmland. Projects thus can seek to provide multiple benefits for both the social and ecological systems with some foresight and planning.
3 Policies Encouraging, Enabling, or Inhibiting Multi-Benefit Projects
While maintaining—or restoring—hydrologically connected floodplains may yield diverse social-ecological benefits, public policies are not always supportive of floodplain reconnection. Table 1 summarizes some key policies in Europe (Germany) and the United States (California), that shape floodplain management and river restoration.
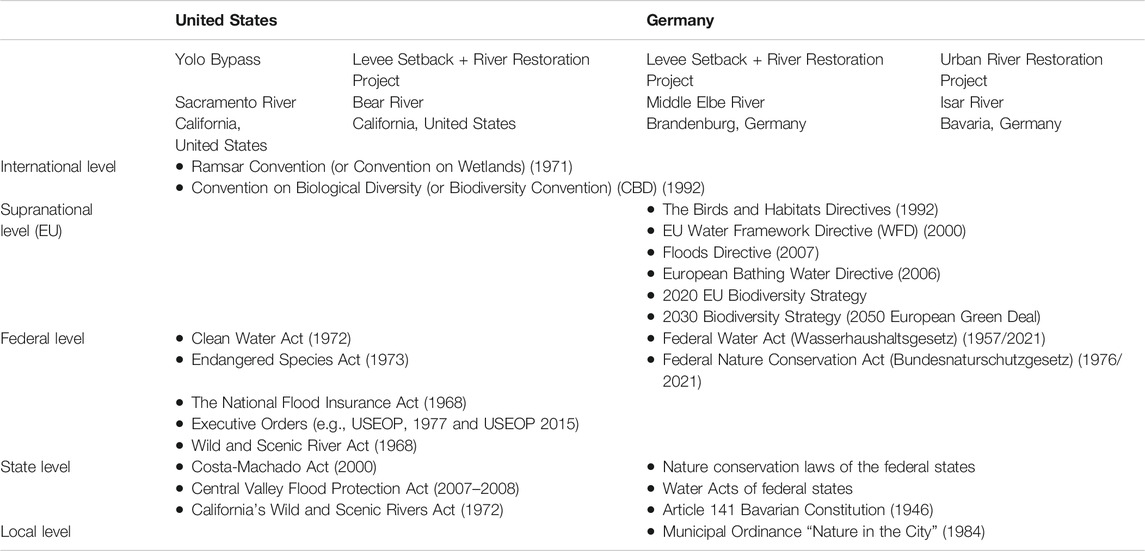
TABLE 1. Main policies and legislation affecting the different case studies. Policies at a state and local level affecting the case studies are discussed in Section 4.
European environment policy and law were developed in the 1970s (EP (European Parliament), 2021). However, the turning point in the policy framework for managing floodplains came with three directives directly related to freshwater ecosystems in the European Union, which are legally binding for EU member states. The Birds and Habitats Directives (EEC (European Economic Community), 1992) aim to protect Europe’s most valuable habitats and species through protecting areas and implementing restoration measures. The EU Water Framework Directive, or WFD (EC (European Commission), 2000) builds the EU-wide basis for integrated water resources management. It aims to achieve a “good ecological status” for all natural water bodies based on biological and chemical quality elements, following extensive monitoring and assessment programs. The main aim of the Floods Directive (EC (European Commission), 2007) is to reduce the negative consequences of flooding on human health, economic activities, the environment and cultural heritage, and measures undertaken under this directive must be consistent with the objectives of the WFD (e.g., Evers and Nyberg 2013, EEA (European Environment Agency), 2016. The current Bathing Water Directive (Council Directive 76/160/EEC, 1975), revised 2006 (EC (European Commission), 2006) requires standardized monitoring and management of bathing waters. It requires that the public be informed regarding bathing water quality, potential health risks, and public health recommendations, mostly aligning with the WFD as the overall framework for water management.
The European Commission has now presented its 2030 Biodiversity Strategy, a component of the European Green Deal. These policy initiatives aim to make Europe climate neutral by 2050, transitioning to a clean, circular economy, while simultaneously restoring biodiversity and cutting pollution. Targets include expansion of protected areas to at least 30% of European land and seas, and restoring at least 25,000 km of rivers. Conflicts and trade-offs persist between biodiversity protection and policies supporting economic growth and food security (Rouillard et al., 2018; van Rees et al., 2021).
In contrast, the United States lacks such comprehensive and integrative legislation for rivers and floodplains. Rather, the US policy framework for floodplains can best be viewed as a “patchwork”, consisting principally of the Clean Water Act (1972) and its protections for wetlands; Endangered Species Act (1973) protections for listed riverine and floodplain-dependent species; the National Flood Insurance Act (1968) and subsequent Federal Emergency Management Agency (FEMA) programs, which influence floodplain land use; and various Executive Orders (e.g., USEOP (United States, Executive Office of the President), 1977 and USEOP (United States, Executive Office of the President and Barbour), 2015) that discourage development on floodplains. One fundamental challenge is that policy at the national level to prevent building and rebuilding in floodplains is easily usurped at the local level, where land use decisions are actually made, usually to allow additional building, even in flood prone lands (Pinter, 2005; Kondolf and Lopez-Llompart, 2018).
4 Multi-Benefit Projects in California and Germany
Behind every river restoration project there is a story, an alignment of events—political momentum, innovative thinking, and a cultural change—that made it happen. These are the stories of four multi-benefit projects that incorporate river restoration (benefits to ecosystems) into their flood risk reduction strategy (benefits to society), and in some cases provided additional social benefits such as recreation and agriculture.
4.1 Yolo Bypass, California, United States
4.1.1 Flood Bypasses in the Sacramento River System
Once vast and productive, floodplains of the Central Valley in California have been largely disconnected from riverine floods by construction of levees and flow regulation by dams, such that they no longer provide essential ecological functions (Garone, 2011; Whipple et al., 2012). It is estimated that only 5% of historical riparian habitat remains in the Central Valley (Vaghti and Greco, 2007), largely contained within narrow corridors along the river inside extensive levee systems. Most wetland and floodplain habitat was drained and replaced with farms and cities in the mid-1800s. The habitat losses and associated floodplain disconnectivity have had dramatic impacts to migratory bird and native fish populations, which have lost access to the shallow, productive slow-moving floodplain habitats that provide ideal conditions for foraging, rest, spawning, and rearing (Moyle, 2002; Moyle et al., 2007; Garone, 2011).
In response to repeated devastating floods in the 19th and early 20th century, a system of “flood bypasses” were designed to convey floodwaters around cities in the Sacramento Valley. In the lower Sacramento River, two wide sections of managed floodplain (bounded by levees) were set aside as flood bypasses (the Sutter and Yolo bypasses) to convey the majority of flow during large floods downstream to the Sacramento-San Joaquin Delta and then out through the San Francisco-Bay estuary to the Pacific Ocean (Kelley, 1998) (Figure 3). Designed to flood only in large floods and graded to quickly drain water from the system, the bypass floodplains are inundated less frequently and for shorter durations than the original floodplains were historically.
The 20th century also brought an extensive network of levees along major rivers, coupled with the construction of large, multi-purpose reservoirs on virtually all rivers draining to the Central Valley (Mayer et al., 2018). However, the bypass projects remain an essential feature of the region’s flood risk management program. Over time, the bypasses have also offered a variety of additional ecosystem and economic benefits beyond flood risk reduction. For example, during the dry-season, the bypass floodplains are intensively farmed, while managed winter and spring flooding provides foraging habitat for waterbirds (Strum et al., 2013), rearing habitat for native fish (Katz et al., 2017), and groundwater recharge as flood waters remain on fields or move slowly downstream (Maples et al., 2019).
4.1.2 Yolo Bypass: Flood Risk Management and Nature Conservation Components
The largest of the Central Valley bypasses, Yolo Bypass is a 66 km long, 4.8 km wide area of floodplain now bounded by levees, which receives water on its upstream (north) end from the Sacramento River and the Sutter Bypass, and discharges into the Sacramento-San Joaquin Delta (Figure 3).
Flow into the upstream end of the bypass is controlled by the Fremont Weir, a fixed concrete sill. In addition, water is diverted into the bypass from the Sacramento River to the east via the Sacramento Weir, which is equipped with 48 manually-operated gates (Figure 3C). Yolo Bypass is an example of a “floodplain bypass,” a type of flood diversion with typically high land coverage requirements, long residence times, and high potential for ecological benefits. Other examples of such bypasses, their benefits, and issues surrounding their operation are described by Serra-Llobet et al. (2021). Yolo Bypass protects the City of Sacramento from floods by accommodating up to 14,000 m3/s of the Sacramento River flow, four times the capacity of the mainstem river channel as it passes Sacramento (Sommer et al., 2001). The Bypass typically floods in two years out of three (Delta Stewardship Council, 2021). Most of the 240 km2 of land in the Yolo Bypass is privately owned farmland, which grows a variety of crops during the dry season when the floodplain is largely dewatered, except for perennial ponds and a single tidal channel (Figure 4). Rice farming is particularly well suited to the clayey soils and wet conditions. The remaining 65 km2 of the bypass land is in the Yolo Bypass Wildlife Area, which provides inundated habitat for birds and native fish (Sommer et al., 2001; Sommer, 2002; Harrell and Sommer, 2003).
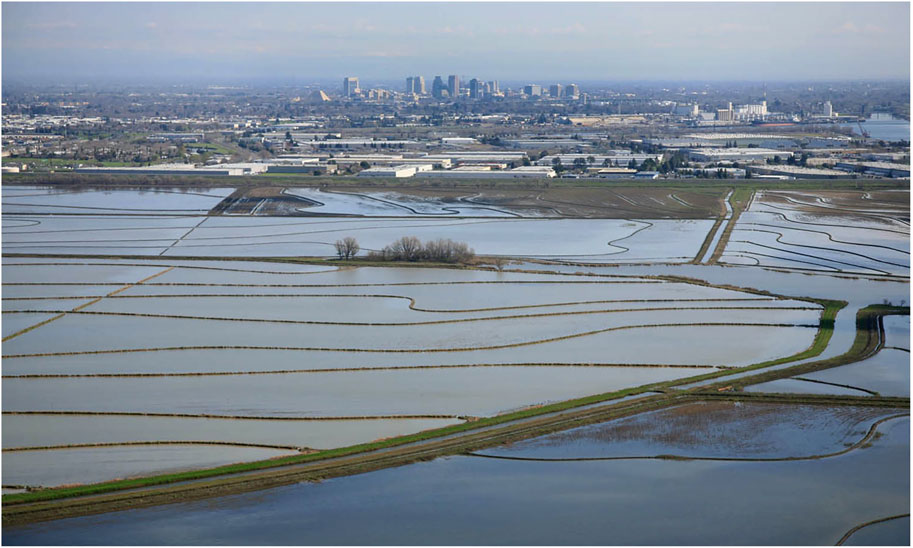
FIGURE 4. Yolo Bypass floodplain and view of downtown Sacramento, 2007 (courtesy of California Department of Water Resources).
The Yolo Bypass has been studied extensively in relation to its functional ecosystem connection to the Delta. When the floodplain bypass is inundated, adult salmon use it to migrate upstream to spawning grounds, and young salmon successfully use the floodplain bypass for rearing during downstream migration to the Delta and Pacific Ocean (Sommer et al., 2001; Sommer et al., 2005). However, managed inundation of portions of the bypass during drier years without large floods has been shown to provide similar rearing habitat conditions and corresponding similar growth rates to those measured under natural flood conditions (Katz et al., 2017). When these highly productive floodwaters saturated with phytoplankton and zooplankton are then pumped from the managed floodplains to the river, juvenile fish rearing in the river channel show increased growth rates as well (Jeffres et al., 2020). Thus, despite limited direct connectivity between the Yolo bypass and the adjacent Sacramento River channel, the bypass provides multiple benefits for native species and downstream ecosystems, as well as flood risk reduction. Furthermore, the Yolo Bypass Wildlife Area also provides recreational (bird watching and hunting) and educational (school visits) opportunities.
