- 1Department of Food Science and Technology, University of Patras, Agrinio, Greece
- 2Department of Material Science and Engineering, University of Ioannina, Ioannina, Greece
- 3Laboratory of Food Chemistry, Department of Chemistry, National and Kapodistrian University of Athens Zografou, Athens, Greece
Introduction: Nowadays, the bioeconomy and sustainability trends drive the food sector to replace chemical preservatives with bioactive compounds recovered from biomass such as essential oils (EOs) and their derivatives. Moreover, nanotechnology trend drives food sector in the nanoencapsulation of such bioactive compounds in novel edible formulations such as nanoemulsions to enhance their controlled release properties, their bioactivity and their biocompatibility.
Methods: In this study the development and characterization of novel casein/lecithin (CSN/LCN) based nanoemulsions (NEs) with carvacrol (CV), cinnamaldehyde (CI), citral (CT), and eugenol (EG) as nanoencapsulated oil phase is presented as well as the application of such NEs as active coatings for the preservation of fresh pork tenderloin.
Results and discussion: DLS measurements as well as TEM images showed an average particle size distribution of 20–40 nm for all obtained NEs, while z-potential results indicate their physicochemical stability. All the obtained NEs needed at least 5 times less amount of EOs derivatives to scavenge 50% of radicals and demonstrated a higher antibacterial activity against Escherichia coli and Listeria monocytogenes compared to free EOs derivatives. Pork fresh tenderloin meat treated with NEs demonstrated 30% higher efficacy in obtained lipid oxidation values than uncoated pork meat samples and 12% higher efficacy in obtained lipid oxidation values than pork meat samples treated with EOs derivatives. From a microbiological point of view NEs manage to extend pork tenderloin meat shelf life by 6 days compared to uncoated pork tenderloin meat and by 3 days compared to tenderloin pork meat coated with free EOs derivatives. Lab* colorimetry analysis as well as sensory analysis revealed that such edible CSN/LCN based NEs succeeded to preserve the texture, appearance, and color of pork tenderloin meat in higher acceptance limits compared to the corresponding characteristics of pork tenderloin meat coated with free EOs derivatives. Overall, the current study suggests novel CSN/LCN-based edible NEs as a promising technology that can be used as edible active coatings in the meat food industry.
1 Introduction
The demand for extending food shelf-life and spoilage prevention has increasingly led the field of food technology to alter their scientific interest to innovative antimicrobial and antioxidant systems which are able to support the food preservation (Pavoni et al., 2020). Concurrently, the growing emphasis on sustainability and the principle of the circular economy is steering the shift away from synthetic food additives towards the use of naturally abundant antimicrobial and antioxidant agents. These natural agents are key to preventing spoilage and extending food shelf life by inhibiting harmful microorganisms, aligning food safety with environmental values. Reflecting this trend, the worldwide demand for essential oils (EOs) reached 247 kilotons. According to Grand View Research (2024), the EO market is projected to grow at an annual rate of 7.5% until 2027, primarily due to the increasing popularity of natural product consumption according to. EOs and their derivatives can be used with meat and meat products, and several EOs have been deemed safe for consumption with a Generally Regarded as Safe (GRAS) status by the Food and Drug Administration (FDA) (Cohen et al., 2021). Based on their chemical composition, EOs can slow down oxidative reactions, prevent the formation of free radicals, and inhibit microbial growth in both in vitro and in vivo food preservation tests. This suggests that EOs have the potential to be used as partial or complete alternatives to conventional chemical preservatives (da Silva et al., 2022).
EOs are derived from various plant species and their structural configuration constitutes has made them known for their antimicrobial properties. The main metabolites of EOs include terpenes, phenylpropanoids, aldehydes, esters, alcohols, and ketones (Bhavaniramya et al., 2019). There is no specific mechanism of action in microbial cells due to the diversity of chemical constituents. However, one of the most reported mechanisms involves increased cell membrane permeability, which can lead to leakage of cytoplasmic content and cell death (Burt, 2004). Studies have shown that EOs and their derivatives can increase electrolyte loss in Aspergillus flavus, increase extracellular nucleic acid levels in viable Escherichia coli and Staphylococcus aureus cells, and reduce the enzymatic activity of ATPase (Kedia et al., 2015). EOs can also inhibit DNA transcription and translation for protein synthesis, which influences bacterial DNA replication (Xu et al., 2016).
Specifically, monoterpenoid derivatives of EOs such as citral (C10H16O), carvacrol (C10H14O) and phenylpropenes including eugenol (C10H12O2), and cinnamaldehyde (C9H8O) constitute potent bioactive compounds, each exhibiting unique well documented antioxidant and antimicrobial properties (Baschieri et al., 2017; Gao et al., 2020; Cao et al., 2021). Citral, is characterized as a linear monoterpene aldehyde, which constitutes the major component of lemongrass EOs, accounting for over 85% of their composition. Citral’s source is citrus fruits such as limes and lemons and is a combination of neral and geranial isomers. The FDA has given it GRAS status, making it a popular citrus-based flavoring agent in consumer products (Gutiérrez-Pacheco et al., 2023). Furthermore, eugenol, the major component of clove oil, is metabolized into glucuronides and sulphates and because of the damage is caused in the microbial cell walls and membranes it is considered as a valuable antimicrobial agent (Goñi et al., 2016; Gutiérrez-Pacheco et al., 2023). Eugenol it is also considered as GRAS (Gooderham et al., 2020). The main sources of carvacrol are oregano and thyme, and during the metabolic pathway, hydroxycarvacrols occur, targeting bacterial cell membranes and ATPase activities, along with their capacity to donate hydrogen atoms to stabilize free radicals (Imran et al., 2022). Carvacrol is also considered as GRAS (Magiet al 2015). On the other hand, the main component of cinnamon bark oil is cinnamaldehyde, which metabolizes into cinnamic acid and its antimicrobial efficacy is achieved by disrupting microbial cell functions and providing electron donation to reduce oxidative stress (Mehta et al., 2015). Cinnamaldehyde is also considered as GRAS (Adams et al., 2004).
Recently, EOs derivatives such as thymol, carvacrol (CV), citral (CT), eugenol (E.G.,) cinnamaldehyde (CI) have been widely investigated for their antimicrobial and antioxidant effects in food preservation (Falleh et al., 2020; Angane et al., 2022). Indeed, numerous studies have indicated that CV nanoemulsions (ΝΕs) have a stronger effect against several food related pathogens when they are nanoencapsulated (Cardoso et al., 2023; Felício et al., 2020). In their study, Song et al. (2024) indicated that CT NEs in 0.15 mg/mL in combination with ultrasound, was able to decrease the number of Sh. flexneri by 8.55 logCFU/mL while, Luciano et al. (2023) provided promising results as their CI NE in 0.6 μL/mL was able to demonstrate bactericidal effects against Listeria monocytogenes and improved the sensory properties of fresh-cut melon and papaya during 7 days storage. Badr, Badawy, and Taktak (2022) in their study reported that CT NEs outperformed in terms of antioxidant and antibacterial effects against Salmonella typhimurium and S. aureus. Finally in accordance with (Fu et al., 2022), nanoformulated, E.G., even at a concentration of 0.02 mg/μL-1, strong effects were observed against E. coli and S aureus.
On the other hand, the composition of meat nutrients, its high water activity (aw) levels and its high pH levels provide a great environment for the microbial proliferation. Even when processing techniques are applied to eliminate microbial counts, factors like storage temperature and oxygen exposure may significantly affect microbial growth (Doulgeraki et al., 2012). Thus, EOs and their derivatives are suggested as potential antimicrobial agents in meat and meat products (Jayasena and Jo 2013; Ojeda-Piedra et al., 2022). However, the direct application of EOs in foods is not suggested due to their ability to directly affect the flavors, aromas and color, which can deteriorate the sensory characteristics of food products and make them undesirable in certain contexts. Moreover, the high concentration of bioactive compounds in EOs increases the probability and severity of occurrence of toxicity or allergic reactions, necessitating strict control over their use to ensure consumer safety (Rao, Chen, and McClements, 2019).
To tackle these obstacles, alternative methods such as nanoencapsulation have led to the synthesis of o/w NEs with an oil droplet size of less than 200 nm (Ojeda-Piedra et al., 2022). The benefits of the increased surface have led to improved antioxidant and antimicrobial properties, by simultaneously eliminating the negative aspects of free EO forms (Barradas and de Holanda e Silva, 2021). In particular, by decreasing the droplet size, the active additive becomes optically clearer, more stable, and less prone to volatility. Simultaneously, due to the structure that is formed, increased solubility and controlled release of the beneficial bioactive compounds are achieved. NEs also improve antimicrobial action by facilitating the transport of bioactive EO constituents across cell membranes and increasing the surface area of contact with specific targets. Various studies have shown that NEs, including those based on casein, have increased the shelf life of meat and meat products (Pan et al., 2014; Liao et al., 2021; Zaharioudakis et al., 2023). In particular, Stratakos and Grant (2018) explored various interventions against pathogenic E. coli in raw beef, finding that NEs containing carvacrol or thyme EOs resulted in significant bacterial reductions and progressively decreased viable numbers during refrigerated storage, suggesting potential shelf-life extension (Stratakos and Grant, 2018). Meanwhile, Liu & Liu (2020) developed thyme EO and thymol chitosan NEs (TEO-CSs and T-CSs), which not only showed strong antibacterial properties against S. aureus and E. coli but also effectively extended the shelf life of refrigerated pork to more than 6 days (Liu and Liu, 2020).
This study follows a recent study where casein (CSN) and lecithin (LCN) were shown to be promising edible and biobased surfactants and cosurfactants, respectively, for the encapsulation of EO derivatives such as CV (Zaharioudakis et al., 2023; X; Wang and Zhao, 2022). Here we present the development and characterization of novel NEs based on CSN/LCN with CV, CT, EG and CI as nanoencapsulated oil phase. In this way, the current study seeks to establish CSN as a novel biobased surfactant and LCN as a novel biobased co-surfactant for formulating edible NEs, thereby enhancing their stability and biocompatibility. Moreover, the present research deals with the application of such CSN/LCN based NEs with CV, CT, EG and CI nanoencapsulated oil phase as novel edible active coatings to extend the shelf life of pork tenderloin. Specific innovative points of the current study are: To the best of our knowledge the development and characterization of such CV- CSN/LCN, EG- CSN/LCN, CI- CSN/LCN, CT- CSN/LCN NEs, as well as the application of such NEs as active edible coatings in preservation of fresh tenderloin pork meat, are reported for the first time in the world literature.
2 Materials and methods
2.1 Materials
Cinnamaldehyde (CAS No. 14371-10-9), citral (CAS No. 5392-40-5), eugenol (CAS No. 97-53-0), carvacrol (CAS No. 499-75-2) sodium caseinate (CAS No. 9005-46-3), L-α-lecithin, soybean (CAS No. 8002-43-5), were purchased from Sigma-Aldrich Co. (3050 Spruce Street, St. Louis, MO 63103, United States; Tel.: 314-771-5765).
Müeller–Hinton agar plates, Müeller–Hinton broth, Tryptic Soy Agar (TSA), and sterile swabs and forceps were also acquired from Sigma-Aldrich Co. (3050 Spruce Street, St. Louis, MO 63103, United States; Tel.: 314-771-5765). Pure Gram-positive bacterial cul-tures of Listeria monocytogenes and E. coli were obtained from the Institute of Technology of Agricultural Products, ELGO-DEMETER, Lykovryssi, Greece.
2.2 Preparation of NE samples
In Table 1, the components and quantities utilized for the formulation of NEs containing CV, CI, CT, EG, as well as their code names, are presented.
