- 1Instituto de Investigaciones en Ciencia y Tecnología de Materiales, Facultad de Ingeniería, Universidad Nacional de Mar del Plata-Consejo Nacional de Investigaciones Científicas y Técnicas, Mar del Plata, Argentina
- 2Grupo de Investigación en Ingeniería en Alimentos, Facultad de Ingeniería, Universidad Nacional de Mar del Plata-Consejo Nacional de Investigaciones Científicas y Técnicas, Mar del Plata, Argentina
One of the main challenges facing the fresh-cut produce industry is maintaining the quality and food safety of these products to ensure both consumer satisfaction, which includes not further compromising the environment by following the global trend toward positive environmental footprints and the spirit of circular economy. Bio-based polymer blend films have emerged as a novel alternative to replace traditional plastics due to their biodegradability, sustainability, and environmental friendliness characteristics. In this work, we evaluated the properties of gelatin/chitosan composite films and coatings incorporated with thyme essential oil (TEO) in free form and microencapsulated in β-cyclodextrin. The incorporation of free and encapsulated TEO enhance the blocking properties of the films against UV–visible light (opacity values of 5.25 and 7.54 for 4% TEO content, respectively) and provides excellent in vitro antimicrobial and antioxidant properties. Films incorporated with encapsulated TEO showed improvement in tensile strength and water vapor permeability, with respect to both, neat composite films and films incorporated with free TEO, while films incorporated with free thyme essential oil exhibited enhanced elongation at break. Encapsulation can slow down the release rate of TEO from the films allowing the bioactive to remain active for much longer time. After 12 days of refrigerated storage (5°C), minimally processed kiwi-fruit coated with film forming solutions incorporated with encapsulated TEO showed reduced weight loss (4.2% reduction showed by kiwi slices coated with Ch-gel-TEO-βCD-4% formulation vs. 7.5% reduction exhibited by kiwi slices stored without coating), enhanced antioxidant properties (53% increment for kiwi slices coated with Ch-gel-TEO-βCD-4% compared to uncoated ones), as well as, significant reductions in the total mesophilic aerobic bacteria and yeast and mold counts (reductions of 3.37 and 2.93 Log CFU g−1, respectively, for kiwi slices coated with Ch-gel-TEO-βCD-4% formulation compared to kiwi slices stored without coating). The sensory acceptability kiwifruit coated with encapsulated TEO was the highest among tested samples, with no adverse effects on flavor and general acceptability. These results provide unique insights into new bio-packaging materials for the development of novel health foods materials with improved nutritional quality and sensory attributes, superior antimicrobial activity and extended shelf life.
1 Introduction
The traditional way of delaying food spoilage by using polyethylene and polystyrene based packaging has worsened environmental pollution (Yan et al., 2024; Eranda et al., 2024). The slow degradation of these traditional petroleum-derived packaging materials in natural environments constitutes a major obstacle to achieving global sustainable development objectives (Eranda et al., 2024). Food packaging plays a vital role in protecting food from spoilage factors such as light, oxygen and moisture, which ultimately prolongs the shelf life of food (Eranda et al., 2024; Xu et al., 2001). It is therefore urgent to find a compromise solution that allows us to maintain or even improve the current level of protection of food provided by traditional packaging, but without further compromising our environment (Salmas et al., 2022).
Among the sustainable replacements to the traditional non-biodegradable packaging that have emerged in recent years, edible packaging is regarded as an ecological, innovative and biodegradable alternative for preserving food (Yan et al., 2024; Kulawik et al., 2024). Edible packaging is defined as a thin layer that covers the surface of food and can be consumed as part of food products, so it must be made from natural ingredients such as proteins, polysaccharides, lipids, etc. of animal and plant origin (Yan et al., 2024; Kulawik et al., 2024; Sekarina et al., 2023). In addition to the various advantages of natural biopolymers that include ecological properties, safety, biodegradability and multi-functionality (Teymoorian et al., 2024), these substrates can be combined to produce packaging materials with properties that exceed those of the individual components or can be enriched with a wide range of bioactive substances that also impart biological properties such as prebiotic, probiotic, antioxidant, antimicrobial, and other health-promoting properties (Yan et al., 2024; Kulawik et al., 2024; Jridi et al., 2014).
Among these sustainable raw materials, the most commonly used protein is gelatin, a collagen product extracted from porcine and bovine wastes, as well as animal and fish skin and bones, which is considered a safe and consumer-acceptable edible packaging material due to its good film-forming properties (Yan et al., 2024; Jridi et al., 2014). Gelatin exhibits good barrier properties against oxygen and aroma transfer, although poor barrier properties against water vapor transfer due to its hydrophilic nature (Sekarina et al., 2023; Jridi et al., 2014; Ramziia et al., 2018). Furthermore, chitosan, a polysaccharide obtained by deacetylation of chitin, has various advantages as matrix material, such as non-toxicity, biocompatibility, and antioxidant and antibacterial activity (Yan et al., 2024; Sekarina et al., 2023; Teymoorian et al., 2024; Ramziia et al., 2018). This natural carbohydrate poses also a positive charge and is soluble at a pH below 6.5, due to the protonation of their constituting amino groups. However, chitosan films also have disadvantages, among the main ones being their poor mechanical and water barrier characteristics (Salmas et al., 2022; Kulawik et al., 2024; Teymoorian et al., 2024).
On the other hand, various studies have shown that the combined use of these two natural matrices can compensate for the deficiencies of their individual films, improving the poor mechanical and barrier properties, while incorporating antimicrobial and antioxidant activity and enhancing freshness retention properties compared to those that would be obtained from films formed by a single constituent (Yan et al., 2024; Salmas et al., 2022; Kulawik et al., 2024; Wang et al., 2021). Moreover, studies demonstrated that the additional incorporation of active molecules into these composite films leads to further enhancement of the antioxidant and bacteriostatic properties and, therefore, improves their food preservation capacity (Yan et al., 2024; Eranda et al., 2024; Sekarina et al., 2023; Teymoorian et al., 2024; Ramziia et al., 2018). According to Díaz-Montes and Castro-Muñoz (2021a), Díaz-Montes and Castro-Muñoz (2021b) and Kulawik et al. (2024), carbohydrates are generally used to form the backbone of the polymeric packaging matrix, while the addition of proteins improve the active transport of bioactive substances and gas vapor permeability.
Essential oils (EOs) are very attractive components for the manufacture of active edible films (Teymoorian et al., 2024; Ansorena et al., 2016): they are natural, non-toxic, and generally recognized as safe (GRAS) and possess multitude functional properties such as antioxidative, antifungal, antibacterial, etc. (Mahmood et al., 2023; Moradi et al., 2020). However, their incorporation into edible films is difficult due to their high volatility and low solubility in aqueous solutions, and their effects usually do not last over time due to their sudden release from film matrices (Teymoorian et al., 2024). Encapsulation of essential oils using natural substances is a way to address these limitations that not only improves their solubility and stability, but also masks their unexpected flavors (Mahmood et al., 2023; Moradi et al., 2020; Hossen et al., 2024; Zhang et al., 2018; Erceg et al., 2023). Taking advantage of the fact that the internal cavity of β-cyclodextrins (β-CDs) has the ability to trap hydrophobic essential oil droplets without denaturing their native compounds (Hossen et al., 2024), in this work we selected them to prepare TEO/β-CD inclusion complexes that were further incorporated into composite gelatin-chitosan films.
Minimally processed fruit, also known as fresh-cut fruit, is the processing of fruit that involves washing, peeling and slicing before packaging, using low temperatures for storage in order to avoid losing its freshness and nutritional value (Sekarina et al., 2023). However, minimal processing of fresh fruits usually causes unavoidable damage, which accelerates cellular respiration favoring undesirable enzymatic reactions that results in damage to the structural integrity and loss of quality of the processed fruit (Dias et al., 2023; Gomes et al., 2023). Therefore, it is a major challenge faced by the fresh-cut industries to maintain the quality of fresh-cut produce to ensure consumer satisfaction and efficient marketing (Guroo et al., 2017; Khan et al., 2021). Additionally, according Khan et al. (2021), studies on the impact of antioxidant packaging on the quality of fresh and minimally processed fruits and vegetables during their shelf life are still scarce. Therefore, the present work was performed in order to find a multifunctional bio-packaging system (chitosan-gelatin-TEO/βCD-TEO) that would enhance nutritional status of food by incorporating active ingredients beneficial to health while remaining antimicrobial and having sufficient mechanical integrity. Hence, film properties related with food packaging applications, such as tensile behavior, opacity and water vapor permeability and in vitro antimicrobial and antioxidant activities and stability over time were evaluated. The film-forming solutions were then applied as a coating to minimally processed kiwifruit, a fruit originally from Asia that is very popular due to its sensory and nutritional characteristics, and the performance of the different coatings regarding weight loss, color, growth of mesophilic aerobic bacteria, yeast and molds and sensory quality attributes during refrigerated storage was evaluated. To the best of our knowledge, the combination of these postharvest treatments (chitosan-gelatin coatings, thyme essential oil active compounds, encapsulation in β-CD) in minimally processed kiwifruit during refrigerated storage is here reported for the first time. These findings provide unique insights into novel bio-packaging materials for the development of new healthy food materials with improved nutritional quality and sensory attributes, superior antimicrobial activity and extended shelf life.
2 Materials and methods
2.1 Materials
Chitosan (Ch) (deacetylation degree 90%, Mv = 1.61 × 105 g/mol) was supplied by PARAFARM, Mar del Plata, Argentina. Glycerol (Gly), used as plasticizer and glacial acetic, used to dissolve chitosan, were purchased from Biopack (Argentina). Gelatin (adhesiveness ≥ 15%) and thyme essential oil (TEO), extracted from the plant Thymus vulgaris, were purchased in local shops (Mar del Plata, Buenos Aires, Argentina); β-cyclodextrin (β-CD) (Cavamax W7 food grade) was provided by Wacker Biochem, Eddyville, IA, USA.
2.2 Methods
2.2.1 Microencapsulation of TEO in β-cyclodextrins
TEO:β-CD microcapsules were obtained by the co-precipitation methodology, as described in Marcovich et al. (2024), which was applied until reaching a TEO:β-CD ratio of 8:92 (%, w/w). This ratio was the one presenting the highest encapsulation efficiency (EE) according to a preliminary study in which different weight ratios of TEO to β-CD were tested. The obtained suspension was filtered and the TEO:β CD inclusion complexes were then dried in a convection oven (50°C, 24 h) and finally stored in a desiccator containing silica-gel at 25°C.
2.2.2 Preparation of films
Chitosan (Ch) film-forming solutions (2% w/v) were prepared according to a previous work with slight modifications (Tomadoni et al., 2019). 2 g of chitosan powder was dissolved in a dilute acetic acid solution (1%v/v) by magnetic stirring (TS-1000, Zhejiang, China) at room temperature (23 ± 2°C). The same weight of gelatin (Gel) was added to distilled water (2% w/v) at 60°C and stirred until complete dissolution. Chitosan and gelatin solutions were mixed in equal proportions and stirred for 1 h at 60°C after the addition of glycerol as plasticizer in the amount of 30% w/w (based on total solid content). Films were prepared by the casting technique: 15 mL of the different film forming solutions were poured into Petri dishes and dried at 40°C until constant weight.
Active films were prepared as described above, but including into the forming solution free or microencapsulated TEO. Two different concentrations of free and microencapsulated TEO were tested, which corresponds to a content of 1.17 and 2.34 g of thymol per liter of solution, as thymol is the main active compound of the essential oil. In this sense, three types of film forming solutions (FFSs) were prepared as follows: (1) Ch-gel (Control film), (2) free TEO concentrations of 2 and 4% (w/v) labeled as Ch-gel-TEO-2 and 4% and (3) TEO:β-CD microcapsules with the same final thymol concentration (main TEO constituent) as the free form (labeled as Ch-gel-β-CD-TEO-2 and 4%).
2.3 Films characterization
2.3.1 Thickness
A digital micrometer (Fowler XTRA MIC II, range 0–1″ (0–25 mm), precision = 0.00005″) was used to measure the film thickness. Five different points were measured for each film and averaged.
2.3.2 Mechanical properties
Films specimens cut from the molded plates according to ASTM D1708-93 (ASTM, 1993) were conditioned for 96 h at 23°C and 50 ± 5% RH before performing tensile mechanical tests. Then they were tested at room temperature (25 ± 2°C) and 5 mm/min crosshead speed in a Universal test apparatus (Zhiqu, ZQ-990LB, China). The tensile strength (TS) and elongation at break (EAB) were calculated as described in ASTM D638-94b (ASTM, 1994).
2.3.3 Opacity
It was evaluated on rectangular film strips, that were placed directly into the cell of an UV–Vis spectrophotometer (Shimadzu 1601 PC, Tokyo, Japan), as the absorbance measured at 600 nm divided by sample thickness (mm). Three values were taken for each sample and the results are presented as mean value ± standard deviation.
