- 1Food Industry Development Department, Teagasc Food Research Centre, Dublin, Ireland
- 2Environment and Life Sciences Research Center, Kuwait Institute for Scientific Research, Safat, Kuwait
- 3KUNRF, Kuwait University, Kuwait City, Kuwait
Polysaccharide-based packaging and its ever-increasing applications in response to the need for sustainable and safe packaging have prompted researchers to investigate the development of antimicrobial biopolymer-based packaging for shelf-life enhancement of food samples. A film made by blending chitosan and pectin had good mechanical properties, that is, a tensile strength of 7.43 MPa and an elongation at break of 23.033%. To produce active packaging, thyme essential oil (TEO) was added to the blend at concentrations of 10%, 15%, and 20%. The developed film was characterized for its techno-functional and antimicrobial properties. Further the developed films were employed in an active food packaging system for milk cake. Films with added TEO showed significant enhancements in elongation at break (28.22%) and water vapor barrier properties (30.08 g/h.m2). Additionally, the microstructure of the film was also improved, as demonstrated by scanning electron microscopy (SEM) and atomic force microscopy (AFM) measurements. Fourier transform infrared spectroscopy (FTIR) indicated the interaction of blended matrix (chitosan and pectin) and TEO as observed from the formation of hydrogen bonds with the addition of TEO owing to the OH functional groups. The use of TEO in pectin and chitosan-based film and its application for milk cake packaging showed delayed microbiological contamination and hardness of milk cake samples. The shelf life of milk cake packaged in pectin and chitosan blend film with 20% TEO was enhanced by > 10 days.
1 Introduction
The upsurge in people’s awareness regarding the side effects of petroleum-based products combined with their desire for a better lifestyle has necessitated the development of novel biodegradable and less harmful alternatives to typical plastic-based packaging materials in recent decades (Leal Filho et al., 2019). In this circumstance, the environmental benefits of these biodegradable packaging materials outweigh the shortcomings they accrue to industrial production and demand the creation of an effective design strategy for their intended food packaging applications. This decade has witnessed the emergence of numerous biopolymers, including polysaccharide-based (starch, pectin, alginate, carrageenan, and gum), protein-based (seed protein, lentil protein, whey protein, and silk fibroin), and lipid-based (beeswax, vegetable oil, and essential oils) biopolymers as novel biodegradable forms of packaging materials (Zhao et al., 2021; Hosseini et al., 2023; Sudheer et al., 2023).
Recently, the design and fabrication of bio-inspired anionic and cationic blended materials, such as gelatin and pectin blend, pectin and chitosan blend, kappa-carrageenan, chitosan, and gelatin blend films, have encouraged greater attention to food packaging as they have effective light barrier and moisture barrier properties with excellent mechanical strength to package the product. Natural polysaccharides such as pectin and chitosan offer an inclusive spectrum of functional properties due to their anionic and cationic properties that propose a greater interaction and intermolecular bindings (Espitia et al., 2014). Apple pectin has remarkable gel characteristics. Industrially, pectin is extracted from two main sources: apple pomace and citrus peel (Cui et al., 2023). While chitosan is the second-largest naturally occurring renewable polymer substance in nature and has weak mechanical qualities due to the limited number of active adsorption sites, it must be modified to increase its properties (Huang et al., 2023). The film prepared from a chitosan and pectin blend shows improved mechanical and transparency properties (Younis and Zhao, 2019; Mulla et al., 2023). Moreover, chitosan and its derivatives also have antimicrobial properties that are dependent on a wide range of internal and external variables, including pH, the kind of microorganism, whether metal cations were present or absent, pKa, the molecular weight (Mw), and the degree of chitosan deacetylation (DD) (Kong et al., 2010). To create packaging for food or medical purposes that can prevent the growth of microorganisms, it is essential to include natural or generally recognized as safe (GRAS) antimicrobial agents. Our previous research has shown that a blend of chitosan and pectin (1:2 ratio) has excellent techno-functional properties (Mulla et al., 2023). Therefore, we have used this combination to produce an antimicrobial film.
Antimicrobial packaging is a novel category of active packaging where antimicrobial agents, mostly essential oils (EOs) and nanoparticles (NPs), are embedded to inhibit spoilage and pathogenic microorganisms (Qin et al., 2024). Active packaging uses a unique combination of advances in food processing, food safety, materials science, and packaging to fulfill consumer demand for fresh and safe products. Furthermore, the research and development of packaging materials/technologies are gradually moving toward “green” and “active” packaging. A recent mega project, NANOPACK, an EU-funded initiative, has used oregano essential oil in a combination of nanomaterials for the development of its antimicrobial packaging (Sagar et al., 2022).
In addition, there is a growing interest in developing sustainable, biodegradable antimicrobial packaging materials. Although the chitosan- and pectin-based films have improved thermomechanical properties, they have certain disadvantages when reinforced with the essential oils or nanoparticles used for shelf life extension, including the release of active ingredients, lack of antibacterial effect, and ease of product sealing. Therefore, this study aimed to develop a pectin- and chitosan-based antimicrobial film using TEO as an active agent at different concentrations and examine its physical, structural, mechanical, and antibacterial properties. The milk cake preservation effect of the developed active film was also evaluated by analyzing the quality of the milk cake in terms of bacterial growth, textural properties, and weight loss after it was wrapped with pectin and chitosan-based active films.
2 Materials and methods
2.1 Materials
Medium molecular weight chitosan powder [deacetylated chitin, Poly (D-glucosamine)] obtained from shrimp shells, pectin from apple (P-2157), and thyme essential oil were purchased from Sigma-Aldrich (St. Louis, MO, United States). Glycerol was bought from SD Fine Chemicals, Mumbai, India, and glacial acetic acid was bought from VWR Chemicals, France. All materials and chemicals used in the experiment were of analytical-grade quality.
2.1.1 Microbial media and strains
Two strains, Culti-loops® of Gram-negative Salmonella enterica sv Typhimurium (ATCC 14028) and Lyfo Disk pellets® of Gram-positive Listeria monocytogenes (ATCC 19114), were purchased from Remel Europe Limited (Dartford, Kent, United Kingdom) and MediMark Europe (Grenoble, CEDEX, France), respectively. Agar Muller–Hinton (MHA) and tryptic soy broth (TSB) were purchased from TM Media in Bhiwadi, India, and Conda Laboratories (Torrejón de Ardoz, MD, Spain) provided the brain–heart infusion broth (BHIB). Oxoid (Basingstoke, HM, United Kingdom) provided the polymyxin acriflavin lithium chloride ceftazidime aesculin mannitol (PALCAM) agar base, xylose lysine deoxycholate agar (XLD), and PALCAM selective supplements.
2.2 Chitosan/pectin blend preparation
The chitosan and pectin blend film was prepared by solution casting as described by Mulla et al. (2023). Analysis of the rheological properties of the film-forming solutions and the thermal, optical, mechanical, and barrier properties of the developed film suggested that 2% pectin and 1% chitosan solution in a 1:2 ratio performed more effectively and produced a film with better mechanical and barrier qualities than the other ratios tested; hence, this ratio was selected to make an antimicrobial film in this work. Initially, 2% pectin solution was prepared in distilled water and then stirred on a magnetic stirrer at 60°C till it was clear (∼60 min). The calcium chloride solution (2%) was then mixed with the clear pectin solution (2%) until the weight ratio of pectin to calcium chloride (4:1) was maintained. Next, the chitosan solution (1%) made in 1% acetic acid was added to the mixture of pectin and calcium chloride.
In our previous study, we observed that the incorporation of 17% cinnamon oil (CIN) in the PLA-based packaging material in combination with high-pressure treatment at 300 MPa could reduce the population of the pathogen in the chicken samples to a safe level during 21 days of refrigerated storage (Ahmed et al., 2017). Therefore, TEO concentrations of 10%, 15%, and 20% were selected for incorporation in the chitosan and pectin-based film in this work.
TEO was added to the mixture of chitosan, pectin, and calcium chloride at concentrations of 10%, 15%, and 20%, respectively, and agitated for 2 h on a magnetic stirrer. For uniform mixing, the chitosan, pectin, calcium chloride, and TEO mixture was homogenized for 0.5 min at 13,000 rpm using a homogenizer. Finally, the mixture was degassed for 30 min in a sonicating bath (Branson 3510-DTH, CT, United States) before casting. The prepared solutions were cast onto a rimmed silicone resin plate (5 × 5 cm2), and the films were solidified and held at a constant temperature of 60°C ± 0.2°C for 4 h to facilitate peeling. The resulting blend films were stored for further analysis at 50% relative humidity and 25°C. The chitosan and pectin blend film with TEO was denoted as CS/P/TEO.
