- 1Facultad de Industrias Alimentarias, Universidad Nacional Agraria La Molina, Lima, Peru
- 2Departamento de Química, Universidad Nacional de Colombia, Bogotá, Colombia
- 3Facultad de Farmacia y Bioquímica, Departamento Académico de Farmacología, Bromatología y Toxicología, Universidad Nacional Mayor de San Marcos, Lima, Peru
Many countries face the problem of agroindustrial waste elimination, which is linked to environmental damage caused by improper waste management. These wastes contain bioactive compounds often discarded due to a lack of knowledge. The objective of this study was to analyze five types of agroindustrial waste from Peru, including avocado (Persea americana) seeds, lucuma (Pouteria lucuma) seeds, grape (Vitis vinifera) pomace, mango (Mangifera indica) peel, and cocoa (Theobroma cacao) pods. This study includes proximal analysis, determination of total phenolic compound content, analysis of phenolic compounds by UHPLC– Q/Orbitrap/ESIMS/MS (Ultrahigh-performance liquid chromatography-electrospray ionization Q-Orbitrap mass spectrometry), and the evaluation of antioxidant, antimicrobial, and antifungal activities of different samples. The results showed that lucuma seeds had the highest protein content of 6.59 g/100 g DW. Mango peels showed the highest fat content of 4.89 g/100 g DW, cocoa pods had the highest crude fiber content of 26.91 g/100 g DW, and cocoa pods exhibited the highest ash content of 7.14 g/100 g DW. The highest carbohydrate content was found in avocado seeds at 85.85 g/100 g DW. Mango peels (56.66 mg GAE/g DW), avocado seeds (56.35 mg GAE/g DW), and cocoa pods (51.52 mg GAE/g DW) extracts showed the highest phenolic compound contents. Mango peel extract exhibited the highest antioxidant activities evaluated by the ABTS (995.01 μmol TEAC/g DW), DPPH (953.54 μmol TEAC/g DW), and FRAP (1661.13 μmol TEAC/g DW) assays. Extracts from cocoa pods, grape pomace, and lucuma seeds showed inhibitory activity on the growth of Staphylococcus aureus and Escherichia coli. In contrast, the extract of lucuma seeds was the only one that showed antifungal activity over Penicillium digitatum. As a result, several phenolic compounds were detected in each, indicating their potential use in developing functional foods and food preservation, among other applications.
1 Introduction
The lack of knowledge on the potential of agroindustrial waste as a source of compounds with antioxidant, antifungal, and antimicrobial activities resulted in their disposal without subsequent reutilization. It is estimated that the fruit industry processes approximately 882 million tons annually, and this tends to increase due to awareness about the benefits for the good health of the consumption of this type of food, so its demand will increase both in fresh as prosecuted (Shahbandeh, 2022). At a global level, the commercialization of fruits generates a large amount of waste throughout the production chain, during harvest, transportation, storage, processing, marketing, and consumption, which could be used as raw material for other products and thus respond to responsible consumption and production, one of the 17 sustainable development goals (SDGs) to transform the world (Saval, 2012). Sosa-Martínez et al. (2023) highlighted that the increase in environmental pollution stems from industrialization and inadequate waste management, varying according to the region and its economy. Furthermore, population growth entails a greater demand for food and, consequently, a larger generation of agro-industrial and food processing waste. In the absence of precise measurements for food waste, it is estimated that nearly 30% of all food produced globally is wasted (FAO, 2018). One of the greenhouse gases, CO2-equivalent, generated 1.6 billion tons in 2016, with an anticipated increase to 2.6 billion by 2050 (Kaza et al., 2018). Castro-Muñoz et al. (2021) emphasize that most waste originates from transforming raw materials such as fruits, vegetables, tubers, and cereals into processed products, including preserved fruits, vegetables, and beverages. Agro-industrial waste can adversely affect aquatic life, soil quality, surface, groundwater contamination, and phytotoxicity, and lead to colored and odorous waters (Nayak and Bhushan, 2019; Castro-Muñoz et al., 2021).
The waste generated by agribusiness has a varied composition rich in nutrients, including biopolymers (polysaccharides, fibers) and bioactive compounds (Santos et al., 2021; Kee et al., 2022). These wastes can be used in various sectors, including biomaterials, biopolymers, bioenergy, pharmaceutical, biofertilizer, and biopesticide sectors (Vaz Júnior, 2020; Leong and Chang, 2022). Wastes with high carbon, macro, and micronutrient content are especially useful in generating energy and producing biofertilizers, biogas, and biofuels (Yaashikaa et al., 2022). Furthermore, bioactive compounds have potential applications in the nutraceutical, cosmetic, and food industries (Fernández-Ochoa et al., 2021). These wastes can also produce ethanol, essential oils, and enzymes through various technologies (Shirahigue and Ceccato-Antonini, 2020). In this context, it could be indicated that there is a wide variety of applications for reusing this waste.
In recent years, Peru has become one of the countries where agroindustrial has grown steadily, so much so that it ranks ninth in 2021 as a supplier of fruits to the world (Staff, 2022); being the fruits of avocado (Persea americana), mango (Mangifera indica), grape (Vitis vinifera), cocoa (Theobroma cacao), and lucuma (Pouteria lucuma), those of greatest commercial importance.
The Peruvian agroindustry produces a large amount of waste, among which the seeds, peel, and pomace stand out from the fruits. The cocoa pod is the main by-product of the cocoa industry and constitutes between 67 and 76% of the weight of the fruit. This waste represents an important and challenging environmental and economic opportunity since 10 tons of wet pods are generated for every ton of dry cocoa bean (Campos-Vega et al., 2018). According to the statistical reports of Ministerio de Agricultura y Riego del Perú (MINAGRI, 2023), during the year 2022 the agricultural production of avocado (Persea americana) was 862,505 tons, with 279,855 tons destined for the domestic market, generating approximately 67,000 tons of seed; of mango (Mangifera indica) was 494,277 tons, with 239,106 tons destined for the domestic market, generating as waste an approximate of 83,000 tons of seed and 156,106 tons of peel; of cocoa (Theobroma cacao) was 170,300 tons, which is processed to market to the domestic and foreign markets, where cocoa pods are the majority waste, generating approximately 130,000 tons; of lucuma (Pouteria lucuma) was 12,933 tons, of which 1,682 tons of seed were generated as waste; and the production of grapes (Vitis vinifera) was 919,200, with a supply in the domestic market of 525,411, producing approximately 150,000 tons of grape pomace as waste. Waste negatively affects the environment due to its high concentration of organic matter and its inadequate final disposal.
Currently, agroindustrial waste is a topic of great interest, not only to mitigate environmental impacts but also to give them added value and improve the economy. Agroindustrial waste contains bioactive compounds that possess antioxidant and antimicrobial properties, including phenolic compounds found in the fruit’s edible portion and its residue (Shahidi and Ambigaipalan, 2015; Albuquerque et al., 2019). It is essential to understand the properties of waste produced from diverse agroindustrial procedures in order to effectively determine its application, such as energy production, pharmaceuticals, food, or agriculture (Cabrera Rodríguez et al., 2016).Thus, mango peels are a rich source of polyphenols, anthocyanins, and carotenoids that exhibit antioxidant activity (Ajila et al., 2007; Masibo and He, 2009), also of vitamins, enzymes, and dietary fiber, which can be beneficial for human health (Berardini et al., 2005; Ajila et al., 2007). Mangiferin stands out as a xanthone with various biological activities, all attributed mainly to its antioxidant capacity (Muruganandan et al., 2005; Barreto et al., 2008; Noratto et al., 2010). Cocoa pods are the main by-product of the cocoa industry (up to 76%) and are a source of dietary fiber, pectin, antioxidant compounds, minerals, and theobromine (Campos-Vega et al., 2018). The industrialization of grapes has grape pomace as a by-product, composed of seeds, peel, and stems, which is rich in different phenolic compounds (such as flavonoids, anthocyanins, and proanthocyanins) and dietary fiber components, which could have benefits for the health of people, such as antioxidant, anti-inflammatory and hypoglycemic activity, among others (Caponio et al., 2022). Of the total phenolic compounds in the fresh fruit, the grape seed provides 60 to 70%, the peel 28 to 35%, and the pulp 10% (Shi et al., 2003). Lucuma is a fruit processed before being consumed in ice cream, juices, and confectionery products and marketed as pulp and flour. Pouteria lucuma seeds exhibit antioxidant and antiulcer activity (Guerrero-Castillo et al., 2019). Rojo et al. (2010) reported that the main components of lucuma oil are: linoleic acid (38.9%), oleic acid (27.9%), palmitic acid (18.6%), stearic acid (8.9%), and γ-linolenic acid (2.9%); Compounds have skin regenerative properties and promote wound healing. It has also been reported to contain phytosterols (stigmasterol and β-sitosterol) that have properties to reduce low-density lipoprotein cholesterol (Racette et al., 2015), amyrin with possible anti-inflammatory properties (Melo et al., 2011), betulin that reduces atherosclerotic plaques (Tang et al., 2011) and tocopherols that exhibit antioxidant properties (Guerrero-Castillo et al., 2021). Avocado is consumed worldwide; its most important residue is the seed, which constitutes 13 to 18% of the weight of the whole fruit. The seed is an important source of phytochemicals that include flavonoids, tannins, saponins, phenols, phytates, and alkaloids, among others (Wang et al., 2010; Bahru et al., 2019). It has been reported that avocado seed extracts have bioactive properties such as antihyperglycemic, anticancer, antihypercholesterolemic, antioxidant, anti-inflammatory, and anti-neurogenerative effects (Bangar et al., 2022). Anaka et al. (2009) reported that aqueous extracts of avocado seeds had a lowering effect on blood pressure and heart rate. It has also been found that the seeds have insecticidal, fungicidal, and antimicrobial activities (Cid-Pérez et al., 2021).
In this context, using phytochemical compounds with bioactive properties in food and pharmacological products can help prevent their oxidation or deterioration and improve nutritional and health benefits. The circular economy model emphasizes looking at agroindustrial waste as an important source of functional compounds.
In Latin America and the Caribbean, agricultural production significantly contributes to the economy, generating a substantial amount of agro-industrial waste that poses a notable environmental challenge (CEPAL-FAO, 2017). Sosa-Martínez et al. (2023) have recently highlighted the emerging exploration of productive applications for these agroindustrial and food processing wastes. Ferreira et al. (2023) propose that the effective reuse of this waste could mitigate its environmental impact. This research emphasizes the relevance of characterizing the waste’s composition because it can vary considerably depending on the region of origin. This study analyzes the chemical composition and phenolic content of waste materials (mango peels, grape pomace, cocoa pods, avocado seeds, and lucuma seeds) and reports their antioxidant, antimicrobial, and antifungal activities. Additionally, the phenolic compound profiles of these five agri-food wastes were examined, aiming to identify other functionalities of these byproducts that are significant to the Peruvian economy.
2 Materials and methods
2.1 Raw material
Avocado (Persea americana) seeds Hass variety was obtained from the Don Carlos Farm in the Quilmaná district, the province of Cañete, Lima, Peru. Fermented grape pomace (Vitis vinifera) Quebranta variety was provided by a company dedicated to producing Pisco (alcoholic beverage), located in the province of Cañete, Lima, Peru. Lucuma seeds (Pouteria lucuma) seda variety was obtained from the Topara farm in Chincha, Ica, Peru. The cocoa pod (Theobroma cacao) of the CCN51 variety was provided from a company dedicated to the production of chocolate located in the province of Tocache, San Martín, Peru. The Kent variety mango peel (Mangifera indica) was obtained from the Tungasuca – Cieneguillo farm, Sullana province, Piura, Peru.
Avocado seeds (AS), grape pomace (GP), lucuma seeds (LS), cocoa pod (CP), and mango peels (MP) by-products were immediately collected after their production, then transported to the laboratory and stored frozen. Subsequently, all samples were lyophilized and stored at −20°C until analysis.
2.2 Reagents
Acetonitrile (HPLC-MS grade), formic acid (HPLC-MS grade), Folin–Ciocalteu 2 N reagent, gallic acid, 2,2′-azinobis-3-ethyl benzothiazoline-6-sulphonic acid (ABTS), 2,2- diphenyl-1-picrylhydrazyl (DPPH), 6-hydroxy-2,5,7,8-tetramethylchromane-2-carboxylic acid (Trolox), 2,4,6-tri(2-pyridyl)-S-triazine (TPTZ), Macozeb were purchased to Sigma Chemicals Co. (St. Louis, MO, USA), Muller-Hinton agar (HiMedia, India), Sabouraud dextrose was purchased to Merck Chemicals Co (Germany). All other chemicals used were analytical grade.
2.3 Proximate analysis
The methods of the Association of Official Analytical Chemists (2005) were used to determine the moisture content (method 984.25), ash (method 940.26), crude protein (method 920.152), crude fiber (method 920.169), and crude fat (method 963.15). Carbohydrate content was determined by the difference.
2.4 Preparation of phenolic-rich extracts
The extracts to determine total phenolic content (TPC), hydrophilic (H-AC), and lipophilic antioxidant capacities (L-AC) were obtained as follows:
Avocado seeds: The extracts were obtained using the methodology proposed by Gómez et al. (2014) with slight modifications. 250 mg of sample was mixed with 10 mL of hexane under stirring for 30 min at room temperature. The mixture was centrifuged at 2700 g for 10 min at 4°C. The supernatant (lipophilic extract) was recovered and stored at −20°C until use. The residual cake was used to obtain the hydrophilic extract, and 10 mL of 50% (v/v) ethanol was added. The extraction was carried out with stirring for 30 min at room temperature, then centrifuged at 2700 g for 15 min. The supernatant was recovered and stored at −20°C until use.
Grape pomace: The extracts were obtained using the methodology proposed by Garcia et al. (2010) with slight modifications. One gram of sample was subjected to extraction with 5 mL of hexane, under stirring for 7 h at room temperature. It was centrifuged at 2700 g for 10 min at 4°C to recover the supernatant (lipophilic extract) and stored at −20°C until analysis. The residual cake was mixed with 5 mL of methanol under stirring for 7 h at room temperature, then centrifuged at 2700 g for 15 min. The supernatant (hydrophilic extract) was recovered and stored at −20°C until use.
Lucuma seeds: The extracts were obtained using the methodology proposed by Guerrero-Castillo et al. (2019) with slight modifications. One gram of sample was mixed with 5 mL of hexane under stirring for 3 h at room temperature. Then, it was centrifuged at 2700 g for 10 min at 4°C, and the supernatant (lipophilic extract) was recovered. The residual cake was mixed with 5 mL of methanol under stirring for 3 h. It was then centrifuged at 2700 g for 15 min. The supernatant (hydrophilic extract) was recovered, and both extracts were stored at −20°C until use.
