- 1Division of Environment Science, ICAR-Indian Agricultural Research Institute, New Delhi, India
- 2International Rice Research Institute, New Delhi, India
Introduction: Global food security is challenged by the increasing levels of air pollutants like ozone (O3) through their impacts on crop productivity. The present study was conducted to quantify the interactive effect of elevated ozone (O3) and carbon dioxide (CO2), on different rice varieties in northern India.
Methods: An experiment was conducted in Genetic H field, Environment science, IARI for two consecutive years (2020 and 2021) during the kharif season, to quantify the impact of elevated O3 and CO2 interaction on productivity, and plant N in three rice varieties (Pusa basmati 1121, Nagina 22, IR64 Drt1) under different nitrogen (N) management practices. Rice crop was grown in Free Air Ozone-Carbon dioxide Enrichment rings (FAOCE) rings with two levels of O3 (elevated 60 ±10ppb and ambient) and two levels of CO2 (elevated, 550±25 ppm and ambient) concentration and their interaction with two N fertilizer treatments i.e., 100% RDN (recommended dose of N) and 125% RDN.
Results and discussion: Elevated O3 significantly decreased physiological parameters like photosynthesis rate, stomatal conductance and transpiration rate of the crop. Grain yield reduced by 7.2-7.5%, in Pusa Basmati 1121 and from 6.9-9% in IR64 Drt1 varieties in elevated O3 treatment as compared to ambient treatment. Yield reduction in Nagina 22 variety was not significant in elevated O3 treatment. Elevated CO2 concentration of 550 ppm was able to fully compensate the yield loss in Nagina 22 variety and partially compensate (3.9-8.0%) in Pusa Basmati 1121 and IR64 Drt1 varieties. Grain N concentration in rice varieties decreased by 10.8-14.7% during first year and by 7.8-20.6% during second year in elevated O3 plus CO2 interaction treatment than ambient. Grain N uptake also decreased (13.2-17.1% in first year and 4.5-22.8% in second year) in elevated O3 plus CO2 interaction treatment as compared to ambient. Application of additional 25% of recommended dose of N improved grain N concentration, grain N uptake as well as available N of soil as compared to 100% RDN treatment in elevated O3 plus CO2 interaction treatment. Additional 25% N dose could help in sustaining rice productivity and quality under elevated O3 and CO2 condition.
Introduction
Air pollution is only one of several environmental issues that threaten food production. Tropospheric ozone (O3) is one of the major air pollutants harmful to plants (Feng et al., 2015). The O3 pollution is expected to worsen in the future due to a warmer climate, depending on the location, and increased anthropogenic emissions of O3 precursors.
Following the Industrial Revolution, the percentage of ground-level O3 has been steadily rising, reaching 35–40 ppb globally. In the 21st century, it may continue to rise more rapidly in developing countries with fast-growing economies (Proietti et al., 2016). The tropospheric O3 level might exceed 70 ppb by 2050, according to the Environmental Protection Agency, if worldwide emissions of O3 precursors continue at their current rate (Pfister et al., 2014). Tropospheric O3 concentrations are increasing at a higher rate in tropical regions due to favorable conditions for O3 formation (Agrawal et al., 2003; Tiwari et al., 2008). Following the mid-1990s, the decadal increase in tropospheric O3 levels has been 2–14% in the tropics, 2–7% in northern mid-latitude areas, and 8–14% in the South Asian region (IPCC, 2021). Tropospheric O3 is created as a secondary pollutant in the atmosphere through photochemical interactions between volatile organic compounds (VOCs) and nitrogen oxides (NOx) in the presence of sunlight (Lefohn et al., 2017). Due to increased economic growth accompanied by higher NOx and VOC emissions, tropospheric O3 levels are rising and are expected to continue to do so across the Asian region, leading to more crop losses (Ashmore, 2005). The O3 concentration ranged from 45 to 65 ppb across the Indian region (David and Nair, 2013; Deb Roy et al., 2009). In most regions of the world, ozone is now the most significant air pollutant that has a detrimental effect on the growth and productivity of crops. Tropospheric O3 is also a greenhouse gas (GHG) and contributes to climate change (Feng et al., 2019). Exposure to O3 leads to stomatal closure, which limits carbon dioxide (CO2) and water uptake by plants, further compromising photosynthetic efficiency and causing reduced growth and decreased grain filling in crop plants (Feng and Kobayashi, 2009). Several studies have quantified the impact of O3 on crop development and yield (Ainsworth, 2008; Mills et al., 2018; Tai et al., 2014; Yadav et al., 2021). There are reports of reduced photosynthesis rates, leaf senescence, and changes in assimilate partitioning in plants due to exposure to elevated levels of O3 (Mina et al., 2016; Tomer et al., 2015). Increased concentrations of surface O3 diminish carbon storage in vegetation, leading to reduced growth and yield of crops (Tai et al., 2014). On the other hand, elevated atmospheric CO2 concentrations lead to the accumulation of carbon, thereby increasing crop growth and also reducing plant nitrogen (N) and protein content (Abebe et al., 2016; Chakrabarti et al., 2020; Raj et al., 2019). The global average concentration of CO2 has increased from 280 ppm to 409.7 ppm (NOAA, ESRI, 2019). An increase in atmospheric CO2 concentrations improves the growth and productivity of crop plants (Bhatia et al., 2012; Singh et al., 2013; Deryng et al., 2016).
The world’s most important food crop, rice, is vulnerable to a variety of contaminants, especially air pollutants such as tropospheric O3. Global food security is likely to suffer if the productivity of rice declines. Elevated tropospheric O3 levels cause stress to rice plants during both the vegetative and reproductive stages, affecting their physiology, yield, and grain quality. According to Xia et al. (2021), major food crops, such as rice, wheat, and maize, are less tolerant to elevated O3 than trees, such as spruce, silver fir, and pine. Elevated O3 levels can alter physiological processes in crop plants, leading to changes in crop morphology and reduced crop growth (Bhatia et al., 2013).
Some reports elevated O3 levels reduced yield by 11.4–12.3% compared to ambient levels in rice crops (Bhatia et al., 2011). Pandey et al. (2018) observed that grain yield and grain N content in wheat decreased under elevated O3 concentrations. Along with reduced plant growth and productivity, higher O3 levels also affect grain quality in crops as it is a strong oxidant and reduces important physiological processes in plants (Avnery et al., 2011; Broberg et al., 2015). Some researchers have reported that protein content in crops gets negatively affected by elevated O3, but to a lesser extent than grain yield (Broberg et al., 2015; Grünhage et al., 2012). The interactive effect of elevated O3 and CO2 on crop growth, yield, and plant nutrient content will differ from the individual effect of O3. Phothi et al. (2016) reported that elevated CO2 levels would mitigate the negative impact of elevated tropospheric O3 in rice crops. A simultaneous increase in both O3 and CO2 concentrations could nullify the negative effects of elevated O3 on the ecosystem (Bhatia et al., 2012). Therefore, the negative effects of elevated tropospheric O3 would be overestimated if the impact of elevated CO2 concentrations is not considered (Xia et al., 2021).
Crop productivity is affected by the availability of N supplied to plants through various sources, including inorganic fertilizers. The interaction between O3 and CO2 will also affect the nutrient levels in crops (Phothi et al., 2016). As crop growth is reduced under elevated O3 conditions (Pleijel et al., 2014), the demand and uptake of nutrients will also get altered under such situations, thereby reducing total nutrient content in plants. A study comprising data analysis of peer-reviewed literature depicted that the translocation of nitrogen from straw and leaves to grain in wheat crops was negatively affected by O3. As N fertilizer use efficiency is reduced under elevated O3 conditions, the risk of N losses from agroecosystems increases (Broberg et al., 2017). Elevated O3 also affects soil microbial function and N transformation in the soil (Chen et al., 2015; Wu et al., 2016), which could, in turn, affect nutrient availability and uptake in plants. As crop productivity is often limited by nitrogen, N management becomes very crucial, especially under changing climatic conditions (Bhatia et al., 2010). A study by Singh et al. (2009) showed that the application of 1.5 times of recommended NPK can help ameliorate the negative effects of elevated tropospheric O3 in mustard crops.
Estimating the effects of crop loss caused by tropospheric O3 is crucial for nations, such as India, that are experiencing rapid urbanization and population growth. A few studies have investigated the interactive effects of elevated O3 and CO2 on crop plants. However, studies on elevated O3 and CO2 interactions with rice productivity and plant N are very limited. In addition, most studies were conducted earlier using open-top chambers (OTCs). It is imperative to conduct studies on the interaction between elevated levels of O3 and CO2 in open-field conditions, as such research is rarely reported. There is a need for a better understanding of cultivar-specific responses to elevated O3 and CO2 interactions to identify suitable adaptation options in rice under ozone stress conditions. In addition, N management strategies need to be formulated to sustain crop productivity and quality under elevated O3 and CO2 conditions. The hypothesis is that elevated O3 and CO2 can negatively affect rice productivity and quality. An additional N dose may counteract the harmful effects by improving growth, yield, and plant N uptake under elevated O3 and CO2 conditions. Therefore, the following study was conducted using a free air ozone and carbon dioxide enrichment (FAOCE) facility under open-field conditions (1) to quantify the yield and N uptake in rice varieties under the interaction of elevated O3 and CO2 and (2) to investigate the effect of an increased N dose on the yield and plant N in rice under elevated O3 and CO2 conditions.
