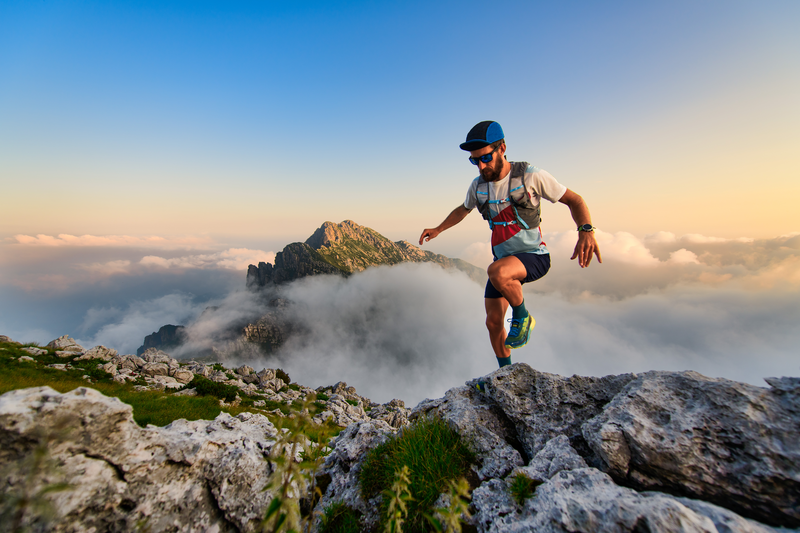
94% of researchers rate our articles as excellent or good
Learn more about the work of our research integrity team to safeguard the quality of each article we publish.
Find out more
ORIGINAL RESEARCH article
Front. Sustain. Food Syst. , 25 September 2024
Sec. Waste Management in Agroecosystems
Volume 8 - 2024 | https://doi.org/10.3389/fsufs.2024.1433129
Dairy farming is a notable source of nitrogen (N) emissions, impacting both atmospheric and aquatic ecosystems, thus necessitating a detailed analysis of nutrient dynamics to curtail nutrient wastage. However, N flow variability and its environmental ramifications differ markedly among dairy farms, and a holistic understanding of these differences is lacking in Inner Mongolia, the biggest dairy production province in China. Utilizing data from 187 dairy farms and employing the NUFER-farm model, this study assessed N flows, N use efficiency (NUE), and N losses across four predominant dairy farming systems in Inner Mongolia. These systems include traditional pastoral dairy farms (PF), smallholder dairy farms with croplands (SF), industrial landless farms (IDF), and coupled dairy cattle and cropland-intensive farms (CDF). Our findings indicate considerable differences in N flows, NUE, and losses among the systems. On average, N deposition and N fertilizer were the primary N sources for PF and SF, respectively, whereas IDF and CDF derived over 90% of their N inputs from purchased feeds. PF and SF recycled all available manure N on-farm, whereas IDF and CDF recycled only approximately 36% of the total available manure N. N losses constituted 39–72% of total N outputs, with ammonia emissions accounting for 68–73% of total N losses across all farm types. In particular, PF had a higher N loss per kilogram of dairy product than other systems. Farm-level NUE ranged from 17 to 35%, with manure management practices showing significant variability, underscoring the potential for enhanced strategies to reduce N losses through improved manure treatment.
The livestock production system accounts for 33% of the world’s arable land dedicated to livestock feed production and consumes 32% of the global agricultural water supply (FAO, 2006). In addition, livestock production has significantly altered nitrogen (N) flows, thereby generating enormous environmental pressures. Ammonia (NH3) is the dominant pathway of N emission to the air (Herrero et al., 2016; Gerber et al., 2013; Wei et al., 2018), and the release of N into aquatic ecosystems has also accelerated the water eutrophication (Biagini and Lazzaroni, 2018; Ministry of Ecology and Environment, 2020). For instance, livestock supply chains accounted for 82% of total agricultural ammonia emissions and 73% of nitrogen emissions into water bodies in the European Union (Leip et al., 2015). In response to these challenges, scientists have suggested many pathways to enhance the sustainability of livestock production systems, such as recycling nutrients from livestock waste back into the land, implementing emission mitigation measures for manure management, integrating crop–livestock systems, establishing tax policies, and optimization of feed rations (Spiegal et al., 2020; Hou et al., 2015; Ramankutty et al., 2018; Ardern et al., 2022; Ma et al., 2024).
With the increasing demands for milk consumption, the dairy production system has become more and more intensive and therefore threatens resources and the environment. Global dairy production contributed approximately one-third to NH3 emission from livestock production (Uwizeye et al., 2020), 10% of the global eutrophication potential, and 6% of acidification potential (Rufí-Salís et al., 2020), especially increased the risk of local nitrogen pollution (Finzi et al., 2020; Theobald et al., 2015). Therefore, it is crucial to understand the N flows of dairy production and further mitigate N-related environmental pollution from the dairy production system.
China was the third largest dairy production country in the world (FAO, 2016), accompanied by the industrial and intensive development of the dairy industry. There is still a vast diversity in dairy farming systems in China, including traditional, grassland-based, collectively owned, and industrialized (Bai et al., 2013). Regional-scale analysis showed that intensification (the proportion of dairy farms in collective or industrialized systems) contributed to the increase in nitrogen use efficiency (NUE) and the decrease in N losses and GHG emissions per kilogram of milk production from 1980 to 2010 in China (Zhang et al., 2017). However, the management of the dairy production system, often characterized by inefficiencies, was still recognized as a pressing issue, highlighted by Bai et al. (2016, 2018) and Zhang et al. (2019).
China has enacted new legislation to enhance the management and utilization of livestock nutrients, focusing on industrial-scale farms (Ministry of Agriculture and Rural Affairs of the State Standards Commission and Ministry of Ecology and Environment, 2023). The dairy production system, in particular, is under scrutiny due to its growing demand for milk and its negative environmental impacts (Bai et al., 2018). Consequently, the dairy sector faces substantial sustainability challenges that require innovative solutions to harmonize environmental stewardship with production demands. However, there is limited information on the up-to-date N management in different dairy farm systems. Insight into the differences in N management among dairy farming systems can help to improve the sustainability of China’s dairy industry.
