- 1ICAR-Central Soil Salinity Research Institute (CSSRI), Karnal, India
- 2ICAR-Indian Institute of Maize Research (IIMR), Ludhiana, India
Soil provides essential nutrients for plant growth, but excess salts hinder development, making crops more vulnerable under climate change conditions. Soil microorganisms play a significant role in nutrient cycling. However, limited information is available on microbial behavior/community changes, and functional diversity in different soil types (normal, sodic, and highly sodic) and cropping systems [rice-wheat (RW); rice-wheat-mungbean (RWMb); maize-wheat-mungbean (MWMb)] and management practices in the north-western Indo-Gangetic Plains of India. We investigated the influence of different soil types on physical and chemical properties at the surface level (0–15 cm) in relation to soil microbial population, activities, and functional diversity, focusing on community-level physiological profiling (CLPP) under different agriculture systems. Seven treatment combinations of soil types, cropping systems, and management practices were evaluated. Soil pHs were found to be lower in zero-till (ZT)-based sodic soil than in conventional-till (CT) sodic soil. Soil organic carbon (SOC) (0.91 and 0.90%) content and available N (154.46 and 132.74 kg ha−1) were higher with the ZT-based system under normal (N) soils (ZT-RWMb-N and ZT-MWMb-N) than in CT-based normal soil (0.67 and 121.04 kg ha−1). Similarly, higher SOC and N (0.85 and 76.11 kg ha−1) were found under ZT management in sodic soils (S) than under CT management (0.73% and 121.05 kg ha−1). Substrate utilization (amino acids, amines, carbohydrates, carboxylic acids, phenolic compounds, and polymers) increased with the incubation period. During 0–120 h of incubation, the highest utilization of amino acids, amines, carboxylic acids, phenolic compounds, and polymers was observed for ZT-MWMb-S soils, while the lowest was recorded for ZT-MWMb-N soils. Under high salt conditions, soil enzymatic activities (dehydrogenase, acid phosphatase, alkaline phosphatase, etc.) declined significantly compared to normal soils, affecting soil chemical and physical conditions. Microbial population and enzyme activities decreased with increasing salt stress across all cropping systems. These findings suggest that adopting efficient crop management practices can help mitigate the adverse effects of soil salinity on microbial diversity and soil health, thereby enhancing sustainable agricultural productivity in salt-affected regions.
1 Introduction
The escalation of salt-affected soils (SASs) over time is well-documented, with climate change further exacerbating this phenomenon. These soils pose significant challenges to crop production, leading to substantial agricultural yield losses and threatening food security. SASs encompass approximately 20% of cultivated land and 33% of irrigated agricultural lands worldwide (Srivastava and Kumar, 2015). The extent of salt-affected areas is expected to increase even further by 2050 due to the aggregating effects of climate change (Central Soil Salinity Research Institute, 2014). Salt concentration profoundly influences soil structure, infiltration rate, nutrient availability, and soil biodiversity, thereby regulating the microbial populations and enzymatic activities, which ultimately influence plant metabolic activities and growth (Wang et al., 2020; Mukhopadhyay et al., 2021; Choudhary et al., 2023). Globally, agriculture is facing the multiple challenges of meeting the food demands of a growing population from limited land resources. Addressing salinity issues and maximizing the productive use of SAS is imperative. In South Asia, saline areas are typically rain-fed, characterized by mono-cropping during the rainy season (July to October). The high evaporative demand and subsequent salt flux toward surface soil during the dry winter season restrict the feasibility of incorporating a second crop into the rotation. Experiments have demonstrated that in conjunction with conservation tillage, mulching, and deficit irrigation, the intensive cropping systems beyond rain-fed mono-cropping can increase profitability, improve soil health, and increase overall food production (Li et al., 2013; Mosaffa and Sepaskhah, 2019; Mutsamba et al., 2020).
The Indo-Gangetic Plains (IGP) of South Asia, spanning over 13.5 million hectares (Mha), are pivotal in sustaining the livelihoods of over 400 million people through the rice-wheat (RW) agricultural system (Kumar et al., 2018). Conventional tillage (CT) or farmer practices in the IGP have resulted in the overexploitation of groundwater, soil, and energy resources, impeding sustainable agricultural biodiversity and crop productivity (Choudhary et al., 2018a). Conservation agriculture (CA), built on minimum soil disturbance, crop rotation, and crop residue retention as soil cover, emerges as a sustainable approach to crop production. CA conserves soil and water resources while reducing input costs (Margenot et al., 2017; Jat et al., 2018, 2022) and also mitigates soil sodicity to some extent in the IGP (Jat et al., 2022). Maize and wheat crops are well-fitted and responsive to CA-based practices (Jat et al., 2020; Pooniya et al., 2021). A great potential exists to enhance both the yields and sustainability in the maize-wheat rotation (MWR) by integrating CA-based management practices. Research indicates that these practices contribute to improved initial crop establishment with greater input efficiency. Moreover, they create opportunities for timely seeding of both crops within the rotation, thereby leading to sustained yields without compromising the degradation of the natural resources (Jat et al., 2019b; Pooniya et al., 2022). The adoption of best management practices significantly improves crop yields while minimizing production costs and sodicity simultaneously (Suhas et al., 2017; Wang et al., 2017; Hawes et al., 2018; Jat et al., 2019b, 2022).
Under different research trials in reclaimed or partially alkali/sodic soils, CA practices showed potential for enhanced soil quality and reduced fertilizer nutrient use (Rezapour et al., 2013; Jat et al., 2018, 2019a,b, 2022; Choudhary et al., 2018b, 2020). Not only management practices but salt type and amount also influence soil properties and soil quality (Choudhary et al., 2023; Jat et al., 2023). To maintain the sustainability of the cropping system, especially under SASs, it is necessary to investigate how all of the soil properties under sodic soil interact with one another under different agriculture management practices. To know the effect of different management practices and other related factors on soil quality and health, studies of soil enzyme activities are used as these are very sensitive to any change in soil or its surrounding environment (Sumner and Naidu, 1998; Singh, 2016). The effect of any additive, amendment, or salt can be observed on soil biological properties before it is noticed on soil chemical or physical properties. Salt stress can be reduced by adopting CA-based practices in SASs. Changes in soil properties resulting from the adoption of CA practices can be studied by examining the microbial activities of that soil. Different CA-based management practices, such as crop residue mulching and efficient management practices, have the potential to increase crop yields with a lower risk of root zone salt stress. This is due to reduced salt loading, decreased evaporation, and improved water distribution. In the current scenario of climate change, it is necessary to develop alternative cropping systems with matching agronomic practices having a net effect of salt leaching for sustained productivity. Several studies have been conducted in isolation on the effect of agriculture management practices (Choudhary et al., 2022b; Khan et al., 2023; Mondaca et al., 2024) and also on the effect of salt concentration (Choudhary et al., 2023; Zhang et al., 2024) on soil microbial properties but the study on the agriculture management practices under SASs and their effect on soil functional diversity is untouched. Keeping agriculture management practices, different salt levels, and their effect on soil microbial properties especially on microbial functional diversity (CLPP) in mind the following objectives were set for this study: (1) to observe the effect of agriculture management practices (crop rotation, tillage, and residue management) on soil microbial community composition under normal and sodic soils; (2) to assess the relationship between soil chemical properties [EC, pH, organic carbon (OC), N, P, and K] and soil biological properties under normal and sodic soils.
2 Materials and methods
2.1 Experimental details and soil sampling
A study was conducted at the experimental farm of ICAR-Central Soil Salinity Research Institute, Karnal (29° 42″20.7′ N latitude, 76° 57″19.79′ E longitude), India (243 m above MSL). This region has a sub-tropical climate characterized by three distinct seasons, i.e., summer/Zaid with a hot and dry spell from April to June, a wet summer spell from July to September, and a cool and dry winter spell from October to March. The annual rainfall is 670 mm, 70–80% of which occurs from June to September through the southwest monsoon. The soil of the experimental field was silty loam in texture. The experiment consists of seven portfolios of different soil types (normal, sodic, and highly sodic) with varied management practices under three cropping systems [RW, rice-wheat-mungbean (RWMb), and maize-wheat-mungbean (MWMb)]. The details of these management scenarios are presented in Table 1. The experiment was conducted in a completely randomized block design (RCBD) with three replications. In this study, a portfolio of management practices has been evaluated under different management systems.
