- 1Reproductive Immunology and Pathology, Institute of Animal Reproduction and Food Research Polish Academy of Science, Olsztyn, Poland
- 2Gamete and Embryo Biology, Institute of Animal Reproduction and Food Research Polish Academy of Science, Olsztyn, Poland
- 3Physiology and Toxicology, Institute of Animal Reproduction and Food Research Polish Academy of Science, Olsztyn, Poland
- 4CIISA- Center for Interdisciplinary Research in Animal Health, Faculty of Veterinary Medicine, University of Lisbon, Lisbon, Portugal
- 5AL4AnimalS-Associate Laboratory for Animal and Veterinary Sciences, Lisbon, Portugal
Introduction: Prostaglandins (PG) are important regulators of the myometrial contractility in mammals. Endometrosis, a condition characterized by morphological changes in the equine endometrium, also affects endometrial secretory function. However, it remains unclear whether and how endometrosis affects myometrial function.
Methods: This study investigated: (i) mRNA transcription of genes encoding specific enzymes responsible for PG synthesis, such as prostaglandin—endoperoxide synthase (PTGS2), PGE2 synthase (PTGES), PGF2α synthase (PTGFS) and PG receptors: PGE2 receptors (PTGER1- 4), and PGF2α receptor (PTGFS) in equine myometrium and, (ii) the effects of PGE2 and PGF2α on myometrial contractile activity, during endometrosis in mares. The myometria used in experiments 1 and 2 were collected from mares in the mid-luteal (n = 23) and follicular (n = 20) phases of the estrous cycle, according to the histological classification of the endometrium (Kenney and Doig categories I, IIA, IIB, and III).
Results: In experiment 1, changes in mRNA transcription of PG synthase or PG receptors in the myometrium during the course of endometrosis were determined using qPCR. During the mid-luteal phase, myometrial mRNA transcription of PTGES increased in mares with endometrial category IIB compared to category I. However, myometrial mRNA transcription of PTGER1 decreased during the progression of endometrosis compared to category I. During the follicular phase, mRNA transcription of PTGER1 and PTGER2 increased in mares with endometrial categories III or IIA, respectively. In addition, mRNA transcription of PTGFS increased in mares with endometrium category IIA compared to category I. In experiment 2, the force of myometrial contractions was measured using an isometric concentration transducer. In the follicular phase, PGE2 decreased the force of contractions in mares with endometrial categories IIA, IIB, and III compared to the respective control groups. Prostaglandin F2α increased the force of myometrial contractions in mares with category IIA endometrium, whereas it decreased in category IIB compared to the respective control groups.
Discussion: We concluded that in the progression of endometrosis there are changes in the myometrial transcription of mRNA encoding PG synthases and receptors, particularly PTGER1 and PTGER2. Mares with endometrosis had abnormal myometrial contractile responses to PG. These findings suggest that myometrial function may be compromised during the progression of endometrosis.
Introduction
Endometrosis is a chronic degenerative condition that affects the uterus of mares, leading to significant economic losses in the equine industry (1–3). The main hallmarks of endometrosis are fibrotic changes in the endometrium, collagen formation around the endometrial glands and/or in the stroma, cystic dilation, as well as dilation of lymphatic vessels (4–8). There have been several studies on the pathogenesis of endometrosis and the secretory function of the endometrium during this condition including secretion and actions of prostaglandins (PG) (8–13). However, little is currently known about the myometrial changes that occur in endometrosis. Hanada et al. (14) observed histopathological changes in equine myometrium in relation to the stage of endometrosis. These changes include the degeneration of smooth muscle cells and lymphatic lacunae in the tissue's vascular layer.
The myometrium's primary function is its contractile activity, responsible for transporting sperm or gametes in the equine reproductive tract, and involved in mating, parturition, and uterine involution (15–19). Insufficient myometrial activity can cause uterine clearance issues that are associated with post-breeding endometritis (20, 21). This can lead to infertility and implantation failure in mares (22). Moreover, there is evidence that endometrosis and endometritis are linked processes (23, 24).
Prostaglandins regulate myometrial contractile activity in mares throughout the estrous cycle (25–28) and during the time period of embryo mobility (27, 29–33). Equine myometrium has recently been shown to be an additional source of PG (34).
Prostaglandin synthesis starts with converting arachidonic acid (AA) into prostaglandin H2 (PGH2) by prostaglandin-endoperoxide synthases (PTGS2). Prostaglandin F2α synthase (PTGFS) and PGE2 synthase (PTGES) catalyze the production of prostaglandin F2α (PGF2α) or prostaglandin E2 (PGE2), respectively (35). Prostaglandins interact with membrane-bound receptors in the equine endometrium (36). Prostaglandin F2α receptor (PTGFR) binds PGF2α, while PGE2 receptor (PTGER) has four subtypes: PTGER1, PTGER2, PTGER3, and PTGER4 (36). According to Wanggren et al. (37), PTGER1 and PTGER3 induce smooth muscle contraction, whereas PTGER2 and PTGER4 induce smooth muscle relaxation. Previous studies highlighted mRNA transcription changes of PG synthase and PG concentration in equine endometrium across endometrosis stages (9, 10, 13). However, the expression status of PG synthases and receptors in myometrium during endometrosis remains unexplored.
We hypothesize that mRNA transcription of PG synthase and PG receptor in equine myometrium is disrupted during endometrosis establishment. Additionally, this study aims to evaluate the differences in myometrial contractile activity mediated by PGE2 or PGF2α in mares with endometrosis during the mid-luteal and follicular phases of the estrous cycle.
Materials and methods
Animals and material collection
Uterine samples (n = 43) were collected post-mortem from Polish cold-blood mares with ovarian cyclicity, weighing 500 ±100 kg and ranging from 2 to 20 years of age during the breeding season (between April and June) at a local abattoir (Rawicz, Poland). The mares were confirmed to be clinically healthy by an official government Veterinary inspector, and by referral to historical health records for each animal. The animals were slaughtered as part of routine meat production protocols at a local abattoir. The slaughter process was conducted in accordance with European legislation (EFSA, AHAW/04-027) to ensure the elimination of pain and suffering. All material collection procedures were approved by The Local Ethics Committee for Experiments on Animals in Olsztyn, Poland (Agreement No. 51/2011). The phase of the estrous cycle was determined based on progesterone (P4) analysis and macroscopic observation of the ovaries (38). Hence, the mid-luteal phase is characterized by a well-developed corpus luteum (CL) associated with 15–20 mm follicles. In contrast, the follicular phase is characterized by the absence of an active CL, the presence of follicles larger than 35 mm in diameter.
Myometrial samples were taken from all uteri from which the endometrium was obtained. The endometrium was washed with cold sterile RNAse-free saline solution and placed into 4% buffered paraformaldehyde (POCH, Gliwice, Poland, #432173111) for histological analysis after hematoxylin-eosin staining (38). The endometria were classified retrospectively into categories I, IIA, IIB, or III based on the Kenney and Doig classification (1), which considers fibrosis, inflammatory infiltrates, and the degree of dilatation of endometrial glands and lymphatic vessels.
Myometrium was excised from the endometrium and perimetrium of the uterine horns that were ipsilateral to the CL (mid-luteal phase of the estrous cycle) or the growing follicle (follicular phase of the estrous cycle). The excised myometrium was then washed with cold, sterile, RNAse-free saline solution and stored in RNAlater (#AM7021; Invitrogen) at −80°C for further qPCR analyses (experiment 1). To measure myometrial activity in experiment 2, a 3–4 mm wide and 6–7 mm long myometrium was excised and cut in the direction of the longitudinal muscle. Each myometrial strip was washed with cold sterile RNAase-free saline solution and placed into 2 mL of aerated physiologic salt solution (PSS, pH 7.4) at 4°C with 95% air and 5% CO2 until measuring myometrial contractility (39). The measurement of myometrial contractility was carried out before histopathological categorization of the endometrium, according to Kenney and Doig (1), to obtain the appropriate number of samples in each experimental group.
