Corrigendum: Food security under compound shocks: Can Lebanon produce its own Mediterranean food basket?
- 1Texas A&M Energy Institute, Texas A&M University, College Station, TX, United States
- 2Department of Biological and Agricultural Engineering, Texas A&M University, College Station, TX, United States
- 3Institute for Science, Technology, and Public Policy, Bush School of Government and Public Service, College Station, TX, United States
- 4Faculty of Agricultural and Food Sciences, American University of Beirut, Beirut, Lebanon
- 5Agriculture and Agri-Food Canada, Lethbridge Research and Development Centre, Lethbridge, AB, Canada
- 6Land and Water Division, Food and Agriculture Organization of the United Nations (FAO), Rome, Italy
- 7Zachry Department of Civil Engineering, Texas A&M University, College Station, TX, United States
As Lebanon faces compound challenges, a looming food security crisis is rapidly approaching, much of which could be attributed to the lack of long-term planning for sustainability in its agricultural sector. The disconnect between decision-makers within the agricultural sector, and other interconnected sectors is exacerbated by the lack of integrative national platforms and methodologies for quantifying the trade-offs associated with possible interventions. This study aims to: (1) identify and quantify the critical interconnections between water, energy, nutrition, and food systems in Lebanon; (2) develop a framework to quantify the trade-offs associated with adopting interventions within current water, energy, and agriculture portfolios and practices; (3) evaluate producers' perceptions toward their willingness to implement proposed changes in crop production, renewable energy, and water reuse. Findings show that investing in locally producing Lebanon's needs of broad beans, lentils, chickpeas, and peas, results in cost savings, increased nutritional value in the locally produced basket, and reduced reliance on foreign markets. In turn, this comes at additional water, energy, land and carbon footprints which needs to be accounted for. Given the uncertainty of future currency conversion rates, it becomes more critical to identify a strategic food basket that could be produced locally to reduce reliance on imports. Conclusions from this study can play a role in informing policymaking and planning in Lebanon, which could be adapted and replicated in other countries in the MENA Region.
1. Introduction
Water, energy, and food securities are tightly interconnected, and have direct implications on human health and wellbeing (Mohtar and Daher, 2012, 2016; Giampietro et al., 2013; Howells et al., 2013; FAO, 2014; Liu et al., 2017). Addressing the challenges facing these resource systems needs to be grounded in an understanding of their interconnections, which need to be reflected in the way they are managed (Daher and Mohtar, 2015). Trade-off analysis tools can play a critical role in catalyzing cross-sectoral dialogue between the stakeholders who regulate, manage, and consume these resource systems (Daher et al., 2019). Such dialogue enhances the processes of integrative planning, supporting the implementation of the United Nations Sustainable Development Goals.
The EAT-Lancet Commission, in its effort toward balanced nutritious diets and sustainable food systems, has proposed a list of recommendations for healthy diets. It suggests substantial dietary shifts where the global consumption of fruits, vegetables, nuts and legumes will have to almost double, and consumption of foods such as red meat and sugar will have to be reduced by more than 50% (Willett et al., 2019). The Mediterranean Diet (MD), rich in plant-based foods and with fewer animal source foods aligns with the EAT-Lancet diet recommendations, and confers both human health and environmental benefits (Naja et al., 2011, 2012, 2013, 2018; Hwalla, 2015). Naja et al. (2018), reported that one of the two main dietary patterns in Lebanon, identified as the Lebanese-Mediterranean pattern, had a lower water use and greenhouse gas emissions (GHG) associated with it compared to the “Western” pattern which is the other main dietary pattern in the country. A study by Vanham et al. (2021) compared the water footprint of the MD and the EAT-Lancet diet (Willett et al., 2019) in nine countries around the Mediterranean accounting for the food intake requirements per gender and age. They reported that the EAT-Lancet diet and the MD (MEDIT as described in Bach-Faig et al., 2011) reduce the water footprint by 17–48 and 4–35%, respectively compared to the reference, which was defined as the current dietary intake based on FAO data. An 11% reduction in GHG production was also reported by Batlle-Bayer et al. (2019) when shifting the Spanish current eating habits to the MD. Vanham et al. (2021) noted that the EAT-Lancet diet is more optimized for human health and environmental indicators that the traditional MD diet. Similar to a developing trend in the Mediterranean region, Lebanon's diet is shifting away from a concentration of pulses, vegetables and fruits toward animal products (Markantonis et al., 2019) which ultimately increases the pressure on water resources.
The impact of relying heavily on plant-based diets differs between regions, which depends on their availability of water, energy and land resources. Given the scarcity of water and arable land in arid and semi-arid regions, over which different sectors compete, our research question asks about the sustainability of producing more of the plant-based Mediterranean diet. We also ask about the ways in which alternative water and energy sources could play a role in affecting the sustainability of this diet. A gap exists in frameworks and tool that provided a system-of-systems perspective that evaluate trade-offs for decisions made across water, energy, and food sectors in Lebanon. This study used a water-energy-food system-of-systems assessment to evaluate the sustainability of such a diet in a Mediterranean country, Lebanon, with specific aims to: (1) identify and quantify the critical interconnections between water, energy, and food systems in Lebanon; (2) develop a nexus framework to assess the trade-offs associated with adopting interventions within the current water, energy, and agriculture portfolios and practices; (3) evaluate farmer perceptions and willingness to implement proposed interventions. The study outcomes will inform policy and decision makers on issues such as sustainable development of the agricultural sector, energy and water subsidy and pricing, and import/export and trade policies. The framework will play an important role in catalyzing cross-sectoral dialogue for tradeoffs evaluation among stakeholders including farmers, rural communities, consumers, industry, and market/supply chains. The developed framework allows for scaling the analysis to broader scopes or other arid and semi-arid regional geographic areas.