The NEs coatings containing 2.5% of each derivative—carvacrol (CV), cinnamaldehyde (CI), citral (CT), and eugenol (EG)—were prepared by mixing the designated quantities (as outlined in Table 1) of distilled water, lecithin, casein, along with each EO, employing a high-speed ultrasonic homogenizer at 15,000 rpm and at ambient temperature for a duration of 20 min (Xue and Zhong, 2014) (see Supplementary Figure S1).
2.3 Physicochemical characterization of obtained NEs
2.3.1 Dynamic light scattering (DLS) measurements and contact angle measurements
Dynamic Light Scattering (DLS) analyses were performed utilizing a sophisticated dual-angle size and molecular analysis system (Zetasizer Nano ZS, Malvern, UK), functioning at a 633 nm wavelength with a helium-neon laser at the conventional 173° angle. A square-section glass cuvette facilitated these analyses. These procedures aimed to determine the solutions’ hydrodynamic diameter and polydispersity index at a controlled temperature of 37°C, employing the Einstein-Stokes equation for calculating the hydrodynamic radius (RH).
Where kB (J/K) is the Boltzmann constant, T (K) is the absolute temperature, η (N·s·m−2) is the solvent’s dynamic viscosity, and D (m2·s −1) is the diffusion coefficient. The concentration of the solutions was approximately 0.1 wt%.
Investigations into the wetting properties of various coating samples, including NECV, NECI, NECT and, NEEG, were conducted using a contact angle meter (OCA 25, DataPhysics Instruments GmbH, Germany). For these tests, solutions of specific concentrations were spin-coated on silicon wafers at conditions set to achieve a uniform thickness in the range of 40–50 nm, following initial wafer treatment with piranha solution (a mix of sulfuric acid and hydrogen peroxide at a 3:1 ratio). Measurement involved applying 4 µL deionized water droplets at a steady rate of 0.5 μL/s to the wafer surfaces. To ensure reliability, three distinct measurements were made on various sections of the same wafer, calculating an average value for the results. The consistency across measurements was confirmed by a maximum deviation of ±2°, highlighting the uniformity in film deposition achieved under the specified conditions.
2.3.2 Z-potential characterization of NEs
A NanoBrook Omni series (Brookhaven Instruments Corporation, 750 Blue Point Road, Holtsville, NY, United States 11742) size and zeta potential analyzer equipped with a solvent-resistant electrode used to determine zeta potential of the NEs. The NEs were diluted 100 times with deionized water to reduce the multiple scattering effect, and tested at 25°C with an equilibrium of 120 s.
2.3.3 Transmission electron microscope (TEM) images of NEs
The transmission electron microscope (TEM) study of samples deposited (drop cast method) on carbon coated copper grids (CF300-CU-UL, carbon square mesh, CU, 300 mesh from Electron Microscopy Science) was performed using the instrument JEM HR-2100, JEOL Ltd., Tokyo, Japan operated at 200 kV in bright-field mode.
2.4 Determination of antioxidant activity of NEs
2.4.1 Antioxidant activity of NEs with 2,2-diphenyl-1-picrylhydrazyl (DPPH) assay
The preparation of the DPPH ethanolic solution was made according to the methodology recommended by (Kechagias et al., 2023). A specific quantity of DPPH 30 ppm was solubilized in ethanol (250 mL) to make a 2.16 mM DPPH. The solution was submitted to mixing process, using vortex in the absence of light exposure, resulting in a a deep purple solution and a stable pH of approximately 7.02 ± 0.01. To maintain the stability of the solution, the solution was kept at 4°C for over 2 h before it is used for the experiment. Finally serial dilutions were necessary to be performed to create a calibration curve.
The examination of the correlation between DPPH absorbance and its concentration, was performed plotting a calibration graph. An initial ethanol-based DPPH solution was serially diluted using ethanol to prepare five different concentrations ranging from 0 to 60 mg/L (ppm). The absorbance of these diluted DPPH solutions in ethanol was then measured at wavelength λmax of 517 nm using a Jasco V-530 UV/VIS Spectrometer (Kechagias et al., 2023). After using the absorbance data collected, a linear calibration curve was constructed following a previously described method, allowing for the quantitative analysis of antioxidant capacity (Zaharioudakis et al., 2023).
The assessment of the necessary concentration for achieving 50% antioxidant effectiveness (EC50) of the newly developed NE coatings NECV, NECI, NECT, NEEG, and the pure EOs themselves, varying volumes of each coating (20, 40, 60, 80, and 100 μL) were incorporated, in triplicate, into a mixture containing 2.8 mL of a 30 ppm DPPH solution in ethanol and 0.2 mL of a sodium acetate buffer (CH3COONa·3H2O). For comparative analysis, a control sample consisting solely of the DPPH solution and the buffer was also prepared. Following a 1-h reaction period, deemed adequate for reaching equilibrium, absorbance levels were recorded at 517 nm for both the experimental and control coatings. Afterwards using a proper equation, it was possible to identify the percentage of DPPH• radical reduction at equilibrium for each coating and through this procedure the determination of the antioxidant capacity of each sample was possible.
The diminished percentage of remaining DPPH• at equilibrium inversely is correlated with the increased antioxidant efficacy of the substance being examined (Zaharioudakis et al., 2023). Following this principle, the antioxidant activity percentages derived from the coatings NECV, NECI, NECT, NEEG, were illustrated graphically against the respective volumes of coatings used. The performance of the examined coatings was evaluated using the equations derived from the graphs. Utilizing these linear equations, the EC50,DPPH values, indicating the concentration at which 50% antioxidant activity is observed, were precisely calculated for each sample. Finally, the antioxidant effectiveness and quantitative assessment of each NE was measured.
2.4.2 Antioxidant activity of o NEs with 2,2′-Azino-bis(3-ethylbenzothiazoline-6-sulfonic acid) diammonium salt (ABTS) assay
First an ABTS stock solution with 7 mM concentration was prepared by adding 900.6 mg of ABTS in 250 mL deionized water under vigorous stirring. For the preparation of 2.45 mM potassium persulfate stock solution 0.0662 g potassium persulfate was dissolved with phosphate buffer solution pH = 6.8 and diluted in volumetric flask of 100.0 mL. The preparation of ABTS solution was done by mixing an equal volume of a 7 mM ABTS stock solution with a 2.45 mM potassium persulfate solution. The mixture was then stored in the dark at room temperature for 12–16 h. The ABTS solution was diluted with 10 mmol/L phosphate-buffered saline (PBS, pH 7.4) to an absorbance of 0.70 ± 0.02 at 734 nm. Then, 20, 40, 60, 80 and 100 μL of each NE sample solution was added to 3 mL of the diluted ABTS solution. The absorbance of the mixture was immediately measured at 734 nm after a 1-h incubation in the dark at room temperature, Control sample was prepared without adding NE solution. The % ABTS scavenging activity was calculated according to the equation:
Then, the obtained antioxidant activity values (ABTS) of each NE sample were plotted as a function of the volume used, and the obtained linear equations were used to determine the EC50,ABTS values for each NE sample.
2.5 Antibacterial activity test of NEs
2.5.1 Inhibition zone tests
The antimicrobial properties of the examined NEs, including NECV, NECI, NECT, and NEEG alongside their respective pure EOs, were evaluated through the well diffusion method against two significant foodborne pathogens: E. coli O157 and Listeria monocytogenes. These strains were obtained from the Institute of Technology of Agricultural Products, ELGO DEMETER, in Lykovryssi, Greece. To begin with, the examined strains were cultivated in Müeller Hinton Broth at 37°C for 24 h to achieve a bacterial concentration of 10^7 to 10^8 CFU mL^-1. Subsequently, the bacterial cultures were spread to Müller-Hinton agar plates by using sterile cotton swabs. To ensure even bacterial distribution, the plates were rotated every 60°. A sterile cork borer was used, sanitized previously with alcohol and flame, and 6-mm wells were punctured into the agar, which were then filled with 100 μL of each treatment. Once the wells were filled, the agar plates were left at 37 °C to incubate overnight. After the incubation period, the sizes (mm) of the transparent areas surrounding each well were measured using a digital calliper to assess the effectiveness of the various treatments against the examined pathogens. The clear zones indicated the regions where the bacterial growth was successfully inhibited by the coatings.
2.5.2 Minimum inhibition concentration (MIC) tests
The Minimal inhibitory concentration (MIC) is identified as the least quantity of an antimicrobial agent needed to prevent visible microbial growth, indicating bacteriostatic capabilities without detailed analysis of the microbial viability. Cultures of Listeria monocytogenes, E. coli was grown in Müeller-Hinton broth to achieve a bacterial concentration of 106 CFU/mL. The antimicrobial efficacy of examined NEs and their free EOs, was assessed across a range of concentrations: 1,000, 500, 250, 125, 62.5, 31.25, 15.625 μg/mL. Later, serial dilutions of microbial cultures were prepared, and the antimicrobial agents were incorporated at each specified concentration for examination. The mixtures were then homogenized using a vortex mixer and incubated at 37 °C for a 24-h period. The control sample consisted of the microbial cultures without any coating, and it was also evaluated at a fixed concentration of 250 μg/mL. The presence of turbidity was monitored as an indication of microbial proliferation, with further confirmation obtained via culturing and colony enumeration. The procedure was performed in triplicate to ensure the reliability of the results.
2.6 Application of NE as active coatings in fresh pork tenderloin meat
All the obtained NEs as well as their free EOs derivatives are applied as active coatings to preserve fresh pork tenderloin meat. The coating of each pork tenderloin meat with NEs or their free EOs derivatives was performed with the method of dip coating (see Supplementary Figure S2). Fresh pork tenderloin slices were obtained from a local meat processing facility and quickly transported to the laboratory. 30 g of tenderloin pork were weighed for each of the five treatment groups: Uncoated, NECV, NECI, NECT, and NEEG. Afterwards, every pork tenderloin coating was submerged in 25 mL of the appropriate treatment solution to ensure that the concentration of the coated solution was consistent across all samples (see Supplementary Figure S2). After being immersed, the pork tenderloin slices were carefully sealed in a specialized membrane and kept at a temperature of 4 °C for a period of 9 days. During the storage period, various analyses were performed at regular intervals to assess the effectiveness of the applied treatments in preserving pork tenderloin. These analyses included pH measurements, Lab* analysis, lipid oxidation assessments, as well as sensory evaluations.
2.7 Physicochemical properties of pork tenderloin meat
The physicochemical properties of pork tenderloin coatings, including pH, color values (Lab), and thiobarbituric acid reactive substances (TBARs), were analyzed at specific intervals: days 0, 3, 6, and 9.
2.7.1 pH analysis
The pH values of the pork tenderloin meat coatings were measured using a portable pH meter fitted with a penetration electrode and a temperature sensor (pH-Star, Matthäus GmbH, Poettmes, Germany). Prior to each set of measurements, the pH meter was calibrated using pH standard solutions of 4.0 and 7.0, and temperature-adjusted to match the meat coating temperature of 4 °C. The entire procedure was conducted in triplicate, and for each treatment group, ten separate pH readings were taken to ensure accuracy and reliability, as per the methods (Janisch, Krischek, and Wicke, 2011).
2.7.2 Lab* analysis
The alterations in the CIELAB color parameters (L*, a*, and b*) of pork tenderloin meat over a period of 9 days under refrigerated storage were assessed using a LS171 colorimeter from Linshang Company. Prior to conducting the measurements, the colorimeter was calibrated with a white standard plate in accordance with methods cited in studies (Hernandez et al., 2016). Color evaluations were conducted directly on the surface of the tenderloin meat coatings, with each treatment group comprising three separate portions. For each of these portions, five discrete readings were taken to capture a robust assessment of the color. The total color differences (ΔE) were calculated using the equation (Roy Choudhury, 2015):
In this equation, L*0, a*0, b*0 denote the initial color parameters of the pork tenderloin meat at day 0 post-treatment. L*, a*, b* represent the respective color parameters at different time points during the 9-day refrigerated storage at 4 °C.