Cocoa pods: The extracts were obtained using the methodology proposed by Sotelo et al. (2015) with slight modifications. One gram of sample was subjected to extraction with 10 mL of hexane, under stirring for 6 h at room temperature. Then, it was centrifuged at 2700 g for 10 min at 4°C, and the supernatant (lipophilic extract) was recovered. The residual cake was used to obtain the hydrophilic extract, and 15 mL of 50% (v/v) ethanol acidified with 1% (v/v) HCl was added. The extraction was carried out with stirring for 6 h at room temperature. It was then centrifuged at 2700 g for 15 min. The supernatant was recovered and stored at −20°C until use.
Mango peels: The extracts were obtained using the methodology proposed by Lizárraga-Velázquez et al. (2018) with slight modifications. 0.5 g of sample was mixed with 5 mL of hexane under stirring for 7 h at room temperature. Then, it was centrifuged at 2700 g for 10 min at 4°C, and the supernatant (lipophilic extract) was recovered. The residual cake was mixed with 5 mL of 70% (v/v) ethanol under stirring for 3 h, and then was centrifuged at 2700 g for 15 min. The supernatant (hydrophilic extract) was recovered, and both extracts were stored at −20°C until analysis.
2.5 Determination of total phenolic content and antioxidant capacity
The Folin–Ciocalteu method described by Singleton and Rossi (1965) was used for total phenolic content, with gallic acid as the standard. The extracts were mixed with diluted Folin–Ciocalteu reagent and 7.5% sodium carbonate. The absorbance was measured at 755 nm in a spectrophotometer (Genesys 20, Thermo Scientific, United States) after incubation for 30 min at room temperature. Results were expressed as mg gallic acid equivalents (GAE) per g of dry weight (DW).
The ABTS assay was performed according to Re et al. (1999) with slight modifications. Hydrophilic or lipophilic extracts (150 μL) were mixed with 2,850 μL of ABTS solution. The mixture was incubated for 1 h in the dark. Methanol or dichloromethane was used as a control. The absorbance of the mixture was measured at 734 nm. Antioxidant capacity was calculated as Trolox Equivalent Antioxidant Capacity (TEAC), μmol Trolox per g of DW. A standard curve of Trolox was used (0.05–1 mM). The IC50 of the antioxidant capacity was determined using the ABTS method as described by Velderrain-Rodríguez et al. (2021); the results were expressed in mg/ml.
The DPPH assay was determined according to Brand-Williams et al. (1995), with slight modifications. Hydrophilic or lipophilic extracts (150 μL) were mixed with 2,850 μL of DPPH solution. The mixture was incubated in the dark for 30 min. Methanol or dichloromethane was used as a control. The absorbance of the mixture was measured at 515 nm. Antioxidant capacity was calculated as Trolox Equivalent Antioxidant Capacity (TEAC), μmol Trolox per g of DW. A standard curve of Trolox was used (0.05–1 mM).
The FRAP test was performed according to Benzie and Strain (1996) with slight modifications. The hydrophilic extracts (150 μL) were mixed with 2,850 μL of FRAP solution. The mixture was incubated for 2.5 h in the dark. Methanol was used as a control. The absorbance of the mixture was measured at 593 nm. The antioxidant capacity was calculated as Trolox Equivalent Antioxidant Capacity (TEAC), μmol Trolox per g of DW. A standard curve of Trolox was used (0.05–1 mM).
2.6 Determination of antimicrobial and antifungal activities
For these tests, bacterial strains of Staphylococcus aureus (ATCC 25923) and Escherichia coli (ATCC 25922) from the Departamento de Bacteriología de la Universidad Peruana Cayetano Heredia (Lima, Peru) and fungal strains Aspergillus niger (061471010) and Penicillium digitatum (SSFC 36296) of the Departamento de Micología del Instituto Nacional de Salud (Lima, Peru) and the Department of Microbiology and Phytopathology of the Smart & Sustainable Food (SSF) Research Center of the company Bio Natural Solutions LTD (Lima, Peru), were used, respectively.
The antimicrobial and antifungal activities of the hydrophilic extracts were determined using the disk diffusion method, following the methodology described by Santos and De Aquino Santana (2019) with some modifications. Before evaluating the antimicrobial and antifungal activities, the organic solvents were removed from hydrophilic extracts under vacuum. The conditions for evaporation varied depending on the extraction solvent: for methanol, the process was conducted at a pressure of 337 mbar and temperature of 40°C, and for ethanol, at 175 mbar and 40°C. Before the antimicrobial assessment, the residues were dissolved in water at 20 mg/mL.
Microorganisms were collected using a swab and then plated on Muller-Hinton agar plates. After that, sterile filter paper disks of 6 mm diameter were taken and 20 μL of each of the extracts was added to the filter paper disks using sterile tweezers. The disks were placed on the agar surface and incubated at 35°C for 24 h. Water was used as a negative control, and Mancozeb as a positive control. In the case of fungi, they were sown on Sabouraud dextrose agar; previously, a well was made with a Pasteur pipette to place 80 μL of each extract. They were incubated at 26°C for 7 days. After incubation, the diameter (mm) of the inhibition zone (IZ), including the diameter of the disk, was measured with a caliper. All experiments were performed in triplicate. The results are expressed as the average diameter in mm ± standard deviation.
2.7 Analysis of phenolic compounds by UHPLC – Q/Orbitrap/ESIMS/MS
The composition of phenolic compounds of the residues mentioned in section 2.1 was analyzed by UHPLC – Q/Orbitrap/ESIMS/MS. The assay was performed according to Cabanillas et al. (2021) with slight modifications. For this purpose, the extracts were prepared as follows: 30 mg of each perfectly homogenized residue were weighed in a clean, dry vial. 5 mL of a MeOH-H2O mixture (8:2) was added to each vial, and the mixture was shaken in ultrasound equipment (150 W, 0.7 A) for 20 min. The resulting solutions were filtered using a hydrophilic PVDF filter (0.22 μm x 25 mm, Millipore brand, United States) into an UHPLC vial and then stored at −20°C until analysis by UHPLC – Q/Orbitrap/ESIMS/MS.
A non-targeted analysis of phenolic compounds was done by using a Thermo Scientific Dionex Ultimate 3,000 UHPLC system (Thermo Fisher Scientific, San Jose, CA, United States) equipped with a quaternary Series RS pump and a Thermo Scientific Dionex Ultimate 3,000 Series TCC-3000RS column compartments with a Thermo Fisher Scientific Ultimate 3,000 SeriesWPS-3000RS autosampler (Thermo Fisher Scientific) and a rapid separations PDA detector controlled by Chromeleon 7.2 Software (Thermo Fisher Scientific, Waltham, MA, United States, and Dionex SoftronGmbH division of Thermo Fisher Scientific) hyphenated with a Thermo high-resolution Q Exactivefocus mass spectrometer (Thermo Fisher Scientific, San Jose, CA, United States).
Mass spectra were acquired using electrospray ionization (ESI) in negative (−2.5 Kv) and positive (+3.2 Kv) modes, source temperature 300°C (positive mode) and 250°C (negative mode), the temperature of desolvation gas was 400°C in both modes, sheath gas pressure (35 arbitrary units) and auxiliary gas (10 arbitrary units). MS spectra were acquired over a mass range of m/z 130–1,500. Protonated [M + H]+ molecules were fragmented in a high-energy collisional dissociation (HCD) cell with different voltages maintained at 35 o 45 V; Nitrogen (N2) was used as collision gas.
Liquid chromatography was performed using a Luna© Omega C18 column (1.6 μm, 150 × 2.1 mm, Phenomenex, United States) operated at 40°C. The detection wavelengths were λ 254, 280, 320, and 440 nm, and PDA was recorded from λ 200 to 800 nm for peak characterization. Mobile phases were 1% formic acid aqueous solution (A) and acetonitrile with 1% formic acid aqueous solution (B). The gradient program was: 10% B for 1 min, 10–100% B for 18 min, 100-10%B in 2 min, and 10%B for 5 min. The flow rate was 0.25 mL/min, and the injection volume was 10 μL. Q Exactive 2.0 SP 2, XCalibur 4.3, and Trace Finder software (Thermo Fisher Scientific and Dionex Softron GmbH Part of Thermo Fisher Scientific) were used for UHPLC-mass spectrometer control and data processing, respectively. Base peak (BPI) chromatograms were extracted at both low and high energy, allowing the identification of compounds. For the low-energy spectra, the mass of pseudomolecular ions [M-H]− was calculated from the elemental composition (mass error < 5 ppm). Fragmentation patterns were obtained and analyzed for the high-energy spectra, and the libraries of NIST (National Institute of Standards and Technology), GNPS (The Global Natural Product Social Molecular Networking), and PubChem were consulted to identify the compounds.
2.8 Statistical analysis
The results were expressed as the mean ± standard deviation (SD) of three repetitions. Analysis of variance (ANOVA) was used to compare means, followed by the Tukey test to evaluate differences between groups (p < 0.05). Data were analyzed using Statgraphics Centurion XV (Stat Point Technologies, Inc., Warrenton, VA, United States).
2.9 Multivariate analysis
The Hierarchical Clustering on Principal Components (HCPC) method was used to generate hierarchical groupings, using the Euclidean distance and Ward’s method, in the principal components (PCA) obtained from the analysis of a data matrix that consisted of 6 variables and 5 agroindustrial waste (6 × 5). The data were automatically scaled as a form of pre-processing and were displayed in 3 dimensions through the dendrogram in the first two dimensions of the PCA. Additionally, the heat map of the Pearson correlations of the variables under study was constructed. The multivariate analysis was carried out with the free software R using the FactoMineR package (Lê et al., 2008).
3 Results and discussion
3.1 Proximate analysis
The results of the proximal analysis of the five residues expressed in percentage (dry weight) are presented in Table 1. The moisture, protein, and carbohydrate contents of the five samples presented significant differences (p < 0.05). In contrast, crude fat content, on avocado seed, grape pomace, and lucuma seed did not present significant differences (p > 0.05). Regarding crude fiber content, lucuma seeds and mango peels did not present significant differences (p > 0.05), but there were significant differences (p < 0.05) between the other samples. Likewise, the ash content in avocado seeds and grape pomace were statistically similar (p > 0.05), while lucuma seed, mango peel, and cocoa pods presented significant differences (p < 0.05). Additionally, the humidity of the waste ranged between 47.04% (lucuma seeds) and 80.94% (cocoa pods); protein between 2.73 g/100 g DW (mango peels) and 6.59 g/100 g DW (lucuma seeds); fat between 1.10 g/100 g DW (cocoa pods) and 4.89 g/100 g DW (mango peels); crude fiber between 2.87 g/100 g DW (avocado seeds) and 26.91 g/100 g DW (cocoa pods); ash between 1.59 g/100 g DW (lucuma seeds) and 7.14 g/100 g DW (cocoa pods) and, finally, carbohydrates between 59.79 g/100 g DW (cocoa pods) and 85.85 g/100 g DW (avocado seeds). The high moisture content of the cocoa pod is close to that reported by Campos-Vega et al. (2018) at 80.2% and by Antwi et al. (2019) at 79.70%. The protein content, crude fiber, and crude fat were similar to those reported by Campos-Vega et al. (2018), with values of 5.9, 22.6, and 1.2%, respectively. The ash content, however, was within the range of 6.99–7.40% reported by other authors (Chan and Choo, 2013; Nazir et al., 2016; Antwi et al., 2019), but it was lower than the 16.70% reported by Kilama et al. (2019). These variations can be attributed to several factors, including the specific treatment processes used on the cocoa pods (Campos-Vega et al., 2018), as well as differing pedo-climatic conditions and cultivation practices in various regions (Adjin-Tetteh et al., 2018).
The mango peel reported a higher crude fat and ash content compared to that reported by Marcillo-Parra et al. (2021) of 1.48 and 2.82%, while the protein content was less than 4.04% (Marcillo-Parra et al., 2021) for the Kent variety; In comparison to other varieties, the protein content is in the range reported by Serna and Torres-León (2015) in the Keiit (1.66%) and Tommy Atkins (3.88%) varieties, the moisture content is similar to that reported in the sugar variety of 74.65% (Sánchez-Camargo et al., 2019).
The moisture content of the avocado seed (Table 1) is within that reported by King-Loeza et al. (2023), with values from 60.83 to 70.54% for different qualities of Hass avocado; other authors reported lower values from 49.81% (Amado et al., 2019) to 52.68. Regarding protein content, they were higher than that indicated by Saavedra et al. (2017) at 2.51%, Amado et al. (2019) at 2.84% and Siol and Sadowska (2023) at 3.4%, the crude fat content was close to that reported by Siol and Sadowska (2023) at 3.2%, but lower than that reported by Saavedra et al. (2017) at 1.11% and Amado et al. (2019) at 2.26%; The ash content was higher than that reported by Saavedra et al. (2017) at 1.15%, Amado et al. (2019) at 0.89. Concurring to Siol and Sadowska (2023) and Cid-Pérez et al. (2021), the nutritional composition of avocado seeds varies depending on various factors such as the origin, crop variety, state of maturity, season, postharvest, environmental, and growth conditions. In this particular case, the difference in the nutritional composition of the residues can be attributed to the origin of the Hass variety.
Grape pomace has a high carbohydrate content (Table 1) at 80.29 g/100 g DW, which would have fructose (0.9 to 6.1 g/100 g) and glucose (0.7 to 5.1 g/100 g) as its main constituents (Abouelenein et al., 2023). The protein content of grape pomace was lower than that reported by Kohajdová et al. (2018) and Abouelenein et al. (2023), being 8 and 10.72%, respectively. The crude fat content at 3.67 g/100 g DW could present a fatty acid profile rich in polyunsaturated/monounsaturated fatty acids and poor in saturated fatty acids (Bordiga et al., 2019; Kim et al., 2020); it presents a mineral content appreciable and would be composed mainly of calcium, magnesium and potassium (Abouelenein et al., 2023), the chemical composition of grape pomace varies depending on various factors such as the cultivar., climatic and agronomic conditions, and the winemaking technology adopted (Kohajdová et al., 2018). In this sense, grape seed turns out to be an interesting by-product to be used in diets that contain ingredients rich in energy and proteins (Khiaosa-ard et al., 2015), improving the nutritional quality and functional properties of the final product.
Lucuma seed has considerable and significant values of crude fiber and protein. However, there are few studies on this agroindustrial waste (Pouteria lucuma). In this sense, when comparing with other varieties of Pouteria, it is observed that the protein content was higher than that reported by Do et al. (2023) (1.12 g/100 g DW). The fat content was similar to that reported by Da Costa et al. (2010) and Do et al. (2023), being 4.62 and 6.27 g /100 g DW, respectively, who worked with the Pouteria variety campechiana. The ash content would be composed of potassium, manganese, and iron, as indicated by Aguiar et al. (2019) in Pouteria elegans. The fatty acid content would be composed of linoleic acid, oleic acid, and palmitic acid (Rojo et al., 2010) and tocopherols, cyclic and non-cyclic triterpenoids (Guerrero-Castillo et al., 2021).
