- 1College of Resources and Environment, Yangtze University, Wuhan, China
- 2Key Laboratory of Exploration Technologies for Oil and Gas Resources, Ministry of Education, Yangtze University, Wuhan, China
- 3Research Institute of Petroleum Exploration and Development, PetroChina Tarim Oilfield Company, Korla, China
Seasonal delta is a sandy fan-shaped sedimentary system formed by seasonal river flooding in continental basins. Modern sedimentary finds that seasonal deltas mainly develop in an arid-semi-arid climate environment with gentle terrain. However, the study of seasonal deltas in ancient rock records is still insufficient, leading to misinterpretations as other sedimentary systems, which poses challenges to the correct understanding of the origin and spatial distribution of sand body deposits. In this study, the red bed deposits of the Cretaceous Baxigai Formation in the west of the Tabei Uplift were selected as the research object. Based on core observation and description, a comprehensive analysis of the sedimentary environment, sedimentary characteristics and sedimentary model of the Baxigai Formation was carried out using data such as well-logging and analytical testing, combined with modern depositional characteristics. Research shows that the sedimentary sequence of the Baxigai Formation red bed developed a seasonal delta sedimentary system in the arid climate. The seasonal delta deposits provide an opportunity to understand the influencing of river flow and total sedimentary loading vary greatly on the sedimentary model, sedimentary mechanism, and distribution of sand bodies in arid climates. The sedimentary process of the seasonal delta in the Baxigai Formation was controlled by the dual-stage sedimentation process in an arid paleoclimate. During the flood period, the supercritical flow sedimentary structures are widely developed in the major distributary channels, and the distributary channels migrate and bifurcate frequently. On the delta plain, crevasse channels are formed through the avulsion of major distributary channels. During the dry period, due to the consumption of water seepage and evaporation transfer, terminal distributary channels are abandoned, crevasse channels are not developed, and the sediment is exposed. The density of the distributary channels is reduced, and the total sedimentary loading is low. The distributary channels sand body is the skeleton sand body of the seasonal delta. This study is of great significance for accurately explaining the formation process of seasonal deltas and provides a useful reference for further exploration and deployment of oil and gas reservoirs and paleogeographic reconstruction in the study area.
1 Introduction
Seasonal deltas are formed by the seasonal river recharge, driven by ephemeral and high-energy flood events, and mainly develop in arid-semi-arid climate environments with gentle terrain (Liu B. B. et al., 2019; Gugliotta et al., 2016). Essential characteristics of seasonal delta: 1) The sediment are mainly red bed deposits, and there is no apparent foreset bed in the profile; 2) The river is controlled by seasonal precipitation, the river discharge and total sedimentary loading variation greatly and the supercritical flow sedimentary structure is widely developed in the deposits; 3) The delta is dominated by rivers action and the center of the basin lacks a large catchment area (Liu Y. F. et al., 2019; Zhang et al., 2018; Li et al., 2021). Although there are many examples of seasonal deltas deposition in an arid environment, in the continental basin sedimentology, the research on the sedimentary origin, distribution, and sedimentary model of seasonal deltas sand bodies in arid environments are not thorough enough, and many seasonal delta sedimentary systems in arid climate may not be correctly explained in ancient rock records. Delta reservoir formation theory has made significant achievements in guiding hydrocarbon exploration in continental basins (Yang et al., 2003; Fu et al., 2013). The Tabei Uplift is one of the Tarim Basin’s most favorable oil and gas enrichment areas, rich in oil and gas resources (Liu B. B. et al., 2019; Huang et al., 2018). In recent years, with the discovery of low-amplitude structural oil and gas reservoirs such as YM463 and YM46, two lithological oil and gas reservoirs YD7 and YM467, the Baxigai Formation, as an essential reservoir system in the west of the Tabei Uplift, have gradually attracted attention and have become one of the critical exploration targets (Ma et al., 2022; Luo et al., 2021). However, the structural background of the Baxigai formation in the study area is complex. The characteristics of the sand body are deep burial, thin thickness, regionalization, and complex lateral changes; moreover, the region is affected by the climate, the depositional environment is complex, and the sand body depositional types are diverse. At present, the understanding of the depositional mechanism and depositional pattern of the sand body of the Baxigai formation is insufficient, and a single depositional mode cannot well explain the complex depositional mechanism of the sand body in the study area. In recent years, Many scholars have conducted in-depth research on the Cretaceous Baxigai Formation in the Kuqa Piedmont and Tabei Uplift, mainly analyzing the distribution of sedimentary facies in large areas. However, there are few studies on the Baxigai Formation in the west of the Tabei Uplift, and only the Kapushaliang group is studied as a whole group. Previous studies have not fully paid attention to the control effect of the Cretaceous paleoclimate on the red-bed sand bodies of the Baxigai Formation, and insufficient understanding of the depositional characteristics, depositional environment, and depositional models in the red-bed sand bodies of the Baxigai Formation, resulting in unclear distribution rules and sedimentary boundaries of sand bodies in this area, which seriously restricts the process of oil and gas resource exploration in the Baxigai Formation in the study area (Hong et al., 2016). studied the sedimentary characteristics of the Baxigai Formation in the Xinhe-Sandaoqiao area and concluded that this set of formations is a braided river delta-lake sedimentary system (Wu et al., 2022). studied the Mesozoic sedimentary evolution characteristics of the Tabei Uplift. They concluded that deltas, braided river deltas, alluvial fan or fan delta sedimentary combinations, and beach bar deposits were mainly developed at the end of the Kapusaliang Group (Luo et al., 2021). concluded that the Baxigai formation on the southern slope of the Kuqa Depression developed the inner front-outer front-shallow lake subfacies of a river-controlled shallow water delta (Gao et al., 2014). researched the sedimentary paleoenvironment and model through field outcrops and geochemical analysis. They concluded that the Cretaceous Baxigai formations in the Kuqa Piedmont belt are wide and shallow lakes with large areas in an arid climate. In the Cretaceous Baxigai Formation deposition period, the study area has a hot and dry climate, gentle terrain, and conditions for seasonal delta development, making it an ideal area for the development of seasonal deltas.
This paper takes the red bed deposits of the Cretaceous Baxigai Formation in the western Tabei Uplift as the research object. Through core, well logging, geochemical analysis, and other data, and based on the regional geological background, a systematic study was conducted on the sedimentary environment and sedimentary characteristics of the Baxigai Formation. Based on the principle of historical comparison and combined with the sedimentary characteristics of modern seasonal delta sand bodies in arid climate conditions, the sedimentary types and characteristics, distribution rules, and sedimentary patterns of seasonal deltas of the Cretaceous Baxigai formation in the western Tabei Uplift are discussed. It provides a valuable reference for the reconstruction of paleogeography in the study area.
2 Geological setting
The Tarim Basin is located in northwest China and is the largest petroleum-bearing basin on land in China, covering an area of approximately 560,000 square kilometers (He C. F. et al., 2023; Wu et al., 2019). The basin is rhombus-shaped in plane and is surrounded by the Tianshan Mountains, the Kunlun Mountains, and the Altun Mountains (Figure 1A). It is a large superposition composite basin composed of Paleozoic craton basins and Meso-Cenozoic foreland basins, with a complex tectonic evolution history of ancient continental crust basement and multiple subsidence and Uplift (Du et al., 2023). Proterozoic-Cenozoic Formation are developed from bottom to top in the basin, with a maximum sedimentary thickness of more than 10.000 m (Tian, 2019). The Tarim Basin can be divided into seven first-level structural units, namely, the Kuqa Depression, the Tabei Uplift, the Northern Depression Belt, the Central Uplift Belt, the Southwestern Depression Belt, the Southeastern Uplift, and the Southeastern Depression Belt, from north to south (Figure 1B) (Jia, 1997). The Tabei Uplift is located in the northern part of the Tarim Basin, spreading in an east-west direction. It is adjacent to the Kuqa Depression in the north and the Northern Depression Belt in the south and consists of the Wensu Bulge, the Yingmaili Low Bulge, the Luntai Bulge, the Lunan Low Bulge, and the Korla Bulge (Li et al., 2012; He et al., 2008; Sun et al., 2020; Li F. J. et al., 2019). The Tabei Uplift is a long-term development of the Paleozoic craton “residual paleo-uplift” with the characteristics of basement uplift (Su et al., 2020). The Uplift was finalized after the late Hercynian movement in the late Paleozoic. At the end of the Permian to the early Triassic, the Tabei uplift was defined as the front Uplift of the foreland basin in the Kuqa depression (Li et al., 2012). Since the Cretaceous period, the Tabei Uplift gradually evolved into a north-dipping gentle slope and was affected by multiple tectonic movements; a concave and convex structural pattern has been formed, and the Tabei Uplift gradually declined (Liu Y. F. et al., 2019; Li J. G. et al., 2015; Xu et al., 2016). Currently, it is mainly covered by the Quaternary strata. The study area is located in the west of Tabei Uplift, which mainly includes the Yingmaili low Uplift, the west of Luntai Uplift, and part of the Lunnan low uplift, and it is a key area for oil and gas exploration in the Tarim Basin at present (Figure 1C).
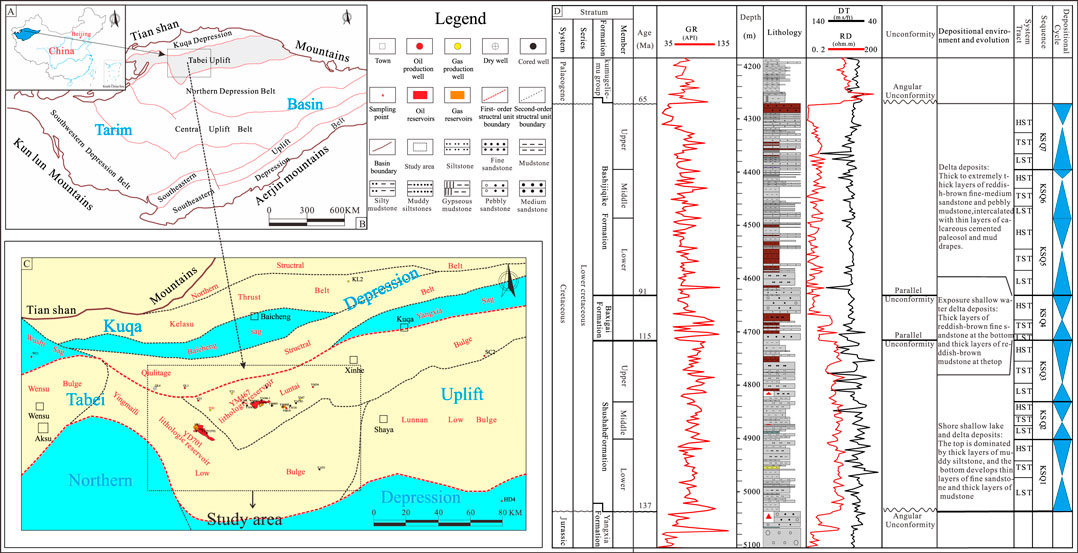
FIGURE 1. Geological background of the western Tabei Uplift (A) Location map of the Tarim Basin in NW China (modified from Wang et al., 2023). (B) The tectonic location of the study area (modified from Zhao et al., 2022). (C) Map showing geological details of the study area, including the location of oil and gas field, cored wells, and partial key wells (modified from Xu et al., 2016). (D) Well YM10 Stratigraphic framework and sedimentary background of the interval of interest (modified from Xia et al., 2019; He et al., 2013; Liu Y. F. et al., 2019).
