- 1College of Marine Sciences, Ningde Normal University, Ningde, Fujian, China
- 2College of Biological Science and Engineering, Ningde Normal University, Ningde, Fujian, China
- 3Industry and University Research Cooperation Demonstration Base in Fujian Province, Ningde, Fujian, China
Introduction: Probiotics can improve immune responses and regulate the ecosystem of microorganisms in the gastrointestinal tract.
Methods: Three primary models, including the Reparameterized Gompertz, Huang, and Baranyi and Roberts models were evaluated and developed to investigate the effects of Laetiporus sulphureus polysaccharides (LSP) on the growth of Lactobacillus plantarum, Streptococcus thermophilus, Clostridium tyrobutyricum, and Bifidobacterium adolescentis.
Results: The Huang model and Reparameterized Gompertz model were suitable for describing the growth of C. tyrobutyricum, S. thermophilus, B. adolescentis, and L. plantarum. LSP could increase the population of B. adolescentis in the fluid environment of the stomach in vitro.
Discussion: These results may support the further development of LSP as a functional food or food additive that has the ability to preserve digestive tract health.
1 Introduction
In recent decades, the gastrointestinal tract has been regarded as the human body's biggest immunological organ. The gastrointestinal tract has a diverse microbiota that is emerged as a critical target for the treatment of disease (Xu et al., 2023; Li et al., 2024). Experimental evidence has shown that metabolic disorders including hypertension, insulin resistance, obesity, hyperglycemia, and hyperlipidemia, etc. are closely associated with gut microbiota (Shao et al., 2022; Zhang H. et al., 2023; Ouyang et al., 2024; Li et al., 2024. Makki et al. (2018) found that a high fat diet could reduce microbial diversity and change the gut microbial metabolism, leading to the development of metabolic syndrome. According to Li et al. (2019), the gut microbiota of the high fat diet-induced rats was modulated in order to prevent hyperlipidemia and hypercholesterolemia. It suggested that improving gastrointestinal tract health might be a new strategy for the treatment of diseases.
Probiotics are the dominant microbiota in the gastrointestinal tract and beneficial to improve the digestion of food. Lactobacillus plantarum, Clostridium tyrobutyricum, Bifidobacterium adolescentis, and Streptococcus thermophilus are important bacteria in the gastrointestinal tract. L. plantarum is a facultative anaerobic gram-positive bacterium. It is reported that L. plantarum can improve intestinal inflammation, preventing the occurrence or aggravation of diseases, and it also can be used to treat mental illness and improve cognitive functions (Toshimitsu et al., 2016; Rudzki et al., 2019; Hu et al., 2020; Zhang Z. et al., 2023; Zeng et al., 2023). C. tyrobutyricum is an obligate anaerobic gram-positive bacterium. Luo et al. (2024) suggested that C. tyrobutyricum may alleviate obesity symptoms by improving lipid metabolism and intestinal health, as well as regulating intestinal microbiota. B. adolescentis is a gram-positive and non-motile bacterium, which has been shown to alleviate anxiety/depression and treat colitis (Kim et al., 2019; Ma et al., 2021; Sharma et al., 2023). S. thermophilus is considered as a probiotic, which is safe and can survive in the human gastrointestinal tract and exhibited health benefits on its host (Uriot et al., 2017). It is widely used in the production of dairy products (Che et al., 2019; Demirci et al., 2024).
Several studies have demonstrated that mushroom polysaccharides are the effective components in the regulation of gut microbiota, as they promote the growth of beneficial microorganisms: Agaricus bisporus polysaccharides could enhance the abundance of beneficial bacteria during the stimulating gastrointestinal digestion and gut microbiota fermentation (Fu et al., 2023). Lv et al. (2019) found that Ganoderma lucidum polysaccharides had the potential to increase total short chain fatty acids and butyric acid while regulating gut microbiota. On the other hand, they decrease the population of pathogens: a study performed in 2018 showed that chitosan obtained from G. lucidum spore powder has the antimicrobial activity for E. coli and S. aureus (Zhu et al., 2018).
Laetiporus sulphureus (L. sulphureus) is an edible and medicinal mushroom belonging to the phylum of Basidiomycota. It has already been documented that L. sulphureus contains various bioactive compounds such as lectin, polysaccharides, triterpenoids, and phenolic compounds, etc. (Wang et al., 2018; Lu et al., 2023; Jen et al., 2024). Polysaccharides are the vital bioactive substances of L. sulphureus. Interestingly, a number of studies have demonstrated the various health promoting properties of L. sulphureus polysaccharides (LSP) including anti-inflammatory (Lu et al., 2023), anti-cancer (Jen et al., 2024), hepatoprotective, antioxidant activity (Zhao et al., 2019), and hypoglycemic activity (Hwang and Yun, 2010). Many reports have shown that modeling experimental data using kinetic models is one of the effective ways to study the effects of prebiotic compounds on probiotics (Altieri et al., 2016; Bernal-Castro et al., 2019; Montes et al., 2024; Wang et al., 2023). However, there was limited investigation into the impact of LSP on gut microbiota or application of any mathematical model.
In general, in order to further develop the application of LSP in promoting microbial growth, the aim of this study was (1) to investigate the effects of LSP on the growth of C. tyrobutyricum, S. thermophilus, B. adolescentis, and L. plantarum, (2) combining with kinetic models to select the accurate mathematical models for describing the growth of C. tyrobutyricum, S. thermophilus, B. adolescentis, and L. plantarum, (3) to evaluate the efficiency of LSP in increasing the survival of C. tyrobutyricum, S. thermophilus, B. adolescentis, and L. plantarum at simulated gastric and intestinal condition. These researches provide a theoretical basis for the development of functional food, health products and other related fields. In addition, they provided a new idea for the exploitation and utilization of LSP.
2 Materials and methods
2.1 Materials and media
The fruiting body of L. sulphureus (dried mushroom) was obtained from Changbaishan Co., Ltd. (Jilin, China). Reinforced Clostridium medium (RCM) broth, RCM agar medium, tryptone peptone yeast extract (TPY) broth, TPY agar medium, modified Chalmers (MC) broth, MC agar medium, and De-Man Rogosa Sharpe (MRS) broth, MRS agar medium were purchased from Hopebio Co., Ltd. (Qingdao, China).
2.2 Preparation of LSP
The dried powder of L. sulphureus was mixed with distilled water (1:20, w/v) at 80°C for 3 h. The supernatant was collected by filtration through a Buchner funnel and precipitated by two volumes of ethanol (95%, v/v) at 4°C for 12 h. The precipitate was obtained by centrifugation (4,000 rpm, 20 min), and then deproteinated with Sevag reagent (n-butanol: chloroform = 1:4, v/v). LSP was harvested after freeze-drying (FDU-2110, Eyela Co., Ltd., Tokyo Japan), which was used for further research.