2. Overview of the current water, energy, and agricultural status in Lebanon
Lebanon faces serious water scarcity issues that hamper its economic and social development. Building a sustainable economic and environmental future in Lebanon requires a paradigm shift that acknowledges the nexus between the water, energy, and food systems to simultaneously address prevailing water issues, food insecurity and natural hazards. Lebanon faces various constraints such as access to water, energy, nutritious food, and health care. These gaps are expected to increase with continuing demographic and climate change. The highly interlinked resources systems carry high risks and great vulnerabilities. Ensuring food security is a national priority which should be approached through a multi-sectoral lens, which branches beyond the agricultural sector, since unilateral, thematic-based, disciplinary approaches have failed to address the deep environmental and societal issues that are currently being faced.
Lebanon is considered to be in a relatively favorable position as far as rainfall and water resources (FAO, 2008), however, Lebanon's amount of renewable water has significantly dropped from more than 1,000 cubic meters/year/person to around 700 cubic meters/year/person (Machayekhi et al., 2017). The total cultivated land area in Lebanon is about 231,000 ha (Agriculture Census, 2010); the Beqaa Valley represents 42% of the agricultural areas in Lebanon (Machayekhi et al., 2017) where a multiplicity of grains, potatoes, stone fruits, vegetables, grapevine, and feed crops are grown (Haydamous and El Hajj, 2016). The Akkar and North Lebanon constitute 26% of the cultivated area growing cereal crops, pulses, vegetables and fruit trees including olives. South Lebanon constitutes about 22% of the agricultural area producing citrus, olives, bananas, cereals, and industrial crops such as tobacco. Mount Lebanon, covering about 9% of the cultivated area focuses on vegetable production especially under greenhouses in the coastal areas, and fruit trees in the mountains. Most farms in Lebanon are small farm holdings that do not exceed 1 hectare. The concentration of agricultural activity in the Beqaa and Akkar imposes a high demand for water, energy, and land resources. Overall, 60% of water in Lebanon is directed to agriculture; agriculture in the Beqaa consumes 86% of Beqaa's available water resources (rivers, springs, and underground aquifers), and in Akkar with over 45% of its cultivated land being irrigated, uses most of its available water resources from rivers, springs and groundwater (World Bank, 2003; MoA, 2010; El Amine et al., 2018). Despite relying on some rivers in agricultural areas such as Litani, Al-Kabir or Al-Bared rivers, and 100 s of springs, farmers rely largely (~80%) on groundwater pumping through public and private wells. Nevertheless, water availability remains a primary challenge: the diminishing quantity and quality of water is a major stressor. Available water resources are threatened by decreasing precipitation, pollution, uncontrolled pumping, and wastewater seepage (El-Kareh et al., 2018).
As for energy, while the agriculture sector uses diesel oil for operations, such as pumping water, drying grains, supplying greenhouses and for traction vehicles, this energy use represents <9% of Lebanon's total energy demand (MoE/UNDP/GEF, 2016). The 2017 EDL (Electricité du Liban) rate of electricity generation was 15 TWh, 96% of which comes from fossil fuels, 3% from hydropower plants, and 0.35% from photovoltaic (PV) panels. Almost all the fuel for energy is imported; this places Lebanon at risk due to dependency on external primary energy resources. The energy for agricultural production is divided between diesel (70%) and gasoline (30%). Decisions related to the type of crops grown in Lebanon are the foundation for quantifying the interconnections across the resource systems considered. Current water and energy portfolios for agricultural production in Lebanon are considered.
3. Methodology
3.1. Choice of crops, water and energy options
The Mediterranean diet is rich in legumes, vegetables, nuts, and fruits, many of which have beneficial health effects while having a smaller environmental footprint. The case study focuses on selected crops from the Mediterranean diet that may be produced in Lebanon. Some crops such as parsley or pine nuts, though part of the diet, were not included because their consumption and production are minor and detailed data about their production and trade is not available. A list of 33 crops including cereals (wheat and corn), vegetables (tomato, cucumber, zucchini, lettuce, potato, onion, garlic, pepper), fruits (apple, apricot, peaches, citrus fruits, banana, cherries, grapes, melon, watermelon, strawberries), nut trees (almond, walnut), olives, and pulses (peas, beans, chickpeas, lentils) that are commonly consumed in the Lebanese diet and consistent with EAT-Lancet recommendations (Willett et al., 2019) was developed for this study. The crops highlighted in red have low self-sufficiency i.e., they are being mostly imported; these crops have low irrigation requirements and high nutritional value (Figure 1). These crops, which are mostly pulses, are classified as staple Mediterranean crops that go into the making of many traditional dishes, in addition to wheat which is also considered a staple food. Figure 2 shows the crops that have low irrigation requirements, are high in protein and caloric value, and have potential to increase their acreage because they are produced on a small percentage of the agricultural land such as lentils, beans, almonds, and walnuts for example. Scatterplots for irrigation vs. calories, yield vs. protein content, and yield vs. calories showed a similar group of crops on low input-high nutritional value.