The Tabei Uplift has superior conditions for the two-way convergence of dual-stage petroleum-bearing systems of Marine Cambrian-Ordovician and continental Triassic-Jurassic and is very rich in oil and gas resources (Zhang et al., 2010). The Triassic, Jurassic, and Cretaceous strata were developed in the study area in the Mesozoic period (Li J. L. et al., 2019). The Cretaceous mainly developed the Lower Cretaceous strata; the Upper Cretaceous strata were denuded and had an angular unconformity contact with the underlying Jurassic and the overlying Palaeogene. The Lower Cretaceous strata are a set of clastic rock depositional systems, and the Kapushaliang Group (Shushanhe Formation and Baxigai Formation) and Bashijiqike Formation developed from bottom to top (Figure 1D). The overall thickness of the Shushanhe Formation is between 50 and 350 m. It is a set of fine-grained clastic rock deposits, the top is dominated by thick layers of muddy siltstone, and the bottom develops thin layers of fine sandstone and thick layers of mudstone, and the development of Shore shallow lake and Delta facies (Xia et al., 2019; He B. Z. et al., 2023). The Bashijiqike Formation is a delta sedimentary system composed of thick to extremely thick layer of reddish-brown fine-medium sandstone and pebbly mudstone, intercalated with thin layers of calcareous cemented paleosol and mud drapes (Liu B. B. et al., 2019). Paleo-uplift were weak in activities, and the accommodation was sustained in the deposition period of the Baxigai Formation (Zhao et al., 2022). Influenced by regional tectonic movement, the paleogeographic pattern of the southeast high and northwest bottom was formed in the study area, which controlled the distribution of sedimentary facies (Su et al., 2020).
3 Materials and methods
This research was based on cores, well logs, 3D seismic data, and analyzed test (X-ray diffraction whole rock mineral analysis, Particle size analysis, and microelement analysis) data of the Baxigai Formation in the northwestern Tarim Basin (see Figure 1C for cored well location). The data used in this study were obtained from the Institute of Exploration and Development, PetroChina Tarim Oilfield Company. Detailed descriptions, photographs, and sediment particle size analysis from 242 m of core records in 15 cored wells that penetrated the Baxigai Formation were taken for identification of lithofacies and interpretation of sedimentary environments. The core observation and description tasks were conducted in the core library of Tarim Oilfield. Well logs from a total of 160 wells (including exploration wells and oil and gas production wells) and well-logging data were in LAS format in this study. 3D seismic data that cover the central part of the study area (3,174 km2) were studied for identification Paleogeomorphology in the depositional period of the Baxigai Formation by horizon flatting of a SE—NW direction seismic profile (approximately 71 km long), the 3D seismic surveys were in SEG-Y format. X-ray diffraction whole rock analysis data comes from 66 samples in 7 boreholes and is mainly used to analyze Baxigai formation sandstone types. Identify single-well sedimentary microfacies of cored wells through core observation and description combined with well logs and particle size analysis data. The microelement analysis data samples were from Well YM19 and Well YD704H, with a total of 7 samples (see Figure 1C for sample locations). It is mainly used to analyze the palaeoclimate and palaeoenvironment in the deposition period of the Baxigai Formation. The PerkinElmer inductively coupled plasma mass spectrometer NexION was used as the test instrument for microelement analysis, and the accuracy of over 5 percent adopts the “Methods for Chemical Analysis of silicate Rocks”(GB/T 14506.30–2010). Modern satellite images from Google Earth software were used for Modern analog to improve understanding of the development pattern of the distributary channels in arid climates. Calibration of gamma ray log data with core interpretations was used to establish electro facies that were used to identify sedimentary facies in non-cored wells.
The core depth was corrected based on the GR curve, analyzing the formation color, special minerals, and sedimentary structure characteristics and using the standard chemical parameters from microelement analysis to explain the sedimentary environment of the Baxigai formation. Based on lithology, sedimentary structure, and other related factors, lithofacies classifications of the Baxigai formation were conducted, and by combining the lithofacies classification, well logs, and particle size analysis, single well sedimentary interpretations were conducted in cored wells. Using core interpretation and GR well log curve characteristics, well log facies schemes are established to identify single-well sedimentary microfacies in non-cored wells. By counting the sandstone thickness of 160 wells in the Baxigai Formation, sand body thickness contours are drawn, and combined with the well-tie profile of sedimentary microfacies comparison, the plane distribution of sand body sedimentation microfacies is obtained. Based on the principle of historical comparison method and combined with the comprehensive analysis of the sedimentary characteristics of modern sedimentary high-definition satellite images (including sedimentary background, sedimentary origin, sedimentary morphology, sedimentary scale, etc.) The channel development models in arid climate conditions was extracted from it, and based on this, an accurate sedimentary interpretation of the Baxigai Formation in the study area was performed. Combining paleotopography, provenance, paleoclimate, and hydrodynamic changes and based on the plane distribution of sand body sedimentation microfacies, a two-stage sedimentation model of seasonal delta sand bodies in arid climate conditions was proposed.
4 Results
4.1 Sedimentary environment analysis
4.1.1 Lithology characteristics
The thickness of the Cretaceous Baxigai Formation in the west of Tabei Uplift is between 40 and 60 m, the shaliness gradually increases from bottom to top (Figure 2). Through the statistical analysis of sandstone samples, the results show that The sandstone types of the Baxigai Formation in the study area are mainly lithic feldspar sandstone, followed by feldspar sandstone and feldspar lithic sandstone. The quartz volume fraction is 35%–74%, the average volume fraction is 55%, the feldspar volume fraction is 14%–48.7%, the average volume fraction is 28%, the lithic fragment volume fraction is 6%–35.2%, the average volume fraction is 17% (Figure 3). Overall, the grain size of the red bed sandstone in the Baxigai Formation is mainly medium-fine, well sorted, mainly sub-angular to sub-rounded, with high structural maturity and medium composition maturity.
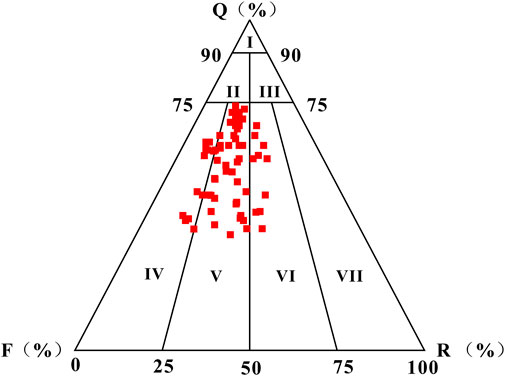
FIGURE 3. Sandstone classification triangle diagram of the Baxigai Formation (66 samples from 7 wells). Classification standard reference (Zhu Xiaomin, 2010). I: quartz sandstone; II: feldspar quartz sandstone; III: lithic quartz sandstone; IV: feldspar sandstone; V: lithic feldspar sandstone; VI: feldspar lithic sandstone; VII: lithic sandstone.
4.1.2 Formation color and special mineral
Mudstone color is divided into autogenetic color and secondary color. Autogenetic color depends on the color of authigenic minerals during the sedimentary process of clayey deposits and its early diagenetic process, and it is an essential symbol for restoring the ancient depositional environment and reflecting the depth of the water body (Fu et al., 2023; Sun et al., 2015). The colors of the Baxigai Formation mudstone in the study area are mainly brown and reddish-brown. The Phenomena such as mud cracks caused by drought exposure, interbedded between oxidized and reduced colors mudstone, and mutation contact between oxidized and reduced colors mudstone can be seen in the Baxigai Formation (Figures 4A–I), which is because the deposits in this area are caused by seasonal changes in climate and cyclical changes in runoff (flooding and drying periods). In the flood period, the climate was relatively humid, the runoff increased sharply, the water body in the sedimentary area was deep, and mudstone was deposited by the fine-grained suspended matter brought by the flood, showing a gray-green color in a weakly reductive sedimentary environment. In the dry period, the climate was dry, with less rain, the water body in the sedimentary area was shallow or even dry, the mudstone color was reddish brown, and a part of the mudstone was exposed on the earth’s surface. From the above mudstone color and bedding plane structure, it is observed that the mudstone is exposed intermittently.
Reduced color sand bodies are commonly found in the oxidized formation (red bed) of the Baxigai Formation in the study area, and the colors of the sandy sediment in the Baxigai Formation are mainly reddish-brown and gray-green. Reddish-brown sandstone (red bed) does not contain oil and gas, reflecting primary sedimentary characteristics (Figure 4J). The origin of gray-green sandstone is a reduction reaction between oxidizing minerals and hydrocarbon substances caused by oil and gas filling after sandy sediments diagenesis, which makes the mineral autogenetic color transformation. However, due to the low physical properties of calcareous nodules, oil and gas are difficult to invade and retain mineral autogenetic color.
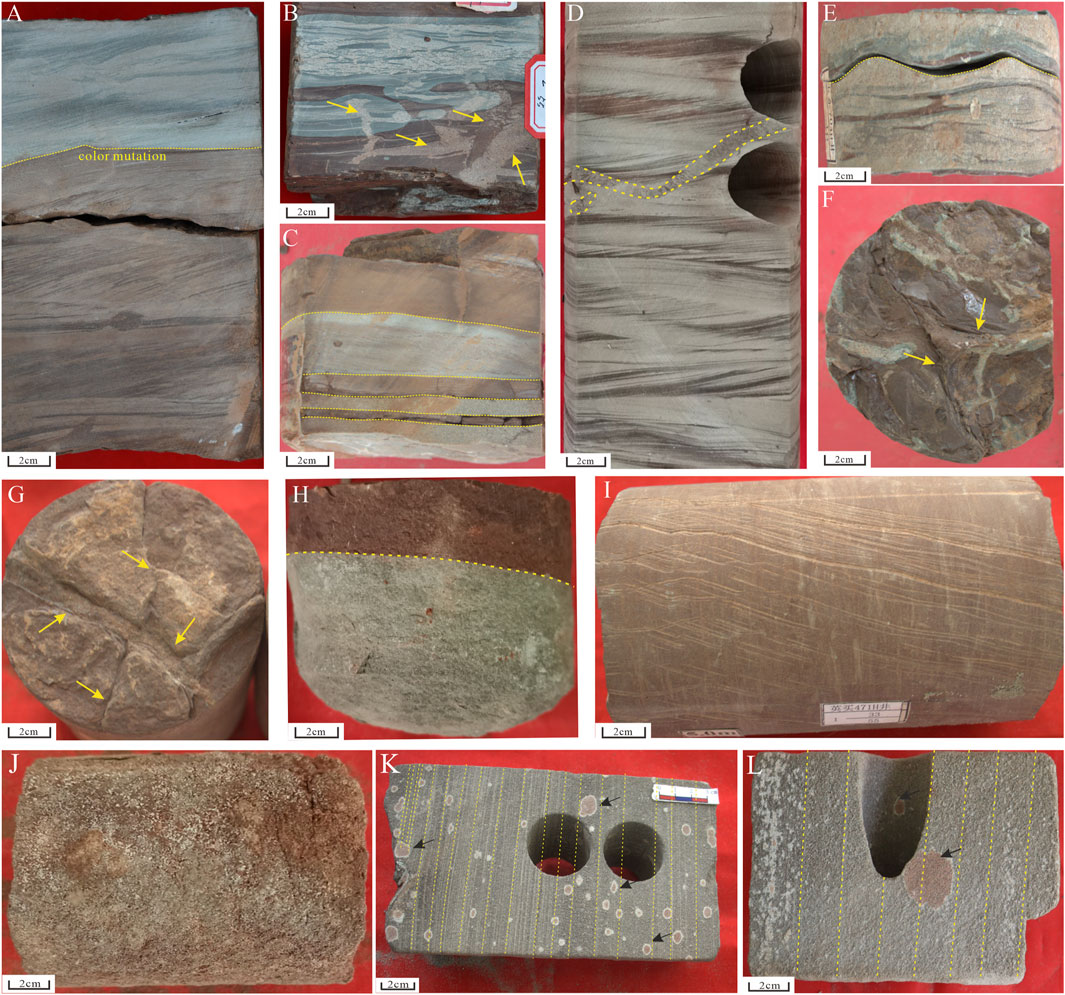
FIGURE 4. Core pictures showing characters in climate-sensitive deposits of the Baxigai Formation. (A) WellYD701, 4975.28 m, Ascending cross-bedding silty mudstone with color mutation; (mutation boundary surface mark by yellow arrow) (B) WellYD701, 4978.5 m, Reddish brown-grey green mudstone alternating with bioturbation (mark by yellow arrow); (C) WellYD704, 4975.63 m, Reddish brown mudstone-gray green siltstone sandstone interbedded; (D) WellYD704, 4970.43 m, Ascending cross-bedding muddy siltstone, black lamina at the bottom and brown lamina at the top with bioturbation (mark by yellow lines); (E) WellYD704, 4975.30 m, Light red and gray-green muddy siltstone interbedded deposit; (F) WellYD704, 4979.18 m, Mud cracks (mark by yellow lines); (G) WellYM19, 4999.8 m, Mud cracks (mark by yellow lines); (H) WellYM46-1, 5166.7 m, Mudstone color change from gray-green to reddish brown; (I) WellYM471, 5201.25 m, reddish brown mudstone; (J) WellYM701, 4995 m, Massive bedding, fine sandstone with salt efflorescence; (K) WellYM471, 5201.65 m, Calcareous nodules (mark by black arrow) in the parallel bedding very fine sandstone; (L) WellYM468, 4998.5 m, Calcareous nodules (mark by black arrow) in the parallel bedding medium sandstone.