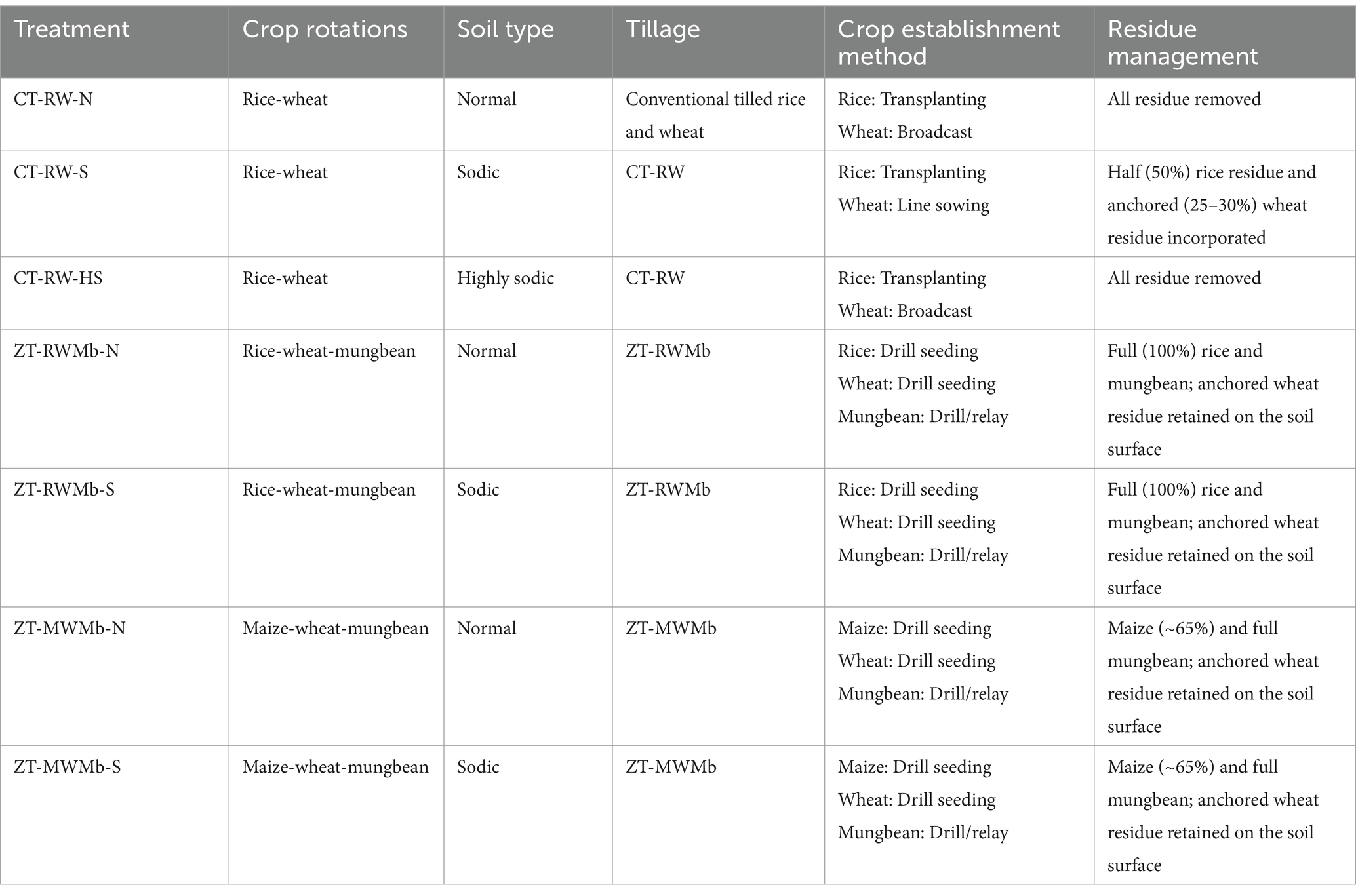
Table 1. Cropping system, tillage, crop establishment method, and residue management protocols under different scenarios.
In this study, soil samples were collected from these management scenarios at 0–15 cm soil depths after the harvest of rice in 2021 (after 9 years of zero tillage). The samples were categorized into seven groups based on the management practices and sodicity levels, as soil samples were collected from CT-RW as normal (CT-RW-N), sodic (CT-RW-S), and highly sodic (CT-RW-HS); from ZT-RWMb as normal (ZT-RWMb-N) and sodic (ZT-RWMb-S); and from ZT-MWMb as normal (ZT-MWMb-N) and sodic (ZT-MWMb-S). The details of management protocols are given in Table 1. Soil samples were divided into two parts. One part is for soil biological analysis, in which fresh soil samples were kept in a refrigerator at 4°C until the completion of analysis. The second part was air-dried in the shade, ground through a 2 mm size sieve, and then stored in plastic boxes for analysis of soil chemical properties.
2.2 Analysis of soil physicochemical and biological parameters
2.2.1 Analysis of chemical parameters
The standard procedures were used to determine the chemical characteristics of the soil samples. The electrical conductivity (ECe) of the extract of saturated paste and the soil pHs was measured by the procedure (USSL, 1954). The potassium (K+) content in a soil saturation extract was measured by a flame photometer (Walkley and Black, 1934; Bhargava, 2003). Oxidizable OC was quantified by the wet oxidation method (Subbiah and Asija, 1956). The available nitrogen (N) in soil was determined by the alkaline permanganate method (Olsen et al., 1954). The available phosphorus (0.5 M NaHCO3 extractable P) in soil was determined colorimetrically following the ascorbic acid reductant method as outlined by Lindsay and Norvell (1978). Available (DTPA-extractable) Fe, Mn, Zn, and Cu of the soil samples were estimated by using an atomic absorption spectrophotometer (AAS) following the method (Tabatabai and Bremner, 1969).
2.2.2 Analysis of biological parameters
The collected soil samples were examined for enzyme activities, microbial colony counts, and CLPP. The dehydrogenase activity was calculated in accordance with Moeskops et al. (2010) description. Phosphatase activities in soils were assayed by the method described by Garau et al. (2007) which involves the determination of p-nitrophenol released by incubation at 37°C for 1 h of 1 g soil with 0.25 mL of toluene, 4 mL of universal buffer, and 1 mL of 5 Mm substrate. For bacterial and fungal colony counts, the serial dilution method was used as described (Choudhary et al., 2018a). For the CLPP analysis, 10 g of soil was homogenized in a rotary shaker after being suspended in 100 mL of 50 mM phosphate buffer (pH 7.0). After the suspension had settled, the supernatant was transferred into tubes. After that, the tubes were centrifuged twice for 5 min at 5000 rpm at room temperature. From the tubes, 150 μL of supernatant was inoculated into 96 wells of BiOLOG Eco Plates (BiOLOG, Hayward, CA, United States) and kept in the dark at room temperature. The absorbance was measured with a microplate reader using the Biolog Micro Station every 24 h for 120 h at 590 nm wavelength. The 31 carbon sources and the control well (water) were in triplicate in the 96 well systems of the BiOLOG Eco Plates. For CLPP, different parameters were calculated by using the optical density (OD) values of the wells of BiOLOG Eco Plates (Garau et al., 2007; Feigl et al., 2017), with the following equations (1–6):
where ODi is the measured corrected OD value of each substrate containing well and N is the number of substrates; here, the N value is 31.
where ODi is the measured corrected OD value of the substrates within the substrate category and N is the number of substrates in the category.
where Pi is calculated by using ODi value divided by ΣODi value.
where ODi value is more or equal to 0.15 after 120 h.
where ni refers to the relative absorbance value for each of the C source wells by subtracting the absorbance value of the control well.
2.3 Statistical analysis
The analysis of variance (ANOVA) method was used by R software to statistically analyze the experiment data among the various soil types (Gomez and Gomez, 1984). Tukey’s honestly significant difference (HSD) at the 5% level of significance was used to compare the treatment means. Principal component analysis (PCA) and correlations matrix between various soil chemical characteristics, AWCD, and enzyme activity in different types of soil were examined by using R software for the existence of a significant positive association throughout the whole dataset.