Inner Mongolia, the largest dairy production province in China, accounted for 18% of total milk production or 6.0 million tons of milk in 2021 (Inner Mongolia Bureau of Statistics, 2021). Among all the livestock production systems in Inner Mongolia, the dairy production system has experienced the vastest transition. In recent years, the dairy industry in Inner Mongolia has been developing rapidly, with large-scale dairy farms adopting modern technologies for breeding, feeding, and milking, which have led to an increase in milk production and posed important effects on local nitrogen emissions and N deposition. Meanwhile, pastoral area traditional grassland-based and semi-pastoral area dairy production systems still coexist with modern high-production intensive systems. There are diversified ecosystems, including grassland, forest, river, and desert ecosystems, which are sensitive to environmental changes, especially nitrogen input changes (Li, 2022). With the increase in milk consumption and resource and environmental constraints faced by milk exporting countries, China would possibly become more milk sufficient in the future (Bai et al., 2018). Therefore, evaluating the N flows of typical dairy farming systems is very important for understanding N management, insight into more sustainable dairy production pathways, and ecosystem protection. Although nutrient balances for grassland-based milk production systems have been studied both for intensive farming systems and extensive milk production systems (Ramírez and Reheul, 2009; Treacy et al., 2008; Haas et al., 2007; Doole, 2015; Aarons et al., 2023), the two systems have not yet been compared for Inner Mongolia.
Farm-gate nutrient balances are recognized as a valuable tool for assessing the environmental pollution risks associated with agricultural practices and gauging the sustainability of nutrient use (Oenema et al., 2003). This methodology has been extensively applied to evaluate nutrient management and flows within various farming systems (Gourley et al., 2012; Hou et al., 2012; Buckley et al., 2015). Nevertheless, the primary constraint of the farm-gate approach neglects internal nutrient flows between the sectors within the farm system, treating the farm as a “black box” (Öborn et al., 2005; Koesling et al., 2017; Reimer et al., 2020; Quemada et al., 2020). Whole-farm models could get rid of constraints by considering the nutrient exchange between the components within a farm (Modin-Edman et al., 2007; del Prado et al., 2016). As a representative whole-farm model, the NUFER (Nutrient Flows in food chains, Environment, and Resource use) model can estimate N and P fluxes in the food chain from crop production to human consumption (Ma et al., 2010). Currently, the NUFER at different scales was developed to assess the losses of N and P to water and air (Ma et al., 2012; Wang et al., 2018; Bai et al., 2016; Zhao et al., 2017). For instance, NUFER-farm was developed to evaluate the nutrient flows of crop–livestock production systems at the farm level (Zhao et al., 2017).
Based on the above motivation, this study posits that the dairy production systems in Inner Mongolia, encompassing a diverse range from traditional grassland-based to modern intensive systems, exhibit significant variations in nutrient management practices and environmental impacts. However, these variations need to be clarified. This study aimed to quantify N flows, use efficiencies, and losses across predominant dairy farming systems in Inner Mongolia using a whole-farm model to elucidate variations in nitrogen management and environmental impacts among these systems. Furthermore, we conclude with suggestions for nutrient pollution control in dairy production to provide insights into the sustainability of the dairy industry.
The research was conducted in Inner Mongolia, located in northern China (Figure 1). This region, characterized by its vast expanses of grasslands and deserts, spans a diverse range of altitudes, with elevations ranging from approximately 500 m to 2,600 m above mean sea level. There are approximately 216 million ha of natural grassland and 4.95 million ha of managed grassland. It contributes approximately one-fifth of the total milk production in China (Inner Mongolia Bureau of Statistics, 2021). The dairy production system in Inner Mongolia was characterized by a mix of traditional smallholder (herd size<120 LU per farm) and modern intensive farming practices (herd size≥120 LU per farm), all of which were registered in the government regulatory system. According to land management, traditional smallholder dairy farms could be classified into smallholder dairy farms with croplands (SF) and traditional pastoral dairy farms without croplands (PF), while intensive dairy farms were grouped into industrial landless farms (IDF) and coupled dairy cattle and cropland intensive farms (CDF). In order to fully reveal the actual situation of dairy production in Inner Mongolia, we randomly selected 187 typical dairy farms to interview, including 93 PSs, 51 SFs, 34 IDFs, and 9 CDFs. The spatial distribution of these dairy farms is shown in Figure 1. Through face-to-face interviews from 2020 to 2021, detailed information about farm area, herd structure, milk yield of dairy cows, feeds, manure management (housing, storage, and treatment), and related farm nutrient management in the dairy production system was acquired. The categories of data gathered and the sources of the data are outlined in Supplementary Table S1 and Figure 1. Through face-to-face interviews from 2020 to 2021, detailed information about farm area, herd structure, and milk yield of dairy cows, feeds, manure management (housing, storage, and treatment), and related farm nutrient management in dairy production systems was acquired. The categories of data gathered and the sources of the data are outlined in Supplementary Table S1.
Figure 1. Study area and locations of farmer survey sites: (A) average precipitation of Inner Mongolia in 2021; (B) net primary productivity of Inner Mongolia in 2021; PF, SF, IDF, and CDF indicate traditional pastoral dairy farms, smallholder dairy farms with croplands, industrial landless farms, and coupled dairy cattle and cropland intensive farms, respectively. Data source: Geographic remote sensing ecological network platform.
Based on on-farm cattle herd sizes, the interviewed 187 dairy farms were first categorized into small-scale family dairy farms and large-scale intensive dairy farms. They were further classified according to land management types: traditional pastoral dairy farms (no croplands, PF), smallholder dairy farms with croplands (SF), industrial landless farms (IDF), and coupled dairy cattle and cropland intensive farms (CDF). Detailed descriptions are provided in the Supplementary materials.
The main characteristics of these farm types are presented in Table 1, and the detailed characteristics of dairy cattle manure management are shown in Supplementary Table S3. In brief, PF has a large area of grassland and a small number of dairy cattle, while there were small numbers of dairy herds but large areas of croplands and grassland in SF. Dairy production was relatively large in IDF but without any connection to the on-farm crop and grassland. The dairy herd was also large in CDF and generally coupled with cropland and grassland. IDF and CDF have generally adopted high feed and herd management technologies, compared to PF and SF. More detailed descriptions of the four farm types were provided in the Supplementary materials.
The NUFER-farm model has been widely adapted for both farm and regional scales to measure N flows within crop–livestock systems and regional food chains in different regions (Ma et al., 2010; Bai et al., 2013; Zhao et al., 2017; Meng et al., 2022). The study updated the NUFER-farm model for an improved understanding of N flows and NUE, N losses during dairy production in the four typical dairy farming systems in Inner Mongolia in 2021. We redefined the system boundary (added farm-own grassland) to establish specific N inputs and outputs for four dairy farming systems at both the herd and the whole-farm levels (Figure 2). In addition, the local parameters about nitrogen contents and emission factors, etc., were also used to update the model.
Figure 2. Nitrogen flows in smallholder dairy farms with croplands system. The dashed line refers to the boundary of the system; solid arrows refer to the nutrient inputs (on the left-hand side), outputs, and losses (on the right-hand side) of the system. The dotted arrows refer to the internal nitrogen flows. Losses include NH3 emission, N2O emission, denitrification, runoff, leaching, erosion, and manure N discharge to surface water.