2.3 Bacterial culture and preparation
Clostridium tyrobutyricum (ATCC25755), Bifidobacterium adolescentis (ATCC15703), Streptococcus thermophilus (ATCC14485), and Lactobacillus plantarum (ATCC8014) were purchased from Guangdong Microbial Culture Collection Center. The bacterial cultures were harvested after 3 consecutive transfers. One day before the experiment, 100 μL of each culture (C. tyrobutyricum, B. adolescentis, S. thermophilus, and L. plantarum) was individually transferred to 10 mL of RCM, TPY, MC, and MRS broth and held at 37°C in an anaerobic box (Mitsubishi Gas Chemical Company Incorporated; Tokyo, Japan) for 24 h.
2.4 Bacterial growth
The 100 μL of each overnight culture (C. tyrobutyricum, B. adolescentis, S. thermophilus, and L. plantarum) was separately added into 10 mL of RCM, TPY, MC, and MRS broth containing 0% (Control), or 2% of LSP in sterile centrifuge tubes. Each tube was mixed evenly and anaerobically kept for 24 h at 37°C. Each tube was serially diluted and enumerated. Additionally, the bacteria's concentrations were transformed to the natural base, or logarithm of base 10, and stated as Ln CFU/mL or lg CFU/mL.
2.5 Primary models
To select the most suitable kinetic model to describe the growth of C. tyrobutyricum, B. adolescentis, S. thermophilus, and L. plantarum, three primary models were chosen. Huang model (Equations 1, 2, Huang, 2013), Baranyi and Roberts model (Equations 3, 4, Baranyi and Roberts, 1995), and Reparameterized Gompertz model (Equation 5, Zwietering et al., 1990) were used in this research. The Huang model is expressed as
The variables in this equation are: t presents the time point (h), Y(t) presents the natural logarithm of microorganism count (ln CFU/g), λ presents the lag phase duration (h), Ymax presents the bacterial count (ln CFU/g) at the stationary phase, Y0 presents the initial microorganisms count (ln CFU/g), μmax presents the maximum specific growth rate (h−1), B(t) is the transfer function and α is a constant (α = 4) that defines the transition from the lag phase to the exponential phase of a growth curve (Huang, 2013).
The Baranyi and Roberts model is expressed as
The physiological state of the microorganism, h0, is equals to λ × μmax. Ymax, Y0, Y(t), and μmax, are specified as in the Huang model. Establishing the h0 averaged value. A(t) is the transfer function. Next, use a fixed h0 value to estimate Y0, μmax, and Ymax.
The Reparameterized Gompertz model is expressed as
t, λ, Y(t), Ymax, μmax, and Y0 are specified as in the Huang model.
2.6 Simulated gastrointestinal condition
2.6.1 Effect of LSP on simulated gastric fluids in vitro
Simulated gastric fluids were prepared as described by Kenari and Razavi (2022) with slight modification. Briefly, the phosphate buffered saline (0.1 mol/L, pH = 7.4) was used as solvent to obtain RCM broth. The pH values of the RCM broth were adjusted to 3 using hydrochloric acid (8 mol/L). After sterilization, 0.5 g of pepsin (Solarbio Life Sciences Co., Ltd. Bejing, China) was added into 100 mL of RCM broth to obtained the simulated gastric fluid of RCM broth. The simulated gastric fluids of TPY, MC and MRS broths were obtained by using the method as described as the simulated gastric fluid of RCM broth. The 100 μL of each overnight culture (C. tyrobutyricum, B. adolescentis, S. thermophilus, and L. plantarum) was separately added into 10 mL of simulated gastric fluids containing 0% (Control) or 2% of LSP. Each tube was collected at 0 and 3 h of digestion. After digestion, the samples were serially diluted and enumerated on RCM, TPY, MC and MRS agar media to enumerate C. tyrobutyricum, B. adolescentis, S. thermophilus, and L. plantarum. The bacterial concentrations were also converted to the logarithm of base 10 or natural base, recorded as Log10 CFU/mL or Ln CFU/mL.
2.6.2 Effect of LSP on simulated intestinal fluids in vitro
Simulated intestinal fluids were prepared by the method of Razavi et al. (2020). For the intestinal digestion, the phosphate buffered saline (0.1 mol/L, pH = 7.4) was used as a solvent to obtain RCM broth. The pH values of the RCM broth were adjusted to 7 using hydrochloric acid (8 mol/L). After sterilization, 1 g of trypsin (Solarbio Life Sciences Co., Ltd. Bejing, China) was added into 100 mL of RCM broth to obtained the simulated intestinal fluid of RCM broth. The simulated intestinal fluids of TPY, MC and MRS broths were obtained by using the method as described as the simulated intestinal fluid of RCM broth. The 100 μL of each overnight culture (C. tyrobutyricum, B. adolescentis, S. thermophilus, and L. plantarum) was separately added into 10 mL of simulated intestinal fluids containing 0% (Control) or 2% of LSP. Each tube was collected at 0 and 3 h of digestion. After digestion, the samples were serially diluted and enumerated on RCM, TPY, MC, and MRS agar media to enumerate C. tyrobutyricum, B. adolescentis, S. thermophilus, and L. plantarum. The bacterial concentrations were also converted to the logarithm of base 10 or natural base, recorded as Log10 CFU/mL or Ln CFU/mL.
2.7 Statistical analysis
The growth curves of C. tyrobutyricum, B. adolescentis, S. thermophilus, and L. plantarum were investigated by the Integrated Pathogen Modeling Program (IPMP) established by the United States Department of Agriculture (USDA, Huang, 2013). The specific growth rates, maximum bacterial concentration, confidence intervals, lag phase duration, and data analysis were acquired from IPMP analysis. Three replication of each experiments were conducted. One-way ANOVA was used to examine the acquired data. Graphpad Prism 9 was used for the statistical analyses.
3 Results and discussion
3.1 Effects of LSP on the growth of microbial
Recent research has demonstrated that polysaccharides have the capacity to modulate gut microbiota and the balance of microbial metabolites (Yin et al., 2020; Xue et al., 2020). Certain species of gut microbiota might acquire energy and carbon sources via the breakdown of polysaccharides for their own proliferation (Ze et al., 2012). Tong et al. (2020) reported that Ganoderma lucidum polysaccharides could increase the relative abundances of beneficial bacteria (Ruminococcus, Oscillibacter, Bifidobacterium, Prevotella, Alloprevotella, Paraprevotella, and Alistipes). The effects of LSP on the population of C. tyrobutyricum, B. adolescentis, S. thermophilus, and L. plantarum were shown in Figure 1. The LSP at 0, 0.5, 1, and 2% concentrations exhibited the population of C. tyrobutyricum of 8.41 ± 0.05, 8.55 ± 0.10, 8.68 ± 0.01, and 8.66 ± 0.06 lg CFU/mL, respectively. The LSP showed a higher population of C. tyrobutyricum than 0% of LSP. Moreover, there was no significant difference in the population of C. tyrobutyricum among 0.5, 1, and 2% of LSP (p > 0.05). The LSP at 0, 0.5, 1, and 2% concentrations exhibited the population of B. adolescentis of 7.49 ± 0.02, 7.70 ± 0.03, 8.26 ± 0.06, and 8.28 ± 0.03 lg CFU/mL, respectively. The LSP exhibited an increasing population of B. adolescentis at higher concentrations. Compared to 0% of LSP, 1% of LSP could significantly increase the population of B. adolescentis and reach the maximum population of B. adolescentis (p < 0.05). There was no significant difference between 1% of LSP and 2% of LSP with regard to their population of B. adolescentis (p > 0.05). Simultaneously, the population of S. thermophilus, and L. plantarum was not increased by LSP (p > 0.05). The results suggested that 1% of LSP could increase the population of C. tyrobutyricum and B. adolescentis. Therefore, 1% of LSP was selected for further research.