3 Results
3.1 Soil chemical properties
Various soil chemical parameters, such as saturated soil pH (pHs), electric conductivity (ECe), OC, and available nutrients (N, P, K, and micronutrients), were assessed across seven treatments. These treatments involved a combination of two types of soils (normal and sodic) and two tillage practices (ZT and CT) in RW, RWMb, and MWMb systems (Table 1). The highest pHs (10.19) and ECe (2.49) were observed in CT-RW-HS soil, followed by CT-RW-S soil (9.75 and 2.20). Conversely, the lowest values of pHs (7.21) and ECe (1.22) were observed in ZT-RWMb-N and CT-RW-N soils, respectively (Table 2). Significant differences in soil chemical properties, such as OC, available N, P, K, and micronutrients (Cu, Zn, Fe, and Mn), were observed among different management practices and soil types. The OC content was found to be higher in ZT-RWMb-N (0.91%) and ZT-MWMb-N (0.90%), which were at par with ZT under sodic soils (0.86 and 0.85%), while the lowest OC was recorded by CT-RW-HS soil (0.61%) (Table 3). A noticeable result was observed for available N, available P, and available Cu2+. Available N increased by 82, 74, and 84%, available P by 42, 22, and 21%; and available Cu2+ was found 50, 51, and 108% in CT-RW-N, ZT-RWMb-N, and ZT-MWMb-N, respectively, compared to the corresponding sodic soil treatments.
The maximum concentration of micronutrients (Fe2+, Zn2+, and Mn2+) was recorded in CT-RW-HS soil, except Cu2+, which was the lowest at 2.15 mg kg−1. Cu2+ concentration varied from 2.15 to 5.85 mg kg−1, with the highest found in CT-RW-N soil (5.85 mg kg−1) (Table 3). The concentration of Zn2+ varied between 2.03 and 2.64 mg kg−1 in surface soil, with the maximum found in CT-RW-HS soil (2.64 mg kg−1), which was at par with the remaining treatments. Despite responding to the application of Zn to the rice, all soils exhibited Zn2+ content above the critical limit (0.74 mg kg−1). However, the lowest concentration of Mn2+ (0.26 mg kg−1) was observed in ZT-MWMb-S soil, which was at par with ZT-RWMb-N and CT-RW-N soil.
3.2 Soil biological properties
Significant differences in enzyme activities were observed between various salt-affected and normal soils (Table 4). Acid phosphatase (ACP) activity was found to be highest in ZT-RWMb-N (190.94 μg p-NP g−1 soil h−1), which was at par with ZT-MWMb-N soil (173.27 μg p-NP g−1 soil h−1). ACP activity was 16 and 13% higher in ZT-based normal soils than in ZT-based sodic soils, respectively. Alkaline phosphatase (ALP) activity was found to be the highest (310.89 μg p-NP g−1 soil h−1) in CT-RW-HS. Excluding highly sodic soils, ALP activity was 70% higher in CT-RW-N, 14% higher in ZT-RWMb-N, and 81% higher in ZT-MWMb-N than in their respective sodic soil counterparts. Similarly, dehydrogenase enzyme activity (DHA) was also found to be higher in normal soils than in sodic soils. It was approximately five times higher in ZT-based normal soils than in ZT-based sodic soils. The highest DHA activity was recorded in ZT-MWMb-N (32.78 μg TPF g−1 soil 24 h−1), followed by ZT-RWMb-N (31.66 μg TPF g−1 soil 24 h−1) and CT-RW-N soil (30.63 μg TPF g−1 soil 24 h−1) (Table 4).
Higher microbial colony counts for both bacteria and fungi (Table 4) were observed in normal soils than in sodic soils. The bacterial population was 5.9, 5.8, and 6.6 times higher in CT-RW-N, ZT-RWMb-N, and ZT-MWMb-N, respectively, than in their corresponding sodic soil treatments. Similarly, the fungal population was 11.2, 12.4, and 19.6 times higher in CT-RW-N, ZT-RWMb-N, and ZT-MWMb-N, respectively, than in their sodic soil treatments. The lowest bacterial (7.89 × 105 CFU) and fungal (2.69 × 105 CFU) populations were observed in highly sodic soils.
Significant differences in AWCD were observed among normal, highly sodic, and sodic soils for days 1, 2, 3, 4, and 5 (Figure 1). AWCD increased with incubation time, but this increase was lowest for ZT-MWMb-N and highest for ZT-MWMb-S. On the last day of incubation, AWCD values for CT-RW-N and ZT-RWMb-N were either similar to or higher than those of their sodic soil treatments. However, this trend was not observed for ZT-MWMb-N, which had exceptionally low AWCD values, while ZT-MWMb-S had exceptionally high values. AWCD for different substrates also showed significant differences among soils (Figure 2). The utilization of all substrates (amino acids, amines, carbohydrates, carboxylic acids, phenolic compounds, and polymers) increased over the incubation period. During 0–120 h of incubation, substrate utilization was highest in ZT-MWMb-S and lowest in ZT-MWMb-N soils. Different indices, such as the Shannon diversity index (H), substrate richness (SR), and Shannon evenness (E), were derived from AWCD (Table 5). The SR was higher in CT-RW-S, CT-RW-HS, ZT-RWMb-N, and ZT-MWMb-S soil and lowest in ZT-MWMb-N.
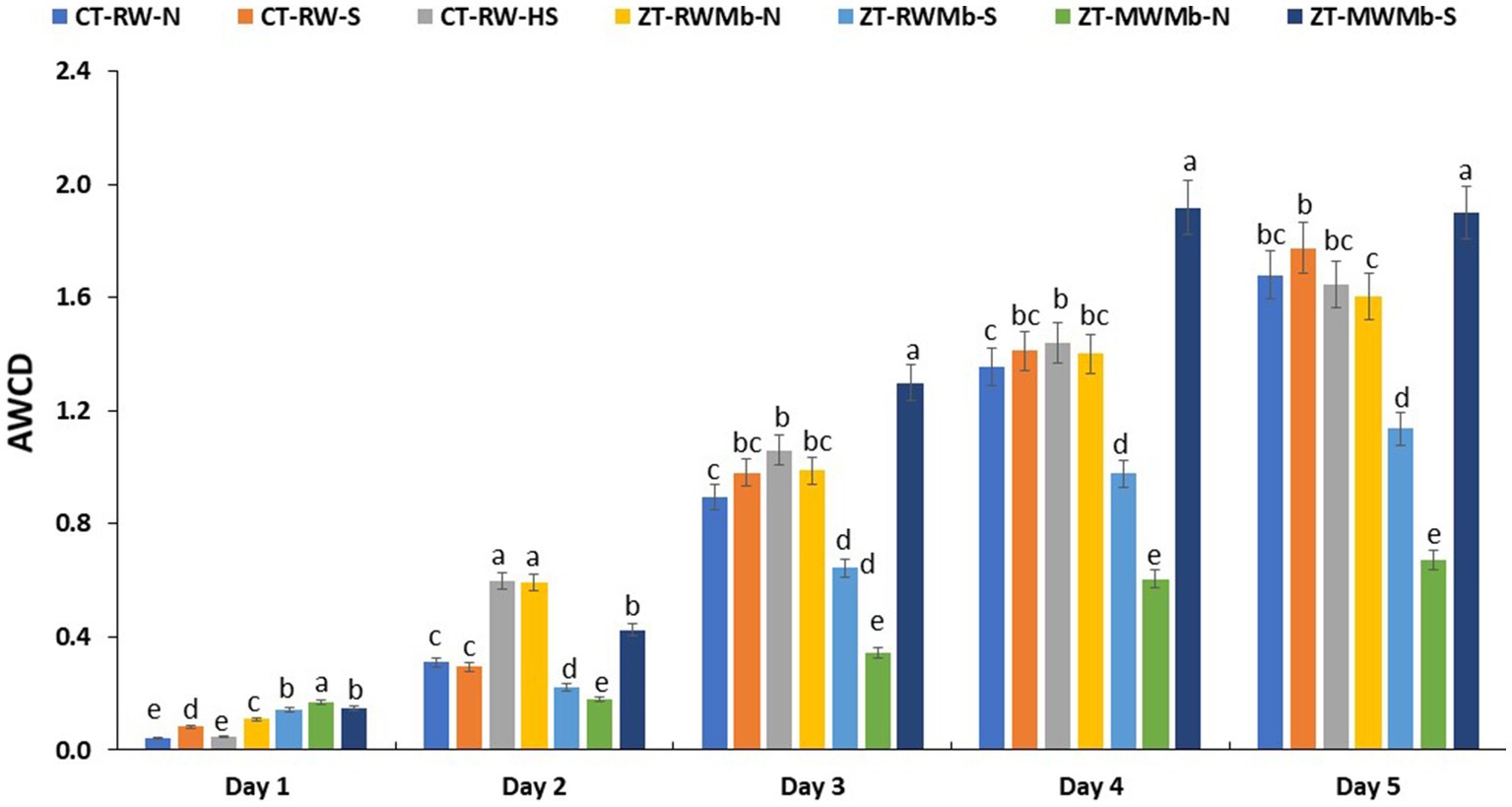
Figure 1. Average well color development (AWCD) of different soils (sodic and normal) in the surface layer.