Four dairy farming systems studied here included crop (grass) production, dairy cattle production, and manure management (Figure 2). In the present study, smallholder dairy farms with croplands, integrating crop planting, natural grassland, and dairy breeding within their production systems, were utilized as the prototype for this study to assess N flows, NUE, and N losses on farms. Figure 2 shows the farm system boundary and the primary N flows within such a farming system. Within smallholder dairy farms with croplands, the crop production section comprises cultivated fields and natural grassland (Figure 2). The cattle production section encompasses dairy cattle (calves, heifers, and lactating cows). The manure management component encompasses dairy cattle housing and storage and the manure treatment process (as depicted in Supplementary Figure S4).
N entered into the crop (grass) production sector (cultivated field and grassland) through natural processes, such as N fixation and atmospheric deposition, and agricultural practices such as the use of seeds, chemical fertilizers, livestock manure, and irrigation. N entered the dairy cattle production sector by obtaining feeds and live cattle while N exited the farm boundaries by means of the exportation of crop products, crop residues (mainly maize straw in our study), grass and dairy cattle products (mainly live cattle and milk), and manure, as well as losses through ammonia (NH3), nitrous oxide (N2O), denitrification, runoff, leaching, erosion, and the direct discharge of N from untreated manure into surface water. Within a smallholder dairy farm with croplands, there were internal nitrogen transfers, as part of the dairy cattle feeds were sourced from cropland and grassland, and dairy manure was used as an organic fertilizer source from the manure management sector (Figure 2).
Amounts of N fixation and deposition were obtained by multiplying field acreage by the rates of these processes (Table 2 and Supplementary Table S9). Flows of N via synthetic fertilizers, cattle manure, seeds, irrigation water, crop residues, feeds, crop products, grass and cattle products, and livestock body weight gains were calculated by multiplying their amounts by their respective N content (Supplementary Tables S4–S9). Dairy cattle excretion of N was estimated by subtracting the amount contained in cattle products and body weight gains from intakes (Ma et al., 2010; Supplementary Table S4). Equations were used to quantify N use efficiency, and N losses are given in Table 2 and Supplementary Box S1. Parameters for N loss factors and N deposition rate were derived from literature data (Ma et al., 2010; Liu et al., 2013) and the NUFER-farm model (Zhao et al., 2017). The relevant data are given in Supplementary Tables S4–S9.
N budgets in farms were calculated using a “bottom-up” approach for different management levels of each farm. First, budgets were calculated for each field (cropland and grassland) and a dairy cattle herd, with the constraint that the total input was equal to the total output. These budgets were then aggregated to field or cattle production and further integrated into a whole-farm budget. Farm N balances were calculated as the difference between total input and output in main products and byproducts. The N losses from the dairy production system were estimated using the method of NUFER (Supplementary Box S1). Soil type, precipitation, and slope were considered in the EFs of N leaching, runoff, and erosion determination. The detailed information on emission factors can be seen in Ma et al. (2010). To accommodate various farm types and sizes, inputs and outputs of N were expressed in kg N per kg dairy cattle product N (N content of milk, meat, and sold cattle). NUE for different farm systems was defined as the proportion of the total N inputs as N outputs in the main products (Table 2). Figure 3 outlines the dairy farming system and main N flows at the farm level. Table 2 and Supplementary Box S1 illustrate the equations used to quantify N flows, calculate NUE, and determine N losses to the environment.
Figure 3. Nitrogen flows of four different dairy farming systems (kg N per kilogram of product N). (A) Traditional pastoral dairy farms, (B) smallholder dairy farms with croplands, (C) industrial landless farms, and (D) coupled dairy cattle and cropland intensive farms. The black arrows represent nitrogen inputs, the green arrows represent nitrogen cycling, the orange arrows represent the accumulation of nitrogen, the red arrows represent nitrogen losses, and the purple arrows represent nitrogen outputs. The box with the black broken line indicates the farm boundary. The thickness of each arrow is positively related to the size of the flow.
N use efficiencies at both the herd and whole-farm levels and N losses were compared among farm types by Tamhane’s T2 test (equal variances not assumed) in one-way ANOVA using SPSS 19.0 (SPSS Inc., Chicago, IL). Specifically, comparisons were made between all farm types in dairy production and the manure management chain. Differences were considered significant when P was <0.05.
N inputs displayed a large variance across four different types of farms (Figure 3). First, the total external N inputs varied from 6.0 to 25.0 kg per kg of N in products (milk and meat). Second, N deposition in PF (which only had open grassy fields) and synthetic fertilizer in SF (which were mainly dominated by croplands) accounted for approximately 70% of their total N inputs on average. In contrast, more than 90% of the total N inputs came from purchased feeds for IDF and CDF. Finally, differences in planting structures resulted in a large variation of synthetic fertilizer inputs among SF. Therefore, the variation of the total N input within SF was larger than other farm types (Table 1).
N losses were the largest proportion of N outputs for all the farm types, which accounted for an average of 46% ~ 76% of the total N outputs. Moreover, grass and cattle products contributed almost equally to the output of N as products in PF, while crop products were the dominant N output in SF, accounting for 71% of total N output on average. Furthermore, manure N output to surrounding croplands was twice more than cattle products N in IDF and CDF. Additionally, approximately 64% of available manure N was exported outside of the farm in CDF (Figure 3 and Supplementary Table S2).
The substantial differences in N recycling among PF, SF, and CDF, which was mainly due to the much higher livestock densities in CDF than those in PF and SF (Figure 3, Table 1, and Supplementary Table S2). We observed that all the available livestock manure N was recycled in PF and SF, while only 36% of available livestock manure N was recycled in CDF. For the grass and crop products, the recycle ratio was 93, 51, and 100%, with the contributions to the total feed N consumption averaging 83, 72, and 16% for PF, SF, and CDF, respectively. These results showed that the livestock densities in PF and SF were generally low but very high in CDF.
The NUE was estimated at the herd and farm levels. At the herd level, the mean values of NUE in PF, SF, IDF, and CDF were 6, 8, 17, and 15%, respectively (Figure 4). This demonstrated that the dairy production in farms with intensive breeding systems (IDF and CDF) was significantly (p < 0.05) more efficient than those in the small-scale traditional farms (PF and SF). Moreover, the differences in NUE between IDF and CDF were not significant, but significant differences were found between PF and SF. Furthermore, the variations of NUE between IDF and CDF were larger than those between PF and SF. The differences in dairy productivity partly contributed to the variations of NUE at the herd level (Supplementary Figure S5 and Table 1).
Figure 4. The distribution of N use efficiency in different types of farms at herd level (left) and farm level (right). PF, traditional pastoral dairy farms; SF, Smallholder dairy farms with croplands; IDF, industrial landless farms; CDF, coupled dairy cattle and cropland intensive farms; a–d indicate the significant differences between farm types at 0.05 level.