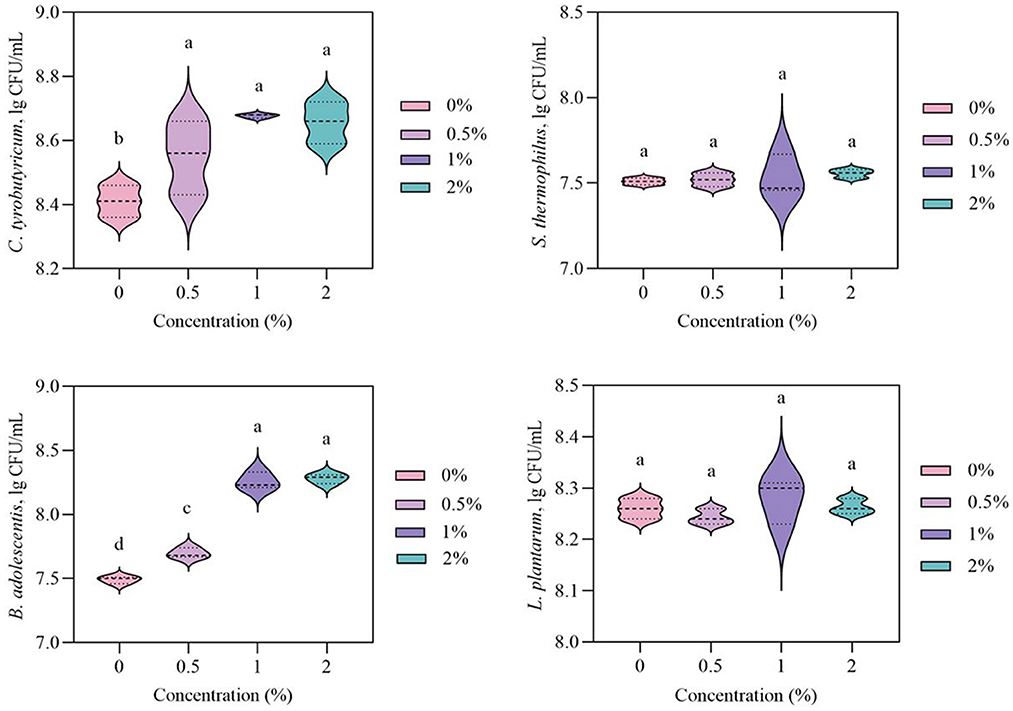
Figure 1. Effects of polysaccharides from Laetiporus sulphureus on the growth of Lactobacillus plantarum, Streptococcus thermophilus, Clostridium tyrobutyricum, and Bifidobacterium adolescentis. Values marked by different letters are significantly different at p < 0.05; values marked by the same letter are not significantly different at p > 0.05.
The effects of LSP on the growth of C. tyrobutyricum, B. adolescentis, S. thermophilus, and L. plantarum were displayed in Figure 2. The population of C. tyrobutyricum, B. adolescentis, S. thermophilus, and L. plantarum increased with increasing incubation time. Compared to the control group, LSP could increase the population of B. adolescentis at 11–24 h, while increasing the population of C. tyrobutyricum at 5–24 h. The result suggested that LSP exhibited an impact on increasing the population of C. tyrobutyricum and B. adolescentis in vitro. A similar phenomenon was found by Li et al. (2019) in which Ganoderma lucidum polysaccharides could increase the relative abundance of Bifidobacterium in rats. Furthermore, the population of S. thermophilus and L. plantarum in the control group and LSP group showed no significant difference. It suggests that LSP could not increase the population of S. thermophilus and L. plantarum in vitro.
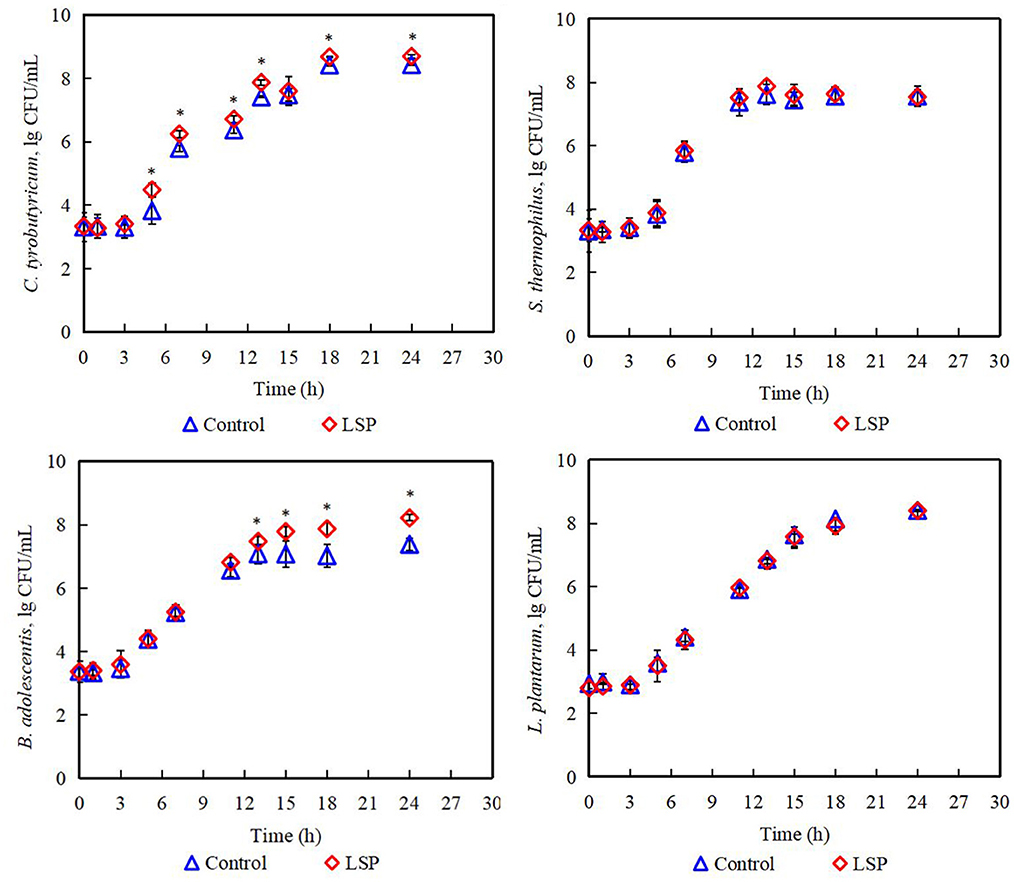
Figure 2. Population of Lactobacillus plantarum, Streptococcus thermophilus, Clostridium tyrobutyricum, and Bifidobacterium adolescentis. Control: 0% of Laetiporus sulphureus polysaccharides, LSP: 2% of Laetiporus sulphureus polysaccharides. *Significant difference at p < 0.05.