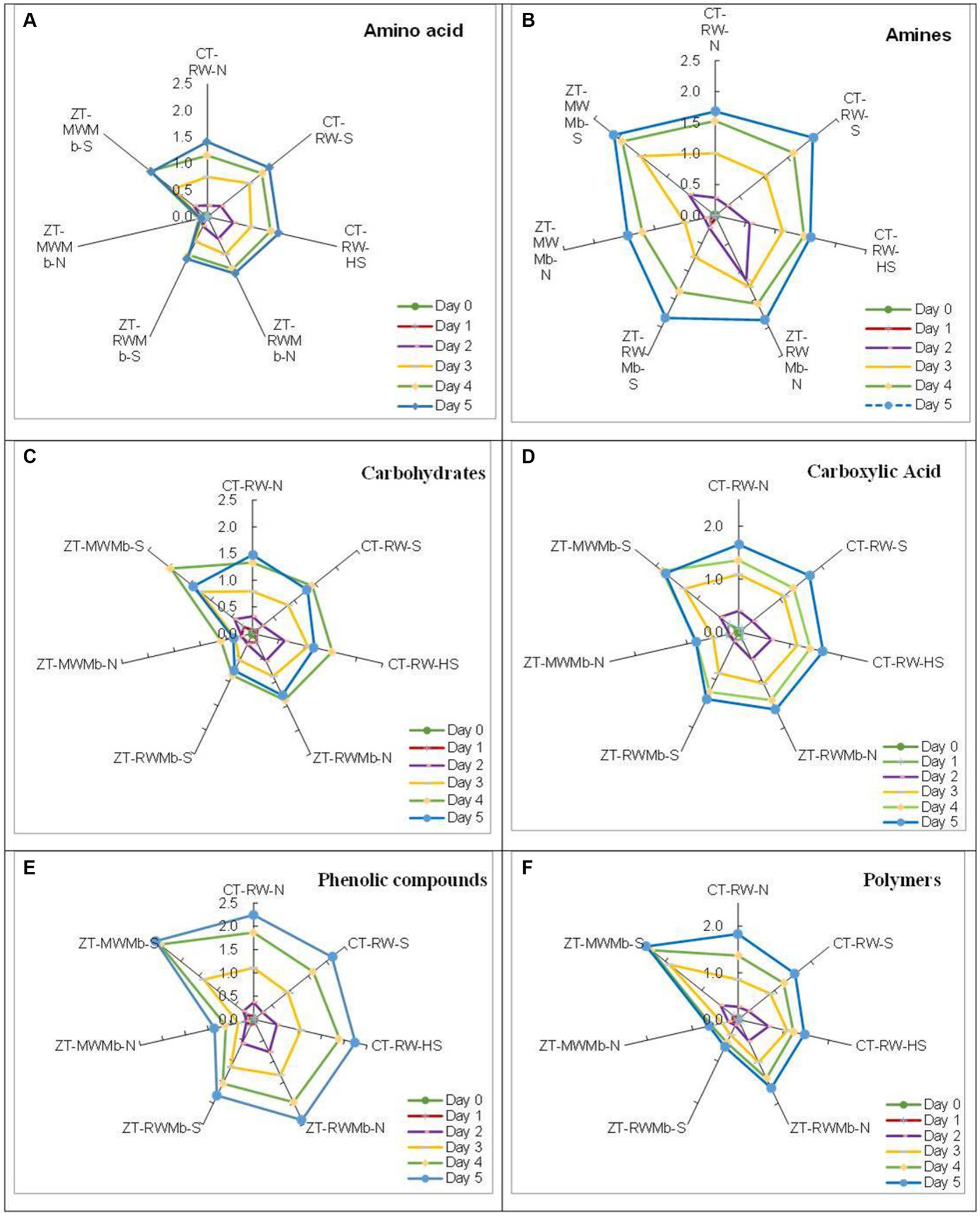
Figure 2. Average well color development (AWCD) for substrates: (A) amino acids, (B) amines, (C) carbohydrates, (D) carboxylic acid, (E) phenolic compounds, and (F) polymers in the surface layer of sodic and normal soil at different time intervals.
3.3 Multivariate analysis
The PCA was used to identify which variables best explained the observed differences in soil properties, AWCD, and enzyme activity across different types of soil. From the PCA of 21 variables, two principal components (PCs) with eigenvalues >0.9 were extracted, accounting for 77.3% of the variance (Figure 3). The first component (PC-1) explained 59.1% of the variation, while the second component (PC-2) accounted for 18.2%. Since most of the data information described by PC-1 (59.1% loadings) was related to soil properties (ECe, pH, available K, Mn, and Zn), AWCD (amino acid, phenolic compounds, amines, carboxylic acids, polymers, and carbohydrates) allowed differentiation between different soil types. However, PC-2 (18.2%) selected the soil properties (OC, available N, P, and Cu), bacteria, fungi, and enzymes (ACP, ALP, and dehydrogenase) in the minimum dataset. The correlation results showed a strong and positive correlation between soil properties. However, enzyme activities were strongly correlated with AWCD (phenolic compound and polymers) (Figure 3). During correlation analysis between soil physicochemical properties and enzyme activities, the impact of soil sodicity on soil properties was more pronounced. All the chemical parameters such as pHs, ECe, OC, available N, P, K, and micronutrients influence the enzyme activities under different soils, which affect soil biological activities and functional diversity as indicated by PCA analysis.
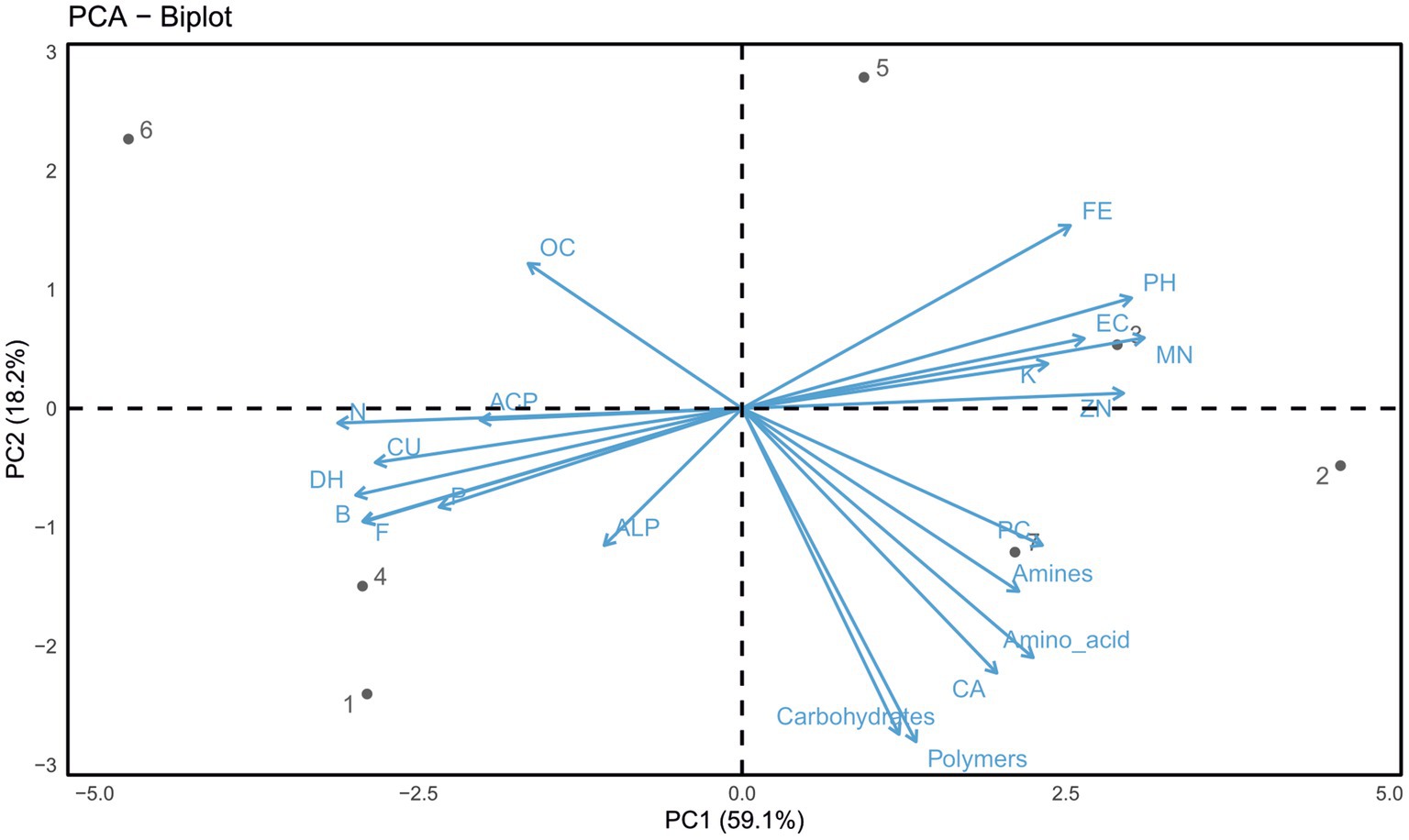
Figure 3. Principal component analysis of different (sodic and normal) soils in surface layer. *Soil physical properties, chemical properties, biological properties, AWCD, and different enzymes are represented in these figures. pHs, soil pH in saturated extract; ECe, electrical conductivity of the saturated soil extract; OC, soil organic carbon; N, nitrogen; P, phosphorus; K, potassium; Cu, available copper; Zn, available zinc; Fe, available iron; Mn, available manganese; ACP, acidic phosphatase; ALP, alkaline phosphatase; DHA, dehydrogenase.