The results indicate a predominance of carbohydrates, whose specific composition, inferred from previous research, suggests a significant presence of starches and pectins. For example, previous studies on Pouteria lucuma, Pouteria campechiana, and avocado seeds report high starch contents, reaching up to 91.2% of total carbohydrates (Cari, 2018; Tesfaye et al., 2018; Álvarez-Yanamango et al., 2020; Li et al., 2021). Regarding pectin, studies on mango peels, cocoa pods, and grape pomace highlight its abundance and usefulness in food and pharmaceutical applications (Rodríguez, 1995; Ajila and Rao, 2013; Lu et al., 2018; Freitas et al., 2020; Delgado-Ospina et al., 2021; Spinei and Oroian, 2023). In addition, it contributes to a greater understanding of the potential of agroindustrial waste, not only as a source of carbohydrates but also in terms of its protein, crude fiber, crude fat, and ash content. This comprehensive approach facilitates exploring varied applications, ranging from food to bioplastics and pharmaceutical manufacturing. Recovering agro-industrial waste contributes to environmental and economic sustainability, reducing the need for new raw materials and opening new markets for the agri-food industry. This approach supports the circular economy and innovation, optimizing waste management and generating economic benefits. In this context, this study lays the foundation for future research on more efficient extraction methods and the comprehensive use of this waste, emphasizing transforming what was previously considered waste into valuable resources.
3.2 Total phenolic content and antioxidant capacity
The total phenolic content (TPC) in Table 2 is significantly different across the by-products (p < 0.05). There was no significant difference in TPC between grape pomace and lucuma seeds (p > 0.05), nor between mango peel, cocoa pod, and avocado seed (p > 0.05); however, significant differences (p < 0.05) were observed between these two groups. The TPC values ranged from 4.39 ± 0.05 mg GAE/g DW in lucuma seeds to 56.66 ± 5.77 mg GAE/g DW in mango peels. The by-products with the highest TPC were mango peels, avocado seeds, and cocoa pods.
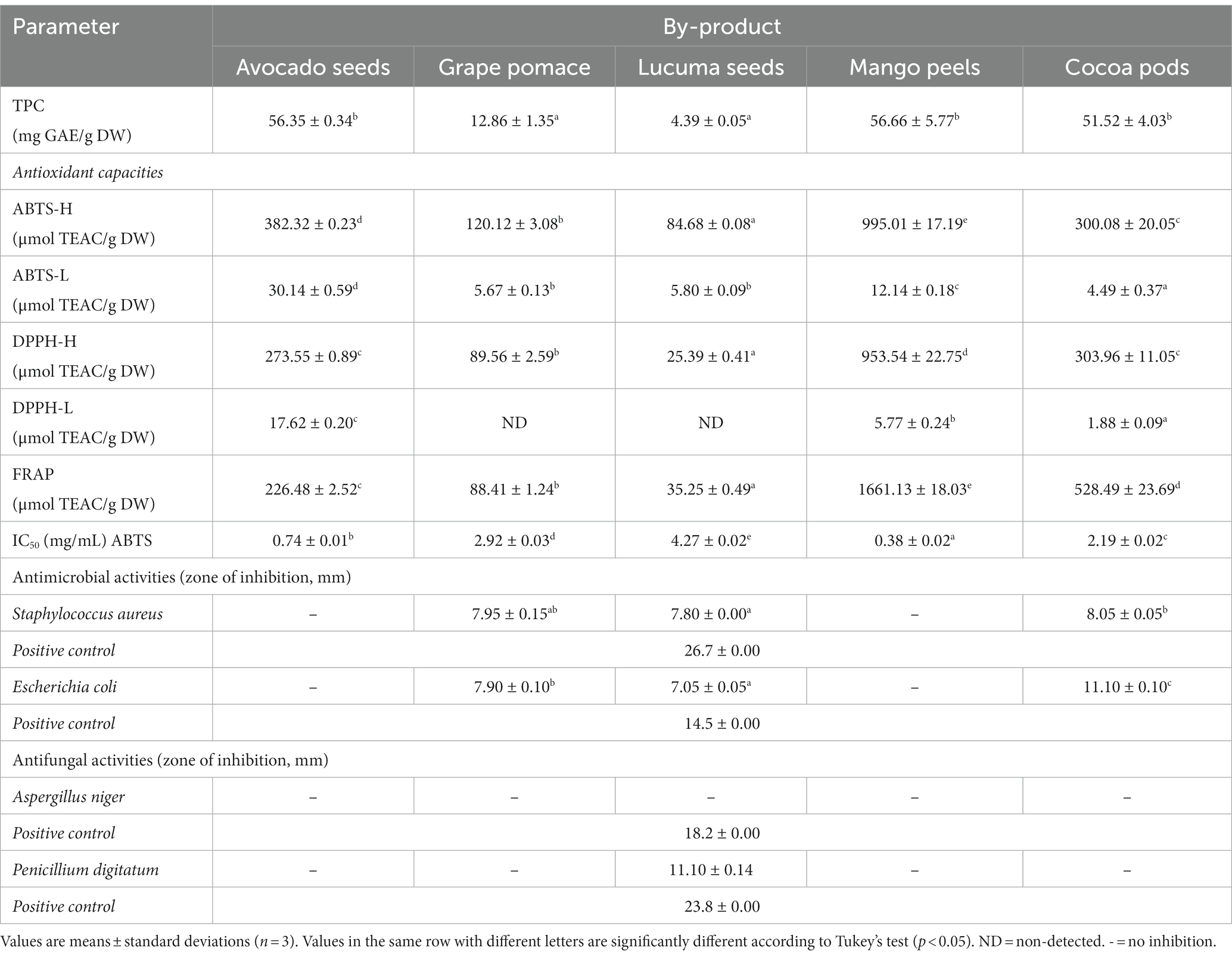
Table 2. Total phenolic content, hydrophilic (H-AC), lipophilic antioxidant capacities (L-AC), antimicrobial and antifungal activities of some Peruvian crops agrifood by-products.
The value found of phenolic compounds in mango peel (56.66 ± 5.77 mg GAE/g DW) was higher than that reported by Serna and Torres-León (2015) when they evaluated mango peel of the Tommy variety (35.88 mg GAE/g DW) and Keitt (46.71 mg GAE/g DW), but similar to the values reported by Marcillo-Parra et al. (2021) at 51.49 mg GAE/g DW in Kent variety. Regarding avocado seeds, the content of phenolic compounds (56.35 ± 0.34 mg GAE/g DW) was higher than that reported by Cid-Pérez et al. (2021) in avocado seeds of the Criolla variety (30.25 mg GAE/g DW), and the Nariño variety (18.2 GAE/g dry seed) (Rosero et al., 2019). The value of phenolic compounds found in cocoa pods (51.52 ± 4.03 mg GAE/g DW) was similar to that reported by Abdul Karim et al. (2014) from 45.60–56. 50 mg GAE/g DW, and higher than that reported by Sotelo et al. (2015) (16.40–23.01 mg GAE/g DW).
The TPC of grape pomace (12.86 mg GAE/g DW) was higher than that reported by Alvarez-Yanamango et al. (2019) (11.45 GAE/g DW) and De la Cruz-Azabache et al. (2023) (1.58 mg GAE/g DW) after the fermentation process to obtain pisco from the Quebranta variety. However, it was lower than that reported by Cisneros-Yupanqui et al. (2020) (38.86 mg GAE/g DW) and Abouelenein et al. (2023) in red grape pomace (41.23–48.3 mg GAE/g DW) and white grape (44.7 mg GAE/g DW). It has been reported that the TPC (total phenolic content) values in wine by-products can be influenced by various factors such as the region where the grapes are produced, the fermentation techniques used, the climate, the genotype of the grapes (Di Stefano et al., 2022), the drying process carried out on the grape pomace (Solari-Godiño et al., 2017), and the distillation technology used (Cisneros-Yupanqui et al., 2022), since generally a batch distillation requires a longer exposure time to high temperatures than the continuous system (Bordiga et al., 2019), generating a significant loss of TPC that could be related to some degradation or polymerization reactions (Spigno et al., 2007). The differences found in the literature can also be attributed mainly to the presence of anthocyanins, flavonols, hydroxycinnamic acid derivatives from the peels, and resveratrol, flavan-3-ols, and proanthocyanidins from the seeds (Rebello et al., 2013). About lucuma seed, the value of phenolic compounds found (4.39 ± 0.05 mg GAE/g DW) was lower than that reported by Guerrero-Castillo et al. (2019) (8.99 mg GAE/g DW), who did the extraction for 9 days, unlike the present study (3 h). On the other hand, Kong et al. (2013) carried out the extraction of bioactive compounds from the seed of the Pouteria variety campechiana with three different solvents (water, 70% ethanol, 70% methanol), finding TPC values that varied from 2.5 to 23.04 mg GAE/g DW, which would indicate that the extraction technique affects the final TPC content, and the optimization of bioactive compound extraction processes is necessary.
The results of the hydrophilic antioxidant capacity using the ABTS, DPPH, and FRAP methods and lipophilic by the ABTS and DPPH methods are shown in Table 2. The ABTS-H assay revealed significant variations (p < 0.05) across all by-products. In contrast, the DPPH-H assay showed no significant difference (p > 0.05) between avocado seed and cocoa pod, while significant differences were observed among the remaining by-products. Furthermore, the FRAP assay demonstrated significant variations (p < 0.05) in all the evaluated by-products.
The range of hydrophilic antioxidant capacity by the ABTS method varies from 84.68 ± 0.08 to 995.01 ± 17.19 01 μmol TEAC/g DW in lucuma seeds and mango peels, respectively. Similar behavior was recorded in the DPPH (25.39 ± 0.41–953.54 ± 22.75 μmol TEAC/g DW) and FRAP (35.25 ± 0.49–1661.13 ± 18.03 μmol TEAC/g DW) methods. The antioxidant capacity of mango peels (995.01 μmol /g DW) by the ABTS method was higher than that reported by Marcillo-Parra et al. (2021) at 499 μmol TEAC /g DW in mango variety Kent. The value of the antioxidant capacity of avocado seeds found in this study was lower than that reported by Saavedra et al. (2017) applying the DPPH method (1,135 μmol TEAC/g DW). In cocoa pods, the antioxidant capacity value found in this study was higher than that reported by Sotelo et al. (2015) applying the ABTS method (229.61 μmol TEAC/g DW) and FRAP (169.4 μmol TEAC/g DW). The research findings on grape pomace indicate that the antioxidant capacity of ABTS (120.12 ± 3.08 μmol TEAC/g DW), DPPH (89.56 ± 2.59 μmol TEAC/g DW), and FRAP (88.41 ± 1.24 μmol TEAC/g DW) was lower than the levels reported by Barriga-Sánchez et al. (2022) for Black Burgundy grape pomace obtained from Chincha and Ica cities of Peru, of ABTS (425.67–448.84 μmol TEAC/g DW) and DPPH (266.35–269.12 μmol TEAC/g DW). Finally, the value of the antioxidant capacity of lucuma seed found in this study was lower than that reported by Guerrero-Castillo et al. (2019) applying the FRAP method (272.50 μmol TEAC/g DW).
No significant differences were observed between grape pomace and lucuma seeds (p > 0.05) during the measurement of the lipophilic antioxidant capacity (Table 2) in the ABTS-L assay. As for the DPPH-L method, significant differences (p < 0.05) were noted among avocado seeds, mango peels, and cocoa pods. The ABTS method varied from 4.49 ± 0.37 to 30.14 ± 0.59 TEAC/g DW in cocoa pods and avocado seeds, respectively. The DPPH method had similar behavior, varying from 1.88 ± 0.09 to 17.62 ± 0.20 TEAC/g DW; with this last method, grape pomace, and lucuma seed did not report lipophilic antioxidant capacity. Avocado seeds showed the highest values in lipophilic antioxidant capacity by both ABTS and DPPH methods.
The different values of antioxidant capacity obtained by the ABTS, DPPH, and FRAP methods of the five residues studied can be attributed to the chemical structure of the radical molecules, their mechanism of action, and the type of phenolic compound present in each residue (Ferreira-Santos et al., 2024), as well as varieties, stages of maturity and extraction methods (Chen et al., 2011).
Using the ABTS method, the IC50 values exhibited significant differences (p < 0.05) among all agrifood by-products (Table 2). The lowest IC50 values were in the extracts of mango peels (0.38 ± 0.02 mg/mL) and avocado seeds (0.74 ± 0.01 mg/mL). These results indicate that mango seeds and avocado seeds extracts have greater antioxidant capacity. Kuganesan et al. (2017) and Velderrain-Rodríguez et al. (2021) reported lower IC50 values in extracts of mango peels (0.19 mg/mL) and avocado seeds (0.32 mg/mL) by the ABTS method, respectively. Umamahesh et al. (2016) determined IC50 using the ABTS method in aqueous extracts of mango peels of five varieties from India, with values between 28.29 and 84.88 μg/mL, highlighting the Sindhura variety for showing better antioxidant capacity and higher content of phenolic compounds (87.38 ± 0.43 mg GAE/g). For their part, Guerrero-Castillo et al. (2019) determined IC50 using the ABTS method in methanolic extracts of lucuma seed (66.97 μg/mL), a value lower than that reported in the present investigation. Regarding grape pomace, Veskoukis et al. (2012) reported IC50 values of 5.5 mg/mL, higher than what was found in this study, which would indicate a lower antioxidant capacity concerning the residue studied.
3.3 Multivariate analysis
Mango peels, avocado seeds, and cocoa pods exhibited the highest values of antioxidant capacity by the three methods, ABTS, DPPH, and FRAP, showing a good correlation with the order of TPC results. By applying Pearson correlation coefficient, high positive correlations were found between the hydrophilic antioxidant capacity and the content of phenolic compounds by the ABTS (r = 0.71), DPPH (r = 0.70), and FRAP (r = 0.62) method. This fact suggests that the antioxidant capacity mainly depends on the phenolic content in these by-products. In order to identify similarities among the various agro-industrial wastes used in the study, a multivariate analysis was carried out that includes hierarchical grouping (Ward’s method and Euclidean distance) in the first two principal components of the PCA (Figure 1A) and the PCA biplot (Figure 1B). Pearson correlation coefficient analysis was performed to elucidate the correlation between the total polyphenol content and the bioactivities data sets of agro-industrial waste extracts (Figure 1C). As a result of the multivariate analysis, the first two principal components describe 95.2% of the total variance of the data (Dim 1 = 74.1% and Dim 2 = 21.1%). Hierarchical clustering evaluates data according to its homogeneity and heterogeneity, grouping them according to the degree of similarity (Ferreira et al., 2019).