Myometrial samples were linked to the endometrium that was previously assigned according to Kenney and Doig endometrial histopathological grading (1), in conjunction with an assessment of the phase of the estrous cycle. The same myometrial were used for both experiment 1 and experiment 2.
Experimental procedures
Experiment 1. Myometrial PG synthases and PG receptors mRNA transcription at different stages of mare's endometrosis.
Myometrial samples were retrospectively assigned to endometrial categories I, IIA, IIB, or III according to the Kenney and Doig classification system (1). Myometria from the mid-luteal phase (n = 6 for category I, n = 6 for category IIA, n = 5 for category IIB, n = 6 for category III); and the follicular phase (n = 5 for category I, n = 6 for category IIA, n = 5 for category IIB, n = 4 for category III) of the estrous cycle were used for the determination of PTGS2, PTGES, PTGFS, PTGER1, PTGER2, PTGER3, PTGER4, and PTGFR mRNA transcription at different stages of mare endometrosis. Myometrial samples were taken from the same uteri for experiments 1 and 2.
Experiment 2. The effects of PGE2 and PGF2α on myometrial contractile activity from different categories of mare endometrium.
Myometrial samples used in this experiment were from the same uteri as those used in experiment 1. Myometrial tissues from the mid-luteal (n = 6 for category I, n = 6 for category IIA, n = 5 for category IIB, n = 6 for category III) and follicular phases of the estrous cycle (n = 5 for category I, n = 6 for category IIA, n = 5 for category IIB, n = 4 for category III) were utilized to determine the effect of PGE2 and PGF2α on myometrial contractile activity at different categories of mare endometrium. Myometrial strips were treated with PGE2 (Cayman Chemical, Ann Arbor, MI, USA #14010) or PGF2α (Cayman Chemical, Ann Arbor, MI, USA #16010) at increasing doses of 10−8M, 10−7M, and 10−6M for 5 min. The concentrations of PGE2 and PGF2α were chosen based on a preliminary study carried out by us, and previous studies by Word et al. (40), Rigby et al. (28), and Chioss et al. (41). The force of myometrial contractions was measured using an isometric concentration transducer (HSE Type 372, HSE Schuler Organ bath apparatus, March-Hugstetten, Germany).
RNA extraction, cDNA synthesis, and real-time qPCR
Total RNA was extracted from myometrial tissues using the TRI Reagent® (T9424-200 ML, Sigma Aldrich, Germany) following the manufacturer's instructions. RNA content and purity were evaluated using a NanoDrop 1000 Spectrophotometer (Thermo Fisher Scientific, ND-1000, Wilmington, DE, USA). The A260/280 absorbance ratio for all samples was ~2.0, and the 260/230 absorbance ratio ranged between 1.8 and 2.0. The RNA was reverse transcribed into cDNA using the Reverse Transcription Kit (Qiagen, Hilden, Germany, #205311) at a concentration of 1.5 μg.
Real-time PCR was performed using TaqMan Universal Master Mix II (4440049; Applied Biosystems, Foster City, CA, USA) on a Viia7 system (Applied Biosystems, Waltham, Massachusetts, USA) in 384-well plates. All samples were run in duplicates. To measure mRNA transcription of analyzed genes, i.e., PTGS2 (cat. no. Ec03467558_m1), PTGES (cat. no. Ec04321097_s1), PTGFS (cat.no. Ec03468273_m1), PTGER1 (cat.no. Ec07038494_m1), PTGER2 (cat. no. Ec03469468_m1), PTGER3 (cat.no. Ec07094974_m1), PTGER4 (cat.no. Ec03397708_g1), PTGFR (cat. no. Ec03467807_m1) in relation to reference genes, i.e., ubiquitin conjugating enzyme E2 (UBE2B) (cat. no. Ec07038512), ribosomal protein L32 (RPL32) (cat. no. Ec06951800_m1), and succinate dehydrogenase complex flavoprotein subunit A (SDHA) (cat. no. Ec03470487_m1), Single Tube TaqMan Gene Expression Assays (Life Technologies Thermo Fisher Scientific) were used. The reaction mixture for the qPCR assay consisted of 5 μL TaqMan Universal PCR Master Mix, 0.5 μL TaqMan probe, 3 μL cDNA (2.5 ng) and 1.5 μL nuclease-free water to a final volume of 10 μL. As a negative control, nuclease-free water was used instead of template cDNA. cDNA amplification was performed under the following conditions: initial denaturation for 10 min at 95°C, followed by 40 cycles of 15 s at 95°C and 1 min at 60°C. Data were analyzed using the method described previously by Zhao and Fernald (42) using the equation R0 = 1/(1 + E)Ct, where E is the average gene efficiency and Ct is the number of cycles at the threshold. Relative gene expression was calculated as R0 target gene/ R0 reference gene and expressed in arbitrary units. The stability of used reference genes was tested and confirmed using NormFinder software (43).
Measurement of myometrial contractility
Figure 1 depicts the experimental protocol for measuring uterine contractility. Each myometrial strip was individually attached to the base of the chambers using the HSE Schuler Organ bath apparatus. The strip was then tied to the isometric contraction transducer HSE Type 372, using a stationary hook and surgical silk. The chambers contained Krebs-Ringer's solution (KRS) with a pH of 7.4 and a volume of 10 mL. The solution was composed of NaCl (120.3 mM), KCl (5.9 mM), CaCl2 (2.5 mM), MgCl2 (1.2 mM), NaH2PO4 (1.2 mM), NaHCO3 (15.5 mM), and glucose (11.5 mM), as described by Wrobel et al. (39). The baths were constantly oxygenated with a mixture of 95% O2 and 5% CO2 and were maintained at a temperature of 38.5°C. All preparations were allowed to equilibrate for 90 min. During the pre-incubation period, we observed spontaneous and regular contractions of the myometrial strips, which we refer to as the stabilization period. The viability and usefulness of smooth muscle tissue isometric contractions were measured every 2 s for 5 min before (basal contractions) and after the application of oxytocin (OT), as previously described (39, 44). To serve as a positive control, we used OT treatment at a concentration of 1 μM (Sigma Aldrich, #04375). Within 5 min, OT clearly stimulated myometrial contraction, which was then stabilized (data not shown). To reassess tissue functionality, the same dose of OT was used as before. The tissues in the chambers were rinsed three times using KRS after OT stimulation. Only results where the difference in response to OT stimulation (1 μM) at the beginning and end of the study was < 20% were included in the statistical analysis.
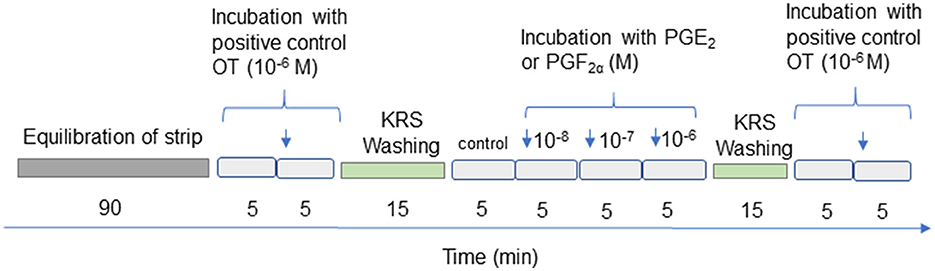
Figure 1. Diagram showing treatment of the myometrial strips. OT, oxytocin; PGE2, prostaglandin E2, PGF2α, prostaglandin F2α. Concentrations of the examined substances are expressed in moles (M).
Myometrial contractility was measured every 2 s for 5 min in response to PGE2 or PGF2α at doses of 10−8M, 10−7M, and 10−6M.
