- Natural Resources Institute Finland (Luke), Jokioinen, Finland
Crop rotation and soil tillage are among key factors impacting cropping system productivity, pest management and soil health. To assess their role in northern cropping systems, we quantified the effects of crop rotation on spring wheat yield in different tillage systems based on a long-term (2005–2017) field experiment in southwestern Finland. In addition, effects of crop rotation on weeds, plant pathogens, and pest insects were assessed. Three types of crop rotation were compared: monoculture (spring wheat), 2-year rotation (spring wheat—turnip rape—spring wheat—barley) and 4-year rotation (spring wheat—turnip rape—barley—pea) under no-tillage and plowing. A diversified crop rotation improved spring wheat yield by up to 30% in no-tillage and by 13% under plowing compared with monoculture. Overall, the yield quantity and quality differences between crop rotations were higher in no-tillage plots than in plowed plots. The occurrence of weed species in spring wheat before herbicide control was highest in the four-year crop rotation and lowest in the wheat monoculture. For plant diseases, wheat leaf blotch disease severity, mainly caused by Pyrenophora tritici-repentis, was lowest in the most diverse crop rotation. On average, wheat leaf blotch disease severity was 20% less when wheat was grown every fourth year compared with wheat monoculture. The effect of crop rotation on stem and root diseases became apparent after 6 years of rotation and the disease index was lowest in the most diverse crop rotation. Neither rotation nor tillage affected the control need of wheat midge (Sitodiplosis mosellana). Based on our results, diverse crop rotations including cereals, oilseed crops, and legumes increase yield and reduce plant disease severity of spring wheat in Finland, with the magnitude being larger in no-tillage systems.
Introduction
Northern cropping systems represent mostly Boreal zone located agroecosystems characterized by high seasonal variation leading to a short, intense growing season, and somewhat restricted selection of crops to grow. The yields are in general lower and less land area is suitable for annual crop production in the Boreal zone than other climatic regions; typically, <10% of land area is used for agriculture in north European boreal regions (Trnka et al., 2011). While the harsh winter provides some protection against major pests, climate change is predicted to increase the incidence of extreme weather events and potential pest outbreaks in northern regions (Hakala et al., 2011). Therefore, the potential for crop diversification should be considered as a strategy to prevent emerging pest problems and improve crop resilience also in the North (Altieri et al., 2015). In the future, Northern cropping systems with their already challenging production conditions are faced with increasing adaptation needs due to alterations in precipitation, temperature, and snow coverage along with the socioeconomic changes brought about by climate change (Peltonen-Sainio, 2012). This emphasizes the need to pay even more attention to the maintenance of healthy and viable soil that can sustain yielding under various biotic and abiotic crop stresses.
Planning of crop rotation and determining soil management techniques are among the most important yearly decisions that every farmer makes. Both these practices can contribute to climate change mitigation and adaptation of field cropping (Paustian et al., 2016) and impact pest management (e.g., Skellern and Cook, 2018), which links these decisions to proactive building of adaptive capacity and resilience at farm scale. To better understand the effect of crop rotation and soil management on cropping system productivity and resilience, we need long-term studies combining an analysis of the productivity component (i.e., yield quantity and quality) and the pest regulation component (incidence of pathogens, weeds, and insect pests).
In addition to the need to adapt to the changes caused by climate change in northern agriculture, modern cropping systems are currently faced with the need to develop alternatives to chemical pesticides. In 2020, the European Commission published a new biodiversity strategy that aims to halt the loss of biodiversity by 2030 (EU, 2020a), setting concrete goals for the EU food system. For example, the use of pesticides and the risks they pose must be decreased by 50%. Thus, reducing the use of chemical plant protection products is one of the key objectives of EU agricultural policy. Earlier, the European Commission adopted Directive 2009/128/EC on the sustainable use of plant protection products (EU, 2009). The aim of the directive is to reduce the risks from plant protection products to human health and the environment. A key factor in achieving this goal is to promote the introduction of the IPM (Integrated Pest Management) tool. IPM aims at healthy growth and a balanced field ecosystem and encourages the use of biological methods to control pests (Vasileiadis et al., 2011).
Crop rotation is among the recommended measures to prevent pest damage in IPM production (EU, 2009). Crop rotation affects the presence of host-specific pests by disturbing their life cycle. Pest management is based on prevention, in which the crop rotation aims to create unfavorable conditions for the pest and favorable conditions for the crop. However, it is not always that vegetational diversification reduces pest incidence (Ratnadass et al., 2012) and crop diversity may also serve as a source of new pests (Keesing et al., 2010).
It is broadly recognized that crop rotation often increases the crop yield (Berzsenyi and Gyorffy, 1997; Sieling et al., 2005) and provides for more sustainable crop production (Bullock, 1992; Bailey et al., 2001; Mamolos and Kalburtji, 2001). Crop rotation can also support ecological intensification, i.e., maximizing productivity per unit area while minimizing environmental impacts (Bommarco et al., 2013). Well-designed crop rotation can contribute to multiple ecosystem services through maintaining soil structure and microbial activity, improving nutrient use efficiency, and providing pest regulation services, with examples available from organic agriculture (Barbieri et al., 2017). By supporting and utilizing ecosystem services more effectively via crop diversification, agrobiodiversity (i.e., diversity at multiple levels of the agroecosystems) can be enhanced (Duru et al., 2015). Thus, overall temporal crop diversification using crop rotation represents an established, yet nonetheless increasingly important strategy to break pest and pathogen cycles, regulate nutrient dynamics, and improve yields via beneficial pre-crop effects (Sieling and Christen, 2015). Considering cropping system productivity in the long term, the aim of crop rotation is to modify the growing environment so that it meets the requirements of successful crop production of different plant species (Klein Haneveld and Stegeman, 2005).
Even though there is ample evidence on the benefits of crop rotation in general, there are knowledge gaps especially for combined effects of crop rotation on yield and on efficacy for controlling weeds, plant diseases and pest insects in spring cereal production in North European conditions. This lack of information became relevant in the early 2000's when no-tillage cultivation became more common in Finland and there was evidence that the shift from tillage to reduced or no-tillage cultivation may cause changes in the incidence of pest species and their abundance (Paulitz, 2006). Tillage influences insect pests variably, depending on their life history strategies, i.e., growth, reproduction, survival, and dispersal (Stinner and House, 1990; Andersen, 1999). Different soil management and cultivation techniques also favor different weed species (Fonteyne et al., 2020). Non-tillage can also impact soil organic carbon sequestration as well as disease suppression and microbial biomass in soil (Palojärvi et al., 2020).
Finland belongs to the northernmost agricultural countries in Europe. In Finland, cereal-based crop rotations dominate active crop production (Peltonen-Sainio and Jauhiainen, 2019). The total area allocated to agricultural crops is currently 2.3 million hectares and in 2020, almost half of the area was sown to spring sown cereals: spring barley (Hordeum vulgare) (458,000 ha), oats (Avena sativa) (348,000 ha), and spring wheat (Triticum aestivum) (210,000 ha) (Luke, 2020a). Forage grasses are cultivated on the other half of the area, while oil and protein crops cover a minor part of the cultivated area. In the past 20 years, spring wheat cultivation area in Finland has increased ca. 70% while the area allocated to oilseed crops has decreased by 43% (Supplementary Figure 1).
The change from dairy farming and intensive grass production to cereal-dominated crop production has affected the occurrence of pest species (weeds, plant diseases, and insect pests) in Finnish fields. The most common weed species in spring cereal fields are Viola arvensis (field pansy), Stellaria media (chickweed), Galeopsis ssp. (hemp-nettle), Galium spurium (false cleavers), and Lamium purpureum (red dead-nettle) (Salonen et al., 2011). For plant diseases in spring wheat, the most common are seed- and straw-borne leaf spot diseases, stagonospora blotch (Parastagonospora nodorum) and tan spot (Pyrenophora tritici-repentis), the incidence of which has increased during recent years together with the increased area of reduced tillage, especially in wheat monoculture (Jalli et al., 2011, 2020). The main insect pests that damage spring wheat in Finland are orange wheat blossom midge (Sitodiplosis mosellana), the bird cherry-oat aphid (Rhopalosiphum padi), and occasionally the frit fly (Oscinella frit). The frequency and amplitude of pest outbreaks vary considerably in time and space, and the growing conditions play an important role in their occurrence (Kurppa, 1990).
Based on a long-term data, if no plant protection practices are used, weeds cause on average a 200 kg/ha yield reduction and leaf blotch diseases a 500 kg/ha yield reduction in Finnish spring wheat production. In addition to reduced crop yield, pests also decrease crop nutrient use efficiency significantly (Kauppi et al., 2021), which hampers the crop's ability to grow (Di and Cameron, 2002; Meisinger and Delgado, 2002).
The reasoning underlying establishment of the long-term trial presented in this study was the practical concern in Finnish cereal production in the early 2000's: how the growth of spring wheat, a crop having common pests, leaf blotch diseases and wheat midge, overwintering in soil or plant debris, and with cultivation being widely based on cereal monoculture, will be affected by the changes in tillage systems. There was also interest in revealing whether more diverse cropping system could help to reduce the possible risks of moving to no-tillage. Thus, the main objective of this study was to find out how different crop rotations affect spring wheat yield and the incidence of weeds, plant pathogens and insect pests common in Finnish spring wheat production in different tillage systems. Conclusions are based on the results gathered over the 13 years of the field study.
Materials and Methods
Location and Growing Conditions
A long-term field trial was conducted at research station fields of Luke, Natural Resources Institute Finland, in Jokioinen (60°49'N, 23°28'E) in southwest Finland in 2005–2017. Finland, one of the northernmost high latitude European countries, represents Boreal climate zone and typical northern growing conditions (Metzger et al., 2005). Soil type in the trial area was sandy clay with pH 6.1–6.5 and 7.2 mg/l phosphorous in soil. Before 2005, the main crops in the field were forage grass, spring wheat, spring barley and oats and before 2003, the field was plowed annually.
