Corrigendum: Effects of varied nutrient regimes on soil health and long-term productivity in a rice–wheat system: insights from a 29-year study in the mollisols of the Himalayan Tarai region
- 1Department of Soil Science, G. B. Pant University of Agriculture and Technology, Pantnagar, India
- 2Department of Agronomy, G. B. Pant University of Agriculture and Technology, Pantnagar, Uttarakhand, India
- 3Department of Agricultural and Food Engineering, Indian Institute of Technology Kharagpur, Kharagpur, India
- 4Department of Agronomy, Dr. Rajendra Prasad Central Agriculture University, Pusa, India
- 5Department of Botany, D.S.B. Campus, Kumaun University, Nainital, India
- 6Department of Biology, College of Science, Taif University, Taif, Saudi Arabia
- 7Department of Physical Sport Science, College of Education, Princess Nourah bint Abdulrahman University, Riyadh, Saudi Arabia
- 8Division of Soil Science, Bangladesh Wheat and Maize Research Institute, Dinajpur, Bangladesh
The maintenance of sustainability and quantification of soil health in the rice–wheat system in the Himalayan tarai region is of utmost importance, and a long-term study can properly demonstrate what needs to be done to achieve this. The current study was conducted after the completion of a 29-year crop cycle in the rice–wheat system in 2015 at Pantnagar. Since the beginning of the experiment in 1984, various NPK combinations with or without Zn and farmyard manure (FYM) applications were maintained in a fixed layout along with an absolute control plot without any external nutrition. FYM at 5 mg ha−1 and Zn at 5 kg ha−1 were applied in only rice, and NPK-chemical fertilizers were applied both in rice and wheat. The results revealed that the application of N at 120 kg ha−1 + P at 40 kg ha−1 + K at 40 kg ha−1 + FYM at 5 mg ha−1 + Zn at 5 kg ha−1 (NPK + FYM + Zn) resulted in the maximum attainment of long-term system productivity and the sustainable yield index (SYI), which were 22% higher than those with NPK application. NPK + FYM + Zn and NPK + FYM also improved the soil's overall physical, chemical, and biological parameters. Soil organic carbon, dehydrogenase activity, soil available P and K, phosphate solubilizing bacteria, and actinomycetes were found to be the most important soil quality parameters in Mollisols. From this study, it can be concluded that the application of the recommended NPK along with FYM and Zn can improve soil health and sustain the system productivity of the rice–wheat system in Mollisols of the Himalayan tarai region.
1. Introduction
The rice–wheat cropping system is an immensely important cropping system in India, especially in the famous Indo–Gangetic plains, (IGP) which cover approximately 12.3 Mha (Kar et al., 2021).
Being cereal-intensive, this system is known to deplete NPK and zinc to the tune of approximately 650 kg ha−1 and 0.5–1.0 kg ha−1, respectively, from soil annually (Shah et al., 2011). The continuous practice of this system with excessive chemical fertilizers since the era of the green revolution in India has led to several soil–environment problems, including multi-nutrient deficiencies, loss of soil organic carbon (SOC), environmental pollution, and so on. All these problems have contributed to a yield stagnation of the system (Yadav and Kumar, 2009; Kar et al., 2021; Pramanick et al., 2022). Despite being one of the most heavily fertilized cropping systems in India, it has been repeatedly marked as suffering from poor nutrient management strategies, injudicious application, and a loss in the efficiency of resource use (Kar et al., 2021; Kumar et al., 2021; Laik et al., 2021b; Lakshmi et al., 2021). Long-term application of imbalanced nutrition to the rice–wheat system should be reviewed with respect to soil quality vis-à-vis system productivity. Many previous studies have recommended that a complete organic mode of nutrient management is a plausible option that can improve productivity and soil health (Laik et al., 2021a). However, relying solely on organic nutrient management presents a significant challenge due to the limited organic availability, prompting scientists to explore potential alternatives. Long-term application of farm yard manure (FYM), along with mineral NPK in tropical and sub-tropical soil, can enhance the labile soil organic matter pools (Laik et al., 2021a). Thus, the incorporation of FYM in the nutrient management modules might be a good option in the rice–wheat cropping system. Indian soil is also becoming deficient in Zn, which can be reclaimed through the sequential application of Zn in the rice–wheat system (Lakshmi et al., 2021). The current study was conducted in one of the most fertile lands of India, viz., the Mollisols of the Himalayan tarai region. These soils are known for their high organic matter content and, thus, higher nutrient availability for crops. However, several studies have reported that intensive cultivation of cereal-based cropping has resulted in multi-nutrient deficiencies in these soils, which include universal nitrogen deficiency along with deficiencies of potassium, sulfur, and zinc in widespread areas with the rice–wheat cropping systems for a significant period of time with no or limited organic inputs (Sharma et al., 2014).
Soil quality evaluation is crucial for understanding factors that contribute to crop production in different ecologies (Kumar et al., 2021; Mahapatra and Dey, 2022). A comprehensive soil quality assessment considering the maximum number of soil properties of any soil is much more acceptable for representing soil quality than considering any single important parameter. Traditionally, soil quality is characterized by its physical and chemical attributes (Bünemann et al., 2018). However, recent studies have shown that soil-biological attributes, especially in tropical and sub-tropical soils, are the most sensitive parameters in determining soil quality (Kumar et al., 2021; Laik et al., 2021a). Soil's bio-physico-chemical properties need to be evaluated properly to assess the influence of organic additions on overall soil quality (Min et al., 2003). A soil quality index (SQI) is a useful tool for interpreting data from various soil measures and assessing whether land-use management practices have the desired impacts on production, soil health, and environmental protection (Vasu et al., 2016). Despite the importance of the SQI, the SQI for the rice–wheat system in Mollisol soil has not been quantified properly. Therefore, the quantification of soil quality parameters for the rice–wheat system in Mollisols can provide valuable insights for future research and crop producers, helping to achieve optimal soil management and higher production.
Long-term studies have been recognized as a crucial tool for understanding these impacts. Previous studies conducted in India have investigated the effects of long-term fertilization and manure on soil biological characteristics and the SQI in various agricultural systems and climatic circumstances (Saha et al., 2008; Bedi et al., 2009; Kumar et al., 2021). However, these scientific findings are not enough for the predominant rice–wheat systems in the Mollisols of the Himalayan tarai region, which are considered one of the most fertile soils in the country.
This experiment is distinctive in that it evaluates all soil quality criteria in the Mollisols of the Himalayan tarai region with a special emphasis on the rice–wheat system. This initiative acknowledges the importance of soil biological properties, particularly in tropical and sub-tropical soils, in determining soil quality, whereas earlier studies mainly focused on the physical and chemical characteristics of the soil. In this study, we aimed to construct an SQI unique to the rice–wheat system in the Mollisols by considering a wide variety of soil characteristics. The current study aimed to investigate the impact of long-term nutrient management schedules on soil quality parameters and crop productivity in the Himalayan tarai region of IGP. Furthermore, the project highlights the importance of long-term studies to understand the impacts of nutrient management practices on soil quality and crop productivity. While previous research has investigated similar aspects in different agricultural systems and climatic conditions, there is a lack of specific studies on the rice–wheat systems in the Mollisols of the Himalayan tarai region. Comprehensive soil quality parameters in relation to long-term nutrient management practices in this region have not been studied in detail. This study is expected to provide useful and systematic information on the long-term impact of nutrient schedules on soil quality parameters, soil functions, and crop performance, contributing to the current knowledge gap on the subject.
2. Materials and methods
2.1. Experimental site
The present experiment was conducted after the completion of the 29th cycle of rice–wheat cropping, which was initiated in June 1986. This study was conducted at the Borlaug Crop Research Farm of Pantnagar University, India (29° N, 79°29' E, and 244 m above MSL). The research site was in a sub-humid and sub-tropical zone with hot and humid summers and cold winters. The prevailing weather conditions during the current experimental period are reflected in Figure 1. The origin of the experimental soil was alluvial. Study soil falls under the order Mollisols and is classified under the sub-group Aquic hapludoll (Deshpande et al., 1971). The soil texture was clay-loam, which contained sand, silt, and clay of 32, 39, and 29%, respectively. The soil was medium-fine granular in the structure with good water-holding capability. In 1986, when the long-term experiment was initiated, the soil at the experimental site had a pH of 7.8, a cation exchange capacity (CEC) of 20.0 cmol (p +) kg−1, and an electrical conductivity of 0.12 dS m−1. The soil was high in organic carbon (1.2%, or 2688 kg ha−1), with 243 kg ha−1 of available nitrogen (alkaline KMnO4 method), 20.0 kg ha−1 of available phosphorus (NaHCO3 extracted), 222 kg ha−1 of available potassium (NH4OAC extracted), and 0.8 kg ha−1 of available zinc (DTPA extracted).
2.2. Experimental design and treatments
The field experiment was laid permanently in the rainy season of 1986 in a randomized complete block design (RCBD) with four replications and 10 treatments, viz., absolute control (no application of any fertilizers); 120 kg ha−1 N (N); 120 kg ha−1 N + 40 kg ha−1 P (NP); 120 kg ha−1 N + 40 kg ha−1 K (NK); 120 kg ha−1 N + 40 kg ha−1 P + 40 kg ha−1 K (NPK), NPK + FYM (farm yard manure) at 5 mg ha−1 (NPK + FYM), NPK + 5 kg ha−1 Zn (NPK + Zn); NPK + FYM + 5 kg ha−1 Zn (NPK + FYM + Zn); 150 kg ha−1 N + 40 kg ha−1 P + 40 kg ha−1 K (125% N + PK); and 150 kg ha−1 N + 80 kg ha−1 P + 40 kg ha−1 K + 5 kg ha−1 Zn (150%N + 200%P + K + Zn). The base treatments were determined based on prevailing fertilization recommendations (NP) and farmers' practices (N). Other treatments, including K and Zn in nutrient schedules, were formulated using crop demand studies and analyzing crop deficiencies. Higher levels of N and P were also included due to the economic response of the system and based on some farmers' practice of over-fertilizing the crop. For the integrated mode, nutrient substitution with FYM has been studied alone and in combination with Zn supplementation. The nutrient sources used for the treatments were commercial-grade urea (46% N), single super phosphate (16% P), fertilizer-grade KCl (60% K), and ZnSO4.7H2O (21% Zn). Farmyard manure (FYM) was applied at 5 mg ha−1 on a dry weight basis only in the case of rice cultivation at the time of final land preparation. The nutrient contents of FYM were 0.3–0.5% N, 0.1–0.2% P, and 0.3–0.75% K on a dry weight basis. Full doses of P, K, and half of the N in each treatment were applied as basal. The rest of the N was applied in two equal splits. Zn was applied only to rice during secondary tillage operations. Field preparation and establishment techniques for rice and wheat were conventional (puddling-transplanting in rice and conventional cross-harrowing in wheat). The rice variety Pant Dhan 4 was transplanted in the second week of July and harvested in the first week of November. Wheat (variety UP 2425) was sown in the second fortnight of November.