4.1.3 Drivers and Wider Applicability
Although recognized today for the multiple benefits it provides, the Yolo Bypass was constructed in the 1930s with a single purpose: flood risk reduction. The US Congress authorized the US Army Corps of Engineers to acquire private land and flowage easements needed to build and operate the Bypass to manage floodwaters of the Sacramento River. Nature conservation was not a factor in its implementation, and of course the term ecosystem restoration had not entered public consciousness at that point. The habitat values of the Bypass were recognized by scientists in the late 20th century, and today the management goals of the Bypass have expanded to include habitat management and restoration in addition to its primary purpose of flood management (DWR (California Department of Water Resources), 2017). This current, multipurpose, version of the Yolo Bypass is considered a model of a well-managed social-ecological system: It is characterized by public-private partnership, and it allows wildlife, flood risk reduction, and agriculture to co-exist adjacent to a major urban region. Its potential to provide greater inundated floodplain habitat with more natural patterns of inundation has been recognized in planning documents, and studies are underway to further these concepts, including testing new ways of adapting rice cultivation in the bypass to be more compatible with fish habitat (Sudduth and Lund, 2016), lowering the Fremont Weir so that the bypass is inundated more frequently and for longer periods, and improving a fish ladder to allow upstream migrating adults to pass Fremont Weir as flows decrease. Documentation of the remarkable ecological value of the inundated bypass has helped to shepherd a new emphasis on floodplain restoration throughout the Sacramento-San Joaquin Valley (Johnson, 2017) and stimulated efforts to understand how to manage flooded fields on the “dry side” of the levee to better support fish and aquatic food webs when flood waters are subsequently pumped into the adjacent river channel (Jeffres et al., 2020). All such flood bypasses are subject to challenges, such as deposition of sediment within the bypass itself, or in the mainstem downstream of the diversion point, or both, and thus require maintenance and adaptive management. While the Yolo Bypass can be considered a clear ‘win-win’ for both ecosystem and flood risk management, replication may face challenges such as intensified agriculture and extensive urban development of floodplain areas with the result that locating and securing a sufficiently large footprint for a flood bypass at this scale may be judged economically infeasible in many areas. However, utilizing the principles of Yolo Bypass in some riverine and deltaic systems to create smaller bypasses may be feasible, and in many cases, these may offer ecological benefits (Serra-Llobet et al., 2021).
4.2 Bear River Levee Setback and Floodplain Restoration Project, California, United States
4.2.1 Levee Setbacks (Dike Relocation)
Levees built close to river channels prevent floodwaters from spreading out across the floodplain, thus greatly reducing the cross-sectional area available to convey flood waters and deepening flow in between levees. This exposes the “wet” side of the levee to higher stages and higher velocity flows, increasing the risk of levee erosion and overtopping. In much of the United States, budgets have not been sufficient to maintain levees, and the backlog of maintenance and repairs has resulted in the country’s levee system receiving a grade of “D”—meaning poor, at risk—from the American Society of Civil Engineers in their 2021 Report Card for America’s Infrastructure (ASCE (American Society of Civil Engineers), 2021). Moreover, by reducing the area of floodplain that is hydrologically connected to the river, levees set close to the channel starve floodplain ecosystems of the water, sediment, and fluvial energy they require to flourish.
Levees can be repositioned further away from the channel (i.e., “setback levees”) to reduce risks of erosion and flooding, to restore floodplain ecosystems, or both (Zhu and Lund, 2009). At The Nature Conservancy’s Cosumnes River Preserve in California’s Central Valley, levees were intentionally breached to promote restoration of floodplain forests, and a low setback levee was built to separate adjacent rice fields from the expanded active floodplain. Subsequent research has shown that, in addition to rapid growth of willows and cottonwoods, the reconnected areas provide otherwise rare shallow, flooded areas that are used by native fish during the spring (Jeffres et al., 2008; Opperman et al., 2017).
Other levee setback projects have been driven primarily by flood-risk reduction objectives, with floodplain restoration as a co-benefit. Here we present the example of the Bear River Levee Setback Project on the Feather and Bear Rivers in California.
4.2.2 Drivers and Wider Applicability
Native Americans had numerous settlements along the riverbanks of the Feather and the Bear Rivers when the Gold Rush started in California (Jones and Stokes, 2005). These river channels were flanked by natural levees (berms of sandy sediment deposits from floodwaters), on which Native American settlements were located. During the mid-19th-century Gold Rush, hydraulic mining produced massive quantities of debris that were transported downstream through the river systems, filling the channels with sediment and causing rivers to overflow and deposit sediment across the river bottomlands. European settlers built artificial levees on top of the natural levees, both to reduce the frequency of flooding low-lying lands behind the levee, and to constrict the river channel, deepening the flow and thereby flushing the sediment from hydraulic mining. However, the levees were overtopped and breached multiple times, flooding the expanding cities of Maryville and Yuba City. As noted by Mayer et al. (2018), in response to numerous levee failures along the Feather River from 1920 to 1934, the levees were set back and enlarged to accommodate greater flows. Devastating floods in 1955, which killed 38 people in Yuba City, led to creation of the Yuba County Water Agency and construction of New Bullard’s Bar Dam (1970). In addition, as part of the California State Water Project, Oroville Dam was completed in 1968. A third dam (Marysville Dam) was planned to provide additional flood storage, such that all three dams could work together as a system to reduce the peak flows in the Feather and Yuba Rivers (Willis et al., 2011); however, this third dam was never built, in part because of environmental concerns.
In floods of 1986 and 1997, levees broke along the Yuba, Feather, and Bear Rivers, causing flooding in Linda and Olivehurst, south of Marysville and east of the Feather River (Hutton et al., 2019) (Figure 5). After the 1997 flood, the Yuba County Water Agency launched a feasibility study for 500-year flood protection for the area, funded in part by the California Department of Water Resources (DWR) under the Costa-Machado Water Act of 2000 (Reinhardt, 2005). Completed in 2003, the study identified several potential levee set back projects, mostly along the Feather River, as well as some other projects. The main driver for these projects was to lower flood stage in urban and urbanizing areas. The confluence of the Bear and Feather Rivers was identified as a bottleneck, so setting the levees back would allow water to spread and thereby lower the stage. Developers were already building in the Plumas Lake area, south of Olivehurst. The new urban area was protected by a deficient levee system. The developers sought to improve the levees, so the communities could join the National Flood Insurance Program (NFIP) and be eligible for subsidized flood insurance and post-disaster aid.
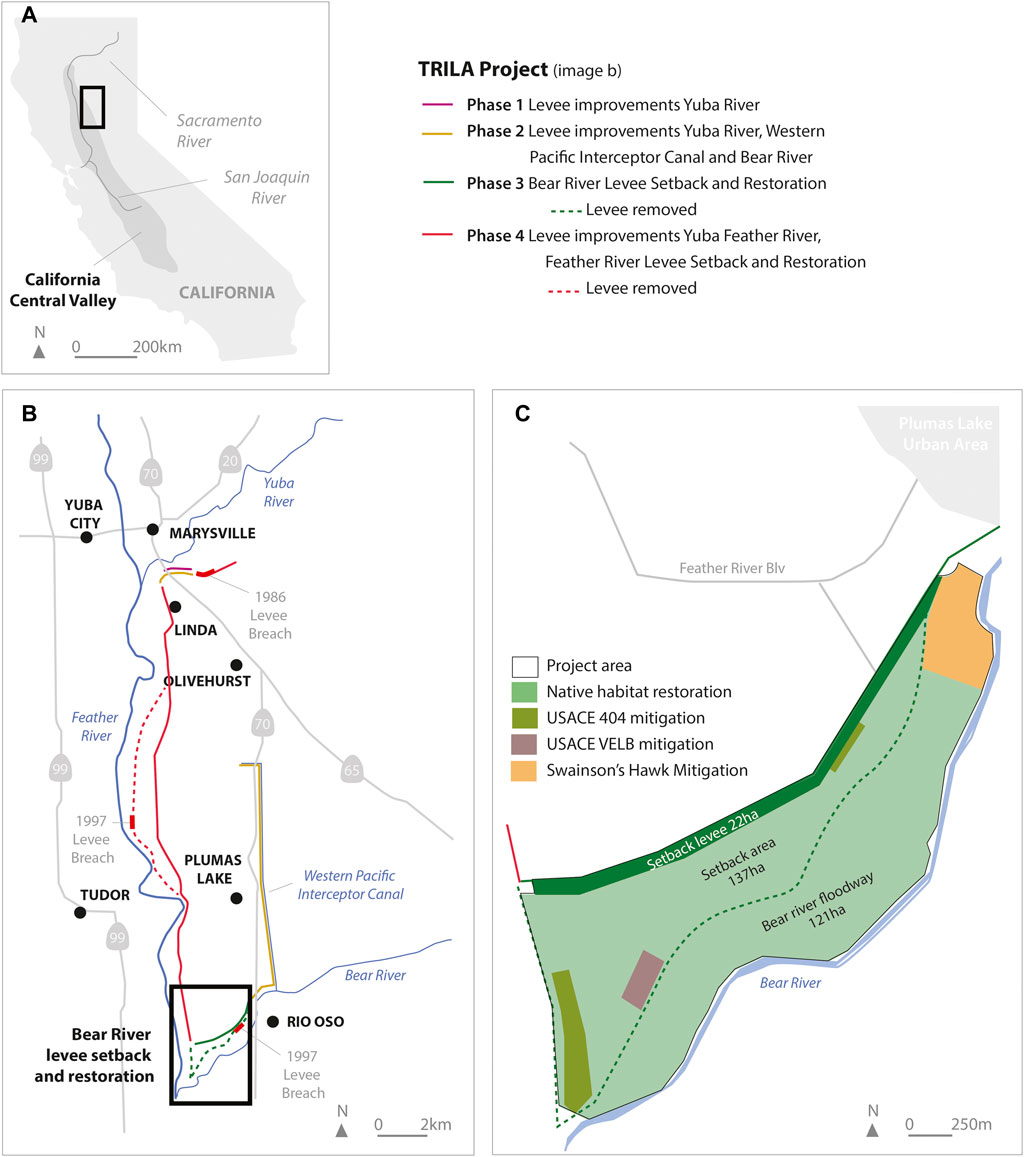
FIGURE 5. Bear River Levee Setback Project, Yuba and Sutter Counties, California. (A) Location Map, (B) Regional Map, and (C) Bear River Levee Setback and Floodplain Restoration Project.
In 2004, Yuba County and Reclamation District 784 formed a joint powers authority (the Three Rivers Levee Improvement Authority, TRLIA), responsible for constructing and financing levees in the Reclamation District 784 service area that would provide 200-year protection. The “Three Rivers Project” had four phases (Figure 5B). Phase 1 (2004) and 2 (2005–2006) were levee improvements, while phase 3 and 4 also included levee setbacks. Phase 3 was the Bear River Levee Setback Project (2005–2006) and Phase 4 the Feather River Levee Setback (2007–2010). The Bear River Levee Setback Project was initially referred to as the Feather-Bear Rivers Levee Setback Project. Alternatives were evaluated that relocated different lengths of the Feather River levee along with 3 km of the Bear River levee. The less costly alternative, with less impact on the Feather River levee, was selected and renamed as the current “Bear River” Levee Setback Project, although it still affected part of the Feather River levee (Figure 5B). The local share of the project funding was supplied by a group of local developers in advance of building in the protected area. The Costa-Machado Act of 2000 promoted multi-purpose projects that involved an ecological component. To comply with this requirement, the Bear River Levee Setback Project included components to restore fish, wildlife, and riparian habitat.
The Hurricane Katrina disaster in 2005 focused the nation’s attention on other major urban areas that are highly vulnerable to flooding. In particular, the flooding of New Orleans brought national attention to Sacramento, which was seen as one of the most vulnerable United States cities because of its high residual risk (from levee beach or overtopping). The public awareness created a momentum that resulted in approval of bonds to improve the levee systems in California and for the legislature to enact a set of reforms in 2007 that included an enhanced flood protection standard for urban areas of the Central Valley, maps showing 100- and 200-year flood hazard areas, and programs to set back some levees and strengthen others (Mayer et al., 2018). The new legislation, the Central Valley Flood Protection Act (2007–2008), also reinforced the idea of a multi-benefit project as a condition to receive state funding.
Three big flood bonds were linked to the Central Valley Flood Protection Act (2007–2008), which promoted incorporation of ecosystem benefits into the flood risk reduction projects. California Proposition 1E—Flood Control (2006) required projects to incorporate multiple beneficial uses into flood projects. The Water Quality, Supply, and Infrastructure Improvement Act of 2014 (Proposition 1) authorized $7.545 billion to fund ecosystem and watershed protection and restoration, water supply infrastructure projects, including surface and groundwater storage, and drinking water protection. Finally, California Proposition 68—Natural Resources Bond (2018) also promoted incorporating ecosystem elements through programs that included drought preparedness, water quality, and habitat enhancement and climate resiliency. Social equity, recreation, and cultural legacy issues were also addressed (CNRA (California Natural Resource Agency), 2015).
DWR was charged with awarding these funds, and the agency prioritized multi-benefit projects for funding. As per the Central Valley Flood Protection Act, DWR developed the California Central Valley Flood Protection Plan, which is periodically updated (2008, 2017, 2022). The plan calls for integration of ecosystem functions into flood risk reduction projects. The plan only affects projects from the State Plan of Flood Control, managed by DWR, which include the Feather and Bear River levees.
To qualify for this bond funding for a flood risk reduction project, local agencies were required to show ecological benefits. TRLIA hired River Partners, a NGO with a strong track record in river restoration projects, to develop the ecological component of the levee setback area. The Bear River Levee Setback Project is considered the first of its kind in California to incorporate ecosystem restoration with flood risk reduction (Figure 5C). The project had certain environmental impacts to wetlands and to habitat for the Valley Elderberry Longhorn Beetle (Desmocerus californicus dimorphus). Consequently, certain areas of the floodplain were designated to mitigate those impacts by creating seasonal wetlands and planting elderberry shrubs. Swainson’s hawk (Buteo swainsoni) foraging habitat was also integrated into the grasslands area to satisfy mitigation under the California Endangered Species Act (CESA).