2.7.3 Lipid oxidation TBARS
The procedure for TBARS measurement was carried out following the method described by Shahidi (1990). Briefly, a 2 g sample of meat was combined with 5 mL of 10% (W/V) TCA solution. This mixture was vortexed for 2 min before the addition of 5 mL of a 0.02 M aqueous solution of 2-thiobarbituric acid, followed by another 2 min of vortex. After centrifugation, the supernatant was collected for color development and the measurement of TBA-MDA absorbance (see Supplementary Figure S3). An absorbance conversion factor of 3.6 was used to calculate TBA numbers (Shahidi, 1990).
2.8 Evaluation of bacterial counts in fresh pork tenderloin coatings
Fresh pork tenderloin treatments were submitted to assessment for mesophilic bacteria on days 0, 3, 6, and 9 during storage period at 4°C. Initially a 10 g pork sample aseptically transferred to a stomacher bag, followed by homogenization with 90 mL of peptone water. Subsequently, a serial dilution (1:10 ratio) was performed. For mesophilic bacteria quantification, 0.1 mL from the diluted samples was spread onto PCA agar plates using a metallic sterilized cell spreader and then were incubated at 37°C for 48 h.
2.9 Sensory evaluation of stored fresh tenderloin meat
Sensory analysis was performed on pork tenderloin treatments to evaluate the main characteristics. For this reason, a panel of 15 trained assessors with expertise in meat and sensory evaluation participated in this procedure. The evaluation employed a five-point hedonic scale to rate attributes such as color, appearance, odor, texture, and overall acceptability, with a rating of five indicating “like extremely” and one indicating “dislike extremely.” Acceptability was defined with a threshold score of 3.5, following the methodology proposed by Hasani-Javanmardi et al. (2021). Each sample was submitted to triplicate assessments per replicate, resulting in a total of six evaluations per treatment group (see Supplementary Figure S4).
2.10 Statistical analysis
For the purposes of the statistical analysis, the average results of each experiment were recorded in triplicate. The software was used refers to IBM SPSS version 22 (IBM Armonk, NY, USA), the statistical analyses were performed. After checking for homogeneity of data, an alpha level of p < 0.05 and due to the low number of samples, Kruskal Wallis test was preferred as a tool for the examination of the significance of the resulting difference.
3 Results
3.1 Particle size-Z potential and TEM images characterization of NE coatings
The droplet size was analyzed as illustrated in Figure 1.
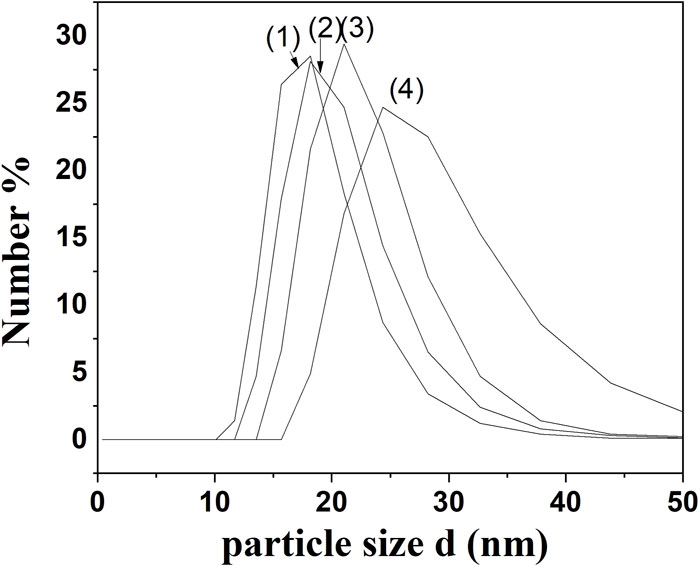
Figure 1. Comparative Particle Size Distribution Analysis from DLS Measurements for (1) NECV, (2) NECI (3) NECT, and (4) NEEG edible nanocoatings.
As it is obtained in Figure 1 all obtained NEs were characterized by droplet sizes in the range of 20–40 nm. More specifically, the mean droplet size distribution across the samples ranged from 18.13 to 24.4 nm. NECΤ exhibited the smallest droplet size distribution at 18.13, followed by NECΑ at 18.24 nm, NEEG at 21.36 nm, and NECI, which had a droplet size of 24.4 nm. The formation of relatively small particles is attributed to the low molecular weight (15–26 kDa), diminutive particle size (40–150 nm) of the casein micelles, and the swift adsorption capability of sodium caseinate at the interface (Rezaei, Fathi, and Jafari, 2019; Peng et al., 2020). The findings are in accordance with other studies (Lu et al., 2018; Rezaei et al., 2019; Nagaraju et al., 2021; Das et al., 2023).
The zeta potential is a major factor that defines the stability of NEs. All tested NEs showed negative zeta potential values ranged between −42.81 and −57.38 mV. These negative zeta potential values of all NEs imply their stability, NECT noting the highest negative charge and NECI the least. This is attributed to the non-ionic to the negative charges of NEs originated from the adsorption of negative ions on the surface of oil droplets, and also due to the presence of functional groups in chemical constituents of NEs such as hydroxyl group (–OH) and carboxyl (C = O) groups (Perumal et al., 2021; Xu et al., 2024). The Z potential values reported here are equal to the Z potential values reported recently for vanilla-cinnamon oil NEs nanoformulated with sodium casein as surfactant and TWEEN-80 as co surfactant (Xu et al., 2024).
In Figure 2 representative TEM images of all obtained NEs are shown. At is observed in all cases the final oil droplet size is in the range of 20–40 nm. The resultant from TEM images nanodroplets size is in accordance with the results presented by mean particle size distribution of DLS measurements.
3.2 Antioxidant activity EC50
Table 2 presents the results of the mean calculated EC50 values for the examined treatments with DPPH and ABTS method.
With a first glance it is obtained that both DPPH and ABTS methods resulted in similar antioxidant activity values with the same trend among the examined NEs. Lower EC50 values are obtained for DPPH method compared to ABTS values because DPPH has a greater affinity with hydrophobic compounds such as EOs and their derivatives (Floegel et al., 2011; Liu et al., 2022). Thus, EC50,DPPH values are considered as more reliable for such NEs. The outcome of the EC50,DPPH analysis indicates that the examined nanoformulated EOs (NECT, NECV, NEEG, NECI) outperformed the efficiency of the free EOs (FCT, FCV. FEG, FCI). In particular, while the free forms exhibit EC50,DPPH quantities ranging from 398.96 to 506.38 μL, the encapsulated forms needed significantly lower quantities (between 61,03 and 99.75) to achieve a 50% radical reduction. More analytically, NECV and NEEG demonstrated over 6 times higher antioxidant effectiveness against its free form with only 61.03 and 73.11 μL, while NECT and NECI, with 96.18 and 99.75, respectively, needed 5 times less quantity to scavenge 50% of the radical than FCT and FCI.
These findings are in accordance with Nagaraju P. G. et al. (2021) as their study revealed that the encapsulated form of clove oil showed superior scavenging effect, leading to 80% DPPH radical formation compared to 40% of the free form. A previous study reported that because of the comparatively simple abstraction of atomic hydrogen from these functional groups, these compounds demonstrate remarkable antioxidant activity (Lazaridis et al., 2024).
Overall, NEs appear to control the release of the antioxidant components leading to higher antioxidant properties. Thus, their use in the field of food additives, pose NEs to be a great potential as alternative preservatives in the food packaging.
3.3 MIC
The antibacterial activity of NEs against E. coli and L. monocytogenes was assessed through the determination of the MIC values and the analysis results are listed in Table 3.
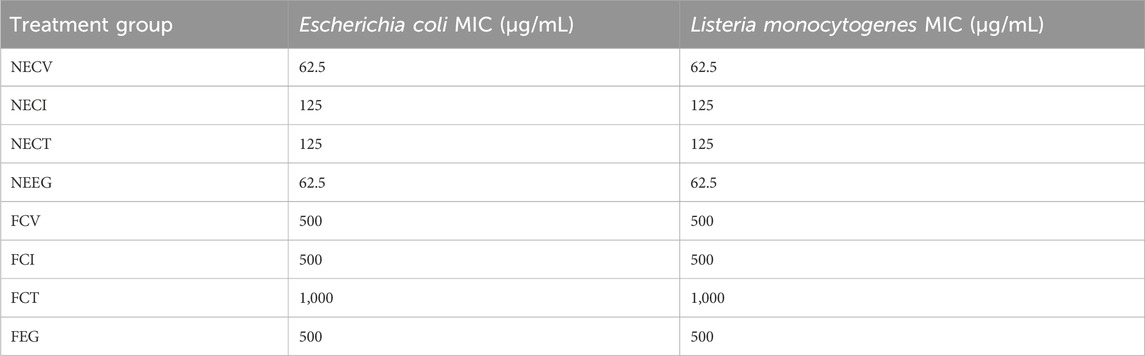
Table 3. MIC analysis results of the examined NEs and free EOs derivatives on Escherichia coli and Listeria monocytogenes.
For both E. coli and L. monocytogenes, NE forms of CV and, E.G., exhibited remarkably low MIC values of 62.5 μg/mL, demonstrating their strong bacteriostatic properties. Similarly, nanoemulsified forms of NECI and NECT showed MIC values of 125 μg/mL against both pathogens.
On the other hand, the free forms of these compounds required much higher concentrations, with MIC values of 500 μg/mL against both E. coli and L. monocytogenes. Notably, the free form of CT exhibited the highest MIC value of 1,000 μg/mL, indicating the least antibacterial effect among the examined free oils.
Various studies also claim that NEs appear to have remarkably higher antibacterial effect against the examined pathogens (Sun et al., 2021; Wang et al., 2022; Zaharioudakis et al., 2023). The explanation for these properties is related to their enhanced solubility, the controlled release of the compounds and the interaction with bacteria cells (Feng et al., 2022). Feng et al. (2022) in their study noticed that the main reason that geraniol NE demonstrated higher antibacterial effectiveness is due to the disruption of the cell membrane of bacteria. Similarly, Negi and Kesari, (2022) encapsulated CV and lemongrass EOs into chitosan nanoparticles leading to significantly improved antibacterial effectiveness against E. coli and Listeria monocytogenes because of the enhanced bacterial cell membrane interaction, providing a controlled release mechanism, and increasing miscibility in aqueous media chitosan nanoparticle encapsulation of antibacterial EOs. According to Sakanaka et al. (2005) casein showed scavenging activity against radicals such as DPPH.
3.4 Well diffusion zone
The antibacterial activity of NEs and free EOs derivatives against E. coli and Listeria monocyte genes was quantitatively assessed through the well diffusion method. The zones of inhibition, measured in millimeters (mm), reflect the efficacy of each treatment. The results, presented as the mean ± standard deviation (SD), are summarized in Table 4. Representative images of observed diffusion zones for all tested NEs as well as the free EOs derivatives are shown in Figure 3.
