- 1Animal Husbandry in the Tropics and Subtropics, Universität Kassel and Georg-August-Universität Göttingen, Steinstrasse, Witzenhausen, Germany
- 2ICAR-National Institute of Animal Nutrition and Physiology, Adugodi, Bengaluru, India
- 3Animal Breeding, Department of Animal Breeding and Genetics, Justus Liebig Universität Gieβen, Stephanstraße, Gießen, Germany
Low- and middle-income countries (LMICs) are rapidly urbanizing, leading to a high demand for high-quality animal products. Production increase is seen as a key to meeting this demand and reducing the global environmental impact of low-yielding dairy production system (DPS) often found in LMICs. Therefore, the present study assesses the relationship between enteric methane emissions and different dairy production strategies, taking DPS in the rural–urban interface of Bengaluru, an Indian megacity, as a case study. Twenty-eight dairy farms, evenly distributed across four DPS, were monitored for 1 year (eight visits at 6-week intervals). Following IPCC 2006 guidelines and a Tier 2 approach, enteric methane emissions from dairy cattle were calculated as carbon dioxide equivalents (CO2 eq). Dairy producers in ExtDPS, an extensive DPS found throughout the rural–urban interface of Bengaluru, fed their dairy cattle a high-quality diet, partly based on organic wastes from markets or neighbors, achieving 9.4 kg energy-corrected milk (ECM) per cow and day. Dairy producers in Semi-ADPS, a semi-intensive and rural DPS, fed an average quality diet and achieved the lowest milk production (7.9 kg ECM cow−1 day−1; p < 0.05). Dairy producers in Semi-BDPS, another semi-intensive and rural DPS, relied on average quality but more abundant feedstuffs and achieved a production of 10.0 kg ECM cow−1 day−1. A similar milk yield (10.1 kg ECM cow−1 day−1) was achieved by IntDPS, an intensive and rural DPS. The intensity of enteric methane emissions was the highest in Semi-BDPS (1.38 kg CO2-eq kg−1 ECM; p < 0.05), lowest in ExtDPS (0.79 kg CO2-eq kg−1 ECM; p < 0.05), and intermediate in semi-ADPS and IntDPS. The results highlight the close relationship between the intensity of enteric methane emissions and the intensification strategies chosen by dairy producers based on locally available resources. They also underline the importance of region- and system-specific environmental assessments of production systems in LMICs.
1 Introduction
The rapid urbanization in low- and middle-income countries (LMICs) over the last decades (United Nations, 2018a) is now a major driver of change in local resources available to dairy producers: on the one hand, urbanization affects land and labor availability since land competition and job opportunities increase as cities grow (Satterthwaite et al., 2010). On the other hand, urbanization, through improved rural–urban linkages, also eases access of dairy producers to inputs such as concentrate feeds and high-yielding cattle genotypes, as well as markets, which are key factors for a production increase (Duncan et al., 2013). Along with the rapid urbanization in West Africa and Asia since the 1990s and driven by urban consumers’ demand for daily provision of a highly perishable and nutritionally important product (Schlecht et al., 2019), up to one-third of global milk is now produced by urban and peri-urban dairy production systems (DPS) in LMICs (United Nations, 2018a; FAO, 2020). Social-ecological dynamics of urban and peri-urban DPS have been documented in various countries, such as Burkina Faso and Ghana (Dossa et al., 2015; Roessler et al., 2016), Benin (Yassegoungbe et al., 2022), Ethiopia (D’Haene and D’Haese, 2019), Egypt (Daburon et al., 2017), and Bengaluru, India (Reichenbach et al., 2021a). In Bengaluru, the urban and peri-urban space even offers new sources of feedstuffs, such as organic fruit and vegetable wastes from markets or neighbors, and green fodder collected from the surroundings of Bengaluru’s numerous urban and peri-urban lakes (Prasad et al., 2019).
In LMICs, milk productivity is usually low and DPS are perceived as having a disproportionately high global environmental impact in comparison to high-yielding DPS because of the strong correlation between emission intensity of greenhouse gases (GHG) and production (Gerber et al., 2011, 2013). Enteric fermentation (EF) is often the largest contributor to emission intensity of livestock products (Gerber et al., 2013; Ndung'u et al., 2022). Low-yielding DPS, however, have a large mitigation potential, whereby common strategies for production increase often focus on improved feeds, feeding, and animal genetics that increase production while reducing emissions, therefore doubling as mitigation options (Gerber et al., 2013). Diet quality and the genetic makeup of cows are defined through the production strategy chosen by dairy producers according to the resources available to them. In LMICs, resource availability is, however, frequently fluctuating, and especially forage availability, both quantitative and qualitative, can constrain dairy production during the dry season or a drought (Lukuyu et al., 2015). Another major constraint is high ambient temperatures during the dry season and the associated risk of heat stress in dairy cows, which negatively impacts milk production (West, 2003). The challenging dairy production environment in LMICs thus limits the adoption of high-yielding cattle genotypes as part of dairy producers’ production strategy because these breeds are often non-native and more susceptible to local environmental constraints (Burrow, 2012).
To assess potential GHG mitigation options in such systems, the present study therefore aims to analyze the relationship between the intensity of enteric methane emissions and farmers’ dairy production strategies, taking DPS in the rural–urban interface (RUI) of Bengaluru, an Indian megacity, as the case study. Not only is India rapidly urbanizing but it also has the largest dairy herd in the world, characterized, however, by low production. Hence, the contribution of Indian dairy production to global GHG emissions is considered being disproportionately high but with a large mitigation potential (Gerber et al., 2011, 2013).
In Bengaluru’s RUI, four DPS with different production strategies have recently been documented (Reichenbach et al., 2021a). The present study thus asks the following question: Does the intensity of enteric methane emissions of these four Indian DPS that coexist within the same rapidly urbanizing environment differ according to their production strategy? To answer this question, we assessed the intensity of enteric methane emissions per unit of milk produced by four clusters of seven dairy farms each that represented the four DPS within Bengaluru’s RUI as documented by Reichenbach et al. (2021a).
2 Materials and methods
2.1 Research area and dairy production systems
Bengaluru is the capital of the southern Indian state of Karnataka and one of the emerging megacities of the recent decades: Its population grew at an average annual rate of 4% between 2000 and 2018 and is currently over 10 million (United Nations, 2018b). Bengaluru has a hot semi-arid climate with a dry season (March–May) followed by the monsoon season (June–October) and winter (November–February). Monthly temperatures range from 18.5°C to 29.5°C and annual rainfall reaches 948 mm (average for 2013–2017, weather station data of the University of Agricultural Sciences Bangalore, GKVK campus). The administrative district of Bengaluru Urban has a bovine population of 145,000 head (National Dairy Development Board, 2015). To assess dairy production strategies in Bengaluru’s RUI, two research transects were established along a northern and a southern urban-to-rural gradient (Figure 1; Hoffmann et al., 2017) and 337 dairy farms were surveyed (Reichenbach et al., 2021a). The genotypes found in Bengaluru’s RUI included Holstein Friesian (HF, 54% of all cattle in the survey) and Jersey (15%) cattle, further referred to as exotic as opposed to native genotypes (10%; mostly Bos indicus Hallikar, a draught breed from the State of Karnataka). Crossbreeds (21%) were either (i) exotic × exotic (first generation), (ii) exotic × native (first generation), or (iii) multi-generation crosses.