3.2 Mathematical modeling
The Huang, Reparameterized Gompertz, and Baranyi and Roberts models were used to evaluate microbial inactivation, survival, and growth in reaction to surrounding environment (Akkermans et al., 2018; Tashiro and Yoshimura, 2019). The commonly reported primary models include the Huang model, Reparameterized Gompertz model, logistic model, and Baranyi and Roberts model (Jia et al., 2020). The growth data of C. tyrobutyricum, B. adolescentis, S. thermophilus, and L. plantarum were analyzed by Huang, Reparameterized Gompertz, and Baranyi and Roberts models. The growth curves of C. tyrobutyricum, B. adolescentis, S. thermophilus, and L. plantarum all presented lag phase, exponential phase, and stationary phase (Figures 3, 4).
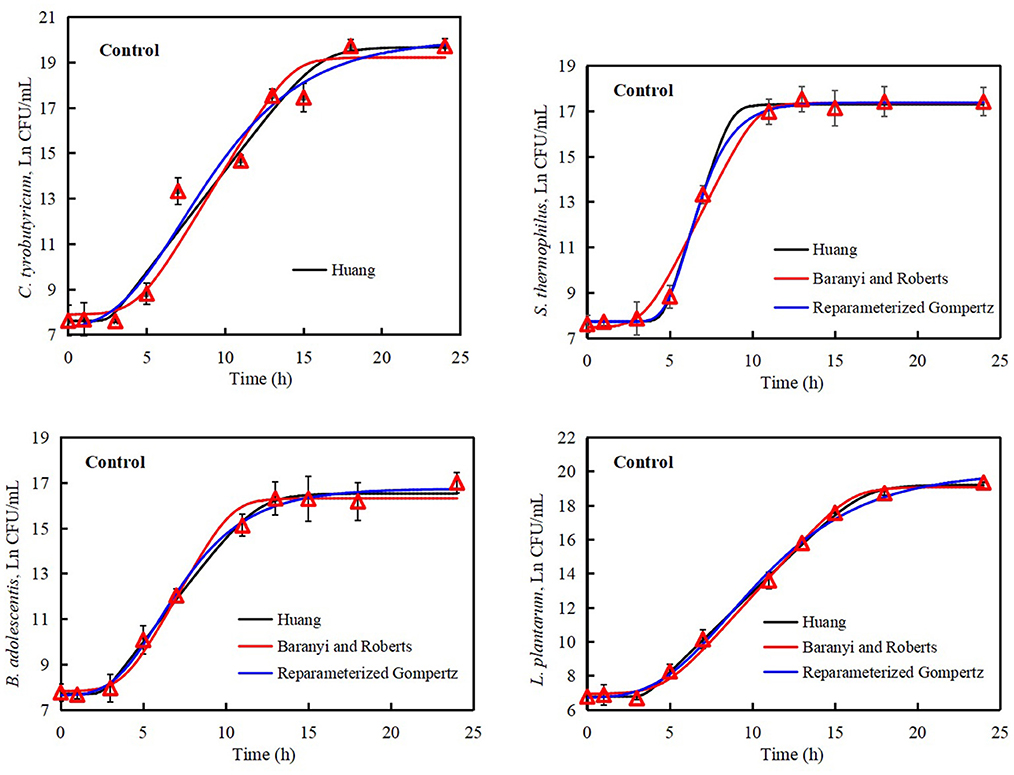
Figure 3. Growth curves of Lactobacillus plantarum, Streptococcus thermophilus, Clostridium tyrobutyricum, and Bifidobacterium adolescentis. Solid black line: Huang model, solid red line: Baranyi and Roberts model, solid blue line: Reparameterized Gompertz model, triangle: observed growth data.
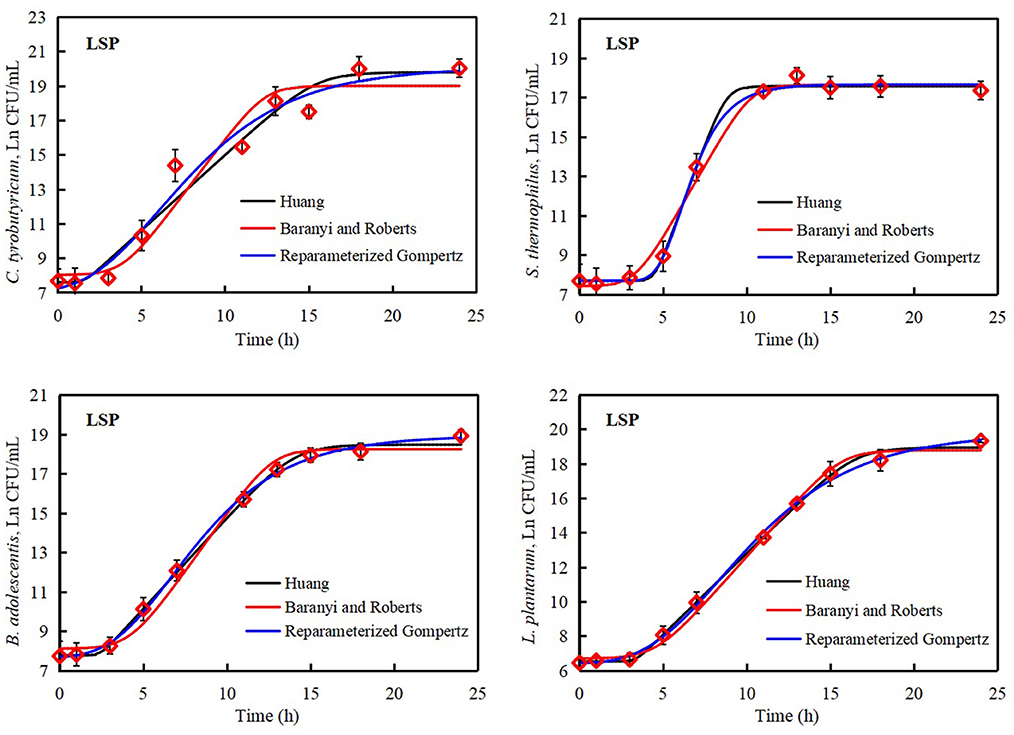
Figure 4. Growth curves of Lactobacillus plantarum, Streptococcus thermophilus, Clostridium tyrobutyricum, and Bifidobacterium adolescentis treated with polysaccharides of Laetiporus sulphureus. Solid black line: Huang model, solid red line: Baranyi and Roberts model, solid blue line: Reparameterized Gompertz model, diamond: observed growth data.