2.3 Determination of film properties and characterization
2.3.1 Thickness, moisture, and transparency value
A digital micrometer (MCD-1″PXF, Mituyoto Corp., Kawasaki-shi, Japan) was used to measure the thickness of each film at 10 random points (selected from previous experiments). The micrometer has a sensitivity of 0.001 mm. The moisture content of the films was determined according to the method of Valizadeh et al. (2019). The films were kept in an electric oven at 105°C for 24 h and weighed before and after drying. The moisture content was calculated as a percentage of the initial weight. The transparency values of the films were determined by using a spectrophotometer (Shimadzu UV-1800, Kyoto, Japan) according to the method of Mulla et al. (2023).
The transparency value (TV) was calculated following Eq. 1:
where T600 is the fractional transmittance at 600 nm, and x is the film thickness (mm). Larger transparency values indicate lower film transparency.
2.3.2 Color
Using a CIE colorimeter (Hunter Associates Laboratory, Inc., Reston, VA, United States), the hues of the films were assessed in terms of L* (lightness), a* (redness/greenness), and b* (yellowness/blueness) values. Eq. 2 was used to determine the total color difference (E*).
2.3.3 Mechanical properties
A Texture Analyzer TA.XTplus (Stable Micro Systems, United Kingdom) with a 50 N load cell outfitted with tensile grips (A/TG type) (D882, ASTM, 2002) was used to determine the tensile strength (TS) and elongation at break (EAB) measurements of the films. The cross-head speed was 50 mm/min, and the grip separation was 30 mm. Ten samples from each type of film were used to perform TS and EAB testing.
2.3.4 Measurement of the water vapor transmission rate (WVTR)
The WVTRs of the prepared films were analyzed using the gravimetric cup method as described by Hoque et al. (2022) with slight modifications. Small cups (50 mL capacity; 100% RH) were filled with 25 mL deionized water for the measurement, and the rims were covered with 5-cm-diameter circle films and a rubber gasket to keep the films in place. The cups were placed in a desiccator with dry silica gel. The cups were weighed twice daily for 10 days to determine how much water was lost through the film. To determine the rate at which water vapor is transmitted through a film, we calculated the slope (g/h) of the line by plotting the water loss against time for each day or every 24 h. Next, we divided the slope by the area of the film to obtain the water vapor transmission rate, which is expressed as g/h.m2.
2.3.5 Attenuated total reflectance-Fourier-transform infrared (ATR-FTIR) spectroscopy
A Thermo Scientific, Madison, Wisconsin, United States, Nicolet iS5 FTIR spectrometer was used for the FTIR analysis. A complete set of 32 scans with a resolution of 4 cm−1 were collected in the absorption mode between 4,000 cm−1 and 550 cm−1.
2.3.6 Scanning electron microscopy
The surface structure of the prepared film samples was observed using a scanning electron microscopy at an accelerating voltage of 10 kV. To make the samples conductive, they were coated with gold using sputtering and connected to brass stubs using adhesive carbon tape. Finally, photographs were taken at a magnification of 240×. The scanning electron microscope used was a Quanta 400 model manufactured by FEI in Eindhoven, Netherlands.
2.3.7 Atomic force microscopy
The morphology and roughness of the film samples were evaluated using an Agilent 5,500 atomic force microscope (Agilent Technologies, United States). To perform topography analysis of essential oil in the chitosan matrix, we used super sharp silicon cantilevers and the intermittent constant mode (ACAFM-tapping mode).
2.3.8 Antimicrobial properties of chitosan/pectin/thyme essential oil films
2.3.8.1 Antimicrobial confirmation test
The antimicrobial efficiency of the CS/P/TEO films against S. Typhimurium (Gram-negative) and L. monocytogenes (Gram-positive) was assessed using the liquid culture test (Ahmed et al., 2019). The test was conducted in a test tube containing 10 mL TSB and 0.1 mL of each inoculum (S. Typhimurium and L. monocytogenes). After the strain concentration was maintained at 1 × 108 colony-forming units (CFU)/mL, a film disc (0.4 g) was put into the test tube. The test tubes were shaken for 7 days at 25οC at a rate of 200 rpm. At regular intervals (1 day and 7 days), aliquots of 1 mL were taken from the test tubes, serially diluted with buffered peptone water, and then spread onto BHIA plates. Plates with the inoculum were then incubated for 24 h at 37°C, during which time the cell concentrations were measured as colony-forming units (CFU). The chitosan/pectin (1:2) film was used as a standard film.
2.3.8.2 Confirmation test with milk cake
The effectiveness of CS/P/TEO films in preventing microbial growth was tested in a food model (milk cake) by measuring the total plate count (TPC) and yeast and mold count. The experiment was carried out following the method described by Singh et al. (2021). Nutrient agar was used for the standard plate count (SPC), while potato dextrose agar was used for yeast and mold counts. Freshly made milk cake samples weighing 10 g each were wrapped in two sets of CS/P and CS/P/TEO films and stored in separate polyethylene terephthalate (PET)-based specimen boxes (8 cm × 5.5 cm x 3.2 cm) at room temperature (25°C ± 1°C). To prevent contamination from the film, the films were sterilized under UV radiation for 1 min before use. Control samples were kept in rectangular PET specimen boxes without wrapping in a film at room temperature (25°C ± 1°C). At different time intervals during storage (days 0, 5, 10, 15, and 20), wrapped and unwrapped samples were retrieved and examined for TPC, yeast, and mold count.
2.4 Changes in texture profile of milk cake
Ten grams of milk cake samples was selected and divided into three groups: one group was packaged in CS/P film, one was packaged in CS/P/TEO film, and the third group was unwrapped and kept in specimen boxes. These samples were then subjected to a hardness measurement test using a Texture Analyzer TA-XTPlus Texture Analyzer (Stable Micro Systems, Surrey, United Kingdom) with a P-6 probe. The height of each milk cake sample was reduced to 50% of its original 45.3 mm height. Hardness is defined as the maximum resistance to cylinder penetration at a depth of 20 mm, and the value was given as the maximum force (kg). The hardness was directly estimated from the force-time curve using the Exponent 32 program (StableMicro Systems Ltd., Surrey, United Kingdom).
2.5 Statistical analysis
Each test was run three times (n = 3) and evaluated in triplicate. Numerous samples from various lots were used in the experiments. The Statistical Package for Social Science (SPSS 17.0 for Windows, SPSS Inc., Chicago, IL, United States) was used to conduct the statistical analysis.
3 Results and discussion
3.1 Film thickness, moisture content, color, and transparency value
The average thickness of the blended pectin and chitosan film with and without EO varied from 0.185 mm to 0.192 mm (Table 1). The incorporation of TEO at various concentrations had no significant effect on the thickness of the blended films up to 15% TEO incorporation; however, 20% incorporation showed marginally higher values than the control films (Xie et al., 2021). Similarly, there was no significant variation in the thickness of nano chitosan and pectin-based biodegradable active packaging films due to the addition of fennel essential oil (Sadadekar et al., 2023). The moisture content of the films ranged from 14.00% to 14.85%. The TEO-incorporated films exhibited no significant variation in moisture content. The findings from Aitboulahsen et al. (2020) observed similar results from gelatin and pectin-based films combined with EO. No significant difference in moisture content could be due to equivalent moisture evaporation from the developed films under the same drying temperature and time.