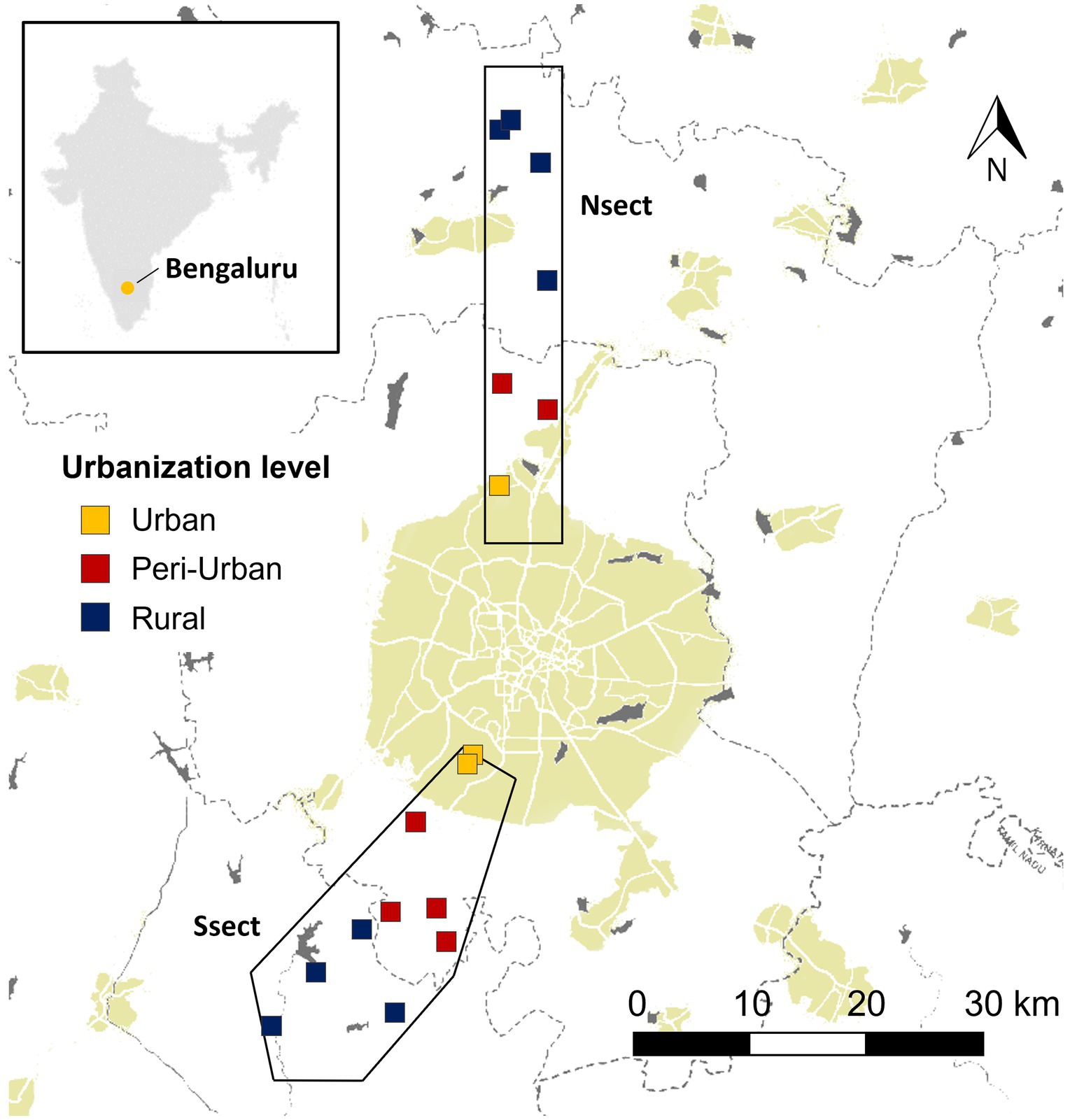
Figure 1. Map showing the location of Bengaluru within India, Bengaluru’s rural–urban interface, the northern (Nsect) and southern (Ssect) research transects and the location of the 17 settlements in which dairy farms were selected. Colors represent the urbanization level of the settlements.
Across the 337 surveyed dairy farms, Reichenbach et al. (2021a) identified four DPS based on their spatial distribution within Bengaluru’s RUI, their feeding strategy, and herd management (Table 1). The first was a DPS found along the whole RUI of Bengaluru and named as ExtDPS because of the dairy producers’ minimum expenditure in labor and capital. In ExtDPS, dairy cattle were sent to pasture and fed forages that were not produced on the dairy farm: Some forages were bought but were more often collected from public grounds or markets such as discarded fruits or vegetables, further referred to as organic wastes. Most dairy producers in ExtDPS kept herds with exotic cattle next to crossbreeds or native ones. The production strategy of ExtDPS was most obviously impacted by urbanization as their reliance on external resources, such as organic wastes and public grounds for pasture, was a direct adaption strategy to the dwindling availability of agricultural land to cultivate forages (Reichenbach et al., 2021a). Two semi-intensive DPS were found in rural areas; in both, dairy cattle were sent to pasture and fed forages produced on the dairy farm. However, these two DPS differed in their breeding strategy: in Semi-ADPS, dairy producers kept mostly exotic cattle and for replacement relied on dairy cattle born on farm. By opposition, in Semi-BDPS, most dairy producers kept mixed genotypes and had dynamic herd management with frequent buying, selling, or both, of dairy cattle. These two DPS were characterized as semi-intensive because of the dairy producers’ higher expenditure on labor and capital, i.e., forage cultivation and high-yielding genotypes. Located in rural areas was the most intensive DPS named IntDPS, in which dairy cattle were not sent to pasture but fed exclusively on-farm with self-cultivated forages. Most dairy producers in IntDPS kept herds with exotic cattle only. Dairy producers in this DPS therefore had the highest labor and capital expenditure of all four DPS. Located in the immediate rural periphery of the city, the latter three DPS benefited from the network of dairy cooperatives and improved rural–urban linkages throughout Greater Bengaluru: established to supply the demand of urban consumers, it provided rural dairy producers with easier access to markets and production inputs, notably concentrated feeds and exotic genotypes, thereby supporting an increase in dairy production (Reichenbach et al., 2021a).
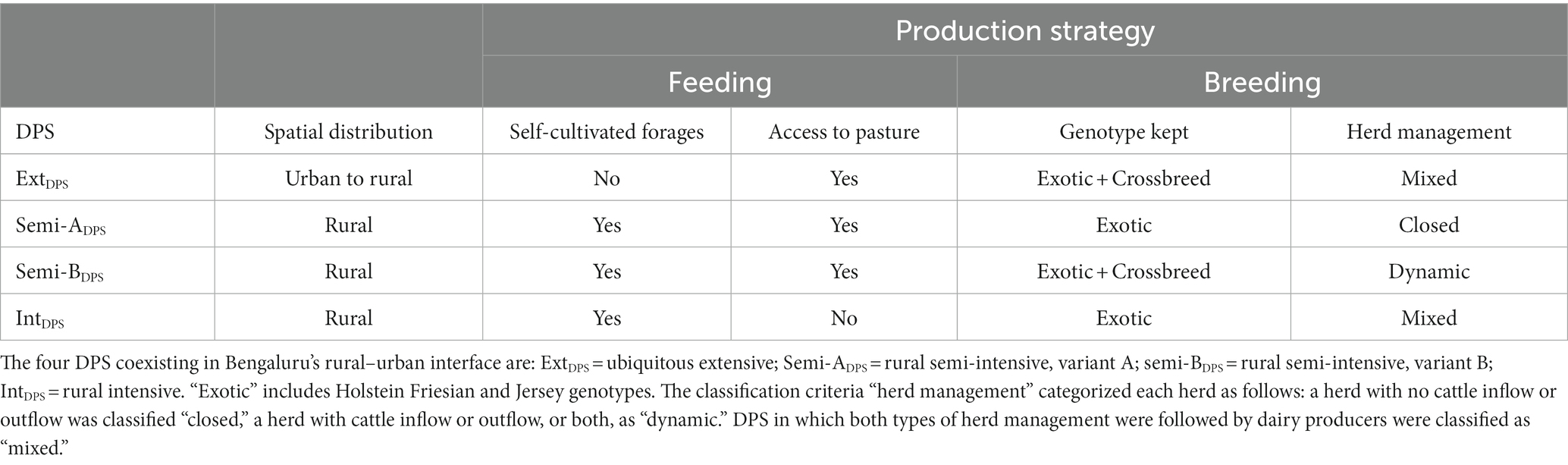
Table 1. Classification criteria and main characteristics of the four dairy production systems (DPS) within Bengaluru’s rural–urban interface.