Annual weather conditions during growing seasons varied considerably in 2005–2017 in Jokioinen (Supplementary Table 1). The long-term (1981–2010) effective temperature sum in May–September in Jokioinen is 1,269° and cumulative precipitation 316 mm. The coolest season was in 2017, with an effective temperature sum of 1,136 and the warmest in 2006, with an effective temperature sum of 1,540. Also, year 2006 was the driest season, with cumulative precipitation of 207 mm while in 2011, the cumulative precipitation was highest, with 414 mm of rain.
Trial Design
In the field experiment, three types of crop rotation were compared: CR1 spring wheat monoculture, CR2 2-year rotation (spring wheat—spring turnip rape (Brassica rapa subsp. oleifera)—spring wheat—spring barley) and CR4 4-year rotation [spring wheat—spring turnip rape—spring barley—field pea (Pisum sativum)] (Table 1). All crop rotation treatments were sown both in no-tillage and plowed soil and formed 16 treatments in total. Each treatment had four replicates.
The plot size was 6 ×15 m. Sowing time ranged between May 5th to July 30th and harvest between August 12th and September 28th (Supplementary Table 1). Tillage plots were plowed in autumn (October, except in 2008 November) and tilled to 5 cm depth with a combined power harrow before sowing. The plowed plots were sown with combined drill seeding machines with 12.5 cm crop rows. Fertilizers were placed between every second crop rows (25 cm apart). No-tillage plots were directly sown to 3–5 cm depth with a combined drill-seeding machine with double disk coulters that placed seed and fertilizer in the same 15 cm crop row. Seeds were sown at 650 seeds/m2 for spring wheat, 500 seeds/m2 for spring barley, 9.6 kg seeds/ha for spring turnip rape and 110 seeds/m2 for field pea. The fertilizer levels followed the EU-agricultural support council directive (EU, 2020b): 120 nitrogen (N) kg/ha for spring wheat, 90 N kg/ha for spring barley, 120 N kg/ha for spring turnip rape, and 50 N kg/ha for field pea (Supplementary Table 2). After pea, the amount of N-fertilizer was reduced by 10 kg/ha.
Plant Protection Treatments
In cereals, weeds were controlled annually with herbicides at tillering stage BBCH 20–24 (Bleiholder et al., 1997). The product was chosen according to the weed species. In addition, no-tillage plots were treated with glyphosate in spring before sowing, except in 2014–2016, when both the no-tillage and plowed plots were treated with glyphosate after the harvest. Glyphosate concentration ranged from 3 to 7 l/ha based on the weed pressure.
For spring wheat and spring barley, fungicide-dressed seed was used to control seed-borne diseases. No foliar fungicide treatments were applied for any of the crops.
Turnip rape seed was coated with neonicotinoids to control flea beetles (Phyllotreta sp.). Insecticides were used when control thresholds were exceeded in different crops. Flea beetles and pollen beetles (Meligethes aeneus) were controlled in turnip rape annually with sprayed insecticides (pyrethroids and neonicotinoid), while pea moths (Cydia nigricana) were controlled with pyrethroids in peas only in the years 2009–2011. In cereals, bird cherry-oat aphid was sprayed with pyrethroids in 2005.
Field Observations
Before herbicide treatments (BBCH 20–24) the number of weeds was counted by species from five 0.1 m2 subsample areas in each plot. For data analyses, weed species were divided into five groups: Group1, common spring germinating weeds in cereal fields (Viola arvense, Chenopodium album); Group2, other spring germinating weeds (Galeopsis, Galium spurium, Lamium purpureum); Group3 weeds germinating both in spring and autumn (Stellaria media, Fumaria officinalis, Taraxacum officinale); Group4 grasses and biennial plants (Agropyron repens, Poa annua, Sonchus arvensis, Cirsium arvense), and Group5, the remaining species.
The occurrence of plant diseases was evaluated twice per growing season. For stem and root disease assessments, a random sample of 30 plants was collected from each spring wheat plot at milk ripening stage (BBCH 75–77). Stems and roots of the plants were rinsed with water and the symptoms were assessed. The plants were divided into five groups (A–E) according to the severity of the symptoms and a disease index was calculated from the number of the plants in different groups. Disease index = (B+2*C+3*D+4*E)*100/4*(A+B+C+D+E) where A = number of plants with no symptoms; B = small spot on coleoptile; C = more attack on coleoptile and some on roots; D = severe attack on coleoptile and roots, plants depressed, and E = dead plants. After observations, stems were dried and stored at room temperature for further pathogen analyses.
Cereal leaf diseases were assessed at early stem elongation stage (BBCH 30–31) and at milk ripening stage (BBCH 75–78). All visible leaf diseases were assessed. The disease assessments were carried out as percentage coverage of the top four green leaves by each individual disease. The severity of tan spot and stagonospora blotch was combined into a single value for leaf blotch diseases.
Fusarium species were analyzed both from stems and from the harvested yield. The stems with visual symptoms and 100 seeds per plot yield were incubated on selective agar medium containing pentachloronitrobenzene (PCNB) at room temperature (22°C) and the growing hyphae were isolated on potato dextrose (PDA) medium for identification. The Fusarium cultures were identified microscopically.
Insect pests were monitored with yellow sticky traps in May–June. Control thresholds were evaluated by counting number of aphids, flea beetles and pollen beetles in the field and the number of pea moths collected in pheromone traps. The occurrence of wheat midges was evaluated at soft dough stage (BBCH 85) from 25 spikes per spring wheat plot, and the numbers of kernels damaged by orange wheat blossom midges were counted. Percentages of infected plants, damaged tillers and damaged kernels were used in the statistical analyses of insect occurrence.
The trials were harvested using a plot combine. The plot yield (40 m2) was dried and cleaned before further analyses. The grain yield, 1,000 seed weight and hectoliter weight were transformed to 14% moisture content. The grain nutrient uptake (kg/ha) of spring wheat was estimated by multiplying the nutrient average concentration N 21.6 g/kg and P 3.7 g/kg (Luke, 2020b) with their corresponding grain yield (kg/ha).
Statistical Methods
Statistical modeling was based on an incomplete block design in which 16 treatments were divided into two incomplete blocks of eight field plots, separately within each of four replications. The values of response variables were measured annually during 2005–2017 and values from the same field plot were statistically correlated. This correlation was taken into account in the selected model:
where yijklm is observed value of the response variable, μ is intercept, repi is the random effect of ith replication (i = 1,…,4), block(rep)ij is random effect of jth incomplete block within replication (j = 1,2), rotationk is fixed effect of kth rotation (k = 1,…,8), tillagel is effect of lth tillage system (l = 1,2), rotation*tillagekl is rotation-by-tillage interaction effect, error1 is between subject error variance (i.e., variance between field plots), yearm is fixed effects of mth year (m = 2005,…,2017), rep*yearim is random effect of year-by-replication interaction, rep*block(rep)ijm is random effect of incomplete block-by-year interaction, rotation*yearkm is rotation-by-year interaction, tillage*yearlm is tillage-by-year interaction, rotation*tillage*yearklm is rotation-by-tillage-by-year interaction and finally εijklm is residual error. Seed yield consists of two main components: number of seeds per hectare, and single seed weight. Only seed yield and single seed weight (1,000 seed weight) were measured. The number of seeds per hectare was calculated as a ratio of two measured variables. The result was presented as number of seeds per m2.
The model was fitted using SAS/MIXED with the REML-estimation method. This model assumes that the data follow the normal probability distribution, and the variances of the populations are equal. These assumptions were checked using scatter plots of residuals and predicted values. Some variables were not normally distributed, also error variance varied among years. Transformations were used to ensure assumptions: loge for total number of weeds, for number of weeds in Groups 2–5, and for total number of monocotyledonous weeds (grass-type weeds) and square-root transformation for damaged kernels and for number of weeds in Group1.
After modeling, the results were presented so that eight rotations were categorized as three different types of rotation: spring wheat monoculture CR1, two-year rotation CR2 and four-year rotation CR4. Data were gathered from several years and cultivated crops varied from rotation to rotation in most of years. Only years having spring wheat in all three different types of rotation (CR1, CR2, CR4) were included to the statistical modeling: 2007, 2008, 2010, 2011, 2012, 2014, 2015, and 2016. In addition, years were combined into three periods: Period1 (2007, 2008), Period2 (2010, 2011, 2012) and Period3 (2014, 2015, 2016). Statistical tests and estimates were calculated for three periods and three rotations using estimate and contrast commands in SAS/MIXED. However, original statistical tests of treatments (rotation, tillage and their interaction) and year-by-treatments interaction were presented also (F-tests). The statistical model was fitted using version 9.4 of SAS/MIXED.
Results
Spring Wheat Yield
The spring wheat plot yield in 2007–2017 ranged between 1,314 (no-tillage CR1 in 2015) and 5,842 (plowed CR4 in 2015) kg/ha (Figure 1). The average spring wheat yield over all treatments and years was 4,046 kg/ha. In the most diverse crop rotation (CR4), the average spring wheat yield was on average 21% higher than the yield in the wheat monoculture CR1 (p < 0.001). In CR2, where wheat was cultivated every second year, the average yield was 15% higher than in the wheat monoculture (p < 0.001).