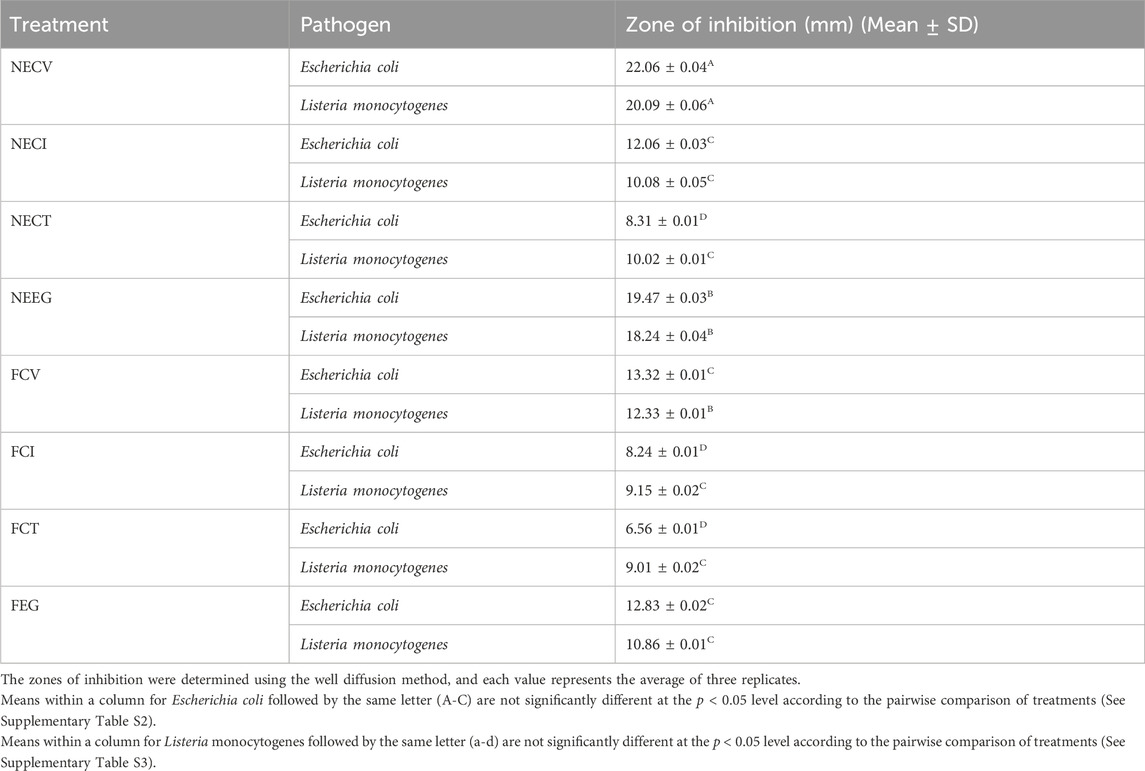
Table 4. Mean inhibition zone results of the examined NEs and free EOs derivatives on E. coli and L. monocytogenes.
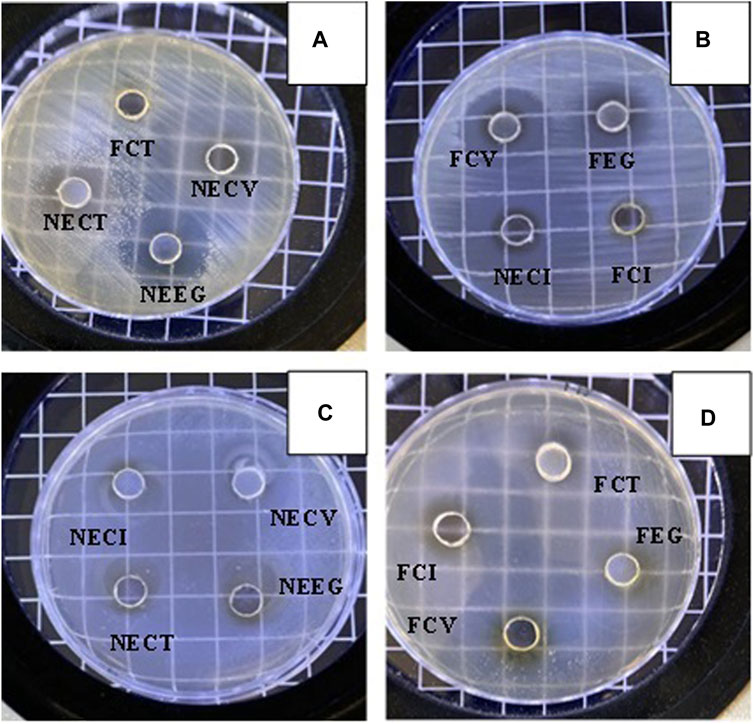
Figure 3. Representative images of diffusion zones observed for all NEs and free EOs derivatives. (A) FCT, NECV, NECT, NEEG, (B) FCV, FEG, NECI, FCI, (C) NECI, NECV, NECT, NEEG and (D) FCT, FCI, FEG, FCV.
The analysis of the diffusion zones reveals that nanoencapsulated EOs exhibit a higher antimicrobial activity compared to their free forms. In particular, NECV demonstrated the most significant (p < 0.05) effect against both E. coli (22.06 mm) and Listeria monocytogenes (20.09 mm). Findings indicate enhanced efficacy of NEs in delivering active compounds more effectively, leading to significantly higher diffusion thereby superior antimicrobial activity. Moreover, the antimicrobial effect between NECT and NECV is considered as significant (p < 0.05) regards S. aureus, while NECV and FCT difference was statistically significant regards to E. coli.
Among the free oils, FCV demonstrated superior antimicrobial activity, with zones of inhibition measuring 13.32 mm against E. coli and 12.33 mm against L. monocytogenes. This suggests that even in its free form, CV retains a noteworthy capacity to inhibit bacterial growth.
When comparing the effectiveness against Gram-positive (Listeria monocytogenes) and Gram-negative (E. coli) bacteria, it is observed that the nanoemulsified forms, particularly NECV and NEEG, maintain a high level of activity against both types of bacteria, indicating a noticeable antimicrobial potential. However, the slightly larger zones of inhibition against E. coli suggest a marginally increased susceptibility of Gram-negative bacteria to these treatments.
Various studies indicate that the results are in accordance with their findings, meaning that the privilege of nanoformulated EOs like the examined of the current study have higher capability of diffusion (Tastan et al., 2016; Saddiq and Khayyat, 2010; Gago et al., 2019; Jiménez et al., 2018; Qureshi et al., 2022; et al., 2022). CV, a natural antimicrobial agent, can be delivered as a NE due to its low solubility. It effectively reduces microorganisms, including E. coli and Pichia pastoris, and can control the safety of fresh-cut vegetables when combined with acidic electrolysed water (Jiménez et al., 2018).
Regarding CT against Listeria monocytogenes various studies investigated the impact of CT on listeria growth and cell damage in Listeria innocua and Listeria monocytogenes, revealing that CT’s antilisterial activity depends on the composition of lemongrass EO, while it is mentioned that may there is a disruption to the fatty acid and peptidoglycan biosynthesis (Silva-Angulo et al., 2015; Lu et al., 2018; Budiati et al., 2020). Another study evaluated the antimicrobial activity of six plant EOs against Listeria monocytogenes. Lemongrass oil showed the highest antimicrobial activity, followed by turmeric and orange peel oil (Silva-Angulo et al., 2015). The study evaluated E. coli BJ4’s resistance to CT in a buffer system, focusing on CT concentration, treatment medium pH, storage time, and initial inoculum size. Results showed that initial inoculum size significantly impacted CT efficacy, and cell envelope damage was a key factor in CT inactivation (Saddiq and Khayyat, 2010; Somolinos et al., 2010).
3.5 Physicochemical properties of pork tenderloin meat
3.5.1 pH analysis
The results of the pH analysis are listed in Table 5 below.
The results from the pH analysis during the 9-day of storage at 4°C, demonstrate that nanoemulsified treatments exhibited a more controlled increase in pH, indicating better preservation capabilities compared to the free treatments and the uncoated control. Among the NE treatments, NECI shows the most controlled increase, with the pH gradually increasing from 5.61 to 5.81, suggesting its superior effectiveness in maintaining meat freshness over time. The uncontrolled pΗ change leads to rapid microbial growth on meat and spoilage, thus nanoencapsulated EOs seem to constitute a potential alternative to tackle this challenge. Indeed, the difference in pH values for the uncoated meat sample was statistically significant at 5% among the examined treatments, while pure oils demonstrated no statistical changes until day 6 (See Supplementary Tables S4–S7).
In contrast, the free EOs derivatives treatments, specifically FCV and FCI, demonstrated a more rapid pH increase, reaching 6.15 and 6.16, respectively, by day 9. Although these treatments still offer some level of preservation compared to the uncoated sample, their efficacy is overshadowed by the nanoemulsified variants. FCT showed relatively better control compared to others, with a final pH of 5.93, indicating it as the better option within the free forms for controlling pH over time. The uncoated exhibited the highest increase in pH, moving from 5.68 to 6.41, which underscores the effectiveness of both EO formulations effectiveness.
Previous studies have also found that NE treatments managed to control the pH change in meat during storage time (A. K. Das et al., 2020; Karthik et al., 2017; Dghais et al., 2023). The oxygen reduction, and the energy transfer constitute the main reasons which explain this effectiveness regards the prevention of damage to meat products (Ujilestari et al., 2023).
3.5.2 Lipid oxidation
In Figure 4, the results of the TBARS analysis are graphically presented.
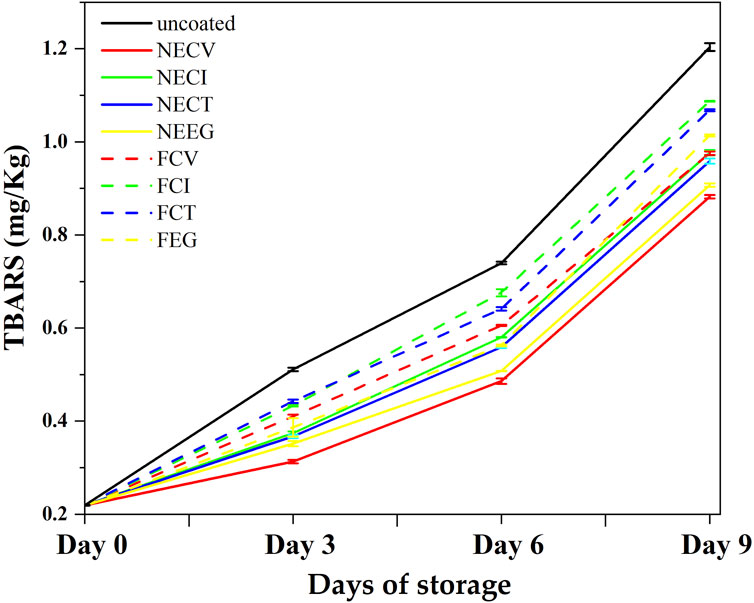
Figure 4. Mean TBARS values plots as a function of day of storage of pork tenderloin meat a coated with NECV, NECI, NECT, NEEG, FCV, FCI, FCT, and free EOs derivatives as well as uncoated pork tenderloin meat.
The TBARS results, appeared NEs to have higher capabilities in controlling lipid oxidation in pork tenderloin meat compared to the free EOs derivatives over the 9-day examined period of storage. Among the NE treatments, NECV demonstrated a notable ability to control lipid oxidation increase, starting from 0.219 and increasing to 0.882 mg MDA/kg meat by day 9. This result suggests that NECV effectively decreases the growth rate of the lipid oxidation on meat compared to other treatments. Similarly, NEEG demonstrates a remarkable performance (p < 0.05) with a final TBARS value below 1.00, indicating its antioxidative properties (see Supplementary Tables S8–S10).
At day 3 NECV showed statistically significant differences among FCT and uncoated treatment, while NEEG showed a significant difference with uncoated treatment (See Supplementary Table S7). Specifically, NECV indicated significant difference between NEEG, free EOs derivatives and uncoated samples. On the other hand, the free EOs derivatives formulations exhibit a less effective control over lipid oxidation, but still their contribution is statistically significant compare to the uncoated sample (p < 0.05). This result confirms that EOs can play a crucial role to the control of lipid oxidation. More analytically, FCI and FCT demonstrated the highest TBARS values by day 9, at 1.087 and 1.068 mg MDA/kg meat, respectively. These values are significantly higher than those observed for NE treatments, highlighting the superior antioxidative efficacy of NEs in protecting pork tenderloin meat from lipid peroxidation. The uncoated control sample experiences the highest rate of lipid oxidation, culminating in a TBARS value of 1.204, further emphasizing the protective role of both nanoformulations and free EOs derivatives treatments.