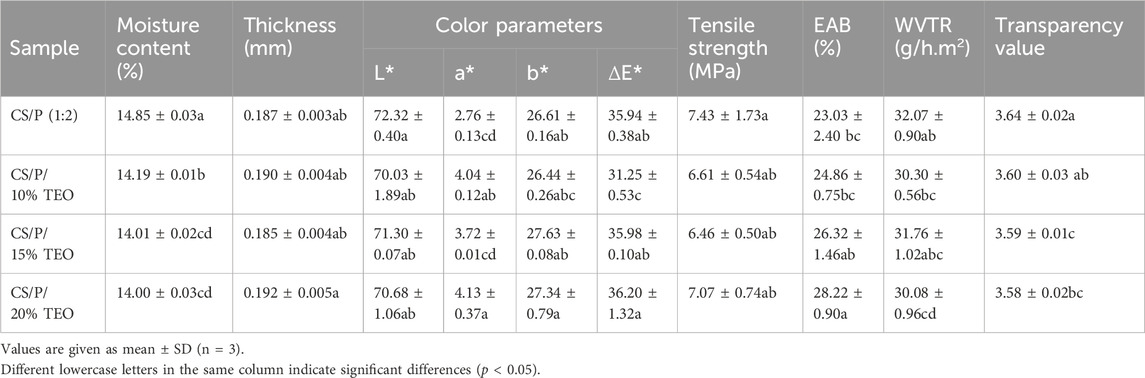
Table 1. Thickness, color, mechanical and barrier properties of thyme essential oil-infused chitosan and pectin blend films.
The color values of pectin and chitosan blend films infused with different concentrations of TEO are shown in Table 1. The results demonstrated a substantial variation in L* value due to TEO incorporation, whereas no significant difference in TEO-loaded chitosan and pectin-based films was observed due to the increase in TEO concentration. Furthermore, the a* value increases from 2.76 to 4.13, indicating an enhancement in the redness of films, while a modest increase from 26.61 to 27.34 in the b* value indicates the yellowness due to the incorporation of TEO. This change could be due to the carotenoids and chlorophyll pigments present in the TEO. The transparency value of the films ranged from 3.64 to 3.58. The transparency value of TEO-loaded chitosan and pectin-based films was slightly less than the pectin and chitosan blend film without TEO, showing improved film transparency. Incorporating TEO resulted in films that were more transparent than the control. Essential oils, known for their clarity and transparency, might have enhanced the film transparency. A similar decrease in the transparency value of gelatin-based film due to the incorporation of EO was observed earlier by Ahmed et al. (2012).
3.2 Fourier-transform infrared (FTIR) spectroscopy
Figure 1A shows the FTIR spectra of neat chitosan and pectin films. The chitosan film spectrum has a broad band at 3357 cm−1, which is attributed to OH stretching vibrations that overlap with the amino group ─NH stretching vibrations. A band at 2932 cm−1 represents C–H stretching. The peak located at 1647 cm−1 was associated with an amide-I stretch, and the peak at 1563 cm−1 represents the deformation of N–H stretching planes (Ren et al., 2017). The pectin film spectrum shows a broad band at 3301 cm−1 corresponding to O–H stretching vibrations resulting from intramolecular hydrogen bonding between monomers of pectin. A band at 2935 cm−1 corresponds to the C–H stretching vibrations of the methylene group. The absorption bands at 1737 cm−1 and 1607 cm−1 were attributed to the C=O component of an ester group and asymmetric stretching vibrations of carboxyl groups present in the pectin (Priyadarshi et al., 2021; Sood and Saini, 2022). Figure 1B depicts the FTIR spectra of the pectin and chitosan blend film with TEO (10%, 15%, and 20%) and without TEO. The “with TEO” spectrum showed a broad band of OH stretching at ∼3430 cm−1 and three characteristic peaks at 2959 cm−1, 2925 cm−1, and 2870 cm−1 corresponding to the C-H stretching vibration of aliphatic CH2 bonds (Del Toro-Sa´nchez et al., 2010; Essifi et al., 2022). NH bending, C−O stretching, and wagging of CH and CH2 group vibrations are depicted at 1515 cm−1, 1289 cm−1, and 813 cm−1, respectively. Similar observations were stated earlier for TEO by Scacchetti et al. (2017) and Ahmed et al. (2022).
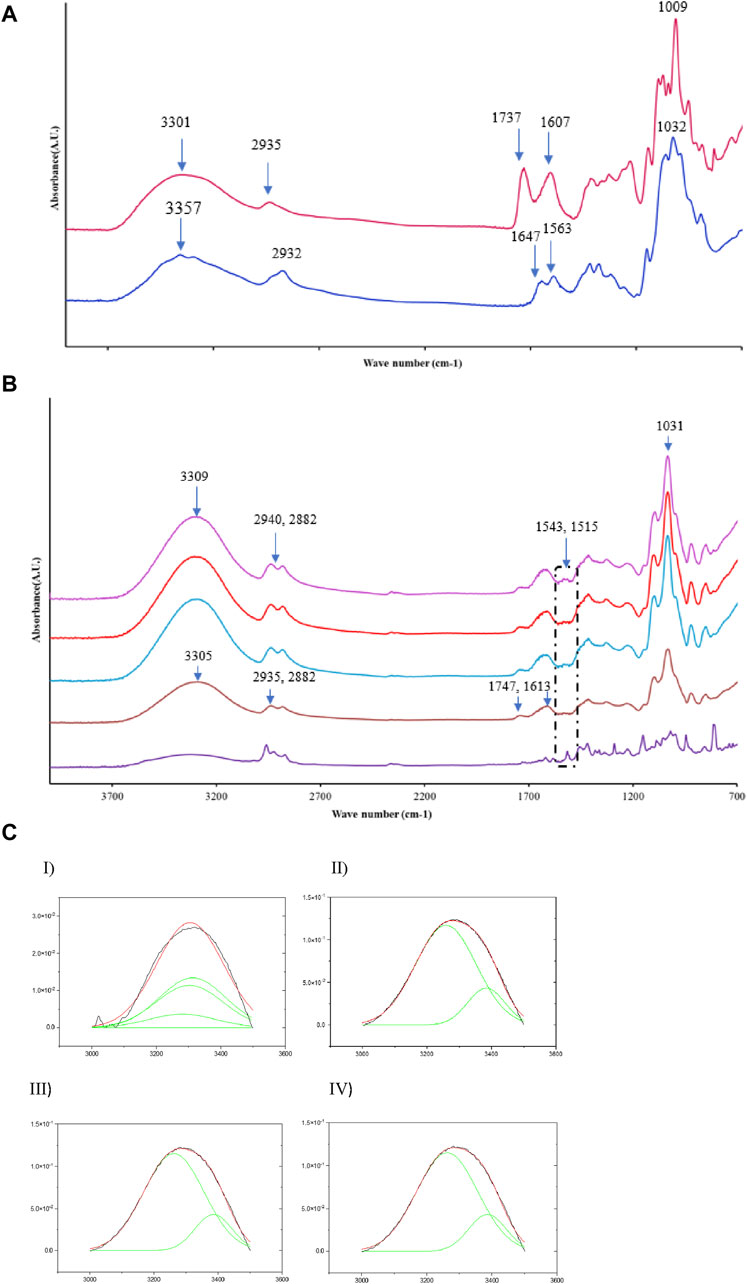
Figure 1. (A) FTIR spectra of neat pectin and chitosan film. (B) FTIR spectra of thyme oil-infused pectin and chitosan blend films { Thyme oil, CS/P (1:2), CS/P (1:2)-TEO 10%, CS/P (1:2)/TEO 15%, and CS/P (1:2)/TEO 20%}. (C) Deconvolution of FTIR peak (3000–3500 cm−1) I) TEO, II) CS/P (1:2), III) CS/P (1:2)/TEO 10%, IV) CS/P (1:2)/TEO 20%.
The FTIR spectra of the pectin and chitosan blend films show an absorption spectrum at 3305 cm−1. This shift could be due to hydrogen bonding between the pectin and chitosan matrix (Athanasopoulou et al., 2024). Another shift was observed in the bands associated with the C=O and–COO vibrations to higher sides at 1747 cm−1 and 1613 cm−1, respectively, compared to pristine pectin film (i.e., 1737 cm−1 and 1607 cm−1) (Santos et al., 2022). The spectrum for the pectin and chitosan blended film with TEO showed a shift in the band at 3305 cm−1 to 3309 cm−1. This shift could be due to the potential interaction between the (–OH and–COOH) bonds of pectin and the –OH bonds of TEO (Almasi et al., 2020). Interestingly, the films with TEO showed new minor peaks at 1543 cm−1 and 1515 cm−1. These bands could be attributed to the TEO’s aromatic ring C=C skeleton vibrations (Topala and Tataru, 2016; Antunes et al., 2021). These bands indicate the interaction of essential oil and blended film matrix.