4.2.3 Bear River Levee Setback Project: The Flood Risk Management and Restoration Components
The levee setback project on the Bear River at its confluence with the Feather River was selected by engineers as the most effective option for reducing flood risk that was being caused by a “bottleneck” at the confluence, as high flows on the Feather River would cause floodwaters on the Bear River to back up between the narrow levees and cause flooding upstream. The project included setting back nearly 3 km of levee along the Bear River and removing a levee along the Feather River at the confluence to reconnect 240 ha of floodplain habitat. This design increased conveyance and is modeled to lower flood stages by 1 m during major floods, reducing flood risk along the lower Bear River (Williams et al., 2009). While the project now allows flooding of 240 ha that were previously laterally disconnected from the channel, it is not clear if this entire area can be considered restored “process space” (Ciotti et al., 2021), because relatively high flows are needed to activate the surface, meaning that it would be rarely subject to geomorphically competent flows, and because the vegetation maintenance (e.g., frequent mowing) required in the grassy areas diverge from natural processes.
Two features of the Bear River setback project illustrate the opportunities and constraints of integrating floodplain restoration into a flood-management project. First, the vegetation restoration plan was designed to be consistent with the primary purpose of the project—reducing flood risk by increasing conveyance. Although most of the project area was allowed to grow into forest, to ensure that the target conveyance is maintained through the overall project, a portion of the setback area is maintained as a grassland with low hydraulic roughness (and also providing distinct habitat features). Second, hydraulic modeling indicates that most of the reconnected floodplain would be inundated only during a 2-year flood, and thus it would not provide the frequent, long duration inundated habitat that has been shown to be important for native fish (Williams et al., 2009). Further, when the project area was inundated, a corner of it could become a stranding hazard for fish. To address the stranding hazard, and to increase the extent of frequently flooded habitat, a low wetland feature (the “floodplain swale”) (Figure 6) was added to ensure that portions of the reconnected floodplain experienced longer duration flooding and to drain the potentially problematic area (Williams et al., 2009).
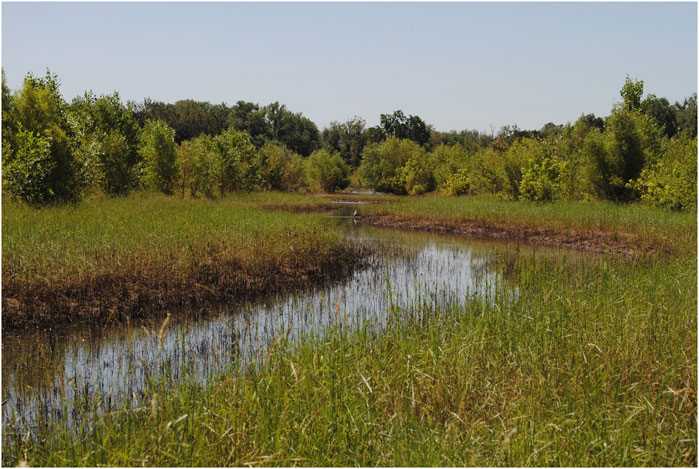
FIGURE 6. Floodplain Swale in the restoration area of the Bear River Levee Setback project, 2016 (Courtesy of River Partners).
The goals of floodplain restoration and flood-risk reduction can equally drive a levee setback project, and a project intended to achieve multiple benefits can diversify the funding sources available to support it.
4.3 Elbe River Levee Setback and Floodplain Restoration Project, Germany
4.3.1 Elbe River Levee Setback and Floodplain Restoration Project: Drivers
The Elbe river is the second largest lowland river in Germany. Having no dams downstream from the Czech Republic until the sluice of Geesthacht seperating the tidal Elbe section. It has a largely unmodified flooding regime and a relatively natural floodplain landscape (Scholten et al., 2005). It has some of the largest floodplain habitat complexes in Germany and has great importance for resident and migratory birds. During the Cold War, this reach of the Elbe River was the border zone between East and West Germany. This prevented most development and thereby protected important natural features of this wetland landscape, part of the “European Green Belt” extending along the former iron curtain from Finland to Bulgaria. However, intensifying agriculture has resulted in the loss of forest in this naturally wooded landscape. Thus, a levee setback project restoring natural flooding conditions to an area of the floodplain offered opportunities to re-establish hard- and softwood floodplain forest, considered the most endangered habitat types in Europe.
With about 80% of the Elbe’s formerly active floodplain area lost to diking since the 19th century (BfN & BMU (Federal Agency for Nature Conservation & Federal Ministry for the Environment, Nature Conservation and Nuclear Safety), 2021), floodplain functions, such as flood attenuation, water quality improvement, and floodplain biodiversity, have been lost. Loss of floodplain storage has increased flood risk, and large floods occurring within the last 2 decades have increased awareness of the need to enlarge river and floodplain conveyance capacity.
Over the past three centuries, the Elbe floodplain in the project area (north-central Germany, half-way between Hamburg and Berlin) (Figures 7A,B) has been transformed from a naturally wooded landscape into one dominated by large-scale agriculture. Although floodplain forests are protected by the EU Habitat Directive (EEC (European Economic Community), 1992) and are the most species-rich forest type in central Europe, they have become increasingly endangered and considerably reduced in area.
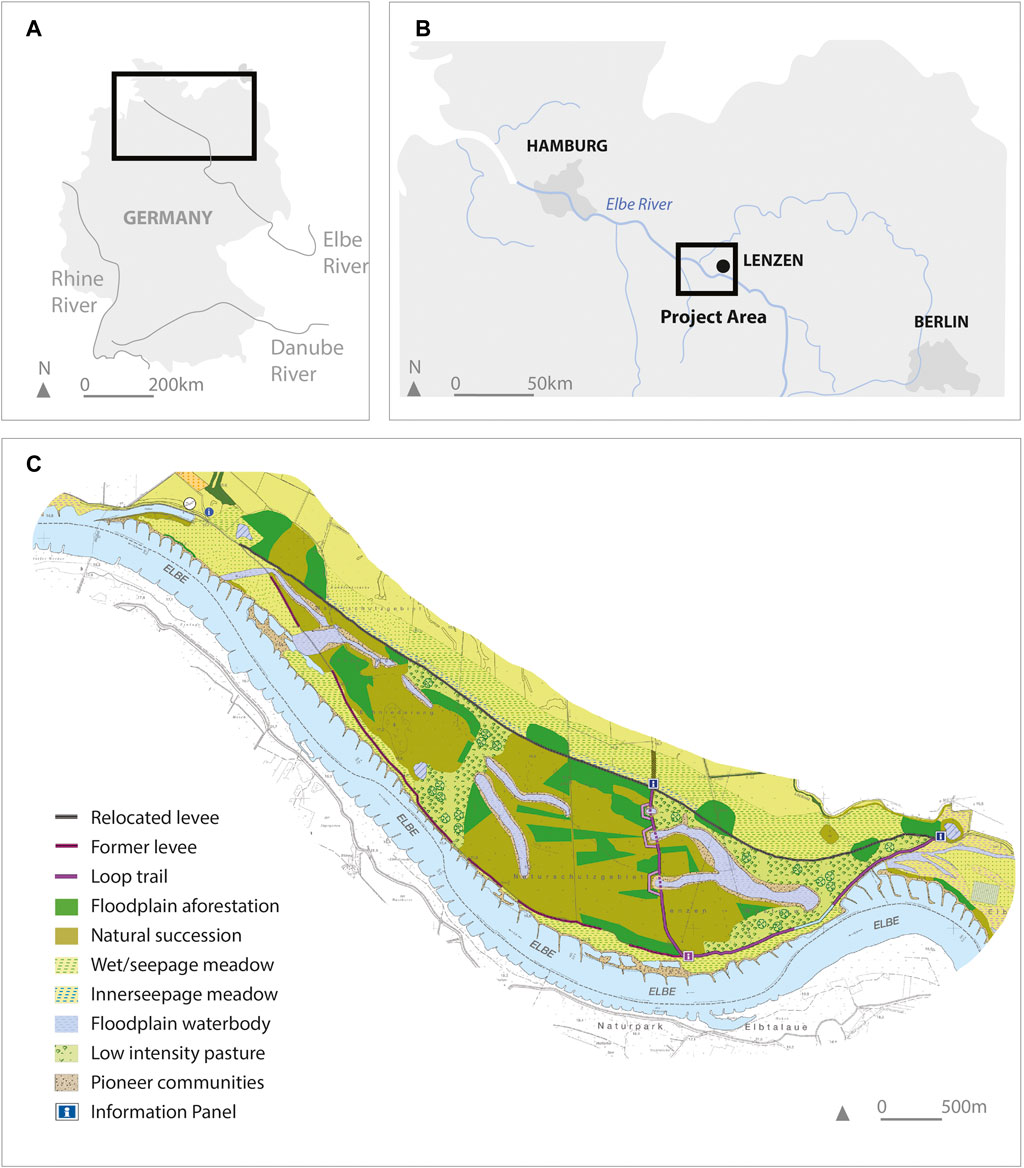
FIGURE 7. Elbe River Levee Setback Project, Germany. (A) Location Map, (B) Regional Map, and (C) Elbe River Levee Setback Restoration Project.
Many ecological and biological processes in floodplains depend on flooding dynamics. In response to loss of floodplain forest because of lateral disconnection by levees, there is increasing interest in relocating levees to reinstate a natural flooding regime as an effective way to re-establish floodplain forests, now an important conservation priority.
More than ten levee relocation projects have been realized along the Middle Elbe (BfN & BMU (Federal Agency for Nature Conservation & Federal Ministry for the Environment, Nature Conservation and Nuclear Safety), 2021). The levee setback near Lenzen (among the larger projects) originated just after the fall of the iron curtain through a local initiative of the administration of the UNESCO-Biosphere Reserve “Flusslandschaft Elbe-Brandenburg,” a section of the 400 km2 biosphere reserve covering five German states along the Elbe River. Its aim was to improve the ecological state of a lowland floodplain, and restoring floodplain forest. A European Union-funded LIFE-project first purchased the floodplain area, and then the federal conservation program “chance.natur” implemented the relocation of 7.4 km of levee to reconnect 420 ha of former floodplain in 2008 (Damm, 2016) (Figure 8).
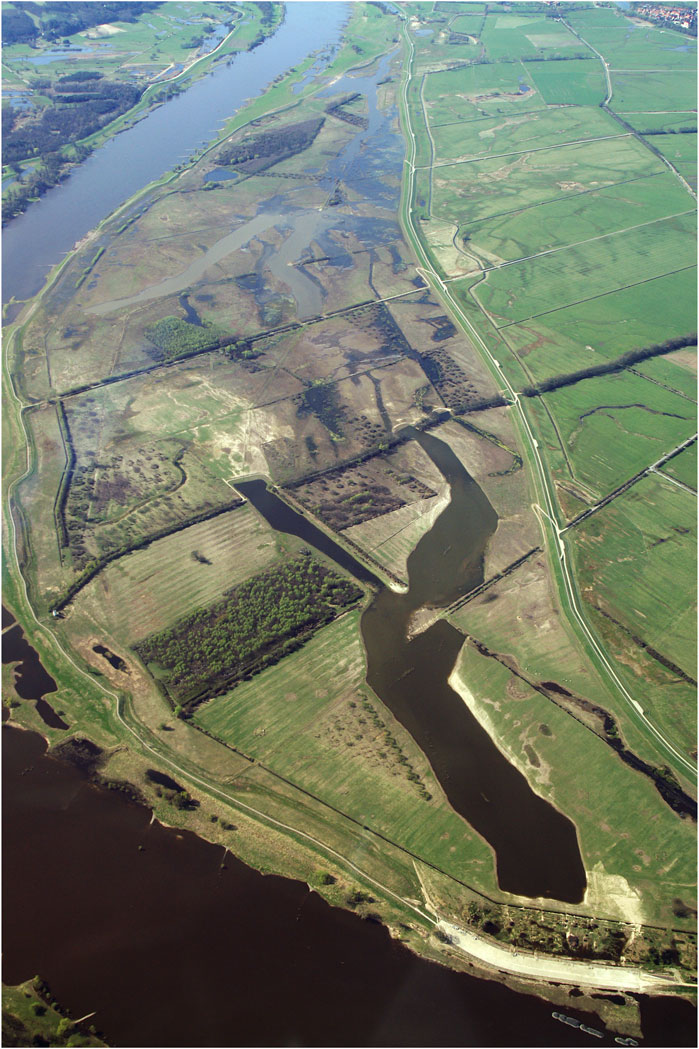
FIGURE 8. Aerial view of the Middle Elbe River Levee Setback and River Restoration Project in the Lenzen Area (Germany) (courtesy of Katharina Nabel).
Despite some early opposition against the project, which had its origins in the difficulties of the German reunification process in this former border region, the local agro-holding company supported the project from the beginning and was essential in gaining increased public acceptance (Warner and Damm, 2019). Moreover, the anticipated benefits from flood risk reduction proved to be a convincing argument regionally, more so than conservation and other environmental benefits.