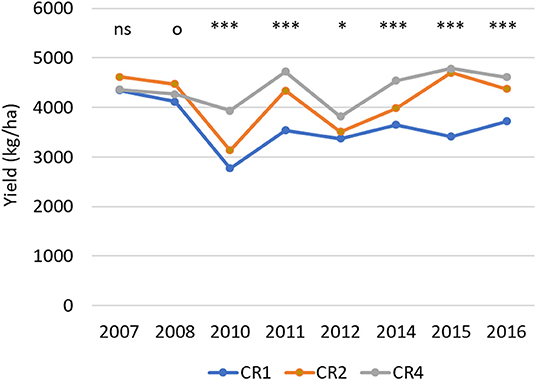
Figure 1. The average spring wheat yield (kg/ha) in different years and crop rotations. CR1, wheat monoculture; CR2, wheat every 2nd year; CR4, wheat every 4th year. Statistically significant differences between yields: ns = not significant, op < 0.10, *p < 0.05, ***p < 0.001.
Differences in the spring wheat yield among three different crop rotations were apparent already during Period 1 and increased further in Period 2 and Period 3 (Table 2). The yield differences among the tested crop rotations were higher in the no-tillage than in the plowed treatments. In both tillage systems, the yield was lowest in wheat monoculture for all time periods except during Period 1 when no-tillage CR4 plots had the lowest yield. When plowed, the yield of CR4 plots was, on average, 13% higher than in the monoculture plots, whereas a 30% yield difference was reached in the no-tillage plots. The wheat yields in monoculture (CR1) were, on average, 14% lower in the no-tillage plots than in plowed plots. In crop rotations CR2 and CR4, there was no significant difference in the average wheat yield between the no-tillage and plowed plots.
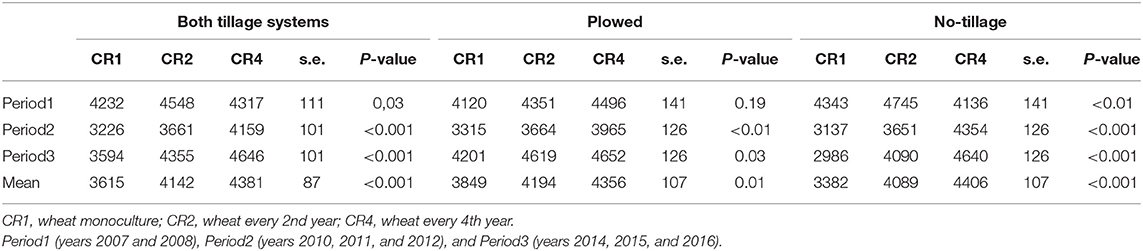
Table 2. The average spring wheat yield (kg/ha) in different tillage systems, time periods, and crop rotations.
Crop rotation affected positively the produced seeds (1,000 seeds/m2), hectoliter weight and thousand seed weight (Figure 2). The effects were more significant in no-tillage plots, except for thousand seed weight, which was positively affected by crop rotation also in plowed plots (Table 3). The effect of crop rotation on the number of seeds per square meter increased over time. During the last period (Period3), the number of seeds per square meter was 50% higher in CR4 than in CR1. When plowed, the corresponding difference was 7%.
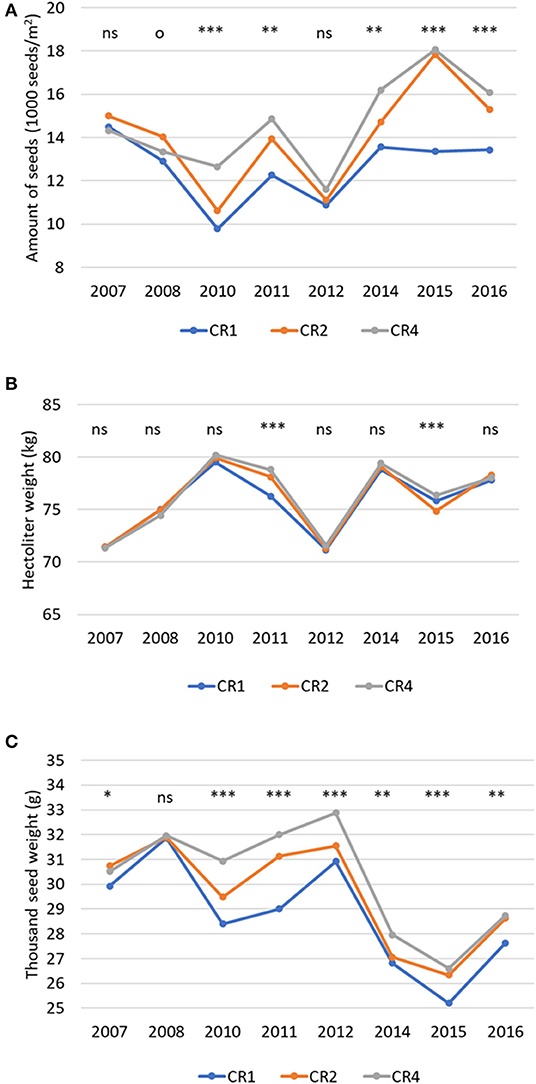
Figure 2. The average number of produced seeds (1,000 seeds/m2) (A), the hectoliter weight (kg) (B), and 1,000 seed weight (g) (C) in spring wheat in different years and crop rotations. CR1, wheat monoculture; CR2, wheat every 2nd year; CR4, wheat every 4th year. Statistically significant differences: ns = not significant, op < 0.10, *p < 0.05, **p < 0.01, ***p < 0.001.
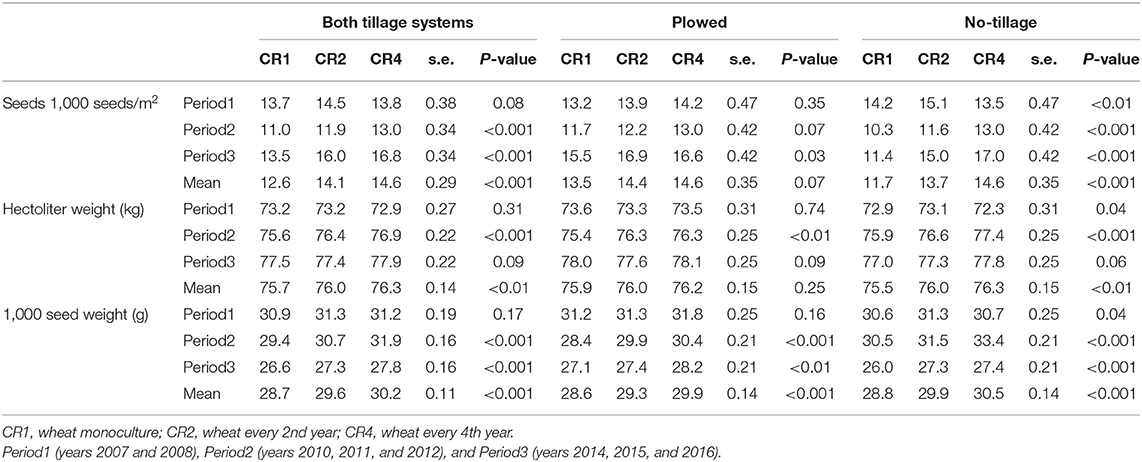
Table 3. The average number of produced seeds (1,000 seeds/m2), the hectoliter weight (kg), and 1,000 seed weight (g) in spring wheat in different tillage systems, time periods, and crop rotations.
The spring wheat grain nutrient uptake (kg/ha) in grain yield was estimated based on the spring wheat yield results. Over the two tillage systems the nitrogen uptake in different crop rotations and time periods was 60–86 kg/ha and phosphorous uptake 10–15 kg/ha (Table 4). Crop rotation had a statistically significant effect on nutrient uptake in Periods 2 and 3. The average nitrogen uptake in plowed plots in CR4 was 14% higher and the average phosphorous uptake 17% higher than in the CR1 plots. In no-tillage plots, the corresponding figures were 30 and 27%, respectively.
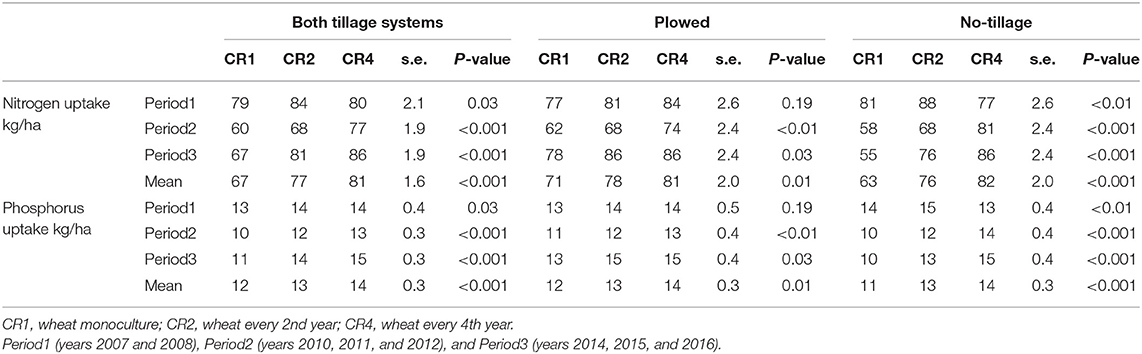
Table 4. The average spring wheat nitrogen and phosphorus uptake in grain yield (kg/ha) in different tillage systems, time periods, and crop rotations.
Occurrence of Weeds
In 2007, at the time of the first observations, the weed density in spring wheat plots was quite even in all the crop rotations, 80–115 plants/m2. The main weed species in the trial were G. spurium, L. purpureum, S. media, F. officinalis, and Trileurospermun inodorum. Among years, there were significant differences in the number of weeds germinated (Figure 3). In spring wheat monoculture (CR1), the weed density ranged between 58 and 200, in CR2 between 117 and 357, and in CR4 between 96 and 416 plants/m2.