2.2 Data collection
Twenty-eight dairy farms, seven per DPS, were selected from the 337 farms surveyed in Bengaluru’s RUI (Supplementary Table S1) and visited eight times at 6-week intervals over 1 year (June 2017 to May 2018). Figure 1 shows the location of the 17 settlements from which dairy farms were selected. Dairy cattle on each farm were categorized as either lactating cow, dry cow (not lactating, pregnant, or not pregnant), heifer (pregnant or inseminated at least once), young cattle, and calf (<3 months old). Non-productive cattle refers to all categories of cattle except lactating cows. Focusing on the feeding strategy of the dairy farm, the variables quantified at each visit for each dairy cattle followed Schlecht et al. (2019) and included 24 h feedstuff offer (considered equivalent to daily feed intake on the farm as refusals were often difficult to quantify due to feeding practices), pasture time, nutritional value of per-farm samples of all offered feedstuffs, concentrations of dry matter (DM), digestible organic matter determined in vitro (DOM) and therefrom derived metabolizable energy (ME; Menke et al., 1979), crude protein (CP), neutral detergent fiber (NDF) and acid detergent fiber (ADF); analysis of 321 representative feed samples (see Supplementary Table S2 for average quality of feedstuffs), daily milk production (kg milk cow−1 day−1 = daily milk offtake + correction for direct calf consumption based on indirect calf consumption records; Reichenbach et al., 2021b), fat and protein content of milk (Lactoscan Milk Analyzer, Softrosys Technologies, Bengaluru, India), and heart girth of the animal (HG; in cm). Gross energy intake (GE) was calculated from ME intake according to Hales (2019). Feed intake at pasture was estimated from actual grazing time manually observed on pasture and an intake rate of 8 g DM per kg metabolic weight and hour (Ayantunde et al., 2002; Reichenbach et al., 2021b). DOM of pasture biomass was based on the analysis of 32 representative samples of non-cultivated grasses (collected by dairy producers from public grounds for on-farm feeding) in urban areas where cows had also access to organic household or market wastes dumped in the streets and three representative samples of vegetables and fruit mixes. Individual body weight (BW), metabolic weight, and daily weight gain (Δ kg BW day−1), were calculated based on an HG to BW regression (kg BW = 3.867 + 2.98 × log10 (HG); 569 weight records; R2 = 0.9846; Grund, 2018; see Supplementary Table S3 for body weight and daily weight gain values). The date of last calving was asked for each cow at the first visit and new calving dates were recorded during the monitoring year. Dry period and lactation duration were calculated as averages for each DPS.
Using the same dataset, Reichenbach et al. (2021b) had calculated ME and CP supply levels (undersupplied, adequately supplied, and oversupplied) for lactating cows, dry cows, and heifers. The supply levels of ME and CP were comparable and followed a similar pattern, i.e., lactating cows, dry cows, and heifers in ExtDPS were adequately supplied on average, but there was a wide variation from animal to animal. In contrast, there was adequate to oversupply in the three other DPS. Interestingly, regardless of DPS, lactating cows were on average adequately supplied, while dry cows and heifers were almost systematically oversupplied.
2.3 Computation of livestock-related greenhouse gas emissions
Following the IPCC (2006) guidelines and a Tier 2 approach, livestock-related GHG emissions per unit of milk were calculated for each dairy farm in CO2-eq of CH4 (28 kg CO2-eq kg−1 CH4) from EF. To account for the herd management strategy of each DPS, the calculated emission intensity included the CH4 emissions due to enteric fermentation of all lactating cows and non-productive cattle, and therefore, consolidated at the farm level, all emissions were first calculated per cattle based on its diet. Our calculations were based on 855 nutritional and 478 production records from 147 dairy cattle. The emission intensity factor related to enteric fermentation was calculated based on the IPCC equation 10.21 using the standard methane conversion factor Ym = 6.5%. The functional unit to which all emissions were allocated was 1 kg of energy-corrected milk (ECM; 1 kg ECM = kg milk × (0.25 + 0.122 fat % + 0.077 protein %); Sjaunja et al., 1990). CH4 emissions, milk production, and intensity of enteric methane emissions per unit of milk were first calculated per visit, with each visit representing a 42-day cycle. Multiple visits per dairy farm during the same season (from one visit to a maximum of four) were considered as repeated measurements (nindividual = 214; nrepeated = 82). CH4 emissions, milk production, and intensity of enteric methane emissions in CO2-eq per unit of milk were then calculated per year (n = 24); four dairy farms, one per DPS, were excluded because they stopped dairy production during the monitoring year.
2.4 Statistical analysis of results
All variables (Y) were analyzed using linear mixed-effects models (lmer) followed by post hoc pairwise comparison (Bonferroni adjustment) in R (RStudio version 4.2+). In the main model, dairy farm nested in season was included as a random effect to correct for repeated measurements: Yij = DPSi + farm:seasonj + eij.
Thereby, DPS comprises factors such as cattle genotype, herd management, feeding strategy, and location (Table 1; see Reichenbach et al., 2021a for details). Results are presented as arithmetic mean and standard deviation (±), unless specified otherwise. Significance was declared at a p-value of <0.05 for all analyses. As the number of dairy cattle varied within and between farms at each visit and thus season, cumulative numbers per DPS and visit are presented in Supplementary Table S4.
3 Results
3.1 Characteristics of dairy farms
A dairy herd within Bengaluru’s RUI typically included 2 ± 1.2 lactating cows (n = 28). The number of non-productive dairy cattle, however, differed between DPS, with dairy producers in Semi-BDPS having larger herds than any other DPS (3 ± 2.0 additional non-productive dairy cattle; p < 0.05; Table 2). Lactating cows in ExtDPS had an intermediate average milk yield and protein content (9.4 kg ECM cow−1 day−1 with 3.10% protein) and were milked longer (414 days) than cows in any other DPS (Table 2). The most productive cows were found in Semi-BDPS (10.0 kg ECM cow−1 day−1 on average) and in IntDPS (10.1 kg ECM cow−1 day−1 on average; lactation length of 356 days for both DPS), with the latter also having a higher milk protein content (3.12%). Milk yield in Semi-ADPS was the lowest (7.9 kg ECM cow−1 day−1 on average; p < 0.05), with a protein content of 3.02% and a lactation length of 359 days. Yearly milk production per farm averaged 5,684 kg ECM in Semi-ADPS, 6,353 kg ECM in ExtDPS, 9,399 kg ECM in IntDPS, and 10,079 kg ECM in Semi-BDPS (Table 2).
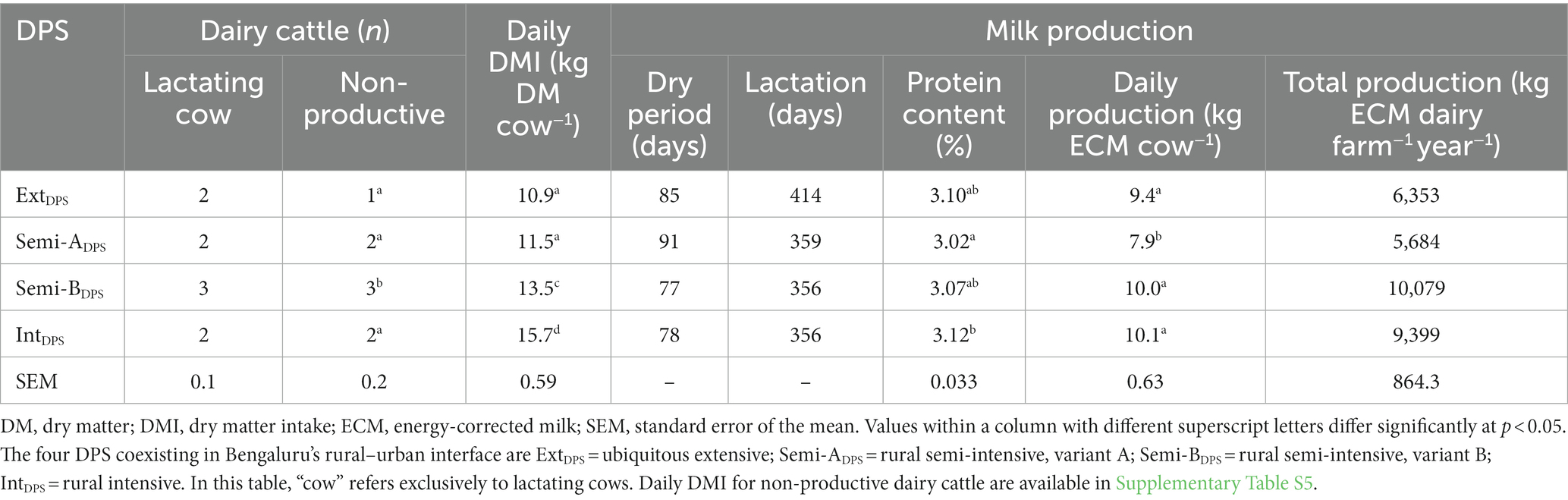
Table 2. Main characteristics of dairy farms (n = 28) per dairy production system (DPS) within Bengaluru’s rural–urban interface.