4 Discussion
High soil pH (˃8.5) and low EC (<4 dSm−1) are the characteristics of sodic soils due to the presence of carbonate (CO32−) and bicarbonate (HCO3−) of sodium (Na+). Soils with pHs higher than 8.5, exchangeable sodium percentage greater than 15, and sodium adsorption ratio (SAR) of more than 13 are considered sodic soils (Filho et al., 2020). With the adoption of CA-based management, soil pH was found to decline, it may be the effect of the decomposition of crop residues recycled over the years (Gura and Mnkeni, 2019). Soil pH was reported to be lower under CA-based management systems, supporting the previous studies (Jat et al., 2018; Sithole and Magwaza, 2019). The lower pH in CA might be due to the accumulation of soil organic matter (SOM), which increases the electrolyte concentration and decreases soil pH (Dhar et al., 2014). The inclusion of a legume crop (mungbean) between rice and wheat and the incorporation of its residues also facilitates the reduction in soil pH, as reported by many researchers (Du et al., 2010; Islam et al., 2019). Husson et al. (2018) found that CA-based management systems tend to maintain favorable EC and pH for better plant growth.
During the study, the higher quantity of crop residue additions (both above as well as below ground) and their slow decomposition due to less soil disturbance in CA-based treatments might have caused higher OC and available N in the soil under ZT-RWMb-N and ZT-MWMb-N (Dikgwatlhe et al., 2014; Sinha et al., 2019; Jat et al., 2022). CA-based practices, particularly conservation tillage, are reported to cause greater accumulation of nutrients than CT (Lopez-Fando and Pardo, 2009; Rai et al., 2022). This impact of CA practices on soil chemical properties was observed in sodic soils, but it was less pronounced than in normal soils (Table 2). SASs had very poor organic matter content due to restricted plant growth, resulting in a low supply of organic input to the soil (Wong et al., 2009). These soils are also prone to leaching, erosion, and dispersion and, therefore, contain less OC (Soni et al., 2021). Lower available N in sodic soils as compared to normal soils is linked to the leaching of nutrients due to the poor soil structure of sodic soils (Qadir and Schubert, 2002; Qadir et al., 2003). As reported earlier (Chhabra and Thakur, 2000; Ghafoor et al., 2004), the availability of P was found to be lower in sodic soils because most of the soil P is found in the form of soluble sodium phosphate compounds (NaH2PO4) that are absorbed by the plants, causing a reduction in plant growth and crop yields due to excess Na uptake even with P fertilization. The high concentration of calcium in the soil results in the precipitation of insoluble calcium phosphate compounds for a short time and decreases P availability (Marchuk et al., 2016). Whereas, the availability of K content was found to be significantly higher in sodic soils, which indicates that Na+ has been replaced by K+ and contributed to soil sodicity (Morita and Nemoto, 1995; Jat et al., 2022).
The soil’s chemical and biological properties were found to be closely related to each other; both were found to be higher in normal soils than in sodic soils. The oxidizable OC plays an important role in enhancing microbial activity in SASs (Soni et al., 2021). In addition, more organic inputs through crop residue recycling regulated the soil structure by protecting it from erosion. Variations in OC coupled with the salt composition of the soil solution (cations and anions concentration) in the different SASs compared to normal soil regulated the enzymes’ activities and functional diversity of microorganisms. The activity of most of the enzymes found in the soil has a higher concentration of available carbon (Dick et al., 1996). The soil is subjected to changes due to the application of amendments, agricultural management practices, and exposure to weather adversity, and these changes influence the activity of enzymes such as ACP, ALP, and dehydrogenase. Dehydrogenase is an important and sensitive indicator of the metabolic state of soil microorganisms (Bastida et al., 2006; Moeskops et al., 2010). Its activity is used to indicate soil quality under different agricultural management practices against salinity stress, heavy metal pollution, and many other biotic and abiotic stresses (Singh et al., 2013; Curyło and Telesiński, 2020; Choudhary et al., 2022a). Microbial properties such as enzyme activities and populations were found to be higher in normal soils than in sodic soils (Table 4), which reflects the toxic effects of sodic soils on microbial properties. The negative correlation of enzyme activities with soil sodicity parameters was reported by many researchers (Singh et al., 2015; Choudhary et al., 2023). Plant growth is restricted under sodic soils, leading to low OC and nitrogen, which ultimately decreases microbial activities (Rietz and Haynes, 2003). In sodic soils, not only the availability of organic matter but also metabolic energy, which is required for microbial assimilation, is reduced, which leads to the lyses of microbial cells and reduced microbial activities (Sumner and Naidu, 1998). Sodicity has a negative effect on microbial biomass carbon, which ultimately declines microbial activities (Garcia et al., 1994). However, enzyme activities and microbial biomasses increased with the application of different amendments in sodic soils (Shaaban et al., 2023). lower microbial growth and biomass resulted in reduced enzyme activities (Gao et al., 2022). The low availability of SOM, nutrients, high pH, and poor soil physical properties of sodic soils create unfavorable conditions for the growth of microbes. Feigl et al. (2017) found that soil chemical properties such as pH, EC, C/N, Na, and K are the main factors that determine the relative abundance of bacterial and fungal communities. Ion toxicity under sodic soils obstructs microbial growth and activities, resulting in low microbial colony counts (Sumner and Naidu, 1998; Singh et al., 2015; Choudhary et al., 2023).
Different agriculture management practices, soil types, and vegetation affect soil microbial functional diversities in various ways (Rutgers et al., 2016; Kumar et al., 2017; Zhang et al., 2018; Choudhary et al., 2023). The functional diversity of community-level physiological profiles of microbes was studied using the concept of utilization of different types of substrates and color development using Biolog ECO-plates (Sasse et al., 2018). Microbial communities are highly sensitive to soil properties such as salt stress, which can be studied by AWCD (Rutgers et al., 2016; Choudhary et al., 2023). The substrate utilization depicts the metabolic activity of active microbial communities. The pattern of AWCD and utilization of substrate in ZT-based MWMb practices was different compared to other practices, as these were very low in normal soil and high in sodic soil. This pattern may be observed due to the difference in crop rotation. Different types of crops (plants) have different rhizobiome compositions as there are differences in their root exudates and rhizodeposits (Jensen et al., 2005). The chemical composition of residue varies with plant species, leading to differences in chemical energy and nutrient release during the decomposition of crop residue (Chhabra, 2004; Redin et al., 2014). Different crop rotations also lead to different times of harvesting. Since the study was conducted after the harvest of rice crops, maize harvesting had already been completed approximately a month ago. Differences in AWCD, substrate utilization, and SR in ZT-MWMb-N and ZT-MWMb-S may be the result of the effect of sodicity on soil microbiota and residue decomposition.