2.3.4 Water vapor permeability (WVP)
Film samples were pre-conditioned for at least 4 days in an environmental chamber kept at room temperature (25 ± 2°C) and 65% relative humidity (RH). The water vapor permeability of the films was measured using a WVP tester (Labstone, B-31E, China) by the weighing method. Film simples were attached to containers with 5 mL of water and the edges were sealed. Then the containers were placed in a desiccator at 38°C and 10% RH. Weight was recorded every hour for 24 h. Water vapor permeability of the films was calculated as indicated in Equation 1:
Where Δm and Δt are the weight difference and the elapsed time for mass change, respectively, A and x are the exposed area (m2) and the thickness (m, measured with a caliper) of the film sample, respectively, and ΔP is the difference of vapor pressures (Pa) inside and outside the film sample that were calculated based on the container and desiccator relative humidities.
2.3.5 In vitro antioxidant capacity and stability of the films
Pieces of films (0.5 g) were immersed in 10 mL of pure methanol and submitted to constant shaking for 24 h at room temperature and under dark conditions. Subsequently, the flasks were sonicated (Ultrasound chamber PS-30A, RoHs, Jiangsu, China) for 20 min. The obtained extracts were further used to determine the antioxidant properties evaluated as the scavenging ability of 2,2-diphenyl-1-picrylhydrazyl (DPPH) free radicals at 517 nm (Marcovich et al., 2024). The inhibition percentage indicates the capacity of the antioxidant to reduce the DPPH radical and is calculated according to Equation 2:
Where A0 is the absorbance of the control group (without the film sample), Ai is the absorbance of the resulting DPPH solution (with the film sample). The stability of antioxidant activity of the films was further evaluated by analyzing total antioxidant capacity (DPPH radical scavenging activity) at time 0 and after 8 days.
2.3.6 In vitro antimicrobial activity and stability of the film forming solutions
The antimicrobial activity was determined according to the method reported in Wu et al. (2023) using the referent Gram (−) bacteria strain Escherichia coli (ATCC 25922). The bacteria were incubated overnight in Mueller Hinton (MH) broth, then centrifuged at 1,300 × g for 10 min and suspended with 0.01 mol/L phosphate buffered saline (PBS) to prepare bacterial suspensions. Then the OD600 of the suspensions was adjusted to 0.1. Subsequently, bacterial suspension was exposed to the different film forming solutions (volume ratio 2/8) and 100 μL of the mixture was added and spread uniformly on the plate, and incubated at 37°C. Microbial activity was measured at 12, 24, 48, 72, and 96 h through the colony count method, to evaluate the stability of the film forming formulations. The inhibition ratio was calculated as indicated in Equation 3:
Where the Nc and Nx represent the microbial concentrations of control and film forming solutions containing free and microencapsulated TEO, respectively.
2.4 Food preservation application
2.4.1 Plant material
Fresh Kiwifruit (A. deliciosa cv. Hayward) were obtained from a local market. They were selected for uniformity in shape and size, discarding defected fruit. The ripening stage of kiwifruit corresponds to soluble solids content of 8.6 ± 2.2%. The kiwifruits were washed with tap water and sanitized with chlorinated water (200 mg/L) for 5 min at 15°C and rinsed with tap water, drained for 10 min, then hand peeled and sliced into 8 mm thickness with a stainless-steel slicer. The slices were randomly distributed among treatments.
2.4.2 Coating application
Slices were immersed for 10 min at ambient temperature in the different film forming solutions. Slices immersed in distilled water were used as control samples. After treatment, slices were air dried for 15 min and then placed in standard polypropylene food grade containers (15 × 9 × 5 cm) (Cotnyl SA, Buenos Aires, Argentina) of 25 μm of thickness (with O2 and CO2 permeabilities of 38 and 230 cm3 mm m−2 d−1 atm−1, respectively, measured at 23°C and 0% RH, and a water vapor permeability of 6 g m−2 d−1; determined at 38°C and 90% HR). Trays were covered with a lid of the same material, sealed with parafilm without initial atmosphere modification, and stored at 5°C for 12 days. It is important to remark that this packaging process did not affect the texture or quality of the samples. The experiments were performed in three independent replicates. At days 0, 2, 4, 6, 8, and 12 of storage, samples were taken for subsequent analysis.
2.4.3 Weight loss
The weight of individual bags was recorded immediately after the treatment (day 0) and at the different sampling times. The percentage weight loss relative to initial weight was calculated by weighing simples at the beginning (0 day) and at the end of each storage Interval. The difference between the initial and final weight of the slices was the total percentage weight loss. The results were expressed as the percentage loss of the initial weight (%).
2.4.4 Color
The flesh color of kiwifruit slices was determined using a colorimeter (LoviBond, RT500, Neu-Isenburg, Germany). The a* parameter indicates chromaticity on a green (−) to red (+) axis and was measured at 10 random areas for each treatment and storage time.
2.4.5 Antimicrobial activity
Kiwifruit slices were mixed with phosphate buffer solution (0.1 mol L−1, pH = 7.2) and homogenized with a Stomacher 400 Circulator Homogenizer. The enumeration and differentiation of microorganisms were performed according to Viacava et al. (2022) by using the following culture media and culture conditions: mesophilic aerobic bacteria were counted on Plate Count Agar (PCA) incubated at 30–32°C for 48–72 h, and yeast and molds were counted in Yeast-Glucose-Chloramphenicol (YGC) medium incubated at 25°C for 5 days. All culture mediums were from Britania, Buenos Aires, Argentina. Microbial counts were performed by triplicate and expressed as log colony forming units (CFU) per gram of fresh weight (Log CFU/g).
2.4.6 DPPH radical scavenging activity
Kiwifruit slices were processed with a kitchen processor for 2 min. Two samples of 2 g each were extracted with 10 mL of ethanol 80% v/v containing citric acid (1% w/v) in an ultrasonic bath for 30 min at 18°C. Afterwards, the homogenate was centrifuged at 10,000 × g and 4°C for 15 min. The supernatant was collected and the precipitate was extracted again with 10 mL of the same solvent, under the previously described conditions. The two supernatants were combined and filtered using Whatman filter paper #1. The final extract was stored at −20°C to be used in the determination of antioxidant activity by DPPH method. Antioxidant activity using the DPPH (2,2-diphenyl 1-picrylhydrazyl) radical was determined based on the methodology described by Viacava et al. (2022). Each sample extract (10 μL) was mixed with 140 μL of an ethanolic DPPH solution (100 μmol L−1) in a microplate. The mixtures were allowed to stand at ambient temperature in the dark for 60 min. The absorbance was measured at 517 nm on a microplate reader device of 300 μL capacity (Biotek, Synergy HT, Winooski, VT, USA). DPPH radical scavenging activity was expressed as inhibition percentage (% inhibition).
2.4.7 Organoleptic quality: overall visual quality (OVQ)
Overall visual quality (OVQ) was evaluated by a sensory panel comprised of 9 trained judges. They were workers and students of our research group with sensory evaluation experience in fruits and vegetables quality. Each sample was presented one at a time in random order to the judges who made independent evaluations. Quality parameters of coated and non-coated kiwifruit including color, texture, smell and visual appearance were analyzed and quantified with a 5-point scale: 5 = excellent, 4 = good, 3 = fair, 2 = poor and 1 = extremely poor. The average of the indices was used as an estimation of OVQ. The limit of acceptance was three indicating that an average OVQ score below 3 was deemed to indicate end of shelf life. Days 4, 8, and 12 of storage were selected to perform the sensory evaluation (Viacava et al., 2022).
2.5 Statistical analysis
Both, the significance of the differences in the physical and mechanical properties of the active films as well the effect of the application of edible coatings to fresh-cut kiwifruit was evaluated through analysis of variance (ANOVA) using statistical software SPSS for Windows, version 19.0 (SPSS Inc., Chicago, IL, USA). Differences between mean values were evaluated using the HSD Tukey test with a 95% confidence interval.
3 Results and discussion
3.1 Physical and mechanical characterization of the active films
At pH above the isoelectric point of gelatin (pH = 4.5–5.2), the solubilized protein is negatively charged, while below pH = 6.2–6.5, chitosan is positively charged. Under these conditions, which were met in this work, gelatin and chitosan can form polyelectrolyte complexes (Eranda et al., 2024). Gelatin is a typical polyampholyte and its charged carboxyl groups (COO−) can interact ionically with the oppositely charged amine groups (NH3+) of chitosan chains under acidic conditions. Furthermore, a significant number of polar groups contained in gelatin chains, such as –COOH, –OH and –NH2, can set up hydrogen bonds with the –NH2 and –OH groups corresponding to chitosan (Eranda et al., 2024; Chen et al., 2008; Sionkowska et al., 2004; Voronko et al., 2016). Therefore, the physical and mechanical properties of gelatin-chitosan composite films have been found to be superior to those of pure gelatin films (Jridi et al., 2014; Hosseini et al., 2013) and/or pure chitosan (Erceg et al., 2023), although the chemical nature of the biopolymeric raw materials (ex. chitosan molecular weight and degree of deacetylation, gelatin origin, etc.) certainly influences the performance of the composite films (Liu et al., 2012; Gómez-Estaca et al., 2011). Furthermore, the inclusion of active molecules such as free or microencapsulated thyme essential oil, further affects the mechanical and physical performance of the resulting composite active films. To determine how the inclusion of TEO affects the performance of the gelatin-chitosan active films, several properties were measured. The results obtained are presented in Table 1.
It is noticed that the thickness of the composite films increases with the addition of TEO in both forms (free and microencapsulated). While the difference is not statistically significant when a low amount of free essential oil is incorporated (2 wt.%), it becomes statistically significant for the highest content or when TEO is added microencapsulated. Certainly, the hydrophobic nature of TEO coupled with its insolubility in aqueous solutions, as well as the oligomeric nature and surface characteristics of β-cyclodextrins-TEO inclusion complexes (consisting in a hydrophilic outer surface with an hydrophobic inner cavity that enables the trapping of TEO) could affect the strong and diverse interactions developed between protein and carbohydrate molecular chains leading, therefore, to a slight increase in the thickness of the resulting films (i.e., looser membrane matrix). However, most probably, this effect can be attributed to the slight increase in the total solids content of the film forming emulsions due to incorporation of free or microencapsulated TEO, which were added “in excess” to the pure chitosan-gelatin film forming solution. These results are consistent with those reported by Ramziia et al. (2018).
Incorporation of active substances such as essential oils or other biocompatible biomolecules like cyclodextrins can play an important role in mechanical properties of the films (Wang et al., 2021; Erceg et al., 2023). The effect of essential oil is rather variable and is dependent on the specific interactions between oil components and polymer matrix (Wang et al., 2021; Bonilla et al., 2018). In the present case free TEO reduces the tensile strength while increasing the elongation at break of the gelatin-chitosan based films. This could be credited to the decreasing cohesion forces within bio-polymers that are replaced by the weaker polymer-essential oil interactions, but also to the heterogeneities and discontinuities that this hydrophobic compound could introduce into film microstructure (Ramziia et al., 2018; Wang et al., 2021; Bonilla et al., 2018; Haghighi et al., 2019), by acting as plasticizing agent. The mechanical behavior of the films containing β-cyclodextrins-TEO inclusion complexes, on the other hand, is the opposite: the tensile strength increases while the elongation at break decreases as the amount of this inclusion increases. This could be addressed to the formation of a denser and more stable polymeric network that could be attributed to the secondary interactions such as electrostatic forces and hydrogen bonding developed between the biopolymers and the inclusion complex, as reported in related works (Erceg et al., 2023; Kan et al., 2019). Similar results were also reported by Salmas et al. (2022) for their edible active films based on thymol-rich halloysite nanostructures and chitosan/polyvinyl alcohol gels.
Regarding transparency or opacity, films with high light transparency are desirable for displaying the food packed in them (Yan et al., 2024; Jridi et al., 2014). However, food packed in highly transparent films is also exposed to ultraviolet light, which can accelerate the oxidation process, thus reducing its quality and shorten its shelf life (Ramziia et al., 2018; Wang et al., 2021; Liu et al., 2024). As expected, the inclusion of the active agent in gelatin-chitosan composite films leads to an increase in opacity, which is greater when microencapsulated TEO rather than free TEO is incorporated. This effect is generally credited to light scattering by essential oil droplets distributed throughout the polymeric network that results in the reduction of the transmission of light through the film (Wang et al., 2021; Liu et al., 2024). Denser or more compact film matrices, such as those containing β-cyclodextrins-TEO inclusion complexes, in which the spacing between polymer chains should have been reduced, could also lead to an increase in opacity by allowing less light to pass through the film (Ghaderi et al., 2019). The increment in film opacity due to the incorporation of the bioactive compounds in our gelatin-chitosan based films improved light barrier properties, as was also reported in related works (Ramziia et al., 2018; Xu et al., 2021), making them interesting alternatives for protecting light-sensitive foods from spoilage.