3.2 Quality of feedstuffs and diets
Common green forages (27% DM) fed in Bengaluru’s dairy farms included African tall maize (Zea mays L.), hybrid Napier grass (Pennisetum glaucum × P. purpureum), and a wide range of wild (non-cultivated) grasses collected from public grounds and, in urban and peri-urban areas, from the surroundings of Bengaluru’s numerous lakes. Green forages had a DOM of 59% and contained 16.7 MJ GE kg−1 DM. On average, green forage contributed to more than half of the daily dry matter intake (DMI) of dairy cattle during the monsoon season (Figure 2). However, the availability of green forages decreased during winter and especially in the dry season, leading to an increased dietary share of dry forages (90% DM) during these two seasons. Dry forages were commonly straw of finger millet (Eleusine coracanal L., locally referred to as ragi) and occasionally dried maize (straw without cobs). Dry forages were rich in fiber (708 g NDF kg−1 DM). At 54%, DOM of dry forages was therefore the lowest of all types of feedstuffs, and their average GE content was 16.5 MJ kg−1 DM. Organic wastes (21% DM) – carrot tops, vegetable or fruit wastes from markets, fields, or neighbors – were fed mostly by dairy producers in ExtDPS and of good nutritional value (68% DOM, 17.3 MJ GE kg−1 DM). Dairy producers also used a large variety of commercial concentrate feeds (89% DM): wheat flour, with or without bran, corn flour, pelleted commercial feed, chickpea husks (Cicer arietinum L., referred to as Bengal gram), and groundnut cake. They offered these concentrate feeds individually or mixed several ones and usually added salt or a commercial mineral mixture. Overall, concentrate feeds had the highest DOM (77%) and GE content (17.6 MJ kg−1 DM) among all types of feedstuffs. The contribution of concentrate feeds to daily DMI of dairy cattle was constant across seasons (Figure 2). Generally, lactating cows received the highest share of concentrate feeds, followed by dry cows and heifers (Supplementary Table S5). The nutritional value of biomass ingested during pasturing was similar to the one of green forages (58% DOM, 16.8 MJ GE kg−1 DM). However, the nutritional value of biomass ingested during pasturing in urban areas was higher than in peri-urban and rural areas (68% DOM, 17.3 MJ GE kg−1 DM) because it also included organic wastes, to which cows had access in the streets. During the dry season, there was a small but significant drop in the contribution of pasture biomass to daily DMI (Figure 2).
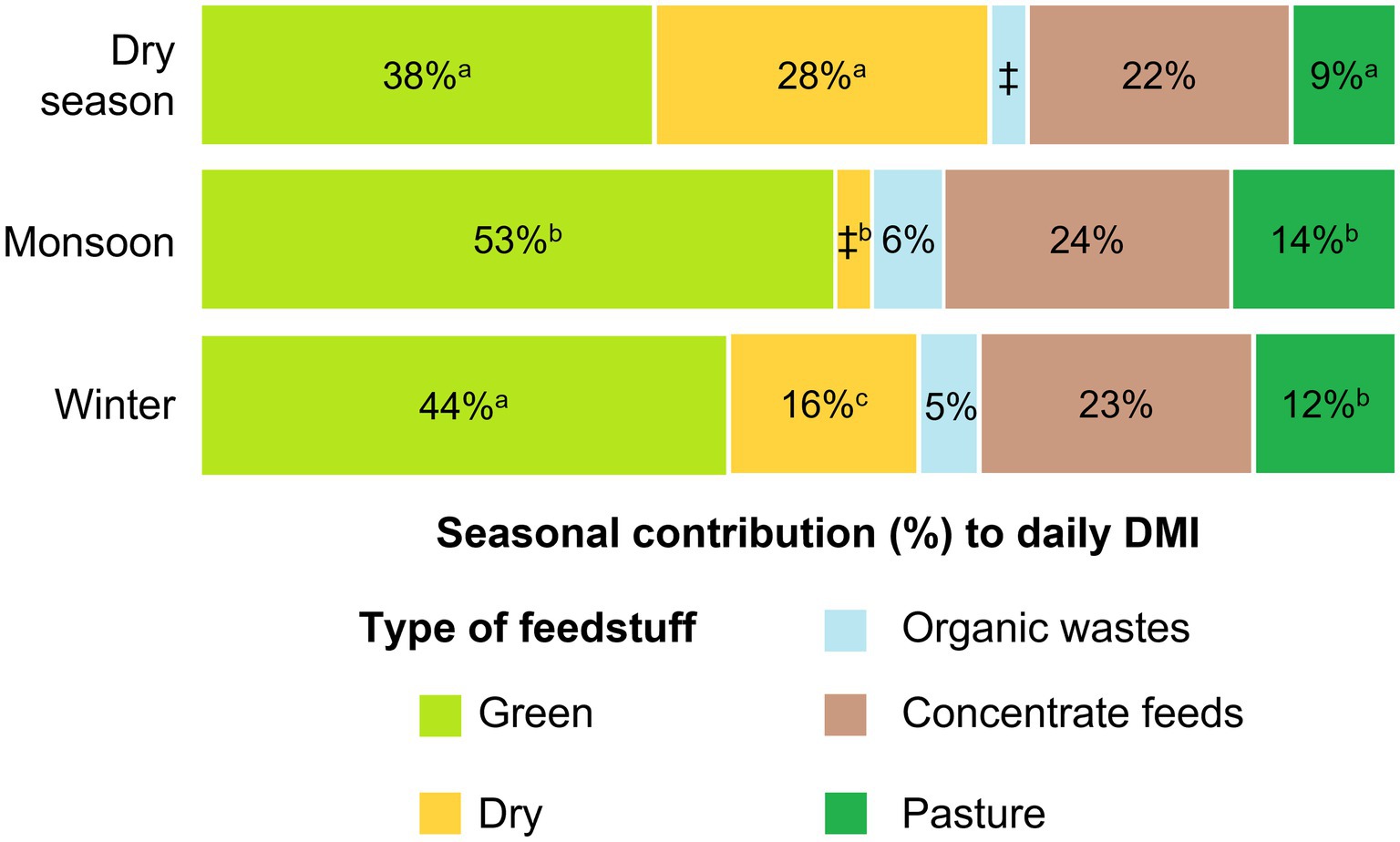
Figure 2. Seasonal contribution (%) of each type of feedstuff to total daily dry matter intake (DMI) of all dairy cattle from dairy farms located within Bengaluru’s rural–urban interface. Percentage per feedstuff with different superscript letters differ significantly across seasons. ‡ = 3%.
Despite differences between dairy producers in the use of feedstuffs and seasonal variation in the contribution of different types of feedstuffs to total daily DMI, there were few differences in average diet DOM (Table 3). Season affected the average diet DOM of ExtDPS, which was lower in the winter than in the dry season (p < 0.05). During the dry season and the monsoon, the average diet DOM of ExtDPS was also higher than in any other DPS (p < 0.05). Details on diet quality (weighted average DOM), feedstuffs’ contribution (%) to DMI and daily DMI (kg DM cattle−1 day−1) per category of dairy cattle and DPS are given in Supplementary Table S5.