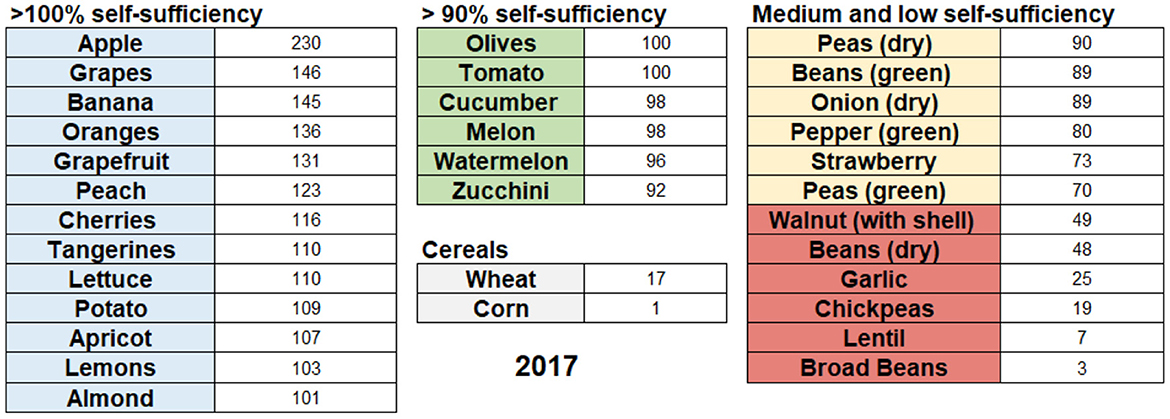
Figure 1. Lebanon's baseline (2017) self-sufficiency (SS) levels (%) for the selected crops; SS is the local production of a crop that satisfies the local Lebanese consumption (<100% means that a crop needs to be partially imported to meet market needs).
3.2. Overarching interconnections framework: Scenario inputs and outputs
A conceptual representation of the interconnections between water, energy, and food systems which are considered in this study is outlined in Figure 3. Decisions made within each of the resource systems, has an impact on others. Decisions related to the type of crops grown are the building foundation for quantifying the interconnections across the resource systems being considered. This section introduces the Water-Energy-Food (WEF) Nexus scenario evaluation structure. It outlines (1) scenario inputs and outputs, (2) a sample of the background data needs, (3) and method for evaluating stakeholder preferences.
3.2.1. Scenario inputs and outputs
The scenario inputs include the self-sufficiency ratios per crop (with 2017 ratios used as base scenario), water sources (ratios of water sources for irrigation which can be from groundwater, surface water, or treated wastewater), energy sources (ratios of energy sources including gasoline, diesel, wind and solar), currency conversion rate (ranging from official rate of 1USD = 1,500 LBP in 2017 to between 3,000 LBP and 10,000 LBP to the US Dollar). Evaluating these scenarios is done according to a list of outputs including irrigated water (m3, representing net irrigation needs), land requirement (ha, representing total land required to produce different ratios of selected crops locally), energy requirement (kJ, representing energy for pumping, treating, and conveying the different water sources Ew, in addition to energy for agricultural production including for harvesting, tillage, planting, and spraying Ea), cost (Lebanese pounds, representing the net cost of locally producing, importing, and exporting the identified list of food products), environmental impact (ton CO2, representing the emissions associated with different scenarios based on choice of energy sources), nutrition (kcal, grams protein, fats, carbs, fiber, and sugar representing the nutritional value of the locally produced crops), and a reliance index (an indicator representing the proportion of food imports in the nationally consumed basket). A detailed description and equations for the outlined inputs and outputs are provided in Appendix I.
3.2.2. A sample of local data needs
FAO databases, MoA census report, USDA Food Composition Database, local weather data, local crop water requirements and others were collected from the survey questions and other published work. Examples of this data can be found in Appendix II.
3.2.3. Evaluating stakeholder preferences: Farmers' survey
In addition to the tight interconnectedness between the physical resource systems, stakeholders, with different preferences and decision-making power, also interact. As scenarios are evaluated, these preferences will be critical in driving the multi-sectoral dialogue about future trade-offs. Given the emphasis of this study on evaluating decisions and practices made at the farm level, a survey was conducted with 200 farmers in the Beqaa Valley, in an effort to learn about their willingness to shift to different crops, alternative water sources, and alternative energy sources on their farms. We were also interested to learn about farmers' priorities to minimize water, energy, land, emissions, cost, and maximize nutritional value, as they made those decisions. Insights from evaluating these preferences could be used to predict the responsiveness to different scenarios and to inform policy incentives. The survey was approved by AUB's Internal Review Board (IRB). The survey could be found in Appendix III.
According to the 2010 Agriculture census, the total farmed area in the Beqaa is about 99,274 ha and the average size of holdings is 2.9 ha resulting in an estimate of 34,085 holdings or farmers. Assuming a response rate of 80–85%, the representative sample size was calculated to be 200–245 farmers, with confidence interval of 95 and 5% margin of error. Farmers selected to participate in the survey were chosen based on contacts that the researchers have from previous projects with the American University of Beirut (AUB) and the Advancing Research Enabling Communities Center (AREC). Snowballing was used as a method to identify other farmers in the Beqaa.
4. Results
4.1. Scenario evaluation
This section includes scenarios using the developed evaluation framework. The goal is to highlight the trade-offs associated with different scenarios as the water, energy, and agricultural portfolios are changed relative to 2017 which was selected as a base year.
4.1.1. Base year scenario 2017
Table 1 shows the self-sufficiency (SS) ratios of the selected crops, water sources, energy sources, and currency conversion rate in 2017.