2.3. System productivity and sustainability parameters
The current experiment comprised two different crops, viz., rice and wheat, which not only have different kinds of yield potential but also fetch a differential price at the market. Thus, the productivity of the system was calculated in terms of rice equivalent yield (REY) using the following formula (Kar et al., 2021):
To calculate the long-term system productivity, the average system productivity over the years was considered. The SYI was calculated with the following formula (Kumar et al., 2021):
where stands for average productivity, σ stands for the standard deviation in the system productivity, and Ymax is the maximum witnessed system productivity.
In this manuscript, the current system's productivity has been calculated with the data from the 2015–16 season. While long-term system productivity was calculated using the average of 29 years' system productivity values, changes between long-term and current system productivity were expressed as percentage changes.
2.4. Soil quality parameters
A composite soil sample from each plot was collected using a bucket auger from 0 to 15 cm depth after harvesting wheat (the end of one rice–wheat cycle) in 2015. The soil samples were collected randomly from five spots in each plot and mixed into one composite sample. For chemical analysis, soil samples were processed by shade drying and passed through a 2-mm sieve. For microbiological and enzymatic assays, the collected soil sample was stored at a low temperature of 0–4°C for 1 week. In the current experiment, soil physical parameters such as bulk density (BD), soil chemical parameters, such as organic carbon (OC), available nitrogen (alkaline KMnO4 method), phosphorus (NaHCO3 extracted), and available potassium (NH4OAC extracted), different soil biological parameters such as soil dehydrogenase activity (DHA) and phosphatase activity, viz. alkaline phosphatase (ALK) and acid phosphatase (ACP), and potentially mineralizable nitrogen (PMN) were determined during the year 2015. The bulk density (BD) of soil was determined using a core sampler with a core of 5 cm height and 5 cm diameter. The mass of the collected soil in the core was measured and divided by the volume of the core to obtain the BD in mg m−3 (McKenzie et al., 2002). The ground, air-dried soil samples were passed through a 0.2-mm sieve to analyze soil organic carbon (SOC). SOC was determined through the modified Walkley and Black method (Jackson, 1973) using 1N K2Cr2O7, conc. H2SO4, orthophosphoric acid, sodium fluoride, and ferrous ammonium sulfate solution. Available (mineralizable) nitrogen (N) in the soil was determined by using the alkaline permanganate (KMnO4-N) method (Subbiah and Asija, 1956). Phosphorus was estimated using sodium bicarbonate as an extractant (Olsen et al., 1954). Color development was measured using a spectrophotometer (Double Beam Spectrophotometer AU2703, Systronics (India) Ltd., India) at 720 nm wavelength with known standards. Available potassium (exchangeable + water-soluble K) in soil was determined using a neutral normal ammonium acetate solution using a flame photometer (Systronics (India) Ltd., India) (Jackson, 1973). The soil dehydrogenase activity was estimated using the method described by Casida et al. (1964). Approximately 6 g of soil sample was saturated with 1.0 ml of freshly prepared 3% triphenyltetrazolium chloride (TTC) and incubated for 24 h in the dark. After incubation, methanol was added to stop enzyme activity, and the absorbance of the filtered aliquot was read at the 485 nm wavelength of the spectrophotometer. These results were expressed as μg TPF g−1 soil d−1. The soil phosphatase activity was assayed in 1 g of soil saturated with 4 ml of modified universal buffer (MUB) and 1 ml of p-nitrophenolphosphate, followed by incubation at 37°C for 1 h. After incubation, 1 ml of 0.5 M CaCl2 and 4 mL of NaOH were added, and the contents were filtered through a Whatman No. 1 filter paper. The amount of p-nitrophenol in the sample was determined at 400 nm, and the enzyme activity was expressed as μg p-NP g−1 soil h−1 (Tabatabai and Bremner, 1969). For measuring potentially mineralizable N (PMN), the methods described by Benedetti and Sebastiani (1996) were used. To analyze PMN, 50 g of soil, air-dried and sieved through a 2-RAM screen, was mixed with quartz sand in a 1:1 ratio (sand particle size, 0.2–0.8 mm) and incubated in a Buchner funnel (outer diameter 13 cm) at 60% water-holding capacity and at 30°C for 30 weeks. The mineral N in the soil was leached before incubation by adding 900 ml of CaSO4 solution and 100 ml of nutrient solution without N (0.002M CaSO4.2H2O, 0.005M Ca(H2PO4), 0.0025M K2SO4, 0.002M MgSO4). NO -N and NH4 + -N concentrations were measured periodically (after 2, 4, 8, 12, 16, 22, and 30 weeks) using an autoanalyzer (Vapodest, Gerhardt, Germany). At the end of the incubation period, exchangeable NH4 + -N was extracted using a 2N KC1 solution following the Bremner (1965) procedure. For microbial counts, collected moist samples were stored at 4°C for less than a month until required for analysis. The pour method and surface spread plate count methods were followed to count the population of the soil microbial community. Thornton's agar medium (Thornton, 1992), Jensen (1930) agar medium, and Martin (1950) Rose Bengal streptomycin agar medium (Martin, 1950) were used to count the total number of viable bacteria, actinomycetes, and fungi, respectively.
2.5. Soil quality index
For the determination of the SQI, a four-step method, as indicated by Masto et al. (2007), was adopted. These four steps included goal setting, selection of the minimum data set (MDS), indicator scoring, and computation of the SQI based on relative scoring and weights. In the current study, the SQI was calculated to correlate soil indicators with SYI and the system's productivity. A total of 15 soil parameters, viz. pH, BD, OC, N, P, K, bacterial count (BAC), fungi count (FUN), actinomycetes count (ACT), Azospirillum, phosphorus solubilizing bacteria (PSB), ALK, ACP, DHA, and PMN, were taken into account, of which only six parameters, viz., OC, DHA, P, K, PSB, and ACT, were selected for the MDS based on their performance in the principal component analysis (PCA), and redundancy in attribute selection was reduced using the procedure described by Andrews et al. (2002b). Principal components (PCs) were extracted based on Eigenvalues of >1 and variation explained by individual PCs of >5% criteria (Andrews et al., 2002a,b).
Further, the number of parameters was reduced using correlation analysis, and factors within the same PC showing significant correlation were identified and simplified. From a wide range of soil quality parameters, the SQI was calculated to properly address the soil quality status in the experimental field so that it can be linked well with the system's productivity and sustainability. The SQI was calculated based on the minimum data set (MDS), which was composed of four principal components (PCs) that had Eigenvalues of >1.0, as presented in Table 1. PCs with higher Eigenvalues were considered better describers of soil quality. Principal components were extracted from a total of 15 soil bio-physico-chemical parameters of importance. The variability in the parameters found in PC 1, PC2, PC3, and PC4 were 49.99, 8.23, 7.24, and 5.83%, respectively. The total variation explained by all four PCs was 71.28%. The factors with the highest absolute loadings and factors lying within 10% of the highest loading factors were retained for the MDS from each PC. If a single factor was selected for multiple PCs, it was assigned to the PC with the highest loading. For PCs, where multiple factors were selected, highly correlated factors (i.e., redundant) were eliminated, and only factors with the highest loading were kept for MDS, whereas factors that were not significantly correlated were kept (Supplementary Figure S1). Thus, the selected indicators were grouped using the soil function to determine whether their higher value was considered ‘good' or ‘bad.' The parameters in the MDS for the current study were characterized as ‘more is better.' For indicators that fell under the ‘more is better' category, each observation was divided by the highest observed value so that it received a score of 1. In contrast to the ‘less is better' indicators, each observation was divided by the lowest value so that the lowest observed value received a score of 1. A non-linear scoring function was used for scoring the parameters used in calculating the SQI, which was previously used by Kumar et al. (2021):
Where, X stands for the value of the indicator, A signifies the baseline value of the soil property where the score is 0.5, and b is the slope.
After the scoring of the variables, weights based on PCA results were assigned. Finally, the SQI was calculated using the following formula, as indicated by Mukherjee and Lal (2014):
Where, n stands for the number of variables in the MDS, Si is the score for soil parameters, and the PCA-derived weighing factor is Wi.
2.6. Statistical analysis
The ANOVA method for RCBD was followed to statistically analyze all the data. Fisher's LSD method (least significant difference) was adopted to differentiate the treatment means at 5% probability (p ≤ 0.05), as stated in the study by Gomez and Gomez (1976). All the statistical computations were conducted using SPSS software version 25 (IBM Corp, 2017) and the R statistical package.
