- 1College of Energy Engineering, Xi’an University of Science and Technology, Xi’an, China
- 2State Key Laboratory of Eco-hydraulics in Northwest Arid Region, Xi’an University of Technology, Xi’an, China
In the process of solid backfill mining, the leaching of heavy metal ions from the gangue backfill body in the mined-out area can pose potential risk of polluting water resources in the mine. Accordingly, based on the environment of the gangue backfill body, the migration model of heavy metal ions from the gangue backfill body was established to reveal the pollution mechanism of water resources by the gangue backfill body in the mined-out area. The main factors that affect the migration of heavy metal ions were analyzed, and prevention and control techniques for the leaching and migration of heavy metal ions from gangue backfill bodies were proposed. Research showed that the heavy metal ions in gangue backfill bodies were subjected to the coupled action of seepage, concentration, and stress and then driven by water head pressure and gravitational potential energy to migrate downward along the pore channels in the floor, during which mine water served as the carrier. The migration distance of heavy metal ions increased with time. According to the migration rate, the migration process can be subdivided into three phases: the rapid migration phase (0–50 years), the slow migration phase (50–125 years), and the stable phase (125–200 years). It was concluded that the leaching concentration of heavy metal ions, the particle size of gangue, the permeability of floor strata, and the burial depth of coal seams were the main influencing factors of the migration of heavy metal ions. From the two perspectives of heavy metal ion leaching and migration, prevention and control techniques for the leaching and migration of heavy metal ions from gangue backfill bodies were proposed to protect water resources in mining area. The present study is of great significance to realizing utilization of solid waste in mines and protecting the ecological environment.
Introduction
China’s sustainable and rapid economic development has raised increasing demands for coal resources. Accompanied by constantly enhanced coal mining intensity, a great deal of gangue was abandoned and piled on the ground to form a gangue mountain (Zhang, et al., 2020; Yang, et al., 2021; Zhang, et al., 2021). According to incomplete statistics, gangue accumulation in China has exceeded six billion tons and has increased steadily at a speed of 0.68 billion tons per year, occupying approximately 13,000 hm3 of land. This can cause serious waste of land resources and water pollution (Zhang, 2019; Zhang, et al., 2020; Wang, 2022). Meanwhile, because of the high carbon content in gangue, large amounts of harmful gases such as CO, CO2, and SO2 can be emitted after spontaneous combustion or slow oxidation of gangue, which then act as new carbon emission sources (Wang, et al., 2016; An, 2017; Liu, et al., 2022). In addition, the processing of waste gangue has also increased the economic cost of coal resource enterprises. In particular, the Dongqu Mine, owned by Xishan Coal & Electricity Group in China, annually produces approximately 150,0000 tons of waste gangue, which processing (at a current processing cost of 27.0 yuan per ton) would amount to about 40.5 million yuan, strongly affecting the enterprise’s economic benefit (Liu, 2018; Liu, et al., 2020; Zhang, et al., 2020), Figure 1 shows the environmental damage caused by gangue accumulation in China. As China has paid increasing attention to environmental protection, China‘s coal industry has entered the stage of green mining. Moveover, the development of China‘s coal industry is divided into three phases, as shown in Figure 2. The 1970s and 1980s were in the efficient mining phase, China began to implement the fully-mechanized mining of coal resources; in the 1990s, for the safety and efficient mining phase, the theory of key stratum was proposed by Qian Minggao et al. (1996) to solve the safety problem of coal mining. Since the beginning of the 21st century, it is the green mining phase, the green technology of coal mining was proposed by Qian Minggao et al. (2003). This had also led to the ground treatment and emissions of waste gangue being controlled strictly. Solid backfill mining, as an environmentally friendly mining technique, can effectively utilize ground and underground gangue while controlling the movement of overlying strata (Zhang, et al., 2018; Zhang, et al., 2021; Lai, et al., 2022). However, after the large-scale backfill of gangue into the mined-out area, heavy metal ions from the gangue can be heavily leached and migrate under long-term leaching in mine water, posing a particular potential risk to the pollution of water resources in mines. Therefore, the prevention and management of water pollution in mines induced by gangue backfill bodies in mined-out regions have become a problem that urgently needs to be addressed.
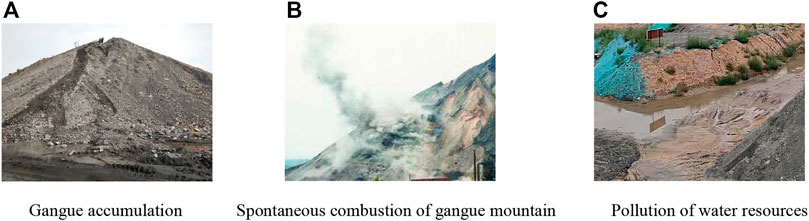
FIGURE 1. The environmental damage caused by gangue accumulation in China. (A) Gangue accumulation (B) Spontaneous combustion of gangue mountain (C) Pollution of water resources.