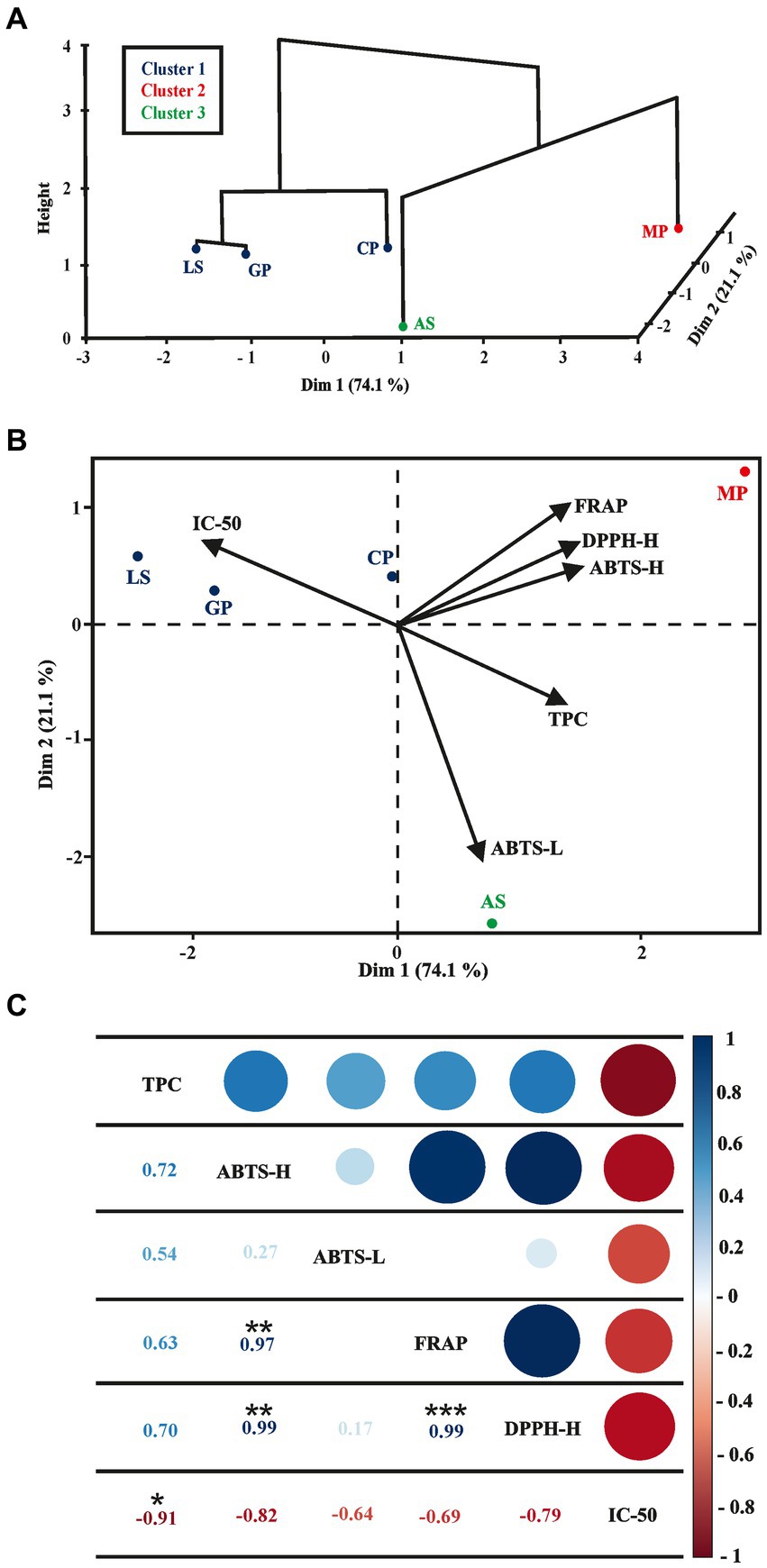
Figure 1. Multivariate analysis of the data sets of agroindustrial waste extracts: lucuma seeds (LS), cocoa pods (CP), grape pomace (GP), mango peels (MP), and avocado seeds (AS). (A) Hierarchical clustering in the first two principal components of the PCA (HCPC). (B) biplot of the first two principal components of the PCA. (C) correlation between the content of total polyphenols, antioxidant activity (ABTS-H, ABTS-L, FRAP, and DPPH-H) and the IC50.
The trends of the correlations observed through PCA were confirmed from the projection of the dendrogram created with the application of HCPC using Ward’s method to obtain the minimum variance between the vectors that make up each group, in addition to the Euclidean distances, to verify similarities between samples (Fernandes Serra Moura et al., 2021).
Ward’s hierarchical clustering algorithm indicates the formation of three groups. The essence of this algorithm lies in minimizing the increase in inertia when two samples are combined. This iterative process continues until the increase in inertia is as minimal as possible (Kassambara, 2017). The clustering quality was assessed using the silhouette coefficient, which ranges from −1 to 1. A positive value indicates good clustering quality, while a negative value suggests a sample might be incorrectly grouped (Gilbert et al., 2020). For all cases, values greater than zero were obtained, confirming the appropriateness of the group formations. The formation of three groups of agroindustrial waste is distinguished. Group 1 comprises lucuma seed, grape pomace, and cocoa pods; Group 2 is for the avocado seed, and Group 3 is for the mango peel. This variation demonstrated that agroindustrial waste has intrinsic chemical characteristics, presenting variations in concentrations compared to other species due to climatic factors, growing conditions, and harvest time (Fernandes Serra Moura et al., 2021). Mango peel (Figure 1B) obtained the highest values of antioxidant capacity measured by FRAP, DPPH-H, and ABTS-H and the lowest IC50 value (opposite position in the PCA biplot); these results indicate a beneficial negative correlation: higher phenolic content corresponds to lower IC50 values, signifying enhanced antioxidant capacity (Figure 1C). Phenolic compounds have received significant interest in recent years. Consuming vegetables with a high concentration of these bioactive compounds can reduce the risk of developing various diseases due to their antioxidant activity (Fernandes Serra Moura et al., 2021). Figure 1C shows the correlation analysis between TPC-vs-IC50 (r = −0.91, value of p <0.05), ABTS-H vs. FRAP (r = 0.97, value of p <0.01), ABTS-H vs. DPPH-H (r = 0.99, value of p < 0.05) and FRAP vs. DPPH-H (r = 0.99, value of p <0.001). Furthermore, the content of total polyphenols and the antioxidant activities measured by the ABTS-H, ABTS-L, FRAP, and DPPH-H methods are negatively correlated with the IC50 value (r = −0.91, −0.82, −0.64, −0.69 and − 0.79, respectively), evidencing the inverse relationship.
These results are comparable with those reported by Khiya et al. (2021) and Li et al. (2009), who studied the correlation of various response variables, including DPPH, ABTS, reducing power, with the content of total polyphenols, including ferulic acid and caffeic acid, finding r values between 0.659 and 0.911. They also found that the IC50 value decreases when the polyphenol content increases. This could be because the results show that a higher content of total polyphenols had a higher antioxidant capacity, so a lower content of the extract will be required to achieve an inhibitory effect of 50% (IC50). This same reason explains the negative correlation between DPPH, ABTS, and FRAP with IC50.
3.4 Antimicrobial and antifungal activities
The results of the antimicrobial and antifungal activities of the extracts of phenolic compounds, in general, showed significant differences (p < 0.05). A significant difference (p < 0.05) is observed in the inhibition zone of Staphylococcus aureus between the cocoa pod (8.05 ± 0.05 mm) and the lucuma seed (7.80 ± 0.00 mm). However, each did not present a significant difference with grape pomace (7.95 ± 0.15). In the case of Escherichia coli, there was no zone of inhibition for avocado seed or mango peel. But there was a significant difference between the other agroindustrial waste (p < 0.05), varying between 7.05 ± 0.05 mm (lucuma seed) and 11.10 ± 0.10 mm (cocoa pod). Furthermore, none of the residues presented potential antifungal activity against Aspergillus niger, and only the lucuma seed caused inhibition against Penicillium digitatum (11.10 ± 0.14). The negative controls, in all the extracts, did not generate a halo of inhibition, and in the case of the positive controls, the average per microorganism is shown (Table 2).
The extracts obtained from cocoa pods, grape pomace, and lucuma seeds have been found to possess antimicrobial properties against colonies of Staphylococcus aureus and Escherichia coli.
The cocoa pod extract can inhibit the growth of Staphylococcus aureus and Escherichia coli at concentrations of 6.25 mg/mL (Sotelo and Alvis, 2018) and from 5 mg/mL to 50 mg/mL (Sotelo and Alvis, 2018; Diniardi et al., 2020), respectively, when a concentration of 20 mg/mL was used of extracts obtained.
The antimicrobial activity of grape pomace on S. aureus and E. coli colonies was similar to previous studies (Mendivil, 2015; Kuganesan et al., 2017). This activity is related to the presence of phenolic compounds (Nitta et al., 2002) and their content of flavonoids (flavanols and flavonols) and non-flavonoid compounds (phenolic acids and stilbenes) (Friedman, 2014). The antimicrobial activity of flavanols may be related to the ability to bind to the peptidoglycan membrane of bacteria, altering its functionality (Yoda et al., 2004). Katalinić et al. (2010) reported that the peel of 14 grape varieties showed antimicrobial activity against Gram positive (Staphylococcus aureus and Bacillus cereus) and Gram negative bacteria (Escherichia coli O157:H7, Salmonella infantis, and Campylobacter coli). Besides, Oliveira et al. (2013) obtained a grape pomace extract using supercritical fluids. They found better antimicrobial properties against Gram positive (S. aureus and B. cereus) than Gram negative (E. coli and P. aeruginosa). Furthermore, Gaafar et al. (2019) demonstrated the antimicrobial effect of aqueous extracts of 2 varieties of grape pomace (red and white) against B. subtilis, E. coli, P. aeruginosa, S. aureus, A. niger, A. flavus, S. cerevisiae, and C. albicans. While it is true that the exact mechanism of antimicrobial activity is not known, Hassan et al. (2019) suggest that in the case of Gram positive bacteria, the compounds present in the extract, such as phenolic acids, stilbenes, flavonols, anthocyanins, and flavanols, can generate the inactivation of bacterial exoenzymes, chelation of essential metals such as iron or deprivation of nutrients such as tannins, or the permeabilization, rupture and disintegration of external membranes, and the reduction of pH and inhibition of proton exchangers. It is known that the main compounds responsible for antimicrobial activity are flavonoids, mainly due to the presence of gallic acid and vanillic acid (Silva et al., 2018). However, curiously, in our study, these compounds were not identified, so there is possibly a synergistic effect between the different phenolic compounds identified, such as Isorhamnetin-3-O-glucoside or kaempferol and its derived compounds that could be responsible for the antimicrobial activity and antifungal (Rashed et al., 2014; Periferakis et al., 2022). Likewise, Taguri et al. (2004) mention that the degree of polymerization and the number of hydroxyl groups could directly affect the antimicrobial activity of each phenolic compound.
The lucuma seed extract exhibited antimicrobial activity against S. aureus and E. coli and antifungal activity against Penicillium digitatum, as well as the essential oil of P. campechiana seeds, which had potent antimicrobial activity against both Gram positive and Gram negative bacteria (Ma et al., 2019; Do et al., 2023). According to Maria et al. (2012), the catechins present in the lucuma seed (Table 2), such as gallocatechin (epimerized form), tend to have a more significant antagonistic effect against S. aureus and E. coli, followed by epigallocatechin, which inhibits growth and causes the death of S. aureus (Kitichalermkiat et al., 2020), caused by the union of hydrogen bonds with the membrane proteins of S. aureus (Zhao et al., 2001; Madhan et al., 2007), which affects its functions, such as decreasing glucose uptake or its protection against external stress (Kitichalermkiat et al., 2019). Likewise, Jeon et al. (2014) mentioned that epicatechin-3-gallate present in lucuma seed has antimicrobial activity against E. coli, and naringenin has a greater inhibition effect for S. aureus than for E. coli (Duda-Madej et al., 2022).
The extracts of avocado seeds and mango peel did not present antimicrobial and antifungal activity. Other researchers point out that Hass avocado seed extract (Romaní et al., 2017), avocado seed (Kupnik et al., 2023), and mango peel (Lozoya-Castillo et al., 2018) inhibit the microbial growth of S. aureus and E. coli. This difference can be attributed to the extraction conditions. In the case of avocado seed, Romaní et al. (2017) used a 96% ethanolic macerate of avocado seed powder followed by fractionation with ethyl acetate, finding a minimum inhibitory concentration of 10 mg/mL. Sotelo and Alvis (2018) reported a minimum inhibition concentration of 50 mg/mL for E. coli O157:H7 and 6.25 mg/mL for S. aureus ATCC 29213, showing that the type of extraction solvent and its concentration influence the antimicrobial capacity. For Sunder et al. (2011), the best solvent for the extraction of antibacterial compounds is methanol. Ilozue et al. (2014) point out that a greater antimicrobial capacity is obtained with methanolic extracts and a lower capacity with petroleum ether, mainly affected by the solubility of the polyphenolic compounds. However, other factors, such as physiological variations, environmental conditions, geographical variation, genetic factors, and extraction conditions, must be considered (Pérez et al., 2015).
Aspergillus niger colonies showed resistance to the five extracts used in this research. Scroccarello et al. (2021) point out that the A. niger strain can survive in extreme conditions and develop resistance against antifungal agents. A. niger can degrade polyphenolic compounds to obtain a potent bioactive molecule such as ellagic acid (Sepúlveda et al., 2016), evidencing its resistance. About the results obtained, possibly A. niger, being a resistant strain, followed the metabolic route that uses polyphenolic compounds to produce hexahydroxydiphenic acid, which is considered an intermediate molecule in the release of ellagic acid, which has important and desirable properties beneficial to health.
The interest in replacing synthetic antimicrobials and antifungals has led to the search for compounds of plant origin that can inhibit foodborne pathogens, prolong their shelf life, and reduce economic losses (Singh et al., 2018). Antimicrobial and antifungal activity is assigned to phytochemical compounds such as polyphenols. The mechanism of action is due to the change in the function of cell membranes and the consequent slowing of growth and inhibition of microorganisms’ reproduction (Singh et al., 2018; Kupnik et al., 2023). Moreover, seeds and agribusiness waste extracts also have many health-related bioactive properties, such as antihyperglycemic, anticancer, antihypercholesterolemic, antioxidant, anti-inflammatory, and antineurogenerative effects, which can be used to formulate or fortify foods (Kupnik et al., 2023; Sławińska and Olas, 2023; Sorrenti et al., 2023). For further details on the microbiological results, please refer to the Supplementary material.