At the farm level, the average NUE was the highest with large variations for SF (mean value: 35%), which was highly positively related to the percentage of on-farm cropland areas (Supplementary Figure S7). The large amount of crop products exported with low on-farm livestock densities partially contributed to the high NUE at the farm level for SF. The mean NUE at the farm level in PF, CDF, and SF showed lower levels (20, 17, and 17%, respectively) (Figure 4). No significant differences between them. NUE of traditional pastoral area farms (PF) was highly negatively related to the amount of on-farm N deposition (Supplementary Figure S6). Large variations in NUE at the farm level were also found among CDF and PF, which were mainly attributed to the discrepancies in on-farm livestock densities and dairy productivity.
Averagely, SF displayed the highest N losses per unit of N output as the product (Figure 5A), while the lowest N losses occurred on IDF and CDF. The largest loss of N occurred in the form of ammonia volatilization, which accounted for 68, 73, 70, and 71% of the total N loss for PF, SF, IDF, and CDF, respectively. Cattle manure NH3 volatilization was the dominant source of NH3 emissions for PF, IDF, and CDF (Figure 5A), while chemical fertilizer and manure application in croplands, as well as dropped cattle manure N during grazing, contributed most of the NH3 emissions in SF. Poor manure management was the main reason (Supplementary Table S3). De-nitrification was the second-largest source of N loss, representing 22, 12, 19, and 20% of the total N loss for PF, SF, IDF, and CDF, respectively. The N losses via N2O emission, N runoff and leaching, and direct discharge were relatively small.
Figure 5. Average N surpluses and their fates for all farm types. (A) NH3_manure, Denitrification_manure, and N2O_manure represent ammonia emission, denitrification N, and nitrous oxide emissions in feedlots, respectively, including N losses in housing, storage, and manure treatment stages. NH3_soil, Denitrification_ soil, and N2O_ soil represent ammonia emission, denitrification N, and N2O emission in the field, which include dropped manure N losses during grazing dairy cattle, chemical and manure fertilizer N losses after applied in cropland; (B) Average N losses and their fates in manure management sector for all farm types; PF, traditional pastoral dairy farms; SF, smallholder dairy farms with croplands; IDF, industrial landless farms; CDF, coupled dairy cattle and cropland intensive farms; a-d indicate the significant differences between farm types at 0.05 level.
Manure management-related N losses accounted for 90, 49, 100, and 89% of total N losses in PF, SF, IDF, and CDF, respectively (Figure 5). Compared to traditional pastoral dairy farms and smallholder dairy farms with poor manure management practices, intensive farms exhibited lower N losses from the manure management chain (Figure 5B, Supplementary Table S3). N losses during cattle housing, storage sector, and treatment process accounted for 36–45%, 26–33%, and 24–30% of total N losses, respectively.
The herd-level NUE (17 and 15% on average) of IDF and CDF in Inner Mongolia were much higher than the estimations (11%) by Bai et al. (2013) for the entire China. This dissimilarity is partially attributed to advancements in dairy production efficiency over the past decade, and the aspect that we did farm surveys and examined 43 farms (34 intensive and 9 coupled dairy cattle and cropland farms), while Bai et al. (2013) modeled a typical dairy farm for entire China. Our herd-level NUE of IDF and CDF were similar to that of intensive dairy farms in the North China Plain as estimated by Tan et al. (2022). Milk productivity and feed consumption in our study were all higher than those found by Tan et al. (2022). However, our herd-level NUE were lower than the level of NUE of the United States of America (18–33%) (Powell et al., 2006), European Union (19%) (Oenema et al., 2011), and much lower than the efficiencies of the Netherlands (23–26% in 2015) (Oenema and Oenema, 2021), which means that there is still improvement potential of dairy production in Inner Mongolia. The lower NUE in PF and SF were explained by high feed consumption and lower milk yield per head (Table 1 and Supplementary Figure S5), which agreed with Gourley et al. (2012), who also reported low conversion of N into milk and meat. Additionally, short lactation periods were also related to the low NUE at the herd level in PF and SF (Table 1). Compared to PF, SF had a higher NUE at the herd level, which might be explained by a higher rate of live cattle sales (Table 1).
Farm-level NUE was defined as the ratio of total N products (via sold alive cattle and milk, crop products, output crop residues, and grass) divided by the input of N. The average NUE in PF was much lower than grassland-based dairy farms in the Netherlands (37–45%) (Oenema and Oenema, 2022) and also lower than the mean of grassland-based dairy farms in Ireland (25%) and also lower than similar dairy production system in Germany (25–61%) (Mihailescu et al., 2015; Löw et al., 2020), which might attribute to the higher grass yield, management level, and nutrient utilization in EU (Oenema and Oenema, 2021; Powell et al., 2007). The livestock density played a significant role in determining the farm-level nutrient use efficiency (NUE) in SF and CDF. In SF, where on-farm livestock density was relatively low, we observed a notably positive correlation between farm-level NUE and on-farm cropland proportions (Supplementary Figure S7). Conversely, there was a significant negative relationship in CDF between farm-level NUE and herd-level NUE (Supplementary Figure S8). Purchased feeds mainly contributed to the variations of N inputs for IDF and CDF. The wide adoption of improved technologies and management might be the main reason for the minor variations among IDF and CDF. Akert et al. (2020) observed an increase in farm NUE for commercial dairy farms from full grazing to part-time grazing with substantial concentrate feed input. Similar to the present study, the farm NUE decreased as the use of concentrates increased. This suggests that differing livestock management strategies can influence NUE outcomes within different farming contexts. In particular, pasture-based livestock production systems, as indicated by previous studies (Burchill et al., 2016), tend to exhibit low NUE levels (<30%), resulting in substantial surpluses that are susceptible to loss.
Due to the low livestock density and high-yield croplands, 53% of total N losses in SF came from the high level of chemical fertilizer N and manure application, which was similar to the estimation for the crop-based dairy farms in the North China Plain (Zhao et al., 2017). The losses of N mainly occurred in the manure management chain for PF and CDF, which might be explained by the natural grassland (no fertilization) and very high livestock density (limited cropland), respectively. Mean N losses in IDF and CDF (3.23 kg and 4.12 kg N per product N) were higher than in the western USA (2.8 kg N per kg animal product) (Spears et al., 2003).
A formal prohibition on manure discharge into surface waters has been in effect since 2014, so there was very small manure discharge in all the interviewed farms. Ammonia volatilization was the largest pathway of N losses for all farms, which has consistently been recognized as the key air pollutant contributing to the acidification of ecosystems, increased oceanic N deposition contributing to eutrophication (Smith and Schindler, 2009; Xu et al., 2016; Liu et al., 2023). Almost all the interviewed farms stored the manure without cover, and manure treatment was mainly composting or storing in oxidation ponds, resulting in high NH3 emissions. Therefore, advanced manure treatment methods must be employed in the future.