Calcareous nodules are authigenic mineral aggregates composed of calcium carbonate, generally formed by evaporation or leaching in arid-semi-arid regions, and are an essential indicator of paleoclimate analysis (Xing et al., 2019). The calcareous nodules of the Baxigai Formation in the study area are reddish-brown, mainly oval, distributed along the bed, and foam strongly after dropping dilute hydrochloric acid with a concentration of 5%, and they are generally deposited in fine sandstones (Figures 4K, L). These nodules have not cut through the bedding, indicating that they are products of the syngenesis period. Based on the above analyses, it is believed that the study area was in an oxidizing environment with hot and arid paleoclimate conditions during the sedimentary period of the Baxigai Formation.
4.1.3 Sedimentary structure
The primary sedimentary structure type indicates the hydrodynamic mechanisms, deposition rate, and sedimentary environment characteristics in sediment formation. Core observation shows that the Baxigai formation in the study area developed a multi-stage superimposed channels sand body. The deposits are mainly fine sandstone and siltstone, and Sedimentary structures reflecting event sedimentation, such as lag deposit fine conglomerate (Figure 5A), positive graded bedding (Figures 5D, E), and gravel imbricated arrangement (Figure 5F), can be seen locally. Lag deposits: distributed along the scour surface, mainly coarse sandstone and fine conglomerate, with mixed particle sizes and poor sorting, the particles are mainly sub-round, followed by sub-angular, and roundness is medium, reflecting a longer transport distance. Graded bedding: distributed at the bottom of the channels sand bodies, from bottom to top, the particle size changes from coarse to fine. Parallel bedding and massive bedding develops in the upper part of the graded bedding, mainly medium sandstone and fine sandstone, reflected in hydrodynamic conditions with extreme transporting capacity, and the transported clastic particles are rapidly unloaded and deposited in a short time. Gravel imbricated arrangement: gravel is intraclasts (muddy clast) formed by diversion or lateral migration of the river bed to erode muddy deposits at the bottom of the river, landslides, or deposition after short-term transport, which reflects hydrodynamic conditions with strong transporting capacity.
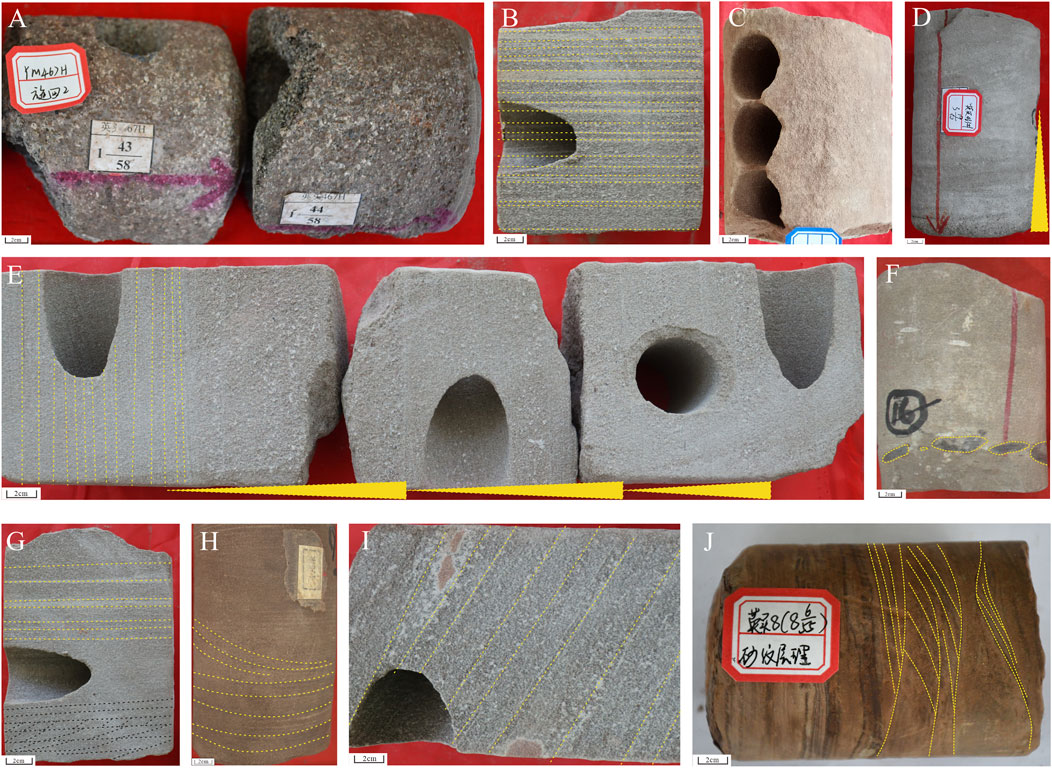
FIGURE 5. Typical sedimentary structure characteristics in the Baxigai Formation. (A) WellYM467, 5180.2 m, Lag deposit fine conglomerate; (B) WellYM468, 5002.45 m, Parallel bedding medium sandstone; (C) WellYM16, 5005 m, Massive bedding fine sandstone; (D) WellYM463, 5104.5 m, Positive graded bedding (mark by yellow triangle); (E) WellYM468, 4998.5 m, Multi-stage lag deposits, The particle size gradually becomes finer from bottom to top and parallel bedding (mark by yellow lines) at the top; (F) WellYT4, 5566.6 m, Massive bedding fine sandstone with gray-green muddy clast, gravel imbricated arrangement (mark by yellow lines); (G) WellYM468, 4999.7 m, Medium sandstone parallel bedding (mark by yellow lines) and low angle cross-bedding (mark by black lines); (H) WellYT4, 5562.42 m, small trough cross-bedding fine sandstone; (I) WellYM468, 5000 m, Oblique bedding medium sandstone; (J) WellYM8, 5252.1 m, Ripple cross-bedding muddy siltstone.
The upper flow regime sedimentary structures are an essential connection between sedimentary records and climate responses and are commonly found in seasonal river deposits (Tan et al., 2018). In the sandstone of the Baxigai Formation, there are not only normal fluvial sedimentary structures but also a series of upper flow regime sedimentary structures. The basic characteristic of upper flow regime is the dynamics of shallow water and rapid flow, which mainly develops after the flow velocity rapidly increases to a certain peak in a short period, and the preservation of upper flow regime sedimentary structures also requires the rapid decline of the flow velocity. The upper flow regime sedimentary structures of the Baxigai Formation mainly include parallel bedding, massive bedding, etc. (Figures 5B, C). Parallel bedding is mainly well developed in fine sandstone, and in hydrodynamic conditions with strong transporting capacity, it is also common in gravelly coarse sandstone and medium sandstone. High deposition rate results in a positive graded of particle size in parallel bedding. Massive bedding is mainly developed in thick layers of fine sandstone, reflecting rapid deposition in high-energy, strong hydrodynamic conditions. The lower flow regime sedimentary structures of the Baxigai Formation mainly include low angle cross-bedding, small trough cross-bedding, oblique bedding, and ripple cross-bedding (Figures 5G–J). They are mostly developed in fine sandstone and represent slow or subcritical flow, a slow-flowing hydrodynamic force dominated by the traction current of one-way water flow, consistent with normal river sedimentary structure characteristics.
The sandstone sedimentation of the Baxigai Formation in the study area is not only affected by the lower flow regime flow mechanism but also develops many distinctive sedimentary structures controlled by the upper flow regime flow mechanism, which reflects the coexistence of seasonal flood events and normal sedimentation in the Baxigai Formation.
4.1.4 Geochemical characteristics
Trace elements in sedimentary rocks are not only controlled by their own physical and chemical properties but also greatly affected by paleoclimate and paleoenvironments. Therefore, the relative content and ratio of trace elements can be used to restore paleoclimate and paleoenvironments (Nesbitt and Young, 1982; Yang et al., 2006). Trace elements such as V, U, Mo, Cr, and Co are redox sensitive elements and are often used as an identification index of the paleooxygen phase (Fan et al., 2019). In this paper, two chemical parameters, V/(V+Ni) and V/Cr, are selected to distinguish the paleo sedimentary environment of the Baxigai Formation through the analysis, calculation, and statistics of the existing sample data. V/(V+Ni) >0.77, 0.6–0.77, and <0.6 indicate reduction environment, weak oxidation-weak reduction environment and oxidation environment; V/Cr >4.25, 2.0–4.25, and <2 indicate reduction environment, weak oxidation-weak reduction environment and oxidation environment (He et al., 2013; Russell and Morford, 2001; Li F. J. et al., 2019; Jones and Manning, 1994).
In the Baxigai Formation, the value of V/(V+Ni) is 0.02–0.36, and the average value is 0.14; the value of V/Cr is 0.01–0.39, and the average value is 0.13. Based on the results of the above analysis, it can be seen that the sedimentary water body in the study area during the sedimentary period of the Baxigai Formation mainly manifests an oxidation environment.
The Sr/Ba ratio is a commonly used identification index for judging the paleosalinity of water body in sedimentary areas. Sr/Ba <0.6, 0.6–1.0, and >1 indicate freshwater, semi-salt, and saltwater deposition (Wang et al., 1979). The statistical analysis of the Baxigai Formation samples in the study area shows that the Sr/Ba ratio is 1.34–5.00, and the average value is 3.32. It can be inferred that the sedimentary water body of the study area in the Baxigai Formation sedimentary period was a saltwater environment, indicating that the evaporation of water was greater than the rainfall in the sedimentary area, and the climate was dry and hot.