3.5 Phenolic compound analysis
Figure 2 shows the total ion chromatogram (TIC) resulting from UHPLC– Q/Orbitrap/ESIMS/MS analysis in the negative mode of phenolic-rich extracts from the five agrifood by-products. The qualitative and quantitative profile is very different among them. Table 3 summarizes the identification of phenolic compounds based on their MS and MS/MS spectra, considering the fragmentation patterns of mass spectra recorded in the NIST (National Institute of Standards and Technology), GNPS (The Global Natural Product Social Molecular Networking), and PubChem comparing with information previously reported in the literature.
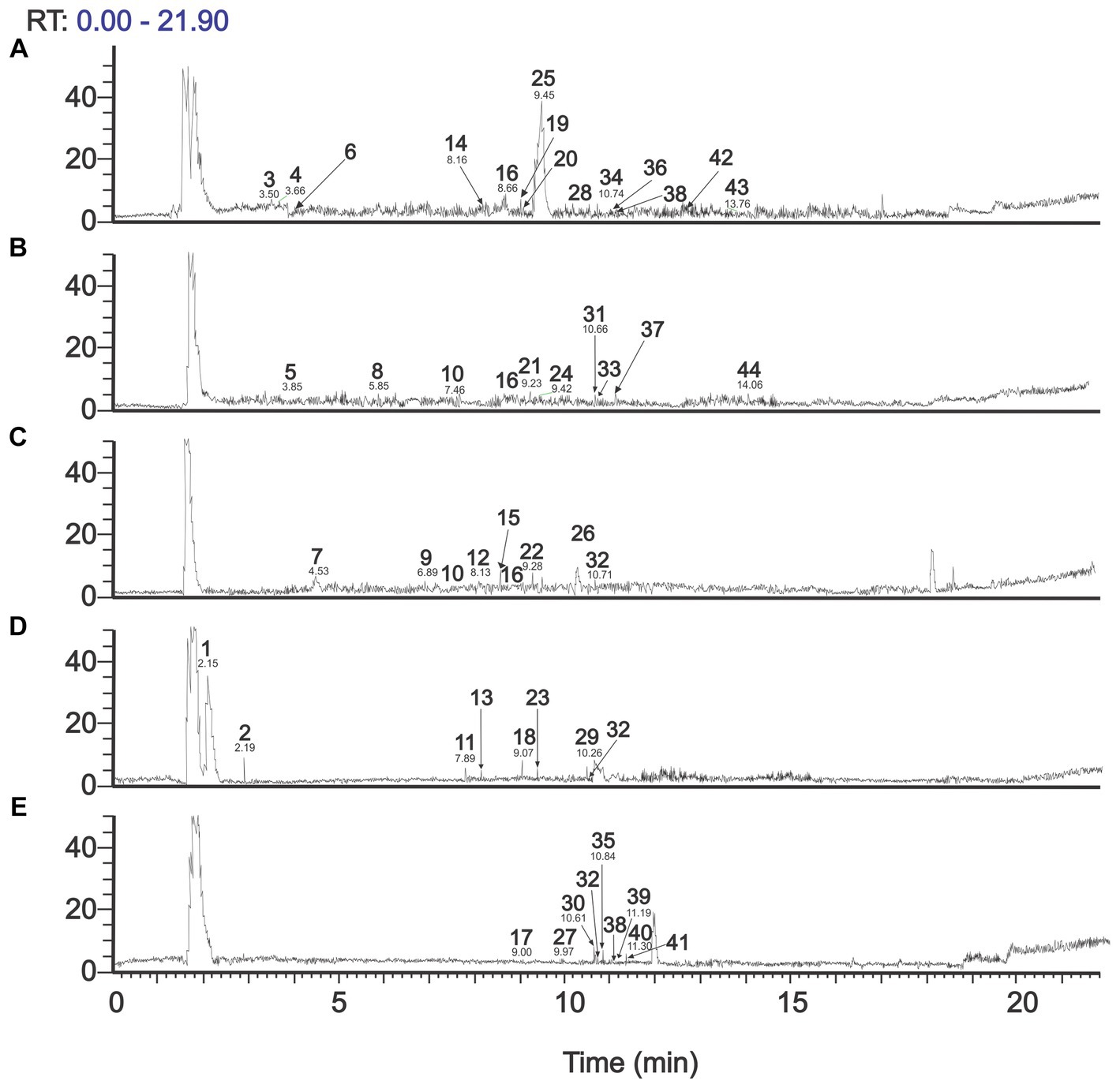
Figure 2. Total ion chromatogram obtained by UHPLC– Q/Orbitrap/ESIMS/MS (negative mode) of (A) Cocoa pods, (B) Lucuma seeds, (C) Avocado seeds, (D) Mango peels, and (E) Grape pomace. Numbers corresponding with those of Table 3.
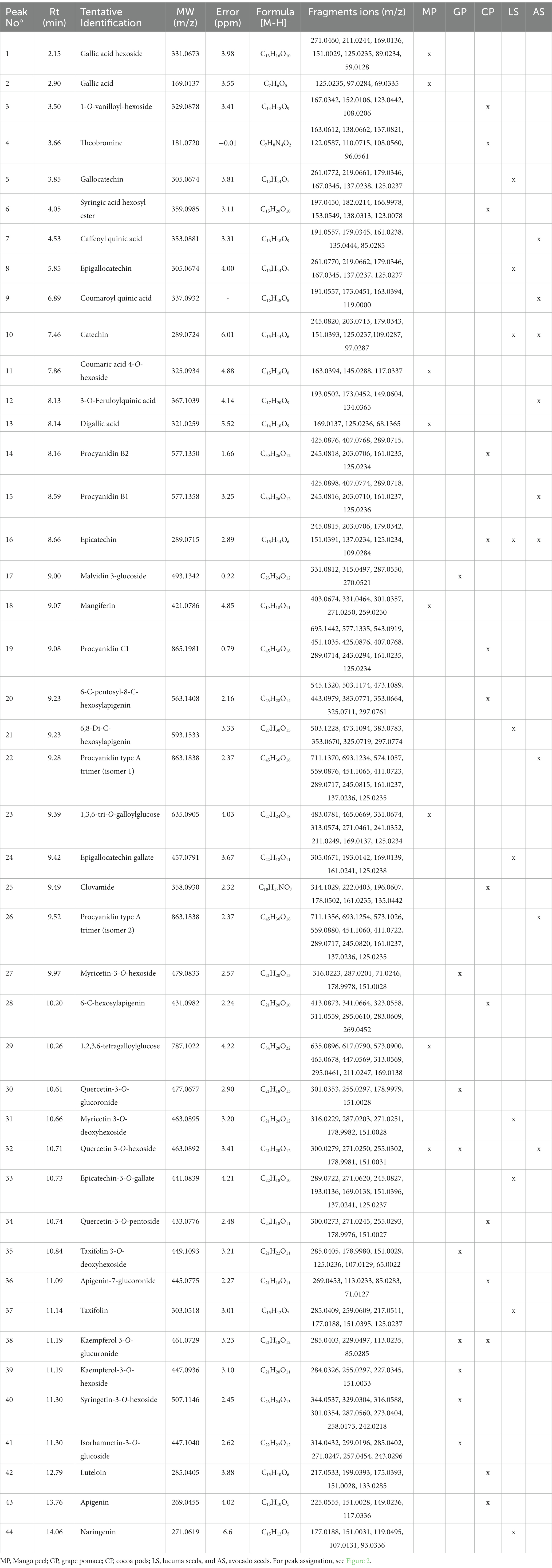
Table 3. Phenolic compounds identified in the extracts of Agrifood by-products by UHPLC– Q/Orbitrap/ESIMS/MS analysis.
One of the phenolic compounds identified in mango peels is mangiferin (Peak 18), with similar findings in Spanish varieties such as Keiit, Sensación, and Gomera (Dorta et al., 2014), which was quantified in significant quantities in mango peel of the Tommy Atkins variety, compared to the Haden varieties (Berardini et al., 2005; Marcillo-Parra et al., 2021). This compound, identified as a xanthone, presents various biological activities, such as antiviral, anticancer, antidiabetic, immunomodulatory, hepatoprotective, and analgesic, all attributed mainly to its antioxidant capacity (Muruganandan et al., 2005; Barreto et al., 2008; Noratto et al., 2010). Furthermore, the mangiferin content in mango peel can vary from 1 to 4 times more than the content in the pulp (Berardini et al., 2005; Ajila et al., 2007).
The cocoa pods compounds identified as theobromine (Peak 4) and epicatechin (Peak 16) are similar to what was reported by Valadez-Carmona et al. (2017), those identified in cocoa pods catechin, quercetin, (−)- epicatechin, gallic, coumaric, and protocatechuic acids, among others. The antioxidant capacity is superior to other cocoa by-products, such as the bean shell and mucilage. Likewise, clovamine (Peak 25) was identified as a possible bioactive compound with anti-inflammatory activity in human cells (Zeng et al., 2011).
Among the compounds identified in grape pomace are malvidin 3-glucoside (Peak 17), quercetin-3-O-glucoronide (Peak 30), kaempferol-3-O-glucuronide (Peak 38), like what was reported in grape pomace from five varieties of grapes from Turkey, where the content of phenolic compounds differs according to the variety, highlighting that red grapes have a higher content of phenolic compounds compared to white grapes (Sagdic et al., 2011). Likewise, Cui et al. (2023) identified 19 compounds in the free extracts of grape pomace, which dominated the gallic and syringic acids, followed by catechin, 3,4-dihydrobenzoic acid, vanillic acid, and epicatechin. It has been reported that grape seed procyanidins have beneficial health properties such as antidiabetic, anti-obesity, antimicrobial, cardioprotective, anti-neurodegenerative, anti-osteoarthritis, anticancer, and cardio and eye-protective properties (Unusan, 2020).
Among the phenolic compounds identified in lucuma seeds include gallocatechin (Peak 5), epicatechin (Peak 16), and catechin (Peak 10). For their part, Guerrero-Castillo et al. (2019) identified gallocatechin, catechin, epicatechin, taxifolin, myricetin, quercetin, eriodictyol chalcone, apigenin, and naringenin.
Among the compounds identified in avocado seed, there is catechin (Peak 10), epicatechin (Peak 16), quercetin 3-O-hexoside (Peak 32), procyanidin type A trimer (isomers 1 and 2) (Peak 26), results similar to those reported by Kosińska et al. (2012) and Rosero et al. (2019). The antioxidant activity of phenolic compounds shows their potential to slow aging processes, reduce oxidative stress, and protect against diseases, among others. Findings show that catechins and epicatechins contribute 38% of all antioxidant activity of all fresh fruit (Wang et al., 2010). The presence of caffeic acid in avocado seed extracts prevents disorders of the cells of the nervous system by inhibiting the action of enzymes that participate in disorders of nerve cells, preventing antidegenerative diseases such as Alzheimer’s (Oboh et al., 2016).
The diversity of phenolic compounds in extracts from mango peel, cocoa pod, grape pomace, lucuma seed, and avocado seed is associated with the antioxidant, antimicrobial, and antifungal capacities reported in this study. This finding opens new research opportunities within a circular economy context, focusing on using eco-friendly and efficient methods that minimize environmental impacts. Specifically, Mir-Cerdà et al. (2023) indicated that the applications of these extracts while those with lower purity have potential in the cosmetics industry and in packaging manufacturing. Review the Supplementary material for more details on the phenolic compounds’ results.
4 Conclusion
According to the results of this study, lucuma seeds were notable for their protein content, cocoa pods for crude fiber and mineral contents, mango peels for their crude fat, and avocado seeds for their carbohydrate content. Mango peel, cocoa pods, and avocado seed extracts exhibited the highest phenolic compound contents, which showed a strong and positive correlation with their antioxidant capacity and an inverse correlation with the IC50 values, with mango peel extract exhibiting a high antioxidant capacity. Lucuma seeds exhibited bactericidal and antifungal effects against Staphylococcus aureus, Escherichia coli, and Penicillium digitatum, while cocoa pod and grape pomace extracts were effective against Staphylococcus aureus and Escherichia coli. Furthermore, in this study, the main phenolic compounds, which could be responsible for their bioactive properties, were identified in each by-product. The results obtained from the agro-industrial wastes studied here provide valuable information on the composition and functional properties, sparking interest in future research exploring their reuse and food applications.
Data availability statement
The original contributions presented in the study are included in the article/Supplementary material, further inquiries can be directed to the corresponding authors.
Author contributions
MI: Conceptualization, Methodology, Supervision, Writing – review & editing, Investigation, Project administration, Validation, Writing – original draft. IB-P: Conceptualization, Data curation, Investigation, Methodology, Supervision, Writing – review & editing. GP-I: Data curation, Formal analysis, Methodology, Software, Writing – review & editing. LC-A: Formal analysis, Investigation, Writing – original draft. CO: Data curation, Supervision, Writing – review & editing. J-D-CV-A: Data curation, Writing – original draft. CV-D-L-C: Data curation, Writing – original draft.
Funding
The author(s) declare that financial support was received for the research, authorship, and/or publication of this article. This research was supported by Programa Nacional de Ciencia y Estudios Avanzados (PROCIENCIA), a non-profit organization that promotes research development in Peru (Contract No. 162–2020).
Acknowledgments
We thank the valuable support of Cinthya Huaman Alvino for her support in the laboratory during the research and Vivero Topara (Chincha, Ica, Peru) for supplying Pouteria lucuma fruits.
Conflict of interest
The authors declare that the research was conducted in the absence of any commercial or financial relationships that could be construed as a potential conflict of interest.
Publisher’s note
All claims expressed in this article are solely those of the authors and do not necessarily represent those of their affiliated organizations, or those of the publisher, the editors and the reviewers. Any product that may be evaluated in this article, or claim that may be made by its manufacturer, is not guaranteed or endorsed by the publisher.