3. Results
3.1. System productivity and sustainability
In the current experiment, the system's performance was evaluated using rice equivalent yields in both the current cycle and the long term. The effect of different long-term nutrient management treatments on the current system's productivity was significant. The highest system productivity was recorded with NPK + FYM + Zn and NPK + FYM (10.5 mg ha−1). The use of NPK + FYM even out yielded 150%N + 200%P + K + Zn, resulting in a 9.3% extra yield with fewer inputs. Adding FYM with NPK resulted in a 22.1% enhancement in system yield after 29 years (Table 2). A similar trend was observed for long-term system productivity. The same treatments were also found to result in the highest SYI than others, indicating the stability of high-yielding ability over a long duration. All the treatments resulted in lower system yields than the initial system productivity levels in the current year. However, the quanta of reduction ranged between 3.2 and 5.9% for treatments with NPK + FYM and NPK + FYM + Zn. Using NPK alone resulted in a 25.9% reduction in system productivity over the years. Treatments with higher chemical inputs, such as 125%N + PK and 150%N + 200%P + K + Zn, also resulted in 11.5 and 10.5% reductions in system productivity in the long run.
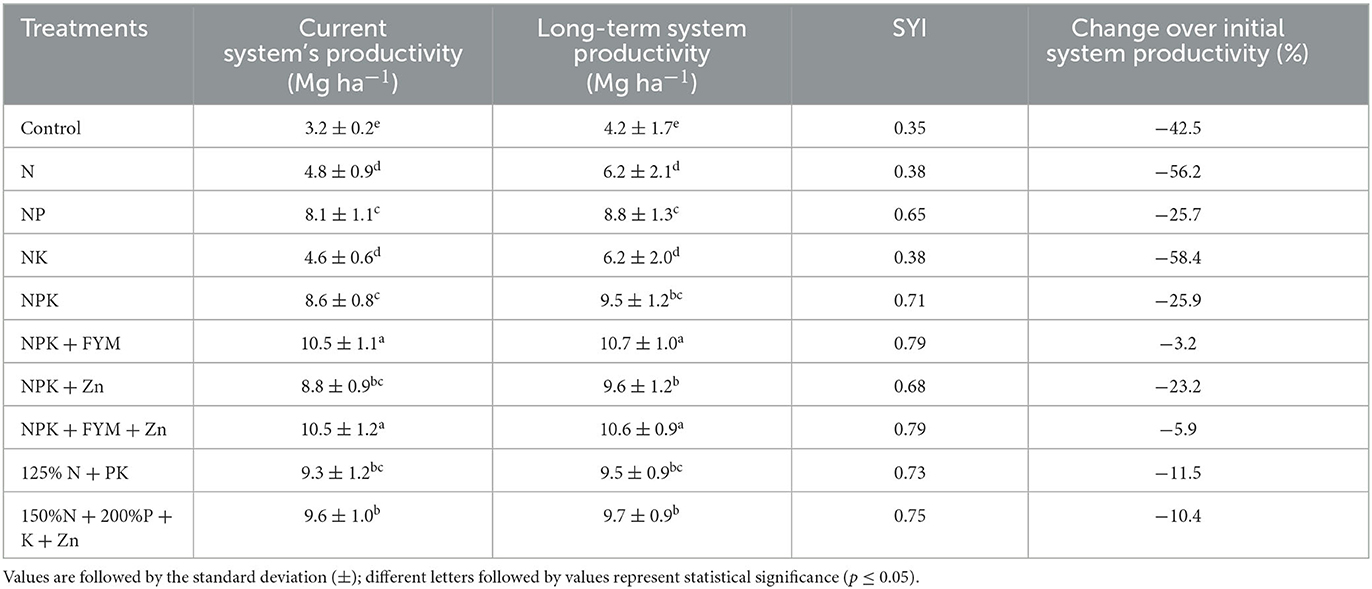
Table 2. Effect of long-term fertility treatments on current and long-term system productivity, SYI, and change over initial system productivity.
The curve was plotted to understand the system's performance in different treatments over a temporal scale and a time series (Figure 2). Fluctuations between replications under different fertilization regimes in each year are shown in Supplementary Table S1. Figure 2 shows that only after four cycles did the performance of insufficiently fertilized plots (N, NK) decline.
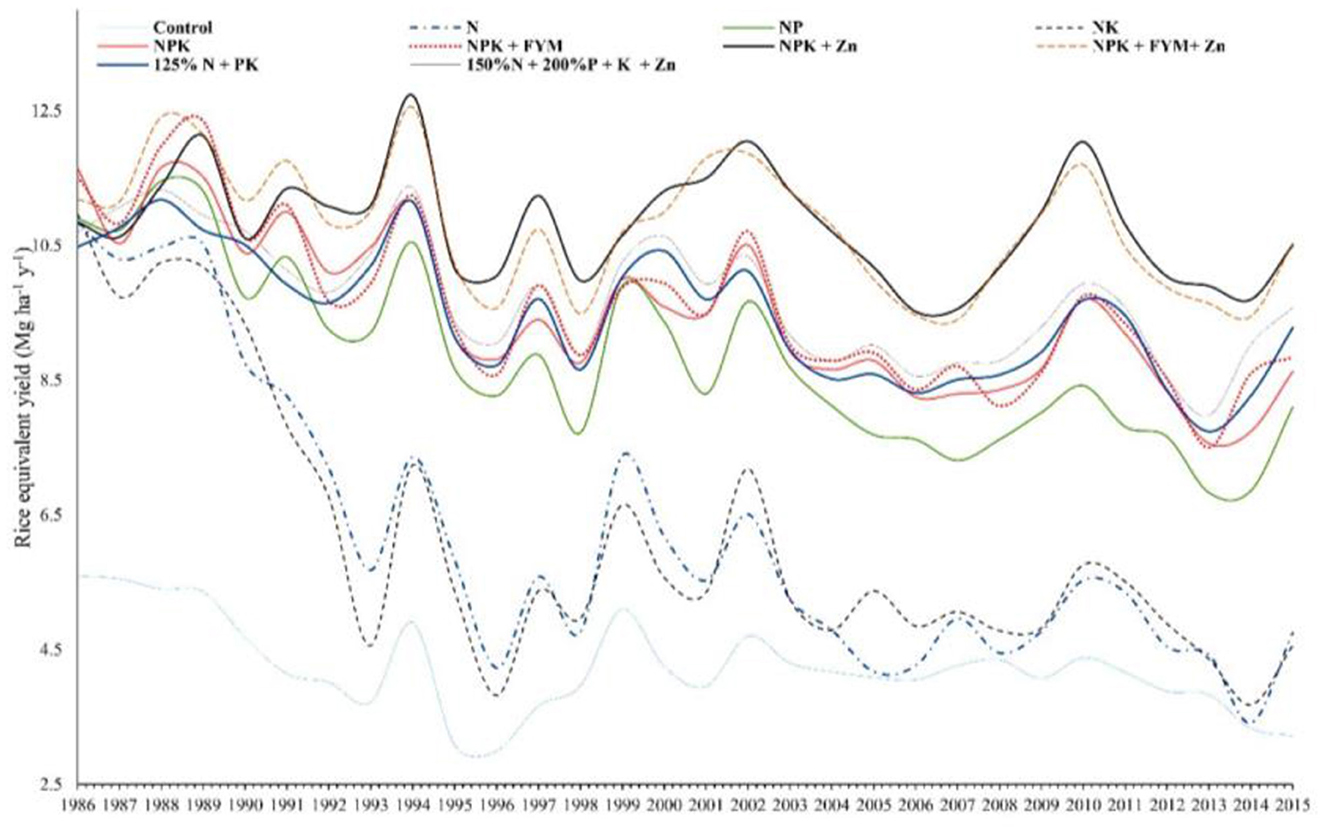
Figure 2. Time-series plot of system productivity from 1986 to 2015 under different fertility regimes [treatments are abbreviated as 120 kg ha-1 N (N), 120 kg ha-1 N + 40 kg ha-1 P (NP), 120 kg ha-1 N + 40 kg ha-1 K (NK), 120 kg ha-1 N + 40 kg ha-1 P + 40 kg ha-1 K (NPK), NPK + FYM (farm yard manure) at 5 mg ha-1 (NPK + FYM), NPK + 5 kg ha-1 Zn (NPK + Zn), NPK + FYM + 5 kg ha-1 Zn (NPK + FYM + Zn), 150 kg ha3 N + 40 kg ha-1 P + 40 kg ha-1 K (125% N + PK), and 150 kg ha-1 N + 80 kg ha-1 P + 40 kg ha-1 K + 5 kg ha-1 Zn (150%N + 200%P + K + Zn)].
The plots with high inorganic inputs, viz. 125%N + PK and 150%N + 200%P + K + Zn, resulted in higher system performance in the initial 3 years, after which the performance started declining, and after 6 years, it stabilized but at a lower level than the initial phase.
Treatments involving integrated nutrient inputs, namely NPK + FYM and NPK + FYM + Zn, initially performed poorly compared to high nitrogen treatments. However, after a period of 5 years, these treatments demonstrated a significant improvement and reached a stable and enhanced yield level.
3.2. Soil physical and chemical parameters
The effect of the different nutrient management regimes on bulk density and soil organic carbon after long-term experimentation was found to be significant (Figure 3). After the completion of the 29th cycle of rice–wheat cropping, treatments with FYM in combination with inorganic fertilizers were recorded to have the lowest BD of soil at 0–15 cm depth. All other treatments resulted in a significant increase in the bulk density from the initial value of 1.26 mg m−3 in 1986. NPK + FYM and NPK + FYM + Zn resulted in statistically similar bulk densities even after extensive rice–wheat cropping, in which puddling had been regularly carried out on rice crops. Among inorganic fertilizer-only treatments, 150%N + 200%P + K + Zn resulted in the lowest BD of 1.31 mg m−3. The BD recorded in control and N-only plots were approximately 7.9 and 6.2% higher than the BD recorded in NPK + FYM (Figure 3). At the end of 29 years of rice–wheat crop rotation, NPK + FYM + Zn and NPK + FYM applications were found to have statistically similar organic carbon levels as compared to the initial years (Figure 3).