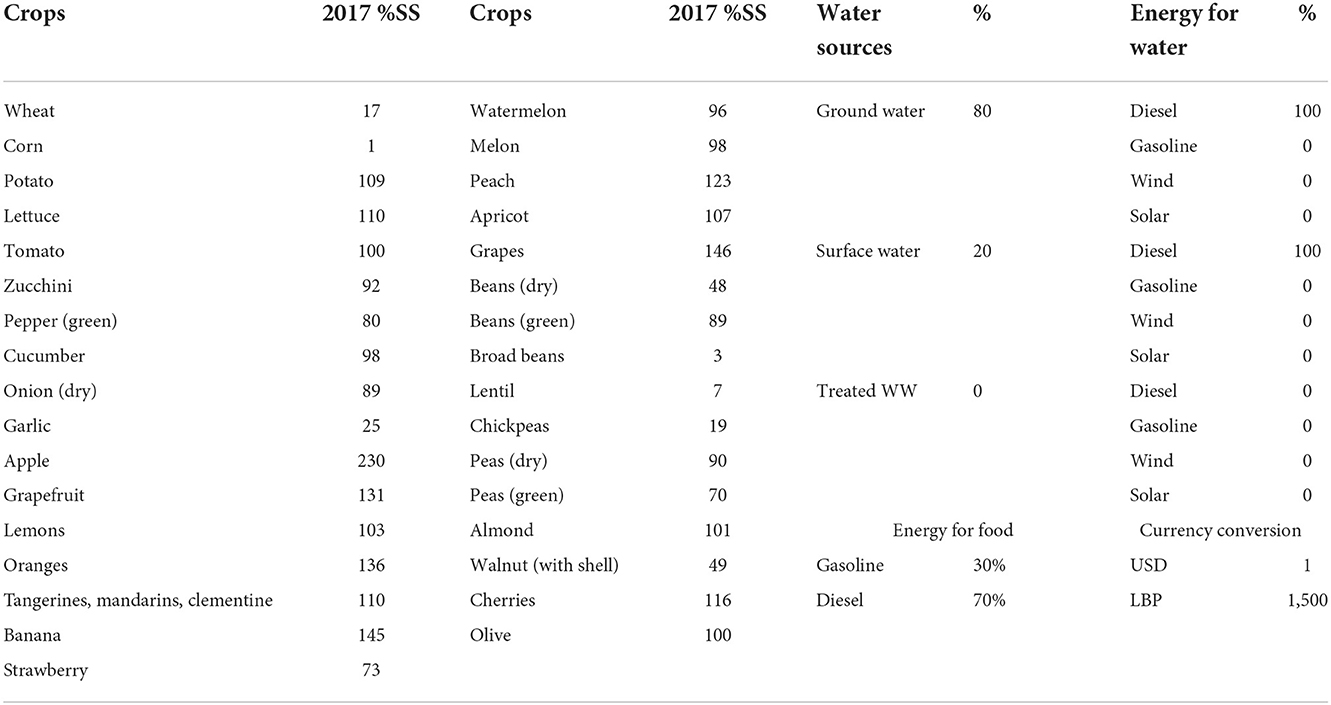
Table 1. The self-sufficiency ratios (%SS), water sources, energy sources, and currency conversion in 2017.
According to the developed evaluation framework, Table 2 outlines the outputs for the base case scenario. After establishing the different resource requirements and outputs for the base scenario, the following will explore the impact of making different interventions relative to this base year, by changing the self-sufficiency of different crops and changing water and energy sources under different currency conversion rates (Table 3).
4.1.2. Scenario A: Nutrition-centric
The current self-sufficiency of beans, chickpeas, and peas is low despite their high nutritional content and low irrigation needs. Therefore, this scenario explores the impact of increasing the self-sufficiency of these crops to 100%. The energy and water sources and ratios, as well as the currency conversion rates are kept as 2017 values. The analysis of this scenario shows that around 12% more water and energy, and 16% more land are required for achieving full self-sufficiency for beans, lentils, chickpeas, and peas (Figure 4). The arable land is estimated to be around 209,072 ha (LUC, 2017). This scenario exceeds the arable limit by 10%. This additional land requirement could be achieved by restoring degraded lands or unexploited lands, which does come at a cost that need to be further accounted for. Alternatively, improved management practices can have the potential for increasing productivity of already utilized lands and contributing to bridging this land gap. A 1.2% cost savings is noted. This is attributed to the difference in cost incurred for local production vs. import. Given that the main source of energy in the base scenario is diesel, this scenario produces 12% more emissions. Producing these crops also contributes to an increase of 6% in locally produced kcal of the overall consumed basket. Further, this also results in reducing the reliance on imports by 2.8%.
Key trade-offs: By investing in growing our need of beans, lentils, chickpeas, and peas locally, we see cost savings, increased nutritional value in the locally produced basket, and reduced reliance on foreign markets. In return, this comes at additional water, energy, land and carbon footprint which needs to be accounted for.
4.1.3. Scenario B1: Shifting from export to more local production
This scenario explores the possibility of reducing the local production of crops that currently exceed full self-sufficiency (SS > 100%) and are currently exported. This includes potatoes, lettuce, apple, grapefruit, citrus fruits, banana, grapes, apricots, peach, almonds, and cherries. Similar to Scenario A, Scenario B1 considers increasing the self-sufficiency of beans, lentils, chickpeas, and peas to 100%. Water and energy sources and ratios, as well as currency conversion are the same as 2017.
In this scenario, we notice a decrease in water and energy requirements, initially allocated to locally produced and exported crops in the base scenario. One of the key challenges of fully growing beans, lentils, chickpeas and peas is their low yield compared to other crops. Despite reducing the self-sufficiency of many of the currently produced and exported crops, this scenario still requires a 9.1% increase in land, compared to 2017 (Figure 5). Innovation in breeding to produce higher yielding varieties would improve these estimates. The reduction in energy requirement comes with a reduction in the carbon footprint. The increase in nutritional value is similar to that in scenario A but with a greater decrease in reliance on imports. This additional decrease compared to scenario A is attributed to limiting imports of some crops to 100% SS, which are also no longer exported according to this scenario. This analysis shows a 4% decrease in cost, this decrease is coming from replacing imported pulses by locally produced ones. This decrease in cost was slightly higher than the losses due to stop of export, hence the 4% decrease.
Key trade-offs: By reallocating resources from crops currently produced above the local full self-sufficiency and exported, into producing other low self-sufficiency, high nutrition, low resource intensive crops, we can have lower reliance on foreign markets while having water, energy, cost and emission savings. Given the low yield of such crops, the other key trade-off will be more land allocation for agriculture.