Supplementary material
The Supplementary material for this article can be found online at: https://www.frontiersin.org/articles/10.3389/fsufs.2024.1341895/full#supplementary-material
References
Abdul Karim, A., Azlan, A., Ismail, A., Hashim, P., Abd Gani, S. S., Zainudin, B. H., et al. (2014). Phenolic composition, antioxidant, anti-wrinkles, and tyrosinase inhibitory activities of cocoa pod extract. BMC Complement. Altern. Med. 14:381. doi: 10.1186/1472-6882-14-38
Abouelenein, D., Mustafa, A. M., Caprioli, G., Ricciutelli, M., Sagratini, G., and Vittori, S. (2023). Phenolic and nutritional profiles, and antioxidant activity of grape pomaces and seeds from Lacrima di Morro d’Alba and Verdicchio varieties. Food Biosci. 53:102808. doi: 10.1016/j.fbio.2023.102808
Adjin-Tetteh, M., Asiedu, N., Dodoo-Arhin, D., Karam, A., and Amaniampong, P. N. (2018). Thermochemical conversion and characterization of cocoa pod husks, a potential agricultural waste from Ghana. Ind. Crop. Prod. 119, 304–312. doi: 10.1016/j.indcrop.2018.02.060
Aguiar, J. P. L., Da Silva, E. P., Pereira, R. C., Nagahama, D., and Da Souza, F. (2019). The aromatic and nutritional profile of an Amazonian autochthonous species, Caramuri Pouteria elegans (a.DC.) Baehni. Int. J. Food Prop. 22, 1242–1249. doi: 10.1080/10942912.2019.1640248
Ajila, C. M., Naidu, K. A., Bhat, S. G., and Rao, U. J. S. P. (2007). Bioactive compounds and antioxidant potential of mango peel extract. Food Chem. 105, 982–988. doi: 10.1016/j.foodchem.2007.04.052
Ajila, C., and Rao, P. (2013). Mango peel dietary fibre: composition and associated bound phenolics. J. Funct. Foods 5, 444–450. doi: 10.1016/j.jff.2012.11.017
Albuquerque, M. A. C., Levit, R., Beres, C., Bedani, R., Moreno de LeBlanc, A., Saad, S. M. I., et al. (2019). Tropical fruit by-products water extracts as sources of soluble fibers and phenolic compounds with potential antioxidant, anti-inflammatory, and functional properties. J. Funct. Foods 52, 724–733. doi: 10.1016/j.jff.2018.12.002
Álvarez-Yanamango, E., Huanqui, G. C., and Huayta, F. (2020). Recovery and characterization of Lucuma seed starch (Pouteria lucuma) with potential industrial application. In: Proceedings of the LACCEI international multi-conference for engineering, education and technology. S.L., Latin American and Caribbean consortium of engineering institutions.
Alvarez-Yanamango, E., Vietti-Guzmán, F., Napan, L., Huayta, F., and Cueva, R. (2019). Recovery of phenolic compounds from grape residues from the production of Pisco quebranta (No. 1063). Proceedings of the LACCEI international Multi-conference for Engineering, Education and Technology.
Amado, D. A. V., Detoni, A. M., De Carvalho, S. L. C., Torquato, A. S., Martin, C. A., Tiuman, T. S., et al. (2019). Tocopherol and fatty acids content and proximal composition of four avocado cultivars (Persea americana mill). Acta Aliment. 48, 47–55. doi: 10.1556/066.2019.48.1.6
Anaka, O. N., Ozolua, R. I., and Okpo, S. O. (2009). Effect of the aqueous seed extract of Persea americana mill (Lauraceae) on the blood pressure of Sprague- dawley rats. Afr. J. Pharm. Pharmacol 3, 485–490.
Antwi, E., Engler, N., Nelles, M., and Schüch, A. (2019). Anaerobic digestion and the effect of hydrothermal pretreatment on the biogas yield of cocoa pods residues. Waste Manag. 88, 131–140. doi: 10.1016/j.wasman.2019.03.034
Association of Official Analytical Chemists . (2005). Official methods of analysis of AOAC international. 18th Edn. Washington, DC: Association of Official Analytical Chemists.
Bahru, T. B., Tadele, Z. H., and Ajebe, E. G. (2019). A review on avocado seed: functionality, composition, antioxidant and antimicrobial properties. Chem. Sci. Int. J. 1–10, 1–10. doi: 10.9734/CSJI/2019/v27i230112
Bangar, S. P., Dunno, K., Dhull, S. B., Kumar Siroha, A., Changan, S., Maqsood, S., et al. (2022). Avocado seed discoveries: chemical composition, biological properties, and industrial food applications. Food Chem. 16:100507. doi: 10.1016/j.fochx.2022.100507
Barreto, J. C., Trevisan, M. T. S., Hull, W. E., Erben, G., De Brito, E. S., Pfundstein, B., et al. (2008). Characterization and quantitation of polyphenolic compounds in bark, kernel, leaves, and peel of mango (Mangifera indica L.). J. Agric. Food Chem. 56, 5599–5610. doi: 10.1021/jf800738r
Barriga-Sánchez, M., Hiparraguirre, H. C., and Rosales-Hartshorn, M. (2022). Chemical composition and mineral content of black Borgoña (Vitis labrusca L.) grapes, pomace and seeds, and effects of conventional and non-conventional extraction methods on their antioxidant properties. Food Sci. Technol. 42:e120021. doi: 10.1590/fst.120021
Benzie, I. F. F., and Strain, J. J. (1996). The ferric reducing ability of plasma (FRAP) as a measure of “antioxidant power”: the FRAP assay. Anal. Biochem. 239, 70–76. doi: 10.1006/abio.1996.0292
Berardini, N., Fezer, R., Conrad, J., Beifuss, U., Carle, R., and Schieber, A. (2005). Screening of mango (Mangifera indica L.) cultivars for their contents of flavonol O- and xanthone C-glycosides, anthocyanins, and pectin. J. Agric. Food Chem. 53, 1563–1570. doi: 10.1021/jf0484069
Bordiga, M., Travaglia, F., and Locatelli, M. (2019). Valorisation of grape pomace: an approach that is increasingly reaching its maturity – a review. Int. J. Food Sci. Technol. 54, 933–942. doi: 10.1111/ijfs.14118
Brand-Williams, W., Cuvelier, M. E., and Berset, C. (1995). Use of a free radical method to evaluate antioxidant activity. LWT Food Sci. Technol. 28, 25–30. doi: 10.1016/S0023-6438(95)80008-5
Cabanillas, B., Espichán, F., Estrada, R., Neyra, E., and Rojas, R. (2021). Metabolomic profile and discrimination of white quinoa seeds from Peru based on UHPLC-HRMS and multivariate analysis. J. Cereal Sci. 101:103307. doi: 10.1016/j.jcs.2021.103307
Cabrera Rodríguez, C., León Fernández, V., Montano Pérez, A. C., and Dopico Ramírez, D. (2016). Caracterización de residuos agroindustriales con vistas a su aprovechamiento. Centro Azúcar 43, 27–35.
Campos-Vega, R., Nieto-Figueroa, K. H., and Oomah, B. D. (2018). Cocoa (Theobroma cacao L.) pod husk: renewable source of bioactive compounds. Trends Food Sci. Technol. 81, 172–184. doi: 10.1016/j.tifs.2018.09.022
Caponio, G., Noviello, M., Calabrese, F., Gambacorta, G., Giannelli, G., and De Angelis, M. (2022). Effects of grape pomace polyphenols and in vitro gastrointestinal digestion on antimicrobial activity: recovery of bioactive compounds. Antioxidants 11:567. doi: 10.3390/antiox11030567
Cari, M. (2018). Extracción y caracterización de almidón de las semillas de lúcuma (Pouteria lúcuma) (Tesis de pregrado). Lima, Perú: Universidad Peruana Unión.
Castro-Muñoz, R., Díaz-Montes, E., Gontarek-Castro, E., Boczkaj, G., and Galanakis, C. M. (2021). A comprehensive review on current and emerging technologies toward the valorization of bio-based wastes and by products from foods. Compr. Rev. Food Sci. Food Saf. 21, 46–105. doi: 10.1111/1541-4337.12894
CEPAL-FAO . (2017). La bioeconomía: oportunidades y desafíos para el desarrollo rural, agrícola y agroindustrial en América Latina y el Caribe. El boletín CEPAL/FAO/IICA, No. 16. Available at: https://hdl.handle.net/11362/51.
Chan, S. Y., and Choo, W. S. (2013). Effect of extraction conditions on the yield and chemical properties of pectin from cocoa husks. Food Chem. 141, 3752–3758. doi: 10.1016/j.foodchem.2013.06.097
Chen, Y. C., Lin, J. T., Liu, S. C., Lu, P. S., and Yang, D. J. (2011). Composition of flavonoids and phenolic acids in lychee (Litchi chinensis Sonn.) flower extracts and their antioxidant capacities estimated with human LDL, erythrocyte, and blood models. J. Food Sci. 76, C724–C728. doi: 10.1111/j.1750-3841.2011.02164.x
Cid-Pérez, T. S., Hernández-Carranza, P., Ochoa-Velasco, C. E., Ruiz-López, I. I., Nevárez-Moorillón, G., and Ávila-Sosa, R. (2021). Avocado seeds (Persea americana cv. Criollo sp.): lipophilic compounds profile and biological activities. Saudi J. Biol. Sci. 28, 3384–3390. doi: 10.1016/j.sjbs.2021.02.087
Cisneros-Yupanqui, M., Rizzi, C., Mihaylova, D., and Lante, A. (2022). Effect of the distillation process on polyphenols content of grape pomace. Eur. Food Res. Technol. 248, 929–935. doi: 10.1007/s00217-021-03924-6
Cisneros-Yupanqui, M., Zagotto, A., Alberton, A., Lante, A., Zagotto, G., Ribaudo, G., et al. (2020). Study of the phenolic profile of a grape pomace powder and its impact on delaying corn oil oxidation. Nat. Prod. Res. 36, 455–459. doi: 10.1080/14786419.2020.1777414
Cui, W., Wang, Y., Sun, Z., Cui, C., Li, H., Luo, K., et al. (2023). Effects of steam explosion on phenolic compounds and dietary fiber of grape pomace. LWT Food Sci. Technol. 173:114350. doi: 10.1016/j.lwt.2022.114350
Da Costa, T., Wondracek, D. C., Lopes, R. M., Vieira, R. F., and Ferreira, F. R. (2010). Composição de carotenoides em canistel (Pouteria campechiana (Kunth) Baehni). Rev. Bras. Frutic. 32, 903–906. doi: 10.1590/S0100-29452010005000083
De la Cruz-Azabache, M., Borja-Borja, M. G., Cosi-Cutipa, R., Espinoza-Meza, L., Quispe-Churata, E., and Lauro-Huacanca, J. (2023). Chemical composition and bioactive compounds of the peel and seed of grapes Quebranta (Vitis vinifera L.) obtained in the pre-fermentation stage of the elaboration of Pisco. 21 st LACCEI International Multi-Conference for Engineering, Education, and Technology: “Leadership in Education and Innovation in Engineering in the Framework of Global Transformations: Integration and Alliances for Integral Development”.
Delgado-Ospina, J., Molina-Hernández, J. B., Chaves-López, C., Romanazzi, G., and Paparella, A. (2021). The role of fungi in the cocoa production chain and the challenge of climate change. J. Fungi 7:202. doi: 10.3390/jof7030202
Di Stefano, V., Buzzanca, C., Melilli, M. G., Indelicato, S., Mauro, M., Vazzana, M., et al. (2022). Polyphenol characterization and antioxidant activity of grape seeds and skins from sicily: a preliminary study. Sustain. For. 14:6702. doi: 10.3390/su14116702
Diniardi, E. M., Argo, B. D., and Wibisono, Y. (2020). Antibacterial activity of cocoa pod husk phenolic extract against Escherichia coli for food processing. IOP Conf. Ser. Earth Environ. Sci. 475:012006. doi: 10.1088/1755-1315/475/1/012006
Do, V. T., Suhartini, W., Phan, C. U., Zhang, Z., Goksen, G., and Lorenzo, J. M. (2023). Nutritional value, phytochemistry, health benefits, and potential food applications of Pouteria campechiana (Kunth) Baehni: a comprehensive review. J. Funct. Foods 103:105481. doi: 10.1016/j.jff.2023.105481
Dorta, E., González, M., Lobo, M. G., Sánchez-Moreno, C., and De Ancos, B. (2014). Screening of phenolic compounds in by-product extracts from mangoes (Mangifera indica L.) by HPLC-ESI-QTOF-MS and multivariate analysis for use as a food ingredient. Food Res. Int. 57, 51–60. doi: 10.1016/j.foodres.2014.01.012
Duda-Madej, A., Stecko, J., Sobieraj, J., Szymańska, N., and Kozłowska, J. (2022). Naringenin and its derivatives—health-promoting phytobiotic against resistant bacteria and fungi in humans. Antibiotics 11:1628. doi: 10.3390/antibiotics11111628
FAO . (2018). The future of food and agriculture: Trends and challenges, the future of food and agriculture: Trends and Challenges. Available at: https://www.fao.org/.
Fernandes Serra Moura, H., de Souza Dias, F., Beatriz Souza e Souza, L., Magalhães, B. E. A. d., de Aragão Tannus, C., Correia de Carvalho, W., et al. (2021). Evaluation of multielement/proximate composition and bioactive phenolics contents of unconventional edible plants from Brazil using multivariate analysis techniques. Food Chem. 363:129995. doi: 10.1016/j.foodchem.2021.129995
Fernández-Ochoa, Á., Leyva-Jiménez, F. J., Pimentel-Moral, S., Del Villegas-Aguilar, M., Alañón, M. E., Segura-Carretero, A., et al. (2021). “Revalorisation of agro-industrial wastes into high value-added products” in Sustainable bioconversion of waste to value added products. ed. A. Inamuddin (Berlin: Springer), 229–246.
Ferreira, D. C. M., Dos Santos, P. N., Santos, F. H., Molina, G., and Pelissari, F. M. (2023). Sustainability approaches for agrowaste solution: biodegradable packaging and microbial polysaccharides bio-production. Sustainability approaches for agrowaste solution: biodegradable packaging and microbial polysaccharides bio-production. Sci. Total Environ. 886:922. doi: 10.1016/j.scitotenv.2023.163922
Ferreira, S. L. C., Silva Junior, M. M., Felix, C. S. A., Da Silva, D. L. F., Santos, A. S., Santos Neto, J. H., et al. (2019). Multivariate optimization techniques in food analysis – a review. Food Chem. 273, 3–8. doi: 10.1016/j.foodchem.2017.11.114
Ferreira-Santos, P., Nobre, C., Rodrigues, R. M., Genisheva, Z., Botelho, C., and Teixeira, J. A. (2024). Extraction of phenolic compounds from grape pomace using ohmic heating: chemical composition, bioactivity, and bioaccessibility. Food Chem. 436:137780. doi: 10.1016/j.foodchem.2023.137780
Freitas, C., Sousa, R., and Dias, M. (2020). Extraction of pectin from passion fruit peel. Food Eng. Rev. 12, 460–472. doi: 10.1007/s12393-020-09254-9
Friedman, M. (2014). Antibacterial, antiviral, and antifungal properties of wines and winery by-products in relation to their flavonoid content. J. Agric. Food Chem. 62, 6025–6042. doi: 10.1021/jf501266s
Gaafar, A. A., Asker, M. S., Ali, M. A., and Salama, Z. A. (2019). The effectiveness of the functional components of grape (Vitis vinifera) pomace as antioxidant, antimicrobial, and antiviral agents. Jordan J. Biol. Sci. 12:11.
Garcia, L., Verde, J., Castro, R., Chávez, A., Oranday, A., Núñez, A., et al. (2010). Actividad biológica de un extracto de orujo de uva mexicana. Rev. Mex. Cien. Farm. 41, 28–36.