Lipid oxidation refers to a natural developing phenomenon in meat which occurs mainly because of the unsaturated fatty acids exposure to the oxygen, which may cause meat deterioration (Domínguez et al., 2019). The factors which affect the growth rate of TBARS values may be endogenous such as pro-oxidants which are known for their ability to accelerate the evolution of this phenomenon, unsaturation of fatty acids or/and exogenous factors like light exposure, temperature and storage conditions that contribute to the acceleration of this process (D. Wang et al., 2023). Various antioxidants, both natural and synthetic, have been studied to be able to control lipid oxidation rate by neutralizing free radicals. Previous studies have reported the enhanced effectiveness of citrus NEs regards the lipid oxidation inhibition during the meat quality preservation over time (Sakanaka et al., 2005; Uçar, 2020; Lakshmayya et al., 2023; Medeleanu et al., 2023; Ujilestari et al., 2023) in their study supported that casein demonstrated high antioxidant activity against lipid oxidation in ground beef homogenates.
3.5.3 Lab* colorimetry analysis
The values of Lab* colorimetry analysis of pork tenderloin meat over a 9-day of storage period coated with NEs, free EOs derivatives and uncoated are listed in Table 6. As it is observed the nanoemulsified treatments managed to control better the change of color parameters in meat compared to free EOs treatments as well as uncoated treatment. This result demonstrates that NEs show a mild mode of effect on pork color which is one of the main quality characteristics of pork. Previous study reported that the meat color turns to brown because of the presence of metmyoglobin (Hernández et al., 2016).
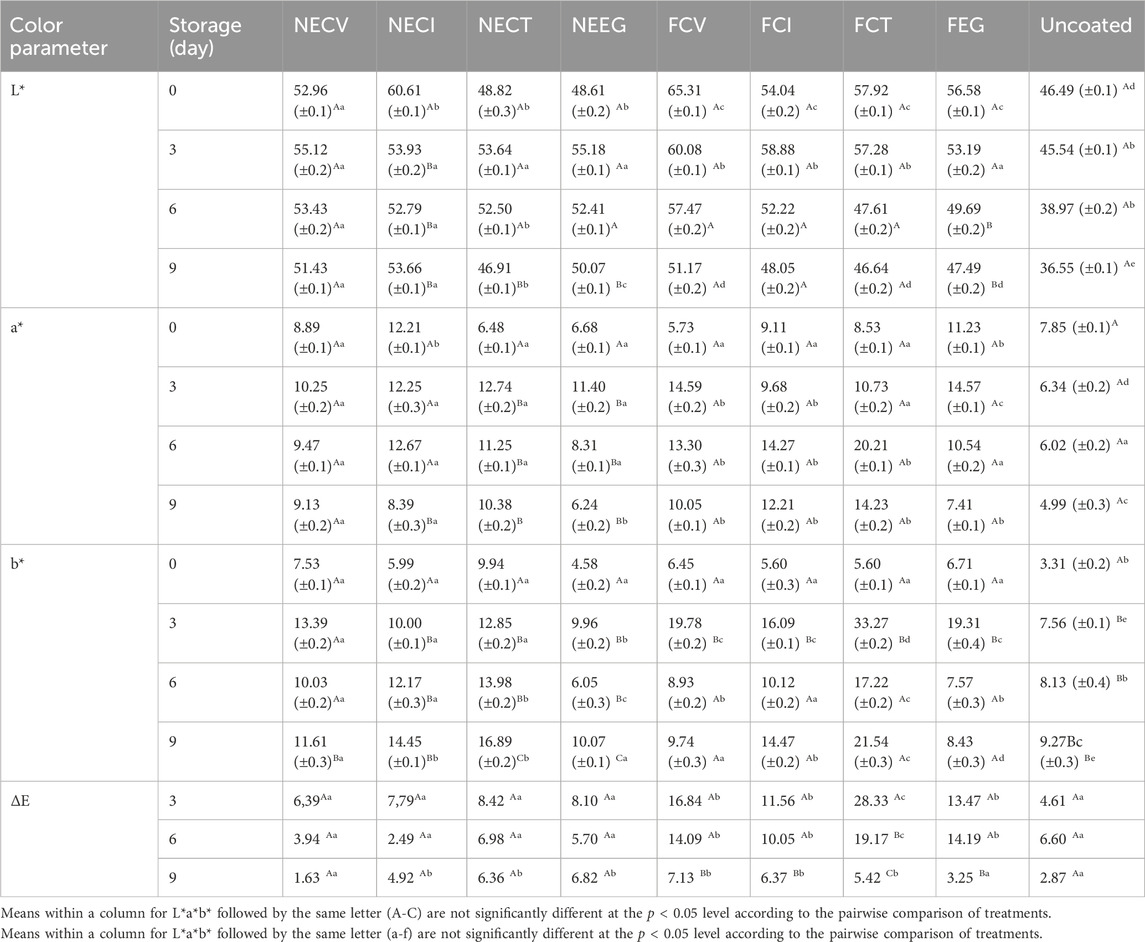
Table 6. Lab* colorimetry analysis pork tenderloin meat over time coated with NEs and free EOs derivatives.
Let us begin with, L* value (see Table 6) which represents the lightness and has been reported to be related to changes in chemical state of myoglobin (Jafarinia et al., 2022). As it is shown in Table 6 L* value was significantly less affected by NEEG, NECT and NECI than FCI and FCV treatments during the examined period. L* value regards NECV increased rapidly on day 3–55.12 from almost 53 on day 0, while it followed a gradual downtrend to 51.43 by the end of the examined period. Notwithstanding the fact that the NECI initial L* value is determined to be 60.61, which is significantly higher than that of NECT and NEEG, it experienced a rapid fall by the end of the examination period to 53.66, meaning the highest NE value for day 9. Even though, free EOs treatments such as FCV, FCT and FEG experienced a much higher L* value the day 0 compared to nanoformulations, the values plunged to lower levels of their NEs, reflecting the direct affection for the meat quality. Simultaneously, the a* values (see Table 6) of the free oil meat treatments experienced a statistically significant (p < 0.05) drop compared to nanoformulated and uncoated meat samples until the end of the examined period, but on day3 the values increased due to retardation of myoglobin oxidation by the EO (Zhang et al., 2016). Indeed, nanoformulated oils managed to maintain the value decline more effectively than their free forms. Furthermore, b* values of free EO treatments escalated to significantly higher levels than those of NEs. Specifically, free oils affected the meat significantly (p < 0.05) compared to NEs and uncoated samples by the ninth day of storage. Overall, NE demonstrates that no significant changes were noticed between NE treatments and uncoated pork tenderloin meat. Findings turn caseinate NEs into a great potential to extend the shelf life of meat, affecting in a gentle way its color.
The results of this study are in accordance with relevant studies which indicate that EOs are able to control more efficiently the discoloration rate during storage (Ghaderi-Ghahfarokhi et al., 2017; Zaharioudakis et al., 2023). EOs’ impact on meat color is attributed to their ability to reduce myoglobin and lipid oxidation (Semenova et al., 2019).
3.6 Total variable counts (TVC) analysis
In Figure 5 the mean TVC values of pork tenderloin meat coated with NEs and free EOs derivatives are plotted as a function of days of storage for comparison.
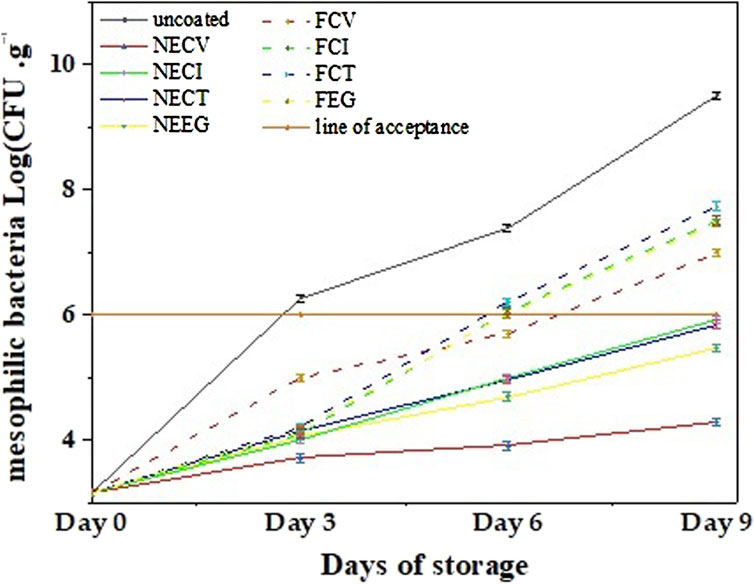
Figure 5. Growth of total mesophilic bacteria (Log CFU/gr^-1) of pork tenderloin samples coated with NEs and free EOs derivatives over 9 days of storage at 4°C.
TVC results for pork tenderloin meat (see Figure 5), indicate that NEs managed to more effectively control the microbial counts growth compared to the free EOs derivatives (see Figure 5 and (see Supplementary Tables S11–S22).
To begin with, NECV treatment demonstrated the highest antimicrobial effectiveness due to the capability of nanoencapsulated CV which demonstrated a slight increase to 4.29 log CFU/g^-1 for the examined period. On day 3 uncoated pork meat sample has a higher TVC value than the lower limit of acceptance which is six log CFU/g^-1 (Zhang et al., 2017). On day 6 samples coated with free EOs except the FCV sample exceeded the 6-log acceptance limit while on day 9 all NE coated pork samples have TVC values lower than the lower acceptance limit. In other words, it is evident that pork samples coated with free EOs derivatives succeed in extending the shelf life of pork tenderloin meat by 3 days compared to uncoated pork samples, while pork samples coated with edible NAs achieved a 6-day shelf life extension of pork tenderloin meat compared to the uncoated pork sample and a 3-day shelf life extension compared to the pork samples coated with free EOs derivatives.
Previous study reported that using adding EO to sausages increased the shelf life to 18 days regards the mesophilic counts (Ajourloo et al., 2021). Moreover Skandamis & Nychas, (2001) reported that during aerobic storage of minced meat, the TVC reached its highest levels within 6 days, with Pseudomonas spp. being the dominant microorganism and shelf life extended by 5 and 12 days. Oregano EO influenced microbial counts by reducing growth rates, and spoilage.
Another study investigated the impact of thyme and oregano EOs, along with modified atmosphere packaging, on fresh lamb meat shelf life during storage at 4°C. They found thyme oil and MAP to notably extend preservation, leading to a microbial counts reduction of up to 2.8 log cfu/g on day 9. Their findings indicated a shelf life of 7 days for air-packaged samples, 9–10 days for those containing 0.1% TEO, and 21–22 days for MAP-packaged samples. (Karabagias et al., 2011).
In terms of NEs, research conducted by Ameur et al. (2022) focused on seafood shelf life in terms of mesophilic bacteria growth. Their study demonstrated that grape seed and cinnamon based NEs appeared to be significantly more effective against total mesophilic and psychrophilic bacteria counts in mullet fillets, suggesting a potential for these NEs to prevent spoilage.
Lastly our previous study evaluated the effectiveness of CV formulations in inhibiting mesophilic bacteria growth in minced meat over a 9-day storage period at 4°C. Results showed that CV microemulsions and NEs inhibited the growth of mesophilic bacteria by 3 days. The samples with no coating experienced a significant increase in mesophilic bacteria levels, while all CV-based treatments-maintained growth within acceptable limits. The NE coating controlled microbial proliferation more effectively than free CV, leading to reductions by one log and 1.2 logs on Days 6 and 9 (Zaharioudakis et al., 2023).
3.7 Sensory analysis
The results from sensory analysis are illustrated in Figure 6 for comparison. Initially, all samples, irrespective of the treatment, were rated optimally with a score of 5.00 across appearance, odor, color, texture, and overall acceptability, setting a uniform benchmark for fresh meat quality.