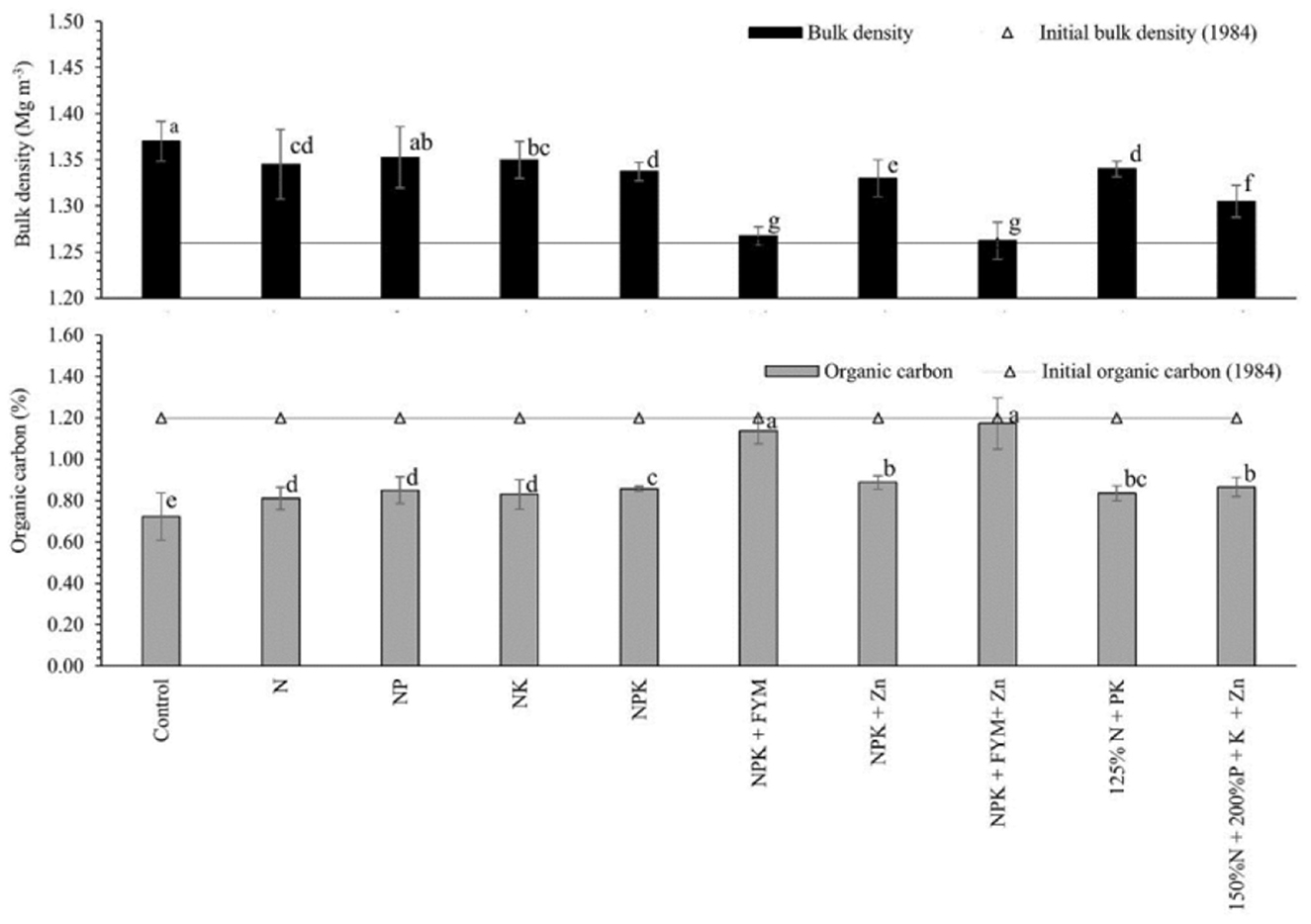
Figure 3. Effect of different long-term nutrient management on bulk density of soil and soil organic carbon content [different letters within the treatment pairs followed at the top of the bars represent statistical significance by Fisher's LSD test (p ≤ 0.05)].
All the other treatments resulted in a notable reduction in soil organic carbon content. The lowest OC content (0.72%) was recorded for control plots, whereas the highest OC was recorded with NPK + FYM and NPK + FYM + Zn. The use of 150%N + 200%P + K + Zn resulted in a reduction of OC in a 0–15 cm soil profile. Such reductions in OC in inorganically fertilized plots demonstrate the need for supplementary organic inputs to the system to maintain the OC. Treatments such as N, NP, and NK resulted in a reduction in OC by 28.9, 25.4, and 27.2% than NPK + FYM. Additional FYM applications with NPK showed a 32.5% increase in the OC compared to the OC under the application of NPK alone.
Concerning soil chemical status, the highest amounts of soil-available N, P, and K after wheat harvest were recorded from NPK + FYM and NPK + FYM + Zn-applied plots. Except for NPK + FYM, NPK + FYM + Zn, 125%N + PK, and 150%N + 200%P + K + Zn, all other treatments resulted in significantly lower soil available N than the initial years (Figure 4). Figure 4 shows that the application of 125% and 150% N resulted in lower available N in the soil than in treatments where an integrated nutrient management approach was adopted. The amount of available K in soil was observed to be similar in treatments where regular addition of K was performed (Figure 4). In treatments where K was not applied, such as control, N, and NP, a significant reduction in the amount of soil K available was observed. Soil available P was found to be the maximum under NPK + FYM and NPK + FYM + Zn, and these two best-performing treatments were found to be comparable concerning soil available P in 150%N + 200%P + K + Zn applied plots (Figure 4). Soil P availability was found to be considerably lower where organic manure or a higher percentage of P fertilizer was not applied.
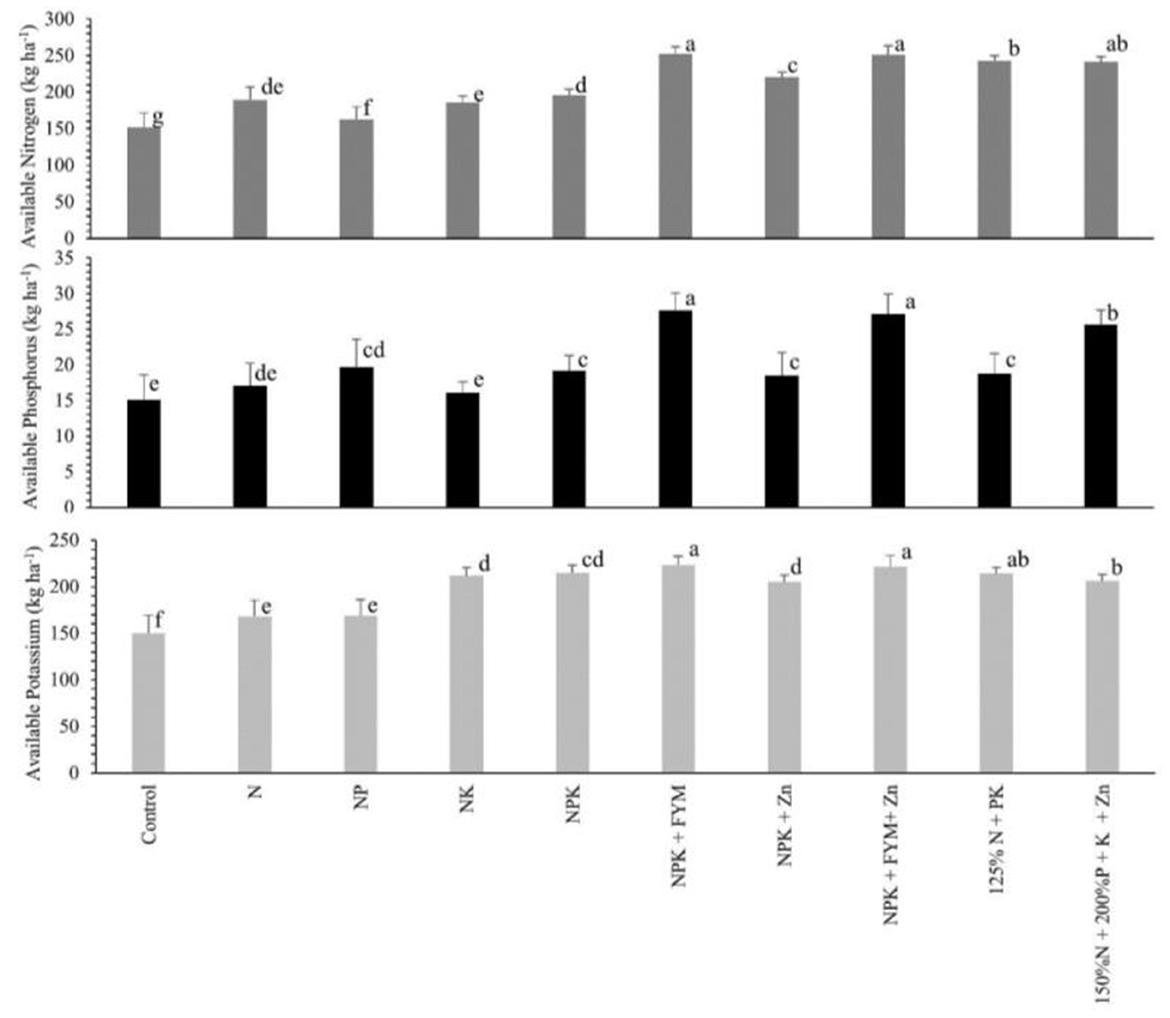
Figure 4. Effect of different long-term nutrient management on available nitrogen, phosphorus, and potassium in soil [different letters within the treatment pairs followed at the top of the bars represent statistical significance by Fisher's LSD test (p ≤ 0.05)].
3.3. Soil biological parameters
The dehydrogenase activity (DHA) at 0–15 cm soil depths under the rice–wheat cropping system differed significantly among all the treatments. The treatment of receiving no nutrients extraneously for 29 years resulted in the lowest DHA activity (Table 3).