The peak in the 3500 cm−1 to 3000 cm−1 region was extracted and then deconvoluted using the curve fitting method to estimate the extent of hydrogen bonding within the film formulations. The extent of interaction was determined by estimating the peak areas. Table 3 and Figure 1C shows that the area under the deconvoluted curves for TEO-infused films increased marginally compared to the control chitosan and pectin blend film. This shows the formation of hydrogen bonds with the addition of TEO owing to the OH functional groups (Agarwal et al., 2020).
3.3 Mechanical properties and water vapor transmission rate
The tensile strength (TS) and the percent elongation at break (EAB) of pectin and chitosan blended films with and without TEO are listed in Table 1. The mass ratio of chitosan and pectin (1:2) film showed the best tensile strength (7.43 ± 1.73 MPa) and elastic strength (23.03 ± 2.40 MPa), indicating that the optimum composite ratio of chitosan and pectin could form the best composite structure, as reported in our previous study (Mulla et al., 2023). The blended film without TEO showed higher tensile strength properties because of the harmonious effect of the cationic polymer, chitosan, and the anionic polymer, pectin, that built a strong network and enhanced the TS of the blended matrix. Similar findings were stated earlier by Zarandona Rodríguez et al. (2023) for a pectin and chitosan matrix. However, the values for TS and EAB differ from the present findings, which could be due to the preparation methods and sources of raw pectin and chitosan. When TEO was added at increasing concentrations, the films showed a drop in tensile strength and an increase in the EAB value. This indicates that beyond a particular concentration of 15%, TEO creates defect sites for TS. The tensile strength was primarily reduced due to the weakening of the film network, most likely as a result of EO’s incompatibility with hydrophilic biopolymer chains. These findings suggest that at excess EO concentration, the intermolecular repulsive forces are stronger, causing an imbalance of particles that hinders the interaction between polymer matrices, which influences its mechanical properties. This can be clearly observed in SEM and atomic force microscopy (AFM) images (Sebaaly et al., 2021). The plasticizing effect of TEO weakens the intermolecular interaction between pectin and chitosan polymer chains, causing discontinuities within the chitosan and pectin-based network that enhances EAB value and decreases stiffness or TS. Similar observations were made by other researchers for essential oil-added films (Norcino et al., 2020; Santos et al., 2022).
It is imperative to determine the water vapor transmission rate (WVTR) of pectin and chitosan blended films because biodegradable films have very high WVTR compared to fossil fuel-based polymer materials. The reinforcement of TEO marginally decreased the WVTR of the pectin and chitosan blended films from 32.07 g/h.m2 to 30.08 g/h.m2. A significant difference in WVTR was noticed between the control film and the film infused with 20% TEO. However, no significant difference was observed between the films infused with 10% and 15% EO, which may be due to the low availability of polar groups for the interaction with water molecules. In a recent study, Santos et al. (2022) observed a similar reduction in WVTR of the pectin film after adding garlic EO. The polar group of the hydrophilic polymer interacts with water molecules, and due to van der Waals interaction, the water is trapped, thereby reducing the WVTR in EO-infused pectin and chitosan blend films.
3.4 Antimicrobial properties
It has been shown that EOs are effective in fighting various types of pathogens. As a result, it is expected that such properties will persist even when they are in contact with packaging materials. The antimicrobial efficacy of the films produced was assessed in vitro against food-borne pathogens such as S. Typhimurium and L. monocytogenes (as shown in Figures 2A, B). The control pectin and chitosan blend film demonstrated no antibacterial activity against selected pathogens on the first and seventh days of incubation. This result could be due to the lower positive charge density of the pectin and chitosan blend, which is associated with the weaker antimicrobial activity against selected pathogens (Kong et al., 2010). The positive charge of chitosan is associated with the degree of deacetylation (DD) or degree of substitution (DS) of the chitosan or its derivatives (Kong et al., 2010). TEO incorporation in the CS/P matrix revealed antibacterial action against both the pathogens S. Typhimurium and L. monocytogenes on the first and seventh day of incubation. A greater concentration of TEO in the CS/P blend film and incubation time was effective in inhibiting the selected pathogens. For the film containing 20% TEO, the decrease was greater on the first day (up to 3.4 log CFU/mL), and complete suppression of S. Typhimurium was observed on the seventh day. Similarly, Ahmed et al. (2022) observed greater inhibition of S. Typhimurium for TEO inclusion complexes of β and γ cyclodextrin. Boskovic et al. (2017) discussed similar results and stated that TEO has a strong anti-Salmonella effect due to its high phenolic content. TEO showed greater inhibitory activity against Gram-negative and Gram-positive bacteria due to the presence of thymol, ρ-cymene, and γ-terpinene (Ben Jabeur et al., 2017; Aksit et al., 2022). The inhibitory effect of the films against test microorganisms is controlled by the amount of antibacterial agents (thymol and carvacrol) released from and present on the film surface. The mode of antimicrobial action is typically a release of antimicrobial agents from the EO to penetrate the semipermeable bacterial membrane and then react with the cytoplasm or cellular protein, resulting in the disruption of cytoplasmic membrane functions such as proton motive forces and active transport (Marino et al., 1999; Aljabeili et al., 2018).
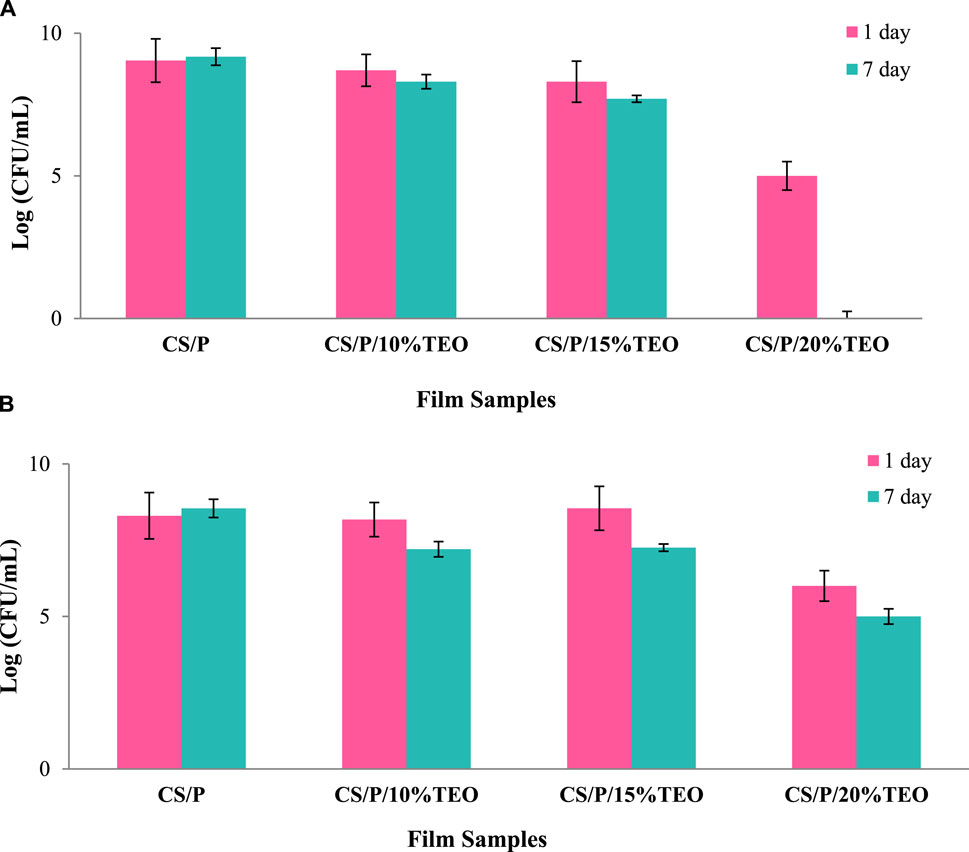
Figure 2. In vitro antibacterial activities of CS/PE-based film with TEO against (A) S. Typhimurium and (B) L. monocytogenes after incubation for 1 day and 7 days.