Industrialization of agriculture has often led to a separation of crop and livestock sub-systems, thus recoupling is seen as a crucial step toward sustainable agricultural management (Ramankutty et al., 2018). Integrating silage maize-dairy farming systems is the most practical way to increase NUE and mitigate N losses for intensive dairy farms in China (Huang et al., 2021). Scenario analysis indicates that changing the intensive farm’s current open oxidation ponds and open yard manure storage to covered basin storage, combined with solid–liquid separation, would increase NUE by 12% at the farm level and decrease N losses (Tan et al., 2022). Coupling dairy cattle feedlots with constructed wetlands could reduce greenhouse gas (carbon dioxide, methane, and nitrous oxide) emissions by 24%, ammonia emissions by 14%, and N discharge into the water by 29%, compared to the semi-coupled systems (Fan et al., 2018). Therefore, the government promotes collaboration between livestock and crop production to utilize livestock manure. In this study, 100% of SF and 89% of CDF integrated dairy breeding with crop cultivation on farms, demonstrating a strong trend toward sustainable agricultural practices and resource optimization in these regions. Dairy production was inefficient in SF, but highly efficient in CDF, while only 36% of available livestock manure N was recycled on farms in CDF.
The NUFER-farm model is a whole-farm model used to describe the nutrient utilization efficiency and flows within typical dairy farming systems, including nutrient movements within the farm. It reveals existing issues in nutrient management, such as high NUE in intensive farming and imbalances in crop and livestock integration within farms. It emphasizes the need for better integration of cropping and livestock systems at the regional level.
However, it should be noted that there was some uncertainty in the NUFER-farm model. For example, our estimation of N flows and N emission factors were based on interviews of farmers, literature, and field monitoring in Inner Mongolia, such as the NH3 and N2O emission factors of maize, which will lead to uncertainty in the calculated N loss to the air. Future research on the NUFER-farm model should prioritize refining emission factors through localized field studies, implementing long-term monitoring to capture seasonal and annual variations in nitrogen dynamics, and integrating remote sensing with modeling to improve spatial representation. Additionally, gathering detailed data on farmers’ practices and behaviors will help refine model assumptions, while validation against independent datasets and sensitivity analyses will pinpoint critical parameters affecting nitrogen loss calculations. Evaluating the effectiveness of agricultural policies and management interventions will further enhance the model’s utility in promoting sustainable farming practices and environmental management in the region.
Looking to the future of Inner Mongolia’s dairy industry, the application or development of advanced technologies to mitigate N losses and the implementation of integrated crop–livestock approaches are critical steps toward achieving sustainable development. For grassland-based dairy farming systems (PF and some of SF in this study), managing grazing patterns and intensities to optimize grass utilization can reduce the N losses of deposition N and dropped manure N. Increasing dairy cattle production levels are also necessary pathways. Mitigating N losses from the cattle housing stage requires changes in floor type, manure collection technology, and frequency. In addition, it is necessary to educate farmers about best management practices for grazing systems, promote awareness of the environmental impacts of N losses, and provide guidance on sustainable practices. By combining these strategies and tailoring them to the specific characteristics of the grazing system, farmers can effectively reduce N losses and promote sustainable and environmentally friendly practices.
For other dairy farm types, it is necessary to call for manure storage with solid–liquid separation (Tan et al., 2022) and encourage large-scale dairy farms to apply advanced manure treatment technologies, such as manure acidification (Zhang et al., 2019) and continuous-closed composting technology combined with NH3 recovery technology (Wang et al., 2021), to reduce N losses. Dietary optimization with reduced crude protein input (Zhang et al., 2019), the most economical option, is also recommended. With the continual scaling of dairy production and the demand for delivering organic fertilizer, we argue that it is necessary to pay more attention to integrating crop and livestock farms to reduce manure N losses. The significant differences between dairy farms in the effectiveness of measures reflect that single-measure policies may not be effective. Instead, farm-specific technologies and measures will be needed, targeted to the specific characteristics and management level of the farms.
This study conducted an in-depth evaluation of nitrogen (N) dynamics within four distinct dairy farming systems in Inner Mongolia, assessing N flows, use efficiencies, and losses. The study identified substantial differences in N management practices among the four dairy farming systems, with traditional pastoral dairy farms (PF) and smallholder dairy farms with croplands (SF) primarily relying on N deposition and N fertilizers, respectively. In contrast, industrial landless farms (IDF) and coupled dairy cattle and cropland intensive farms (CDF) predominantly utilized purchased feeds, contributing to over 90% of their N inputs. While PF and SF recycled all available manure N on-farm, CDF recycled only 36% of their available manure N, indicating a significant gap in recycling efficiency. A substantial proportion of total N outputs, ranging from 46 to 76%, was emitted into the environment, with ammonia (NH3) emissions being the primary contributor. IDF and CDF exhibited significantly lower N losses, with values of 3.23 kg and 4.12 kg N per kg product N, respectively. Intensive farming systems (IDF and CDF) demonstrated higher herd-level N use efficiency (NUE), averaging 17 and 15%, respectively. At the farm level, SF showed the highest NUE (35%), and IDF and CDF were the lowest (both at 17%). We recommend the application of mitigation measures that consider the variability within and between dairy farming systems. It emphasizes the importance of considering the unique characteristics of natural grasslands in agricultural practices. The findings suggest that policy interventions should aim to optimize N management, promote best practices, and integrate crop–livestock systems to enhance N use efficiency and reduce environmental impact.
The raw data supporting the conclusions of this article will be made available by the authors, without undue reservation.
MQ: Conceptualization, Data curation, Formal analysis, Investigation, Methodology, Writing – original draft, Writing – review & editing. ZZ: Conceptualization, Data curation, Formal analysis, Investigation, Methodology, Writing – original draft, Writing – review & editing. JB: Conceptualization, Data curation, Formal analysis, Investigation, Writing – review & editing. NW: Data curation, Investigation, Writing – review & editing. BG: Investigation, Methodology, Supervision, Writing – review & editing. YY: Data curation, Investigation, Writing – review & editing. BB: Data curation, Formal analysis, Investigation, Writing – review & editing. LS: Data curation, Investigation, Methodology, Writing – review & editing. YX: Data curation, Investigation, Writing – review & editing. FY: Conceptualization, Data curation, Formal analysis, Methodology, Supervision, Validation, Writing – review & editing.
The author(s) declare that financial support was received for the research, authorship, and/or publication of this article. This study was supported by the Science and Technology Cooperation Agreement of the GuangXi Paper Mulberry Feed Engineering Technology Research Center (202205512010149) and the Youth Innovation Fund of Inner Mongolia Agriculture and Animal Husbandry (no. 2023QNJJM04).