Materials and methods
Experimental site
A field experiment was conducted during the kharif season (July–October) for two consecutive years, i.e., 2020 and 2021, inside free air O3 and CO2 enrichment (FAOCE) rings located at the experimental farm of the-Indian Agricultural Research Institute (ICAR), New Delhi (28°35’N and 77°12′E) India. The mean temperatures during the cropping season were 29.5°C in the first and 29.1°C in the second year of the study. Three different rice varieties, Pusa Basmati 1121, Nagina 22, and IR64 Drt1, were grown in crates inside the free air ozone and carbon dioxide enrichment (FAOCE) facility. Pusa Basmati 1509 is a popular basmati variety of rice in the northwestern Indo-Gangetic Plain (IGP). It is known for its long, slender grains and distinct aroma. IR64 DRT1 is a high-yielding, drought-tolerant rice variety that is resistant to major pests and diseases and can be grown in different agro-climatic conditions. Nagina 22 is a short-duration rice variety adaptable to various abiotic stresses and also resistant to pests and diseases. The rice varieties were grown under ambient and elevated (60 ppb) O3 levels, as well as ambient and elevated (550 ± 25 ppm) CO2 concentrations. The ambient CO2 concentration ranged from 398 to 421 ppm and from 404 to 420 ppm in 2020 and 2021, respectively. The elevated CO2 concentration ranged from 518 to 570 ppm and from 526 to 575 ppm in the FAOCE rings in 2020 and 2021, respectively. The 5 m diameter FAOCE rings were effective in exposing the crops to elevated O3 and CO2 under natural field conditions. Air mixed with CO2 was supplied at the canopy level through perforated pipes with laser-drilled holes (0.3 mm in diameter) inside the ring from commercial-grade compressed 30 kg pure CO2 gas cylinders. The release of CO2 inside the rings was controlled by the opening and closing of the solenoid valves depending on the wind speed and direction (Yadav et al., 2021). Ozone generators were used to convert atmospheric oxygen (O2) into O3, which was then released inside the rings with the help of flappers through a common duct placed perpendicular to the rings (Yadav et al., 2019). Air samples were drawn from the center of the rings, and the CO2 concentration was measured using a CO2 analyzer (NDIR, Topak United States), while the O3 concentration was measured using an O3 analyzer (2B Technologies). The CO2 and O3 concentrations in the rings were automatically logged in the computer by microprocessors through digital input and output modules on a real-time basis. The crops were exposed to elevated CO2 and O3 for 7 h (10.00 am to 17.00 pm). Four rings were used for the study: (1) ambient CO2 and O3, (2) ambient O3 and elevated CO2, (3) elevated O3 and ambient CO2, and (4) elevated O3 and elevated CO2. Rice seedlings (30 days old) were transplanted into crates (42 cm × 63 cm) filled with 40 kg of soil inside the FAOCE rings during the second week of July. The crops were fertilized with two doses of N: the recommended dose of N (RDN) (120 kg ha−1) and 125% of the recommended dose of N (150 kg ha−1). Phosphorus (P) and potassium (K) were applied at the rate of 60 kg ha−1. Half of the N dose and the total amount of P and K were applied at the time of transplanting. The remaining half of the N dose was applied in two equal splits at the maximum tillering and flowering stages of the crops. In total, there were 24 treatments, each with three replications.
Measurements of the crop growth parameters
Gas exchange parameters, such as photosynthesis rate, stomatal conductance, and transpiration rate, were measured using the Portable Photosynthesis System IRGA (LI-6400XT, LiCOR, United States) at the maximum tillering stage of the crops. The observations were recorded on physiologically active, fully expanded leaves exposed to the sun between 9:00 AM to 11:00 AM. The flow rate of input air was set at 300 μmol s−1, and photosynthetically active radiation (PAR) was set at 1,000 μmol m−2 s−1. The reference CO2 concentration was maintained at 410 ppm. Ten readings were recorded for each observation in each treatment. The number of tillers per hill was also counted for each treatment. The plant samples were harvested at maturity and air-dried, and the weights of the grain and straw were recorded. The number of grains per panicle was counted after harvesting the crops for each treatment.
Soil and plant sample collection and analysis
Soil samples were collected at the flowering stage. Available N in the soil was estimated using the Subbiah and Asija (1956) method. The soil was distilled with alkaline KMnO4 (potassium permanganate) and NaOH (sodium hydroxide) solutions, and the amount of liberated NH3 (ammonia) was estimated by titration with standard H2SO4 (sulphuric acid). The plant samples were collected after harvesting the crops. The rice grains were separated from the straw biomass, and the samples were dried in an oven at 65 ± 2°C for 72 h. The nitrogen concentration in the grain and straw samples was determined using the method described by Jackson (1956). The plant samples were digested using concentrated H2SO4 and a digestion mixture in a micro Kjeldahl digestion block. The digested samples were distilled with NaOH, and the liberated NH3 was absorbed in H3BO3 (boric acid) and then titrated against standard H2SO4. The grain N uptake (mg plant−1) was then calculated by multiplying the grain weight/plant by the grain N concentration, as described by Cowan et al. (2021).
Statistical analysis
The experiment was a factorial, completely randomized design with 24 treatments, each having three replicates. Four factorial (CO2 level, O3 level, variety, and N dose) analysis of variance (ANOVA) was carried out using SAS (ver. 9.3) statistical package (SAS Institute Inc., CA, United States). Tukey’s honestly significant difference (HSD) test at the 5% level of significance was performed to check if the differences were statistically significant.
Results
Effect of elevated O3 and CO2 on the rice physiology
The photosynthesis rates varied among the different rice varieties, ranging from 23.1 to 36.1 μmol CO2 m−2 s−1 in the first year and from 23.2 to 35.1 μmol CO2 m−2 s−1 in the second year under the recommended N dose (Figures 1a,b). Pusa Basmati 1121 exhibited a significant decrease in the photosynthesis rates, dropping from 30.3 to 26.5 μmol CO2 m−2 s−1 in the first year and from 31.1 to 27.9 μmol CO2 m−2 s−1 in the second year. In the case of IR64 Drt1, the photosynthesis rate was significantly lower under the elevated O3 levels compared to the ambient levels in the second year. Conversely, there was no notable reduction in the photosynthesis rates for the Nagina 22 variety over both years under the elevated O3 conditions. The elevated CO2 concentration exhibited a positive impact on the photosynthesis rates of all rice varieties. However, the interactive treatment of elevated O3 and CO2 still maintained the photosynthesis rates similar to those under the ambient condition. The application of additional N positively influenced the photosynthesis rates across all treatments. When 25% additional N was applied, the photosynthesis rates in the elevated O3 treatment became comparable to those in the ambient treatment.
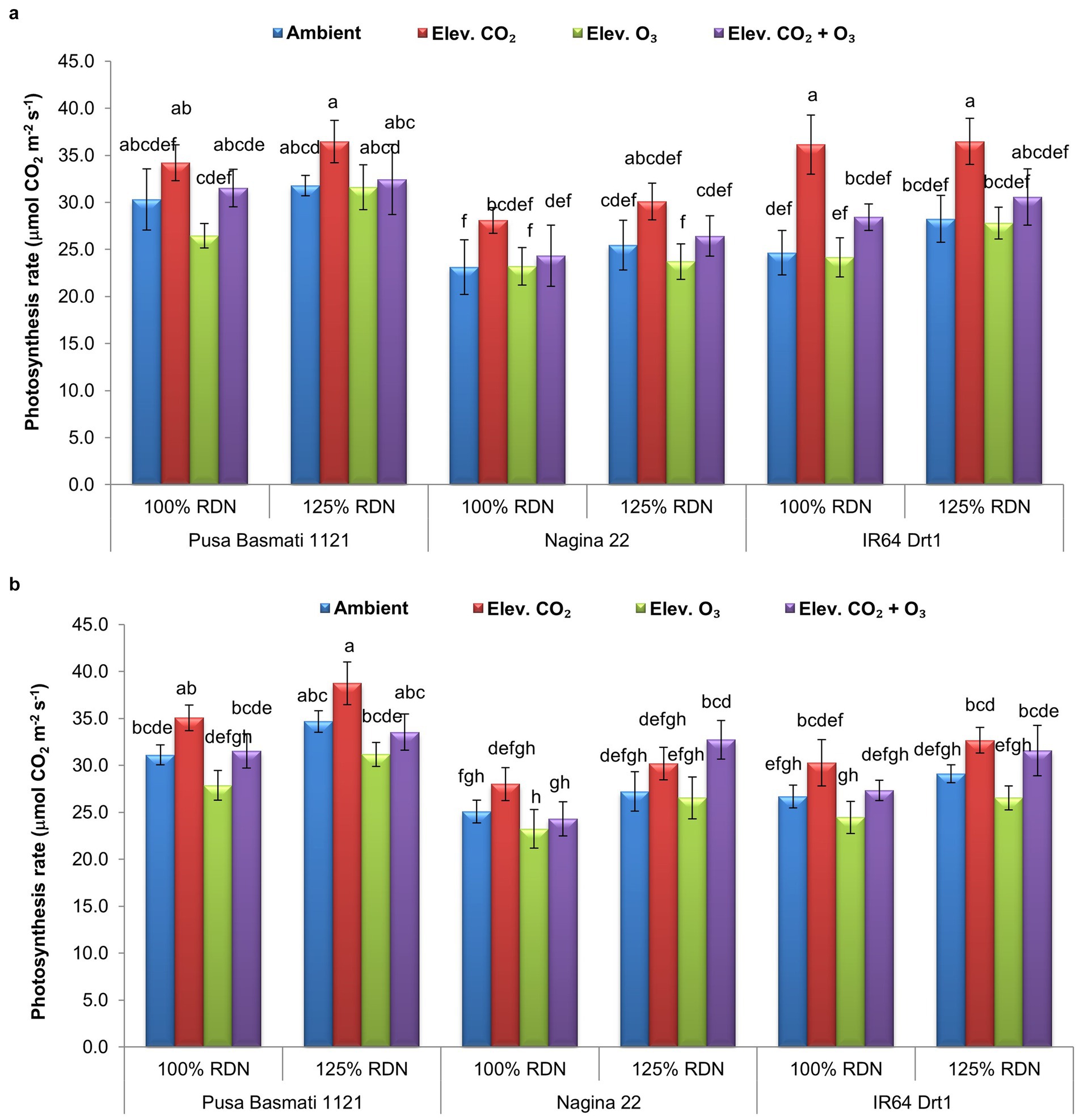
Figure 1. Photosynthesis rates (μmol CO2 m−2 s−1) in the rice varieties under the elevated O3 and CO2 conditions during the (a) first and (b) second year. Columns with different letters are significantly different (p ≤ 0.05). The error bars represent the standard deviation of the data, indicating the variability around the mean.