3.5 Morphological analysis
Scanning electron microscopy (SEM) primarily provides high-resolution, two-dimensional images of sample surfaces, while AFM is significant due to its ability to provide high-resolution, three-dimensional surface topography and mechanical property measurements in a non-destructive manner; these techniques were selected to analyze the chitosan and pectin-based film matrices. Figures 3A–D compare SEM cross-section micrographs and AFM 3D pictures of pectin and chitosan blend films with TEO. The chitosan and pectin blend film without TEO showed an even surface. The addition of TEO caused observable changes in the film structure, which can be seen in Figures 3B–D. SEM results showed a smooth and irregular pattern with a whitish aspect on the surface of TEO-incorporated films. A smooth surface was observed for an edible film made from chitosan and native glutinous rice starch incorporated with EO (Venkatachalam et al., 2023). Because of the small size and excellent integration into the polymer matrix, oil droplets were hardly noticeable in the film containing 10% TEO. The films containing higher concentrations of TEO (15% and 20%) showed an irregular structure on the surface that could be due to the higher collision frequency between the oil droplets at greater concentrations (Hosseini et al., 2015). The morphology results of the developed films support the tensile results. This shows that the mechanical and barrier properties are greatly influenced by the structural arrangement of the constituents in the film-forming dispersion.
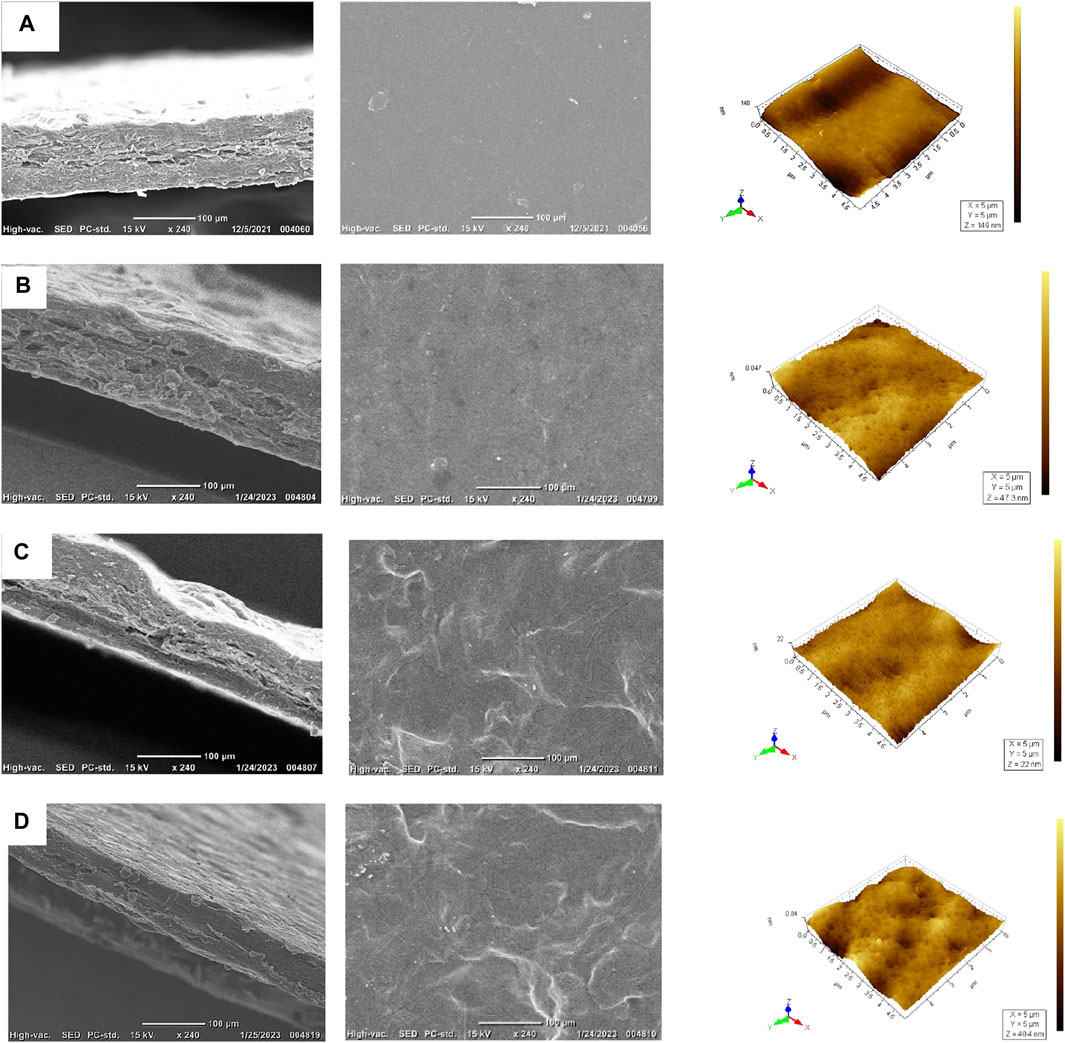
Figure 3. AFM 3D images and scanning electron micrographs of chitosan and pectin blend films with thyme essential oil (5 µm2 × 5 µm2): (A) CS/P, (B) CS/P/10% TEO, (C) CS/P/15% TEO, and (D) CS/P/20% TEO.
AFM was conducted to investigate the change in topography and roughness of the film due to the incorporation of TEO in chitosan and pectin blended films (Figure 3; Table 2). The films prepared without TEO showed the highest roughness values. Adding TEO reduced the roughness; however, further incorporation (15% and 20%) affected the smoothness and increased roughness. Among all TEO-incorporated films, the 10% TEO-incorporated film showed the least root mean square height (Sq) of 6.01 nm, while the incorporation of TEO above 10% concentration increased the Sq value to 13.4 nm. The increased roughness of the TEO-incorporated chitosan and pectin-blend films reveals the ideal TEO concentration for a harmonious intermolecular interaction between the polymer matrix and TEO components. Similar results were observed earlier for edible chitosan-zein films with EO (Escamilla-García et al., 2017).
3.6 Investigation of the quality properties of milk cake packaged with active film
3.6.1 Weight loss of milk cake
Monitoring weight loss during packaging is crucial to mitigating decreases in shelf life. The control samples, which were kept in a specimen box without any packaging, exhibited the highest weight loss, as observed in Figure 4. It is important to consider the loss of sample weight during packaging as one of the significant factors in preserving the product’s shelf life. Milk cake samples packaged in the pectin and chitosan blended film without TEO showed comparable weight loss to control samples, which could be due to the film’s water vapor transpiration rate that allows dehydration of samples at a greater speed (Wang et al., 2021). The film with a 20% TEO concentration exhibited the least weight loss, which could be due to less moisture evaporation during storage owing to the TEO-infused film’s low water vapor transpiration rate compared to other selected films.
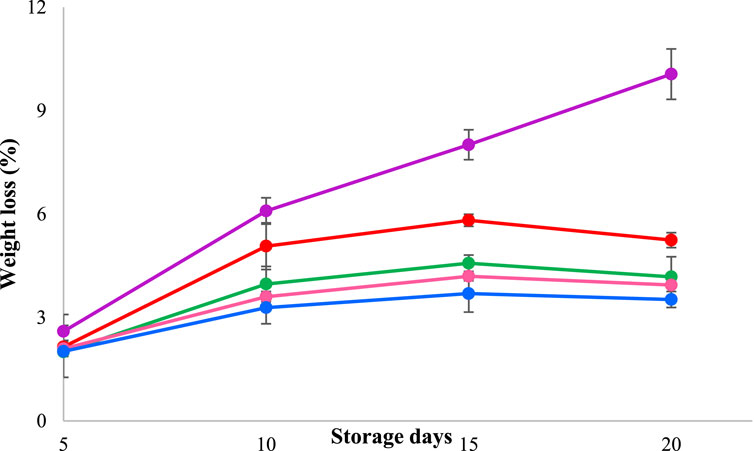
Figure 4. The weight loss of milk cake samples wrapped in CS/PE-based film with TEO (
3.6.2 Hardness of milk cake
The hardness of milk cake is one of the important quality characteristics for its acceptance by consumers; therefore, the hardness of milk cake was studied for 20 days at room temperature. Figure 5 illustrates the mean hardness values of milk cake packed in chitosan and pectin blended film with and without TEO. In the present study, throughout the storage, the hardness of the control milk cake and milk cake packaged in pectin and chitosan blend film without TEO was considerably greater (p < 0.05) than that of the milk cake packaged in the blended film containing TEO. The hardness of the milk cake increased over the 20 days, possibly due to moisture loss. Figure 1 shows a direct correlation between the weight loss of milk cake samples and their hardness, as hardness increases with the weight loss of the samples. Regardless of the packaging methods used, Londhe et al., 2012 noticed a progressive rise in the hardness of brown peda samples and associated it with a gradual loss of moisture over time.