Gilbert, N., Mewis, R. E., and Sutcliffe, O. B. (2020). Classification of fentanyl analogues through principal component analysis (PCA) and hierarchical clustering of GC–MS data. Forensic Chem. 21:100287. doi: 10.1016/j.forc.2020.100287
Gómez, F., Sánchez, S., Iradi, M., Azman, N., and Almajano, M. (2014). Avocado seeds: extraction optimization and possible use as antioxidant in food. Antioxidants 3, 439–454. doi: 10.3390/antiox3020439
Guerrero-Castillo, P., Reyes, S., Acha, O., Sepulveda, B., and Areche, C. (2021). Agro-industrial waste seeds from Peruvian Pouteria lucuma as new source of phytosterols. LWT Food Sci. Technol. 144:111259. doi: 10.1016/j.lwt.2021.111259
Guerrero-Castillo, P., Reyes, S., Robles, J., Simirgiotis, M. J., Sepulveda, B., Fernandez-Burgos, R., et al. (2019). Biological activity and chemical characterization of Pouteria lucuma seeds: a possible use of an agricultural waste. Waste Manag. 88, 319–327. doi: 10.1016/j.wasman.2019.03.055
Hassan, Y. I., Kosir, V., Yin, X., Ross, K., and Diarra, M. S. (2019). Grape pomace as a promising antimicrobial alternative in feed: a critical review. J. Agric. Food Chem. 67, 9705–9718. doi: 10.1021/acs.jafc.9b02861
Ilozue, N. M., Ikezu, U. P., and Ugwu, P. C. (2014). Antimicrobial and phytochemical screening of the seed extracts of Persea americana (AVOCADO PEAR). IOSR J. Pharm. Biol. Sci. 9, 23–25. doi: 10.9790/3008-09262325
Jeon, J., Kim, J., Lee, C., Oh, C., and Song, H. (2014). The antimicrobial activity of (−)-Epigallocatehin-3-Gallate and green tea extracts against Pseudomonas aeruginosa and Escherichia coli isolated from skin wounds. Ann. Dermatol. 26, 564–569. doi: 10.5021/ad.2014.26.5.564
Kassambara, A. (2017). Practical guide to cluster analysis in R: unsupervised machine learning (Vol. 1). Sthda. Available at: https://xsliulab.github.io/Workshop/2021/week10/r-cluster-book.pdf (Accessed January 15, 2024).
Katalinić, V., Možina, S. S., Skroza, D., Generalić, I., Abramovič, H., Miloš, M., et al. (2010). Polyphenolic profile, antioxidant properties and antimicrobial activity of grape skin extracts of 14 Vitis vinifera varieties grown in Dalmatia (Croatia). Food Chem. 119, 715–723. doi: 10.1016/j.foodchem.2009.07.019
Kaza, S., Yao, L. C., Bhada-Tata, P., and Van Woerden, F. (2018). What a waste 2.0: a global snapshot of solid waste management to 2050. Washington, DC: World Bank.
Kee, S. H., Ganeson, K., Rashid, N. F. M., Yatim, A. F. M., Vigneswari, S., Amirul, A. A. A., et al. (2022). A review on biorefining of palm oil and sugar cane agro-industrial residues by bacteria into commercially viable bioplastics and biosurfactants. Fuel 321:124039. doi: 10.1016/j.fuel.2022.124039
Khiaosa-ard, R., Metzler-Zebeli, B. U., Ahmed, S., Muro-Reyes, A., Deckardt, K., Chizzola, R., et al. (2015). Fortification of dried distillers grains plus solubles with grape seed meal in the diet modulates methane mitigation and rumen microbiota in Rusitec. J. Dairy Sci. 98, 2611–2626. doi: 10.3168/jds.2014-8751
Khiya, Z., Oualcadi, Y., Gamar, A., Berrekhis, F., Zair, T., and Hilali, F. E. (2021). Correlation of total polyphenolic content with antioxidant activity of hydromethanolic extract and their fractions of the Salvia officinalis leaves from different regions of Morocco. J. Chem. 2021, 1–11. doi: 10.1155/2021/8585313
Kilama, G., Lating, P. O., Byaruhanga, J., and Biira, S. (2019). Quantification and characterization of cocoa pod husks for electricity generation in Uganda. Energy Sustain. Soc. 9:22. doi: 10.1186/s13705-019-0205-4
Kim, T. K., Yong, H. I., Jung, S., Kim, Y. B., and Choi, Y. S. (2020). Effects of replacing pork fat with grape seed oil and gelatine/alginate for meat emulsions. Meat Sci. 163:108079. doi: 10.1016/j.meatsci.2020.108079
King-Loeza, Y., Ciprián-Macías, D. A., Cardador-Martínez, A., Martín-del-Campo, S. T., Castañeda-Saucedo, M., and Ramírez-Anaya, J. P. (2023). Functional composition of avocado (Persea americana mill. Var Hass) pulp, extra virgin oil, and residues is affected by fruit commercial classification. J. Agric. Food Res. 12:100573. doi: 10.1016/j.jafr.2023.100573
Kitichalermkiat, A., Katsuki, M., Sato, J., Sonoda, T., Masuda, Y., Honjoh, K., et al. (2020). Effect of epigallocatechin gallate on gene expression of Staphylococcus aureus. J. Glob. Antimicrob. Resist. 22, 854–859. doi: 10.1016/j.jgar.2020.06.006
Kitichalermkiat, A., Kurahachi, M., Nonaka, A., Nakayama, M., Shimatani, K., Shigemune, N., et al. (2019). Effects of epigallocatechin Gallate on viability and cellular proteins of Staphylococcus aureus. Food Sci. Technol. Res. 25, 277–285. doi: 10.3136/fstr.25.277
Kohajdová, V. K. Z., Karovičová, J., and Lauková, M. (2018). Physical, textural and sensory properties of cookies incorporated with grape skin and seed preparations. Polish J. Food Nutr. Sci. 68, 309–317. doi: 10.2478/pjfns-2018-0004
Kong, K. W., Khoo, H. E., Prasad, N. K., Chew, L. Y., and Amin, I. (2013). Total phenolics and antioxidant activities of Pouteria campechiana fruit parts. Sains Malaysia. 42, 123–127.
Kosińska, A., Karamac, M., Estrella, I., Hernández, T., Bartolomé, B., and Dykes, G. A. (2012). Phenolic compound profiles and antioxidant capacity of Persea americana mill. Peels and seeds of two varieties. J. Agric. Food Chem. 60, 4613–4619. doi: 10.1021/jf300090p
Kuganesan, A., Thiripuranathar, G., Navaratne, A. N., and Paranagama, P. A. (2017). Antioxidant and anti-inflammatory activities of peels, pulps and seed kernels of three common mango (Mangifera indica) varieties in sri. Int. J. Pharm. Sci. Res. 8, 70–78. doi: 10.13040/IJPSR.0975-8232.8(1).70-78
Kupnik, K., Primožič, M., Kokol, V., Knez, Ž., and Leitgeb, M. (2023). Enzymatic, antioxidant, and antimicrobial activities of bioactive compounds from avocado (Persea americana L.) seeds. Plan. Theory 12:1201. doi: 10.3390/plants12051201
Lê, S., Josse, J., and Husson, F. (2008). FactoMineR: an R package for multivariate analysis. J. Stat. Softw. 25, 1–18. doi: 10.18637/jss.v025.i01
Leong, Y. K., and Chang, J. S. (2022). Valorization of fruit wastes for circular bioeconomy: current advances, challenges, and opportunities. Bioresour. Technol. 359:127459. doi: 10.1016/j.biortech.2022.127459
Li, X., Wu, X., and Huang, L. (2009). Correlation between antioxidant activities and phenolic contents of radix Angelicae sinensis (Danggui). Molecules 14, 5349–5361. doi: 10.3390/molecules14125349
Li, B., Zhang, Y., Zhang, Y., Zhang, Y., Xu, F., and Zhu, K. (2021). A novel underutilized starch resource–Lucuma nervosa a.DC seed and fruit. Food Hydrocoll. 120:106934. doi: 10.1016/j.foodhyd.2021.106934
Lizárraga-Velázquez, C., Hernández, C., González-Aguilar, G., and Heredia, J. (2018). Effect of hydrophilic and lipophilic antioxidants from mango peel (Mangifera indica L. cv. Ataulfo) on lipid peroxidation in fish oil. CyTA 16, 1095–1101. doi: 10.1080/19476337.2018.1513425
Lozoya-Castillo, D. L., Castillo-Hernández, S. L., Hernández-Marín, D. A., Rivas-Morales, C., and Sánchez-García, E. (2018). Evaluación de la actividad antimicrobiana, antiinflamatoria y antioxidante de subproductos de Opuntia ficus-indica y Mangifera indica 3, 139–144.
Lu, F., Rodriguez-Garcia, J., Van Damme, I., Westwood, N. J., Shaw, L., and Robinson, J. S. (2018). Valorisation strategies for cocoa pod husk and its fractions. Curr. Opin. Green Sustain. Chem. 14, 80–88. doi: 10.1016/j.cogsc.2018.07.007
Ma, J. S., Sheng, M. A., Wang, L. X., and Liu, H. (2019). Analysis and evaluation of nutritional components of Pouteria campechiana seeds. Chin. J. Trop. Crops 40, 166–170.
Madhan, B., Krishnamoorthy, G., Rao, J. R., and Nair, B. U. (2007). Role of green tea polyphenols in the inhibition of collagenolytic activity by collagenase. Int. J. Biol. Macromol. 41, 16–22. doi: 10.1016/j.ijbiomac.2006.11.013
Marcillo-Parra, V., Anaguano, M., Molina, M., Tupuna-Yerovi, D. S., and Ruales, J. (2021). Characterization and quantification of bioactive compounds and antioxidant activity in three different varieties of mango (Mangifera indica L.) peel from the Ecuadorian region using HPLC-UV/VIS and UPLC-PDA. NFS J. 23, 1–7. doi: 10.1016/j.nfs.2021.02.001
Maria, J., Mandal, A. K., Rajesh, J., and Natarajan, S. (2012). Antioxidant and antimicrobial activity of individual catechin molecules: a comparative study between gallated and epimerized catechin molecules. Res. J. Biotechnol. 7, 5–8.
Masibo, M., and He, Q. (2009). Mango bioactive compounds and related nutraceutical properties—a review. Food Rev. Intl. 25, 346–370. doi: 10.1080/87559120903153524
Melo, C. M., Morais, T. C., Tomé, A. R., Brito, G. A. C., Chaves, M. H., Rao, V. S., et al. (2011). Anti-inflammatory effect of α,β-amyrin, a triterpene from Protium heptaphyllum, on cerulein-induced acute pancreatitis in mice. Inflamm. Res. 60, 673–681. doi: 10.1007/s00011-011-0321-x
Mendivil, N. (2015). Efecto antibacteriano de extractos de orujo y escobajo sobre Helicobacter pylori y Escherichia coli [Tesis de maestría]. Universidad de Chile. Available at: https://repositorio.uchile.cl/handle/2250/151487.
MINAGRI . (2023). SIEA–BI. Available at: https://siea.midagri.gob.pe/portal/siea_bi/index.html.
Mir-Cerdà, A., Nuñez, O., Granados, M., Sentellas, S., and Saurina, J. (2023). An overview of the extraction and characterization of bioactive phenolic compounds from Agri-food waste within the framework of circular bioeconomy. Trends Anal. Chem. 161:116994. doi: 10.1016/j.trac.2023.116994
Muruganandan, S., Srinivasan, K., Gupta, S., Gupta, P. K., and Lal, J. (2005). Effect of mangiferin on hyperglycemia and atherogenicity in streptozotocin diabetic rats. J. Ethnopharmacol. 97, 497–501. doi: 10.1016/j.jep.2004.12.010
Nayak, A., and Bhushan, B. (2019). An overview of the recent trends on the waste valorization techniques for food wastes. J. Environ. Manag. 233, 352–370. doi: 10.1016/j.jenvman.2018.12.041
Nazir, N., Novelina, N., Juita, E., Amelia, C., and Fatli, R. (2016). Optimization of pre-treatment process of cocoa pod husk using various chemical solvents. Int. J. Adv. Sci. Eng. Inf. Technol. 6, 403–409. doi: 10.18517/ijaseit.6.3.848
Nitta, T., Arai, T., Takamatsu, H., Inatomi, Y., Murata, H., Iinuma, M., et al. (2002). Antibacterial activity of extracts prepared from tropical and subtropical plants on methicillin-resistant Staphylococcus aureus. J. Health Sci. 48, 273–276. doi: 10.1248/jhs.48.273
Noratto, G. D., Bertoldi, M. C., Krenek, K., Talcott, S. T., Stringheta, P. C., and Mertens-Talcott, S. U. (2010). Anticarcinogenic effects of polyphenolics from mango (Mangifera indica) varieties. J. Agric. Food Chem. 58, 4104–4112. doi: 10.1021/jf903161g
Oboh, G., Odubanjo, V. O., Bello, F., Ademosun, A. O., Oyeleye, S. I., Nwanna, E. E., et al. (2016). Aqueous extracts of avocado pear (Persea americana mill.) leaves and seeds exhibit anti-cholinesterases and antioxidant activities in vitro. J. Basic Clin. Physiol. Pharmacol. 27, 131–140. doi: 10.1515/jbcpp-2015-0049
Oliveira, D. A., Salvador, A. A., Smânia, A., Smânia, E. F., Maraschin, M., and Ferreira, S. R. (2013). Antimicrobial activity and composition profile of grape (Vitis vinifera) pomace extracts obtained by supercritical fluids. J. Biotechnol. 164, 423–432. doi: 10.1016/j.jbiotec.2012.09.014
Pérez, R., Leos, C., Oranday, A., Hernández Luna, E., Sanchez, E., and Rivas, C. (2015). Efecto in vitro en la inhibición del proceso de nucleación en litiasis renal, capacidad de captura de radicales libres, actividad antimicrobiana y tóxica del extracto metanólico de Berberis trifoliata. Rev. Mex. Cien. Farm. 46, 71–76.