Stomatal conductance in the rice varieties decreased in the presence of the elevated O3 and elevated O3 plus CO2 treatments compared to the ambient treatment. In the treatments with the recommended N application, stomatal conductance ranged from 0.31 to 0.42 m mol H2O m−2 s−1 in Pusa Basmati 1121, 0.29 to 0.31 m mol H2O m−2 s−1 in Nagina 22, and 0.33 to 0.41 m mol H2O m−2 s−1 in IR64 Drt1 under the ambient conditions (Figures 2a,b). However, stomatal conductance decreased in the elevated O3 plus CO2 treatment, ranging from 0.24 to 0.30 m mol H2O m−2 s−1 in Pusa Basmati 1121, 0.22 to 0.24 m mol H2O m−2 s−1 in Nagina 22, and 0.29 to 0.30 m mol H2O m−2 s−1 in IR64 Drt1. Furthermore, the elevated O3 and elevated O3 plus CO2 treatments significantly reduced transpiration rates in the Pusa Basmati 1121 and IR64 Drt1 varieties. Over the two-year study, the transpiration rates in Pusa Basmati 1121 ranged from 12.3 to 16.4 m mol H2O m−2 s−1 in the ambient treatment, while decreasing to 9.5 from 11.9 H2O m−2 s−1 in the elevated O3 treatment (Figures 3a,b). Similarly, in the IR64 Drt1 variety, the transpiration rates ranged from 14.5 to 15.0 m mol H2O m−2 s−1 in the ambient treatment, but in the elevated O3 treatment, they ranged from 9.5 to 11.8 m mol H2O m−2 s−1.
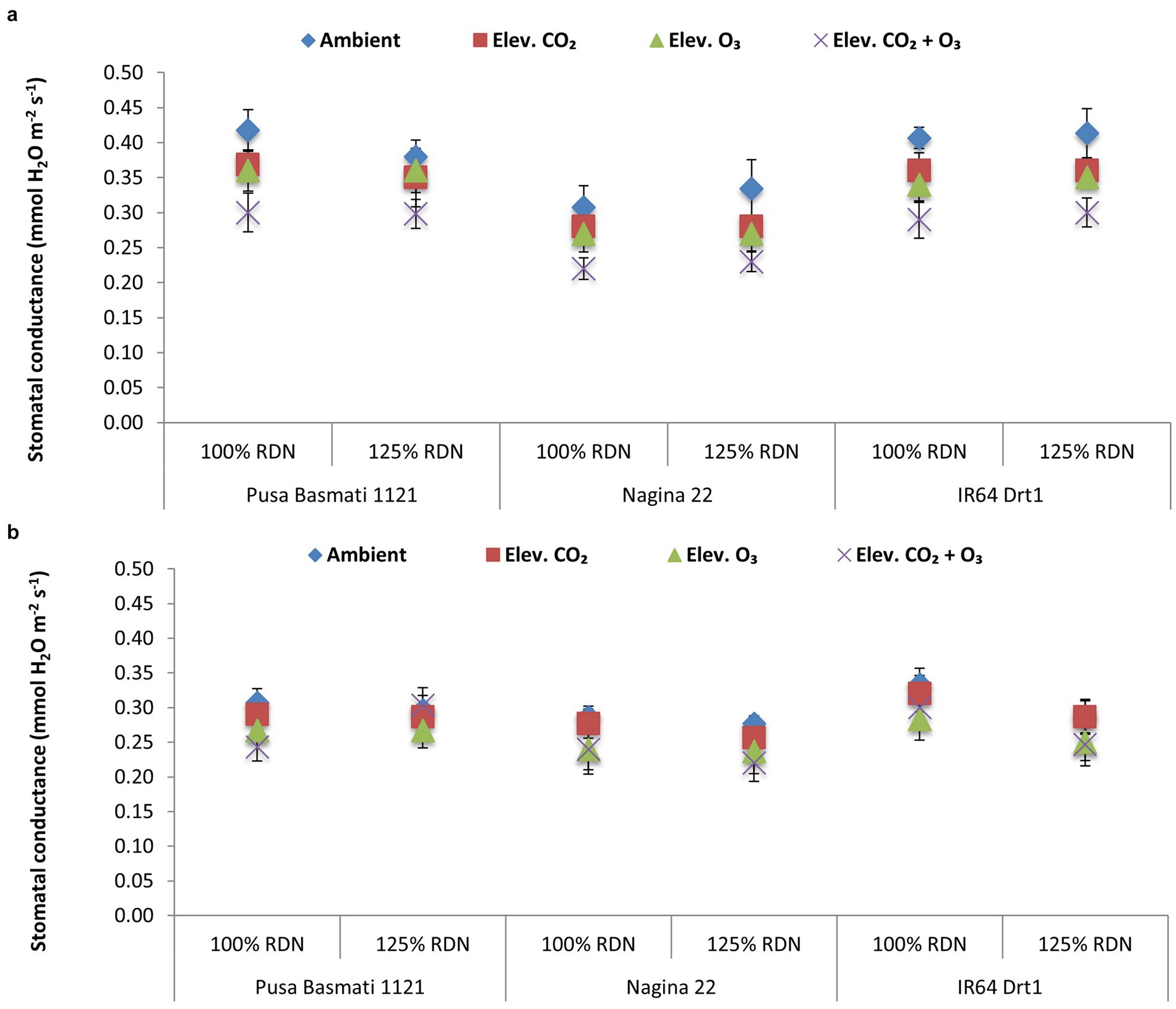
Figure 2. Stomatal conductance (mmol H2O m−2 s−1) in the rice varieties under the elevated O3 and CO2 conditions during the (a) first and (b) second year. The error bars represent the standard deviation of the data, indicating the variability around the mean.