Scholars have conducted a great deal of research on the environmental pollution induced by waste gangue accumulation and the gangue backfill materials of solid backfill mining. Concerning ecological pollution caused by the pile-up of gangue, Shang et al. (2022) measured and analyzed the contents of heavy metals in the soil around gangue mountains and found a high enrichment degree of heavy metals and severe pollution in soil. Based on mathematical statistics, Li et al. (2022) analyzed the distribution characteristics of heavy metals in the environment and soil around the gangue mountains. They concluded that the content of heavy metals dropped with increasing distance from gangue heaps. Employing a leaching test, Liu et al. (Liu, et al., 2005; Liu, et al., 2014) examined the effect of gangue accumulation on the surrounding water and found that the leaching water from gangue destroyed the ecological system of surface water, seriously affecting the subsistence of aquatic organisms. Concerning gangue backfill materials in solid backfill mining, Li et al. (Li, et al., 2020; Li, et al., 2020) measured the bearing compressive characteristics of gangue backfill materials and obtained the instant and creep compression deformation rules of gangue backfill materials during the loading process. By combining numerical simulations and compression tests, Zha et al. (2009) analyzed the effect of the non-linear deformation characteristics of gangue backfill bodies on strata movement and the impact of the grading characteristics of backfill gangue on compressive performance. Huang et al. (Huang, et al., 2019; Huang, et al., 2021) established the fractional viscoelastic creep model of the gangue backfill body and the fractal viscoelastic creep foundation beam model, they analyzed the secular deformation characteristics of the roof under solid backfill mining. However, pollution of water resources in mines induced by the gangue backfill body of solid backfill mining has been poorly investigated and still needs further exploration.
On that basis, this study analyzed the environment of the gangue backfill body, established the migration model of heavy metal ions from the gangue backfill body, and investigated the migration rules of heavy metal ions at different times. Finally, the water pollution mechanism of gangue backfill bodies in mined-out areas and the main influencing factors that affect the migration of heavy metal ions from gangue backfill bodies are revealed to propose prevention and control techniques for the leaching and migration of heavy metal ions. The present study is of great engineering and reference significance for the prevention and control of water resource pollution in mines, the development of backfill mining techniques, and the reasonable utilization of waste gangue in mines.
Analysis of water resource pollution mechanism induced by gangue backfill body in the mined-out area
During the solid backfill mining process, heavy metal ions from the gangue backfill body are constantly leached and migrate downward under the long-term leaching action of mine water, which can threaten the environmental safety of water resources in the mine. Accordingly, this study aimed to establish a migration model of heavy metal ions from gangue backfill bodies and explore water resource pollution mechanisms.
Governing equations
(1) Basic assumptions
For the simplification of the calculation process, before the establishment of the migration model, the following basic assumptions can be made according to the migration characteristics of heavy metal ions:
1) Both floor strata and coal pillar are saturated porous elastomers with isotropic properties.
2) Heavy metal ions migrate with mine water as the carrier.
3) By ignoring convection, only the diffusion of heavy metal ions in the porous medium is considered.
4) Seepage is a kind of transient motion that satisfies generalized Darcy’s law.
(2) Governing equation of the seepage field
Based on the above basic assumptions, heavy metal ions migrate from the gangue backfill body with mine water as the carrier. The mass conservation equation of seepage can be written as:
where t is the time; ε is porosity; ρ is fluid density; Qm is a the mass source term; and u is the flow rate of the fluid.
According to Darcy’s law, the seepage under gravitational potential energy and head pressure of fluid can be written as:
where κ is permeability, μ is dynamic viscosity of fluid, p is pressure, g is gravitational acceleration.
(3) Governing equation of the concentration field
The floor strata and coal pillar can be regarded as a saturated porous elastomer with isotropic properties. The migration of heavy metal ions from the gangue backfill body should be combined with the fluid flow velocity in the governing equation of the seepage field. The following expression should be satisfied:
where ci is the concentration of heavy metal ions, J is the diffusion flux, u is the Darcy velocity, Ri is the reaction rate expression, Si is any source sink term.
Heavy metal ions migrate mainly in the format of molecular diffusion. The migration obeys Fick’s second law, and the diffusion flux J can be written as:
Where DF,i is the diffusion coefficient of fluid, τF,i is tortuosity.
(4) Governing equation of the stress field
Based on elastic mechanical theory, the properties of floor strata and coal pillars can change under the stress of the surrounding rock. The governing equation of the stress field can be written as follows:
where ρ′ is the density of porous media, v is the displacement, Fv is the volume force, and σ is the stress of surrounding rock.
The floor strata at a larger burial depth is subjected to greater stress on the surrounding rock, leading to a change in the permeability of the floor strata. The floor strata is mainly composed of sandstone in coal-measurement strata, and the relation between permeability and stress can be described as (He, et al., 2004):
where κ is permeability (unit: 10−3 μm2); σ is surrounding rock stress (unit MPa).