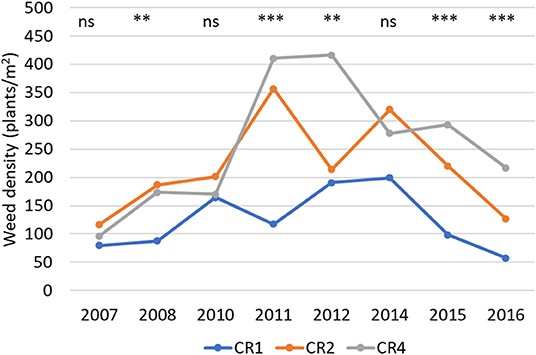
Figure 3. The average weed density (plants/m2) in spring wheat in different years and crop rotations. CR1, wheat monoculture; CR2, wheat every 2nd year; CR4, wheat every 4th year. Statistically significant differences: ns = not significant, **p < 0.01, ***p < 0.001.
In different crop rotations, the weed density was affected by the tillage type (p < 0.001). The effect of crop rotation on weed density was visible already at Period1 and the differences were more significant in no-tillage than in plowed environments (Table 5). The average number of weeds in plowed plots was 154 and in no-tillage plots 201 plants/m2.
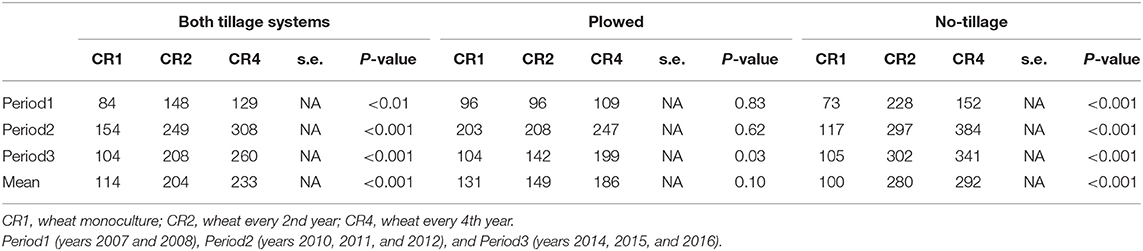
Table 5. The average density of weeds (plants/m2), in spring wheat in different tillage systems, time periods, and crop rotations.
The effect of crop rotation and tillage system varied among different weed species groups (Table 6). Except in Group4 (A. repens, P. annua, S. arvensis, and C. arvense) the weed density was lowest in CR1 and highest in CR4. The density of Group3 weeds (S. media, F. officinalis, and T. officinale) was higher in plowed plots and the density of Group 4 and Group 5 (other weeds) in no-tillage plots.
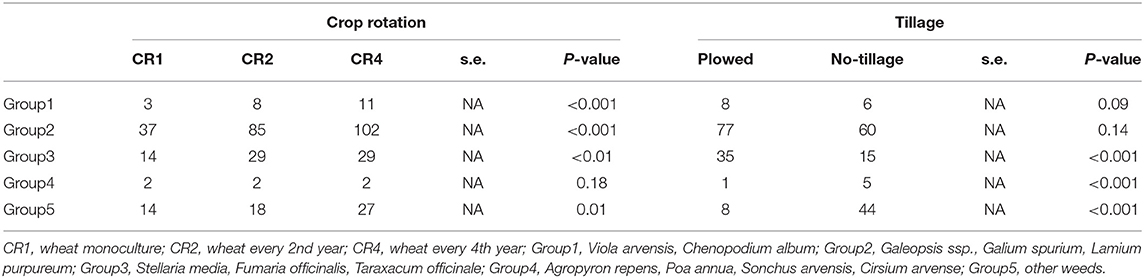
Table 6. The average density of weeds (plants/m2), in spring wheat in different weed groups, tillage systems, and crop rotations.
Plant Disease Severity
The stem and root disease severity in spring wheat was at a low level and decreased over time (Figure 4). The disease index in different treatments ranged between 2.9 (plowed CR4 in 2015) and 39.6 (no-tillage CR1 in 2008) during the trial period when the maximum potential value was 100. The average stem and root disease indexes were highest in the CR1 plots and lowest in CR4 plots, both in no-tillage and plowed environments, except during Period1 when the differences were not statistically significant. The average disease severity was slightly higher in no-tillage (21.4) than in plowed (19.6) plots (p < 0.001) (Table 7). The main causal agents of the stem and root infections were Fusarium culmorum and F. avenaceum. The incidence of other Fusarium species was minor. No take-all (Gaeumannomyces graminis) was identified on stems.
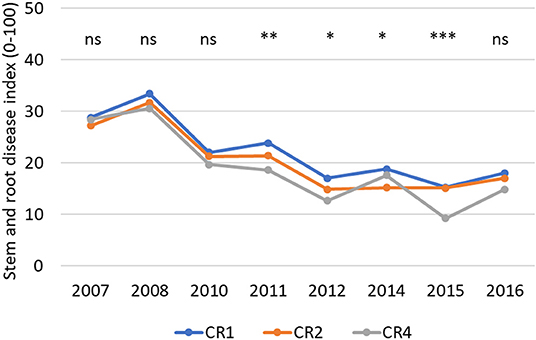
Figure 4. The average stem and root disease index (0–100) in spring wheat in different years and crop rotations. CR1, wheat monoculture; CR2, wheat every 2nd year; CR4, wheat every 4th year. Statistically significant differences: ns = not significant, *p < 0.05, **p < 0.01, ***p < 0.001.
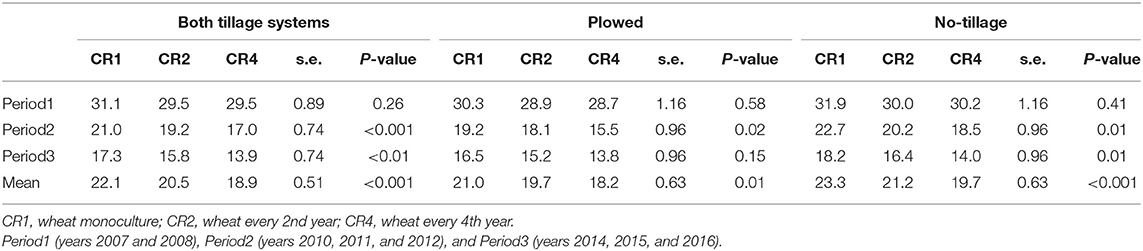
Table 7. The average stem and root disease index (0–100) in spring wheat in different tillage systems, time periods, and crop rotations.
The dominant leaf spot disease on spring wheat was tan spot, which was visible in no-tillage CR1 plots soon after emergence. Also, some stagonospora blotch was detected at BBCH 75. The observations on leaf spot diseases were combined into a single value—leaf blotch disease. Low levels of powdery mildew (Blumeria graminis) were observed occasionally and it was distributed evenly across the trial plots (no data shown).
The average leaf blotch disease severity at BBCH 75 was quite even during the trial period, except in 2008, when it was lower and in 2011, when it was higher than average (Figure 5), and no major differences in disease severity were observed between the two tillage systems. The average leaf blotch severity was highest in CR1 and lowest in CR4 in all time periods and both tillage systems (Table 8). On average, leaf blotch disease severity was 15% lower in CR2 than CR1 and 20% lower in CR4 than CR1.
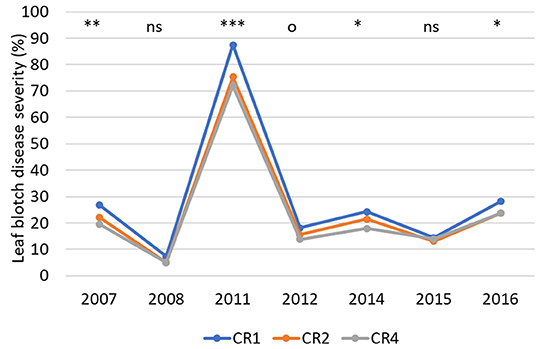
Figure 5. The average leaf blotch disease severity (%) in spring wheat in different years and crop rotations. CR1, wheat monoculture; CR2, wheat every 2nd year; CR4, wheat every 4th year. Statistically significant differences: ns = not significant, op < 0.10, *p < 0.05, **p < 0.01, ***p < 0.001.
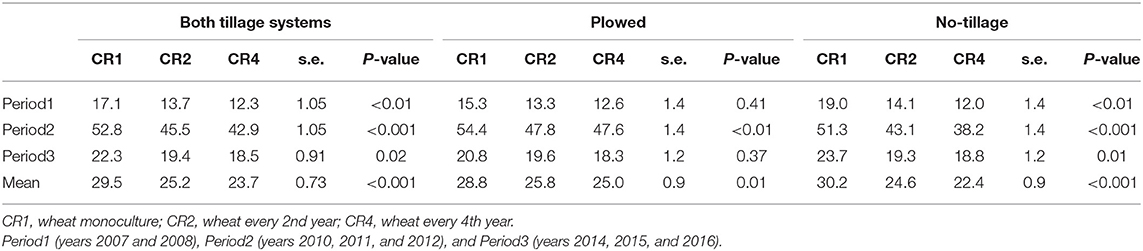
Table 8. The average leaf blotch disease severity (%) in spring wheat in different tillage systems, time periods, and crop rotations.
The incidence of Fusarium species in spring wheat yield was analyzed in 2011–2017. The incidence varied from 42% in 2015 to 100% in 2012. The main fungal species detected were F. avenaceum (23% seeds infected), F. poae (17% seeds infected), and F. graminearum (14% seeds infected). The incidence of F. avenaceum was highest in CR4 and lowest in CR1 (p < 0.01). In contrast, the incidence of F. poae was highest in CR1 and lowest in CR4 (p < 0.01). Crop rotation had no effect (p = 0.40) on total Fusarium incidence on wheat seeds.
Insect Pests' Prevalence
Occurrence of wheat midges varied widely among years, but no chemical control of midges was needed. Crop rotation and tillage had no effect on the amount of kernels damaged by wheat midge (p = 0.76 and p = 0.42, respectively), but some differences were found in individual years. In 2015, the amount of kernels damaged by wheat midge was highest in spring wheat monoculture (CR1) and lowest in the most diverse crop rotation (CR4) (Table 9).