Statistical analysis
For each statistical analysis, a Gaussian distribution was tested using the D'Agostino & Pearson normality test (GraphPad Prism 10.2.1 software; GraphPad, San Diego, CA, USA). In experiment 1, a two-way ANOVA followed by Sidak‘s multiple comparison test (GraphPad Prism) was used. Gene expression differences in the myometrium of mares with Kenney and Doig categories I, IIA, IIB, and III endometria were assessed between the mid-luteal and follicular phases of the estrous cycle (different superscript letters) and between categories IIA, IIB, and III compared with category I in each phase of the estrous cycle (asterisk). In experiment 2, we conducted a statistical analysis of the force of myometrial contraction using two-way ANOVA for repeated measures, followed by the Sidak‘s multiple comparison test (GraphPad). The mean contraction force values (mN) ± SEM were calculated based on measurements collected every 2 s over a 5-min period. Results were considered significant at P < 0.05.
Results
Experiment 1. The determination of PG synthases and PG receptors mRNA transcription in equine myometrium at different stages of endometrosis.
In mares with endometrium category IIA, PTGS2 mRNA transcription was lower in the myometrium during the mid-luteal stage compared to the follicular phase of the estrous cycle (P < 0.05; Figure 2A).
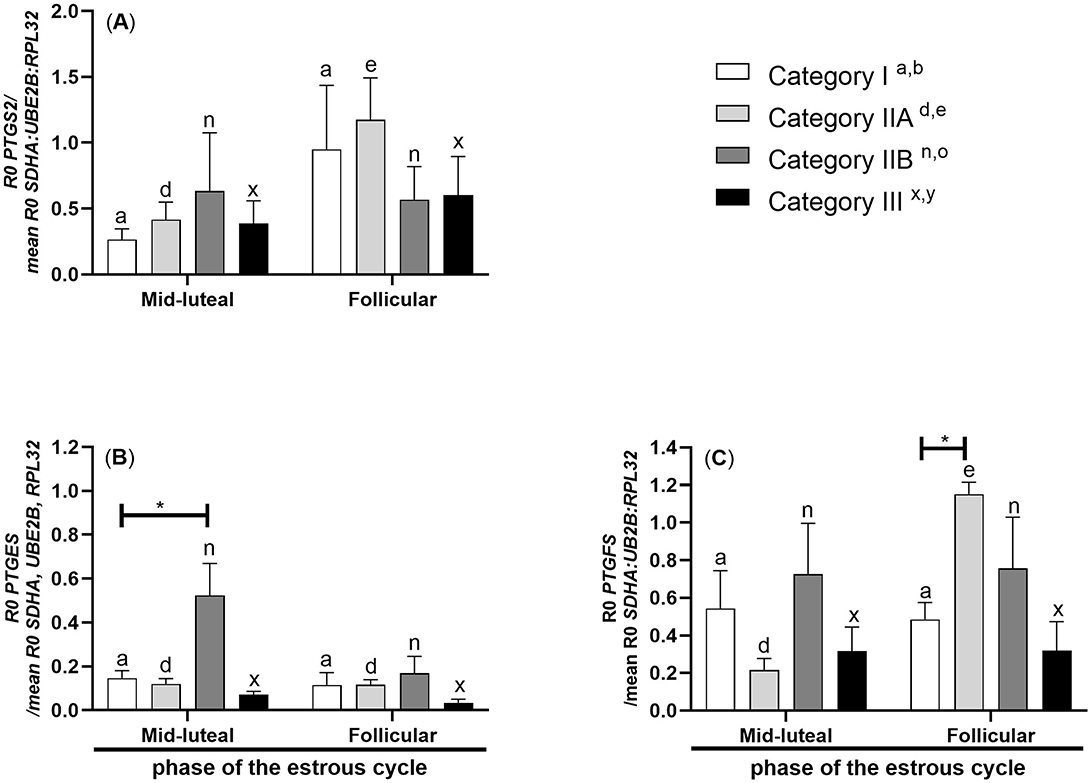
Figure 2. Myometrial mRNA transcription of PG synthases in mares with endometrosis at different stages of the estrous cycle. mRNA transcription of (A) PTGS2, (B) PTGES, and (C) PTGFS in mares with category I (n = 6), IIA (n = 6), IIB (n = 5), and III (n = 6) endometrium during the mid-luteal phase or with category I (n =5), IIA (n = 6), IIB (n = 5), and III (n = 4) endometrium during the follicular phase of the estrous cycle. Bars represent mean ± SEM. The superscript indicates statistical differences in myometrium in categories Ia, b, IIAd, e, IIBn, o, and IIIx, y between mid-luteal and follicular phases. The asterisk indicates statistical differences in mRNA transcription of PG synthase in myometria compared to category I, within the mid-luteal or follicular phase of the estrous cycle (*P < 0.05).
mRNA transcription of PTGES was found to be upregulated in the myometrium of mares with endometrium category IIB during the mid-luteal stage of the estrous cycle compared to mares with endometrium category I (P < 0.05; Figure 2B).
In mares with endometrium category IIA, PTGFS mRNA transcription was lowered in the myometrium during the mid-luteal phase of the estrous cycle compared to the myometrium during the follicular phase of the estrous cycle (P < 0.05; Figure 2C). mRNA transcription of PTGFS increased in the myometrium of mares with endometrium category IIA during the follicular phase of the estrous cycle compared to mares with endometrium category I (P < 0.001; Figure 2C).
In mares with endometrium category III, PTGER1 mRNA transcription was lowered in the myometrium during the mid-luteal phase of the estrous cycle compared to the follicular phase (P < 0.05; Figure 3A). mRNA transcription of PTGER1 was decreased in the myometrium of mares with endometrium categories IIA, IIB, and III in the mid-luteal phase of the estrous cycle compared to the myometrium of mares with endometrium category I (P < 0.05; P < 0.01; P < 0.01, respectively; Figure 3A). While during the follicular phase, its mRNA transcription was increased in the myometrium of mares with endometrium category III compared to the myometrium of mares with endometrium category I (P < 0.001; Figure 3A).