Establishment and validation of the migration model of heavy metal ions from gangue backfill body
(1) Establishment of the model
Based on the technological characteristics of solid backfill mining, the migration model of heavy metal ions from gangue backfill body was established with COMSOL Multiphysics. The governing equations of the seepage, concentration, and stress fields can be solved with three specific modules: Darcy’s law interface, the transport of diluted species in the porous media interface, and the solid mechanics interface. The model should be simplified considering the coupling among the seepage, concentration, and stress fields. Some size parameters of the model are described below. The overall size of the model is 400.0 m × 300.0 m × 44.0 m, the working face in solid backfill mining is 300.0 m × 200.0 m × 4.0 m, the depth of the floor strata is 40.0 m, and the width of the boundary coal pillar is 50 m. Finally, the established model was then divided into tetrahedral meshes, and the size was calibrated with fluid dynamics. Figure 3 shows the model details.
Based on the detailed condition of the environment of the backfill body, it can be assumed that a particular volume of mine water was filled in the mined-out region and that the gangue backfill body was soaked by mine water. Accordingly, the water head pressure was 4.0 m. By taking Mn ions as the research object, the leaching concentration was 3.2 × 10–5 mol/L. The floor is composed of sandstone in terms of lithology, and the burial depth of the coal seam was 300.0 m. Accordingly, the migration of heavy metal ions from the gangue backfill body at different times can be simulated.
(2) Validation of the time step and the mesh
The solution precision of the migration model of heavy metal ions from gangue backfill body depends on the appropriate time step and mesh number. A time step that is too large can reduce the calculation precision, and if the time step is too small, the calculation time is too long (Tan, et al., 2022; Zhang, et al., 2022; Liu, et al., 2023), however, the mesh number is the opposite. Therefore, the time step was set as three values: 1, 5, and 25 years, the mesh number was set as three values: 85490, 205955 and 389112. The migration distances were calculated respectively for 25, 50, 75, 100, 125, 150, 175, and 200 years of operation, as shown in Figure 4.
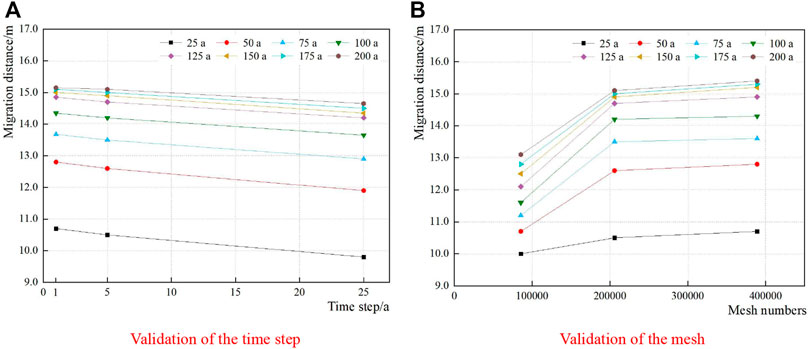
FIGURE 4. Validation of the time step and the mesh. (A) Validation of the time step (B) Validation of the mesh.
The average calculation errors were as small as 5.3 and 1.0% when the time step was reduced from 25 to 10 to 1 year. The average calculation errors were as small as 15.8 and 1.5% when the mesh number was reduced from 85,490 to 205,955 to 389,112. The average error at the time step of 25 years and the mesh number of 85,490 exceeded 5.0%. At the time step of 1 year and the mesh number of 389,112, the calculation time was too long. In conclusion, the time step was set as 5 years and the mesh number was set as 205,955 for the model solution.
Analysis of the pollution of the underlying aquifer during the solid backfill mining process
Figure 5 displays the distribution cloud charts of the concentration of Mn ions at different times. Overall, the concentration of Mn ions showed identical distribution patterns, which was highest at the interface between the gangue backfill body and the floor and infinitely close to the leaching concentration of Mn ions. Moreover, the concentration of Mn ions dropped gradually with the floor depth, suggesting a gradually weakened migration ability of heavy metal ions with the floor depth. The farthest point of the migration distance of heavy metal ions was always near the position below the center of the backfill mining working face. This is because some heavy metal ions on two sides of the working face can migrate toward the center, leading to a further migration distance of heavy metal ions at the center.