4.3.2 Elbe River Levee Setback Project: The Flood Risk Management and Restoration Components
The project originated primarily with a conservation focus in the Biosphere Reserve. A 2002 catastrophic flood on the Elbe drew attention to the area and added flood protection to the public agenda. During subsequent large floods, the Federal Waterways Engineering and Research Institute (BAW) conducted field measurements showing the beneficial effects of levee setback on flood peak reduction and thus flood risk (Alexy and Faulhaber, 2011; Faulhaber, 2013). These benefits were subsequently confirmed by other independent investigations by the Federal Institute of Hydrology (BfG) (Promny et al., 2014). These scientific validations of nearly 50 cm local decrease of flood peak level and its effect on a city 25 km upstream at a 4,000 m3/s discharge helped promote levee setbacks on a national scale.
Furthermore, the project has established about 80 ha of floodplain forest, adding to the area reforested by the preceding LIFE-project. In addition, 45 ha of shallow waterbodies were excavated to restore aquatic and semiaquatic habitat as well as to supply levee construction material (Figure 6C). Investigations evaluating the project’s success have documented the return of many waterfowl species and other birds, as well as a diversity of habitat types. As one of the first large levee setbacks in Germany, the project is still cited as a successful example of synergistic implementation of conservation and flood risk reduction objectives (Scholz et al., 2012b; Thieken et al., 2016; Veidemane, 2019; Pieck, 2020; Schindler et al., 2021).
4.4 Isar River Restoration Project in Munich, Germany
4.4.1 Isar River Restoration Project in Munich: Drivers
The Isar is an alpine river that descends from the Austrian Alps, through multiple glacial moraines, onto the gravel plain of Munich. Because of its steep drop, the Isar was heavily exploited for hydroelectric power production, with 37 hydropower plants built by the mid-20th century. On the upper and middle Isar, power plants are built on side canals diverting most of the Isar water. For example, in Munich, the Großhesselohe weir (a few km upstream of Munich) had essentially unrestricted rights to divert water into a side canal to supply the hydroelectric power plant Isarwerk 1, and as a result, after 1907, the Isar river channel in Munich was dry during base-flow months, except for sewage and “urban slobber,” and during major floods when water spilled from upstream dams (Döring and Binder, 2010). Shortly after the second world war, the Bavarian Parliament added Article 141 to the Bavarian Constitution outlining the state’s obligation to protect the social functions of rivers and lakes for recreational use. In response, the Sylvenstein Reservoir (1954–1959) (Figures 9A,B) was constructed to provide a minimum flow in the upper Isar for recreational uses and to support fish habitats. Later the dam was refitted to generate power and to reduce risk of floods. However, the minimum flows released by the dam were not sufficient to dilute the pollution from urban areas such as Bad Tölz, especially during the dry season (Döring and Binder, 2010).
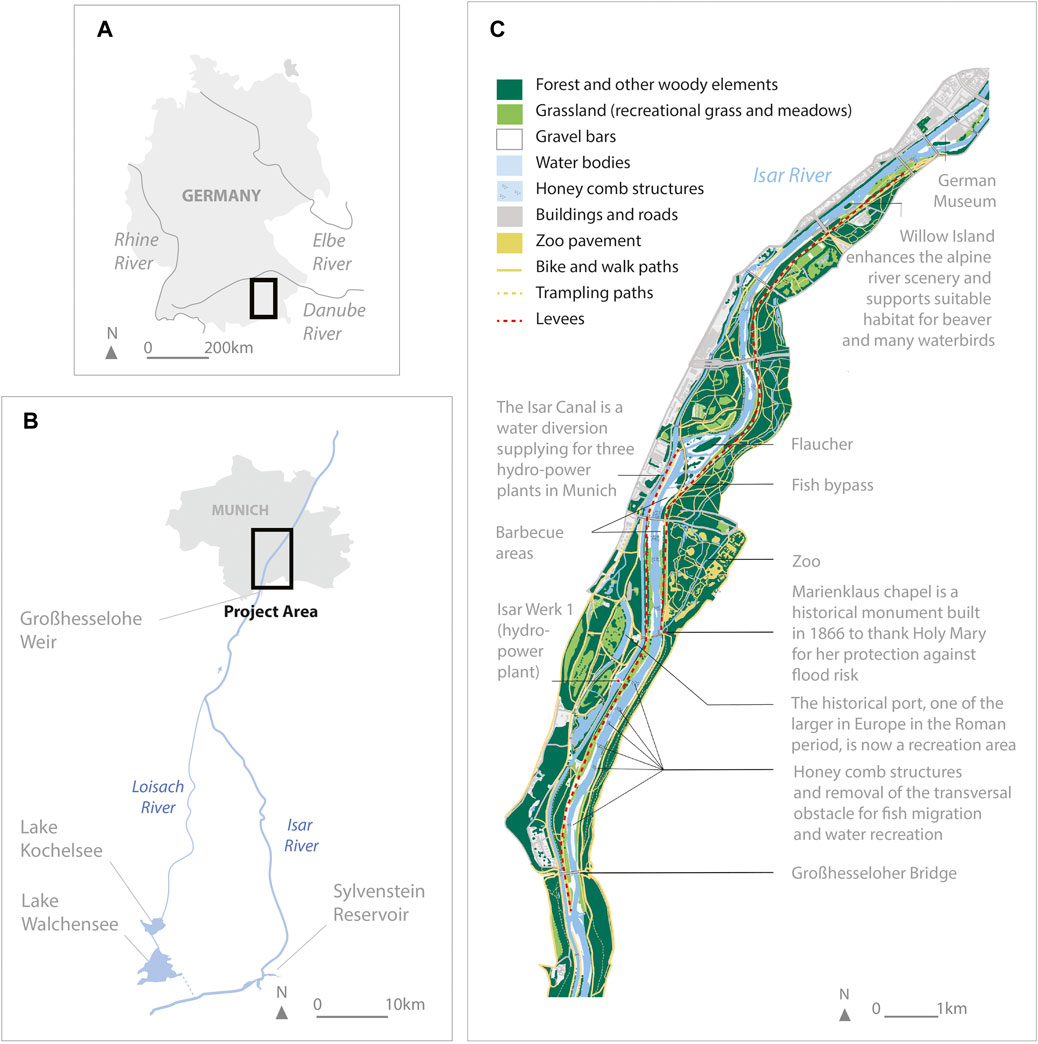
FIGURE 9. Isar River Restoration Project in Munich, Germany. (A) Location Map, (B) Regional Map, and (C) Isar Restoration Project.
As the environmental movement gathered steam throughout western democracies in the 1960s–1970s, the Bavarian State Ministry for the Environment and the Environmental Protection Department of Munich came into being. Growing public pressure on local and regional water agencies to achieve good water quality for recreational uses was backed up by the European Bathing Water Directive (1975, revised 2006), requiring improved water quality. In 1984, the Parliament extended Article 141 of the constitution to secure not only social functions but also nature conservation, and Munich adopted a municipal ordinance “Nature in the city” (“Natur in der Stadt”) supporting the restoration of the Isar River (Rossano, 2016). In 1987, the Bavarian Water Act required minimum water flows for ecosystems functions, which led to negotiations among the agencies, energy providers, and NGOs. An interdisciplinary effort involving experts from forestry, hydraulics, biology, ecology, river morphology, and landscape architecture developed a plan to restore the Isar, the “Isarplan,” reinforced by the 1992 European Fauna and Flora Directive and the 2000 EU Water Framework Directive (Zingraff-Hamed et al., 2019).
A parallel objective was flood risk management. The 100-year flood was estimated to be 1,150 m3/s, but the existing channel and floodplain capacity (prior to flooding urban areas) was only 800 m3/s. As hard engineering measures, such as flood walls close to the channel and upstream dam construction, had negative effects on the riverscape and its biodiversity, there was strong pressure from the civil society on the Water Agency (responsible for flood control) to find new kinds of solutions to manage flood risk (Zingraff-Hamed et al., 2019). In response, the Bavarian State government urged the water agency to find a solution that would satisfy multiple objectives. The water agency and city of Munich began intensive consultation with NGOs and civil society, a level of public involvement that was later identified as a key factor for the success of the project (Schaufuß, 2016), even though it required a long planning process.
4.4.2 Isar River Restoration Project in Munich: Flood Risk Management and Restoration Components
The Isarplan is an 8-km-long river restoration in an urban context (i.e., within the city of Munich), starting from the southern city border to the inner city (Figure 9C). It started 80 years after the channelization of the Isar River for hydro-electrical production and flood protection. The project illustrates that river and floodplain restoration is possible even in densely populated areas (Kondolf, 2012). Initiated as a collaboration between the city of Munich and the state of Bavaria, the Isarplan had three main objectives: 1) improvement of flood risk reduction, 2) restoration of aquatic habitats, and 3) enhancement of recreational access and quality.
Flood risk was primarily reduced by giving more space to the river and thus increasing its conveyance capacity through the city. Overall, the width of the river was increased from about 50 to 90 m, and in reaches with low floodplain such as Flaucher, the width over which active river processes can occur was increased further. Levees were set back from the active channel and raised in height. The project was thus also seen as a form of adaptation to climatic change and the increased precipitation predicted for the catchment.
Ecologically oriented habitat improvements were primarily achieved by re-establishing longitudinal and lateral connectivity and by re-activating morphological processes that provide direct benefits for gravel-dependent species such as the Danube salmon (Hucho hucho), which was found to spawn on gravel beds of the restored river. Rock walls and concrete embankments stabilizing the banks were removed and the gravel banks allowed to erode, softening and making irregular the bank edges and contributing gravel to the river’s sediment load. Straight concrete weirs extending across the channel posed barriers to fish migration and recreational rafting. These were removed and replaced with multiple, irregular rock steps that accommodated the same elevation drop but over a longer channel length, and which provided pathways for fish migration. Because Sylvenstein Reservoir traps all of the river’s natural sand and gravel load, the Isar was sediment starved flowing into Munich, so a gravel mixture was added to the river to augment its sediment load. Gravel bars built up in response both to the wider river corridor (and thus greater depositional opportunities across the channel), and to the increased gravel supply. The result is large gravel bars (befitting the alpine sources of the river) that serve wildlife and human recreation (Figure 10).
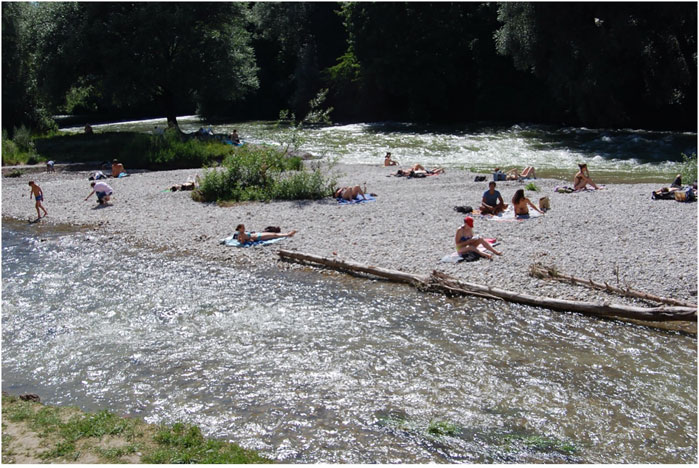
FIGURE 10. Gravel added to the river is transported downstream and redeposited in large gravel bars that provide habitat for fish and wildlife, as well as recreational opportunities for the urban population, July 2012 (Photograph by Kondolf).
A key restoration component was treatment of sewage effluent from upstream towns, notably Bad Tölz, where a tertiary sewage treatment plant with UV disinfection was installed in 2000, providing tertiary-treated clean water in place of the contaminated effluent formerly released. The improved water quality better supported ecosystem recovery, and also permitted human contact with the water, thereby enhancing the desirability of recreation along the river margins and in the river itself.
Recreational benefits were created by making the river more accessible to the urban population and by allowing recreational use of the wider gravel banks, e.g., for sunbathing and barbecues (Figure 10) (Zingraff-Hamed et al., 2018). It also included establishing public spaces and river access features that did not contribute to either restoration or flood risk management, but which increased the acceptance of the restoration measures by the local population. Elements of the landscape design contributing to public use could not jeopardize flood protection or nature conservation, and vice-versa. For example, picnic areas with toilets could not be built anywhere that would impact flood conveyance, no vegetation could be removed from the levees, and restored habitats were expected to be resilient to flooding.
The project was realized over a construction period of 11 years from 2000–2011 with a budget of around 35 million EUR, transforming the canalized river bed into a wider and more dynamic, naturally looking system with a greater structural richness.
Today, to visit the Isar on a summer weekend is to witness thousands of local residents enjoying the sun on gravel bars in mid-channel as well as along channel margins, against backdrops that range from natural riparian forest to imposing urban buildings. With the improved water quality, residents of all ages can interact with the water, from swimming and wading, to enjoying barbeques on the gravel bars and river banks. The Isarplan received the first German award for river development (“Gewässerentwicklungspreis”) in 2007.
5 Discussion
To date, projects that address both flood risk management and ecosystem restoration in California and Germany have had encouraging success overall, but each situation is unique, not only in physical and ecological setting, but equally important, in terms of political and institutional context. In this discussion, we first draw some salient points from comparison of the four case studies, then we review the enablers and barriers for multi-benefit projects and how these are illustrated by the four case study projects.