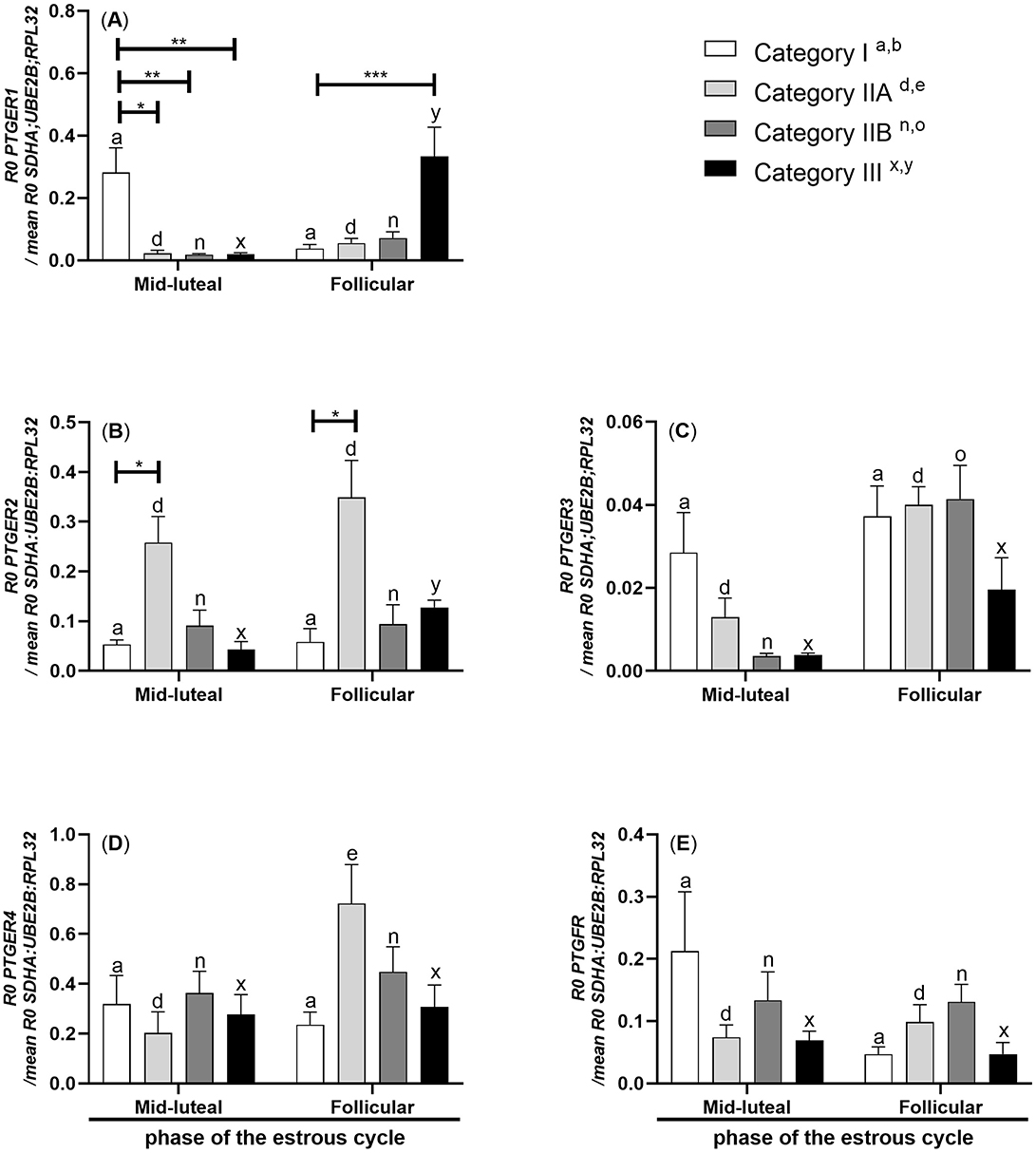
Figure 3. Myometrial mRNA transcription of PG receptors in mares with endometrosis at different stages of the estrous cycle. mRNA transcription of (A) PTGER1, (B) PTGER2, (C) PTGER3, (D) PTGER4, and (E) PTGFR in mares with category I (n = 6), IIA (n = 6), IIB (n = 5), and III (n = 6) endometrium during the mid-luteal or with category I (n =5), IIA (n = 6), IIB (n = 5), and III (n = 4) endometrium during the follicular phase of the estrous cycle. Bars represent mean ± SEM. The superscript indicates statistical differences in myometrium in categories Ia, b, IIAd, e, IIBn, o, and IIIx, y between mid-luteal and follicular phases. The asterisk indicates statistical differences in mRNA transcription of PG receptors in myometria compared to category I, within the mid-luteal or follicular phase of the estrous cycle (*P < 0.05; **P < 0.01; ***P < 0.001).
In mares with endometrium category III, PTGER2 mRNA transcription was lowered in the myometrium during the mid-luteal phase of the estrous cycle compared to the follicular phase (P < 0.05; Figure 3B). mRNA transcription of PTGER2 was increased in the myometrium of mares with endometrium category IIA in both phases of the estrous cycle compared to myometrium of mares with endometrium category I (P < 0.05; Figure 3B).
In mares with endometrium category IIB, PTGER3 mRNA transcription was lowered in the myometrium during the mid-luteal phase of the estrous cycle compared to the myometrium during the follicular phase (P < 0.01; Figure 3C).
In mares with endometrium category IIA, PTGER4 mRNA transcription was lowered in the myometrium during the mid-luteal phase compared to the follicular phase of the estrous cycle (P < 0.05; Figure 3D).
There were no statistically significant differences in the mRNA transcription of PTGFR in the mare myometrium between different stages of endometrosis and phases of the estrous cycle (P > 0.05; Figure 3E).
Experiment 2. The effect of prostaglandin E2 and prostaglandin F2α on myometrial contractile activity from different categories of mare's endometrium.
There were no statistically significant differences in the force of contractions of control and PGE2 stimulated stripes from mares with category I endometrium (P > 0.05; Figure 4A). Prostaglandin E2 at a dose of 10−6M was found to downregulate the force of myometrial contractions in mares with endometrium category IIA during the mid-luteal phase of the estrous cycle, compared with the control group (P < 0.05; Figure 4B). While, during the follicular phase of the estrous cycle, PGE2 at a dose of 10−7 and 10−6M decreased the force of contractions in mares with endometrium category IIA (P < 0.05; Figure 4B), IIB (P < 0.05, Figure 4C), and III (P < 0.05, Figure 4D), compared with respective control groups. Moreover, in mares with category IIA endometrium, the force of myometrial contraction was significantly higher in both the control and PGE2 (10−8M) treated groups during the follicular phase compared to the respective groups in the mid-luteal phase of the estrous cycle (P < 0.001; Figure 4B).
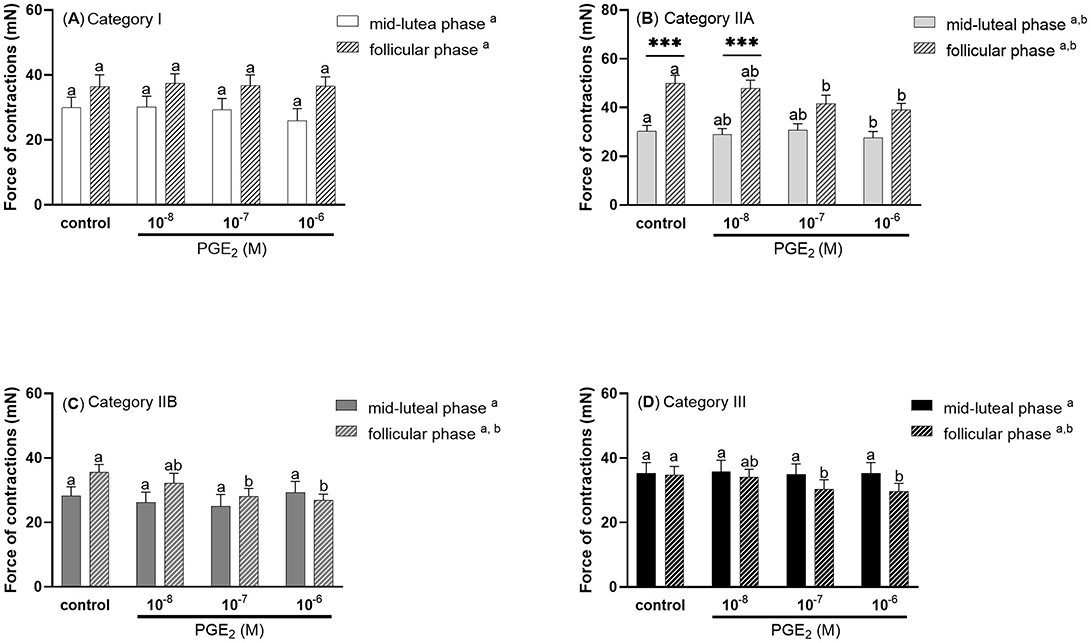
Figure 4. The force (mean ± SEM) of myometrial contraction of control and PGE2-stimulated (10−8M, 10−7M, 10−6M; patterned bars) strips from mares with categories of endometrium: (A) I, (B) IIA, (C) IIB, and (D) III collected at mid-luteal phase (n = 6 for category I, n = 6 for category IIA, n = 5 for category IIB, n = 6 for category III) or follicular phase (n = 5 for category I, n = 6 for category IIA, n = 5 for category IIB, n = 4 for category III) of the estrous cycle. The asterisk indicates statistical differences in the myometrial contractile force of the PGE2-stimulated myometrial strip compared to the respective control group (***P < 0.001) in each category of endometrium during mid -luteal or follicular phase of the estrous cycle. Different superscript letters (a, b) indicate statistical significance in the force of the myometrial contraction within control groups or PGE2 -stimulated myometrial strips between mid-luteal phase vs. follicular phase of the estrous cycle (P < 0.05).