5 Conclusion
The salts available in soil impacted its physical, chemical, and biological properties. Rising salt concentrations led to major nutrient imbalances and low OC content in various types of soils, negatively affecting the sustainability and quality of soil systems. The study concluded that an increase in salt load adversely affected soil physicochemical and biological conditions, leading to reduced soil functions. By adopting crop rotation, tillage, and residue-based agriculture management practices, the negative effects of salt load can be mitigated to some extent. Soil pHs was found to decrease and EC increased under CA-based management. Soil OC, N, biological activities, and microbial populations were also found to increase under CA-based management practices. Soil AWCD values increased over time; however, the highest levels for amino acids, amines, carboxylic acids, phenolic compounds, and polymers were recorded in ZT-MWMb-S soils, while the lowest was in ZT-MWMb-N soils. Bacteria and fungi populations, enzymatic activities (e.g., ACP, ALP, and dehydrogenase), and metabolic activities declined with increasing stress from sodicity, with the highest populations and activities observed in normal soil. Therefore, research on the interrelationship between soil physicochemical and biological properties is crucial before recommending or adopting any land use, reclamation strategies, or nutrient management practices to ensure long-term crop productivity and soil sustainability. Management of agriculture practices under a specific agroecosystem has important implications for nutrient availability to plants. Upon decomposition, crop residues release nutrients, helping to conserve externally applied nutrients while improving overall soil quality and carbon enrichment.
Data availability statement
The original contributions presented in the study are included in the article/supplementary material, further inquiries can be directed to the corresponding author.
Author contributions
TP: Data curation, Formal analysis, Investigation, Writing – original draft, Writing – review & editing. MC: Conceptualization, Investigation, Supervision, Writing – original draft, Writing – review & editing. MK: Data curation, Formal analysis, Writing – review & editing, Writing – original draft. BD: Data curation, Formal analysis, Writing – review & editing, Writing – original draft. HJ: Conceptualization, Funding acquisition, Project administration, Supervision, Writing – review & editing, Writing – original draft.
Funding
The author(s) declare that no financial support was received for the research, authorship, and/or publication of this article.
Acknowledgments
The authors acknowledge the ICAR-Central Soil Salinity Research Institute (CSSRI PME cell reference No. Research Article/7/2024) for providing opportunities to work in salt-affected soils.
Conflict of interest
The authors declare that the research was conducted in the absence of any commercial or financial relationships that could be construed as a potential conflict of interest.
Publisher’s note
All claims expressed in this article are solely those of the authors and do not necessarily represent those of their affiliated organizations, or those of the publisher, the editors and the reviewers. Any product that may be evaluated in this article, or claim that may be made by its manufacturer, is not guaranteed or endorsed by the publisher.
References
Bastida, F., Moreno, J. L., Hernández, T., and García, C. (2006). Microbiological degradation index of soils in a semiarid climate. Soil Biol. Biochem. 38, 3463–3473. doi: 10.1016/j.soilbio.2006.06.001
Bhargava, G. P. (2003). Training manual for undertaking studies on genesis of sodic/alkali soils. Karnal: CSSRI, 111.
Central Soil Salinity Research Institute (2014). Vision 2050. Pragmatic assessment of the agricultural production and food demand scenario of India by the year 2050. Karnal: Central Soil Salinity Research Institute.
Chhabra, R. (2004). Classification of salt-affected soils. Arid Land Res. Manag. 19, 61–79. doi: 10.1080/15324980590887344
Chhabra, R., and Thakur, N. P. (2000). “Long-term study on phosphorus fertilization for rice-wheat cropping system in an alkali soils in the Indo-Gangetic plains” in Long-term soil fertility experiments in rice-wheat cropping systems. ed. I. P. Abrol (New Delhi: Rice-Wheat Consortium), 31–39.
Choudhary, M., Chandra, P., Dixit, B., Nehra, V., Choudhary, U., and Choudhary, S. (2022a). Plant growth-promoting microbes: role and prospective in amelioration of salt stress. Commun. Soil Sci. Plant Anal. 53, 1692–1711. doi: 10.1080/00103624.2022.2063316
Choudhary, M., Datta, A., Jat, H. S., Yadav, A. K., Gathala, M. K., Sapkota, T. B., et al. (2018a). Changes in soil biology under conservation agriculture based sustainable intensification of cereal systems in indo-Gangetic plains. Geoderma 313, 193–204. doi: 10.1016/j.geoderma.2017.10.041
Choudhary, M., Jat, H. S., Datta, A., Sharma, P. C., Rajashekar, B., and Jat, M. L. (2020). Topsoil bacterial community changes and nutrient dynamics under climate-smart agriculture based agri-food systems. Front. Microbiol. 11:1812. doi: 10.3389/fmicb.2020.01812
Choudhary, M., Jat, H. S., Datta, A., Yadav, A. K., Sapkota, T. B., Mondal, S., et al. (2018b). Sustainable intensification influences soil quality, biota, and productivity in cereal-based agroecosystems. Appl. Soil Ecol. 126, 189–198. doi: 10.1016/j.apsoil.2018.02.027
Choudhary, M., Jat, H. S., Jat, M. L., and Sharma, P. C. (2022b). Climate-smart agricultural practices influence the fungal communities and soil properties under major agri-food systems. Front. Microbiol. 13:986519. doi: 10.3389/fmicb.2022.986519
Choudhary, M., Jat, H. S., Mukhopadhyay, R., Manish, K., Tanuja, P., Anshul, P., et al. (2023). Functional diversity and behavioral changes of microbial communities under salt affected soils. Appl. Soil Ecol. 190:105017. doi: 10.1016/j.apsoil.2023.105017
Curyło, K., and Telesiński, A. (2020). Use of phosphatase and dehydrogenase activities in the assessment of calcium peroxide and citric acid effects in soil contaminated with petrol. Open Life Sci. 15, 12–20. doi: 10.1515/biol-2020-0002
Dhar, D., Datta, A., Basak, N., Paul, N., Badole, S., and Thomas, T. (2014). Residual effect of crop residues on growth, yield attributes and soil properties of wheat under rice-wheat cropping system. Indian J. Agric. Res. 48, 373–378. doi: 10.5958/0976-058X.2014.01317.1
Dick, R. P., Breakwell, D. P., and Turco, R. F. (1996). “Soil enzyme activities and biodiversity measurements as integrative microbiological indicators” in Methods for assessing soil quality (Madison, WI: American Society of Agronomy), 247–271.
Dikgwatlhe, S. B., Chen, Z., Lal, R., Zhang, H., and Chen, F. (2014). Changes in soil organic carbon and nitrogen as affected by tillage and residue management under wheat-maize cropping system in the North China Plain. Soil Tillage Res. 144, 110–118. doi: 10.1016/j.still.2014.07.014
Du, Z., Ren, T., and Hu, C. (2010). Tillage and residue removal effects on soil carbon and nitrogen storage in the North China Plain. Soil Sci. Soc. Am. J. 74, 196–202. doi: 10.2136/sssaj2009.0048
Feigl, V., Ujaczki, É., Vaszita, E., and Molnár, M. (2017). Influence of red mud on soil microbial communities: application and comprehensive evaluation of the Biolog EcoPlate approach as a tool in soil microbiological studies. Sci. Total Environ. 595, 903–911. doi: 10.1016/j.scitotenv.2017.03.266
Filho, F. G., Silva Dias, N., Suddarth, S. R. P., Ferreira, J. F. S., Anderson, R. G., Fernandes, C. S., et al. (2020). Reclaiming tropical saline606 sodic soils with gypsum and cow manure. Water 12:57. doi: 10.3390/w12010057
Gao, J., Zhao, Q., Chang, D., Ndayisenga, F., and Yu, Z. (2022). Assessing the effect of physicochemical properties of saline and sodic soil on soil microbial communities. Agriculture 12:782. doi: 10.3390/agriculture12060782
Garau, G., Castaldi, P., Santona, L., Deiana, P., and Melis, P. (2007). Influence of red mud, zeolite and lime on heavy metal immobilization, culturable heterotrophic microbial populations and enzyme activities in a contaminated soil. Geoderma 142, 47–57. doi: 10.1016/j.geoderma.2007.07.011
Garcia, C., Hernandez, T., and Costa, F. (1994). Microbial activity in soils under Mediterranean environmental conditions. Soil Biol. Biochem. 26, 1185–1191. doi: 10.1016/0038-0717(94)90142-2
Ghafoor, A., Qadir, M., and Murtaza, G. (2004). Salt-affected soils: principles of management. Urdu Bazar, Lahore: Allied Book Centre.