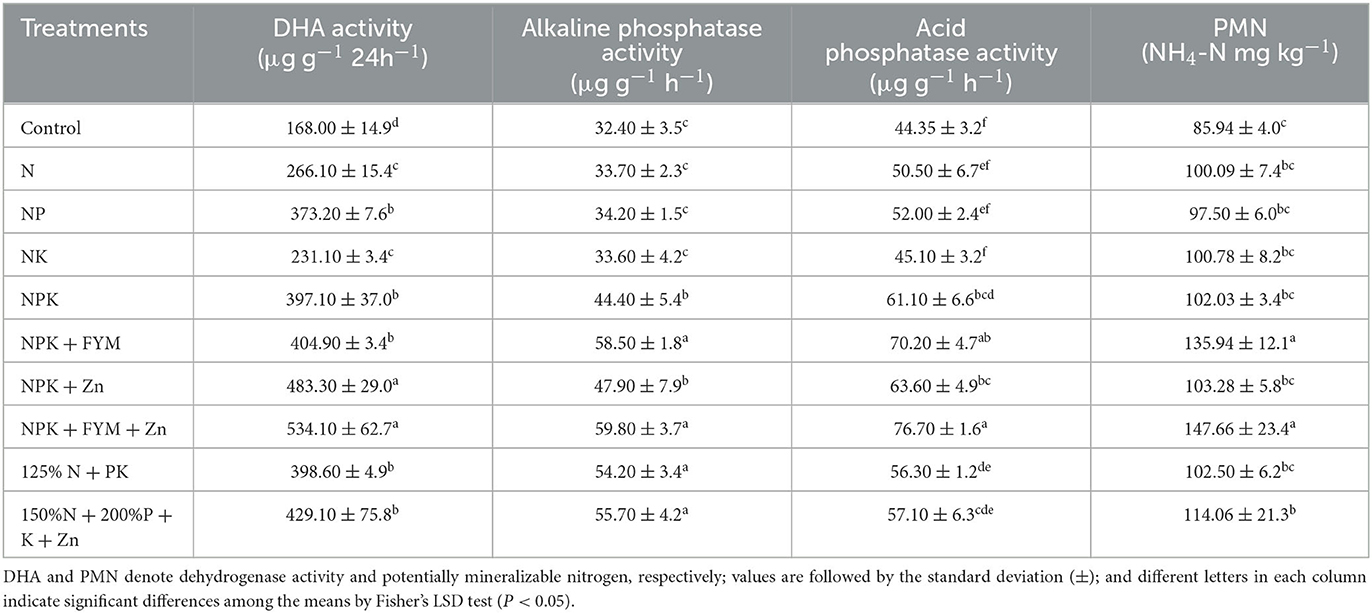
Table 3. Soil enzymatic activities under different long-term nutrition regimes in the rice–wheat cropping system.
However, the highest DHA activity was obtained due to the combined use of chemical fertilizer and FYM compared to control and other inorganic inputs. The application of Zn was observed to stimulate DHA activity. The combined use of FYM and Zn with NPK fertilizers resulted in the highest DHA activity, which was 31.9% higher than NPK + FYM, where no chemical Zn fertilizers were applied. The effect of different long-term nutrient management treatments on the soil's acid phosphatase (ACP) and alkaline phosphatase (ALK) activity was found to be significant. The highest ACP and ALK activity in the 0–15 cm soil layer was recorded under NPK + FYM + Zn, being statistically at the same level as NPK + FYM. In the case of ACP, the activity of ACP in 125%N + PK and 150%N + 200%P + K + Zn was also found to be statistically similar to that of the best-performing treatment. In both cases, the lowest ACP and ALK activities were detected in unfertilized plots, and insufficiently fertilized plots such as N, NP, and NK were also observed to perform similarly. All other treatments resulted in significantly higher activity of both phosphatases. The highest value of potentially mineralizable nitrogen (PMN) was estimated in NPK + FYM + Zn as being at par with NPK + FYM, and these two treatments were found to be significantly superior to all other treatments. The use of FYM with NPK resulted in 33.2% more PMN than NPK alone. The lowest PMN was recorded in the unfertilized control. The application of 50% additional N over the recommended N fertilizer did not show any significant increase in PMN.
3.4. Soil quality index
In PC-1, OC, available N, and P were loaded, out of which only OC was retained, as a high correlation was observed between OC and N (r = 0.74) and OC and P (r = 0.74). In PC-2, K and PSB were retained as those factors were not highly correlated (r = 0.46). DHA and PMN were selected in PC-3; however, only DHA was retained due to the presence of a significant correlation between DHA and PMN (r = 0.58). In PC-4, ACT and K were selected, and both factors were retained due to non-significant correlations (r = 0.37). Finally, six soil quality attributes, viz., OC, DHA, P, K, PSB, and ACT, were selected for the MDS in the Mollisols of the study region to calculate the SQI.
For the computation of SQI, parameters that are most sensitive, easy to measure, and responsive to soil, crop, and climatic variations are preferred. In the current experiment, MDS contained OC, which has been identified as a very important soil quality indicator, followed by DHA. Soil chemical parameters such as available P and K were also found to be important parameters determining the SQI. Other included parameters were populations of PSBs and actinomycetes. The parameters were scored based on threshold values, and different parameters were noted from various sources presented in Table 4. The factors and their respective scores have been depicted in Supplementary Figure S2.
The SQI for the current experiment ranged from 0.58 to 0.79, depending on the treatments. The highest SQI (0.79) was observed in NPK + FYM + Zn, which was statistically at par with NPK + FYM (0.77). All other treatments resulted in significantly lower SQI than those treatments. SQI in NPK + FYM was 7.8% lower in NPK-only treatments (Figure 5). The use of higher chemical inputs also resulted in lower SQI than those in which regular organic matter was added. The lowest SQI (0.58) was recorded for the control. The average contribution of factors in the SQI across the treatments was to the tune of 75.4, 6.0, 4.4, 5.8, 4.3, and 4.0% for OC, DHA, P, K, PSB, and ACT, respectively. An x-y plot between SQI and system productivity (both current and long-term average) and SYI showed a direct relationship with a significantly high coefficient of determinations (Figure 6). The long-term system productivity has been expressed as SQI with a regression equation (2) with R2 = 0.86, whereas the relationship between the current system's productivity and the SQI was expressed in equation (3) with R2 = 0.83. The long-term SYI had also been observed to share a similar relationship, which can be expressed in equation 4 (R2 = 0.74).
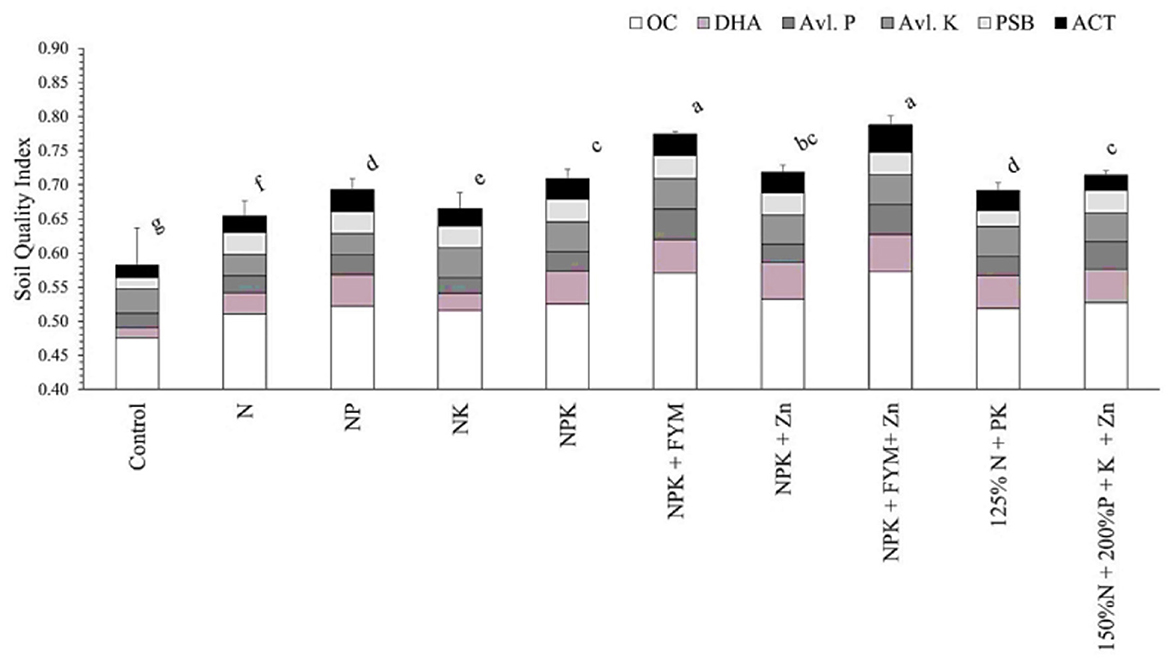
Figure 5. The SQI under different long-term fertility treatments [different letters within the treatment pairs followed at the top of the bars represent statistical significance using Fisher's LSD test (p ≤ 0.05)].