Prostaglandin F2α at doses 10−7 and 10−6M upregulated the force of myometrial contractions in mares with endometrium category IIA, in the follicular phase of the estrous cycle (P < 0.05; Figure 5B), while it decreased the force of myometrial contractions in mares with category IIB endometrium (P < 0.05; Figure 5C), compared with respective controls. In mares with category I or IIA endometrium, the force of myometrial contraction was significantly higher in the control and PGF2α (10−8M, 10−7M, 10−6M) treated groups during the follicular phase compare to the respective groups in the mid-luteal phase of the estrous cycle (P < 0.01; Figures 5A, B). There were no statistically significant differences in the force of contractions of control and PGE2α stimulated stripes from mares with category III endometrium (P > 0.05; Figure 5D).
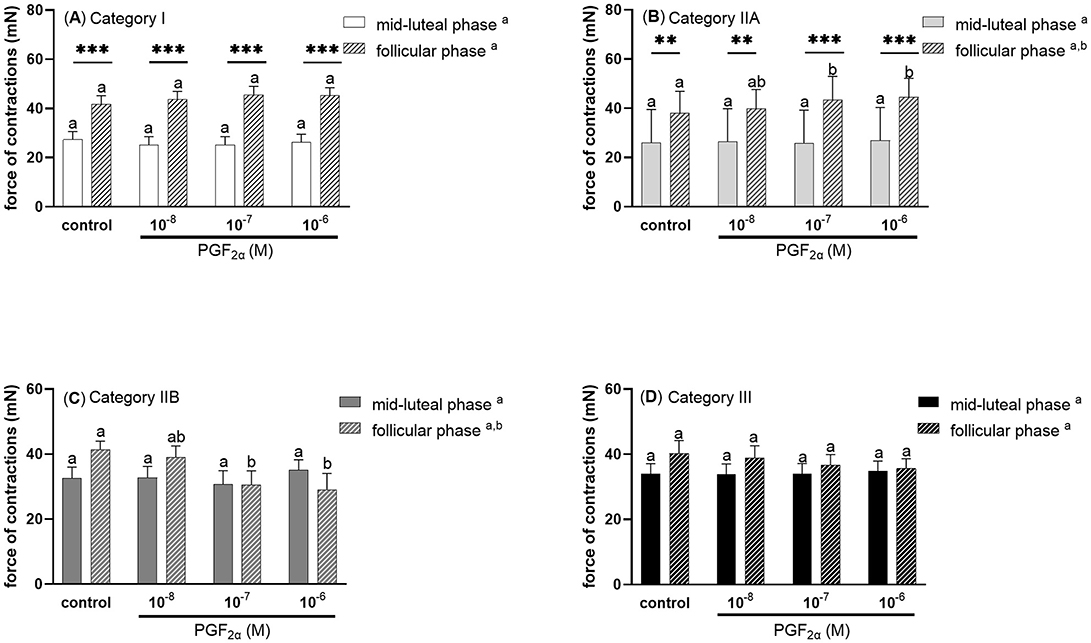
Figure 5. The force (mean ± SEM) of myometrial contraction of control and PGF2α-stimulated (10−8M, 10−7M, 10−6M; patterned bars) strips from mares with categories of endometrium: (A) I, (B) IIA, (C) IIB, and (D) III collected at mid-luteal phase (n = 6 for category I, n = 6 for category IIA, n = 5 for category IIB, n = 6 for category III) or follicular phase (n = 5 for category I, n = 6 for category IIA, n = 5 for category IIB, n = 4 for category III) of the estrous cycle. The asterisk indicates statistical differences in the myometrial contractile force of PGF2α-stimulated myometrial strip compared to the respective control group (**P < 0.01, ***P < 0.001) in each category of endometrium during mid -luteal or follicular phase of the estrous cycle. Different superscript letters (a, b) indicate statistical significance in the force of the myometrial contraction within control groups or PGF2α -stimulated myometrial strips between mid-luteal phase vs. follicular phase of the estrous cycle (P < 0.05).
Discussion
Histological changes in the myometrium of mares with endometrosis and reduced myometrial contractile activity (14, 45) may contribute to subfertility or infertility observed in mares with this condition (1, 8, 46). To better understand the underlying mechanisms of myometrial contractile dysfunction associated with equine endometrosis, it is essential to investigate the regulatory pathways of PG secretion and action in the myometrium, as well as the effect of PG on myometrial contractile activity.
Therefore, in this study, we clarified the mRNA transcription of PG synthases and PG receptors in the myometrium of mares with different endometrial categories, classified according to the Kenney and Doig system. Additionally, we examined the myometrial response to PG across different endometrial categories. To the best of our knowledge, this study is the first to identify disruptions in the PG synthesis pathway in the myometrium of mares during progression of endometrosis. These alterations in PG synthase mRNA transcription may affect the local uterine microenvironment, potentially impairing myometrial function during the estrous cycle.
Our results revealed that myometrial mRNA transcription of PG receptors PTGER1, PTGER2, PTGER3, and PTGER4 remained unchanged during the mid-luteal and follicular phases of the estrous cycle in mares with a category I endometrium. This is consistent with the findings of Silva et al. (47), who also reported no significant differences in PTGER2 and PTGER4 mRNA transcription between preovulatory estrus, late diestrus, and pregnancy, in both equine endometrium and the myometrium. In our study, in mares during progression of endometrosis, notable alternations in PGE2 receptor mRNA transcription in the myometrium was observed. Specifically, mRNA transcription of PTGER2 was significantly elevated in the myometrium of mares with category IIA endometrium during both phases of the estrous cycle compared to mares with category I endometrium. While, myometrial PTGER4 transcription decreased in mare with category IIA endometrium during the mid-luteal phase of the estrous cycle. Additionally, mRNA transcription of PTGER1 was significantly altered in the myometrium of mares with endometrial categories IIA, IIB and III compared to mares with category I endometrium, particularly during the mid-luteal phase of the estrous cycle. During the follicular phase, myometrial mRNA transcription of PTGER1 increased in mares with category III endometrium compared to mares with category I endometrium. These findings suggest that receptor- specific changes in transcription are associated with the progression of endometrosis in mares. Interestingly, we found that myometrial mRNA transcription of PGFR remained unchanged across all endometrosis categories. However, alterations in myometrial contractility in response to PGF2α were observed during the follicular phase of the estrous cycle, indicating that activity of the myometrium may not be solely attributable to changes in the expression of PG receptors alone. This suggests that additional factors, such as alterations in molecular signaling pathways activated by PG, may contribute to the observed contractile dysfunction. The limitation of this study is that post-transcriptional analysis of PG synthase and its receptors was not performed. The mRNA levels do not always correlate directly with protein expression. Therefore, it can be postulated that discrepancies between the transcription level and the myometrial contraction patterns may be explained by different posttranscriptional changes in their expression within the myometrium during the progression of endometrosis. This should be the direction of future studies.
The role of the myometrium is mainly related to its motility and its proper regulation is essential for optimal reproductive performance in mare (18). In present study, reduced contractile activity was observed in response to PGE2 in myometrium of mares with category IIA, IIB, and III endometria compared to respective control groups, particularly during the follicular phase of the estrous cycle. Myometrium of mares with category IIB endometrium also exhibited reduced contractility in response to PGF2α despite unchanged myometrial PGFR mRNA transcription. These findings suggest that myometrial contractile activity during endometrosis compared to healthy mare is reduced in response to PG. In veterinary practice PGF2α or oxytocin (OT) is commonly administered post-breeding to stimulate uterine contractions and clear intraluminal fluid. Mares with delayed uterine clearance or chronic endometritis exhibit diminished responsiveness to PGF2α relative to healthy mares (14, 28, 48, 49). This diminished response has been linked to a contractile defect in the myometrium, particularly in older mares and those with advanced endometrosis, who are prone to persistent breeding-induced endometritis (50). We can assume that the mares in our study with category IIB endometrium had a reduced myometrial contractile response to PGF2α, suggesting that traditional therapies may be less effective in these cases. This reduced myometrial contractile function could contribute to poor uterine clearance and persistence of infections, negatively impacting fertility. The age range of the mares used in our study was between 2 and 20 years. The wide age range of the animals was chosen to provide a cross-sectional view of endometrial fibrosis at different stages of its progression. As noted by Ebert et al. (51), endometrosis tends to increase with age, affecting 32% of mares under 5 years of age and 93% of mares over 20 years of age. By including mares across this wide age range, our study aimed to capture different stages of the condition. The progression of endometrosis may also be influenced by other age-related changes in the myometrium. Studies in other species have shown that advanced age is associated with increased collagen deposition in the endometrium and myometrium, leading to impaired uterine function (52, 53). In mares, older age and repeated uterine infections can result in endometrosis, which in turn contributes to subfertility or reproductive failure (8, 24, 54).