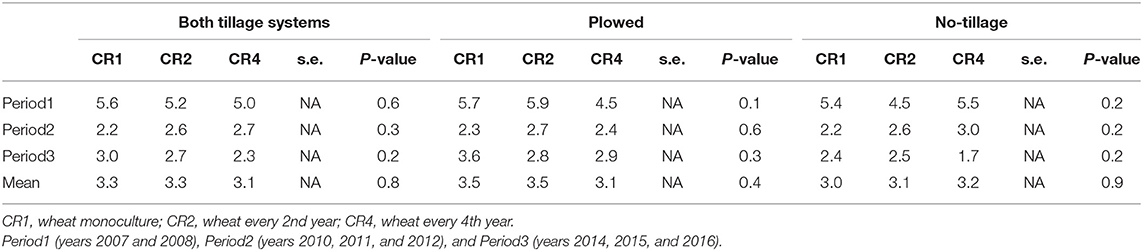
Table 9. The average amount of kernels damaged by wheat midge in spring wheat in different tillage systems, time periods, and crop rotations.
The abundance of other insect pests, including flea beetles, frit fly, and aphids, varied among growing seasons. Their numbers in yellow sticky traps were higher in plowed plots in several years but the difference among rotations was not monitored (Table 10). Aphids achieved the threshold for insecticide treatment in 2005. Abundance of flea beetles was especially high in 2007. Frit fly risk was high when sowing date was late. However, minor damage was attributable to these insect pests.
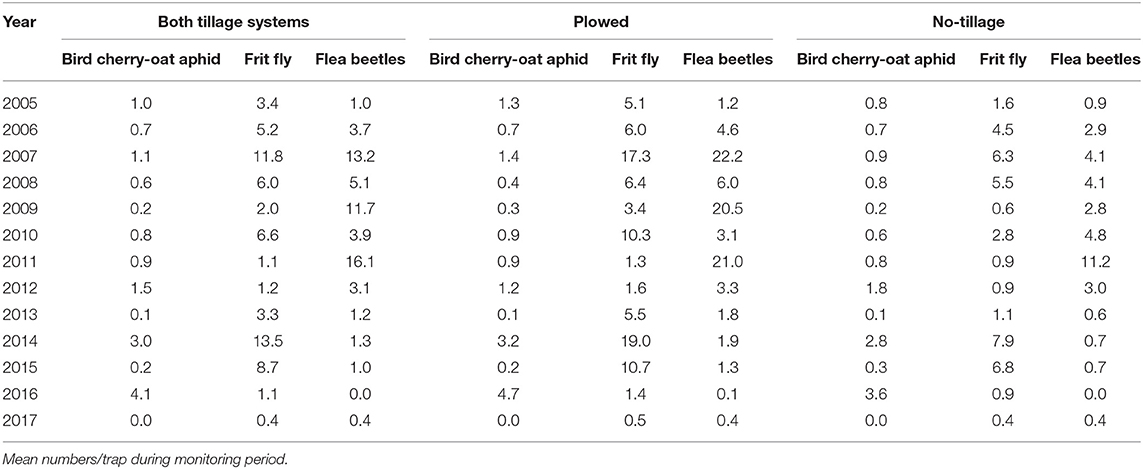
Table 10. Abundance of pest insects in spring wheat monitored with yellow sticky traps in 2005–2017.
Discussion
Our long-term study made it possible to estimate the short- and long-term effects of different crop rotations on spring wheat growth and pest occurrence under northern European conditions. Crop rotations, as well as pre-crop values, are difficult to model and considerable replication is needed. The effects observed also vary greatly depending e.g., on the growing conditions. For example, variation in weather events impacts crop growth and soil processes that contribute to the yield results (Sieling and Christen, 2015). Both short- and long-term effects can be mutually confounded, and long-term studies are needed to separate effects.
In our study, the same rotations were repeated several times, which allowed the identification of the true impact of rotations: most differences were based on pre-crop values of different crops in the first cycle (short term effect). In the later cycles, increased differences between rotations were the true long-term effects of rotations. In addition, we used eight different rotations, but rotations were compressed into three different types in the statistical analysis. This compression increased the number of replications and allowed one to see beyond the data and make wider statistical inference.
Effect of Crop Rotation on Spring Wheat Yield
The systemic effects of crop rotation were visible since 2010, after 5 years of the rotation experiment. A diversified crop rotation improved spring wheat yield most, when combined with no-tillage. Under plowing, the yield increase by rotation was also significant, but lower compared with no-tillage. The phenomenon was similar both in low and high yield level years. According to Berzsenyi and Gyorffy (1997) the yield-increasing effect of crop rotation is negatively correlated with the ratio of wheat in the sequence, which was the case in our study also. The yield differences between CR4, CR2, and CR1 were consistently higher in no-tillage plots than plowed plots mainly due to lower yield levels in CR1 in no-tillage plots. The results are in line with other crop rotation trials incorporating wheat (Berzsenyi et al., 2000; López-Bellido et al., 2001; Soon and Clayton, 2002; Sieling et al., 2005; Smith et al., 2008; Wozniak, 2020) even though most of the previous research was carried out with winter wheat rather than spring wheat.
The largest benefits in terms of yield were found using the most diverse crop rotation, having spring wheat every fourth year. In general, inclusion of crops from various families (cereals, oilseeds, and legumes) in rotations and lengthening the rotation cycle adds agroecological complexity through increased variation in shoot and root characteristics, biological and chemical traits, and crop management. In the current study, legumes were included only in CR4, where pea was a pre-crop for wheat. Pea has as good pre-crop value (Babublicová, 2016) and may increase wheat yield by 40% compared with wheat monoculture (Stevenson and van Kessel, 1996). In our study, the pre-crop value was not analyzed as such. However, the fertilizing effect of pea was considered by decreasing the nitrogen fertilizer level by 30 kg/ha when spring wheat was grown after pea. The differences in yield levels in different crop rotations might have been even larger if the nitrogen levels were same despite the pre-crop. According to Stevenson and van Kessel (1996), 92% of the yield advantage in the pea–wheat rotation is attributed to non-N rotation benefit.
Seed number is, in most cases, the major yield component that determines yield (Peltonen-Sainio et al., 2007). According to Galantini et al. (2000) crop rotation has a positive effect on spike number, but not number on spikelets per spike. In our study, the number of seeds per unit area was significantly higher in CR4 than in CR1. This may have resulted from positive changes in soil texture and microbial biomass (Bullock, 1992; Chan and Heenan, 1996; Govaerts et al., 2007) as well as decreased disease pressure via crop rotation (Bockus, 1992; Paulitz et al., 2010).
The average spring wheat yield in the trial was 4,050 kg/ha, which was close to the average spring wheat yield on Finnish farmers' fields in 2019, 4,300 kg/ha (Luke, 2020c). However, weather conditions, especially precipitation, greatly influenced the yield: the lowest yield, on average, was achieved in 2010 when the precipitation was below and the effective temperature sum above the long-term average. The highest yield was achieved in 2015, when weather conditions were very close to the long-term average. Weather conditions relating to different plant growth stages in particular affect the yield formation: for example, drought at the flowering stage decreases the number of fertile florets (Rajala et al., 2009).
Effect of Crop Rotation on Weeds
Weeds grow best in the presence of crops with the same growth requirements (Liebman and Dyck, 1993). In our trial, the weed population was greatest in a diverse crop rotation, with residual effects from previous weed management that may persist for up to 2 years depending on the rotation (Légère and Stevenson, 2002). The main reason for the increased weed density in the more diverse crop rotation was the lack of effective weed control methods in turnip in the no-tillage system. During the implementation of the experiment, wheat monoculture had effective herbicide treatment every year while in CR2 and CR4 there was no herbicide available for turnip rape in the no-tillage system. The weed control in field pea with the active ingredients available (bentazone and aclonifen) was not as effective as weed control in wheat. Crop rotation may decrease the density of perennial weeds compared with monoculture (Chamanabad et al., 2009), but in our trial the efficacy could not be measured because of the glyphosate treatments in spring before sowing or in autumn after the harvest.
The most common weeds in the current trial, G. spurium, L. purpureum, S. media, F. officinalis, and T. inodorum, represent the typical weeds growing on Finnish cereal fields (Salonen et al., 2011). In monoculture plots, the mean weed density before herbicide treatment was 114 weeds/m2 and it was double in CR4 plots while the average weed density in Finnish spring cereal fields is 160 weeds/m2 (Salonen et al., 2011). The phenomenon of higher weed density in CR2 and CR4 compared with CR1 was visible already at Period1, and especially in no-tillage plots. Liebman and Dyck (1993) reported contrasting findings in their literature survey where in 21 cases out of 27 there were fewer weeds in crop rotations than in monoculture. In several studies, crop rotation is reported to be an effective tool to control weeds (Mertens et al., 2002; Zawislak and Kostrzewska, 2005; Fonteyne et al., 2020). However, Bárberi and Cascio (2001) reported that crop rotation only slightly decreased major weed species abundance when tillage had a large negative influence on weed seedbank size, as was the case in our study where plowing was a more effective tool to control weeds than crop rotation.
Effect of Crop Rotation on Plant Diseases
According to Kennedy and Huseth (2020), pest and pathogen infestations are associated with phylogenetic distance of crop plants and related native plants. In our trial, wheat leaf blotch disease damage was greatest in spring wheat monoculture and the differences were more significant in no-tillage than in plowed plots. The most common leaf spot disease in the trial was tan spot, even though in more humid years stagonospora blotch was detected on the upper leaves of the crop. The most significant source of tan spot infection is plant debris from the previous growing season, in which case the crop rotation plays the greatest role in its control (Krupinsky et al., 2002). Moreover, no-tillage is a major factor increasing the severity of tan spot compared with plowing (Jørgensen and Olsen, 2007), as observed in our trial also. On farmers' fields and on larger plots, the differences in final leaf blotch disease level might even be larger. Tan spot development is also affected by weather conditions during the growing season (Jørgensen et al., 2020), and in our trial the infection pressure was most severe in 2011, which was the season with high precipitation and an effective temperature sum above the long-term average.