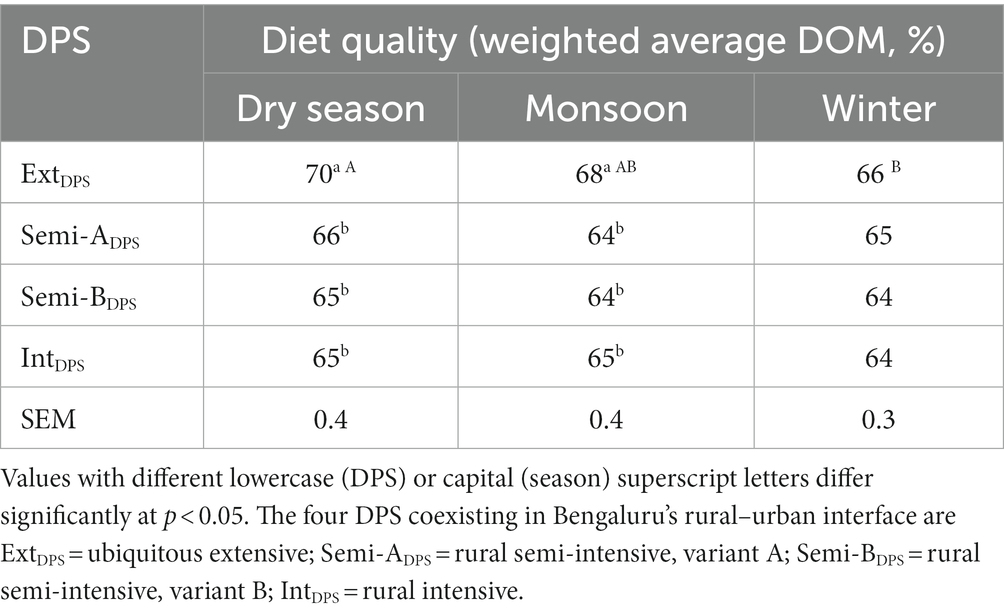
Table 3. Seasonal variation in diet quality based on the weighted average digestible organic matter (DOM, %) per dairy production system (DPS) within Bengaluru’s rural–urban interface.
3.3 Livestock-related emissions of dairy production systems
3.3.1 Enteric methane emissions
The amount of enteric methane that was released per dairy farm over a 42-day cycle was impacted by DPS: at all times, emissions were high in Semi-BDPS and low in ExtDPS as well as Semi-ADPS, while intermediate values were obtained for IntDPS (Table 4).
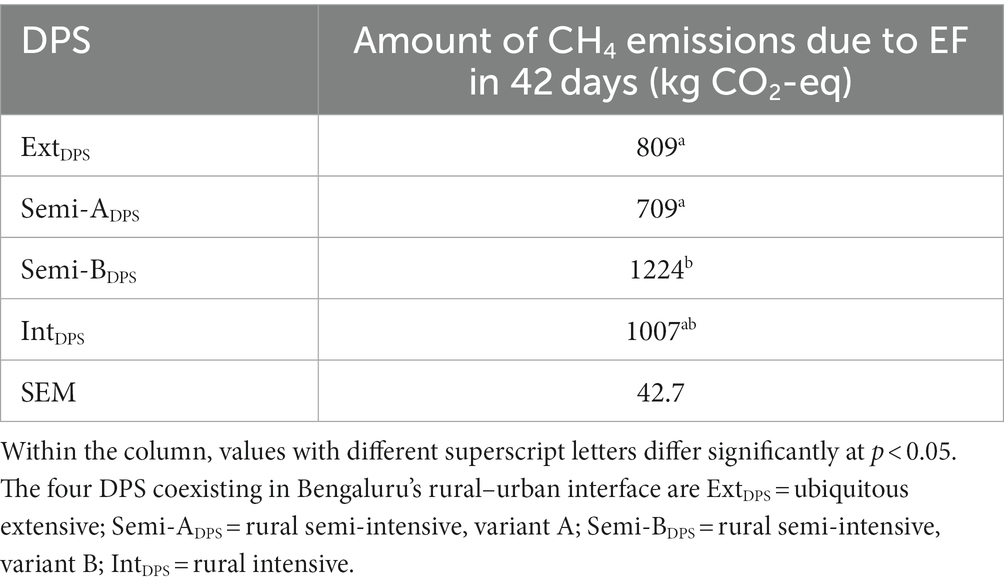
Table 4. Amount of methane (CH4) emitted due to enteric fermentation (EF) expressed in carbon dioxide equivalents (kg CO2-eq) over a 6-week period (42-day cycle) for each dairy production system (DPS) in Bengaluru’s rural–urban interface.
3.3.2 Intensity of enteric methane emissions per unit of milk and per DPS
Despite variations within and between DPS (Figure 3), there was no significant difference in intensity of enteric methane emissions per unit of milk over a 42-day cycle milk due to the large variation within all DPS except Semi-ADPS. Interestingly, there was a trend of Semi-BDPS to (negatively) impact emission intensity (p = 0.06).
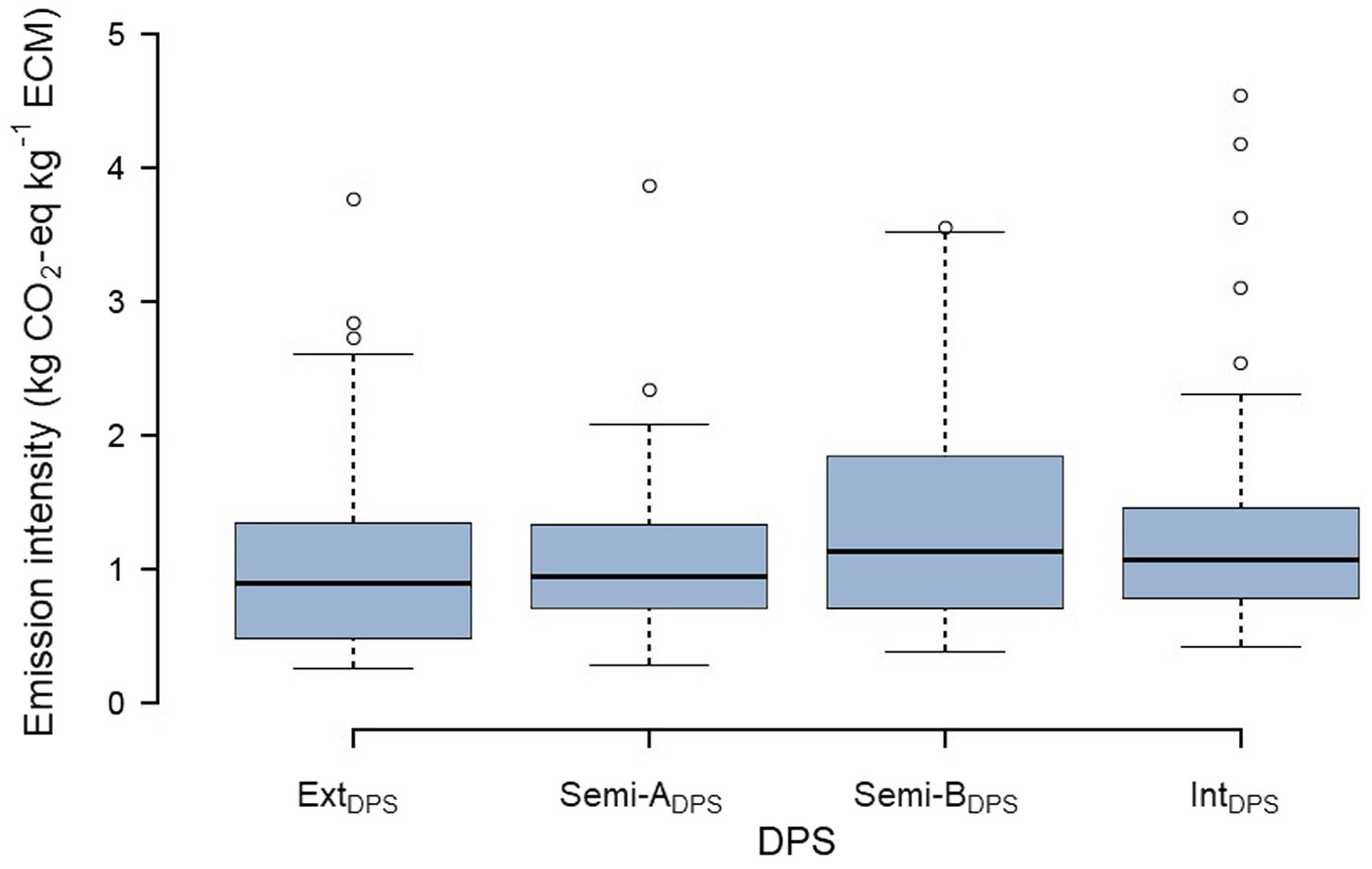
Figure 3. Intensity of enteric methane emissions, expressed as carbon dioxide equivalents (CO2-eq) per kg of energy corrected milk (ECM), during a 6-week period per dairy production system (DPS) within Bengaluru’s rural–urban interface. The four DPS coexisting in Bengaluru’s rural–urban interface are ExtDPS = ubiquitous extensive; Semi-ADPS = rural semi-intensive, variant A; Semi-BDPS = rural semi-intensive, variant B; IntDPS = rural intensive.