Beyond PG receptor expression, other molecular mechanisms may contribute to the myometrial contractile dysfunction observed in endometrosis. Rigby et al. (28) suggested that age-related changes in receptor-second messenger signaling pathways, particularly those downstream of intracellular Ca2+, may affect myometrial contractility. Indeed, smooth muscle contraction is regulated by several cellular mechanisms, including Ca2+/calmodulin-dependent myosin light chain kinase, Ca2+-independent regulatory light chain phosphorylation, myosin phosphatase inhibition, and actin filament-associated proteins (55–57). Moreover, changes in uterine blood flow, vascular structure, nerve supply, and increased collagen deposition may also play significant roles in reducing the tension-generating capacity of myometrial cells (4, 53, 58).
Examination of PG signaling pathways in the equine myometrium during reproductive pathologies could provide further insights into the broader roles of PG in reproductive disorders. Previous studies have shown that inflammation disrupts PG signaling in the equine uterus during endometritis (24, 59). In pregnancy-related conditions such as placentitis, inflammatory responses significantly alter PG signaling and contraction-associated pathways, such as OXTR, PTGS2 and connexin-43 (Cx43), affecting pregnancy outcomes (60, 61). Our recent study has shown that the myometrial mRNA transcription of OXTR remained unchanged between the Kenney and Doig endometrial categories and the phases of the estrous cycle (62). Therefore, these results suggest that the reduced myometrial activity is not related to reduced OXTR expression, but may be due to other processes mentioned above, such as age-related changes in receptor-second messenger signaling mechanisms downstream of intracellular Ca2+ release, as suggested by Rigby et al. (28). Given the importance of PG signaling in these pathological conditions, potential alterations in Cx43 expression could impair myometrial contractility in mares with endometrosis. Connexin-43 is essential in the myometrium, where it forms gap junctions that facilitate coordinated uterine contractions, a process important for effective labor (63). However, further study is necessary to clarify the molecular mechanisms contributing to myometrial dysfunction in mares with endometrosis.
In conclusion, our study demonstrates that myometrial contractile activity is altered in mares with endometrosis, in response to PG during the mid- luteal and follicular phases of the estrous cycle. Changes in myometrial mRNA transcription of PG synthases and receptors, such as PTGER1 and PTGER2, were observed during endometrosis progression in mares. However, these alterations alone do not fully explain the disrupted myometrial contractile activity. The dysfunction likely involves complex interactions between multiple signaling pathways activated by PG in both the endometrium and myometrium. In addition, changes in the structure of the myometrium observed in mares during endometrosis may be related to the reduced tension-generating capacity of myometrial cells. Further research is needed to fully elucidate the molecular mechanisms underlying myometrial dysfunction in endometrosis and to identify potential therapeutic targets.
Data availability statement
The original contributions presented in the study are included in the article/supplementary material, further inquiries can be directed to the corresponding author/s.
Ethics statement
Ethical approval was not required for the study involving animals in accordance with the local legislation and institutional requirements because slaughterhouse material collection.
Author contributions
KP-T: Conceptualization, Formal analysis, Methodology, Visualization, Writing – original draft, Writing – review & editing. AS-M: Formal analysis, Writing – review & editing. ED: Formal analysis, Investigation, Writing – review & editing. AJ: Formal analysis, Investigation, Visualization, Writing – review & editing. AW: Formal analysis, Investigation, Writing – review & editing. MW: Formal analysis, Writing – review & editing. GF-D: Supervision, Writing – review & editing. DS: Conceptualization, Formal analysis, Funding acquisition, Methodology, Supervision, Writing – review & editing.
Funding
The author(s) declare financial support was received for the research, authorship, and/or publication of this article. This work was supported by National Science Centre in Poland, grant number 2011/02/A/NZ5/00338. The present work was financed as part of a bilateral Polish-Portuguese research project under the NAWA and FCT agreement (NAWA project no. BPN/BPT/2021/1/00026/U/00001). The authors confirm that the funders had no role in the study design, data collection and analysis, decision to publish, preparation of the manuscript or selection of this journal.
Acknowledgments
The authors wish to thank K. Jankowska and W. Krzywiec (Institute of Animal Reproduction and Food Research, Polish Academy of Science, Olsztyn, Poland), for technical support in the experiments.
Conflict of interest
The authors declare that the research was conducted in the absence of any commercial or financial relationships that could be construed as a potential conflict of interest.
The author(s) declared that they were an editorial board member of Frontiers, at the time of submission. This had no impact on the peer review process and the final decision.
Publisher's note
All claims expressed in this article are solely those of the authors and do not necessarily represent those of their affiliated organizations, or those of the publisher, the editors and the reviewers. Any product that may be evaluated in this article, or claim that may be made by its manufacturer, is not guaranteed or endorsed by the publisher.
References
1. Kenney RM, Doig PA. Equine endometrial biopsy. In:Morrow DA, , editor. Current Therapy in Theriogenology. Philadelphia, PA: W.B. Saunders (1986). p. 723–9.
2. Buczkowska J, Kozdrowski R, Nowak M, Raś A, Mrowiec J. Endometrosis—Significance for horse reproduction, pathogenesis, diagnosis, and proposed therapeutic methods. Pol J Vet Sci. (2014) 17:547–54. doi: 10.2478/pjvs-2014-0083
3. Schöniger S, Schoon HA. The healthy and diseased equine endometrium: a review of morphological features and molecular analyses. Animals. (2020) 10:625. doi: 10.3390/ani10040625
4. Kenney RM. Cyclic and pathologic changes of the mare endometrium as detected by biopsy, with a note on early embryonic death. J Am Vet Med Assoc. (1978) 172:241–62.
5. Kenney RM. The etiology, diagnosis, and classification of chronic degenerative endometritis. In:Hughes JP, , editor. Workshop on Equine Endometritis. Volume 125. New York, NY: Newmarket Press (1992). 186 p.