The mean square error (MSE), root mean square error (RMSE), and akaike information criterion (AIC) are important to evaluate the accuracy of the primary models. The smaller value of MSE, RMSE, and AIC, indicated the higher accuracy of the model. For Huang, Baranyi and Roberts, and Reparameterized Gompertz models, the MSE of C. tyrobutyricum in control group were 0.969, 1.309, and 0.977 Ln CFU/mL, respectively, while LSP showed the MSE of C. tyrobutyricum of 1.292, 1.904, and 1.048 Ln CFU/mL, respectively (Table 1). There were no significant differences in the values of MSE, RSME, and AIC among Huang, Baranyi and Roberts, and Reparameterized Gompertz models, indicating that Huang, Baranyi and Roberts, and Reparameterized Gompertz models were suitable for describing the growth of C. tyrobutyricum.
For Huang, Baranyi and Roberts, and Reparameterized Gompertz models, the AIC of S. thermophilus in the control group were −12.358, −1.994, and −17.508, respectively, and MSE were 0.040, 0.236, and 0.024 Ln CFU/mL, respectively. Simultaneously, LSP showed the AIC of −5.590, −0.920, and −5.555, respectively, while MSE of 0.078, 0.236, and 0.078 Ln CFU/mL, respectively. Compared to Baranyi and Roberts model, Huang model and Reparameterized Gompertz model had the lower MSE, RMSE, and AIC values in B. adolescentis, S. thermophilus, and L. plantarum, indicating that Huang model and Reparameterized Gompertz model were more suitable for describing the growth of B. adolescentis, S. thermophilus, and L. plantarum than Baranyi and Roberts model. This was consistent with the results of Wei et al. (2021), in which the Reparameterized Gompertz model was suitable for describing the growth of Lactococcus lactis in Flammulina velutipes fruiting bodies. Therefore, the Reparameterized Gompertz model was suitable for describing the growth of lactic acid bacteria.
Compared to control group, the lag time of microbial (C. tyrobutyricum, B. adolescentis, S. thermophilus, and L. plantarum) decreased while LSP added. The maximum population of B. adolescentis obtained from the Huang model, Baranyi and Roberts model, and Reparameterized Gompertz model of 18.479, 18.252, and 18.940 Ln CFU/mL, respectively, while control exhibited the maximum population of B. adolescentis of 16.530, 16.318, and 16.746 Ln CFU/mL, respectively. The results suggested that LSP exhibited a higher maximum population of B. adolescentis than the control group.
3.3 Effects of LSP on microbial in simulated gastric fluid
Simulated gastrointestinal conditions can be used to study the composition of intestinal microorganisms and understand the relationship between intestinal health and disease. Experimental evidence has shown that prebiotics have health-promoting properties by increasing the survivability of bacteria traveling through the gastrointestinal tract (Chow, 2002; Khalf et al., 2010). It has been suggested that prebiotics contribute to improving probiotic proliferation in the intestine and modulate the composition of gut microbiota community structure (Zhao et al., 2019; Xue et al., 2020). It is a vital characteristic for probiotics to have the gastrointestinal tolerance, which is related to the type and the fermentation ability of probiotics (Shi et al., 2019). Table 2 presented the survival of C. tyrobutyricum, B. adolescentis, S. thermophilus, and L. plantarum in simulated gastric fluid. In the present study, the decreasing population of C. tyrobutyricum was observed in control and LSP groups under simulated gastric fluid condition for 3 h. The population of C. tyrobutyricum in control group was 4.39 ± 0.17 lg CFU/mL while that of LSP group was 4.77 ± 0.10 lg CFU/mL under simulated gastric fluid conditions for 3 h. S. thermophilus was not found after 3 h of digestion in simulated gastric fluid. It suggested that the S. thermophilus might not survive in simulated gastric fluid. Meanwhile, there was no significant difference between control and LSP at the same condition concerning to their population of L. plantarum. Over 3 h gastric digestion, the population of B. adolescentis in the LSP group (5.24 ± 0.04 lg CFU/mL) was significantly higher than the control group (4.70 ± 0.25 lg CFU/mL, p < 0.05). There was no significant difference between 0 and 3 h concerning to the population of B. adolescentis in the LSP group (p > 0.05). The results indicated that LSP could prevent the decrease of the population of B. adolescentis in simulated intestinal fluid. It can also be seen from the different performances of L. plantarum and B. adolescentis in simulated gastric fluid that the effects of LSP on probiotics may be strain specific rather than species or genera based, as suggested by Nobre et al. (2018). Therefore, the results showed evidence that LSP could enhance the population of B. adolescentis and C. tyrobutyricum in simulated gastric fluid conditions.
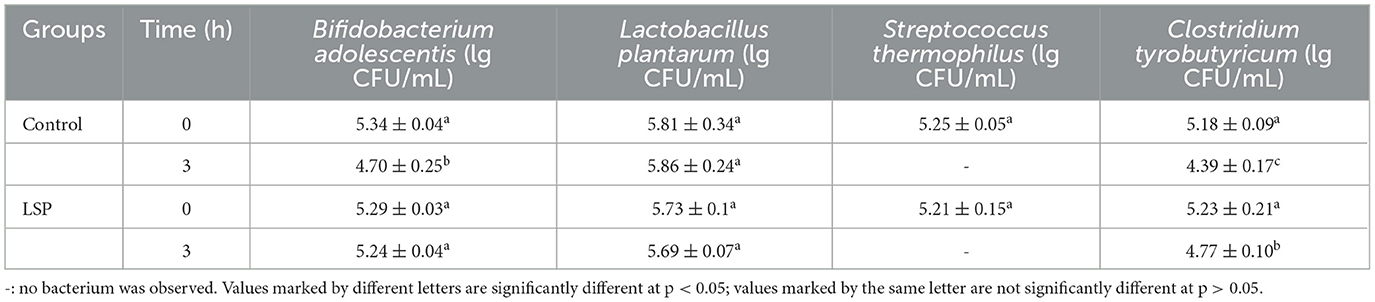
Table 2. Survival of Lactobacillus plantarum, Streptococcus thermophilus, Clostridium tyrobutyricum, and Bifidobacterium adolescentis in simulated gastric fluid treated with polysaccharides of Laetiporus sulphureus.