The Sr/Cu ratio responds sensitively to changes in paleoclimate and has a good indication effect. Sr/Cu between 1.3 with 5.0 indicates a warm and humid climate, and Sr/Cu>5.0 indicates a hot and dry climate (Ding et al., 2021). The Sr/Cu ratio of the Baxigai Formation is 2.05–42.32, with an average value of 14.10, and 5 samples are >5.0, indicating that the sedimentary period of Baxigai Formation in the study area was dominated by a hot and dry, with a few periods are humid and warm climate, reflecting the paleoclimate feature of alternating dry and wet.
4.2 Sedimentary facies and their spatial distribution of the Baxigai Formation seasonal delta
4.2.1 Sedimentary facies description and interpretation
Sedimentary facies and their sedimentary characteristics can be identified from core descriptions and particle size analysis to build seasonal delta sedimentary systems in an arid climate. The delta plain and the delta front depositional environments were identified in the seasonal delta sedimentary system.
4.2.1.1 Delta plain
The delta plain is located above the water surface and is mainly controlled by rivers, not affected by lakes, and has been in an oxidizing environment for a long time. The delta plain in the study area can be divided into upper delta and lower delta plain according to the intensity of river action, sand body thickness, and deposit particle size, mainly developing distributary channels, crevasse channels, Floodplains, and interdistributary bays.
4.2.1.1.1 Distributary channels
There are obvious scour marks at the bottom of the distributary channels sand body, and there is a phenomenon of repeated erosion of multiple stages river channels. The thick layer of massive sandstone is enriched with a large amount of muddy clast and rip-up mud clast, and some of the lag deposit pebbly sandstone layers can be seen (Figures 6A–D). Massive bedding, positive graded bedding, parallel bedding, and low angle cross-bedding are developed above the scour surface (Figures 6E–G). The sediments are mainly medium sandstone and fine sandstone, with a small amount of coarse sandstone and pebbly sandstone. The channels is dominated by vertical aggradation, and multi-stage superimposed channels sand body develops in a positive rhythmic depositional sequence. The thickness of the stage channels sand body is between 4 and 8 m, and the cumulative thickness is up to 45 m.
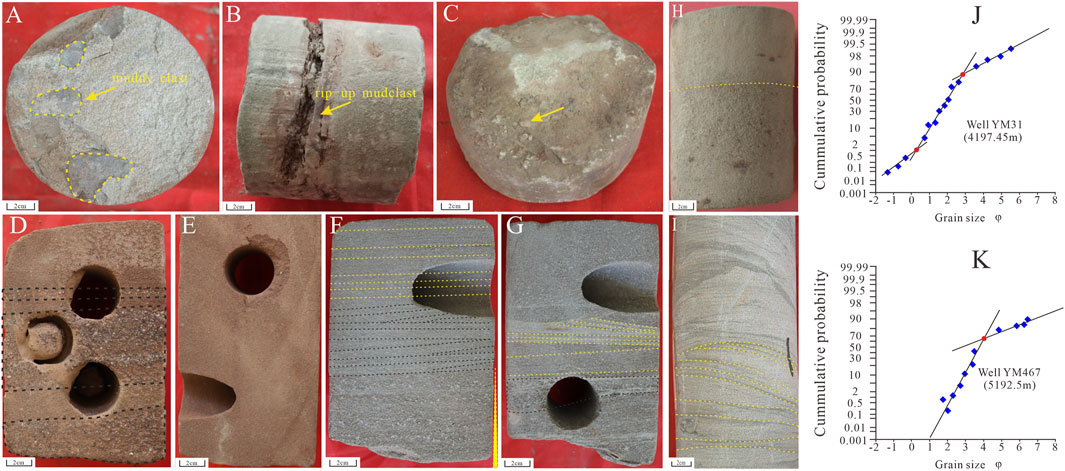
FIGURE 6. Typical sedimentary structure characteristics and cumulative grain-size probability distribution curves of core samples in distributary channels deposits in the Baxigai Formation. (A) WellYM31, 4174.25 m, Grayish green muddy clast (mark by yellow lines) in massive bedding medium sandstone; (B) WellYM31, 4196.5 m, Brownish rip-up mud clast deposits (arrow) in massive bedding fine sandstone; (C) WellYM31, 4197.95 m, Lag deposits (arrow) in medium sandstone; (D) WellYM468, 5002.7 m, Multi-stage lag deposits pebbly sandstone layer (mark by black lines); (E) WellYM468, 5001.3 m, Massive bedding fine sandstone; (F) WellYM468, 4999.8 m, Parallel bedding at the top (mark by yellow lines), low angle cross-bedding (mark by black lines), and positive graded bedding at the bottom (mark by yellow triangle); (G) WellYM468, 4998.6 m, Low angle cross-bedding (mark by yellow lines), lag deposits (mark by black lines); (H) WellYM46-1, 5173.5 m, Erosional surface between fine sandstone and underlying coarse sandstone; (I) WellYM46-1, 5176.1 m, Ripple cross-bedding very fine sandstone; (J) Well YM31, 4197.45 m; (K) WellYM467, 5192.5 m.
The cumulative grain-size probability distribution curves are mainly three-stage and two-stage (Figures 6J, K). The deposits are mainly transported by jumps, and the ratio of traction load to suspended load is high. Taking wellYM31 and wellYM467 as examples, wellYM31: the rolling population <2%, the jump population >88%, and the suspended population <10%. YM467: the rolling population is not developed, the jump population >70%, and the suspension population <30%.
In the process of the distributary channels developing forward, the terrain slope gradually slows down, the distributary channels extends and diverts downstream, the density of the channels increases, and the scale decreases. With the increase of provenance distance, the average particle size of distributary channels sediments decreases, and the ratio of traction load to suspended load changes (Figures 6J, K). The sedimentary structure characteristics change accordingly (Figures 6H, I), and the sand body’s connectivity gradually worsens. In an arid climate, the hydrodynamic mechanisms of the distributary channels changes periodically with seasonality due to intermittent flood events. In the flood period, distributary channels have extremely high flow intensity, and the upper flow regime is the major sedimentation transport mechanisms. The sediment transported by running water is mainly carried by bed sand load, and the distributary channels sand body is relatively developed. In the dry period, due to the transmission consumption, the runoff decreases, the flow velocity slows down, the channels transport capacity changes and the total sedimentary loading reduces.
4.2.1.1.2 Crevasse channels
Seasonal channels and extreme flood events are easily produced with highly fluctuating discharge in semi-arid climates (Zaman et al., 2012). Hyper-concentrated flows can be generated during flood events (Stuart et al., 2014). Crevasse channels are formed by bifurcation or crevasse processes of delta plain distributary channels during flood periods. The crevasse channels in the Baxigai Formation is controlled by high-energy transient water flow during the flood period, and the crevasse channels are exposed after the flood stage, with mudstone fill deposits in the dry period and turn to red color deposits.
The sediment of the crevasse channels is composed of brown, reddish-brown fine sandstone, very fine sandstone, and siltstone. The sediment developed massive bedding, parallel bedding, low angle cross-bedding, drainage structure, etc (Figures 7A–F). Muddy clast and rip-up mud clast occur at the bottom of crevasse channels succession. The thickness of the sand body in the crevasse channels is mainly 2–4.5 m, and the scale of the sand body is smaller than that of the distributary channels. The cumulative grain-size probability distribution curves are mainly two-stage, and sediments are mainly transported by suspension. Taking wellYM8 as an example, the jump population <40%, and the suspension population >60%. The shaliness in the sandstone is relatively high, and the particle size of the sand body in the crevasse channels is finer than that in the distributary channels (Figure 7G).
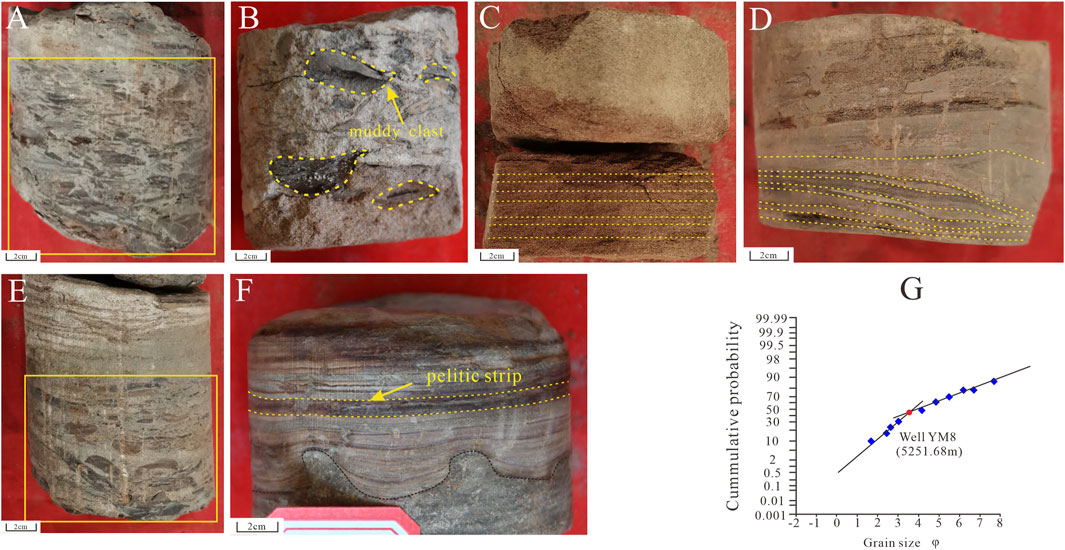
FIGURE 7. Typical sedimentary structure characteristics of the crevasse channels and cumulative grain-size probability distribution curves of the crevasse channels. (A) WellYM8, 5250.62 m, Massive bedding Siltstone with rip-up mud clast and muddy clast (marked with rectangle); (B) WellYM8, 5250 m, Massive bedding fine sandstone with muddy clast (mark by yellow lines); (C) WellYM8, 5248.73 m, Parallel bedding very fine sandstone; (D) WellYM8, 5249.24 m, Low angle cross-bedding siltstone; (E) WellYM8, 5250.28 m, Siltstone with rip-up mud clast and muddy clast (marked with rectangle); (F) WellYM8, 5252.69 m, Drainage structure, fine sandstone at the bottom (mark by black lines) and muddy siltstone at the top with pelitic strip (mark by yellow lines); (G) YM8, 5246.2 m, Cumulative grain-size probability distribution curves.
4.2.1.1.3 Floodplains
The flood plain is a stagnant depression between distributary channels in the upper delta plain and has weak hydrodynamic forces. Since flood events are often accompanied by channels migration and crevasse diversion, frequent flood events make Floodplains smaller in size. The fine-grained suspended load brought by flowing during the flood period is the most important supply method of Floodplains sediments, mainly composed of reddish-brown mudstone intercalated with thin silty mudstone, and the bedding structure is not developed.
4.2.1.1.4 Interdistributary bays
Interdistributary bays are mainly developed in low-lying areas between distributary channels in the lower delta plain, with weak hydrodynamic, and the sediment are composed of suspended muddy sediments formed during the flood period. The sediment are mainly composed of brown and reddish-brown mudstone and dark brown mudstone with laminated siltstone and silty mudstone. Mud cracks (Figure 4G), plant roots (Figure 8A), salt film (Figure 8B), wave bedding (Figure 8C), and biological fossils of dark brown mudstone (Figure 8D) in periodic wetting-reducing conditions are developed in interdistributary areas. The redox changes in interdistributary bay sediments of the upper delta plain are affected by seasonal runoff. In the flood period, the low-lying areas were small stagnant lakes with dark brown mudstone sediments and preserved biological fossils. Due to sufficient source supply in the flood period, isolated thin sandy sediments are formed. The sediment is mostly siltstone, the thickness of the sand body is generally less than 1 m, and it is often interbedded with mudstone to form a uniform rhythm. In the dry period, the water body shrinks, the sediment is exposed to dry air, and the salt film can be seen in some mudstone, which is an evaporite facies.