6. Kenney RM. Chronic degenerative endometritis (CDE) (endometrosis). Equine Vet J. (1993) 25:184–93. doi: 10.1111/j.2042-3306.1993.tb02940.x
7. Flores JM, Rodríguez A, Sánchez J, Gómez-Cuétara C, Ramiro F. Endometrosis in mares: incidence of histopathological alterations. Reprod Dom Anim. (1995) 30:61–5. doi: 10.1111/j.1439-0531.1995.tb00606.x
8. Hoffmann C, Ellenberger C, Mattos RC, Aupperle H, Dhein S, Stief B, et al. The equine endometrosis: new insights into the pathogenesis. Anim Reprod Sci. (2009) 111:261–78. doi: 10.1016/j.anireprosci.2008.03.019
9. Szóstek AZ, Siemieniuch MJ, Lukasik K, Galvão AM, Ferreira-Dias GM, Skarzynski DJ. mRNA transcription of prostaglandin synthases and their products in the equine endometrium in the course of fibrosis. Theriogenology. (2012) 78:768–76. doi: 10.1016/j.theriogenology.2012.03.024
10. Gajos K, Kozdrowski R, Nowak M, Siemieniuch MJ. Altered secretion of selected arachidonic acid metabolites during subclinical endometritis relative to estrous cycle stage and grade of fibrosis in mares. Theriogenology. (2015) 84:457–66. doi: 10.1016/j.theriogenology.2015.03.038
11. Szóstek-Mioduchowska AZ, Baclawska A, Rebordão MR, Ferreira-Dias G, Skarzynski DJ. Prostaglandins effect on matrix metallopeptidases and collagen in mare endometrial fibroblasts. Theriogenology. (2020) 153:74–84. doi: 10.1016/j.theriogenology.2020.04.040
12. Rebordão MR, Amaral A, Fernandes C, Silva E, Lukasik K, Szóstek-Mioduchowska A, et al. Enzymes present in neutrophil extracellular traps may stimulate the fibrogenic PGF2α pathway in the mare endometrium. Animals. (2021) 11:2615. doi: 10.3390/ani11092615. doi: 10.3390/ani11092615
13. Byron M, Lection J, Foster RA, Chenier T, Wagner B, Diel de Amorim M. Prostaglandin-related genes are differentially expressed in equine endometrium with different biopsy grade, degrees of inflammation, and fibrosis. Theriogenology. (2024) 1:151–6. doi: 10.1016/j.theriogenology.2024.07.014
14. Hanada M, Maeda Y, Oikawa MA. Histopathological characteristics of endometrosis in 436 thoroughbred mares in Japan: results from 50 necropsy cases. J Equine Sci. (2014) 25:45–52. doi: 10.1294/jes.25.45
15. Ginther OJ. Internal regulation of physiological processes through local venoarterial pathways: a review. J Anim Sci. (1974) 39:550–64. doi: 10.2527/jas1974.393550x
16. Ginther OJ. Mobility of the early equine conceptus. Theriogenology. (1983) 19:603–11. doi: 10.1016/0093-691X(83)90180-2
17. Stout TA, Allen WR. Role of prostaglandins in intrauterine migration of the equine conceptus. Reproduction. (2001) 121:771–5. doi: 10.1530/rep.0.1210771
18. Hirsbrunner G, Reist M, Couto SS, Steiner A, Snyder J, Vanleeuwen E, et al. An in vitro study on spontaneous myometrial contractility in the mare during estrus and diestrus. Theriogenology. (2006) 65:517–27. doi: 10.1016/j.theriogenology.2005.05.048
19. Troedsson MHT. Problems after breeding. J Equine Vet Sci. (2008) 28:635–9. doi: 10.1016/j.jevs.2008.10.011
20. Troedsson MH. Uterine clearance and resistance to persistent endometritis in the mare. Theriogenology. (1999) 52:461–71. doi: 10.1016/S0093-691X(99)00143-0
21. Christoffersen M, Troedsson M. Inflammation and fertility in the mare. Reprod Domest Anim. (2017) 52:4–20. doi: 10.1111/rda.13013
22. Aguilar HN, Mitchell BF. Physiological pathways and molecular mechanisms regulating uterine contractility. Hum Reprod Update. (2010) 16:725–44. doi: 10.1093/humupd/dmq016
23. Liepina E, Antane V. Endometrial histological changes and pregnancy rates in mares impaired cervical drainage. In: Proceedings of 29th International Scientific Conference Animals. Health. Food Hygiene. Jelgava (2010). p. 73–8.
24. Katila T, Ferreira-Dias G. Evolution of the concepts of endometrosis, post breeding endometritis, and susceptibility of mares. Animals. (2022) 12:779. doi: 10.3390/ani12060779
25. Griffin PG, Ginther OJ. Uterine contractile activity in mares during the estrous cycle and early pregnancy. Theriogenology. (1990) 34:47–56. doi: 10.1016/0093-691X(90)90576-F
26. Troedsson MHT, Liu IKM, Ing M, Pascoe J. Smooth muscle electrical activity in the oviduct, and the effect of oxytocin, prostaglandin F2α, and prostaglandin E2 on the Myometrium and the oviduct of the cycling mare1. Biol Reprod. (1995) 52:475–88. doi: 10.1093/biolreprod/52.monograph_series1.475
27. Gastal MO, Gastal EL, Torres CA, Ginther OJ. Effect of PGE2 on uterine contractility and tone in mares. Theriogenology. (1998) 50:989–99. doi: 10.1016/S0093-691X(98)00202-7
28. Rigby SL, Barhoumi R, Burghardt RC, Colleran P, Thompson JA, Varner DD, et al. Mares with delayed uterine clearance have an intrinsic defect in myometrial function. Biol Reprod. (2001) 65:740–7. doi: 10.1095/biolreprod65.3.740
29. Leith GS, Ginther OJ. Characterization of intrauterine mobility of the early equine conceptus. Theriogenology. (1984) 22:401–8. doi: 10.1016/0093-691X(84)90460-6
30. Watson ED, Sertich PL. Prostaglandin production by horse embryos and the effect of co-culture of embryos with endometrium from pregnant mares. J Reprod Fertil. (1989) 87:331–6. doi: 10.1530/jrf.0.0870331
31. Weber JA, Freeman DA, Vanderwall DK, Woods GL. Prostaglandin E2 hastens oviductal transport of equine embryos. Biol Reprod. (1991) 45:544–6. doi: 10.1095/biolreprod45.4.544
32. Vanderwall DK, Woods GL, Weber JA, Lichtenwainer AB. PGE2 secretion by the conceptus and binding by non-pregnant endometrium in the horse. Equine Vet J. (1993) 15:24–7. doi: 10.1111/j.2042-3306.1993.tb04818.x
33. Castro T, Jacob JC, Domingues RR, Ginther OJ. Local embryo-mediated changes in endometrial gene expression during embryo mobility in mares. Theriogenology. (2022) 182:78–84. doi: 10.1016/j.theriogenology.2022.01.032
34. Piotrowska-Tomala KK, Jonczyk AW, Skarzynski DJ, Szóstek-Mioduchowska AZ. Luteinizing hormone and ovarian steroids affect in vitro prostaglandin production in the equine myometrium and endometrium. Theriogenology. (2020) 153:1–8. doi: 10.1016/j.theriogenology.2020.04.039
35. Simmons DL, Botting RM, Hla T. Cyclooxygenase isozymes: the biology of prostaglandin synthesis and inhibition. Pharmacol Rev. (2004) 56:387–437. doi: 10.1124/pr.56.3.3
36. Atli MO, Kurar E, Kayis SA, Aslan S, Semacan A, Celik S, et al. Evaluation of genes involved in prostaglandin action in equine endometrium during estrous cycle and early pregnancy. Anim Reprod Sci. (2010) 122:124–32. doi: 10.1016/j.anireprosci.2010.08.007
37. Wanggren K, Lalitkumar PG, Stavreus-Evers A, Stabi B, Gemzell-Danielsson K. Prostaglandin E2 and F2 receptors in the human fallopian tube before and after mifepristone treatment. Mol Hum Reprod. (2006) 12:577–85. doi: 10.1093/molehr/gal058
38. Roberto da Costa RP, Serrão PM, Monteiro S, Pessa P, Silva JR, Ferreira-Dias G. Caspase-3-mediated apoptosis and cell proliferation in the equine endometrium during the oestrous cycle. Reprod Fertil Dev. (2007) 19:925–32. doi: 10.1071/RD06159
39. Wrobel MH, Grzeszczyk M, Młynarczuk J, Kotwica J. The adverse effects of aldrin and dieldrin on both myometrial contractions and the secretory functions of bovine ovaries and uterus in vitro. Toxicol Appl Pharmacol. (2015) 285:23–31. doi: 10.1016/j.taap.2015.03.005
40. Word RA, Kamm KE, Casey ML. Contractile effects of prostaglandins, oxytocin, and endothelin-1 in human myometrium in vitro: refractoriness of myometrial tissue of pregnant women to prostaglandins E2 and F2 alpha. J Clin Endocrinol Metab. (1992) 75:1027–32. doi: 10.1210/jcem.75.4.1400867
41. Chioss G, Costantine MM, Bytautiene E, Betancourt A, Hankins GDV, Saade GR, et al. In vitro myometrial contractility profiles of different pharmacological agents used for induction of labor. Am J Perinatol. (2012) 29:699–704. doi: 10.1055/s-0032-1314891
42. Zhao S, Fernald RD. Comprehensive algorithm for quantitative real-time polymerase chain reaction. J Comput Biol. (2005) 12:1047–64. doi: 10.1089/cmb.2005.12.1047
43. Andersen CL, Jensen JL, Ørntoft TF. Normalization of real-time quantitative reverse transcription-PCR data: a model-based variance estimation approach to identify genes suited for normalization, applied to bladder and colon cancer data sets. Cancer Res. (2004) 64:5245–50. doi: 10.1158/0008-5472.CAN-04-0496
44. Wrobel M, Kaminski K, Kotwica J. In vitro effects of polychlorinated biphenyls(PCBs) on the contractility of bovine myometrium from the periovulatory stage of the estrous cycle. Reprod Biol. (2005) 5:303–19.