The yearly intensity of enteric methane emissions confirmed this tendency, with Semi-BDPS having a significantly higher intensity of enteric methane emissions per unit of milk (1.38 kg CO2-eq kg−1 ECM) than ExtDPS (0.79 kg CO2-eq kg−1 ECM; p < 0.05; Figure 4). Intermediate intensities were calculated for Semi-ADPS (1.04 kg CO2-eq kg−1 ECM) and IntDPS (1.21 kg CO2-eq kg−1 ECM).
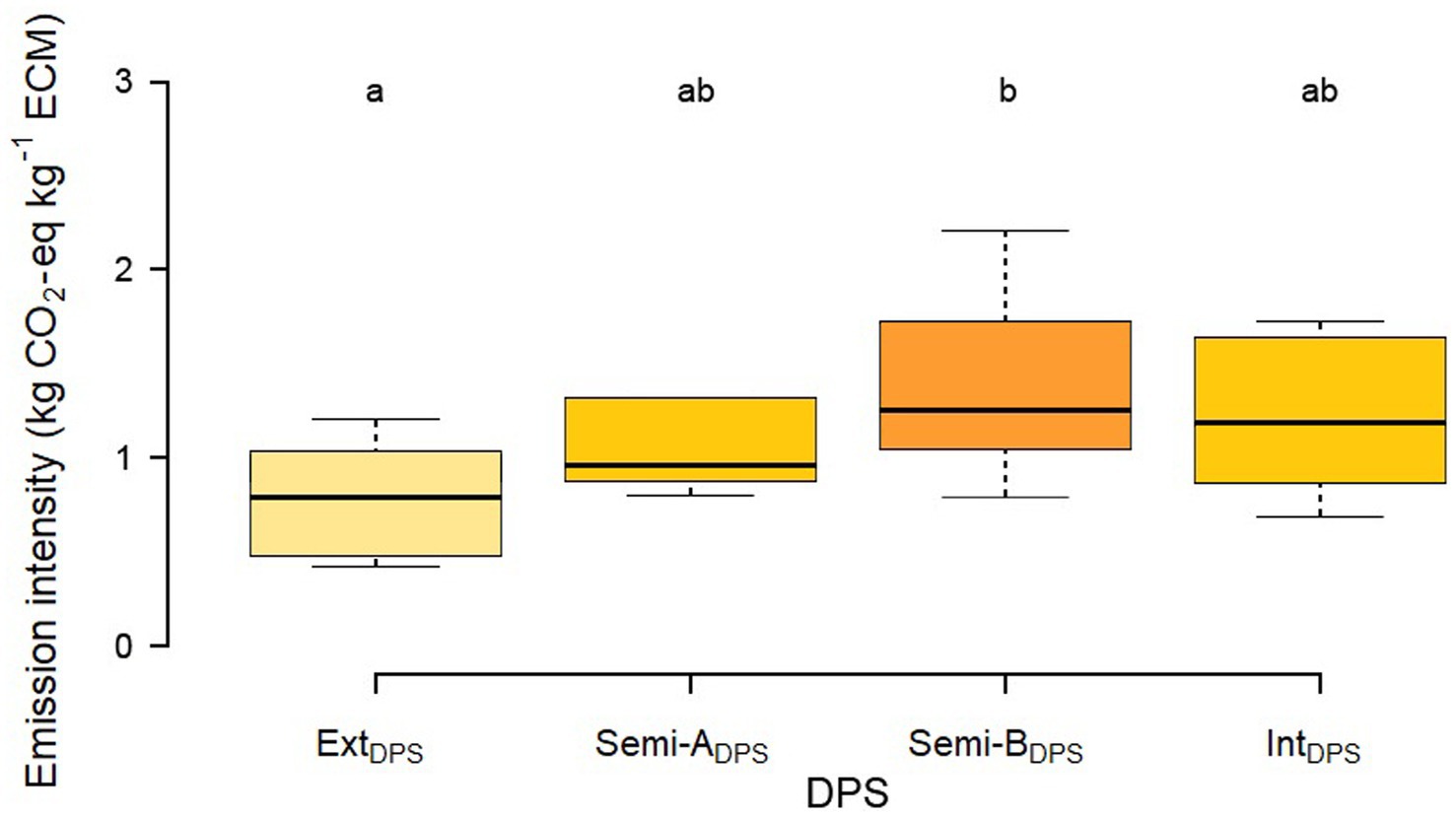
Figure 4. Intensity of enteric methane emissions, expressed as carbon dioxide equivalents (CO2-eq) per kg of energy corrected milk (ECM) during 1-year period per dairy production system (DPS) within Bengaluru’s rural–urban interface. Boxplots with different superscript letters differ significantly across DPS. The four DPS coexisting in Bengaluru’s rural–urban interface are: ExtDPS = ubiquitous extensive; Semi-ADPS = rural semi-intensive, variant A; Semi-BDPS = rural semi-intensive, variant B; IntDPS = rural intensive.
4 Discussion
Quantifying the emission intensity of enteric methane per unit of milk from Bengaluru’s DPS provides interesting insights into its relationship with the production strategies chosen by dairy producers based on the resources available to them within the RUI of an emerging megacity. In particular, two findings are interesting: (a) during a 42-day cycle, variation within all DPS except Semi-ADPS was large, indicating potential for mitigation of emissions at the farm level; (b) on an annual basis, differences in emission intensities existed, but were relatively small, even non-existent, despite different production strategies.
The production strategies adopted by Bengaluru’s DPS were primarily based on feeding management, namely, reliance on self-cultivated forages or pasture use or both, implying that dairy producers not sending their cattle to pasture required more means of production (land and labor) for their activity, while the others took advantage of local resources available for free or at a cheap price (organic wastes, green forages from public grounds; Reichenbach et al., 2021a). Based on DOM data, feeding strategies across Bengaluru’s DPS can roughly be distinguished as follows: feeding a good quality diet and using pasture (ExtDPS), feeding an average quality diet and using pasture (Semi-ADPS), or oversupplying a diet of average quality, with or without using pasture (Semi-BDPS and IntDPS). The production strategy of ExtDPS resulted in the lowest intensity of enteric methane emissions per unit of milk for this most extensive DPS, which was extensive in its use of labor and capital (Reichenbach et al., 2021a), as well as conventional forages (Reichenbach et al., 2021b) but relied on atypical feedstuffs (banana peel, cabbage leaves, and field bean leaves) of good quality in addition to concentrate feeds, that were also used in all other DPS. Although energy and crude protein supply to the animals were variable and frequently implied undersupply of dairy cows, at system-level ExtDPS had the best feed conversion efficiency among Bengaluru’s four DPS (Reichenbach et al., 2021b). At the same time, ExtDPS was also relying the most on cheap but external resources. Whereas reliance on external inputs is a common characteristic of intensive DPS in high-income countries, in Bengaluru, reliance on external inputs was the result of a production strategy based on the highly flexible use of several locally available low-cost but quality feed resources by dairy producers as an adaptation to their urbanizing environment and the subsequent decrease of land and labor availability. In consequence, for Bengaluru’s ExtDPS, the reliance on external resources was not a limiting factor for milk production but an enabling one: without access to organic wastes or green forages on public grounds, dairy producers in ExtDPS would have to stop their activity. At this stage of knowledge, it is interesting to note that such a production strategy can exist as a complement to more “traditional” strategies to increase production without leading to too large a difference in emission intensity, and can even have a favorable outcome. Therefore, the consequences of such a strategy, for the longer-term economic sustainability or rather, vulnerability, of the DPS, the scalability of such a minimal expenditure strategy, as well as for its upstream emissions need to be further investigated.