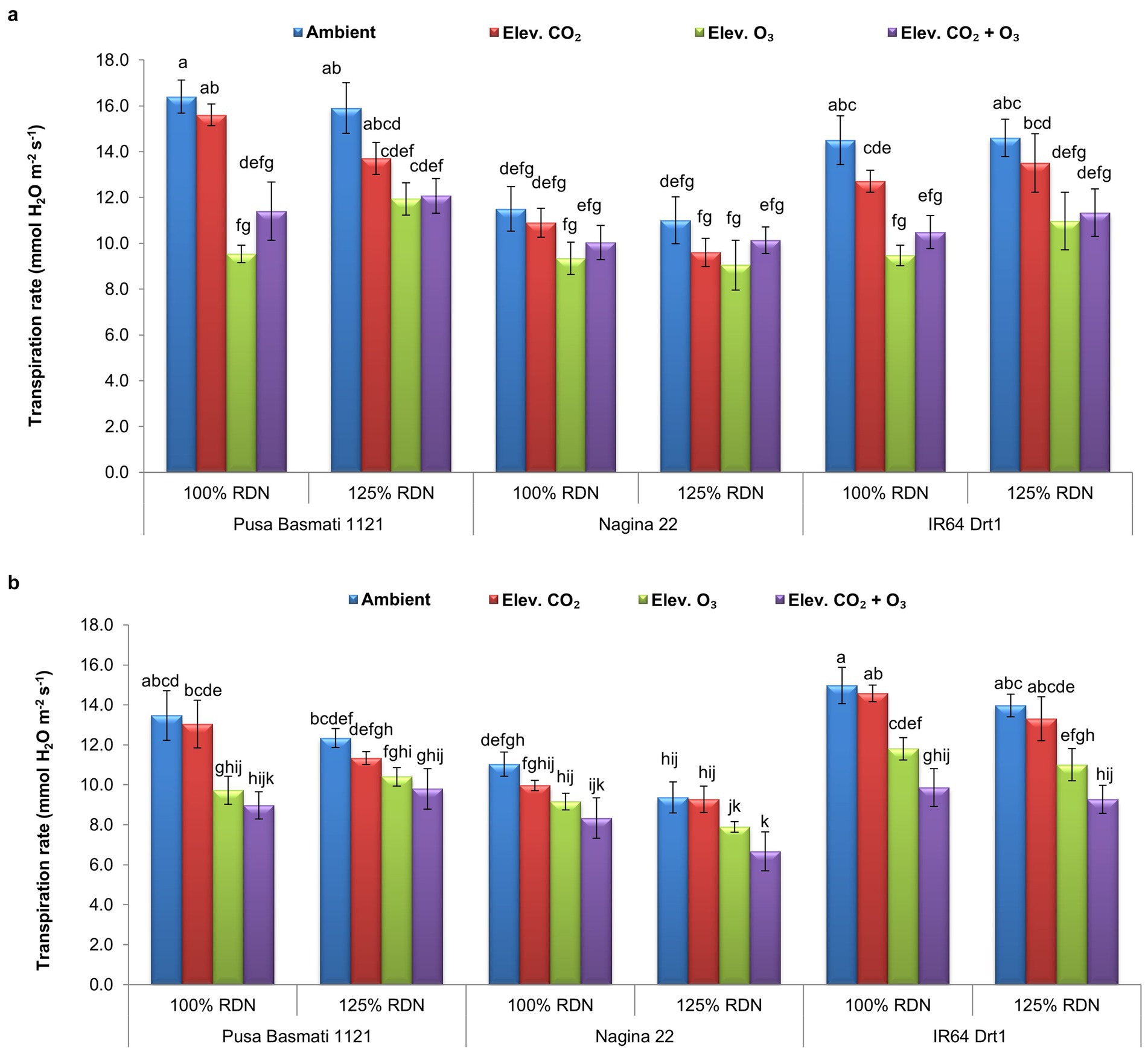
Figure 3. Transpiration rates (mmol H2Om−2 s−1) in the rice varieties under the elevated O3 and CO2 conditions during the (a) first and (b) second year. Columns with different letters are significantly different (p ≤ 0.05). The error bars represent the standard deviation of the data, indicating the variability around the mean.
Effect of elevated O3 and CO2 on rice yield
The elevated O3 level significantly decreased the grain yield of the Pusa Basmati 1121 and IR64 Drt1 varieties in both years of the study. The grain yield was reduced by 7.2–7.5%, in Pusa Basmati 1121 and by 6.9–9% in IR64 Drt1 in the elevated O3 treatment as compared to the ambient treatment (Figure 4). In the Nagina 22 variety, there was a reduction in the yield by 4.4% in the elevated O3 treatment compared to the ambient treatment during the first year. However, in the second year, there was no reduction in the grain yield of this variety under the elevated O3 condition. Nagina 22 is a stress-tolerant variety, which is why an increase in the O3 levels had little to no effect on it. In the present study, the reduction in the grain yield in the elevated O3 treatment was attributed to a lower number of panicles per hill and fewer grains per panicle under the elevated O3 condition. Under the ambient condition, the number of grains per panicle ranged from 101 to 111 among the different rice varieties, while in the elevated O3 treatment, the number of grains per panicle ranged from 95 to 101 (Table 1). The number of panicles per hill also decreased in the elevated O3 treatment compared to the ambient treatment. In the ambient treatment, the number of panicles per hill ranged from 14 to 17, while in the elevated O3 treatment, it decreased from 15 to 13.
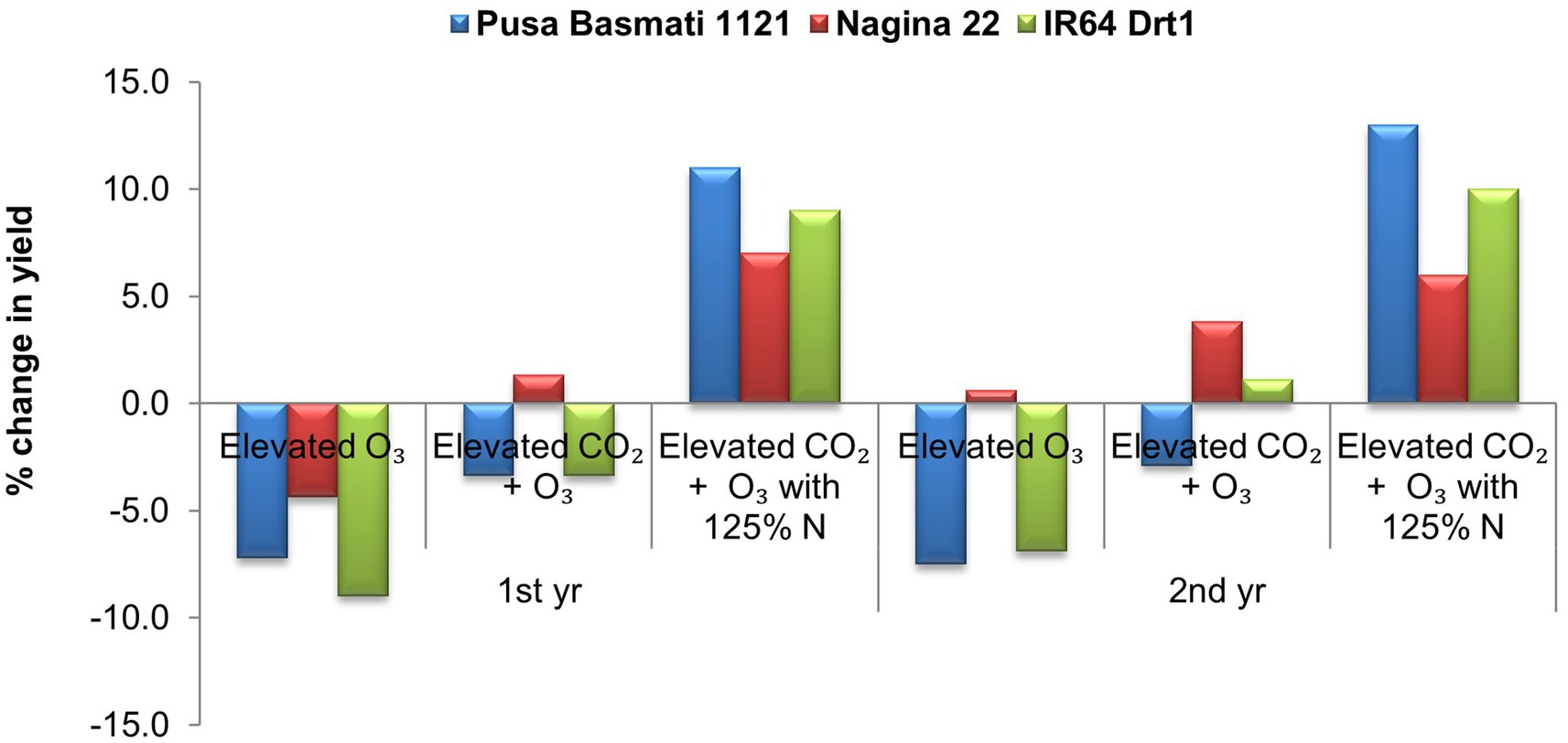
Figure 4. Percent change in the yield in the rice varieties under the elevated O3 and CO2 conditions.
In the elevated O3 plus elevated CO2 treatment, the grain yield was 2.9–3.4% lower than in the ambient treatment for the Pusa Basmati 1121 variety. This shows that the increased CO2 concentration of 550 ppm was able to compensate for 3.9–4.6% yield loss in the Pusa Basmati 1121 variety. In the IR64 Drt1 variety, the grain yield in the elevated O3 plus elevated CO2 treatment was 3.4% lower than in the ambient during the first year of the study. However, during the second year, the yield was 1.1% higher than in the ambient treatment for this variety. The Nagina 22 variety recorded higher yields in the elevated O3 plus elevated CO2 treatment compared to the ambient treatment in both years. The application of a higher N dose also helped in preventing the yield loss in the rice varieties under the elevated O3 concentration. In the treatment with elevated O3 plus CO2, along with 125% of the RDN, the grain yield of the Pusa Basmati 1121 variety increased by 11–13% compared to the ambient treatment (Figure 4). Similarly, in the IR64 Drt1 variety, the yield increased by 9–10%, and in the Nagina 22 variety, it increased by 6–7% in this treatment.
The grain number per panicle also increased in the elevated CO2 plus elevated O3 treatment compared to that in the elevated O3 treatment. The elevated CO2 level increased the photosynthesis rates of the crops, which led to greater biomass accumulation and subsequently more grains, resulting in higher crop yields. The application of a higher dose of N also increased the grain number in all three rice varieties, which led to higher crop yields. In the elevated O3 treatment, the application of a higher N dose also helped in increasing the panicle number and grain number, thereby increasing the grain yield to a certain extent.