The severity of stem and root diseases remained low in the trial. On average, the disease severity was slightly higher in a no-tillage compared with a plowed environment. The stem and root diseases were mainly caused by F. culmorum and F. avenaceum species. No take-all or Rhizoctonia root rot (Rhizoctonia solani) was detected in the trial area. The effects of crop rotation were visible 6 years after the establishment of the trial. The root and stem disease indexes were highest in wheat monoculture and lowest in CR4, both in plowed and no-tillage environments. This observation is supported by Palojärvi et al. (2020), who demonstrated the effect of tillage system and crop rotation on soil microbial communities and the general disease suppression. There is no known cultivar resistance to stem and root diseases in Nordic spring wheat cultivars and the possibilities for chemical control are limited. Therefore, the importance of crop rotation and tillage methods, and their interaction, is emphasized. Effective weed control and straw waste treatments also reduce the risk (Paulitz, 2006).
Effect of Crop Rotation on Wheat Midges
Wheat midges are important pests of spring wheat: infestations of 30, 60, and 90% reduce the yield of spring wheat by 40–65 and 79%, respectively (Olfert et al., 1985). Considered as a whole, crop rotation and tillage had no effect on wheat midge damage in the current study. However, the amount of seeds damaged by wheat midge differed (CR1>CR4) in an individual year. In Finland, risk of orange wheat blossom midge is concentrated in the main cropping areas of wheat, where barley and oats are also commonly cropped. Because pupae of wheat midges survive in soil for several years, high frequency of wheat cropping in combination with no-tillage can increase the risk of damage. For example, Elliot et al. (2002) reported that a population of wheat midge was 2.2 times higher in no-tillage conditions than in tilled systems. However, the coincidence of midge flight peak and ear emergence of wheat and favorable egg-laying conditions, are important factors, and the risk varies widely annually.
Overall, only if insect species are host-specific and their dispersal ability is low, is crop rotation a powerful tool to control them (Coaker, 1987). However, reliable estimation of the effect of crop rotation on mobile insect pests at the scale of experimental plots is difficult to verify and larger plots are needed. Furthermore, in the case of insect pests, an area-wide pest management system and crop rotation on a regional scale is often needed (Chandler and Faust, 1998; Sexon and Wyman, 2005; Huusela-Veistola and Jauhiainen, 2006).
Crop Rotation Strategy
Considering crop rotation as a strategy to support several agroecosystem functions or services (e.g., Duru et al., 2015; Wozniak, 2019), our results suggest that higher yields were mostly accompanied by beneficial belowground interactions such as improved nutrient dynamics and soil functions over the rotational cycles. A recent meta-analysis on impacts of agricultural diversification on yield and other ecosystem services concluded that crop diversification had mostly a positive impact on soil fertility, crop yield, nutrient cycling and pest control (Tamburini et al., 2020). However, for yield in particular, high context specificity was established. In the same analysis, reduced tillage had a predominantly positive impact on soil fertility and nutrient cycling, a neutral or negative impact on yield and climate and water regulation, and a negative impact on pest control (Tamburini et al., 2020).
The positive effect of crop rotation on crop nutrient uptake (Venkatesh et al., 2017) was also verified in our study: spring wheat had better N and P uptake in CR2 and CR4 than in CR1 with both tillage conditions, the effect being even higher in no-tillage conditions as also reported by Malhi and Lemke (2007). Higher nutrient balance increases the risk of nutrient leaching (Uusitalo et al., 2015; Turtola et al., 2017) which can be prevented with proper crop rotation (Kayser et al., 2010), tillage method (Wang et al., 2015) and successful crop protection (Delin et al., 2008; Kauppi et al., 2021).
Crop rotation planning for northern agriculture involves considering multiple objectives, including suitability for a particular farm and locality, and the economic benefits (Peltonen-Sainio et al., 2019). Both crop diversification and reduced soil tillage represent potential efforts toward more agrobiodiverse and climate-resilient agriculture (Altieri et al., 2015). One challenge in using these strategies is the potential trade-off between high yield and biodiversity and soil preservation (e.g., Pittelkow et al., 2015), which was also evidenced here by the reduction in yield in the no-tillage treatment compared with plowed spring wheat. However, the results showed that using crop rotation in no-tillage systems can help to solve this problem, at least partly.
Conclusions
Long-term experiments are valuable for assessing the impacts of crop rotation cycle on production and environmental benefits, as well as potential constraints from diversification. Such information is essential when designing more resilient cropping systems for northern agriculture. Based on our results, there are yield gains from diverse crop rotations that include cereals, oilseeds, and legumes in northern spring wheat production, with the magnitude being larger in reduced tillage systems. Assessing the yield quantity and quality, presence of weeds, plant diseases and insect pests as a whole, can contribute to understanding aspects of temporal diversification on cropping system performance in the long run. To get the best output from crop diversification in cereal production, particular attention should be paid to the health of each plant species in the crop rotation, especially if the pest control effects of the soil tillage are excluded.
Data Availability Statement
The original contributions presented in the study are included in the article/Supplementary Material, further inquiries can be directed to the corresponding author/s.
Author Contributions
LJ, EH, and HJ designed the experiment. MJ, EH, and HJ conducted the experiments and contributed to field data collections. LJ and MN analyzed the data. All authors prepared the manuscript, participated in the writing process, and approved the manuscript before its submission.
Funding
The work has been funded by Ministry of Agriculture and Forestry of Finland (Makera) grants, by the Finnish Cultural Foundation through Shared waters project (2019–2021), and by Luke's (previously MTT Agrifood Research Finland) own funding.
Conflict of Interest
The authors declare that the research was conducted in the absence of any commercial or financial relationships that could be construed as a potential conflict of interest.
Acknowledgments
We warmly acknowledge the technical staff at Luke Jokioinen for their valuable and skilled assistance in the field, laboratory and data processing. Special thanks are given to Niko Jalava, Matti Eskola, Anne Muotila, Leena Ruokonen, Auli Kedonperä, Jaana Grahn, Päivi Koski, and Marjo Segerstedt. Senior scientist Päivi Parikka is acknowledged for Fusarium species identifications and Jonathan Robinson for language revision.
Supplementary Material
The Supplementary Material for this article can be found online at: https://www.frontiersin.org/articles/10.3389/fsufs.2021.647335/full#supplementary-material
References
Altieri, M. A., Nicholls, C. I., Henao, A., and Lana, M. A. (2015). Agroecology and the design of climate change -resilient farming systems. Agron. Sustain. Dev. 35, 869–890. doi: 10.1007/s13593-015-0285-2
Andersen, A. (1999). Plant protection in spring cereal production with reduced tillage. II. Pests and beneficial insects. Crop. Prot. 18, 651–657. doi: 10.1016/S0261-2194(99)00071-X
Babublicová, M. (2016). Enhancing of winter wheat productivity by the introduction of field pea into crop rotation. Agriculture 62, 101–110. doi: 10.1515/agri-2016-0011
Bailey, K. L., Gossen, B. D., Lafond, G. P., Watson, P. R., and Derksen, D. A. (2001). Effect of tillage and crop rotation on root and foliar diseases of wheat and pea in Saskatchewan from 1991 to 1998: univariate and multivariate analyses. Can. J. Plant Sci. 81, 789–803. doi: 10.4141/P00-152
Bárberi, P., and Cascio, B. L. (2001). Long-term tillage and crop rotation effects on weed seedbank size and composition. Weed Res. 41, 325–340. doi: 10.1046/j.1365-3180.2001.00241.x
Barbieri, P., Pellerin, S., and Nesme, T. (2017). Comparing crop rotations between organic and conventional farming. Sci. Rep. 7:13761. doi: 10.1038/s41598-017-14271-6
Berzsenyi, Z., and Gyorffy, B. (1997). Effect of crop-rotation and fertilization on wheat yields and yield stability in long-term experiments. Novenytermeles 46, 145–161.
Berzsenyi, Z., Gyorffy, B., and Lap, D. (2000). Effect of crop rotation and fertilization on maize and wheat yields and yield stability in a long-term experiment. Eur. J. Agron. 13, 225–244. doi: 10.1016/S1161-0301(00)00076-9
Bleiholder, H., Feller, C., Hess, M., Meier, U., van den Boom, T., Lancashire, P. D., et al. (1997). Compendium of Growth Stage Identification Keys for Mono- and Dicotyledonous Plants-Extended BBCH Scale, eds M. Enz and Ch. Dachler. Novartis.
Bockus, W. W. (1992). Effects of crop rotation and residue management practices on severity of tan spot of winter wheat. Plant Dis. 76, 633–636. doi: 10.1094/PD-76-0633
Bommarco, R., Kleijn, D., and Potts, S. G. (2013). Ecological intensification: harnessing ecosystem services for food security. Trends Ecol. Evol. 28, 230–238. doi: 10.1016/j.tree.2012.10.012
Bullock, D. G. (1992). Crop rotation. Crit. Rev. Plant Sci. 11, 309–326. doi: 10.1080/07352689209382349
Chamanabad, H. R. M., Ghorbani, A., Asghari, A., Tulikov, A. M., and Zargarzadeh, F. (2009). Long-term effects of crop rotation and fertilizers on weed community in spring barley. Turk. J. Agric. For. 33, 315–323. doi: 10.3906/tar-0712-47
Chan, K. Y., and Heenan, D. P. (1996). The influence of crop rotation on soil structure and soil physical properties under conventional tillage. Soil Tillage Res. 37, 113–125. doi: 10.1016/0167-1987(96)01008-2
Chandler, L. D., and Faust, R. M. (1998). Overview of area-wide management of insects. J. Agric. Urban Entomol. 15, 319–325.