3.4 Effects of LSP on microbial in simulated intestinal fluid
Survival of C. tyrobutyricum, B. adolescentis, S. thermophilus, and L. plantarum in simulated intestinal fluid was illustrated in Table 3. The population of B. adolescentis in the LSP group was 5.24 ± 0.06 lg CFU/mL, which was significantly higher than the control group (5.01 ± 0.09 lg CFU/mL) under simulated intestinal fluid condition for 3 h (p < 0.05). Compared to 0 h, the population of B. adolescentis in the control group significantly decreased after 3 h digestion in simulated intestinal fluid condition (p < 0.05). Consequently, there was no significant difference between 0 and 3 h with regard the population of B. adolescentis in the LSP group (p > 0.05). In the meantime, there was no significant difference in the population of C. tyrobutyricum, S. thermophilus, and L. plantarum between the control group and the LSP group. The results suggested that LSP had a protective effect on B. adolescentis. However, the LSP did not affect on the population of L. plantarum, S. thermophilus and C. tyrobutyricum in simulated intestinal fluid conditions.
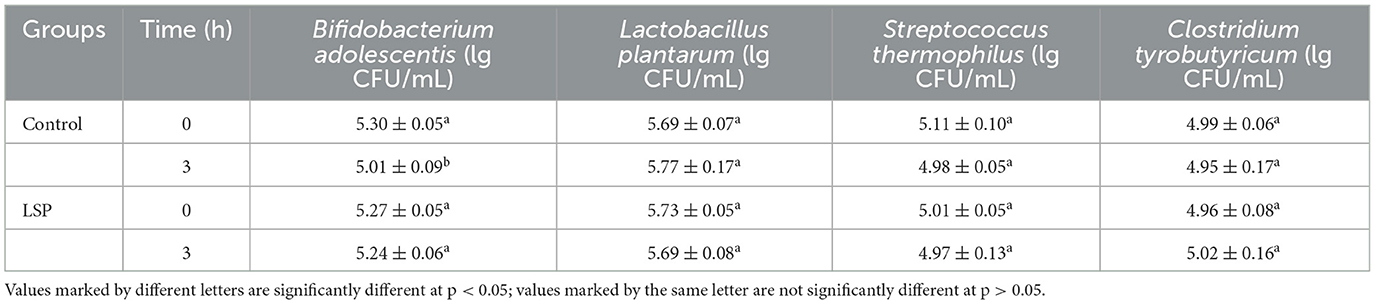
Table 3. Survival of Lactobacillus plantarum, Streptococcus thermophilus, Clostridium tyrobutyricum, and Bifidobacterium adolescentis in simulated intestinal fluid treated with polysaccharides of Laetiporus sulphureus.
4 Conclusions
In summary, the obtained results demonstrated that LSP could increase the population of C. tyrobutyricum, S. thermophilus, B. adolescentis, and L. plantarum in vitro. The developed Huang model and Reparameterized Gompertz model could be suitable for describing the growth of C. tyrobutyricum, S. thermophilus, B. adolescentis, and L. plantarum with or without LSP treatment, respectively. Additionally, LSP could improve the population of B. adolescentis and C. tyrobutyricum in simulated gastric fluid conditions. Therefore, this study can provide some theoretical bases and ideas for the investigation of the biological activity of LSP. Future research can explore the effect of LSP on pathogens or combine with other prebiotics to evaluate the characteristics of LSP as natural prebiotic supplements in food products.
Data availability statement
The original contributions presented in the study are included in the article/supplementary material, further inquiries can be directed to the corresponding authors.
Author contributions
XZ: Data curation, Formal analysis, Writing – original draft. QW: Conceptualization, Data curation, Investigation, Methodology, Software, Supervision, Visualization, Writing – original draft, Writing – review & editing. SL: Funding acquisition, Supervision, Writing – review & editing.
Funding
The author(s) declare financial support was received for the research, authorship, and/or publication of this article. This work was supported by Natural Science Foundation of Fujian Province, China [grant number: 2022J05275] and Scientific Research Foundation of Ningde Normal University [grant number: 2023T02].
Conflict of interest
The authors declare that the research was conducted in the absence of any commercial or financial relationships that could be construed as a potential conflict of interest.
Publisher's note
All claims expressed in this article are solely those of the authors and do not necessarily represent those of their affiliated organizations, or those of the publisher, the editors and the reviewers. Any product that may be evaluated in this article, or claim that may be made by its manufacturer, is not guaranteed or endorsed by the publisher.
References
Akkermans, S., Logist, F., and Van Impe, J. F. (2018). Parameter estimations in predictive microbiology: statistically sound modelling of the microbial growth rate. Food Res. Int. 106, 1105–1113. doi: 10.1016/j.foodres.2017.11.083
Altieri, C., Iorio, M. C., Bevilacqua, A., and Sinigaglia, M. (2016). Influence of prebiotics on Lactobacillus reuteri death kinetics under sub-optimal temperatures and pH. Int. J. Food Sci. Nutr. 67, 92–98. doi: 10.3109/09637486.2015.1136905
Baranyi, J., and Roberts, T. A. (1995). Mathematics of predictive food microbiology. Int. J. Food Microbiol. 26, 199–218. doi: 10.1016/0168-1605(94)00121-L
Bernal-Castro, C. A., Díaz-Moreno, C., and Gutiérrez-Cortés, C. (2019). Inclusion of prebiotics on the viability of a commercial Lactobacillus casei subsp. rhamnosus culture in a tropical fruit beverage. J. Food Sci. Technol. Mysore 56, 987–994. doi: 10.1007/s13197-018-03565-w
Che, H. Q., Zhang, H., Tian, Y. J., Lai, P. F. H., Xia, Y. J., Wang, S. J., et al. (2019). Exopolysaccharide from Streptococcus thermophilus as stabilizer in fermented dairy: binding kinetics and interactions with casein of milk. Int. J. Biol. Macromol. 140, 1018–1025. doi: 10.1016/j.ijbiomac.2019.08.146
Chow, J. (2002). Probiotics and prebiotics: a brief overview. J. Renal Nutr. 12, 76–86. doi: 10.1053/jren.2002.31759
Demirci, A., Ocak, E., and Ceylan, Z. (2024). A new nanobiotechnology material for yogurt samples: characterized nanotubes- Streptococcus thermophilus and Lactobacillus delbrueckii bulgaricus. Food Biosci. 59:104178. doi: 10.1016/j.fbio.2024.104178
Fu, C. J., Ye, K., Ma, S., Du, H. J., Chen, S. G., Liu, D. H., et al. (2023). Simulated gastrointestinal digestion and gut microbiota fermentation of polysaccharides from Agaricus bisporus. Food Chem. 418:135849. doi: 10.1016/j.foodchem.2023.135849
Hu, T., Song, J. J., Zeng, W. Y., Li, J., Wang, H. W., Zhang, Y., et al. (2020). Lactobacillus plantarum LP33 attenuates Pb-induced hepatic injury in rats by reducing oxidative stress and inflammation and promoting Pb excretion. Food Chem. Toxicol. 143:111533. doi: 10.1016/j.fct.2020.111533