Water vapor barrier is a key feature since it limits the passage of water vapor through the polymeric membrane (Wang et al., 2021; Bonilla et al., 2018; Amjadi et al., 2019) and thus, it can be considered as a measure of the ability of the film to protect food against microbial growth under humid conditions thus extending the shelf life and guaranteeing the quality of food during storage (Wang et al., 2021; Bonilla et al., 2018; Ghaderi et al., 2019; Chen H.-Z. et al., 2020).
Wang et al. (2021), who reviewed the physical properties of chitosan-gelatin edible films indicated that blend films exhibited the lowest water vapor permeability in comparison with bilayer chitosan-gelatin or pure chitosan films, because the covalent and hydrogen interactions between chitosan and gelatin network reduced the availability of the hydrophilic groups, leading finally to a decrease in the water affinity. According our results, the incorporation of free or microencapsulated TEO further reduced the WVP of the active films. As indicated above, the hydrophobic nature of the thyme essential oil and the final microstructure developed by the films incorporated with free TEO, consisting in oil droplets dispersed throughout the polymeric matrix that act as obstacles to the passage of water vapor, could be the cause of the decrease in this property with the concentration of free TEO. In the same way, the densification of the gelatin-chitosan network credited to the incorporation of β-cyclodextrins-TEO inclusion complexes reduces the free volume of the composite polymeric matrix, thus affecting the rate of diffusion of water molecules through the film, which should slow down. These results are consistent with those reported be Rezaee et al. (2018), although there are other examples in literature that inform that incorporation of β-cyclodextrin increased the WVP of the chitosan-gelatin film (Wang et al., 2021; Erceg et al., 2023). Moreover, there are also reports indicating that the inclusion of plant extracts or oils into gelatin-chitosan based films could impose negative or positive effects on their WVP properties (Ramziia et al., 2018; Kan et al., 2019; Lin et al., 2019). The increase in permeability associated to the inclusion of essential oils into chitosan-gelatin films was explained by considering that the presence of oil droplets in these films led to a weakening of intermolecular interactions between polymer molecules, resulting in an open structure that facilitates the water vapor transfer across the films (Haghighi et al., 2019). Kan et al. (2019), on the other hand, noticed that the WVP first decreased and then increased with the increasing Crataegus pinnatifida fruit extract content and credited this effect to that at low loads, the extract could disperse well in the film matrix thereby retarding effectively the passage of water vapor while for higher loads (>6 wt.%) the extract aggregated and disrupted the compact film matrix.
3.2 In vitro antioxidant capacities and antimicrobial activities of the films
The results of the in vitro oxidation resistance of the films measured by the DPPH method are shown in Figure 1 and Supplementary Table S1. Results show that at day zero, DPPH antioxidant capacity of the Ch-gel films increased with the addition of TEO, being higher when TEO is incorporated in its free form. Moreover, the higher the TEO concentration, the higher is the antioxidant capacity of the film which is due to the good antioxidant activity of the polyphenols present in the essential oil. The antioxidant activity of TEO has been well demonstrated and is attributed to the structure of TEO constituents; it has been demonstrated that TEO is composed of a variety of phenolic mono terpenes, such as thymol and carvacrol, with antioxidant properties (Marcovich et al., 2024; Pilozo et al., 2024). The initial DPPH free radical scavenging ability of the films with free TEO was 55 and 70% for Ch-gel-TEO-2% and Ch-gel-TEO-4%, respectively and for films with encapsulated TEO 36 and 45% for Ch-gel-βCD-TEO-2% and Ch-gel-βCD-TEO-4%, respectively.
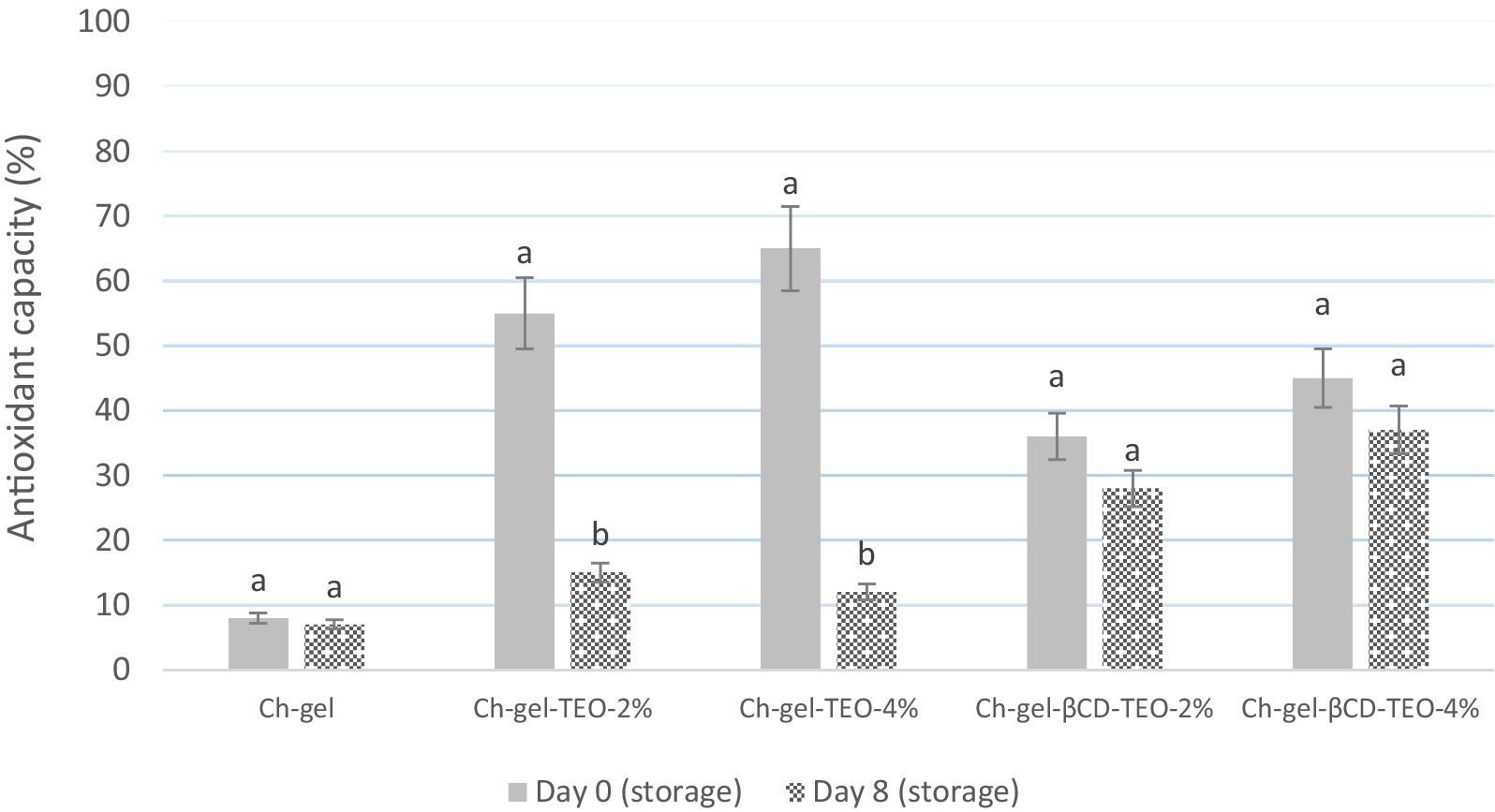
Figure 1. In vitro antioxidant capacity of the active Ch-gel films measured by the DPPH method. Reported values correspond to the mean and the error bars represent the standard deviation associated with each LSMEAN. Different letters denote a significant difference between treatment mean values (p < 0.05).
After 8 days, the antioxidant capacity of films with free TEO experienced a sharp decrease, probably due to the instability and volatility of unencapsulated TEO (p ≤ 0.05) reaching an antioxidant capacity of 15 and 12%, for Ch-gel-TEO-2% and Ch-gel-TEO-4%, respectively. The decrease rate of antioxidant capacity of Ch-gel-βCD-TEO films was much lower than that of Ch-gel-TEO films, indicating that microencapsulation delays the release rate of TEO while maintaining a high effective concentration over a long-term exposure time compared with its free form. The encapsulation of TEO in β-CD reduce the influence of external factors during the preparation process, thus improving the stability of TEO and extending its activity (Marcovich et al., 2024; Wen et al., 2020). It has been shown that films in which the active agent is encapsulated have higher antioxidant activity than films in which the active agent is directly added (Liu et al., 2024), which was attributed to the higher retention of the incorporated TEO into these complex films (Marcovich et al., 2024). The active substances show low inactivation and high stability during preparation and drying under the protection of microcapsules (Rajapaksha and Shimizu, 2021). Wu et al. (2018) found that the in vitro antioxidant activity of films based on fish gelatin Incorporated with curcumin (active agent) directly added decreased significantly after 90 days of storage, while the antioxidant activity of films with β-Cyclodextrin/curcumin microcapsules did not decrease significantly. Similar results to those presented in this work were reported by Mirsharifi et al. (2023) when determining the antioxidant activity of encapsulated thyme essential oil incorporated in almond gum/polyvinyl alcohol/chitosan composite films and Oskouei et al. (2024) using thyme essential oil loaded nanostructured lipid carriers.
Antimicrobial performance is one of the most crucial factors for extending the shelf life of food products. Previous studies (Marcovich et al., 2024; Liu et al., 2024) reported that TEO could damage the integrity of cell membranes, which leads to the changes in cell membrane permeability and the leakage of intracellular components. Considering the potentiality of thyme essential oil as antimicrobial, the in vitro antimicrobial activity of film forming solutions was tested against E. coli. This strain was selected due to the fact that fruits and vegetables are highly susceptible to E. coli contamination both in the field and during processing (Liu et al., 2024).
The antimicrobial activity results of Ch-gel film forming solutions (FFSs) incorporated with free and microencapsulated TEO against E. coli is presented in Figure 2 and Supplementary Table S2. Data suggested that Ch-gel-FFS presented an initial 12% inhibition ratio against E. coli, a low value when compared to the Ch-gel coatings enriched with free and microencapsulated TEO. These results are in agreement with those reported by Muñoz-Nuñez et al. (2024) that informed relatively low activity of chitosan-based films against E. coli.
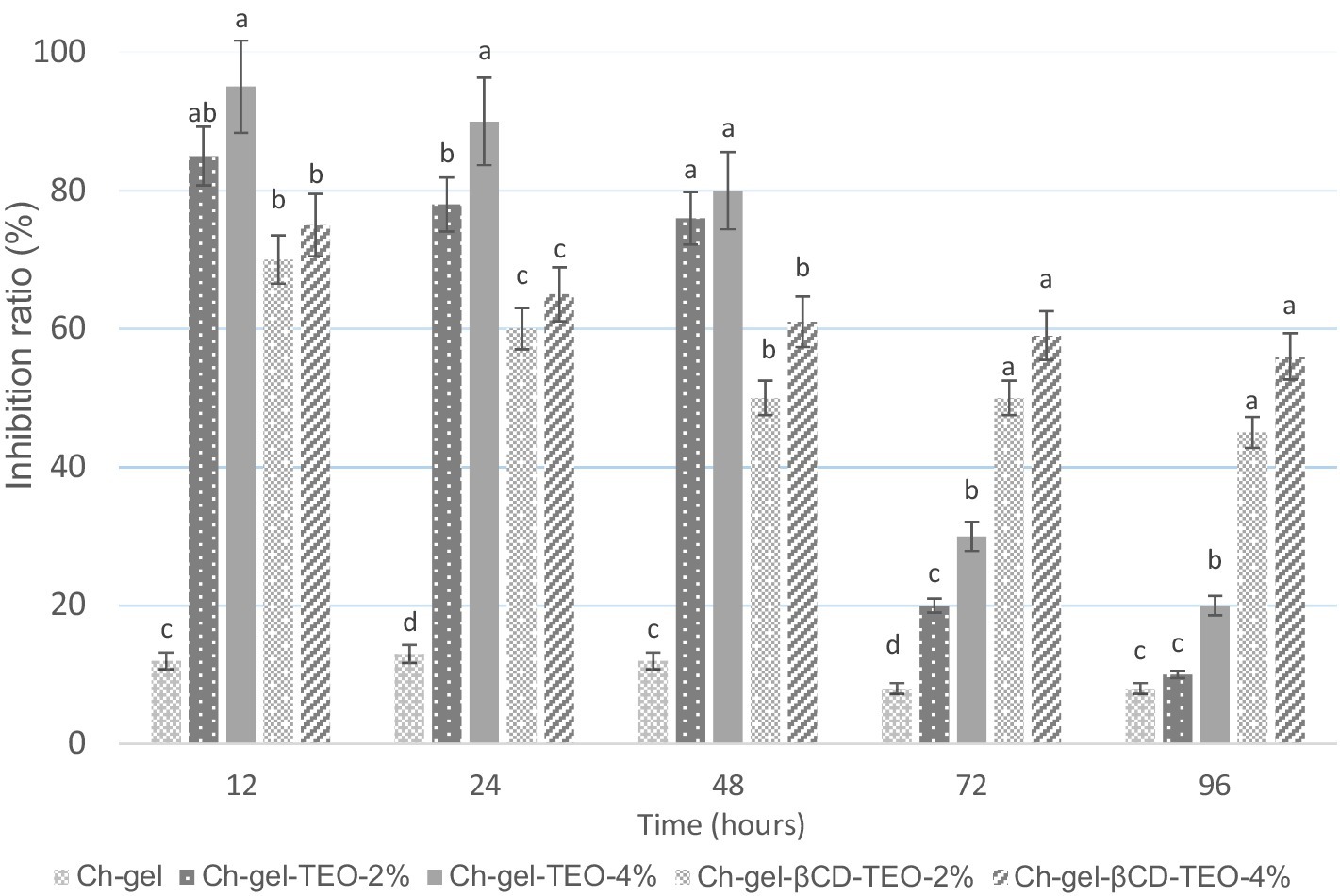
Figure 2. Inhibition ratio of film forming solutions (FFS) of Ch-gel containing free and encapsulated TEO against E. coli. Reported values correspond to the mean and the error bars represent the standard deviation associated with each LSMEAN. Different letters indicate significant differences between samples for each storage time.