Coaker, T. H. (1987). “Cultural methods: the crop,” in Integrated Pest Management, eds A. J. Burn, T. J. Coaker, and P. C. Jepson (London: Academic Press), 69–88.
Delin, S., Nyberg, A., Lindén, B., Ferm, M., Torstensson, G., Lerenius, C., et al. (2008). Impact of crop protection on nitrogen utilisation and losses in winter wheat production. Eur. J Agron. 28, 361–370. doi: 10.1016/j.eja.2007.11.002
Di, H. J., and Cameron, K. C. (2002). Nitrate leaching in temperate agroecosystems: sources, factors and mitigating strategies. Nutr. Cycling Agroecosyst. 46, 237–256. doi: 10.1023/A:1021471531188
Duru, M., Therond, O., Martin, G., Martin-Clouaire, R., Magne, M. A., Justes, E., et al. (2015). How to implement biodiversity-based agriculture to enhance ecosystem services: a review. Agron. Sustain. Dev. 35, 1259–1281. doi: 10.1007/s13593-015-0306-1
Elliot, B., Mann, L., Braun, M., and Olfert, O. (2002). Integrated Management of Crop Pests–Abundance of Wheat Midge and Its Parasite in Different Management Systems. Soils and Crops Workshop. Available online at: http://hdl.handle.net/10388/9735 (accessed December 28, 2020).
EU (2009). Establishing a Framework for Community Action to Achieve the Sustainable Use of Pesticides. Available online at https://eur-lex.europa.eu/legal-content/EN/ALL/?uri=celex%3A32009L0128 (accessed November 19, 2020).
EU (2020a). Biodiversity Strategy for 2030. Available online at https://eur-lex.europa.eu/legal-content/EN/TXT/?qid=1590574123338&uri=CELEX:52020DC0380 (Accessed November 19, 2020).
EU (2020b). The Common Agricultural Policy at a Glance: The Common Agricultural Policy Supports Farmers and Ensures Europe's Food Security. Available online at https://ec.europa.eu/info/food-farming-fisheries/key-policies/common-agricultural-policy/cap-glance_en (Accessed December 28, 2020).
Fonteyne, S., Singh, R. G., Goaverts, B., and Verhulst, N. (2020). Rotation, mulch and zero tillage reduce weeds in a long-term conservation agriculture trial. Agronomy 10:962. doi: 10.3390/agronomy10070962
Galantini, J. A., Landriscini, M. R., Iglesias, J. O., Miglierina, A. M., and Rosell, R. A. (2000). The effects of crop rotation and fertilization on wheat productivity in the Pampean semiarid region of Argentina: 2. Nutrient balance, yield and grain quality. Soil Tillage Res. 53, 137–144. doi: 10.1016/S0167-1987(99)00097-5
Govaerts, B., Mezzalama, M., Unno, Y., Sayre, K. D., Luna-Guido, M., Vanherck, K., et al. (2007). Influence of tillage, residue management, and crop rotation on soil microbial biomass and catabolic diversity. Appl. Soil Ecol. 37, 18–30. doi: 10.1016/j.apsoil.2007.03.006
Hakala, K., Hannukkala, A., Huusela-Veistola, E., Jalli, M., and Peltonen-Sainio, P. (2011). Pests and diseases in a changing climate a major challenge for Finnish crop production. Agric. Food Sci. 20, 3–14. doi: 10.2137/145960611795163042
Huusela-Veistola, E., and Jauhiainen, L. (2006). Expansion of pea cropping increases the risk of pea moth (Cydia nigricana; Lep., Tortricidae) infestation. J. Appl. Entomol. 130, 142–149. doi: 10.1111/j.1439-0418.2006.01047.x
Jalli, M., Kaseva, J., Andersson, B., Ficke, F., Nistrup-Jørgensen, L., Ronis, A., et al. (2020). Yield increases due to fungicide control of leaf blotch diseases in wheat and barley as a basis for IPM decision-making in the Nordic-Baltic region. Eur. J. Plant Pathol. 158, 315–333. doi: 10.1007/s10658-020-02075-w
Jalli, M., Laitinen, P., and Latvala, S. (2011). The emergence of cereal fungal diseases and the incidence of leaf spot diseases in Finland. Agric. Food Sci. 20, 67–73. doi: 10.2137/145960611795163015
Jørgensen, L. N., Matzen, N., Ficke, A., Nielsen, G. C., Jalli, M., Ronis, A., et al. (2020). Validation of risk models for control of leaf blotch diseases in wheat in the Nordic and Baltic countries. Eur. J. Plant Pathol. 157, 599–613. doi: 10.1007/s10658-020-02025-6
Jørgensen, L. N., and Olsen, L. V. (2007). Control of tan spot (Drechslera tritici-repentis) using cultivar resistance, tillage methods and fungicides. Crop Prot. 26, 1606–1616. doi: 10.1016/j.cropro.2007.01.009
Kauppi, K., Rajala, A., Huusela-Veistola, E., Kaseva, J., Ruuttunen, P., Jalli, H., et al. (2021). Impact of pests on cereal grain and nutrient yield in Boreal growing conditions. Agronomy 11:592. doi: 10.3390/agronomy11030592
Kayser, M., Müller, J., and Isselstein, J. (2010). Nitrogen management in organic farming: comparison of crop rotation residual effects on yields, N leaching and soil conditions. Nutr. Cycl. Agroecosyst. 87, 21–31. doi: 10.1007/s10705-009-9309-0
Keesing, F., Belden, L. K., Daszac, P., Dobson, A., Harvell, D., Holt, R. D., et al. (2010). Impacts of biodiversity on the emergence and transmission of infectious diseases. Nature 468, 647–652. doi: 10.1038/nature09575
Kennedy, G. G., and Huseth, A. S. (2020). Pest pressure relates to similarity of crops and native plants. Proc. Natl. Acad. Sci. U. S. A. 117:945117. doi: 10.1073/pnas.2020945117
Klein Haneveld, W. K., and Stegeman, A. W. (2005). Crop succession requirements in agricultural production planning. Eur. J. Oper. Res. 166, 406–429. doi: 10.1016/j.ejor.2004.03.009
Krupinsky, J. M., Bailey, K. L., McMullen, M. P., Gossen, B. D., and Turkington, T. K. (2002). Managing plant disease risk in diversified cropping systems. Agron. J. 94, 198–209. doi: 10.2134/agronj2002.0198
Kurppa, S. (1990). Insect Pest Damage, Predicting and Control in Finnish Cereal Cultivation During 1980's. (PhD Thesis). Faculty of Agriculture and Forestry, University of Helsinki, Helsinki, Finland, 53.
Légère, A., and Stevenson, E. C. (2002). Residual effects of crop rotation and weed management on a wheat test crop and weeds. Weed Sci. 50, 101–111. doi: 10.1614/0043-1745(2002)050[0101:REOCRA]2.0.CO;2
Liebman, M., and Dyck, E. (1993). Crop rotation and intercropping strategies for weed management. Ecol. Appl. 3, 92–122. doi: 10.2307/1941795
López-Bellido, L., López-Bellido, R., Castillo, J., and López-Bellido, F. (2001). Effects of long-term tillage, crop rotation and nitrogen fertilization on bread-making quality of hard red spring wheat. Field Crops Res. 72, 197–210. doi: 10.1016/S0378-4290(01)00177-0
Luke (2020a). Official Statistics of Finland (OSF): Utilised Agricultural Area. Helsinki: Natural Resources Institute Finland. Available online at https://stat.luke.fi/en/utilised-agricultural-area (accessed November 21, 2020).
Luke (2020b). Official Statistics of Finland (OSF): Feed Tables. Helsinki: Natural Resources Institute Finland. Available online at https://portal.mtt.fi/portal/page/portal/Rehutaulukot/feed_tables_english (accessed November 26, 2020).
Luke (2020c). Official Statistics of Finland (OSF): Yield of the Main Crop. Helsinki: Natural Resources Institute Finland. Available at http://statdb.luke.fi/PXWeb/pxweb/en/LUKE/LUKE__02%20Maatalous__04%20Tuotanto__14%20Satotilasto/01_Viljelykasvien_sato.px/table/tableViewLayout1/?rxid=69c91bf6-f37a-4386-97bb-3c510b782811 (accessed November 16, 2020).