We are grateful to Jin Xinpeng at the Institute of Genetic and Developmental Biology, Chinese Academy of Sciences for suggestions on data analysis.
The authors declare that the research was conducted in the absence of any commercial or financial relationships that could be construed as a potential conflict of interest.
All claims expressed in this article are solely those of the authors and do not necessarily represent those of their affiliated organizations, or those of the publisher, the editors and the reviewers. Any product that may be evaluated in this article, or claim that may be made by its manufacturer, is not guaranteed or endorsed by the publisher.
The Supplementary material for this article can be found online at: https://www.frontiersin.org/articles/10.3389/fsufs.2024.1433129/full#supplementary-material
Aarons, S. R., Gourley, C. J. P., and Powell, J. M. (2023). Estimating excreted nutrients to improve nutrient management for grazing system dairy farms. Animals 13:1404. doi: 10.3390/ani13081404
Akert, F. S., Dorn, K., Frey, H., and Hofstetter, P. (2020). Farm-gate nutrient balances of grassland-based milk production systems with full-or part-time grazing and fresh herbage indoor feeding at variable concentrate levels. Nutr. Cycl. Agroecosyst. 117, 383–400. doi: 10.1007/s10705-020-10072-y
Ardern, J., O’Connor, D., and Shaw, J. (2022). Pragmatic proposal to reduce agricultural emissions and enhance exports and economy. Available at: https://www.beehive.govt.nz/release/pragmatic-proposal-reduce-agricultural-emissions-and-enhance-exports-and-economy (Accessed March 28, 2024)
Bai, Z. H., Lee, M. R., Ma, L., Ledgard, S., Oenema, O., Velthof, G. L., et al. (2018). Global environmental costs of China’s thirst for milk. Glob. Chang. Biol. 24, 2198–2211. doi: 10.1111/gcb.14047
Bai, Z. H., Ma, L., Jin, S. Q., Ma, W. Q., Velthof, G. L., Oenema, O., et al. (2016). Nitrogen, phosphorus, and potassium flows through the manure management chain in China. Environ. Sci. Technol. 50, 13409–13418. doi: 10.1021/acs.est.6b03348
Bai, Z. H., Ma, L., Oenema, O., Chen, Q., and Zhang, F. S. (2013). Nitrogen and phosphorus efficiencies in dairy production in China. J. Environ. Qual. 42, 990–1001. doi: 10.2134/jeq2012.0464
Biagini, D., and Lazzaroni, C. (2018). Eutrophication risk arising from intensive dairy cattle rearing systems and assessment of the potential effect of mitigation strategies. Agric. Ecosyst. Environ. 266, 76–83. doi: 10.1016/j.agee.2018.07.026
Buckley, C., Wall, D. P., Moran, B., and Murphy, P. N. C. (2015). Developing the EU farm accountancy data network to derive indicators around the sustainable use of nitrogen and phosphorus at farm level. Nutr. Cycl. Agroecosyst. 102, 319–333. doi: 10.1007/s10705-015-9702-9
Burchill, W., Lanigan, G. J., Li, D., Williams, M., and Humphreys, J. (2016). A system N balance for a pasture-based system of dairy production under moist maritime climatic conditions. Agric. Ecosyst. Environ. 220, 202–210. doi: 10.1016/j.agee.2015.12.022
del Prado, A., Corre, W. J., Gallejones, P., Pardo, G., Pinto, M., del Hierro, O., et al. (2016). NUTGRANJA 2.0: a simple mass balance model to explore the effects of different management strategies on nitrogen and greenhouse gases losses and soil phosphorus changes in dairy farms. Mitig. Adapt. Strat. G.L. 21, 1145–1164. doi: 10.1007/s11027-014-9598-8
Doole, G. J. (2015). Efficient mitigation of nitrogen leaching in pasture-based dairy systems. Nutr. Cycl. Agroecosyst. 101, 193–209. doi: 10.1007/s10705-015-9669-6
Fan, X., Chang, J., Ren, Y., Wu, X., Du, Y. Y., Xu, R. H., et al. (2018). Recoupling industrial dairy feedlots and industrial farmlands mitigates the environmental impacts of milk production in China. Environ. Sci. Technol. 52, 3917–3925. doi: 10.1021/acs.est.7b04829
FAO (2016). Food and agricultural organization, Rome. Available at: http://faostat.fao.org/site/291/default.aspx (Accessed March 25, 2024)
FAO (2006). “Livestock’s long shadow: environmental issues and options,” in Food and agriculture organization/livestock environment and development paper. Rome: FAO, 101–111.
Finzi, A., Mattachini, G., Lovarelli, D., Riva, E., and Provolo, G. (2020). Technical, economic, and environmental assessment of a collective integrated treatment system for energy recovery and nutrient removal from livestock manure. Sustain. For. 12:2756. doi: 10.3390/su12072756
Gerber, P. J., Steinfeld, H., Henderson, B., Mottet, A., Opio, C., Dijkman, J., et al. (2013). Tackling climate change through livestock. A global assessment of emissions and mitigation opportunities. Rome: Food and Agriculture Organization of the United Nations (FAO).