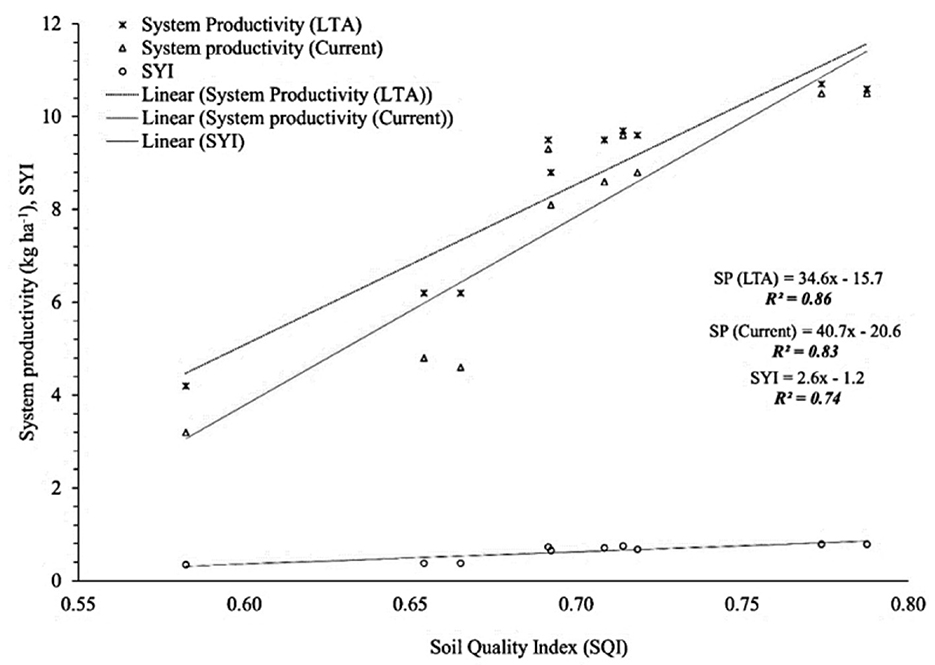
Figure 6. Linear relationship of current and long-term system productivity and the SYI with the SQI.
Where, x is the SQI.
Thus, the SQI was found to be linearly correlated with the current system's productivity and with long-term system productivity. System sustainability in terms of the SYI was also found to be well correlated with the SQI.
Extraction Method: Principal component analysis. PC stands for the principal component. Parameters are abbreviated as bulk density (BD), soil organic carbon (OC), available nitrogen (N), available phosphorus (P), available potassium (K), total bacterial count (FUN), total actinomycetes count (ACT), total azotobacter count (AZO), total phosphate solubilizing bacteria (PSB), alkaline phosphatase activity (ALK), and acid phosphatase activity (ACP).
4. Discussion
NPK + FYM + Zn- and NPK + FYM-based treatments improved system productivity and the SYI. These nutrient management options even out yielded 150%N + 200%P + K + Zn. These trends might be explained by the fact that the use of inorganic nutrient inputs without any additional organic matter addition may have resulted in systemic deterioration in soil health over a long period due to continuous cropping (Yang et al., 2020). Moreover, the application of balanced NPK coupled with organic input through FYM and the addition of micro-nutrient Zn resulted in better soil health by increasing SOC, lowering the BD, and improving the soil enzymatic activities, which ultimately helped in making the necessary soil nutrients available to the plant, resulting in a sustainable yield in the long run (Kumar et al., 2021; Laik et al., 2021a). This study also found significant correlations between system productivity, SOC, available N, DHA, ACP, and so on.
Only after four cycles did the yield of insufficiently fertilized plots (N, NK) decline, which might be explained by the mining of soil nutrients due to insufficient or ill-balanced nutrition, specifically in an exhaustive system such as the rice-wheat system (Biswas and Naher, 2019). The plots with a high inorganic input showed higher yields only up to the initial 3-year cycle. Afterward, the yield declined. Higher doses of nutrients cannot sustain productivity in the long run due to the continuous depletion of soil carbon and other micronutrients and secondary nutrients and poor soil health (Hubanks et al., 2018). The nutrient regime with balanced inorganic + organic nutrients showed a comparatively poor yield to high nitrogen treatments. However, these integrated approaches stabilized the yield, reaching a significantly improved level only after 5 years.
After 14 years, these treatments significantly diverged from all other treatments and continued to outperform others. Similar trends were also observed by Mangaraj et al. (2022).
Transplanted rice, followed by a conventionally grown wheat system, is a resource-intensive and soil-damaging system, especially due to the destruction of soil structure due to puddling and tillage operations (Alam et al., 2014; Bhatt et al., 2016). Such intensive tillage operations on a long-term basis have significantly increased the soil bulk density, which was also observed in this study except under NPK + FYM + Zn and NPK + FYM, where soil BD was found to be almost similar even after 29 years. An integrated nutrient management mode with the regular addition of organic matter might have stabilized the soil structure to an extent where a change in the BD was greatly buffered (Ramesh et al., 2019). Thus, the BD was found to be almost similar under NPK + FYM and NPK + FYM + Zn, comparing the BD at the initiation of this long-term study. However, BD increased considerably in all other nutrient management regimes.
Soil organic carbon is central to soil health, quality, and sustainability in agroecosystems (Page et al., 2020). The experimental plots were previously forest lands and, thus, initially had a very high organic carbon content of 1.2%, which is rather rare in the cultivated lands of sub-tropical India. SOC was found to be almost at the same level even after 29 years of the rice–wheat cropping system under nutrient management with NPK + FYM and NPK + FYM + Zn. However, the SOC level was found to be considerably lower in all other nutrient management regimes. These results are consistent with the findings by Patel et al. (2018). Laik et al. (2021a) reported that long-term application of FYM with mineral N fertilizer in sub-tropical soil can improve the labile organic matter pools and SOC levels in the soil. Not only the external application of organic matter but also better root biomass and plant growth may contribute to improved soil organic matter, especially in the 0–15 cm soil depth (Ismail-Meyer et al., 2018).
In this study, it was observed that the application of 125% and 150% N resulted in lower levels of the available N in soil than that of under-integrated nutrient management. It might be due to the high losses of N under heavy fertilization (Hou et al., 2021). Treatments with higher fertilization rates without any organic matter also result in lower factor productivity and bear a high risk of becoming unsustainable over time (Meemken and Qaim, 2018). The higher N-availability in the FYM-applied plot might be attributed to higher soil-microbial activity through the FYM application, which facilitates N-mineralization (Hagemann et al., 2016). In this study, a higher correlation between soil available N and DHA was also found. Besides, the addition of organic matter as a source of nutrients also improves soil bio-physico-chemical health, which in turn results in the better availability of nutrients (Bot and Jose, 2005). The production of many organic acids during organic matter decomposition could facilitate phosphate solubilization (Alori et al., 2017). Improvement in the soil's available plant nutrients with the combined use of chemical fertilizers and FYM was also reported by many researchers (Patel et al., 2018). The amount of available K in soil was observed to be similar in treatments where regular addition of K was carried out. This may be justified by the fact that most of the soil K is not heavily dependent upon soil organic matter but depends on the soil mineral composition (Singh et al., 2021).
The soil DHA is an important index indicating the activities of soil microbes (Wolinska and Stepniewsk, 2012). DHA activity was also found to be higher when the addition of FYM was supplemented with the seasonal application of Zn, which might be due to the fact that Zn is a cofactor of many dehydrogenase enzymes and that the availability of Zn with proper carbon-nitrogen balance may have resulted in higher DHA activity (Mathpal et al., 2015). The treatments that have received no external inputs resulted in the lowest microbial activity due to low available nitrogen in the soil and lower microbial activity due to low root activity in crops. In the current study, a higher correlation between soil available N and DHA was found, which justifies this result. The phosphatase activity was also found to be increasing with an increased supply of inorganic nitrogen and phosphates (Widdig et al., 2019). Higher soil phosphatase activity is dependent on the addition of inorganic nutrients. In particular, phosphates had also been noted by Masto et al. (2006). The combined application of organic and inorganic fertilizers provides sufficient carbon and nitrogen sources in the soil and thus raises the activity of soil enzymes (Masto et al., 2006). Potentially mineralizable nitrogen (PMN) is an excellent parameter that can indicate the actual capability of soil microbes to mineralize the organic-residue-bound N into an available NH4 + form for plants (Osterholz et al., 2017). The PMN is strongly related to soil microbial biomass, SOC, and soil organic nitrogen (Osterholz et al., 2017). Increased levels of PMN indicate higher soil biological activity, which may be stimulated by organic matter added to the soil (Masunga et al., 2016). Thus, the nutrient management regimes (NPK + FYM and NPK + FYM + Zn) increased the SOC, soil available N, etc., and also increased the PMN compared to other treatments in this long-term study. A higher, more significant correlation between PMN and SOC was also found in this study.
In the current experiment, MDS contains OC, which has been identified as a very important soil quality indicator in Mollisols and central to the soil's physical, chemical, and biological health by many researchers (Wienhold et al., 2005; Courtney and Mullen, 2008; Bünemann et al., 2018). Soil chemical parameters such as available P and K are also of great importance, especially in the cereal-based cropping systems (Das et al., 2014). Concerning soil biological parameters, DHA was found to be the most important demonstration of other soil microbial activities (Zhang et al., 2010). Integrated nutrient management with regular FYM addition to the soil can thus improve SQI by favorably altering the soil's physical, chemical, and biological parameters, which ultimately resulted in higher system performance in the current year after a 29-year study. Only chemical input, even at high dosage, cannot sustain yield performance, resulting in poor soil quality due to continuous cropping (Röös et al., 2018).
By correlating system productivity (both present and long-term average) with the SYI, the SQI's accuracy was shown. High coefficients of determination were observed in the study to show a direct link between SQI and system performance. The SYI, the present system's productivity, and the long-term system's productivity were all linearly connected to the SQI. Furthermore, the selected soil parameters in the MDS align with their importance in assessing soil quality in the Mollisols. Organic carbon (OC) is a crucial indicator of soil health, and available phosphorus (P) and potassium (K) are essential for the cereal-based cropping systems. The soil biological parameter, dehydrogenase activity (DHA), reflects soil microbial activity. By combining chemical fertilizers with farmyard manure (FYM) and zinc, we found that the integrated nutrient management approach positively affected soil health and system productivity. The SQI used in this study offered an accurate and thorough evaluation of the soil quality criteria, their scoring, and their weighted contribution to the overall index. The SQI's relationship to system productivity and sustainability emphasizes the need to use it to assess soil health and provide the best nutrient management strategies for long-term agricultural sustainability.