The inhibition ratios of Ch-gel FFSs with free TEO varies between 85 and 98% at 12 h, 78–95 at 24 h, 76–80 at 48 h, 20–30 at 72 h, and 10–20 at 96 h, for Ch-gel-TEO-2% and Ch-gel TEO-4%, respectively, indicating that free TEO is rapidly released into the medium achieving ideal inhibition toward E. coli only during the first 24–48 h. As can be seen from Figure 2, the inhibition of free TEO experienced a sharp decrease over time, which is attributed to the instability of thyme essential oil itself. On the other hand, as higher is the concentration of TEO in the films, higher is the antimicrobial activity against E. coli. The antimicrobial activity of thyme essential oil has been well demonstrated and is directly related to the synergistic effect of its main components thymol and carvacrol (Binazir et al., 2024). The mechanism of action of TEO is related to the lipophilic characteristics of these major compounds, which attach themselves to the phospholipid bilayer of the cell membrane, increasing its permeability, therefore causing damage to the cellular enzymatic system by altering the cytoplasmic structure (Yammine et al., 2023). The antimicrobial activity of thyme essential oil against E. coli was also found in the starch based composite films incorporated with thyme oil microemulsion and microcapsules developed by Yuan et al. (2021), as well as in previous works on active wheat gluten films obtained by compression molding (Ansorena et al., 2016; Marcovich et al., 2024) or chitosan edible coatings (Viacava et al., 2022).
On the other hand, the inhibition ratio of the Ch-gel FFS containing encapsulated TEO decreased from 70–75% to 55–61% as time proceeded for Ch-gel-βCD-TEO-2% and Ch-gel-βCD-TEO-4%, respectively. β-cyclodextrin significantly limited the initial release of TEO, resulting in a poor antibacterial performance in the initial period in comparison with FFSs incorporated with free TEO. The antibacterial activity tended to be stable (approximately 55%) after 48 h, indicating that the antimicrobial effect is more sustained when TEO is incorporated as an inclusion complex compared to adding free TEO directly into the Ch-gel film forming solution. Results confirms that microencapsulation delay the liberation rate of TEO maintaining rather good inhibition performance in a prolonged exposure time compared with its free form. Similar results with those found in this work were reported for fish gelatin films incorporated with sulfobutyl ether, either in its free form or incorporated as a β-cyclodextrin inclusion complex, against Pseudomona aeruginosa (Wu et al., 2023) and chitosan-gelatin edible coatings with β-cyclodextrin/lemongrass essential oil inclusion complex against Penicillium aurantiogriseum (Erceg et al., 2023).
3.3 Food preservation application
3.3.1 Weight loss
Figure 3 and Supplementary Table S3 show the weight loss of kiwifruit slices during refrigerated storage. As expected, weight loss of all kiwi samples increased continuously with storage time. After 12 days of storage kiwi slices stored without a coating (control C) exhibited the highest weight loss highlighting the important role that coating plays as a water barrier between the kiwi slices and the environment throughout the storage period. As indicated in related papers, the weight loss of minimally processed fresh food is due to loss of moisture or leakage of juice and during storage mainly due to respiration and transpiration of the produce, and has a great impact on the product appearance, production efficiency and consumer acceptance (Sekarina et al., 2023; Wu et al., 2023). Among all Ch-gel coatings tested, the weight loss of coated kiwi with encapsulated TEO was always lower than that of free TEO, which might be due to the lower WVP value of Ch-gel-TEO-βCD coatings. Furthermore, the weight loss of samples packed with Ch-gel-TEO-βCD-4% was the lowest among all tested coatings throughout the storage period.
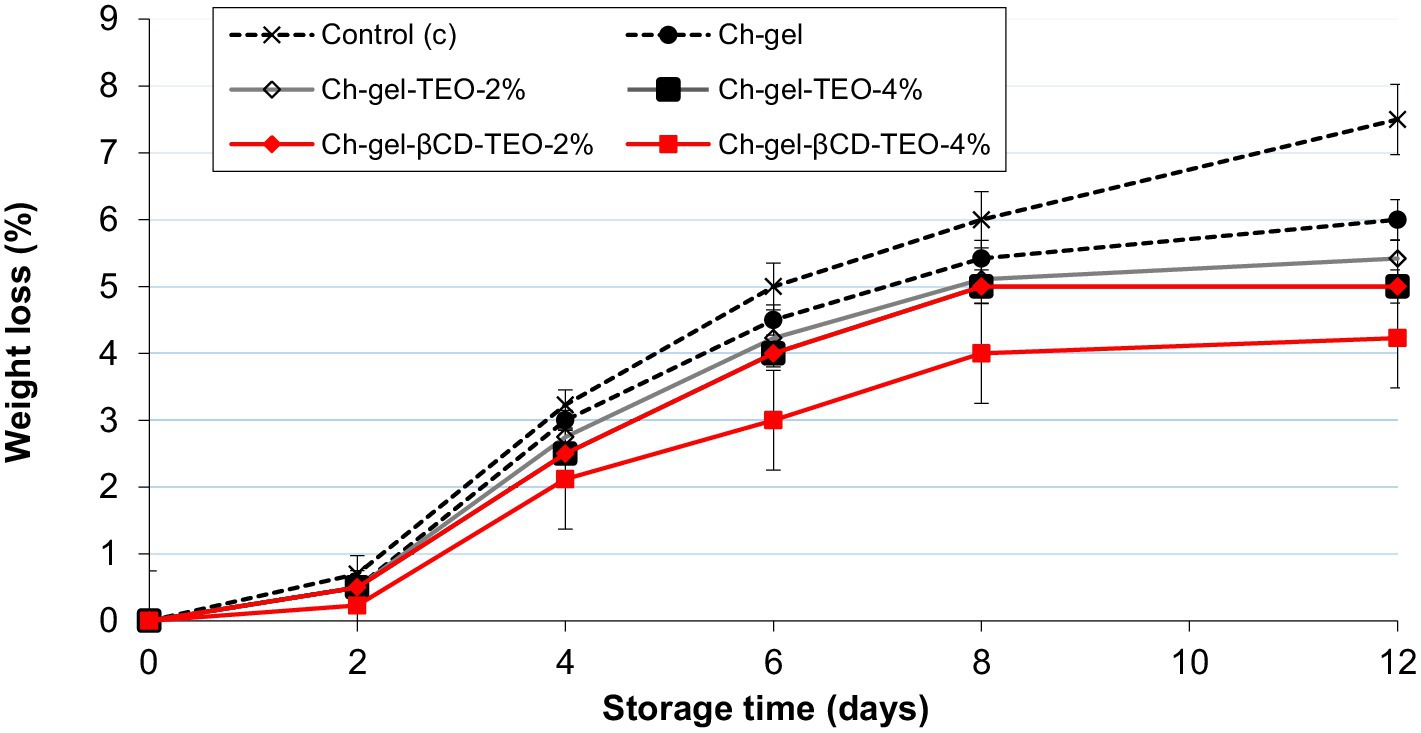
Figure 3. Weight loss of kiwifruit slices coated with different FFSs during storage. Reported values correspond to the mean and the error bars represent the standard deviation associated with each LSMEAN.
According to Sekarina et al. (2023), the decrease in fruit weight is caused by the fruit respiration, a mechanism that converts sugar into CO2 and H2O, followed by water evaporation (transpiration). Our results indicate that the Ch-gel-coated samples decelerate the mass loss of fresh-cut kiwifruits throughout the storage period. The semi-permeable film formed on the fruit surface by the Ch-gel coating acts as a physical barrier to gas and water exchange, thereby reducing the respiration and transpiration rates through the fruit surfaces. The results achieved in this study are consistent with those of Zhao et al. (2023), who reported that the incorporation of green tea microcapsules in chitosan-gelatin coatings had a favorable effect on weight loss of strawberries during storage and in agreement with those reported by Sekarina et al. (2023) for their edible chitosan—fish skin gelatine coatings containing black tea extract applied to minimally processed papaya during refrigerated storage.
3.3.2 Color
The color and texture of fresh-cut fruits and vegetables directly affect the buying intention of consumers. The green color of ‘Hayward’ kiwifruit slices is attributed to the presence of chlorophyll (Passafiume et al., 2020) and the a* value represents the color from green to red (the greater the absolute value, the deeper the color). The initial a* value of the kiwifruit slices was −7.11 ± 0.35 similar to the value reported by Allegra et al. (2016). As illustrated in Figure 4 and Supplementary Table S4, the a* parameter continued to increase with storage time for all treatments. No significant differences in color were detected among samples until day 4 of storage, but from day 4 and until the end of storage, kiwifruit slices coated with TEO microcapsules had greener color and lower a* value compared to the other treatments. The application of all the other treatments underwent a markedly and significant color change from green to yellowish/brownish which continued until the end of storage as a result of ripening. Samples from the control group (Control and Ch-gel without TEO) exhibited signs of decay, including darker color and juice leakage, as will be discussed later (Section 3.3.5, Figure 5).
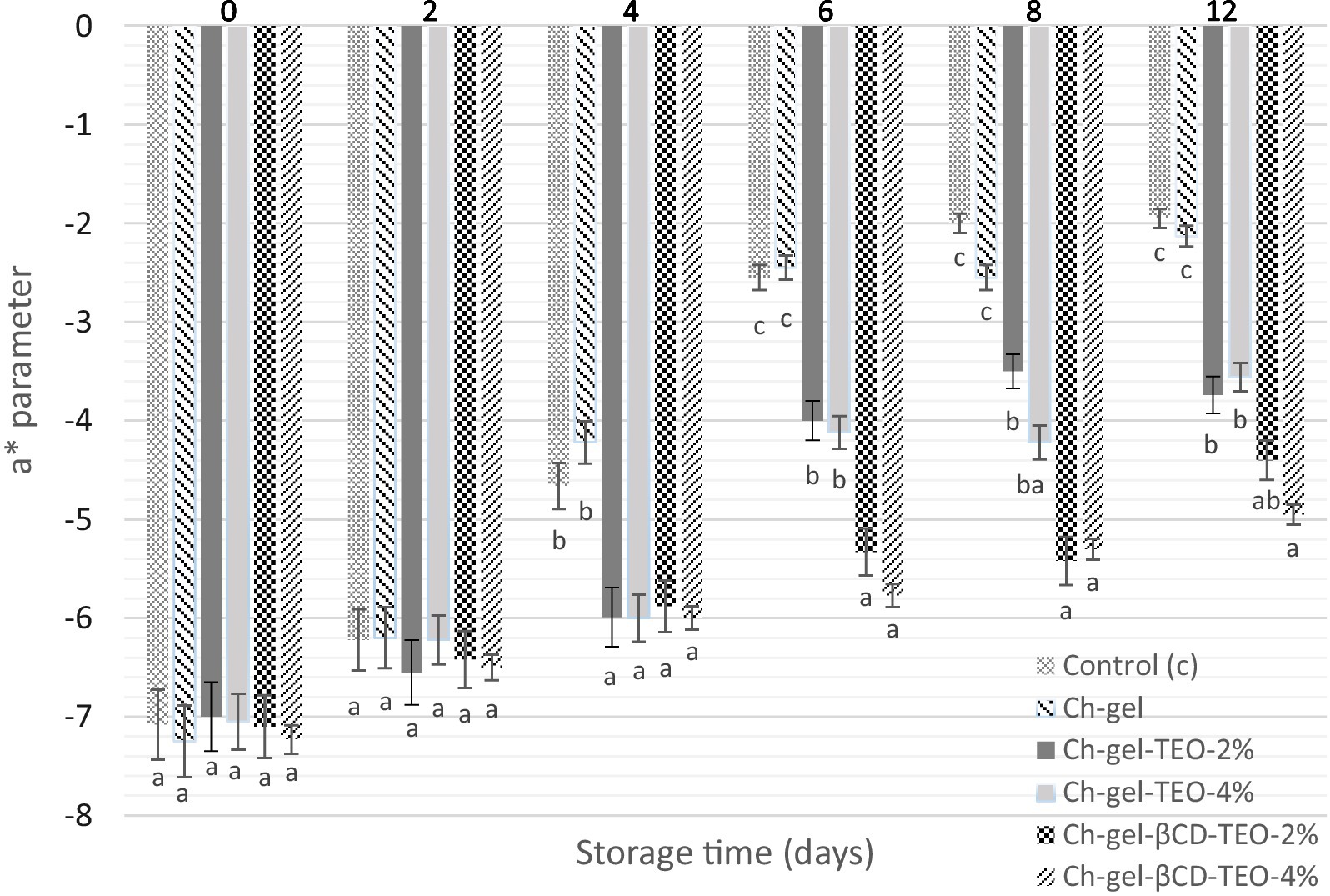
Figure 4. Evolution of a* parameter of coated and uncoated kiwifruit slices during storage. Different letters indicate significant differences between samples for each storage time.