4.1.4. Scenario B2: Shifting from export to more local production under currency fluctuation
The difference between scenario B1 and B2 is in the currency conversion rate of the USD to the Lebanese Pound. This scenario explores a conversion rate of 1 USD = 4,000 LPB (compared to 1 USD = 1,500 LBP in 2017). To do this assessment, import costs and export revenues were calculated according to the new rate.
This scenario highlights the impact of the new currency conversion rate on the cost indicator. It is no surprise that given the 4,000/1,500 = 2.67-fold increase of the conversion rate there is an overall cost increase relative to 2017 (Figure 6). To do this assessment, import costs and export revenues were calculated according to the new rate. Given that many of the primary resources used on the farm are imported, it was assumed that the increase in local production costs will be equal to 50% of the increase between the 2017 and the new rate. Given the uncertainty of future currency conversion rates, it becomes more critical to identify a strategic food basket that could be produced locally, to reduce reliance on foreign markets.
Since 2019, the Lebanese Pound has lost more than 90% of its value, where 1 USD is being exchanged for 39,600 LP on the black market in 2022, compared to 1500 LP in 2019. The World Bank has described this financial crisis as one of the worst in world history (World Bank, 2022). Table 4 shows the percentage change in the demonstrated scenario cost under different LBP/USD black market conversion rates over the past years of the financial crisis. This situation makes it even more critical to develop an agricultural strategy that reduces reliance on foreign markets while improving food security and nutrition outcomes through evidence-based resource allocation.
Key trade-offs: The financial crises and the resulting currency fluctuation amplifies the trade-off between increasing the county's reliance on local production vs. import.
4.1.5. Scenario C: Scenario A + renewable energy + treated water + currency fluctuation
Scenario C explores the potential of diversifying the water and energy portfolios, as it builds on the main components of Scenario A above. This scenario includes shifting from using diesel as a main energy source for pumping water on farms to solar energy. It also explores the impact of using treated wastewater as part of the irrigation portfolio.
Similar to scenario A, this scenario requires additional water and land, and provides additional nutrition and reduced reliance. Through shifting to lower energy intensive water sources (surface water and treated water) this scenario shows around 13% energy savings (Figure 7). By shifting toward a greater use of solar energy for water pumping, conveyance, and treatment, 55% of emissions are reduced. Due to the new currency conversion rate, we again see a major increase of 94% in the net cost. Given the lower $/kwh of producing energy from solar compared to diesel, producing more locally becomes more competitive, compared to import. This assessment does not currently include initial investment costs for adopting these new water and energy portfolio options. Future development of this framework would expand the cost function of the tool to include initial investment costs.
Key trade-offs: Energy requirements, carbon emissions, and cost of local production could be reduced by shifting to less energy intensive water sources for irrigation and renewable energy, making local production more competitive compared to import.
4.1.6. Scenario D: Scenario B2 + renewable energy + treated water + currency fluctuation
Scenario D builds on Scenario B2 while diversifying water and energy portfolios under currency conversion change. Similar to the trends in scenario C, this scenario shows greater energy savings and emissions reduction, as well as water preservation by supplementing 20% of irrigation water from treated wastewater (Figure 8).
Key trade-offs: The key message lies in the potential of mitigating some of the negative trade-offs and improving resource savings by exploring new water and energy options for agriculture, making local production more competitive.
4.2. Survey findings about willingness to accept
In an effort to learn about farmers' willingness to shift to different crops, alternative water sources, and alternative energy sources, we conducted an in-person survey with 200 farmers in the Beqaa Valley. We were also interested to learn about farmers' priorities to minimize water, energy, land, emissions, cost, and maximize nutritional value, as they made those decisions.
The overall results from the surveys showed that:
• The average land size of the surveyed farmers was 5.8 ha.
• 38.5% of the farmers reported their main income being from agriculture
• Land ownership: 57% owned and invested, 37% owned by farmer, 3.5% invested
The ranking related to decisions on the willingness of farmers to use alternative energy, grow different crops or use alternative irrigation sort are shown in Figure 9 and it clearly shows that these shifts would be mainly driven by the profit increase before saving on energy or water resources. The least important driver to change for the farmers was the reduction of emissions and improving nutritional value of their diets/crops.
Asked to rank options or changes that the farmers are more likely to adopt also revealed that the main incentive for change is profit which, in the Beqaa, is usually manifested in the use of alternative energy sources since energy in farming is one of the most expensive inputs. Specifically, the results of the survey revealed that farmers will most likely: (1) Use alternative energy sources as priority, (2) Grow different agricultural products as a second option, and (3) Use alternative irrigation sources (treated wastewater). Selecting treated wastewater as the least likely change to adopt shows that although farmers are somewhat willing to adopt wastewater reuse as an irrigation source, they would still be more willing to change their energy source or grow different crops than convert to using treated wastewater. Such insights are valuable in evaluating the potential adoptability of a scenario. According to the survey, farmers indicated willingness to grow different crops motivated by profit (weight = 6), energy savings (weight = 5; En), land savings (weight = 4; L), water savings (weight = 3; W), emissions reduction (weight = 2; Em), nutritional value (weight = 1; N). This could be translated to the following:
A more favorable scenarios is one that minimize cost, energy, land, water, emissions, and maximizes nutrition which is reflected in the equation above. The higher the sustainability index, the more favorable a given scenario is for adoption, for the perspective of a specific stakeholder group.
If we take scenarios A and B1 from the farmer's perspective, for example,
Different stakeholder groups could have different sets of priorities and preferences which need to be accounted for. Given the developed trade-off evaluation for scenarios A and B1, combined with farmer preferences, Scenario B1 appears to be more favorable. This case might be different for a consumer vs. water provider vs. policy maker. One way of reflecting these diverse perspectives could be achieved though multi-stakeholder dialogue sessions, during which the analytics and preferences of different actors drive a dialogue about trade-offs associated with alternative pathways forward.