5. Conclusion
The purpose of the current study was to assess the effects of various long-term nutrient management techniques on the yield and soil health of the rice-wheat cropping system in the Mollisols of the Himalayan tarai region. The application of chemical nitrogen, phosphorus, and potassium fertilizers coupled with farm yard manure and zinc led to the highest long-term system productivity and the SYI, highlighting the significance of integrated fertilizations. The results also showed that the integrated application of chemical fertilizers such as NPK with farm yard manure could also resist the decline in system productivity as compared to the long-term use of chemical fertilizers alone. Under the combined application of NPK fertilizers with farm yard manure and zinc, the highest levels of soil enzymatic activity and potentially mineralizable nitrogen were found, demonstrating the beneficial effects of organic inputs and zinc fertilization on soil health. In addition, farm yard manure application in an integrated mode with chemical fertilizers enhanced soil organic carbon and available NPK compared to chemical fertilization alone.
Interestingly, neither crop yield nor soil health was improved by applying 50% more nitrogen or 100% more phosphorus supplied through chemical fertilizers over time. According to the study, a long-term improvement in soil health, the SQI, and system sustainability depend on balanced chemical fertilization coupled with regular organic inputs. Together, the findings indicate that it is feasible to increase and sustain the productivity of the rice-wheat cropping system and overall soil quality by applying NPK at 120:40:40 kg ha−1 in both rice and wheat, as well as additional applications of 5 mg ha−1 FYM + 5 kg ha−1 Zn in rice in the Mollisols of the Himalayan tarai region. These results strongly support integrating chemical fertilization with organic inputs to promote system sustainability over time.
Data availability statement
The original contributions presented in the study are included in the article/Supplementary material, further inquiries can be directed to the corresponding authors.
Author contributions
MB, DS, KR, NP, and PD led the research, planned, supervised, conducted field experiments, read, and edited the manuscript. MB, HJ, BP, MK, and PD collected soil/plant samples, performed the chemical analysis, wrote the initial draft of the manuscript, and prepared figures and tables. KR, RC, BP, HJ, AG, AA, AH, and NP supervised the project and reviewed, read, and edited the manuscript with significant contributions. BP, MK, AG, AA, AH, and PD performed the statistical analysis. All authors contributed to the article and approved the submitted version.
Funding
This research was funded by the G. B. Pant University of Agriculture and Technology, Pantnagar−263145, India, and partially funded by the Princess Nourah bint Abdulrahman University Researchers Supporting Project Number (PNURSP2023R65), Princess Nourah bint Abdulrahman University, Riyadh, Saudi Arabia.
Acknowledgments
The authors of this study are grateful to the Director of the Experimentation Station, Pantnagar, who provided all the necessary funds and facilities to conduct this study. The authors would also like to extend their sincere appreciation to Princess Nourah bint Abdulrahman University Researchers Supporting Project Number (PNURSP2023R65), Princess Nourah bint Abdulrahman University, Riyadh, Saudi Arabia, for supporting the current research.
Conflict of interest
The authors declare that the research was conducted in the absence of any commercial or financial relationships that could be construed as a potential conflict of interest.
Publisher's note
All claims expressed in this article are solely those of the authors and do not necessarily represent those of their affiliated organizations, or those of the publisher, the editors and the reviewers. Any product that may be evaluated in this article, or claim that may be made by its manufacturer, is not guaranteed or endorsed by the publisher.
Supplementary material
The Supplementary Material for this article can be found online at: https://www.frontiersin.org/articles/10.3389/fsufs.2023.1206878/full#supplementary-material
References
Alam, M. K., Islam, M. M., Salahin, N., and Hasanuzzaman, M. (2014). Effect of tillage practices on soil properties and crop productivity in wheat–mungbean–rice cropping system under subtropical climatic conditions. Sci World J. 4, 1–15. doi: 10.1155/2014/437283
Alori, E. T., Glick, B. R., and Babalola, O. O. (2017). Microbial phosphorus solubilization and its potential for use in sustainable agriculture. Front. Microbiol. 8, 971. doi: 10.3389/fmicb.2017.00971
Andrews, S., Karlen, D., and Mitchell, J. A. (2002b). Comparison of soil quality indexing methods for vegetable production systems in northern California. Agric. Ecosyst. Environ. 90, 25–45. doi: 10.1016/S0167-8809(01)00174-8
Andrews, S., Mitchell, J. P., Mancinelli, R., Karlen, D. L., Hartz, T. K., Horwath, W. R., et al. (2002a). On-farm assessment of soil quality in California's central valley. Agron J 94, 12. doi: 10.2134/agronj2002.0012
Bedi, P., Dubey, Y. P., and Datt, N. (2009). Microbial properties under rice–wheat cropping sequence in acid Alfisol. J. Indian Soc. Soil Sci. 57, 373–377. doi: 10.1556/AAgr.57.2009.3.5
Benedetti, A., and Sebastiani, G. (1996). Determination of potentially mineralizable nitrogen in agricultural soil. Biol Fertil. Soils 21, 114–120. doi: 10.1007/BF00336002
Bhatt, R., Kukal, S. S., Busari, M. A., Arora, S., and Yadav, M. (2016). Sustainability issues on rice–wheat cropping system. Int Soil Water Conserv. Res. 4, 64–74. doi: 10.1016/j.iswcr.2015.12.001
Biswas, J. C., and Naher, U. A. (2019). “Soil nutrient stress and rice production in Bangladesh,” in Advances in Rice Research for Abiotic Stress Tolerance, eds M Hasanuzzaman, M Fujita, K Nahar (London: Woodhead Publishing), 431–445.
Bot, A., and Jose, B. (2005). The Importance of Soil Organic Matter, The Importance of Soil Organic Matter. Rome: FAO.
Bremner, J. M. (1965). Nitrogen availability index. Methods of soil analysis. Agronomy 9, 1324–1341.
Bünemann, E. K., Bongiorno, G., Bai, Z., Creamer, R. E., De Deyn, G., de Goede, R., et al. (2018). Soil quality – A critical review. Soil Biol Biochem 120, 105–125. doi: 10.1016/j.soilbio.2018.01.030
Casida, L. E., Klein, D. A., and Santoro, T. (1964). Soil dehydrogenase activity. Soil Sci. 98, 371–376. doi: 10.1097/00010694-196412000-00004
Courtney, R. G., and Mullen, G. J. (2008). Soil quality and barley growth as influenced by the land application of two compost types. Bioresour Technol. 99, 2913–2918. doi: 10.1016/j.biortech.2007.06.034
Das, B., Chakrabarti, K., Tripathi, S., and Chakraborty, A. (2014). Review of some factors influencing jute fiber quality. J. Nat0 Fibers 11, 268–281. doi: 10.1080/15440478.2014.880103
Deshpande, S. B., Fehrenbacher, J. B., and Ray, B. W. (1971). Mollisols of tarai region of Uttar Pradesh, northern India, 2. Genesis and classification. Geoderma 6, 195–201. doi: 10.1016/0016-7061(71)90006-1
Hagemann, N., Harter, J., and Behrens, S. (2016). Elucidating the Impacts of Biochar Applications on Nitrogen Cycling Microbial Communities. Biochar Application: Essential soil Microbial Ecology. New York, NY: Elsevier.
Hou, P., Jiang, Y., Yan, L., Petropoulos, E., Wang, J., Xue, L., et al. (2021). Effect of fertilization on nitrogen losses through surface runoffs in Chinese farmlands: a meta-analysis. Sci. Total Environ. 793, 148554. doi: 10.1016/j.scitotenv.2021.148554
Hubanks, H. L., Deenik, J. L., and Crow, S. E. (2018). Getting the Dirt on Soil Health and Management. Reference Module in Earth, Systems, and Environmental Sciences. Amsterdam: Elsevier.
Ismail-Meyer, K., Stolt, M. H., and Lindbo, D. L. (2018). “Soil organic matter,” in Interpretation of Micromorphological Features of Soils and Regoliths, eds G Stoops, V Marcelino, F Mees (Amsterdam: Elsevier), 471–512.
Jensen, H. L. (1930). Actinomycetes in Danish soils. Soil Sci. 30, 59–77. doi: 10.1097/00010694-193007000-00005
Kar, S., Pramanick, B., Brahmachari, K., Saha, G., Mahapatra, B. S., Saha, A., et al. (2021). Exploring the best tillage option in rice based diversified cropping systems in alluvial soil of eastern India. Soil Till Res 205, 104761. doi: 10.1016/j.still.2020.104761
Kumar, M., Mitra, S., Mazumdar, S. P., Majumdar, B., Saha, A. R., Singh, S. R., et al. (2021). Improvement of soil health and system productivity through crop diversification and residue incorporation under jute-based different cropping systems. Agronomy 11, 1622. doi: 10.3390/agronomy11081622
Laik, R., Kumara, B. H., Pramanick, B., and Singh, S. K. (2021a). Labile soil organic matter pools are influenced by 45 years of applied farmyard manure and mineral nitrogen in the wheat–pearl millet cropping system in the sub-tropical condition. Agronomy 11, 2190. doi: 10.3390/agronomy11112190
Laik, R., Singh, S. K., Pramanick, B., Kumari, V., Nath, D., Dessoky, E. S., et al. (2021b). Improved method of boron fertilization in rice (Oryza sativa L.)–mustard (Brassica juncea L.) cropping system in upland calcareous soils. Sustainability 13, 5037. doi: 10.3390/su13095037
Lakshmi, P. V., Singh, S. K., Pramanick, B., Kumar, M., Laik, R., Kumari, A., et al. (2021). Long term zinc fertilization in calcareous soils improves wheat (Triticum aestivum L.) productivity and soil zinc status in the rice-wheat cropping system. Agronomy 11, 1306. doi: 10.3390/agronomy11071306
Mahapatra, B. S., and Dey, P. (2022). “Integrated Management Practices for Incremental Wheat Productivity, in New Horizons in Wheat and Barley Research Singapore, ed Singh GP. Singapore: Springer Nature.