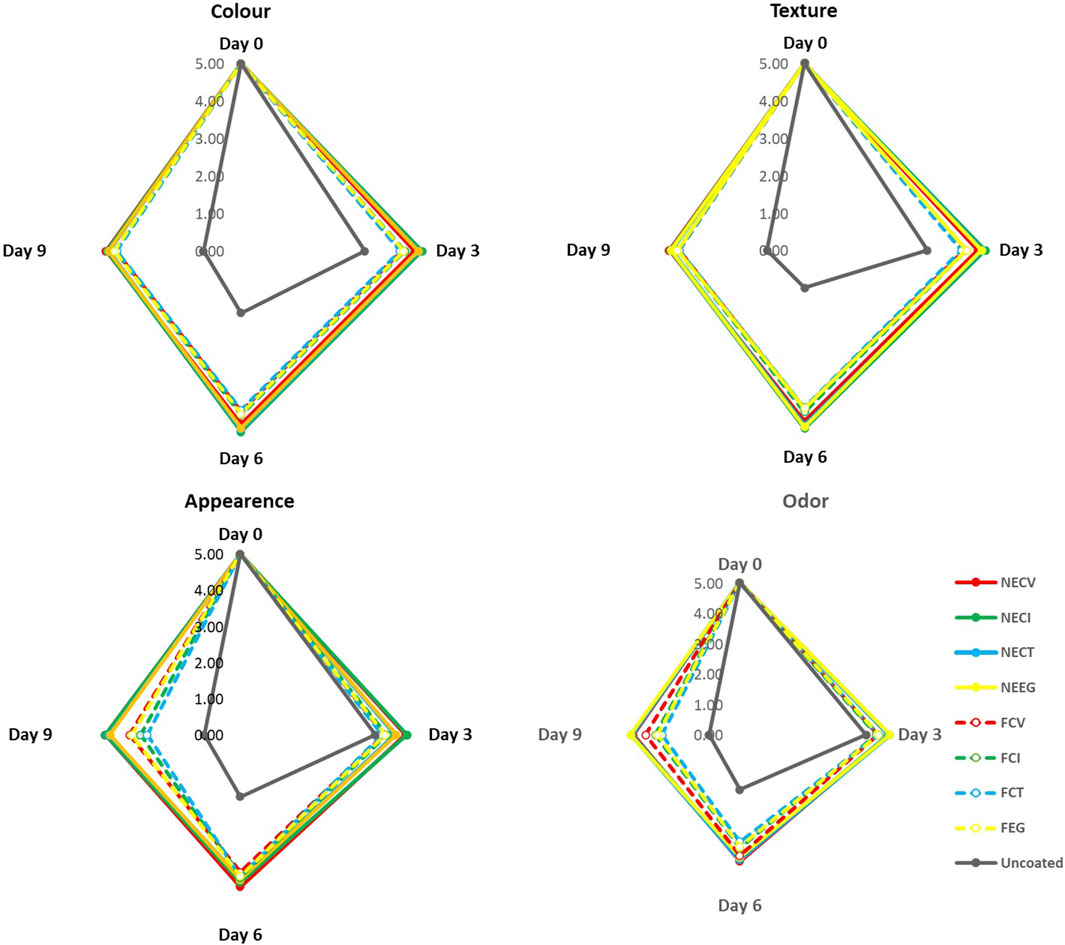
Figure 6. Sensory analysis οn (Α) Color, (B) Appearance, (C) Texture, (D) Odor over 9-day of storage at 4°C of pork tenderloin meat samples coated with NEs and free EOs derivatives.
It is well documented that sensory properties including texture, appearance, color, and odor make up the most critical factors when consumers show their preference in purchasing a food product (Giannakas et al., 2023). The deterioration of odor is related to various compounds which enable the growth of several microorganisms including dimethyl sulfide/disulfide which are sourced by the appearance of P. spp. οr diacetyl and 3-methylbutanol which are generated by homofermentative LAB, Enterobacteriaceae, or Brochothrix thermosphacta (Hematizad et al., 2021).
In the passage of storage period, nanoformulated treatments exhibited statistically significant score across the 9 days, with NECV and NECI particularly demonstrating their ability to retard the deterioration of meat qualities. By day 6 and 9, the score of the nanoencapsulated EO treatments was above the acceptance threshold of 3.5. Notwithstanding the fact that the free oil treatments scored significantly lower than NE treatments, they still constitute a necessary alternative since they appear to have statistically significant results against the uncoated (0 < 0.05), extending the acceptance threshold of 3.5–6 days (See Supplementary Table S17). Among the NEs, the CT based demonstrated statistically lower score in terms of appearance and texture, while in case of NECI color noted significant lower score than the CV NE.
Overall, sensory analysis results revealed a remarkable superiority of NEs treatments in preserving meat quality against the free EOs derivatives treatments. NECV and NECI appear as the most effective treatments to control the deterioration of the main sensory characteristics due to their ability to control the microbial growth and the gentle effect on the main organoleptic characteristics.
4 Discussion
As shown in the results section above, the development of new edible NEs based on EOs derivatives such as CV, CI, CT and, EG using CSN and LCN as biobased surfactant and co-surfactant respectively was achieved. Physicochemical characterization of such new edible NEs by DLS measurements and TEM images showed a nanoparticle distribution in the range of 20–40 nm, while Z-potential measurements showed that such derived NEs are physicochemically stable. All NEs showed much higher antioxidant activity and antibacterial activity than free EOs derivatives as shown by antioxidant activity measurements by both DPPH and ATBS method and by MIC and diffusion zone antibacterial experiments respectively. The higher antioxidant and antibacterial activity of all NEs compared to free EOs derivatives was depicted in the lipid oxidation and total mesophilic bacteria growth rates of fresh pork tenderloin meat coated with all edible NEs. Thus, all fresh pork tenderloin meat samples coated with edible NAs showed much lower rates of increase in lipid oxidation compared to fresh pork tenderloin meat samples coated with free EOs derivatives and uncoated fresh pork tenderloin meat sample. Moreover, as it was shown by TVC results free EOs derivatives succeed to extend the pork tenderloin meat shelf-life by 3 days compared to uncoated pork meat sample while the pork meat samples coated with edible NEs succeeded to extend the shelf-life of pork tenderloin meat by 6 days compared to uncoated sample and by 3 days compared to the samples coated with free EOs derivatives. In addition, it must be considered that as it was revealed by Lab* colorimetry analysis as well as sensory analysis such edible CSN/LCN based NEs succeeded to preserve the texture, appearance, and color of pork tenderloin meat in more acceptance limits in comparison with the corresponding characteristics of pork tenderloin meat coated with free EOs derivatives. The results reported here are similar to these reported recently (Sun et al., 2021; Wang et al., 2022). Wang et al. (2022) treated pork meat with thymol and thyme oil chitosan based NEs. Compared to the control group, the treatment groups inhibited lipid oxidation and microbial growth in pork and significantly extended the shelf-life of fresh pork (Wang et al., 2022). Sun et al. (2021) also prepared chitosan based NE edible coatings for pork meat patties loaded with fennel essential oil/cinnamaldehyde and shown that the quality of control pork meat patties gradually declined during storage and completely corrupted on the sixth day (Sun et al., 2021). On the contrary the samples coated by the NE had the lowest TVC including the number of mold and yeast, TBARS value and TVB-N content (Sun et al., 2021). The study concluded that NE coating maintained the moisture state, flavor and texture of the samples and extending the shelf life from 6 days to 10 days (Sun et al., 2021). Overall, the current study concludes that such novel CSN/LCN-based edible NEs are a promising technology that can be used as edible active coatings in the meat food industry. Using the well-known adage that a picture is worth a thousand words, we have illustrated the most important results of this study in the following Figure 7.
5 Conclusion
The development, characterization, and application of CSN/LCN based edible NEs with encapsulated CV, CI, CT, and, EG as oil phase was explored in this study. DLS measuraments as well as TEM images showed a mean particle size distribution of 20–40 nm for all obtained NEs while z potential results suggest their physicochemical stability. NEs, were evaluated for their preservation capabilities against pork tenderloin meat spoilage over a 9-day storage period at a temperature of 4°C ± 1°C compared to free EOs derivatives. Overall NEs exhibited superior effects on the meat, especially those containing CV and, EG. Nanoformulated EOs had a statistically significant advantage over free EOs derivatives forms due to the nanoencapsulated and controlled release bioactivity. The calculated EC50 values both with DPPH and ABTS method revealed that NEs needed at least 5 times less quantity of EOs derivatives to scavenge the 50% of radicals compared to free EOs derivatives. The results from antibacterial tests including MIC and agar well diffusion methods, demonstrated a higher antibacterial activity of NEs against E. coli and L. monocytogenes compared to free EOs derivatives because of NE’s enhanced solubility, controlled release of their bioactive compounds and their ability to disrupt the cell membrane of bacteria. When NEs were applied as active edible coatings to the pork tenderloin meat, they managed to control the pH level change more efficiently compared to free EOs derivatives coated pork tenderloin meat samples. This is an index than NEs succeed to control the microbial growth. Simultaneously, regarding calculated TBARS values, NEs demonstrated 30% higher efficacy compared to uncoated pork meat samples and 12% higher efficacy compared to pork meat samples coated with EOs derivatives. This result indicates NE’s enhanced antioxidant effectiveness. Concerning the mesophilic bacteria growth, NEs exhibited superior antimicrobial effectiveness at coated pork tenderloin meat by retarding the bacterial spoilage, maintaining the microbial population below the lower acceptance limit of six log CFU/g^-1 for 9 days of storage. From a microbiological point of view, NEs succeed to extend pork tenderloin meat shelf life for 6 days compared to uncoated pork tenderloin meat and for 3 days compared to tenderloin pork meat coated with free EOs derivatives. Regarding Lab* colorimetry values, NEs managed not to significantly change the color of coated pork tenderloin, and also managed to counteract the discoloration of coated tenderloin pork meat. Overall, this research study revealed all the benefits of NEs using a wide range of techniques to confirm their properties. CSN/LCN based NEs seem capable of producing stable NEs and even increasing their antioxidant/antibacterial effectiveness in meat food products. Prospects of this study will be (1) the development and characterization of CSN/LCN based NEs with encapsulated mixtures of CV-CI and EU-CI to study the synergistic effect between encapsulated EOs derivatives in food preservation and (2) the development, characterization, and application in food preservation of novel nanogels with encapsulated such EOs derivatives.
Data availability statement
The original contributions presented in the study are included in the article/Supplementary Material, further inquiries can be directed to the corresponding authors.
Author contributions
KZ: Validation, Data curation, Investigation, Writing–original draft. CES: Methodology, Project administration, Supervision, Validation, Visualization, Writing–review and editing. NDA: Data curation, Investigation, Methodology, Validation, Writing–review and editing. EK: Data curation, Investigation, Validation, Writing–original draft. AL: Validation, Visualization, Writing–review and editing. VKK: Data curation, Methodology, Validation, Writing–original draft. AK-M: Data curation, Methodology, Writing–review and editing. DM: Methodology, Writing–review and editing. NEZ: Data curation, Methodology, Writing–review and editing. AA: Methodology, Writing–review and editing, Validation. CP: Methodology, Project administration, Supervision, Writing–review and editing. AEG: Methodology, Project administration, Supervision, Validation, Writing–review and editing.
Funding
The author(s) declare that no financial support was received for the research, authorship, and/or publication of this article.
Conflict of interest
The authors declare that the research was conducted in the absence of any commercial or financial relationships that could be construed as a potential conflict of interest.
The author(s) declared that they were an editorial board member of Frontiers, at the time of submission. This had no impact on the peer review process and the final decision.
Publisher’s note
All claims expressed in this article are solely those of the authors and do not necessarily represent those of their affiliated organizations, or those of the publisher, the editors and the reviewers. Any product that may be evaluated in this article, or claim that may be made by its manufacturer, is not guaranteed or endorsed by the publisher.