FIGURE 8. Core images showing sedimentary characteristics of interdistributary bays in delta plain (A) WellYM8, 5239.13 m, Plant roots (mark by yellow arrow); (B) WellYM8, 5237.98 m, Salt film; (C) WellYM701, 4998.6 m, Wave bedding silty mudstone; (D) WellYD701, 4969.6 m, Biological fossils (mark by yellow lines).
4.2.1.2 Delta front
The delta front develops in the transition positions between the delta plain and the shallow lakes and is characterized by dual control by river and lake interaction. Two types of sedimentary facies were developed in the delta front: terminal distributary channels and subaqueous interdistributary bays.
4.2.1.2.1 Terminal distributary channels
The terminal distributary channels develop as subaqueous extensions of distributary channels on the lower delta plain that are under the influence of high inertia-driven flow passes during flooding periods. In the dry period, due to the consumption of flow percolation and evaporation transfer, most of the river water will disappear along the path above the shoreline of arid lake basins or in the deserts, the terminal distributary channels dry up, and the sediment will be exposed. The hydrodynamic force of the distributary channels is weak, and the sediment is mainly composed of very fine sandstone and siltstone. Pelitic strips and mudstone interlayers are developed in the sandstone. The deposits develop parallel bedding, Ripples, low angle cross-bedding, ripple cross-bedding, etc. (Figures 9A–D). The thickness of the terminal distributary channels sand body is usually less than 2.5 m.
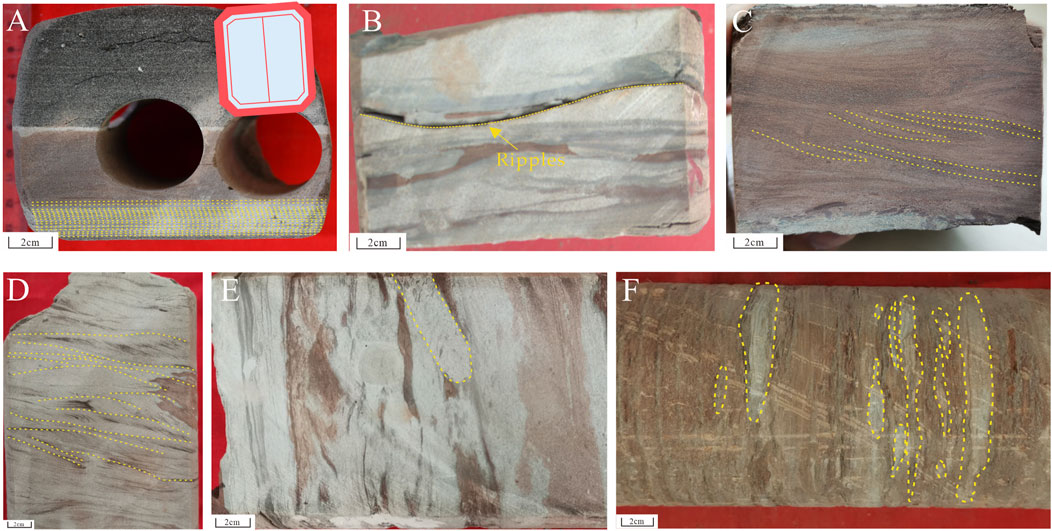
FIGURE 9. (A) WellYM467H, 5180.25 m, Parallel bedding, very fine sandstone (mark by yellow lines); (B) WellYD704H, 4977.85 m, Ripples siltstone; (C) WellYD704H, 4967.34 m, Low angle cross bedding muddy siltstone (mark by yellow lines); (D) WellYD704H, 4970.82 m, Ripple cross-bedding siltstone (mark by yellow lines); (E) WellYD704H, 4974 m, Silty mudstone bioturbation (mark by yellow lines); (F) WellYD704H, 4972.53 m, Lenticular bedding (mark by yellow lines).
4.2.1.2.2 Subaqueous interdistributary bays
The subaqueous interdistributary bays are relatively developed in the delta front subfacies of the study area, and sedimentation is mainly suspended sedimentation, reflecting a weak hydrodynamic environment. Sediments are deposited between terminal distributary channels consisting of gray-green mudstone and reddish-brown mudstone. Mud cracks (Figure 4F), bioturbation, and lenticular bedding (Figures 9E, F) are common in subaqueous interdistributary bays deposits. The variation of shallow lake water levels controlled by seasonal runoff leads to periodic exposure of sediments in the subaqueous interdistributary bays.
4.2.2 Distribution of different sedimentary facies
From the flattened seismic profile, the paleotopography of the study area was gentle in the sedimentary period of the Baxigai Formation, the average gradient of the study area is low (Figure 10). Determine the type of distributary channels based on the detailed sedimentary interpretation and description in cored wells of the Baxigai Formation. The distributary channels types were identified in non-cored wells mainly through electro facies of gamma ray log curves (Figure 11). From the single-well sedimentary microfacies and the well-tie profile of sedimentary microfacies comparison along the provenance (Figure 12), the boundaries of the upper delta plain, lower delta plain, and delta front were roughly divided. Combined with provenance, paleotopography along the provenance, and sand body thickness contours, the plane distribution of sand body sedimentation microfacies is obtained. In the deposition period of the Baxigai Formation, the study area was in an oxidizing environment of an arid climate. The clastic particle mainly came from the southeast of the study area and entered the study area through several water channels, extended to the northwest, and continued to bifurcate, forming a large fan-shaped accumulation body. The delta plain in the study area extends far to the northwest and mainly develops major distributary channels and crevasse channels. The delta front is relatively underdeveloped and mainly develops terminal distributary channels. The thickness of the sand body in the study area also shows a clear trend of thinning from southeast to northwest (Figure 13).
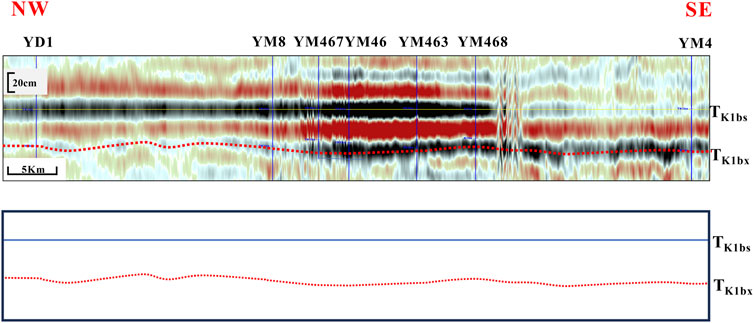
FIGURE 10. Seismic profile (flattened by the bottom of the overlying Bashijiqike formation) showing the paleotopography of the Baxigai Formation from SE to NW in the study area. The average gradient is 0.065%. Note the position of the profile on Figure 13.
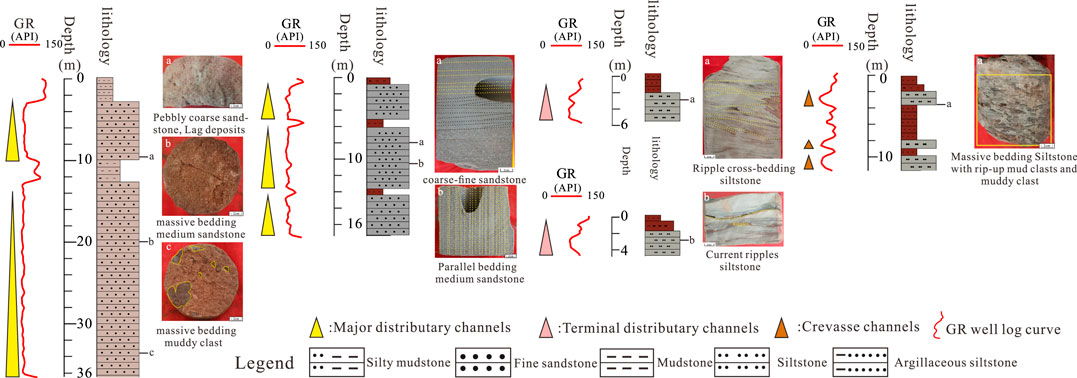
FIGURE 11. Well log facies scheme of the major distributary channels, crevasse channels, and terminal distributary channels deposits of the Baxigai formation in the study area. The box-shaped or Serrate box-shaped with low-amplitude well logs indicate the major distributary channels; The serrate bell-shaped logs with low-amplitude well logs indicate the crevasse channels; finger-shaped logs with low-amplitude or symmetrically serrate-shaped indicate the terminal distributary channels.
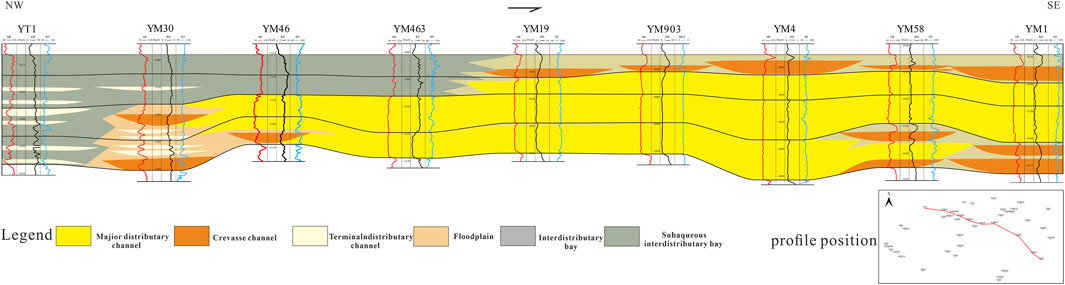
FIGURE 12. Well-tie profile of sedimentary microfacies comparison in the Baxigai Formation. Note the position of the profile on Figure 13.
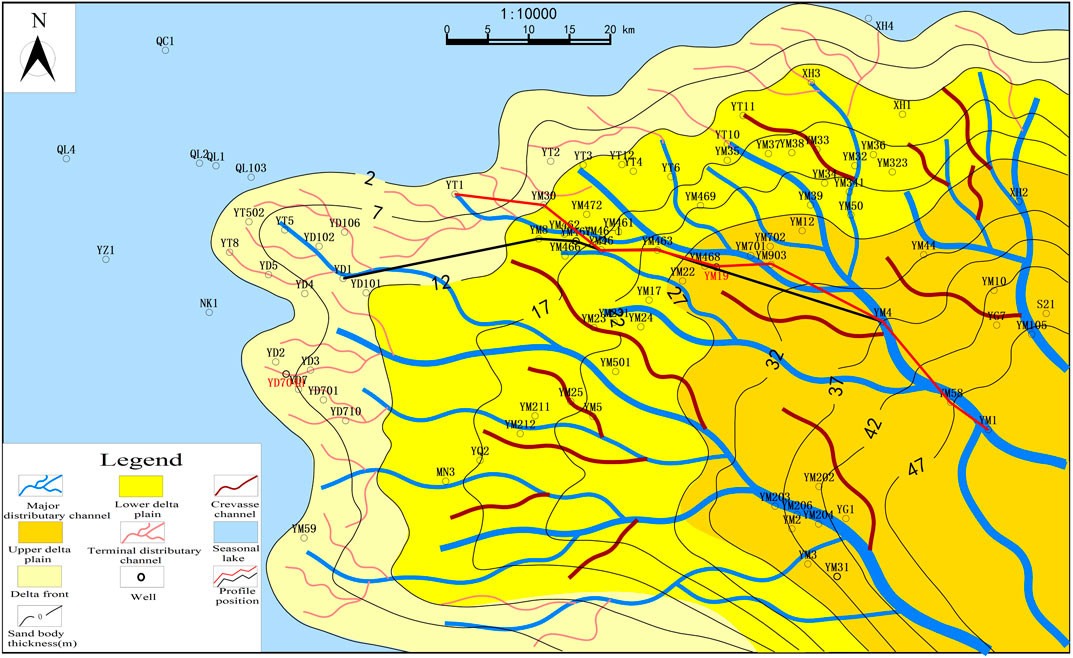
FIGURE 13. Plane distribution of sand body sedimentation microfacies of Cretaceous Baxigai Formation in western Tabei Uplift, (The well locations marked in red are sampling wells).