Malhi, S. S., and Lemke, R. (2007). Tillage, crop residue and N fertilizer effects on crop yield, nutrient uptake, soil quality and nitrous oxide gas emissions in a second 4-yr rotation cycle. Soil Tillage Res. 96, 269–283. doi: 10.1016/j.still.2007.06.011
Mamolos, A. P., and Kalburtji, K. L. (2001). Significance of allelopathy in crop rotation. J. Crop Prod. 4, 197–218. doi: 10.1300/J144v04n02_06
Meisinger, J. J., and Delgado, J. A. (2002). Principles for managing nitrogen leaching. J. Soil Water Conserv. 57, 485–498. Available online at: https://www.jswconline.org/content/jswc/57/6/485.full.pdf
Mertens, S. K., van den Bosch, F., and Heesterbeek, J. A. P. (2002). Weed populations and crop rotations: exploring dynamics of a structured periodic system. Ecol. Appl. 12, 1125–1141. doi: 10.1890/1051-0761(2002)012[1125:WPACRE]2.0.CO;2
Metzger, M. J., Bunce, R. G. H., Jongman, R. H. G., Mücher, C. A., and Watkins, J. W. (2005). A climatic stratification of the environment of Europe. Glob. Ecol. Biogeogr. 14, 549–563. doi: 10.1111/j.1466-822X.2005.00190.x
Olfert, O. O., Mukerji, M. K., and Doane, J. F. (1985). Relationship between infestations levels and yield loss caused by wheat midge, Sitodiplosis mosellana (Géhin) (Diptera: Cecidomyiidae), in spring wheat in Saskatchewani. Can. Entomol. 117, 593–598. doi: 10.4039/Ent117593-5
Palojärvi, A., Kellock, M., Parikka, P., Jauhiainen, L., and Alakukku, L. (2020). Tillage system and crop sequence affect soil disease suppressiveness and carbon status in Boreal climate. Front. Microbiol. 2020:534786. doi: 10.3389/fmicb.2020.534786
Paulitz, T. C. (2006). Low input no-till production in the Pacific Northwest of the U.S.: the challenges of root diseases. Eur. J. Plant Pathol. 115, 271–281. doi: 10.1007/s10658-006-9023-6
Paulitz, T. C., Schroeder, K. L., and Schillinger, W. F. (2010). Soilborne pathogens of cereals in an irrigated cropping system: effects of tillage, residue management, and crop Rotation. Plant Dis. 94, 61–68. doi: 10.1094/PDIS-94-1-0061
Paustian, K., Lehmann, J., Ogle, S., Reay, D., Robertson, G. P., and Smith, P. (2016). Climate-smart soils. Nature 532, 49–57. doi: 10.1038/nature17174
Peltonen-Sainio, P. (2012). “Crop production in a northern climate,” in Proceedings of a Joint FAO/OECD Workshop, Building Resilience to Climate Change in the Agriculture Sector, eds A. Meybeck, J. Lankoski, S. Redfern, N. Azzu, and V. Gitz, 183–216. Available online at: http://www.fao.org/agriculture/crops/news-events-bulletins/detail/en/item/134976/ (accessed December 28, 2020).
Peltonen-Sainio, P., and Jauhiainen, L. (2019). Unexploited potential to diversify monotonous crop sequencing at high latitudes. Agric. Syst. 174, 73–82. doi: 10.1016/j.agsy.2019.04.011
Peltonen-Sainio, P., Jauhiainen, L., Honkavaara, E., Wittke, S., Karjalainen, M., and Puttonen, E. (2019). Pre-crop values from satellite images for various previous and subsequent crop combinations. Front. Plant Sci. 10:462. doi: 10.3389/fpls.2019.00462
Peltonen-Sainio, P., Kangas, A., Salo, Y., and Jauhiainen, L. (2007). Grain number dominates grain weight in temperate cereal yield determination: evidence based on 30 years of multi-location trials. Field Crops Res. 100, 179–188. doi: 10.1016/j.fcr.2006.07.002
Pittelkow, C. M., Liang, X., Linquist, B. A., van Groenigen, K. J., Lee, J., Lundy, M. E., et al. (2015). Productivity limits and potentials of the principles of conservation agriculture. Nature 517, 365–368. doi: 10.1038/nature13809
Rajala, A., Hakala, K., Mäkelä, P., Muurinen, S., and Peltonen-Sainio, P. (2009). Spring wheat response to timing of water deficit through sink and grain filling capacity. Field Crops Res. 114, 263–271. doi: 10.1016/j.fcr.2009.08.007
Ratnadass, A., Fernandes, P., Avelino, J., and Habib, R. (2012). Plant species diversity for sustainable management of crop pests and diseases in agroecosystems: a review. Agron. Sustain. Dev. 32, 273–303. doi: 10.1007/s13593-011-0022-4
Salonen, J., Hyvönen, T., and Jalli, H. (2011). Composition of weed flora in spring cereals in Finland - a fourth survey. Agric. Food Sci. 20, 245–261. doi: 10.2137/145960611797471534
Sexon, D. L., and Wyman, J. A. (2005). Effect of crop rotation distance in populations of Colorado potato beetle (Coleoptera: Chrysomelidae): development of area wide Colorado potato beetle pest management strategies. J. Econ. Entomol. 98, 716–724. doi: 10.1603/0022-0493-98.3.716
Sieling, K., and Christen, O. (2015). Crop rotation effects on yield of oilseed rape, wheat and barley and residual effects on the subsequent wheat. Arch. Agron. Soil Sci. 61, 1531–1549. doi: 10.1080/03650340.2015.1017569
Sieling, K., Stahl, C., Winkelmann, C., and Christen, O. (2005). Growth and yield of winter wheat in the first 3 years of a monoculture under varying N fertilization in NW Germany. Eur. J. Agron. 22, 71–84. doi: 10.1016/j.eja.2003.12.004
Skellern, M. P., and Cook, S. M. (2018). The potential of crop management practices to reduce pollen beetle damage in oilseed rape. Arthropod Plant Interact. 12, 867–879. doi: 10.1007/s11829-017-9571-z
Smith, R. G., Gross, K. L., and Robertson, G. P. (2008). Effects of crop diversity on agroecosystem function: crop yield response. Ecosystems 11, 355–366. doi: 10.1007/s10021-008-9124-5
Soon, Y. K., and Clayton, G. W. (2002). Eight years of crop rotation and tillage effects on crop production and N fertilizer use. Can. J. Soil Sci. 82, 165–172. doi: 10.4141/S01-047
Stevenson, F. C., and van Kessel, C. (1996). The nitrogen and non-nitrogen rotation benefits of pea to succeeding crops. Can. J. Plant Sci. 76, 735–745. doi: 10.4141/cjps96-126
Stinner, B. R., and House, G. J. (1990). Arthropods and other invertebrates in conservation-tillage agriculture. Annu. Rev. Entomol. 35, 299–318. doi: 10.1146/annurev.en.35.010190.001503
Tamburini, G., Bommarco, R., Wanger, T. C., Kremen, C., van der Heijden, M. G. A., Liebman, M., et al. (2020). Agricultural diversification promotes multiple ecosystem services without compromising yield. Sci. Adv. 6:aba1715. doi: 10.1126/sciadv.aba1715
Trnka, M., Olesen, J. E., Kersebaum, K. C., Skjelvåg, A. O., Eitzinger, J., Seguin, B., et al. (2011). Agroclimatic conditions in Europe under climate change. Glob. Change Biol. 17, 2298–2318. doi: 10.1111/j.1365-2486.2011.02396.x
Turtola, E., Salo, T., Miettinen, A., Iho, A., Valkama, E., Rankinen, K., et al. (2017). Hyötyä taseista-Ravinnetaseiden tulkinta ympäristön ja viljelyn hyödyksi. Luonnonvara- ja biotalouden tutkimus 15/2017. Helsinki: Luonnonvarakeskus.
Uusitalo, R., Närvänen, A., Kaseva, A., Launto-Tiuttu, A., Heikkinen, J., Joki-Hieskala, P., et al. (2015). Conversion of dissolved phosphorus in runoff by ferric sulfate to a form less available to algae: field performance and cost assessment. AMBIO 44, 286–296. doi: 10.1007/s13280-014-0622-8
Vasileiadis, V. P., Sattin, M., Otto, S., Veres, A., Pálinkás, Z., Ban, R., et al. (2011). Crop protection in European maize-based cropping systems: current practices and recommendations for innovative Integrated Pest Management. Agric. Syst. 104, 533–540. doi: 10.1016/j.agsy.2011.04.002
Venkatesh, M. S., Hazra, K. K., Ghosh, P. K., Khuswah, B. L., Ganeshamurthy, A. N., Ali, M., et al. (2017). Long–term effect of crop rotation and nutrient management on soil–plant nutrient cycling and nutrient budgeting in Indo-Gangetic plains of India. Arch. Agron. Soil Sci. 63, 2007–2022. doi: 10.1080/03650340.2017.1320392
Wang, H., Guo, Z., Shi, Y., Zhang, Y., and Yu, Z. (2015). Impact of tillage practices on nitrogen accumulation and translocation in wheat and soil nitrate-nitrogen leaching in drylands. Soil Tillage Res. 153, 20–27. doi: 10.1016/j.still.2015.03.006
Wozniak, A. (2019). Effect of crop rotation and cereal monoculture on the yield and quality of winter wheat grain and on crop infestation with weeds and soil properties. Int. J. Plant Prod. 13, 177–182. doi: 10.1007/s42106-019-00044-w
Wozniak, A. (2020). Effect of cereal monoculture and tillage systems on grain yield and weed infestation of winter durum wheat. Int. J. Plant Prod. 14, 1–8. doi: 10.1007/s42106-019-00062-8
Keywords: Triticum aestivum, crop sequence, biodiversity, plant protection, weeds, insects, plant diseases, no-tillage
Citation: Jalli M, Huusela E, Jalli H, Kauppi K, Niemi M, Himanen S and Jauhiainen L (2021) Effects of Crop Rotation on Spring Wheat Yield and Pest Occurrence in Different Tillage Systems: A Multi-Year Experiment in Finnish Growing Conditions. Front. Sustain. Food Syst. 5:647335. doi: 10.3389/fsufs.2021.647335
Received: 29 December 2020; Accepted: 31 May 2021;
Published: 05 July 2021.
Edited by:
Adrian Unc, Memorial University of Newfoundland, CanadaReviewed by:
Hao Wang, Northwest A and F University, ChinaMary Barbercheck, Pennsylvania State University (PSU), United States
Copyright © 2021 Jalli, Huusela, Jalli, Kauppi, Niemi, Himanen and Jauhiainen. This is an open-access article distributed under the terms of the Creative Commons Attribution License (CC BY). The use, distribution or reproduction in other forums is permitted, provided the original author(s) and the copyright owner(s) are credited and that the original publication in this journal is cited, in accordance with accepted academic practice. No use, distribution or reproduction is permitted which does not comply with these terms.
*Correspondence: Marja Jalli, bWFyamEuamFsbGlAbHVrZS5maQ==