Effect of elevated O3 and CO2 on the plant N
The responses of the grain N concentration (%) in the rice varieties to the elevated CO2 and O3 treatments are shown in Figure 5a. In the 100% recommended N applied treatments, the grain N concentration ranged from 1.20–1.36% under the ambient condition, with a mean value of 1.30%. The grain N concentration significantly decreased in the elevated CO2 and elevated O3 plus CO2 interaction treatment compared to the ambient treatment. In the elevated O3 plus CO2 interaction treatment, the grain N concentration ranged from 1.07–1.18%, with a mean value of 1.13%. The application of an additional 25% of N improved the grain N concentration in both years. When the additional N dose was applied, the grain N concentration ranged from 1.25–1.33% in the elevated O3 plus CO2 interaction treatments, with a mean value of 1.29%. The grain N concentration was higher in the Pusa Basmati 1121 and IR64 Drt1 varieties than in the Nagina 22 variety.
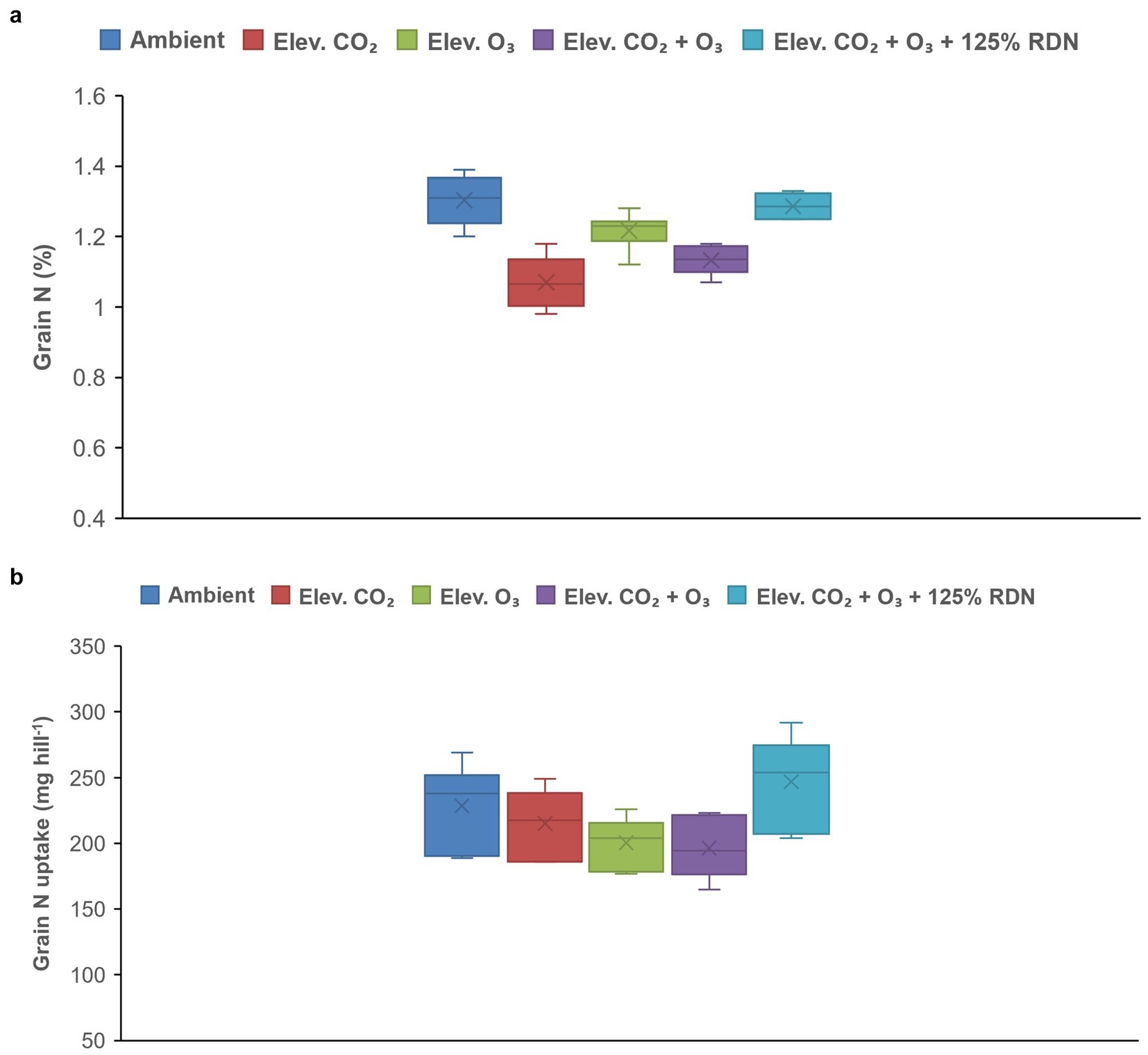
Figure 5. (a) Grain nitrogen concentration (%) and (b) grain N uptake (mg hill−1) in the rice varieties under the elevated O3 and CO2 conditions. The bottom and top of the whiskers denote the first and third quartiles, respectively. The line inside the box represents the median line.
Elevated O3 also reduced the grain N uptake of the rice varieties compared to the ambient treatment. In the ambient treatment, the grain N uptake ranged from 189 to 269 mg hill−1, with a mean value of 229 mg hill−1 in the treatment with the 100% RDN (Figure 5b). In the elevated O3 plus CO2 interaction treatment, the grain N uptake decreased and ranged from 165 to 223 mg hill−1, with a mean value of 196 mg hill−1. The application of a higher N dose increased the grain N uptake in the rice varieties. In the elevated O3 plus CO2 interaction treatment, the grain N uptake was even higher than in the ambient treatment. It ranged from 204 to 292 mg hill−1, with a mean value of 247 mg hill−1. The grain N uptake was greater in the Pusa Basmati 1121 and IR64 Drt1 varieties than in the Nagina 22 variety. The application of an additional 25% of N increased the grain N uptake by 30.2–40.8% in Pusa Basmati 1121, by 15.3–22.3% in Nagina 22, and by 21.7% in the IR64 Drt1 variety of the rice crops (Table 2).
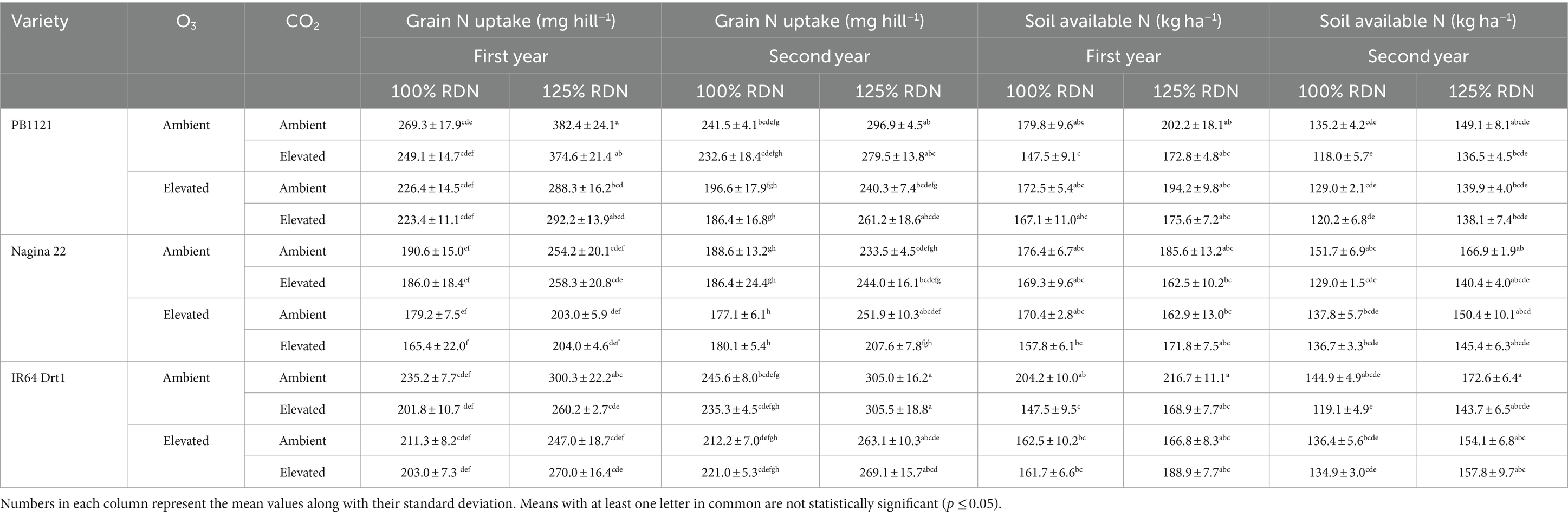
Table 2. Effect of the elevated CO2, O3, and N treatments on the grain N uptake and soil available N of the rice varieties.
Effect of elevated O3 and CO2 on the soil available N
In the ambient treatment, the soil available N ranged from 176.4–204.2 kg ha−1 in the first year and from 135.2–151.7 kg ha−1 in the second year among the different rice varieties with the recommended dose of the N application (Table 2). In the elevated O3 plus CO2 interaction treatment, the soil available N was lower than in the ambient treatment in both years of the study. The soil available N was also found to be lower in the elevated CO2 treatment compared to the ambient treatment. The application of a higher dose of N increased the available N content of the soil. In the elevated O3 plus CO2 interaction treatment, the application of an additional 25% of N increased the soil available N by 5.3–14.9% in Pusa Basmati 1121, by 6.3–9.0% in Nagina 22 and by 16–16.9% in IR64 Drt1 of the rice crops.