The semi-intensive and intensive strategies of Semi-BDPS and IntDPS resulted in an oversupply of their cattle with feed (Reichenbach et al., 2021b), and their intensity of enteric methane emissions per unit of milk was thus crippled by “unnecessary” CH4 emissions. National emission intensities computed according to IPCC (2006) are usually based on estimated daily DMI derived from national dairy cattle requirements and average diet (quality). In view of feeding inefficiencies previously quantified in Bengaluru’s RUI (Reichenbach et al., 2021b), the environmental impact of Bengaluru’s DPS based on estimated DMI would potentially differ from the present computations that are largely based on quantified DMI (Goopy et al., 2018). This underlines the importance of detailed assessments of ruminant production systems in LMICs, which might emit less greenhouse gasses than projected by gross assessments based on literature (Goopy et al., 2018).
To a lesser extent, the production strategy of Bengaluru’s DPS also relied on breeding management, namely, the use of exotic genotypes and cattle inflow and outflow within the herd (Reichenbach et al., 2021a). Crossbreeding of local genotypes with high-yielding exotic genotypes is a common strategy in tropical countries to increase production (Widi et al., 2015), which potentially decreases emission intensity due to the general relationship between (increasing) production at the cow level and (decreasing) emission intensity as highlighted by Gerber et al. (2011). In the case of smallholder beef production in central Java, Indonesia, the global environmental impact of farms with crossbred animals was however similar to those with unimproved cattle: The productivity gain due to crossbreeding was offset by the additional emissions related to the additional feed resources needed to fatten the crossbreds (Widi et al., 2015). In the context of Bengaluru, no direct relationship between cattle genetics and intensity of enteric methane emissions could be detected between systems keeping mixed herds and those keeping mostly exotic cows. Inadequate feeding (Reichenbach et al., 2021b), indiscriminate crossbreeding, and heat stress are common in Bengaluru’s RUI (Pinto et al., 2020), thus potentially hindering the full expression of exotic genotypes’ production potential and limiting breeding as a strategy for production increase. Dairy producers in Semi-BDPS tended to buy and sell dairy cattle at a higher rate than producers in other DPS and kept a higher number of unproductive dairy cattle (Reichenbach et al., 2021a), which contributed to the large variation in emissions from one visit to the next and a higher intensity of enteric methane emissions.
5 Conclusion
Given the observed variability in feed availability, both quantitative and qualitative, across DPS, better feeding strategies are a promising mitigation option for Bengaluru DPS, i.e., providing feed in adequate quantity and quality according to the expected requirements of dairy cattle in a consistent manner (Hermansen and Kristensen, 2011). Targeted feeding would promote animal welfare and resource efficiency, reduce the intensity of enteric methane emissions, and improve the rearing of female offspring on the farm by reducing the age at first calving. Using the network of dairy cooperatives, information on feed quality and dairy cow requirements could be easily disseminated to a large number of smallholder dairy farmers. In the longer term, mitigation options should take a broader system approach, e.g., addressing low reproductive rate and genetic production potential in parallel. Bengaluru’s case study sheds light on DPS in a rapidly urbanizing environment with minor differences in emission intensities depending on the production strategy chosen by the dairy producers, which is largely based on the locally available resources. The study thus contributes to the still limited but growing body of literature on the environmental impacts of livestock production systems in urbanizing regions of India, with strong parallels to similarly transforming regions across the LMICs. Given that millions of small-scale producers in LMICs are suspected of contributing disproportionately to global dairy GHG emissions, this study highlights the importance of a careful local assessment of system-specific GHG emissions, as dairy farms could be more emission-efficient than assumed, even at intermediate levels of production and with the implementation of a limited set of strategies to increase production. The local perspective therefore also challenges the case for a unique pathway to emission mitigation in LMICs. Despite different production strategies, emission intensities varied little between DPS, highlighting that diverse production strategies can be environmentally friendly when based on locally available feed resources such as, in India, forages on public grounds or organic wastes, which can contribute to more sustainable livestock production in the context of circular food systems.
Data availability statement
All relevant data used to calculate the intensity of enteric methane emissions for different dairy production systems in Bengaluru are presented in detail in the article and the supplementary material; further data requests can be directed to the corresponding author.
Ethics statement
Ethical review and approval was not required for this animal study because all data were collected from dairy cows without directly disturbing them (monitoring feed offer and pasturing) and as part of the normal operation of the dairy farm, such as milk production. Only heart girth was measured with a tape directly on the cow, which caused little discomfort to the cow. Each dairy farmer’s oral consent to participate with his/her cows in our study was obtained before the start of the 1-year monitoring period and renewed at each visit. Oral consent was preferred to written consent because of the low literacy level of some dairy producers.
Author contributions
MR: conceptualization, investigation, formal analysis, writing – original draft, and writing – review and editing. AM: formal analysis, writing – original draft. AP: investigation. RB: conceptualization and funding acquisition. PKM: coordination of lab analyses and computations. SK: supervision. ES: conceptualization, funding acquisition, supervision, and writing – review and editing. All authors contributed to the article and approved the submitted version.
Funding
This work was supported by the German Research Foundation (DFG) through grant number SCHL 587/6-1 (ES) and by the Department of Biotechnology of India (DBT) through grant number BT/IN/German/DFG/14/BVCR/2016 (RB).
Acknowledgments
The authors deeply thank all the dairy producers and their families for their time and for repeatedly welcoming us during a whole year. The authors thank Hedwig Schröter and our research assistants in Bengaluru for their commitment and diligent field work. This research was conducted as part of a doctoral thesis by M. Reichenbach titled “Dairy production in an urbanizing environment. A system approach in Bengaluru, India” (doi: 10.17170/kobra-202010221984) within the project A03 of the DFG-funded Research Unit FOR2432/1.
Conflict of interest
The authors declare that the research was conducted in the absence of any commercial or financial relationships that could be construed as a potential conflict of interest.
Publisher’s note
All claims expressed in this article are solely those of the authors and do not necessarily represent those of their affiliated organizations, or those of the publisher, the editors and the reviewers. Any product that may be evaluated in this article, or claim that may be made by its manufacturer, is not guaranteed or endorsed by the publisher.
Supplementary material
The Supplementary material for this article can be found online at: https://www.frontiersin.org/articles/10.3389/fsufs.2023.1204218/full#supplementary-material
References
Ayantunde, A. A., Fernández-Rivera, S., Hiernaux, P. H., van Keulen, H., and Udo, H. M. (2002). Day and night grazing by cattle in the Sahel. J. Range Manag. 55, 144–149. doi: 10.2307/4003350
Burrow, H. (2012). Importance of adaptation and genotype × environment interactions in tropical beef breeding systems. Animal 6, 729–740. doi: 10.1017/S175173111200002X
D’Haene, E., and D’Haese, M. (2019). Milk in the city: profiles and development paths for urban dairy holders in Ethiopia. Dev. South. Afr. 37, 644–660. doi: 10.1080/0376835X.2019.1689099
Daburon, A., Alary, V., Ali, A., El Sorougy, M., and Tourrand, J.F., (2017). Urban farms under pressure: Cairo’s dairy producers, Egypt. Toward sustainable relations between agriculture and the city (Eds. C.-T. Soulard, C. Perrin, and É. Valette), Springer, Cham, Switzerland, 73–88
Dossa, L. H., Sangaré, M., Buerkert, A., and Schlecht, E. (2015). Intra-urban and peri-urban differences in cattle farming systems of Burkina Faso. Land Use Policy 48, 401–411. doi: 10.1016/j.landusepol.2015.06.031
Duncan, A. J., Teufel, N., Mekonnen, K., Singh, V. K., Bitew, A., and Gebremedhin, B. (2013). Dairy intensification in developing countries: effects of market quality on farm-level feeding and breeding practices. Animal 7, 2054–2062. doi: 10.1017/S1751731113001602
FAO (2020). Production systems. Rome, Italy: Food and Agricultural Organization of the United Nations. Available at: http://www.fao.org/dairy-production-products/production/production-systems/en/ (Accessed March 04, 2020).