45. Troedsson MH, Liu IK, Crabo BG. Sperm transport and survival in the mare. Theriogenology. (1998) 1:905–15. doi: 10.1016/S0093-691X(98)00040-5
46. Aresu L, Benali S, Giannuzzi D, Mantovani R, Castagnaro M, Falomo ME. The role of inflammation and matrix metalloproteinases in equine reproduction. J Vet Sci. (2005) 13:171–7. doi: 10.4142/jvs.2012.13.2.171
47. Silva ES, Scoggin KE, Canisso IF, Troedsson MH, Squires EL, Ball BA. Expression of receptors for ovarian steroids and prostaglandin E2 in the endometrium and myometrium of mares during estrus, diestrus and early pregnancy. Anim Reprod Sci. (2014) 151:169–81. doi: 10.1016/j.anireprosci.2014.11.001
48. von Reitzenstein M, LeBlanc MM. Aberrations in uterine contractile patterns in mares with delayed uterine clearance after administration of detomidine and oxytocin. Theriogenology. (2002) 58:887–98. doi: 10.1016/S0093-691X(02)00847-6
49. Troedsson MH, Liu IK, Ing M, Pascoe J, Thurmond M. Multiple site electromyography recordings of uterine activity following an intrauterine bacterial challenge in mares susceptible and resistant to chronic uterine infection. J Reprod Fertil. (1993) 99:307–13. doi: 10.1530/jrf.0.0990307
50. Woodward EM, Christoffersen M, Campos J, Squires EL, Troedsson MH. Susceptibility to persistent breeding-induced endometritis in the mare; relationship to endometrial biopsy score and age, and variations between seasons. Theriogenology. (2012) 78:495–501. doi: 10.1016/j.theriogenology.2012.02.028
51. Ebert A, Schoon D, Schoon HA. Age-related endometrial alterations in mares—biopsy findings of the last 20 years In:Rackwitz R, Pees M, Aschenbach, JR, Gäbel G, , editors. Leipziger Blaue Hefte, 7th Leipzig Veterinary Congress, 8th International Conference on Equine Reproductive Medicine. Berlin: Lehmanns Media GmbH (2014). p. 230–2.
52. Maurer RR, Foote RH. Uterine collagenase and collagen in young and aging rabbits. J Reprod Fertil. (1972) 30:301–4. doi: 10.1530/jrf.0.0300301
53. Mulholland J, Jones CJP. Characteristics of uterine aging. Microsc Res Tech. (1993) 25:148–68. doi: 10.1002/jemt.1070250207
54. Lehmann J, Ellenberger C, Hoffmann C, Bazer FW, Klug J, Allen WR, et al. Morpho-functional studies regarding the fertility prognosis of mares suffering from equine endometrosis. Theriogenology. (2011) 76:1326–36. doi: 10.1016/j.theriogenology.2011.06.001
55. Marston SB, Pritchard K. Thin filament control of uterine smooth muscle. In:Carsten ME, Miller JD, , editors. Uterine Function Molecular and Cellular Aspects. New York, NY: Plenum Press (1990). p. 99–119.
56. Tansey MG, Luby-Phelps K, Kamm KE, Stull JT. Ca(2+)-dependent phosphorylation of myosin light chain kinase decreases the Ca2+ sensitivity of light chain phosphorylation within smooth muscle cells. J Biol Chem. (1994) 1:9912–20. doi: 10.1016/S0021-9258(17)36969-7
57. Zhang WC, Peng YJ, Zhang GS, He WQ, Qiao YN, Dong YY, et al. Myosin light chain kinase is necessary for tonic airway smooth muscle contraction. J Biol Chem. (2010) 285:5522–31. doi: 10.1074/jbc.M109.062836
58. Guna-Smith Z, Woessner JF. Content of the collagen and elastin crosslinks pyridinoline and the desmosines in the human uterus in varius reproductive states. Am J Obstet Gynecol. (1985) 153:92–5. doi: 10.1016/0002-9378(85)90602-7
59. Nash DM, Sheldon IM, Herath S, Lane EA. Markers of the uterine innate immune response of the mare. Anim Reprod Sci. (2010) 119:31–9. doi: 10.1016/j.anireprosci.2009.11.008
60. El-Sheikh Ali H, Legacki EL, Loux SC, Esteller-Vico A, Dini P, Scoggin KE, et al. Equine placentitis is associated with a downregulation in myometrial progestin signaling. Biol Reprod. (2019) 101:162–76.
61. El-Sheikh Ali H, Boakari YL, Loux SC, Dini P, Scoggin KE, Esteller-Vico A, et al. Transcriptomic analysis reveals the key regulators and molecular mechanisms underlying myometrial activation during equine placentitis. Biol Reprod. (2020) 26:1306–25. doi: 10.1093/biolre/ioaa020
62. Silva BCE, Drzewiecka EM, Piotrowska-Tomala K, Alpoim-Moreira J, Sadowska A, Kowalik MK, et al. The alteration in myometrial mRNA transcription of the regulatory genes of DNA methylation in mare with endometrosis. Reprod Biol. (2024) 22:100962. doi: 10.1016/j.repbio.2024.100962
Keywords: prostaglandin E2, prostaglandin F2α, contractions, myometrium, mare, endometrosis
Citation: Piotrowska-Tomala KK, Szóstek-Mioduchowska AZ, Drzewiecka EM, Jonczyk AW, Wójtowicz A, Wrobel MH, Ferreira-Dias G and Skarzynski DJ (2024) Prostaglandin pathways in equine myometrium regulations: endometrosis progression. Front. Vet. Sci. 11:1479508. doi: 10.3389/fvets.2024.1479508
Received: 12 August 2024; Accepted: 11 November 2024;
Published: 05 December 2024.
Edited by:
Kirsten E. Scoggin, University of Kentucky, United StatesReviewed by:
Roman Dabrowski, University of Life Sciences of Lublin, PolandHossam El-Sheikh Ali, University of Kentucky, United States
Mohammed Ahmed Elmetwally, Mansoura University, Egypt
Copyright © 2024 Piotrowska-Tomala, Szóstek-Mioduchowska, Drzewiecka, Jonczyk, Wójtowicz, Wrobel, Ferreira-Dias and Skarzynski. This is an open-access article distributed under the terms of the Creative Commons Attribution License (CC BY). The use, distribution or reproduction in other forums is permitted, provided the original author(s) and the copyright owner(s) are credited and that the original publication in this journal is cited, in accordance with accepted academic practice. No use, distribution or reproduction is permitted which does not comply with these terms.
*Correspondence: Dariusz J. Skarzynski, ZC5za2Fyenluc2tpJiN4MDAwNDA7cGFuLm9sc3p0eW4ucGw=