Supplementary material
The Supplementary Material for this article can be found online at: https://www.frontiersin.org/articles/10.3389/frfst.2024.1400224/full#supplementary-material
References
Adams, T. B., Cohen, S. M., Doull, J., Feron, V. J., Goodman, J. I., Marnett, L. J., et al. (2004). The FEMA GRAS assessment of cinnamyl derivatives used as flavor ingredients. Food Chem. Toxicol. 42 (2), 157–185. doi:10.1016/j.fct.2003.08.021
Ajourloo, M., Khanjari, A., Misaghi, A., Akhondzadeh Basti, A., Kamkar, A., Yadegar, F., et al. (2021). Combined effects of Ziziphora clinopodioides essential oil and lysozyme to extend shelf life and control Listeria monocytogenes in Balkan-style fresh sausage. Food Sci. Nutr. 9, 1665–1675. doi:10.1002/fsn3.2141
Ameur, A., Bensid, A., Ozogul, F., Ucar, Y., Durmus, M., Kulawik, P., et al. (2022). Application of oil-in-water nanoemulsions based on grape and cinnamon essential oils for shelf-life extension of chilled flathead mullet fillets. J. Sci. Food Agric. 102, 105–112. doi:10.1002/jsfa.11336
Badr, M. M., Badawy, M. E. I., and Taktak, N. E. M. (2022). Preparation, characterization, and antimicrobial activity of cinnamon essential oil and cinnamaldehyde nanoemulsions. J. Essent. Oil Res. 34, 544–558. doi:10.1080/10412905.2022.2107100
Barradas, T. N., and de Holanda e Silva, K. G. (2021). Nanoemulsions of essential oils to improve solubility, stability and permeability: a review. Environ. Chem. Lett. 19, 1153–1171. doi:10.1007/s10311-020-01142-2
Baschieri, A., Ajvazi, M. D., Tonfack, J. L. F., Valgimigli, L., and Amorati, R. (2017). Explaining the antioxidant activity of some common non-phenolic components of essential oils. Food Chem. 232, 656–663. doi:10.1016/j.foodchem.2017.04.036
Budiati, T., Wibisono, Y., Pambayun, R. A., Fahrezy, M. F., Ariyani, R., Kurniawati, E., et al. (2020). Inhibition of Listeria monocytogenes by natural antimicrobial. IOP Conf. Ser. Earth Environ. Sci. 411, 012042. doi:10.1088/1755-1315/411/1/012042
Cao, J., Liu, H., Wang, Y., He, X., Jiang, H., Yao, J., et al. (2021). Antimicrobial and antivirulence efficacies of citral against foodborne pathogen Vibrio parahaemolyticus RIMD2210633. Food control. 120, 107507. doi:10.1016/j.foodcont.2020.107507
Cohen, S. M., Eisenbrand, G., Fukushima, S., Gooderham, N. J., Peter Guengerich, F., Hecht, S. S., et al. (2021). FEMA GRAS assessment of natural flavor complexes: origanum oil, thyme oil and related phenol derivative-containing flavoring ingredients. Food Chem. Toxicol. 155 (September), 112378. doi:10.1016/j.fct.2021.112378
Das, A. K., Nanda, P. K., Bandyopadhyay, S., Banerjee, R., Biswas, S., and McClements, D. J. (2020). Application of nanoemulsion-based approaches for improving the quality and safety of muscle foods: a comprehensive review. Compr. Rev. Food Sci. Food Saf. 19, 2677–2700. doi:10.1111/1541-4337.12604
Das, S., Chaudhari, A. K., Singh, V. K., Dwivedy, A. K., and Dubey, N. K. (2023). Angelica archangelica essential oil loaded chitosan nanoemulsion as edible coating for preservation of table grape fruit against Botrytis cinerea contamination and storage quality deterioration. Postharvest Biol. Technol. 205, 112482. doi:10.1016/j.postharvbio.2023.112482
Dghais, S., Ben Jemaa, M., Chouchen, M., Jallouli, S., Ksouri, R., and Falleh, H. (2023). Nano-emulsification of cinnamon and curcuma essential oils for the quality improvement of minced meat beef. Foods 12, 235. doi:10.3390/foods12020235
Domínguez, R., Pateiro, M., Gagaoua, M., Barba, F. J., Zhang, W., and Lorenzo, J. M. (2019). A comprehensive review on lipid oxidation in meat and meat products. Antioxidants 8, 429. doi:10.3390/antiox8100429
Doulgeraki, A. I., Ercolini, D., Villani, F., and Nychas, G.-J. E. (2012). Spoilage microbiota associated to the storage of raw meat in different conditions. Int. J. Food Microbiol. 157, 130–141. doi:10.1016/j.ijfoodmicro.2012.05.020
Feng, X., Feng, K., Zheng, Q., Tan, W., Zhong, W., Liao, C., et al. (2022). Preparation and characterization of geraniol nanoemulsions and its antibacterial activity. Front. Microbiol. 13, 1080300. doi:10.3389/fmicb.2022.1080300
Feng, X., Feng, K., Zheng, Q., Tan, W., Zhong, W., Liao, C., et al. (2022). Preparation and characterization of geraniol nanoemulsions and its antibacterial activity. Front. Microbiol. 13 (November), 1080300. doi:10.3389/fmicb.2022.1080300
Floegel, A., Kim, D.-Ok, Chung, S.-J., Koo, S. I., and Chun, O. K. (2011). Comparison of ABTS/DPPH assays to measure antioxidant capacity in popular antioxidant-rich US foods. J. Food Compos. Analysis 24 (7), 1043–1048. doi:10.1016/j.jfca.2011.01.008
Gago, C. M. L., Artiga-Artigas, M., Antunes, M. D. C., Faleiro, M. L., Miguel, M. G., and Martín-Belloso, O. (2019). Effectiveness of nanoemulsions of clove and lemongrass essential oils and their major components against Escherichia coli and Botrytis cinerea. J. Food Sci. Technol. 56, 2721–2736. doi:10.1007/s13197-019-03762-1
Gao, S., Liu, G., Li, J., Chen, J., Li, L., Li, Z., et al. (2020). Antimicrobial activity of lemongrass essential oil (cymbopogon flexuosus) and its active component citral against dual-species biofilms of Staphylococcus aureus and Candida species. Front. Cell Infect. Microbiol. 10, 603858. doi:10.3389/fcimb.2020.603858
Ghaderi-Ghahfarokhi, M., Barzegar, M., Sahari, M. A., Ahmadi Gavlighi, H., and Gardini, F. (2017). Chitosan-cinnamon essential oil nano-formulation: application as a novel additive for controlled release and shelf life extension of beef patties. Int. J. Biol. Macromol. 102, 19–28. doi:10.1016/j.ijbiomac.2017.04.002
Giannakas, A. E., Karabagias, V. K., Moschovas, D., Leontiou, A., Karabagias, I. K., Georgopoulos, S., et al. (2023). Thymol@activated carbon nanohybrid for low-density polyethylene-based active packaging films for pork fillets’ shelf-life extension. Foods 12, 2590. doi:10.3390/foods12132590
Goñi, M. L., Gañán, N. A., Strumia, M. C., and Martini, R. E. (2016). Eugenol-loaded LLDPE films with antioxidant activity by supercritical carbon dioxide impregnation. J. Supercrit. Fluids 111, 28–35. doi:10.1016/j.supflu.2016.01.012
Gooderham, N. J., Cohen, S. M., Eisenbrand, G., Fukushima, S., Peter Guengerich, F., Hecht, S. S., et al. (2020). FEMA GRAS assessment of natural flavor complexes: clove, cinnamon leaf and west Indian bay leaf-derived flavoring ingredients. Food Chem. Toxicol. 145 (November), 111585. doi:10.1016/j.fct.2020.111585
Gutiérrez-Pacheco, M. M., Torres-Moreno, H., Flores-Lopez, M. L., Velázquez Guadarrama, N., Ayala-Zavala, J. F., Ortega-Ramírez, L. A., et al. (2023). Mechanisms and applications of citral’s antimicrobial properties in food preservation and pharmaceuticals formulations. Antibiot. (Basel) 12, 1608. doi:10.3390/antibiotics12111608
Hasani-Javanmardi, M., Fallah, A. A., and Abbasvali, M. (2021). Effect of safflower oil nanoemulsion and cumin essential oil combined with oxygen absorber packaging on the quality and shelf-life of refrigerated lamb loins. LWT 147, 111557. doi:10.1016/j.lwt.2021.111557
Hematizad, I., Khanjari, A., Basti, A. A., Karabagias, I. K., Noori, N., Ghadami, F., et al. (2021). In vitro antibacterial activity of gelatin-nanochitosan films incorporated with Zataria multiflora Boiss essential oil and its influence on microbial, chemical, and sensorial properties of chicken breast meat during refrigerated storage. Food Packag. Shelf Life 30, 100751. doi:10.1016/j.fpsl.2021.100751
Hernández, B., Sáenz, C., Alberdi, C., and Diñeiro, J. M. (2016). CIELAB color coordinates versus relative proportions of myoglobin redox forms in the description of fresh meat appearance. J. Food Sci. Technol. 53, 4159–4167. doi:10.1007/s13197-016-2394-6
Jafarinia, S., Fallah, A. A., and Dehkordi, S. H. (2022). Effect of virgin olive oil nanoemulsion combined with ajowan (Carum copticum) essential oil on the quality of lamb loins stored under chilled condition. Food Sci. Hum. Wellness 11, 904–913. doi:10.1016/j.fshw.2022.03.013
Janisch, S., Krischek, C., and Wicke, M. (2011). Color values and other meat quality characteristics of breast muscles collected from 3 broiler genetic lines slaughtered at 2 ages. Poult. Sci. 90, 1774–1781. doi:10.3382/ps.2010-01073
Jiménez, M., Domínguez, J. A., Pascual-Pineda, L. A., Azuara, E., and Beristain, C. I. (2018). Elaboration and characterization of O/W cinnamon (Cinnamomum zeylanicum) and black pepper (Piper nigrum) emulsions. Food Hydrocoll. 77, 902–910. doi:10.1016/j.foodhyd.2017.11.037
Karabagias, I., Badeka, A., and Kontominas, M. G. (2011). Shelf life extension of lamb meat using thyme or oregano essential oils and modified atmosphere packaging. Meat Sci. 88, 109–116. doi:10.1016/j.meatsci.2010.12.010
Karthik, P., Ezhilarasi, P. N., and Anandharamakrishnan, C. (2017). Challenges associated in stability of food grade nanoemulsions. Crit. Rev. Food Sci. Nutr. 57, 1435–1450. doi:10.1080/10408398.2015.1006767
Lakshmayya, N. S. V., Mishra, A. K., Mohanta, Y. K., Panda, J., Naik, B., Mishra, B., et al. (2023). Essential oils-based nano-emulsion system for food safety and preservation: current status and future prospects. Biocatal. Agric. Biotechnol. 53, 102897. doi:10.1016/j.bcab.2023.102897
Lazaridis, D. G., Karabagias, V. K., Karabagias, I. K., Andritsos, N. D., and Giannakas, A. E. (2024). Physicochemical and phytochemical characterization of green coffee, cinnamon clove, and nutmeg EEGO, and aroma evaluation of the raw powders. Eur. Food Res. Technol. 250, 83–96. doi:10.1007/s00217-023-04367-x
Liao, W., Gharsallaoui, A., Dumas, E., Ghnimi, S., and Elaissari, A. (2021). Effect of carrier oil on the properties of sodium caseinate stabilized O/W nanoemulsions containing Trans-cinnamaldehyde. LWT 146, 111655. doi:10.1016/j.lwt.2021.111655
Liu, T., Gao, Z., Zhong, W., Fu, F., Li, G., Guo, J., et al. (2022). Preparation, characterization, and antioxidant activity of nanoemulsions incorporating lemon essential oil. Antioxidants 11 (4), 650. doi:10.3390/antiox11040650