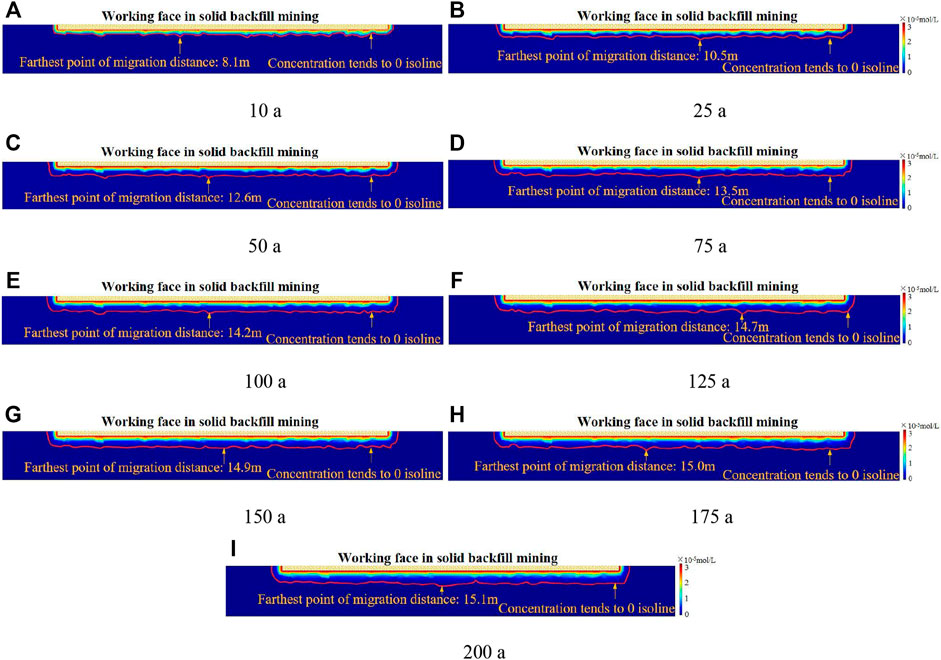
FIGURE 5. The distribution cloud charts of the concentration of Mn ions at different times. (A) 10 a (B) 25 a (C) 50 a (D) 75 a (E) 100 a (F) 125 a (G) 150 a (H) 175 a (I) 200 a.
Figure 6 shows the histograms of the migration distances of Mn ions at different times. Overall, the migration distance of Mn ions increased with time. The migration distances of Mn ions at 10, 25, 50, 75, 100, 125, 150, 175, and 200 years were 8.1, 10.5, 12.6, 13.5, 14.2, 14.7, 14.9, 15.0, and 15.1 m, respectively. Based on the migration velocity of heavy metal ions, migration can be subdivided into three phases: the rapid migration phase (0–50 years), the slow migration phase (50–125 years), and the stable phase (125–200 years). The migration distance increased by 4.5 m as time increased from 10 to 50 years, with an increasing rate of 55.6%. In the second slow migration phase, the migration distance increased by 2.1 m and 16.7%. Finally, in the last stable phase (125–200 years), the migration distance increased by 0.4 m and 2.7%, respectively.
Based on the analysis of the migration rules of heavy metal ions after different times, it can be concluded that heavy metal ions were driven by the water head pressure and gravitational potential energy and then migrated constantly downward along the pore channels in the floor strata, during which mine water served as the carrier. With prolonged time, the migration distance of heavy metal ions increased constantly; however, on account of the concentration gradient, the migration ability weakened gradually, and the increasing trend of migration distance decreased. Conclusively, at a migration distance that is too large, heavy metal ions can enter the underlying aquifer, posing a certain pollution risk to water resources in mines.
Main controlling factors of the migration of heavy metal ions from gangue backfill body
Multiple factors codetermine the migration of heavy metal ions from gangue backfill bodies. Accordingly, by combining the environment of the gangue backfill body and the pollution mechanism of water resources by the gangue backfill body in the mined-out area, this study started from two perspectives—leaching and migration of heavy metal ions - and identified four main controlling factors that affect the migration of heavy metal ions. Figure 7 depicts the environment where the gangue backfill body is located.
Leaching concentration of heavy metal ions
The migration of heavy metal ions in the floor strata is subjected to the leaching concentration of heavy metal ions. The leaching concentration of heavy metal ions can be regarded as the fundamental factor that affects the migration of heavy metal ions from gangue backfill bodies. After being heavily leached, heavy metal ions can migrate downward along the floor. At a higher leaching concentration of heavy metal ions, the concentration gradient is more significant in migration, accompanied by enhanced migration ability. Accordingly, the migration rate can be accelerated, and the migration distance increases, posing a certain pollution risk to the underlying aquifer.
The particle size of gangue
During the solid backfill mining process, gangue particle size can determine the backfill quality and the final backfilling performance, which is connected with the leaching of heavy metal ions from the gangue backfill body. Under the same environmental conditions, gangue with smaller particle sizes shows a larger specific surface area, corresponding to a larger contact area with mine water. After the long-term leaching of mine water, a more rapid reaction occurred on the gangue surface. Therefore, the leaching concentration and migration distance of heavy metal ions increased.
Permeability of floor strata
The permeability of the floor strata is also an essential factor that affects the migration of heavy metal ions from the gangue backfill body and is even the main factor. After leaching of the gangue backfill body, heavy metal ions constantly migrated downward along the pore channels in the floor strata with mine water as the carrier. On the other hand, the permeability of the floor strata is an essential factor that reflects the number of pores in the floor strata and can directly determine the migration distance of heavy metal ions. A greater number of pores can be found at a higher permeability of the floor strata, and more channels can be provided for the migration of heavy metal ions, thereby leading to a larger migration distance.