5 Discussion
5.1 Modern sedimentological demonstration in arid climate
Research on modern arid-semi-arid regions has revealed several basic commonalities: arid and hot environments, flat terrain, extreme flood events, intense evaporation and infiltration, and so on (Zhang et al., 2018; Donselaar et al., 2013). Rivers in arid-semi-arid areas are mostly seasonal rivers, with sedimentary records reflecting oxidation and seasonal river activities (Li et al., 2021; Gao et al., 2015). Today, regions such as Xinjiang and Inner Mongolia in western China have an arid-semi-arid climate environment, with many seasonal rivers (Chen et al., 2020; Zhang et al., 2020). Take the Daihai Lake area of Inner Mongolia as an example; the Bantanzi River is a typical seasonal river sedimentary system in the northern part of the Daihai Lake, and the hydrodynamic mechanism changes periodically with the season. In the flood period, the upper flow regime is the primary sedimentary transport process, and a large amount of deposits can be transported. In the dry period, the lower flow regime is the primary sedimentary transport process, and the river transport capacity is extremely low (Tan et al., 2018). The study area had an arid climate in the Baxigai formation sedimentary period, and combined with the widely developed Supercritical flow sedimentary structures, the authors believes that in the Baxigai sedimentary period, the study area also developed seasonal rivers. Modern examples of seasonal delta deposition can be found in arid-semi-arid sedimentary environments (Li M. et al., 2015; Zan et al., 2022; Gondwe et al., 2021). These sedimentary examples help us to understand the depositional mechanisms and depositional pattern of sand bodies in seasonal delta depositional systems. The Okavango Delta and Amu Darya river Delta are modern examples of seasonal delta deposition in the Baxigai Formation in an arid climate. The Okavango Delta region has an arid-semi-arid climate. Rainfall is mainly concentrated in summer, usually with rainstorms and short duration. The average annual rainfall is 490 mm, and the average annual evaporation is 2,172 mm. The runoff of the Okavango River is controlled by rainfall of the Angola highlands and has seasonal changes, with the maximum runoff occurring in March-April (McCarthy et al., 2005; Tan, 2011). The Amu Darya river Delta has a warm temperate continental climate. The average annual precipitation in the lower reaches of the Amu Darya river is less than 100 mm, and evaporation is about 2000 mm, with the maximum runoff occurring in July-August. The river water in the Amu Darya river Basin is mainly supplied by melting water from mountain ice and snow and winter and spring rainfall in the upper reaches of the mountains, it is a snow-glacier fed river (Su et al., 2021; Reyisha, 2019; Conrad et al., 2007). Affected by seasonal runoff, the Okavango Delta and Amudarya river Delta areas change during the flood and dry seasons (Figures 14A–D). Seasonal changes in the Okavango Delta and Amu Darya river Delta result in different sedimentary models. In the flood period, the delta area extends, the distributary channels enters a high-flow stage, the total sedimentary loading is high, and the major distributary channels forms crevasse channels by frequent avulsion processes, and terminal distributary channels develop (Figures 14E, G). In the dry period, the total sedimentary loading of the distributary channels decreases with the decrease in runoff, and the river transport capacity is extremely low. The water level of the major distributary channels decreases and the scale decreases. The terminal distributary channels and the crevasse channels dry up, and the deposits are exposed to the dry air (Figures 14F, H).
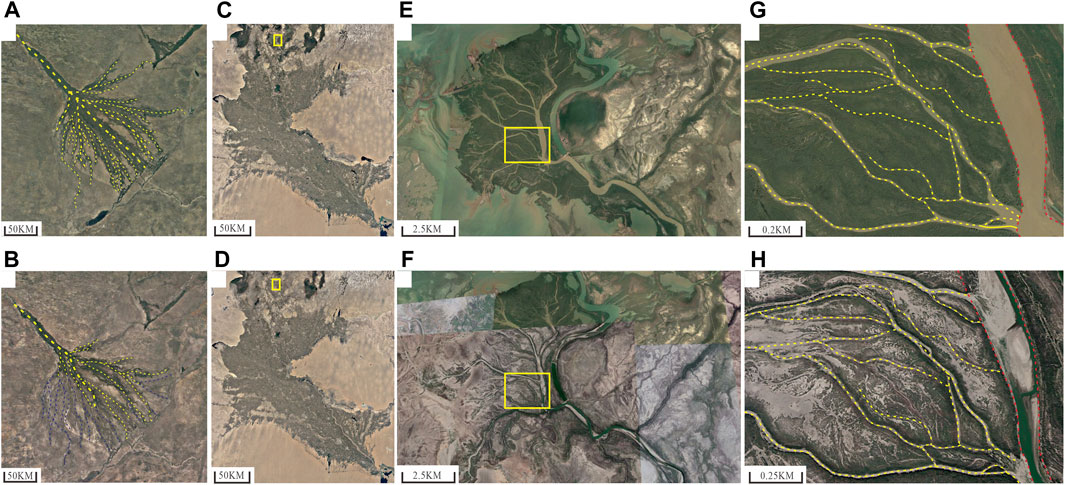
FIGURE 14. Satellite image from the Google Earth software showing seasonal changes of Delta (A) Okavango delta. In flood season 17 March 2018; active channels (mark by yellow lines); (B) Okavango delta in dry season 25 September 2018; active channels (mark by yellow lines); seasonal channels (mark by blue lines); (C) Amu Darya river delta in flood season 1 July 2018; (D) Amu Darya river delta in dry season 1 March 2018; (E) Part of Amu Darya river delta see location in the yellow rectangle of image (C); (F) Part of Amu Darya river delta see location in the yellow rectangle of image (D); (G) Major distributary channels and crevasse channels in flood season see location in the yellow rectangle of image (E) Major distributary channels (mark by red lines), crevasse channels (mark by yellow lines); (H) Major distributary channels and crevasse channels in dry season see location in the yellow rectangle of image (F), major distributary channels (mark by red lines), crevasse channels (mark by yellow lines).
5.2 Sedimentary model of the seasonal delta in an arid climate
The sand body depositional mechanisms in semi-arid-arid climates with total sedimentary loading variation greatly are quite different from the conventional model (Donselaar et al., 2013; Zhang et al., 2018) The red bed sedimentary sequence of the Baxigai Formation records changes in the total sedimentary loading variation greatly and seasonal flooding events with periodic exposure of sediments in an arid climate. The widely developed supercritical flow sedimentary structures have paleoclimate characteristics indicating strong seasonal changes. In the sedimentary period of the Baxigai Formation, the study area has gentle terrain, and the deposits come from the southeastern part of the basin (Luo et al., 2021; Chen et al., 2012). The climate was hot and arid, and the hydrodynamic mechanisms had seasonal periodic change. Combining regional background geological and based on the sedimentary characteristics and sedimentary facies types of the Baxigai Formation in the study area, considering the changes in the hydrodynamic mechanisms of the current seasonal rivers in arid climate and the seasonal periodic changes of the total sedimentary loading and by the morphology and sand body distribution characteristics of current seasonal deltas in an arid climate, the sedimentary model of seasonal deltas of the Baxigai Formation in an arid climate was established (Figure 15). This model explains the depositional process and distribution of seasonal delta sand body in an arid climate. The development of the delta has gone through dual-stage depositional processes, and the depositional characteristics of different stages are obviously different.
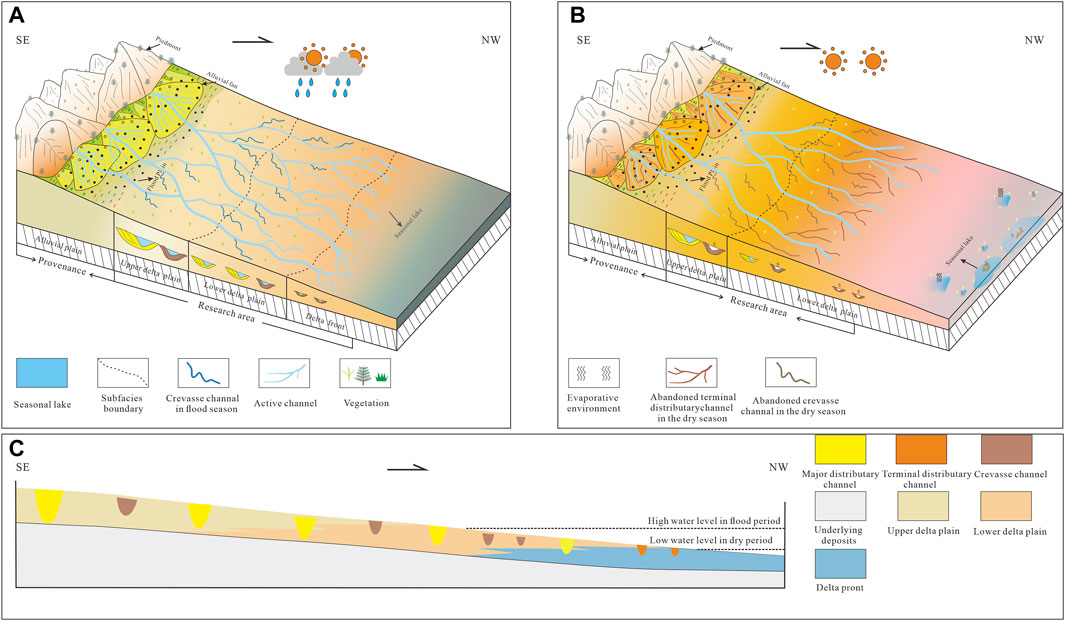
FIGURE 15. Sedimentary models for an arid climate seasonal delta of the Cretaceous Baxigai Formation in the west of the Tabei Uplift. (A) Flood period; (B) dry period (C) showing the sedimentary transition from the delta plain to the delta front.
In the high discharge stage of the flood period, The delta plain developed a distributary channels network composed of relatively stable major distributary channels and short-lived periodic crevasse channels that are formed by the avulsion process from major distributary channels. Supercritical flow sedimentary structures, such as parallel bedding, massive bedding, rip-up mud clast, etc., are widely developed in the major distributary channels. The major transport mechanism of sediments is the upper flow regime, and the total sedimentary loading is high. The delta progradation towards the basin’s center forms terminal distributary channels at the delta front. During severe flood periods, a small amount of terminal distributary channels sand bodies were deposited underwater (Figure 15A). In the low discharge stage of the dry period, the channels takes the lower flow regime as the major transport mechanism, and the lower flow regime sedimentary structure generally exists in the major distributary channels deposits. Due to the consumption of water seepage and evaporation transfer, the terminal distributary channels and crevasse channels are exposed, with mudstone fill deposits, and turn to red color deposits. The density of the distributary channels is reduced, the total sedimentary loading is low, and the major distributary channels in the delta plain becomes the principal sand transport channels (Figure 15B). Controlled by the avulsion, bifurcation process, and influence of climate factors the scale of the major distributary channels and crevasse channels decreased from the upper delta plain to the lower delta plain (Figure 15C).