5. Discussions
The Mediterranean-style plant-based diet which is recommended from a nutritional and health perspective and shown to have a moderate environmental footprint (Naja et al., 2019), was assessed for sustainability of production in Lebanon. Resources that limit agricultural production in Lebanon are primarily water, followed by energy cost and land area. Although Lebanon produces some of the crops that constitute a Mediterranean diet, it still relies heavily on importing crops which are thought to be cheaper to import than to produce. However, the compound shocks that have face the county in the past few years, particularly its historic financial crisis, have reduced the country's ability to import food (Daher et al., 2021, 2022). That is in addition to disruptions in food supply chains resulting from the Russia-Ukraine war, two countries from which Lebanon imports 70–90% of its wheat, and secures over 25% of total calories through wheat-based products or sunflower oil (Ben Hassen and El Bilali, 2022; IFPRI, 2022). Shifting toward more local food production will have an impact on water, energy, and land resource systems (Mortada et al., 2018; Karnib and Alameh, 2020). Evaluating the impact of such decision requires a systems approach to quantify the different trade-offs and impact or resource systems that are associate with it. Building on this, we explored multiple scenarios that aim to produce more of the Mediterranean diet crops locally, which improve the nutrition of the locally produced food basket, and explore the use of alternative water and energy sources as available in Lebanon.
The framework and scenario analysis developed for this study shows that within the scope of the investigated food basket, Lebanon has the potential to be more food and nutrition secure by exploring beyond zero-sum game solutions, supported by the following three-tier approach: (1) reallocate within the existing resources pie, (2) expand the existing resources pie, and (3) create an environment that provides the necessary incentives for allowing the reallocation and expansion of the resources pie. First, by strategically reallocating resources from producing crops that exceed full self-sufficiency, to less resource intensive and nutrition rich crops with low self-sufficiency (beans, lentils, chickpeas, for example), we can have a lower reliance on foreign markets while having water, energy, cost, and emission savings. A trade-off exists between allocating resources to produce more food for local consumption vs. producing more for export. The uncertainty of future currency conversion rates amplifies the advantage of higher self-sufficiency as a key contributing factor to improved food security. Second, expanding the existing potential of resources could be done by improving efficiencies and management practices in current operations in one hand, and exploring synergies across different sectors in another. There is a need for expanding research for improving the yields and productivity of highly nutritious crops with low irrigation requirement by looking at better varieties, cropping patterns, technologies, and breeding. Intercropping or understory cropping systems could be used to minimize land use or restoration of marginal degraded land. That is in addition to improving irrigation efficiencies. This would be catalyzed by better metering and accounting for water use on farms. Investing in renewable energy expansion on farms and exploring the potential of treated water for agriculture, have the potential to improve the competitiveness of local production compared to import. Specializing in high-value cash crops for export can further play a role in expanding financial returns into the sector. Third, it is critical to create an environment with the necessary incentives for farmers to grow new crops, switch to alternative energy and water sources, invest in new more efficient technologies while accounting for their preferences and willingness to change practices on their farms. For any change to be adopted there needs to be a long-term agricultural strategy and policies set by the government, which will need to consider responding to trade risks and value chain disruptions (Al-Saidi and Hussein, 2021). Building private-public partnerships to support the investments needed could facilitate the adoption some of these solutions. It was obvious that farmers' interests and willingness to change are primarily driven by maximizing profit, followed by reduced energy use then water use. The least important driver for change from the farmers' perspective is improving environmental conditions and improving diet quality.
Questions remain about the interlinkages between agricultural production and any changes that occur therein, with other sectors that use the same resources. The feedback from farmers implies that there are avenues related to trade, import/export, markets, and general strategies that still need assessment. The framework and analytics developed in this study should be used to catalyze an evidence-based multi-stakeholder dialogue to guide policies and strategies in agriculture.
6. Conclusions
Given the uncertainty of future currency conversion rates, it becomes more critical to identify a strategic food basket that could be produced locally, to reduce reliance on foreign markets. Moving forward with WEF framework analysis, it is important to account for spatio-temporal distribution, soil suitability maps, and variability and its roles in making these trade-off decisions. This allows the development of this framework into a scalable tool that use customized WEF analytics to address questions at the country and regional levels. Using these analytics could play a role in engaging multi-stakeholders and catalyzing cross-sectoral dialogue around tradeoffs and future pathways and development strategies. This could be facilitated through engagement workshops geared at “gamifying” the developed analytics to drive that trade-off dialogue. Integrative agricultural strategies need to account for barriers to implementation that might results from existing farmer preferences. Understanding the preferences and perspectives of the broader group of cross-sectoral stakeholders would allow for a better evaluation of possible interventions and policy changes. On the technical side, improvement in the analytics can be made through the improvement of functions in the current evaluation including cost assessment of different scenarios which currently only look at the difference between the cost of local production and import on one hand and revenues from export on another. Further study and analysis of existing incentive structures and their impact on current farmer preferences are also needed, without omitting the urgent need for reliable country and basin level data on water accounting, water resources, agronomic practices, energy use, food consumption, and other relevant parameters.
Author's note
This study quantifies the trade-offs associated with producing more of Lebanon's food basket locally, under different food production, water, and energy scenarios in light of the country's currency devaluation. This study aims to: (1) Identify and quantify the critical interconnections between water, energy, nutrition, and food systems in Lebanon. (2) Develop a framework to quantify the trade–offs associated with adopting interventions within current water, energy, and agriculture portfolios and practices. (3) Evaluate producers' perceptions of their willingness to implement proposed changes in crop production, renewable energy, and water reuse. Findings from this study can play a role in informing policymaking and planning in Lebanon, as the country works to implement the UN Sustainable Development Goals. All authors contributed to the article and approved the submitted version.
Data availability statement
The original contributions presented in the study are included in the article/Supplementary material, further inquiries can be directed to the corresponding author.