5.1 Lessons From Case Studies
5.1.1 Yolo Bypass
The largest floodplain area of our case studies (240 km2), Yolo Byapss was established over 8 decades ago for flood risk reduction only and initially managed with only this in mind. As the ecological value of the bypass became widely recognized, management evolved to enhance the ecological benefits of the Bypass. When inundated, the Bypass functions as a natural floodplain in accommodating floodwaters and providing habitat for migratory birds and fish. Rather than “restoration,” the Yolo Bypass can be viewed as preserving a functioning area of floodplain, even though its course is separated from the main channel of the Sacramento River. The agriculture practiced on most of the Bypass, rice cultivation, is already adapted to frequent inundation, and modifications to make agriculture still more compatible with juvenile fish use of inundated areas are being tested in an adaptive management framework (Jeffres et al., 2020).
5.1.2 Bear River Levee Setback
The Bear River levee setback differs from the Yolo Bypass in that it restored flood inundation to an area that had been cut off from flooding by levees and used primarily for farming. At 240 ha, its area was only one percent of the area of the Yolo Bypass, but the cost of floodplain land in the 21st century was much greater than in the 1930s when the Yolo Bypass was created. The motivation for the Bear River levee setback was to allow more development on the floodplains of the Yuba, Bear, and Feather rivers, by providing protection against the 200-year flood. However, to qualify for funding required substantive ecological restoration. The joint powers authority hired qualified consultants who designed a successful restoration component to the levee setback. These restoration measures are now recognized as a model for restoration in the context of a levee setback. However, ironically, there are more houses now exposed to the residual risk of floods greater than the 200-year design standard, or to flooding from levee breach (as occurred in 1986).
5.1.3 Middle Elbe River Levee Setback
The Middle Elbe River Levee Setback originated with nature conservation goals. The 420-ha area of floodplain reconnected via the levee setback had been acquired as part of a UNESCO Biosphere Reserve to restore floodplain forest, but at the time levees prevented the floodplain inundation needed to support a healthy riparian forest. However, by increasing the conveyance capacity of this river reach, modeling showed that the levee setback reduced flood levels in urban areas upstream by 50 cm, which generated more broad-based support for the project than would have been the case with the ecological objectives alone. Thus, the Bear and Elbe River projects can be seen as inverse of one another in terms of original motivations, although each ended up as projects benefiting both flood risk reduction and ecological restoration.
5.1.4 Isar River Restoration in Munich
The Isar is unique among the four case studies in that it did not restore extensive areas of floodplain but was focused more on the river channel and adjacent floodplain areas within a large city, with a footprint of under 100 ha over its 8 km length. However, it illustrates the multi-benefit approach and a highly collaborative planning process that occurred over more than a decade. The project was motivated by long-time public dissatisfaction with highly degraded river conditions through Munich in the 20th century, caused in large measure by hydroelectric plants diverting the entire flow of the Isar upstream of Munich, such that residual flows through the city were composed mostly of municipal effluent and other contaminated urban sources. With expiration of water use for hydroelectric production, and with revisions to the Bavarian Water Law calling for restoration of the Isar, the city and state launched a collaborative planning process involving scientists from many disciplines, NGOs, industry, and civil society, resulting in the Isarplan to increase flood conveyance capacity, restore ecosystem functions, and improve open-space access to the river.
5.2 Enablers and Barriers for Multi-Benefit Projects
Our four case studies illustrate enablers and barriers to multi-benefit projects as complex social-ecological systems. Although there could be many ways to categorize these enabling factors and barriers, we present one way to “slice the apple” in Figure 11. Physical setting is key as it determines whether the topography is suitable and whether there is sufficient land available (i.e., not already built out). Perceptions of floods refers to the fact that floods are still widely viewed as a threat only, without appreciation for their important role in river ecology. Scientific knowledge is growing about the benefits of inundated floodplains, but it needs to be better integrated in flood risk management and planning, and better communicated to the public and to decision makers. Education is needed so that the public and decision makers can better understand the nature of flood risks and also appreciate the beneficial role of floods in rivers. The policy framework determines many of the incentives and largely defines the realm of the possible in river and floodplain management. Many policies set in the 19th and early 20th centuries were designed to encourage economic growth above all else and would effectively preclude many restoration measures if not revised. Funding opportunities often track the policy framework, as some funding programs are available to support multi-benefit projects (as illustrated by the Bear and Elbe River examples). Leadership and collaboration is a factor that emerged from our review of the four case studies. While these projects now seem very practical solutions to both flood risk management and ecological restoration challenges, they resulted from an alignment of multiple enabling factors and all required dedicated perseverance to be ultimately achieved. We expand on these factors below.
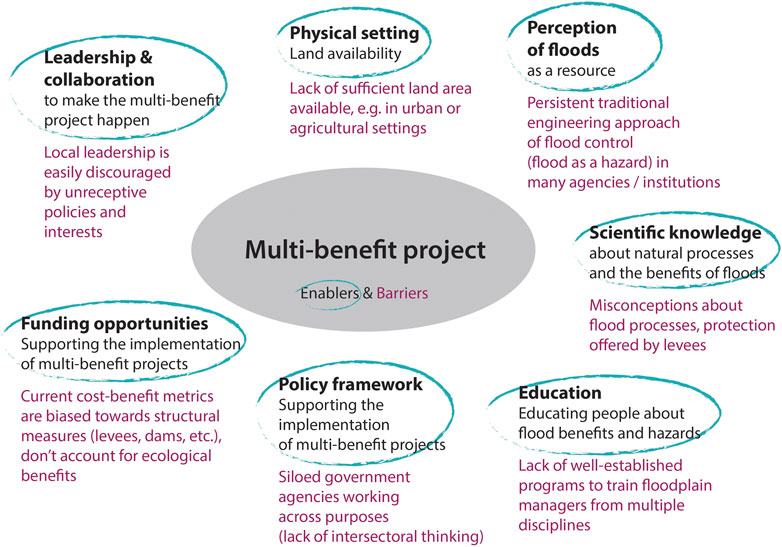
FIGURE 11. Enablers and barriers for the realization of multi-benefit projects as learned from the four case studies.
5.2.1 Physical Setting
Both Yolo Bypass and Middle Elbe Levee Setbacks were possible because of land available. In the case of Yolo, the land was set aside in the 1930s for flood conveyance. In the case of Elbe, land availability was increased by the former socialist system’s large-scale agriculture and property structure and a window of opportunity opening due to the severe economic changes of the post-cold-war period. To purchase developed land to devote to flood conveyance on this scale would in many cases be cost prohibitive, even assuming landowners were willing to sell (in many cases they might not) (Seher and Löschner, 2017). Thus, it is important to take socioeconomic and ecological trade-offs into account in floodplain management (Auerswald et al., 2019): in densely populated areas it is often impossible to provide the space needed to give more room to the rivers and their floodplains. This makes a spatially prioritized approach necessary (Geist, 2015).
5.2.2 Perceptions of Floods, Scientific Knowledge, Education
All four case studies illustrate the evolution of perception of floods from viewing floods purely as hazards to recognizing their beneficial aspects for ecosystems. While the perception of floods as a resource was not current when the Yolo Bypass was created in the 1930s, management of the Bypass has evolved to optimize its value to fish and wildlife, while still supporting agriculture over most of its area. On the Isar, the poor condition of the river (from contamination and lack of frequent floods to rejuvenate the bed and build natural gravel bars typical of an alpine river) in the 20th century led to widespread discontent and created public support for a comprehensive program to restore dynamic river processes. The engagement of a multi-disciplinary scientific team ensured that current understanding of river behavior was considered in the design of the project. Perhaps the most effective framework by which to integrate scientific knowledge into multi-benefit projects is through an adaptive management process, in which uncertainty is explicitly acknowledged and accounted for (Holling, 1978). A key feature of the approach is that restoration actions need not wait until “perfect” knowledge of the system is achieved, as this is unlikely in any case, and because many human-natural process are non-stationary. Instead, adaptive management rather allows for “learning by doing” through pilot projects (Healey, 1998). As “a systemic approach for linking learning with implementation to facilitate ongoing improvement in natural resources management” (Roux et al., 2022), adaptive management allows for restoration to be undertaken in a manner that maximizes learning potential, e.g., pilot projects to test the system response to specific types of interventions, which then provide objective feedback to decision makers as they move forward with a restoration program. This approach is illustrated on the Yolo Bypass, where modifications to internal levees in agricultural lands have been made to test potential improvements to water circulation to benefit juvenile fish (Katz et al., 2017; Jeffres et al., 2020).
As adaptive management has evolved, the concept of strategic adaptive management has emerged, which recognizes that stakeholders commonly hold different views of floods and the social-ecological system of the river and floodplain, and calls for an initial step of “co-creating” a vision and objectives for the project (Geist 2015; Roux et al., 2022). This highlights the need to expand the perception of floods from hazard only to also a resource for riverine ecology. With anticipated changes in hydrology anticipated from climate change, it becomes increasingly important to better value the socioeconomic functions and services of floodplains, and to integrate these aspects into conservation and restoration planning, as exemplified in the Isar River restoration. We also need better metrics to capture ecosystem values for evaluating benefits and tradeoffs (Geist, 2011; Geist and Hawkins, 2016). To fill this gap, new tools are now being developed and tested, such as the River Ecosystem Services index (RESI) (Hornung et al., 2019).
Education is needed not only in the public realm but also for managers to better understand the range of options available, rather than always defaulting to structural solutions. As succinctly summarized by the renown American geographer Gilbert F. White: “Floodplain managers at all levels of government have an uneven degree of knowledge about the diverse strategies and measures (both structural and non-structural) that constitute floodplain management. Effective management must draw upon a variety of disciplines, but in the United States there is no well-established program to train floodplain managers from multiple disciplinary perspectives. Commonly, lack of familiarity with the full range of approaches biases the selection of solutions for specific flood problems towards structural solutions. This hinders the development of comprehensive floodplain management, and impedes balancing of the dual objectives of flood vulnerability reduction and natural values protection” (White et al., 1992). These challenges (or barriers to achieve multi-benefit projects) articulated 3 decades ago resonate with debates that transpire today.
5.2.3 Policy Framework and Funding Opportunities
As described above, the EU has policies in place that require a strategic approach to flood risk management and coordination with ecological protection and restoration. The United States lacks such an overarching framework for managing flood risk and the ecological status of rivers. Actual management reflects siloed authorities and mandates. There are limitations inherent in the institutional structures, legal regulations and different views of floods (as a resource or as a hazard) in both the United States and Germany. In the United States, funding seems to come primarily from flood risk management and ecological benefits are either inadvertent (Yolo Bypass) or because a levee setback was found to be the most efficient way to achieve flood risk reduction goals and satisfy ecological restoration requirements (Bear River). Moreover, the Government of California is advanced in terms of environmental protection, whereas many other parts of the country are hostile to any controls on building houses on floodplains, such as the state of Missouri, which in 2004 adopted legislation prohibiting local governments from imposing any requirements stricter than the minimum national standards, thereby contributing to extensive floodplain development near St Louis (Pinter, 2005). In Germany, European and national legislation increasingly require integrated planning e.g., requesting compliance of EU Water Framework Directive, Habitat-Fauna-Flora Directive, Floods Directive and national Water and Conservation Law.
The balance between flood risk management and ecosystem restoration objectives varies widely among the case studies. The Yolo Bypass was built over 80 years ago with the sole objective of managing floods, but today inadvertently yields spectacular ecological benefits. Today, integration of the Central Valley Conservation Strategy with the Central Valley Flood Protection Plan has allowed for the identification of new large-scale projects that will alter the existing levee systems of the Central Valley (i.e., Yolo Bypass expansion) to provide both flood risk reduction and ecosystem restoration (DWR (California Department of Water Resources), 2017), with the former generally driving the latter, as illustrated by the Bear River levee setback. By contrast, the Middle Elbe River levee setback idea originated as an ecological restoration, but by documenting the flood risk management benefits, the project gained broader support. Like all projects discussed, the Middle Elbe River project exemplifies that creating “win-win” situations greatly increase project acceptance on all levels, thus making a project more likely to be realized (Schindler et al., 2014). The Isar restoration in Munich was motivated by both public pressure for environmental improvement of the river and better public access, and the need to increase the river’s flood conveyance capacity within the city of Munich. Nearly a decade of negotiations led to a balance among these goals that increased public acceptance of the project. It bears noting that despite the success of the Isarplan, such restoration cannot make biological community structures return to “natural states” especially in a restricted urban setting (Geist and Hawkins, 2016). Given the partly artificial structure of most floodplains and given the many dams that are in place, this commonly requires a flow management strategy to optimize functionality and benefits for target species in these reconciled ecosystems (e.g., Opperman et al., 2017; Pander et al., 2019).
It is notable that none of the case studies resulted from implementation of a top-down comprehensive basin-wide plan of the sort that might result from the kind of strategic adaptive management described by Roux et al. (2022). Rather, each resulted from a unique alignment of factors that made the specific project possible, but whose replicability elsewhere would depend upon physical settings, institutional and policy frameworks, funding opportunities, etc. (Figure 11).