Gomez, K. A., and Gomez, A. A. (1984). Statistical procedures for agricultural research. New York: John Wiley & Sons.
Gura, I., and Mnkeni, P. N. S. (2019). Crop rotation and residue management effects under no till on the soil quality of a Haplic Cambisol in Alice, Eastern Cape, South Africa. Geoderma 337, 927–934. doi: 10.1016/j.geoderma.2018.10.042
Hawes, C., Alexander, C. J., Begg, G. S., Iannetta, P. P. M., Karley, A. J., Squire, G. R., et al. (2018). Plant responses to an integrated cropping system designed to maintain yield whilst enhancing soil properties and biodiversity. Agronomy 8:229. doi: 10.3390/agronomy8100229
Husson, O., Brunet, A., Babre, D., Charpentier, H., Durand, M., and Sarthou, J. P. (2018). Conservation agriculture systems alter the electrical characteristics (Eh, pH and EC) of four soil types in France. Soil Tillage Res. 176, 57–68. doi: 10.1016/j.still.2017.11.005
Islam, M. M., Urmi, T. A., Rana, M. S., Alam, M. S., and Haque, M. M. (2019). Green manuring effects on crop morpho-physiological characters, rice yield and soil properties. Physiol. Mol. Biol. Plants 25, 303–312. doi: 10.1007/s12298-018-0624-2
Jat, H. S., Choudhary, M., Datta, A., Kakraliya, S. K., McDonald, A. J., Jat, M. L., et al. (2022). Long-term conservation agriculture helps in the reclamation of sodic soils in major agri-food systems. Land Degrad. Dev. 33, 2423–2439. doi: 10.1002/ldr.4321
Jat, H. S., Choudhary, K. M., Nandal, D. P., Yadav, A. K., Poonia, T., Singh, Y., et al. (2020). Conservation agriculture-based sustainable intensification of cereal systems leads to energy conservation, higher productivity and farm profitability. Environ. Manag. 65, 774–786. doi: 10.1007/s00267-020-01273-w
Jat, H. S., Datta, A., Choudhary, M., Sharma, P. C., Yadav, A. K., Choudhary, V., et al. (2019a). Climate smart agriculture practices improve soil organic carbon pools, biological properties and crop productivity in cereal-based systems of North-West India. Catena 181:104059. doi: 10.1016/j.catena.2019.05.005
Jat, H. S., Datta, A., Choudhary, M., Yadav, A. K., Choudhary, V., Sharma, P. C., et al. (2019b). Effects of tillage, crop establishment and diversification on soil organic carbon, aggregation, aggregate associated carbon and productivity in cereal systems of semi-arid Northwest India. Soil Tillage Res. 190, 128–138. doi: 10.1016/j.still.2019.03.005
Jat, H. S., Datta, A., Sharma, P. C., Kumar, V., Yadav, A. K., Choudhary, M., et al. (2018). Assessing soil properties and nutrient availability under conservation agriculture practices in a reclaimed sodic soil in cereal-based systems of North-West. Arch. Agron. Soil Sci. 64, 531–545. doi: 10.1080/03650340.2017.1359415
Jat, M. L., Gathala, M. K., Choudhary, M., Sharma, S., Jat, H. S., Gupta, N., et al. (2023). Conservation agriculture for regenerating soil health and climate change mitigation in smallholder systems of South Asia. Adv. Agron. 181, 183–277. doi: 10.1016/bs.agron.2023.05.003
Jensen, L. S., Salo, T., Palmason, F., Breland, T. A., Henriksen, T. M., and Stenberg, B. (2005). Influence of biochemical quality on C and N mineralisation from a broad variety of plant materials in soil. Plant Soil 273, 307–326. doi: 10.1007/s11104-004-8128-y
Khan, M. H., Liu, H., Zhu, A., Khan, M. H., Hussain, S., and Cao, H. (2023). Conservation tillage practices affect soil microbial diversity and composition in experimental fields. Front. Microbiol. 14:1227297. doi: 10.3389/fmicb.2023.1227297
Kumar, V., Jat, H. S., Sharma, P. C., Gathala, M. K., Malik, R. K., Kamboj, B. R., et al. (2018). Can productivity and profitability be enhanced in intensively managed cereal systems while reducing the environmental footprint of production? Assessing sustainable intensification options in the breadbasket of India. Agric. Ecosyst. Environ. 252, 132–147. doi: 10.1016/j.agee.2017.10.006
Kumar, U., Shahid, M., Tripathi, R., Mohanty, S., Kumar, A., Bhattacharyya, P., et al. (2017). Variation of functional diversity of soil microbial community in sub-humid tropical rice-rice cropping system under long-term organic and inorganic fertilization. Ecol. Indic. 73, 536–543. doi: 10.1016/j.ecolind.2016.10.014
Li, S. X., Wang, Z. H., Li, S. Q., Gao, Y. J., and Tian, X. H. (2013). Effect of plastic sheet mulch, wheat straw mulch, and maize growth on water loss by evaporation in dryland areas of China. Agric. Water Manag. 116, 39–49. doi: 10.1016/j.agwat.2012.10.004
Lindsay, W. L., and Norvell, W. A. (1978). Development of DTPA soil test for zinc, iron, manganese and copper. Soil Sci. Soc. Am. J. 42, 421–428. doi: 10.2136/sssaj1978.03615995004200030009x
Lopez-Fando, C., and Pardo, M. T. (2009). Changes in soil chemical characteristics with different tillage practices in a semi-arid environment. Soil Tillage Res. 104, 278–284. doi: 10.1016/j.still.2009.03.005
Marchuk, S., Churchman, J., and Rengasamy, P. (2016). Possible effects of irrigation with wastewater on the clay mineralogy of some Australian clayey soils: laboratory study. Soil Sci. 54:857. doi: 10.1071/SR14373
Margenot, A. J., Paul, B. K., Sommer, R. R., Pulleman, M. M., Parikh, S. J., Jackson, L. E., et al. (2017). Can conservation agriculture improve phosphorus (P) availability in weathered soils? Effects of tillage and residue management on soil P status after 9 years in a Kenyan Oxisol. Soil Tillage Res. 166, 157–166. doi: 10.1016/j.still.2016.09.003
Moeskops, B., Buchan, D., Sleutel, S., Herawaty, L., Husen, E., Saraswati, R., et al. (2010). Soil microbial communities and activities under intensive organic and conventional vegetable farming in West Java, Indonesia. Appl. Soil Ecol. 45, 112–120. doi: 10.1016/j.apsoil.2010.03.005
Mondaca, P., Celis-Diez, J. L., Díaz-Siefer, P., Olmos-Moya, N., Montero-Silva, F., Molina, S., et al. (2024). Effects of sustainable agricultural practices on soil microbial diversity, composition, and functions. Agric. Ecosyst. Environ. 370:109053. doi: 10.1016/j.agee.2024.109053
Morita, S., and Nemoto, K. (1995). “Morphology and anatomy of rice roots with special reference to coordination in organo-and histogenesis” in Structure and function of roots (Dordrecht: Springer), 75–86.
Mosaffa, H. R., and Sepaskhah, A. R. (2019). Performance of irrigation regimes and water salinity on winter wheat as influenced by planting methods. Agric. Water Manag. 216, 444–456. doi: 10.1016/j.agwat.2018.10.027
Mukhopadhyay, R., Sarkar, B., Jat, H. S., Sharma, P. C., and Bolan, N. S. (2021). Soil salinity under climate change: challenges for sustainable agriculture and food security. J. Environ. Manag. 280:111736. doi: 10.1016/j.jenvman.2020.111736
Mutsamba, E. F., Nyagumbo, I., and Mupangwa, W. (2020). Forage and maize yields in mixed crop-livestock farming systems: enhancing forage and maize yields in mixed crop-livestock systems under conservation agriculture in sub-humid Zimbabwe. NJAS - Wagening. J. Life Sci. 92, 100317, 1–100310. doi: 10.1016/j.njas.2019.100317
Olsen, B. C., Cole, C. V., Watenabe, F. S., and Dean, L. A. (1954). Estimation of available phosphorus by extraction with sodium bicarbonate. USDA Circular No. 939. Washington, DC: US Government Printing Office, 19.