Huang, L. H. (2013). Optimization of a new mathematical model for bacterial growth. Food Control 32, 283–288. doi: 10.1016/j.foodcont.2012.11.019
Hwang, H. S., and Yun, J. W. (2010). Hypoglycemic effect of polysaccharides produced by submerged mycelial culture of Laetiporus sulphureus on streptozotocin-induced diabetic rats. Biotechnol. Bioprocess Eng. 15, 173–181. doi: 10.1007/s12257-009-0160-6
Jen, C.-I., Lu, M.-K., Lai, M.-N., and Ng, L.-T. (2024). Sulfated polysaccharides of Laetiporus sulphureus fruiting bodies exhibit anti-breast cancer activity through cell cycle arrest, apoptosis induction, and inhibiting cell migration. J. Ethnopharmacol. 321:117546. doi: 10.1016/j.jep.2023.117546
Jia, Z., Peng, Y. B., Yan, X. T., Zhang, Z. Y., Fang, T., and Li, C. C. (2020). One-step kinetic analysis of competitive growth of Salmonella spp. and background flora in ground chicken. Food Control 117:107103. doi: 10.1016/j.foodcont.2020.107103
Kenari, R. E., and Razavi, R. (2022). Encapsulation of bougainvillea (Bougainvillea spectabilis) flower extract in Urtica dioica L. seed gum: characterization, antioxidant/antimicrobial properties, and in vitro digestion. Food Sci. Nutr. 10, 3436–3443. doi: 10.1002/fsn3.2944
Khalf, M., Dabour, N., Kheadr, E., and Fliss, I. (2010). Viability of probiotic bacteria in maple sap products under storage and gastrointestinal conditions. Bioresour. Technol. 101, 7966–7972. doi: 10.1016/j.biortech.2010.05.053
Kim, H. I., Kim, J.-K., Kim, J.-Y., Jang, S.-E., Han, M. J., and Kim, D.-H. (2019). Lactobacillus plantarum LC27 and Bifidobacterium longum LC67 simultaneously alleviate high-fat diet-induced colitis, endotoxemia, liver steatosis, and obesity in mice. Nutr. Res. 67, 78–89. doi: 10.1016/j.nutres.2019.03.008
Li, L., Guo, W.-L., Zhang, W., Xu, J.-X., Qian, M., Bai, W.-D., et al. (2019). Grifola frondosa polysaccharides ameliorate lipid metabolic disorders and gut microbiota dysbiosis in high-fat diet fed rats. Food Funct. 10, 2560–2572. doi: 10.1039/C9FO00075E
Li, Y. L., Zhou, E. R., Yu, Y. H., Wang, B., Zhang, L., Lei, R. Y., et al. (2024). Butyrate attenuates cold-induced hypertension via gut microbiota and activation of brown adipose tissue. Sci. Total Environ. 943:173835. doi: 10.1016/j.scitotenv.2024.173835
Lu, M.-K., Jen, C.-I., Chao, C.-H., Hsu, Y.-C., and Ng, L.-T. (2023). SPS, a sulfated galactoglucan of Laetiporus sulphureus, exhibited anti-inflammatory activities. Int. J. Biol. Macromol. 226, 1236–1247. doi: 10.1016/j.ijbiomac.2022.11.237
Luo, Y. Q., Jin, Y. Y., Wang, H. D., Wang, G., Lin, Y. Y., Chen, H. H., et al. (2024). Effects of Clostridium tyrobutyricum on lipid metabolism, intestinal barrier function, and gut microbiota in obese mice induced by high-fat diet. Nutrients 16:23. doi: 10.3390/nu16040493
Lv, X.-C., Guo, W.-L., Li, L., Yu, X.-D., and Liu, B. (2019). Polysaccharide peptides from Ganoderma lucidum ameliorate lipid metabolic disorders and gut microbiota dysbiosis in high-fat diet-fed rats. J. Funct. Foods 57, 48–58. doi: 10.1016/j.jff.2019.03.043
Ma, T., Jin, H., Kwok, L.-Y., Sun, Z. H., Liong, M.-T., and Zhang, H. P. (2021). Probiotic consumption relieved human stress and anxiety symptoms possibly via modulating the neuroactive potential of the gut microbiota. Neurobiol. Stress 14:294. doi: 10.1016/j.ynstr.2021.100294
Makki, K., Deehan, E. C., Walter, J., and Backhed, F. (2018). The impact of dietary fiber on gut microbiota in host health and disease. Cell Host Microbe 23, 705–715. doi: 10.1016/j.chom.2018.05.012
Montes, Y. M. G., Calle, E. R. V., Terán, S. G. S., García, M. R. C., Nájera, J. C. R., and Vera, M. R. L. (2024). Growth kinetics of Lactococcus lactis and Lactobacillus casei in liquid culture medium containing as prebiotics inulin or fructose. J. Sci. Food Agric. 104, 1258–1270. doi: 10.1002/jsfa.13032
Nobre, C., Sousa, S. C., Silva, S. P., Pinheiro, A. C., Coelho, E., Vicente, A. A., et al. (2018). In vitro digestibility and fermentability of fructo-oligosaccharides produced by Aspergillus ibericus. J. Funct. Food 46, 278–287. doi: 10.1016/j.jff.2018.05.004
Ouyang, J., Li, X. P., Liu, C. W., Lu, D. M., Ouyang, J., Zhou, F., et al. (2024). Junshanyinzhen tea extract prevents obesity by regulating gut microbiota and metabolic endotoxemia in high-fat diet fed rats. Food Sci. Hum. Wellness 13, 2036–2047. doi: 10.26599/FSHW.2022.9250169
Razavi, R., Kenari, R. E., Farmani, J., and Jahanshahi, M. (2020). Fabrication of zein/alginate delivery system for nanofood model based on pumpkin. Int. J. Biol. Macromol. 165, 3123–3134. doi: 10.1016/j.ijbiomac.2020.10.176
Rudzki, L., Ostrowska, L., Pawlak, D., Malus, A., Pawlak, K., Waszkiewicz, N., et al. (2019). Probiotic Lactobacillus Plantarum 299v decreases kynurenine concentration and improves cognitive functions in patients with major depression: a double-blind, randomized, placebo controlled study. Psychoneuroendocrinology 100, 213–222. doi: 10.1016/j.psyneuen.2018.10.010
Shao, W. M., Xiao, C., Yong, T. Q., Zhang, Y. F., Hu, H. P., Xie, T., et al. (2022). A polysaccharide isolated from Ganoderma lucidum ameliorates hyperglycemia through modulating gut microbiota in type 2 diabetic mice. Int. J. Biol. Macromol. 197, 23–38. doi: 10.1016/j.ijbiomac.2021.12.034
Sharma, S., Bhatia, R., Devi, K., Rawat, A., Singh, S., Bhadada, S. K., et al. (2023). A synbiotic combination of Bifidobacterium longum Bif10 and Bifidobacterium breve Bif11, isomaltooligosaccharides and finger millet arabinoxylan prevents dextran sodium sulphate induced ulcerative colitis in mice. Int. J. Biol. Macromol. 231:123326. doi: 10.1016/j.ijbiomac.2023.123326