Discussion
Tropospheric O3 is a secondary air pollutant generated through photochemical reactions among precursors such as nitrogen oxides (NOx), volatile organic compounds (VOCs), and carbon monoxide (CO), primarily released from anthropogenic activities (Broberg et al., 2015). Several researchers have reported the substantial impact of elevated O3 levels on crop productivity and quality (Singh et al., 2018; Yadav et al., 2019). The results from the current study showed that elevated O3 reduced the photosynthesis rates of the Pusa Basmati 1121 and IR64 Drt1 varieties of the rice crop, while the elevated O3 plus CO2 treatment was able to maintain the photosynthesis rates of the crops. The elevated O3 and elevated O3 plus CO2 treatments significantly reduced the stomatal conductance and transpiration rates of the Pusa Basmati 1121 and IR64 Drt1 varieties. Previous studies have also indicated that elevated O3 could hinder photosynthetic carbon acquisition in crops (Guidi et al., 2001; Morgan et al., 2003; Singh et al., 2009; Bhatia et al., 2012). There are reports that elevated CO2 concentrations reduce stomatal conductance and transpiration rates in rice crops (Maity et al., 2023; Zhang et al., 2022). The elevated O3 level substantially decreased the yield of the Pusa Basmati 1121 and IR64 Drt1 varieties. In contrast, the Nagina 22 variety demonstrated comparatively less or no yield reduction. Nagina 22 is known for its stress tolerance, and the results indicate that the increase in the O3 concentration had minimal to no effect on its yield. Such yield losses under the O3 exposure occurred due to the reduction in photosynthesis, which reduced the supply of assimilates required for reproductive development and seed growth (Feng et al., 2010). Tatsumi et al. (2019) also reported that brown rice yield was significantly reduced under elevated O3 concentrations. A similar decrease in grain yield with exposure to elevated O3 concentrations was reported for wheat cultivars in north India (Daripa et al., 2016; Yadav et al., 2019). In our study, an elevated CO2 concentration of 550 ppm was able to partially mitigate the reduction in the grain yield due to increased O3 exposure in the Pusa Basmati 1121 and IR64 Drt1 varieties, while it fully compensated for the yield reduction in the Nagina 22 variety. Elevated CO2 has a fertilization effect on crops, which has helped compensate for yield losses in different rice varieties. The yield loss in the Nagina 22 variety, which is stress-tolerant, was already less than the other varieties. Therefore, the reduction in the yield in Nagina 22 was fully compensated under the elevated CO2 plus O3 treatment. Various researchers have earlier reported the beneficial effects of increased CO2 levels in terms of increasing yields in different crops (Dey et al., 2017; Kobayashi et al., 1999; Pramanik et al., 2018; Sanyal et al., 2023). Bhatia et al. (2021) observed that a high CO2 concentration of 554 ppm was able to counter the harmful impacts of O3 exposure on yield and nutrient content in chickpeas. A study conducted with rice showed that elevated CO2 could counter yield reduction due to O3 exposure by more than 40% (Kumar et al., 2021).
The application of a 25% higher dose of N increased the photosynthesis rates and different yield parameters, such as the number of panicles and the number of grains per panicle, in the rice crops, thereby increasing the yield to a certain extent under the elevated O3 concentration. According to Chen et al. (2018), decreased photosynthesis in nitrogen-deficient leaves increases the yield sensitivity of crops to high O3 concentrations. Therefore, the increased dose of N had a positive effect on the yield parameters of the rice crops even under the elevated O3 condition. The beneficial impact of higher N doses was also reported by Raj et al. (2019), who observed that increased atmospheric CO2 concentrations and higher N doses synergistically contributed to an increase in the panicle number, thereby improving the yield of rice crops.
The elevated O3 plus CO2 interaction decreased the grain N concentration and N uptake in the rice varieties compared to the ambient condition. As O3 is a strong oxidant, it harmfully affects important physiological functions in plants, which leads to reduced quality in crops (Avnery et al., 2011). Similar findings have been reported by earlier researchers, who found that protein content in plants is negatively affected by increased O3 (Broberg et al., 2015; Grünhage et al., 2012). Broberg et al. (2017) reported that O3 impairs the N translocation from straw to grain, thereby reducing the N use efficiency of crops. In addition, the applied N fertilizer is used less efficiently under elevated O3, leading to adverse effects on grain protein content in crops. Under elevated CO2 conditions, higher photosynthesis rates lead to greater carbon assimilation in rice, thereby reducing plant N concentrations due to the dilution effect (Kim et al., 2001). Reports indicate that protein content and N content in plants, especially cereal crops, decrease under elevated CO2 conditions (Abebe et al., 2016; Chakrabarti et al., 2020; Raj et al., 2019).
Soil available N is also negatively affected by elevated O3 and CO2 treatment, which may be attributed to increased N losses from crop fields under elevated O3 (Broberg et al., 2017). Under O3 exposure, plant photosynthesis and grain filling duration are shortened (Gelang et al., 2000), which reduces the nutrient uptake period and increases the likelihood of greater N loss from the soil. Earlier studies (Chakrabarti et al., 2020; Maity et al., 2020) have also reported a decrease in soil available N under elevated CO2 conditions due to increased crop growth resulting in higher N demand by the crop. As plant photosynthesis is hampered under increased O3 concentrations, reduced photosynthates in the roots affect the root system and various soil processes (Chen et al., 2008). This may further affect soil N transformation rates (Wu et al., 2016), thereby lowering the available N status in the soil. The application of a higher N dose increased both the grain N uptake and soil available N in rice. As the crop growth was greater under the higher N doses, this generated more aboveground and belowground biomass of the crops. This might have led to increased microbial activity in the rhizosphere, leading to enhanced availability of N to the plants.
The study showed that although the negative effects of elevated O3 on the rice yield were negated by the elevated CO2 concentration, the adverse effect of elevated O3 on the grain N content could not be compensated for. The response of rice to elevated CO2 may be limited when nitrogen levels are sub-optimal. However, the decrease in plant N could be alleviated to a certain extent by applying higher doses of N (Maity et al., 2020). Our study showed that the application of the 25% RDN improved both the grain N concentration and grain N uptake, as well as soil available N, in the rice crops under the elevated O3 and CO2 interaction.
Conclusion
The grain yield of the rice varieties decreased under the elevated O3 condition. An elevated CO2 concentration of 550 ppm was able to compensate for the yield loss by 3.9–4.6% in the Pusa Basmati 1121 rice variety and by 4.6–8.0% in the IR64 Drt1 variety. Although elevated CO2 was able to compensate for the yield loss due to elevated O3, the N content in the rice grains was further reduced in the elevated O3 plus CO2 treatment. The application of an additional 25% of the recommended dose of N improved the grain N uptake by 15.3–40.8% in the different rice varieties compared to the 100% RDN in the elevated O3 plus CO2 interaction treatment. The study shows that nitrogen in rice grains and soil available N will decrease under elevated O3 plus CO2 conditions. An elevated CO2 concentration of 550 ppm will be able to compensate for yield loss to a certain extent, but grain quality will further deteriorate in the elevated O3 plus CO2 treatment. The application of an additional 25% of the recommended dose of N could help in sustaining rice yield and also maintaining plant and soil N under elevated O3 and CO2 conditions in the future. However, the response of rice varieties to elevated O3 and CO2 might vary with different climate types. Therefore, the findings of the study could be further improved by testing different N fertilizer formulations to suggest the best N management options for the sustaining productivity and quality of rice under elevated O3 and CO2 conditions.
Data availability statement
The raw data supporting the conclusions of this article will be made available by the authors, without undue reservation.
Author contributions
BC: Formal analysis, Investigation, Project administration, Visualization, Writing – original draft, Writing – review & editing. SS: Funding acquisition, Project administration, Writing – review & editing. AM: Funding acquisition, Project administration, Writing – review & editing. SK: Investigation, Writing – original draft. VK: Methodology, Writing – original draft. SB: Conceptualization, Writing – review & editing. AB: Conceptualization, Formal analysis, Writing – review & editing, Project administration, Supervision.
Funding
The author(s) declare that financial support was received for the research, authorship, and/or publication of this article. The authors acknowledged the funding provided by the ICAR-IRRI project, which supported this work and the publication of the manuscript. The facilities for carrying out this study were funded and maintained by the National Innovations in Climate Resilient Agriculture, NICRA project (IARI-12-115).
Acknowledgments
The authors would like to thank the ICAR-Indian Agricultural Research Institute, New Delhi, for providing the facilities for conducting the study.
Conflict of interest
The authors declare that the research was conducted in the absence of any commercial or financial relationships that could be construed as a potential conflict of interest.
Publisher’s note
All claims expressed in this article are solely those of the authors and do not necessarily represent those of their affiliated organizations, or those of the publisher, the editors and the reviewers. Any product that may be evaluated in this article, or claim that may be made by its manufacturer, is not guaranteed or endorsed by the publisher.