Burial depth of the coal seam
The burial depth of the coal seam can also impose a certain effect on the migration of heavy metal ions from the gangue backfill body. However, compared with the permeability of the rock strata, the burial depth of the coal seam can indirectly affect the permeability of the floor strata by affecting the stress on the surrounding rock, which can further affect the migration of heavy metal ions from the gangue backfill body. Based on the theory of rock mechanics, the floor strata was subjected to higher stresses at a deeper burial depth of the coal seam. Accordingly, pores in the floor strata were constantly closed, and the permeability constantly dropped, decreasing both the migration channels of heavy metal ions and the migration distance.
Prevention and control technique for leaching and migration of heavy metal ions from gangue backfill body
Based on the above analyses of the migration mechanism of heavy metal ions and the main controlling factors, this study started from two perspectives—prevention and control of both leaching and migration of heavy metal ions—and the prevention and control technique for leaching and migration of heavy metal ions from gangue backfill bodies was proposed, as follows.
Gangue surface solidification technique
During the solid backfill mining process, gangue, as a backfill material, can be thrown to the mined-out area by the throw gangue machine; then, after long-term leaching in mine water, many heavy metal ions are leached. Accordingly, this study started from the prevention and control of the leaching of heavy metal ions and proposed the gangue surface solidification technique for reducing the contact area between gangue and mine water, as shown in Figure 8.
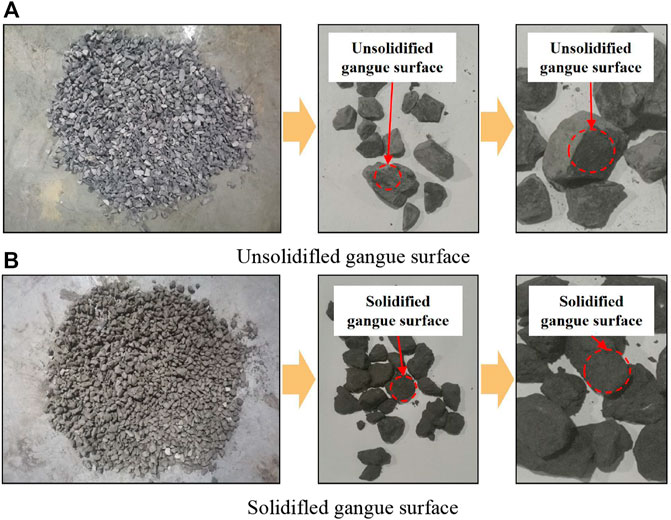
FIGURE 8. Comparison diagram of gangue surface before and after solidified. (A) Unsolidifled gangue surface (B) Solidifled gangue surface.
The gangue surface solidification technique refers to spraying the prepared cement onto the surface of gangue during the throwing process of gangue by the throw gangue machine; accordingly, gangue can be coated by cement to establish a separation barrier between gangue and mine water, thereby leading to a decline in the contact area between gangue and mine water. Heavy metal ions can thus be solidified into gangue to lower the leaching of heavy metal ions.
Control technique of gangue particle size
According to the above analysis of the main controlling factors of the migration of heavy metal ions, gangue particle size is a core factor that affects the filling ratio in the solid backfill mining process. Meanwhile, gangue particle size is also essential in determining heavy metal ions’ leaching. The gangue before and after fragmentation is compared in Figure 9. After crushing into small gangue fragments with small sizes, the surface area can increase obviously, and the contact between gangue and mine water also increases, which can increase the leaching concentration of heavy metal ions and the migration distance. Therefore, the gangue particle size control technique was proposed to reduce heavy metal ions’ leaching concentration while maintaining the filling ratio.
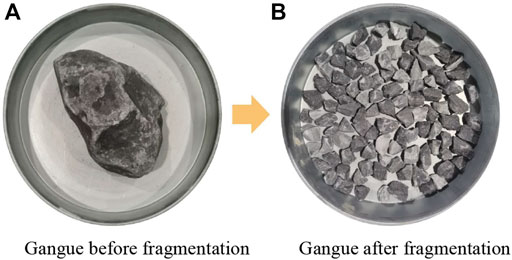
FIGURE 9. Comparison diagram before and after gangue fragmentation. (A) Gangue before fragmentation (B) Gangue after fragmentation.
The particle size control technique attempts to choose gangue with large particle sizes as the backfill material while ensuring that the filling ratio satisfies the requirements. Accordingly, both the specific surface area of the gangue backfill body and the contact area between the gangue backfill body and mine water can be reduced, which can inhibit the leaching of heavy metal ions and lower the migration distance, thereby achieving an effective balance between the backfill efficiency and pollution prevention of water resources.