Liu, T., and Liu, L. (2020). Fabrication and characterization of chitosan nanoemulsions loading thymol or thyme essential oil for the preservation of refrigerated pork. Int. J. Biol. Macromol. 162, 1509–1515. doi:10.1016/j.ijbiomac.2020.07.207
Lu, W.-C., Huang, D.-W., Wang, C.-C. R., Yeh, C.-H., Tsai, J.-C., Huang, Y.-T., et al. (2018). Preparation, characterization, and antimicrobial activity of nanoemulsions incorporating citral essential oil. J. Food Drug Analysis 26, 82–89. doi:10.1016/j.jfda.2016.12.018
Magi, G., Marini, E., and Facinelli, B. (2015). Antimicrobial activity of essential oils and carvacrol, and synergy of carvacrol and erythromycin, against clinical, erythromycin-resistant group A streptococci. Front. Microbiol. 6 (March), 165. doi:10.3389/fmicb.2015.00165
Medeleanu, M. L., Fărcaș, A. C., Coman, C., Leopold, L., Diaconeasa, Z., and Socaci, S. A. (2023). Citrus essential oils – based nano-emulsions: functional properties and potential applications. Food Chem. X 20, 100960. doi:10.1016/j.fochx.2023.100960
Mehta, S. K., Gowder, S. J. T., Mehta, S. K., and Gowder, S. J. T. (2015). “Members of antioxidant machinery and their functions,” in Basic principles and clinical significance of oxidative stress (London, UK: IntechOpen). doi:10.5772/61884
Nagaraju, P. G., Sengupta, P., Chicgovinda, P. P., and Rao, P. J. (2021b). Nanoencapsulation of clove oil and study of physicochemical properties, cytotoxic, hemolytic, and antioxidant activities. J. Food Process Eng. 44, e13645. doi:10.1111/jfpe.13645
Nagaraju, P. G. P. S., Dubey, T., Chinnathambi, S. C. P. P., Rao, P. J., and C G, P. P. (2021a). Influence of sodium caseinate, maltodextrin, pectin and their Maillard conjugate on the stability, in vitro release, anti-oxidant property and cell viability of eugenol-olive oil nanoemulsions. Int. J. Biol. Macromol. 183, 158–170. doi:10.1016/j.ijbiomac.2021.04.122
Negi, A., and Kesari, K. K. (2022). Chitosan nanoparticle encapsulation of antibacterial essential oils. Micromachines 13, 1265. doi:10.3390/mi13081265
Pan, K., Chen, H., Davidson, P. M., and Zhong, Q. (2014). Thymol nanoencapsulated by sodium caseinate: physical and antilisterial properties. J. Agric. Food Chem. 62, 1649–1657. doi:10.1021/jf4055402
Peng, S., Zhou, L., Cai, Q., Zou, L., Liu, C., Liu, W., et al. (2020). Utilization of biopolymers to stabilize curcumin nanoparticles prepared by the pH-shift method: caseinate, whey protein, soy protein and gum Arabic. Food Hydrocoll. 107, 105963. doi:10.1016/j.foodhyd.2020.105963
Perumal, A. B., Li, X., Su, Z., and He, Y. (2021). Preparation and characterization of a novel green tea essential oil nanoemulsion and its antifungal mechanism of action against magnaporthae oryzae. Ultrason. Sonochemistry 76 (August), 105649. doi:10.1016/j.ultsonch.2021.105649
Qureshi, K. A., Mohammed, S. A. A., Khan, O., Ali, H. M., El-Readi, M. Z., and Mohammed, H. A. (2022). Cinnamaldehyde-based self-nanoemulsion (CA-SNEDDS) accelerates wound healing and exerts antimicrobial, antioxidant, and anti-inflammatory effects in rats’ skin burn model. Molecules 27, 5225. doi:10.3390/molecules27165225
Rao, J., Chen, B., and McClements, D. J. (2019). Improving the efficacy of essential oils as antimicrobials in foods: mechanisms of action. Annu. Rev. Food Sci. Technol. 10, 365–387. doi:10.1146/annurev-food-032818-121727
Rezaei, A., Fathi, M., and Jafari, S. M. (2019). Nanoencapsulation of hydrophobic and low-soluble food bioactive compounds within different nanocarriers. Food Hydrocoll. 88, 146–162. doi:10.1016/j.foodhyd.2018.10.003
Saddiq, A. A., and Khayyat, S. A. (2010). Chemical and antimicrobial studies of monoterpene: citral. Pesticide Biochem. Physiology 98, 89–93. doi:10.1016/j.pestbp.2010.05.004
Sakanaka, S., Tachibana, Y., Ishihara, N., and Juneja, L. R. (2005). Antioxidant properties of casein calcium peptides and their effects on lipid oxidation in beef homogenates. J. Agric. Food Chem. 53, 464–468. doi:10.1021/jf0487699
Semenova, A. A., Nasonova, V. V., and Tunieva, Е. K. (2019). The effect of essential oils on the color stability of minced meat. IOP Conf. Ser. Earth Environ. Sci. 333, 012098. doi:10.1088/1755-1315/333/1/012098
Shahidi, F. (1990). The 2-thiobarbituric acid (TBA) methodology for the evaluation of warmed-over flavour and rancidity in meat products. Proc. 36th Int. Congr. Meat Sci. Technol. 3, 1008–1014.
Silva-Angulo, A. B., Zanini, S. F., Rosenthal, A., Rodrigo, D., Klein, G., and Martínez, A. (2015). Comparative study of the effects of citral on the growth and injury of Listeria innocua and Listeria monocytogenes cells. PLOS ONE 10, e0114026. doi:10.1371/journal.pone.0114026
Skandamis, P. N., and Nychas, G.-J. E. (2001). Effect of oregano essential oil on microbiological and physico-chemical attributes of minced meat stored in air and modified atmospheres. J. Appl. Microbiol. 91, 1011–1022. doi:10.1046/j.1365-2672.2001.01467.x
Somolinos, M., García, D., Condón, S., Mackey, B., and Pagán, R. (2010). Inactivation of Escherichia coli by citral. J. Appl. Microbiol. 108, 1928–1939. doi:10.1111/j.1365-2672.2009.04597.x
Stratakos, A. C., and Grant, I. R. (2018). Evaluation of the efficacy of multiple physical, biological and natural antimicrobial interventions for control of pathogenic Escherichia coli on beef. Food Microbiol. 76, 209–218. doi:10.1016/j.fm.2018.05.011
Sun, Y., Zhang, M., Bhandari, B., and Bai, B. (2021). Nanoemulsion-based edible coatings loaded with fennel essential oil/cinnamaldehyde: characterization, antimicrobial property and advantages in pork meat patties application. Food control. 127 (September), 108151. doi:10.1016/j.foodcont.2021.108151
Tastan, Ö., Ferrari, G., Baysal, T., and Donsì, F. (2016). Understanding the effect of formulation on functionality of modified chitosan films containing carvacrol nanoemulsions. Food Hydrocoll. 61, 756–771. doi:10.1016/j.foodhyd.2016.06.036
Uçar, Y. (2020). Antioxidant effect of nanoemulsions based on citrus peel essential oils: prevention of lipid oxidation in trout. Eur. J. Lipid Sci. Technol. 122, 1900405. doi:10.1002/ejlt.201900405
Ujilestari, T., Febrisiantosa, A., Sholikin, M. M., Wahyuningsih, R., and Wahyono, T. (2023). Nanoemulsion application in meat product and its functionality: review. J. Anim. Sci. Technol. 65, 275–292. doi:10.5187/jast.2022.e120
Wang, D., Xiao, H., Lyu, X., Chen, H., and Wei, F. (2023). Lipid oxidation in food science and nutritional health: a comprehensive review. Oil Crop Sci. SI:Lipid Sci. Health 8, 35–44. doi:10.1016/j.ocsci.2023.02.002
Wang, L., Liu, T., Liu, L., Liu, Y., and Wu, X. (2022). Impacts of chitosan nanoemulsions with thymol or thyme essential oil on volatile compounds and microbial diversity of refrigerated pork meat. Meat Sci. 185 (March), 108706. doi:10.1016/j.meatsci.2021.108706
Wang, X., and Zhao, Z. (2022). Improved encapsulation capacity of casein micelles with modified structure. J. Food Eng. 333, 111138. doi:10.1016/j.jfoodeng.2022.111138
Xu, F., Shi, Y., Li, B., Liu, C., Zhang, Y., and Zhong, J. (2024). Characterization, stability and antioxidant activity of vanilla nano-emulsion and its complex essential oil. Foods 13 (5), 801. doi:10.3390/foods13050801
Xu, J.-G., Liu, T., Hu, Q.-P., and Cao, X.-M. (2016). Chemical composition, antibacterial properties and mechanism of action of essential oil from clove buds against Staphylococcus aureus. Molecules 21, 1194. doi:10.3390/molecules21091194
Zaharioudakis, K., Kollia, E., Leontiou, A., Moschovas, D., Karydis-Messinis, A., Avgeropoulos, A., et al. (2023). Carvacrol microemulsion vs. Nanoemulsion as novel pork minced meat active coatings. Nanomater. (Basel) 13, 3161. doi:10.3390/nano13243161
Zhang, J., Wang, Y., Pan, D.-D., Cao, J.-X., Shao, X.-F., Chen, Y.-J., et al. (2016). Effect of black pepper essential oil on the quality of fresh pork during storage. Meat Sci. 117, 130–136. doi:10.1016/j.meatsci.2016.03.002
Zhang, Le, Liu, A., Wang, W., Ye, R., Liu, Y., Xiao, J., et al. (2017). Characterisation of microemulsion nanofilms based on Tilapia fish skin gelatine and ZnO nanoparticles incorporated with ginger essential oil: meat packaging application. Int. J. Food Sci. Technol. 52 (7), 1670–1679. doi:10.1111/ijfs.13441
Keywords: caseinate nanoemulsions, active edible coatings, citral, eugenol, carvacrol, cinnamaldehyde, pork tenderloin meat
Citation: Zaharioudakis K, Salmas CE, Andritsos ND, Kollia E, Leontiou A, Karabagias VK, Karydis-Messinis A, Moschovas D, Zafeiropoulos NE, Avgeropoulos A, Proestos C and Giannakas AE (2024) Carvacrol, citral, eugenol and cinnamaldehyde casein based edible nanoemulsions as novel sustainable active coatings for fresh pork tenderloin meat preservation. Front. Food. Sci. Technol. 4:1400224. doi: 10.3389/frfst.2024.1400224
Received: 13 March 2024; Accepted: 31 May 2024;
Published: 20 June 2024.
Edited by:
Vandana Chaudhary, Lala Lajpat Rai University of Veterinary and Animal Sciences, IndiaReviewed by:
Swarup Roy, Lovely Professional University, IndiaRafael Gavara, Spanish National Research Council (CSIC), Spain
Copyright © 2024 Zaharioudakis, Salmas, Andritsos, Kollia, Leontiou, Karabagias, Karydis-Messinis, Moschovas, Zafeiropoulos, Avgeropoulos, Proestos and Giannakas. This is an open-access article distributed under the terms of the Creative Commons Attribution License (CC BY). The use, distribution or reproduction in other forums is permitted, provided the original author(s) and the copyright owner(s) are credited and that the original publication in this journal is cited, in accordance with accepted academic practice. No use, distribution or reproduction is permitted which does not comply with these terms.
*Correspondence: Aris E. Giannakas, YWdpYW5uYWthc0B1cGF0cmFzLmdy; Constantinos E. Salmas, a3NhbG1hc0B1b2kuZ3I=; Charalampos Proestos, aGFycHJvQGNoZW0udW9hLmdy