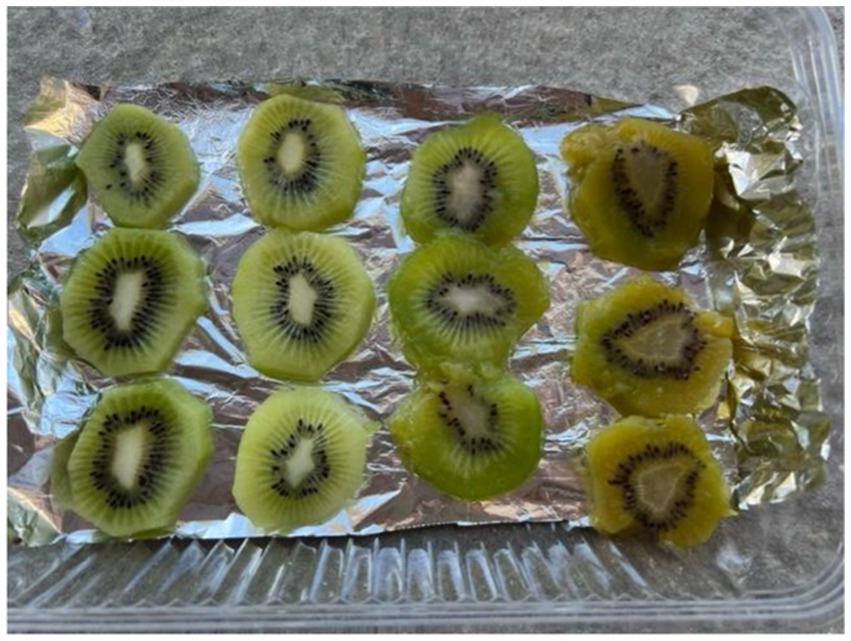
Figure 5. Changes in appearance of kiwifruit slices with different treatments at the end of storage at 5°C. From left to right: Ch-gel-free TEO; Ch-gel-encapsulated TEO; Ch-gel; Control.
Results indicate that Ch-gel-TEO-βCD-2% and Ch-gel-TEO-βCD-4% can significantly delay the change in kiwifruit slices color during the evaluated storage period, as well as delay the progression of senescence and browning. In agreement with our findings, Liu et al. (2024) reported that alginate-based edible coatings incorporated with magnoliae essential oil/β-cyclodextrin inclusion complex delay color change of Kyoho grapes after 9 days of storage.
3.3.3 Antimicrobial activity
Microbial safety is one of the significant factors to be considered in order to maintain commercial marketability and preservation of fresh cut produce. Fresh cut fruits, especially kiwi, a fruit that has become popular worldwide due to its sensory and nutritional properties (i.e., contains high levels of bioactive compounds such as vitamin C and E, flavonoids, carotenoids and minerals) (Guroo et al., 2017), are good substrates for microbial growth. This is mainly due to the large cutting surface they exhibit: they are highly exposed to the filtration of water and nutrients from damaged tissues, which in turn make them extremely sensitive to putrefaction by microorganisms (Manzoor et al., 2021) Therefore, bacterial growth serves as an important indicator to measure fruit freshness and quality loss during storage. Figure 6A and Supplementary Table S5 show the changes of total mesophilic counts on slices coated with different coatings during storage at 5°C. The initial microbial aerobic count in kiwifruit slices was 2.1 ± 0.2 Log CFU g−1. Similar results were reported by Manzoor et al. (2021) and Li et al. (2017). In general, and for all treatments an increase of total mesophilic counts was observed as time increased. In particular, kiwifruit with no coating showed the highest microbial load increase (p < 0.05) throughout storage (final mesophilic counts of 9.02 ± 0.4 Log CFU g−1), indicating a positive effect of the coating on microbial growth. The application of the Ch-gel coating reduced microbial load approximately by 0.5 Log during the first 6 days of storage compared to control, reaching to the same microbial load at the end of storage. The addition of TEO into Ch-gel coatings (both free and microencapsulated) significantly reduced (p < 0.05) the growth of mesophilic counts when compared to Ch-gel, throughout the storage period. However, there are important differences regarding the level and duration of the protection offered by the two different active coatings.
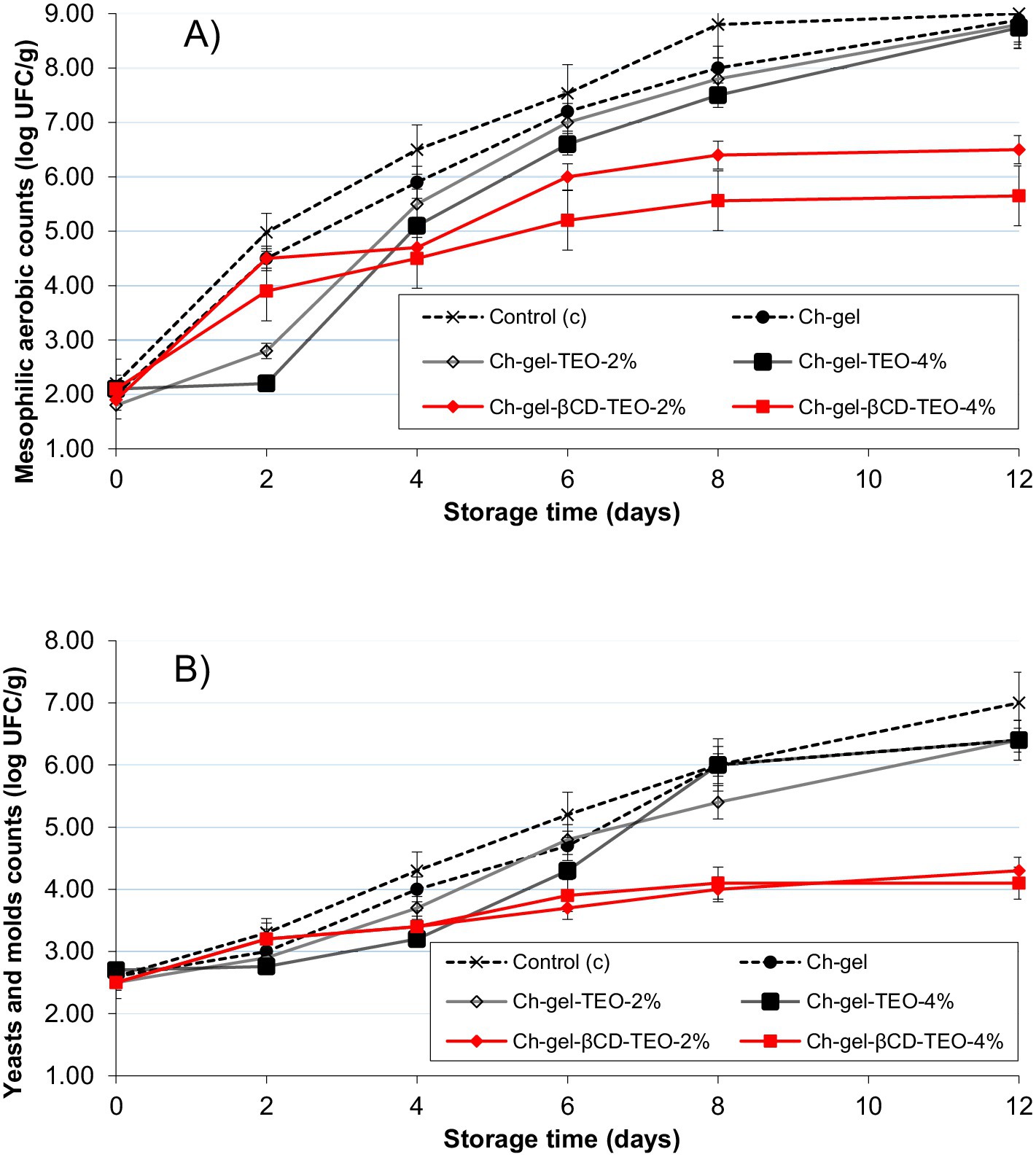
Figure 6. Antimicrobial activity in kiwifruit slices coated with different film forming solutions during refrigerated storage. (A) Mesophilic aerobic bacteria; (B) yeast and molds.
Microbial populations in coated kiwifruit with free TEO (Ch-gel-TEO) grew slower during the first 4–6 days of storage compared to control samples (C and Ch-gel) and coated samples incorporated with encapsulated TEO (Ch-gel-βCD-TEO). However, this treatment was not effective in controlling this population at the end of storage period, reaching at day 8 almost the same microbial load than samples treated with the Ch-gel coating alone. This observed behavior is in agreement with the in vitro antimicrobial activity of films, indicating that free TEO releases quickly from the coating to the kiwifruit slices.
On the other hand, a bacteriostatic effect on mesophilic bacteria can be noticed on samples coated with encapsulated TEO from day 8 of storage. As expected, the higher the microcapsules concentration in the blend, the lower the aerobic counts. Significant log reductions (p < 0.05) of 1.8 and 3.11 Log CFU g−1 in the mesophilic population (compared to control) were achieved at the end of storage period in slices coated with Ch-gel-βCD-TEO-2% and Ch-gel-βCD-TEO-4% formulations, respectively. The significant differences found between different coating treatments (Ch-gel, Ch-gel-TEO and Ch-gel-βCD-TEO) and the control group (C) (p < 0.05) confirm that both, TEO concentration and encapsulation are significant factors affecting antimicrobial activity of Ch-gel blends.
The behavior of yeast and molds during storage (Figure 6B and Supplementary Table S6) showed similar trend as that of total mesophilic counts analysis. A delay in the initial growth was observed in coated kiwifruit treated with free TEO and in this case, significant differences in yeast and molds counts were detected among both TEO concentrations (Ch-gel-TEO-2% and Ch-gel-TEO-4%) between days 2 and 8 of storage. However, these differences disappeared from day 8 onwards, so no significant differences in yeast and molds growth were detected between both treatment levels at the end of storage period.
The incorporation of TEO microcapsules into Ch-gel coatings, on the other hand, led to a significant additional reduction (p < 0.05) in the yeast and molds growth of kiwi slices during storage compared to samples coated with free TEO. It should be noted that, contrary to mesophilic counts analysis, no significant differences were found in yeast and molds counts during storage between slices treated with Ch-gel-βCD-TEO-2% and Ch-gel-βCD-TEO-4% coatings. The incorporation of encapsulated TEO in Ch-gel coatings significantly reduced yeast and molds counts throughout storage, showing a reduction at the end of storage of 1.2 and 1.5 Log for Ch-gel-βCD-TEO-2% and Ch-gel-βCD-TEO-4% treated samples, respectively, when compared to coatings incorporated with free TEO.