6 Conclusion
(1) The Cretaceous Baxigai Formation in the west of Tabei Uplift is dominated by red bed deposits. Bioturbation and plant rhizome fossils are developed in the mudstone, and mud cracks can be seen in the mudstone layer. Supercritical flow sedimentary structures such as parallel bedding and massive bedding are developed in the sandstone, and some sandstones develop calcareous nodules. Through the geochemical analysis of the core, it is concluded that the sedimentary environment of the Baxigai Formation in the study area is mainly arid and oxidized, and the sand body deposits have seasonal characteristics.
(2) The red bed sedimentary sequence of the Baxigai Formation records the seasonal delta sedimentary system with total sedimentary loading variation greatly in an arid climate. The sedimentary process of the seasonal delta sand body in an arid climate is controlled by the dual-stage of the seasonal delta with flood and dry periods. The identification of sedimentary facies characteristic of seasonal river discharge variations is different. In the flood period, The deposits in distributary channels of the delta plain develop supercritical flow sedimentary structures, and the distributary channels migrate and bifurcate frequently. On the delta plain, crevasse channels are formed by the avulsion process of major distributary channels, and the delta progrades toward the center of the basin. In the dry period, The major distributary channels had relatively small scale. Terminal distributary channels and crevasse channels are exposed, with mudstone fill deposits, and turn to red color deposits. The major distributary channels sand bodies in the delta plain area are identified as framework sand bodies of seasonal deltas in arid paleoclimate, and the sedimentary scale of sand bodies is large and relatively thick. The scale of the sand body in the crevasse channels and terminal distributary channels is small, and the thickness of the sand body is thin.
(3) Combining paleotopography, provenance, paleoclimate, and hydrodynamic changes and based on the plane distribution of sand body sedimentation microfacies, combined with the sedimentary characteristics of the Baxigai Formation and referring to modern sedimentary examples, the dual-stage sedimentary model of the seasonal delta sand body with total sedimentary loading variation greatly in an arid climate was established. This dual-stage sedimentary model provides a new perspective on the development patterns of distributary channels in a lacustrine seasonal delta.
Data availability statement
The original contributions presented in the study are included in the article/Supplementary Material, further inquiries can be directed to the corresponding authors.
Author contributions
TW: Conceptualization, Methodology, Writing–original draft. YH: Conceptualization, Methodology, Writing–original draft. BL: Methodology, Writing–review and editing. WH: Project administration, Writing–review and editing. XG: Writing–review and editing, Formal Analysis. LD: Writing–review and editing, Project administration. JW: Formal Analysis, Writing–review and editing.
Funding
The author(s) declare financial support was received for the research, authorship, and/or publication of this article. “This research was funded by (Scientific Research Foundation of Yangtze University), grant number (8021003201)” and “The APC was funded by (8021003201).”
Acknowledgments
The authors wish to thank the Research Institute of Petroleum Exploration and Development, PetroChina Tarim Oilfield Company for providing various data for this study. We are grateful to Li Zhang, Associate Professor, Yangtze University for her constructive feedback that helped improve the quality of this manuscript. We are also greatly appreciate reviewers and editors for their constructive comments for their precious advice.
Conflict of interest
Author BL was employed by PetroChina Tarim Oilfield Company.
The remaining authors declare that the research was conducted in the absence of any commercial or financial relationships that could be construed as a potential conflict of interest.
Publisher’s note
All claims expressed in this article are solely those of the authors and do not necessarily represent those of their affiliated organizations, or those of the publisher, the editors and the reviewers. Any product that may be evaluated in this article, or claim that may be made by its manufacturer, is not guaranteed or endorsed by the publisher.
References
Chen, G., Huang, Z. B., Zhang, H. L., Zhang, R. H., and Yan, X. F. (2012). Provenance analysis of clastic rocks in the cretaceous Bashijiqike Formation at Kuqa depression. Nat. Gas. Geosci. 23 (6), 1025–1033. Available at: http://www.nggs.ac.cn/CN/Y2012/V23/I6/1025.
Chen, X. J., Wang, X. Q., and Lu, Q. (2020). Soil moisture content and soil water lnfiltration in seasonal watercourse in arid area. Arid. Zone Res. 37 (01), 97–104. doi:10.13866/j.azr.2020.01.11
Conrad, C., Dech, S. W., Hafeez, M., Lamers, J., Martius, C., and Strunz, G. (2007). Mapping and assessing water use in a Central Asian irrigation system by utilizing MODIS remote sensing products. Irrig. Drain. Syst. 21, 197–218. doi:10.1007/s10795-007-9029-z
Ding, J. H., Zhang, J. C., Shi, G., Shen, B. J., Tang, X., and Yang, Z. X. (2021). Sedimentary environment and organic matter accumulation for the Longtan Formation shale in xuancheng area. Acta Sedimentol. Sin. 39 (02), 324–340. doi:10.14027/j.issn.1000-0550.2020.056
Donselaar, M. E., Gozalo, M. C. C., and Moyano, S. (2013). Avulsion processes at the terminus of low-gradient semi-arid fluvial systems: lessons from the Río Colorado, Altiplano endorheic basin, Bolivia. Sediment. Geol. 283, 1–14. doi:10.1016/j.sedgeo.2012.10.007
Du, D. D., Li, H. H., Yan, L., Zhou, D. Y., Cao, Y. H., Yang, M., et al. (2023). Deformation migration and three major geological events in Tarim Basin and their geological significance. Chin. J. Geol. 58 (02), 379–397. doi:10.12017/dzkx.2023.024
Fan, M. M., Bu, J., Zhao, X. Y., Kang, B., Li, W. H., and Zhang, W. G. (2019). Geochemical characteristics and environmental implications of trace elements of Yanchang Formation in southeastern Ordos Basin. J. Northwest Univ. Sci. Ed. 49 (4), 633–642.
Fu, J. H., Li, S. X., and Liu, X. Y. (2013). Geological theory and practice of petroleum exploration in the Ordos Basin. Nat. Gas. Geosci. 24 (6), 1091–1101. Available at: http://www.nggs.ac.cn/CN/Y2013/V24/I6/1091.
Fu, W. J., Zhang, C. M., Ji, D. S., Lou, L., Liu, J. L., and Wang, X. L. (2023). Sedimentary characteristics of shallow water delta of middle jurassic toutunhe Formation in southern anjihai river outcrops, junggar basin. Lithol. Reserv. 35 (4), 145–160. doi:10.12108/yxyqc.20230414
Gao, Z. Y., Feng, J. R., Zhou, C. M., Cui, J. G., Li, X. P., Zhao, X. S., et al. (2014). Arid climate seasonal rivers deposition: a case of lower cretaceous in kuche river outcrop. Acta Sedimentol. Sin. 32 (06), 1060–1071.
Gao, Z. Y., Zhou, C. M., Feng, J. R., Cui, J. G., Guo, M. L., and Wu, H. (2015). Distribution of a large area of sand body formation mechanism:ephemeral streams in arid climate. Acta Sedimentol. Sin. 33 (03), 427–438. doi:10.14027/j.cnki.cjxb.2015.03.001
Gondwe, M. J., Murray-Hudson, M., Mazrui, N. M., Moses, O., Mosimanyana, E., and Mogobe, O. (2021). A review of the limnology of the Okavango Delta, Botswana. Afr. J. Aquat. Sci. 46 (3), 251–273. doi:10.2989/16085914.2021.1882931
Gugliotta, M., Kurcinka, C. E., Dalrymple, R. W., Flint, S. S., and Hodgson, D. M. (2016). Decoupling seasonal fluctuations in fluvial discharge from the tidal signature in ancient deltaic deposits: an example from the Neuquén Basin, Argentina. J. Geol. Soc. Lond. 173 (1), 94–107. doi:10.1144/jgs2015-030
He, B. Z., Jiao, C. L., Liu, R. H., Cao, Z. C., Cai, Z. H., Lan, M. J., et al. (2023). The paleotectonic and paleogeography of Neoproterozoic in the Tarim Basin and the prediction of favorable deep source rock areas. Earth Sci. Front. 30 (4), 19–42. doi:10.13745/j.esf.sf.2022.10.18
He, C. F., Zhang, X., Tian, J. C., Xia, Y. T., Yang, Y. R., Chen, J., et al. (2023). Sedimentary facies characteristics and sedimentary model of thin sand bodies of Lower Cretaceous Shushanhe Formation in Xinhe area,northern Tarim Basin. Lithol. Reserv. 35 (01), 120–131. doi:10.12108/yxyqc.20230111
He, D. F., Zhou, X. Y., Yang, H. J., Guan, S. W., and Zhao, C. J. (2008). Formation mechanism and tectonic types of intracratonic paleo-uplifts in the Tarim basin. Earth Sci. Front. 15 (2), 207–221. doi:10.3321/j.issn:1005-2321.2008.02.024
He, Z. W., Yang, R. D., Gao, J. B., Cheng, W., and Huang, J. G. (2013). Geological and geochemical characteristics of manganese-bearing rock series of yangjiawan manganese deposit, songtao county, guizhou province. Geoscience 27 (03), 593–602. Available at: http://www.geoscience.net.cn/CN/Y2013/V27/I3/593.
Hong, C. J., Kang, R. D., Zhou, F. F., Fei, J. W., and Fang, X. L. (2016). Sedimentary characteristics of cretaceous Baxigai Formation in xinhe-sandaoqiao area. Xinjiang Geol. 34 (02), 230–234.
Huang, S. Y., Yang, W. J., Lu, Y. H., Zhang, K., Zhao, Q., and Fan, S. (2018). Geological conditions, resource potential and exploration direction of natural gas in Tarim Basin. Nat. Gas. Geosci. 29 (10), 1497–1505. doi:10.11764/j.issn.1672-1926.2018.09.012
Jia, C. Z. (1997). Structural characteristics and oil and gas in Tarim basin. China. Beijing: Petroleum Industry Press.
Jones, B., and Manning, D. A. C. (1994). Comparison of geochemical indices used for the interpretation of palaeoredox conditions in ancient mudstones. Chem. Geol. 111 (1-4), 111–129. doi:10.1016/0009-2541(94)90085-X
Li, F. J., Lei, Y. L., Gong, G. L., Zhang, H., Shen, F., Jing, X. G., et al. (2019). Geochemical characteristics and sedimentary environment analysis from datangpo Formation in neoproterozoic nanhua system, southwestern hunan province-an example from zhaodong manganese ore deposits in jingzhou county, hunan province. China. Earth Sci. 44 (10), 3484–3494. doi:10.3799/dqkx.2019.075
Li, J. G., Bristow, C. S., Luthi, S. M., and Donselaar, M. E. (2015). Dryland anabranching river morphodynamics: Río Capilla, Salar de Uyuni, Bolivia. Geomorphology 250, 282–297. doi:10.1016/j.geomorph.2015.09.011
Li, J. L., Wu, G. K., Lin, C. S., Liu, Y. F., Liu, J. Y., Sun, Q., et al. (2019). Restriction of unconformity triangle zone on stratigraphic traps in the slope area with paleo-uplift: a case study of the Cretaceous in the West North Tarim Uplift. J. Northeast Petroleum Univ. 43 (02), 68–78.