5.2.4 Leadership and Collaboration
All successful projects evinced strong leadership and collaboration among stakeholders. Strong leadership is essential during all steps of floodplain restoration from the initiation and planning through the steps of adaptive management decision making and communication of the project outcomes. After an initial idea for a floodplain restoration project, deciding on the specific objectives, planning and evaluation of alternatives, as well as acquiring funding, all require integrating and balancing different stakeholder views and decision-making on multiple levels. In contrast to past disciplinary approaches, a social-ecological system approach with a broader stakeholder group requires even greater integrative leadership capabilities to successfully nurture a collaborative approach. For the Isarplan, collaborative planning was led by the local government. The successful collaboration relied on the long-term trust built up among stakeholders, nurtured by forward-looking authorities that allowed the project to be realized over a long period of time (Zingraff-Hamed et al., 2019). On the other hand, initiation of projects involving development of leadership can gain momentum directly after major floods, as illustrated on the Elbe River. Successful leadership is not limited only to the first steps of floodplain restoration projects, but is needed in communicating the outcomes. This is particularly important for disseminating knowledge to other potential projects. In the Bear River Levee setback, the collaborative approach between TRLIA, GEI Consultants (engineers), and River Partners (ecologists) was key to integrate hydraulic goals with habitat restoration. Finally, the Yolo Bypass Wildlife Area Restoration project received national attention as a model for collaborative restoration as an example of public-private collaboration of the Yolo Basin Foundation and California Department of Fish and Game, engaging federal, state, and local government officials with management responsibilities in the Bypass, and landowners to assess and ultimately achieve restoration (Salcido, 2012.)
6 Conclusion
Flood policy—at least on the aspirational level—is shifting from flood “control” to a new view that integrates ecosystem components and functionality as part of social-ecological systems. While there are still conflicting policies that make the implementation of this new, more integrative type of project challenging, the experience in California and Germany demonstrates the potential for such multi-benefit projects to offer new synergies (EEA (European Environment Agency), 2016; CRS (Congressional Research Service), 2020).
Projects that combine both flood risk reduction and preservation or restoration of ecosystem functions are still relatively uncommon. Our analysis of four well-documented but very different cases, two in California and two in Germany, show how multi-benefit projects in different contexts can find their window of opportunity. Such multi-benefit floodplain projects may become more frequent with the concept of Nature-based Solutions (NbS) now being adopted in many countries to promote more sustainable approaches to managing nature. The International Union for Conservation of Nature (IUCN) defines NbS as “actions to protect, sustainably manage, and restore natural or modified ecosystems, that address societal challenges effectively and adaptively, simultaneously providing human wellbeing and biodiversity benefits” (IUCN (International Union for Conservation of Nature), 2021). The multi-benefit projects documented here would all arguably qualify as “nature-based solutions” in that they preserve or restore natural riverine processes and simultaneously provide societal benefits (flood risk reduction).
The urgent need for climate change adaption was evidenced by the severe flooding in north-western Germany in July 2021 (184 causalities and 30 billion Euro in damages), which was estimated to have been intensified by a factor of 1.2–9 due to climate change (Kreienkamp et al., 2021). The increasing number of such extreme events argues for a shift in our management approaches, which now rely on statistical values such as 100-year flooding frequencies drawn from historical flood records, which may no longer hold true. Conventional management approaches typically place levees as close to the river as possible while still meeting the 100-year flood minimum flow capacity, leaving no room for error, nor accommodation for climate change induced increases in floods. Moreover, the conventional view of floods strictly as a risk does not account for the beneficial aspects of floods for ecology, water quality, and water supply (Galloway, 2005). Floodplain restoration will be more and more accepted by planners and the general public if its wider functions and benefits to human societies and economies are better stressed. For instance, including increased flood resilience, temperature buffering effects, and recreational benefits into planning and communication will likely motivate potential funders to engage in such action.
Author Contributions
AS-L, SJ, JG, and GMK contributed to conception and design of the study and wrote the first draft of the manuscript. AS-L, GMK, CD, MS, JL, JO, SY, JC, and AZ-H contributed to develop the case studies. AS-L, SJ, JG, AP, ES, contributed to the policy sections. TG, BE, RS, and the rest of the authors contributed to the manuscript revision, read, and approved the submitted version.
Funding
This paper results from a collaboration supported by DAAD (German Academic Exchange Service), the Institute of European Studies (UC Berkeley), the Institute of International Studies (UC Berkeley), and the Beatrix Farrand Fund of the Department of Landscape Architecture & Environmental Planning (UC Berkeley). This publication was made possible in part by support from the Berkeley Research Impact Initiative of the UC Berkeley Library. SJ acknowledges funding through the German Federal Ministry of Education and Research (BMBF; 01LN1320A and 02WGR1430A), and the Leibniz Association for the project Freshwater Megafauna Futures. The research of AZ-H was supported by the PHUSICOS project, H2020 Grant Agreement No. 776681.
Conflict of Interest
The authors declare that the research was conducted in the absence of any commercial or financial relationships that could be construed as a potential conflict of interest.
Publisher’s Note
All claims expressed in this article are solely those of the authors and do not necessarily represent those of their affiliated organizations, or those of the publisher, the editors and the reviewers. Any product that may be evaluated in this article, or claim that may be made by its manufacturer, is not guaranteed or endorsed by the publisher.
Acknowledgments
The project benefited from insights provided by Greg Farley and Heidi Hall of the California Department of Water Resources (DWR), Helen Swagerty of River Partners and Alberto Pujol of GEI Consultants. We also thank two anonymous reviewers for insightful comments, which greatly improved the paper.
References
Ahearn, D. S., Viers, J. H., Mount, J. F., and Dahlgren, R. A. (2006). Priming the Productivity Pump: Flood Pulse Driven Trends in Suspended Algal Biomass Distribution across a Restored Floodplain. Freshw. Biol 51 (8), 1417–1433. doi:10.1111/j.1365-2427.2006.01580.x
Alexy, M., and Faulhaber, P. (2011). Hydraulische Wirkung der Deichrückverlegung Lenzen an der Elbe. Wasserwirtschaft 12 (12), 17–22. ISSN 0043–0978.
ASCE (American Society of Civil Engineers) (2021). 2021 Report Card for America’s Infrastructure. Reston, Virginia: American Society of Civil Engineers. Retrieved from: https://infrastructurereportcard.org/wp-content/uploads/2020/12/2021-IRC-Executive-Summary-1.pdf (Accessed August 6, 2021).
Auerswald, K., Moyle, P., Seibert, S. P., and Geist, J. (2019). HESS Opinions: Socio-Economic and Ecological Trade-Offs of Flood Management - Benefits of a Transdisciplinary Approach. Hydrol. Earth Syst. Sci. 23, 1035–1044. doi:10.5194/hess-23-1035-2019
BfN & BMU Federal Agency for Nature Conservation & Federal Ministry for the Environment, Nature Conservation and Nuclear Safety (2021). Status Report on Floodplains 2021 Floodplains in Germany. Bonn-Bad-Godesbeg. Available at: https://www.bfn.de/sites/default/files/2021-12/Endversion_AZB_2021_eng_bf_Web_0.pdf (Accessed September 2, 2021).
Ciotti, D. C., Mckee, J., Pope, K. L., Kondolf, G. M., and Pollock, M. M. (2021). Design Criteria for Process-Based Restoration of Fluvial Systems. BioScience 71 (8), 831–845. doi:10.1093/biosci/biab065
CNRA (California Natural Resource Agency) (2015). Bond Accountability. Available at: http://bondaccountability.resources.ca.gov/p68.aspx (Accessed September 10, 2021).
Council Directive 76/160/EEC (1975). Concerning the Quality of Bathing Water. Brussels: European Commission. Available at: http://eur-lex.europa.eu/LexUriServ/LexUriServ.do?uri=CELEX:31976L0160:EN: HTML (Accessed August 2, 2009).
CRS (Congressional Research Service) (2020). Flood Risk Reduction from Natural and Nature-Based Features: Army Corps of Engineers Authorities. CRS Report 46328. Available at: https://crsreports.congress.gov/product/pdf/R/R46328.
D'Elia, A. H., Liles, G. C., Viers, J. H., and Smart, D. R. (2017). Deep Carbon Storage Potential of Buried Floodplain Soils. Sci. Rep. 7 (1), 8181. doi:10.1038/s41598-017-06494-4
Damm, C. (2016). The Lenzener Elbtalaue Large-Scale Conservation Project - Pilot Project Actions and Experience / Naturschutzgroßprojekt Lenzener Elbtalaue - Umsetzung und Erfahrungen eines Pilotprojekts. Natur und Landschaft 91 (8), 359–365. doi:10.17433/8.2016.50153404.359-365
Delta Stewardship Council (2021). Functional Flows: Yolo Bypass Inundation. Delta Plan Performance Measures, Delta Stewardship Council. Available at: https://viewperformance.deltacouncil.ca.gov/pm/functional-flows-yolo-bypass-inundation (Accessed September 14, 2021).
Döring, N., and Binder, W. (2010). “Die neue Isar/1 -Renaturierung, kulturelle Öffnung und Ideen-Fluß,” in Geschichtliches Wie Literarisches. Editor R. Sartori (Munich, Germany: buch & media).
DWR (California Department of Water Resources) (2017). Central Valley Flood Protection Plan. Available at: https://water.ca.gov/-/media/DWR-Website/Web-Pages/Programs/Flood-Management/Flood-Planning-and-Studies/Central-Valley-Flood-Protection-Plan/Files/2017-CVFPP-Update-FINAL_a_y19.pdf (Accessed September 7, 2021).
EC (European Commission) (2000). Directive 2000/60/EC of the European Parliament and of the Council Establishing a Framework for the Community Action in the Field of Water Policy. Available at: https://eur-lex.europa.eu/eli/dir/2000/60/oj (Accessed April 10, 2021).
EC (European Commission) (2006). Directive 2006/7/EC of the European Parliament and of the Council Concerning the Management of Bathing Water Quality and Repealing Directive 76/160/EEC. Available at: https://eur-lex.europa.eu/legal-content/EN/TXT/?uri=celex%3A32006L0007 (Accessed April 10, 2021).
EC (European Commission) (2007). Directive 2007/60/EC of the European Parliament and of the Council of 23 October 2007 on the Assessment and Management of Flood Risks. Available at: https://eur-lex.europa.eu/legal-content/EN/TXT/?uri=celex:32007L0060 (Accessed: April 10, 2021).
EEA (European Environment Agency) (2010). Ecosystem Accounting and the Cost of Biodiversity Losses — the Case of Coastal Mediterranean Wetlands. Technical report No 3/2010. Luxembourg, Luxembourg: EEA, Office for Official Publications of the European Union.
EEA (European Environment Agency) (2016). Flood Risks and Environmental Vulnerability. Exploring the Synergies between Floodplain Restoration, Water Policies and Thematic Policies. EEA Report No 1/2016. Available at: https://www.eea.europa.eu/publications/flood-risks-and-environmental-vulnerability.
EEC (European Economic Community) (1992). 1992 Council Directive 92/43/EEC of 21 May 1992 on the Conservation of Natural Habitats and of Wild Fauna of the European Union, L 206 (Published on 22 July 1992). Available at: https://eur-lex.europa.eu/legal-content/EN/TXT/?uri=CELEX:31992L0043 (Accessed April 3, 2021).
EP (European Parliament) (2021). Fact Sheets on the European Union - Environment Policy: General Principles and Basic Framework. Available at: https://www.europarl.europa.eu/factsheets/en/sheet/71/environment-policy-general-principles-and-basic-framework (Accessed September 12, 2021).
Erős, T., and Bányai, Z. (2020). Sparing and Sharing Land for Maintaining the Multifunctionality of Large Floodplain Rivers. Sci. Total Environ. 728, 138441. doi:10.1016/j.scitotenv.2020.138441
Evers, M., and Nyberg, L. (2013). Coherence and Inconsistency of European Instruments for Integrated River basin Management. Int. J. River Basin Manage. 11 (2), 139–152. doi:10.1080/15715124.2013.811416
Faulhaber, P. (2013). “Zusammenschau und Analyse von Naturmessdaten”. In: Die Deichrückverlegung bei Lenzen an der Elbe. BAW-Mitteilungen 97, 109–134.
FEMA (Federal Emergency Management Agency) (2021). Building Community Resilience with Nature-Based Solutions. Available at: https://www.fema.gov/sites/default/files/documents/fema_riskmap-nature-based-solutions-guide_2021.pdf.
Florsheim, J. L., and Mount, J. F. (2002). Restoration of Floodplain Topography by Sand-Splay Complex Formation in Response to Intentional Levee Breaches, Lower Cosumnes River, California. Geomorphology 44 (1–2), 67–94. doi:10.1016/S0169-555X(01)00146-5
Galloway, G. E. (2005). Corps of Engineers Responses to the Changing National Approach to Floodplain Management since the 1993 Midwest Flood. Universities Counc. Water Resour. J. Contemp. Water Res. Educ. 130, 5–12. Available at: https://opensiuc.lib.siu.edu/cgi/viewcontent.cgi?referer=&httpsredir=1&article=1064&context=jcwre (Accessed April 9, 2021). doi:10.1111/j.1936-704X.2005.mp130001002.x
Galloway, G. E., and Lewis, E. L. (2012). “A White Paper: The Need for a Unified National Program for Floodplain Management in the 21st Century,” in Report Prepared for the Federal Interagency Floodplain Management Task Force (Bethesda, MD: Abt Associates).