Ethics statement
The studies involving human participants were reviewed and approved by American University of Beirut IRB protocol ID: SBS-2019-0490. Written informed consent for participation was not required for this study in accordance with the national legislation and the institutional requirements.
Author contributions
All authors listed have made a substantial, direct, and intellectual contribution to the work and approved it for publication.
Funding
This study is part of a project that was supported by the United Nations Food and Agriculture Organization (UN-FAO) Pilot study on Evapotranspiration and Trade-offs in the Water Energy Food Nexus in Bekaa Valley in Lebanon. The study was also partially supported by the National Science Foundation under Grant Addressing Decision Support for Water Stressed FEW Nexus Decisions Numbered 1739977.
Acknowledgments
The authors would like to acknowledge the work of three research assistants, Ms. Reem Khattar, Mr. Ali Olliek, and Mr. Haydar Sleiman, who contributed to data and survey collections, data analysis, and literature review.
Conflict of interest
The authors declare that the research was conducted in the absence of any commercial or financial relationships that could be construed as a potential conflict of interest.
Publisher's note
All claims expressed in this article are solely those of the authors and do not necessarily represent those of their affiliated organizations, or those of the publisher, the editors and the reviewers. Any product that may be evaluated in this article, or claim that may be made by its manufacturer, is not guaranteed or endorsed by the publisher.
Supplementary material
The Supplementary Material for this article can be found online at: https://www.frontiersin.org/articles/10.3389/fsufs.2022.969248/full#supplementary-material
References
Al-Saidi, M., and Hussein, H. (2021). The water-energy-food nexus and covid-19: toward a systematization of impacts and responses. Sci. Total Environ. 779, 146529. doi: 10.1016/j.scitotenv.2021.146529
Bach-Faig, A., Berry, E. M., Lairon, D., Reguant, J., Trichopoulou, A., Dernini, S., et al. (2011). Mediterranean diet pyramid today. Science and cultural updates. Public Health Nutri. 14, 2274–2284. doi: 10.1017/S1368980011002515
Batlle-Bayer, L., Bala, A., Garcia-Herrero, I., Lemaire, E., Song, G., Aldaco, R., et al. (2019). The Spanish dietary guidelines: a potential tool to reduce greenhouse gas emissions of current dietary patterns. J. Clean. Prod. 213, 588–598. doi: 10.1016/j.jclepro.2018.12.215
Ben Hassen, T., and El Bilali, H. (2022). Impacts of the russia-ukraine war on Global Food Security: Towards more sustainable and resilient food systems? Foods 11, 2301. doi: 10.3390/foods11152301
Daher, B., Hamie, S., and Pappas, K. (2022). Examining Lebanon's resilience through a water-energy-food nexus lens. Front. Sustain. Food Syst. 6, 748343. doi: 10.3389/fsufs.2022.748343
Daher, B., Hamie, S., Pappas, K., and Nahidul Karim, M. (2021). Toward resilient water-energy-food systems under shocks: understanding the impact of migration, pandemics, and natural disasters. Sustainability 13, 9402. doi: 10.3390/su13169402
Daher, B., and Mohtar, R. H. (2015). Water-energy-food (WEF) nexus tool 2.0 guiding integrative resource planning and decision-making. Water Int. 40:748–71. doi: 10.1080/02508060.2015.1074148
Daher, B., Mohtar, R. H., Pistikopoulos, E. N., Portney, K. E., Kaiser, R., Saad, W., et al. (2019). Developing socio-techno-econo-political (STEP) solutions for addressing resource nexus hotspots. Sustainability 10, 512. doi: 10.3390/su10020512
El Amine, Y., Farajalla, N., Chehade, A., and Acaf, Y. (2018). Water (in)security in the Bekaa: Introducing an Integrative Lens to the Lebanese Water Sector. Beirut: Policy Brief #4, AUB Policy Institute (Issam Fares Institute for Public Policy and International Affairs).
El-Kareh, J., El Hajj, R., Farajalla, N., and Chehade, A. (2018). Water Conflict in the Bekaa: Assessing Predisposition and Contributing Factors. Beirut: Policy Brief #3, AUB Policy Institute (Issam Fares Institute for Public Policy and International Affairs).
FAO (2008). Lebanon report in Irrigation in the Middle East region in figures—AQUASTAT Survey 2008 (Water Report). p. 34.
FAO (2014). The Water-Energy-Food Nexus. A New Approach in Support of Food Security and Sustainable Agriculture. Rome: FAO.
Giampietro, M., Aspinall, R. J., Bukkens, S. G. F., Benalcazar, J. C., Diaz-Maurin, F., Flammini, A., et al. (2013). An Innovative Accounting Framework for the Food-Energy-Water Nexus—Application of the MuSIASEM Approach to Three Case Studies (Environment and Natural Resources Working Paper No. 56.). FAO, Rome. p. 81.
Haydamous, P., and El Hajj, R. (2016). Lebanon's Agricultural Sector Policies: Considering Inter-regional Approaches to Adaptation to Climate Change. Beirut: Policy Brief #2, AUB Policy Institute (Issam Fares Institute for Public Policy and International Affairs).
Howells, M., Hermann, S., Welsch, M., Bazilian, M., Segerström, R., Alfstad, T., et al. (2013). Integrated analysis of climate change, land use, energy and water strategies. Nat. Climate Change 3, 621–626. doi: 10.1038/nclimate1789
Hwalla, N. (2015). Sustainable Food Consumption in Arab Countries. Available online at: http://www.afedonline.org/uploads/conferences_media/Hwalla.pdf
IFPRI (2022). One of the world's worst economic collapses, now compounded by the Ukraine crisis: What's next for Lebanon? Food Security Portal. Available online at: https://www.foodsecurityportal.org/node/1989#:~:text=Like%20other%20countries%20in%20the,based%20products%20or%20sunflower%20oil (accessed November 21, 2022).