Pooniya, V., Zhiipao, R. R., Niraj, B., Jat, S. L., Kumar, D., Parihar, C. M., et al. (2021). Six years of conservation agriculture and nutrient management in maize-mustard rotation: impact on soil properties, system productivity and profitability. Field Crop Res. 260:108002. doi: 10.1016/j.fcr.2020.108002
Pooniya, V., Zhiipao, R. R., Niraj, B., Kumar, D., Shivay, Y. S., Babu, S., et al. (2022). Conservation agriculture based integrated crop management sustains productivity and economic profitability along with soil properties of the maize-wheat rotation. Sci. Rep. 12:1962. doi: 10.1038/s41598-022-05962-w
Qadir, M., and Schubert, S. (2002). Degradation processes and nutrient constraints in sodic soils. Land Degrad. Dev. 13, 275–294. doi: 10.1002/ldr.504
Qadir, M., Steffens, D., Yan, F., and Schubert, S. (2003). Sodium removal from a calcareous saline-sodic soil through leaching and plant uptake during phytoremediation. Land Degrad. Dev. 14, 301–307. doi: 10.1002/ldr.558
Rai, A. K., Basak, N., Soni, P. G., Kumar, S., Sundha, P., Narjary, B., et al. (2022). Bioenergy sorghum as balancing feedback loop for intensification of cropping system in salt-affected soils of the semi-arid region: energetics, biomass quality and soil properties. Eur. J. Agron. 134:126452. doi: 10.1016/j.eja.2021.126452
Redin, M., Recous, S., Aita, C., Dietrich, G., Skolaude, A. C., Ludke, W. H., et al. (2014). How the chemical composition and heterogeneity of crop residue mixtures decomposing at the soil surface affects C and N mineralization. Soil Biol. Biochem. 78, 65–75. doi: 10.1016/j.soilbio.2014.07.014
Rezapour, S., Taghipour, A., and Samadi, A. (2013). Modifications in selected soil attributes as influenced by long-term continuous cropping in a calcareous semiarid environment. Nat. Hazards 69, 1951–1966. doi: 10.1007/s11069-013-0786-8
Rietz, D. N., and Haynes, R. J. (2003). Effects of irrigation-induced salinity and sodicity on soil microbial activity. Soil Biol. Biochem. 35, 845–854. doi: 10.1016/S0038-0717(03)00125-1
Rutgers, M., Wouterse, M., Drost, S. M., Breure, A. M., Mulder, C., and Stone, D. (2016). Monitoring soil bacteria with community-level physiological profiles using Biolog ECO-plates in the Netherlands and Europe. Appl. Soil Ecol. 97, 23–35. doi: 10.1016/j.apsoil.2015.06.007
Sasse, J., Martinoia, E., and Northen, T. (2018). Feed your friends: do plant exudates shape the root microbiome? Trends Plant Sci. 23, 25–41. doi: 10.1016/j.tplants.2017.09.003
Shaaban, M., Wu, Y., Núñez-Delgado, A., Kuzyakov, Y., Peng, Q. A., Lin, S., et al. (2023). Enzyme activities and organic matter mineralization in response to application of gypsum, manure and rice straw in saline and sodic soils. Environ. Res. 224:115393. doi: 10.1016/j.envres.2023.115393
Singh, K. (2016). Microbial and enzyme activities of saline and sodic soils. Land Degrad. Dev. 27, 706–718. doi: 10.1002/ldr.2385
Singh, K., Singh, B., and Singh, R. R. (2013). Effect of land rehabilitation on physicochemical and microbial properties of a sodic soil. Catena 109, 49–57. doi: 10.1016/j.catena.2013.05.006
Singh, K., Trivedi, P., Singh, G., Singh, B., and Patra, D. D. (2015). Effect of different leaf litters on carbon, nitrogen and microbial activities of sodic soils. Land Degrad. Dev. 27, 1215–1226. doi: 10.1002/ldr.2313
Sinha, A. K., Ghosh, A., Dhar, T., Bhattacharya, P. M., Mitra, B., Rakesh, S., et al. (2019). Trends in key soil parameters under conservation agriculture-based sustainable intensification farming practices in the Eastern Ganga Alluvial Plains. Soil Res. 57, 883–893. doi: 10.1071/SR19162
Sithole, N. J., and Magwaza, L. S. (2019). Long-term changes of soil chemical characteristics and maize yield in no-till conservation agriculture in a semi-arid environment of South Africa. Soil Tillage Res. 194:104317. doi: 10.1016/j.still.2019.104317
Soni, P. G., Basak, N., Rai, A. K., Sundha, P., Narjary, B., Kumar, P., et al. (2021). Deficit saline water irrigation under reduced tillage and residue mulch improves soil health in sorghum-wheat cropping system in semi-arid region. Sci. Rep. 11, 1880–1813. doi: 10.1038/s41598-020-80364-4
Srivastava, P., and Kumar, R. (2015). Soil salinity: a serious environmental issue and plant growth promoting bacteria as one of the tools for its alleviation. Saudi J. Biol. Sci. 22, 123–131. doi: 10.1016/j.sjbs.2014.12.001
Subbiah, B. V., and Asija, G. L. (1956). A rapid procedure for the estimation of available nitrogen in soils. Curr. Sci. 22, 123–131.
Suhas, P. W., Anantha, K. H., and Garg, K. K. (2017). Soil properties, crop yield, and economics under integrated crop management practices in Karnataka, Southern India. World Dev. 93, 43–61. doi: 10.1016/j.worlddev.2016.12.012
Sumner, M. E., and Naidu, R. (1998). Sodic soils: distribution, properties, management, and environmental consequences. Oxford: Oxford University Press.
Tabatabai, M. A., and Bremner, J. M. (1969). Use of p-nitrophenyl phosphate for assay of soil phosphatase activity. Soil Biol. Biochem. 1, 301–307. doi: 10.1016/0038-0717(69)90012-1
USSL (1954) in Diagnosis and improvement of saline and alkali soils USDA handbook. ed. L. A. Richards (Washington, DC: US Department of Agriculture), 60.
Walkley, A., and Black, I. A. (1934). An examination of the Degtjareff method for determining soil organic matter, and a proposed modification of the chromic acid titration method. Soil Sci. 37, 29–38. doi: 10.1097/00010694-193401000-00003
Wang, D., Haung, J., Nie, L., and Wang, F. (2017). ICM practices for maximizing grain yield of doubled-season rice crop. Sci. Rep. 7:38982. doi: 10.1038/srep38982
Wang, S., Sun, L., Ling, N., Zhu, C., Chi, F., Li, W., et al. (2020). Exploring soil factors determining composition and structure of the bacterial communities in saline-alkali soils of Songnen Plain. Front. Microbiol. 10:2902. doi: 10.3389/fmicb.2019.02902
Wong, V. N. L., Greene, R. S. B., Dalal, R. C., and Murphy, B. W. (2009). Soil carbon dynamics in saline and sodic soils: a review. Soil Use Manag. 26, 2–11. doi: 10.1111/j.1475-2743.2009.00251.x
Zhang, G., Bai, J., Zhai, Y., Jia, J., Zhao, Q., Wang, W., et al. (2024). Microbial diversity and functions in saline soils: a review from a biogeochemical perspective. J. Adv. Res. 59:129. doi: 10.1016/j.jare.2023.06.015
Keywords: crop management practices, enzymatic activities, microbial community, salt-affected soils, soil health, sustainable agriculture
Citation: Poonia T, Choudhary M, Kakraliya M, Dixit B and Jat HS (2024) The influence of soil types and agricultural management practices on soil chemical properties and microbial dynamics. Front. Sustain. Food Syst. 8:1412198. doi: 10.3389/fsufs.2024.1412198
Edited by:
Subhash Babu, Division of Agronomy, ICAR-Indian Agricultural Research Institute, IndiaReviewed by:
Sunanda Biswas, Division of Soil Science and Agricultural Chemistry, Indian Agricultural Research Institute (ICAR), IndiaMalu Ram Yadav, Sri Karan Narendra Agriculture University, India
Copyright © 2024 Poonia, Choudhary, Kakraliya, Dixit and Jat. This is an open-access article distributed under the terms of the Creative Commons Attribution License (CC BY). The use, distribution or reproduction in other forums is permitted, provided the original author(s) and the copyright owner(s) are credited and that the original publication in this journal is cited, in accordance with accepted academic practice. No use, distribution or reproduction is permitted which does not comply with these terms.
*Correspondence: Madhu Choudhary, bWFkaHVjc3NyaUBnbWFpbC5jb20=; bWFkaHUuY2hvdWRoYXJ5QGljYXIuZ292Lmlu