Li, M., Tang, L. J., Qi, L. X., Huang, T. Z., Zhen, S. J., and Tian, Y. J. (2015). Differential tectonic evolution and its controlling on hydrocarbon accumulation in the south slope of Tabei Uplift. Nat. Gas. Geosci. 26 (2), 218–228. doi:10.11764/j.issn.1672-1926.2015.02.0218
Li, X. B., Liu, H. Q., Dong, X. Q., Wang, Y. T., Long, L. W., Wen, L. H., et al. (2021). The concept of fluvial fans in an arid environment: a new explanation of the origin of “sand-filled basins” in the yanchang formation, ordos basin. Acta Sedimentol. Sin. 39 (05), 1208–1221. doi:10.14027/j.issn.1000-0550.2020.077
Li, Y. J., Yang, H. J., Zhang, G. Y., Zheng, D. M., Liu, Y. X., Zhao, Y., et al. (2012). Redivision of the tectonic units of Tabei rise in Tarim Basin, NW China. Acta Petrol. Sin. 28 (8), 2466–2478.
Liu, B. B., Tan, C. P., Yu, X. H., Shan, X., and Li, S. L. (2019). Evolution model of a modern delta fed by a seasonal river in Daihai Lake, North China: determined from ground-penetrating radar and trenches. Front. Earth Sci-Prc 13, 262–276. doi:10.1007/s11707-018-0740-x
Liu, Y. F., Xia, H., Sun, Q., Lin, C. S., Zhao, H. T., and Li, H. (2019). Sequence stratigraphy and depositional evolution of the Bashijiqike Formation in the western Tabei uplift, Tarim Basin. Nat. Gas. Geosci. 30 (1), 62–73. Available at: http://www.nggs.ac.cn/EN/Y2019/V30/I1/62.
Luo, H. Y., Chen, J., Zhang, X. Q., Meng, X. X., Zhao, F. Q., Wu, S. j., et al. (2021). Sedimentary characteristics of fluvial dominated shallow water delta front and its control lithologic reservoir: a case study of Baxigai Formation in south slope of Kuqa Depression. Lithol. Reserv. 33 (5), 70–80. doi:10.12108/yxyqc.20210507
Ma, H. Q., Xia, Y. T., Zhao, Y. Q., Han, Q., and Qiao, G. L. (2022). Reservoir characteristics and controlling factors of cretaceous Baxigai Formation in xinhe-sandaoqiao area. Xinjiang Geol. 40 (02), 236–241.
McCarthy, J., Gumbricht, T., and McCarthy, T. S. (2005). Ecoregion classification in the Okavango Delta, Botswana from multitemporal remote sensing. Int. J. Remote Sens. 26 (19), 4339–4357. doi:10.1080/01431160500113583
Nesbitt, H. W., and Young, G. M. (1982). Early Proterozoic climates and plate motions inferred from major element chemistry of lutites. Nature 299, 715–717. doi:10.1038/299715a0
Reyisha, J. L. L. (2019). Study on the impacts of climate change and human activitieson runoff in the Amu Darya river, Central Asia. dissertation/master’s thesis. Urumchi (China): Xinjiang Normal University.
Russell, A. D., and Morford, J. L. (2001). The behavior of redox-sensitive metals across a laminated-massive-laminated transition in Saanich Inlet, British Columbia. Mar. Geol. 174 (1-4), 341–354. doi:10.1016/S0025-3227(00)00159-6
Stuart, J. Y., Mountney, N. P., McCaffrey, W. D., Lang, S. C., and Collinson, J. D. (2014). Prediction of channel connectivity and fluvial style in the flood-basin successions of the Upper Permian Rangal coal measures (Queensland). AAPG Bull. 98 (2), 191–212. doi:10.1306/06171312088
Su, Y. Y., Li, Y. P., Liu, Y. R., Huang, G. H., Jia, Q. M., and Li Y, F. (2021). An integrated multi-GCMs Bayesian-neural-network hydrological analysis method for quantifying climate change impact on runoff of the Amu Darya River basin. Int. J. Climatol. 41 (5), 3411–3424. doi:10.1002/joc.7026
Su, Z., Liu, Y. F., Han, J. F., Yang, S. W., Liu, B., Lai, P., et al. (2020). Application of ultra-deep sandstone reservoirs prediction technology under seismic facies controlled in Yudong Block of Tabei Uplift, Tarim Basin. Nat. Gas. Geosci. 31 (2), 295–306. doi:10.11764/j.issn.1672-1926.2019.10.012
Sun, C. Y., Hu, M. Y., Hu, Z. G., Xue, D., and Liu, S. Y. (2015). Sedimentary characteristics of shallow water delta of Quan-4 member in Xingcheng area, Songliao Basin. Lithol. Reserv. 27 (03), 66–74. doi:10.3969/j.issn.1673-8926.2015.03.010
Sun, D. Q., Li, W. H., Lu, S. F., Liu, X. P., He, T. H., Zhu, P. F., et al. (2020). Reservoir characteristics and controlling factors of Shushanhe Formation in Yingmaili area of Tabei uplift. J. Northeast Petroleum Univ. 44 (06), 82–93. doi:10.3969/j.issn.2095-4107.2020.06.009
Tan, C. P., Yu, X. H., Liu, B. B., Xu, L., Li, S. L., Feng, S. Q., et al. (2018). Sedimentary structures formed under upper-flow-regime in seasonal river system: a case study of Bantanzi River, Daihai Lake, Inner Mongolia. J. Palaeogeogr. 20 (06), 929–940.
Tan, T. (2011). Ground water resources exploitation and protection in Okavango delta under the condition of climate change. dissertation/master’s thesis. Wuhan (China): China University of Geosciences, Wuhan.
Tian, J. (2019). Petroleum exploration achievements and future targets of Tarim Basin. XingJiang Pet. Geol. 40 (01), 1–11. doi:10.7657/XJPG20190101
Wang, C., Yan, C. J., Zhu, Z. J., Li, S. H., Lv, D. C., Wang, X. X., et al. (2023). Interpretation of sand body architecture in complex fault block area of craton basin: case study of TIII in sangtamu area, Tarim Basin. Energies 16 (8), 3454. doi:10.3390/en16083454
Wang, Y. Y., Guo, W. Y., and Zhang, G. D. (1979). Application of some geochemical indicators in determining of sedimentary environment of the Funing Group Jinhu depression, Jiangsu Province. J. Tongji Univ. Nat. Sci. (2), 51–60.
Wu, G. K., Lin, C. S., Liu, Y. F., Liu, J. Y., Yang, X. Z., and Li, H. (2019). Characteristics of major unconformities and paleo-geomorphology during the Mesozoic key transformation stages in Kuqa-Tabei area. Oil Gas. Geol. 40 (04), 763–777. doi:10.11743/ogg20190407
Wu, G. K., Zhang, Z. M., Lin, C. S., Tian, N. X., Zuo, X. J., and Li, H. (2022). Evolution of mesozoic sedimentary fill in the Tabei uplift region, Tarim Basin. Oil Gas. Geol. 43 (4), 845–858. doi:10.11743/ogg20220409
Xia, H., Lin, C. S., Liu, Y. F., Li, H., Sun, Q., Zhao, H. T., et al. (2019). A research on relative lacustrine level changes of Cretaceous Shushanhe Formation in the Yingmaili area of Tarim Basin. Nat. Gas. Geosci. 30 (11), 1579–1589. doi:10.11764/j.issn.1672-1926.2019.08.001
Xing, Z. F., Lin, J., Zhou, H., Li, D., Qi, Y. A., and Zheng, W. (2019). Palaeoclimate changes revealed by sedimentary records of the upper permian-lower triassic strata in yiyang area, western henan province, China. Geol. J. China Univ. 25 (3), 401–411. Available at: https://geology.nju.edu.cn/EN/Y2019/V25/I3/401.
Xu, G. F., Lin, C. S., Liu, Y. F., and Sun, Q. (2016). Evolution of palaeo-uplift and its controlling on sedimentation of Kapushaliang group of early cretaceous in western Tabei uplift. Earth Sci. 41 (04), 619–632. doi:10.3799/dqkx.2016.051
Yang, H., Fu, J. H., and Yu, J. (2003). Oil reservoir enrichment models of large delta systems and application of exploration techniques in Shanbei area. Acta Petrol. Sin. 24 (03), 6–10. doi:10.7623/syxb200303002
Yang, S. Y., Li, C. X., and Cai, J. G. (2006). Geochemical compositions of core sediments in eastern China: implication for Late Cenozoic palaeo-environmental changes. Palaeogeogr. Palaeoclimatol. Palaeoecol. 229 (4), 287–302. doi:10.1016/j.palaeo.2005.06.026
Zaman, M. A., Rahman, A., and Haddad, K. (2012). Regional flood frequency analysis in arid regions: a case study for Australia. J. Hydrol. 475, 74–83. doi:10.1016/j.jHydrol.2012.08.054
Zan, C. J., Liu, T., Huang, Y., Bao, A. M., Yan, Y. Y., Ling, Y. N., et al. (2022). Spatial and temporal variation and driving factors of wetland in the Amu Darya River Delta, central asia. Ecol. Indic. 139, 108898. doi:10.1016/j.ecolind.2022.108898
Zhang, B., Cui, J., Gu, Q. Y., Zhu, Y. G., and Su, J. (2010). Oil origin classification in composite hydrocarbon accumulation play in the western Tabei Uplift and its geological significance. Acta Petrol. Sin. 31 (01), 55–60+67. doi:10.7623/syxb201001009
Zhang, C. M., Zhu, R., Guo, X. G., Wang, X. L., Yin, T. J., and Yuan, R. (2020). Arid fluvial fandelta-fluvial fan transition: implications of huangyangquan fan area. Earth Sci. 45 (05), 1791–1806. doi:10.3799/dqkx.2019.165
Zhang, L., Bao, Z. D., Dou, L. X., Zang, D. S., Mao, S. W., Song, J., et al. (2018). Sedimentary characteristics and pattern of distributary channels in shallow water deltaic red bed succession: a case from the Late Cretaceous Yaojia formation, southern Songliao Basin, NE China. J. Petrol Sci. Eng. 171, 1171–1190. doi:10.1016/j.petrol.2018.08.006
Keywords: seasonal delta, sedimentary environment, sedimentary model, Baxigai Formation, Tarim Basin
Citation: Wang T, Hu Y, Li B, He W, Gao X, Dou L and Wang J (2024) Sedimentary characteristics and model of seasonal deltaic sandstone: a case study on the continental red bed from the Cretaceous Baxigai Formation, Tarim Basin, NW China. Front. Earth Sci. 12:1348336. doi: 10.3389/feart.2024.1348336
Received: 02 December 2023; Accepted: 18 January 2024;
Published: 13 February 2024.
Edited by:
Wenguang Wang, Northeast Petroleum University, ChinaReviewed by:
Shengli Li, China University of Geosciences, ChinaYang Wang, Chang’an University, China
Mingyang Wei, Southwest Petroleum University, China
Copyright © 2024 Wang, Hu, Li, He, Gao, Dou and Wang. This is an open-access article distributed under the terms of the Creative Commons Attribution License (CC BY). The use, distribution or reproduction in other forums is permitted, provided the original author(s) and the copyright owner(s) are credited and that the original publication in this journal is cited, in accordance with accepted academic practice. No use, distribution or reproduction is permitted which does not comply with these terms.
*Correspondence: Wenxiang He, hwx@yangtzeu.edu.cn; Luxing Dou, luxingdou@yangtzeu.edu.cn