Gourley, C. J. P., Dougherty, W. J., Weaver, D. M., Aarons, S. R., Awty, I. M., Gibson, D. M., et al. (2012). Farm-scale nitrogen phosphorus potassium and sulfur balances and use efficiencies on Australian dairy farms. Anim. Prod. Sci. 52, 929–944. doi: 10.1071/AN11337
Gu, B., Leach, A. M., Ma, L., Galloway, J. N., Chang, S. X., and Chang, J. (2013). Nitrogen footprint in China: food, energy, and nonfood goods. Environ. Sci. Technol. 47, 9217–9224. doi: 10.1021/es401344h
Haas, G., Deittert, C., and Kopke, U. (2007). Farm-gate nutrient balance assessment of organic dairy farms at different intensity levels in Germany renew. Agric. Food Syst. 22, 223–232. doi: 10.1017/S1742170507001780
Herrero, M., Henderson, B., Havlik, P., Thornton, P. K., Conant, R. T., Smith, P., et al. (2016). Greenhouse gas mitigation potentials in the livestock sector. Nat. Clim. Chang. 6, 452–461. doi: 10.1038/nclimate2925
Hou, Y., Gao, Z., Heimann, L., Roelcke, M., Ma, W., and Nieder, R. (2012). Nitrogen balances of smallholder farms in major cropping systems in a peri-urban area of Beijing China. Nutr. Cycl. Agroecosyst. 92, 347–361. doi: 10.1007/s10705-012-9494-0
Hou, Y., Velthof, G., and Oenema, O. (2015). Mitigation of ammonia, nitrous oxide and methane emissions from manure management chains: a meta-analysis and integrated assessment. Glob. Chang. Biol. 21, 1293–1312. doi: 10.1111/gcb.12767
Huang, X. L., Shi, B. Y., Wang, S., Yin, C. B., and Fang, L. N. (2021). Mitigating environmental impacts of milk production via integrated maize silage planting and dairy cow breeding system: a case study in China. J. Clean. Prod. 309:127343. doi: 10.1016/j.jclepro.2021.127343
Koesling, M., Hansen, S., and Azzaroli, B. M. (2017). Variations in nitrogen utilisation on conventional and organic dairy farms in Norway. Agric. Syst. 157, 11–21. doi: 10.1016/j.agsy.2017.06.001
Leip, A., Billen, G., Garnier, J., Grizzetti, B., Lassaletta, L., Reis, S., et al. (2015). Impacts of European livestock production: nitrogen, Sulphur, phosphorus and greenhouse gas emissions, land-use, water eutrophication and biodiversity. Environ. Res. Lett. 10:115004. doi: 10.1088/1748-9326/10/11/115004
Liu, L., Xu, W., Wen, Z., Liu, P., Xu, H., Liu, S., et al. (2023). Modeling global oceanic nitrogen deposition from food systems and its mitigation potential by reducing overuse of fertilizers. PNAS 120, 1–8. doi: 10.1073/pnas.2221459120
Liu, X., Zhang, Y., Han, W., Tang, A., Shen, J., Cui, Z. L., et al. (2013). Enhanced nitrogen deposition over China. Nature 494, 459–462. doi: 10.1038/nature11917
Li, Y. S. (2022). Dry atmospheric deposition of nitrogen into Inner Mongolia. Thesis. Inner Mongolia Agricultural University, Hohhot, China
Löw, P., Karatay, Y. N., and Osterburg, B. (2020). Nitrogen use efficiency on dairy farms with different grazing systems in northwestern Germany. Environ. Res. Commun. 2:105002.
Ma, L., Ma, W. Q., Velthof, G. L., Wang, F. H., Qin, W., Zhang, F. S., et al. (2010). Modeling nutrient flows in the food chain of China. J. Environ. Qual. 39, 1279–1289. doi: 10.2134/jeq2009.0403
Ma, L., Velthof, G. L., Wang, F. H., Qin, W., Zhang, W. F., Liu, Z., et al. (2012). Nitrogen and phosphorus use efficiencies and losses in the food chain in China at regional scales in 1980 and 2005. Sci. Total Environ. 434, 51–61. doi: 10.1016/j.scitotenv.2012.03.028
Ma, Y. F., Hou, Y., Zhang, T., Zhu, X. Q., Fang, Q. C., and Oenema, O. (2024). Decreasing environmental footprints of dairy production systems through optimization of feed rations and origins. Glob. Chang. Biol. 461:142637. doi: 10.1016/j.jclepro.2024.142637
Meng, F. L., Wang, M. R., Strokal, M., Kroeze, C., Ma, L., Li, Y. N., et al. (2022). Nitrogen losses from food production in the North China plain: a case study for Quzhou. Sci. Total Environ. 816:151557. doi: 10.1016/j.scitotenv.2021.151557
Mihailescu, E., Ryan, W., Murphy, P. N. C., Casey, I. A., and Humphreys, J. (2015). Economic impacts of nitrogen and phosphorus use efficiency on nineteen intensive grass-based dairy farms in the south of Ireland. Agric. Syst. 132, 121–132. doi: 10.1016/j.agsy.2014.09.008
Ministry of Agriculture and Rural Affairs of the State Standards Commission and Ministry of Ecology and Environment (2023). The guiding opinions on promoting the construction of a standard system for the utilization of livestock and poultry waste resources. Available at: https://www.samr.gov.cn/zw/zfxxgk/fdzdgknr/bzjss/art/2023/art_96258038062f4dcba1bdd3bffcfc283a.html (Accessed March 28, 2024)
Ministry of Ecology and Environment (2020). Bulletin of the second National Census of pollution sources. Available at at: https://www.mee.gov.cn/xxgk2018/xxgk/xxgk01/202006/t20200610_783547.html (Accessed March 25, 2024)
Modin-Edman, A.-K., O’born, I., and Sverdrup, H. (2007). FARMFLOW—A dynamic model for phosphorus mass flow, simulating conventional and organic management of a Swedish dairy farm. Agric. Syst. 94, 431–444. doi: 10.1016/j.agsy.2006.11.007
Öborn, I., Modin-Edman, A.-K., Bengtsson, H., Gustafson, G. M., Salomon, E., Nilsson, S. I., et al. (2005). A system approach to assess farm-scale nutrient and trace element dynamics: a case study at the Ojebyn dairy farm. Ambio 34, 301–310. doi: 10.1579/0044-7447-34.4.301
Oenema, J., and Oenema, O. (2021). Intensification of grassland-based dairy production and its impacts on land nitrogen and phosphorus use efficiencies. Front Agricult. Sci. Eng. 8, 130–147. doi: 10.15302/J-FASE-2020376
Oenema, J., and Oenema, O. (2022). Unraveling feed and nutrient use efficiencies in grassland-based dairy farms. Front. Sustainable. Food Syst. 6:846561. doi: 10.3389/fsufs.2022.846561
Oenema, J., van Keulen, H., Schils, R. L. M., and Aarts, H. F. M. (2011). Participatory farm management adaptations to reduce environmental impact on commercial pilot dairy farms in the Netherlands. NJAS Wageningen J. Life Sci. 58, 39–48. doi: 10.1016/j.njas.2010.08.001
Oenema, O., Kros, H., and de Vries, W. (2003). Approaches and uncertainties in nutrient budgets: implications for nutrient management and environmental policies. Eur. J. Agron. 20, 3–16. doi: 10.1016/S1161-0301(03)00067-4
Powell, J., Jackson-Smith, D. B., McCrory, D. F., Saam, H., and Mariola, M. (2007). Nutrient management behavior on Wisconsin dairy farms. Agron. J. 99, 211–219. doi: 10.2134/agronj2006.0116