Karnib, A., and Alameh, A. (2020). Technology-oriented approach to quantitative assessment of water–energy–food nexus. Int. J. Energ. Water Res. 4, 189–197. doi: 10.1007/s42108-020-00061-w
Liu, J., Yang, H., Cudennec, C., Gain, A. K., Hoff, H., Lawford, R., et al. (2017). Challenges in operationalizing the water–energy–food nexus. Hydrol. Sci. J. 11, 1714–1720. doi: 10.1080/02626667.2017.1353695
Machayekhi, D., Pierpaoli, M., and Cancelliere, G. (2017). Domestic Water in the Bekaa Valley, Lebanon: Demand, Access and Institutional Aspects. London: International Institute for Environment and Development.
Markantonis, V., Reynaud, A., Karabulut, A., El Hajj, R., Altinbilek, D., Awad, I. M., et al. (2019). Can the implementation of the Water-Energy-Food Nexus support economic growth in the Mediterranean region? The current status and the way forward. Front. Environ. Sci. 7, 84. doi: 10.3389/fenvs.2019.00084
Ministry of Agriculture. (2010). Agriculture Census 2010. Beirut: Ministry of Agriculture. Available online at: http://www.agriculture.gov.lb/Statistics-and-Studies/Comprehensive-Agricultural-Statistics/statistics-2010 (accessed December 08, 2022).
Mohtar, R. H., and Daher, B. (2012). “Water, energy, and food: the ultimate nexus”, in Encyclopedia of Agricultural, Food, and Biological Engineering Second Edition, ed. D. R. Heldman, C. I. Moraru. (Abingdon, UK: Taylor and Francis). p. 1–15. doi: 10.1081/E-EAFE2-120048376
Mohtar, R. H., and Daher, B. (2016). Water-Energy-Food Nexus Framework for Facilitating Multi-Stakeholder Dialogue. Milton Park: Water International. doi: 10.1080/02508060.2016.1149759
Mortada, S., Abou Najm, M., Yassine, A., and El Fadel, M. (2018). Towards sustainable water-food nexus: an optimization approach. J. Cleaner Prod. 178, 408–418. doi: 10.1016/j.jclepro.2018.01.020
Naja, F., Hwalla, N., Itani, L., Salem, M., Azar, S. T., Zeidan, M. N., et al. (2012). Dietary patterns and odds of type 2 diabetes in Beirut, Lebanon: a case–control study. Nutr. Metab. 9, 111. doi: 10.1186/1743-7075-9-111
Naja, F., Itani, L., Hamade, R., and Chamieh, M. (2019). Mediterranean diet and its environmental footprints amid nutrition transition: the case of Lebanon. Sustainability 11, 6690. doi: 10.3390/su11236690
Naja, F., Jomaa, L., Itani, L., Zidek, J., El Labban, S., Sibai, A. M., et al. (2018). Environmental footprints of food consumption and dietary patterns among Lebanese adults: a cross-sectional study. Nutr. J. 17, 85. doi: 10.1186/s12937-018-0393-3
Naja, F., Nasreddine, L., Itani, L., Adra, N., Sibai, A., Hwalla, N., et al. (2013). Association between dietary patterns and the risk of metabolic syndrome among Lebanese adults. Eur. J. Clin. Nutr. 52, 97–105. doi: 10.1007/s00394-011-0291-3
Naja, F., Nasreddine, L., Itani, L., Chamieh, M. C., Adra, N., Sibai, A. M., et al. (2011). Dietary patterns and their association with obesity and sociodemographic factors in a national sample of Lebanese adults. Public Health Nutr. 14, 1570. doi: 10.1017/S136898001100070X
Vanham, D., Guenther, S., Ros-Baro, M., and Bach-Faig, A. (2021). Which diet has the lower water footprint in Mediterranean countries. Resour. Conserv. Recy. 171, 105631. doi: 10.1016/j.resconrec.2021.105631
Willett, W., Rockström, J., Loken, B., Springmann, M., Lang, T., Vermeulen, S., et al. (2019). Food in the anthropocene: the EAT–lancet commission on healthy diets from sustainable food systems. Lancet 393, 447–492. doi: 10.1016/S0140-6736(18)31788-4
World Bank (2022). The World Bank In Lebanon. Available online at: https://www.worldbank.org/en/country/lebanon/overview (accessed November 13, 2022).
Keywords: water-energy-food nexus, trade-offs analysis, farmer perceptions, sustainable development, Beqaa Valley, Lebanon
Citation: Daher B, Bachour R, Yanni SF, Koo-Oshima S and Mohtar RH (2022) Food security under compound shocks: Can Lebanon produce its own Mediterranean food basket? Front. Sustain. Food Syst. 6:969248. doi: 10.3389/fsufs.2022.969248
Received: 14 June 2022; Accepted: 30 November 2022;
Published: 22 December 2022.
Edited by:
Yanjun Shen, University of Chinese Academy of Sciences, ChinaReviewed by:
Sophia Ghanimeh, Qatar University, QatarMarta Rivera-Ferre, Universitat de Vic - Universitat Central de Catalunya, Spain
Copyright © 2022 Daher, Bachour, Koo-Oshima and Mohtar, and His Majesty the King in Right of Canada, as represented by the Minister of Agriculture and Agri-Food Canada for the contribution of Sandra F. Yanni. This is an open-access article distributed under the terms of the Creative Commons Attribution License (CC BY). The use, distribution or reproduction in other forums is permitted, provided the original author(s) and the copyright owner(s) are credited and that the original publication in this journal is cited, in accordance with accepted academic practice. No use, distribution or reproduction is permitted which does not comply with these terms.
*Correspondence: Rabi H. Mohtar, bW9odGFyQHRhbXUuZWR1; bW9odGFyQGF1Yi5lZHUubGI=