Gerber, P.J., Steinfeld, H., Henderson, B., Mottet, A., and Opio, C., (2013). Tackling climate change through livestock: A global assessment of emissions and mitigation opportunities. Food and Agriculture Organization of the United Nations (FAO), Rome
Gerber, P. J., Vellinga, T., Opio, C., and Steinfeld, H. (2011). Productivity gains and greenhouse gas intensity in dairy systems. Livest. Sci. 139, 100–108. doi: 10.1016/j.livsci.2011.03.012
Goopy, J. P., Onyango, A. A., Dickhoefer, U., and Butterbach-Bahl, K. (2018). A new approach for improving emission factors for enteric methane emissions of cattle in smallholder systems of East Africa-results for Nyando, Western Kenya. Agric. Syst. 161, 72–80. doi: 10.1016/j.agsy.2017.12.004
Grund, J.-N. , (2018). Biometric characteristics of dairy cattle in Karnataka. Master’s thesis. Georg-August-Universität Göttingen, Germany
Hales, K. E. (2019). Relationships between digestible energy and metabolizable energy in current feedlot diets. Trans. Animal Sci. 3, 945–952. doi: 10.1093/tas/txz073
Hermansen, J. E., and Kristensen, T. (2011). Management options to reduce the carbon footprint of livestock products. Anim. Front. 1, 33–39. doi: 10.2527/af.2011-0008
Hoffmann, E., Jose, M., Nölke, N., and Möckel, T. (2017). Construction and use of a simple index of urbanisation in the rural–urban interface of Bangalore. India. Sustainability 9:2146. doi: 10.3390/su9112146
IPCC (Ed.) (2006). 2006 IPCC guidelines for national greenhouse gas inventories. Institute for Global Environmental Strategies, Hayama, Japan, 1 online resource, vol. 5.
Lukuyu, B. A., Ravichandran, T., Maass, B., and Laswai, G. (2015). Enhancing livestock productivity through feed and feeding interventions in India and Tanzania ILRI Project Report. Nairobi, Kenya: International Livestock Research Institute (ILRI).
Menke, K. H., Raab, L., Salewski, A., Steingass, H., Fritz, D., and Schneider, W. (1979). The estimation of the digestibility and metabolizable energy content of ruminant feedingstuffs from the gas production when they are incubated with rumen liquor in vitro. J. Agric. Sci. 93, 217–222.
National Dairy Development Board . (2015). Dairying in Karnataka: a statistical profile 2015. Anand, Gujarat, India. Available at: https://www.nddb.coop/sites/default/files/pdfs/NDDB-Karnataka_10-March-2015.pdf.
Ndung'u, P. W., Takahashi, T., du Toit, C. J. L., Robertson-Dean, M., Butterbach-Bahl, K., and McAuliffe, G. A. (2022). Farm-level emission intensities of smallholder cattle (Bos indicus; B. indicus–B. taurus crosses) production systems in highlands and semi-arid regions. Animal 16:100445. doi: 10.1016/j.animal.2021.100445
Pinto, A., Yin, T., Reichenbach, M., Bhatta, R., and Schlecht, E. (2020). Phenotypic dairy cattle trait expressions in dependency of social-ecological characteristics along rural–urban gradients. Sustainability 12:9021. doi: 10.3390/su12219021
Prasad, C. S., Anandan, S., Gowda, N. K. S., Schlecht, E., and Buerkert, A. (2019). Managing nutrient flows in Indian urban and peri-urban livestock systems. Nutr. Cycl. Agroecosyst. 115, 159–172. doi: 10.1007/s10705-018-9964-0
Reichenbach, M., Pinto, A., König, S., Bhatta, R., and Schlecht, E. (2021a). Dairy production in an urbanizing environment – typology and linkages in the megacity of Bengaluru, India. PLoS One 16:e0255791. doi: 10.1371/journal.pone.0255791
Reichenbach, M., Pinto, A., Malik, P. K., and Bhatta, R. (2021b). Dairy feed efficiency and urbanization – a system approach in the rural-urban interface of Bengaluru, India. Livest. Sci. 253:104718. doi: 10.1016/j.livsci.2021.104718
Roessler, R., Mpouam, S., Muchemwa, T., and Schlecht, E. (2016). Emerging development pathways of urban livestock production in rapidly growing West African cities. Sustainability 8:1199. doi: 10.3390/su8111199
Satterthwaite, D., McGranahan, G., and Tacoli, C. (2010). Urbanization and its implications for food and farming. Phil. Trans. R. Soc. Biol. Sci. 365, 2809–2820. doi: 10.1098/rstb.2010.0136
Schlecht, E., Plagemann, J., Mpouam, S. E., Sanon, H. O., and Sangaré, M. (2019). Input and output of nutrients and energy in urban and peri-urban livestock holdings of Ouagadougou. Burkina Faso. Nutrient Cycl. Agroecosyst. 115, 201–230. doi: 10.1007/s10705-019-09996-x
Sjaunja, L. O., Baevre, L., Junkkarinen, L., Pedersen, J., and Setäla, J. (Eds.) (1990). A nordic proposal for an energy corrected milk (ECM) formula: 27th Session of the International Committee for Recording and Productivity of Milk Animals. Paris, France: ICRPM.
United Nations , (2018a). Map: Growth rate of urban agglomerations by size class. United Nations, Department of Economic and Social Affairs Population Division. Available at: https://population.un.org/wup/Maps/ (accessed 5th March 2020)
United Nations , (2018b). The World’s cities in 2018: Data booklet. United Nations, Department of Economic and Social Affairs, Population Division, 34
West, J. W. (2003). Effects of heat-stress on production in dairy cattle. J. Dairy Sci. 86, 2131–2144. doi: 10.3168/jds.S0022-0302(03)73803-X
Widi, T. S. M., Udo, H. M. J., Oldenbroek, K., and Budisatria, I. G. S. (2015). Is cross-breeding of cattle beneficial for the environment? The case of mixed farming systems in Central Java, Indonesia. Animal Gen. Resources 57, 1–13. doi: 10.1017/S2078633615000259
Keywords: cattle, emission intensity, greenhouse gas, India, urbanization
Citation: Reichenbach M, Mech A, Pinto A, Malik PK, Bhatta R, König S and Schlecht E (2024) Differences in enteric methane emissions across four dairy production systems in the urbanizing environment of an Indian megacity. Front. Sustain. Food Syst. 7:1204218. doi: 10.3389/fsufs.2023.1204218
Edited by:
Arif Reza, Stony Brook University, United StatesReviewed by:
Ashish Kumar Das, Jashore University of Science and Technology, BangladeshUmair Ali Toor, King Abdullah University of Science and Technology, Saudi Arabia
Copyright © 2024 Reichenbach, Mech, Pinto, Malik, Bhatta, König and Schlecht. This is an open-access article distributed under the terms of the Creative Commons Attribution License (CC BY). The use, distribution or reproduction in other forums is permitted, provided the original author(s) and the copyright owner(s) are credited and that the original publication in this journal is cited, in accordance with accepted academic practice. No use, distribution or reproduction is permitted which does not comply with these terms.
*Correspondence: Marion Reichenbach, dHJvcGFuaW1hbHNAdW5pLWthc3NlbC5kZQ==