Grouting sealing technique for floor
Based on the analysis results of the pollution of the underlying aquifer during the solid backfill mining process, it can be found that heavy metal ions, as the pollution source, mainly migrate in pore and fracture channels inside the floor strata. Accordingly, a floor grouting sealing technique was proposed from the perspective of the prevention and control of the migration of heavy metal ions, to change the floor permeability. During the solid backfill mining process, many fractures appear in floor strata under mining-induced dynamic loads, which can enhance the permeability of floor strata. The increase in pore channels required for migrating heavy metal ions can lead to a rise in migration distance. The floor grouting sealing technique aims to drill and inject slurry into the fractures in the floor for sealing to reduce the permeability of the floor strata and inhibit the migration of heavy metal ions.
To explore the prevention and control effect of the migration of heavy metal ions with floor grouting sealing, the distributions of the concentration of Mn ions before and after grouting sealing were analyzed, as shown in Figure 10. After grouting and sealing, the permeability of the floor strata deceased, and the migration distance of Mn ions was 5.6 m, which was reduced by 63.0% compared with the value before floor grouting and sealing (15.1 m). Therefore, floor grouting and sealing techniques can effectively prevent the migration of heavy metal ions from gangue backfill bodies.
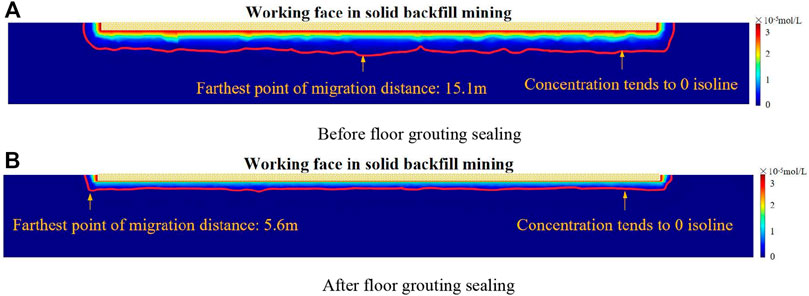
FIGURE 10. The distribution cloud diagram of Mn ion concentration before and after floor grouting sealing. (A) Before floor grouting sealing (B) After floor grouting sealing.
Discussion
Based on previous research results, once the migration distance of heavy metal ions exceeds the thickness of the isolating protective layer during the solid backfill mining process, heavy metal ions enter the underlying aquifer and cause pollution to water resources in the mine. The isolating protective layer refers to the strata between the backfill working face and the underlying aquifer, as shown in Figure 11.
Therefore, according to the position relation among the isolating protective layer, the underlying aquifer, and the backfill working face, the following formula allows one to judge whether the gangue backfill body polluted the underlying aquifer or not:
If Eq. 7 is satisfied, heavy metal ions fail to enter the underlying aquifer, and prevention and control measures should not be adopted; otherwise, heavy metal ions will migrate toward the underlying aquifer and cause particular pollution to water resources in mines. Some prevention and control techniques for the leaching and migration of heavy metal ions should be adopted to prevent the leaching and migration of heavy metal ions, improve the environment in the working face, and achieve the goal of water pollution control in the mine.
Conclusion
Based on the results obtained, the following conclusions were drawn:
1) In the solid backfill mining process, heavy metal ions in the gangue backfill body were subjected to the coupling action of seepage, concentration, and stress and then driven by water head pressure and gravitational potential energy to migrate downward along the pore channels in the floor strata, during which mine water served as the carrier. Thus can pose a potential pollution risk to water resources in mines.
(2) The migration distance of heavy metal ions increased with time. According to the migration rate, the whole migration process can be divided into three phases: the rapid migration phase (0–50 years), the slow migration phase (50–125 years), and the stable phase (125–200 years).
3) It can be concluded that the main influencing factors of the migration of heavy metal ions are the leaching concentration of heavy metal ions, the particle size of gangue, the permeability of the floor strata, and the burial depth of the coal seam. Moreover, from the two perspectives of heavy metal ion leaching and migration, prevention and control techniques for the leaching and migration of heavy metal ions from gangue backfill bodies were proposed to protect water resources in mining area.
4) By combining the location relation among the isolating protective layer, the underlying aquifer and the backfill working face, and the migration distance of heavy metal ions, it can be judged whether the underlying aquifer was polluted by heavy metal ions to determine whether to adopt the prevention and control technique for leaching and migration for preventing the leaching and migration of heavy metal ions. Zhang and Cao, 2021.
Data availability statement
The original contributions presented in the study are included in the article/supplementary material, further inquiries can be directed to the corresponding author.
Author contributions
YZ and YL conceived of the research. YZ analyzed the data and wrote the paper. JL, RZ, WH, LT, PW, and LB participated in the design of the study and verified the results. All authors have read and approved the final manuscript.
Funding
This research was funded by the National Natural Science Foundation of China (No. 52004201 and No. 51874284), and China Postdoctoral Science Foundation (No. 2020M683677XB and No. 2021T140551).