Garone, P. F. (2011). The Fall and Rise of the Wetlands of California’s Great Central Valley. Berkeley and Los Angeles: University of California Press. ISBN: 9780520355576. Available at: https://www.ucpress.edu/book/9780520355576/the-fall-and-rise-of-the-wetlands-of-californias-great-central-valley (Accessed July 14, 2021).
Geist, J., and Hawkins, S. J. (2016). Habitat Recovery and Restoration in Aquatic Ecosystems: Current Progress and Future Challenges. Aquat. Conserv: Mar. Freshw. Ecosyst. 26, 942–962. doi:10.1002/aqc.2702
Geist, J. (2011). Integrative Freshwater Ecology and Biodiversity Conservation. Ecol. Indicators 11, 1507–1516. doi:10.1016/j.ecolind.2011.04.002
Geist, J. (2015). Seven Steps towards Improving Freshwater Conservation. Aquat. Conserv: Mar. Freshw. Ecosyst. 25, 447–453. doi:10.1002/aqc.2576
Grosholz, E., and Gallo, E. (2006). The Influence of Flood Cycle and Fish Predation on Invertebrate Production on a Restored California Floodplain. Hydrobiologia 568 (1), 91–109. doi:10.1007/s10750-006-0029-z
Harrell, W. C., and Sommer, T. R. (2003). “Patterns of Adult Fish Use on California’s Yolo Bypass Floodplain,” in California Riparian Systems: Processes and Floodplain Management, Ecology, and Restoration, 88–93.
Healey, M. C. (1998). “Paradigms, Policies and Prognostication About the Management of Watershed Ecosystems,” In River Ecology and Management: Lessons from the Pacific Coastal Ecoregion. Editors R. J. Naiman, and R. Bilby. New York, NY: Springer, 662–681. doi:10.3390/su10061747
Heine, R. A., and Pinter, N. (2012). Levee Effects upon Flood Levels: an Empirical Assessment. Hydrol. Process. 26 (21), 3225–3240. doi:10.1002/hyp.8261
Hoagland, B., Schmidt, C., Russo, T. A., Adams, R., and Kaye, J. (2019). Controls on Nitrogen Transformation Rates on Restored Floodplains along the Cosumnes River, California. Sci. Total Environ. 649, 979–994. doi:10.1016/j.scitotenv.2018.08.379
Holling, C. S. (1978). Adaptive Environmental Assessment and Management. New York, NY: John Wiley & Sons.
Hornung, L. K., Podschun, S. A., and Pusch, M. (2019). Linking Ecosystem Services and Measures in River and Floodplain Management. Ecosyst. People 15 (1), 214–231. doi:10.1080/26395916.2019.1656287
Hutton, N. S., Tobin, G. A., and Montz, B. E. (2019). The Levee Effect Revisited: Processes and Policies Enabling Development in Yuba County, California. J. Flood Risk Manage. 12, e12469. doi:10.1111/jfr3.12469
IUCN (International Union for Conservation of Nature) (2021). Nature-Based Solutions. Available at: https://www.iucn.org/theme/nature-based-solutions.
Jeffres, C. A., Holmes, E. J., Sommer, T. R., and Katz, J. V. E. (2020). Detrital Food Web Drives Aquatic Ecosystem Productivity in a Managed Floodplain. Plos One 15 (9), e0216019. doi:10.1371/journal.pone.0216019
Jeffres, C. A., Opperman, J. J., and Moyle, P. B. (2008). Ephemeral Floodplain Habitats Provide Best Growth Conditions for Juvenile Chinook salmon in a California River. Environ. Biol. Fish. 83 (4), 449–458. doi:10.1007/s10641-008-9367-1
Johnson, M. (2017). Cosumnes River Provides Model for Floodplain Restoration in California. Water Deeply. 2017, April 19. Available at: https://www.newsdeeply.com/water/articles/2017/04/19/cosumnes-river-provides-model-for-floodplain-restoration-in-california (Accessed July 14, 2021).
Jones and Stokes (2005). “Archaeological Testing and Evaluation Report for the Bear-Feather River Setback Levee, Yuba County, California,” in Prepared for Three Rivers Levee Improvement Authority Jones and Stokes: Sacramento, CA Marysville, CA.
Katz, J. V. E., Jeffres, C., Conrad, J. L., Sommer, T. R., Martinez, J., Brumbaugh, S., et al. (2017). Floodplain Farm fields Provide Novel Rearing Habitat for Chinook salmon. PLOS ONE 12 (6), e0177409. doi:10.1371/journal.pone.0177409
Kelley, R. (1998). Battling the Inland Sea: Floods, Public Policy, and the Sacramento Valley. Berkeley, California: University of California Press.
Klein, C. A. (2019). The National Flood Insurance Program at Fifty: How the Fifth Amendment Takings Doctrine Skews Federal Flood Policy. Geo. Envtl. L. Rev. 31, 285. Available at https://scholarship.law.ufl.edu/facultypub/868/.
Kondolf, G. M. (2012). “The espace de liberté and restoration of fluvial process: When can the river restore itself and when must we intervene?,” in River Conservation and Management. Editors P. Boon, and P. Raven (Chichester: John Wiley & Sons), 225–242. doi:10.1002/9781119961819.ch18:
Kondolf, G. M., and Lopez-Llompart, P. (2018). National-Local Land-Use Conflicts in Floodways of the Mississippi River System. AIMS Environ. Sci. 5 (1), 47–63. doi:10.3934/environsci.2018.1.47
Kreienkamp, F., Philip, S. Y., Tradowsky, J. S., Kew, S. F., Lorenz, P., Arrighi, J., et al. (2021). Rapid Attribution of Heavy Rainfall Events Leading to the Severe Flooding in Western Europe during July 2021. Available at: https://www.worldweatherattribution.org/wp-content/uploads/Scientific-report-Western-Europe-floods-2021-attribution.pdf (Accessed December 09, 2021).
Lake, P. S., Bond, N., and Reich, P. (2007). Linking Ecological Theory with Stream Restoration. Freshw. Biol 52, 597–615. doi:10.1111/j.1365-2427.2006.01709.x
Limm, M. P., and Marchetti, M. P. (2009). Juvenile Chinook salmon (Oncorhynchus T) Growth in Off-Channel and Main-Channel Habitats on the Sacramento River, CA Using Otolith Increment Widths. Environ. Biol. Fish. 85 (2), 141–151. doi:10.1007/s10641-009-9473-8
Lulloff, A. (2013). The Floodway Encroachment Standard: Minimizing Cumulative Adverse Impacts. ASFPM. Available at: https://s3-us-west-2.amazonaws.com/asfpm-library/FSC/General/ASFPM_The_Floodway_Encroachment_Standard_Minimizing_Cumulative_Adverse_Impacts_Lulloff_2013.pdf (Accessed May 21, 2021).
Maples, S. R., Fogg, G. E., and Maxwell, R. M. (2019). Modeling Managed Aquifer Recharge Processes in a Highly Heterogeneous, Semi-confined Aquifer System. Hydrogeology J. 27, 2869–2888. doi:10.1007/s10040-019-02033-9
Mayer, R., Washburn, T., Cain, J., and Serra-Llobet, A. (2018). “Managing Floods in Large River Basins in the USA: The Sacramento River,” in Managing Flood Risk: Innovative Approaches from Big Floodplain Rivers and Urban Streams. Editors A Serra-Llobet, G. M Kondolf, K Schaefer, and N Scott (Cham: Palgrave Macmillan), 43–73. doi:10.1007/978-3-319-71673-2_3
M. G. Anderson, D. E. Walling, and P.D. Bates (Editors) (1996). Floodplain Processes (Chichester, United Kingdom: Wiley). ISBN: 978-0-471-96679-1.
Moyle, P. B. (2002). Inland Fishes of California: Revised and Expanded. Berkeley, CA: University of California Press. ISBN: 9780520227545. Available at: https://www.ucpress.edu/book/9780520227545/inland-fishes-of-california (Accessed June 6, 2021).
Moyle, P. B., Crain, P. K., and Whitener, K. (2007). Patterns in the Use of a Restored California Floodplain by Native and Alien Fishes. San Francisco Estuary Watershed Sci. 5 (3), 1–27. doi:10.15447/sfews.2007v5iss5art1
Moyle, P. B., Crain, P. K., Whitener, K., and Mount, J. F. (2003). Alien Fishes in Natural Streams: Fish Distribution, Assemblage Structure, and Conservation in the Cosumnes River, California, U.S.A. Environ. Biol. Fishes 68 (2), 143–162. doi:10.1023/b:ebfi.0000003846.54826.a6
Nichols, A. L., and Viers, J. H. (2017). Not all Breaks Are Equal: Variable Hydrologic and Geomorphic Responses to Intentional Levee Breaches along the Lower Cosumnes River, California. River Res. Applic 33 (7), 1143–1155. doi:10.1002/rra.3159
Opperman, J. J., Galloway, G. E., Fargione, J., Mount, J. F., Richter, B. D., and Secchi, S. (2009). Sustainable Floodplains through Large-Scale Reconnection to Rivers. Science 326 (5959), 1487–1488. doi:10.1126/science.1178256
Opperman, J. J., Luster, R., McKenney, B. A., Roberts, M., and Meadows, A. W., (2010). Ecologically Functional Floodplains: Connectivity, Flow Regime, and Scale1, 46, 211–226. doi:10.1111/j.1752-1688.2010.00426.x
Opperman, J. J., Moyle, P. B., Larsen, E. W., Florsheim, J. L., and Manfree, A. D. (2017). Floodplains: Processes and Management for Ecosystem Services. Oakland, CA: Univ of California Press.
Pander, J., Knott, J., Mueller, M., and Geist, J. (2019). Effects of Environmental Flows in a Restored Floodplain System on the Community Composition of Fish, Macroinvertebrates and Macrophytes. Ecol. Eng. 132, 75–86. doi:10.1016/j.ecoleng.2019.04.003
Pander, J., Mueller, M., and Geist, J. (2018). Habitat Diversity and Connectivity Govern the Conservation Value of Restored Aquatic Floodplain Habitats. Biol. Conservation 217, 1–10. doi:10.1016/j.biocon.2017.10.024
Perosa, F., Gelhaus, M., Zwirglmaier, V., Arias-Rodriguez, L., Zingraff-Hamed, A., Cyffka, B., et al. (2021). Integrated Valuation of the Co-Benefits of Nature-Based Solutions for Flood Risk with TESSA: An Example of Floodplain Restoration Measures at the Local Scale. Sustainability 13 (3), 1482. doi:10.3390/su13031482
Pieck, S. K. (2020). Conserving Novel Ecosystems and Layered Landscapes along the Inter-German Border. Landscape Res. 45 (3), 346–358. doi:10.1080/01426397.2019.1623183
Pinter, N. (2005). One Step Forward, Two Steps Back on U.S. Floodplains. Science 308, 207–208. doi:10.1126/science.1108411
Promny, M., Hammer, M., and Busch, N. (2014). Untersuchungen zur Wirkung der Deichrückverlegung Lenzen auf das Hochwasser vom Juni 2013 an der unteren Mittelelbe. Korrespondenz Wasserwirtschaft 6 (7), 344–349. doi:10.3243/kwe2014.06.004
Pugliese, F., Caroppi, G., Zingraff-Hamed, A., Lupp, G., and Giugni, M. (2020). Nature-Based Solutions (NBSs) Application for Hydro-Environment Enhancement. The Case Study of the Isar River (DE). Environ. Sci. Proc. 2 (30), 2030. doi:10.3390/environsciproc2020002030
Reinhardt, R. (2005). Letter to Charles K. McClain, Executive Director of the Three Rivers Levee Improvement Authority. Correspondence from Ric Reinhardt of MBK Enginners regarding levee improvements in Reclamation District 784.
Rossano, F. (2016). Isar Plan: The Wild as the New Urban? Contour 1 (2), 1–10. Available at: https://contourjournal.org/index.php/contour/article/view/58.
Rouillard, J., Lago, M., Abhold, K., Roeschel, L., Kafyeke, T., Klimmek, H., et al. (2018). Protecting and Restoring Biodiversity across the Freshwater, Coastal and Marine Realms: Is the Existing EU Policy Framework Fit for Purpose? Env. Pol. Gov. 28, 114–128. doi:10.1002/eet.1793
Roux, D. J., Novellie, P., Smit, I. P. J., de Kraker, J., Culloch-Jones, S. M., Dziba, L. E., et al. (2022). Appraising Strategic Adaptive Management as a Process of Organizational Learning. J. Environ. Manage. 301, 113920. doi:10.1016/j.jenvman.2021.113920
Salcido, R. E. (2012). Success and Continued Challenges of the Yolo Bypass Wildlife Area: A Grassroots Restoration. Ecol. LQ 39 (4), 1085. doi:10.15779/Z38B541
Schaufuß, D. (2016). Isar-Plan – Water Management Plan and Restoration of the Isar River. Munich, Germany: City of Munich.