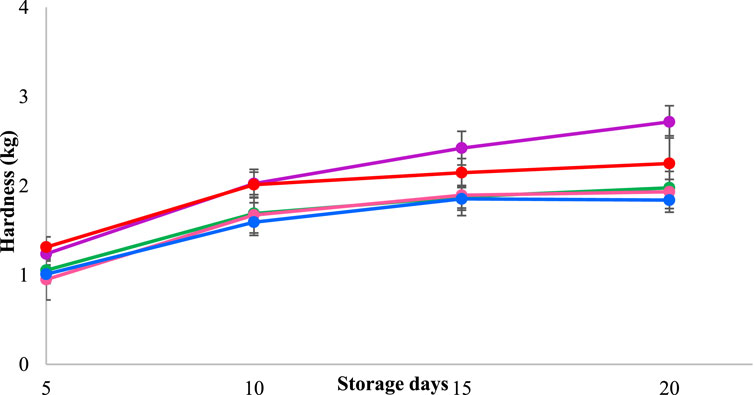
Figure 5. The hardness of milk cake samples wrapped in CS/P-based film with TEO (
3.6.3 Microbial quality of milk cake
Initial microbial load is an important criterion for determining the shelf life of milk products. United States and European Regulation (E.G.) no. 853/2004 states that the Pasteurized Milk Ordinance grade “A” milk should not contain more than 100,000 CFU/mL (USPHS/FDA, 2016). The milk cake samples were analyzed for microbial contamination by observing the TPC and yeast and mold count for 20 days (Figure 6). The initial total plate count population of yeasts and mold were 2.344 log10 cfu/g and 3.70 log10 cfu/g, respectively. Control and milk cake samples packaged in pectin and chitosan film without TEO showed a steep increase in the microbial count with the progression of storage. Meanwhile, the milk cake samples packaged in pectin and chitosan film with TEO showed an increase in count till the fifth day of storage, while a further increase in the storage period reduced the microbial count. Over the 20-day period, the CFU count in milk cake samples packaged in TEO-loaded films was acceptable and did not reach the critical limit. Similarly, the shelf life of meat packaged in the chitosan film infused with TEO was prolonged by ∼10 days compared to meat samples packaged in chitosan film without TEO (Gaba et al., 2022). Among all films, those with 20% TEO exhibited the strongest antimicrobial activity and reduced the TPC and yeast and mold counts to 2.29 CFU/mL and 2.09 log10 CFU/mL, respectively, on the 20th day of storage. TEO components such as carvacrol, thymol, and phenolic compounds are responsible for pathogen inactivation. These compounds efficiently prevent the proper functioning of pathogen cells by increasing the permeability of the cell wall by penetrating through the periplasm, accessing the deeper part of the cells, and releasing the cellular constituents like nucleic acids, which ultimately lead to the loss of pathogens (Liu et al., 2021). Liu et al. (2021) found that TEO and thymol can hinder the formation of biofilms by restricting the movement of bacteria. Additionally, the ability of TEO and thymol to permeate the pathogen cell membrane was found to be concentration-dependent. Hence, the pectin and chitosan blend film containing 20% TEO showed greater inhibition till the 20th day of packaging as compared to the films containing lower TEO concentrations.
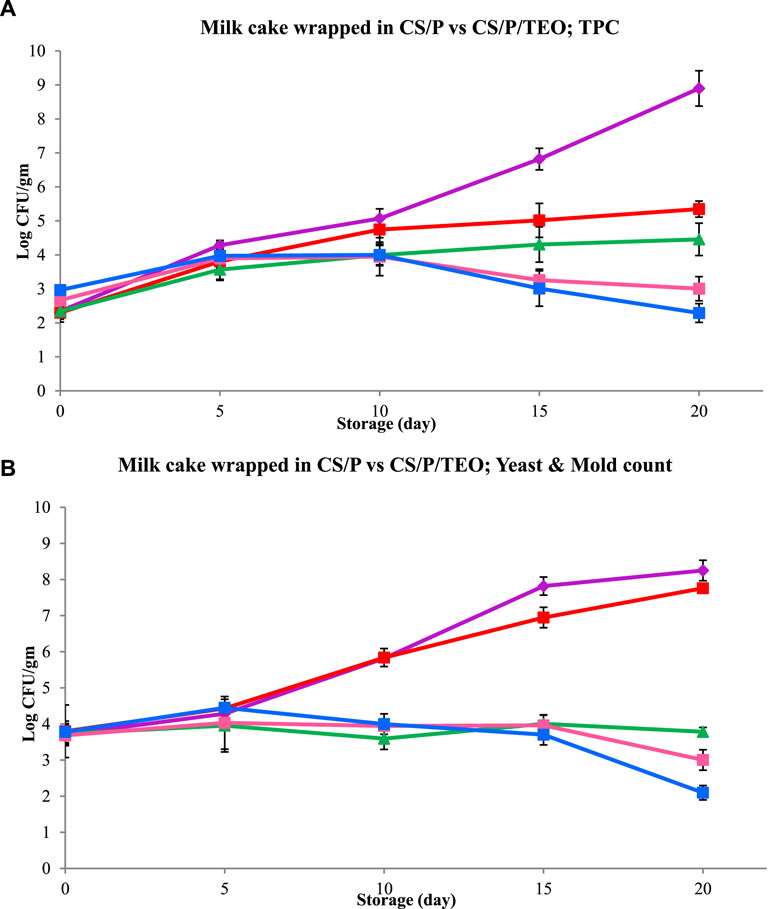
Figure 6. (A) The total plate count and (B) yeast and mold count of milk cake samples wrapped in the CS/PE-based film with TEO (
4 Conclusion
By using a solution casting technique, a biodegradable chitosan and pectin blended film containing TEO (10%, 15%, and 20%) was developed. The mechanical strength and flexibility of the blend were significantly impacted by the addition of EOs, as indicated by the TS and EAB values. Furthermore, TEO also improved the blend film’s structural and antimicrobial properties. TEO improved the antimicrobial properties of chitosan and pectin blend films against S. Typhimurium and L. monocytogenes, as revealed by FTIR spectra. On the seventh day of storage, the chitosan and pectin film containing 20% TEO demonstrated total suppression of S. Typhimurium. Compared to other chosen films, the milk cake samples wrapped in chitosan and pectin blend films with 20% TEO showed an extended shelf life compared to control samples at room temperature.
The antimicrobial packaging developed in this study offers significant benefits for the environment, society, and human health. Environmentally, these biodegradable (pectin and chitosan) materials reduce plastic waste and pollution, utilizing renewable resources with lower carbon footprints. For society, the developed packaging enhances food safety by inhibiting microbial growth, extending shelf life, and reducing food waste, which contributes to food security. It also lowers reliance on synthetic preservatives, thus minimizing public health risks associated with chemical additives. For individuals, it promotes safer food consumption through natural antimicrobial agents, preserving nutritional quality and reducing exposure to toxic substances. This innovative packaging film not only supports sustainable practices but also fosters environmental awareness and encourages responsible consumer behavior, aligning with global efforts toward a more sustainable future.
Data availability statement
The original contributions presented in the study are included in the article/Supplementary Material, further inquiries can be directed to the corresponding author.
Author contributions
MM: conceptualization, methodology, and writing–original draft. JA: resources, supervision, and writing–review and editing. AV: methodology and writing–original draft. SP: supervision and writing–review and editing. MR: data curation, Software, and writing–original draft.
Funding
The author(s) declare that no financial support was received for the research, authorship, and/or publication of this article.
Acknowledgments
The authors express their gratitude to the Nanotechnology Research Center, Kuwait University, for providing AFM facilities for the work.
Conflict of interest
The authors declare that the research was conducted in the absence of any commercial or financial relationships that could be construed as a potential conflict of interest.
Publisher’s note
All claims expressed in this article are solely those of the authors and do not necessarily represent those of their affiliated organizations, or those of the publisher, the editors, and the reviewers. Any product that may be evaluated in this article, or claim that may be made by its manufacturer, is not guaranteed or endorsed by the publisher.