Powell, J. M., Jackson-Smith, D. B., McCrory, D. F., Saam, H., and Mariola, M. (2006). Validation of feed and manure data collected on Wisconsin dairy farms. J. Dairy Sci. 89, 2268–2278. doi: 10.3168/jds.S0022-0302(06)72298-6
Quemada, M., Lassaletta, L., Jensen, L. S., Godinot, O., Brentrup, F., Buckley, C., et al. (2020). Exploring nitrogen indicators of farm performance among farm types across several European case studies. Agric. Syst. 177:102689. doi: 10.1016/j.agsy.2019.102689
Ramankutty, N., Mehrabi, Z., Waha, K., Jarvis, L., Kremen, C., Herrero, M., et al. (2018). Trends in global agricultural land use: implication for environmental health and food security. Annu. Rev. Plant Biol. 69, 789–815. doi: 10.1146/annurev-arplant-042817-040256
Ramírez, E., and Reheul, D. (2009). Statistical modelling of nitrogen use efficiency of dairy farms in Flanders. Agron. Sustain. Dev. 29, 339–352. doi: 10.1051/agro/2008065
Reimer, M., Möller, K., and Hartmann, T. E. (2020). Meta-analysis of nutrient budgets in organic farms across Europe. Org. Agr. 10, 65–77. doi: 10.1007/s13165-020-00300-8
Rufí-Salís, M., Petit-Boix, A., Villalba, G., Ercilla-Montserrat, M., Sanjuan-Delmás, D., Parada, F., et al. (2020). Identifying eco-efcient year-round crop combinations for rooftop greenhouse agriculture. Int. J. Life Cycle Assess. 25, 564–576. doi: 10.1007/s11367-019-01724-5
Smith, V. H., and Schindler, D. W. (2009). Eutrophication science: where do we go from here? Trends Ecol. Evolution 24, 201–207. doi: 10.1016/j.tree.2008.11.009
Spears, R. A., Kohn, R. A., and Young, A. J. (2003). Whole-farm nitrogen balance on western dairy farms. J. Dairy Sci. 86, 4178–4186. doi: 10.3168/jds.S0022-0302(03)74033-8
Spiegal, S., Kleinman, P. J. A., Endale, D. M., Bryant, R. B., Dell, C., Goslee, S., et al. (2020). Manuresheds: advancing nutrient recycling in US agriculture. Agric. Syst. 182:102813. doi: 10.1016/j.agsy.2020.102813
Tan, M. X., Hou, Y., Zhang, L., Shi, S. L., Long, W. T., Ma, Y. F., et al. (2022). Nutrient use efficiency of intensive dairy farms in China-current situation and analyses of options for improvement. Agric. Syst. 203:103495. doi: 10.1016/j.agsy.2022.103495
Theobald, E. J., Ettinger, A. K., Burgess, H. K., DeBey, L. B., Schmidt, N. R., Froehlich, H. E., et al. (2015). Global change and local solutions: tapping the unrealized potential of citizen science for biodiversity research. Biol. Conserv. 181, 236–244. doi: 10.1016/j.biocon.2014.10.021
Treacy, M., Humphreys, J., McNamara, K., Browne, R., and Watson, C. J. (2008). Farm-gate nitrogen balances on intensive dairy farms in the south west of Ireland. Ir. J. Agric. Food Res. 47, 105–117.
Uwizeye, A., de Boer, I. J. M., Opio, C. I., Schulte, R. P. O., Falcussi, A., Tempio, G., et al. (2020). Nitrogen emissions along global livestock supply chains. Nat. Food. 1, 437–446. doi: 10.1038/s43016-020-0113-y
Wang, H. D., Zhao, Z. Q., Winiwarter, W., Bai, Z. H., Wang, X., Fan, X. W., et al. (2021). Strategies to reduce ammonia emissions from livestock and their cost-benefit analysis: A case study of Sheyang county. Environ. Pollut. 290:118045. doi: 10.1016/j.envpol.2021.118045
Wang, M. R., Ma, L., Strokal, M., Ma, W. Q., Liu, X. J., and Kroeze, C. (2018). Hotspots for nitrogen and phosphorus losses from food production in China: a county-scale analysis. Environ. Sci. Technol. 52, 5782–5791. doi: 10.1021/acs.est.7b06138
Wei, S., Bai, Z. H., Chadwick, D., Hou, Y., Qin, W., Zhao, Z. Q., et al. (2018). Greenhouse gas and ammonia emissions and mitigation options from livestock production in peri-urban agriculture: Beijing - a case study. J. Clean. Prod. 178, 515–525. doi: 10.1016/j.jclepro.2017.12.257
Xu, W., Wu, Q. H., Liu, X. J., Tang, A. H., Dore, A., and Heal, M. (2016). Characteristics of ammonia acid gases and PM2.5 for three typical land-use types in the North China plain. Environ. Sci. Pollut. Res. 23, 1158–1172. doi: 10.1007/s11356-015-5648-3
Zhang, N. N., Bai, Z. H., Luo, J. F., Ledgard, S., Wu, Z. G., and Ma, L. (2017). Nutrient losses and greenhouse gas emissions from dairy production in China: lessons learned from historical changes and regional differences. Sci. Total Environ. 598, 1095–1105. doi: 10.1016/j.scitotenv.2017.04.165
Zhang, N. N., Bai, Z. H., Winiwarter, W., Ledgard, S., Lou, J. F., Liu, J., et al. (2019). Reducing Ammonia emissions from dairy cattle production via cost-effective manure management techniques in China. Environ. Sci. & Technol. 53, 11840–11848. doi: 10.1021/acs.est.9b04284
Keywords: dairy farm, nitrogen use efficiency, nitrogen flows, nitrogen losses, nitrogen management
Citation: Qili M, Zhao Z, Bao J, Wu N, Gou B, Ying Y, Bilige B, Sun L, Xue Y and Yang F (2024) Nitrogen use efficiencies, flows, and losses of typical dairy farming systems in Inner Mongolia. Front. Sustain. Food Syst. 8:1433129. doi: 10.3389/fsufs.2024.1433129
Received: 21 June 2024; Accepted: 03 September 2024;
Published: 25 September 2024.
Edited by:
José Martinez, Institut National de Recherche pour l’Agriculture, l’Alimentation et l’Environnement (INRAE), FranceReviewed by:
Hatirarami Nezomba, University of Zimbabwe, ZimbabweCopyright © 2024 Qili, Zhao, Bao, Wu, Gou, Ying, Bilige, Sun, Xue and Yang. This is an open-access article distributed under the terms of the Creative Commons Attribution License (CC BY). The use, distribution or reproduction in other forums is permitted, provided the original author(s) and the copyright owner(s) are credited and that the original publication in this journal is cited, in accordance with accepted academic practice. No use, distribution or reproduction is permitted which does not comply with these terms.
*Correspondence: Zhanqing Zhao, enpxZ3Jhc3NAMTYzLmNvbQ==; Fuyu Yang, eWZ1eXVAMTI2LmNvbQ==
Disclaimer: All claims expressed in this article are solely those of the authors and do not necessarily represent those of their affiliated organizations, or those of the publisher, the editors and the reviewers. Any product that may be evaluated in this article or claim that may be made by its manufacturer is not guaranteed or endorsed by the publisher.
Research integrity at Frontiers
Learn more about the work of our research integrity team to safeguard the quality of each article we publish.