Schindler, M., Donath, T. W., Terwei, A., and Ludewig, K. (2021). Effects of Flooding Duration on the Occurrence of Three Hardwood Floodplain Forest Species Inside and Outside a Dike Relocation Area at the Elbe River. Int. Rev. Hydrobiol. 2021, 1–8. doi:10.1002/iroh.202002078
Schindler, S., Sebesvari, Z., Damm, C., Euller, K., Mauerhofer, V., Schneidergruber, A., et al. (2014). Multifunctionality of Floodplain Landscapes: Relating Management Options to Ecosystem Services. Landscape Ecol. 29 (2), 229–244. doi:10.1007/s10980-014-9989-y
Scholten, M., Anlauf, A., Büchele, B., Faulhaber, P., Henle, K., Kofalk, S., et al. (2005). The River Elbe in Germany - Present State, Conflicting Goals, and Perspectives of Rehabilitation. Arch. Hydrobiol. Suppl. 155 Large Rivers 15 (1-4), 579–602. doi:10.1127/lr/15/2003/579
Scholz, M., Kasperidus, H. D., Schulz-Zunkel, C., and Mehl, D. (2012b). Betrachtung von Auenfunktionen vor und nach der Deichrückverlegung bei Lenzen. Auenreport Spezial 2012, 85–91.
Scholz, M., Mehl, D., Schulz-Zunkel, C., Kasperidus, H. D., Born, W., and Henle, K. (2012a). “Ökosystemfunktionen von Flussauen (Ecosystem Functions of Floodplain) – Analyse und Bewertung von Hochwasserretention, Nährstoffrückhalt, Kohlenstoffvorrat, Treibhausgasemissionen und Habitatfunktion,” in Naturschutz und Biologische Vielfalt (Bundesamt für Naturschutz/Landwirtschaftsverlag), 124, 258.
Scown, M. W., Thoms, M. C., and De Jager, N. R. (2015). Floodplain Complexity and Surface Metrics: Influences of Scale and Geomorphology. Geomorphology 245, 102–116. doi:10.1016/j.geomorph.2015.05.024
Seher, W., and Löschner, L. (2017). “Anticipatory Flood Risk Management–Challenges for Land Policy,” in Land Ownership and Land Use Development. Editors E. Hepperle, R. Dixon-Gough, R. Mansberger, J. Paulsson, J. Hernik, and T. Kalbro (Zurich: VDF at ETH), 370.
Serra-Llobet, A., Kondolf, G. M., Magdaleno, F., and Keenan-Jones, D. (2021). Flood Diversions and Bypasses: Benefits and Challenges. WIREs Water 9, e1562. doi:10.1002/wat2.1562
Serra-Llobet, A., Tourment, R., Montané, A., and Buffin-Belanger, T. (2022). Managing Residual Flood Risk behind Levees: Comparing USA, France, and Quebec (Canada). J. Flood Risk Manage. e12785. doi:10.1111/jfr3.12785
Sommer, T. R. (2002). The Aquatic Ecology of the Yolo Bypass Floodplain: Evaluation at the Species and Landscape Scales. [Dissertation]. Davis (CA): University of California at Davis.
Sommer, T. R., Harrell, W. C., and Nobriga, M. L. (2005). Habitat Use and Stranding Risk of Juvenile Chinook Salmon on a Seasonal Floodplain. North Am. J. Fish. Manage. 25 (4), 1493–1504. doi:10.1577/M04-208.1
Sommer, T. R., Nobriga, M. L., Harrell, W. C., Batham, W., and Kimmerer, W. J. (2001). Floodplain Rearing of Juvenile Chinook Salmon: Evidence of Enhanced Growth and Survival. Can. J. Fish. Aquat. Sci. 58 (2), 325–333. doi:10.1139/f00-245
Steger, K., Fiener, P., Marvin-DiPasquale, M., Viers, J. H., and Smart, D. R. (2019). Human-induced and Natural Carbon Storage in Floodplains of the Central Valley of California. Sci. Total Environ. 651, 851–858. doi:10.1016/j.scitotenv.2018.09.205
Strum, K. M., Reiter, M. E., Hartman, C. A., Iglecia, M. N., Kelsey, T. R., and Hickey, C. M. (2013). Winter Management of California's rice fields to Maximize Waterbird Habitat and Minimize Water Use. Agric. Ecosyst. Environ. 179, 116–124. doi:10.1016/j.agee.2013.08.003
Sudduth, G., and Lund, J. (2016). Multi-Purpose Optimization for Reconciliation Ecology on an Engineered Floodplain: Yolo Bypass. Calif. San Francisco Estuary Watershed Sci. 14 (1), 5. Available at: https://creativecommons.org/licenses/by/4.0/4.0. doi:10.15447/sfews.2016v14iss1art5
Thieken, A. H., Kienzler, S., Kreibich, H., Kuhlicke, C., Kunz, M., Mühr, B., et al. (2016). Review of the Flood Risk Management System in Germany after the Major Flood in 2013. Ecol. Soc. 21 (2), 51. Available at: http://www.jstor.org/stable/26270411. doi:10.5751/es-08547-210251
Thoms, M. C., Delong, M. D., Flotemersch, J. E., and Collins, S. E. (2017). Physical Heterogeneity and Aquatic Community Function in River Networks: A Case Study from the Kanawha River Basin, USA. Geomorphology 290, 277–287. doi:10.1016/j.geomorph.2017.02.027
Thoms, M. C. (2003). Floodplain-River Ecosystems: Lateral Connections and the Implications of Human Interference. Geomorphology 56, 335–349. doi:10.1016/S0169-555X(03)00160-0
Tockner, K., and Stanford, J. A. (2002). Riverine Flood plains: Present State and Future Trends. Envir. Conserv. 29, 308–330. doi:10.1017/S037689290200022X
Trowbridge, W. B. (2007). The Role of Stochasticity and Priority Effects in Floodplain Restoration. Ecol. Appl. 17 (5), 1312–1324. Available at: http://watershed.ucdavis.edu/pdf/Trowbridge-2007.pdf. doi:10.1890/06-1242.1
USEOP United States, Executive Office of the President (1977). Executive Order 11988: Floodplain Management. Available at: https://www.archives.gov/federal-register/codification/executive-order/11988.html (Accessed June 6, 2021).
USEOP United States, Executive Office of the President (2015). Executive Order 13690: Establishing a Federal Flood Risk Management Standard and a Process for Further Soliciting and Considering Stakeholder Input. Available at: https://obamawhitehouse.archives.gov/the-press-office/2015/01/30/executive-order-establishing-federal-flood-risk-management-standard-and-.
Vaghti, M. G., and Greco, S. E. (2007). “Chapter 16 Riparian Vegetation of the Great Valley,” in Terrestrial Vegetation of California. Editors M. G. Barbour, T. Keeler-Wolf, and A. A. Schoenherr. 3rd edition (Berkeley, Los Angeles, London: University of California Press), 313–338. ISBN: 9780520249554. Available at: https://www.ucpress.edu/book/9780520249554/terrestrial-vegetation-of-california-3rd-edition.
van Rees, C. B., Waylen, K. A., Schmidt-Kloiber, A., Thackeray, S. J., Kalinkat, G., Martens, K., et al. (2021). Safeguarding Freshwater Life Beyond 2020: Recommendations for the New Global Biodiversity Framework from the European Experience. Conservation Lett. 14 (1), e12771. doi:10.1111/conl.12771
Veidemane, K. (2019). “Commentary: Reflection on Governance Challenges in Large-Scale River Restoration Actions,” in Nature-Based Flood Risk Management on Private Land. Editors T. Hartmann, L. Slavíková, and S McCarthy (Cham: Springer). doi:10.1007/978-3-030-23842-1_20
Ward, J. V., Tockner, K., and Schiemer, F. (1999). Biodiversity of Floodplain River Ecosystems: Ecotones and Connectivity. Regul. Rivers: Res. Mgmt. 15, 125–139. doi:10.1002/(sici)1099-1646(199901/06)15:1/3<125::aid-rrr523>3.0.co;2-e
Warner, B., and Damm, C. (2019). “Relocation of Dikes: Governance Challenges in the Biosphere Reserve "River Landscape Elbe-Brandenburg",” in Nature-Based Flood Risk Management on Private Land: Disciplinary Perspectives on a Multidisciplinary Challenge. Editors T Hartmann, L Slavikova, and S McCarthy (Cham: Springer), 171–180. doi:10.1007/978-3-030-23842-1_18
Whipple, A. A., Grossinger, R. M., Rankin, D., Stanford, B., and Askevold, R. A. (2012). Sacramento-San Joaquin Delta Historical Ecology Investigation: Exploring Pattern and Process. Richmond, CA: Prepared for the California Department of Fish and Game and Ecosystem Restoration Program. A Report of SFEI-ASC’s Historical Ecology Program, SFEI-ASC Publication 672, San Francisco Estuary Institute-Aquatic Science Center. Available at: https://www.sfei.org/documents/sacramento-san-joaquin-delta-historical-ecology-investigation-exploring-pattern-and-proces (Accessed June 6, 2021).
White, G. F., Burby, R. J., Galloway, G. E., Goddard, J. E., Gosselink, J. G., Owen, H. J., et al. (1992). “Action Agenda for Managing the Nation's Floodplains,” in A Review of Floodplain Management in the United States: An Assessment Report (Boulder, Colorado: National Review Committee. Special Publication 25, Natural Hazards Research and Applications Information Center, Institute of Behavioral Science).
White, G., Kates, R., and Burton, I. (2001). Knowing Better and Losing Even More: the Use of Knowledge in Hazards Management. Glob. Environ. Change B: Environ. Hazards 3, 81–92. doi:10.1016/S1464-2867(01)00021-3
Williams, P. B., Andrews, E., Opperman, J. J., Bozkurt, S., and Moyle, P. B. (2009). Quantifying Activated Floodplains on a Lowland Regulated River: its Application to Floodplain Restoration in the Sacramento Valley. San Francisco Estuary Watershed Sci. 7 (1), 1–25. doi:10.15447/sfews.2009v7iss1art4
Willis, A., Lund, J., Townsley, E., and Faber, B. (2011). Climate Change and Flood Operations in the Sacramento Basin, California. San Francisco Estuary Watershed Sci. 9 (3), 1–18. doi:10.15447/sfews.2011v9iss2art3
Wohl, E., Castro, J., Cluer, B., Merritts, D., Powers, P., Staab, B., et al. (2021). Rediscovering, Reevaluating, and Restoring Lost River-Wetland Corridors. Front. Earth Sci. 9, 653623. doi:10.3389/feart.2021.653623
Yarnell, S. M., Petts, G. E., Schmidt, J. C., Whipple, A. A., Beller, E. E., Dahm, C. N., et al. (2015). Functional Flows in Modified Riverscapes: Hydrographs, Habitats and Opportunities. Bioscience 65, 963–972. doi:10.1093/biosci/biv102
Zhu, T., and Lund, J. R. (2009). Up or Out? - Economic-Engineering Theory of Flood Levee Height and Setback. J. Water Resour. Plann. Manag. ASCE 135 (2), 90–95. doi:10.1061/(asce)0733-9496(2009)135:2(90)
Zingraff-Hamed, A., Martin, J., Lupp, G., Linnerooth-Bayer, J., and Pauleit, S. (2019). Designing a Resilient Waterscape Using a Living Lab and Catalyzing Polycentric Governance. Landscape Architecture Front. 7 (3), 12–31. doi:10.15302/J-LAF-1-020003
Keywords: floodplain management, flood risk management, California Central Valley, United States, Germany, multi-benefit, river restoration, nature-based solutions
Citation: Serra-Llobet A, Jähnig SC, Geist J, Kondolf GM, Damm C, Scholz M, Lund J, Opperman JJ, Yarnell SM, Pawley A, Shader E, Cain J, Zingraff-Hamed A, Grantham TE, Eisenstein W and Schmitt R (2022) Restoring Rivers and Floodplains for Habitat and Flood Risk Reduction: Experiences in Multi-Benefit Floodplain Management From California and Germany. Front. Environ. Sci. 9:778568. doi: 10.3389/fenvs.2021.778568
Received: 17 September 2021; Accepted: 31 December 2021;
Published: 16 March 2022.
Edited by:
Avril C. Horne, The University of Melbourne, AustraliaReviewed by:
Martin Thoms, University of New England, AustraliaRui Manuel Vitor Cortes, University of Trás-os-Montes and Alto Douro, Portugal
Copyright © 2022 Serra-Llobet, Jähnig, Geist, Kondolf, Damm, Scholz, Lund, Opperman, Yarnell, Pawley, Shader, Cain, Zingraff-Hamed, Grantham, Eisenstein and Schmitt. This is an open-access article distributed under the terms of the Creative Commons Attribution License (CC BY). The use, distribution or reproduction in other forums is permitted, provided the original author(s) and the copyright owner(s) are credited and that the original publication in this journal is cited, in accordance with accepted academic practice. No use, distribution or reproduction is permitted which does not comply with these terms.
*Correspondence: Anna Serra-Llobet, YW5uYXNlcnJhbGxvYmV0QGJlcmtlbGV5LmVkdQ==