References
Abebe, A., Pathak, H., Singh, S. D., Bhatia, A., Harit, R. C., and Kumar, V. (2016). Growth, yield and quality of maize with elevated atmospheric carbon dioxide and temperature in north–West India. Agric. Ecosyst. Environ. 218, 66–72. doi: 10.1016/j.agee.2015.11.014
Agrawal, M., Singh, B., Rajput, M., Marshall, F., and Bell, J. N. B. (2003). Effect of air pollution on peri-urban agriculture: a case study. Environ. Pollut. 126, 323–329. doi: 10.1016/S0269-7491(03)00245-8
Ainsworth, E. A. (2008). Rice production in a changing climate: a metaanalysis of responses to elevated carbon dioxide and elevated ozone concentration. Glob. Change Biol. 14, 1642–1650. doi: 10.1111/j.1365-2486.2008.01594.x
Ashmore, M. R. (2005). Assessing the future global impacts of ozone on vegetation. Plant Cell Environ. 28, 949–964. doi: 10.1111/j.1365-3040.2005.01341.x
Avnery, S., Mauzerall, D. L., Liu, J., and Horowitz, L. W. (2011). Global crop yield reductions due to surface ozone exposure: 1. Year 2000 crop production losses and economic damage. Atmos. Environ. 45, 2284–2296. doi: 10.1016/j.atmosenv.2010.11.045
Bhatia, A., Ghosh, A., Kumar, V., Tomer, R., Singh, S. D., and Pathak, H. (2011). Effect of elevated tropospheric ozone on methane and nitrous oxide emission from rice soil in North India. Agric. Ecosyst. Environ. 144, 21–28. doi: 10.1016/j.agee.2011.07.003
Bhatia, A., Kumar, V., Kumar, A., Tomer, R., Singh, B., and Singh, S. D. (2013). Effect of elevated ozone and carbon dioxide interaction on growth and yield of maize. Maydica 58, 291–298.
Bhatia, A., Mina, U., Kumar, V., Tomer, R., Kumar, A., Chakrabarti, B., et al. (2021). Effect of elevated ozone and carbon dioxide interaction on growth, yield, nutrient content and wilt disease severity in chickpea grown in northern India. Heliyon 7:e06049. doi: 10.1016/j.heliyon.2021.e06049
Bhatia, A., Pathak, H., Aggarwal, P. K., and Jain, N. (2010). Trade-off between productivity enhancement and global warming potential of rice and wheat in India. Nutr. Cycl. Agroecosys. 86, 413–424. doi: 10.1007/s10705-009-9304-5
Bhatia, A., Tomer, R., Kumar, V., Singh, S. D., and Pathak, H. (2012). Impact of tropospheric ozone on crop growth and productivity–a review. J. Sci. Indust. Res. 71, 97–112.
Broberg, M. C., Feng, Z. Z., Xin, Y., and Pleijel, H. (2015). Ozone effects on wheat grain quality – a summary. Environ. Pollut. 197, 203–213. doi: 10.1016/j.envpol.2014.12.009
Broberg, M. C., Uddling, J., Mills, G., and Pleijel, H. (2017). Fertilizer efficiency in wheat is reduced by ozone pollution. Sci. Total Environ. 607-608, 876–880. doi: 10.1016/j.scitotenv.2017.07.069
Chakrabarti, B., Singh, S. D., Bhatia, A., Kumar, V., and Harit, R. C. (2020). Yield and nitrogen uptake in wheat and chickpea grown under elevated carbon dioxide level. Natl. Acad. Sci. Lett. 43, 109–113. doi: 10.1007/s40009-019-00816-y
Chen, C. T., Lee, C. L., and Yeh, D. M. (2018). Effects of nitrogen, phosphorus, potassium, calcium, or magnesium deficiency on growth and photosynthesis of eustoma. Hortic. Sci. 53, 795–798. doi: 10.21273/HORTSCI12947-18
Chen, Z., Wang, X., Feng, Z., Xiao, Q., and Duan, X. (2008). Impact of elevated O3 on soil microbial community function under wheat crop. Water Air Soil Pollut. 198, 189–198.
Chen, Z., Wang, X., and Shang, H. (2015). Structure and function of rhizosphere and non-rhizosphere soil microbial community respond differently to elevated ozone in field planted wheat. J. Environ. Sci. (China) 32, 126–134. doi: 10.1016/j.jes.2014.12.018
Cowan, N., Bhatia, A., Drewer, J., Jain, N., Singh, R., Tomer, R., et al. (2021). Experimental comparison of continuous and intermittent flooding of rice in relation to methane, nitrous oxide and ammonia emissions and the implications for nitrogen use efficiency and yield. Agric. Ecosyst. Environ. 319:107571. doi: 10.1016/j.agee.2021.107571
Daripa, A., Bhatia, A., Ojha, S., Tomer, R., Chattaraj, S., Singh, K. P., et al. (2016). Chemical and natural plant extract in ameliorating negative impact of tropospheric ozone on wheat crop: a case study in a part of semiarid north West India. Aerosol Air Qual. Res. 16, 1742–1756. doi: 10.4209/aaqr.2014.10.0263
David, L. M., and Nair, P. R. (2013). Tropospheric column O3 and NO2 over the Indian region observed by ozone monitoring instrument (OMI): seasonal changes and long-term trends. Atmos. Environ. 65, 25–39. doi: 10.1016/j.atmosenv.2012.09.033
Deb Roy, S., Beig, G., and Ghude, S. D. (2009). Exposure plant response of ambient ozone over the tropical Indian region. Atmos. Chem. Phys. 9, 5253–5260. doi: 10.5194/acp-9-5253-2009
Deryng, D., Elliott, J., Folberth, C., Müller, C., Pugh, T. A. M., Boote, K. J., et al. (2016). Regional disparities in the beneficial effects of rising CO2 concentrations on crop water productivity. Nat. Clim. Chang. Lett. 6, 786–790. doi: 10.1038/nclimate2995
Dey, S. K., Chakrabarti, B., Prasanna, R., Singh, S. D., Purakayastha, T. J., Datta, A., et al. (2017). Productivity of mungbean (Vigna radiata) with elevated carbon dioxide at various phosphorus levels and cyanobacteria inoculation. Legum. Res. 40, 497–505.
Feng, Z., Hu, E., Wang, X., Jiang, L., and Liu, X. (2015). Ground-level O3 pollution and its impacts on food crops in China: a review. Environ. Pollut. 199, 42–48. doi: 10.1016/j.envpol.2015.01.016
Feng, Z., and Kobayashi, K. (2009). Assessing the impacts of current and future concentrations of surface ozone on crop yield with meta-analysis. Atmos. Environ. 43, 1510–1519. doi: 10.1016/j.atmosenv.2008.11.033
Feng, Z. Z., Kobayashi, K., Li, P., Xu, Y. S., Tang, H. Y., Guo, A. H., et al. (2019). Impacts of current ozone pollution on wheat yield in China as estimated with observed ozone, meteorology and day of flowering. Atmos. Environ. 217:116945.
Feng, Z. Z., Pang, J., Kobayashi, K., Zhu, J., and Ort, D. R. (2010). Differential responses in two varieties of winter wheat to elevated ozone concentration under fully open-air field conditions. Glob. Change Biol. doi: 10.1111/j.1365-2486.2010.02184.X
Gelang, J., Pleijel, H., Sild, E., Danielsson, H., Younis, S., and Sellden, G. (2000). Rate and duration of grain filling in relation to flag leaf senescence and grain yield in spring wheat (Triticum aestivum) exposed to different concentrations of ozone. Physiol. Plant. 110, 366–375. doi: 10.1111/j.1399-3054.2000.1100311.x
Grünhage, L., Pleijel, H., Mills, G., Bender, J., Danielsson, H., Lehmann, Y., et al. (2012). Updated stomatal flux and flux-effect models for wheat for quantifying effects of ozone on grain yield, grain mass and protein yield. Environ. Pollut. 165, 147–157. doi: 10.1016/j.envpol.2012.02.026
Guidi, L., Nali, C., Lorenzini, G., Filppi, F., and Soladatini, G. F. (2001). Effect of chronic ozone fumigation on the photosynthetic process of poplar clones showing different sensitivity. Environ. Pollut. 113, 245–254. doi: 10.1016/S0269-7491(00)00194-9
IPCC (2021). “Climate change 2021: the physical science basis” in Contribution of working group I to the sixth assessment report of the intergovernmental panel on climate change. eds. V. Masson-Delmotte, P. Zhai, A. Pirani, S. L. Connors, C. Péan, and S. Berger, et al. (Cambridge, United Kingdom and New York, NY, USA: Cambridge University Press).
Jackson, M. L. (1956). Soil chemical analysis - advanced course. Madison, WI: Published by the author, Dep. of Soil Science, Univ. of Wisconsin.
Kim, H. Y., Lieffering, M., Miura, S., Kobayashi, K., and Okada, M. (2001). Growth and nitrogen uptake of CO2-enriched rice under field conditions. New Phytol. 150, 223–229. doi: 10.1046/j.1469-8137.2001.00111.x
Kobayashi, K., Okada, M., and Kim, H. Y. (1999). “The free air CO2 enrichment (FACE) with rice in Japan” in World food security and crop production technologies for tomorrow. eds. T. Horie, S. Geng, T. Amano, T. Inamura, and T. Shiraiwa (Kyoto University, Kyoto, Japan: Graduate School of Agriculture), 213–215.