Mangaraj, S., Paikaray, R. K., Maitra, S., Pradhan, S. R., Garnayak, L. M., Satapathy, M., et al. (2022). Integrated nutrient management improves the growth and yield of rice and greengram in a rice–greengram cropping system under the coastal plain agro-climatic condition. Plants 11, 142. doi: 10.3390/plants11010142
Martin, J. P. (1950). Rose Bengal agar base. Soil Sci 69, 215. doi: 10.1097/00010694-195003000-00006
Masto, E. R., Chhonkar, P. K., Singh, D., and Patra, A. K. (2006). Changes in soil biological and biochemical characteristics in a long-term field trial on a sub-tropical Inceptisol. Soil Biol Biochem 38, 1577–1582. doi: 10.1016/j.soilbio.2005.11.012
Masto, R. E., Chhonkar, P. K., Purakayastha, T. J., Patra, A. K., and Singh, D. (2008). Soil quality indices for evaluation of long-term land use and soil management practices in semi-arid sub-tropical India. Land Degrad Dev. 19, 516–529. doi: 10.1002/ldr.857
Masto, R. E., Chhonkar, P. K., Singh, D., and Patra, A. K. (2007). Soil quality response to long-term nutrient and crop management on a semi-arid Inceptisol. Agric Ecosyst Environ 118, 130–142. doi: 10.1016/j.agee.2006.05.008
Masunga, R. H., Uzokwe, V. N., Mlay, P. D., Odeh, I., Singh, A., Buchan, D., et al. (2016). S. Nitrogen mineralization dynamics of different valuable organic amendments commonly used in agriculture. Appl. Soil Ecol. 101, 185–193. doi: 10.1016/j.apsoil.2016.01.006
Mathpal, B., Srivastava, P. C., Shankhdhar, D., and Shankhdhar, S. C. (2015). Improving key enzyme activities and quality of rice under various methods of zinc application. Physiol. Mol. Biol. Plants 21, 567–572. doi: 10.1007/s12298-015-0321-3
McKenzie, N., Coughlan, K., and Cresswell, H. (2002). Soil Physical Measurement and Interpretation for Land Evaluation. Collingwood, VIC: CISRO Publishing.
Meemken, E. M., and Qaim, M. (2018). Organic agriculture, food security, and the environment. Annu. Rev. Resour. Econ. 10, 39–63. doi: 10.1146/annurev-resource-100517-023252
Min, D. H., Islam, K. R., Vough, L. R., and Weil, R. (2003). Dairy manure effects on soil quality properties and carbon sequestration in alfalfa-orchard grass systems. Comm. Soil Sci. Plant Anal. 34, 781–799. doi: 10.1081/CSS-120018975
Mukherjee, A., and Lal, R. (2014). Comparison of soil quality index using three methods. PLoS ONE 9, e105981. doi: 10.1371/journal.pone.0105981
Olsen, S. R., Cole, C. V., Watanabe, F. S., and Dean, L. (1954). Estimation of Available Phosphorus in Soil by Extraction With Sodium Carbonate. Washington: USDA.
Osterholz, W. R., Rinot, O., Shaviv, A., Linker, R., Liebman, M., Sanford, G., et al. (2017). Predicting gross nitrogen mineralization and potentially mineralizable nitrogen using soil organic matter properties. Soil Sci. Soc. Am. J. 81, 1115–1126. doi: 10.2136/sssaj2017.02.0055
Page, K. L., Dang, Y. P., and Dalal, R. C. (2020). The ability of conservation agriculture to conserve soil organic carbon and the subsequent impact on soil physical, chemical, and biological properties and yield. Front. Sustain Food Syst. 4, 31. doi: 10.3389/fsufs.2020.00031
Patel, G., Dwivedi, B. S., Dwivedi, A. K., Thakur, R., and Singh, M. (2018). Long-term effect of nutrient management on soil biochemical properties in a vertisol under soybean–wheat cropping sequence. J. Indian Soc. Soil Sci. 66, 215. doi: 10.5958/0974-0228.2018.00027.0
Pramanick, B., Kumar, M., Naik, B. M., Kumar, M., Singh, S. K., Maitra, S., et al. (2022). Long-term conservation tillage and precision nutrient management in maize–wheat cropping system: effect on soil properties, crop production, and economics. Agronomy 12, 2766. doi: 10.3390/agronomy12112766
Ramesh, T., Bolan, N. S., Kirkham, M. B., Wijesekara, H., Kanchikerimath, M., Srinivasa Rao, C., et al. (2019). Soil organic carbon dynamics: Impact of land use changes and management practices: a review. Adv Agron 156, 1–107. doi: 10.1016/bs.agron.2019.02.001
Röös, E., Mie, A., Wivstad, M., Salomon, E., Johansson, B., Gunnarsson, S., et al. (2018). Risks and opportunities of increasing yields in organic farming. A review. Agron Sustain Dev. 38, 14. doi: 10.1007/s13593-018-0489-3
Saha, S., Prakash, V., Kundu, S., Kumar, N., and Mina, B. L. (2008). Soil enzymatic activity as affected by long term application of farmyard manure and mineral fertilizer under a rainfed soybean–wheat system in N-W Himalaya. Eur. J. Soil Biol. 44, 309–315. doi: 10.1016/j.ejsobi.2008.02.004
Shah, Z., Ahmad, R., and Rahman, H. U. (2011). Sustaining rice-wheat system through the management of legumes I: effect of green manure legumes on rice yield and soil quality. Pak J. Bot. 43, 1569–1574.
Sharma, V., Hussain, S., Sharma, K. R., and Arya, V. M. (2014). Labile carbon pools and soil organic carbon stocks in the foothill Himalayas under different land use systems. Geoderma 234, 81–87. doi: 10.1016/j.geoderma.2014.04.039
Singh, V. K., Gautam, P., Nanda, G., Dhaliwal, S. S., Pramanick, B., Meena, S. S., et al. (2021). Soil test based fertilizer application improves productivity, profitability and nutrient use efficiency of rice (Oryza sativa L.) under direct seeded condition. Agronomy 11, 1756. doi: 10.3390/agronomy11091756
Subbiah, B. V., and Asija, G. L. A. (1956). rapid procedure for estimation of available N in soils. Curr Sci 25, 259–260.
Tabatabai, M. A., and Bremner, J. M. (1969). Use of p-nitrophenyl phosphate for assay of soil phosphatase activity. Soil Biol. Biochem. 1, 301–307. doi: 10.1016/0038-0717(69)90012-1
Thornton, H. G. (1992). On the development of a standardised agar medium for counting soil bacteria, with especial regard to the repression of spreading colonies. Ann. Appl. Biol 9, 241–274. doi: 10.1111/j.1744-7348.1922.tb05958.x
Vasu, D., Singh, S. K., Ray, S. K., Duraisami, V. P., Tiwary, P., Chandran, P., et al. (2016). Soil quality index (SQI) as a tool to evaluate crop productivity in semi-arid Deccan plateau, India. Geoderma 282, 70–79. doi: 10.1016/j.geoderma.2016.07.010
Velmurugan, A., Krishan, G., Dadhwal, V. K., Kumar, S., Swarnam, T., Saha, S., et al. (2009). Harmonizing soil organic carbon estimates in historical and current data. Curr Sci. 97, 554–558.
Widdig, M., Schleuss, P. M., Weig, A. R., Guhr, A., Biederman, L. A., Borer, E. T., et al. (2019). Nitrogen and phosphorus additions alter the abundance of phosphorus-solubilizing bacteria and phosphatase activity in grassland soils. Front Environ. Sci. 7, 185. doi: 10.3389/fenvs.2019.00185
Wienhold, B. J., Varvel, G. E., and Doran, J. W. (2005). Quality of Soil. Encyclopedia of Soils in the Environment. London: Academic Press, 349–353.
Wolinska, A., and Stepniewsk, Z. (2012). Dehydrogenase Activity in the Soil Environment. Dehydrogenases. London: InTech Open.
Yadav, D. S., and Kumar, A. (2009). Long-term effect of nutrient management on soil health and productivity of rice (Oryza Sativa)–wheat (Triticum aestivum) system. Indian J. Agron 54, 15–23. Available online at: https://www.indianjournals.com/ijor.aspx?target=ijor:ija&volume=54&issue=1&article=003
Yang, T., Siddique, K. H. M., and Liu, K. (2020). Cropping systems in agriculture and their impact on soil health-A review. Glob Ecol Conserv. 23, e01118. doi: 10.1016/j.gecco.2020.e01118
Keywords: integrated nutrient management, rice-wheat cropping system, soil quality index, sustainable yield index, system productivity
Citation: Bhatt MK, Singh DK, Raverkar KP, Chandra R, Pareek N, Dey P, Pramanick B, Joshi HC, Kumar M, Gaber A, Alsuhaibani AM and Hossain A (2023) Effects of varied nutrient regimes on soil health and long-term productivity in a rice–wheat system: insights from a 29-year study in the mollisols of the Himalayan Tarai region. Front. Sustain. Food Syst. 7:1206878. doi: 10.3389/fsufs.2023.1206878
Received: 16 April 2023; Accepted: 29 May 2023;
Published: 27 June 2023.
Edited by:
Arnab Majumdar, Jadavpur University, IndiaReviewed by:
Reshu Chauhan, National Botanical Research Institute (CSIR), IndiaOwais Ali Wani, Sher-e-Kashmir University of Agricultural Sciences and Technology of Kashmir, India
Heba Elbasiouny, Al-Azhar University, Egypt
Copyright © 2023 Bhatt, Singh, Raverkar, Chandra, Pareek, Dey, Pramanick, Joshi, Kumar, Gaber, Alsuhaibani and Hossain. This is an open-access article distributed under the terms of the Creative Commons Attribution License (CC BY). The use, distribution or reproduction in other forums is permitted, provided the original author(s) and the copyright owner(s) are credited and that the original publication in this journal is cited, in accordance with accepted academic practice. No use, distribution or reproduction is permitted which does not comply with these terms.
*Correspondence: Biswajit Pramanick, Ymlzd2FqaXQmI3gwMDA0MDtycGNhdS5hYy5pbg==; Akbar Hossain, YWtiYXJob3NzYWlud3JjJiN4MDAwNDA7Z21haWwuY29t