Periferakis, A., Periferakis, K., Badarau, I. A., Petran, E. M., Popa, D. C., Caruntu, A., et al. (2022). Kaempferol: antimicrobial properties, sources, clinical, and traditional applications. Int. J. Mol. Sci. 23:15054. doi: 10.3390/ijms232315054
Racette, S. B., Lin, X., Ma, L., and Ostlund, R. E. (2015). Natural dietary Phytosterols. J. AOAC Int. 98, 679–684. doi: 10.5740/jaoacint.SGERacette
Rashed, K., Ćirić, A., Glamočlija, J., Calhelha, R. C., Ferreira, I. C., and Soković, M. (2014). Antimicrobial and cytotoxic activities of Alnus rugosa L. aerial parts and identification of the bioactive components. Ind. Crop. Prod. 59, 189–196. doi: 10.1016/j.indcrop.2014.05.017
Re, R., Pellegrini, N., Proteggente, A., Pannala, A., Yang, M., and Rice-Evans, C. (1999). Antioxidant activity applying an improved ABTS radical cation decolorization assay. Free Radic. Biol. Med. 26, 1231–1237. doi: 10.1016/S0891-5849(98)00315-3
Rebello, L. P. G., Lago-Vanzela, E. S., Barcia, M. T., Ramos, A. M., Stringheta, P. C., Da-Silva, R., et al. (2013). Phenolic composition of the berry parts of hybrid grape cultivar BRS Violeta (BRS Rubea× IAC 1398-21) using HPLC–DAD–ESI-MS/MS. Food Res. Int. 54, 354–366. doi: 10.1016/j.foodres.2013.07.024
Rodríguez, P. (1995). Obtención y caracterización de pectina a partir de desechos indrustriales del mango (cascara). Rev. Colomb. Cien. Químico Farm. 1995, 29–35.
Rojo, L. E., Villano, C. M., Joseph, G., Schmidt, B., Shulaev, V., Shuman, J. L., et al. (2010). Original contribution: wound-healing properties of nut oil from Pouteria lucuma. J. Cosmet. Dermatol. 9, 185–195. doi: 10.1111/j.1473-2165.2010.00509.x
Romaní, L., Enciso, E., Cárdenas, V., and Condorhuamán, Y. M. (2017). Actividad antibacteriana de compuestos fenólicos de semillas de Persea americana Mill. “Palta hass” frente a Escherichia coli. Cienc. Invest. 20, 19–22. doi: 10.15381/ci.v20i2.14806
Rosero, J. C., Cruz, S., Osorio, C., and Hurtado, N. (2019). Analysis of phenolic composition of by-products (seeds and peels) of avocado (Persea americana mill.) cultivated in Colombia. Molecules 24:3209. doi: 10.3390/molecules24173209
Saavedra, J., Córdova, A., Navarro, R., Díaz-Calderón, P., Fuentealba, C., Astudillo-Castro, C., et al. (2017). Industrial avocado waste: functional compounds preservation by convective drying process. J. Food Eng. 198, 81–90. doi: 10.1016/j.jfoodeng.2016.11.018
Sagdic, O., Ozturk, I., Ozkan, G., Yetim, H., Ekici, L., and Yilmaz, M. T. (2011). RP-HPLC–DAD analysis of phenolic compounds in pomace extracts from five grape cultivars: evaluation of their antioxidant, antiradical and antifungal activities in orange and apple juices. Food Chem. 126, 1749–1758. doi: 10.1016/j.foodchem.2010.12.075
Sánchez-Camargo, A. D. P., Gutiérrez, L. F., Vargas, S. M., Martinez-Correa, H. A., Parada-Alfonso, F., and Narváez-Cuenca, C.-E. (2019). Valorisation of mango peel: proximate composition, supercritical fluid extraction of carotenoids, and application as an antioxidant additive for an edible oil. J. Supercrit. Fluids 152:104574. doi: 10.1016/j.supflu.2019.104574
Santos, T. R. J., and De Aquino Santana, L. C. L. (2019). Antimicrobial potential of exotic fruits residues. S. Afr. J. Bot. 124, 338–344. doi: 10.1016/j.sajb.2019.05.031
Santos, F. H., Siqueira, L. E., Cardoso, G. P., Molina, G., and Pelissari, F. M. (2021). Antioxidant packaging development and optimization using agroindustrial wastes. J. Appl. Polym. Sci. 138, 1–13. doi: 10.1002/app.50887
Saval, S. (2012). Aprovechamiento de residuos agroindustriales: Pasado, presente y futuro. Biotecnologia 16, 14–46.
Scroccarello, A., Molina Hernandez, J., Della Pelle, F., Ciancetta, J., Ferraro, G., Fratini, E., et al. (2021). Effect of phenolic compounds-capped AgNPs on growth inhibition of aspergillus Niger. Colloids Surf. B: Biointerfaces 199:111533. doi: 10.1016/j.colsurfb.2020.111533
Sepúlveda, L., De la Cruz, R., Buenrostro, J. J., Ascacio-Valdés, J. A., Aguilera-Carbó, A. F., Prado, A., et al. (2016). Effect of different polyphenol sources on the efficiency of ellagic acid release by aspergillus Niger. Rev. Argent. Microbiol. 48, 71–77. doi: 10.1016/j.ram.2015.08.008
Serna, L., and Torres-León, C. (2015). Agro-industrial potential of peels of mango (Mangifera indica) Keitt and Tommy Atkins. Acta Agron. 64, 110–115. doi: 10.15446/acag.v64n2.43579
Shahbandeh, M. (2022). Statista–the statistics portal. Statista. Available at: https://www.statista.com/aboutus/our-research-commitment/1239/m-shahbandeh.
Shahidi, F., and Ambigaipalan, P. (2015). Phenolics and polyphenolics in foods, beverages and spices: antioxidant activity and health effects – a review. J. Funct. Foods 18, 820–897. doi: 10.1016/j.jff.2015.06.018
Shi, J., Yu, J., Pohorly, J. E., and Kakuda, Y. (2003). Polyphenolics in grape seeds—biochemistry and functionality. J. Med. Food 6, 291–299. doi: 10.1089/109662003772519831
Shirahigue, L. D., and Ceccato-Antonini, S. R. (2020). Agro-industrial wastes as sources of bioactive compounds for food and fermentation industries. Ciên. Rural 50:857. doi: 10.1590/0103-8478cr20190857
Silva, V., Igrejas, G., Falco, V., Santos, T. P., Torres, C., Oliveira, A. M., et al. (2018). Chemical composition, antioxidant and antimicrobial activity of phenolic compounds extracted from wine industry by-products. Food Control 92, 516–522. doi: 10.1016/j.foodcont.2018.05.031
Singh, B., Singh, J. P., Kaur, A., and Singh, N. (2018). Antimicrobial potential of pomegranate peel: a review. Int. J. Food Sci. Technol. 54, 959–965. doi: 10.1111/ijfs.13964
Singleton, V. L., and Rossi, J. A. (1965). Colorimetry of total phenolics with phosphomolybdic-phosphotungstic acid reagents. Am. J. Enol. Vitic. 16, 144–158. doi: 10.5344/ajev.1965.16.3.144
Siol, M., and Sadowska, A. (2023). Chemical composition, physicochemical and bioactive properties of avocado (Persea americana) seed and its potential use in functional food design. Agriculture 13, 1–13. doi: 10.3390/agriculture13020316
Sławińska, N., and Olas, B. (2023). Selected seeds as sources of bioactive compounds with diverse biological activities. Nutrients 15:187. doi: 10.3390/nu15010187
Solari-Godiño, A., Lindo-Rojas, I., and Pandia-Estrada, S. (2017). Determination of phenolic compounds and evaluation of antioxidant capacity of two grapes residues (Vitis vinifera) of varieties dried: Quebranta (red) and Torontel (white). Cogent Food Agric. 3:1361599. doi: 10.1080/23311932.2017.1361599
Sorrenti, V., Burò, I., Consoli, V., and Vanella, L. (2023). Recent advances in health benefits of bioactive compounds from food wastes and by-products: biochemical aspects. Int. J. Mol. Sci. 24:2019. doi: 10.3390/ijms24032019
Sosa-Martínez, J. D., Montañez, J., Contreras-Esquivel, J. C., Balagurusamy, N., Gadi, S. K., and Morales-Oyervides, L. (2023). Agroindustrial and food processing residues valorization for solid-state fermentation processes: a case for optimizing the co-production of hydrolytic enzymes. J. Environ. Manag. 347:119067. doi: 10.1016/j.jenvman.2023.119067
Sotelo, L., and Alvis, A. (2018). Extracción de compuestos con actividad antimicrobiana a partir de subproductos del cacao. Alimentos Hoy 26:26.
Sotelo, L., Alvis, A., and Arrázola, G. (2015). Evaluación de epicatequina, teobromina y cafeína en cáscaras de cacao (Theobroma cacao L.), determinación de su capacidad antioxidante. Rev. Colomb. Cien. Hortíc. 9:124. doi: 10.17584/rcch.2015v9i1.3751
Spigno, G., Tramelli, L., and De Faveri, D. M. (2007). Effects of extraction time, temperature and solvent on concentration and antioxidant activity of grape marc phenolics. J. Food Eng. 81, 200–208. doi: 10.1016/j.jfoodeng.2006.10.021
Spinei, M., and Oroian, M. (2023). Structural, functional and physicochemical properties of pectin from grape pomace as affected by different extraction techniques. Int. J. Biol. Macromol. 224, 739–753. doi: 10.1016/j.ijbiomac.2022.10.162
Staff, F. (2022). Perú se posicionó como el noveno proveedor de frutas más grande del mundo en 2021. Forbes Perú. Available at: https://forbes.pe/negocios/2022-10-05/peru-se-posiciono-como-el-noveno-proveedor-de-frutas-mas-grande-del-mundo-en-2021/.
Sunder, J., Singh, D. R., Sakthivel, J., and Kundu, A. (2011). Antibacterial activity in solvent extract of different parts of Morinda citrifolia plant. J. Pharm. Sci. Res. 3:807.
Taguri, T., Tanaka, T., and Kouno, I. (2004). Antimicrobial activity of 10 different plant polyphenols against bacteria causing food-borne disease. Biol. Pharm. Bull. 27, 1965–1969. doi: 10.1248/bpb.27.1965
Tang, J.-J., Li, J.-G., Qi, W., Qiu, W.-W., Li, P.-S., Li, B.-L., et al. (2011). Inhibition of SREBP by a small molecule, betulin, improves hyperlipidemia and insulin resistance and reduces atherosclerotic plaques. Cell Metab. 13, 44–56. doi: 10.1016/j.cmet.2010.12.004
Tesfaye, T., Gibril, M., Sithole, B., Ramjugernath, D., Chavan, R., Chunilall, V., et al. (2018). Valorisation of avocado seeds: extraction and characterisation of starch for textile applications. Clean Techn. Environ. Policy 20, 2135–2154. doi: 10.1007/s10098-018-1597-0
Umamahesh, K., Sivudu, S. N., and Reddy, O. V. S. (2016). Evaluation of antioxidant activity, total phenolics and total flavonoids in peels of five cultivars of mango (Mangifera indica) fruit. J. Med. Plants Stud. 4, 200–203.
Unusan, N. (2020). Proanthocyanidins in grape seeds: an updated review of their health benefits and potential uses in the food industry. J. Funct. Foods 67:103861. doi: 10.1016/j.jff.2020.103861
Valadez-Carmona, L., Plazola-Jacinto, C. P., Hernández-Ortega, M., Hernández-Navarro, M. D., Villarreal, F., Necoechea-Mondragón, H., et al. (2017). Effects of microwaves, hot air and freeze-drying on the phenolic compounds, antioxidant capacity, enzyme activity and microstructure of cacao pod husks (Theobroma cacao L.). Innovative Food Sci. Emerg. Technol. 41, 378–386. doi: 10.1016/j.ifset.2017.04.012
Vaz Júnior, S. (2020). Aproveitamento de resíduos agroindustriais - uma abordagem sustentável. embrapa agroenergia, Brasília, DF. Available at: http://www.infoteca.cnptia.embrapa.br/infoteca/handle/doc/1126255.
Velderrain-Rodríguez, G. R., Quero, J., Osada, J., Martín-Belloso, O., and Rodríguez-Yoldi, M. J. (2021). Phenolic-rich extracts from avocado fruit residues as functional food ingredients with antioxidant and antiproliferative properties. Biomol. Ther. 11:977. doi: 10.3390/biom11070977
Veskoukis, A. S., Kyparos, A., Nikolaidis, M. G., Stagos, D., Aligiannis, N., Halabalaki, M., et al. (2012). The antioxidant effects of a polyphenol-rich grape pomace extract in vitro do not correspond in vivo using exercise as an oxidant stimulus. Oxidative Med. Cell. Longev. 2012, 1–14. doi: 10.1155/2012/185867
Wang, W., Bostic, T. R., and Gu, L. (2010). Antioxidant capacities, procyanidins and pigments in avocados of different strains and cultivars. Food Chem. 122, 1193–1198. doi: 10.1016/j.foodchem.2010.03.114
Yaashikaa, P. R., Kumar, P. S., and Varjani, S. (2022). Valorization of agro-industrial wastes for biorefinery process and circular bioeconomy: a critical review. Bioresour. Technol. 343:126126. doi: 10.1016/j.biortech.2021.126126
Yoda, Y., Hu, Z.-Q., Zhao, W.-H., and Shimamura, T. (2004). Different susceptibilities of Staphylococcus and gram-negative rods to epigallocatechin gallate. J. Infect. Chemother. 10, 55–58. doi: 10.1007/s10156-003-0284-0
Zeng, H., Locatelli, M., Bardelli, C., Amoruso, A., Coisson, J. D., Travaglia, F., et al. (2011). Anti-inflammatory properties of clovamide and Theobroma cacao phenolic extracts in human monocytes: evaluation of respiratory burst, cytokine release, NF-κB activation, and PPARγ modulation. J. Agric. Food Chem. 59, 5342–5350. doi: 10.1021/jf2005386
Keywords: phenolic compounds, antioxidant capacity, antimicrobial activity, antifungal activity, circular economic, agroindustrial wastes, multivariate analysis
Citation: Inga M, Betalleluz-Pallardel I, Puma-Isuiza G, Cumpa-Arias L, Osorio C, Valdez-Arana J-D-C and Vargas-De-La-Cruz C (2024) Chemical analysis and bioactive compounds from agrifood by-products of peruvian crops. Front. Sustain. Food Syst. 8:1341895. doi: 10.3389/fsufs.2024.1341895
Edited by:
Paula Jauregi, Centro Tecnológico Experto en Innovación Marina y Alimentaria (AZTI), SpainReviewed by:
Rodolfo Ramos-González, National Council of Science and Technology (CONACYT), MexicoMaria Simona Chi, University of Agricultural Sciences and Veterinary Medicine of Cluj-Napoca, Romania
Copyright © 2024 Inga, Betalleluz-Pallardel, Puma-Isuiza, Cumpa-Arias, Osorio, Valdez-Arana and Vargas-De-La-Cruz. This is an open-access article distributed under the terms of the Creative Commons Attribution License (CC BY). The use, distribution or reproduction in other forums is permitted, provided the original author(s) and the copyright owner(s) are credited and that the original publication in this journal is cited, in accordance with accepted academic practice. No use, distribution or reproduction is permitted which does not comply with these terms.
*Correspondence: Marianela Inga, marianelainga@lamolina.edu.pe; Indira Betalleluz-Pallardel, ipb@lamolina.edu.pe