In the present study, among all tested coatings, Ch-gel-βCD-TEO exhibited the most effective inhibitory control on mesophilic bacteria, and yeast and mold growth. These results indicate a possible synergy between the blend chitosan-gelatin and the TEO-βCD inclusion complex on kiwifruit, which allowed the highest antimicrobial impact. Nieddu et al. (2014) reported that cyclodextrins increase solubility of essential oils through their inclusion in the CD hydrophobic cavity. Thus, the increased solubility of TEO may improve its antimicrobial efficiency probably due to an enhanced access of the oil to the sites of antimicrobial action. The increased solubility of the TEO due to its entrapment in the β-cyclodextrin cavity, in combination with the inclusion complex incorporation into the Ch-gel matrix, may have slowed down the release behavior of TEO, positively influencing its antimicrobial efficiency during storage. The results obtained were consistent with that obtained from Liu et al. (2024) who also found a prolonged antimicrobial effect of thyme essential oil on cherry tomato when using soy protein isolate (SPI) as a carrier and Volić et al. (2022) who reported that encapsulated TEO in alginate-whey protein concentrate carriers was more effective at lower concentrations and for longer periods than free oil for food preservation. In addition, Chen et al. (2019) demonstrated that films made from ethylene vinyl alcohol copolymer (EVOH) incorporated with free and β-Cyclodextrin inclusion complexes of citral and trans-cinnamaldehyde were able to extended the shelf life of beef about 4 days at 4°C.
Maximum permitted levels of 7.7 and 6 Log CFU g−1 for aerobic mesophilic and yeast and mold counts, respectively, is considered acceptable at any point in the shelf life of a fruit product as established by IFST (1999). Based on this regulation, the applied Ch-gel films incorporated with encapsulated TEO, lead to a significant improvement of shelf life of minimally processed kiwifruit.
3.3.4 Antioxidant capacity
Figure 7 and Supplementary Table S7 show the evolution during storage of the antioxidant activity of control and coated kiwi slices determined with DPPH technique. The incorporation of TEO into Ch-gel coating (both free and microencapsulated) caused a significant additional increment (p < 0.05) in the DPPH of kiwifruit slices throughout storage compared to samples coated without TEO. Furthermore, the higher the concentration of TEO in the coating (both free and microencapsulated), the higher the increment of the antioxidant capacity.
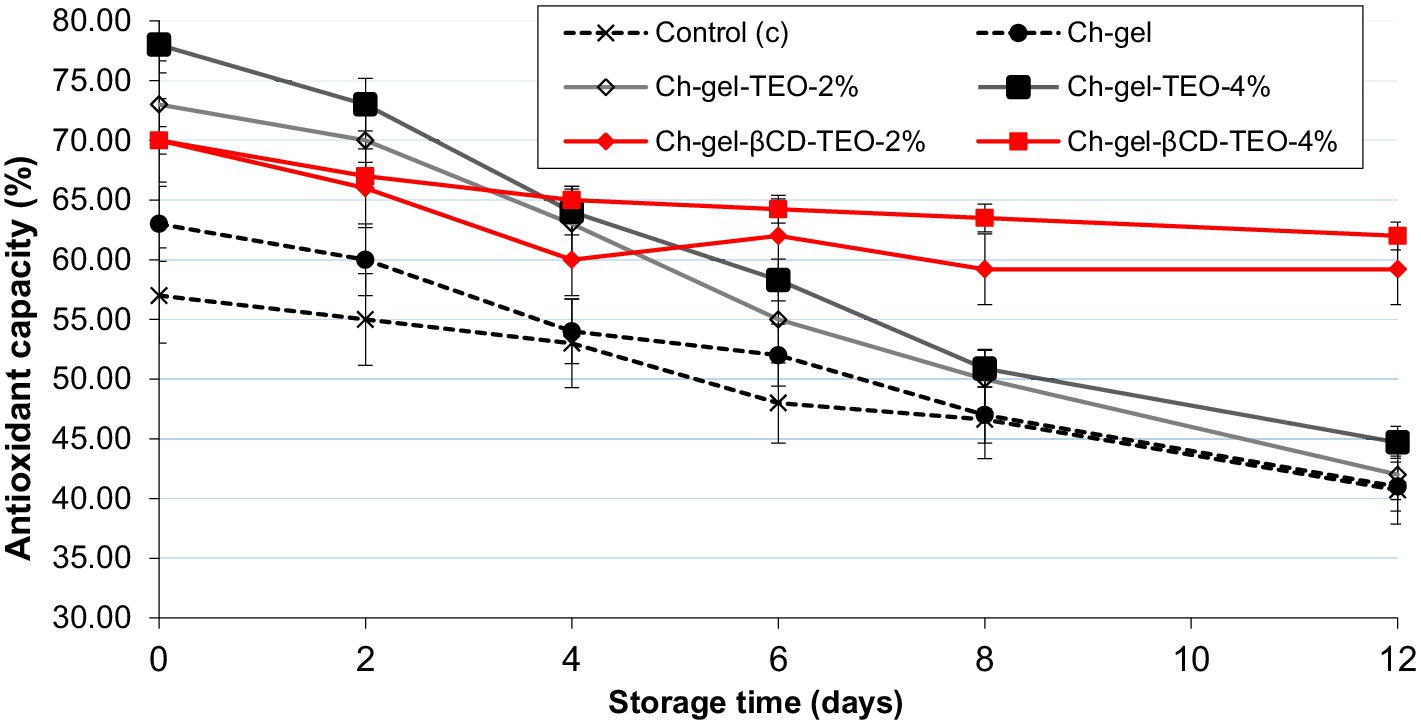
Figure 7. Antioxidant capacity of kiwifruit slices coated with different film forming solutions during refrigerated storage.
During the first 4 days of storage, a significant higher radical scavenging activity was initially observed in samples coated with Ch-gel incorporated with free TEO, with increments between 15 and 34% with regard to control samples (C and Ch-gel) as the TEO concentration increased. However, the antioxidant activity of these samples decreased with time, with no significant differences at the end of storage compared to control. As expected, these results are in agreement with previous findings on in vitro antioxidant capacity of film enriched with free TEO.
On the other hand, samples coated with Ch-gel-TEO-βCD exerted during the first 4 days a lower increase in the DPPH scavenging activity than coated samples with free TEO. However, a significant increase in the antioxidant capacity was observed from day 4 until the end of storage, with final increments (p < 0.05) of 30 and 45% for kiwi slices coated with Ch-gel-TEO-βCD-2% and Ch-gel-TEO-βCD-4%, respectively, compared to control samples (C). The application of these films enhances antioxidant activity of kiwi slices throughout the storage and achieves, at the end of storage, radical scavenging values even higher than those of the fresh product.
Results confirm that the incorporation of thyme essential oil into Ch-gel coatings increases the polyphenols content and the antioxidant activity of the minimally processed kiwifruit. Similarly, other authors also reported that the antioxidant activity of fruit and vegetables using coatings with EOs was higher than in those coated without EOs or uncoated (Yousuf et al., 2021; Sánchez-González et al., 2011). These results are attributed to the presence of phenolic monoterpenes with antioxidant properties coming from the thyme essential oil (Sánchez-González et al., 2011). Incorporation of TEO microcapsules into Ch-gel matrix provides a long-term enhancement of phytochemical quality when compared to coatings with free oil. This difference could be ascribed to the ability of the coating material to release the entrapped bioactive compounds gradually in time, which is improved by encapsulation of the active component. From the results obtained for kiwi slices coated with microencapsulated TEO, it is evident that the release of the TEO antioxidant phenolics from the β-CD capsules it is more gradual and extended over time. Similar results to those found in this work were reported by Chen Z. et al. (2020) when determining the antioxidant activity of PVA-starch active films incorporated with encapsulated lemongrass oil in β-cyclodextrin, by Zhao et al. (2023) when incorporating green tea essential oil microcapsules in gelatin/chitosan films for strawberry preservation and by Viacava et al. (2022) using chitosan edible coatings with TEO-βCD for fresh cut carrot preservation.
3.3.5 Sensory quality attributes of kiwifruit slices during storage
Figure 8 shows the evolution of the quality parameters of control and kiwifruit slices subjected to different coatings at days 4 (Figure 8A), 8 (Figure 8B), and 12 (Figure 8C) of storage at 5°C. After 4 days, kiwi samples coated with free TEO presented a very intense aroma of thyme essential oil, being this aroma perception stronger in samples coated with the highest TEO concentration (Ch-gel-TEO-4%). The persistence of this strong aroma continued throughout the entire storage and affected the organoleptic quality of kiwi coated with free TEO. On the other hand, the use of microencapsulated TEO in the Ch-gel coating delayed the release of encapsulated TEO compounds avoiding the presence of TEO aroma on kiwi samples and as a result they did not affect kiwifruit odor and general acceptability. The intense aroma in thyme oil is attributed to the presence of a great number of volatile compounds with strong odor present in the oil that mask the natural flavor of fruits and vegetables (Yousuf et al., 2021). Liguori et al. (2024) evaluated the sensory quality of mandarin coated with gellan-based edible coatings enriched with oregano essential oil. Sensory analyses indicated that oregano essential oil led to a decrease in the overall preference of samples. Residual aromatic herbal taste was detected after 2 weeks of storage. Regarding kiwifruit, Benítez et al. (2015) indicated that the incorporation of aloe vera to chitosan coatings lead to a lower acceptance of kiwifruit slices in comparison with the chitosan coating alone while Li et al. (2017) reported higher sensory quality of fresh cut kiwifruit slices coated with alginate edible coatings incorporated with poly-ε-lysine compared to uncoated samples.
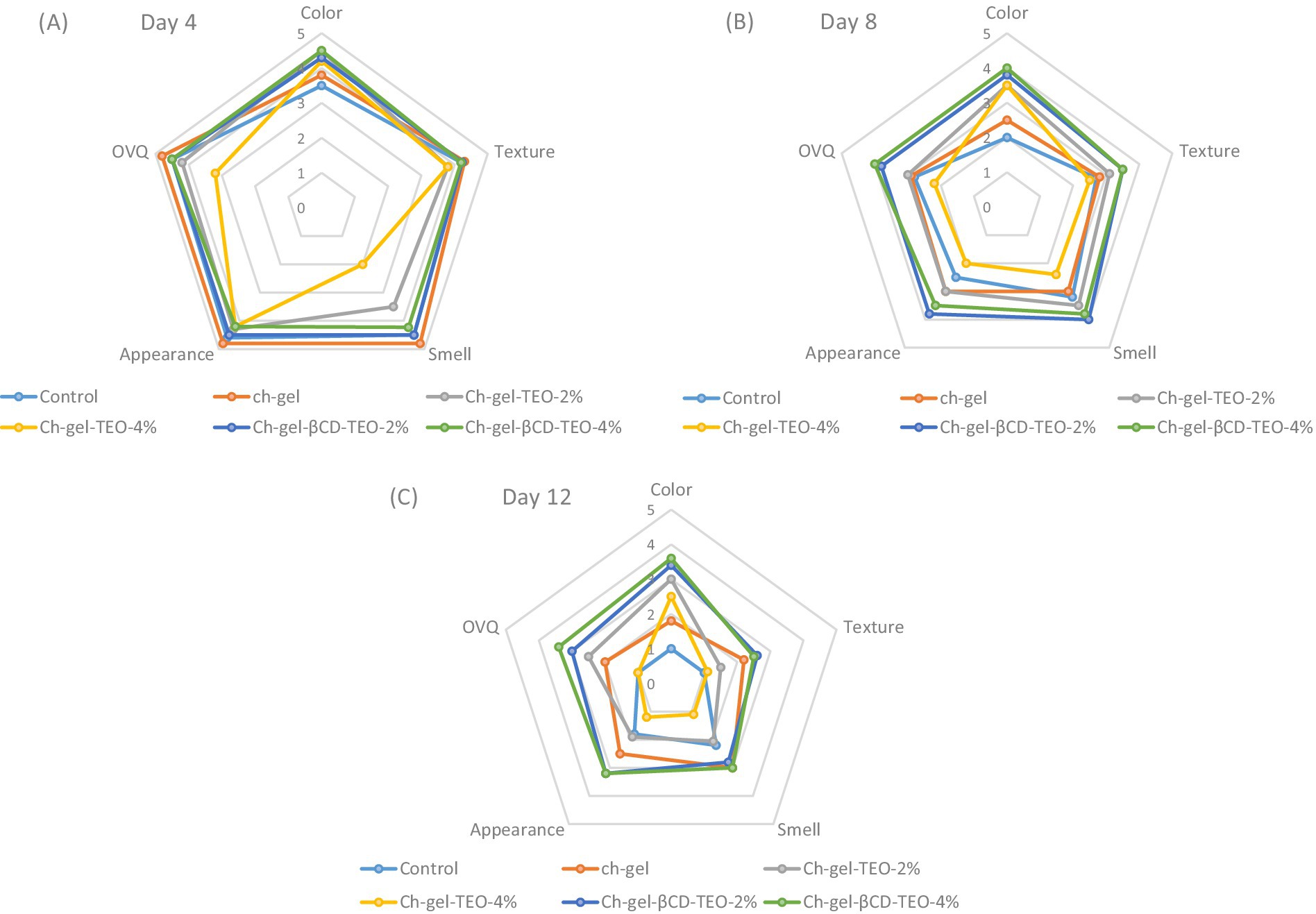
Figure 8. Effect of storage time and different film forming solutions on sensorial quality parameters of kiwifruit slices evaluated at (A) 4, (B) 8 and (C) 12 days of storage.