Supplementary material
The Supplementary Material for this article can be found online at: https://www.frontiersin.org/articles/10.3389/frfst.2024.1356582/full#supplementary-material
References
Agarwal, S., Hoque, M., Bandara, N., Pal, K., and Sarkar, P. (2020). Synthesis and characterization of tamarind kernel powder-based antimicrobial edible films loaded with geraniol. Food Packag. Shelf Life 26, 100562. doi:10.1016/j.fpsl.2020.100562
Ahmad, M., Benjakul, S., Prodpran, T., and Agustini, T. W. (2012). Physico-mechanical and antimicrobial properties of gelatin film from the skin of unicorn leatherjacket incorporated with essential oils. Food Hydrocoll. 28 (1), 189–199. doi:10.1016/j.foodhyd.2011.12.003
Ahmed, J., Mulla, M., and Arfat, Y. A. (2017). Application of high-pressure processing and polylactide/cinnamon oil packaging on chicken sample for inactivation and inhibition of Listeria monocytogenes and Salmonella Typhimurium, and post-processing film properties. Food control. 78, 160–168. doi:10.1016/j.foodcont.2017.02.023
Ahmed, J., Mulla, M., Jacob, H., Luciano, G., Bini, T. B., and Almusallam, A. (2019). Polylactide/poly (ε-caprolactone)/zinc oxide/clove essential oil composite antimicrobial films for scrambled egg packaging. Food Packag. shelf life 21, 100355. doi:10.1016/j.fpsl.2019.100355
Aitboulahsen, M., Chairi, H., Laglaoui, A., Zantar, S., Abdelhay, A., Bakkali, M., et al. (2020). Gelatin/pectin-based film incorporated with essential oils: functional characteristics and shelf life extension of tilapia fillets under refrigeration. J. food Saf. 40 (3), e12774. doi:10.1111/jfs.12774
Aksit, H., Bayar, Y., Simsek, S., and Ulutas, Y. (2022). Chemical composition and antifungal activities of the essential oils of Thymus species (Thymus pectinatus, Thymus convolutus, Thymus vulgaris) against plant pathogens. J. Essent. Oil Bear. Plants 25 (1), 200–207. doi:10.1080/0972060X.2022.2043189
Aljabeili, H. S., Barakat, H., and Abdel-Rahman, H. A. (2018). Chemical composition, antibacterial and antioxidant activities of thyme essential oil (Thymus vulgaris). Food Nutr. Sci. 9 (5), 433–446. doi:10.4236/fns.2018.95034
Almasi, H., Azizi, S., and Amjadi, S. (2020). Development and characterization of pectin films activated by nanoemulsion and Pickering emulsion stabilized marjoram (Origanum majorana L.) essential oil. Food Hydrocoll. 99, 105338. doi:10.1016/j.foodhyd.2019.105338
Antunes, J. C., Tavares, T. D., Teixeira, M. A., Teixeira, M. O., Homem, N. C., Amorim, M. T. P., et al. (2021). Eugenol-containing essential oils loaded onto chitosan/polyvinyl alcohol blended films and their ability to eradicate Staphylococcus aureus or Pseudomonas aeruginosa from infected microenvironments. Pharmaceutics 13 (2), 195. doi:10.3390/pharmaceutics13020195
Athanasopoulou, E., Bigi, F., Maurizzi, E., Karellou, E. I. E., Pappas, C. S., Quartieri, A., et al. (2024). Synthesis and characterization of polysaccharide-and protein-based edible films and application as packaging materials for fresh fish fillets. Sci. Rep. 14 (1), 517. doi:10.1038/s41598-024-51163-y
Ben Jabeur, M., Somai-Jemmali, L., and Hamada, W. (2017). Thyme essential oil as an alternative mechanism: biofungicide-causing sensitivity of Mycosphaerella graminicola. J. Appl. Microbiol. 122 (4), 932–939. doi:10.1111/jam.13408
Boskovic, M., Djordjevic, J., Ivanovic, J., Janjic, J., Zdravkovic, N., Glisic, M., et al. (2017). Inhibition of Salmonella by thyme essential oil and its effect on microbiological and sensory properties of minced pork meat packaged under vacuum and modified atmosphere. Int. J. Food Microbiol. 258, 58–67. doi:10.1016/j.ijfoodmicro.2017.07.011
Candido, G. S., Natarelli, C. V. L., Carvalho, E. E. N., and Oliveira, J. E. (2022). Bionanocomposites of pectin and pracaxi oil nanoemulsion as active packaging for butter. Food Packag. Shelf Life 32, 100862. doi:10.1016/j.fpsl.2022.100862
Cui, J., Zhang, L., Wang, J., Zhao, S., Zhao, C., Liu, D., et al. (2023). Study on the relationship between primary structure/spatial conformation and gel properties of pectins from different varieties. Food Hydrocoll. 144, 109055. doi:10.1016/j.foodhyd.2023.109055
Escamilla-García, M., Calderón-Domínguez, G., Chanona-Pérez, J. J., Mendoza-Madrigal, A. G., Di Pierro, P., García-Almendárez, B. E., et al. (2017). Physical, structural, barrier, and antifungal characterization of chitosan–zein edible films with added essential oils. Int. J. Mol. Sci. 18 (11), 2370. doi:10.3390/ijms18112370
Espitia, P. J. P., Du, W. X., de Jesús Avena-Bustillos, R., Soares, N. D. F. F., and McHugh, T. H. (2014). Edible films from pectin: physical-mechanical and antimicrobial properties-A review. Food Hydrocoll. 35, 287–296. doi:10.1016/j.foodhyd.2013.06.005
Essifi, K., Hammani, A., Berraaouan, D., El Bachiri, A., Fauconnier, M. L., and Tahani, A. (2022). Montmorillonite nanoclay based formulation for controlled and selective release of volatile essential oil compounds. Mater. Chem. Phys. 277, 125569. doi:10.1016/j.matchemphys.2021.125569
Gaba, A. B. M., Hassan, M. A., Abd EL-Tawab, A. A., Abdelmonem, M. A., and Morsy, M. K. (2022). Protective impact of chitosan film loaded oregano and thyme essential oil on the microbial profile and quality attributes of beef meat. Antibiotics 11 (5), 583. doi:10.3390/antibiotics11050583
Gao, H. X., He, Z., Sun, Q., He, Q., and Zeng, W. C. (2019). A functional polysaccharide film forming by pectin, chitosan, and tea polyphenols. Carbohydr. Polym. 215, 1–7. doi:10.1016/j.carbpol.2019.03.029
Hoque, M., Sarkar, P., and Ahmed, J. (2022). Preparation and characterization of tamarind kernel powder/ZnO nanoparticle-based food packaging films. Industrial Crops Prod. 178, 114670. doi:10.1016/j.indcrop.2022.114670
Hosseini, S. F., Mousavi, Z., and McClements, D. J. (2023). Beeswax: a review on the recent progress in the development of superhydrophobic films/coatings and their applications in fruits preservation. Food Chem. 424, 136404. doi:10.1016/j.foodchem.2023.136404
Hosseini, S. F., Rezaei, M., Zandi, M., and Farahmandghavi, F. (2015). Bio-based composite edible films containing Origanum vulgare L. essential oil. Industrial Crops Prod. 67, 403–413. doi:10.1016/j.indcrop.2015.01.062
Huang, K. X., Zhou, L. Y., Chen, J. Q., Peng, N., Chen, H. X., Gu, H. Z., et al. (2023). Applications and perspectives of quaternized cellulose, chitin and chitosan: a review. Int. J. Biol. Macromol. 242, 124990. doi:10.1016/j.ijbiomac.2023.124990
Kong, M., Chen, X. G., Xing, K., and Park, H. J. (2010). Antimicrobial properties of chitosan and mode of action: a state of the art review. Int. J. food Microbiol. 144 (1), 51–63. doi:10.1016/j.ijfoodmicro.2010.09.012
Leal Filho, W., Saari, U., Fedoruk, M., Iital, A., Moora, H., Klöga, M., et al. (2019). An overview of the problems posed by plastic products and the role of extended producer responsibility in Europe. J. Clean. Prod. 214, 550–558. doi:10.1016/j.jclepro.2018.12.256
Liu, T., Kang, J., and Liu, L. (2021). Thymol as a critical component of Thymus vulgaris L. essential oil combats Pseudomonas aeruginosa by intercalating DNA and inactivating biofilm. LWT-Food Sci. Technol. 136, 110354. doi:10.1016/j.lwt.2020.110354