Shi, Y. J., Cui, X. Y., Gu, S. S., Yan, X., Li, R., Xia, S., et al. (2019). Antioxidative and probiotic activities of lactic acid bacteria isolated from traditional artisanal milk Cheese from Northeast China. Probiotics Antimicrob. Proteins 11, 1086–1099. doi: 10.1007/s12602-018-9452-5
Tashiro, T., and Yoshimura, F. (2019). A neo-logistic model for the growth of bacteria. Phys. A 525, 199–215. doi: 10.1016/j.physa.2019.03.049
Tong, A. J., Hu, R. K., Wu, L. X., Lv, X. C., Li, X., Zhao, L. N., et al. (2020). Ganoderma polysaccharide and chitosan synergistically ameliorate lipid metabolic disorders and modulate gut microbiota composition in high fat diet-fed golden hamsters. J. Food Biochem. 44:13109. doi: 10.1111/jfbc.13109
Toshimitsu, T., Mochizuki, J., Ikegami, S., and Itou, H. (2016). Identification of a Lactobacillus plantarum strain that ameliorates chronic inflammation and metabolic disorders in obese and type 2 diabetic mice. J. Dairy Sci. 99, 933–946. doi: 10.3168/jds.2015-9916
Uriot, O., Denis, S., Junjua, M., Roussel, Y., Dary-Mourot, A., and Blanquet-Diot, S. (2017). Streptococcus thermophilus: from yogurt starter to a new promising probiotic candidate? J. Funct. Foods 37, 74–89. doi: 10.1016/j.jff.2017.07.038
Wang, H., Li, H. S., Li, Z. K., Feng, L., and Peng, L. (2023). Evaluation of prebiotic activity of stellariae radix polysaccharides and its effects on gut microbiota. Nutrients 15:12. doi: 10.3390/nu15224843
Wang, Y. F., Wu, B. Y., Shao, J. J., Jia, J. X., Tian, Y. Q., Shu, X., et al. (2018). Extraction, purification and physicochemical properties of a novel lectin from Laetiporus sulphureus mushroom. LWT Food Sci. Technol. 91, 151–159. doi: 10.1016/j.lwt.2018.01.032
Wei, Q., Pan, X. Y., Li, J., Jia, Z., Fang, T., and Jiang, Y. J. (2021). Isolation and molecular identification of the native microflora on Flammulina velutipes fruiting bodies and modeling the growth of dominant microbiota (Lactococcus lactis). Front. Microbiol. 12:664874. doi: 10.3389/fmicb.2021.664874
Xu, L., Wang, R., Liu, Y. N., Zhan, S. N., Wu, Z. F., and Zhang, X. (2023). Effect of tea polyphenols on the prevention of neurodegenerative diseases through gut microbiota. J. Funct. Foods 107:669. doi: 10.1016/j.jff.2023.105669
Xue, Z. H., Ma, Q. Q., Chen, Y., Lu, Y. P., Wang, Y. J., Jia, Y. N., et al. (2020). Structure characterization of soluble dietary fiber fractions from mushroom Lentinula edodes (Berk.) Pegler and the effects on fermentation and human gut microbiota in vitro. Food Res. Int. 129:108870. doi: 10.1016/j.foodres.2019.108870
Yin, C. M., Noratto, G. D., Fan, X. Z., Chen, Z. Y., Yao, F., Shi, D. F., et al. (2020). The impact of mushroom polysaccharides on gut microbiota and its beneficial effects to host: a review. Carbohydr. Polym. 250:116942. doi: 10.1016/j.carbpol.2020.116942
Ze, X. L., Duncan, S. H., Louis, P., and Flint, H. J. (2012). Ruminococcus bromii is a keystone species for the degradation of resistant starch in the human colon. ISME J. 6, 1535–1543. doi: 10.1038/ismej.2012.4
Zeng, Z. B., Huang, Z. H., Yue, W., Nawaz, S., Chen, X. Z., and Liu, J. (2023). Lactobacillus plantarum modulate gut microbiota and intestinal immunity in cyclophosphamide-treated mice model. Biomed. Pharmacother. 169:115812. doi: 10.1016/j.biopha.2023.115812
Zhang, H., Zhang, Y. H., Mu, T., Cao, J. X., Liu, X. X., Yang, X. B., et al. (2023). Response of gut microbiota and ileal transcriptome to inulin intervention in HFD induced obese mice. Int. J. Biol. Macromol. 225, 861–872. doi: 10.1016/j.ijbiomac.2022.11.151
Zhang, Z., Li, J. H., Jiang, S. M., Xu, M., Ma, T., Sun, Z. H., et al. (2023). Lactobacillus fermentum HNU312 alleviated oxidative damage and behavioural abnormalities during brain development in early life induced by chronic lead exposure. Ecotoxicol. Environ. Saf. 251:114543. doi: 10.1016/j.ecoenv.2023.114543
Zhao, R. Q., Cheng, N. H., Nakata, P. A., Zhao, L. Y., and Hu, Q. H. (2019). Consumption of polysaccharides from Auricularia auricular modulates the intestinal microbiota in mice. Food Res. Int. 123, 383–392. doi: 10.1016/j.foodres.2019.04.070
Zhu, L. F., Yao, Z. C., Ahmad, Z., Li, J. S., and Chang, M. W. (2018). Synthesis and evaluation of herbal chitosan from Ganoderma lucidum spore powder for biomedical applications. Sci. Rep. 8:12. doi: 10.1038/s41598-018-33088-5
Keywords: Laetiporus sulphureus, polysaccharides, kinetic model, gastrointestinal probiotics, in vitro digestion
Citation: Zhu X, Lin S and Wei Q (2024) Evaluation of polysaccharides from Laetiporus sulphureus on the growth of gastrointestinal probiotics and in vitro digestion. Front. Sustain. Food Syst. 8:1470426. doi: 10.3389/fsufs.2024.1470426
Received: 25 July 2024; Accepted: 29 November 2024;
Published: 18 December 2024.
Edited by:
Robin Chandra Boro, Assam Agricultural University, IndiaReviewed by:
Vandana Gulati, University of New England, AustraliaRictika Das, Ministry of Science and Technology, India
Copyright © 2024 Zhu, Lin and Wei. This is an open-access article distributed under the terms of the Creative Commons Attribution License (CC BY). The use, distribution or reproduction in other forums is permitted, provided the original author(s) and the copyright owner(s) are credited and that the original publication in this journal is cited, in accordance with accepted academic practice. No use, distribution or reproduction is permitted which does not comply with these terms.
*Correspondence: Shan Lin, MTA0OTIxNDkwM0BxcS5jb20=; Qi Wei, d2VpcWk5MTAyQDE2My5jb20=