During storage and for all samples, a decrease in sensory characteristics was detected by the panelists. In particular, the disappearance of the green bright color and a decrease in texture (turgid loss) were observed. The panelists gave higher color and texture scores to the samples treated with Ch-gel coatings incorporated with encapsulated TEO. In fact, Ch-gel-TEO-βCD coated samples maintained their light green color throughout the storage, as evidenced in Figure 5. Panelists perceived a slightly whitish appearance, probably due to the white color of the βCD, which did not negatively affect organoleptic quality of these samples.
The color of kiwifruit flesh is mainly due to the presence of some pigments, as chlorophyll and carotenoids. Obtained results suggested that the Ch-gel-TEO-βCD coating maintained the typical bright green color of kiwifruit by preventing chlorophyll oxidative degradation. These results are in agreement with the trend of the parameter a*. Also, kiwifruit coated with Ch-gel-TEO-βCD had the lowest loss of texture throughout the storage period which may be due to the barrier effect of this coating. The lower WVP of Ch-gel-TEO-βCD coatings act as a barrier to water transfer, retarding dehydration and maintaining firmness during fruit shelf life. Evaluation of sensory quality attributes of kiwifruit showed that only coated kiwifruit samples with encapsulated TEO maintained OVQ scores above the marketability threshold throughout storage.
4 Conclusion
Innovative bio-based chitosan/gelatine blend films incorporated with thyme essential oil in free form and microencapsulated in β-cyclodextrin were developed as potential active food packaging coatings. The physical and functional properties of the films produced were evaluated. Films incorporated with encapsulated TEO showed improvement in mechanical properties and water vapor permeability, with respect to both, neat composite films and films incorporated with free TEO. Moreover, the incorporation of encapsulated TEO to the blend can slow down the release rate of TEO from the films imparting excellent in vitro antimicrobial and antioxidant properties. Developed active films were tested as a functional coating on minimally processed kiwifruit. After 12 days of storage at 5°C, coated kiwifruit incorporated with encapsulated TEO showed reduced weight loss, enhanced antioxidant properties, as well as, significant reductions in the total mesophilic aerobic bacteria and yeast and mold counts throughout the storage. This study also found that the sensory acceptability of kiwifruit coated with biobased film forming solutions enriched with encapsulated TEO was higher than that of the coated with FFS enriched with free TEO, since the former did not show adverse effects on flavor and general acceptability. These results revealed the potential of gelatin/chitosan blend incorporated with thyme oil microcapsules to be used as an active food packaging material.
Data availability statement
The raw data supporting the conclusions of this article will be made available by the authors without undue reservation.
Author contributions
NM: Conceptualization, Formal analysis, Funding acquisition, Methodology, Resources, Supervision, Validation, Writing – original draft, Writing – review & editing, Data curation, Investigation, Project administration, Software, Visualization. MA: Conceptualization, Data curation, Formal analysis, Funding acquisition, Investigation, Methodology, Project administration, Resources, Validation, Visualization, Writing – original draft, Writing – review & editing, Software, Supervision.
Funding
The author(s) declare that no financial support was received for the research and/or publication of this article.
Conflict of interest
The authors declare that the research was conducted in the absence of any commercial or financial relationships that could be construed as a potential conflict of interest.
Generative AI statement
The authors declare that no Gen AI was used in the creation of this manuscript.
Publisher’s note
All claims expressed in this article are solely those of the authors and do not necessarily represent those of their affiliated organizations, or those of the publisher, the editors and the reviewers. Any product that may be evaluated in this article, or claim that may be made by its manufacturer, is not guaranteed or endorsed by the publisher.
Supplementary material
The Supplementary material for this article can be found online at: https://www.frontiersin.org/articles/10.3389/fsufs.2025.1571114/full#supplementary-material
References
Allegra, A., Inglese, P., Sortino, G., Settanni, L., Todaro, A., and Liguori, G. (2016). The influence of Opuntia ficus-indica mucilage edible coating on the quality of ‘Hayward’ kiwifruit slices. Postharvest Biol. Technol. 120, 45–51. doi: 10.1016/j.postharvbio.2016.05.011
Amjadi, S., Emaminia, S., Davudian, S. H., Pourmohammad, S., Hamishehkar, H., and Roufegarinejad, L. (2019). Preparation and characterization of gelatin-based nanocomposite containing chitosan nanofiber and ZnO nanoparticles. Carbohydr. Polym. 216, 376–384. doi: 10.1016/j.carbpol.2019.03.062
Ansorena, M. R., Zubeldía, F., and Marcovich, N. E. (2016). Active wheat gluten films obtained by thermoplastic processing. LWT Food Sci. Technol. 69, 47–54. doi: 10.1016/j.lwt.2016.01.020
ASTM. (1993). ASTM D1708-93: Standard test method for tensile properties of plastics by use of microtensile specimens.
Benítez, S., Achaerandio, I., Pujolà, M., and Sepulcre, F. (2015). Aloe vera as an alternative to traditional edible coatings used in fresh-cut fruits: a case of study with kiwifruit slices. LWT Food Sci. Technol. 61, 184–193. doi: 10.1016/j.lwt.2014.11.036
Binazir, E., Ghanbarzadeh, B., Hanifian, S., Gharekhani, M., Kafil, H. S., and Falcone, P. M. (2024). Development and optimization of thyme-pennyroyal essential oils based active nanostructured lipid carrier (NLC) containing saffron extract with antioxidant and antimicrobial properties. Food Biosci. 61:104992. doi: 10.1016/j.fbio.2024.104992
Bonilla, J., Poloni, T., Lourenço, R. V., and Sobral, P. J. (2018). Antioxidant potential of eugenol and ginger essential oils with gelatin/chitosan films. Food Biosci. 23, 107–114. doi: 10.1016/j.fbio.2018.03.007
Chen, H., Li, L., Ma, Y., Mcdonald, T. P., and Wang, Y. (2019). Development of active packaging film containing bioactive components encapsulated in β-cyclodextrin and its application. Food Hydrocoll. 90, 360–366. doi: 10.1016/j.foodhyd.2018.12.043
Chen, Z., Mo, X., He, C., and Wang, H. (2008). Intermolecular interactions in electrospun collagen–chitosan complex nanofibers. Carbohydr. Polym. 72, 410–418. doi: 10.1016/j.carbpol.2007.09.018
Chen, H.-Z., Zhang, M., Bhandari, B., and Yang, C.-H. (2020). Novel pH-sensitive films containing curcumin and anthocyanins to monitor fish freshness. Food Hydrocoll. 100:105438. doi: 10.1016/j.foodhyd.2019.105438
Chen, Z., Zong, L., Chen, C., and Xie, J. (2020). Development and characterization of PVA-starch active films incorporated with β-cyclodextrin inclusion complex embedding lemongrass (Cymbopogon citratus) oil. Food Packag. Shelf Life 26:100565. doi: 10.1016/j.fpsl.2020.100565
Dias, J. V. B., Costa, W. K. A., de Sousa Melo, D., de Oliveira, K. Á. R., Batista, A. U. D., de Souza, E. L., et al. (2023). Probiotic and synbiotic edible coatings: effects on Lacticaseibacillus casei viability and general quality of minimally processed fruit during storage, food. Bioscience 56:103144. doi: 10.1016/j.fbio.2023.103144
Díaz-Montes, E., and Castro-Muñoz, R. (2021a). Edible films and coatings as food-quality preservers: an overview. Food. 10:249. doi: 10.3390/foods10020249
Díaz-Montes, E., and Castro-Muñoz, R. (2021b). Trends in chitosan as a primary biopolymer for functional films and coatings manufacture for food and natural products. Polymers 13:767. doi: 10.3390/polym13050767
Eranda, D. H. U., Chaijan, M., Panpipat, W., Karnjanapratum, S., Cerqueira, M. A., and Castro-Muñoz, R. (2024). Gelatin-chitosan interactions in edible films and coatings doped with plant extracts for biopreservation of fresh tuna fish products: a review. Int. J. Biol. Macromol. 280:135661. doi: 10.1016/j.ijbiomac.2024.135661
Erceg, T., Šovljanski, O., Stupar, A., Ugarković, J., Aćimović, M., Pezo, L., et al. (2023). A comprehensive approach to chitosan-gelatine edible coating with β-cyclodextrin/lemongrass essential oil inclusion complex—characterization and food application. Int. J. Biol. Macromol. 228, 400–410. doi: 10.1016/j.ijbiomac.2022.12.132
Ghaderi, J., Hosseini, S. F., Keyvani, N., and Gómez-Guillén, M. C. (2019). Polymer blending effects on the physicochemical and structural features of the chitosan/poly (vinyl alcohol)/fish gelatin ternary biodegradable films. Food Hydrocoll. 95, 122–132. doi: 10.1016/j.foodhyd.2019.04.021
Gomes, B. A. F., Alexandre, A. C. S., de Andrade, G. A. V., Zanzini, A. P., de Barros, H. E. A., and Costa, P. A. (2023). Recent advances in processing and preservation of minimally processed fruits and vegetables: a review–part 2: physical methods and global market outlook, food chemistry. Advances 2:100304. doi: 10.1016/j.focha.2023.100304
Gómez-Estaca, J., Gómez-Guillén, M., Fernández-Martín, F., and Montero, P. (2011). Effects of gelatin origin, bovine-hide and tuna-skin, on the properties of compound gelatin–chitosan films. Food Hydrocoll. 25, 1461–1469. doi: 10.1016/j.foodhyd.2011.01.007
Guroo, I., Wani, S., Wani, S., Ahmad, M., Mir, S., and Masoodi, F. (2017). A review of production and processing of kiwifruit. J. Food Process. Technol. 8:1000699. doi: 10.4172/2157-7110.1000699
Haghighi, H., Biard, S., Bigi, F., De Leo, R., Bedin, E., Pfeifer, F., et al. (2019). Comprehensive characterization of active chitosan-gelatin blend films enriched with different essential oils. Food Hydrocoll. 95, 33–42. doi: 10.1016/j.foodhyd.2019.04.019
Hosseini, S. F., Rezaei, M., Zandi, M., and Ghavi, F. F. (2013). Preparation and functional properties of fish gelatin–chitosan blend edible films. Food Chem. 136, 1490–1495. doi: 10.1016/j.foodchem.2012.09.081
Hossen, M. A., Shimul, I. M., Sameen, D. E., Rasheed, Z., Tanga, W., Chen, M., et al. (2024). Chitosan/gelatin coating loaded with ginger essential oil/β-cyclodextrin inclusion complex on quality and shelf life of blueberries. Int. J. Biol. Macromol. 279:135026. doi: 10.1016/j.ijbiomac.2024.135026
Jridi, M., Hajji, S., Ayed, H. B., Lassoued, I., Mbarek, A., Kammoun, M., et al. (2014). Physical, structural, antioxidant and antimicrobial properties of gelatin–chitosan composite edible films. Int. J. Biol. Macromol. 67, 373–379. doi: 10.1016/j.ijbiomac.2014.03.054
Kan, J., Liu, J., Yong, H., Liu, Y., Qin, Y., and Liu, J. (2019). Development of active packaging based on chitosan-gelatin blend films functionalized with Chinese hawthorn (Crataegus pinnatifida) fruit extract. Int. J. Biol. Macromol. 140, 384–392. doi: 10.1016/j.ijbiomac.2019.08.155
Khan, M. R., Di Giuseppe, F. A., Torrieri, E., and Sadiq, M. B. (2021). Recent advances in biopolymeric antioxidant films and coatings for preservation of nutritional quality of minimally processed fruits and vegetables. Food Packag. Shelf Life 30:100752. doi: 10.1016/j.fpsl.2021.100752
Kulawik, P., Jamróz, E., Tkaczewska, J., Vlčko, T., Zając, M., Guzik, P., et al. (2024). Application of antimicrobial chitosan-furcellaran-hydrolysate gelatin edible coatings enriched with bioactive peptides in shelf-life extension of pork loin stored at 4 and− 20 °C. Int. J. Biol. Macromol. 254:127865. doi: 10.1016/j.ijbiomac.2023.127865