Acknowledgments
We would like to extend my gratitude to the College of Energy Engineering Xi’an University of Science and Technology China for providing us the platforms to complete this project. We thank the academic editors and reviewers for their kind suggestions and valuable comments.
Conflict of interest
The authors declare that the research was conducted in the absence of any commercial or financial relationships that could be construed as a potential conflict of interest.
Publisher’s note
All claims expressed in this article are solely those of the authors and do not necessarily represent those of their affiliated organizations, or those of the publisher, the editors and the reviewers. Any product that may be evaluated in this article, or claim that may be made by its manufacturer, is not guaranteed or endorsed by the publisher.
References
An, Y. L. (2017). Environmental behaviors of the whole life cycle of coal and their effects on land resources. [dissertation/doctoral’s thesis]. [Xuzhou, China]: China University of Mining and Technology.
He, Y. L., and Yang, L. Z. (2004). Testing study on variational characteristics of rockmass permeability under loading-unloading of confining pressure. Chin. J. Rock Mech. Eng., (3): 415–419. doi:10.3321/j.issn:1000-6915.2004.03.010
Huang, P., Spearing, S., Ju, F., Vishwanath, K., Wang, Z. W., and Ning, P. (2019). Control effects of five common solid waste backfilling materials on in situ strata of gob. Energies, 12(1): 154. doi:10.3390/en12010154
Huang, P., Zhang, J. X., Guo, Y. M., Li, M., and Zhang, Q. (2021). Viscoelastic effect of deep gangue bacfill body and time-dependent defrmation characteristics of roof in deep mining. J. China Univ. Min. Technol., 50(3): 489–497. doi:10.13247/j.cnki.jcumt.001280
Lai, X. P., Zhang, L. M., Zhang, Y., Shan, P. F., Wan, P. F., and Mu, K. W. (2022). Research of the backfill body compaction ratio based on upward backfill safety mining of the close-distance coal seam group. Geofluids, vol. 2022, 8418218. doi:10.1155/2022/8418218
Li, J. F. (2022). Study on temporal and spatial characteristics of heavy metal pollution in coal gangue piles in mining area. Multipurp. Util. Mineral Resour., (2): 181–186. doi:10.3969/jissn.1000-6532.2022.02.032
Li, M., Zhang, J. X., Meng, G. H., Gao, Y., and Li, A. L. (2020). Testing and modeling creep compression of waste rocks for backfill with different lithologies. Int. J. Rock Mech. Min. Sci., 125: 1–10. doi:10.1016/j.ijrmms.2019.104170
Li, M., Zhang, W. Q., Li, A. L., Zhu, C. L., Song, W. J., and Hu, Q. (2020). Experimental study on time-dependent compressive deformation of gangue backfilling materials. J. Min. Saf. Eng., 37(1): 147–154. doi:10.13545/j.cnki.jmse.2020.01.016
Liu, J. G., Li, X. W., and He, T. (2020). Application status and prospect of backfill mining in Chinese coal mines. J. China Coal Soc., 45(1): 141–150. doi:10.13225/j.cnki.jccs.YG19.1063
Liu, J., Xue, Y., Fu, Y., Yao, K., and Liu, J. Q. (2023), Numerical investigation on microwave-thermal recovery of shale gas based on a fully coupled electromagnetic, heat transfer, and multiphase flow model. Energy,263: 126090. doi:10.1016/j.energy.2022.126090
Liu, L., Wang, S. M., Zhu, M. B., Zhang, B., Hou, D. Z., Xu, C., et al. (2022). CO2 storage-cavern construction and storage method based on functional backfill. J. China Coal Soc., 47(3): 1072–1086. doi:10.13225/j.cnki.jccs.xr21.1558
Liu, Z. B., and Su, H. M. (2014). Release of arsenic from coal and coal gangue. Environ. Prot. Chem. Industry, 34(2): 101–104. doi:10.3969/j.issn.1006-1878.2014.02.002
Liu, Z. B., Zhang, Y. J., and Wang, J. (2005). Research of influence of leaching water of gangue on environments. J. Liaoning Tech. Univ. Nat. Sci., (2): 280–283. doi:10.3969/j.issn.1008-0562.2005.02.041
Lu, J. B. (2018). Gangue filling technology and economic benefit analysis in Dongqu coal mine. Shanxi Metall., 41(6): 137–138+165. doi:10.16525/j.cnki.cn14-1167/tf.2018.06.50
Qian, M. G., Miao, X. X., and Xu, J. L. (1996). Theoretical study of key stratum in ground control. J. China Coal Soc., 21(3): 2–7. :doi:10.13225/j.cnki.jccs.1996.03.001
Qian, M. G., Xu, J. L., and Miao, X. X. (2003). Green technique in coal mining. J. China Univ. Min. Technol., 32(4): 5–10. doi:10.3321/j.issn:1000-1964.2003.04.001