Kumar, R., Bhatia, A., Chakrabarti, B., Kumar, V., Tomer, R., Sharma, D. K., et al. (2021). Effect of elevated ozone and carbon dioxide on growth and yield of rice (Oryza sativa). Indian J. Agric. Sci. 91, 1607–1611. doi: 10.56093/ijas.v91i11.118540
Lefohn, A. S., Malley, C. S., Simon, H., Wells, B., Xu, X., Zhang, L., et al. (2017). Responses of human health and vegetation exposure metrics to changes in ozone concentration distributions in the European Union, United States, and China. Atmos. Environ. 152, 123–145. doi: 10.1016/j.atmosenv.2016.12.025
Maity, P. P., Chakrabarti, B., Bhatia, A., Kumar, S. N., Purakayastha, T. J., Chakraborty, D., et al. (2023). Co-elevation of atmospheric CO2 and temperature affect instantaneous and intrinsic water use efficiency of rice varieties. J. Agrometeorol. 25, 404–409. doi: 10.54386/jam.v25i3.2243
Maity, P. P., Chakrabarti, B., Purakayastha, T. J., Bhatia, A., Saha Das, N. D., Jatav, R. S., et al. (2020). Do elevated CO2 and temperature affect organic nitrogen fractions and enzyme activities in soil under rice crop? Soil Res. 58, 400–410. doi: 10.1071/SR19270
Mills, G., Sharps, K., Simpson, D., Pleijel, H., Broberg, M., Uddling, J., et al. (2018). Ozone pollution will compromise efforts to increase global wheat production. Glob. Change Biol. 24, 3560–3574. doi: 10.1111/gcb.14157
Mina, U., Fuloria, A., and Aggarwal, R. (2016). Effect of ozone and antioxidants on wheat and its pathogen—Bipolaris sorokininana. Cereal Res. Comm. 44, 594–604. doi: 10.1556/0806.44.2016.039
Morgan, P. B., Ainsworth, E. A., and Long, S. P. (2003). How does elevated ozone impact soybean? A meta analysis of photosynthesis, growth and yield. Plant Cell Environ. 26, 1317–1328. doi: 10.1046/j.0016-8025.2003.01056.x
Pandey, A. K., Ghosh, A., Agrawal, M., and Agrawal, S. B. (2018). Effect of elevated ozone and varying levels of soil nitrogen in two wheat (Triticum aestivum L.) cultivars: growth, gas-exchange, antioxidant status, grain yield and quality. Ecotoxicol. Environ. Saf. 158, 59–68. doi: 10.1016/j.ecoenv.2018.04.014
Pfister, G. G., Walters, S., Lamarque, J. F., Fast, J., Barth, M. C., Wong, J., et al. (2014). Projections of future summertime ozone over the US. J. Geophys. Res. Atmos. 119, 5559–5582. doi: 10.1002/2013JD020932
Phothi, R., Umponstira, C., Sarin, C. S. W., and Nabheerong, N. (2016). Combining effects of ozone and carbon dioxide application on photosynthesis of Thai jasmine rice (Oryza sativa L.) cultivar Khao Dawk Mali 105. Aus. J. Crop Sci. 10, 591–597. doi: 10.21475/ajcs.2016.10.04.p7595x
Pleijel, H., Danielsson, H., Simpson, D., and Mills, G. (2014). Have ozone effects on carbon sequestration been overestimated? A new biomass response function for wheat. Biogeosciences 11, 4521–4528. doi: 10.5194/bg-11-4521-2014
Pramanik, P., Chakrabarti, B., Bhatia, A., Singh, S. D., Mridha, N., and Krishnan, P. (2018). Effect of elevated carbon dioxide on soil hydrothermal regimes and growth of maize crop (Zea mays L.) in semi-arid tropics of indo-Gangetic Plains. Environ. Monit. Assess. 190:661. doi: 10.1007/s10661-018-6988-5
Proietti, C., Anav, A., De Marco, A., Sicard, P., and Vitale, M. (2016). A multi-sites analysis on the ozone effects on gross primary production of European forests. Sci. Total Environ. 556, 1–11. doi: 10.1016/j.scitotenv.2016.02.187
Raj, A., Chakrabarti, B., Pathak, H., Singh, S. D., Mina, U., and Purakayastha, T. J. (2019). Growth, yield and nitrogen uptake in rice crop grown under elevated carbon dioxide and different doses of nitrogen fertilizer. Indian J. Exp. Biol. 57, 181–187.
Sanyal, S., Chakrabarti, B., Bhatia, A., Kumar, S. N., Purakayastha, T. J., Kumar, D., et al. (2023). Response of aestivum and durum wheat varieties to elevated CO2 and temperature under OTC condition. J. Agrometeorol. 25, 498–502. doi: 10.54386/jam.v25i4.2366
Singh, P., Agrawal, M., and Agrawal, S. B. (2009). Evaluation of physiological, growth and yield responses of a tropical oil crop (Brassica campestris L. var. Kranti) under ambient ozone pollution at varying NPK levels. Environ. Pollut. 157, 871–880. doi: 10.1016/j.envpol.2008.11.008
Singh, S. D., Chakrabarti, B., Muralikrishna, K. S., Chaturvedi, A. K., Kumar, V., Mishra, S., et al. (2013). Yield response of important field crops to elevated air temperature and CO2 levels. Indian J. Agri. Sci. 83, 1009–1012.
Singh, E., Rai, R., Pandey, B., and Agrawal, M. (2018). Development of resistance in two wheat cultivars against constant fumigation of ozone. Proc. Natl. Acad. Sci. India Sect. B Biol. Sci. 88, 1121–1134. doi: 10.1007/s40011-017-0849-9
Subbiah, B., and Asija, G. L. (1956). A rapid procedure for estimation of available nitrogen in soils. Curr. Sci. 25, 259–260.
Tai, A. P. K., Val Martin, M., and Heald, C. L. (2014). Threat to future global food security from climate change and ozone air pollution. Nat. Clim. Chang. 4, 817–821. doi: 10.1038/nclimate2317
Tatsumi, K., Abiko, T., Kinose, Y., Inagaki, S., and Izuta, T. (2019). Effects of ozone on the growth and yield of rice (Oryza sativa L.) under different nitrogen fertilization regimes. Environ. Sci. Pollut. Res. Int. 26, 32103–32113. doi: 10.1007/s11356-019-06358-6
Tiwari, S., Rai, R., and Agrawal, M. (2008). Annual and seasonal variations in tropospheric ozone concentrations around Varanasi. Int. J. Remote Sens. 29, 4499–4514. doi: 10.1080/01431160801961391
Tomer, R., Bhatia, A., Kumar, V., Kumar, A., Singh, R., Singh, B., et al. (2015). Impact of elevated ozone on growth, yield and nutritional quality of two wheat species in northern India. Aerosol Air Qual. Res. 15, 329–340. doi: 10.4209/aaqr.2013.12.0354
Wu, H., Li, Q., Lu, C., Zhang, L., Zhu, J., Dijkstra, F. A., et al. (2016). Elevated ozone effects on soil nitrogen cycling differ among wheat cultivars. Appl. Soil Ecol. 108, 187–194. doi: 10.1016/j.apsoil.2016.08.015
Xia, L., Lam, S. K., Kiese, R., Chen, D., Luo, Y., van Groenigen, K. J., et al. (2021). Elevated CO2 negates O3 impacts on terrestrial carbon and nitrogen cycles. One Earth 4, 1752–1763. doi: 10.1016/j.oneear.2021.11.009
Yadav, A., Bhatia, A., Yadav, S., Kumar, V., and Singh, B. (2019). The effects of elevated CO2 and elevated O3 exposure on plant growth, yield and quality of grains of two wheat cultivars grown in North India. Heliyon 5:e02317. doi: 10.1016/j.heliyon.2019.e02317
Yadav, A., Bhatia, A., Yadav, S., Singh, A., Tomer, R., Harit, R., et al. (2021). Growth, yield and quality of maize under ozone and carbon dioxide interaction in north West India. Aerosol Air Qual. Res. 21:200194. doi: 10.4209/aaqr.2020.05.0194
Keywords: elevated O3, elevated CO2, N management, plant nitrogen, rice variety
Citation: Chakrabarti B, Sharma S, Mishra AK, Kannojiya S, Kumar V, Bandyopadhyay SK and Bhatia A (2024) Application of additional dose of N could sustain rice yield and maintain plant nitrogen under elevated ozone (O3) and carbon dioxide (CO2) condition. Front. Sustain. Food Syst. 8:1477210. doi: 10.3389/fsufs.2024.1477210
Edited by:
Aliza Pradhan, National Institute of Abiotic Stress Management (ICAR), IndiaReviewed by:
Khushboo Rani, Indian Institute of Soil Science (ICAR), IndiaArjun Tayade, Central Institute for Cotton Research (ICAR), India
Copyright © 2024 Chakrabarti, Sharma, Mishra, Kannojiya, Kumar, Bandyopadhyay and Bhatia. This is an open-access article distributed under the terms of the Creative Commons Attribution License (CC BY). The use, distribution or reproduction in other forums is permitted, provided the original author(s) and the copyright owner(s) are credited and that the original publication in this journal is cited, in accordance with accepted academic practice. No use, distribution or reproduction is permitted which does not comply with these terms.
*Correspondence: Sheetal Sharma, c2hlZXRhbGhwa3Z2QGdtYWlsLmNvbQ==; Arti Bhatia, YXJ0aS5iaGF0aWFAaWNhci5nb3YuaW4=