Li, S., Zhang, L., Liu, M., Wang, X., Zhao, G., and Zong, W. (2017). Effect of poly-ε-lysine incorporated into alginate-based edible coatings on microbial and physicochemical properties of fresh-cut kiwifruit. Postharvest Biol. Technol. 134, 114–121. doi: 10.1016/j.postharvbio.2017.08.014
Liguori, G., Greco, G., Salsi, G., Garofalo, G., Gaglio, R., Barbera, M., et al. (2024). Effect of the gellan-based edible coating enriched with oregano essential oil on the preservation of the ‘Tardivo di Ciaculli’mandarin (Citrus reticulata Blanco cv. Tardivo di Ciaculli). Front. Sustain. Food Syst. 8:1334030. doi: 10.3389/fsufs.2024.1334030
Lin, L., Gu, Y., and Cui, H. (2019). Moringa oil/chitosan nanoparticles embedded gelatin nanofibers for food packaging against listeria monocytogenes and Staphylococcus aureus on cheese. Food Packag. Shelf Life 19, 86–93. doi: 10.1016/j.fpsl.2018.12.005
Liu, Z., Ge, X., Lu, Y., Dong, S., Zhao, Y., and Zeng, M. (2012). Effects of chitosan molecular weight and degree of deacetylation on the properties of gelatine-based films. Food Hydrocoll. 26, 311–317. doi: 10.1016/j.foodhyd.2011.06.008
Liu, Q., Han, R., Yu, D., Wang, Z., Zhuansun, X., and Li, Y. (2024). Characterization of thyme essential oil composite film based on soy protein isolate and its application in the preservation of cherry tomatoes. LWT 191:115686. doi: 10.1016/j.lwt.2023.115686
Liu, Y., Weng, L., Dai, S., Liu, M., Guo, X., and Meng, F. (2024). Alginate-based edible coating with magnoliae essential oil/β-cyclodextrin inclusion complex for Kyoho grape preservation. LWT 210:116874. doi: 10.1016/j.lwt.2024.116874
Mahmood, K., Kamilah, H., Karim, A. A., and Ariffin, F. (2023). Enhancing the functional properties of fish gelatin mats by dual encapsulation of essential oils in β-cyclodextrins/fish gelatin matrix via coaxial electrospinning. Food Hydrocoll. 137:108324. doi: 10.1016/j.foodhyd.2022.108324
Manzoor, S., Gull, A., Wani, S. M., Ganaie, T. A., Masoodi, F. A., Bashir, K., et al. (2021). Improving the shelf life of fresh cut kiwi using nanoemulsion coatings with antioxidant and antimicrobial agents. Food Biosci. 41:101015. doi: 10.1016/j.fbio.2021.101015
Marcovich, N.-E., Hernández, M.-F., and Ansorena, M.-R. (2024). Multifunctional films based on wheat gluten and microencapsulated thyme essential oil. J. Renew. Mater. 1–10. doi: 10.32604/jrm.2024.055151
Mirsharifi, S. M., Sami, M., Jazaeri, M., and Rezaei, A. (2023). Production, characterization, and antimicrobial activity of almond gum/polyvinyl alcohol/chitosan composite films containing thyme essential oil nanoemulsion for extending the shelf-life of chicken breast fillets. Int. J. Biol. Macromol. 227, 405–415. doi: 10.1016/j.ijbiomac.2022.12.183
Moradi, S., Barati, A., Tonelli, A. E., and Hamedi, H. (2020). Chitosan-based hydrogels loading with thyme oil cyclodextrin inclusion compounds: from preparation to characterization. Eur. Polym. J. 122:109303. doi: 10.1016/j.eurpolymj.2019.109303
Muñoz-Nuñez, C., Hevilla, V., Blázquez-Blázquez, E., Zagora, J., Placha, D., Muñoz-Bonilla, A., et al. (2024). Functionalization of chitosan films using essential oils by supercritical CO2 impregnation method for various applications. Carbohydr. Polym. Technol. Appl. 8:100559. doi: 10.1016/j.carpta.2024.100559
Nieddu, M., Rassu, G., Boatto, G., Bosi, P., Trevisi, P., Giunchedi, P., et al. (2014). Improvement of thymol properties by complexation with cyclodextrins: in vitro and in vivo studies. Carbohydr. Polym. 102, 393–399. doi: 10.1016/j.carbpol.2013.10.084
Oskouei, H. D., Almasi, H., Zeynali, F., Hamishehkar, H., and Amjadi, S. (2024). Improving oxidative stability of flaxseed oil by Zein-basil seed gum electrospun nanofibers activated by thyme essential oil loaded nanostructured lipid carriers. Appl. Food Res. 4:100616. doi: 10.1016/j.afres.2024.100616
Passafiume, R., Gaglio, R., Sortino, G., and Farina, V. (2020). Effect of three different aloe vera gel-based edible coatings on the quality of fresh-cut “Hayward” kiwifruits. Food. 9:939. doi: 10.3390/foods9070939
Pilozo, G., Villavicencio-Vásquez, M., Chóez-Guaranda, I., Murillo, D. V., Pasaguay, C. D., Reyes, C. T., et al. (2024). Chemical, antioxidant, and antifungal analysis of oregano and thyme essential oils from Ecuador: effect of thyme against Lasiodiplodia theobromae and its application in banana rot. Heliyon 10:e31443. doi: 10.1016/j.heliyon.2024.e31443
Rajapaksha, S. W., and Shimizu, N. (2021). Development and characterization of functional starch-based films incorporating free or microencapsulated spent black tea extract. Molecules 26:3898. doi: 10.3390/molecules26133898
Ramziia, S., Ma, H., Yao, Y., Wei, K., and Huang, Y. (2018). Enhanced antioxidant activity of fish gelatin–chitosan edible films incorporated with procyanidin. J. Appl. Polym. Sci. 135:45781. doi: 10.1002/APP.45781
Rezaee, M., Askari, G., EmamDjomeh, Z., and Salami, M. (2018). Effect of organic additives on physiochemical properties and anti-oxidant release from chitosan-gelatin composite films to fatty food simulant. Int. J. Biol. Macromol. 114, 844–850. doi: 10.1016/j.ijbiomac.2018.03.122
Salmas, C. E., Giannakas, A. E., Moschovas, D., Kollia, E., Georgopoulos, S., Gioti, C., et al. (2022). Kiwi fruits preservation using novel edible active coatings based on rich thymol halloysite nanostructures and chitosan/polyvinyl alcohol gels. Gels 8:823. doi: 10.3390/gels8120823
Sánchez-González, L., Vargas, M., González-Martínez, C., Chiralt, A., and Chafer, M. (2011). Use of essential oils in bioactive edible coatings: a review. Food Eng. Rev. 3, 1–16. doi: 10.1007/s12393-010-9031-3
Sekarina, A., Munawaroh, H., Susanto, E., Show, P., and Ningrum, A. (2023). Effects of edible coatings of chitosan-fish skin gelatine containing black tea extract on quality of minimally processed papaya during refrigerated storage. Carbohydr. Polym. Technol. Appl. 5:100287. doi: 10.1016/j.carpta.2023.100287
Sionkowska, A., Wisniewski, M., Skopinska, J., Kennedy, C. J., and Wess, T. J. (2004). Molecular interactions in collagen and chitosan blends. Biomaterials 25, 795–801. doi: 10.1016/S0142-9612(03)00595-7
Teymoorian, M., Moghimi, R., Hosseinzadeh, R., Zandi, F., and Lakouraj, M. M. (2024). Fabrication the emulsion-based edible film containing Dracocephalum kotschyi Boiss essential oil using chitosan–gelatin composite for grape preservation. Carbohydr. Polym. Technol. Appl. 7:100444. doi: 10.1016/j.carpta.2024.100444
Tomadoni, B., Ponce, A., Pereda, M., and Ansorena, M. R. (2019). Vanillin as a natural cross-linking agent in chitosan-based films: optimizing formulation by response surface methodology. Polym. Test. 78:105935. doi: 10.1016/j.polymertesting.2019.105935
Viacava, G. E., Cenci, M. P., and Ansorena, M. R. (2022). Effect of chitosan edible coatings incorporated with free or microencapsulated thyme essential oil on quality characteristics of fresh-cut carrot slices. Food Bioprocess Technol. 15, 768–784. doi: 10.1007/s11947-022-02783-7
Volić, M., Đurović, S., Micić, D., Pećinar, I., Pantić, M., Bugarski, B., et al. (2022). Biopolymer-based powders with encapsulated thyme oil: characterization and comparison with free oil regarding thermal stability and antimicrobial activity. Food Biosci. 50:102100. doi: 10.1016/j.fbio.2022.102100
Voronko, N. G., Derkach, S. R., Kuchina, Y. A., and Sokolan, N. I. (2016). The chitosan–gelatin (bio) polyelectrolyte complexes formation in an acidic medium. Carbohydr. Polym. 138, 265–272. doi: 10.1016/j.carbpol.2015.11.059
Wang, H., Ding, F., Ma, L., and Zhang, Y. (2021). Edible films from chitosan-gelatin: physical properties and food packaging application. Food Biosci. 40:100871. doi: 10.1016/j.fbio.2020.100871
Wen, H., Hsu, Y.-I., Asoh, T.-A., and Uyama, H. (2020). Antioxidant activity and physical properties of pH-sensitive biocomposite using poly (vinyl alcohol) incorporated with green tea extract. Polym. Degrad. Stab. 178:109215. doi: 10.1016/j.polymdegradstab.2020.109215
Wu, J., Song, G., Huang, R., Yan, Y., Li, Q., Guo, X., et al. (2023). Fish gelatin films incorporated with cinnamaldehyde and its sulfobutyl ether-β-cyclodextrin inclusion complex and their application in fish preservation. Food Chem. 418:135871. doi: 10.1016/j.foodchem.2023.135871
Wu, J., Sun, X., Guo, X., Ji, M., Wang, J., Cheng, C., et al. (2018). Physicochemical, antioxidant, in vitro release, and heat sealing properties of fish gelatin films incorporated with β-cyclodextrin/curcumin complexes for apple juice preservation. Food Bioprocess Technol. 11, 447–461. doi: 10.1007/s11947-017-2021-1
Xu, D., Chen, T., and Liu, Y. (2021). The physical properties, antioxidant and antimicrobial activity of chitosan–gelatin edible films incorporated with the extract from hop plant. Polym. Bull. 78, 3607–3624. doi: 10.1007/s00289-020-03294-1
Xu, S., Chen, X., and Sun, D.-W. (2001). Preservation of kiwifruit coated with an edible film at ambient temperature. J. Food Eng. 50, 211–216. doi: 10.1016/S0260-8774(01)00022-X
Yammine, J., Gharsallaoui, A., Fadel, A., Mechmechani, S., Karam, L., Ismail, A., et al. (2023). Enhanced antimicrobial, antibiofilm and ecotoxic activities of nanoencapsulated carvacrol and thymol as compared to their free counterparts. Food Control 143:109317. doi: 10.1016/j.foodcont.2022.109317
Yan, J., He, S., Chen, L., Chen, H., Ouyang, K., and Wang, W. (2024). Effect of gelatin-chitosan-Cyclocarya paliurus flavonoids edible coating film on the preservation of chilled beef. LWT 199:116138. doi: 10.1016/j.lwt.2024.116138
Yousuf, B., Wu, S., and Siddiqui, M.W., Incorporating essential oils or compounds derived thereof into edible coatings: effect on quality and shelf life of fresh/fresh-cut produce, Trends Food Sci. Technol., 108 (2021) 245–257, doi: 10.1016/j.tifs.2021.01.016.
Yuan, L., Feng, W., Zhang, Z., Peng, Y., Xiao, Y., and Chen, J. (2021). Effect of potato starch-based antibacterial composite films with thyme oil microemulsion or microcapsule on shelf life of chilled meat. LWT 139:110462. doi: 10.1016/j.lwt.2020.110462
Zhang, Y., Zhang, H., Wang, F., and Wang, L.-X. (2018). Preparation and properties of ginger essential oil β-cyclodextrin/chitosan inclusion complexes. Coatings 8:305. doi: 10.3390/coatings8090305
Zhao, W., Yu, L., Gu, Y., Ma, W., Wang, K., Liang, J., et al. (2023). Preparation of dual-functional packaging films containing green tea essential oil microcapsules for strawberry preservation: excellent barrier properties and antioxidant activity. Food Biosci. 56:103279. doi: 10.1016/j.fbio.2023.103279
Keywords: active films, thyme essential oil, minimally processed, kiwi fruit, bio-based polymers
Citation: Marcovich NE and Ansorena MR (2025) Development and characterization of sustainable active films incorporated with free and microencapsulated thyme essential oil for kiwi-fruit preservation. Front. Sustain. Food Syst. 9:1571114. doi: 10.3389/fsufs.2025.1571114
Edited by:
M. Leonor Faleiro, University of Algarve, PortugalReviewed by:
Aris E. Giannakas, University of Patras, GreeceA. D. Srikanth Tangirala, Acharya N. G. Ranga Agricultural University, India
Copyright © 2025 Marcovich and Ansorena. This is an open-access article distributed under the terms of the Creative Commons Attribution License (CC BY). The use, distribution or reproduction in other forums is permitted, provided the original author(s) and the copyright owner(s) are credited and that the original publication in this journal is cited, in accordance with accepted academic practice. No use, distribution or reproduction is permitted which does not comply with these terms.
*Correspondence: María Roberta Ansorena, cmFuc29yZW5hQGZpLm1kcC5lZHUuYXI=