Shang, Y., and Sang, N. (2022). Pollution characteristics and phytotoxicity of heavy metals in the soil around coal gangue accumulation area. Environ. Sci., 43(7): 3773–3780. doi:10.13227/j.hjkx.202106016
Tan, S. F., Li, J. W., Zhang, Z. H., He, Y., and Wang, N. F. (2022). Simulation study on the influence of spin on ignition process of two-phase in solid rocket motor. J. Aerosp. Power, 37(7): 1503–1515. doi:10.13224/j.cnki.jasp.20210327
Wang, S. B., Luo, K. L., Wang, X., and Sun, Y. Z. (2016). Estimate of sulfur, arsenic, mercury, fluorine emissions due to spontaneous combustion of coal gangue: An important part of Chinese emission inventories. Environ. Pollut., 209: 107–113. doi:10.1016/j.envpol.2015.11.026
Wang, Y. T. (2022). Status and prospect of harmless disposal and resource comprehensive utilization of solid waste of coal gangue. Coal Geol. Explor., 50(10): 54–66. doi:10.12363/issn.1001-1986.21.11.0614
Yang, K., Wei, Z., Zhao, X. Y., He, X., Zhang, J. Q., and Ji, J. S. (2021). Theory and technology of green filling of solid waste in underground mine at coal power base of Yellow River Basin. J. China Coal Soc., 46(S2): 925–935. doi:10.13225/j.cnki.jccs.st21.0284
Zha, J. F., Guo, G. L., Liu, Y. X., and Wu, B. (2009). The noninear features of waste deformation and its impaction on strata movement. J. China Coal Soc., 34(8): 1071–1075.
Zhang, H., Zhang, Q., Zuo, X., Wu, Z. Y., Fan, W. C., Liu, H. J., et al. (2021). Design and application of mining-sparating-backfilling system for mining ecological and environmental protection. J. China Univ. Min. Technol., 50(3): 548–557. doi:10.13247/j.cnki.jcumt.001287
Zhang, Q., Miao, W. P., Liu, Q. S., Li, C., and Zhang, W. F. (2022). Research on pitch control strategy and aerodynamic performance of vertical axis wind turbine. Acta Energiae Solaris Sin., 43(10): 296–303. doi:10.19912/j.0254-0096.tynxb.2021-0234
Zhang, Q., Yang, K., Zhang, H., Xia, K. Q., and Zhang, Q. (2021). Research on weakening law and quantitative characterization of strata behavior in solid filling mining. J. China Univ. Min. Technol. 50(3): 479–488. doi:10.13247/j.cnki.jcumt.001279
Zhang, Y., and Cao, S. G. (2021). Control of water-flowing fracture development with solid backfill mining: Designing a backfill body compression ratio for water resources protection. Mine Water Environ., 40(4): 877–890. doi:10.1007/s10230-021-00821-y
Zhang, Y., Cao, S. G., Lai, X. P., Shan, P. F., Zhang, N., Zhao, C. Z., et al. (2020). Analysis on the mechanical properties of water-conducting fractures development during the shortwall block mining. J. China Coal Soc., 45(S2): 551–560. doi:10.13225/j.cnki.jccs.2019.1833
Zhang, Y., Cao, S. G., and Wang, J. J. (2018).Mechanisms of the development of water-conducting fracture zone in overlying strata during shortwall block backfill mining: A case study in northwestern China. Environ. Earth Sci., 77(14): 543. doi:10.1007/s12665-018-7726-6
Zhang, Y., Cao, S. G., Zhang, N., and Zhao, C. Z. (2020). The application of short-wall block back fill mining to preserve surface water resources in Northwest China. J. Clean. Prod., vol. 261, 121232. doi:10.1016/j.jclepro.2020.121232
Keywords: solid backfill mining, gangue backfill bodies, migration of heavy metal ions, pollution of water resources, environmental protection
Citation: Zhang Y, Liu Y, Liu J, Zhan R, He W, Tong L, Wan P and Bai L (2023) Control mechanism of the migration of heavy metal ions from gangue backfill bodies in mined-out areas. Front. Earth Sci. 10:1090799. doi: 10.3389/feart.2022.1090799
Received: 06 November 2022; Accepted: 25 November 2022;
Published: 18 January 2023.
Edited by:
Fangtian Wang, China University of Mining and Technology, ChinaReviewed by:
Defu Zhu, Taiyuan University of Technology, ChinaWenlong Shen, Henan Polytechnic University, China
Copyright © 2023 Zhang, Liu, Liu, Zhan, He, Tong, Wan and Bai. This is an open-access article distributed under the terms of the Creative Commons Attribution License (CC BY). The use, distribution or reproduction in other forums is permitted, provided the original author(s) and the copyright owner(s) are credited and that the original publication in this journal is cited, in accordance with accepted academic practice. No use, distribution or reproduction is permitted which does not comply with these terms.
*Correspondence: Yun Zhang, emhhbmd5dW5AY3VtdC5lZHUuY24=