Londhe, G., Pal, D., and Raju, P. N. (2012). Effect of packaging techniques on shelf life of brown peda, a milk-based confection. LWT-Food Sci. Technol. 47 (1), 117–125. doi:10.1016/j.lwt.2011.12.025
Marino, M., Bersani, C., and Comi, G. (1999). Antimicrobial activity of the essential oils of Thymus vulgaris L. measured using a bioimpedometric method. J. food Prot. 62 (9), 1017–1023. doi:10.4315/0362-028X-62.9.1017
Mulla, M. Z., Ahmed, J., Vahora, A., and Pathania, S. (2023). Effect of pectin incorporation on characteristics of chitosan based edible films. J. Food Meas. Charact. 17 (6), 5569–5581. doi:10.1007/s11694-023-02047-8
Nisar, T., Wang, Z. C., Yang, X., Tian, Y., Iqbal, M., and Guo, Y. (2018). Characterization of citrus pectin films integrated with clove bud essential oil: physical, thermal, barrier, antioxidant and antibacterial properties. Int. J. Biol. Macromol. 106, 670–680. doi:10.1016/j.ijbiomac.2017.08.068
Norcino, L. B., Mendes, J. F., Natarelli, C. V. L., Manrich, A., Oliveira, J. E., and Mattoso, L. H. C. (2020). Pectin films loaded with copaiba oil nanoemulsions for potential use as bio-based active packaging. Food Hydrocoll. 106, 105862. doi:10.1016/j.foodhyd.2020.105862
Priyadarshi, R., Kim, S. M., and Rhim, J. W. (2021). Pectin/pullulan blend films for food packaging: effect of blending ratio. Food Chem. 347, 129022. doi:10.1016/j.foodchem.2021.129022
Qin, C., Li, Z., Zhang, J., Meng, H., and Zhu, C. (2024). Preparation, physicochemical properties, antioxidant, and antibacterial activities of quaternized hawthorn pectin films incorporated with thyme essential oil. Food Packag. Shelf Life 41, 101235. doi:10.1016/j.fpsl.2023.101235
Sadadekar, A. S., Shruthy, R., Preetha, R., Kumar, N., Pande, K. R., and Nagamaniammai, G. (2023). Enhanced antimicrobial and antioxidant properties of Nano chitosan and pectin based biodegradable active packaging films incorporated with fennel (Foeniculum vulgare) essential oil and potato (Solanum tuberosum) peel extracts. J. Food Sci. Technol. 60 (3), 938–946. doi:10.1007/s13197-021-05333-9
Sánchez-González, L., Cháfer, M., González-Martínez, C., Chiralt, A., and Desobry, S. (2011). Study of the release of limonene present in chitosan films enriched with bergamot oil in food simulants. J. Food Eng. 105 (1), 138–143. doi:10.1016/j.jfoodeng.2011.02.016
Santos, V., Lorevice, M. V., Baccarin, G. S., Medeiros, F., Aouada, F., and de Moura, M. R. (2022). Combining chitosan nanoparticles and garlic essential oil as additive fillers to pectin edible films for primary rice containing.
Scacchetti, F. A. P., Pinto, E., and Soares, G. M. B. (2017). Functionalization and characterization of cotton with phase change materials and thyme oil encapsulated in beta-cyclodextrins. Prog. Org. coatings 107, 64–74. doi:10.1016/j.porgcoat.2017.03.015
Sebaaly, C., Trifan, A., Sieniawska, E., and Greige-Gerges, H. (2021). Chitosan-coating effect on the characteristics of liposomes: a focus on bioactive compounds and essential oils: a review. Processes 9 (3), 445. doi:10.3390/pr9030445
Singh, N., Chawla, R., and Sivakumar, S. (2021). Studying the properties of edible packaging for milk cake and its effect during refrigerated storage. J. Packag. Technol. Res. 5, 29–40. doi:10.1007/s41783-020-00102-z
Sood, A., and Saini, C. S. (2022). Red pomelo peel pectin based edible composite films: effect of pectin incorporation on mechanical, structural, morphological and thermal properties of composite films. Food Hydrocoll. 123, 107135. doi:10.1016/j.foodhyd.2021.107135
Sudheer, S., Bandyopadhyay, S., and Bhat, R. (2023). Sustainable polysaccharide and protein hydrogel-based packaging materials for food products: a review. Int. J. Biol. Macromol. 248, 125845. doi:10.1016/j.ijbiomac.2023.125845
Topala, C. M., and Tataru, L. D. (2016). ATR-FTIR Study of thyme and rosemary oils extracted by supercritical carbon dioxide. Rev. Chim. Buchar. 67, 842–846.
U.S. Public Health Service/Food and Drug Administration (USPHS/FDA) (2016). Grade “A” pasteurized milk ordinance. Washington, DC: FDA.
Valizadeh, S., Naseri, M., Babaei, S., Hosseini, S. M. H., and Imani, A. (2019). Development of bioactive composite films from chitosan and carboxymethyl cellulose using glutaraldehyde, cinnamon essential oil and oleic acid. Int. J. Biol. Macromol. 134, 604–612. doi:10.1016/j.ijbiomac.2019.05.071
Venkatachalam, K., Rakkapao, N., and Lekjing, S. (2023). Physicochemical and antimicrobial characterization of chitosan and native glutinous rice starch-based composite edible films: Influence of different essential oils incorporation. Membranes 13 (2), 161. doi:10.3390/membranes13020161
Wang, X., Sun, Y., Liu, Z., Huang, X., Yi, F., Hou, F., et al. (2021). Preparation and characterization of chitosan/zein film loaded with lemon essential oil: effects on postharvest quality of mushroom (Agaricus bisporus). Int. J. Biol. Macromol. 192, 635–643. doi:10.1016/j.ijbiomac.2021.10.068
Xie, Q., Zheng, X., Li, L., Ma, L., Zhao, Q., Chang, S., et al. (2021). Effect of curcumin addition on the properties of biodegradable pectin/chitosan films. Molecules 26 (8), 2152. doi:10.3390/molecules26082152
Younis, H. G., and Zhao, G. (2019). Physicochemical properties of the edible films from the blends of high methoxyl apple pectin and chitosan. Int. J. Biol. Macromol. 131, 1057–1066. doi:10.1016/j.ijbiomac.2019.03.096
Zarandona, I., Correia, D. M., Moreira, J., Costa, C. M., Lanceros-Mendez, S., Guerrero, P., et al. (2023). Magnetically responsive chitosan-pectin films incorporating Fe3O4 nanoparticles with enhanced antimicrobial activity. Int. J. Biol. Macromol. 227, 1070–1077. doi:10.1016/j.ijbiomac.2022.11.286
Keywords: chitosan, pectin, thyme essential oil, antibacterial, atomic force microscopy, water vapor, transmission rate
Citation: Mulla MFZ, Ahmed J, Vahora A, Pathania S and Rashed MS (2024) Characterization of biopolymers based antibacterial films enriched with thyme essential oil and their application for milk cake preservation. Front. Food. Sci. Technol. 4:1356582. doi: 10.3389/frfst.2024.1356582
Received: 15 December 2023; Accepted: 01 July 2024;
Published: 08 August 2024.
Edited by:
Ashish Rawson, National Institute of Food Technology, Entrepreneurship, and Management, Thanjavur (NIFTEM-T), IndiaReviewed by:
Sonia Kundu, Maulana Abul Kalam Azad University of Technology, IndiaSumit Kumar, Jain University, India
A. D. Srikanth Tangirala, Acharya N. G. Ranga Agricultural University, India
Copyright © 2024 Mulla, Ahmed, Vahora, Pathania and Rashed. This is an open-access article distributed under the terms of the Creative Commons Attribution License (CC BY). The use, distribution or reproduction in other forums is permitted, provided the original author(s) and the copyright owner(s) are credited and that the original publication in this journal is cited, in accordance with accepted academic practice. No use, distribution or reproduction is permitted which does not comply with these terms.
*Correspondence: Mehraj Fatema Z. Mulla, TWVocmFqRmF0ZW1hLk11bGxhQHRlYWdhc2MuaWU=, bWVocmFqZmF0ZW1hbUBnbWFpbC5jb20=